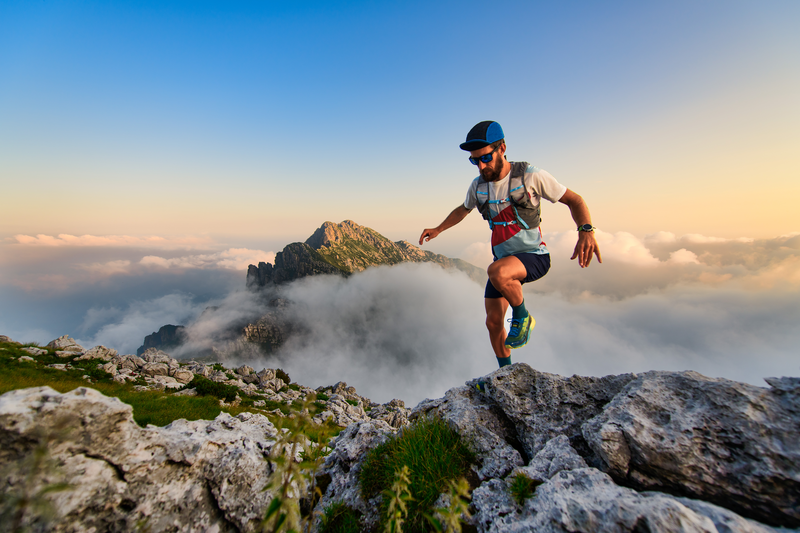
95% of researchers rate our articles as excellent or good
Learn more about the work of our research integrity team to safeguard the quality of each article we publish.
Find out more
ORIGINAL RESEARCH article
Front. Mar. Sci. , 06 October 2021
Sec. Marine Evolutionary Biology, Biogeography and Species Diversity
Volume 8 - 2021 | https://doi.org/10.3389/fmars.2021.688316
This article is part of the Research Topic Biodiversity and Distribution of Benthic Invertebrates - From Taxonomy to Ecological Patterns and Global Processes View all 16 articles
Deep-water emergence (DWE) is the phenomenon where marine species normally found at great depths (i.e., below 200 m), can be found locally occurring in significantly shallower depths (i.e., euphotic zone, usually shallower than 50 m). Although this phenomenon has been previously mentioned and deep-water emergent species have been described from the fjord regions of North America, Scandinavia, and New Zealand, local or global hypotheses to explain this phenomenon have rarely been tested. This publication includes the first literature review on DWE. Our knowledge of distribution patterns of Chilean marine invertebrates is still very scarce, especially from habitats below SCUBA diving depth. In our databases, we have been gathering occurrence data of more than 1000 invertebrate species along the Chilean coast, both from our research and from the literature. We also distributed a list of 50 common and easily in situ-identifiable species among biologically experienced sport divers along the Chilean coast and recorded their sighting reports. Among other findings, the analysis of the data revealed patterns from 28 species and six genera with similar longitudinal and bathymetric distribution along the entire Chilean coast: along the Chilean coast these species are typically restricted to deep water (>200 m) but only in some parts of Chilean Patagonia (>39°S–56°S), the same species are also common to locally abundant at diving depths (<30 m). We found 28 of these ‘deep’ species present in shallow-water of North Patagonia, 32 in Central Patagonia and 12 in South Patagonia. The species belong to the phyla Cnidaria (six species), Mollusca (four species), Arthropoda (two species) and Echinodermata (16 species). We ran several analyses comparing depth distribution between biogeographic regions (two-way ANOVA) and comparing abiotic parameters of shallow and deep sites to search for correlations of distribution with environmental variables (Generalized Linear Models). For the analyses, we used a total of 3328 presence points and 10635 absence points. The results of the statistical analysis of the parameters used, however, did not reveal conclusive results. We summarize cases from other fjord regions and discuss hypotheses of DWE from the literature for Chilean Patagonia.
Vertical changes in the physical and chemical properties of the ocean divide the benthic environment into six depth zones: (1) the intertidal, which is exposed during low tide; (2) the littoral or photic zone (0–200 m) which extends from the high tide line down to about 200 m at the edge of the continental shelf and includes the euphotic zone (0–50 m) and the oligophotic zone (50–200 m); the sublittoral or deep-sea, which is lightless and cold, and is sub-dived into (3) the archibenthic zone (200–1000 m) at the continental slope, which is often oxygen-deficient and also called dysphotic, mesophotic or twilight zone (there is <1% of light left, but it is not enough for primary production); (4) the bathybenthic zone or bathyal (1000–4000 m), which is aphotic, includes most of the continental rise and usually has a greater oxygen-content than the overlaying layer; (5) the abyssobenthic zone or abyssal (4000–6000 m), which includes the deep ocean floor and has little nutrient import; and (6) the hadobenthic zone or hadal (>6000 m) which is usually found in deep-sea trenches (Sutton, 2013; Pinet, 2019).
The deep-sea was considered an azoic environment until the mid-nineteenth century when Norwegian naturalist Michael Sars listed hundreds of invertebrate species collected from the deep-sea. Nowadays it is known as the largest biome on earth, distinct from other ecosystems and comparably diverse (Ramirez-Llodra et al., 2010). The origin and possible antiquity of the surprisingly diverse modern deep-sea fauna has been debated since the beginning of deep-sea research. Based on material collected during the HMS Challenger Expedition from 1872 to 1876, Murray (1895) concluded that the animals recovered from the deep-sea were similar to the taxa found in shallow-water high-latitude habitats, and thus the origin of this life was in shallow waters. Subsequently, papers discussed the two possible pathways explaining the origin of deep-sea species: shallow-water submergence versus an origin in deep water. Most studies compared the southern ocean littoral fauna to deep-sea fauna. Examples for both pathways have been published. Research that supports the DWE pathway include those by Hessler and Thistle (1975) that suggests an in situ evolution for parasseloidean deep-sea isopods, also Thuy and Schulz (2012) described an ancient echinoderm assemblage from the NE Atlantic deep-sea and Quattro et al. (2001) showed the upper bathyal zone of the North Atlantic to be an active site of population differentiation for mollusks. In support of the shallow-water submergence pathway, Riehl and Kaiser (2012) described a deep-sea isopod originating from the Antarctic shelf. Some relationships between shallow and deep-water fauna, however, could not be explained by either scenario, e.g., for the echinoid genus Sterechinus (Dıaz et al., 2010) and for foraminiferans (Lipps and Hickman, 1982); and for some groups of deep-sea octopuses, both pathways have been shown (Strugnell et al., 2011). Discussions on the origin of deep-sea fauna are ongoing.
Deep-water benthic research in Chile started with the Challenger (1872–1876) and Lund University Chile Expeditions (1948/49). Since then, advances in the knowledge of deep-sea benthos have been sparse, including mainly occasional samples of US research vessels transiting to Antarctica between the 1960s and early 1980s (e.g., R/V Hero and USNS Eltanin), as well as surveys onboard the R/V Anton Bruun in 1965–1966, to mention a few (reviewed in Sievers-Czischke, 2018). Major national expeditions started in the 1960s, mainly with the MarChile program, highlighting benthic studies at the shelf break and upper slope in northern Chile during the MarChile II expedition in 1962 (Gallardo, 1963). However, the first comprehensive bathymetric and latitudinal study of the upper-bathyal benthos (∼200 to 2000 m depth) was performed during the Oceanographic Expedition PUCK (R/V Sonne, SO-156 cruise) in 2001 (Quiroga et al., 2009; Sellanes et al., 2010). In this cruise, the emphasis was put on studying the role of the SE Pacific Oxygen Minimum Zone (OMZ) in shaping the patterns of benthic assemblages along the Chilean continental margin.
Methane-seep environments were discovered in 2003 and later studied on the upper slope off Concepción (∼36°S, ∼800 m depth), leading to the description of many new species and new records of existing species for the area (see Sellanes et al., 2004, 2008). Subsequent findings of methane seep communities include areas from off the Taitao Peninsula (∼45°S, ∼500 m depth) (Zapata-Hernández et al., 2014), to off El Quisco (∼33°S; ∼300 m depth) (Krylova et al., 2014), and more recently off Huasco (∼28°S, ∼1500 m depth) (Kobayashi and Araya, 2018).
Benthic surveys conducted using remotely operated vehicles (ROVs), are quite scarce on the continental slope off Chile. An advantage of using ROVs is that benthic communities can be observed in situ before being altered by the sampling procedure, and characteristics of the living animal are lost. Probably the most important ROV survey was on the R/V Sonne cruise SO-210, in which the ROV Kiel 6000 was used (Treude et al., 2011). Another ROV survey led to the first report of antipatharian black corals on the shelf break off La Higuera (∼29°S, 70–107 m depth) (Gorny et al., 2018). Other methods of benthic studies in the area include studying species collected as bycatch from deep-water Patagonian Toothfish and crustacean fisheries (e.g., Ibáñez et al., 2006, 2011, 2012, 2016; Araya, 2013, 2016).
The deep-water emergence (DWE) hypothesis has been mentioned in the literature since 1970 (Hessler, 1970). Since this phenomenon is more common in higher latitudes, it has also been called “polar emergence” or “high-latitude emergence.” Hessler (1970) and Hessler et al. (1979) argued that specialized deep-sea isopods evolved in situ and their presence at shallow high latitudes is a result of subsequent emergence. In the following decades, other deep-water species have been described from shallow waters in several places. Some Chilean species with deep-water records off northern and central Chile as well as Patagonia are well known in shallow waters of Chilean Patagonia (Häussermann and Försterra, 2009). Other deep-water species have been described in shallow waters of New Zealand, Alaska, and Norway (summarized in this paper), from nearshore marine caves in the Mediterranean and the Atlantic (e.g., Grigg, 1965; Stock and Vermeulen, 1982) and from the turbid waters between Trinidad and Venezuela (Warner, 1981).
In the present paper, we list 28 species and six genera from the phyla Cnidaria, Mollusca, Arthropoda, and Echinodermata that have been recorded in deep waters between 200 and 1800 m off the coast of Chile, restricted to these deep waters in central and northern Chile, while in addition, present at diving depth in fjords and channels of Chilean Patagonia. Biogeographically there is a break at about 30°S, which separates the Peruvian Province (north of ∼30°S) from the Intermediate Area (∼30°S to ∼40–42°S) (Tellier et al., 2009). Chilean Patagonia is part of the Magellan Province and is subdivided into North Patagonia (NP; ∼40–42°S to Golfo de Penas, ∼47–48°S), Central Patagonia (CP; ∼47–48°S to Straight of Magellan, ∼53–54°S) and South Patagonia (SP; ∼53–54°S to Cape Horn, 56°S). We summarize the latitudinal and bathymetric distribution of the listed species and genera, their type of larvae and mode of reproduction, as far as it is known. We compared depth distribution between biogeographic regions and searched for correlations between distribution and environmental variables. Our paper also includes the first review on the DWE phenomenon: we list deep-water emergent species described in the literature from other parts of the world and summarize and discuss the hypotheses used to explain this phenomenon.
The PAtagonia MArine DAtabase (PAMADA) includes nearly 2,000 identified benthic species and more than 20,000 presence points collected during 43 SCUBA expeditions and six ROV expeditions at more than 500 study sites during the years 1997 and 2020, and from the literature. The Biological Collection of Universidad Católica del Norte UCN (SCBUCN) currently keeps more than 6,000 samples of preserved marine organisms, mainly from the continental slope and oceanic islands of Chile, as well as from seamounts within the Chilean Exclusive Economic Zone (Figure 1 and Supplementary Figure 1). The SCBUCN database is available through the Global Biodiversity Information Facility (Sellanes, 2018) and for the current study, 144 of these records were used. During data searches to complement the PAMADA database from SCBUCN and OBIS, V. Häussermann (see also Häussermann, 2006) detected some species with unusual distribution patterns which led to an extensive search for species present in both shallow waters of Chilean Patagonia and deep waters off Central and Northern Chile. To complete the existing Patagonian shallow-water records (a coastline of > 100,000 km) for the Central and Northern Chilean coast, we distributed a list of common and easily in situ-identifiable species, including the 28 species and six genera, among biologically experienced sport divers along the Chilean coast between Bahía Mansa (40.77°S; 73.84°W) and Caldera (27.05°S; 70.82°W) (a coastline of 1500 km). We used data from the 24 dive sites they regularly visit. Christian Ibáñez added data from 40 intertidal sites along the exposed coast of North and Central Chile. We could not find any diving biologists or sports divers who dive at sites north of 27°S.
Figure 1. (A) Sampling sites. Black points represent dive sites and blue points intertidal study sites. (B–D) Sites of invertebrate presence data along the Chilean coast from both PAMADA and SCBUCN (total 3331 points). (B) Presence data <50 m depth. (C) Presence data 50–200 m depth. (D) Presence data >200 m depth.
We compared the depth distribution of 34 genera between biogeographic regions (Peruvian Province and Intermediate Area to Magellan Province) by means of two-way ANOVA using R ver. 4.0.5 (R Core Team, 2021).
We also tested the correlations of abiotic factors (where data were available) with species presence data. The sea bottom temperature (SBT), sea bottom salinity (SBS), molecular dissolved oxygen at bottom (SBO), primary productivity (PP), Chlorophyll-a, silicate and current velocity were obtained from the BioOracle v2 (Assis et al., 2018) based on monthly averages from 2000 to 2017. Environmental variables were extracted from each presence/absence point. The BioOracle web platform1 (Tyberghein et al., 2012; Assis et al., 2018) was used to obtain these data using QGIS (QGIS Development Team, 2012).
Three Generalized Linear Models (GLM) with a binomial distribution error type were run to establish the existence of linear patterns between the presence/absence data and the predictor variables (depth, temperature, salinity, primary production, chlorophyll-a, oxygen, silicate, current velocity). The first GLM was run with all presence/absence data (>13,000 records). To detect the common predictors responsible for shallow water presence points in the south and deep water presence points in the north, we ran two more GLMs, one for those records shallower than 50 m and one with those records deeper than 200 m. The predictor variables were set over 250 models (for each GLM), executed using the “glmulti” function of the ‘GLMULTI’ package (Calcagno and de Mazancourt, 2010) implemented in the software R, this function examines all possible combinations of predictor variables, and the best model for each analysis was selected based on Bayesian information criterion (BIC). To establish which predictor variables contribute to a total variance, an ANOVA was used over the GLM coefficients. The most important variable for each GLM was obtained based on the t-statistic, using the “varImp” function from the “caret” package (Kuhn, 2008) implemented in the software R.
Based on the data search described above, we compiled a list of 28 species and six genera that are restricted to deep waters off Central and Northern Chile but reach into shallow water in Chilean Patagonia, a pattern first mentioned in Häussermann (2006). The listed taxa are from the phyla Cnidaria (six species, four genera), Mollusca (four species, one genus), Arthropoda (two species, one genus) and Echinodermata (16 species) and include three sea anemones (Cnidaria, Actiniaria), three stony corals (Cnidaria, Scleractinia), three gorgonians and one soft coral (Cnidaria, Alcyonacea), three snails (Mollusca, Gastropoda), one mussel (Mollusca, Bivalvia), one chiton (Mollusca, Polyplacophora), two crabs (Arthropoda, Decapoda), one sea spider (Arthropoda, Pycnogonida), six starfish (Echinodermata, Asteroidea), five brittle stars (Echinodermata, Ophiuroidea), two sea urchins (Echinodermata, Echinoidea), two sea cucumbers (Echinodermata, Holothuroidea) and one feather star (Echinodermata, Crinoidea) (Table 1). The search resulted in a total of 3331 presence (Supplementary Table 1) and 10842 absence points. For typical distributions of some selected deep-water emergent species, see Figure 2. In addition to these 28 species and six genera we found more species that present a eurybathic distribution within Chilean Patagonia (from the deep-sea to diving depth) but so far have not been observed north of Patagonia. These species are typical members of deep-water genera, which within the Chilean fiord region extend into shallow water: the sea anemones Bolocera kerguelensis (25–485 m; possibly 3329 m at 38.10°S if the suggested synonymy with B. patens is correct) and Isotealia antarctica (25–420 m) (Häussermann and Försterra, 2005), the gorgonian Convexella magelhaenica (12–1666 m) (Häussermann et al., 2016) and the hydrocoral Errina antarctica (18–771 m) (Häussermann and Försterra, 2007b).
Table 1. List of deep-water emergent species from Chilean Patagonia: geographic and bathymetric distribution (bd) for North and Central Chile (N-C), North Patagonia (NP), Central Patagonia (CP), and South Patagonia (SP) main citation for Chile and first citation of deep-water emergence (DWE), typical deep-water species, type of reproduction and larvae.
Figure 2. Depth distribution of six typical deep-water emergent species with above-average number of records throughout Chile and appearance in shallow water of North Patagonia (A–D) and Central Patagonia (E–F), sorted by appearance from north to south. A trend is visible with the upper depth distribution limit (deep-water emergence phenomenon) rising toward the south. (A) Cosmasterias lurida (Asteroidea), (B) Gorgonocephalus chilensis (Ophiuroidea), (C) Acesta patagonica (Bivalvia); (D) Solaster regularis (Asteroidea); (E) Ophiura lymani (Ophiuroidea); (F) Florometra magellanica (Crinoidea).
Actinostola intermedia (=A. chilensis) and Hormathia pectinata (described down to 1220 m) are sea anemone species from typical deep-water genera; along the Chilean coast they have been collected between 30 and 34°S from 350 to 400 m depth and between 34 and 36°S from 744 to 936 m. In Chilean Patagonia, south of 41°S, both are regularly found below 20 and 25 m, respectively (H. pectinata to 53°S, A. chilensis to 55°S). Single specimens of A. intermedia were even found at 9 m depth, and H. pectinata at 12 m. A. intermedia inhabits current-exposed sites and can also be found in groups of up to 15–25 individuals. H. pectinata is rarely found in water shallower than 30 m, specimens were found on near vertical walls with little sedimentation. Dactylanthus antarcticus (described down to 610 m) was sighted on a video from the SONNE expedition in 700 m depth at 36°S. South of 45°S it is regularly found below 20 m depth in channels, becoming more abundant south of 49°S.
The cosmopolitan stony corals Desmophyllum dianthus (described down to 2460 m depth) and the recently described Caryophyllia huinayensis have been collected at about 750 m depth at 36.4°S. Desmophyllum dianthus was also collected at 35.5°S from 1100 to 1300 m, and C. huinayensis at 29.4°S from 256 to 276 m. Both species live on near vertical to overhanging rocky walls in Chilean Patagonia, south of 41°S, with little sediment (D. dianthus below 8 m down to 52.8°S, C. huinayensis below 11 m depth down to 53.4°S). D. dianthus was also found on a shipwreck at the exposed coast slightly north of the fjord region at 40.6°S in 11 m depth. The recently described Tethocyathus endesa was collected at 36.5°S in 240 m depth in a methane seep area. It is described from Patagonian fjords between 42°S and 48.4°S below 11 m depth on rocky substratum, withstands some sedimentation and is rarely found south of 47°S (Taitao Peninsula). All three species belong to the family Caryophylliidae.
Specimens of the gorgonian genus Swiftia were collected between 29 and 38°S from 320 to 906 m and in NP (42–46°S) between 20 and 31 m. Specimens of the primnoid gorgonian genus Thouarella were collected at 20 and 36°S from 761 and 960 m respectively, and between 41.6 and 53.4°S while diving below 18 m. South of 50°S there are nine deep records, the deepest at 53°S from 1666 and 1920 m. The two records south of 53.4°S were from 269 and 1666 m. The gorgonian genus Acanthogorgia has one record from 940 m (36.3°S) and was found diving below 18–20 m between 45.3 and 53°S. The two records further south (53.1 and 53.8°S) were 1920 and 269 m. The soft coral genus Alcyonium has 14 records between 29.5 and 36.4°S from 250 to 764 m, and was found between 41.6°S (common south of 45.4°S) and 55°S while diving below 12 m. The record at 56°S is from 1800 m.
The deep-water bivalve Acesta patagonica (described down to 820 m) was found between 31°S and 34°S from 280 to 450 m and at 36.4°S from 751 to 763 m depth. At 38.8°S it was found in 137 m and at 42.5°S below 88 m depth (with two records from 20 to 30 m in Comau Fjord), associated with the scleractinian coral banks of D. dianthus. Further records lie south of 45°S with shallow-water specimens being more common between 48 and 49°S where it was found diving below 15 m depth; we have no records south of 53.4°S (Figure 2C).
The gastropod Fusitriton magellanicus has 12 records between 30 and 39°S where it was found from 200 to 960 m. South of 42°S in Patagonia it is found below 5 m, being more common in shallow water south of 48°S. Capulus ungaricoides is a poorly known species, it was found twice between 30 and 32°S at 300 and 450 m depth, at 41°S in 212 m depth, at 53°S in 522 m depth, and at 42.5 and 49°S in 30 m depth.
The chiton Stenosemus exaratus is a deep-water inhabiting species described down to 2580 m depth; most Chilean records are between 36 and 52°S from approximately 700–900 m depth; in Chilean Patagonia the species was collected at three sites in the intertidal and shallow subtidal.
Specimens of the gastropod genus Trophon have eight records between 30°S and 34.5°S from 280 to 450 m between (one record at 30°S is from 88 m), and can be found south of 39.9°S (down to 55°S) within diving depths. The southernmost record at 55.8°S is from 115 m depth.
The decapod crab Pagurus comptus was collected once at 30°S at 400 m depth; it is common below 5 m depth throughout Chilean Patagonia. The cosmopolitan deep-sea spider Colossendeis macerrima (described from 141 to 4000 m) was collected at 26.7°S from 1500 to 1800 m depth and at 45.9°S in 510 m depth. In Chilean Patagonia it was found during a diving expedition at 48.5°S in 18 m depth.
Specimens of the decapod genus Lithodes have two records at 31.9°S and 36.4°S in 108 m and 769 m depth and can be found south of 41.5°S down to 55.3°S within diving depths.
The sea stars Lophaster stellans and Solaster regularis (both Family Solasteridae) have been collected in Northern and Central Chile between 29 and 33°S from 260 to 420 m depth and between 32 and 39°S from 376 to 850 m. Both have been collected between 39 and 42°S in the littoral zone below diving depth (L. stellans at 39°S in 73 m and S. regularis at 42.5°S in 146 m) and appear within diving depths south of approximately 44°S, becoming much more abundant in shallow water between 48 and 50°S while there is only one (L. stellans) or no record (S. regularis) south of 53°S (Figure 2D). Labidiaster radiosus was found at 35.5°S in 1300 m depth. It is known from shallow water of Patagonia south of 42.6°S. Cosmasterias lurida presents 10 records between 29 and 37°S from 200 to 400 m depth. At 38.7°S it was collected in 140 m and at 39.1°S from 36 to 90 m. Between 41°S and 55°S it is very common, especially in fjords and inner channels from 5 m down to 460 m depth (Figure 2A). Henricia studeri has three records between 29 and 33°S from 256 to 450 m, it is known from shallow water south of 41°S with the shallowest record being from 5 m depth. Poraniopsis echinaster has 25 records between 26 and 39°S from 200 to 450 m. It was also found four times at 29, 30, and 33°S at the lower limit of diving depth from 25 to 40 m. It is very common in shallow water south of 41.6°S, with the shallowest record being from 5 m depth.
The feather star Florometra magellanica has 10 records between 19 and 37°S from 275 to 949 m depth. Between 45 and 47°S it was collected from 574 to 687 m; south of 47°S it was found regularly within diving depths (Figure 2F). The sea cucumber Bathyplothes moseleyi has two records at 32°S (580, 960 m). it was found at 42°S in 454 m and between 48 and 53.4°S within diving depths. The sea cucumber Psolus squamatus was found once at 31°S in 400 m depth and is common between 42 and 48°S within diving depths.
The brittle star Gorgonocephalus chilensis (Family Gorgonocephalidae) has 25 records between 35 and 39°S from 354 to 990 m depth. Between 41.7 and 53.8°S it was regularly seen in shallow water, being more abundant south of 48°S (Figure 2B). Astrotoma agassizi (Family Gorgonocephalidae) was found 30 times between 18.7 and 38.2°S from 327 to 1300 m and six times between 42 and 43°S from 85 to 860 m. Between 41.7 and 53°S there are 12 records from diving depth, most from 30 to 35 m, the shallowest being 20 m at 48.6°S. The brittle stars Ophiura lymani and Ophiocten aminitum belong to the family Ophiuridae. Ophiura lymani was collected 15 times between 31.7 and 43°S from 212 to 940 m, O. amitinum four times between 22.8 and 36.5°S from approximately 200–1300 m. Both species were found regularly in shallow water between approximately 47.8°S and 53.7/53.8°S, respectively, and were not found in shallow water south of 54°S; O. lymani being more common (25 records versus 11 records) (Figure 2E). Ophiomitrella chilensis has four records between 33°S and 39°S from 344 m to 843 m and was collected at 41°S in 100 m and at 42°S between 25 and 70 m.
The sea urchins Arbacia dufresnei has eleven records between 28.4 and 38.9°S from 223 to 1300 m and two recent records at 30°S from 55 and 60 m. In Chilean Patagonia south of 40.6°S it is very common from the intertidal to diving depths. Tripylaster philippi was found four times between 35.5 and 37.8°S from 714 to 1300 m, between 41.7 and 42.1°S from 230 to 460 m, and eight times between 42.3 and 54.9°S within diving depths.
Seven of the 34 deep-water species/genera from Northern and Central Chile appear at diving depth between 39.9°S (Valdivia) and 41.5°S (the northern end of the fjord region), further 16 species/genera appear between 41.6 and 43°S (the region around Chiloé Island, corresponding to the northern section of NP), six between 43 and 47°S (the Guaitecas and Chonos Archipelago, corresponding to the southern section of NP), and five between 47°S and 49°S (CP) (Table 2 and Figure 2). This pattern results in 28 deep-water emergent species being present in NP (12 Echinodermata, nine Cnidaria, five Mollusca, two Arthropoda), 32 in CP (15 Echinodermata, nine Cnidaria, five Mollusca, three Arthropoda) and 12 in SP (five Echinodermata, three Cnidaria, two Gastropoda, two Arthropoda). Although also recorded in NP, the species Lophaster stellans, Labidiaster radiosus, Solaster regularis (all Echinodermata), and Dactylanthus antarcticus and the genera Thouarella and Acanthogorgia (all Cnidaria) were found only rarely in NP but were common in CP. This results in 22 common deep-water emergent species/genera in diving depth in NP; 32 in CP and 12 in SP (Table 2).
Table 2. Deep-water emergent species within diving depths in Chilean Patagonia (nl, northern limit; sl, southern limit), sorted by appearance from north to south, including summary of their presence in North Patagonia (NP), Central Patagonia (CP), and South Patagonia (SP), and in fjords, channels and at the exposed coast.
Twenty-eight of the 34 deep-water emergent species/genera have been registered from fjords, 34 from channels and only 11 from the exposed coast: one specimen of the coral Desmophyllum dianthus, however, was registered once at the exposed coast at 40°S (Table 2).
Single specimens of the species Acesta patagonica and Tripylaster philippi were found in Comau Fjord, a comparably well-studied fjord in NP, while specimens were only recorded more frequently south of 46 – 47°S. The species (Ophiomitrella chilensis), which was recorded in shallow water of NP, but not in CP, has only nine records in Patagonia. Colossendeis macerrina (Arthropoda) was only recorded once with diving depths in CP (Försterra et al., 2013). For one species (Porianopsis echinaster) around 29/30°S and 33°S four shallow-water records (25–40 m) were found during the last years, however most records are from sites deeper than 293 m. Two shallow-water records from Arbacia dufresnei (55 and 60 m) come from 29.8 and 30°S; all other northern records are from depth greater than 223 m. North of Chilean Patagonia, the genus Trophon has one record at 88 m (30°S), all other records lie between 280 and 450 m. The three stony coral species Desmopyhllum dianthus, Caryophyllia huinayensis, and Tethocyathus endesa, described for Chilean Patagonia, are much more common in NP than in CP. In NP they form dense banks in the fjords of the Los Lagos Region (Försterra and Häussermann, 2003) and a patch in the Pitipalena Fjord. The genus Swiftia was only found within diving depths in NP.
Twenty-eight deep-water emergent species have been described from other fjord regions, from near-shore marine caves and turbid waters (Table 3): in New Zealand fjords, the black coral Antipathes fiordensis was found at depths as shallow as 5 m (Grange, 1985), the hydrocoral Errina novezelandiae below 15 m (Grange et al., 1981), and some deep-water fish species (Perciformes, Scorpaeniformes) below 6–15 m (Roberts, 2001; Wing and Jack, 2013). On the western Canadian shelf, the hexactinellid sponge Aphrocallistes vastus can be found as shallow as 10 m depth (Leys et al., 2004; Austin et al., 2007), in Alaska the hexactinellid sponge Heterchone calyx below 22 m. In south-eastern Alaskan fjords, the octocoral Primnoa pacifica occurs as shallow as 25 m (Stone et al., 2005), and two Sebastes (Scorpaeniformes) species were described below 6 and 20 m, respectively (Stone and Mondragon, 2018). In Norwegian fjords, the stony coral Lophelia pertusa inhabits the sill inside Trondheimsfjorden below 39 m (Mortensen and Fosså, 2006), and two Alcyonacea can be found as shallow as 25–32 m (Strömgren, 1970, 1971). Examples of DWE are described from nearshore marine caves in the Mediterranean and Atlantic (e.g., Grigg, 1965; Stock and Vermeulen, 1982) and from the turbid waters between Trinidad and Venezuela (Warner, 1981).
Table 3. List of deep-water emergent species from other parts of the world: geographic and bathymetric distribution, main citation and first citation of deep-water emergence (DWE), typical deep-water species, type of reproduction and larvae.
A variety of mainly physical factors have been proposed in the literature to explain the phenomenon of DWE into shallower waters, such as low light levels, and low temperature (Table 4).
Table 4. Literature overview on deep-water emergence theories from different regions: North America (Alaska and British Columbia), Chilean Patagonia, New Zealand, Scandinavia (Norway, Sweden), caves and turbid waters (Trinidad).
Although not discussed inter-regionally, several hypotheses of DWE were mentioned independently in the literature for the different regions. The factors or combinations of factors used to explain this phenomenon differ between regions (Table 4). The factor “low light levels” was mentioned in eight publications for all regions, followed by “low temperature” which was mentioned in five publications for all regions except New Zealand. Factors such as “less wave action” were mentioned in three publications for three regions. The following factors were only mentioned for two regions each: “less interspecific competition” (Alaska, New Zealand), “stenohaline conditions” (Alaska, Norway), “low sedimentation rates” (British Columbia, New Zealand), “upwelling of cold deep water” (New Zealand, Sweden) and “narrow continental shelf” (Alaska, Sweden). Other factors were mentioned for one region only: “low nutrient levels” (caves), “high levels of dissolved silica (important for Hexactinellidae)” (British Columbia), “tidewater glaciers (resulting in deep-sea like conditions)” (Alaska) and “fjord bathymetry (e.g., sills, resulting in deep-sea like conditions” (Alaska).
Our analysis, based on the best available information, indicates that marine invertebrates inhabiting the Chilean coast have a wide bathymetric distribution, but exhibit marked depth preferences or depth distribution limits that differ across latitudes. Most of the listed species occurred only at deep-sea depths north of 39°S but extended into shallower water south of 39°S (Figure 3). In the same way, all studied genera show the same significant pattern (P < 0.001, Table 5 and Supplementary Table 2) of deeper distribution at northern locations, mostly down to 500 m depth, and reaching significantly shallower at southern locations. Most of the genera in the data set did not reveal significant positive or negative coefficients related to depth occurrences (Supplementary Table 2). Only 9 of 33 genera (27%) showed positive associations with depth at northern sites. Similarly, 11 of 33 genera (33%) showed negative coefficients related to depth at southern localities (Supplementary Table 2).
Figure 3. Average depth of analyzed species and genera north (“North” corresponding to 18–40°S) and south of 40°S (“South,” corresponding to 40–56°S). A: genera with significant positive coefficient for depth in “North”; B: genera with significant negative coefficient for depth in “South”; AB: genera with pattern A and B.
Table 5. Two-way ANOVA results for comparison of mean bathymetric distribution by genus and biogeographic regions.
Using the entire data set, the GLM revealed significant differences in species presence/absence (P < 0.001) related to depth, temperature, primary production, silica, salinity and oxygen but with a low deviance (<12%, Tables 6, 7 and Supplementary Figure 2), and temperature was considered the most important variable for this model. The GLM that only used records up to 50 m depth followed a similar pattern, revealing significant differences in species presence/absence related to depth, primary production (Supplementary Figure 3), temperature (Supplementary Figure 4), salinity (Supplementary Figure 5), oxygen (Supplementary Figure 6), and velocity, with low deviance explained (<11%, Table 7), and temperature was the most important variable (Table 6). In both GLMs, temperature is inversely related to presence probability, in other words, presence probability is high at lower temperatures, and declines when temperatures increases. The GLM with records deeper than 200 m depth revealed a significant effect of depth, silica (Supplementary Figure 7), salinity, oxygen and clorophyll-a on the presence/absence of species, with an explained deviance close to 25% (Table 7). In this case, oxygen is considered to be the most important variable and is inversely related to presence probability (Table 6).
Table 6. Coefficients and most important variable for the best GLM of each data (all data; <50 m; >200 m).
In general, the statistical analyses searching for correlations between species distribution and seven environmental parameters (Supplementary Figure 8) suggest that several of these factors (primary production, salinity, oxygen and temperature, see Table 6 and Supplementary Figures 3–7, 8A,B,D,E) were significantly associated to the bathymetric distribution of the studied taxa of benthic invertebrates, but temperature was the most important environmental driver (Supplementary Figure 8D). In the intertidal areas of northern and central Chile species composition, abundance and diversity are influenced by temperature and salinity (Broitman et al., 2001, 2011; Rivadeneira et al., 2002; Ibáñez et al., 2019). This study shows the same pattern at deep-sea communities (below the OMZ), where bottom temperature and salinity have a strong influence on bathymetric distribution. The sea surface temperature is the most conspicuous gradient along the Chilean coast ranging from 19.5 to 6.9°C, however bottom temperature in the top 100 m is similar at northern and southern localities (mean 10.5 and 9.7°C, respectively); a similar pattern is observed at 500 m depth (mean 4.1 and 3.8°C, respectively). Salinity shows little variation at latitudinal and bathymetric scales along the exposed coast but may have strong gradients within the fjords and channels where a Low Salinity Layer (LSL) and further haloclines may be present with surface salinities below 10 psu (see Häussermann and Försterra, 2009). The OMZ has a powerful effect on the bathymetric distribution of benthic communities along the Chilean coast (Thiel et al., 2007). In the southern fjords and channels the high oxygen concentration in shallow water promotes the occurrence of deep-water species, living below the OMZ at northern localities (>200 m). Nevertheless, the results of the analyses do not allow us to conclude if the parameters that show significant correlations with distribution represent minimum or sufficient conditions for triggering DWE, and the negative correlation of oxygen levels with presence probability seems counter-intuitive (Supplementary Figure 2). It is possible that at least some of the detected correlations are indirect effects of other parameters we have not looked at so far.
Most species mentioned in the literature for DWE and 18 of the genera mentioned in this article for Chile belong to typical deep-water genera (Table 1) and are only at some special locations found in shallow water. Therefore at least for most, if not all of the analyzed species, we are observing DWE and not shallow-water submergence.
Low light level is the most frequently mentioned factor hypothesized to trigger DWE (Table 4). In some regions of DWE, these low light levels are caused by cave habitats (e.g., in the Mediterranean), light absorbing tannin-stained surface water (e.g., in New Zealand) and/or high particle load in the upper column either from glaciers, rivers or other sources. Looking at light attenuation (Supplementary Figure 9), areas with lower water clarity coincide with areas with strong DWE (inner fjords and channels); see also turbid estuarine plume in Aysén Fjord (Figure 3 in Cáceres, 2004). But values fluctuate strongly within the fjords and channels of Patagonia. Due to the extremely high precipitation in the Chilean fjord region (more than 5000 mm between 42 and 51°S, see Alvarez-Garreton et al., 2019, and even to 6700 mm, see Häussermann and Försterra, 2009), tannin-stained run-off in most fjords is so diluted that the light-absorbing effect of the surface layer is negligible and average visibility within the Chilean fjords and channels is generally even higher than along large sections of the exposed coast. The highest values of light-absorbing turbidity are often measured in summer when radiation is at maximum and lowest in winter when cloud coverage and low sun angles reduce radiation. This has a leveling effect throughout the year and reduces longer phases of low light conditions at the surface. In some Chilean fjords on the other hand (e.g., Tempano Fjord; 48°43′07′′S; 74°14′19.3′′W) with extremely turbid glacial run-off or tannin-stained surface layer (e.g., Seno Farquhar; 48°29′18.7′′S; 74°12′25.7′′W), the low-salinity surface layer blocks most of the light causing night-like conditions below. Nevertheless, the number of deep-water emergent species in these fjords are not higher than in neighboring fjords with clear water and high light levels (e.g., Paso Schlucht; 48°8′3.84′′S; 74°47′25.44′′S) where deep-water gorgonians can even be seen through the surface.
Kregting and Gibbs (2006) showed that salinity rather than light levels controlled the upper limit of black corals in Doubtful Sound, New Zealand and proved a hypothesis wrong that was used for decades to explain DWE in NZ fjords.
Thus any correlation between light levels and DWE is either a coincidence, or low light is a factor that could be enhancing conditions for DWE but is not enough to trigger DWE.
The wave energy (Supplementary Figure 10) to a large extent has a negative correlation with DWE and suggests a connection. While wave energy decreased from the exposed coast and islands in the west toward the protected channels and fjords in the east, the number of deep-water emergent species is higher in the protected areas (e.g., none of the listed anthozoans were recorded in shallow water of the exposed Patagonian coast). The current velocity data (Supplementary Figure 8G) decreases from northern and central Chile toward Patagonia, but the resolution is not high enough to see differences between areas within Patagonia. Although most fjord shores are indeed protected from open ocean waves, there are many examples of areas with several deep-water emerging species present despite their exposure to waves and surge (e.g., Isla Millar at the entrance to Penas Gulf 47°58′45.4′′S, 74°40′47.0′′W). In fact, in most fjord regions, some fjords and channels can be found that act as funnels for wave action from the ocean with a pronounced discharge of wave energy at their entrance, but which still exhibit DWE. Since a hypothesized connection fails to explain the exceptions, again, low wave energy may create favorable but alone does not provide sufficient conditions for DWE. Most DWE takes place in depths where wave energy is negligible anyway. Only in special locations (e.g., in caves) where deep-water emergent species reach into extremely shallow depths, the absence of wave energy may play a role.
For DWE in Mediterranean caves, Iliffe et al. (1984) proposed low nutrient levels to be the cause. While this factor may be valid for the studied caves, there are many examples of high-nutrient habitats that show strong DWE. In fact, fjord water due to strong terrestrial runoff is generally rich in nutrients and thus highly productive (Iriarte et al., 2014). For the Comau fjord in Chilean Patagonia, high nutrient levels have been hypothesized to be the main reason why the deep-water emergent coral species Desmophyllum dianthus can thrive in shallow water with Aragonite-corrosive pH levels as low as 7.4 (Höfer et al., 2018; Martínez-Dios et al., 2020). Interestingly, based on data from BioOracle, chlorophyll-a (Supplementary Figures 3, 8F) show the highest levels for the inner fjords and channels while primary production (Supplementary Figure 8E) shows a different, more patchy, and seemingly more erratic pattern. The maps reveal strong fluctuations in both parameters, which makes it hard to hypothesize any correlation with DWE.
Since DWE is also called high-latitude emergence, the phenomenon is often correlated with comparably low average water temperatures. Nevertheless, DWE can also be found in regions that do not have low temperatures at all. In the Caribbean Sea between Trinidad and Venezuela, several examples of DWE are described (Tables 3, 4) while the average temperature in shallow water is significantly above deep-sea values. The same is valid for Mediterranean caves. Average water temperature in Comau fjord (42°30′S) in southern Chile, in depths where 14 deep-water emergent species can be found, varies throughout the year between 8 and 12°C (Häussermann and Försterra, 2009), which is not colder than average water temperatures from the exposed coast at the same latitude where less deep-water emerging species were observed (e.g., only the sea urchin Arbacia dufresnei down to 15 m at Puñihuil, 41°55.743′S; 74°02.202′W and a total of eight species at the three sites down to 30 m around Guafo Island, 43°36.269′S; 74°42.911′W: the echinoderms A. dufresnei, Cosmasterias lurida, Poraniopsis echinaster, Labidiaster radiosus, Henricia sp., the gastropod Fusitriton magellanicus and the decapods Pagurus comptus and Lithodes santolla). These temperatures are significantly higher than those from the deep-sea environments where these species are normally found (Supplementary Figure 8D). The temperature data (Supplementary Figures 4, 8D) reveal that along the Chilean coast shallow-water temperature is gradually and significantly decreasing from north to south, with shallow-water temperatures approaching deep-water values from northern latitudes at the southern tip of South America. The statistical analyses (Tables 6, 7) suggest a negative correlation of the occurrence of the selected species with temperature (Supplementary Figure 2). But the number of deep-water emergent species is highest in CP, followed by NP while fewer species were recorded in SP (Table 2). The temperature hypothesis fails to explain this pattern, which again suggests that low temperatures may be an enhancing factor or even a necessary requirement for DWE, but alone are not sufficient to trigger DWE.
Low sedimentation rates have been proposed to allow for DWE in British Columbia by Leys et al. (2004) and in New Zealand fjords by Grange and Singleton (1988). While this may be the case for the studied areas, in other regions, DWE was explained by light absorption from highly turbid water off Trinidad (Table 4) with a consequently high sedimentation load. Furthermore, even in extremely high sediment-loaded glacial fiords of Alaska (Stone and Mondragon, 2018) and Chilean Patagonia (own data, e.g., Tempano Fjord) DWE was observed. Nevertheless, sedimentation indeed may be a stressor for passive suspension feeders (e.g., the listed anthozoans), which are absent from highly sediment-laden places. In Tempano Fjord, however, the bivalve Acesta patagonica was recorded at a slightly overhanging wall at the unusual shallow depth of 15 m.
Cairns (1995) proposed the upwelling of colder deep-water was triggering DWE in New Zealand Fjords. The same was suggested by Wisshak et al. (2005) for DWE off the Swedish coast. While temperature alone has already been shown to be an insufficient factor for causing DWE, the upwelling of deep-water could in fact transport larvae from deeper habitats into shallower zones. Most fjords show internal circulations that are driven by an outflow of low-saline surface water and a density-driven inflow of deep salty water (Stigebrandt, 2012). Nevertheless, for significant larvae transport into shallow water, the deep water must come directly from deep-water supply site populations. Within the Chilean fjord region, there are many examples of fjords where extended and comparably shallow bays and gulfs lie between the deeper ocean and the fjords (Supplementary Figure 1). Actually, within the Chilean fjord region the only location with a narrow shelf is off Peninsula Taitao (∼47°S), where relatively few deep-water emerging species were present (Supplementary Figure 1 and Table 3). It is highly unlikely that the larvae transport from the deep-sea into the shallow water of these fjords is stronger than at upwelling zones along the exposed Chilean coast where no or less DWE is observed. In addition, the hypothesis suggests that the shallow-water populations are sinks that depend on the constant supply of larvae from the deep. However, several studies show reproductive success of the deep-water emergent species in the shallow habitats (Rossin et al., 2017; Feehan et al., 2019), which suggests that these populations can sustain themselves. Stone et al. (2005) suggest that the deep-water emergent tree coral population in Alaskan fjords is a disjunct population with little connection to tree coral populations outside the fjords.
The highest abundance of glass sponges in British Columbia was correlated with fjords that exhibited high dissolved silicate, low light, temperatures between 9 and 10°C and low suspended sediments (Leys et al., 2004). While high levels of silica are in fact typical for fjord water (Silva, 2008), especially in areas with high glacial freshwater run off (Cuevas et al., 2019), and may be beneficial or even indispensable for glass sponges, silica is of minor to no importance for other deep-water emerging species. Silica concentration on the other hand affects phytoplankton communities and thus may have an effect on the food web (Cuevas et al., 2019) and consequently also on benthic species composition. However, the maps of silica concentrations within the fjord region based on BioOracle data do not coincide with the pattern of DWE (Supplementary Figures 7, 8C), which is probably due to a lack of spatial resolution of the data.
Stone and Mondragon (2018) proposed tidewater glaciers and typical fjord bathymetry (e.g., sills) to be triggers for DWE of tree corals in Alaskan fjords. Many fjords in Chilean Patagonia have no sill, or at least no pronounced sill (Pickard and Stanton, 1980). In addition, due to the large inland icefields located in CP, the fjords of CP, and only some fjords of SP, possess glaciers at their heads, while there are no glaciers reaching the ocean in NP (Häussermann and Försterra, 2009). However, DWE in Patagonia is also present in channels. Again, these factors coincide with DWE in many fjords but not in all fjords and fail to explain DWE outside of fjords.
Grange and Singleton (1988) and Stone et al. (2005) proposed low interspecific competition for promoting DWE in Alaskan and New Zealand fjords, respectively. In Chilean fjords, average benthic coverage and species diversity are higher than at the exposed coast north of Patagonia (Fernández et al., 2000; Häussermann and Försterra, 2009). In fact, the additional presence of deep-water emerging species is one factor to explain the extremely high benthic diversity in Chilean fjords. Here, the deep-water emerging species live intermingled with typical shallow-water species and form part in different stages of succession after disturbance (own data).
Stable salinity in zones with DWE was proposed to mimic deep-water conditions and thus create a suitable habitat for deep-water emergent species (Table 4). While salinity levels are a key factor for marine species distribution (Broitman et al., 2001), places with stable salinity are much more frequent than places with DWE. At the same time, Kregting and Gibbs (2006) could show that the deep-water emergent black corals in Doubtful Sound, New Zealand, withstand drops in salinity down to 20 psu when the exposure to low salinity is restricted to less than 6 h (a medium diurnal tide cycle) and when they are followed by salinities above 32 psu. Chilean fjords generally possess a superficial LSL with salinities down to approximately 2–10 psu and a thickness of up to 10 m (Häussermann and Försterra, 2009). But even below this LSL, fluctuations in salinity are present and stronger than in most marine regions without DWE: at three sites in Comau and Reñihué Fjords (NP) at depth where Desmophyllum dianthus was habitat-forming, monthly average salinities varied between 25 and 31.5 psu during a 13 months cycle, and spikes at the site Morro Gonzalo in Reñihué Fjord (42°32′46′′S; 72°37′7′′W) at 27 m even reached 10 psu (Feehan et al., 2019). So stable salinity alone, although a possibly enhancing factor, cannot explain the occurrence of DWE.
In Chilean Patagonia, the deepest reach of the LSL sharply marks the highest extension of many deep-water emerging species [the sea star Cosmasterias lurida (Figure 2A) regularly migrates close to the surface in fjords but was regularly found dead in large numbers after strong rainfall events when the LSL grows faster than the star fish can retreat into safe higher saline depths]. However, data from the superficial LSLs are not present in the BioOracle database and thus not available for statistical analyses and thus the magnitude of the LSL is not revealed by the salinity data (Supplementary Figures 5, 7B). In addition, this LSL is very dynamic and is varying on several time scales since its depth, horizontal extension, and time of persistence depend on precipitation, glacial run-off, currents and mixing events. Hence, low salinity may explain the upper limitation for DWE in some areas. On the other hand, the magnitude of DWE along the Chilean fjord region with high numbers of species in NP and CP shows a positive correlation with precipitation which peaks between 42 and 51°S (Figure 4 and Table 2). The latter determines the magnitude of the LSL. In addition, most deep-water emergent species are found in channels (34) followed by fjords (28), with least species at the exposed coast (11) (Table 2), where salinity is highest. Since DWE takes place below the LSL, the positive effect of low a LSL on DWE, if present, must be indirect. One effect of a LSL is the suppression of macroalgae. This suggests that the lack of dense kelp forests may have a positive influence on the phenomenon of DWE. In fact, most areas with DWE worldwide have in common a lower abundance or the absence of macroalgae (fjords, caves and turbid waters, see Table 4). The reasons for that may vary between regions, and besides low salinity, low light levels may also cause a scarcity of macroalgae. The presence or absence of macroalgae on the other hand may have a significant influence on the primary production regime, the quality and quantity of food for filter- and detritus-feeders and the annual dynamics in their availability. However, the role of kelp as a trophic resource is still not well understood (Miller and Page, 2012). How far the conditions created by the absence of macroalgae may allow deep-water species to emerge into shallow water remains to be studied. Unfortunately, our own macro-algae data set is relatively poor, does not include abundances, and sampling-effort was biased (Supplementary Figure 11) and the models for macroalgae-presence in the Chilean fjord region have too many false positives, due to the presence of Ulva species and shore vegetation to make them useful for statistical analyses (see Mora-Soto et al., 2020). Nevertheless, the positive correlation of precipitation, and the negative correlation of the abundance of macroalgae with DWE is eye-catching and justifies hypothesizing a connection.
Figure 4. Mean annual precipitation of Chilean Patagonia 1980–2015, R. Garreaud (personal communication), see Alvarez-Garreton et al. (2019).
None of the factors hypothesized in the literature to explain DWE alone seems to be able to explain this phenomenon in all areas around the world where it occurs. This leaves several alternative explanations or combinations of those: (1) In each region where DWE occurs, unique factors or combinations of factors cause this phenomenon, (2) not one single factor, but a combination of widely common factors is triggering DWE or (3) there are other factors that have not been looked at so far that cause or significantly contribute to DWE. (4) There is not one factor or a combination of factors that trigger DWE for a variety of species, but each species or phylum requires its own specific set of factors to deep-water emerge that are only met in specific locations. For example, the mentioned anthozoans, ophiurids, the holothurian, the crinoid, the bivalve and the pycnogonid are restricted to fjords and channels, while some of the mentioned sea stars, gastropods and decapods can also be found at more exposed sites. Fjord regions are very diverse in their near-shore oceanographic conditions (Pickard and Stanton, 1980). As a consequence, a high number of strong gradients interfere within comparably small areas, producing a large number of possible combinations of parameters (Häussermann and Försterra, 2009). This increases the probability that certain combinations of factors are met that are required for certain species to deep-water emerge. As a result, in these regions, a comparably high number of deep water emerging species can be found in a given area. (5) Rather than looking for DWE-triggering factors, we would need to look for the absence of DWE-inhibiting factors. This latter hypothesis is based on the assumption that factors or factor combinations enabling DWE are widely common, but do not have an effect due to single or few inhibiting factors. These inhibiting factors may be difficult to detect since the coastal marine systems are often dynamic and the “knock-out” criteria are most likely rare extreme or peak values of otherwise unproblematic factors (e.g., drops in oxygen after die offs of algae blooms or sedimentation peaks near river mouths after extreme weather events that occur only very sporadically).
Although the statistical analyses for the Chilean coast show some significant influence of some factors on the presence of the mentioned species, by looking at the distribution maps, the correlation of the analyzed factors with the magnitude of DWE within Patagonia is not completely clear, or at least there seem to be too many mismatches to justify a rule. This basically rules out the possibility that a single factor is responsible for the frequent DWE in Chilean Patagonia. We could neither find combinations of the mentioned factors that would work to explain the depth-dependent pattern. This suggests that the factors that showed statistical significance rather represent minimum prerequisites for DWE than actual triggers for this phenomenon. For example salinities that do not drop below certain minima, lower temperatures and less wave action most likely make it easier for deep-water species to persist in shallow water. Upwelling and larval support from deep-water populations are also factors that help to establish and/or maintain shallow-water populations. However, none of these factors alone nor the combination of them seem to be the crucial trigger for DWE.
Eight records of deep-water species from Central and Northern Chile are from depths shallower than 100 m; four from the sea star Poraniopsis echinaster (29, 30, and 33°S) in 20–30 m depth, two from the sea urchin Arbacia dufresnei (30°S) in 55 and 60 m depth, one from the sea star Cosmasterias lurida in 30 m depth (30°S), and one from the gastropod Trophon sp. in 88 m depth (30°S). All records are from the last decade, which for records below diving depths (30 m) can be explained by the time frame these ROV surveys have taken place. However, it is interesting that three of the five records from diving depths are from the last year. Since all mentioned records are situated in known upwelling areas (around 30°S and around 33°S) (Aravena et al., 2014) and upwelling is getting stronger due to climate change (Bakun et al., 2015), it is possible that these species appeared and will become more abundant in shallow water with climate change advancing. The southern distribution limits of other species from Central and Northern Chile are moving southward (e.g., the sea star Heliaster helianthus was originally described to Valparaiso, approximately 32.5°S, and is now frequently seen in Concepcion, approximately 37°S), and also within Patagonia species described from further north are expending southward (e.g., the sea stars Patiria chilensis and Meyenaster gelatinosus formerly described to 44°S were spotted down to 53 and 50°S, respectively, the temperate sunfish Mola mola has been observed at 47°S, tuna at 50°S, etc.; our own observations). Thus, one should expect to see important changes in the composition of benthic species of Chilean Patagonia with advancing climate change. In particular, it would be interesting if to note if, with a general southward shift of habitats and distributions, the phenomenon of DWE also shifts southward.
The dataset from deep-water habitats along the Chilean coast is still extremely poor, with only nine of 34 species having more than 10 records, and only three having more than 20 records. The poor sampling in deep-water habitats, in general, leads to biased and statistically non-significant analyses. Taking into account a coastline of more than 100,000 km, the data we have on Patagonian shallow-water habitats are also poor; gaps are located especially between 50° and 52°S and 54–56°S. In addition, even species that are well-known by local divers along the Northern and Central Chilean coast are lacking published records in the literature, thus more and continuous species inventories along the entire Chilean coast are needed.
Since many of the abiotic data available for Chilean Patagonia are derived from satellite imagery and/or models, the resolution in the finely structured maize of the Chilean Fjord Region is poor and does not reflect the diversity and patchiness of the small-scale mosaic of oceanographic conditions (Supplementary Figures 3–8).
(1) Twenty-eight species and six genera show a distinct distribution pattern with deep-water records off northern and central Chile and a continuous rise of the shallowest records into diving depths along Chilean Patagonia. On a north-south gradient, the highest number of deep-water emergent species in the upper 30 m can be found in CP (32), followed by NP (28) and SP (12), while on an east–west gradient, most species occur in channels (34) followed by fjords (28) and the exposed coast (11).
(2) None of the factors proposed in the literature alone, nor combinations of them, can satisfyingly explain the phenomenon of DWE in some places and its absence in other places, where the same combination of abiotic factors are present.
(3) Besides some basic requirements, most likely, DWE requires a combination of factors and this combination may vary between areas and or species. There may be factors involved that have not been hypothesized so far. The fresh-water input and associated absence of macroalgae may be one of them. More studies of this phenomenon are needed to understand the observed patterns.
(3) Through the phenomenon of DWE climate change may not only cause latitudinal but also bathymetric species shifts.
(4) More sampling in deep and poorly known areas and inventorying efforts, even in better-known areas, are strongly recommended to be able to carry out significant analyses to understand phenomena like DWE. More data of abiotic environmental variables, especially from the inner fjords and channels, are required for statistically sound analyses. The data provided, and analysis carried out can only be the first step toward a better knowledge of Chilean benthic invertebrates.
The data analyzed in this study is subject to the following licenses/restrictions: The database of the collection of the Universidad Catolica del Norte is available at GBIF (Sellanes, 2018). The PAMADA database includes data from other scientists who do not agree with their publication. Requests to access these datasets should be directed to VH, di5oYXVzc2VybWFubkBnbWFpbC5jb20=.
VH and GF: design of the project, collection of data, analysis and interpretation of data, and writing of the manuscript. SB: preparation of data and discussion of the manuscript. CC: preparation and analysis of data, writing of the manuscript. CC and CI: preparation and analysis of data and writing of the manuscript. JS: collection of data and writing of the manuscript. AT and JE: background research, collection of data, and discussion of the manuscript. FB: collection of data and discussion of the manuscript. All authors: contributed to the article and approved the submitted version.
This research was supported by the Fondecyt project 1201717 to GF, Fondecyt project 1161699 to VH, and Fondecyt project 1181153 to JS and CI. CC was supported by the ANID project “ANID-PCHA/Doctorado Nacional/2019-21191261”.
The authors declare that the research was conducted in the absence of any commercial or financial relationships that could be construed as a potential conflict of interest.
All claims expressed in this article are solely those of the authors and do not necessarily represent those of their affiliated organizations, or those of the publisher, the editors and the reviewers. Any product that may be evaluated in this article, or claim that may be made by its manufacturer, is not guaranteed or endorsed by the publisher.
Many thanks to all the former assistants, volunteers, and employees who supported the work at Huinay Scientific Field Station, and to the Huinay Foundation.
The Supplementary Material for this article can be found online at: https://www.frontiersin.org/articles/10.3389/fmars.2021.688316/full#supplementary-material
Supplementary Figure 1 | Bathymetry map of Chile showing 50 and 200 m isobaths. (A) Chile, (B) North Patagonia, (C) Central Patagonia, (D) South Patagonia. The bathymetry of fjords and channels is very poor, thus many deep areas are not shown; e.g. Comau fjord, NP (left of blue asterisk) reaches nearly 500 m depth and Messier Channel, CP (orange asterisk) reaches 1300 m depth.
Supplementary Figure 2 | Relationship between each variable and presence probability calculated during GLM analysis (A) complete dataset. (B) <50 m; (C) >200 m.
Supplementary Figure 3 | Spatial variation of mean Chlorophyll-a concentration at different depths. (A) surface and (B) bottom waters.
Supplementary Figure 4 | Spatial variation of mean temperature at different depths. (A) Surface and (B) bottom waters.
Supplementary Figure 5 | Spatial variation of mean salinity at different depths. (A) surface and (B) bottom waters.
Supplementary Figure 6 | Spatial variation of mean dissolved molecular oxygen concentration at different depths. (A) Bottom and (B) Surface waters.
Supplementary Figure 7 | Spatial variation of silica concentration at different depths. (A) Surface and (B) bottom waters.
Supplementary Figure 8 | Latitudinal variation of parameters along the Chilean coast (18–56°S) at two depths. The blue line represents surface water while the orange line represents deep water (>200 m). The points are the mean for each latitudinal band while the whiskers represent minimum and maximum values at each latitudinal band. (A) oxygen, (B) salinity, (C) silica, (D) temperature, (E) primary production, (F) chlorophyll a. (G) current velocity.
Supplementary Figure 9 | Turbidity (Kd_490) for Chilean Patagonia based on southern hemisphere winter average for Kd_490 for the year 2009, as derived from NASA MODIS Aqua and Terra Satellites. Darker areas indicate a higher incidence of Kd_490 and thus a smaller attenuation depth and lower clarity of ocean water (Zhong-Ping et al., 2005).
Supplementary Figure 10 | Wave Exposure Model for Chilean Patagonia, showing areas of high (red) to low (blue) energy wave action. Wave exposure is a derived product of wave fetch and wave energy, that calculates the wind energy as a function of wind speed average occurrence and direction, and wave fetch as a derivative of distance and direction of energy to the coastline, with the maximum wave fetch set at 200 km. Model produced by M. T. Burrows, Department of Ecology, Scottish Association for Marine Science (Burrows et al., 2008).
Supplementary Figure 11 | Presence of macroalgae based on Huinay Fiordos Expeditions. Abundances of macroalgae strongly rise with elevated surface salinity values which, in Patagonia, can be found along the exposed coast and in some channels, but usually not inside fjords (for model, see https://biogeoscienceslaboxford.users.earthengine.app/view/kelpforests).
Supplementary Table 1 | Presence points of the 28 species and six genera used during the present study: number of presence points in: North and Central Chile (N-C: < 40°S); North Patagonia (NP: 40–47/48°S), Central Patagonia (CP: 47/48–53/54°S), and South Patagonia (SP: 53/54–56°S).
Supplementary Table 2 | Relationship of the occurrence probability with depth, site and genus. SE, standard error; z, Z-statistics; P, p-value.
Alvarez-Garreton, C., Mendoza, P., Boisier, J. P., Addor, N., Galleguillos, M., Zambrano-Bigiarini, M., et al. (2019). The CAMELS-CL dataset: catchment attributes and meteorology for large sample studies – Chile dataset. Hydrol. Earth Syst. Sci. 22, 5817–5846. doi: 10.5194/hess-22-5817-2018
Andres, H. V. (1975). Nicippe buchi, n. sp., ein Pardaliscide aus einem Lavatunnel auf Lanzarote (Amphipoda, Crustacea). Mitteilungen aus dem Hamburgischen Zool. Museum Institut 72, 91–95.
Aravena, G., Broitman, B., and Stenseth, N. C. (2014). Twelve years of change in coastal upwelling along the central-northern coast of Chile: spatially heterogeneous responses to climatic variability. PLoS One 9:e90276. doi: 10.1371/journal.pone.0090276
Araya, J. F. (2013). A new species of aeneator finlay, 1926 (Mollusca, Gastropoda, Buccinidae) from northern Chile, with comments on the genus and a key to the Chilean species. ZooKeys 89–101. doi: 10.3897/zookeys.257.4446
Araya, J. F. (2016). New records of deep-sea sea spiders (Chelicerata: Pycnogonida) in the southeastern Pacific. Mar. Biodiversity 46, 725–729. doi: 10.1007/s12526-015-0416-7
Assis, J., Tyberghein, L., Bosch, S., Verbruggen, H., Serrão, E. A., and De Clerck, O. (2018). Bio-ORACLE V2.0: extending marine data layers for bioclimatic modelling. Glob. Ecol. Biogeogr. 27, 277–284. doi: 10.1111/geb.12693
Austin, W. C., Conway, K. W., Barrie, J. V., and Krautter, M. (2007). Growth and morphology of a reef-forming glass sponge, Aphrocallistes vastus (Hexactinellida), and implications for recovery from widespread trawl damage. Por. Res. Biod. Inn. Sustain. 2007, 139–145.
Bakran-Petricioli, T., Vacelet, J., Zibrowius, H., Petricioli, D., and Chevaldonné, P. (2007). New data on the distribution of the “deep-sea” sponges Asbestopluma hypogea and Oopsacas minuta in the Mediterranean Sea. Mar. Ecol. 28, 10–23. doi: 10.1111/j.1439-0485.2007.00179.x
Bakun, A., Black, B. A., Bograd, S. J., Garcia-Reyes, M., Miller, A. J., Rykaczewski, R. R., et al. (2015). Anticipated effects of climate change on coastal upwelling ecosystems. Curr. Climate Change Rep. 1, 85–93. doi: 10.1007/s40641-015-0008-4
Boury-Esnault, N., and Vacelet, J. (1994). “Preliminary studies on the organization and development of a hexactinellid sponge from a Mediterranean cave, Oopsacas minuta,” in Sponges in Time and Space, eds R. W. M. van Soest, Th.M.G. van Kempen, and J.-C. Braekman, (Rotterdam: Balkema).
Breedy, O., Cairns, S., and Häussermann, V. (2015). A new alcyonian octocoral (Cnidaria, Anthozoa, Octocorallia) from Chilean fjords. Zootaxa 3919, 327–334. doi: 10.11646/zootaxa.3919.2.5
Broitman, B. R., Navarrete, S. A., Smith, F., and Gaines, S. D. (2001). Geographic variation of southeastern Pacific intertidal communities. Mar. Ecol. Prog. Series 224, 21–34. doi: 10.3354/meps224021
Broitman, B. R., Veliz, F., Manzur, T., Wieters, E. A., Finke, G. R., Fornes, P. A., et al. (2011). Geographic variation in diversity of wave exposed rocky intertidal communities along central Chile. Revista Chilena de Historia Nat. 84, 143–154. doi: 10.4067/s0716-078x2011000100011
Brown, R. R., Davis, C. S., and Leys, S. P. (2017). Clones or clans: the genetic structure of a deep-sea sponge, Aphrocallistes vastus, in unique sponge reefs of British Columbia, Canada. Mol. Ecol. 26, 1045–1059. doi: 10.1111/mec.13982
Burrows, M.T., Harvey, R., and Robb, L. (2008). Wave exposure indices from digital coastlines and the prediction of rocky shore community structure. Mar. Ecol. Prog. Ser. 353, 1–12. doi10.3354/meps07284
Cáceres, M. (2004). Surface features of the circulation in fjords of southern Chile observed in ERS and LANDSAT images. Gayana (Concepción) 68, 71–76. doi: 10.4067/S0717-65382004000200014
Cairns, S., Häussermann, V., and Försterra, G. (2005). A review of the Scleractinia (Cnidaria: Anthozoa) of Chile, with the description of two new species. Zootaxa 1018, 15–46. doi: 10.11646/zootaxa.1018.1.2
Cairns, S. D. (1994). Scleractinia of the temperate North Pacific. Smiths. Contrib. Zool. 557, 1–150.
Cairns, S. D. (1995). The Marine Fauna of New Zealand: Scleractinia (Cnidaria: Anthozoa). New Zealand: New Zealand Oceanographic Institute Memoir.
Cairns, S. D., Gerhswin, L. A., Brook, F., Pugh, P. R., Dawson, E. W., Ocaña, V. O., et al. (2009). Phylum Cnidaria; Corals, MEDUSAE, HYDROIDS, MYXOZOA. New Zealand Inventory of Biodiversity. Kingdom Animalia: Radiata,.
Cairns, S. D., and Häussermann, V. (in press). A new species of Thouarella (Anthozoa: Octocorallia: Primnoidae) from off Chile. Spixiana.
Calcagno, V., and de Mazancourt, C. (2010). glmulti: an R package for easy automated model selection with (generalized) linear models. J. Statist. Softw. 34, 1–29.
Catalán, J., Merino-Yunnissi, C., Martínez, A., Sellanes, J., and Ibáñez, C. M. (2020). New records of crinoids (Echinodermata: Crinoidea) in the continental slope of Chile. Rev. Biol. Mar. Oceanogr. 55, 68–72. doi: 10.22370/rbmo.2020.55.1.2395
Choy, E., Watanabe, K., Williams, B., Stone, R., Etnoyer, P., Druffel, E., et al. (2020). Understanding growth and age of red tree corals (Primnoa pacifica) in the North Pacific Ocean. PLoS One 15:e0241692. doi: 10.1371/journal.pone.0241692
Cuevas, L. A., Tapia, F. J., Iriarte, J. L., González, H. E., Silva, N., and Vargas, C. A. (2019). Interplay between freshwater discharge and oceanic waters modulates phytoplankton size-structure in fjords and channel systems of the Chilean Patagonia. Prog. Oceanography 173, 103–113. doi: 10.1016/j.pocean.2019.02.012
Dahm, C. (1999). Ophiuroids (Echinodermata) of southern Chile and the Antarctic: taxonomy, biomass, diet and growth of dominant species. Sci. Mar. 63(Suppl. 1), 427–432.
Dıaz, J.-P., Feral, B., David, T., and Saucede, E. P. (2010). Evolutionary pathways among shallow and deep-sea echinoids of the genus Sterechinus in the Southern Ocean. Deep. Sea Res. II. 58, 205–211. doi: 10.1016/j.dsr2.2010.10.012
Feehan, K. A., Waller, R. G., and Häussermann, V. (2019). Highly seasonal reproduction in deep-water emergent Desmophyllum dianthus (Scleractinia: Caryophylliidae) from the Northern Patagonian Fjords. Mar. Biol. 166, 1–13.
Fernández, M., Jaramillo, E., Marquet, P. A., Moreno, C. A., Navarrete, S. A., Ojeda, P. F., et al. (2000). Diversity, dynamics and biogeography of Chilean benthic nearshore ecosystems: an overview and guidelines for conservation. Revista Chilena de Historia Natural 73, 797–830.
Försterra, G., and Häussermann, V. (2003). First report on large scleractinian (Cnidaria: Anthozoa) accumulations in cold-temperate shallow water of south Chilean fjords. Zool. Verhandelingen 345, 117–128.
Försterra, G., Häussermann, V., Melzer, R. R., and Weis, A. (2013). A deep water pycnogonid close to the beach: Colossendeis macerrima Hoek, 1881 spotted at 18 m in the Chilean fjords. Spixiana 36:20.
Gallardo, V. A. (1963). Notas sobre la densidad de la fauna bentónica en el sublitoral del norte de Chile. Gayana 10, 3–15.
Gorny, M., Easton, E. E., and Sellanes, J. (2018). First record of black corals (Antipatharia) in shallow coastal waters of northern Chile by means of underwater video. Latin Am. J. Aquatic Res. 46, 457–460. doi: 10.3856/vol46-issue2-fulltext-20
Grange, K. R. (1985). Distribution, standing crop, population structure, and growth rates of black coral in the southern fiords of New Zealand. New Zealand J. Mar. Freshwater Res. 19, 467–475. doi: 10.1080/00288330.1985.9516111
Grange, K. R. (1990). Antipathes fiordensis, a new species of black coral (Coelenterata: Antipatharia) from New Zealand. New Zealand J. Zool. 17, 279–282. doi: 10.1080/03014223.1990.10422603
Grange, K. R., Singleton, R. I., Richardson, J. R., Hill, P. J., and Main, W. D. (1981). Shallow rock-wall biological associations of some southern fiords of New Zealand. New Zealand J. Zool. 8, 209–227. doi: 10.1080/03014223.1981.10427963
Grange, K. R., and Singleton, R. J. (1988). Population structure of black coral. Antipathes aperta, in the southern fiords of New Zealand. New Zealand J. Zool. 15, 481–489. doi: 10.1080/03014223.1988.10422628
Hartmann-Schröder, G. (1974). Die Unterfamilie Macellicephalinae Hartmann-Schröder, 1971. Mittheilungen aus dem Hamburgischen Zoologischen Museum und Institut 71, 75–85.
Häussermann, V. (2004). The sea anemone genus Actinostola Verrill, 1883: variability and utility of traditional taxonomic features; and a re-description of Actinostola chilensis McMurrich, 1904. Pol. Biol. 28, 26–28. doi: 10.1007/s00300-004-0637-x
Häussermann, V. (2006). Biodiversity of Chilean sea anemones (Cnidaria: Anthozoa): distribution patterns and zoogeographic implications, including new records for the fjord region. Latin Am. J. Aquatic Res. 34, 23–35.
Häussermann, V., and Försterra, G. (2005). Distribution patterns of Chilean shallow-water sea anemones (Cnidaria: Anthozoa: Actiniaria, Corallimorpharia); with a discussion of the taxonomic and zoogeographic relationships between the actinofauna of the South East Pacific, the South West Atlantic and the Antarctic. Sci. Mar. 69, 91–102. doi: 10.3989/scimar.2005.69s291
Häussermann, V., and Försterra, G. (2007a). “Large assemblages of cold-water corals in Chile: a summary of recent findings and potential impacts,” in Conservation and Adaptive Management of Seamount and Deep-sea Coral Ecosystems, eds R. Y. George and S. D. Cairns (Miami: Rosenstiel School of Marine and Atmospheric Science, University of Miami), 324.
Häussermann, V., and Försterra, G. (2007b). Extraordinary abundance of hydrocorals (Cnidaria, Hydrozoa, Stylasteridae) in shallow water of the Patagonian fjord region. Pol. Biol. 30, 487–492. doi: 10.1007/s00300-006-0207-5
Häussermann, V., and Försterra, G. (2009). Marine Benthic Fauna of Chilean Patagonia. Santiago: Nature in Focus.
Häussermann, V., Försterra, G., and Cairns, S. D. (2016). New record of the primnoid gorgonian Primnoella delicatissima Kükenthal, 1909 for Chilean waters. Spixiana 39, 147–148.
Hessler, R. R. (1970). High-latitude emergence of deep-sea isopods. U. S. Antarctic Res. J. 5, 133–134.
Hessler, R. R., and Thistle, D. (1975). On the place of origin of deep-sea lsopods. Mar. Biol. 32, 155–165. doi: 10.1007/bf00388508
Hessler, R. R., Wilson, G. D., and Thistle, D. (1979). The deep-sea isopods: a biogeographic and phylogenetic overview. Sarsia 64, 67–75. doi: 10.1080/00364827.1979.10411365
Höfer, J., González, H. E., Laudien, J., Schmidt, G. M., Häussermann, V., and Richter, C. (2018). All you can eat: the functional response of the cold-water coral Desmophyllum dianthus feeding on krill and copepods. PeerJ 6:e5872. doi: 10.7717/peerj.5872
Ibáñez, C. M., Pardo-Gandarillas, M. C., Párraga, D., Ziruelello, M., and Sellanes, J. (2011). Cefalópodos recolectados en el talud continental de Chile. Amici Molluscarum 19, 37–40.
Ibáñez, C. M., Pardo-Gandarillas, M. C., Peña, F., Gleadall, I. G., Poulin, E., and Sellanes, J. (2016). Phylogeny and biogeography of Muusoctopus (Cephalopoda: Enteroctopodidae). Zool. Scripta 45, 494–503. doi: 10.1111/zsc.12171
Ibáñez, C. M., Pardo-Gandarillas, M. C., Poulin, E., and Sellanes, J. (2012). Morphological and molecular description of a new record of Graneledone (Cephalopoda, Octopodidea) in the southeastern Pacific ocean. Revista de Biol. Mar. y Oceanografía 47, 439–450. doi: 10.4067/s0718-19572012000300007
Ibáñez, C. M., Sepúlveda, R. D., and Chong, J. (2006). A new species of Benthoctopus Grimpe 1921 (Cephalopoda: Octopodidae) from the southeastern Pacific Ocean. Proc. Biol. Soc. Washington 119, 355–364. doi: 10.2988/0006-324x(2006)119[355:ansobg]2.0.co;2
Ibáñez, C. M., Waldisperg, M., Torres, F. I., Carrasco, S. A., Sellanes, J., Pardo-Gandarillas, M. C., et al. (2019). Environmental and ecological factors mediate taxonomic composition and body size of polyplacophoran assemblages along the Peruvian Province. Sci. Rep. 9, 1–11.
Iliffe, T. M., Wilkens, H., Parzefall, J., and Williams, D. (1984). Marine lava cave fauna: composition, biogeography, and origins. Science 225, 309–311. doi: 10.1126/science.225.4659.309
Iriarte, J. L., Pantoja, S., and Daneri, G. (2014). Oceanographic processes in Chilean fjords of Patagonia: from small to large-scale studies. Prog. Oceanogr. 129, 1–7. doi: 10.1016/j.pocean.2014.10.004
Kobayashi, G., and Araya, J. F. (2018). Southernmost records of Escarpia spicata and Lamellibrachia barhami (Annelida: Siboglinidae) confirmed with DNA obtained from dried tubes collected from undiscovered reducing environments in northern Chile. PLoS One 13:e0204959. doi: 10.1371/journal.pone.0204959
Kregting, L. T., and Gibbs, M. T. (2006). Salinity controls the upper depth limit of black corals in Doubtful Sound, New Zealand. New Zealand J. Mar. Freshwater Res. 40, 43–52. doi: 10.1080/00288330.2006.9517402
Krylova, E. M., Sellanes, J., Valdes, F., and D’Elía, G. (2014). Austrogena: a new genus of chemosymbiotic bivalves (Bivalvia; Vesicomyidae; Pliocardiinae) from the oxygen minimum zone off central Chile described through morphological and molecular analyses. Systematics Biodiversity 12, 225–246. doi: 10.1080/14772000.2014.900133
Kuhn, M. (2008). Building predictive models in R using the caret package. J. Statist. Softw. 28, 1–26.
Leys, S. P., Mackie, G. O., and Reiswig, H. M. (2007). The biology of glass sponges. Adv. Mar. Biol. 52, 1–145. doi: 10.1016/s0065-2881(06)52001-2
Leys, S. P., Wilson, K., Holeton, C., Reiswig, H. M., Austin, W. C., and Tunnicliffe, V. (2004). Patterns of glass sponge (Porifera, Hexactinellida) distribution in coastal waters of British Columbia, Canada. Mar. Ecol. Prog. Ser. 283, 133–149. doi: 10.3354/meps283133
Lipps, J. H., and Hickman, C. S. (1982). Origin, age and evolution of Antarctic and Deep-Sea Faunas. The Environment of the Deep-Sea. Englewood Cliffs, NJ: Prentice-Hall.
Manso, C. (2010). Deep-water Ophiuroidea (Echinodermata) from off Chile in the Eastern South Pacific. Biota Neotropica (Brasil) 2: 185–199.
Martínez-Dios, A., Pelejero, C., López-Sanz, A., Sherrell, R., Ko, S., Häussermann, V., et al. (2020). Effects of low pH and feeding on calcification rates of the cold-water coral Desmophyllum dianthus. PeerJ 8:e8236. doi: 10.7717/peerj.8236
Miller, R. J., and Page, H. M. (2012). Kelp as a trophic resource for marine suspension feeders: a review of isotope-based evidence. Mar. Biol. 159, 1391–1402. doi: 10.1007/s00227-012-1929-2
Mora-Soto, A., Palacios, M., Macaya, E. C., Gómez, I., Huovinen, P., Pérez-Matus, A., et al. (2020). A high-resolution global map of giant kelp (Macrocystis pyrifera) forests and intertidal green algae (Ulvophyceae) with Sentinel-2 Imagery. Remote Sens. 12:694. doi: 10.3390/rs12040694
Mortensen, P. B., and Fosså, J. H. (2006). “Species diversity and spatial distribution of invertebrates on deep-water Lophelia reefs in Norway,” in Proceedings of 10th International Coral Reef Symposium, (Okinawa).
Mortensen, P. B., Hovland, T., Fosså, J. H., and Furevik, D. M. (2001). Distribution, abundance and size of Lophelia pertusa coral reefs in mid-Norway in relation to seabed characteristics. J. Mar. Biol. Ass. 81, 581–597. doi: 10.1017/s002531540100426x
Mortensen, T. (1952). Echinoidea and Ophiuroidea. Reports of the Lund University Chile Expedition 1948-49, 3. Lunds Universitets årsskrift N.F. avd. 47, 1–22. doi: 10.1080/00364827.1970.10411156
Murray, J. (1895). A Summary of the Scientific Results Obtained at the Sounding, Dredging and Trawling Stations of HMS Challenger. London: HM Stationery Office.
Opresko, D. M. (2001). Revision of the Antipatharia (Cnidaria: Anthozoa). Part I. Establishment of a new family, Myriopathidae. Zool. Meded. 75, 343–370.
Opresko, D. M., Nuttall, M. F., and Hickerson, E. L. (2016). Black corals of the flower garden banks national marine sanctuary. Gulf Mexico Sci. 33:5.
Opresko, D. M., and Sanchez, J. A. (2005). Caribbean shallow-water black corals (Cnidaria: Anthozoa: Antipatharia). Caribbean J. Sc. 41, 492–507.
Orr, J. W., and Blackburn, J. E. (2004). The dusky rockfishes (Teleostei: Socrpaeniformes) of the North Pacific Ocean: resurrection of Sebastes variabilis (Pallas, 1814) and a redescription of Sebastes ciliatus (Tilesius, 1813). Fish. Bull. 102, 328–348.
Orr, J. W., and Hawkins, S. (2008). Species of the rougheye rockfish complex: resurrection of Sebastes melanostictus (Matsubara, 1934) and a redescription of Sebastes aleutianus (Jordan and Evermann, 1898)(Teleostei: Scorpaeniformes). Fish. Bull. 106, 111–134.
Osorio, C. (1968). Lima (Acesta) patagonica (Dall), en Chile (Mollusca Lamellibranchiata, Limidae). Noticiario Mensual del Museo Nacional de Historia Natural, Chile 143, 3–5.
Paulin, C. D. (1989). Redescription of Helicolenus percoides (Richardson) and H. barathri (Hector) from New Zealand (Pisces, Scorpaenidae). J. Roy. Soc. New Zealand 19, 319–325. doi: 10.1080/03036758.1989.10427185
Paulin, C., Stewart, A., Roberts, C., and McMillan, P. (1989). New Zealand Fish: a Complete Guide. Wellington: Museum of New Zealand. National Museum of New Zealand Miscellaneous Series No. 19.
Pickard, G., and Stanton, B. (1980). “Pacific fjords-A review of their water characteristics,” in Proceedings of the Conference on Fjord Oceanography, Victoria, 1979. New York, NY: Plenum Press.
Pinet, P. R. (2019). “The physiography of the Ocean Floor,” in Invitation to Oceanography. Burlington, MA: Jones & Bartlett Learning.
QGIS Development Team (2012). QGIS Geographic Information System. Open Source Geospatial Foundation Project. Available online at: http://qgis.osgeo.org (accessed March 31, 2021).
Quattro, J., Chase, M., Rex, M., Greig, T., and Etter, R. (2001). Extreme mitochondrial DNA divergence within populations of the deep-sea gastropod Frigidoalvania brychia. Mar. Biol. 139, 1107–1113. doi: 10.1007/s002270100662
Quiroga, E., Sellanes, J., Arntz, W. E., Gerdes, D., Gallardo, V. A., and Hebbeln, D. (2009). Benthic megafaunal and demersal fish assemblages on the Chilean continental margin: the influence of the oxygen minimum zone on bathymetric distribution. Deep Sea Res. Part II: Top. Stud. Oceanography 56, 1112–1123. doi: 10.1016/j.dsr2.2008.09.010
R Core Team (2021). R A Language and Environment for Statistical Computing. Vienna: R Foundation for Statistical Computing.
Ramirez-Llodra, E., Brandt, A., Danovaro, R., Mol, B. D., Escobar, E., German, C. R., et al. (2010). Deep, diverse and definitely different: unique attributes of the world’s largest ecosystem. Biogeosciences 7, 2851–2899. doi: 10.5194/bg-7-2851-2010
Riehl, T., and Kaiser, S. (2012). Conquered from the deep sea? a new deep-sea isopod species from the Antarctic shelf shows pattern of recent colonization. PLoS One 7:e49354. doi: 10.1371/journal.pone.0049354
Rivadeneira, M. M., Fernández, M., and Navarrete, S. A. (2002). Latitudinal trends of species diversity in rocky intertidal herbivore assemblages: spatial scale and the relationship between local and regional species richness. Mar. Ecol. Prog. Ser. 245, 123–131. doi: 10.3354/meps245123
Roberts, C. D. (1989). A revision of New Zealand and Australian orange perches (Teleostei; Serranidae) previously referred to Lepidoperca pulchella (Waite) with description of a new species of Lepidoperca from New Zealand. J. Nat. Hist. 23, 557–589. doi: 10.1080/00222938900770321
Roberts, C. D. (2001). Survey of deepwater emergence in New Zealand fiord fishes. NZ J. Mar. Freshwater Res. 35:661.
Roberts, J. M., Wheeler, A., Freiwald, A., and Cairns, S. (2009). Cold-water Corals: the Biology and Geology of Deep-sea Coral Habitats. Cambridge: Cambridge University Press.
Rogers, A. D. (1999). The Biology of Lophelia pertusa (Linnaeus 1758) and other deep-water reef-forming corals and impacts from human activities. Int. Rev. Hydrobiol. 84, 315–406. doi: 10.1002/iroh.199900032
Rossin, A. M., Waller, R. G., and Försterra, G. (2017). Reproduction of the cold-water coral Primnoella chilensis (Philippi, 1894). Continental Shelf Res. 144, 31–37. doi: 10.1016/j.csr.2017.06.010
Russell, B. C. (1988). Revision of the labrid genus Pseudolabrus and allied genera. Rec. Aust. Mus. Suppl. 9, 1–72. doi: 10.3853/j.0812-7387.9.1988.95
Schwabe, E., and Sellanes, J. (2010). Revision of Chilean bathyal chitons (Mollusca: Polyplacophora) associated with cold-seeps, including description of a new species of Leptochiton (Leptochitonidae). Org. Divers. Evol. 10, 31–55. doi: 10.1007/s13127-009-0002-6
Sellanes, J. (2018). Base de Datos de la Sala de Colecciones Biológicas de la Universidad Católica del Norte (SCBUCN). Version 1.2. Universidad Católica del Norte. Occurrence Dataset. Available online at: https://doi.org/10.15468/d3auf9 (accessed August 6, 2021).
Sellanes, J., Neira, C., Quiroga, E., and Teixido, N. (2010). Diversity patterns along and across the Chilean margin: a continental slope encompassing oxygen gradients and methane seep benthic habitats. Mar. Ecol. 31, 111–124. doi: 10.1111/j.1439-0485.2009.00332.x
Sellanes, J., Quiroga, E., and Gallardo, V. A. (2004). First direct evidence of methane seepage and associated chemosynthetic communities in the bathyal zone off Chile. marine biological association of the United Kingdom. J. Mar. Biol. Assoc. U K 84:1065. doi: 10.1017/s0025315404010422h
Sellanes, J., Quiroga, E., and Neira, C. (2008). Megafauna community structure and trophic relationships at the recently discovered Concepción Methane Seep Area, Chile, 36 S. ICES J. Mar. Sci. 65, 1102–1111. doi: 10.1093/icesjms/fsn099
Sievers-Czischke, H. (2018). “La oceanografía en Chile: historia de un desarrollo imperativo,” in Servicio Hidrográfico y Oceanográfico de la Armada de Chile, Valparaíso, 266.
Silva, N. (2008). “Dissolved oxygen, pH, and nutrients in the austral Chilean channels and fjords,” in Progress in the Oceanographic Knowledge of Chilean Interior Waters, from Puerto Montt to Cape Horn, eds N. Silva and S. Palma (Valparaíso: Comité Oceanográfico Nacional - Pontificia Universidad Católica de Valparaíso), 37–43.
Simpson, A. W., Eckelbarger, J., and Watling, L. (2005). “Some aspects of the reproductive biology of Paramuricea placomus (Octocorallia) from the Gulf of Maine. Integr. Comp. Biol. 45,” in Proceedings of the 3rd International Symposium on Deep-sea Coral, (Miami).
Stigebrandt, A. (2012). “Hydrodynamics and Circulation of Fjords,” in Encyclopedia of Lakes and Reservoirs. Encyclopedia of Earth Sciences Series, eds L. Bengtsson, R. W. Herschy, and R. W. Fairbridge (Dordrecht: Springer), doi: 10.1007/978-1-4020-4410-6_247
Stock, J. H., and Vermeulen, J. J. (1982). A representative of the mainly abyssal family Pardaliscidae (Crustacea, Amphipoda) in cave waters of the Caicos Islands. Bijdragen tot de Dierkunde 52, 3–12. doi: 10.1163/26660644-05201002
Stone, R. P., Andrews, A., and Mondragon, J. (2005). “Deepwater emergence of red tree coral (Primnoa sp.) in Glacier Bay, Alaska,” in Proceedings of the 3rd International Symposium on Deep Water Corals, (Miami, FL).
Stone, R. P., and Mondragon, J. (2018). Deep-sea Emergence of Red Tree Corals (Primnoa pacifica) in Southeast Alaska Glacial Fjords. Washington, DC: NOAA.
Strömgren, T. (1970). Emergence of Paramuricea placomus (L.) and Primnoa resedaeformis (Gunn.) in the inner part of Trondheimsfjorden (Western coast of Norway). Det Kongelige Norske Videnskabers Selskabs Skrifter 4, 1–6.
Strömgren, T. (1971). Vertical and horizontal distribution of Lophelia pertusa (Linné) in Trondheimsfjordan on the West Coast of Norway. K. Nor. Vidensk. Selsk. Skr. 6, 1–9.
Strugnell, J. M., Cherel, Y., Cooke, I. R., Gleadall, I. G., Hochberg, F. G., Ibáñez, C. M., et al. (2011). The Southern Ocean: source and sink? Deep Sea Res. Part II: Top. Stud. Oceanography 58, 196–204.
Sutton, T. T. (2013). Vertical ecology of the pelagic ocean: classical patterns and new perspectives. J. Fish Biol. 83, 1508–1527. doi: 10.1111/jfb.12263
Taylor, M. L., Cairns, S. D., Agnew, D. J., and Rogers, A. D. (2013). A revision of the genus Thouarella Gray, 1870 (Octocorallia: Primnoidae), including an illustrated dichotomous key, a new species description, and comments on Plumarella Gray, 1870 and Dasystenella, Versluys, 1906. Zootaxa 3602, 1–105. doi: 10.11646/zootaxa.3602.1.1
Tellier, F., Meynard, A. P., Correa, J. A., Faugeron, S., and Valero, M. (2009). Phylogeographic analyses of the 30° S south-east Pacific biogeographic transition zone establish the occurrence of a sharp genetic discontinuity in the kelp Lessonia nigrescens: vicariance or parapatry? Mol. Phylogenet. Evol. 53, 679–693. doi: 10.1016/j.ympev.2009.07.030
Thiel, M., Castilla, J. C., Fernández, M., and Navarrete, S. (2007). The Humboldt current system of northern and central Chile. oceanographic processes, ecological interactions and socioeconomic feedback. Ocean. Mar. Biol. Annu. Rev. 45, 195–344. doi: 10.1201/9781420050943.ch6
Thuy, B., and Schulz, H. (2012). The oldest representative of a modern deep-sea ophiacanthid brittle-star clade from Jurassic shallow-water coral reef sediments. Acta Palaeontologica Polonica 58, 525–531.
Treude, T., Kiel, S., Linke, P., Peckmann, J., and Goedert, J. L. (2011). Elasmobranch egg capsules associated with modern and ancient cold seeps: a nursery for marine deep-water predators. Mar. Ecol. Prog. Series 437, 175–181. doi: 10.3354/meps09305
Tyberghein, L., Verbruggen, H., Pauly, K., Troupin, C., Mineur, F., and De Clerck, O. (2012). Bio-ORACLE: a global environmental dataset for marine species distribution modelling. Global Ecol. Biogeography 21, 272–281. doi: 10.1111/j.1466-8238.2011.00656.x
Vacelet, J., and Boury-Esnault, N. (1996). A new species of carnivorous sponge (Demospongiae: Cladorhizidae) from a mediterranean cave. Bull. Inst. R. Sci. Nat. Belg. 66, 109–115.
van der Ham, J. L. (2002). Addition to the description of Spelaeonicippe provo (Amphipoda, Pardaliscidae). Crustaceana 75, 1271–1274. doi: 10.1163/156854002321518199
van Ofwegen, L., Häussermann, V., and Försterra, G. (2007). The genus Alcyonium (Octocorallia: Alcyonacea: Alcyoniidae) in Chile. Zootaxa 1607, 1–19. doi: 10.11646/zootaxa.1607.1.1
Wagner, D., Brugler, M. R., Opresko, D. M., France, S. C., Montgomery, A. D., and Toonen, R. J. (2010). Using morphometrics, in situ observations and genetic characters to distinguish among commercially valuable Hawaiian black coral species; a redescription of Antipathes grandis Verrill, 1928 (Antipatharia: Antipathidae). Invert. Syst. 24, 271–290. doi: 10.1071/is10004
Waller, R. G., Stone, R. P., Mondragon, J., and Clark, C. E. (2011). “Reproduction of red tree corals in the southeastern Alaskan fjords: implications for conservation and population turnover,” in Proceedings of the American Academy of Underwater Sciences 30th Scientific Symposium, (Dauphin Island, AL), 29.
Warner, G. F. (1981). Species descriptions and ecological observations of black corals (Antipatharia) from Trinidad. Bull. Mar. Sci. 31, 147–163.
Weingartner, T., Eisner, L., Eckert, G. L., and Danielson, S. (2009). Southeast Alaska: oceanographic habitats and linkages. J. Biogeogr. 36, 387–400. doi: 10.1111/j.1365-2699.2008.01994.x
Wilkens, H., Parzefall, J., and Ribowski, A. (1990). Population biology and larvae of the anchialine crab Munidopsis polymorpha (Galatheidae) from Lanzarote (Canary Islands). J. Crust. Biol. 10, 667–675. doi: 10.2307/1548411
Wing, S. R., and Jack, L. (2013). Marine reserve networks conserve biodiversity by stabilizing communities and maintaining food web structure. Ecosphere 4, 1–14.
Wisshak, M., Freiwald, A., Lundälv, T., and Gektidis, M. (2005). “The physical niche of the bathyal Lophelia pertusa in a non-bathyal setting: environmental controls and palaeoecological implications,” in Cold-water Corals and Ecosystems, eds A. Freiwald and J. M. Roberts (Berlin: Springer).
Zapata-Hernández, G., Sellanes, J., Thurber, A. R., and Levin, L. A. (2014). Trophic structure of the bathyal benthos at an area with evidence of methane seep activity off southern Chile (45° S). J. Mar. Biol. Assoc. U K. 94, 659–669. doi: 10.1017/s0025315413001914
Keywords: Norway, British Columbia, Alaska, New Zealand, Chile, fjord region, marine invertebrates, deep-water emergence
Citation: Häussermann V, Ballyram SA, Försterra G, Cornejo C, Ibáñez CM, Sellanes J, Thomasberger A, Espinoza JP and Beaujot F (2021) Species That Fly at a Higher Game: Patterns of Deep–Water Emergence Along the Chilean Coast, Including a Global Review of the Phenomenon. Front. Mar. Sci. 8:688316. doi: 10.3389/fmars.2021.688316
Received: 30 March 2021; Accepted: 19 July 2021;
Published: 06 October 2021.
Edited by:
Marcos Rubal, University of Porto, PortugalReviewed by:
Clara F. Rodrigues, University of Aveiro, PortugalCopyright © 2021 Häussermann, Ballyram, Försterra, Cornejo, Ibáñez, Sellanes, Thomasberger, Espinoza and Beaujot. This is an open-access article distributed under the terms of the Creative Commons Attribution License (CC BY). The use, distribution or reproduction in other forums is permitted, provided the original author(s) and the copyright owner(s) are credited and that the original publication in this journal is cited, in accordance with accepted academic practice. No use, distribution or reproduction is permitted which does not comply with these terms.
*Correspondence: Vreni Häussermann, di5oYXVzc2VybWFubkBnbWFpbC5jb20=
†These authors have contributed equally to this work
Disclaimer: All claims expressed in this article are solely those of the authors and do not necessarily represent those of their affiliated organizations, or those of the publisher, the editors and the reviewers. Any product that may be evaluated in this article or claim that may be made by its manufacturer is not guaranteed or endorsed by the publisher.
Research integrity at Frontiers
Learn more about the work of our research integrity team to safeguard the quality of each article we publish.