- 1Department of Geography, Memorial University of Newfoundland, St. John's, NL, Canada
- 2Riparia, Montréal, QC, Canada
- 3Civic Laboratory for Environmental Action Research, Memorial University of Newfoundland, St. John's, NL, Canada
Shoreline surveys are an accessible and common method for monitoring plastic pollution in aquatic environments. Their results are critical to well-informed pollution mitigation efforts. Here, we show that three environmental variables: (1) coarse sediment, (2) accumulations of organic material, and (3) snow and ice are dramatically underrepresented by existing shoreline plastic pollution research efforts. We reviewed 361 published shoreline surveys, encompassing 3,284 sample sites, and found that only 4% of sites included coarse sediment, only one study described sampling organic material for plastic, and only 2.5% of sites are sampled in the presence of ice or snow. The relative absence of these environmental variables may stem from the tailoring of shoreline survey guidelines to a narrow range of shoreline environments. These three features influence plastic deposition and retention on shorelines, and their underrepresentation signals a need to recalibrate research efforts towards better methodological reporting, and regional representation and relevance.
Introduction
As an inexpensive and accessible method of monitoring both micro- and macro-plastic pollution in marine and freshwater environments, shoreline surveys have become a common research method internationally. The widespread use of this research technique, often guided by standard protocols, is illustrated by the breadth of environments that have been examined for plastic debris characterisation and distribution. These environments include: popular tourist beaches (Retama et al., 2016; da Silva et al., 2018), remote islands (Andrades et al., 2018; Hipfner et al., 2018; Rech et al., 2018), mangrove forests (Cordeiro and Costa, 2010; Martin et al., 2019), mudflats (Lo et al., 2018; Phuong et al., 2018), industrial harbours (Abu-Hilal and Al-Najjar, 2004; Muller-Karanassos et al., 2019), and Arctic and Antarctic shorelines (Convey et al., 2002; Eriksson et al., 2013; Bergmann et al., 2017).
Shoreline surveys have some of the longest standing standardised methods in the field of plastic pollution research. As early as 1984, the United Nations Educational, Scientific and Cultural Organisation (UNESCO) released a manual detailing a standard methodology for monitoring tar balls on beaches [IOC (Intergovernmental Oceanographic Commission), 1984]. Following the success of this manual, the United States' National Oceanic and Atmospheric Administration (NOAA) released its first Marine Debris Survey Manual in 1992 (Ribic et al., 1992). In the years since, several intergovernmental and environmental organisations have provided standardised guidelines in an effort to improve comparability of shoreline debris data and allow for the compilation of global assessments of plastic distribution and abundance (Cheshire et al., 2009; OSPAR Commission, 2010; Opfer et al., 2012; European Commission, 2013; Lippiatt et al., 2013). Several reviews have made significant progress in collating individual studies from around the world as a way of gaining global insights on the problem of plastic pollution on marine and freshwater shorelines with the ultimate goal of identifying hotspots of pollution and enabling effective mitigation strategies (Hidalgo-Ruz et al., 2012; Ivar do sul and Costa, 2014; Van Cauwenberghe et al., 2015; Horton et al., 2017; Serra-Gonçalves et al., 2019). The challenge of combining the methodologies and results of shoreline surveys from around the world has led most of these authors to call for more rigorous standardisation of techniques for collecting, extracting, and reporting on plastics in environmental samples.
It is amidst these calls for even greater standardisation that this paper aims to make a timely intervention. While standardisation helps to ensure consistently commensurable data across disparate contexts, they are never able to manage all possibilities (Bowker and Star, 1999), or landscapes. New research indicates that landscape features common to shoreline environments may affect how and where plastics accumulate upon deposition. Among these landscape features are: (1) sediment grain size, (2) accumulation of organic material at the tidelines, and (3) the presence of ice and snow. The inclusion of diverse shoreline landscapes in plastic pollution research, therefore, is crucial to our understanding of this ubiquitous pollutant and the ability to manage it. This review asks two questions; first, to what degree are these landscape features represented in shoreline plastic pollution surveys; and second, what are the likely explanations for the significant underrepresentation of sites with coarse sediments, organic material, and winter precipitation?
Recent scholarship reveals the important role diverse landscape features play in shaping the incidence and distribution of shoreline plastics. Researchers have demonstrated that coarse sediment (e.g., pebbles, gravel, cobble, boulders) are more efficient at burying plastic particles than fine sediment (e.g., sand) in the laboratory (Efimova et al., 2018; Chubarenko et al., 2020). Preliminary results from field surveys on rocky shorelines demonstrate that plastic debris can become trapped within coarse sediments in these environments (McWilliams et al., 2018; Weideman et al., 2020). In the laboratory, coarse sediment also generates plastic particles (particularly microplastics) through mechanical fragmentation between 5 and 145 times more effectively than sand (Chubarenko et al., 2020). Large organic material may also function to trap plastics on shorelines, as these often appear together in accumulation zones on shorelines, typically at the tidelines (Hoellein et al., 2014; Corcoran et al., 2017; Lazcano et al., 2020). Microplastics can adhere strongly to the surface of seaweed (Gutow et al., 2016; Sundbæk et al., 2018), such that the removal of plastic and other anthropogenic contaminants has become a standard component of pre-processing procedures in commercial seaweed aquaculture operations (Raikova et al., 2019). While the impact of snow and ice on shoreline plastic accumulation has not yet been fully investigated, both snow and sea ice have been identified as important sinks for microplastics in other contexts (Obbard et al., 2014; Peeken et al., 2018; Bergmann et al., 2019; Kelly et al., 2020). High concentrations of microplastics found in snow and ice are released seasonally following snow and ice melt, a process that has been observed in surface waters (Ory et al., 2020; Uurasjärvi et al., 2020; Von Friesen et al., 2020). There is a significant body of emerging research confirming the importance of landscape features in shaping shoreline plastic deposition.
The purpose of this paper is to assess the extent to which published shoreline surveys reflect three diverse shoreline landscape features which have a demonstrated impact on the distribution and accumulation of plastic pollutants: (1) coarse sediment, (2) accumulations of organic material, and (3) snow and ice. The results of our analysis point to the need for recalibration of research efforts in the field of marine plastic pollution in a way that further enables our shared goal of highlighting “where the most urgent actions are required to better understand the impacts of marine debris, enabling more effective mitigation policies to be developed.” (Serra-Gonçalves et al., 2019; p. 12159). To this end, we conducted a systematic literature review to examine how the three landscape features identified above are accounted for within the methodologies of published shoreline studies from 1977 to 2019. This analysis of over 350 shoreline studies, spanning over 40 years of research and encompassing over 3,000 individual sample sites around the world, is representative of the state of knowledge in this area.
Methods
Search Criteria
We conducted a systematic literature review for all English-language shorelines studies published in peer reviewed venues between 1977 and September 2019. We defined a shoreline survey as any original research study that collected count or concentration data in the field for anthropogenic debris, including plastics (of any size), on a marine or freshwater shoreline. Any shoreline influenced by a tide (including estuaries) or characterised by salt water (including enclosed seas) was categorised as a marine sample site. Freshwater shorelines, such as lakeshores and riverbanks, were not tidally influenced, meaning they did not include estuarine river mouths. The search was performed in Web of Science, a publisher-neutral repository that is home to over 170 million records covering over 34,600 journals. The following three searches were run: (1) (“marine plastic” OR “marine debris” OR “plastic pollution” OR litter OR plastics) AND (shoreline OR beach OR snow OR ice), (2) Microplastic, (sediment OR “beach” OR “seaweed” OR “algae” OR “wrack” OR “snow” OR “ice”), and (3) (“Marine plastic” OR “marine debris” OR “plastic pollution” OR litter OR plastics), (seaweed OR wrack OR algae). The results of these searches were initially screened for broad-scale relevance based on title and abstract. Following the initial screening, full text articles were screened based on the following inclusion criteria: (1) original research, (2) shoreline survey area with exposure at low tide, (3) research results included plastic density estimates, (4) not a single-item survey. Throughout the literature review we also noted any shoreline survey articles that were referenced within this literature but not collected by our searches. All articles included in this review were from the peer reviewed literature, with the exception of one Master's thesis. For full PRISMA flow diagram depicting the literature screening process, see Supplementary Figure 1.
For each publication, we extracted the coordinates for each available sample site and information relevant to the following categories: (1) type of shoreline survey, (2) sediment type, (3) organic materials, (4) sampling frequency and mentions of snow or ice presence during the survey period. Where individual sample sites within a study could not be extracted, the entire study was treated as a single sample site (n = 18 studies).
Sample Site
The constitution of an individual sample site followed delineations provided by the authors for each study. This means that the sample sites referred to in this review ranged in size from a quadrat area of 0.0625 m2 to a whole beach. Where no coordinates were provided but a map and/or place names were presented, approximate coordinates were obtained using Google Maps (google.ca/maps/). Where exact GPS coordinates could not be identified, either because no sample site information was provided by the article (n = 18), or because the site could not be confidently located based on the descriptors provided (n = 11), sites were not included in any visualisations of geographic distribution.
Survey Type
For the purposes of this study, we categorised the reviewed surveys into one or both of two categories: (1) “surface surveys” that quantify plastics sitting on the beach surface, and (2) “buried surveys” that quantify plastics buried beneath the beach surface. Surveys were considered to address buried plastics if researchers described removing, raking, or sieving the sediment to any depth for plastic detection. Plastic size classes included in each survey type were not standardised and were in some cases not explicitly reported. Surface surveys typically investigated plastics to a minimum size of what could be seen by eye from standing height (generally 2–2.5 cm), but the minimum size detected by this survey type ranges from 1 mm to 5 cm. Microplastics (< 5mm) or plastics not captured by surface surveys (<2cm) were the most common target of buried surveys, and the lower limit of plastic detected by these surveys range from 0.2 μm to 5 cm.
Sediment Type
Beach sediment type was classified according to the following categories: (1) sand, (2) coarse sediment, (3) unknown, and (4) other. Sediment type was categorised wherever possible according to the terminology used by the authors of each survey. Any sample sites described as being characterised by sediment coarser than sand (e.g., pebbles, cobbles, gravel, boulders, or bedrock) were classified as “coarse sediment.” Where sediment grain sizes were provided, sample sites were categorised as predominated either by sand (<2mm) or coarse sediment (>2mm) according to the Udden-Wentworth scale for grain size (Chesworth, 2008). Where sample sites were described as being a mix of both sand and coarse sediment, they were counted in both of these categories. The “unknown” classification covered all cases where sediment type could not be inferred for individual sample sites in a study. This occurred where: (a) no mention of sediment type was made in the report, or (b) authors indicated that sample sites covered a range of sediment types, but did not describe sediment type per individual sample site, or give any indication of the number of non-sandy sites. In order to account for these cases of coarse sediment sampling, we analysed sediment data not just for sample sites, but for studies as a whole. The “other” category was created to account for non-sedimentary shorelines (i.e., mangroves). Coarse sediment (particularly gravel and pebble) is typically not problematic with existing surface survey protocols, but it does not suit the suggested sieving method promoted in the most commonly cited protocols for buried surveys. We therefore analysed sediment type not only for all sample sites, but also within buried surveys alone in order to determine whether the representation of coarse sediment beaches was influenced by survey type.
Organic Material
Organic material was defined as any biological material of natural origin. In the marine environment, this material most often took the form of stranded seaweed or kelp detritus, or driftwood. In the freshwater environment, organic material included algae, leaves, feathers or grass. Information regarding organic materials was most often provided within sampling and sample processing protocols, and was therefore not site-specific. As a result, information on organic material was collected per study rather than sample site. Documenting stranded organic materials present during surveys was done using the following organic procedures categories: (1) explicit targeting of a tideline, (2) organic material on shoreline, (3) organics removal, (4) organic material in sediment samples, and (5) samples of stranded organic materials. Details on how surveys were classified into these categories are provided in Table 1. The term “tideline” refers to any reference to a natural accumulation zone of organic material on a shoreline, encompassing “wrackline,” “strandline” and other interchangeable terms, as well as accumulation zones in environments that are not tidally influenced (e.g., lakes and rivers).
Survey Frequency
The following survey frequency categories were considered: (1) single survey, (2) daily, (3) weekly to bimonthly, (4) seasonal, (5) annual, and (6) other repeated. Sites that were revisited anywhere from 1 week later to 2 months later were classified as “weekly to bimonthly.” Surveys were considered seasonal if they covered more than one season. Each sample site classified as “seasonal” was given a ranking (0.5, 0.75, or 1) based on the seasonal regime of its region. For example, a survey that crossed two seasons would be assigned a value of 1 (100% of seasons covered) if it took place in a region that experiences only two seasons (such as wet and dry). Whereas, a similar survey crossing two seasons would be assigned a value of 0.5 (50% of seasons covered) if it occurred in a region that experiences four temperate seasons (such as spring, summer, fall and winter). The “other repeated” category was used to account for studies that visited a site more than once but did not fit into any of the other frequency categories (i.e., more than 1 year between visits). A survey could qualify for more than one of the above categories. For example, a monthly survey that continued for 2 years would be counted as “weekly to bimonthly,” “seasonal,” “annual,” and “other repeated.” Where a study explicitly described revisiting sample sites from a previously published work already included in this review, the survey frequency classification for those sample sites was determined based on the most recent publication.
Results
Sample Sites
This review captured 361 published shoreline studies from 1977 to 2019, yielding 3,284 individual sample sites (Figure 1). Freshwater sample sites accounted for 3.6% (n = 118) of these, stemming from 20 studies (5.5% of studies). Surveys of the shoreline surface were more common (62% of sample sites; n = 2,047) than surveys of buried plastics (43% of sample sites; n = 1,406). A total of 5% of all sample sites (n = 167) were subjected to both survey types.
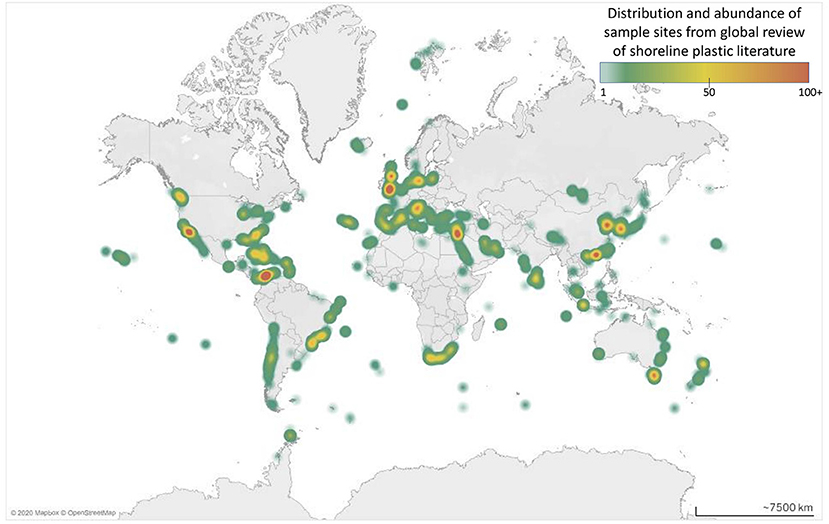
Figure 1. Global distribution heat map of sample sites from a literature review of shoreline plastic pollution encompassing 361 shoreline studies and 3,284 individual sites investigated for macro- and/or micro-plastics on the shoreline surface and/or buried within the shoreline sediment. Regions hosting over 100 sample sites are indicated in red. Only sample sites with sufficient location data provided within the report are included (n = 3,257).
Sediment Type
Sediment type was not reported for 39% of all sample sites (n = 1,274). Of the sample sites for which sediment type was reported (n = 2,010), 90% (n = 1,816) of these were sandy beaches and only 4% (n = 89) were described as coarse sediment beaches (Figure 2A). When the analysis is restricted only to surveys of buried plastics, sediment type was not reported for 14% (n = 194) of sample sites. For those buried plastic surveys that reported sediment type (n = 1,212; pattern fill in Figure 2A), 3% (n = 37) of sample sites were described as containing any amount of coarse sediment (i.e., including both “coarse” and “mix” sediment sites), 97% were dominated by sand (n = 1,175), and 0.2% (n = 3) of buried plastic sample sites or samples were described as being dominated by coarse sediment.
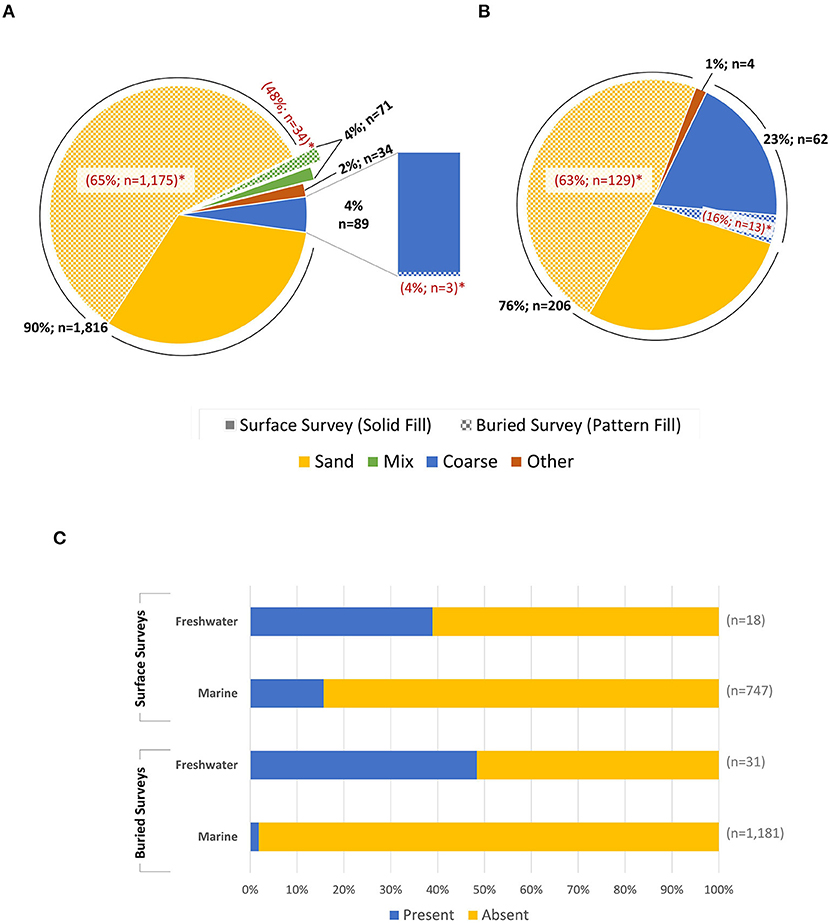
Figure 2. (A) Dominant sediment composition of sample sites for which sediment information was provided, broken down by shoreline survey type; (B) Incidence of coarse sediment in studies that referenced sediment type, broken down by survey type (studies including one or more coarse sediment or mixed sediment sample sites were included in the “Coarse” category). [*Buried survey proportions in red are calculated according to the respective sample size for each sediment type, not the population as a whole]; (C) Proportional representation of the presence of coarse sediment in marine and freshwater sample sites, separated by survey type. Sites for which sediment type was not provided, or sediment was classified as “other” are not included.
Several studies (n = 17) sampled at least one coarse sediment shoreline, but did not provide site-specific sediment information and were therefore not included in the analysis of sediment type per survey site. In order to account for these sample sites, the inclusion of coarse sediment sample sites per study was also analysed (Figure 2B), with a finding that 23% (n = 62) of studies included at least one sample site containing coarse sediment. When the analysis is restricted only to surveys of buried plastics, sediment type was not referred to in 15% (n = 25) of studies. For those buried plastic studies that referenced sediment type (n = 142; pattern fill in Figure 2B), 91% (n = 129) surveyed exclusively on sandy shorelines, and 9% (n = 13) included at least one sample site with some coarse sediment. Coarse sediment was more common in freshwater sample sites than for sample sites in the marine environment (Figure 2C). Among surface surveys, 39% (n = 7) of freshwater sites contained coarse sediment, compared to 16% (n = 117) of marine sample sites. Among buried surveys, coarse sediment was again better represented on freshwater shorelines (48%; n = 15) than in the marine environment (2%; n = 22). Relative to sand, coarse sediment is underrepresented in surveys for plastics both on the surface and buried within shorelines, and a failure to report sediment type for individual sample sites was relatively common, particularly in the case of surface surveys.
Organic Material
Information on the presence of organic material and the methodologies related to it was typically provided per study rather than for individual sample sites. Of the 361 reviewed studies, 48% (n = 174) described targeting a tideline, strandline, wrackline, or other accumulation zone of organic material on the shoreline (hereafter referred to as “tideline” inclusively). These descriptions of tideline sampling, where organic accumulations typically occur, were more common for buried surveys (60%; n = 101) than for surface surveys (38%; n = 73) (Figure 3A). Although targeting natural accumulation zones for organic material (i.e., tidelines) was common in buried surveys, natural debris was mentioned in 25% (n = 25) of buried surveys where a tideline was targeted, and only one study described extracting plastics buried within the organic matrix itself. More commonly, microplastics were extracted from sediment samples that contained quantities of organic material small enough (often microscopic) to be dissolved by acid digestion (“Sediment Processing” in Figure 3A). Mentions of an organic accumulation zone in the sample area was more common for marine studies (50%; n = 169) than for those that included freshwater sample sites (25%; n = 5), but the presence of organic material during site characterisation or sample collection was noted more often in studies that investigated freshwater sample sites (30%; n = 6) than in those that strictly sampled marine shorelines (16%; n = 57) (Figure 3B). Despite the prevalence of tideline-targeted sampling in both surface and buried plastic survey methodologies, and the likelihood of encountering both organic material and anthropogenic debris in the area, very few studies mention the presence or nature of the organic debris, and only one study described methods for extracting plastics from organic material.
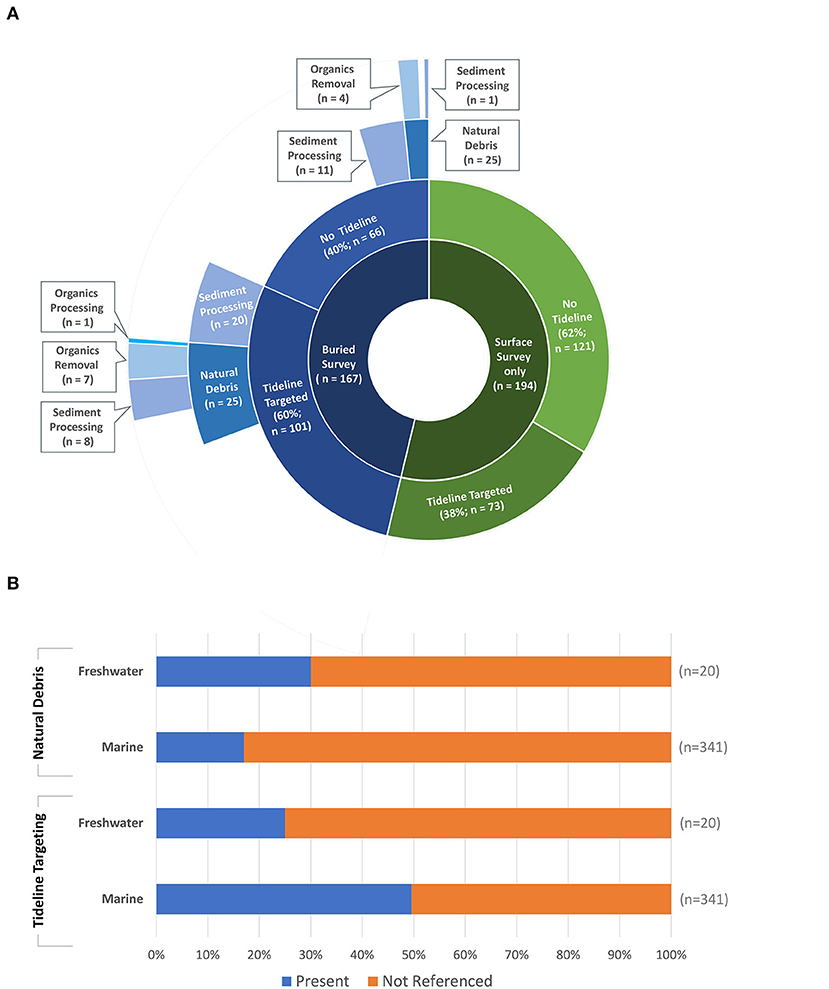
Figure 3. (A) Methodological breakdown of tideline targeting and treatment of organic material in 361 studies. Some studies included both buried and surface surveys, these studies were counted under the “Buried Survey” category. [A single study can qualify for more than one category where multiple treatments of organic materials were employed]; (B) Acknowledgement of tideline targeting or natural debris at sample sites from marine and freshwater studies. Any study that included at least one freshwater sample site was counted as a freshwater study. See Table 1 for more information on the classification system for treatment of organic material referred to here.
Seasonality and Snow and Ice
A total of 31% (n = 1,025) of all sample sites were subject to repeat sampling in the same location. Most of the repeatedly sampled sites captured more than one season (62% of repeat sample sites; n = 639), while 43% (n = 438) of revisited sample sites covered the full complement of seasons for their region (Figure 4). None of the freshwater sample sites were monitored across a full complement of seasons for their region. Most seasonally surveyed sample sites are outside of regions of snow accumulation (Figure 4). The presence of snow or ice was mentioned for 0.8% (n = 26) of all 3,284 sample sites. Mentions of snow and ice were more common for freshwater sample sites (14%; n = 16) than for marine sample sites (0.3%; n = 10). All 26 sample sites stemmed from five studies (1% of studies). Three of these studies mentioned snow and ice as a justification for not conducting winter sampling, one study sampled during the winter season but avoided areas of snow cover, and one study sampled during the presence of snow and ice (as evidenced by photos within the publication) but described no protocol adaptations. While many shoreline surveys were designed to capture seasonal variations, very few of these took place in regions frequently subjected to snow and ice precipitation, and none describe protocols for surveying in the presence of snow and ice.
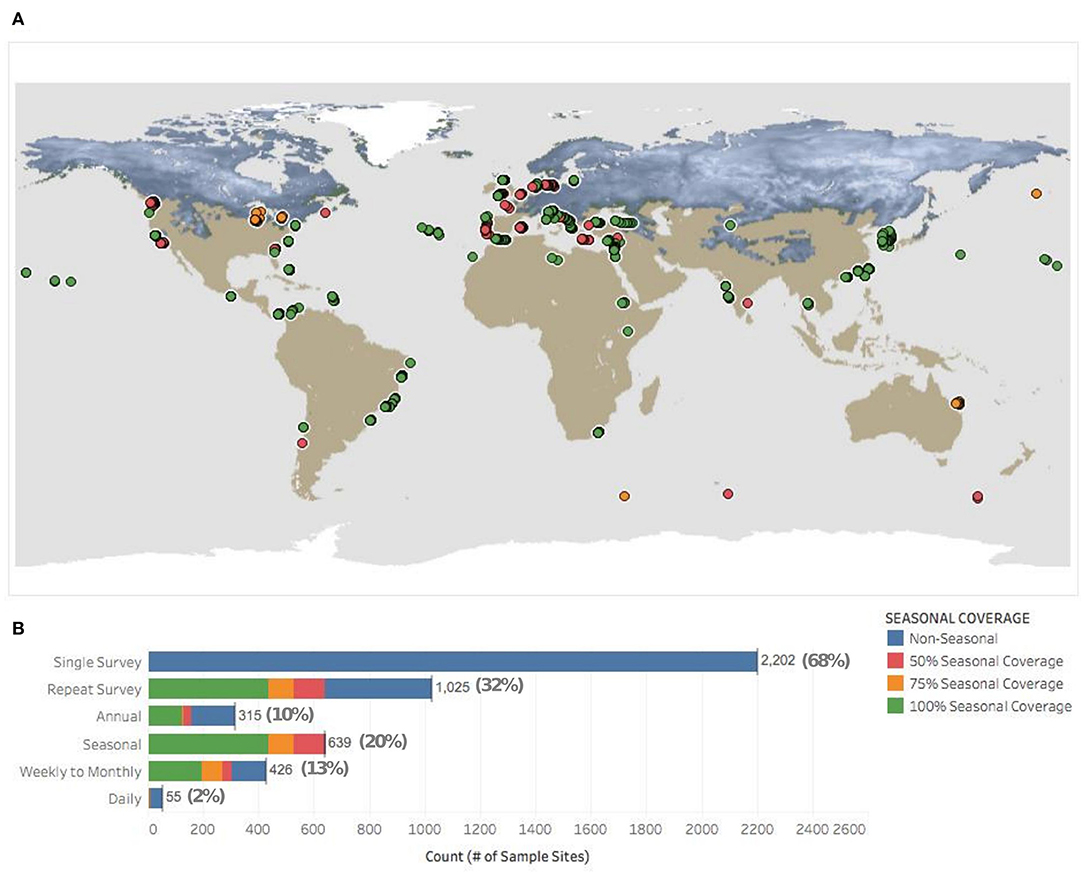
Figure 4. (A) Distribution of survey sites sampled in more than one season overlaid on NASA Earth Observations snow water equivalent map layer; (B) Counts of sample sites subject to different survey frequencies. Proportions are calculated from total number of sample sites. A single sample site can qualify for more than one frequency category (i.e., “Weekly to Monthly” and “Seasonal”). Map source: NASA Earth Observations (NEO).
Discussion
In answer to the first question posed by this paper on the degree to which diverse landscapes features are represented in the published literature, our findings reveal that the state of knowledge in shoreline plastic debris research focuses overwhelmingly on shoreline landscapes that are sandy and free of organic material or winter precipitation. Globally, roughly 70% of ice-free shorelines are not sandy (Luijendijk et al., 2018), while within plastic pollution research only 5% of sample sites were characterised by sediment that is coarser than sand. Moreover, although stranded organic debris is an important feature of any shoreline where the coastal waters are characterised by kelp forests or other macroalgae colonies (Orr et al., 2005; Krumhansl and Scheibling, 2012), only 18% of studies mention its presence and only one study describes any methods for locating plastics buried within stranded organic material. According to the National Snow & Ice Data Centre, snow covers 46 million square kilometres of land annually [NSIDC (National Snow and Ice Data Centre), 2020] mostly in the Northern Hemisphere, yet only 1% of studies make mention of ice or snow, and none include methods for locating plastics in the presence of ice or snow on shorelines, besides avoiding areas of snow/ice cover.
Next, we take up the second question posed by this paper: what are possible explanations for the acute underrepresentation of sites with coarse sediments, organic material, and winter precipitation in plastic pollution research? When sediment type was described, 94% of sample sites in this review were composed of sand. This value was even higher among sample sites surveyed for buried plastics, where 99.7% of sample sites were described as containing sandy sediment. Although some widely-used guidelines for surveying marine plastics include gravel and pebble beaches among their recommended site selection criteria (Cheshire et al., 2009; Opfer et al., 2012; Lippiatt et al., 2013), protocols for monitoring microplastics are only applicable to sandy beaches. For example, protocols require running sand samples through fine mesh sieves to extract plastic particles (impossible when the shoreline comprises sediment coarser than the sieve mesh size) [European Commission, 2013; Lippiatt et al., 2013; GESAMP (Joint Group of Experts on the Scientific Aspects of Marine Environmental Protection), 2019].
The emphasis in existing protocols on sandy beaches may explain, in part, why researchers describe seeking out sandy beaches even when these are not representative of the prevailing landscape in the region. Nachite et al. (2019), for example, selected 14 sandy beaches for their study in Morocco, despite acknowledging that only 20% of beaches in the country are made up of sand. Likewise, Kunz et al. (2016) selected four sandy beaches for their survey in Taiwan, despite the fact that the sampled region in the country “is mostly made up of rocky shores, and sandy beaches occur only in several relatively small and isolated areas” (Kunz et al., 2016, pp. 127–128). Others describe choosing sandy beaches specifically because of an anticipated inability to sample for small or buried plastics on coarse substrate (Ryan, 1987; Slip and Burton, 1992; Davis and Murphy, 2015; Stolte et al., 2015).
The European Commission's marine debris protocol document states “Sandy beaches are the easiest to survey, but pebbly and rocky beaches can be included in the assessment programme. However, it must be noted that the results from such beaches will not be comparable to sandy beaches as there will be an underestimation of small items on pebbly beaches and accumulation processes will be different (especially on rocky coastlines)” (European Commission, 2011, p. 13). In the literature examined here, when researchers did attempt to compare shorelines of different sediment types the results were often significant, but mixed. Debris density was sometimes reported to be higher on coarse sediment when compared to sand (Kuo and Huang, 2014; Hardesty et al., 2017; Brennan et al., 2018; Ríos et al., 2018), but the trend was not consistent across coarse sediment categories. For example, Brennan et al. (2018) found the highest debris densities on pebble shorelines, followed by sand, while rock platforms and boulder beaches revealed the lowest densities. In contrast, Hardesty et al. (2017) found the highest debris densities on boulder shorelines, followed by sand, with lower densities on rock slabs and gravel shorelines. Schmuck et al. (2017) found macro-debris densities to be significantly higher on sandy shorelines than on rocky or mixed sediment shorelines.
More consistently, researchers are finding that debris size and composition varies significantly between sandy and coarse sediment shorelines (Moore et al., 2001; Thiel et al., 2013; Giovacchini et al., 2018; Ríos et al., 2018). These differences could begin to explain the lack of consistency described above. The results of comparative studies of sandy and coarse sediment beaches will be influenced by: (1) the types of anthropogenic debris reported in overall debris densities, since non-plastic debris such as glass and metal have been reported to be more abundant on coarse sediment shorelines (Moore et al., 2001; Thiel et al., 2013; Kuo and Huang, 2014), and (2) the debris size categories under investigation, since large debris is reported to be more common on coarse sediment (Giovacchini et al., 2018; Ríos et al., 2018) and small debris can become buried in the interstitial spaces of these environments (Giovacchini et al., 2018; McWilliams et al., 2018). The potential effect of debris burial is not accounted for by any of the above listed comparative studies, which are all surveys of surface debris.
Clearly, more investigation into the role that coarse sediment shorelines play in accumulating (and/or generating) plastic debris is needed, but the inclusion of coarse sediment beaches in existing assessment programs is easier said than done due to logistical constraints. McWilliams et al. (2018, p. 1) conclude that “standardised protocols for shoreline marine debris studies are not developed for the rocky and icy shores that characterise locations such as Newfoundland, Canada, or indeed, much of the coastline found in high latitudes.” The findings of this review, namely the vast underrepresentation of non-sandy shorelines, likely indicates that researchers around the globe struggle to apply these protocols in their own geographies, and may, as a result, choose to avoid coarse sediment in their surveys.
The same sieving protocol which proves unsuitable for investigating buried plastics in coarse sediment is similarly unsuitable for any organic debris large enough to resist sieving (>5 mm). This is particularly problematic for tideline areas covered in large quantities of stranded seaweed which can become incorporated into the sediment up to 30 cm depth, especially in coarser sediment (Orr et al., 2005). This may, in part, explain why plastic buried or entangled within large organic debris was only investigated at 1% of all sample sites, despite the fact that 60% of buried plastic survey studies reviewed here targeted a tideline for sampling; a sampling strategy which is explicitly recommended in guideline documents [European Commission, 2013; Lippiatt et al., 2013; GESAMP (Joint Group of Experts on the Scientific Aspects of Marine Environmental Protection), 2019].
These logistical reasons may be why some researchers document avoiding shoreline areas with large organic deposits (Blumenröder et al., 2017; Edo et al., 2019; Leads and Weinstein, 2019), while others report moving organic material out of the way in order to sample the sand below (Wessel et al., 2016; Abidli et al., 2018; Hansen and Gross, 2019). However, given that high quantities of recently deposited plastic debris can be expected within these mounds of stranded organic matter (Thornton and Jackson, 1998; Gutow et al., 2016; Rodrigues et al., 2019; Lazcano et al., 2020), their avoidance may result in an underestimation of plastic pollution density particularly given the strong association between deposits of organic material and plastic debris, which has been noted by several researchers within this corpus (Thornton and Jackson, 1998; Velander and Mocogni, 1998; Viehman et al., 2011; Dippo, 2012; Zhou et al., 2018; Rodrigues et al., 2019).
Snow and ice act as sinks, concentrating microplastics until temperatures rise and plastics are released in meltwater (Ory et al., 2020; Uurasjärvi et al., 2020; Von Friesen et al., 2020). Although a full complement of seasons is universally recommended for shoreline monitoring programs in key monitoring guides [European Commission, 2013; Lippiatt et al., 2013; GESAMP (Joint Group of Experts on the Scientific Aspects of Marine Environmental Protection), 2019), there are no guidelines for monitoring shorelines in the presence of snow and ice in these same documents. A recent shoreline study highlights this contradiction: “According to protocol, OSPAR beaches are surveyed at 3-month intervals annually (winter, spring, summer and autumn). However, due to harsh winter conditions with ample snow, none of the Norwegian OSPAR beaches are registered more than twice a year” (Falk-Andersson et al., 2019, p.366). This discrepancy in protocols may explain why only 1% of the studies reviewed here mention snow or ice. Since the completion of this review, one recent study in Finland sampled snow, ice, and sediment near freshwater shorelines. The authors, however, note a critical problem: “Regarding snow samples, it was not possible to make any data comparison, as there are no published data on the occurrence of [microplastics] in a snow matrix” (Scopetani et al., 2019, p.14).
The three landscape features we have identified–sediment grain size, organic material and snow/ice–often intersect, compounding the challenges of conducting shoreline surveys where they occur. Coarse sediment beaches are more likely to accumulate large quantities of stranded organic materials like seaweed detritus than their finer sediment counterparts (Orr et al., 2005). Seaweed and kelp colonies are commonly found in the waters adjacent to rocky shorelines (Rodríguez, 2003; Krumhansl and Scheibling, 2012). Most of these colonies require cold, nutrient-rich waters, causing a peak algal biomass to occur between 45° and 60° N latitude (Steneck and Johnson, 2014), a region characterised by northern temperate or subarctic climates with marked seasonality and cold winters that give rise to snow and ice. The avoidance of any one of these environmental features individually may hinder the appearance of the other two in shoreline plastic pollution research. Alternatively, the inclusion of one feature may often lead to the appearance of all three, making it incredibly challenging to apply standard plastics monitoring techniques in these regions.
Here, we have demonstrated that existing plastic pollution research efforts are not addressing diverse shoreline landscapes proportionately. Based on the overall figures presented above, methodological comments made by individual researchers in published work, and the content of the shoreline survey guidelines themselves, we find that the nature of standardised methods in this field may be contributing to an overrepresentation of those landscape features for which protocols are readily available (i.e., sandy beaches free of organic debris, snow or ice). While standardised methods are critical for the global comparability of data, according to Bowker and Star (1999), they also tend to limit and delegitimize realities and representations thereof to which the standards cannot be applied (see above quote from the European Commission). Global syntheses which do not account for this underrepresentation are likely to generate a very partial view of shoreline plastic pollution. To fulfil the purposes of shoreline pollution research beyond the important goal of comparability (in this case, global synthesis, but also regional policy information, activism, community engagement and countless other place-based outcomes), we must prioritise the creation of knowledge that is representative of the region and landscape about which it speaks in the methods we employ.
In light of the need to balance the diverse and critical goals of plastic pollution research on shorelines, we make the following recommendations. First, in agreement with other reviewers in this field, we recommend more detailed methodological reporting. Site information including GPS coordinates, sediment type, surrounding landscape features and other environmental influences are critical to the comparability and interpretation of data across regions (Cowger et al., 2020). Second, we recommend that more attention is paid to diverse landscapes and the regionally appropriate representation thereof in published shoreline studies. This must necessarily be facilitated by protocol guideline documents that acknowledge variation in plastic accumulation across substrates (including but not limited to coarse sediment, organic deposits and ice and snow) and seek to include more diverse landscapes in the protocols they provide. Finally, we recommend acknowledging and legitimising the outcomes of shoreline surveys beyond comparability and global synthesis to make room for regionally specific and place-based priorities. We do not make this recommendation lightly, but with the confident knowledge that addressing the problem of plastic pollution will require flexibility, creativity and reflexivity.
Data Availability Statement
The original contributions presented in the study are included in the article/Supplementary Material, further inquiries can be directed to the corresponding author.
Author Contributions
JM conducted the original review for this paper and initially noted the central finding of this paper. MB, JM, and JA extracted methodological data per sample site. MB and JM wrote each draught and integrated insight and comments provided by JA, CM, and ML. ML provided critical insight and direction, and assisted JM with data analysis and design of figures and tables. This paper was conceptually a joint effort by all authors.
Acknowledgements
We acknowledge that this research was conducted on the unceded, unsurrendered ancestral Lands of the Mi'Kmaq and Beothuk peoples. We would also like to acknowledge the Inuit of Nunatsiavut and NunatuKavut and the Innu of Nitassinan, and their ancestors, as the original peoples of Labrador. Thank you to France Liboiron, Alexandra Hayward, and Cherish Blackmore, who were with us in the field and in the laboratory when we first identified these gaps, and without whom we never could have overcome the challenges of conducting shoreline research on our own rocky shorelines.
Funding
This work was jointly funded by the Ocean Frontier Institute (OFI), and the Department of Fisheries and Oceans Canada (DFO). Research Portal numbers for these Grants [DFO: RP-20200063 and OFI: RP-20191801].
Conflict of Interest
The authors declare that the research was conducted in the absence of any commercial or financial relationships that could be construed as a potential conflict of interest.
Supplementary Material
The Supplementary Material for this article can be found online at: https://www.frontiersin.org/articles/10.3389/fmars.2021.689108/full#supplementary-material
References
Abidli, S., Antunes, J. C., Ferreira, J. L., Lahbib, Y., Sobral, P., and El Menif, N. T. (2018). Microplastics in sediments from the littoral zone of the north Tunisian coast (Mediterranean Sea). Estuar. Coast. Shelf Sci. 205, 1–9. doi: 10.1016/j.ecss.2018.03.006
Abu-Hilal, A. H., and Al-Najjar, T. (2004). Litter pollution on the Jordanian shores of the Gulf of Aqaba (Red Sea). Mar. Environ. Res. 58, 39–63. doi: 10.1016/j.marenvres.2003.12.003
Andrades, R., Santos, R. G., Joyeux, J.-C., Chelazzi, D., Cincinelli, A., and Giarrizzo, T. (2018). Marine debris in Trindade Island, a remote island of the South Atlantic. Mar. Pollut. Bull. 137, 180–184. doi: 10.1016/j.marpolbul.2018.10.003
Bergmann, M., Lutz, B., Tekman, M. B., and Gutow, L. (2017). Citizen scientists reveal: marine litter pollutes Arctic beaches and affects wild life. Mar. Pollut. Bull. 125, 535–540. doi: 10.1016/j.marpolbul.2017.09.055
Bergmann, M., Müzel, S., Primpke, S., Tekman, M. B., Trachsel, J., and Gerdts, G. (2019). White and wonderful? microplastics prevail in snow from the Alps to the Arctic. Sci. Adv. 5:eaax1157. doi: 10.1126/sciadv.aax1157
Blumenröder, J., Sechet, P., Kakkonen, J. E., and Hartl, M. G. J. (2017). Microplastic contamination of intertidal sediments of Scapa Flow, Orkney: a first assessment. Mar. Pollut. Bull. 124, 112–120. doi: 10.1016/j.marpolbul.2017.07.009
Bowker, G. C., and Star, S. L. (1999). Sorting Things Out: Classification and Its Consequences. Cambridge, MA: MIT Press.
Brennan, E., Wilcox, C., and Hardesty, B. D. (2018). Connecting flux, deposition and resuspension in coastal debris surveys. Sci. Total Environ. 644, 1019–1026. doi: 10.1016/j.scitotenv.2018.06.352
Cheshire, A. C., Adler, E., Barbière, J., Cohen, Y., Evans, S., Jarayabhand, S., et al. (2009). UNEP/IOC Guidelines on Survey and Monitoring of Marine Litter. UNEP Regional Seas Reports and Studies, 186 (IOC Technical Series No. 83). Nairobi: UNEP.
Chesworth, W. (2008). “Wentworth scale” in Encyclopedia of Soil Science. Encyclopedia of Earth Sciences Series, ed W. Chesworth (Dordrecht: Springer). p. 830.
Chubarenko, I., Efimova, I., Bagaeva, M., Bagaev, A., and Isachenko, I. (2020). On mechanical fragmentation of single-use plastics in the sea swash zone with different types of bottom sediments: Insights from laboratory experiments. Mar. Pollut. Bull. 150:110726. doi: 10.1016/j.marpolbul.2019.110726
Convey, P., Barnes, D., and Morton, A. (2002). Debris accumulation on oceanic island shores of the Scotia Arc, Antarctica. Polar Biol. 25, 612–617. doi: 10.1007/s00300-002-0391-x
Corcoran, P., Jazvac, K., and Ballent, A. (2017). “Plastics and the anthropocene,” in Encyclopedia of the Anthropocene, eds D. Dellasala and M. Goldstein (Oxford: Elsevier Science and Technology), 163–170.
Cordeiro, C. A. M. M., and Costa, T. M. (2010). Evaluation of solid residues removed from a mangrove swamp in the São Vicente Estuary, SP, Brazil. Mar. Pollut. Bull. 60, 1762–1767. doi: 10.1016/j.marpolbul.2010.06.010
Cowger, W., Booth, A. M., Hamilton, B. M., Thaysen, C., Primpke, S., Munno, K., et al. (2020). Reporting guidelines to increase the reproducibility and comparability of research on microplastics. Appl. Spectroc. 74, 1066–1077. doi: 10.1177/0003702820930292
da Silva, L. M., Castro, R. O., Sales, A. S., and de Araújo, F. V. (2018). Marine debris on beaches of Arraial do Cabo, RJ, Brazil: an important coastal tourist destination. Mar. Pollut. Bull. 130, 153–158. doi: 10.1016/j.marpolbul.2018.03.026
Davis, W., and Murphy, A. G. (2015). Plastic in surface waters of the Inside Passage and beaches of the Salish Sea in Washington State. Mar. Pollut. Bull. 97, 169–177. doi: 10.1016/j.marpolbul.2015.06.019
Dippo, B. M. (2012). Microplastics in the coastal environment of west Iceland (Master's thesis). University of Akureyri, Ísafjörð*ur, Iceland. Available online at: https://skemman.is/ (accessed March 31, 2021).
Edo, C., Tamayo-Belda, M., Martínez-Campos, S., Martín-Betancor, K., González-Pleiter, M., Pulido-Reyes, G., et al. (2019). Occurrence and identification of microplastics along a beach in the Biosphere Reserve of Lanzarote. Mar. Pollut. Bull. 143, 220–227. doi: 10.1016/j.marpolbul.2019.04.061
Efimova, I., Bagaeva, M., Bagaev, A., Kileso, A., and Chubarenko, I. P. (2018). Secondary microplastics generation in the sea swash zone with coarse bottom sediments: laboratory experiments. Front. Mar. Sci. 5:313. doi: 10.3389/fmars.2018.00313
Eriksson, C., Burton, H., Fitch, S., Schulz, M., and van den Hoff, J. (2013). Daily accumulation rates of marine debris on sub-Antarctic island beaches. Mar. Pollut. Bull. 66, 199–208. doi: 10.1016/j.marpolbul.2012.08.026
European Commission (2011). Marine Litter: Technical Requirements for the Implementation of MSFD Requirements. JRC Scientific and Technical Reports. MSFD GES Technical Subgroup on Marine Litter.
European Commission (2013). Guidance on Monitoring of Marine Litter in European Seas. Luxembourg: Joint Research Centre.
Falk-Andersson, J., Berkhout, B. W., and Abate, T. G. (2019). Citizen science for better management: Lessons learned from three Norwegian beach litter data sets. Mar. Pollut. Bull. 138, 364–375. doi: 10.1016/j.marpolbul.2018.11.021
GESAMP (Joint Group of Experts on the Scientific Aspects of Marine Environmental Protection) (2019). Guidelines for the Monitoring and Assessment of Plastic Litter in the Ocean. London: IMO/FAO/UNESCO-IOC/UNIDO/WMO/IAEA/UN/UNEP/ENDP/ISA Joint Group of Experrts on the Scientific Aspects of Marine Environmental Protection.
Giovacchini, A., Merlino, S., Locritani, M., and Stroobant, M. (2018). Spatial distribution of marine litter along italian coastal areas in the Pelagos sanctuary (Ligurian Sea–NW Mediterranean Sea): a focus on natural and urban beaches. Mar. Pollut. Bull. 130, 140–152. doi: 10.1016/j.marpolbul.2018.02.042
Gutow, L., Eckerlebe, A., Giménez, L., and Saborowski, R. (2016). Experimental evaluation of seaweeds as a vector for microplastics into marine food webs. Environ. Sci. Technol. 50, 915–923. doi: 10.1021/acs.est.5b02431
Hansen, R. A., and Gross, A. (2019). Determination of microplastics in coastal beach sediments along Kattegat Sea, Denmark. Teor. Prikl. Ekol. 2, 75–82. doi: 10.25750/1995-4301-2019-2-075-082
Hardesty, B. D., Lawson, T. J., van der Velde, T., Lansdell, M., and Wilcox, C. (2017). Estimating quantities and sources of marine debris at a continental scale. Front. Ecol. Environ. 15, 18–25. doi: 10.1002/fee.1447
Hidalgo-Ruz, V., Gutow, L., Thompson, R. C., and Thiel, M. (2012). Microplastic in the marine environment: a review of the methods used for identification and quantification. Environ. Sci. Technol. 46, 3060–3075. doi: 10.1021/es2031505
Hipfner, J.M., Lok, E.K., Jardine, C., Studholme, K.R., Lebeau, A.C., Wright, K.G., et al. (2018). Beach-cast debris surveys on Triangle Island, British Columbia, Canada indicate the timing of arrival of 2011 Tohoku tsunami debris in North America. Mar. Pollut. Bull. 136, 407–413. doi: 10.1016/j.marpolbul.2018.09.041
Hoellein, T., Rojas, M., Pink, A., Gasior, J., and Kelly, J. (2014). Anthropogenic litter in urban freshwater ecosystems: distribution and microbial interactions. PLoS ONE 9:e98485. doi: 10.1371/journal.pone.0098485
Horton, A. A., Walton, A., Spurgeon, D. J., Lahive, E., and Svendsen, C. (2017). Microplastics in freshwater and terrestrial environments: evaluating the current understanding to identify the knowledge gaps and future research priorities. Sci. Total Environ. 586, 127–141. doi: 10.1016/j.scitotenv.2017.01.190
IOC (Intergovernmental Oceanographic Commission) (1984). Manual for Monitoring Oil and Dissolved/Dispersed Petroleum Hydrocarbons in Marine Waters and on Beaches. Intergovernmental Oceanographic Commission Manuals and Guides, 13. Paris: UNESCO.
Ivar do sul, J. A., and Costa, M. F. (2014). The present and future of microplasticpollution in the marine environment. Environ. Pollut. 185, 352–364. doi: 10.1016/j.envpol.2013.10.036
Kelly, A., Lannuzel, D., Rodemann, T., Meiners, K. M., and Auman, H. J. (2020). Microplastic contamination in east Antarctic sea ice. Mar. Pollut. Bull. 154:111130. doi: 10.1016/j.marpolbul.2020.111130
Krumhansl, K. A., and Scheibling, R. E. (2012). Production and fate of kelp detritus. Mar. Ecol. Prog. Ser. 467, 281–302. doi: 10.3354/meps09940
Kunz, A., Walther, B. A., Löwemark, L., and Lee, Y.-C. (2016). Distribution and quantity of microplastic on sandy beaches along the northern coast of Taiwan. Mar. Pollut. Bull. 111, 126–135. doi: 10.1016/j.marpolbul.2016.07.022
Kuo, F.-J., and Huang, H.-W. (2014). Strategy for mitigation of marine debris: analysis of sources and composition of marine debris in northern Taiwan. Mar. Pollut. Bull. 83, 70–78. doi: 10.1016/j.marpolbul.2014.04.019
Lazcano, R. F., Vincent, A. E. S., and Hoellein, T. J. (2020). Trash dance: anthropogenic litter and organic matter co-accumulate on urban beaches. Geosci. J. 10:335. doi: 10.3390/geosciences10090335
Leads, R. R., and Weinstein, J. E. (2019). Occurrence of tire wear particles and other microplastics within the tributaries of the Charleston Harbor Estuary, South Carolina, USA. Mar. Pollut. Bull. 145, 569–582. doi: 10.1016/j.marpolbul.2019.06.061
Lippiatt, S., Opfer, S., and Arthur, C. (2013). Marine Debris Monitoring and Assessment: Recommendations for Monitoring Debris Trends in the Marine Environment. Silver Spring, MD: NOAA Marine Debris Program.
Lo, H.-S., Xu, X., Wong, C.-Y., and Cheung, S.-G. (2018). Comparisons of pollution between mudflats and sandy beaches in Hong Kong. Environ. Pollut. 236, 208–217. doi: 10.1016/j.envpol.2018.01.031
Luijendijk, A., Hagenaars, G., Ranasinghe, R., Baart, F., Donchyts, G., and Aarninkhof, S. (2018). The state of the world's beaches. Sci. Rep. 8:6641. doi: 10.1038/s41598-018-24630-6
Martin, C., Almahasheer, H., and Duarte, C. M. (2019). Mangrove forests as traps for marine litter. Environ. Pollut. 247, 499–508. doi: 10.1016/j.envpol.2019.01.067
McWilliams, M., Liboiron, M., and Wiersma, Y. (2018). Rocky shoreline protocols miss microplastics in marine debris surveys (Fogo Island, Newfoundland and Labrador). Mar. Pollut. Bull. 129, 480–486. doi: 10.1016/j.marpolbul.2017.10.018
Moore, S. L., Gregorio, D., Carreon, M., Weisberg, S. B., and Leecaster, M. K. (2001). Composition and distribution of beach debris in Orange County, California. Mar. Pollut. Bull. 42, 241–245. doi: 10.1016/S0025-326X(00)00148-X
Muller-Karanassos, C., Turner, A., Arundel, W., Vance, T., Lindeque, P. K., and Cole, M. (2019). Antifouling paint particles in intertidal estuarine sediments from southwest England and their ingestion by the harbour ragworm, Hediste diversicolor. Environ. Pollut. 249, 163–170. doi: 10.1016/j.envpol.2019.03.009
Nachite, D., Maziane, F., Anfuso, G., and Williams, A. T. (2019). Spatial and temporal variations of litter at the Mediterranean beaches of Morocco mainly due to beach users. Ocean Coast. Manag. 179:104846. doi: 10.1016/j.ocecoaman.2019.104846
NSIDC (National Snow Ice Data Centre) (2020). All About Snow. Boulder, CO: National Snow and Ice Data Center. Available online at: https://nsidc.org/cryosphere/snow (accessed February 1, 2020).
Obbard, R. W., Sadri, S., Wong, Y. Q., Khitun, A. A., Baker, I., and Thompson, R. C. (2014). Global warming releases microplastic legacy frozen in Arctic Sea ice. Earths Future 2, 315–320. doi: 10.1002/2014EF000240
Opfer, S., Arthur, C., and Lippiatt, S. (2012). NOAA Marine Debris Shoreline Survey Field Guide. Silver Spring, MD: NOAA Marine Debris Program.
Orr, M., Zimmer, M., Jelinski, D. E., and Mews, M. (2005). Wrack deposition on different beach types: spatial and temporal variation in the pattern of subsidy. Ecology 86, 1496–1507. doi: 10.1890/04-1486
Ory, N. C., Lehmann, A., Javidpour, J., Stöhr, R., Walls, G. L., and Clemmesen, C. (2020). Factors influencing the spatial and temporal distribution of microplastics at the sea surface–a year-long monitoring case study from the urban Kiel Fjord, southwest Baltic Sea. Sci. Total Environ. 736:139493. doi: 10.1016/j.scitotenv.2020.139493
OSPAR Commission (2010). Guideline for Monitoring Marine Litter on the Beaches in the OSPAR Maritime Area. London: OSPAR Commission.
Peeken, I., Primpke, S., Beyer, B., Gütermann, J., Katlein, C., Krumpen, T., et al. (2018). Arctic sea ice is an important temporal sink and means of transport for microplastic. Nat. Commun. 9:1505. doi: 10.1038/s41467-018-03825-5
Phuong, N. N., Poirier, L., Lagarde, F., Kamari, A., and Zalouk-Vergnoux, A. (2018). Microplastic abundance and characteristics in French Atlantic coastal sediments using a new extraction method. Environ. Pollut. 243, 228–237. doi: 10.1016/j.envpol.2018.08.032
Raikova, S., Knowles, T. D. J., Allen, M. J., and Chuck, C. J. (2019). Co-liquefaction of macroalgae with common marine plastic pollutants. ACS Sustain. Chem. Eng. 7, 6769–6781. doi: 10.1021/acssuschemeng.8b06031
Rech, S., Thiel, M., Pichs, Y. J. B., and García-Vazquez, E. (2018). Travelling light: fouling biota on macroplastics arriving on beaches of remote Rapa Nui (Easter Island) in the South Pacific Subtropical Gyre. Mar. Pollut. Bull. 137, 119–128. doi: 10.1016/j.marpolbul.2018.10.015
Retama, I., Jonathan, M. P., Shruti, V. C., Velumani, S., Sarkar, S. K., Roy, P. D., et al. (2016). Microplastics in tourist beaches of Huatulco Bay, Pacific coast of southern Mexico. Mar. Pollut. Bull. 113, 530–535. doi: 10.1016/j.marpolbul.2016.08.053
Ribic, C. A., Dixon, T. R., and Vining, I. (1992). Marine Debris Survey Manual. NOAA Technical Report NMFS 108. Washington, DC: U.S. Department of Commerce.
Ríos, N., Frias, J. P. G. L., Rodríguez, Y., Carriço, R., Garcia, S. M., Juliano, M., et al. (2018). Spatio-temporal variability of beached macro-litter on remote islands of the North Atlantic. Mar. Pollut. Bull. 133, 304–311. doi: 10.1016/j.marpolbul.2018.05.038
Rodrigues, A., Oliver, D. M., McCarron, A., and Quilliam, R. S. (2019). Colonisation of plastic pellets (nurdles) by E. coli at public bathing beaches. Mar. Pollut. Bull. 139, 376–380. doi: 10.1016/j.marpolbul.2019.01.011
Rodríguez, S. R. (2003). Consumption of drift kelp by intertidal populations of the sea urchin Tetrapygus niger on the central Chilean coast: possible consequences at different ecological levels. Mar. Ecol. Prog. Ser. 251, 141–151. doi: 10.3354/meps251141
Ryan, P. G. (1987). The origin and fate of artefacts stranded on islands in the African sector of the Southern Ocean. Environ. Conserv. 14, 341–346. doi: 10.1017/S0376892900016854
Schmuck, A. M., Lavers, J. L., Stuckenbrock, S., Sharp, P. B., and Bond, A. L. (2017). Geophysical features influence the accumulation of beach debris on Caribbean islands. Mar. Pollut. Bull. 121, 45–51. doi: 10.1016/j.marpolbul.2017.05.043
Scopetani, C., Chelazzi, D., Cincinelli, A., and Esterhuizen-Londt, M. (2019). Assessment of microplastic pollution: occurrence and characterisation in Vesijärvi lake and Pikku Vesijärvi pond, Finland. Environ. Monit. Assess. 191:652. doi: 10.1007/s10661-019-7843-z
Serra-Gonçalves, C., Lavers, J. L., and Bond, A. L. (2019). Global review of beach debris monitoring and future recommendations. Environ. Sci. Technol. 53, 12158–12167. doi: 10.1021/acs.est.9b01424
Slip, D. J., and Burton, H. R. (1992). Accumulation of fishing debris, plastic litter, and other artefacts, on Heard and Macquarie Islands in the Southern Ocean. Environ. Conserv. 18, 249–254. doi: 10.1017/S0376892900022177
Steneck, R. S., and Johnson, C. R. (2014). “Kelp forests: dynamic patterns, processes, and feedbacks” in Marine Community Ecology and Conservation, eds M. D. Bertness, J. F. Bruno, B. R. Silliman, and J. J. Stacchowicz (Sunderland, MA: Sinauer Associates), 315–336.
Stolte, A., Forster, S., Gerdts, G., and Schubert, H. (2015). Microplastic concentrations in beach sediments along the German Baltic coast. Mar. Pollut. Bull. 99, 216–229. doi: 10.1016/j.marpolbul.2015.07.022
Sundbæk, K. B., Koch, I. D. W., Villaro, C. G., Rasmussen, N. S., Holdt, S. L., and Hartmann, N. B. (2018). Sorption of fluorescent polystyrene microplastic particles to edible seaweed Fucus vesiculosus. J. Appl. Phycol. 30, 2923–2927. doi: 10.1007/s10811-018-1472-8
Thiel, M., Hinojosa, I. A., Miranda, L., Pantoja, J. F., Rivadeneira, M. M., and Vásquez, N. (2013). Anthropogenic marine debris in the coastal environment: a multi-year comparison between coastal waters and local shores. Mar. Pollut. Bull. 71, 307–316. doi: 10.1016/j.marpolbul.2013.01.005
Thornton, L., and Jackson, N. L. (1998). Spatial and temporal variations in debris accumulation and composition on an estuarine shoreline, Cliffwood Beach, New Jersey, USA. Mar. Pollut. Bull. 9, 705–711. doi: 10.1016/S0025-326X(98)00041-1
Uurasjärvi, E., Hartikainen, S., Setälä, O., Lehtiniemi, M., and Koistinen, A. (2020). Microplastic concentrations, size distribution, and polymer types in the surface waters of a northern European lake. Water Environ. Res. 92, 149–156. doi: 10.1002/wer.1229
Van Cauwenberghe, L. V., Devriese, L., Galgani, F., Robbens, J., and Janssen, C. R. (2015). Microplastics in sediments: a review of techniques, occurrence, and effects. Mar. Environ. Res. 111, 5–17. doi: 10.1016/j.marenvres.2015.06.007
Velander, K., and Mocogni, M. (1998). Maritime litter and sewage contamination at Cramond Beach Edinburgh–a comparative study. Mar. Pollut. Bull. 38, 385–389. doi: 10.1016/S0025-326X(97)00204-X
Viehman, S., Pluym, J. L. V., and Schellinger, J. (2011). Characterization of marine debris in North Carolina salt marshes. Mar. Pollut. Bull. 62, 2771–2779. doi: 10.1016/j.marpolbul.2011.09.010
Von Friesen, L. W., Granberg, M. E., Pavlova, O., Magnusson, K., Hassellöv, M., and Gabrielsen, G. W. (2020). Summer sea ice melt and wastewater are important local sources of microlitter to Svalbard waters. Environ. Int. 139:105511. doi: 10.1016/j.envint.2020.105511
Weideman, E. A., Perold, V., Omardien, A., Smyth, L. K., and Ryan, P. G. (2020). Quantifying temporal trends in anthropogenic litter in a rocky intertidal habitat. Mar. Pollut. Bull. 160:111543. doi: 10.1016/j.marpolbul.2020.111543
Wessel, C. C., Lockridge, G. R., Battiste, D., and Cebrian, J. (2016). Abundance and characteristics of microplastics in beach sediments: Insights into microplastic accumulation in northern Gulf of Mexico estuaries. Mar. Pollut. Bull. 109, 178–183. doi: 10.1016/j.marpolbul.2016.06.002
Keywords: plastic pollution, marine debris, marine pollution, shoreline, environmental monitoring
Citation: Melvin J, Bury M, Ammendolia J, Mather C and Liboiron M (2021) Critical Gaps in Shoreline Plastics Pollution Research. Front. Mar. Sci. 8:689108. doi: 10.3389/fmars.2021.689108
Received: 31 March 2021; Accepted: 10 June 2021;
Published: 07 July 2021.
Edited by:
Mónica Noemí Gil, CONICET Center for the Study of Marine Systems (CESIMAR), ArgentinaReviewed by:
Patricia Corcoran, Western University, CanadaRodrigo Hernandez-Moresino, Centro para el Estudios de Sistemas Marinos (CESIMAR, CCT CONICET-CENPAT), Argentina
Copyright © 2021 Melvin, Bury, Ammendolia, Mather and Liboiron. This is an open-access article distributed under the terms of the Creative Commons Attribution License (CC BY). The use, distribution or reproduction in other forums is permitted, provided the original author(s) and the copyright owner(s) are credited and that the original publication in this journal is cited, in accordance with accepted academic practice. No use, distribution or reproduction is permitted which does not comply with these terms.
*Correspondence: Jessica Melvin, jmelvin@mun.ca