- 1Department of Biological Oceanography, Center for Scientific Research and Higher Education of Ensenada (CICESE), Ensenada, Mexico
- 2Department of Biological Sciences, California State University, Long Beach, CA, United States
- 3Monterey Bay Aquarium, Monterey, CA, United States
Knowledge about top predators’ trophic ecology is crucial for defining their role in ecosystems, understanding habitat preferences, characterizing life stage-specific feeding habits, and evaluating their interaction with fisheries. In the northeastern Pacific, white sharks (Carcharodon carcharias) occupy coastal habitats during the early life stages, including Bahía Sebastián Vizcaíno (BSV) in Mexico, which is a known nursery area. Although BSV presumably provides high prey abundance, the trophic ecology of immature white sharks is poorly understood. Carbon and nitrogen bulk stable isotope analyses (SIA) were used to explore the trophic relationship of early life stages with their potential prey and to infer dietary overlap with sympatric sharks, while SIA of amino acids were used to estimate trophic position. Muscle samples from young white sharks and inshore demersal prey commonly found in their stomach contents were sampled. Demersal prey and literature-derived isotope ratios for pelagic and offshore species were incorporated into mixing models with a Bayesian framework to estimate their contribution to white shark tissues. Nearshore demersal prey had the highest contribution for all life stages (between 35 and 47%), consistent with previous reports based on gut content analysis. The contribution of pelagic (between 26 and 37%) and offshore (between 14 and 32%) prey was smaller and suggests potential periodic changes in foraging grounds and the presence of a maternal-derived isotopic signature. A high contribution of demersal prey indicates a high level of interaction with local fisheries that target those species and catch white sharks incidentally and is consistent with immature shark movement patterns. Isotope ratios of two sympatric sharks, smooth hammerhead Sphyrna zygaena and copper sharks Carcharhinus brachyurus, were used to estimate the overlap in isotopic niche space. Immature white sharks had the smallest isotopic niche, while the highest was for copper sharks. Overlap was greatest between white sharks and hammerheads (∼45%), while overlap with copper sharks was limited (<20%), suggesting less potential for competition. Trophic position estimates were similar to those previously reported for the species. These results highlight the importance of coastal demersal prey heavily targeted by local fisheries for the diet of young white sharks and support the importance of BSV as a nursery habitat.
Introduction
Most shark species are considered top predators occupying high trophic levels and their presence or absence influences food web structure (Cortés, 1999; Ferretti et al., 2010; Heupel et al., 2014). Some species are highly migratory and move between coastal and pelagic habitats, that are used throughout ontogeny for nursery, feeding, mating, or parturition (Heupel et al., 2007; Block et al., 2011; Domeier and Nasby-Lucas, 2013; Carlisle et al., 2015a; Nasby-Lucas et al., 2019; Nosal et al., 2019). Ontogenetic habitat changes are commonly accompanied by switches in their diet (Malpica-Cruz et al., 2013; Carlisle et al., 2015b; Estupiñán-Montaño et al., 2019; Matich et al., 2019). So, characterizing the trophic relationships of sharks throughout their life cycle is needed for understanding their ecological role in each ecosystem they inhabit.
Adult white sharks (Carcharodon carcharias) are distributed mostly in offshore pelagic waters, including oceanic islands (Klimley et al., 1992; Domeier and Nasby-Lucas, 2007; Jorgensen et al., 2010; Francis et al., 2015; Boldrocchi et al., 2017; Skomal et al., 2017; Moro et al., 2020), while juveniles occupy coastal areas as nurseries (Bruce and Bradford, 2012; Harasti et al., 2017; Curtis et al., 2018; Bruce et al., 2019). In the northeast Pacific, newborn, young of the year, and juvenile white shark occupy coastal areas until they become sub-adults and can migrate between spatially separate nurseries (Klimley et al., 1992; Dewar et al., 2004; Weng et al., 2007, 2012; Lowe et al., 2012; Oñate-González et al., 2017; White et al., 2019). Coastal nurseries harbor high food abundance and provide shelter from predators, allowing young white sharks to grow faster, which is crucial for their juvenile survival and recruitment to adult population growth (Mollet and Cailliet, 2002; Heupel et al., 2007).
The white shark is a top predator that exhibits ontogenetic changes in its feeding habits and habitat use (Estrada et al., 2006; Carlisle et al., 2012; Kim et al., 2012; French et al., 2018). Whereas the feeding preferences of adults have been more widely studied (Estrada et al., 2006; Hussey et al., 2012), dietary information for the early life stages is more limited and suggest a more benthic-oriented foraging (Santana-Morales et al., 2012; Grainger et al., 2020). Juveniles shift their diet from feeding on smaller bony fishes and elasmobranchs (mainly rays) to larger fishes as they grow. After becoming sub-adults, white sharks start to expand their diet to include marine mammals (Tricas and McCosker, 1984; Estrada et al., 2006; Hussey et al., 2012; Malpica-Cruz et al., 2013). In the northeast Pacific, adult white sharks spend between six and eight months of the year in offshore areas (Domeier and Nasby-Lucas, 2008; Jorgensen et al., 2010) where their feeding habits are poorly understood.
Bahía Sebastián Vizcaíno (BSV) bay is located on the western coast of the Baja California Peninsula, Mexico. BSV is a natal nursery area for white sharks born locally as well as young sharks born in the Southern California Bight, United States, that migrate south covering approximately 600 km (Oñate-González et al., 2017; White et al., 2019). In Mexican waters, the species has been under protection since 2007 and the targeted catch is prohibited (DOF, 2007, 2010, 2014). However, they are caught incidentally in the nearshore areas of BSV as well as within Ojo de Liebre Lagoon (OLL), a semi-enclosed hypersaline shallow estuary located within the bay system (García-Rodríguez and Sosa-Nishizaki, 2020). These catches occur when local artisanal fishers target high-value species that are part of the white shark’s diet (Santana-Morales et al., 2012; García-Rodríguez and Sosa-Nishizaki, 2020).
In addition to the direct mortality caused by incidental catches, the potential competition between artisanal fisheries and young white sharks (and other shark species) for prey could have a negative effect on regional conservation efforts. For top predators like turtles, birds, and marine mammals, it has been well documented that competition could have adverse effects on their abundance by limiting prey’s spatial availability (Huss et al., 2014; Hilborn et al., 2017; Hansson et al., 2018). For example, a switch in the diet resulting from prey overfishing has been reported for the spiny dogfish Squalus acanthias (Koen Alonso et al., 2002). Also, high overlap between target fishery species and shark prey has been documented for the spiny dogfish (Lucifora et al., 2009). In sand tiger sharks (Carcharias taurus), spatial overlap between prey and fisheries increased their vulnerability to overfishing (Gračan et al., 2017). Changes in prey availability due to spatial overlap with fisheries in nursery areas could decrease the benefits these provide for the early life stages of sharks, potentially changing their diets, limiting their growth, and affecting juvenile survival.
Limitation in prey availability due to fisheries could increase the competition between shark species with similar dietary preferences. Interspecific competition between young white sharks and sympatric shark species that inhabit BSV, like smooth hammerheads (Sphyrna zygaena) or Carcharhinids, is possible. Previous studies have reported resource partitioning in communal areas to reduce interspecific competition, which could be associated with changes in the distribution and habitat use of sympatric shark species with high dietary overlap (Bethea et al., 2004; Papastamatiou et al., 2006; Kinney et al., 2011). The description of the trophic relationships between immature white sharks, their prey, and the level of dietary overlap with sympatric species that may compete for feeding resources lend insight into their ecological role in the nursery habitat provided by BSV.
Quantitative diet composition estimates are based on stomach content analysis, providing a basis for understanding a species’ prey spectrum and its overall trophic ecology, including trophic level (Cortés, 1999; Bizzarro et al., 2017). While it can provide a snapshot of what a shark has eaten most recently, stomach content analysis has several constraints, including that it is labor-intensive and highly invasive or lethal, soft-bodied prey can be under-represented in the analysis due to the digestive process, and the results can have limited spatial resolution unless extensive sampling efforts are implemented. It can, however, provide a snapshot of what a shark has eaten most recently. This approach often requires a very high sample size to quantify diet variation across a population and still can lead to a limited interpretation of their trophic ecology (Hussey et al., 2012; Munroe et al., 2018).
Bulk stable isotope analysis (SIA) of soft tissues provides information that complements that obtained through stomach content analysis by providing integrated spatial-temporal insight into trophic relationships and energy fluxes in marine ecosystems (Michener and Kaufman, 2007; Boecklen et al., 2011). Isotopic ratios can also be used to characterize the isotopic niche, which is reflective of a consumer’s trophic niche and can be used to estimate the overlap in prey resources between populations, life stages, or species (Jackson et al., 2011; Hette-Tronquart, 2019; Marshall et al., 2019).
Isotope turnover rates should be considered when interpreting SIA ratios in sharks (Kim et al., 2012; Malpica-Cruz et al., 2012). Soft, metabolically active tissues reflect the isotopic composition of the prey consumed over time; tissues with higher isotope turnover rates (i.e., blood) reflect a shorter feeding period than those with lower turnover rates (i.e., muscle) (Logan and Lutcavage, 2010; Hussey et al., 2012; Malpica-Cruz et al., 2012). Isotopic equilibrium between the isotopic composition of muscle tissue in sharks is reached after several months or even years, with younger, faster growing individuals reaching equilibrium faster, after about a threefold biomass gain (Logan and Lutcavage, 2010; Kim et al., 2012; Malpica-Cruz et al., 2012).δ13C values provide information regarding the dominant sources of primary production, which vary among habitat types, for example between benthic and pelagic food webs. Thus, they can be used to trace shark habitat shifts, particularly between coastal and oceanic ecosystems, as well as to distinguish between benthic vs. pelagic feeding (Finlay and Kendall, 2007; Munroe et al., 2018). Due to a consistent enrichment in 15N between a consumer and its assimilated diet, δ15N values have been used to characterize ontogenetic shifts in trophic level (Peterson and Fry, 1987; Post, 2002). Given prior knowledge regarding a consumer’s feeding habits and adequate characterization of the spatial and temporal variability in the isotopic baseline (Phillips et al., 2014), SIA are also useful for estimating the contribution of different sources to a consumer’s diet (Parnell et al., 2013). However, characterization of the isotopic baseline can be challenging, particularly for highly migratory species.
Recently, the use of compound-specific stable isotope analysis of amino acids (CSIA-AA) has been proposed as a complementary method to bulk SIA. “Source” AAs do not exhibit a substantial enrichment in 15N between consumers and their foods, and thus reflect the isotopic baseline, while “trophic” AA exhibit a high and consistent enrichment that is conductive to estimate trophic level (Chikaraishi et al., 2009; O’Connell, 2017). CSIA-AA are advantageous because a single tissue contains both kinds of AA, allowing for the calculation of trophic position and inferring of foraging patterns without the need for an independent characterization of an isotopic baseline (Chikaraishi et al., 2010; Hoen et al., 2014; Munroe et al., 2018). The canonical source and trophic AAs are phenylalanine (Phe) and glutamic acid (Glu), respectively (McMahon and McCarthy, 2016).
To understand the trophic ecology of the early life stages of white sharks in the BSV-OLL nursery, we used bulk SIA of shark muscle and putative prey items to estimate the contribution of different prey groups to their diet and assess ontogenetic dietary shifts related to movements between habitats. CSIA of the canonical source and trophic AA were used to estimate trophic level. We also evaluate the level of dietary overlap with sympatric shark species that feed within the system, which provides insight into the potential for competition with local fisheries and sympatric species for local prey. The study provides information that can improve the environmentally sound management and conservation strategies in the area.
Methods
Sample Collection
Muscle samples (1 cm3) from the dorsal area of white sharks were collected between 2013 to 2017, either within BSV or off the northwestern coast of the Baja California peninsula, termed outside of BSV (OBSV) hereafter (Figure 1, Table 1 and Supplementary Material). Samples were obtained opportunistically from white sharks caught incidentally by artisanal fisheries and from individuals tagged within BSV. Artisanal fisheries operate in coastal areas, so incidental catches of white sharks occur near landing sites (Figure 1). Individuals tagged within BSV were sampled along the southern coast of the Bay and near the mouth of the Ojo de Liebre lagoon (Figure 1). For all sharks sampled inside BSV, total length (TL) and sex were recorded. Based on their size, sharks were divided into three life stages: newborn (NB; less than 150 cm TL), young of the year (YOY; between 151 and 175 cm TL), and juveniles (JUV; larger than 175 cm TL). Although sharks sampled OBSV were not measured because local fishers provided samples, they estimated their size >200 cm TL and were therefore categorized as juveniles.
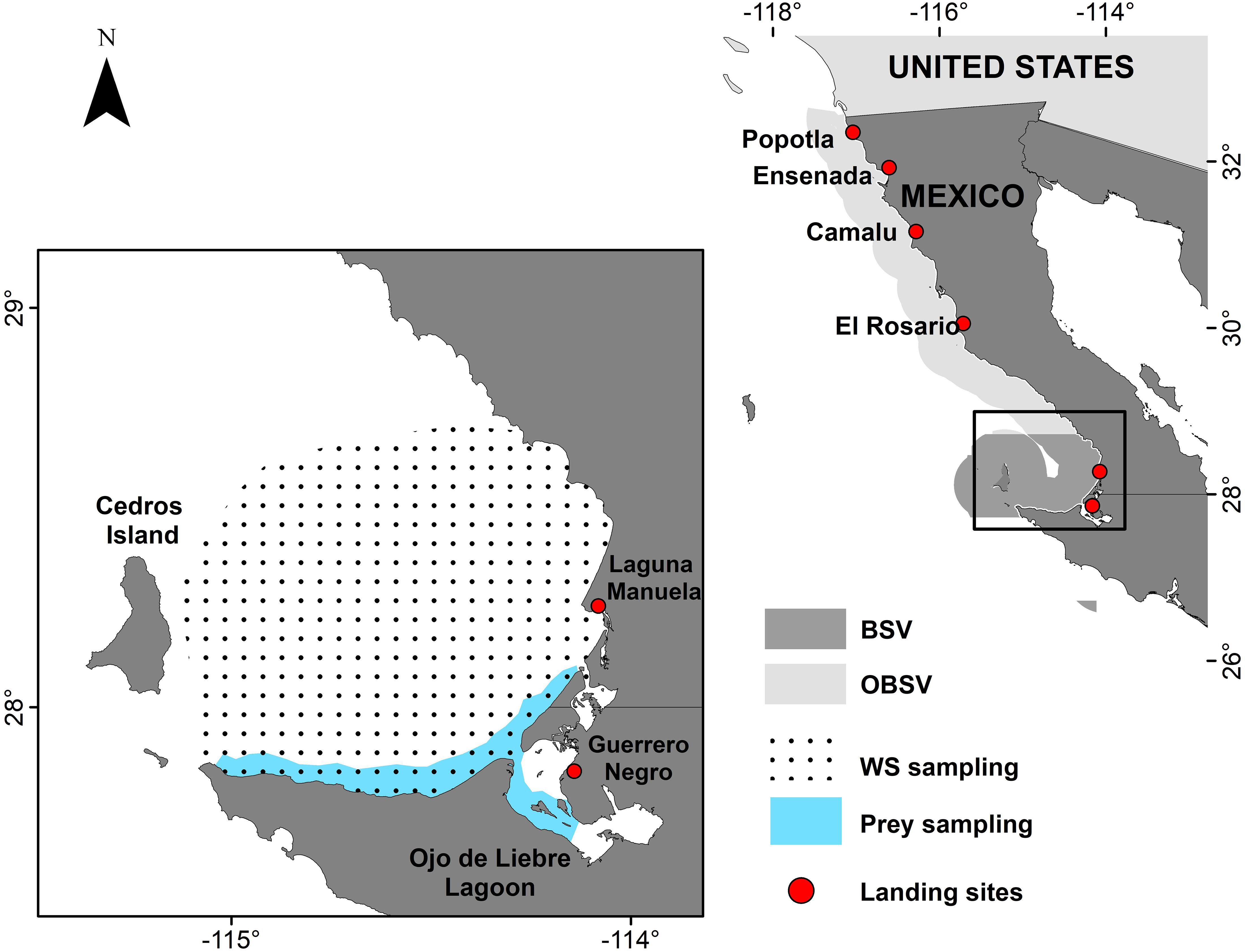
Figure 1. Areas of the Baja California peninsula where white shark muscle samples were collected. BSV, Bahía Sebastián Vizcaíno; OBSV, Outside Bahía Sebastián Vizcaíno. Red points indicate landing sites where white sharks incidentally caught were sampled. Pointed area refers to the places where white sharks were collected inside BSV. The blue area indicates regions where prey inside BSV were collected. Prey samples from Tamburin et al. (2019, 2020) where collected in the pointed area.
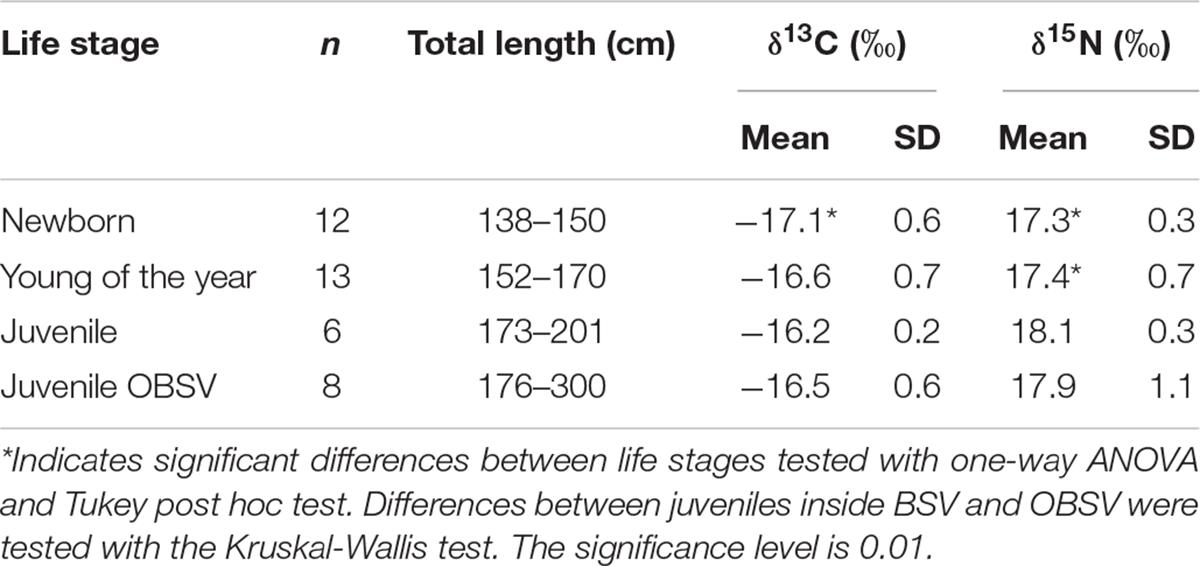
Table 1. Isotopic values for different life stages of immature white sharks collected in Bahía Sebastián Vizcaíno and the northern part of the Baja California peninsula (OBSV: Outside Bahía Sebastián Vizcaíno).
Based on the analysis of small white sharks captured incidentally in BSV, Malpica-Cruz et al. (2013) inferred that blood and muscle tissues were in isotopic equilibrium with local prey given the similarity in isotope ratios between tissues. Our interpretation of the stable isotope ratios of immature white sharks sampled in BSV is based on this assumption. Larger sharks sampled outside BSV move along the west coast of the Baja California peninsula (Weng et al., 2012), and may exhibit a different isotopic composition than those from BSV, reflecting distinct food sources or isotopic baselines.
Muscle tissue (1 cm3) from demersal fish and rays (n = 72) previously reported as prey for juvenile white sharks in BSV by Santana-Morales et al. (2012) were collected from fishing activities during the summer of 2016. Potential prey were caught near the mouth of OLL and along BSVs southern coast (Figure 1), and included white seabass (Atractoscion nobilis), California halibut (Paralichthys californicus), California bat ray (Myliobatis californica), California butterfly ray (Gymnura marmorata), diamond stingray (Hypanus dipterururs), and shovelnose guitarfish (Pseudobatos productus) (Table 2). Shovelnose guitarfish was selected because it is the most abundant demersal elasmobranch in the area, as well as potential prey for white sharks. Muscle tissue from smooth hammerheads (S. zygaena) and copper sharks (Carcharhinus brachyurus) was also collected opportunistically in BSV during the summer of 2016 to examine the level of niche overlap with white sharks.
Sample Preparation for Bulk Isotope and CSIA-AA Analysis
All samples were lipid and urea extracted (white seabass and halibut were only lipid extracted) as previously recommended for bulk SIA (Li et al., 2016; Carlisle et al., 2017) by following the methods of Kim and Koch (2012) and Carlisle et al. (2017). Briefly, tissue samples were soaked in petroleum ether and mixed with a vortex mixer. After letting the samples dry uncapped for 8 h, they were centrifuged for 10 min, rinsed in 10 ml of DIW and mixed again. Samples were then centrifuged after 30 min and were dried in an oven at 60°C for 24 h. Subsequently, 0.8–1.2 mg were ground to a fine powder and placed in tin capsules for shipment to the Stable Isotope Facility at the University of California Davis (SIA-UCD) using a PDZ Europa ANCA-GSL elemental analyzer interfaced to a PDZ Europa 20-20 isotope ratio mass spectrometer (Sercon Ltd., Cheshire, United Kingdom). Analytical precision for laboratory internal standards, bovine liver, glutamic acid, enriched alanine and nylon 6, was 0.06, 0.06, 0.06, and 0.05‰ for δ15N, respectively, and 0.06, 0.10, 0.07, and 0.08‰ for δ13C, respectively.
For CSIA-AA analysis, white shark samples were dried in an oven for 24 h at 60° as recommended by Hoen et al. (2014). Between 7 and 10 mg of ground dry sample was weighed and stored in pre-combusted 5 ml glass vials. Samples were analyzed at SIF-UCD with a Trace Ultra GC gas chromatography coupled to Thermo Delta V Plus through a GC IsoLink, following protocols described by Yarnes and Herszage (2017). Each sample was analyzed in triplicate. Precision for Phe and Glu were 0.30 and 0.40‰ respectively.
Stable isotope values are expressed in standard delta notation (δ). For δ15N values, atmospheric nitrogen was used as a standard, while the Vienna Pee Dee Belemnite (VPDB) standard was used for δ13C. Units are in parts per thousand (‰):
where R is the isotope ratio (15N:14N or 13C:12C).
Differences in mean δ15N and δ13C between life stages (NB vs. YOY vs. Juveniles) were tested using one-way ANOVA analysis with a Tukey post-hoc test, the data met the assumptions of normality and homogeneity of variances Differences in isotopic composition of juvenile white sharks sampled inside and outside of BSV were examined using a Kruskal-Wallis test because the assumption of normality was not met, as determined with a Shapiro-Wilk test.
Trophic Relationships Between White Sharks and Potential Prey
To estimate the relative contribution of potential prey caught inside BSV to the diet of immature white sharks sampled within the system, prey were categorized into three groups of species depending on their predominant habitat: demersal, pelagic, and offshore. The potential prey sampled in this study (see Section “Sample Collection”) were categorized to a demersal habitat (Table 2). All these species are caught with bottom-set gillnets in BSV, supporting their use of demersal habitats in the region (García-Rodríguez and Sosa-Nishizaki, 2020). In addition, the stable isotope ratios of the six species were very similar, and thus similar prey were grouped for mixing models, as recommended by Parnell et al. (2013) and Phillips et al. (2014). For the pelagic and offshore habitats, we used the δ13C and δ15N values published by Tamburin et al. (2019, 2020) for potential prey of immature white sharks sampled in the BSV region (Table 2). White shark samples from Tamburin et al. (2019, 2020) were collected between 2015 and 2017, and we assume their prey samples were also collected during those years. Overall means ± standard deviation (SD) for each habitat category were used as inputs for the isotope mixing models.
Bayesian mixing models (Parnell et al., 2013) were used to estimate the contribution of each prey category to the diet of immature white sharks. Analyses were performed using the simmr package (Parnell, 2016) in R. Models were run with uninformed priors, four Markov Chain Monte Carlo (MCMC) chains, with 1,000 burn-in and 10,000 iterations. We used the empirically derived trophic enrichment factors (TEF) reported for shark muscle estimated in controlled feeding experiments by Kim et al. (2012) and Malpica-Cruz et al. (2012). TEF values from those studies were averaged for their use in mixing models (Δδ13C = 1.3 ± 0.5‰; Δδ15N = 3.0 ± 0.9‰). Prey contributions were estimated separately for each life stage (newborns, young of the year, and juveniles). Results are reported as the percent contribution of each prey group with 97.5% credibility intervals.
Isotopic Niche Overlap With Sympatric Species
The isotopic niche was estimated as the standard ellipse area (SEAc; Jackson et al., 2011). Smooth hammerheads were categorized based on their size (Table 3). Size categories were selected to match the sizes of the immature white sharks: newborns (60–85 cm TL), small juvenile (103–147 cm TL) that have similar sizes to newborn white sharks, and large juveniles (151–183 cm TL), which overlap with YOY white sharks. The isotopic niche overlap between the life stages of white sharks (newborn, YOY, and juvenile caught inside and outside BSV) and between sympatric shark species was estimated using the SIBER package in R.
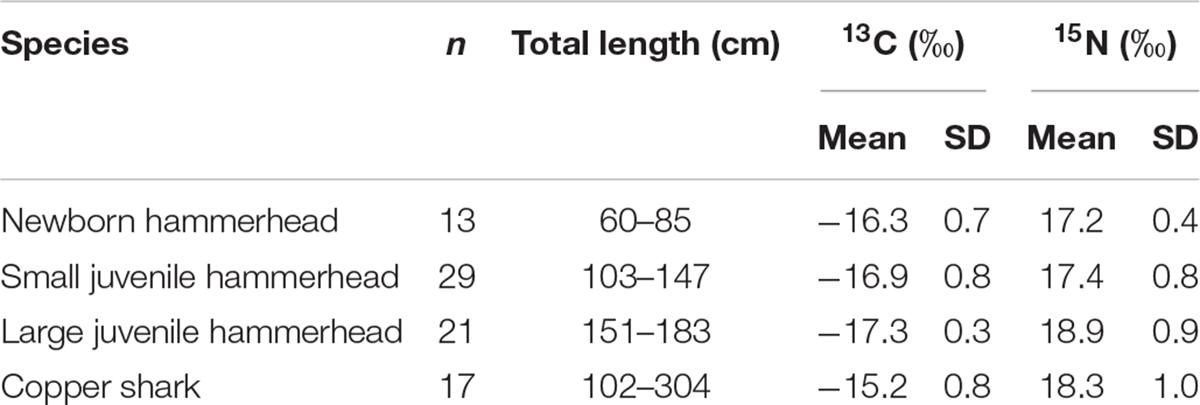
Table 3. Mean isotopic values for size classes of hammerheads and copper sharks on Bahía Sebastián Vizcaíno.
Estimates of Trophic Position
Trophic position was estimated using CSIA-AA and the equation proposed by Hoen et al. (2014). This equation uses a trophic enrichment factor (TEF, the trophic discrimination per trophic level between trophic and source AA calculated based on the isotope discrimination of Glu and Phe) that accounts for differences in AA isotope discrimination between grazers and carnivores, which is relevant for high trophic level sharks:
Where δ15Nglu (the canonical trophic AA) and δ15Nphe (the canonical source AA) are the isotopic compositions of Glu and Phe in the consumer tissues. β is the difference between the δ15N values of trophic and source AAs in primary producers (3.4 ± 0.9‰, Chikaraishi et al., 2009), TEFherbivore is equal to 7.6‰ and the TEFcarnivore is equal to 2.8‰, as reported for sharks in controlled experiments (Hoen et al., 2014).
Results
Muscle tissue samples from 31 immature white sharks were collected inside BSV. Based on their size, 12 were categorized as newborns, 13 as YOY, and six as juveniles. Eight additional samples were collected from juveniles outside BSV. Isotope ratios for white sharks sampled in BSV ranged from −17.8 to −15.5‰ for δ13C (−16.7 ± 0.6‰ mean ± SD) and from 16.4 to 18.7‰ for δ15N (17.5 ± 0.6‰). Muscle samples from YOY and juveniles had slightly higher δ13C (0.5 and 0.8‰) and δ15N values (0.2 and 0.8‰) compared with those of NB (Figure 2 and Table 1). There were significant differences in mean δ15N values between NB and YOY compared with juveniles (one-way ANOVA, p = 0.02, and p = 0.03) but not between NB and YOY (p = 0.91). There were significant differences in mean δ13C values between NB and the other life stages (p = 0.01). We did not find significant differences in the mean C or N isotopic composition for juveniles caught within and outside BSV (Kruskal-Wallis, p = 0.2 and p = 0.7, respectively).
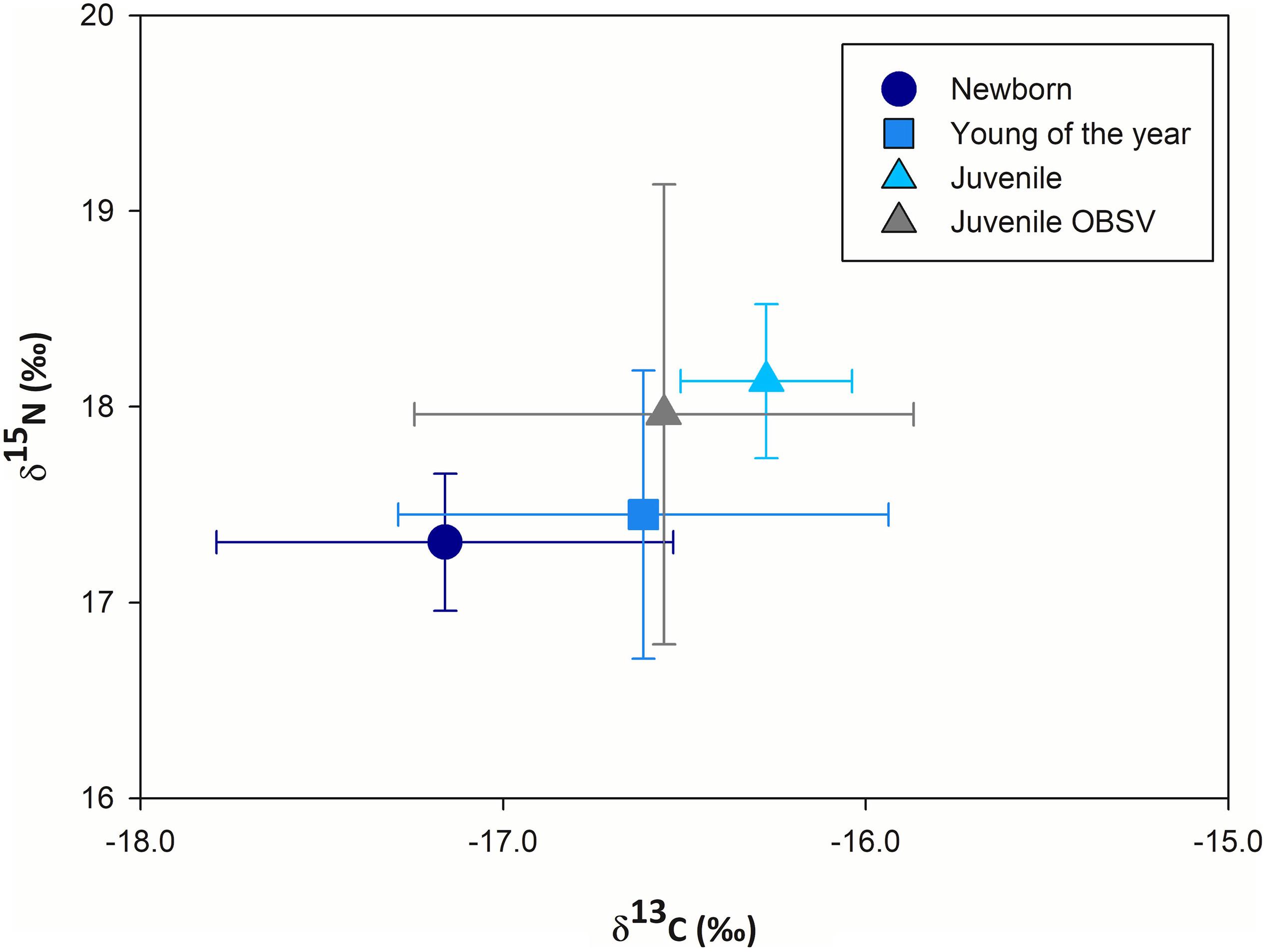
Figure 2. Mean carbon and nitrogen stable isotope ratios (±SD) measured in the muscle tissues of immature white sharks sampled in Bahía Sebastián Vizcaíno and the northern part of the Baja California peninsula. Isotopic values are reported as a function of life stage: newborn (<150 cm TL), young of the year (between 151 and 170 cm TL), and juveniles (<171 cm TL). TL, total length.
The overall variability in prey from the demersal habitat was small (SD = 0.8‰ for both δ15N and δ13C); Table 2). Muscle tissue from demersal prey showed intraspecific variability in δ15N and δ13C values. White seabass and diamond stingray had higher δ15N values (0.3 and 2.9‰ more enriched in 15N) than the other demersal species sampled (Table 2). The mean isotopic composition of the prey from the demersal habitat was enriched in 13C (0.8‰) compared with those from the pelagic habitat. However, prey from the pelagic habitat were slightly enriched in 15N compared with demersal prey (0.2‰). Compared to prey from the offshore habitat, demersal species were enriched in both 13C and 15N (2.46 and 3.75‰; Table 2).
Bayesian mixing models indicate that demersal prey species provide the highest percentage contribution to muscle in NB (35.2%; CI: 7.0–64.7%), YOY (47.1%; CI: 10.1–75.15%), and juvenile white sharks (47.5%; CI: 10.8–81.0%) (Figure 3), followed by prey from offshore habitat in NB (32.6%; CI: 5.9–62.6%) and YOY (26.7%; CI: 9.6–41.9%) and by the pelagic habitat in juveniles (37.8%; CI: 6.7–75.3%).
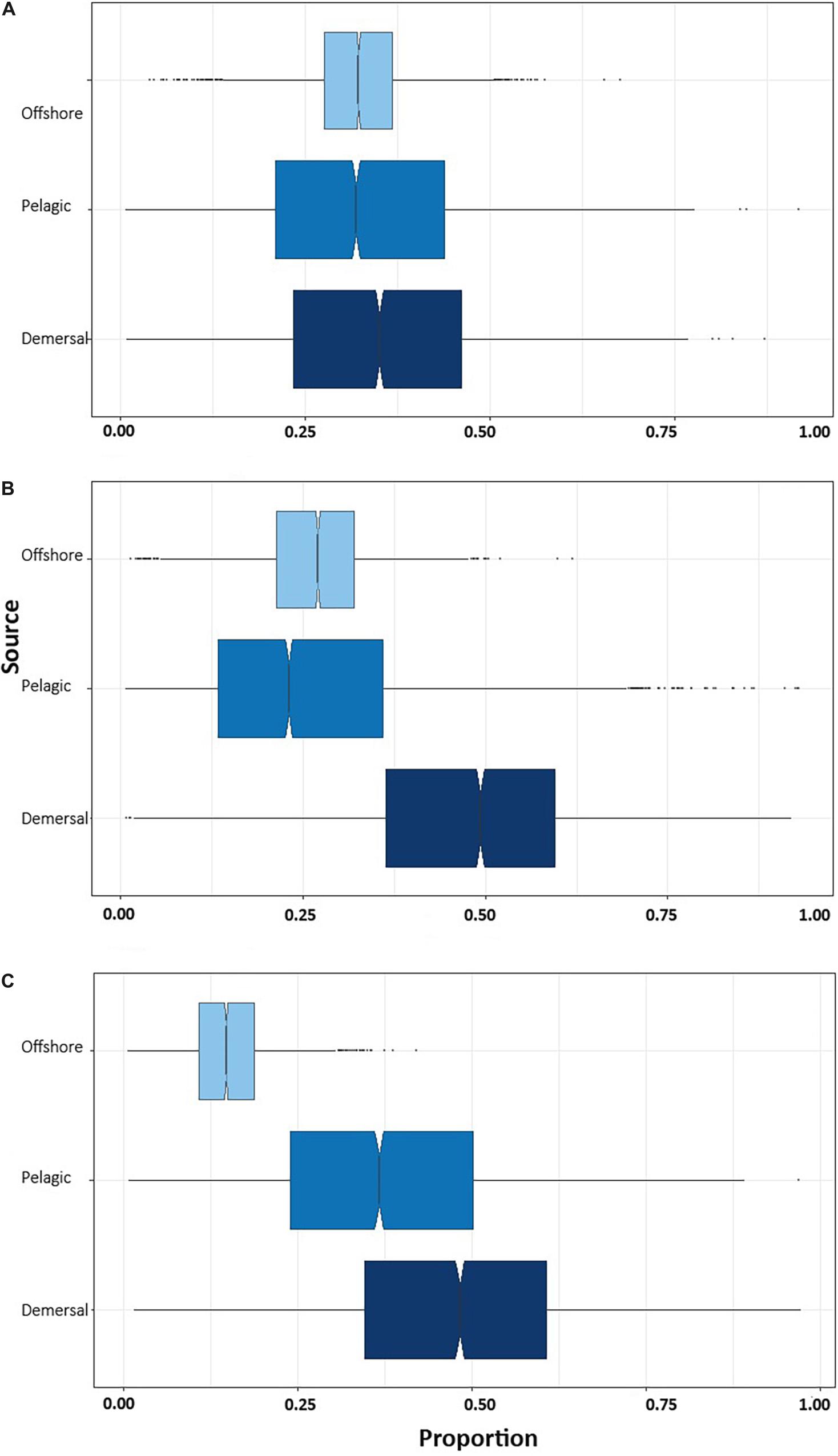
Figure 3. Contribution of prey from different habitats to the isotopic composition of the muscle tissue of immature white sharks sampled in Bahía Sebastián Vizcaíno. (A) Newborn, (B) young of the year (C) juvenile white sharks.
Isotopic niche analysis showed that juveniles from OBSV had the broadest isotopic niche (SEAc = 2.45) of all white shark groups, followed by BSV YOY (SEAc = 1.51), NB (SEAc = 0.65), and juveniles (SEAc = 0.38; Figure 4). The highest overlap between isotopic niches was between YOY and juveniles OBSV (57.4%), while juveniles sampled in BSV had the smallest overlap with the other groups (<25%) (Figure 4).
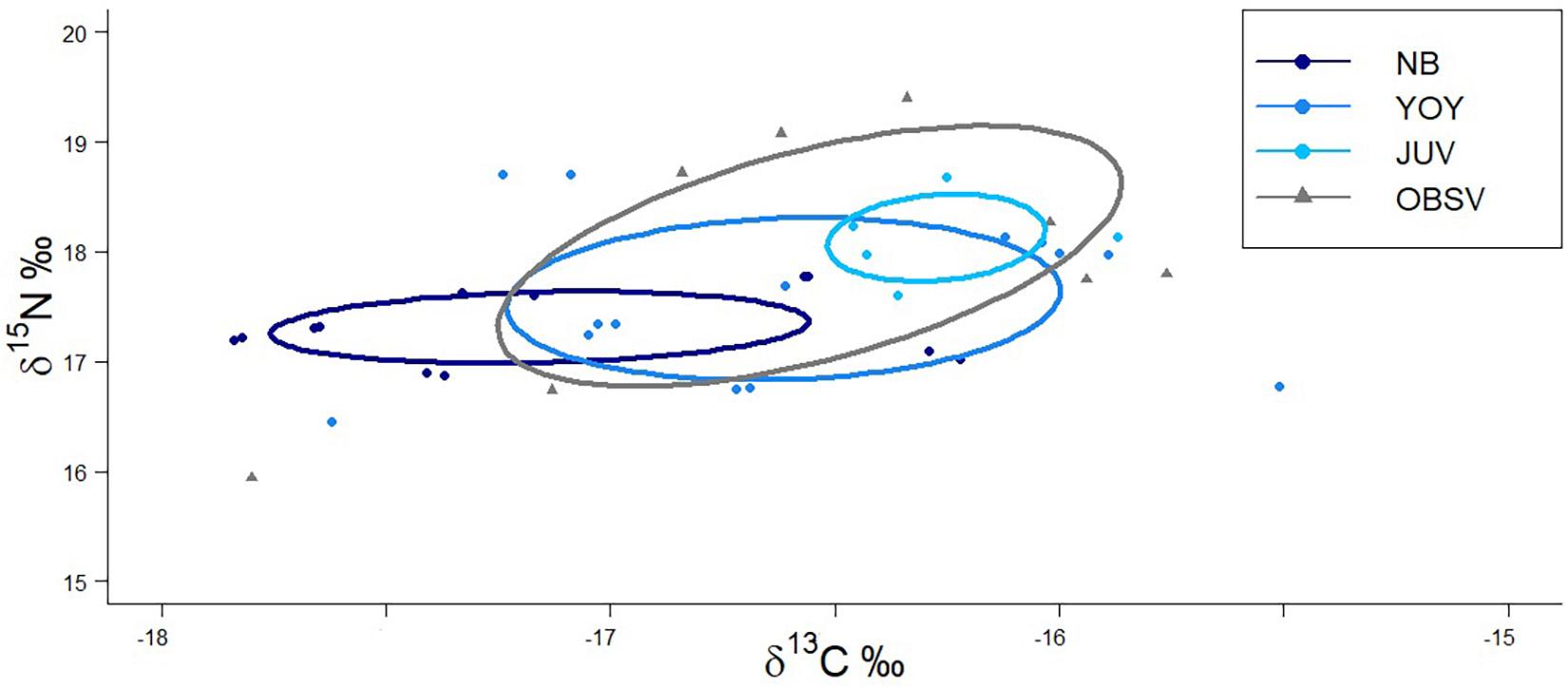
Figure 4. Standardized ellipse of isotopic niches of immature white sharks sampled along the western coast of the Baja California peninsula. NB, newborn; YOY, young of the year; JUV, juvenile collected within Bahía Sebastián Vizcaíno; OBSV, juveniles outside Bahía Sebastián Vizcaíno. Points indicate individual values from immature white sharks sampled.
Regarding sympatric shark species, in smooth hammerheads, δ15N values ranged from 14.9 to 20.1‰ and δ13C values from −14.2 to −18.0‰ (Table 3). Mean δ15N values from larger hammerheads were higher compared with conspecific newborns (1.7‰) and small juveniles (1.5‰). For δ13C, mean values from larger hammerheads were slightly lower than for the other groups (1.0‰ and 0.4‰) (Figure 5 and Table 3). For copper sharks, δ15N values ranged from 16.5 to 20.4‰ and δ13C between −17.1 to −14.4‰ (Table 3). Muscle tissue from copper sharks had higher δ13C values compared with hammerheads (by 1.1 to 2.1‰). For δ15N values, copper sharks also had higher values, except for large hammerheads that were slightly higher (0.6‰) than copper sharks.
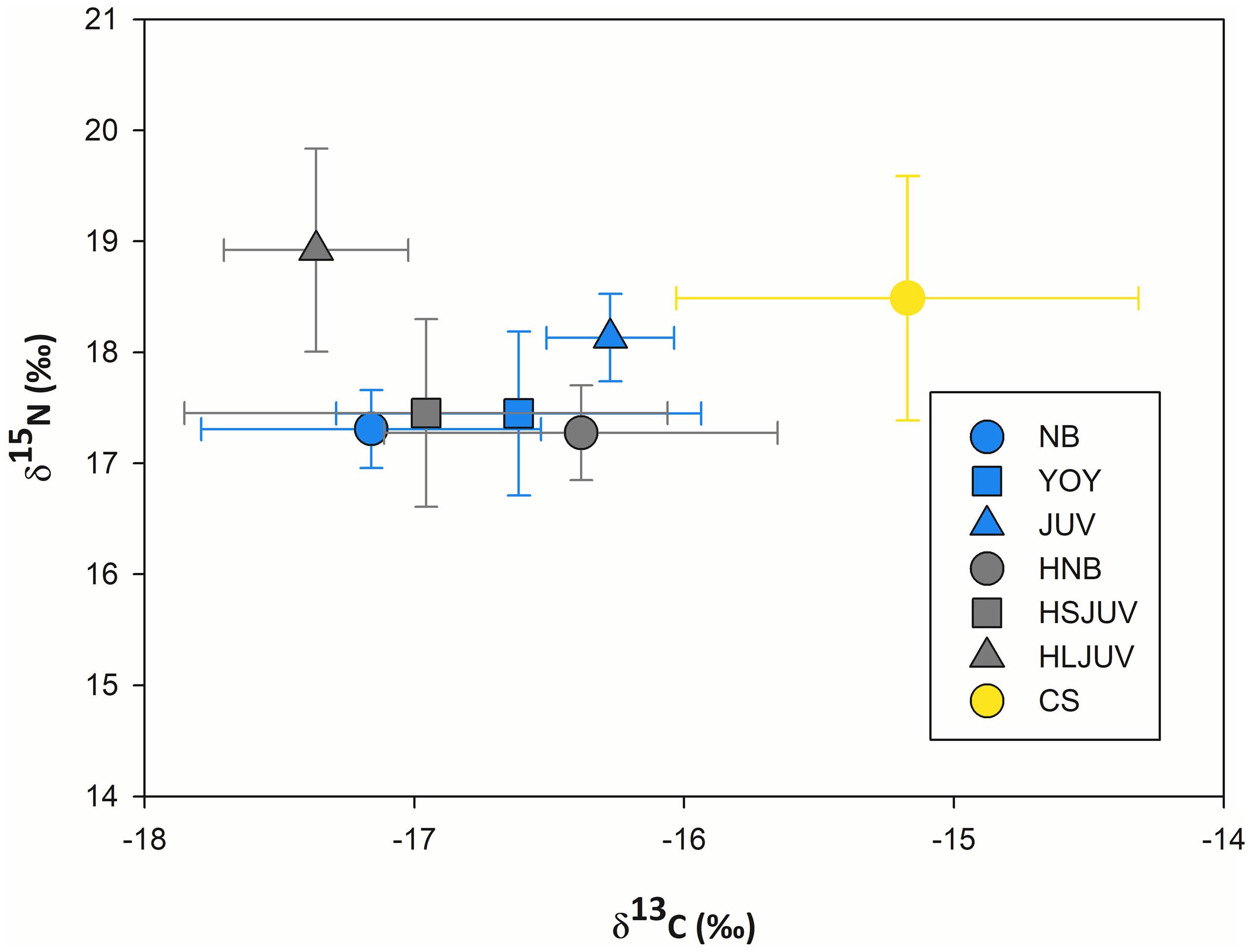
Figure 5. Mean carbon and nitrogen stable isotope ratios (±SD) measured in the muscle of immature white sharks, smooth hammerheads and copper sharks from Bahía Sebastián Vizcaíno and the northern part of the Baja California peninsula. NB = newborn white sharks, YOY = young of the year white sharks, JUV = juvenile white sharks, HNB = newborn hammerheads, HSJUV = small juvenile hammerheads, HLJUV = large juvenile hammerheads and CS = copper shark.
SIBER results showed that immature white sharks had the smallest isotopic niche of all sympatric shark species, while copper sharks had the largest. The overlap between immature white sharks and hammerheads was higher (45.27%) than with copper sharks (15.92%). A higher overlap was found when comparing life stages. The most extensive overlap was estimated between YOY and newborn hammerheads (55.17%), followed by the overlap between YOY and small juvenile hammerheads (54.69%) and the overlap between NB and newborn hammerheads (48.48%). The overlap with copper sharks was higher for YOY (27.55%) than for NB and juveniles from BSV (8.92 and 12.03%, respectively) (Figure 6).
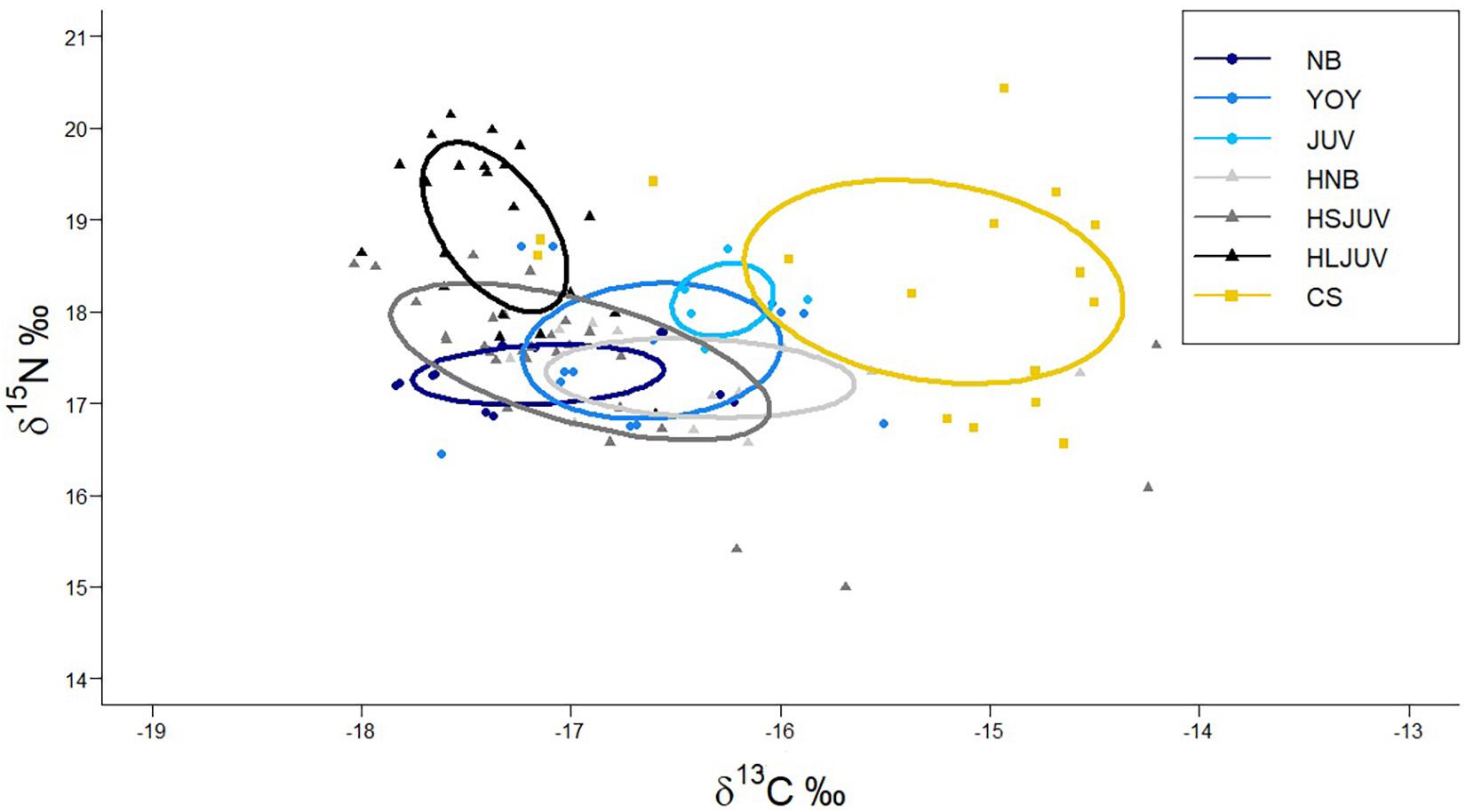
Figure 6. Isotopic niches of different life stages of different size classes of immature white sharks, smooth hammerhead sharks and copper sharks sampled in Bahía Sebastián Vizcaíno. NB, newborn white sharks; YOY, young of the year white sharks; JUV, juvenile white sharks; HNB, newborn hammerheads; HSJUV, small juvenile hammerheads; HLJUV, large juvenile hammerheads; CS, copper shark.
Ten sharks were sampled for CSIA-AA of δ15N inside (n = 7) and outside (n = 3) of BSV. Glutamic acid values ranged from 25.5 to 31.0‰, and phenylalanine ranged from 9.2 to 12.8‰ (Table 4). The trophic position estimated was higher for sharks outside BSV (5.3) than for the ones sampled within the Bay (4.3; Table 4).
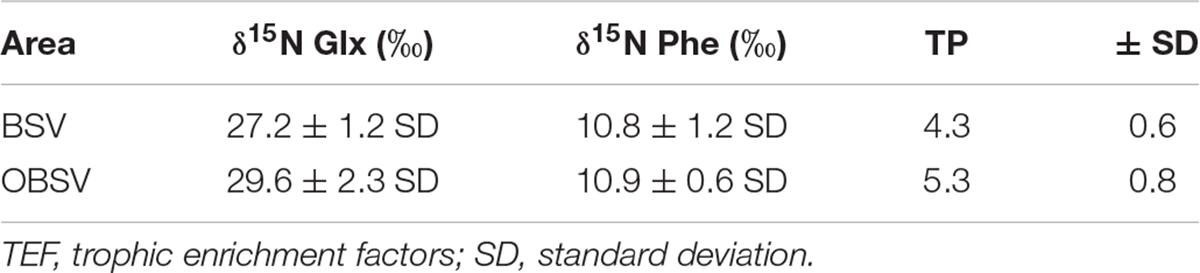
Table 4. Trophic position (TP) for immature white sharks from Bahía Sebastián Vizcaíno and the northern part of the Baja California peninsula.
Discussion
Differences in δ15N values found between immature white shark life stages indicate a size-based shift in feeding, and the and δ13C values of NB likely reflect the maternal contribution. In the northeastern Pacific, adult white sharks are known to feed in offshore areas, including oceanic islands like Guadalupe island (Jorgensen et al., 2010; Hoyos-Padilla et al., 2016), where the isotopic baseline is depleted in 13C relative to coastal and benthic habitats (Carlisle et al., 2012; Malpica-Cruz et al., 2013). Pregnant females move from these offshore areas to parturition grounds in coastal areas in Mexico and the US (Domeier and Nasby-Lucas, 2013; Oñate-González et al., 2015), which are enriched in 13C due to the predominance of benthic production (Carlisle et al., 2012; Allen et al., 2013; Tamburin et al., 2020) and higher δ13C phytoplankton in coastal areas compared to offshore waters (Vokhshoori et al., 2014). Our results show that newborn shark muscle tissue was depleted in 13C compared with the following life stages. Given that very young white sharks are known to feed on benthic prey (Tricas and McCosker, 1984; Grainger et al., 2020), these results suggest the prevalence of 13C-depleted maternal signature transferred to embryos during their gestation, as reported for other shark species with matrotrophic reproductive strategies (Estrada et al., 2006; McMeans et al., 2009; Olin et al., 2011, 2018). By the time sharks reached the YOY stage in our study, this maternal influence seems to have been diluted through the incorporation of new tissues during growth, leading to isotopic values similar to those of juveniles (Malpica-Cruz et al., 2013; Tamburin et al., 2020). For tissues of rapidly growing young sharks, exogenous feeding is reflected faster than in older sharks due to rapid growth and a threefold gain in mass (Malpica-Cruz et al., 2012). In immature white sharks, the change in isotopic composition that reflects active foraging has been reported to occur, for muscle tissue, at sizes between 150 and 170 cm TL (Malpica-Cruz et al., 2013), like our data indicate.
Immature white sharks are known to primarily use coastal habitats, sometimes covering long distances (hundreds of kilometers) between nurseries (Dewar et al., 2004; Harasti et al., 2017; Curtis et al., 2018; Bruce et al., 2019; Anderson et al., 2021). We did not find significant differences in the mean δ13C or δ15N between juvenile sharks caught inside and outside BSV. These results suggest that immature white sharks, born in BSV or migrating from southern California, United States toward BSV (Weng et al., 2007, 2012; White et al., 2019), are feeding on prey with similar isotopic values along the western coast of the Baja California peninsula. However, considerable variability in δ15N was observed for sharks outside BSV. This variability is due to an enrichment in 15N in larger juveniles (around 300 cm TL), which could be related to a shift in diet to feed on larger prey in coastal areas, like large bony fishes and marine mammals, as has been previously reported for the species (Carlisle et al., 2012; Hussey et al., 2012).
Results from mixing models indicated that white shark muscle reflected demersal prey as the main contributor for all life stages. These results are similar to what has been reported for the diet of juvenile white sharks based on stomach contents analysis (Tricas and McCosker, 1984; Grainger et al., 2020), including samples from BSV where the most common items usually found are rays (mostly bat rays; Santana-Morales et al., 2012; pers. obs.). However, the relative contribution of demersal prey changed between life stages.
For juvenile white sharks, the contribution of pelagic prey (37.8%) was higher than for newborns and YOY (32.2 and 26.2%, respectively). Although previous feeding studies of immature white sharks report a diet dominated by demersal species, they also report the presence of pelagic prey like sardines, mackerel, pelagic sharks, and cephalopods, which become more important in juveniles larger than 185 cm TL (Tricas and McCosker, 1984; Hussey et al., 2012). In BSV, pelagic prey like mackerel Scomber japonicus and squid (order Teuthoidea) have been found in the stomach contents of immature white sharks (Santana-Morales et al., 2012).
BSV is a critical feeding and nursery region for a large variety of pelagic and demersal species (Hernández-Rivas et al., 2000), suggesting that it could sustain high prey availability for immature white sharks. Preliminary tagging results in BSV show that immature white sharks move from very nearshore areas (including the Ojo de Liebre Lagoon) to areas farther from the coastline but still within BSV, where they might feed on those pelagic species (García-Rodíguez, 2020). In addition, pelagic prey could move to inshore areas seasonally, where their availability to immature white sharks may be higher. The higher contribution of demersal and pelagic prey to the isotopic composition of immature white sharks confirms the function of BSV as a nursery for this species, which provides important feeding grounds.
A higher contribution of prey from offshore habitats for neonates (32.6%) and YOY (26.2%) than for juveniles (14.9%) was also estimated, suggesting that the maternal isotopic composition may still have been detectable in some of these smaller sharks but diluted by the time sharks are juveniles, as biomass gain dilutes the maternal signature present at birth and metabolic turnover accelerates the rate at which isotopic equilibrium is reached (Herzka, 2005). Based on mass-length relationships reported for immature white sharks (Logan et al., 2018), immature white sharks born at 138 cm TL double their mass by the time they reach 160–165 cm TL. Previous studies have reported that immature white sharks in the region reflect exogenous feeding in muscle tissues between 150 and 170 cm TL (Malpica-Cruz et al., 2013). A three-fold increase in weight, which is necessary for approximating equilibrium following a dietary shift, would be reached at 190 cm. Hence, juveniles should reflect little or none of the maternally derived isotopic composition.
This smaller contribution of offshore prey estimated for juveniles could be related to movements and foraging in offshore areas. According to satellite telemetry, in the Northeast Pacific, juvenile white sharks commonly select very nearshore habitats (<30 km from the coast; White et al., 2019); however, a few individuals have been shown to move occasionally to offshore areas as far as 1,350 km from the mainland coast in the United States and Mexico (Weng et al., 2012; White et al., 2019).
Although mixing models provide insight into feeding habitats and ontogenetic shifts in feeding, in our study SIA was not useful for discriminating between specific prey that contribute to the diet of immature white sharks. Given the protected status of this species, direct sampling of sharks for stomach contents is not feasible, although gut content analysis of incidental captures could provide some insight into their feeding preferences, as it has in the past (Santana-Morales et al., 2012).
One caveat in our study is the temporal mismatch between white shark and prey samples. Ideally, consumer and sources should be collected at the same time to limit temporal variability (Phillips et al., 2014). As is the case for other top predators, white sharks are not abundant (Huveneers et al., 2018), so sample collection is mostly opportunistic. Due to the small sample size, we could not control for year and life stage in our statistical analyses. However, isotopic values for white sharks were similar to those previously reported for the species within BSV (Malpica-Cruz et al., 2013), suggesting that temporal variability is limited in younger sharks.
Tamburin et al. (2020) sampled muscle tissue (n = 12) for bulk SIA from immature white sharks in OLL and other areas inside BSV between 2015 and 2018. They also sampled potential prey representing different habitats where these sharks could be foraging (including prey from Guadalupe Island). These authors found a higher contribution of offshore prey to the isotopic muscle composition from neonates and YOY. They associate it with the prevalence of a maternally derived isotopic signature. They also estimated higher proportional contribution of inshore pelagic species to tissues of juvenile white sharks, and suggested that these prey were a more important dietary component than reported in previous studies (Santana-Morales et al., 2012; Malpica-Cruz et al., 2013). In contrast, we found a higher contribution from demersal prey to the isotopic composition of immature white sharks, consistent with the more benthic foraging previously reported (Tricas and McCosker, 1984; Hussey et al., 2012; Grainger et al., 2020). Their demersal prey species differed from those analyzed in our study. They did not include batoids other than the bat ray, nor demersal bony fishes like white seabass and halibut, which are the target species of fisheries where immature white sharks are incidentally caught and which have been reported in their stomach contents (Santana-Morales et al., 2012; García-Rodríguez and Sosa-Nishizaki, 2020). Hence, demersal prey may not have been fully representative of the shark’s food sources. Also, differences in the trophic discrimination factors could contribute to the differences between studies (Phillips et al., 2014). Tamburin et al. (2020) used the values reported by Kim et al. (Δδ13C = 1.7 ± 0.5‰; Δδ15N = 3.7 ± 0.4‰; 2012) while we averaged those values with the ones reported by Malpica-Cruz et al. (Δδ13C = 1.0‰; Δδ15N = 2.3‰; 2012). The TDFs from both studies are based on controlled feeding experiments on young leopard sharks and should be considered valid. In addition, many of the samples collected by Tamburin et al. (2020) were from white sharks sampled inside the Ojo de Liebre lagoon during March. According to preliminary acoustic telemetry data, March is when juveniles (larger than 175 cm TL) enter into this habitat (García-Rodíguez, 2020). These juvenile white sharks could be migrating between the Southern California Bight and BSV and could be feeding on coastal pelagic species with different isotopic compositions during these habitat transitions, as the higher variability in the isotopic composition of sharks caught outside of BSV suggests.
In our study, all of the demersal prey samples were collected near the Ojo de Liebre Lagoon’s mouth, which is a high-intensity fishing area where most of the incidental catches of immature white sharks occur (García-Rodríguez and Sosa-Nishizaki, 2020). Based on information from satellite transmitters, White et al. (2019) predicted that juvenile individuals select shallow areas (>1,000 m deep), close to the shore (<30 km from the coast) and surface waters between 14 and 24°C. Based on their characterization of the habitat characteristics suitable for juveniles, BSV, including the Ojo de Liebre lagoon, is suitable year-round for immature white sharks. Other coastal areas along the western coast of the Baja California peninsula also appear to provide suitable habitats during parts of the year. Coastal habitats near lagoons like San Ignacio and San Quintin Bay are suitable mostly during summer and summer-fall, respectively (White et al., 2019), and incidental catches of immature white sharks have been reported. Rochín-Alamillo (2011) documented incidental catches during summer in San Ignacio lagoon, and a juvenile white shark tagged in BSV with a satellite transmitter moved near the San Quintin Bay, where it was incidentally caught during summer (Benson et al., 2018). Some immature white sharks seem to enter these lagoon and estuary habitats to take advantage of seasonal prey availability (Harasti et al., 2017). Coastal lagoons along the Baja California peninsula and their inlet areas present a high abundance of known prey (Danemann and de la Cruz-Agüero, 1993; De la Cruz-Agüero et al., 1994; Rosales-Casián, 1996) and seasonal environmental suitability (White et al., 2019) for immature white sharks. This suitability and the confirmed presence of white sharks incidentally caught when fishers target their prey (Rochín-Alamillo, 2011), suggest that these coastal lagoons and their inlet areas could provide benefits commonly associated with the known nursery areas from the Southern California Bight and BSV.
Anecdotal knowledge gathered from artisanal fishers indicates that demersal prey sampled in this study, like shovelnose guitarfishes and diamond stingray, are found in BSV waters throughout the year, while California bat rays, butterfly rays, and demersal bony fishes like California halibut and white seabass are present seasonally (García-Rodríguez and Sosa-Nishizaki, 2020). The availability of potential prey throughout the year supports the importance of BSV as a nursery habitat.
Some of the demersal prey species are targeted by the local artisanal fishery in BSV during summer, when most immature white sharks are caught (Santana-Morales et al., 2012; Oñate-González et al., 2017; García-Rodríguez and Sosa-Nishizaki, 2020). Landings of California halibut, white seabass, and rays represent around 30% of the total yearly landings from bony fishes in the area (CONAPESCA, 2018), and are therefore an important component of local fisheries. Changes in fishing effort directed at these demersal species might change the white shark’s susceptibility to being incidentally caught. In addition, intense fishing activities could influence immature white sharks behavior, such as their feeding habits, movement patterns, and habitat shifts. Hence, future studies should monitor potential prey distribution, fishing effort, and white sharks.
The comparison of SEAc between life stages showed that juveniles outside BSV have a broader isotopic niche, which is likely due to feeding during their movements between California and Baja California (Weng et al., 2007, 2012; White et al., 2019). YOY and NB likely have smaller isotopic niches because their activities are restricted to BSV. Surprisingly, juveniles inside BSV had the narrowest isotopic niche, although this could be due to the small sample size (n = 6; Jackson et al., 2011), which may not be sufficiently representative of this life stage. The smaller sample size we obtained from juvenile white sharks is mostly due to the lower susceptibility of this life stage to be incidentally caught in demersal bottom nets compared with newborns and YOYs (Oñate-González et al., 2017; García-Rodríguez and Sosa-Nishizaki, 2020). While there was partial overlap in the isotopic niche between all life stages, the highest was between juveniles caught OBSV and YOY from BSV. Sharks sampled outside BSV could have been born in BSV or in the Southern California Bight. However, as they increase in size, they can move between nurseries and spend some months of the year in BSV, where they could feed on similar habitats or on resources with similar isotopic composition of the YOY.
Comparisons of the isotopic niches between sympatric species showed that immature white sharks and smooth hammerheads had a 45% overlap. This overlap was higher for YOY white sharks and small juvenile hammerheads (103–147 cm), suggesting they feed on prey with similar isotopic composition. Juvenile smooth hammerheads feed mostly on squid, small pelagic fishes like scombrids and benthic bony fishes (Smale, 1991; Ochoa-Díaz, 2009), which are also prey for juvenile white sharks collected in BSV (Santana-Morales et al., 2012).
In contrast, there was a limited (15%) isotopic niche overlap between white sharks and copper sharks. Based on what has been reported for other regions, copper sharks in BSV may be feeding on many of the same pelagic species consumed by white sharks, like sardines, mackerels, skipjacks, and tunas (Smale, 1991; Santana-Morales et al., 2012). However, copper sharks are only caught in late spring and summer, when immature white sharks are caught incidentally (Oñate-González et al., 2017; García-Rodríguez and Sosa-Nishizaki, 2020). The temporal overlap between species is thus limited. In addition, copper sharks have a more tropical distribution than white sharks. Some of the copper sharks we sampled were also larger than white sharks and spanned the adult stages, which could contribute to a broader isotopic niche.
A partial overlap in the isotopic niche was reported between juvenile white sharks and juvenile shortfin makos Isurus oxyrinchus in BSV (Tamburin et al., 2019). However, makos have been reported to feed on more pelagic prey like Pacific saury Cololabis saira and jumbo squid Dosidicus gigas off the California coast (Preti et al., 2012). The partial overlap with makos and the higher overlap between the smaller life stages of immature white sharks and juvenile smooth hammerheads found in this study does not necessarily mean that they are competing for resources, since resource partitioning and spatial segregation could avoid competition (Bethea et al., 2004; Kinney et al., 2011). The potential for competition for resources among sympatric species highlights the importance of monitoring the population trends of prey in addition to those of sympatric sharks.
The trophic position calculated for sharks sampled in BSV (4.3) was similar to those previously reported for white sharks of similar sizes based on stomach content or bulk stable isotope analysis (Cortés, 1999; Estrada et al., 2006; Hussey et al., 2012). The trophic position estimated for white sharks caught OBSV was higher than that for BSV (5.3 vs 4.3), and higher than reported previously for the species. White sharks caught OBSV are likely moving between different habitats and could be feeding on prey with a higher TP than those in BSV, or on a different isotopic baseline. Alternatively, this could indicate that Hoen et al.’s (2014) TEF is unsuitable for large sharks due to size-related changes in the nutritional characteristics of the prey (such as protein or lipid content and quality; Nuche-Pascual et al., 2021). Some of the OBSV sharks were large juveniles (∼300 cm TL), and nearing the size when a shift in diet occurs and individuals start feeding on bigger prey, like marine mammals. These results highlight the importance of empirical studies examining the factors influencing AA TEFs for shark tissues.
Conclusion
Stable isotope analysis provided new information about the trophic ecology and complexity of immature white sharks in the northeastern Pacific and new insights into how their trophic habits are related to habitat shifts through their ontogeny. Our results support the importance of BSV as a nursery and foraging ground for sharks born within BSV and California and indicate that they may be sharing prey with sympatric species. We found that the most important prey for white sharks caught within BSV are those heavily targeted by coastal fisheries, which likely increases their susceptibility to being incidentally caught. However, SIA could not reveal the specific prey that white sharks are feeding in the region, so complementary methods should be used in the future. Also, research should focus on comprehending predator-prey relationships between white sharks and their prey to understand how changes in prey abundance and distribution could influence the early life stages of white sharks.
Data Availability Statement
The raw data supporting the conclusions of this article will be made available by the authors, without undue reservation.
Ethics Statement
Ethical review and approval was not required for the animal study because Animals were sampled under permits SGPS/DGVS/04180/13, SGPS/DGVS/06243/14, SGPS/DGVS/06777/15, SGPS/DGVS/06294/16, SGPS/DGVS/07290/17, issued by the General Direction of WIldlife from the National Ministry of Environment and Natural Resources.
Author Contributions
EG-R, SH, OS-N, and CL conceived the study and designed the study. EG-R collected and processed samples, analyzed the data, wrote the original draft, and prepared figures. All authors contributed equally to manuscript revisions and approved the final draft.
Funding
This research was funded by the Monterey Bay Aquarium, the Alliance WWF- Fundación Carlos Slim and the National Commission of Natural Protected Areas (CONANP) through the Conservation Program for Threatened Species (PROCER). EG-R held a scholarship (#237075) from CONACYT. The funder was not involved in the study design, collection, analysis, interpretation of data, the writing of this article or the decision to submit it for publication.
Conflict of Interest
The authors declare that the research was conducted in the absence of any commercial or financial relationships that could be construed as a potential conflict of interest.
Publisher’s Note
All claims expressed in this article are solely those of the authors and do not necessarily represent those of their affiliated organizations, or those of the publisher, the editors and the reviewers. Any product that may be evaluated in this article, or claim that may be made by its manufacturer, is not guaranteed or endorsed by the publisher.
Acknowledgments
We thank the members of the Fisheries Ecology Laboratory from CICESE that took part in the sampling collection; Beatriz Cordero and Abelardo Campos from CICESE’s Aquaculture Department and Carmen Rodriguez from the CICESE’s Biological Oceanography Department for helping in sample preparation; the Biosphere Reserve of El Vizcaíno for the support with permits. Many thanks to all the fishermen from the Baja California peninsula that supported the sampling collection, especially Edgar Zepeda and Rodrigo Guzmán.
Supplementary Material
The Supplementary Material for this article can be found online at: https://www.frontiersin.org/articles/10.3389/fmars.2021.687738/full#supplementary-material
References
Allen, C. D., Lemons, G. E., Eguchi, T., LeRoux, R. A., Fahy, C. C., Dutton, P. H., et al. (2013). Stable isotope analysis reveals migratory origin of loggerhead turtles in the southern California bight. Mar. Ecol. Prog. Ser. 472, 275–285. doi: 10.3354/meps10023
Anderson, J. M., Burns, E. S., Meese, E. N., Farrugia, T. J., Stirling, B. S., White, C. F., et al. (2021). Interannual nearshore habitat use of young of the year white sharks off southern California. Front. Mar. Sci. 8:645142. doi: 10.3389/fmars.2021.645142
Benson, J. F., Jorgensen, S. J., O’Sullivan, J. B., Winkler, C., White, C. F., Garcia-Rodriguez, E., et al. (2018). Juvenile survival, competing risks, and spatial variation in mortality risk of a marine apex predator. J. Appl. Ecol. 55, 2888–2897. doi: 10.1111/1365-2664.13158
Bethea, D. M., Buckel, J. A., and Carlson, J. K. (2004). Foraging ecology of the early life stages of four sympatric shark species. Mar. Ecol. Prog. Ser. 268, 245–264. doi: 10.3354/meps268245
Bizzarro, J. J., Carlisle, A. B., Smith, W. D., and Cortés, E. (2017). “Diet composition and trophic ecology of northeast Pacific ocean sharks,” in Northeast Pacific Shark Biology, Research and Conservation (Part A), Adv. Mar. Biol, Vol. 77, eds S. E. Larson and D. Lowry (London: Academic Press), 111–148. doi: 10.1016/bs.amb.2017.06.001
Block, B. A., Jonsen, I. D., Jorgensen, S. J., Winship, A. J., Shaffer, S. A., Bograd, S. J., et al. (2011). Tracking apex marine predator movements in a dynamic ocean. Nature 475, 86–90. doi: 10.1038/nature10082
Boecklen, W. J., Yarnes, C. T., Cook, B. A., and James, A. C. (2011). On the use of stable isotopes in trophic ecology. Annu. Rev. Ecol. Evol. Syst. 42, 411–440. doi: 10.1146/annurev-ecolsys-102209-144726
Boldrocchi, G., Kiszka, J., Purkis, S., Storai, T., Zinzula, L., and Burkholder, D. (2017). Distribution, ecology, and status of the white shark, Carcharodon carcharias, in the Mediterranean Sea. Rev. Fish Biol. Fish. 27, 515–534. doi: 10.1007/s11160-017-9470-5
Bruce, B., Harasti, D., Lee, K., Gallen, C., and Bradford, R. (2019). Broad-scale movements of juvenile white sharks Carcharodon carcharias in eastern Australia from acoustic and satellite telemetry. Mar. Ecol. Prog. Ser. 619, 1–15. doi: 10.3354/meps12969
Bruce, B. D., and Bradford, R. W. (2012). “Habitat use and spatial dynamics of juvenile white sharks, Carcharodon carcharias, in eastern Australia,” in Global Perspectives on the Biology and Life History of White Shark, ed. M. L. Domeier (Boca Raton, FL: CRC Press), 225–254.
Carlisle, A. B., Goldman, K. J., Litvin, S. Y., Madigan, D. J., Bigman, J. S., Swithenbank, A. M., et al. (2015a). Stable isotope analysis of vertebrae reveals ontogenetic changes in habitat in an endothermic pelagic shark. Proc. R. Soc. B Biol. Sci. 282:20141446. doi: 10.1098/rspb.2014.1446
Carlisle, A. B., Kim, S. L., Semmens, B. X., Madigan, D. J., Jorgensen, S. J., Perle, C. R., et al. (2012). Using stable isotope analysis to understand the migration and trophic ecology of northeastern pacific white sharks (Carcharodon carcharias). PLoS One 7, e30492. doi: 10.1371/journal.pone.0030492
Carlisle, A. B., Litvin, S. Y., Hazen, E. L., Madigan, D. J., Goldman, K. J., Lea, R. N., et al. (2015b). Reconstructing habitat use by juvenile salmon sharks links upwelling to strandings in the California Current. Mar. Ecol. Prog. Ser. 525, 217–228. doi: 10.3354/meps11183
Carlisle, A. B., Litvin, S. Y., Madigan, D. J., Lyons, K., Bigman, J. S., Ibarra, M., et al. (2017). Interactive effects of urea and lipid content confound stable isotope analysis in elasmobranch fishes. Can. J. Fish. Aquat. Sci. 74, 419–428. doi: 10.1139/cjfas-2015-0584
Chikaraishi, Y., Ogawa, N. O., Kashiyama, Y., Takano, Y., Suga, H., Tomitani, A., et al. (2009). Determination of aquatic food-web structure based on compound-specific nitrogen isotopic composition of amino acids. Limnol. Oceanogr. Methods 7, 740–750. doi: 10.4319/lom.2009.7.740
Chikaraishi, Y., Ogawa, N. O., and Ohkouchi, N. (2010). “Further evaluation of the trophic level estimation based on nitrogen isotopic composition of amino acids,” in Earth, Life, and Isotopes, eds. N. Ohokouchi, I. Tayasu, and K. Koba (Kyoto: Kyoto University Press), 37–51.
CONAPESCA (2018). Base De Datos De Avisos De Arribo Pesqueros. Available online at: https://www.plataformadetransparencia.org.mx [accessed June 20, 2019]
Cortés, E. (1999). Standardized diet compositions and trophic levels of sharks. ICES J. Mar. Sci. 56, 707–717. doi: 10.1006/jmsc.1999.0489
Curtis, T. H., Metzger, G., Fischer, C., Mcbride, B., Mccallister, M., and Winn, L. J. (2018). First insights into the movements of young-of-the-year white sharks (Carcharodon carcharias) in the western north Atlantic ocean. Sci. Rep. 8, 1–8. doi: 10.1038/s41598-018-29180-5
Danemann, G. D., and de la Cruz-Agüero, J. (1993). Ictiofauna de laguna San Ignacio, Baja California sur, México. Cienc. Mar. 19, 333–341. doi: 10.7773/cm.v19i3.936
De la Cruz-Agüero, J., Galván-Magaña, F., Abitia-Cárdenas, L. A., Rodríguez-Romero, J., and Gutiérrez-Sánchez, F. J. (1994). Lista sistematica de los peces marinos de Bahía Magdalena, Baja California sur (México). Cienc. Mar. 20, 17–31.
Dewar, H., Domeier, M., and Nasby-Lucas, N. (2004). Insights into young of the year white shark, Carcharodon carcharias, behavior in the Southern California Bight. Environ. Biol. Fishes 70, 133–143. doi: 10.1023/B:EBFI.0000029343.54027.6a
DOF (2007). Norma Oficial Mexicana NOM-029-PESC-2006: pesca responsable de tiburónes y rayas. especificaciones para su aprovechamiento. Diario Oficial Federación 1, 60–102.
DOF (2010). Norma Oficial Mexicana NOM-059-ECOL-2001:protección ambiental-especies nativas de México de flora y fauna silvestres-categorías de riesgo y especificaciones para su inclusión, exclusión o cambio-Lista de especies en riesgo. Diario Oficial Federación 1, 1–77.
DOF (2014). Acuerdo por el que se establece veda permanente para la pesca de tiburón blanco (Carcharodon carcharias) en aguas de jurisdicción federal de los Estados Unidos Mexicanos. Diario Oficial Federación 1, 14–16.
Domeier, M. L., and Nasby-Lucas, N. (2007). Annual re-sightings of photographically identified white sharks (Carcharodon carcharias) at an eastern Pacific aggregation site (Guadalupe Island, Mexico). Mar. Biol. 150, 977–984. doi: 10.1007/s00227-006-0380-7
Domeier, M. L., and Nasby-Lucas, N. (2008). Migration patterns of white sharks Carcharodon carcharias tagged at Guadalupe Island, Mexico, and identification of an eastern Pacific shared offshore foraging area. Mar. Ecol. Prog. Ser. 370, 221–237. doi: 10.3354/meps07628
Domeier, M. L., and Nasby-Lucas, N. (2013). Two-year migration of adult female white sharks (Carcharodon carcharias) reveals widely separated nursery areas and conservation concerns. Anim. Biotelemetry 1:2. doi: 10.1186/2050-3385-1-2
Estrada, J. A., Rice, A. N., Natanson, L. J., and Skomal, G. B. (2006). Use of isotopic analysis of vertebrae in reconstructiong ontogenetic feeding ecology in white sharks. Ecology 87, 829–834. doi: 10.1890/0012-9658(2006)87[829:uoiaov]2.0.co;2
Estupiñán-Montaño, C., Galván-Magaña, F., Sánchez-González, A., Elorriaga-Verplancken, F. R., Delgado-Huertas, A., and Páez-Rosas, D. (2019). Dietary ontogeny of the blue shark, Prionace glauca, based on the analysis of δ13C and δ15N in vertebrae. Mar. Biol. 166:101. doi: 10.1007/s00227-019-3550-0
Ferretti, F., Worm, B., Britten, G. L., Heithaus, M. R., and Lotze, H. K. (2010). Patterns and ecosystem consequences of shark declines in the ocean. Ecol. Lett. 13, 1055–1071. doi: 10.1111/j.1461-0248.2010.01489.x
Finlay, J. C., and Kendall, C. (2007). “Stable isotope tracing of temporal and spatial variability in organic matter sources to freshwater ecosystems,” in Stable Isotopes in Ecology and Environmental Science, eds R. H. Michener and K. Lajtha (Oxford, UK: Blackwell Publishing Ltd), 283–333. doi: 10.1002/9780470691854.ch10
Francis, M. P., Duffy, C., and Lyon, W. (2015). Spatial and temporal habitat use by white sharks (Carcharodon carcharias) at an aggregation site in southern New Zealand. Mar. Freshw. Res. 66, 900–918. doi: 10.1071/MF14186
French, G. C. A., Rizzuto, S., Stürup, M., Inger, R., Barker, S., van Wyk, J. H., et al. (2018). Sex, size and isotopes: cryptic trophic ecology of an apex predator, the white shark Carcharodon carcharias. Mar. Biol. 165:102. doi: 10.1007/s00227-018-3343-x
García-Rodíguez, E. (2020). Importance of Ojo de Liebre lagoon and Vizcaino Bay in the Early Life Stages of White Sharks:Ecological and Fishery Issues. Doctoral thesis. Ensenada, BC: Centro de Investigación Científica y de Educación Superior de Ensenada, Baja California.
García-Rodríguez, E., and Sosa-Nishizaki, O. (2020). Artisanal fishing activities and their documented interactions with juvenile white sharks inside a nursery area. Aquat. Conserv. Mar. Freshw. Ecosyst. 30, 903–914. doi: 10.1002/aqc.3300
Gračan, R., Zavodnik, D., Krstinić, P., Dragičević, B., and Lazar, B. (2017). Feeding ecology and trophic segregation of two sympatric mesopredatory sharks in the heavily exploited coastal ecosystem of the Adriatic Sea. J. Fish Biol. 90, 167–184. doi: 10.1111/jfb.13158
Grainger, R., Peddemors, V. M., Raubenheimer, D., and Machovsky-capuska, G. E. (2020). Diet composition and nutritional niche breadth variability in juvenile white sharks (Carcharodon carcharias). Front. Mar. Sci. 7:422. doi: 10.3389/fmars.2020.00422
Hansson, S., Bergström, U., Bonsdorff, E., Härkönen, T., Jepsen, N., Kautsky, L., et al. (2018). Competition for the fish – fish extraction from the Baltic Sea by humans, aquatic mammals, and birds. ICES J. Mar. Sci. 75, 999–1008. doi: 10.1093/icesjms/fsx207
Harasti, D., Lee, K., Bruce, B., Gallen, C., and Bradford, R. (2017). Juvenile white sharks Carcharodon carcharias use estuarine environments in south-eastern Australia. Mar. Biol. 164:58. doi: 10.1007/s00227-017-3087-z
Hernández-Rivas, M., Jimenez-Rosenberg, S. P., Funes-Rodríguez, R., and Saldierna-Martinez, R. (2000). “El centro de actividad biológica de la bahía de Sebastián Vizcaíno, una primera aproximación,” in BAC: Centros De Actividad Biológica Del Pacífico Mexicano, eds D. Lluch-Belda, J. F. Elorduy-Garay, S. Lluch-Cota, and G. Ponce Diaz (La Paz: Centro de Investigaciones Biológicas del Noroeste), 65–68.
Herzka, S. Z. (2005). Assessing connectivity of estuarine fishes based on stable isotope ratio analysis. Estuar. Coast. Shelf Sci. 64, 58–69. doi: 10.1016/j.ecss.2005.02.006
Hette-Tronquart, N. (2019). Isotopic niche is not equal to trophic niche. Ecol. Lett. 22, 1987–1989. doi: 10.1111/ele.13218
Heupel, M., Knip, D., Simpfendorfer, C., and Dulvy, N. (2014). Sizing up the ecological role of sharks as predators. Mar. Ecol. Prog. Ser. 495, 291–298. doi: 10.3354/meps10597
Heupel, M. R., Carlson, J. K., and Simpfendorfer, C. A. (2007). Shark nursery areas: concepts, definition, characterization and assumptions. Mar. Ecol. Prog. Ser. 337, 287–297. doi: 10.3354/meps337287
Hilborn, R., Amoroso, R. O., Bogazzi, E., Jensen, O. P., Parma, A. M., Szuwalski, C., et al. (2017). When does fishing forage species affect their predators? Fish. Res. 191, 211–221. doi: 10.1016/j.fishres.2017.01.008
Hoen, D. K., Kim, S. L., Hussey, N. E., Wallsgrove, N. J., Drazen, J. C., and Popp, B. N. (2014). Amino acid 15N trophic enrichment factors of four large carnivorous fishes. J. Exp. Mar. Bio. Ecol. 453, 76–83. doi: 10.1016/j.jembe.2014.01.006
Hoyos-Padilla, E. M., Klimley, A. P., Galván-Magaña, F., and Antoniou, A. (2016). Contrasts in the movements and habitat use of juvenile and adult white sharks (Carcharodon carcharias) at Guadalupe Island, Mexico. Anim. Biotelemetry 4, 1–14. doi: 10.1186/s40317-016-0106-7
Huss, M., de Roos, A. M., Van Leeuwen, A., and Gårdmark, A. (2014). Facilitation of fisheries by natural predators depends on life history of shared prey. Oikos 123, 1071–1080. doi: 10.1111/oik.00839
Hussey, N. E., MacNeil, M. A., Olin, J. A., McMeans, B. C., Kinney, M. J., Chapman, D. D., et al. (2012). Stable isotopes and elasmobranchs: tissue types, methods, applications and assumptions. J. Fish Biol. 80, 1449–1484. doi: 10.1111/j.1095-8649.2012.03251.x
Huveneers, C., Apps, K., Becerril-García, E. E., Bruce, B., Butcher, P. A., Carlisle, A. B., et al. (2018). Future research directions on the “elusive”white shark. Front. Mar. Sci. 5:455. doi: 10.3389/fmars.2018.00455
Jackson, A. L., Inger, R., Parnell, A. C., and Bearhop, S. (2011). Comparing isotopic niche widths among and within communities: SIBER - stable isotope Bayesian Ellipses in R. J. Anim. Ecol. 80, 595–602. doi: 10.1111/j.1365-2656.2011.01806.x
Jorgensen, S. J., Reeb, C. A., Chapple, T. K., Anderson, S., Perle, C., Van Sommeran, S. R., et al. (2010). Philopatry and migration of Pacific white sharks. Proc. R. Soc. B Biol. Sci. 277, 679–688. doi: 10.1098/rspb.2009.1155
Kim, S. L., del Rio, C. M., Casper, D., and Koch, P. L. (2012). Isotopic incorporation rates for shark tissues from a long-term captive feeding study. J. Exp. Biol. 215, 2495–2500. doi: 10.1242/jeb.070656
Kim, S. L., and Koch, P. L. (2012). Methods to collect, preserve, and prepare elasmobranch tissues for stable isotope analysis. Environ. Biol. Fishes 95, 53–63. doi: 10.1007/s10641-011-9860-9
Kinney, M. J., Hussey, N. E., Fisk, A. T., Tobin, A. J., and Simpfendorfer, C. A. (2011). Communal or competitive? Stable isotope analysis provides evidence of resource partitioning within a communal shark nursery. Mar. Ecol. Prog. Ser. 439, 263–276. doi: 10.3354/meps09327
Klimley, A. P., Anderson, S. D., Pyle, P., and Henderson, R. P. (1992). Spatiotemporal patterns of white shark (Carcharodon carcharias) predation at the south Farallon Islands, California. Copeia 1992, 680–690. doi: 10.2307/1446143
Koen Alonso, M., Alberto Crespo, E., Aníbal García, N., Noemí Pedraza, S., Ariel Mariotti, P., and Judith Mora, N. (2002). Fishery and ontogenetic driven changes in the diet of the spiny dogfish, Squalus acanthias, in Patagonian waters, Argentina. Environ. Biol. Fishes 63, 193–202. doi: 10.1023/A:1014229432375
Li, Y., Zhang, Y., Hussey, N. E., and Dai, X. (2016). Urea and lipid extraction treatment effects on δ15N and δ13C values in pelagic sharks. Rapid Commun. Mass Spectrom. 30, 1–8. doi: 10.1002/rcm.7396
Logan, J. M., and Lutcavage, M. E. (2010). Stable isotope dynamics in elasmobranch fishes. Hydrobiologia 644, 231–244. doi: 10.1007/s10750-010-0120-3
Logan, R. K., White, C. F., Winkler, C., Jorgensen, S. J., O’Sullivan, J. B., Lowe, C. G., et al. (2018). An evaluation of body condition and morphometric relationships within southern California juvenile white sharks Carcharodon carcharias. J. Fish Biol. 93, 842–849. doi: 10.1111/jfb.13785
Lowe, C. G., Blasius, M. E., Jarvis, E. T., Mason, T. J., Goodmanlowe, G. D., and O’Sullivan, J. B. (2012). “Historic fisheries interactions with white sharks in the southern California Bigh,” in Global Perspectives on the Biology and Life History of the White Shark, ed. M. L. Domeier (Boca Raton, FL: CRC Press), 169–186.
Lucifora, L. O., García, V. B., and Escalante, A. H. (2009). How can the feeding habits of the sand tiger shark influence the success of conservation programs? Anim. Conserv. 12, 291–301. doi: 10.1111/j.1469-1795.2009.00247.x
Malpica-Cruz, L., Herzka, S. Z., Sosa-Nishizaki, O., and Escobedo-Olvera, M. A. (2013). Tissue-specific stable isotope ratios of shortfin mako (Isurus oxyrinchus) and white (Carcharodon carcharias) sharks as indicators of size-based differences in foraging habitat and trophic level. Fish. Oceanogr. 22, 429–445. doi: 10.1111/fog.12034
Malpica-Cruz, L., Herzka, S. Z., Sosa-Nishizaki, O., Lazo, J. P., and Trudel, M. (2012). Tissue-specific isotope trophic discrimination factors and turnover rates in a marine elasmobranch: empirical and modeling results. Can. J. Fish. Aquat. Sci. 69, 551–564. doi: 10.1139/f2011-172
Marshall, H. H., Inger, R., Jackson, A. L., McDonald, R. A., Thompson, F. J., and Cant, M. A. (2019). Stable isotopes are quantitative indicators of trophic niche. Ecol. Lett. 22, 1990–1992. doi: 10.1111/ele.13374
Matich, P., Kiszka, J. J., Heithaus, M. R., Le Bourg, B., and Mourier, J. (2019). Inter-individual differences in ontogenetic trophic shifts among three marine predators. Oecologia 189, 621–636. doi: 10.1007/s00442-019-04357-5
McMahon, K. W., and McCarthy, M. D. (2016). Embracing variability in amino acid δ15N fractionation: mechanisms, implications, and applications for trophic ecology. Ecosphere 7, 1–26. doi: 10.1002/ecs2.1511
McMeans, B. C., Olin, J. A., and Benz, G. W. (2009). Stable-isotope comparisons between embryos and mothers of a placentatrophic shark species. J. Fish Biol. 75, 2464–2474. doi: 10.1111/j.1095-8649.2009.02402.x
Michener, R. H., and Kaufman, L. (2007). “Stable isotope ratios as tracers in marine food webs: an update,” in Stable Isotopes in Ecology and Environmental Science, eds R. Michener and K. Lajtha (Oxford, UK: Blackwell Publishing Ltd), 238–282. doi: 10.1002/9780470691854.ch9
Mollet, H. F., and Cailliet, G. M. (2002). Comparative population demography of elasmobranch using life history tables, Leslie matrixes and stage-based models. Mar. Freshw. Res. 53, 503–516. doi: 10.1071/MF01083
Moro, S., Jona-Lasinio, G., Block, B., Micheli, F., De Leo, G., Serena, F., et al. (2020). Abundance and distribution of the white shark in the Mediterranean Sea. Fish Fish. 21, 338–349. doi: 10.1111/faf.12432
Munroe, S. E. M., Meyer, L., and Heithaus, M. (2018). “Dietary biomarkers in shark foraging and movement ecology,” in Shark Research: Emerging Technologies and Applications for the Field and Laboratory, eds J. Carrier, M. Heithaus, and C. Simpfendorfer (Boca Raton, FL: CRC Press), 1–24.
Nasby-Lucas, N., Dewar, H., Sosa-Nishizaki, O., Wilson, C., Hyde, J. R., Vetter, R. D., et al. (2019). Movements of electronically tagged shortfin mako sharks (Isurus oxyrinchus) in the eastern North Pacific Ocean. Anim. Biotelemetry 7, 1–26. doi: 10.1186/s40317-019-0174-6
Nosal, A. P., Cartamil, D. P., Wegner, N. C., Lam, C. H., and Hastings, P. A. (2019). Movement ecology of young-of-the-year blue sharks Prionace glauca and shortfin makos Isurus oxyrinchus within a putative binational nursery area. Mar. Ecol. Prog. Ser. 623, 99–115. doi: 10.3354/meps13021
Nuche-Pascual, M. T., Ruiz-Cooley, R. I., and Herzka, S. (2021). A meta-analysis of amino acid δ15N trophic enrichment factors in fishes relative to nutritional and ecological drivers. Ecosphere e03570. doi: 10.1002/ecs2.3570
Ochoa-Díaz, M. R. (2009). Espectro Trófico Del Tiburón Martillo Sphyrna Zygaena (Linnaeus, 1758) En Baja California Sur: Aplicación De Δ13c Y Δ15n. Ph.D. thesis. La Paz: Centro Interdisciplinario de Ciencias Marinas.
O’Connell, T. C. (2017). ‘Trophic’ and ‘source’ amino acids in trophic estimation: a likely metabolic explanation. Oecologia 184, 317–326. doi: 10.1007/s00442-017-3881-9
Olin, J. A., Hussey, N. E., Fritts, M., Heupel, M. R., Simpfendorfer, C. A., Poulakis, G. R., et al. (2011). Maternal meddling in neonatal sharks: implications for interpreting stable isotopes in young animals. Rapid Commun. Mass Spectrom. 25, 1008–1016. doi: 10.1002/rcm.4946
Olin, J. A., Shipley, O. N., and McMeans, B. C. (2018). Stable isotope fractionation between maternal and embryo tissues in the bonnethead shark (Sphyrna tiburo). Environ. Biol. Fishes 101, 489–499. doi: 10.1007/s10641-018-0715-5
Oñate-González, E. C., Rocha-Olivares, A., Saavedra-Sotelo, N. C., and Sosa-Nishizaki, O. (2015). Mitochondrial genetic structure and matrilineal origin of white sharks, Carcharodon carcharias, in the northeastern Pacific: implications for their conservation. J. Hered. 106, 347–354. doi: 10.1093/jhered/esv034
Oñate-González, E. C., Sosa-Nishizaki, O., Herzka, S. Z., Lowe, C. G., Lyons, K., Santana-Morales, O., et al. (2017). Importance of Bahia Sebastian Vizcaino as a nursery area for white sharks (Carcharodon carcharias) in the northeastern Pacific: a fishery dependent analysis. Fish. Res. 188, 125–137. doi: 10.1016/j.fishres.2016.12.014
Papastamatiou, Y., Wetherbee, B., Lowe, C., and Crow, G. (2006). Distribution and diet of four species of carcharhinid shark in the Hawaiian Islands: evidence for resource partitioning and competitive exclusion. Mar. Ecol. Prog. Ser. 320, 239–251. doi: 10.3354/meps320239
Parnell, A. C. (2016). simmr: A Stable Isotope Mixing Model. https://CRAN.R-project.org/package=simmr.
Parnell, A. C., Phillips, D. L., Bearhop, S., Semmens, B. X., Ward, E. J., Moore, J. W., et al. (2013). Bayesian stable isotope mixing models. Environmetrics 24, 387–399. doi: 10.1002/env.2221
Peterson, B. J., and Fry, B. (1987). Stable isotopes in ecosystem studies. Annu. Rev. Ecol. Syst. 18, 293–320. doi: 10.1016/0198-0254(88)92720-3
Phillips, D. L., Inger, R., Bearhop, S., Jackson, A. L., Moore, J. W., Parnell, A. C., et al. (2014). Best practices for use of stable isotope mixing models in food-web studies. Can. J. Zool. 92, 823–835. doi: 10.1139/cjz-2014-0127
Post, D. M. (2002). Using stable isotopes to estimate trophic position: models, methods, and assumptions. Ecology 83, 703–718. doi: 10.1890/0012-9658(2002)083[0703:USITET]2.0.CO;2
Preti, A., Soykan, C. U., Dewar, H., Wells, R. J. D., Spear, N., and Kohin, S. (2012). Comparative feeding ecology of shortfin mako, blue and thresher sharks in the California Current. Environ. Biol. Fishes 95, 127–146. doi: 10.1007/s10641-012-9980-x
Rochín-Alamillo, A. (2011). Composición y Abundancia De Elasmobranquios En El Complejo Lagunar San Ignacio, Baja California Sur. Ph.D. thesis. La Paz: Centro Interdisciplinario de Ciencias Marinas.
Rosales-Casián, J. A. (1996). Ictiofauna de la bahía de San Quintín, Baja California, México, y su costa adyacente. Cienc. Mar. 22, 443–458. doi: 10.7773/cm.v22i4.875
Santana-Morales, O., Sosa-Nishizaki, O., Escobedo-Olvera, M., Onate-Gonzalez, E., O’Sullivan, J., and Cartamil, D. (2012). “Incidental catch and ecological observations of juvenile white sharks, Carcharadon carcharias, in western Baja california, Mexico. conservation implications,” in Global Perspectives on the Biology and Life History of the White Shark, ed. M. L. Domeier (Boca Raton, FL: CRC Press), 187–198.
Skomal, G. B., Braun, C. D., Chisholm, J. H., and Thorrold, S. R. (2017). Movements of the white shark Carcharodon carcharias in the north Atlantic ocean. Mar. Ecol. Prog. Ser. 580, 1–16. doi: 10.3354/meps12306
Smale, M. J. (1991). Occurrence and feeding of three shark species, Carcharhinus brachyurus, Carcharhinus obscurus and Sphyrna zygaena, on the eastern Cape coast of South Africa. South Afr. J. Mar. Sci. 11, 31–42. doi: 10.2989/025776191784287808
Tamburin, E., Elorriaga, F. R., Colombo, V., Montaño, E., Madigan, D. J., Sánchez, A., et al. (2020). New insights into the trophic ecology of young white sharks (Carcharodon carcharias) in waters off the Baja California Peninsula, Mexico. Mar. Biol. 167:55. doi: 10.1007/s00227-020-3660-8
Tamburin, E., Kim, S., Elorriaga-Verplancken, F., Madigan, D., Hoyos-Padilla, M., Sánchez-González, A., et al. (2019). Isotopic niche and resource sharing among young sharks (Carcharodon carcharias and Isurus oxyrinchus) in Baja California, Mexico. Mar. Ecol. Prog. Ser. 613, 107–124. doi: 10.3354/meps12884
Tricas, T. C., and McCosker, J. E. (1984). Predatory behavior of the white shark (Carcharodon carcharias), with notes on its biology. Proc. Calif. Acad. Sci. 43, 221–238.
Vokhshoori, N. L., Larsen, T., and McCarthy, M. D. (2014). Reconstructing δ13C isoscapes of phytoplankton production in a coastal upwelling system with amino acid isotope values of littoral mussels. Mar. Ecol. Prog. Ser. 504, 59–72. doi: 10.3354/meps10746
Weng, K. C., O’Sullivan, J. B., Lowe, C. G., Winkler, C. E., Dewar, H., and Block, B. A. (2007). Movements, behavior and habitat preferences of juvenile white sharks Carcharodon carcharias in the eastern Pacific. Mar. Ecol. Prog. Ser. 338, 211–224. doi: 10.3354/meps338211
Weng, K. C., Sullivan, J. B. O., Lowe, C. G., Winkler, C. E., Blasius, M. E., Locke-Smith, K. A., et al. (2012). “Back to the wild release of juvenile white sharks from the monterey bay aquarium,” in Global Perspectives on the Biology and Life History of the White Shark, ed. M. L. Domeier (Boca Raton, FL: CRC Press), 419–446.
White, C. F., Lyons, K., Jorgensen, S. J., O’Sullivan, J., Winkler, C., Weng, K. C., et al. (2019). Quantifying habitat selection and variability in habitat suitability for juvenile white sharks. PLoS One 14:e0214642. doi: 10.1371/journal.pone.0214642
Keywords: white shark, trophic ecology, stable isotope analyses, nursery area, demersal foraging, amino acids
Citation: García-Rodríguez E, Herzka SZ, Sosa-Nishizaki O, Lowe CG and O’Sullivan JB (2021) Stable Isotope Analysis of Juvenile White Sharks Inside a Nursery Area Reveals Foraging in Demersal-Inshore Habitats and Trophic Overlap With Sympatric Sharks. Front. Mar. Sci. 8:687738. doi: 10.3389/fmars.2021.687738
Received: 30 March 2021; Accepted: 28 May 2021;
Published: 10 August 2021.
Edited by:
J. Marcus Drymon, Mississippi State University, United StatesReviewed by:
Simona Alessandra Ceriani, Florida Fish and Wildlife Research Institute, United StatesRichard Reina, Monash University, Australia
Copyright © 2021 García-Rodríguez, Herzka, Sosa-Nishizaki, Lowe and O’Sullivan. This is an open-access article distributed under the terms of the Creative Commons Attribution License (CC BY). The use, distribution or reproduction in other forums is permitted, provided the original author(s) and the copyright owner(s) are credited and that the original publication in this journal is cited, in accordance with accepted academic practice. No use, distribution or reproduction is permitted which does not comply with these terms.
*Correspondence: Sharon Z. Herzka, c2hlcnprYUBjaWNlc2UubXg=; Oscar Sosa-Nishizaki, b3Nvc2FAY2ljZXNlLm14
†ORCID: Emiliano García-Rodríguez, orcid.org/0000-0002-9078-1300; Sharon Z. Herzka, orcid.org/0000-0001-7091-7656; Oscar Sosa-Nishizaki, orcid.org/0000-0002-3043-768X