- 1Instituto de Ecología, A. C., Xalapa, Mexico
- 2Instituto de Ingeniería, Universidad Nacional Autónoma de México, Ciudad de México, Mexico
- 3Beach and Dune Systems (Beads) Lab, College of Science and Engineering, Flinders University, Bedford Park, SA, Australia
The impact of storms on coastal dunes and beaches and the effects they induce in topography and plant communities are natural processes that contribute to maintaining natural coastal dynamics. However, because coasts are often densely populated, these phenomena are perceived as major threats to human property. To protect human assets sustainably, nature-based defenses have emerged as an option. Coastal dunes act as natural buffers that mitigate the extent of erosion and inland flooding, and their resistance depends on the biogeomorphological feedback between the plants and the dunes. This study aimed to evaluate the effect of one winter storm on beach and dune topography and the plant communities, and to explore the effect of plants in mitigating erosion on beaches with different geomorphological features. The effects on plant communities were evaluated by comparing diversity and plant cover before and after the storm. Later, the role of plants in conferring dune resistance against erosion was examined by measuring erosion on the exposed face of the dunes considering plant cover and plant richness. The results did not show significant differences in plant diversity and plant cover between pre-and post-storm conditions, but turnover of species was recorded. The dune building species were not affected but inland species disappeared. Erosion was reduced when the dunes were higher and, furthermore, plant cover was negatively correlated with erosion on these dunes. The results showed a reduced impact of the storm on the plant communities, which is important as it facilitates the recovery of dunes by the dune-building species and protects them in a subsequent storm. The novelty of this study is that: (a) it demonstrates the species-specific role of plants in mitigating dune erosion in field conditions; (b) it shows the interaction between plant-related features and geomorphological variables in promoting dune resistance to erosion, and (c) it explores the immediate effect of a winter storm on the plant community and dune-building species.
Introduction
Tropical cyclones and winter storms are major drivers in shaping coastal geomorphology through energetic waves, storm surges, set-up, and strong winds which may induce dramatic changes (Coch, 1994; Castelle et al., 2015; Masselink et al., 2016). On wave-dominated sandy coasts, these natural, episodic phenomena are frequently associated with considerable erosion on the beach and dunes (Vellinga, 1982; Carter, 2013; Davidson et al., 2020). After a storm episode, the beach face is lowered and cut back, and a vertical scarp is often seen, due to the loss of sand volume on the dry beach (Hesp, 2002; Carter, 2013; Castelle et al., 2015). However, this sand will return and accumulate on the beach and dunes when calm conditions prevail if the sediment budget is not affected by natural or human processes, and the beach-surfzone has no net sediment deficit (Carter, 2013; Davidson-Arnott et al., 2018). In brief, the coastal response, including the volume of sand that will be eroded during a storm, depends on storm-related features, the cluster of storms (Karunarathna et al., 2014), the synchronicity of environmental variables (e.g., tide, wind direction; Guisado-Pintado and Jackson, 2018, 2019), and factors such as dune height (Pries et al., 2008), the slope of the beach and dunes (Vellinga, 1982), the mechanical characteristics of the bed material (e.g., type of sediment, diameter, and sand density; Overton et al., 1994), the sand volume stored on the beach and dunes (Sigren et al., 2018), the compaction of the foredune (Nishi and Kraus, 1997), the presence of a berm and sand bars (Figlus et al., 2011), the length of foredune ridge (Houser, 2013), and the width of the beach and dunes (Saye et al., 2005; Pries et al., 2008; Davidson et al., 2020).
Besides the geomorphological features of the coast, the impact of storms is also relevant for plant communities and coastal risk management. Plant communities are commonly disturbed by the environmental conditions associated with storms, such as heavy precipitation, flooding, high salinity concentrations, accretion, and erosion (Wolner et al., 2013; Brantley et al., 2014; Cheplick, 2016). The effects of storms on plant communities depend on the spatial and temporal scale of the storm, its magnitude, storm clustering, and the species-specific responses of the plants growing in the different dune habitats (foredunes, interdunes, and back dunes; Judd and Sides, 1983; Gornish and Miller, 2010; Miller et al., 2010; Miller, 2015). Some of these effects include a reduction in plant species richness and diversity (Judd and Sides, 1983; Miller et al., 2010; Cheplick, 2016), species turnover (Synder and Boss, 2002; Miller et al., 2010), changes in species dominance, and their distribution in the dune habitats (Gornish and Miller, 2010), and changes in plant cover or abundance (Cheplick, 2016).
Moreover, because of the biogeomorphological feedback between dunes and plants, the geomorphological responses of the beaches and dunes during storm (but also during dune development and the recovery of the dune) depend on the response of the plant communities and the species-specific response (Stallins and Parker, 2003; Stallins, 2005; Wolner et al., 2013; Brantley et al., 2014). The close interaction between dune plants and coastal dunes occurs because plants entrap, collect, and stabilize sediment, and thus shape dune topography (Moreno-Casasola, 1986; Hesp, 1991, 2002; Hesp et al., 2019). By acting as sediment traps, plants accumulate sand, accelerate accretion, build dunes, withhold sand, and help mitigate erosion. Dune geomorphology depends on factors such as plant zonation (Doing, 1985; Maun and Perumal, 1999; Durán and Moore, 2013), plant density and height (Hesp, 2002; Hesp et al., 2019), and plant architecture (Zarnetske et al., 2012; Charbonneau et al., 2016, 2021). Consequently, different dune types shaped by distinct species could differ in their resistance to erosion (Charbonneau et al., 2021). In addition to burial by sand, plants on the backshore and incipient dunes are also tolerant to salinity (Maun, 1998), so they are likely to survive and recover after the impact of storms, which bring sand movement and flooding with salt water. The resistance and tolerance of plants to storm conditions is of relevance because if dune-building species are harmed, the recovery of the dunes (growth and development) could be arrested and bring about changes in dune morphology. In consequence, storm protection might decrease and the recovery of dunes after the storm could be arrested. Inland vulnerability after subsequent storms may thus increase.
In terms of coastal risk management, when storms are likely to damage human infrastructure and result in the loss of human lives, the above-mentioned biogeomorphological system generated through the dynamic interaction between plants and dunes, becomes highly relevant. This is especially true for coastal green infrastructure solutions whose premise is to protect exposed human interests from ocean hazards, and potentially from sea-level rise, by working with natural processes (Temmerman et al., 2013; Spalding et al., 2014; Chávez et al., 2021). Coastal dunes are often the first natural terrestrial defense against storm impact. Dunes help to reduce the landward extent of erosion, overwashing, the impact of waves (swash, collision, overwash, and inundation; Sallenger, 2000), and the degree of damage to property, by dissipating wave energy, and reducing/preventing floods (Sigren et al., 2018). The protective role of the dunes lies in their natural ability to adjust to the natural disturbances in the littoral (including storm impacts) by changing their morphological configuration (their relative position and form) migrating landward or seaward in response to wind, waves, and sea-level rise (Davidson-Arnott, 2005; Saye et al., 2005).
In addition to the role of plants in promoting the development of these natural barriers, they can also help reduce beach and dune erosion during storms. Laboratory studies have shown that erosion on vegetated dunes is reduced or slowed down, in comparison with dunes without vegetation (Kobayashi et al., 2013; Silva et al., 2016; Figlus et al., 2017; Bryant et al., 2019). It has also been demonstrated that their roots contribute to strengthening the cohesiveness of the sand (Sigren et al., 2014; De Battisti and Griffin, 2020), while the above-ground plant parts slow wave uprush, and reduce wave overtopping and overwashing (Silva et al., 2016; Figlus et al., 2017; Feagin et al., 2019), displace the wave breaking point seawards and deaccelerate the undertow close to the shoreline (Mendoza et al., 2017). Furthermore, Charbonneau et al. (2017) demonstrated that the reduction of erosion is species-specific. These findings are supported by Maximiliano-Cordova et al. (2019), while Odériz et al. (2020) determined that the spatial location of the plants on the dune affects the erosion patterns, as well as the mass, density, and depth of the roots (Davidson et al., 2020). To our knowledge, only three studies performed in field conditions have tested the effect of plants in conferring dune resistance to wave-induced erosion. Lindell et al. (2017) observed that when plants were removed, wave erosion was greater than that on dunes where the vegetation remained, while Charbonneau et al. (2017) and Biel et al. (2017) documented species-specific dune resistance. Nevertheless, there is a large information gap concerning field conditions which demonstrate how protection offered by plants could depend on the plant- and geomorphological-related features that vary between beaches.
In brief, the impact of even a single storm event is a complex process because it may affect the topography and morphology of the beach and dunes as well as the plant community developing in this environment. In addition, in field conditions, as revealed in findings from laboratory experiments, plants can have a negative impact on erosion and play a protective role. Based on the above, this study aims to: (I) analyze how beach and dune profiles were affected by one winter storm; (II) analyze the impact of this storm on the plant communities established on the beach, the backshore and dunes; (III) examine how pre-existing morphological conditions of the beach-dune complex affect erosion; and (IV) explore whether plant cover and plant species richness help mitigate beach erosion in different dune and beach geomorphologies. Our working hypotheses were: (a) Plant cover and diversity decrease because of the impact of the storm, which also induces changes in species composition and dominance of the dune-building species. (b) Pre-existing beach and dune geomorphological conditions determine storm-induced erosion. Beaches with higher dunes are less likely to be eroded and overwashed than those that are flatter. (c) Plants help mitigate beach and dune erosion, which is expected to decrease with increasing plant cover and species richness. Ultimately, our premise was that the interaction between plants and geoforms modifies beach and dune response to the impact of storms.
Materials and Methods
Study Sites
Three beaches located in the central region of the Gulf of Mexico, on the coast of Veracruz (Mexico), were studied: Riachuelos, Navarro, and La Mancha (Figure 1). The climate is warm and sub-humid (Martínez et al., 2014) with mean yearly temperatures ranging from 22 to 25°C, and total annual precipitation fluctuating between 1,200 and 1,650 mm (Infante-Mata et al., 2011). The rainy season is from August to September, coinciding with the season of tropical cyclones (Martínez et al., 2014). Winter storms, known locally as “nortes,” occur from November to February and can generate storm surges exceeding 1.5 m and extreme winds of 80 to 100 km/h (Ramírez and Reséndiz, 2002). Both of these phenomena produce energetic waves (Psuty et al., 2009) and can consequently induce dramatic changes in the topography of the beach and dunes.
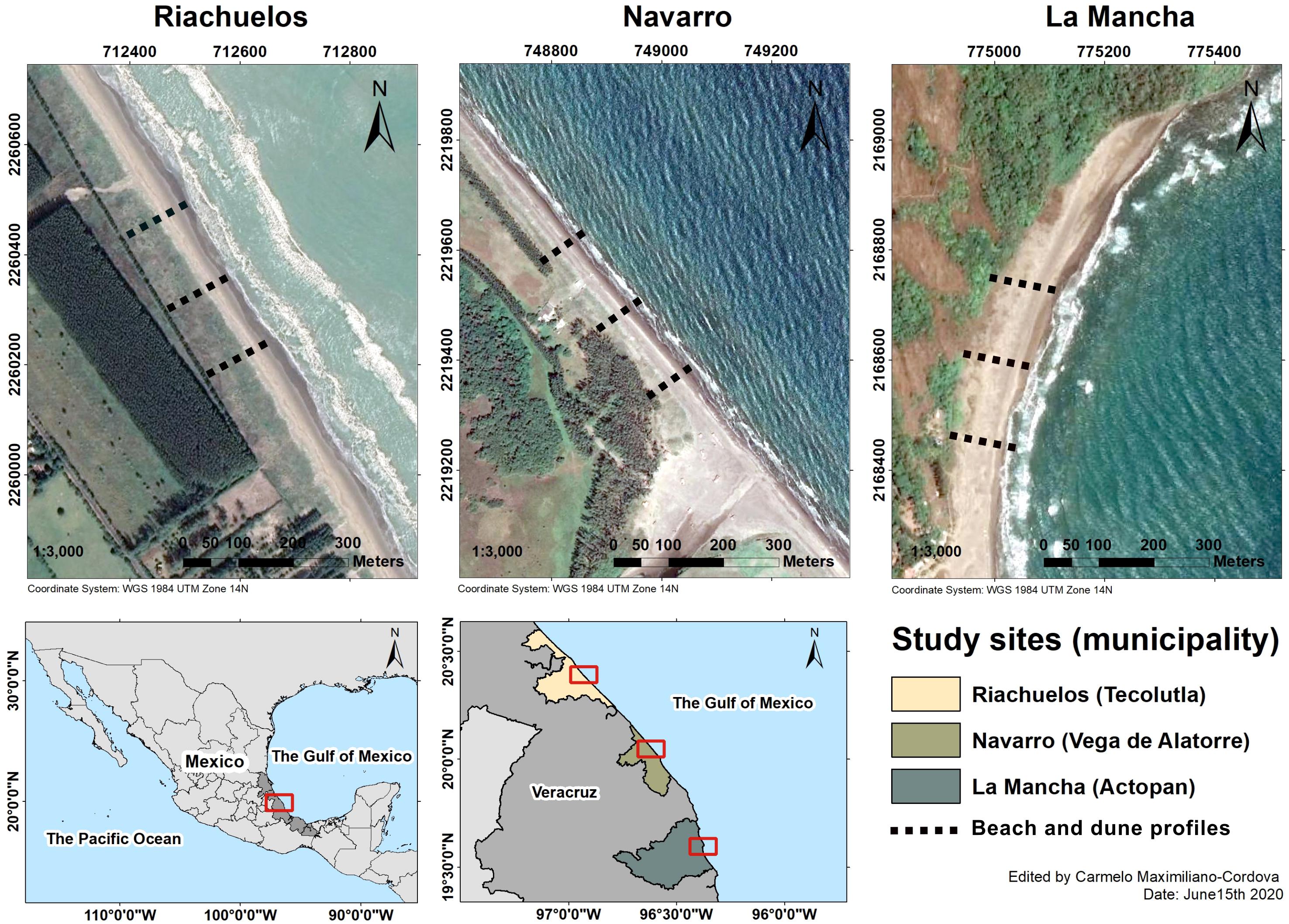
Figure 1. Location of the study sites in the state of Veracruz, Mexico. Images based on satellite images from Google Earth.
The three beaches are fed by sand from at least one of the two most important rivers on the central coast of Veracruz. Riachuelos receives sediment from the Tecolutla River while Navarro and La Mancha receive sediments from the Tecolutla and Filobobos rivers. In addition, sediment from adjacent lagoons such as “El Llano” and “La Mancha” is supplied to the beach-dune system of La Mancha. On the central coast of Veracruz, the along-shore sediment is transported from north to south, and its distribution on the coast depends on waves and currents. Aeolian transport is also important, contributing to the alongshore sediment supply, especially in embayed coasts, such as La Mancha (Psuty et al., 2009). Therefore, well-developed dune systems are likely to be found in the study area.
The three beaches are different in terms of their geomorphological features, as well as the dominant vegetation growing on the beach and coastal dunes (Table 1). Incipient dunes generated by the creeping vine species Ipomoea pes-caprae and the succulent Sesuvium portulacastrum are formed on the beach at Riachuelos and La Mancha, with established foredunes behind them. At both sites, the incipient dunes are developed behind the high tide line and do not form a continuous ridge parallel to the coast. At Navarro, there is a well-formed continuous foredune ridge formed by the grass Panicum amarum and no incipient dunes are found here. Instead, a second well-formed foredune is developed behind the first one. These geomorphological differences are associated with the dominant plant species growing at each location (in addition to other biophysical variables). They are relevant in determining the response of the beach and dunes to the impact of storms. For instance, a continuous dune ridge is expected to be more resistant to the impact of waves than isolated dunes, which are more susceptible to scarping and erosion (Claudino-Sales et al., 2008). Therefore, the effects of the storm on beach and dune topography, the plant communities, and the effect of the plants in creating dune resistance versus erosion, were explored on different beaches, with different geomorphologies and plant dominance. For simplicity, from here on, although different, both types of dunes are referred to as “dunes.”
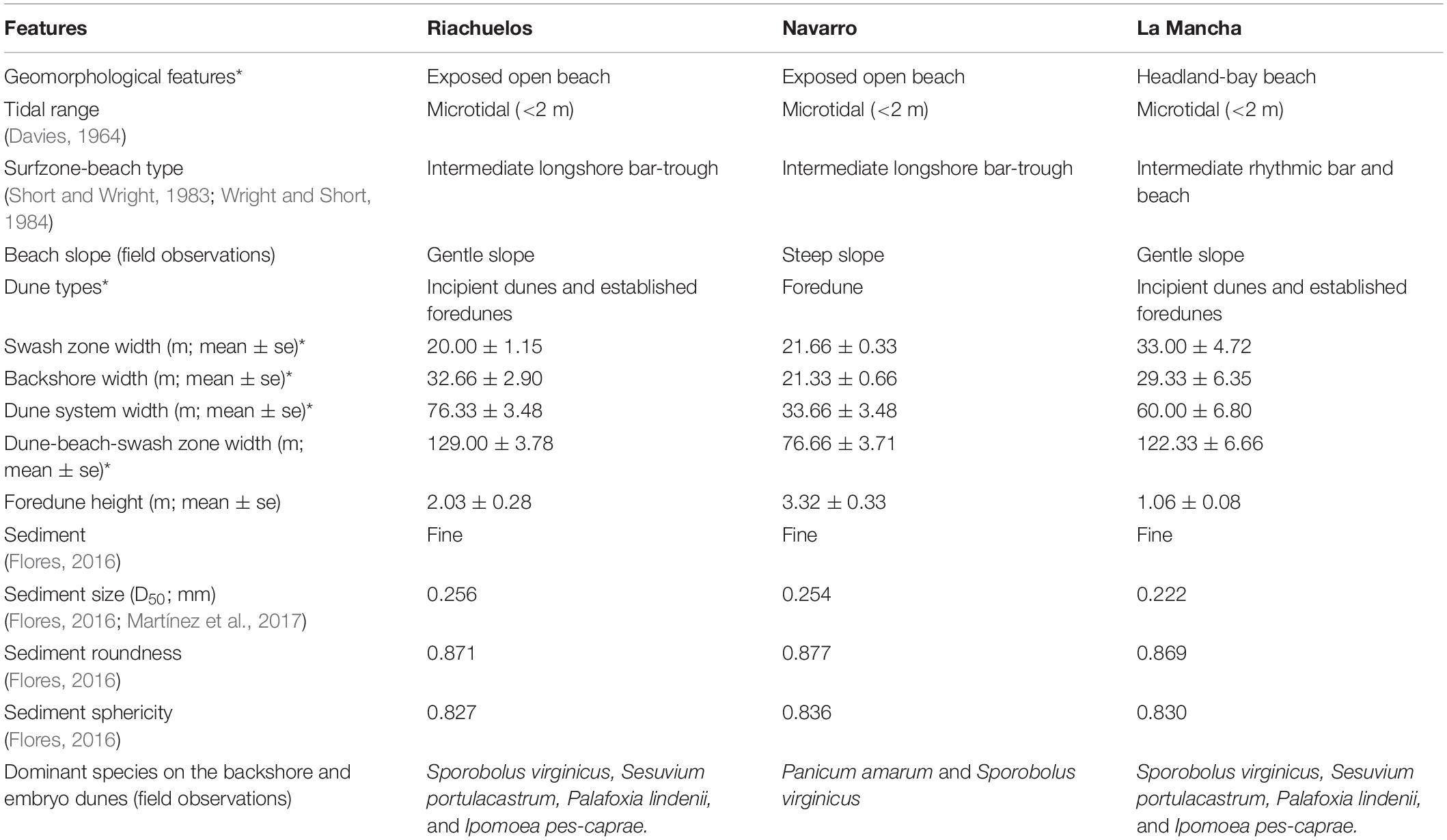
Table 1. Main geomorphological attributes of the beaches studied (* geomorphological features estimated from field surveys performed before the storm).
Storm Conditions
This study focuses on the impacts of a single winter storm event which took place in 2019. Winter storms are milder than tropical cyclones and therefore less destructive. As winter storms occur shortly after the rainy season, the vegetation is fully developed, plant cover is likely to be at a maximum, and plants can more effectively protect the backshore and dunes from erosion. In turn, tropical cyclones occur during the summer, when the vegetation is recovering from the spring-time drought, so they are likely to be less effective in providing protection.
We studied the effects of winter storm Number 12, which arrived at the Gulf of Mexico on November 12th, 2019. Even though 11 winter storms had previously occurred in the Gulf of Mexico, this was the first of the season to affect the coast of Veracruz. According to the reanalysis of ERA5 (Copernicus Climate Change Service (C3S), 2017), the storm had sustained winds of up to 15.5 m/s, significant waves heights of up to 4.5 m, with a mean wave period of 9 s, and induced 27.95 mm of precipitation. The winds had a predominant Northwest direction, and were mainly moderate in velocity (Figure 2A). The prevailing waves arrived from the north and were high (Figure 2B). It is important to mention that the lack of bathymetry for the three beaches means that the description of the wave propagation during the storm could not be more accurate.
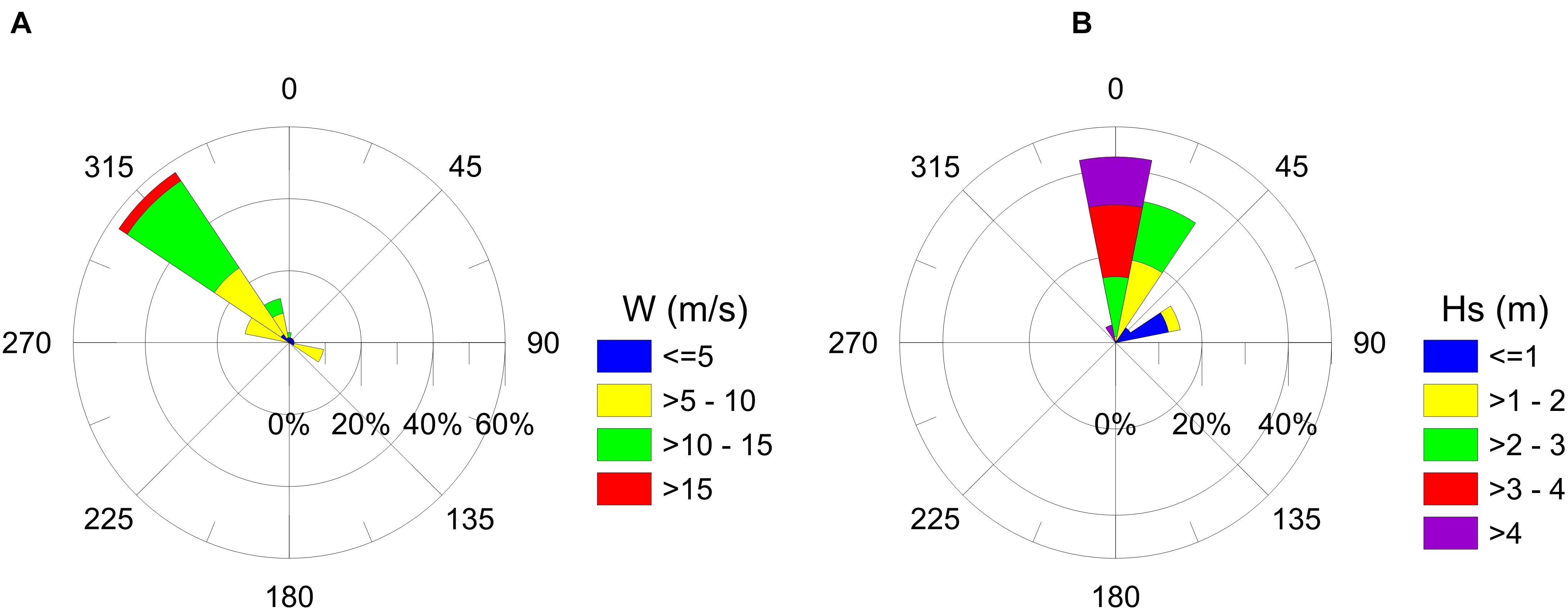
Figure 2. (A) Wind velocity and (B) significant wave height roses associated to the Winter Storm Number 12 (November 12–15, 2019).
Fieldwork
The topography and vegetation of the beach and dunes were monitored before and after the impact of a single winter storm, in September and November 2019, respectively. At each study site, three transects were established, perpendicular to the coastline (Figure 1). The length of the transects varied depending on the width of the beach, the number of dune ridges, and the limit of private properties at the back of the beach. The transects in Riachuelos and Navarro were set from the toe of the second dune ridge toward the ocean, while in La Mancha they were set from the toe of the stabilized foredune toward the ocean (Figure 3A). On each transect, three control points were marked to facilitate their relocation in the post-storm monitoring. The first marker was placed at the beginning of the dune-ocean transect and was considered as the zero reference point (x = 0 and y = 0 coordinates). The second marker was placed in the middle of the transects and the third in the swash zone of the beach. All three points were georeferenced with a GPS (Garmin GPSMAP®64) and the first and the second points were also marked with aluminum stakes.
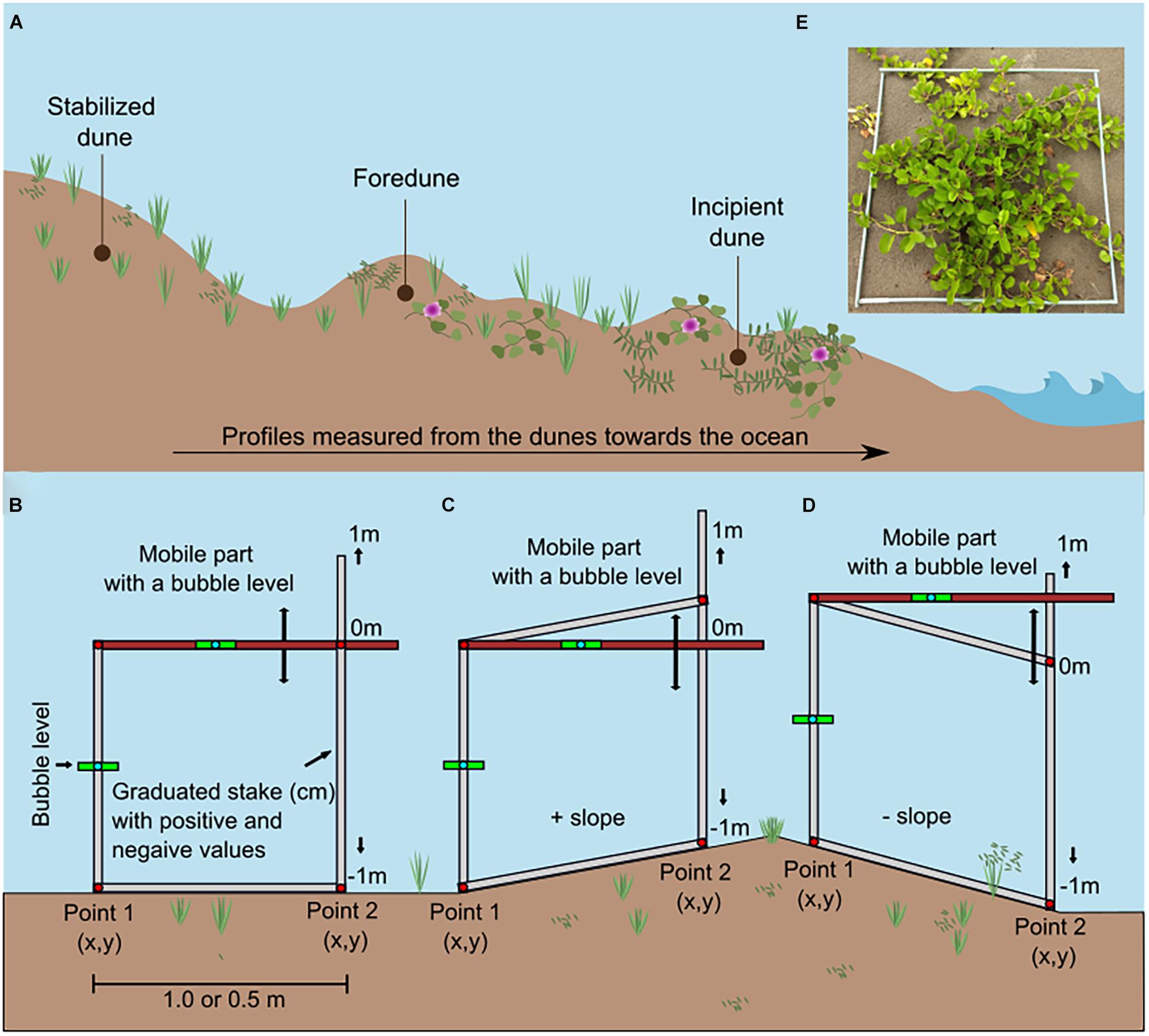
Figure 3. (A) Beach and dune profile showing the direction in which the profiles were measured from the foredune to the swash zone. (B) A self-made instrument to measure changes in height (y) between two points separated by a known distance (x). Each time that the user advances, in one-m segments (or 0.5 m segments), changes in height can be measured. Because of the direction in which the profiles were measured (from the dunes to the beach), positive slopes were mainly associated with protected faces of the dune (C), and negative slopes with the exposed face (D). (E) Picture showing one of the plots where vegetation was sampled.
Monitoring of Topography (Beach and Dune Profile)
The pre- and post-storm beach and dune profiles were measured along the three transects set on each beach, from the dune toward the ocean (Figure 3A), beginning at a previously set point of a known height (our x = 0 and y = 0 coordinates), with two inclinometers made in the laboratory (Figure 3B; Emery, 1961; Krause, 2004). The instruments measured changes in topography between two points set at distances of either 0.5 or 1 m, depending on the topography (Figures 3B–D).
The zero-level of the sand surface used as the x = 0 and y = 0 (height and distance) reference point to measure changes in topography was set based on a 1 m aluminum stake which was marked every 1 cm, with positive and negative numbers, from the zero-line drawn in the middle of the stake. The stake was buried in the sand so that the zero-line coincided with the level of the sand. Following the storm, the topography was measured again, erosion and accretion were registered, and the reference point was adjusted so that the changes in topography could be assessed similar to Davidson-Arnott and Law (1996).
Vegetation Monitoring
Plots (2 × 2 m) were established at two meter intervals to monitor the vegetation along each transect (Figure 3E). The first plot was at the x = 0, y = 0 coordinates used to measure the topography, and subsequent plots were placed at two-meter intervals with the last plot being located at the seaward-most limit of vegetation on the backshore. The corners of each plot were georeferenced and three plots per transect were marked with aluminum stakes to locate them precisely in the post-storm monitoring.
The number of plots monitored per beach and per transect varied, depending on the beach width and the distribution of plants on the foredunes and backshore. Before the storm, 52 plots were monitored at Riachuelos, 24 at Navarro and, 47 at La Mancha. After the storm 54, 24, and 40 plots were monitored, respectively. In each plot, every vascular plant species was identified and the percentage of plant cover per species, the total percentage of plant cover, and the percentage of bare sand were estimated visually (Martínez et al., 2001, 2019). For each plot, the visual percent cover estimations were performed by the same person to avoid bias. When species were unknown, three samples were collected for identification in the herbarium at the Institute of Ecology (XAL), in Xalapa, Veracruz.
Data Analyses
Topography
The beach profiles measured, as explained above, were processed and analyzed with ArcMap 10.5. The x, y coordinates from each transect were projected as points, transformed into lines, and then into a single polygon, by joining the lines with a straight, horizontal line drawn from the first to the last point. This line was set at a constant height and serves as the baseline of the polygon. Then, the “union” tool of ArcMap was used to overlap the pre-and post-storm profiles. After the overlap, new polygons were created for each pair of profiles, producing new polygons showing accretion, erosion, and no changes in the topography. The area of the new polygons was calculated with the “calculate geometry” tool of ArcMap and then the volume of sand eroded or accreted was calculated for (i) each entire profile, (ii) the frontal dune, backshore, and beach of each profile (measured from the base of the lee slope of the dune to the lowest seaward elevation surveyed) and, (iii) each two-meter segment where the plot to monitor vegetation had been placed.
Beach volume was calculated based on the area of the polygon and by assuming that changes measured in the profile were the same in the adjacent 0.25 m on each side of the transect (0.5 m in total). Thus, changes in the volume were calculated as Eq. 1.
where V = volume, Ab = base area (polygon area) and, h = height (0.5 m). For the three areas where the volume was calculated, net erosion was estimated by subtracting the total accretion from the total erosion. In the case of the erosion on the entire profile and erosion on the frontal dune and beach, an analysis of variance was performed to see whether the amount of erosion varied between the beaches. The analysis was run in R Studio (R version 3.6.1 “Action of the Toes”; R core Team, 2019).
In addition, for each profile, the maximum height (m) of the first incipient, or foredune, was calculated as the vertical distance from the sea level to the crest of the dune, and with the x, y coordinates, the following variables were calculated: width of the backshore (m), slope of the backshore and the stoss slope of the dune. The slopes were calculated as the slope of the line (Eq. 2) and then they were expressed as angle (θ) in sexagesimal degrees (Eq. 3).
where x1 and y1 represent the coordinates of the first point of the profile (the beginning) and x2 and y2 represent the coordinates of the second point (the end) of the segment.
It is important to clarify that since the profiles were measured from the dunes toward the ocean, positive values refer to the lee face of the foredunes (the higher the positive number, the steeper the slope on the lee face of the dunes) and negative slopes were measured on the stoss face of the dune (the greater the negative numbers, the steeper the slope in the exposed faces of the embryo dunes). However, for the statistical analysis in which the slopes were considered, the absolute values of the slopes were used. Finally, values close to 0 indicate a gentle or flat slope.
Assessing the Effect of the Winter Storm on the Plant Community
The effect of the winter storm on the plant community was determined based on changes in plant cover, plant diversity, and species dominance. For each sampled plot, and at each observation date, the percentage of species cover was converted into m2 (Martínez et al., 2001, 2019) as Eq. 4.
Then, plant cover per species (m2), total plant cover (m2), and species richness were used to evaluate: (i) the changes in plant cover, (ii) plant diversity, and (iii) the Relative Importance Value (RIV).
Plant cover
Two paired sample t-tests were performed (i) to look for differences in overall plant cover between pre- and post-storm conditions at each site by considering all the plots located on the dunes and backshore, and (ii) to explore the changes in seaward vegetation established on the dunes closest to the ocean (incipient dunes for Riachuelos and La Mancha and foredunes for Navarro). To achieve this, first, we added total plant cover per transect so that for each beach, we had three plant cover values before the storm and three after the storm, which were compared with a t-test for each site. Then, we focused the analyses on the three vegetated plots located closest to the ocean (per transect) and followed the same procedure that was used for the complete transects. The tests were run in R studio (R version 3.6.1 “Action of the Toes”; R core Team, 2019).
Alpha-diversity
Alpha diversity was evaluated using the concept of “effective numbers of species” (Jost, 2006; Eq. 5), a method that is equivalent to Hill’s number (Hill, 1973). This method has mathematical properties that accurately capture the diversity concept, the replication principle is met and the magnitude of the differences in diversity between two communities can be interpreted adequately (Jost, 2006; Cultid-Medina and Escobar, 2016).
Where:
qD is the diversity of the community according to the chosen diversity index (Jost, 2006). It depends on the proportional abundance per species (Pi) and the exponent q (Jost, 2006; Cultid-Medina and Escobar, 2016). The exponent and superscript q is called the “order of diversity” and indicates the sensitivity to common and rare species (sensitivity to species abundance). For the study, the q values used were: 0 (°D) which is the species richness, 1 (1D) which is the common species (Shannon diversity), and 2 (2D) which is the number of dominant species (Simpson diversity; Jost, 2006).
The values of the three orders of diversity were measured before and after the storm and were compared. Because the methods established by Jost (2006) and Hill (1973) were used, the comparison is only possible if the sample coverage is the same in the two communities (Chao and Jost, 2012). Therefore, it is necessary to know the sample coverage, which is a measure indicating the proportion of the statistical population represented by the species sampled (Eq. 6; Chao and Jost, 2012). Thus, before estimating and comparing diversity, sample coverage (m) was calculated.
Where f1 and f2 are the numbers of singletons and doubletons, respectively, and n is the abundance of the sample. The values of sample coverage range from 0 (minimal completeness) to 100 (maximum completeness). When the completeness value is close to 100, and it is similar to the species assemblages to be compared, then diversity values (qD) can be compared directly (Chao and Jost, 2012; Cultid-Medina and Escobar, 2016). Finally, comparisons between the different orders of diversity (0, 1, and 2) were performed, considering the overlap between the confidence intervals (CI) at 95% (Cumming et al., 2007). Data analyses were performed with the iNEXT library (Hsieh et al., 2016) in R studio (R version 3.6.1 “Action of the Toes”; R core Team, 2019).
Beta-diversity
For each site, beta-diversity (variations in species composition) was evaluated as the total dissimilarity between two species assemblages at two-time steps (the pre-and post-storm; Baselga, 2010). The total dissimilarity was computed as the Jaccard Index (βjac; Eq. 7). Additionally, its two partition components, species turnover (βjtu; Eq. 8), and nestedness (βjne; Eq. 9) were considered (Baselga, 2010, 2012; Baselga and Orme, 2012).
Jaccard dissimilarity (Eq. 7):
Turnover component of Jaccard dissimilarity (Eq. 8):
Nestedness-resultant component of Jaccard dissimilarity (Eq. 9):
where:
a is the number of shared species in both sites (in this study, on both dates), b is the number of species present on the first date but not on the second, and c is the number of species present on the second date but not on the first (Jaccard, 1912; Baselga, 2012). The index ranges from 0 (null dissimilarity) to 1 (complete dissimilarity; Baselga, 2012). The analysis was run with the betapart library (Baselga and Orme, 2012) in R studio (R version 3.6.1 “Action of the Toes”; R core Team, 2019).
Relative importance value
Relative importance value was calculated per site by adding relative frequency (the number of plots where each species was observed, divided by the total number of plots) and relative cover (the total cover per species, divided by the total plant cover). The result was divided by 2 to obtain a value ranging from 0 (very scarce) to 1 (very abundant), which facilitates comparisons between species and sites or dates (Brower and Zar, 1977).
Pre-Existing Conditions and Beach-Dune Erosion After the Winter Storm
First, we explored the effect of pre-existing morphological conditions on beach and dune erosion by incorporating the geomorphological parameters measured in the field, and calculated from the field surveys, into a Principal Component Analysis (PCA). With this, we explored the relative contribution of each variable to the geomorphological variability between the three beaches. We also looked for possible correlated variables. From this prior analysis we intended to select the most relevant variables, to statistically assess the variables most related with erosion. Furthermore, to show that the most relevant variable can vary greatly on the beaches, an analysis of variance was performed to compare the beaches studied. Once the variables were selected, we ran a Generalized Linear Mixed-Effects Model (GLME) to evaluate the net erosion on the entire beach profile, as a function of the most relevant geomorphological variables. In this model, the variable site (beach identity) was used as a random component.
Second, to see whether plants mitigated erosion, linear regression analyses were performed using the information from the vegetated plots located closest to the ocean. This decision was made because it has been demonstrated that plant cover is positively correlated with the distance from the beach. Then, to avoid errors of comparison, plots that were directly impacted by storm surges and waves, and had reduced vegetation cover, were not compared with the plots further inland that had higher vegetation cover. Thus, at Riachuelos and La Mancha, three plots per transect were considered, whereas at Navarro only two plots per transect were analyzed because the beach is narrower. The plots selected were all on the stoss slope of the dunes and backshore.
The following variables were evaluated for each beach with the linear regressions: plant cover, plant richness, and the overlap cover index. This index was estimated, as the plant cover is only the cover visible in a 2D aerial view. When dunes (in this case the plots) are covered by one or more species that do not overlap, plant cover is a good proxy to study its effects on mitigating erosion. In this case, the maximum plant cover value is ≤4 m2, because this is the maximum area of each plot. However, when two or more species coexist and overlap in a plot, plant cover is stratified, since the plant cover of taller species could be on top of the plant cover of shorter species. The total plant cover of all species found in a plot could be more than 4 m2 because of the juxtaposition of species. When this occurs, the cover could be highly relevant in reducing the erosion induced by waves. Therefore, a value for this stratification (overlap) was calculated (Eq. 10) to test its possible effects in reducing erosion.
Where:
Total plant cover = the addition of plant cover per species in the plot.
Overlap = the addition of plant cover and bare sand (if it was present) minus the total area of the plot (4 m2).
Two is a constant number, used only to magnify the resulting values.
The analyses were run in R Studio (R version 3.6.1 “Action of the Toes”; R core Team, 2019), the PCA was run with the “rda” library of the “Vegan” package (Oksanen et al., 2019), and the GLME was run with the “glmer” function of the “lme4” package (Bates et al., 2015). Finally, the analysis of variance and the linear regressions were performed with the “lm” function.
Results
Erosion Induced by a Single Winter Storm Event
The impact of the storm varied between the sites. Based on field observations after the storm and analyses of the beach and dune profiles, washover penetration was documented at Riachuelos and La Mancha, where the dominant regime of wave impact, based on Sallenger (2000), was swash and overwash, but only over the incipient dunes. However, in La Mancha, there was lateral variation in the storm impacts, and collision regime was also observed. At Navarro, there was no evidence of washover penetration, and only the collision regime was observed on the foredunes (Figures 4–6).
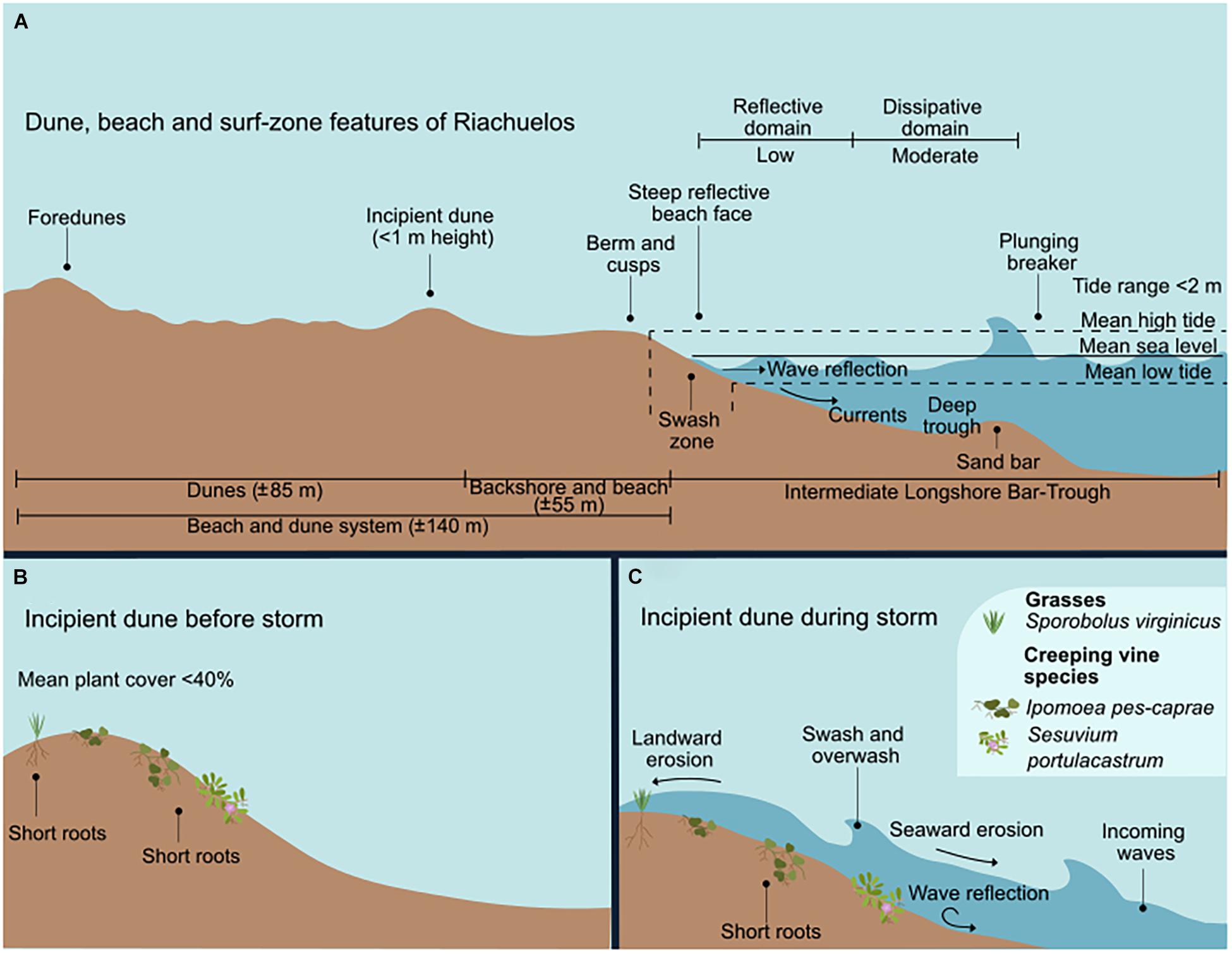
Figure 4. The surf-zone and dunes of Riachuelos. (A) Dune, beach, and surf-zone features. (B) The incipient dunes and species before the storm. (C) The incipient dune during the storm, showing the wave-induced erosion.
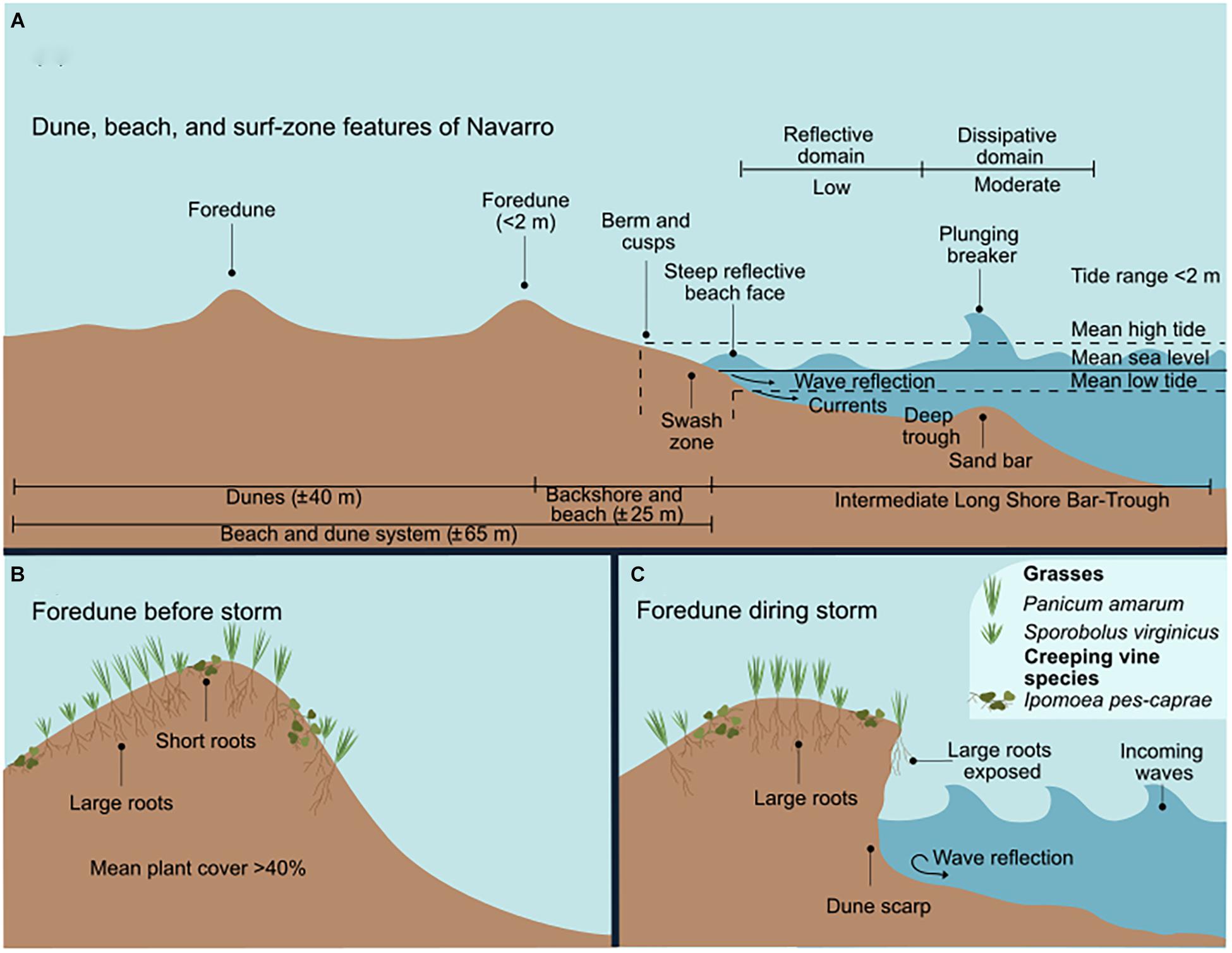
Figure 5. The surf-zone and foredunes of Navarro. (A) Dune, beach, and surf-zone features. (B) The foredunes and species before the storm. (C) The foredunes during the storm, showing the wave-induced erosion.
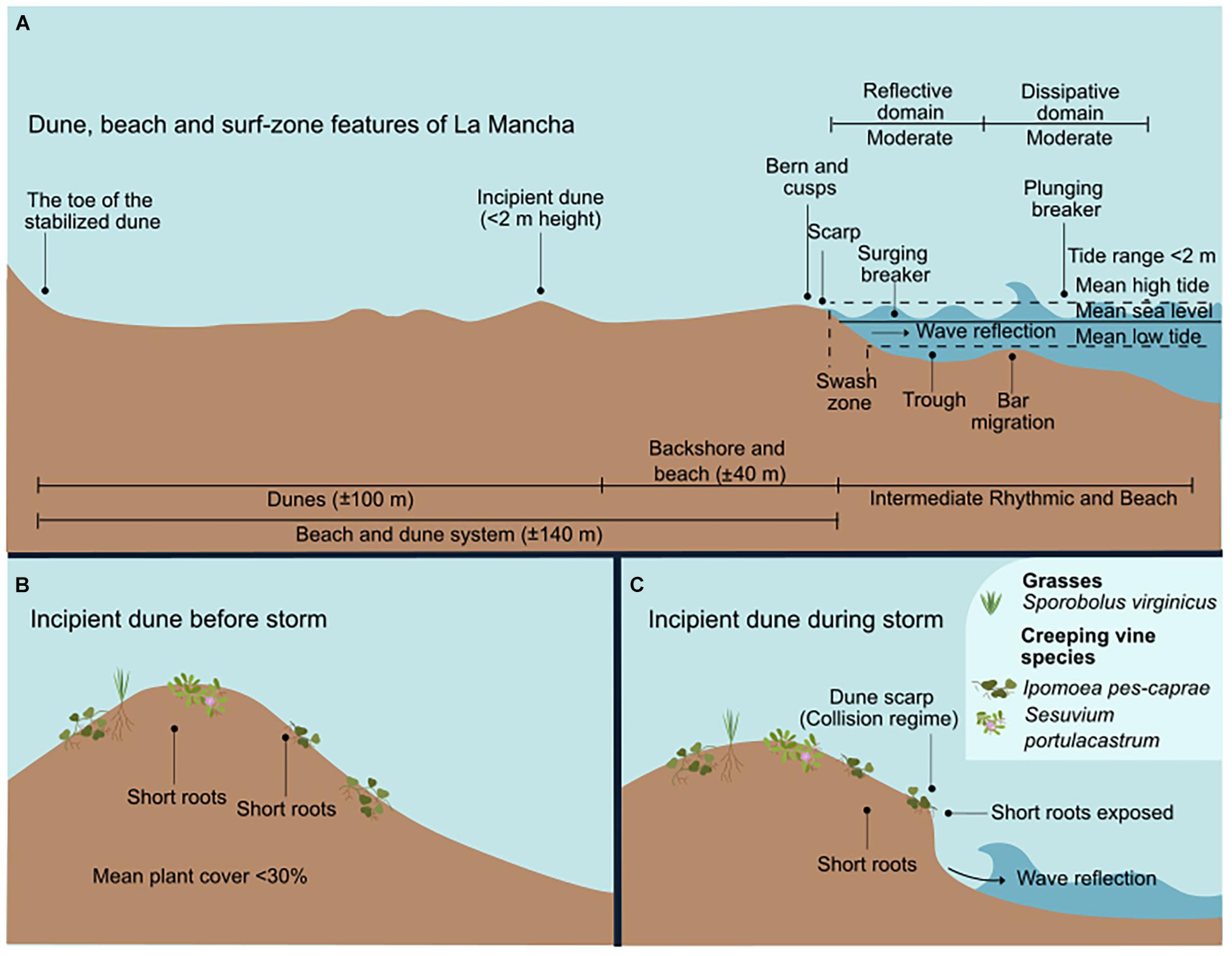
Figure 6. The surf-zone and dunes of La Mancha. (A) Dune, beach, and surf-zone features. (B) The incipient dunes and species before the storm. (C) The incipient dunes during the storm, showing the wave-induced erosion.
Erosion was the dominant process taking place during the storm along each transect. No net accretion (income of sand to the system) was documented (Figure 7), and the accretion was only of the sand stored on the dunes, repositioned along the transects. Only in La Mancha, profile 1, was accretion observed on the backshore, whereas on the other beaches accretion took place on the back of the dunes. At Riachuelos erosion occurred not only on the stoss slope of the foredunes and backshore, but also on the back of the dunes (Figure 7). At Navarro, erosion mainly occurred on the stoss slope of the foredune, and slight accretion was observed on the back of the foredunes (Figure 7). At La Mancha, erosion took place on the backshore, and incipient dunes (Figure 7).
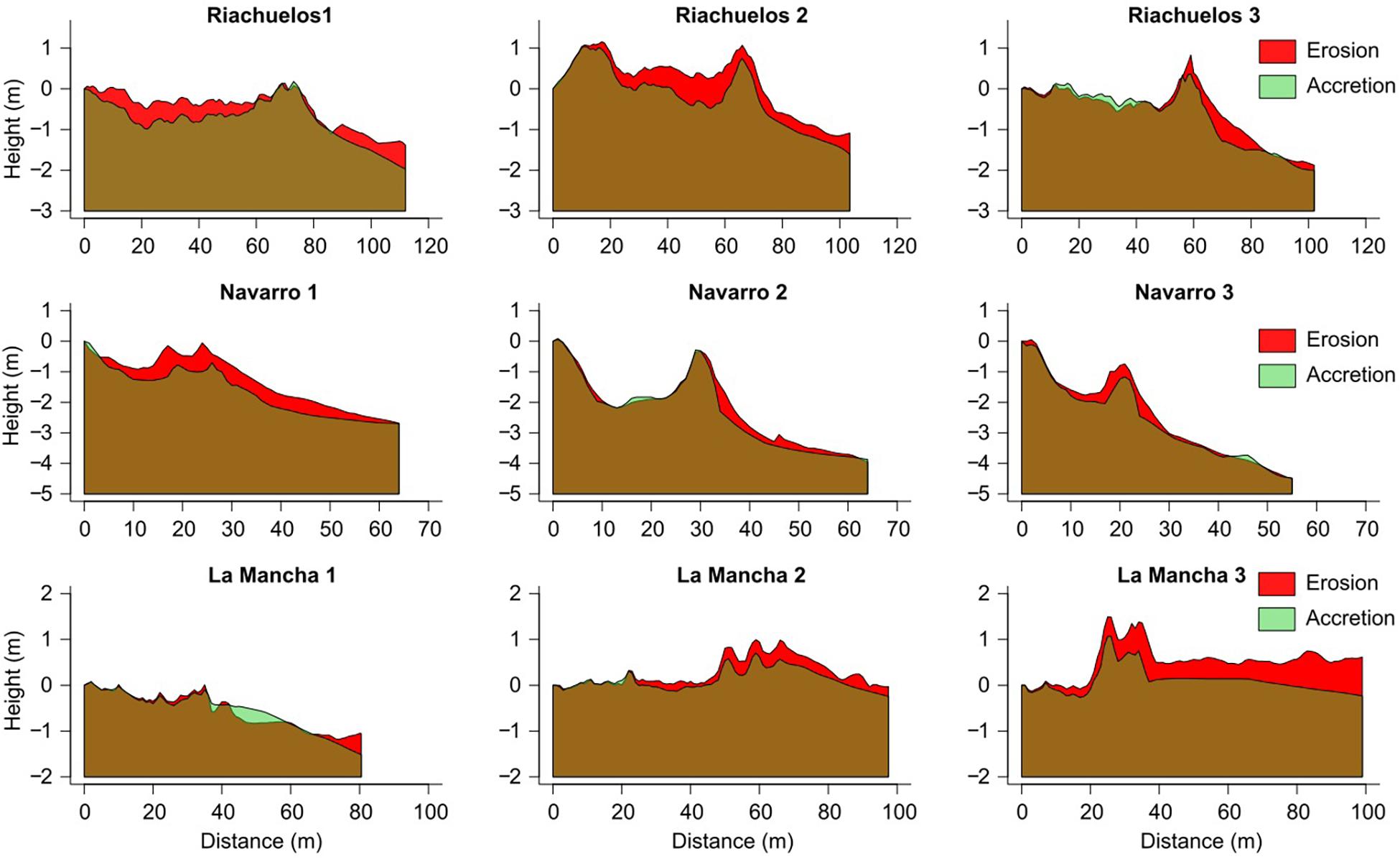
Figure 7. Pre-and post-storm profiles on Riachuelos, Navarro, and La Mancha. Note that scale in axes y and x is not the same for all the beaches because of the differences in length and height of the beach and dune systems.
In terms of the total net erosion (the sum of the erosion taking place on all three transects for each beach), Riachuelos had the highest value, followed by La Mancha, and then Navarro. Nonetheless, the analysis of variance (P ≤ 0.05) did not show any statistical differences for the erosion taking place on the three beaches (F value = 0.44; P = 0.66; Figure 8A). The same trend was seen for the net erosion taking place on the dunes closest to the ocean (F value = 0.16; P = 0.84; Figure 8B).
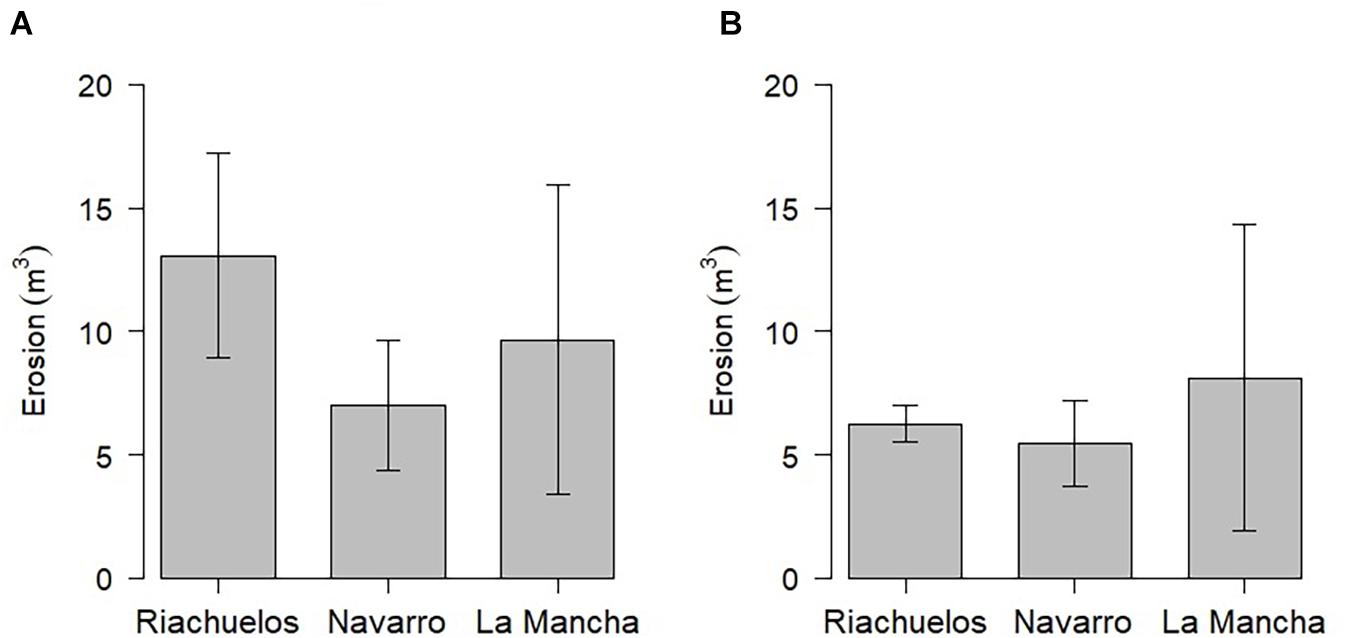
Figure 8. Erosion at the study sites: (A) mean erosion on the three transects; (B) mean erosion on the frontal dunes (from the lee slope to the windward slope) and the lowest seaward point surveyed.
Response of the Plant Community to the Impact of the Winter Storm
Plant Cover
There was no clear impact of the storm on the vegetation. Plant cover increased in Riachuelos and Navarro, but decreased in La Mancha, although these differences were not statistically significant (Table 2). The same trends were observed on the seaward plots (Table 2).
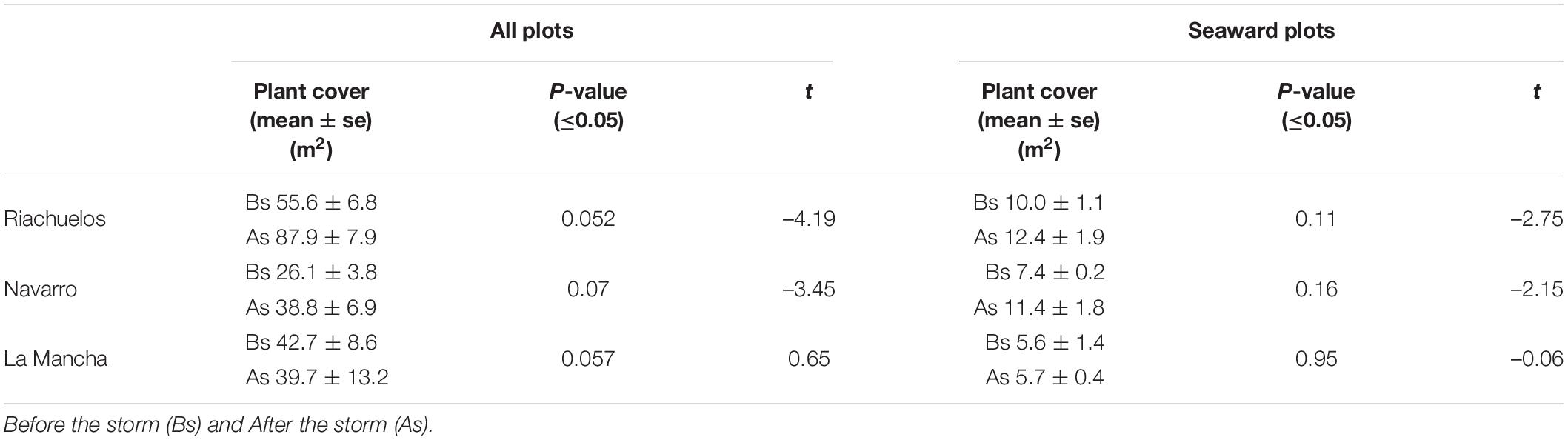
Table 2. Mean plant cover, p-value, and t-test value for all the plots on the three beaches, and in the frontal plots.
Alpha Diversity
A total of 32 species were recorded on all the beaches in the two sampling periods, with 28 species occurring in both periods. There were variations between the sites: 18 species were recorded in Riachuelos, 15 in Navarro, and 24 in La Mancha. On the three beaches, the sample coverage (evaluated as part of the diversity analysis to make the comparisons between the two sampling periods) was ≥96%. Thus, diversity comparisons of each order of diversity were done directly, and no extrapolation or interpolation methods were necessary (Chao and Jost, 2012). In Riachuelos, we observed a slight increase in the three orders of diversity. Species richness (0D) increased from 16 to 18 before and after the storm, respectively. The number of common species (1D) increased from 10 to 11 and the dominant species (2D) from 8 to 9. The species that appeared after the storm, prompting the increase in species richness were the inland grass Cenchrus echinatus and forb Cyperus rotundus. In contrast, no changes were observed in Navarro (Figure 9A). Before and after the storm, species richness was 14, the number of common species was 10, and the dominant species 9. Finally, in La Mancha, the three orders of diversity decreased after the storm. Species richness (0D) was the order with the greatest change, since it decreased from 21 to 17; while the common and dominant species varied from 12 to 11 and from 9 to 8, respectively. The inland species Bidens pilosa, Commelina erecta, Euphorbia dioica, and the beach plant Oenothera drummondii are the four species that disappeared after the storm. Despite the slight changes observed in Riachuelos and Navarro, the analyses performed with the 95% CI did not reveal significant differences for any order of diversity (°D, 1D, and 2D) in any of the beaches studied (Figure 9A), which means that in terms of alpha diversity, the plant communities were not significantly affected by the winter storm.
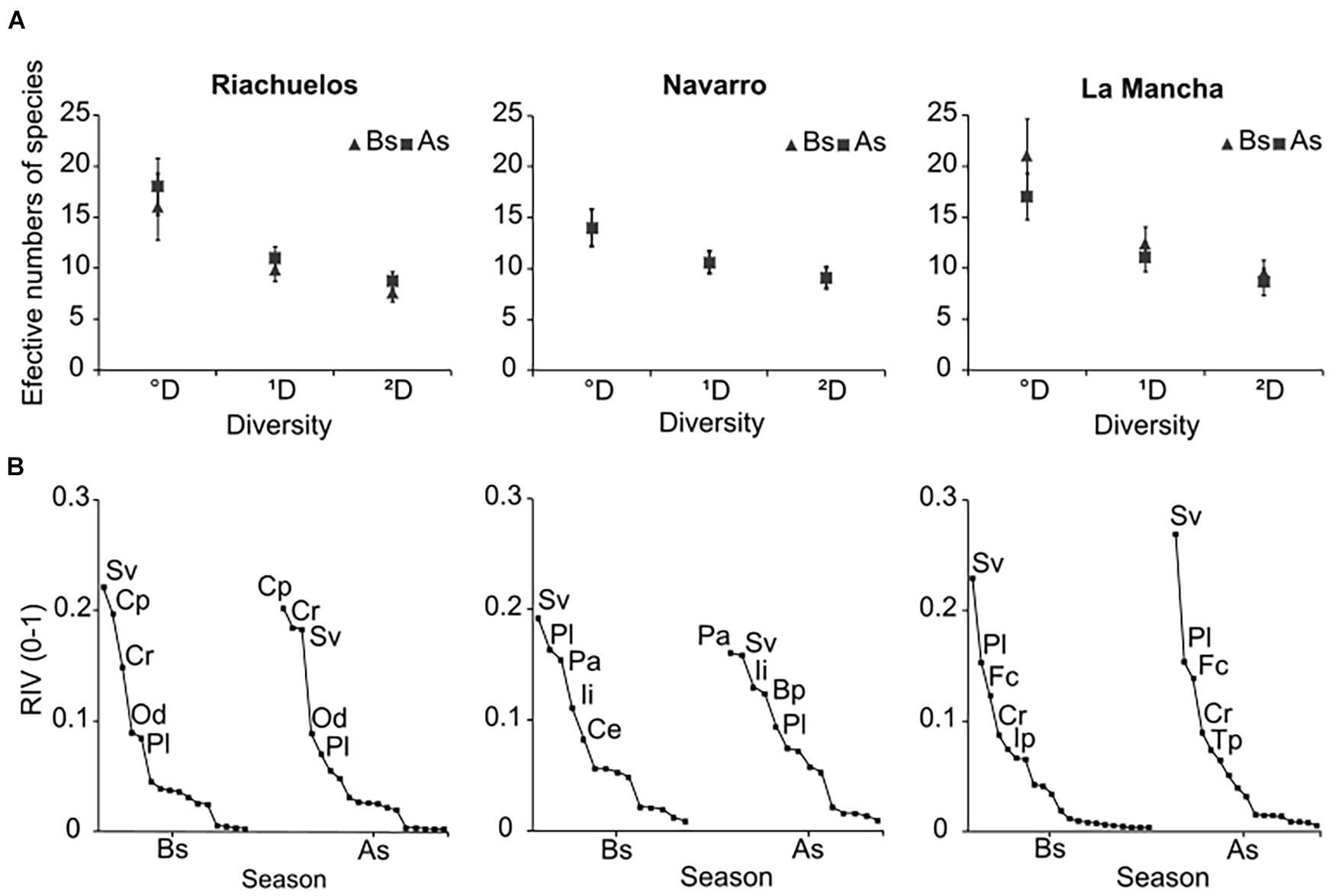
Figure 9. (A) Plant diversity on the three beaches before (Bs) and after the storm (As). Species richness (0D), the number of common species (1D), and the dominant species (2D) in the community were evaluated. Error bars correspond to the 95% CI. (B) Relative Importance Value (RIV) on the three beaches before (Bs) and after the storm (As). Only the five most dominant species on each beach are shown. Letters next to the point denote the name of the species: Sv (Sporobolus virginicus), Cp (Croton punctatus), Cr (Canavalia rosea), Od (Oenothera drummondii), Pl (Palafoxia lindenii), Pa (Panicum marum), Ii (Ipomoea imperati), Ce (Cenchrus echinatus), Bp (Bidens pilosa), Fc (Fimbristylis cymosa), Ip (Ipomoea pes-caprae), and Tp (Trachypogon plumosus).
There were, however, slight differences in the RIV between pre- and post-storm conditions. On the three beaches, the grass Sporobolus virginicus was the dominant species before the storm. However, after it, this species became the third and second most dominant species in Riachuelos and Navarro, respectively, being replaced by Croton punctatus and P. amarum, respectively, (Figure 9B). In La Mancha, S. virginicus continued to be the most dominant species and its dominance slightly increased after the storm. Of the three beaches, La Mancha had the fewest changes in species ranking (Figure 9B). Concerning the species with geomorphological relevance being considered dune-building plants, in Riachuelos, we observed that some had lower RIV (Ipomoea pes-caprae and S. virginicus) after the storm, while others (Palafoxia lindenii) remained unchanged. In contrast with Riachuelos, in Navarro Ipomoea pes-caprae and S. portulacastrum remained unchanged and the RIV of P. lindenii decreased. Furthermore, on this beach, the dune building and sand stabilizer grass, P. amarum, was the most dominant species after the storm, replacing S. virginicus. In La Mancha, as was previously mentioned, no major changes were observed and the two important dune building species (S. virginicus and P. lindenii) remained the most dominant. Finally, and coinciding with our observations at Riachuelos, in La Mancha the importance value of Ipomoe pes-caprae also fell.
Beta Diversity
The Jaccard index was used to show changes in species composition on the three beaches, after the winter storm. The Jaccard dissimilarity (Bjac) in Riachuelos (0.11), Navarro (0.13), and La Mancha (0.41) showed that La Mancha had the greatest dissimilarity values between the pre- and post-storm species assemblages, while Riachuelos and Navarro had lower values, closer to 0 which indicates less dissimilarity. The turnover component (βjtu) in Riachuelos was zero while Navarro (0.13) and La Mancha (0.30) had almost similar values to those for the total dissimilarity. Regarding the nestedness component (βjne), Riachuelos had the same value (0.11) that the total dissimilarity, while Navarro had a zero value and La Mancha 0.11. These results indicate that changes in species assemblages were site-dependent. In Riachuelos, the arrival of two new species was recorded, however, no species disappeared (nestedness), while in Navarro changes were due to species turnover and the number of species did not vary between pre-and post-storm species assemblages. Finally, in La Mancha, dissimilarities due to species turnover and nestedness were observed, however, the first was dominant.
Species turnover before and after the storm varied between sites. In Ricahuelos, the two new species found after the storm were C. echinatus and C. rotundus. In Navarro, Erigeron longipes was present before the event but absent after it, while Palafoxia texana appeared after the storm. In La Mancha, E. longipes, B. pilosa, C. erecta, O. drummondii, Lantana camara and Schizachyrium scoparium were observed before the storm but were absent after it. The species present after the storm, but absent before it, were Parthenium hysterophorus, Macroptilium atropurpureum and C. rotundus.
Pre-Existing Conditions Related to Beach-Dune Erosion and the Role of Plants
The PCA shows differences between the geomorphological features of the beaches studied. Axis 1 and Axis 2 accounted for 70 and 17% of the total data variability, respectively, (Figure 10A). Dune height, the stoss slope of the dune and slope of the backshore appear on the positive extreme of Axis 1. A strong correlation was found between dune height and the slope of the backshore (r2 = 0.85), and dune height and the stoss slope of the dune (r2 = 0.76). Beach width was associated with Axis 2 and no strong correlations were observed with dune height (r2 = –0.35), backshore width (r2 = –0.56), or the stoss slope of the dune (r2 = –0.53).
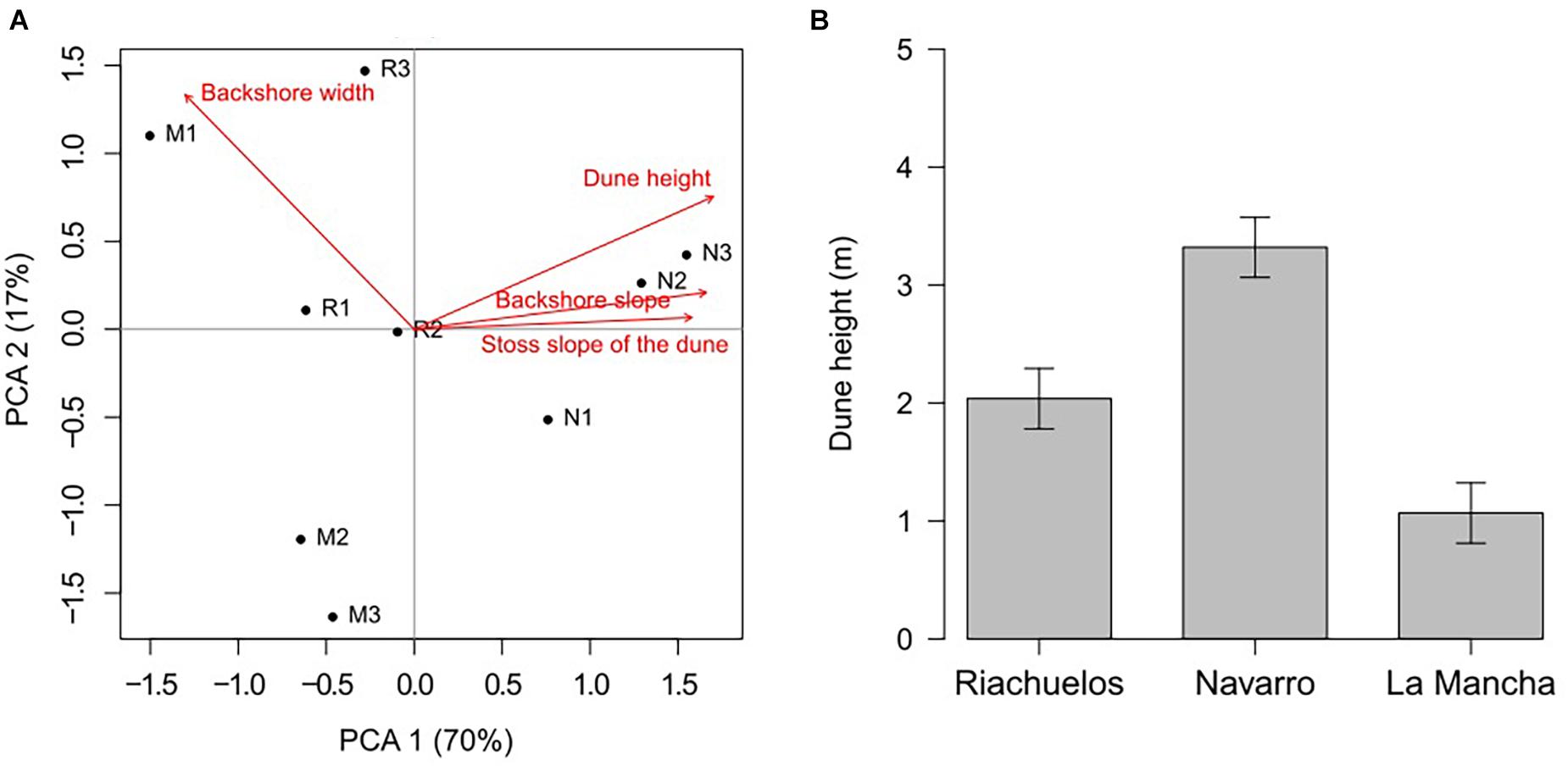
Figure 10. (A) Geomorphological variables included in the Principal Component Analyses. (B) Mean height of the frontal dunes on the three beaches (incipient dunes for Riachuelos and La Mancha, and foredunes at Navarro).
In the biplot, the points that corresponded to Navarro were located on the positive extreme of Axis 1, while the points from Riachuelos and La Mancha were located in the center of the plot and toward the negative extreme of the same axis (Figure 10A). This shows that the beach at Navarro is narrower, and the dunes are higher, with steeper exposed face slopes, compared to Riachuelos and La Mancha. These results coincide with the field observations and the metrics shown in Table 1. Finally, the analysis of variance to compare the dune heights of the beaches (the variable with most contribution and associated correlation with the other geomorphological variables) also shows that they were statistically different for the beaches, with Navarro having the highest dunes and La Mancha the lowest (P = 0.002; Figure 10B).
Based on this information and the correlation analyses, dune height and backshore width were selected as good predictors to evaluate the effect of the geomorphological variables in erosion using the GLME. The results showed a significant effect of dune height (Chisq = 20.51, P < 0.001), backshore width (Chisq = 58.81, P < 0.001) and the interaction of both variables (Chisq = 9.39, P = 0.002). Erosion was greater on the beaches fronted by lower dunes (Figure 11A), and narrower beaches also tended to lose more sediment (Figure 11B). The interaction of both variables indicates that narrower beaches with higher dunes are less prone to erosion, however, in some cases when beach width increased, the erosion was also less.
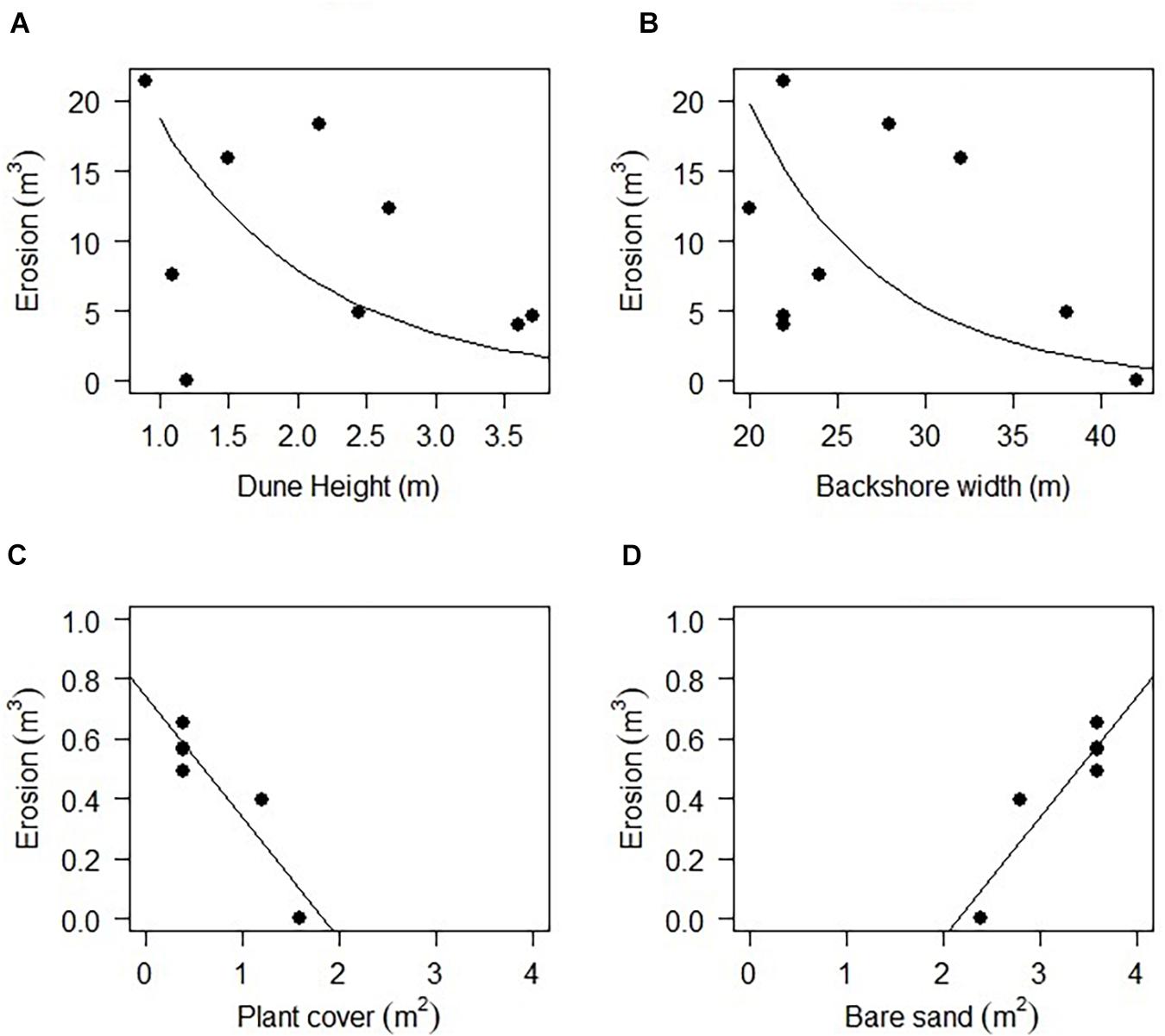
Figure 11. Erosion as a function of (A) dune height, (B) backshore width, (C) plant cover and (D) bare sand.
Finally, the linear regressions performed to explore the effect of plant cover, plant richness and the overlap cover index in mediating erosion, only showed a significant negative correlation between plant cover and erosion in Navarro (r2 = 0.84, P = 0.009; Figure 11C) and a significant positive correlation between bare sand and erosion (r2 = 0.84, P = 0.009; Figure 11D). On this beach, severe erosion occurred when plant cover was reduced on the exposed face of the dunes.
Discussion
This study is novel because it: (a) demonstrates the species-specific role of plants in mitigating dune erosion in field conditions; (b) shows the interaction between plant-related features and geomorphological variables in mitigating dune erosion, and (c) explores the immediate effect of a winter storm on beach and dune topography, the plant community, and dune-building species. This is relevant because previous work has focused on laboratory experiments and seldom explored the interaction between plant-related features with geomorphological attributes after exposure to a storm.
Our results are partially in accordance with our working hypotheses. We expected that: (a) plant cover and diversity would decrease after the impact of the storm and species dominance would change; (b) pre-existing beach and dune geomorphological conditions would determine storm-induced erosion; and (c) plants would help mitigate beach and dune erosion. We found that the winter storm did not have a significant impact on plant cover and diversity, but species composition and dominance varied for pre- and post-storm conditions. Beach and dune erosion did not vary significantly among sites, although it was less intense at Playa Navarro, where the highest dunes were observed. The correlation between erosion and plant cover was significantly negative only in Navarro.
Impacts of the Storm on Beach and Dune Morphology
Coinciding with previous findings, our results show that pre-existing conditions (dune and beach geomorphology before the storm) are relevant in determining the run-up, and the outcome of the storm on the beach and dune topography (Pries et al., 2008). Erosion was lower on the beaches with higher frontal dunes, but higher on narrower beaches (Vellinga, 1982; Saye et al., 2005; Pries et al., 2008; Keijsers et al., 2014). In Navarro, the narrowest beach with the highest dunes, erosion was induced through collision regime, and the resistance to flooding from the storm surge was conferred because the higher foredune ridge prevented inland flooding (Sallenger, 2000). In contrast, in Riachuelos and La Mancha, the lower dunes were more likely to be overwashed and inundated, although the width of the beach helped to reduce the effect of the storm surge (Keijsers et al., 2014) and consequent destruction of the incipient dunes. These results coincide with the previous findings of Houser et al. (2008) who observed that areas with lower dunes are more prone to overwashing, while the well-developed foredunes tend to be eroded on the exposed face.
Impacts of the Winter Storm on the Beach and Dune the Plant Communities
One of the most relevant characteristics of nature-based defense for coastal protection is that natural ecosystems are capable of recovering naturally after disturbances, such as storms. Indeed, storms are natural events that are part of the natural dynamics of the coasts. Although natural, the stress induced by winter storms and tropical cyclones (e.g., hurricanes) on the coast (e.g., inundation, high salinity concentration, overwash, scarping and erosion, and burial) may have positive or negative impacts on plant communities (Rodgers et al., 2009; Miller, 2015). Consequently, we hypothesized that plant cover and alpha diversity would decrease after the impact of the winter storm. Additionally, we also expected to observe changes in species composition and dominance, with the most tolerant species becoming dominant after the storm.
Species richness was not altered by the storm, although species composition did change. Most of the species involved in species turnover were inland species (e.g., B. pilosa and E. dioica), with low incidence, abundance, and plant cover, and which were negatively affected by the storm. In turn, the abundance of dune builder plants increased after the storm. These results coincide with previous findings by Miller et al. (2010), who demonstrated that beach and dune species are highly resistant to storm conditions. These authors also recorded species turnover after a storm, mostly owing to the arrival of grasses and forbs. They noted that (i) major changes in plant cover on the incipient dunes are more related to temperature and drought, rather than to the action of waves, and (ii) species with higher occurrence are more likely to respond positively to storms. In accordance with this, the present study shows that in the three study sites, plant cover of the most dominant dune-building species (for example, Canavalia rosea, C. punctatus, Ipomoea pes-caprae, S. virginicus, and P. lindenii), increased after the storm, probably due to the rain brought by the storm, but also perhaps because of a positive response to burial by sand (Martínez et al., 2002) in the overwash areas where accretion occurred. It is important to mention that the coast of Veracruz faced a severe drought in the year when the study took place. Thus, when the rain from the winter storm reached the coast, it was beneficial, rather than harmful, for the plants (personal field observations).
Furthermore, the fact that the dune building species Ipomoea pes-caprae, P. lindenii, S. virginicus, S. portulacastrum, and P. amarum were little or not affected by the storm is relevant because, besides being effective dune builders (Devall, 1992; Stallins, 2002; Lonard and Judd, 2011; Lonard et al., 2013), these species are known to help mitigate wave-induced dune erosion (Silva et al., 2016; Feagin et al., 2019; Maximiliano-Cordova et al., 2019). This means that these species maintain their protective role by building dunes and by resisting the impact of the storm. However, this also depends on factors such as the sediment budget and the storm incidence.
Pre-Existing Conditions and the Effect of Plants in Mitigating Erosion
Experiments previously performed in wave flume conditions have shown the effectiveness of vegetation in mitigating erosion induced by storm waves (e.g., Kobayashi et al., 2013; Sigren et al., 2014; Silva et al., 2016; De Battisti and Griffin, 2020). However, to date, only the studies conducted by Charbonneau et al. (2017), Lindell et al. (2017), and Biel et al. (2017) have demonstrated the protective role of plants in field conditions. To contribute to this field, the aim and novelty of the present study were to explore the effect of plant-related features (plant cover, overlap cover index, and plant richness), in mitigating erosion in different beaches with varying morphological features, in order to understand under what conditions plants confer dune resistance against erosion. This study shows that plant richness and the overlap cover did not affect erosion, except on one beach, where plant cover was negatively correlated with erosion on the exposed face of the dunes.
The differences in erosion observed at the three study sites and the protective role of vegetation may be associated with beach-dune morphology (Sallenger, 2000; Saye et al., 2005; Pries et al., 2008) as well as the dominant species, because protection may be species- and site-specific (Charbonneau et al., 2017; Maximiliano-Cordova et al., 2019). Our results show that the dominant species varied between beaches. The incipient dunes of La Mancha and Riachuelos were covered by the creeping vine Ipomoea pes-caprae, while the foredunes of Navarro were covered by the tall grass P. amarum. Even though experimental evidence has demonstrated the efficiency of both species in mitigating beach and dune erosion in laboratory conditions (Silva et al., 2016; Figlus et al., 2017; Maximiliano-Cordova et al., 2019), it is possible that in field conditions, the architecture of P. amarum is better at mitigating erosion as its plant cover increases because the dunes that develop around this plant are higher and the plants effectively trap sediment that would otherwise be eroded. Nevertheless, there is a threshold in the protective effect beyond which, erosion will not be mitigated (Odériz et al., 2020).
The mechanisms involved in the protective role of vegetation are diverse. Previous laboratory experiments have demonstrated that dune plants primarily reduce the velocity of beach erosion by attenuating wave swash and run-up with their stems and leaves (Mendoza et al., 2017; Feagin et al., 2019), but they do not compensate for the sediment deficit of the submerged beach (Silva et al., 2016; Odériz et al., 2020). Feagin et al. (2019) observed that plant roots also attenuated waves and reduced erosion. Furthermore, Mendoza et al. (2017) explored the mechanisms through which plant cover decreased dune erosion. Their findings show that where plants were present, the wave breaking point was displaced seawards, and bed velocities close to the shoreline were lower, associated with a reduction of the wave energy reflected on the beach. Both phenomena help explain the protective role of vegetation on the beach, which provides a slight, but relevant, contribution to the resilience and resistance of the beach profile.
Hydrodynamic changes are species-specific (Bouma et al., 2013), and hence, plant cover and architecture will play a differential role in modifying the impact of waves. For instance, I. pes-caprae mitigates wave impact and provides dune resistance (Silva et al., 2016; Feagin et al., 2019; Maximiliano-Cordova et al., 2019), but when plant cover increases, the results are unpredictable (Silva et al., 2016). In some cases, the maximum plant cover of this species could induce an increase in the coefficients of wave reflection, and as a consequence, induce greater dune erosion (personal observations). Instead, P. amarum mitigates turbulence as plant cover increases (Figlus et al., 2017). In laboratory conditions, it was demonstrated that P. amarum and the similar Ammophila arenaria were more efficient in mitigating erosion than forb species because of differences in their aerial architecture and below-ground biomass (Figlus et al., 2017; De Battisti and Griffin, 2020). These trends probably apply to our results. For example, I. pes-caprae has long branches that extend over the surface of the sand and abundant shallow adventitious roots that grow a few centimeters below the surface (Devall, 1992). In turn, P. amarum is a tall grass with dense and compact roots. The plant architecture and below-ground roots of both help mitigate the impact of storms, and retain sediment, thus reducing erosion.
Finally, the protective role of plants is the result of reciprocal interaction between plants and dunes which converts them into a dynamic bio-shield that determines the extent of the storm-induced effects. Indeed, beach and dune resistance is an accumulative process that depends on the biogeomorphological feedback between the plants and the dunes (Stallins and Parker, 2003; Durán and Moore, 2013; Charbonneau et al., 2021). In brief, our results show that plant cover can help mitigate erosion. However, this protective role is species- and site-dependent; influenced by local geomorphological characteristics.
Caveats of the Study
Spatio-Temporal Dynamics
In temperate coasts, it is possible that the abundance and diversity of plant species vary over time because of seasonal changes during which annual species may be present or absent, and plant cover varies drastically, depending on the weather conditions. Unlike temperate coasts, in the tropics there are almost no noticeable seasonal changes. For instance, annual plants are absent; all species are perennial, and thus, they do not disappear seasonally. This means that without disturbances that cause major disruptions, plants remain present even during the dry season, although their cover may decrease. Also, there is no successional species turnover on the beach and incipient dunes because of the recurrent disturbance events which repeatedly set vegetation trends back to early pioneer stages. Therefore, in our case, a spatio-temporal relationship is likely to occur simultaneously, between the state of the vegetation and geomorphological changes, because plant cover is highest when storms occur (Martínez et al., 2001).
One Single Storm
It is also important to acknowledge the limitations of a study with only one single storm event. It is certainly necessary to explore the cumulative effects of subsequent storms with different intensities, as well as the time elapsed between them, because this affects the possibility of plant recovery after the disturbance events. In addition, long-term studies will contribute to the understanding of the dynamic interaction between plants and geoforms. We also lacked information on the bathymetry of the study sites, and this would have been useful to describe the impact regime of the waves during the storm and the redistribution of the sediment along the surf zone.
Number of Replicates
The significant correlations between different environmental variables (dune height, backshore width, plant cover, and bare sand) and observed erosion were performed with a relatively reduced number of replicates. This was due to the fact that we chose the plots closest to the ocean to test the impact of storm-induced waves on the dunes, with and without vegetation, because the impact of waves decreases inland. Certainly, these results would be strengthened with a greater number of replicates, both within each beach and by adding more beaches. Nevertheless, the general trends are statistically significant and reveal interesting trends in field conditions which coincide with laboratory experiments, as well as the relatively small number of field observations performed previously (Feagin et al., 2019).
Applicability Worldwide
The study was only performed in three sites (three beaches), all located on the Gulf of Mexico. Nevertheless, the results can be extended worldwide, specifically to sandy coasts with vegetated dunes, and which are exposed to storms (winter storms or tropical cyclones; see for example, Costanza et al., 2021; Castelle et al., 2015; Masselink et al., 2016). While sandy beaches are heterogeneous, and dune-building plant species vary between regions. Nevertheless, sediment dynamics and the tolerance of dune building plants to salinity and sand movement is similar between species (Maun, 1998). Therefore, the response of the beach-dune system to the impact of storms is likely to be parallel in coastal regions beyond the Gulf of Mexico. Thus, in tropical and temperate latitudes, the interaction between geomorphological features, plants and storms are likely to share similarities. Nevertheless, further field observations are necessary to confirm this assumption.
Conclusion
This study aimed to evaluate the impact of a single winter storm on beach and dune morphology and plant communities, and to explore whether plants help in reducing wave-induced erosion in field conditions. The results show that: (1) erosion and accretion occurred on the three beaches studied, but that the patterns depend on the local geomorphological attributes and pre-existing conditions. Erosion was less intense where dunes were higher, although the beach was narrower (Navarro). On the other hand, wider beaches with more gentle slopes and shorter incipient dunes (Riachuelos and La Mancha) and were more eroded. (2) The impact of the winter storm on the plant communities established on the backshore and dunes was most often observed on typical inland plant species. Dune-building plants were not only tolerant to the storm, but they also increased their plant cover, demonstrating their ability to tolerate harsh storm conditions. (3) The protective role of plant-cover and species richness in mitigating erosion was site-dependent, and was only significant where erosion was less intense, which coincided with higher dunes and a narrower beach. The results from this study show that in field conditions, plants can confer dune resistance. However, this protective role is not linear because it is site- and species-specific, and depends on pre-existing geomorphological conditions and the plant species growing on the beach and dunes. Finally, concerning the applicability of this study, our results help understand the circumstances under which plants contribute to mitigating dune erosion. This is relevant as there is growing global interest in protecting coasts and mitigating erosion by means of ecosystem-based solutions.
Data Availability Statement
The raw data supporting the conclusions of this article will be made available by the authors, without undue reservation.
Author Contributions
CM-C conceived the manuscript, collected field data, performed the statistical analyses, wrote the first draft, and edited the manuscript. MM conceived, reviewed, and edited the manuscript. RS reviewed and edited the manuscript and oversaw the project administration. PH reviewed and edited the manuscript. RG performed the statistical analyses, reviewed, and edited the manuscript. RL helped with data curation and analysis. All authors contributed to the article and approved the submitted version.
Funding
The present research has been developed under the framework of CEMIE-Océano (Mexican Centre for Innovation in Ocean Energy), project FSE-2014-06-249795 financed by CONACYT-SENER-Sustentabilidad Energética. CM-C received a graduate studies scholarship from CONACYT (584584).
Conflict of Interest
The authors declare that the research was conducted in the absence of any commercial or financial relationships that could be construed as a potential conflict of interest.
Publisher’s Note
All claims expressed in this article are solely those of the authors and do not necessarily represent those of their affiliated organizations, or those of the publisher, the editors and the reviewers. Any product that may be evaluated in this article, or claim that may be made by its manufacturer, is not guaranteed or endorsed by the publisher.
Acknowledgments
We are thankful to Valeria Chávez for her contribution to the acquisition of ERA-5 data to perform the analysis of wave and wind conditions during the storm. Andrea Perez-Campos contributed to the design of the supplementary material.
References
Baselga, A. (2010). Partitioning the turnover and nestedness components of beta diversity. Glob. Ecol. Biogeogr. 19, 134–143. doi: 10.1111/j.1466-8238.2009.00490.x
Baselga, A. (2012). The relationship between species replacement, dissimilarity derived from nestedness, and nestedness. Glob. Ecol. Biogeogr. 21, 1223–1232. doi: 10.1111/j.1466-8238.2011.00756.x
Baselga, A., and Orme, C. L. (2012). Betapart: an R package for the study of beta diversity. Methods Ecol. Evol. 3, 808–812. doi: 10.1111/j.2041-210X.2012.00224.x
Bates, D., Mächler, M., Bolker, B. M., and Walker, S. C. (2015). Fitting linear mixed-effects models using lme4. J. Stat. Softw. 67, 1–48. doi: 10.18637/jss.v067.i01
Biel, R. G., Hacker, S. D., Ruggiero, P., Cohn, N., and Seabloom, E. W. (2017). Coastal protection and conservation on sandy beaches and dunes: context-dependent tradeoffs in ecosystem service supply. Ecosphere 8:e01791. doi: 10.1002/ECS2.1791
Bouma, T. J., Temmerman, S., van Duren, L. A., Martini, E., Vandenbruwaene, W., Callaghan, D. P., et al. (2013). Organism traits determine the strength of scale-dependent bio-geomorphic feedbacks: a flume study on three intertidal plant species. Geomorphology 180–181, 57–65. doi: 10.1016/j.geomorph.2012.09.005
Brantley, S. T., Bissett, S. N., Young, D. R., Wolner, C. W. V., and Moore, L. J. (2014). Barrier island morphology and sediment characteristics affect the recovery of dune building grasses following storm-induced overwash. PLoS One 9:104747. doi: 10.1371/journal.pone.0104747
Brower, J. E., and Zar, J. H. (1977). Field and Laboratory Methods for General Ecology. Dubuque: Wm. C. Brown Company Publishers.
Bryant, D. B., Anderson Bryant, M., Sharp, J. A., Bell, G. L., and Moore, C. (2019). The response of vegetated dunes to wave attack. Coast. Eng. 152:103506. doi: 10.1016/j.coastaleng.2019.103506
Carter, R. W. G. (2013). Coastal Environmets: An Introduction to the Physical, Ecological and Cultural Systems of Coastlines. Available online at: https://www.elsevier.com/books/coastal-environments/carter/978-0-08-050214-4 (accessed February 26, 2021)
Castelle, B., Marieu, V., Bujan, S., Splinter, K. D., Robinet, A., Sénéchal, N., et al. (2015). Impact of the winter 2013-2014 series of severe Western Europe storms on a double-barred sandy coast: beach and dune erosion and megacusp embayments. Geomorphology 238, 135–148. doi: 10.1016/j.geomorph.2015.03.006
Chao, A., and Jost, L. (2012). Coverage-based rarefaction and extrapolation: standardizing samples by completeness rather than size. Ecology 93, 2533–2547. doi: 10.1890/11-1952.1
Charbonneau, B. R., Dohner, S. M., Wnek, J. P., Barber, D., Zarnetske, P., and Casper, B. B. (2021). Vegetation effects on coastal foredune initiation: wind tunnel experiments and field validation for three dune-building plants. Geomorphology 378:107594. doi: 10.1016/j.geomorph.2021.107594
Charbonneau, B. R., Wnek, J. P., Langley, J. A., Lee, G., and Balsamo, R. A. (2016). Above vs. belowground plant biomass along a barrier island: implications for dune stabilization. J. Environ. Manage. 182, 126–133. doi: 10.1016/j.jenvman.2016.06.032
Charbonneau, B. R., Wootton, L. S., Wnek, J. P., Langley, J. A., and Posner, M. A. (2017). A species effect on storm erosion: invasive sedge stabilized dunes more than native grass during Hurricane Sandy. J. Appl. Ecol. 54, 1385–1394. doi: 10.1111/1365-2664.12846
Chávez, V., Lithgow, D., Losada, M., and Silva-Casarin, R. (2021). Coastal green infrastructure to mitigate coastal squeeze. J. Infrastruct. Preserv. Resil. 2, 1–12. doi: 10.1186/s43065-021-00026-1
Cheplick, G. P. (2016). Changes in plant abundance on a coastal beach following two major storm surges. J. Torrey Bot. Soc. 143, 180–191. doi: 10.3159/TORREY-D-15-00030
Claudino-Sales, V., Wang, P., and Horwitz, M. H. (2008). Factors controlling the survival of coastal dunes during multiple hurricane impacts in 2004 and 2005: santa Rosa barrier island, Florida. Geomorphology 95, 295–315. doi: 10.1016/J.GEOMORPH.2007.06.004
Coch, N. K. (1994). Geologic effects of hurricanes. Geomorphology 10, 37–63. doi: 10.1016/0169-555X(94)90007-8
Copernicus Climate Change Service (C3S) (2017). ERA5: Fifth Generation of ECMWF Atmospheric Reanalyses of the Global Climate. Copernicus Climate Change Service Climate Data Store (CDS), 2018/12. Available online at: https://cds.climate.copernicus.eu/cdsapp#W/home (accessed June 18, 2020).
Costanza, R., Anderson, S. J., Sutton, P., Mulder, K., Mulder, O., Kubiszewski, I., et al. (2021). The global value of coastal wetlands for storm protection. Glob. Environ. Chang. 70:102328. doi: 10.1016/J.GLOENVCHA.2021.102328
Cultid-Medina, C. A., and Escobar, F. (2016). Assessing the ecological response of dung beetles in an agricultural landscape using number of individuals and biomass in diversity measures. Environ. Entomol. 45, 310–319. doi: 10.1093/EE/NVV219
Cumming, G., Fidler, F., and Vaux, D. L. (2007). Error bars in experimental biology. J. Cell Biol. 177, 7–11. doi: 10.1083/jcb.200611141
Davidson, S. G., Hesp, P. A., and Silva, G. M. (2020). Controls on dune scarping. Prog. Phys. Geogr. Earth Environ. 44, 923–947. doi: 10.1177/0309133320932880
Davidson-Arnott, R. G. D. (2005). Conceptual model of the effects of sea level rise on sandy coasts. J. Coast. Res. 21, 1166–1172. doi: 10.2112/03-0051.1
Davidson-Arnott, R. G. D., Hesp, P. A., Ollerhead, J., Walker, I., Bauer, B., Delgado-Fernandez, I., et al. (2018). Sediment budget controls on foredune height: comparing simulation model results with field data. Earth Surf. Process. Landforms 43, 1798–1810. doi: 10.1002/esp.4354
Davidson-Arnott, R. G. D., and Law, M. N. (1996). Measurement and prediction of long-term sediment supply to coastal foredunes. J. Coast. Res. 12, 654–663.
Davies, J. L. (1964). A morphogenic approach to world shorelines. Zeitschrift für Geomorphol. 8, 127–142. doi: 10.1127/zfg/mortensen/8/1964/127
De Battisti, D., and Griffin, J. N. (2020). Below-ground biomass of plants, with a key contribution of buried shoots, increases foredune resistance to wave swash. Ann. Bot. 125, 325–334. doi: 10.1093/aob/mcz125
Devall, M. S. (1992). The biological flora of coastal dunes and wetlands. 2. Ipomoea pes-caprae (L.) Roth. J. Coast. Res. 8, 442–456.
Doing, H. (1985). “Coastal fore-dune zonation and succession in various parts of the world,” in Ecology of Coastal Vegetation, eds W. G. Beeftink, J. Rozema, and A. H. L. Huiskes (Dordrecht: Springer), 65–75. doi: 10.1007/978-94-009-5524-0_7
Durán, O., and Moore, L. J. (2013). Vegetation controls on the maximum size of coastal dunes. Proc. Natl. Acad. Sci. U.S.A. 110, 17217–17222. doi: 10.1073/pnas.1307580110
Emery, K. O. (1961). A simple method of measuring beach profiles. Limnol. Oceanogr. 6, 90–93. doi: 10.4319/lo.1961.6.1.0090
Feagin, R. A., Furman, M., Salgado, K., Martínez, M. L., Innocenti, R. A., Eubanks, K., et al. (2019). The role of beach and sand dune vegetation in mediating wave run up erosion. Estuar. Coast. Shelf Sci. 219, 97–106. doi: 10.1016/j.ecss.2019.01.018
Figlus, J., Kobayashi, N., Gralher, C., and Iranzo, V. (2011). Wave overtopping and overwash of dunes. J. Waterw. Port Coast. Ocean Eng. 137, 26–33. doi: 10.1061/(ASCE)WW.1943-5460.0000060
Figlus, J., Sigren, J. M., Power, M. J., and Armitage, A. R. (2017). Physical model experiment investigating interactions between different dune vegetation and morphology changes under wave impact. Proc. Coast. Dyn. 470–480.
Flores, P. (2016). Evaluación de la Opresión Costera y sus Consecuencias en las Playas Arenosas y Dunas Costeras de Veracruz. Master’s Thesis. Xalapa, Mexico: Instituto de Ecología, A. C.
Gornish, E. S., and Miller, T. E. (2010). Effects of storm frequency on dune vegetation. Glob. Chang. Biol. 16, 2668–2675. doi: 10.1111/j.1365-2486.2009.02144.x
Guisado-Pintado, E., and Jackson, D. W. T. (2018). Multi-scale variability of storm Ophelia 2017: the importance of synchronised environmental variables in coastal impact. Sci. Total Environ. 630, 287–301. doi: 10.1016/j.scitotenv.2018.02.188
Guisado-Pintado, E., and Jackson, D. W. T. (2019). Coastal impact from high-energy events and the importance of concurrent forcing parameters: the cases of storm Ophelia (2017) and storm Hector (2018) in NW Ireland. Front. Earth Sci. 7:190. doi: 10.3389/feart.2019.00190
Hesp, P. A. (1991). Ecological processes and plant adaptations on coastal dunes. J. Arid Environ. 21, 165–191. doi: 10.1016/s0140-1963(18)30681-5
Hesp, P. A. (2002). Foredunes and blowouts: initiation, geomorphology and dynamics. Geomorphology 48, 245–268. doi: 10.1016/S0169-555X(02)00184-8
Hesp, P. A., Dong, Y., Cheng, H., and Booth, J. L. (2019). Wind flow and sedimentation in artificial vegetation: field and wind tunnel experiments. Geomorphology 337, 165–182. doi: 10.1016/j.geomorph.2019.03.020
Hill, M. O. (1973). Diversity and evenness: a unifying notation and its consequences. Ecology 54, 427–432. doi: 10.2307/1934352
Houser, C. (2013). Alongshore variation in the morphology of coastal dunes: implications for storm response. Geomorphology 199, 48–61. doi: 10.1016/j.geomorph.2012.10.035
Houser, C., Hapke, C., and Hamilton, S. (2008). Controls on coastal dune morphology, shoreline erosion and barrier island response to extreme storms. Geomorphology 100, 223–240. doi: 10.1016/j.geomorph.2007.12.007
Hsieh, T. C., Ma, K. H., and Chao, A. (2016). iNEXT: an R package for rarefaction and extrapolation of species diversity (Hill numbers). Methods Ecol. Evol. 7, 1451–1456. doi: 10.1111/2041-210X.12613
Infante-Mata, D., Moreno-Casasola, P., Madero-Vega, C., Castillo-Campos, G., and Warner, B. G. (2011). Floristic composition and soil characteristics of tropical freshwater forested wetlands of Veracruz on the coastal plain of the Gulf of Mexico. For. Ecol. Manage. 262, 1514–1531. doi: 10.1016/j.foreco.2011.06.053
Jaccard, P. (1912). The distribution of the flora in the alpine zone. New Phytol. 11, 37–50. doi: 10.1111/j.1469-8137.1912.tb05611.x
Judd, F. W., and Sides, S. L. (1983). The effect of Hurricane Allen on the near-shore vegetation of South Padre Island. Southwest. Nat. 28, 365–369. doi: 10.2307/3670799
Karunarathna, H., Pender, D., Ranasinghe, R., Short, A. D., and Reeve, D. E. (2014). The effects of storm clustering on beach profile variability. Mar. Geol. 348, 103–112. doi: 10.1016/j.margeo.2013.12.007
Keijsers, J. G. S., Poortinga, A., Riksen, M. J. P. M., and Maroulis, J. (2014). Spatio-temporal variability in accretion and erosion of coastal foredunes in the Netherlands: regional climate and local topography. PLoS One 9:e91115. doi: 10.1371/journal.pone.0091115
Kobayashi, N., Gralher, C., and Do, K. (2013). Effects of woody plants on dune erosion and overwash. J. Waterw. Port Coast. Ocean Eng. 139, 466–472. doi: 10.1061/(asce)ww.1943-5460.0000200
Krause, G. (2004). The “Emery-Method” revisited: performance of an inexpensive method of measuring beach profiles and modifications. J. Coast. Res. 20, 340–346. doi: 10.2112/1551-5036(2004)20[340:teroai]2.0.co;2
Lindell, J., Fredriksson, C., and Hanson, H. (2017). Impact of dune vegetation on wave and wind erosion: a case study at Ängelholm Beach, South Sweden. Vatten Tidskr. för vattenvård/J. Water Manag. Res. 73, 39–48.
Lonard, R. I., and Judd, F. W. (2011). The biological flora of coastal dunes and wetlands: panicum amarum S. Elliott and Panicum amarum S. Elliott var. amarulum (A.S. Hitchcock and M.A. Chase) P. Palmer. J. Coast. Res. 27, 233–242. doi: 10.2112/JCOASTRES-D-09-00129.1
Lonard, R. I., Judd, F. W., and Stalter, R. (2013). The biological flora of coastal dunes and wetlands: sporobolus virginicus (C. Linnaeus) K. Kunth. J. Coast. Res. 29, 706–716. doi: 10.2112/JCOASTRES-D-12-00139.1
Martínez, M. L., Moreno-Casasola, P., Espejel, I., Jimenéz-Orocio, O., Infante-Mata, D., and Rodríguez-Revelo, N. (eds) (2014). Diagnóstico de las Dunas Costeras de México. Guadalajara: CONAFOR.
Martínez, M. L., Silva, R., Lithgow, D., Mendoza, E., Flores, P., Martínez, R., et al. (2017). Human Impact on coastal resilience along the coast of Veracruz. Mexico. J. Coast. Res. 2017, 143–153. doi: 10.2112/SI77-015.1
Martínez, M. L., Vázquez, G., and Colón, S. S. (2001). Spatial and temporal variability during primary succession on tropical coastal sand dunes. J. Veg. Sci. 12, 361–372. doi: 10.2307/3236850
Martínez, M. L., Vázquez, G., Favila, M. E., and Álvarez-Molina, L. (2019). Variation in different measures of diversity during primary succession on a tropical coastal dune. Plant Ecol. Divers. 12, 489–505. doi: 10.1080/17550874.2019.1628114
Martínez, M. L., Vázquez, G., White, D. A., Thivet, G., and Brengues, M. (2002). Effects of burial by sand and inundation by fresh-and seawater on seed germination of five tropical beach species. Can. J. Bot. 80, 416–424. doi: 10.1139/b02-027
Masselink, G., Castelle, B., Scott, T., Dodet, G., Suanez, S., Jackson, D., et al. (2016). Extreme wave activity during 2013/2014 winter and morphological impacts along the Atlantic coast of Europe. Geophys. Res. Lett. 43, 2135–2143. doi: 10.1002/2015GL067492
Maun, M. A. (1998). Adaptations of plants to burial in coastal sand dunes. Can. J. Bot. 76, 713–738. doi: 10.1139/b98-058
Maun, M. A., and Perumal, J. (1999). Zonation of vegetation on lacustrine coastal dunes: effects of burial by sand. Ecol. Lett. 2, 14–18. doi: 10.1046/j.1461-0248.1999.21048.x
Maximiliano-Cordova, C., Salgado, K., Martínez, M. L., Mendoza, E., Silva, R., Guevara, R., et al. (2019). Does the functional richness of plants reduce wave erosion on embryo coastal dunes? Estuaries Coasts 42, 1730–1741. doi: 10.1007/s12237-019-00537-x
Mendoza, E., Odériz, I., Martínez, M. L., and Silva, R. (2017). Measurements and modelling of small scale processes of vegetation preventing dune erosion. J. Coast. Res. 77, 19–27. doi: 10.2112/si77-003.1
Miller, T. E. (2015). Effects of disturbance on vegetation by sand accretion and erosion across coastal dune habitats on a barrier island. AoB Plants 7:lv003. doi: 10.1093/aobpla/plv003
Miller, T. E., Gornish, E. S., and Buckley, H. L. (2010). Climate and coastal dune vegetation: disturbance, recovery, and succession. Plant Ecol. 206, 97–104. doi: 10.1007/s11258-009-9626-z
Moreno-Casasola, P. (1986). Sand movement as a factor in the distribution of plant communities in a coastal dune system. Vegetatio 65, 67–76. doi: 10.1007/BF00044876
Nishi, R., and Kraus, N. C. (1997). “Mechanism and calculation of sand dune erosion by storms,” in Coastal Engineering 1996, ed. L. E. Billy (New York, NY: American Society of Civil Engineers), 3034–3047.
Odériz, I., Knöchelmann, N., Silva, R., Feagin, R. A., Martínez, M. L., and Mendoza, E. (2020). Reinforcement of vegetated and unvegetated dunes by a rocky core: a viable alternative for dissipating waves and providing protection? Coast. Eng. 158:103675. doi: 10.1016/j.coastaleng.2020.103675
Oksanen, J., Blanchet, F. G., Friendly, M., Kindt, R., Legendre, P., McGlinn, D., et al. (2019). vegan: Community Ecology Package. Available online at: https://cran.r-roject.org/package=vegan (accessed March 5, 2020).
Overton, M. F., Pratikto, W. A., Lu, J. C., and Fisher, J. S. (1994). Laboratory investigation of dune erosion as a function of sand grain size and dune density. Coast. Eng. 23, 151–165. doi: 10.1016/0378-3839(94)90020-5
Pries, A. J., Miller, D. L., and Branch, L. C. (2008). Identification of structural and spatial features that influence storm-related dune erosion along a barrier-island ecosystem in the Gulf of Mexico. J. Coast. Res. 24, 168–175. doi: 10.2112/06-0799.1
Psuty, N. P., Martínez, M. L., López-Portillo, J., Silveira, T. M., García-Franco, J. G., and Rodríguez, N. A. (2009). Interaction of alongshore sediment transport and habitat conditions at Laguna La Mancha, Veracruz, Mexico. J. Coast. Conserv. 13, 77–87. doi: 10.1007/s11852-009-0060-0
R core Team (2019). R: A Language and Environment for Statistical Computing. Available online at: https://www.r-project.org/ (accessed March 5, 2010).
Ramírez, M. G., and Reséndiz, I. N. R. (2002). Seguimiento de nortes en el litoral del Golfo de México en la temporada 1999-2000. Rev. Geográfica 131, 5–19.
Rodgers, J. C., Murrah, A. W., and Cooke, W. H. (2009). The impact of Hurricane katrina on the coastal vegetation of the Weeks Bay Reserve, Alabama from NDVI data. Estuaries Coasts 32, 496–507. doi: 10.1007/s12237-009-9138-z
Saye, S. E., van der Wal, D., Pye, K., and Blott, S. J. (2005). Beach-dune morphological relationships and erosion/accretion: an investigation at five sites in England and Wales using LIDAR data. Geomorphology 72, 128–155. doi: 10.1016/j.geomorph.2005.05.007
Short, A. D., and Wright, L. D. (1983). “Physical Variability of Sandy Beaches,” in Sandy Beaches as Ecosystems, eds A. McLachlan and E. Theuns (Berlin: Springer), 133–144. doi: 10.1007/978-94-017-2938-3_8
Sigren, J. M., Figlus, J., and Armitage, A. R. (2014). Coastal sand dunes and dune vegetation: restoration, erosion, and storm protection. Shore Beach 82, 5–12.
Sigren, J. M., Figlus, J., Highfield, W., Feagin, R. A., and Armitage, A. R. (2018). The effects of coastal dune volume and vegetation on storm-induced property damage: analysis from hurricane Ike. J. Coast. Res. 34, 164–173. doi: 10.2112/jcoastres-d-16-00169.1
Silva, R., Martínez, M. L., Odériz, I., Mendoza, E., and Feagin, R. A. (2016). Response of vegetated dune-beach systems to storm conditions. Coast. Eng. 109, 53–62. doi: 10.1016/j.coastaleng.2015.12.007
Spalding, M. D., Ruffo, S., Lacambra, C., Meliane, I., Hale, L. Z., Shepard, C. C., et al. (2014). The role of ecosystems in coastal protection: adapting to climate change and coastal hazards. Ocean Coast. Manag. 90, 50–57. doi: 10.1016/j.ocecoaman.2013.09.007
Stallins, J. A. (2002). Dune plant species diversity and function in two barrier island biogeomorphic systems. Plant Ecol. 165, 183–196. doi: 10.1023/A:1022224216705
Stallins, J. A. (2005). Stability domains in barrier island dune systems. Ecol. Complex. 2, 410–430. doi: 10.1016/j.ecocom.2005.04.011
Stallins, J. A., and Parker, A. J. (2003). The influence of complex systems interactions on barrier Island dune vegetation pattern and process. Ann. Assoc. Am. Geogr. 93, 13–29. doi: 10.1111/1467-8306.93102
Synder, R. A., and Boss, C. L. (2002). Recovery and stability in barrier island plant communities. J. Coast. Res. 18, 530–536.
Temmerman, S., Meire, P., Bouma, T. J., Herman, P. M., Ysebaert, T., and De Vriend, H. J. (2013). Ecosystem-based coastal defence in the face of global change. Nature 504, 79–83. doi: 10.1038/nature12859
Vellinga, P. (1982). Beach and dune erosion during storm surges. Coast. Eng. 6, 361–387. doi: 10.1016/0378-3839(82)90007-2
Wolner, C. W. V., Moore, L. J., Young, D. R., Brantley, S. T., Bissett, S. N., and McBride, R. A. (2013). Ecomorphodynamic feedbacks and barrier island response to disturbance: insights from the Virginia Barrier Islands, Mid-Atlantic Bight, USA. Geomorphology 199, 115–128. doi: 10.1016/j.geomorph.2013.03.035
Wright, L. D., and Short, A. D. (1984). Morphodynamic variability of surf zones and beaches: a synthesis. Mar. Geol. 56, 93–118. doi: 10.1016/0025-3227(84)90008-2
Keywords: coastal erosion, vegetation, foredunes, plant cover, plant diversity, storms, erosion mitigation
Citation: Maximiliano-Cordova C, Martínez ML, Silva R, Hesp PA, Guevara R and Landgrave R (2021) Assessing the Impact of a Winter Storm on the Beach and Dune Systems and Erosion Mitigation by Plants. Front. Mar. Sci. 8:734036. doi: 10.3389/fmars.2021.734036
Received: 30 June 2021; Accepted: 03 August 2021;
Published: 24 August 2021.
Edited by:
Juan Jose Munoz-Perez, University of Cádiz, SpainReviewed by:
Raul Martell-Dubois, National Commission for the Knowledge and Use of Biodiversity (CONABIO), MexicoPatricia Lopez-Garcia, University of Cádiz, Spain
Copyright © 2021 Maximiliano-Cordova, Martínez, Silva, Hesp, Guevara and Landgrave. This is an open-access article distributed under the terms of the Creative Commons Attribution License (CC BY). The use, distribution or reproduction in other forums is permitted, provided the original author(s) and the copyright owner(s) are credited and that the original publication in this journal is cited, in accordance with accepted academic practice. No use, distribution or reproduction is permitted which does not comply with these terms.
*Correspondence: Carmelo Maximiliano-Cordova, cmcordova14@gmail.com