- 1Tyndall Centre for Climate Change Research, University of East Anglia, Norwich, United Kingdom
- 2School of Engineering, University of Southampton, Southampton, United Kingdom
- 3Bureau de Recherches Géologiques et Minières, Orléans, France
- 4Department of Civil, Environmental and Construction Engineering, University of Central Florida, Orlando, FL, United States
- 5CITG, Delft, Netherlands
- 6Bournemouth, Christchurch and Poole Council, Bournemouth, United Kingdom
- 7Department of Agriculture and Environmental Sciences, University of Rostock, Rostock, Germany
- 8School of Geography, Faculty of Humanities and Social Sciences, Queen Mary University of London, London, United Kingdom
- 9Department of Civil, Environmental and Construction Engineering and National Center for Integrated Coastal Research, University of Central Florida, Orlando, FL, United States
- 10Department of Estuarine and Delta Systems, NIOZ Royal Netherlands Institute for Sea Research, Yerseke, Netherlands
- 11Coastal Partners, Havant Borough Council, Havant, United Kingdom
Populated coastal areas worldwide have a legacy of numerous solid waste disposal sites. At the same time, mean sea level is rising and likely to accelerate, increasing flooding and/or erosion. There is therefore concern that landfill sites located at and near the coast pose a growing risk to the environment from the potential release of liquid and solid waste materials. This paper aims to assess our present understanding of this issue as well as research and practice needs by synthesizing the available evidence across a set of developed country cases, comprising England, France, Germany, the Netherlands, and the United States (Florida). Common insights gained here include: (1) a lack of data and limited appreciation of waste release from coastal landfill as a potential problem; (2) recognition of the scale and diversity of coastal landfill waste within a range of generic settings (or situations); and (3) a lack of robust protocols that allow the impact of different categories of waste release to the coast to be assessed in a consistent and evidence-based manner, most particularly for solid waste. Hence, a need for greater understanding of the following issues is identified: (1) the amount, character and impact of waste that could be released from landfill sites; (2) the acceptability and regulation of waste eroding from coastal landfills; (3) present and future erosion rates at landfill sites suggesting the need for more monitoring and relevant predictive tools; (4) the full range of possible management methods for dealing with waste release from landfills and the science to support them; and (5) relevant long-term funding mechanisms to address this issue. The main focus and experience of current management practice has been protection/retention, or removal of landfills, with limited consideration of other feasible solutions and how they might be facilitated. Approaches to assess and address solid waste release to the marine/coastal environment represent a particular gap. Lastly, as solid waste will persist indefinitely and sea levels will rise for many centuries, the long timescale of this issue needs wider appreciation and should be included in coastal and waste policy.
Introduction
Our historic use of the coastal zone for the disposal of solid wastes has left a significant legacy, with a large (but unknown) number of landfills worldwide. In Europe alone, it is estimated that there are 350,000–500,000 landfills in total containing wide-ranging wastes – industrial, commercial, domestic, hazardous, and liquid sludge (Hogland et al., 2011). Around 90% of these pre-date modern European legislation pertaining to waste control and landfill (EURELCO, 2019) and significant numbers are located in coastal and alluvial areas prone to flooding and/or erosion (Wille, 2018). In 2019, the US Government Accountability Office reported that nationally at least 945 US “Superfund” waste sites, many of which are municipal solid waste landfills, face increasing risks from climate change effects including rising seas (Grandoni and Dennis, 2019). There is also increasing evidence that extreme flood and erosion events result in the release of large volumes of toxic material to adjacent waters. For example, 13 toxic waste sites in Texas were flooded by Hurricane Harvey in 2017 (USEPA, 2017), while storm-induced failure of the Fox River historic landfill in New Zealand polluted hundreds of kilometers of coastline (JonoB, 2019). In the developed world, improved regulations for landfills combined with waste minimization hopefully mean that new coastal landfill sites are limited or absent, but in the developing world it is almost certain that the volume and legacy of waste in vulnerable coastal areas continues to grow (e.g., Zalasiewicz et al., 2016).
At the same time, we are experiencing a significant global rise in mean sea levels due to human-induced climate change: stringent climate mitigation as proposed in the Paris Agreement will slow but not stop this rise which will continue for centuries (Oppenheimer et al., 2019). Sea-level rise (SLR) will cause a significant increase in a range of coastal hazards, including more flooding and erosion of landfills unless there is appropriate adaptation. There is therefore growing concern that such waste could be released at an increasing rate and pose a significant risk to the coastal and marine environment over the coming decades.
Importantly, while all potential consequences of the release of soluble and solid wastes to the marine environment are unknown, they are of significant concern (Chen et al., 2020). Where legacy or eroded wastes have been examined, organic and inorganic contaminants [e.g., lead, mercury and poly-aromatic hydrocarbons (PAHs)] are present at levels that could cause significant harm to the marine environment (e.g., Pope et al., 2011; Brand and Spencer, 2020), and whilst soluble contaminants released may be much lower (O’Shea et al., 2018; Brand and Spencer, 2020) elevated concentrations of emerging contaminants of concern have been identified in historical landfill leachate decades after disposal (Propp et al., 2021). In addition, a range of other solid waste materials could be released including asbestos, plastics and composite waste materials (e.g., batteries) that would cause physical damage to marine biota through ingestion, abrasion, and entanglement, as well as a reduction in amenity value for people using the coast for recreation. Even without considering SLR, Chen et al. (2020) have estimated that the cumulative plastic waste inputs to the ocean will continue growing due to mismanaged plastic waste (e.g., in open dumps and some landfills) in coastal areas.
Approaches to assessing and managing historical landfills have been considered in a number of countries but appear to vary widely. For example, in the United States, coastal landfills in some states have been ranked with respect to their vulnerability to climate change including SLR, tidal flooding, erosion and increased precipitation to assist the prioritization of remediation efforts (e.g., ADEC, 2015; Asher, 2019). Belgium is considering the possibility of eliminating landfills (through waste relocation and/or landfill mining) in areas prone to flooding (Wille, 2018). However, such national analyses are unusual. A series of recent papers have analyzed this issue for England (Brand et al., 2018; Wadey et al., 2019; Beaven et al., 2020; Nicholls et al., 2020). Given that coastal waste and landfill and the effects of SLR and climate change are a universal problem, and best management practices are lacking, more international exchange of these experiences could be beneficial.
Uncontrolled release of solid wastes to the coast by erosion would seem to be unacceptable, as evidenced by widespread scientific consensus that release of plastics into the marine environment is damaging to marine and human life (Eriksen et al., 2014; Bergmann et al., 2017). There is also widespread public/emotional concern over the issue (Dunn et al., 2020). In addition, waste has a long timescale – many solid wastes are persistent, potentially over geological timescales (Zalasiewicz et al., 2016). While solid wastes may be modified by leaching and degradation within a landfill, the risks associated with the release of solid waste are a long-term problem which will persist and become more widespread because of SLR.
Hence, coastal landfills and the threats of waste release will pose ongoing coastal management questions over the coming decades and longer. This raises fundamental questions such as should waste release be avoided at all costs, or is there an acceptable rate of release, depending on the nature of the waste material? If release is unacceptable how can this be prevented in terms of remediation and/or protection in perpetuity (e.g., Bardos et al., 2020) or relocation of the waste material outside areas subject to flooding and/or erosion? Funding for these issues will generally fall on public funds requiring a budget item that was not appreciated when the landfills were active and is still not fully appreciated today. While there is some guidance on coastal landfill management (Cooper et al., 2013; Nicholls et al., 2018b), there is an absence of protocols that allow the impact of different categories of waste release to the sea to be assessed in a consistent and evidence-based manner, especially for solid wastes. This hinders strategic action and planning on this important issue.
The aim of this paper is to assess the implications of the release of waste materials from landfills into the marine environment, including erosion of solid waste and migration of leachates, and set our current understanding into a wider context, including research and development needs. We use the available evidence and experience in developed country settings, based on case studies in Europe (England, Germany, Netherlands, and France) (Figure 1) and the United States (Florida). First, it presents a generic typology of coastal landfills and waste release that is applicable across all the sites considered. It then reviews the evolution of landfills in the EU, setting the scene for the European case studies that follow. Then the status of landfills with respect to present and future flooding and erosion in each jurisdiction, including linking the analysis to the landfill and release typology. These case studies are heterogeneous by nature, reflecting different levels of awareness, analysis to date and policy responses in each country. This is followed by a cross-nation synthesis and an assessment of the status of coastal landfill management approaches for erosion and flooding. Finally the generic lessons are summarized, including research and development needs.
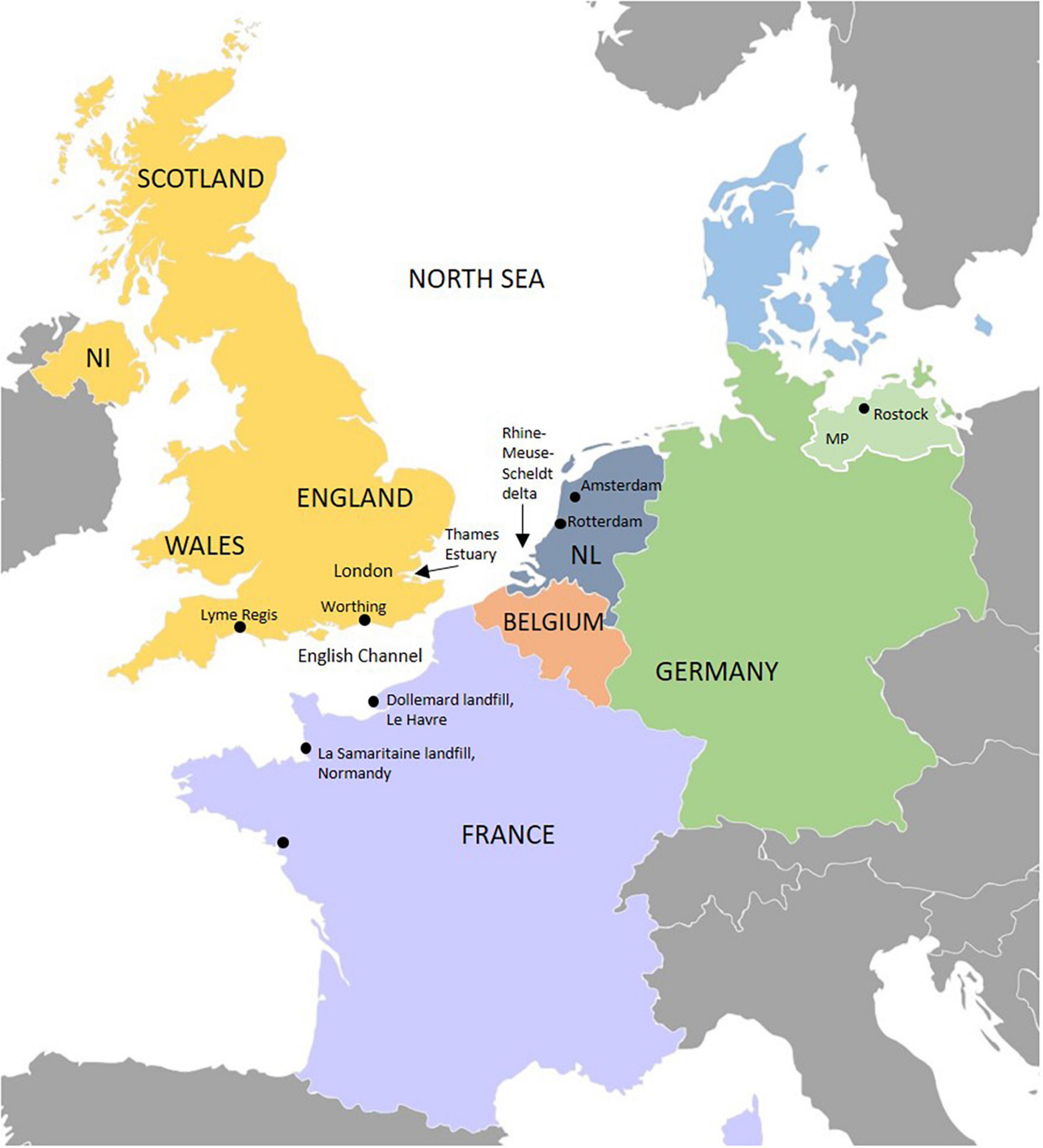
Figure 1. Locations of the case studies in Europe. NI, Northern Ireland; NL, Netherlands; MP, Mecklenburg-West Pomerania.
A Generic Typology of Coastal Landfills and Waste Release
CIRIA guide C718 (Cooper et al., 2013) provides generic guidance on the identification and management of landfill sites and areas of land contamination located on eroding or low-lying coastlines drawing on United Kingdom experience. The guide identified four main situations where wastes or materials could be released from a landfill by erosion or sea flooding (Beaven et al., 2020). Here we expand these to nine situations (A to I) that are broadly applicable across all the case studies considered in the paper. These include the geomorphological setting where the landfill is located and resulting hazards of concern – erosion and/or flooding – and also the presence or absence of coastal protection – undefended versus defended landfill sites (Figure 2). It also recognizes two special cases (H and I) that are relevant to the overall discussion of coastal landfills and waste release. The typology emphasizes the importance of coastal protection on future outcomes, whether the defense is built specifically to protect the waste or not.
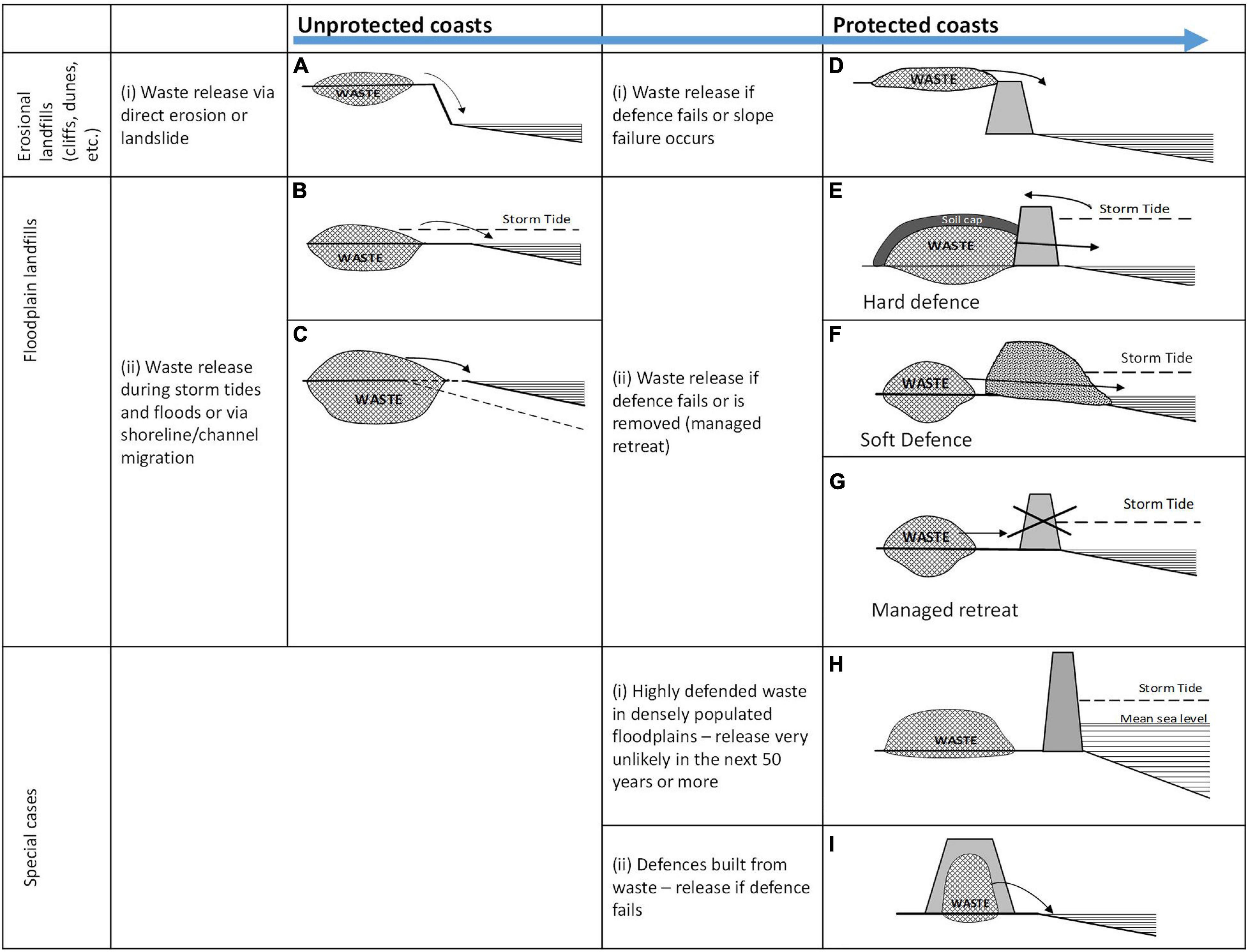
Figure 2. The potential situations recognized in this paper where landfill materials can be released to the sea. The situations labelled (A) to (I) are described in the text.
Explaining Figure 2 in detail, situations A and D occur on erosional coasts where flooding is not a consideration and landfills are situated, for example, on cliffs or in sand dunes. Here, waste can be released by episodic (e.g., individual storms) or chronic erosion. In general, SLR will exacerbate erosion although this is a complex process (e.g., for dunes, de Winter and Ruessink, 2017; for beaches Ranasinghe, 2016; Toimil et al., 2020; for cliffs, Walkden and Dickson, 2008). Landfill is also found widely in coastal floodplains. On undefended coasts (situation B and C), waste can be eroded during episodic events linked to extreme flood events and high tides. Waste release will be more of an issue under ongoing erosion as the shoreline and/or associated channels progressively migrate landward, a process which will be increased by SLR. On coasts with hard defenses, landfill releases depend on the nature and integrity of the defenses. Wastes defended by engineered structures (situation E) can be released by extreme events exceeding (or over-topping) the defenses, defense deterioration, or event-induced structure failure (ranging from minor exposure of waste to complete failure of large sea wall sections). Soft defenses, whether natural or artificial (e.g., beach nourishment), can stop or retard waste release (situation F) although they may still be subject to event-induced failure. The duration of release in these cases will depend on the management response. Deliberate defense abandonment (managed retreat) or removal of structures (situation G) is being actively debated and considered in many locations (Nicholls et al., 2013; CCC, 2018; Siders et al., 2019), although the presence of waste sites often constrains decisions about how best to manage the coast given SLR and in some situations, new defenses are planned to defend waste at sites where otherwise retreat might be preferred (Wadey et al., 2019; Beaven et al., 2020). Two special cases are identified. Many coastal landfills around major urban areas (e.g., London, Netherlands, German North Sea Coast) are protected to such a high standard that landfill erosion is unlikely in the next few decades (situation H). The legacy of this waste will persist into the future as sea levels continue rising and, to avoid release, protection will be needed forever. There are also cases where coastal defenses themselves contain waste, so defense failure will release waste material (situation I) as is the case on the Thames estuary (Brand and Spencer, 2020).
This typology is used to compare the different sites across our case studies, including considering risks, long-term management options and their implications.
Landfilling Within the European Union
Landfills in member states of the EU (which included England and the United Kingdom prior to January 2020) have been subject to the same European Directives that harmonize the regulation, financing, and certain design and operating requirements of landfill. Important Directives include the Waste Framework Directive, which originated in 1975 (EEC, 1975; latest amendment in 2006), and the Landfill Directive of 1999 [as amended in 2018 (EU, 2018)]. The Waste Framework Directive required the consistent regulation of waste disposal across member states, and the use of permits to ensure that waste disposal does not endanger health or harm the environment. Important provisions of the Landfill Directive control the type of wastes suitable for different classes of landfill, stipulate some minimum landfill engineering and technical requirements, and introduced the concept of landfill aftercare during which the operator of the landfill remains responsible for the contents of the site for however, long the site could pose a hazard. The reliance on landfill as a disposal route for wastes in Europe has decreased since the implementation of the Waste Framework Directive and, especially since the 1990s, a major emphasis on waste prevention, recycling, and processing of waste using alternative waste treatment technologies. Many countries have used landfill tax to reduce landfill use (e.g., Scharff, 2014). The landfill tax charge varies considerably across member states, but often now represents the largest component of cost for the disposal of waste in landfill. These policies, together with the change in the nature of wastes produced by society, mean that the composition of waste in landfills has changed over time.
European Directives are implemented through national legislation, which has obviously differed between member states. However, in broad terms landfills across Europe fall into two distinct categories:
(1) Landfills that closed prior to 1996, especially those prior to 1975, which will generally no longer have a permit and are usually referred to as legacy landfills. These are likely to make up the vast majority of the estimated 350,000+ landfills (Hogland et al., 2011). Most of these historical or legacy landfills have no leachate or gas management, or impermeable liners and there is evidence that during historical waste decomposition leachate has been released to the surrounding sub-surface environment where natural attenuation has resulted in localized ‘hot spots’ of sediment contamination (Njue et al., 2012; O’Shea et al., 2018).
(2) Post-Landfill Directive sites that have been constructed to high engineering standards, and will have permits to allow for ongoing long-term regulation. Landfill Directive sites are generally larger than legacy sites.
An English Perspective on Coastal Landfills
There are approximately 20,000 legacy or historical landfills in England. Around 1,200 historical landfills in England are located in coastal settings within the tidal flood zone (0.5% annual probability of coastal flooding) with many clustered around estuaries, large cities and industrial centers (Brand et al., 2018). They have a strong association with designated and environmentally sensitive areas because these areas were once considered low value land and hence suitable for waste disposal. Approximately 3,400 ha of landfill is at risk of flooding (0.1% probability or greater) (CCC, 2018). Many of these historical landfill sites are defended and in south east England there are a number of waste-filled coastal defenses such as along parts of the Thames estuary (Situation I, Figure 2).
Wadey et al. (2019) analyzed in detail the Central English Channel Coast (Lyme Regis to Worthing, Figure 1), a region where there is a concentration of coastal landfill sites which contain a mixture of waste types. By intersecting flood and erosion hazard data with historical landfill locations, they identified 144 historical coastal landfills covering 22 km2 and occupying 86 km of shoreline length. About 89 sites are considered at risk of coastal erosion today (i.e., the landfill intersects with the present shoreline), while 136 landfills are in the coastal floodplain. As sea levels rise and the shoreline retreats, these sites are increasingly at risk of leakage, along with new sites further inland. Most landfills are in relatively low energy sites, mainly estuaries, with less than a quarter of landfills at sites exposed to larger waves. The predominant land use for these low energy sites is recreation and open space, with some commercial and/or industrial activity. However, about 6,500 residential properties are located on areas of historical landfill, with 4,400 of these in Portsmouth where substantial new defenses are being built over the next 10 years. The majority of the landfills are located on publicly owned land (local authority) and through Shoreline Management Plans (SMP) have a ‘Hold the Line’ (HTL) policy (Beaven et al., 2020). This is aspirational, however, as central government funding is not guaranteed unless there are additional benefits, particularly protection of other properties. As a regional illustration of the scale of potential costs involved, removal of all 144 threatened landfills to ‘safe’ locations is estimated to cost at least £4.3 billion (€4.9 billion; 2019 prices) based on landfill tax, with additional and substantial costs for excavation and transport. Alternatively, to defend the 80 ‘higher priority’ sites which are at risk of flood and coastal erosion over the next 100 years, would cost roughly £150 million (€170 million; 2019 prices), an order of magnitude less than the £1.3 billion (€1.5 billion; 2019 prices) estimate to remove this same waste.
Wadey et al. (2019) also assessed seven locations containing 13 distinct landfill areas in more detail (Figure 3A and Table 1). The survey underlined the complexity for future management with most landfills being situated behind natural and/or artificial defenses with a desire to continue to protect them, but no certainty of funding being available. Detailed analysis for three of these sites, also drawing on Beaven et al. (2020), illustrate the challenges (Figure 3).
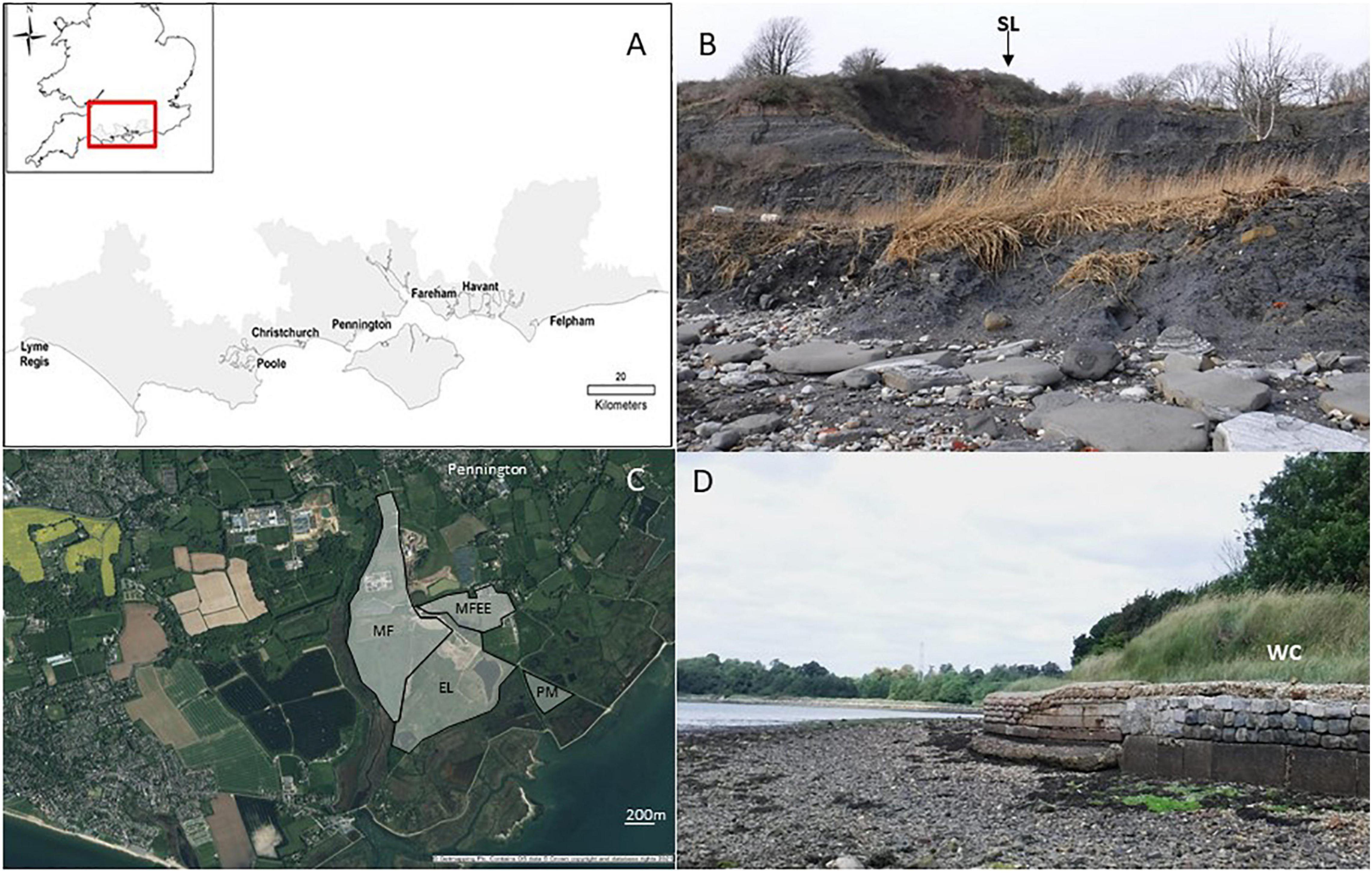
Figure 3. (A) Location of study sites in England, highlighted is the region assessed by Wadey et al. (2019) and the locations of the case study areas referred to in Column 1 of Table 1. (B) View from the beach below Spittles Lane landfill site (SL), Lyme Regis. (C) Aerial photograph showing the location of Pennington Marshes landfill (PM), and the larger more landward landfill complex at Pennington comprising three landfills –EL, Efford Landfill; MF, Manor Farm Landfill; and MFEE, Manor Farm Eastern Extension. © Getmapping Plc. Contains OS data. © Crown copyright and database rights 2021. (D) View showing the informal coastal defenses of Wicor Cams landfill (WC) and foreshore, Fareham. Photographs B and D courtesy of Anne Stringfellow.
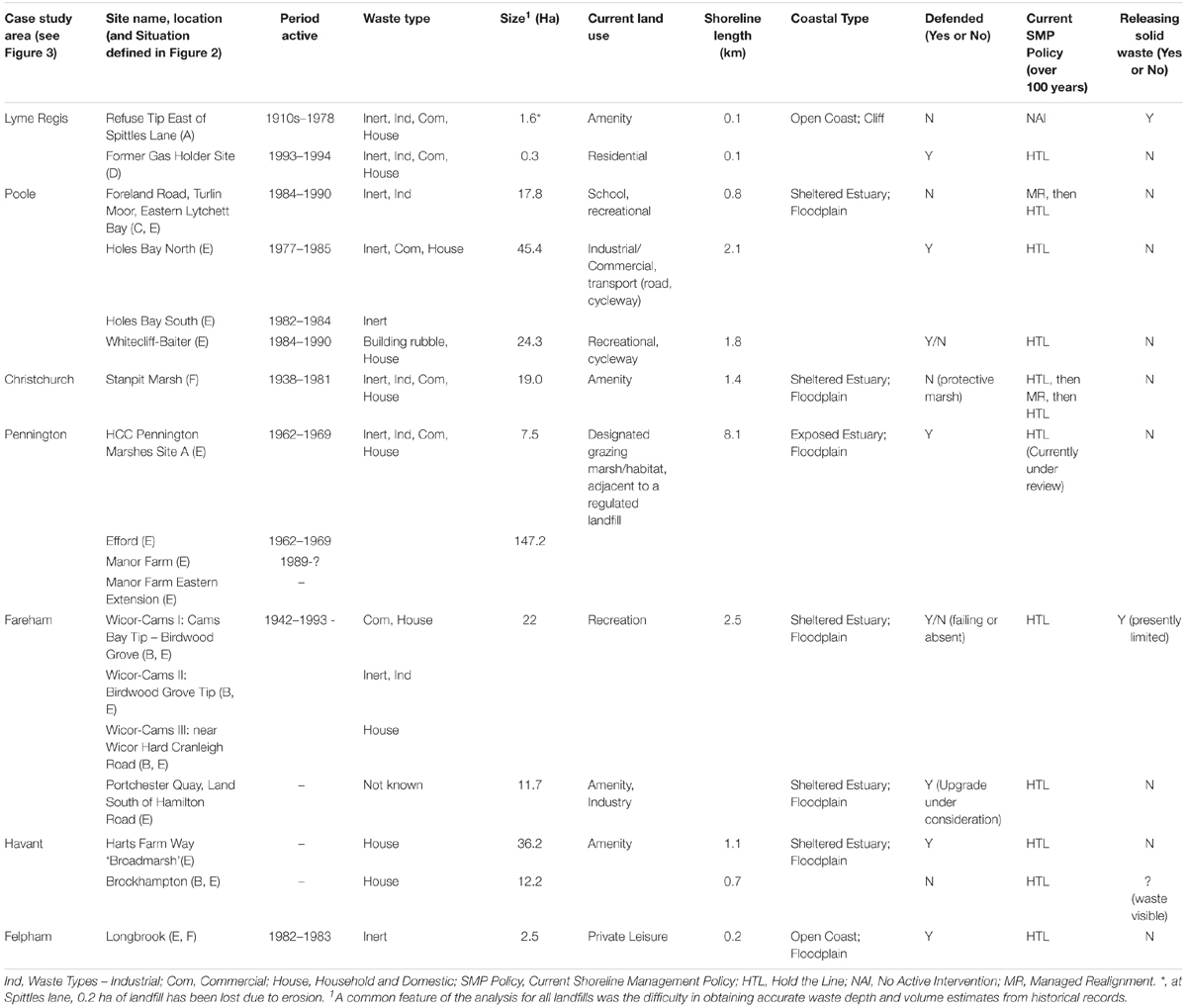
Table 1. Selected case studies of coastal landfill sites on the central south coast of England [adapted from Wadey et al. (2019) with additional information for Spittles Lane, Pennington and Wicor Cams from Nicholls et al. (2018b) and Beaven et al. (2020)].
The Spittles Lane landfill site at Lyme Regis (Figure 3B) is located on a 50 m high eroding cliff top where the cliff is prone to large-scale landslides, weathering, and surface and toe erosion (situation A, Figure 2). Average retreat rates are uncertain and in the range 0.3–3 m/year at present (Beaven et al., 2020). In 2008, a 400 m long stretch of cliff collapsed, releasing waste from the landfill to the cliff face and ultimately the beach below. The release of waste raised concerns of potential contamination and pollution. While an assessment found no significant contamination of controlled waters, lead and PAHs were found in the sediments together with fragments of asbestos or asbestos-containing materials which were attributed to the waste. Frequent beach inspections were established due to the risk assessment, and waste materials removed as necessary to prevent harm to beach users. No protection is planned at the site, so it is almost certain that the whole landfill (estimated to be 50,000 tonnes; Nicholls et al., 2018b) will erode into the sea over decades or longer (timescales are highly uncertain). Management options are limited due to the active erosion. Removal of the in situ waste and geotechnical stabilization of the site is one approach that could be considered, but these works could destabilize the cliff and increase the risk of further landslides limiting full consideration of the approach. Hence, monitoring and removal of these items at beach level appear to be the only practical steps available to limit harm to beach users. Given the long-term outlook of complete release of the landfill to the marine environment over many decades or longer, this approach will need to be sustained. The potential for adverse public reaction after a major waste release should also be considered.
There are four historical and authorized (permitted) landfills at Pennington to the southwest of Lymington, Hampshire (situation E, Figure 2). These sites lie on low-lying land (collectively covering some 500 ha) adjacent to important designated habitat and landward of substantial coastal defenses exposed to high energy wave action. The most seaward historical landfill – Pennington Marshes landfill – occupies a triangle of land to the south-east of the main landfill complex (Figure 3C). SLR is reducing the level of protection offered by the seawall, while the protective saltmarsh fronting the seawall is also rapidly eroding and has completely disappeared on much of the frontage. The SMP policy is HTL for the next 100 years. To prevent flooding, the seawall will need to be raised and widened. Capital costs were estimated to range from £42 to £97.5 million (€48 to €111 million) under low to extreme high SLR scenarios (up to 1.5 m rise by 2100) (Nicholls et al., 2018b), with additional maintenance costs. Alternative management options include ‘Managed Realignment’ and the removal of the Pennington Marshes landfill. The former option would allow the sea to access the currently protected zone expanding the intertidal area, although habitat compensation would still be required showing the complexity within these choices. A new seawall could be relocated inland on higher ground, but the main, much larger Pennington landfill complex (Figure 3C) would still need to be defended to prevent erosion of waste. The capital cost of this option is reduced by 20–50%. If a ‘No Active Intervention’ policy was adopted the seawall would eventually breach. Pollution could be mitigated by removing the Pennington Marshes landfill, costing £10 to £21 million (€11 to €24 million), depending on the rate of landfill tax incurred (Nicholls et al., 2018b).
The Wicor Cams landfill complex, Fareham (situation E, Figures 2, 3D) is situated on a low wave energy, estuarine environment adjacent to designated habitat. There are three landfills (Table 1); the last closed in 1993, and the site has been restored to recreational open space. The landfills are partly protected by informal coastal defense structures such as concrete sandbags or rock, while some landfill waste is unprotected and visible. The preferred SMP policy is HTL. With SLR, erosion is likely to release landfill without upgrading defenses. Defense costs were estimated to be up to £3 million (€3.4 million), although there are no clear funding avenues to implement the preferred SMP policy. An alternative approach would be to remove the landfill. Cost estimates range from £70 to £140 million (€80 to €160 million) depending on the rate of landfill tax paid, meaning that the removal of waste is not financially viable (Nicholls et al., 2018b).
These national, regional and local assessments of the historical coastal landfill in England indicate that it is, and will continue to be, a major issue which requires significant investment. Currently, there is insufficient funding to either manage these issues using conventional approaches or develop and test innovative management approaches.
A French Perspective on Coastal Landfills
About 1,000 French municipalities are located close to the coast, and each of them owns at least one landfill. A first inventory of old landfills was financed by the French Environment Agency (ADEME) in the 2000s in order to assess the potential risks for ground and surface water, for the environment (e.g., impacts on landscape) and for human security (e.g., potential for landslides, etc.), as well as to assess the need for rehabilitation works. All these sites are in the BASIAS database (Basias-Georisques, 2021), the inventory of old industrial activities in France. Rehabilitation typically consists of preventing infiltration of rainwater by adding impermeable layers, conducting geotechnical works where needed, and landscaping. Hence, the wastes remain in situ. However, as in other countries, this national inventory remains incomplete. For example, the exact landfill location is often unknown, which prevents assessment of those located in erosion or flood prone areas. Furthermore, the surface area and/or volume of wastes, and their nature (household, inert, industrial, asbestos, etc.) are often poorly documented.
Coastal erosion and major storm surge events such as Xynthia (2010) (e.g., Lumbroso and Vinet, 2011) have exposed a few historical landfills on the French coast (Ouest France, 2014; La Presse de la Manche, 2020). These extreme events led public authorities to conduct emergency works such as reinforcement and waste containment, and then assess and ultimately rehabilitate the situation. Yet there is no specific consideration of the effects of SLR on coastal landfills over the 21st century and beyond. Adaptation in France is currently limited to informing coastal risk prevention plans with the impacts of a 60 cm SLR scenario by 2100 in order to prevent further urbanization of hazard-prone areas (Le Cozannet et al., 2017). A specific guide for coastal landfill is being prepared by BRGM and the Environment Ministry.
While coastal landfill erosion and flooding is expected to occur at multiple locations in the future, it is also already happening at a few sites. Two case examples are outlined here: (a) La Samaritaine landfill, Lingreville, and (b) Dollemard landfill, Le Havre, both in the Normandy region (Figure 1) and reflecting situation A (Figure 2). Waste release by chronic erosion, the willingness of the authorities to restore the coastal landscape, and societal pressure for restoration meant that removal of the landfill was the preferred solution in both cases.
La Samaritaine landfill (EPF Normandie, 2018) was located close to the shoreline in sand dunes (Figure 4) near the natural harbor of La Venlée in Lingreville municipality on the west coast of the Cherbourg Peninsula. It was a municipal landfill from the 1960s to the 1980s, then decommissioned and buried with sand in the 1990s. The landfill was then forgotten, not even recorded in the national database of landfills, until waste was uncovered due to chronic erosion of about 2 m/year of the sandy shoreline in the 2010s (Figure 4A). In November 2016, a storm accelerated the erosion of the waste and a temporary riprap and waste containment were installed in an attempt to prevent further release (Figure 4B) (La Presse de la Manche, 2020). To find a permanent solution, the owner of the site, the French Coastal Conservation agency, supervised the removal of 14,000 m3 of waste mixed with sand from November 2017 to February 2018 (Figure 4C) (Ouest France, 2018). In this case, the ultimate aim was to restore the recreational and landscape value of the site, which is classified as Natura-2000. The main rehabilitation work involved sifting the sand for reuse in situ (4,000 tonnes; Les Champs Jouault, 2019), conducting a post-excavation diagnosis, sending the wastes to an inland landfill (12,652 tonnes; Les Champs Jouault, 2019) and restoring the site by filling with clean sand. The main difficulties encountered during the rehabilitation were adverse weather conditions, as a winter storm accelerated the erosion and rainfall slowed the sifting of the sand. Furthermore, asbestos was discovered in the waste requiring additional processing. The cost of this rehabilitation work was about €1.6 million, which was funded by public agencies and the administration (EPF Normandie, 2018). This includes the French landfill tax at €35/tonne in 2020.
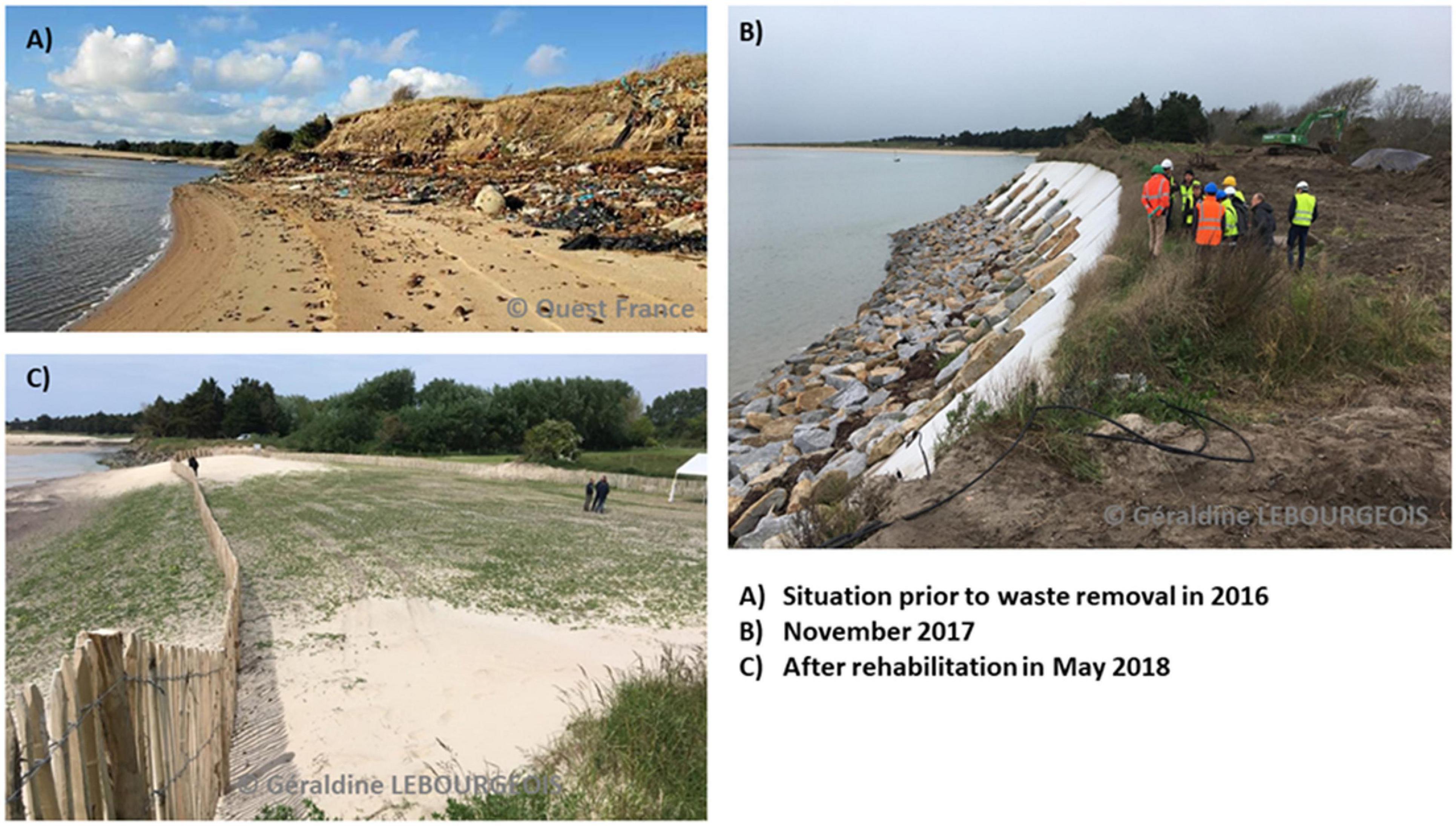
Figure 4. La Samaritaine landfill (A) prior to waste removal in 2016, (B) during waste excavation (November 2017) with erosion being stopped by riprap and a geotextile, (C) the restored site (May 2018). Photography (A) courtesy of Ouest-France archives, (B,C) courtesy of Geraldine Lebourgeois/La Presse de la Manche.
The Dollemard landfill is part of a set of landfills which have been receiving construction and demolition waste since the 1960s. It is located north of Le Harve on top of 90 m high eroding cliffs composed of chalk and other materials (Figure 5). As at Spittles Lane (England), retreat of the cliff top allowed waste to be progressively released to an unstable vegetated slope at the toe of the cliff, which is difficult to access. This unstable slope is eroding at approximately 1–2 m/year, so the waste ultimately reaches the beach/sea. The total amount of waste is estimated to be 200,000 m3 (SCE, 2012), predominantly comprising inert waste (concrete, stone, brick), mixed with other wastes such as metals (steel bars), plastic and rubber. The volume of plastic and metals migrating from the unstable slope to the beach is estimated at about 30 m3/year, and this causes visual pollution on the coast, plastic pollution in the sea, as well as risks of injury for walkers (SCE, 2012). These issues were identified in the 1980s, but landfilling of waste continued until 2000 (SCE, 2012). Since 2009, the Le Havre municipality has funded an association to conduct cleaning operations at beach level (1–2 tons/year, €22,000/year). The scenarios available to manage this landfill in the long term include: (1) removal of the landfill, including excavation on the top of the cliff, transportation and treatment of all wastes (as at La Samaritaine), (2) confinement of the wastes with riprap or other coastal defenses, and (3) continue regular manual cleaning along the shoreline over the next 40 years. The costs of the removal action were estimated at €20 million (including landfill tax) over a 10–15 years work span, against €5 million for waste confinement, and €2 million for sustained cleaning. Despite its higher costs, the waste removal option has been chosen (Le Parisien, 2020) funded by the French Environment Agency ADEME (70% of the budget), City Council (20%) and the Water Agency (10%). A demonstration of the feasibility of waste removal and treatment was conducted in 2020 (Figure 6). Equipment and personnel reached the site by barge, wastes were removed from the cliff in big-bags by helicopter, transported by truck to a waste treatment center, separated into recyclable waste (mostly metals and inerts) and the residual waste landfilled. This demonstration also provided an improved assessment of waste characteristics.
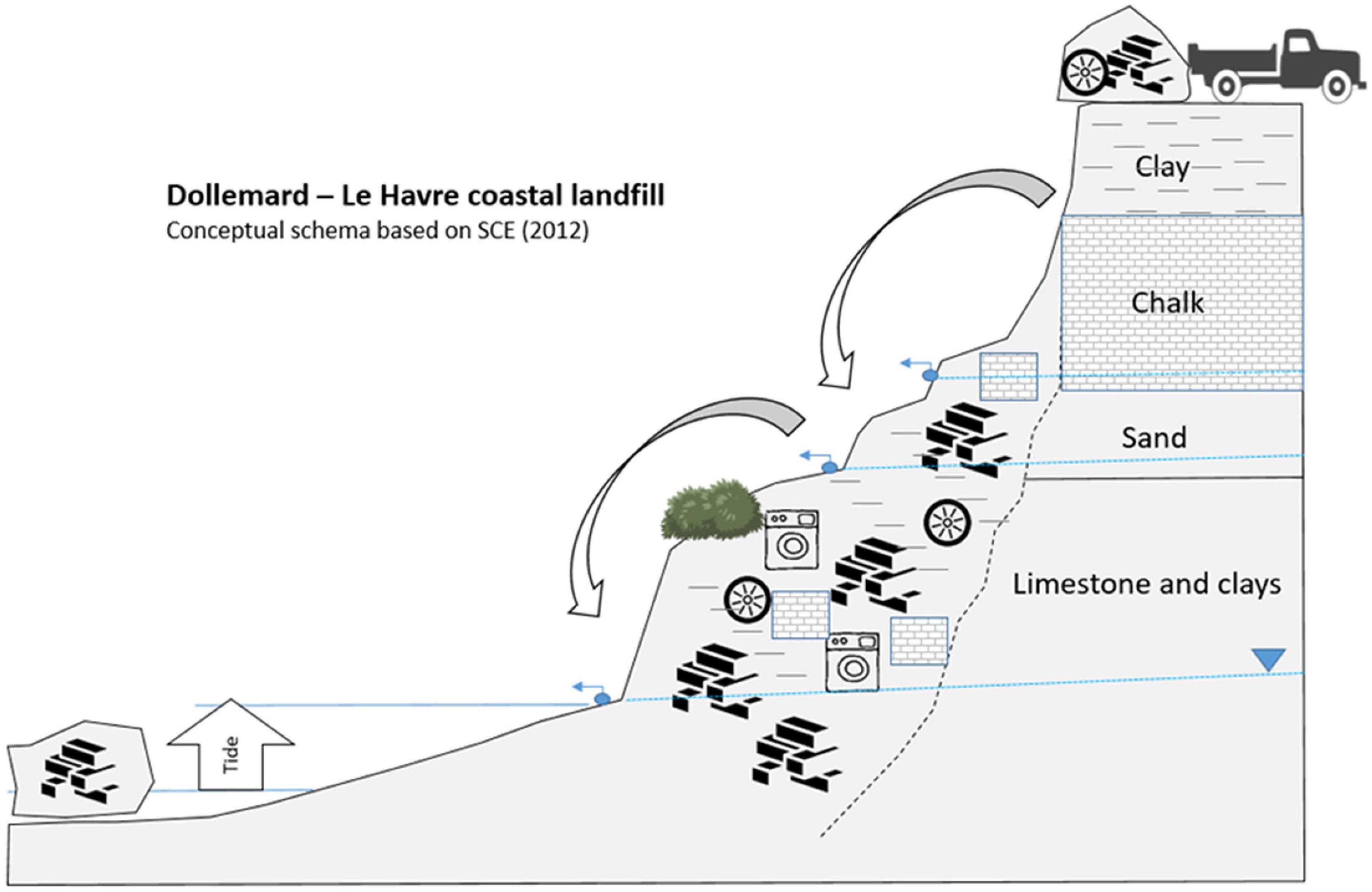
Figure 5. Conceptual cross-section of the Dollemard landfill in Le Havre (France). Adapted from SCE (2012).
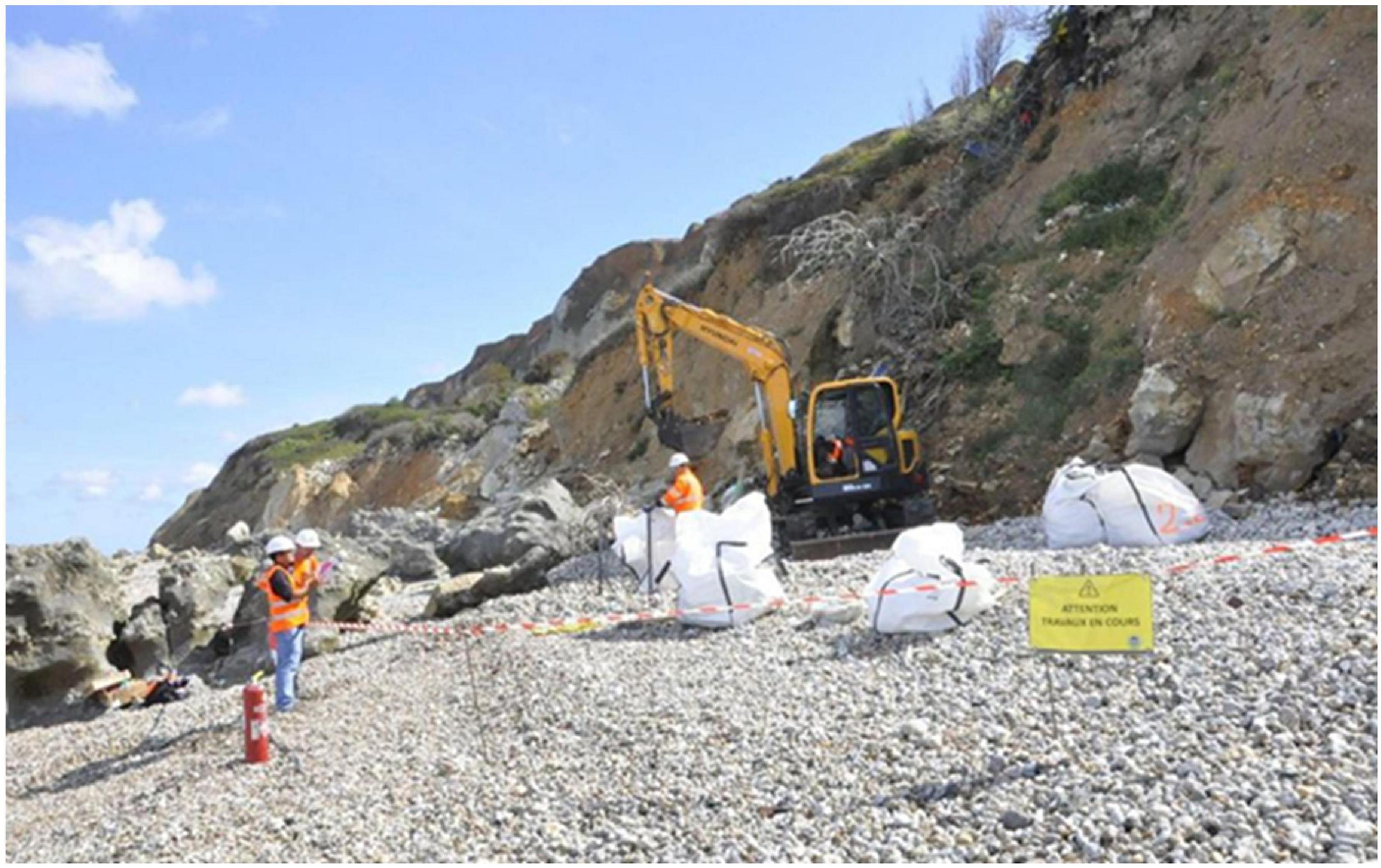
Figure 6. Trial waste removal at the Dollemard landfill (summer 2020). Photograph courtesy of Le Havre council.
In both these cases, the total amount and the nature of waste was not precisely known until the landfill began to rapidly erode (around 2 m/year). Funding of the remediation was difficult, especially at La Samaritaine, as no organization was willing to fund the entire costs. There, the 2016 storm and the potential for greater release of waste to the sea was key to stimulating a decision, and the operations were ultimately funded by a partnership of state agencies (Water Agency), the region, the department and the municipalities. Although removal was the selected option for both case studies, removal is not a national policy in France and other options were considered for the Dollemard case. These case studies highlight the need to anticipate better where and when other French coastal landfills might be flooded or eroded as sea level rises, so that more proactive management approaches can be followed.
A German Perspective on Coastal Landfills
Currently, 1,027 landfills are in active operation in Germany, of which 849 are landfills for excavated soil or inert waste and 213 sites receive hazardous wastes (DESTATIS, 2021). These numbers contrast with the vast legacy of nearly 67,000 inactive (i.e., former) waste disposal sites (LABO, 2018). Germany comprises a federal republic of 16 states, each being responsible for its own waste management. Five federal states (Hamburg, Bremen, Lower-Saxony, Schleswig-Holstein, Mecklenburg-West Pomerania) are potentially affected by coastal flooding, erosion and SLR. There is no systematic assessment on the number or size of active or inactive landfills directly on Germany’s coastline and few active landfills are situated near the coast. Further, unlike in England and France, there are no reports of landfill waste being released to the marine environment. The extensive and continuous flood defense systems along the North Sea coast suggest that waste release is unlikely (Sterr, 2008), while on the Baltic coast there is more variability in terms of both geomorphology and presence of coastal defenses (van der Pol et al., 2021). For legacy sites, targeted information on the number close to the coast is also not available; although more information could be harvested from GIS data on location and local topography, which is available for some states. Readily available data, published on the websites of the respective five federal states, report the overall number of landfill sites as:
• Hamburg: No specific data on old waste deposits, but 1,099 confirmed contaminated sites (Hamburg, 2021)
• Bremen: 141 old waste deposits (Bauumwelt Bremen, 2021)
• Lower Saxony: 10,508 old waste deposits (LBEG Niedersachsen, 2021)
• Schleswig-Holstein: 3,023 old waste deposits (Schleswig-Holstein, 2021)
• Mecklenburg-West Pomerania: 2,400 old waste deposits (Regierung MV, 2021).
Note that in Germany the risk of flooding and the effects of erosion on landfills may also arise along rivers where climate change may increase peak flows. In Austria for example, Laner et al. (2009) showed that around 30% of the old waste deposits are located in areas prone to river flooding once every 200 years, indicating the potential scale of the problem.
As an exemplar, we consider the Hanseatic City of Rostock (Figure 1), which is one of 294 counties in Germany. With around 200,000 inhabitants and a land area of around 181 km2, Rostock is the largest city in Mecklenburg-West Pomerania (MV) and is located where the Warnow (the largest river in MV) drains into the Baltic Sea. There are no active landfill sites. However, the inventory of potentially harmful soil changes, contaminated sites, and suspected contaminated sites for Rostock contains more than 3,550 historical sites, including 350 locations where waste and harmful substances have been deposited (e.g., landfills, including illegal sites). The majority of these sites have been assessed and 57 landfills are defined as contaminated, or sites that are still suspected of being contaminated.
We investigated the flood risk for these historical landfills. Landfills potentially flooded in a 200-year event (2.3 m water level; StALU MM, 2012) today are identified considering local topography assuming a simple first-order bathtub method and assuming that the existing coastal defenses are removed. As all the relevant landfills are inside the flood protected areas, they are all presently protected to design level or even higher with heights between 2.8 and 3.0 m. Mean SLR projections by 2100 associated with two Representative Concentration Pathways, RCP4.5 and RCP8.5 emission scenarios (Church et al., 2013), are added linearly to estimate the 200-year return levels by 2100 (2.78 and 3.07 m, respectively) and again potentially flooded landfills are identified using the same assumptions. Today, nine individual sites (16% of the contaminated locations) in Rostock are below the 200-year return water level. Under the RCP4.5 and RCP8.5 scenarios, current design levels are exceeded and the number of potentially affected landfills increases to 26 and 27 sites (64 and 47%), respectively. Assuming defenses continue to be upgraded for SLR, the risks to 2100 still appear low.
More generally, around 70% of the German Baltic Sea coast is affected by erosion, with recession rates of 34 m in 100 years, on average (Gurwell, 2008). All the landfills investigated here are situated at least 300 m inland of the open coast, so erosion is not expected to affect them appreciably over the coming decades. Nonetheless, given the long timescales of landfill waste degradation, more detailed assessments are worthwhile considering sites where release is most likely, as well as exploring the long-term implications for coastal management and defense.
A Perspective on Coastal Waste and Landfills From the Netherlands
The Netherlands is located in the low-lying delta of the Rhine, Meuse and Scheldt rivers which flow into the North Sea (Figure 1). The elevation of around half of the Netherlands’ surface area does not exceed 1 m above mean sea level and around one third of the country lies below sea level. This region is also the most densely populated area in north-western Europe, home to the megalopolis formed by the county’s main cities including Amsterdam and Rotterdam (Dutch: Randstad), and protected from flooding by a system of dike rings (Priemus, 2018). For centuries, land reclamation, continued pumping of water, and heightening of strategic areas have enabled human settlement and economic activities (Van Koningsveld et al., 2008; de Moel et al., 2011).
Next to the rise in mean sea level, threats of flooding induced by climate change include increased storm surges and increased river discharge (Katsman et al., 2011). Effects are aggravated by land subsidence rates of up to 5–8 mm/year (Hoogland et al., 2012). However, intrusion of saltwater, resulting from SLR in combination with lower river discharge during summer, is currently projected to threaten freshwater supplies from ground and surface waters more than direct flooding (Kwadijk et al., 2010). Coastal defenses in the Netherlands include the six key storm surge barriers such as the Eastern Scheldt and the Maeslant Barrier, dikes, flood protection walls, but also the coastal dune belt. Protection against coastal erosion is based on the principle of dynamic preservation of the sediment balance (Van Koningsveld and Mulder, 2004) by adding sand nourishment of the order of 12M m3 annually. Options for adaptation to SLR in the Netherlands include additional/upgraded storm surge barriers, strengthening of dikes and dams, enhanced sand nourishment, increased pumping, construction of flood-proof buildings, land reclamation, and planned (or managed) retreat (Haasnoot et al., 2020).
A direct result of the dense settlement and the intensive economic activity in the Dutch delta is the multitude of former or legacy landfills, which are defined as landfills that ceased operation before September 1996. The base of most of these sites is located below current sea level and approximately 25% are in direct contact with the groundwater as backfills, for example, of sand or gravel pits. Post-medieval coastal defense structures in the Netherlands usually do not contain waste materials, although the filling of waste into concrete blocks for coastal defense structures has been considered in the recent past (Land+Water en Milieumarkt, 1994). The location of Dutch waste disposal sites therefore mostly corresponds to the situation depicted by Figure 2, situation H. A specific legal framework for legacy landfills is missing and competent authorities act on the basis of the Soil Protection Act and provincial environmental regulations. Information on type and volume of landfilled material is not available at a national level as records reside with the provincial or municipal authorities (Lieten, 2018) and, for around 10% of these legacy sites, information on their specific location is missing. In the 1990s, the Dutch government commissioned the NAVOS project (Dutch: Advies Nazorg Voormalige Stortplaatsen, “Advice on aftercare of former landfills”), which carried out a comprehensive inventory of the estimated 4,000–6,000 Dutch legacy sites (Tauw B V, 2016; Lieten, 2018). These sites, of which half are smaller than 0.6 ha, comprise an estimated surface area of 8,000 ha and possess neither bottom nor surface sealing systems. The absence of sealing components effectively means that the waste body has been leached for decades. The objective of the study was to assess the related environmental impacts, regulatory deficiencies and societal problems in order to estimate the scope, organization and financial implications of necessary aftercare or remediation measures. The assessment was based on historical data of waste composition, visual inspection of the cover soil, quality of the groundwater in the sites’ vicinity and size of the affected plume based on a limited number of monitoring wells positioned on an assumed principal groundwater flow direction.
With respect to the condition of the landfill cover, it was found that in about 90% of cases the cover did not fulfill the regulatory thickness requirements of the Soil Protection Act. With respect to groundwater, the study concluded that for 75% of the sites the downstream groundwater quality was degraded, restricting its use, for example, for agricultural purposes. However, it was also seen that in some provinces, metal contamination in groundwater was more related to the elevated background geological conditions than to the landfill site. Furthermore, investigations into the natural attenuation potential concluded that in 70% of the cases the contamination was not spreading. Hence, it was judged that those landfills did not pose a significant environmental risk. In the remaining 30% of cases, further monitoring and possibly remediation measures were deemed necessary.
There are approximately 70 modern engineered landfills, with 19 of these sites still in operation (Lieten, 2018). All these landfills are constructed on elevated areas, meeting the distance between the bottom liner and the location-specific highest free groundwater table as required in the European Landfill Directive. Not all, or not all individual landfill cells, however, are equipped with a combination base liner [high-density polyethylene (HDPE) membrane underlain by a mineral sealing layer].
The Dutch sustainable landfill project (iDS), enabled by a Green Deal between the Dutch competent authorities and Dutch landfill operators, comprises full-scale pilot stabilization projects by aeration and leachate recirculation at three closed landfills (Lammen et al., 2019; Dutch Foundation for Sustainable Landfilling, 2021). This project is at the forefront of international efforts to solve the long-term legacy issues relating to leachate and landfill gas emissions but does not consider the excavation and relocation of solid waste.
Experience with landfill mining in the Netherlands is limited. The projects carried out were motivated by property redevelopment, by gain in landfill capacity and by the need for remediation including installation of a bottom lining system, rather than risks posed by flooding or coastal erosion. However, the main hurdles to economically successful mining projects were found to be contamination with asbestos, availability of effective mining and separation techniques and the need to pay landfill tax for re-disposal of the excavated non-reusable or recyclable wastes (Lieten, 2018).
In view of the large uncertainties related to prediction of SLR, the Netherlands follow the adaptive Delta program 2021 (National Delta Programme, 2020), initiated in 2010 and updated annually, which strives to keep the Netherlands as the ‘best protected river delta in the world’. Every 6 years, short- and long-term strategies for coastal and flood defense are reviewed and adapted based on predefined tipping points. The Delta program aims at: (1) protecting the country against flooding, now and in the future; (2) ensuring sufficient freshwater supplies; and (3) climate-proofing the country’s spatial planning using the full range of adaptation options. Continued waste and landfill management in compliance with European, national and provincial regulations, are inevitably included in these aims and the corresponding actions, although not explicitly addressed in the Delta program. Therefore, it is not foreseen that SLR will motivate excavation, treatment or re-disposal of wastes in order to minimize environmental impact. This would only be needed if a planned retreat strategy is ultimately implemented triggered by large and rapid rises in sea level (cf. Olsthoorn et al., 2008; Haasnoot et al., 2020). To prepare for such an eventuality, the most obvious step would be to re-visit the assessment of the risk to the groundwater quality emanating from legacy landfills and from modern landfills lacking a bottom liner in light of increased saltwater intrusion. Possibly, the outcome might suggest future investment in surface sealing systems. The country’s engineered landfills that are protected by dual-component bottom liners pose no concern in this respect. The clay component in the mineral layer may suffer from dispersion effects due to exchange of polyvalent cations with sodium; the functionality of the second component, the HDPE liner, should however, not be affected by increased groundwater salinity. In the unlikely event of a severe flooding disaster occurring because of failing flood defenses, the contribution of the former landfills in the region to environmental damages will be relatively small compared to those caused by all other anthropogenic contaminant sources.
A Florida Perspective on Coastal Waste
Similar to the European examples discussed in the previous sections, thousands of active and inactive landfills are located in coastal counties around the United States, which is where nearly 40% of the population reside (NOAA, 2013). Amongst all 50 states, Florida is often considered most vulnerable to the effects of SLR with several population hotspots like Miami and Tampa/St. Petersburg listed globally in the top 10 among large coastal cities in terms of present and future flood risk (Hallegatte et al., 2013). Therefore, we focus here on Florida, but the general conclusions apply in similar ways to other U.S. coastal regions (as shown for Texas; Kiaghadi et al., 2020).
In addition to a high population density along its coastline, Florida’s low-lying topography and geology, comprising porous limestone on top of bedrock, make the state susceptible to flood impacts. High-tide (or nuisance) flooding events already occur regularly in coastal cities like Miami and the number of events and places affected are projected to increase substantially even under moderate SLR scenarios (Sweet et al., 2018). Florida also lies in the paths of tropical cyclones, which can produce dangerous storm surges and waves and associated flooding and beach erosion, as experienced, for example, during Hurricanes Irma (in 2017) and Michael (in 2018).
Design and construction of municipal solid waste and hazardous waste landfills in the U.S. is regulated by Subtitle D and C, respectively, of the Resource Conservation and Recovery Act (RCRA) initially passed in 1989. Subtitle D requires composite liners with a minimum of 61 cm of 10–7 cm/s clay underlying a 1.5 mm HDPE membrane. The U.S. Environmental Protection Agency (USEPA) states that “landfills in 100-year floodplains must demonstrate that the unit will not restrict the flow of the 100-year flood, reduce the temporary water storage capacity of the floodplain, or result in washout of solid waste so as to pose a hazard to human health and the environment.” However, prior to the RCRA, few if any regulations on landfill location existed. The legacy landfills in coastal areas are therefore of particular concern in terms of being affected by SLR, coastal flooding and erosion.
The Florida Department of Environmental Protection (FDEP) has been delegated by the USEPA to regulate landfills in the state following RCRA Subtitle D. Because of the geological conditions outlined above, the excavation depths for landfills are relatively shallow and they are elevated, instead of below ground. Hence, landfills in Florida are often landmarks and high points in the landscape. The FDEP Solid Waste Facility Locator shows over 10,000 sites in the state, including closed (the vast majority) and active municipal solid waste and construction and demolition debris landfills (95 at present), transfer stations, tire dumps, etc. This also includes disaster debris management sites used as intermediate staging areas in the wake of natural or man-made disasters. Hurricane Irma in 2017, for example, created up to 22M m3 of debris across the state of Florida, as estimated by the U.S. Army Corps of Engineers (Florida Counties Foundation, 2021). Hurricane Maria in the same year produced 4.7M m3 of debris (Kennedy and Migaki, 2017) overloading Puerto Rico’s existing landfills, so that soccer fields and grounds of closed public schools had to be used as interim storage sites; the heavy rain also left many landfills uncovered. These examples highlight that dealing with natural disaster debris is a recurring issue (as outlined in USEPA, 2019) that will escalate as the frequency and magnitude of these events is likely to increase due to SLR and global warming in the case of tropical storms.
In the FDEP database, we identified 8,082 individual locations in terms of latitude and longitude information (sometimes multiple facilities or components are at the same location). Of these, 3,026 are located within the Federal Emergency Management Agency’s (FEMA) 100-year flood hazard zone, where the chance of experiencing flooding is at least 1% in any given year; 97 are in the V zone and 2,929 in the A zone. Sites located in the A zone may be affected by inland flooding or coastal flooding (or both), while the V zone is a particular case within the A zone, designating higher risk areas from storm surge flooding and wave impacts, potentially leading to erosion. Focusing only on the 473 sites categorized as Class I, II, or III municipal landfills [classified based on the amount of solid waste received daily, following Florida Administrative Code Rule 62-701.340(3)], 143 are within FEMA’s 100-year flood hazard zone (3 in the V zone, 140 in the A zone).
While FEMA’s flood zones are derived from extensive hydrodynamic numerical modeling and provide useful information on contemporary flood risk for given exceedance probabilities, the information is often insufficient to identify facilities threatened by flooding (Kiaghadi et al., 2020). Importantly, the effects of SLR are not included and it is also unclear which locations in the A zone are at risk from coastal versus inland flooding. Hence, we used a simple first-order bathtub approach with hydrologic connectivity to identify landfills that are at risk from coastal flooding resulting from extreme storm tides under present conditions and with different SLR scenarios. Note that this approach excludes velocity reduction due to bottom friction and therefore the flood extent could be overestimated. On the other hand, the extreme water level on the coast could be underestimated because wave action is not considered. A digital elevation model (NOAA, 2001) and extreme sea-level information derived with the latest version of the Global Tide and Surge Model (Muis et al., 2020) for the period 1979 to 2017 was used. The extreme sea-level data was bias corrected using tide gauge information and inverse distance weighting as described in Arns et al. (2015). Return periods (RPs) and associated water levels are derived with annual maxima and a Generalized Extreme Value distribution as well as peaks-over-threshold (using the 99th percentile) with a Generalized Pareto Distribution; the root mean squared error between empirical and theoretical distributions is used to select the best approach for a given grid point along the coast. For SLR scenarios, we consider a uniform rise of 0.5 m as a low-end scenario, 0.62 and 0.81 m as the average of the 50th percentile SLR by 2100, derived by Kopp et al. (2014) for the Florida coastline under RCP4.5 and RCP8.5 emissions respectively, and 1.5 m as a high-end scenario.
We estimate that 1,099 landfills are located within the present 100-year coastal flood zone (see Figure 7, left); this number increases to 1,642 by 2100 under the RCP8.5 scenario (see Figure 7, right) and reaches 2,454 when considering the 1,000 year return level and high-end SLR scenario. The results for a range of different return levels (10-, 50-, 100-, and 1000-years) and the four SLR scenarios are summarized in Table 2.
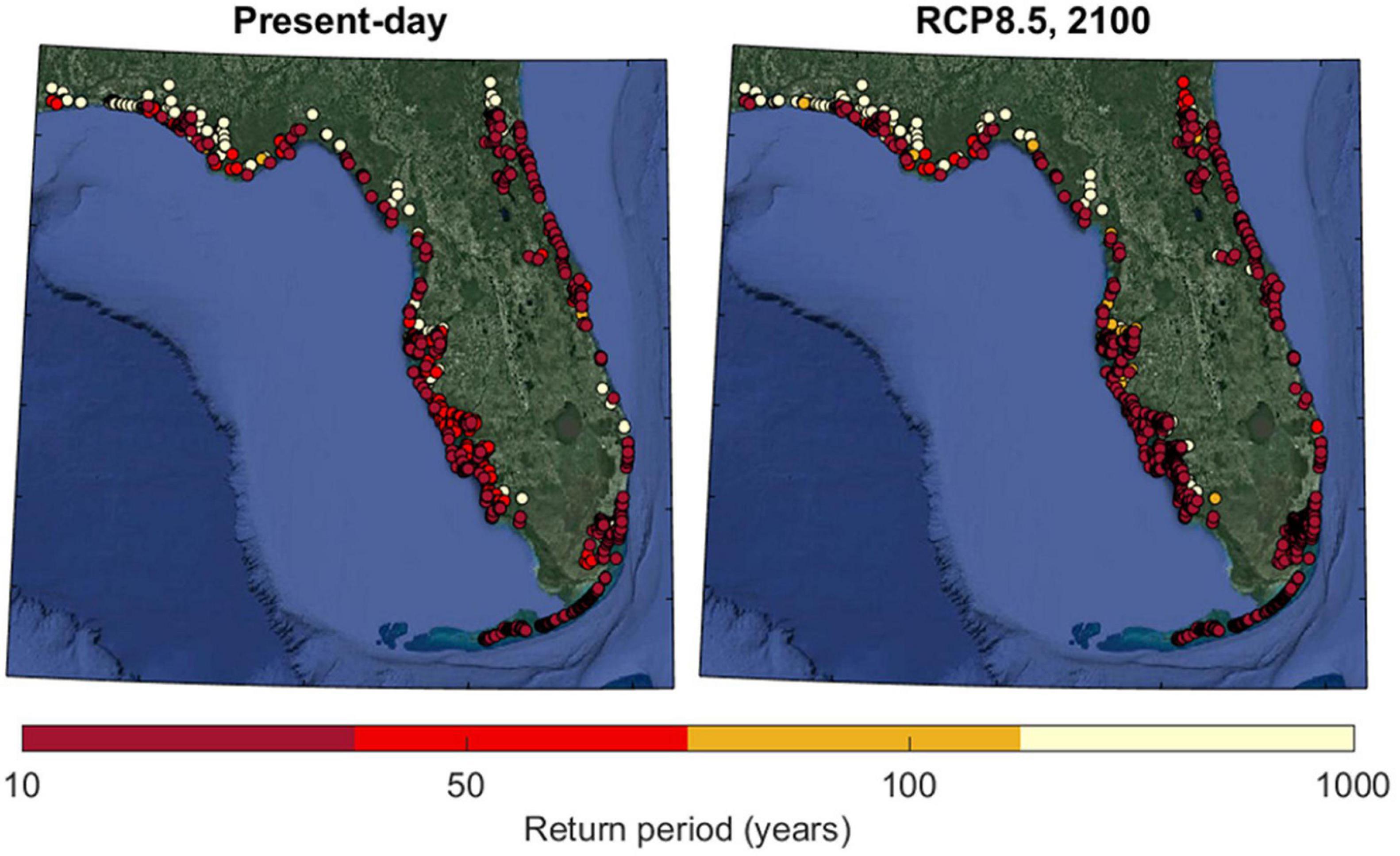
Figure 7. Landfills across Florida located in the floodplain associated with different return periods (see color bar) under present sea level conditions (left) and with future SLR, considering the RCP8.5 scenario (right).
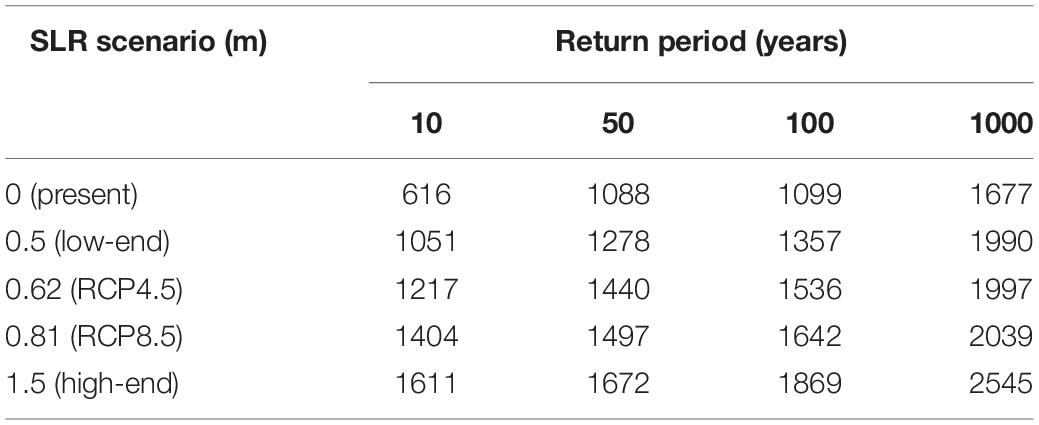
Table 2. Number of landfills located in the coastal floodplain associated with different return periods and SLR projections.
As an indicator for erosion potential, we use the coastal vulnerability index (CVI) (Thieler and Hammar-Klose, 2000). This is preferred over shoreline change rates derived from satellites and/or beach surveys (e.g., Kratzmann et al., 2017; Luijendijk et al., 2018) as it excludes the influence of the regular beach nourishment of Florida beaches (Elko et al., 2021); our goal is to combine information on flood potential with information on erosion potential and compare it with landfill locations. For example, of the 1,099 landfills located in the present 100-year coastal flood zone, 87 are located near a coastline with a low CVI, 592 with a moderate CVI, and 420 with a high CVI (Figure 8). Results for other return periods and under present-day sea level conditions are summarized in Table 3.
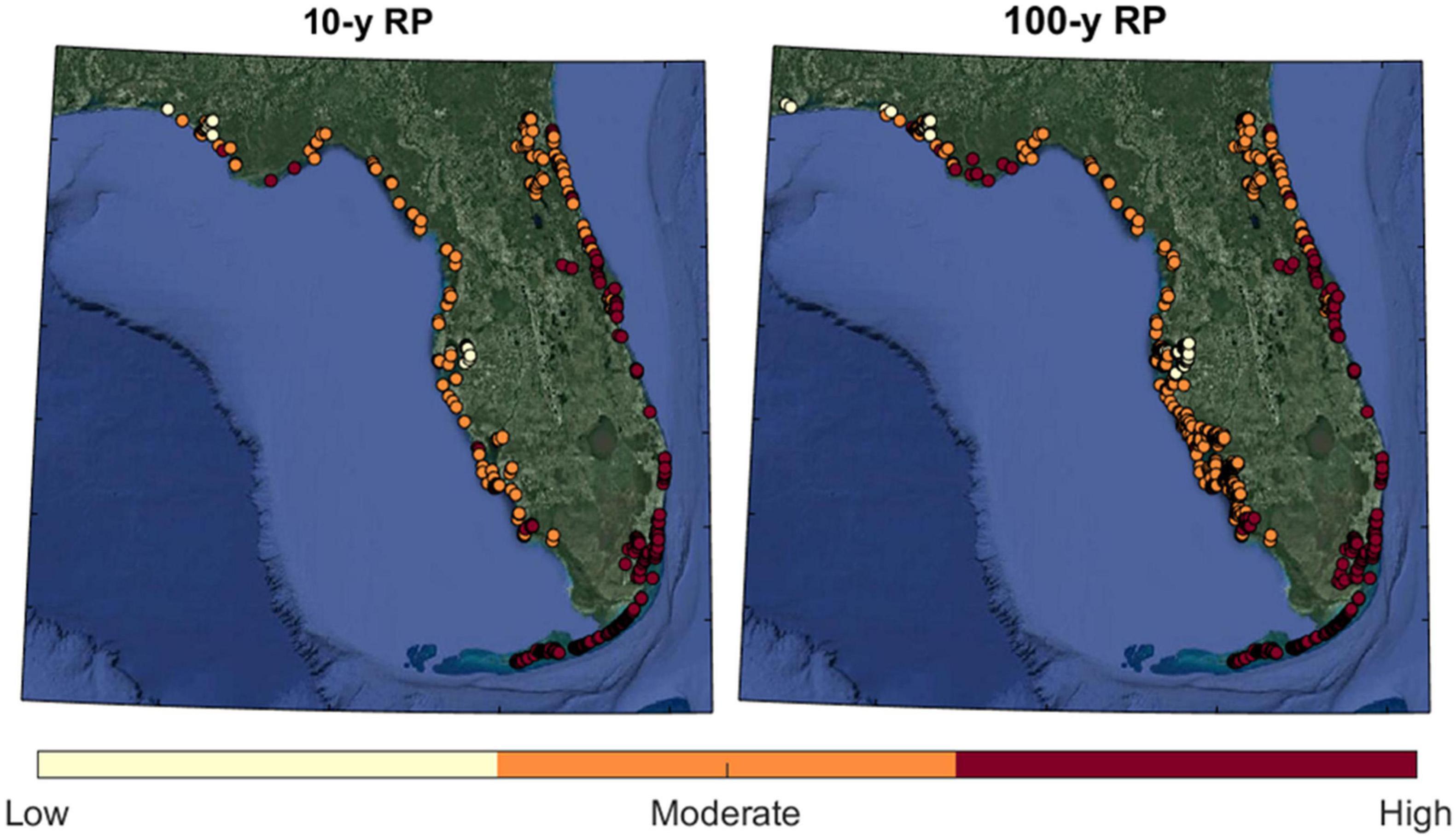
Figure 8. Coastal vulnerability index (CVI) for coastline stretches with landfills located in the 10-year coastal flood plain (left) and 100-year coastal floodplain (right).
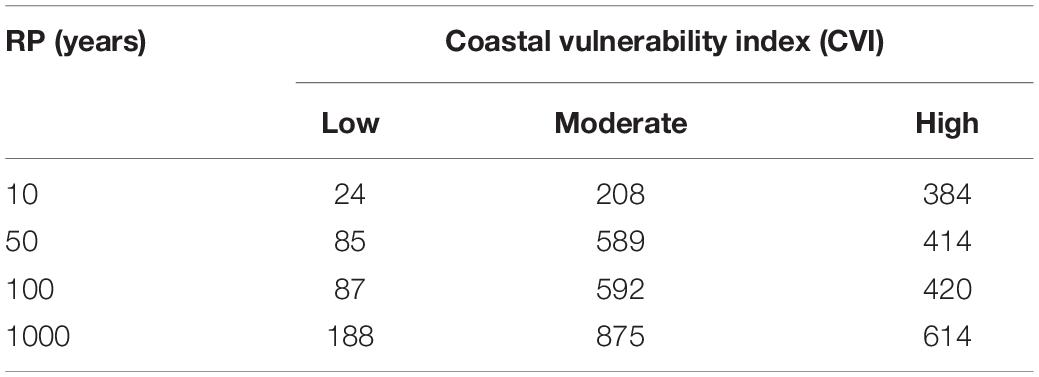
Table 3. Number of landfills located near a coastline with low, moderate, or high coastal vulnerability index (CVI) under water levels associated with different return periods.
These results highlight that landfills along the entire Florida coast are exposed to SLR, especially on the east coast, from Cape Canaveral to the Keys, where a high CVI amplifies the threat of flooding and erosion. Figures 9A,B show two examples from South Florida, the South Dade landfill in Miami and Long Key landfill in Monroe County, both located at the water’s edge. Another example is Virginia Key off the coast of Miami, where one of the city’s biggest parks is being built on an old landfill (Figure 9C). The landfill opened in 1960, was closed in 1977 and capped in 1980. The cap has not been maintained and has been eroded by rainfall events to as little as several centimeters thick in places. Now, 60 cm of lime-rock fill, in combination with two pumps and a deep injection well to remove contaminated groundwater, will be installed (Viglucci, 2017). Being built up to 9 m high, the risk for overtopping is relatively low but being located on a barrier island the base of the site is highly exposed to future impacts from SLR, surges and wave impacts. In Key West, the Stock Island landfill (Figure 9D) collected waste from 1930 until its closure in 1990 (released from long-term care in 2016), growing to 27 m above sea level and occupying a 7.3 ha parcel (for comparison, the highest natural elevation across the entire Florida Keys is about 6 m). The landfill has passed testing for compliance with federal regulations, but does not have a liner, raising concerns about contamination of the surrounding soil. However, removing the landfill to road level and transporting the waste to other facilities has an estimated cost of $70 to $190 million (€60 to €160 million) and this has not been pursued (Blinckmann, 2018). All examples shown in Figure 9 can be classified as situations B and C in Figure 2.
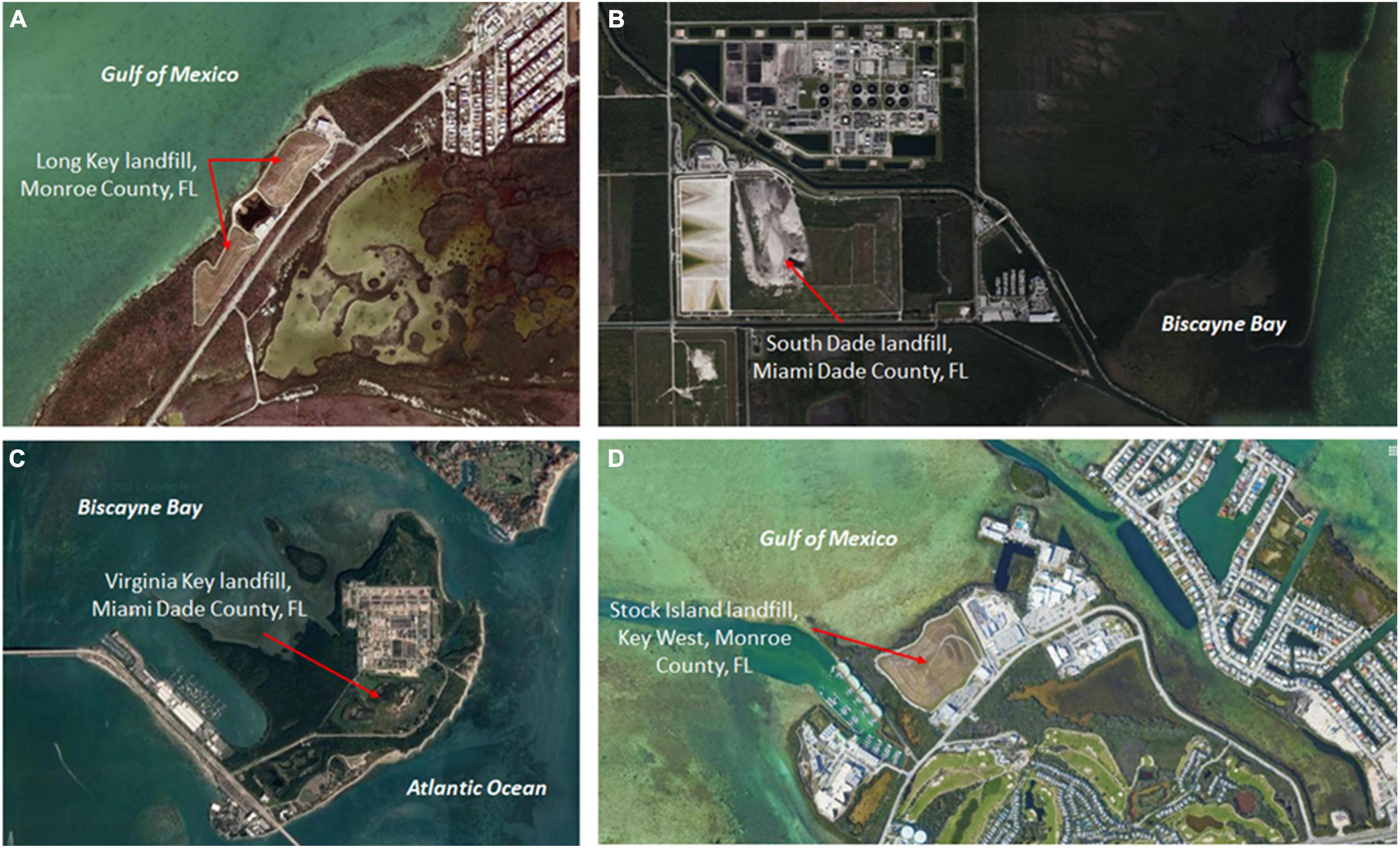
Figure 9. Aerial photos of (A) the Long Key landfill in Monroe County, FL, next to the Gulf of Mexico, (B) the South Dade landfill in Miami, FL, next to Biscayne Bay, (C) the Virginia Key landfill in Miami Dade County, FL, between Biscayne Bay and the Atlantic Ocean, and (D) the Stock Island landfill in Key West, Monroe County, FL, next to the Gulf of Mexico (source: Google Earth).
The results highlight the existing risk of flooding and erosion from storm surges and waves for coastal landfills across Florida, and by implication nationally. This risk will increase as sea level continues to rise and exacerbates beach erosion, which is constantly battled in Florida by recurring beach nourishment in support of the thriving tourism industry which is one of the largest contributors of the state’s GDP (Elko et al., 2021). In the long-term, nourishment costs will increase as frequency is increased and sand availability is expected to decline. Ultimately, and without additional adaptation measures being implemented (e.g., building dunes, using sand fences or vegetation as sand traps, providing accommodation space for beach/dune migration), this may lead to shoreline retreat exposing some landfills to erosion. As experience of landfill reclamation grows globally (Jones et al., 2013), in the United States (USEPA, 1997) and in Florida (e.g., Jain et al., 2013), new options may arise (see section “Synthesis of the National Case Studies and Management Options for Historical Coastal Landfill”). Overall, the awareness of climate impacts on coastal landfills is increasing in the United States. Citizen concerns have been raised regarding the Key West landfill as discussed, but even in Alaska a report has identified solid waste management sites as vulnerable to flooding and proposed action plans for those of most concern (ADEC, 2015).
Synthesis of the National Case Studies and Management Options for Historical Coastal Landfill
Table 4 summarizes the preceding case studies. This emphasizes the scale of the problem that exists today; there are thousands of coastal landfills that are of concern under present conditions and waste release events are already documented in England, France, and the United States. SLR (and maybe wider climate change) will increase this number, but are certainly not creating them alone. The wide range of situations where landfill releases can occur is apparent, and coastal adaptation choices are critical in future outcomes. For example, the expected abandonment of large lengths of defenses in England over the next century (CCC, 2018) has profound implications for landfill release beyond the magnitude of SLR. Without recognition of the problem, substantial release of waste into the sea would appear inevitable. The long timescale inherent in the management of landfill sites is also apparent, with potential consequences growing over time.
The management responses to these actual and potential landfill problems are wide-ranging and, in many instances untested. Building on earlier experience of Cooper et al. (2013); Nicholls et al. (2018b), Wadey et al. (2019); Beaven et al. (2020), and the results of this paper (see above sections), Table 5 summarizes available options and current experience. These approaches draw on the source-pathway-receptor model used for controlling pollution risks (Watts, 1998). Beyond ‘do nothing’ and simple ‘inspection and surveillance,’ several distinct options can be selected over time to manage a coastal landfill, e.g., starting with ‘reactively removing released waste’ after a storm, proactively ‘remove the source’ of waste, ‘break the pathway’ between the source and the receptor, and finally ‘remove the receptor.’ While there is much experience of protection (break the pathway), and experience is growing for other options, such as waste relocation in France, or landfill mining in Belgium (Winterstetter et al., 2018), many gaps in knowledge are apparent which hinder the full range of these options being applied in an evidence-based manner. The large differences in cost between break the pathway (protect) and waste removal identified in the English case studies are noteworthy and suggest protection would be preferred. However, as the waste needs to be protected in perpetuity, the time dimension also needs to be considered and developing affordable, acceptable and more permanent solutions is essential. The lack of a method to assess the harm from waste release is a major limitation as it hinders the definition of (1) acceptable (if any) flux of release of different types of solid wastes into the marine environment, and (2) potential standards for landfill mining to remove the more hazardous materials. It is also noteworthy that landfill taxes can hinder relocation of waste, which was not part of its original intention: waiving or greatly reducing the tax could facilitate more use of this approach.
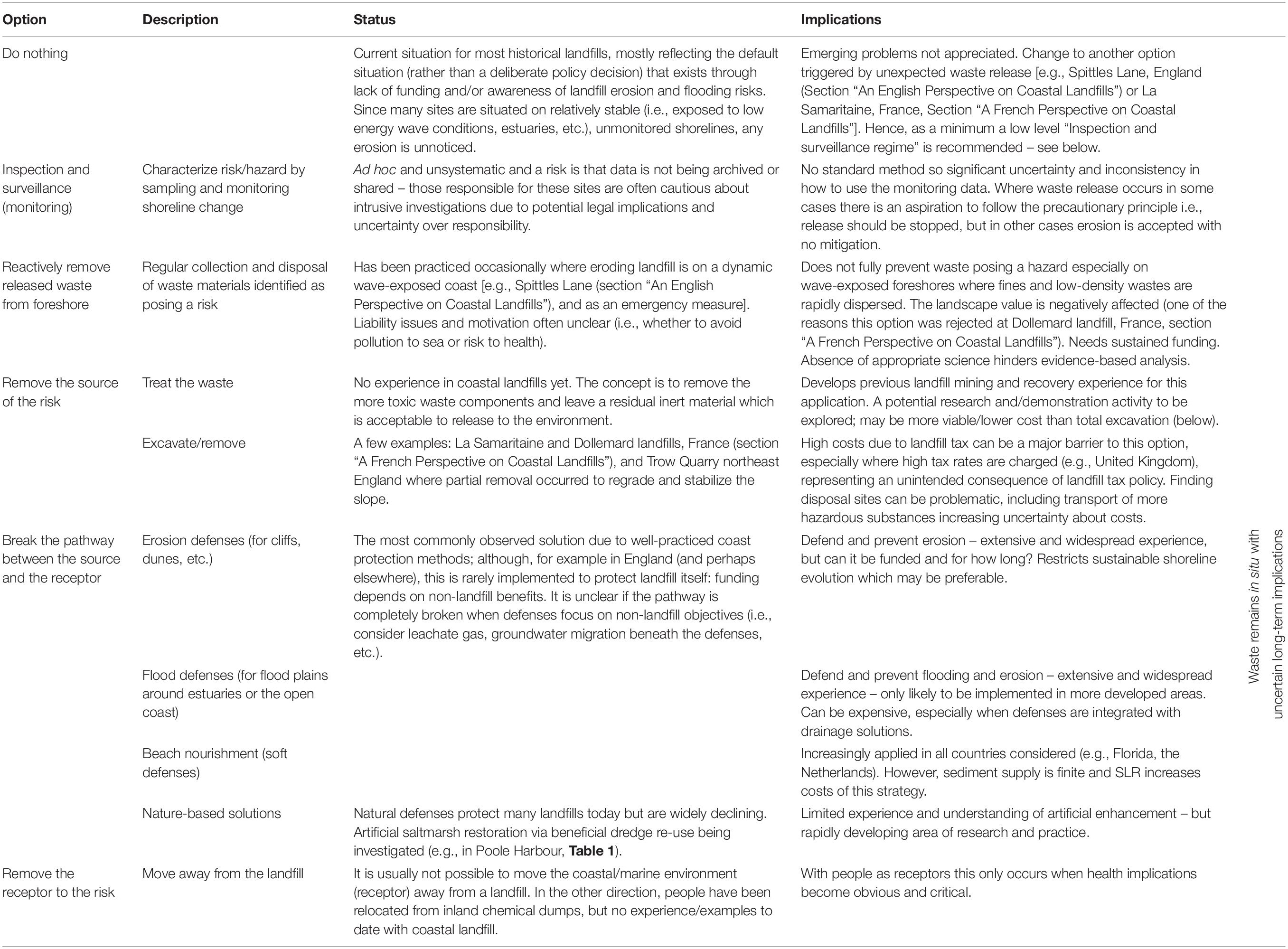
Table 5. Summary of potential historical coastal landfill management options from this analysis, using the source-pathway-receptor terminology for pollution risk (see also Cooper et al., 2013; Wadey et al., 2019).
Discussion
Based on the case studies discussed here, and the wider assessment of the status of coastal historical landfills, five main issues emerge which resonate with earlier assessments in England (Nicholls et al., 2018b; Wadey et al., 2019). First, the potential scale and implications of waste release to the coastal and marine environment is not sufficiently understood or appreciated. Hence, it remains important to develop a better understanding of the amount, character (biological, physical, and chemical) and potential impact on human health and the environment of waste release from coastal landfill sites for the countries considered and by implication, globally. While some national or regional situations have been assessed, as reviewed here, these are incomplete and have significant gaps. The case studies also highlight the large uncertainties at individual sites and that basic information such as the depth and volume of waste in historical landfills is often missing. A fundamental gap is the lack of methodology and suitable protocols to characterize waste in modern and historical landfills which captures waste heterogeneity and assesses the potential impact of solid wastes release into the marine environment. There is also a need to develop appropriate sampling protocols linked to the outcomes of any new waste characterization review. Landfills are currently being managed without this important information.
Second, there is a need to better address the regulation of solid wastes eroding from coastal landfills. To date, there has been only limited investigation of the hazards associated with the release of these waste materials to the marine environment. Current projections of waste release into the ocean are not compliant with the Sustainable Development Goals (Chen et al., 2020). This raises the following questions: are all such releases unacceptable, or is there an acceptable rate of discharge of certain solid wastes under specific circumstances? How can the limited funding available be used most effectively to manage/address the risk of waste release to the ocean from landfill erosion and flooding?
Third, while flooding and groundwater flushing of coastal landfills can generally be assessed to some degree, there are significant gaps in information and understanding on present and future release of waste due to erosion, including the impact of SLR. To understand this a wide range of factors beyond climate change need to be considered for each landfill site, including: (1) the dominant coastal hazard; (2) the coastal geomorphology/setting; (3) present and future coastal adaptation; (4) adjacent land use, including habitat designations; and (5) the waste and landfill properties (cf. Beaven et al., 2020). While systematic coastal monitoring data are becoming more widely available from national and regional monitoring activities like the Channel Coastal Observatory1 and satellite-based observations in more data-sparse areas (e.g., Luijendijk et al., 2018), this problem requires more focus on local changes around coastal landfill sites. Small erosional changes can release significant amounts of waste materials as shown in the two French case studies. In addition, there is a need for more analysis of future geomorphic change in the diverse range of settings where coastal waste is found, especially estuarine settings where landfill sites are concentrated. It should be noted that changes in designated coastal habitats are often linked to, and significant for, coastal landfills. This suggests the need for an integrated climate service that takes information on SLR and climate change and other coastal data to translate these changes into threats to all human activities at the coast. In this regard coastal landfills could be seen as part of coastal infrastructure from this climate service perspective. Climate services have mainly focused on either the land, the sea, or climate change, without much focus at the coastal interface. A new core climate service for coastal adaptation to SLR is being developed as part of the European research project CoCliCo. There is a strong focus on flooding and erosion, but downstream services assessing cascading impacts of SLR, such as risks associated with landfills, could be developed as well.
Fourth, there is a need to develop more pragmatic and cost-effective remediation options that facilitate action rather than encouraging ‘kicking the can down the road’, which would seem to characterize many historical responses. In the case studies, identified problems are generally being ignored, but in the cases where it cannot be ignored, the main solution is to protect or relocate the landfills which are both expensive options. What other options are available (Table 5) such as landfill mining to remove the most toxic and unsightly materials and allow the residual more inert materials to erode? The role of landfill taxes in shaping these solutions should also be noted as the high costs attached to moving landfill are having effects that the original proposers did not have in mind. In general, the menu of proactive measures needs to be enhanced and developed.
Lastly, the widespread lack of funding hinders progress across these issues as these costs are not appreciated or considered in national budgets for coastal and environmental management. In highly developed flood-prone areas like the Netherlands, the German North Sea coast and the Thames Estuary in England, high and extensive defenses already protect most landfills so this is less of a consideration today. Elsewhere funding is a real concern. In England, whilst HTL is the stated policy around most landfill sites, funding is limited and unless protection of other properties from flood and coastal erosion risk is also a benefit, the proposed policy often cannot be delivered (CCC, 2018; Wadey et al., 2019). This is increasingly recognized in policy circles (Stratton, 2019) and a national steering group has been established to champion these challenges to government in England. In France and the United States, funding is also raised as a challenge. Landfill mining experience in Belgium (Winterstetter et al., 2018) shows that the main benefit is the enhanced land value. However, mining has yet to be explored for coastal landfills (Table 5). To ensure long-term safety, dedicated funding is required to assess and proactively manage landfills rather than reacting to crises such as sudden waste release in a stormy year, as illustrated by La Samaritaine landfill, France. The appropriate scale of funding is open to debate.
This paper has focused on the current management challenges. The timescale of leachate release from modern landfills is already well-known as a concern that may last for centuries (Belevi and Baccini, 1989; Laner et al., 2012), and the concept of landfills acting as “final sinks” for wastes on a geological scale has also been recognized (e.g., Brunner, 2013). However, as far as we are aware, this paper is the first to acknowledge the geological scale of the problems that may arise from the release of solid waste to the environment through long-term erosion processes. As SLR will also continue for many centuries even if the Paris Agreement is fully implemented (Nicholls et al., 2018a; Oppenheimer et al., 2019), these problems will persist and worsen far into the future, reinforcing the need for research to improve scientific understanding and policy in this area.
The management measures for coastal landfills (Table 5) also need to be set in the wider context of coastal adaptation planning where a variety of strategies are available, including advance, protect, accommodate and planned retreat and growing interest in nature-based approaches (Oppenheimer et al., 2019; Hinkel and Nicholls, 2020). There are strong advocates for large-scale retreat (Siders et al., 2019) and the extent to which this occurs has significant implications for coastal landfill release and management (Figure 2). Using a benefit-cost analysis approach, Lincke and Hinkel (2018) conclude that 13% of the world’s coast by length is worth protecting against SLR over the 21st century irrespective of the scenario and discount rate uncertainties, while 65% of the world’s coast by length is never worth protecting and some form of retreat is likely. In terms of assets, the 13% of coast contains 96% of assets and any protection will therefore benefit many coastal landfill sites (situation H in Figure 2). If coastal defenses are deliberately abandoned or fail, large amounts of waste would be released, but as argued from the Netherlands perspective, in this situation other sources of anthropogenic material may dwarf the contribution of the historical landfill sources considered here. Nonetheless, further analysis of the implications of coastal landfill within strategic coastal adaptation planning would be prudent, especially when managed retreat is being considered.
Conclusion
Based on this analysis, the potential release of liquid and solid wastes from coastal landfill is an important threat which will worsen with SLR. Improved guidance is required to support risk assessment in relation to the long (geological) timescales of landfill sites, supported where necessary by new research and development activities to provide the required scientific understanding and evidence. This includes improved methods to characterize waste in landfills in terms of the potential impact of different categories of solid waste if released into the coastal and marine environment. The magnitude, transport, and impact of solid wastes need more consideration to develop appropriate assessment methods, including ranking sites for action. There is also a need to understand better the behavior of waste-associated contaminants in historical landfill materials and the likely response to leaching across the range of salinities from fresh to fully ocean conditions. Furthermore, there is a need to better identify both the site characteristics of coastal landfills and the potential costs (over different time frames) of the range of adaptation measures available to avoid adverse impacts.
Where protect (HTL) is the preferred adaptation policy for coastal landfills, the costs and benefits beyond avoided erosion and flood damage need to be considered. This is not arguing for universal defense, but rather asking what are the appropriate resources to plan and implement a response. Funding mechanisms need to be reviewed and coastal societies need to ask what level of current and future funding is appropriate for this issue. It should be noted that where landfill tax has been implemented, this may be a major cost impediment to removing historical landfill material to less vulnerable locations. This was not its original intention. A number of other remediation approaches are possible, but they are at various stages of development and providing a comprehensive menu requires science-based evidence that is not yet available. The cases considered in this paper are drawn from European and U.S. situations, but they are transferable more widely: while the context will vary, the fundamental issues appear generic. In conclusion, managing the legacy of coastal landfills over the next century (and beyond) poses a significant challenge to coastal societies, and our scientific tools to analyze these problems and the policies that are applied require significant enhancement.
Author Contributions
RN, RB, and AS conceived the manuscript, produced the overall manuscript framework, discussion and conclusions and together with KS. MW, and SC wrote the England section. DM and GL wrote the French section. TW, DR, VS, and AE wrote the Florida section. JG and TH wrote the Netherlands section. AA wrote the German section. All the authors completed and reviewed the final manuscript.
Funding
RN, RB, and AS were supported by the UK’s Natural Environment Research Council’s (NE/N012909/1). DM was supported by the support policy-makers project from the French Environment Ministry and he also thanks the support of C. Cousin (BRGM). TW acknowledges support by the National Science Foundation (Grant Number 1854896). KS acknowledges support from the Environment Agency and from the UK’s Natural Environment Research Council (NE/I018212/1). This publication was supported by PROTECT. RN and GL received funding from the European Union’s Horizon 2020 Research and Innovation Program under grant agreement No. 869304, PROTECT contribution number 22.
Conflict of Interest
The authors declare that the research was conducted in the absence of any commercial or financial relationships that could be construed as a potential conflict of interest.
Publisher’s Note
All claims expressed in this article are solely those of the authors and do not necessarily represent those of their affiliated organizations, or those of the publisher, the editors and the reviewers. Any product that may be evaluated in this article, or claim that may be made by its manufacturer, is not guaranteed or endorsed by the publisher.
Acknowledgments
Susan Hanson is thanked for reviewing an earlier draft of this manuscript.
Footnotes
References
ADEC (2015). Waste Erosion Assessment and Review (WEAR). Available online at: https://dec.alaska.gov/eh/solid-waste/wear-project/ (accessed May 12, 2021).
Arns, A., Wahl, T., Haigh, I. D., and Jensen, J. (2015). Determining return water levels at ungauged coastal sites: a case study for northern Germany. Ocean Dyn. 65, 539–554. doi: 10.1007/s10236-015-0814-1
Asher, C. (2019). “Climate change and cleanup: a vulnerability assessment and adaptation strategy for Washington State’s contaminated sites,” in Proceedings of Coastal Sediments 2019, (Singapore: World Scientific), 2247–2259.
Bardos, P., Spencer, K. L., Ward, R. D., Maco, B. H., and Cundy, A. B. (2020). Integrated and sustainable management of post-industrial coasts. Front. Environ. Sci. 8:86. doi: 10.3389/fenvs.2020.00086
Basias-Georisques (2021). Inventaire Historique Des Anciens Sites Industriels et Activités de Service. Available online at: https://www.georisques.gouv.fr/risques/basias/donnees#/ (accessed April 13, 2021).
Bauumwelt Bremen (2021). Contaminated Sites and Harmful Soil Changes. Available online at: https://www.bauumwelt.bremen.de/umwelt/boden_und_altlasten/altlasten_und_schaedliche_bodenveraenderungen-24962#abs_804786 (accessed August 8, 2021).
Beaven, R. P., Stringfellow, A. M., Nicholls, R. J., Haigh, I. D., Kebede, A. S., and Watts, J. (2020). Future challenges of coastal landfills exacerbated by sea level rise. Waste Manag. 105, 92–101. doi: 10.1016/j.wasman.2020.01.027
Belevi, H., and Baccini, P. (1989). Long-term behavior of municipal solid waste landfills. Waste Manag. Res. 7, 43–56. doi: 10.1016/0734-242X(89)90007-4
Bergmann, M., Tekman, M. B., and Gutow, L. (2017). Sea change for plastic pollution. Nature 544, 297–297. doi: 10.1038/544297a
Blinckmann, H. (2018). Key West City Commission Debates Landfill. Available online at: https://keysweekly.com/42/key-west-city-commission-debates-landfill/ (accessed January 26, 2021).
Brand, J. H., and Spencer, K. L. (2020). Will flooding or erosion of historic landfills result in a significant release of soluble contaminants to the coastal zone? Sci. Tot. Environ. 724, 138150. doi: 10.1016/j.scitotenv.2020.138150
Brand, J. H., Spencer, K. L., O’Shea, F. T., and Lindsay, J. E. (2018). Potential pollution risks of historic landfills on low-lying coasts and estuaries. WIREs Water 5:e1264. doi: 10.1002/wat2.1264
Brunner, P. H. (2013). Cycles, spirals and linear flows. Waste Manag. Res. 31, 1–2. doi: 10.1177/0734242X13501152
CCC (2018). Managing The Coast in a Changing Climate. Available online at: https://www.theccc.org.uk/publication/managing-the-coast-in-a-changing-climate/ (accessed May 12, 2021).
Chen, D. M.-C., Bodirsky, B. L., Krueger, T., Mishra, A., and Popp, A. (2020). The world’s growing municipal solid waste: trends and impacts. Environ. Res. Lett. 15:074021. doi: 10.1088/1748-9326/ab8659
Church, J. A., Clark, P. U., Cazenave, A., Gregory, J. M., Jevrejeva, S., Levermann, A., et al. (2013). “Sea level change,” in Climate Change 2013: The Physical Science Basis. Contribution of Working Group I to the Fifth Assessment Report of the Intergovernmental Panel on Climate Change, eds T. F. Stocker, D. Qin, G.-K. Plattner, M. Tignor, S. K. Allen, J. Boschung, et al. (Cambridge, MA: Cambridge University Press).
Cooper, N. J., Bower, G., Tyson, R., Flikweert, J. J., Rayner, S., and Hallas, A. (2013). Guidance on the Management of Landfill Sites and Land Contamination or Eroding or Low-Lying Coastlines. London: CIRIA.
de Moel, H., Aerts, J. C. J. H., and Koomen, E. (2011). Development of flood exposure in the Netherlands during the 20th and 21st century. Glob. Environ. Change 21, 620–627. doi: 10.1016/j.gloenvcha.2010.12.005
de Winter, R. C., and Ruessink, B. G. (2017). Sensitivity analysis of climate change impacts on dune erosion: case study for the Dutch Holland coast. Clim. Change 141, 685–701. doi: 10.1007/s10584-017-1922-3
DESTATIS (2021). Waste Disposal - Number of Landfills and Input. Wiesbaden: Statistisches Bundesamt.
Dunn, M. E., Mills, M., and Veríssimo, D. (2020). Evaluating the impact of the documentary series Blue Planet II on viewers’ plastic consumption behaviors. Conserv. Sci. Pract. 2:e280. doi: 10.1111/csp2.280
Dutch Foundation for Sustainable Landfilling (2021). Introducing Sustainable Landfill programme (iDS). Available online at: https://duurzaamstorten.nl/en/#stortbeheer (accessed April 12, 2021).
EEC (1975). Council directive 75/442/EEC of 15 July 1975 on waste (OJ L 194, 25.7.1975, p.39-41), 75/442/EEC. Available online at: https://www.eea.europa.eu/policy-documents/council-directive-75-442-eec (accessed May 14, 2021).
Elko, N., Briggs, T. R., Benedet, L., Robertson, Q., Thomson, G., Webb, B. M., et al. (2021). A century of U.S. beach nourishment. Ocean Coast. Manag. 199:105406. doi: 10.1016/j.ocecoaman.2020.105406
EPF Normandie (2018). Fin Des Travaux De Renaturation De L’ Ancienne Décharge De “La Samaritaine. Available online at: https://www.robindesbois.org/wp-content/uploads/decharge-Lingreville-conservatoire-du-Littoral-communique-mai2018.pdf (accessed April 10, 2021).
Eriksen, M., Lebreton, L. C. M., Carson, H. S., Thiel, M., Moore, C. J., Borerro, J. C., et al. (2014). Plastic pollution in the world’s oceans: more than 5 trillion plastic pieces weighing over 250,000 Tons Afloat at Sea. PLoS One 9:e111913. doi: 10.1371/journal.pone.0111913
EU (2018). Directive (EU) 2018/850 of the European Parliament and of the Council of 30 May 2018 Amending Directive 1999/31/EC on the Landfill of Waste. Available online at: https://www.eea.europa.eu/policy-documents/directive-eu-2018-850-amending (accessed May 14, 2021).
EURELCO (2019). Data Launched on the Landfill Situation in the EU-28. Available online at: https://eurelco.org/infographic/ (accessed March 23, 2019).
Florida Counties Foundation (2021). Hurricane Irma - Debris. Available online at: http://factor.fl-counties.com/hurricane-irma-debris (accessed January 26, 2021).
Grandoni, D., and Dennis, B. (2019). 60% of Superfund Sites Could Be Hit by Climate Change, New Government Report Finds. Available online at: https://www.washingtonpost.com/climate-environment/2019/11/18/about-percent-superfund-sites-could-be-hit-by-climate-change-new-government-report-finds/ (accessed May 13, 2021).
Gurwell, B. (2008). Coastal protection along the Baltic Sea coast - MecklenburgVorpommern. Available online at: https://izw.baw.de/die-kueste/0/k074114.pdf (accessed May 12, 2021).
Haasnoot, M., Kwadijk, J., van Alphen, J., Le Bars, D., van den Hurk, B., Diermanse, F., et al. (2020). Adaptation to uncertain sea-level rise; how uncertainty in Antarctic mass-loss impacts the coastal adaptation strategy of the Netherlands. Environ. Res. Lett. 15:034007. doi: 10.1088/1748-9326/ab666c
Hallegatte, S., Green, C., Nicholls, R. J., and Corfee-Morlot, J. (2013). Future flood losses in major coastal cities. Nat. Clim. Change 3, 802–806. doi: 10.1038/nclimate1979
Hamburg (2021). Processing of Contaminated Sites and Surface Recycling: Decades of Industrial Use Have Left Traces in the Soil and Groundwater. Available online at: https://www.hamburg.de/altlasten/135322/altlasten-allgemein (accessed August 8, 2021).
Hinkel, J., and Nicholls, R. J. (2020). Responding to Sea Level Rise. Available online at: https://www.nae.edu/228938/Responding-to-Sea-Level-Rise (accessed May 14, 2021).
Hogland, W., Hogland, M., and Marques, M. (2011). “Enhanced landfill mining: material recovery, energy utilization and economics in the EU (Directive) perspective,” in Proceedings International Academic Symposium on Enhanced Landfill Mining, Houthalen-Helchteren, 233–247.
Hoogland, T., van den Akker, J. J. H., and Brus, D. J. (2012). Modeling the subsidence of peat soils in the Dutch coastal area. Geoderma 171-172, 92–97. doi: 10.1016/j.geoderma.2011.02.013
Jain, P., Townsend, T. G., and Johnson, P. (2013). Case study of landfill reclamation at a Florida landfill site. Waste Manag. 33, 109–116. doi: 10.1016/j.wasman.2012.09.011
Jones, P. T., Geysen, D., Tielemans, Y., Van Passel, S., Pontikes, Y., Blanpain, B., et al. (2013). Enhanced landfill mining in view of multiple resource recovery: a critical review. J. Clean. Product. 55, 45–55. doi: 10.1016/j.jclepro.2012.05.021
JonoB (2019). Compromised Landfills at Risk During Extreme Weather. Office of the Prime Minister’s Chief Science Advisor. Available online at: https://www.pmcsa.ac.nz/2019/11/05/compromised-landfills-at-risk-during-extreme-weather/ (accessed May 14, 2021).
Katsman, C. A., Sterl, A., Beersma, J. J., van den Brink, H. W., Church, J. A., Hazeleger, W., et al. (2011). Exploring high-end scenarios for local sea level rise to develop flood protection strategies for a low-lying delta—the Netherlands as an example. Clim. Change 109, 617–645. doi: 10.1007/s10584-011-0037-5
Kennedy, M., and Migaki, L. (2017). After Maria, Puerto Rico Struggles Under the Weight of Its Own Garbage. Available online at: https://www.npr.org/sections/thetwo-way/2017/12/14/570927809/after-maria-puerto-rico-struggles-under-the-weight-of-its-own-garbage (accessed February 11, 2021).
Kiaghadi, A., Govindarajan, A., Sobel, R. S., and Rifai, H. S. (2020). Environmental damage associated with severe hydrologic events: a LiDAR-based geospatial modeling approach. Nat. Hazards 103, 2711–2729. doi: 10.1007/s11069-020-04099-1
Kopp, R. E., Horton, R. M., Little, C. M., Mitrovica, J. X., Oppenheimer, M., Rasmussen, D. J., et al. (2014). Probabilistic 21st and 22nd century sea-level projections at a global network of tide-gauge sites. Earth’s Future 2, 383–406. doi: 10.1002/2014EF000239
Kratzmann, M. G., Himmelstoss, E. A., and Thieler, E. R. (2017). National Assessment of Shoreline Change – A GIS Compilation of Updated Vector Shorelines and Associated Shoreline Change Data for the Southeast Atlantic Coast: Data Release. New York, NY: U.S. Geological Survey.
Kwadijk, J. C. J., Haasnoot, M., Mulder, J. P. M., Hoogvliet, M. M. C., Jeuken, A. B. M., van der Krogt, R. A. A., et al. (2010). Using adaptation tipping points to prepare for climate change and sea level rise: a case study in the Netherlands. WIREs Clim. Change 1, 729–740. doi: 10.1002/wcc.64
La Presse de la Manche (2020). La mer met au Jour Une Ancienne Décharge : Des Travaux Lancés En Urgence Sur Une Plage Du Cotentin. Available online at: https://actu.fr/normandie/vicq-sur-mer_50142/la-mer-met-jour-une-ancienne-decharge-travaux-lances-urgence-sur-une-plage-cotentin_33303189.html (accessed May 12, 2021).
LABO (2018). Daten / Informationssysteme. Bund/Länder-Arbeitsgemeinschaft Bodenschutz (LABO). Available online at: https://www.labo-deutschland.de/Veroeffentlichungen-Daten-Informationssysteme.html (accessed January 14, 2021).
Lammen, H., Van Zomeren, A., Dijkstra, J. J., and Comans, R. N. J. (2019). “Sustainable landfill management: solid waste sampling and geochemical characterization prior to (an)aerobic stabilization of three old landfills,” in Proceedings of the Seventeenth International Waste Management and Landfill Symposium, (London: International Waste Working Group).
Laner, D., Crest, M., Scharff, H., Morris, J. W. F., and Barlaz, M. A. (2012). A review of approaches for the long-term management of municipal solid waste landfills. Waste Manag. 32, 498–512. doi: 10.1016/j.wasman.2011.11.010
Laner, D., Fellner, J., and Brunner, P. H. (2009). Flooding of municipal solid waste landfills–an environmental hazard? Sci. Tot. Environ. 407, 3674–3680. doi: 10.1016/j.scitotenv.2009.03.006
LBEG Niedersachsen (2021). Old deposits. Landesamt fur Bergbau, Energie und Geologie, Niedersachsen, Germany. Available online at: https://www.lbeg.niedersachsen.de/boden_grundwasser/altlasten/altablagerungen/altablagerungen-927.html (accessed August 8, 2021).
Le Cozannet, G., Nicholls, R. J., Hinkel, J., Sweet, W. V., McInnes, K. L., Van de Wal, R. S. W., et al. (2017). Sea level change and coastal climate services: the way forward. J. Mar. Sci. Eng. 5:49. doi: 10.3390/jmse5040049
Le Parisien (2020). Au Havre, Premiers Tests Pour Nettoyer la Décharge de Dollemard. Available online at: https://www.leparisien.fr/environnement/au-havre-premiers-tests-pour-nettoyer-la-decharge-de-dollemard-26-08-2020-8373354.php (accessed May 12, 2021).
Les Champs Jouault (2019). Installation de Stockage de Déchets Ultimes Non Dangereux en mode Bioréacteur CUVES (50). Rapport annuel d’exploitation 2018. Available online at: https://www.manche.gouv.fr/content/download/48163/336961/file/Rapport%202020_ISDUND%20Bioreacteur%20-%20SAS%20Les%20Champs%20Jouault.pdf (accessed May 12, 2021).
Lieten, S. H. (2018). Landfill management in the Netherlands. Witteveen+Bos Raadgevende ingenieurs B.V. Available online at: https://www.interregeurope.eu/fileadmin/user_upload/tx_tevprojects/library/file_1534506068.pdf (accessed May 12, 2021).
Lincke, D., and Hinkel, J. (2018). Economically robust protection against 21st century sea-level rise. Glob. Environ. Change 51, 67–73. doi: 10.1016/j.gloenvcha.2018.05.003
Luijendijk, A., Hagenaars, G., Ranasinghe, R., Baart, F., Donchyts, G., and Aarninkhof, S. (2018). The state of the world’s beaches. Sci. Rep. 8:6641. doi: 10.1038/s41598-018-24630-6
Lumbroso, D. M., and Vinet, F. (2011). A comparison of the causes, effects and aftermaths of the coastal flooding of England in 1953 and France in 2010. Nat. Hazards Earth Syst. Sci. 11, 2321–2333. doi: 10.5194/nhess-11-2321-2011
Muis, S., Apecechea, M. I., Dullaart, J., de Lima Rego, J., Madsen, K. S., Su, J., et al. (2020). A high-resolution global dataset of extreme sea levels, tides, and storm surges, including future projections. Front. Mar. Sci. 7:263. doi: 10.3389/fmars.2020.00263
National Delta Programme (2020). Delta Programme 2021. Available online at: https://english.deltaprogramma.nl/ (accessed May 14, 2021).
Nicholls, R. J., Beaven, R., Stringfellow, A., Spencer, K. L., Tarrant, O., and Cope, S. (2020). “Coastal landfills, rising sea levels and shoreline management: a challenge for the 21st century,” in Coastal Management 2019, eds N. Hardiman and T. Ice (London: Insitution of Civil Engineers), 391–404.
Nicholls, R. J., Brown, S., Goodwin, P., Wahl, T., Lowe, J., Solan, M., et al. (2018a). Stabilization of global temperature at 1.5°C and 2.0°C: implications for coastal areas. Philos. Trans. R. Soc. A Math. Phys. Eng. Sci. 376:20160448. doi: 10.1098/rsta.2016.0448
Nicholls, R. J., Stringfellow, A., and Beaven, R. P. (2018b). Guidance on the Management of Landfill Sites and Land Contamination Or Eroding or Low-Lying Coastlines - Supplementary Guide. London: CIRIA.
Nicholls, R. J., Townend, I. H., Bradbury, A. P., Ramsbottom, D., and Day, S. A. (2013). Planning for long-term coastal change: experiences from England and Wales. Ocean Eng. 71, 3–16. doi: 10.1016/j.oceaneng.2013.01.025
Njue, C. N., Cundy, A. B., Smith, M., Green, I. D., and Tomlinson, N. (2012). Assessing the impact of historical coastal landfill sites on sensitive ecosystems: a case study from Dorset, Southern England. Estuar. Coast. Shelf Sci. 114, 166–174. doi: 10.1016/j.ecss.2012.08.022
NOAA (2001). U.S. Coastal Relief Model Vol.3 - Florida and East Gulf of Mexico. National Geophysical Data Center.
NOAA (2013). Population trends from 1970 to 2020, National Coastal Population Report. Available online at: https://coast.noaa.gov/digitalcoast/training/population-report.html (accessed January 26, 2021).
Olsthoorn, X., van der Werff, P., Bouwer, L. M., and Huitema, D. (2008). Neo-Atlantis: the Netherlands under a 5-m sea level rise. Clim. Change 91, 103–122. doi: 10.1007/s10584-008-9423-z
Oppenheimer, M., Glavovic, B., Hinkel, J., van der Wal, R., Magnan, A. K., Abd-Elgawad, A., et al. (2019). Sea Level Rise and Implications for Low Lying Islands, Coasts and Communities. Available online at: https://www.ipcc.ch/srocc/home/ (accessed May 12, 2021).
O’Shea, F. T., Cundy, A. B., and Spencer, K. L. (2018). The contaminant legacy from historic coastal landfills and their potential as sources of diffuse pollution. Mar. Pollut. Bull. 128, 446–455. doi: 10.1016/j.marpolbul.2017.12.047
Ouest France (2014). Après la Tempête, à La Torche on Trouve De Tout. Available online at: https://www.ouest-france.fr/bretagne/apres-la-tempete-la-torche-trouve-de-tout-1844585 (accessed May 12, 2021).
Ouest France (2018). Lingreville. La Décharge Sauvage de la Samaritaine, C’est Du Passé. Available online at: https://www.ouest-france.fr/normandie/manche/lingreville-la-decharge-sauvage-de-la-samaritaine-c-est-du-passe-5762681 (accessed May 12, 2021).
Pope, N. D., O’Hara, S. C. M., Imamura, M., Hutchinson, T. H., and Langston, W. J. (2011). Influence of a collapsed coastal landfill on metal levels in sediments and biota—a portent for the future? J. Environ. Monit. 13, 1961–1974. doi: 10.1039/C0EM00741B
Priemus, H. (2018). How housing, infrastructure and water determined the spatial structure of the Randstad. Eur. Plan. Stud. 26, 546–570. doi: 10.1080/09654313.2017.1402867
Propp, V. R., De Silva, A. O., Spencer, C., Brown, S. J., Catingan, S. D., Smith, J. E., et al. (2021). Organic contaminants of emerging concern in leachate of historic municipal landfills. Environ. Pollut. 276:116474. doi: 10.1016/j.envpol.2021.116474
Ranasinghe, R. (2016). Assessing climate change impacts on open sandy coasts: a review. Earth Sci. Rev. 160, 320–332. doi: 10.1016/j.earscirev.2016.07.011
Regierung MV (2021). Contaminated Sites Situation in MV. Mecklenberg-Vorpommern Ministerium fur Landwirtschaft und Umwelt. Available online at: https://www.regierung-mv.de/Landesregierung/lm/Umwelt/Boden/Altlastenbearbeitung/Altlastensituation-in-MV (accessed August 8, 2021).
SCE (2012). Diagnostic Environnemental et Propositions de Solutions de Gestion des Décharges de Dollemard. Available online at: https://www.lehavre.fr/sites/default/files/atoms/files/diagnostic_environnemental_et_propositions_de_solutions_de_gestion_des_decharges_de_dollemard_-_tranche_conditionnelle_definition_de_solutions_doptimisation_de_gestion.pdf (accessed April 12, 2021).
Scharff, H. (2014). Landfill reduction experience in The Netherlands. Waste Manag. 34, 2218–2224. doi: 10.1016/j.wasman.2014.05.019
Schleswig-Holstein (2021). Legacy: Contaminated Sites. Schleswig-Holstein. Available online at: https://www.schleswig-holstein.de/DE/Fachinhalte/G/grundwasser/gefaehrdungspotenzialeAltlasten.html (accessed August 8, 2021).
Siders, A. R., Hino, M., and Mach, K. J. (2019). The case for strategic and managed climate retreat. Science 365:761. doi: 10.1126/science.aax8346
StALU MM (2012). Coastal Protection Regulations Mecklenburg-Western Pomerania. Staatliches Amt für Landwirtschaft und Umwelt Mittleres Mecklenburg. Available online at: http://www.stalu-mv.de/mm/Themen/K%C3%BCstenschutz/Regelwerk-K%C3%BCstenschutz-Mecklenburg%E2%80%93Vorpommern/ (accessed August 8, 2021).
Sterr, H. (2008). Assessment of vulnerability and adaptation to sea-level rise for the coastal zone of Germany. J. Coast. Res. 24, 380–393.
Sweet, W. V., Dusek, G., Obeysekera, J. T. B., and Marra, J. J. (2018). Patterns and Projections of High Tide Flooding Along the US Coastline Using A Common Impact Threshold. NOAA Center for Operational Oceanographic Products and Services. Available online at: https://tidesandcurrents.noaa.gov/publications/techrpt86_PaP_of_HTFlooding.pdf (accessed May 12, 2021).
Thieler, E. R., and Hammar-Klose, E. S. (2000). National Assessment of Coastal Vulnerability To Sea-Level Rise; Preliminary Results for the US Pacific Coast (Report No. 2000-178). Available online at: https://www.usgs.gov/centers/whcmsc/science/national-assessment-coastal-vulnerability-sea-level-rise?qt-science_center_objects=0#qt-science_center_objects (accessed May 12, 2021).
Toimil, A., Camus, P., Losada, I. J., Le Cozannet, G., Nicholls, R. J., Idier, D., et al. (2020). Climate change-driven coastal erosion modelling in temperate sandy beaches: methods and uncertainty treatment. Earth Sci. Rev. 202:103110. doi: 10.1016/j.earscirev.2020.103110
USEPA (2017). Hurricane Harvey. Available online at: https://response.epa.gov/site/site_profile.aspx?site_id=12353. (accessed June 12, 2019).
USEPA (2019). Planning for Natural Disaster Debris. Available online at: https://www.epa.gov/sites/production/files/2019-04/documents/final_pndd_guidance_0.pdf (accessed May 12, 2021).
van der Pol, T., Hinkel, J., Merkens, J., MacPherson, L., Vafeidis, A. T., Arns, A., et al. (2021). Regional economic analysis of flood defence heights at the German Baltic Sea coast: a multi-method cost-benefit approach for flood prevention. Clim. Risk Manag. 32:100289. doi: 10.1016/j.crm.2021.100289
Van Koningsveld, M., and Mulder, J. P. M. (2004). Sustainable coastal policy developments in the Netherlands. A systematic approach revealed. J. Coast. Res. 20, 375–385.
Van Koningsveld, M., Mulder, J. P. M., Stive, M. J. F., Van Der Valk, L., and Van Der Weck, A. W. (2008). Living with sea-level rise and climate change: A case study of the Netherlands. J. Coast. Res. 24, 367–445.
Viglucci, A. (2017). Say bye to Virginia Key’s toxic old landfill, and hi to Miami’s biggest park. Available online at: https://www.miamiherald.com/news/local/community/miami-dade/article156328394.html (accessed January 26, 2021).
Wadey, M. P., Kermode, T., Cope, S., and Neill, S. (2019). Landfill Sites at Risk of Flooding and Erosion within the SCOPAC Area. Southern Coastal Group and SCOPAC. Available online at: https://scopac.org.uk/research/scopac-landfills-study/ (accessed May 12, 2021).
Walkden, M., and Dickson, M. (2008). Equilibrium erosion of soft rock shores with a shallow or absent beach under increased sea level rise. Mar. Geol. 251, 75–84. doi: 10.1016/j.margeo.2008.02.003
Wille, E. (2018). “Flooding risks at old landfill sites: linear economy meets climate change,” in Proceedings of the 4th International Symposium on Enhanced Landfill Mining, New York, NY, 361–365.
Winterstetter, A., Wille, E., Nagels, P., and Fellner, J. (2018). Decision making guidelines for mining historic landfill sites in Flanders. Waste Manag. 77, 225–237. doi: 10.1016/j.wasman.2018.03.049
Keywords: landfill, waste, erosion, flood, sea-level rise
Citation: Nicholls RJ, Beaven RP, Stringfellow A, Monfort D, Le Cozannet G, Wahl T, Gebert J, Wadey M, Arns A, Spencer KL, Reinhart D, Heimovaara T, Santos VM, Enríquez AR and Cope S (2021) Coastal Landfills and Rising Sea Levels: A Challenge for the 21st Century. Front. Mar. Sci. 8:710342. doi: 10.3389/fmars.2021.710342
Received: 16 May 2021; Accepted: 30 August 2021;
Published: 30 September 2021.
Edited by:
Ivica Vilibic, Ruđer Bošković Institute, CroatiaReviewed by:
Patrick Barnard, United States Geological Survey (USGS), United StatesJoy Jacqueline Pereira, National University of Malaysia, Malaysia
Copyright © 2021 Nicholls, Beaven, Stringfellow, Monfort, Le Cozannet, Wahl, Gebert, Wadey, Arns, Spencer, Reinhart, Heimovaara, Santos, Enríquez and Cope. This is an open-access article distributed under the terms of the Creative Commons Attribution License (CC BY). The use, distribution or reproduction in other forums is permitted, provided the original author(s) and the copyright owner(s) are credited and that the original publication in this journal is cited, in accordance with accepted academic practice. No use, distribution or reproduction is permitted which does not comply with these terms.
*Correspondence: Robert J. Nicholls, cm9iZXJ0Lm5pY2hvbGxzQHVlYS5hYy51aw==
†ORCID: Robert J. Nicholls, orcid.org/0000-0002-9715-1109; Richard P. Beaven, orcid.org/0000-0002-1387-8299; Daniel Monfort, orcid.org/0000-0003-2038-4139; Thomas Wahl, orcid.org/0000-0003-3643-5463; Julia Gebert, orcid.org/0000-0002-7805-5373; Arne Arns, orcid.org/0000-0002-3709-2514; Kate L. Spencer, orcid.org/0000-0001-6621-6945; Victor M. Santos, orcid.org/0000-0002-4458-0890; Alejandra R. Enríquez, orcid.org/0000-0001-7836-6748