- 1The Nature Conservancy, Caribbean Division, Punta Cana, Dominican Republic
- 2Laboratorio de Ecología Experimental, Departamento de Estudios Ambientales, Universidad Simón Bolívar, Caracas, Venezuela
- 3Department of Marine Sciences, University of Puerto Rico, Mayaguez, Puerto Rico
- 4Wetland and Aquatic Research Center, United States Geological Survey, Baton Rouge, LA, United States
For several decades, white plagues (WPDs: WPD-I, II and III) and more recently, stony coral tissue loss disease (SCTLD) have significantly impacted Caribbean corals. These diseases are often difficult to separate in the field as they produce similar gross signs. Here we aimed to compare what we know about WPD and SCTLD in terms of: (1) pathology, (2) etiology, and (3) epizootiology. We reviewed over 114 peer-reviewed publications from 1973 to 2021. Overall, WPD and SCTLD resemble each other macroscopically, mainly due to the rapid tissue loss they produce in their hosts, however, SCTLD has a more concise case definition. Multiple-coalescent lesions are often observed in colonies with SCTLD and rarely in WPD. A unique diagnostic sign of SCTLD is the presence of bleached circular areas when SCTLD lesions are first appearing in the colony. The paucity of histopathologic archives for WPDs for multiple species across geographies makes it impossible to tell if WPD is the same as SCTLD. Both diseases alter the coral microbiome. WPD is controversially regarded as a bacterial infection and more recently a viral infection, whereas for SCTLD the etiology has not been identified, but the putative pathogen, likely to be a virus, has not been confirmed yet. Most striking differences between WPD and SCTLD have been related to duration and phases of epizootic events and mortality rates. While both diseases may become highly prevalent on reefs, SCTLD seems to be more persistent even throughout years. Both transmit directly (contact) and horizontally (waterborne), but organism-mediated transmission is only proven for WPD-II. Given the differences and similarities between these diseases, more detailed information is needed for a better comparison. Specifically, it is important to focus on: (1) tagging colonies to look at disease progression and tissue mortality rates, (2) tracking the fate of the epizootic event by looking at initial coral species affected, the features of lesions and how they spread over colonies and to a wider range of hosts, (3) persistence across years, and (4) repetitive sampling to look at changes in the microbiome as the disease progresses. Our review shows that WPDs and SCTLD are the major causes of coral tissue loss recorded in the Caribbean.
Introduction
Coral reef ecosystems worldwide are facing natural and human-induced stressors resulting in significant declines in coral cover and diversity, and elevated coral extinction risks (Carpenter et al., 2008; Jackson et al., 2014). Declines in average live coral cover are variable across bioregions, but are particularly evident in the Caribbean, where different studies have found 20 to 80% loss in just a few decades (Gardner et al., 2003; Bruno and Selig, 2007; Jackson et al., 2014). In the Caribbean for example, the emergence, prevalence, incidence, and virulence of coral reef diseases during the past four decades appear to be unprecedented (Aronson and Precht, 2001; Weil and Rogers, 2011; Woodley et al., 2016a). Outbreaks of black band, white band (WBD), white plague (WPD), and Caribbean yellow band disease (CYBD) during the late 1900s and early 2000s, significantly impacted populations of the most important branching and massive foundational species (Acropora spp., Orbicella spp., Pseudodiploria spp., Diploria labyrinthiformis, Colpophyllia spp., Siderastrea siderea, Montastraea, etc.), killing large colonies, some more than 500–1,000 years old, in a short period of time. The causes are complex and possibly synergistic, but diseases and bleaching events associated with changing climate, and some synergistic effects of local human stressors, are thought to be the major drivers of reef deterioration (Harvell et al., 1999, 2002, 2004, 2009; Hoegh-Guldberg et al., 2007; Cróquer and Weil, 2009; Weil and Rogers, 2011).
The first report of a white disease that produced significant coral mortalities occurred in Florida in 1975 and was referred to as WPD. Later, the term WPD-I was adopted to acknowledge the first epizootic event of a white syndrome in the Caribbean. It mainly affected the plating coral Mycetophyllia ferox leading to fears of it disappearing from some areas in the Florida Keys (Dustan, 1977). During a second WPD epizootic event 10 years later, colonies of M. ferox were unaffected, whereas massive Orbicellas and 10 other species suffered extensive mortalities (Dustan, 1999), suggesting resistant Mycetophyllia colonies or a different causative agent. A third and more virulent outbreak of WPD occurred in the Florida Keys in 1995 mainly affecting populations of Dichocoenia stokesii, but also 16 additional species over 2 years. This disease was named white plague disease type 2 (WPD-II; Richardson, 1998; Richardson et al., 1998b). In the late 1990s and early 2000s, a fourth more virulent epizootic was termed WPD-III and affected mostly Orbicella spp. and Colpophyllia natans in Florida, the United States Virgin Islands, Puerto Rico, and Venezuela during the next 10 years, and it is still present even if not as prevalent as before (Richardson and Aronson, 2002; Weil et al., 2002, 2009; Cróquer et al., 2005; Miller et al., 2009; Bastidas et al., 2012). During this period, the names WPD III and WPD II were used without consistent criteria; and they were even exchanged until the pathogen of WPD-II was described by Denner et al. (2003). However, the presumed putative pathogen Aurantimonas coralicida was not found in analyses of diseased tissue samples from different localities over the years, further indicating inconsistency for the pathogen identification (Sunagawa et al., 2009); thus, the term WPD-III was discarded and only WPD (type I and II) currently adopted as valid.
One new disease, stony coral tissue loss disease (SCTLD), emerged in Florida in 2014, following sediment re-suspension from large sediment plumes near “ground zero” (i.e., a dredging operation at Miami Harbor) and after Summer-Fall high thermal anomalies that led to extensive bleaching across the Florida Reef Tract (Weil et al., 2006; Work and Aeby, 2006; Manzello, 2015; Miller et al., 2016; Precht et al., 2016; Walton et al., 2018; Gintert et al., 2019; Precht, 2021). Often new disease outbreaks occur following a change in the host-parasite relationship, the introduction of a novel pathogen(s), the release of otherwise innocuous pathogens when resuspension of sediment occurs, the emergence of newly evolved pathogens and/or changes in environmental conditions that alter the microbiome/host physiological equilibrium, fostering increased pathogen virulence, transmissibility, host susceptibility, and coral mortality (Daszak et al., 2000, 2001; Harvell et al., 2009; Weil and Rogers, 2011; Aeby et al., 2019; Vega Thurber et al., 2020). However, for decades we have assumed that coral diseases are always infectious, which has not been rigorously proven for every tissue loss disease described in the Caribbean. Changes in habitat phenology (i.e., the specific characteristics of the habitats where evolution of morphological, physiological and behavioral adaptations and life history traits of animals occur), for instance, can lead to starvation and death, thermal stress can lead to bleaching and shifts in environmental and climatic conditions can drive non-infectious death in corals.
Stony coral tissue loss disease shares similar signs with WPD-II for both produce rapid tissue loss on their coral hosts (Richardson et al., 1998b; Weil and Rogers, 2011; Miller et al., 2016). This has produced uncertainty when attempting to identify the disease in the field. Although SCTLD is accepted as a new disease based on specific pathological and epizootiological traits (e.g., virulence, dispersion, and persistence), it is still not known if this disease represents a more virulent form of former WPD outbreaks. In this report we compare what is known in terms of pathology, etiology and epizootiology of SCTLD and WPD-II based on a detailed review of the literature. We aimed to summarize distinctive characteristics, patterns and traits reported in the literature for both diseases and other researchers experience in the Caribbean.
Materials and Methods
We conducted a literature review to compare STCLD and white plague diseases (WPD I, II, and III) on different web browsers: (1) Google scholar, (2) Dimensions, and (3) the data base created by Montilla et al.(2019; Figure 1). Keywords for this search included: coral diseases, Caribbean, WPD-I II and III, white syndromes, pathology, histology, histopathology, etiology, epizootiology, outbreak, epizootic, treatments, shifts on coral host, modeling, and environmental drivers. The review included all papers published in peer-reviewed journals from 1971 to June 2021. We also included relevant information from reports published in various web sites (e.g., AGRRA, Reef Resilience Network, GCFI, NOAA and Florida Department of Environmental Protection). After eliminating duplicates, only 585 papers were screened from consulted sources, of which over 127 were included because we were focused on comparing what is known about the pathology, etiology, and epizootiology of WPD and SCTLD (Figure 1). In this review, other “white syndromes” such as WBD, white pox, and patchy necrosis were not included because they are only known to affect Acroporids; and these species are not regarded as susceptible to WPD and SCTLD.
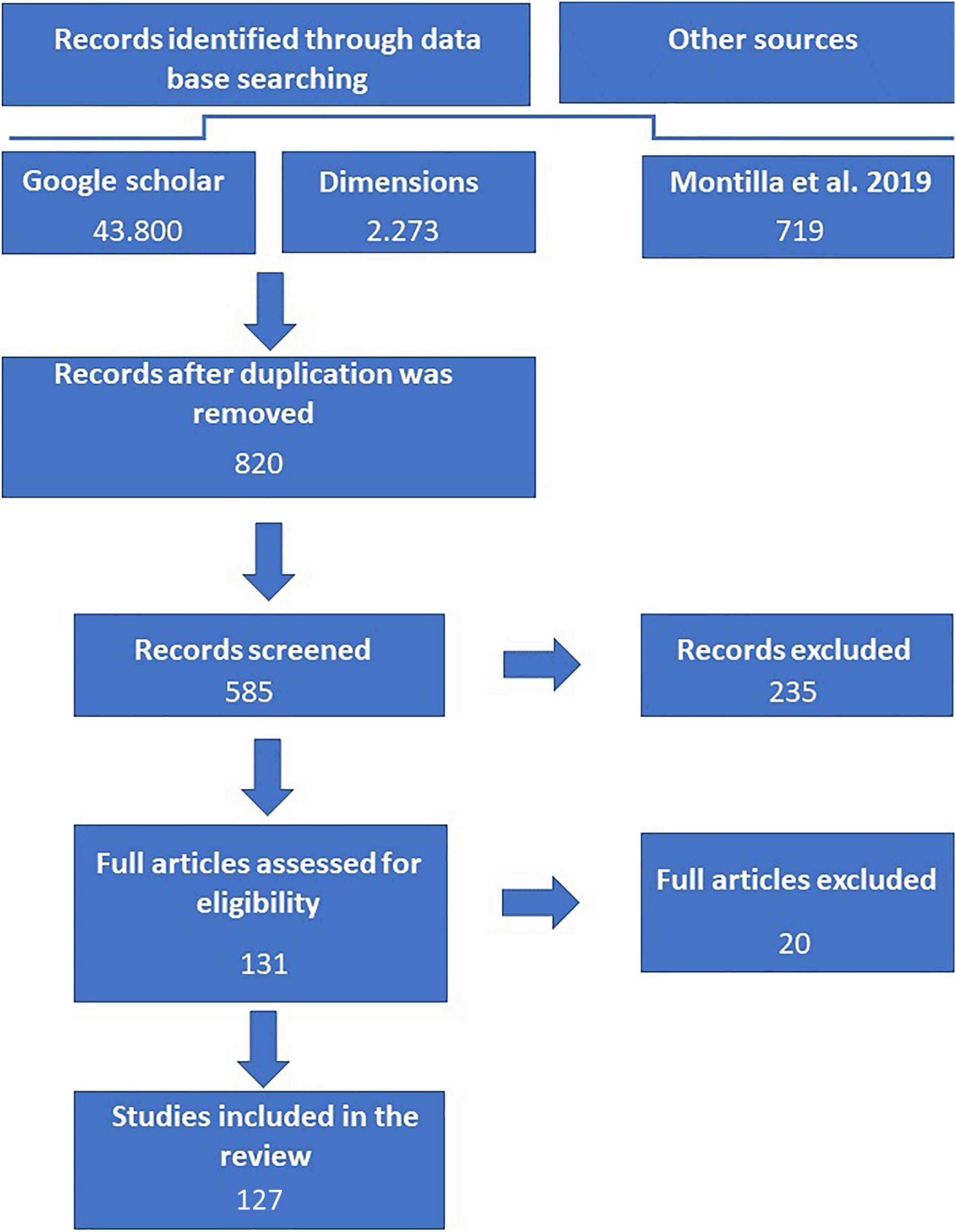
Figure 1. Prisma diagram showing the workflow conducted for the literature review. Three data bases were consulted. In addition, local and regional monitoring data bases (e.g., AGRRA) were consulted for reports and news (not displayed in the figure).
Results
Pathology to Decipher Etiology
Coral pathology is the systematic description of tissue changes associated with the disease at the gross and microscopic levels in attempts to detect causes of lesions, characterize host response as well as mechanism leading to disease (Porter et al., 2001; Bythell et al., 2002; Work and Aeby, 2006; Work et al., 2008; Woodley et al., 2016b). Stony coral tissue loss results in variable degrees of acute to subacute tissue loss in a wide number of coral species in a broad expanse of Florida and the Caribbean (Aeby et al., 2019). The presence of a bleached border separating apparently healthy tissues seems to be a distinctive characteristic of the disease and disease lesions, although these signs are not always similar among species or across regions (Aeby et al., 2019). However, the bleached circular area is a clear and often consistent macroscopic sign across many coral species in areas where lesions are just beginning to appear (Figures 2A–J). Furthermore, lesions always have acute (recent and localized) or subacute (long-lasting and dispersed) areas of tissue loss from sloughing (Figures 2K,L), resulting in patches of coalescent stark white areas of newly denuded skeleton that are rapidly covered by turf algae, macroalgae, and sediments (Alvarez-Filip et al., 2019; Sharp W. et al., 2020; Figures 2A–L).
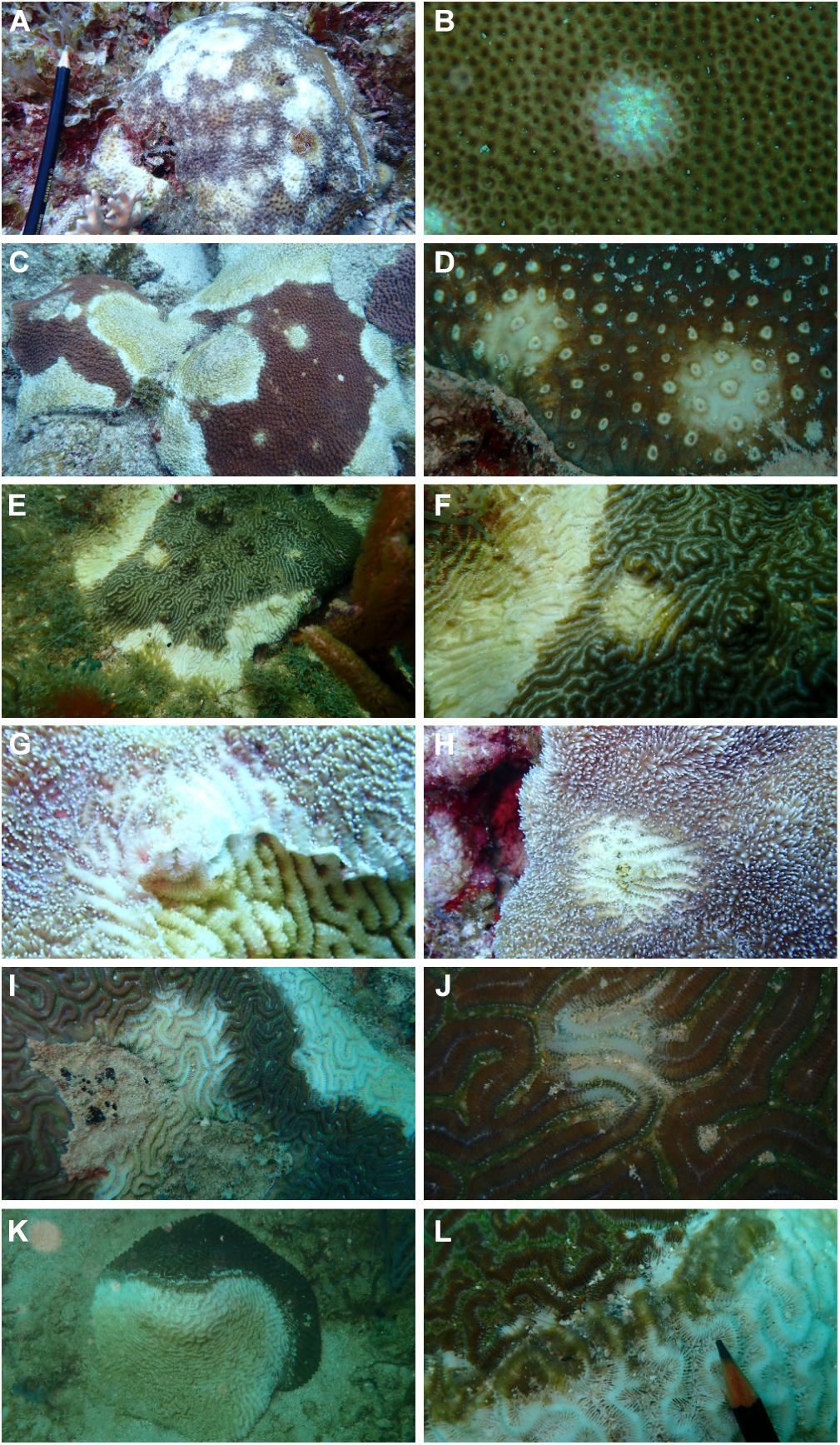
Figure 2. Examples of consistent differentiating signs on different species of corals affected by Stony Coral Tissue Loss Disease (SCTLD). A multifocal bleached round area is observed in areas where the lesion is apparently initiating. This blotch was never seen or reported for WPD. Multifocal coalescent infection on Siderastrea siderea (A) and a close up of a bleached small lesion (B), Montastraea cavernosa (C,D), Pseudodiploria strigosa (E,F) and Dendrogyra cylindrus (G,H) and Diploria labyrinthiformis (I,J). A front of sloughing tissue between apparently healthy and recently exposed skeleton suggest rapid mortality is ongoing on Pseudodiploria strigosa (K,L). Photos taken at Carriles, Sanama, Dominican Republic in March 2021.
White plague diseases (WPD I, II, and III) produce regular bands, and sometimes lesions that may or may not coalesce and advance across the colony (Figures 2A–I). While the more virulent WPD-III was later discarded as a new syndrome and regarded as a new WPD-II epizootic event, no convincing evidence has ever been advanced for any of the WPD types. Yet, in the literature two major distinctions between WPD II- and the original WPD I description are made. First, WPD II generally begins at the base of the colony, or in any depression in the center of massive corals bearing sediments deposited in the contact area and/or in portions of colonies that are in contact with the substrate (Figure 3A) and occasionally in the center where there is not a depression (Figure 3C). The disease then advances upward or outward from these focal points (Figures 3D–I). When originally described, WPD-1 consisted of a sharp white band separating exposed skeleton from apparently healthy tissues on massive corals, also a common trait of SCTLD (Figures 3, 4). However, such distinctions were based on anecdotal observations rather than rigorous compared histopathology to establish statistical association between lesion development on different coral hosts across geographies. Secondly, WPD II was significantly more virulent, with rates of advance 2-to-3-fold higher (0.3–3.0 cm/day) than WPD I, and affected a larger number of hosts (up to 41 reported for WPD-II) but particularly Orbicella faveolata and O. annularis (Miller et al., 2009; Weil and Rogers, 2011; Peters, 2016). Nevertheless, relying only on virulence to separate diseases may not be an accurate criterium because rates of tissue loss may be seasonal, climate-related, and/or depend on host response rather than being associated with different etiology. For example, rabies may kill some species faster than others (Fisher et al., 2018), yet it is the same etiology.
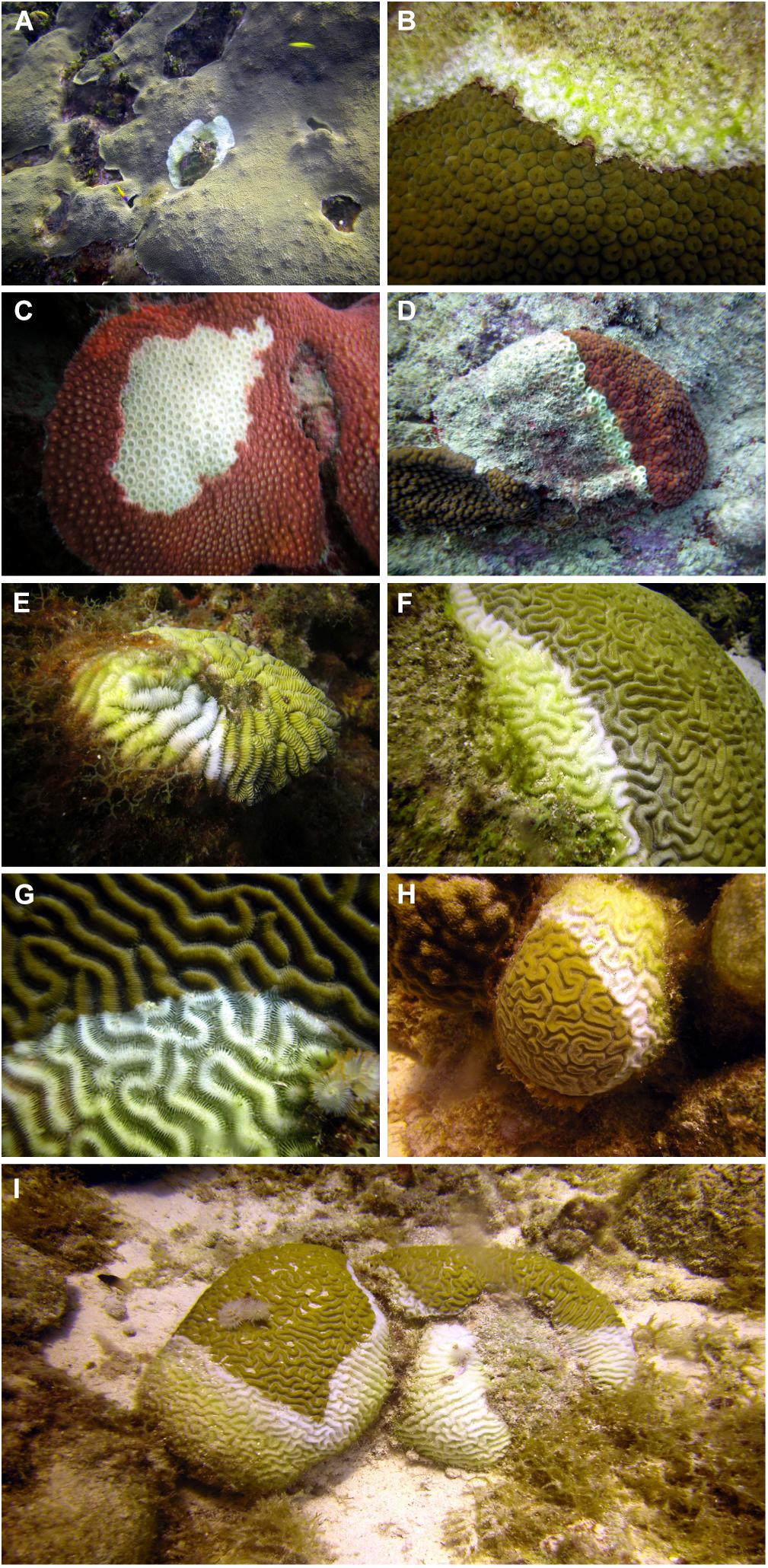
Figure 3. Photos of classic description of WPD-II lesions in different localities of the Caribbean. (A) Focal lesion starting from a depression of an Orbicella faveolata in Cayman Islands (Summer of 2006) and on the center of a Montastraea cavernosa (B) in Curacao (Summer 2005). (C) Secondary algal growth behind active WPD-II active front affecting Montastraea cavernosa in Curacao in 2006. Examples of focal lesions starting at the base of colonies and moving upward in Montastraea cavernosa (D: Bermuda, Summer 2006), Meandrina meandrites (E: Curacao, Summer 2005), Pseudodiploria strigosa (F, G: Curacao, Summer 2006) and Diploria labyrinthiformis (H: Curacao, Summer 2006). Two P. strigosa colonies close to each other showing a lesion starting from the base and moving upward the colonies (I: Curacao: Summer 2006). Photos A. Croquer.
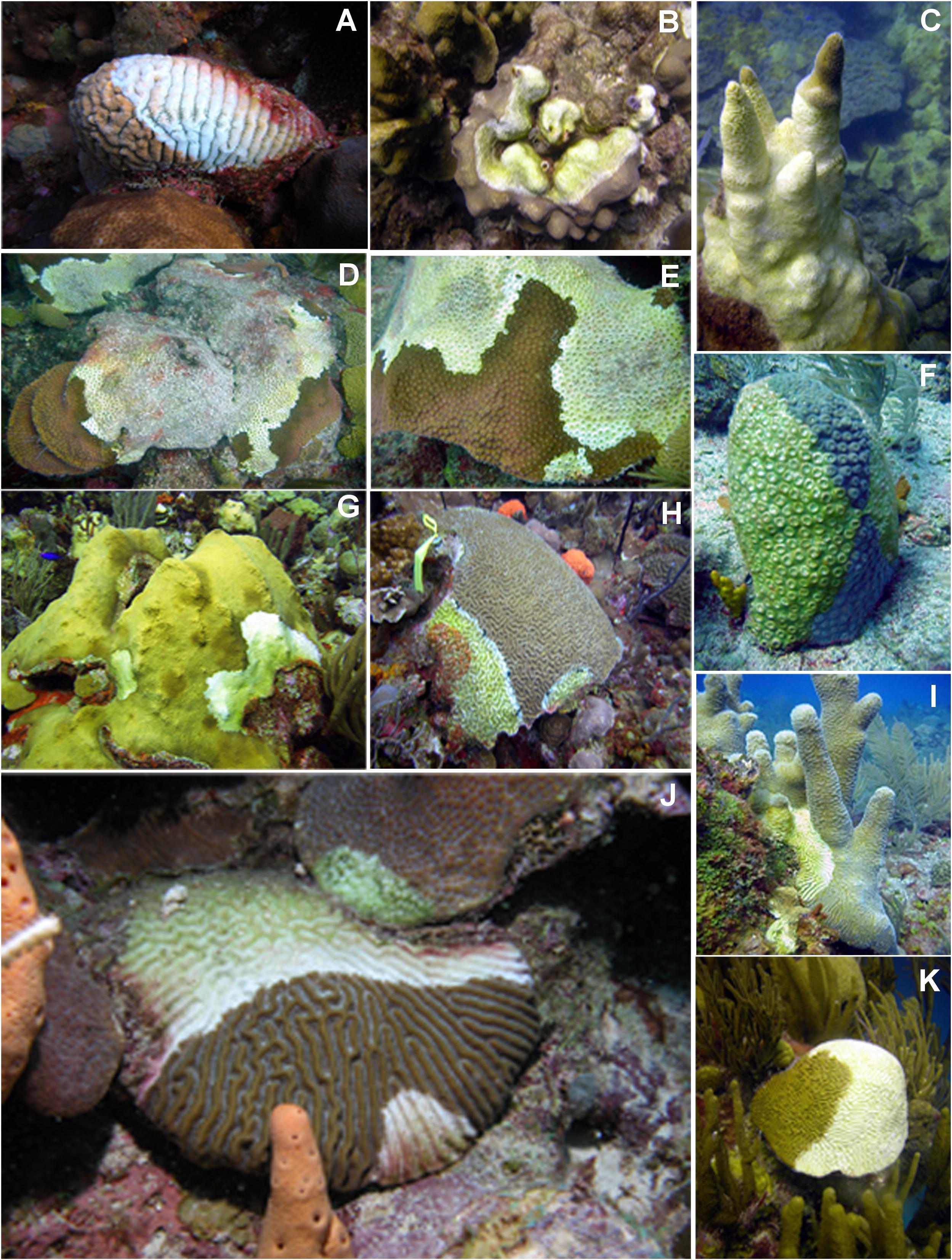
Figure 4. Photos showing colonies of reef-building coral species with WPD-II between 2004 and 2007 in different localities of the Caribbean. Cases having multifocal and focal lesions which not always started from the base of the colony. (A) Meandrina meandrites with fast moving WPD-II in La Parguera in 2005; (B) Orbicella faveolata in Mona Island in 2004; (C) Dendrogyra cylindrus with virulent WPD-II in Curacao in 2005; (D) Montastraea cavernosa in Desecheo in 2005; (E) M. cavernosa with two focal infections and (F) fast mortality in Bermuda in 2005; (G) O. faveolata in La Parguera in 2006. (H) Colpophyllia natans with two focal infections in La Parguera in 2006; (I) D. cylindrus with virulent WPD-II infection in Cayman Islands in 2005; (J) Pseudodiploria strigosa with two WPD-II fast advancing infections and contagion to O. franksi in La Parguera 2006: (K); P. strigosa with a highly virulent WPD-II in Bermuda in 2005. Photos E. Weil.
Common features of the macroscopic lesions of SCTLD and WPD as well as many other diseases are the rapid loss of tissues, which leaves denuded skeleton quickly colonized by opportunistic organisms (Figure 2). However, lesions of SCTD are reported to advance faster than WPD II (Aeby et al., 2019; Alvarez-Filip et al., 2019; Estrada-Saldívar et al., 2021), just as this disease was reported to move faster than WPD I. Another sign that has been regarded as unique for SCTLD, is the bleaching front and/or spots (Aeby et al., 2019; Landsberg et al., 2020; Figures 2–4), nevertheless, the bleaching front was noticed in some cases in corals affected by WPD II and WBD (Pantos et al., 2003; Bythell et al., 2004). The unique feature that appears to be exclusive to SCTLD is the multifocal, coalescent lesions that spread across the affected colonies (Aeby et al., 2019) and a front of sloughing tissue (Supplementary Material), whereas WPD starts from the colony base and progresses upward in a concentric ring or elongated band (Weil et al., 2002; Sutherland et al., 2004; Weil et al., 2006; Bruckner, 2016b; Richardson, 2016; Figures 3A–F). Nevertheless, multifocal lesions were also described as an “occasional” condition for WPD-II (Richardson et al., 1998b), and the condition was observed in different geographic localities in the 2000s (Weil and Croquer pers. Observations; Figures 4G–J). Thus, the distinction between macroscopic signs of SCTLD and WPD is challenging. This is because the “case definition (i.e., standardized descriptive terms applied to classify diseased individuals showing clinical and subclinical signs of a disease)” for SCTLD in Florida was established with a more structured veterinary framework (Work and Aeby, 2006; Work et al., 2008; Aeby et al., 2019) compared to WPD (Richardson et al., 1998b). Specifically, the use of systematic histologic description of the disease in multiple species was never conducted for WPD as it was done for black band disease (BBD; Miller et al., 2011; Miller and Richardson, 2012) and SCTLD (Landsberg et al., 2020).
The most comprehensive microscopic examination of tissues exists mainly for SCTLD with relatively few to no such studies for WPD-I and WPD-II. Microscopically, SCTLD is characterized multifocal lytic necrosis originating in the gastrodermis of the basal body wall and extending to the calicodermis and overlying gastrodermis and epidermis with necrosis and degeneration of zooxanthellae (Landsberg et al., 2020; Thome et al., 2021). Also, other alterations such as peripheral nuclear chromatin condensation; cytoplasmic vacuolation accompanied by deformation, swelling, or atrophy; swollen accumulation bodies; prominent pyrenoids; and degraded chloroplasts have been reported (Landsberg et al., 2020). Tissular damages are detectable in apparently healthy tissue, further indicating tissue might be affected before the macroscopic signs are noticeable (Landsberg et al., 2020).
However, there are histological changes that are not present in every host species. For example, Montastraea cavernosa and Pseudodiploria strigosa show a series of different histopathological signs (see Landsberg et al., 2020 for detailed description). Coccoid-like or coccobacilloid-like Gram-neutral bacteria reminiscent of microorganisms are occasionally associated with SCTLD lesions; suggesting these bacteria and other microorganisms are secondary colonizers (Thome et al., 2021). Thus, SCTLD is thought to be the result of disruption of host–symbiont physiology leading to cell death and sloughing of tissues from the skeleton. It is unclear whether this disruption starts with the symbiont or the host cell housing the zooxanthellae (Landsberg et al., 2020), and the cause of the zooxanthellae pathology remains to be elucidated.
On the other hand, histological studies addressing microscopic changes at the tissue level for corals with WPD are less detailed. In 1995, TEM of bleached tissues associated with WPD-II revealed degenerated coral tissue with the gram negative, rod-shaped bacteria and remnants of zooxanthellae in D. stokesii, Dendrogyra cylindrus, and D. labyrinthiformis (Richardson et al., 1998b). These findings were later confirmed (Denner et al., 2003; Bythell et al., 2004; Sutherland et al., 2004; Bruckner, 2016b). Proteomic analysis of WPD-II tissues showed the expression of proteins playing a role in innate immunity, cytoskeletal integrity, cell adhesion, oxidative stress and chemical defense (Daniels et al., 2015), responses that have been also reported for bleached Caribbean reef builders (Pinzón et al., 2015). Recently, Thome et al. (2021) found high levels of phenoloxidase (two orders of magnitude higher PO activity than P. strigosa affected by BBD) and antibacterial activity in diseased corals during an epizootic event that resembled both WPD-II and SCTLD. Meta-proteomic studies conducted for a white syndrome similar to WPD-II affecting Mussismilia braziliensis revealed shifts toward proteins involved with nitrogen fixation (Garcia et al., 2016). However, no systematic descriptions of WPD at the light microscopic level are available making it difficult to say how it compares to SCTLD other than presence of tissue loss.
Experimental Confirmation of Etiology
Etiology is the study of disease causation through statistical inference (Thrusfield, 2016b). The cause of SCTLD is currently unknown and Koch’s postulates have not been fulfilled because to date, no evidence of an infectious etiology exists. In SCTLD, potential causes of coral-algal symbiosis dysfunction are hypothesized to include toxicosis, a pathogen of zooxanthellae not visible at the light microscopic level, or some sort of aberrant host response (Landsberg et al., 2020). Recent experiments also show co-infection with the well-known pathogen Vibrio coralliitycus exacerbates SCTLD lesions, with varying degrees of co-infection being used to explain differences in mortality rates across affected species (Ushijima et al., 2020); however, no evidence of bacteria has been associated with SCTLD lesions at the microscopic level. As such, these Vibrio spp. are likely contaminants or detrital microbiota.
As for WPD, no pathogen was ever confirmed for WPD-I (Richardson et al., 1998b; Bythell et al., 2004), whereas A. coralicida was controversially identified as the primary pathogen of WPD-II (Denner et al., 2003; Richardson, 2016) with no evidence at the cellular level that this organism was responsible for tissue damage. Furthermore, subsequent tests failed to isolate the same pathogen (Sunagawa et al., 2009). Thus, etiology of WPD-II remains unknown. A few studies have reported small circular ssDNA viruses and phages associated with WPD-II tissues from Orbicella annularis combining next generation sequencing with TEM (Soffer et al., 2014, 2015); however, no convincing evidence was shown that these viruses led to cell death in corals at the microscopic level. Thus, both WPD-II and STCLD have been hypothesized to be potentially caused by viruses and/or a consortium of bacteria, but so far there is no convincing evidence directly associating cell death with any of the putative pathogens. Opportunistic (i.e., secondary invaders) such as saprophytic ciliates have been found associated with WPD (Weil, 2004; Cróquer and Weil, 2009) but their role was not firmly established. Ciliates found in SCTLD histological preparations have been regarded as incidental due to the lack of association with cell pathology (Landsberg et al., 2020).
Molecular tools have also been used for the identification of coral pathogens. In the past, there were some attempts to develop specific probes for A. coralicida (Richardson et al., 2005). Furthermore, Vibrios associated with WPD-II have also been used as diagnostic tools using qPCR which wrongly assumes the presence of this bacteria alone suffices to infer causation (Tonon et al., 2017). Additionally, electron microscopy has been incorporated to evaluate the pathology of WPD-II (Soffer et al., 2014) assuming the disease is infectious and produced by viruses and bacteria. However, the systematic approach proposed by Work and Meteyer (2014) to establish disease causation was never adopted to establish the etiology of WPD-II. To our knowledge, attempts to detect potential pathogens in SCTLD tissues using specific probes have only been attempted in the United States Virgin Islands with identification of four potential bacteria strains (Algicola, Cohaesibacter, Thalassobius, and Vibrio) consistently associated with SCTLD (Becker et al., 2021). However, SCTLD, WPD-II and other rapid loss diseases have all been shown to trigger significant changes in the coral microbiome in Caribbean and Indo Pacific corals which lead to coral death (Pantos et al., 2003; Sunagawa et al., 2009; Cárdenas et al., 2012; Roder et al., 2014; Meyer et al., 2019; Rosales et al., 2020). Recent findings suggest that Rhobacteriales and Rhizobiales are associated with SCTLD and sediments could be a source of transmission for these bacteria (Rosales et al., 2020). Yet, to our knowledge no one has shown whether the altered microbiome is the cause and/or the consequence of the disease.
Epizootiology: Occurrence, Spread, Effects of Disease on Coral Hosts and Maintenance on Coral Populations
Epizootiology is the study of a disease in populations of animals (Thrusfield, 2016a). The epizootiology of coral diseases, often with unknown etiology, has been focused on describing occurrence (i.e., origin and geographical distribution, host ranges, prevalence, and incidence), establishing mechanisms of transmission and maintenance of infection (i.e., host susceptibility, virulence of pathogens, and environmental drivers) and finally; the characterization of the spread of infection in the host population (i.e., description of spatial and temporal trends).
Reports show that WPD-I, WPD-II, WPD-III, and STCLD were all first described along the Florida reef tract in different years (Dustan, 1977; Richardson et al., 1998b; Bruckner, 2016b; Precht et al., 2016; Aeby et al., 2019; Kramer et al., 2020). SCTLD has a faster dispersion (7–10 km in 2 years) compared to WPD-II (Weil et al., 2019). WPD-II has been reported from north western-Atlantic locations (e.g., Bermuda), all the way south to Brazil, and across the wider Caribbean assuming all these reports identified the same disease (Weil et al., 2000; Weil, 2004; Cróquer et al., 2005; Weil et al., 2006; Cróquer and Weil, 2009; Weil and Cróquer, 2009; Weil and Rogers, 2011; Bruckner, 2016a,b). From 1995 to 2012, WPD-II outbreaks were documented in the Bahamas (Voss and Richardson, 2006), Venezuela (Croquer et al., 2003), United States Virgin Islands (Miller et al., 2009); Colombia (Sánchez et al., 2010), Puerto Rico (Weil et al., 2009), Turks and Caicos (Heres et al., 2021), and many other countries with prevalence ranging from 15 to 60% (Sutherland et al., 2004; Weil and Rogers, 2011; Smith et al., 2013; Bruckner, 2016b).
Since first described in 2014, SCTLD spread across the United States Caribbean region in 4 years, with cases reported across 18 locations, specifically in Jamaica (late 2017, Lang, personal communication) and later in Mexico, Saint Maarten, the United States Caribbean and United States Virgin Islands (Brandt et al., 2021), the Dominican Republic (Irazabal and Rodriguez, 2019), Turks and Caicos Islands, Belize, Saint Eustatius, and Puerto Rico, The Bahamas, the Cayman Islands, Guadeloupe, St. Lucia and Honduras (Kramer et al., 2020)1. The most deadly events have been reported for Mexico (Thome et al., 2021), the Mesoamerican Reef System, The USVI, and the Flroida Reef Tract (i.e., 40 to 50% live coral loss) with prevalence levels similar to the ones reported for WPD-II and in some cases, much higher than disease baseline levels expected for Caribbean reefs (Ruiz-Moreno et al., 2012; Aeby et al., 2019; Alvarez-Filip et al., 2019; Precht, 2019; Weil et al., 2019; Neely and Lewis, 2020; Brandt et al., 2021; Estrada-Saldívar et al., 2021). This is likely because of the more persistent nature of SCTLD.
Results so far indicate that SCTLD kills corals faster than WPD-II. Reported linear tissue mortality rates for SCTLD range from 3.6 to 5.3 cm2 day–1 as lesions quickly coalesce (Aeby et al., 2019) or 3–4 cm/day as colonies die within weeks or months (AGRRA2), with fatality (i.e., entire colony mortality) varying from 73 to nearly 100% within a few months depending on location and species (Aeby et al., 2019; Thome et al., 2021). On the other hand, rates of tissue mortality for for WPD-II are extremely variable ranging from 0.1 to 2 day 1 and 1-4 cm week or month depending on coral species, time of the year and location with fatality ranging from 7 to 38% over of a 12-month period and eventually 60 to 100% mortality (Bruckner and Bruckner, 1997; Richardson, 1998; Richardson et al., 1998a,b; Bruckner, 2002; Cróquer et al., 2005; Weil et al., 2009; Bruckner, 2016b; Richardson, 2016). As for the range of hosts, SCTLD and WPD-II are presumably the two Caribbean coral diseases with the broadest host ranges, both affecting between 22 (SCTLD) to 42 (WPD-II) coral species and sharing more than 45% of their hosts. The most susceptible species for both diseases are overall the same important reef builders in the region, particularly the O. faveolata, O. annularis, O. franksi, M. cavernosa, Eusmilia fastigiata, S. siderea, C. natans, Pseudodiploria spp., D. labyrinthiformis, Stephanocoenia intersepta, D. cylindrus, and Meandrina spp. among others. While there is a strong overlapping on host ranges, susceptibility to both diseases is slightly different, with Orbicella spp. being more susceptible to WPD-II (Nugues, 2002; Sutherland et al., 2004; Weil, 2004; Weil and Cróquer, 2009; Richardson, 2016), and Dendrogyra cylindrus, Meandrina ssp., M. cavernosa, S. siderea, and E. fastigiata being particularly prone to get SCTLD in the field (Walton et al., 2018; Aeby et al., 2019; Alvarez-Filip et al., 2019; Kramer et al., 2020; Brandt et al., 2021; Costa et al., 2021; Estrada-Saldívar et al., 2021). Furthermore, a recent study indicates that Orbicella annularis, C. natans and S. siderea are highly susceptible to SCTLD in the laboratory (Meiling et al., 2021). Lastly, SCTLD prevalence is positively, significantly but moderately correlated with coral species diversity and richness across epidemic zones in the United States Virgin Islands (Costa et al., 2021). Similarly, outbreaks of WPD-II often occurred in reefs with high species richness such as Los Roques, Venezuela (Croquer et al., 2003; Cróquer et al., 2005) and La Parguera, Puerto Rico (Weil et al., 2009). However, in the past, the correlation between disease prevalence, coral diversity and evenness has been shown to be moderate to low and non-statistically significant (Ward et al., 2006).
We found no records documenting the mechanism of transmission for WPD-I. However, controlled experimentation determined that WPD-II disease can be transmitted through multiple mechanisms, including water transport and predation by Coralliophila abbreviata, whereas fire worms did not transmit the disease (Clemens and Brandt, 2015). However, studies my Miller et al. (2014) demonstrated that fire worms exacerbate mortality of Acropora cervicornis showing tissue-loss like diseases. Furthermore, contact with macroalgae such as Halimeda spp. was regarded as a pathogen reservoir that triggers WPD-II (Nugues et al., 2004). Similarly, SCTLD is apparently transmitted horizontally from diseased to susceptible hosts and ballast waters have been hypothesized as a potential vector of the disease (Aeby et al., 2019; Meiling et al., 2021; Rosenau et al., 2021). Nevertheless, the probability of transmission differs across species. For example, C. natans develop lesions more rapidly than M. cavernosa (ca 5 days; Aeby et al., 2019). Recently, 8 coral species housed with lesioned D. labyrinthiformis developed lesions (Meiling et al., 2021).
To our knowledge only two attempts to model WPD-II epizootic dynamics have been attempted. Using spatially explicit models, Brandt and McManus (2009) showed that WPD-II has a limited dispersion capacity as probability of transmission decreases with coral cover decline. Furthermore, the distance between WPD-II diseased and susceptible coral hosts is a key factor for transmission and permanence of WPD-II. Zvuloni et al. (2015) showed that the probability of spread of WPD-II is driven by the spatial distribution of susceptible hosts and sea surface temperature anomalies. Spatial epidemiology models suggest that SCTLD is a waterborne disease as distance between affected and susceptible corals does not affect the potential of spread, whereas larger surface area of colonies enhances the probability of infection (Muller et al., 2020). SCTLD dispersion is independent of coral colony density with lack of a positive association between a colony showing signs of SCTLD and the condition of, or distance to, its neighboring colonies (Sharp W. C. et al., 2020).
Temporal variation in the prevalence of WPD-II and SCTLD seems to differ, with clear seasonal behavior for the former and a more persistent pattern in the latter (Weil, 2004; Rogers, 2010; Aeby et al., 2019; Paul et al., 2019). However, in Puerto Rico WPD-II epizootic events lasted 8 years (Weil et al., 2009). SCTLD epizootics occur in three different phases: (1) invasion (i.e., 1–7 months), (2) outbreak (i.e., 3–12 months), and (3) endemic which occur in a 12–48-month period (see text footnote 1). During the initial phase, prevalence is low, acute lesions (i.e., short-lasting) are rare, and most susceptible corals start dying. The outbreak phase is when the disease spreads out from most susceptible to less susceptible species, the prevalence peaks and acute lesions become frequent. Finally, during the endemic phase, prevalence declines because of high fatality (Thome et al., 2021) (see text footnote 1) and the concomitant reduction of susceptible host populations; acute and chronic (i.e., long-lasting) lesions may persist and the coral community collapses with significant loss of live coral cover (Neely, 2018) (see text footnote 1). In the Florida Reef Track these long-lasting and persistent epizootic events have been responsible for the recent population decline of the endangered D. cylindrus (Neely and Lewis, 2020). In the United States Virgin Islands, SCTLD progression has been shown to slow down with the accumulation of heat stress and the concomitant bleaching events (Meiling et al., 2020).
As for treatments, we found that WPD-II corals had only been treated by shading diseased colonies (Muller and van Woesik, 2009). On the other hand, different approaches to treat corals with SCTLD have been employed since it was first described in 2014. The use of antibiotics and probiotics are the most common type of intervention, both considered justified because of the devastating effects STCLD is having across reefs (Figures 5A–F) and the concomitant rapid decline of Caribbean reef building corals (Alvarez-Filip et al., 2019; Precht, 2019; Voss et al., 2019; Landsberg et al., 2020; Neely and Lewis, 2020) in spite of the fact that no convincing data exist that bacteria are responsible for SCTLD. Moreover, limited consideration seems to be given to adverse long-term collateral effects dumping antibiotics onto reefs might have on other biota as current assessments are based on short-term studies. According to AGRRA’s web site and related reports from different institutions shared in the site3, these treatments have been attempted in less than ten coral species in Caribbean countries (Neely et al., 2020; Shilling et al., 2021) which represents about 50% of the coral species that are susceptible to SCTLD. Thus, approaches to control SCTLD and WPD-II have been completely different, with a series of biological (e.g., use of antibiotics) and mechanical (e.g., carving narrow trenches in front of the diseased-looking tissues, removal of colonies, and cryopreservation) in situ interventions attempted for the former and only shading for the latter. For both diseases, treatments have been shown to control mortality in individual colonies, with 70 to 85% (Voss et al., 2019; Neely et al., 2020; Meiling et al., 2021) and in some cases up to 95% success halting of lesions after application of Base 2B plus amoxicill specifically for SCTLD (Shilling et al., 2021).
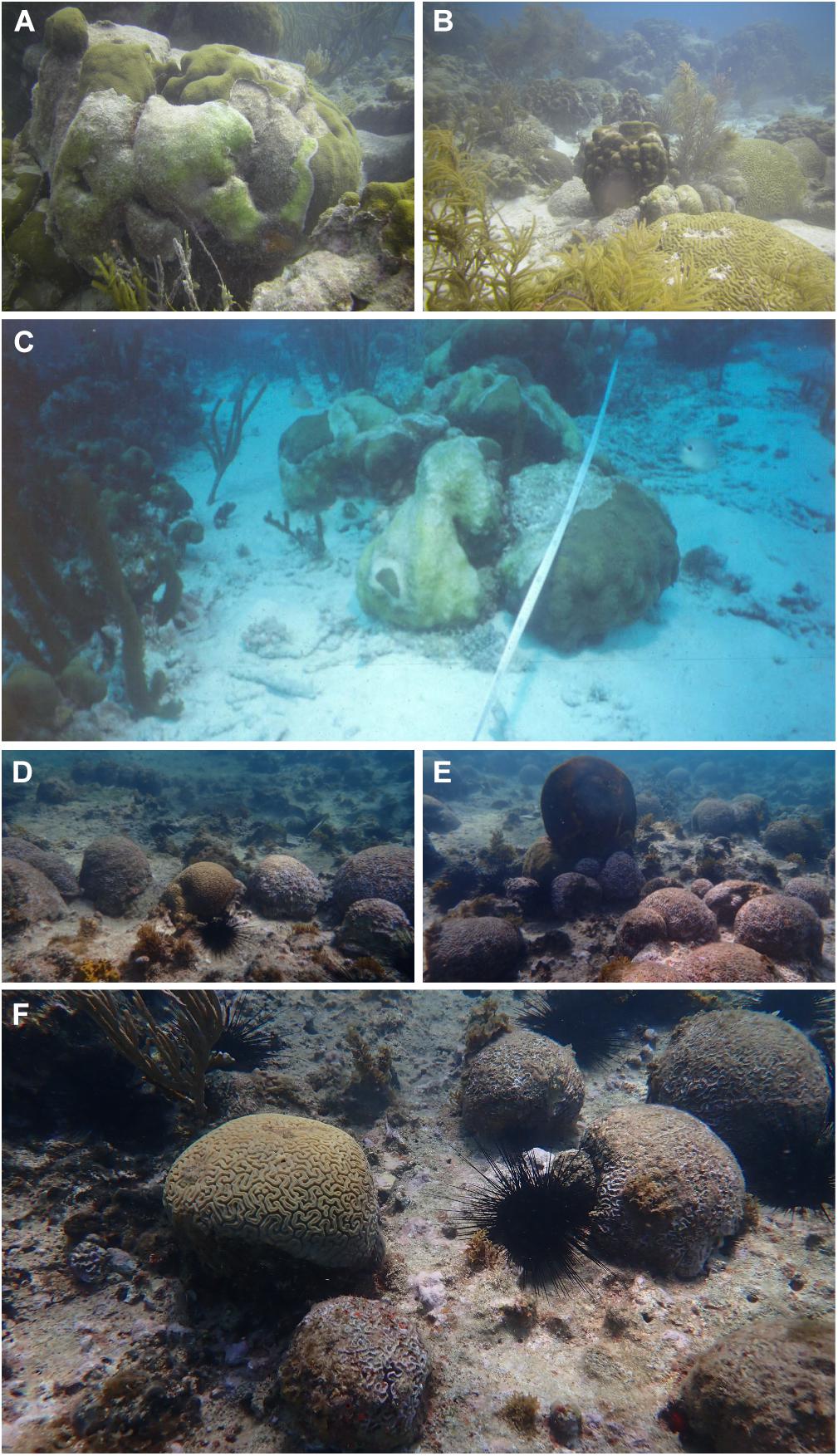
Figure 5. Examples of devastating effects of WPD-II and SCTLD on coral communities after epizootic events. (A) Active WPD-II multifocal infection on Orbicella faveolata and recent mortality during an epizootic event along the eastern barrier of Los Roques in 2009. (B) Arrows pointing dead colonies of Orbicella annularis and Pseudodiploria strigosa at the eastern barrier of Los Roques during a WPD-II epizootic event. (C) Orbicella annularis colony showing active WPD-II lesion during an epizootic event in Madrizqui, Los Roques in 2002. This event significantly reduced live coral cover in this site. (D–F) SCTLD mortality recorded at Piedra Miguelito (Sosua), Dominican Republic. The disease was first reported in 2020 and photos were taken in April 2021. Photos A. Croquer.
Discussion
Our analysis shows that in most cases, distinction between SCTLD and WPD-II is impossible based on a mere description of macroscopic signs (Brandt et al., 2021; Table 1). While not specifically noted at the time, careful review of historical photos taken to document WPD-II epizootic events shows multifocal and coalescent lesions that did not start from the base of the colony. For these unusual cases, it is impossible to assert if colonies were affected by WPD-II or SCTLD. The focal point moving from the base (interphase with sediment) to the tip of the colony was a descriptor of the disease affecting mostly D. stokesii colonies in Florida, and most but not all of the 40 plus host-species, or all colonies within a single species, later affected after WPD-II dispersed across the Caribbean. Thus, the lack of consistency of macroscopic signs across species and geographies make this gross sign unreliable to distinguish between WPD-II and SCTLD; and therefore they both should be regarded as rapid loss diseases as quoted by Brandt et al. (2021). Until more systematic observations of lesions by species and progression of lesions over time can be assessed, ideally along with microscopic pathology as microbiomes change, definitions of WPDs will remain vague and non-specific. For SCTLD and WPD there is a need to go beyond light microscopy and rule out the possibility of infectious agents not visible on light microscopy using tools such as TEM. Getting a clearer understanding of whether host cells or zooxanthellae die first is critical.
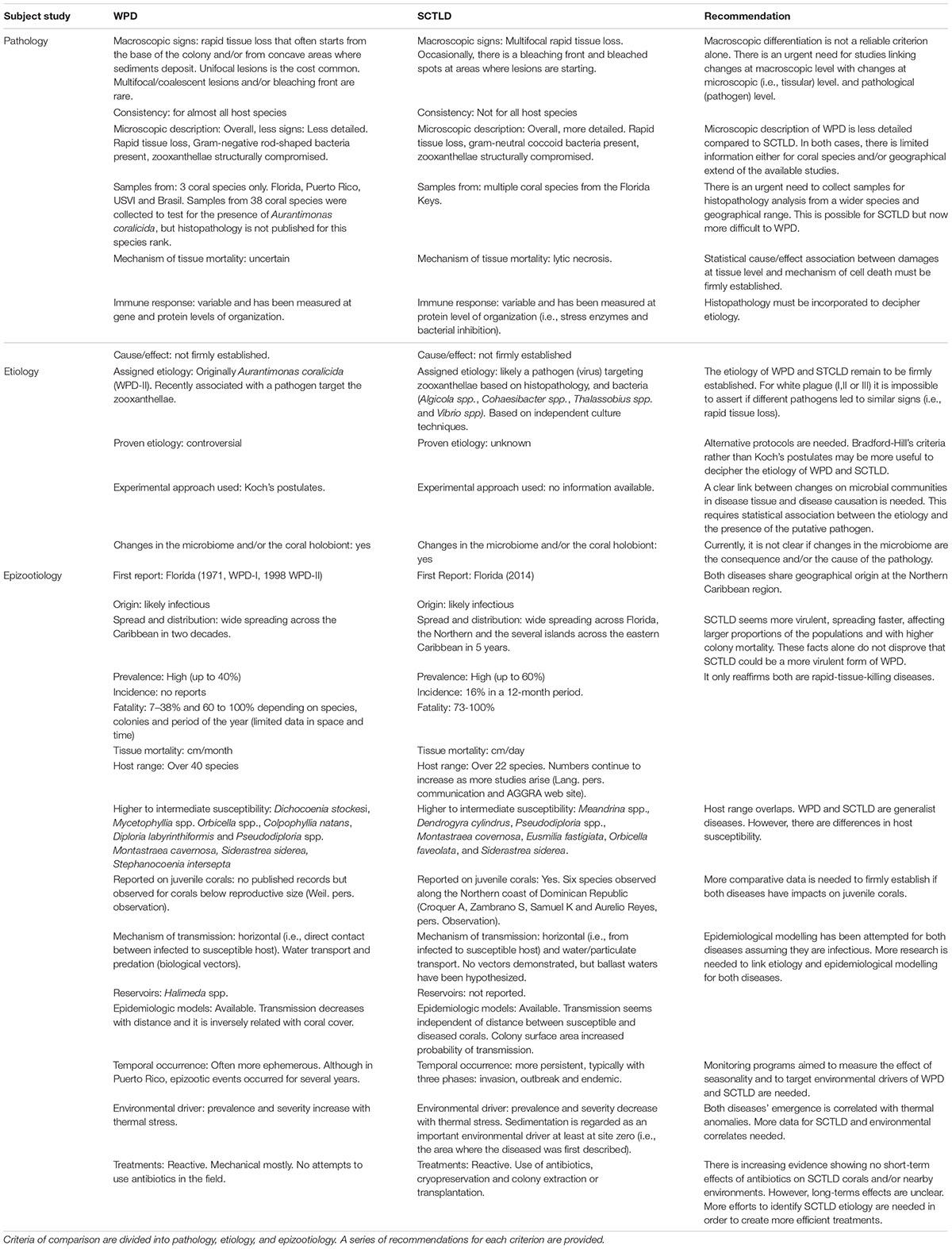
Table 1. Summary of similarities and differences between WPD and SCTLD based on literature review including information displayed in web pages cited along the text. Author credits are already cited and acknowledged throughout the text.
Likewise, microscopic description of WPD-II (histopathology) was not done with the same level of detail as the one presented for SCTLD by Landsberg et al. (2020). For instance, when WPD-II was first described, necrosis was assumed as the mechanism of tissue mortality, which is the case for SCTLD (Landsberg et al., 2020; Thome et al., 2021). Following Bradford-Hill criteria (Fedak et al., 2015), the relevant question to decipher diseases showing rapid tissue loss (e.g., WPD and SCTLD) should not be whether there is necrosis or not, but why/how is the tissue mortality happening. As such, the value of histopathology is not only descriptive, but can also be used to understand plausible mechanisms of cell death (i.e., health response when exposed to the agent; Table 1). Adopting such comparative approaches might be useful to confidently tell managers why corals are or are not dying. We also found that damages to the zooxanthellae have been described for both diseases, with the presence of virus-like particles, being a common feature in diseased tissues (Soffer et al., 2014; Landsberg et al., 2020; Thierry Work, USGS written communication). For the specific case of SCTLD, effects on zooxanthellae are expressed not only structurally (i.e., damage on thylakoid membranes), but also functionally (i.e., photosynthetic performance; Aeby et al., 2019; Landsberg et al., 2020). Functional disruption of zooxanthellae was never investigated for WPD-I and WPD-II. Therefore, more histopathological research is needed to characterize and confirm differences between these diseases at the microscopic level. However, such studies must rely on accurate discrimination between WPD-II and SCTLD in the field, particularly in places where both diseases co-occur. Until such comparative studies between WPD and SCTLD are available, we propose to use tissue loss to any sign suggesting rapid coral tissue sloughing. From that point, the next step must be to collect samples and then examine at the tissue tissular level for a more accurate case definition and thereby disease differentiation.
This is an ongoing effort that recently started throughout a cooperation between AGRRA (JC Lang), the USGS (A Hawthorn and T Work), The University of Tennessee College of Veterinary Medicine (M Denins) Red Arrecifal Dominicana (Someira Zambrano), The Nature Conservancy Caribbean and other organizations.
Etiology as Diagnostic Criteria
Characterizing the etiology of a coral disease is not a simple task because it is difficult to collect samples without contamination, and variability in survey and sample collection methods may confound comparative results (Weil and Rogers, 2011). Furthermore, there are still huge gaps in understanding coral pathogenesis at cellular levels. In consequence, in contrast to other animals, we are far behind in using host response as a diagnostic tool for coral diseases. Additionally, most marine bacteria, including pathogens, are difficult to culture or have never been cultured, making their identification, laboratory manipulation, and testing of Koch’s postulates difficult. Even if a putative pathogen is identified, testing which environmental variable or driver is responsible for its emergence is extremely difficult (Weil and Rogers, 2011; Woodley et al., 2016b). In fact, because of the limited number of cases where these postulates had been fulfilled for coral diseases in the past, recent approaches suggest complementing Koch’s postulates with histopathology (Work and Meteyer, 2014), molecular genomics (Pollock et al., 2010, 2011; Bourne et al., 2015), and immunological tools (Palmer et al., 2008) for a better inference of coral disease causality. Thus, a more balanced approach aimed to tease out infections from non-infections guided by careful examination of coral cells is of paramount importance. A common feature of SCTLD and WPD-II is the capacity of producing significant shifts in the microbiome of their coral hosts. Furthermore, opportunistic co-infections often exacerbate SCTLD lesions (Ushijima et al., 2020). Likewise, opportunistic microorganisms (ciliates) were often described for WPD-II potentially increasing the disease development across the Caribbean as these organisms feed on decaying tissues (Cróquer and Weil, 2009). Thus, regardless of the overwhelming literature showing significant shifts in the microbiome of WPD, SCTLD and healthy corals, this alone does not prove causality (Table 1). Thus, the etiology of rapid tissue loss diseases remains unknown and/or poorly understood. Ultimately identifying the pathogens is a key step to developing highly specific probes which are extremely valuable tools to discriminate among syndromes that produce similar signs. In the future, this must be a priority if definitive differentiation between diseases with similar signs is intended. While recent advances have identified in situ biomarkers for at least four potential pathogens likely associated with SCTLD (Becker et al., 2021), specificity and sensibility of these probes must be tested for consistency across different geographies and more coral species susceptible to SCTLD.
Epizootiology
Both WPD-II and SCTLD became epizootic. The former dispersed all over the wider Caribbean, including Bermuda, in a few years, and the latter is on its way to do the same (Weil and Rogers, 2011; Kramer et al., 2019, AGGRA). The major differences between WPD-II and SCTLD are relative to their spatial and temporal trends. SCTLD has a well-documented epidemiological behavior with three clear phases since the disease arrives and infects a few colonies, quickly turns into an outbreak, and then becomes endemic in the site for years when coral cover is reduced (Table 1). However, WPD-II was usually documented during the outbreak phase, while the initial infections and endemic phases were probably regarded as background disease prevalence before and after the outbreak, respectively. Thus, apparent epizootiological differences might be the result of methodological documentation of both diseases. Clearer criteria to decide what is enzootic (i.e., a persistent disease that occurs at low levels of prevalence in a population) and epizootic (i.e., the outbreak phase of a disease) are required, particularly for corals affected by WPD. This necessarily requires the existence of long-term and geographically extended data to check for baseline levels of disease prevalence as proposed by Ruiz-Moreno et al. (2012).
The literature indicates that SCTLD and WPD-II have had devastating effects on coral communities (Alvarez-Filip et al., 2019; Neely and Lewis, 2020; Estrada-Saldívar et al., 2021). While both diseases share a wide range of susceptible hosts, WPD-II had largest impacts on Orbicella spp. Pseudodiploria spp., Colpophyllia spp., S. siderea, and Mycetophyllia spp. populations (Weil, 2004; Miller et al., 2009; Weil et al., 2009; Weil and Rogers, 2011), and SCTLD is having striking consequences on populations of meandrinid corals (Aeby et al., 2019; Neely and Lewis, 2020; Brandt et al., 2021; Table 1). To our knowledge, Kaplan–Meir survivorship curves (KMSC) were never developed for WPD-II, but mortality risk for this disease was high and often determined by the size of the colony, with larger colonies having more chances to survive the initial infection (Weil and Croquer Pers. Observation). However, KMSC from a Mexican Caribbean case study showed low probability of survival within an 11-month period on P. strigosa colonies having signs similar to SCTLD. This study showed that probability of survivorship was independent of colony size (Thome et al., 2021) as reported in the Florida keys (Aeby et al., 2019). As mentioned before, rates of tissue mortality for WPD-II and SCTLD vary from several cm per month in the former to a few cm per day in the latter (Aeby et al., 2019). Differences in tissue mortality rates and virulence may explain the lack of size-dependent survivorship on corals affected by SCTLD. Therefore, tagging colonies (healthy and diseased) to determine incidence, rates of tissue mortality, and lethality (Aeby et al., 2019; Thome et al., 2021) is essential to better define how tissue loss diseases affect different species of corals and their behavior over time. Photogrammetry is a useful tool that has been used in various Caribbean locations (e.g., United States Virgin Islands and the Bahamas) to determine the fate of SCTLD-affected corals (Meiling et al., 2020).
Continuous monitoring programs with clear goals (e.g., detecting disease outbreaks, outlining procedures to collect samples for comparative histopathology and host response) provide an effective way to gather information to differentiate between WPD-II and SCTLD. However, this imposes a series of challenges; first, continuous monitoring plans aimed to follow-up reef health indicators and individual colonies require logistics, time and resources that are not available across all Caribbean countries. Particularly important is to adopt a holistic approach to better decipher the disease dynamics in corals. The idea is to integrate modern paradigms that consider multiple and variable interactions among the three major players in an epizootic event: the host, its associated microbiome, and the environment (Tracy et al., 2020; Vega Thurber et al., 2020). Secondly, permanent monitoring programs not only depend on resources but also in building capacity to increase the number of observers with standardized protocols. In this regard, the contributions of well-established networks such as AGRRA, GCFI (Gulf Caribbean Fisheries Institute), the Reef Resilient Network, the Marine Park Service Inventory and Monitoring Program and NOAA have been critical to coordinate data collection, training, workshops; and to increase accessibility of data that are being collected across the Caribbean.
In the past coral diseases have been treated using mechanical (e.g., barriers to separate diseased from apparently healthy tissues, vacuuming the pathogen, etc.); chemical (e.g., use of antibiotics); and biological interventions such as removal of potential vectors, phage therapy and more recently, the use of probiotics (Efrony et al., 2009; Teplitski and Ritchie, 2009; Sweet et al., 2014; Randall et al., 2018). However, treating diseased corals is challenging for several reasons. First, even if the “default” assumption of infectiveness would be true, most coral diseases have unknown etiology (Randall et al., 2018), often involving consortia of microorganisms (Mera and Bourne, 2018) which hampers the use of specific antibiotics to control the bacterial disease progression compared with other syndromes affecting wildlife. Therefore, treatments developed to control coral diseases are usually “blind” and do not discern between pathogenic (mucus and tissues), and opportunistic microorganisms or co-infection which are common of coral disease (Tracy et al., 2021). Secondly, the indiscriminate use antibiotics (e.g., amoxicillin) might be risky because in the long term, no one knows how these are affecting the microbiome associated with the mucus and coral tissues, or in nearby organisms or microhabitats (Voss et al., 2019). Such studies are very important because today we know that the coral microbiome is highly complex, with responsive, individual and core microbiomes having different community structure, and perhaps function inside coral hosts (Hernandez-Agreda et al., 2018).
Interventions developed so far are reactive and limited in space and time, often targeting a low number of colonies (ca 8–20) throughout short periods of time (weeks or months; e.g., Aeby et al., 2015; Randall et al., 2018), or a large number of colonies on particular reefs (Table 1). Treatments have been used to promote recovery of individual colonies, but fail to prevent disease from spreading across reefs and therefore sometimes more than one treatment is required on the same individual colonies (Neely et al., 2020), likely because treatments do not control direction or speed of currents or other key environmental drivers that trigger epizootic events. While we understand the urgency for saving diseased colonies to reduce the local impacts of SCTLD in the short term, the use of antibiotics may induce artificial selection for specific bacteria to the detriment of others that might be beneficial for the coral holobiont (Sweet et al., 2014). A broader scale approach, while challenging, could address the source of problems such as climate change, coastal pollution and habitat destruction that are the ultimate cause of the rapid decline of modern coral reefs.
Author Contributions
AC conducted the review. AC, EW, and CR wrote and revised the manuscript. AC and EW provided images. All authors contributed equally to revise the manuscript.
Funding
The authors would like to thank the Department of Marine Sciences, University of Puerto Rico for partial funding support and NSF-RAPID grant # 2000863 to EW for partial funding. This work was also supported by TNC grant FY21-G-CEBSE-CoralDR-012021.
Conflict of Interest
The authors declare that the research was conducted in the absence of any commercial or financial relationships that could be construed as a potential conflict of interest.
Publisher’s Note
All claims expressed in this article are solely those of the authors and do not necessarily represent those of their affiliated organizations, or those of the publisher, the editors and the reviewers. Any product that may be evaluated in this article, or claim that may be made by its manufacturer, is not guaranteed or endorsed by the publisher.
Acknowledgments
We would like to thank Thierry Work for his thoughtful review which significantly contributed to improve the quality of this manuscript. We acknowledge Red Arrecifal Dominicana for providing images of SCTLD in Galeras and Samana Bay. The Nature Conservancy Caribbean and Centro para el Ecodesarrollo de la Bahia de Samana y Su Entorno (CEBSE) for supporting the coral disease action plan in the Dominican Republic. Aurelio Reyes, Samuel King, and Someira Zambrano for field assistance during Samana field trip. Two reviewers for their comments and feedback to improve our manuscript. We also like to thank Francisco Nunez, TNC Director for the Central Caribbean for supporting the SCTLD action plan in the Dominican Republic and to Ivan Cano for its support to organize the literature review.
Supplementary Material
The Supplementary Material for this article can be found online at: https://www.frontiersin.org/articles/10.3389/fmars.2021.709544/full#supplementary-material
The Supplementary Video is available at: https://figshare.com/s/7e2734b89e0a08 f9fdd9.
Footnotes
- ^ https://www.agrra.org/wp-content/uploads/2021/02/MPAConnect-SCTLD-monitoring-roving-diver-surveys-JLang-Jan-2021.pdf
- ^ https://www.agrra.org/coral-disease-outbreak/
- ^ www.agrra.org/experimental-interventions
References
Aeby, G. S., Ushijima, B., Campbell, J. E., Jones, S., Williams, G. J., Meyer, J. L., et al. (2019). Pathogenesis of a tissue loss disease affecting multiple species of corals along the florida reef tract. Front. Mar. Sci. 6:678. doi: 10.3389/fmars.2019.00678
Aeby, G. S., Work, T. M., Runyon, C. M., Shore-Maggio, A., Ushijima, B., Videau, P., et al. (2015). First record of black band disease in the Hawaiian archipelago: response, outbreak status, virulence, and a method of treatment. PLoS One 10:e0120853. doi: 10.1371/journal.pone.0120853
Alvarez-Filip, L., Estrada-Saldívar, N., Pérez-Cervantes, E., Molina-Hernández, A., and González-Barrios, F. J. (2019). A rapid spread of the stony coral tissue loss disease outbreak in the Mexican Caribbean. PeerJ 7:e8069. doi: 10.7717/peerj.8069
Aronson, R. B., and Precht, W. F. (2001). White-band disease and the changing face of Caribbean coral reefs. Hydrobiologia 460, 25–38. doi: 10.1023/A:1013103928980
Bastidas, C., Bone, D., Croquer, A., Debrot, D., Garcia, E., Humanes, A., et al. (2012). Massive hard coral loss after a severe bleaching event in 2010 at Los Roques, Venezuela. Rev. Biol. Trop. 60, 29–37. doi: 10.15517/rbt.v60i0.19843
Becker, C. C., Brandt, M., Miller, C. A., and Apprill, A. (2021). Stony coral tissue loss disease biomarker bacteria identified in corals and overlying waters using a rapid field-based sequencing approach. bioRxiv [preprint] 3. doi: 10.1101/2021.02.17.431614
Bourne, D. G., Ainsworth, T. D., Pollock, F. J., and Willis, B. L. (2015). Towards a better understanding of white syndromes and their causes on Indo-Pacific coral reefs. Coral Reefs 34, 233–242. doi: 10.1007/s00338-014-1239-x
Brandt, M. E., and McManus, J. W. (2009). Dynamics and impact of the coral disease white plague: insights from a simulation model. Dis. Aquat. Org. 87, 117–133. doi: 10.3354/dao02137
Brandt, M. E., Ennis, R. S., Meiling, S. S., Townsend, J., Cobleigh, K., Glahn, A., et al. (2021). The emergence and initial impact of stony coral tissue loss disease (SCTLD) in the United States Virgin Islands. Front. Mar. Sci. 8:715329. doi: 10.3389/fmars.2021.715329
Bruckner, A. (2002) Priorities for the Effective Management of Coral Diseases. NOAA Technical Memorandum, NMFS-OPR-22. Silver Spring, MD
Bruckner, A., and Bruckner, R. (1997) Outbreak of coral disease in Puerto Rico. Coral Reefs 16, 260.
Bruckner, A. W. (2016a). “History of coral disease research,” in Diseases of Coral, eds S. B. Galloway, C. M. Woodley, A. W. Bruckner, and J. W. Porter (Hoboken, NJ: Wiley Blackwell), 52–84.
Bruckner, A. W. (2016b). “White Syndromes of Western Atlantic Reef-Building Corals,” in Diseases of Coral, eds S. B. Galloway, C. M. Woodley, A. W. Bruckner, and J. W. Porter (Hoboken, NJ: Wiley Blackwell), 316–332.
Bruno, J. F., and Selig, E. R. (2007). Regional decline of coral cover in the Indo-Pacific: timing, extent, and subregional comparisons. PLoS One 2:e00711. doi: 10.1371/journal.pone.0000711
Bythell, J. C., Barer, M. R., Cooney, R. P., Guest, J. R., O’Donnell, A. G., Pantos, O., et al. (2002). Histopathological methods for the investigation of microbial communities associated with disease lesions in reef corals. Lett. Appl. Microbiol. 34, 359–364. doi: 10.1046/j.1472-765X.2002.01097.x
Bythell, J., Pantos, O., and Richardson, L. (2004). “White plague, white band, ~ and other “white” diseases,” in Coral Health and Disease, eds E. Rosenberg and Y. Loya (Berlin: Springer)
Cárdenas, A., Rodriguez-R, L. M., Pizarro, V., Cadavid, L. F., and Arévalo-Ferro, C. (2012). Shifts in bacterial communities of two caribbean reef-building coral species affected by white plague disease. ISME J. 6, 502–512. doi: 10.1038/ismej.2011.123
Carpenter, K. E., Abrar, M., Aeby, G., Aronson, R. B., Banks, S., Bruckner, A., et al. (2008). One-third of reef-building corals face elevated extinction risk from climate change and local impacts. Science 321, 560–563. doi: 10.1126/science.1159196
Clemens, E., and Brandt, M. E. (2015). Multiple mechanisms of transmission of the Caribbean coral disease white plague. Coral Reefs 34, 1179–1188. doi: 10.1007/s00338-015-1327-6
Costa, S. V., Hibberts, S. J., Olive, D. A., Budd, K. A., Long, A. E., Meiling, S. S., et al. (2021). Diversity and disease: the effects of coral diversity on prevalence and impacts of stony coral tissue loss disease in Saint Thomas, U.S. Virgin Islands. Front. Mar. Sci. 8:682688. doi: 10.3389/fmars.2021.682688
Cróquer, A., and Weil, E. (2009). Spatial variability in distribution and prevalence of Caribbean scleractinian coral and octocoral diseases. II. Genera-level analysis. Dis. Aquat. Org. 83, 209–222. doi: 10.3354/dao02012
Cróquer, A., Weil, E., Zubillaga, A. L., and Pauls, S. M. (2005). Impact of a white plague-II outbreak on a coral reef in the archipelago Los Roques National Park, Venezuela. Caribb. J. Sci. 41, 815–823.
Daniels, C. A., Baumgarten, S., Yum, L. K., Michell, C. T., Bayer, T., Arif, C., et al. (2015). Metatranscriptome analysis of the reef-building coral Orbicella faveolata indicates holobiont response to coral disease. Front. Mar. Sci. 2:62. doi: 10.3389/fmars.2015.00062
Daszak, P., Cunningham, A. A., and Hyatt, A. D. (2000). Emerging infectious diseases of wildlife - Threats to biodiversity and human health. Science 287, 443–449. doi: 10.1126/science.287.5452.443
Daszak, P., Cunningham, A. A., and Hyatt, A. D. (2001). Anthropogenic environmental change and the emergence of infectious diseases in wildlife. Acta Trop. 78, 103–116. doi: 10.1016/S0001-706X(00)00179-0
Denner, E. B. M., Smith, G. W., Busse, H. J., Schumann, P., Narzt, T., Polson, S. W., et al. (2003). Aurantimonas coralicida gen. nov., sp. nov., the causative agent of white plague type II on Caribbean scleractinian corals. Int. J. Syst. Evol. Microbiol. 53, 1115–1122. doi: 10.1099/ijs.0.02359-0
Dustan, P. (1977). Vitality of reef coral populations off Key Largo, Florida: recruitment and mortality. Environ. Geol. 2, 51–58. doi: 10.1007/BF02430665
Dustan, P. (1999). Coral reefs under stress: sources of mortality in the Florida keys. Nat. Resour. Forum 23, 147–155.
Efrony, R., Atad, I., and Rosenberg, E. (2009). Phage therapy of coral white plague disease: properties of phage BA3. Curr. Microbiol. 58, 139–145. doi: 10.1007/s00284-008-9290-x
Estrada-Saldívar, N., Quiroga-garcía, B. A., Pérez-cervantes, E., and Alvarez-filip, L. (2021). Effects of the stony coral tissue loss disease outbreak on coral communities and the benthic composition of cozumel reefs. Front. Mar. Sci. 8:632777. doi: 10.3389/fmars.2021.632777
Fedak, K. M., Bernal, A., Capshaw, Z. A., and Gross, S. (2015). Applying the Bradford Hill criteria in the 21st century: how data integration has changed causal inference in molecular epidemiology. Emerg. Themes Epidemiol. 12, 1–9. doi: 10.1186/s12982-015-0037-4
Fisher, C. R., Streicker, D. G., and Schnell, M. J. (2018). The spread and evolution of rabies virus: conquering new frontiers. Nat. Rev. Microbiol. 16, 241–255. doi: 10.1038/nrmicro.2018.11
Garcia, G. D., Santos, E., de, O., Sousa, G. V., Zingali, R. B., Thompson, C. C., et al. (2016). Metaproteomics reveals metabolic transitions between healthy and diseased stony coral Mussismilia braziliensis. Mol. Ecol. 25, 4632–4644. doi: 10.1111/mec.13775
Gardner, T. A., Côté, I. M., Gill, J. A., Grant, A., and Watkinson, A. R. (2003). Long-term region-wide declines in Caribbean corals. Science 301, 958–960. doi: 10.1126/science.1086050
Gintert, B. E., Precht, W. F., Fura, R., Rogers, K., Rice, M., Precht, L. L., et al. (2019). Regional coral disease outbreak overwhelms impacts from a local dredge project. Environ. Monit. Assess. 191:630. doi: 10.1007/s10661-019-7767-7
Harvell, C. D., Kim, K., Burkholder, J. M., Colwell, R. R., Epstein, P. R., Grimes, D. J., et al. (1999). Emerging marine diseases - Climate links and anthropogenic factors. Science 285, 1505–1510. doi: 10.1126/science.285.5433.1505
Harvell, C. D., Mitchell, C. E., Ward, J. R., Altizer, S., Dobson, A. P., Ostfeld, R. S., et al. (2002). Climate warming and disease risks for terrestrial and marine biota. Science 296, 2158–2162. doi: 10.1126/science.1063699
Harvell, D., Altizer, S., Cattadori, I. M., Harrington, L., and Weil, E. (2009). Climate change and wildlife diseases: when does the host matter the most? Ecology 90, 912–920. doi: 10.1890/08-0616.1
Harvell, D., Aronson, R., Baron, N., Connell, J., Dobson, A., Ellner, S., et al. (2004). The rising tide of ocean diseases: unsolved problems and research priorities. Front. Ecol. Environ. 2:375–382. doi: 10.1890/1540-92952004002[0375:TRTOOD]2.0.CO;2
Heres, M. M., Farmer, B. H., and Hertler, H. (2021). Ecological consequences of stony coral tissue loss disease in the Turks and Caicos Islands. Coral Reefs 40, 609–624. doi: 10.1007/s00338-021-02071-4
Hernandez-Agreda, A., Leggat, W., Bongaerts, P., Herrera, C., and Ainsworth, T. D. (2018). Rethinking the coral microbiome: simplicity exists within a diverse microbial biosphere. mBio 9, 1–14. doi: 10.1128/mBio.00812-18
Hoegh-Guldberg, O., Mumby, P. J., Hooten, A. J., Steneck, R. S., Greenfield, P., Gomez, E., et al. (2007). Coral reefs under rapid climate change and ocean acidification. Science (New York, N.Y.) 318, 1737–1742. doi: 10.1126/science.1152509
Irazabal, I., and Rodriguez, M. A. (2019). First Report of Stony Coral Tissue Loss Disease in the Domincan Republic. Available online at: https://www.agrra.org/wp-content/uploads/2019/03/First-report-of-SCTLD-in-the-Dominican-Republic.pdf
Jackson, J. B. C., Donovan, M. K., Cramer, K. L., and Lam, W. (2014). Status and Trends of Caribbean Coral Reefs: 1970-2012. Global Coral Reef Monitoring Network. Gland: IUCN, 958.
Kramer, P. R., Roth, L. A., and Lang Judith, C. (2020). Atlantic and Guld Rapid Reef Assessment. Available online at: https://oref.maps.arcgis.com/apps/opsdashboard/index.html#/54b5df5c111b4fcc986e300c6aea63a3
Kramer, P. R., Roth, L., and Lang, J. (2019). Map of Stony Coral Tissue Loss Disease Outbreak in the Caribbean. Available online at: www.agrra.org
Landsberg, J. H., Kiryu, Y., Peters, E. C., Wilson, P. W., Perry, N., Waters, Y., et al. (2020). Stony coral tissue loss disease in Florida is associated with disruption of host–Zooxanthellae physiology. Front. Mar. Sci. 7:576013. doi: 10.3389/fmars.2020.576013
Manzello, D. P. (2015). Rapid recent warming of coral reefs in the Florida keys. Sci. Rep. 5:16762. doi: 10.1038/srep16762
Meiling, S. S., Muller, E. M., Lasseigne, D., Rossin, A., Veglia, A. J., Macknight, N., et al. (2021). Variable species responses to experimental stony coral tissue loss disease (SCTLD) exposure. Front. Mar. Sci. 8:670829. doi: 10.3389/fmars.2021.670829
Meiling, S., Muller, E. M., Smith, T. B., and Brandt, M. E. (2020). 3D photogrammetry reveals dynamics of stony coral tissue loss disease (SCTLD) lesion progression across a thermal stress event. Front. Mar. Sci. 7:597643. doi: 10.3389/fmars.2020.597643
Mera, H., and Bourne, D. G. (2018). Disentangling causation: complex roles of coral-associated microorganisms in disease. Environ. Microbiol. 20, 431–449. doi: 10.1111/1462-2920.13958
Meyer, J. L., Castellanos-Gell, J., Aeby, G. S., Häse, C. C., Ushijima, B., and Paul, V. J. (2019). Microbial community shifts associated with the ongoing stony coral tissue loss disease outbreak on the florida reef tract. Front. Microbiol. 10:2244. doi: 10.3389/fmicb.2019.02244
Miller, A. W., and Richardson, L. L. (2012). Fine structure analysis of black band disease (BBD) infected coral and coral exposed to the BBD toxins microcystin and sulfide. J. Invert. Pathol. 109, 27–33. doi: 10.1016/j.jip.2011.09.007
Miller, A. W., Blackwelder, P., Al-Sayegh, H., and Richardson, L. L. (2011). Fine-structural analysis of black band disease infected coral reveals boring cyanobacteria and novel bacteria. Dis. Aquat. Org. 93, 179–190. doi: 10.3354/dao02305
Miller, J., Muller, E., Rogers, C., Waara, R., Atkinson, A., Whelan, K. R. T., et al. (2009). Coral disease following massive bleaching in 2005 causes 60% decline in coral cover on reefs in the US Virgin Islands. Coral Reefs 28, 925–937. doi: 10.1007/s00338-009-0531-7
Miller, M. W., Karazsia, J., Groves, C. E., Griffin, S., Moore, T., Wilber, P., et al. (2016). Detecting sedimentation impacts to coral reefs resulting from dredging the Port of Miami, Florida USA. PeerJ 4:e2711. doi: 10.7717/peerj.2711
Miller, M. W., Marmet, C., Cameron, C. M., and Williams, D. E. (2014). Prevalence, consequences, and mitigation of fireworm predation on endangered staghorn coral. Mar. Ecol. Prog. Ser. 516, 187–194. doi: 10.3354/meps10996
Montilla, L. M., Ascanio, A., Verde, A., and Croquer, A. (2019). Systematic review and meta-analysis of 50 years of coral disease research visualized through the scope of network theory. PeerJ 7:e7041. doi: 10.7717/peerj.7041
Muller, E. M., and van Woesik, R. (2009). Shading reduces coral-disease progression. Coral Reefs 28, 757–760. doi: 10.1007/s00338-009-0504-x
Muller, E. M., Sartor, C., Alcaraz, N. I., and van Woesik, R. (2020). Spatial epidemiology of the stony-coral-tissue-loss disease in Florida. Front. Mar. Sci. 7:163. doi: 10.3389/fmars.2020.00163
Neely, K. L., and Lewis, C. L. (2020). Rapid population decline of the Pillar coral Dendrogyra cylindrus along the Florida Reef Tract. bioRxiv [preprint] 8. doi: 10.1101/2020.05.09.085886
Neely, K. L., Macaulay, K. A., Hower, E. K., and Dobler, M. A. (2020). Effectiveness of topical antibiotics in treating corals affected by Stony Coral Tissue Loss Disease. PeerJ 8:e9289. doi: 10.7717/peerj.9289
Nugues, M. M. (2002). Impact of a coral disease outbreak on coral communities in St. Lucia: what and how much has been lost? Mar. Ecol. Prog. Ser. 229, 61–71. doi: 10.3354/meps229061
Nugues, M. M., Smith, G. W., Van Hooidonk, R. J., Seabra, M. I., and Bak, R. P. M. (2004). Algal contact as a trigger for coral disease. Ecol. Lett. 7, 919–923. doi: 10.1111/j.1461-0248.2004.00651.x
Palmer, C. V., Mydlarz, L. D., and Willis, B. L. (2008). Evidence of an inflammatory-like response in non-normally pigmented tissues of two scleractinian corals. Proc. R. Soc. B Biol. Sci. 275, 2687–2693. doi: 10.1098/rspb.2008.0335
Pantos, O., Cooney, R. P., Le Tissier, M. D. A., Barer, M. R., O’Donnell, A. G., and Bythell, J. C. (2003). The bacterial ecology of a plague-like disease affecting the Caribbean coral Montastrea annularis. Environ. Microbiol. 5, 370–382. doi: 10.1046/j.1462-2920.2003.00427.x
Paul, V. J., Ushijima, B., and Aeby, G. (2019). Progress Report: Studies of the Ecology and Microbiology of Florida’s Coral Tissue Loss Diseases. Miami, FL: Florida DEP, 1–3.
Peters, E. (2016). “Anatomy,” in Diseases of Coral, eds S. B. Galloway, C. M. Woodley, A. W. Bruckner, and J. W. Porter (Hoboken, NJ: Wiley Blackwell), 85–107.
Pinzón, J. H., Kamel, B., Burge, C. A., Harvell, C. D., Medina, M., Weil, E., et al. (2015). Whole transcriptome analysis reveals changes in expression of immune-related genes during and after bleaching in a reef-building coral. R. Soc. Open Sci. 2:140214. doi: 10.1098/rsos.140214
Pollock, F. J., Morris, P. J., Willis, B. L., and Bourne, D. G. (2010). Detection and quantification of the coral pathogen vibrio coralliiytkus by real-time PCR with taqman fluorescent probes. Appl. Environ. Microbiol. 76, 5282–5286. doi: 10.1128/AEM.00330-10
Pollock, F. J., Morris, P. J., Willis, B. L., and Bourne, D. G. (2011). The urgent need for robust coral disease diagnostics. PLoS Pathog. 7:e1002183. doi: 10.1371/journal.ppat.1002183
Porter, J. W., Dustan, P., Jaap, W. C., Patterson, K. L., Kosmynin, V., Meier, O. W., et al. (2001). Patterns of spread of coral disease in the Florida Keys. Hydrobiologia 460, 1–24. doi: 10.1023/A:1013177617800
Precht, W. (2019). Failure to respond to a coral disease outbreak: potential costs and consequences. PeerJ Preprints 7:e27860v2. doi: 10.7287/peerj.preprints.27860
Precht, W. (2021). Failure to respond to a coral disease epizootic in Florida: causes and consequences. Rethink. Ecol. 6, 1–47. doi: 10.3897/RETHINKINGECOLOGY.6.56285
Precht, W. F., Gintert, B. E., Robbart, M. L., Fura, R., and Van Woesik, R. (2016). Unprecedented disease-related coral mortality in Southeastern Florida. Sci. Rep. 6:31374. doi: 10.1038/srep31374
Randall, C. J., Whitcher, E. M., Code, T., Pollock, C., Lundgren, I., Hillis-Starr, Z., et al. (2018). Testing methods to mitigate Caribbean yellow-band disease on Orbicella faveolata. PeerJ 6:e4800. doi: 10.7717/peerj.4800
Richardson, L. L. (1998). Coral diseases: what is really known? Trends Ecol. Evol. 13, 438–443. doi: 10.1016/S0169-5347(98)01460-8
Richardson, L. L. (2016). “Aurantimonas coralicida—white plague Type II,” in Diseases of Coral, eds C. M. Woodley, C. Downs, A. W. Bruckner, J. W. Porter, and S. B. Galloway (Hoboken, NJ: Wiley Blackwell), 231–235.
Richardson, L. L., Goldberg, W. M., Carlton, R. G., and John, C. H. (1998a). Coral disease outbreak in the Florida Keys: plague Type II. Rev. Biol. Trop. 46, 187–198.
Richardson, L. L., Goldberg, W. M., Kuta, K. G., Aronson, R. B., Smith, G. W., Ritchie, K. B., et al. (1998b). Florida’s mystery coral-killer identified. Nature 392, 557–558. doi: 10.1038/33302
Richardson, L. L., Mills, D. E. K., Remily, E. R., and Voss, J. D. (2005). Development and field application of a molecular probe for the primary pathogen of the coral disease white plague type II. Rev. Biol. Trop. 53, 1–10. doi: 10.15517/rbt.v53i1.26590
Richardson, L. L., and Aronson, R. B. (2002). “Infectious diseases of reef corals,” in Proceedings of the Ninth International Coral Reef Symposium, 2, 1225–1230. Available online at: http://www.coremap.or.id/downloads/ICRS9th-Coral_Disease1.pdf
Roder, C., Arif, C., Bayer, T., Aranda, M., Daniels, C., Shibl, A., et al. (2014). Bacterial profiling of White Plague Disease in a comparative coral species framework. ISME J. 8, 31–39.
Rogers, C. S. (2010). Words matter: recommendations for clarifying coral disease nomenclature and terminology. Dis. Aquat. Organ. 91, 167–175. doi: 10.3354/dao02261
Rosales, S. M., Clark, A. S., Huebner, L. K., Ruzicka, R. R., and Muller, E. M. (2020). Rhodobacterales and Rhizobiales are associated with stony coral tissue loss disease and its suspected sources of transmission. Front. Microbiol. 11:681. doi: 10.3389/fmicb.2020.00681
Rosenau, N. A., Gignoux-Wolfsohn, S., Everett, R. A., Miller, A. W., Minton, M. S., and Ruiz, G. M. (2021). Considering commercial vessels as potential vectors of stony coral tissue loss disease. Front. Mar. Sci. 8:709764. doi: 10.3389/fmars.2021.709764
Ruiz-Moreno, D., Willis, B. L., Page, A. C., Weil, E., Cróquer, A., Vargas-Angel, B., et al. (2012). Global coral disease prevalence associated with sea temperature anomalies and local factors. Dis. Aquat. Org. 100, 249–261. doi: 10.3354/dao02488
Sánchez, J. A., Herrera, S., Navas-Camacho, R., Rodríguez-Ramírez, A., Herron, P., Pizarro, V., et al. (2010). White plague-like coral disease in remote reefs of the Western Caribbean. Rev. Biol. Trop. 58, 145–154. doi: 10.15517/rbt.v58i1.20031
Sharp, W. C., Shea, C. P., Maxwell, K. E., Muller, E. M., and Hunt, J. H. (2020). Evaluating the small-scale epidemiology of the stony-coral -tissue-loss-disease in the middle Florida Keys. PLoS One 15:e0241871. doi: 10.1371/journal.pone.0241871
Sharp, W., Maxwell, K., Smith, K., and Hunt, J. (2020). Investigating the Ongoing Coral Disease Outbreak in the Florida Keys: Continued SCTLD Monitoring at Middle and Lower Florida Keys, Experimental Coral Restoration of SCTLD-Susceptible Coral Species, and Assessing the Prevalence of SCTLD on Intermediate Ree. Tallahassee, FL: Florida Department of Environmental Protection
Shilling, E. N., Combs, I. R., and Voss, J. D. (2021). Assessing the effectiveness of two intervention methods for stony coral tissue loss disease on Montastraea cavernosa. Sci. Rep. 11:8566. doi: 10.1038/s41598-021-86926-4
Smith, S. R., Sarkis, S., Murdoch, T. J., Weil, E., Croquer, A., Bates, N. R., et al. (2013). “Threats to coral reefs of Bermuda,” in Coral Reefs of the United Kingdom Overseas Territories, ed. C. Sheppard (Dordrecht: Springer), 173–188.
Soffer, N., Brandt, M. E., Correa, A. M. S., Smith, T. B., and Thurber, R. V. (2014). Potential role of viruses in white plague coral disease. ISME J. 8, 271–283. doi: 10.1038/ismej.2013.137
Soffer, N., Zaneveld, J., and Vega Thurber, R. (2015). Phage-bacteria network analysis and its implication for the understanding of coral disease. Environ. Microbiol. 17, 1203–1218. doi: 10.1111/1462-2920.12553
Sunagawa, S., Desantis, T. Z., Piceno, Y. M., Brodie, E. L., Desalvo, M. K., Voolstra, C. R., et al. (2009). Bacterial diversity and white Plague disease-associated community changes in the caribbean coral montastraea faveolata. ISME J. 3, 512–521. doi: 10.1038/ismej.2008.131
Sutherland, K. P., Porter, J. W., and Torres, C. (2004). Disease and immunity in Caribbean and Indo-Pacific zooxanthellate corals. Mar. Ecol. Prog. Ser. 266, 273–302. doi: 10.3354/meps266273
Sweet, M. J., Croquer, A., and Bythell, J. C. (2014). Experimental antibiotic treatment identifies potential pathogens of white band disease in the endangered Caribbean coral Acropora cervicornis. Proc. R. Soc. B Biol. Sci. 281:20140094. doi: 10.1098/rspb.2014.0094
Teplitski, M., and Ritchie, K. (2009). How feasible is the biological control of coral diseases? Trends Ecol. Evol. 24, 378–385. doi: 10.1016/j.tree.2009.02.008
Thome, P. E., Rivera-Ortega, J., Rodríguez-Villalobos, J. C., Cerqueda-García, D., Guzmán-Urieta, E. O., García-Maldonado, J. Q., et al. (2021). Local dynamics of a white syndrome outbreak and changes in the microbial community associated with colonies of the scleractinian brain coral Pseudodiploria strigosa. PeerJ 9:e10695. doi: 10.7717/peerj.10695
Thrusfield, M. (2016a). “Epidemiology,” in Diseases of Coral, eds C. M. Woodley, C. Downs, A. W. Bruckner, J. W. Porter, and S. B. Galloway (Hoboken, NJ: Wiley Blackwell), 28–51.
Thrusfield, M. (2016b). “Etiology,” in Diseases of Coral, eds C. M. Woodley, C. Downs, A. W. Bruckner, J. W. Porter, and S. B. Galloway (Hoboken, NJ: Wiley Blackwell), 16–27.
Tonon, L. A. C., Thompson, J. R., Moreira, A. P. B., Garcia, G. D., Penn, K., Lim, R., et al. (2017). Quantitative detection of active vibrios associated with white plague disease in Mussismilia braziliensis corals. Front. Microbiol. 8:2272. doi: 10.3389/fmicb.2017.02272
Tracy, A. M., Weil, E., and Burge, C. A. (2021). Ecological factors mediate immunity and parasitic co-infection in Sea Fan Octocorals. Front. Immunol. 11:608066. doi: 10.3389/fimmu.2020.608066
Tracy, A. M., Weil, E., and Harvell, C. D. (2020). Warming and pollutants interact to modulate octocoral immunity and shape disease outcomes. Ecol. Appl. 30:e02024. doi: 10.1002/eap.2024
Ushijima, B., Meyer, J. L., Thompson, S., Pitts, K., Marusich, M. F., Tittl, J., et al. (2020). Disease diagnostics and potential coinfections by Vibrio coralliilyticus during an ongoing coral disease outbreak in Florida. Front. Microbiol. 11:569354. doi: 10.3389/fmicb.2020.569354
Vega Thurber, R., Mydlarz, L. D., Brandt, M., Harvell, D., Weil, E., Raymundo, L., et al. (2020). Deciphering coral disease dynamics: integrating host, microbiome, and the changing environment. Front. Ecol. Evol. 8:575927. doi: 10.3389/fevo.2020.575927
Voss, J. D., and Richardson, L. L. (2006). Coral diseases near Lee Stocking Island, Bahamas: patterns and potential drivers. Dis. Aquat. Org. 69, 33–40. doi: 10.3354/dao069033
Voss, J. D., Shilling, E. N., and Combs, I. R. (2019). Intervention and Fate Tracking for Corals Affected by Stony Coral Tissue Loss Disase in the Northern Florida Reef Tract. Miami, FL: Florida DEP. 1–23. Available online at: https://floridadep.gov/sites/default/files/Voss%20SEFL%20Disease%20Intervention%202019_FDEP%20FINAL%20Report.Fully508compliant.pdf.
Walton, C. J., Hayes, N. K., and Gilliam, D. S. (2018). Impacts of a regional, multi-year, multi-species coral disease outbreak in Southeast Florida. Front. Mar. Sci. 5:323. doi: 10.3389/fmars.2018.00323
Ward, J. R., Rypien, K. L., Bruno, J. F., Harvell, C. D., Jordán-Dahlgren, E., Mullen, K. M., et al. (2006). Coral diversity and disease in Mexico. Dis. Aquat. Org. 69, 23–31. doi: 10.3354/dao069023
Weil, E. (2004). “Coral diseases in the wider Caribbean,” in Coral Health and Disease, ed. E. Rosenberg (Berlin: Springer Berlin Heidelberg), 35–68.
Weil, E., and Cróquer, A. (2009). Spatial variability in distribution and prevalence of Caribbean scleractinian coral and octocoral diseases. I. Community-level analysis. Dis. Aquat. Org. 83, 195–208. doi: 10.3354/dao02011
Weil, E., and Rogers, C. S. (2011). “Coral reef diseases in the Atlantic-Caribbean,” in Coral Reefs: An Ecosystem in Transition, eds Z. Dubinsky and N. Stambler (Dordrecht: Springer). doi: 10.1007/978-94-007-0114-4_27
Weil, E., Croquer, A., and Urreiztieta, I. (2009). Temporal variability and impact of coral diseases and bleaching in La Parguera, Puerto Rico from 2003-2007. Caribb. J. Sci. 45, 221–246. doi: 10.18475/cjos.v45i2.a10
Weil, E., Hernández-Delgado, E. A., Gonzalez, M., Williams, S., Suleimán-Ramos, S., Figuerola, M., et al. (2019). Spread of the new coral disease “SCTLD” into the Caribbean: implications for Puerto Rico. Reef Encounter 34, 38–43.
Weil, E., Smith, G., and Gil-Agudelo, D. L. (2006). Status and progress in coral reef disease research. Dis. Aquat. Org. 69, 1–7. doi: 10.3354/dao069001
Weil, E., Urreiztieta, I., and Garzón-Ferrera, J. (2000). “Geographic variability in the incidence of coral and octocoral diseases in the wider Caribbean,” in Proceedings of the 9th International Coral Reef Symposium, Bali, Vol. 2, 1231–1237.
Weil, E., Urreiztieta, I., and Garzón-Ferreira, J. (2002). “Geographic variability in the incidence of coral and octocoral diseases in the wider Caribbean,” in Proceedings of the 9th International Coral Reef Symposium, Vol. 2, Bali, 1231–1237.
Woodley, C. M., Downs, C., Bruckner, A. W., Porter, J. W., and Galloway, S. B. (2016a). Diseases of Coral. Hoboken, NJ: Wiley Blackwell.
Woodley, C. M., Harley, R. A., Nicholson, J. H., and Reynolds, T. L. (2016b). “Pathology,” in Diseases of Coral, eds C. M. Woodley, C. Downs, A. W. Bruckner, J. W. Porter, and S. B. Galloway (Hoboken, NJ: Wiley Blackwell), 4–15.
Work, T. M., and Aeby, G. S. (2006). Systematically describing gross lesions in corals. Dis Aquat Organ. 70, 155–160.
Work, T. M., Richardson, L. L., Reynolds, T. L., and Willis, B. L. (2008). Biomedical and veterinary science can increase our understanding of coral disease. J. Exp. Mar. Biol. Ecol. 362, 63–70. doi: 10.1016/j.jembe.2008.05.011
Work, T., and Meteyer, C. (2014). To understand coral disease, look at coral cells. EcoHealth 11, 610–618. doi: 10.1007/s10393-014-0931-1
Keywords: coral disease, stony coral tissue loss disease, white plague, white plague type II, pathology, epizootiology
Citation: Cróquer A, Weil E and Rogers CS (2021) Similarities and Differences Between Two Deadly Caribbean Coral Diseases: White Plague and Stony Coral Tissue Loss Disease. Front. Mar. Sci. 8:709544. doi: 10.3389/fmars.2021.709544
Received: 14 May 2021; Accepted: 25 August 2021;
Published: 18 October 2021.
Edited by:
Les Kaufman, Boston University, United StatesReviewed by:
Adán Guillermo Jordán-Garza, Universidad Veracruzana, MexicoPaul Carl Sikkel, Arkansas State University, United States
Copyright © 2021 Cróquer, Weil and Rogers. This is an open-access article distributed under the terms of the Creative Commons Attribution License (CC BY). The use, distribution or reproduction in other forums is permitted, provided the original author(s) and the copyright owner(s) are credited and that the original publication in this journal is cited, in accordance with accepted academic practice. No use, distribution or reproduction is permitted which does not comply with these terms.
*Correspondence: Aldo Cróquer, YWxkby5jcm9xdWVyQHRuYy5vcmc=