- Department of Aquaculture, Bangladesh Agricultural University, Mymensingh, Bangladesh
The use of nanotechnology in food production systems is being investigated globally, though there is limited research on its effect on fish nutrition. Therefore, this study aimed to identify the effects of silica nanoparticles (NPs) on the nutrition and physiology of tilapia, Oreochromis niloticus. Four isonitrogenous diets (300 g/kg crude protein) with NPs (0, 1, 2, and 3 mg/kg diet) were fed to fish (6.52 ± 0.20 g) in a recirculatory aquaculture system for 56 days. Throughout the study period, the effects of silica NP on survival rate, blood cell count, hemoglobin (Hb) level, condition factor (CF), and final product composition (except lipid content) were insignificant. However, growth performance and feed efficiency increased with an increasing level of silica NP, up to 2 mg/kg, and then decreased. This increase was due to the highest apparent protein digestibility and dry matter digestibility when fish were fed silica NP at 2 mg/kg. However, fish at the early stage showed better performance in all dietary groups than in later. Blood glucose (BG) content and histology of the kidney revealed that fish were stressed when a 3 mg/kg silica NP was used and they adapted through excessive excretion via expanded glomeruli. Though no significant effect on villi length was observed, silica NP increased the surface area widening the villi of the gut along with the number of goblet cells in the intestine significantly, when supplemented at a level of 2 mg/kg. The bioaccumulation of silica shows that incorporating silica NP in the fish feed will not compromise human health safety upon consumption. Although silica NP at 1 mg/kg and 3 mg/kg yielded some improvements to growth and final product quality, a 2 mg/kg silica NP generated the best results in all measured parameters.
Introduction
Increased access to multidisciplinary knowledge and the worldwide availability of low-cost compliances have made the global aquaculture industry one of the fastest-growing and irreplaceable animal protein sectors (Belton and Thilsted, 2014; FAO, 2016; Abd El-Naby et al., 2019). This intensified sector has been proved to be a significant contributor to food security, especially for developing Asian and African countries including Bangladesh (Gui et al., 2018; Chan et al., 2019; Hasan et al., 2021c). Inputs for aquaculture include feed, seed, and water, of which feed accounts for nearly 60–70% of the total production costs (Yuan et al., 2017; Yang et al., 2019; Nguyen et al., 2020a). In aquaculture operations, the quality of feed is a fundamental requirement that must be met (Ahmed, 2007; Singha et al., 2020). Besides the quality, digestibility of nutrients also impacts water quality, disease outbreak (Heal et al., 2021), total yield, and, consequently, the business profitability (Guo et al., 2020; Hassaan et al., 2020a; Kong et al., 2020; Nguyen et al., 2020a). While the sustainable use of feed is a challenge to the aquaculture industry, many strategies have been implemented, including the replacement of fishmeal (Perez-Velazquez et al., 2019; Li et al., 2020), the use of byproducts (Irm et al., 2020), selective breeding (Carlberg et al., 2018), minimizing nutrient waste (de Verdal et al., 2018), and, most recently, the use of feed additives including probiotics (Haque et al., 2021b; Hasan et al., 2021a), and nanoparticles (NPs) (Abd El-Naby et al., 2019; Rathore et al., 2020).
Over the last decade, Tilapia (Oreochromis niloticus), the global aquatic chicken, has gained its popularity throughout the world (Abdel-Tawwab et al., 2020) for its compatible characteristics, such as easy to produce seed (Barman and Little, 2011), quick response to artificial feeds (Ahmed et al., 2014; Ogello et al., 2014), a wide range of environmental tolerance (Singha et al., 2020), short crop cycle (Francis and Esa, 2016), nutritive values and larger edible portion with no intermuscular bone (Moesch et al., 2016), and high resistance to physical and biological hazards (Al-Deriny et al., 2020; Chaput et al., 2020; Foysal et al., 2020; Naiel et al., 2020). In the context of the increased feed price because of the increasing cost against the limited protein source, such as fish meal (Nguyen et al., 2020a; Pianesso et al., 2020) and a recession in the farmgate price of tilapia due to supply outstripping the national demand and export barriers for developing countries like Bangladesh (Uddin et al., 2019; Bashar et al., 2021; Haque et al., 2021a; Hasan et al., 2021b), recently dealing with this species in semi-intensive, intensive, and super-intensive commercial culture systems have become a great challenge (Kabir et al., 2019). Moreover, due to the lower nutrient digestion capability of tilapia, nutrients, e.g., protein, end up as metabolic waste, like NH3 (Crab et al., 2007). These waste metabolites not only increase feed costs by increasing the feed conversion ratio (FCR) but also make the fish more susceptible to pathogens (Kent et al., 2009; Hasan and Haque, 2020; Hasan et al., 2020) through deterioration of water quality. Therefore, finding a way of increasing nutrient digestibility and absorption in O. niloticus can be a multifaceted solution for a range of issues existing in aquaculture, which could help to make the industry sustainable.
Due to unique physicochemical properties, NPs are being acknowledged by the food production industries for their medical and nutritional uses (Vidya et al., 2016; Khosravi-Katuli et al., 2017; Kumar et al., 2018; Rodriguez et al., 2018; Bashar et al., 2019; Thangapandiyan and Monika, 2020). NPs can benefit aquaculture production by enhancing the bioactivity of molecules, including micronutrients (Xu et al., 2018; Shah and Mraz, 2020), and enabling tissue-specific applications of disease treatments with no consequences to human health (Jennings et al., 2016). The high specific surface area of NPs facilitates the absorption of micronutrients from the intestine to the bloodstream in terrestrial and aquatic animals and makes them suitable for use as feed additives (Huang et al., 2015; Khosravi-Katuli et al., 2017; Pieszka et al., 2019). Along with other NPs, silica (silicon dioxide) NP can be used for its outstanding optical properties, adsorption capacity, low toxicity, biocompatibility (Bitar et al., 2012), thermal stability, and low production cost (Priyadarsini et al., 2018). In the aquaculture industry, silica NP has been reported to favor fish welfare through easing drug administration and reducing the risk of disease outbreak even in case of huge crowding (Khosravi-Katuli et al., 2017). In addition, silica in nanoform is found to be effective in wastewater treatment (Jarvie et al., 2009), controlling microbial load (Huang et al., 2015), and stimulating diatom growth in aquaculture systems.
Despite the huge potential of silica NP in aquaculture, studies on its use as feed additives for finfish, like tilapia, have not been carried out. Therefore, to make the aquaculture production more sustainable from the nutritional consideration without averting human health safety upon consumption, the current experiment was carried out to investigate the effects of different levels of silica NPs on growth performance, feed utilization, blood physiology, histology, and muscle composition of and bioaccumulation in tilapia.
Materials and Methods
Ethical Issues
The design and execution of the experiment were approved by the ethical committee of the Bangladesh Agricultural University Research System (Approval No.: 2021/44/BAU). Fish were fed, handled, sampled, harvested, and sacrificed, maintaining proper care and welfare by all the mentioned authors.
Experimental Site and Culture Design
To conduct the 8-week experiment, a Recirculatory Aquaculture System with 12 fiberglass tanks (0.8 × 0.5 × 0.5 m) arranged in a two-tier system was developed in the Faculty of Fisheries, BAU, Mymensingh, Bangladesh. Continuous aeration and a water depth of 0.4 m were maintained throughout the feeding trial. To avoid the circulation of silica NP leaching from the feed and fecal content of different treatments, the inlet and outlet pipes of every biofilter were fitted with a 1-mm thick ceramic filter (0.01 μm pore size) covered by cheesecloth (double layered) of the 1-μm mesh size. Three tanks were designated as control (T0) and every treatment (T1, T2, and T3) had three replications.
Silica NP
Highly pure, magnetically activated, and 100% natural silica NP, composed of more than 98% of silicon dioxide (SiO2), 0.08% Al2O3, 0.05% Fe2O3, and CaO, 0.5% K2O, and 0.10% TiO2, was collected from Ceresco Nutrition Ltd., Canada. The size of the ethanol-extracted silica NP ranges from 100 nm to 400 nm with an average active density of 2.03 ± 0.56 g/cm3 (3.08 × 1016 NP per gram).
Diet Formulation
Four isonitrogenous (300 g/kg crude protein) (Nguyen et al., 2020b) experimental diets containing different levels of silica NPs were formulated according to the square method of Pearson (Wagner and Stanton, 2012), with locally available fish feed ingredients (Table 1). Silica NP was incorporated into diets at levels of 0 mg/Kg (T0), 1 mg/Kg (T1), 2 mg/kg (T2), and 3 mg/Kg (T3). As an innate marker, 0.05% Cr2O3 was incorporated into diets for the further determination of the digestibility of tilapia according to Austreng (1978). The proximate compositions of the ingredients and diets were analyzed before and after the formulation of diets (Table 1), respectively, according to AOAC (2005) (for details, as shown in section Proximate Composition of Fish Muscle).
All feed ingredients were milled (in powder form) and mixed thoroughly using a mixer machine to ensure homogeneous mixing of ingredients and silica NP. Then, double distilled water was added to make the dough, and pellets were prepared through a pelletizer (0.5 mm diameter). After drying for 4 days, pellets were stored in polythene bags at −20°C until feeding tilapia.
Experimental Fish
Male O. niloticus (GIFT strain) fry, weighing 6.52 g (± 0.20 g), from the same breeding stock, were procured from Reliance Hatchery Ltd., Mymensingh, Bangladesh. There was no sign of diseases and/or abnormalities. After collection, the fry was acclimatized for 10 days in the experimental system, following the method adopted by Zhang et al. (2019). During the acclimation period, a constant oxygen supply was maintained and fish were fed with a control diet. After 10 days, fish were randomly assigned to the replication tanks, at a density of 188 per cubic meter (30 fish per 160 L tank). To understand how the age of fish affects silica NP utilization, two sampling stages were considered: the first stage (0–28 days) and the second stage (28–56 days). At the second stage, the number of fish in replications of different treatments reduced and varied between 22 and 24 because of different mortality rates and immolation for histological and hematological studies after the first stage. To ensure evenness among the treatments, the number of fish was adjusted to 21 (132 per cubic meter) in each tank.
Feeding Trials and Data Collection
After final stocking, all groups of fish were fed with their respective diet, at a level close to the apparent satiation, as defined by Simon et al. (2019) twice daily (09:00 and 15:00). In each sampling trip (14 days), at least 50% of fish from each replication tank was sampled randomly and weighed individually with an analytical balance (Model: AS 310.X2 Plus). Fish were fasted for 6 h before and after sampling. Mortality was observed daily, and dead fish were removed to calculate the survival rate.
Growth and Feed Utilization Parameters
Growth indices were calculated according to the following formulae (Aanyu et al., 2018, 2020; Hassaan et al., 2020a):
1. Weight gain (g) = final weight (g)−initial weight(g)
2. Percentweightgain (%) = (weightgain (g))/(initial weight (g)) × 100
3. Specific growth rate (% per day) = (Ln (final weight)−Ln (initial weight))/(study period (day)) × 100
4. Daily growth coefficient (% per day) = (final weight (g)ˆ.33−initial weight(g)ˆ.33)/(study period (day)) × 100
5. Condition factor = (final weight (g))/(final length (cm)ˆ3)
6. Survival rate (%) = (final number)/(initial number) × 100
Feed utilization parameters were calculated from the following formulae (Aanyu et al., 2018, 2020):
1. Food conversion ratio = (dry feed fed (kg))/(live weightgain (kg))
2. Protein efficiency ratio = (total weight gain (g))/(proteinintake (g))
3. Dry matter digestibility (% DMD) = 100−100 ((% Cr2O3in diet)/(% Cr2O3 in feces))
4. Protein digestibility (% APD) = 100−100 (% Cr2O3 inDiet)/(% Cr2O3 in feces) × (% CP in feces)/(% CP in diet))
For the estimation of %APD and %DMD, five fish from each replication tank were sacrificed after 28 days (first stage) and 56 days (second stage) of feeding. In both stages, to avoid nutrient and marker leaching into the water, the fecal content was collected from the marginal gut, close to the anal region, following the method of Austreng (1978). Feces were extracted from each of the five fish sacrificed, weighed, and dried individually (thereby 15 replications for each treatment) in a hot-air oven (106°C). Fifty milligrams of each dried sample was digested with 5 ml of concentrated nitric acid (20 min) and then 3 ml of perchloric acid (until they turned reddish) in a micro-Kjeldahl flask, keeping in an electrothermal heater at 170°C. After cooling, double distilled water was added to make the volume to be exactly 100 ml and transferred to a spectrophotometer cuvette. The Cr2O3 contents in diet and feces were measured by the optical density at 440 nm (Fenton and Fenton, 1979) using a spectrophotometer (T60UV, PG Instrument, UK) according to the following formula:
where Y = optical density
Cr2O3 % was calculated by the following formula:
The crude protein contents of the feed and feces were estimated according to AOAC (2005) (as shown in section Proximate Composition of Fish Muscle for details).
Blood Physiology
In both stages of the experiment, five fish (later used for the digestibility study) from each replication were anesthetized randomly with MS-222 (15 μg/L) after sampling, and blood samples were collected from the caudal vein to determine the level of hemoglobin (Hb), blood glucose (BG), red blood cells (RBCs), and white blood cells (WBCs). Immediately after blood collection, the Hb level (g/dl) and BG level (mg/dl) were determined by a digital EasyMate® GHB meter (Model ET 232, Bioptic Technology Inc., Taiwan) using Hb and glucose strips, respectively. WBCs and RBCs were counted using a Neubauer hemocytometer (Blaxhall and Daisley, 1973) placed under a light microscope (Olympus IX71) fitted with a Zeiss camera (Axiocam ERc 5s).
Histology
At the end of the feeding trial, five fish from each replication were necropsied to pick out organs of interest: e.g., liver, kidney, and intestine in the Fish Disease Laboratory, Department of Aquaculture, BAU. Intestines were defined and divided into foregut, midgut, and hindgut, according to Giorgini et al. (2018) and only the hindgut was used for the histomorphological study. The organs were fixed in a 10% buffered formalin, and an automatic tissue processor was used for the histological process (Naiel et al., 2020). Gut (5 μm), liver (4 μm), and kidney (4 μm) sections were cut with a microtome machine. Histological slides stained with hematoxylin (CI 75290) and eosin (Y, CI 45380; 0.1% alcoholic solution) and mounted with Canada balsam (C-1795; Sigma-Aldrich) were observed under a light microscope (Olympus IX71) at X100 magnification. The images of the histological slides were prepared with a fixed Zeiss camera and were analyzed with Image J (version 14.0) software.
Proximate Composition of Fish Muscle
At the end of the feeding trial, six fish from each replication were sacrificed to determine the proximate composition of the muscle. Moisture, ash, crude protein, and crude lipid contents were determined according to AOAC (2005). The total nitrogen content was determined using the micro-Kjeldahl analysis (method 945.01) and multiplied by the conversion factor (6.25) to translate it into the total crude protein content. Crude fat, ash, and moisture content were estimated by Soxhlet extraction (method 920.39C), by calcination in a muffle furnace at 550°C for 5 h (method 942.05), and by drying in a hot-air oven at 105°C (method 950.01), respectively. The crude fiber content (only for feed samples) was estimated with Fiber Tech (Tulin equipment, India) following the calcination in a muffle furnace.
Silica Bioaccumulation in Fish Muscle
To quantify the amount of silica persisting in fish muscle, at the end of the feeding trial, six fish from each replication were analyzed in the Bangladesh Council of Scientific and Industrial Research (BCSIR) laboratory by inductively coupled plasma-optical emission spectrophotometry (ICP-OES) determination method, as defined by Hauptkorn et al. (2001). Samples were digested with 25% tetramethylammonium hydroxide and distilled water for 30 min at 120°C under an 800-W microwave power. ICP-OES measurements of silicon contents were conducted at a 251.66-nm wavelength. Silica in muscle was determined by multiplying the silicon content with a conversion factor of 2.142 [as silica contains 46.69% (Merry, 2017)]. The bioaccumulation factor of silica, from the feed to muscle, was calculated according to the following formula [adapted from Gobas (2001)]:
Statistical Analysis
The data were analyzed using SPSS (Version 23.0, IBM, Armonk, NY) and presented as mean ± SD. A one-way ANOVA was used to determine the significance of different levels of silica NPs on the measured responses. To specify the differences among the treatments, a multiple range test of Tukey, at a 5% significance level, was performed when a significant influence of silica NP was observed. A p < 0.05 was considered to be statistically significant.
Results
Growth Performance and Feed Efficiency
The growth performance of fish fed with experimental and control diets is presented in Table 2. The results show that silica had a significant effect (P < 0.05) on all growth parameters measured, except percent weight gain (PWG) in the second stage and CF in the first stage. T2 had the most significant effect (p < 0.001) on weight gain (WG), length gain (LG), and daily growth coefficient (DGC) in both stages, while T2 had the most significant effect on PWG and specific growth rate (SGR) in only the first stage. The influence of the silica NP supplementation at 3 mg/kg on WG, SGR, PWG, and DGC was highly significant (P < 0.001) for the first 28 days, but no significant influence was found after 56 days. The survival rate throughout the feeding trial, in all the treatments, was above 90% with no significant differences between treatments (P > 0.05).
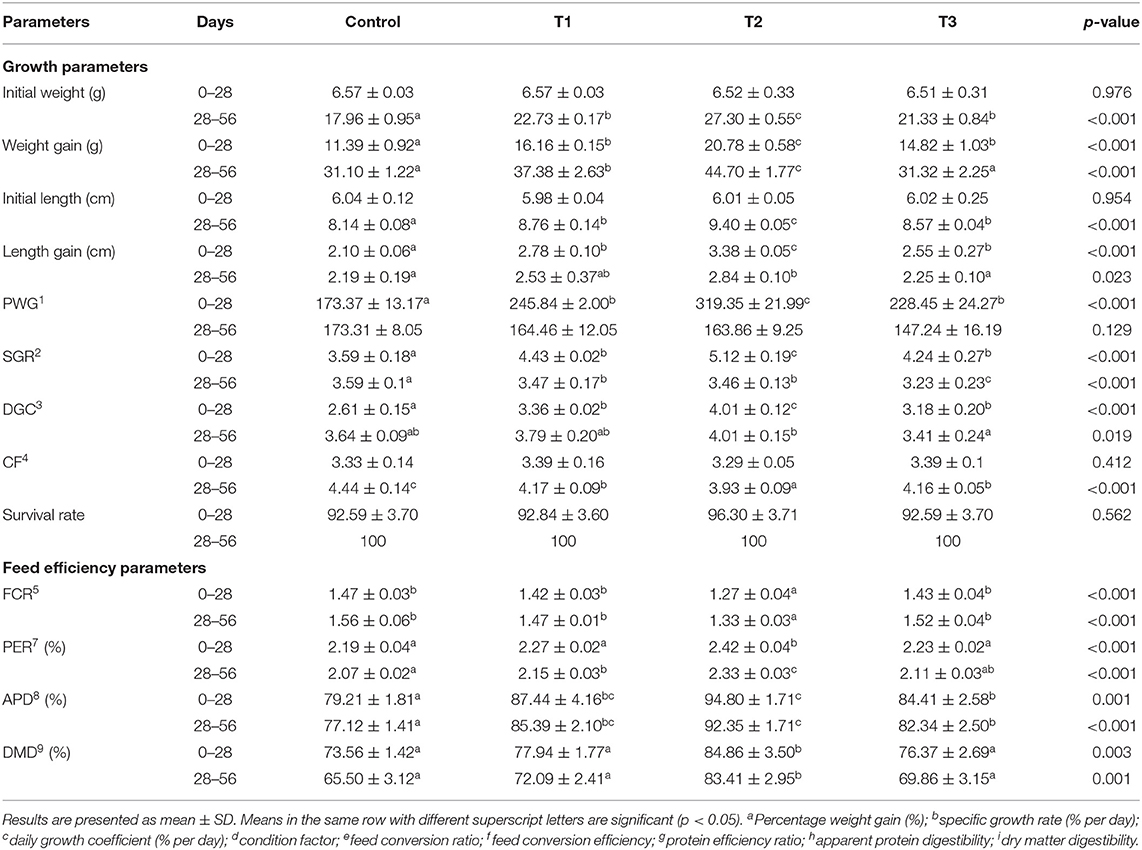
Table 2. The effects of silica nanoparticle (NP) on growth performance and feed efficiency of experimental tilapia, Oreochromis niloticus, after 28 and 56 days of feeding trial.
The lowest FCR was found in fish from T2 (P < 0.001) in both stages (Table 2). PER in T2 was the highest in both stages (P < 0.001), while in T3, a significant effect on PER was observed only in the second stage. Though the effects of silica NP on DMD on both sampling days were not significant, except T2 (P = 0.003, 0.001), a significant difference was found between all the treatments for APD (P < 0.001), with T2 causing the most significant increase in both stages.
Blood Physiology
Silica NP, at all treatment levels, had no significant effect on Hb, RBC, and WBC on either sampling date. Silica NP supplementation at 3 mg/kg (T3) showed a significant effect on the BG level of tilapia in both sampling stages (P = 0.001 and 0.006, respectively) (Table 3). The BG level was found to increase with the increasing dietary concentration of silica NP.
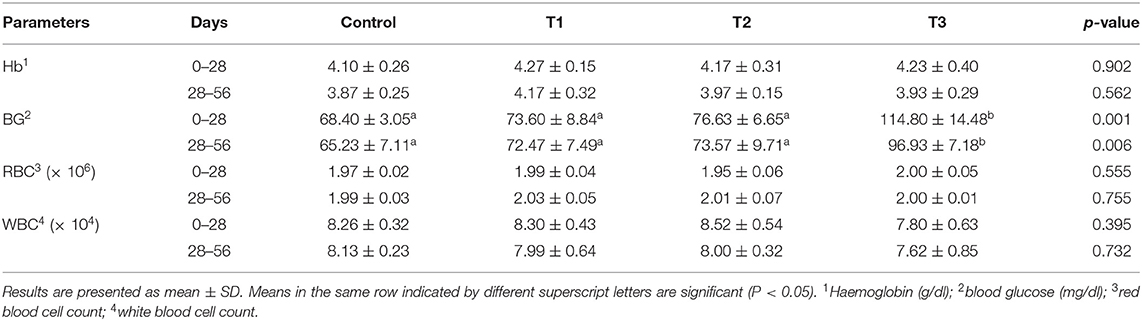
Table 3. Hematological parameters of tilapia, O. niloticus, fed with control and experimental diets after 28 or 56 days of feeding trial.
Histomorphology of Midgut, Liver, and Kidney
Data generated from the histological observations of the hindgut revealed that the villi width and villi surface area increased significantly (P < 0.001) by silica NP at 2 mg/kg (Table 4). Dietary silica NP had no significant effect on villi length (P > 0.05); however, the longest villi were observed in the tilapia from control. Silica NP at 2 mg/kg significantly increased the number of goblet cells (P = 0.007), ~2-folds compared to the control (Table 4).

Table 4. Histomorphological data of hindgut collected from tilapia, O. niloticus, after 56 days of feeding trial with silica NPs.
The normal kidney structure was observed in tilapia fed with silica NP at 0 mg/kg (control) and 1 mg/kg (T1), while slightly widened and extremely widened glomeruli were found in tilapia fed with silica NP at 2 mg/kg (T2) and 3 mg/kg (T3), respectively (Figures 1A–D). No structural aberration was found in liver histology in all treatments. However, with the increase of dietary silica NP, a substantial amount of fat deposition was observed in liver sections (Figures 1F–H), with the highest amount found in T3.
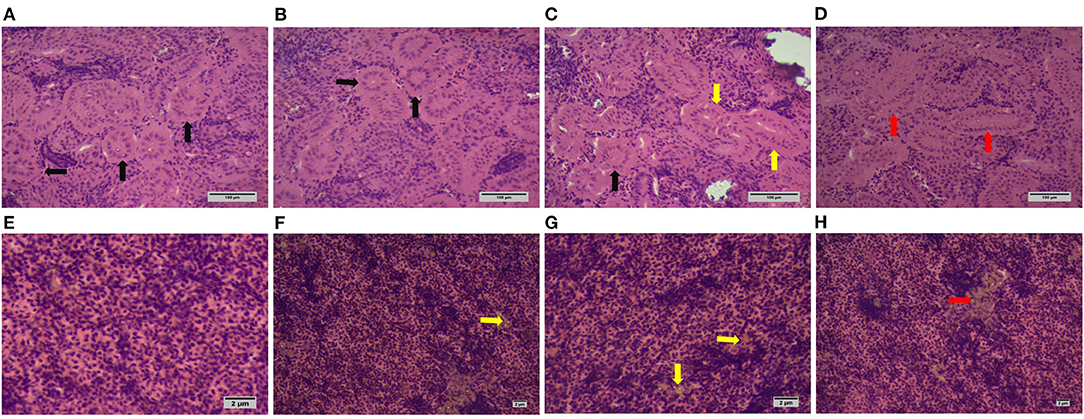
Figure 1. Histoarchitecture of kidney and liver of experimental Oreochromis niloticus (H & E; X100 magnification; scale bar: 100 μm for kidney and 1 μm for liver samples). The normal glomerular structure is indicated by black arrows observed in control (A) and T1 (B); both normal (black arrow) and slight widened (yellow arrow) structures are found in T2 (C); extremely widened glomerulus is indicated by red arrows observed in T3 (D). (E) indicates normal liver histology from control fish with no fat deposition; liver sections from T1 (F) and T2 (G) showed a slight fat deposition (yellow arrows), while T3 (H) experienced a higher deposition (red arrow).
Proximate Composition of Tilapia Muscle and Silica NP Bioaccumulation
Moisture, ash, and crude protein contents of experimental tilapia at the final harvest showed no significant differences between the dietary groups (Table 5). However, the crude lipid content increased with increasing levels of silica NP supplementation. The effect of silica NP on the crude lipid content was significant, irrespective of the incorporation rate (P < 0.05), and the highest content was observed in T3 (Table 5). The highest silica levels and bioaccumulation of silica NP were found in T3, where the experimental diet was supplemented with 3 mg/kg silica NP.
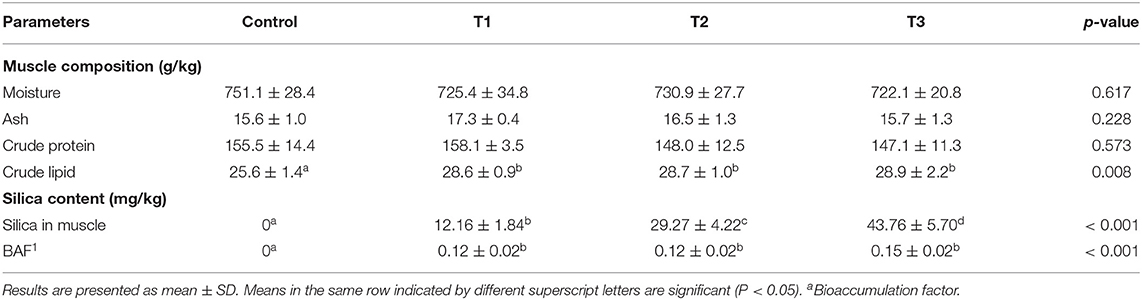
Table 5. Final muscle composition of experimental tilapia in the recirculatory aquaculture system (RAS) and bioaccumulation of silica NPs.
Discussion
To the best of the knowledge, this is the first study to investigate the effects of silica NP on feed utilization, growth performance, blood physiology, and digestive morphology of O. niloticus. The notable outcomes have been achieved in terms of growth performance and feed utilization without compromising human health safety.
Control fish reared with a low proteinous basal diet at a higher stocking density (almost 6-folds than the traditional pond farming system practiced in Bangladesh) showed better growth performance compared to other culture systems like pond-based intensive and semi-intensive cultures (Kabir et al., 2019; Dawood et al., 2020f), raceway culture, tank and aquaria cultures (El-Naby et al., 2019; Abd El-Naby et al., 2020), and even biofloc culture (da Silva et al., 2018; Martins et al., 2019). Controlled environmental conditions and nitrogenous waste maintenance in RAS might ensure optimum welfare for fish and ensure larger spillover advantages of surpassed yield. The growth performances favored from silica NP experienced in this study are far superior to the available findings in the growing literature (Aanyu et al., 2020; Amer et al., 2020; Dawood et al., 2020a,f; Jiang et al., 2020; Wardani et al., 2020) on tilapia, irrespective of culture systems and diets. The supplementation of NPs like nanochitosan (Abd El-Naby et al., 2019, 2020), nanoselenium (Dawood et al., 2020f; Rathore et al., 2020), nanoselenium with Vit-C (Dawood et al., 2020e), and nanozeolite (Hassaan et al., 2020b) in tilapia have resulted in poorer growth performances than the findings presented in this study. The improved growth performance as seen in this study occurred because silica in nanoform may function as the nutrient carrier (especially for amino acids). These hauled nutrients could have further contributed to increasing the digestion and absorption of nutrient molecules through the controlled encapsulation and release of nutrients from the gastrointestinal tract to the bloodstream (Bahabadi et al., 2017). This increase could also be correlated with the amelioration of DNA and RNA syntheses and an improvement in gut microorganisms, which are supported by NPs (Onuegbu et al., 2018); however, this warrants a further investigation with silica NPs. The survival rate corresponds to those shown in tilapia fed with chitosan NP (Abd El-Naby et al., 2019) and nanoselenium (Dawood et al., 2020a) for the first 28 days. In the later stage, no mortality in any of the treatments, including the control, is in agreement with Abd El-Naby et al. (2019) and Rathore et al. (2020).
The FCR found in the present study is far lower than the results obtained from feeding tilapia with nanoselenium and vitamin E (Dawood et al., 2020e), nanoselenium (Abd El-Kader et al., 2020; Rathore et al., 2020), and nanozeolite (Hassaan et al., 2020b). Similar results were obtained in tilapia fed with chitosan NP supplemented with vitamin C (Naiel et al., 2020) and with only chitosan NP (Abd El-Naby et al., 2019). In the case of T1 and T3, although the growth parameters are significantly different, the FCR was not significant compared to the control. This indicates a high level of feed intake by fish in the T1 and T3 treatments but poorer growth outcomes following the ingestion. The highest PER in this study is similar to those seen in chitosan NP-treated tilapia (Abd El-Naby et al., 2019, 2020); however, it is greater than the findings of PER documented by Rathore et al. (2020) from nanoselenium in tilapia. Fish fed with silica NP demonstrated improved growth performance in tilapia, compared to the control of the current experiment and to other previously mentioned studies. This supports the recommendation of silica NP as one of the best feed additives for O. niloticus.
Digestibility data also demonstrate the effect of silica NP on improving growth performance. Digestive enzymes hydrolyze proteins, carbohydrates, and lipids into smaller parts for absorption through the microvilli of the fish intestine (García-Meilán et al., 2016). Tilapia, an omnivorous fish, comparatively possesses an inactive stomach for protein digestion. Digestion of protein eminently takes place in the gut just after the stomach, with the action of hydrochloric acid, and due to size limitations, only smaller peptides and amino acids are permitted to access through the gut wall to be absorbed and become available for growth (Wu et al., 2009). The water inside the gut remains inactive and, in general, has no direct role, except facilitating gut microbiota and maintaining homeostasis (Laforenza, 2012; Giatsis et al., 2015); however, in the presence of silica NP, water molecules within the gut may become activated (Figure 2A) with the influence of a higher infrared emissivity of silica NP (Faisal et al., 2021). A similar indication with Tourmaline (Borosilicate minerals) NP concluded that radiation emissivity from NP can modulate the structure of water clusters into smaller molecules through breaking hydrogen bonds (Sun et al., 2010). Furthermore, the magnetic treatment of silica NP may have a role in the activation of water molecules through creating magnetic resonance. These activated molecules are reported to facilitate a multitude of reactions, including protein metabolism and immune response (Sun et al., 2010). In the digestion process, ionized water molecules as nucleophiles act on the peptide carbonyl group of the ingested protein (Berg et al., 2002), resulting in smaller peptides and free amino acids, suitable for absorption through the gut epithelium into the blood (Figures 2B,C). Enzymatic hydrolysis of protein is largely influenced by the availability of water required for hydration (Butré et al., 2014). Furthermore, the presence of catalytic water is assumed to pledge the enzyme-substrate inhibition through cleaving the peptide bonds of the substrate tying to outside the active sites (Butré et al., 2014). Activated water molecules may also regenerate the enzymes, enabling them to work again within the shortest possible time, as clued by Berg et al. (2002). These evidences clearly disclose that silica NP might accelerate the digestibility of feed and, hence, the growth performance of fish, while the subsequent decrease in nutrient loss results in lower FCR (Figure 2D).
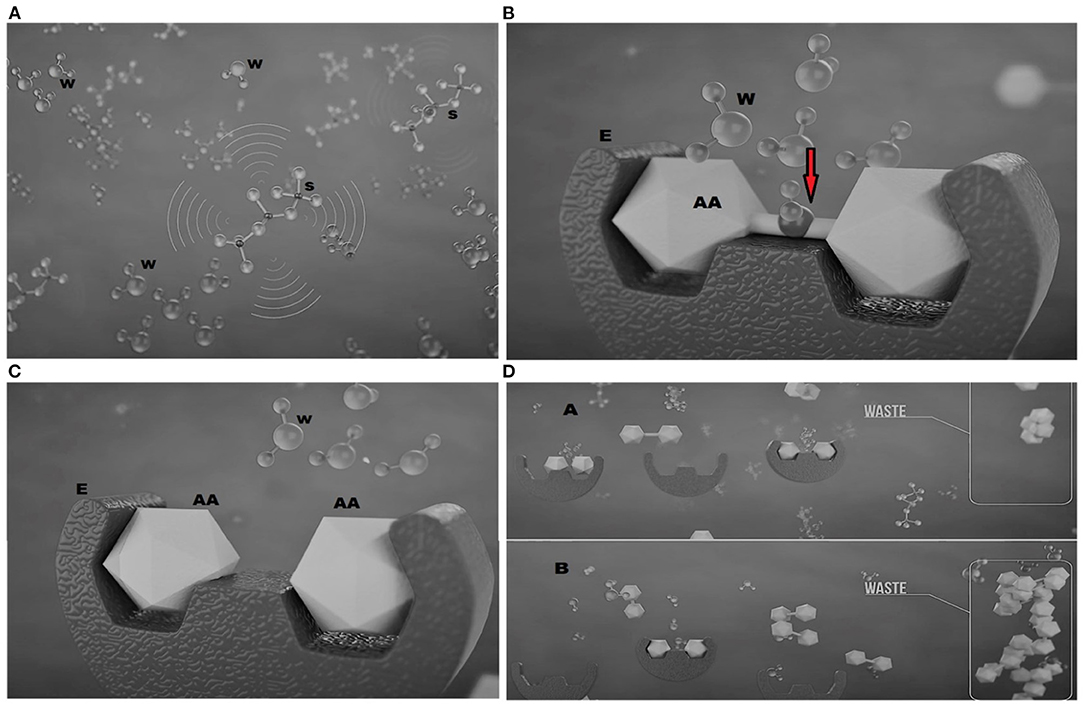
Figure 2. Mechanisms of silica nanoparticles (NPs) responsible for the increased digestibility in tilapia fish (source: Ceresco Nutrition Ltd.) showing (A) silica NP(S) activating water molecules (W) inside the gut of fish; (B) activated water molecules (W) splitting (red arrow) the amino acid (AA) when it binds to a specific enzyme (E); (C) cleaved amino acids (AAs) suitable for absorption through the gut; and (D) the reduction in waste in the presence of silica NP (A) compared to that without (B).
Blood physiology data of the experimental fish explain why the growth performance of experimental O. niloticus decreased while we incorporated silica at a level of 3 mg/Kg diet. BG is an indicator of stress syndrome, with increased levels providing a biomarker of stress levels in fish (Dawood et al., 2020b,c,d). The BG level of fish fed with 3 mg/kg silica was significantly high, indicating physiological stress due to the high level of silica and rendering that they are not able to use the nutrients properly to acquire growth. Generally, in this state of stress, fish continuously try to respond physiologically, be resistant to the stress, and/or restore homeostasis. These stress responses are energetically costly (Rodnick and Planas, 2016; Schreck and Tort, 2016): consequently, energy might be diverted to maintenance, rather than being available for growth. This explains the lower growth performance in T3, even though the digestibility of protein was still significantly greater than the control. As a defensive agent, WBCs circulate in the bloodstream searching for foreign particles and proliferating when an exogenous particle is identified. There were no significant changes in the Hb level and WBC count throughout the study, indicating that silica NP was not present in the blood and, therefore, no residual effect.
A larger surface area of intestinal villi facilitates nutrient absorption in fish, providing a greater surface area for enzymes for reactions to occur (Dawood et al., 2020a). Though silica NP did not enlarge the villi significantly, it did widen the villi, therefore significantly increasing the surface area. The enhanced surface area enabled the increased absorption of nutrients. Furthermore, the mucus-producing goblet cells have numerous roles in the digestive system, including prevention of gut wall damage and antibacterial action (Pirarat et al., 2015) and maintaining intestinal homeostasis (Junqueira and Carneiro, 2013). Increasing numbers of goblet cells with silica NP treatment may confirm the advantages of silica NP to intestinal health and the gut microbiota of fish that promotes the activities of mucus-secreting goblet cells. The kidney of fish plays an important role in RBC production, osmoregulation, and excretion of waste metabolites. The widened glomerular structure in freshwater fish indicates a high filtration rate for maintaining osmoregulation and excreting detrimental and ionic substances (Oguz, 2015). This reveals the adaptation measures of experimental tilapia against the stress when challenged with silica NP at 3 mg/kg. This adaptation might be accomplished through the excretion of silica NP via the enlarged kidney tubules, and this broadening of glomeruli was associated with the increasing level of silica NP in feed. This is also in line with the expansion of glomeruli observed by Hussain et al. (2019) in freshwater fishes coping against different environmental pollutants. Similar adaptation measures against arsenic in Channa punctata (Roy and Bhattacharya, 2006) also corroborate the reasoning for enlarged kidney tubules. However, the normal kidney structure in the control and T1 and the nearly normal and slight widening in T2 indicate that there was no such extreme pressure on the fish excretory system below 2 mg/kg dietary silica NP.
Lipids in fish play a crucial role as a source of energy and for the provision of essential fatty acids, necessary for fish growth and development (Kim et al., 2012). However, fish prefer to consume energy from protein, more specifically from the amino acids (Walton and Cowey, 1982; Wu et al., 2020), while lipids are known to be stored in the liver and muscle by fish and to spare the role of proteins (Kim et al., 2012; Zhang et al., 2019) when they are sourced inadequately or fish need to adapt physiologically through energetic cost (McCue, 2013). Silica NP undoubtedly increased digestibility and absorption of nutrients including proteins and lipids. However, due to proper feeding regimes, very little sparing might be required by lipids throughout the study period, and the higher growth performance could have resulted mainly from the absorption of proteins (amino acids). This allowed a greater portion of lipids to remain unused and deposited in the muscle.
Though crystalline silica is considered as a class-1 carcinogenic, amorphous silica in nanoform was non-carcinogenic in rats and mice up to 2,500 mg/kg and 7,500 mg/kg body weights, respectively (Younes et al., 2018). When it is available in food, drugs, and beverages, more than 50% silica in the form of silicon is filtered by the kidney in humans (Kelsay et al., 1979) and the remaining residue disperses through the skin, aorta, bone, and other parts of the body (Carlisle, 1981). Moreover, if it exists in the blood, as silicic acid, it has no adverse effect on human physiology as it does not bind to proteins (De Araújo et al., 2016). In fact, in favorable concentrations, silica plays an important biological role in bone, brain, nerve, skin, and memory health. It also benefits patients with diabetes by stimulating insulin secretion from the pancreas (Jugdaohsingh, 2007). However, the US Food and Drug Administration has defined 2% by the weight of food as the maximum limit for human consumption, when silica is used as additives (FDA, 2019). The results presented in this study show that only a low level of silica NP accumulated in fish muscle, and therefore, the BAF data show that incorporating silica NP into fish diets will not adversely impact human health upon consumption of the fish.
Conclusion
These results have verified the clear advantages of silica NP on growth performance, feed utilization, and the final product quality in the experimental fish. Bioaccumulation study strongly approved a much higher amount of silica NP to be incorporated into feed without averting human health safety; however, growth performance and hematological and histological findings apprehend the limit to 2 mg/Kg for obtaining the highest possible payback from tilapia. On the other hand, the question of maintaining proper dose during manufacturing and feeding is apposite because of the narrow effective range as suggested in this study. The industrial bulk production of feed using appropriate binders to minimize the leaching of silica NP, paradoxically, could knock down the contradiction of accommodating the required dose. Conclusively, silica NP as an input of nanotechnology can be applied as novel feed additives to improve the rate of digestion, as well as improve absorption in the production of O. niloticus without making human health safety questioned. Much progress has been made through this experiment, and hopefully, it will make a big sense to widen the gateway of future investigation. It still possesses some limitations on which basis, this publication warrants furthermore studies delving the insights from molecular and physiological prospects.
Data Availability Statement
The raw data supporting the conclusions of this article will be made available by the first author (AB), without undue reservation.
Ethics Statement
The animal study was reviewed and approved by Bangladesh Agricultural University Research System.
Author Contributions
AB: conceptualization, methodology, investigation, writing—original draft, visualization, and fund acquisition. NH: formal analysis, data curation, writing—original draft, and visualization. MHa: conceptualization, validation, writing—review, and editing. MR: methodology, investigation, and visualization. MHo: methodology, validation, resources, and supervision. All authors contributed to the article and approved the submitted version.
Funding
As part of the MSc dissertation of first author (AB), this research work was funded by Bangladesh Agricultural University (Grant No. 2020/44/BAU) and National Science and Technology (NST) Fellowship grant (Grant No. MOST/MS/572).
Conflict of Interest
The authors declare that the research was conducted in the absence of any commercial or financial relationships that could be construed as a potential conflict of interest.
Publisher's Note
All claims expressed in this article are solely those of the authors and do not necessarily represent those of their affiliated organizations, or those of the publisher, the editors and the reviewers. Any product that may be evaluated in this article, or claim that may be made by its manufacturer, is not guaranteed or endorsed by the publisher.
Acknowledgments
The authors express their gratitude to Professor Dr. Tanvir Rahman, Department of Aquaculture, BAU, for his laboratory assistance during histology and hematology. The authors are also grateful to Ceresco Nutrition Ltd. for permitting to use of their artwork in developing figures for this manuscript.
Supplementary Material
The Supplementary Material for this article can be found online at: https://www.frontiersin.org/articles/10.3389/fmars.2021.706179/full#supplementary-material
Figure 1. Kidney of control fish (T0).
Figure 2. Kidney of fish treated with 1 mg/kg SiNP (T1).
Figure 3. Kidney of fish treated with 2 mg/kg SiNP (T2).
Figure 4. Kidney of fish treated with 3 mg/kg SiNP (T4).
Figure 5. Liver of control fish (T0).
Figure 6. Liver of fish treated with 1 mg/kg SiNP (T1).
Figure 7. Liver of fish treated with 2 mg/kg SiNP (T2).
Figure 8. Liver of fish treated with 3 mg/kg SiNP (T3).
References
Aanyu, M., Betancor, M. B., and Monroig, O. (2018). Effects of dietary limonene and thymol on the growth and nutritional physiology of Nile tilapia (Oreochromis niloticus). Aquaculture 488, 217–226. doi: 10.1016/j.aquaculture.2018.01.036
Aanyu, M., Betancor, M. B., and Monroig, Ó. (2020). The effects of combined phytogenics on growth and nutritional physiology of Nile tilapia Oreochromis niloticus. Aquaculture 519:734867. doi: 10.1016/j.aquaculture.2019.734867
Abd El-Kader, M. F., Fath El-Bab, A. F., Abd-Elghany, M. F., Abdel-Warith, A.-W. A., Younis, E. M., and Dawood, M. A. O. (2020). Selenium nanoparticles act potentially on the growth performance, hemato-biochemical indices, antioxidative, and immune-related genes of European seabass (Dicentrarchus labrax). Biol. Trace Elem. Res. 199, 3126–3313. doi: 10.1007/s12011-020-02431-1
Abd El-Naby, A. S., Al-Sagheer, A. A., Negm, S. S., and Naiel, M. A. E. (2020). Dietary combination of chitosan nanoparticle and thymol affects feed utilization, digestive enzymes, antioxidant status, and intestinal morphology of Oreochromis niloticus. Aquaculture 515:734577. doi: 10.1016/j.aquaculture.2019.734577
Abd El-Naby, A. S., Khattaby, A. E. R. A., Samir, F., Awad, S. M. M., and Abdel-Tawwab, M. (2019). Stimulatory effect of dietary butyrate on growth, immune response, and resistance of Nile tilapia, Oreochromis niloticus against Aeromonas hydrophila infection. Anim. Feed Sci. Technol. 254:114212. doi: 10.1016/j.anifeedsci.2019.114212
Abdel-Tawwab, M., Adeshina, I., and Issa, Z. A. (2020). Antioxidants and immune responses, resistance to Aspergilus flavus infection, and growth performance of Nile tilapia, Oreochromis niloticus, fed diets supplemented with yeast, Saccharomyces serevisiae. Anim. Feed Sci. Technol. 263:114484. doi: 10.1016/j.anifeedsci.2020.114484
Ahmed, G. U., Upala, S. R., Hasan, M. T., and Hasan, N. (2014). Comparative study on growth performance between Vietnam koi and Thai koi in mini ponds. J. Bangladesh Agric. Univ. 12, 405–409. doi: 10.22004/ag.econ.211262
Ahmed, N. (2007). “Economics of aquaculture feeding practices: Bangladesh,” in Economics of Aquaculture Feeding Practices in Selected Asian Countries, ed M. R. Hasan (Rome: FAO Fisheries Technical Paper. No. 505).
Al-Deriny, S. H., Dawood, M. A. O., Elbialy, Z. I., El-Tras, W. F., and Mohamed, R. A. (2020). Selenium nanoparticles and spirulina alleviate growth performance, hemato-biochemical, immune-related genes, and heat shock protein in Nile Tilapia (Oreochromis niloticus). Biol. Trace Elem. Res. 198, 661–668. doi: 10.1007/s12011-020-02096-w
Amer, S. A., Ahmed, S. A. A., Ibrahim, R. E., Al-Gabri, N. A., Osman, A., and Sitohy, M. (2020). Impact of partial substitution of fish meal by methylated soy protein isolates on the nutritional, immunological, and health aspects of Nile tilapia, Oreochromis niloticus fingerlings. Aquaculture 518:734871. doi: 10.1016/j.aquaculture.2019.734871
AOAC (2005). Official Methods of Analysis. eds W. Horwitz and G. W. Latimer (Maryland: Association of Official Analytical Chemists (AOAC) International).
Austreng, E. (1978). Digestibility determination in fish using chromic oxide marking and analysis of contents from different segments of the gastrointestinal tract. Aquaculture 13, 265–272. doi: 10.1016/0044-8486(78)90008-X
Bahabadi, M. N., Delavar, F. H., Mirbakhsh, M., Niknam, K., and Johari, S. A. (2017). Assessment of antibacterial activity of two different sizes of colloidal silver nanoparticle (cAgNPs) against Vibrio harveyi isolated from shrimp Litopenaeus vannamei. Aquac. Int. 25, 463–472. doi: 10.1007/s10499-016-0043-8
Barman, B. K., and Little, D. C. (2011). Use of hapas to produce Nile tilapia (Oreochromis niloticus L.) seed in household foodfish ponds: A participatory trial with small-scale farming households in Northwest Bangladesh. Aquaculture 317, 214–222. doi: 10.1016/j.aquaculture.2011.04.005
Bashar, A., Heal, R. D., Hasan, N. A., and Haque, M. M. (2021). Effect of COVID-19 on shrimp aquaculture in Bangladesh. SSRN Electron. J. 31:7896. doi: 10.2139/ssrn.3867896
Bashar, A., Islam, M. R., Zarin, I., Rohani, M. F., Haque, M. M., and Hossain, M. S. (2019). “Nutritional Intervention of Oreochromis niloticus the application of Silica nano-particles in Recirculatory Aquaculture System (RAS),” in Abstract Book (Biennial International Conference-2019) (Mymensingh: Fisheries Society of Bangladesh).
Belton, B., and Thilsted, S. H. (2014). Fisheries in transition: Food and nutrition security implications for the global South. Glob. Food Sec. 3, 59–66. doi: 10.1016/j.gfs.2013.10.001
Berg, J. M., Tymoczko, J. L., and Stryer, L. (2002). Biochemistry. 5th edition. Proteases: Facilitating a Difficult Reaction. New York, NY: W H Freeman.
Bitar, A., Ahmad, N. M., Fessi, H., and Elaissari, A. (2012). Silica-based nanoparticles for biomedical applications. Drug Discov. Today 17, 1147–1154. doi: 10.1016/j.drudis.2012.06.014
Blaxhall, P. C., and Daisley, K. W. (1973). Routine haematological methods for use with fish blood. J. Fish Biol. 5, 771–781. doi: 10.1111/j.1095-8649.1973.tb04510.x
Butré, C. I., Wierenga, P. A., and Gruppen, H. (2014). Influence of water availability on the enzymatic hydrolysis of proteins. Process Biochem. 49, 1903–1912. doi: 10.1016/j.procbio.2014.08.009
Carlberg, H., Nilsson, J., Brännäs, E., and Alanär,ä, A. (2018). An evaluation of 30 years of selective breeding in the Arctic charr (Salvelinus alpinus L.) and its implications for feeding management. Aquaculture 495, 428–434. doi: 10.1016/j.aquaculture.2018.06.008
Carlisle, E. M. (1981). Silicon: A requirement in bone formation independent of vitamin D1. Calcif. Tissue Int. 33, 27–34. doi: 10.1007/BF02409409
Chan, C. Y., Tran, N., Pethiyagoda, S., Crissman, C. C., Sulser, T. B., and Phillips, M. J. (2019). Prospects and challenges of fish for food security in Africa. Glob. Food Sec. 20, 17–25. doi: 10.1016/j.gfs.2018.12.002
Chaput, D. L., Bass, D., Alam, M. M., Hasan, N., Al Stentiford, G. D., van Aerle, R., et al. (2020). The Segment Matters: Probable Reassortment of Tilapia Lake Virus (TiLV) Complicates Phylogenetic Analysis and Inference of Geographical Origin of New Isolate from Bangladesh. Viruses 12:258. doi: 10.3390/v12030258
Crab, R., Avnimelech, Y., Defoirdt, T., Bossier, P., and Verstraete, W. (2007). Nitrogen removal techniques in aquaculture for a sustainable production. Aquaculture 270, 1–14. doi: 10.1016/j.aquaculture.2007.05.006
da Silva, M. A., de Alvarenga, É. R., Alves, G. F., Manduca, L. G., and Turra, E. M. (2018). Crude protein levels in diets for two growth stages of Nile tilapia (Oreochromis niloticus) in a biofloc system. Aquac. Res. 49, 2693–2703. doi: 10.1111/are.13730
Dawood, M. A. O., Eweedah, N. M., Khalafalla, M. M., and Khalid, A. (2020a). Evaluation of fermented date palm seed meal with Aspergillus oryzae on the growth, digestion capacity and immune response of Nile tilapia (Oreochromis niloticus). Aquac. Nutr. 26, 828–841. doi: 10.1111/anu.13042
Dawood, M. A. O., Magouz, F. I., Mansour, M., Saleh, A. A., Asely, A. M., El Fadl, S. E., et al. (2020b). Evaluation of yeast fermented poultry by-product meal in Nile Tilapia (Oreochromis niloticus) feed: effects on growth performance, digestive enzymes activity, innate immunity, and antioxidant capacity. Front. Vet. Sci. 6:516. doi: 10.3389/fvets.2019.00516
Dawood, M. A. O., Metwally, A. E. S., El-Sharawy, M. E., Ghozlan, A. M., Abdel-Latif, H. M. R., Van Doan, H., et al. (2020c). The influences of ferulic acid on the growth performance, haemato-immunological responses, and immune-related genes of Nile tilapia (Oreochromis niloticus) exposed to heat stress. Aquaculture 525:735320. doi: 10.1016/j.aquaculture.2020.735320
Dawood, M. A. O., Moustafa, E. M., Gewaily, M. S., Abdo, S. E., AbdEl-kader, M. F., SaadAllah, M. S., et al. (2020d). Ameliorative effects of Lactobacillus plantarum L-137 on Nile tilapia (Oreochromis niloticus) exposed to deltamethrin toxicity in rearing water. Aquat. Toxicol. 219:105377. doi: 10.1016/j.aquatox.2019.105377
Dawood, M. A. O., Zommara, M., Eweedah, N. M., and Helal, A. I. (2020e). Synergistic effects of selenium nanoparticles and vitamin e on growth, immune-related gene expression, and regulation of antioxidant status of Nile Tilapia (Oreochromis niloticus). Biol. Trace Elem. Res. 195, 624–635. doi: 10.1007/s12011-019-01857-6
Dawood, M. A. O., Zommara, M., Eweedah, N. M., and Helal, A. I. (2020f). The evaluation of growth performance, blood health, oxidative status and immune-related gene expression in Nile tilapia (Oreochromis niloticus) fed dietary nanoselenium spheres produced by lactic acid bacteria. Aquaculture 515:734571. doi: 10.1016/j.aquaculture.2019.734571
De Araújo, L. A., Addor, F., and Campos, P. M. B. G. M. (2016). Use of silicon for skin and hair care: An approach of chemical forms available and efficacy. An. Bras. Dermatol. 91, 331–335. doi: 10.1590/abd1806-4841.20163986
de Verdal, H., Vandeputte, M., Mekkawy, W., Chatain, B., and Benzie, J. A. H. (2018). Quantifying the genetic parameters of feed efficiency in juvenile Nile tilapia Oreochromis niloticus. BMC Genet. 19:105. doi: 10.1186/s12863-018-0691-y
El-Naby, F. S. A., Naiel, M. A. E., Al-Sagheer, A. A., and Negm, S. S. (2019). Dietary chitosan nanoparticles enhance the growth, production performance, and immunity in Oreochromis niloticus. Aquaculture 501, 82–89. doi: 10.1016/j.aquaculture.2018.11.014
Faisal, A. M., Salaün, F., Giraud, S., Ferri, A., Chen, Y., and Wang, L. (2021). Far-infrared emission properties and thermogravimetric analysis of ceramic-embedded polyurethane films. Polymers. 13, 1–9. doi: 10.3390/polym13050686
FAO (2016). The State of World Fisheries and Aquaculture 2016 (SOFIA): Contributing to Food Security and Nutrition for All. Rome: Food and Agriculture Organization
FDA (2019). CFR - Code of Federal Regulations Title 21. Department of Health and Human Services, USFDA, Maryland, USA. Available online at: https://bit.ly/30JvCRh (sccessed August 10, 2020).
Fenton, T. W., and Fenton, M. (1979). An improved procedure for the determination of chromic oxide in feed and feces. Can. J. Anim. Sci. 59, 631-−634. doi: 10.4141/cjas79-081
Foysal, M. J., Alam, M., Kawser, A. Q. M. R., Hasan, F., Rahman, M. M., Tay, C. Y., et al. (2020). Meta-omics technologies reveals beneficiary effects of Lactobacillus plantarum as dietary supplements on gut microbiota, immune response and disease resistance of Nile tilapia (Oreochromis niloticus). Aquaculture 520:734974. doi: 10.1016/j.aquaculture.2020.734974
Francis, N. O., and Esa, Y. (2016). A review of production protocols used in producing economically viable monosex tilapia. J. Fish. Aquat. Sci. 11, 1–11. doi: 10.3923/jfas.2016.1.11
García-Meilán, I., Ordóñez-Grande, B., Machahua, C., Buenestado, S., Fontanillas, R., and Gallardo, M. A. (2016). Effects of dietary protein-to-lipid ratio on digestive and absorptive processes in sea bass fingerlings. Aquaculture 463, 163–173. doi: 10.1016/j.aquaculture.2016.05.039
Giatsis, C., Sipkema, D., Smidt, H., Heilig, H., Benvenuti, G., Verreth, J., et al. (2015). The impact of rearing environment on the development of gut microbiota in tilapia larvae. Sci. Rep. 5, 1–15. doi: 10.1038/srep18206
Giorgini, E., Randazzo, B., Gioacchini, G., Cardinaletti, G., Vaccari, L., Tibaldi, E., et al. (2018). New insights on the macromolecular building of rainbow trout (O. mykiss) intestine: FTIR Imaging and histological correlative study. Aquaculture 497, 1–9. doi: 10.1016/j.aquaculture.2018.07.032
Gobas, F. A. P. C. (2001). “Assessing bioaccumulation factors of persistent organic pollutants in aquatic food-chains,” in Persistent Organic Pollutants, ed S. Harrad (Boston, MA: Springer). doi: 10.1007/978-1-4615-1571-5_6
Gui, J. F., Tang, Q., Li, Z., Liu, J., and De Silva, S. S. (2018). Aquaculture in China: Success Stories and Modern Trends. Chichester: John Wiley & Sons Ltd.). doi: 10.1002/9781119120759
Guo, J., Huang, Y., Salze, G., Roy, L. A., and Davis, D. A. (2020). Use of plant-based protein concentrates as replacement for fishmeal in practical diets for the Pacific white shrimp (Litopenaeus vannamei) reared under high stocking density and low salinity conditions. Aquac. Nutr. 26, 225–232. doi: 10.1111/anu.12982
Haque, M. M., Alam, M. M., Hoque, M. S., Hasan, N. A., Nielsen, M., Hossain, M. I., et al. (2021a). Can Bangladeshi pangasius farmers comply with the requirements of aquaculture certification? Aquac. Rep. 21:100811. doi: 10.1016/j.aqrep.2021.100811
Haque, M. M., Hasan, N. A., Eltholth, M. M., Saha, P., Mely, S. S., Rahman, T., et al. (2021b). Assessing the impacts of in-feed probiotic on the growth performance and health condition of pangasius (Pangasianodon hypophthalmus) in a farm trial. Aquac. Rep. 20:100699. doi: 10.1016/j.aqrep.2021.100699
Hasan, N. A., and Haque, M. M. (2020). Dataset of white spot disease affected shrimp farmers disaggregated by the variables of farm site, environment, disease history, operational practices, and saline zones. Data Br. 31:105936. doi: 10.1016/j.dib.2020.105936
Hasan, N. A., Haque, M. M., Bashar, A., Hasan, M. T., Faruk, M. A. R., and Ahmed, G. U. (2021a). Effects of dietary Papaveraceae extract on growth, feeding response, nutritional quality and serum biochemical indices of striped catfish (Pangasianodon hypophthalmus). Aquac. Rep. 21:100793. doi: 10.1016/J.AQREP.2021.100793
Hasan, N. A., Haque, M. M., Hinchliffe, S. J., and Guilder, J. (2020). A sequential assessment of WSD risk factors of shrimp farming in Bangladesh: looking for a sustainable farming system. Aquaculture 526:735348. doi: 10.1016/j.aquaculture.2020.735348
Hasan, N. A., Heal, R. D., Bashar, A., Bablee, A. L., and Haque, M. M. (2021b). Impacts of COVID-19 on the finfish aquaculture industry of Bangladesh: A case study. Mar. Policy 130:104577. doi: 10.1016/j.marpol.2021.104577
Hasan, N. A., Heal, R. D., Bashar, A., and Haque, M. M. (2021c). Face masks: protecting the wearer but neglecting the aquatic environment? - A perspective from Bangladesh. Environ. Challenges 4:100126. doi: 10.1016/j.envc.2021.100126
Hassaan, M. S., Mohammady, E. Y., Soaudy, M. R., Palma, J., Shawer, E. E., and El-Haroun, E. (2020a). The effect of dietary sericite on growth performance, digestive enzymes activity, gut microbiota and haematological parameters of Nile tilapia, Oreochromis niloticus (L.) fingerlings. Anim. Feed Sci. Technol. 262:114400. doi: 10.1016/j.anifeedsci.2020.114400
Hassaan, M. S., Nssar, K. M., Mohammady, E. Y., Amin, A., Tayel, S. I., and El-Haroun, E. R. (2020b). Nano-zeolite efficiency to mitigate the aflatoxin B1 (AFB1) toxicity: Effects on growth, digestive enzymes, antioxidant, DNA damage and bioaccumulation of AFB1 residues in Nile tilapia (Oreochromis niloticus). Aquaculture 523:735123. doi: 10.1016/j.aquaculture.2020.735123
Hauptkorn, S., Pavel, J., and Seltner, H. (2001). Determination of silicon in biological samples by ICP-OES after non-oxidative decomposition under alkaline conditions. Anal. Bioanal. Chem. 370, 246–250. doi: 10.1007/s002160100759
Heal, R. D., Hasan, N. A., and Haque, M. M. (2021). Increasing disease burden and use of drugs and chemicals in Bangladesh shrimp aquaculture: a potential menace to human health. Mar. Pollut. Bull. 171:112796. doi: 10.1016/j.marpolbul.2021.112796
Huang, S., Wang, L., Liu, L., Hou, Y., and Li, L. (2015). Nanotechnology in agriculture, livestock, and aquaculture in China. A review. Agron. Sustain. Dev. 35, 369–400. doi: 10.1007/s13593-014-0274-x
Hussain, B., Fatima, M., Al-Ghanim, K. A., and Mahboob, S. (2019). Environmentally induced nephrotoxicity and histopathological alternations in Wallago attu and Cirrhinus mrigla. Saudi J. Biol. Sci. 26, 752–757. doi: 10.1016/j.sjbs.2019.02.003
Irm, M., Taj, S., Jin, M., Luo, J., Andriamialinirina, H. J. T., and Zhou, Q. (2020). Effects of replacement of fish meal by poultry by-product meal on growth performance and gene expression involved in protein metabolism for juvenile black sea bream (Acanthoparus schlegelii). Aquaculture 528:735544. doi: 10.1016/j.aquaculture.2020.735544
Jarvie, H. P., Al-Obaidi, H., King, S. M., Bowes, M. J., Lawrence, M. J., Drake, A. F., et al. (2009). Fate of silica nanoparticles in simulated primary wastewater treatment. Environ. Sci. Technol. 43, 8622–8628. doi: 10.1021/es901399q
Jennings, S., Stentiford, G. D., Leocadio, A. M., Jeffery, K. R., Metcalfe, J. D., Katsiadaki, I., et al. (2016). Aquatic food security: insights into challenges and solutions from an analysis of interactions between fisheries, aquaculture, food safety, human health, fish and human welfare, economy and environment. Fish Fish. 17, 893–938. doi: 10.1111/faf.12152
Jiang, M., Ma, L., Shao, H., Wu, F., Liu, W., Tian, J., et al. (2020). Dietary vitamin E requirement of sub-adult genetically improved farmed tilapia strain of Nile tilapia (Oreochromis niloticus) reared in freshwater. Aquac. Nutr. 26, 233–241. doi: 10.1111/anu.12983
Jugdaohsingh, R. (2007). Silicon and Bone Health in Journal of Nutrition, Health and Aging (Europe PMC Funders). Available online at: /pmc/articles/PMC2658806/?report=abstract (accessed August 10, 2020).
Junqueira, L. C., and Carneiro, J. (2013). Histologia Básica. 12th ed. Rio de Janeiro: Guanabara Koogan.
Kabir, K. A., Verdegem, M. C. J., Verreth, J. A. J., Phillips, M. J., and Schrama, J. W. (2019). Effect of dietary protein to energy ratio, stocking density and feeding level on performance of Nile tilapia in pond aquaculture. Aquaculture 511:634200. doi: 10.1016/j.aquaculture.2019.06.014
Kelsay, J. L., Behall, K. M., and Prather, E. S. (1979). Effect of fiber from fruits and vegetables on metabolic responses of human subjects. II. Calcium, magnesium, iron, and silicon balances. Am. J. Clin. Nutr. 32, 1876–1880. doi: 10.1093/ajcn/32.9.1876
Kent, M. L., Feist, S. W., Harper, C., Hoogstraten-Miller, S., Law, J., Mac, Sánchez-Morgado, J. M., et al. (2009). Recommendations for control of pathogens and infectious diseases in fish research facilities. Comp. Biochem. Physiol. C Toxicol. Pharmacol. 149, 240–248. doi: 10.1016/j.cbpc.2008.08.001
Khosravi-Katuli, K., Prato, E., Lofrano, G., Guida, M., Vale, G., and Libralato, G. (2017). Effects of nanoparticles in species of aquaculture interest. Environ. Sci. Pollut. Res. 24, 17326–17346. doi: 10.1007/s11356-017-9360-3
Kim, K. D., Lim, S. G., Kang, Y. J., Kim, K. W., and Son, M. H. (2012). Effects of dietary protein and lipid levels on growth and body composition of juvenile far eastern catfish Silurus asotus. Asian-Austr. J. Anim. Sci. 25, 369–374. doi: 10.5713/ajas.2011.11089
Kong, W., Huang, S., Yang, Z., Shi, F., Feng, Y., and Khatoon, Z. (2020). Fish feed quality is a key factor in impacting aquaculture water environment: evidence from incubator experiments. Sci. Rep. 10:187. doi: 10.1038/s41598-019-57063-w
Kumar, N., Krishnani, K. K., and Singh, N. P. (2018). Effect of dietary zinc-nanoparticles on growth performance, anti-oxidative and immunological status of fish reared under multiple stressors. Biol. Trace Elem. Res. 186, 267–278. doi: 10.1007/s12011-018-1285-2
Laforenza, U. (2012). Water channel proteins in the gastrointestinal tract. Mol. Aspects Med. 33, 642–650. doi: 10.1016/j.mam.2012.03.001
Li, Y., Kortner, T. M., Chikwati, E. M., Belghit, I., Lock, E. J., and Krogdahl, Å. (2020). Total replacement of fish meal with black soldier fly (Hermetia illucens) larvae meal does not compromise the gut health of Atlantic salmon (Salmo salar). Aquaculture 520:734967. doi: 10.1016/j.aquaculture.2020.734967
Martins, G. B., da Rosa, C. E., Tarouco, F. M, and Robaldo, R. B. (2019). Growth, water quality and oxidative stress of Nile tilapia Oreochromis niloticus (L.) in biofloc technology system at different pH. Aquac. Res. 50, 1030–1039. doi: 10.1111/are.13975
McCue, M. D. (2013). Comparative Physiology of Fasting, Starvation, and Food Limitation. Berlin: Springer-Verlag. doi: 10.1007/978-3-642-29056-5
Merry, M. (2017). How to Calculate Silicon From Silica. Sciencing. Available online at: https://sciencing.com/calculate-silicon-silica-7548918.html (accessed December 8, 2020).
Moesch, A., Meurer, F., Zadinelo, I. V., Carneiro, W. F., da Silva, L. C. R., and dos Santos, L. D. (2016). Growth, body composition and hepatopancreas morphology of Nile tilapia fingerlings fed crude glycerol as a replacement for maize in diets. Anim. Feed Sci. Technol. 219, 122–131. doi: 10.1016/j.anifeedsci.2016.05.009
Naiel, M. A. E., Ismael, N. E. M., Abd El-hameed, S. A. A., and Amer, M. S. (2020). The antioxidative and immunity roles of chitosan nanoparticle and vitamin C-supplemented diets against imidacloprid toxicity on Oreochromis niloticus. Aquaculture 523:735219. doi: 10.1016/j.aquaculture.2020.735219
Nguyen, L., Dinh, H., and Davis, D. A. (2020a). Efficacy of reduced protein diets and the effects of indispensable amino acid supplements for Nile tilapia Oreochromis niloticus. Anim. Feed Sci. Technol. 268:114593. doi: 10.1016/j.anifeedsci.2020.114593
Nguyen, L., Salem, S. M. R., Salze, G. P., Dinh, H., and Davis, D. A. (2020b). Optimizing amino acid balance in diets for Nile tilapia Oreochromis niloticus. Aquaculture 515:734566. doi: 10.1016/j.aquaculture.2019.734566
Ogello, E. O., Munguti Jonathan, Sakakura, Y., and Hagiwara, A. (2014). Complete replacement of fish meal in the diet of Nile tilapia (Oreochromis niloticus L.) grow-out with alternative protein sources. Int. J. Adv. Res. 2, 962–978.
Oguz, A. R. (2015). A histological study of the kidney structure of Van fish (Alburnus tarichi) acclimated to highly alkaline water and freshwater. Mar. Freshw. Behav. Physiol. 48, 135–144. doi: 10.1080/10236244.2015.1004838
Onuegbu, C. U., Aggarwal, A., and Singh, N. B. (2018). ZnO nanoparticles as feed supplement on growth performance of cultured African catfish fingerlings. J. Sci. Ind. Res. 77, 213–218. Retrieved from: http://nopr.niscair.res.in/handle/123456789/44151
Perez-Velazquez, M., Gatlin, D. M., González-Félix, M. L., García-Ortega, A., de Cruz, C. R., Juárez-Gómez, M. L., et al. (2019). Effect of fishmeal and fish oil replacement by algal meals on biological performance and fatty acid profile of hybrid striped bass (Morone crhysops ♀ × M. saxatilis ♂). Aquaculture 507, 83–90. doi: 10.1016/j.aquaculture.2019.04.011
Pianesso, D., Adorian, T. J., Mombach, P. I., Dalcin, M. O., Loebens, L., Telles, Y. B., et al. (2020). Nutritional assessment of linseed meal (Linum usitatissimum L.) protein concentrate in feed of silver catfish. Anim. Feed Sci. Technol. 265:114517. doi: 10.1016/j.anifeedsci.2020.114517
Pieszka, M., Bederska-Łojewska, D., Szczurek, P., and Pieszka, M. (2019). The membrane interactions of nano-silica and its potential application in animal nutrition. Animals 9:1041. doi: 10.3390/ani9121041
Pirarat, N., Boonananthanasarn, S., Laddawan Katagiri, T., and Maita, M. (2015). Effect of activated charcoal-supplemented diet on growth performance and intestinal morphology of Nile Tilapia (Oreochromis niloticus). Thai J. Vet. Med. 45, 113–119. Retrieved from: https://he01.tci-thaijo.org/index.php/tjvm/article/view/32140
Priyadarsini, S., Mukherjee, S., and Mishra, M. (2018). Nanoparticles used in dentistry: a review. J. Oral Biol. Craniofacial Res. 8, 58–67. doi: 10.1016/j.jobcr.2017.12.004
Rathore, S. S., Murthy, H. S., Mamun, M. A., Al Nasren, S., Rakesh, K., Kumar, B. T. N., et al. (2020). Nano-selenium supplementation to ameliorate nutrition physiology, immune response, antioxidant system and disease resistance against aeromonas hydrophila in monosex Nile Tilapia (Oreochromis niloticus). Biol. Trace Elem. Res. 199, 3073–3088. doi: 10.1007/s12011-020-02416-0
Rodnick, K. J., and Planas, J. V. (2016). “The stress and stress mitigation effects of exercise: cardiovascular, metabolic, and skeletal muscle adjustments” in Fish Physiology, eds C. B. Schreck, L. Tort, A. P. Farrell, and C. J. Brauner (Cambridge: Academic Press-Elsevier). doi: 10.1016/B978-0-12-802728-8.00007-2
Rodriguez, Y. E., Laitano, M. V., Pereira, N. A., López-Zavala, A. A., Haran, N. S., and Fernández-Gimenez, A. V. (2018). Exogenous enzymes in aquaculture: Alginate and alginate-bentonite microcapsules for the intestinal delivery of shrimp proteases to Nile tilapia. Aquaculture 490, 35–43. doi: 10.1016/j.aquaculture.2018.02.022
Roy, S., and Bhattacharya, S. (2006). Arsenic-induced histopathology and synthesis of stress proteins in liver and kidney of Channa punctatus. Ecotoxicol. Environ. Saf. 65, 218–229. doi: 10.1016/j.ecoenv.2005.07.005
Schreck, C. B., and Tort, L. (2016). “The concept of stress in fish” in Fish Physiology, eds C. B. Schreck, L. Tort, A. P. Farrell, and C. J. Brauner (Cambridge: Academic Press-Elsevier) doi: 10.1016/B978-0-12-802728-8.00001-1
Shah, B. R., and Mraz, J. (2020). Advances in nanotechnology for sustainable aquaculture and fisheries. Rev. Aquac. 12, 925–942. doi: 10.1111/raq.12356
Simon, C. J., Blyth, D., Ahmad Fatan, N., and Suri, S. (2019). Microbial biomass (NovacqTM) stimulates feeding and improves the growth performance on extruded low to zero-fishmeal diets in tilapia (GIFT strain). Aquaculture 501, 319–324. doi: 10.1016/j.aquaculture.2018.11.052
Singha, K. P., Shamna, N., Sahu, N. P., Sardar, P., Harikrishna, V., Thirunavukkarasar, R., et al. (2020). Optimum dietary crude protein for culture of genetically improved farmed tilapia (GIFT), Oreochromis niloticus (Linnaeus, 1758) juveniles in low inland saline water: effects on growth, metabolism and gene expression. Anim. Feed Sci. Technol. 271:114713. doi: 10.1016/j.anifeedsci.2020.114713
Sun, S., Wei, C. D., and Liu, Y. X. (2010). Characterization and water activation behavior of tourmaline nanoparticles. J. Nanosci. Nanotechnol. 10, 2119–2124. doi: 10.1166/jnn.2010.2087
Thangapandiyan, S., and Monika, S. (2020). Green synthesized zinc oxide nanoparticles as feed additives to improve growth, biochemical, and hematological parameters in freshwater fish labeo rohita. Biol. Trace Elem. Res. 195, 636–647. doi: 10.1007/s12011-019-01873-6
Uddin, M. T., Goswami, A., Rahman, M. S., and Dhar, A. R. (2019). How can governance improve efficiency and effectiveness of value chains? An analysis of pangas and tilapia stakeholders in Bangladesh. Aquaculture 510, 206–215. doi: 10.1016/j.aquaculture.2019.05.055
Vidya, H., Kumara Swamy, B. E., and Schell, M. (2016). One step facile synthesis of silver nanoparticles for the simultaneous electrochemical determination of dopamine and ascorbic acid. J. Mol. Liq. 214, 298–305. doi: 10.1016/j.molliq.2015.12.025
Wagner, J., and Stanton, T. L. (2012). Formulating Rations With the Pearson Square. Colorado, CO: Colorado State University.
Walton, M. J., and Cowey, C. B. (1982). Aspects of intermediary metabolism in salmonid fish. Comp. Biochem. Physiol. – Part B Biochem. 73, 59–79. doi: 10.1016/0305-0491(82)90201-2
Wardani, W. W., Alimuddin, A., Zairin, M., Setiawati, M., Nuryati, S., and Suprayudi, M. A. (2020). Evaluation of cysteamine supplementation in red tilapia (Oreochromis sp.) diet: Serum insulin and somatostatin, IGF-1 and GLUT4 genes expression, growth performance, and robustness against stress. Aquaculture 528:735514. doi: 10.1016/j.aquaculture.2020.735514
Wu, T., Sun, L. C., Du, C. H., Cai, Q. F., Zhang, Q. B., Su, W. J., et al. (2009). Identification of pepsinogens and pepsins from the stomach of European eel (Anguilla anguilla). Food Chem. 115, 137–142. doi: 10.1016/j.foodchem.2008.11.077
Wu, W., Ji, H., Yu, H., Sun, J., and Zhou, J. (2020). Effect of refeeding dietary containing different protein and lipid levels on growth performance, body composition, digestive enzyme activities and metabolic related gene expression of grass carp (Ctenopharyngodon idellus) after overwinter starvation. Aquaculture 523:735196. doi: 10.1016/j.aquaculture.2020.735196
Xu, W., Huang, K., Jin, W., Luo, D., Liu, H., Li, Y., et al. (2018). Catalytic and anti-bacterial properties of biosynthesized silver nanoparticles using native inulin. RSC Adv. 8, 28746–28752. doi: 10.1039/c8ra03386b
Yang, P., Lai, D. Y. F., Yang, H., Tong, C., Lebel, L., Huang, J., et al. (2019). Methane dynamics of aquaculture shrimp ponds in two subtropical estuaries, Southeast China: dissolved concentration, net sediment release, and water oxidation. J. Geophys. Res. Biogeosciences 124, 1430–1445. doi: 10.1029/2018JG004794
Younes, M., Aggett, P., Aguilar, F., Crebelli, R., Dusemund, B., Filipič, M., et al. (2018). Re-evaluation of silicon dioxide (E 551) as a food additive. EFSA J. 16:5088. doi: 10.2903/j.efsa.2018.5088
Yuan, Y., Yuan, Y., Dai, Y., and Gong, Y. (2017). Economic profitability of tilapia farming in China. Aquac. Int. 25, 1253–1264. doi: 10.1007/s10499-017-0111-8
Zhang, H., Luo, Y., Lu, D. L., Jiao, J. G., Li, L. Y., Qin, J. G., et al. (2019). Diacylglycerol oil reduces fat accumulation and increases protein content by inducing lipid catabolism and protein metabolism in Nile tilapia (Oreochromis niloticus). Aquaculture 510, 90–99. doi: 10.1016/j.aquaculture.2019.05.035
Keywords: silica nanoparticle, tilapia (Oreochromis Nilotica), recirculatory aquaculture system, digestibility, nanotechnology in fish nutrition, bioaccumulation
Citation: Bashar A, Hasan NA, Haque MM, Rohani MF and Hossain MS (2021) Effects of Dietary Silica Nanoparticle on Growth Performance, Protein Digestibility, Hematology, Digestive Morphology, and Muscle Composition of Nile Tilapia, Oreochromis Niloticus. Front. Mar. Sci. 8:706179. doi: 10.3389/fmars.2021.706179
Received: 06 May 2021; Accepted: 19 July 2021;
Published: 17 August 2021.
Edited by:
Min Gu, Shandong University, ChinaReviewed by:
Mohamed Hassaan, Benha University, EgyptFrancisco Javier Moyano, University of Almeria, Spain
Copyright © 2021 Bashar, Hasan, Haque, Rohani and Hossain. This is an open-access article distributed under the terms of the Creative Commons Attribution License (CC BY). The use, distribution or reproduction in other forums is permitted, provided the original author(s) and the copyright owner(s) are credited and that the original publication in this journal is cited, in accordance with accepted academic practice. No use, distribution or reproduction is permitted which does not comply with these terms.
*Correspondence: Md. Sazzad Hossain, c2F6emFkYmF1QGdtYWlsLmNvbQ==
†These authors have contributed equally to this work and share first authorship