- 1Department of Marine Biology, Leon H. Charney School of Marine Sciences, University of Haifa, Haifa, Israel
- 2Morris Kahn Marine Research Station, The Leon H. Charney School of Marine Sciences, University of Haifa, Haifa, Israel
- 3The Interuniversity Institute of Marine Sciences, Eilat, Israel
- 4Central Caribbean Marine Institute, Little Cayman, Cayman Islands
Coral recruitment represents a key element for coral reef persistence and resilience in the face of environmental disturbances. Studying coral recruitment patterns is fundamental for assessing reef health and implementing appropriate management strategies in an era of climate change. The FluorIS system has been developed to acquire high resolution, wide field-of-view (FOV) in situ images of coral recruits fluorescence and has proven successful in shallow reef environments. However, up to now, its applicability to mesophotic coral ecosystems remains unknown due to the complexity of the system and the limited time available when working at mesophotic depth. In this study we optimized the FluorIS system by utilizing a single infrared-converted camera instead of the bulkier regular dual-camera system, substantially reducing the system complexity and significantly decreasing the acquisition time to an average of 10 s for a set of 3 images. Moreover, the speed-FluorIS system is much more economical, decreasing the cost of the full set-up by roughly 40% compared to the original dual-camera system. We tested the utility of the speed-FluorIS by surveying coral recruits across shallow and mesophotic reefs of the Red Sea (Gulf of Eilat) and Bermuda, two of the most northerly reefs in the world with markedly different substrate and topography, and demonstrate that the modified system enables fast imaging of fluorescence to study coral recruitment patterns over a broader range of depths and reef topographies than previous fluorescence methods. Our single-camera system represents a valuable, non-invasive and rapid underwater tool which will help standardize surveys and long-term monitoring of coral recruits, contributing to our understanding of these vital and delicate early life stages of corals.
Introduction
Over the last three decades, coral reef ecosystems have been suffering massive declines due to both local (e.g., pollution, nutrient enrichment, overfishing, and sedimentation) and global stressors (global warming, ocean acidification, and sea level rise) (Dustan and Halas, 1987; Hoegh-Guldberg and Bruno, 2010; D’Angelo and Wiedenmann, 2014). The resilience of these ecosystems under the pressure of rapid environmental change heavily relies on successful recruitment of juvenile corals. The presence of new coral recruits is a strong indicator of the health of the reef (Glassom et al., 2004; Baird et al., 2006). Thus, knowledge and monitoring of recruitment processes assist the implementation of conservation and management actions to preserve coral reef ecosystems (Glassom et al., 2004; Martinez and Abelson, 2013).
Coral recruitment patterns are commonly quantified by examination of artificial settlement tiles that are deployed on the reef and subsequently inspected in the lab using microscopes under UV light. This method, however, does not allow continuous monitoring of recruitment dynamics, as the tiles need to be taken out for inspection (Mundy, 2000; Soong et al., 2003; Field et al., 2007). As an alternative, in situ visual surveys are also conducted (Miller et al., 2000; Soong et al., 2003; Martinez and Abelson, 2013), but they can be very time-consuming. Coral recruits in fact are very small and often cryptic at settlement, which makes them very difficult to detect via normal census techniques (Baird et al., 2006). Fluorescence census techniques instead have increasingly been used as they make coral recruits much easier and faster to detect than under standard white light census (Piniak et al., 2005). Fluorescence techniques depend on the high abundance of fluorescent pigments in many coral species (Papina et al., 2002). Most scleractinian corals contain two primary groups of fluorophores in their tissues. The first major group is comprised of photosynthetic pigments, mainly chlorophyll-a, from the coral endosymbiotic dinoflagellate algae (Symbiodiniaceae family) (Warner et al., 2010; LaJeunesse et al., 2018), and the second group includes GFP-like fluorescent proteins (FPs) found in the coral host tissue (Alieva et al., 2008). Recently, the FluorIS system was developed to acquire high resolution, wide field-of-view (FOV) in situ images of coral recruits fluorescence during daytime (Zweifler et al., 2017), enabling simultaneous imaging of GFP and chlorophyll-a with a single excitation source. Such a system has been shown to be successful in shallow reef environments (Zweifler et al., 2017), however, its application to mesophotic ecosystems is highly challenging, due to the time limits and technical constraints imposed by non-recreational deep diving.
Ranging from 30 m to approximately 150 m depth, mesophotic coral ecosystems (MCEs) comprise a diverse abundance of habitat-building taxa including corals, crustose coralline algae, macroalgae, and sponges (Kahng et al., 2016). In view of the large-scale degradation affecting shallow reef systems, MCEs have gained substantial interest as they appear to be protected from many of the local and global impacts affecting shallow-water coral reefs (Hoegh-Guldberg et al., 2007; Lesser et al., 2009; Bongaerts et al., 2010; Kahng et al., 2010). Even though MCEs are not immune to the impacts of disturbance (Bongaerts et al., 2013; Appeldoorn et al., 2016; Smith et al., 2016), they have not experienced the same degree of decline as their shallow-water counterparts (Bak et al., 2005). Therefore, it is hypothesized that coral populations at depths greater than 30 m may serve as a refuge for coral reef species and provide a source for recruits, genetic diversity, and repopulation of shallow regions via larval exchange (termed the Deep Reef Refuges Hypothesis “DRRH”; Bongaerts et al., 2010; Lesser et al., 2018). Despite their inferred importance, data on recruitment patterns of MCEs are sparse, mainly due to the difficulties in accessing these habitats that lie beyond recreational SCUBA diving limits (Pyle, 2019), which poses increased logistical challenges. MCEs have been studied in only few areas around the world, making it difficult to obtain generalizable knowledge of the processes regulating their structure and dynamics, such as recruitment (Turner et al., 2017). If MCEs are in fact an important life-boat for coral survival, it is critical to develop and adopt broad, ecosystem-wide approaches that allow to have better understanding of recruitment dynamics in these deeper reefs worldwide. Some technological advances have enabled access to study MCEs, such as technical SCUBA diving, but pose significant challenges in terms of the amount of time that can be spent underwater.
To overcome these challenges and facilitate the assessment of coral recruitment dynamics at mesophotic depths, we optimized the FluorIS system by utilizing a single infrared-converted camera instead of the bulkier regular dual-camera system (Treibitz et al., 2015). We thus significantly reduced the system complexity and the time consumed for completing the underwater survey, while also broadening the types of terrain shapes and slopes that can be surveyed. With this faster, more flexible and easier to handle system we surveyed coral recruits across shallow and mesophotic reefs of the Red Sea (Gulf of Eilat) and Bermuda, two of the northern-most coral reefs in the world. These two locations are characterized by different substrate topographies and reef structures (Kahng et al., 2010; Murdoch and Murdoch, 2016). In Bermuda, cover of reef-building scleractinian corals declines with depth, although both shallow and mesophotic reefs maintain similar topographic complexity (Murdoch and Murdoch, 2016). In Eilat, branching structures in shallow waters become encrusting or plate-like at mesophotic depths, and this flattening creates a relatively low complexity and low rugosity of reef structure on MCEs (Kahng et al., 2010). The existence of such variations in reef structure across depths, and in general between reefs located in different geographical areas, represents a potential challenge for the development of an underwater survey system capable of efficiently detecting coral recruits with a standardized methodology.
With our improved system we provide a valuable, non-invasive and rapid underwater tool to investigate coral reproductive ecology in situ across wide depth ranges and different terrain shapes, significantly increasing the speed of coral recruit counts underwater. This new system ultimately allows us to obtain a deeper understanding of coral recruitment patterns across depths, which is of upmost importance for developing suitable reef conservation and management strategies aiming at protecting these ecosystems in the face of increasing local and global stressors.
Materials and Methods
Imaging System
We modified the two cameras FluorIS system (Zweifler et al., 2017) to enable a wider spectrum capability and faster working volume in a one camera system, that we named speed-FlorIS (Figure 1). Specifically, similar to Treibitz et al. (2015), we removed the Infrared Cut filter (ICF), which is regularly located on top of the camera sensor, by applying an infrared conversion to the camera (Life Pixel, United States). The converted camera (we used Nikon D850) can capture a spectrum of 200-1200 nm, which improves the ability to record red fluorescence of chlorophyll-a, while giving the surveyor another layer of information that can be used for identification purposes, compared to a non-converted camera that can record only 200–700 nm. However, we replaced the use of a dome port with a flat port which allowed us to place the barrier filter out of the housing, this modification allowed us to attach or detach the different filters rapidly underwater and to use a single camera system. A barrier filter is needed to block the blue UV light from the strobes and to let only the emission of fluorescence light to be seen by the sensor. When the barrier filter is removed, the camera acts as a wide spectrum camera, and can record ambient or white light (reflectance, or strobe light).
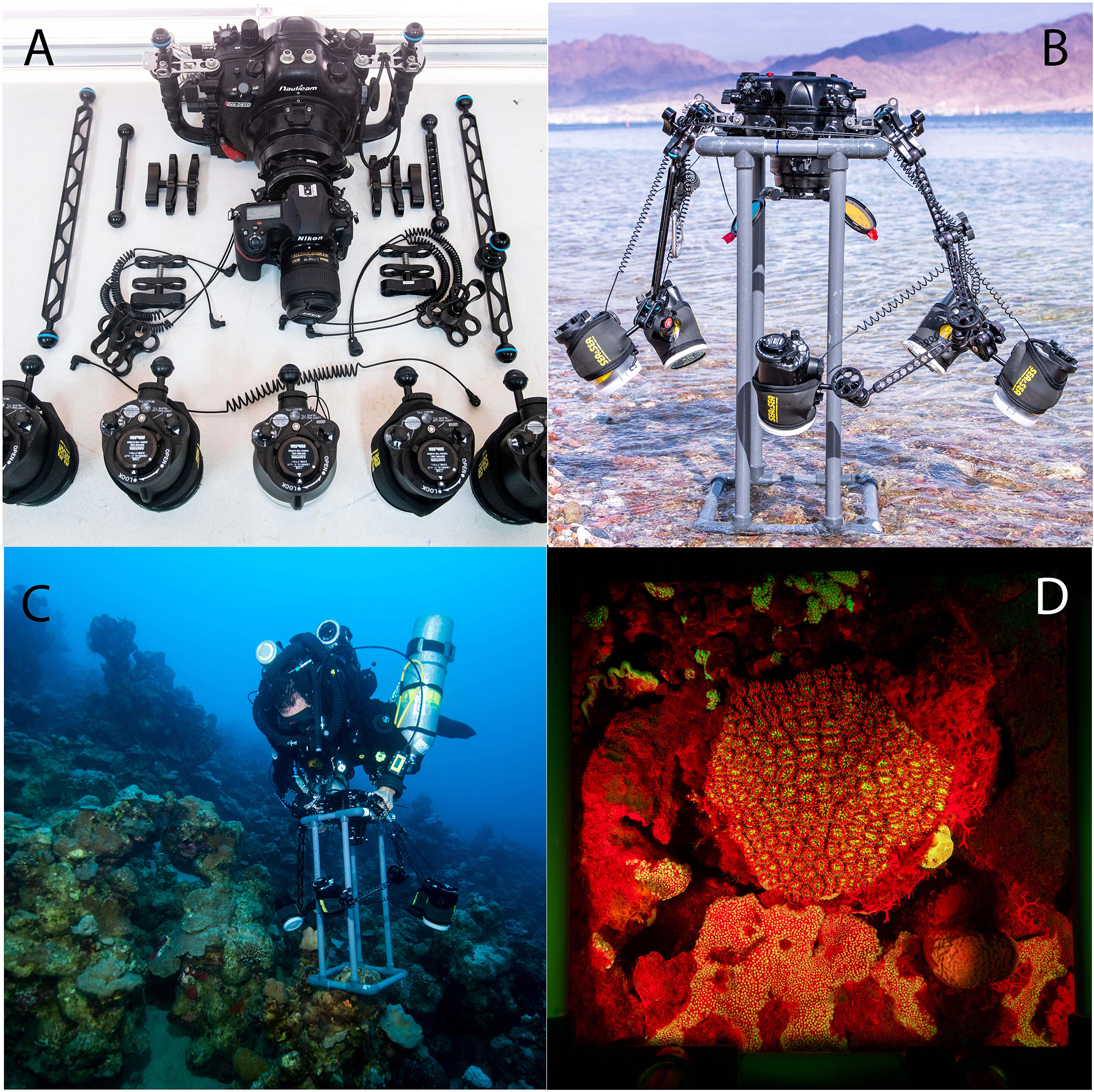
Figure 1. (A) Complete gear set of Nauticam housing, Nikon D850 camera with 35 mm lens, strobes and arms before assembly. (B) The complete set assembled on the quadrat before diving. (C) A diver equipped with closed circuit rebreather and the speed-FlorIS at 45 m in Bermuda. (D) An example of stony coral’s image taken in Bermuda at daytime at 45 m with the fluorescence excitation strobe without subtraction.
The camera was fitted with a Nikon 35 mm 1.8 lens and housed within a Nauticam NAD850 housing with a set of five Sea & Sea YS-D2 underwater strobes with a guide number of 32 (Figure 2). The original FluorIS used the same type of lens, as it is considered to be a very sharp prime lens with wide FOV and relatively no aberrations or optical distortion. In addition, this is the widest FOV lens that is suitable to use with a flat port, which is necessary for the ability to change filters underwater and is the fundamental condition of using a one camera system.
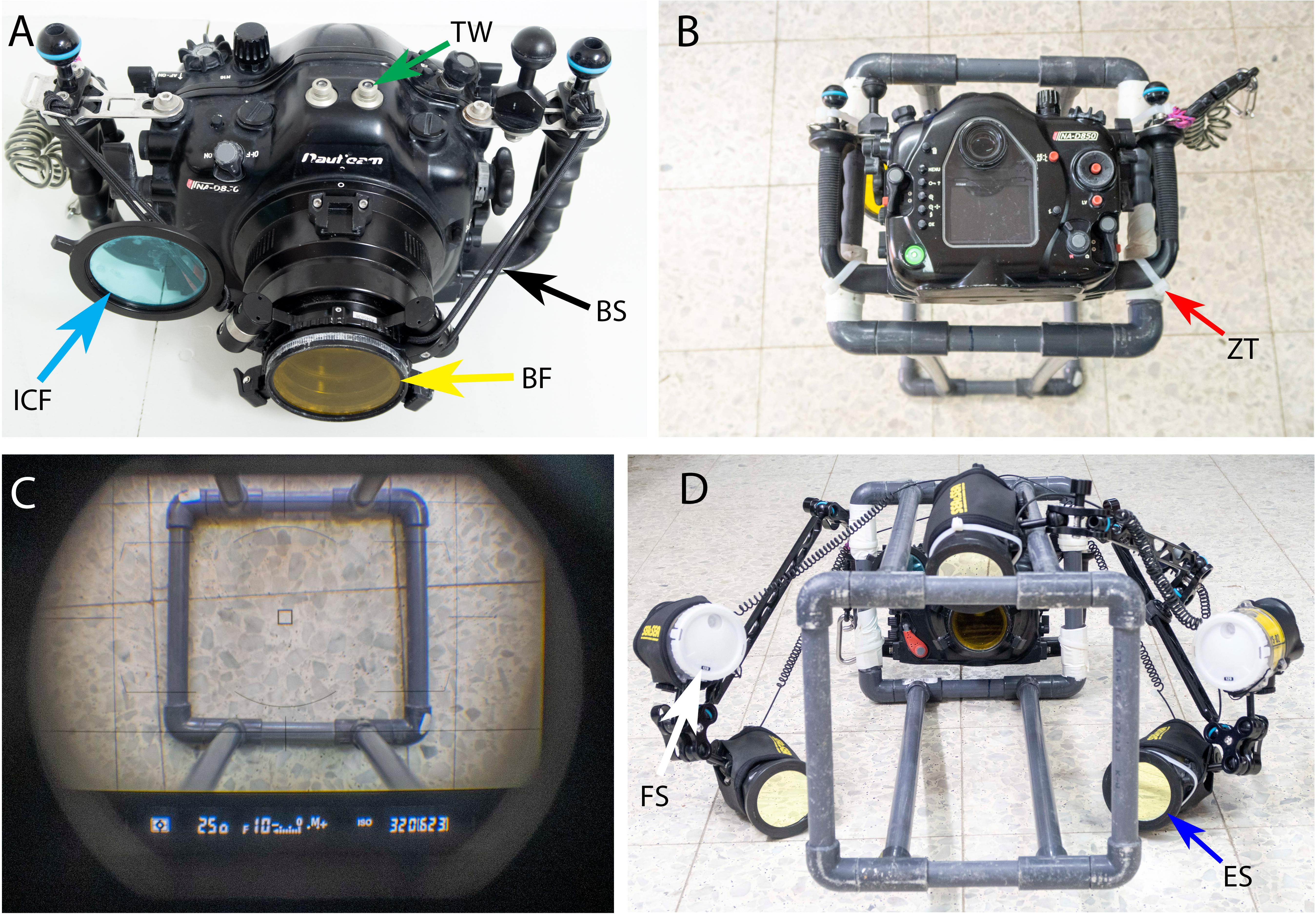
Figure 2. (A) The Nauticam NAD850 camera housing with Nauticam double filter holder for a Barrier Filter (BF-yellow arrow) and Infrared Cut Filter (ICF-blue arrow). See the Bungee Straps (BS-black arrow) added to the setup that helps to retract the filter from the port in one finger touch and the Trigger Window (TW-green arrow) on the upper side of the housing. (B) The housing is mounted on the quadrat frame with Zip-Ties (ZT-red arrow). (C) A look via the camera viewfinder to verify if the camera is centered with the quadrat and the shooting parameters are correct. (D) The speed-FluorIS is mounted and ready to use with five strobes, 3 Excitation Strobes (ES-blue arrow) and 2 Full Spectrum (FS-white arrow) strobes.
Three of the strobes were covered with excitation filters (Schott-BG39 and Night-Sea filters on top of each other) to give an excitation light of 385–500 nm, and the other two strobes, with 120° diffusers on, were unfiltered for white light (full spectrum). When shooting a fluorescence and reflectance image, the Full Spectrum (FS) set is disabled (turned off) and the barrier filter is on, and when shooting a FS image, the excitation strobes are off, and the barrier filter is removed from the camera port. The camera triggers the strobes through two sets of dual fiber optic cables (Nauticam, United States). One set is triggering the excitation strobes, and the other set is triggering the FS strobes. To trigger the 3rd excitation strobe, we connect it with a single fiber optic cable to one of the excitation strobes triggered directly by the camera. By alternating between the two fiber optic cables and holding them in front of the housing synchronization window we can trigger the different strobe sets. Such modification enables the system to record the fluorescence, reflectance and FS image using one camera. The camera housing is equipped with a Nauticam Macro Port 60 in front of the camera lens and with a Nauticam double filter holder. We used two filters: one barrier filter (Tiffen yellow 12) to block the blue excitation light from the filtered strobes (all wavelengths below 510 nm), and a second external ICF filter, replacing the one removed from the camera sensor, for FS reflectance images (see Table 1 for a full list of the equipment used). The ICF blocks any wavelength above 750 nm. However, we found that the difference between images taken with or without the ICF was minor and, if needed, could be easily adjusted in a post-processing software with the white balance tool as demonstrated in Figure 3 and Supplementary Movie 1.
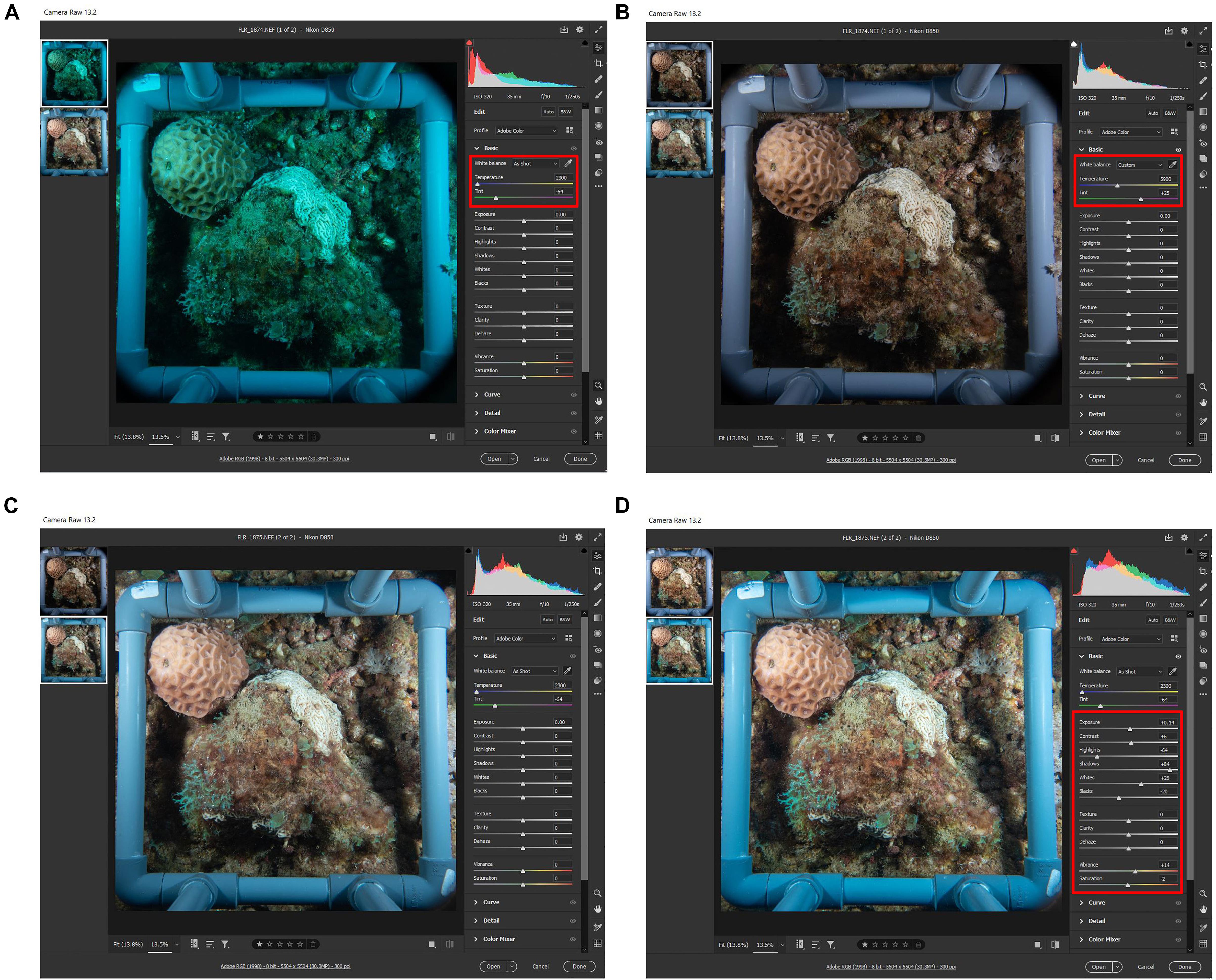
Figure 3. Demonstrating the irrelevance of the Infrared Cut Filter. (A) Raw image taken with ICF on the camera port. (B) The same image from A after white balance (WB) correction in post processing using adobe camera raw (Adobe, United States). (C) Raw image taken without ICF shows the correct colors without the need of WB correction. (D) The same image from C after automatic editing tool using adobe camera raw (Adobe, United States). Red box indicates the command setting.
Building the Quadrat and the Support Structure
A custom-made 25 × 25 cm quadrat (standard quadrat size used in ecological monitoring programs) was constructed to enable rapid imaging of the same area (Figure 1). In order to find the best working distance to get the highest possible resolution for the 25 × 25 cm quadrat, we performed test shots in a 500 L water tank (80 W × 120 L × 60 H cm): we submerged all the setup and checked on the camera Viewfinder (VF) for a distance that showed all the corners of the quadrat. Once the right distance was found, we measured it from the quadrat plane to the tray handles plane, to later build the spacer rods at a determined length of 65 cm. The structure was built using 25 mm-diameter PVC pipes and its parts glued together to create a strong and solid structure that can hold the camera and strobes on land and underwater in a position that enables the diver to take multiple images at the same camera position, without damaging the reef and with minimal addition to its weight (∼ 1 pound) and drag. The quadrat frame was attached to the camera housing using zip-ties (Figure 2B), facing down at a distance of ∼65 cm measured from the quadrat plane to the sensor plane.
Study Sites
Both the Gulf of Eilat and Bermuda are situated at ∼30°N, however, environmental conditions differ significantly, as Eilat is within an enclosed oligotrophic bay, whereas Bermuda is an oceanic island situated in the oligotrophic Sargasso Sea.
In Bermuda, two study sites in the Bermuda platform that varied in environmental conditions were selected (Figure 4): a shallow reef (∼5 m depth; 32.45733N, 64.83475W) and a mesophotic reef (∼45 m depth; 32.49145N, 64.85449W), located at 4.3 km from each other. Temperatures at shallow reefs sites in Bermuda vary from 22.8 to 29.5°C, and from 22.2 to 27.8°C at the mesophotic reef (Goodbody-Gringley et al., 2015). The clarity of oceanic water surrounding Bermuda greatly extends the euphotic zone, where the 10% photosynthetically active radiation (PAR) depth varies seasonally from ∼30 to ∼60 m (Siegel et al., 1995). Macroalgal cover up to 45 m depth is low mainly because of the slope and storm wave action. Fish richness, abundance and biomass increase with depth from the shallow to the mesophotic zone, with community structure strongly changing across depths (Pinheiro et al., 2016).
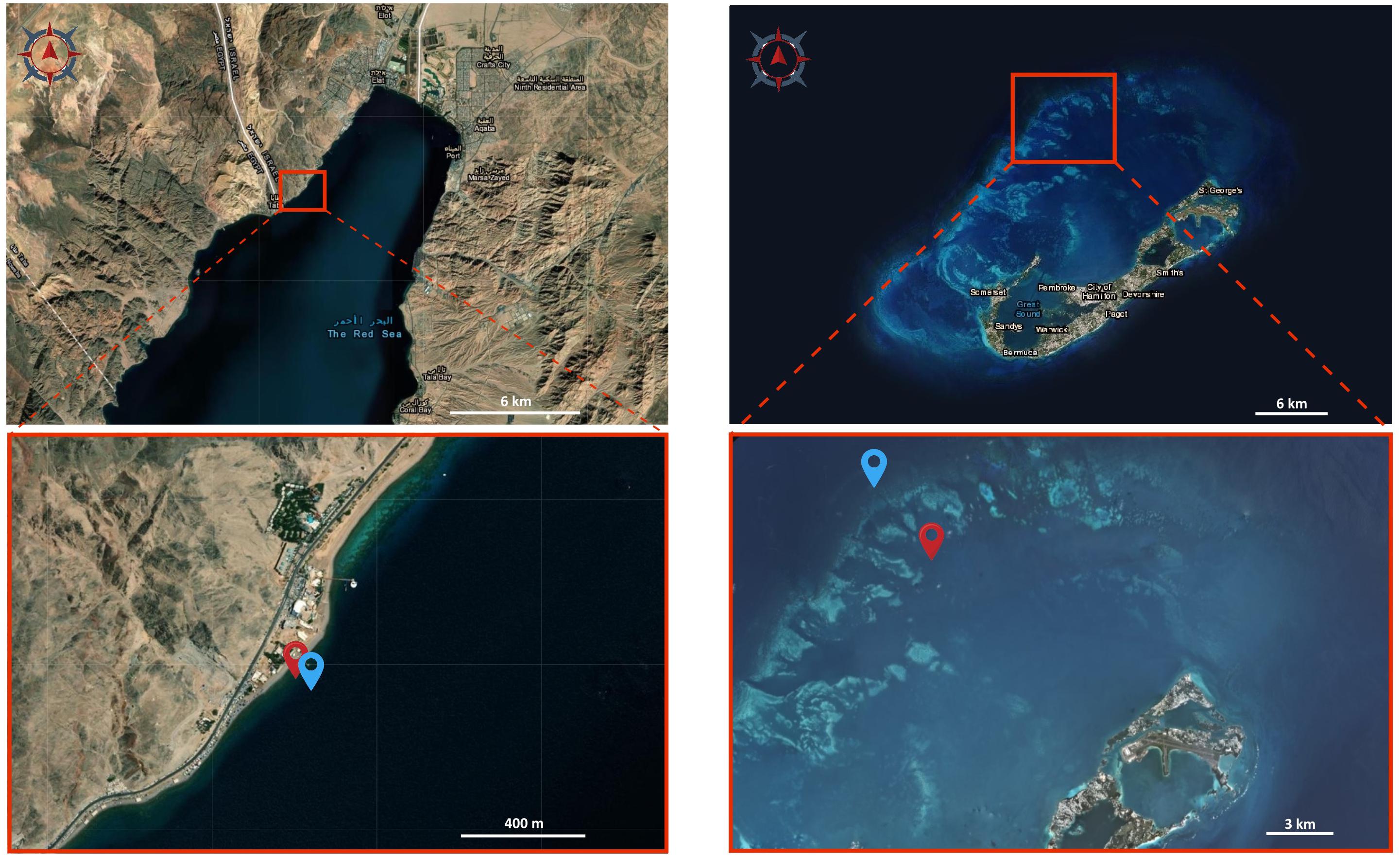
Figure 4. Satellite images of the study sites. Images of Eilat (left) and Bermuda (right) showing the sampling locations. Red locators in the bottom images indicate the shallow reefs, blue locators indicate the mesophotic reefs. Image source: Earthexplorer.usgs.gov.
In Eilat, the survey was conducted in the shallow (∼5 m depth) and mesophotic (∼45 m depth) reefs adjacent to the Interuniversity Institute (IUI) for Marine Sciences (29.50221N, 34.91660E) (Figure 4), located at a distance of around 100 m from each other. Annual monitoring at these reefs sites records temperatures that range from 20.7 to 29.8°C in the shallow reef and 20.9–27.8°C at 40 m depth (National Monitoring Program, Eilat). Light attenuation varies significantly with the annual cycle, with the depths of 6% PAR varying between 30–60 m depth (Dishon et al., 2012). Fleshy algae flourish at mesophotic depths and are sometimes present on top of reef structures at a higher coverage than in shallower environments. Reef fish assemblages reveal a change along the depth gradient of the Red Sea due to the steep bathymetry of the region. The abundance, biomass, and density of the most common herbivorous fish decreased along the depth gradient in the Gulf, potentially leading to reduced grazing pressure on MCEs (Brokovich et al., 2010).
Testing the Imaging Method in the Field
In order to find the right camera settings, test shots with the system were made on the reef adjacent to the Interuniversity Institute (IUI) of Eilat. Repetitive co-located images with and without filters of different stony corals and algae were captured during daytime at depths of approximately 6 m and 40 m. To capture two sequential shots with the shortest interval possible, the camera was set to shoot in continuous mode of 7 Frames Per Second (FPS), which is the highest frame rate possible for this camera model. During image post-processing, the Image Calculator function of the FIJI software (Schindelin et al., 2012) was used for subtracting the fluorescence effect in daylight, in order to assess the quality of the shots.
Survey of Coral Recruits Using the Speed-FlorIS
Once found the optimal camera settings with the test shots, co-located reflectance and fluorescence image-pairs were captured during daytime to survey coral recruits among the shallow and mesophotic reefs of Eilat (April 2019) and Bermuda (July 2019). Image locations were chosen randomly at each depth (5 and 45 m) in both sites. In Eilat, 46 random locations were selected for the shots at 5 m and 36 were selected at 45 m. In Bermuda, 17 random locations were selected at 5 m and 21 were selected at 45 m.
During image post-processing, the FIJI software was employed to subtract the strobes-off images from the strobes-on images, using the Image Calculator function. Coral recruits between 1 mm and 2 cm of diameter expressing FP fluorescence were counted using the Cell Counter plugin in FIJI. Reflectance FS images under white light illumination were used to verify that the fluorescent organisms detected in the fluorescence images corresponded to coral recruits by shape.
Statistical Analysis
Recruit count data were tested for normality (Shapiro-Wilk test) and homogeneity of variance (Brown–Forsythe test). As the assumption of normality was violated, a non-parametric Mann-Whitney test was used to compare recruit numbers between depths at the same location. Significant groups have a value of P ≤ 0.05. The GraphPad Prism software version 8.0.2 (GraphPad Inc.) was used to perform the statistical tests. Results are presented as mean ± standard error.
All raw data used in this study are accessible through the electronic notebook1.
Results
Camera Settings Optimization
Setting the camera to shoot at the highest frame rate (i.e., 7 FPS) does not provide enough recharging time to the excitation strobes, that with the highest output (GN32) can fire at less than 1.5 s between shots. As a result, the first image of each set is full power fluorescent shoot, and the subsequent shoot is reflectance (ambient) light (Figure 5). If ambient light is absent or weak, for example at depth or at sunrise and sunset, we found that there is no need to make a reflectance image for subtraction, since the fluorescence is strong enough (Figure 1D). However, at shallow depth and with strong light during the day, there is an advantage in using the software developed with the FluorIS (Zweifler et al., 2017) or with FIJI software for subtracting and emphasizing the fluorescence effect in daylight (Figure 5). The result of the subtraction is a fluorescence image excited solely by the blue strobes (Figure 5D). Alternatively, the system can be used without the need for subtracting by eliminating as much ambient light as possible with few basic adjustments and considerations before and during the dive. First, the exposure value of the camera should be set to a value that minimizes ambient light, allowing at the same time as much strobe light as the sensor can record. To accomplish that, we found that the shutter needs to be set to the highest synchronization speed allowed by the camera. With the Nikon D850 this corresponds to 1/250th of a second (note that each camera model can present a different value for synchronization speed). The aperture of the lens has to be set to a value that allows a good depth of field and sharpness but gives enough strobe light to penetrate to the sensor. We found that such aperture value corresponds to f10. The sensor sensitivity was set to a final value of ISO320, so to summarize, the ultimate exposure parameters were set to 1/250th, f10, ISO320. Using these settings, we reduced the acquisition time to an average of 10 s for a set of 3 images produced on each quadrat.
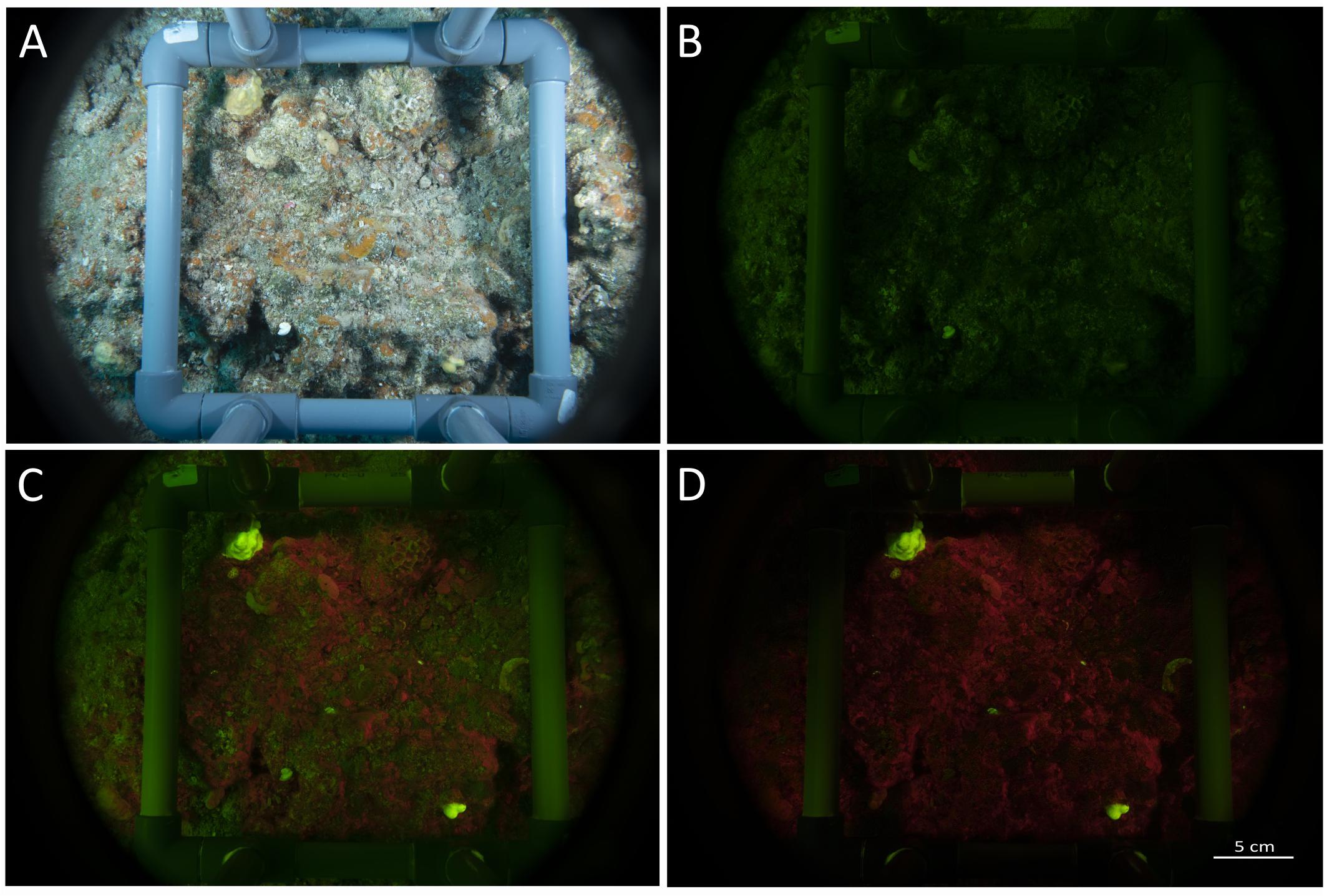
Figure 5. Images set taken with the speed-FlorIS. (A) Reflectance image taken under white illumination, (B) ambient image (blue strobes off), (C) fluorescence image (blue strobes on), and (D) fluorescence image after subtracting image (B) from image (C) using Fiji software (Schindelin et al., 2012).
Another two points that are recommended when removing the ICF and using a barrier filter is to set the camera White Balance (WB) to 2300K or less and to set the tint to green. This setting can be done in the camera WB menu, or by adjusting a preset WB, or in post processing if the images file format is set to RAW. We found that these two points allow complete control on the final picture.
Because light underwater fades rapidly with increasing distance from the source, it is crucial to position the strobes as close as possible to the subject without the strobe body appearing in the image. To achieve this, we used 40 cm Nauticam light mounting arms that can hold the strobes to the housing handles and give the flexibility to move and lock the strobes in the desired position (Figure 2D). However, we observed that the use of the excitation filters significantly reduces the strobes power, therefore it is crucial to set them on full output and to place the strobe approximately 20 cm from the target. The two FS strobes can instead be place in a larger distance or set on lower output, as their power is not affected by filters. Finally, the surveyor’s body can be used to block the ambient light on the subject. Even with the exposure value mentioned above and maximal excitation strobe output, the intensity of direct sunlight at noon in tropic areas is strong enough to overcome the fluorescence emission and to be undetected by the camera. To avoid this, the surveyor should plan the dives to early morning or afternoon, but if diving at noon time, the surveyor can hover right above the quadrat and shade it with the diving gear and body, so that the intensity of the ambient light in the image drops dramatically and a good reflectance image can be easily captured.
Changes in Reef Structure With Depth and Coral Recruits Survey
The topography of the sampling sites differed significantly across depths in each geographic location (Figures 6, 7). In Eilat, scattered stony coral colonies with mainly branching morphology in the shallow reef are replaced by encrusting or plate-like colonies in the mesophotic reef, which leads to a flattening of the reef structure with increasing depth (Figure 6A). Differently in Bermuda, the high density of stony and soft corals substantially declines at mesophotic depths, where a non-coral dominated reef with a high topographic complexity is predominant (Figure 7A).
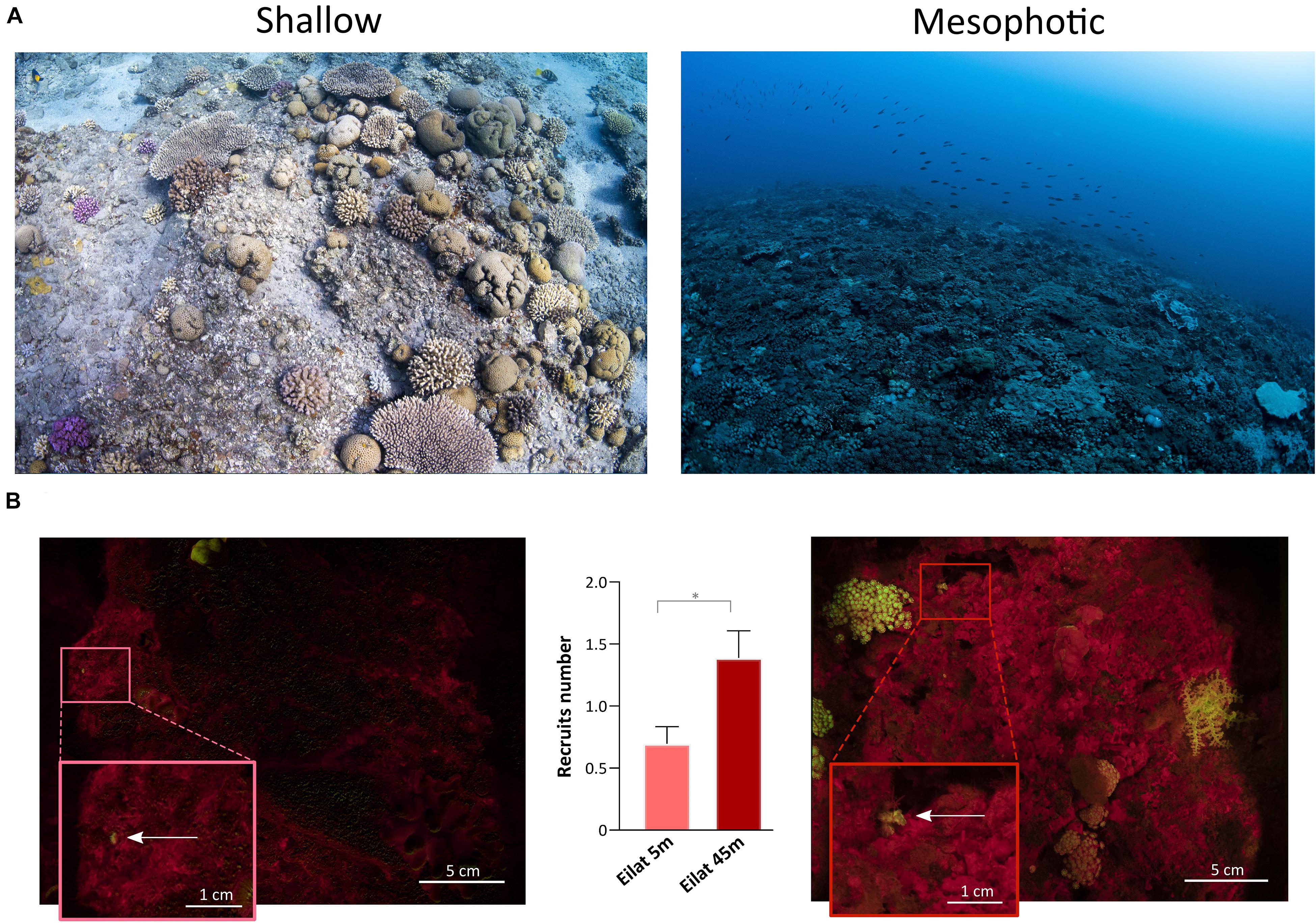
Figure 6. Shallow and mesophotic reefs in Eilat and detection of coral recruits. (A) Images of the sampling sites at the shallow (left) and mesophotic (right) reefs in Eilat. (B) Fluorescence images of the quadrat deployed in the shallow (left) and mesophotic (right) reefs of Eilat. The arrows within the insets point to coral recruits. The central graph shows the number of recruits identified in the shallow (n = 46 quadrats) and mesophotic (n = 36 quadrats) reefs of Eilat. Values are shown as means ± SEM. Asterisk indicates statistical difference (Mann-Whitney test, P < 0.05).
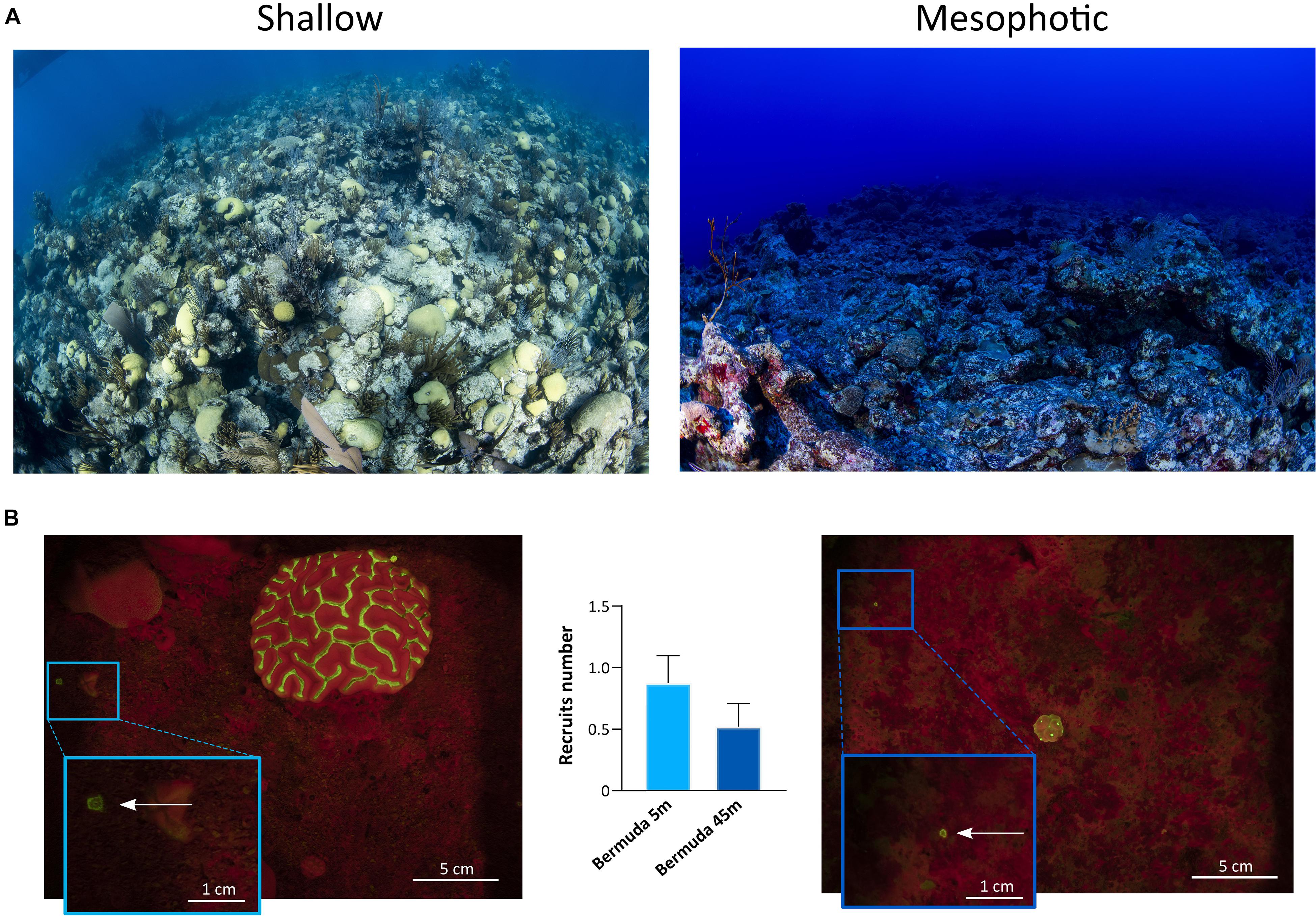
Figure 7. Shallow and mesophotic reefs in Bermuda and detection of coral recruits. (A) Images of the sampling sites at the shallow (left) and mesophotic (right) reefs in Bermuda. (B) Fluorescence images of the quadrat deployed in the shallow (left) and mesophotic (right) reefs of Bermuda. The arrows within the insets point to coral recruits. The central graph shows the number of recruits identified in the shallow (n = 17 quadrats) and mesophotic (n = 21 quadrats) reefs of Bermuda. Values are shown as means ± SEM.
In Eilat and Bermuda, the total distance that we sampled corresponded to around 100 m in both shallow and mesophotic reefs. Considering the total time needed to complete each dive, we estimated that we employed a maximum of 1 min to sample each single quadrat. Using the speed-FluorIS system we recorded wide FOV fluorescence images of coral recruits from 1 mm to 2 cm in diameter (Figures 6B, 7B). In Eilat, we found significantly higher numbers of recruits in the randomly placed 25 × 25 cm quadrat at 45 m compared to 5 m (Mann-Whitney, P = 0.009) (Figure 6B). In Bermuda instead, we found no significant difference between the shallow and mesophotic reefs (Mann-Whitney, P > 0.05) (Figure 7B).
Discussion
The development of the FluorIS system has demonstrated the biases of in situ visual surveys of coral recruits, by providing a higher precision and standardized method to accurately assess coral recruitment (Zweifler et al., 2017). Such a system eases and speeds up the detection of small and highly cryptic coral recruits (Figures 6B, 7B), overcoming the limitations of non-standardized and labor-intensive visual recruit counts. Here we show that the speed-FluorIS system greatly facilitates high sensitivity in situ fluorescence imaging of coral ecosystems, especially by eliminating the excess weight and encumbrance of a double-camera system and by reducing the time needed to acquire all sets of images. In fact, the use of speed-FlorIS significantly reduces the acquisition time to an average of 10 s for a set of 3 images, reducing the overall time employed to sample a single quadrat to 1 min. This time frame is significantly shorter compared to the sampling time of the original FluorIS system, which corresponds to ∼ 6 min per quadrat (Zweifler et al., 2017; data not shown). As survey depth increases, the dive complexity increases as well. For example, technical dives are needed to go beyond recreational dive limits, which require heavier and bulkier equipment and are strictly limited in time. Therefore, conducting underwater surveys with speed-FluorIS brings several advantages for the diver, including safety, ease of navigation, reducing fatigue, and simplifying the logistics of conducting fluorescent surveys in the field. Moreover, the speed-FluorIS system is much more economical compared to the original dual-camera system, reducing the cost of the full set-up of about 40% (Table 1).
In this study, we demonstrate that speed-FluorIS enables a fast imaging of fluorescence to study coral recruitment patterns over a broader range of depths and reef topographies than previous fluorescence methods (Figures 6, 7; Schmidt-Roach et al., 2008; Roth and Knowlton, 2009; Hsu et al., 2014; Zweifler et al., 2017; Ramesh et al., 2019), providing a standardized survey methodology that can be applied in different sites across the world. Solely based on the results of our survey (Figures 6B, 7B), it is difficult to estimate recruitment dynamics in the shallow and mesophotic reefs of Eilat and Bermuda, considering that coral recruitment greatly varies between seasons and years (Jouval et al., 2019, and references therein). Further long-term surveys would significantly improve the resolution and accuracy of temporal and spatial data, revealing the full picture of coral recruitment patterns and assessing the true status of the reef. Moreover, environmental data such as light, temperature, nutrients, algae and fish abundance of the sampling sites also need to be assessed, as they have a strong influence on coral recruitment patterns (Brokovich et al., 2010; Kramer et al., 2019; Loya et al., 2019; Stefanoudis et al., 2019).
In mesophotic reefs, in situ surveys of recruitment patterns have mostly been limited to the northern Red Sea and Western Australia, where artificial settlement tiles have been employed to count new coral recruits (Turner et al., 2018; Kramer et al., 2019; Shlesinger and Loya, 2021). Several studies have evaluated in situ coral recruitment patterns in the shallow reefs of Eilat and Bermuda through visual underwater identification of coral recruits on settlement tiles or on the natural substrate (Smith, 1992; Glassom et al., 2004; Abelson et al., 2005; Glassom and Chadwick, 2006; Martinez and Abelson, 2013; Shlesinger and Loya, 2016; Guerrini et al., 2020). Although being performed on the same site, these surveys revealed high variation in coral recruit numbers. Inconsistent findings in recruitment studies using different methods have been attributed to the differences in substrate, method of attachment, and duration and depth of tile deployment (Harriott and Fisk, 1988; Mumby, 1999; Glassom et al., 2004; Abelson et al., 2005). In addition, the use of settlement tiles of different sizes may also affect abundances of coral recruits, which in turn will affect the number of individuals per unit area (Birkeland et al., 1981). Thus, studies on coral recruitment based on this methodology can be difficult to compare, especially since the type and preparation of recruitment tiles greatly affect which organisms will settle on them (Brandt et al., 2019). Such lack of a standardized methodology hinders the comparison of recruitment patterns between reef sites worldwide (Glassom et al., 2004; Zweifler et al., 2017). Moreover, the labor-intensive demands of executing visual surveys and direct counts of recruits in the field make it difficult to generalize findings to broader geographic extents and across wide depth distributions (Shlesinger and Loya, 2016).
Fluorescent proteins fluorescence has been widely used for better detection of coral recruits by divers and in images (Piniak et al., 2005; Baird et al., 2006; Schmidt-Roach et al., 2008; Roth and Knowlton, 2009). However, FPs fluorescence alone might not be enough to identify young corals, since FPs fluorescence intensity greatly varies among species (Eyal et al., 2015), with some corals showing very weak or no FPs signal (Baird et al., 2006; Roth et al., 2010). Moreover, there are other organisms besides corals that contain fluorescent pigments, such as algae, sponges, and worms. Therefore, accurate identification is only possible by comparing each coral recruit identified in the fluorescence image to a high-resolution FS image, as we did in this study, which can also facilitate taxonomic identification (Baird et al., 2006).
The speed-FluorIS that we propose here represents an easy-to-use and non-invasive method that will help standardize surveys and long-term monitoring of coral recruits. In the future, speed-FluorIS can be applied via underwater vehicles for rapid and automated surveys. The recorded data could be uploaded to create universal and easily accessible databases that contribute to our understanding of the vital and delicate early life stages of corals. Importantly, if MCEs are in fact important lifeboats for coral survival as increasingly advocated (Bongaerts et al., 2010; Lesser et al., 2018), it is imperative to accurately investigate coral recruitment dynamics of these deeper reefs. Coral recruitment has been long identified as a key process in the ability of reefs to recover from disturbances (Hughes et al., 2007, 2010; Ritson-Williams et al., 2009). Therefore, understanding the recruitment process is essential for developing suitable reef conservation and management strategies to protect these vital ecosystems.
Data Availability Statement
The original contributions presented in the study are included in the article/Supplementary Material, further inquiries can be directed to the corresponding authors.
Author Contributions
HN, GG-G, and TM designed the research. HN, GG-G, SM, SE, AC, and TM performed the underwater surveys. FS and HN carried out the image analysis and recruit counts. HN, FS, GG-G, and TM wrote the manuscript. All authors contributed to improving, revision and approval of the manuscript.
Funding
This project has received funding from the joint United States National Science Foundation and United States – Israel Binational Science Foundation (NSF #1937770 to GG-G; BSF #2019653 to TM) the ASSEMBLE Plus consortium for an access grant (ref. SR16022018108e1) to the Interuniversity Institute for Marine Sciences in Eilat and a Grant-in-Aid from the Bermuda Institute of Ocean Sciences (BIOS).
Conflict of Interest
The authors declare that the research was conducted in the absence of any commercial or financial relationships that could be construed as a potential conflict of interest.
Publisher’s Note
All claims expressed in this article are solely those of the authors and do not necessarily represent those of their affiliated organizations, or those of the publisher, the editors and the reviewers. Any product that may be evaluated in this article, or claim that may be made by its manufacturer, is not guaranteed or endorsed by the publisher.
Acknowledgments
We would like to thank T. Triebitz, A. Avni, and A. Meri-Esh for technical support. Additional thank go to Eran Rozen, diving safety officer of the Morris Kahn Research Station, and the IUI staff.
Supplementary Material
The Supplementary Material for this article can be found online at: https://www.frontiersin.org/articles/10.3389/fmars.2021.709175/full#supplementary-material
Footnotes
References
Abelson, A., Olinky, R., and Gaines, S. (2005). Coral recruitment to the reefs of Eilat, Red Sea: temporal and spatial variation, and possible effects of anthropogenic disturbances. Mar. Pollut. Bull. 50, 576–582. doi: 10.1016/j.marpolbul.2005.02.021
Alieva, N. O., Konzen, K. A., Field, S. F., Meleshkevitch, E. A., Hunt, M. E., Beltran-Ramirez, V., et al. (2008). Diversity and evolution of coral fluorescent proteins. PLoS One 3:e2680. doi: 10.1371/journal.pone.0002680
Appeldoorn, R., Ballantine, D., Bejarano, I., Carlo, M., Nemeth, M., Otero, E., et al. (2016). Mesophotic coral ecosystems under anthropogenic stress: a case study at Ponce, Puerto Rico. Coral Reefs 35, 63–75. doi: 10.1007/s00338-015-1360-5
Baird, A. H., Salih, A., and Trevor-Jones, A. (2006). Fluorescence census techniques for the early detection of coral recruits. Coral Reefs 25, 73–76. doi: 10.1007/s00338-005-0072-7
Bak, R. P. M., Nieuwland, G., and Meesters, E. H. (2005). Coral reef crisis in deep and shallow reefs: 30 years of constancy and change in reefs of Curacao and Bonaire. Coral Reefs 24, 475–479. doi: 10.1007/s00338-005-0009-1
Birkeland, C., Rowley, D., and Randall, R. H. (1981). Coral recruitment patterns at Guam. Proc. 4th Int. Coral Reef Symp. 2, 339–344.
Bongaerts, P., Muir, P., Englebert, N., Bridge, T. C. L., and Hoegh-Guldberg, O. (2013). Cyclone damage at mesophotic depths on Myrmidon Reef (GBR). Coral Reefs 32, 935–935. doi: 10.1007/s00338-013-1052-y
Bongaerts, P., Ridgway, T., Sampayo, E. M., and Hoegh-Guldberg, O. (2010). Assessing the ‘deep reef refugia’ hypothesis: focus on Caribbean reefs. Coral Reefs 29, 309–327. doi: 10.1007/s00338-009-0581-x
Brandt, M. E., Olinger, L. K., Chaves-Fonnegra, A., Olson, J. B., and Gochfeld, D. J. (2019). Coral recruitment is impacted by the presence of a sponge community. Mar. Biol. 166:49. doi: 10.1007/s00227-019-3493-5
Brokovich, E., Ayalon, I., Einbinder, S., Segev, N., Shaked, Y., Genin, A., et al. (2010). Grazing pressure on coral reefs decreases across a wide depth gradient in the Gulf of Aqaba, Red Sea. Mar. Ecol. Prog. Ser. 399, 69–80. doi: 10.3354/meps08354
D’Angelo, C., and Wiedenmann, J. (2014). Impacts of nutrient enrichment on coral reefs: new perspectives and implications for coastal management and reef survival. Curr. Opin. Environ. Sustain. 7, 82–93. doi: 10.1016/j.cosust.2013.11.029
Dishon, G., Dubinsky, Z., Fine, M., and Iluz, D. (2012). Underwater light field patterns in subtropical coastal waters: a case study from the Gulf of Eilat (Aqaba). Isr. J. Plant Sci. 60, 265–275. doi: 10.1560/IJPS.60.1-2.265
Dustan, P., and Halas, J. C. (1987). Changes in the reef-coral community of Carysfort reef, Key Largo, Florida: 1974 to 1982. Coral Reefs 6, 91–106. doi: 10.1007/BF00301378
Eyal, G., Wiedenmann, J., Grinblat, M., D’Angelo, C., Kramarsky-Winter, E., Treibitz, T., et al. (2015). Spectral diversity and regulation of coral fluorescence in a mesophotic reef habitat in the Red Sea. PLoS One 10:e0128697. doi: 10.1371/journal.pone.0128697
Field, S. N., Glassom, D., and Bythell, J. (2007). Effects of artificial settlement plate materials and methods of deployment on the sessile epibenthic community development in a tropical environment. Coral Reefs 26, 279–289. doi: 10.1007/s00338-006-0191-9
Glassom, D., and Chadwick, N. (2006). Recruitment, growth and mortality of juvenile corals at Eilat, northern Red Sea. Mar. Ecol. Prog. Ser. 318, 111–122. doi: 10.3354/meps318111
Glassom, D., Zakai, D., and Chadwick-Furman, N. E. (2004). Coral recruitment: a spatio-temporal analysis along the coastline of Eilat, northern Red Sea. Mar. Biol. 144, 641–651. doi: 10.1007/s00227-003-1243-0
Goodbody-Gringley, G., Marchini, C., Chequer, A. D., and Goffredo, S. (2015). Population structure of Montastraea cavernosa on Shallow versus Mesophotic Reefs in Bermuda. PLoS One 10:e0142427. doi: 10.1371/journal.pone.0142427
Guerrini, G., Yerushalmy, M., Shefy, D., Shashar, N., and Rinkevich, B. (2020). Apparent recruitment failure for the vast majority of coral species at Eilat, Red Sea. Coral Reefs 39, 1715–1726. doi: 10.1007/s00338-020-01998-4
Harriott, V., and Fisk, D. (1988). Recruitment patterns of scleractinian corals: a study of three reefs. Mar. Freshw. Res. 39, 409–416. doi: 10.1071/MF9880409
Hoegh-Guldberg, O., and Bruno, J. F. (2010). The impact of climate change on the world’s marine ecosystems. Science 328, 1523–1528. doi: 10.1126/science.1189930
Hoegh-Guldberg, O., Mumby, P. J., Hooten, A. J., Steneck, R. S., Greenfield, P., Gomez, E., et al. (2007). Coral reefs under rapid climate change and ocean acidification. Science 318, 1737–1742. doi: 10.1126/science.1152509
Hsu, C. M., de Palmas, S., Kuo, C. Y., Denis, V., and Chen, C. A. (2014). Identification of scleractinian coral recruits using fluorescent censusing and DNA barcoding techniques. PLoS One 9:e107366. doi: 10.1371/journal.pone.0107366
Hughes, T. P., Graham, N. A. J., Jackson, J. B. C., Mumby, P. J., and Steneck, R. S. (2010). Rising to the challenge of sustaining coral reef resilience. Trends Ecol. Evol. 25, 633–642. doi: 10.1016/j.tree.2010.07.011
Hughes, T. P., Rodrigues, M. J., Bellwood, D. R., Ceccarelli, D., Hoegh-Guldberg, O., McCook, L., et al. (2007). Phase shifts, herbivory, and the resilience of coral reefs to climate change. Curr. Biol. 17, 360–365. doi: 10.1016/j.cub.2006.12.049
Jouval, F., Latreille, A. C., Bureau, S., Adjeroud, M., and Penin, L. (2019). Multiscale variability in coral recruitment in the mascarene islands: from centimetric to geographical scale. PLoS One 14:e0214163. doi: 10.1371/journal.pone.0214163
Kahng, S., Copus, J. M., and Wagner, D. (2016). “Mesophotic coral ecosystems,” in Marine Animal Forests, eds S. Rossi, L. Bramanti, A. Gori, and C. Orejas (Cham: Springer), 1–22. doi: 10.1007/978-3-319-17001-5_4-1
Kahng, S. E., Garcia-Sais, J. R., Spalding, H. L., Brokovich, E., Wagner, D., Weil, E., et al. (2010). Community ecology of mesophotic coral reef ecosystems. Coral Reefs 29, 255–275. doi: 10.1007/s00338-010-0593-6
Kramer, N., Eyal, G., Tamir, R., and Loya, Y. (2019). Upper mesophotic depths in the coral reefs of Eilat, Red Sea, offer suitable refuge grounds for coral settlement. Sci. Rep. 9:2263. doi: 10.1038/s41598-019-38795-1
LaJeunesse, T. C., Parkinson, J. E., Gabrielson, P. W., Jeong, H. J., Reimer, J. D., Voolstra, C. R., et al. (2018). Systematic revision of symbiodiniaceae highlights the antiquity and diversity of coral endosymbionts. Curr. Biol. 28, 2570–2580.e6. doi: 10.1016/j.cub.2018.07.008
Lesser, M. P., Slattery, M., and Leichter, J. J. (2009). Ecology of mesophotic coral reefs. J. Exp. Mar. Biol. Ecol. 375, 1–8. doi: 10.1016/j.jembe.2009.05.009
Lesser, M. P., Slattery, M., and Mobley, C. D. (2018). Biodiversity and functional ecology of mesophotic coral reefs. Annu. Rev. Ecol. Evol. Syst. 49, 49–71. doi: 10.1146/annurev-ecolsys-110617-062423
Loya, Y., Puglise, K. A., and Bridge, T. C. L. (eds) (2019). Mesophotic Coral Ecosystems, Vol. 12. New York, NY: Springer. doi: 10.1007/978-3-319-92735-0
Martinez, S., and Abelson, A. (2013). Coral recruitment: the critical role of early post-settlement survival. ICES J. Mar. Sci. 70, 1294–1298. doi: 10.1093/icesjms/fst035
Miller, M. W., Weil, E., and Szmant, A. M. (2000). Coral recruitment and juvenile mortality as structuring factors for reef benthic communities in Biscayne National Park, USA. Coral Reefs 19, 115–123. doi: 10.1007/s003380000079
Mumby, P. (1999). Can Caribbean coral populations be modelled at metapopulation scales? Mar. Ecol. Prog. Ser. 180, 275–288. doi: 10.3354/meps180275
Mundy, C. N. (2000). An appraisal of methods used in coral recruitment studies. Coral Reefs 19, 124–131. doi: 10.1007/s003380000081
Murdoch, T. J. T., and Murdoch, J. M. H. (2016). Murdoch TJT, Murdoch JMH (2016) Baseline Condition of the Coral Reefs and Fishes Across Three Depth Zones of the Forereef of Bermuda. BREAM: Bermuda Reef Ecosystem Analysis and Monitoring Programme Report, Bermuda Zoological Society, Flatts Bermuda. BBP-2016-237. Flatts: Bermuda Aquarium, Museum and Zoo. doi: 10.13140/RG.2.2.22260.35204
Papina, M., Sakihama, Y., Bena, C., van Woesik, R., and Yamasaki, H. (2002). Separation of highly fluorescent proteins by SDS-PAGE in Acroporidae corals. Comp. Biochem. Physiol. Part B Biochem. Mol. Biol. 131, 767–774. doi: 10.1016/S1096-4959(02)00025-8
Pinheiro, H. T., Goodbody-Gringley, G., Jessup, M. E., Shepherd, B., Chequer, A. D., and Rocha, L. A. (2016). Upper and lower mesophotic coral reef fish communities evaluated by underwater visual censuses in two Caribbean locations. Coral Reefs 35, 139–151. doi: 10.1007/s00338-015-1381-0
Piniak, G. A., Fogarty, N. D., Addison, C. M., and Kenworthy, W. J. (2005). Fluorescence census techniques for coral recruits. Coral Reefs 24, 496–500. doi: 10.1007/s00338-005-0495-1
Pyle, R. L. (2019). “Advanced technical diving,” in Mesophotic Coral Ecosystems, Vol. 12, eds Y. Loya, K. A. Puglise, and T. C. L. Bridge (Cham: Springer), 959–972. doi: 10.1007/978-3-319-92735-0_50
Ramesh, C. H., Koushik, S., Shunmugaraj, T., and Murthy, M. R. (2019). A rapid in situ fluorescence census for coral reef monitoring. Reg. Stud. Mar. Sci. 28:100575. doi: 10.1016/j.rsma.2019.100575
Ritson-Williams, R., Arnold, S., Fogarty, N., Steneck, R. S., Vermeij, M., and Paul, V. J. (2009). New perspectives on ecological mechanisms affecting coral recruitment on reefs. Smithson. Contrib. Mar. Sci. 38, 437–457. doi: 10.5479/si.01960768.38.437
Roth, M., and Knowlton, N. (2009). Distribution, abundance, and microhabitat characterization of small juvenile corals at Palmyra Atoll. Mar. Ecol. Prog. Ser. 376, 133–142. doi: 10.3354/meps07787
Roth, M. S., Latz, M. I., Goericke, R., and Deheyn, D. D. (2010). Green fluorescent protein regulation in the coral Acropora yongei during photoacclimation. J. Exp. Biol. 213, 3644–3655. doi: 10.1242/jeb.040881
Schindelin, J., Arganda-Carreras, I., Frise, E., Kaynig, V., Longair, M., Pietzsch, T., et al. (2012). Fiji: an open-source platform for biological-image analysis. Nat. Methods 9, 676–682. doi: 10.1038/nmeth.2019
Schmidt-Roach, S., Kunzmann, A., and Martinez Arbizu, P. (2008). In situ observation of coral recruitment using fluorescence census techniques. J. Exp. Mar. Biol. Ecol. 367, 37–40. doi: 10.1016/j.jembe.2008.08.012
Shlesinger, T., and Loya, Y. (2016). Recruitment, mortality, and resilience potential of scleractinian corals at Eilat, Red Sea. Coral Reefs 35, 1357–1368. doi: 10.1007/s00338-016-1468-2
Shlesinger, T., and Loya, Y. (2021). Depth-dependent parental effects create invisible barriers to coral dispersal. Commun. Biol. 4:202. doi: 10.1038/s42003-021-01727-9
Siegel, D. A., Michaels, A. F., Sorensen, J. C., O’Brien, M. C., and Hammer, M. A. (1995). Seasonal variability of light availability and utilization in the Sargasso Sea. J. Geophys. Res. 100, 8695–8713. doi: 10.1029/95JC00447
Smith, S. R. (1992). Patterns of coral recruitment and post-settlement mortality on Bermuda’s reefs: comparisons to Caribbean and pacific reefs. Am. Zool. 32, 663–673. doi: 10.1093/icb/32.6.663
Smith, T. B., Gyory, J., Brandt, M. E., Miller, W. J., Jossart, J., and Nemeth, R. S. (2016). Caribbean mesophotic coral ecosystems are unlikely climate change refugia. Glob. Change Biol. 22, 2756–2765. doi: 10.1111/gcb.13175
Soong, K., Chen, M., Chen, C., Dai, C., Fan, T., Li, J., et al. (2003). Spatial and temporal variation of coral recruitment in Taiwan. Coral Reefs 22, 224–228. doi: 10.1007/s00338-003-0311-8
Stefanoudis, P. V., Rivers, M., Smith, S. R., Schneider, C. W., Wagner, D., Ford, H., et al. (2019). Low connectivity between shallow, mesophotic and rariphotic zone benthos. R. Soc. Open Sci. 6:190958. doi: 10.1098/rsos.190958
Treibitz, T., Neal, B. P., Kline, D. I., Beijbom, O., Roberts, P. L. D., Mitchell, B. G., et al. (2015). Wide Field-of-view fluorescence imaging of coral reefs. Sci. Rep. 5:7694. doi: 10.1038/srep07694
Turner, J. A., Babcock, R. C., Hovey, R., and Kendrick, G. A. (2017). Deep thinking: a systematic review of mesophotic coral ecosystems. ICES J. Mar. Sci. 74, 2309–2320. doi: 10.1093/icesjms/fsx085
Turner, J. A., Thomson, D. P., Cresswell, A. K., Trapon, M., and Babcock, R. C. (2018). Depth-related patterns in coral recruitment across a shallow to mesophotic gradient. Coral Reefs 37, 711–722. doi: 10.1007/s00338-018-1696-8
Warner, M. E., Lesser, M. P., and Ralph, P. J. (2010). “Chlorophyll fluorescence in reef building corals,” in Chlorophyll a Fluorescence in Aquatic Sciences: Methods and Applications, eds D. J. Suggett, O. Prášil, and M. A. Borowitzka (Dordrecht: Springer), 209–222. doi: 10.1007/978-90-481-9268-7_10
Keywords: coral recruitment, ecological monitoring, fluorescence, underwater imaging, underwater survey tool
Citation: Nativ H, Scucchia F, Martinez S, Einbinder S, Chequer A, Goodbody-Gringley G and Mass T (2021) In situ Estimation of Coral Recruitment Patterns From Shallow to Mesophotic Reefs Using an Optimized Fluorescence Imaging System. Front. Mar. Sci. 8:709175. doi: 10.3389/fmars.2021.709175
Received: 13 May 2021; Accepted: 12 July 2021;
Published: 30 July 2021.
Edited by:
James Davis Reimer, University of the Ryukyus, JapanReviewed by:
Tyler Burton Smith, University of the Virgin Islands, US Virgin IslandsChuki Hongo, Nanki Kumano Geopark Center, Japan
Copyright © 2021 Nativ, Scucchia, Martinez, Einbinder, Chequer, Goodbody-Gringley and Mass. This is an open-access article distributed under the terms of the Creative Commons Attribution License (CC BY). The use, distribution or reproduction in other forums is permitted, provided the original author(s) and the copyright owner(s) are credited and that the original publication in this journal is cited, in accordance with accepted academic practice. No use, distribution or reproduction is permitted which does not comply with these terms.
*Correspondence: Gretchen Goodbody-Gringley, Z2dvb2Rib2R5QHJlZWZyZXNlYXJjaC5vcmc=; Tali Mass, dG1hc3NAdW5pdi5oYWlmYS5hYy5pbA==
†Present address: Stephane Martinez, Centre Scientifique de Monaco, Coral Ecophysiology Team, Monaco, Monaco