- Marine Ecology Department, Faculty of Biology and Chemistry, University of Bremen, Bremen, Germany
In the Mediterranean Sea, the fleshy red alga Phyllophora crispa forms dense mats of up to 15 cm thickness, mainly located on rocky substrates in water depths below 20 m. Because of the observed density of these mats and some first observations, we hypothesize that P. crispa is a yet undescribed ecosystem engineer that provides a multitude of ecological niches for associated organisms along small-scale environmental gradients. Therefore, we conducted an in-situ pilot study in the Western Mediterranean Sea to assess potential influence of the algae mats on the key environmental factors water movement, temperature and light intensity. We comparatively and simultaneously measured in P. crispa mats, in neighboring Posidonia oceanica seagrass meadows, on neighboring bare rocky substrates without algae mats, and in the directly overlying water column. We used several underwater logging sensors and gypsum clod cards. Findings revealed that P. crispa significantly reduced water movement by 41% compared to the overlying water column, whereas water movement was not affected by P. oceanica meadows and bare rocky substrates. Surprisingly, P. crispa increased the water temperature by 0.3°C relative to the water column, while the water temperature in P. oceanica and on bare rocky substrates was reduced by 0.5°C. Light intensity inside the red algae mats was reduced significantly by 69% compared to the water column. This was similar to measured light reduction of 77% by P. oceanica. These findings highlight the strong influence of the dense red algae mats on some key environmental factors. Their influence is obviously similar or even higher than for the well-known seagrass ecosystem engineer. This may be a factor that facilitates associated biodiversity similarly as described for P. oceanica.
Introduction
Ecosystem engineers are defined as organisms that control the availability of resources either directly or indirectly by their ability to change the physical state of abiotic or biotic material (Jones et al., 1994, 1997). Therefore, ecosystem engineers can create or modify habitats (Crooks, 2002) by affecting environmental parameters, such as light, temperature, pH, oxygen and availability of substrate. Jones et al. (1994, 1997) define two types of bioengineering organisms: (1) autogenic engineers which modify their environment by endogenous processes, such as growth (e.g., trees) and (2) allogenic engineers which modify other living or non-living material in their surrounding (e.g., beaver).
Corals are a prominent example of structural engineers, a subgroup of autogenic engineers, which physically create substrate, control resources and shape their environment (Jones et al., 1997; Berke, 2010). For example, the metabolism of both corals and their symbiotic algae (i.e., respiration and photosynthesis) cause oxygen micro-gradients near reefs (Shashar et al., 1993) that can affect a range of associated species (Vogel, 1981; Atkinson, 1992). Mangroves are another example of structural ecosystem engineers that create habitat for numerous aquatic species (Robertson and Blaber, 1992; Kandasamy and Bingham, 2001). The complex structure of their roots provides shelter from predators (Laegdsgaard and Johnson, 2001; Macia et al., 2003). Moreover, kelp algae modify their surroundings’ physical state through their morphology in coastal environments worldwide (Jones et al., 1997). Aggregations of such macroalgae form a unique habitat for marine organisms (Christie et al., 2003) by dampening water currents (Jackson, 1997; Gaylord et al., 2007) and diminishing light (Pearse and Hines, 1979; Reed and Foster, 1984; Stewart et al., 2008).
In the Mediterranean Sea, a member of the seagrass family Posidoniaceae, the endemic Posidonia oceanica (Delile, 1813) acts as a well-known biodiversity hotspot (Montefalcone et al., 2007; Pergent et al., 2014) and an autogenic ecosystem engineer. It forms wide meadows that can, among others, affect the hydrodynamics of currents and waves (Gambi et al., 1990; Bouma et al., 2005). Further, P. oceanica promotes sediment stability by buffering resuspension (Gacia et al., 1999; Terrados and Duarte, 2000; Gacia and Duarte, 2001).
Another potential habitat-building species in the Mediterranean Sea is the fleshy red alga Phyllophora crispa (Dixon, 1964). In water depths below 20 m, the alga forms discontinuous mats of at least 5 cm thickness, mainly on rocky substrates, which can cover large parts of the seafloor (Bonifazi et al., 2017). A massive development of these red-algae mats lead to it becoming a dominant benthic habitat around the Island of Giglio since 2012 (Bonifazi et al., 2017).
Bonifazi et al. (2017) provided a first description of the associated fauna of P. crispa, and its role as a habitat forming species at the Island of Giglio, Italy, and came to the conclusion that biodiversity of mobile and sessile invertebrates associated with the mats is high. A reason for this finding is likely that P. crispa mats create a range of micro-niches by influencing key environmental parameters such as water current exposure, temperature and light availability. Because of the observed density of these mats and some first observations, we hypothesize that P. crispa is a yet undescribed ecosystem engineer that provides a multitude of ecological niches for associated organisms along small-scale environmental gradients. This study thus aimed to investigate the potential role of P. crispa as an ecosystem engineer and answer the following research question: What are the differences in water movement, temperature and light availability between P. crispa mats, P. oceanica meadows and rocky substrates without any overlying mats? To answer this question, we conducted a series of comparative and simultaneous in-situ measurements in the Mediterranean Sea.
Materials and Methods
Study Site and Habitat Characterization
The study was performed in September and October 2019 on the Island of Giglio, Tuscany, Italy. All observational surveys were conducted in situ via scuba-diving at a depth of 28–30 m at the dive site “Punta del Morto” (42°23′22.2″N 10°53′24.3″E), which is located at the northern coast of Giglio. The study area was on the west side of the dive site, where, starting at a depth of 20 m, granite blocks cover most parts of the seafloor. In the Mediterranean Sea, the occurrence of small patches of bare granitic rock at water depths of 30 m is common. Additionally, dense Posidonia oceanica seagrass meadows, characterized by straight, single leaves, and dense P. crispa mats of robust, branched, and entangled structure are present at the study site. All three habitats (i.e., P. crispa mats of at least 5 cm thickness, P. oceanica seagrass meadows of at least 20 cm height, bare rocky substrate; Supplementary Figure 1) were found at the same water depth in an area of about 250 m2. Limiting the study to this one sampling site allowed for a direct comparison by quantifying environmental conditions within these three habitats, as we expect that all habitats experienced identical external conditions (e.g., temperature, salinity, insolation, hydrodynamics, etc.). The rhizome part of P. oceanica, above and below ground, was not included in this study.
Environmental Parameters
Effects on water movement relative to the water column by P. crispa and the other two ecosystem types were measured simultaneously using the gypsum dissolution technique (Eckman et al., 1989; Duggins et al., 1990). While this method is not appropriate to measure absolute water flow, it is well suitable for relative comparison of water movement (Jokiel and Morissey, 1993; Thompson and Glenn, 1994; Evans and Abdo, 2010). Gypsum of smooth consistency (Quick-mix gips, toom # 3050388, CaSO4) was poured into muffin cups. Air bubbles were removed with continuous finger snapping for 30 s. After a drying period of 24 h at air temperature, gypsum balls were taken out of the cups and dried to constant weight in an oven at 60°C before being glued to plastic cards avoiding gaps between both the cards and the gypsum balls. Then, clod cards were numbered and weighed. Clod cards were placed at heights of 0 cm (seafloor), 1 cm (within P. crispa mats), 5 cm (algae-water interface) and 20 cm (above the algal mats) by attaching them to a 30 cm long metal rod with cable ties (Supplementary Figure 2). Replication was done primarily temporal, with rods being deployed at four different time points. A total of eleven rods was placed in P. crispa at all four timepoints. The same set-up was used at one timepoint on rocky substrates, resulting in two replicates. Since seagrass meadows grow higher than the red algae mats, a metal rod of 80 cm length was used and an additional clod card was placed 30 cm from the ground. This setup allowed selecting the same positions that were used for P. crispa and rocky substrates: 0 cm (seafloor), 1 cm (within P. oceanica meadow), 5 cm (within P. oceanica meadow), 20 cm (meadow-water interface) and 30 cm (above the meadow). A total of three rods was deployed at three different time points in P. oceanica, resulting in a replication of three. The size of the gypsum balls was similar for all environments. The set-ups were prepared before deploying in situ and stayed in the water for 6–7 days after deployment. The set-ups were then transported out of the water, clod cards were carefully removed from the metal stick, rinsed with fresh water and placed on a drying rack. Then, clod cards were dried in an oven at 60°C until a constant dry weight was reached. The relative weight loss of gypsum balls [presented as a reduction of daily average weight loss of gypsum balls relative to the overlaying water (%)] was used as an indicator for the strength of relative water movement. We used this relative approach to exclude effects of habitat volume differences on the results. Calculations were made as follows:
reduction of daily average weight loss relative to the overlaying water
with w1 = weight before water exposure, wl = total weight loss [g], t = time in the water [days], wlw = weight loss at position [% day–1], wlp = weight loss at position [% day–1].
For light and temperature measurements, the same set-up as for the water movement measurements was used simultaneously in P. crispa, P. oceanica and on rocky substrates using Onset HOBO Pendant Data Loggers (part # UA-002-64, accuracy temperature: ± 0.53°C, light unit: lux). These loggers are designed for measurements of relative light intensity differences, as they are not accurate for measuring absolute light intensities (Ali et al., 2016). For P. oceanica, the loggers were positioned at 0, 1, 5, 20, 35, and 55 cm distance from the seafloor to increase the vertical resolution of measurements. A total of seven rods were placed in P. crispa mats at four time points, a total of three rods in P. oceanica meadows at three time points, and a total of eight rods were placed at four time points on rocky substrates. Data loggers were exchanged and placed alternately in different habitats and in different heights above the seafloor for each observation to avoid any systematic error. Data collection intervals were set at 15 s, and loggers collected data for five consecutive days. The resulting replication for temperature and light observations in the different habitats is shown in Supplementary Table 1. Loggers were read out with the program “HOBOware” (version 3.7.17).
This study was conducted simultaneously in the three investigated habitats, which means that all habitats experienced identical environmental conditions. Our findings therefore reveal differences in environmental factors resulting directly from the different characteristics of the investigated habitats.
Statistical Analysis
Statistical analysis was conducted using the statistic program “R” (version 3.5.1) and the package “car” (Fox and Weisberg, 2011). One-Way-ANOVA was used to calculate significant differences between data sets when the sample size was three or higher. Due to the small sample size, differences regarding weight loss of gypsum balls on rocky substrates were calculated with a t-test. Differences were considered as significant at p-values below 0.05.
For temperature and light analysis, data points used for calculations correspond to the mean of single data points over a period of 5 min [data point5 min = mean (20 ×data point15 s)]. Light data of light hours, from 6 am to 8 pm, was used for the analysis.
Results
Water Movement
The weight loss of gypsum clod cards was used as an indirect measurement of water movement. Overall, results show a reduction in weight loss of gypsum clod cards relative to the water column by 41 ± 8% in P. crispa mats, 25 ± 16% in Posidonia oceanica seagrass meadows, and 13 ± 8% on the rocky substrates (Figure 1). Only in P. crispa mats, the weight loss was significantly different among heights above seafloor (Supplementary Table 2A). Gypsum balls placed on the substrate surface inside the P. crispa mats showed a significantly lower weight loss of 41 ± 8% compared to the gypsum balls in the overlaying open water (ANOVA: F1,21 = 26.28; p ≤ 0.001).
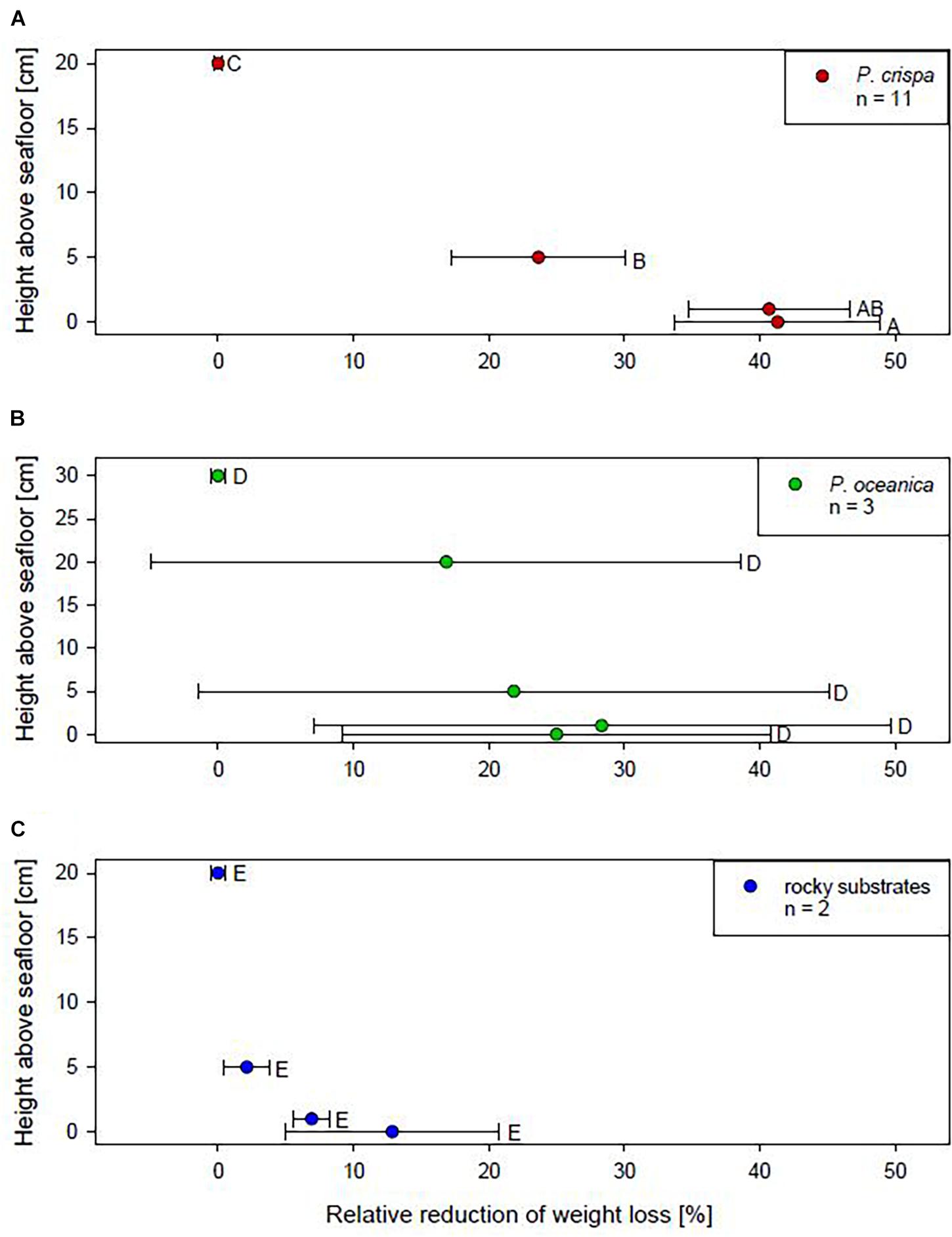
Figure 1. Reduction of daily average weight loss of gypsum balls in Phyllophora crispa (A), Posidonia oceanica (B) and on rocky substrates (C) relative to the overlaying water (0%). Displayed are mean values and standard error. n = number of rods deployed in situ. No significant differences are indicated by the same letter, significant differences by different letters.
Temperature
The daily temperature cycle was similar in all three habitats, as increasing temperatures from morning (6 am) to evening (6 pm) were observed. Then, temperatures decreased until midnight and remained stable until the morning (Figure 2). The temperature in P. crispa mats ranged from a minimum of 18.6 to a maximum of 20.4°C, from 17.2 to 19.3°C in P. oceanica, and from 18.2 to 20.0°C on rocky substrates. Furthermore, temperature stratifications varied within the investigated habitats. Temperatures at the bottom of P. crispa mats (0 cm) were significantly higher than those measured at 1, 5, and 20 cm distance from the seafloor (Supplementary Table 2B), which all were in a similar range (Figure 2A). For P. oceanica, the temperatures observed at the seafloor were significantly (ANOVA: F1,575 = 156.98; p ≤ 0.001) lower than temperatures found 35 and 55 cm above the seafloor (Figure 2B). On rocky substrates, temperatures measured at the seafloor (i.e., 0 cm) were significantly lower compared to the other positions (Figure 2C). The water temperature was 0.31°C higher at the seafloor compared to the water column for P. crispa. For P. oceanica and the bare rocky substrates, the water temperature was 0.5°C lower at the seafloor compared to the water column, respectively.
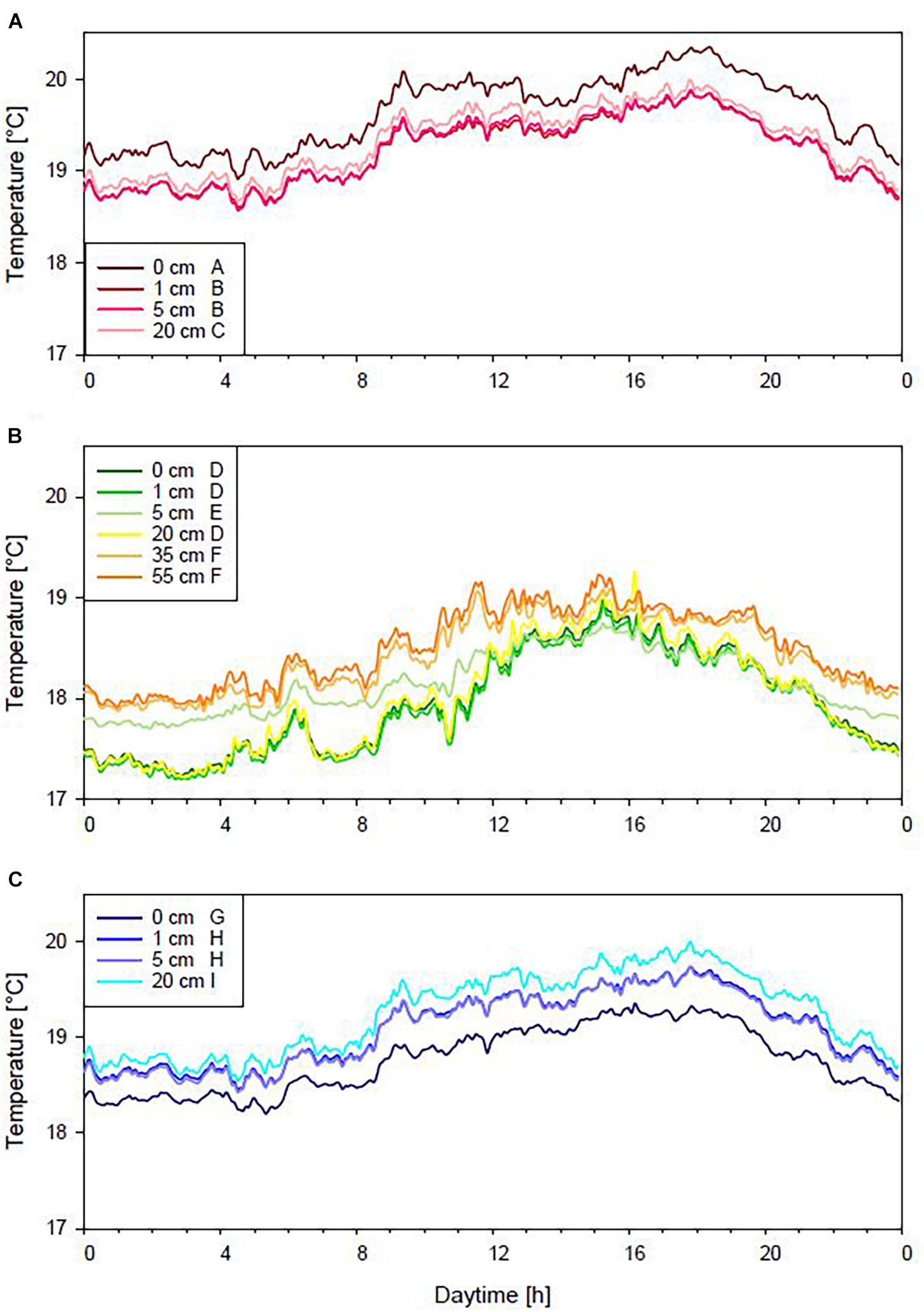
Figure 2. Average temperature in Phyllophora crispa (A), Posidonia oceanica (B) and on rocky substrates (C). Displayed are mean values. No differences are indicated by the same letter, significant differences by different letters.
Light Intensity
Light stratification was different for the three investigated habitats (Figure 3). In P. crispa mats (Figure 3A), light intensity was consistent at 0 cm and 1 cm above the seafloor, i.e., within the mat, while the light intensity was twofold higher at 5 and 20 cm above the seafloor (Supplementary Table 2C). Close to the seafloor, a relative light intensity of 31 ± 0.3% compared to the overlying water was recorded in this habitat. Similar light values were recorded for P. oceanica (Figure 3B) 0, 1, and 5 cm above the seafloor. Higher light intensities occurred 20 cm above the seafloor, while the highest light values were noted 35 cm and 55 cm above the seafloor. Lowest values of minimum 23 ± 0.2% light intensity relative to the overlying water were recorded close to the seafloor in seagrass. On rocky substrates (Figure 3C), light intensity showed an irregular increase with increasing distance from the seafloor. A reduction of light intensity in the absence of plant canopies, on rocky substrates, was less intense, i.e., 58 ± 0.3% relative to the logger placed furthest away from the seafloor at 20 cm height. Overall, a reduction in light intensity from the highest to the lowest position of 69 ± 0.3% for P. crispa, 77 ± 0.2% for P. oceanica and 43 ± 0.3% for rocky substrates was noted.
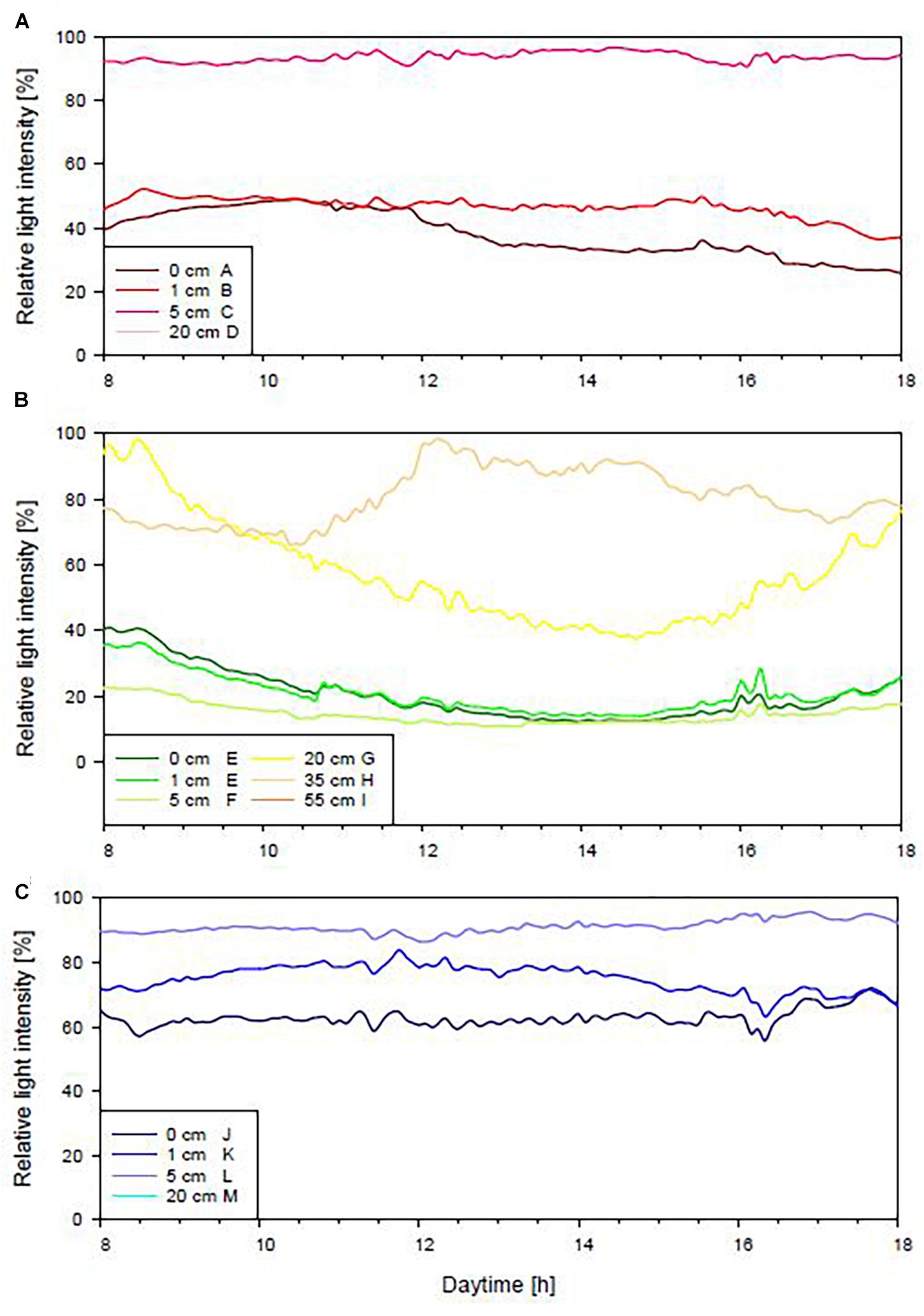
Figure 3. Relative light intensity compared to loggers placed above canopy [%] in Phyllophora crispa (A), Posidonia oceanica (B) or at similar water depth on rocky substrates (C). Displayed are mean values observed during light hours from 8 am to 6 pm. References (100%) are included in the legend for the display of statistical differences. No statistical differences are indicated by the same letter, significant statistical differences by different letters.
Discussion
It has recently been shown that the red alga P. crispa harbors many associated organisms in the north-western part of the Mediterranean Sea (Bianchi et al., 2010; Casoli et al., 2016, 2019; Bonifazi et al., 2017). In this study, we investigated the influence of P. crispa on the environmental factors water movement, temperature and light intensity. Furthermore, we related our finding of P. crispa to neighboring Posidonia oceanica meadows and bare rocky substrates. Our results indicate a potential role of P. crispa as an autogenic ecosystem engineer.
Characteristics of Investigated Habitats
The water movement was reduced by 41% at the deepest part of the P. crispa mats compared to the water movement 20 cm above the seafloor. Studies on soft-sediment macro algae canopies with a similar structure found similar results, showing reduced water flow and mixing in the lower part of the canopy (Kregting et al., 2011). Both, rocky substrates and P. oceanica meadows showed a less intense decrease (13 and 25%, respectively) in relative water movement toward the substrate, which was not statistically significant, potentially due to low replication. Previous studies (Koch and Gust, 1999; Arumugam et al., 2013; Bacci et al., 2016) found significant reductions of water currents inside seagrass meadows that were located at a water depth of 10 m (e.g., Peterson et al., 2004; Bouma et al., 2005; Koch et al., 2006). However, investigated seagrass meadows in the present study were located at a depth of 28 m, in which current is usually weaker than in more shallow areas, which hypothetically explain the absence of statistically significant effects. Water movement in the water column directly above P. crispa and P. oceanica was similar to rocky substrates, which indicates that the differences between the three investigated habitats are likely caused by the lack of topography of the rocky substrates compared to the structural complexity of the plant canopy habitats as shown for i.e., seagrass (Fonseca et al., 1982; Gambi et al., 1990). Structural complexity or heterogeneity of a habitat is characterized by the spatial arrangement and diversity of substratum types (McCormick, 1994) and has previously been shown to correlate with lowered water flow (Gorman and Karr, 1978; Willis et al., 2005) and the degree of protection from predation (Hereu et al., 2004). A stronger reduction of water movement was observed in the rather thin P. crispa mats over a shorter distance, compared to the P. oceanica meadows, suggesting a higher structural complexity of the thalli, which have a relatively high surface area within a small volume compared to seagrass leaves.
Temperatures were significantly higher at the bottom of the algae mats, on average by 0.31°C. Although observed differences were within the range of accuracy of the data logger, all replicates showed similar differences. Since a temperature gradient was observed on the bare rocky substrates with decreasing temperatures toward the seafloor, the observed differences in P. crispa likely do not originate from potential temperature differences within the water column. It has been shown for seagrass, that their leaves retain organic matter from the water (Bacci et al., 2016). As the structural complexity seems higher in P. crispa mats, the filter capacity may also be higher, resulting in potentially high microbial activity. Habitat complexity also promotes micro-scale heterogeneity (Bell et al., 2001; Ondiviela et al., 2018) and additionally provides a large surface area for high microbial activity. It has been shown that P. crispa enlarges the seafloor surface by a factor of 4.9 (YC El-Khaled et al., unpublished data), which could potentially lead to high microbial activity. High rates of microbial replication and activity have been observed to create a heat output in laboratory experiments (Djamali et al., 2012). Potential warming underneath the algae mat could therefore likely be a result of the intense respiration activity of associated (micro) organisms.
Temperatures in P. oceanica meadows and on rocky substrates decreased with decreasing distance to the seafloor. The combination of less self-shading, limited water exchange and enhanced microbial activity could cause the temperature differences in P. crispa mats as opposed to P. oceanica meadows and rocky substrates (Table 1; Arumugam et al., 2013). Although an increase in temperature as indicated for P. crispa could not be observed for seagrass leaves, it may potentially occur in the rhizomes, as water current reduction is expected, and the habitat structure becomes more complex (Buia et al., 2000).
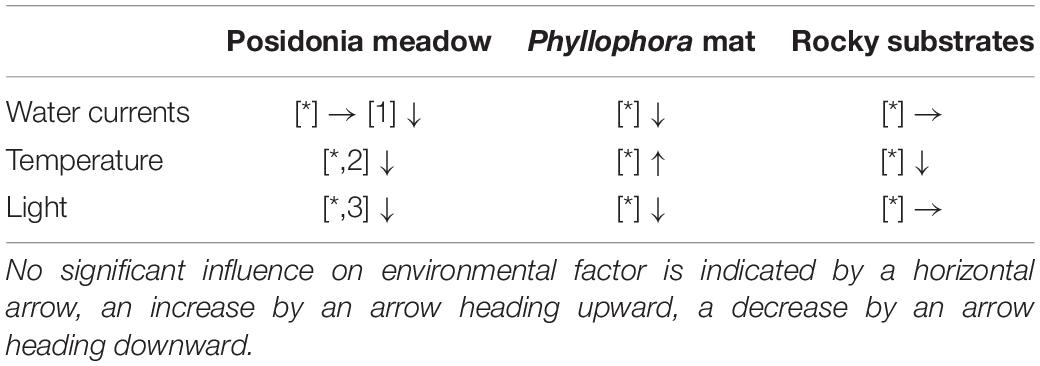
Table 1. Environmental gradients in different habitats observed in this study [*] and previous reports by [1] Bacci et al. (2016), [2] Arumugam et al. (2013), [3] Dalla Via et al. (1998).
Overall, a statistically significant gradient in light intensity occurred on rocky substrates, and in the observed plant canopy habitats of P. crispa and P. oceanica. Light intensity gradients observed on rocky substrate were presumably the same as in the open water for all substrates. P. crispa mats decreased the light intensity by 69% from the overlaying water to the bottom of the mat, which is a strong environmental light gradient across the relatively thin mat of about 5 cm thickness. For P. oceanica meadows, a decrease in light intensity of 77% compared to the overlaying water was observed. Previous studies also showed strong light gradients in habitats of macrophyte dominance, i.e., P. oceanica meadows, of up to 70% and confirm self-shading effects (Dalla Via et al., 1998). These structures reduced the incidence of the blue, green and red wavelengths by, respectively, 57–58%, 61–63%, and 51–53% within the seagrass stands, additionally to light attenuation of the water column. A third interconnected light gradient occurs due to direct shading of macrophyte structures caused by epiphytes (Riedl, 1966; Dalla Via et al., 1998), which can reach a thickness of up to 6 cm on P. crispa mats (Bonifazi et al., 2017). In combination with self-shading induced by the entangled thalli of the alga and horizontally lying blades, this can explain the exceptional strong light gradient.
Potential Relevance for Ecosystem Functions
Based on several studies, lowered water movement inside P. crispa mats potentially promotes sediment trapping and reduces resuspension, as described for seagrass meadows (Gacia et al., 1999; Gacia and Duarte, 2001; Hendriks et al., 2008). Subsequently, this could affect the associated biodiversity, as large amounts of sediment trapped within P. crispa mats provide a heterogeneous habitat for infauna species and offer building material and food in form of organic and inorganic matter for tube-building species (Prathep et al., 2003). Previously, the global occurrence of algal turfs has been related to stressful habitats (Hay, 1981; Benedetti-Cecchi et al., 2001; Piazzi and Cinelli, 2001) and their structural characteristic of sediment trapping (Piazzi and Cinelli, 2001). However, the coast of Giglio island is affected by sediment deposition, which consists of fine terrigenous particles controlled by rainfall and larger particles derived from erosion of granite rocks (Bonifazi et al., 2017). Sedimentary processes in this area are therefore a natural scenario rather than the result of anthropogenic stressors, such as pollution (Bonifazi et al., 2017).
Moreover, the community composition can be affected by reduced water movement within the algae mat. A similar pattern has been observed for the eelgrass Zostera marina (L.), where lowered water movement enhances the accumulation of planktonic organisms, such as invertebrate larvae and protozoans (Eckman, 1987). The settlement of invertebrate larvae is greatly affected by small-scale fluid dynamics (Koehl, 2007) and thus by changing these parameters, P. crispa mats consequently change the community composition. Reduced water movement could favor the settlement of e.g., certain bryozoan species (Ryland, 1970; McKinney and Jackson, 1991) and other sessile invertebrates. Experimental studies have shown that low water turbulence and velocity enhance the settlement of some species of e.g., hydroids (Mullineaux and Garland, 1993), bryozoans (Mullineaux and Garland, 1993; Qian et al., 1999, 2000), barnacles (Mullineaux and Butman, 1991; Mullineaux and Garland, 1993), bivalves (Judge and Craig, 1997), and polychaetes (Qian et al., 1999), while it decreases the settlement of other species of the same taxa (Mullineaux and Garland, 1993; Judge and Craig, 1997; Qian et al., 1999). In general, we expect a high number of settling invertebrate larvae in P. crispa, as low water movement increases the acceptance of surfaces by post-settled larvae and therefore the attaching of certain species, e.g., barnacles (Mullineaux and Butman, 1991; Jonsson et al., 2004; Larsson and Jonsson, 2006). Further, high numbers of mobile organisms, particularly larvae and juveniles, may be attracted to this habitat as it offers protection from water turbulences.
Observed higher temperatures at the bottom of P. crispa mats could potentially indicate a thermal insulation effect of P. crispa. However, future studies are required to confirm this hypothesis. While many studies focused on the effect of temperature on algae growth (e.g., Butterwick et al., 2004; Baker et al., 2007; Martin and Gattuso, 2009), the effects of algae on the habitat’s temperature are so far unstudied. Studies of another important ecosystem engineer, however, report similar effects on temperature: shallow water scleractinians increased water temperatures in their microenvironment, due to solar radiation and water holding capacities by surface heterogeneity (Jimenez et al., 2008; Ong et al., 2017). While solar radiation plays only a minor role in the deep layer of P. crispa mats, we showed that water movement is low in the algae mats, which could indicate a long water residence time and explain a similar effect on the thermal microenvironment of this habitat.
P. crispa provides a habitat that differs in light intensity to bare rocky substrates, as the plant structures create shade. The alga’s phylloids hinder light to reach the seafloor, which limits requirements for primary production within the red algae mats. Light availability is most important for photoautotrophic organisms and therefore an important factor for overall oxygen availability. Hence, in seagrass meadows, oxygen availability generally increases with the distance to the rhizome (Borum et al., 2007). Other macroalgae mats have been shown to inhabit a high abundance of microphytobenthos along a vertical gradient, which is a food source for associated organisms (Shadrin et al., 2019, 2021; Prazukin et al., 2020) and causes high phototrophic activity. In the present study, we found a 1.1-fold stronger light gradient over a shorter distance in P. crispa mats, which implies that the layer of high phototrophic activity is in closer proximity to the deeper layer of heterotrophic activity. This could lead to higher oxygen availability for heterotrophic organisms, especially heterotrophic microbes, and hence fuel the potential warming effect of microbial activity inside the mats. High microbe abundance is beneficial for suspension feeders, such as certain Porifera (Larsen and Riisgård, 1994), ascidians (Jørgensen et al., 1984; Riisgård, 1998), bryozoans (e.g., Ryland, 1976; Grünbaum et al., 1998; Lisberg and Petersen, 2000), bivalves (e.g., Beninger, 2000; Riisgård and Larsen, 2000; Silverman et al., 2000), cnidarians (Miglietta et al., 2000), crustaceans (Trager et al., 1990; Riisgård, 2015), and polychaetes (Emlet, 1990; Hansen, 1993), that feed on microbes. The aforementioned active benthic filter feeders create their own currents, e.g., by using their cilia or muscles (Jørgensen, 1966; Riisgård and Larsen, 2000), and are therefore not affected by the lowered water movement inside P. crispa mats.
Ecological Implications
Phyllophora crispa widely covers rocky substrates and increases in abundance with increasing water depth beyond 25 m (Sagarra and François, 2015; Bonifazi et al., 2017). Loss of P. oceanica meadows and rocky substrate communities threaten the overall high biodiversity in the Mediterranean Sea (Coll et al., 2010). P. crispa potentially recolonizes these degraded habitats following the concept of algal phase shifts. The importance of P. crispa as an autogenic ecosystem engineer was stated by Zaitsev (2008) in the Black Sea, where P. crispa is a key species along the north-western rocky coasts. More than 100 species of invertebrates and 40 species of fish, some of which are endangered or rare (Shcherbak et al., 1994), inhabited the P. crispa mats in this area. This associated community benefits from the algal habitat as a food source, substratum for spawning, material for the construction of nests, or shelter from predators (Zaitsev, 2008). Provision of food or protection for rich associated communities are also characteristics of other algal habitats, such as Cladophora mats (Shadrin et al., 2019, 2021; Prazukin et al., 2020) or Cystoseira beds (Mineur et al., 2015).
The term “ecosystem engineer” is often associated with large-scale bioengineers, such as corals, mangroves, and seagrasses, which create extensive contiguous habitats covering wide areas (e.g., Copper, 1994; Kandasamy and Bingham, 2001; Duarte, 2002). However, referring to Jones et al. (1994, 1997), scale is not a parameter defining “ecosystem engineers.” Bivalves for example, such as mussels or oysters, are well-recognized structural small-scale bioengineers that form dense beds or reefs (Ruesink et al., 2005; Bouma et al., 2009), which create habitats of smaller volume and act mainly on a local scale (Kelaher and Castilla, 2005). Their shells provide substrate for other organisms to colonize (Padilla, 2010), alter the local water flow regime (Widdows et al., 2009), as well as the local dynamics of sediment (Padilla, 2010). This study showed that P. crispa influences its environment in a similar way, i.e., by reducing water movement and creating habitat. Despite reaching heights of only about 15 cm, this red alga therefore acts as a small-scale ecosystem engineer, forming dense but thin mats, that can cover areas of up to 10,000 km2, as shown for the Black Sea (Zaitsev, 2008).
Our study showed that key environmental parameters are shaped by P. crispa, thus a complex and valuable habitat is likely provided in the Mediterranean Sea. As the complexity of a habitat increases biodiversity (Russ, 1979) and P. crispa mats show highly complex structures and environmental gradients, they could act as a biodiversity hotspot in the Mediterranean Sea, similar to the Black Sea, as already indicated by the pilot study of Bonifazi et al. (2017). A total of 99 non-colonial invertebrate taxa were collected in P. crispa mats in the Mediterranean Sea, while polychaetes were the most abundant group, followed by Mollusca and Crustacea (Bonifazi et al., 2017). Additionally, that study reports 37 taxa of colonial invertebrates, which were dominated by Bryozoa and Porifera, but also included Tunicata and Anthozoa. Further, Bonifazi et al. (2017) state that P. crispa mats significantly increase species richness and abundance of sessile filter feeders in contrast to shallower areas without the red algae mats. This could be the result of enhanced invertebrate larvae settlement due to reduced water movement in P. crispa mats observed in this study, which has been previously shown for seagrass meadows (Eckman, 1987). The dominance of sessile filter feeders within P. crispa mats observed (Bonifazi et al., 2017) strengthens our hypothesis of high oxygen availability due to high microphytobenthos abundance in the mats that enhances overall microbial abundance resulting in a higher food availability for active filter feeders.
This study provides the first attempt to investigate the potential role of the red alga P. crispa as an autogenic ecosystem engineer, which may harbor high associated biodiversity. Our results indicate the possibility that P. crispa provides resources for associated organisms by retaining sediment, providing food, and creating habitat. While we were able to show that P. crispa serves a habitat by influencing the key environmental factors water movement and light intensity, its effect on temperature needs to be studied further.
Future studies should investigate the diversity of infauna in Mediterranean P. crispa mats to understand their role as potential biodiversity hotspots and also confirm the abundance of P. crispa in other regions of the Mediterranean coast. Further, studies on the turnover rate of P. crispa mats are needed to understand the ability to generate refuge for organisms, which may suffer from habitat loss due to anthropogenic pressure and climate change.
Data Availability Statement
The raw data supporting the conclusions of this article will be made available by the authors, without undue reservation.
Author Contributions
NS, YE-K, FR, and CW: conceptualization, methodology, investigation, and writing—review and editing. NS: formal analysis, writing—original draft preparation, and visualization. CW: supervision. All authors have read and agreed to the published version of the manuscript.
Conflict of Interest
The authors declare that the research was conducted in the absence of any commercial or financial relationships that could be construed as a potential conflict of interest.
Publisher’s Note
All claims expressed in this article are solely those of the authors and do not necessarily represent those of their affiliated organizations, or those of the publisher, the editors and the reviewers. Any product that may be evaluated in this article, or claim that may be made by its manufacturer, is not guaranteed or endorsed by the publisher.
Acknowledgments
We would like to thank Jenny Tuček and the “Institut für Marine Biologie” (IfMB) for hosting us on Giglio island and providing logistics and equipment. We would also like to thank Reiner and Regina Krumbach [Campese Diving Center (CDC)] for the support throughout our study. We would also further like to thank Alice G. Bianchi, Beltran Montt, and Susann Roßbach for their support in data collection, as well as Ulrich Merkel for logistical support.
Supplementary Material
The Supplementary Material for this article can be found online at: https://www.frontiersin.org/articles/10.3389/fmars.2021.721626/full#supplementary-material
References
Ali, A. S., Zanzinger, Z., Debose, D., and Stephens, B. (2016). Open source building science sensors (OSBSS): a low-cost arduino-based platform for long-term indoor environmental data collection. Build Environ. 100, 114–126. doi: 10.1016/j.buildenv.2016.02.010
Arumugam, R., Kannan, R. R. R., Saravanan, K. R., Thangaradjou, T., and Anantharaman, P. (2013). Hydrographic and sediment characteristics of seagrass meadows of the Gulf of Mannar Marine Biosphere Reserve, South India. Environ. Monit. Assess. 185, 8411–8427. doi: 10.1007/s10661-013-3183-6
Atkinson, M. J. (1992). Productivity of ENEWETAK atoll reef flats predicted from mass transfer relationships. Cont. Shelf Res. 12, 799–807. doi: 10.1016/0278-4343(92)90045-L
Bacci, T., Penna, M., Rende, S. F., Trabucco, B., Gennaro, P., Bertasi, F., et al. (2016). Effects of costa concordia shipwreck on epiphytic assemblages and biotic features of Posidonia oceanica canopy. Mar. Pollut. Bull. 109, 110–116. doi: 10.1016/j.marpolbul.2016.06.012
Baker, J. W., Grover, J. P., Brooks, B. W., Urena-Boeck, F., Roelke, D. L., Errera, R., et al. (2007). Growth and toxicity of Prymnesium parvum (Haptophyta) as a function of salinity, light, and temperature. J. Phycol. 43, 219–227. doi: 10.1111/j.1529-8817.2007.00323.x
Bell, S. S., Brooks, R. A., Robbins, B. D., Fonseca, M. S., and Hall, M. O. (2001). Faunal response to fragmentation in seagrass habitats: implications for seagrass conservation. Biol. Conserv. 100, 115–123. doi: 10.1016/S0006-3207(00)00212-3
Benedetti-Cecchi, L., Pannacciulli, F., Bulleri, F., Moschella, P. S., Airoldi, L., Relini, G., et al. (2001). Predicting the consequences of anthropogenic disturbance: large-scale effects of loss of canopy algae on rocky shores. Mar. Ecol. Prog. Ser. 214, 137–150. doi: 10.3354/meps214137
Beninger, P. G. (2000). A critique of premises and methods in a recent study of particle capture mechanisms in bivalves. Limnol. Oceanogr. 45, 1196–1199. doi: 10.4319/lo.2000.45.5.1196
Berke, S. K. (2010). Functional groups of ecosystem engineers: a proposed classification with comments on current issues. Integr. Comp. Biol. 50, 147–157. doi: 10.1093/icb/icq077
Bianchi, C. N., Morri, C., and Navone, A. (2010). I popolamenti delle scogliere rocciose sommerse dell’area marina protetta di tavolara punta coda cavallo (Sardegna nord-orientale). Sci. Rep. Port Cros. Natl. Park 24, 39–85.
Bonifazi, A., Ventura, D., Gravina, M. F., Lasinio, G. J., Belluscio, A., and Ardizzone, G. D. (2017). Unusual algal turfs associated with the rhodophyta Phyllophora crispa: benthic assemblages along a depth gradient in the central Mediterranean sea. Estuar. Coast. Shelf Sci. 185, 77–93. doi: 10.1016/j.ecss.2016.12.013
Borum, J., Sand-Jensen, K., Binzer, T., Pedersen, O., and Greve, T. M. (2007). “Oxygen movement in seagrasses,” in Seagrasses Biology, Ecology and Conservation, eds A. W. D. Larkum, J. R. Orth, and C. M. Duarte (Dordrecht: Springer), 255–270. doi: 10.1007/1-4020-2983-7_10
Bouma, T. J., de Vries, M. B., Low, E., Peralta, G., Tanczos, C., van de Koppel, J., et al. (2005). Trade-offs related to ecosystem engineering: a case study on stiffness of emerging macrophytes. Ecology 86, 2187–2199. doi: 10.1890/04-1588
Bouma, T. J., Olenin, S., Reise, K., and Ysebaert, T. (2009). Ecosystem engineering and biodiversity in coastal sediments: posing hypotheses. Helgol. Mar. Res. 63, 95–106. doi: 10.1007/s10152-009-0146-y
Buia, M. C., Gambi, M. C., and Zupo, V. (2000). Structure and functioning of Mediterranean seagrass ecosystems: an overview. Biol. Mar. Mediterr. 7, 167–190.
Butterwick, C., Heaney, S. I., and Talling, J. F. (2004). Diversity in the influence of temperature on the growth rates of freshwater algae, and its ecological relevance. Freshw. Biol. 50, 291–300. doi: 10.1111/j.1365-2427.2004.01317.x
Casoli, E., Bonifazi, A., Ardizzone, G., and Gravina, M. F. (2016). How algae influence sessile marine organisms: the tube worms case of study. Estuar. Coast. Shelf Sci. 178, 12–20. doi: 10.1016/j.ecss.2016.05.017
Casoli, E., Bonifazi, A., Ardizzone, G., Gravina, M. F., Russo, G. F., Sandulli, R., et al. (2019). Comparative analysis of mollusc assemblages from different hard bottom habitats in the central tyrrhenian sea. Diversity 11:74. doi: 10.3390/d11050074
Christie, H., Jørgensen, N. M., Norderhaug, K. M., and Waage-Nielsen, E. (2003). Species distribution and habitat exploitation of fauna associated with kelp (Laminaria hyperborea) along the Norwegian coast. J. Mar. Biol. 83, 687–699. doi: 10.1017/S0025315403007653h
Coll, M., Piroddi, C., Steenbeek, J., Kaschner, K., Lasram, F. B. R., Aguzzi, J., et al. (2010). The biodiversity of the Mediterranean sea: estimates, patterns, and threats. PLoS One 5:e11842. doi: 10.1371/journal.pone.0011842
Copper, P. (1994). Ancient reef ecosystem expansion and collapse. Coral Reefs 13, 3–11. doi: 10.1007/BF00426428
Crooks, J. A. (2002). Characterizing ecosystem-level consequences of biological invasions: the role of ecosystem engineers. OIKOS 97, 153–166. doi: 10.1034/j.1600-0706.2002.970201.x
Dalla Via, J., Sturmbauer, C., Schönweger, G., Sötz, E., Mathekowitsch, S., Stifter, M., et al. (1998). Light gradients and meadow structure in Posidonia oceanica: ecomorphological and functional correlates. Mar. Ecol. Prog. Ser. 163, 267–278. doi: 10.3354/meps163267
Delile, A. R. (1813). Description de l’Égypte Pour Recueile et des Observations et des Recherches Qui Ont été Faites en Égypte Pendant L’expédition de L’armée Française, Publié par les Orderes de Sa Majesté l’Empereur Napoléon le Grand. Histoire Naturelle. Tom. Second. Paris: Imprimerie Impériale, 1–144.
Dixon, P. S. (1964). Taxonomic and nomenclatural notes on the Florideae. IV. Botan. Notiser. 117, 56–78.
Djamali, E., Nulton, J. D., Turner, P. J., Rohwer, F., and Salamon, P. (2012). Heat output by marine microbial and viral communities. J. Non-Equilib. Thermodyn. 37, 291–313. doi: 10.1515/jnetdy-2011-0235
Duarte, C. M. (2002). The future of seagrass meadows. Environ. Conserv. 29, 192–206. doi: 10.1017/S0376892902000127
Duggins, D. O., Eckman, J. E., and Sewell, A. T. (1990). Ecology of understory kelp environments. II. effects of kelps on recruitment of benthic invertebrates. J. Exp. Mar. Biol. Ecol. 143, 27–45. doi: 10.1016/0022-0981(90)90109-P
Eckman, J. E. (1987). The role of hydrodynamics in recruitment, growth, and survival of Argopecten irradians (L.) and Anomia simplex (D’Orbigny) within eelgrass meadows. J. Exp. Mar. Biol. Ecol. 106, 165–191. doi: 10.1016/0022-0981(87)90154-7
Eckman, J. E., Duggins, D. O., and Sewell, A. T. (1989). Ecology of understory kelp environments. I. effects of kelps on flow and particle transport near the bottom. J. Exp. Mar. Biol. Ecol. 129, 173–187. doi: 10.1016/0022-0981(89)90055-5
Emlet, R. (1990). Flow fields around ciliated larvae: effects of natural and artificial tethers. Mar. Ecol. Prog. Ser. 63, 211–225. doi: 10.3354/meps063211
Evans, S. N., and Abdo, D. A. (2010). A cost-effective technique for measuring relative water movement for studies of benthic organisms. Mar. Freshw. Res. 61, 1327–1335. doi: 10.1071/MF10007
Fonseca, M. S., Fisher, J. S., Zieman, J. C., and Thayer, G. W. (1982). Influence of the seagrass zostera marina (L.) on current flow. Estuar. Coast. Shelf Sci. 15, 351–364. doi: 10.1016/0272-7714(82)90046-4
Fox, J., and Weisberg, S. (2011). An R Companion to Applied Regression, 2nd Edn. Thousand Oaks CA: Sage.
Gacia, E., and Duarte, C. M. (2001). Sediment retention by a Mediterranean Posidonia oceanica meadow: the balance between deposition and resuspension. Estuar. Coast. Shelf Sci. 52, 505–514. doi: 10.1006/ecss.2000.0753
Gacia, E., Granata, T. C., and Duarte, C. M. (1999). An approach to the measurement of particle flux and sediment retention within seagrass (Posidonia oceanica) meadows. Aquat. Bot. 65, 255–269. doi: 10.1016/S0304-3770(99)00044-3
Gambi, M. C., Nowell, A. R. M., and Jumars, P. A. (1990). Flume observations on flow dynamics in Zostera marina (eelgrass) beds. Mar. Ecol. Prog. Ser. 61, 159–169. doi: 10.3354/meps061159
Gaylord, B., Rosman, J. H., Reed, D. C., Koseff, J. R., Fram, J., MacIntyre, S., et al. (2007). Spatial patterns of flow and their modification within and around a giant kelp forest. Limnol. Oceanogr. 52, 1838–1852. doi: 10.4319/lo.2007.52.5.1838
Gorman, O. T., and Karr, J. R. (1978). Habitat structure and stream fish communities. Ecology 59, 507–515. doi: 10.2307/1936581
Grünbaum, D., Eyre, D., and Fogelson, A. (1998). Functional geometry of ciliated tentacular arrays in active suspension feeders. J. Exp. Biol. 201, 2575–2589. doi: 10.1242/jeb.201.18.2575
Hansen, B. (1993). Aspects of feeding, growth and stage development by trochophora larvae of the boreal polychaete Mediomastus fragile (Rasmussen)(Capitellidae). J. Exp. Mar. Biol. Ecol. 166, 273–288. doi: 10.1016/0022-0981(93)90224-C
Hay, M. E. (1981). The functional morphology of turf-forming seaweeds: persistence in stressful marine habitats. Ecology 62, 739–750. doi: 10.2307/1937742
Hendriks, I. E., Sintes, T., Bouma, T. J., and Duarte, C. M. (2008). Experimental assessment and modelling evaluation of the effects of the seagrass Posidonia oceanica on flow and particle trapping. Mar. Ecol. Prog. Ser. 356, 163–173. doi: 10.3354/meps07316
Hereu, B., Zabala, M., Linares, C., and Sala, E. (2004). The effects of predator abundance and habitat structural complexity on survival of juvenile sea urchins. Mar. Biol. 146, 293–299. doi: 10.1007/s00227-004-1439-y
Jackson, G. A. (1997). Currents in the high drag environment of a coastal kelp stand of California. Cont. Shelf Res. 17, 1913–1928. doi: 10.1016/S0278-4343(97)00054-X
Jimenez, I. M., Kühl, M., Larkum, A. W. D., and Ralph, P. J. (2008). Heat budget and thermal microenvironment of shallow-water corals: do massive corals get warmer than branching corals? Limnol. Oceanogr. 53, 1548–1561. doi: 10.4319/lo.2008.53.4.1548
Jokiel, P. L., and Morissey, J. I. (1993). Water motion on coral reefs: evaluation of the ‘clod card’ technique. Mar. Ecol. Prog. Ser. 93, 175–181. doi: 10.3354/meps093175
Jones, C. G., Lawton, J. H., and Shachak, M. (1994). Organisms as ecosystem engineers. OIKOS 689, 373–386. doi: 10.1007/978-1-4612-4018-1_14
Jones, C. G., Lawton, J. H., and Shachak, M. (1997). Positive and negative effects of organisms as physical ecosystem engineers. Ecology 78, 1946–1957. doi: 10.2307/2265935
Jonsson, P. R., Berntsson, K. M., and Larsson, A. I. (2004). Linking larval supply to recruitment: flow-mediated control of initial adhesion of barnacle larvae. Ecology 85, 2850–2859. doi: 10.1890/03-0565
Jørgensen, C. B., Kiørboe, T., Møhlenberg, F., and Riisgård, H. U. (1984). Ciliary and mucus net filter feeding, with special reference to fluid mechanical characteristics. Mar. Ecol. Prog. Ser. 15, 283–292. doi: 10.3354/meps015283
Judge, M. L., and Craig, S. F. (1997). Positive flow dependence in the initial colonization of a fouling community: results from in situ water current manipulations. Mar. Biol. Ecol. 210, 209–222. doi: 10.1016/S0022-0981(96)02691-3
Kandasamy, K., and Bingham, B. L. (2001). Biology of mangroves and mangrove ecosystems. Adv. Mar. Biol. 40, 81–251. doi: 10.1016/S0065-2881(01)40003-4
Kelaher, B. P., and Castilla, J. C. (2005). Habitat characteristics influence macrofauna communities in coralline turf more than mesoscale coastal upwelling on the coast of northern Chile. Estuar. Coast. Shelf Sci. 63, 155–165. doi: 10.1016/j.ecss.2004.10.017
Koch, E. W., Ackerman, J. D., Verduin, J., and van Keulen, M. (2006). “Fluid dynamics in seagrass ecology–from molecules to ecosystems,” in Seagrasses: Biology, Ecology and Conservation, eds A. W. D. Larkum, R. J. Orth, and C. M. Duarte (Dordrecht: Springer), 193–225. doi: 10.1007/1-4020-2983-7_8
Koch, E. W., and Gust, G. (1999). Water flow in tide- and wave-dominated beds of the seagrass Thalassia testudinum. Mar. Ecol. Prog. Ser. 184, 63–72. doi: 10.3354/meps184063
Koehl, M. R. A. (2007). Mini review: hydrodynamics of larval settlement into fouling communities. Biofouling 23, 357–368. doi: 10.1080/08927010701492250
Kregting, L. T., Stevens, C. L., Cornelisen, C. D., Pilditch, C. A., and Hurd, C. L. (2011). Effects of a small-bladed macroalgal canopy on benthic boundary layer dynamics: implications for nutrient transport. Aquat. Biol. 14, 41–56. doi: 10.3354/ab00369
Laegdsgaard, P., and Johnson, C. (2001). Why do juvenile fish utilise mangrove habitats? J. Exp. Mar. Biol. Ecol. 257, 229–253. doi: 10.1016/S0022-0981(00)00331-2
Larsen, P. S., and Riisgård, H. U. (1994). The sponge pump. J. Theor. Biol. 168, 53–63. doi: 10.1006/jtbi.1994.1087
Larsson, A. I., and Jonsson, P. R. (2006). Barnacle larvae actively select flow environments supporting post-settlement growth and survival. Ecology 87, 1960–1966. doi: 10.1890/0012-9658(2006)87[1960:blasfe]2.0.co;2
Lisberg, D., and Petersen, J. K. (2000). Clearance capacity of electra bellula (Bryozoa) in seagrass meadows of Western Australia. J. Exp. Mar. Biol. Ecol. 62, 225–236. doi: 10.1016/S0022-0981(99)00147-1
Macia, A., Abrantes, K. G. S., and Paula, J. (2003). Thorn fish Terapon jarbua (Forskål) predation on juvenile white shrimp Penaeus indicus h. milne edwards and brown shrimp Metapenaeus monoceros (Fabricius): the effect of turbidity, prey density, substrate type and pneumatophore density. J. Exp. Mar. Biol. Ecol. 291, 29–56. doi: 10.1016/S0022-0981(03)00097-2
Martin, S., and Gattuso, J. (2009). Response of coralline algae to ocean acidification and evaluated temperatures. Glob. Change Biol. 15, 2089–2100. doi: 10.1111/j.1365-2486.2009.01874.x
McCormick, M. (1994). Comparison of field methods for measuring surface topography and their associations with a tropical reef fish assemblage. Mar. Ecol. Prog. Ser. 112, 87–96. doi: 10.3354/MEPS112087
McKinney, F. K., and Jackson, J. B. (1991). Bryozoan Evolution. Chicago, IL: University. of Chicago Press.
Miglietta, M., Delia Tommasa, L., Denitto, E., Gravili, C., Pagliara, P., Bouillon, J., et al. (2000). Approaches to the ethology of hydroids and medusae (Cnidaria, Hydrozoa). Sci. Mar. 64, 63–71. doi: 10.3989/scimar
Mineur, F., Arenas, F., Assis, J., Davies, A. J., Engelen, A. H., Fernandes, F., et al. (2015). European seaweeds under pressure: consequences for communities and ecosystem functioning. J. Sea Res. 98, 91–108. doi: 10.1016/j.seares.2014.11.004
Montefalcone, M., Morri, C., Peirano, A., Albertelli, G., and Bianchi, C. N. (2007). Substitution and phase shift within the Posidonia oceanica seagrass meadows of NW Mediterranean sea. Estuar. Coast. Shelf Sci. 75, 63–71. doi: 10.1016/j.ecss.2007.03.034
Mullineaux, L. S., and Butman, C. A. (1991). Initial contact, exploration, and attachment of barnacle (Balanus amphitrite) cyprids settling in flow. Mar. Biol. 110, 93–103. doi: 10.1007/BF01313096
Mullineaux, L. S., and Garland, E. D. (1993). Larval recruitment in response to manipulated field flows. Mar. Biol. 116, 667–683. doi: 10.1007/BF00355484
Ondiviela, B., Fernández, L., Puente, A., García-Castrillo, G., and Juanes, J. A. (2018). Characterization of a resilient seagrass meadow during a decline period. Sci. Mar. 82, 67–75.
Ong, R. H., King, A. J. C., Kaandorp, J. A., Mullins, B. J., and Caley, M. J. (2017). The effect of allometric scaling in coral thermal microenvironments. PLoS One 12:e0184214.
Padilla, D. K. (2010). Context-dependent impacts of a non-native ecosystem engineer, the pacific oyster Crassostrea gigas. Integr. Comp. Biol. 50, 213–225. doi: 10.1093/icb/icq080
Pearse, J. S., and Hines, A. H. (1979). Expansion of a central California kelp forest following the mass mortality of sea urchins. Mar. Biol. 51, 83–91. doi: 10.1007/BF00389034
Pergent, G., Bazairi, H., Bianchi, C., Boudouresque, C., Buia, M., Calvo, S., et al. (2014). Climate change and Mediterranean seagrass meadows: a synopsis for environmental managers. Mediterr. Mar. Sci. 15, 462–473. doi: 10.12681/mms.621
Peterson, C. H., Luettich, R. A., Micheli, F., and Skilleter, G. A. (2004). Attenuation of water flow inside seagrass canopies of differing structure. Mar. Ecol. Prog. Ser. 268, 81–92. doi: 10.3354/meps268081
Piazzi, L., and Cinelli, F. (2001). Distribution and dominance of two introduced turfforming macroalgae on the coast of Tuscany, Italy, Northwestern Mediterranean sea in relation to different habitats and sedimentation. Bot. Mar. 44, 509–520. doi: 10.1515/BOT.2001.057
Prathep, A., Marrs, R., and Norton, T. (2003). Spatial and temporal variations in sediment accumulation in an algal turf and their impact on associated fauna. Mar. Biol. 142, 381–390. doi: 10.1007/s00227-002-0940-4
Prazukin, A., Shadrin, N., Balycheva, D., Firsov, Y., Lee, R., and Anufriieva, E. (2020). Cladophora spp. (Chlorophyta) modulate environment and create a habitat for microalgae in hypersaline waters. Eur. J. Phycol. 56, 231–243. doi: 10.1080/09670262.2020.1814423
Qian, P. Y., Rittschof, D., and Sreedhar, B. (2000). Macrofouling in unidirectional flow: miniature pipes as experimental models for studying the interaction of flow and surface characteristics on the attachment of barnacle, bryozoan and polychaete larvae. Mar. Ecol. Prog. Ser. 207, 109–121. doi: 10.3354/meps207109
Qian, P. Y., Rittschof, D., Sreedhar, B., and Chia, F. S. (1999). Macrofouling in unidirectional flow: miniature pipes as experimental models for studying the effects of hydrodynamics on invertebrate larval settlement. Mar. Ecol. Prog. Ser. 191, 141–151. doi: 10.3354/meps191141
Reed, D. C., and Foster, M. S. (1984). The effects of canopy shading on algal recruitment and growth in a giant kelp forest. Ecology 65, 937–948. doi: 10.2307/1938066
Riedl, R. (1966). Biologie der Meereshöhlen: Topographie, Faunistik und Ökologie eines unterseeischen Lebensraumes. Hamburg: Paul Parey.
Riisgård, H. U. (1998). Filter feeding and plankton dynamics in a Danish fjord: a review of the importance of flow, mixing, and density-driven circulation. J. Environ. Manag. 53, 195–207. doi: 10.1006/jema.1998.0205
Riisgård, H. U. (2015). “Filter-feeding mechanisms in crustaceans,” in Life Styles and Feeding Biology, Vol. 2 in the Natural History of Crustaceans, eds M. Thiel and L. Watling (Oxford: Oxford University Press), 418–463.
Riisgård, H. U., and Larsen, P. S. (2000). A comment on experimental techniques for studying particle capture in filter-feeding bivalves. Limnol. Oceanogr. 45, 1192–1195. doi: 10.4319/lo.2000.45.5.1192
Robertson, A. I., and Blaber, S. J. M. (1992). “Tropical mangrove ecosystems,” in Plankton, Epibenthos and Fish Communities, eds A. I. Robertson and D. M. Alongi (Washington DC: American Geophysical Union), 173–224.
Rossbach, F. I., Casoli, E., Beck, M., and Wild, C. (2021). Mediterranean red macro algae mats as habitat for high abundances of serpulid polychaetes. Diversity 13:265. doi: 10.3390/d13060265
Ruesink, J. L., Lenihan, H. S., Trimble, A. C., Heiman, K. W., Micheli, F., Byers, J. E., et al. (2005). Introduction of non-native oysters: ecosystem effects and restoration implications. Ann. Rev. Ecol. Evol. Syst. 36, 643–689. doi: 10.1146/annurev.ecolsys.36.102003.152638
Russ, G. (1979). “The relationship of fish grazing to bryozoan and ascidian communities at Portsea Pier, Victoria, Australia (Preliminary report),” in Advances in Bryozoology, eds G. P. Larwood and M. B. Abbott (New York, NY: Academic Press), 295–296.
Ryland, J. S. (1976). Physiology and ecology of marine bryozoans. Adv. Mar. Biol. 14, 285–443. doi: 10.1016/S0065-2881(08)60449-6
Sagarra, E. B., and François, T. L. (2015). Marine Wildlife of the Mediterranean. Madrid: Gallocanta ediciones.
Shadrin, N., Balycheva, D., and Anufriieva, E. (2021). Microphytobenthos in the hypersaline water bodies, the case of bay Sivash (Crimea): is salinity the main determinant of species composition? Water 13:1542. doi: 10.3390/w13111542
Shadrin, N., Kolesnikova, E., Revkova, T., Latushkin, A., Chepyzhenko, A., Drapun, I., et al. (2019). Do separated taxa react differently to a long-term salinity increase? The meiobenthos changes in Bay Sivash, largest hypersaline lagoon worldwide. Knowl. Manag. Aquat. Ecosyst. 420:36. doi: 10.1051/kmae/2019028
Shashar, N., Cohen, Y., and Loya, Y. (1993). Extreme diel fluctuations of oxygen in diffusive boundary layers surrounding stony corals. Biol. Bull. 185, 455–461. doi: 10.2307/1542485
Shcherbak, M. M., Monchenko, V. I., Ermolenko, V. M., Movchan, Y., and Peklo, O. M. (1994). Red Data Book of Ukraine. Animal Kingdom. Kyiv: Ukrainian Encyclopedia.
Silverman, H., Lynn, J. W., and Dietz, T. H. (2000). In vitro studies of particle capture and transport in suspension-feeding bivalves. Limnol. Oceanogr. 45, 1199–1203. doi: 10.4319/lo.2000.45.5.1199
Stewart, H. L., Fram, J. P., Reed, D. C., Williams, S. L., Brzezinski, M. A., MacIntyre, S., et al. (2008). Differences in growth, morphology, and tissue carbon and nitrogen of Macrocystis pyrifera within and at the outer edge of a giant kelp forest in California, USA. Mar. Ecol. Prog. Ser. 375, 101–112. doi: 10.3354/meps07752
Terrados, J., and Duarte, C. M. (2000). Experimental evidence of reduced particle resuspension within a seagrass (Posidonia oceanica L.) meadow. J. Exp. Mar. Biol. Ecol. 243, 45–53. doi: 10.1016/S0022-0981(99)00110-0
Thompson, T., and Glenn, E. (1994). Plaster standards to measure water motion. Limnol. Oceanogr. 39, 1768–1779. doi: 10.4319/LO.1994.39.7.1768
Trager, G. C., Hwang, J. S., and Strickler, J. R. (1990). Barnacle suspension-feeding in variable flow. Mar. Biol. 105, 117–127. doi: 10.1007/BF01344277
Widdows, J., Pope, N., Brinsley, M., Gascoigne, J., and Kaiser, M. J. (2009). Influence of self-organized structures on near-bed hydrodynamics and sediment dynamics within a mussel (Mytilus edulis) bed in the Menai Strait. J. Exp. Mar. Biol. Ecol. 379, 92–100. doi: 10.1016/j.jembe.2009.08.017
Willis, S. C., Winemiller, K. O., and Lopez-Fernandez, H. (2005). Habitat structural complexity and morphological diversity of fish assemblages in a Neotropical floodplain river. Oecologia 142, 284–295. doi: 10.1007/s00442-004-1723-z
Keywords: macroalgae, seagrass, Posidonia oceanica, phytal habitat, hard-bottom communities, environmental gradients
Citation: Schmidt N, El-Khaled YC, Roßbach FI and Wild C (2021) Fleshy Red Algae Mats Influence Their Environment in the Mediterranean Sea. Front. Mar. Sci. 8:721626. doi: 10.3389/fmars.2021.721626
Received: 07 June 2021; Accepted: 10 August 2021;
Published: 31 August 2021.
Edited by:
Stelios Katsanevakis, University of the Aegean, GreeceReviewed by:
Nickolai Shadrin, A. O. Kovalevsky Institute of Marine Biological Research, RussiaBeata Messyasz, Adam Mickiewicz University in Poznań, Poland
Copyright © 2021 Schmidt, El-Khaled, Roßbach and Wild. This is an open-access article distributed under the terms of the Creative Commons Attribution License (CC BY). The use, distribution or reproduction in other forums is permitted, provided the original author(s) and the copyright owner(s) are credited and that the original publication in this journal is cited, in accordance with accepted academic practice. No use, distribution or reproduction is permitted which does not comply with these terms.
*Correspondence: Neele Schmidt, neele.schmidt@ebc.uu.se
†Present address: Neele Schmidt, Animal Ecology, Department of Ecology and Genetics, Uppsala University, Uppsala, Sweden