- 1CAS Key Laboratory of Marine Ecology and Environmental Sciences, Institute of Oceanology, Chinese Academy of Sciences, Qingdao, China
- 2Laboratory for Marine Ecology and Environmental Science, Qingdao National Laboratory for Marine Science and Technology, Qingdao, China
- 3University of Chinese Academy of Sciences, Beijing, China
- 4Center for Ocean Mega-Science, Chinese Academy of Sciences, Qingdao, China
- 5Key Laboratory of Science and Engineering for Marine Ecology and Environment, The First Institute of Oceanography, Ministry of Natural Resources, Qingdao, China
The genus Alexandrium is one of the major harmful algal blooms (HABs)-forming dinoflagellate group and at least half of ~40 described species have been reported to produce paralytic shellfish toxins (PSTs). The potentially harmful species Alexandrium insuetum has been reported from many countries of Asia and Europe, and to have paralytic shellfish poisoning toxicity, but no mortality of marine animals was observed during its bloom. Therefore, it is ecologically important to characterize the possible toxicity and toxins of this organism. In this study, based on the establishment of two clonal cultures through cyst germination collected from the Yellow Sea, we identified A. insuetum from China as the first record via light microscopy (LM) and scanning electron microscopy (SEM) observations and phylogenetic analyses. The cultures of A. insuetum were further observed to be toxic to finfish and zooplankton and deleterious to rotifer eggs via laboratory bioassays. The exposure bioassays using rotifer (Brachionus plicatilis), brine shrimp (Artemia salina), and larval finfish (Oryzias melastigma) demonstrated that A. insuetum caused significant lethal effects on finfish and zooplankton species. Rotifer bioassays using cell-free culture medium, heat-treated cultures, and water, methanol, and trichloromethane extracts of algal cells revealed that A. insuetum produced heat-labile, water-soluble toxin(s) that could be excreted from A. insuetum cells and steadily accumulated in the medium during the growth phases. Hatching success of rotifer eggs was also found to be seriously affected by the exposure to A. insuetum. Importantly, ultra-high performance liquid chromatography-tandem mass spectrometry [UPLC (or LC)-MS-MS] analyses suggest the above-described toxicity of A. insuetum was caused by neither PSTs nor spiroimines (13-desmethyl spirolide C and gymnodimine). Collectively, our findings demonstrated the novel toxicity to finfish and zooplankton in A. insuetum, which is ecologically important in not only possibly contributing to population dynamics and even the formation of HABs of the species, but also affecting the on-the-spot survival and the reproduction potency of marine animals. The present work is believed to set a cornerstone for the monitoring and risk assessment of the species along the coastal waters of China and for understanding the general ecology of A. insuetum.
Introduction
Dinoflagellates are not only important primary producers and grazers of aquatic ecosystems, but also responsible for the most harmful algal blooms (HABs) (Hallegraeff et al., 2021). The dinoflagellate genus Alexandrium Halim, one of the most important genera responsible for causing HABs, has negative impacts on marine ecosystems, economy, and human health worldwide (Anderson, 1998; Anderson et al., 2012). Many Alexandrium species have been proved to produce toxins, e.g., paralytic shellfish toxins (PSTs, saxitoxin and its derivatives), spiroimines (spirolides and gymnodimines), goniodomins, and lytic compounds or allelochemicals (Cembella et al., 2000; Ichimi et al., 2002; Nascimento et al., 2005; Hsia et al., 2006; Tang et al., 2007; Espina et al., 2016; Blossom et al., 2019; Mertens et al., 2020; Shang et al., 2021). Furthermore, at least more than a half of Alexandrium species have been shown having a resting cyst stage in their life histories, which can be associated with seeding recurrent HABs and geographic expansion of populations (Anderson et al., 2005, 2012; Bravo et al., 2006; Nagai et al., 2009; Gu et al., 2013; Tang et al., 2016, 2021). The range, frequency, and intensity of HAB events caused by Alexandrium species have been increasing worldwide during the past decades (Hosoi-Tanabe and Sako, 2006; Anderson et al., 2012; Zou et al., 2014; Yu and Liu, 2016).
In the coastal waters of China, about 11 Alexandrium species have been documented, including Alexandrium affine, Alexandrium andersonii, Alexandrium catenella, Alexandrium fraterculum, Alexandrium leei, Alexandrium minutum, Alexandrium ostenfeldii, Alexandrium pacificum, Alexandrium pseudogonyaulax, Alexandrium tamiyavanichii, and Alexandrium tamutum (Gu, 2011; Gu et al., 2013; Huang et al., 2021; Tang et al., 2021; Xu et al., 2021). In Chinese coastal waters, large-scale HABs caused by species of this genus have been reported frequently since 2000. For instance, recurrent blooms of toxic Alexandrium spp. have been detected from 2001 to 2006 in East China Sea (Zhou and Zhu, 2006; Yu and Liu, 2016). Many blooms that were attributed to A. tamarense (but possibly either A. catenella or A. pacificum; see John et al., 2014; Fraga et al., 2015), a PSTs-producing species, have been also observed in the coastal waters of Dalian, Yantai, Zhoushan, and Xiamen, China, causing massive wild and caged fish kills (Zhou and Zhu, 2006; Zou et al., 2014; Yu and Liu, 2016). It is essential to identify Alexandrium species accurately, as this genus includes many hard-to-differentiate and toxic species that cause diverse impacts in aquaculture, human health, and marine ecosystems (Bravo et al., 2006).
Alexandrium insuetum was first described by Balech (1985) from Korea, and it has been then reported from the coastal areas of Korea (Shin et al., 2014), Japan (Yuki and Yoshimatsu, 1990; Kim et al., 2005), Greece (Nikolaidis et al., 2005; Spatharis et al., 2007), France (Guillou et al., 2002), and Tunis (Daly Yahia-Kefi et al., 2001). Both toxic or nontoxic strains of A. insuetum have been reported (Adachi et al., 1994; Lilly et al., 2005) and paralytic shellfish poisoning (PSP) toxicity was also detected in a strain isolated from Uchinoumi, Japan, using high performance liquid chromatography (HPLC)-fluorometric method (Sako et al., 2004), whereas many other strains have not been subjected to toxins analysis (Guillou et al., 2002; Kim et al., 2002; Spatharis et al., 2007; Shin et al., 2014). In April 2003, an A. insuetum bloom (max. 2.54 × 106 cells·L−1) was reported for the first time in Amvrakikos Bay, Greece, which caused brownish water discoloration but without death of marine organisms being observed, and blooms of this species reoccurred and lasted for 3 weeks in the following year (Nikolaidis et al., 2005). During the winter of 2004–2005, co-occurrence of A. insuetum (max. 1.4 × 105 cells·L−1) with Pseudo-nitzschia calliantha (Bacillariophyceae) was observed and developed into dense blooms in the Aegean Sea, Greece (Spatharis et al., 2007), but no death of marine animals were reported. Alexandrium insuetum has been generally considered to be a potentially harmful species (Sako et al., 2004; Spatharis et al., 2007; Shin et al., 2014), but the characterization of its toxicity and toxins has never been well-accomplished.
Vegetative cells of A. insuetum are solitary unless two daughter cells in division, oval in shape, and also characterized by highly reticulated cell surface and presence of a ventral pore located on the first apical (1′) plate (Balech, 1985; Yuki and Yoshimatsu, 1990; Shin et al., 2014). Alexandrium insuetum is morphologically similar to A. minutum and A. tamutum (Lilly et al., 2005) in all having a small cell size and a posterior sulcal (sp) plate wider than its length (Balech, 1989; Montresor et al., 2004; Shin et al., 2014). Moreover, A. insuetum is phylogenetically close to A. minutum in terms of forming the minutum/insuetum group (Lilly et al., 2005; John et al., 2014; Shin et al., 2014). Compared with most species of the A. minutum/insuetum group, however, A. insuetum has a feature that the pore plate (Po) and the 1′ plate are completely disconnected (Lilly et al., 2005; Shin et al., 2014). It has been speculated that there have been only a few records of this species in the literature due to the remarkable morphological and phylogenetic similarities between A. insuetum and A. minutum (Lilly et al., 2005; Spatharis et al., 2007; Shin et al., 2014). Thus, more detailed observations on the isolates of this species from different geographic regions are needed for accurate identification of the species group in the future.
In this study, we established two clonal cultures of A. insuetum via germinating resting cysts collected from the coast of Lianyungang, China, and examined their morphology via light microscopy (LM) and scanning electron microscopy (SEM) and phylogeny based on the large subunit (LSU) rDNA sequences. More importantly, we characterized their toxicity to marine animals (rotifer, brine shrimp, and finfish) via laboratory bioassays and ultra-high performance liquid chromatography-tandem mass spectrometry [UPLC (or LC)-MS-MS] analyses for their toxin profiles. Our results are believed to set a cornerstone for the monitoring and risk assessment of the species along the coastal waters of China and important knowledge for the general ecology of A. insuetum.
Materials and Methods
Sediment Sample Collection and Clonal Culture Establishment
Surface sediment samples (0–2 cm) were collected from the coast of the Yellow Sea, China (34.78° N, 119.45° E; water depth: 10 m), on March 22, 2016, using a grab corer, and stored in the dark at 4°C until further treatment. Cyst assemblage was concentrated using a protocol of sodium polytungstate (SPT) gradient centrifugation (Bolch, 1997). The single cysts were washed several times using sterile seawater, then inoculated into the individual wells of 24-well tissue culture plates (Corning, USA) filled with 2 mL f/2-Si medium (Guillard, 1975) and a final concentration of 2% antibiotic solution (a mixture of 10,000 IU. Penicillin and 10,000 μg·mL−1 streptomycin; Solarbio, China). Two clonal cultures of A. insuetum (strains AILYG12 and AILYG23) were established by transferring vegetative cells into fresh f/2-Si medium using micropipettes after cysts germination. All cultures were maintained in the incubator at 21°C, light intensity of ~100 μmol photons m−2·s−1, and a12:12 h light: dark cycle.
Light Microscopy and Scanning Electron Microscopy
Cysts and vegetative cells were observed and photographed using an inverted microscope (IX73, Olympus, Japan) and a light microscope (BX53, Olympus, Japan) equipped with a digital camera (DP80, Olympus, Japan). Vegetative cells were stained with SYBR Green (Solarbio, Beijing, China), viewed and photographed for chlorophyll-induced red autofluorescence and SYBR Green-induced green fluorescence of nucleus. Cells sizes for 50 live cells at the mid-exponential growth phase were measured at ×200 magnification using a DP80 digital camera (Olympus, Tokyo, Japan). For plate pattern identification, live cells were stained with calcofluor white and examined using the epifluorescence microscope (BX53, Olympus, Japan) with a UV filter set (Fritz and Triemer, 1985).
For SEM observation, vegetative cells at mid-exponential growth stage were fixed with glutaraldehyde (2.5% final concentration) for 40–50 min, then gently filtered onto an 11-μm pore size Millipore nylon membrane, dehydrated in an acetone series (10%, 30%, 50%, 70%, 90%, and three times in 100%, 15 min for each step), critical point-dried with liquid CO2 (EM CPD300, Leica, Austria), sputter-coated with gold (EM ACE200, Leica, Austria), and observed using a S-3400 N SEM (Hitachi, Japan; Hu et al., 2020).
DNA Extraction, PCR Amplification, and Sequencing
Total DNA was extracted from the cells using a plant DNA extraction kit (Tiangen, China) following the protocol of the manufacturer. About 1,400 bp of LSU rDNA sequence was amplified using the primer set, D1R (F: 5′-ACCCGCTGAATTTAAGCATA-3′) (Scholin et al., 1994) and 28-1483R (R: 5′-GCTACTACCACCAAGATCTGC-3′) (Daugbjerg et al., 2000), and an ~1,800 bp of the small subunit (SSU) rDNA was amplified using primers EukA (forward, 5′-ACCTGGTTGATCCTGCCAGT-30) and EukB (reverse, 5′-TCACCTACGGAAACCTTGT-30) (Medlin et al., 1988). The PCR reactions were conducted in a total volume of 20 μL, containing 7 μL ddH2O, 10 μL High Fidelity (HiFi) PCR SuperMix (Transgen, China), 1 μL of each PCR primer, and 1 μL DNA template. The PCR protocol was as follows: initial denaturation at 94°C for 5 min, followed by 35 cycles at 94°C for 30 s, 54°C for 30 s, and 72°C for 2 min, and extension for 10 min at 72°C. PCR-amplified products were confirmed by 1.0% agarose gel electrophoresis. The amplicons were purified with agarose gel DNA fragment recovery kit (GENEray, China) and ligated into the pMD-19T vector (TaKaRa, Japan), and then transformed into Escherichia coli DH5α (Biomed, China), the positive cloning were sequenced (TSINGKE, China). New sequences were deposited in GenBank with accession numbers MZ489186 and MZ489188 (LSU rDNA), and MZ489187 (SSU rDNA).
Phylogenetic Analysis
Large subunit rDNA gene sequences of A. insuetum and other closely related Alexandrium species were used for phylogenetic analysis. A sequence of Lingulodinium polyedra (accession No. EF613357) was used as an out-group. Sequence alignments were performed using the MAFFT v7.475 with the default settings (Katoh et al., 2019) (http://mafft.cbrc.jp/alignment/server/) and then modified with BioEdit v7.2.5 (Hall, 1999). The final alignment of LSU rDNA sequences included 1,725 characters, including gaps introduced from alignment. The general-time reversible model with estimated proportion of invariant sites and gamma-distributed rate variation among sites (GTR+I+G) substitution model was selected as the best-fit model using jModelTest 2.1.4 (Darriba et al., 2012) based on the Akaike information criterion (Akaike, 1974). Phylogenetic trees were constructed using Bayesian inference (BI) and maximum likelihood (ML) analyses. The BI analysis was performed with MrBayes 3.2.6 (Ronquist et al., 2012) using the best-fitting substitution model (GTR+ I + G). Four independent Markov chain Monte Carlo simulations were run simultaneously for 10 million generations and trees were sampled every 1,000 generations. The initial 25% of trees were discarded as burn-in, and convergence was judged based on the average SD of split frequencies (all <0.01). The remaining trees were used to generate a consensus tree and calculate posterior probabilities for all branches using a majority-rule consensus approach. ML analysis was conducted with RaxML v7.2.6 (Stamatakis, 2006) using the model GTR + I + G, and node support was assessed with 1,000 bootstrap replicates. FigTree v1.4.4 was used to visualize consensus tree.
Exposure Experiments of Marine Animals (Rotifer, Brine Shrimp, and Finfish)
Experiment 1: Bioassays of Rotifer and Brine Shrimp With the Live Cell Culture and the Cell Lysate
In order to test the toxicity of A. insuetum culture, exposure experiments of marine zooplankton (rotifer, Brachionus plicatilis; and brine shrimp, Artemia salina) were conducted using the live cell culture and cell lysates of A. insuetum. The resting eggs of rotifer B. plicatilis (L-type) and brine shrimp Ar. salina were bought from Ningbo Futian Biotechnology Co., Ltd (China) and Boaifeng Biological Products Co., Ltd (China), respectively, and 2-day-old neonates were used in this study. Cell lysates were prepared by sonicating the live cell culture on ice at 250 W for 60 × 10 s with a high energy JY92-II sonicator (Scientz Biotechnology Co., Ltd., China), microscopic examination confirmed no intact cells present. Test animals were exposed to a series of densities of live cell cultures and cell lysates (7,000, 4,000, 2,000, and 1,000 cells·mL−1, respectively), which were both obtained from diluting the initial culture (7,000 cells·mL−1, stationary phase) using f/2-Si medium. The bioassays were conducted in 24-well culture plates, with one test animal and 2 mL prediluted culture added to each well (n = 24). The f/2-Si medium and Isochrysis galbana (strain T-ISO, nontoxic prey) were used as negative controls. Test animals were observed every 12 h within a period of 120 h, and the death of the animal was recorded when a lack of movement. Dissolved oxygen (DO) levels of culture medium were measured at the beginning of the experiment and immediately after animal death, or at the end of the experiment.
Experiment 2: Fish Bioassays in the Live Cell Culture and Cell-Free Culture Medium
For fish bioassay, experiments were performed in six-well tissue culture plates (Labselect, China) with transwell polycarbonate membrane inserts (8.0 μm pore size) that divided each well into two portions using larval medaka Oryzias melastigma to measure the toxicity of live culture and cell-free culture medium to finfish. The larval fish was purchased from Qingdao Feiruite Biotechnology Co., Ltd., China. A volume of 6 mL culture of A. insuetum (4,000, 6,000, and 8,000 cells·mL−1, respectively) was added into the insert and the membrane with pore size of 8.0 μm would allow the cell-free culture medium to seep out the insert, but prevent A. insuetum cells (>10 μm) moving out the insert, meanwhile, we examined that no cells passed through the membrane during the experiment under a light microscope (IX73, Olympus, Japan). Three- to five-day-old juveniles (3–4 mm in length) were placed into the inserts (live cell culture) and the outer compartments (cell-free culture medium) when the culture mediums of two compartments maintained osmotic balance (about 1:1 in volume for inside and outside; one fish for each compartment, 10 replicates for each treatment). The f/2-Si medium and I. galbana (T-ISO) were used as negative controls. The state of the finfish was monitored every 3 h within the first 24 h and then observed every 12 h for a period of 96 h. Death time was recorded once the heart stopped beating. The DO levels were measured before the addition of fish and the time of fish death.
Experiment 3: Bioassays of Rotifer With Cell-Free Culture Mediums at Different Growth Phases
The toxicity of cell culture at the initial, exponential, and stationary growth phases were examined. Algal cultures collected at day 3, 6, and 9 were used in this experiment (2,840, 4,410, and 7,625 cells·mL−1, respectively). The cell-free culture medium was prepared by gravity filtration of algal culture through a 5-μm pore size nylon membrane (Millipore, USA) and the filtrate was further filtered through a syringe filter of 0.22-μm pore size (Jinteng Experiment Equipment Co., Ltd., China), no intact cells were observed in the cell-free culture medium with a light microscope (IX73, Olympus, Japan). Exposure bioassays of rotifer were conducted as described above in experiment 1 (n = 24).
Experiment 4: Characterizing the Chemical Nature of A. insuetum Toxin(s)
To further determine the chemical nature of toxin(s) produced by A. insuetum, toxin(s) were extracted using three solvents, i.e., sterile filtered seawater enriched with f/2-Si medium, methanol, and trichloromethane. Cells at exponential phases were harvested by centrifuging and removal of supernatant, cell pellets were suspended in 600 μL solvents and extracted by reciprocal shaking at 6 m s−1 with lysing matrix E (MP Biomedicals, USA) for 60 s (30 s for each time) in a MP FastPrep tissue homogenizer (MP Biomedicals, USA). The extracts were then centrifuged at 12,000 rpm for 5 min, and the resultant supernatants (600 μL) were used as the toxin extracts. For water and methanol extracts, the supernatants were filled with fresh f/2-Si culture medium to volume of 60 mL (1% final concentration); for trichloromethane extracts, the supernatants were evaporated and the residue redissolved in 300 μL dimethylsulfoxide (DMSO) for further exposure bioassays (60 mL final volume, 0.5% of DMSO). Therefore, 240,000, 420,000, 600,000, 3,000,000, and 6,000,000 cells were used in each treatment, corresponding to the equivalent cell concentration of 4,000, 7,000, 10,000, 50,000, and 100,000 cells·mL−1, respectively. Exposure bioassays of rotifer were also conducted as described in experiment 1 to examine the toxicity of these extracts (n = 12), mortalities were calculated every 12 h for a period of 60 h. Fresh f/2-Si culture medium, with and without addition of 1% methanol or 0.5% DMSO, nontoxic to rotifer, were used as negative controls.
To test the heat stability of toxin(s) from A. insuetum, exposure experiments of rotifer were also conducted using both heat-killed cell cultures and heated water extracts. The culture mediums were prepared by heating the cell cultures (10,000 cells·mL−1) or water extracts (equ. 10,000 cells·mL−1) in a 100°C water bath for 30 min, and then cooling to room temperature before testing. The same cell cultures and water extracts of A. insuetum without heating were used as positive controls, f/2-Si medium and I. galbana (T-ISO) culture were used as negative controls. The bioassays were conducted in 24-well culture plates (one rotifer for each well, n = 24) for a period of 96 h, and the state of rotifer was monitored every 12 h. DO levels were also detected during the bioassays.
Influence of A. insuetum on the Hatching of Rotifer Eggs
Eggs produced by rotifers were found not being able to hatch success when exposed to the culture of A. insuetum in the exposure experiment 1 and 2 above, thus, exposure bioassays of rotifer eggs to cell-free culture mediums and whole-cell cultures at different growth stages were conducted to examine the influence of toxic A. insuetum on the hatching success of healthy eggs. The rotifer eggs were purchased from Ningbo Futian Biotechnology Co., Ltd, China. The hatching experiments were performed in 24-well culture plates, with one resting egg in each well (n = 24). Each well-contained 2 mL of test culture medium, which included live cell culture or cell-free culture medium of A. insuetum at different growth phases (3, 6, and 9 d after inoculation, respectively). Cell-free culture mediums were acquired followed procedure in experiment 3 above. The cell culture and cell-free culture medium of I. galbana (T-ISO), and f/2-Si medium were set as the controls. The hatching of neonates was conducted at 21°C with an irradiance of 100 μmol photons m−2·s−1 and a12:12 h light: dark photoperiod. The resting eggs were checked every 12 h, hatching rates were calculated for the 72 h-incubation experiments.
Analyses of PSTs and Spirolides
For the analysis of PSTs, aliquots of 200 mL culture at the mid-exponential, late exponential, and stationary growth phases were collected by centrifugation at 5,000 rpm for 5 min. Cell pellets were suspended in 10 mL acetic acid (0.1 M), extracted with a high energy JY92-II DN ultrasonic probe (Ningbo Scientz Biotechnology Co., Ltd., China) on ice bath, then centrifuged at 12,000 rpm at 4 °C for 15 min. The extracts were filtered through a syringe filter of 0.22 μm pore size and stored at −20°C until analysis. PSP toxins were analyzed by UPLC-MS-MS method using the Agilent 1290 Infinity II UPLC coupled to an Agilent 6470 triple quadrupole mass spectrometry system. The detailed procedures of the chromatographic separation and mass detection for PSTs were shown in Supplementary Material 1.
For the analysis of toxin 13-desmethyl spirolide C (SPX1), the major component of spirolides in A. ostenfeldii and A. peruvianum (Sleno et al., 2004; Borkman et al., 2012), and eight common lipophilic algal toxins (LMATs), including gymnodimine (GYM), okadaic acid (OA), dinophysistoxin (DTX1, DTX2), azaspiracids (AZA1, AZA2, and AZA3), and pectenotoxin (PTX2) were also detected in A. insuetum. The toxin extraction and determination procedure followed that in Gao et al. (2016) and Wang et al. (2021), aliquots of 200 mL culture were collected by centrifugation, followed by homogenization with 6 mL methanol for 5 min (400 W, 2 s/5 s work-rest cycles) using a ultrasonic treatment (Ningbo Scientz Biotechnology Co., Ltd., China), and then ultrasonic-assisted extraction in an ice-cold bath (60% power for 30 min). The extracts were centrifuged at 12,000 rpm for 5 min. Then, the supernatant was transferred to a clean tube and methanol was added to 10 mL and filtered through a 0.22 μm syringe filter. Three replicates were performed in this study. The toxin extracts were stored at −20°C and further analyzed by online solid phase extraction (SPE) coupled with LC-MS/MS method (Wang et al., 2021). The detailed information was shown in Supplementary Material 1.
Statistics
Differences among treatments were generally analyzed using t-test or One-way ANOVA followed by least significant difference (LSD) post-hoc test in the toxicity experiments. The regression analysis of fish bioassays was performed by linear regression using IBM Statistical Product and Service Solutions (SPSS) Statistics 22. Significance level was set at p < 0.05 in all cases unless otherwise indicated.
Results
Morphological and Molecular Confirmation of A. insuetum
Two cysts of A. insuetum were both spherical with a diameter of 28.6 and 29.9 μm, respectively, had globular and granular content and a prominent orange-red accumulation body (Figures 1A,C). Cyst wall was slightly thick and smooth relatively (Figures 1A,C). The two resting cysts were germinated after 14 (Figures 1A,B) and 5 (Figures 1C,D) days' incubation, and the archeopyle was chasmic (Figures 1B,D). Vegetative cell of A. insuetum was very small in size, 11–25 μm long (15.93 ± 2.44 μm, n = 50) and 10–17 μm wide (13.39 ± 1.70 μm, n = 50). Cells were always solitary, had a slightly conical epitheca and round hypotheca (Figures 2, 3). The nucleus was located in the hypocone of cell (Figure 2B), and numerous rod-like chloroplasts were located almost throughout the cell (Figure 2E). The cell surface was very rough and highly reticulated, both on the epitheca and the hypotheca (Figure 3). The plate formula is: Po, 4′, 6″, 6C, 5″′, 2″′′ (Figures 2, 3). The first apical plate (1′) was rhomboidal in outline with a complete disconnection to the apical pore plate (Po) (Figure 3). The Po was drop-shaped, with the apical pore comma-shaped on the center, and small pores on the plate margin (Figures 3C,G–I). The sulcal plate (sp) was wider than its length with the irregular pentagon, attachment pore was absent on the Sp plate (Figure 3A).
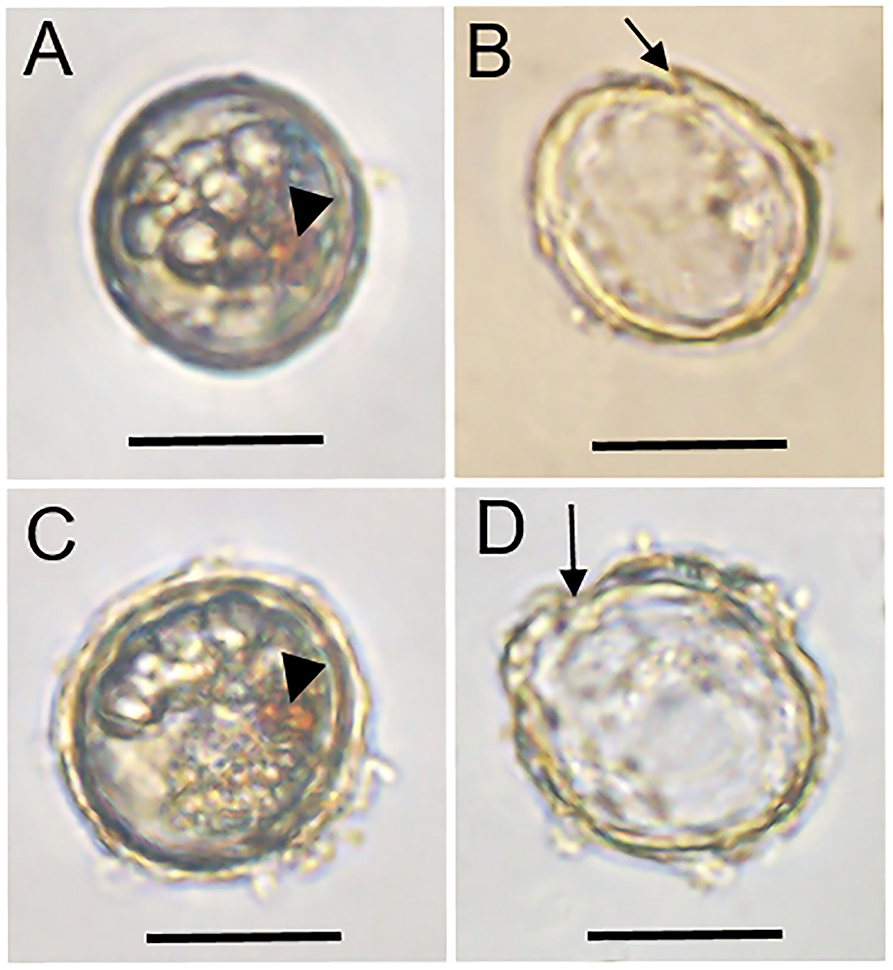
Figure 1. Light microscopy micrographs of two living (A,C) and the corresponding empty (B,D) cysts of Alexandrium insuetum. Arrowheads indicate the red accumulation body, the archeopyle (arrow) appeared after cyst germination. Scale bars = 20 μm.
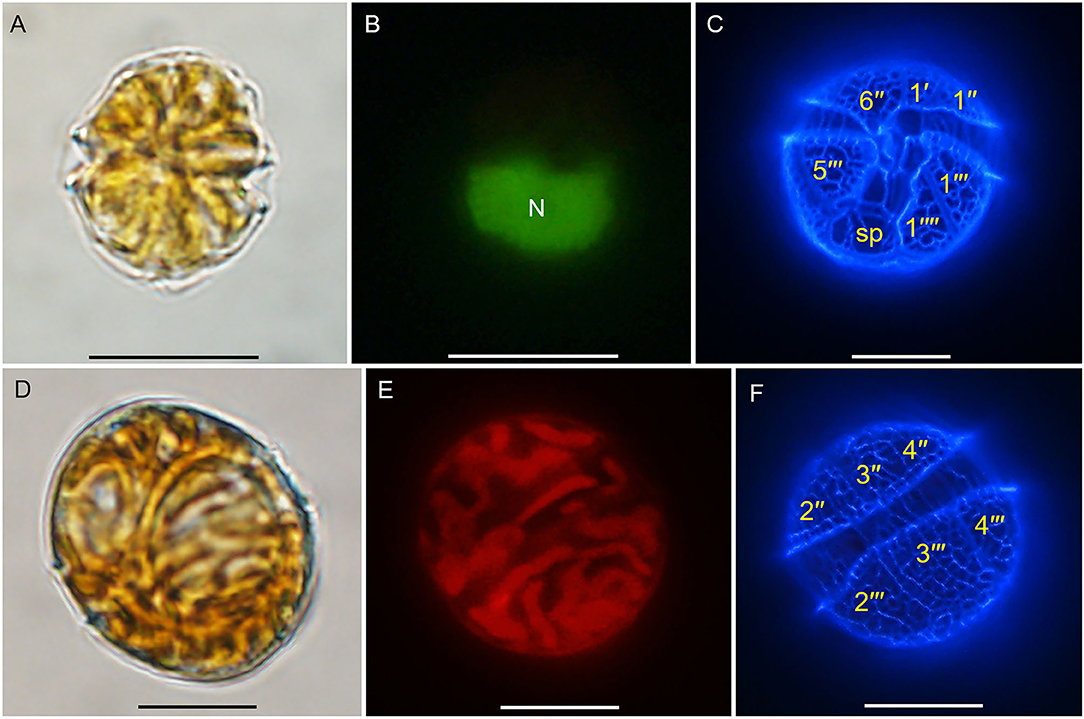
Figure 2. Light microscopy micrographs of Alexandrium insuetum strain AILYG12. (A) Ventral view. (B) SYBR green-stained cell showing the nucleus (N). (C) Ventral view of a calcofluor white-stained cell showing the plate pattern. (D) Dorsal view. (E) Epifluorescence image of vegetative cell showing the chloroplasts. (F) Dorsal view of a calcofluor white-stained cell showing the plate pattern. Scale bars = 10 μm. sp, posterior sulcal plate.
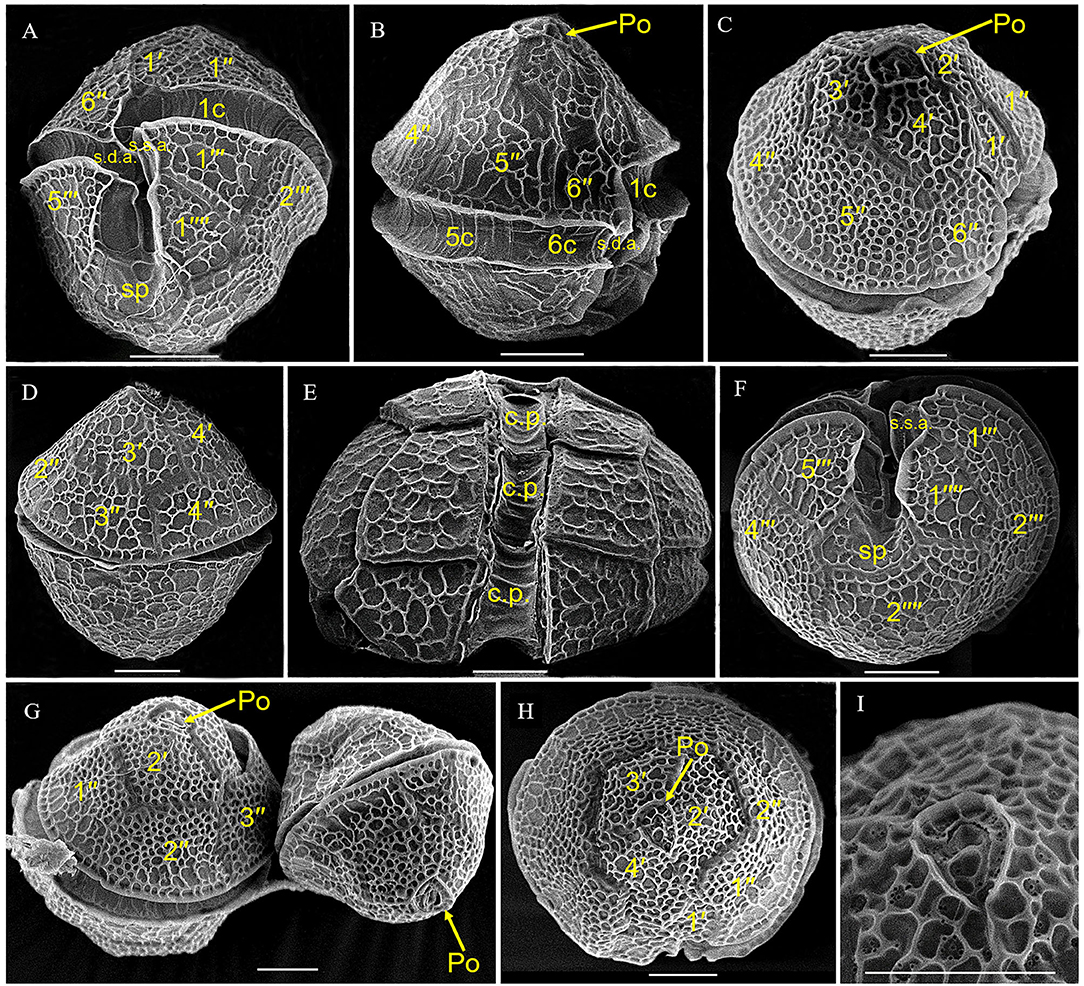
Figure 3. Scanning electron microscopy micrographs of Alexandrium insuetum strain AILYG12. (A,B) Ventral view showing the 1′ and sp. (D) Dorsal view. (E) Dorsal view showing the cingular plates (c.p.). (F) Antapical view showing the sp. (C,G,H) Apical view showing the epitheca. (I) Detail of the apical pore complex. s.d.a., right anterior sulcal plate; s.s.a., left anterior sulcal plate; sp, posterior sulcal plate; Po, apical pore plate; c.p., cingular plate.
One SSU rDNA and two partial LSU rDNA sequences of A. insuetum were obtained from our clonal cultures established from resting cyst germination. We compared the SSU rDNA sequence of the Chinese isolate (strain AILYG12; 1,800 bp, GenBank accession No. MZ489187) with that of other strains of A. insuetum in the National Center for Biotechnology Information (NCBI) database and found it was 99.8% (1,797 bp/1,800 bp) and 99.8% (1,754 bp/1,757 bp) identical to the Japanese strains AI104 and CCMP2082 (GenBank accession Nos. AB088298, JF521630). We also compared our 1,487 bp LSU rDNA sequence (strain AILYG12; accession No. MZ489186) of A. insuetum with other strains and found that it was 99.7% (1,483/1,487 bp), 99.4% (712/716 bp), and 99.6% (673/676 bp), 99.6% (1,186/1,191 bp), 97.9% (702/717 bp) identical to the Japanese strains CCMP2082, AI104, and D-155-B-1 (GenBank accession Nos. JF521630, AB088249, AY962834), Korean strain LMBE-C7 (GenBank accession No. KF752546), French strain X6 (GenBank accession No. AF318233), respectively. Phylogenetic analyses using BI and ML generated similar phylogenetic trees (Figure 4). All sequences of A. insuetum separated from the other Alexandrium species and grouped together with a higher support (0.74 PP). The well-resolved A. insuetum clade included strains from China, Japan, Korea, and France (Figure 4). Our isolates and the isolates from Japan and Korea formed a coherent clade with maximal support (1.00 PP), and a sister group with the French isolates (0.74 PP; Figure 4).
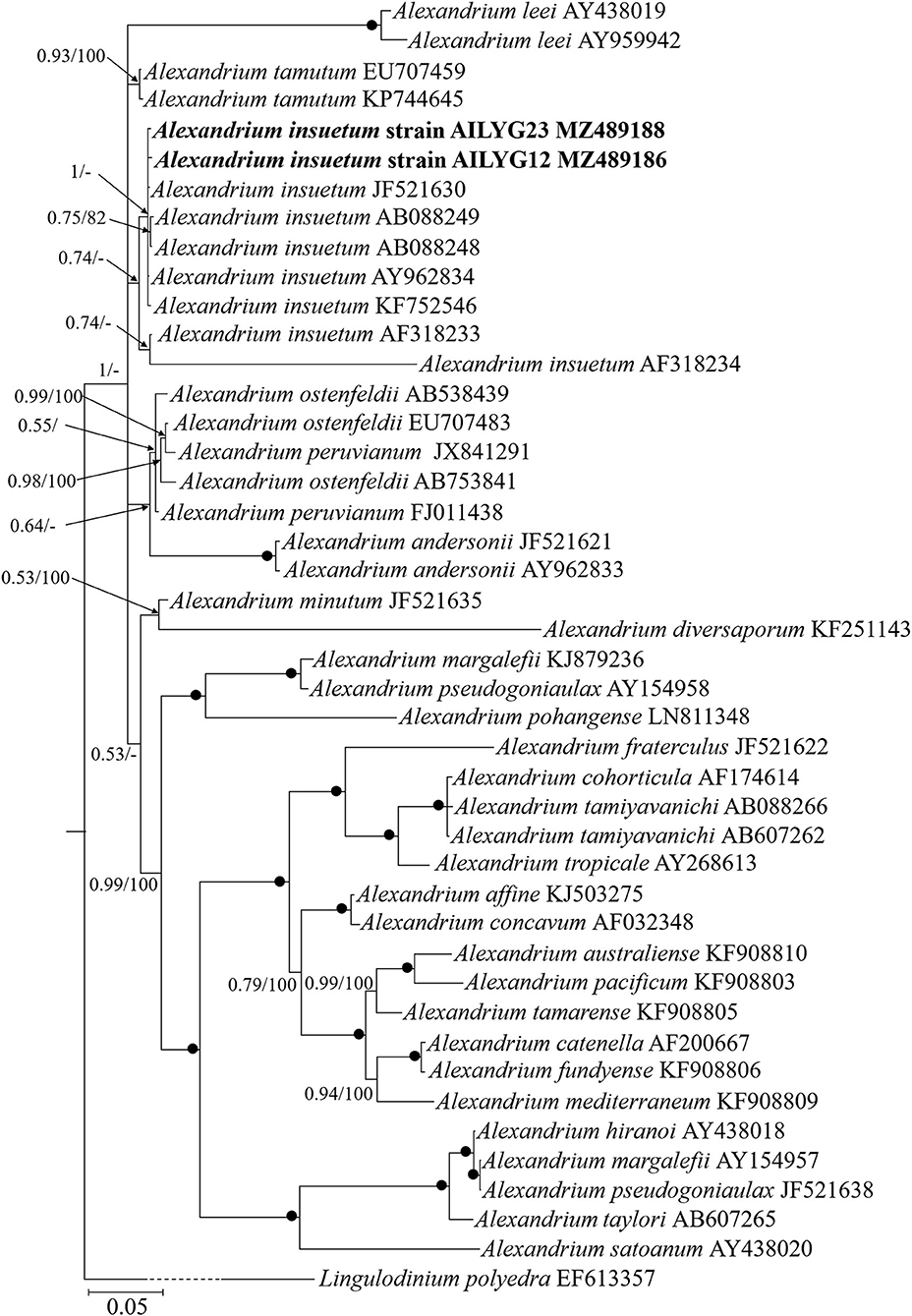
Figure 4. Molecular phylogeny of Chinese strain of Alexandrium insuetum inferred from partial large subunit rDNA sequences based on Bayesian inference (BI) analysis with Lingulodinium polyedra (EF613357) as the outgroup. New sequences of A. insuetum are indicated in bold. Numbers on branches are statistical support values (left, Bayesian posterior probabilities; right, maximum likelihood (ML) bootstrap support values). Bootstrap values >50% and posterior probabilities (pp) above 0.5 are shown. Black circles (•) indicate maximal support (pp = 1.00 in BI and bootstrap support = 100% in ML, respectively). All branches are drawn to scale.
Toxicity of A. insuetum to Rotifer, Brine Shrimp, and Finfish
Both the whole cell culture and cell lysate of A. insuetum exhibited strong toxicity to rotifer and brine shrimp (Figure 5). In the exposure bioassays of rotifer, the rotifers B. plicatilis exposed to the live cell culture showed significantly higher mortality compared with those exposed to the sonicated cell lysate at the same cell concentration, with 38–75% mortality observed in the live cell cultures at the cell densities of 1,000–7,000 cells·mL−1 within 120 h, but only 21–46% rotifer kills observed in the treatment of cell lysates with equivalent cell densities (p < 0.05, paired t-test; Figure 5A). Relatively low mortalities (4–21%) of rotifer exposed to the whole cell cultures (cell densities: 1,000, 2,000, 4,000, 7,000 cells·mL−1) were observed in the first 60 h, then it reached to 38–75% at the end of the experiments (120 h; Figure 5A). Mortalities of rotifer exposed to the cell lysates were mainly observed in the first 60 h (55–100%), but death of rotifer was not observed in the sonicated cultures of 1,000 and 7,000 cells·mL−1 after 60 h (Figure 5A). The DO levels were 80–90% of the saturated DO concentrations at 21°C and no rotifer died in the negative controls within 120 h. The surviving rotifers exposed to culture mediums could feed on A. insuetum and were able to produce eggs during the bioassays, but no successful hatch was observed (Supplementay Figure 1). For brine shrimp bioassays, the mortalities were significantly higher than that of rotifer exposed to the same culture medium with same cell densities at the 120 h exposure (p < 0.05, paired t-test; Figure 5B). Mortalities of brine shrimps at 120 h exposure were 83–96 and 79–83% for the treatments of live cells and cell lysates, respectively, and mortality of brine shrimp exposed to cell culture was slightly higher than that in the cell lysate with the same cell density (p = 0.06, paired t-test; Figure 5B). A 17% mortality of brine shrimp was observed in the f/2-Si medium while no death was present in the algal culture of I. galbana, and the DO levels were above 7.30 mg·L−1 in the course of bioassays, indicating the brine shrimps in the medium control died as a result of starvation (Figure 5B).
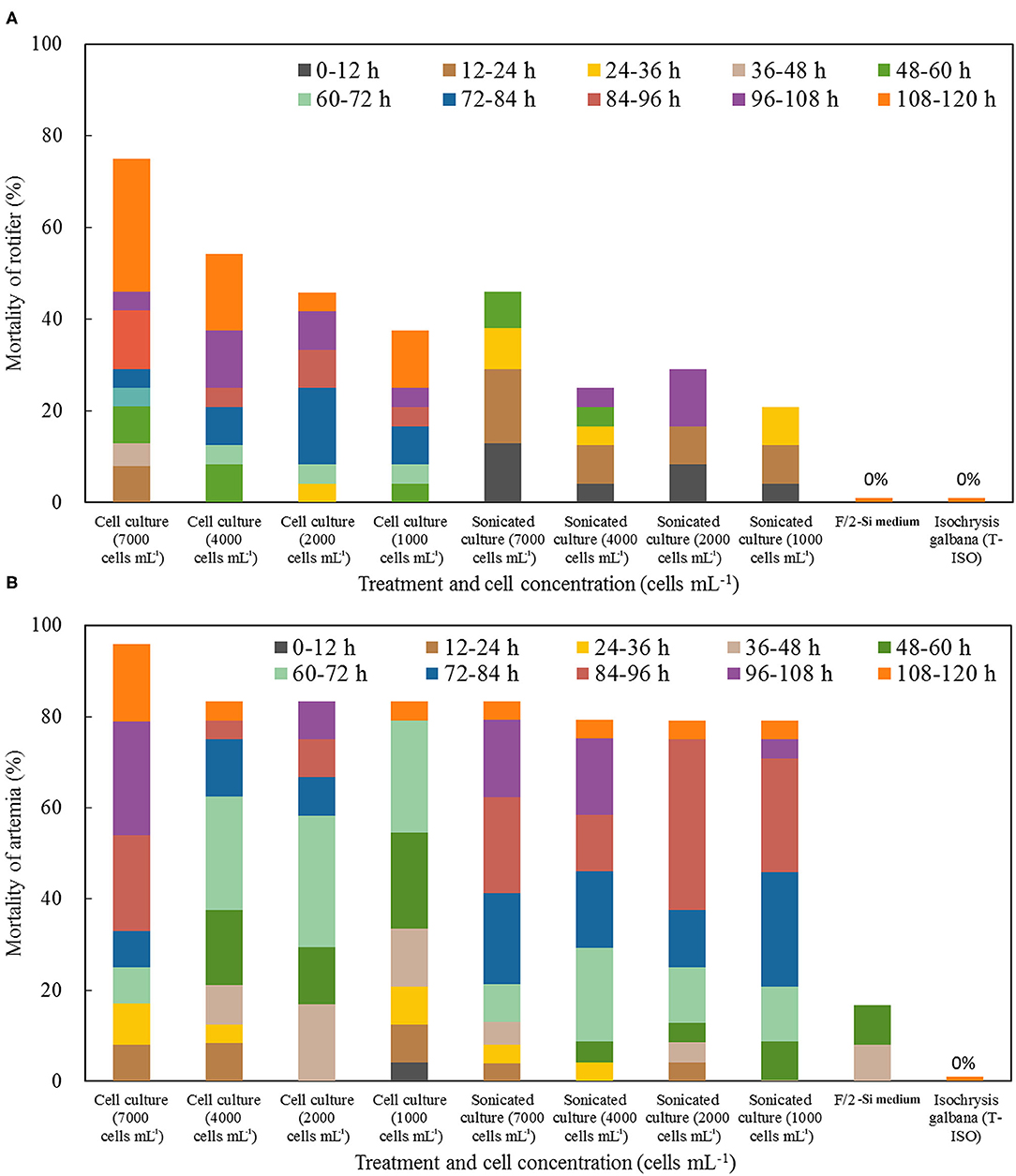
Figure 5. Mortality of rotifer Brachionus plicatilis (A) and brine shrimp Artemia salina (B) treated with a density range of live cells and cell lysates of Alexandrium insuetum within 120 h. F/2-Si medium and Isochrysis galbana (T-ISO, nontoxic) were used as negative controls.
In the fish bioassays using culture plates with inserts, fish mortality was observed only in the side with live cells (inside the inserts) within 24 h, with 10, 50, and 90% fish mortality in the treatments with the initial cell densities of 4,000, 6,000, and 8,000 cells·mL−1, respectively (Table 1). Finfish mortality (M) was positively correlated with the cell density (D) of A. insuetum within 96 h [Regression equation: M = 0.022D−79.481, R2 = 0.981 (n = 17, p < 0.01)], but the time of fish death (T) was negatively correlated with the density (D) of algal culture within 24 h [Regression equation: T = −0.001D + 18.158, R2 = 0.921 (n = 15, p < 0.01)]. However, all fish survived in the bioassays using cell-free culture medium within 48 h, only 10% mortality was observed at 96 h exposure with a cell density of 6,000 cells·mL−1, and no fish died in the negative controls. It was possible that the toxin content of cell-free culture medium with all cell concentration we used was not lethal to the larval fish. The DO levels measured immediately after fish death were in the range of 7.29–7.44 mg L−1. The lethal effect of A. insuetum algal culture on finfish larvae was observed with an inverted epifluorescence microscope with a filter for blue excitation (450–480 nm), cells of A. insuetum (red in micrographs) were observed in the intestine and near the gills (Figure 6).
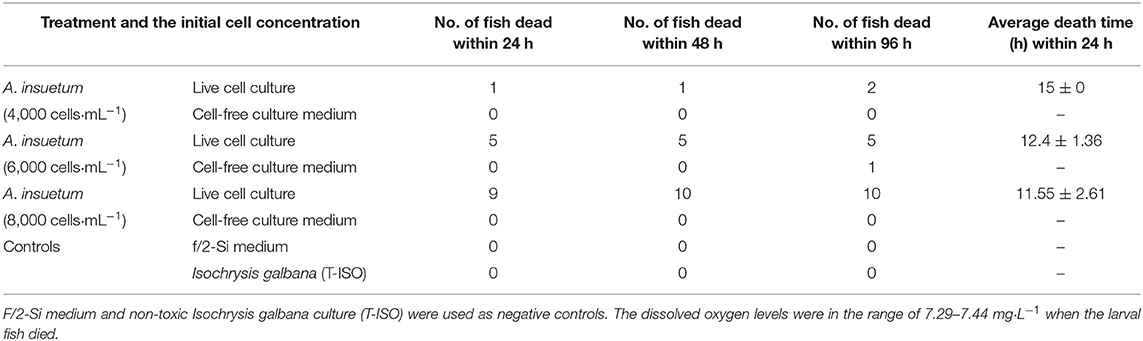
Table 1. Fish bioassays using partitioned culture plates showing the toxicity of live cell culture and cell-free culture medium within 96 h.
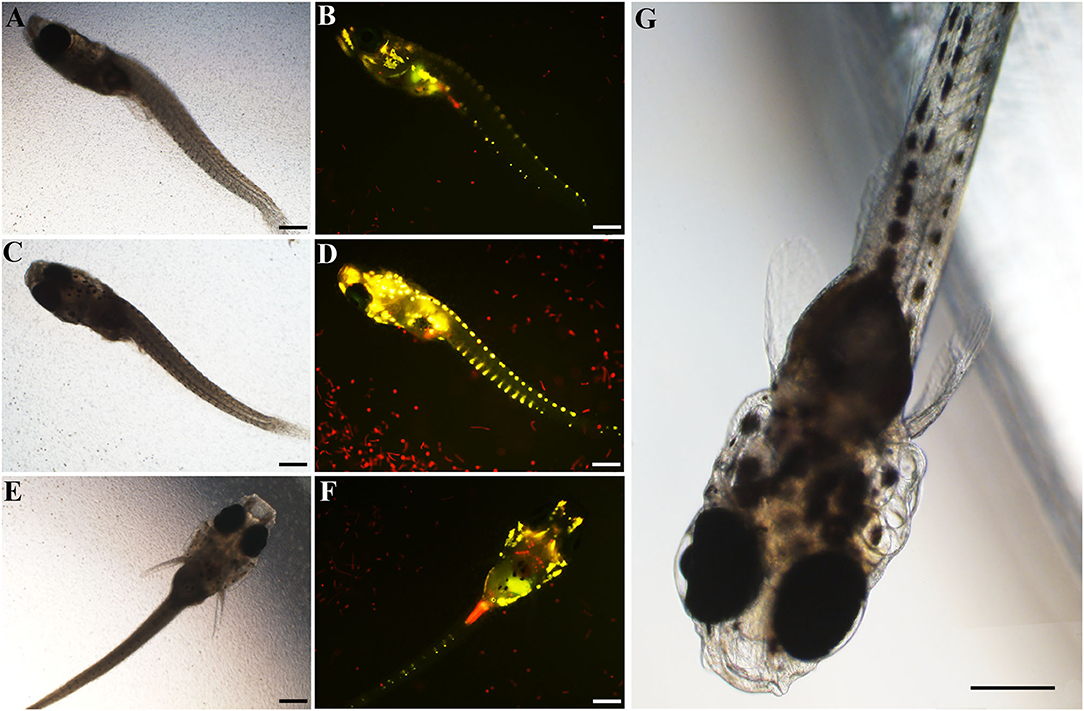
Figure 6. The finfish marine medaka Oryzias melastigma used in the fish bioassays exposed to live cell culture of 16,000 cells·mL−1, bright field light microscopic micrographs (A,C,E) of the dead fish, and corresponding epifluorescent micrographs (B,D,F). Normal larval finfish O. melastigma was showed in micrograph (G). Scale bars = 200 μm.
Toxicity of Cell-Free Culture Mediums of the Cultures at Different Growth Phases
The results of exposure experiments on two zooplankton species showed the toxicity was increased in the cell-free culture medium with the incubation time (Figure 7). The cell-free culture media of day 3, 6, and 9 caused 29, 41, and 54% mortalities of rotifer within 120 h, respectively, contrasting to 0 and 8% mortalities in the negative controls of I. galbana culture and f/2-Si medium. Higher mortality of brine shrimp was also observed at the 120 h exposure to cell-free culture medium as compared to the mortality of rotifer exposed to the culture medium at the same cell densities, with 54% rotifer mortality and 88% brine shrimp mortality observed at 120 h exposure to the cell-free culture medium collected on the day 9, but the difference was that death of rotifer mainly observed within the first 96 h, however, most brine shrimp kills were occurred after the initial 72 h.
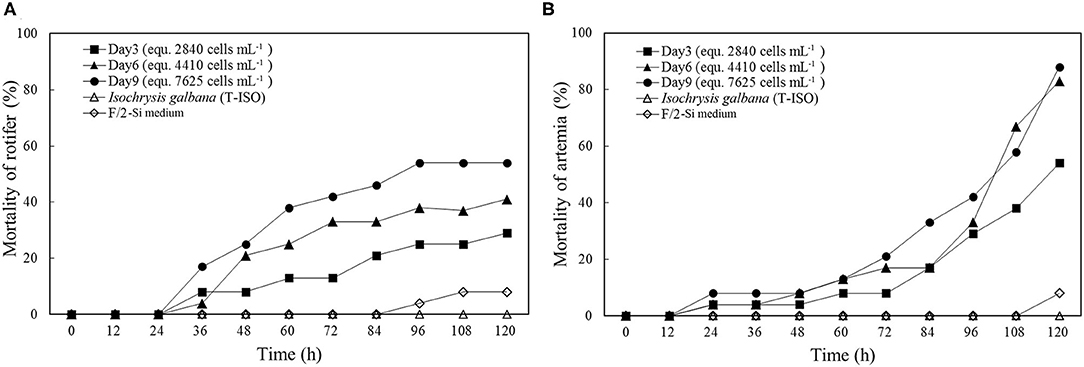
Figure 7. Mortality of rotifer (A) and brine shrimp (B) in the bioassays using cell-free culture mediums at the initial, exponential, and stationary growth phases (Day 3, 6, and 9, respectively). F/2-Si medium and Isochrysis galbana (strain T-ISO) were used as negative controls.
Characterization of the Chemical Nature of Toxin(s)
The rotifer bioassays using the crude toxin extracts that were extracted using solvents with different polarities demonstrated that the rotifer mortalities caused by water extract were significantly higher than those caused by methanol extract at the equivalent cell densities (p < 0.05, paired t-test; Figure 8A). The methanol extract caused higher mortalities of rotifer than did by the trichloromethane extract at the equivalent cell densities (p < 0.05, paired t-test; Figure 8A). Lethal effect of the trichloromethane extract was observed only if the equivalent cell densities were above 10,000 cells·mL−1 (p < 0.05, One-way ANOVA; Figure 8A).
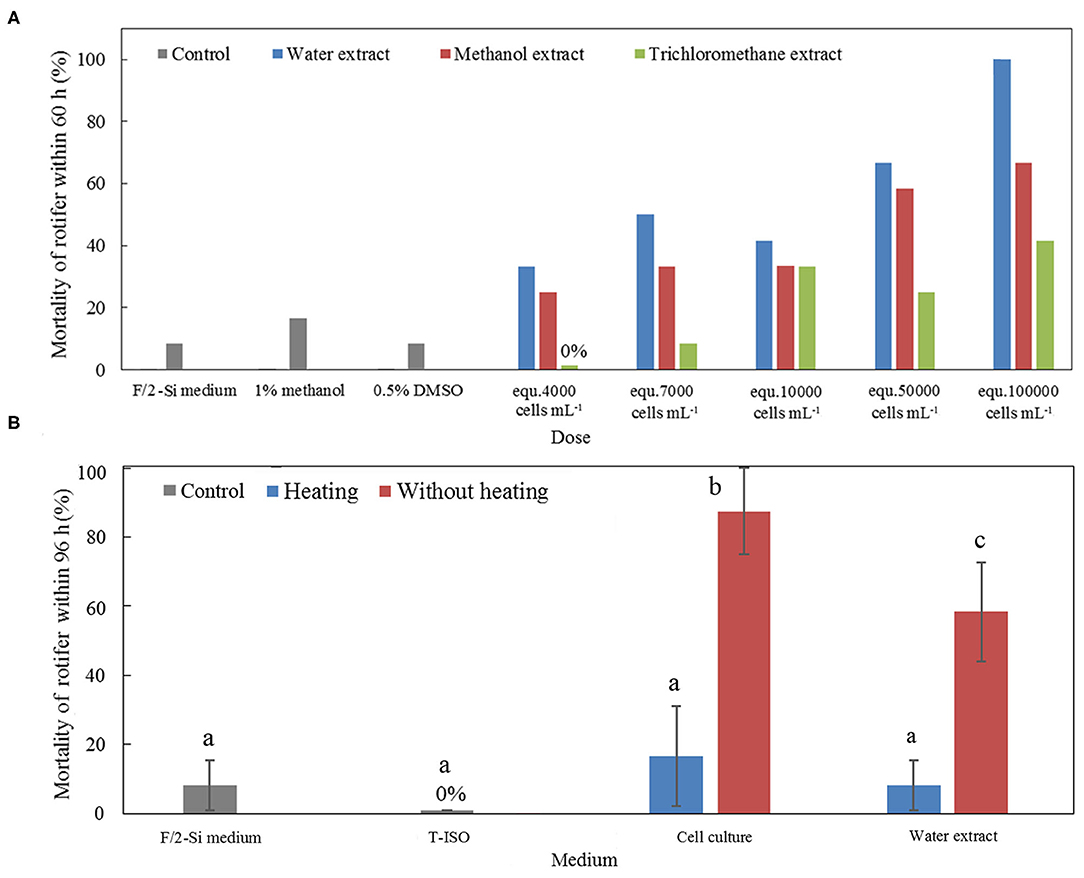
Figure 8. (A) Mortality of rotifer in the bioassays of toxin extracts using three solvents with different polarities (water, methanol, and trichloromethane). F/2-Si culture medium, with and without addition of 1% methanol or 0.5% dimethylsulfoxide, were used as negative controls. (B) Mortality of rotifer in the bioassays using both heat-killed cell cultures and heated water extracts. The same cell cultures and water extracts of A. insuetum without heating were used as positive controls, f/2-Si medium and Isochrysis galbana algal culture (T-ISO) were used as negative controls. The error bars indicate ±1 SD, and different superscript letters (a, b, and c) showed significant differences between different treatments (p < 0.05, ANOVA).
The heat stability of the toxins of A. insuetum was tested via exposing rotifers to the whole live culture and the water extract of algal cells with or without heat pretreatment. The rotifers exposed to the whole culture and the water extract without heat treatment exhibited significantly higher mortalities compared with that exposed to the whole culture and the water extract with heat treatment (p < 0.05, One-way ANOVA; Figure 8B). In addition, there was no significant difference in rotifer mortality among the heat-treated whole culture, heat-treated water extract, and the negative controls (i.e. fresh culture medium; p < 0.05, One-way ANOVA; Figure 8B).
Effect of A. insuetum on Hatching of the Rotifer Eggs
Since in the experiments of exposing rotifer to the whole live culture, cell lysate, and cell-free culture medium, rotifers that survived were observed being capable of producing eggs (Supplementary Figure 1), hatching experiments of healthy rotifer eggs were performed using the whole live culture and cell-free culture medium. Hatching success of healthy eggs was observed to be significantly influenced by the live cell cultures and cell-free culture media of A. insuetum (Figure 9). The hatching rate of eggs was significantly reduced in the cell-free culture medium on day 9 compared with that in the controls (p < 0.05, One-way ANOVA; Figure 9), where 70.8 and 91.7% of eggs successfully hatched in the cell-free culture medium and the fresh culture medium (control), respectively (Figure 9). The hatching rates of eggs that were exposed to the whole live culture on day 3 and day 6 were 75 and 70.83%, respectively, significantly lower than that in the fresh culture medium control (p < 0.05, One-way ANOVA; Figure 9).
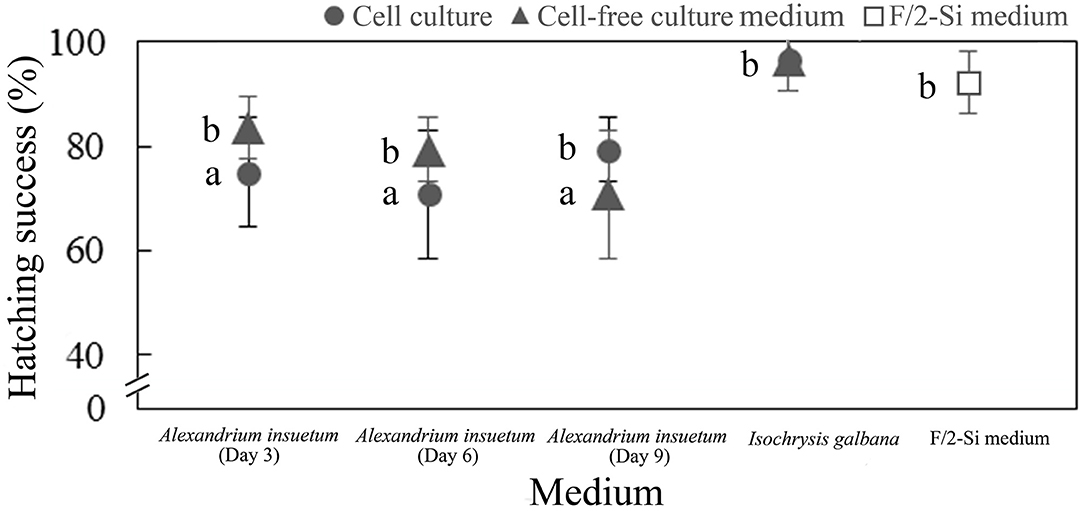
Figure 9. Hatching success of rotifer resting eggs in the live cell culture (circle) and cell-free culture medium (triangles) at different growth stages (Day 3, 6, and 9) within 72 h. F/2-Si medium and Isochrysis galbana (T-ISO) were used as negative controls. Data were shown as mean values ± SDs. The a and b letters next to bars indicated the post-hoc tests between the treatments with different letters denoting significant difference (p < 0.05, ANOVA).
Analyses of PSTs and Spirolide
PSP toxins and nine lipophilic marine algal toxins (SPX1, GYM, OA, DTX1, DTX2, AZA1, AZA2, AZA3, and PTX2) were detected in A. insuetum strain AILYG12 (Supplementary Figures 2, 3). The 13 derivatives of PSTs (C1, C2, GTX1, GTX4, GTX2, GTX3, GTX5, dcGTX2, dcGTX3, STX, dcSTX, NEO, and dcNEO) were not detected or under the detection limit in A. insuetum (Supplementary Figure 2) with UPLC–MS–MS analysis. The online SPE coupled with LC-MS/MS analysis showed no indicative peaks of any lipophilic marine algal toxins (Supplementary Figure 3), including 13-desmethyl spirolide C (SPX1), which had been detected from some strains of A. ostenfeldii (Cembella et al., 2000; MacKinnon et al., 2006; Paredes-Banda et al., 2018) and A. peruvianum (Borkman et al., 2012; Tomas et al., 2012).
Discussions
Morphological and Molecular Characterization
To monitor the dynamics of the toxic Alexandrium blooms and the spread of the toxic population, it is extremely important to identify the species/strains accurately (Bravo et al., 2006; Anderson et al., 2012). While the morphology of our isolate is almost identical to the original species descriptions of A. insuetum (Balech, 1985), the cell size of our isolates was smaller than that of the isolates from Japan, Korea, and France (Yuki and Yoshimatsu, 1990; Spatharis et al., 2007; Shin et al., 2014). A. insuetum is characteristic in small cell size, highly reticulated thecal plates, a disconnection between Po and 1′ plate, and the absence of the attachment pore on the Sp plate, which would allow for A. insuetum to be differentiated from other Alexandrium species (Lilly et al., 2005; Shin et al., 2014; Kim et al., 2017). Cyst morphology of A. insuetum was first described by Shin et al. (2014), which is a spherical, 20–25 μm in diameter, and of granular content and three or four red accumulation bodies. This description somewhat differs from our observations in cyst size and general appearance as described above. The significant difference in cyst morphology of A. insuetum between the Chinese and Korean strains suggests that it could be extremely difficult to accurately identify the cysts of Alexandrium species based on their morphological features only.
In the phylogenetic tree, while all strains of A. insuetum formed a clade with high support, the clade, however, divides into a sub-clade of Asian strains and a sub-clade of French strains, which is in accordance with the results conducted by Lilly et al. (2005) and Shin et al. (2014). The clustering and separation of Asian and French strains agree with their geographic distance, indicating that there may be intraspecific variations in LSU rDNA sequences and even other traits among different geographic populations of A. insuetum.
Toxicity of A. insuetum and Its Ecological Implication
Our results clearly showed that A. insuetum exhibited significant toxicity to rotifer, brine shrimp, and finfish. Mortality of rotifer exposed to live cell culture was significantly higher than that exposed to cell lysate and cell-free culture medium, and the death of rotifer was mainly occurred in the last 60 h when exposed to the whole-cell culture, whereas most of the mortalities happened in the first 60 h of bioassays using the cell lysate and cell-free culture medium. Similarly, the whole live culture showed significantly higher toxicity to fish than did the cell-free culture medium and the epifluorescence LM micrographs of dead fish showed the presence of algal cells in the gut, suggesting that the toxins of A. insuetum could be accumulated along with the test animals ingesting algal cells during the bioassays, which explains the higher mortality of test animals caused by the whole live culture than that by the cell-free culture medium and cell lysate. Moreover, a number of algal cells appeared near the finfish gills (see Figure 6F), which could be another reason for the fish death. A. insuetum with the same or equivalent cell density showed variable lethal effects among the different test animals, which exhibited different sensitivity to the toxins among various groups of animals and, in this work, an order of sensitivity (e.g., brine shrimp, rotifer, and the larval fish). Considering that the test animals differ in body size, body structure, feeding mechanism, and physiological character, we are not able to provide a convincing explanation for their differences in sensitivity, but we believe that the complete identification of toxin(s) will facilitate the intoxication mechanisms in these different animals. In addition, the higher mortality of test animals (rotifer and brine shrimp) caused by the “older” cell-free culture medium indicates that toxins of A. insuetum could be continuously released into and accumulated in the culture medium and the toxins may be stable at least for days under room temperatures.
Some Alexandrium species may expand their populations to form HABs by suppressing micro-zooplankton (Wang et al., 2003; Lin et al., 2015). For instance, the marine copepod Acartia tonsa displayed reproductive impairment when feeding on A. catenella alone (Abdulhussain et al., 2021). Our results also revealed that hatching success of rotifer eggs was reduced by A. insuetum. More importantly, the eggs produced by the rotifers that had survived the exposure to either the whole live culture or cell-free culture medium of A. insuetum exhibited no hatching success at all, suggesting that the sublethal toxicity of A. insuetum to the rotifers led to the production of unhealthy eggs. Therefore, the ecological consequences of toxin production in A. insuetum could be viewed from two aspects: while the A. insuetum-caused mortality in marine animals will reduce the grazing pressure and thus enhance the growth of A. insuetum, on the other hand, the toxicity could affect marine animals not only in on-the-spot survival but also in the reproduction potency as reflected in the no or lowered hatching success of rotifer eggs, which will subsequently influence the population recruitment of the animals.
Characterization of Toxin(s) Produced by A. insuetum
Our work clearly demonstrated that the live cell culture, cell-free culture medium, and crude toxin extracts exhibited lethal effects on rotifers. Taken these results together, the toxins produced by A. insuetum were both intra- and extracellular, that is, the toxins were not only present in algal cells but also continuously released into the surrounding medium. Furthermore, the mortality of rotifer exposed to the extracts using water, methanol, and trichloromethane indicates that the toxin(s) is (are) of high polarity (more easily dissolve in water and methanol). We also found that the toxins were not heat stable.
Within the genus Alexandrium, PSP toxins have been considered as the most important toxins because they are produced by a number of well-known species and have potent toxicity to human health (Anderson et al., 2012), e.g., A. catenella, A. tamarense, A. pacificum, and A. minutum are responsible for the majority of PSP events worldwide (Anderson et al., 2012; Zou et al., 2014; Gu et al., 2021). Spirolides, a group of macrocyclic imines, have been isolated from some strains of A. ostenfeldii (Hu et al., 1995; Cembella et al., 2000; Cembella, 2001; MacKinnon et al., 2006; Paredes-Banda et al., 2018) and A. peruvianum (Borkman et al., 2012; Tomas et al., 2012). In addition, A. hiranoi and A. monilatum have been reported to produce goniodomins (Espina et al., 2016). Our previous studies demonstrated that A. leei also produces potent allelochemicals (affect phytoplankton) and ichthyotoxins that are hydrophilic, heat-stable, non-PSTs, and non-spirolides (Tang et al., 2007; Shang et al., 2021). In this study, analyses by UPLC-MS-MS and SPE-LC-MS/MS demonstrated that the toxins produced by A. insuetum were also neither PSTs nor spiroimines (13-desmethyl spirolide C and gymnodimine), but are heat unstable, suggesting that the toxins of A. leei and A. insuetum are different. However, PSP toxin has once been detected from a strain of A. insuetum isolated from Uchinoumi, Japan (Sako et al., 2004), but without the quantity of specific toxins, while non-toxic strains have also been reported for the isolates from Ofunato Bay and Shodoshima, Japan (Adachi et al., 1994; Lilly et al., 2005). These findings, thus, indicate that toxicity and toxin profiles may vary among different strains and geographic populations of A. insuetum.
Conclusions
This work represents the first characterization of the morphology, molecular phylogeny, and toxicology of A. insuetum from Chinese coastal water. The Chinese isolate exhibited significant lethal effects on rotifer, brine shrimp, and finfish, and also impacted the hatching success of rotifer eggs. Moreover, chemical characterization and toxin analyses demonstrated that the toxin(s) of A. insuetum is (are) hydrophilic, heat-labile, non-PSTs, and nonspiroimines. Therefore, A. insuetum may produce novel toxins that are different from those well-known, PSTs-producing Alexandrium species, and the toxicity to marine animals may not only contribute to the survival and HABs formation of A. insuetum, but also negatively impact the on-spot survival and the population recruitment of marine animals (rotifers in particular, as evidenced in the current work). Therefore, further investigations for the toxin(s) identification are highly desired.
Data Availability Statement
The datasets presented in this study are included in the article/Supplementary Material, further inquiries can be directed to the corresponding author.
Author Contributions
XS, ZH, and YT contributed to the conception and design of the study. XZ isolated the algal cultures of Alexandrium insuetum. ZH and XZ performed the LM and SEM microscopic images. XZ conducted the PCR reactions. ZH analyzed the rDNA sequence and constructed the phylogenetic tree. JC and SH performed the PSP and the lipophilic marine algal toxins analysis. LS, YD, and ZC helped with the design of laboratory bioassays. XS conducted the toxicity bioassays, extracted the lipophilic marine algal toxins, performed the statistical analyses, and wrote the primary draft manuscript. XZ, SH, LS, ZC, JC, and ZH revised the draft manuscript. YT perceived the project, designed the experiments, and revised the draft manuscript. All authors contributed to manuscript revision, read, and approved the submitted version.
Funding
This work was financially supported by the Science & Technology Basic Resources Investigation Program of China (2018FY100200), the National Science Foundation of China (Nos. 41976134 and 41776125), and the Key Deployment Project of Centre for Ocean Mega-Research of Science, Chinese Academy of Sciences (Grant No. COMS2019Q09).
Conflict of Interest
The authors declare that the research was conducted in the absence of any commercial or financial relationships that could be construed as a potential conflict of interest.
Publisher's Note
All claims expressed in this article are solely those of the authors and do not necessarily represent those of their affiliated organizations, or those of the publisher, the editors and the reviewers. Any product that may be evaluated in this article, or claim that may be made by its manufacturer, is not guaranteed or endorsed by the publisher.
Acknowledgments
We express our gratitude to Dr. Yuanyuan Sun from CAS Key Laboratory of Experimental Marine Biology, Institute of Oceanology, Chinese Academy of Sciences for assistance with SEM sample preparation.
Supplementary Material
The Supplementary Material for this article can be found online at: https://www.frontiersin.org/articles/10.3389/fmars.2021.735752/full#supplementary-material
References
Abdulhussain, A. H., Cook, K. B., Turner, A. D., Lewis, A. M., Bibby, T. S., and Mayor, D. J. (2021). The influence of the toxin-producing dinoflagellate, Alexandrium catenella (1119/27), on the survival and reproduction of the marine copepod, Acartia tonsa, during prolonged exposure. Front. Mar. Sci. 8:652225. doi: 10.3389/fmars.2021.652225
Adachi, M., Sako, Y., and Ishida, Y. (1994). Restriction fragment length polymorphism of ribosomal DNA internal transcribed spacer and 5.8S regions in Japanese Alexandrium species (Dinophyceae). J. Phycol. 30, 857–863. doi: 10.1111/j.0022-3646.1994.00857.x
Akaike, H. T. (1974). A new look at the statistical model identification. IEEE Trans. Autom. Control 19, 716–723. doi: 10.1109/TAC.1974.1100705
Anderson, D. M. (1998). Physiology and Bloom Dynamics of Toxic Alexandrium Species, With Emphasis on Life Cycle Transitions. Berlin: Springer.
Anderson, D. M., Alpermann, T. J., Cembella, A. D., Collos, Y., Masseret, E., and Montresor, M. (2012). The globally distributed genus Alexandrium: multifaceted roles in marine ecosystems and impacts on human health. Harmful Algae 14, 10–35. doi: 10.1016/j.hal.2011.10.012
Anderson, D. M., Stock, C. A., Keafer, B. A., Bronzino Nelson, A., Thompson, B., McGillicuddy, D. J., et al. (2005). Alexandrium fundyense cyst dynamics in the Gulf of Maine. Deep Sea Res. Part II 52, 2522–2542. doi: 10.1016/j.dsr2.2005.06.014
Balech, E. (1985). The Genus Alexandrium or Gonyaulax of the Tamarensis Group. New York, NY: Elsevier Science Publishing.
Balech, E. (1989). Redescription of Alexandrium minutum Halim (Dinophyceae) type species of the genus Alexandrium. Phycologia 28, 206–211. doi: 10.2216/i0031-8884-28-2-206.1
Blossom, H. E., Markussen, B., Daugbjerg, N., Krock, B., Norlin, A., and Hansen, P. J. (2019). The cost of toxicity in microalgae: direct evidence from the dinoflagellate Alexandrium. Front. Microbiol. 10:1065. doi: 10.3389/fmicb.2019.01065
Bolch, C. J. S. (1997). The use of sodium polytungstate for the separation and concentration of living dinoflagellate cysts from marine sediments. Phycologia 36, 472–478. doi: 10.2216/i0031-8884-36-6-472.1
Borkman, D. G., Smayda, T. J., Tomas, C. R., York, R., Strangman, W., and Wright, J. L. C. (2012). Toxic Alexandrium peruvianum (Balech and de Mendiola) Balech and Tangen in Narragansett Bay, Rhode Island (USA). Harmful Algae 19, 92–100. doi: 10.1016/j.hal.2012.06.004
Bravo, I., Garcés, E., Diogène, J., Fraga, S., Sampedro, N., and Figueroa, R. I. (2006). Resting cysts of the toxigenic dinoflagellate genus Alexandrium in recent sediments from the Western Mediterranean coast, including the first description of cysts of A. kutnerae and A. peruvianum. Eur. J. Phycol. 41, 293–302. doi: 10.1080/09670260600810360
Cembella, A. D. (2001). Association of the gonyaulacoid dinoflagellate Alexandrium ostenfeldii with spirolide toxins in size-fractionated plankton. J. Plankton Res. 23, 1413–1419. doi: 10.1093/plankt/23.12.1413
Cembella, A. D., Lewis, N. I., and Quilliam, M. A. (2000). The marine dinoflagellate Alexandrium ostenfeldii (Dinophyceae) as the causative organism of spirolide shellfish toxins. Phycologia 39, 67–74. doi: 10.2216/i0031-8884-39-1-67.1
Daly Yahia-Kefi, O., NÉZan, É., and Daly Yahia, M. N. (2001). Sur la présence du genre Alexandrium halim (Dinoflagellés) dans la baie de Tunis (Tunisie). Oceanol. Acta 24, 17–25. doi: 10.1016/S0399-1784(01)00076-7
Darriba, D., Taboada, G. L., Doallo, R., and Posada, D. (2012). jModelTest 2: more models, new heuristics and parallel computing. Nat. Methods 9:772. doi: 10.1038/nmeth.2109
Daugbjerg, N., Hansen, G., Larsen, J., and Moestrup, Ø. (2000). Phylogeny of some of the major genera of dinoflagellates based on ultrastructure and partial LSU rDNA sequence data, including the erection of three new genera of unarmoured dinoflagellates. Phycologia. 39, 302–317. doi: 10.2216/i0031-8884-39-4-302.1
Espina, B., Cagide, E., Louzao, M. C., Vilarino, N., Vieytes, M. R., Takeda, Y., et al. (2016). Cytotoxicity of goniodomin A and B in non contractile cells. Toxicol. Lett. 250–251, 10–20. doi: 10.1016/j.toxlet.2016.04.001
Fraga, S., Sampedro, N., Larsen, J., Moestrup, Ø., and Calado, A. J. (2015). Arguments against the proposal 2302 by John and al. to reject the name Gonyaulax catenella (Alexandrium catenella). Taxon 64, 634–635. doi: 10.12705/643.15
Fritz, L., and Triemer, R. E. (1985). A rapid simple technique utilizing calcofluor white M2R for the visualization of dinoflagellate thecal plates. J. Phycol. 21, 662–664. doi: 10.1111/j.0022-3646.1985.00662.x
Gao, L., Wang, Y., Chen, J., Wang, S., Shi, X., Shi, H., et al. (2016). Determination of eight typical lipophilic algae toxins in marine microalgae powder using high-performance liquid chromato-graphy-ion trap mass spectrometry. Mar. Sci. 40, 113–119. doi: 10.11759/hykx20151225002
Gu, H. (2011). Morphology, phylogenetic position, and ecophysiology of Alexandrium ostenfeldii (Dinophyceae) from the Bohai Sea, China. J. Syst. Evol. 49, 606–616. doi: 10.1111/j.1759-6831.2011.00160.x
Gu, H., Wu, Y., Lü, S., Lu, D., Tang, Y. Z., and Qi, Y. (2021). Emerging harmful algal bloom species over the last four decades in China. Harmful Algae 2021:102059. doi: 10.1016/j.hal.2021.102059
Gu, H., Zeng, N., Liu, T., Yang, W., Müller, A., and Krock, B. (2013). Morphology, toxicity, and phylogeny of Alexandrium (Dinophyceae) species along the coast of China. Harmful Algae 27, 68–81. doi: 10.1016/j.hal.2013.05.008
Guillard, R. R. L. (1975). “Culture of marine invertebrate animals,” in Culture of Phytoplankton for Feeding Marine Invertebrates, eds W. L. Smith and M.H. Chanley (New York, NY: Plenum Press), 26–60. doi: 10.1007/978-1-4615-8714-9_3
Guillou, L., Nezan, E., Cueff, V., Erard-Le Denn, E., Cambon-Bonavita, M. A., Gentien, P., et al. (2002). Genetic diversity and molecular detection of three toxic dinoflagellate genera (Alexandrium, Dinophysis, and Karenia) from French coasts. Protist 153, 223–238. doi: 10.1078/1434-4610-00100
Hall, T. A. (1999). BioEdit: a user-friendly biological sequence alignment editor and analysis program for windows 95/98/NT. Nucleic Acids Symp. Ser. 41, 95–98.
Hallegraeff, G. M., Anderson, D. M., Belin, C., Bottein, M.-Y. D., Bresnan, E., Chinain, M., et al. (2021). Perceived global increase in algal blooms is attributable to intensified monitoring and emerging bloom impacts. Commun. Earth Environ. 2, 1–10. doi: 10.1038/s43247-021-00178-8
Hosoi-Tanabe, S., and Sako, Y. (2006). Genetic differentiation in the marine dinoflagellates Alexandrium tamarense and Alexandrium catenella based on DNA-DNA hybridization. Plankton Bethos Res. 1, 138–146. doi: 10.3800/pbr.1.138
Hsia, M. H., Morton, S. L., Smith, L. L., Beauchesne, K. R., Huncik, K. M., and Moeller, P. D. R. (2006). Production of goniodomin A by the planktonic, chain-forming dinoflagellate Alexandrium monilatum (Howell) Balech isolated from the Gulf Coast of the United States. Harmful Algae 5, 290–299. doi: 10.1016/j.hal.2005.08.004
Hu, T., Curtis, J. M., Oshima, Y., Quilliam, M. A., Walter, J. A., Watson-Wright, W. M., et al. (1995). Spirolides B and D, two novel macrocycles isolated from the digestive glands of shellfish. J. Chem. Soc. Chem. Commun. 1995, 2159–2161. doi: 10.1039/c39950002159
Hu, Z., Li, Z., Deng, Y., Iwataki, M., Luo, Z., Wang, J., et al. (2020). Morphology, ultrastructure, and molecular phylogeny of the unarmoured dinoflagellate Kirithra sigma sp. nov. (Ceratoperidiniaceae, Dinophyceae). Phycologia 59, 385–396. doi: 10.1080/00318884.2020.1771660
Huang, H., Xu, Q., Gibson, K., Chen, Y., and Chen, N. (2021). Molecular characterization of harmful algal blooms in the Bohai Sea using metabarcoding analysis. Harmful Algae 106:102066. doi: 10.1016/j.hal.2021.102066
Ichimi, K., Suzuki, T., and Ito, A. (2002). Variety of PSP toxin profiles in various culture strains of Alexandrium tamarense and change of toxin profile in natural A. tamarense population. J. Exp. Mar. Biol. Ecol. 273, 51–60. doi: 10.1016/S0022-0981(02)00137-5
John, U., Litaker, R. W., Montresor, M., Murray, S., Brosnahan, M. L., and Anderson, D. M. (2014). Formal revision of the Alexandrium tamarense species complex (Dinophyceae) taxonomy: the introduction of five species with emphasis on molecular-based (rDNA) classification. Protist 165, 779–804. doi: 10.1016/j.protis.2014.10.001
Katoh, K., Rozewicki, J., and Yamada, K. D. (2019). MAFFT online service: multiple sequence alignment, interactive sequence choice and visualization. Brief. Bioinform. 20, 1160–1166. doi: 10.1093/bib/bbx108
Kim, C. J., Kim, C. H., and Sako, Y. (2005). Development of molecular identification method for genus Alexandrium (Dinophyceae) using whole-cell FISH. Mar. Biotechnol. 7, 215–222. doi: 10.1007/s10126-004-0424-2
Kim, E. S., Li, Z., Oh, S. J., Yoon, Y. H., and Shin, H. H. (2017). Morphological identification of Alexandrium species (Dinophyceae) from Jinhae-Masan Bay, Korea. Ocean Sci. J 52, 427–437. doi: 10.1007/s12601-017-0031-6
Kim, K., Yoshida, M., Fukuyo, Y., and Kim, C. (2002). Morphological observation of Alexandrium tamarense (Lebour) Balech, A. catenella (Whedon et Kofoid) Balech and one related morphotype (Dinophyceae) in Korea. Algae 17, 11–19. doi: 10.4490/ALGAE.2002.17.1.011
Lilly, E. L., Halanych, K. M., and Anderson, D. M. (2005). Phylogeny, biogeography, and species boundaries within the Alexandrium minutum group. Harmful Algae 4, 1004–1020. doi: 10.1016/j.hal.2005.02.001
Lin, J., Yan, T., Zhang, Q., and Zhou, M. (2015). Impact of several harmful algal bloom (HAB) causing species, on life history characteristics of rotifer Brachionus plicatilis Müller. Chin. J. Oceanol. Limn. 34, 642–653. doi: 10.1007/s00343-016-5065-6
MacKinnon, S. L., Walter, J. A., Quilliam, M. A., Cembella, A. D., Leblanc, P., Burton, I. W., et al. (2006). Spirolides isolated from Danish strains of the toxigenic dinoflagellate Alexandrium ostenfeldii. J. Nat. Prod. 69, 983–987. doi: 10.1021/np050220w
Medlin, L., Elwood, H. J., Stickel, S., and Sogin, M. L. (1988). The characterization of enzymatically amplified eukaryotic 16S-like rRNA-coding regions. Gene 71, 491–499. doi: 10.1016/0378-1119(88)90066-2
Mertens, K. N., Adachi, M., Anderson, D. M., Band-Schmidt, C. J., Bravo, I., Brosnahan, M. L., et al. (2020). Morphological and phylogenetic data do not support the split of Alexandrium into four genera. Harmful Algae 98:101902. doi: 10.1016/j.hal.2020.101902
Montresor, M., John, U., Beran, A., and Medlin, L. K. (2004). Alexandrium tamutum sp. nov. (Dinophyceae): a new nontoxic species in the genus Alexandrium. J. Phycol. 40, 398–411. doi: 10.1111/j.1529-8817.2004.03060.x
Nagai, S., Nishitani, G., Takano, Y., Yoshida, M., and Takayama, H. (2009). Encystment and excystment under laboratory conditions of the nontoxic dinoflagellate Alexandrium fraterculus (Dinophyceae) isolated from the Seto Inland Sea, Japan. Phycologia 48, 177–185. doi: 10.2216/08-43.1
Nascimento, S. M., Purdie, D. A., Lilly, E. L., Larsen, J., and Morris, S. (2005). Toxin profile, pigment composition, and large subunit rDNA phylogenetic analysis of an Alexandrium minutum (Dinophyceae) strain isolated from the Fleet Lagoon, United Kingdom. J. Phycol. 41, 343–353. doi: 10.1111/j.1529-8817.2005.03088.x
Nikolaidis, G., Koukaras, K., Aligizaki, K., Heracleous, A., Kalopesa, E., Moschandreou, K., et al. (2005). Harmful microalgal episodes in Greek coastal waters. J. Biol. Res. 3, 77–85.
Paredes-Banda, P., García-Mendoza, E., Ponce-Rivas, E., Blanco, J., Almazán-Becerril, A., Galindo-Sánchez, C., et al. (2018). Association of the toxigenic dinoflagellate Alexandrium ostenfeldii with spirolide accumulation in cultured mussels (Mytilus galloprovincialis) from northwest Mexico. Front. Mar. Sci. 5:491. doi: 10.3389/fmars.2018.00491
Ronquist, F., Teslenko, M., van der Mark, P., Ayres, D. L., Darling, A., Hohna, S., et al. (2012). MrBayes 3.2: efficient Bayesian phylogenetic inference and model choice across a large model space. Syst. Biol. 61, 539–542. doi: 10.1093/sysbio/sys029
Sako, Y., Hosoi-Tanabe, S., and Uchida, A. (2004). Fluorescence in situ hybridization using rRNA-targeted probes for simple and rapid identification of the toxic dinoflagellates Alexandrium tamarense and Alexandrium catenella. J. Phycol. 40, 598–605. doi: 10.1111/j.1529-8817.2004.03035.x
Scholin, C. A., Herzog, M., Sogin, M., and Anderson, D. M. (1994). Identification of group-and strain-specific genetic markers for globally distributed Alexandrium (Dinophyceae). II. Sequence analysis of a fragment of the LSU rRNA gene. J. Phycol. 30, 999–1011. doi: 10.1111/j.0022-3646.1994.00999.x
Shang, L., Xu, Y., Leaw, C. P., Lim, P. T., Wang, J., Chen, J., et al. (2021). Potent allelopathy and non-PSTs, non-spirolides toxicity of the dinoflagellate Alexandrium leei to phytoplankton, finfish and zooplankton observed from laboratory bioassays. Sci. Total Environ. 780:146484. doi: 10.1016/j.scitotenv.2021.146484
Shin, H. H., Baek, S. H., Li, Z., Han, M. S., Oh, S. J., Youn, S. H., et al. (2014). Resting cysts, and effects of temperature and salinity on the growth of vegetative cells of the potentially harmful species Alexandrium insuetum Balech (Dinophyceae). Harmful Algae 39, 175–184. doi: 10.1016/j.hal.2014.07.012
Sleno, L., Windust, A. J., and Volmer, D. A. (2004). Structural study of spirolide marine toxins by mass spectrometry. Part I. Fragmentation pathways of 13-desmethyl spirolide C by collision-induced dissociation and infrared multiphoton dissociation mass spectrometry. Anal. Bioanal. Chem. 378, 969–976. doi: 10.1007/s00216-003-2297-z
Spatharis, S., Danielidis, D. B., and Tsirtsis, G. (2007). Recurrent Pseudo-nitzschia calliantha (Bacillariophyceae) and Alexandrium insuetum (Dinophyceae) winter blooms induced by agricultural runoff. Harmful Algae 6, 811–822. doi: 10.1016/j.hal.2007.04.006
Stamatakis, A. (2006). RAxML-VI-HPC: maximum likelihood-based phylogenetic analyses with thousands of taxa and mixed models. Bioinformatics 22, 2688–2690. doi: 10.1093/bioinformatics/btl446
Tang, Y. Z., Gu, H., Wang, Z., Liu, D., Wang, Y., Lu, D., et al. (2021). Exploration of resting cysts (stages) and their relevance for possibly HABs -causing species in China. Harmful Algae 2021:102050. doi: 10.1016/j.hal.2021.102050
Tang, Y. Z., Hu, Z. X., and Deng, Y. Y. (2016). Characteristic life history (resting cyst) provides a mechanism for recurrence and geographic expansion of harmful algal blooms of dinoflagellates: a Rev. Stud. Mar. Sin. 51, 132–154. doi: 10.12036/hykxjk20160730001
Tang, Y. Z., Kong, L., and Holmes, M. J. (2007). Dinoflagellate Alexandrium leei (Dinophyceae) from Singapore coastal waters produces a water-soluble ichthyotoxin. Mar. Biol. 150, 541–549. doi: 10.1007/s00227-006-0396-z
Tomas, C. R., van Wagoner, R., Tatters, A. O., White, K. D., Hall, S., and Wright, J. L. C. (2012). Alexandrium peruvianum (Balech and Mendiola) Balech and Tangen a new toxic species for coastal North Carolina. Harmful Algae 17, 54–63. doi: 10.1016/j.hal.2012.02.011
Wang, J., Chen, J., He, X., Hao, S., Wang, Y., Zheng, X., et al. (2021). Simple determination of six groups of lipophilic marine algal toxins in seawater by automated on-line solid phase extraction coupled to liquid chromatography-tandem mass spectrometry. Chemosphere 262:128374. doi: 10.1016/j.chemosphere.2020.128374
Wang, L., Yan, T., Tan, Z., and Zhou, M. (2003). Effects of Alexandrium tamarense and Prorocentrum donghaiense on rotifer Brachionus plicatilis population. Chin. J. Appl. Ecol. 14, 1151–1155.
Xu, Y., He, X., Li, H., Zhang, T., Lei, F., Gu, H., et al. (2021). Molecular identification and toxin analysis of Alexandrium spp. in the Beibu Gulf: first report of toxic A. tamiyavanichii in Chinese coastal waters. Toxins 13:161. doi: 10.3390/toxins13020161
Yu, R., and Liu, D. (2016). Harmful algal blooms in the coastal waters of China: current situation, long-term changes and prevention strategies. Bull. Chinese Acad. Sci. 31, 1167–1174. doi: 10.1007/978-3-319-70069-4_15
Yuki, K., and Yoshimatsu, S. (1990). New record of Alexandrium insuetum Balech (Dinophyceae) from Japan with some supplementary observations on thecal morphology. Bull. Plankton Soc. Jpn. 36, 121–126.
Zhou, M., and Zhu, M. (2006). Progress of the project “ecology and oceanography of harmful algal blooms in China”. Adv. Earth Sci. 21, 673–679. doi: 10.1016/S1002-0160(06)60035-0
Keywords: harmful algal blooms (HABs), Alexandrium insuetum, morphology, phylogeny, novel toxicity, fish kill
Citation: Song X, Zhai X, Hao S, Shang L, Deng Y, Chai Z, Chen J, Hu Z and Tang YZ (2021) Novel Non-paralytic Shellfish Toxin and Non-spirolide Toxicity to Finfish, Brine Shrimp, and Rotifer Observed in a Culture of the Dinoflagellate Alexandrium insuetum Isolated From the Coastal Water of China. Front. Mar. Sci. 8:735752. doi: 10.3389/fmars.2021.735752
Received: 05 July 2021; Accepted: 25 August 2021;
Published: 28 September 2021.
Edited by:
Cristiana Moreira, University of Porto, PortugalReviewed by:
Annalisa Zaccaroni, University of Bologna, ItalyPaula Mariela González, University of Buenos Aires, Argentina
Copyright © 2021 Song, Zhai, Hao, Shang, Deng, Chai, Chen, Hu and Tang. This is an open-access article distributed under the terms of the Creative Commons Attribution License (CC BY). The use, distribution or reproduction in other forums is permitted, provided the original author(s) and the copyright owner(s) are credited and that the original publication in this journal is cited, in accordance with accepted academic practice. No use, distribution or reproduction is permitted which does not comply with these terms.
*Correspondence: Zhangxi Hu, zhu@qdio.ac.cn; Ying Zhong Tang, yingzhong.tang@qdio.ac.cn