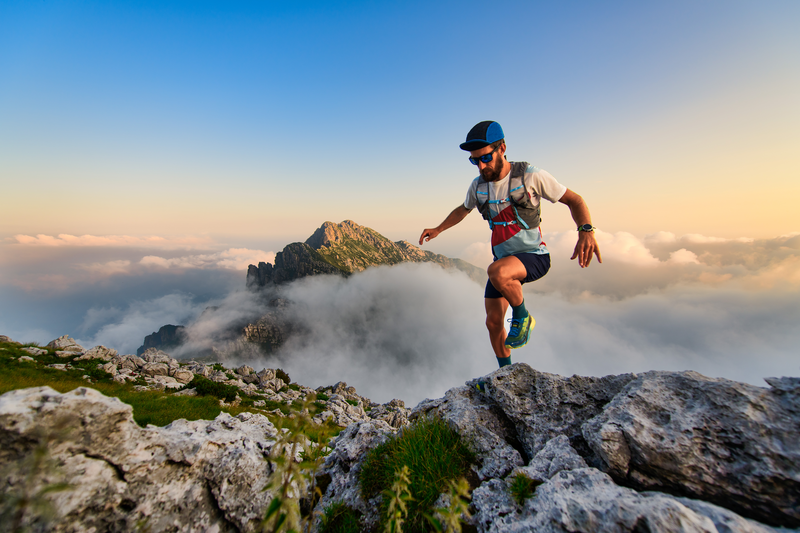
94% of researchers rate our articles as excellent or good
Learn more about the work of our research integrity team to safeguard the quality of each article we publish.
Find out more
ORIGINAL RESEARCH article
Front. Mar. Sci. , 16 September 2021
Sec. Marine Fisheries, Aquaculture and Living Resources
Volume 8 - 2021 | https://doi.org/10.3389/fmars.2021.732970
This article is part of the Research Topic Biological and Ecological Studies on Marine Ichthyoplankton View all 14 articles
Ichthyoplankton assemblages and their relationship with environmental variables are investigated in waters off the Pearl River Estuary in spring and autumn of 2019. Of 80 ichthyoplankton taxa identified using DNA barcode and morphological methods, 61 are identified to species. The most abundance families (Carangidae, Trichiuridae, Mullidae, and Scombridae) account for 61.34% of the horizontal total catch in spring, while Menidae and Carangidae are the most abundant families identified in autumn, accounting for 89.72% of the horizontal total catch. Cluster analysis identifies three species assemblages in spring, and four in autumn based on horizontal trawls. Relationships between assemblage structure and environmental variables (in situ and remote sensed) are determined by canonical correspondence analysis. Ichthyoplankton assemblage structure appears to be strongly influenced by sea level anomalies, salinity, water depth, temperature at 10 m depth, and distance from shore. We demonstrate the efficacy of using DNA barcode to identify ichthyoplankton, and suggest how these data can be used to protect fish spawning grounds in waters off the Pearl River Estuary.
Information on the composition, and spatial and temporal distributions of ichthyoplankton assemblages is of value because it can be used to identify fish spawning sites and reproductive seasons, and appropriate areas and strategies for their protection (Potter et al., 1990; Raynie and Shaw, 1994; Oliveira and Ferreira, 2010; Makrakis, 2019). Ichthyoplankton–the early life stages of fish–undergo dynamic changes in morphology, physiological capability, behavior, ecological role, and habitat as they develop. Their natural mortality rate is high (Fuiman, 2003). Being a key life cycle stage, the survival rate of these fish eggs and larvae affects their recruitment and assemblage dynamics (Chambers and Trippel, 1997; Cao et al., 2007). The formation of ichthyoplankton assemblages is regulated by the physical and biological processes, and their distributions are considered to be more important to the survival of larvae than absolute larval abundance (Hewitt, 1981). Because ichthyoplankton assemblage distribution can be correlated with adult spawning phases and biotic/abiotic variables, those conditions suitable for adult reproduction, and mechanisms that affect planktonic early life stages, can be identified (Doyle et al., 1993; Whitfield, 1999; Hare et al., 2001; Mesa et al., 2016).
Ranked as the 13th or 14th largest river in the world and 2nd largest river in China, the Pearl River, has an annual runoff exceeding 33.6 × 109 m3 (Ou et al., 2007). This river discharges into the South China Sea (SCS) across the Pearl River Delta floodplain, and in doing so transports abundant nutrients into the sea (Lu et al., 2007; Chen et al., 2008). The terrain of the Pearl River Estuary is complex, and the interlaced river network empties into the northern SCS through eight distributaries. This geographic complexity, coupled with variable salinity and high nutrient input, render the Pearl River Estuary and adjacent aquatic environments variable, changeable, and high productive (Zhang et al., 2011; Zhu et al., 2019). Waters of and off the Pearl River Estuary provide habitat for 542 species of fish (287 species in the estuary area, and 330 species in coastal and offshore areas) (Li, 2008). This species richness is more than 36% of the total number of fish species (nearly 1500) reported from the northern SCS (Zhan, 1998; Li, 2008; Sun and Chen, 2013). This high richness and the fish yield from these waters from depths greater than 30 m render this area one of the most important fishing grounds in the SCS (Wang, 2012; Cai et al., 2018). However, fish resources in this region have decreased dramatically in recent decades, largely because of anthropogenic disturbance and climate change (Zeng et al., 2019; Zhou et al., 2019). Meanwhile, the Indo-Pacific humpback dolphin, Sousa Chinese Osbeck, 1765, Indo-Pacific finless porpoise Neophocaena phocaenoides (Cuvier, 1829), Eden’s Whale, Balaenoptera edeni Anderson, 1879, Bryde’s whale, B. edeni Olsen, 1913, and humpback whale Megaptera novaeangliae Borowski, 1781, which mainly prey on fishes, have all been reported from waters off the Pearl River Estuary (Jefferson et al., 2012; Peilie, 2012; Lin et al., 2019). Sadly Bryde’s and humpback whales have disappeared from these waters, and the population of Indo-Pacific humpback dolphin has continuously declined (Hung et al., 2006; Peilie, 2012; Lin et al., 2019). A shortage of food is considered to be one of the main reasons for these disappearances and population declines (Li et al., 2020).
Understanding the spatial distributions of fish is necessary to understand marine ecosystem dynamics. The Pearl River Estuary and its offshore waters are important spawning, feeding, and nursing ground in the northern SCS (Zhan, 1998; Li et al., 2000; Wang and Lin, 2006; Xiao et al., 2013). Accordingly, understanding how the distributions of ichthyoplankton are linked to environmental variables can provide valuable information to assist with spatial, temporal, and/or ecosystem-based approaches to fishery management (Hsieh C. H. et al., 2010; Laman et al., 2017). However, despite the importance of these waters to fish stocks, due to the difficulties identifying early life stages of fish, only Xiao et al. (2013) has studied the ichthyoplankton fauna from them, wherein the abundance of 113 identified taxa was reported. Anthropogenic disturbance, climate change, and fisheries over-exploitation have all contributed to serious declines in fishery resources in the waters of and off the Pearl River Estuary (Duan et al., 2009; Wang et al., 2016; Zeng et al., 2019; Zhou et al., 2019). Because of this there is a pressing need to investigate the status of fish spawning grounds, and ichthyoplankton assemblages in this area, so that more effective management and/or protection of these resources can be achieved.
Precise identification of species in ichthyoplankton samples is important to understand species reproductive biology, preferred spawning time, and spawning grounds (Baumgartner et al., 2004; Cao et al., 2007; Claydon et al., 2014; Zhang et al., 2015). However, accurately identifying fish eggs and larvae to species is difficult, probably for which reason there have been few empirical studies on ichthyoplankton in the SCS (Li K. Z. et al., 2014; Huang et al., 2017; Hou et al., 2021). DNA barcode, which delimits species using molecular maker (e.g., cytochrome c oxidase subunit I, COI), have been widely applied to facilitate the identification of fish eggs and larvae (Valdez-Moreno et al., 2010; Frantine-Silva et al., 2015; Hubert et al., 2015; Leyva-Cruz et al., 2016; Burrows et al., 2018; Kerr et al., 2020; Chen et al., 2021; Hou et al., 2021). For instance, Leyva-Cruz et al. (2016) identified 42 taxa of fish eggs collected from the waters surrounding Banco Chinchorro in the Mexican Caribbean. Burrows et al. (2018) applied the DNA barcode to identify fish eggs in the Gulf of Mexico, and delimited 709 eggs to 62 species. Kerr et al. (2020) successfully identified 564 fish eggs to 89 taxa collected from northwestern Cuba and across the Florida Straits using DNA barcode. Therefore, it has been demonstrated that DNA barcode can effectively identify fish eggs and larvae, and illustrated the potentiality in the study of ichthyoplankton assemblage and ecology.
To identify fish spawning grounds in waters off the Pearl River Estuary we undertook ichthyoplankton surveys in the spring and autumn of 2019. Our aims were to: (i) identify fish eggs and larvae using DNA barcodes and morphology, and develop a morphological database for the ichthyoplankton in this area, (ii) describe the ichthyoplankton assemblages structure in spring and autumn, and (iii) reveal relationships between environmental variables and ichthyoplankton assemblage structure. By evaluating the influence of human activities on recruitment of fishery resources, the management and conservation of these species and their spawning grounds in waters off the Pearl River Estuary can be improved.
Surveys occurred at 12 stations in waters off the Pearl River Estuary (112.50–114.50°E, 20.00–21.50°N) in spring (April) and autumn (October) of 2019 (Figure 1). Ichthyoplankton samples were collected by zooplankton net (0.8 m diameter, 2.7 m long, 505 μm mesh, with cod-end container mesh of 400 μm) at stations over seabed depths exceeding 30 m. Four zooplankton net deployments were made at each station: two simultaneous horizontal tows at fixed depth (15 min at 1.5–2.2 knots), and two vertical hauls (∼sea bed to the surface, hauled at 1.5 m/s). To preserve useful morphological characteristics of fish eggs and larval specimens, and to ensure that we could extract quality COI sequences from samples, we (upon collection) immediately preserved ichthyoplankton samples in 4% neutral formalin for 2 h, then filtered this out and transferred fixed samples to ∼75% ethanol.
In the waters off the PER, several oceanographic events occurred, i.e., salinity front, coastal current, river plume, and water masses etc. (Dong et al., 2004; Su, 2004; Li et al., 2018), and these events were evaluated for possible effects on the ichthyoplankton assemblages. Temperature and salinity were considered as key variables in the oceanographic events. In the present study, the surveyed water was at the sea side off the salinity front (>32 PSU, the offshore boundary of the river plume; Ou et al., 2007). Thus, environmental variables included surface sea water temperature (SST, °C), sea water temperature at 10 m (T-10, °C), sea water temperature at 40 m (T-40, °C), sea surface salinity (SSS, PSU) were considered in the present study. In addition, sea level anomaly (SLA, mm), water depth (m), and offshore distance (km) were also adopted (He et al., 2014, 2016). SST and water depth were measured in situ. Satellite remote-sensed T-10, T-40, and SSS and SLA data were downloaded from HYCOM model data1, for which temporal resolution was 1 days, and spatial resolution was 1 km. SLA data were derived from sea surface elevation data of HYCOM, then calculated using the Google Earth Engine, a cloud platform by mean processing algorithm. Minimum offshore distance was calculated in R version 4.0.5 (R Core Team, 2021) using the Sp package (Pebesma and Bivand, 2005).
Ichthyoplankton were identified in the laboratory by combined the molecular and morphological analyses. Briefly, ichthyoplankton specimens were first identified by cytochrome c oxidase subunit I (COI) to the lowest taxonomic level using a combined local DNA barcode library (Hou et al., 2018) and the Barcode of Life Data (BOLD) system, and the blast search criteria similar to those used in Hubert et al. (2015). Because formalin-fixation can reduce the success rate of DNA extraction and sequence amplification after 10 days (Hou et al., 2021), especially for fish eggs, we quickly picked out the ichthyoplankton specimen beneath a stereomicroscope and randomly selected 15 eggs/larvae in each horizontal and vertical trawl to be DNA extracted and amplified for the first time. If possible we performed this 2 or 3 times for each sample if a sample contained >60/90 specimens. In the 3 times, the success rate of DNA extraction and sequence amplification reduced sharply to below 20%, after which we stopped. For the purpose of developing a morphological database for the ichthyoplankton in the study area, each specimen was photographed by a Zeiss microscope (Axioplan 2 imaging E) before the DNA extraction (Figure 2). For the specimens that failed in the DNA extraction were identified by morphology following Okiyama (1989) and Shao et al. (2001).
Figure 2. Photographs of the specimens that were identified to species level by COI sequences off Pearl River Estuary. Egg stages, i.e., (1) Ophichthus erabo, GDYH4502, 4.102 mm; (2) Trachinocephalus myops, GDYH4477, 1.265 mm; (3) Oxyporhamphus micropterus, GDYH5679, 2.622 mm; (4) Seriolina nigrofasciata, GDYH6025, 1.264 mm; (5) Psenopsis anomala, GDYH4493, 1.046 mm; (6) Coryphaena hippurus, GDYH4501, 1.593 mm; (7) Nemipterus virgatus, GDYH6061, 0.727 mm; (8) Auxis thazard, GDYH4497, 1.066 mm; (9) Auxis rochei, GDYH5571, 1.007 mm; (10) Tentoriceps cristatus, GDYH4597, 1.674 mm; (11) Uranoscopus oligolepis, GDYH8442, 1.862 mm; (12) Heteromycteris japonicus, GDYH4481, 1.309 mm; larval stages, i.e., (13) Encrasicholina punctifer, GDYH8199, 14.133 mm; (14) Ostorhinchus kiensis, GDYH8267, 12.468 mm; (15) Caranx ignobilis, GDYH8296, 8.153 mm; (16) Decapterus macrosoma, GDYH5565, 4.252 mm; (17) Elagatis bipinnulata, GDYH8295, 8.941 mm; (18) Selar crumenophthalmus, GDYH8440, 3.644 mm; (19) Seriola dumerili, GDYH5583, 4.297 mm; (20) Seriola rivoliana, GDYH8519, 4.207 mm; (21) Nemipterus bathybius, GDYH8463, 7.310 mm; (22) Nemipterus virgatus, GDYH6066, 4.368 mm; (23) Auxis rochei, GDYH5969, 6.176 mm; (24) Terapon jarbua, GDYH8191, 7.725 mm; (25) Pristigenys niphonia, GDYH8518, 4.857 mm; (26) Trichiurus brevis, GDYH8198, 7.139 mm; (27) Upeneus japonicus, GDYH5575, 3.989 mm; (28) Arnoglossus tenuis, GDYH5949, 5.459 mm; (29) Psettina hainanensis, GDYH6000, 9.746 mm; (30) Engyprosopon multisquama, GDYH5869, 4.783 mm; (31) Lagocephalus spadiceus, GDYH8268, 4.527 mm; (32) Diaphus watasei, GDYH5965, 5.790 mm. The measured length for eggs is egg diameter, for larvae is total length.
Species density (abundance per tow) was standardized to catch per unit effort. Taxa were assigned to different ecological guilds using habitat data for adult fish species in Fishbase2. An index of relative importance (IRI) was calculated for each species or taxon using the following function (Zhu et al., 2002; Ren et al., 2016):
IRI = N% × F% × 10,000
Where N% and F% are the relative abundance of the total catch and frequency of occurrence. Accordingly, each species or taxon was then defined as dominant (IRI ≥ 100), common (10 ≤ IRI < 100), or rare (IRI < 10).
Species accumulation curves were used to assess if our sampling effort was sufficient to describe ichthyoplankton species richness. The classic method “random” was used as an accumulator function to determine the means and standard deviations of species accumulation curves from random subsampling of the data without replacement (Gotelli and Colwell, 2001). The species accumulation curves were fitted in the “vegan” package implemented in R version 4.0.5 (Oksanen et al., 2020; R Core Team, 2021).
Species/taxa which accounted for more than 1% of the total catch in averaged horizontal tows were used in seasonal assemblage structure analysis. Data were first square root transformed and standardized to enhance the weighting of rare species (Clarke, 1993), and then clustered using Bray-Curtis similarity (Primer Software, Version 5.2). A non-parametric ANOSIM analysis was performed to examine differences within clusters in seasons (Clarke and Warwick, 2001). SIMPER analysis was used to calculate the contribution of each taxon to the Bray-Curtis similarity within clusters, and dissimilarity among clusters (Clarke and Warwick, 2001). Canonical correspondence analysis (CCA) was used to elucidate relationships between environmental variables and ichthyoplankton assemblages (CCA, CANOCO Software, Version 4.5). A category of sample coded “no fish” was created to prevent CANOCO from eliminating samples containing no ichthyoplankton (Grothues and Cowen, 1999). Down-weighting of rare species was performed. A forward selection was applied to test the statistical significance of environmental variables by a Monte Carlo permutation test (999 permutations) (Ye et al., 2015). Variation inflation factor (VIF) was used to test for collinearity between independent environment variables, with an upper cutoff of 10 (indicating excessive multicollinearity) (Graham, 2003; Kutner et al., 2004). Ordination diagrams of the CCA results were plotted to demonstrate associations between environmental variables and species. The length of a vector of a given variable on the CCA plot indicates the importance of that variable, with any variable having a correlation coefficient ≥ |0.4| is considered to be biologically important (Rakocinski et al., 1996; Akin et al., 2005). Species plotted closer to any vector with a longer distance from the origin have a stronger relationship with that vector (Ren et al., 2016). Species located near the origin either do not show a strong relationship to any of the variables, or they are found at average values of environmental variables (Elliott, 1998; Akin et al., 2005).
Of 4769 ichthyoplankton specimens collected, 2867 were from spring and 1902 from autumn. In all, 931 fish eggs and 229 larvae were selected for photography and DNA extraction, from which high-quality COI sequences were obtained from 308 fish eggs (33.1% of samples) and 125 larvae (54.6% of samples). A total of 75 taxa were identified using BOLD and local DNA barcode libraries (Supplementary Table 1). Among the 75 taxa identified by COI sequences, 61 were identified to species (referred to 50 genera, 33 families, and 10 orders), 5 were identified to genus, and 9 could not be identified. Of these, 38 taxa identified from eggs were attributed to species (comprising 31 genera, 20 families, and 8 orders), and 35 larvae were identified to species (comprising 29 genera, 21 families, and 7 orders) (Supplementary Table 1). In all, 80 taxa were identified by morphological and molecular analyses (75 taxa by COI sequences, 4 by morphology, and 1 taxon that are unidentified) (Supplementary Tables 1, 2). Our species accumulation curves were non-asymptotic, indicating substantially more taxa would have been encountered with increased sampling effort in spring and autumn (Figure 3).
Figure 3. Species accumulation curves of ichthyoplankton off Pearl River Estuary. (A) Spring; (B) autumn. Light blue area indicates 95% confidence intervals. Yellow boxes are the interquartile ranges, the central bold mark on each box indicates the median, and the bottom and top edges of the box indicate the 25th and 75th percentiles, respectively. The whiskers extend to the most extreme data points not considered outliers, and the outliers are plotted individually using the “+” symbol.
In spring, in horizontal net tows, 11 taxa were categorized as dominant, contributing 82.46% to the total abundance in these samples. In vertically hauled net tows, nine taxa were dominant, contributing 77.18% to vertical samples. In autumn, in horizontal net tows, five taxa were dominant, contributing 92.92% to the total abundance of species in these samples. In vertically hauled net samples, three species were dominant, contributing 79.52% to these samples (Table 1 and Supplementary Table 2). Among these taxa, Terapon jarbua (Forsskål, 1775) was dominant in both horizontal and vertical trawls during spring and autumn.
The mean total abundance in spring was 91.7 ind./net in horizontal tows and 27.75 ind./net in vertical hauls. These values were higher than those in autumn: 68.88 ind./net (horizontal tows) and 10.38 ind./net (vertical hauls). However, One-way ANOVA indicated that there are no significant difference for the abundance in horizontal and vertical tows between spring and autumn (Fspring = 0.19, Fautumn = 2.08, P > 0.05). In the present study, we took horizontal samples to examine differences within clusters in seasons. In spring, ichthyoplankton composition was not very similar, and there was significant variation among clusters (ANOSIM, R > 0.41, P < 0.05). In autumn, ichthyoplankton composition was not very similar, however, there was not significant variation among most clusters (ANOSIM, R > 0.54), and average dissimilarities among clusters in each seasonal survey were high (SIMPER, average dissimilarity ≥58.29% Table 2). The most dominant characteristics taxa in each cluster calculated by SIMPER were unidentified Gobiidae, Decapterus maruadsi (Temminck and Schlegel, 1843) and Upeneus japonicus (Houttuyn, 1782) in spring, and Decapterus macrosoma Bleeker, 1851, Mene maculata (Bloch and Schneider, 1801), and T. jarbua in autumn (Supplementary Table 3). In spring, there were three main clusters of taxa comprising 18 categories of ichthyoplankton, each represented more than 1% of the total abundance (I to III, Figures 4A,B). In autumn, there were four clusters indicated by six categories of ichthyoplankton (Figures 4C,D).
Table 2. The comparison of the assemblage structure according to one-way ANOSIM and SIMPER analysis.
Figure 4. Relationships between station clusters, larval assemblages for ichthyoplankton off Pearl River Estuary. (A) Station clusters in spring, (B) Mapping of the clusters of stations in spring. (C) Station clusters in autumn, (D) Mapping of the clusters of stations in autumn. □ indicates not occured in the station, and ■ indicates occurred in the station. Symbols indicate the membership to clusters.
Environmental variables off the PER are shown in Supplementary Table 4. Because a VIF test indicated that SST and T-40 showed multicollinearity with other environmental variables, we excluded them from subsequent analysis, leaving us with five variables (SLA, SSS, T-10, Depth, and Offshore distance) for CCA analysis. Monte Carlo permutation tests indicated that four (excepting SLA) of these variables contributed significantly to explaining ichthyoplankton assemblage structure (P < 0.05). According to the rule of correlation coefficient ≥ |0.4|, SLA was evaluated to be biologically important and used in CCA analysis (Supplementary Table 5). Eigenvalues were 0.413 (CCA1), 0.295 (CCA2), 0.132 (CCA3), and 0.095 (CCA4). In brief, the first and second CCA axes accounted for 41.6 and 29.8% of the variance in the species-environment relationship, respectively, with the correlation efficiencies of the first two axes being 0.878 and 0.921, respectively (Table 3). The sum of all canonical eigenvalues (0.992) only equaled 33.09% of the unconstrained eigenvalues (2.998), showing the restrictive effect of building environmental relationships into the CCA model. Results obtained from the first two axes were plotted to explain species–environment relationships. The first axis was highly correlated with T-10 and distinguished autumn sampling stations. The second axis was highly correlated with SSS, SLA, and water depth, and distinguished spring sampling stations (Figure 5A). The ordination diagram of the first two axes with the scores for environmental variables and species showed that most species scattered near the origin, which indicated average values in relation to environmental variables for these species. However, some species were outliers, e.g., Pristigenys niphonia (Cuvier, 1829), Istiophorus platypterus (Shaw, 1792), and Elagatis bipinnulata (Quoy and Gaimard, 1825), which was separated from the majority of species along the first axis, showing mainly a negative correlation with SSS; Euthynnus affinis (Cantor, 1849), Ophisurus serpens (Linnaeus, 1758), Tentoriceps cristatus (Klunzinger, 1884), and Unidentified Gobiidae, which were separated from most species along the second axis, showing mainly a positive correlation with SLA and a negative correlation with water depth and offshore distance (Figure 5B).
Table 3. Results of CCA based on ichthyoplankton abundance collected off the Pearl River Estuary in spring and autumn, 2019.
Figure 5. Canonical correspondence analysis ordination plots for ichthyoplankton assemblages and environmental variables off Pearl River Estuary. (A) Biplot of sampling stations. The circles represent the stations in spring, and gray represent stations in autumn. (B) Biplot of species.
We identified 75 taxa using DNA barcodes, combining the BOLD system with a local DNA barcode library. Of these taxa, 61 were identified to species, including 38 taxa from eggs, and 35 taxa from larvae; we could not attribute 11 egg or 6 larval COI sequences (3.93% of all sequences) to any taxon, indicating incompleteness of regional barcode libraries (Supplementary Table 1). Our study demonstrates the importance of DNA barcoding in studies involving ichthyoplankton identification, especially their egg stages. However, for sequencing large numbers of ichthyoplankton samples this technology is expensive and time consuming, and its application would be impracticable for large spawning-ground surveys. To obtain species-level information from ichthyoplankton using molecular approaches, it proved indispensable to first differentiate morphotypes based on their morphology. Thus, developing a morphological database together with a corresponding DNA barcode library will facilitate identification of fish early life stages (Hubert et al., 2015; Hou et al., 2021). In the present study, we develop a preliminary morphological database of 75 ichthyoplankton taxa for these waters, layed the foundation for the morphological identification in the waters off the PER (Figure 2). Compared to the previous study, we improved the preserved method, transferred from the 4% neutral formalin to ∼75% ethanol, which can ensure that we can obtain a certain proportion of COI sequences of both fish larvae and fish eggs, although the morphology quality of larvae is not good as those preserved in formalin solution (Hou et al., 2021). In addition, the present study also represents the first attempt by using the DNA Barcode to provide more detailed information regarding the species composition, occurrence of spawning site and spawning seasons for ichthyoplankton off the PER. However, the present surveys effort were not robust estimates of species richness due to the lack of asymptote in the species accumulation curves (Figure 3), indicating that adding more survey stations would added many more ichthyoplankton species.
Because we surveyed only in spring (April) and autumn (October), we are limited to discussing these two sampling events. The 33 ichthyoplankton taxa that occur in both spring and autumn samples account for 41.25% of all species (80 taxa) that we report from this area. This suggests that many fish species spawn in both spring and autumn. Most species also have spawning peaks in spring, which corresponds to subtropical monsoon characteristics in the northern SCS and adjacent waters (Tzeng et al., 2002; Hsieh et al., 2005; Hsieh H. Y. et al., 2010; Xiao et al., 2013; Li K. Z. et al., 2014). Earlier analyses of fish spawning times were based largely on monitoring gonad development of fishes in the northern SCS (i.e., begin at gonadal maturity stage IV); such an approach is also expensive and requires considerable manpower and logistical resources (Chen et al., 2002, 2003). While spawning periods could be inferred from earlier monitoring work, the spawning locations of fishes remained unknown. Thus, that information we present from this region provides clear evidence of the spatial and temporal distribution of ichthyoplankton in spring and autumn, and likely presence of spawning grounds nearby.
To protect spawning fish stocks and juveniles, a 2-month (June and July) moratorium on fishing in the SCS was implemented from 1999 to 2008; and extended to 77 days from 16 May to 1 August from 2009 to 2016; since 2017 it was even extended to 3.5 months (1 May to 16 August). Our study indicates that the moratorium in effect from 2017 does not protect spawning fish in this region in spring. For 1 month prior to closure, fishers off the Pearl River Estuary could be catching spawning adults, which intuitively would affect recruitment and exacerbate resource declines. Within Pearl River, a fishing moratorium extends from 1 March to 30 June–a closure which coincides with the spawning periods of freshwater fish, effectively increasing their recruitment (e.g., Megalobrama hoffmanni Herre and Myers, 1931) (Li Y. F. et al., 2014). Our data for offshore waters support advancing the fisheries moratorium in waters off the Pearl River Estuary by at least 1 month to April to better protect fishery resources.
Because we used a zooplankton net to collect drifting and suspended eggs, many eggs (e.g., demersal eggs of sea catfish, mouth-hatched eggs such as those of cardinalfish, and adhesive eggs) were not sampled. Accordingly the composition of our ichthyoplankton assemblages is somewhat biased, and not representative of the entire fish community known from these waters. However, we do report several unexpected and most important taxa, such as commercially important Scombridae species [Thunnus tonggol (Bleeker, 1851), E. affinis (Cantor, 1849), Auxis thazard (Lacepède, 1800), Auxis rochei (Risso, 1810)], and Nemipteridae species [Nemipterus virgatus (Houttuyn, 1782) and Nemipterus bathybius Snyder, 1911], the most caught fish taxon Decapterus spp. in the northern SCS, and the new species Strophidon tetraporus sp. nov. (Huang et al., 2020). The spawning grounds of these species are not well known, mainly because of difficulties experienced with accurate identification of their eggs and larvae, and lack of any illustrated handbooks. Additionally, benthopelagic fish (e.g., Diaphus watasei Jordan and Starks, 1904, and Cyclothone sp.) were identified from COI sequences, indicating that waters off the Pearl River Estuary are affected by continental shelf surface water mixing (Zhang et al., 2011).
Understanding relationships between ichthyoplankton assemblages and physical and biological processes is becoming increasingly important for ecosystem-based fisheries management. Physical process such as the estuaries (Zhang et al., 2015), ocean currents (Doyle et al., 2002; Sassa et al., 2007; Mullaney et al., 2011; Thompson et al., 2014), water mass (Grothues and Cowen, 1999; Hare et al., 2001; Espinosa-Fuentes and Flores-Coto, 2004; Muhling et al., 2010), monsoons (Hsieh et al., 2005; Hsieh H. Y. et al., 2010) can influence the distributions and survival of fish eggs and larvae, and define the structure and diversity of their assemblages. In the present study, the suite of environmental variables that we examined were significantly associated with ichthyoplankton assemblage structure. The first CCA axis (significantly influenced by SSS and T-10) represents a spatial gradient from the distance off the Pearl River Estuary. Increased salinity and temperature at 10 m depth were associated with changes in ichthyoplankton assemblage structure. Salinity and temperature affect fish reproductive activity and the spatial and temporal distribution of ichthyoplankton assemblages (Whitfield, 1999; Fuiman, 2003).
Salinity is inversely related to egg diameter through its influence on the width of the perivitelline space, and it also affects the hatching process and development rate (Fuiman, 2003). Temperature, a potent environmental regulator of fish physiology, can affect production and activity of hatching enzymes, and is strongly, negatively correlated with the duration of incubation (Fuiman, 2003). Thus, temperature and salinity drive reproductively mature fishes to appropriate habitat for spawning. In the nearshore and offshore waters of Pearl River Estuary, the salinity gradually increases from upstream to downstream, ranging (multi-year) 0.03–33.08 PSU through dry and wet seasons (Jia et al., 2011), meanwhile the sanility front discriminate the nearshore waters (with a salinity of <26, the nearshore boundary of the plume, Wong et al., 2003) and offshore waters (with a salinity of >32, the offshore boundary of the plume, Ou et al., 2007). The horizontal structure and shapes of Pearl River buoyant plume varied among monthly and annually, which are driven by the river discharge in different months and years (Ou et al., 2007; Li et al., 2018). This variability provides varied habitat for many (542) fish species (Wang and Lin, 2006; Zhu et al., 2019), which may also accordingly affect the spatial distribution and ichthyoplankton assemblages for this area. In the present study, the surveyed water was at the sea side off the salinity front (>32 PSU), the dominant characteristics taxa in clusters were associate with high salinities and temperature, i.e., Selar crumenophthalmus (Bloch, 1793), D. macrosoma Bleeker, 1851, A. thazard and A. rochei in spring, and M. maculata and Coryphaena hippurus Linnaeus, 1758 in autumn (Table 1 and Supplementary Table 3).
Biological processes, i.e., spawning behavior of adults, duration of the incubation period, metamorphosis, interspecific competition, and predation may also affect ichthyoplankton assemblages (Harden Jones, 1968; Mason and Brandt, 1996; Miller, 2002; Olivar et al., 2010). Fish have evolved a wide range of life-history strategies in response to the environments, and the offspring must be produced at a specific time and locations, delivered to appropriate nursery grounds to survive and grow, and then mature to join the reproductive population at the appropriate place (Harden Jones, 1968). The interspecific competition for zooplankton prey may exist in the larval stages and lead to reduce growth and survival of competitors’ larvae, which may disrupt the assemblages. Meanwhile, the predators can reduce the relative larval populations. In the nearshore and offshore waters of PRE, the abundant nutrients transported by river discharges produce high productivity of zooplankton (Li et al., 2006), which attract large number of fishes to spawn and nurse in the coastal waters, i.e., the D. maruadsi (South China Sea Fisheries Research Institute, 1966). As the D. maruadsi was the dominant prey for genus Trichiurus fishes (Yan et al., 2011), the prey availability induced the formation of predators’ spawning ground, and then affected the ichthyoplankton assemblages.
Furthermore, variation in species distribution explained by the first four CCA axes was 31.2%, indicating that other factors influence the distribution and composition of ichthyoplankton assemblages off the Pearl River Estuary. The effect of other variables (e.g., chlorophyll-a, and phytoplankton and zooplankton concentration) on ichthyoplankton assemblage structure should be examined in subsequent investigations.
Our analysis of ichthyoplankton assemblages indicates that waters off the Pearl River Estuary are important spawning grounds for many commercially important species. We believe that consideration should be given to protecting this area.
The original contributions presented in the study are included in the article/Supplementary Material, further inquiries can be directed to the corresponding author/s.
The animal study was reviewed and approved by Guangdong Ocean University.
GH and HZ analyzed the data and completed the first draft. GH, YC, and JW performed the field survey. GH, JW, LL, YC, and JL conducted the laboratory experiments. CP and HZ provided the guidance on data analysis and structure. All authors contributed to the article and approved the submitted version.
This study was supported by Fund of Southern Marine Science and Engineering Guangdong Laboratory (Zhanjiang) (ZJW-2019-08), the National Natural Science Foundation of China (Nos. 31702347 and 42090044), Youth Innovation Promotion Association CAS (No. 2020211), Undergraduate Innovation and Entrepreneurship Training Program of Guangdong Province (S202010566005), and the Startup Project of Guangdong Ocean University (R19006).
The authors declare that the research was conducted in the absence of any commercial or financial relationships that could be construed as a potential conflict of interest.
All claims expressed in this article are solely those of the authors and do not necessarily represent those of their affiliated organizations, or those of the publisher, the editors and the reviewers. Any product that may be evaluated in this article, or claim that may be made by its manufacturer, is not guaranteed or endorsed by the publisher.
We would like to thank the editor and reviewers for their constructive comments on our manuscript. We also thank numerous members on the survey ship for their help.
The Supplementary Material for this article can be found online at: https://www.frontiersin.org/articles/10.3389/fmars.2021.732970/full#supplementary-material
Akin, S., Buhan, E., Winemiller, K. O., and Yilmaz, H. (2005). Fish assemblage structure of Koycegiz Lagoon–Estuary, Turkey: spatial and temporal distribution patterns in relation to environmental variation. Estuar. Coast. Shelf. Sci. 64, 671–684. doi: 10.1016/j.ecss.2005.03.019
Baumgartner, G., Nakatani, K., Gomes, L., Bialetzki, A., and Sanches, P. (2004). Identification of spawning sites and natural nurseries of fishes in the upper Paraná River. Brazil. Environ. Biol. Fish. 71, 115–125. doi: 10.1007/s10641-004-0098-z
Burrows, M., Browing, J. S., Breitbart, M., and Murawski, S. A. (2018). DNA barcoding reveals clear delineation between spawning sites for neritic versus oceanic fishes in the Gulf of Mexico. Fish. Oceanogr. 28, 228–239. doi: 10.1111/fog.12404
Cai, Y., Xu, S. N., Chen, Z. Z., Xu, Y. W., Jiang, Y., et al. (2018). Current status of community structure and diversity of fishery resources in offshore northern South China Sea. South China Fish. Sci. 14, 10–18. doi: 10.3969/j.issn.2095-0780.2018.02.002
Cao, W., Chang, J., and Duan, Z. (2007). Fish Resources of Early Life Stages in Yangtze River. Beijing: China Waterpower Press.
Chambers, R. C., and Trippel, E. A. (1997). “Early life history and recruitment: legacy and challenges,” in Early Life History and Recruitment in Fish Populations (London: Chapman and Hall), 515–549. doi: 10.1007/978-94-009-1439-1_19
Chen, C., Wang, S. L., Lu, X. X., Zhang, S. R., Lui, H. K., Tseng, H. C., et al. (2008). Hydrogeochemistry and greenhouse gases of the Pearl River, its estuary and beyond. Quat. Int. 186, 79–90. doi: 10.1016/j.quaint.2007.08.024
Chen, G. B., Li, Y. Z., and Chen, P. M. (2002). Spawning ground of Nemipterus bathybius in northern continental shelf waters of South China Sea. J. Zhanjiang. Ocean. Uni. 22, 20–25.
Chen, G. B., Li, Y. Z., and Chen, P. M. (2003). A study on spawning ground of blue mackerel scad (Decapterus Maruadsi) in continental shelf waters on northern South China Sea. J. Trop. Oceanogr. 22, 22–28.
Chen, W. T., Li, C., Yang, J. P., Zhu, S. L., Li, J., Li, Y., et al. (2021). Temporal species-level composition of larvae resources in the lower Pearl River drainage and implications for species’ reproductive cycles. Gene 776:145351. doi: 10.1016/j.gene.2020.145351
Clarke, K. R. (1993). Non-parametric multivariate analyses of changes in community structure. Austral. Ecol. 18, 117–143. doi: 10.1111/j.1442-9993.1993.tb00438.x
Clarke, K. R., and Warwick, R. (2001). Changes in marine communities: an approach to statistical analysis and interpretation. Mt. Sinai. J. Med. 40, 689–692. doi: 10.2337/diacare.26.7.2005
Claydon, J., Mccormick, M. I., and Jones, G. P. (2014). Multispecies spawning sites for fishes on a low-latitude coral reef: spatial and temporal patterns. J. Fish. Biol. 84, 1136–1163. doi: 10.1111/jfb.12355
Dong, L. X., Su, J. L., Wong, L. A., Cao, Z. Y., and Chen, J. C. (2004). Seasonal variation and dynamics of the Pearl River plume. Cont. Shelf Res. 24, 1761–1777. doi: 10.1016/j.csr.2004.06.006
Doyle, M. J., Mier, K. L., Busby, M. S., and Brodeur, R. D. (2002). Regional variation in springtime ichthyoplankton assemblages in the northeast Pacific Ocean. Prog. Oceanogr. 53, 247–281. doi: 10.1016/S0079-6611(02)00033-2
Doyle, M. J., Morse, W. W., and Kendall, A. W. Jr. (1993). A Comparison of larval fish assemblages in the temperate zone of the northeast pacific and northwest atlantic oceans. Bull. Mar. Sci. 53, 588–644. doi: 10.1080/07055900.1993.9649474
Duan, L. J., Li, S. Y., Liu, Y., Moreau, J., and Christensen, V. (2009). Modeling changes in the coastal ecosystem of the Pearl River Estuary from 1981 to 1998. Ecol. Model. 220, 2802–2818. doi: 10.1016/j.ecolmodel.2009.07.016
Elliott, S. M. (1998). Environmental influences on the fish assemblage of the humber estuary. UK. Estuar. Coast. Shelf. Sci. 46, 175–184. doi: 10.1006/ecss.1997.0268
Espinosa-Fuentes, M. L., and Flores-Coto, C. (2004). Cross-shelf and vertical structure of ichthyoplankton assemblages in continental shelf waters of the Southern Gulf of Mexico. Estuar. Coast. Shelf. Sci. 59, 333–352. doi: 10.1016/j.ecss.2003.09.006
Frantine-Silva, W., Sofia, S., Orsi, M., and Almeida, F. (2015). DNA barcoding of freshwater ichthyoplankton in the neotropics as a tool for ecological monitoring. Mol. Ecol. Resour. 15, 1226–1237. doi: 10.1111/1755-0998.12385
Fuiman, L. A. (2003). “Special considerrations of fish eggs and larvae,” in Fishery Science: The Unique Contributions of Early Life Stages, eds L. A. Fuiman and R. G. Werner (Hoboken, NJ: Blackwell Science), 1–32.
Gotelli, N. J., and Colwell, R. K. (2001). Quantifying biodiversity: procedures and pitfalls in the measurement and comparison of species richness. Ecol. Lett. 4, 379–391. doi: 10.1046/j.1461-0248.2001.00230.x
Graham, M. H. (2003). Confronting multicollinearity in ecological multiple regression. Ecology 84, 2809–2815. doi: 10.1890/02-3114
Grothues, T. M., and Cowen, R. K. (1999). Larval fish assemblages and water mass history in a major faunal transition zone. Cont. Shelf. Res. 19, 1171–1198. doi: 10.1016/S0278-4343(99)00010-2
Hare, J. A., Fahay, M. P., and Cowen, R. K. (2001). Springtime ichthyoplankton of the slope region off the north-eastern United States of America: larval assemblages, relation to hydrography and implications for larval transport. Fish. Oceanogr. 10, 164–192.
He, L., Li, G. S., Li, K., and Shu, Y. Q. (2014). Estimation of regional sea level change in the Pearl River Delta from tide gauge and satellite altimetry data. Estuar. Coast. Shelf Sci. 141, 69–77. doi: 10.1016/j.ecss.2014.02.005
He, X. Q., Xu, D. F., Bai, Y., Pan, D. L., Chen, C. T. A., Chen, X., et al. (2016). Eddy-entrained Pearl River plume into the oligotrophic basin of the South China Sea. Cont. Shelf Res. 124, 117–124. doi: 10.1016/j.csr.2016.06.003
Hewitt, R. (1981). The value of pattern in the distribution of young fish. Rapports et Proce′s-Verbaux des Re′unions 178, 229–236.
Hou, G., Chen, W. T., Lu, H. S., Cheng, F., and Xie, S. G. (2018). Developing a DNA barcode library for perciform fishes in the South China Sea: species identification, accuracy and cryptic diversity. Mol. Ecol. Resour. 18, 137–146. doi: 10.1111/1755-0998.12718
Hou, G., Chen, Y. Y., Wang, S. J., Chen, W. T., and Zhang, H. (2021). Formalin-fixed fish larvae could be effectively identified by DNA barcodes: a case study on thousands of specimens in South China Sea. Front. Mar. Sci. 8:634575. doi: 10.3389/fmars.2021.634575
Hsieh, C. H., Chen, C. S., and Chiu, T. S. (2005). Composition and abundance of copepods and ichthyoplankton in Taiwan Strait (western North Pacific) are influenced by seasonal monsoons. Mar. Freshw. Res. 56, 153–161. doi: 10.1071/MF04058
Hsieh, C. H., Yamauchi, A., Nakazawa, T., and Wang, W. F. (2010). Fishing effects on age and spatial structures undermine population stability of fishes. Aquat. Sci. 72, 165–178. doi: 10.1007/s00027-009-0122-2
Hsieh, H. Y., Lo, W. T., Liu, D. C., and Su, W. C. (2010). Influence of hydrographic features on larval fish distribution during the south-westerly monsoon in the waters of Taiwan, western North Pacific Ocean. J. Fish. Biol. 76, 2521–2539. doi: 10.1111/j.1095-8649.2010.02643.x
Huang, D. L., Zhang, X., Jiang, Z. J., Zhang, J. P., Arbi, I., Jiang, X., et al. (2017). Seasonal fluctuations of ichthyoplankton assemblage in the northeastern south china sea influenced by the kuroshio intrusion. J. Geophys. Res. Oceans 122, 7253–7266. doi: 10.1002/2017JC012906
Huang, W. C., Mohapatra, A., Thu, P. T., Chen, H. M., and Liao, T. Y. (2020). A review of the genus strophidon (anguilliformes: muraenidae), with description of a new species. J. Fish Biol. 97, 1462–1480. doi: 10.1111/jfb.14514
Hubert, N., Espiau, B., Meyer, C., and Planes, S. (2015). Identifying the ichthyoplankton of a coral reef using DNA barcodes. Mol. Ecol. Resour. 15, 57–67. doi: 10.1111/1755-0998.12293
Hung, C., So, M. K., Connell, D. W., Fung, C. N., Lam, M., Nicholson, S., et al. (2006). A preliminary risk assessment of organochlorines accumulated in fish to the Indo-Pacific humpback dolphin (Sousa chinensis) in the northwestern waters of Hong Kong. Chemosphere 144, 190–196. doi: 10.1016/j.envpol.2005.12.028
Jefferson, T. A., Hung, S. K., Robertson, K. M., and Archer, F. I. (2012). Life history of the Indo-Pacific humpback dolphin in the Pearl River Estuary, southern China. Mar. Mamm. Sci. 28, 84–104. doi: 10.1111/j.1748-7692.2010.00462.x
Jia, H., Xie, J., Wu, S., and He, G. (2011). Temproral and spatial variations of salinity in peral river estuary in recent years. Trans. Oceanol. Limnol. 2, 142–146.
Kerr, M., Browning, J., Bønnelycke, E. M., Zhang, Y., Hu, C., Armenteros, M., et al. (2020). DNA barcoding of fish eggs collected off northwestern Cuba and across the Florida Straits demonstrates egg transport by mesoscale eddies. Fish. Oceanogr. 29, 340–348. doi: 10.1111/fog.12475
Kutner, M., Nachtsheim, C., and Neter, J. (2004). Applied Linear Regression Models. NewYork, NY: McGraw-Hill/Irwin.
Laman, E. A., Rooper, C. N., Turner, K., Rooney, S., Cooper, D. W., and Zimmermann, M. (2017). Using species distribution models to describe essential fish habitat in Alaska. Can. J. Fish. Aquat. Sci. 75, 1230–1255. doi: 10.1139/cjfas-2017-0181
Leyva-Cruz, E., Vásquez-Yeomans, L., Carrillo, L., and Valdez-Moreno, M. (2016). Identifying pelagic fish eggs in the southeast Yucatan Peninsula using DNA barcodes. Genome 59, 1117–1129. doi: 10.1139/gen-2015-0151
Li, K. Z., Yin, J. Q., Huang, L. M., and Lin, Z. J. (2014). Seasonal variations in diversity and abundance of surface ichthyoplankton in the northern south china sea. Acta Oceanol. Sin. 33, 145–154. doi: 10.1007/s13131-014-0533-3
Li, K. Z., Yin, J. Q., Huang, L. M., and Tan, Y. H. (2006). Spatial and temporal variations of mesozooplankton in the Pearl River estuary. China. Estuar. Coast. Shelf S 67, 543–552. doi: 10.1016/j.ecss.2005.12.008
Li, Q. P., Zhou, W. W., Chen, Y. C., and Wu, Z. C. (2018). Phytoplankton response to a plume front in the northern South China Sea. Biogeosciences 15, 2551–2563. doi: 10.5194/bg-15-2551-2018
Li, S. H., Lin, M. L., Caruso, F., Dong, L. J., Lin, W. Z., Rosso, M., et al. (2020). Cetaceans under threat in South China Sea. Science 368, 1074–1075. doi: 10.1126/science.abc7557
Li, Y. (2008). Study on Fish Community Strucure in the Pearl River Estuary Waters. China: Guangdong Ocean university.
Li, Y. F., Li, X. X., Yang, J., Sovan, L., Shuai, F. M., and Li, J. (2014). Effect fo Pearl River closed fishing on Megalobrama hoffmanni recruitment stock. J. Fish. China 38, 503–509.
Li, Y. Z., Chen, G. B., and Sun, D. R. (2000). Analysis of the composition of fishes in the Pearl River estuarine waters. J. Fish. China 24, 312–317.
Lin, M., Xing, L., Fang, L., Huang, S. L., Yao, C. J., Turvey, S. T., et al. (2019). Can local ecological knowledge provide meaningful information on coastal cetacean diversity? a case study from the northern South China Sea. Ocean. Coast. Manag. 172, 117–127. doi: 10.1016/j.ocecoaman.2019.02.004
Lu, X. X., Zhang, S. R., Xie, S. P., and Ma, P. K. (2007). Rapid channel incision of the lower Pearl River (China) since the 1990s as a consequence of sediment depletion. Hydrol. Earth. Syst. Sci. 11, 1897–1906. doi: 10.5194/hess-11-1897-2007
Makrakis, S. (2019). Tributaries as biodiversity preserves: An ichthyoplankton perspective from the severely impounded Upper Paraná River. Aquat. Conserv: Mar. Freshw. Ecosyst. 29, 258–269. doi: 10.1002/aqc.3037
Mason, D. M., and Brandt, S. B. (1996). Effect of alewife predation on survival of larval yellow perch in an embayment of Lake Ontario. Can. J. Fish. Aqua. Sci. 53, 1609–1617. doi: 10.1139/cjfas-53-7-1609
Mesa, M. L., Mesa, G. L., Catalano, B., and Jones, C. D. (2016). Spatial distribution pattern and physical-biological interactions in the larval notothenioid fish assemblages from the Bransfield Strait and adjacent waters. Fish. Oceanogr. 25, 624–636. doi: 10.1111/fog.12178
Miller, T. J. (2002). “Assemblages, communities, and species interactions,” in Fishery Science: The Unique Contributions of Early Life Stages, eds L. A. Fuiman and R. G. Werner (Hoboken, NJ: Blackwell Science), 183–205.
Muhling, B. A., Beckley, L. E., Koslow, J. A., and Pearce, A. F. (2010). Larval fish assemblages and water mass structure off the oligotrophic south-western Australian coast. Fish. Oceanogr. 17, 16–31. doi: 10.1111/j.1365-2419.2007.00452.x
Mullaney, T. J., Miskiewicz, A. G., Baird, M. E., Burns, P., and Suthers, I. M. (2011). Entrainment of larval fish assemblages from the inner shelf into the East Australian current and into the western Tasman Front. Fish. Oceanogr. 20, 434–447. doi: 10.1111/j.1365-2419.2011.00594.x
Oksanen, J., Blanchet, F. G., Friendly, M., Kindt, R., and Wagner, H. H. (2020). Package ‘vegan’: Community Ecology Package. R package Version 2.5-6. https://github.com/vegandevs/.
Olivar, M. P., Emelianov, M., and Villate, F. (2010). The role of oceanographic conditions and plankton availability in larval fish assemblages off the Catalan coast (NW Mediterranean). Fish. Oceanogr. 19, 209–229. doi: 10.1111/j.1365-2419.2010.00538.x
Oliveira, E., and Ferreira, E. (2010). Spawning areas, dispersion and microhabitats of fish larvae in the Anavilhanas Ecological Station, rio Negro, Amazonas State, Brazil. Neotrop. Ichthyol. 6, 559–566. doi: 10.1590/s1679-62252008000400003
Ou, S. Y., Zhang, H., Wang, D. X., and He, J. (2007). Horizontal characteristics of buoyant plume off the Pearl River Estuary during summer. J. Coast Res. 50, 652–657.
Pebesma, E. J., and Bivand, R. S. (2005). Classes and Methods for Spatial Data: The sp Package [CP/OL]. Available online at: http://ftp.yz.yamagata-u.ac.jp/pub/cran/web/packages/sp/
Potter, I. C., Beckley, L. E., Whitfield, A. K., and Lenanton, R. (1990). Comparisons between the roles played by estuaries in the life cycles of fishes in temperate western Australia and southern Africa. Environ. Biol. Fishes. 28, 143–178. doi: 10.1007/BF00751033
Rakocinski, C. F., Lyczkowski-Shultz, J., and Richardson, S. L. (1996). Ichthyoplankton assemblage structure in mississippi sound as revealed by canonical correspondence analysis. Estuar. Coast. Shelf. Sci. 43, 237–257. doi: 10.1006/ecss.1996.0067
Raynie, R. C., and Shaw, R. F. (1994). Ichthyoplankton abundance along a recruitment corridor from offshore spawning to estuarine nursery ground. Estuar. Coast. Shelf. Sci. 39, 421–450. doi: 10.1006/ecss.1994.1074
Ren, P., He, H., Song, Y. Q., Cheng, F., and Xie, S. G. (2016). The spatial pattern of larval fish assemblages in the lower reach of the Yangtze River: potential influences of river-lake connectivity and tidal intrusion. Hydrology 766, 365–379. doi: 10.1007/s10750-015-2471-2
Sassa, C., Kawaguchi, K., and Taki, K. (2007). Larval mesopelagic fish assemblages in the Kuroshio–Oyashio transition region of the western north pacific. Mar. Biol. 150, 1403–1415.
Shao, K.-T., Yang, J.-S., Chen, K.-C., and Lee, Y.-S. (2001). An Idenfification Guide of Marine Fish Eggs From Taiwan. Institute of Zoology Academia Sinica. Taibei: Taiwan Power Company.
South China Sea Fisheries Research Institute (1966). The investigation report of fishery resources by bottom trawl survey in Northern South China Sea. Guangzhou: South China Sea Fisheries Research Institute.
Su, J. L. (2004). Overview of the South China Sea circulation and its influence on the coastal physical oceanography outside the Pearl River Estuary. Cont. Shelf Res. 24, 1745–1760. doi: 10.1016/j.csr.2004.06.005
Sun, D. R., and Chen, Z. (2013). Fish Categories Books in the South China Sea. Beijing: Maritime Press.
Thompson, A. R., Auth, T. D., Brodeur, R. D., Bowlin, N. M., and Watson, W. (2014). Dynamics of larval fish assemblages in the California current system: a comparative study between Oregon and southern California. Mar. Ecol. Prog. 506, 193–212. doi: 10.3354/meps10801
Tzeng, W. N., Wang, Y. T., and Chang, C. W. (2002). Spatial and temporal variations of the estuarine larval fish community on the west coast of Taiwan. Mar. Freshw. Res. 53, 419–430. doi: 10.1071/MF01136
Valdez-Moreno, M., Vásquez-Yeomans, L., Elías-Gutiérrez, M., Ivanova, N. V., and Hebert, P. D. (2010). Using DNA barcodes to connect adults and early life stages of marine fishes from the Yucatan Peninsula, Mexico: potential in fisheries management. Mar. Freshw. Res. 61, 655–671. doi: 10.1071/MF09222
Wang, D., and Lin, Z. J. (2006). Spatial and temporal variations of fish commun ity structure in the PearlRiver Estuary waters. South China Fish. Sci. 2, 37–45. doi: 10.3969/j.issn.2095-0780.2006.04.007
Wang, Y. (2012). Fishery policy exploration in the Pearl River Estuary based on an ecosim model. Ecol. Modell. 230, 34–43. doi: 10.1016/j.ecolmodel.2012.01.017
Wang, Y., Hu, J., Pan, H., Li, S., and Failler, P. (2016). An integrated model for marine fishery management in the Pearl River Estuary: linking socio-economic systems and ecosystems. Mar. Policy 64, 135–147. doi: 10.1016/j.marpol.2015.11.014
Whitfield, A. K. (1999). Ichthyofaunal assemblages in estuaries: a South African case study. Rev. Fish. Biol. Fish. 9, 151–186. doi: 10.1023/A:1008994405375
Wong, L. A., Chen, J. C., Xue, H., Dong, L., Guan, W., and Su, J. (2003). A model study of the circulation in the Pearl River Estuary and its adjacent coastal waters: 2 sensitivity experiments. J. Geophys. Res. 108, 249–260. doi: 10.1029/2002JC001451
Xiao, Y. Z., Wang, R., Zheng, Y. J., and Wei, H. E. (2013). Species composition and abundance distribution of ichthyoplankton in the Pearl River Estuary. J. Trop. Oceanogr. 32, 80–87. doi: 10.3969/j.issn.1009-5470.2013.06.012
Yan, Y., Hou, G., Chen, J., Lu, H., and Jin, X. (2011). Feeding ecology of hairtail trichiurus margarites and largehead hairtail trichiurus lepturus in the Beibu Gulf, the South China Sea. China. J. Ocean. Limnol. 29, 174–183. doi: 10.1007/s00343-011-0004-z
Ye, S., Lin, M., Li, L., Liu, J., Song, L., and Li, Z. (2015). Abundance and spatial variability of invasive fishes related to environmental factors in a eutrophic Yunnan Plateau lake, Lake Dianchi, southwestern China. Environ. Biol. Fish. 98, 209–224. doi: 10.1007/s10641-014-0252-9
Zeng, Z. Z., Cheung, W. W., Li, S. Y., Hu, J. T., and Wang, Y. (2019). Effects of climate change and fishing on the Pearl River Estuary ecosystem and fisheries. Rev. Fish Biol. Fisher. 29, 861–875. doi: 10.1007/s11160-019-09574-y
Zhan, H. (1998). Study on fish community structure in the Zhujiang estuary and adjacent waters. Acta Oceanol. Sin. 20, 91–97.
Zhang, H., Xian, W., and Liu, S. (2015). Ichthyoplankton assemblage structure of springs in the Yangtze Estuary revealed by biological and environmental visions. PeerJ 3:e1186. doi: 10.7717/peerj.1186
Zhang, Y., Xia, H. Y., Qian, L. B., and Zhu, P. L. (2011). Analysis on hydrological characteristics off the Pearl River Estuary in summer and winter of 2006. J. Trop. Oceanogr. 30, 20–28.
Zhou, L., Wang, G., Kuang, T., Guo, D., and Li, G. (2019). Fish assemblage in the Pearl River Estuary: spatial-seasonal variation, environmental influence and trends over the past three decades. J. Appl. Ichthyol. 35, 884–895. doi: 10.1111/jai.13912
Zhu, J., Zheng, Q., Hu, J., Lin, H., Chen, D., Chen, Z., et al. (2019). Classification and 3-D distribution of upper layer water masses in the northern South China Sea. Acta Oceanol. Sin. 38, 126–135. doi: 10.1007/s13131-019-118-2
Keywords: ichthyoplankton, DNA barcode, assemblage structure, spawning ground conservation, Pearl River Estuary
Citation: Hou G, Wang J, Liu L, Chen Y, Pan C, Lin J and Zhang H (2021) Assemblage Structure of the Ichthyoplankton and Its Relationship With Environmental Factors in Spring and Autumn off the Pearl River Estuary. Front. Mar. Sci. 8:732970. doi: 10.3389/fmars.2021.732970
Received: 29 June 2021; Accepted: 16 August 2021;
Published: 16 September 2021.
Edited by:
JInghui Fang, Yellow Sea Fisheries Research Institute, Chinese Academy of Fishery Sciences (CAFS), ChinaReviewed by:
Shuyang Ma, Ocean University of China, ChinaCopyright © 2021 Hou, Wang, Liu, Chen, Pan, Lin and Zhang. This is an open-access article distributed under the terms of the Creative Commons Attribution License (CC BY). The use, distribution or reproduction in other forums is permitted, provided the original author(s) and the copyright owner(s) are credited and that the original publication in this journal is cited, in accordance with accepted academic practice. No use, distribution or reproduction is permitted which does not comply with these terms.
*Correspondence: Hui Zhang, emhhbmdodWlAcWRpby5hYy5jbg==
Disclaimer: All claims expressed in this article are solely those of the authors and do not necessarily represent those of their affiliated organizations, or those of the publisher, the editors and the reviewers. Any product that may be evaluated in this article or claim that may be made by its manufacturer is not guaranteed or endorsed by the publisher.
Research integrity at Frontiers
Learn more about the work of our research integrity team to safeguard the quality of each article we publish.