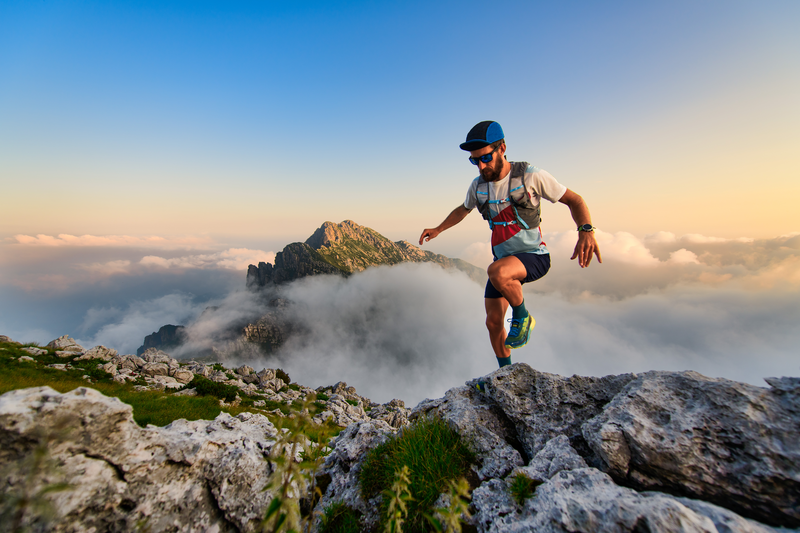
94% of researchers rate our articles as excellent or good
Learn more about the work of our research integrity team to safeguard the quality of each article we publish.
Find out more
ORIGINAL RESEARCH article
Front. Mar. Sci. , 09 December 2021
Sec. Deep-Sea Environments and Ecology
Volume 8 - 2021 | https://doi.org/10.3389/fmars.2021.713022
This article is part of the Research Topic Recent and Emerging Innovations in Deep-Sea Taxonomy to Enhance Biodiversity Assessment and Conservation View all 15 articles
With the accelerating development of direct and indirect anthropogenic threats, including climate change and pollution as well as extractive industries such as deep-sea mining, there is an urgent need for simple but effective solutions to identify conservation priorities for deep-sea species. The International Union for Conservation of Nature (IUCN) Red List of Threatened Species is an effective and well-recognized tool to promote the protection of species and presents an opportunity to communicate conservation threats to industry, policy makers, and the general public. Here, we present the Vent Red List for molluscs: a complete global assessment of the extinction risk of all described molluscs endemic to hydrothermal vents, a habitat under imminent threat from deep-sea mining. Of the 184 species assessed, 62% are listed as threatened: 39 are Critically Endangered, 32 are Endangered, and 43 are Vulnerable. In contrast, the 25 species that are fully protected from deep-sea mining by local conservation measures are assessed as Least Concern, and a further 45 species are listed as Near Threatened, where some subpopulations face mining threats while others lie within protected areas. We further examined the risk to faunas at specific vent sites and biogeographic regions using a relative threat index, which highlights the imperiled status of vent fields in the Indian Ocean while other vent sites within established marine protected areas have a high proportion of species assessed as Least Concern. The Vent Red List exemplifies how taxonomy-driven tools can be utilized to support deep-sea conservation and provides a precedent for the application of Red List assessment criteria to diverse taxa from deep-sea habitats.
As a vast and relatively unexplored ecosystem, the deep sea presents unique conservation challenges. The heterogeneity of deep-sea habitats makes it difficult to identify representative systems for area-based conservation (Van Dover et al., 2018), variability in governance across Exclusive Economic Zones (EEZ) and Areas Beyond National Jurisdiction (ABNJ) engenders inconsistency in global deep-sea management (Gjerde et al., 2008), and restricted biological knowledge limits the capacity to understand the impact of threatening events to deep-sea taxa (Danovaro et al., 2017). As industrial interest and commercial exploitation begins to accelerate faster than biological discovery, we face an increasingly urgent need for simple but effective tools to protect deep-sea species.
While the seafloor is home to incredible life, it is also characterized by large quantities of commercially valuable minerals including polymetallic sulfides at hydrothermal vents, manganese nodules on abyssal plains, and cobalt-rich ferromanganese crusts on seamounts (Hein et al., 2013; Petersen et al., 2016). The mining of these deep-sea environments and their resources is now being widely considered, and in some cases instigated (Okamoto et al., 2019), as demand for industrially important metals grows and technological capabilities improve (Sharma, 2011, 2015). Despite several studies concluding that mining will have an adverse and often irreversible impact on local deep-sea biodiversity (e.g., Gollner et al., 2017; Van Dover et al., 2017; Niner et al., 2018; Simon-Lledó et al., 2019), very few conservation measures have been implemented to date.
Of the deep-sea habitats threatened by mining, hydrothermal vents harbor the highest density of life (Van Dover et al., 2018). These are very small environments, occupying only ∼ 50 km2 of the seafloor, globally (Sigwart et al., 2017), yet each hydrothermal vent site is characterized by a multitude of unique species, hosting a relative biomass to that of coral reefs or tropical rainforests (Van Dover, 2000). As well as being highly insular and distinct in biodiversity from the surrounding benthos, hydrothermal vent communities also exhibit high levels of endemism and regional variation in species composition, with many endemics having only limited connectivity with other local vent sites (Rogers et al., 2012; Yahagi et al., 2019). Molluscs represent one of the dominant groups in vent habitats, with good global coverage and a large degree of vent-endemism (Wolff, 2005; Chapman et al., 2019). As important members of the vent community, they inhabit an array of niches including hosting endosymbiotic bacteria in specialized organs (Chen et al., 2018a), forming dense aggregations that provide substrate for other species (Laming et al., 2018; Sun et al., 2020), and exhibit unique ecological traits (e.g., Chen et al., 2018b).
Taxonomically driven solutions should be central to deep-sea conservation initiatives (Glover et al., 2018). A transparent and reliable evaluation of the conservation status of vent-endemic species would enable clear communication of threats to diverse stakeholders. The International Union for Conservation of Nature (IUCN) Red List of Threatened Species (hereafter, the Red List) is an internationally recognized taxon-based conservation tool that informs global policies by providing the most comprehensive and rigorous information available on species extinction risk (Rodrigues et al., 2006). The Red List allows for consistent assessment of extinction risk for any animal, plant, or fungal taxon through the use of standardized criteria, and its application has been widely successful in raising awareness of threats and ensuring the protection of species in other systems (Betts et al., 2020).
The Red List uses five categories with escalating risk that imply a higher expectation of extinction. Species with adequate data that are not threatened can fall into two categories: Least Concern, typically including widespread taxa or taxa not affected by threats, or Near Threatened, with taxa that are close to qualifying for a threatened category but do not meet all criteria (IUCN, 2012). Species that are threatened are subdivided into the following categories: Vulnerable, Endangered, and Critically Endangered, where taxa face a high, very high, or extremely high risk of extinction, respectively (IUCN, 2012). To be listed within a threatened category, species must meet the requirements and specific thresholds of at least one of the IUCN Red List Criteria: comprising criteria A and C which use data on population sizes and declines, criterion B which uses the geographic range of the species to estimate extinction risk, criterion D which is applicable to species with very restricted populations, and criterion E which uses quantitative analysis to assess probability of extinction (IUCN, 2012).
Deep-sea mining is already a recognized potential threat to vent ecosystems, as extensively discussed in recent literature (Van Dover et al., 2017; Miller et al., 2018; Niner et al., 2018), but it remains uncertain whether mining poses a sufficient threat to risk the total global extinction of vent species. The Red List presents an opportunity to assess the extent to which deep-sea mining would imperil individual vent-endemic species across different global sites (Sigwart et al., 2019). Furthermore, assessments of entire taxonomic groups are most effective as they allow for comprehensive comparison of threat (e.g., Carpenter et al., 2008). This study therefore aimed to assess the extinction risk of all molluscs endemic to hydrothermal vents using the Red List criteria. The resulting Vent Red List provides a universally recognized assessment of the threat of deep-sea mining for vent molluscs. All of these assessments have been reviewed and are published on the global IUCN Red List of species (IUCN, 2021). We further compare the distribution of species at risk to illustrate the relative threat levels to vent-endemic taxa across different biogeographic regions and regulatory areas.
We used species records from existing studies (Wolff, 2005; Chapman et al., 2019) and expert knowledge to compile a complete list of all mollusc species described to date (up to 2021) endemic to active hydrothermal vent environments. As Red List assessments are taxon-specific, only named species were included in this study. Species were considered endemic where they had only been recorded at hydrothermal vents at time of assessment. Species that are also known from other deep-sea habitats, as well as vent-peripheral species (including all cephalopods), were excluded from this list, leaving a total of 184 vent-endemic molluscs (Supplementary Material 1). These species span five different mollusc classes: Gastropoda, Bivalvia, Monoplacophora, Polyplacophora, and Solenogastres.
We assessed the extinction risk of each species using the Red List criteria (IUCN, 2012), following IUCN guidelines and the method presented by Thomas et al. (2021). All assessments were made using Red List criteria B and/or D2 (Table 1), which use geographic distribution data and information about continuing declines (criterion B) or plausible future threats causing extreme declines (criterion D2) to determine extinction risk. Nearly all vent species are lacking data to calculate population sizes and trends or run extinction risk models required for the other criteria (Thomas et al., 2021). Literature reviews were conducted for each species and data collected included the name, location, depth, biogeographic region, and local jurisdiction of the vent fields from which each species is known. Each assessment is therefore based on the best available published distribution data available at the time and may be subject to revisions as new data become available. Vent field names and coordinates listed in the literature were compared and aligned with the InterRidge Vents Database to ensure consistent nomenclature for sites (Beaulieu and Szafranski, 2020).
Table 1. Application of the IUCN Red List categories and criteria to hydrothermal vent-endemic molluscs, based on IUCN guidelines (IUCN, 2012) and definitions listed in Thomas et al. (2021).
As the primary anthropogenic threat to vent-endemic species (Van Dover, 2014), deep-sea mining informed the criteria B and D2 requirements of continuing declines and plausible future threats for the Vent Red List assessments (Table 1). The local mining threat for each locality was determined based on regional seabed management objectives and the regulatory frameworks at sites within the range of each species (Thomas et al., 2021). Hydrothermal vents in EEZs are regulated by national governments and those in ABNJ are regulated by the International Seabed Authority (ISA) (Thompson et al., 2018). Key considerations for threat assessment included the implementation of deep-sea mining licenses, marine protected areas (MPAs), and mining moratoria. For example, continuing decline was inferred in areas of exploratory mining contracts signed by the ISA or in the EEZs of nations that have granted mining licenses (criterion B); plausible future threat was considered in areas where there are no regulations in place to protect from future deep-sea mining (criterion D2) (Thomas et al., 2021).
To illustrate the global distribution of extinction risk to vent species, all assessment data were amalgamated to produce a list of hydrothermal vent fields with the number of species assessed under each Red List category at each site (Supplementary Material 2). As with previous studies (e.g., Tingley et al., 2019), the five Red List categories were then assigned a sequential ranked risk score (Least Concern = 1, Near Threatened = 2, Vulnerable = 3, Endangered = 4, Critically Endangered = 5), and the number of species in each category were multiplied by its risk score and summed, to produce a total assessment of threat to all species at that vent field (Eq. 1).
where i is each Red List category.
To reduce bias between sites with varying species richness, a standardized Threat Score was calculated by dividing the Ranked Sum by the total number of species across all categories at that site (Eq. 2).
For example, at the Menez Gwen vent field on the Mid-Atlantic Ridge, there are eight species, of which six species were assessed as Least Concern (risk score = 1) and two Near Threatened (risk score = 2). Thus, the Ranked Sum is (6∗1)+(2∗2) = 10, resulting in a Threat Score of 10/8 = 1.25 for that vent field.
Of the 184 vent-endemic mollusc species assessed for the Vent Red List, 114 (62%) are assessed as threatened (listed as Vulnerable, Endangered or Critically Endangered) by deep-sea mining, and a further 45 (24.4%) are listed as Near Threatened (Table 2, Figure 1, and Supplementary Material 1). Only 13.6% of species are listed as Least Concern, under the protection of MPAs. Sufficient data were available to complete assessments of extinction risk for all species and none are listed as Data Deficient. The majority of the molluscs assessed are highly restricted within their respective biogeographic regions (Figure 1A), with over 60% of species known only from one or two hydrothermal vent fields (Figure 2).
Table 2. Current IUCN Red List status for all 184 hydrothermal vent-endemic mollusc species described to date, by biogeographic region.
Figure 1. Global distribution of threat to hydrothermal vent-endemic mollusc species from deep-sea mining, based on IUCN Red List assessments. (A) Map showing the species richness of vent-endemic molluscs described to date at the hydrothermal vent fields included in this study, where light green denotes low species richness and dark green denotes high species richness. Dashed shapes denote the different biogeographic regions for hydrothermal vents (Rogers et al., 2012): (i) Mid-Atlantic Ridge, (ii) Mid-Cayman Spreading Center, (iii) East Scotia Ridge, (iv) Indian Ocean, (v) Northeast Pacific, (vi) Northern East Pacific Rise, vii: Southern East Pacific Rise, (viii) Northwest Pacific, (ix) Southwest Pacific. (B) Map showing the locality and extent of different seabed regulatory areas relevant to the hydrothermal vent fields included within this study, including exploratory polymetallic sulfide mining licenses granted by the International Seabed Authority, Marine Protected Areas, and countries’ Exclusive Economic Zones. Dashed mid blue line denotes the Antarctic Treaty boundary. Insets are included for areas with smaller details. (C) Map showing the scale of mining threat to each hydrothermal vent field included within this study, where 1 denotes a low Threat Score associated with Least Concern Red List assessments, and 5 denotes a high Threat Score associated with Critically Endangered assessments. Insets are included for areas with high density of vent fields.
Figure 2. Frequency distribution of the range of global vent-endemic mollusc species; histogram based on the numbers of hydrothermal vent sites in the range of species assessed in the Vent Red List.
Generally, mollusc species richness is low at individual sites: of the 110 vent fields listed in this study, only 35 host five or more vent-endemic mollusc species, with over half hosting only one or two species (Figure 1A). Individual hydrothermal vent fields in the Northern East Pacific Rise have the greatest recorded species richness for vent-endemic molluscs, with the 13N, 21N and 9 50’N East Pacific Rise vent fields hosting 32, 28, and 28 species, respectively (Supplementary Material 2). The Mid-Atlantic Ridge has the greatest collective diversity, with an average species richness of 7.13 across its vent fields. The Northeast Pacific ridges and Southwest Pacific basins also have relatively high species richness, while the Indian Ocean and East Scotia Ridge vents have the lowest collective species richness across the nine biogeographic regions (Figure 1A).
The assignment of different Red List categories is dependent on local regulatory frameworks, with the threat level varying across different countries’ EEZs, ISA mining license areas, and designated MPAs (Figure 1B; Thomas et al., 2021). The Threat Score illustrates the overall extinction risk for the endemic mollusc species at each vent field and is indicative of the threat posed by deep-sea mining to the area (Figure 1C). Globally, over half of vent fields have a Threat Score ≥ 3, signifying that hydrothermal vent species in those areas are at a high extinction risk. Individually, vent fields in the Indian Ocean and Northwest Pacific have the maximum Threat Score of 5, indicative of the Critically Endangered status assigned to species at these sites, whereas all vent fields on the East Scotia Ridge have the minimum Threat Score of 1, representative of assessments of Least Concern (Figure 1C).
Among the nine major biogeographical regions examined (Figure 1A), Indian Ocean vent molluscs are under the greatest extinction risk, with 100% of species listed in threatened categories, including 60% as Critically Endangered (Table 2 and Figure 1C). This coincides with the distribution of ISA mining licenses across vent sites along the Central and Southwest Indian Ridges in the Indian Ocean (Figure 1B). Species at Northwest and Southwest Pacific vents, where there is a varying threat level across different countries’ EEZs (Figures 1B,C), are also at high risk, with 77.8 and 95.7% listed in threatened categories, respectively (Table 2). The individual vent fields with the highest Threat Scores in these regions lie within the Japan and Papua New Guinea EEZs, where deep-sea mining licenses have been granted, whereas vent fields protected by the Marianas Trench Marine National Monument have a lower Threat Score (Figure 1C). Mid-Atlantic Ridge vent molluscs have the greatest spread of extinction risk, with 30% of species listed in threatened categories, 30% as Near Threatened, and 40% as Least Concern (Table 2). This corresponds with the incidence of both ISA mining licenses and the Azores’ vent-specific MPAs along the Mid-Atlantic Ridge (Figure 1B).
The three East Pacific biogeographic regions have the greatest proportion of species assessed as Near Threatened, each with over 50% of species located both within and outside MPAs implemented by Mexico and Canada (Table 2 and Figure 1). There are no active mining licenses and as a consequence there are no species in the East Pacific assessed as Endangered or Critically Endangered (Table 2). Nonetheless, several vent fields in this region, especially along the Southern East Pacific Rise, lie in ABNJ without protection from deep-sea mining (Figure 1B). Species located in areas that have significant protection from deep-sea mining are consistently assessed at lowest risk; for example, all four East Scotia Ridge vent molluscs are assessed as Least Concern as a result of the Antarctic Treaty and the South Georgia and the South Sandwich Islands MPA (Figure 1B).
The Vent Red List is indicative of the unique biodiversity and threat profile of each hydrothermal vent field and biogeographic region, and can be used to effectively communicate and drive the conservation of these remarkable deep-sea habitats. The importance of mining licenses as a controlling factor in the determination of Red List status underlines the threat of potential mining to the conservation of vent-endemic species.
The distribution of threats to hydrothermal vents is closely tied to the regulation within each geographic area. In ABNJ, hydrothermal vents within ISA mining license areas along the Mid-Atlantic and Indian Ocean Ridges (Miller et al., 2018) have a higher Threat Score than those at the Northern and Southern East Pacific Rise, with Indian Ocean vent species exhibiting the greatest proportion of threatened Red List assessments. This is influenced by the overlap in species ranges with a variety of regulatory areas: species found at sites along the Mid-Atlantic Ridge have ranges across a mosaic of ABNJ and EEZs with differing protections. Threats to vent sites within individual EEZs are highly variable and dependent on current national regulation of the seabed. Vent fields that lie within the territorial waters of countries that have granted mining licenses, such as Japan (Okamoto et al., 2019) and Papua New Guinea (Hoagland et al., 2010), generally exhibit high Threat Scores. Conversely, vent species in countries and regions that have endeavored to preserve portions of the seabed with MPAs, such as the Azores (Calado et al., 2011; Abecasis et al., 2015) and Mexico (Menini and Van Dover, 2019), are at a much lower risk of extinction.
While this study is focused on vent-endemic molluscs, our results are representative of the global distribution of mining threat for all vent-endemic taxa. Deep-sea species tend to be very data-limited, therefore future assessments of extinction risk for other vent taxa, including crustaceans and polychaetes, are expected to be reliant on the same Red List criteria as the Vent Red List (Thomas et al., 2021). Vent species exhibit high endemicity, and the primary anthropogenic threat is regionally controlled, thus the overall proportion of Red List categories for hydrothermal vent environments is unlikely to vary significantly with the addition of new taxa. The Red List is a species-based conservation tool, yet in this case it provides a comprehensive and reliable illustration of the geographic distribution of threats to a specific deep-sea habitat.
Taxonomy-driven tools such as the Red List can cultivate deep-sea conservation; the application of Red List assessments to vent-endemic molluscs provides a precedent for other deep-sea taxa and habitats (Glover et al., 2018; Sigwart et al., 2019). Red List assessments are easily understood by a wide range of stakeholders (Rodrigues et al., 2006; Betts et al., 2020), and have the potential to provide an alternative perspective to ecosystem-based management approaches and enhance deep-sea conservation initiatives beyond hydrothermal vents.
The cobalt-rich ferromanganese crusts of seamounts, for example, are a target of five mining exploration licenses granted by the ISA to Brazil, China, Japan, Russia, and the South Korea (Miller et al., 2018; ISA, 2021). Seamounts are characterized by high productivity (Clark et al., 2010) and are rich in long-lived corals that likely take decades to millennia to recover from disturbance (Gollner et al., 2017; Watling and Auster, 2017). Likewise, gas hydrate deposits that occur in conjunction with cold seeps, another important chemosynthetic habitat in the deep sea that hosts hundreds of similarly endemic species to vents (Wolff, 2005; Suess, 2018), are also under increasing consideration for commercial extraction (Chong et al., 2016; Miller et al., 2018). The insular nature, relatively well-documented global distribution, and imminent mining threat to both these habitats mean it is viable to apply a Red List approach to assess these hotspots of deep-sea biodiversity (Thomas et al., 2021).
One potential limitation to our Red List approach, as with the majority of deep-sea research, is the underlying lack of observational data for different vent populations. None of the species included in this study were assessed as Data Deficient, yet it is recognized that the distribution and range of some mollusc species at hydrothermal vents is not fully known and requires further research. The global summary of endemic molluscs at hydrothermal vent sites illustrates overall low species richness across the majority of vent fields, compared to a few species-rich sites. This may be indicative of the paucity of baseline biodiversity knowledge for deep-sea habitats (Glover et al., 2018); however, this could also be a feature of the local and regional heterogeneity seen in vent communities arising from geographic variation in tectonic activity and vent geochemistry (Van Dover, 2000; Thaler and Amon, 2019). In the face of accelerating threats, assessments of extinction risk can only be based on the best available data at the time (IUCN, 2016), and although data are lacking for many groups (Glover et al., 2018), the Vent Red List demonstrates that there is sufficient information to assess even relatively data-poor species using Red List criteria (Thomas et al., 2021).
Specimen collection and taxonomic research are fundamental to deep-sea conservation, and present a bottleneck to the application of taxon-based conservation tools (Glover et al., 2018). This can be especially problematic where there is ongoing research that could result in taxonomic revisions, such as the study of species complexes. For example, among Lepetodrilus species on the East Pacific Rise, genetic evidence from multiple studies delineate separate lineages that are currently included within nominal species (Johnson et al., 2008; Matabos and Jollivet, 2019). Assessing a species complex as a single taxon could artificially lower the threat category in a Red List assessment because the taxon represents an over-estimation of combined abundance and range for several species. Taxonomic research and timely updates following reviews are, therefore, of the utmost importance to ensure accurate measures of extinction risk. Ongoing research can be integrated into Red List assessment text and taxa can be reassessed as frequently as required (IUCN, 2012). Furthermore, based on current findings, seabed management and mining regulation appears to have a greater impact on Red List assessment outcomes than the distribution data for individual species (Thomas et al., 2021).
A more concerning trend that has recently emerged is the potential North/South divide in the extent of biological research and deep-sea mining prospects (Thaler and Amon, 2019). We found a similar pattern in this study, with the Indian Ocean having the greatest proportion of threatened Red List assessments despite exhibiting one of the lowest collective species richness counts. The high proportion of Critically Endangered assessments at the Indian Ocean vent fields is indicative of their biodiversity uniqueness as species can only be assessed as Critically Endangered under Red List criterion B if they are known from a single location (Thomas et al., 2021). In fact, along with the Southwest Pacific, the Indian Ocean hosts the greatest proportion of locally endemic species known only from a single hydrothermal vent field. Further research is however required to determine whether this is simply a factor of low sampling effort in the Southern Hemisphere.
While entire vent biotas are threatened in biogeographic regions that have no protection from deep-sea mining, such as the Indian Ocean, the threat is significantly reduced in regions that straddle different regulatory areas, like the Mid-Atlantic Ridge. Despite several ISA mining licenses issued for the Mid-Atlantic Ridge, no vent fields in this region have a Threat Score greater than three as a result of the protection provided by the Azores MPA network (Abecasis et al., 2015). This highlights the importance of implementing conservation measures like MPAs to reduce extinction risk, yet at present, other than the South Georgia and South Sandwich Islands MPA, all other vent-related MPAs are located in the northern hemisphere. In an ecosystem that has well-documented, distinct faunal communities across different biogeographic regions, the preservation of areas representative of each region is essential.
The conservation of hydrothermal vent habitats and their unique fauna requires action to lower the extinction risk of vent species. While the incorporation of new data to the Vent Red List has potential to influence assessment outcome and lead to Red List category change, this would only constitute a non-genuine change under IUCN regulations (IUCN Standards and Petitions Committee, 2019); i.e., improved knowledge on the species rather than a true change in extinction risk. Furthermore, given the relatively broad category thresholds of the Red List criteria, the addition of new species distribution data does not always affect assessment result (Thomas et al., 2021). Consequently, to improve the extinction risk of vent species, real conservation measures would need to be implemented that mitigate the threat of deep-sea mining.
One conservation method that has potential to safeguard hydrothermal vents from deep-sea mining is the implementation of MPAs (Menini and Van Dover, 2019). Globally, 70 vent-endemic mollusc species were assessed as Least Concern or Near Threatened, based on the protection afforded by MPAs, either to the entire, or a proportion of the population. These assessment outcomes demonstrate the effectiveness of MPAs to protect individual species against the threat of extinction. However, the presence of an MPA alone does not always constitute protection and both assessment and real conservation outcomes are dependent on whether the threat is sufficiently mitigated by implementation of the MPA (Edgar et al., 2014). For example, while bottom fishing is prohibited within New Zealand’s Kermadec Benthic Protected Area, there is no active legislation against other commercial activities like seafloor mining, so vent sites are left unprotected (Van Dover et al., 2011). Furthermore, even MPAs that include specific regulation for the protection of hydrothermal vents are at times too small to afford protection from nearby threats. Research predicts that mining sediment plumes can spread up to 70 km (Luick, 2012; Miller et al., 2018), therefore hydrothermal vents within this proximity, protected or not, could possibly be impacted. For example, the Endeavor Hydrothermal Vents MPA in Canada encompasses five hydrothermal vents of interest, but is within 70 km of nearby unprotected vents. Likewise, proximity to inactive vent deposits that are also potential mining targets (Van Dover, 2019) should be considered. Therefore, it is imperative that MPAs are planned with reference to all vents in an area, including inactive deposits, and are established including a generous buffer zone, as a precautionary measure.
With increasing demand for metals to support renewable energy technologies, blanket MPAs may not be the most realistic approach for policy makers. A more measured approach may be to implement a moratorium on deep-sea mining to allow for further research into the biodiversity, ecology, connectivity, and resilience of vent communities (Glover et al., 2018; Van Dover et al., 2018). Several countries (Kakee, 2020) and, more recently, large corporations (No Deep Seabed Mining, 2021), have declared their support for a mining moratorium until sufficient advances have been made to inform environmentally sound mining legislation. The Red List assessments presented in this study provide a global overview of mining threat at hydrothermal vents and support a precautionary approach for deep-sea conservation, including the implementation of a deep-sea mining moratorium.
Whether in the form of MPAs or moratoria, we have an international obligation to protect hydrothermal vents from anthropogenic threats, and the Red List is a valuable conservation tool to help inform such policy decisions. In this case, the application of the Red List criteria to all known vent-endemic molluscs highlights the variation in mining threat across global biogeographic regions and the impact of jurisdiction status, with vent fields in mining license areas (e.g., Indian Ocean) exhibiting a greater threat level than those in protected areas (e.g., Azores MPA). The Vent Red List conveys the very real extinction risk that deep-sea mining poses to vent-endemic species to a wide audience and provides a new platform to ensure the conservation of this unique deep-sea habitat.
The original contributions presented in the study are included in the article/Supplementary Material, further inquiries can be directed to the corresponding author/s.
ET and JS conceived and designed the research. ET, AM, NH, and JS collected the data and completed IUCN Red List assessments. MB and MS facilitated and reviewed IUCN Red List assessments. ET analyzed the data and led the manuscript development. All authors contributed to writing and have approved the manuscript.
This work was partially supported by the Mohamed bin Zayed Species Conservation Fund grant (182518473). ET was supported by the studentship awarded by the Faculty of Medicine, Health and Life Sciences, Queen’s University Belfast. NH was supported by the funding from the Canada Research Chairs Program. MB was supported by the grant from the Rufford Foundation. JS was supported by the Hong Kong Branch of Southern Marine Science and Engineering Guangdong Laboratory (Guangzhou). Publication of this study was supported by the Marine Institute under the Marine Research Programme with the support of the Irish Government.
The views expressed in this paper do not necessarily reflect those of IUCN. Also, the designation of geographical entities, and the presentation of the material, do not imply the expression of any opinion whatsoever on the part of IUCN concerning the legal status of any country, territory, or area, or of its authorities, or concerning the delimitation of its frontiers or boundaries.
The authors declare that the research was conducted in the absence of any commercial or financial relationships that could be construed as a potential conflict of interest.
All claims expressed in this article are solely those of the authors and do not necessarily represent those of their affiliated organizations, or those of the publisher, the editors and the reviewers. Any product that may be evaluated in this article, or claim that may be made by its manufacturer, is not guaranteed or endorsed by the publisher.
We thank the colleagues who have supported this study, especially Chong Chen, Caroline Pollock, Sarah Helyar, and Amanda Bates. We thank the two reviewers for providing valuable comments to improve this manuscript.
The Supplementary Material for this article can be found online at: https://www.frontiersin.org/articles/10.3389/fmars.2021.713022/full#supplementary-material
Abecasis, R. C., Afonso, P., Colaço, A., Longnecker, N., Clifton, J., Schmidt, L., et al. (2015). Marine conservation in the azores: evaluating marine protected area development in a remote island context. Front. Mar. Sci. 2:104. doi: 10.3389/fmars.2015.00104
Beaulieu, S. E., and Szafranski, K. (2020). InterRidge Global Database of Active Submarine Hydrothermal Vent Fields, Version 3.4. Available online at: http://vents-data.interridge.org (accessed June 7, 2019).
Betts, J., Young, R. P., Hilton-Taylor, C., Hoffmann, M., Rodríguez, J. P., Stuart, S. N., et al. (2020). A framework for evaluating the impact of the IUCN red list of threatened species. Conserv. Biol. 34, 632–643. doi: 10.1111/cobi.13454
Calado, H., Ng, K., Lopes, C., and Paramio, L. (2011). Introducing a legal management instrument for offshore Marine protected areas in the Azores—the Azores Marine Park. Environ. Sci. Policy 14, 1175–1187. doi: 10.1016/j.envsci.2011.09.001
Carpenter, K. E., Abrar, M., Aeby, G., Aronson, R. B., Banks, S., Bruckner, A., et al. (2008). One-third of reef-building corals face elevated extinction risk from climate change and local impacts. Science 321, 560–563. doi: 10.1126/science.1159196
Chapman, A. S. A., Beaulieu, S. E., Colaço, A., Gebruk, A. V., Hilario, A., Kihara, T. C., et al. (2019). sFDvent: a global trait database for deep-sea hydrothermal-vent fauna. Glob. Ecol. Biogeogr. 28, 1538–1551. doi: 10.1111/geb.12975
Chen, C., Linse, K., Uematsu, K., and Sigwart, J. D. (2018a). Cryptic niche switching in a chemosymbiotic gastropod. Proc. R. Soc. B 285:20181099. doi: 10.1098/rspb.2018.1099
Chen, C., Marsh, L., and Copley, J. T. (2018b). Is it sex in chains? Potential mating stacks in deep-sea hydrothermal vent snails. Plankton Benthos Res. 13, 25–27. doi: 10.3800/pbr.13.25
Chong, Z. R., Yang, S. H. B., Babu, P., Linga, P., and Li, X.-S. (2016). Review of natural gas hydrates as an energy resource: prospects and challenges. Appl. Energy 162, 1633–1652. doi: 10.1016/j.apenergy.2014.12.061
Clark, M. R., Rowden, A. A., Schlacher, T., Williams, A., Consalvey, M., Stocks, K. I., et al. (2010). The ecology of seamounts: structure, function, and human impacts. Annu. Rev. Mar. Sci. 2, 253–278. doi: 10.1146/annurev-marine-120308-081109
Danovaro, R., Corinaldesi, C., Dell’Anno, A., and Snelgrove, P. V. R. (2017). The deep-sea under global change. Curr. Biol. 27, 461–465. doi: 10.1016/j.cub.2017.02.046
Edgar, G. J., Stuart-Smith, R. D., Willis, T. J., Kininmonth, S., Baker, S. C., Banks, S., et al. (2014). Global conservation outcomes depend on Marine protected areas with five key features. Nature 506, 216–220. doi: 10.1038/nature13022
Gjerde, K. M., Dotinga, H., Hart, S., Molenaar, E. J., Rayfuse, R., and Warner, R. (2008). Regulatory and Governance Gaps in the International Regime for the Conservation and Sustainable Use of Marine Biodiversity in Areas beyond National Jurisdiction. Gland: IUCN.
Glover, A. G., Wiklund, H., Chen, C., and Dahlgren, T. G. (2018). Managing a sustainable deep-sea ‘blue economy’ requires knowledge of what actually lives there. eLife 7:e41319.
Gollner, S., Kaiser, S., Menzel, L., Jones, D. O. B., Brown, A., Mestre, N. C., et al. (2017). Resilience of benthic deep-sea fauna to mining activities. Mar. Environ. Res. 129, 76–101. doi: 10.1016/j.marenvres.2017.04.010
Hein, J. R., Mizell, K., Koschinsky, A., and Conrad, T. A. (2013). Deep-ocean mineral deposits as a source of critical metals for high- and green-technology applications: comparison with land-based resources. Ore Geol. Rev. 51, 1–14. doi: 10.1016/j.oregeorev.2012.12.001
Hoagland, P., Beaulieu, S., Tivey, M. A., Eggert, R. G., German, C., Glowka, L., et al. (2010). Deep-sea mining of seafloor massive sulfides. Mar. Policy 34, 728–732. doi: 10.1016/j.marpol.2009.12.001
ISA (2021). Minerals: Cobalt-Rich Ferromanganese Crusts, International Seabed Authority. Available online at: https://www.isa.org.jm/exploration-contracts/cobalt-rich-ferromanganese (accessed July 14, 2021).
IUCN Standards and Petitions Committee (2019). Guidelines for Using the IUCN Red List Categories and Criteria. Version 14. Available online at: http://www.iucnredlist.org/documents/RedListGuidelines.pdf (accessed January 24, 2019).
IUCN (2016). Guidelines for Appropriate Uses of IUCN Red List Data. Version 3.0. Available online at: https://www.iucnredlist.org/resources/guidelines-for-appropriate-uses-of-red-list-data (accessed May 18, 2021).
IUCN (2021). The IUCN Red List of Threatened Species. Version 2021-3. Available online at: https://www.iucnredlist.org
Johnson, S. B., Warén, A., and Vrijenhoek, C. (2008). DNA barcoding of Lepetodrilus limpets reveals cryptic species. J. Shellfish Res. 27, 43–51. doi: 10.2983/0730-8000(2008)27[43:dbollr]2.0.co;2
Kakee, T. (2020). Deep-sea mining legislation in Pacific Island countries: from the perspective of public participation in approval procedures. Mar. Policy 117:103881. doi: 10.1016/j.marpol.2020.103881
Laming, S. R., Gaudron, S. M., and Duperron, S. (2018). Lifecycle ecology of deep-sea chemosymbiotic mussels: a review. Front. Mar. Sci. 5:282. doi: 10.3389/fmars.2018.00282
Luick, J. L. (2012). Physical Oceanographic Assessment of the Nautilus EIS for the Solwara 1 Project. Available online at: http://www.deepseaminingoutofourdepth.org/wp-content/uploads/EIS-Review-FINAL-low-res.pdf (accessed January 10, 2013).
Matabos, M., and Jollivet, D. (2019). Revisiting the Lepetodrilus elevatus species complex (Vetigastropoda: Lepetodrilidae), using samples from the Galápagos and Guaymas hydrothermal vent systems. J. Mollus. Stud. 85, 154–165. doi: 10.1093/mollus/eyy061
Menini, E., and Van Dover, C. L. (2019). An atlas of protected hydrothermal vents. Mar. Policy 108:103654. doi: 10.1016/j.marpol.2019.103654
Miller, K. A., Thompson, K. F., Johnston, P., and Santillo, D. (2018). An overview of seabed mining including the current state of development, environmental impacts, and knowledge gaps. Front. Mar. Sci. 4:418. doi: 10.3389/fmars.2017.00418
Niner, H. J., Ardron, J. A., Escobar, E. G., Gianni, M., Jaeckel, A., Jones, D. O. B., et al. (2018). Deep-sea mining with no net loss of biodiversity—an impossible aim. Front. Mar. Sci. 5:53. doi: 10.3389/fmars.2018.00053
No Deep Seabed Mining (2021). Business Statement Supporting a Moratorium on Deep Seabed Mining. Available online at: https://www.noseabedmining.org/ (accessed April 19, 2021).
Okamoto, N., Shiokawa, S., Kawano, S., Yamaji, N., Sakurai, H., and Kurihara, M. (2019). “First lifting test for seafloor massive sulphides in the Okinawa Trough in the EEZ of Japan,” in Paper Presented at the 29th International Ocean and Polar Engineering Conference, (Honolulu, HI).
Petersen, S., Krätschell, A., Augustin, N., Jamieson, J., Hein, J. R., and Hannington, M. D. (2016). News from the seabed – geological characteristics and resource potential of deep-sea mineral resources. Mar. Policy 70, 175–187. doi: 10.1016/j.marpol.2016.03.012
Rodrigues, A. S. L., Pilgrim, J. D., Lamoreux, J. F., Hoffmann, M., and Brooks, T. M. (2006). The value of the IUCN Red List for conservation. Trends Ecol. Evol. 21, 71–76. doi: 10.1016/j.tree.2005.10.010
Rogers, A. D., Tyler, P. A., Connelly, D. P., Copley, J. T., James, R., Larter, R. D., et al. (2012). The discovery of new deep-sea hydrothermal vent communities in the Southern Ocean and implications for biogeography. PLoS Biol. 10:e1001234. doi: 10.1371/journal.pbio.1001234
Sharma, R. (2011). Deep-sea mining: economic, technical, technological, and environmental considerations for sustainable development. Mar. Technol. Soc. J. 45, 28–41. doi: 10.4031/MTSJ.45.5.2
Sharma, R. (2015). Environmental issues of deep-sea mining. Procedia Earth Planet. Sci. 11, 204–211. doi: 10.1016/j.proeps.2015.06.026
Sigwart, J. D., Chen, C., and Marsh, L. (2017). Is mining the seabed bad for mollusks? The Nautilus 131, 43–50.
Sigwart, J. D., Chen, C., Thomas, E. A., Allcock, A. L., Böhm, M., and Seddon, M. (2019). Red listing can protect deep-sea biodiversity. Nat. Ecol. Evol. 3:1134. doi: 10.1038/s41559-019-0930-2
Simon-Lledó, E., Bett, B. J., Huvenne, V. A. I., Köser, K., Schoening, T., Greinert, J., et al. (2019). Biological effects 26 years after simulated deep-sea mining. Sci. Rep. 9:8040. doi: 10.1038/s41598-019-44492-w
Suess, E. (2018). “Marine cold seeps: background and recent advances,” in Hydrocarbons, Oils and Lipids: Diversity, Origin, Chemistry and Fate, ed. H. Wilkes (Cham: Springer International Publishing), 1–21. doi: 10.1007/978-3-319-54529-5_27-1
Sun, J., Zhou, Y., Chen, C., Kwan, Y. H., Sun, Y., Wang, X., et al. (2020). Nearest vent, dearest friend: biodiversity of Tiancheng vent field reveals cross-ridge similarities in the Indian Ocean. R. Soc. Open Sci. 7:200110. doi: 10.1098/rsos.200110
Thaler, A. D., and Amon, D. (2019). 262 voyages beneath the sea: a global assessment of macro- and megafaunal biodiversity and research effort at deep-sea hydrothermal vents. PeerJ 7:e7397. doi: 10.7717/peerj.7397
Thomas, E. A., Böhm, M., Pollock, C., Chen, C., Seddon, M., and Sigwart, J. D. (2021). Assessing the extinction risk of insular, understudied marine species. Conserv. Biol. doi: 10.1111/cobi.13854
Thompson, K. F., Miller, K. A., Currie, D., Johnston, P., and Santillo, D. (2018). Seabed mining and approaches to governance of the deep seabed. Front. Mar. Sci. 5:480. doi: 10.3389/fmars.2018.00480
Tingley, R., Macdonald, S. L., Mitchell, N. J., Woinarski, J. C. Z., Meiri, S., Bowles, P., et al. (2019). Geographic and taxonomic patterns of extinction risk in Australian squamates. Biol. Conserv. 238:108203. doi: 10.1016/j.biocon.2019.108203
Van Dover, C. (2000). The Ecology of Deep-Sea Hydrothermal Vents. Princeton, NJ: Princeton University Press.
Van Dover, C. L. (2014). Impacts of anthropogenic disturbances at deep-sea hydrothermal vent ecosystems: a review. Mar. Environ. Res. 102, 59–72. doi: 10.1016/j.marenvres.2014.03.008
Van Dover, C. L. (2019). Inactive sulfide ecosystems in the deep sea: a review. Front. Mar. Sci. 6:461. doi: 10.3389/fmars.2019.00461
Van Dover, C. L., Ardron, J. A., Escobar, E., Gianni, M., Gjerde, K. M., Jaeckel, A., et al. (2017). Biodiversity loss from deep-sea mining. Nat. Geosci. 10, 464–465. doi: 10.1038/ngeo2983
Van Dover, C. L., Arnaud-Haond, S., Gianni, M., Helmreich, S., Huber, J. A., Jaeckel, A. L., et al. (2018). Scientific rationale and international obligations for protection of active hydrothermal vent ecosystems from deep-sea mining. Mar. Policy 90, 20–28. doi: 10.1016/j.marpol.2018.01.020
Van Dover, C., Smith, C. R., Ardron, J., Arnaud-Haond, S., Beaudoin, Y., Bezaury-Creel, J., et al. (2011). Environmental Management of Deep-Sea Chemosynthetic Ecosystems: Justification of and Considerations for A Spatially Based Approach. Technical Study No 9. Kingston: International Seabed Authority.
Watling, L., and Auster, P. J. (2017). Seamounts on the high seas should be managed as vulnerable marine ecosystems. Front. Mar. Sci. 4:14. doi: 10.3389/fmars.2017.00014
Wolff, T. (2005). Composition and endemism of the deep-sea hydrothermal vent fauna. Cah. Biol. Mar. 46, 97–104. doi: 10.1007/978-3-319-45340-8_4
Keywords: deep-sea mining, IUCN Red List, hydrothermal vents, conservation, molluscs
Citation: Thomas EA, Molloy A, Hanson NB, Böhm M, Seddon M and Sigwart JD (2021) A Global Red List for Hydrothermal Vent Molluscs. Front. Mar. Sci. 8:713022. doi: 10.3389/fmars.2021.713022
Received: 21 May 2021; Accepted: 30 September 2021;
Published: 09 December 2021.
Edited by:
Greg W. Rouse, University of California, San Diego, United StatesReviewed by:
Damianos Chatzievangelou, Jacobs University Bremen, GermanyCopyright © 2021 Thomas, Molloy, Hanson, Böhm, Seddon and Sigwart. This is an open-access article distributed under the terms of the Creative Commons Attribution License (CC BY). The use, distribution or reproduction in other forums is permitted, provided the original author(s) and the copyright owner(s) are credited and that the original publication in this journal is cited, in accordance with accepted academic practice. No use, distribution or reproduction is permitted which does not comply with these terms.
*Correspondence: Elin A. Thomas, ZXRob21hczA3QHF1Yi5hYy51aw==
Disclaimer: All claims expressed in this article are solely those of the authors and do not necessarily represent those of their affiliated organizations, or those of the publisher, the editors and the reviewers. Any product that may be evaluated in this article or claim that may be made by its manufacturer is not guaranteed or endorsed by the publisher.
Research integrity at Frontiers
Learn more about the work of our research integrity team to safeguard the quality of each article we publish.