- 1Key Laboratory of Applied Marine Biotechnology, Ningbo University, Chinese Ministry of Education, Ningbo, China
- 2Collaborative Innovation Center for Zhejiang Marine High-Efficiency and Healthy Aquaculture, Ningbo, China
Demand from consumers for small quantities of live swimming crab Portunus trituberculatus is rising with the development of e-commerce. However, it is challenging to keep P. trituberculatus alive post-capture. In this study, a transport bag containing oxygen and seawater (24 ppt) was used to investigate the survival and metabolic changes of P. trituberculatus during transport. The results showed that more than 80% P. trituberculatus could survive at least 24 h in the transport bag. The ability of the crabs to survive may be attributed to adaptive metabolism, as suggested by the switch from an aerobic to an anaerobic pathway for energy generation and a decline in amino acid metabolism, nucleic acid metabolism, and osmoregulation. Our findings suggest that the transport bag could effectively extend the post-capture survival time of P. trituberculatus. Metabolic adaptation – especially energy homeostasis – is crucial for crab survival during transport. Our study provides a promising method for the transport of live P. trituberculatus.
Highlights
– A transport bag was designed for P. trituberculatus.
– Changes in energy metabolism, amino acid metabolism, nucleic acid metabolism, and osmoregulation occurred during crab transport.
– Metabolic adaptation is crucial for crab survival during transport.
Introduction
Seafood is perishable, and its postmortem freshness reduces quickly (Grassi et al., 2019). Live crabs are appreciated in China because of their taste (Lu et al., 2015). The swimming crab P. trituberculatus (Crustacea, Decapoda, and Brachyura) is a commercially important fishery species in China, with a yield of 113,810 tons in 2019 (China Fishery Statistical Yearbook, 2020). However, the post-capture survival of the swimming crab is poor because they are a water-breathing species and are prone to suffer from stress because of factors such as handling, cannibalism, overcrowding, temperature fluctuation, low humidity, and oxygen (Fotedar and Evans, 2011; Dong et al., 2019). Although various tanks, containers, and vehicles equipped with aeration facilities are available for holding P. trituberculatus during transport, the mortality remains high, mainly because of unavoidable air exposure and temperature fluctuation (Lu et al., 2015, 2016; Dong et al., 2019). As a result, it is hard to transport live P. trituberculatus over long distances (Lu et al., 2015). Nevertheless, with the development of e-commerce, consumers demand comparatively small quantities of P. trituberculatus, which necessitates long-distance transport. Therefore, it is necessary to develop a suitable method for the transport of live P. trituberculatus.
Accumulating evidence shows that the post-capture survival rate of crabs depends on the species and holding conditions. For example, the green mud crab Scylla paramamosain can survive without water for 36 h at 25°C (Gu et al., 2017), while the southern king crab Lithodes santolla can tolerate up to 30 h at 12°C (Urbina et al., 2013). Chinese mitten crab Eriocheir sinensis and the stone king crab Paralomis granulosa could survive without water only for 16 and 18 h, respectively (Romero et al., 2011; Bao et al., 2019). In addition, adverse environmental conditions such as suboptimal temperature also can lead to physiological effects on crabs. For example, temperature fluctuation and air exposure lead to abrupt changes in levels of energy-related metabolites such as glucose, lactate, and adenine nucleotides in hemolymph and muscle of P. trituberculatus (Lu et al., 2015, 2016). Because air exposure reduces the moisture of gills and leads to the accumulation of fatal metabolites in the hemolymph of P. trituberculatus, >90% of P. trituberculatus cannot survive for >24 h under air exposure even at the optimal temperature (Dong et al., 2019). Therefore, we made the following assumptions: (1) water is indispensable for long-distance transport of P. trituberculatus, and (2) metabolic modulation is vital for the survival of P. trituberculatus during live transport.
In this context, we designed a transport bag containing oxygen and seawater to investigate whether the survival of P. trituberculatus during transport could be improved. Furthermore, the metabolic changes over transport time in each of four tissues were investigated to obtain further insight into the survival mechanisms of P. trituberculatus. The present study aimed to provide an alternative method for small-scale, long-distance transport of live P. trituberculatus.
Materials and Methods
Experimental Animal, Rearing, and Sampling
One hundred adult male P. trituberculatus weighing 187.5 ± 37.5 g (mean ± SD) were purchased from a local crab farm in Ningbo, China, in November 2018. Only male crabs were used in the present study to avoid physiological differences caused by gender. After the crabs were transferred to the laboratory, they were kept in an aerated recycling seawater system (salinity 24 ppt) for 1 week. Crabs were fed with fresh manila clam Ruditapes philippinarum once daily at 18:00. Residual food and feces were removed, and approximately 20% seawater was exchanged each morning.
To investigate the survival time, crabs were randomly distributed into four groups (25 crabs per group). The crabs were starved for 24 h before the experiment. A plastic box (17.5 cm × 13.5 cm × 7.5 cm) with holes on the bottom and top and a plastic bag (30 cm × 17 cm) equipped with an air-tight type air tap were used to hold one live crab (Figure 1). First, a crab was placed into the plastic box to prevent the plastic bag from being punctured by the crab’s hard shell. Next, the plastic box containing the crab was placed into the plastic bag containing 0.7 L seawater (salinity 24 ppt). Then, the air was extracted from the bag, and the bag was sealed with a heat sealer. Finally, 4.8 L of oxygen was added into the bag through the air tap, and the tap was closed to prevent gas leakage. After packaging, all crabs were transferred to 12°C with one replicate group per incubator. The temperature was set based on the results of Dong et al. (2019). The crabs were starved during the experiment. The number of dead crabs was recorded every 12 h. Mortality was determined based on the somatic response, as Barrento et al. (2011) and Lu et al. (2016) described and discarded.
To measure the metabolomics changes during transport, two live crabs per group were randomly collected at 0, 1, 2, and 3 days for nuclear magnetic resonance (NMR) analysis (eight crabs in total per time point). The crabs were removed from the bags, anesthetized with iced seawater, and sacrificed to collect tissues, including gills, hepatopancreas, muscle, and intestine. All the samples were frozen in liquid nitrogen and stored at −80°C until analysis.
Nuclear Magnetic Resonance Measurements
Metabolite Extraction From Crab Tissues
Each tissue sample (approximately 100 mg) was treated twice with an ice-cold aqueous methanol extraction solution (2:1, v/v) using a TissueLyser (QIAGEN, Hilden, Germany) at 20 Hz for 90 s. The resultant supernatants were pooled and lyophilized. The lyophilized powder was reconstituted in 600 μL of phosphate buffer (K2HPO4/NaH2PO4, 0.1 M, pH 7.46) containing 50% deuterated water (D2O) and 0.001% sodium 3-trimethylsilyl [2,2,3,3-2D4] propionate (TSP). Here, D2O and TSP were, respectively, used as a field lock signal and an internal quantitative reference for NMR analysis. Following 10 min of centrifugation (6037.2 × g, 4°C), 550 μL of supernatant from each sample were transferred into an NMR tube (5 mm).
Nuclear Magnetic Resonance Spectroscopy
All NMR spectra were acquired at 298 K on a Bruker Avance III 600 MHz spectrometer (Bruker Biospin, Germany) equipped with an inverse cryogenic probe according to the procedure described in a previous study (Ye et al., 2016). In brief, one-dimensional 1H NMR spectra were acquired using a standard noesygppr1d pulse sequence with recycle delay time of 2 s and mixing time of 100 ms. The 90° pulse length was set to approximately 10 μs, and 64 transients were collected into 32,768 data points for each 1H NMR spectrum with a spectral width of 20 ppm. Several two-dimensional NMR spectra were recorded for the NMR signal assignment for gill, hepatopancreas, muscle, and intestine extracts, including 1H–1H COSY, 1H–1H TOCSY, 1H–13C HSQC, and 1H–13C HMBC.
Data Analyses
The survival rate was analyzed using a log-rank (Mantel–Cox) test with the Kaplan–Meier approach (GraphPad Prism 5, GraphPad Software, Inc., La Jolla, CA, United States). The phase and baseline of all 1H NMR spectra were corrected using TOPSPIN software (V2.0, Bruker Biospin) with chemical shifts referenced to the TSP peak as δ 0.00. The spectral region δ 0.8–9.2 without residual water and methanol signals was bucketed into bins with equal width of 0.004 ppm (2.4 Hz) for all tissue samples. Wet weight normalization was conducted for each bucketed region of gill, hepatopancreas, muscle, and intestine extracts.
Multivariate data analysis was conducted on the normalized NMR data using SIMCA-P+ (12.0, Umetrics, Umeå, Sweden) as previously described (Ye et al., 2014). In brief, principal component analysis (PCA) was performed to provide groupings, trends, and outliers of all tissue samples. Subsequently, orthogonal projection to latent structures with discriminant analysis (OPLS-DA) was conducted using unit-variance scaled NMR data. The quality of all OPLS-DA models was evaluated by sevenfold cross-validation with CV-ANOVA (p < 0.05) (Eriksson et al., 2008). After back-transformation of the NMR data, color-coded loading plots of OPLS-DA were generated to mine information on the metabolites that significantly contributed to intergroup differences using a MATLAB script (V7.1, The MathWorks Inc., Natick, MA, United States). Herein, the absolute value of correlation coefficient (| r|) > 0.666 for a metabolite was statistically significant based on Pearson correlation analysis. The changing rate of lactate in four tissues with transport time was calculated as (Ci−C0)/C0, where Ci represents the lactate concentration in the tissues at day 1, 2, and 3 and C0 represents the lactate concentration in the tissues at day 0.
Results
Survival Rate During Transport
To investigate the survival of P. trituberculatus in the transport bag, the survival rates at 12°C were recorded at different time points (Figure 2). More than 80% crabs could survival the first day, and then a sharp decline was observed on day 2, during which the survival rate dropped below 40% (Figure 2).
Metabolite Changes During Transport
Untargeted 1H NMR spectra for low-molecular-weight metabolites in crab tissues were acquired to investigate the metabolic changes in gills, hepatopancreas, muscle, and intestine when P. trituberculatus were kept in the oxygen-filled bag containing seawater at 12°C. The assigned metabolites in the crab tissues are listed in Supplementary Table 1 with 1H and 13C NMR data. In multivariate data analysis, PCA score plots based on the first and second principal components (PC1 and PC2) were obtained from all 1H NMR spectral data obtained from aqueous methanol extracts of the four tissues (Figure 3). The PCA results differentiated the intestinal tissue and the other three tissues in PC1 (Figure 3A). Notably, six outliers were observed for the intestinal extracts in this PCA plot. However, these intestine samples were still included in subsequent analyses as the distinct metabolomic profile of the intestine compared with that of the other three tissues may result in these outliers. This view was supported by the plot in Figure 3E, where only one sample was outside the confidence ellipse. Furthermore, in Figure 3A, minor clustering was observed among gill, hepatopancreas, and muscle. Although tissue type played a dominant role in separating crab metabolic profiles, transport time still played an essential role in the metabolite changes in some tissues. The metabolite changes in the gill, muscle, and intestine displayed a time-dependent pattern (Figures 3B,D,E), whereas no apparent clustering was observed among the four hepatopancreas samples at different sampling times (Figure 3C).
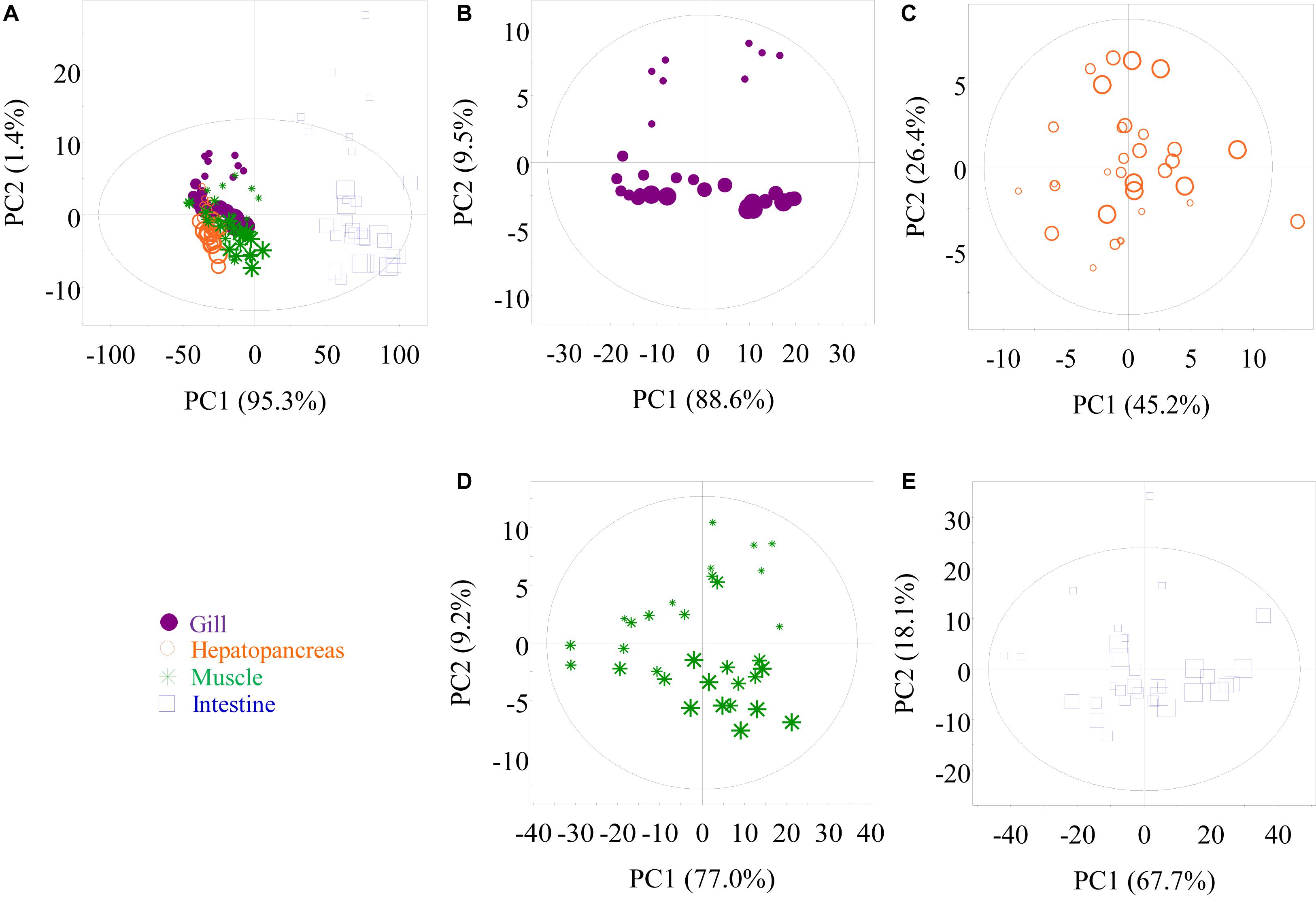
Figure 3. Principal component analysis score plots of nuclear magnetic resonance data, respectively, obtained from methanolic extracts of all tissues (A), gill (B), hepatopancreas (C), muscle (D), and intestine (E) samples of P. trituberculatus during transport. G0, G1, G2, and G3: gills of crabs at day 0, 1, 2, and 3, dot size 40, 32, 24, and 16, respectively; H0, H1, H2, and H3: hepatopancreas of crabs at day 0, 1, 2, and 3, dot size 40, 32, 24, and 16, respectively; M0, M1, M2, and M3: muscle of crabs at day 0, 1, 2, and 3, dot size 40, 32, 24, and 16, respectively; I0, I1, I2, and I3: intestine of crabs at day 0, 1, 2, and 3, dot size 40, 32, 24, and 16, respectively.
Pairwise comparison via OPLS-DA was conducted with sevenfold cross-validation of the 1H NMR spectral data from the transported crabs compared with their control counterparts. A total of 12 OPLS-DA models were constructed for the four tissue samples. However, only eight OPLS-DA models were of good quality, as evaluated based on high values of prediction indicators R2X and Q2 but low p-values in CV-ANOVA (Figures 4, 5 and Supplementary Figures 1, 2). No significant difference was observed in the 1H NMR spectral data for the four tissue types between day 0 and day 1, which indicates that the significant metabolomic differences occurred after one transport day. In comparison with the control crabs (day 0), crabs at day 2 showed a significant elevation in the levels of gill lactate and a significant depletion in the levels of gill glutamate, glutamine, arginine, glycine, taurine, betaine, trehalose, trigonelline, histamine, choline-O-sulfate (COS), uridine monophosphate (UMP), and adenosine monophosphate (AMP) (Figure 4). Moreover, crabs at day 3 showed a significant elevation in the levels of gill lactate and succinate and a significant depletion in the levels of gill glutamine, UMP, 2-pyridinemethanol, histamine, AMP, and trehalose, when compared with the control crabs. For hepatopancreas and muscle, the number of metabolites with significantly altered levels was considerably lower than that of the gill. In detail, crabs at day 2 showed significant elevation in alanine levels in the hepatopancreas, whereas crabs at day 3 showed a significant elevation in lactate levels and a significant depletion in AMP levels in the hepatopancreas, when compared with the control hepatopancreas samples (Supplementary Figure 1). Simultaneously, a significant depletion in the levels of trimethylamine-N-oxide (TMAO) and trigonelline in the muscle of crabs at day 2 and a significant elevation in lactate levels and succinate in the muscle of crabs at day 3 was observed when compared with the control muscle samples (Supplementary Figure 2). Intestine presented a higher number of metabolites with significantly altered levels than hepatopancreas and muscle. In the crabs at day 2, intestinal lactate, succinate, and 2-pyridinemethanol levels were significantly higher, and glutamate, glutamine, glucose, trehalose, and COS levels were significantly lower in controls (Figure 5). For crabs at day 3, intestinal lactate and inosine significantly increased, and ATP significantly decreased. These metabolic changes suggest that tissue-specific metabolic modulation occurs in P. trituberculatus during live transport.
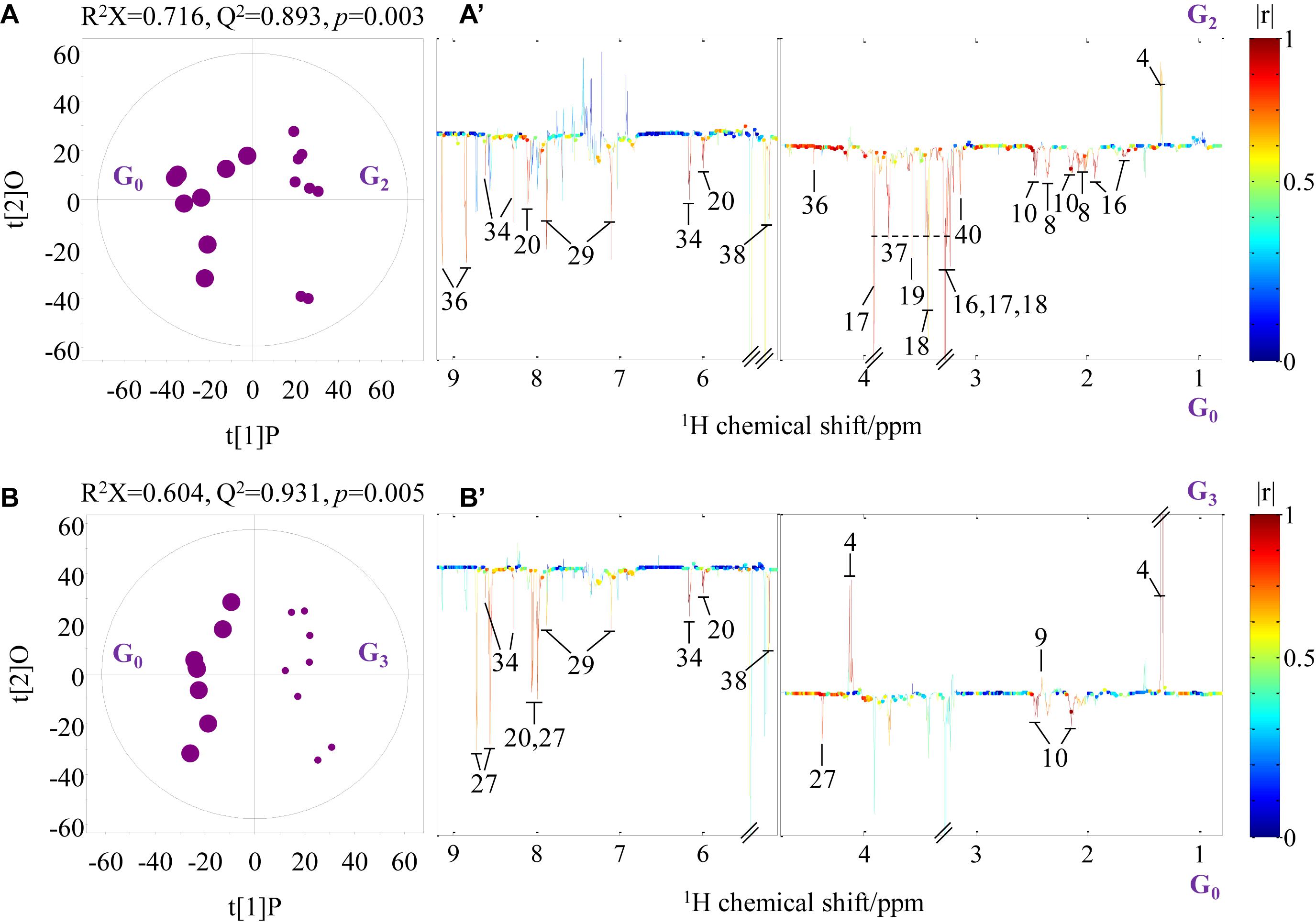
Figure 4. Orthogonal projection to latent structures with discriminant analysis scores (A,B) and coefficient-coded loading plots (A’,B’) for the gill models discriminating crabs at day 0 (G0, dot size 40), crabs at day 2 (G2, dot size 24), and crabs at day 3 (G3, dot size 16). Metabolites: 4: lactate; 8: glutamate; 9: succinate; 10: glutamine; 16: arginine; 17: betaine; 18: taurine; 19: glycine; 20: uridine monophosphate; 27: 2-pyridinemethanol; 29: histamine; 34: adenosine monophosphate; 36: trigonelline; 38: trehalose; 40: choline-O-sulfate.
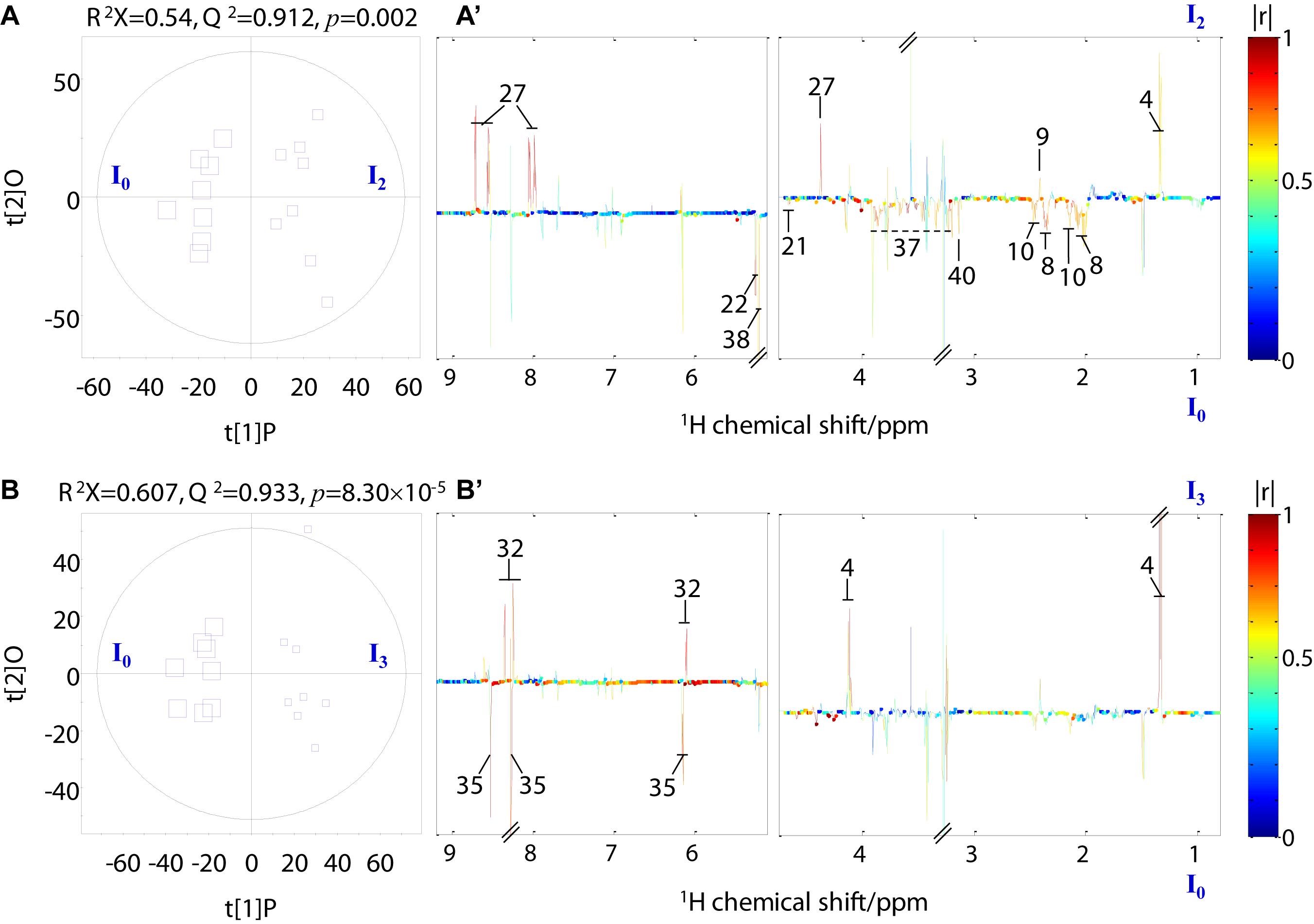
Figure 5. Orthogonal projection to latent structures with discriminant analysis scores (A,B) and coefficient-coded loading plots (A’,B’) for the intestine models discriminating crabs at day 0 (I0, square size 40), crabs at day 2 (I2, square size 24), and crabs at day 3 (I3, square size 16). Metabolites: 4: lactate; 9: succinate; 27: 2-pyridinemethanol; 8: glutamate; 10: glutamine; 21: glucose; 32: inosine; 35: ATP; 38: trehalose; 40: choline-O-sulfate.
The changes in the concentration of this organic acid in the four tissue types with time compared with their control counterparts were calculated to examine the temporal dynamics of lactate concentration. Overall, a gradual elevation in the lactate level was observed in all tissues throughout the experiment (Figure 6). The highest accumulation of lactate was found in the gill and muscle tissues at day 3, increased by 1172.4 and 908.8%, respectively, relative to their control counterparts; hepatopancreas and intestine, respectively, showed 427.7 and 273.2% increases.
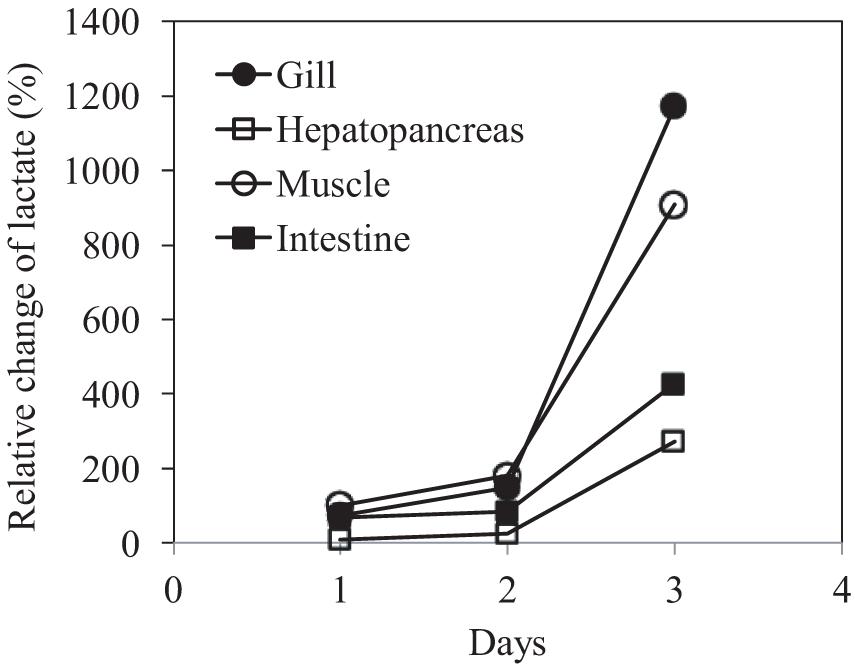
Figure 6. Relative changes in lactate concentration in the gills, hepatopancreas, muscle, and intestine of P. trituberculatus during transport.
Discussion
Freshness plays a crucial role in crab quality, reducing rapidly after crab death (Wang et al., 2018). In the present study, 80% of the crabs kept in the transport bag were able to survive for 24 h, which is considerably longer than those under air exposure even at the optimal temperature (10°C; <12 h) (Lu et al., 2015, 2016; Dong et al., 2019). Our results confirm that air exposure is a detrimental stressor for P. trituberculatus post-capture. Although an array of evidence has indicated the adverse effects of air exposure on P. trituberculatus (Lu et al., 2015; Dong et al., 2019) and other crab species such as porcelain crabs (Stillman and Somero, 1996), Carcinus maenas, Necora puber (Durand and Regnault, 1998), Cancer pagurus (Barrento et al., 2011, 2012), Neohelice granulata (Geihs et al., 2013, 2014), L. santolla (Urbina et al., 2013), and C. maenas (Simonik and Henry, 2014), a dry or semi-dry approach remains prevalent, for economic reasons, in the transport of live crabs. Our study shows that water-bathed P. trituberculatus survive much longer than air-exposed individuals, offering the possibility of long-distance transport of P. trituberculatus.
Although most swimming crabs could survive 1 day in the transport bag, mortality rose sharply on day 2. Previous studies have suggested that loss of energy homeostasis results in crab mortality (Lu et al., 2015, 2016). Our results showed that gills exhibited the most significant metabolic change among the four tissue types studied, which is predictable, as the gills are the gas/solute exchange organs of P. trituberculatus; they also play an essential role in osmoregulation, acid–base regulation, waste excretion, and immune and antioxidative responses (Burnett et al., 2006; Lorenzon et al., 2008; Romero et al., 2011; Dong et al., 2019). A significant elevation in lactate levels and succinate together with a significant depletion in the levels of 12 metabolites suggests that gill metabolism changed profoundly after crab storage in the transport bag for 2 days.
Notably, we observed a >10-fold increase in gill lactate levels relative to controls. As a well-known end-product of anaerobic respiration in crustaceans (Spicer et al., 1990), lactate is often observed to accumulate in stressed crabs. For example, lactate accumulation has been reported in the hemolymph of C. pagurus during air exposure or water transport (Lorenzon et al., 2008; Barrento et al., 2011) and in P. trituberculatus under temperature fluctuation or air exposure (Lu et al., 2015, 2016). The gills of crustaceans engage anaerobic pathways under hypoxic stress (McMahon, 2001; Racotta et al., 2002). In light of these observations, severe hypoxia in the gills may occur after 3-day transport, as indicated by the significantly elevated gill succinate level, an essential metabolite in the tricarboxylic acid cycle (TCA). Succinate accumulation may be associated with a branched, non-cyclic TCA cycle where succinate is produced reductively under hypoxia (Rosenqvist et al., 1973). However, lactate accumulation could be an adaptive response to a stressful situation (Barton, 2002), as lactate can act as a positive effector in increasing the oxygen affinity of hemocyanin (Morris et al., 1986; Bridges, 2001). Moreover, lactate also acts as the predominant gluconeogenic precursor (Jenssen et al., 1993). In this study, it is not surprising that no significant changes were observed in gill glucose and ATP levels. In addition, the gill AMP level decreased markedly from day 2 in the transport bag. AMP is a direct agonist of AMP-activated protein kinase, which is involved in energy-sensing (Hardie, 2003), and AMP depletion further confirms that the ATP levels in the gills were not low. Taken together, energy homeostasis seems to be maintained in the gills of P. trituberculatus after 3-day transport, although the pathway of energy metabolism has been modified.
In addition to energy-related metabolites, we also detected a significant decrease in glutamine, taurine, betaine, and UMP levels in gills. Taurine and betaine are well recognized as osmoregulators in aquatic animals under stress (Carr et al., 1996; Avella et al., 2009; Ye et al., 2013). Therefore, the significantly decreased levels of these four metabolites strongly indicate a change in amino acid metabolism, osmoregulation, and nucleic acid metabolism in the gill. It seems like a positive metabolic modulation in the gill for crabs to survive longer.
Significantly altered lactate levels and AMP were also observed in the hepatopancreas of P. trituberculatus, indicating an adaptive switch from aerobic to anaerobic metabolism for energy generation. The hepatopancreas is an essential organ in crabs, with multiple functions, including digestion, metabolism, and stress resistance (Wang et al., 2014; Huang et al., 2015; Sun et al., 2015). However, the number of metabolites with significantly altered levels was considerably lower in the hepatopancreas than in the gills, indicating a less severe metabolic change in this organ during transport.
Muscle tissues showed a significant alteration in levels of only four metabolites during the experiment. Among them, two energy-related metabolites – lactate and succinate – were significantly elevated after 3-day transport, indicating an adaptive modulation in the strategy for energy generation. Concomitantly, levels of two compatible osmolytes – TMAO (Yancey et al., 2002) and trigonelline (Hammer et al., 2012) – significantly decreased at day 2. Accumulation of TMAO and trigonelline was observed in the muscle of C. maenas exposed to a high seawater CO2 level (Hammer et al., 2012) and P. trituberculatus exposed to low salinity (Ye et al., 2014). Therefore, we speculate that osmotic balance is altered in the muscle during transport.
Aside from the gills, the intestine presented the most significant metabolic change in the studied P. trituberculatus tissues. The significantly altered levels of lactate and succinate in the intestine suggest an adaptive regulation of energy generation. However, the significantly decreased intestine ATP level at day 3, which was not found in other tissues, may imply an energy imbalance in the intestine. The intestine of P. trituberculatus harbors vast bacteria (Zeng et al., 2016; Shi et al., 2019). Consequently, the energy demand of the intestine may be higher than that of the other three tissues tested here. Although proteins and lipids can be an energy source for crustaceans (Barclay et al., 1983; Pascual et al., 2006), starvation before and during transport likely leads to the energy deficiency in the intestine of P. trituberculatus. In addition, we also observed increased 2-pyridinemethanol levels and decreased glutamate, glutamine, trehalose, and COS levels at day 2 and increased inosine levels in the intestine at day 3. Trehalose and COS are involved in antioxidation and osmoregulation (Hanson et al., 1991; Benaroudj et al., 2001). These observations indicate the perturbed amino acid metabolism, nucleic acid metabolism, and osmoregulation in P. trituberculatus intestine during the transport process.
Conclusion
The transport bag could effectively extend the post-capture survival time for P. trituberculatus at the laboratory scale. Their ability to survive may be attributed to adaptive metabolism, as suggested by the switch from an aerobic to an anaerobic pathway for energy generation, and a decline in amino acid metabolism, nucleic acid metabolism, and osmoregulation. Our study provides a promising method for live transport of P. trituberculatus. Further research should be carried out under commercial transport conditions.
Data Availability Statement
The raw data supporting the conclusions of this article will be made available by the authors, without undue reservation.
Author Contributions
CS and FP performed the research. YY and CW designed the protocol. FP analyzed the data. CS and YY wrote the manuscript. All authors participated in the revision of this manuscript by providing comments and editing.
Funding
This work was supported by the National Natural Science Foundation of China (Grant Nos. 41776164 and 31972783), the special research funding from the Marine Biotechnology and Marine Engineering Discipline Group in Ningbo University (Grant No. 422004582), 2025 Technological Innovation for Ningbo (Grant No. 2019B10010), and the K. C. Wong Magna Fund of Ningbo University.
Conflict of Interest
The authors declare that the research was conducted in the absence of any commercial or financial relationships that could be construed as a potential conflict of interest.
Publisher’s Note
All claims expressed in this article are solely those of the authors and do not necessarily represent those of their affiliated organizations, or those of the publisher, the editors and the reviewers. Any product that may be evaluated in this article, or claim that may be made by its manufacturer, is not guaranteed or endorsed by the publisher.
Supplementary Material
The Supplementary Material for this article can be found online at: https://www.frontiersin.org/articles/10.3389/fmars.2021.724156/full#supplementary-material
References
Avella, M., Ducoudret, O., Pisani, D. F., and Poujeol, P. (2009). Swelling activated transport of taurine in cultured gill cells of sea bass: physiological adaptation and pavement cell plasticity. Am. J. Physiol. Regul. Integr. Comp. Physiol. 296, R1149–R1160.
Bao, J., Xing, Y. N., Jiang, H. B., and Li, X. D. (2019). Identification of immune-related genes in gills of Chinese mitten crabs (Eriocheir sinensis) during adaptation to air exposure stress. Fish Shellfish Immunol. 84, 885–893.
Barclay, M. C., Dall, W., and Smith, D. M. (1983). Changes in lipid and protein during starvation and the moulting cycle in the tiger prawn Penaeus esculentus Hawell. J. Exp. Mar. Biol. Ecol. 68, 229–244. doi: 10.1016/0022-0981(83)90055-2
Barrento, S., Marques, A., Vaz-Pires, P., and Nunes, M. L. (2011). Cancer pagurus (Linnaeus, 1758) physiological responses to simulated live transport: influence of temperature, air exposure and AQUI-S®. J. Therm. Biol. 36, 128–137. doi: 10.1016/j.jtherbio.2010.12.006
Barrento, S., Marques, A., Vaz-Pires, P., and Nunes, M. L. (2012). Physiological changes during simulated live transport of Cancer pagurus and recovery in holding tanks. Aquacult. Res. 43, 1415–1426. doi: 10.1111/j.1365-2109.2011.02943.x
Barton, B. A. (2002). Stress in fishes: a diversity of responses with particular reference to changes in circulating corticosteroids. Integ. Comp. Biol. 42, 517–525. doi: 10.1093/icb/42.3.517
Benaroudj, N., Lee, N. H., and Goldberg, A. L. (2001). Trehalose accumulation during cellular stress protects cells and cellular proteins from damage by oxygen radicals. J. Biol. Chem. 276, 24261–24267. doi: 10.1074/jbc.m101487200
Bridges, C. R. (2001). Modulation of haemocyanin oxygen affinity: properties and physiological implications in a changing world. J. Exp. Biol. 204, 1021–1032. doi: 10.1242/jeb.204.5.1021
Burnett, L. E., Holman, J. D., Jorgensen, D. D., Ikerd, J. L., and Burnett, K. G. (2006). Immune defense reduces respiratory fitness in Callinectes sapidus, the Atlantic blue crab. Biol. Bull. 211, 50–57. doi: 10.2307/4134577
Carr, W. E. S., Netherton, J. C., Gleeson, R. A., and Derby, C. D. (1996). Stimulants of feeding behavior in fish; analyses of tissues of diverse marine organisms. Biol. Bull. 190, 149–160.
China Fishery Statistical Yearbook (2020). Bureau of Fisheries of the Ministry of Agriculture, and Rural Affairs of the People’s Republic of China. Beijing: China Agriculture Press.
Dong, Z., Mao, S., Chen, Y., Ge, H., Li, X., Wu, X., et al. (2019). Effects of air-exposure stress on the survival rate and physiology of the swimming crab Portunus trituberculatus. Aquaculture 500, 429–434. doi: 10.1016/j.aquaculture.2018.10.049
Durand, F., and Regnault, M. (1998). Nitrogen metabolism of two portunid crabs, Carcinus maenas and Necora puber, during prolonged air exposure and subsequent recovery: a comparative study. J. Exp. Biol. 201, 2515–2528. doi: 10.1242/jeb.201.17.2515
Eriksson, L., Trygg, J., and Wold, S. (2008). CV-ANOVA for significance testing of PLS and OPLS (R) models. J. Chemom. 22, 594–600. doi: 10.1002/cem.1187
Fotedar, S., and Evans, L. (2011). Health management during handling and live transport of crustaceans: a review. J. Invertebr. Pathol. 106, 143–152. doi: 10.1016/j.jip.2010.09.011
Geihs, M. A., Maciel, F. E., Vargas, M. A., Cruz, B. P., and Nery, L. E. M. (2013). Effects of hypoxia and reoxygenation on the energetic metabolism of the crab Neohelice granulate (Decapoda, Varunidae). J. Exp. Mar. Biol. Ecol. 445, 69–78. doi: 10.1016/j.jembe.2013.03.019
Geihs, M. A., Vargas, M. A., and Nery, L. E. M. (2014). Damage caused during hypoxia and reoxygenation in the locomotor muscle of the crab Neohelice granulata (Decapoda: Varunidae). Comp. Biochem. Physiol. A Mol. Integr. Physiol. 172, 1–9. doi: 10.1016/j.cbpa.2014.02.010
Grassi, S., Beneditte, S., Opizzio, M., Nardo, D. E., and Buratti, S. (2019). Meat and fish freshness assessment by a portable and simplified electronic nose system (Mastersense). Sensors 19:3225. doi: 10.3390/s19143225
Gu, W.-B., Zhou, Z.-K., Tu, D.-D., Zhou, Y.-L., and Shu, M.-A. (2017). Molecular cloning and characterization of an inhibitor of apoptosis protein in the mud crab Scylla paramamosain: its potential anti-apoptosis role under air exposure stress. Fish. Sci. 83, 715–723. doi: 10.1007/s12562-017-1103-3
Hammer, K. M., Pedersen, S. A., and Størseth, T. R. (2012). Elevated seawater levels of CO2 change the metabolic fingerprint of tissues and hemolymph from the green shore crab Carcinus maenas. Comp. Biochem. Physiol. 7, 292–302. doi: 10.1016/j.cbd.2012.06.001
Hanson, A. D., Rathinasabapathi, B., Chamberlin, B., and Gage, D. A. (1991). Comparative physiological evidence that β-alanine betaine and choline-O-sulfate act as compatible osmolytes in halophytic Limonium species. Plant Physiol. 97, 1199–1205. doi: 10.1104/pp.97.3.1199
Hardie, D. G. (2003). Minireview: the AMP-activated protein kinase cascade: the key sensor of cellular energy status. Endocrinology 144, 5179–5183. doi: 10.1210/en.2003-0982
Huang, S., Wang, J., Yue, W., Chen, J., Gaughan, S., Lu, W., et al. (2015). Transcriptomic variation of hepatopancreas reveals the energy metabolism and biological processes associated with molting in Chinese mitten crab, Eriocheir sinensis. Sci. Rep. 5:14015.
Jenssen, T., Nurjhan, N., Consoli, A., and Gerich, J. E. (1993). Dose-response effects of lactate infusions on gluconeogenesis from lactate in normal man. Eur. J. Clin. Investig. 23, 448–454. doi: 10.1111/j.1365-2362.1993.tb00789.x
Lorenzon, S., Giulianini, P. G., Libralato, S., Martinis, M., and Ferrero, E. A. (2008). Stress effect of two different transport systems on the physiological profiles of the crab Cancer pagurus. Aquaculture 278, 156–163. doi: 10.1016/j.aquaculture.2008.03.011
Lu, Y. L., Wang, F., and Dong, S. L. (2015). Energy response of swimming crab Portunus trituberculatus to thermal variation: implication for crab transport method. Aquaculture 441, 64–71. doi: 10.1016/j.aquaculture.2015.02.022
Lu, Y. L., Zhang, D., Wang, F., and Dong, S. L. (2016). Hypothermal effects on survival, energy homeostasis and expression of energy-related genes of swimming crabs Portunus trituberculatus during air exposure. J. Therm. Biol. 60, 33–40. doi: 10.1016/j.jtherbio.2016.06.007
McMahon, B. R. (2001). Respiratory and circulatory compensation to hypoxia in crustaceans. Respir. Physiol. 128, 349–364. doi: 10.1016/s0034-5687(01)00311-5
Morris, S., Tyler-Jones, R., and Taylor, W. (1986). The regulation of the haemocyanin oxygen affinity during emersion of the crayfish Austropotamobius pallipes: I. An in vitro investigation of the interactive effect of calcium and L-lactate on oxygen affinity. J. Exp. Biol. 121, 315–326. doi: 10.1242/jeb.121.1.315
Pascual, C., Sanchez, A., Zenteno, E., Cuzon, G., Gabriela, G., Brito, R., et al. (2006). Biochemical, physiological and immunological changes during starvation in juveniles of Litopenaeus vannamei. Aquaculture 251, 416–429. doi: 10.1016/j.aquaculture.2005.06.001
Racotta, I. S., Palacios, E., and Mendez, L. (2002). Metabolic responses to short and long-term exposure to hypoxia in white shrimp (Penaeus vannamei). Mar. Freshw. Behav. Physiol. 35, 269–275.
Romero, M. C., Tapella, F., Sotelano, M. P., Ansaldo, M., and Lovrich, G. A. (2011). Oxidative stress in the subantarctic false king crab Paralomis granulosa during air exposure and subsequent re-submersion. Aquaculture 319, 205–210. doi: 10.1016/j.aquaculture.2011.06.041
Rosenqvist, H., Kasula, H., Reunanen, O., and Nurmikko, V. (1973). The 4-aminobutyrate pathway and 2-oxoglutarate dehydrogenase in Escherichia coli. Acta Chem. Scand. 27, 3091–3100. doi: 10.3891/acta.chem.scand.27-3091
Shi, C., Xia, M., Li, R., Mu, C., Zhang, L., Liu, L., et al. (2019). Vibrio alginolyticus infection induces coupled changes of bacterial community and metabolic phenotype in the gut of swimming crab. Aquaculture 499, 251–259. doi: 10.1016/j.aquaculture.2018.09.031
Simonik, E., and Henry, R. P. (2014). Physiological responses to emersion in the intertidal green crab, Carcinus maenas (L.). Mar. Freshw. Behav. Physiol. 47, 101–115. doi: 10.1080/10236244.2014.905001
Spicer, I. J., Hill, A. D., Taylor, A. C., and Strang, R. H. (1990). Effect of aerial exposure on concentrations of selected metabolites in the blood of the Norwegian lobster Nephrops norvegicus (Crustacea: Nephropidae). Mar. Biol. 105, 129–135. doi: 10.1007/bf01344278
Stillman, J., and Somero, G. (1996). Adaptation to temperature stress and aerial exposure in congeneric species of intertidal porcelain crabs (genus Petrolisthes): correlation of physiology, biochemistry and morphology with vertical distribution. J. Exp. Biol. 199, 1845–1855. doi: 10.1242/jeb.199.8.1845
Sun, M., Li, Y. T., Liu, Y., Lee, S. C., and Wang, L. (2015). Transcriptome assembly and expression profiling of molecular responses to cadmium toxicity in hepatopancreas of the freshwater crab Sinopotamon henanense. Sci. Rep. 6:19405.
Urbina, M. A., Paschke, K., Gebauer, P., Cumillaf, J. P., and Rosas, C. (2013). Physiological responses of the southern king crab, Lithodes santolla (Decapoda: Lithodidae), to aerial exposure. Comp. Biochem. Physiol. A Mol. Integr. Physiol. 166, 538–545. doi: 10.1016/j.cbpa.2013.08.006
Wang, W., Wu, X., Liu, Z., Zheng, H., and Cheng, Y. (2014). Insights into hepatopancreatic functions for nutrition metabolism and ovarian development in the crab Portunus trituberculatus: gene discovery in the comparative transcriptome of different hepatopancreas stages. PLoS One 9:e84921. doi: 10.1371/journal.pone.0084921
Wang, Y., Shi, Z., Wang, X., Zhao, L., and Li, F. (2018). The quality evaluation of alive and dead Chinese mitten crab (Eriocheir sinensis). J. Chin. Inst. Food Sci. Technol. 18, 244–254.
Yancey, P. H., Blake, W. R., and Conley, J. (2002). Unusual organic osmolytes in deep-sea animals: adaptations to hydrostatic pressure and other perturbants. Comp. Biochem. Physiol. A Mol. Integr. Physiol. 133, 667–676. doi: 10.1016/s1095-6433(02)00182-4
Ye, Y., An, Y., Li, R., Mu, C., and Wang, C. (2014). Strategy of metabolic phenotype modulation in Portunus trituberculatus exposed to low salinity. J. Agric. Food Chem. 62, 3496–3503. doi: 10.1021/jf405668a
Ye, Y., Xia, M., Mu, C., Li, R., and Wang, C. (2016). Acute metabolic response of Portunus trituberculatus to Vibrio alginolyticus infection. Aquaculture 463, 201–208. doi: 10.1016/j.aquaculture.2016.05.041
Ye, Y., Zhang, L., Yang, R., Luo, Q., Chen, H., Yan, X., et al. (2013). Metabolic phenotypes associated with high-temperature tolerance of Porphyra haitanensis strains. J. Agric. Food Chem. 61, 8356–8363. doi: 10.1021/jf402749a
Keywords: Portunus trituberculatus, transport, energy metabolism, metabolomics, survival
Citation: Shi C, Ye Y, Pei F, Mu C and Wang C (2021) Survival and Metabolic Modulation of Swimming Crab Portunus trituberculatus During Live Transport. Front. Mar. Sci. 8:724156. doi: 10.3389/fmars.2021.724156
Received: 12 June 2021; Accepted: 20 September 2021;
Published: 12 October 2021.
Edited by:
Menghong Hu, Shanghai Ocean University, ChinaReviewed by:
Wei Jiang, Zhejiang Ocean University, ChinaBaoquan Gao, Yellow Sea Fisheries Research Institute, Chinese Academy of Fishery Sciences (CAFS), China
Yunliang Lu, Qingdao Agricultural University, China
Xiaowen Long, Dali University, China
Copyright © 2021 Shi, Ye, Pei, Mu and Wang. This is an open-access article distributed under the terms of the Creative Commons Attribution License (CC BY). The use, distribution or reproduction in other forums is permitted, provided the original author(s) and the copyright owner(s) are credited and that the original publication in this journal is cited, in accordance with accepted academic practice. No use, distribution or reproduction is permitted which does not comply with these terms.
*Correspondence: Yangfang Ye, eWV5YW5nZmFuZ0BuYnUuZWR1LmNu; Chunlin Wang, d2FuZ2NodW5saW5AbmJ1LmVkdS5jbg==