- 1CAS Key Laboratory of Marine Ecology and Environmental Sciences, Institute of Oceanology, Chinese Academy of Sciences, Qingdao, China
- 2Qingdao National Laboratory for Marine Science and Technology, Qingdao, China
- 3Center for Ocean Mega-Science, Chinese Academy of Sciences, Qingdao, China
- 4CAS Engineering Laboratory for Marine Ranching, Institute of Oceanology, Chinese Academy of Sciences, Qingdao, China
- 5University of Chinese Academy of Sciences, Beijing, China
- 6Tianjin Key Laboratory of Aqua-Ecology and Aquaculture, Fisheries College, Tianjin Agricultural University, Tianjin, China
Metamorphosis is a critical developmental event in mollusks, and neuroendocrine system plays an essential role in this process. Rapana venosa is an economically important shellfish in China, but the artificial technology of R. venosa aquaculture is limited by metamorphosis. As a carnivorous gastropod, food habit transition makes the mechanism of R. venosa metamorphosis more complex. To investigate the changes in the neuroendocrine system and to reveal its role in regulating the food habit transition and metamorphosis of R. venosa, we cloned the cDNA sequences encoding 5-hydroxytryptamine receptor (Rv-5HTR), nitric oxide synthetase (Rv-NOS) and cholecystokinin receptor (Rv-CCKR), and investigated their expression by quantitative real-time PCR analysis, and explore the spatio-temporal changes of 5-HT protein expression using Immunohistochemical (IHC) analysis. The expression of the three geens was significantly increased in the early intramembrane veliger stage, which indicates that the three genes are related to the development of digestive system. Additionally, expression of the three genes was decreased after metamorphosis, while Rv-NOS and Rv-CCKR were increasingly expressed in competent larvae, which may help the larvae find suitable environments and promote digestive system development for metamorphosis, and the result of 5-HT IHC analysis also reflects the development of neuroendocrine system. Furthermore, results show that CCK can effect the expression of digestive enzyme, NOS and 5-HT receptor. Finally, based on the present results, we hypothesized that CCK and CCK receptor may be critical regulatory factors of food habit transition and metamorphosis. These results might provide information on the development of neuroendocrine system of R. venosa, and new insight into the regulation of the food habit transition and metamorphosis of gastropods.
Introduction
Metamorphosis is a critical developmental event in the biphasic life cycle in mollusks that is evolutionarily crucial because it develops independently in different clades (Hadfield et al., 2000; Huan et al., 2015). Extensive morphological and physiological changes occur during metamorphosis but are accompanied by high mortality (Harding, 2006; Huan et al., 2015). Therefore, premetamorphosis recruitment and postmetamorphosis survival control mollusk population dynamics. To date, the mechanism of metamorphosis has been most well studied in bivalves (Coon et al., 1985; Satuito et al., 1999; Yang et al., 2007; Gireesh and Cherukara, 2008; Yang B. et al., 2015; Niu et al., 2016; Moore and Bringolf, 2018), and in gastropods, studies are mainly focused on abalone and some herbivorous snails (Barlow and Truman, 2010; Li et al., 2018). Numerous neuroactive compounds, including g-aminobutyric acid (GABA), various catecholamines, serotonin (5-HT), and nitric oxide (NO) are associated with metamorphic processes in a variety of mollusks, which has been extensively studied (Leise et al., 2001), and the neuroendocrine system plays an important role in the regulation of metamorphosis.
The veined rapa whelk (Rapana venosa), a native to temperate Asian waters (Harding, 2006), is an economically important gastropod snail in China due to its high economic and medicinal value (Dolashka et al., 2011; Nesterova et al., 2011; Olga et al., 2015; Voelter et al., 2016). Unfortunately, R. venosa is highly threatened, and its population has dramatically declined in recent years (Wei et al., 1999; Yang et al., 2007). Although artificial aquaculture was started early (Yuan, 1992; Yang et al., 2007), the large-scale culture of this species is seriously limited by its high mortality during metamorphosis. As a carnivorous gastropod, R. venosa needs to undergo a food habit transition from herbivorous to carnivorous during metamorphosis, which is different from the metamorphosis of lifelong herbivory gastropods and makes the mechanism of metamorphosis more complex. Our group has performed some studies on the metamorphosis of R. venosa, including studies of the differences in transcriptome, proteome, metabolome, digestive enzyme and microorganisms before and after metamorphosis (Song et al., 2016a,b,c; Yang et al., 2020a,b), as well as the effect of bait induction on the rate of metamorphosis (Yu et al., 2020). Song et al. (2016a) mentioned that the expression of the NOS and 5-HT receptors decreased after metamorphosis (Song et al., 2016a). However, there is no further study on the regulation of the neuroendocrine system on the metamorphosis of R. venosa.
Bather (1921) showed that the presence of conspecific adults or useful food sources could trigger metamorphosis in marine invertebrates, and our previous study suggested that oysters can significantly improve the metamorphosis rate of larvae (Yu et al., 2020). In this process, oysters were inducers, as well as food sources; these results may suggest a link between the digestive system and metamorphosis and that the neuroendocrine system regulates metamorphosis by regulating the digestive system, which is very likely to occur in carnivorous gastropods. Many studies have indicated that food can increase digestive enzyme activity (Ou and Liu, 2007; Genodepa et al., 2018). Kinouchi et al. (2012) also indicated that the consumption of large protein molecules can promote the development of pancreatic digestive functions in rats, and cholecystokinin (CCK) is an important agent in this process. CCK is a brain-gut peptide with a variety of physiological functions that regulate the secretion of trypsin and the growth of the pancreas; as well as a neurotransmitter in the central and enteric nervous systems. Many studies have suggested the regulatory effect of CCK on the digestive system in the vertebrates, such as rats (Gibbs et al., 2012), porcines (Bugge et al., 2018), fish (Himick and Peter, 1994; Liu et al., 2014; Navarro-Guillén et al., 2017), and also a few studies in invertebrate, Aplysia californica, Nereis diversicolor, and Crassostrea gigas (Vigna et al., 1984; Dhainaut-Courtois et al., 1985; Schwartz et al., 2018). Furthermore, He et al. (2015) indicated that increased CCK expression after food habit transition might suggest a regulatory effect of CCK on the food habit transition of carp, and leptin, insulin and NOS were also described as being related to the food habit transition. These results may show that the regulation of the neuroendocrine system plays a vital role in digestive system development, food habit transition, and even metamorphosis in larvae. Meanwhile, NOS has been suggested to play an indispensable role in the regulation of feeding behavior in Lymnaea stagnalis (Kawai et al., 2011) and Aplysia (Jacklet, 1995). Moreover, Tripathi et al. (2015) have showed that the NOS signal transduction pathway is downstream of CCK receptor, and the NO has been indicated that can activate cGMP synthesis in the crab stomatogastric nervous system and regulate the digestive fuction in crab (Scholz et al., 1996). Additionally, NOS also plays a role in the regulation of 5-HT on metamorphosis, and 5-HT has also been indicated has effect on feeding behavior in goldfish, rat and chickens (Pedro et al., 1998; Vry and Schreiber, 2000; Zendehdel et al., 2012). Therefore, NOS, 5-HT and CCK are likely to be the key mediators of the process of the food habit transition oyster-induced metamorphosis of R. venosa.
A previous study indicated that significant changes occur in the nervous system during the metamorphosis of R. venosa. The expression of the NOS and 5-HT receptor was found to decrease after metamorphosis (Song et al., 2016a), but no further study has examined their roles in the metamorphosis of R. venosa, and whether the neuroendocrine system regulates metamorphosis by regulating the digestive system is unknown. In the present study, we aimed to examine the development of the neuroendocrine system by obtaining cDNA sequences and determining the expression profile of 5-HT receptor, NOS and CCK receptor gene mRNA, and observed the spatiotemporal expression characteristics of 5-HT by immunohistochemical (IHC) analysis. Therefore, the present study can provide new insight into the mechanism of gastropod metamorphosis from the perspective of the neuroendocrine and digestive systems.
Materials and Methods
Larval Rearing and Sample Preparation
A pair of adult R. venosa (2 year old) as parents, and the spawn, intramembrane larvae and planktonic larvae of R. venosa were cultured according to the methods described by Yang et al. (2007) at Blue Ocean Co., Ltd (Laizhou, Shandong, China). Planktonic larvae were cultured in 3 m × 5 m × 1.5 m cement pools at 24–26°, at a density of 0.5/ml. The mixture of Platymonas subcordiformis, Isochrysis galbana, and Chlorella vulgaris was used as a diet (15 × 104 cell/ml, three or four times) for the Planktonic larvae. Intramembrane larval samples were collected from seven major developmental stages: the cleavage stage (c), the blastula stage (b), the gastrulae stage (g), the trochophore stage (t), the early intramembrane veliger stage (ev), the middle intramembrane veliger stage (mv), and the later intramembrane veliger stage (lv). Planktonic larval and postlarval samples were collected from five major developmental stages: the one-spiral whorl stage (V-I), the two-spiral whorl stage (V-II), the three-spiral whorl stage (V-III), the four-spiral whorl stage (competent larva, V-IV), and the postlarval stage (J). The samples were collected based on the methods described in our previous study (Yang et al., 2020a), and each developmental stage was sampled in triplicate and sextuplicate and stored at −80°C for qRT-PCR, respectively, and each sample contains 30 larvae. For the immunohistochemistry (IHC) assays, larvae at different developmental stages were washed in PBS, and 7.5% MgCl2 solution was slowly added to completely anesthetize the larvae. Then, the samples were fixed at room temperature for 2–6 h using 4% paraformaldehyde solution, washed again in PBS, transferred to 70% ethanol and stored at −20°C for use.
CCK Induction Assays
The larvae reached the four-spiral stage (shell height >1,250 μm, competent larvae) were used for the CCK induction assays (CCK peptide: T510159, Shanghai). The following controls and treatments were included in the assays: (1) control group without CCK polypeptide (control, C), and (2) treatment group with CCK polypeptide (1 mg/L) (CCK induction, K), each group with three biological repeats, each tank (393 mm × 282 mm × 223 mm) contained 80 larvae. We randomly collected three samples from each tank at 2 h (early stage, e) and 12 h (later stage, l) after treatment, and each sample contains 30 larvae, then washed them three times with PBS. We obtained a total of 12 samples, which were divided into four groups, namely Ce, Cl, Ke, and Kl, which were used to analyze the mRNA expression of critical genes related to metamorphosis, including carboxypeptidase, cellulase, 5-HTR, and NOS. All samples were stored at -80°C until use.
All procedures involved in the animal collection, rearing and dissection were conducted following the Guideline of Ethical Regulations of Animal Welfare of the Institute of Oceanology, Chinese Academy of Sciences (IOCAS 2013.3). Our study protocols were approved by the Animal Welfare Committee of the IOCAS.
Cloning of the Full-Length cDNA and Sequence Analysis
Total RNA was extracted from all the larval samples using the RNA Isolation Kit (Tiangen, China) following the manufacturer’s protocol, and the quality was determined by a NanoDrop spectrophotometer (Thermo Scientific, United States) and gel electrophoresis. First-strand cDNA (5′- and 3′-RACE-ready cDNAs) synthesis and rapid amplification of the cDNA ends (RACE) were performed by using the SMARTerTM RACE cDNA amplification kit (Clontech, United States). The primers for RACE were designed based on expressed sequence tags in the transcriptome library of R. venosa (Song et al., 2016a) using Primer Premier 5 software (Supplementary Table 1). The 5′ ends of the 5-HT receptor, NOS, and CCK receptor genes were amplified with the primers 5HTR-5′-GSPn, NOS-5′-GSPn and CCKR-5′-GSPn, respectively, and a universal primer mix (UPM) (Supplementary Table 1). The 5′-RACE-ready cDNA was used as the template. The 3′ ends of the three genes were amplified using 5HTR-3′-GSPn and NOS-3′-GSPn, and UPM and 3′RACE-ready cDNA were used as the templates. Confirmation of the full-length cDNAs by polymerase chain reaction (PCR) was conducted as follows: 94°C for 2 min; 35 cycles of 94°C for 30 s; 50–60°C for 30 s; 72°C for 1 min; and 72°C for 10 min (Eppendorf, Germany). The PCR products were inserted into the pMD19-T vector (Takara, Japan) and transformed into JM109 competent cells (Takara, Japan) according to the manufacturer’s instructions, and ten positive clones were selected to confirm the nucleotide sequences by TsingKe Biological Technology (Beijing, China). The sequences were analyzed and assembled by DNAStar software to obtain the full-length cDNA sequence.
The full-length cDNA and predicted protein sequences were analyzed using National Center for Biotechnology Information BLAST programs1. The molecular masses and theoretical isoelectric points of the putative proteins were predicted using ExPasy Compute pI/Mw software2. The transmembrane structure of 5-HT receptor was predicted by TMHMM. The sequences of the three genes from different species were obtained from GenBank databases. Then, DNAMAN software and the ClustalW sequence alignment program3 were used to perform multiple alignments, and MEGA 7.0 was used to construct phylogenetic trees with the neighbor-joining method.
Quantitative Real-Time PCR (qRT-PCR)
Total RNA was extracted from all the larval samples using the RNA Isolation Kit (Tiangen, China) to analyze the mRNA expression of the 5-HT receptor, NOS, CCK receptor, carboxy genes during early stages of development. The qRT-PCR primers were designed based on the full-length cDNA sequences of the three genes, and the 60S ribosomal protein L28 (RL28-F, RL28-R) was used as a housekeeping gene for within-experiment signal normalization (Song et al., 2017; Supplementary Table 1). First-strand cDNA was synthesized for qRT-PCR using reverse transcriptase (Takara). The SYBR Green real-time PCR assay (2 × SYBR Green qPCR Mix, Sparkjade) was used with an Eppendorf Mastercycler® ep realplex (Eppendorf, Hamburg, Germany) for the qRT-PCR analysis. The 10-μl reaction mix volume was prepared, containing 10 μl of 2 × SYBR Green qPCR Mix (Sparkjade, China), 0.2 μl each of 10 pmol the forward and reverse primers, 0.6 μl (100 ng) of cDNA and 4 μl sterile deionized water. Amplifications were performed according to the manufacturer’s instructions. Standard curves were generated with cDNA template dilutions of 10, 102, 103, 104, and 105. qRT-PCRs were performed using the following thermocycler program: 95°C for 2 min and 40 cycles of 95°C for 15 s and 60°C for 30 s. The relative gene expression was calculated using the 2–ΔΔ Ct method.
Immunohistochemical (IHC) Analysis of 5-HT
The samples used for the immunohistochemical analysis of 5-HT in R. venosa were washed in PBS three times, for 10 min each time. An 8% EDTA solution was used to dissolve the shell of the larvae, and the soaking time varied from 1 to 12 h depending on the thickness of the larval shell at the different stages. The larvae were washed with PBS three times again, and then, blocking solution (0.25% BSA, 0.03% NaN3, 1% Triton X-100 and PBS) was added overnight at 4°C. After that, the samples were incubated for 2 h at room temperature with primary antibodies (1:800 in PBS) and washed three times with PBS for 1 h each. Then, the samples were incubated with Alexa 594-conjugated goat anti-rat IgG and Alexa 488-conjugated goat anti-rabbit IgG (1:1,000 in PBS) for 12–24 h at 4°C and then washed three times with PBS for 10 min each. Next, a suitable amount of the samples was transferred to slides to generate slide specimens, which were sealed with 80% glycerol. The specimens were examined and imaged with a fluorescence microscope (Nikon ECLIPSE 90i), and the images were processed with Adobe Photoshop CS3. Negative controls for all the IHC tests were included. The primary antibodies used for IHC were 5-HT (Serotonin) Rabbit Antibody (ImmunoStar, 20080)4 and anti-α-tubulin (Sigma, T6793). The secondary antibodies used for IHC analysis were Alexa 594-conjugated goat anti-rat IgG (Jackson Lab, United States) and Alexa Fluor® 488-conjugated goat anti-rabbit IgG (Jackson Lab, United States). Negative control slides for all the IHC tests were included.
Data Analysis
The results of the qRT-PCR analysis of 5-HT receptor, NOS and CCK receptor were analyzed by using one-way ANOVA with Tukey’s test and LSD test with a significance level of P < 0.05, and the normality and equality of variances were assessed by Levene’s test before performing ANOVA. All the statistics were conducted using SPSS 19.0 software (SPSS Inc., United States), and the results were expressed in (Means ± SEM). Different superscript letters indicate significant differences (P < 0.05). The heatmaps in Figure 3 were made using the pheatmap package in R with Euclidean distance.
Results
Identification and Analysis of the 5-HT Receptor, NOS and CCK Receptor Genes
The full-length cDNA sequences of 5-HT receptor, NOS and CCK receptor were cloned from a cDNA library generated from an R. venosa larval developmental sample mixture and named Rv-5HTR, Rv-NOS, and Rv-CCKR, respectively. The full-length cDNA of Rv-5HTR is 1,741 bp in length, comprising a 47-bp 5′ UTR, a 212-bp 3′ UTR, and a 1,482-bp ORF encoding 493 amino acids (Supplementary Figure 1A), and contains a serotonin receptor subtype 7 domain (Supplementary Figure 2) (GenBank Accession No. MW383250). The calculated molecular mass and isoelectric point of the predicted Rv-5HTR protein are 54.26 kDa and 9.33, respectively. The full-length cDNA of Rv-NOS is 3,813 bp in length, comprising a 28-bp 5′ UTR, a 38-bp 3′ UTR, and a 3,747-bp ORF encoding 1248 amino acids, and contains a PDZ domain, a NOS eukaryotic oxygenase domain and a ferredoxin-reductase (FNR)-like C-terminal domain (Supplementary Figure 1B) (GenBank Accession No. MW383249). The calculated molecular mass and isoelectric point of the predicted Rv-NOS protein are 138.82 kDa and 6.12, respectively. Then, the full-length cDNA of Rv-CCKR is 3,630 bp in length, comprising a 105-bp 5′ UTR, a 2418-bp 3′ UTR, and a 1290-bp ORF encoding 429 amino acids, and contains a CCK receptor domain (Supplementary Figure 1C) (GenBank Accession No. MW383248). The calculated molecular mass and isoelectric point of the predicted Rv-CCKR protein are 48.86 kDa and 9.80, respectively.
Homology and Phylogenetic Analysis of the Rv-5HTR, Rv-NOS, and Rv-CCKR Genes
The protein sequences of Rv-5HTR, Rv-NOS and Rv-CCKR from different species were used for homology and phylogenetic analyses. A BLAST search showed that the predicted amino acid sequences of Rv-5HTR had the highest sequence identity with that from Pomacea canaliculata (XP_025094540.1, 79.08%), followed by that from Aplysia californica (XP_005102984.1, 67.43%), Haliotis discus hannai (QEZ90776.1, 63.79%), Mizuhopecten yessoensis (XP_021371611.1, 59.68%), Octopus sinensis (XP_036367596.1, 53.21%), Bombyx mori (AIZ66402.1, 42.28%), Homo sapiens (AAF35842.1, 41.48%), Mus musculus (NP_032334.2, 39.44%), and Danio rerio (NP_001116793.1, 41.10%) (Figure 1A). The Rv-NOS shared higher similarity with that from other species and had extremely high sequence identity with that from Stramonita haemastoma (CBV37021.3, 95.83%), followed by that from P. canaliculata (XP_025092594.1, 73.86%), A. californica (NP_001191470.1, 70.38%), Crassostrea virginica (XP_022339299.1, 58.20%), Octopus vulgaris (QHX41539.1, 62.99%), Bos taurus (XP_024833521.1, 50.16%), H. sapiens (NP_000611.1, 49.84%), M. musculus (NP_032738.1, 57.72%), and D. rerio (XP_005165110.1, 57.93%) (Figure 1B). Rv-CCKR had the highest sequence identity with that from P. canaliculata (XP_025088086.1, 67.37%), followed by that from O. vulgaris (XP_029642692.2, 51.52%), M. yessoensis (VDI47157.1, 52.70%), C. virginica (XP_034300900.1, 47.86%), H. sapiens (NP_795344.1, 41.69%), M. musculus (NP_033957.1, 40.48%), Drosophila melanogaster (NP_001097023.1, 48.46%), and D. rerio (XP_017213239.1, 42.09%) (Figure 1C). To further elucidate the evolutionary relationships of the three genes from R. venosa with those of other species, we chose >10 species for phylogenetic analyses and constructed phylogenetic trees by N-J methods. The phylogenetic tree indicated that both Rv-5HTR and Rv-CCK were first clustered with those of P. canaliculata, while Rv-NOS was first clustered with that of S. haemastoma and was far from that of vertebrates. All three genes were clustered with those of mollusks, which are closely related to gastropods, and significantly separated from those of vertebrates (Figure 2).
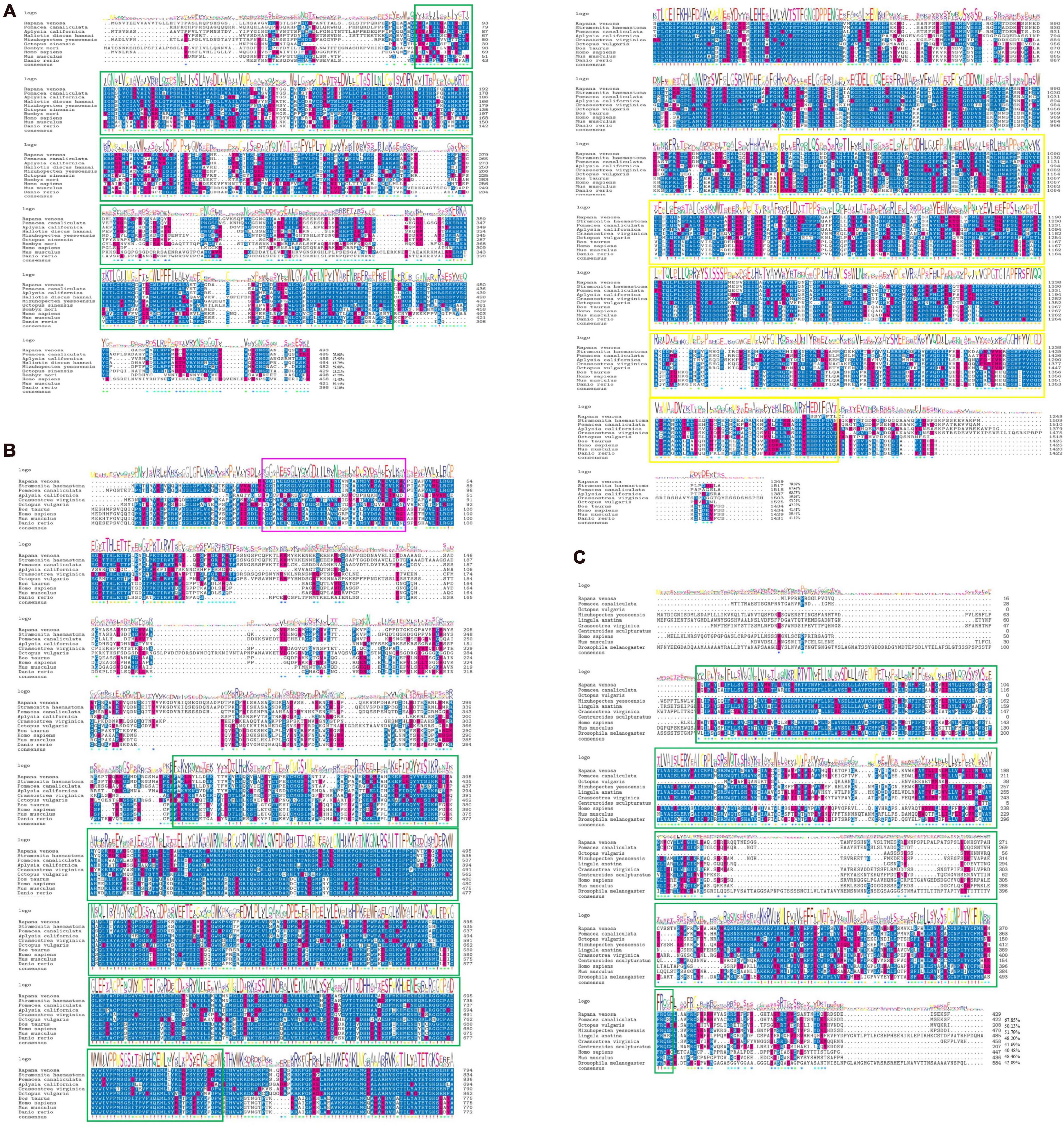
Figure 1. Multiple alignment based on Rv-5HTR (A), Rv-NOS (B), and Rv-CCKR (C) deduced amino acid sequences from Rapana venosa and other species. Rv-5HTR: serotonin receptor subtype domain 7, denoted in green box; Rv-NOS: PDZ domain denoted in pink box, NOS eukaryotic oxygenase domain denoted in green box and ferredoxin-reductase (FNR) like C-terminal domain denoted in yellow box; Rv-CCKR: CCK receptors domain denoted in green box. Additionally, the logo shows how conservative motif is in each position. The higher the letter, the more conservative the position. Different amino acids in the same position will scale according to its frequency. (5-HT receptor: GenBank Accession No. MW383250; NOS: GenBank Accession No. MW383249; CCK receptor: GenBank Accession No. MW383248) (The proteins used in the analysis were showed in Supplementary Table 2).
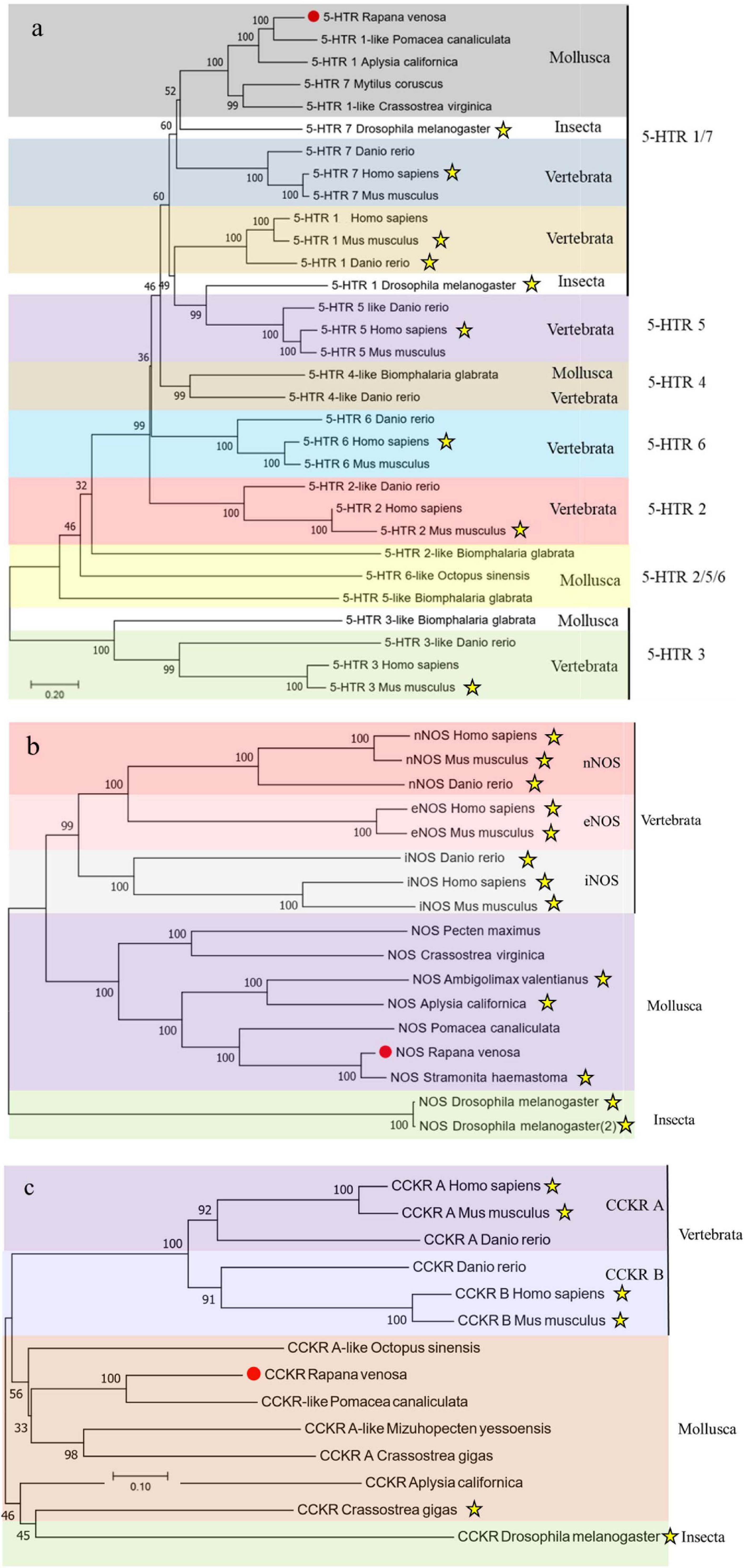
Figure 2. Phylogenetic trees based on Rv-5HTR (a), Rv-NOS (b), and Rv-CCKR (c) deduced amino acid sequences from Rapana venosa and other species. The trees were constructed based on the multiple sequence generated by Clustal X and aligned using the neighbor-joining method in MEGA 7.0. The genes which have been pharmacologically proven were highlighted with asterisk, which has been listed in Supplementary Table 3.
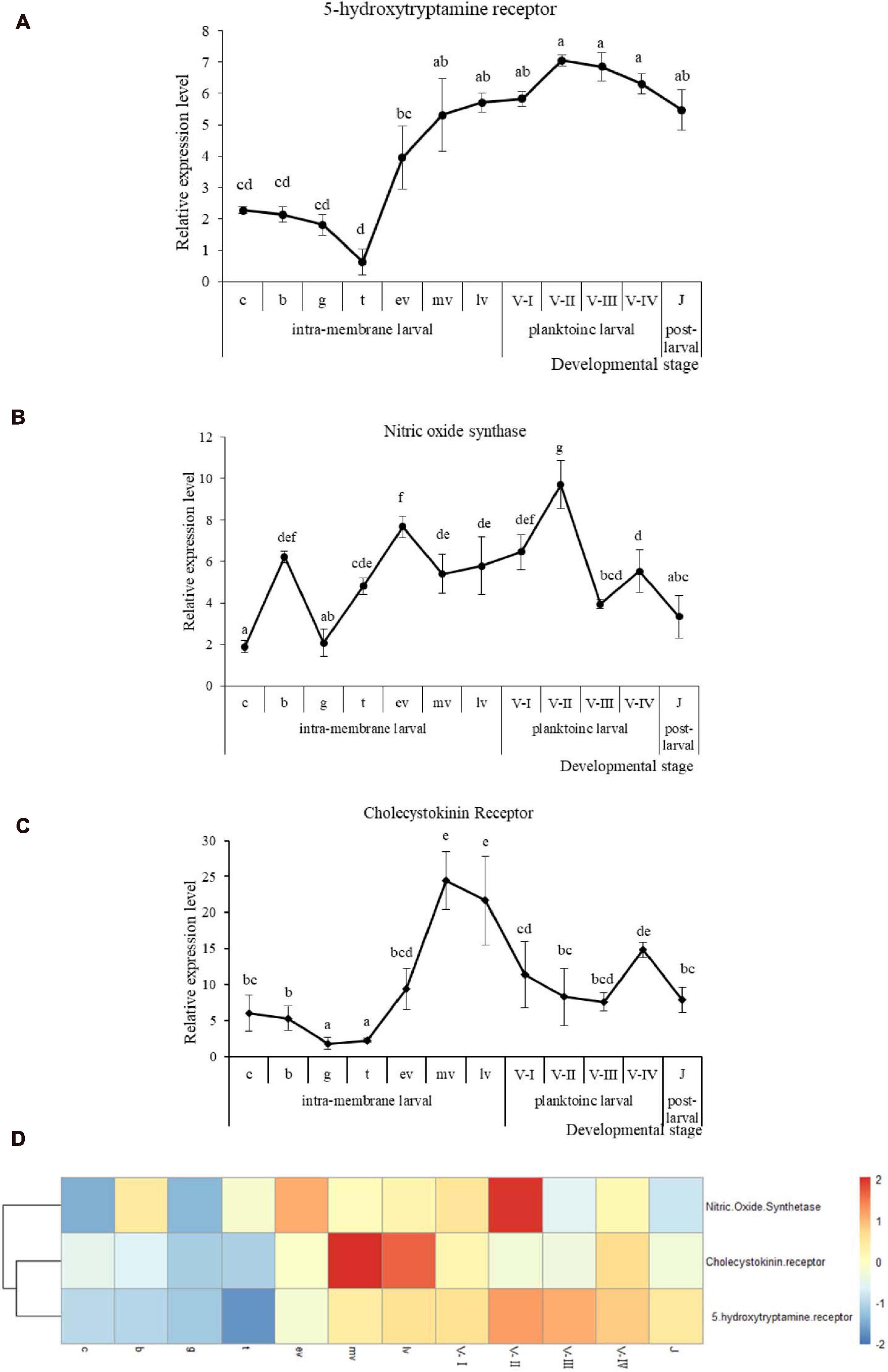
Figure 3. qRT-PCR analysis of Rv-5HTR (A), Rv-NOS (B), and Rv-CCKR (C) mRNA expression and the heatmap (D) of three genes expression during Rapana venosa larval development (mean ± SEM, n = 3). Different superscript letters indicate significant differences (P < 0.05). [the cleavage stage (c), the blastula stage (b), the gastrulae stage (g), the trochophore stage (t), the early intramembrane veliger stage (ev), the middle intramembrane veliger stage (mv), and the later intramembrane veliger stage (lv). the one-spiral whorl stage (V-I), the two-spiral whorl stage (V-II), the three-spiral whorl stage (V-III), the four-spiral whorl stage (competent larva, V-IV), and the postlarval stage (J)].
Gene Expression of Rv-5HTR, Rv-NOS, and Rv-CCKR During Development
Quantitative real-time PCR was performed to detect the mRNA expression levels of the three genes at different developmental stages. The mRNA expression of Rv-5HTR was high in the cleavage stage (c) when the larvae had just hatched, then significantly decreased and was low in the early intramembrane larval stage (Figure 3A) (P < 0.05). The high expression reappeared in the early intramembrane veliger stage (ev) and into the planktonic larval stage, was maintained a high level throughout this stage, and a significant decrease occurred in the postlarval stage (J). Additionally, the expression of Rv-NOS greatly fluctuated throughout the whole early stage of development. Rv-NOS reached the first peak in the blastula stage (b), then significantly decreased in the gastrulae stage (g), significantly increased in the trochophore stage (t), significantly increased again in the early intramembrane veliger stage (ev) and reached the second peak. Then, the expression level slightly decreased and remained at this level until the two-spiral whorls stage (V-II), when it increased again, reached the third peak, sharply decreased in the three-spiral whorls stage (V-III), and increased again in the four-spiral layer (competent larva, V-IV), when it reached the fourth peak before significantly decreasing at postlarval stage (J) (Figure 3B). The expression of Rv-CCKR was low in the early intramembrane larval stage, then significantly increased in the early intramembrane veliger stage (ev), reached a peak in the middle intramembrane veliger stage (mv), continuously decreased until the four-spiral whorl stage (competent larva, V-IV), increased again and significantly decreased in the postlarval stage (J) (Figure 3C). The expression patterns of the three genes were slightly similar (Figure 3D), especially those of Rv-5HTR and Rv-CCKR, which were clustered, and all three genes first increased in the early intramembrane veliger stage (ev) and decreased in the postlarval stage (J) (P < 0.05).
Immunohistochemical (IHC) Analysis of 5-HT Throughout the Whole Early Developmental Stage
The results of the immunohistochemical analysis of 5-HT showed in Figure 4. We didn’t observed positive immune signals in the cleavage stage (c), blastocyst stage (b) and gastrula stage (g) (Figures 4a–c). Two pairs of positive immune signals (Figure 4d, top organ, profile figure, only one signal is visible, as indicated by a white arrow) were observed at the base of the velum and the future eye site during the trochophore larval stage (t). The number of immune-positive signals increased to 5 in the early intramembrane veliger stage (ev), and these signals indicated the ganglia between the parietal and lateral ganglia of the parietal organs (Figure 4e, white arrow). The 5-HT immune-positive signal extended to both cerebral nerve fibers in the middle intramembrane veliger (mv) (Figure 4f, hollow arrow). Positive signals began to appear in the lower foot primordia of the later intramembrane veliger larvae (lv) (Figure 4g, white arrow), and a strong signal appeared at the velum (Figure 4g, white arrow). Furthermore, the immunohistochemical analysis of the four-spiral whorl stage larvae (competent larvae, V-IV) revealed a complex neural network in the mantle (Figure 4h, hollow arrow), foot (Figure 4h, white arrow) and velum (Figure 4h, blue arrow). The three major nerves in the velum (Figure 4i, white arrow) and the receptors in the base of the ciliate (Figure 4i, hollow arrow) were clearly connected in a complex nerve network.
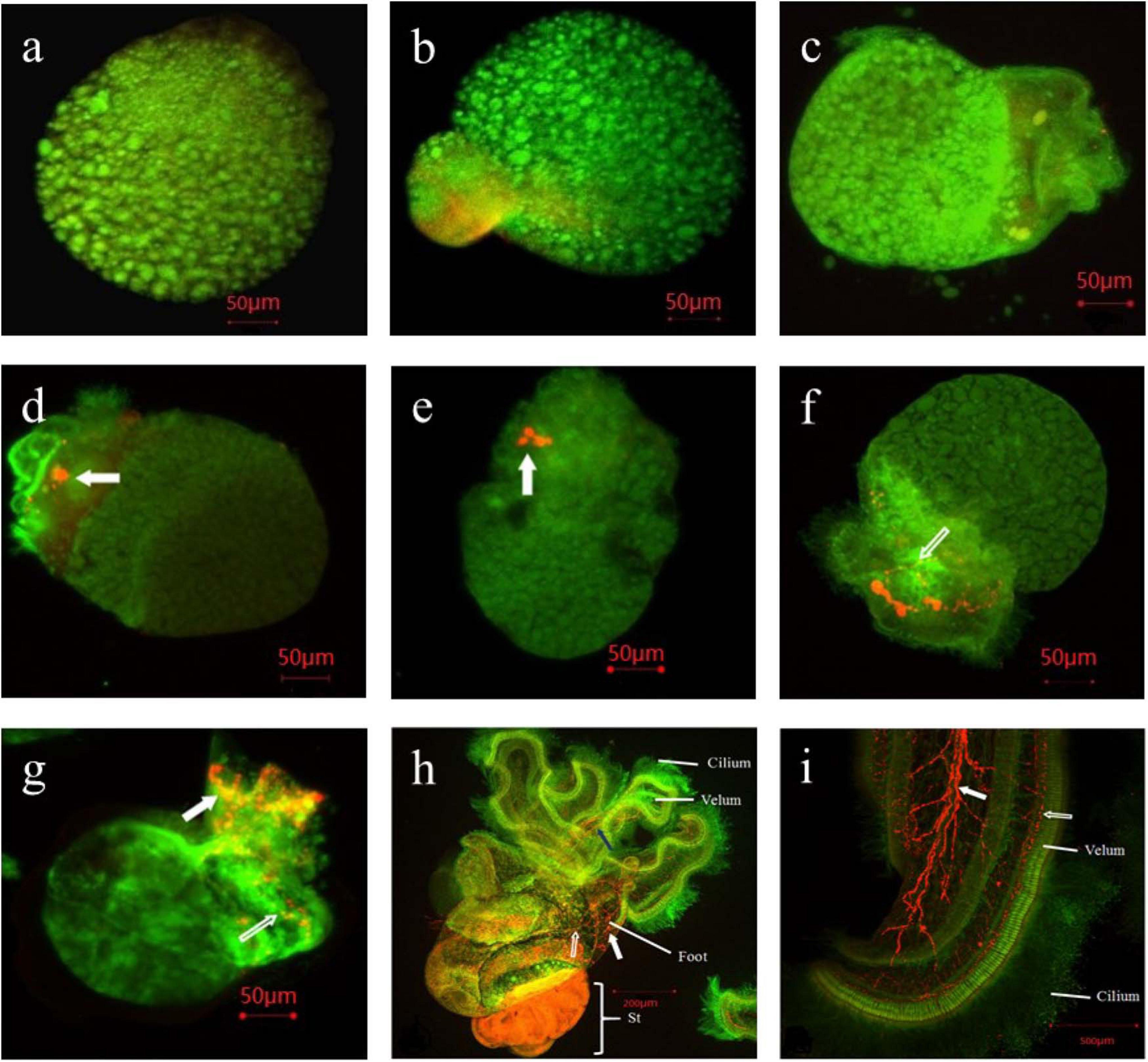
Figure 4. Immunoreactivity of 5-HT and α-tublin in early development stage of Rapana venosa. [(a). cleavage; (b). blastula; (c). gastrulae; (d). late trochophore; (e). early intra-membrane veliger; (f). middle intra-membrane veliger; (g). later intra-membrane veliger; (h). competent larval stage; (i). velum of competent larvae]. No positive immune signals in the cleavage stage (a), blastula stage (b) and gastrulae stage (c). Two pairs of positive immune signals in late trochophore stage [(d), top organ, profile figure, only one signal is visible, as indicated by a white arrow]. Immune-positive signals increased to 5 in early intra-membrane veliger stage (e). The 5-HT immune-positive signal extended to both cerebral nerve fibers in the middle intramembrane veliger stage [(f), hollow arrow]. Positive signals began to appear in the lower foot primordia of the later intramembrane veliger larvae, and a strong signal appeared at the velum [(g), white arrow]. The immunohistochemical analysis revealed a complex neural network in the mantle in four-spiral whorl stage larvae (competent larvae, V-IV) [(h), hollow arrow], foot [(h), white arrow] and velum [(h), blue arrow]. The three major nerves in the velum [(i), white arrow] and the receptors in the base of the ciliate [(i), hollow arrow] were clearly connected in a complex nerve network.
Changes in Critical Gene Expression Induced by CCK
To assess the digestive and neuroendocrine systems of larvae induced by CCK, the mRNA expression of carboxypeptidase, cellulase, 5-HTR and NOS was detected (Figure 5). The expression of the carboxypeptidase gene was significantly increased in both the early and later stages in competent larvae induced by oysters, while cellulase was significantly decreased in the later stage (P < 0.05). The expression of 5-HT receptor increased significantly, while the expression of NOS decreased significantly in both the early and later stage in competent larvae induced by oysters (P < 0.05).
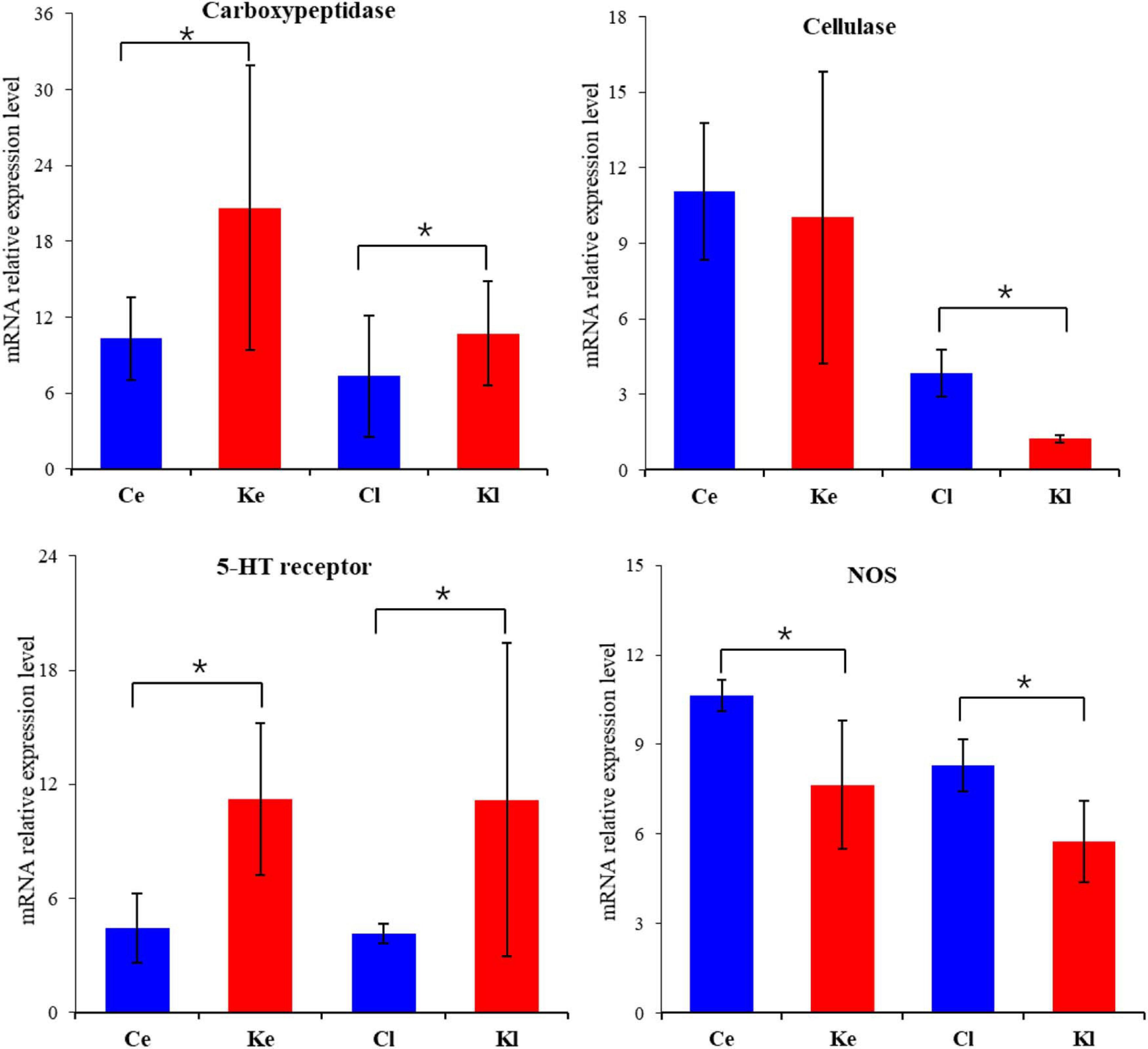
Figure 5. mRNA expression of critical genes (Carboxypeptidase, Cellulase, 5-HT receptor and NOS) in Competent larva induced by CCK (mean ± SE, n = 3). (Different superscript letters indicate significant differences, *P < 0.05). [Ce: Control (early, 2 h), Cl: Control (later, 12 h), Ke: CCK Induction (early, 2 h), Kl: CCK Induction (later, 12 h)].
Discussion
Metamorphosis, the transition of free-swimming larvae to benthic and often sessile and attached juveniles, is one of the most distinctive life-changing events for many molluscan species, and the neuroendocrine system plays a key role in metamorphosis and has been extensively studied in herbivorous gastropods and bivalves. 5-HT and NO have been widely confirmed to regulate metamorphosis in I. obsolete, Phestilla sibogae, Haliotis asinine and a variety of bivalve species (Satuito et al., 1999; Leise et al., 2001; Croll, 2006; Yang et al., 2013; Ueda and Degnan, 2014; Zhu et al., 2020). However, the mec hanism by which metamorphosis is regulated in carnivorous gastropods may be more complicated due to the accompanying food habit transition, but little is known about this phenomenon. Therefore, to further understand the regulatory mechanism of R. venosa, the development and changes in the neuroendocrine system, especially those related to the digestive system during the early developmental stage, deserve more attention. This study elucidated the mechanism of neuroendocrine system development in R. venosa through an integrated approach of localization of the Rv-5HTR protein in the larva and examination of the molecular-metabolic responses of 5-HT, NOS, and CCK in order to further investigate the regulatory effect of the neuroendocrine system on the food habit transition and metamorphosis of R. venosa.
5-HT is one of the evolutionarily oldest neurotransmitters and performs its various physiological functions through seven 5-HT receptor subfamilies (5-HT 1–7) (Hannon and Hoyer, 2008); 5-HT exerts a significant effect on the development and metamorphosis of marine invertebrate larvae (Leise et al., 2001; Sahoo and Khandeparker, 2018). In the present study, Rv-5HTR, which may belongs to serotonin receptor subtype 7, one of the 14 vertebrates serotonin receptors, is a member of the class A GPCRs and is activated by the neurotransmitter 5-HT. We found that the different subtypes of 5-HTR evolved independently in vertebrates, which has been not clear in invertebrates since the few studies. Previous studies indicated that the most prominent 5-HT cells during larval development were the five apical cells, and Hadfield et al. (2000) found that 5-HT was first expressed in apical organs during the development of gastropod larvae and was the main neurotransmitter in the apical organs. Our results showed that the 5-HT increased in the early intramembrane veliger stage (ev) both in the results of qRT-PCR and IHC, which may be the critical stage in the initial development of the digestive system in R. venosa. These results may reveal the regulation of 5-HT in the early development of R. venosa and suggest a relationship between 5-HT and the digestive system; a previous study also reported that 5-HT and its receptors play important roles in the regulation of gastrointestinal and endocrine functions (Berger et al., 2009).
Furthermore, 5-HT has been reported to significantly increase the rate of metamorphosis in Ilyanassa obsoleta by soaking or injection (Couper and Leise, 1996; Leise et al., 2001). Hadfield et al. (2000) indicated that 5-HT and 5-HTR in the apical organ can facilitate metamorphosis by sensing external chemical signals, and 5-HT is the key factor of signal transduction and metamorphosis initiation. We also observed the complex neural network based on the localization of the 5-HT protein in the competent larval stage of R. venosa (Figure 4h) and the velum of competent larvae (Figure 4i), and the receptors in the base of the ciliate network were particularly clear. Croll (2006) indicated that early development of serotonergic pedal neurons may reflect the need to regulate the activities of large numbers of ciliated cells found on the foot, which has been demonstrated in adults of a variety of gastropod species (Audesirk et al., 1979; Syed and Winlow, 1989). On the other hand, Glebov et al. (2014) reported that the expression level of 5-HT receptor gene was downregulated during the transition from the premetamorphic to metamorphic stages in Helisoma trivolvis; similarly, the expression level of Rv-5HTR was decreased after metamorphosis in the postlarval stage (J) in the present study, and Yang Z. et al. (2015) indicated that 5-HT did not significantly induce the metamorphosis of R. venosa. These results may suggest that 5-HT and Rv-5HTR may not be positive regulators of metamorphosis in R. venosa. Therefore, the regulatory mechanism of 5-HT and Rv-5HTR requires further exploration.
Nitric oxide is an important molecular messenger that plays a critical role in the nervous systems of both vertebrates and invertebrates (Snyder and Bredt, 1992; Colasanti and Venturini, 1998), and Leise et al. (2001) indicated that NO played an antagonistic role in the 5-HT mediated pathway of larval metamorphosis, and that NO was produced by NOS catalyzing the five-electron heme-based oxidation of the guanidine nitrogen of L-arginine to L-citrulline. In the present study, the Rv-NOS gene contains a PDZ domain, a NOS eukaryotic oxygenase domain and a ferredoxin-reductase (FNR)-like C-terminal domain. The PDZ domain is often found in a variety of eumetazoan signaling molecules and may be responsible for specific protein-protein interactions. The NOS eukaryotic oxygenase domain also has a C-terminal electron-supplying reductase region, which is homologous to cytochrome P450 reductase and binds to NADH, FAD, and FMN. We found that Rv-NOS was tightly clustered with the gene from S. haemastoma in the phylogenetic tree (Figure 2b), and the sequence similarity of homologous alignment was as high as 95.83%; this result indicated that the two species were closely related. In S. haemastoma, the mRNA transcripts mainly expressed in the central nervous system and peripheral structures which involved in sensory organs, such as the osphradium, cephalic tentacles, and buccal/esophageal tissues (Brown et al., 2004; Cioni et al., 2011); this result has also been confirmed in A. californica (Moroz, 2010). The present results showed that the expression of NOS was increased in both trochophore larvae (t) and the early intramembrane veliger stage (ev), which are critical periods for the development of velum and the digestive system. These results may suggest that NOS plays an important role in the olfactory and digestive systems in R. venosa and that NOS dramatically changes during the metamorphosis of R. venosa (Song et al., 2016a; Yang et al., 2020a,b). In addition, we found that both the mRNA expression levels of NOS were decreased in the postlarval stage (J) compared to the competent larval stage (V-IV), which may further confirm the hypothesis that NOS plays a negative regulatory role (Froggett and Leise, 1999). Additionally, Sahoo and Khandeparker (2018) indicated that the inhibitory effects of NO and NOS may enhance the probability of locating a suitable substratum by delaying metamorphosis and the dispersal capability of the larvae to increase gene flow and enhance individual vigor, which may explain the increase in the mRNA expression of Rv-NOS in the competent larvae (V-IV) in our results. However, Ueda and Degnan (2014) found that NOS and NO may be positive regulators of the initiation of metamorphosis in some gastropods, such as H. asinine. The signaling pathways of NOS and NO have extensive functions, and their regulation during the metamorphosis of R. venosa requires further study.
Tripathi et al. (2015) demonstrated that CCK stimulates NOS, which has also been confirmed by Moustafa et al. (2011), while we found the inhibition of CCK on NOS in R. venosa. The Rv-CCKR gene contains a CCK receptor domain and is a member of the class A family of seven-transmembrane G-proteins, which are abundant in pancreatic acinar cells. CCK is a gastrointestinal regulatory hormone that is implicated in the regulation of digestion and appetite control by stimulating pancreatic enzyme secretion, gallbladder contraction, and gut motility satiety and inhibit acid secretion from the stomach via CCK receptor (Tripathi et al., 2015; Rehfeld, 2017). In the present result, we also found that CCK can decreased the expression of Carboxypeptidase in R. venosa, and increased the expression of Cellulase, which may suggest that CCK can promote the food habit transition of R. venosa. In addition, CCK is also known to stimulate pancreatic growth in vitro and in vivo in mice, rats, or hamsters (Cordelier et al., 1999), which may potentially explain the increased expression of Rv-CCKR in the early intramembrane veliger stage (ev), which is the critical stage of digestive system development in R. venosa. Additionally, Schwartz et al. (2018) indicated that plausible role of Cragi-CCK signaling in the regulation of feeding in in the oyster Crassostrea gigas. He et al. (2015) reported that CCK was increasingly expressed in grass carp after food habit transition, while in the present study, the expression of Rv-CCKR was significantly decreased, the difference may due to the different type of food habit transition, and the significant changes suggest that Rv-CCKR and CCK are involved in food habit transition during metamorphosis. Additionally, the CCK can increase the expression of 5-HT receptor, and Cooper and Dourish (1990) have also indicated that the 5-HT and CCK are synergistic and interdependent in the regulation of feeding.
Conclusion
Here, we show the changes of 5-HT and NOS during food habit transition in metamorphosis of R. venosa. Moreover, we found CCK is an important factor that regulates the development and function of the digestive system, which may further suggest a close relationship between food habit transition and metamorphosis. By exploring the changes in critical genes during the early stages of larval development, the developmental process of the neuroendocrine system was initially identified; by further exploring the regulation of CCK, we reveal the relationship between digestive system and neuroendocrine system. This study provides new insight for studying the process of metamorphosis in carnivorous gastropods.
Data Availability Statement
The datasets presented in this study can be found in online repositories. The names of the repository/repositories and accession number(s) can be found in the article/Supplementary Material.
Author Contributions
TZ conceived and designed the experiments. M-JY and HS conducted the experiments. M-JY, JF, and HS analyzed the data. Z-LY, ZH, CZ, PS, JL, and X-LW contributed reagents, materials, and analytical tools. M-JY wrote the manuscript. All authors contributed to the article and approved the submitted version.
Funding
This research was supported by the National Natural Science Foundation of China (Grant Nos. 31972814 and 32002409), the Natural Science Foundation of Shandong Province (Grant No. ZR2019BD003), the China Post-doctoral Science Foundation (Grant No. 2019M652498), the Major Scientific and Technological Innovation Project of Shandong Provincial Key Research and Development Program (2019JZZY020708), the Earmarked Fund for Modern Agro-Industry Technology Research System (CARS-49), the Industry Leading Talents Project of Taishan Scholars (Recipient: TZ, Grant No. LJNY201704), the “Double Hundred” Blue Industry Leader Team of Yantai (Recipient: TZ), and the Creative Team Project of the Laboratory for Marine Ecology and Environmental Science, Qingdao National Laboratory for Marine Science and Technology (no. LMEESCTSP-2018-1). The funders had no role in the study design, data collection and analysis, decision to publish or preparation of the manuscript.
Conflict of Interest
The authors declare that the research was conducted in the absence of any commercial or financial relationships that could be construed as a potential conflict of interest.
Supplementary Material
The Supplementary Material for this article can be found online at: https://www.frontiersin.org/articles/10.3389/fmars.2021.690282/full#supplementary-material
Footnotes
- ^ http://www.ncbi.nlm.nih.gov/BLAST/
- ^ http://www.expasy.org/tools/pi_tool.html
- ^ http://www.ebi.ac.uk/clustalW
- ^ https://www.immunostar.com/shop/antibody-catalog/5-ht-serotonin-rabbit-antibody/
References
Audesirk, G., McCaman, R. E., and Willows, A. O. D. (1979). The role of serotonin in the control of pedal ciliary activity by identified neurons in Tritonia diomedea. Comp. Biochem. Physiol. Part C Comp. Pharmacol. 62, 87–91. doi: 10.1016/0306-4492(79)90104-7
Bard, J. A., Zgombick, J., Adham, N., Vaysse, P., and Weinshank, R. L. (1993). Cloning of a novel human serotonin receptor (5-HT7) positively linked to adenylate cyclase. J. Biol. Chem. 268, 23422–23426. doi: 10.1016/s0021-9258(19)49479-9
Barlow, L. A., and Truman, J. W. (2010). Patterns of serotonin and SCP immunoreactivity during metamorphosis of the nervous system of the red abalone, haliotis rufescens. Dev. Neurobiol. 23, 829–844. doi: 10.1002/neu.480230705
Bather, F. A. (1921). Studies of the development and larval forms of echinoderms. Nature 108, 459–460. doi: 10.1038/108459a0
Berger, M., Gray, J. A., and Roth, B. L. (2009). The expanded biology of serotonin. Annu. Rev. Med. 60, 355–366. doi: 10.1146/annurev.med.60.042307.110802
Bhattarai, P., Cosacak, M. I., Mashkaryan, V., Demir, S., and Kizil, C. (2020). Neuron-glia interaction through serotonin-bdnf-ngfr axis enables regenerative neurogenesis in alzheimer’s model of adult zebrafish brain. PLoS Biol. 18:e3000585. doi: 10.1371/journal.pbio.3000585
Brown, K. M., Mcdonough, M., and Richardson, T. D. (2004). Intraspecific life history variation in the southern oyster drill, Stramonita haemastoma: patterns and causes. J. Shellfish Res. 23, 149–155.
Bugge, A., Jansen, P. G., Maria, L., Sanni, S. J., and Clausen, T. R. (2018). Cloning and characterization of the porcine gastrin/cholecystokinin type 2 receptor. Eur. J. Pharmacol. 833, 357–363. doi: 10.1016/j.ejphar.2018.06.020
Celniker, S. E., Wheeler, D. A., Kronmiller, B., and Joseph, W. C. (2002). Finishing a whole-genome shotgun: release 3 of the drosophila melanogaster euchromatic genome sequence. Genome Biol. 3, 1–14.
Charest, A., Wainer, B. H., and Albert, P. R. (1993). Cloning and differentiation-induced expression of a murine serotonin1a receptor in a septal cell line. J. Neurosci. Offic. J. Soc. Neurosci. 13, 51–64.
Cioni, C., Patti, M. C. B. D., Venturini, G., Modica, M. V., Scarpa, F., Oliverio, M., et al. (2011). Cellular, biochemical, and molecular characterization of nitric oxide synthase expressed in the nervous system of the prosobranch Stramonita haemastoma (gastropoda, neogastropoda). J. Comp. Neurol. 520, 364–383. doi: 10.1002/cne.22729
Colasanti, M., and Venturini, G. (1998). Nitric oxide in invertebrates. Mol. Neurobiol. 17, 157–174. doi: 10.1007/bf02802029
Coon, S. L., Bonar, D. B., and Weiner, R. M. (1985). Induction of settlement and metamorphosis of the pacific oyster, crassostrea gigas (thunberg), by l-dopa and catecholamines. J. Exp. Mar. Biol. Ecol. 94, 211–221. doi: 10.1016/0022-0981(85)90059-0
Cooper, S. J., and Dourish, C. T. (1990). Multiple cholecystokinin (CCK) receptors and CCK-monoamine interactions are instrumental in the control of feeding. Physiol. Behav. 48, 849–857. doi: 10.1016/0031-9384(90)90239-z
Cordelier, P., Esteve, J. P., Rivard, N., Marletta, M., Vaysse, N., Susini, C., et al. (1999). The activation of neuronal no synthase is mediated by g-protein betagamma subunit and the tyrosine phosphatase shp-2. FASEB J. 13, 2037–2050. doi: 10.1096/fasebj.13.14.2037
Couper, J. M., and Leise, E. M. (1996). Serotonin injections induce metamorphosis in larvae of the gastropod mollusc ilyanassa obsoleta. Biol. Bull. 191, 178–186. doi: 10.2307/1542921
Croll, R. P. (2006). Development of embryonic and larval cells containing serotonin, catecholamines, and Fmrfamide-related peptides in the gastropod mollusc Phestilla sibogae. Biol. Bull. 211, 232–247. doi: 10.2307/4134546
Dhainaut-Courtois, N., Dubois, M. P., Tramu, G., and Masson, M. (1985). Occurrence and coexistence in Nereis diversicolor O.F. Müller (Annelida Polychaeta) of substances immunologically related to vertebrate neuropeptides. Cell Tissue Res. 242, 97–108.
Dolashka, P., Moshtanska, V., Borisova, V., Dolashki, A., Stevanovic, S., Dimanov, T., et al. (2011). Antimicrobial proline-rich peptides from the hemolymph of marine snail Rapana venosa. Peptides 32, 1477–1483. doi: 10.1016/j.peptides.2011.05.001
Froggett, S. J., and Leise, E. M. (1999). Metamorphosis in the marine snail Ilyanassa obsoleta, yes or NO? Biol. Bull. 196, 57–62. doi: 10.2307/1543167
Geller, D. A., Lowenstein, C. J., Shapiro, R. A., Nussler, A. K., Silvio, M. D., Wang, S. C., et al. (1993). Molecular cloning and expression of inducible nitric oxide synthase from human hepatocytes. Proc. Natl. Acad. Sci. USA 90, 3491–3495.
Genodepa, J., Zeng, C., and Southgate, P. C. (2018). Changes in digestive enzyme activities and nutrient utilization during embryonic development and starvation of newly hatched larvae of the mud crab, scylla serrata. Aquaculture. 493, 137–143. doi: 10.1016/j.aquaculture.2018.04.053
Gibbs, J., Young, R. C., and Smith, G. P. (2012). Cholecystokinin decreases food intake in rats. J. Comp. Physiol. Psychol. 5, 284–290. doi: 10.1002/j.1550-8528.1997.tb00305.x
Gireesh, R., and Cherukara, P. G. (2008). Effects of diet, stocking density and environmental factors on growth, survival and metamorphosis of clam, paphia malabarica (chemnitz) larvae. Aquacult. Res. 39, 928–933. doi: 10.1111/j.1365-2109.2008.01951.x
Glebov, K., Voronezhskaya, E. E., Khabarova, M. Y., Ivashkin, E., Nezlin, L. P., et al. (2014). Mechanisms underlying dual effects of serotonin during development of Helisoma trivolvis (Mollusca). BMC Dev. Biol. 14:14. doi: 10.1186/1471-213X-14-14
Gnanapandithen, K., Chen, Z., Kau, C. L., Gorczynski, R. M., and Marsden, P. A. (1996). Cloning and characterization of murine endothelial constitutive nitric oxide synthase. Biochim. Biophys. Acta Gene Struct. Express. 1308, 103–106. doi: 10.1016/0167-4781(96)00098-x
Hadfield, M. G., Meleshkevitch, E. A., and Boudko, D. Y. (2000). The apical sensory organ of a gastropod veliger is a receptor for settlement cues. Biol. Bull. 198, 67–76. doi: 10.2307/1542804
Hall, A. V., Antoniou, H., Wang, Y., Cheung, A. H., and Marsden, P. A. (1994). Structural organization of the human neuronal nitric oxide synthase gene (nos1). J. Biol. Chem. 269, 33082–33090. doi: 10.1016/s0021-9258(20)30099-5
Hannon, J., and Hoyer, D. (2008). Molecular biology of 5-HT receptors. Behav. Brain Res. 195, 198–213. doi: 10.1016/j.bbr.2008.03.020
Harding, J. M. (2006). Growth and development of veined rapa whelk Rapana venosa veligers. J. Shellfish Res. 25, 941–946. doi: 10.2983/0730-8000(2006)25[941:gadovr]2.0.co;2
He, S., Liang, X. F., and Li, L. (2015). Transcriptome analysis of food habit transition from carnivory to herbivory in a typical vertebrate herbivore, grass carp Ctenopharyngodon idella. BMC Genomics 16:15. doi: 10.1186/s12864-015-1217-x
Himick, B. A., and Peter, R. E. (1994). CCK/gastrin-like immunoreactivity in brain and gut, and CCK suppression of feeding in goldfish. Am. J. Physiol. Regul. Integ. Comp. Physiol. 267(3 Pt 2), 841–851.
Huan, P., Wang, H., and Liu, B. (2015). A label-free proteomic analysis on competent larvae and juveniles of the pacific oyster Crassostrea gigas. PLoS One 10:e0135008. doi: 10.1371/journal.pone.0135008
Jacklet, J. W. (1995). Nitric oxide is used as an orthograde cotransmitter atidentified histaminergic synapses. J. Neurophysiol. 74, 891–895. doi: 10.1152/jn.1995.74.2.891
Kauserud, H., Lie, M., Stensrud, O., and Ohlson, M. (2005). Molecular characterization of airborne fungal spores in boreal forests of contrasting human disturbance. Mycologia 97, 1215–1224. doi: 10.3852/mycologia.97.6.1215
Kawai, R., Kobayashi, S., Fujito, Y., and Ito, E. (2011). Multiple subtypes of serotonin receptors in the feeding circuit of a pond snail. Zool. Sci. 28, 517–525. doi: 10.2108/zsj.28.517
Kinouchi, T., Koyama, S., Harada, E., and Yajima, T. (2012). Large molecule protein feeding during the suckling period is required for the development of pancreatic digestive functions in rats. Am. J. Physiol. Regul. 303, R1268–R1276.
Kohen, R., Metcalf, M. A., Khan, N., Druck, T., Huebner, K., Lachowicz, J. E., et al. (2010). Cloning, characterization, and chromosomal localization of a human 5-ht6 serotonin receptor. J. Neurochem. 66, 47–56. doi: 10.1046/j.1471-4159.1996.66010047.x
Kone, B. C., Schwöbel, J., Turner, P., Mohaupt, M. G., and Cangro, C. B. (1995). Role of nf-kappa b in the regulation of inducible nitric oxide synthase in an mtal cell line. Am. J. Physiol. 269(5 Pt 2), F718.
Lay, J. M., Jenkins, C., Friis-Hansen, L., and Samuelson, L. C. (2000). Structure and developmental expression of the mouse cck-b receptor gene. Biochem. Biophys. Res. Commun. 272, 837–842. doi: 10.1006/bbrc.2000.2875
Leise, E. M., Thavaradhara, K., Durham, N. R., and Turner, B. E. (2001). Serotonin and nitric oxide regulate metamorphosis in the marine snail Ilyanassa obsoleta. Am. Zool. 41, 258—267.
Li, J. Q., Wang, M. L., Fang, J. G. F., Liu, X. L., Xue, S. Y., Mao, Y. Z., et al. (2018). A comparison of offspring growth and survival among a wild and a selected strain of the pacific abalone (Haliotis discus hannai) and their hybrids. Aquaculture 495, 721–725. doi: 10.1016/j.aquaculture.2018.06.071
Liu, L., Liang, X. F., Li, J., Yuan, X., Zhou, Y., and He, Y. (2014). Feed intake, feed utilization and feeding-related gene expression response to dietary phytic acid for juvenile grass carp (Ctenopharyngodon idellus). Aquaculture 42, 201–206. doi: 10.1016/j.aquaculture.2013.12.044
Matsuo, R., Misawa, K., and Ito, E. (2008). Genomic structure of nitric oxide synthase in the terrestrial slug is highly conserved. Gene 415, 74–81. doi: 10.1016/j.gene.2008.02.021
Matthews, B. B., Santos, G. D., Crosby, M. A., Emmert, D. B., and Gelbart, W. M. (2015). Gene model annotations for drosophila melanogaster: impact of high-throughput data. G3 Genes Genomes Genetics 5, 1721–1736. doi: 10.1534/g3.115.018929
Moore, A. P., and Bringolf, R. B. (2018). Effects of nitrate on freshwater mussel glochidia attachment and metamorphosis success to the juvenile stage. Environ. Pollut. 242, 807–813. doi: 10.1016/j.envpol.2018.07.047
Moroz, L. L. (2010). Localization of putative nitrergic neurons in peripheral chemosensory areas and the central nervous system of aplysia californica. J. Comp. Neurol. 495, 10–20. doi: 10.1002/cne.20842
Moroz, L. L., Edwards, J. R., Puthanveettil, S. V., Kohn, A. B., Ha, T., Heyland, A., et al. (2006). Neuronal transcriptome of aplysia: neuronal compartments and circuitry. Cell 127, 1453–1467. doi: 10.1016/j.cell.2006.09.052
Moustafa, A., Sakamoto, K. Q., and Habara, Y. (2011). A fundamental role for no-plc signaling pathway in mediating intracellular Ca2+ oscillation in pancreatic acini. Nitric Oxide 24, 139–150. doi: 10.1016/j.niox.2011.02.001
Mural, R. J., Adams, M. D., Myers, E. W., Smith, H. O., Miklos, G. L., Wides, R., et al. (2002). A comparison of whole-genome shotgun-derived mouse chromosome 16 and the human genome. Science 296, 1661–1667. doi: 10.1126/science.1069193
Nadaud, S., Bonnardeaux, A., Lathrop, M., and Soubrier, F. (1994). Gene structure, polymorphism and mapping of the human endothelial nitric oxide synthase gene. Biochem. Biophys. Res. Commun. 198, 1027–1033. doi: 10.1006/bbrc.1994.1146
Navarro-Guillén, C., Rnnestad, I., Jordal, A. E. O., Moyano, F. J., and Yúfera, M. (2017). Involvement of cholecystokinin (CCK) in the daily pattern of gastrointestinal regulation of Senegalese sole (Solea senegalensis) larvae reared under different feeding regimes. Comp. Biochem. Physiol. A Mol. Integ. Physiol. 203, 126–132. doi: 10.1016/j.cbpa.2016.09.003
Nesterova, N. V., Zagorodnya, S. D., Moshtanska, V., Dolashka, P., Baranova, G. V., Golovan, A. V., et al. (2011). Antiviral activity of hemocyanin isolated from marine snail Rapana venosa. Antiviral Res. 90, A38–A38.
Niswender, C. M., Sanders-Bush, E., and Emeson, R. B. (1998). Identification and characterization of RNA editing events within the 5-HT2C receptor. Ann. N. Y. Acad. Sci. 861, 38–48. doi: 10.1111/j.1749-6632.1998.tb10171.x
Niu, D., Wang, F., Xie, S., Sun, F., Wang, Z., Peng, M., et al. (2016). Developmental transcriptome analysis and identification of genes involved in larval metamorphosis of the razor clam, Sinonovacula constricta. Mar. Biotechnol. 18, 168–175. doi: 10.1007/s10126-016-9691-y
Olga, A., Lilia, Y., Rada, S., Stefan, S., Pavlina, D., and Draga, T. (2015). Changes in the gene expression profile of the bladder cancer cell lines after treatment with Helix lucorum and Rapana venosa hemocyanin. Offic. J. Balkan Union Oncol. 20:180.
Ou, Y., and Liu, Z. (2007). Effects of starvation and refeeding on digestive enzyme activity of lutjanus sebae juveniles. Acta Oceanol. Sin. 29, 86–91.
Pedro, N. D., Pinillos, M. L., Valenciano, A. I., Alonso-Bedate, M., and Delgado, M. J. (1998). Inhibitory effect of serotonin on feeding behavior in goldfish: involvement of crf. Peptides 19, 505–511. doi: 10.1016/s0196-9781(97)00469-5
Poon, K. L., Richardson, M., Lam, C. S., Khoo, H. E., and Korzh, V. (2003). Expression pattern of neuronal nitric oxide synthase in embryonic zebrafish. Gene Expres. Patt. 3, 463–466. doi: 10.1016/s1567-133x(03)00063-2
Regulski, M., and Tully, T., (1995). Molecular and biochemical characterization of dnos: a drosophila ca2+/calmodulin-dependent nitric oxide synthase. Proc. Natl. Acad. Sci. U.S.A. 92, 9072–9076. doi: 10.1073/pnas.92.20.9072
Rehfeld, J. F. (2017). Cholecystokinin-from local gut hormone to ubiquitous messenger. Front. Endocrinol. 8:47. doi: 10.3389/fendo.2017.00047
Sahoo, G., and Khandeparker, L. (2018). Nitric oxide-serotonin interplay in the cyprid metamorphosis of Balanus amphitrite (cirripedia, thoracica). Int. Biodeter. Biodegrad. 127, 95–103. doi: 10.1016/j.ibiod.2017.11.018
Satuito, C. G., Natoyama, K., Yamazaki, M., Shimizu, K., and Fusetani, N. (1999). Induction of metamorphosis in the pediveliger larvae of the mussel mytilus galloprovincialis by neuroactive compounds. Fish. Sci. 65, 384–389. doi: 10.2331/fishsci.65.384
Scholz, N. L., Goy, M. F., Truman, J. W., and Graubard, K. (1996). Nitric oxide and peptide neurohormones activate cGMP synthesis in the crab stomatogastric nervous system. J. Neurosci. Offic. J. Soc. Neurosci. 16, 1614–1622. doi: 10.1523/jneurosci.16-05-01614.1996
Schwartz, J., Dubos, M. P., Pasquier, J., Zatylny-Gaudin, C., and Favrel, P. (2018). Emergence of a cholecystokinin/sulfakinin signalling system in lophotrochozoa. Sci. Rep. 8:16424.
Snyder, S. H., and Bredt, D. S. (1992). Biological roles of nitric oxide. Am. Sci. 266, 68–71,74–67.
Song, H., Dang, X., He, Y. Q., Tao, Z., and Wang, H. Y. (2017). Selection of housekeeping genes as internal controls for quantitative rt-pcr analysis of the veined rapa whelk (Rapana venosa). PeerJ 5:e3398. doi: 10.7717/peerj.3398
Song, H., Yu, Z. L., Sun, L. N., Xue, D. X., Zhang, T., and Wang, H. Y. (2016a). Transcriptomic analysis of differentially expressed genes during larval development of Rapana venosa by digital gene expression profiling. G3 Genes Genomes Genetic 6, 2181–2193. doi: 10.1534/g3.116.029314
Song, H., Wang, H. Y., and Zhang, T. (2016b). Comprehensive and quantitative proteomic analysis of metamorphosis-related proteins in the veined rapa whelk, Rapana venosa. Int. J. Mol. Sci. 17:924. doi: 10.3390/ijms17060924
Song, H., Zhang, T., Yu, Z. L., et al. (2016c). Metabolomic analysis of competent larvae and juvenile veined rapa whelks (Rapana venosa). Mar. Biol. 163:145.
Stasiv, Y. (2001). The drosophila nitric-oxide synthase gene (dnos) encodes a family of proteins that can modulate nos activity by acting as dominant negative regulators. J. Biol. Chem. 276, 42241–42251. doi: 10.1074/jbc.m105066200
Syed, N. I., and Winlow, W. (1989). Morphology and electrophysiology of neurons innervating the ciliated locomotor epithelium in Lymnaea stagnalis. Comp. Biochem. Physiol. Part A Physiol. 93A, 633–644. doi: 10.1016/0300-9629(89)90024-8
Takata, Y., Takiguchi, S., Kataoka, K., Funakoshi, A., Miyasaka, K., and Kono, A. (1997). Mouse cholecystokinin type-a receptor gene and its structural analysis. Gene 187, 267–271. doi: 10.1016/s0378-1119(96)00765-2
Tate, S., Gray, J., Denyer, J., Stolz, M., Foord, S., and Lee, M. (1993). Cloning and expression of the human temporal cortex cholecystokinin b receptor. Neuropeptides 24:241. doi: 10.1016/0143-4179(93)90263-a
Tripathi, S., Flobak, Å, Chawla, K., Baudot, A., Bruland, T., Thommesen, L., et al. (2015). The gastrin and cholecystokinin receptors mediated signaling network: a scaffold for data analysis and new hypotheses on regulatory mechanisms. BMC Syst. Biol. 9:40. doi: 10.1186/s12918-015-0181-z
Ueda, N., and Degnan, S. M. (2014). Nitric oxide is not a negative regulator of metamorphic induction in the abalone Haliotis asinine. Front. Mar. Sci. 1:21. doi: 10.3389/fmars.2014.00021
Venter, J. C. (2001). The sequence of the human genome (vol 292, pg 1304, 2001). Science 292, 1838–1838.
Vigna, S. R., Morgan, J. L., and Thomas, T. M. (1984). Localization and characterization of gastrin/cholecystokinin-like immunoreactivity in the central nervous system of Aplysia californica. J. Neurosci. 4, 1370–1377. doi: 10.1523/jneurosci.04-05-01370.1984
Voelter, W., Stevanovic, S., Velkova, L., Floetenmeyer, M., Beeumen, J. V., Dolashki, A., et al. (2016). Antimicrobial activity of molluscan hemocyanins from helix and Rapana snails. Curr. Pharmaceut. Biotechnol. 17, 263–267. doi: 10.2174/1389201016666150907113435
Vry, J. D., and Schreiber, R. (2000). Effects of selected serotonin 5-HT(1) and 5-HT(2) receptor agonists on feeding behavior: possible mechanisms of action. Neurosci. Biobehav. Rev. 24, 341–353. doi: 10.1016/s0149-7634(99)00083-4
Weerth, A. D., Pisegna, J. R., Huppi, K., et al. (2010). Molecular cloning, functional expression, and chromosomal localization of the human cholecystokinin type A receptor. Biochem. Biophys. Res. Commun. 194, 811–818. doi: 10.1006/bbrc.1993.1894
Wei, L. P., Qiu, S. Y., Wang, B. G., Sun, X. F., and Wang, X. D. (1999). Studies on the reproductive biology of Rapana Venosa. Shuichan Xuebao 23, 150–155.
Yang, B., Li, L., Pu, F., You, W., Huang, H., and Ke, C. (2015). Molecular cloning of two molluscan caspases and gene functional analysis during Crassostrea angulate (fujian oyster) larval metamorphosis. Mol. Biol. Rep. 42, 963–975. doi: 10.1007/s11033-014-3833-y
Yang, D. Z., Zhou, Y. B., Guan, Z. C., and Zhao, F. F. (2007). Technique for industrial breeding in Rapana venosa Valenciennes. Fish. Sci. 26, 237–239.
Yang, J. L., Li, S. H., Li, Y. F., et al. (2013). Effects of neuroactive compounds, ions and organic solvents on larval metamorphosis of the mussel Mytilus coruscus. Aquaculture 399, 106–112. doi: 10.1016/j.aquaculture.2013.02.039
Yang, M. J., Song, H., Yu, Z. L., Bai, Y. C., Hu, Z., Hu, N., et al. (2020a). Expression and activity of critical digestive enzymes during early larval development of the veined rapa whelk, Rapana venosa (Valenciennes, 1846). Aquaculture 519:734722. doi: 10.1016/j.aquaculture.2019.734722
Yang, M. J., Song, H., Yu, Z. L., Hu, Z., Zhou, C., Wang, X. L., et al. (2020b). Changes in symbiotic microbiota and immune responses in early development stages of Rapana venosa (valenciennes, 1846) provide insights into immune system development in gastropods. Front. Microbiol. 11:1265. doi: 10.3389/fmicb.2020.01265
Yang, Z., Yu, H., Yu, R., and Li, Q. (2015). Induced metamorphosis in larvae of the veined rapa whelk Rapana venosa using chemical cues. Mar. Biol. Res. 11, 1–8.
Yu, Z. L., Yang, M. J., Song, H., Hu, Z., Zhou, C., Wang, X. L., et al. (2020). Settlement and metamorphosis of Rapana venosa (Gastropoda: Muricidae) with implications for artificial culture. J. Oceanol. Limnol. 38, 249–259. doi: 10.1007/s00343-019-9107-8
Zendehdel, M., Taati, M., Jonaidi, H., and Amini, E. (2012). The role of central 5-HT(2c) and NMDA receptors on LPS-induced feeding behavior in chickens. J. Physiol. Sci. 62, 413–419. doi: 10.1007/s12576-012-0218-7
Zhang, Y., Dijkman, P. M., Zou, R., Zandl-Lang, M., and Kudryashev, M. (2021). Asymmetric opening of the homopentameric 5-ht 3a serotonin receptor in lipid bilayers. Nat. Commun. 12:1074.
Keywords: Rapana venosa, metamorphosis, neuroendocrine system, food habit transition, digestive system
Citation: Yang M-J, Feng J, Song H, Yu Z-L, Shi P, Liang J, Hu Z, Zhou C, Wang X-L and Zhang T (2021) The Characteristic of Critical Genes in Neuroendocrine System and Their Regulation on Food Habit Transition and Metamorphosis of Veined Rapa Whelk Rapana venosa (Valenciennes, 1846). Front. Mar. Sci. 8:690282. doi: 10.3389/fmars.2021.690282
Received: 02 April 2021; Accepted: 07 June 2021;
Published: 16 July 2021.
Edited by:
Youji Wang, Shanghai Ocean University, ChinaReviewed by:
Liqiang Zhao, Guangdong Ocean University, ChinaJin-Long Yang, Shanghai Ocean University, China
Xian De Liu, Jimei University, China
Copyright © 2021 Yang, Feng, Song, Yu, Shi, Liang, Hu, Zhou, Wang and Zhang. This is an open-access article distributed under the terms of the Creative Commons Attribution License (CC BY). The use, distribution or reproduction in other forums is permitted, provided the original author(s) and the copyright owner(s) are credited and that the original publication in this journal is cited, in accordance with accepted academic practice. No use, distribution or reproduction is permitted which does not comply with these terms.
*Correspondence: Tao Zhang, dHpoYW5nQHFkaW8uYWMuY24=