- Tjärnö Marine Laboratory, Department of Marine Sciences, University of Gothenburg, Gothenburg, Sweden
Climate change leads to multiple effects caused by simultaneous shifts in several physical factors which will interact with species and ecosystems in complex ways. In marine systems the effects of climate change include altered salinity, increased temperature, and elevated pCO2 which are currently affecting and will continue to affect marine species and ecosystems. Seaweeds are primary producers and foundation species in coastal ecosystems, which are particularly vulnerable to climate change. The brown seaweed Fucus vesiculosus (bladderwrack) is an important foundation species in nearshore ecosystems throughout its natural range in the North Atlantic Ocean and the Baltic Sea. This study investigates how individual and interactive effects of temperature, salinity, and pCO2 affect F. vesiculosus, using a fully crossed experimental design. We assessed the effects on F. vesiculosus in terms of growth, biochemical composition (phlorotannin content, C:N ratio, and ∂13C), and susceptibility to the specialized grazer Littorina obtusata. We observed that elevated pCO2 had a positive effect on seaweed growth in ambient temperature, but not in elevated temperature, while growth increased in low salinity at ambient but not high temperature, regardless of pCO2-level. In parallel to the statistically significant, but relatively small, positive effects on F. vesiculosus growth, we found that the seaweeds became much more susceptible to grazing in elevated pCO2 and reduced salinity, regardless of temperature. Furthermore, the ability of the seaweeds to induce chemical defenses (phlorotannins) was strongly reduced by all the climate stressors. Seaweeds exposed to ambient conditions more than doubled their phlorotannin content in the presence of grazers, while seaweeds exposed to any single or combined stress conditions showed only minor increases in phlorotannin content, or none at all. Despite the minor positive effects on seaweed growth, the results of this study imply that climate change can strongly affect the ability of fucoid seaweeds to induce chemical defenses and increase their susceptibility to grazers. This will likely lead to widespread consequences under future climate conditions, considering the important role of F. vesiculosus and other fucoids in many coastal ecosystems.
Introduction
The complex effects of climate change generate significant stress that is currently affecting and will continue to affect marine ecosystems in the future (Teagle and Smale, 2018). When CO2 and other greenhouse gases accumulate in the atmosphere due to anthropogenic activity, they capture infrared radiation, resulting in increased air and water temperature globally. This has caused a 0.1°C increase in mean sea surface temperature per decade during the last 40 years (Taboada and Anadón, 2012) and temperatures are projected to continue to rise 2–5°C by the end of this century (IPCC, 2013). Changes in global temperature are also predicted to alter evaporation and increase precipitation, with the net effect of decreasing salinity in coastal areas (Jenkins, 1999; Wentz et al., 2007). This has already occurred, for example in the Baltic Sea where the salinity in surface water has decreased by –0.005 to –0.014 psu per year from 1982 to 2016 (Liblik and Lips, 2019) and is projected to decrease by 4 psu at the entrance to the Baltic Sea before the end of the century (Eilola et al., 2009; Kinnby et al., 2020). Furthermore, the Baltic Sea area has already experienced a documented abnormal increase in temperature which averages 0.5–1°C per decade (HELCOM, 2013). Additionally, approximately 30% of the carbon emitted into the atmosphere is absorbed by the ocean (Sabine et al., 2004). When CO2 dissolves in saltwater, the carbonate system of the seawater is altered, resulting in a decrease in pH known as ocean acidification (Sabine et al., 2004) and also increasing the carbon availability for primary production. Atmospheric CO2-concentrations have increased from pre-industrial average of 280 μatm to approximately 400 μatm and are predicted to reach 1,000 μatm by the end of this century, with a corresponding decrease of 0.14–0.35 units in mean ocean pH (Cornwall and Hurd, 2016). Taken together, changes to these three factors—temperature, salinity, and pCO2—represent the most powerful climate change related effects on marine systems (Solomon, 2007) and are fundamental to oceanic organisms and ecosystems (Harley et al., 2006; Takolander et al., 2017).
The co-occurring changes of multiple environmental variables may lead to additive or synergistic interactive effects on marine nearshore ecosystems under future climate change scenarios. Seaweeds are primary producers and foundation species in these coastal ecosystems, which are systems that are particularly vulnerable to climate change (Harley et al., 2006; Levitus et al., 2009). Several recent studies have investigated the interactive effects of climate change stressors on seaweeds, and the most commonly combined climate stressors are increased temperature and pCO2 (e.g., Olischläger and Wiencke, 2013; Kram et al., 2016; Mensch et al., 2016; Celis-Plá et al., 2017; Johnson et al., 2017; Roth-Schulze et al., 2018). In general, these studies show that the effect of increased temperature on growth vary from positive (Olischläger and Wiencke, 2013; Roth-Schulze et al., 2018), to neutral (Kram et al., 2016; Celis-Plá et al., 2017; Johnson et al., 2017), or negative (Kram et al., 2016; Mensch et al., 2016). Increased pCO2 may alleviate the negative effect of low or high temperature (i.e., an interactive effect; Olischläger and Wiencke, 2013; Kram et al., 2016; Roth-Schulze et al., 2018) or has a general positive effect on growth (Mensch et al., 2016; Celis-Plá et al., 2017; Johnson et al., 2017). The effect of increased temperature combined with decreased salinity on seaweed growth show either a general negative effect of increased temperature and no effect of salinity (Takolander et al., 2017), or general positive effect of temperature and a negative effect of salinity (Monteiro et al., 2021). Taken together, results from factorial experiments combining two different climate stressors show variable results (maybe with the exception that elevated pCO2 is generally positive for seaweed growth). While experiments combining two climate stressors remain valuable, there are to our knowledge no previous studies on seaweeds that have simultaneously investigated effects of predicted increases in both temperature and pCO2, simultaneously with a decrease in salinity.
The seaweed Fucus vesiculosus (bladderwrack) is a foundation species in nearshore ecosystems throughout its natural range in the North Atlantic Ocean, and it is the only fucoid that has colonized almost the entire Baltic Sea (Bergström et al., 2005; Lima et al., 2007). It provides habitat and food for invertebrates, fish, and other algal species in rocky intertidal areas in both sheltered and exposed rocky shorelines (Bergström et al., 2005; Forslund and Kautsky, 2013). Evidence from previous studies show that changes to a single environmental factor can have negative (reduced salinity, Nygård and Dring, 2008; Connan and Stengel, 2011a; increased temperature, Mensch et al., 2016) or positive (reduced salinity, Rugiu et al., 2018; Kinnby et al., 2021) effect on growth. Changes to multiple climate factors can cause tissue necrosis (reduced salinity and increased temperature, Takolander et al., 2017), reduce growth (reduced salinity and increased temperature, Rugiu et al., 2018) or mitigate the effects of changes to a single variable (increased temperature and elevated pCO2, Mensch et al., 2016). Moreover, growth responses to climate change have also been observed to vary over small geographic areas (Kinnby et al., 2020) and over seasons (Wahl et al., 2020).
Changes to climate variables can also affect the biochemical composition such as the ratio of carbon to nitrogen in tissue (C:N ratio), the isotopic signature of carbon in the tissue (∂13C), and secondary metabolites such as phlorotannins. Changes to the C:N ratio could affect the nutritional value and thus the attraction of grazers (Takolander et al., 2019). Phlorotannins are a group of polyphenolic metabolites that can occur in high concentrations in brown seaweeds, especially in fucoids. These compounds have several functions, including UV protection and chemical defense against grazers (Pavia et al., 1997; Toth and Pavia, 2007), and have been experimentally shown in F. vesiculosus to decrease when exposed to lower salinity (Connan and Stengel, 2011b; Kinnby et al., 2020) and elevated pCO2 (Kinnby et al., 2021). Climate change induced alterations in chemical defenses and overall biochemical composition of seaweed biomass can have consequences for ecological interactions such as grazing pressure on the seaweeds. In a recent study it was shown that the palatability of F. vesiculosus was reduced under warming and even more under the combined effects of warming and acidification (Raddatz et al., 2017).
This study investigates individual and interactive effects of changes in temperature, salinity, and pCO2 on F. vesiculosus, using a fully crossed experimental design. By exposing seaweeds to the projected changes in average salinity, temperature, and pCO2 in the study area (–4 psu, +5°C, and +700 μatm; Eilola et al., 2009; Solomon, 2007; Cornwall and Hurd, 2016; Kinnby et al., 2020) by year 2100 we assess the effects on growth of F. vesiculosus, measured as area, biochemical composition (tissue phlorotannin content, C:N ratio, and ∂13C), as well as the susceptibility of the seaweed to a common grazer, the snail Littorina obtusata. We hypothesize that these three climate change factors will affect growth of the seaweeds, more specifically that we would see an enhanced growth under elevated pCO2 but also that there would be antagonistic effects from different combinations of these climate variables. Furthermore, we hypothesize that the tissue C:N ratio will increase and ∂13C values will decrease as seaweeds transition from active to passive carbon uptake, which is expected under elevated pCO2 conditions. Finally, we hypothesize that phlorotannin content of the seaweeds will decrease under projected climate change conditions resulting in a higher susceptibility to grazing.
Materials and Methods
Sampling and Experimental Set-Up
Eighty individuals of F. vesiculosus were scraped off of their substrate and collected on the Swedish west coast near Tjärnö Marine Laboratory (TML, 58°52′36.4″N 11°6′42.84″E). Individuals weighed on average 11.5 g (± 0.6, 95% CI) and were kept under ambient salinity, temperature, and pCO2 conditions (Table 1) in flow-through seawater (0.3 L/min) for 7 days. After this acclimation period the seaweeds were randomly assigned to their individual experimental tanks (i.e., 1 individual/1 L tank). Salinity treatments were either “control” (23 ± 1 psu) using ambient surface salinity, or “experimental” (19 ± 1 psu). Temperature treatments were either “control” (15°C) using ambient surface water temperature, or “experimental” (20°C). To obtain lower salinity the flow-through seawater was diluted with freshwater, and the temperature was increased using submersible heaters. Experimental pCO2 treatments were aerated with control (400 μatm) or treatment (1,100 μatm) air which was regulated using solenoid valves and pH computers (Aqua Medic) (Kinnby et al., 2021). The pCO2 was monitored using a LI-850 CO2/H2O gas analyzer (Li-Cor). The CO2 analyzer was calibrated with custom mixed gas, 970 ppm (Linde Gas AB, Sweden). Temperature, salinity, pHNBS, and pCO2 were all measured in the experimental tanks. The pH was recorded using HANNA instruments pH electrode HALO probe (HI-1102) calibrated with NBS pH 4.01, 7.01, and 10.01 standards (HANNA instruments) before each measurement. Total alkalinity was estimated from salinity using long-term salinity:alkalinity relationship data for Tjärnö (r = 0.94; data obtained from Swedish Meteorological and Hydrological Institute)1 (Eriander et al., 2016) and pHT was calculated from the temperature, salinity, pCO2, and total alkalinity using CO2calc (Robbins et al., 2010; Table 1). All experimental treatments were fully crossed in an orthogonal design, i.e., seven treatments and one ambient control, with 10 replicates per treatment. The growth experiment ran for 7 weeks, followed by a 2-week long grazing experiment using the same seaweed individuals to investigate the susceptibility to grazing following the various treatments.
Seaweed Growth
Each algal thallus was weighed and photographed at the beginning and at the end of the experiment. Growth was assessed as percent increase in the thallus area from the photographs analyzed in ImageJ (Schneider et al., 2012) and as percent increase in wet weight during the experiment. The results for both area and weight were quantitatively similar and so only area is presented.
Grazing Experiment
The susceptibility to grazing of the F. vesiculosus thalli grown in the different treatments was measured in feeding trials using the specialist grazer Littorina obtusata. The feeding experiment was performed using a total of 160 containers (200 ml) with constant seawater flow from the treatments. Five individuals of L. obtusata were placed in half of the containers. To control for autogenic changes in mass (growth) during the experiment that was not caused by the grazing of the snails, each container with seaweed pieces and herbivores was paired with a control container without herbivores containing a piece of thallus from the same individual of seaweed (n = 10). The wet weight of all seaweed pieces was determined at the start and at the end of the 14-day long experiment by using a standard blotting procedure, and the wet-weight change of each seaweed piece was calculated by subtracting the weight at the end of the experiment from the starting weight. The herbivore consumption of seaweeds exposed to different treatments was determined by subtracting the weight change in the autogenic control containers from the wet weight change of the grazed seaweeds.
Biochemical Composition
Following the grazing experiment all seaweeds were frozen at –60°C. Newly grown seaweed tissue from apical tips was used for all biochemical analyses. The frozen tissue was lyophilized and homogenized to a fine powder.
Samples for elemental analysis (2–3 mg/sample) were sent to the Stable Isotope Facility at University of California Davis for analysis. C:N ratio and ∂13C were analyzed using an elemental analyzer interfaced to a continuous flow isotope ratio mass spectrometer (IRMS).
For phlorotannin analysis, seaweed powder was extracted in 70% acetone. The total phlorotannin content was quantified colorimetrically using the Folin-Ciocalteu method (van Alstyne, 1995), with phloroglucinol (1,3,5-trihydroxybenzene, art. 7069; Merck, Darmstadt, Germany) as a standard. The apical tissue used in the consumption experiment, i.e., both grazed and ungrazed tissue, was analyzed separately for phlorotannin content. An increase in phlorotannin content in grazed compared to ungrazed tissue of the same individual indicates an induced chemical defense as a result of grazing. The difference in phlorotannin content is presented as% dw (dry weight).
Statistical Analysis
The main and interactive effects of the three factors (temperature, salinity, and pCO2) on growth measured as area and weight, C:N ratio, ∂13C, difference in phlorotannin content between grazed and ungrazed tissue, as well as susceptibility to grazing were examined using three-way ANOVAs with temperature (2 levels), salinity (2 levels), and pCO2 (2 levels) as fixed, orthogonal factors. Before analysis the assumptions of normality and homogeneity of variance were visually checked with Q-Q plots and data were log-transformed when required (i.e., growth data for area and weight). Significant differences between means were further analyzed post-hoc with SNK-tests (Student-Newman-Keuls test). All analyses were performed in RStudio (version 1.3.1093).
Results
Seaweed Growth
The three-way ANOVA showed that there was a main effect of salinity as well as an interaction between temperature and pCO2 on growth measured as area (Table 2 and Figures 1A,B). At ambient temperature seaweed individuals grew significantly more under elevated pCO2 than at ambient pCO2 (SNK, p < 0.05), however exposure to increased temperature mitigated this effect under elevated pCO2 (Table 2 and Figure 1A). Individuals of seaweeds exposed to lowered salinity grew more than individuals grown in ambient salinity (SNK, p < 0.05; Table 2 and Figure 1B). Although the effects of climate factors on growth variables were statistically significant, the magnitude of the changes was small.
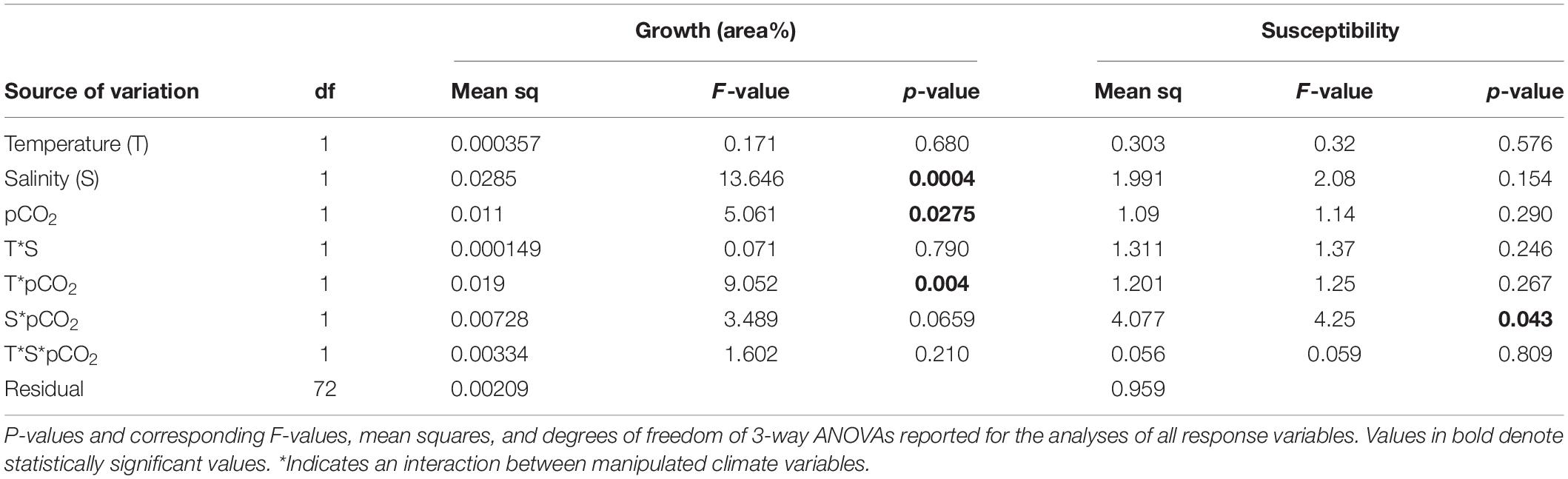
Table 2. Summary of effects of temperature (ambient and high), salinity (ambient and low), and pCO2 (ambient and elevated) for growth on Fucus vesiculosus and susceptibility to Littorina obtusata.
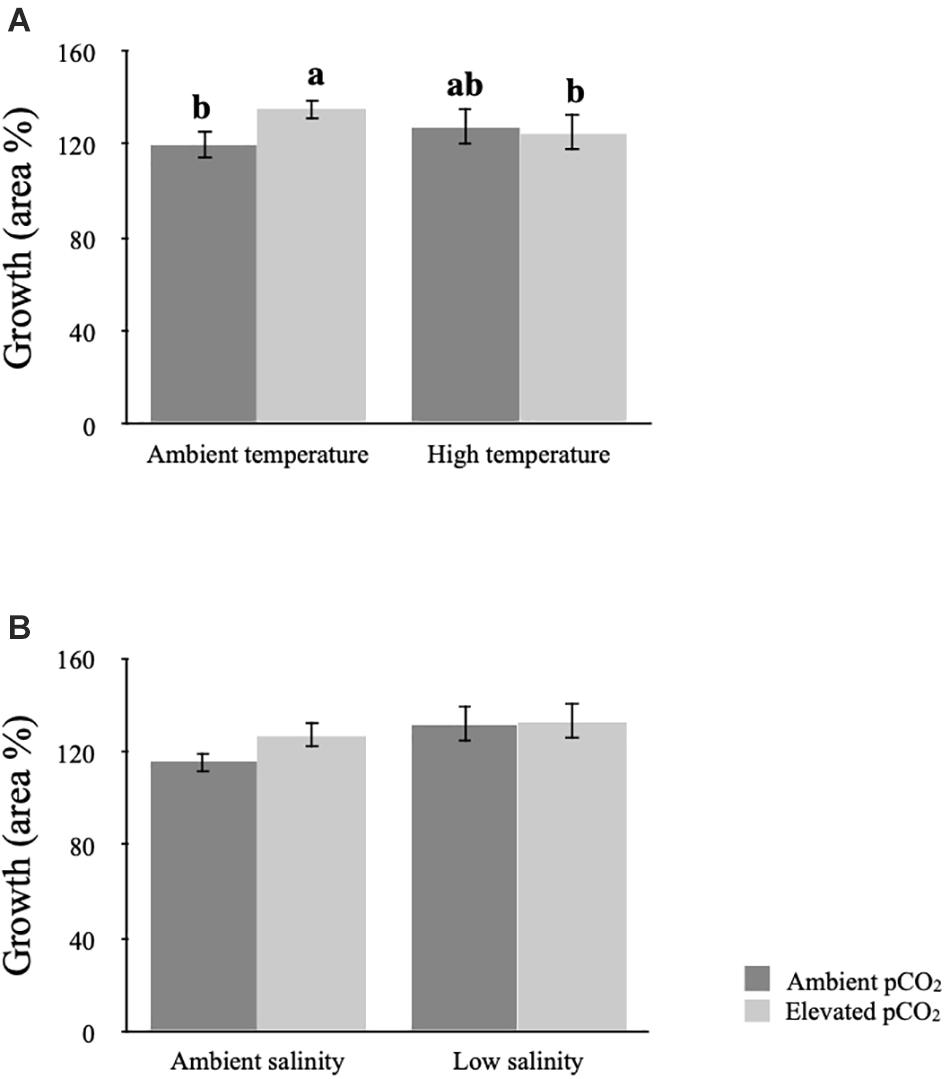
Figure 1. Effects on growth measured as area in Fucus vesiculosus exposed to different levels of temperature (ambient and high, A), salinity (ambient and low, B), and pCO2 (ambient and elevated) as fixed orthogonal factors. Letters denote statistically significant post-hoc groupings (SNK post-hoc test, p < 0.05). Values are means ± 95% CI, n = 20. Letters are missing in (B) because the interaction term was not statistically significant in the ANOVA (p = 0.066).
Susceptibility of F. vesiculosus to Littorina obtusata
Statistical analysis of consumption data from the grazing experiment showed that there was a significant interaction between salinity and pCO2. The SNK-tests showed that at ambient pCO2 the seaweeds grown in low salinity became 83% more susceptible to grazing compared to ambient salinity (Table 2 and Figure 2). Moreover, when exposed to elevated pCO2 seaweeds in ambient salinity became 74% more susceptible to grazing by L. obtusata (Table 2 and Figure 2).
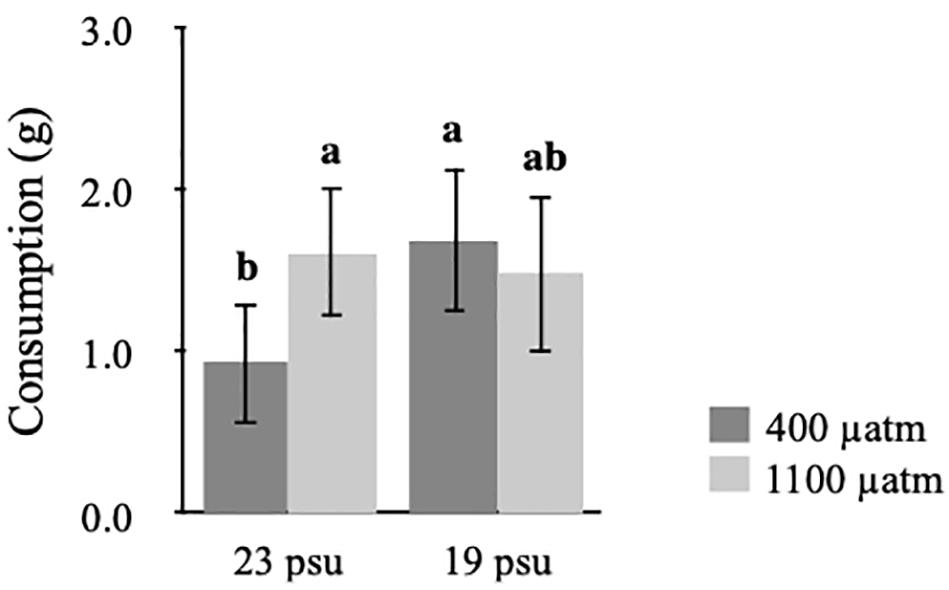
Figure 2. Susceptibility, measured as consumption, of Fucus vesiculosus to Littorina obtusata after exposure to different levels of temperature (ambient and high), salinity (ambient and low), and pCO2 (ambient and elevated) as fixed, orthogonal factors. Letters denote statistically significant post-hoc groupings (SNK post-hoc test, p < 0.05). Values are means ± 95% CI, n = 20.
Biochemical Composition
We found no statistically significant effect of any of the climate factors on C:N ratio, which was on average 21.2 between/overall treatments (Table 3). However, we found main effects of pCO2 and salinity on ∂13C values (Table 3). There was a shift in ∂13C value from an average of –15.8 (±0.6, 95% CI) to –17.9 (±0.7, 95% CI) in seaweeds grown in ambient compared to elevated pCO2 conditions, and from an average of –15.6 (±0.5, 95% CI) to –18.1 (±0.7, 95% CI) in seaweeds grown in ambient compared to low salinity.
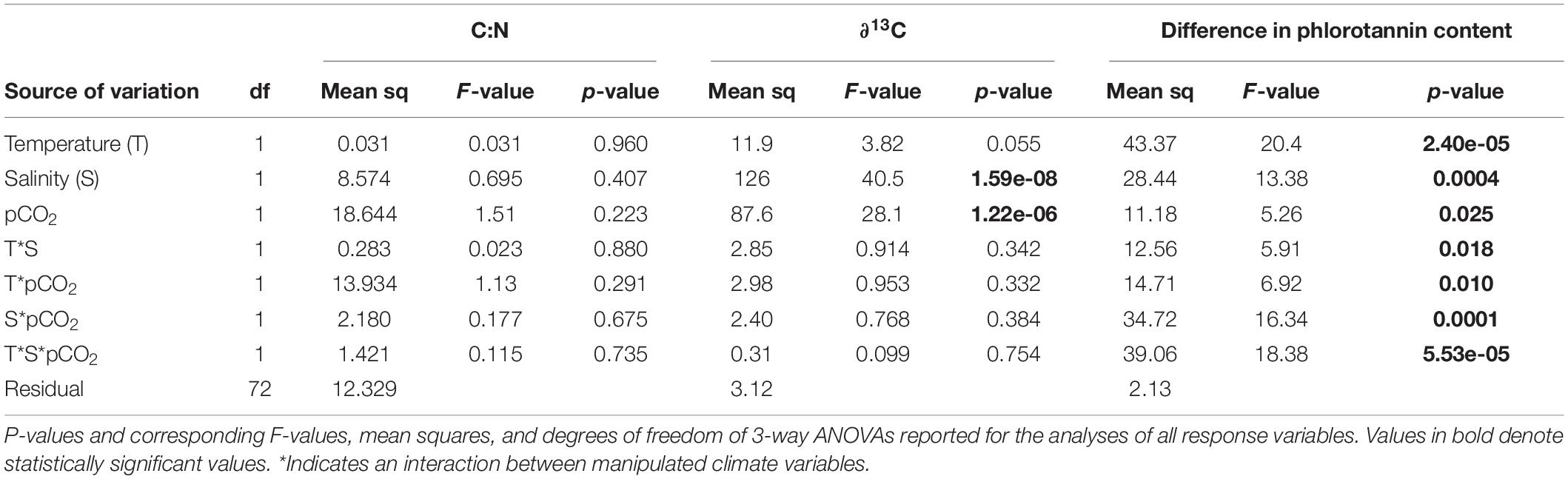
Table 3. Summary of effects of temperature (ambient and high), salinity (ambient and low), and pCO2 (ambient and elevated) on biochemical composition of Fucus vesiculosus.
Analysis of the difference in phlorotannin content between grazed and ungrazed tissue (from the same individual) of seaweeds showed that there was a statistically significant three-way interaction between salinity, temperature, and pCO2 (Table 3 and Figure 3). In the seaweeds grown under ambient conditions we found the most pronounced change in phlorotannin content, with an increase from 3.5% dw (± 0.5, 95%CI) in ungrazed tissue to 8.0% dw (±2.4, 95% CI) in grazed tissue. None of the other treatments, showed the same increase in phlorotannin content after grazing (Figure 3, Supplementary Table 1, and Supplementary Figure 1). The difference in phlorotannin content between grazed and ungrazed tissue was 39–61% lower in all the treated seaweeds compared to seaweeds exposed to ambient conditions (Figure 3, Supplementary Table 1, and Supplementary Figure 1).
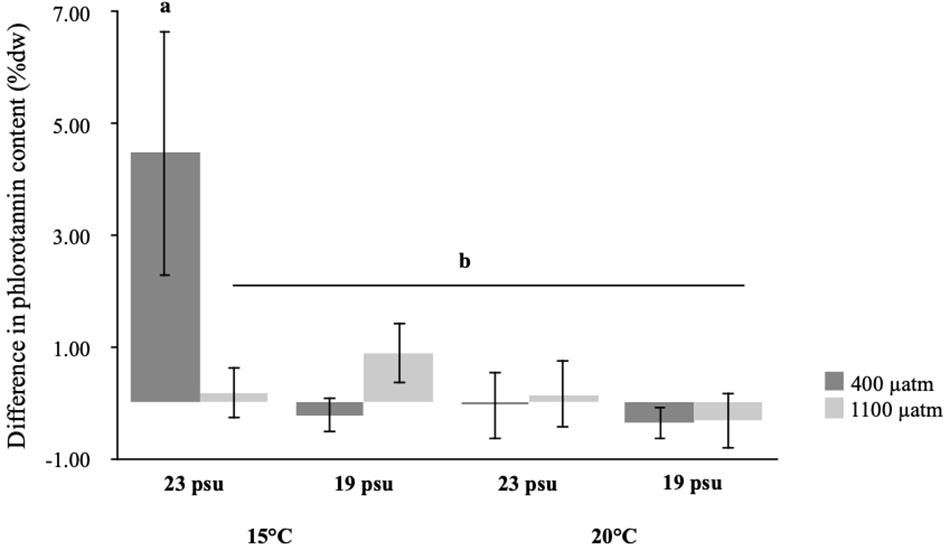
Figure 3. Difference in phlorotannin content of the same individual of Fucus vesiculosus between ungrazed and grazed tissue by Littorina obtusata after exposure to different levels of temperature (ambient and high), salinity (ambient and low), and pCO2 (ambient and elevated) as fixed, orthogonal factors. Letters denote statistically significant post-hoc groupings (SNK post-hoc test, p < 0.05). Values are means ± 95% CI, n = 10.
Discussion
We found both individual and combined effects of changes in temperature, salinity, and pCO2 on Fucus vesiculosus growth, biochemical composition, and susceptibility to grazing by one of its most important grazers, the snail Littorina obtusata. Although the effects on F. vesiculosus growth were small, there were statistically significant interaction effects. Overall, the effects of increased pCO2 and decreased salinity on seaweed growth were positive, but increased temperature counteracted the positive effect of pCO2. In parallel, increased pCO2 and decreased salinity caused a strong reduction in the seaweeds’ ability to induce chemical defenses (phlorotannins), and greatly increased the susceptibility to grazing. Together, these results imply that climate change may lead to a future net decline in habitat-forming F. vesiculosus populations, which may have large negative consequences on associated organisms that depend on this habitat forming species for shelter and food.
The treatment levels in our experiments followed the projected changes in salinity, temperature and pCO2 in the study area (–4 psu, +5°C, and +700 μatm) due to climate change by the end of this century (Eilola et al., 2009; IPCC, 2013; Cornwall and Hurd, 2016; Kinnby et al., 2020). In the grazing experiment with L. obtusata, we found that F. vesiculosus individuals exposed to reduced salinity and elevated pCO2 became significantly more susceptible to grazing, regardless of temperature. In one of the few other studies on the effects of climate change on the grazing susceptibility in fucoids, Raddatz et al. (2017) found that the susceptibility of F. vesiculosus showed a tendency to become less palatable to the crustacean grazer Idotea baltica when exposed to warming in summer, but not in other seasons. As recognized by the authors they used pellets of ground, freeze-dried F. vesiculosus, which means that physical properties (e.g., toughness) were destroyed during processing (in addition, water-soluble compounds, such as phlorotannins, will leak out). Susceptibility to grazing in F. vesiculosus and other fucoids can be affected by the morphology (e.g., toughness) and/or nutrient content (e.g., nitrogen content) of the thallus, as well as the presence of chemical defense compounds (e.g., phlorotannins). Changes in tissue toughness in F. vesiculosus were found by Rothäusler et al. (2017) in response to reduced salinity and warming, as well as by Kinnby et al. (2021) who found that seaweeds became more fragile under elevated pCO2. However, none of these previous studies found a correlation between tissue toughness and susceptibility to grazing (Rothäusler et al., 2017; Kinnby et al., 2021). Raddatz et al. (2017) found a weak negative correlation between susceptibility to grazing and C:N ratio in F. vesiculosus, however, this was not related to changes in climate variables (pCO2 or temperature). The C:N ratio of seaweeds exposed to elevated pCO2 is predicted to increase thereby becoming less nutritional and attractive to grazers. However, there are varied results in the literature; the C:N ratio was found to be reduced under elevated pCO2 (Gutow et al., 2014) but in another study the C:N ratio was found to increase at high temperature only to be strongly reduced at even higher temperatures (Graiff et al., 2015). Furthermore, the C:N ratio has been found to increase under elevated pCO2 during the winter but not during the summer (Takolander et al., 2019) suggesting a light or temperature dependence of the carbon metabolism of F. vesiculosus. We did not find a difference in C:N ratio of F. vesiculosus in response to changes in salinity, temperature, or pCO2, indicating that our experimental treatments of the seaweeds did not change their nutritional content.
Phlorotannins function as grazer deterrents in fucoid seaweeds (Pavia and Toth, 2000; Haavisto et al., 2010), but to date there are only a handful of studies investigating the effects of climate variables on phlorotannin production. Both reduced salinity and increased pCO2 caused a general decrease in phlorotannin content in F. vesiculosus (Kinnby et al., 2020, 2021). In addition to constitutive production, fucoid seaweeds can also increase production of phlorotannins in response to grazing, which leads to stronger resistance against further grazing (induced resistance, reviewed in Pavia et al., 2012). Inhibition of induced resistance (measured as changes in susceptibility to grazers) in F. vesiculosus have been found in response to changes in temperature (Weinberger et al., 2011; but see Rothäusler et al., 2017), although in these previous studies phlorotannins were not measured. Our results show that under ambient climate conditions, grazing by L. obtusata induces a strong increase in phlorotannins in F. vesiculosus, while single or combined changes in salinity, temperature and pCO2 inhibited induction. A key result of this study is therefore that the loss of phlorotannin induction in F. vesiculosus exposed to predicted changes in climate variables may explain why seaweeds became much more susceptible to grazing.
In contrast to the positive effect of reduced salinity on growth found in this study, several previous studies found decreased growth in response to various salinity reductions (Nygård and Dring, 2008; Connan and Stengel, 2011a; Kinnby et al., 2020). These contradictory results may in part be explained by the small salinity manipulation in the present study (from 23 to 19 psu) compared to Nygård and Dring (2008) and Connan and Stengel (2011a), who decreased salinity with 20 psu (from 35 to 15 psu). In contrast, the salinity decrease in Kinnby et al. (2020) was of the same magnitude as in the present study (4 psu), but from a lower ambient salinity level (4 psu decrease from the native salinity: 18, 14, 10 psu, from six populations along a salinity gradient). F. vesiculosus naturally occurs in a wide range of salinities, can be locally adapted to varying salinities (Kinnby et al., 2020) and has been reported to grow in salinities as low as 3.5 psu (e.g., Rinne and Salovius-Laurén, 2020). Therefore, the low salinity treatment in Kinnby et al. (2020) possibly approached the minimum salinity level required for continued growth in these F. vesiculosus populations.
We did not find any negative effects of a 5°C temperature increase (from 15 to 20°C) on F. vesiculosus growth in our study; rather there was a tendency toward higher growth under high temperature at ambient pCO2. In contrast, an increase in temperature from an already high ambient summer temperature (>26°C) had large negative effects on F. vesiculosus (Graiff et al., 2015; Takolander et al., 2017), even though an increase from lower (5–15°C) spring temperature levels enhanced growth (Graiff et al., 2015). Together, these results show that both the magnitude of change in climate variables, as well as the levels from which the variables are changed, have a clear impact on the growth response of the studied species. Since F. vesiculosus populations in the Skagerrak and Baltic Sea are adapted to substantial variations in salinity and temperature, changes to these variables will have the largest effects on seaweed populations already exposed to conditions close to their physiological limits, e.g., during summer heatwaves (Graiff et al., 2015, but see Saha et al., 2019) or in low salinity populations in the Baltic Sea (Kinnby et al., 2020). This could be further investigated in future studies using an experimental set-up that simulates fluctuating treatments and extreme events like heatwaves.
The positive effects of increased pCO2 under ambient temperature and salinity corroborate previous findings by Kinnby et al. (2021) who demonstrated increased growth, and Graiff et al. (2015) who found a tendency toward increased growth, in F. vesiculosus in response to elevated pCO2 (but see Gutow et al., 2014). Altered growth in response to changes in pCO2 could possibly be explained by changes in the carbon uptake strategy of the seaweed (Hepburn et al., 2011). F. vesiculosus has an active uptake of HCO3–, a so-called carbon concentrating mechanism (CCM; Surif and Raven, 1990), which is reflected by the carbon isotope composition of the seaweed tissue (Maberly et al., 1992). Since CO2 (aq) is a more isotopically depleted source of carbon than HCO3–, the carbon isotope signature of seaweeds assimilating CO2 will be reduced. In our study, we found that elevated pCO2, as well as reduced salinity, led to reduced ∂13C values. The latter results may be explained by the fact that the proportion of HCO3– to CO2 (aq) increases with increasing salinity. The reduction in ∂13C values suggests a partial transition from active to passive carbon uptake (as suggested by van der Loos et al., 2019), which agrees with the increased growth we observed at reduced salinity and elevated pCO2 at ambient temperature in the present study.
In comparison to the relatively small changes caused by the climate variables on seaweed growth in this study, the effects on grazing susceptibility were substantial (≈70–80% increase in grazing). In natural populations, this means that any positive effects caused by climate change factors on seaweed growth rate would be overshadowed by the negative effects of increased biomass loss to grazers. Littorinid snails, and in particular, L. obtusata are common and abundant grazers in fucoid seaweed stands (Pavia and Toth, 2000; Wikström et al., 2006) and exert a strong grazing pressure which significantly reduces the net growth of the seaweeds (Toth and Pavia, 2006). The closely related snail Littorina littorea has been shown to reduce its grazing when exposed to elevated pCO2 but at the same time increase condition index (Kinnby et al., 2021), and other littorinid species have been shown to alter their behavior due to temperature stress (reviewed in Ng et al., 2017) which would result in changes to grazing patterns. F. vesiculosus is a foundation species in intertidal areas and provides habitat and food for a number of invertebrates, fish, and other algal species in both sheltered and exposed rocky shorelines (Bergström et al., 2005; Forslund and Kautsky, 2013). Therefore, an impaired ability to induce defenses toward grazers in this foundation rockweed, or secondary effects due to the climate change responses of other interacting species, could potentially have large consequences for the biodiversity and functioning of rocky shore communities (c.f. Wahl et al., 2020).
Data Availability Statement
The raw data supporting the conclusions of this article will be made available by the authors, without undue reservation.
Author Contributions
AK, GT, and HP conceived the study. AK executed fieldwork, experiment, and laboratory analyses. AK analyzed the data and wrote the manuscript with input from all authors.
Funding
This work was funded by the Swedish Research Council VR and Formas through a Linnaeus grant to the Centre for Marine Evolutionary Biology (CeMEB; http://cemeb.science.gu.se, 217-2008-1719 awarded to HP). The funders had no role in study design, data collection and analysis, decision to publish, or preparation of the manuscript.
Conflict of Interest
The authors declare that the research was conducted in the absence of any commercial or financial relationships that could be construed as a potential conflict of interest.
Acknowledgments
We are grateful to Kerstin Johannesson (University of Gothenburg) for valuable comments on the manuscript as well as Joel White (University of Gothenburg) and Gunnar Cervin (University of Gothenburg) for help with the experiments.
Supplementary Material
The Supplementary Material for this article can be found online at: https://www.frontiersin.org/articles/10.3389/fmars.2021.688406/full#supplementary-material
Footnotes
References
Bergström, L., Tatarenkov, A., Johannesson, K., Jonsson, R. B., and Kautsky, L. (2005). Genetic and morphological identification of Fucus radicans Sp. Nov. (Fucales, Phaeophyceae) in the brackish Baltic Sea. J. Phycol. 41, 1025–1038. doi: 10.1111/j.1529-8817.2005.00125.x
Celis-Plá, P., Martinez, B., Korbee, N., Hall-Spencer, J., and Figueroa, F. (2017). Ecophysiological responses to elevated CO2 and temperature in Cystoseira tamariscifolia (Phaeophyceae). Clim. Change 142, 67–81. doi: 10.1007/s10584-017-1943-y
Connan, S., and Stengel, D. B. (2011a). Impacts of ambient salinity and copper on brown algae: 1. interactive effects on photosynthesis, growth, and copper accumulation. Aquat. Toxicol. 104, 94–107. doi: 10.1016/j.aquatox.2011.03.015
Connan, S., and Stengel, D. B. (2011b). Impacts of ambient salinity and copper on brown algae: 2. interactive effects on phenolic pool and assessment of metal binding capacity of phlorotannin. Aquat. Toxicol. 104, 1–13. doi: 10.1016/j.aquatox.2011.03.016
Cornwall, C. E., and Hurd, C. L. (2016). Experimental design in ocean acidification research: problems and solutions. ICES J. Mar. Sci. 73, 572–581. doi: 10.1093/icesjms/fsv118
Eilola, K., Meier, H. E. M., and Almroth, E. (2009). On the dynamics of oxygen, phosphorus and cyanobacteria in the Baltic Sea; a model study. J. Mar. Syst. 75, 163–184. doi: 10.1016/j.jmarsys.2008.08.009
Eriander, L., Wrange, A. L., and Havenhand, J. N. (2016). Simulated diurnal pH fluctuations radically increase variance in—but not the mean of—growth in the barnacle Balanus improvisus. ICES J. Mar. Sci. 73, 596–603. doi: 10.1093/icesjms/fsv214
Forslund, H., and Kautsky, L. (2013). Reproduction and reproductive isolation in Fucus radicans (Phaeophyceae). Mar. Biol. Res. 9, 321–326. doi: 10.1080/17451000.2012.731694
Graiff, A., Liesner, D., Karsten, U., and Bartsch, I. (2015). Temperature tolerance of western Baltic Sea Fucus vesiculosus – growth, photosynthesis and survival. J. Exp. Mar. Biol. Ecol. 471, 8–16. doi: 10.1016/j.jembe.2015.05.009
Gutow, L., Rahman, M. M., Bartl, K., Saborowski, R., Bartsch, I., and Wiencke, C. (2014). Ocean acidification affects growth but not nutritional quality of the seaweed Fucus vesiculosus (Phaeophyceae, Fucales). J. Exp. Mar. Biol. Eco. 453, 84–90. doi: 10.1016/j.jembe.2014.01.005
Haavisto, F., Valikangas, T., and Jormalainen, V. (2010). Induced resistance in a brown alga: phlorotannins, genotypic variation and fitness costs for the crustacean herbivore. Oecologia 162, 685–695. doi: 10.1007/s00442-009-1494-7
Harley, C. D., Randall Hughes, A., Hultgren, K. M., Miner, B. G., Sorte, C. J., Thornber, C. S., et al. (2006). The impacts of climate change in coastal marine systems. Ecol. Lett. 9, 228–241. doi: 10.1111/j.1461-0248.2005.00871.x
HELCOM (2013). Accidents and Response. Compilation on Ship Accidents in the Baltic Sea Area. Available online at: http://www.helcom.fi/shipping/accidents/en_GB/accidents
Hepburn, C. D., Pritchard, D. W., Cornwall, C. E., McLeod, R. J., Beardall, J., Raven, J. A., et al. (2011). Diversity of carbon use strategies in a kelp forest community: implications for a high CO2 ocean. Glob. Change Biol. 17, 2488–2497. doi: 10.1111/j.1365-2486.2011.02411.x
IPCC. (2013). “Climate change 2013: the physical science basis,” in Contribution of Working Group I to the Fifth Assessment Report of the Intergovernmental Panel on Climate Change, eds T. F. Stocker, D. Qin, G.-K. Plattner, M. Tignor, S. K. Allen, J. Boschung, et al. (Cambridge: Cambridge University Press).
Jenkins, A. (1999). The impact of melting ice on ocean waters. J. Phys. Oceanogr. 29, 2370–2381. doi: 10.1175/1520-04851999029<2370:Tiomio<2.0.Co;2
Johnson, M. D., Comeau, S., Lantz, C. A., and Smith, J. E. (2017). Complex and interactive effects of ocean acidification and temperature on epilithic and endolithic coral-reef turf algal assemblages. Coral Reefs 36, 1059–1070. doi: 10.1007/s00338-017-1597-2
Kinnby, A., Jonsson, P. R., Ortega-Martinez, O., Topel, M., Pavia, H., Pereyra, R. T., et al. (2020). Combining an ecological experiment and a genome scan show idiosyncratic responses to salinity stress in local populations of a seaweed. Front. Mar. Sci. 7:470. doi: 10.3389/fmars.2020.00470
Kinnby, A., White, J. C. B., Toth, G. B., and Pavia, H. (2021). Ocean acidification decreases grazing pressure but alters morphological structure in a dominant coastal seaweed. PLoS One 16:e0245017. doi: 10.1371/journal.pone.0245017
Kram, S. L., Price, N. N., Donham, E. M., Johnson, M. D., Kelly, E. L. A., Hamilton, S. L., et al. (2016). Variable responses of temperate calcified and fleshy macroalgae to elevated pCO2 and warming. ICES J. Mar. Sci. 73, 693–703. doi: 10.1093/icesjms/fsv168
Levitus, S., Antonov, J. I., Boyer, T. P., Locarnini, R. A., Garcia, H. E., and Mishonov, A. V. (2009). Global ocean heat content 1955-2008 in light of recently revealed instrumentation problems. Geophys. Res. Lett. 36:L07608. doi: 10.1029/2008GL037155
Liblik, T., and Lips, U. (2019). Stratification has strengthened in the Baltic Sea - an analysis of 35 years of observational data. Front. Earth Sci. 7:174. doi: 10.3389/feart.2019.00174
Lima, F. P., Ribeiro, P. A., Queiroz, N., Hawkins, S. J., and Santos, A. M. (2007). Do distributional shifts of northern and southern species of algae match the warming pattern? Glob. Change Biol. 13, 2592–2604. doi: 10.1111/j.1365-2486.2007.01451.x
Maberly, S. C., Raven, J. A., and Johnston, A. M. (1992). Discrimination between 12C and 13C by marine plants. Oecologia 91, 481–492.
Mensch, B., Neulinger, S. C., Graiff, A., Pansch, A., Kunzel, S., Fischer, M. A., et al. (2016). Restructuring of epibacterial communities on Fucus vesiculosus forma mytili in response to elevated pCO2 and increased temperature levels. Front. Microbiol. 7:434. doi: 10.3389/fmicb.2016.00434
Monteiro, C., Li, H. R., Diehl, N., Collen, J., Heinrich, S., Bischof, K., et al. (2021). Modulation of physiological performance by temperature and salinity in the sugar kelp Saccharina latissima. Phycol. Res. 69, 48–57. doi: 10.1111/pre.12443
Ng, T. P., Lau, S. L., Seuront, L., Davies, M. S., Stafford, R., Marshall, D. J., et al. (2017). Linking behaviour and climate change in intertidal ectotherms: insights from littorinid snails. J. Exp. Mar. Biol. Ecol. 492, 121–131. doi: 10.1016/j.jembe.2017.01.023
Nygård, C. A., and Dring, M. J. (2008). Influence of salinity, temperature, dissolved inorganic carbon and nutrient concentration on the photosynthesis and growth of Fucus vesiculosus from the Baltic and Irish Seas. Eur. J. Phycol. 43, 253–262. doi: 10.1080/09670260802172627
Olischläger, M., and Wiencke, C. (2013). Ocean acidification alleviates low-temperature effects on growth and photosynthesis of the red alga Neosiphonia harveyi (Rhodophyta). J. Exp. Bot. 64, 5587–5597. doi: 10.1093/jxb/ert329
Pavia, H., Baumgartner, F., Cervin, G., Enge, S., Kubanek, J., Nylund, G. M., et al. (2012). Chemical Defences Against Herbivores. Oxford: Oxford University Press, 210–235.
Pavia, H., Cervin, G., Lindgren, A., and Åberg, P. (1997). Effects of UV-B radiation and simulated herbivory on phlorotannins in the brown alga Ascophyllum nodosum. Mar. Ecol. Progr. Ser. 157, 139–146.
Pavia, H., and Toth, G. (2000). Inducible chemical resistance to herbivory in the brown seaweed Ascophyllum nodosum. Ecology 81, 3212–3225.
Raddatz, S., Guy-Haim, T., Rilov, G., and Wahl, M. (2017). Future warming and acidification effects on anti-fouling and anti-herbivory traits of the brown alga Fucus vesiculosus (Phaeophyceae). J. Phycol. 53, 44–58. doi: 10.1111/jpy.12473
Rinne, H., and Salovius-Laurén, S. (2020). The status of brown macroalgae Fucus spp. and its relation to environmental variation in the Finnish marine area, northern Baltic Sea. Ambio 49, 118–129. doi: 10.1007/s13280-019-01175-0
Robbins, L., Hansen, M. E., Kleypas, J. A., and Meylan, S. C. (2010). CO2calc- A User-Friendly Carbon Calculator for Windows, Mac OS X, and iOS (iPhone) Florida Shelf Ecosystems Response to Climate Change Project CO2calc: A User-Friendly Seawater Carbon Calculator for Windows, Mac OS X, and iOS (iPhone).
Rothäusler, E., Haavisto, F., and Jormalainen, V. (2017). Is the future as tasty as the present? Elevated temperature and hyposalinity affect the quality of Fucus (Phaeophyceae, Fucales) as food for the isopod Idotea balthica. Mar. Biol. 164:207. doi: 10.1007/s00227-017-3237-3
Roth-Schulze, A. J., Thomas, T., Steinberg, P., Deveney, M. R., Tanner, J. E., Wiltshire, K. H., et al. (2018). The effects of warming and ocean acidification on growth, photosynthesis, and bacterial communities for the marine invasive macroalga Caulerpa taxifolia. Limnol. Oceanogr. 63, 459–471. doi: 10.1002/lno.10739
Rugiu, L., Manninen, I., Rothäusler, E., and Jormalainen, V. (2018). Tolerance and potential for adaptation of a Baltic Sea rockweed under predicted climate change conditions. Mar. Environ. Res. 134, 76–84. doi: 10.1016/j.marenvres.2017.12.016
Sabine, C. L., Feely, R. A., Gruber, N., Key, R. M., Lee, K., Bullister, J. L., et al. (2004). The oceanic sink for anthropogenic CO2. Science 305, 367–371. doi: 10.1126/science.1097403
Saha, M., Barboza, F. R., Somerfield, P. J., Al-Janabi, B., Beck, M., Brakel, J., et al. (2019). Response of foundation macrophytes to near-natural simulated marine heatwaves. Glob. Change Biol. 26, 417–430. doi: 10.1111/gcb.14801
Schneider, C. A., Rasband, W. S., and Eliceiri, K. W. (2012). NIH Image to ImageJ: 25 years of image analysis. Nat. Methods 2012, 671–675.
Solomon, S. (2007). “IPCC (2007): climate change the physical science basis,” in Proceedings of the Agu Fall Meeting Abstracts, Vol. 2007, San Francisco, CA, U43D-01.
Surif, M. B., and Raven, J. A. (1990). Photosynthetic gas-exchange under emersed conditions in eulittoral and normally submersed members of the Fucales and the Laminariales - interpretation in relation to C-Isotope ratio and N-Isotope and water-use efficiency. Oecologia 82, 68–80. doi: 10.1007/Bf00318535
Taboada, F. G., and Anadón, R. (2012). Patterns of change in sea surface temperature in the North Atlantic during the last three decades: beyond mean trends. Clim. Change 115, 419–431. doi: 10.1007/s10584-012-0485-6
Takolander, A., Cabeza, M., and Leskinen, E. (2019). Seasonal interactive effects of pCO2 and irradiance on the ecophysiology of brown macroalga Fucus vesiculosus L. Eur. J. Phycol. 54, 380–392. doi: 10.1080/09670262.2019.1572226
Takolander, A., Leskinen, E., and Cabeza, M. (2017). Synergistic effects of extreme temperature and low salinity on foundational macroalga Fucus vesiculosus in the northern Baltic Sea. J. Exp. Mar. Biol. Ecol. 495, 110–118. doi: 10.1016/j.jembe.2017.07.001
Teagle, H., and Smale, D. A. (2018). Climate-driven substitution of habitat-forming species leads to reduced biodiversity within a temperate marine community. Divers. Distrib. 24, 1367–1380. doi: 10.1111/ddi.12775
Toth, G. B., and Pavia, H. (2006). Artificial wounding decreases plant biomass and shoot strength of the brown seaweed Ascophyllum nodosum (Fucales, Phaeophyceae). Mar. Biol. 148, 1193–1199. doi: 10.1007/s00227-005-0167-2
Toth, G. B., and Pavia, H. (2007). Induced herbivore resistance in seaweeds: a meta-analysis. Ecology 95, 425–434. doi: 10.1111/j.1365-2745.2007.01224.x
van Alstyne, K. L. (1995). Comparison of three methods for quantifying brown algal polyphenolic compounds. J. Chem. Ecol. 21, 45–58. doi: 10.1007/BF02033661
van der Loos, L. M., Schmid, M., Leal, P. P., McGraw, C. M., Britton, D., Revill, A. T., et al. (2019). Responses of macroalgae to CO2 enrichment cannot be inferred solely from their inorganic carbon uptake strategy. Ecol. Evol. 9, 125–140. doi: 10.1002/ece3.4679
Wahl, M., Werner, F. J., Buchholz, B., Raddatz, S., Graiff, A., Matthiessen, B., et al. (2020). Season affects strength and direction of the interactive impacts of ocean warming and biotic stress in a coastal seaweed ecosystem. Limnol. Oceanogr. 65, 807–827. doi: 10.1002/lno.11350
Wentz, F. J., Ricciardulli, L., Hilburn, K., and Mears, C. (2007). How much more rain will global warming bring? Science 317, 233–235.
Weinberger, F., Rohde, S., Oschmann, Y., Shahnaz, L., Dobretsov, S., and Wahl, M. (2011). Effects of limitation stress and of disruptive stress on induced antigrazing defense in the bladder wrack Fucus vesiculosus. Mar. Ecol. Prog. Ser. 427, 83–94. doi: 10.3354/meps09044
Keywords: ocean acidification, warming, freshening, salinity, temperature, Fucus vesiculosus, grazers, macroalgae
Citation: Kinnby A, Toth GB and Pavia H (2021) Climate Change Increases Susceptibility to Grazers in a Foundation Seaweed. Front. Mar. Sci. 8:688406. doi: 10.3389/fmars.2021.688406
Received: 30 March 2021; Accepted: 24 May 2021;
Published: 17 June 2021.
Edited by:
Aleksandra M. Lewandowska, University of Helsinki, FinlandReviewed by:
Veijo Jormalainen, University of Turku, FinlandCamilla Gustafsson, University of Helsinki, Finland
Copyright © 2021 Kinnby, Toth and Pavia. This is an open-access article distributed under the terms of the Creative Commons Attribution License (CC BY). The use, distribution or reproduction in other forums is permitted, provided the original author(s) and the copyright owner(s) are credited and that the original publication in this journal is cited, in accordance with accepted academic practice. No use, distribution or reproduction is permitted which does not comply with these terms.
*Correspondence: Alexandra Kinnby, alexandra.kinnby@marine.gu.se