- 1IPMA – Portuguese Institute for Sea and Atmosphere, Olhão, Portugal
- 2CCMAR – Centre of Marine Sciences, University of Algarve, Faro, Portugal
- 3DINARA – Dirección Nacional de Recursos Acuáticos, Laboratorio de Recursos Pelágicos, Montevideo, Uruguay
- 4National Marine Fisheries Service, Southeast Fisheries Science Center, Panama City, FL, United States
- 5National Marine Fisheries Service, Northeast Fisheries Science Center, Narragansett Laboratory, RI, United States
- 6Departamento de Pesca e Aquicultura, Universidade Federal Rural de Pernambuco, Recife, Brazil
- 7Instituto Español de Oceanografía, Fuengirola, Spain
- 8CICMAR – Centro de Investigación y Conservación Marina, Canelones, Uruguay
The shortfin mako is one of the most important shark species caught in Atlantic Ocean pelagic fisheries. Given increasing concerns for the stock status of the species, the present study was designed to fill gaps in the knowledge of habitat use and movement patterns of shortfin mako in the Atlantic Ocean. From 2015 to 2019, 53 shortfin makos were tagged with pop-up satellite archival tags within the North, Central, and Southwest Atlantic Ocean, with successful transmissions received from 34 tags. Generally, sharks tagged in the Northwest and Central Atlantic moved away from tagging sites showing low to no apparent residency patterns, whereas sharks tagged in the Northeast and Southwest Atlantic spent large periods of time near the Canary Archipelago and Northwest Africa, and over shelf and oceanic waters off southern Brazil and Uruguay, respectively. These areas showed evidence of site fidelity and were identified as possible key areas for shortfin mako. Sharks spent most of their time in temperate waters (18–22°C) above 90 m; however, data indicated the depth range extended from the surface down to 979 m, in water temperatures ranging between 7.4 and 29.9°C. Vertical behavior of sharks seemed to be influenced by oceanographic features, and ranged from marked diel vertical movements, characterized by shallower mean depths during the night, to yo-yo diving behavior with no clear diel pattern observed. These results may aid in the development of more informed and efficient management measures for this species.
Introduction
The shortfin mako, Isurus oxyrinchus, is a pelagic shark with a circum-global distribution. It occurs from the surface down to at least 1,064 m depth and is occasionally found in coastal waters where the continental shelf is narrow (Compagno, 2001; Stevens, 2008; Abascal et al., 2011; Mucientes-Sandoval et al., 2012; Rogers et al., 2015; Vaudo et al., 2017; Francis et al., 2019). It is among the fastest fishes of the sea and is an ambush predator that feeds on pelagic prey such as cephalopods and teleosts (Stillwell and Kohler, 1982; Compagno, 2001; Maia et al., 2006; Díez et al., 2015). The shortfin mako is one of the most important shark species caught by pelagic longline and gillnet fisheries targeting mainly swordfish and tunas in the Atlantic Ocean, second only to blue shark, Prionace glauca, in terms of catches (Campana et al., 2005; Camhi et al., 2008; Mejuto et al., 2009; Coelho et al., 2012). Although they are usually captured as bycatch, shortfin makos were nearly always retained because of their highly prized meat and fins (Camhi et al., 2008; Dulvy et al., 2008; Stevens, 2008; Rigby et al., 2019). The species aggressiveness and power made it one of the most desirable game fishes in the world for recreational anglers (Stevens, 2008). Since their inclusion in 2019 on CITES Appendix II it is possible that discards have increased. However, like many other pelagic sharks, populations of shortfin mako have a limited capacity to resist, and rebound from, high fishing pressure on account of their life history characteristics (Barker and Schluessel, 2005; Dulvy et al., 2008). In all, the shortfin mako is considered one of the shark species at greatest risk of overexploitation in the Atlantic Ocean owing to its low productivity and high susceptibility to pelagic fisheries (Simpfendorfer et al., 2008; Cortés et al., 2010, 2015).
Recent findings indicate that increasing fishing pressure in recent decades has seriously impacted the shortfin mako populations in the Atlantic Ocean. The latest stock assessment carried out in 2017 by the International Commission for the Conservation of Atlantic Tunas (ICCAT, the inter-governmental organization responsible for the management and conservation of pelagic sharks in the Atlantic) showed that the South Atlantic stock had a 32% probability of being overfished and a 42% probability of experiencing overfishing, while for the North Atlantic stock there was a combined 90% probability of the stock being in an overfished state and experiencing overfishing (Anon, 2017). Given the increasing concerns for its stock status, the shortfin mako was recently declared globally Endangered by the IUCN Red List of Threatened Species (Rigby et al., 2019) and also included in 2019 in the CITES Appendix II list, which bans international trade unless proven to be legal and sustainable (CITES, 2019). The current unsustainable fishing mortality levels and population declines bring to light the importance of focusing research efforts on ecological and biological aspects of this species. Animal tracking has become one of the major tools used to understand the ecology and behavior of a variety of marine species, providing essential information for management planning (e.g., Shillinger et al., 2008; Stevens et al., 2010; Graham et al., 2012; Wilson et al., 2015; Ketchum et al., 2020).
Shifts in oceanographic conditions and seasonal variations of highly productive areas are believed to lead large oceanic fishes like sharks to undertake long-distance migrations in search of food and mating grounds (e.g., Weng et al., 2007; Skomal et al., 2009; Francis et al., 2015). Elucidating these movements (i.e., use of space and activity patterns) is therefore fundamental to understand the behavior of a species and its population structure, as well as to define essential habitats, with an aim to implement effective management measures (Camhi et al., 2008; Rogers et al., 2015; Vaudo et al., 2016). Considering the likely possibility of sharks as key-elements in oceanic ecosystems, knowledge of whether they are moving within regions that might be undergoing different types and levels of fishing activity is essential to predict fishing impacts on their populations and throughout the food web. However, even though these issues are of great importance, studying long-term movements of pelagic sharks can be challenging mostly because of the highly migratory nature of the species and the complex logistics involved in this type of studies (Vaudo et al., 2016).
In recent years, satellite tagging has been increasingly used to study a wide range of marine species, including large pelagic fishes (Hammerschlag et al., 2011; Abascal et al., 2015; Wilson et al., 2015; Santos and Coelho, 2018). For the Atlantic Ocean, these studies have provided important information on spatial ecology of several pelagic shark species including Lamniform sharks such as the porbeagle, Lamna nasus (e.g., Pade et al., 2009; Saunders et al., 2011; Biais et al., 2017; Skomal et al., 2021) and the basking shark, Cetorhinus maximus (e.g., Skomal et al., 2009; Doherty et al., 2017; Braun et al., 2018). In contrast, and despite its historical importance for commercial and recreational fisheries, studies on movement patterns and habitat use of shortfin mako in the Atlantic Ocean are still scarce. In the Atlantic Ocean, satellite tagging data come almost exclusively from shortfin makos tagged in the northwestern area. The most comprehensive study to date was carried out by Vaudo et al. (2017), in which long-term horizontal movements and seasonal distributions were analyzed for 26 shortfin makos. This study reported region-specific movements as well as the species capacity for making long-distance and highly directional excursions. In an earlier study, Vaudo et al. (2016) investigated the vertical movements of eight shortfin makos which occupied a wide range of temperatures and depths, although movements seemed to be highly influenced by ocean temperature. Additionally, a study by Loefer et al. (2005) analyzed data from only one adult male that revealed a diel pattern of vertical movement defined by greater mean depths and larger depth ranges during daytime. Again, vertical movements seemed to be influenced by sea-surface temperatures. More recently, a study by Gibson et al. (2021) reported movement patterns of mostly mature shortfin makos tagged in the northwestern Gulf of Mexico, which seemed to differ by sex. Males made extensive migrations toward the Caribbean Sea and the northeastern United States Atlantic coast, while the lone female demonstrated high fidelity to the Gulf of Mexico coastal shelf. Additionally, a study by Coelho et al. (2020) reported movements of two shortfin makos tagged outside of the northwestern area. These sharks, tagged in the tropical Northeast Atlantic in the Cabo Verde islands region, used areas along the western African continental shelf. In Queiroz et al. (2016, 2019), the Gulf Stream, the North Atlantic Current/Labrador Current convergence zone, the Azores islands, the mid-Atlantic Ridge area, and the upwelling zone off Northwest Africa were identified as high use areas for the shortfin mako.
Here we address these information gaps by presenting the most extensive study to date on habitat use and movements of shortfin mako tagged with PSATs in the Atlantic Ocean. By joining efforts with various research teams working in the Atlantic, we were able to tag a large set of specimens across a wide area. Specific objectives of this study include (1) analyzing horizontal movements and spatial distribution of shortfin mako in the Atlantic Ocean, (2) investigating vertical habitat utilization, and (3) discussing the use of oceanic vs. continental shelf and slope areas by this species in the Atlantic Ocean.
Materials and Methods
Tagging Procedure
Tagging took place across a wide area of the Atlantic Ocean, between 2015 and 2019, integrated within the ICCAT Shark Research and Data Collection Programme (SRDCP). The main tagging areas were the Northwest, Northeast, Central, and Southwest Atlantic, with the tagging conducted onboard Uruguayan research vessels, and vessels from the Portuguese, Brazilian, Spanish, and United States pelagic longline fleets (Figure 1). The tag deployment was opportunistic when sharks were captured during regular fishing operations and was carried out by scientific fisheries observers and scientists. Sharks were either hoisted alongside the vessel or brought on board for tagging. An umbrella-type nylon dart (Domeier dart) was used to insert the tag laterally to the dorsal musculature below the first dorsal fin base, using the methodology described by Howey-Jordan et al. (2013). Sharks were tagged and released immediately upon capture, without anesthesia, by cutting the gangion line as close as possible to the mouth or removing the hook when possible. Before tag attachment, tags were tested and programmed to record information for periods between 30 and 180 days (see Supplementary Material). In addition, during the tagging operation, animals were sexed, and measurements in fork length (FL) taken to the nearest cm. Date and time were recorded for each individual release, and the geographic tagging location (latitude and longitude) was determined by global positioning system (GPS). All shortfin mako tagging was performed in accordance with protocols approved by the ICCAT Standing Committee on Research and Statistics. A total of 53 tags were deployed and two models of PSATs were used: MiniPAT (39) and sPAT tags (14) (see Supplementary Material) built by Wildlife Computers.
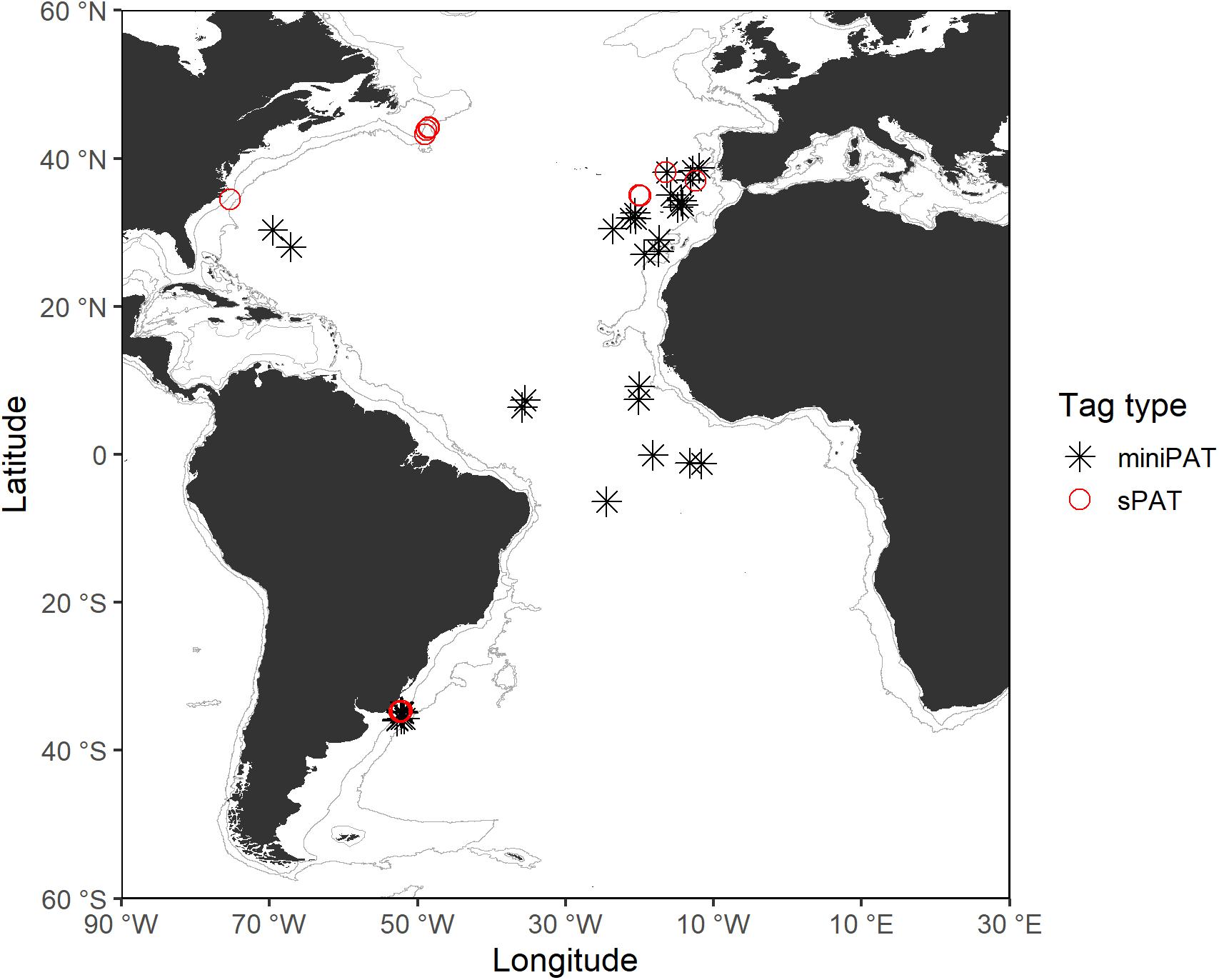
Figure 1. Tagging locations of shortfin makos tagged with PSATs in the Atlantic Ocean during this study. Gray lines represent the boundaries of continental and insular margins (140–3,500 m depth).
Data Analysis
Geographic positions at tagging were determined by GPS, while the pop-up locations of transmitting tags were established as the first point of transmission with an Argos satellite. In order to investigate movement patterns, the most probable tracks between tagging and pop-up locations were calculated from miniPATs light level data using astronomical algorithms provided by the tag manufacturer (Pedersen et al., 2011; Wildlife Computers, 2018). For the miniPATs, the daily locations were calculated based on the light levels recorded and using state-space statistical models (GPE3 software, processed through the tag manufacturer web portal). The miniPATs provide observations on twilight, temperature and dive depth, and the state-space modeling approach uses those observations and the corresponding reference data, along with a simple diffusion-based movement model, to generate time-discrete gridded probability surfaces throughout the deployment. The corresponding oceanographic reference data used were from the NOAA Optimum Interpolation SST V2 High Resolution Dataset for the sea surface temperature provided by NOAA/OAR/ESRL PSD, Boulder, CO, United States, from their web site at http://www.esrl.noaa.gov/psd/, and from the NOAA ETOP01 Global Relief Model, “Bedrock” version, for bathymetry (Amante and Eakins, 2009). The grids used were 0.25 × 0.25° of latitude × longitude. From those probability surfaces, the most likely animal locations for a given day/time were derived. In the case of sPATs, light sensors are not optimized for geolocation. Therefore, the distances traveled by the sharks tagged with sPATs were measured in straight lines between the tagging and the pop-up locations.
In order to assess the habitat use among different bathymetric areas, two main areas were considered, namely the continental margins vs. oceanic areas. The continental margins include both the shelf and slope areas, with the shelf defined as the areas where bottom depth is less than 140 m, and the slope defined as the areas wherever bottom depth ranges between 140 and 3,500 m. The oceanic area was considered as that where bottom depths exceed 3,500 m (IFREMER, 2009). The habitat use was then calculated as the number of occurrences recorded within each of those bathymetric areas. Additionally, the time spent within national EEZs vs. international waters was calculated by considering the number of recordings obtained for each area. The time spent within each bathymetric area (continental margins vs. oceanic area) was also calculated for the different size classes (110–140, 141–175, and 176–220 cm FL).
For sharks tagged with miniPATs, vertical habitat use was investigated by calculating the percentage of time-at-depth and time-at-temperature, separately for daytime and night-time. Sunset and sunrise were calculated using the library “RAtmosphere” in R, and took into account the date (Julian day), latitude and longitude (Teets, 2003). Time-at-depth and time-at-temperature data were aggregated into 30 m and 2°C bins, respectively, based on the above analyses. These data were subsequently expressed as a fraction of the total time of observation for each shark, and the fractional data bins averaged across all sharks within each category.
Mortality events were assigned in cases where depth profiles showed individuals rapidly sinking through the water column to depths greater than 1,700 m, thus initiating the tag safety release mechanism, earlier than 30 days after release. Depredation events were assigned in cases where no light changes were detected during a period of several days, with temperature profiles showing sudden increase in temperature that remained above the temperature values measured before ingestion, regardless of depth, until the tag was expelled/regurgitated (see Supplementary Material). In these cases, the tracks were estimated excluding all data collected after the depredation event, and the subsequent depth and temperature data were not considered in the habitat use analyses.
Depth and temperature data were tested for normality with Kolmogorov–Smirnov tests with Lilliefors correction and for homogeneity of variances with Levene tests. Given the lack of normality in the data and the heterogeneity of variances, time-at-depth and time-at-temperature were compared between the daily period (daytime vs. night-time), and bathymetric areas (continental margins vs. oceanic area) with non-parametric k-sample permutation tests. For this, a Monte Carlo approach was used with the data randomized and re-sampled 9,999 times to build the expected distribution of the differences under a random distribution, which was then used to determine the significance of the differences in the time-at-depth and time-at-temperature for the sample. Time-at-depth and time-at-temperature were also compared among size classes (110–140, 141–175, and 176–220 cm FL) with non-parametric Kruskal–Wallis tests.
Statistical analyses for this paper were carried out using the R language (R Core Team, 2020), with the following additional libraries: “car” (Fox and Weisberg, 2019), “ggplot2” (Wickham, 2016), “grid” (R Core Team, 2020), “maps” (Becker et al., 2013), “maptools” (Bivand and Lewin-Koh, 2020), “ncdf4” (Pierce, 2019), “nortest” (Gross and Ligges, 2015), “perm” (Fay and Shaw, 2010), “plotrix” (Lemon, 2006), “raster” (Hijmans, 2020), “scales” (Wickham and Seidel, 2020), “RColorBrewer” (Neuwirth, 2014), and “shapefiles” (Stabler, 2013). The analysis of habitat use among different bathymetric areas and distances traveled was carried out using QGIS 3.10.14 (QGIS.org, 2021).
Results
PSAT Tagging
Of the 53 tags deployed, only 16 tags detached on their scheduled pop-up date, which represented a premature release rate of ≈70%. Three tags failed to transmit any kind of data and 16 tags detached from the shark earlier than 20 days after being deployed. Premature release of these tags resulted largely from mortality events (14) but also from unknown causes (2). Additionally, two tags suffered early tag detachment (37 and 58 days after tag deployment) and were determined to be ingested by other fish, most probably as a result of a depredation attempt on the tagged sharks. Data collected with the 16 tags that suffered premature release earlier than 20 days after being deployed were excluded from the analysis. For the remaining 34 tags, a total of 1,877 tracking days was registered (1,578 tracking days for miniPATs and 299 tracking days for sPATs, with mean tracking duration of 66 and 30 days for miniPATs and sPATs, respectively) (see Supplementary Material).
Horizontal Movements and Spatial Distribution
The estimated most likely tracks of the 34 sharks monitored showed that shortfin makos ranged widely between 77°W–13°E and 44°N–41°S, and traveled approximate distances ranging from 19 to 8,931 km (see Figures 2, 3 and Supplementary Material).
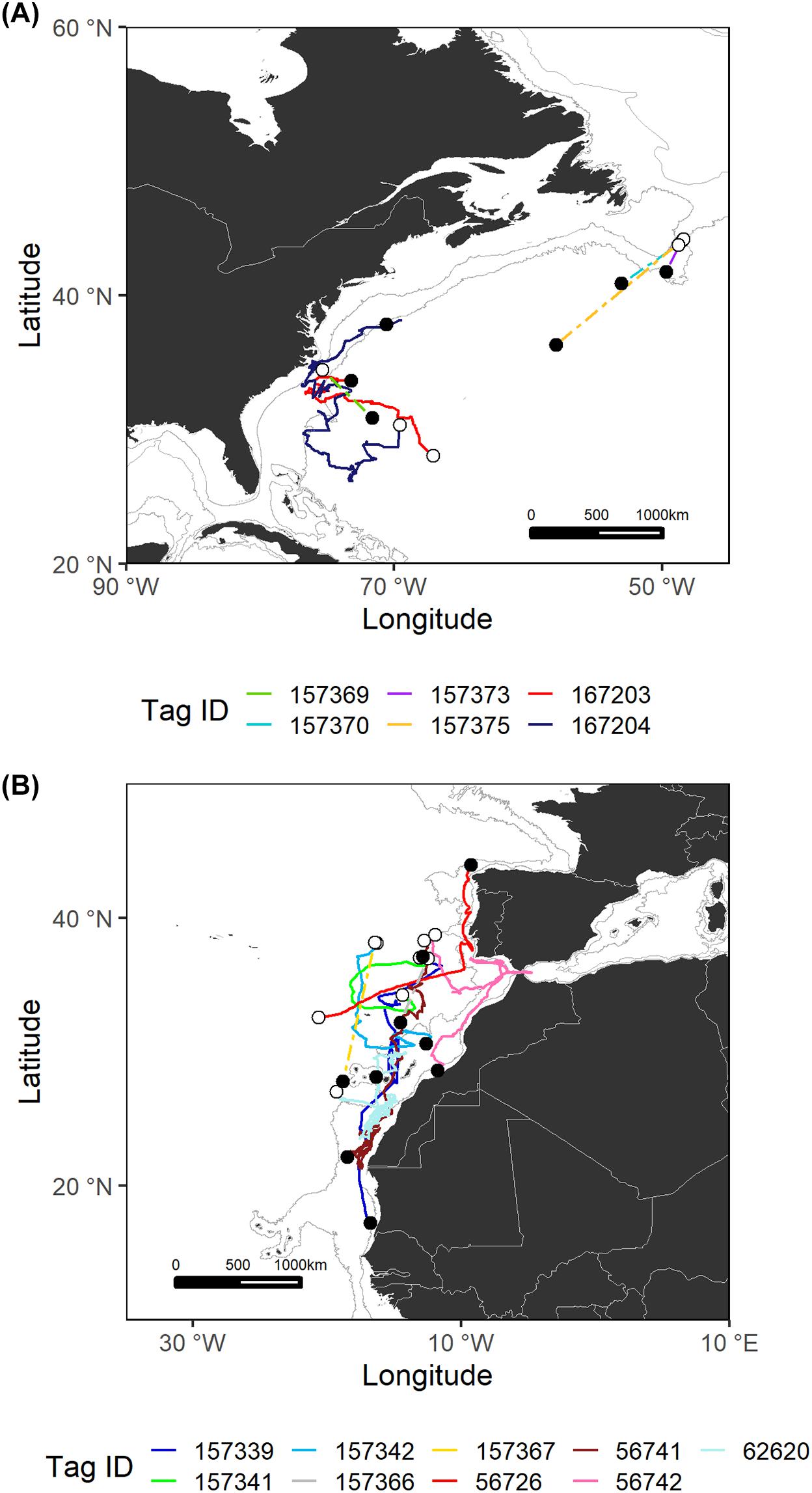
Figure 2. Estimated most likely tracks for each shortfin mako tagged in the Northwest Atlantic (A) and Northeast Atlantic (B) regions. The tagging locations are represented with open circles and the pop-up locations with black circles. Note that for sPATs only straight line tracks are shown (dashed lines). Gray lines represent the boundaries of continental and insular margins (140–3,500 m depth).
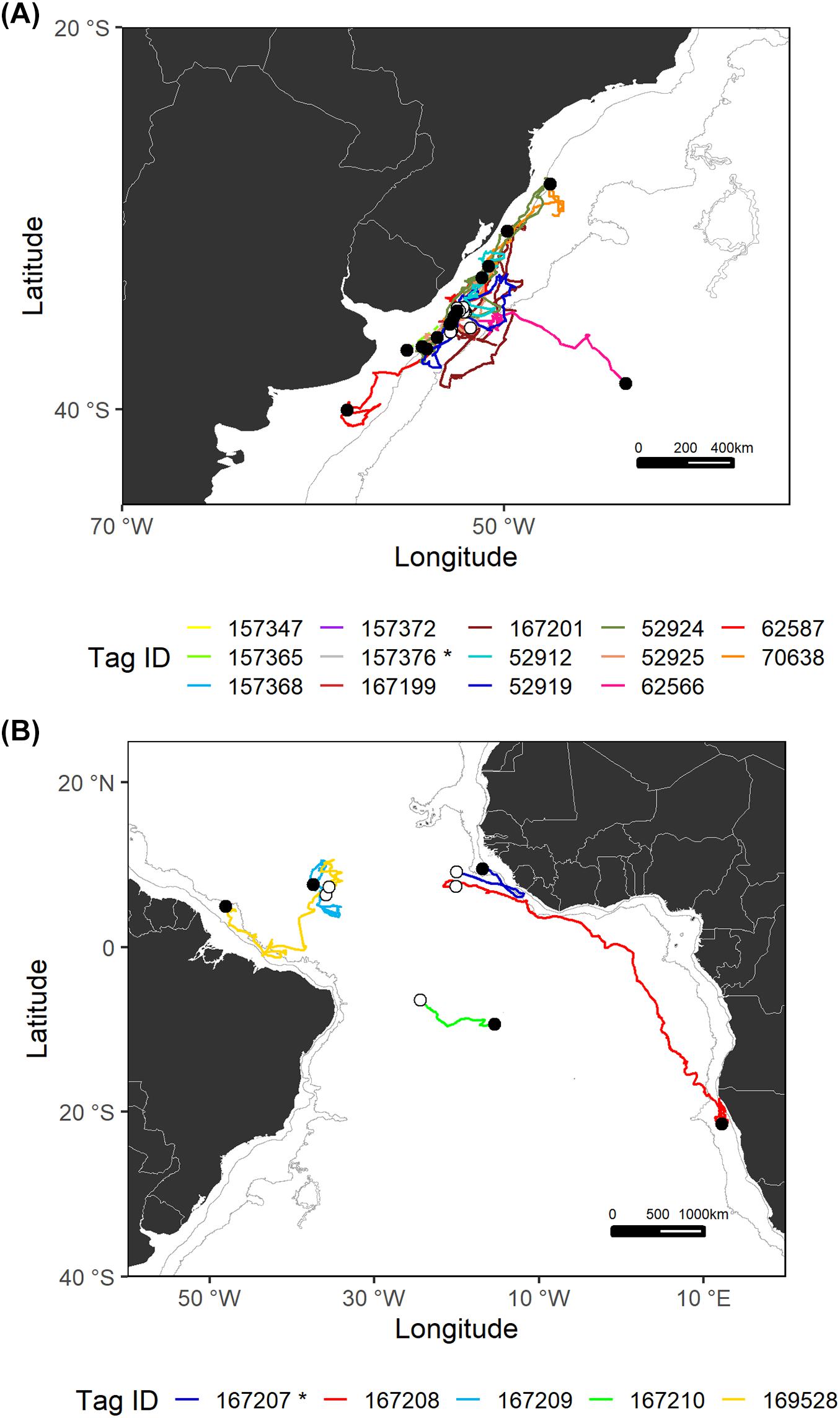
Figure 3. Estimated most likely tracks for each shortfin mako tagged in the Southwest Atlantic (A) and Central Atlantic (B) regions. The tagging locations are represented with open circles and the pop-up locations with black circles. The asterisk (*) in the legend indicates mature sharks. Note that for sPATs only straight line tracks are shown (dashed lines). Gray lines represent the boundaries of continental and insular margins (140–3,500 m depth).
Shortfin makos tagged with sPATs in the temperate Northwest Atlantic followed general southern trajectories (Figure 2). Distances traveled by these specimens were measured in straight lines between the tagging and pop-up locations and ranged from 278 to 1,191 km. The two shortfin makos tagged with miniPATs followed distinct paths. The shortfin mako with tag ID 167203 traveled more than 2,400 km, swam westward toward the shelf and slope waters off the northeastern United States and subsequently turned east into oceanic waters. Shark 167204 initially swam southward and then shifted its course moving north to continental shelf areas and approaching the United States eastern coast for a total of 5,684 km traveled.
In the Northeast Atlantic, the movement patterns recorded were diverse (Figure 2). Most shortfin makos tagged in the temperate Northeast Atlantic swam to southern tropical areas, while two of them (tag IDs 157341 and 56726) remained in temperate waters, moving toward higher latitudes. Shark 56726 was tagged in open waters and subsequently directed its movement toward the southwestern Portuguese coast, moving north afterwards, in an excursion that seemed to be oriented toward the Gulf of Biscay. Shark 157341 traveled about 1,500 km in offshore waters, following a general northward direction. The remaining shortfin makos made extensive journeys toward the shelf and slope waters of the Canary archipelago and the West African coast. Through the entire tracks, these sharks covered total distances ranging from 561 to 5,315 km.
In the Southwest Atlantic, sharks were tagged within the continental slope off Uruguay and subsequently tended to stay in the same general area, except for one specimen (tag ID 62566) that ventured east toward international waters after spending an initial period moving around within the same area (Figure 3). Shortfin makos tagged in this region appeared to reveal site fidelity and periods of residency concentrated near and within the continental margin. Specifically, these residency periods occurred over the continental shelf, slope, and adjacent waters off southern Brazil, Uruguay, and northern Argentina. Generally, resident behavior was followed and/or preceded by oscillatory patterns of movements parallel to the continent.
In general, sharks tagged in the central region of the Atlantic Ocean made long-distance movements from oceanic waters toward continental areas (Figure 3). Two of the longest movements recorded in this study were made by shortfin makos tagged in the central region, over a 4-month period. Shark 167208 swam south, crossing the Gulf of Guinea and reaching the Namibian EEZ where it remained for 4 weeks until the tag popped-up. This shark crossed hemispheres, in a trans-equatorial highly directional movement covering a total distance of more than 8,900 km. Shark 169528 swam 6,780 km, moving west and nearing the continental slope off northern Brazil.
The vast majority of analyzed tracks belong to juvenile sharks of both sexes; only two mature sharks were monitored. These sharks were presumed to be mature based on published 50% size-at-maturity data (males: 182 cm FL, females: 280 cm FL; Natanson et al., 2020). Both were male, tagged in the Southwest (tag ID 157376) and Central (tag ID 167207) Atlantic regions and followed the general trend to move within/toward the continental shelf.
Generally, sharks tagged in this study spent most of their time (≈56%) swimming within the shelf and slope areas. They spent more than 80% of their time inside national waters, crossing 17 different EEZs. In terms of high-use areas, probabilities of distribution of tagged shortfin makos were higher closer to the West African continental shelf and around the Canary archipelago and the south coastal region of the Iberian Peninsula. Similarly, the continental shelf and adjacent waters off Uruguay and south Brazil were also highly used areas where sharks tended to stay for longer periods (Figures 4, 5). This contrasts with oceanic waters of the Central Atlantic Ocean, where shortfin makos were also present but seemed to be mostly traveling and not staying for longer periods in any specific areas. The use of oceanic vs. continental shelf and slope areas varied among sharks of different size classes. Smaller sharks (110–140 cm FL) spent ≈56% of their time within shelf/slope areas, while medium-sized sharks (141–175 cm FL) spent ≈80% of their time within shelf/slope areas, and larger sharks (176–220 cm FL) swam mostly in oceanic waters, spending ≈28% of their time within shelf/slope areas. However, these differences may be related to the tagging locations of sharks.
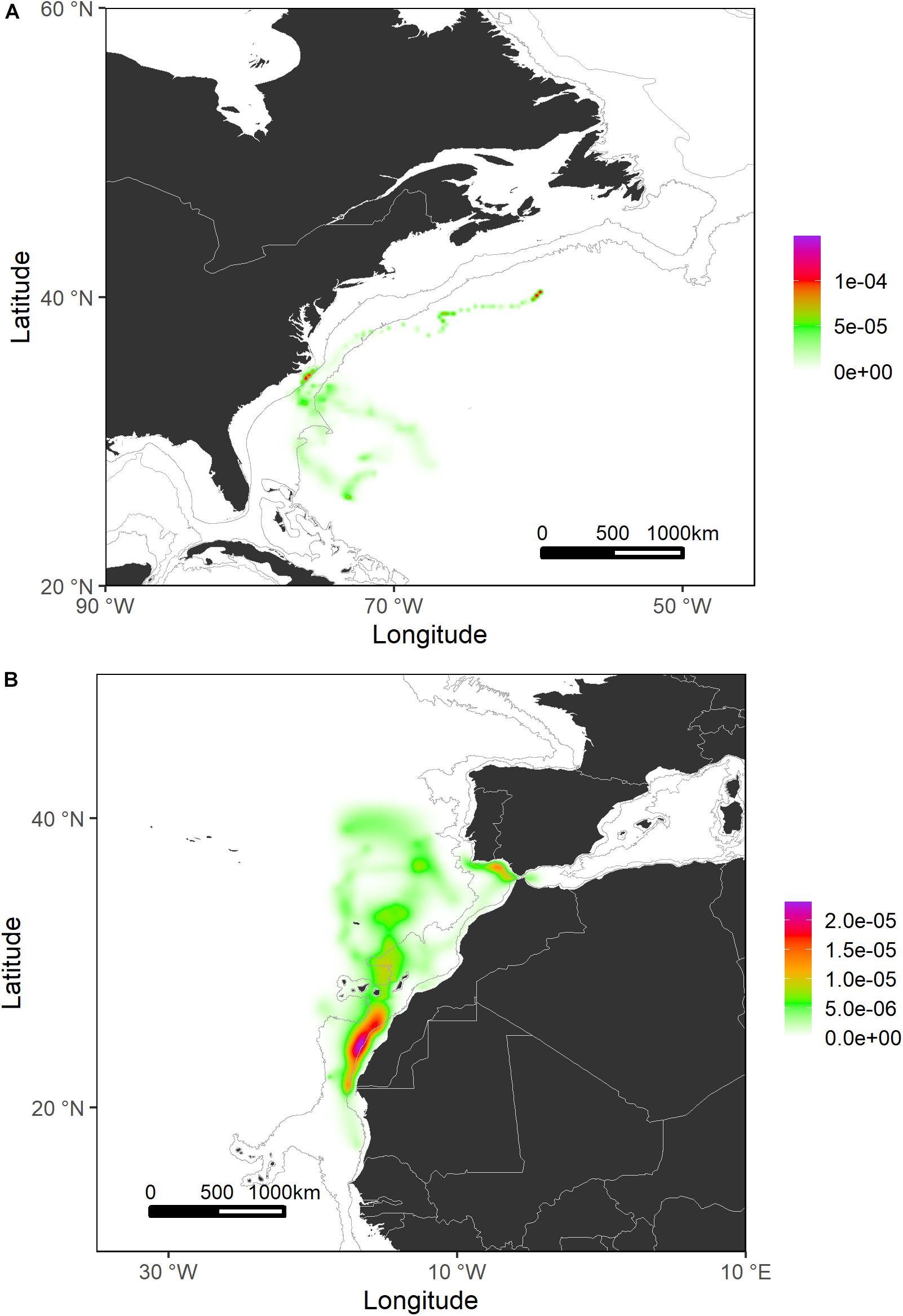
Figure 4. Probability surfaces of the spatial distribution of shortfin makos tagged in the Northwest Atlantic (A) and Northeast Atlantic (B) regions. Gray lines represent the boundaries of continental and insular margins (140–3,500 m depth).
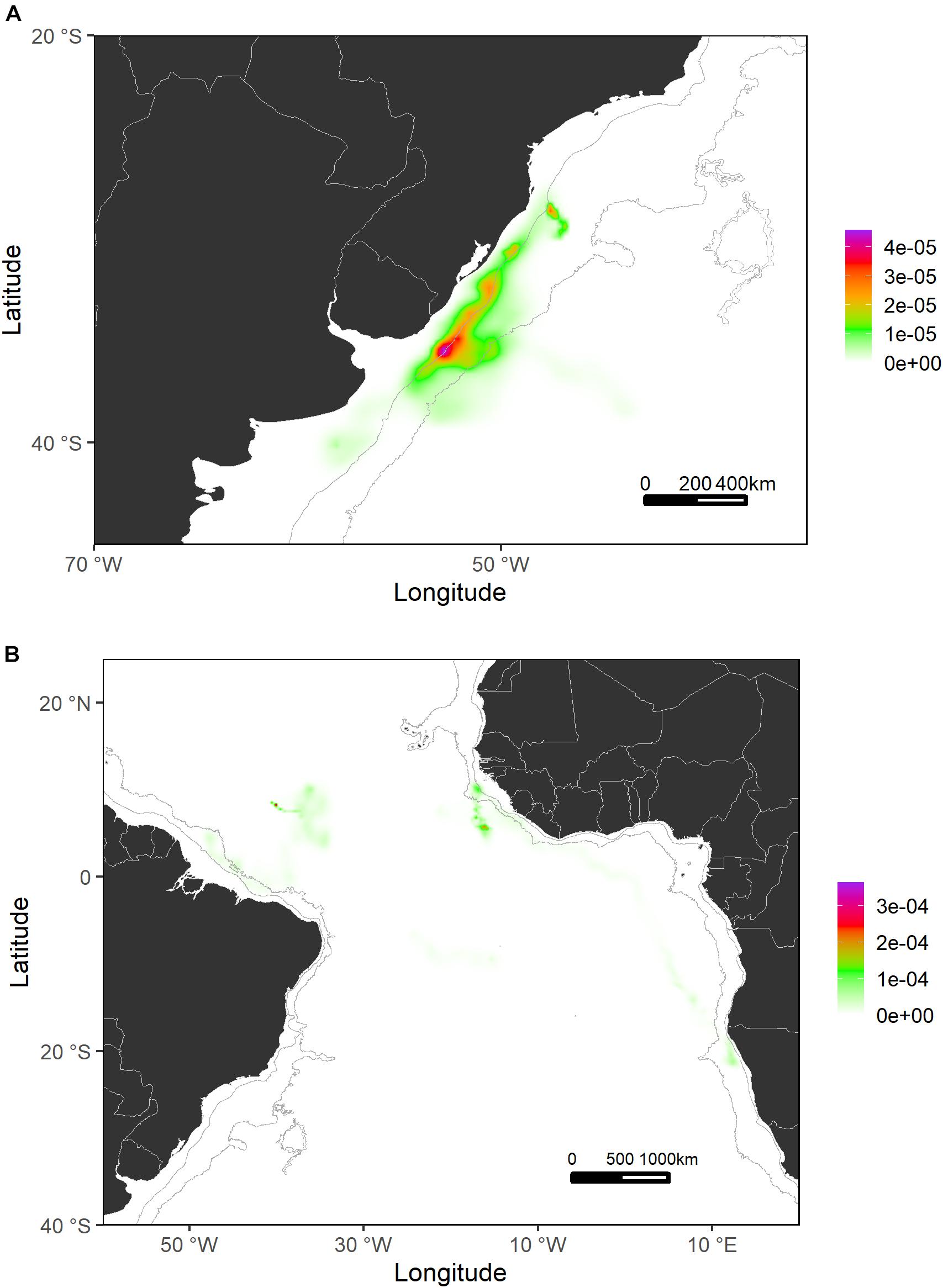
Figure 5. Probability surfaces of the spatial distribution of shortfin makos tagged in the Southwest Atlantic (A) and Central Atlantic (B) regions. Gray lines represent the boundaries of continental and insular margins (140–3,500 m depth).
Vertical Habitat Use
The tagged shortfin makos swam through a depth range from the surface down to 979.5 m, in water temperatures that ranged between 7.4 and 29.9°C. However, sharks spent the largest proportion of their time in depths above 90 m, in water temperatures ranging from 18 to 22°C (mean depth = 68.5 m, SD = 78.1 m; mean temperature = 19.4°C, SD = 3.1°C) (Figure 6). These vertical habitat preferences were displayed during both day and night-time, although significant differences were found when comparing habitat use between day and night (depth: permutation test differences = 21.7 m, p-value < 0.001; temperature: permutation test differences = −1.1°C, p-value < 0.001). Differences in vertical habitat use between day and night were less notable when sharks swam in shelf and slope areas (daytime: mean depth = 63.6 m, SD = 87.8 m; night-time: mean depth = 49.1 m, SD = 41.1 m) compared to when they used oceanic waters, where they occupied greater mean depths during daytime and night-time (daytime: mean depth = 121.1 m, SD = 97.5 m; night-time: mean depth = 72.3 m, SD = 65.4 m) (Figure 7). Vertical habitat use varied among sharks of different size classes (Kruskal–Wallis tests, p-value < 0.001). Smaller sharks (≤140 cm FL) spent more time in slightly cooler waters than larger individuals (110–140 cm FL: mean temperature = 19.0°C, min = 7.4°C, max = 28.1°C; 141–175 cm FL: mean temperature = 19.7°C, min = 7.5°C, max = 25.2°C; 176–220 cm FL: mean temperature = 19.7°C, min = 8.1°C, max = 29.9°C) (Figures 8–10). Larger shortfin makos (≥176 cm FL) occupied greater mean depths (110–140 cm FL: mean depth = 83.1 m, min = 0.5 m, max = 490.5 m; 141–175 cm FL: mean depth = 58.7 m, min = 0 m, max = 979 m; 176–220 cm FL: mean depth = 90.4 m, min = 0 m, max = 740 m) compared to smaller- and especially medium-sized specimens (Figures 8–10). These differences may be related to the tagging locations of sharks.
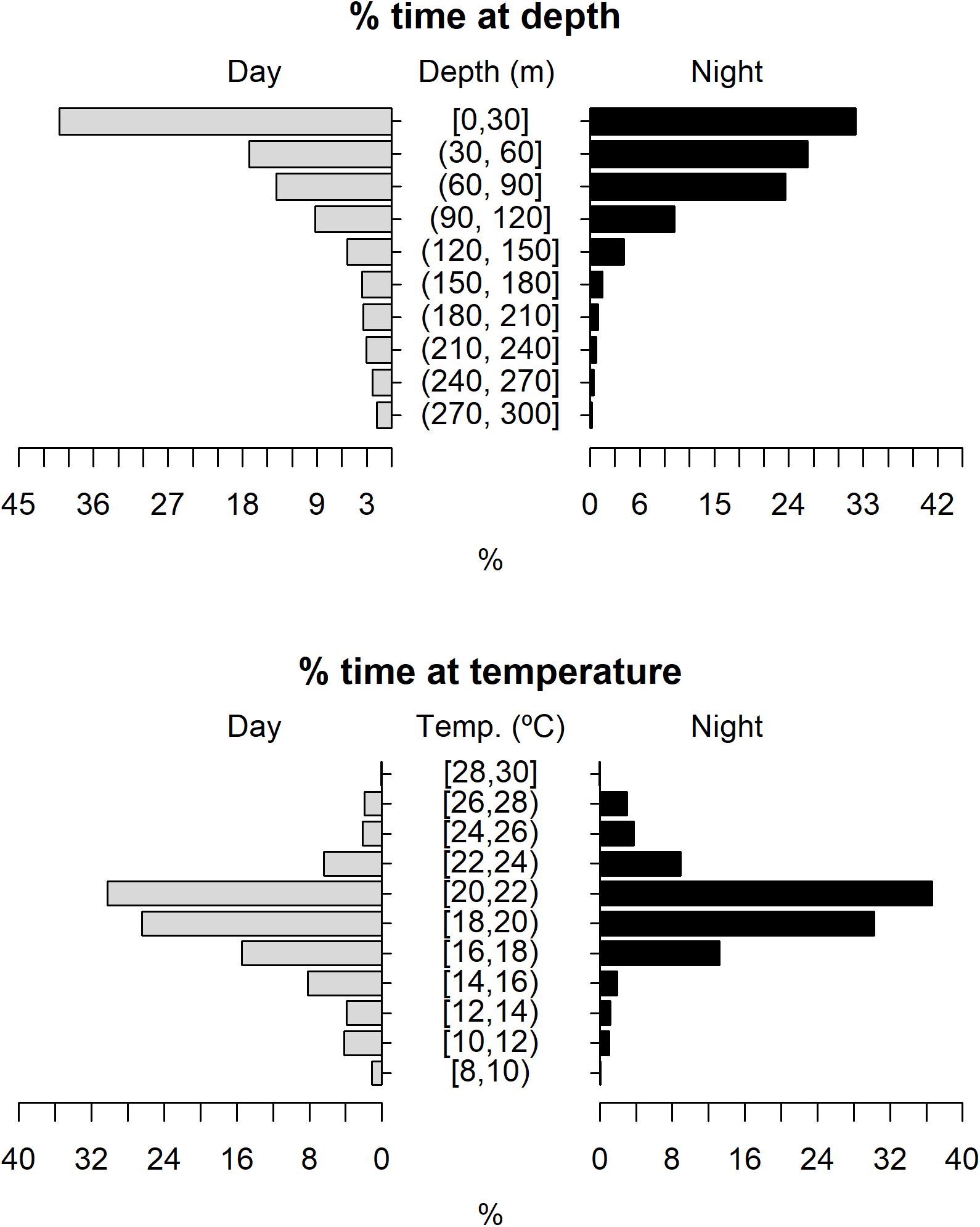
Figure 6. Vertical habitat use of shortfin makos for daytime and night-time in terms of depth and temperature. Depth classes are categorized in 30 m intervals and temperature classes in 2°C intervals.
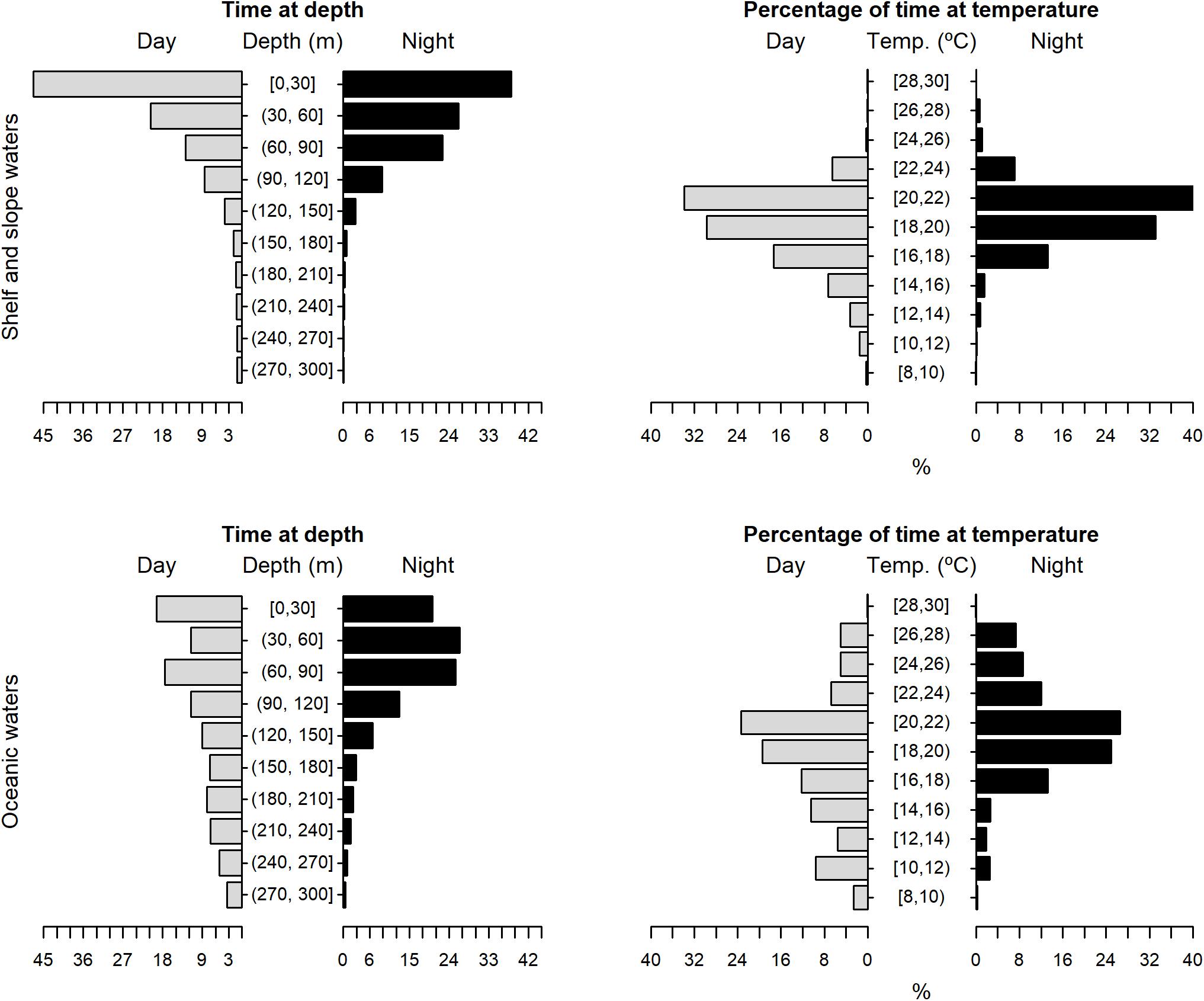
Figure 7. Vertical habitat use of shortfin makos when using shelf and slope waters (top plots) vs. oceanic waters (bottom plots), for daytime and night-time in terms of depth and temperature. Depth classes are categorized in 30 m intervals and temperature classes in 2°C intervals.
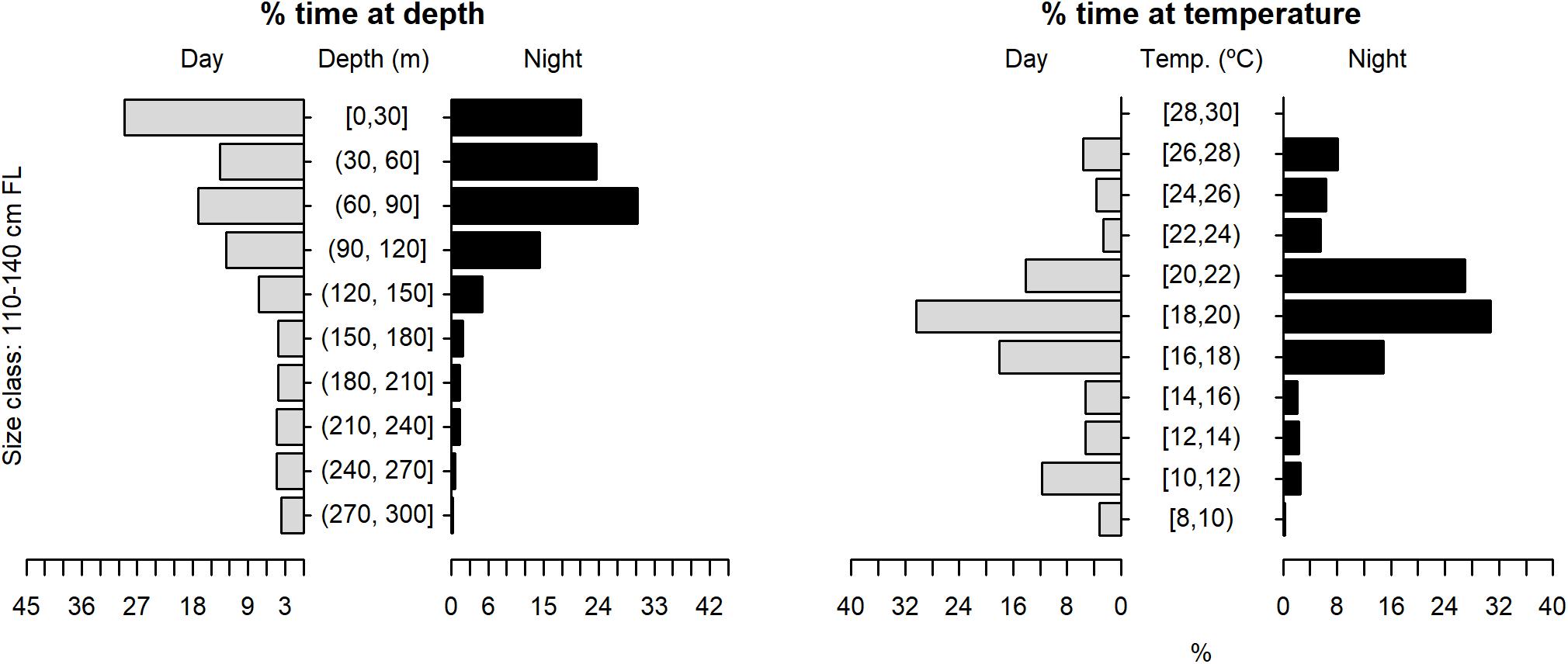
Figure 8. Vertical habitat use of shortfin makos ranging in size from 110 to 140 cm FL, for daytime and night-time in terms of depth and temperature. Depth classes are categorized in 30 m intervals and temperature classes in 2°C intervals.
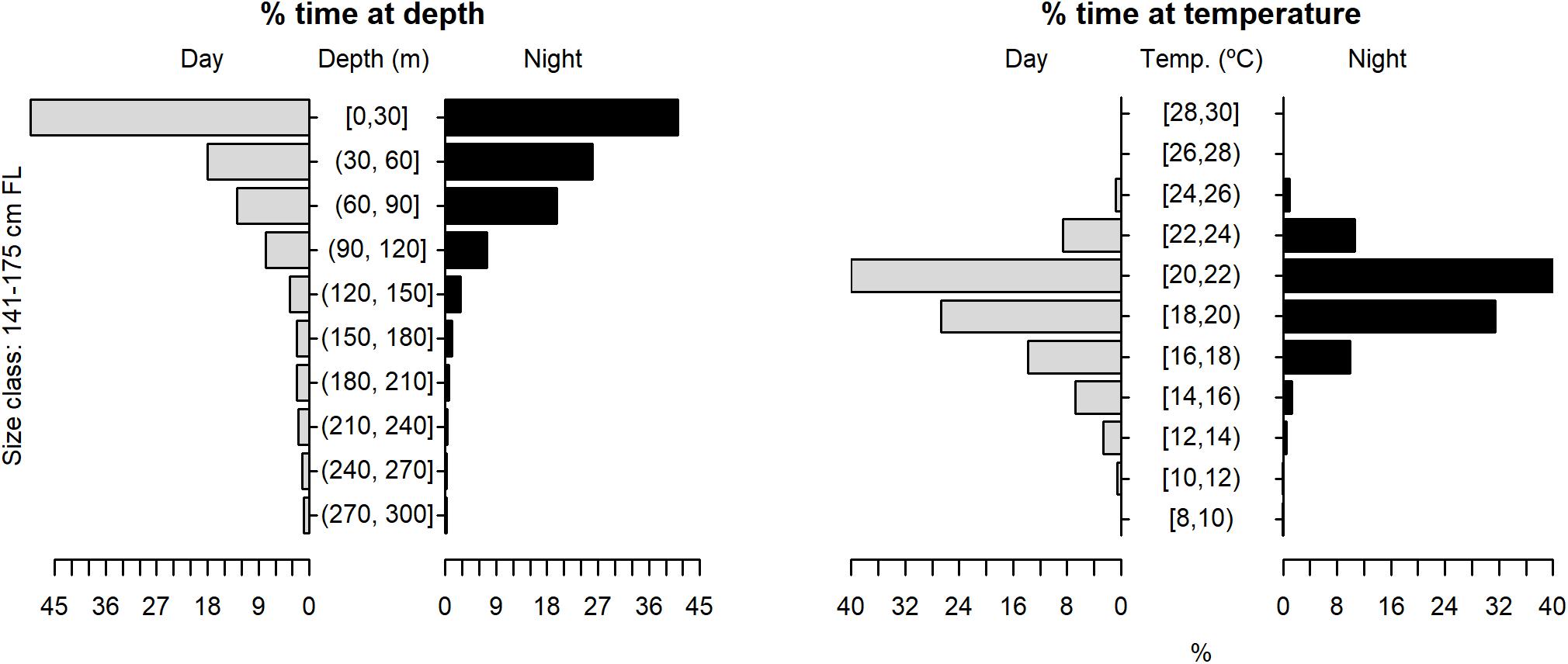
Figure 9. Vertical habitat use of shortfin makos ranging in size from 141 to 175 cm FL, for daytime and night-time in terms of depth and temperature. Depth classes are categorized in 30 m intervals and temperature classes in 2°C intervals.
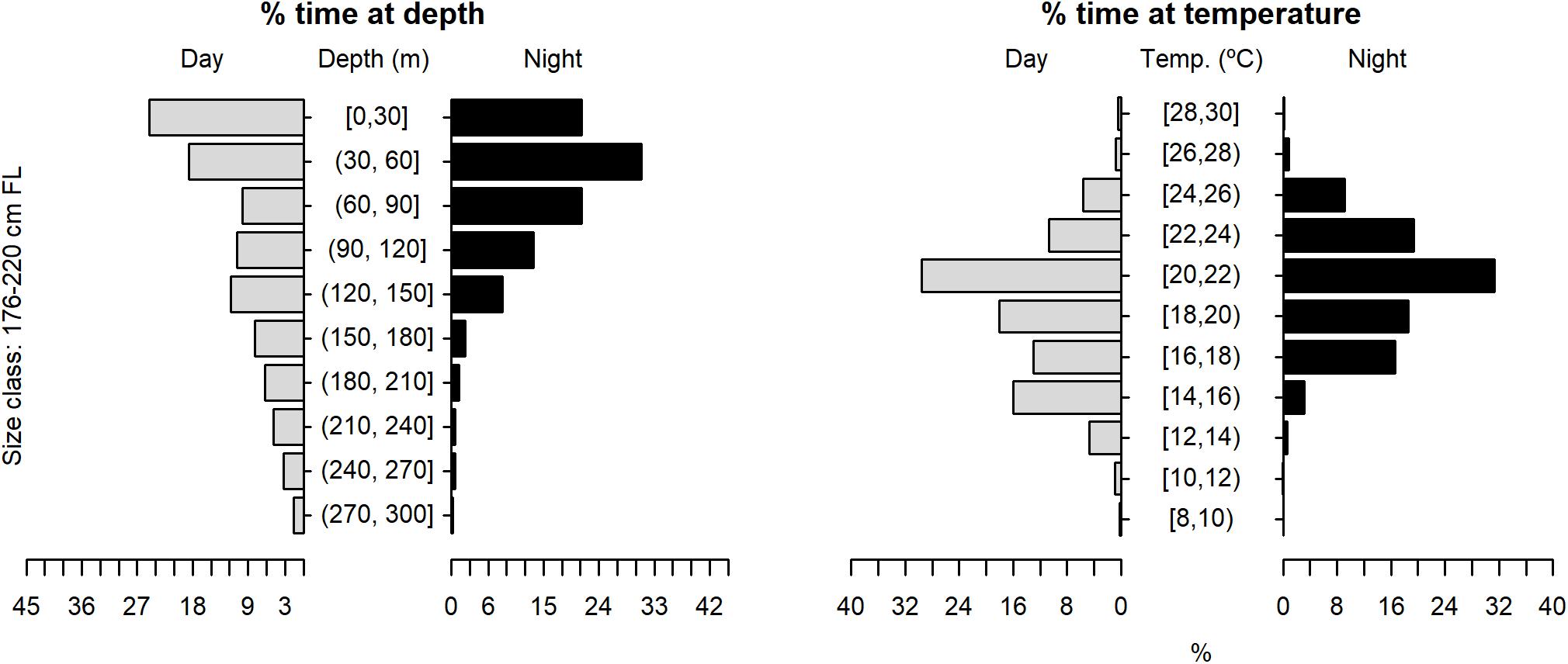
Figure 10. Vertical habitat use of shortfin makos ranging in size from 176 to 220 cm FL, for daytime and night-time in terms of depth and temperature. Depth classes are categorized in 30 m intervals and temperature classes in 2°C intervals.
Diel behavior varied considerably among sharks. While some individuals exhibited a clear diel cyclicity defined by shallower mean depths during the night and greater mean depths during daily hours, most sharks showed an oscillatory swimming behavior characterized by continuous movements up and down the water column (yo-yo diving) with some occasional deep dives followed by rapid ascents (Figure 11). Diel vertical movements were exhibited by sharks that spent greater periods of time in oceanic waters, namely those tagged in the Central and Northwest Atlantic. Sharks that mostly swam in shelf and slope waters, like the shortfin makos tagged in the Southwest and Northeast regions of the Atlantic, tended to demonstrate oscillatory swimming behavior.
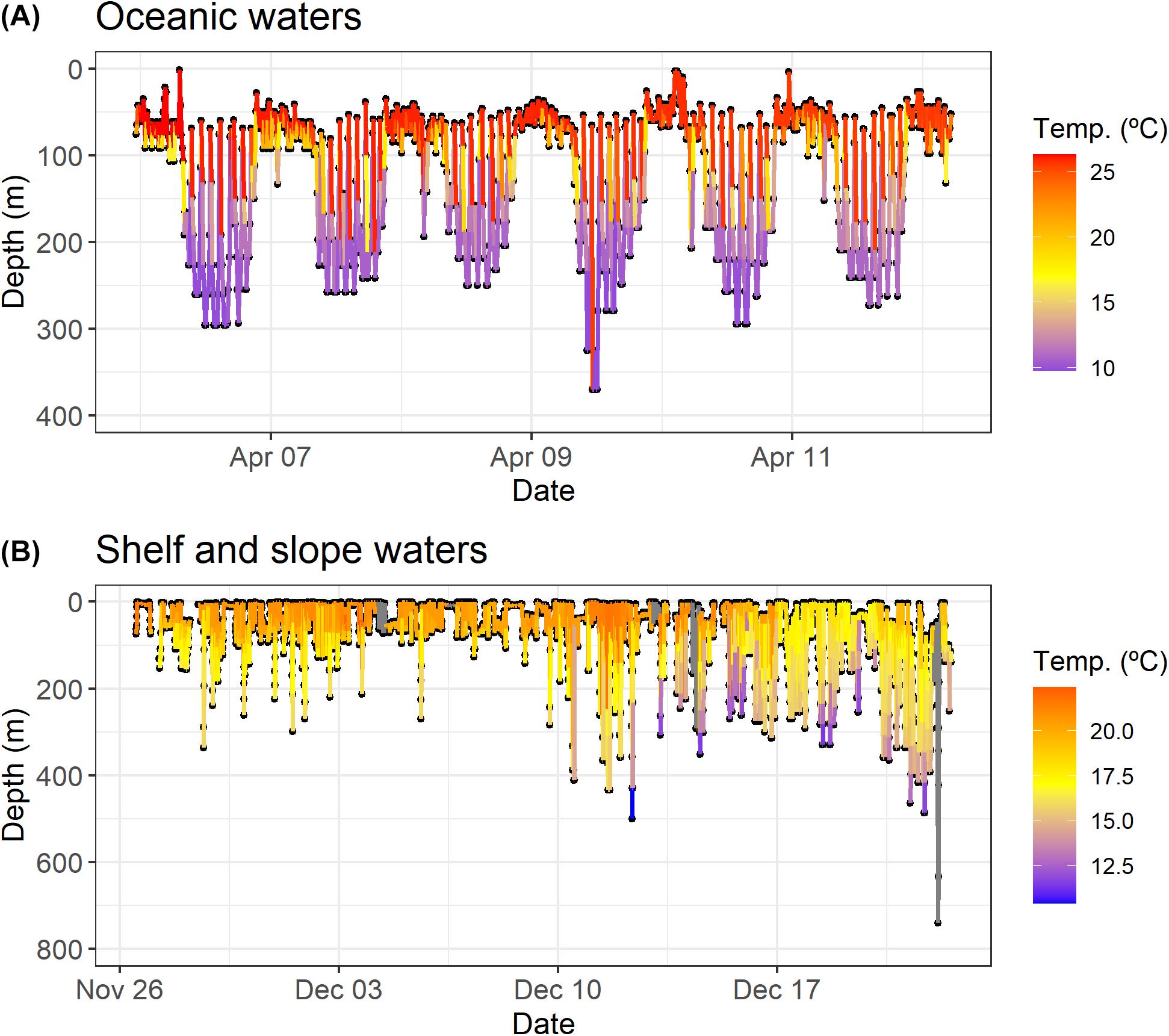
Figure 11. Details of diving behavior profiles of shortfin makos. The plot on the top (A) (shark with tag ID 169528) represents the diel vertical behavior. The plot on the bottom (B) (shark with tag ID 52925) shows the oscillatory diving (yo-yo diving) behavior, with occasional deep dives.
Discussion
Worldwide, overfishing has had severe effects on marine ecosystems (Stevens et al., 2000; Myers et al., 2007; Ferretti et al., 2010; Pershing et al., 2010). Direct targeting and incidental bycatch of apex fish predators by commercial fishing fleets has negatively impacted their stocks, including those of pelagic sharks, in most regions of the world (Dulvy et al., 2008; Pacoureau et al., 2021). Due to their life history traits that result in low intrinsic rates of population increase, poor management, and low conservation priority, pelagic sharks are among the most vulnerable species to overexploitation (Camhi et al., 2008; Dulvy et al., 2008; Cortés et al., 2010). Increasing fishing pressure on shortfin makos in recent decades has raised concerns over their conservation, with the latest projections indicating alarming signs of population depletion in the North Atlantic Ocean (Anon, 2017). For this reason, understanding habitat preferences and identifying migratory routes that might provide insight on the existence of possible critical habitats, such as mating grounds and nursery areas, or areas that may be considered as separate fisheries management units, is crucial to ensure effective management measures and successful conservation strategies.
Here we present the most extensive record of movements of shortfin makos tagged with PSATs in the Atlantic Ocean to date, by tagging juvenile specimens of both sexes and two mature males. No mature females were tagged, and sex was undetermined for seven specimens. The main constraint in the present study was the premature detachment of PSATs. Of the 53 tags deployed, only 16 detached on their scheduled pop-up date, representing a premature release rate of ≈70%. This percentage is close to the average rate of 66% found in a review of shark satellite tagging studies by Hammerschlag et al. (2011). The causes of early tag detachment and tag failure are still not well understood and there may be several explanations including biofouling, mechanical failure of critical tag components, human error, battery failure, and tag damage (Hays et al., 2007; Musyl et al., 2011). Additionally, as one of the fastest sharks, premature release in shortfin mako may be caused by high-burst swimming events that could weaken the anchorage and cause early detachment of the tag. Hammerschlag et al. (2011) stated that the method used for PSAT deployment, which consists of inserting the tag in the shark skin using a dart anchor, is highly conducive to tag shedding, which may explain the high premature release rate of this type of tags. By contrast, SPOT tags (smart position and temperature tags) have demonstrated the capacity to stay attached to sharks for much longer periods. These tags are usually mounted to the dorsal fin of sharks using bolts and nuts, and as such are less conducive to rapid shedding. When comparing studies on shortfin mako movements, studies that used SPOT tags (e.g., Vaudo et al., 2017; Francis et al., 2019; Gibson et al., 2021) generally reported much longer tracks than studies using PSATs (e.g., Stevens et al., 2010; Abascal et al., 2011; Vaudo et al., 2016). Here we used the same Domeier umbrella anchor used in some of the tags deployed in Vaudo et al. (2016). In that study, two out of four tags suffered premature release, although mean tracking days (132 days) was higher than that reported in our study (30–66 days). In Vaudo et al. (2016), the remaining tags were attached using a stainless-steel M dart and all suffered premature release (mean tracking days = 15). Abascal et al. (2011), which used the same stainless-steel M dart, stated that premature detachment of tags was found to be the main problem in the study (premature release rate of 87.5%; mean tracking days = 53). In Stevens et al. (2010), of seven PSATs deployed either on blue, shortfin mako, thresher, or bigeye thresher sharks, only one reached its pop-off date (mean tracking days = 58). Those tags were attached using a stainless-steel Floy tag-anchor. High premature release rates of PSAT tags and consequent short tracking periods limit the ability to determine seasonal patterns of habitat use, contrary to SPOT tags which frequently report tracking periods of >1 year and therefore can be used to assess movement seasonality. However, since SPOT tags are not equipped with depth sensors, they are not suitable for examining vertical behavior of fish. Similarly to what was reported for several large pelagic species (Kerstetter et al., 2004; Hoolihan et al., 2014; Cosgrove et al., 2015), two early tag detachments detected in this study were probably the result of depredation events by other sharks, as indicated by sudden increases in temperature which remained stable regardless of depth, and low light level values which demonstrate that the tag was in darkness.
Our data confirmed the wide geographic distribution of shortfin mako in the Atlantic Ocean (Compagno, 2001; Cortés et al., 2010). Shortfin makos tagged in this study moved in multiple directions, usually traveling long distances between oceanic waters and waters within the continental shelf and slope. These extensive movements are consistent with previous studies that also demonstrated the highly migratory nature of this species (Casey and Kohler, 1992; Abascal et al., 2011; Vaudo et al., 2017; Queiroz et al., 2019; Coelho et al., 2020; Gibson et al., 2021). A previous conventional tag-and-recapture study by Casey and Kohler (1992) suggested that movements of shortfin mako in the Northwest Atlantic Ocean were seasonal and largely influenced by sea surface temperature. In Vaudo et al. (2017) shortfin makos displayed considerable variability in movements, with seven sharks tagged off the United States making long-distance, highly directional southern excursions into less productive subtropical/tropical waters before returning north. More recently, Gibson et al. (2021) reported high use of the Gulf of Mexico area and extensive seasonal migrations of mature male shortfin makos toward the Caribbean Sea and the northeastern United States Atlantic coast beginning in the late summer-early fall. These movements differed from those undertaken by the two shortfin makos in our study tagged with miniPATs in the Northwest Atlantic (tag IDs 167203 and 167204), which did not approach the Gulf of Mexico region and followed general north-oriented movements toward shelf and slope waters off eastern United States starting in late winter. Our data revealed that for shortfin makos tagged in the Northeast and Central Atlantic regions, except for a couple of sharks, horizontal movements tended to be oriented toward shelf and slope waters. Coelho et al. (2020) obtained similar results, with most shortfin makos tagged in the Cabo Verde EEZ traveling toward areas closer to the African shelf. Finally, sharks tagged in the Southwest area off Uruguay appeared to demonstrate fidelity to the more nutrient-rich waters of the Subtropical Convergence Zone. This area is characterized by the confluence of two water masses with contrasting features: the warm Brazil Current and the cold Malvinas/Falkland Current. In addition to this water-mixing process, discharges from the Rio de la Plata make the Subtropical Convergence Zone a highly productive ecosystem with capacity to sustain high trophic levels (sharks, tunas, seabirds, sea turtles, and marine mammals) (Acha et al., 2004; Domingo et al., 2009; Jiménez et al., 2011; Pons and Domingo, 2013; Passadore et al., 2014; Gaube et al., 2017). The reasons for these horizontal movements are not entirely known but may suggest an ability to discriminate among areas of particular significance for foraging or reproduction purposes (Heupel et al., 2007; Petersen et al., 2007; Block et al., 2011).
Generally, shortfin makos tracked in the present study made excursions toward the continental shelf and slope. The shelf and slope waters of the Subtropical Convergence Zone, the Canary archipelago and the northwestern African continental shelf seemed to be areas of particular importance for the species. Previous studies reported the aggregation of immature sharks around these areas, suggesting that they may serve as nursery grounds. Coelho et al. (2018) documented high density of smaller-sized blue sharks in temperate Southwest waters off southern Brazil and Uruguay, which would represent one of the main nursery grounds for that species in the South Atlantic Ocean. For shortfin mako, Vooren et al. (2005) and Costa et al. (2002) reported the presence of neonates and pregnant females off southern Brazil. Moreover, the hypothesis of a nursery ground off Northwest Africa and Portugal is supported by the occurrence of neonate and young-of-the-year shortfin makos reported in Maia et al. (2007) and Natanson et al. (2020). Here, the presence of two neonates tagged off the Canary archipelago (tag IDs 62420 and 62621) reinforces the importance of this region for parturition. Additionally, horizontal movements might be driven by feeding events. For instance, the shortfin mako with tag ID 167208 swam for more than 8,900 km from the Central Atlantic Ocean toward shelf and slope waters off northern Namibia, remaining in the area for nearly 1 month until tag detachment. This region is included within the Benguela marine ecosystem, one of the most productive marine systems in the world, which attracts many top predators seeking food (Petersen et al., 2007; Santos and Coelho, 2018). Interestingly, this shark moved from northern to southern hemisphere, which may question the current North–South Atlantic (separated by the 5°N) division of stocks used for all pelagic sharks by ICCAT.
Tagged shortfin makos spent their time from the surface down to 979.5 m depth, in temperature ranges of 7.4–29.9°C, although most of the time they were in depths above 90 m, in water temperatures ranging from 18 to 22°C. When sharks used oceanic environments they occupied greater mean depths during daytime and night-time. Information on habitat preference of shortfin mako in the Atlantic Ocean is limited; however, our results are consistent with those previously reported for this species. In Loefer et al. (2005), tag data of one specimen captured off the southeastern United States indicated a depth range of 0–556 m in temperatures between 10.4 and 28.6°C. Vaudo et al. (2016) reported that shortfin makos tagged off the northeastern United States and Gulf of Mexico experienced temperatures between 5.2 and 31.1°C, swimming through a depth range from near the surface down to 866 m. In Gibson et al. (2021) shortfin makos frequented a wide range of sea surface temperatures ranging from 10.0 to 31.0°C. Casey and Kohler (1992) indicated that in the North Atlantic the preferred surface water temperature of shortfin mako appeared to lie in a narrow range between 17 and 22°C. Body size has been suggested to influence the vertical distribution of shortfin mako, with larger sharks showing a greater tolerance to cooler waters than smaller individuals (Vaudo et al., 2016). In this study, mean temperature recorded for smaller individuals was slightly lower than those recorded for medium- and larger-sized sharks. Moreover, smaller individuals spent more time in cooler waters compared to medium and larger-sized sharks, revealing they are able to occupy a broad range of thermal habitats.
In terms of diel movement patterns, we found that swimming behavior differed among sharks. Sharks tagged in open waters of the Central and Northwest regions tended to exhibit diel vertical movements, characterized by shallower mean depths during the night and greater mean depths during daytime. Vaudo et al. (2016) obtained similar results, with sharks showing diel diving behavior with deeper dives occurring primarily during daytime. In Loefer et al. (2005), the specimen tagged also demonstrated a diel pattern of vertical movement defined by greater mean depths and larger depth ranges during daylight hours. The results of both studies suggested that vertical movements of shortfin mako were strongly influenced by water temperatures, which appeared to be the major influence on the movement ecology of the species. The differences between day and night behavior have also been described as a foraging strategy to locate and remain near prey (Sepulveda et al., 2004; Vetter et al., 2008).
Most sharks tagged in the present study, namely those tagged in the Southwest and Northeast Atlantic regions which mostly used shelf and slope waters, showed a vertical oscillatory swimming behavior. These sharks constantly moved up and down in the water column, with some occasional deep dives followed by rapid ascents. In the Southeastern Pacific Ocean, a study on environmental preferences of shortfin mako by Abascal et al. (2011) reported that sharks did not exhibit any clear diel cyclicity, but mean vertical distribution was deeper during daytime. These sharks also displayed bounce dives, which the authors associated with feeding events. Deep diving behavior has been suggested to be related with foraging ecology of other pelagic sharks, including blue shark, tiger shark, Galeocerdo cuvier, scalloped hammerhead, Sphyrna lewini, bigeye thresher, Alopias superciliosus, and oceanic whitetip, Carcharhinus longimanus (Stevens et al., 2010; Nakamura et al., 2011; Hoffmayer et al., 2013; Howey-Jordan et al., 2013; Coelho et al., 2015). Additionally, the most widely proposed reason for this behavior has been attributed to warming the body after heat loss during descents into cooler water (Klimley et al., 2002). However, like other lamnid sharks, the shortfin mako is an endothermic species that is able to maintain body temperatures above the surrounding seawater temperature (Carey et al., 1981). Other functions proposed for yo-yo diving are related to energy conservation through a fly-glide swimming strategy, orientation, and the use of chemical and magnetic information to guide migrations (Klimley et al., 2002; Iosilevskii et al., 2012).
Conclusion
Overall, our findings for the shortfin mako confirmed its capacity to inhabit temperate and tropical waters, over broad depth and temperature ranges. Nevertheless, sharks spent most of their time in depths above 90 m, in water temperatures ranging from 18 to 22°C. Our results suggested two different patterns of diving activity which seemed to be influenced by the proximity to the continents. Individuals that spent greater periods of time in oceanic waters demonstrated diel cyclicity, staying in shallower waters during the night, while sharks that swam in shelf and slope waters tended to display yo-yo diving behavior with some occasional deep dives. Furthermore, satellite-tagged shortfin makos showed considerable variability in horizontal movements, but generally tended to move toward or remain over shelf and slope waters of the Southwest Atlantic Subtropical Convergence Zone, the Canary archipelago and West Africa. These movements challenge the typical view of shortfin makos being mostly oceanic nomads and hint at the importance of these continental margin areas for the species. Despite the existing relationship between habitat use and tagging locations, it is interesting to highlight that sharks spent most of their time in national EEZ waters moving across the jurisdictional management borders of several nations and the high seas, which emphasizes the necessity for international coordination efforts to effectively apply the required management actions. Moreover, the evidence of shark movements across hemispheres may question the current north–south division of stocks in the Atlantic. Finally, the apparent residency of those sharks in some areas, such as in the Southwest Atlantic, might help to better delineate and refine management unit areas for the species. This should be done in tandem with ongoing population genetic studies being carried out within ICCAT, whose preliminary results have suggested, for example, a unique genetic structure of shortfin makos off Uruguay in the Southwest Atlantic (ICCAT, 2018).
The results of this study provide a better understanding of shortfin mako movements and activity patterns in the Atlantic Ocean and confirm that satellite tagging is an important tool that can provide valuable information on spatial ecology of data-limited species. We expect that similar studies will continue to be carried out to elucidate ecological aspects and determine the drivers of movement patterns of these animals, as well as to provide insight on movement differences between sexes and/or maturity stages, ultimately contributing to the provision of sustainable management and conservation measures.
Data Availability Statement
Data from ICCAT tags used in the paper can be made available upon request to ICCAT Secretariat, following the rules and procedures for the protection, access to, and dissemination of data compiled by ICCAT. Data from other tags used in the paper can be made available upon reasonable request to the authors.
Ethics Statement
The animal study was reviewed and approved by ICCAT Standing Committee on Research and Statistics.
Author Contributions
AD, EC, and RC contributed to the conception and design of the study. AD, JC, LN, PT, DM, PM, FH, FM, JO, and RC organized and led the fieldwork, and undertook data collection. CS, RC, PM, and PL undertook analyses. CS led the writing of the manuscript with revisions and assistance from all other authors. All authors contributed to the final article and approved the submitted version.
Funding
This study was carried out as a part of a collaborative work conducted by the ICCAT Shark Species Group integrated in the ICCAT Shark Research and Data Collection Program (SRDCP). This work was funded by ICCAT as a part of the regular budget and by contributions from individual Contracting Parties, particularly the European Union through the EU Grant Agreements with ICCAT on “Strengthening the scientific basis for decision-making in ICCAT” and the “ICCAT Science envelope.” Additional satellite tags were acquired by Project “MAKOWIDE – A wide scale inter-hemispheric and inter-disciplinary study aiming the conservation of the shortfin mako shark in the Atlantic Ocean (Ref: FAPESP/19740/2014),” funded by FCT (Portuguese Foundation for Science and Technology), and there was also support from FCT through project UIDB/04326/2020. CS was supported by an FCT Doctoral grant (Ref: SFRH/BD/139187/2018). The authors thank NOAA/NMFS/SEFSC for providing funds to publish this article as open access.
Conflict of Interest
The authors declare that the research was conducted in the absence of any commercial or financial relationships that could be construed as a potential conflict of interest.
Publisher’s Note
All claims expressed in this article are solely those of the authors and do not necessarily represent those of their affiliated organizations, or those of the publisher, the editors and the reviewers. Any product that may be evaluated in this article, or claim that may be made by its manufacturer, is not guaranteed or endorsed by the publisher.
Acknowledgments
We are grateful to all fishery observers and longline skippers from the Nations involved in this study. We would like to dedicate this study to FH, who passed away during the late stage revision process of this manuscript. The premature departure of our colleague FH leaves the fisheries and marine biology and ecology community poorer. His legacy and dedication to research will always be remembered.
Supplementary Material
The Supplementary Material for this article can be found online at: https://www.frontiersin.org/articles/10.3389/fmars.2021.686343/full#supplementary-material
References
Abascal, F. J., Mejuto, J., Quintans, M., García-Cortés, B., and Ramos-Cartelle, A. (2015). Tracking of the broadbill swordfish, Xiphias gladius, in the central and eastern North Atlantic. Fish. Res. 162, 20–28. doi: 10.1016/j.fishres.2014.09.011
Abascal, F. J., Quintans, M., Ramos-Cartelle, A., and Mejuto, J. (2011). Movements and environmental preferences of the shortfin mako, Isurus oxyrinchus, in the southeastern Pacific Ocean. Mar. Biol. 158, 1175–1184. doi: 10.1007/s00227-011-1639-1
Acha, E. M., Mianzan, H. W., Guerrero, R. A., Favero, M., and Bava, J. (2004). Marine fronts at the continental shelves of austral South America. Physical and ecological processes. J. Mar. Syst. 44, 83–105. doi: 10.1016/j.jmarsys.2003.09.005
Amante, C., and Eakins, B. W. (2009). ETOPO1 1 Arc-Minute Global Relief Model: Procedures, Data Sources and Analysis. NOAA Technical Memorandum NESDIS NGDC-24. Washington, D.C: NOAA National Geophysical Data Center. doi: 10.7289/V5C8276M
Anon (2017). Report of the 2017 ICCAT Shortfin mako assessment meeting. Madrid: International Commission for the Conservation of Atlantic Tunas.
Barker, M. J., and Schluessel, V. (2005). Managing global shark fisheries: suggestions for prioritizing management strategies. Aquat. Conserv. Mar. Freshwater Ecosyst. 15, 325–347. doi: 10.1002/aqc.660
Becker, R. A., Wilks, A. R., Brownrigg, R., and Minka, T. P. (2013). Maps: Draw Geographical Maps, R Package Version 2.3-6. Available online at: http://CRAN.R-project.org/package=maps (accessed February 26, 2021)
Biais, G., Coupeau, Y., Séret, B., Calmettes, B., Lopez, R., Hetherington, S., et al. (2017). Return migration patterns of porbeagle shark (Lamna nasus) in the Northeast Atlantic: implications for stock range and structure. ICES J. Mar. Sci. 74, 1268–1276. doi: 10.1093/icesjms/fsw233
Bivand, R., and Lewin-Koh, N. (2020). Maptools: Tools for Handling Spatial Objects. R package version 1.0-2. Available online at: https://CRAN.R-project.org/package=maptools (accessed February 26, 2021).
Block, B. A., Jonsen, I. D., Jorgensen, S. J., Winship, A. J., Shaffer, S. A., Bograd, S. J., et al. (2011). Tracking apex marine predator movements in a dynamic ocean. Nature 475, 86–90. doi: 10.1038/nature10082
Braun, C. D., Skomal, G. B., and Thorrold, S. R. (2018). Integrating archival tag data and a high-resolution oceanographic model to estimate basking shark (Cetorhinus maximus) movements in the Western Atlantic. Front. Mar. Sci. 5:25. doi: 10.3389/fmars.2018.00025
Camhi, M., Pikitch, E. K., and Babcock, E. A. (2008). Sharks of the Open Ocean: Biology, Fisheries and Conservation. Oxford: Blackwell Publishing.
Campana, S. E., Marks, L., and Joyce, W. (2005). The biology and fishery of shortfin mako sharks (Isurus oxyrinchus) in Atlantic Canadian waters. Fish. Res. 73, 341–352. doi: 10.1016/j.fishres.2005.01.009
Carey, F. G., Teal, J. M., and Kanwisher, J. W. (1981). The visceral temperature of mackerel sharks (Lamnidae). Physiol. Zool. 54, 334–344.
Casey, J. G., and Kohler, N. E. (1992). Tagging studies on the shortfin mako shark (Isurus oxyrinchus) in the western North Atlantic. Aust. J. Mar. Freshwater Res. 43, 45–60. doi: 10.1071/MF9920045
CITES (2019). Summary Record of the Twelfth Session for Committee I. Available online at: https://cites.org/sites/default/files/eng/cop/18/Com_I/SR/E-CoP18-Com-I-Rec-12-R1.pdf (accessed October 25, 2020).
Coelho, R., Fernandez-Carvalho, J., Lino, P. G., and Santos, M. N. (2012). An overview of the hooking mortality of elasmobranchs caught in a swordfish pelagic longline fishery in the Atlantic Ocean. Aquat. Living Resour. 25, 311–319. doi: 10.1051/alr/2012030
Coelho, R., Fernandez-Carvalho, J., and Santos, M. N. (2015). Habitat use and diel vertical migration of bigeye thresher shark: overlap with pelagic longline fishing gear. Mar. Environ. Res. 112, 91–99. doi: 10.1016/j.marenvres.2015.10.009
Coelho, R., Macías, D., de Urbina, J. O., Martins, A., Monteiro, C., Lino, P. G., et al. (2020). Local indicators for global species: pelagic sharks in the tropical northeast Atlantic, Cabo Verde islands region. Ecol. Indic. 110:105942. doi: 10.1016/j.ecolind.2019.105942
Coelho, R., Mejuto, J., Domingo, A., Yokawa, K., Liu, K. M., Cortés, E., et al. (2018). Distribution patterns and population structure of the blue shark (Prionace glauca) in the Atlantic and Indian Oceans. Fish. Fish. 19, 90–106. doi: 10.1111/faf.12238
Compagno, L. J. V. (2001). Sharks of the World. An annotated and Illustrated Catalogue of Shark Species Known to Date. Bullhead, Mackerel and Carpet Sharks (Heterodontiformes, Lamniformes and Orectolobiformes), Vol. 2. Rome: FAO, 109–115.
Cortés, E., Arocha, F., Beerkircher, L., Carvalho, F., Domingo, A., Heupel, M., et al. (2010). Ecological risk assessment of pelagic sharks caught in Atlantic pelagic longline fisheries. Aquat. Living Resour. 23, 25–34. doi: 10.1051/alr/2009044
Cortés, E., Domingo, A., Miller, P., Forselledo, R., Mas, F., Arocha, F., et al. (2015). Expanded ecological risk assessment of pelagic sharks caught in Atlantic pelagic longline fisheries. Collect. Vol. Sci. Pap. ICCAT 71, 2637–2688.
Cosgrove, R., Arregui, I., Arrizabalaga, H., Goni, N., and Neilson, J. D. (2015). Predation of pop-up satellite archival tagged albacore (Thunnus alalunga). Fish. Res. 162, 48–52. doi: 10.1016/j.fishres.2014.09.003
Costa, F. E. S., Braga, F. M. S., Arfelli, C. A., and Amorim, A. F. (2002). Aspects of the reproductive biology of the Shortfin mako, Isurus oxyrinchus (elasmobranchii Lamnidae), in the southeastern region of Brazil. Braz. J. Biol. 62, 239–248. doi: 10.1590/S1519-69842002000200007
Díez, G., Soto, M., and Blanco, J. M. (2015). Biological characterization of the skin of shortfin mako shark Isurus oxyrinchus and preliminary study of the hydrodynamic behaviour through computational fluid dynamics. J. Fish. Biol. 87, 123–137. doi: 10.1111/jfb.12705
Doherty, P. D., Baxter, J. M., Gell, F. R., Godley, B. J., Graham, R. T., Hall, G., et al. (2017). Long-term satellite tracking reveals variable seasonal migration strategies of basking sharks in the north-east Atlantic. Sci. Rep. 7:42837. doi: 10.1038/srep42837
Domingo, A., Rios, M., and Pons, M. (2009). Distribucion espacio temporal, composición de tallas y relaciones ambientales del atún Aleta Amarilla (Thunnus albacares) en el Atlántico SW. Col. Vol. Sci. Pap. ICCAT 64, 999–1010.
Dulvy, N. K., Baum, J. K., Clarke, S., Compagno, L. J., Cortés, E., Domingo, A., et al. (2008). You can swim but you can’t hide: the global status and conservation of oceanic pelagic sharks and rays. Aquat. Conserv. Mar. Freshwater Ecosyst. 18, 459–482. doi: 10.1002/aqc.975
Fay, M. P., and Shaw, P. A. (2010). Exact and asymptotic weighted logrank tests for interval censored data: the interval R package. J. Stat. Softw. 36, 1–34.
Ferretti, F., Worm, B., Britten, G. L., Heithaus, M. R., and Lotze, H. K. (2010). Patterns and ecosystem consequences of shark declines in the ocean. Ecol. Lett. 13, 1055–1071. doi: 10.1111/j.1461-0248.2010.01489.x
Fox, J., and Weisberg, S. (2019). An {R} Companion to Applied Regression, 3rd Edn. Thousand Oaks, CA: Sage.
Francis, M. P., Holdsworth, J. C., and Block, B. A. (2015). Life in the open ocean: seasonal migration and diel diving behaviour of Southern Hemisphere porbeagle sharks (Lamna nasus). Mar. Biol. 162, 2305–2323. doi: 10.1007/s00227-015-2756-z
Francis, M. P., Shivji, M. S., Duffy, C. A., Rogers, P. J., Byrne, M. E., Wetherbee, B. M., et al. (2019). Oceanic nomad or coastal resident? Behavioural switching in the shortfin mako shark (Isurus oxyrinchus). Mar. Bio. 166, 1–16. doi: 10.1007/s00227-018-3453-5
Gaube, P., Barceló, C., McGillicuddy, D. J. Jr., Domingo, A., Miller, P., Giffoni, B., et al. (2017). The use of mesoscale eddies by juvenile loggerhead sea turtles (Caretta caretta) in the southwestern Atlantic. PLoS One 12:e0172839. doi: 10.1371/journal.pone.0172839
Gibson, K. J., Streich, M. K., Topping, T. S., and Stunz, G. W. (2021). New insights into the seasonal movement patterns of Shortfin Mako sharks in the Gulf of Mexico. Front. Mar. Sci. 8:5. doi: 10.3389/fmars.2021.623104
Graham, R. T., Witt, M. J., Castellanos, D. W., Remolina, F., Maxwell, S., Godley, B. J., et al. (2012). Satellite tracking of manta rays highlights challenges to their conservation. PloS One 7:e36834. doi: 10.1371/journal.pone.0036834
Gross, J., and Ligges, U. (2015). nortest: Tests for Normality. R Package Version 1.0-4. Available online at: https://CRAN.R-project.org/package=nortest (accessed February 26, 2021).
Hammerschlag, N., Gallagher, A. J., and Lazarre, D. M. (2011). A review of shark satellite tagging studies. J. Exp. Mar. Biol. Ecol. 398, 1–8. doi: 10.1016/j.jembe.2010.12.012
Hays, G. C., Bradshaw, C. J. A., James, M. C., Lovell, P., and Sims, D. W. (2007). Why do Argos satellite tags deployed on marine animals stop transmitting? J. Exp. Mar. Bio. Ecol. 349, 52–60. doi: 10.1016/j.jembe.2007.04.016
Heupel, M. R., Carlson, J. K., and Simpfendorfer, C. A. (2007). Shark nursery areas: concepts, definition, characterization and assumptions. Mar. Ecol. Prog. Ser. 337, 287–297. doi: 10.3354/meps337287
Hijmans, R. J. (2020). raster: Geographic Data Analysis and Modeling. R package version 3.4-5. Available online at: https://CRAN.R-project.org/package=raster (accessed February 26, 2021).
Hoffmayer, E. R., Franks, J. S., Driggers, W. B., and Howey, P. W. (2013). Diel vertical movements of a scalloped hammerhead, Sphyrna lewini, in the northern Gulf of Mexico. B. Mar. Sci. 89, 551–557. doi: 10.5343/bms.2012.1048
Hoolihan, J. P., Wells, R. J. D., Luo, J., Falterman, B., Prince, E. D., and Rooker, J. R. (2014). Vertical and horizontal movements of yellowfin tuna in the Gulf of Mexico. Mar. Coast. Fish. 6, 211–222. doi: 10.1080/19425120.2014.935900
Howey-Jordan, L. A., Brooks, E. J., Abercrombie, D. L., Jordan, L. K., Brooks, A., Williams, S., et al. (2013). Complex movements, philopatry and expanded depth range of a severely threatened pelagic shark, the oceanic whitetip (Carcharhinus longimanus) in the western North Atlantic. PloS One 8:e56588. doi: 10.1371/journal.pone.0056588
ICCAT (2018). Report of the 2018 ICCAT Intersessional Meeting of the Shark Species Group. Madrid: ICCAT–International Commission for the Conservation of Atlantic Tunas.
IFREMER (2009). Continental Margins Between 140m and 3500m Depth. Available online at: https://www.marineregions.org/ (accessed February 22, 2021)
Iosilevskii, G., Papastamatiou, Y. P., Meyer, C. G., and Holland, K. N. (2012). Energetics of the yo-yo dives of predatory sharks. J. Theor. Biol. 294, 172–181. doi: 10.1016/j.jtbi.2011.11.008
Jiménez, S., Domingo, A., Abreu, M., and Brazeiro, A. (2011). Structure of the seabird assemblage associated with pelagic longline vessels in the southwestern Atlantic: implications for bycatch. Endang. Species Res. 15, 241–254. doi: 10.3354/esr00378
Kerstetter, D. W., Polovina, J., and Graves, J. E. (2004). Evidence of shark predation and scavenging on fishes equipped with pop-up satellite archival tags. Fish. Bull. 102:750.
Ketchum, J. T., Hoyos-Padilla, M., Aldana-Moreno, A., Ayres, K., Galván-Magaña, F., Hearn, A., et al. (2020). Shark movement patterns in the Mexican Pacific: a conservation and management perspective. Adv. Mar. Biol. 85, 1–37. doi: 10.1016/bs.amb.2020.03.002
Klimley, A. P., Beavers, S. C., Curtis, T. H., and Jorgensen, S. J. (2002). Movements and swimming behavior of three species of sharks in La Jolla Canyon. California. Environ. Biol. Fishes 63, 117–135. doi: 10.1023/A:1014200301213
Loefer, J. K., Sedberry, G. R., and McGovern, J. C. (2005). Vertical movements of a shortfin mako in the western North Atlantic as determined by pop-up satellite tagging. Southeast. Nat. 4, 237–246.
Maia, A., Queiroz, N., Cabral, H. N., Santos, A. M., and Correia, J. P. (2007). Reproductive biology and population dynamics of the shortfin mako, Isurus oxyrinchus Rafinesque, 1810, off the southwest Portuguese coast, eastern North Atlantic. J. Appl. Ichthyol 23, 246–251. doi: 10.1111/j.1439-0426.2007.00849.x
Maia, A., Queiroz, N., Correia, J. P., and Cabral, H. (2006). Food habits of the shortfin mako, Isurus oxyrinchus, off the southwest coast of Portugal. Environ. Biol. Fish. 77, 157–167. doi: 10.1007/s10641-006-9067-7
Mejuto, J., García-Cortés, B., Ramos-Cartelle, A., De la Serna, J. M., González-González, I., and Fernández-Costa, L. (2009). Standardized catch rates for the blue shark (Prionace glauca) and shortfin mako (Isurus oxyrinchus) caught by the Spanish surface longline fleet in the Atlantic Ocean during the period 1990-2007. Collect. Vol. Sci. Pap. ICCAT 64, 1509–1521.
Mucientes-Sandoval, G., Queiroz, N., Humphries, N. E., Saborido, F., and Sims, D. W. (2012). Movements, Behaviour And Habitat Preferences of Pop-Up Satellite Tracked Shortfin Mako Sharks (Isurus oxyrinchus) in the North Atlantic. Vancouver, CA: World Congress of Herpetology, 497–498.
Musyl, M. K., Domeier, M. L., Nasby-Lucas, N., Brill, R. W., McNaughton, L. M., Swimmer, J. Y., et al. (2011). Performance of pop-up satellite archival tags. Mar. Ecol. Prog. Ser. 433, 1–28. doi: 10.3354/meps09202
Myers, R. A., Baum, J. K., Shepherd, T. D., Powers, S. P., and Peterson, C. H. (2007). Cascading effects of the loss of apex predatory sharks from a coastal ocean. Science 315, 1846–1850. doi: 10.1126/science.1138657
Nakamura, I., Watanabe, Y. Y., Papastamatiou, Y. P., Sato, K., and Meyer, C. G. (2011). Yo-yo vertical movements suggest a foraging strategy for tiger sharks Galeocerdo cuvier. Mar. Ecol. Prog. Ser. 424, 237–246. doi: 10.3354/meps08980
Natanson, L. J., Winton, M., Bowlby, H., Joyce, W., Deacy, B., Coelho, R., et al. (2020). Updated reproductive parameters for the shortfin mako (Isurus oxyrinchus) in the North Atlantic Ocean with inferences of distribution by sex and reproductive stage. Fish. Bull 118, 21–36.
Neuwirth, E. (2014). RColorBrewer: Color Brewer Palettes. R package version 1.1-2. Available online at: https://CRAN.R-project.org/package=RColorBrewer (accessed February 26, 2021).
Pacoureau, N., Rigby, C. L., Kyne, P. M., Sherley, R. B., Winker, H., Carlson, J. K., et al. (2021). Half a century of global decline in oceanic sharks and rays. Nature 589, 567–571. doi: 10.1038/s41586-020-03173-9
Pade, N. G., Queiroz, N., Humphries, N. E., Witt, M. J., Jones, C. S., Noble, L. R., et al. (2009). First results from satellite-linked archival tagging of porbeagle shark, Lamna nasus: area fidelity, wider-scale movements and plasticity in diel depth changes. J. Exp. Mar. Biol. Ecol. 370, 64–74. doi: 10.1016/j.jembe.2008.12.002
Passadore, C., Domingo, A., Szephegyi, M., and Secchi, E. R. (2014). Influence of environmental and longline fishing operational variables on the presence of killer whales (Orcinus orca) in south-western Atlantic. J. Mar. Biol. Assoc. U.K. 94:1267. doi: 10.1017/S002531541200166X
Pedersen, M. W., Patterson, T. A., Thygesen, U. H., and Madsen, H. (2011). Estimating animal behavior and residency from movement data. Oikos 120, 1281–1290. doi: 10.1111/j.1600-0706.2011.19044.x
Pershing, A. J., Christensen, L. B., Record, N. R., Sherwood, G. D., and Stetson, P. B. (2010). The impact of whaling on the ocean carbon cycle: why bigger was better. PloS One 5:e12444. doi: 10.1371/journal.pone.0012444
Petersen, S., Nel, D., and Omardien, A. (2007). Towards an Ecosystem Approach to Longline Fisheries in the Benguela: An Assessment of Impacts on Seabirds, Sea Turtles and Sharks. WWF Report Series–2007/Marine/001. Cape Town: World Wild Fund.
Pierce, D. (2019). ncdf4: Interface to Unidata netCDF (Version 4 or Earlier) Format Data Files. R Package Version 1.17. Available online at: https://CRAN.R-project.org/package=ncdf4 (accessed February 26, 2021).
Pons, M., and Domingo, A. (2013). Update of standardized catch rates of shortfin mako, Isurus oxyrinchus, caught by the Uruguayan longline fleet (1982-2010). Collect. Vol. Sci. Pap. ICCAT 69, 1630–1638.
QGIS.org (2021). QGIS Geographic Information System. Available online at: https://qgis.org/en/site/ (accessed February 27, 2021).
Queiroz, N., Humphries, N. E., Couto, A., Vedor, M., da Costa, I., Sequeira, A. M. M., et al. (2019). Global spatial risk assessment of sharks under the footprint of fisheries. Nature 572, 461–466. doi: 10.1038/s41586-019-1444-4
Queiroz, N., Humphries, N. E., Mucientes, G., Hammerschlag, N., Lima, F. P., Scales, K. L., et al. (2016). Ocean-wide tracking of pelagic sharks reveals extent of overlap with longline fishing hotspots. P. Natl. Acad. Sci.U.S.A. 113, 1582–1587. doi: 10.1073/pnas.1510090113
R Core Team (2020). R: A Language and Environment for Statistical Computing. Vienna: R Foundation for Statistical Computing.
Rigby, C. L., Barreto, R., Carlson, J., Fernando, D., Fordham, S., Francis, M. P., et al. (2019). Isurus oxyrinchus. The IUCN Red List of Threatened Species 2019:e.T39341A2903170. Available online at: http://dx.doi.org/10.2305/IUCN.UK.2019-1.RLTS.T39341A2903170.en (accessed October 25, 2020).
Rogers, P. J., Huveneers, C., Page, B., Goldsworthy, S. D., Coyne, M., Lowther, A. D., et al. (2015). Living on the continental shelf edge: habitat use of juvenile shortfin makos Isurus oxyrinchus in the Great Australian Bight, southern Australia. Fish. Oceanogr. 24, 205–218. doi: 10.1111/fog.12103
Santos, C. C., and Coelho, R. (2018). Migrations and habitat use of the smooth hammerhead shark (Sphyrna zygaena) in the Atlantic Ocean. PloS One 13:e0198664. doi: 10.1371/journal.pone.0198664
Saunders, R. A., Royer, F., and Clarke, M. W. (2011). Winter migration and diving behaviour of porbeagle shark, Lamna nasus, in the Northeast Atlantic. ICES J. Mar. Sci. 68, 166–174. doi: 10.1093/icesjms/fsq145
Sepulveda, C. A., Kohin, S., Chan, C., Vetter, R., and Graham, J. B. (2004). Movement patterns, depth preferences, and stomach temperatures of free-swimming juvenile mako sharks, Isurus oxyrinchus, in the Southern California. Bight. Mar. Biol. 145, 191–199. doi: 10.1007/s00227-004-1356-0
Shillinger, G. L., Palacios, D. M., Bailey, H., Bograd, S. J., Swithenbank, A. M., Gaspar, P., et al. (2008). Persistent leatherback turtle migrations present opportunities for conservation. PLoS Biol. 6:e171. doi: 10.1371/journal.pbio.0060171
Simpfendorfer, C., Cortés, E., Heupel, M., Brooks, E., Babcock, E., Baum, J., et al. (2008). An Integrated Approach to Determining the Risk of Overexploitation for Data-Poor Pelagic Atlantic sharks. ICCAT SCRS/2008/140. Madrid: International Commission for the Conservation of Atlantic Tunas.
Skomal, G., Marshall, H., Galuardi, B., Natanson, L., Braun, C. D., and Bernal, D. (2021). Horizontal and vertical movement patterns and habitat use of juvenile porbeagles (Lamna nasus) in the western north Atlantic. Front. Mar. Sci. 8:624158. doi: 10.3389/fmars.2021.624158
Skomal, G. B., Zeeman, S. I., Chisholm, J. H., Summers, E. L., Walsh, H. J., McMahon, K. W., et al. (2009). Transequatorial migrations by basking sharks in the western Atlantic Ocean. Curr. Biol. 19, 1019–1022. doi: 10.1016/j.cub.2009.04.019
Stabler, B. (2013). Shapefiles: Read and Write ESRI Shapefiles. R Package Version 0.7. Available online at: https://CRAN.R-project.org/package=shapefiles (accessed February 26, 2021).
Stevens, J. D. (2008). “The biology and ecology of the shortfin mako shark, Isurus oxyrinchus,” in Sharks of the Open Ocean: Biology, Fisheries and Conservation, eds M. D. Camhi, E. K. Pikitch, and E. A. Babcock (Oxford: Blackwell Publishing), 87–94.
Stevens, J. D., Bonfil, R., Dulvy, N. K., and Walker, P. A. (2000). The effects of fishing on sharks, rays, and chimaeras (chondrichthyans), and the implications for marine ecosystems. ICES J. Mar. Sci. 57, 476–494. doi: 10.1006/jmsc.2000.0724
Stevens, J. D., Bradford, R. W., and West, G. J. (2010). Satellite tagging of blue sharks (Prionace glauca) and other pelagic sharks off eastern Australia: depth behaviour, temperature experience and movements. Mar. Biol. 157, 575–591. doi: 10.1007/s00227-009-1343-6
Stillwell, C. E., and Kohler, N. E. (1982). Food, feeding habits, and estimates of daily ration of the shortfin mako (Isurus oxyrinchus) in the Northwest Atlantic. Can. J. Fish. Aquat. Sci. 39, 407–414. doi: 10.1139/f82-058
Vaudo, J. J., Byrne, M. E., Wetherbee, B. M., Harvey, G. M., and Shivji, M. S. (2017). Long-term satellite tracking reveals region-specific movements of a large pelagic predator, the shortfin mako shark, in the western North Atlantic Ocean. J. Appl. Ecol. 54, 1765–1775. doi: 10.1111/1365-2664.12852
Vaudo, J. J., Wetherbee, B. M., Wood, A. D., Weng, K., Howey-Jordan, L. A., Harvey, G. M., et al. (2016). Vertical movements of shortfin mako sharks Isurus oxyrinchus in the western North Atlantic Ocean are strongly influenced by temperature. Mar. Ecol. Prog. Ser. 547, 163–175. doi: 10.3354/meps11646
Vetter, R., Kohin, S., Preti, A., Mcclatchie, S. A. M., and Dewar, H. (2008). Predatory interactions and niche overlap between mako shark, Isurus oxyrinchus, and jumbo squid, Dosidicus gigas, in the California Current. CalCOFI Rep. 49, 142–156.
Vooren, C. M., Klippel, S., and Galina, A. B. (2005). “Elasmobrânquios das águas costeiras da Plataforma Sul,” in Ações Para a Conservação de Tubarões e Raias no Sul do Brasil, eds C. M. Vooren and S. Klippel (Porto Alegre: Igaré), 113–120.
Weng, K. C., Boustany, A. M., Pyle, P., Anderson, S. D., Brown, A., and Block, B. A. (2007). Migration and habitat of white sharks (Carcharodon carcharias) in the eastern Pacific Ocean. Mar. Biol. 152, 877–894. doi: 10.1007/s00227-007-0739-4
Wickham, H., and Seidel, D. (2020). scales: Scale Functions for Visualization. R package version 1.1.1. Available online at: https://CRAN.R-project.org/package=scales (accessed February 26, 2021).
Wildlife Computers (2018). Location Processing (GPE3 & Fastloc) in the Wildlife Computers Data Portal User Guide. Available online at: https://static.wildlifecomputers.com/Location-Processing-UserGuide.pdf (accessed November 22, 2019).
Keywords: fisheries, shortfin mako, Atlantic Ocean, animal behavior, satellite telemetry, shark, pelagic longline
Citation: Santos CC, Domingo A, Carlson J, Natanson LJ, Travassos P, Macías D, Cortés E, Miller P, Hazin F, Mas F, Ortiz de Urbina J, Lino PG and Coelho R (2021) Movements, Habitat Use, and Diving Behavior of Shortfin Mako in the Atlantic Ocean. Front. Mar. Sci. 8:686343. doi: 10.3389/fmars.2021.686343
Received: 26 March 2021; Accepted: 06 July 2021;
Published: 28 July 2021.
Edited by:
David Wells, Texas A&M University at Galveston, United StatesReviewed by:
Christopher G. Lowe, California State University, Long Beach, United StatesKesley Gibson Banks, Texas A&M University Corpus Christi, United States
Copyright © 2021 Santos, Domingo, Carlson, Natanson, Travassos, Macías, Cortés, Miller, Hazin, Mas, Ortiz de Urbina, Lino and Coelho. This is an open-access article distributed under the terms of the Creative Commons Attribution License (CC BY). The use, distribution or reproduction in other forums is permitted, provided the original author(s) and the copyright owner(s) are credited and that the original publication in this journal is cited, in accordance with accepted academic practice. No use, distribution or reproduction is permitted which does not comply with these terms.
*Correspondence: Catarina C. Santos, catarina.santos@ipma.pt
†Deceased