- 1Bodega Marine Laboratory, Karen C. Drayer Wildlife Health Center, Coastal Marine Science Institute, University of California, Davis, Davis, CA, United States
- 2Bodega Marine Laboratory, California Department of Fish and Wildlife, Bodega Bay, CA, United States
Marine ecosystems are vulnerable to climate driven events such as marine heatwaves yet we have a poor understanding of whether they will collapse or recover. Kelp forests are known to be susceptible, and there has been a rise in sea urchin barrens around the world. When temperatures increase so do physiological demands while food resources decline, tightening metabolic constraints. In this case study, we examine red abalone (Haliotis rufescens) looking at sublethal impacts and their prospects for recovery within kelp forests that have shifted to sea urchin barrens. Abalone are a recreationally fished species that once thrived in northern California’s bull kelp forests but have recently suffered mass mortalities since the 2014–2016 marine heatwave. Quantitative data exist on the health and reproduction of abalone both prior to and after the collapse. The survivors of the mass mortality show a 2-year lag in body and gonad condition indices. After the lag, body and gonad indexes decreased substantially, as did the relationship between shell length and body weight. Production of mature eggs per female declined by 99% (p < 0.001), and the number of eggs per gram of female body weight (2,984/g) declined to near zero (9/g). The number of males with sperm was reduced by 33%, and the sperm abundance score was reduced by 28% (p = 0.414). We observed that these reductions were for mature eggs and sperm while immature eggs and spermatids were still present in large numbers. In the lab, after reintroduction of kelp, weight gains were quickly lost following a second starvation period. This example illustrates how climate-driven declines in foundation species can suppress recovery of the system by impacting body condition and future reproduction of surviving individuals. Given the poor reproductive potential of the remaining abalone in northern California, coupled with ongoing mortality and low kelp abundances, we discuss the need to maintain the fishing moratorium and implement active abalone restoration measures. For fished species, such as abalone, this additional hurdle to recovery imposed by changes in climate is critical to understand and incorporate into resource management and restoration.
Introduction
Climate driven impacts to marine systems are occurring around the world and human mediated warming is the major contributor to these changes (Frölicher and Laufkötter, 2018). Marine heatwaves (MHW) are not only a concern for the future but are currently impacting marine systems and fisheries (Smale et al., 2019; Holbrook et al., 2020). Further, MHW are predicted to increase in intensity and duration (Solomon et al., 2007; Wernberg et al., 2016; Oliver et al., 2018). Some regions and habitats are going to be particularly sensitive to these changes (Hobday and Pecl, 2014), such as large marine ecosystems (Belkin, 2009), coral reefs (Hughes et al., 2017; Fordyce et al., 2019), seagrass meadows (Marbà and Duarte, 2010), and kelp forests (Cannell et al., 2019; Arafeh-Dalmau et al., 2020). The collapse of marine ecosystem structuring species has the potential to impact not only the foundation species but also the structure, function, and services provided by entire ecosystems (Harley et al., 2012; Arafeh-Dalmau et al., 2019; Smale et al., 2019). Climate stressors will impact services such as fisheries directly through mortality yet there will also be sublethal impacts which must be quantified, understood, and incorporated into fisheries management and recovery planning. A warming climate will lead to a narrowing of metabolically suitable habitats in marine systems (Deutsch et al., 2015).
Northern California’s bull kelp (Nereocystis luetkeana) forests are uniquely sensitive to warming due to their species characteristics of being an annual species, needing cool water for growth and survival (Schiel and Foster, 2015), and being at the southern edge of their physiological tolerances. These dense bull kelp forests were, in part, maintained by intense nearshore coastal upwelling in the region (García-Reyes and Largier, 2010). The productivity of this area is due to strong seasonal winds which generate upwelling of cold nutrient-rich waters (García-Reyes and Largier, 2010). However, the intense MHW in 2014–2016 in the Northeast Pacific (Di Lorenzo and Mantua, 2016) impacted this region and there were massive northern shifts in populations (Sanford et al., 2019), reductions in nutrients and productivity (Leising et al., 2015) and changes to the local kelp forest ecosystems. Following the MHW, purple sea urchin (Strongylocentrotus purpuratus) populations exploded and contributed to the collapse of the bull kelp forest across more than 300 km of coastline in northern California (Rogers-Bennett and Catton, 2019; McPherson et al., 2021). This region’s kelp forest was the epicenter of the recreational red abalone (Haliotis rufescens) fishery, which was the last abalone fishery remaining open in California.
The red abalone recreational fishery was a treasured part of the natural heritage of the north coast of California, an important cultural heritage resource for First Nations, as well as a key species in the ecology of the bull kelp forest community. The recreational fishery, worth an estimated >$44 M per year (Reid et al., 2016), was one of the largest in the world where an average of 37,000 fishers removed 260,000 red abalone per year (310 mtons) (CDFW data). Red abalone are the largest species of abalone in the world and there were a select subset of trophy fishers that participated in the fishery. The fishery was one of the best managed fisheries, with annual and daily limits, closed seasons and areas as well as a free diving only regulations. Following the climate driven loss of the kelp forest in 2014, the red abalone population began to experience mass mortalities of up to 80% (Rogers-Bennett et al., 2019). Landings in the fishery declined and both California (CDFW Code section 29.15) and Oregon closed the recreational red abalone fisheries in 2018 (Oregon Department of Fish and Wildlife, 2021).
While mass abalone mortalities have been documented following MHWs (Caputi et al., 2014; Rogers-Bennett et al., 2019), less is known of the lingering impacts of starvation and thermal stress to wild abalone populations. Past studies have identified that warm water conditions lead to decreases in growth (Haaker et al., 1998), body condition, abalone physiology (Morash and Alter, 2016), and reproductive capacity (Vilchis et al., 2005; Rogers-Bennett et al., 2010) in red abalone. Severe impacts to body condition, resulting in body shrinkage has not been observed in the wild, except in the case of disease (Moore et al., 2002). Laboratory experiments examining the effects of increased water temperature and starvation on the body condition and fecundity of red abalone (Rogers-Bennett et al., 2010) highlight the potential for the MHW and collapse of the kelp forest to have additional sublethal impacts on the surviving abalone. Sperm production in male red abalone was shown to decrease with increased temperature and decreased food supply. Female red abalone exposed to warm water had lower egg production and the number of mature eggs produced was markedly reduced by starvation, to the point of full cessation.
Here we examine the health and reproductive fitness of wild red abalone before, during, and after the MHW and collapse of the kelp forest in 2014 in northern California. We use quantitative data that span the periods before, during, and after the kelp forest collapse. We quantify changes in abalone body condition, gonad condition, and fecundity (numbers of mature eggs and sperm). We quantify changes in size-specific body mass and fecundity, as well as sperm production in males and the quantity of premature and degenerating eggs in females. To better understand the potential for these sublethal impacts to delay population recovery, we assess the recovery of body condition in starving abalone 12 weeks after the reintroduction of food. We discuss the implications of these results for abalone population dynamics, fisheries, and conservation.
Materials and Methods
Red abalone samples were compared among three time periods; the first in the Pre-Kelp Forest Collapse (Pre-KFC) time period defined as 2000–2013, the second in the Transition period of 2014–2015, and the third in the Post-KFC period defined as 2016–2018. Red abalone were collected by recreational divers and rock pickers at Van Damme State Park, Mendocino County, California (Figure 1). This site was among the most popular recreational red abalone landing sites in the fishery, especially in the more recent years. Recreational divers and rock pickers typically fished from the intertidal in the nearshore areas out to a depth of 10 m. The fishery was open April through the end of November which was when the samples were collected from recreationally caught abalone (in a few cases, samples for analyses were collected during the closed months). The reliance upon recreationally caught abalone may have introduced some bias in terms of the period of the year, mostly missing the months December through March. Since the effects of the MHW realized by red abalone persisted across years, the actual impact to the data was a discontinuous data set with respect to time. We do not consider this a substantial issue, since the data set is quite large, covers many years overall, and reproduction of red abalone occurs year-round (Rogers-Bennett, pers comm).
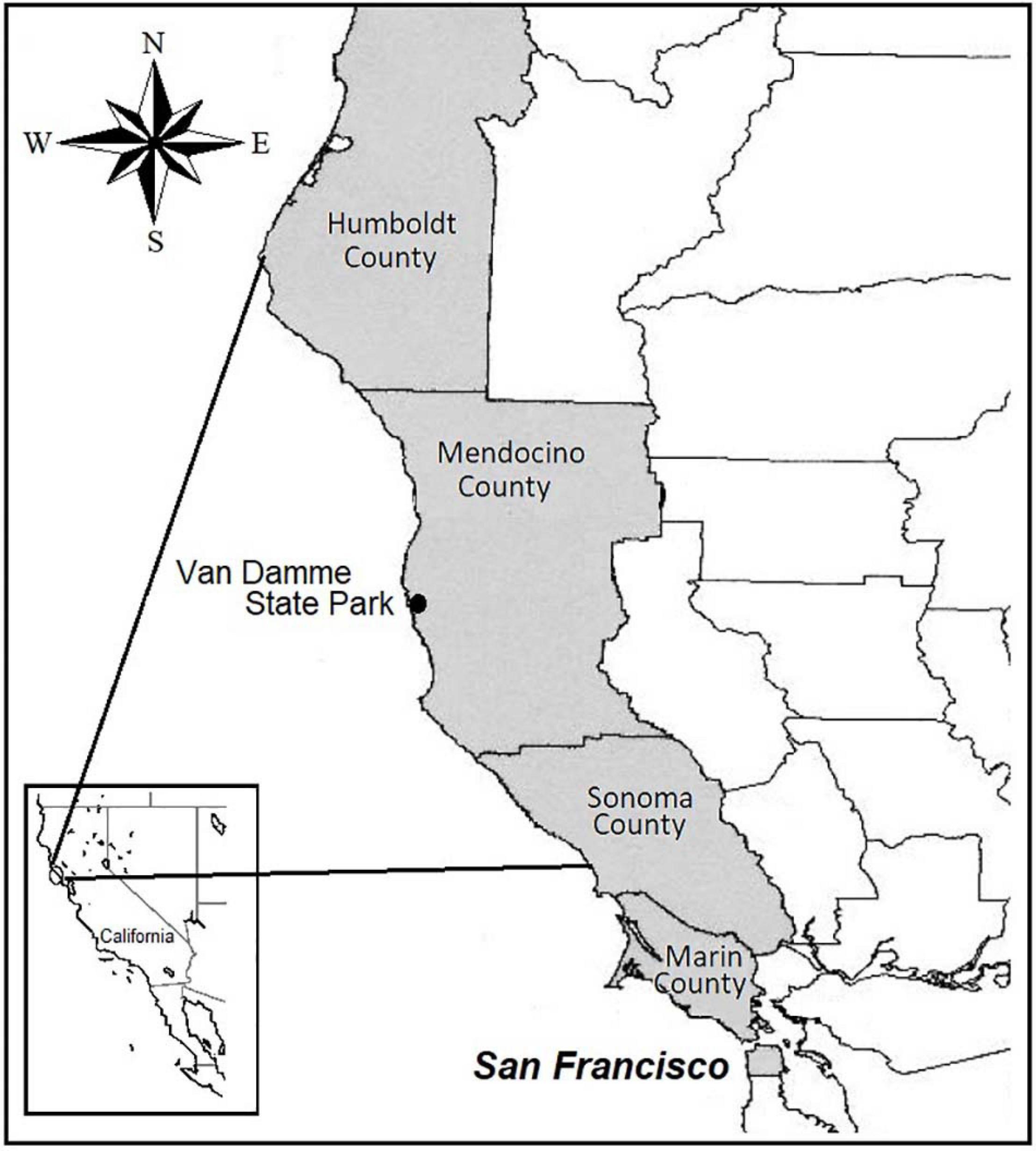
Figure 1. Map of Northern California showing the locations of Van Damme State Park where red abalone were collected for this study.
Additionally, the recreational fishery size limit for red abalone is a minimum shell length of 178 mm; consequently, the bulk of the data include abalone of shell length at least that large. The presence of some much larger specimens provided useful information for large fecund females. Collections by California Department of Fish and Wildlife and Bodega Marine Lab divers during some of the months closed to recreational fishing provided some individuals smaller than 178 mm. The data set was truncated at individuals of 100 mm shell length or larger, as this is the size at which most red abalone are reproductively viable.
We recorded meristic characteristics from 2,121 wild red abalone and obtained gonad samples from 2,079 abalone collected at Van Damme State Park, CA from 2000 to 2017. The suite of measurements collected on each animal included maximum shell length and total weight. The body then was detached from the shell and weighed separately. Sex was visually determined and often confirmed later using histology. The length of the gonadal/digestive gland appendage was measured and the appendage sliced at its midpoint to obtain height and width of the appendage and the inner core. The volume of the gonad was estimated by subtracting the volume of the inner cone (digestive gland) from the outer cone (gonad) as described in Figure 2.4, page 37 of Tutschulte (1976). Samples of the gonad were taken for later histological examination as described in Rogers-Bennett et al. (2004). Shell lengths varied substantially depending on the desired observations for a sampling event, however, all of the observations presented in this paper are from red abalone of 100 mm or longer shell length. Sample sizes for the various metrics are provided in each subheading.
Body and Gonad Condition
Body and gonad indices were calculated for each animal at each sampling date using the following equations:
Body Condition Index = W/[(10 × L)3], where
Gonad Condition Index = EGV/[(10 × L)3], where
It is important to note that while gonad and condition index typically use whole body weight as the metric for the index, in this case, the abalone were starving and losing weight Post-KFC, while shell length was essentially unchanged. This meant that we could no longer index gonads based on body weight. Therefore, for the gonad index, we used the shell length which does not change appreciably during periods of starvation.
The individual indices for each date were averaged and the standard deviation calculated, providing a mean and 95% confidence interval for each date. The means and confidence intervals (as error bars) were plotted by sampling date. Medians of the Pre-KFC and Post-KFC data were used to compare those periods on a broader basis, using the non-parametric Mann-Whitney test in “R” to test for differences.
Sample sizes for the condition indices were: Females–1,066 Pre-KFC and 44 Post-KFC and Males–894 Pre-KFC and 76 Post-KFC. Sample sizes for the gonad indices were: Females–1049 Pre-KFC and 42 Post-KFC and Males–869 Pre-KFC and 77 Post-KFC.
Female Weight-Length Relationship
Weights and corresponding shell lengths for each abalone from Pre-KFC and Post-KFC samplings were plotted as a scatter plot. We fit curves to the length-weight relationships using non-linear (weighted) least squares estimation with the “nls” function in the statistical computing platform “R” for both event periods using the following equation:
W = aLb, where
The coefficient of determination (r2) was derived by linear correlation of the predicted weight () and actual weight (W) for Pre- and Post-KFC periods. Sample size for weight-length relationships in the Pre-KFC sample was 290, and for the Post-KFC was 44. Only females were used in this analysis.
Number of Eggs per Gram Female Body Weight
The number of mature eggs per gonad was calculated by multiplying the estimated gonad volume times the density of eggs per cubic mm of gonad. Histology slides were examined under a compound microscope, and the number of eggs per cubic mm was determined taking care to measure only eggs that could be seen clearly in the 7 μm thick histology tissue slice (for a full description of methods; see Rogers-Bennett et al., 2004). Not all samples contained enough gonad tissue to score or were not quantifiable, resulting in histology results from 290 female red abalone for Pre-KFC and 42 females for Post-KFC.
Each of the observations of total mature egg count was divided by the corresponding animal weight, then quantiles determined for those observations in both Pre- and Post-KFC samples. Boxplots were developed for the two time periods using the boxplot function in the “ggplot2” package for “R,” and outliers were identified. In this method, the box is bound by the 25th and 75th percentiles, the dark horizontal line splitting the box is the median, and the whiskers are the data value lower than or equal to 1.5 times the interquartile range, IQR (75th minus 25th percentile). The bounds of the notch approximate a 95% confidence interval [median + (1.58 × IQR/(√n))]. Values beyond the ends of the whiskers are outliers, plotted individually. Medians of Pre-KFC and Post-KFC data were used to compare the two periods on a broader basis, using the non-parametric Mann-Whitney test in “R” to test for differences.
Total Mature Egg Count Relationship to Shell Length
The relationship of total mature egg count as a function of shell length was plotted for three time periods to help visualize the changes between the Pre-KFC years and the Post-KFC years. Different symbols were used to distinguish the Pre-KFC data from the transition year and Post-KFC time period data. Sample sizes for Pre-KFC and Post-KFC were 292 and 42, respectively (2015, n = 17).
Curves were fit to the Pre-KFC and Post-KFC (2016–2017) data with the same “nls” method in “R” and substituting number of mature eggs for weight in Eq. 3.
Histological Examination of Gonads
Histological examination of gonadal tissues was per (Rogers-Bennett et al., 2004, 2010) and included enumeration of types of oocytes and visual sperm observations. Oocyte numbers were derived from microscopic examination of four fields at 200× magnification and included mature (fully developed), immature (smaller less developed), and necrotic (non-viable degenerating) oocytes.
Sperm evaluations were conducted using a visual scoring, since actual counts are often impractical, and included presence/absence of sperm. An average score was computed for each male abalone examined through the visual observation of 12 fields per slide at 200× magnification. Scoring of each field ranged from 0 to 6: 0 = no sperm in field; 1 = fewer than 100 sperm in field; 2 = 2–4 patches of dispersed sperm; 3 = dispersed sperm along less than 50% of trabecula; 4 = sperm more dispersed along 50 to less than 100% of trabecula; 5 = sperm along all trabecula, but room for more; 6 = sperm so densely packed that germ cells and/or trabecula are obscured (Rogers-Bennett et al., 2010).
Boxplots of the Pre-KFC and Post-KFC fecundity metrics from histology (percent of mature, immature, and necrotic eggs; presence/absence of mature and immature eggs; presence/absence of sperm; sperm score) were done in “R” (same method as for number of eggs per gram) and medians used for determination of percent changes between the time periods. The female abalone sample size was 359 oocyte observations for Pre-KFC (323) and Post-KFC (36). Male abalone sperm observations numbered 307, with 247 for the Pre-KFC period and 60 for the Post-KFC period. As in other analyses above, medians and non-parametric methods were used to compare results on a broader basis.
Feeding/Recovery Trials
We performed 12-week feeding trials on emaciated red abalone exhibiting a range of foot muscle shrinkage from 0 to 2 (a measure of overall condition, 0–3, where 0 is no visible shrinkage). The abalone were divided into two groups (n = 17 in each group); those in which feeding was resumed and a control group in which macroalgal food was withheld. All abalone were fed giant kelp (Macrocystis pyrifera) and dulse (Paleria palmata) ad libitum in two outside opaque and covered cylindrical tanks. However, animals from both treatments were observed feeding on a diatom layer on the sides of the tanks. Each tank was fitted with a single water inflow and a large air bubbler. These tanks have been used to maintain healthy abalone in the past. Tanks were checked daily for abalone health and food and to be cleaned. Condition of starved abalone was compared to abalone fed ad libitum using visual scoring.
Results
Body and Gonad Condition
Sampling to determine abalone health as measured by condition and gonad indices was started in 2000. From 2000 to 2009 kelp forest conditions fluctuated but remained within normal ranges or declines lasted for less than 1 year. Sampling to re-examine the health of red abalone was renewed in 2015, during a major warm water event (2014–2016 warm water blob). Body condition indices for female red abalone from Van Damme Pre-KFC (2000–2013) ranged from 0.0434 to 0.4315 (median of 0.136, n = 1,066) and dropped dramatically to a range of 0.0508–0.2704 (median of 0.107, n = 44) for Post-KFC (2016–2017) samples (Figure 2A), an overall 21% decrease (p < 0.001). Likewise, overall median female gonad condition dropped 94% (p < 0.001) from 394 Pre-KFC to 25 Post-KFC.
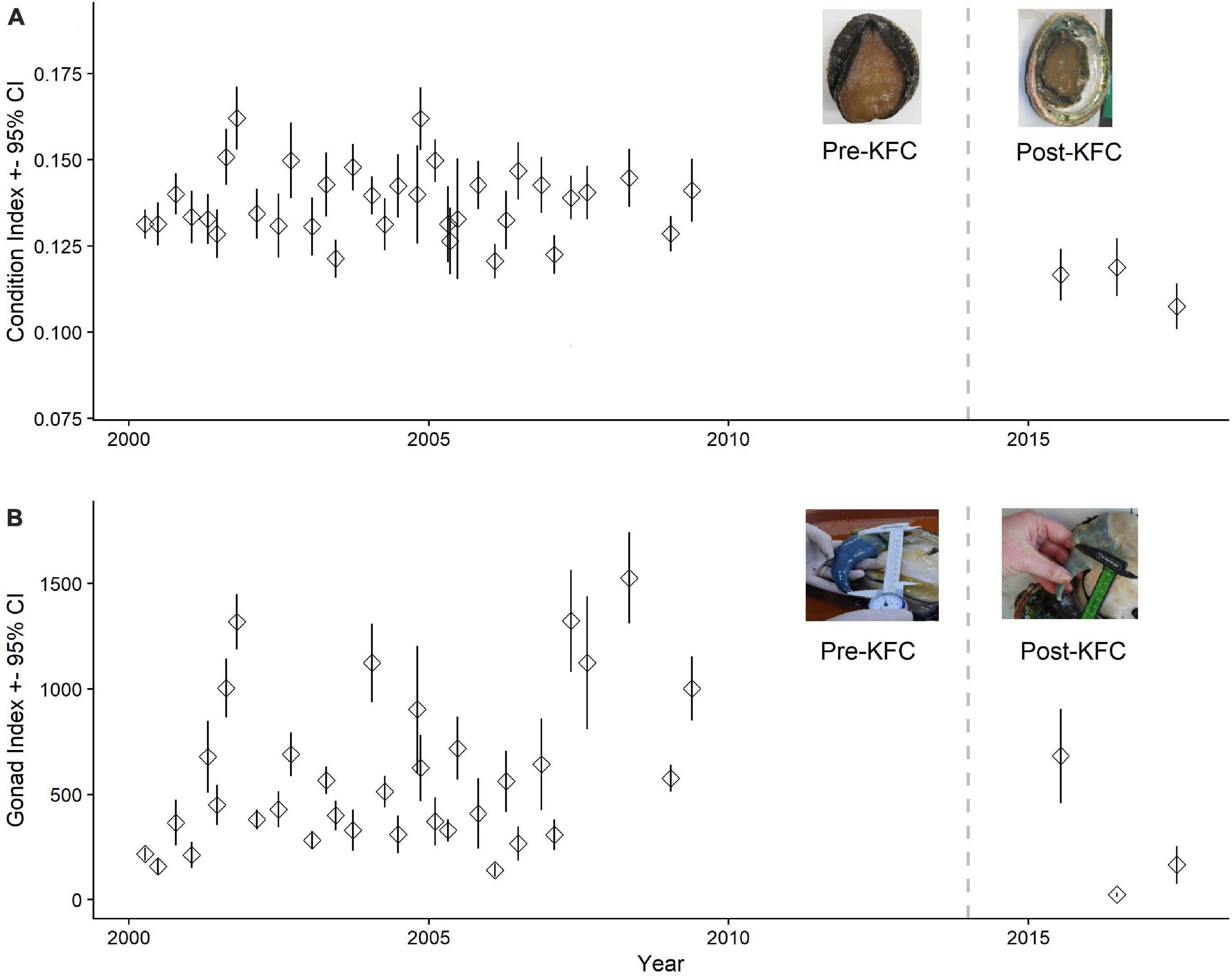
Figure 2. (A) Female red abalone condition and (B) gonad indices with 95% error bars from Van Damme State Park, California. The vertical dashed line at year 2014 separates the Pre-KFC and Post-KFC periods. The inset photos typify body and gonad condition for the two periods. Median female condition index for Pre-KFC was 0.136 (n = 1,066) and gonad index, 394 (n = 1,049). Median female condition index for Post-KFC (2016–2017) was 0.107 (n = 44) and gonad index, 24.9 (n = 42). Condition index = W/[(10 × L)3], where W = body weight in grams and L = shell length in mm. Gonad index = EGV/[(10 × L)3], where EGV = estimated gonad volume in mm3 and L = shell length in mm.
The effects of starvation on female body weight and fecundity were not definitively observed until the 2016 sampling period; these indexes had not yet declined in 2015. Therefore, we did not include the 2015 data in the analysis. Body condition (Figure 2A) declined more slowly following the decline of the kelp forest than did gonad condition (Figure 2B). By 2017, we see body condition decline at Van Damme compared with the previous years, however, even in this year there is a high degree of variance with some abalone retaining high body condition (Figure 2A).
Similarly for the males, body condition declined Post-KFC, but 2015 was a transition year with normal condition and gonad index values (Figures 2A,B) (The lag in the impact to reproduction from the heat wave in 2015 is also described in “Total Mature Eggs Counts Relationship to Shell Length.”). By 2016 and later, we saw a 33% decrease (p < 0.001) in male median body condition from 0.153 (n = 894) to 0.103 (n = 76) (Figure 3A). Median male gonad indices decreased to a larger extent between Pre- and Post-KFC at 87% reduction (p < 0.001) from 194 (n = 869) to 26 (n = 77) (Figure 3B). The percentages of abalone with degraded gonads in the Pre-KFC and Post-KFC samples were similar at 13 and 11%, respectively.
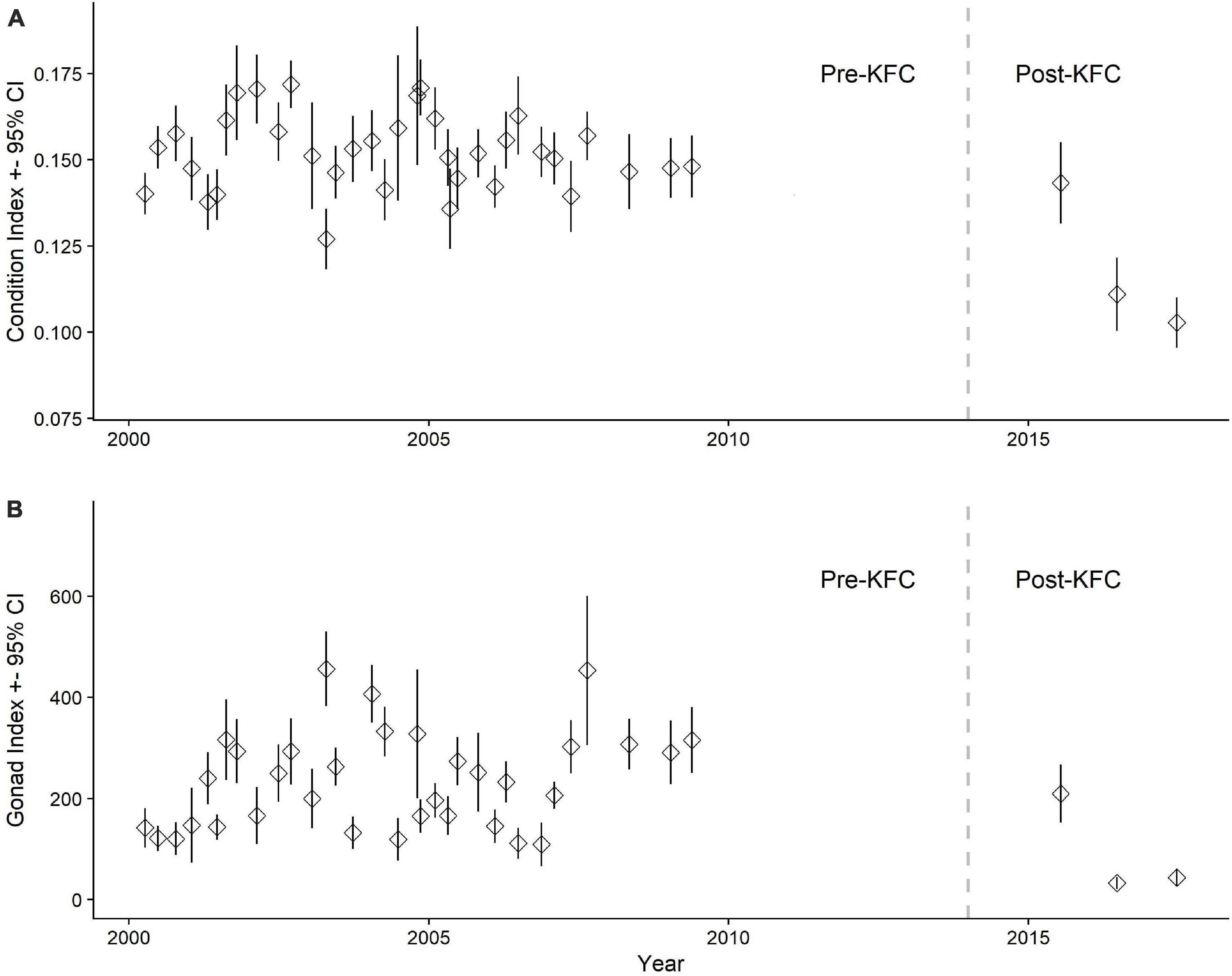
Figure 3. (A) Male red abalone condition and gonad (B) indices with 95% error bars from Van Damme State Park, California. The vertical dashed line at year 2014 separates the Pre-KFC and Post-KFC periods. Median male condition index for Pre-KFC was 0.153 (n = 894) and gonad index, 194 (n = 869). The median male condition index for Post-KFC (2016–2017) was 0.103 (n = 76) and gonad index, 26.5 (n = 77). Condition index = W/[(10 × L)3], where W = body weight in grams and L = shell length in mm. Gonad index = EGV/[(10 × L)3], where EGV = estimated gonad volume in mm3 and L = shell length in mm.
Female Weight-Length Relationship
The fitted curve for weight-length relationships was higher in the Pre-KFC time period (Figure 4) compared with the Post-KFC time period (Figure 4) following the decline in kelp and subsequent red abalone starvation. The relationship from Pre-KFC was W = 0.0001267 × L3.017 (r2 = 0.692; p < 0.001, n = 290), whereas the relationship from Post-KFC was W = 0.000000643 × L3.982 (r2 = 0.640; p < 0.001, n = 44) (Figure 4). The drop in the length-weight relationships in the Post-KFC years is consistent with visual observations of increased incidence of abalone body shrinkage.
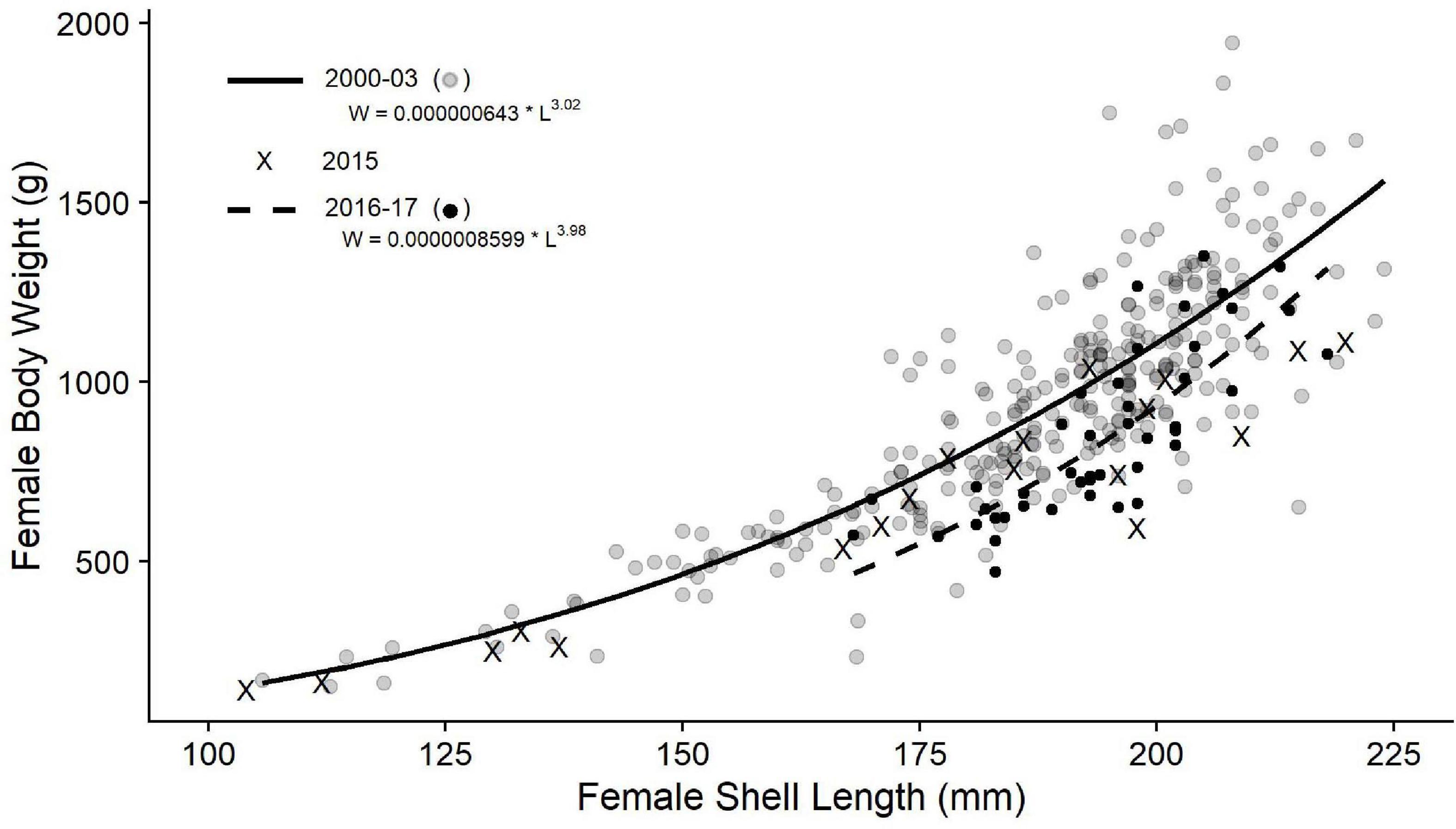
Figure 4. Weight-length relationships of female red abalone from Van Damme, California, from Pre-KFC (2000–2003, solid line) and Post-KFC (2016–2017, dashed line). Equations displayed on the graph are from non-linear least squares run in the “R” statistical package. Sample size, r2, and p are: Pre-KFC–n = 290, r2 = 0.692, p < 0.001; Post-KFC–n = 44, r2 = 0.640, p < 0.001.
Number of Eggs per Gram Female Body Weight
The fecundity of red abalone measured as number of mature eggs per gram of body weight declined in the Post-KFC years following the collapse of the kelp forest and the subsequent starvation of abalone. Abalone in the Post-KFC time period had less than 1% (p < 0.001) of the median fecundity and reproductive potential of red abalone in the Pre-KFC time period (median of 2,984 vs. 9.0) (Figure 5). This reduction is due to decreases in both the gonad volume, which was only 20% of the Pre-KFC value, and egg density of only 46% of the Pre-KFC value. The stark difference in gonad structure and mature egg structure from substantially reduced body and gonad condition following starvation is evident in the photomicrographs of Pre-KFC and Post-KFC in Figure 5.
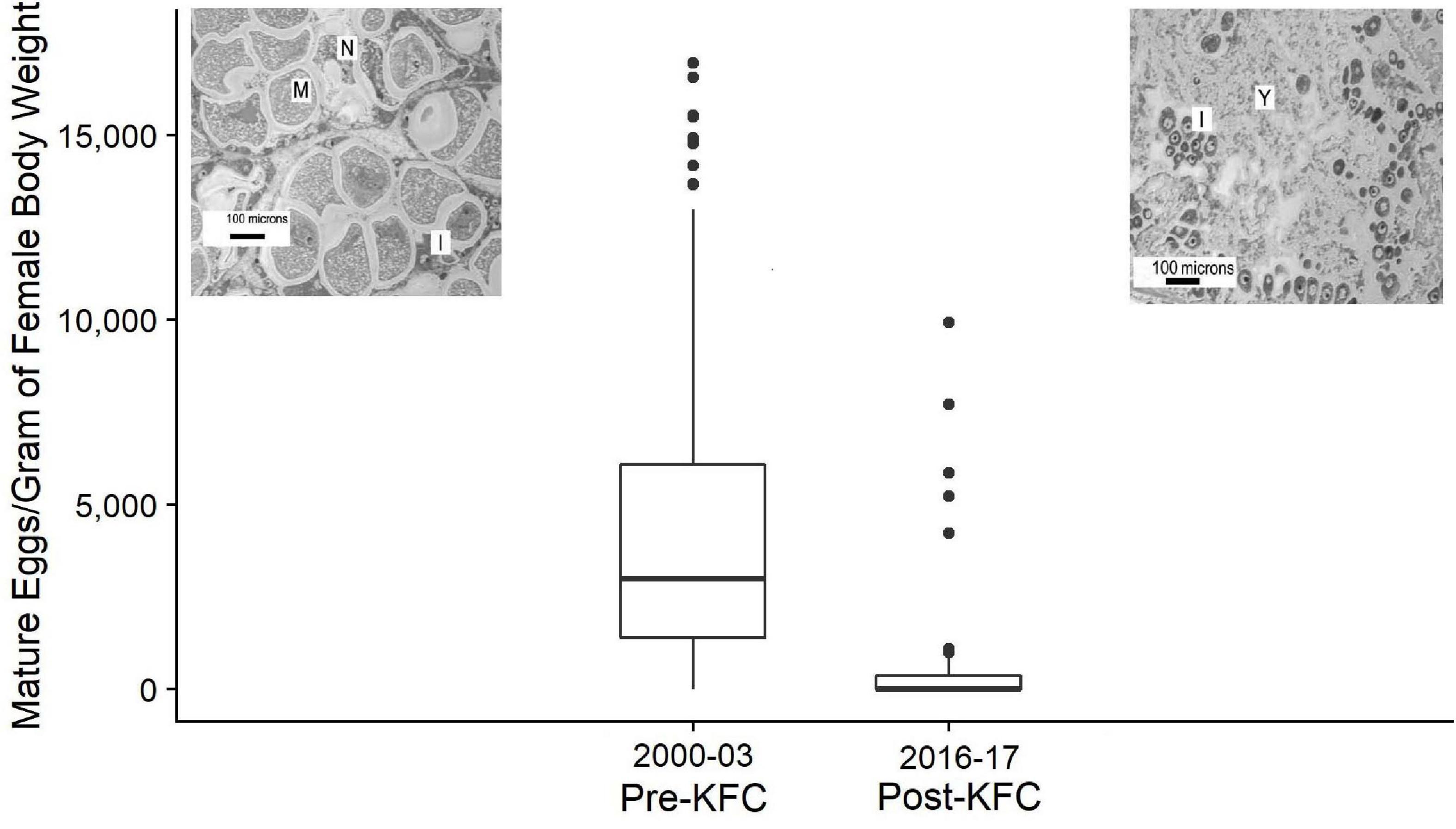
Figure 5. Distributions of the number of mature eggs per gram of mature female body weight, red abalone from Van Damme, California, Pre-KFC and Post-KFC expressed as boxplots. The Pre-KFC median is 2,984 (n = 292), and for Post-KFC is 9.0 (n = 42). Photomicrographs of mature ovaries are from Rogers-Bennett et al. (2010): upper left is a healthy wild red abalone, and upper right is from a starved red abalone (“M” = mature oocytes, “N” = necrotic degenerating oocytes, and “I” = immature oocytes).
Total Mature Egg Count Relationship to Shell Length
The relationship between the total number of mature eggs and abalone shell length prior to the warm water impacts, Pre-KFC, was exponential (non-linear relationship) with egg number increasing as shell length increased (Figure 6, gray circles). The relationship in 2015 was similar and represents a year that was similar to the Pre-KFC years despite the lack of food in the wild. The relationship between the total number of eggs to abalone shell length in the Post-KFC period had declined precipitously. Exponential curve fits to the Pre- and Post-KFC show the dramatic difference (Figure 6). The mid-size and larger females no longer had more than 8 million eggs, whereas prior to this, some females had just under 30 million eggs (Figure 6).
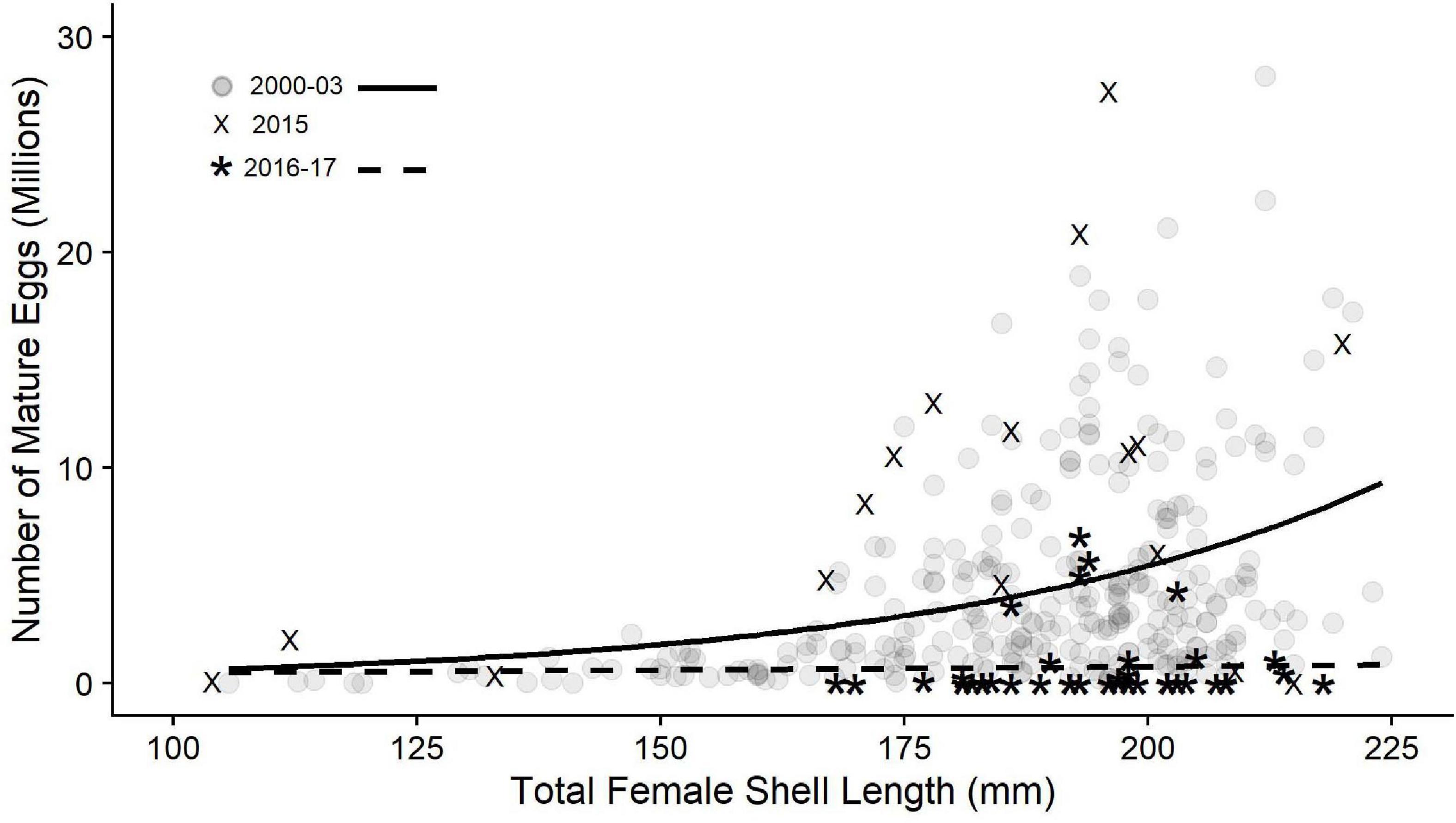
Figure 6. The relationship of number of mature eggs per female to shell length for red abalone from Van Damme, CA, for Pre-KFC (gray dots, solid line, 2000–03, n = 292), transition year 2015 (n = 17), and Post-KFC (2015–17, n = 42). The dashed line is a curve fit to the non-transition Post-KFC data (“X” symbols, 2016–2017). Shell lengths ranged from 105.7 to 224 mm Pre-KFC and from 104 to 220 mm Post-KFC.
While the proportion of abalone with degraded gonads in the Pre-KFC and Post-KFC samples were similar, 100% of Pre-KFC abalone had eggs compared with only 66% in the Post-KFC time period.
Histological Examination of Gonads
Histological examination of female gonads included enumeration of immature eggs (not fully matured as determined by size) and degenerating or necrotic eggs (mature eggs in the process of resorption). Total shell length of abalone ranged from 105.7 to 224 mm for Pre-KFC and 168–218 for Post-KFC. The relative abundance of necrotic eggs as a percentage of the number of total eggs was very low (0–1.6%) in both Pre-KFC and Post-KFC samples. However, the percentages of immature eggs to total eggs increased 40% (p = 1) from Pre-KFC (median = 70%, n = 323) to Post-KFC (median = 98%, n = 36). The median number of immature eggs per female decreased by 80% (p < 0.001). Mature egg production as measured by median number of mature eggs/gram body weight decreased 99.7% (p < 0.001) between the two time periods.
Male gonad histological examination provided presence/absence of sperm, visual sperm scores, and some qualitative sperm densities for 247 males in Pre-KFC and 60 males in Post-KFC. Sperm production of male red abalone was reduced substantially between the Pre- and Post-KFC periods. There was an overall 33% reduction in the percentage of male abalone with sperm from Pre-KFC (86%) to Post-KFC (58%). Visual sperm scores obtained during the histological analysis declined 28% (p = 0.414) between Pre-KFC (1.50) and Post-KFC (1.08).
Feeding/Recovery Trials
A few abalone died during the recovery trial in both treatments. By week 12, 100% of the fed animals had returned to normal body condition (score = 0), while only 50% of the unfed animals had a score of 0. The abalone exhibited improved body conditions after 12 weeks of ad libitum feeding of macroalgae. Surprisingly, the control group of emaciated abalone, maintained under starvation conditions for 12 weeks, partially recovered presumably due to a diatom layer that developed on the tanks and showing clear grazing (radula marks).
Discussion
The case study of red abalone in northern California illustrates how climate stressors may result in ongoing changes to productivity which need to be accounted for in management of natural resources. While the kelp forest collapse in northern California resulted in a dramatic mass mortality of red abalone, less readily observed effects on the health and reproduction of the surviving individuals are important to quantify to understand the recovery potential of the population should the kelp forest return.
Delayed Impacts and Hindered Recovery Potential
The relative body mass and fecundity of the red abalone remained similar to Pre-KFC levels within the first 2 years of the kelp collapse (Figure 6). However, by 2016 both body and gonad condition in both sexes were severely impacted (Figures 2, 3). By 2017, the recreational fishers had learned to select for the fattest (healthiest) abalone they could find (personal observation). Our samples included those larger individuals likely resulting in underestimation of the ongoing estimated impacts of the KFC after 2016.
The results of the feeding trials show that full recovery from the starvation impacts may be slow or not viable, even with ready access to quality food. The abalone in the feeding trials did improve body condition during the 12-week reintroduction of food. In a separate trial, red abalone collected from the wild post-KFC required more than 2 years of feeding in captivity to successfully spawn and generate viable larvae (Boles, 2020).
Surprisingly, the abalone that were maintained with no kelp food during the feeding trials also improved in body condition, potentially due to the availability of diatoms on the sides of the outdoor tanks despite regular cleaning. This result suggests that natural habitats (wild) were so severely food-limited that feeding on laboratory tank biofilms offered a better food source than was available in the wild following the KFC and competition with purple urchins and other herbivores.
Recent satellite imagery confirms that surface coverage of the kelp forests in northern California are still at less than 5% of good years (McPherson et al., 2021), indicating that starvation conditions continue to exist for the vast majority of the red abalone stocks. Being at the southern border of Nereocystis spp., this region and species of kelp may be particularly susceptible to warming (Smale et al., 2019). The stability of these kelp forests is tenuous given that future oceans are predicted to warm even more. MHW impacts are likely to be of increased intensity, duration, and frequency (Frölicher and Laufkötter, 2018), thus making kelp forests particularly susceptible.
Gender-Specific Impacts
The health and reproduction of surviving females and males were both negatively impacted by 2016. The median body condition index for both sexes in aggregate decreased 24% in the post-KFC years (Figures 2A, 3A), significantly impacting the length-weight relationship (Figure 4). The decline in male condition index from 2015 to 2016 was more dramatic than for females. This could be due to additional lipid storage that females gained from the degenerating eggs as they began to starve.
The decrease in female gonad index was dramatic with a 94% drop in 2016 and 2017 (Figure 2B). Histological assessments revealed that while females typically had more than 4,000 eggs per gram of body weight in the pre-KFC years (before 2014), very few females had more than 1,000 eggs per gram after 2015 (Figure 5). Likewise, we observed a 99% decrease (p < 0.001) in medians of total numbers of mature eggs per female. The reduction in reproductive energy from starvation decreased the numbers of mature eggs, so that the few eggs present in the gonad were predominantly immature. This result suggests that while oogenesis was occurring and immature eggs were present, maturation of the eggs was suppressed. In the males, the median percentage of males with sperm decreased by 33% between the pre-KFC and post-KFC years, and the median qualitative sperm abundance score from histological examination of the testes tissue decreased by 28% (p = 0.414).
Comparison With Laboratory Experiments
Similar patterns of gender-specific impacts on gonad condition from starvation and warmer water temperatures have been previously reported from laboratory experiments (Rogers-Bennett et al., 2010), providing additional insights into these recent observations in the wild. In the lab, male abalone gonad condition was more sensitive than females to warmer water temperatures, resulting in total reproductive failure when exposed to temperatures above 16°C for 12 months, regardless of food supply. Similar to the wild, female abalone continued to produce immature eggs, indicating that oogenesis was still occurring, however, egg maturation was substantially reduced under starvation conditions in the lab.
In the wild, the MHW and subsequent KFC produced concurrent warm water and starvation conditions. However, the warming in the lab was more extreme than in the wild, resulting in reproductive impacts that were more pronounced in the laboratory experiments (Rogers-Bennett et al., 2010). The warm temperature treatment in the lab was maintained at a steady 18°C, whereas the wild abalone experienced variable temperatures >12°C, with a record peak of 17.4°C. The decrease in the percentage of male abalone with mature sperm in the warm water laboratory experiments was nearly twice that found in the wild post-KFC. Likewise, the percentage of females with mature eggs decreased under food limitation conditions at nearly twice the rate as in the wild.
Impacts to Big Fecund Females
In the Pre-KFC years, wild female red abalone fecundity (number of mature eggs) increased exponentially with shell length (Figure 6). The importance of highly fecund large females for population productivity has been recognized as a guiding principle for fishery management strategies (Birkeland and Dayton, 2005). For abalone, the reproductive potential of large female abalone inside Marine Protected Areas was a good predictor of recruitment success of newly settled abalone in Baja California Mexico (Rossetto et al., 2013), demonstrating the value of areas that protect large females. For other climate related stressors, such as hypoxic events, abalone recruitment inside MPAs recovered more quickly due to the resilience provided by the large fecund females in the MPAs, even though both areas were impacted by low oxygen (Micheli et al., 2012). In northern California, large female red abalone were protected in a network of Marine Protected Areas along the coast, as well as in habitats >10 m depth due to limited access by most breath-hold abalone divers.
By 2016, the size-specific female fecundity curve had flattened, requiring a critical re-evaluation of population dynamics models and fisheries management assumptions under these new conditions. The environmental conditions impacted fished and protected areas equivalently (Rogers-Bennett and Catton, 2019), undermining the productivity advantages previously gained from the protected areas. The value of the protected areas will be once again realized when kelp forest conditions improve and female fecundity curves recover. Until then, protecting large females, inside and outside of historically protected areas, is important to promote faster recovery once conditions improve.
Climate Change and Natural Resource Management
Long-term monitoring of health and reproduction is critical to informing natural resource management decisions, whether to promote further exploitation or to pursue restoration actions. In the past, short-term warming, such as El Niño events (1 year), have briefly impacted abalone reproduction (Vilchis et al., 2005; Rogers-Bennett et al., 2010), though stocks rebounded quickly without management intervention. In this study, the impacts of a multi-year MHW and subsequent KFC were more long lasting and dramatically more pronounced. Future events, influenced by climate change, are expected to continue to bring more frequent and extreme stressor conditions. The back-to-back and interacting poor environmental conditions brought on by these events exceeded the red abalones’ 1-year resilience period, severely impacting both the health and reproductive potential of the few survivors. The surviving large females are the basis for any resilience the population might have (Rossetto et al., 2013) as well as preserving genetic diversity (Munguía-Vega et al., 2015). In this case, active abalone restoration measures are needed in northern California, including re-establishing robust kelp forest areas, protecting abalone in recovery areas, and stocking of hatchery-reared juveniles. Without the political will to enact active red abalone restoration, we may continue to see the decline of red abalone survivors, exacerbating the challenges to reversing the downward population trajectory.
This case study highlights that climate change can not only bring severe, lethal impacts to populations but that detrimental sublethal effects influencing health, and future recruitment success may linger for several years following the initial climate event. Given these impacts, it will be important to determine the resilience thresholds of exploited species facing climate stress and to reduce other sources of mortality during prolonged recovery periods. Depending on the life history of the target species, exploitation pressure on large adults may undermine future population growth by further reducing the survivors of the mortality event.
For natural resource managers facing climate change impacts, the critical question is whether the resource will be able to recover on its own, or if active restoration intervention is needed. In the absence of historical monitoring data, this question can only be answered with ongoing surveillance after the event, which may require many years or decades to ascertain (Caputi et al., 2019). Population modeling may also aid managers trying to determine future abalone population trajectories following KFC (Hart et al., 2018). Coupling traditional monitoring data of density with life-history knowledge, body and gonad condition, as well as recruitment surveillance data, will help to identify the species and systems that might be at the greatest risk of ongoing collapse. Baseline genetics information can also be used forensically following unexpected mass mortality events by comparing transcriptomes before and after the event to assist in the determination of causal factors (De Wit et al., 2014). Therefore, comprehensive monitoring programs must be established to quantify, not only baseline densities, but also baseline (pre-stressor) health, reproductive capacity, and genetics. This means having surveillance data before impacts occur to be able to recognize how climate stressors have changed productivity. Knowing the severity of the impacts as compared with baseline conditions will be critical to informing effective management decisions of exploited species as well as restoration strategies.
Data Availability Statement
The raw data supporting the conclusions of this article will be made available by the authors, without undue reservation.
Author Contributions
LR-B and RK wrote the first draft of the manuscript. LR-B and CC designed the study. RK worked on the data analysis and creation of the figures. All authors contributed to the manuscript revision, read, and approved the submitted version.
Funding
Funding for this work was provided by the California Department of Fish and Wildlife.
Conflict of Interest
The authors declare that the research was conducted in the absence of any commercial or financial relationships that could be construed as a potential conflict of interest.
Publisher’s Note
All claims expressed in this article are solely those of the authors and do not necessarily represent those of their affiliated organizations, or those of the publisher, the editors and the reviewers. Any product that may be evaluated in this article, or claim that may be made by its manufacturer, is not guaranteed or endorsed by the publisher.
Acknowledgments
We thank Richard Dondanville for his work on red abalone reproduction and egg production. We also thank the California State University COAST summer interns for helping us assemble and compile these data, as well as Katie Sowul for assisting with the feeding and recovery experiment on red abalone. Thanks to all recreational red abalone divers and rock pickers who contributed their abalone for sampling, without whom we could not have done this work.
References
Arafeh-Dalmau, N., Montaño-Moctezuma, G., Martínez, J. A., Beas-Luna, R., Schoeman, D. S., and Torres-Moye, G. (2019). Extreme marine heatwaves alter kelp forest community near its equatorward distribution limit. Front. Mar. Sci. 6:499. doi: 10.3389/fmars.2019.00499
Arafeh-Dalmau, N., Schoeman, D. S., Montaño-Moctezuma, G., Micheli, F., Rogers-Bennett, L., Olguin-Jacobson, C., et al. (2020). Marine heat waves threaten kelp forests. Science 367, 635–635.
Belkin, I. M. (2009). Rapid warming of large marine ecosystems. Prog. Oceanogr. 81, 207–213. doi: 10.1016/j.pocean.2009.04.011
Birkeland, C., and Dayton, P. K. (2005). The importance in fishery management of leaving the big ones. Trends Ecol. Evol. 20, 356–358. doi: 10.1016/j.tree.2005.03.015
Boles, S. E. (2020). Effects of Ocean Acidification on the Growth and Survival in Early-Stage Red Abalone (Haliotis rufescens). Davis, CA: Univeristy of California.
Cannell, B. L., Thomson, P. G., Schoepf, V., Pattiaratchi, C. B., Fraser, M. W. (2019). “Impacts of marine heatwaves,” in Marine Extremes, eds Techera, E. J., and Winter, G. (Milton Park: Routledge), 123–140. doi: 10.4324/9780429491023-8
Caputi, N., Jackson, G., and Pearce, A. (2014). The Marine Heat Wave off Western Australia During the Summer of 2010/11: 2 Years On. Fisheries Research Report No. 250. North Beach, WA: Western Australian Fisheries and Marine Research Laboratories.
Caputi, N., Kangas, M., Chandrapavan, A., Hart, A., Feng, M., Marin, M., et al. (2019). Factors affecting the recovery of invertebrate stocks from the 2011 Western Australian extreme marine heatwave. Front. Mar. Sci. 6:484. doi: 10.3389/fmars.2019.00484
De Wit, P., Rogers-Bennett, L., Kudela, R. M., and Palumbi, S. R. (2014). Forensic genomics as a novel tool for identifying the causes of mass mortality events. Nat. Commun. 5:3652.
Deutsch, C., Ferrel, A., Seibel, B., Pörtner, H. O., and Huey, R. B. (2015). Ecophysiology. Climate change tightens a metabolic constraint on marine habitats. Science 348, 1132–1135. doi: 10.1126/science.aaa1605
Di Lorenzo, E., and Mantua, N. (2016). Multi-year persistence of the 2014/15 North Pacific marine heatwave. Nat. Clim. Chang. 6:1042. doi: 10.1038/nclimate3082
Fordyce, A. J., Ainsworth, T. D., Heron, S. F., and Leggat, W. (2019). Marine heatwave hotspots in coral reef environments: physical drivers, ecophysiological outcomes, and impact upon structural complexity. Front. Mar. Sci. 6:498. doi: 10.3389/fmars.2019.00498
Frölicher, T. L., and Laufkötter, C. (2018). Emerging risks from marine heat waves. Nat. Commun. 9:650.
García-Reyes, M., and Largier, J. (2010). Observations of increased wind-driven coastal upwelling off central California. J. Geophys. Res. Oceans 115:2009JC005576.
Haaker, P. L., Parker, D. O., Barsky, K. C., and Chun, C. S. Y. (1998). Growth of red abalone, Haliotis rufescens (Swainson), at Johnsons Lee, Santa Rosa Island, California. J. Shellfish Res. 17, 747–753.
Harley, C. D., Anderson, K. M., Demes, K. W., Jorve, J. P., Kordas, R. L., Coyle, T. A., et al. (2012). Effects of climate change on global seaweed communities. J. Phycol. 48, 1064–1078.
Hart, A. M., Strain, L. W. S., and Brown, J. (2018). Regulation dynamics of exploited and protected populations of Haliotis roei, and their response to a marine heatwave. ICES J. Mar. Sci. 75, 1924–1939. doi: 10.1093/icesjms/fsy064
Hobday, A. J., and Pecl, G. T. (2014). Identification of global marine hotspots: sentinels for change and vanguards for adaptation action. Rev. Fish Biol. Fish. 24, 415–425. doi: 10.1007/s11160-013-9326-6
Holbrook, N. J., Gupta, A. S., Oliver, E. C., Hobday, A. J., Benthuysen, J. A., Scannell, H. A., et al. (2020). Keeping pace with marine heatwaves. Nat. Rev. Earth Environ. 1, 482–493. doi: 10.1038/s43017-020-0068-4
Hughes, T. P., Kerry, J. T., Álvarez-Noriega, M., Álvarez-Romero, J. G., Anderson, K. D., Baird, A. H., et al. (2017). Global warming and recurrent mass bleaching of corals. Nature 543:373.
Leising, A. W. I., Schroeder, D., Bograd, S. J., Abell, J., Durazo, R., Gaxiola-Castro, G., et al. (2015). State of the California Current 2014-15: impacts of the Warm-Water” Blob”. Calif. Cooperat. Ocean. Fish. Invest. Rep. 56, 31–68.
Marbà, N., and Duarte, C. M. (2010). Mediterranean warming triggers seagrass (Posidonia oceanica) shoot mortality. Glob. Chang. Biol. 16, 2366–2375. doi: 10.1111/j.1365-2486.2009.02130.x
McPherson, M. L., Finger, D. J. I., Houskeeper, H. F., Bell, T. W., Carr, M. H., Rogers-Bennett, L., et al. (2021). Large-scale shift in the structure of a kelp forest ecosystem co-occurs with an epizootic and marine heatwave. Commun. Biol. 4:298.
Micheli, F., Saenz-Arroyo, A., Greenley, A., Vazquez, L., Espinoza Montes, J. A., Rossetto, M., et al. (2012). Evidence that marine reserves enhance resilience to climatic impacts. PLoS One 7:e40832. doi: 10.1371/journal.pone.0040832
Moore, J. D., Finley, C. A., Robbins, T. T., and Friedman, C. S. (2002). Withering syndrome and restoration of southern California abalone populations. Calif. Cooperat. Ocean. Fish. Invest. Rep. 43, 112–117.
Morash, A. J., and Alter, K. (2016). Effects of environmental and farm stress on abalone physiology: perspectives for abalone aquaculture in the face of global climate change. Rev. Aquac. 8, 342–368. doi: 10.1111/raq.12097
Munguía-Vega, A., Sáenz-Arroyo, A., Greenley, A. P., Espinoza-Montes, J. A., Palumbi, S. R., Rossetto, M., et al. (2015). Marine reserves help preserve genetic diversity after impacts derived from climate variability: lessons from the pink abalone in Baja California. Glob. Ecol. Conserv. 4, 264–276. doi: 10.1016/j.gecco.2015.07.005
Oliver, E. C. J., Donat, M. G., Burrows, M. T., Moore, P. J., Smale, D. A., Alexander, L. V., et al. (2018). Longer and more frequent marine heatwaves over the past century. Nat. Commun. 9:1324.
Oregon Department of Fish and Wildlife. (2021). Commission Extends Red Abalone Closure, Delists Borax Lake Chub, News Releases, February 12, 2021. Available online at: https://www.dfw.state.or.us/news/2021/02_feb/021221.asp
Reid, J., Rogers-Bennett, L., Lavín, F., Pace, M., Catton, C., and Taniguchi, I. (2016). The economic value of the recreational red abalone fishery in northern California. Calif. Fish Game 102, 119–130.
Rogers-Bennett, L., and Catton, C. A. (2019). Marine heat wave and multiple stressors tip bull kelp forest to sea urchin barrens. Sci. Rep. 9: 15050.
Rogers-Bennett, L., Dondanville, R. F., and Kashiwada, J. (2004). Size specific fecundity of red abalone (Haliotis rufescens): evidence for reproductive senescence? J. Shellfish Res. 23, 553–560.
Rogers-Bennett, L., Dondanville, R. F., Moore, J. D., and Vilchis, L. I. (2010). Response of red abalone reproduction to warm water, starvation, and disease stressors: implications of ocean warming. J. Shellfish Res. 29, 599–611. doi: 10.2983/035.029.0308
Rogers-Bennett, L., Kashiwada, J. V. I., Taniguchi, K., Kawana, S. K., and Catton, C. A. (2019). Using density-based fishery management strategies to respond to mass mortality events. J. Shellfish Res. 38:411.
Rossetto, M., Leo, G. A. D., Greenley, A., Vazquez, L., Saenz-Arroyo, A., Montes, J. A. E., et al. (2013). Reproductive potential can predict recruitment rates in abalone. J. Shellfish Res. 32, 161–169. doi: 10.2983/035.032.0122
Sanford, E., Sones, J. L., García-Reyes, M., Goddard, J. H., and Largier, J. L. (2019). Widespread shifts in the coastal biota of northern California during the 2014–2016 marine heatwaves. Sci. Rep. 9, 1–14.
Schiel, D. R., and Foster, M. S. (2015). The Biology and Ecology of Giant Kelp Forests. California: University of California Press.
Smale, D. A., Wernberg, T., Oliver, E. C. J., Thomsen, M., Harvey, B. P., Straub, S. C., et al. (2019). Marine heatwaves threaten global biodiversity and the provision of ecosystem services. Nat. Clim. Chang. 9, 306–312. doi: 10.1038/s41558-019-0412-1
Solomon, S., Manning, M., Marquis, M., and Qin, D. (2007). Climate Change 2007-the Physical Science Basis: Working Group I Contribution to the Fourth Assessment Report of the IPCC. Cambridge: Cambridge university press.
Tutschulte, T. C. (1976). The Comparative Ecology of Three Sympatric Abalones. San Diego, CA: Scripps Institution of Oceanography.
Vilchis, L. I., Tegner, M. J., Moore, J. D., Friedman, C. S., Riser, K. L., Robbins, T. T., et al. (2005). Ocean warming effects on growth, reproduction, and survivorship of Southern California abalone. Ecol. Appl. 15, 469–480. doi: 10.1890/03-5326
Keywords: marine heatwave, kelp deforestation, gonad index, red abalone (Haliotis rufescens), recovery
Citation: Rogers-Bennett L, Klamt R and Catton CA (2021) Survivors of Climate Driven Abalone Mass Mortality Exhibit Declines in Health and Reproduction Following Kelp Forest Collapse. Front. Mar. Sci. 8:725134. doi: 10.3389/fmars.2021.725134
Received: 14 June 2021; Accepted: 15 July 2021;
Published: 16 August 2021.
Edited by:
Gretchen E. Hofmann, University of California, Santa Barbara, United StatesReviewed by:
Norman Ragg, Cawthron Institute, New ZealandNick Caputi, Department of Primary Industries and Regional Development of Western Australia (DPIRD), Australia
Copyright © 2021 Rogers-Bennett, Klamt and Catton. This is an open-access article distributed under the terms of the Creative Commons Attribution License (CC BY). The use, distribution or reproduction in other forums is permitted, provided the original author(s) and the copyright owner(s) are credited and that the original publication in this journal is cited, in accordance with accepted academic practice. No use, distribution or reproduction is permitted which does not comply with these terms.
*Correspondence: Laura Rogers-Bennett, rogersbennett@ucdavis.edu