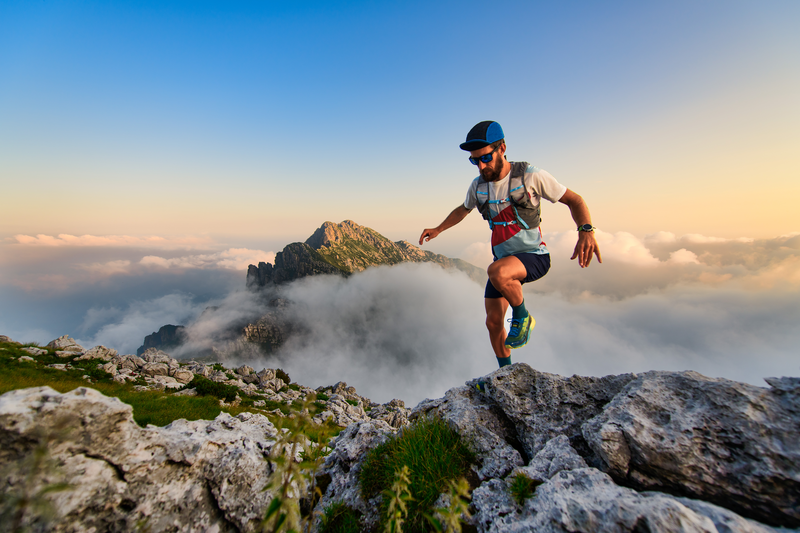
95% of researchers rate our articles as excellent or good
Learn more about the work of our research integrity team to safeguard the quality of each article we publish.
Find out more
ORIGINAL RESEARCH article
Front. Mar. Sci. , 01 September 2021
Sec. Marine Conservation and Sustainability
Volume 8 - 2021 | https://doi.org/10.3389/fmars.2021.694846
This article is part of the Research Topic Marine Conservation: Knowledge, Experience and Tools for Change View all 18 articles
Ensuring the efficacy of Marine Protected Areas (MPAs) requires that adequate management strategies be implemented according to the MPA’s objectives. Within the scope of species conservation, achieving MPA objectives demands understanding of the role played by MPAs for the target species. In 2014, Brazilian stakeholders and experts set the action plan for elasmobranchs’ conservation, which intended to create new protected areas and expand the existing ones. Nevertheless, more than 65% of Brazilian elasmobranch species are threatened by anthropogenic pressures such as fisheries and habitat loss. In addition, their ecological aspects are not well studied, which might jeopardize the success of the proposed actions. To assess the functionality and effectiveness of two no-take MPAs for sixteen demersal species, the Wildlife Refuge of Alcatrazes (WRA) and the Tupinambás Ecological Station (TES), we evaluated the community structure, space-time variations in functional diversity and changes in fishery indicators. Community dynamics were driven by inshore intrusion and time persistent effects of a cold and nutrient-rich water mass, the South Atlantic Central Water, which increased the relative abundance of species, functional groups, and overall diversity. Spatially, the heterogeneity of benthic habitats, due to the action of stronger waves in specific parts of the MPAs, reflects a diverse community of benthic invertebrates, explaining differences in relative abundance and similarities in space use by the functional groups. Regarding effectiveness, the MPAs make up a key network with the surrounding protection areas to support the ecosystem maintenance on the central and northern coast of the São Paulo state. The establishment of the TES has positively influenced the community throughout the years while the recent creation of the WRA may have promoted some improvements in fisheries indicators for a threatened guitarfish. We propose different functions of the Alcatrazes archipelago for each species and suggest some measures to enhance not only elasmobranch conservation but also the MPAs’ effectiveness.
For decades, governments have been using Marine Protected Areas (MPAs) to manage use of ocean resources. MPAs can address socioenvironmental issues by supporting traditional fishing communities, avoiding fisheries depletion and marine habitat degradation, and maintaining ecological services (Halpern, 2003; Fox et al., 2012). They are usually employed as a tool for conservation of critical habitats and dependent organisms, accounting for different requirements through a species’ life stages that can be safeguarded from anthropogenic disturbances (Claudet et al., 2010; Grüss et al., 2011; Wiegand et al., 2011; Knip et al., 2012; Rolim et al., 2019). Since the accomplishment of multiple objectives is challenging and success indicators, such as fishery sustainability, go beyond the MPA boundaries, coordination between MPA design and other management strategies (e.g., measures of control and restriction, networks of reserves, and adaptive management) are mandatory to achieve effectiveness (Fox et al., 2012; Lubchenco and Grorud-Colvert, 2015; Hilborn, 2016).
Previous works have highlighted the importance of adopting multiple strategies, especially when the MPA goals involve long-lived and mobile species like elasmobranchs (Chapman et al., 2005; Wiegand et al., 2011; Knip et al., 2012). For instance, Brazil is home to more than 14% of the worldwide biodiversity of sharks, skates and rays, driving experts and stakeholders to determine that conservation actions including MPAs are needed for the taxon (i.e., The action plan for elasmobranch conservation; ICMBio, 2016a). Currently, at least 65% of the species recorded in Brazilian waters are threatened or have insufficient data (ICMBio, 2016b; IUCN, 2021) and this lack of information might jeopardize the success of conservation and management actions (Gill et al., 2017; Giakoumi et al., 2018).
Among the strategic regions delimited by the action plan (ICMBio, 2016a) two marine reserves call attention: The Wildlife Refuge of Alcatrazes (WRA) and the Tupinambás Ecological Station (TES). They were established three decades apart seeking ecosystems preservation by restriction of human interference (Brazil, 1987, 2016). First, TES was created in 1987 to secure coastal and offshore rock formations, covering two coastal islands in northern São Paulo (i.e., Cabras and Palmas islands) and the islets, shallow flats and submerged pinnacles of the Alcatrazes archipelago. Later, in 2016, the WRA was implemented to shelter a greater area of the archipelago, especially the Alcatrazes island, becoming the largest marine reserve in south and southeastern Brazil. Both are no-take zones and although their delimitations overlap, they have different management plans. TES is the most restrictive, allowing only scientific and educational activities, while the WRA allows supervised visits to general public (ICMBio, 2017; Marconi et al., 2020).
At present, WRA and TES are part of a critical network for biodiversity maintenance that includes adjacent protected areas on the central and northern coasts of the São Paulo state (São Paulo, 2008). It is located at the middle continental shelf, which makes the Alcatrazes archipelago a unique area that interfaces parallel and perpendicular gradients of granulometry and organic matter in relation to the coast (Mahiques et al., 1999, 2004, 2011). Furthermore, it is near temperate and subtropical transition zones, being markedly influenced by mesoscale physical processes that promote seasonal changes in water properties (Castro-Filho et al., 1987; Campos et al., 2000). From late spring through summer, the prevalence of north and northeast winds carries superficial waters offshore, composed by the Coastal and Tropical water masses. This process promotes bottom inshore intrusions of the South Atlantic Central Water (SACW), a colder water mass that stratifies the water column (Castro-Filho et al., 1987).
The archipelago is ecologically important, presenting greater values of species richness, abundance and biomass of fish assemblages compared to the fished areas inshore, as well as the other no-take areas in the region (Gibran and Moura, 2012; Morais et al., 2017; Rolim et al., 2019). These trends reflect a complex ecosystem primarily regulated by top-down effects, with higher heterogeneity of functional groups when compared to the previously mentioned areas (Rolim et al., 2019). The high abundance of larger individuals of fishery target species (e.g., Epinephelidae, Kyphosidae, Carangidae, and Scaridae) suggests a great spillover potential to adjacent zones (Rolim et al., 2019). However, for elasmobranchs, especially the demersal species, the relationship of local species with environmental features is unknown and available information is restricted to community studies that focus mainly in actinopterygians. Approximately seventeen elasmobranch species are reported in the area (Hoff, 2015; ICMBio, 2017; Rolim et al., 2019), which exhibit differences in feeding and reproductive strategies, and behavioral ecology (Lessa et al., 1986; Soares et al., 1992; Vögler et al., 2003; Vooren and Klippel, 2005; Aguiar and Valentin, 2010), highlighting the variety of roles that the WRA and TES MPAs may play according to habitat use by the species.
Obtaining knowledge on the ecology of these species is crucial since fishing pressure and habitat degradation on coastal and inner shelf regions have disturbed the ichthyofauna, resulting in population depletion, diversity loss, and ecosystem unbalancing (Imoto et al., 2016; Dias et al., 2017; Prado et al., 2019; Rolim et al., 2019; Trevizani et al., 2019). Thus, the present study aimed to assess the functionality and effectiveness of the two MPAs to assist the decision making process that involves the conservation of demersal elasmobranchs. Our findings are important not only to understand the local and regional dynamics, but also to enhance policies for species conservation, in order to underlie the MPAs’ management. We hypothesize that those species use the archipelago for distinct purposes, which would reflect in different population structures. Differences in species distributions and in diversity metrics are also expected throughout space and time. Furthermore, we believe that variations in the relative abundance of functional groups as well as of their species, are related to the seasonal dynamics of the environment and to the heterogeneity of habitats. Finally, we expect that the size structure of a threatened guitarfish changes significantly due to the protection of a newer and larger MPA (i.e., the WRA).
The animal study was reviewed and approved by the Ethics Committee of Animal Use of the Oceanographic Institute of the University of São Paulo (CEUA IO-USP) and by the Chico Mendes Institute for Biodiversity Conservation (ICMBio) under the survey permit SISBIO/55824.
Biotic and abiotic data used in this study were obtained from five oceanographic expeditions performed by the following projects: Contributions to the Tupinambás Ecological Station Management Plan: oceanography and marine biodiversity (September/2011), Biotic Integrity of the Alcatrazes Archipelago Ecosystems (January/2014) and Geohabitat of the demersal ichthyofauna of the Alcatrazes region: an environmental assessment (September/2015, December/2018, and July/2019). Position of the oceanographic stations was defined according to the objectives of each project. Thus, they were set at different locations throughout the archipelago, except to 2019, when the 2018 stations were re-sampled (Figure 1). Sampling of sea water and sediments as well as capture of elasmobranchs were carried out at fifty oceanographic stations between 28 and 53 m depth.
Figure 1. Map showing the Alcatrazes Archipelago area, its marine protected areas (MPAs) delimitations [Wildlife Refuge of Alcatrazes (WRA) and Tupinambás Ecological Station (TES)] and oceanographic stations by expedition.
The assessment of temperature and salinity data was performed through different methods. Both variables were directly assessed using a conductivity-temperature-depth probe (CTD) (2011 and 2015) and a multiparameter probe (2018). Samples of bottom water taken by Nansen bottles, in 2014 and 2019, were used to measure temperature and salinity values using reversing thermometers and a refractometer, respectively. The sediment mosaic of the MPA region was characterized from samples collected through a van Veen grab in 2011 (Palóczy et al., 2012) and 2019. The area was classified into five zones, calculated as buffers of 2.5, 5, 7.5, 10, and 12.5 km from the center of Alcatrazes island, according to the home ranges of the caught specimens (or nearest taxa, i.e., genus) (Cartamil et al., 2003; Collins et al., 2007; Farrugia et al., 2011; Tilley et al., 2013). These zones were intended to capture any potential changes in benthic ecology with distance from the Alcatrazes island. Species with relatively small home ranges might have home ranges at a finer scale than these zones, while more wide-ranging species would encompass multiple zones.
Demersal elasmobranch specimens were collected through otter trawl nets (20–21 m in the foot rope, 40–60 mm mesh in the body as well as in the sleeves and 25–30 mm in the cod-end), which were operated from 10 to 20 min at a speed of two knots by the R/Vs Alpha Delphini (IO-USP) and Soloncy Moura (ICMBio). On board, specimens were accommodated in boxes with seawater and information was collected concerning their sex, total length (TL), disc width (DW), and total weight (TW). To ensure correct identification, pictures of each specimen were taken and identification to species level was conducted in accordance with Figueiredo (1977), Viana et al. (2016), and Gomes et al. (2019). After data collecting, all live elasmobranchs were released to the sea. The specimens that did not survive (i.e., less than 5% of the elasmobranch catches) were cooled and brought to the Oceanographic Institute (USP), being stored at the teaching collection. The non-elasmobranch species, such as the actinopterygians, were sacrificed through a solution of 400 mg L–1 of eugenol (Fernandes et al., 2017), cooled and also brought to the Oceanographic Institute to be used as research material in studies of community ecology, reproductive biology and so on.
According to Rossi-Wongtschowski and Paes (1993), the community structure of actinopterygians and elasmobranchs of the northern coast of São Paulo was related to sediment distribution and to the SACW presence. Thus, granulometric and hydrographic analyses were performed, as well as the estimation of calcium carbonate concentrations (CaCO3) of sediment.
In 2014, the refractometer did not operate correctly. Thus, based on the SACW properties, we fixed salinity values at 35.7 to water samples collected at depths where temperature was below 17°C, for this year only. For the whole dataset, values of temperature and salinity of each oceanographic expedition were combined in diagrams and potential densities with pressure equals zero (σθ) were calculated. We set diagrams with isopycnal curves through the oce package (Kelley et al., 2021) using σθ = 25.8 (Stramma and England, 1999; Mémery et al., 2000) as a threshold to identify the SACW presence. To define the sediment type of each oceanographic station, we combined available information about sedimentological parameters of the 2011 samples (Palóczy et al., 2012; Hoff et al., 2015) with data obtained in 2019. Sediment granulometry was determined by application of the sieve-pipette method (Suguio, 1973) to 50 g of the 2019 samples, followed by Folk and Ward (1957) classification. Further, concentrations of CaCO3 were estimated through weight differences after digestion by 10% solution of hydrochloric acid. Gravimetric results were used to characterize the oceanographic stations in accordance with Larsonneur et al. (1982).
Three main factors determine the energy dynamic in the Alcatrazes island surroundings: its Y-shaped morphology, the abrupt change in the bathymetry and the predominance of incident waves from south and southeast. Together, they act as mitigating elements and reflect a more stable environment in the north and toward the coast, due to the indirect incidence of waves as well as energy loss by the decreasing bathymetry. Furthermore, regions are more energetic in the south, with waves varying slightly through the seasons and years (Takase et al., 2021). These factors rule the deposition process in the archipelago, forming sediment features that are sustained over time. Thus, the same characteristics of 2011 and 2019 samples were assumed for unsampled sediments of the other years. Both classifications were applied to the nearest oceanographic stations (Supplementary Table 3) with distances ranging from 0.38 to 3.17 km.
A bibliographic survey was performed to gather information regarding the size at maturity, reproductive strategies, and food items of each species. They were used to classify specimens as juvenile or adult based on the size at first maturity and to identify functional groups through the reproductive and feeding guilds (Supplementary Tables 1, 2). Thus, species were classified into six groups by embryonic feeding method (trophonemata, oviparous, or lecithotrophic) and by trophic category (hyperbenthivorous, infauna consumers, or piscivorous), according to Elliott et al. (2007). Due to spatial variations in terms of biological and ecological aspects we used information of specimens from the closest regions.
To estimate changes in diversity patterns over time, we calculated species richness, the Shannon-Wiener diversity (H’) and the Pielou evenness (J’) for each year (Begon et al., 2006). Due to differences in sampling effort among years, instead of comparing raw species counts, we estimated rarefaction curves and species richness through non-parametric estimators (Chao and Chiu, 2016). Those estimators take into account underestimations in richness due to low sampling effort and differences in detection probability of species, since some species might have not been caught despite being present (Chao and Chiu, 2016). Quantities of juveniles and adults, sex ratios, and frequencies of TL/DW classes were counted for the most abundant species (>25 specimens caught). Deviations from 1:1 of sex ratios and contingency tables of species by life stages were evaluated by chi-square tests (χ2). Distributions of TL/WD frequency classes between sexes were compared by two-sample Kolmogorov-Smirnov tests (Zar, 2009).
Next, to test our hypothesis that species use spatial areas differently, and thus elucidate the roles played by WRA and TES, we conducted a three-step analyses. First, for each oceanographic station, we estimated the Bray-Curtis dissimilarities of the functional group abundances and performed principal coordinate analysis (PCoA) (Borcard et al., 2011) using the “cmdscale” function in R. Then, the relationship of the two first ordination scores and buffers were modeled by smoothed splines fitted using the “ordisurf” function. This function uses generalized additive models (GAMs) to fit non-linear response surfaces of predictor variables to ordinations (Oksanen et al., 2020). Maps of species’ relative abundance by oceanographic expedition were set and compared to the PCoA results to identify spatial-temporal variations in the community composition.
Influences of abiotic features on the relative abundance of species and functional groups were evaluated through generalized linear models (GLMs). Before model fitting, the predictive variables temperature, salinity, depth, year, seasons, the SACW presence, buffers, sediment type, and classes of CaCO3 concentrations were centralized and the collinearity of continuous and ordinal variables were estimated among pairs using the Spearman’s correlation coefficient (Zuur et al., 2009, 2010). Since the SACW presence was correlated (>80%) with temperature, and sediment type was correlated (>0.80) with classes of CaCO3 concentrations, only one of each pair of variables was included in each model. According to Larsonneur et al. (1982), sediments with CaCO3 concentrations above 30% are substantially composed of biogenic sources (i.e., animal and vegetal debris), being classified as litho-bioclastic (from 30 to 50%), bio-lithoclastic (from 50 to 70%) and bioclastic (>70%). Therefore, for model fitting, the sediment variable was set as one of two categories: lithoclastic (up to 30% of CaCO3) and biogenic sediments. Fixed effect models of the count of each species per trawl with the log of swept area (in meters per seconds) as offset term were set up according to prior information about which variables were likely to be relevant for each species (Oddone and Vooren, 2004; Vögler et al., 2008; Menni et al., 2010; Barbini et al., 2011; Palmeira, 2012; Schlaff et al., 2014). Models were fitted using “glm” and “glm.nb” functions with Poisson and Negative Binomial error distributions (Zuur et al., 2009). Alternative models were compared by the second order Akaike information criterion (AICc) with ΔAICc < 2 as a threshold to evaluate them regarding their descriptive capacity (Burnham and Anderson, 2002). If more than one model was ranked as plausible, model averaging was applied and parameters estimates were weighted by the Akaike weights (Wi) (Burnham and Anderson, 2002). To evaluate the model fits, scaled residuals were analyzed through plots generated by the DHARMa package in R (Hartig and Lohse, 2020). DHARMa residuals are estimated as quantiles of one thousand simulated draws from the distribution used to calculate the likelihood corresponding to each observation. Deviations from the expected values of a uniform distribution as well as of variances in relation to predicted values were compared by qq-plots and residual plots, respectively.
Finally, the WRA effect was assessed through changes in size structure over time only for the most common species: the lesser guitarfish, Zapteryx brevirostris (Müller and Henle, 1841). None of the other species had a large enough sample size to calculate these size-based indicators. The TL data of Z. brevirostris were grouped in two periods (2011–2015 and 2018–2019) according to the MPA establishment in 2016 (Brazil, 2016). We set a linear model with interactions between season and time period (TL ∼ period × season) to test whether differences in mean TL are an effect of the MPA creation or due to sampling different seasons (Zar, 2009). Also, indicators of fishery sustainability for each period were estimated. Fishing mortality relative to natural mortality (F/M) and spawning potential ratios (SPR, defined as the spawning stock biomass relative to unfished SSB) are indicators of stock status. They measure how much higher is the mortality experienced by a fished population and how much lower is its potential fecundity (Goodyear, 1993), respectively, compared to unfished conditions. The F/M indicator was calculated under two different methods with different assumptions about selectivity. First, in the mean length method, total mortality (Z) was estimated by the Beverton and Holt (1957) estimator assuming the same catchability of specimens over the minimum fully exploited size (Lc). The second method, length-based spawning potential ratio (LBSPR), assumes that catchability increases logistically with the length of specimens and estimates the logistic parameters as well as the average F/M and SPR that best fits the length-frequency data, assuming variability in length at age (Hordyk et al., 2015).
Life history parameters were required to estimate fishery indicators. However, most of them have not been calculated for Alcatrazes population, so we used values of populations from nearby regions. To estimate Lc and other parameters, such as the mean and variance of natural mortality (M), methods proposed by Babcock et al. (2013, 2018) were implemented (see Supplementary Table 4 for details about parameters and indicators). Uncertainties of parameters’ estimates were obtained by ten thousand Monte Carlo simulations. They were performed with bootstrapped samples of the observed length data and values of the life history parameters drawn from a multivariate normal distribution. Then, the 90% confidence interval (CI) of each indicator was set as the 5 and 95% quantiles of the simulated values (Babcock et al., 2018).
To evaluate whether a difference in mean length should be expected in the before vs. after MPA samples, the necessary time after the establishment of a MPA for the Z. brevirositris population to reach an unfished level of the mean length was assessed considering several selectivity assumptions. Life history values of a fished population (Supplementary Table 4) were used to calculate the numbers (Hilborn and Walters, 1992) and lengths at age (von Bertalanffy growth model, Beverton and Holt, 1957) assuming both natural and fishing mortalities before the WRA, and only natural mortality after its establishment. Then, we calculated the mean length of specimens larger than Lc, which is the mean length that is used for the Beverton-Holt estimator, in each year after the founding of the MPA.
All analyses were performed using the R environment (R Core Team, 2020) through the vegan (Oksanen et al., 2020), SpadeR (Chao et al., 2016), MASS (Ripley et al., 2021), MuMIn (Bartoń, 2020), DHARMa, mvtnorm (Genz et al., 2020), and LBSPR (Hordyk, 2019) packages.
Temperature and salinity diagrams (Supplementary Figure 1) showed that the influence of SACW has changed over the years and across the MPA area. The water mass was detected in all years except 2019, which was characterized by higher values of temperature/salinity and homogeneity in the water column with the majority of temperature records from 22.4 to 23.5°C. Despite the absence of σ0 reference values in 2014, low temperatures (18°C<) were verified by reversing thermometers up to 25 m above the bottom, indicating the presence of SACW. In terms of distribution through the area, the SACW was identified at all oceanographic stations until 2015. Although the 2018 campaign was conducted in summer, the SACW was only detected at oceanographic stations exposed to the open ocean (#08, #09, #10, #11, and #12) and in the area between the Sapata and Alcatrazes islands (#05). These variations in the water mass coverage indicate that the intrusion process was beginning, since the samples were collected at the onset of the season. Sediments of both MPAs were defined by fine grains (fine and very fine sand > 85%) and poor CaCO3 composition (i.e., lithoclastic sediments). However, bio-lithoclastic and bioclastic sediments with large quantities of biogenic CaCO3 (making up by 79% of sediment content) were assessed on patches of coarse and very coarse sand. The distribution of these patches was limited to nearby regions of the Alcatrazes island and especially to the island side that is exposed to open ocean (i.e., the south side). Hydrographic and sedimentological compiled data are presented in Supplementary Table 3.
A total of 562 specimens were recorded, belonging to 16 species of seven families. Species richness across all years was estimated as 16.33–17, depending on the estimation method used, with CIs ranging from 16.02 to 27.05 species (Table 1). Two families, the Trygonorrhinidae and Arhynchobatidae, were the most common, accounting for almost 85% of the elasmobranchs sampled (Supplementary Table 5). Trygonorrhinidae was represented by just one species, Z. brevirostris, which was recorded in 86% of the oceanographic stations and showed the highest number of individuals caught (n = 257; Supplementary Table 5). Following Z. brevirostris, the Rio skate, Rioraja agassizii (Müller and Henle, 1841), made up around 15% of the total sample (n = 81; Supplementary Table 5) and despite its absence in 2015, the species was recorded in 60% of all oceanographic stations.
According to the estimates of diversity, evenness, and species richness, changes in demersal community composition were identified over the time. Overall, the number of observed species and specimens caught were lower (Table 2) in oceanographic expeditions of smaller sampling effort: the summer of 2014 (five trawls) and spring of 2015 (six trawls). However, rarefaction curves did not reach asymptotes (Supplementary Figure 2) and the 95% upper CI limits revealed the potential for greater values of estimated richness (Supplementary Table 5). Diversity and evenness of those oceanographic expeditions were quite similar with higher estimates of the other spring and summer expeditions (2011 and 2018, respectively), which were carried out with a sampling effort almost three times greater (Table 2). In this sense, a trend in diversity and evenness was observed, with estimates increasing through the seasons, from the lowest ones in the winter (2019’ oceanographic expedition) to the highest during the summer (Table 2).
Table 2. Relative abundance, number of species and ecological index estimates by oceanographic expedition of demersal elasmobranchs.
Altogether, lengths were measured for 554 and sexes for 549 specimens, of which 499 were from six species that had a samples size of at least 25 (Supplementary Table 5). Species showed significant differences in the distribution of life stage classes (χ2 = 105.6, df = 5, p < 0.05). The community was mainly composed of adults for Z. brevirostris, R. agassizii, the zipper sand skate, Psammobatis extenta (Garman, 1913) and the groovebelly stingray, Dasyatis hypostigma Santos and Carvalho, 2004. However, for two species of the Arhynchobatidae family, the spotback skate, Atlantoraja castelnaui (Miranda Ribeiro, 1907) and the eyespot skate, Atlantoraja cyclophora (Regan, 1903), the number of juveniles were substantially higher (over 75% of each species abundance). Deviations in sex ratios from 1:1 were verified of both Arhynchobatidae species, with males outnumbered by females (0.33:1, χ2A. castelnaui = 25, df = 1, p < 0.05; 0.4:1, χ2A. cyclophora = 18.37, df = 1, p < 0.05). Concerning the species length ranges, no significant differences among sexes were found for either of these two species (DA. castelnaui = 0.26, p > 0.05; DA. cyclophora = 0.26, p > 0. 05; Figure 2).
Figure 2. Histograms shows the total length or disk width frequency classes of females (purple) and males (green) of (A) Atlantoraja castelnaui, (B) A. cyclophora, (C) Rioraja agassizii, (D) Psammobatis extenta, (E) Zapteryx brevirostris, and (F) Dasyatis hypostigma. Sizes of first maturity taken from the literature are indicated by purple solid (females) and green dashed (males) vertical lines. Donut charts represent sex ratios and proportions of juveniles and adults.
Conversely, for the other two skate populations, more than 70% of collected specimens were adults. Differences in sex ratios were also verified with more females of R. agassizii (0.57:1, χ2 = 7.44, df = 1, p < 0.05) and of P. extenta (0.54:1, χ2 = 8.84, df = 1, p < 0.05). For the latter, TL frequencies did not differ (D = 0.20, p > 0.05), however, females of R. agassizii exhibited larger sizes (D = 0.43, p < 0.05) prevailing in TL classes above 40 cm (Figure 2). The same pattern was observed for Z. brevirostris with more than 70% of the analyzed specimens as adults. The ratio between males and females was equal (0.88:1, χ2 = 0.44, df = 1, p > 0.05) and as for R. agassizii, females were larger than males (D = 0.19, p < 0.05). For D. hypostigma, there were no differences among sex ratios (0.75:1, χ2 = 2.04, df = 1, p > 0.05) and life stage classes were also similar (Supplementary Table 5). Although the majority of males showed smaller sizes (Figure 2), no significant differences were found in DW distributions by sex (D = 0.38, p > 0.05).
The first two axis of the PCoA explained 55.6% of the data variance (PCoA1 = 32.4 and PCoA2 = 23.20), being correlated with distances from the Alcatrazes island (i.e., buffers) as shown by the contour lines (Figure 3). The slight differences in space use by the functional groups appeared to be more related to the species’ trophic categories than to their reproductive modes. While the hyperbenthivorous and infauna consumers were common in regions of intermediate distances, the piscivorous species were mainly caught at the farthest oceanographic stations (i.e., those positively loaded on the PCoA1 and negatively loaded on the PCoA2). Regarding the reproductive guilds, such oceanographic stations were also the most different, being separated even from those of other lecithotrophic species (i.e., negatively loaded on the PCoA1). Fifteen individuals of two shark species, the angular angel shark, Squatina guggenheim Marini, 1936 and the dogfishes, Squalus albicaudus Viana et al., 2016 and Squalus sp. were classified as lecithotrophic and piscivore (Supplementary Table 5). They were caught at seven oceanographic stations that were characterized by low temperatures (μ = 18.1°C), presence of the SACW and predominance of finer grains without biogenic CaCO3.
Figure 3. Principal coordinate analysis (PCoA) results showing the space use configuration by functional groups in relation to distances (km) from the Alcatrazes island. Purple points are the oceanographic stations, initials represent the functional groups that most contributed to dissimilarities among oceanographic stations and the contour lines are the fitted splines of the distances from the Alcatrazes island (i.e., buffers) from closer (blue) to farther (green) zones.
Differences in relative abundances were observed through the archipelago (Figure 4). In general, the functional groups were present in all regions of the archipelago, however, the region that corresponds to the exposed side of the Alcatrazes island showed higher values of relative abundance and was more heterogeneous in terms of species composition than the northwest side. Some species were widely distributed while occurrence of the other ones was occasional and restricted to certain regions. A. castelnaui and A. cyclophora were abundant in the surroundings of the Alcatrazes island and were present through almost the entire sampling period. Similarly, R. agassizii and Z. brevirostris were ubiquitous in terms of space-time occurrence. However, in 2019 a pattern was identified with concentrations of the skate in the northeast and of the guitarfish in the northwest and south regions. Also, in 2019 large groups of D. hypostigma and solitary individuals of the bullnose eagle ray, Myliobatis freminvillei Lesueur, 1824 were observed in the northeast region. Still in the northeast, juveniles of S. albicaudus were recorded in 2018. Congeneric species, such as the cownose rays and the angel sharks, were not caught together in any of the trawls, indicating possible spatial segregation with the exposed region being mainly used by Rhinoptera brasiliensis Müller, 1836 and S. guggenheim and the northwest side by Rhinoptera bonasus (Mitchill, 1815) and Squatina occulta Vooren and Silva, 1991.
Figure 4. Maps of spatial-temporal variation of species relative abundance through the Alcatrazes archipelago. Colors indicate species relative abundance by oceanographic station and pie chart sizes represent the contribution of each oceanographic station to the total catch by expedition. The sides of the archipelago that are exposed to the open ocean and turned to the continent (sheltered) are present in the first map.
According to the most parsimonious models (ΔAICc < 2), temperature and seasons were the predominant variables that explained shifts in abundance of the species and functional groups (Table 3). Except for the trophonemata-hyperbenthivorous (i.e., species that produce lipid-rich liquid through trophonemas to supplement embryo nutrient provision and feed on benthic invertebrates which live above the sediment, respectively), the relative abundance of all groups was inversely related to bottom water temperature (Table 4). Moreover, significant differences between summer and spring were found with higher abundances of oviparous-hyperbenthivorous (i.e., species of which embryos depend solely on the yolk-sac reserves, developing inside encapsulated eggs that were deployed in the environment) and lecithotrophic-infauna consumers (i.e., species of which embryos also feed mainly on the yolk-sac reserves, but develop inside the mother uterus and, in later life’ stages, feed on benthic invertebrates which live in the sediment) in the former season.
Table 4. Estimated parameters of variables from the best models that explain the number of individuals of functional groups.
Similar trends were exhibited by the species (Table 5). For example, the relative abundance of A. castelnaui changed seasonally, with higher values in the summer, the same trend seen for its group (i.e., oviparous-hyperbenthivorous). Increases in A. cyclophora as well as in the most representative species of lecithotrophic-infauna consumers, Z. brevirostris, were related to temperature decrease and, particularly for some skates, salinity had an inverse effect (e.g., P. extenta). Spatial variations were mainly explained by depth and differences among buffers. For the oviparous-hyperbenthivorous group, the number of specimens were higher at farther buffers and increased with depth (Table 4). Overall, the relative abundance of this group, and specifically of A. castelnaui (Table 5), appear to be lower in shallow regions. However, none of the skates varied in relative abundance among buffers and only R. agassizii showed significant differences with CaCO3 content (Table 5). Its lower abundance in biogenic than in lithoclastic sediments might reflect the patterns of the functional group, since oceanographic stations with higher CaCO3 concentrations were found in the vicinity of Alcatrazes island.
Table 5. Estimated parameters of variables from the best models that explain the number of individuals of elasmobranch species.
No significant differences in mean lengths of Z. brevirostris were identified before and after the WRA MPA establishment when season was included in the model (βbefore + summer = 44.89 ± 1.33; βafter + summer = 45.14 ± 0.77, t = −0.19, p = 0.85). On the other hand, there was a significant effect of seasons, with higher mean TL in summer than in spring (Table 6). The number of specimens of sizes above Lc, meaning they were susceptible to fishery harvest, was 168 (before MPA: n = 65, after: n = 103) and the small increase in mean length implied a small decrease in the mean F/M for fish larger than Lc estimated by the Beverton-Holt method although the effect was not significant judging by the overlapping CIs. According to LBSPR, which estimates F/M of fully selected (i.e., large) individuals, assuming a logistic selectivity curve, the mean estimated fishing mortalities increased and CIs of (F/M)LBSPR overlapped, being above the overfishing threshold (>1) (Supplementary Figure 3A). These numbers are not directly comparable because they correspond to fish of different sizes. Nevertheless, large values of either metric can be taken as evidence of overfishing. The CIs of SPR also overlapped, although the mean increased slightly (current SPR > 0.4) (Supplementary Figure 3B). According to our simulation, if fishing was completely eliminated, the mean length of guitarfish larger than Lc would be expected to increase after the WRA establishment, reaching the unfished level in approximately 5 or 6 years depending on the assumed selectivity of the fishery (Supplementary Figure 4).
Table 6. Effects of the Wildlife Refuge of Alcatrazes (WRA) establishment and seasons in mean total length (TL) of Zapteryx brevirostris.
Marine communities are usually characterized by a few predominant species that are continuously present and many other species that have relative low abundances and occasional occurrences associated with natural events (Magurran and Henderson, 2003). Our findings showed such patterns with shifts in the Alcatrazes elasmobranch fauna, and consequently in the MPA’s functional diversity, being driven by thermohaline and chemical changes in the water column through the seasons. The inshore intrusion of the SACW is known for lowering water temperatures, raising primary production in the euphotic zone, and enriching the bottom by the input of particulate organic matter during spring and summer. Therefore, increases in diversity metrics were likely a response of the enhancement of feeding resources, given that the energy surplus advantages the benthic megafauna, and is also a consequence of the expansion of the SACW’s associated species (Pires-Vanin et al., 1993; Castro-Filho and de Miranda, 1998; Muto et al., 2000). On average, 60% of the species caught are temperate species that are probably related to the water mass (Menni and Stehmann, 2000; Menni et al., 2010). Higher abundances of functional groups in spring and summer (e.g., lecithotrophic-infauna consumers and oviparous-hyperbenthivorous) and their increase with a decrease in temperature, indicate the same association with the SACW. Even though variations could be explained by the input of individuals, the reproductive cycle of such species appears to be synchronized to periods of more suitable conditions. Reported peaks on mating, birth or egg-laying of A. cyclophora, A. castelnaui, R. agassizii, Z. brevirostris, the Brazilian guitarfish, Pseudobatos horkelii (Müller and Henle, 1841), and S. occulta, coincides with the timing of the SACW influence (Lessa et al., 1986; Ponz-Louro, 1995; Oddone and Vooren, 2005; Vooren and Klippel, 2005; Oddone et al., 2007, 2008; Colonello et al., 2011, 2012). This could enable energy recovery by females and access to food by the newborns.
On the other hand, when the SACW retreats to deeper zones (>100 m) in autumn and winter, the Tropical Water mass dominates the middle shelf, increasing the temperature and salinity of the water (Castro-Filho and de Miranda, 1998; Campos et al., 2000). Our results show that under the Tropical Water influence, the community became less diverse although some of the recorded species had never been caught before (e.g., R. bonasus and R. brasiliensis). The cownose rays are trophonemata species that display reproductive traits of high energetic demand to improve likelihood of offspring success (Rangel et al., 2020). Seasonal migrations to nursery areas along the coast have been suggested, with parturition from late spring through summer (Rangel et al., 2018). Thus, the recorded specimens might have been caught while foraging for more energetic resources to improve reserves before mating or during gestation (e.g., Rangel et al., 2021). After stronger SACW events, the availability of potential food items, including higher level species of the benthic megafauna, are more abundant on the middle shelf, making Alcatrazes a productive foraging area (Pires-Vanin et al., 1993; De Léo and Pires-Vanin, 2006; Shimabukuro et al., 2016).
Spatially, while the northwest and northeast parts were predominantly characterized by finer grains and poor CaCO3 content, the south (i.e., part exposed to the open ocean), could be distinguished in two regions: the eastern portion, that is similar to the first two, and the western, with presence of coarse sands and higher CaCO3 concentrations. According to Takase et al. (2021), this region is highly influenced by energetic waves which explains the sediment configuration by the displacement of finer grains to the east. Consequently, the heterogeneity of habitats in the exposed part resulted in a more diverse fauna in comparison to the northern area. Higher abundances of oviparous and hyperbenthivorous species at farther offshore and deeper locations might be related to the distribution of preys. For example, organisms of biogenic source such as mollusks, starfishes, and corals, are not part of the R. agassizii diet, which like P. extenta, feeds significantly on small crustaceans of the benthic macrofauna (Soares et al., 1992; Aguiar and Valentin, 2010; Bornatowski et al., 2014). Moreover, brachyuran and portunid crabs are main preys of A. castelnaui and A. cyclophora, respectively (Soares et al., 1992, 2008). Thus, great densities of the macrobenthos on the inner and outer shelf (Pires-Vanin, 2008) and presence of such crabs (e.g., Persephona punctata, Libinia spinosa, Portunus spinimanus, and Callinectes sapidus), which were found in trawls performed at deeper oceanographic stations, would have attracted the skates to those regions, consistent with our findings. Likewise, spatial differences between angel sharks were probably related to the resource distribution. Abundance of infauna invertebrates (e.g., polychaetas), may be higher in the northwest part due to sediment composition and higher levels of organic matter (Hoff et al., 2015). Thus, whereas S. guggenheim are strictly piscivorous, eating demersal, and pelagic species (Vögler et al., 2003), the S. occulta diet, which consists of polychaetas and nematodes, relies on configurations of benthic habitats (Aguiar and Valentin, 2010; Domingos et al., 2021).
Groupings of mature males (i.e., calcified clasper) of R. agassizii and Z. brevirostris, in different parts of the archipelago, suggest formation of shoals for reproductive purposes (Paijmans et al., 2019). Although specific evidence of females’ maturity stage has not been assessed, the majority of recorded specimens in 2019 were bigger than the published size of first maturity (Supplementary Table 1). Those results support the reproductive cycle defined by Oddone et al. (2007) and Colonello et al. (2011). Nevertheless, it is possible that the lesser guitarfishes of tropical waters perform two mating periods, since mature males were also recorded during summer oceanographic expeditions. Colonello et al. (2011) previously highlighted the asynchrony of reproductive females when comparing populations from temperate regions and the northern São Paulo coast (Ponz-Louro, 1995). Catches of D. hypostigma and M. freminvillei at the same oceanographic stations may indicate formation of mixed-species shoals. Despite the fact that both species are hyperbenthivorous and the diet overlap could increase species competition, interspecific associations may also increase foraging efficiency (Paijmans et al., 2019). Stingrays perform foraging traits which expose the benthic fauna (Freitas et al., 2019), facilitating prey catchability.
Overall, dissimilarities regarding the population structures from other Brazilian regions may be related to geographical features, sexual segregation, and ontogenetic changes in habitat use (Schlaff et al., 2014). Despite the substantial presence of adults, most of them were individuals just over the reference size of first maturity. In almost all species, juveniles were present, but only A. castelnaui and A. cyclophora were dominated by them, which will be discussed later. The sex ratio favoring females was similar to what was found with populations of A. castelnaui, from the northern coast of São Paulo (Ponz-Louro, 1995) and of R. agassizii, along the southeastern Brazil (Oddone and Amorim, 2007). In contrast, the sex ratio of the northern state population of P. extenta did not deviate from 1 (Martins et al., 2005) which was not consistent with our findings of a female dominated sex ratio. Furthermore, Martins et al. (2005) found variations in habitat use through the species’ life span. This does not seem to be our case as young juveniles, older juveniles, and adults were found in Alcatrazes. No significant deviations from 1 were found for A. cyclophora in southern and southeastern Brazil (Oddone and Vooren, 2004; Oddone and Amorim, 2007). The evaluated specimens of both studies came from different, and even deeper regions (over 100 m), inside of a wider area, which might have caused those disparities. For Z. brevirostris and D. hypostigma, our results exhibited equal rates between sexes, which agreed with results of the northern guitarfishes evaluated by Ponz-Louro (1995). But, for the last one, no information about population structure was found, pointing out the necessity of efforts to broaden our understanding of the species.
Based on the structure results, evidence of reproductive availability and patterns found in the literature, we propose uses of the MPAs by each elasmobranch although further research regarding species movement ecology is essential to strengthen these conclusions (Supplementary Table 5). Like other insular regions in Brazil (Wetherbee et al., 2007; Aguiar et al., 2009), Alcatrazes is a nursery area specifically used for development by many species. Early life stages, such as neonates, young of the year and/or juveniles were found, supporting this hypothesis. Juveniles of angel sharks and stingrays (genus Hypanus) were found at deeper regions, whereas records of smaller specimens (e.g., Hypanus americanus (Hildebrand and Schroeder, 1928) and S. albicaudus), indicate that younger animals may use sheltered habitats (Aguiar et al., 2009; Farrugia et al., 2011), such as the shallow zones closer to rock formations and low energetic parts in the northeast. However, for two skates, A. castelnaui and A. cyclopohora, the area works not only as nursery, but also as mating place, as indicated by the lower frequencies of adults and seasonal records of mature males. The possibility that records of mature specimens of the other species may have been related to migratory behavior hampers the definition that the area was used only for reproduction by them. Thus, as proposed for cownose rays, which are species of large home ranges and exhibit key areas for population maintenance along the coast (Collins et al., 2007; Rangel et al., 2018), we suppose that the MPAs may be a seasonal feeding ground for M. freminvillei and D. hypostigma.
Connection between the inner and outer shelf may play a critical role in the species’ reproductive success, especially for P. horkelii and the chola guitarfish, Pseudobatos percellens (Walbaum, 1792). Seasonal migrations of P. horkelii, from deeper regions (>100 m) to give birth and mate in coastal zones, is well described by Lessa et al. (1986). This might have been the case of the adult females of both species that were caught in spring and summer. Even though movements of great distances were not reported for P. percellens, embryonic diapause, which is a reproductive trait associated with the migratory behavior of P. horkelii, was proposed for the former species (Rocha and Gadig, 2013). This may suggest that P. percellens also displays such behavior, being consistent with the absence of neonates in our records. Finally, for R. agassizii, P. extenta, and Z. brevirostris, all length classes were collected, indicating their resident status. Nevertheless, their presence may be intermittent, particularly for the skates that were absent in some oceanographic expeditions. According to Martins et al. (2005), abundance fluctuations of P. extenta was observed in the northern coast, being higher in periods when the species were not recorded in Alcatrazes (e.g., 2009’expedition).
Magnitude differences between the methods and uncertainties in fisheries indicators for Z. brevirostris may be caused in part by the small sample size, requiring larger datasets to obtain more precise and accurate results, especially for the LBSPR method. Despite the fact that our results did not find a significant positive effect of the WRA establishment, the decrease in mean F/M(ML) and increase in mean SPR may suggest some improvements in fisheries indicators. Our calculation of the expected time to show an improvement in mean length after MPA establishment suggests that under several possible selectivity patterns in the fishery, the WRA effect could be detectable within a few years of the MPA formation. However, further monitoring is needed to estimate the trends of the Alcatrazes population. The WRA is a novel MPA, for which the management plan was defined in 2017 (ICMBio, 2017), starting its initiatives 1 year before our last sampling campaign. Thus, our short-term evaluation and inconsistent sampling among seasons, might be the reason to the small changes we got in the mean length between periods. Furthermore, Z. brevirostris is a relative long-lived species that exhibits late maturity and low intrinsic rate of population growth (Caltabellotta, 2014; D’Alberto et al., 2019), which would increase the estimated times of recovery relative to more short lived species.
As previously mentioned, parts of the archipelago have been being protected by TES and even before its creation, by the Brazilian Navy, which used to perform tactical exercises, forbidding navigation in the surroundings (Hoff et al., 2015). At that time, the demersal fish community was represented by predominance of sole fishes (e.g., Syacium micrurum, S. papillosum, Citharichthys macrops, and Symphurus jenynsi) and poor diversity of elasmobranchs, with Z. brevirostris as the only one in the records (Paiva-Filho et al., 1989). Nowadays, the archipelago shows a well-structured community, with presence of higher-level predators (Rolim et al., 2019) and the apparent improvement of the Z. brevirostris population, since the great number of recorded specimens is comparable to other studies that were performed in wider areas along the coast (e.g., Marion et al., 2011; Caltabellotta et al., 2019). In this sense, our results provide a useful baseline for further evaluations of causal effects regarding the WRA. Some studies have pointed out the importance of tracking changes in ecological indicators of a MPA throughout time (Edgar et al., 2004, 2011) and between a control site (Villaseńor-Derbez et al., 2018). However, the historical safeguarding of the archipelago, the influence of physical processes (Castro-Filho et al., 1987), the higher complexity of ecological interactions (Rolim et al., 2019) and its great distance from coastal as well as other insular regions, increase the potential sources of variability (Edgar et al., 2014), making difficult the designation of control areas or comparisons with other MPAs.
The relevance of the MPAs for the local ichthyofauna is clear, especially the WRA, which broadened the protection, encompassing the Alcatrazes island and consequently, the essential habitats for elasmobranchs. Furthermore, both areas seem to play pivotal roles for endangered species, as more than 75% of the recorded elasmobranchs are in threatened categories (IUCN, 2021). Both MPAs together encompass an area of approximately 70,000 ha (ICMBio, 2017) which would cover the home ranges of the caught species (see Section “Abiotic Data”). Nevertheless, ontogenetic differences in their requirements may not be provided, so that for some species the archipelago was used only at specific life stages (i.e., non-resident species). Such differences imply movements to specific habitats outside the MPAs boundaries, raising the threats over the species and consequently affecting the efficiency of the protected areas. Chapman et al. (2005) discovered that the lack of connectivity among adjacent habitats was exposing reef and nursery sharks to the fisheries, demanding additional management measures for species conservation, and some Alcatrazes species may experience similar threats.
Similarly, the intense anthropogenic pressure in the surrounding area may compromise such functionality and thus, the effectiveness of TES and WRA. Alcatrazes is placed between two disturbed areas on the São Paulo coast. To southwest, the Santos Port is the largest port in Latin America and the most important industrial hub in Brazil (Luiz-Silva et al., 2002), producing great concentrations of mercury and plastic pellets, that reach adjacent (e.g., Santos Bay) (Siqueira et al., 2005; Ribeiro, 2020) and even farther regions, such as the archipelago. To northwest and closer to Alcatrazes, the São Sebastião Port will be expanded over the Araçá Bay (Angelini et al., 2018), an important nursery place (Contente et al., 2020). Besides the local impacts, its expansion could also affect the vicinities, disturbing the fauna by the carriage of pollutants and increase in underwater noise (Slabbekoorn et al., 2010; Barletta et al., 2016).
In addition, despite fishery activities being concentrated on the inner and middle shelf (Imoto et al., 2016), including inside the less restrictive protected areas (Carneiro et al., 2013), exploration of deeper zones has been increasing in the past decades (Pincinato and Gasalla, 2019). According to Imoto et al. (2016), great amounts of demersal catches were obtained by industrial fleets in those regions, raising the threat over species that use the archipelago seasonally for feeding or for mating, while also using the surrounding fished area. Currently, fishing of threaten elasmobranchs is forbidden or only allowed for subsistence in Brazilian waters (i.e., species classified as VU) (MMA, 2014). Nevertheless, they are still caught as bycatch by fleets that are known to directly impact the demersal fauna, such as gillnets, and otter, double-ring, and pair trawlers. Those activities are controlled in the surroundings of TES and WRA by the management plans of other two protected areas (i.e., Marine Environment Protection Area of the North and Central Coast – APA Norte and APA Centro) (Forestry Foundation, 2019, 2020) and different legislations of federal and state level. Inside the APAs, input measures, such as the restriction of industrial (APA Centro) and even traditional (APA Norte) pair trawlers until the 23.6 m isobath as well as the specification of day periods to operation of beach seines (São Paulo, 2009, 2012), are applied. Nevertheless, the fishing zonation become less restrictive as distance from the coast increases and despite seasonal closures of catfish and shrimp fishing occur from January to March (SUDEPE, 1984) and March to May (IBAMA, 2008), respectively, gillnets remain allowed (IBAMA, 2007).
Thus, based upon the MPAs use by elasmobranchs and the potential connectivity with other protected areas, we recommend that besides the creation/expansion of marine reserves, fishing control measures should be implemented. Temporal closures in winter as well as extension of the pre-existing ones through all summer months, and limitation of effort (Cochrane and Garcia, 2009), could reduce the pressure on species that make reproductive migrations and/or require larger home ranges (e.g., guitarfishes, eagle, and cownoses stingrays). Moreover, economic incentives (i.e., referred to “Seguro Desemprego,” a category of social insurance in Brazil) (Brazil, 2003, 2009, 2015) could be provided to artisanal fishermen during the proposed temporal closures and to those who will not be able to fish or will have to change their techniques due to permanent spatial closures. Last, integrated evaluations of the effectiveness of conservation actions for benthic elasmobranchs and the Alcatrazes ecosystem must consider the associated areas, since they have provide essential services to the ecosystem’s maintenance (Rolim et al., 2019; Contente et al., 2020). If these measures are taken into account, a network with key habitats along the coast (e.g., nursery, reproduction and feeding places) could be developed, assisting the conservation of elasmobranch populations in the southeastern Brazil and consequently, enhancing the WRA and TES efficacy.
The original contributions presented in the study are included in the article/Supplementary Material, further inquiries can be directed to the corresponding author.
The animal study was reviewed and approved by the Ethics Committee of Animal Use of the Oceanographic Institute of the University of São Paulo (CEUA IO-USP) and by the Chico Mendes Institute for Biodiversity Conservation (ICMBio) under the survey permit SISBIO/55824.
TK, JD, and EB conceptualized this study. TK, RG, and JD collected abiotic and biotic samples, with PP, identified functional groups. TK and RG performed the sedimentological analyses. TK, EB, and PP performed statistical analyses and results interpretation. TK prepared the original draft. EB, JD, PP, and RG reviewed the manuscript. All authors contributed to the manuscript elaboration and approved the submitted version.
PP received productivity grants from the National Council for Scientific and Technological Development (CNPq) (Proc. 28447/2018–0) and the Fundação de Amparo à Pesquisa do Estado do Rio de Janeiro (FAPERJ) (E-26/202.607/2019 – 246952). The Coordination for the Improvement of Higher Education Personnel (CAPES) provided national (Finance Code 001) and international (88887.371157/2019-00) scholarships to TK, through the programs PROEX and PrInt, respectively. The University of Miami hosted part of TK’s Ph.D. at The Rosenstiel School of Marine and Atmospheric Sciences (RSMAS) as a visiting student. The Oceanographic Institute of the University of São Paulo and the Chico Mendes Institute for Biodiversity Conservation - Alcatrazes financed oceanographic cruises (fuel, food on board, and vessel personnel).
The authors declare that the research was conducted in the absence of any commercial or financial relationships that could be construed as a potential conflict of interest.
All claims expressed in this article are solely those of the authors and do not necessarily represent those of their affiliated organizations, or those of the publisher, the editors and the reviewers. Any product that may be evaluated in this article, or claim that may be made by its manufacturer, is not guaranteed or endorsed by the publisher.
We are indebted to undergraduate and graduate students, especially to Natasha. T. Hoff, Francesco Chioatto and Giovanna A. Garcia from ECORREP, who had participated in oceanographic expeditions and laboratorial analysis, assisting on data acquisition. We thank the Masters and crews of R/Vs Alpha Delphini and Soloncy Moura for all support provided. We are deeply grateful to José L. Figueiredo and Michel M. de Mahiques, as well as the laboratorial assistants, Michel Gianeti and Edilson Oliveira, for the use of laboratorial facilities, guidance on identification of Pseudobatos sp. specimen’s and sedimentological analyses to TK. We would like to thank the biologist Joana D. Ho and Marcella F. de Almeida for the amazing scientific illustrations and visual content design. This study was possible thanks to the collaboration between the Oceanographic Institute of the University of São Paulo and the Chico Mendes Institute for Biodiversity Conservation - Alcatrazes, which provided logistical and financial support for campaigns and laboratorial analyses. This article is part of TK Ph.D. thesis in Oceanography under supervision of JD (IO – USP) and EB (RSMAS – UM).
The Supplementary Material for this article can be found online at: https://www.frontiersin.org/articles/10.3389/fmars.2021.694846/full#supplementary-material
Aguiar, A. A., and Valentin, J. L. (2010). Biologia e ecologia alimentar de elasmobrânquios (Chondrichthyes: Elasmobranchii): uma revisão dos métodos e do estado da arte no Brasil. Oecol. Aust. 14, 464–489. doi: 10.4257/oeco.2010.1402.09
Aguiar, A. A., Valentin, J. L., and Rosa, R. S. (2009). Habitat use by Dasyatis americana in a south-western Atlantic oceanic Island. J. Mar. Biol. Assoc. U.K. 89, 1147–1152. doi: 10.1017/S0025315409000058
Angelini, R., Contente, R. F., Rossi-Wongtschowski, C. L. D. B., Soares, L. S. H., Schaeffer-Novelli, Y., Lopes, R. M., et al. (2018). Ecosystem modeling as a framework to convert a multi-disciplinary research approach into a useful model for the Araçá Bay (Brazil). Ocean Coast. Manag. 164, 92–103. doi: 10.1016/j.ocecoaman.2018.02.007
Babcock, E. A., Coleman, R., Karnauskas, M., and Gibson, J. (2013). Length-based indicators of fishery and ecosystem status: Glover’s Reef Marine Reserve, Belize. Fish. Res. 147, 434–445. doi: 10.1016/j.fishres.2013.03.011
Babcock, E. A., Tewfik, A., and Burns-Perez, V. (2018). Fish community and single-species indicators provide evidence of unsustainable practices in a multi-gear reef fishery. Fish. Res. 208, 70–85. doi: 10.1016/j.fishres.2018.07.003
Barbini, S. A., Lucifora, L. O., and Hozbor, N. M. (2011). Feeding habits and habitat selectivity of the shortnose guitarfish, Zapteryx brevirostris (Chondrichthyes, Rhinobatidae), off North Argentina and Uruguay. Mar. Biol. Res. 7, 365–377. doi: 10.1080/17451000.2010.515229
Barletta, M., Cysneiros, F. J. A., and Lima, A. R. A. (2016). Effects of dredging operations on the demersal fish fauna of a South American tropical-subtropical transition estuary. J. Fish Biol. 89, 890–920. doi: 10.1111/jfb.12999
Bartoń, K. (2020). MuMIn: Multi-Model Inference. Available online at: https://cran.r-project.org/web/packages/MuMIn/index.html (accessed August 10, 2021).
Begon, M., Townsend, C. R., and Harper, J. L. (2006). Ecology: From Individuals to Ecosystems, 4th Edn. Oxford: Blackwell Publishing.
Beverton, R. J. H., and Holt, S. J. (1957). On the Dynamics of Exploited Fish Populations. London: Chapman and Hall.
Borcard, D., Gillet, F., and Legendre, P. (2011). Numerical Ecology with R, eds R. Gentleman, K. Hornik, and G. G. Parmigiani (New York, NY: Springer). doi: 10.1007/978-1-4419-7976-6
Bornatowski, H., Wosnick, N., Do Carmo, W. P. D., Corrêa, M. F. M., and Abilhoa, V. (2014). Feeding comparisons of four batoids (Elasmobranchii) in coastal waters of southern Brazil. J. Mar. Biol. Assoc. U.K. 94, 1491–1499. doi: 10.1017/S0025315414000472
Brazil (1987). Estações Ecológicas de Carijós, Pirapitinga e Tupinambás. Decreto No94.656 20 Julho 1987. Available online at: http://www.planalto.gov.br/ccivil_03/decreto/1980-1989/1985-1987/D94656.htm (accessed March 14, 2021).
Brazil (2003). Lei No 10.779, de 25 de Novembro de 2003. Available online at: https://www.planalto.gov.br/ccivil_03/leis/2003/L10.779compilado.htm (accessed August 10, 2021).
Brazil (2009). Lei No 11.959, de 29 de Julho de 2009. Available online at: http://www.planalto.gov.br/ccivil_03/_Ato2007-2010/2009/Lei/L11959.htm#art24 (accessed August 10, 2021).
Brazil (2015). Lei No 13.134, de 16 de Junho de 2015. Available online at: http://www.planalto.gov.br/ccivil_03/_ato2015-2018/2015/lei/l13134.htm (accessed August 10, 2021).
Brazil (2016). Refúgio da Vida Silvestre do Arquipélago de Alcatrazes no litoral norte do Estado de São Paulo. Decreto 2 Agosto 2016. Available online at: https://www.icmbio.gov.br/portal/images/stories/biodiversidade/UC-RPPN/decreto_sem_numero_02ago2016_cria_Revis_do_Arquipelogo_de_Alcatrazes.pdf (accessed March 14, 2021).
Burnham, K. P., and Anderson, D. R. (2002). Model Selection and Multimodel Inference: A Practical Information-Theoretic Approach, 2nd Edn. New York, NY: Springer.
Caltabellotta, F. P. (2014). Dinâmica Populacional das raias-violas, Rhinobatos horkelli, Rhinobatos percellens e Zapteryx brevirostris (Chondrichthyes, Rhinobatidae) da Plataforma Continental de São Paulo. Available online at: http://hdl.handle.net/11449/115721 (accessed August 10, 2021).
Caltabellotta, F. P., Siders, Z. A., Murie, D. J., Motta, F. S., Cailliet, G. M., and Gadig, O. B. F. (2019). Age and growth of three endemic threatened guitarfishes Pseudobatos horkelii, P. percellens and Zapteryx brevirostris in the western South Atlantic Ocean. J. Fish Biol. 95, 1236–1248. doi: 10.1111/jfb.14123
Campos, E. J. D., Velhote, D., and Silveira, I. C. A. (2000). Shelf break upwelling driven by Brazil Current cyclonic meanders. Geophys. Res. 27, 751–754.
Carneiro, M. H., de Almeida Kooling, J., Ávila-da-Silva, A. O., Mendonça, J. T., Namora, R. C., and de Miranda, L. V. (2013). A Pesca nas Áreas de Proteção Ambiental Marinha do Estado de São Paulo, Brasil, entre Agosto de 2008 e julho 2009. São Paulo: Instituto de Pesca.
Cartamil, D. P., Vaudo, J. J., Lowe, C. G., Wetherbee, B. M., and Holland, K. N. (2003). Diel movement patterns of the Hawaiian stingray, Dasyatis lata: implications for ecological interactions between sympatric elasmobranch species. Mar. Biol. 142, 841–847. doi: 10.1007/s00227-003-1014-y
Castro-Filho, B. M., and de Miranda, L. B. (1998). “Physical oceanography of the Western Atlatic continental shelf located between 4° N and 34°S—coastal segment (4°W),” in The Seas, eds A. R. Robinson and K. Brink (New York, NY: John Wiley and Sons), 209–251.
Castro-Filho, B. M., de Miranda, L. B., and Miyao, S. Y. (1987). Condições hidrográficas na plataforma continental ao largo de Ubatuba: variações sazonais e em média escala. Bol. Inst. Ocean. 35, 135–151. doi: 10.1590/s0373-55241987000200004
Chao, A. (1984). Nonparametric Estimation of the Scandinavian Journal of Statistics. Scand. J. Stat. 11, 265–270.
Chao, A., and Chiu, C.-H. (2016). Species Richness: Estimation and Comparison. Available online at: http://chao.stat.nthu.edu.tw/wordpress/paper/119.pdf (accessed August 10, 2021).
Chao, A., and Lee, S. M. (1992). Estimating the number of classes via sample coverage. J. Am. Stat. Assoc. 87, 210–217. doi: 10.1080/01621459.1992.10475194
Chao, A., Ma, K. H., Hsieh, T. C., and Chiu, C.-H. (2016). SpadeR: Species-Richness Prediction and Diversity Estimation with R. Available online at: https://rdrr.io/cran/SpadeR/man/SimilarityPair.html (accessed August 10, 2021).
Chapman, D. D., Pikitch, E. K., Babcock, E., and Shiyji, M. S. (2005). Marine reserve design and evaluation using automated acoustic telemetry: a case-study involving coral reef-associated sharks in the mesoamerican Caribbean. Mar. Technol. Soc. J. 39, 42–55. doi: 10.4031/002533205787521640
Chiu, C. H., Wang, Y. T., Walther, B. A., and Chao, A. (2014). An improved nonparametric lower bound of species richness via a modified good-turing frequency formula. Biometrics 70, 671–682. doi: 10.1111/biom.12200
Claudet, J., Osenberg, C. W., Domenici, P., Badalamenti, F., Milazzo, M., Falcón, J. M., et al. (2010). Marine reserves: fish life history and ecological traits matter. Ecol. Appl. 20, 830–839. doi: 10.1890/08-2131.1
Cochrane, K. L., and Garcia, S. M. (eds) (2009). A Fishery Manager’s Guidebook, 2nd Edn. Rome: FAO. doi: 10.1002/9781444316315
Collins, A. B., Heupel, M. R., and Motta, P. J. (2007). Residence and movement patterns of cownose rays Rhinoptera bonasus within a south-west Florida estuary. J. Fish Biol. 71, 1159–1178. doi: 10.1111/j.1095-8649.2007.01590.x
Colonello, J. C., García, M. L., Lasta, C. A., and Menni, R. C. (2012). Reproductive biology of the spotback skate Atlantoraja castelnaui in the south-west Atlantic Ocean. J. Fish Biol. 80, 2405–2419. doi: 10.1111/j.1095-8649.2012.03288.x
Colonello, J. C., García, M. L., and Menni, R. C. (2011). Reproductive biology of the lesser guitarfish Zapteryx brevirostris from the south-western Atlantic Ocean. J. Fish Biol. 78, 287–302. doi: 10.1111/j.1095-8649.2010.02864.x
Contente, R. F., Mancini, P. L., Vaz-dos-Santos, A. M., Soares, L. S. H., Fischer, L. G., Silveira, L. F., et al. (2020). Spatial-seasonal variability of vertebrate assemblages in a Neotropical tidal flat: recommendations for monitoring the potential impacts of port expansion. Reg. Stud. Mar. Sci. 34, 101013. doi: 10.1016/j.rsma.2019.101013
D’Alberto, B. M., Carlson, J. K., Pardo, S. A., and Simpfendorfer, C. A. (2019). Population productivity of shovelnose rays: inferring the potential for recovery. PLoS One 14:e0225183. doi: 10.1371/journal.pone.0225183
De Léo, F. C., and Pires-Vanin, A. M. S. (2006). Benthic megafauna communities under the influence of the South Atlantic Central Water intrusion onto the Brazilian SE shelf: a comparison between an upwelling and a non-upwelling ecosystem. J. Mar. Syst. 60, 268–284. doi: 10.1016/j.jmarsys.2006.02.002
Dias, J. F., da Rocha, M. L. F., Schmidt, T. C., dos, S., Villamarin, B. C., and Morais, D. B. (2017). Ichthyofauna as an environmental quality indicator of the Bertioga channel, São Paulo (Brazil). Braz. J. Oceanogr. 65, 29–43. doi: 10.1590/S1679-87592017125206501
Domingos, J. F., dos, S., Paiva, B., Bruno, C. E. M., and de Amorim, A. F. (2021). Diet of elasmobranchs captured in the fishing of pink shrimp off souther of Brazil. Braz. J. Anim. Environ. Res. 4, 637–658. doi: 10.34188/bjaerv4n1-054
Edgar, G. J., Banks, S. A., Bessudo, S., Cortés, J., Guzmán, H. M., Henderson, S., et al. (2011). Variation in reef fish and invertebrate communities with level of protection from fishing across the Eastern Tropical Pacific seascape. Glob. Ecol. Biogeogr. 20, 730–743. doi: 10.1111/j.1466-8238.2010.00642.x
Edgar, G. J., Bustamante, R. H., Fariña, J. M., Calvopiña, M., Martínez, C., and Toral-Granda, M. V. (2004). Bias in evaluating the effects of marine protected areas: the importance of baseline data for the Galapagos Marine Reserve. Environ. Conserv. 31, 212–218. doi: 10.1017/S0376892904001584
Edgar, G. J., Stuart-Smith, R. D., Willis, T. J., Kininmonth, S., Baker, S. C., Banks, S., et al. (2014). Global conservation outcomes depend on marine protected areas with five key features. Nature 506, 216–220. doi: 10.1038/nature13022
Elliott, M., Whitfield, A. K., Potter, I. C., Blaber, S. J. M., Cyrus, D. P., Nordlie, F. G., et al. (2007). The guild approach to categorizing estuarine fish assemblages: a global review. Fish Fish. 8, 241–268. doi: 10.1111/j.1467-2679.2007.00253.x
Farrugia, T. J., Espinoza, M., and Lowe, C. G. (2011). Abundance, habitat use and movement patterns of the shovelnose guitarfish (Rhinobatos productus) in a restored southern California estuary. Mar. Freshw. Res. 62, 648–657. doi: 10.1071/MF10173
Fernandes, I. M., Bastos, Y. F., Barreto, D. S., Lourenço, L. S., and Penha, J. M. (2017). The efficacy of clove oil as an anaesthetic and in euthanasia procedure for small-sized tropical fishes. Braz. J. Biol. 77, 444–450. doi: 10.1590/1519-6984.15015
Figueiredo, J. L. (1977). Manual de Peixes Marinhos do Sudeste do Brazil I. Introdução cações, Raias e Quimeras. São Paulo: Museu de Zoologia da Universidade de São Paulo.
Folk, R. L., and Ward, W. C. (1957). Brazos River bar: a study in the significance of grain size parameters. J. Sediment. Petrol. 27, 3–26.
Forestry Foundation, S. P. S. (2019). Plano de Manejo da Área de Proteção Ambiental Marina Litoral Centro. São Paulo. Available online at: https://sigam.ambiente.sp.gov.br/sigam3/Repositorio/511/Documentos/APAM_LC/2019.02.26_Plano_Manejo_APAMLC.pdf (accessed August 10, 2021).
Forestry Foundation, S. P. S. (2020). Plano de Manejo da Área de Proteção Ambiental Marinha do Litoral Norte. São Paulo. Available online at: https://www.sigam.ambiente.sp.gov.br/sigam3/Repositorio/511/Documentos/APAM_LN/APAMLN_Plano_de_manejo_CTBio.pdf (accessed August 10, 2021).
Fox, H. E., Mascia, M. B., Basurto, X., Costa, A., Glew, L., Heinemann, D., et al. (2012). Reexamining the science of marine protected areas: linking knowledge to action. Conserv. Lett. 5, 1–10. doi: 10.1111/j.1755-263X.2011.00207.x
Freitas, R. H. A., Aguiar, A. A., Freitas, A. K. C. H. A., Lima, S. M. Q., and Valentin, J. L. (2019). Unravelling the foraging behavior of the southern stingray, Hypanus americanus (Myliobatiformes: Dasyatidae) in a southwestern atlantic MPA. Neotrop. Ichthyol. 17, 1–14. doi: 10.1590/1982-0224-20180131
Genz, A., Bretz, F., Miwa, T., Mi, X., Leisch, F., Scheipl, F., et al. (2020). mvtnorm: Multivariate Normal and t Distributions. Available online at: https://cran.r-project.org/web/packages/mvtnorm/index.html (accessed August 10, 2021).
Giakoumi, S., McGowan, J., Mills, M., Beger, M., Bustamante, R. H., Charles, A., et al. (2018). Revisiting “success” and “failure” of marine protected areas: a conservation scientist perspective. Front. Mar. Sci. 5:223. doi: 10.3389/fmars.2018.00223
Gibran, F. Z., and de Moura, R. L. (2012). The structure of rocky reef fish assemblages across a nearshore to coastal islands’ gradient in Southeastern Brazil. Neotrop. Ichthyol. 10, 369–382. doi: 10.1590/S1679-62252012005000013
Gill, D. A., Mascia, M. B., Ahmadia, G. N., Glew, L., Lester, S. E., Barnes, M., et al. (2017). Capacity shortfalls hinder the performance of marine protected areas globally. Nature 543, 665–669. doi: 10.1038/nature21708
Gomes, U. L., Santos, H. R., Gadig, O. B. F., Signori, C. N., and Vicente, M. M. (2019). Guia para identificação dos Tubarões, Raias e Quimeras do Rio de Janeiro. Rev. Nord. Biol. 27, 171–368. doi: 10.22478/ufpb.2236-1480.2019v27n1.47122
Goodyear, C. P. (1993). Spawning stock biomass per recruit in fisheries management: foundation and current use. Can. Spec. Publ. Fish. Aquat. Sci. 120, 67–81.
Grüss, A., Kaplan, D. M., Guénette, S., Roberts, C. M., and Botsford, L. W. (2011). Consequences of adult and juvenile movement for marine protected areas. Biol. Conserv. 144, 692–702. doi: 10.1016/j.biocon.2010.12.015
Halpern, B. S. (2003). The impact of marine reserves: Do reserves work and does reserve size matter? Ecol. Appl. 13, 117–137.
Hartig, F., and Lohse, L. (2020). DHARMa: Residual Diagnostics for Hierarchical (Multi-Level/Mixed) Regression Models. Available online at: http://florianhartig.github.io/DHARMa/ (accessed August 10, 2021).
Hilborn, R. (2016). Policy: marine biodiversity needs more than protection. Nature 535, 224–226. doi: 10.1038/535224a
Hildebrand, S. F., and Schroeder, W. C. (1928). Fishes of Chesapeake Bay. Bulletin of the Bureau of Fisheries, 43, 1–366.
Hilborn, R., and Walters, C. J. (1992). “Statistical catch-at-age methods,” in Quantitative Fisheries Stock Assessment, eds R. Hilborn and C. J. Walters (Boston, MA: Springer), 369–390. doi: 10.1007/978-1-4615-3598-0_11
Hoff, N. T. (2015). Integridade Biótica dos Ecossistemas na região do Arquipélago dos Alcatrazes, São Sebastião - SP. Master’s dissertation. São Paulo: Instituto Oceanográfico.
Hoff, N. T., Figueira, R. C. L., and Abessa, D. M. S. (2015). Levels of metals, arsenic and phosphorus in sediments from two sectors of a Brazilian Marine Protected Area (Tupinambás Ecological Station). Mar. Pollut. Bull. 91, 403–409. doi: 10.1016/j.marpolbul.2014.10.044
Hordyk, A. (2019). LBSPR: Length-Based Spawning Potential Ratio. Available online at: https://cran.r-project.org/web/packages/LBSPR/index.html (accessed August 10, 2021).
Hordyk, A., Ono, K., Valencia, S., Loneragan, N., and Prince, J. (2015). A novel length-based empirical estimation method of spawning potential ratio (SPR), and tests of its performance, for small-scale, data-poor fisheries. ICES J. Mar. Sci. 72, 217–231. doi: 10.1093/icesjms/fsu004
IBAMA (2007). Instrução Normativa IBAMA No 166 de 18 de JULHO de 2007. Available online at: https://www.icmbio.gov.br/cepsul/images/stories/legislacao/Instrucao_normativa/2007/in_ibama_166_2007_redeemalhe_suspensa_p_ibama_icmbio_7_2008_vigente.pdf (accessed August 10, 2021).
IBAMA (2008). Instrução Normativa IBAMA No 189, de 23 de Setembro de 2008. Available online at: https://www.gov.br/agricultura/pt-br/assuntos/aquicultura-e-pesca/legislacao/defesos/in-ibama-no-189_09_2008.pdf/view (accessed August 10, 2021).
ICMBio (2016a). Avaliação do risco de extinção dos elasmobrânquios e quimeras no Brasil: 2010-2012. Itajaí: ICMBio.
ICMBio (2016b). Sumário Executivo do Plano de Ação Nacional para a conservação dos Tubarões e Raias Marinhos Ameaçados de Extinção. Available online at: https://www.icmbio.gov.br/portal/faunabrasileira/plano-de-acao-nacional-lista/2839-plano-de-acao-nacional-para-a-conservacao-dos-tubaroes (accessed August 10, 2021).
ICMBio (2017). Plano de Manejo da Estação Ecológica Tupinambás e Refúgio de Vida Silvestre do arquipélago de Alcatrazes. Available online at: http://www.icmbio.gov.br/portal/images/stories/plano-de-manejo/plano_de_manejo_esec_tupinambas_revisarquipelogoalcatrazes_vol1.pdf (accessed August 10, 2021).
Imoto, R. D., Carneiro, M. H., and Ávila-da-Silva, A. O. (2016). Spatial patterns of fishing fleets on the Southeastern Brazilian Bight. Lat. Am. J. Aquat. Res. 44, 1005–1018. doi: 10.3856/vol44-issue5-fulltext-12
IUCN (2021). The IUCN Red List of Threatened Species. 2020-3. Available online at: https://www.iucnredlist.org (accessed March 14, 2021).
Kelley, D., Richards, C., and Layton, C. (2021). oce: Analysis of Oceanographic Data package. Available online at: https://cran.r-project.org/package=oce (accessed August 10, 2021).
Knip, D. M., Heupel, M. R., and Simpfendorfer, C. A. (2012). Evaluating marine protected areas for the conservation of tropical coastal sharks. Biol. Conserv. 148, 200–209. doi: 10.1016/j.biocon.2012.01.008
Larsonneur, C., Bouysse, P., and Auffret, J.-P. (1982). The superficial sediments of the English Channel and its Western Approaches. Sedimentology 29, 851–864.
Lessa, R. P., Vooren, C. M., and Lahaye, J. (1986). Desenvolvimento e ciclo sexual das fêmeas, migrações e fecundidade da viola, Rhinobatos horkelii (Müller & Henle, 1841) do Sul do Brasil. Atlântica 8, 5–34.
Lesueur, C. A. (1824). Description of several species of the Linnaean genus Raia, of North America. J. Acad. Nat. Sci. Philadel. 4, 100–121.
Lubchenco, J., and Grorud-Colvert, K. (2015). Making waves: the science and politics of ocean protection. Science 350, 382–383. doi: 10.1126/science.aad5443
Luiz-Silva, W., Matos, R. H. R., and Kristosch, G. C. (2002). Geoquímica e índice de geoacumulação de mercúrio em sedimentos de superfície do estuário de Santos - Cubatão (SP). Quim. Nova 25, 753–756. doi: 10.1590/s0100-40422002000500009
Magurran, A. E., and Henderson, P. A. (2003). Explaining the excess of rare species in natural species abundance distributions. Nature 422, 714–716. doi: 10.1038/nature01547
Mahiques, M. M., Mishima, Y., and Rodrigues, M. (1999). Characteristics of the sedimentary organic matter on the inner and middle continental shelf between Guanabara Bay and Sao Francisco do Sul, southeastern Brazilian margin. Cont. Shelf Res. 19, 775–798. doi: 10.1016/S0278-4343(98)00105-8
Mahiques, M. M., Sousa, S. H. M., Burone, L., Nagai, R. H., Silveira, I. C. A., Figueira, R. C. L., et al. (2011). Radiocarbon geochronology of the sediments of the São Paulo Bight (southern Brazilian upper margin). An. Acad. Bras. Cienc. 83, 817–834. doi: 10.1590/S0001-37652011005000028
Mahiques, M. M., Tessler, M. G., Maria Ciotti, A., Da Silveira, I. C. A., E Sousa, S. H. D. M., Figueira, R. C. L., et al. (2004). Hydrodynamically driven patterns of recent sedimentation in the shelf and upper slope off Southeast Brazil. Cont. Shelf Res. 24, 1685–1697. doi: 10.1016/j.csr.2004.05.013
Marini, T. L. (1936). Revisión de las especies de la familia Squatinidae en las aguas argentinas (S. guggenheim n. sp.). Physis 12, 19–30.
Marconi, M., Giglio, V. J., Pereira-Filho, G. H., and Motta, F. S. (2020). Does quality of scuba diving experience vary according to the context and management regime of marine protected areas? Ocean Coast. Manag. 194:105246. doi: 10.1016/j.ocecoaman.2020.105246
Marion, C., Vaske-Junior, T., Gadig, O., and Martins, I. (2011). Feeding habits of the shortnose guitarfish, Zapteryx brevirostris (Müller and Henle, 1841) (Elasmobranchii, Rhinobatidae) in southeastern Brazil. Braz. J. Biol. 71, 83–89. doi: 10.1590/s1519-69842011000100013
Martins, I. A., Martins, C. L., and Leme, M. H. D. A. (2005). Biological parameters and population structure of Psammobatis extenta in Ubatuba region, north coast of the State of São Paulo, Brazil. J. Mar. Biol. Assoc. U.K. 85, 1113–1118. doi: 10.1017/S0025315405012166
Mémery, L., Arhan, M., Alvarez-Salgado, X. A., Messias, M. J., Mercier, H., Castro, C. G., et al. (2000). The water masses along the western boundary of the south and equatorial Atlantic. Prog. Oceanogr. 47, 69–98.
Menni, R. C., Jaureguizar, A. J., Stehmann, M. F. W., and Lucifora, L. O. (2010). Marine biodiversity at the community level: zoogeography of sharks, skates, rays and chimaeras in the southwestern Atlantic. Biodivers. Conserv. 19, 775–796. doi: 10.1007/s10531-009-9734-z
Menni, R. C., and Stehmann, M. F. (2000). Distribution, environment and biology of batoid fishes off Argentina, Uruguay and Brazil. A review. Rev. Mus. Argentino Cienc. Nat. 2, 69–109.
Miranda Ribeiro, A. M. (1907). Fauna braziliense. Peixes (Desmobrânchios). Arch. Museu Nactl. 14, 137–171.
Mitchill, S. L. (1815). The fishes of New-York, described and arranged. Transact. Liter. Philos. Soc. N. Y. 1, 355–492.
MMA (2014). Portaria No 445, de 17 de Dezembro de 2014. 11. Available online at: https://www.icmbio.gov.br/portal/images/stories/docs-plano-de-acao-ARQUIVO/00-saiba-mais/05_-_PORTARIA_MMA_N%C2%BA_445_DE_17_DE_DEZ_DE_2014.pdf (accessed August 10, 2021).
Morais, R. A., Ferreira, C. E. L., and Floeter, S. R. (2017). Spatial patterns of fish standing biomass across Brazilian reefs. J. Fish Biol. 91, 1642–1667. doi: 10.1111/jfb.13482
Muto, E. Y., Soares, L. S., and Wongtschowski, C. (2000). Demersal fish assemblages off São Sebastião, southeastern Brazil: structure and environmental conditioning factors (summer 1994). Rev. Bras. Oceanogr. 48, 9–27.
Müller, J. (1836). Vergleichende Anatomie der Myxinoiden, der Cyclostomen mit durchbohrtem Gaumen. Erster Theil. Osteologie und Myologie. Abhandlungen der K niglichen Akademie der Wissenschaften, Berlin, 1834, 65–340.
Müller, J., and Henle, F. G. J. (1841). Systematische Beschreibung der Plagiostomen. Berlin: von Veit und Comp, 1–28.
Oddone, M. C., Amorim, A. F., Mancini, P. L., Norbis, W., and Velasco, G. (2007). The reproductive biology and cycle of Rioraja agassizi (Müller and Henle, 1841) (Chondrichthyes: Rajidae) in southeastern Brazil, SW Atlantic Ocean. Sci. Mar. 71, 593–604. doi: 10.3989/scimar.2007.71n3593
Oddone, M. C., and de Amorim, A. F. (2007). Length-weight relationships, condition and population structure of the genus Atlantoraja (Elasmobranchii, Rajidae, Arhynchobatinae) in Southeastern Brazilian waters, SW Atlantic Ocean. J. Northwest Atl. Fish. Sci. 38, 43–52. doi: 10.2960/J.v38.m599
Oddone, M. C., Norbis, W., Mancini, P. L., and Amorim, A. F. (2008). Sexual development and reproductive cycle of the Eyespot skate Atlantoraja cyclophora (Regan, 1903) (Chondrichthyes: Rajidae: Arhynchobatinae), in southeastern Brazil. Acta Adriat. 49, 73–87.
Oddone, M. C., and Vooren, C. M. (2004). Distribution, abundance and morphometry of Atlantoraja cyclophora (Regan, 1903) (Elasmobranchii: Rajidae) in southern Brazil, Southwestern Atlantic. Neotrop. Ichthyol. 2, 137–144. doi: 10.1590/S1679-62252004000300005
Oddone, M. C., and Vooren, C. M. (2005). Reproductive biology of Atlantoraja cyclophora (Regan 1903) (Elasmobranchii: Rajidae) off southern Brazil. ICES J. Mar. Sci. 62, 1095–1103. doi: 10.1016/j.icesjms.2005.05.002
Oksanen, J., Blanchet, F. G., Friendly, M., Kindt, R., Legendre, P., McGlinn, D., et al. (2020). Vegan: Community Ecology Package. Available online at: https://cran.r-project.org/web/packages/vegan/index.html (accessed August 10, 2021).
Paijmans, K. C., Booth, D. J., and Wong, M. Y. L. (2019). Towards an ultimate explanation for mixed-species shoaling. Fish Fish. 20, 921–933. doi: 10.1111/faf.12384
Paiva-Filho, A. M., Schmiegelow, J. M. M., Giannini, R., and Ribeiro-Neto, F. B. (1989). Contribuição ao conhecimento da ictiofauna da região da Ilha de Alcatrazes (SP), Brasil. Relat. Int. Inst. Ocean. 25, 1–6.
Palmeira, A. R. O. (2012). Biologia Reprodutiva da raia Dasyatis guttata (Bloch & Schneider, 1801) (Myliobatiformes: Dasyatidae) no litoral do Pará. Master’s thesis. João Pessoa: Universidade Federal da Paraíba.
Palóczy, A., Sartoretto, J. R., Hoff, N. T., Marques, O. B., Oliveira, R., and Biló, T. C. (2012). ESEC Tupinambás: Relatório de Levantamento de Dados Primários – Meio Físico. São Paulo.
Pincinato, R. B. M., and Gasalla, M. A. (2019). Exploring simple ecological indicators on landings and market trends in the South Brazil Shelf Large Marine Ecosystem. Fish. Manag. Ecol. 26, 200–210. doi: 10.1111/fme.12340
Pires-Vanin, A. M. S. (2008). “Megafauna e macrofauna,” in Oceanografia de um Ecossistema Subtropical: Plataforma de São Sebastião, SP, eds S. Biral, M. Vizentin, and C. F. Fontana (São Paulo: EDUSP), 464.
Pires-Vanin, A. M. S., Rossi-Wongtschwski, C. L. D. B., Aidar, E., Mesquita, H. S. L., Soares, L. S. H., Katsuragawa, M., et al. (1993). Estrutura e Função do Ecossistema de Platafoma Continental do Atlântico Sul Brasileiro: Síntese dos Resultados. São Paulo: Universidade de São Paulo, 217–231.
Ponz-Louro, M. (1995). Estratégias e Táticas Reprodutivas de Elasmobrânquios no Ecossistema de Ubatuba, SP, Brasil. Master’s thesis. São Paulo: Universidade de São Paulo.
Prado, H. M., Schlindwein, M. N., Murrieta, R. S. S., Nascimento, D. R., de Souza, E. P., Cunha Lignon, M., et al. (2019). The valo grande channel in the Cananéia-Iguape estuary-lagoon complex (SP, Brazil): environmental history, ecology, and future perspectives. Ambient. Soc. 22, 1–24. doi: 10.1590/1809-4422ASOC0182R2VU19L4TD
R Core Team (2020). R: A Language and Environment for Statistical Computing. Vienna: R Foundation for Statistical Computing.
Rangel, B. D. S., Hussey, N. E., Niella, Y., Martinelli, L. A., Gomes, A. D. O., and Moreira, R. G. (2020). Neonatal nutritional strategy of a viviparous elasmobranch with extremely low reproductive output. Mar. Ecol. Prog. Ser. 638, 107–121. doi: 10.3354/meps13261
Rangel, B. S., Hammerschlag, N., Sulikowski, J., and Guimarães Moreira, R. (2021). Foraging for nutrients? Dietary and reproductive biomarkers in a generalist apex predator reveal differences in nutritional ecology across life stages. Mar. Ecol. Prog. Ser. 664, 149–163. doi: 10.3354/meps13640
Rangel, B. S., Rodrigues, A., and Moreira, R. G. (2018). Use of a nursery area by cownose rays (Rhinopteridae) in southeastern Brazil. Neotrop. Ichthyol. 16, 1–8. doi: 10.1590/1982-0224-20170089
Regan, C. T. (1903). On a collection of fishes made by Dr. Goeldi at Rio Janeiro. Proc. Zool. Soc. Lond. 2, 59–68.
Ribeiro, V. V. (2020). Índice de Poluição por Pellets (IPP) na Baía de Santos (SP) no inverno de 2019. Rev. Sítio Novo 4:102. doi: 10.47236/2594-7036.2020.v4.i2.102-111p
Ripley, B., Venables, B., Bates, D. M., Hornik, K., Gebhardt, A., and Firth, D. (2021). MASS: Support Functions and Datasets for Venables and Ripley’s MASS. Available online at: https://cran.r-project.org/web/packages/MASS/index.html
Rocha, F., and Gadig, O. B. F. (2013). Reproductive biology of the guitarfish Rhinobatos percellens (Chondrichthyes, Rhinobatidae) from the São Paulo Coast, Brazil, western South Atlantic Ocean. J. Fish Biol. 82, 306–317. doi: 10.1111/j.1095-8649.2012.03493.x
Rolim, F. A., Langlois, T., Rodrigues, P. F. C., Bond, T., Motta, F. S., Neves, L. M., et al. (2019). Network of small no-take marine reserves reveals greater abundance and body size of fisheries target species. PLoS One 14:e0204970. doi: 10.1371/journal.pone.0204970
Rossi-Wongtschowski, C. L. D. B., and Paes, E. T. (1993). Padrões Espaciais e Temporais da Comunidade de Peixes Demersais do Litoral Norte do Estado de São Paulo-Ubatuba, Brasil. (São Paulo: Universidade de São Paulo), 169–188.
São Paulo (2008). Mosaico das Ilhas e Áreas Marinhas Protegidas do Litoral Paulista. Decreto No 53.528 8 Outubro 2008. Available online at: https://www.al.sp.gov.br/repositorio/legislacao/decreto/2008/decreto-53528-08.10.2008.html (Accessed March 14, 2021).
São Paulo (2009). Resolução SMA No 69 de 28 de Setembro de 2009. Available online at: https://www.cetesb.sp.gov.br/licenciamento/documentos/2009_Res_SMA_69.pdf (accessed August 10, 2021).
São Paulo (2012). Resolução SMA N° 51, de 28 de Junho de 2012. Available online at: http://www.mpsp.mp.br/portal/page/portal/cao_urbanismo_e_meio_ambiente/legislacao/leg_estadual/leg_est_resolucoes/Resol-SMA-51-12-uso-rede-pesca-litoral-centro.pdf (accessed August 10, 2021).
Santos, H. R. S., and Carvalho, M. R. (2004). Description of a new species of whiptailed stingray from the southwestern Atlantic Ocean (Chondricthyes, Myliobatiformes, Dasyatidae). Bol. Mus. N. S. Zool. 516, 1–24.
Schlaff, A. M., Heupel, M. R., and Simpfendorfer, C. A. (2014). Influence of environmental factors on shark and ray movement, behaviour and habitat use: a review. Rev. Fish Biol. Fish. 24, 1089–1103. doi: 10.1007/s11160-014-9364-8
Shimabukuro, M., Bromberg, S., and Pires-Vanin, A. M. S. (2016). Polychaete distribution on the southwestern Atlantic continental shelf. Mar. Biol. Res. 12, 239–254. doi: 10.1080/17451000.2015.1131299
Siqueira, G. W., de Santis Braga, E., Pereira, S. F. P., and da Silva, E. (2005). Distribuição do mercúrio em sedimentos de fundo no Estuário de Santos SP/Brasil. Rev. Esc. Minas 58, 309–316. doi: 10.1590/s0370-44672005000400004
Slabbekoorn, H., Bouton, N., van Opzeeland, I., Coers, A., ten Cate, C., and Popper, A. N. (2010). A noisy spring: the impact of globally rising underwater sound levels on fish. Trends Ecol. Evol. 25, 419–427. doi: 10.1016/j.tree.2010.04.005
Soares, L. S. H., Muto, E. Y., Gasparro, M. R., and Rossi-Wongtschwski, C. L. D. B. (2008). “Organização trófica dos peixes,” in Oceanografia de um Ecossistema Subtropical: Plataforma de São Sebastião, SP, eds S. Biral, M. Vizentin, and C. F. Fontana (São Paulo: EDUSP), 464.
Soares, L. S. H., Rossi-Wongtschowski, C. L. D. B., Alvares, L. M. C., Muto, E. Y., and Gasalla, M. L. A. (1992). Grupos tróficos de peixes demersais da plataforma continental interna de Ubatuba, Brasil: I. Chondrichthyes. Bol. Inst. Ocean. 40, 79–85. doi: 10.1590/s0373-55241992000100006
Stramma, L., and England, M. (1999). On the water masses and mean circulation of the South Atlantic Ocean. J. Geophys. Res. 104, 20863–20883.
SUDEPE (1984). Portaria SUDEPE N° 42 de 18 de Outubro de 1984. Available online at: https://www.icmbio.gov.br/cepsul/images/stories/legislacao/Portaria/1984/p_sudepe_42_n_1984_defesobagrerosado_rs_sc_pr_sp.pdf (accessed August 10, 2021).
Takase, L. S., Stein, L. P., Hoff, N. T., and Siegle, E. (2021). Wave climate and power distribution around a rocky island: Alcatrazes, Brazil. Ocean Coast. Res. 69:14.
Tilley, A., López-Angarita, J., and Turner, J. R. (2013). Effects of scale and habitat distribution on the movement of the southern stingray Dasyatis americana on a Caribbean atoll. Mar. Ecol. Prog. Ser. 482, 169–179. doi: 10.3354/meps10285
Trevizani, T. H., Domit, C., Vedolin, M. C., Angeli, J. L. F., and Figueira, R. C. L. (2019). Assessment of metal contamination in fish from estuaries of southern and southeastern Brazil. Environ. Monit. Assess. 191:308. doi: 10.1007/s10661-019-7477-1
Viana, S. T. F., de Carvalho, M. R., and Gomes, U. L. (2016). Taxonomy and morphology of species of the genus Squalus Linnaeus, 1758 from the Southwestern Atlantic Ocean (Chondrichthyes: Squaliformes: Squalidae). Zootaxa 4133, 1–89. doi: 10.11646/zootaxa.4133.1.1
Villaseńor-Derbez, J. C., Faro, C., Wright, M., Martínez, J., Fitzgerald, S., Fulton, S., et al. (2018). A user-friendly tool to evaluate the effectiveness of no-take marine reserves. PLoS One 13:e0191821. doi: 10.1371/journal.pone.0191821
Vögler, R., Milessi, A. C., and Quiñones, R. A. (2003). Trophic ecology of Squatina guggenheim on the continental shelf off Uruguay and northern Argentina. J. Fish Biol. 62, 1254–1267. doi: 10.1046/j.1095-8649.2003.00105.x
Vögler, R., Milessi, A. C., and Quiñones, R. A. (2008). Influence of environmental variables on the distribution of Squatina guggenheim (Chondrichthyes, Squatinidae) in the Argentine-Uruguayan Common Fishing Zone. Fish. Res. 91, 212–221. doi: 10.1016/j.fishres.2007.11.028
Vooren, C. M., and Klippel, S. (2005). Ações para a Conservação de Tubarões e Raias no Sul do Brasil, 1st Edn, eds C. M. Vooren and S. Klippel Porto (Igaré: Alegre).
Vooren, C. M., and Silva, K. G. (1991). On the taxonomy of the angel sharks from southern Brazil, with the description of Squatina occulta sp. n. Rev. Bras. Biol. 51, 589–602.
Walbaum, J. J. (1792). Petri Artedi Sueci Genera Piscium in Quibus Systema Totum Ichthyologiae Proponitur Cum Classibus, Ordinibus, Generum Characteribus, Specierum Diffentiis, Observationibus Plumiris. Ichthyologiae Pars III. Rose, Grypeswaldiae. 723.
Wetherbee, B. M., Gruber, S. H., and Rosa, R. S. (2007). Movement patterns of juvenile lemon sharks Negaprion brevirostris within Atol das Rocas, Brazil: a nursery characterized by tidal extremes. Mar. Ecol. Prog. Ser. 343, 283–293. doi: 10.3354/meps06920
Wiegand, J., Hunter, E., and Dulvy, N. K. (2011). Are spatial closures better than size limits for halting the decline of the North Sea thornback ray, Raja clavata? Mar. Freshw. Res. 62, 722–733. doi: 10.1071/MF10141
Zuur, A. F., Ieno, E. N., and Elphick, C. S. (2010). A protocol for data exploration to avoid common statistical problems. Methods Ecol. Evol. 1, 3–14. doi: 10.1111/j.2041-210x.2009.00001.x
Keywords: Alcatrazes archipelago, management strategies, habitat use, community structure, fishery indicators, elasmobranch conservation, functional diversity
Citation: Karlovic TC, Gomes RR, Paiva PC, Babcock EA and Dias JF (2021) Functionality and Effectiveness of Marine Protected Areas in Southeastern Brazilian Waters for Demersal Elasmobranchs. Front. Mar. Sci. 8:694846. doi: 10.3389/fmars.2021.694846
Received: 13 April 2021; Accepted: 04 August 2021;
Published: 01 September 2021.
Edited by:
Sophia N. Wassermann, University of California, Davis, United StatesReviewed by:
Rachel Amanda Skubel, University of Miami, United StatesCopyright © 2021 Karlovic, Gomes, Paiva, Babcock and Dias. This is an open-access article distributed under the terms of the Creative Commons Attribution License (CC BY). The use, distribution or reproduction in other forums is permitted, provided the original author(s) and the copyright owner(s) are credited and that the original publication in this journal is cited, in accordance with accepted academic practice. No use, distribution or reproduction is permitted which does not comply with these terms.
*Correspondence: Thamíris C. Karlovic, thamykarlovic@usp.br
†These authors have contributed equally to this work and share senior authorship
Disclaimer: All claims expressed in this article are solely those of the authors and do not necessarily represent those of their affiliated organizations, or those of the publisher, the editors and the reviewers. Any product that may be evaluated in this article or claim that may be made by its manufacturer is not guaranteed or endorsed by the publisher.
Research integrity at Frontiers
Learn more about the work of our research integrity team to safeguard the quality of each article we publish.