- 1Global Climate Forum, Berlin, Germany
- 2Division of Resource Economics, Humboldt-Universität zu Berlin, Berlin, Germany
- 3Bureau de Recherches Géologiques et Minières, French Geological Survey, Orléans, France
- 4Institute for Advanced Sustainability Studies, Potsdam, Germany
Climate services are ideally co-developed by scientists and stakeholders working together to identify decisions and user needs. Yet, while climate services have been developed at regional to local scales, relatively little attention has been paid to the global scale. Global climate services involve decisions that rely on climate information from many locations in different world regions, and are increasingly salient. Increasing interconnections in the global financial system and supply chains expose private companies and financial institutions to climate risk in multiple locations in different world regions. Further, multilateral decisions on greenhouse gas emission reduction targets, disaster risk finance or international migration should make use of global scale climate risk assessments. In order to advance global climate service development, we present a typology of decisions relying on global (i.e., non-local) climate risk information. We illustrate each decision type through examples of current practice from the coastal domain drawn from the literature and stakeholder interviews. We identify 8 types of decisions making use of global climate information. At a top-level, we distinguish between “multilateral climate policy decisions,” and “portfolio decisions involving multiple locations.” Multilateral climate policy decisions regard either “mitigation targets” or “multilateral adaptation” decisions. Portfolio decisions regard either “choice of location” or “choice of financial asset” decisions. Choice of location decisions can be further distinguished as to whether they involve “direct climate risks,” “supply chain risks” or “financial network risks.” Our survey of examples shows that global climate service development is more advanced for portfolio decisions taken by companies with experience in climate risk assessment, i.e., (re-)insurers, whereas many multilateral climate policy decisions are at an earlier stage of decision-making. Our typology thus provides an entry-point for global climate service development by pointing to promising research directions for supporting global (non-local) decisions that account for climate risks.
Introduction
Climate services provide stakeholders with “usable” climate information and tools in order to assist decision-making (Hewitt et al., 2012), and are being promoted by leading institutions in the United States and Europe, e.g., National Research Council, the European JPI-Climate, or the global Climate Services Partnership (Brasseur and Gallardo, 2016). Climate services however exhibit “usability gap” (Lemos et al., 2012). Uptake of climate services has been limited by the science-driven, as opposed to demand-driven, climate service development (Lourenço et al., 2016). Indeed, barriers to uptake include low climate risk awareness among some stakeholders, a lack of tailored communication formats of the services, or lack of salience of climate service products (Brasseur and Gallardo, 2016). To overcome these barriers, co-development approaches to climate services that involve stakeholders working directly with scientists to identify decisions, user needs, and thus climate service products needed (Bremer et al., 2019). Indeed, at local and regional scales, climate service co-development has led to seasonal forecasting tools for agricultural sector stakeholders, local flood risk assessment tools (Soares et al., 2018), and coastal risk assessment (Van der Pol et al., 2019).
Yet global climate services have hardly been addressed to date. Global climate services refer to climate information or tools that are non-local, and thus support specific global decisions by delivering coherent information for many locations, regions or countries around the globe. This is a significant gap as there are a number of salient decisions with global dimensions that are exposed to climate risks. Multilateral decisions on greenhouse gas emission mitigation action, disaster risk finance or international migration rely in part on global scale climate risk assessments (Hedlund et al., 2018). Further, decisions on trade policy, food security and macro-economic policy (e.g., sovereign risk) can be usefully informed by global scale information on climate risks (Benzie et al., 2019). More broadly, both the global financial system and global supply chains have become increasingly interconnected, exposing private companies and financial institutions to direct and indirect climate risks in multiple locations around the world (SEI, 2020).
This paper aims to fill the gap in the literature regarding climate services addressing global decisions. We do so by identifying different types of decisions that rely on information on climate risks at the global scale. We thus develop a typology of decisions relying on global, i.e., non-local, climate risk information, and explore the current state-of-the-art in global climate services to address these decisions. Our analysis is aligned with a co-development approach, as we identify global decisions through analysis of real-world decisions through both stakeholder interviews and literature review. Further, we characterize these global decisions and the need for global climate services they give rise to.
Our paper is thus exploratory presenting a typology of global decisions in order to explore the state-of-the-art in the global climate service development. We note that political scientists have studied global governance arrangements and contexts emerging in transnational climate governance for more than a decade (Pattberg and Stripple, 2008; Biermann et al., 2009; Dzebo and Stripple, 2015). Our paper presents a complementary view to the governance one, which describes governance contexts and involve actors. Our paper focuses on describing global decisions and influence of climate risk upon these in order to identify salient climate information needs. Governance contexts also influence these information needs (discussed in section “Characterizing global decisions and decision contexts”), however, understanding these contexts in not the main focus of our analysis.
Our examples of global climate services are mostly drawn from real-world approaches to decision-making incorporating global (i.e., non-local) information on coastal risks and sea-level rise (SLR). We focus on coastal risk and SLR, as one of the most critical areas for climate service development (Kopp et al., 2019), and because this domain is relatively well-developed for aspects important to global decisions, i.e., indirect economic impacts (Parrado et al., 2020) and financial system impacts (Mandel et al., 2021) of coastal flooding. Indeed, SLR is a salient issue because of the severe consequences of high-end SLR for Small Island States (Nurse et al., 2014), and because of the prevalence of population and economic assets in coastal flood plains globally (Lincke and Hinkel, 2018). Further, SLR increases flood exposure key economic hubs of ports and exclusive economic zones (EEZ), which have can have disproportionally large indirect impacts globally due to supply chain linkages (Haraguchi and Lall, 2015). Our typology illustrated with examples from the relatively advanced coastal domain thus enables an assessment of the state-of-the-art in global climate services development, to identify gaps and discuss salient directions of future research for developing global climate services.
Materials and Methods
Approach to Global Climate Services
Climate services have been defined by the WMO Global Framework for Climate Services as “the provision of climate information in such a way as to assist decision-making” (Hewitt et al., 2012). More precisely, under climate service, we understand any effort to deliver information and tools that support addressing the specific decision-problems users face (Hinkel et al., 2019). Global climate services, a sub-set of climate services, deliver climate information and tools at a global scale to support specific global decisions users face. Such global decisions make use of global scale information on climate risk, i.e., coherent information for many locations, regions or countries around the globe.
The aim of this paper is to explore the state-of-the-art in global climate services and our approach to doing so is as follows. First, we build a typology of global decisions requiring climate information. The typology is based on several distinctions regarding the number of actors involved, whether decisions involve mitigation or adaptation, as well as the types of assets and risks at issue. Second, in the spirit of the co-development approach, we identify real-world examples of such decisions mostly from the coastal domain based on stakeholder interactions and literature review. We further characterize the decisions based on decision-analysis and context variables in order to highlight the relevance of global climate and SLR information to the decisions. This further characterization of decisions provides insight into directions for the future development of global climate services by identifying appropriate decision-making methods, and identifying constraints or enablers (e.g., institutions or norms) for global climate service development.
Characterizing Global Decisions and Decision Contexts
Global decisions can be further characterized according to typical decision-analysis variables, e.g., decision objectives, available alternatives, time horizon of options, and presence of uncertainty (Kleindorfer et al., 1993). Characterizing decisions along these variables can inform the choice of appropriate decision-making methods and the climate information needs they give rise to, which in turn is key to climate service development (Hinkel et al., 2019). For example, if a decision involves only costly, long-term and inflexible options, in which there is high risk adversity regarding negative outcomes, it is important to identify options that are effective under a wide range of scenarios, and the climate information needed to do so should be developed as climate services (Lempert et al., 2012). In contrast, when the set of options considered include short-term or flexible options, climate services can focus on monitoring and re-evaluating options over time (Hinkel et al., 2016). For global climate services, examples that involve costly, long-term, inflexible options because climate and SLR information is salient for these decisions (see Section “Results”).
The decision context may also influence global climate service development. Relevant decision context variables include, e.g., risk aversion, legal and regulatory rules, norms and practices. For example, national laws may influence which objectives (e.g., efficiency, equality, loss avoidance, etc.) or which options are deemed appropriate in coastal protection decisions (Bisaro et al., 2020). For instance, in France, coastal planning regulations became stricter following Cyclone Xynthia, leading to more coastal hazard and SLR impact assessments to inform coastal land use planning and set-back zones (Le Cozannet et al., 2017).
Further, for multilateral climate policy decisions, where collective decision-making is required, an important decision context variable is the “empirical status of the decision,” which describes the extent to which a decision-making process has been institutionalized. This variable has an indication on climate services development, as multilateral speculative decisions may require scoping assessments that identify major knowledge gaps or provide rough order of magnitude estimates of impacts. In contrast, institutionalized multilateral decisions require more precise information on climate impacts, for example, on the costs and benefits of the measures being considered. In section “Results,” we highlight in examples any decision context aspects that influence global climate service development, for example, by presenting barriers to supporting a particular decision.
Table 1 lists variables characterizing decisions and their contexts, and describes their influence on climate service development and SLR information needs in the coastal domain. The list is not exhaustive, but reflects those often discussed for adaptation decision-making (Kwadijk et al., 2010; Haasnoot et al., 2012).
Data Collection
Data collection for identifying real-world global decisions was carried out in the INSeaPTION project, a collaborative research project, which had, among others, the aim of co-developing coastal climate services at the global scale. To this end, two workshops (September 2018 and June 2020) were conducted with global stakeholders. The initial workshop (n = 22) included stakeholders from governments (n = 8), and private companies (n = 4), as well as coastal domain experts and researchers (n = 10). Participants were asked to present current decisions they faced that were influenced by climate risks, and these were discussed among the wider group with the aim of distilling key characteristics and information needs. Based on the results of the initial workshop, interview candidates were identified and in-depth semi-structured interviews (n = 6) were conducted to further elaborate decisions and climate services. A literature review, based on suggestions of interviews and workshops, and the domain knowledge of the author group, was then conducted, to further characterize the specific real-world examples and global climate services identified (see Supplementary Materials). Finally, a second workshop was conducted (n = 27) with stakeholders from government (n = 6), private companies (n = 2), and research (n = 19). IN the workshop, the authors of the present paper presented a description of the identified decisions and climate services in order to collect further feedback and validate the description of decisions and climate services developed. The results of this workshop were then integrated into the typology and examples (see Supplementary Materials for details).
Results
A Typology of Global Decisions Making Use of Climate Information
At a top-level, global decisions that make use of climate information involve either: multilateral climate policy decisions – multiple countries deciding on climate policy measures that affect many or all of them; or portfolio decisions involving multiple regions/locations – single decision-making entities (e.g., countries, companies, etc.) exposed to climate risk across many and diverse locations around the world.
Multilateral climate policy decisions are generally public decisions that consider aggregate outcomes, e.g., damages, fatalities, macroeconomic effects, in many different locations. The involvement of multiple countries’ governments is key to the global character of these decisions because when multiple countries are involved and affected by a climate policy decision, information needs increase beyond the local or regional scale. For instance, for a single country deciding on coastal protection options, a local-scale impact assessment may be sufficient. In contrast, for multiple countries considering large-scale geoengineering measures to adapt to SLR, global (or regional/continental) SLR impact assessments would be required.
Portfolio decisions involving multiple regions/locations consider selected physical or financial assets at specific locations or world regions. In contrast to multilateral climate policy decisions, portfolio decisions can have a global character without the involvement of multiple actors in decision-making. Rather, the global distribution of assets, supply chains or financial networks affected by climate impacts give this type of decision its global character. For example, a multinational company designing its global supply chain requires information on climate risks in multiple locations around the globe when selecting its suppliers and transportation hubs.
At a next level, different distinctions are relevant for each of these two top-level decision types. For multilateral climate policy decisions, a subsequent distinction is between decisions involving mitigation (i.e., greenhouse gas emission reduction) and those involving multilateral adaptation. The former generally involves countries weighing up the costs and benefits of different emission pathways with respect to various outcome metrics, e.g., GDP, extreme event damages, fatalities, biodiversity losses, etc. For the latter, i.e., decisions on multilateral adaptation options, several further sub-types can be distinguished based on the stage of the decision-making, and types of measures considered (e.g., insurance versus protection measures) (see section “Choosing mitigation policy options”).
For portfolio decisions, a distinction can be made between decisions that involve choosing physical assets (i.e., direct investments) and those that involve choosing financial assets (e.g., indirect investments) (Bisaro and Hinkel, 2018). Physical assets are tangible and thus can depreciate through wear and tear. Financial assets, in contrast, are not tangible. Thus, a parcel of land and any building on it are physical assets. In contrast, a mortgage on the same parcel of land and building is a financial asset, and derives its value through the stream of revenues it generates, i.e., interest and capital repayments.
For each of these portfolio decision sub-types, a further distinction can be made regarding whether the decision is impacted by direct climate risks, supply chain risks or financial network risks. Direct climate risks refer to direct impacts of climate change, e.g., rising temperatures, droughts, floods, storms, forest fires, etc. Supply chain risks refer to climate impacts that propagate through supply chains thus impacting a physical or financial asset. For example, a company producing and selling cars in Europe may be exposed to climate-related supply chain risks due to its production or transport centers being located in the coastal flood plains in other world regions. Financial network risks only apply to financial assets, and arise due to the risk that the issuer of the financial asset (i.e., the counterparty) cannot meet its obligations due to climate impacts on its balance sheet or due to broader financial system impacts (see section “Choice of financial asset such that financial network risk is minimized”). A prominent example of financial network risk is climate-induced sovereign risk, namely, the risk that a given country’s government bonds will lose their value because the government in question is not able to meet their financial obligations due to climate impacts (Kling et al., 2018).
Combining these distinctions, results in 8 types of global decision influenced by climate risk (see Table 2). We note that there is no one-to-one relationship between decision type and global climate services needed. Global climate service development requires in-depth decision and contextual analysis (together with stakeholders) to choose appropriate decision-making methods and identify climate information needs (Vincent et al., 2018). For each of the decision types, we elaborate real-world examples and describe decision characteristics that make global SLR information relevant to the decision. Further, we describe the current state-of-the-art in terms of global climate services addressing these decisions. In doing so, we identify gaps in current global climate services and some of the barriers identified by stakeholders to addressing these gaps.
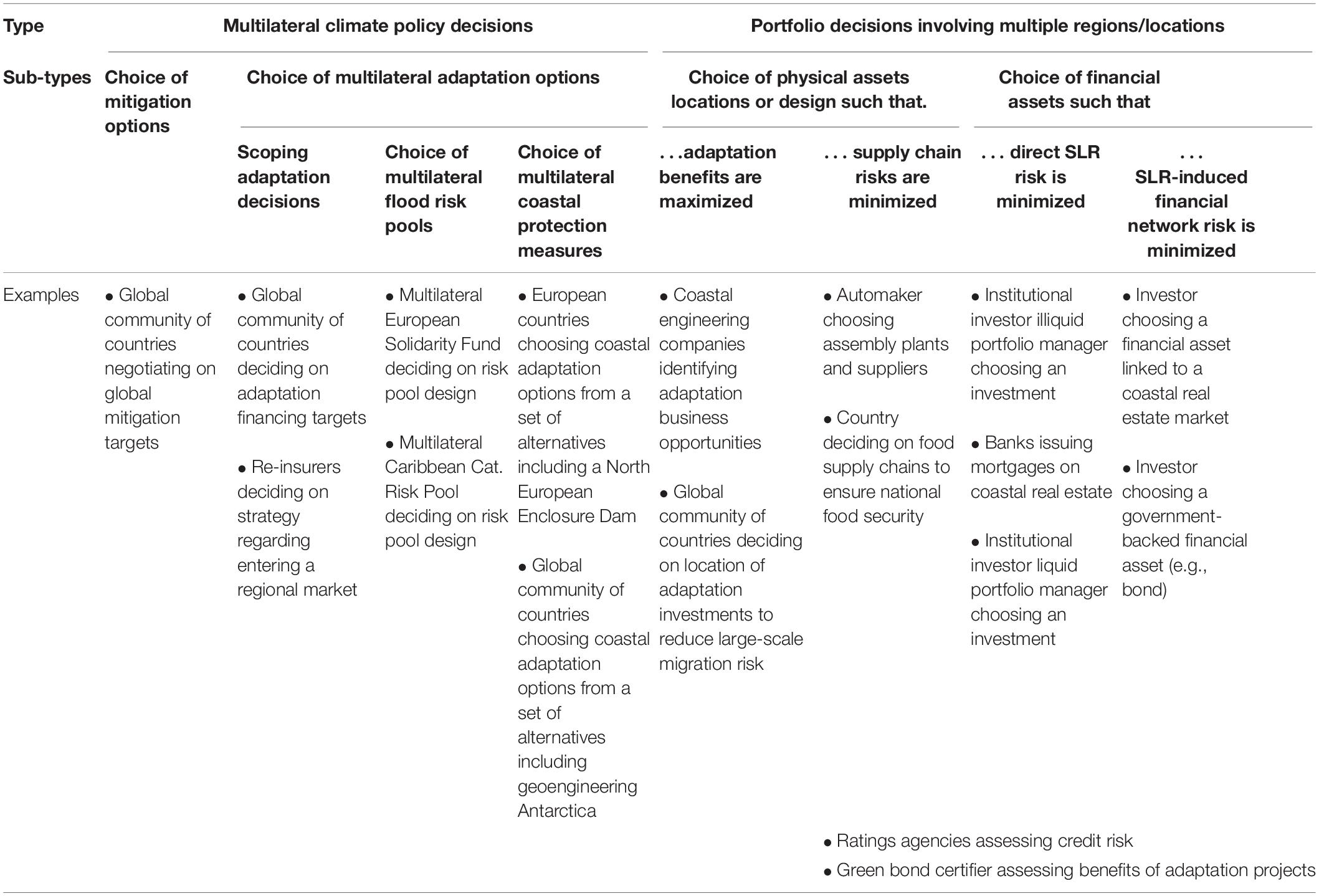
Table 2. A typology of decisions requiring global climate information with examples from the coastal domain.
Table 2 presents the typology of global decisions, which combines each of the three dimensions and presents real-world examples for each, identified mostly from the coastal domain. We note that the decision types are “ideal types” and in practice decisions may encompass more than one decision type. For instance, adaptation and mitigation are complementary responses to climate change. Thus, decisions on mitigation targets depend on the outcomes of decisions on adaptation measures and vice versa. Further, some portfolio decisions may need to consider several types of risk, i.e., direct climate risk, supply chain risk, and financial network risk. Here, we however, discuss each decision type separately. In section “Discussion: global climate service development,” we discuss our results and implications for global climate service development, including examples that address multiple decision types (see Table 2).
Multilateral Climate Policy Decisions
Choosing Mitigation Policy Options
A first type of multilateral climate policy decision involves governments choosing mitigation targets. Generally, this involves countries assessing different emission pathways with respect to various social, economic and environmental outcome metrics, e.g., GDP changes, extreme event damages, fatalities, biodiversity losses, etc.
An example is the collective choice of GHG emission targets involving the global community of states in the international climate negotiations, perhaps the longest established global climate policy decision. The decision context has evolved over time formalized in 1992 by the United Nations Framework Convention on Climate Change (UNFCCC) with the objective to stabilize greenhouse gas concentrations “at a level that would prevent dangerous anthropogenic interference with the climate system” (Article 2 UNFCCC, 1992). More recently, with the 2015 Paris Agreement countries committed to National Determined Contributions toward global goals, which were agreed as “well below” 2 degrees Celsius increase in global mean temperatures over pre-industrial levels.
Global climate services supporting these decisions are provided foremost through the Intergovernmental Panel on Climate Change (IPCC), which synthesizes the scientific knowledge on climate change and its impacts through its Assessment and Special Reports. The IPCC has thus informed the design of international treaties, including the UNFCCC and the Paris Agreement, that specify global GHG targets. For example, an IPCC Special Report assessed the impacts of 1.5°C of global warming, and thus provided support to the decision on the ambitious goal of the Paris Agreement to limit warming to 1.5°C (IPCC, 2018). Another example of global climate services comes from the Intersectoral Impact Modeling Intercomparison Project (ISIMIP) project, a global climate impact modeling effort finding that coastal impacts produce among the highest expected costs of all sectors from climate change (Lange et al., 2020). For the coastal domain specifically, global coastal flood damages by 2100 are much more sensitive to coastal adaptation strategies than to differences in climate and socioeconomic scenarios, showing that long-term adaptation strategies are key complements to any mitigation targets that may be achieved (Hinkel et al., 2014).
Choosing Multilateral Adaptation Options
Overview
A second type of multilateral climate policy decision involves governments choosing multilateral adaptation options. Multilateral adaptation measures are defined as adaptation measures that affect more than one country through direct or indirect impacts (e.g., avoided damages). Multilateral adaptation measures that require global climate services are not often considered in the literature, as adaptation is generally considered a local responsibility (Nalau et al., 2015). While transboundary coastal risk management is taking place in, for example, the low-lying west-European North Sea flood plain (Zagonari, 2013; Kuiper, 2020), these initiatives are largely at local scale.
Sub-types of decisions regarding multilateral adaptation options can be distinguished based on the stage of the decision-process at issue, i.e., scoping versus choosing options. Scoping is a common stage in decision-making aimed at identifying options and generating information on the impacts of these (Kleindorfer et al., 1993). For choosing adaptation options, further sub-types can be distinguished based on whether they involve “soft” options (e.g., flood insurance) or hard structural options (e.g., coastal protection measures).
Scoping Adaptation Decisions
Scoping adaptation decisions is a broad decision type that involves decision-makers seeking to generate information on climate impacts prior to formalizing the decision by specifying decision objectives, criteria and alternatives. Order of magnitude estimates of climate and SLR impacts are often sufficient for scoping decisions. Global (or multi-regional) climate impact assessment that are too coarse in scale for local formal decision-making are often sufficient for generating such estimates (Patt et al., 2005).
A first example involves the global community scoping global adaptation financing targets for support to developing countries. This is a scoping decision because deciding on adaptation financing targets requires an understanding of adaptation objectives and needs in developing countries, which are also not yet clearly defined (UNEP, 2020). While the Paris Agreement includes a global adaptation goal, it does not define a/the level of adaptation needed or metrics for measuring progress toward this goal. Further, Article 2.1c of the Paris Agreement stipulates to make “finance flows consistent with a pathway toward low greenhouse gas emissions and climate-resilient development.” Yet several decades of scholarship have shown that measuring adaptation, or climate-resilient development, is often contested and varies across different national and socio-economic contexts (Adger et al., 2009). Indeed, the 2020 Adaptation Gap Report finds difficulties in estimating the current adaptation finance gap (and thus related adaptation finance targets to fill this gap) because of problems in estimating both current adaptation finance flows as well as current and future adaptation costs (UNEP, 2020).
A global climate service supporting the scoping of this decision in the coastal domain is a recent World Bank global assessment of coastal adaptation infrastructure investment needs (Nicholls et al., 2019). The assessment scopes aggregate investment needs for reducing climate risks under alternative adaptation strategies, such as constant protection levels, constant flood risk, or cost-benefit analysis.
The study shows that there is high uncertainty regarding future SLR impacts. Importantly, for scoping global adaptation financing decisions it shows that the key driver of future adaptation costs is the type of adaptation strategy applied, though other factors also contribute including socio-economic development, national development priorities and SLR scenarios. Such indicative information on global protection needs and costs provides an entry-point for supporting decision-making on global coastal adaptation finance needs, and key knowledge gaps to address.
A second example involves re-insurers taking a strategic decision regarding entering or leaving regional coastal insurance markets. Generally, re-insurance policies (e.g., property, business interruption, etc.) are issued for only 1 year. Thus, climate change information does not play a significant role in re-insurers’ core business decisions of risk pool design or setting re-insurance premiums, because climate change does not significantly influence insured risks on annual time scales. However, strategic decisions regarding entering a particular regional market or sector may involve longer time horizons, e.g., 10–15 years, because of the time and resources needed to establish a risk pool by marketing and selling re-insurance policies. This is a scoping decision because rough estimates of coastal risk and how it is likely to develop over the relevant timescale are sufficient for informing the decision. Whereas subsequent decisions, e.g., on risk pool design, will require a more precise and detailed risk assessment.
A global climate service supporting this decision involves reinsurers current practices of assessing climate change and SLR influence on regional coastal risks. Generally, (re-)insurer risk assessment methodologies apply statistical approaches based on historical data, e.g., storm losses, to support their core business decisions regarding flood risk pool design, and insurance underwriting. However, in order to assess how risks in coastal regions will develop beyond the short-term, (re-)insurers are now considering how to integrate climate change projections into risk assessment methodologies. To this end, one major European reinsurer has an established internal process for reviewing developments in climate science in order to assess whether scientific knowledge on climate change can provide information on how risks are changing. Currently, the scientific evidence, e.g., on the magnitude and direction of changes in hurricane incidence, remains too uncertain for use in medium-term decision-making, and thus they rely on a historical datasets and statistical modeling approaches.
Choice of Multilateral Flood Risk Pool Design
The choice of multilateral flood risk pool design involves a (re-)insurer choosing an appropriate design for a flood risk pool covering multiple countries. When designed appropriately, flood risk pools are an adaptation measure because they provide the insured with incentives for ex ante flood risk reduction, and needed liquidity that can enable faster recovery from disasters (Hudson et al., 2016). Multilateral flood risk pool design must consider, first, capital requirements to ensure solvency in the case of major hazards events (Gurenko, 2006). For instance, the Solvency II Directive requires European (re-)insurers have sufficient capital to cover claims from a 1-in-200 years event. Second, flood risk pool design must consider premium affordability particularly in developing countries, where flood insurance is often heavily subsidized, e.g., through development assistance (Surminski and Oramas-Dorta, 2014).
A first example involves EU Member States deciding on capital allocation to the European Union Solidarity Fund (EUSF). The EUSF is a core disaster financing instrument in the EU, providing a risk pooling mechanism between EU member states that supplements national disaster risk financing, and disperses disaster risk finance to Member States in the case of triggering events, such as, natural hazards. EU Member States must decide on EUSF capital requirements, which determine the level of risk up to which the Fund can provide coverage for. Further, it must decide the contributions (i.e., premiums) of each Member State to participate in the EUSF. These contributions can be differentiated by Member State and thus may include cross-subsidies according to relative exposure or ability-to-pay.
A global climate service is provided by Jongman et al. (2014), who estimate river flood damages for Europe by developing a model that calculates the joint probabilities of flood events across all of Europe’s 1,007 river basins. Further, they estimate damages from these events by including known and estimated flood protection standards in place. They find that the EU Solidarity Fund of €1 billion had a 5% chance of exceedance in 2013, which will rise to 9% in 2050. Thus, capital allocation to EUSF under future climate change will need be increased substantially to maintain the same level of coverage.
A second example is the decision on the design of the risk pool managed by the Caribbean Catastrophic Risk Insurance Facility (CCRIF), which provides disaster risk financing for Caribbean countries. The Caribbean Climate Risk Insurance Facility (CCRIF) has been operating for more than a decade. It is a parametric risk finance instrument, which means that pay-outs are triggered by pre-defined values of climate variables, e.g., extreme water levels, max. wind speed and duration, rather than damages thresholds, which lowers the monitoring cost associated with pay-outs.
Key for the long-term sustainability of CCRIF is an appropriate assessment of the current and future frequency and severity of hurricanes. This is a complex issue as one of its determinants is the Atlantic Multidecadal Variability (AMO) and its two alternating phases, plus the quite complex issue of how climate change is going to impact both the AMO pattern and the respective hurricane dynamics in each of them (O’Reilly et al., 2019). Moreover, organizing a risk pool is completely different a business than re-insurance as it involves building up trust among governments and finding a scheme that is economically and politically sustainable long-term.
Choice of Multilateral Adaptation Measures
The choice of multilateral adaptation measures involves large regional or global-scale adaptation measures that have direct impacts (i.e., reducing climate risk) in multiple countries. Such measures are of a much greater scale than local adaptation measures typically considered in the adaptation literature. Indeed, while many coastal adaptation measures have an international dimension because, for example, they affect sediment transport, related decisions generally are not global because they require only regional scale information. In contrast, multilateral adaptation measures with a global dimension are much larger in terms of engineering works and the geographical extent of their impacts.
A first example involves countries along the North and Baltic Sea Coasts choosing from a set of coastal adaptation measures that include a North European Enclosure Dam (NEED), a measure that entails enclosing the North Sea with 576 km of dams between France, the United Kingdom, and Norway. The NEED would reduce coastal flood and erosion risk along the coast of 14 countries over the long term (100+ years). Alternatives to NEED include another major enclosure of only the Baltic Sea. Finally, countries could also choose to implement coastal adaptation nationally or at sub-national scales through a mix of protection, accommodation and retreat measures, as is the current practice.
A global climate service supporting this decision is provided in the literature by a feasibility and costs assessment of NEED in the context of SLR (Groeskamp and Kjellsson, 2020). NEED appears to be technologically feasible because the maximum depth of sea-floor the dam would cross is ca. 300 m, while currently stationary oil rigs can be built at 500 m depth. Costs are estimated at €300 to €600 billion, including the 40,000 m3 of pumping capacity needed to balance the incoming water flow from rivers in the new enclosure. Total costs annualized over 20 years amount to around 0.2% GDP of countries involved, which may be less than the cost of protection the Netherlands alone for 2 m SLR (Lincke and Hinkel, 2018). NEED would, however, also have major adverse impacts for ecosystems both within and outside the barrier (Groeskamp and Kjellsson, 2020). A similar project has been proposed for the Mediterranean (Gower, 2015), with the caveat, also applicable to NEED, that the proposed solution only addresses SLR and would accelerate marine ecosystem decline and related services.
A second example involves the global community choosing geoengineering measures in Antarctica to slow ice sheet loss and reduce coastal food and erosion risk along the entire global coastline over the long term (100+ years) (Feldmann et al., 2019). This decision affects coastal risk along the entire global coastline. Alternatives to geoengineering Antarctica include geoengineering of Greenland, which also would have impacts of global extent, and other multilateral adaptation measures including those discussed above.
A global climate service supporting this decision is provided by the feasibility and impact assessments of various measures to geoengineer polar glaciers, and particularly those in Antarctica (Moore et al., 2018; Gürses et al., 2019). Various authors have assessed measures designed to slow ice flow in West Antarctica, which is of greatest concern for high-end global sea level rise scenarios. These include constructing islands in front of the glaciers, berms to prevent warm water from flowing underneath glacial sea-ice, and cooling or removing water underneath glaciers. Moore et al. (2018) find that all of these measures appear to be effective in slowing glacial flow into the sea. In contrast, Gürses et al. (2019) apply a global sea-ice ocean model to assess impacts of an underwater wall in West Antarctica to prevent the inflow of warm water underneath sea-ice. They find that warm waters diverted by the sea-wall to other parts of Antarctica reduce its overall effectiveness, with ice loss for Antarctica as a whole being reduced by only 10% with the wall in place. Thus, ambiguity remains regarding the engineering effectiveness of these measures. Further, such a project requires major energy consumption with extremely large environmental impacts (Feldmann et al., 2019), and only addresses SLR and not other climate change impacts (Mora et al., 2017). Future climate service development could focus on further resolving both engineering effectiveness and environmental impact ambiguities and thus scoping decisions to support multilateral decision-making processes (see section “Discussion: global climate service development”).
Portfolio Decisions Involving Multiple Countries
Choice of Physical Asset Location
A first type of portfolio decision involves actors choosing the location of physical assets. This decision type can be distinguished into three sub-types. A first sub-types involves decisions on location that maximize adaptation benefits. A second sub-type involves decisions on location that minimize supply chain risks. A third sub-type involves decisions on location that minimize direct climate risks (Note that for this third sub-type, the decision may involve either the choice of physical or financial assets, see Table 2).
Choice of Physical Asset Location Such That Adaptation Benefits Are Maximized
Decisions on location that maximize adaptation benefits in the coastal domain involve identifying locations in which an adaptation intervention can significantly reduce climate risks compared to alternative locations. It is important to note that for this decision type, location selection is for a prospective asset or adaptation measures, which must be subsequently designed and implemented. Thus, the location decision involves identifying “hot-spots” of climate vulnerability as entry point for deeper analysis to develop specific adaptation measures under various criteria (e.g., cost-efficiency, cost-effectiveness, robustness, etc.).
A first example involves a multinational coastal engineering company that operates at a global scale seeking to identify new business opportunities through scoping coastal impacts and adaptation measures. Coastal engineering companies, whose core business involves dredging and construction, want to identify coastal areas or regions for future business development regarding coastal engineering solutions. Currently, such companies are particularly interested in identifying areas attractive for nature-based solutions (NBS) due to the potential to attract additional investors (e.g., development banks or foundations) to such projects (Kok et al., 2021). Locations that are attractive and feasible for coastal NBS present significant opportunities for partnerships that can attract investment needed to make a project viable. Identifying locations (hot spots) that are attractive for coastal NBS involves identifying locations where the benefit-cost ratio of coastal adaptation projects is likely to be high, and coastal NBS are feasible.
A global climate service supporting this decision is being developed by a multinational coastal engineering company. For this company, their coastal risk assessment experts have developed a global assessment tool, the Climate Risk Overview, that integrates climate, SLR and flood and erosion hazard data with socio-economic data in order to identify coastal locations that are most “at risk,” and thus have high adaptation benefits potential (Van Oord, 2021). The tool also seeks to identify coastal segments well- suited for NBSs, and has been made available in the public domain, as a tool to attract public investment and catalyze public-private partnerships for coastal adaptation.
Choice of Physical Asset Location Such That Supply Chain Risk Is Minimized
Portfolio decisions on physical asset locations may also aim to minimize supply chain risks. A company or government’s supply chain risk is determined by the physical locations of its production and inventory management sites (and those of its suppliers) as well as the structural characteristics of the supply chain. Structural characteristics include the diversity of suppliers, and asset specificity of production processes or locations (i.e., substitutability of assets or production processes) (Haraguchi and Lall, 2015). While supply chain decisions are common, only when they involve physical assets that have high costs, long lifetimes, and high asset specificity (low substitutability) is SLR information relevant. In such cases, supply chains are inflexible and SLR should be considered in decision-making because it is a significant driver of coastal risks over long time horizons (Weaver et al., 2013). This decision type generally involves large companies with global supply chains spanning many countries and world regions. Public actors, however, may also face supply chain decisions regarding the supply of public goods, e.g., food security, vaccines or other pharmaceuticals, etc.
A first example involves an automaker choosing assembly plant and inventory management locations in their supply chain such that SLR risk is minimized. Large automakers cannot entirely avoid coastal risk because, as automobiles are relatively large and heavy, assembly plants and storage facilities are generally located close to ports to minimize overland transportation costs (Haraguchi and Lall, 2015). Moreover, automakers make asset specific supply chain investments and are generally not able to shift key production processes, i.e., assembly and inventory hubs, quickly at low cost to other locations. Indeed, following the 2011 floods in Thailand that severely impacted the auto industry, surveys of affected companies found that most would not change the location of existing facilities due to financial constraints (Haraguchi and Lall, 2015). Automakers must choose supply chain locations such that exposure to SLR is tolerable, while their production processes remain efficient.
Examples of global climate service supporting such decisions are scarce, as the literature largely addresses port and supply chain implications for the perspective of macro-economic impacts. For example, a recent study assesses how global port infrastructure will be affected by SLR under different socio-economic and climate scenarios until 2050 (Hanson and Nicholls, 2020). The authors find that the costs of building new ports to meet global demand due to increasing trade will be much greater than the cost of adapting existing ports. While this provides relevant information regarding SLR exposure of existing ports, for multinational companies making supply chain location decisions global climate services development could be extended by including more precise information on their supply chains.
A second example involves a national government choosing agricultural production and inventory management locations in order to ensure domestic food security. Many developing countries are net food importers, and are thus exposed to supply chain risks from SLR impacts on food transport infrastructure. Moreover, agricultural trade depends on critical transport hubs in ports with exceptional large trade volumes that are exposed to coastal risk. For example, 53% of global wheat, rice, maize and soybean exports pass through two hubs in Brazil and the United States (Bailey and Wellesley, 2017). Food importing countries concerned with food security decision-making should account for coastal risks at major transportation hubs in their food supply chains.
A global climate service supporting this decision is the assessment of “chokepoints” in global food trade (Bailey and Wellesley, 2017). Chokepoints are transportation hubs that pass through significant shares of trade volume in a basic agricultural commodities or food stuff. For food importing countries, food security risks arise because disruption at a chokepoint can directly interrupt imports or indirectly increase global food market prices. Currently, there are three major coastal chokepoints in global agricultural trade: US Gulf Coast Ports, Brazil’s southern ports, and Black Sea ports. For example, Black Sea ports are a major coastal wheat chokepoint, passing through 12% of global wheat trade and 60% of Ukrainian and Russian exports (Bailey and Wellesley, 2017).
Future global climate service development could combine chokepoint analysis with global SLR impact analysis to assess SLR impacts at key chokepoint locations. This could support governments to integrate choke-point analysis and SLR information into food supply chain decisions, and would also be relevant for systemic actors concerned with food security, e.g., development aid organizations, UNCHR, etc. (Hedlund et al., 2018; Benzie et al., 2019).
Choice of Physical Asset Location Such That Direct Sea-Level Rise Risk Is Minimized
Portfolio decisions on physical asset locations may also aim to minimize direct climate risk. This decision type differs from the previous one in that only the direct risk at particular location is considered, and not the role of the asset or location in a broader production process (i.e., supply chain).
For these location decisions, as for other portfolio decision types, SLR information is only useful when the decisions involve physical assets with high costs, long lifetimes, and high asset specificity (low substitutability) (Hinkel et al., 2019). These characteristics mean that location decisions are relatively inflexible, and thus SLR should be considered over the medium to long-term.
We note however that asset specificity is a decision characteristic that depends also on the decision-maker. Consider portfolio decisions on physical real estate assets. While real estate assets can generally be exchanged at low transaction costs (e.g., cost of information collection, negotiation, contracting), the relative importance of these transaction costs (and thus asset specificity) differs for different investors. For instance, for institutional investors (i.e., insurers and pension funds) transaction costs associated with individual real estate transactions may be prohibitively high because of the large volume of assets they hold. They prefer “buy and hold” investment strategies with investment horizons of 10–50 years, over which time SLR information is increasingly relevant (Ameli et al., 2020). In contrast, smaller volume investors (e.g., individual investors or single market real estate companies) may be less constrained by the transaction costs of trading real estate assets, and such transactions may even be part of their core business model and competitive advantage. For these investors, SLR information is less salient because price dynamics in the real estate markets may be affected by other factors that vary on much shorter time scales.
A first example involves an institutional investor, a European re-insurer, choosing physical asset investment locations within their direct investment portfolio. Broadly, re-insurers make direct (illiquid) and indirect (liquid) investments with the objective of managing overall assets and liabilities, including those from the insurance side of their business. For this European re-insurer, direct investments make up around 10% of their equity portfolio, and for these investments they prefer “buy and hold” strategies in choosing physical assets in order to minimize transaction costs, which include physical risk assessments. The time horizon of such direct investments is thus at least 10–15 years, and typically longer. For specific investments, detailed local information is needed to conduct risk assessments and thus global climate information is not strictly required. However, for the illiquid investment portfolio more broadly, the reinsurer assesses their exposure to particular natural catastrophe scenarios, particularly considering the (re-)insurance policies they have issued. Thus, similar to the examples of multilateral risk pools above, re-insurers require assessments of current and future frequency and severity of hurricanes to assess their overall exposure.
A global climate service supporting this decision is generally provided internally by such investors through their own experts making use of experience and datasets from the insurance side of their business. Re-insurers core business involves assessing physical climate-related risks, and thus they make use of this expertise in risk assessments for investment decisions. The investment arm of a major European re-insurer convenes an internal expert group that is involved in due diligence for every major investment decision in physical assets. Further, where relevant, the due diligence process of the re-insurer makes use of historical databases, e.g., on storm or flood events, claims, etc, from the insurance side of business to conduct the physical risk assessments. However, the re-insurer identifies a salient gap in their risk assessments. Their current approaches focus only on historical data sets, whereas equity “buy and hold” investors require assessments of how risks will develop under climate change over much longer time horizons, i.e., 50+ years.
Future global climate service development could focus on addressing this gap in terms of integrating climate change impacts with long-term natural catastrophe modeling. However, generally, re-insurers indicate that they will seek to address this gap internally with their own experts. This is not surprising as private investing is a competitive arena, and improved risk assessments can lead to profitable investments for companies that development them. This raises an interesting issue for global climate service development as supporting private investment decisions may lead to adaptation benefits that are almost entirely private.
Choice of Financial Assets
A second type of portfolio decision involves choosing financial assets. This decision type can be distinguished into two sub-types. A first sub-type involves decisions on financial assets such that direct SLR risks to the underlying physical assets are minimized. A second sub-type involves decisions on financial assets such that climate-induced financial network risks are minimized.
Choice of Financial Assets Such That Direct Physical Risk Is Minimized
A first sub-type of financial asset decisions involves choosing financial assets such that direct SLR risk is minimized. This decision type is similar to that described in section “Choice of physical asset location such that direct SLR risk is minimized.” The risks addressed (i.e., direct physical SLR risk) are the same, only the type of asset and its related decision context differ (see Table 2). One key difference between physical assets and financial assets is that the latter are often tradeable at lower transaction costs compared to physical assets. Financial assets thus exhibit low asset specificity and SLR is generally not salient to such decisions. However, there are some exceptions, when financial asset choice requires considering long time horizons.
A first example is a bank deciding on issuing mortgages on coastal real estate. Mortgages are financial assets tied to underlying physical real estate assets that may be exposed to coastal risk and SLR. Typical mortgage terms in many countries can range up to 30 years or more, time horizons over which SLR information is useful. Mortgage-issuing banks are often concentrated lenders making many loans in few markets, and thus making use of detailed local information, e.g., on environmental amenities and risks, individual credit worthiness, etc. Given their local knowledge and concentrated lending practices, such banks do not need to make use of global SLR information. However, concentrated lenders often make many loans in disaster recovery periods and sell them on to larger more diversified investors (Keenan and Bradt, 2020). There is thus a related decision of an investor choosing mortgage-backed securities containing bundled coastal real estate mortgages. Keenan and Bradt (2020) report information asymmetry between concentrated local lenders who issue mortgages, and then sell them on to large diversified investors. The information asymmetry arises out of the local knowledge of flood risk that local lenders have, and that is not reflected in national flood maps.
A global climate service supporting this decision of large investors choosing bundled mortgage securities is generally provided by regulations such as, those enacted through National Flood Insurance Program flood maps in the US. However, current evidence shows that these maps do not adequately reflect property level flood risks for investment decision, as they are largely only elevation based, and do not incorporate local hydrology, adaptations, etc. (Keenan and Bradt, 2020). Relying on national flood maps can lead to investment decisions based on somewhat arbitrary “bluelines” from national flood maps that increase exposure to SLR. Future global climate service development can address this gap, and information asymmetry between local and more diversified investors, by incorporating SLR information in flood maps.
A second example involves an institutional investor, a re-insurer, choosing a financial asset (e.g., a stock or bond), such that physical risks are minimized. As discussed in section “Choice of physical asset location such that direct SLR risk is minimized,” these decisions are generally taken by the investment arm of reinsurers within the overall framework of seeking to balance assets and liabilities including the insurance side of the business. Decisions involving financial assets are taken by managers of the “liquid” asset portfolio and generally make up a larger share (e.g., 90%) of the overall re-insurer investment portfolio. These managers generally act within an Environment, Social and Governance (ESG) investing framework in which they seek to identify physical risks to the financial assets they invest in, and to the companies issuing them. However, while initiatives such as the Task Force on Climate-Related Financial Disclosures (TCFD) launched in 2015 are driving increased disclosure of both climate policy and physical risks, there are still major gaps, and information on physical climate risks is not available for many financial assets.
A global climate service supporting such decisions is currently provided by third-party data providers and ratings agencies. However, for institutional investors, there are a number of barriers to assessing physical risks associated with financial assets. First, third-party data providers and ratings agencies do not generally focus on physical risks as key performance indicators for financial assets, and thus often do not provide this information at all. Second, companies or governments issuing financial assets are not under pressure to produce data on physical risks, as the focus of disclosure initiatives as to date has been on climate policy risks. Third, producing this information is itself challenging and costly because physical risks to financial assets can change on short time scales. For example, company that issuing a bond may change its production site from an inland to a coastal area, which would require a new assessment of physical risk associated with the bond. Finally, a comprehensive risk assessment would also require information on insurance coverage of a given company, e.g., against Atlantic hurricanes, but such information is generally not publicly available, and coverage may also change annually.
Choice of Financial Asset Such That Financial Network Risk Is Minimized
A second sub-type of financial asset decision involves choosing financial assets such that climate-related financial network risk is minimized. Financial network risks arise with respect to the counterparty in a financial asset (e.g., risks to a bond issuer’s balance sheet) as well as with respect to the financial system more broadly. The latter aspect entails “transition risks,” whereby financial assets are at risk of being dramatically re-priced following a triggering event. While transition risks most often refer to climate policy risks and stranded assets related to the sustainability transition, transition risks may also arise from physical risks. For instance, slow onset SLR combined with a triggering event, e.g., a major flood, could significantly affect perceptions of coastal risk leading to an abrupt mass exit of the coastal real estate market (herd behavior) that threatens financial system stability (Ortega and Taṣpınar, 2018).
A first example involves an institutional investor choosing a financial asset, such as a mortgage-backed security or an equity stake in a real estate investment trust (REIT) and considering financial network or “transition risks,” i.e., the risk that climate change causes a disruptive shift in real estate market conditions. We note that this example is similar to the second example in “Choice of financial assets such that direct physical risk is minimized,” however, here we consider broader financial network risks, rather than only direct physical risks. We note that such transition risks may be particularly acute in real estate markets that have not already priced in SLR exposure (Bakkensen and Barrage, 2017).
A global climate service supporting this decision is provided by recent research analyzing whether SLR is accounted for in real estate prices, and the conditions under which these risks are priced in. Evidence from the US shows that real estate markets are beginning to account for SLR, discounting residential property prices exposed to SLR by 7% compared to non-exposed properties (Bernstein et al., 2019). However, owner-occupant dominated markets may be susceptible to financial network and transition risks. In such markets, SLR risk is less likely to impact real estate prices (Bernstein et al., 2019). Further, owner-occupied dominated markets may be more susceptible to financial network and transition risks, as owner-occupants are more likely to update their beliefs regarding SLR following flood events. Research in the US coastal housing market shows that accounting for changing beliefs, i.e., increased risk perception, after flood events leads to a fourfold increase in expected coastal housing market declines and increased price volatility (Bakkensen and Barrage, 2017).
A second example involves an institutional investor choosing a government bond and seeking to minimize financial network risk. Government bonds are generally attractive to institutional investors as low-risk assets. However, government-backed financial assets, including government bonds, are exposed to financial network risk in the form of sovereign risk, i.e., the risk that a government cannot meet its financial obligations. Climate change can impact sovereign risk, as direct climate impacts can damage a government’s physical assets or increase its liabilities, e.g., through damages to individuals or companies that it has committed to compensate. Thus, assessing sovereign risk involves assessing not only direct climate impacts, but also the resilience of a countries’ financial system, e.g., whether it has disaster risk finance in place, and if so, whether its disaster risk finance is sufficiently sustainable.
To date, global climate service supporting the decision on choosing a government bond though assessing sovereign risk is somewhat lacking. There are effects of ratings agencies or other third-party data providers to assess sovereign risk, but these largely focus on carbon footprint approaches and sovereign risk related to climate policy risks and stranded assets. Physical risks have yet to be systematically incorporated into sovereign risk ratings by ratings agencies. In the scientific literature, there have been efforts to assess financial system resilience under climate change and SLR. For instance, Schinko et al. (2017) assess sovereign risk in Austria posed by natural disasters under climate change until 2050 by comparing projected disaster losses and national disaster risk financing arrangements. They find that sovereign risk will increase as the National Disaster Fund, capitalized at €260 million, approximately covers current expected annual disaster losses, but will be insufficient in the near future as expected losses increase due to climate change and socio-economic development.
Future global climate service development could build on approaches in the scientific literature to provide more differentiated assessment of sovereign risk based on not only direct climate impacts assessed by Schinko et al. (2017), but also climate risks that propagate through the financial system due to international lending arrangements (see Mandel et al., 2021).
Discussion: Global Climate Service Development
Empirical Status of Decisions
Our typology presents eight decision types and real-world examples mostly for the coastal domain together with the current-state of-the-art in global climate services supporting these decisions. We note that the decision types identified, and associated example provide an entry-point for developing global climate services, and that in practice decisions (and decision types) may be combined, leading to synergies or trade-offs between different objectives. We have not been able to address such combinations here. Our discussion illustrates that global decision-making on climate risks is salient, as both public and private stakeholders are becoming increasingly aware of the need to incorporate global scale information on climate risks into their decision-making.
The examples presented further illustrate that both decision-making processes and related global climate service development vary widely in terms of how advanced they are. On one hand, for decisions on multilateral adaptation, we observe that decisions’ “empirical status” (Table 1) is often in early stages. Moreover, because of the absence of institutionalized decision-making processes driving demand for knowledge, scientific knowledge may be lacking for these decisions. For example, multilateral adaptation measures involve geoengineering at a scale that exceeds most engineering works in world history. Decision-making processes on such measures remains highly speculative and often controversial (Leane and McGee, 2019). Global climate services development in such settings can focus on “decision identification” approaches (Kleindorfer et al., 1993) that generate information on likely impacts of particular measures (e.g., costs, effectiveness) and identify knowledge gaps to be addressed at a later stage for more formal decision-analysis. Such global climate services may inform public and policy discussions on whether such measures merit being considered, given the high stakes and risk aversion regarding the decisions.
On the other hand, for portfolio decisions, decision-making is driven by private actors’ own business models and incentives, and multi-actor processes are not generally required. Indeed, portfolio decisions are generally taken in a competitive market environment and create largely private adaptation benefits (Pauw, 2017). Further, complementary to these private incentives are regulations related to climate risk in the financial sector, such as, financial disclosure regulations proposed by the Task Force on Climate Disclosure (Ameli et al., 2020). Examples of portfolio decisions thus show greater consideration of climate risks. For example, most institutional investors, including those interviewed for this paper, consider ESG requirements in their portfolio decisions, which includes considering both climate policy and physical climate risk components. Further, the COVID-19 pandemic has demonstrated the salience of global supply chain risks and is likely to reinforce the considering of global climate information in portfolio decisions.
Private investors that are most advanced in considering global climate risks in portfolio decisions are experienced with climate risks from their core business, i.e., (re-)insurers. For these stakeholders, global climate service development should be complementary to approaches they are developing internally, e.g., integrating climate modeling with historical extreme event data, and focus on co-development tailored to gaps in existing knowledge and data sets in the financial sector. Even for stakeholders not experienced with climate risk analysis, e.g., automakers, co-development approaches are also needed. In this case, combining private supply chain data with climate change impact projections could improve decision-making providing private benefits to the concerned stakeholders, as well as positive indirect benefits for the global economy. Finally, co-development approaches can also support portfolio decisions of governments related to national security, e.g., to minimize climate risks to critical national defense infrastructure. While such decisions are generally highly sensitive and thus difficult to obtain information, there is evidence that governments are beginning to consider climate risks in this context, and may be an important direction for global climate service (co-) development (DRGIS, 2020).
It should be noted that future development of global climate services will be influenced by approaches to respective public and private roles in managing climate risks, and appropriate sharing of costs and benefits of such adaptation. Further, approaches to cost and benefit sharing between governments and the private sector are shaped at the global level by power asymmetries between actors in the Global North and Global South. Such power asymmetries can be manifested both in interactions between governments, as well as, between multinational corporations and developing country governments (Vogel et al., 2019). The salience of power asymmetries for cost and benefit sharing between public and private actors in climate risk management can be illustrated through the issue of private versus open access data. Large multinational companies may make use of data to facilitate their own adaptation, and seek to protect this data on competition grounds. Yet open access to this data, which is often particularly scarce in developing countries, may also facilitate adaptation more broadly with wider collective or public benefits. How such data protection issues are managed in the development of global climate services is strongly shaped by power relations, and attention to such issues is needed to ensure both efficient and equitable adaptation in developing global climate services.
Global Scale Knowledge Gaps and Implications for Global Climate Service Development
In addition to differences in the focus of global climate service development for multilateral and portfolio decisions noted above, other knowledge gaps emerge that cut across multiple decision types and should be addressed by global climate service development.
One knowledge gap involves climate-related financial network risk, which can affect portfolios decisions regarding both physical and financial assets as well as multilateral climate policy decisions. This is because financial network risks can affect individual asset prices, but also financial system stability adding to the overall global costs of climate change (Batten, 2018). While emerging efforts to integrate climate risks into sovereign risk assessments capture financial network risks to an extent, these activities do not address climate-related risk to financial system stability more broadly. A few recent studies have begun to address the question of climate-related risks to financial stability. For example, Lamperti et al. (2019) apply an agent-based macro-economic model linked to climate impact functions finding that climate change may increase the frequency of banking crises and contribute to financial instability through weakening of banks’ balance sheets. Another example is Mandel et al. (2021) who apply a global SLR impact model together with financial system network analysis, finding that direct flood losses can be amplified by a factor 10 in terms of financial losses depending on the centrality in the global financial system and leverage of the country that is directly impacted. While these studies begin to address questions relevant to the global decisions presented here, further global service development should focus on co-developing financial network risk analysis to specific decisions faced by stakeholders, e.g., investors or governments.
Another knowledge gap relates to decisions that address more than one decision type. As noted in section “A typology of global decisions making use of climate information,” real-world decisions often involve more than one decision type, and global climate services to address such decisions are lacking. Prominent examples from the financial services sector address multiple decision types and are promising directions for global climate service development.
One example involves ratings agencies that currently aim to integrate climate risk into their ratings activities. Such credit ratings are in principle comprehensive assessments and thus should consider all types of climate-induced risk (i.e., direct physical, supply chain, and financial network). While ratings agencies are currently directing efforts toward integrating physical climate risk into sovereign risk assessment (Kling et al., 2018), they face several limitations, e.g., lack of data, lack of access to propriety data on productive assets, etc., and do not yet comprehensively assess climate risk.
Another example involves financial services companies certifying “Green Bonds” linked to adaptation projects. Currently, certification decisions are supported by “Green Bond taxonomies,” which define activities and benefit thresholds that qualify a bond for certification (EC, 2019). While conducting detailed local assessments for individual projects is too resource intensive to implement at the scale required for the large and growing Green Bond market (CPI, 2019), Green Bond taxonomy activities could be extended by global scale SLR impact assessments. Such assessments would be particularly valuable outside of the US and Western Europe, where data needed to assess coastal risk is often scarce. Moreover, these assessments could also address all types of climate-induced risk (i.e., physical, supply chain and financial network risk), and the adaptation benefits produced from reducing this risk.
Finally, beyond these specific knowledge gaps, we note that developing “usable” science for global climate services requires co-development between scientists, policy-makers and private companies, and these interfaces are influenced by the norms, institutions and preferences in specific decision contexts (Haasnoot et al., 2020). Of particular relevance for developing such “usable” science at the global level is the global sustainable development agenda, as articulated in international agreements, most prominently through the UNFCCC, Convention on Biodiversity, Sustainable Development Goals and Agenda 2030. Developing global climate services requires understanding and addressing specific decisions and the relevant context in which they are taken, of which the sustainable development agenda makes up a significant part. At the global level, understanding of how to address these specific decisions, within the context of the sustainable development agenda, is underdeveloped (Kopp et al., 2019). Our typology provides a differentiated set of entry-points toward advancing understanding of the governance challenges involved in global climate service development. It is worth noting in the post-COP26 context that such global decisions, because of their transnational and multilateral character, may be particularly challenging to integrate with current calls for locally led adaptation. Resolving such tensions is a salient challenge for the future development of global climate services.
Conclusion
This paper has presented a typology of global decisions affected by climate risk. The aim has been to bring a focus onto global climate services from the perspective of decisions that users are currently facing. This perspective has currently been neglected at the global level in the literature, and this paper contributes to filling this gap. The presented typology shows that there are many salient global decisions currently being addressed by governments and private companies around the world. Further, we found that global climate service development should address very different aspects for multilateral (and generally public) decisions, as compared to portfolio (and largely private) decisions. For the former, scoping assessments and decision identification are needed, in order to advance discussion and research on impacts of large-scale global climate policy measures. For the latter, private investors are already beginning to address climate risk in their own decisions, and global climate service development can further support this through co-developed tailored approaches that respond to the specific decision contexts and knowledge gaps encountered by these stakeholders.
Data Availability Statement
The raw data supporting the conclusions of this article will be made available by the authors, without undue reservation.
Ethics Statement
Ethical review and approval was not required for the study on human participants in accordance with the local legislation and institutional requirements. The patients/participants provided their written informed consent to participate in this study. Written informed consent was obtained from the individual(s) for the publication of any potentially identifiable images or data included in this article.
Author Contributions
AB, JH, and GL designed the research. AB, AH, JH, and GL organized and carried out the research. AB wrote the manuscript, with AH contributing sections of the manuscript. All the authors contributed to revising and editing the manuscript.
Funding
We acknowledge funding from the project INSeaPTION as part of ERA4CS, an ERA-NET initiated by JPI Climate, and funded by BMBF (DE), MINECO (ES), NWO (NL), and ANR (FR) with co-funding by the European Union (Grant 690462). This publication was also supported by PROTECT. This project has received funding from the European Union’s Horizon 2020 Research and Innovation Program under grant agreement no. 869304. This is PROTECT contribution number 24.
Conflict of Interest
The authors declare that the research was conducted in the absence of any commercial or financial relationships that could be construed as a potential conflict of interest.
Publisher’s Note
All claims expressed in this article are solely those of the authors and do not necessarily represent those of their affiliated organizations, or those of the publisher, the editors and the reviewers. Any product that may be evaluated in this article, or claim that may be made by its manufacturer, is not guaranteed or endorsed by the publisher.
Supplementary Material
The Supplementary Material for this article can be found online at: https://www.frontiersin.org/articles/10.3389/fmars.2021.728687/full#supplementary-material
References
Adger, W. N., Dessai, S., Goulden, M., Hulme, M., Lorenzoni, I., Nelson, D. R., et al. (2009). Are there social limits to adaptation to climate change? Clim. Change 93, 335–354. doi: 10.1007/s10584-008-9520-z
Ameli, N., Drummond, P., Bisaro, A., Grubb, M., and Chenet, H. (2020). Climate finance and disclosure for institutional investors: why transparency is not enough. Clim. Change 160, 565–589. doi: 10.1007/s10584-019-02542-2
Bailey, R., and Wellesley, L. (2017). Chokepoints and Vulnerabilities in Global Food Trade. London: Chatham House.
Bakkensen, L. A., and Barrage, L. (2017). Flood Risk Belief Heterogeneity and Coastal Home Price Dynamics: Going Under Water? (Working Paper No. 23854). Cambridge: National Bureau of Economic Research. doi: 10.3386/w23854
Batten, S. (2018). Climate Change And The Macro-Economy: A Critical Review. London: Bank of England.
Benzie, M., Carter, T. R., Carlsen, H., and Taylor, R. (2019). Cross-border climate change impacts: implications for the European Union. Reg. Environ. Change 19, 763–776.
Bernstein, A., Gustafson, M. T., and Lewis, R. (2019). Disaster on the horizon: the price effect of sea level rise. J. Financ. Econ. 134, 253–272. doi: 10.1016/j.jfineco.2019.03.013
Biermann, F., Pattberg, P., Van Asselt, H., and Zelli, F. (2009). The fragmentation of global governance architectures: a framework for analysis. Glob. Environ. Polit. 9, 14–40. doi: 10.1162/glep.2009.9.4.14
Bisaro, A., de Bel, M., Bouwer, L. M., Hinkel, J., Kok, S., Stojanovic, T. A., et al. (2020). Multilevel governance of coastal flood risk reduction: a public finance perspective. Environ. Sci. Policy 12, 203–212. doi: 10.1016/j.envsci.2020.05.018
Bisaro, A., and Hinkel, J. (2018). Mobilising private finance for coastal adaptation: a literature review. Wiley Interdiscip. Rev. Clim. Change 9:e514. doi: 10.1002/wcc.514
Brasseur, G. P., and Gallardo, L. (2016). Climate services: lessons learned and future prospects. Earths Fut. 4, 79–89. doi: 10.1002/2015ef000338
Bremer, S., Wardekker, A., Dessai, S., Sobolowski, S., Slaattelid, R., and van der Sluijs, J. (2019). Toward a multi-faceted conception of co-production of climate services. Clim. Serv. 13, 42–50. doi: 10.1016/j.cliser.2019.01.003
DRGIS (2020). Climate security in the Western Indian Ocean - Septembre 2020. Washington: Direction Générale des relations internatinales et de la strategie.
Dzebo, A., and Stripple, J. (2015). Transnational adaptation governance: an emerging fourth era of adaptation. Glob. Environ. Change 35, 423–435. doi: 10.1016/j.gloenvcha.2015.10.006
Feldmann, J., Levermann, A., and Mengel, M. (2019). Stabilizing the West Antarctic Ice Sheet by surface mass deposition. Sci. Adv. 5:eaaw4132. doi: 10.1126/sciadv.aaw4132
Gower, J. (2015). A sea surface height control dam at the Strait of Gibraltar. Nat. Hazards 78, 2109–2120. doi: 10.1007/s11069-015-1821-8
Groeskamp, S., and Kjellsson, J. (2020). NEED: the Northern European Enclosure Dam for if climate change mitigation fails. Bull. Am. Meteorol. Soc. 101, E1174–E1189.
Gurenko, E. (2006). Earthquake Insurance in Turkey: History of the Turkish Catastrophe Insurance Pool. Washington: World Bank Publications.
Gürses, Ö., Kolatschek, V., Wang, Q., and Rodehacke, C. B. (2019). Brief communication: a submarine wall protecting the Amundsen Sea intensifies melting of neighboring ice shelves. Cryosphere 13, 2317–2324. doi: 10.5194/tc-13-2317-2019
Haasnoot, M., Biesbroek, R., Lawrence, J., Muccione, V., Lempert, R., and Glavovic, B. (2020). Defining the solution space to accelerate climate change adaptation. Reg. Environ. Change 20:37. doi: 10.1007/s10113-020-01623-8
Haasnoot, M., Middelkoop, H., Offermans, A., Beek, E., and van Deursen, W. A. (2012). Exploring pathways for sustainable water management in river deltas in a changing environment. Clim. Change 115, 795–819. doi: 10.1007/s10584-012-0444-2
Hanson, S. E., and Nicholls, R. J. (2020). Demand for Ports to 2050: climate policy, growing trade and the impacts of sea-level rise. Earths Fut. 8:e2020EF001543. doi: 10.1029/2020EF001543
Haraguchi, M., and Lall, U. (2015). Flood risks and impacts: a case study of Thailand’s floods in 2011 and research questions for supply chain decision making. Int. J. Disaster Risk Reduct. 14, 256–272.
Hedlund, J., Fick, S., Carlsen, H., and Benzie, M. (2018). Quantifying transnational climate impact exposure: new perspectives on the global distribution of climate risk. Glob. Environ. Change 52, 75–85. doi: 10.1016/j.gloenvcha.2018.04.006
Hewitt, C., Mason, S., and Walland, D. (2012). The global framework for climate services. Nat. Clim. Change 2, 831–832. doi: 10.1002/wcc.290
Hinkel, J., Bisaro, A., and Swart, R. (2016). Towards a Diagnostic Adaptation Science. Berlin: Springer. doi: 10.1007/s10113-015-0850-x
Hinkel, J., Church, J., Gregory, J., Lambert, E., Le Cozannet, G., Lowe, J., et al. (2019). Meeting user needs for sea-level rise information: a decision analysis perspective. Earths Fut. 7, 320–337. doi: 10.1029/2018ef001071
Hinkel, J., Lincke, D., Vafeidis, A. T., Perrette, M., Nicholls, R. J., Tol, R. S. J., et al. (2014). Coastal flood damage and adaptation costs under 21st century sea-level rise. Proc. Natl. Acad. Sci. U. S. A. 111, 3292–3297. doi: 10.1073/pnas.1222469111
Hudson, P., Botzen, W. J. W., Feyen, L., and Aerts, J. C. J. H. (2016). Incentivising flood risk adaptation through risk based insurance premiums: trade-offs between affordability and risk reduction. Ecol. Econ. 125, 1–13. doi: 10.1016/j.ecolecon.2016.01.015
IPCC (2018). “Summary for Policymakers,” in Global Warming of 1.5°C. An IPCC Special Report on the Impacts of Global Warming of 1.5°C Above Pre-Industrial Levels and Related Global Greenhouse Gas Emission Pathways, in the Context of Strengthening the Global Response to the Threat of Climate Change, Sustainable Development, and Efforts to Eradicate Poverty, eds V. Masson-Delmotte, P. Zhai, H.-O. Pörtner, D. Roberts, J. Skea, P. Shukla, et al. (Geneva: World Meteorological Organization).
Jongman, B., Hochrainer-Stigler, S., Feyen, L., Aerts, J. C., Mechler, R., Botzen, W. W., et al. (2014). Increasing stress on disaster-risk finance due to large floods. Nat. Clim. Change 4, 264–268. doi: 10.1038/nclimate2124
Keenan, J. M., and Bradt, J. T. (2020). Underwaterwriting: from theory to empiricism in regional mortgage markets in the U.S. Clim. Change 162, 2043–2067. doi: 10.1007/s10584-020-02734-1
Kleindorfer, P. R., Kunreuther, H. G., and Schoemaker, P. J. (1993). Decision Sciences: An Integrative Perspective. Cambridge: Cambridge University Press.
Kling, G., Lo, Y. C., Murinde, V., and Volz, U. (2018). Climate Vulnerability and the Cost of Debt (SSRN Scholarly Paper No. ID 3198093). Rochester: Social Science Research Network, doi: 10.2139/ssrn.3198093
Kok, S., Bisaro, A., de Bel, M., Hinkel, J., and Bouwer, L. M. (2021). The potential of nature-based flood defences to leverage public investment in coastal adaptation: cases from the Netherlands, Indonesia and Georgia. Ecol. Econ. 179:106828. doi: 10.1016/j.ecolecon.2020.106828
Kopp, R. E., Gilmore, E. A., Little, C. M., Lorenzo-Trueba, J., Ramenzoni, V. C., and Sweet, W. V. (2019). Usable science for managing the risks of sea-level rise. Earths Fut. 7, 1235–1269. doi: 10.1029/2018EF001145
Kuiper, C. J. (2020). The Regulation of Flood Risk Management of Transboundary Rivers in the Dutch-German Border Area. Available Online at: http://essay.utwente.nl/82390/ (accessed July16, 2021).
Kwadijk, J. C. J., Haasnoot, M., Mulder, J. P. M., Hoogvliet, M. M. C., Jeuken, A. B. M., van der Krogt, R. A. A., et al. (2010). Using adaptation tipping points to prepare for climate change and sea level rise: a case study in the Netherlands. Wiley Interdiscip. Rev. Clim. Change 1, 729–740. doi: 10.1002/wcc.64
Lamperti, F., Bosetti, V., Roventini, A., and Tavoni, M. (2019). The public costs of climate-induced financial instability. Nat. Clim. Change 9, 829–833. doi: 10.1038/s41558-019-0607-5
Lange, S., Volkholz, J., Geiger, T., Zhao, F., Vega, I., Veldkamp, T., et al. (2020). Projecting exposure to extreme climate impact events across six event categories and three spatial scales. Earths Fut. 8:e2020EF001616. doi: 10.1029/2020EF001616
Le Cozannet, G., Nicholls, R. J., Hinkel, J., Sweet, W. V., McInnes, K. L., Van de Wal, R. S. W., et al. (2017). Sea level change and coastal climate services: the way forward. J. Mar. Sci. Eng. 5:49. doi: 10.3390/jmse5040049
Leane, E., and McGee, J. (2019). Anthropocene Antarctica: Perspectives From The Humanities, Law And Social Sciences. London: Routledge. doi: 10.4324/9780429429705
Lemos, M. C., Kirchhoff, C. J., and Ramprasad, V. (2012). Narrowing the climate information usability gap. Nat. Clim. Change 2, 789–794. doi: 10.1038/nclimate1614
Lempert, R., Sriver, R. L., and Keller, K. (2012). Characterizing Uncertain Sea Level Rise Projections to Support Investment Decisions. Sacramento: California Energy Commission.
Lincke, D., and Hinkel, J. (2018). Economically robust protection against 21st century sea-level rise. Glob. Environ. Change 51, 67–73. doi: 10.1016/j.gloenvcha.2018.05.003
Lourenço, T. C., Swart, R., Goosen, H., and Street, R. (2016). The rise of demand-driven climate services. Nat. Clim. Change 6, 13–14.
Mandel, A., Tiggeloven, T., Lincke, D., Koks, E., Ward, P., and Hinkel, J. (2021). Risks on global financial stability induced by climate change: the case of flood risks. Clim. Change 166:4. doi: 10.1007/s10584-021-03092-2
Moore, J. C., Gladstone, R., Zwinger, T., and Wolovick, M. (2018). Geoengineer polar glaciers to slow sea-level rise. Nature 555, 303–305. doi: 10.1038/d41586-018-03036-4
Mora, C., Dousset, B., Caldwell, I. R., Powell, F. E., Geronimo, R. C., Bielecki, C. R., et al. (2017). Global risk of deadly heat. Nat. Clim. Change 7, 501–506. doi: 10.1038/nclimate3322
Nalau, J., Preston, B. L., and Maloney, M. C. (2015). Is adaptation a local responsibility? Environ. Sci. Policy 48, 89–98. doi: 10.1016/j.envsci.2014.12.011
Nicholls, R. J., Hinkel, J., Lincke, D., and van der Pol, T. (2019). Global Investment Costs for Coastal Defense through the 21st Century. Washington: The World Bank. doi: 10.1596/1813-9450-8745
Nurse, L. A., Mclean, R. F., Agard, J., Briguglio, L. P., Duvat-magnan, V., Pelesikoti, N., et al. (2014). “Small islands,” in Climate Change 2014: Impacts, Adaptation, and Vulnerability. Part B: Regional Aspects. Contribution of Working Group II to the Fifth Assessment Report of the Intergovernmental Panel on Climate Change, eds V. R. Barros, C. B. Field, D. J. Dokken, M. D. Mastrandrea, K. J. Mach, T. E. Bilir, et al. (Cambridge: Cambridge University Press), 1613–1654.
O’Reilly, C. H., Zanna, L., and Woollings, T. (2019). Assessing external and internal sources of Atlantic multidecadal variability using models, proxy data, and early instrumental indices. J. Clim. 32, 7727–7745. doi: 10.1175/JCLI-D-19-0177.1
Ortega, F., and Taṣpınar, S. (2018). Rising sea levels and sinking property values: hurricane Sandy and New York’s housing market. J. Urban Econ. 106, 81–100. doi: 10.1016/j.jue.2018.06.005
Parrado, R., Bosello, F., Delpiazzo, E., Hinkel, J., Lincke, D., and Brown, S. (2020). Fiscal effects and the potential implications on economic growth of sea-level rise impacts and coastal zone protection. Clim. Change 160, 283–302. doi: 10.1007/s10584-020-02664-y
Patt, A. G., Klein, R. J. T., and de la Vega-Leinert, A. C. (2005). Taking the uncertainty in climate change vulnerability assessment seriously. Comptes Rendus Geosci. 337, 411–424. doi: 10.1016/j.crte.2004.11.006
Pattberg, P., and Stripple, J. (2008). Beyond the public and private divide: remapping transnational climate governance in the 21st century. Int. Environ. Agreem. 8, 367–388. doi: 10.1007/s10784-008-9085-3
Pauw, W. P. (2017). Mobilising private adaptation finance: developed country perspectives. Int. Environ. Agreem. 17, 55–71. doi: 10.1007/s10784-016-9342-9
Schinko, T., Mechler, R., and Hochrainer-Stigler, S. (2017). A methodological framework to operationalize climate risk management: managing sovereign climate-related extreme event risk in Austria. Mitig. Adapt. Strateg. Glob. Chang. 22, 1063–1086. doi: 10.1007/s11027-016-9713-0
SEI (2020). Climate-Resilient Trade and Production: The Transboundary Effects of Climate Change and Their Implications for EU Member States. Oaks: SEI.
Soares, M. B., Alexander, M., and Dessai, S. (2018). Sectoral use of climate information in Europe: a synoptic overview. Clim. Serv. 9, 5–20. doi: 10.1016/j.cliser.2017.06.001
Surminski, S., and Oramas-Dorta, D. (2014). Flood insurance schemes and climate adaptation in developing countries. Int. J. Disaster Risk Reduct. 7, 154–164. doi: 10.1016/j.ijdrr.2013.10.005
UNFCCC (1992). United Nations Framework Convention On Climate Change. United Nations, FCCC/INFORMAL/84 GE.05-62220 (E) 200705. Bonn: Secretariat of the United Nations Framework Convention on Climate Change, 24.
Van der Pol, T., Hinkel, J., Merkens, J., and Macpherson, L. (2019). A Comparison of Economic Decision-Support Methods for the Efficient and Robust Protection of the German Baltic Sea Coast Against Flooding. Lisbon: ECCA.
Vincent, K., Daly, M., Scannell, C., and Leathes, B. (2018). What can climate services learn from theory and practice of co-production? Clim. Serv. 12, 48–58. doi: 10.1016/j.cliser.2018.11.001
Vogel, C., Steynor, A., and Manyuchi, A. (2019). Climate services in Africa: re-imagining an inclusive, robust and sustainable service. Clim. Serv. 15:100107. doi: 10.1016/j.cliser.2019.100107
Weaver, C. P., Lempert, R. J., Brown, C., Hall, J. A., Revell, D., and Sarewitz, D. (2013). Improving the contribution of climate model information to decision making: the value and demands of robust decision frameworks. Wiley Interdiscip. Rev. Clim. Change 4, 39–60. doi: 10.1002/wcc.202
Keywords: climate services, sea-level rise, adaptation, finance, climate risk
Citation: Bisaro A, Hinkel J, Le Cozannet G, van der Pol T and Haas A (2021) Global Climate Services: A Typology of Global Decisions Influenced by Climate Risk. Front. Mar. Sci. 8:728687. doi: 10.3389/fmars.2021.728687
Received: 29 June 2021; Accepted: 23 November 2021;
Published: 16 December 2021.
Edited by:
João Canning-Clode, Center for Marine Environmental Sciences (MARE), PortugalReviewed by:
Adam Peter Hejnowicz, Newcastle University, United KingdomRachel Gjelsvik Tiller, SINTEF, Norway
Copyright © 2021 Bisaro, Hinkel, Le Cozannet, van der Pol and Haas. This is an open-access article distributed under the terms of the Creative Commons Attribution License (CC BY). The use, distribution or reproduction in other forums is permitted, provided the original author(s) and the copyright owner(s) are credited and that the original publication in this journal is cited, in accordance with accepted academic practice. No use, distribution or reproduction is permitted which does not comply with these terms.
*Correspondence: Alexander Bisaro, c2FuZHkuYmlzYXJvQGdsb2JhbGNsaW1hdGVmb3J1bS5vcmc=