- 1Chicago Zoological Society’s Sarasota Dolphin Research Program, c/o Mote Marine Laboratory & Aquarium, Sarasota, FL, United States
- 2Mote Marine Laboratory & Aquarium, Sarasota, FL, United States
Red tide blooms caused by the toxic dinoflagellate Karenia brevis are natural disturbance events that occur regularly along Florida’s west coast, often resulting in massive fish kills and marine mammal, seabird, and sea turtle mortalities. Limited prior work on the ecological effects of red tides suggests they play an important role in structuring ecosystem dynamics and regulating communities, however specific effects on prey populations and potential alterations to predator-prey interactions are unknown. We surveyed the prey fish assemblage of a top marine predator, the common bottlenose dolphin (Tursiops truncatus), in shallow seagrass habitat in Sarasota Bay, Florida, during 2004–2019, collecting data on prey density, species composition, K. brevis cell densities, and environmental variables. Across eight distinct red tide bloom events, resistance, resilience, and the ecological effects on the prey assemblage varied depending on bloom intensity, season, and frequency. Prey assemblage structure showed significant and distinct short-term shifts during blooms independent of the normal seasonal shifts in prey structure seen during non-bloom conditions. Canonical correspondence analysis indicated a strong influence of K. brevis density on assemblage structure. Blooms occurring primarily in the summer were associated with less initial prey resistance and higher than average annual catch per unit effort (CPUE) 1–3 years following bloom cessation, with bloom frequency prolonging the time needed to reach higher than average annual CPUE. Regardless of season, recovery to pre-bloom prey abundances occurred within 1 year. Sample-based rarefaction and extrapolation indicated significant differences in prey diversity among summer bloom events. This study is a first step in identifying differences in resistance, resilience, and the ecological effects of multiple red tide bloom events of various temporal scales and intensity on a dolphin prey assemblage. Improved understanding of the influence of red tides on estuarine structural dynamics and function can better inform management, and potentially guide mitigation efforts post-bloom.
Introduction
Disturbance events are recognized as playing a crucial role in the formation and maintenance of ecological communities (Connell and Slatyer, 1977; Sousa, 1984; White and Jentsch, 2001; Callaway et al., 2002; Borer et al., 2006; Miller et al., 2011; Steudel et al., 2012; Johnke et al., 2014). Key aspects of disturbance include event type, intensity, timing, duration, spatial extent, distribution, and frequency (Pickett and White, 1985). The severity of a disturbance event depends primarily on its intensity, but varies by disturbance type and the degree of vulnerability of the species affected (Pickett and White, 1985; Miller et al., 2011). Severe disturbance events can affect community composition, resources, and food web structure through physiological stress, mortality, and a reordering of species’ dominance (Ostfeld and Keesing, 2000; Jentsch et al., 2007; Smith, 2011; Boucek and Rehage, 2014; Hoover et al., 2014). Disturbances of moderate severity have been shown to foster maximum species diversity [intermediate disturbance hypothesis (IDH); Connell, 1978] by reducing the effects of competitive dominance (Miller et al., 2011). However, chronic ecological disturbances can negatively impact environmental conditions, resulting in ecosystems with lower resilience to perturbation events, and greater risk of surpassing a tipping point between stable states (Scheffer et al., 2001; Lewis et al., 2021). Much work has focused on the dynamics of single species or guilds of species at the same trophic level, therefore the direct and indirect disturbance effects on the dynamics of populations linked by predator-prey interactions, across different trophic levels, are not as well understood (Commander and White, 2020).
Estuaries along Florida’s west coast are dynamic and highly productive natural habitats that are frequently impacted by harmful algal blooms (HABs) caused by the toxic dinoflagellate Karenia brevis (Steidinger and Ingle, 1972; Brand and Compton, 2007). Known as “red tides,” these natural disturbance events have been recorded as far back as the 1500s in Florida (Schrope, 2008), however data in recent decades suggests a changing disturbance regime, through increased frequency, duration and geographic spread (Smayda and White, 1990; Tester et al., 1991; Viviani, 1992; Tester and Steidinger, 1997; Van Dolah, 2000; Brand and Compton, 2007). K. brevis cells produce a suite of potent neurotoxins known as brevetoxins (PbTx) which cause acute neurologic symptoms in fish, birds, marine mammals, and sea turtles, including mortality (Gunter et al., 1948; Forrester et al., 1977; Baden, 1988; Bossart et al., 1998; Baden and Mende, 1982, reviewed by Landsberg, 2002; Naar et al., 2007). Previous research has documented exposure routes in wildlife (Landsberg, 2002; Flewelling et al., 2005; Naar et al., 2007; Fire, 2007), trophic transfer and vectoring of brevetoxins (Tester et al., 2000; Flewelling et al., 2005; Naar et al., 2007; Fire, 2007), and the persistence of brevetoxins in the ecosystem for up to a year post-bloom (Baden, 1988; Flewelling et al., 2005).
Two major red tide events along the west coast of Florida in 2005 and 2006 provided the most comprehensive assessment of the effects of K. brevis on fish populations and estuarine communities to date. Gannon et al. (2009) used a weight of evidence approach to show consistent patterns of decreases in fish abundance, species density, and shifts in community structure during ichthyotoxic conditions (i.e., red tide bloom conditions toxic to fish) across five different habitats in Sarasota Bay, Florida. Clupeid species (small pelagic schooling fishes) showed a positive association with K. brevis density and were the only group of fishes that did not decline in abundance during red tide conditions in 2005 and 2006. Additionally, Flaherty and Landsberg (2011) found significant declines in the annual recruitment of three important recreational sportfish species in Tampa Bay, Florida, an estuary just north of Sarasota Bay, in 2005 and 2006, despite species-specific subadult and adult abundances remaining consistent with years prior to 2005. Distinct shifts in community structure were detected during ichthyotoxic conditions in 2005 and 2006 that differed from normal seasonal patterns; however, the relationship between seasons remained cyclical. Fish communities returned to their normal seasonal patterns of community structure within 1 year following these severe blooms. Significant ecological effects were not detected in other years with documented red tide blooms. Schrandt and MacDonald (2020) attributed significant changes in community structure in Tampa Bay, Florida in 2005 and 2006 to red tide, however effects were also not detected in other years with bloom events. Walters et al. (2013) found that K. brevis toxicity was the likely cause of adult sand seatrout (Cynoscion arenarius) mortality and a reduction in subsequent spawning activity in Tampa Bay, Florida, in 2005. Indeck et al. (2015) found that ambient noise (snapping shrimp and fish chorusing) was significantly lower Tampa Bay, Florida, in 2005, compared to years in which there were no red tides. Recent ecosystem modeling suggests K. brevis blooms along the west Florida shelf can indeed impose top-down food web effects, causing trophic cascades, as well as changes in community structure and biodiversity (DiLeone and Ainsworth, 2019). These studies suggest a pattern of ecological effects on fish abundance, diversity, and/or community structure due to severe red tides; however, it is unknown how generalizable these results are to red tide blooms of differing intensity, season (timing), duration, and frequency.
In response to a recent severe bloom event in 2018–2019, the first major red tide to occur in the summer months in the Sarasota Bay area since 2006, this study evaluates the population- and community-level effects of red tide on a prey assemblage of a year-round, long-term resident bottlenose dolphin (Tursiops truncatus) community. Expanding upon work by Gannon et al. (2009), we first describe the ichthyotoxic bloom conditions associated with differential changes in prey abundance, prey diversity, and temporary shifts in prey assemblage structure from 2018 to 2019. We then take a unique and comprehensive approach to assess whether these results are consistent across multiple bloom events by examining the hypothesis that bloom severity (i.e., intensity, season, and/or frequency) is associated with differential changes in prey resistance, resilience, and temporary shifts in assemblage structure using a long-term fisheries-independent monitoring dataset compiled during 2004–2019. Resistance describes the ability of a system to remain unchanged by a disturbance event (Waide and Willig, 2012), while resilience refers to the ability of an ecosystem to recover its essential structure and function after a disturbance event (Holling, 1973; Walker et al., 1999). Both concepts are components of what determines ecosystem stability and are often quantified in relation to the normal range of variability within a community (Van Straalen, 2002). Understanding resistance, resilience, and the ecological effects of red tides, including population/community level effects and potential alterations to predator-prey interactions, across multiple bloom events can lend insight into regime patterns, better inform management, and potentially guide mitigation efforts following a severe disturbance event (Stevens et al., 2016).
Materials and Methods
Study Area
This study was conducted in the Sarasota Bay region of central west Florida, in estuarine waters from Passage Key Inlet at the southwestern edge of Tampa Bay (27.5528° N/82.7423° W) southward to Phillippi Creek (27.27096° N/82.53757° W), including the waters of Sarasota, Palma Sola, Roberts Bays, and Anna Maria Sound. K. brevis sampling to characterize the local distribution of red tide bloom events included additional areas south of Phillippi Creek, to Blackburn Bay (27.1176° N/82.4660° W), including the waters of Little Sarasota Bay.
Prey Sampling
We conducted concurrent surveys of K. brevis cell density, environmental variables (temperature, dissolved oxygen (DO), and salinity), and the density of dolphin prey fish species as part of the Chicago Zoological Society’s Sarasota Dolphin Research Program’s (SDRP) long-term seasonal fish monitoring program. Standardized SDRP fish survey methods and procedures have been detailed previously in Gannon et al. (2009), however a brief description of the methods is included below.
Data from fish monitoring surveys in seagrass habitat were used to quantify the relative abundance of a prey assemblage comprised of 10 common dolphin prey species, based on Wells et al. (2013). Assemblage species included pinfish (Lagodon rhomboides), pigfish (Orthopristis chrysoptera), scaled sardine (Harengula jaguana), Atlantic threadfin herring (Opisthonema oglinum), ladyfish (Elops saurus), mullet (Mugil spp.), sheepshead (Archosargus probatocephalus), spot (Leiostomus xanthurus), spotted seatrout (Cynoscion nebulosus), and Gulf toadfish (Opsanus beta). Shallow seagrass beds have been identified as important foraging habitat for resident dolphins in Sarasota Bay (Barros and Wells, 1998; Rossman et al., 2015), therefore sampling effort focused on seagrass habitat exclusively. Fish were caught at randomly selected seagrass habitat sites from June 1, 2004 through September 30, 2019, in the summer (June–September) and winter months (January–March) using a small purse-seine net (183 × 6.6 m, 2.5 cm stretch mesh). In response to high K. brevis cell counts (>100,000 cells l–1) in the study area, additional sampling occurred in the winter months of December in 2004 and November in 2012, and from October through December in 2018. A summary of the fish sampling chronology is detailed in Table 1. Fish were identified to species, measured, counted, and released as possible. Relative abundance data were expressed as catch per unit effort (CPUE: the number of fish caught during each standardized deployment of the purse-seine net).
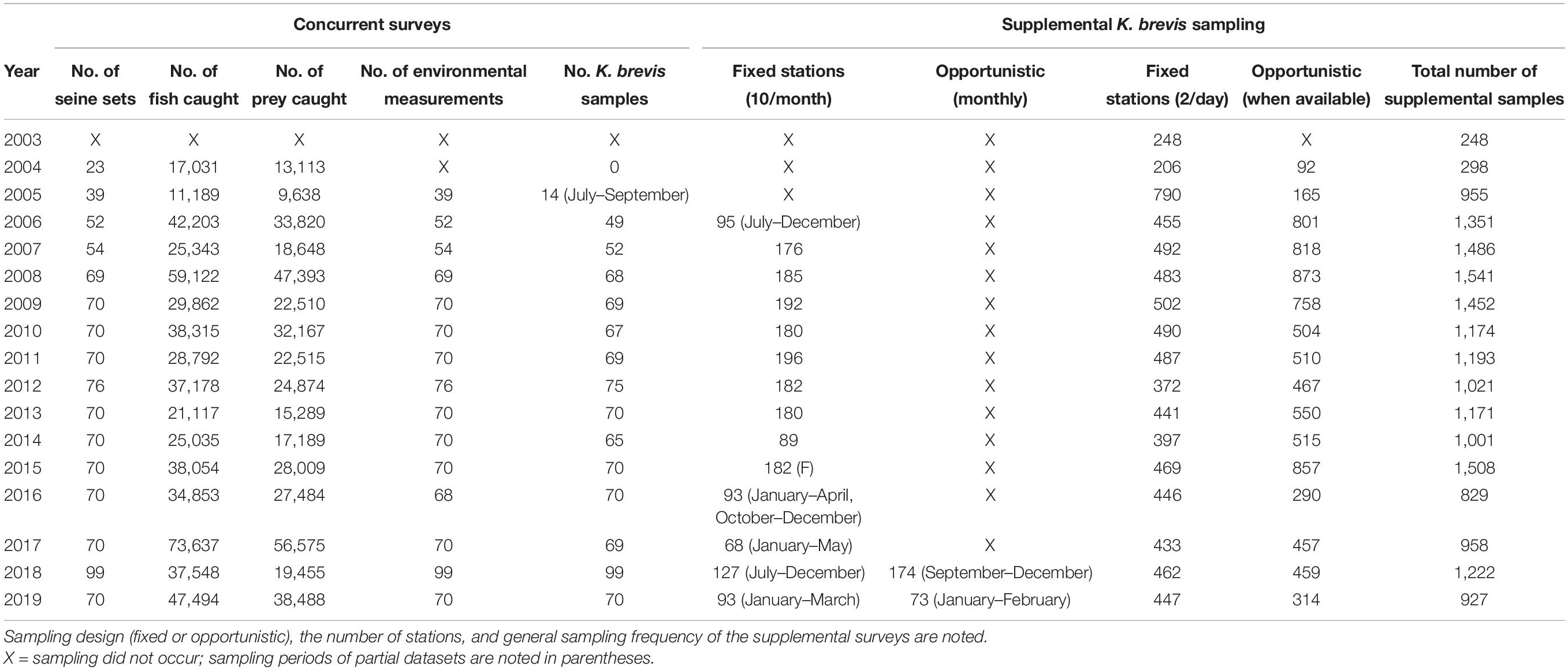
Table 1. Summary of sampling chronology and effort during, (1) concurrent random surveys of fish, environmental variable, and Karenia brevis sampling in the summer and winter months, and (2) supplemental K. brevis sampling throughout the Sarasota Bay study area during 2003–2019.
Karenia brevis Cell Counts and Environmental Variables
We used two independent sampling schemes to assess the spatial and temporal trends in K. brevis abundance. During concurrent SDRP sampling surveys, K. brevis samples were collected at every random fish sampling site, and temperature, salinity, and DO environmental variables were measured with a YSI 85 multiprobe or YSI Pro2030 approximately 15 cm above the bottom. A summary of the K. brevis and environmental variable sampling chronology is detailed in Table 1. These data were used to investigate the relationship between K. brevis density and prey abundance at fine temporal and spatial scales.
Then, in addition to the random K. brevis sampling scheme described above, all available data from several fixed and opportunistic independent sampling schemes were utilized to assess the local regime of red tide bloom events throughout the study area from 2003 through 2019, specifically, the intensity, duration, and the spatial and temporal trends in K. brevis abundance. K. brevis samples were collected on a near-monthly basis at 10 standard monitoring locations distributed throughout the study area, from 2006 through 2019. In 2018 and 2019, additional K. brevis samples were collected at each dolphin sighting location during monthly monitoring surveys. We used K. brevis samples collected year-round on a near-daily basis at two fixed monitoring sites sampled by Mote Marine Laboratory’s Phytoplankton Ecology Program (New Pass channel and City Island Seagrass Flats), from 2003 through 2019 (Figure 1). Opportunistic sites sampled by Mote Marine Laboratory’s Phytoplankton Ecology and Chemical Ecology Programs were included when available within the study area. While fish sampling did not commence until 2004, we include K. brevis data from 2003 to give insight into the dynamics of the fish community in 2004. These data were used to investigate temporal trends at a larger scale, to characterize discrete bloom events over the 17-year sampling period, and to determine if blooms occurred outside of the summer and winter fish sampling seasons. Water sample collection and cell counting procedures were identical for all sampling schemes and followed standard protocols (see Lund et al., 1958; Sournia, 1978; Sellner et al., 2003).
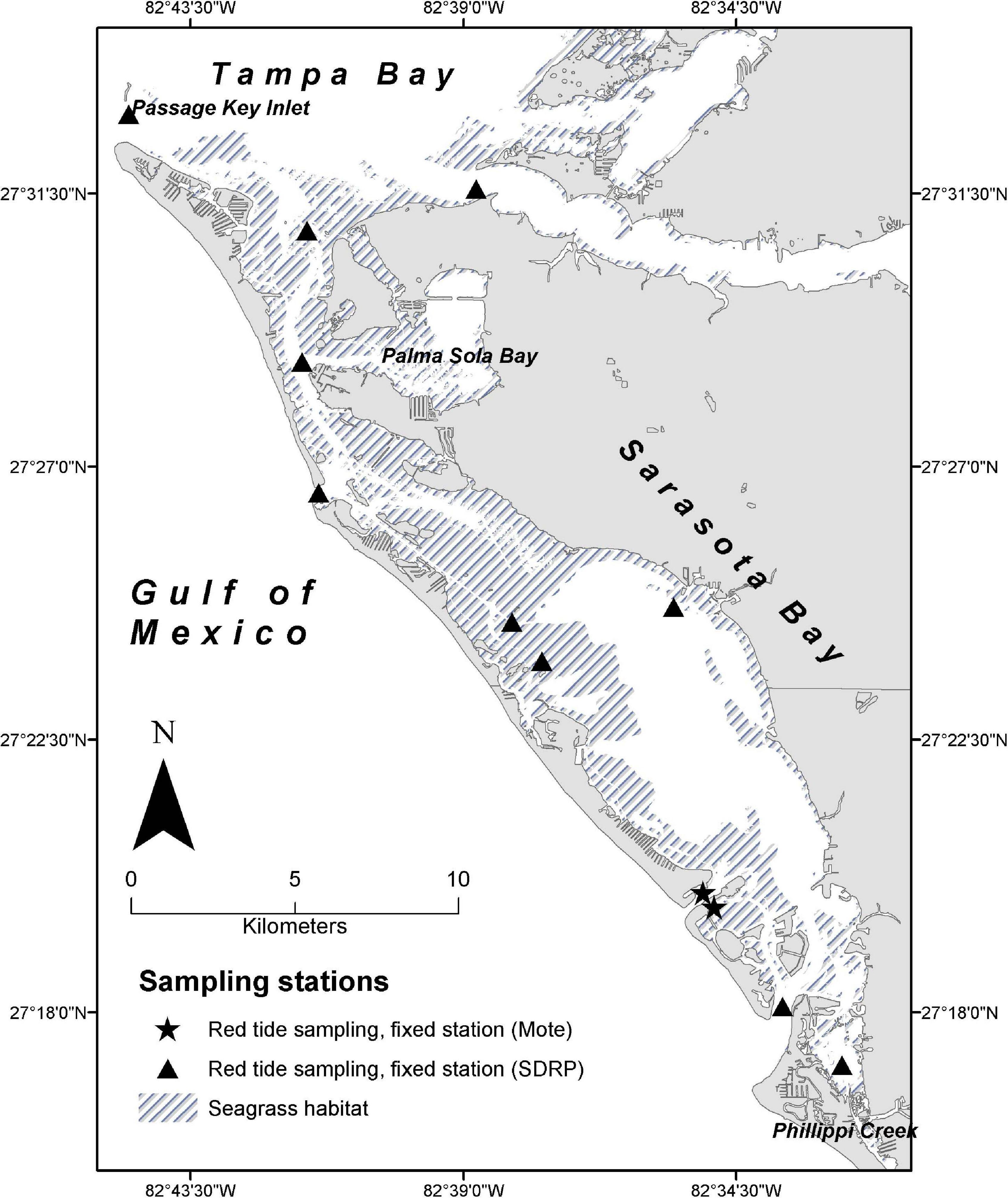
Figure 1. Study area showing locations seagrass habitat and of daily and monthly Karenia brevis fixed monitoring stations sampled by Mote Marine Laboratory (New Pass and City Island Grass Flats) and the Chicago Zoological Society’s Sarasota Dolphin Research Program (SDRP), respectively.
Data Analyses
We first examined prey abundance, species density, diversity, and assemblage structure across the 2018–2019 red tide event to expand upon Gannon et al. (2009), using similar methodology and analyses. Then, to assess whether these response patterns were consistent and generalizable across multiple bloom events, we examined the relationship between bloom severity (i.e., intensity, season, and/or frequency) and differential changes in prey resistance, resilience, and shifts in assemblage structure across the full 17-year sampling period. An overview and summary of the data analyses are detailed in Supplementary Table 1.
Designation of Bloom Periods and Non-bloom Periods
To account for the patchy nature of red tide blooms, fish sampling periods were designated as “red tide” or “non-red tide” based on K. brevis cell counts measured at fish sampling stations only. Sampling periods prior to July 7, 2005 were designated based on K. brevis cell counts from fixed K. brevis sampling stations located throughout the study area. Red tide conditions were defined as occurring during the period when a cell count at a fish sampling station exceeded 100,000 cells l–1 and continued for 30 days beyond the time at which cell counts dropped back down below 100,000 cells l–1. This threshold was chosen based on the cell concentration level typically required for fish kills (Quick and Henderson, 1975; Landsberg and Steidinger, 1998) and has been used extensively in previous work (Gannon et al., 2009; Flaherty and Landsberg, 2011). Following Gannon et al. (2009), a 30-day lag period was incorporated as a conservative approach to account for some lingering effects of red tide on prey assemblage structure. We acknowledge that this approach likely underestimates the full effective duration of each bloom disturbance, as brevetoxins are known to persist in the food web for up to a year post-bloom (Naar et al., 2007). These designations were used to assess the 2018–2019 bloom event and to assess shifts in prey assemblage structure across the 17-year dataset.
2018–2019 Red Tide Bloom Event
Karenia brevis Cell Densities
To quantify changes in abundance, species density, diversity, and structure of the dolphin prey assemblage during the 2018–2019 red tide event, monthly and seasonal K. brevis cell counts were summarized and plotted across time. K. brevis samples collected from April through September were considered summer samples. Samples collected from October to March were considered winter samples in efforts to gauge the effects of the preceding winter season, as seasonal seagrass senescence and loss generally occurs in late September and/or October and many fish species move offshore to spawn during the fall months.
Prey Abundance, Species Density, Diversity, and Community Structure
Catch per unit effort, species density, and Shannon diversity indices (Shannon, 1948) of the prey assemblage were calculated during non-red tide and red tide periods in the summer and winter months. Total and species-specific CPUEs during non-red tide periods were compared to those during red tide periods, using Welsh’s t-tests and non-parametric Mann-Whitney U tests, respectively. Differences between red tide and non-red tide periods regarding species density (the number of species caught per standardized seine set) were assessed using Mann-Whitney U tests. We chose Hutcheson’s two-sample t-tests (Hutcheson, 1970) to evaluate differences in Shannon diversity indices between red tide and non-red tide periods, as a direct comparison with past analyses from Gannon et al. (2009).
Differences in prey assemblage structure related to month, season, and bloom period were analyzed using non-metric multidimensional scaling (NMDS). NMDS is an indirect gradient analysis approach which produces an ordination based on a distance or dissimilarity matrix, by maximizing the rank order correlation; as such the method does not assume linear relationships or a unimodal response. CPUE data were fourth-root transformed to reduce the influence of extremely abundant taxa on subsequent analyses and to allow mid-range and rare species to contribute to the calculation of similarity (Clarke and Warwick, 2001). Data were averaged over month, season, and bloom period (non-bloom or bloom conditions) and an NMDS ordination was constructed using a Bray-Curtis distance matrix. We then used a permutation-based multivariate analog of Levene’s test for homogeneity of variances (Anderson, 2006) to check for homogeneity of dispersions among bloom conditions and seasons, followed by a two-way crossed permutational multivariate analysis of variance (PERMANOVA) at α = 0.05, with bloom condition and season as fixed effects, to test for significant differences in prey assemblage structure. PERMANOVA allows the use of dissimilarity measures to test for significance of specific effects using a permutation test that does not require the data to follow a particular distribution (Anderson et al., 2008). Canonical correspondence analysis (CCA) was chosen to evaluate patterns of association between prey assemblage structure and environmental gradients (temperature, DO, salinity, and K. brevis cell densities measured in situ). CCA is a constrained ordination technique that extracts major gradients among combinations of explanatory variables in a dataset. These analyses examine the effects of a K. brevis red tide on fish abundance, species density, Shannon diversity, and community structure using similar methodology as Gannon et al. (2009).
Multi-Event Response Patterns
Karenia brevis Cell Densities
Bloom intensity, duration, and the spatial and temporal trends of multiple red tide events were summarized across the study area from 2003 through 2019, based on all available K. brevis cell counts and additional bloom period criteria. Categorizing blooms as distinct events across the entire study area can be difficult when blooms move, die-off, reappear multiple times across many months, or when a single cell count occurs above the 100,000 l–1 threshold but at no other location or time thereafter. For these reasons, a distinct red tide bloom event had to have at least two consecutive weeks of K. brevis cell counts above the 100,000 l–1 threshold for ichthyotoxic conditions anywhere in the study area, in addition to the previously described bloom period criteria of commencing when at least one K. brevis cell count exceeded the 100,000 l–1 threshold and persisting for 30 days beyond the time at which cell counts dropped back down below 100,000 cells l–1.
Resistance
Prey resistance, resilience, and assemblage structure across multiple red tide bloom events were examined from 2004 through 2019, as prey sampling did not begin until 2004. Previous work by Gannon et al. (2009) and analysis of species-specific CPUEs in this study (see section “Results”) indicated that the abundance of clupeids (scaled sardines and Atlantic threadfin herring) increased during red tide bloom periods, a trend opposite that of non-clupeid species in the prey assemblage. For this reason and because prey assemblage CPUEs were species-aggregated, clupeids were excluded from analyses of prey resistance and resilience. Following Stevens et al. (2016), resistance of the prey assemblage to red tide bloom events was analyzed by plotting the log-transformed annual CPUE (mean and SE) of the prey assemblage over time.
Resilience
To analyze resilience and prey population recovery post-bloom, each sample was standardized to the mean CPUE in years in which there were no summer red tide events in the study area (2004, 2007–2017, 2019; standardized CPUE = [CPUE/non-red tide event average]). Analysis of resistance indicated that winter red tides had little or no effect on CPUE, therefore CPUEs in years in which winter red tide events occurred were included in the resilience CPUE standardization procedure (see section “Results”). Prey assemblage responses to red tide bloom events were determined by comparing standardized CPUEs between the years with no summer blooms (referred to as non-red tide years) (2004, 2007–2017, 2019) to samples collected during each year with a bloom event and to those collected 1 year following a bloom event. Differences were determined by calculating 95% confidence intervals (CIs) for non-red tide and post-bloom event means from a bootstrapped distribution with 1000 iterations. If CIs of the non-red tide years and the red tide event standardized CPUEs overlapped, the prey assemblage was considered resistant to the red tide event. Recovery was determined as the number of years needed for the CIs around the standardized CPUE to overlap with their respective pre-event CI.
Community Structure
Differences in prey assemblage structure related to year, season, and bloom period (based on K. brevis cell counts measured at fish sampling stations only) from 2004 through 2019 were analyzed using NMDS and PERMANOVA, following tests for homogeneity of dispersions among bloom conditions and seasons. Canonical analysis of principal coordinates (CAP; Anderson and Willis, 2003) ordination was used to aid our interpretation of the PERMANOVA analyses, specifically the bloom condition:season interaction effect. CAP is a form of canonical discriminant analysis, based on any distance measure, that uses principle coordinate axes to search for the vectors in multivariate space that maximize the differences among a priori defined groups (Anderson and Willis, 2003). If significant seasonal or red tide bloom event patterns in prey assemblage structure were determined by PERMANOVA, we used one-way similarity percentage (SIMPER, Clarke, 1993) analysis to determine which prey species contributed most to differences in assemblage structure between red tide conditions and non-red tide conditions within each season. Based on the decomposition of Bray-Curtis dissimilarity index, SIMPER analysis performs pairwise comparisons of groups of sampling units to find the average contribution of each species to the average overall Bray-Curtis dissimilarity. CCA ordination analysis was used to evaluate patterns of association between prey assemblage structure and environmental gradients (temperature, DO, salinity, and K. brevis cell densities measured in situ).
Bloom Severity–Species Richness and Diversity
Analysis of prey resistance, resilience, and assemblage structure in this study indicated that red tide blooms may have greater effects on dolphin prey populations in the summer months (see section “Results”). Therefore, to assess differences in summer bloom severity independently of prey abundance, we used sample-based and coverage-based rarefaction and extrapolation. Sample coverage during each summer bloom period was assessed to validate each comparison of species richness and diversity (Chao and Jost, 2012). Hill’s numbers were chosen because they have been shown to have numerous advantages over other diversity indices (Chao et al., 2014). Hill’s numbers (qD) were calculated to indicate the effective number of species for different orders of q (q = 0, 1, 2; Jost, 2006). 0D is species richness, where the number of species is not sensitive to species abundance, and therefore gives a disproportionate weight to rare species. 1D is the exponential of Shannon entropy, which weights each species according to its proportional abundance in the sample, and therefore it can be interpreted as representing common species. 2D can be interpreted as number of dominant species (Jost, 2006; Díaz-García et al., 2017). All extrapolation curves were plotted using a doubling in sample size (Chao and Jost, 2012). Differences in species richness and diversity between bloom events were determined by calculating 95% CIs for the Hill’s numbers using a bootstrap method with 1000 replications (Chao and Jost, 2012). Significant differences were considered to exist if the 95% CIs did not overlap.
For analyses in which samples could be categorized by red tide or non-red tide conditions (i.e., Welsh’s t-tests, Mann-Whitney U tests, NMDS, PERMANOVA, CAP, and SIMPER), we were able to include all data collected during 2018–2019, and during 2004–2019 for multi-event analyses. For CCA, which require a full suite of data (K. brevis, environmental variables, and fish abundance) for every sample, only data collected from 2018 through 2019 and from 2005 through 2019 for multi-event analyses were included. Prior to analysis, CPUE and K. brevis cell count data were transformed using ln(x + 1). Hutcheson’s two-sample t-tests to evaluate differences in Shannon diversity indices between bloom conditions during 2018–2019 were completed in Past 3.10 (Hammer et al., 2001). All other analyses were completed in R (R Core Team, 2020), with the package Vegan (functions “metaMDS” for NMDS; “betadisper,” “permutest,” and “adonis2” for PERMANOVA; and “capscale” for CAP; Oksanen et al., 2019) to analyze community structure, and the package iNEXT (Chao et al., 2014; Hsieh et al., 2020) to compare species richness and diversity. Figures were produced using the package ggplot2 (Wickham, 2009).
Results
2018–2019 Red Tide Bloom Event
Karenia brevis Cell Densities
Sampling effort is detailed in Table 1. Red tide cell concentrations exceeded 100,000 cells l–1 in the study area from June 7 to June 21, 2018, and then dropped down to background levels throughout July (Figure 2). On August 1, cell concentrations spiked to more than 3 million cells l–1, with maximum concentrations of up to 90 million cells l–1 on August 13. Counts continued to fluctuate until December 7 with counts as low as zero and as high as 82.8 million cells l–1. Cell concentrations then dropped to fewer than 24,000 cells l–1 through much of December only to rise to 122,500 cells l–1 on January 2, 2019, and up to 2.12 million cells l–1 on January 3. Concentrations fluctuated for a brief period but dropped below 100,000 cells l–1 on January 10. Background levels persisted in the study area through January 16.
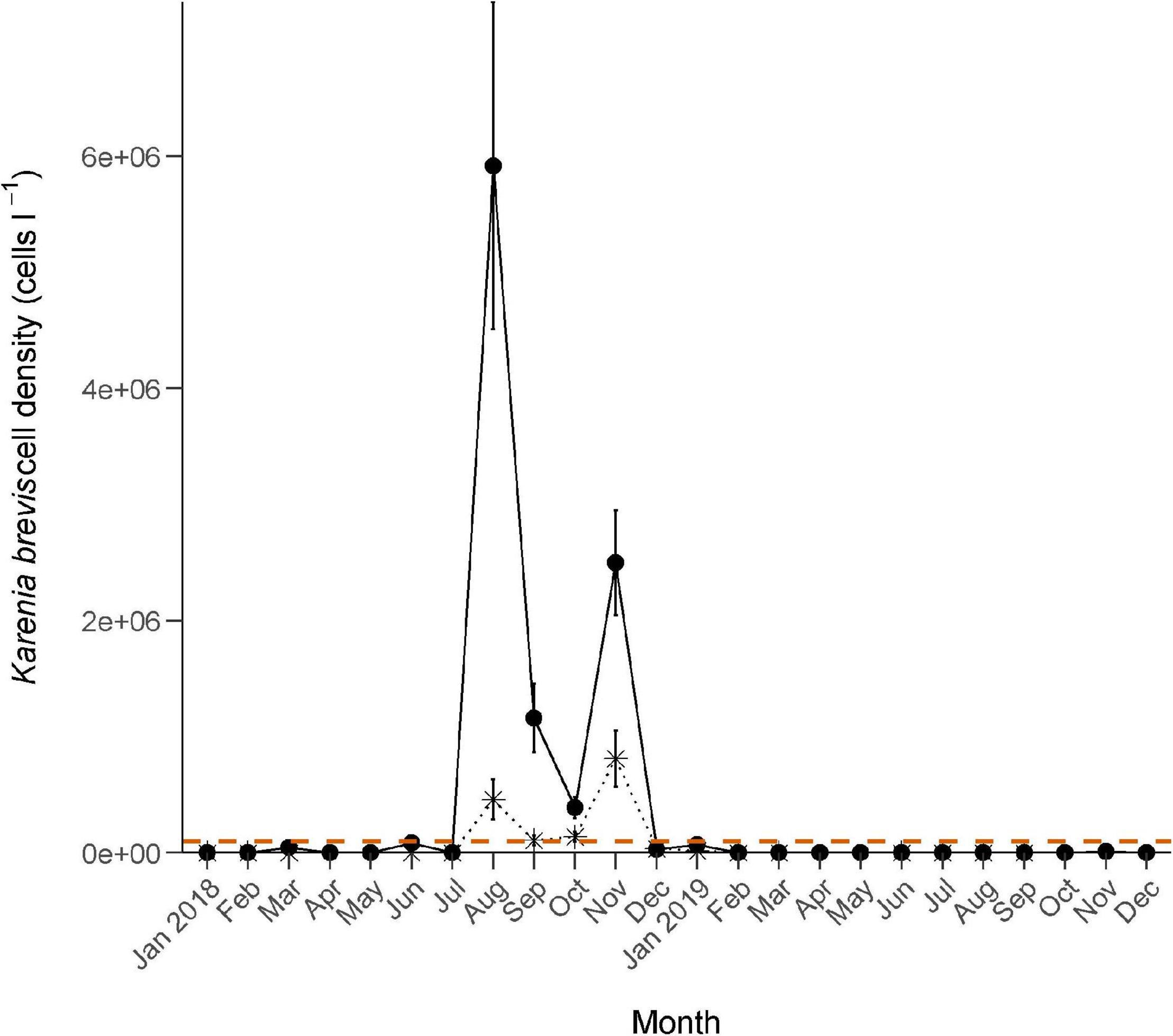
Figure 2. Monthly Karenia brevis cell densities (mean ± se) sampled from January 1, 2018–December 31, 2019. Symbols: • = samples collected anywhere in the Sarasota Bay study area; ✴ = samples collected at fish sampling stations; horizontal dashed line = 100,000 cells l–1 threshold for ichthyotoxic conditions.
At fish sampling stations, cell concentrations did not exceed 100,000 cells l–1 until August 13, 2018 (Figure 2). Counts fluctuated thereafter, with maximum concentrations of up to 2.28 million cells l–1, until December 7. A maximum cell concentration of 90,000 cells l–1 was recorded on January 9, 2019, with concentrations dropping down to background levels or lower (≤4,000 cells l–1) from January 11 to March 31.
The study area was under red tide conditions for 67% of the days between April 1, 2018 and March 31, 2019, 63% of days in the summer months (April–September) and 72% in the winter months (October–March). Based on cell concentrations taken at fish sampling stations, August 13, 2018 through January 7, 2019, were considered “red tide periods”; June 1, 2018 through August 12, 2018, and January 8, 2019 through March 31, 2019 were considered “non-red tide periods.” Mean ± SD of K. brevis cell counts at fish sampling stations were 311,428 ± 72,888 cells l–1 during red tide conditions and 4,466 ± 3,237 cells l–1 during non-red tide conditions.
Prey Abundance
From June 21, 2018 to March 15, 2019 we captured 38,801 fishes from 74 species in 99 purse-seine sets, including 29,995 from the prey assemblage. Seasonally, we captured 20,896 fishes, 16,589 prey fishes, and 66 species in 40 sets in the summer months, and 17,905 fishes, 13,406 prey fishes, and 47 species in 59 sets in the winter months. Prey CPUEs in June and July of 2018 (CPUE = 412.3, SD = 294.0, Median = 352, min = 56, max = 992) were generally comparable to CPUEs during the same months from 2004 through 2019 (CPUE = 505.9, SD = 506.0, Median = 381.0, min = 255, max = 868). In August 2018 CPUE decreased by 66.3%, coinciding with K. brevis cell densities exceeding ichthyotoxic threshold levels at fish sampling sites and directly following an August 1, 2018 spike in K. brevis concentrations within the study area. With clupeid species (Atlantic threadfin herring and scaled sardines) excluded from the analyses, CPUE decreased by 72.3%. Regardless of whether or not clupeid species were included in the analyses, CPUE was significantly lower during summer red tide bloom conditions (August/September) than during non-red tide bloom conditions (June/July) [prey assemblage: t(24) = 3.4623, p = 0.0020; prey assemblage excluding clupeids: t(26.7) = 5.3959, p < 0.0000]. Five species-specific CPUEs (ladyfish, mullet, pigfish, pinfish, and spotted seatrout) were significantly lower during bloom conditions in the summer months (Figure 3A).
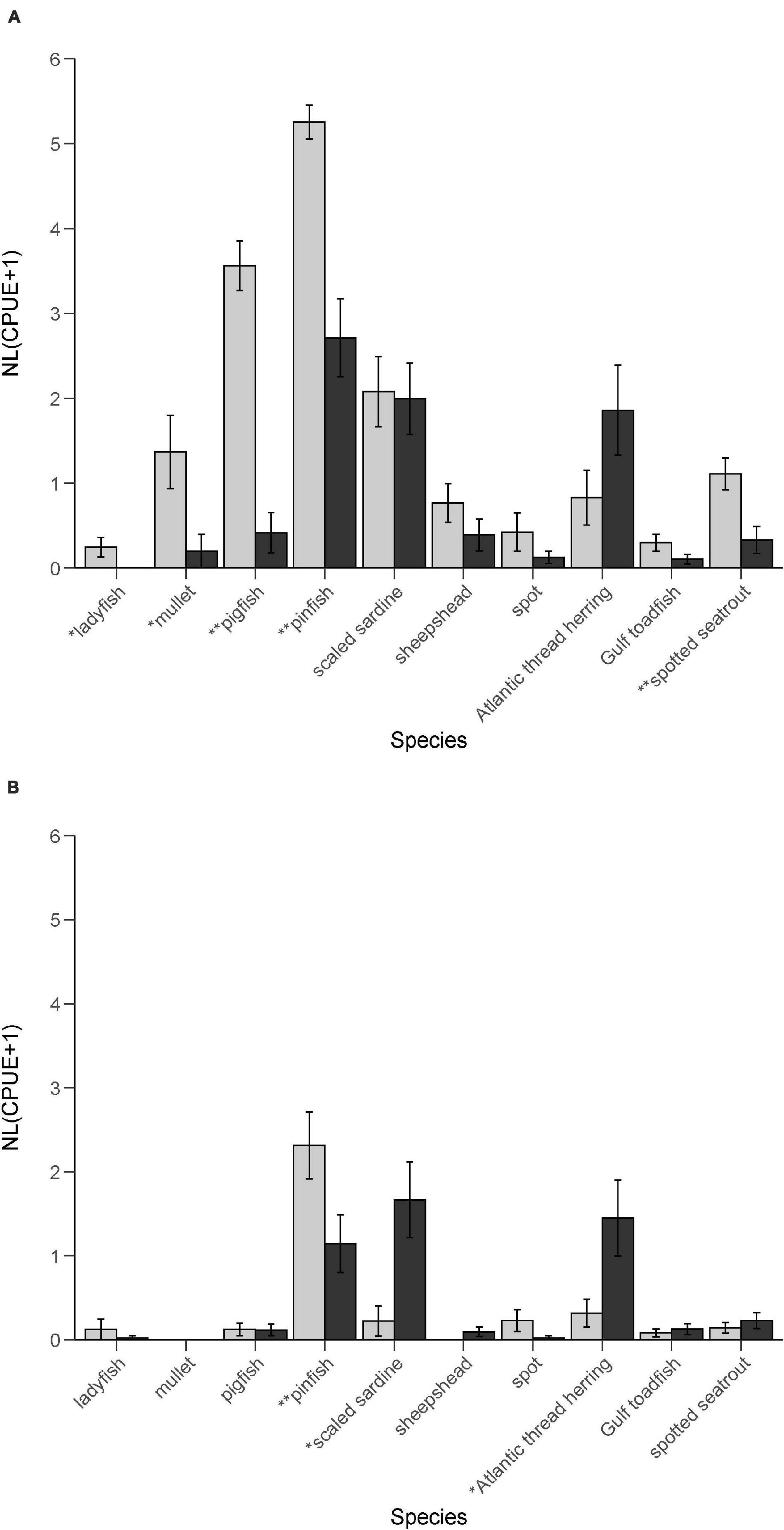
Figure 3. Seasonal catch per unit effort (CPUE ± se) of bottlenose dolphin prey species caught in seagrass habitat during non-bloom (gray bars) and bloom (black bars) conditions, from (A) June–September of 2018 (summer) and from (B) October 2018–March 2019 (winter). *Mann-Whitney U, p < 0.05; **Mann-Whitney U, p < 0.01.
The CPUE of most prey species decreased or remained at low levels without trend through December 2018, as K. brevis concentrations from fish sampling stations and others throughout the study area consistently exceeded ichthyotoxic threshold levels. Clupeid CPUE increased 54.6% in October 2018 before decreasing each month through December 2018. K. brevis cell densities dropped below threshold levels at fish sampling sites in January 2019. CPUE increased 568.1% from January to February, 2019 and 49.1% from February to March 2019. Overall, the increase in clupeid CPUE negated statistically significant changes in CPUE during winter red tide bloom conditions (October/December) compared to non-red tide bloom conditions (January/March) [t(54) = −0.4273, p = 0.6708]. When clupeids were excluded from the analyses, CPUE increased significantly during winter non-red tide bloom conditions [t(56) = 2.0752, p = 0.0425]. Three species-specific CPUEs changed significantly between bloom periods (Figure 3B). Pinfish CPUE was significantly lower during bloom conditions, while scaled sardine and Atlantic thread herring CPUEs were significantly higher.
Species Density and Diversity
Species density was significantly lower during summer bloom conditions (U = 334, n1 = n2 = 20, Median1 = 5.5, Median2 = 3.0, p = 0.0002), however, no significant changes were found between bloom conditions in the winter (U = 431, Median1 = Median2 = 1.0, n1 = 29, n2 = 30, p = 0.9558). Shannon diversity indices were significantly lower during summer red tide bloom conditions [t(16385) = 20.433, p < 0.0001], but significantly higher during winter bloom conditions [t(3962.9) = 16.33, p < 0.0001].
Community Structure
Non-metric multidimensional scaling analysis effectively summarized prey assemblage structure in two dimensions (stress = 0.0361, random restarts = 100, Figure 4) and showed distinct shifts across the 2018–2019 red tide event, mainly by season and bloom period. Samples from summer non-red tide periods were tightly grouped together in the mid left portion of the graphic, resulting in a convex hull that did not overlap with any other group. Summer non-red tide samples were characterized by higher abundances of mullet, spot, pigfish, and ladyfish. Summer red tide samples grouped together in the lower middle portion of the graphic and were most closely associated with higher abundances of Atlantic threadfin herring and scaled sardines. Samples from winter non-red tide periods grouped in the upper mid portion of the graphic, resulting in a convex hull that overlapped slightly with the winter red tide group. Winter red tide samples comprised the most dispersed group, occupying a relatively large portion of the middle and right side of the graphic.
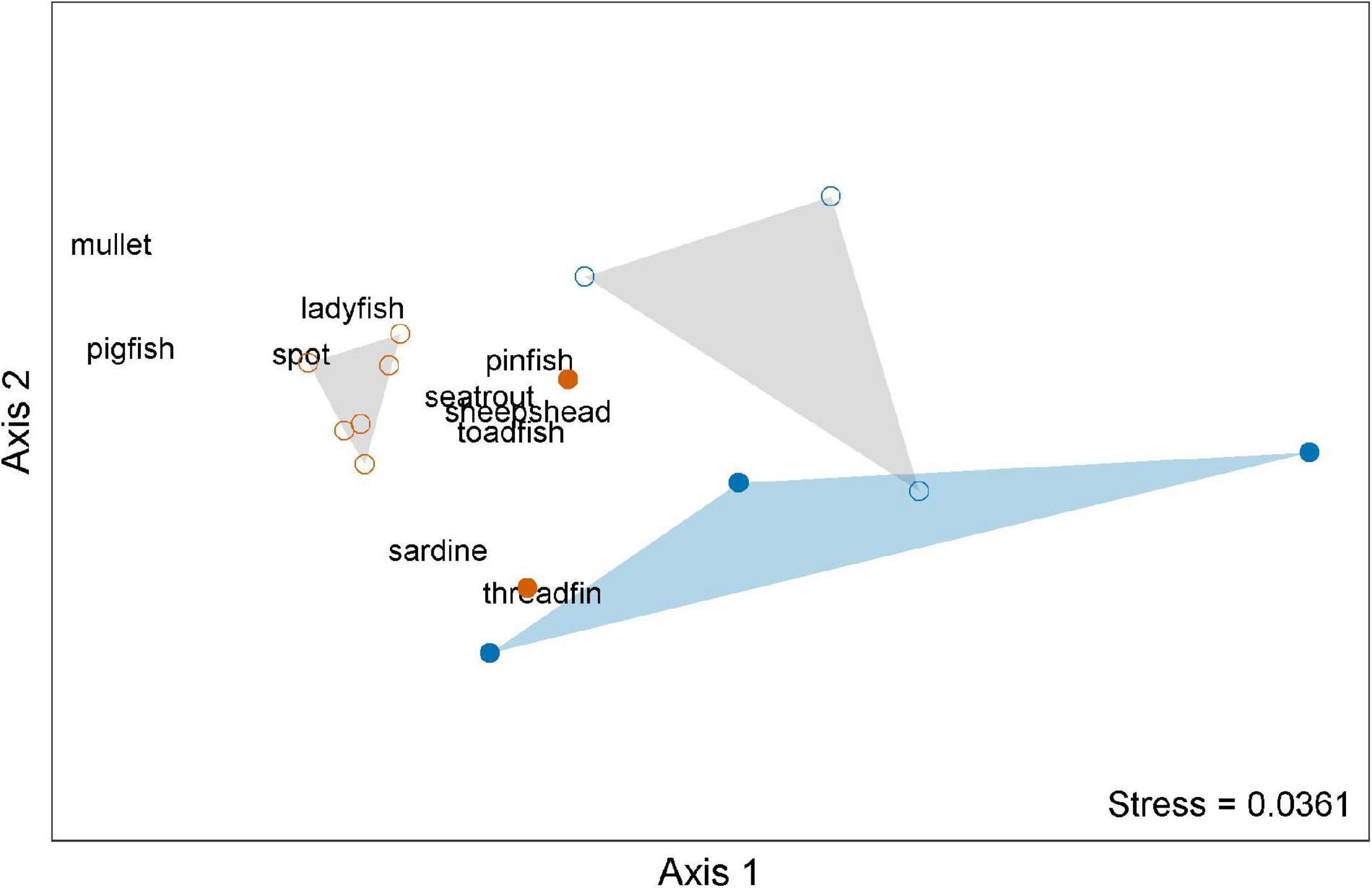
Figure 4. Non-metric multidimensional scaling (NMDS) ordination of prey assemblage structure based on month, season, and bloom condition during red tide events from June 2018 through September 2019. Symbols: = summer red tide conditions;
= summer non-red tide conditions, gray convex hull;
= winter red tide conditions, blue convex hull;
= winter non-red tide conditions, gray convex hull.
Multivariate homogeneity of group dispersion analysis indicated no significant differences in dispersion between bloom periods. However, significant differences in dispersion were found between seasons, indicating greater variation in prey composition in winter than in summer (Table 2). PERMANOVA analysis revealed significant effects of bloom condition and season on prey assemblage composition.
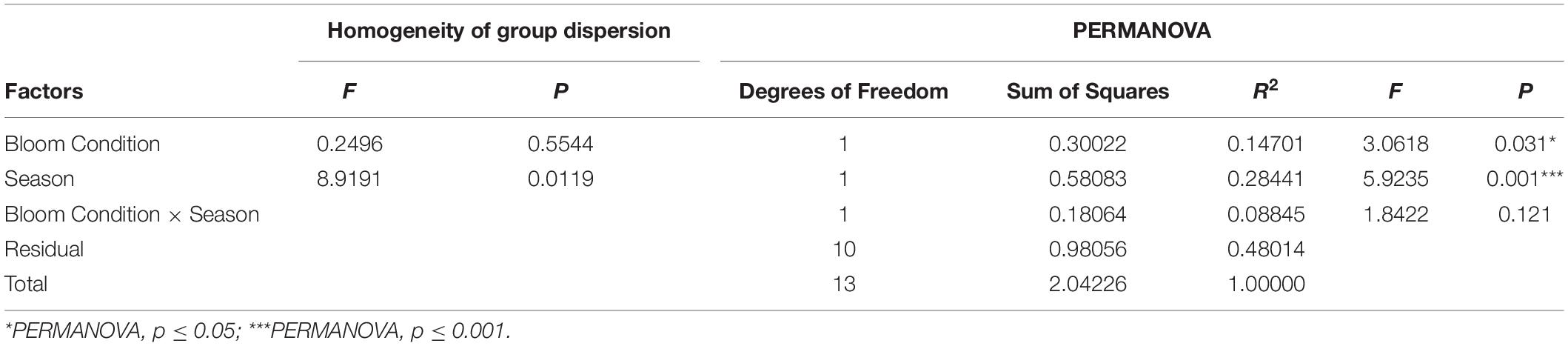
Table 2. Homogeneity of group dispersion and permutational multivariate analysis of variance (PERMANOVA) results of prey community structure in the Sarasota Bay study area from June 2018 to March 2019, in relation to bloom condition and season.
Direct gradient analysis by CCA ordination of the relationships of prey assemblage structure to environmental variables (temperature, DO, and salinity) and K. brevis density at each fish sampling location resulted in first and second axes that explained 21.7 and 14.7% of the variation in prey data, respectively. Biplots indicated a strong association between positive scores on the first axis and salinity, and less strong associations with temperature (positive scores), K. brevis (negative scores), and DO (negative scores) (Figure 5). The second axis was primarily associated with temperature and secondarily with K. brevis and DO. Samples in the summer and winter months of 2018 segregated from each other along the K. brevis cell count gradient, with the K. brevis vector terminating nearest the clupeid species, Atlantic thread herring and scaled sardines.
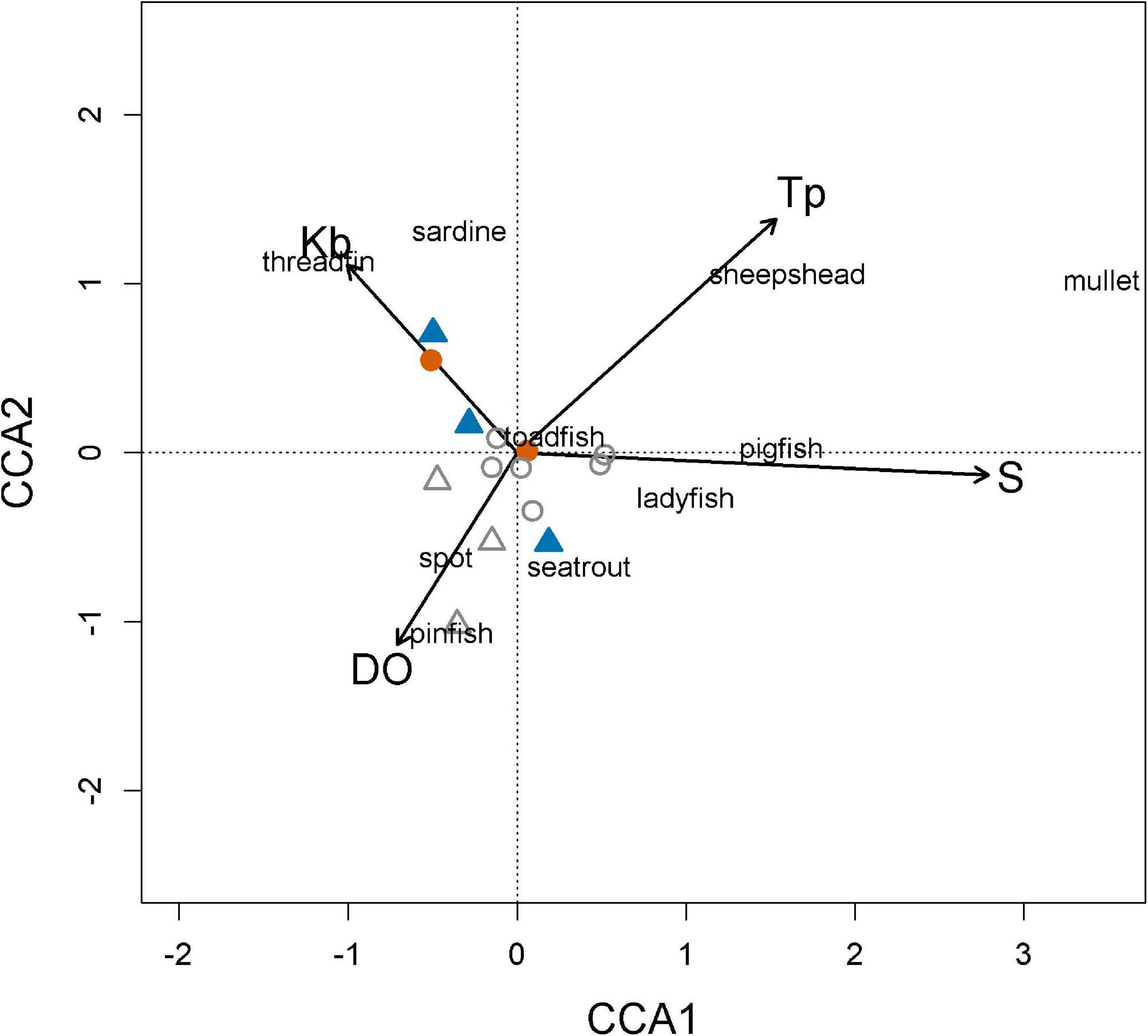
Figure 5. Canonical correspondence analysis (CCA) of the prey assemblage from purse seine catches, from June 2018 to March 2019. Symbols: = summer red tide conditions, (August–September);
= summer non-red tide conditions, (June–July);
= winter red tide conditions, (October–December.); Δ = winter non-red tide conditions, (January–March); environmental vector codes: DO: dissolved oxygen (mg l–1); Tp: temperature (°C); S: salinity (ppt); Kb: Karenia brevis cell density (cells l–1).
Multi-Event Response Patterns
Karenia brevis Cell Densities
Since 2003, eight distinct red tide blooms have affected the Sarasota Bay study area, spanning from 9 to 39 weeks (includes a 30-day lag period following the time at which cell counts dropped below 100,000 cells l–1), with 5–25 consecutive weeks of cell concentrations exceeding 100,000 cells l–1 (Table 3). Most extended blooms crossed multiple seasons; however, based on cell concentrations, severity was not necessarily ubiquitous across seasons within the same year. Overall, one severe bloom occurred primarily in the summer months (2003), four occurred in the winter months (2004, 2013, 2016, and 2017), and three encompassed significant portions of both the summer and winter months (2005–2006, 2006–2007, and 2018–2019). When cell concentrations were analyzed at just the fish sampling stations, one distinct summer bloom emerged (2005–2006), one winter bloom (2016), and one bloom spanning both seasons (2018) (Figure 6).
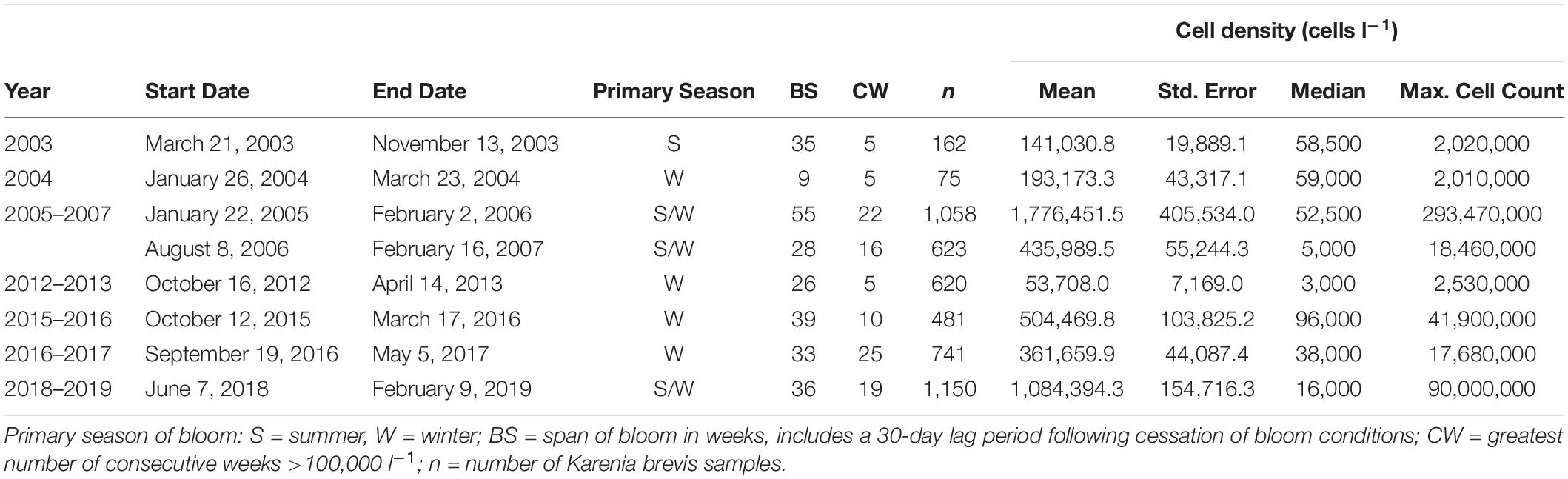
Table 3. Duration and intensity of red tide blooms affecting the Sarasota Bay study area during 2003–2019.
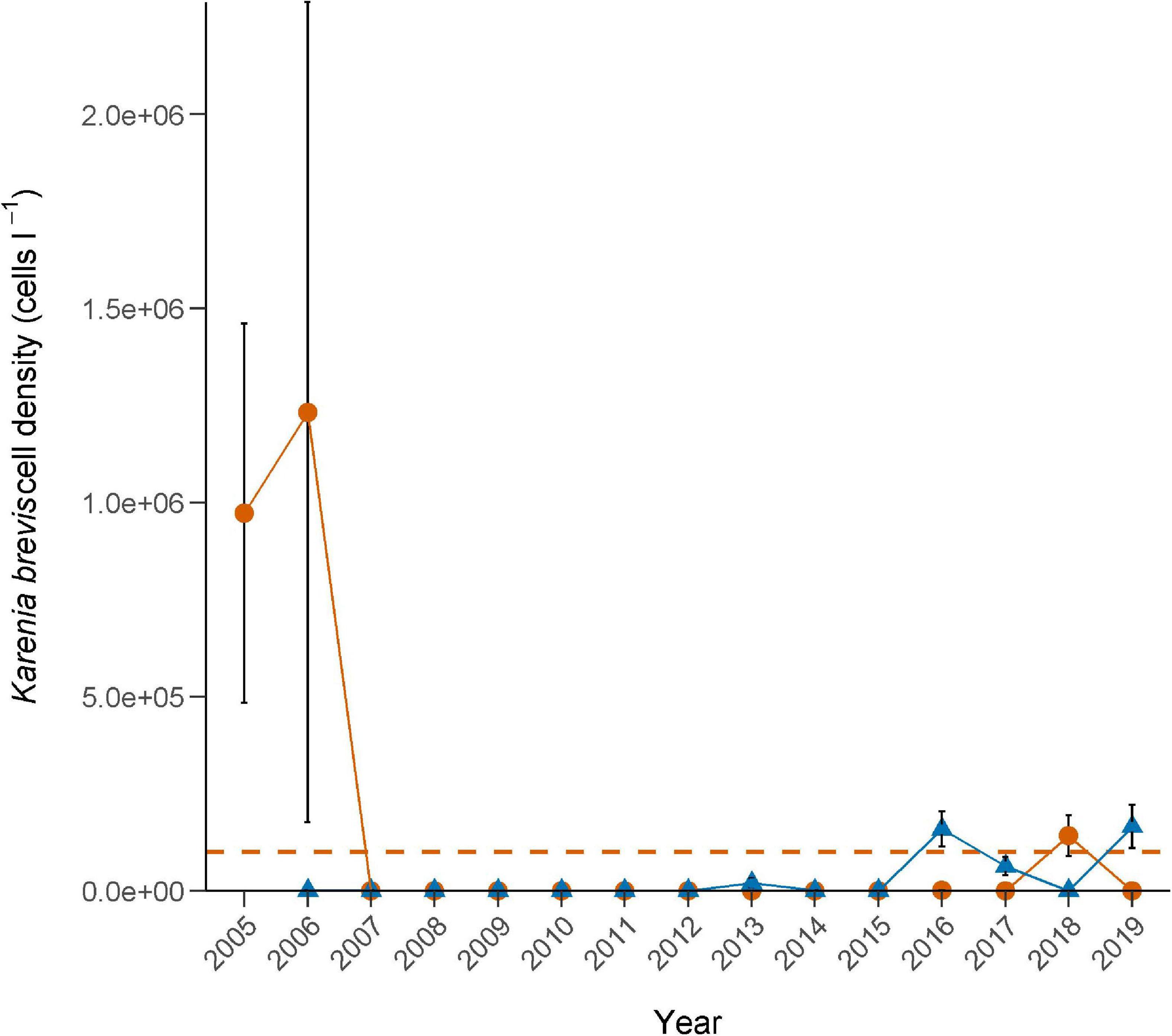
Figure 6. Annual Karenia brevis cell densities (mean ± se) measured at each fish sampling station in the Sarasota Bay study area during 2005–2019. Symbols: = summer months, (April–September);
= preceding winter months, (October–March). Horizontal dashed line = 100,000 cells l–1 threshold for ichthyotoxic conditions.
Resistance
In Sarasota Bay, the initial effects of each bloom event on the CPUE of the eight-species dolphin prey assemblage (excluding Clupeidae spp.) varied by season (Figure 7). Prey assemblage CPUE decreased to all-time or near all-time lows in years with severe summer red tide events (2005/2006 and 2018), and two of the three summer bloom events (2005 and 2006) were preceded by moderate to severe red tide events in the winter months (Table 2). Specifically, standardized CPUEs decreased by 62.9% in 2005 and by 58.6% in 2018, compared to each previous year’s CPUE (Figure 8). CPUE increased from 2005 to 2006 (+76.4%) despite a continued but intermittent bloom; however, the 2006 CPUE showed a decrease of 34.7% when compared to the closest previous summer non-bloom year (2004). In 2005 and 2018, the CI between the non-bloom CPUE and the annual CPUE did not overlap, indicating a clear lack of resistance during years with severe and prolonged summer blooms. The 2006 CI did overlap with the non-bloom CPUE; however, non-bloom conditions occurred from February 2, 2006 through August 8, 2006 making detection of resistance problematic.
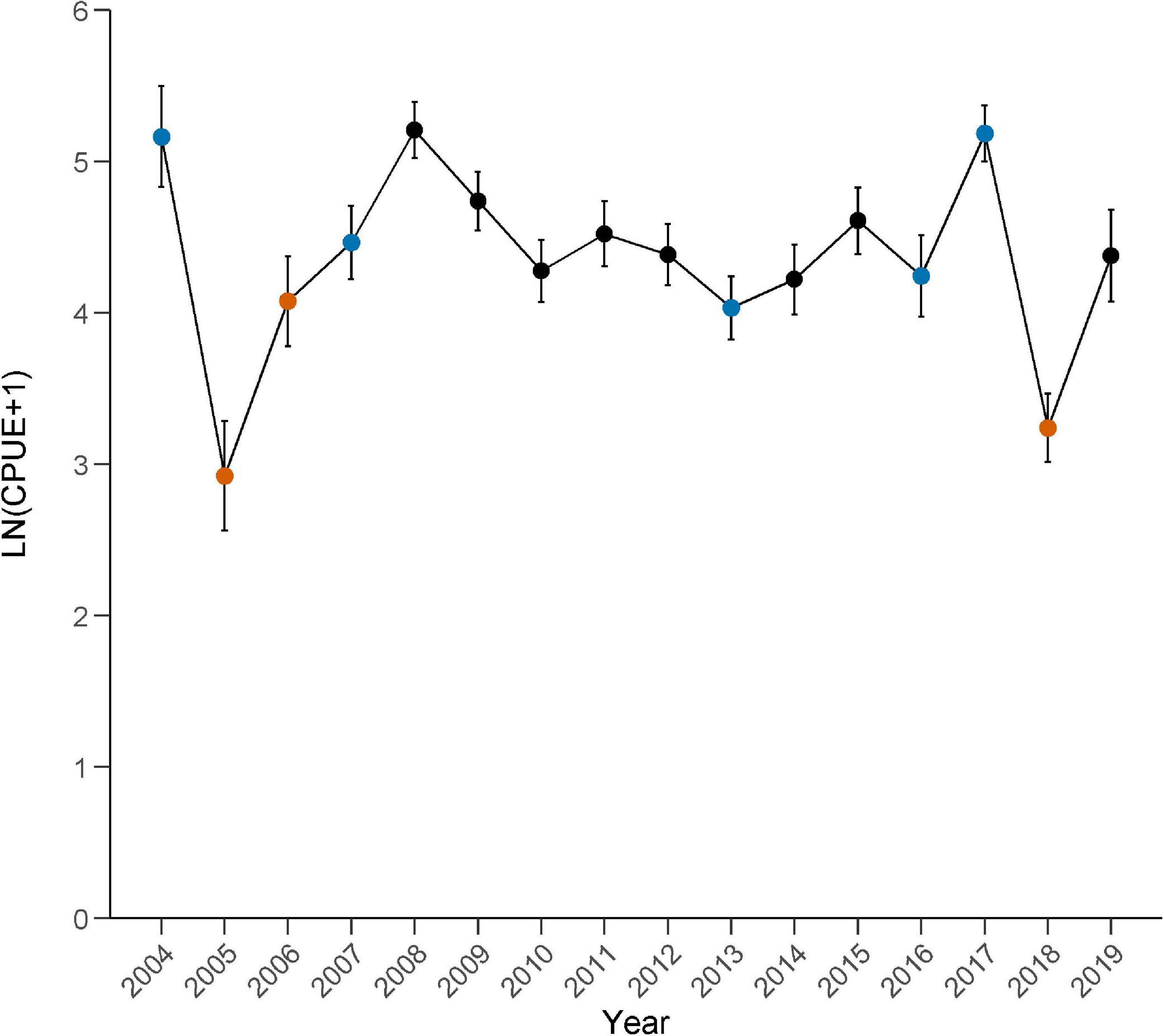
Figure 7. Annual catch per unit of effort (CPUE; mean ± se) of bottlenose dolphin prey species (excluding Clupeidae spp.) caught in the Sarasota Bay study area during 2004–2019. Symbols: = summer red tide years,
= winter red tide years, • = no known red tide disturbance events.
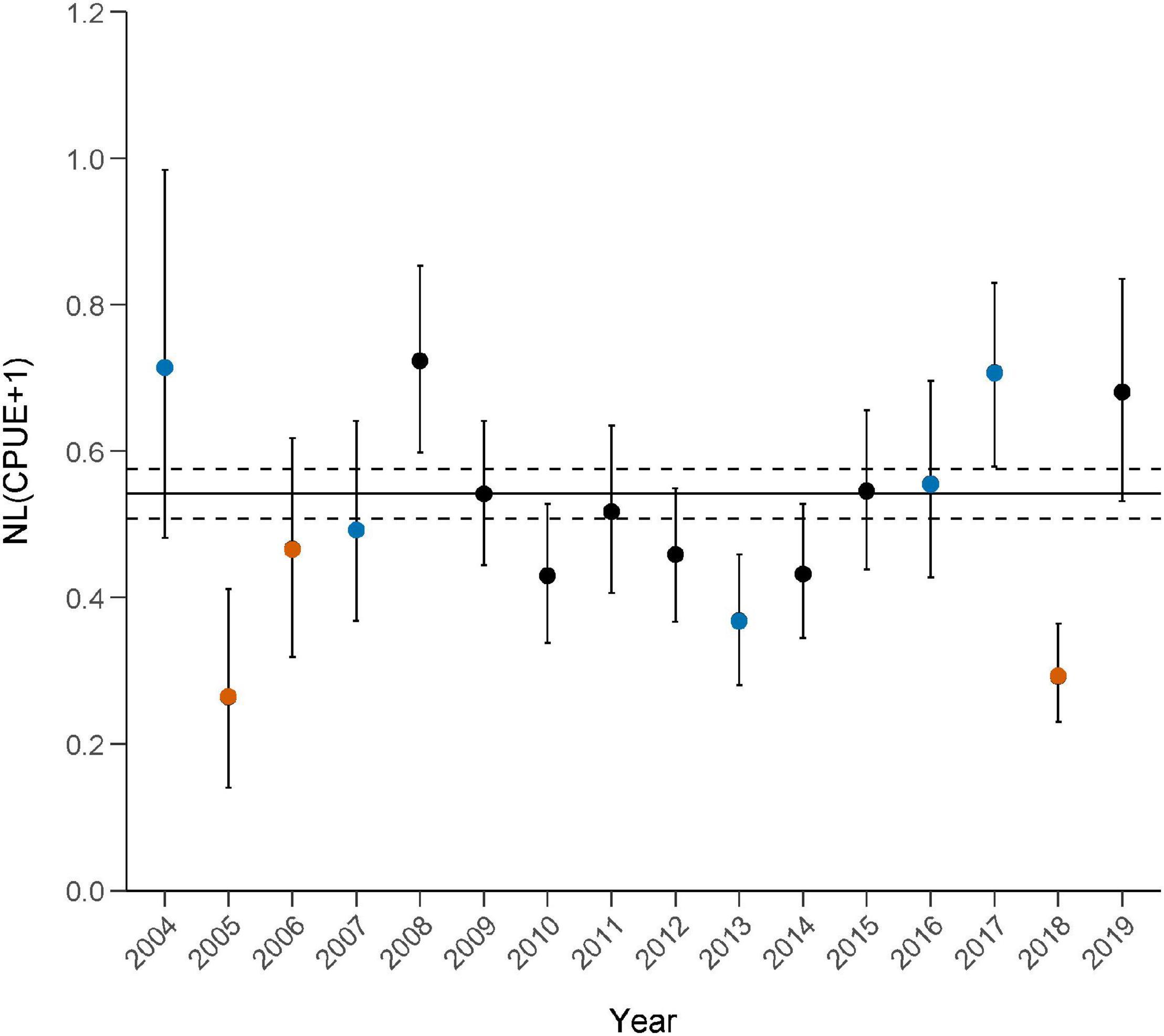
Figure 8. Standardized catch per unit effort (CPUE; mean ± 95% confidence intervals) of 8 bottlenose dolphin prey species combined (excludes Clupeidae spp.) caught during non-severe bloom periods (horizontal line) and in each survey year (circles) between 2004 and 2019. Overlap of 95% CIs between the non-severe bloom periods (horizontal dotted lines) and the CI of an annual CPUE indicates resistance, i.e., no effect of the bloom event. Resilience, or the recovery period post-bloom, is defined as the number of years between the bloom event and the year in which 95% CI overlap. Symbols: = severe summer red tide years,
= winter red tide years, • = no known red tide disturbance events.
Catch per unit effort varied in years with red tide events primarily in the winter months (2004, 2007, 2013, 2016, and 2017) (Figure 7). CPUEs in 2004 and 2017 were at or near all-time highs, while CPUEs in 2007, 2013, and 2016 were low but similar to CPUEs in other years (2009–2015, 2019). Notably, a period of unusually cold temperatures occurred in the first week of January 2010. CPUE in 2010 was low but similar to CPUEs in other years. Standardized CPUEs increased by 5.5% in 2007, 1.8% in 2016, and 27.3% in 2017, but decreased by 19.6% in 2013, compared to the previous year’s CPUE (Figure 8). Prey populations were not sampled in 2003 therefore changes in CPUE from 2003 to 2004 could not be evaluated. In 2004, 2007, and 2016, the CIs between the non-bloom CPUE and the annual CPUE overlapped, indicating resistance during some years with severe winter blooms. Lack of CI overlap between the non-bloom CPUE and annual CPUEs in 2013 and 2017 indicate a lack of resistance, however effects were negative in 2013 (32.1% below non-bloom CPUE) and positive in 2017 (30.3% above non-bloom CPUE.
Resilience
Following years with summer red tides (2005, 2006, and 2018), 95% CIs of each annual standardized CPUE (excluding Clupeidae spp.) overlapped with that of the average non-bloom CI 1 year later (2006, 2007, and 2019; Figure 8). Recovery was also associated with elevated annual CPUEs 1–3 years following a bloom (2008 and 2019). Red tide events in 2003 (based on red tide data throughout study area, see Table 2) and 2018 were followed by near all-time high CPUEs in 2004 and 2019, respectively. Recovery from the 2005 red tide event occurred in 2006, despite additional severe red tide conditions from August 8, 2006 to February 16, 2007. However, CPUE in 2006 remained relatively low, indicating lag effects from brevetoxin or other conditions likely affected prey recovery. CPUE increased slightly (5.2%) from 2006 to 2007, but a significant increase in assemblage abundance was not seen until 2008, when CPUE increased 46.8% (compared to 2007) and the annual CI was significantly greater than the non-bloom CI. Unusually cold temperatures in January of 2010 corresponded with a low, but not statistically significant, annual CPUE. Following winter red tide blooms in 2013, 2016, and 2017, CIs of each standardized CPUE overlapped with the non-bloom CI 1 year later in just one case, 2013–2014. Following the 2016 winter bloom event, 2017 exhibited a significant near all-time high CPUE, while CPUE decreased significantly from 2017 to 2018 due to a severe summer bloom event in 2018. Regardless of season, standardized CPUEs increased each year following a red tide event, unless the following year included a severe summer red tide event.
Community Structure
Dolphin prey assemblage structure across multiple bloom periods could effectively be summarized by an NMDS ordination in three dimensions (stress = 0.0562, random restarts = 100) (Figure 9). The main gradients along which species composition varied were by season and bloom period. Samples from summer non-red tide periods were tightly grouped together in the upper left portion of the graphic, resulting in a convex hull that did not overlap with any other group. Summer non-red tide samples were characterized by higher abundances of mullet, spot, and pigfish. Summer red tide samples grouped together in the lower middle portion of the graphic, resulting in a convex hull that overlapped that of the winter red tide group. Summer red tide samples were most closely associated with higher abundances of Atlantic threadfin herring and scaled sardines. Samples from winter non-red tide periods loosely grouped in the upper right portion of the graphic, resulting in a convex hull that overlapped with the winter red tide group. Winter non-red tide samples were associated with higher abundances of several species, including ladyfish, pinfish, and sheepshead. Group overlap made the association with Gulf toadfish and spotted seatrout difficult to interpret. Winter red tide samples comprised the most disperse group, occupying a relatively large portion of the middle and right side of the graphic. Overlap occurred with the summer red tide and winter non-red tide groups and made the interpretation of species associated with this group problematic.
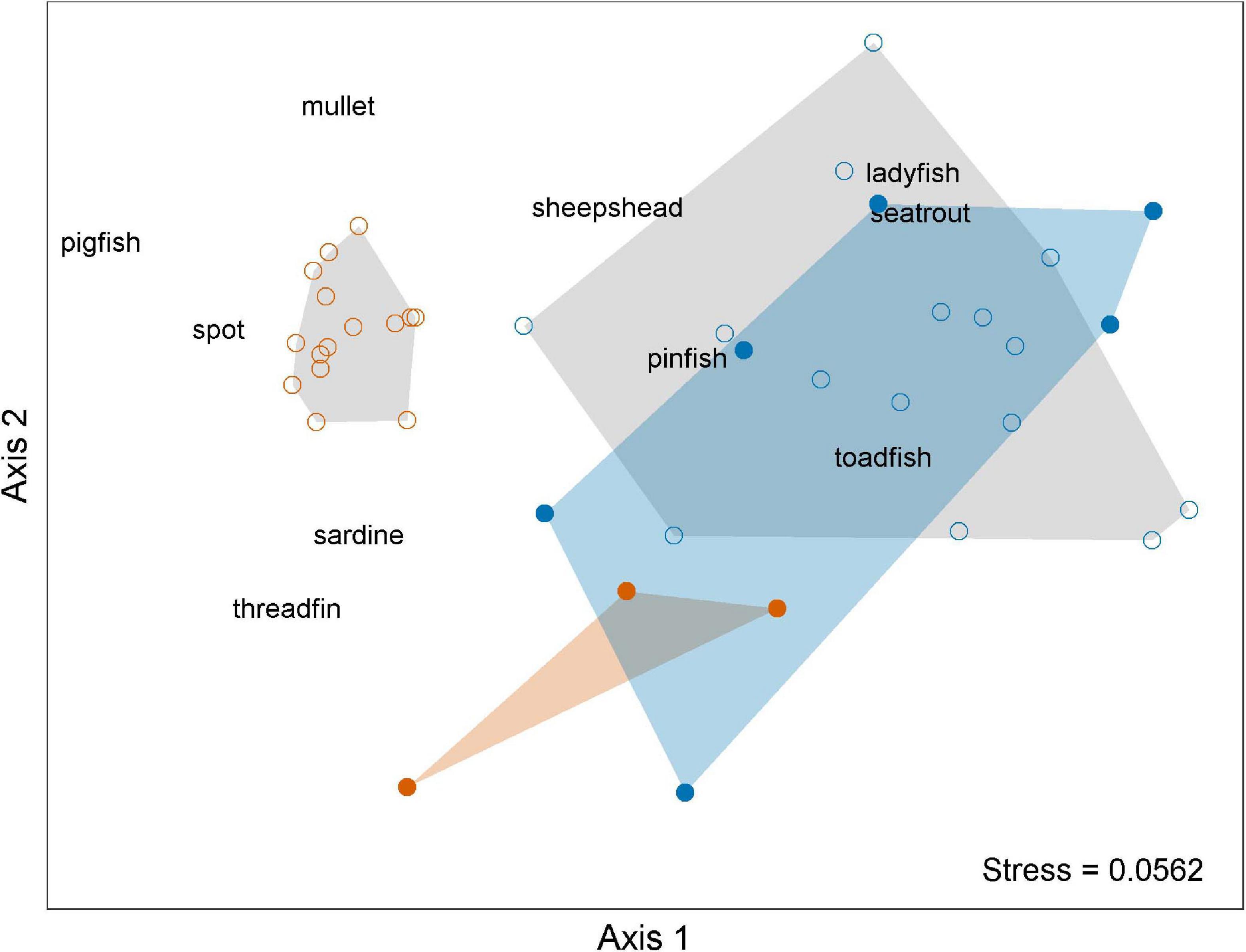
Figure 9. Non-metric multidimensional scaling (NMDS) ordination of prey assemblage structure based on year, season, and bloom condition during red tide events during 2004–2019 in Sarasota Bay, Florida. Symbols: = summer red tide conditions, orange convex hull;
= summer non-red tide conditions, gray convex hull;
= winter red tide conditions, blue convex hull;
= winter non-red tide conditions, gray convex hull.
Multivariate homogeneity of group dispersion analysis indicated no significant differences in dispersion between bloom periods, however significant differences in dispersion were found between seasons, indicating greater variation in prey composition in winter than in summer (Table 4). PERMANOVA analysis revealed significant effects of season, bloom periods, and the season:bloom period interaction on prey assemblage composition. The CAP ordination better explained the significant season:bloom period interaction effect (ANOVA permutation test: F = 12.688, p = 0.001) (Supplementary Figure 1). While sample sites discriminated along CAP2 and summer samples separated from winter samples along CAP1, the degree of separation during non-red tide/red tide conditions in the summer is much greater than seen during the winter. The first and second CAP ordination axes explained between 43.5 and 5.9% of the variation in prey composition data, with a total of 52.0% of the prey abundance data explained by the three canonical axes. SIMPER analysis carried out on the prey assemblage revealed that differences between non-red tide and red tide CPUEs during the summer months were best explained by pigfish (9.7%), pinfish (9.4%), and clupeids (Atlantic threadfin herring [5.0%], scaled sardine [4.3%]; 9.3% total contribution) (Table 5). These 4 species contributed 28.5% to the dissimilarity between non-red tide and red tide CPUEs during the summer months. During the winter months, dissimilarity was best explained by pinfish (7.6%), clupeids (scaled sardine [6.5%] and Atlantic threadfin herring [5.5%]), and spotted seatrout (3.3%), contributing a total of 23.1% to the dissimilarity combined. In the summer months, all non-clupeid prey species decreased in abundance during red tide conditions, while all clupeid species increased in abundance. In the winter, 4 of the 10 prey species increased in abundance during red tide conditions, including scaled sardine, Atlantic threadfin herring, spotted seatrout, and mullet. These results support and lend further insight into the results of the NMDS analysis.
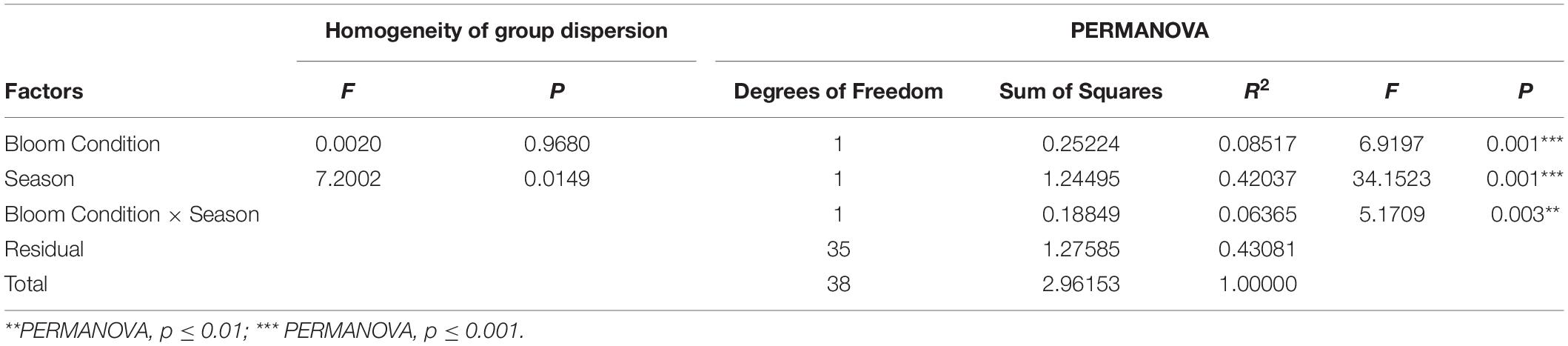
Table 4. Homogeneity of group dispersion and permutational multivariate analysis of variance (PERMANOVA) results of prey community structure in the Sarasota Bay study area, from 2004 to 2019, in relation to bloom condition and season.
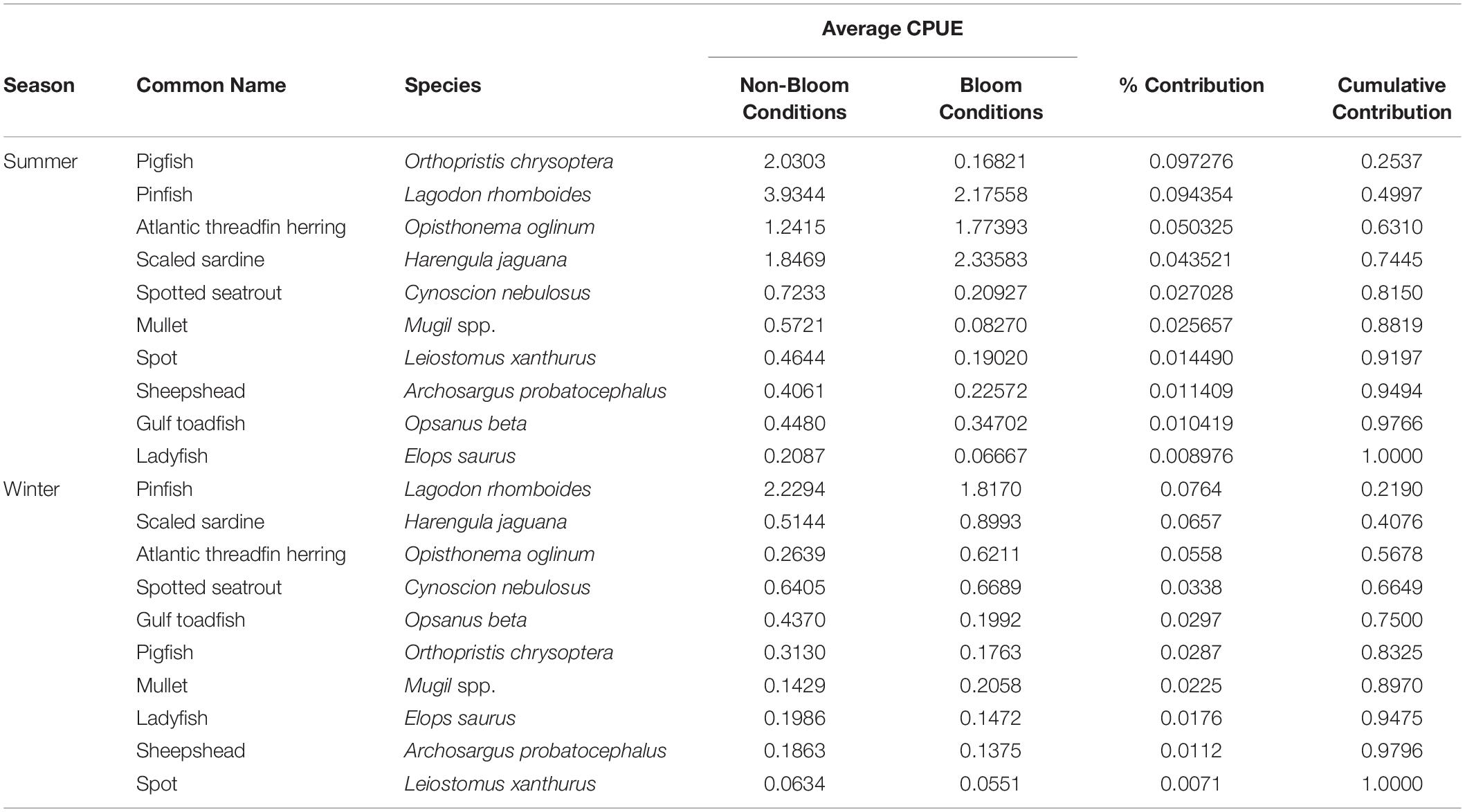
Table 5. Summary of similarity of percentage (SIMPER) analyses comparing the difference in prey assemblage composition between non-bloom and bloom conditions during each season, 2004–2019.
Direct gradient analysis by CCA explored the relationships of prey assemblage structure to environmental variables (temperature, DO, and salinity) and K. brevis density at each fish sampling location. The first and second ordination axes explained between 32.2 and 9.9% of the variation in the prey abundance data. Together, the first three canonical axes explain 44.8% of the prey abundance data. The CCA biplot showed strong associations between the first axis and temperature and DO, reflecting a gradient from sampling sites with lower temperatures, higher DO measures, and increased abundances of Gulf toadfish, spotted seatrout, and ladyfish on the right side of the diagram, to higher temperatures, lower DO measures, and increased abundances of pigfish, spot, and mullet on the left (Figure 10). The second axis was negatively correlated with K. brevis abundance at each of the fish sampling stations; the sites with higher K. brevis densities and associated species (clupeids) appeared at lower scores, while the sites with lower K. brevis densities and associated species (pinfish, pigfish, mullet, spot, sheepshead, ladyfish, and spotted seatrout) were located at the positive end. Based on biplot arrow length, temperature and DO had the greatest influence on prey assemblage composition, directly followed by K. brevis density.
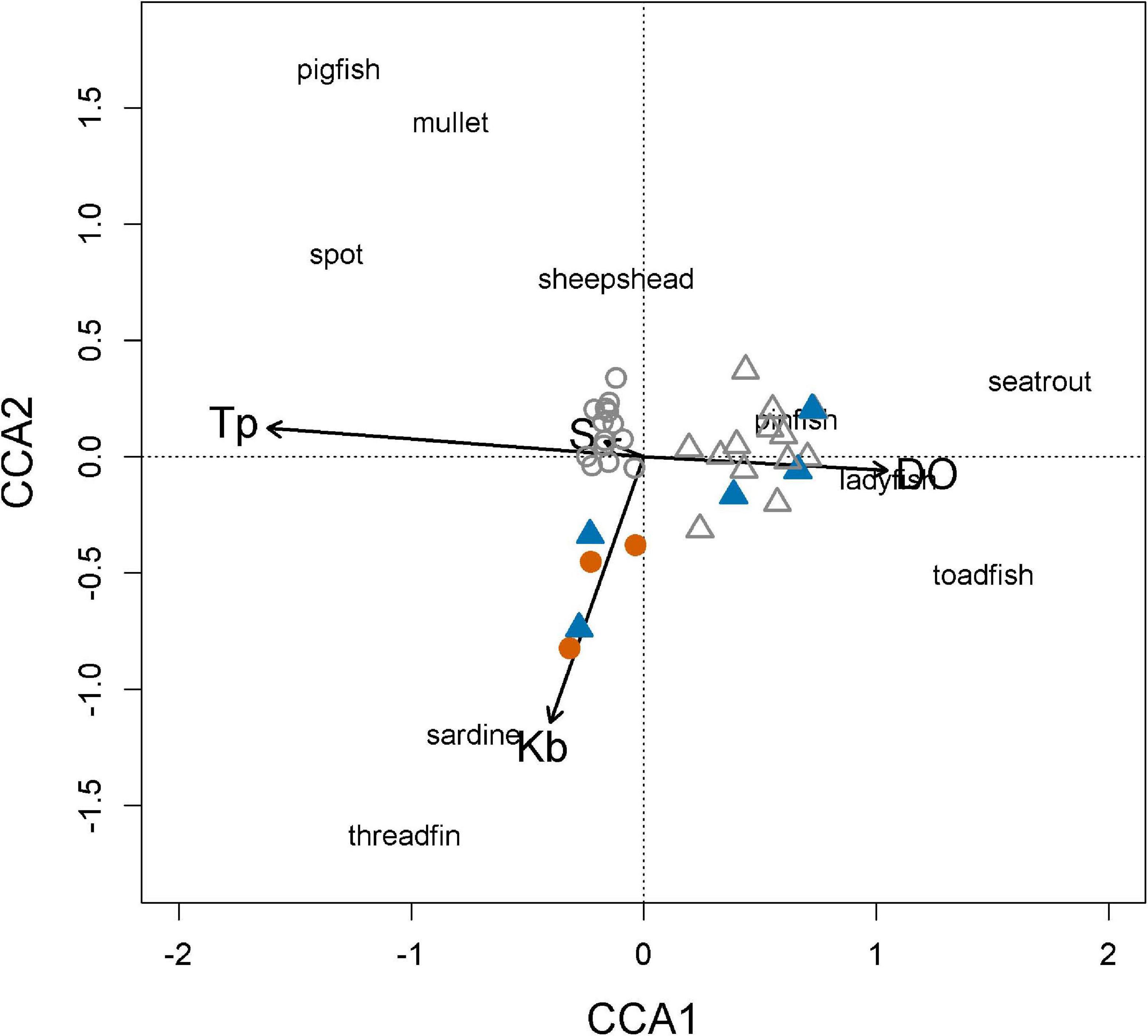
Figure 10. Canonical correspondence analysis (CCA) of the prey assemblage from purse seine catches, from July 2005 through March 2019. Symbols: = summer red tide conditions,
= summer non-red tide conditions,
= winter red tide conditions, Δ = winter non-red tide conditions, environmental vector codes: DO: dissolved oxygen (mg l–1); Tp: temperature (°C); S: salinity (ppt); Kb: Karenia brevis cell density (cells l–1).
Bloom Severity–Species Richness and Diversity
Both the sample- and coverage-based rarefaction and extrapolation curves indicated that assemblage richness and Shannon diversity estimates were significantly different from one another for all but the smallest sample sizes, with the lowest estimate in 2005, followed by 2006, and the highest estimate in 2018 (Supplementary Figure 2A). Simpson diversity was also highest in 2018 and significantly different from 2005 to 2006, however 95% CIs continuously overlapped in 2005 and 2006 indicating Simpson diversity did not differ significantly in those years. Significant differences between the numbers of species detected between bloom years existed at numerous sampling sizes (Supplementary Figure 2B). For each diversity order, coverage-based rarefaction and extrapolation curves indicated that sample coverage (completeness) was above 85% during each year, implying that correcting for sample completeness was not warranted as the lowest coverage, known as the base coverage, did not differ drastically from the highest coverage value (Supplementary Figure 2C).
Discussion
Using data from long-term surveys of K. brevis across the study area, we identified eight distinct red tide blooms that affected the Sarasota Bay study area between 2003 and 2019. During the most recent bloom event (2018–2019), the abundance of a bottlenose dolphin prey assemblage decreased during ichthyotoxic conditions; however, changes in species density, diversity, and assemblage structure exhibited seasonal differences. From 2004 through 2019, resistance, resilience, and the ecological effects of multiple red tide events on the dolphin prey assemblage varied, depending on bloom intensity, season, and frequency. These results improve our understanding of how different bloom events affect the prey base of a year-round, long-term, top marine predator, the bottlenose dolphin.
The northern extent of the 2018–2019 red tide bloom event bordered Sarasota Bay and the very southern parts of Tampa Bay, Florida. This bloom had a patchy distribution; entering the Sarasota Bay estuary in the southern portion of the bay first in June 2018, then spreading northward from August 2018 on. The combination of decreases in prey assemblage abundance, species density, and Shannon diversity at fish sampling stations during ichthyotoxic conditions in the summer months suggests K. brevis had a severe effect on dolphin prey populations. Work by Rycyk et al. (2020) in the Sarasota Bay region supports these findings. Using passive acoustic techniques and data from SDRP’s fish monitoring program, Rycyk et al. (2020) documented a sudden decrease in mean sound spectrum levels in 2018 (June 2018 to October 2018), coincident with decreased non-clupeid and soniferous (i.e., noise-making) fish abundances, decreased fish species density, and increased observations of K. brevis in the Sarasota Bay region. In the winter months, results were less conclusive. Abundance declined during bloom conditions, however species density did not change and the Shannon diversity index value was lower during non-red tide conditions. A lower Shannon diversity index value during non-red tide conditions can be attributed to lower species evenness, as this index takes into account both species density and evenness. These results may indicate less severe K. brevis impacts in the winter months; however, bloom dynamics as well as seasonal dynamics, such as fish movement offshore as part of the normal reproductive cycle, with subsequent recruitment back into the estuary, and ontogenetic changes in habitat use, likely play important roles. In conjunction, shifting top-down or bottom-up forces may influence the population dynamics of dolphin prey. Declines in predatory fish abundances during bloom events, along with compensatory density-dependent reproductive, growth, and survival processes, and/or potential increases in the abundance of seasonal drift macroalgae (providing increased access to shelter and/or resources) may contribute to an increase in dolphin prey abundance during or following a winter bloom event.
From 2018 through 2019, changes in non-clupeid CPUEs ranged between −48.3% (pinfish) to −100% (ladyfish) between non-bloom and bloom periods in the summer months, and between +77.0% (toadfish) to −90.4% (ladyfish) in the winter months (negative percentages indicating lower densities during red tide and positive percentages indicating higher densities during red tide). Changes in clupeid CPUEs ranged between −4.0% (scaled sardine) to +124.2% (Atlantic thread herring) in the summer and between +317.9% (scaled sardine) to +565.1% (Atlantic thread herring) in the winter. On average 299 fewer non-clupeid prey fishes were caught per purse-seine set during summer red tide periods, compared to non-red tide periods when 366 non-clupeid fish were caught on average. Ordination analyses showed a positive association between clupeid abundance and K. brevis density across multiple red tide events (Figures 4, 5, 9, 10). In fact, clupeids have been one of the biggest contributors to the differences in CPUE between red tide and non-red tide periods across multiple bloom events, ranking 3rd highest in the summer months and 2nd highest in the winter months (Table 3). Pigfish contributed the most to differences in CPUE in the summer. Pinfish, the most abundant dolphin prey species in the study area, ranked 2nd in the summer but contributed the most in the winter months. These results indicate a pattern of species-specific responses to red tide events. Dolphins consume a variety of prey species (Barros and Wells, 1998; Wells et al., 2013; Rossman et al., 2015), however previous work has shown that dolphins selectively consume soniferous fish in Sarasota Bay (Berens McCabe et al., 2010). Four of the ten prey species included in this study are soniferous, specifically pigfish, spot, spotted seatrout, and Gulf toadfish. Due to their increased availability during bloom events, clupeids may be an important prey resource for dolphins, as the abundances of other prey species, especially soniferous prey species, decline. However, catching and consuming these smaller, less energy-dense clupeid species, likely comes with an energetic cost for the dolphins.
Across multiple bloom events since 2004, prey assemblage structure showed distinct and significant short-term shifts between red tide and non-red tide conditions independent of the normal seasonal shifts in prey structure seen during non-bloom conditions (Table 4, Figures 4, 9, and Supplementary Figure 1). In the winter months, the structure of the prey assemblage exhibited the greatest variation, and there was a higher degree of overlap in prey composition between red tide and non-red tide conditions. During red tide conditions, assemblage structure exhibited some degree of overlap between summer and winter as well (Figure 9 and Supplementary Figure 1). These results indicate a pattern of altered prey assemblage structure during red tide bloom events. Normal seasonal patterns in prey structure were seen within 1-year post-bloom, suggesting some level of resilience to repeated K. brevis bloom events of varying severity.
From 2018 through 2019, and separately from 2004 through 2019, differences between red tide and non-red tide groups corresponded primarily with the K. brevis cell count vector in the CCA analyses (Figures 5, 10). Vectors representing temperature and DO corresponded as expected between seasons, with the summer months corresponding primarily to higher temperatures and secondarily to higher salinity, and the winter months corresponding to higher DO (Figure 10). These results suggest a pattern of change in prey assemblage structure directly related to the presence of K. brevis or to brevetoxins, and little evidence of hypoxia during bloom conditions.
Previous work by Gannon et al. (2009) and Flaherty and Landsberg (2011) found similar species-specific responses during the 2005/2006 red tide event, and altered patterns of community structure during red tide conditions with little evidence of hypoxic or anoxic conditions. Differential physiological tolerances to brevetoxin or better detection and avoidance of K. brevis and/or brevetoxin patches were cited as possible reasons for differing species-specific responses to red tide events. Flaherty and Landsberg (2011) also documented a return to normal seasonal patterns of community structure within 1-year post-bloom. Our study uses similar methodology to expand on the work of Gannon et al. (2009), however it differs in several ways. Gannon et al. (2009) utilized a 4-year dataset (2004 through 2007) to assess effects of the 2005/2006 red tide events on fish abundance, species richness and diversity, and community structure during the summer months. Our study reexamined these questions with similar methodology to assess the effects of the recent 2018/2019 red tide bloom event, the first major summer bloom since 2006, on select common dolphin prey species across summer and winter seasons. Then, we assessed whether these results were consistent across multiple bloom events, utilizing a 17-year dataset to characterize 8 distinct regional bloom events, and to assess prey resistance, resilience, and assemblage structure. Gannon et al. (2009) determined bloom conditions based on K. brevis cell counts exceeding the 100,000 l–1 threshold anywhere in the study area, and persisting for 30 days beyond the time at which cell counts dropped below 100,000 cells l–1. In contrast, our study uses bloom conditions based on cell counts sampled at fish sampling stations only in an effort to account for the patchy nature of red tide blooms.
Flaherty and Landsberg (2011) utilized an 11-year dataset (1996 through 2006) to assess fish abundance and community structure across several regions defined by salinity and habitat type; however, K. brevis samples were not directly linked to fish sampling sites and sampling was not consistent across all regions and time periods. The authors found significant shifts in community structure in 2005 and 2006, but not in any other year with documented red tide blooms. In contrast, our study found significant effects of season and bloom period on prey assemblage structure across multiple red tide events, with greater variation in the winter months.
Following these studies, we used cell density as a proxy for brevetoxin exposure in prey fish due to the difficulty in sampling free and intracellular brevetoxins in situ. Actual ichthyotoxic conditions at fish sampling sites likely varied based on bloom patch dynamics and the degree to which brevetoxins persist in the environment during and after a bloom. Prey sampling was restricted to the daylight hours, therefore diel variation in DO that might influence changes in prey assemblage structure during bloom conditions was not fully measured (Flaherty and Landsberg, 2011). Despite these noted limitations, strong relationships between K. brevis density and prey abundance were detected, as well as significant shifts in prey assemblage structure between seasons and bloom periods.
Communities that routinely experience disturbance events, like the prey assemblage in our study, often show some level of resistance and resilience to ecological change; however, the severity and scale of the disturbance may influence the degree to which communities are affected (Flaherty and Landsberg, 2011). In our study, the prey assemblage exhibited less resistance to red tides that occurred primarily in the summer months (2005, 2006, and 2018), resulting in two of the three lowest annual CPUEs during this study and a common pattern of decreased prey abundance (Figure 7). Each summer bloom event was also associated with higher than average annual CPUEs 1–3 years following a bloom, with recurrent blooms in 2005 and 2006 resulting in an increased time to higher than average annual CPUE following bloom cessation (Figure 8). Prey resistance in years with winter red tides varied, with two annual CPUEs at all-time or near all-time highs (2004 and 2017), two CPUEs that could be considered average (2007 and 2016), and one annual CPUE that was the 3rd lowest of this study (2013). When compared to the average standardized CPUE across all years, annual standardized CPUEs decreased by 14.0–52.1% in years with summer red tides. Greater variability resulted in declines in CPUE by as much as 32.1%, to increases of as much as 31.6%, in years with winter red tides. Following all but one instance (2017), time to recovery to pre-red tide prey abundances occurred within 1 year, regardless of the season in which the red tide event primarily occurred (Figure 8). These results suggest that bloom frequency and season affect dolphin prey resistance and resilience to red tide events in estuarine waters along the west coast of Florida.
Our study sampled seagrass habitat exclusively because it has been identified as important foraging habitat for resident dolphins in Sarasota Bay (Barros and Wells, 1998; Rossman et al., 2015). Prey movement into other habitats or movement outside of the study area would not have been detected by our methods; however both of these mechanisms presume that dolphin prey fish can detect and avoid patches of K. brevis and/or brevetoxin (Gannon et al., 2009). In contrast, mortality is more likely the cause of changes in prey abundance and shifts in assemblage structure during red tide conditions. In 2018, 100% of fish kills reported to the State of Florida’s Fish and Wildlife Research Institute (FWRI) statewide fish kill database1 in the study area occurred during red tide conditions, and high concentrations of brevetoxins were present in the viscera of dolphin prey collected during bloom conditions (personal communication, S. Fire, October 9, 2020). During 2003–2007, Gannon et al. (2009) documented that 95% of fish kills reported to FWRI’s fish kill database occurred during red tide conditions; in addition, during bloom periods there were high concentrations of brevetoxins measured in fish tissues collected in Sarasota Bay (Fire, 2007; Fire et al., 2008) and standardized fish surveys showed little evidence of habitat compression in non-seagrass habitats. Historically, mass mortalities of fish communities have long been associated with red tides as well, with mortality attributed primarily to direct toxicity and secondarily to anoxia, hypoxia, and/or hydrogen-sulfide poisoning (Simon and Dauer, 1972; Steidinger and Ingle, 1972; Steidinger et al., 1973; Smith, 1975).
Sample-based rarefaction and extrapolation estimates of prey assemblage richness and Shannon diversity were significantly different between each year with a summer red tide bloom event (Supplementary Figure 2A), except at very low sample sizes. Diversity was lowest in 2005, followed by a relatively small increase in 2006. Comparatively, diversity was much higher in 2018. Simpson diversity did not change significantly between 2005 and 2006, indicating similar proportions or dominance of prey species. Following the IDH, these results suggest a more moderate, less severe bloom event in 2018, compared to those in 2005 or 2006. While K. brevis influx into Sarasota Bay occurred in the month of August in 2006 and 2018, lag effects from 2005 may have contributed to significantly lower estimates of prey assemblage diversity in 2006 relative to 2018.
In addition to a consistent pattern of severe impacts of summer red tides on dolphin prey, direct effects on dolphins resulted in the National Marine Fisheries Service declaring a marine mammal Unusual Mortality Event along the central and southwest coast of Florida in each year with a summer red tide event (2005, 2006, and 2018) (NOAA, 2006; NOAA Fisheries, 2018). Indirect effects of red tide events on dolphins may include changes in behavior, sociality, resource utilization (i.e., foraging on different prey species or using different habitats), and/or changes in predator-prey interactions. In 2018, there was an increase in sightings of dolphins in areas of low-salinity or freshwater during red tide conditions, however there was no evidence to suggest that resident Sarasota Bay dolphins moved outside of their long-term community range in efforts to avoid brevetoxin exposure or to access additional food or habitat resources to any great degree (unpublished data).
Declines in the abundance of stingrays (Dasyatidae spp.) following red tide events appear to coincide with increases in the frequency of shark bites on resident dolphins in Sarasota Bay, and increased disappearances of young-of-the-year dolphin calves (unpublished data). Decreases in the abundance of stingrays, in addition to declines in CPUE of prey fish common between sharks and dolphins, including mullet, toadfish (Opsanus spp.), ladyfish, and catfish (Ariidae spp.) (Snelson et al., 1984; Cortés and Gruber, 1990), may result in increased interaction frequency between bottlenose dolphins and their shark predators as they vie for fewer remaining prey fish. Dietary analyses of predatory sharks within the study area would undoubtedly improve our understanding of possible shifts in predator-prey interactions affecting the resident dolphin community in Sarasota Bay.
Potential alterations to predator-prey interactions between free-ranging bottlenose dolphins in Sarasota Bay and their prey were observed in response to the bloom events in 2005 and 2006. McHugh et al. (2011) documented a suite of behavioral changes in juvenile dolphins during bloom conditions, including significantly altered activity budgets, increased sociality, and expanded ranging behavior. The authors hypothesized that the behavioral changes observed would be consistent with a dietary shift toward more pelagic clupeid species as other prey species declined, thereby suggesting widespread ecosystem change due to red tide. In addition, Powell and Wells (2011) and Christiansen et al. (2016) reported a rapid increase in conditioning to human interactions through food provisioning beginning in 2005–2006 resulting, at least in part, from the effects of red tide on fish populations and community structure in Sarasota Bay (see Gannon et al., 2009). Understanding how different red tide events affect the dynamics and persistence of populations linked by predator–prey interactions, including possible temporary alterations in the strength or rate of interspecific interactions within a community without directly changing abundances, is critical for effective management efforts (Commander and White, 2020).
Our study is a first step in identifying differences in resistance, resilience, and the ecological effects of multiple red tide bloom events of various temporal scales and intensity on a dolphin prey assemblage. Red tide blooms in Sarasota Bay demonstrated a great deal of variability in their impacts on the prey fish community. Possible changing disturbance regimes and the complex interactions involved in these processes require the use of long-term monitoring datasets, such as the 17-year dataset used in this study. Advances in K. brevis detection methods and brevetoxin rapid testing techniques are needed to better characterize fine-scale bloom dynamics in relation to environmental variables and ecological changes in nearshore and estuarine waters, and to better determine the relative importance of the major brevetoxin vectors in the water and within living K. brevis cells. Additional work looking at the effects of red tide disturbances on predator-prey linkages, including possible exploitative interactions across trophic levels during bloom conditions and possible alterations in demographic processes that may indirectly change predator and/or prey abundances, is needed to better understand the influence of red tides on estuarine structural dynamics and function, to better inform management, and to potentially guide mitigation efforts post-bloom.
Data Availability Statement
The raw data supporting the conclusions of this article will be made available by the authors, without undue reservation.
Ethics Statement
The animal study was reviewed and approved by Mote Marine Laboratory Institutional Animal Care and Use Committee approvals (06-10-DG1 through 19-10-RW2). Long-term fish sampling was conducted under a series of Special Activity Licenses by the Florida Fish and Wildlife Conservation Commission (numbers 03SR-809 through 19-0809-SR).
Author Contributions
EBM led the prey fish surveys, collected and/or processed the majority of red tide samples collected by the Chicago Zoological Society’s Sarasota Dolphin Research Program, completed statistical analyses, and drafted the manuscript. RW provided input on initial survey design and obtained support for the research. AB, CT, and KW assisted EBM with prey fish surveys. RW, CT, AB, KW, and VP provided feedback on statistical analyses and manuscript drafts. All authors have read and approved the final manuscript.
Funding
Primary funding for long-term fish surveys and data collection in Sarasota Bay has been provided by the Chicago Zoological Society and the Charles and Margery Barancik Foundation. The Batchelor Foundation, Mote Scientific Foundation, NOAA’s Fisheries Service (#NA16FL1355), Harbor Branch Oceanographic Institution’s Protect Wild Dolphins Program (PWD 2004-2018 and PWD 2005-2009), Florida’s State Wildlife Grants Program (SWG05_028), and Florida’s Fish and Wildlife Research Institute (FWCC #04089) provided additional funding for this work.
Conflict of Interest
The authors declare that the research was conducted in the absence of any commercial or financial relationships that could be construed as a potential conflict of interest.
Publisher’s Note
All claims expressed in this article are solely those of the authors and do not necessarily represent those of their affiliated organizations, or those of the publisher, the editors and the reviewers. Any product that may be evaluated in this article, or claim that may be made by its manufacturer, is not guaranteed or endorsed by the publisher.
Acknowledgments
We thank Chicago Zoological Society/Sarasota Dolphin Research Program (CZS/SDRP) staff members, interns, and volunteers who made this work possible by providing logistical support and assistance in the field. Damon Gannon established the CZS/SDRP fish survey program and Janet Gannon developed the CZS/SDRP prey sampling database. We thank Mote Marine Laboratory’s Phytoplankton Ecology Lab for their expertise, use of their equipment, and access to long-term data. We also thank the reviewers whose valuable comments and suggestions helped improve and clarify this manuscript.
Supplementary Material
The Supplementary Material for this article can be found online at: https://www.frontiersin.org/articles/10.3389/fmars.2021.711114/full#supplementary-material
Footnotes
References
Anderson, M. J. (2006). Distance-based tests for homogeneity of multivariate dispersions. Biometrics 62, 245–253. doi: 10.1111/j.1541-0420.2005.00440.x
Anderson, M. J., Gorley, R. N., and Clarke, K. R. (2008). PERMANOVA+ for PRIMER: Guide to Software and Statistical Methods. Plymouth, UK: PRIMER-E Ltd.
Anderson, M. J., and Willis, T. J. (2003). Canonical analysis of principal coordinates: a useful method of constrained ordination for ecology. Ecology 84, 511–525. doi: 10.1890/0012-9658(2003)084[0511:caopca]2.0.co;2
Baden, D. G. (1988). “Public health problems of red tides,” in Handbook of Natural Toxins, vol 3: Marine Toxins and Venoms, ed. A. T. Tu (New York: Marcel Dekker Inc.), 259–277.
Baden, D. G., and Mende, T. J. (1982). Toxicity of two toxins from the Florida red tide marine dinoflagellate, Gymnodinium breve. Toxicon 20, 457–461. doi: 10.1016/0041-0101(82)90009-5
Barros, N. B., and Wells, R. S. (1998). Prey and feeding patterns of resident bottlenose dolphins (Tursiops truncatus) in Sarasota Bay, Florida. J. Mammal. 79, 1045–1059. doi: 10.2307/1383114
Berens McCabe, E. J., Gannon, D. P., Barros, N. B., and Wells, R. S. (2010). Prey selection by resident common bottlenose dolphins (Tursiops truncatus) in Sarasota Bay, Florida. Mar. Biol. 157, 931–942. doi: 10.1007/s00227-009-1371-2
Borer, E. T., Halpern, B. S., and Seabloom, E. W. (2006). Asymmetry in community regulation: effects of predators and productivity. Ecology 87, 2813–2820. doi: 10.1890/0012-9658(2006)87[2813:aicreo]2.0.co;2
Bossart, G. D., Baden, D. G., Ewing, R. Y., Roberts, B., and Wright, S. D. (1998). Brevetoxicosis in manatees (Trichechus manatus latirostris) from the 1996 epizootic: gross, histologic, and immunohistochemical features. Toxicol. Pathol. 26, 276–282. doi: 10.1177/019262339802600214
Boucek, R. E., and Rehage, J. S. (2014). Climate extremes drive changes in functional community structure. Global Change Biol. 20, 1821–1831. doi: 10.1111/gcb.12574
Brand, L. E., and Compton, A. (2007). Long-term increase in Karenia brevis abundance along the southwest Florida coast. Harmful Algae 6, 232–252. doi: 10.1016/j.hal.2006.08.005
Callaway, R. M., Brooker, R. W., Choler, P., Kikvidze, Z., Lortie, C. J., Michalet, R., et al. (2002). Positive interactions among alpine plants increase with stress. Nature 417, 844–848. doi: 10.1038/nature00812
Chao, A., Gotelli, N. J., Hsieh, T. C., Sander, E. L., Ma, K. H., Colwell, R. K., et al. (2014). Rarefaction and extrapolation with Hill numbers: a framework for sampling and estimation in species diversity studies. Ecol. Monogr. 84, 45–67. doi: 10.1890/13-0133.1
Chao, A., and Jost, L. (2012). Coverage-based rarefaction and extrapolation: standardizing samples by completeness rather than size. Ecology 93, 2533–2547. doi: 10.1890/11-1952.1
Christiansen, F., McHugh, K. A., Bejder, L., Siegal, E. M., Lusseau, D., Berens McCabe, E. J., et al. (2016). Food provisioning increases the risk of injury in a long-lived marine top predator. R. Soc. Open Sci. 3:160560. doi: 10.1098/rsos.160560
Clarke, K. R. (1993). Non-parametric multivariate analyses of changes in community structure. Aus. J. Eco. 18, 117–143. doi: 10.1111/j.1442-9993.1993.tb00438.x
Clarke, K. R., and Warwick, R. M. (2001). Change in marine communities: an approach to statistical analysis and interpretation, 2nd Edn. Plymouth, UK: Primer-E Ltd.
Commander, C. J., and White, J. W. (2020). Not all disturbances are created equal: disturbance magnitude affects predator–prey populations more than disturbance frequency. Oikos 129, 1–12. doi: 10.1111/oik.06376
Connell, J. H. (1978). Diversity in tropical rain forests and coral reefs. Science 199, 1302–1310. doi: 10.1126/science.199.4335.1302
Connell, J. H., and Slatyer, R. O. (1977). Mechanisms of succession in natural communities and their role in community stability and organization. Am. Nat. 111, 1119–1144. doi: 10.1086/283241
Cortés, E., and Gruber, S. H. (1990). Diet, feeding habits and estimates of daily ration of young lemon sharks, Negaprion brevirostris (Poey). Copeia 1990, 204–218. doi: 10.2307/1445836
Díaz-García, J. M., Pineda, E., López-Barrera, F., and Moreno, C. E. (2017). Amphibian species and functional diversity as indicators of restoration success in tropical montane forest. Biodivers. Conserv. 26, 2569–2589. doi: 10.1007/s10531-017-1372-2
DiLeone, A. G., and Ainsworth, C. H. (2019). Effects of Karenia brevis harmful algal blooms on fish community structure on the West Florida Shelf. Ecol. Modell. 392, 250–267. doi: 10.1016/j.ecolmodel.2018.11.022
Fire, S. E. (2007). Effects of Karenia brevis blooms on wild coastal bottlenose dolphins (Tursiops truncatus) in Sarasota Bay, Florida. [PhD dissertation]. Santa Cruz, CA: University of California.
Fire, S. E., Flewelling, L. J., Naar, J., Twiner, M. J., Henry, M. S., Pierce, R. H., et al. (2008). Prevalence of brevetoxins in prey fish of bottlenose dolphins in Sarasota Bay, Florida. Mar. Ecol. Prog. Ser. 368, 283–294. doi: 10.3354/meps07643
Flaherty, K. E., and Landsberg, J. H. (2011). Effects of a persistent red tide (Karenia brevis) bloom on community structure and species-specific relative abundance of nekton in a Gulf of Mexico estuary. Estuaries Coast. 34, 417–439. doi: 10.1007/s12237-010-9350-x
Flewelling, L. J., Naar, J. P., Abbott, J. P., Baden, D. G., Barros, N. B., Bossart, G. D., et al. (2005). Red tides and marine mammal mortalities. Nature 435, 755–756. doi: 10.1038/nature435755a
Forrester, D. J., Gaskin, J. M., White, F. H., Thompson, N. P., Quick, J. A. Jr., Henderson, G. E., et al. (1977). An epizootic of waterfowl associated with a red tide episode in Florida. J. Wildl. Dis. 13, 160–167. doi: 10.7589/0090-3558-13.2.160
Gannon, D. P., Berens McCabe, E. J., Camilleri, S. A., Gannon, J. G., Brueggen, M. K., Barleycorn, A. A., et al. (2009). Effects of Karenia brevis harmful algal blooms on nearshore fish communities in southwest Florida. Mar. Ecol. Prog. Ser. 378, 171–186. doi: 10.3354/meps07853
Gunter, G., Williams, R. H., Davis, C. C., and Smith, F. G. W. (1948). Catastrophic mass mortality of marine animals and coincident phytoplankton bloom on the west coast of Florida, November 1946 to August 1947. Ecol. Monogr. 18, 309–324. doi: 10.2307/1948575
Hammer, Ø, Harper, D. A. T., and Ryan, P. D. (2001). PAST: Paleontological statistics software package for education and data analysis. Palaeontol. Electron. 4:9.
Holling, C. S. (1973). Resilience and stability of ecological systems. Annu. Rev. Ecol. Syst. 4, 1–23. doi: 10.1007/978-3-030-54560-4_1
Hoover, D. L., Knapp, A. K., and Smith, M. D. (2014). Contrasting sensitivities of two dominant C 4 grasses to heat waves and drought. Plant Ecol. 215, 721–731. doi: 10.1007/s11258-014-0345-8
Hsieh, T. C., Ma, K. H., and Chao, A. (2020). iNEXT: interpolation and extrapolation for species diversity. R package version 2.0.20. Avaialable Online at: http://chao.stat.nthu.edu.tw/wordpress/software-download/ [Accessed October 20, 2020].
Indeck, K. L., Simard, P., Gowans, S., Lowerre-Barbieri, S., and Mann, D. A. (2015). A severe red tide (Tampa Bay, 2005) causes an anomalous decrease in biological sound. R. Soc. Open Sci. 2:150337. doi: 10.1098/rsos.150337
Jentsch, A., Kreyling, J., and Beierkuhnlein, C. (2007). A new generation of climate-change experiments: events, not trends. Front. Ecol. Environ. 5, 365–374. doi: 10.1890/1540-9295(2007)5[365:angoce]2.0.co;2
Johnke, J., Cohen, Y., de Leeuw, M., Kushmaro, A., Jurkevitch, E., and Chatzinotas, A. (2014). Multiple micro-predators controlling bacterial communities in the environment. Curr. Opin. Biotechnol. 27, 185–190. doi: 10.1016/j.copbio.2014.02.003
Landsberg, J. H. (2002). The effects of harmful algal blooms on aquatic organisms. Rev. Fish. Sci. 10, 113–390. doi: 10.1080/20026491051695
Landsberg, J. H., and Steidinger, K. A. (1998). “A historical review of Gymnodinium breve red tides implicated in mass mortalities of the manatee (Trichechus manatus latirostris) in Florida, USA,” in Harmful Algae, eds B. Reguera, J. Blanco, M. L. Fernandez, and T. Wyatt (Vigo, Spain: Xunta de Galicia and Intergovernmental Oceanographic Commission, UNESCO), 97–100.
Lewis, D. M., Thompson, K. A., MacDonald, T. C., and Cook, G. S. (2021). Understanding shifts in estuarine fish communities following disturbances using an ensemble modeling framework. Ecol. Indic. 126:107623. doi: 10.1016/j.ecolind.2021.107623
Lund, J. W. G., Kipling, C., and LeCren, E. D. (1958). The inverted microscope method of estimating algal numbers and the statistical basis of estimations of counting. Hydrobiologia 11, 143–170. doi: 10.1007/bf00007865
McHugh, K. A., Allen, J. B., Barleycorn, A. A., and Wells, R. S. (2011). Severe Karenia brevis red tides influence juvenile bottlenose dolphin (Tursiops truncatus) behavior in Sarasota Bay, Florida. Mar. Mamm. Sci. 27, 622–643. doi: 10.1111/j.1748-7692.2010.00428.x
Miller, A. D., Roxburgh, S. H., and Shea, K. (2011). How frequency and intensity shape diversity-disturbance relationships. Proc. Natl. Acad. Sci. U.S.A. 108, 5643–5648. doi: 10.1073/pnas.1018594108
Naar, J. P., Flewelling, L. J., Lenzi, A., Abbott, J. P., Granholm, A., Jacocks, H. M., et al. (2007). Brevetoxins, like ciguatoxins, are potent ichthyotoxic neurotoxins that accumulate in fish. Toxicon 50, 707–723. doi: 10.1016/j.toxicon.2007.06.005
NOAA. (2006). Notice: availability of new criteria for designation of marine mammal unusual mortality events (UMEs). Fed. Regist. 71, 75234–75236.
NOAA Fisheries. (2018). 2018-2020 Bottlenose dolphin unusual mortality event in southwest Florida. Available Online at: https://www.fisheries.noaa.gov/southeast/marine-life-distress/2018-2020-bottlenose-dolphin-unusual-mortality-event-southwest (accessed October 10, 2020).
Oksanen, J., Guillaume Blanchet, F., Friendly, M., Kindt, R., Legendre, P., McGlinn, D., et al. (2019). Vegan: Community Ecology Package. R package version 2.5-6. Available Online at: https://CRAN.R-project.org/package=vegan [Accessed October 10, 2020].
Ostfeld, R. S., and Keesing, F. (2000). Pulsed resources and community dynamics of consumers in terrestrial ecosystems. Trends. Ecol. Evol. 15, 232–237. doi: 10.1016/S0169-5347(00)01862-0
Pickett, S., and White, P. (1985). The Ecology of Natural Disturbance and Patch Dynamics. New York: Academic Press, Inc.
Powell, J. R., and Wells, R. S. (2011). Recreational fishing depredation and associated behaviors involving common bottlenose dolphins (Tursiops truncatus) in Sarasota Bay, Florida. Mar. Mamm. Sci. 27, 111–129. doi: 10.1111/j.1748-7692.2010.00401.x
Quick, J. A., and Henderson, G. E. (1975). “Effects of Gymnodinium breve red tide on fishes and birds: a preliminary report on behavior, anatomy, hematology, and histopathology,” in Proceedings of the Gulf Coast regional symposium on diseases of aquatic animals, eds R. L. Ambroski, M. A. Hood, and R. R. Miller (Louisiana Sea Grant: Louisiana State University), 85–113.
R Core Team. (2020). R: A language and environment for statistical computing. Vienna, Austria: R Foundation for Statistical Computing.
Rossman, S., McCabe, E. B., Barros, N. B., Gandhi, H., Ostrom, P. H., Stricker, C. A., et al. (2015). Foraging habits in a generalist predator: sex and age influence habitat selection and resource use among bottlenose dolphins (Tursiops truncatus). Mar. Mamm. Sci. 31, 155–168. doi: 10.1111/mms.12143
Rycyk, A. M., Tyson Moore, R. B., Wells, R. S., McHugh, K. A., Berens McCabe, E. J., and Mann, D. A. (2020). Passive acoustic listening stations (PALS) show rapid onset of ecological effects of harmful algal blooms in real time. Sci. Rep. 10, 1–12. doi: 10.1038/s41598-020-74647-z
Scheffer, M., Carpenter, S., Foley, J. A., Folke, C., and Walker, B. (2001). Catastrophic shifts in ecosystems. Nature 413, 591–596. doi: 10.1038/35098000
Schrandt, M. N., and MacDonald, T. C. (2020). Long-term stability of the faunal community of a subtropical estuary: evaluating disturbances in the context of interannual variability. Estuar. Coast. 43, 347–359. doi: 10.1007/s12237-019-00684-1
Sellner, K. G., Doucette, G. J., and Kirkpatrick, G. J. (2003). Harmful algal blooms: causes, impacts, and detection. J. Ind. Microbiol. Biotechnol. 30, 383–406. doi: 10.1007/s10295-003-0074-9
Simon, J. L., and Dauer, D. M. (1972). A quantitative evaluation of red-tide induced mass mortalities of benthic invertebrates in Tampa Bay, Florida. Environ. Lett. 3, 229–234. doi: 10.1080/00139307209435468
Smayda, T. J., and White, A. W. (1990). “Has there been a global expansion of algal blooms? If so is there a connection with human activities?,” in Toxic Marine Phytoplankton, ed. E. Granelli (New York: Elsevier Scientific Publishing), 516–557.
Smith, G. B. (1975). The 1971 red tide and its impact on certain reef communities in the mid-eastern Gulf of Mexico. Environ. Lett. 9, 141–152. doi: 10.1080/00139307509435843
Smith, M. D. (2011). An ecological perspective on extreme climatic events: a synthetic definition and framework to guide future research. J. Ecol. 99, 656–663. doi: 10.1111/j.1365-2745.2011.01798.x
Snelson, F. F., Mulligan, T. J., and Williams, S. E. (1984). Food habits, occurrence, and population structure of the bull shark, Carcharhinus leucas, in Florida coastal lagoons. Bull. Mar. Sci. 34, 71–80.
Sournia, A. (1978). Phytoplankton Manual. Monographs on oceanographic methodology, Vol. 6. Paris: UNESCO.
Sousa, W. P. (1984). The role of disturbance in natural communities. Annu. Rev. Ecol. Syst. 15, 353–391. doi: 10.1146/annurev.es.15.110184.002033
Steidinger, K. A., Burklew, M. A., and Ingle, R. M. (1973). “The effects of Gymnodinium breve toxin on estuarine animals,” in Marine Pharmacognosy, eds D. F. Martin and G. M. Padilla (New York: Academic Press, Inc), 179–202. doi: 10.1016/b978-0-12-474550-6.50011-7
Steidinger, K. A., and Ingle, R. M. (1972). Observations on the 1971 summer red tide in Tampa Bay, Florida. Environ. Lett. 3, 271–278. doi: 10.1080/00139307209435473
Steudel, B., Hector, A., Friedl, T., Löfke, C., Lorenz, M., Wesche, M., et al. (2012). Biodiversity effects on ecosystem functioning change along environmental stress gradients. Ecol. Lett. 15, 1397–1405. doi: 10.1111/j.1461-0248.2012.01863.x
Stevens, P. W., Blewett, D. A., Boucek, R. E., Rehage, J. S., Winner, B. L., Young, J. M., et al. (2016). Resilience of a tropical sport fish population to a severe cold event varies across five estuaries in southern Florida. Ecosphere 7:e01400. doi: 10.1002/ecs2.1400
Tester, P. A., and Steidinger, K. A. (1997). Gymnodinium breve red tide blooms: initiation, transport and consequences of surface circulation. Limnol. Oceanogr. 45, 1039–1051. doi: 10.4319/lo.1997.42.5_part_2.1039
Tester, P. A., Stumpf, R. P., Vukovich, F. M., Fowler, P. K., and Turner, J. T. (1991). An expatriate red tide bloom: transport, distribution and persistence. Limnol. Oceanogr. 36, 1053–1061. doi: 10.4319/lo.1991.36.5.1053
Tester, P. A., Turner, J. T., and Shea, D. (2000). Vectorial transport of toxins from the dinoflagellate Gymnodinium breve through copepods to fish. J. Plankton Res. 22, 47–61. doi: 10.1093/plankt/22.1.47
Van Dolah, F. M. (2000). Marine algal toxins: origins, health effects, and their increased occurrence. Environ. Health. Perspect. 108, 133–141. doi: 10.1289/ehp.00108s1133
Van Straalen, N. M. (2002). Assessment of soil contamination – a functional perspective. Biodegradation 13, 41–52.
Viviani, R. (1992). “Eutrophication, marine biotoxins, human health,” in Marine coastal eutrophication, eds R. A. Vollenweider, R. Marchetti, and R. Vicyiani (Amsterdam: Elsevier), 631–662. doi: 10.1016/b978-0-444-89990-3.50056-0
Waide, R. B., and Willig, M. R. (2012). “Conceptual overview disturbance, gradients, and ecological response,” in A Caribbean Forest Tapestry, eds N. Brokaw, T. Crowl, A. Lugo, W. McDowell, F. Scatena, R. Waide, et al. (New York: Oxford University Press), 42–71. doi: 10.1093/acprof:osobl/9780195334692.003.0002
Walker, B., Kinzig, A., and Langridge, J. (1999). Plant attribute diversity, resilience, and ecosystem function: the nature and significance of dominant and minor species. Ecosystems 2, 95–113. doi: 10.1007/s100219900062
Walters, S., Lowerre-Barbieri, S., Bickford, J., Tustison, J., and Landsberg, J. H. (2013). Effects of Karenia brevis red tide on the spatial distribution of spawning aggregations of sand seatrout Cynoscion arenarius in Tampa Bay, Florida. Mar. Ecol. Prog. Ser. 479, 191–202. doi: 10.3354/meps10219
Wells, R., McHugh, K. A., Douglas, D. C., Shippee, S., Berens McCabe, E. J., Barros, N. B., et al. (2013). Evaluation of potential protective factors against metabolic syndrome in bottlenose dolphins: feeding and activity patterns of dolphins in Sarasota Bay, Florida. Front. Endocrinol. 4:139. doi: 10.3389/fendo.2013.00139
White, P. S., and Jentsch, A. (2001). The search for generality in studies of disturbance and ecosystem dynamics. Prog. Bot. 62, 399–450. doi: 10.1007/978-3-642-56849-7_17
Keywords: disturbance, red tide, resilience, predator, prey
Citation: Berens McCabe EJ, Wells RS, Toms CN, Barleycorn AA, Wilkinson KA and Palubok VI (2021) Effects of Multiple Karenia brevis Red Tide Blooms on a Common Bottlenose Dolphin (Tursiops truncatus) Prey Fish Assemblage: Patterns of Resistance and Resilience in Sarasota Bay, Florida. Front. Mar. Sci. 8:711114. doi: 10.3389/fmars.2021.711114
Received: 17 May 2021; Accepted: 14 July 2021;
Published: 12 August 2021.
Edited by:
Sophie von der Heyden, Stellenbosch University, South AfricaReviewed by:
Andrew M. Fischer, University of Tasmania, AustraliaEls Vermeulen, University of Pretoria, South Africa
Copyright © 2021 Berens McCabe, Wells, Toms, Barleycorn, Wilkinson and Palubok. This is an open-access article distributed under the terms of the Creative Commons Attribution License (CC BY). The use, distribution or reproduction in other forums is permitted, provided the original author(s) and the copyright owner(s) are credited and that the original publication in this journal is cited, in accordance with accepted academic practice. No use, distribution or reproduction is permitted which does not comply with these terms.
*Correspondence: Elizabeth J. Berens McCabe, emccabe@mote.org