- 1Marine Science Institute, University of the Philippines Diliman, Quezon City, Philippines
- 2Department of Earth Sciences, Natural History Museum, London, United Kingdom
Prolonged thermal stress and high levels of solar irradiance can disrupt the coral-algal symbiosis and cause bleaching and lowered overall fitness that lead to the likely death of the cnidarian host. Adaptive bleaching and acclimatization of corals, which posits bleaching as an opportunity for the coral host to switch its currently susceptible endosymbionts to more stress-tolerant taxa, offers hope for survival of reefs amid rapidly warming oceans. In this study, we explored the diversity and distribution of coral-zooxanthellae associations in the context of geospatial patterns of sea surface temperature (SST) and thermal anomalies across the Philippine archipelago. Thermal clusters based on annual sea surface temperature means and each site’s frequency of exposure to heat stress were described using three-decade (1985–2018) remotely sensed data. Haphazard sampling of 628 coral fragments was conducted in 14 reef sites over 3 years (2015–2018). Using polymerase chain reaction-denaturing gradient gel electrophoresis (PCR-DGGE) fingerprinting and sequencing of the zooxanthellae ITS2 region, we characterized endosymbiont diversity within four reef-building coral families across archipelagic thermal regimes. Consistency in dominant Symbiodiniaceae taxon was observed in Acropora spp., Porites spp., and Heliopora coerulea. In contrast, the family Pocilloporidae (Pocillopora spp., Seriatopora spp., and Stylophora pistillata) exhibited biogeographic variability in zooxanthellae composition, concordant with inferred occurrences of sustained thermal stress. Multivariate analyses identify two broad Pocilloporidae clusters that correspond with mean SST ranges and frequency of exposure to bleaching-level thermal stress which are largely supported by ANOSIM. Differences in zooxanthellae assemblages may reflect host-specific responses to ecological or environmental gradients across biogeographic regions. Such patterns of variability provide insight and support for the adaptability and potential resilience of coral communities in geographically and oceanographically complex regions, especially amidst the increasing severity of global and local-scale stressors.
Introduction
Protozoan dinoflagellates of the family Symbiodiniaceae, colloquially known as zooxanthellae, are a diverse taxon of mostly endosymbiotic unicellular algae in marine invertebrates like corals, mollusks, and sponges (Trench et al., 1981; Berkelmans and van Oppen, 2006; Weisz et al., 2010). Formerly under the genus Symbiodinium and classified into nine clades (A–I; Coffroth and Santos, 2005; Pochon and Gates, 2010), the taxonomy of zooxanthellae was eventually revised into at least seven genera, six of which are novel and largely congruent with their predecessor clades (LaJeunesse et al., 2018). In corals, the symbiotic relationship involves the host providing shelter and inorganic nutrients for the photoautotrophic zooxanthellae which assimilate and export back to the host high concentrations of oxygen for respiration and calcification and other photosynthates like glycerol, glucose, amino acids, and lipids, supplying more than 90% of the host’s metabolic requirements (Muscatine, 1990; Yellowlees et al., 2008; Davy et al., 2012; Fransolet et al., 2012). However, thermal stress and high levels of solar irradiance could disrupt the mutualistic relationship and cause bleaching and reduced fitness that lead to the likely death of the cnidarian host (Hoegh-Guldberg, 1999; Douglas, 2003; Weis, 2008; Wooldridge, 2013). While bleaching is a normal sign of coral stress, the rapid increase in mean global ocean temperatures due to anthropogenic climate change (Peñaflor et al., 2009; Lough et al., 2018), when compounded with prolonged periods of positive thermal anomalies brought by the El Niño Southern Oscillation (ENSO), indisputably leads to mass coral bleaching events that have been more widespread and more intense through the years (Eakin et al., 2016, 2017, 2019; Hughes et al., 2017) and that have ultimately degraded coral reefs around the world (Hoegh-Guldberg et al., 2007; Carpenter et al., 2008). The minor El Niño event in 2014 (with Oceanic Niño Index, or ONI, peaking at 0.66) and the subsequent year’s severe El Niño (peaking at ONI 2.64) kicked off an unprecedented multi-year global coral bleaching which lasted 3 years and affected at least half of the world’s coral reefs (Heron et al., 2016; Bahr et al., 2017; Couch et al., 2017; DeCarlo et al., 2017; Hughes et al., 2017; Le Nohaïc et al., 2017; NOAA, 2021). Although coral populations may respond variably to heat stress due to differences in local conditions (e.g., water flow, turbidity, sedimentation, etc.) and phenotypic plasticity, thermal stress is still predicted to be the major factor that will cause massive coral mortality in the foreseeable future (Guest et al., 2012; McManus et al., 2020; Quimpo et al., 2020; Valino et al., 2021).
Recurring bleaching events have been correlated with signs of acclimatization (i.e., increased tolerance to thermal stress) and lower mortality rates in several coral taxa, even in species expected to be more susceptible to bleaching (e.g., Acropora and Pocillopora spp.; Maynard et al., 2008; Guest et al., 2012). For over two decades, this “natural acclimatization” of corals to thermal stress has offered hope for the survival of reefs amid rapidly warming oceans (Buddemeier and Fautin, 1993; Berkelmans and van Oppen, 2006; Thompson and Van Woesik, 2009; Brown and Cossins, 2011). This has motivated research in identifying potentially resilient coral taxa to gain evolutionary insights, with the end goal of accelerating selection for climate-resistant traits in the holobiont (i.e., the assemblage of coral host and associated microbiota), which will then be useful for coral restoration efforts and engineering reef resilience (van Oppen et al., 2015).
The overall adaptive response of the coral holobiont to high temperatures has been shown to depend on the complex interactions between host- and symbiont-related factors (Abrego et al., 2008; Mieog et al., 2009a; Putnam et al., 2012; Parkinson et al., 2015; Bay et al., 2016). One prominent hypothesis for coral acclimatization centers on the algal endosymbiont and posits coral bleaching as an opportunity for the host to switch its currently susceptible symbionts with more stress-tolerant taxa like Durusdinium (formerly Symbiodinium clade D; LaJeunesse et al., 2018), ending up with a resilient holobiont geared for the conditions that caused the bleaching in the first place (Fautin and Buddemeier, 2004; Berkelmans and van Oppen, 2006). Durusdinium glynnii and D. trenchii zooxanthellae can tolerate a wider range of water temperatures (Mostafavi et al., 2007; Lajeunesse et al., 2014; Silverstein et al., 2017; Wham et al., 2017) and are hypothesized to contribute to the resilience of the coral holobiont (Stat and Gates, 2011; Guest et al., 2016). In many studies, these thermally tolerant zooxanthellae have been reported to reproduce from background concentrations within coral tissues (i.e., “rare biosphere”; Boulotte et al., 2016), eventually overtaking its bleaching-susceptible predecessor, and dominating the coral colony post-bleaching (Jones et al., 2008; Silverstein et al., 2015; Bay et al., 2016; Cunning et al., 2018; Davies et al., 2018). This is demonstrated among surveyed natural populations and within particular coral species where the observed coral-symbiont associations often signify recent thermal regimes or anomalies. Brener-Raffalli et al. (2018), sampling Pocillopora damicornis sensu lato from four thermally variable sites across the Indo-Pacific, reported warmer-site corals (Djibouti and French Polynesia) hosting stress resistant species of zooxanthellae (clade D1) and cooler-site corals (Taiwan and New Caledonia) harboring a less tolerant clade (Cladocopium goreaui, formerly Symbiodinium subclade C1). This reinforces the results of previous studies which also investigated the diversity of coral zooxanthellae assemblages at finer spatial scales across different host species and thermal variabilities and revealed the occurrence of thermally tolerant symbiont lineages in warmer or historically thermally stressed sites (Baker et al., 2013; Stat et al., 2013; Zhou et al., 2017; Baumann et al., 2018). Moreover, it has been demonstrated that certain host-symbiont associations can be more labile than others (e.g., Pocillopora spp. are likelier to be shuffled with a tolerant species than the more stable Porites spp.) (Baker et al., 2013).
The Philippines, an archipelago located at the northern apex of the Coral Triangle, has surrounding waters governed by thermal regimes characterized into four clusters (Peñaflor et al., 2009) largely shaped by variabilities in latitudinal heating and circulation patterns. Philippine reefs have a history of mass coral mortality during previous bleaching events. First documented during the 1997–1998 ENSO (ONI peaking at a 2.40 and crashing to −1.57; NOAA, 2021), a bleaching event was estimated to have caused ∼ 8% coral loss across the country (Licuanan and Gomez, 2000; Arceo et al., 2001; Magdaong et al., 2014). The Bolinao-Anda Reef Complex, a diverse marine ecosystem and vital center for fisheries and aquaculture in northwestern Philippines (Cruz-Trinidad et al., 2011), was one of the worst hit areas in the region where about 80% of corals bleached. Mass coral bleaching incidents were again reported in the Philippines and neighboring Southeast Asian reefs during another significant ENSO event (ONI peaking at 1.56 and down to −1.64) in 2009–2010 (Tun et al., 2010). For 6 months, the region experienced elevated sea surface temperatures (SST) that resulted in an estimated coral mortality of 18%. Recently, Licuanan et al. (2019) reiterated the long-term decline in hard coral cover and health of Philippine reefs and estimated that 30% of shallow-water corals have died over the recent decades, but also reported the limited impact of the 2016–2017 mass bleaching event (based on non-significant change in hard coral cover in most of 101 reef sites from 2015 to 2018). Low bleaching rates that have led to minimal changes in community structure were observed in a few Philippine reefs during the 2016–2017 event which, in conjunction with the interaction of different local environmental factors (Quimpo et al., 2020; Valino et al., 2021), could be a sign that reef response to severe bleaching events can potentially improve.
Corals being exposed to more intense heating anomalies and subjected to shorter recovery periods (Burke et al., 2011; Muñiz-Castillo et al., 2019) have underscored further the importance of understanding the coral holobiont’s adaptability. In this study, we characterized the diversity of Symbiodiniaceae in several coral families across local regions and explored their association with existing thermal gradients and theoretical bleaching occurrences in the Philippine archipelago. Examining the differentiation of coral-zooxanthellae associations across regions allows us to infer how each site’s thermal history may have shaped the symbioses through prolonged exposures to thermal stress (Gierz et al., 2020; Keshavmurthy et al., 2020) and provide insights on the adaptive capacity of coral reefs.
Materials and Methods
Collection of Coral Fragments
Haphazard sampling was conducted in 14 reef sites across the archipelago from July 2015 to May 2018 (Figure 1A). Study sites represent the spatial gradients of SST means sensu Peñaflor et al. (2009) and cover the northern Philippine Sea and Luzon Strait, the West Philippine Sea (the Philippines’ exclusive economic zone in the South China Sea), Sulu Sea, Bohol Sea, and Celebes Sea (Table 1 and Supplementary Table 1). Non-bleached coral fragments (i.e., live and with color; N = 628) from targeted families of varied thermal stress tolerance were collected at 2–10 m depths, except for samples from the off-shore reefs in Bicol Shelf (13–24 m) where no shallow reef was found. The families Acroporidae, Pocilloporidae, Poritidae, and Helioporidae were found to be abundant or potentially resilient (as defined by Walker et al., 2004) in the Bolinao-Anda Reef Complex, northwestern Philippines and, among others were considered candidate taxa for propagation in reef restoration initiatives in the country (Levy et al., 2010; Shaish et al., 2010a,b). As such, these four families were selected for this study. An arbitrary minimum distance of 2 m between colonies was set to minimize sampling clonal ramets. From the center of each adult colony (>5 cm diameter), a 2–4 cm coral nubbin was cut, immediately preserved in salt-saturated DMSO buffer (Gaither et al., 2011), and stored at 4°C. Due to the opportunistic nature of sampling and differences in field campaign objectives, not all species were collected in all of the study sites. Only the genus Pocillopora (Pocillopora acuta and P. verrucosa) was collected from all 14 sites. Two other pocilloporid genera (Seriatopora and Stylophora), poritids (Porites cylindrica or P. lutea) and Heliopora coerulea were collected from six sites, while acroporids (Acropora digitifera, A. millepora, or A. tenuis) were sampled from four (Table 2). All collections were covered by research permits (DA-BFAR Gratuitous Permit Nos. 0102-15, 0150-18, 0153-18, and PCSD Permit Nos. 2015-08 and 2017-09).
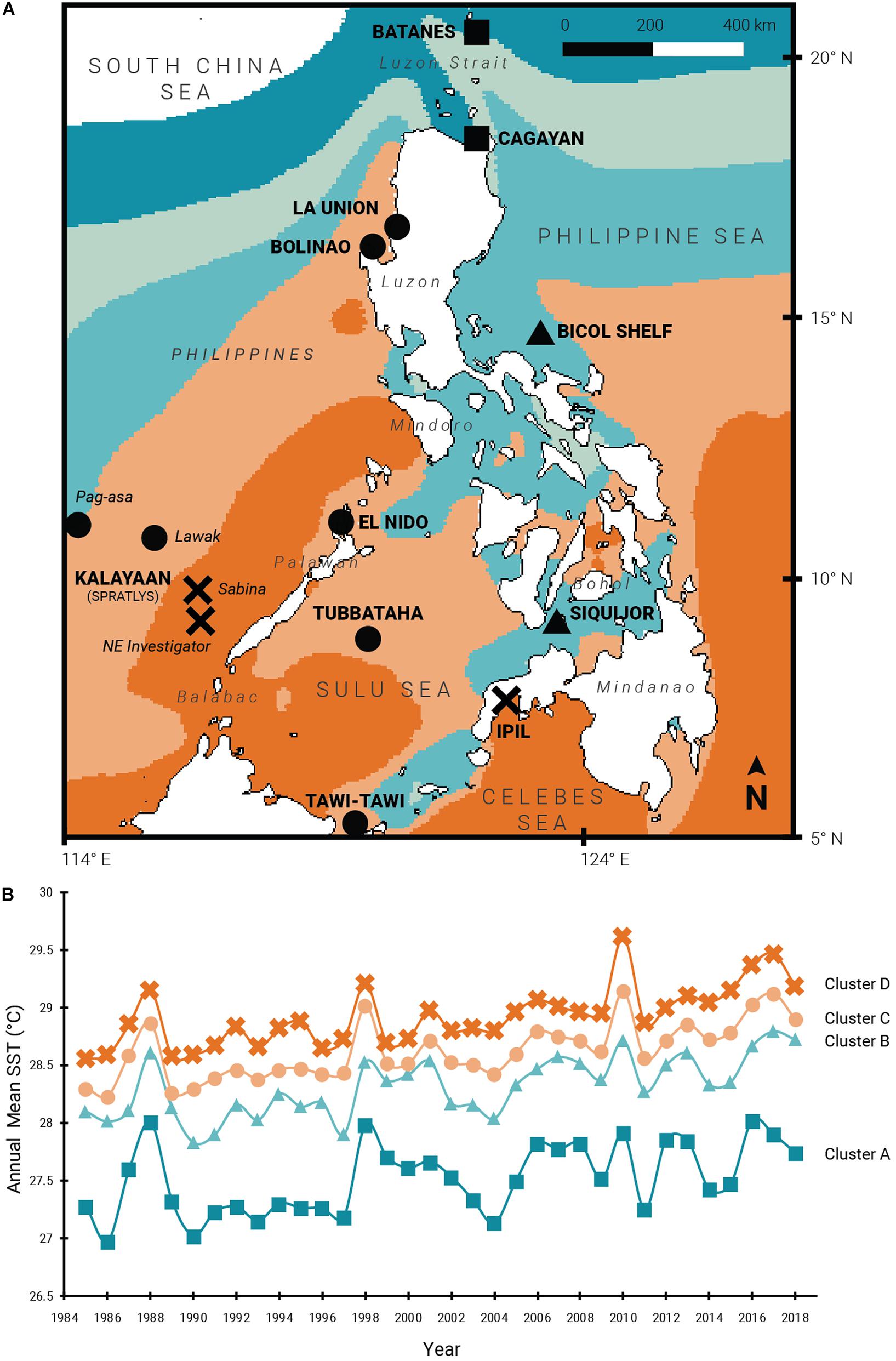
Figure 1. Thermal regimes across the Philippine archipelago. (A) 14 study sites representing four thermal regions clustered using SST data obtained from 1985 to 2018; (B) Annual mean SSTs of clusters A–D from 1985 to 2018. Site symbols correspond to thermal cluster membership: square (cluster A), triangle (B), circle (C), and cross (D).
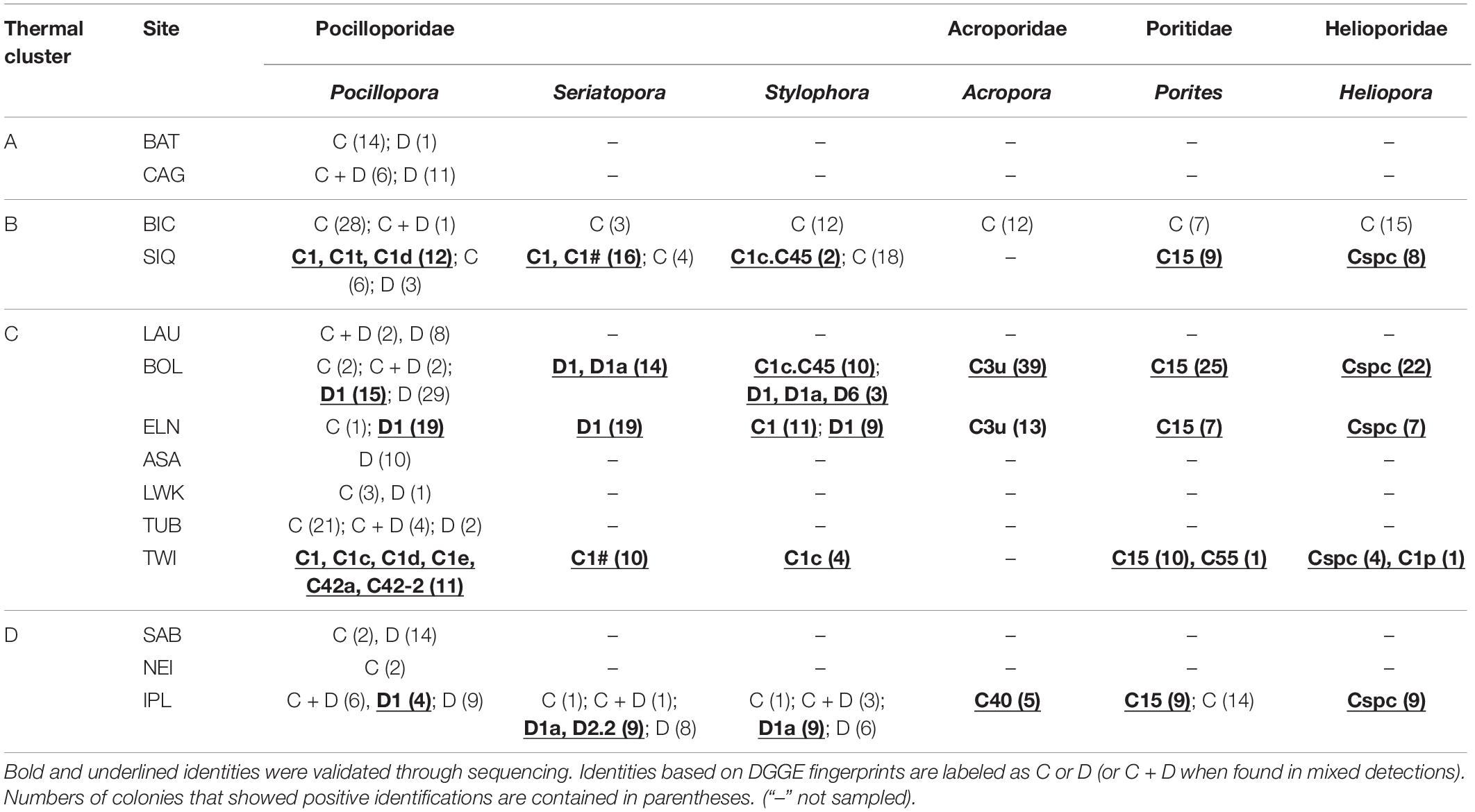
Table 2. Dominant zooxanthellae detected from four coral families across 14 sampling sites clustered according to thermal regime.
PCR-DGGE Fingerprinting
Holobiont DNA was extracted using a modified organic DNA extraction method (Mieog et al., 2009b) employing overnight digestion of coral tissue in CTAB and proteinase K, phase separation using 24:1 chloroform/isoamyl alcohol, precipitation with isopropanol, washing with 70% ethanol, and resuspension with nuclease-free water. GeneJET Genomic DNA purification kits (Thermo Fisher Scientific) were also used following manufacturer’s protocols.
Following the touchdown thermal cycle protocol of LaJeunesse (2002), the zooxanthella-specific primers ITSintfor2 and the GC-attached ITS2clamp were used to amplify the internal transcribed spacer 2 region (ITS2, ∼ 350 bp) for downstream DGGE fingerprinting. PCR amplification of the ITS2 was performed in 15 μL reactions using 0.7 μM of both primers, 1 × PCR buffer, 0.45 μM dNTPs, 1.8 mM MgCl2, 0.23 units of Taq polymerase (Invitrogen), and ∼ 10 ng of template DNA. Thermal cycling protocol had an initial denaturation of 3 min at 92°C; 20 cycles of denaturation for 30 s at 92°C, annealing for 40 s at 62°C (with a drop of 0.5°C per cycle), and extension for 30 s at 72°C; followed by another 20 cycles with a fixed annealing temperature of 52°C and a final extension of 5 min at 72°C.
All ITS2 PCR products were loaded 1:1 with 0.05% bromophenol blue-xylene cyanol solution into an 8% polyacrylamide (37.5:1 acrylamide/bis) denaturing gel with an internal gradient (30–60%) of denaturants (urea and formamide) and were separated by electrophoresis using DCode Universal Mutation Detection System (Bio-Rad) for 18 h at 100 V with a constant tank buffer temperature of 60°C. The acrylamide gels were post-stained with 1 × SYBR Green (Thermo Fisher Scientific) in 1 × TAE buffer for 30 min and photographed under UV illumination.
A subset of prominent bands and heteroduplexes from five initial sites BOL, ELN, SIQ, IPL, and TWI (Table 1) were excised and incubated overnight with 500 μL nuclease-free water. Eluates were used as template for re-amplification of the ITS2 using the oligonucleotide primers ITSintfor2 and ITS2reverse following a similar thermal cycling protocol but without the touchdown cycles (LaJeunesse, 2002). Bidirectional DNA sequencing was performed (First Base Laboratories, Malaysia) and individual ITS2 sequences were manually trimmed, checked, and aligned using Geneious Prime v2020.0.3 (Kearse et al., 2012). For zooxanthellae clade/species identification, sequences were queried through BLAST in a custom database assembled from the curated GeoSymbio database of zooxanthellae taxa (Franklin et al., 2012) and additional unique sequences obtained from GenBank (query: “Symbiodiniaceae” “ITS2”) that were not covered by the former. Genetically identified samples were used as diversity standards in subsequent DGGE runs for the other sites.
Sea Surface Temperatures Clustering and Inferring Stress Occurrences
Thermal regions in the Philippines were recharacterized to extend the dataset (Peñaflor et al., 2009) with more recent SSTs (1985–2018). The daily updated 5 km resolution SST product (CoralTemp) of Coral Reef Watch (CRW) produced from combining and blending geostationary and polar-orbiting environmental satellites (Liu et al., 2014) were averaged using SNAP v7.0.01 binning function. Clustering of similar-value pixels was done to the resulting three-decadal mean SSTs using SAGA k-means grid clustering tool (Peñaflor et al., 2009; Kleypas et al., 2015). Annual mean SSTs in all sites within each cluster were calculated.
Having limited empirical information on actual bleaching and mortality, possible past occurrences can be extrapolated from bleaching- and mortality-level degree-heating weeks (DHW, i.e., a thermal stress index based on the accumulation of SST anomalies) that have reached four to eight consecutive weeks (Liu et al., 2006; McClanahan et al., 2007). These metrics can proxy for sustained thermal stress especially during the ENSO years, when high levels of solar irradiance can be expected in the region (Muñiz-Castillo et al., 2019). Exposure to positive temperature anomalies and theoretical bleaching and mortality experienced by corals in all 14 sites were characterized by obtaining mean mortality-level degree-heating weeks (8 ≤ DHWs) and bleaching-level DHWs (4 ≤ DHWs < 8) during ENSO years (Muñiz-Castillo et al., 2019). The daily 5 km DHW product of CRW comes from positive heat anomalies or the accumulation of heat stress during a 12-week period derived from CoralTemp (Liu et al., 2014).
Statistical Analyses
Patterns for spatial distribution or association with thermal regimes were examined by performing multivariate analyses in the family Pocilloporidae where variability was observed. A dataset representing abundance information (i.e., colony counts for dominant or co-occurring symbiont genera) was produced. To quantify compositional differences of dominant zooxanthellae between populations, Bray-Curtis dissimilarity indices (BCD) were calculated based on both raw and square root-transformed abundance data. Non-metric multidimensional scaling (NMDS) was performed on BCD matrices for all-Pocilloporidae (combined Pocillopora, Seriatopora, and Stylophora) and Pocillopora-only datasets. Sites LWK and NEI were excluded from the analysis due to small sample sizes (N < 5 colonies). While square root transformation on abundance data returned lower BCD values versus non-transformed data, the clustering of populations did not change (Supplementary Figure 2). Likewise, performing NMDS on raw and transformed abundance data produced identical stress values and ordination, thus only the non-transformed data were used for subsequent analysis. Ordination plots were generated to visualize structuring of zooxanthellae diversity based on each of the site’s thermal cluster membership and frequency of exposure to bleaching-level DHWs. Analysis of similarities (ANOSIM) was then used to test for significant differences in zooxanthellae species composition between emergent groupings from the NMDS analysis. All statistical analyses were conducted using the package “vegan” v2.5-7 (Oksanen et al., 2020) in R v4.0.2 (R Core Team, 2021).
Results
Diversity of Dominant Coral Zooxanthellae
All coral samples were evaluated for zooxanthella clade/species/genus diversity using PCR-DGGE fingerprinting. Most profiles displayed the expected one or two prominent bands accompanied by background heteroduplexes (LaJeunesse, 2002). ITS2 sequences from a subset of these bands (N = 217) returned homology to 18 Symbiodiniaceae taxa (Table 2): Cladocopium goreaui (C1), 13 other Cladocopium lineages (C1c, C1c.C45, C1d, C1e, C1p, C1t, C1#, C3u, C15, C40, C42a, C42-2, and Cspc), Durusdinium glynnii (D1), D. trenchii (D1a), and two more Durusdinium clades (D2.2 and D6) (GenBank Accession Nos. MW024153–MW024369).
Sea Surface Temperatures Clustering and Thermal Stress
Geospatial clustering analysis shows five distinct marine SST regions surrounding the Philippines (Figure 1A). Four of these clusters (A–D) cover most circumjacent waters of the Philippines and are described in this study. Over the last three decades, most of the sites experienced above-average temperatures that peak during years with confirmed mass bleaching events (1998, 2010, 2015–2017; Figure 1B and Supplementary Table 2). Clusters A and B recorded lower annual SST means (ranging 26.98–28.02°C and 27.83–28.80°C, respectively) and cover the waters of northern and eastern Luzon, the internal seas of central Philippines, and the Sulu archipelago in the south. Annual mean SST values in these clusters show less fluctuations during the initial 10 years which increased toward the recent decades. In contrast, clusters C and D exhibited higher mean SSTs (28.23–29.15°C and 28.57–29.62°C) with low annual temperature fluctuations (Table 1). Cluster C partially encompasses the western seaboard of Luzon in the West Philippine Sea, the Kalayaan Island Group (Spratly Islands), and the northern section of the Sulu Sea. Cluster D exhibits the highest annual mean SSTs and covers mainly the western Palawan shelf, southwestern Sulu Sea, and the southern and eastern waters off Mindanao.
All collection sites, with the exception of SIQ and TWI, were exposed to bleaching-level DHWs (≥4 DHWs) between 1 and 4 times during ENSO years within the span of the time series and are thus inferred to have experienced bleaching (Figure 2A). One site (ASA) was exposed to one bleaching DHW, seven sites (BOL, ELN, LWK, TUB, SAB, NEI, and IPL) to two, and three sites (BAT, CAG, and LAU) were exposed to three. BIC with an exposure frequency of four had the most frequent bleaching DHWs. Only four sites reached mortality-level DHWs (≥8 DHWs): BIC, SAB, and NEI with one exposure, and CAG with two exposures. No site in Cluster C was exposed to mortality-level DHWs. Coincident with mean SST fluctuations, sites from the cooler clusters A and B have been exposed more frequently to positive heat anomalies compared with the warmer clusters C and D (Figures 1B,2A).
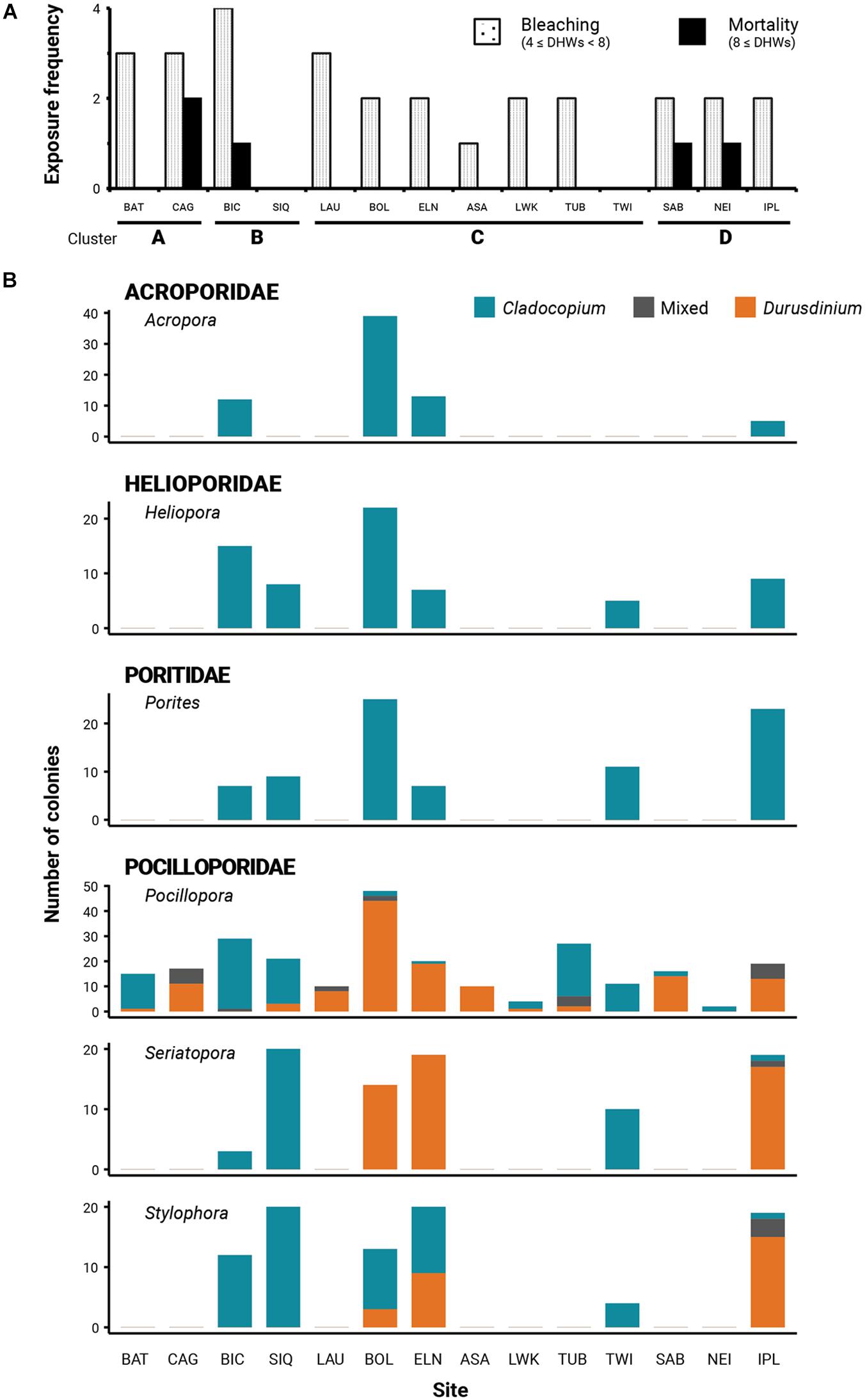
Figure 2. (A) Frequency of site exposure to at least four and at least eight degree-heating weeks (DHW) indicative of prolonged thermal stress and respectively, predictive of coral bleaching and mortality; (B) Relative Cladocopium, Durusdinium, and mixed zooxanthellae occurrences across sites of different thermal histories and coral genera.
Distribution of Coral-Symbiont Associations
Regional consistency in dominant zooxanthella species was observed in Acroporidae, Poritidae, and Helioporidae where each family was dominated by 1–2 clades of Cladocopium (Figure 2B and Table 2). Poritidae samples, regardless of species or site of collection, hosted Cladocopium clade C15. Most Helioporidae samples from five of six sites harbored Cladocopium clade Cspc with one colony detected with clade C1p. Acroporidae from two sites along the western Philippine seaboard hosted Cladocopium clade C3u (BOL and ELN), while colonies sampled from the Celebes Sea (IPL) hosted clade C40.
Pocilloporidae exhibited variation in zooxanthellae composition across sites (Table 2 and Supplementary Table 3), with an emergent pattern of community composition broadly concordant with thermal clusters (Figure 2B). Pocilloporids from SIQ and TWI, falling within SST clusters B and C, respectively, and which have had neither bleaching nor mortality-level DHWs, predominantly host Cladocopium species (e.g., C. goreaui) and clades (e.g., C1c, C1d, C1t, C1#, and C42a). Pocillopora samples from BAT and TUB and all three pocilloporid genera from BIC, likewise, predominantly hosted Cladocopium zooxanthellae. Conversely, pocilloporid communities in BOL, ELN, and IPL were composed of colonies mainly dominated by Durusdinium glynnii or D. trenchii or mixed with Cladocopium symbionts (C + D; Supplementary Figure 1), except for the Stylophora colonies of BOL and ELN where slightly more than half of the colonies were detected only with Cladocopium. Within thermal cluster A, Pocillopora samples from BAT and CAG exhibit contrasting patterns, with colonies from CAG predominantly harboring Durusdinium or a mix of Cladocopium and Durusdinium while all colonies from BAT harbor predominantly Cladocopium, with the exception of one colony which harbors predominantly Durusdinium. Other sites within the West Philippine Sea, all falling under thermal clusters C and D and all having experienced bleaching-level DHWs, consisted of colonies hosting differential proportions of Cladocopium and Durusdinium. Sites LWK and NEI harbored predominantly Cladocopium, while LAU, ASA, and SAB colonies were dominated by Durusdinium.
Multivariate analysis of zooxanthellae composition for the Pocilloporidae reveal two broad groupings largely coinciding with SST rankings (Figure 3). Bray-Curtis dissimilarity indices between populations range from 0.143 (raw) and 0.136 (square root-transformed) to 1 (Supplementary Table 4). Stress values for both Pocillopora-only (0.069) and all-Pocilloporidae (0.012) ordinations are below the recommended threshold value of 0.2, indicating good fit to the original distance matrices (Dexter et al., 2018). The NMDS plots for all Pocilloporidae and for Pocillopora spp. present comparable ordinations differing only in the ordination of 1 site (BOL). NMDS axis 1 delineates two groups reflecting the predominance of Durusdinium (Group 1) and Cladocopium (Group 2). Group 1 consists of sites mostly from thermal clusters C and D (except for CAG from thermal cluster A) while Group 2 consists of sites mostly from thermal clusters A and B (except for TWI from thermal cluster C). Considering both NMDS axes, the ordination plot for all Pocilloporidae delineates four clusters based on clade composition: Subgroup 1A (CAG-LAU-IPL-BOL) predominantly hosting Durusdinium and C + D, Subgroup 1B (ASA-ELN-SAB) predominantly Durusdinium with a lower proportion of Cladocopium, Subgroup 2A (TUB-BIC) predominantly Cladocopium with C + D, and Subgroup 2B (BAT-SIQ-TWI) with predominantly Cladocopium and a lower proportion of Durusdinium. Considering Pocillopora only, the minor difference in subgrouping composition is the ordination of BOL intermediate between all-Pocilloporidae Subgroups 1A and 1B.
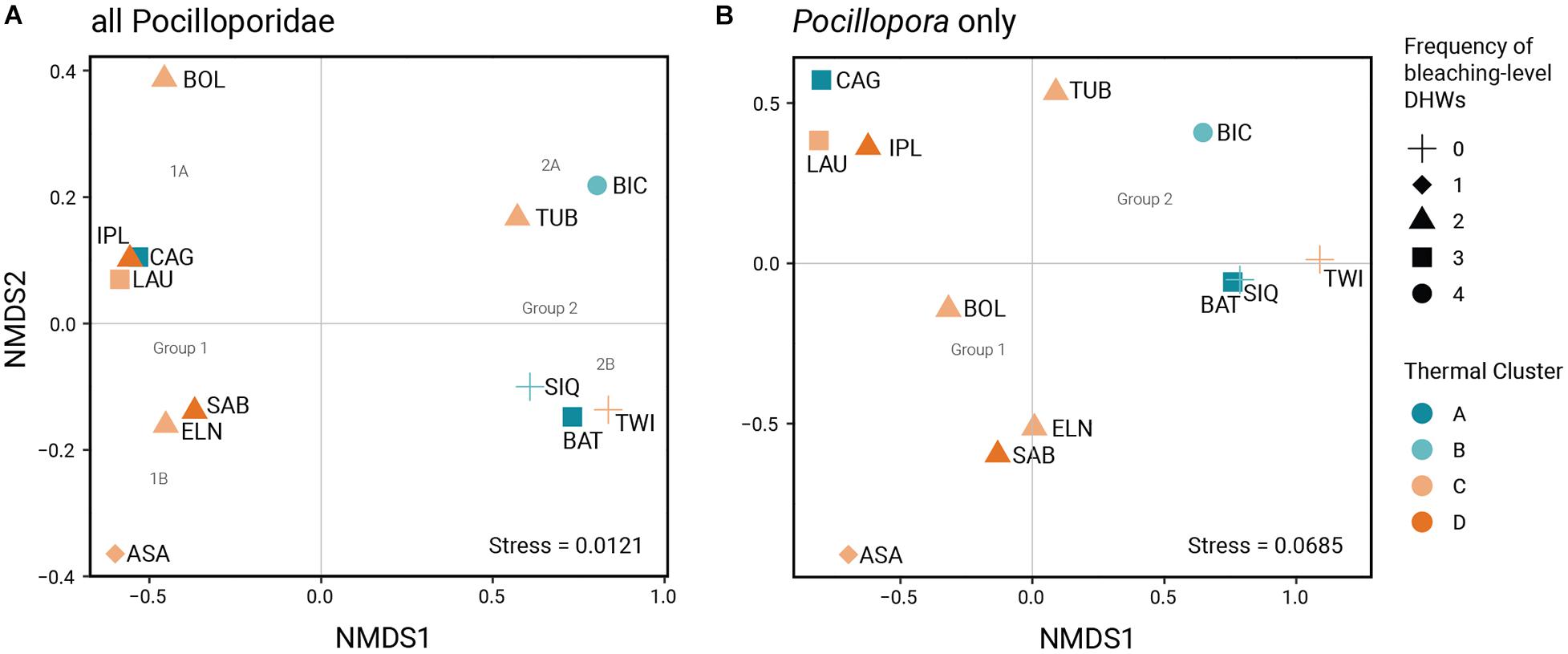
Figure 3. Ordination plots from the non-metric multidimensional scaling analysis for (A) all-Pocilloporidae and (B) Pocillopora-only datasets, which suggest two broad groupings that coincide with thermal cluster and four subgroupings according to symbiont composition.
The difference in zooxanthellae species composition between the two NMDS groups is statistically significant (ANOSIM, R = 0.656, p = 0.007). When divided into four subgroups, the differentiation in species composition remains significant (R = 0.499; p = 0.025). Species composition does not vary significantly when sites are grouped by thermal cluster (R = 0.023; p = 0.372). Grouping according to frequency of exposure to bleaching-level DHWs likewise does not reveal statistically significant differences, although the R value is an order of magnitude higher than when grouping sites according to thermal cluster and the p-value is close to the threshold for significance (R = 0.2912, p = 0.075).
Discussion
Understanding determinants underlying the adaptability of corals can provide valuable insight toward inferring potential responses to climate change-induced environmental stress. Having baseline information on the contemporary diversity and distribution of coral zooxanthellae provides important context regarding the flexibility and specificity of the symbiosis, as well as the potential of corals for acclimatization or adaptability through periods of environmental fluctuations and sustained thermal stress. This study characterized zooxanthellae diversity and coral-endosymbiont associations in several coral taxa across the biogeographic regions of the Philippine archipelago at the northern region of the Coral Triangle, which exhibit varying thermal histories based on analysis of sea surface temperature data covering the past 34 years.
Three-Decadal Thermal History of Philippine Waters
Thermal stress that corals may have experienced can be inferred from historical SST data and recorded heating anomalies (Kayanne, 2016; McManus et al., 2020). The time-averaged thermal clusters described herein represent the net of the interactions of archipelagic and bathymetric complexity of the Philippines, a latitudinal heating gradient, and oceanic-atmospheric systems like the East Asian monsoons. Despite having different spatial resolutions, we observed resemblance of the five thermal regions found in this study with the SST sections identified by Peñaflor et al. (2009) and Kleypas et al. (2015). Thermal clusters A and B, characterized by lower means of daily SSTs (27.3–28.1°C), are largely influenced by the Pacific waters streaming through San Bernardino and Surigao Straits into the relatively shallow basins of central Philippines (Qu et al., 2009; Hurlburt et al., 2011; Lermusiaux et al., 2011) and by the intrusion of Kuroshio waters through the Luzon Strait. These systems, in conjunction with the cooling mechanisms of frequent precipitation (David et al., 2015), high riverine input, bathymetric features (e.g., Sulu archipelago; Han et al., 2009), and the existence of wind-driven upwelling (e.g., Zamboanga Peninsula, Bohol Sea; Cabrera et al., 2011; Villanoy et al., 2011) may have lowered SST signatures in these subregions, possibly buffering extreme warmings especially at local scales (Riegl et al., 2019). On the other hand, clusters C and D are characterized by slightly warmer SST means (28.6–28.9°C). These warmer clusters comprise most of the western and southern Philippines which are largely influenced by the mixed water masses, circulated in the larger South China Sea and which seasonally flow into the Sulu Sea through Mindoro and Balabac Straits (Sprintall et al., 2012), and by the Mindanao Current flowing south of the archipelago toward the Makassar Strait in Indonesia (Gordon et al., 1999). The Mindanao Current and its associated anticyclonic eddy to its north ultimately downwell the surface waters in the relatively shallow Moro Gulf, further warming the northern Celebes (Masumoto et al., 2001). Aggravated by an ENSO event, these existing oceanographic systems could be easily tipped toward conditions that can be too stressful for corals.
Specificity and Adaptability Across Thermal Gradients
All corals evaluated in this study either exclusively or predominantly hosted zooxanthellae taxa of Cladocopium or Durusdinium. This is consistent with previous studies that show Cladocopium and Durusdinium spp. as the main zooxanthellae in hard corals throughout the South China basin using DGGE (Chen et al., 2005; Zhou and Huang, 2011) and more recent approaches using high-throughput sequencing (Chen et al., 2019).
The homogeneity or variability observed among coral family-zooxanthella associations in the present study demonstrates the differential levels of host specificity to certain symbiont taxa (Baker, 2003; Thornhill et al., 2006). While Pocilloporidae were found to host either Cladocopium, Durusdinium, or a mix of both, Acropora and Heliopora coerulea displayed specificity, with each coral species detected with only one or two clades of Cladocopium regardless of the thermal regime or history of sampling location. The widespread Poritidae corals (Porites cylindrica and P. lutea) also exhibited strict preference to Cladocopium C15, a zooxanthella clade previously observed to be relatively stable under thermal stress (LaJeunesse et al., 2003). Porites corals mainly associating with C15 have also been observed in the Gulf of Thailand (Chankong et al., 2020) and across a wide latitudinal range in the South China Sea (Ng and Ang, 2016; Chen et al., 2019; Gong et al., 2019). Previous transcriptomic studies that performed simulated bleaching (Barshis et al., 2013, 2014; Kenkel et al., 2013; Dixon et al., 2015) and transplantation experiments (Palumbi et al., 2014) have demonstrated potential molecular mechanisms for climate resiliency of the coral host itself. Thus, specific host-symbiotic associations suggest successful molecular mechanisms in the coral host that are independent of the symbiont (Drury, 2020). Host genotype has also been shown to positively play a role in tolerance to multiple and simultaneous stressors in Acropora millepora (Wright et al., 2019). However, further research can be done on specifically partnered symbioses, especially on the coral hosts’ and zooxanthellae’s physiological limits to wider ranges of stress conditions. It is also important to note that the symbiont composition presented here likely does not reflect low-abundance (<5–10%) zooxanthellae due to the limits of detection of DGGE-based assays (Thornhill et al., 2006; LaJeunesse et al., 2008; Baker et al., 2013). This limitation could result in an underestimation of the potential contribution of rare biosphere species like Durusdinium spp. (Boulotte et al., 2016) and underscores the advantages of methods like high-throughput sequencing which offer greater sensitivity and resolution in elucidating endosymbiont diversity. For example, multiple Porites lineages along the Saudi Arabian Red Sea have shown exceptionally diverse endosymbionts, following a north-south Cladocopium-Durusdinium gradient (Terraneo et al., 2019), not observed in most other DGGE-only studies.
The geographic variability of zooxanthellae hosted by pocilloporids (Pocillopora, Seriatopora, and Stylophora), in contrast to the consistency of symbionts hosted by Acropora, Heliopora and Porites, provides an interesting model to examine symbiont diversity in the context of varying environmental conditions. Sequencing of sampled DGGE bands from pocilloporids revealed Cladocopium goreaui, Durusdinium glynnii, D. trenchii, and other Cladocopium and Durusdinium clades, which all vary in thermal stress tolerance. Zooxanthellae composition is significantly different among sites, with two broad groups coincident with mean SST ranges and frequency of exposure to bleaching-level thermal stress. Pocilloporids in warmer thermal clusters C and D and the less warm clusters A and B generally differ in the predominance of the thermally tolerant Durusdinium and less tolerant Cladocopium, respectively. There are, however, a few exceptions. For instance, pocilloporids in TWI, despite experiencing higher mean SSTs, were shown to host thermally sensitive Cladocopium symbionts. That TWI had not experienced more-than-four continuous DHWs during the 34-year period (Figure 2A) may account for the observed dominance of Cladocopium instead of Durusdinium. Similarly, the dominance of Cladocopium symbionts in pocilloporids from TUB amidst relatively warmer temperatures, and in BIC which had the most frequent exposure to bleaching-level thermal stress, suggest the influence of other factors (e.g., clouds, reef health) not captured by mean SSTs and thermal anomalies. The highly protected Tubbataha Reefs Natural Park is situated in the middle of Sulu Sea away from anthropogenic stress and is known to host some of the most pristine and healthiest coral reefs in the world (Licuanan et al., 2017). While protection in itself cannot negate the impact of high temperatures to coral reef health, the compounded support by the immense biomass of herbivores, lower levels of localized stress, robust supply of larvae (i.e., connectivity), ample cloud cover, and geographic isolation could have facilitated recovery of stressed reefs in TUB (Selig et al., 2012; Gilmour et al., 2013; Peñaflor, 2015; Licuanan et al., 2019; McManus et al., 2020). Moreover, our findings in BIC imply the potential importance of deep or even turbid reef systems in providing refuge for future climate change scenarios in this specific region (Bongaerts et al., 2010; Sully and van Woesik, 2020). The depth of the sampled reefs in BIC (∼ 26 m) may have prevented surface thermal anomalies to reach the reef, providing more stable ambient temperatures for corals. Additionally, as Cladocopium symbionts are more photosynthetically efficient than Durusdinium spp. (i.e., higher Fv/Fm) in lower temperatures (Silverstein et al., 2017), it might be expected for the former to thrive in deeper reef systems where irradiance levels are much reduced, as was observed in Montastraea cavernosa colonies along a depth gradient in Belize (Eckert et al., 2020). For all these cases, incorporating additional in situ and smaller-scale observations of other environmental factors is essential and should be considered for future studies.
The dominance of Durusdinium zooxanthellae (or their presence in mixed detections) in sites that were previously under thermal stress could indicate a switch to thermally tolerant endosymbionts from a thermally sensitive species like C. goreaui (C1). The observed Durusdinium dominance in historically stressed pocilloporids complements the findings of Baker et al. (2013); Brener-Raffalli et al. (2018), Qin et al. (2019); Chankong et al. (2020), and Poquita-Du et al. (2020) which report Pocillopora’s flexibility and tendency to harbor Durusdinium symbionts with increasing temperatures or recent thermal anomalies. In addition, mixing of zooxanthellae genera was exclusively observed in pocilloporid species. This mixing in conjunction with the geographically varied coral-zooxanthellae associations found in Pocilloporidae could suggest the occurrence of symbiont shuffling or a transition toward the dominance of more thermally tolerant zooxanthellae. The significant difference in zooxanthellae composition between the four NMDS subgroupings, which also correlate with frequency of bleaching-level DHWs, may imply incidences of coral acclimatization among pocilloporids. However, the haphazard sampling in this study does not allow us to fully capture possible fine-scale temporal changes in the zooxanthellae assemblage which may have already transpired within days, weeks, or months after peak stress levels (Claar and Baum, 2019). It is therefore not possible to conclude with certainty whether the co-occurrence or dominance of Durusdinium spp. is a transient switch from Cladocopium spp. or a snapshot of an enduring symbiosis with its coral host.
Implications for the Future of Coral Reefs
Identifying resilient coral communities will be vital in prioritizing areas for conservation (Hoegh-Guldberg et al., 2018) and augmenting levels of protection of such sites against other possible stressors such as overfishing that contribute to the decline of reef health, which will ultimately benefit coastal communities who extensively rely on these critical reef ecosystems. While several studies have predicted that neither level of protection nor bleaching history nor coral taxon may save coral reefs from extremely severe stress as what occurred at the Great Barrier Reef during the 2014–2017 event (Hughes et al., 2017; Eakin et al., 2019), it is also still possible that geographically and oceanographically more complex regions such as archipelagos may shed light on their biogeographic variability as a means for reef adaptability and resilience. Observations on the limited impact of the recent global bleaching event to Philippine reefs (Licuanan et al., 2019) provide interesting avenues for further research on the underlying basis of reef recovery and resilience. The Coral Triangle, a still understudied hotspot of reef biodiversity and geographic and oceanographic complexity will be proven valuable in understanding the potential resilience of coral reefs.
The diversity of symbionts and the flexibility of coral zooxanthellae associations observed in this study, particularly of pocilloporids, suggest that acclimatization of corals through symbiont shuffling is plausible in natural coral communities and could be a sign of hope for the persistence of reefs in the face of global change and uncertainty. The thermally tolerant Durusdinium, however, are known opportunists and harboring them has its trade-offs for the coral host (Little et al., 2004; Jones and Berkelmans, 2010, 2011; Stat and Gates, 2011; Lesser et al., 2013; Sproles et al., 2020). An established coral-Durusdinium symbiosis also does not guarantee bleaching resistance, as prolonged exposure to thermal stress can still result in the death the coral (Claar et al., 2020). Promising new results (Abbott et al., 2021) suggest that a near-equal relative proportion (co-dominance) of Cladocopium and Durusdinium symbionts elevates expression of genes related with productivity (i.e., translation and photosynthesis) in the zooxanthellae and at the same time promotes cellular growth for the coral host. This highlights the potential significance of co-occurring symbiont genera in corals and has important implications for predicting the health of the holobiont and of coral reefs in general.
Only a few studies have conducted long-term monitoring of the recovery and zooxanthella assemblage dynamics in corals and none has captured the net effect of D. trenchii or D. glynnii dominance to the long-term overall health of the coral. Loya et al. (2001) and van Woesik et al. (2011), for example, categorized species found in Okinawa, Japan as bleaching winners or losers in a 14-year interval, classifying all pocilloporid genera (that were considered in this study) as long-term losers due to lack of signs of recovery after two mass bleaching events. On the contrary, Guest et al. (2012) demonstrated a likely adaptive response from the usually susceptible corals Pocillopora and Acropora in Singapore, showing highly improved performance during the same mass bleaching events (1998 and 2010) and indicating what might be holobiont adaptability that is geographically variable. Both studies were not able to consider the diversity of endosymbionts and would have helped explain the difference in the two regions’ post-bleaching performance. Whether Durusdinium can persist to be the dominant symbiont in the coral colony or coral populations years after shuffling also remains in question. Reversion from D. glynnii/D. trenchii back to the coral host’s original symbiont assemblage has been observed in Orbicella annularis and O. franksi in the Florida Keys (Thornhill et al., 2006). Durusdinium symbionts among clonal ramets of O. faveolata have been recently detected, suggesting an intergenerational transmission of bleaching resistance (Manzello et al., 2019). Likewise, vertical transmission of endosymbiotic algae is known for the pocilloporid genera Pocillopora, Seriatopora, and Stylophora (Isomura and Nishihira, 2001), whose brooded larvae have also been shown to exhibit “environmental hardening” and pre-adaptability to thermal stress and acidic environmental conditions (Jiang et al., 2020; Kitchen et al., 2020). Other species like Montipora digitata in the Great Barrier Reef pass on shuffled endosymbionts through spawned gametes as well (Quigley et al., 2019). All these examples and their effect on the overall coral mortality or recovery rates in a population would be interesting to explore in the Philippines or the wider Coral Triangle region, which have been documented to have experienced frequent thermal stress occurrences in the past 30 years. In the broader context, the ability of reefs in the region to withstand future projections of global warming, to be a temporary refuge for genetic resources, or to naturally supply propagules for replenishing impacted reefs can only be predicted and realized with systematic and consistent monitoring of coral survival, investigating its underlying mechanisms, and effectively translating science into policy. Limited information on the factors underlying adaptability of the coral holobiont in the face of a changing environment emphasizes the need for more studies focusing on the Coral Triangle, while equally highlighting the need to act on mitigating anthropogenic stressors negatively impacting ecosystem function, whether global or local, with a heightened sense of urgency and resolve.
Data Availability Statement
The datasets presented in this study can be found in the supplementary material and in online repositories. The names of the repository/repositories and accession number(s) can be found below: NCBI (GenBank Accession Nos. MW024153–MW024369).
Author Contributions
AT, DV, and RR-G contributed to the conception and design of the study. AT conducted sample collection, laboratory work, all zooxanthellae-related analyses, and wrote the first draft of the manuscript. DV assisted in sampling and performed all SST-related methods. DV and RR-G wrote sections of the manuscript. All authors contributed to manuscript revision, read, and approved the submitted version.
Funding
This study was supported by the Philippine Department of Science and Technology – Philippine Council for Agriculture, Aquatic, and Natural Resources Research and Development (DOST-PCAARRD Project Numbers QSR-MR-COR.02.01 and QSR-MR-COR.02.03), Department of Environment and Natural Resources – Biodiversity Management Bureau (DENR-BMB), and University of the Philippines – Marine Science Institute.
Conflict of Interest
The authors declare that the research was conducted in the absence of any commercial or financial relationships that could be construed as a potential conflict of interest.
Publisher’s Note
All claims expressed in this article are solely those of the authors and do not necessarily represent those of their affiliated organizations, or those of the publisher, the editors and the reviewers. Any product that may be evaluated in this article, or claim that may be made by its manufacturer, is not guaranteed or endorsed by the publisher.
Acknowledgments
This paper is dedicated to the late Ronald D. Villanueva whose contribution to the project during its inception has been invaluable. The authors thank Patrick R. Pata and the reviewers LE and RC-T for their helpful comments and suggestions, and acknowledge Hazel O. Arceo, Cesar L. Villanoy, and Maria Lourdes San Diego-McGlone for their support of this study. Eileen Peñaflor and Mariana Soppa shared key knowledge in processing satellite products. Mikhael Tañedo, Romer Albino, Emmeline Jamodiong, David Siquioco, Lovely Heyres, Rhea Luciano, Joey Cabasan, Frederico Sabban, Geminne Manzano, Clairecynth Yu, Joyce Velos, Joseph Garcia, Robert Casauay, Maryjune Cabiguin, Macy A onuevo-Arcega, Ariel Loja, Jerome Genilan, Amabelle Go, Jamie Dichaves, Elaine Saniel, and Miledel Quibilan assisted with field collections. Supporting hard coral data were provided by the DOST-PCAARRD NACRE Program and the DENR-BMB WPS and SECURE Philippine Rise Projects. This is MSI contribution number 486.
Supplementary Material
The Supplementary Material for this article can be found online at: https://www.frontiersin.org/articles/10.3389/fmars.2021.731023/full#supplementary-material
Footnotes
References
Abbott, E., Dixon, G., and Matz, M. (2021). Codominance of two symbiont genera within the same coral host is associated with elevated symbiont productivity and lower host susceptibility to thermal stress. bioRxiv [Preprint]. doi: 10.1101/2021.01.20.427463
Abrego, D., Ulstrup, K. E., Willis, B. L., and van Oppen, M. J. H. (2008). Species-specific interactions between algal endosymbionts and coral hosts define their bleaching response to heat and light stress. Proc. R. Soc. B Biol. Sci. 275, 2273–2282. doi: 10.1098/rspb.2008.0180
Arceo, H. O., Quibilan, M. C., Aliño, P. M., Lim, G., and Licuanan, W. Y. (2001). Coral bleaching in Philippine reefs: coincident evidences with mesoscale thermal anomalies. Bull. Mar. Sci. 69, 579–593.
Bahr, K. D., Rodgers, K. S., and Jokiel, P. L. (2017). Impact of three bleaching events on the reef resiliency of Kāne’ohe Bay, Hawai’i. Front. Mar. Sci. 4:398. doi: 10.3389/fmars.2017.00398
Baker, A. C. (2003). Flexibility and specificity in coral-algal symbiosis: diversity, ecology, and biogeography of Symbiodinium. Annu. Rev. Ecol. Evol. Syst. 34, 661–689. doi: 10.1146/annurev.ecolsys.34.011802.132417
Baker, A. C., McClanahan, T. R., Starger, C. J., and Boonstra, R. K. (2013). Long-term monitoring of algal symbiont communities in corals reveals stability is taxon dependent and driven by site-specific thermal regime. Mar. Ecol. Prog. Ser. 479, 85–97. doi: 10.3354/meps10102
Barshis, D. J., Ladner, J. T., Oliver, T. A., and Palumbi, S. R. (2014). Lineage-specific transcriptional profiles of Symbiodinium spp. Unaltered by heat stress in a coral host. Mol. Biol. Evol. 31, 1343–1352. doi: 10.1093/molbev/msu107
Barshis, D. J., Ladner, J. T., Oliver, T. A., Seneca, F. O., Traylor-Knowles, N., and Palumbi, S. R. (2013). Genomic basis for coral resilience to climate change. Proc. Natl. Acad. Sci. U.S.A. 110, 1387–1392. doi: 10.1073/pnas.1210224110
Baumann, J. H., Davies, S. W., Aichelman, H. E., and Castillo, K. D. (2018). Coral Symbiodinium community composition across the belize mesoamerican barrier reef system is influenced by host species and thermal variability. Microb. Ecol. 75, 903–915. doi: 10.1007/s00248-017-1096-6
Bay, L. K., Doyle, J., Logan, M., and Berkelmans, R. (2016). Recovery from bleaching is mediated by threshold densities of background thermo-tolerant symbiont types in a reef-building coral. R. Soc. Open Sci. 3:160322. doi: 10.1098/rsos.160322
Berkelmans, R., and van Oppen, M. J. H. (2006). The role of zooxanthellae in the thermal tolerance of corals: a “nugget of hope” for coral reefs in an era of climate change. Proc. R. Soc. B Biol. Sci. 273, 2305–2312. doi: 10.1098/rspb.2006.3567
Bongaerts, P., Ridgway, T., Sampayo, E. M., and Hoegh-Guldberg, O. (2010). Assessing the ‘deep reef refugia’ hypothesis: focus on Caribbean reefs. Coral Reefs 29, 309–327. doi: 10.1007/s00338-009-0581-x
Boulotte, N. M., Dalton, S. J., Carroll, A. G., Harrison, P. L., Putnam, H. M., Peplow, L. M., et al. (2016). Exploring the Symbiodinium rare biosphere provides evidence for symbiont switching in reef-building corals. ISME J. 10, 2693–2701. doi: 10.1038/ismej.2016.54
Brener-Raffalli, K., Clerissi, C., Vidal-Dupiol, J., Adjeroud, M., Bonhomme, F., Pratlong, M., et al. (2018). Thermal regime and host clade, rather than geography, drive Symbiodinium and bacterial assemblages in the scleractinian coral Pocillopora damicornis sensu lato. Microbiome 6:39. doi: 10.1186/s40168-018-0423-6
Brown, B. E., and Cossins, A. R. (2011). “The potential for temperature Acclimatisation of reef corals in the face of climate change,” in Coral Reefs: An Ecosystem in Transition, eds Z. Dubinsky and N. Stambler (Dordrecht: Springer), 421–433. doi: 10.1007/978-94-007-0114-4
Buddemeier, R. W., and Fautin, D. G. (1993). Coral bleaching as an adaptive mechanism. Bioscience 43, 320–326. doi: 10.2307/1312064
Burke, L., Reytar, K., Spalding, M., and Perry, A. (2011). Reefs at Risk Revisited. Washington, DC: World Resources Institute.
Cabrera, O. C., Villanoy, C. L., David, L. T., and Gordon, A. L. (2011). Barrier layer control of entraitnment and upwelling in the Bohol Sea, Philippines. Oceanography 24, 130–141. doi: 10.5670/oceanog.2011.10
Carpenter, K. E., Abrar, M., Aeby, G., Aronson, R. B., Banks, S., Bruckner, A., et al. (2008). One-third of reef-building corals face elevated extinction risk from climate change and local impacts. Science 321, 560–563. doi: 10.1126/science.1159196
Chankong, A., Kongjandtre, N., Senanan, W., and Manthachitra, V. (2020). Community composition of Symbiodiniaceae among four scleractinian corals in the eastern Gulf of Thailand. Region. Stud. Mar. Sci. 33:100918. doi: 10.1016/j.rsma.2019.100918
Chen, B., Yu, K., Liang, J., Huang, W., Wang, G., Su, H., et al. (2019). Latitudinal variation in the molecular diversity and community composition of Symbiodiniaceae in Coral From the South China Sea. Front. Microbiol. 10:1278. doi: 10.3389/fmicb.2019.01278
Chen, C. A., Yang, Y. W., Wei, N. V., Tsai, W. S., and Fang, L. S. (2005). Symbiont diversity in scleractinian corals from tropical reefs and subtropical non-reef communities in Taiwan. Coral Reefs 24, 11–22. doi: 10.1007/s00338-004-0389-7
Claar, D. C., and Baum, J. K. (2019). Timing matters: survey timing during extended heat stress can influence perceptions of coral susceptibility to bleaching. Coral Reefs 38, 559–565. doi: 10.1007/s00338-018-01756-7
Claar, D. C., Starko, S., Tietjen, K. L., Epstein, H. E., Cunning, R., Cobb, K. M., et al. (2020). Dynamic symbioses reveal pathways to coral survival through prolonged heatwaves. Nat. Commun. 11:6097. doi: 10.1038/s41467-020-19169-y
Coffroth, M. A., and Santos, S. R. (2005). Genetic diversity of symbiotic dinoflagellates in the genus Symbiodinium. Protist 156, 19–34. doi: 10.1016/j.protis.2005.02.004
Couch, C. S., Burns, J. H. R., Liu, G., Steward, K., Gutlay, T. N., Kenyon, J., et al. (2017). Mass coral bleaching due to unprecedented marine heatwave in Papahānaumokuākea Marine National Monument (Northwestern Hawaiian Islands). PLoS One 12:e0185121. doi: 10.1371/journal.pone.0185121
Cruz-Trinidad, A., Geronimo, R. C., Cabral, R. B., and Aliño, P. M. (2011). How much are the Bolinao-Anda coral reefs worth? Ocean Coast. Manage. 54, 696–705. doi: 10.1016/j.ocecoaman.2011.07.002
Cunning, R., Silverstein, R. N., and Baker, A. C. (2018). Symbiont shuffling linked to differential photochemical dynamics of Symbiodinium in three Caribbean reef corals. Coral Reefs 37, 145–152. doi: 10.1007/s00338-017-1640-3
David, L. T., Borja-Del Rosario, R., Peñaflor, E. L., Cordero-Bailey, K., Villanoy, C. L., Aliño, P. M., et al. (2015). “Developing a Philippine climate-ocean typology as input to national vulnerability assessments,” in Proceedings of the 36th 2015 Asian Conference on Remote Sensing, Quezon, 34–38.
Davies, S. W., Ries, J. B., Marchetti, A., and Castillo, K. D. (2018). Symbiodinium functional diversity in the Coral Siderastrea siderea Is influenced by thermal stress and reef environment, but not ocean acidification. Front. Mar. Sci. 5:150. doi: 10.3389/fmars.2018.00150
Davy, S. K., Allemand, D., and Weis, V. M. (2012). Cell biology of Cnidarian-Dinoflagellate symbiosis. Microbiol. Mol. Biol. Rev. 76, 229–261. doi: 10.1128/mmbr.05014-11
DeCarlo, T. M., Cohen, A. L., Wong, G. T. F., Davis, K. A., Lohmann, P., and Soong, K. (2017). Mass coral mortality under local amplification of 2°c ocean warming. Sci. Rep. 7:44586. doi: 10.1038/srep44586
Dexter, E., Rollwagen-Bollens, G., and Bollens, S. M. (2018). The trouble with stress: a flexible method for the evaluation of nonmetric multidimensional scaling. Limnol. Oceanogr. Methods 16, 434–443. doi: 10.1002/lom3.10257
Dixon, G. B., Davies, S. W., Aglyamova, G. A., Meyer, E., Bay, L. K., and Matz, M. V. (2015). Genomic determinants of coral heat tolerance across latitudes. Science 348, 1460–1462. doi: 10.1126/science.1261224
Douglas, A. E. (2003). Coral bleaching - How and why? Mar. Pollut. Bull. 46, 385–392. doi: 10.1016/S0025-326X(03)00037-7
Drury, C. (2020). Resilience in reef-building corals: the ecological and evolutionary importance of the host response to thermal stress. Mol. Ecol. 29, 448–465. doi: 10.1111/mec.15337
Eakin, C. M., Liu, G., Gomez, A. M., De La Cour, J. L., Heron, S. F., Skirving, W. J., et al. (2016). Global coral bleaching 2014–2017: status and an appeal for observations. Reef Encount. 31, 20–26.
Eakin, C. M., Liu, G., Gomez, A. M., De La Cour, J. L., Heron, S. F., Skirving, W. J., et al. (2017). Ding, dong, the witch is dead (?)—three years of global coral bleaching 2014–2017. Reef Encount. 32, 33–38.
Eakin, C. M., Sweatman, H., and Brainard, R. E. (2019). The 2014–2017 global-scale coral bleaching event: insights and impacts. Coral Reefs 38, 539–545. doi: 10.1007/s00338-019-01844-2
Eckert, R. J., Reaume, A. M., Sturm, A. B., Studivan, M. S., and Voss, J. D. (2020). Depth Influences Symbiodiniaceae associations among Montastraea cavernosa corals on the belize barrier reef. Front. Microbiol. 11:518. doi: 10.3389/fmicb.2020.00518
Fautin, D. G., and Buddemeier, R. W. (2004). Adaptive bleaching: a general phenomenon. Hydrobiologia 530-531, 459–467. doi: 10.1007/s10750-004-2642-z
Franklin, E. C., Stat, M., Pochon, X., Putnam, H. M., and Gates, R. D. (2012). GeoSymbio: a hybrid, cloud-based web application of global geospatial bioinformatics and ecoinformatics for Symbiodinium–host symbioses. Mol. Ecol. Resour. 12, 369–373. doi: 10.1111/j.1755-0998.2011.03081.x
Fransolet, D., Roberty, S., and Plumier, J. C. (2012). Establishment of endosymbiosis: the case of cnidarians and Symbiodinium. J. Exp. Mar. Biol. Ecol. 420-421, 1–7. doi: 10.1016/j.jembe.2012.03.015
Gaither, M. R., Szabó, Z., Crepeau, M. W., Bird, C. E., and Toonen, R. J. (2011). Preservation of corals in salt-saturated DMSO buffer is superior to ethanol for PCR experiments. Coral Reefs 30, 329–333. doi: 10.1007/s00338-010-0687-1
Gierz, S., Ainsworth, T. D., and Leggat, W. (2020). Diverse symbiont bleaching responses are evident from 2-degree heating week bleaching conditions as thermal stress intensifies in coral. Mar. Freshw. Res. 71, 1149–1160. doi: 10.1071/mf19220
Gilmour, J. P., Smith, L. D., Heyward, A. J., Baird, A. H., and Pratchett, M. S. (2013). Recovery of an isolated coral reef system following severe disturbance. Science 340, 69–71. doi: 10.1126/science.1232310
Gong, S., Xu, L., Yu, K., Zhang, F., and Li, Z. (2019). Differences in Symbiodiniaceae communities and photosynthesis following thermal bleaching of massive corals in the northern part of the South China Sea. Mar. Pollut. Bull. 144, 196–204. doi: 10.1016/j.marpolbul.2019.04.069
Gordon, A. L., Susanto, R. D., and Ffield, A. (1999). Throughflow within Makassar Strait. Geophys. Res. Lett. 26, 3325–3328. doi: 10.1029/1999GL002340
Guest, J. R., Baird, A. H., Maynard, J. A., Muttaqin, E., Edwards, A. J., Campbell, S. J., et al. (2012). Contrasting patterns of coral bleaching susceptibility in 2010 suggest an adaptive response to thermal stress. PLoS One 7:e33353. doi: 10.1371/journal.pone.0033353
Guest, J. R., Low, J., Tun, K., Wilson, B., Ng, C. S. L., Raingeard, D., et al. (2016). Coral community response to bleaching on a highly disturbed reef. Sci. Rep. 6:20717. doi: 10.1038/srep20717
Han, W., Moore, A. M., Levin, J., Zhang, B., Arango, H. G., Curchitser, E., et al. (2009). Seasonal surface ocean circulation and dynamics in the Philippine Archipelago region during 2004-2008. Dyn. Atmos. Oceans 47, 114–137. doi: 10.1016/j.dynatmoce.2008.10.007
Heron, S. F., Johnston, L., Liu, G., Geiger, E. F., Maynard, J. A., De La Cour, J. L., et al. (2016). Validation of reef-scale thermal stress satellite products for coral bleaching monitoring. Remote Sens. 8, 1–16. doi: 10.3390/rs8010059
Hoegh-Guldberg, O. (1999). Climate Change, coral bleaching and the future of the world’ s coral reefs. Mar. Freshw. Res. 50, 839–866. doi: 10.1071/MF99078
Hoegh-Guldberg, O., Kennedy, E. V., Beyer, H. L., McClennen, C., and Possingham, H. P. (2018). Securing a long-term future for coral reefs. Trends Ecol. Evol. 33, 936–944. doi: 10.1016/j.tree.2018.09.006
Hoegh-Guldberg, O., Mumby, P. J., Hooten, A. J., Steneck, R. S., Greenfield, P., Gomez, E. D., et al. (2007). Coral reefs under rapid climate change and ocean acidification. Science 318, 1737–1742. doi: 10.1126/science.1152509
Hughes, T. P., Kerry, J. T., Álvarez-Noriega, M., Álvarez-Romero, J. G., Anderson, K. D., Baird, A. H., et al. (2017). Global warming and recurrent mass bleaching of corals. Nature 543, 373–377. doi: 10.1038/nature21707
Hurlburt, H. E., Metzger, J. E., and Sprintall, J. (2011). Circulation in the Philippine Archipelago Simulated by 1/12° and 1/25° Global HYCOM and EAS NCOM. Oceanography 24, 28–47. doi: 10.5670/oceanog.2011.02
Isomura, N., and Nishihira, M. (2001). Size variation of planulae and its effect on the lifetime of planulae in three pocilloporid corals. Coral Reefs 20, 309–315. doi: 10.1007/s003380100180
Jiang, L., Guo, M.-L., Zhang, F., Zhang, Y.-Y., Zhou, G.-W., Lei, X.-M., et al. (2020). Impacts of elevated temperature and pCO2 on the brooded larvae of Pocillopora damicornis from Luhuitou Reef, China: evidence for local acclimatization. Coral Reefs 39, 331–344. doi: 10.1007/s00338-020-01894-x
Jones, A. M., and Berkelmans, R. (2010). Potential costs of acclimatization to a warmer climate: growth of a reef coral with heat tolerant vs. sensitive symbiont types. PLoS One 5:e10437. doi: 10.1371/journal.pone.0010437
Jones, A. M., and Berkelmans, R. (2011). Tradeoffs to thermal acclimation: energetics and reproduction of a reef coral with heat tolerant Symbiodinium Type-D. J. Mar. Biol. 2011:185890. doi: 10.1155/2011/185890
Jones, A. M., Berkelmans, R., van Oppen, M. J. H., Mieog, J. C., and Sinclair, W. (2008). A community change in the algal endosymbionts of a scleractinian coral following a natural bleaching event: field evidence of acclimatization. Proc. R. Soc. B Biol. Sci. 275, 1359–1365. doi: 10.1098/rspb.2008.0069
Kayanne, H. (2016). Validation of degree heating weeks as a coral bleaching index in the northwestern Pacific. Coral Reefs 36, 63–70. doi: 10.1007/s00338-016-1524-y
Kearse, M., Moir, R., Wilson, A., Stones-Havas, S., Cheung, M., Sturrock, S., et al. (2012). Geneious Basic: an integrated and extendable desktop software platform for the organization and analysis of sequence data. Bioinformatics 28, 1647–1649. doi: 10.1093/bioinformatics/bts199
Kenkel, C. D., Goodbody-Gringley, G., Caillaud, D., Davies, S. W., Bartels, E., and Matz, M. V. (2013). Evidence for a host role in thermotolerance divergence between populations of the mustard hill coral (Porites astreoides) from different reef environments. Mol. Ecol. 22, 4335–4348. doi: 10.1111/mec.12391
Keshavmurthy, S., Tee, H. S., Kao, K.-W., Wang, J.-T., and Chen, C. A. (2020). Specificity trumps flexibility—location-based stable associations between Symbiodiniaceae genera and Platygyra verweyi (Scleractinia; Merulinidae). PeerJ 8:e8791. doi: 10.7717/peerj.8791
Kitchen, R. M., Piscetta, M., de Souza, M. R., Lenz, E. A., Schar, D. W. H., Gates, R. D., et al. (2020). Symbiont transmission and reproductive mode influence responses of three Hawaiian coral larvae to elevated temperature and nutrients. Coral Reefs 39, 419–431. doi: 10.1007/s00338-020-01905-x
Kleypas, J. A., Castruccio, F. S., Curchitser, E. N., and Mcleod, E. (2015). The impact of ENSO on coral heat stress in the western equatorial Pacific. Glob. Change Biol. 21, 2525–2539. doi: 10.1111/gcb.12881
LaJeunesse, T. C. (2002). Diversity and community structure of symbiotic dinoflagellates from Caribbean coral reefs. Mar. Biol. 141, 387–400. doi: 10.1007/s00227-002-0829-2
LaJeunesse, T. C., Loh, W. K. W., Van Woesik, R., Hoegh-Guldberg, O., Schmidt, G. W., and Fitt, W. K. (2003). Low symbiont diversity in southern Great Barrier Reef corals, relative to those of the Caribbean. Limnol. Oceanogr. 48, 2046–2054. doi: 10.4319/lo.2003.48.5.2046
LaJeunesse, T. C., Parkinson, J. E., Gabrielson, P. W., Jeong, H. J., Reimer, J. D., Voolstra, C. R., et al. (2018). Systematic revision of Symbiodiniaceae highlights the antiquity and diversity of coral endosymbionts. Curr. Biol. 28, 2570–2580.e6. doi: 10.1016/j.cub.2018.07.008
LaJeunesse, T. C., Reyes-Bonilla, H., Warner, M. E., Wills, M., Schmidt, G. W., and Fitt, W. K. (2008). Specificity and stability in high latitude eastern Pacific coral-algal symbioses. Limnol. Oceanogr. 53, 719–727. doi: 10.4319/lo.2008.53.2.0719
Lajeunesse, T. C., Wham, D. C., Pettay, D. T., Parkinson, J. E., Keshavmurthy, S., and Chen, C. A. (2014). Ecologically differentiated stress-tolerant endosymbionts in the dinoflagellate genus Symbiodinium (Dinophyceae) Clade D are different species. Phycologia 53, 305–319. doi: 10.2216/13-186.1
Le Nohaïc, M., Ross, C. L., Cornwall, C. E., Comeau, S., Lowe, R. J., McCulloch, M. T., et al. (2017). Marine heatwave causes unprecedented regional mass bleaching of thermally resistant corals in northwestern Australia. Sci. Rep. 7:14999. doi: 10.1038/s41598-017-14794-y
Lermusiaux, P. F. J., HaLey, P. J. Jr., Leslie, W. G., Agarwal, A., Logutov, O. G., and Burton, L. J. (2011). Multiscale physical and biological dynamics in the Philippine Archipelago: predictions and processes. Oceanography 24, 70–89. doi: 10.5670/oceanog.2011.05
Lesser, M. P., Stat, M., and Gates, R. D. (2013). The endosymbiotic dinoflagellates (Symbiodinium sp.) of corals are parasites and mutualists. Coral Reefs 32, 603–611. doi: 10.1007/s00338-013-1051-z
Levy, G., Shaish, L., Haim, A., and Rinkevich, B. (2010). Mid-water rope nursery—Testing design and performance of a novel reef restoration instrument. Ecol. Eng. 36, 560–569. doi: 10.1016/j.ecoleng.2009.12.003
Licuanan, W. Y., and Gomez, E. D. (2000). “Philippine coral reefs: status and the role of the academe to improve their management,” in Proceedings of the Ninth International Coral Reef Symposium, Bali, 835–840.
Licuanan, W. Y., Robles, R., and Reyes, M. (2019). Status and recent trends in coral reefs of the Philippines. Mar. Pollut. Bull. 142, 544–550. doi: 10.1016/j.marpolbul.2019.04.013
Licuanan, W. Y., Robles, R., Dygico, M., Songco, A., and van Woesik, R. (2017). Coral benchmarks in the center of biodiversity. Mar. Pollut. Bull. 114, 1135–1140. doi: 10.1016/j.marpolbul.2016.10.017
Little, A. F., van Oppen, M. J. H., and Willis, B. L. (2004). Flexibility in algal endosymbioses shapes growth in reef corals. Science 304, 1492–1494. doi: 10.1126/science.1095733
Liu, G., Heron, S. F., Eakin, C. M., Muller-Karger, F. E., Vega-Rodriguez, M., Guild, L. S., et al. (2014). Reef-scale thermal stress monitoring of coral ecosystems: new 5-km global products from NOAA Coral Reef Watch. Remote Sens. 6, 11579–11606. doi: 10.3390/rs61111579
Liu, G., Strong, A. E., Skirving, W., and Arzayus, L. F. (2006). Overview of NOAA Coral Reef Watch Program’s Near-Real Time Satellite Global Coral Bleaching Monitoring Activities. (Washington, DC: NOAA), 1783–1793.
Lough, J. M., Anderson, K. D., and Hughes, T. P. (2018). Increasing thermal stress for tropical coral reefs: 1871-2017. Sci. Rep. 8:6079. doi: 10.1038/s41598-018-24530-9
Loya, Y., Sakai, K., Yamazato, K., Nakano, Y., Sambali, H., and Van Woesik, R. (2001). Coral bleaching: the winners and the losers. Ecol. Lett. 4, 122–131. doi: 10.1046/j.1461-0248.2001.00203.x
Magdaong, E. T., Fujii, M., Yamano, H., Licuanan, W. Y., Maypa, A., Campos, W. L., et al. (2014). Long-term change in coral cover and the effectiveness of marine protected areas in the Philippines: a meta-analysis. Hydrobiologia 733, 5–17. doi: 10.1007/s10750-013-1720-5
Manzello, D. P., Matz, M. V., Enochs, I. C., Valentino, L., Carlton, R. D., Kolodziej, G., et al. (2019). Role of host genetics and heat-tolerant algal symbionts in sustaining populations of the endangered coral Orbicella faveolata in the Florida Keys with ocean warming. Glob. Change Biol. 25, 1016–1031. doi: 10.1111/gcb.14545
Masumoto, Y., Kagimoto, T., Yoshida, M., Fukuda, M., Hirose, N., and Yamagata, T. (2001). Intraseasonal eddies in the Sulawesi Sea simulated in an ocean General Circulation Model. Geophys. Res. Lett. 28, 1631–1634. doi: 10.1029/2000GL011835
Maynard, J. A., Anthony, K. R. N., Marshall, P. A., and Masiri, I. (2008). Major bleaching events can lead to increased thermal tolerance in corals. Mar. Biol. 155, 173–182. doi: 10.1007/s00227-008-1015-y
McClanahan, T. R., Ateweberhan, M., Ruiz Sebastián, C., Graham, N. A. J., Wilson, S. K., Bruggemann, J. H., et al. (2007). Predictability of coral bleaching from synoptic satellite and in situ temperature observations. Coral Reefs 26, 695–701. doi: 10.1007/s00338-006-0193-7
McManus, L. C., Vasconcelos, V. V., Levin, S. A., Thompson, D. M., Kleypas, J. A., Castruccio, F. S., et al. (2020). Extreme temperature events will drive coral decline in the Coral Triangle. Glob. Change Biol. 26, 2120–2133. doi: 10.1111/gcb.14972
Mieog, J. C., Olsen, J. L., Berkelmans, R., Bleuler-Martinez, S. A., Willis, B. L., and van Oppen, M. J. H. (2009a). The roles and interactions of symbiont, host and environment in defining coral fitness. PLoS One 4:e6364. doi: 10.1371/journal.pone.0006364
Mieog, J. C., van Oppen, M. J. H., Berkelmans, R., Stam, W. T., and Olsen, J. L. (2009b). Quantification of algal endosymbionts (Symbiodinium) in coral tissue using real-time PCR. Mol. Ecol. Resour. 9, 74–82. doi: 10.1111/j.1755-0998.2008.02222.x
Mostafavi, P. G., Fatemi, S. M. R., Shahhosseiny, M. H., Hoegh-Guldberg, O., and Loh, W. K. W. (2007). Predominance of clade D Symbiodinium in shallow-water reef-building corals off Kish and Larak Islands (Persian Gulf, Iran). Mar. Biol. 153, 25–34. doi: 10.1007/s00227-007-0796-8
Muñiz-Castillo, A. I., Rivera-Sosa, A., Chollett, I., Eakin, C. M., Andrade-Gómez, L., McField, M., et al. (2019). Three decades of heat stress exposure in Caribbean coral reefs: a new regional delineation to enhance conservation. Sci. Rep. 9:11013. doi: 10.1038/s41598-019-47307-0
Muscatine, L. (1990). “The role of symbiotic algae in carbon and energy flux in reef corals,” in Ecosystems of the world 25: Coral Reefs, ed. Z. Dubinsky (Amsterdam: Elsevier), 75–87.
Ng, T. Y., and Ang, P. (2016). Low symbiont diversity as a potential adaptive strategy in a marginal non-reefal environment: a case study of corals in Hong Kong. Coral Reefs 35, 941–957. doi: 10.1007/s00338-016-1458-4
NOAA (2021). Cold & Warm Episodes by Season. Available online at: https://origin.cpc.ncep.noaa.gov/products/analysis_monitoring/ensostuff/ONI_v5.php (accessed August 17, 2021).
Oksanen, J., Blanchet, F. G., Friendly, M., Kindt, R., Legendre, P., McGlinn, D., et al. (2020). vegan: Community Ecology Package. Available online at: https://CRAN.R-project.org/package=vegan (accessed August 02, 2021).
Palumbi, S. R., Barshis, D. J., Traylor-Knowles, N., and Bay, R. A. (2014). Mechanisms of reef coral resistance to future climate change. Science 344, 895–898. doi: 10.1126/science.1251336
Parkinson, J. E., Banaszak, A. T., Altman, N. S., LaJeunesse, T. C., and Baums, I. B. (2015). Intraspecific diversity among partners drives functional variation in coral symbioses. Sci. Rep. 5:15567. doi: 10.1038/srep15667
Peñaflor, E. L. (2015). Investigating physical parameters associated with massive coral bleaching events in the Coral Triangle. [dissertation]. (Quezon City, Philippines: University of the Philippines Diliman).
Peñaflor, E. L., Skirving, W. J., Strong, A. E., Heron, S. F., and David, L. T. (2009). Sea-surface temperature and thermal stress in the Coral Triangle over the past two decades. Coral Reefs 28, 841–850. doi: 10.1007/s00338-009-0522-8
Pochon, X., and Gates, R. D. (2010). A new Symbiodinium clade (Dinophyceae) from soritid foraminifera in Hawai’i. Mol. Phylogenet. Evol. 56, 492–497. doi: 10.1016/j.ympev.2010.03.040
Poquita-Du, R. C., Huang, D., Chou, L. M., and Todd, P. A. (2020). The contribution of stress-tolerant endosymbiotic dinoflagellate Durusdinium to Pocillopora acuta survival in a highly urbanized reef system. Coral Reefs 39, 745–755. doi: 10.1007/s00338-020-01902-0
Putnam, H. M., Stat, M., Pochon, X., and Gates, R. D. (2012). Endosymbiotic flexibility associates with environmental sensitivity in scleractinian corals. Proc. R. Soc. B Biol. Sci. 279, 4352–4361. doi: 10.1098/rspb.2012.1454
Qin, Z., Yu, K., Chen, B., Wang, Y., Liang, J., Luo, W., et al. (2019). Diversity of Symbiodiniaceae in 15 Coral Species From the Southern South China Sea: potential relationship with coral thermal adaptability. Front. Microbiol. 10:2343. doi: 10.3389/fmicb.2019.02343
Qu, T., Song, Y. T., and Yamagata, T. (2009). An introduction to the South China Sea throughflow: its dynamics, variability, and application for climate. Dyn. Atmos. Oceans 47, 3–14. doi: 10.1016/j.dynatmoce.2008.05.001
Quigley, K. M., Willis, B. L., and Kenkel, C. D. (2019). Transgenerational inheritance of shuffled symbiont communities in the coral Montipora digitata. Sci. Rep. 9:13328. doi: 10.1038/s41598-019-50045-y
Quimpo, T. J. R., Requilme, J. N. C., Gomez, E. J., Sayco, S. L. G., Tolentino, M. P. S., and Cabaitan, P. C. (2020). Low coral bleaching prevalence at the Bolinao-Anda Reef Complex, northwestern Philippines during the 2016 thermal stress event. Mar. Pollut. Bull. 160:111567. doi: 10.1016/j.marpolbul.2020.111567
R Core Team (2021). R: A Language and Environment for Statistical Computing. Vienna: R Foundation for Statistical Computing.
Riegl, B., Glynn, P. W., Banks, S., Keith, I., Rivera, F., Vera-Zambrano, M., et al. (2019). Heat attenuation and nutrient delivery by localized upwelling avoided coral bleaching mortality in northern Galapagos during 2015/2016 ENSO. Coral Reefs 38, 773–785. doi: 10.1007/s00338-019-01787-8
Selig, E. R., Casey, K. S., and Bruno, J. F. (2012). Temperature-driven coral decline: the role of marine protected areas. Glob. Change Biol. 18, 1561–1570. doi: 10.1111/j.1365-2486.2012.02658.x
Shaish, L., Levy, G., Katzir, G., and Rinkevich, B. (2010a). Coral reef restoration (Bolinao, Philippines) in the face of frequent natural catastrophes. Restor. Ecol. 18, 285–299. doi: 10.1111/j.1526-100X.2009.00647.x
Shaish, L., Levy, G., Katzir, G., and Rinkevich, B. (2010b). Employing a highly fragmented, weedy coral species in reef restoration. Ecol. Eng. 36, 1424–1432. doi: 10.1016/j.ecoleng.2010.06.022
Silverstein, R. N., Cunning, R., and Baker, A. C. (2015). Change in algal symbiont communities after bleaching, not prior heat exposure, increases heat tolerance of reef corals. Glob. Change Biol. 21, 236–249. doi: 10.1111/gcb.12706
Silverstein, R. N., Cunning, R., and Baker, A. C. (2017). Tenacious D: Symbiodinium in clade D remain in reef corals at both high and low temperature extremes despite impairment. J. Exp. Biol. 220, 1192–1196. doi: 10.1242/jeb.148239
Sprintall, J., Gordon, A. L., Flament, P., and Villanoy, C. L. (2012). Observations of exchange between the South China Sea and the Sulu Sea. J. Geophys. Res. Oceans 117, 1–18. doi: 10.1029/2011JC007610
Sproles, A. E., Oakley, C. A., Krueger, T., Grossman, A. R., Weis, V. M., Meibom, A., et al. (2020). Sub-cellular imaging shows reduced photosynthetic carbon and increased nitrogen assimilation by the non-native endosymbiont Durusdinium trenchii in the model cnidarian Aiptasia. Environ. Microbiol. 22, 3741–3753. doi: 10.1111/1462-2920.15142
Stat, M., and Gates, R. D. (2011). Clade D Symbiodinium in Scleractinian Corals: A “Nugget” of hope, a selfish opportunist, an ominous sign, or all of the above? J. Mar. Biol. 2011:730715. doi: 10.1155/2011/730715
Stat, M., Pochon, X., Franklin, E. C., Bruno, J. F., Casey, K. S., Selig, E. R., et al. (2013). The distribution of the thermally tolerant symbiont lineage (Symbiodinium clade D) in corals from Hawaii: correlations with host and the history of ocean thermal stress. Ecol. Evol. 3, 1317–1329. doi: 10.1002/ece3.556
Sully, S., and van Woesik, R. (2020). Turbid reefs moderate coral bleaching under climate-related temperature stress. Glob. Change Biol. 26, 1367–1373. doi: 10.1111/gcb.14948
Terraneo, T. I., Fusi, M., Hume, B. C. C., Arrigoni, R., Voolstra, C. R., Benzoni, F., et al. (2019). Environmental latitudinal gradients and host-specificity shape Symbiodiniaceae distribution in Red Sea Porites corals. J. Biogeogr. 46, 2323–2335. doi: 10.1111/jbi.13672
Thompson, D. M., and Van Woesik, R. (2009). Corals escape bleaching in regions that recently and historically experienced frequent thermal stress. Proc. R. Soc. B Biol. Sci. 276, 2893–2901. doi: 10.1098/rspb.2009.0591
Thornhill, D. J., LaJeunesse, T. C., Kemp, D. W., Fitt, W. K., and Schmidt, G. W. (2006). Multi-year, seasonal genotypic surveys of coral-algal symbioses reveal prevalent stability or post-bleaching reversion. Mar. Biol. 148, 711–722. doi: 10.1007/s00227-005-0114-2
Trench, R. K., Wethey, D. S., and Porter, J. W. (1981). Observations on the symbiosis with zooxanthellae among the tridacnidae (mollusca, bivalvia). Biol. Bull. 161, 180–198. doi: 10.2307/1541117
Tun, K., Chou, L. M., Low, J., Teemin, T., Phongsuwan, N., Setiashi, N., et al. (2010). “A regional overview on the 2010 coral bleaching event in Southeast Asia,” in Status of Coral Reefs in East Asian Seas Region: 2010, (Sumida City: Japan Wildlife Research Center), 9–26.
Valino, D. A. M., Baria-Rodriguez, M. V., Dizon, R. M., and Aliño, P. M. (2021). Responses of Buluan Island turbid fringing reefs, southern Philippines to the 2016 thermal anomaly. Region. Stud. Mar. Sci. 43:101704. doi: 10.1016/j.rsma.2021.101704
van Oppen, M. J. H., Oliver, J. K., Putnam, H. M., and Gates, R. D. (2015). Building coral reef resilience through assisted evolution. Proc. Natl. Acad. Sci. U.S.A. 112, 2307–2313. doi: 10.1073/pnas.1422301112
van Woesik, R., Sakai, K., Ganase, A., and Loya, Y. (2011). Revisiting the winners and the losers a decade after coral bleaching. Mar. Ecol. Prog. Ser. 434, 67–76. doi: 10.3354/meps09203
Villanoy, C. L., Cabrera, O. C., Yñiguez, A., Camoying, M., de Guzman, A., David, L. T., et al. (2011). Monsoon-driven coastal upwelling off Zamboanga peninsula, Philippines Oceanography 24, 156–165. doi: 10.5670/oceanog.2011.12
Walker, B., Holling, C. S., Carpenter, S., and Kinzig, A. (2004). Resilience, Adaptability and Transformability in Social–ecological Systems. Ecol. Soc. 9:5.
Weis, V. M. (2008). Cellular mechanisms of Cnidarian bleaching: stress causes the collapse of symbiosis. J. Exp. Biol. 211, 3059–3066. doi: 10.1242/jeb.009597
Weisz, J. B., Massaro, A. J., Ramsby, B. D., and Hill, M. S. (2010). Zooxanthellar Symbionts shape host sponge trophic status through translocation of carbon. Biol. Bull. 219, 189–197.
Wham, D. C., Ning, G., and LaJeunesse, T. C. (2017). Symbiodinium glynnii sp. nov., a species of stress-tolerant symbiotic dinoflagellates from pocilloporid and montiporid corals in the Pacific Ocean. Phycologia 56, 396–409. doi: 10.2216/16-86.1
Wooldridge, S. A. (2013). Breakdown of the coral-algae symbiosis: towards formalising a linkage between warm-water bleaching thresholds and the growth rate of the intracellular zooxanthellae. Biogeosciences 10, 1647–1658. doi: 10.5194/bg-10-1647-2013
Wright, R. M., Mera, H., Kenkel, C. D., Nayfa, M., Bay, L. K., and Matz, M. V. (2019). Positive genetic associations among fitness traits support evolvability of a reef-building coral under multiple stressors. Glob. Change Biol. 25, 3294–3304. doi: 10.1111/gcb.14764
Yellowlees, D., Rees, T. A. V., and Leggat, W. (2008). Metabolic interactions between algal symbionts and invertebrate hosts. Plant Cell Environ. 31, 679–694. doi: 10.1111/j.1365-3040.2008.01802.x
Zhou, G.-W., and Huang, H. (2011). Low genetic diversity of symbiotic dinoflagellates (Symbiodinium) in scleractinian corals from tropical reefs in southern Hainan Island, China. J. Syst. Evol. 49, 598–605. doi: 10.1111/j.1759-6831.2011.00161.x
Keywords: coral-algal symbiosis, Symbiodiniaceae, coral bleaching, thermal stress, PCR-DGGE fingerprinting, Coral Triangle
Citation: Torres AF, Valino DAM and Ravago-Gotanco R (2021) Zooxanthellae Diversity and Coral-Symbiont Associations in the Philippine Archipelago: Specificity and Adaptability Across Thermal Gradients. Front. Mar. Sci. 8:731023. doi: 10.3389/fmars.2021.731023
Received: 25 June 2021; Accepted: 28 September 2021;
Published: 20 October 2021.
Edited by:
Yehuda Benayahu, Tel Aviv University, IsraelReviewed by:
Leïla Ezzat, École Polytechnique Fédérale de Lausanne, SwitzerlandRafael A. Cabral-Tena, Center for Scientific Research and Higher Education at Ensenada (CICESE), Mexico
Copyright © 2021 Torres, Valino and Ravago-Gotanco. This is an open-access article distributed under the terms of the Creative Commons Attribution License (CC BY). The use, distribution or reproduction in other forums is permitted, provided the original author(s) and the copyright owner(s) are credited and that the original publication in this journal is cited, in accordance with accepted academic practice. No use, distribution or reproduction is permitted which does not comply with these terms.
*Correspondence: Andrew F. Torres, a.torres@nhm.ac.uk