- 1Fisheries College, Jimei University, Xiamen, China
- 2Xiamen Jiakang Feed Co., Ltd., Xiamen, China
- 3College of Ocean and Earth Science, Xiamen University, Xiamen, China
A 56 day feeding trial was conducted to examine the effects of different levels of dietary histamine on growth performance, immune response, and intestinal health of grouper (Epinephelus coioides). Seven isonitrogenous (46%), isolipidic (10%) diets were prepared with histamine supplement levels of 0 (T0), 0.05% (T1), 0.1% (T2), 0.15% (T3), 0.2% (T4), 0.25% (T5), and 0.3% (T6), respectively. The results showed that histamine supplementation had no significant effects on weight gain rate (WGR), specific growth rate (SGR), feed conversion rate (FCR), hepatosomatic index (HSI), and survival rate (SR) at the initial feeding period (day 0–28), but WGR and SGR had negative linear responses to the dietary histamine level at the whole feeding period (day 0–56), and a significant decrease was observed in groups T5 and T6 compared with T0 (P < 0.05). Supplementation of histamine decreased antioxidant capacity, immune response, the contents of serum interleukin-1 beta (IL-1β), intestinal-type fatty acid-binding protein (FABP2) and intestinal trefoil factor (ITF), and caused serious damage of intestine with significantly decreased VH and MFH of grouper, especially in fish fed with diets supplemented with high doses of histamine (0.25 and 0.3%). The intestinal microbial communities in treatments were different clearly with the control (T0), in terms of beta (β)-diversity boxplots and UPGMA phylogenetic tree based on unweighted unifrac distance. At the phylum level, the relative abundance of Fusobacteria was lower in group T0, while the abundance of Firmicutes was significantly lower in groups T5 and T6 (P < 0.05). At the genus level, the relative abundance of uncultured_bacterium_f_Bacteroidales_S24-7_group, uncultured_bacterium_f_Lachnospiraceae, and Ruminiclostridium were significantly higher in the control, while the abundance of Cetobacterium was significantly higher in groups T5 and T6 (P < 0.05). In conclusion, the present study suggested that up to 0.2% of dietary histamine did not result in a remarkable reduction in growth, immune response, and intestinal health; however, 0.25% or more dietary histamine could cause significant negative effects on growth performance, immune response, and intestinal health in E. coioides.
Introduction
With the rapid development of aquaculture industry worldwide in recently years, the quality of fish meal (FM), which served as the most primary protein source in aquatic feeds, has received more and more attention (Li et al., 2018; Wang et al., 2019; Ye et al., 2020; Zhai et al., 2020). High levels of histamine are usually presented in poor quality FM due to difference of original fish species, suboptimal conditions of preparation and transportation, improper storage, and so on (Higgs et al., 1995; Anderson et al., 1997; Visciano et al., 2012), resulting in a series of negative effects in aquatic animals, such as suppression of growth performance, reduction of feed utilization, inflammatory reaction, and intestinal diseases (Aksnes et al., 1997; Aksnes and Mundheim, 1997; Caballero et al., 1999; Tapia-Salazar et al., 2004). Therefore, histamine level is a useful and widely recognized parameter to assess FM quality and also as a vital safety indicator for food (Pike and Hardy, 1997; Tapia-Salazar et al., 2001; Tao et al., 2011; Biji et al., 2016).
The effects of dietary histamine exhibited variation in humans and different animal species. In humans, it was found that high dose of histamine (exceed 0.75 mg/kg body weight) may significantly enhance the risk of poisoning (Doeun et al., 2017). The European Union set regulatory ensures of histamine level below 0.2 g/kg in fresh fish and 0.4 g/kg in seafood products (Visciano et al., 2014). Moreover, suppression of growth, reduction of feed utilization and survival rate (Harry et al., 1975; Zhao et al., 2012), and gizzard lesions (Harry and Tucker, 1976) have been reported in chickens suffering from high doses of dietary histamine. The dietary histamine supplementation exerts detrimental effects in mysis (Neomysis awatschensis and Neomysis japonica Nakazawa) (Yang et al., 2010), rainbow trout (Oncorhynchus mykiss) (Moghaddam et al., 2015), and American eel (Anguilla rostrata) (Zhai et al., 2020). Li et al. (2018) found digestive system damage and liver inflammation in yellow catfish (Pelteobagrus fulvidraco) fed a diet supplemented with 0.1% histamine. Zhao et al. (2016) reported that high levels of histamine (4 g/kg) decreased the activity of digestive enzymes and exerted great damage to the morphology of the intestine and hepatopancreas in Chinese mitten crab (Eriocheir sinensis); however, dietary histamine supplementation had no significant effect on growth performance. In rainbow trout (O. mykiss), digestive tract damage was observed in fish fed diet containing 2 g/kg histamine (Watanabe et al., 1987; Fairgrieve et al., 1994), and dietary supplementation of histamine (2 g/kg) (Fairgrieve et al., 1994) or putrescine (13.3 g/kg) (Cowey and Cho, 1992) decreased feed consumption. Interestingly, Tapia-Salazar et al. (2001) reported that diet supplemented with 1.2–2.4 g/kg histamine/diet had a positive effect on weight gain in blue shrimp (Litopenaeus stylirostris).
Groupers as typical marine carnivorous fish have been widely cultured in several regions of China and Southeast Asia with the development of the intensive aquaculture industry. In China, annual production of groupers reached 183,127 tons in 2019 (China Fishery and Statistics Yearbook, 2020), for its fast growth, rich nutrition, high economic value, and consumer demand. To the best of our knowledge, study on the effects of dietary histamine on the performance of grouper is lacking, and its impact on intestinal health of fish is less reported (Zhao et al., 2016). Therefore, the present study was conducted to evaluate the effects of different doses of dietary histamine on growth performance, immune response, and intestinal health of grouper (Epinephelus coioides).
Materials and Methods
Experimental Diets
A basal diet (T0; non-supplemented with histamine), served as control, was formulated (Table 1) based on nutritional requirements of grouper (E. coioides) as recommended by Yang et al. (2019). The experimental diets were prepared by supplementing the basal diet with 0.05% (T1), 0.1% (T2), 0.15% (T3), 0.2% (T4), 0.25% (T5), and 0.3% (T6) histamine (S20188; histamine [C5H9N3] ≥ 98.0%; 111.15 g/mol; Shanghai yuanye Bio-Technology Co., Ltd., China). All the dietary ingredients were crushed to powders and through 60 mesh sieve and thoroughly mixed using the progressive enlargement method, then added the premixed fish oil, soybean oil and lecithin; subsequently, water with/without histamine was supplemented slowly and kept stirring until forming a dough. After pelletized, the diets were packed in plastic bags and stored at refrigerator (−20°C) until subsequent use. The histamine level in the basal diet was determined to be 158.7 mg/kg.
Animals and Experimental Conditions
The procedures for care and use of animals were approved by the Animal Care and Use Committee of Jimei University, China. The feeding trial was conducted in a recirculating water system and healthy grouper (E. coioides) were obtained from the Haikang Aquaculture Research Base of Dabeilong Aquaculture group. The whole study followed a strict series of rules and regulations promulgated by animal care and use committee of Jimei University, China. After acclimation (2 weeks), 30 fish (29 ± 0.52g; mean ± SE) were randomly allocated to each tank (containing 300 L seawater) and three tanks for each treatment were served as replicate, and thus 21 tanks (seven groups, and three repeats in each group) and 630 fish were used in the feed trial. Fish were hand fed one of seven diets for 56 days to apparent satiation two times daily at 08:30 and 18:30, respectively, and approximately 60% seawater was replaced daily. During the trial period, rearing water environment were monitored daily and maintained in a suitable range (temperature: 20–26°C; salinity: 30–32 g/L, pH: 7.5–8.2, dissolved oxygen [DO]: ≥7.5 mg/L; and total ammonia concentration: <0.2 mg/L).
Sample Collection
To determine the growth performance, 10 fish were randomly collected from each tank (three tanks for each treatment) after starvation for 24 h at days 28 and 56 respectively, thus 30 fish for each treatment were collected at each time point, batchwise anesthetized by 2-phenoxyethanol, and single-weighed for calculation of growth performance. Blood samples were taken from the caudal vein of 10 fish in each tank, immediately transferred into 1.5 mL Eppendorf tubes and held at 4°C overnight. Then, serum was collected and pooled following centrifugation at 10,000 r/min at 4°C for 10 min and stored in 1.5 mL Eppendorf tubes at −80°C for further analysis. Liver and intestine samples were dissected, weighed, and frozen immediately in liquid nitrogen and stored at −80°C for subsequent analysis. Foregut were randomly sampled from four fish in each tank, fixed with Bouin’s fixative solution (75 ml saturated aqueous solution of picric acid, 25 ml formalin, 5 ml glacial acetic acid) for morphological determination. Intestine samples were collected from one fish per tank for intestinal microbiota analysis at day 56.
Measurement of Serum and Liver Biochemical Parameters
The total antioxidant capacity (T-AOC) and activities of alkaline phosphatase (AKP), acid phosphatase (ACP), and superoxide dismutase (SOD) in serum were evaluated spectrophotometrically using commercial kits (Nanjing Jiancheng Bioengineering Institute, Nanjing, China) according to the manufacturer’s instructions as previously described (Hu et al., 2019).
The liver sample was homogenized in ice-cold 0.86% physiological saline (pH: 7.2–7.4; w: v = 1:9), centrifuged at 3,000 rpm at 4°C for 10 min and the supernatant was collected. Protein concentration of the supernatant was determined with Coomassie brilliant blue method described by Hu et al. (2019). Malondialdehyde (MDA) level and activities of glutamic oxaloacetic transaminase (GOT) and glutamic propylic transaminase (GPT) of the supernatant were estimated using commercial kits (Nanjing Jiancheng Bioengineering Institute, Nanjing, China) following the manufacturer protocol.
Serum and Intestinal Inflammatory Factors
Interleukin-1 beta (IL-1β), serum amyloid A (SAA), and C-reactive protein (CRP) in serum were analyzed by enzyme-linked immunosorbent assay (ELISA) kits (Nanjing Jiancheng Bioengineering Institute, Nanjing, China). An ELISA kit was purchased from Shanghai Jianglai Biotechnology Co., Ltd. (Shanghai, China) for determination of fatty acid-binding protein 2 (FABP2) in serum, according to the manufacturer’s instruction.
Intestinal samples were rinsed in 0.86% physiological saline of precooling (pH: 7.2–7.4; w: v = 1:9), homogenized and centrifuged (3,000 rpm, 4°C for 10 min). Protein concentration of the supernatant was measured by Coomassie brilliant blue method. Intestinal trefoil factor (ITF) was estimated by ELISA Kit provided by Shanghai Jianglai Biotechnology Co., Ltd. (Shanghai, China). The content of ITF was expressed as “pg per mg of protein.”
Intestinal Morphology
Analysis of intestinal morphology by making Hematoxylin and Eosin (H&E)- stained sections as described in our previous study (Zhang J. J. et al., 2020). Briefly, the pre-fixed anterior intestinal samples with Bouin’s fixative solution were subsequently dehydrated with a graded series of ethanol and cleared in xylene, then embedded in paraffin, finally sectioned at 6 um thickness. After that, sections were stained using Hematoxylin and Eosin (H&E) and mounted on glass slides. The sections were observed with positive fluorescence microscope (Leica TM 820, Nussloch, Germany), and muscular thickness (MT) and mucosal fold height (MFH) were measured using Image-Pro Plus 6.0 software (Media Cybernetics, Silver Spring, MD, United States) as described in our previous study (Zhang J. J. et al., 2020).
Intestinal Microbiota Analysis
Total DNA of intestinal samples was extracted using a DNA extraction kit, and DNA integrity and quality were measured by electrophoresis on a 1% agarose gel. Then, the V3 + V4 regions of the 16S rRNA gene of intestinal bacteria was amplified with the forward primer 338F (5′-ACTCCTACGGGAGGCAGCA-3′) and the reverse primer 806R (5′-GGACTACHVGGGTWTCTAAT-3′) by Polymerase Chain Reaction (PCR). The PCR product purity and concentration were determined with Nano-Drop®ND-1000 spectrophotometer (Nano-Drop Technologies, Wilmington, DE, United States). Subsequently, high-throughput sequencing was performed on an Illumina HiSeq platform (Beijing Biomarker Biotechnology Co., Ltd., Beijing, China). The sequencing data have been uploaded to GenBank (Accession number, PRJNA718150). Analysis of intestinal microbiota abundance and diversity was performed using BMKCloud1. The detailed protocols were described in our earlier study (Yang et al., 2019).
Calculations and Statistical Analysis
The following formulas were used to calculate growth performances and feed utilization parameters: Weight gain rate (WGR,%) = 100 × (final body weight–initial body weight)/initial body weight; Specific growth rate (SGR,%/d) = 100 × (ln final body weight–ln initial body weight)/days of feeding trial; Feed conversion rate (FCR) = feed intake/(final body weight–initial body weight); Hepatosomatic index (HSI,%) = 100 × (liver weight/body weight); Survival rate (SR,%) = 100 × number of survived fish in sampling/initial number of fish allocated.
All data were subjected by one-way analysis of variance (ANOVA) followed by Duncan’s multiple-range test, which was conducted to examine significant differences among treatments using SPSS statistical package version 22.0 (SPSS Inc., Chicago, United States). The results are presented as mean values followed by the standard error of the mean (Mean ± SE) and significance was declared at the P < 0.05.
Results
Growth Performance
The growth performance of grouper is presented in Table 2. With increasing histamine level, WGR and SGR tend to decrease, while FCR showed an increase trend, but no significant difference was observed at the initial feed period (day 0–28). Compared with group T0, significant increased FCR and decreased WGR and SGR were observed in the groups T5 and T6 at the whole feeding period (day 0–56) (P < 0.05). Neither HSI nor SR statistically differed across dietary treatments at the initial feed period (day 0–28) and the whole feeding period (day 0–56) (P > 0.05), and the SR were above 95.56% in all groups.
Serum Non-specific Immune Parameters
The serum non-specific immune parameters are presented in Table 3. At days 28 and 56, the serum ACP activities in fish fed the histamine supplemented diets were lower than those fed the control diet (T0), and significant difference was observed in fish fed the diet T6 (P < 0.05). Fish fed the diets T4, T5, and T6 at days 28 and 56 showed significant decreased AKP activities compared to those fed the diet T0 (P < 0.05), while fish fed the diet T6 exhibited the lowest AKP activity. Serum SOD activity decreased with the increment of dietary histamine in experimental diets and a significant decrease was observed in the treatment T6 compared with the T0 at days 28 and 56 (P < 0.05). The T-AOC activities in the histamine treatments at day 28 was significantly lower than that in the control (P < 0.05), with the exception of the group T2. T-AOC activity was affected negatively by all histamine treatments at day 56, while significant decrease was only observed in fish fed the diet T6 (P < 0.05).
Liver Biochemical Indices
The results of the liver biochemical parameters are displayed in Table 4. The MDA contents of fish fed the histamine supplemented diets (except for diet T1) at day 28 were significantly higher than those fed the diet T0 (P < 0.05). Meanwhile, compared with the group T0, significantly decreased activities of GOT and GPT were observed in group T6 (P < 0.05). At day 56, the MDA content in liver of fish fed diets T5 and T6 increased statistically compared with the control (P < 0.05). Moreover, GOT and GPT activities in groups T5 and T6 were significantly lower than those in group T0 (P < 0.05).
Inflammation Markers in Serum and Intestine
As can be seen from Table 5, serum amyloid A (SAA) and C-reactive protein (CRP) contents did not vary statistically in all dietary treatments at days 28 and 56. Compared with the control, the serum IL-1β content of fish fed diet T6 increased significantly at day 28 (P < 0.05). At day 56, serum IL-1β contents of fish fed the histamine supplemented diets increased statistically compare with those fed the diet T0 (P < 0.05), while the treatment T6 showed the highest value. In groups T4, T5, and T6, the contents of serum fatty acid-binding protein 2 (FABP2) and intestinal trefoil factor (ITF) increased significantly at day 28 as compared to the control and the highest values exhibited in fish fed diet T6 (P < 0.05). At day 56, the contents of serum FABP2 and intestinal ITF in group T6 were significantly higher than that in the control (P < 0.05).
Intestinal Morphology
Intestinal morphometrical parameters of grouper fed different diets at days 28 and 56 are shown in Table 6. There was a significant reduction of muscular thickness (MT) in fish fed diets T5 and T6 at days 28 and 56 compared with those fed the diet T0 (P < 0.05). In addition, the mucosal fold height (MFH) in groups T4, T5, and T6 at day 28 was lower significantly than the control, while the significant decrease was only observed in group T6 (P < 0.05). The intestine section of fish fed with diet supplemented with histamine displayed an inflammation and damage, characterized by a thin MT, reduced MFH, while the most serious impairment was observed in fish fed diets T5 and T6 with higher doses of histamine (0.25 and 0.3%) (Figures 1, 2).

Table 6. Effects of histamine on foregut muscular thickness and mucosal fold height of grouper (Epinephelus coioides).
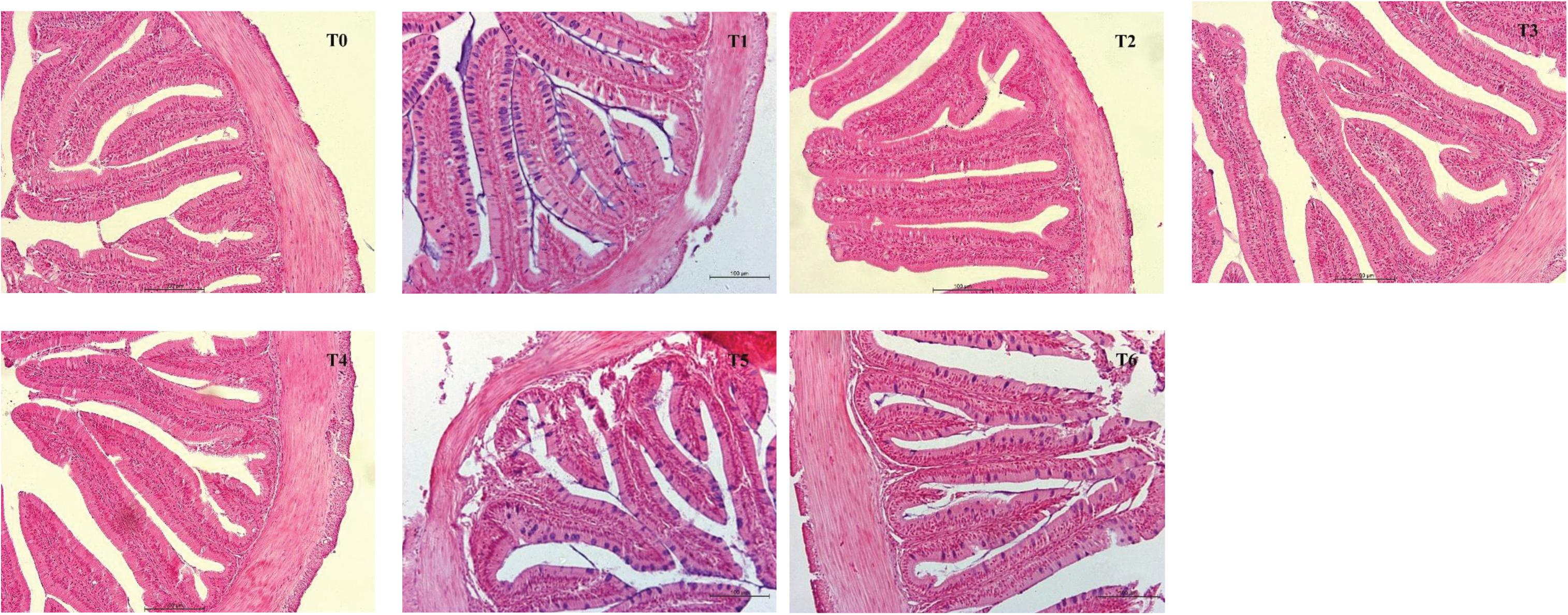
Figure 1. The foregut morphological images from H&E-stained section of E. coioides fed the experimental diets for 28 days (100×).
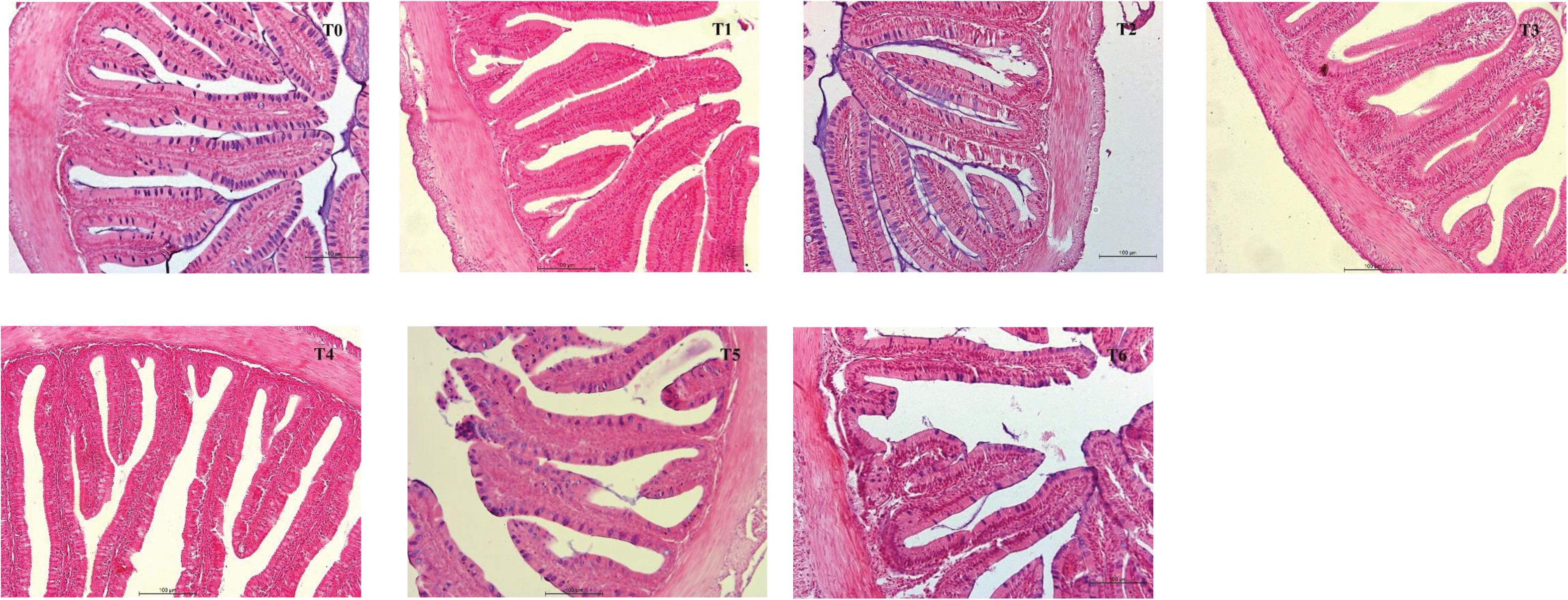
Figure 2. H&E stained anterior intestine morphological sections of E. coioides fed the experimental diets for 56 days (100×).
Intestinal Microbiota
The phylum level analysis demonstrated that Proteobacteria, Firmicutes, Bacteroidetes, Actinobacteria, Fusobacteria, Chloroflexi, and Acidobacteria constituted common predominant bacterial phylum in all groups, and histamine supplemented diets significantly reduced the relative abundance of Firmicutes and Bacteroidetes, significantly increased the relative abundance of Proteobacteria and Fusobacteria, especially in groups T5 and T6 (P < 0.05) (Figure 3A and Table 7). At the genus level, enhanced abundance of Cetobacterium was observed in all treatments (except T3) compared with the control (T0), as well as decreased abundances of Ruminiclostridium and uncultured Bacteroidales_S24-7_group and Lachnospiraceae, while significant changes were observed in high histamine groups (0.25 and 0.3%) (P < 0.05) (Figure 3B and Table 7).
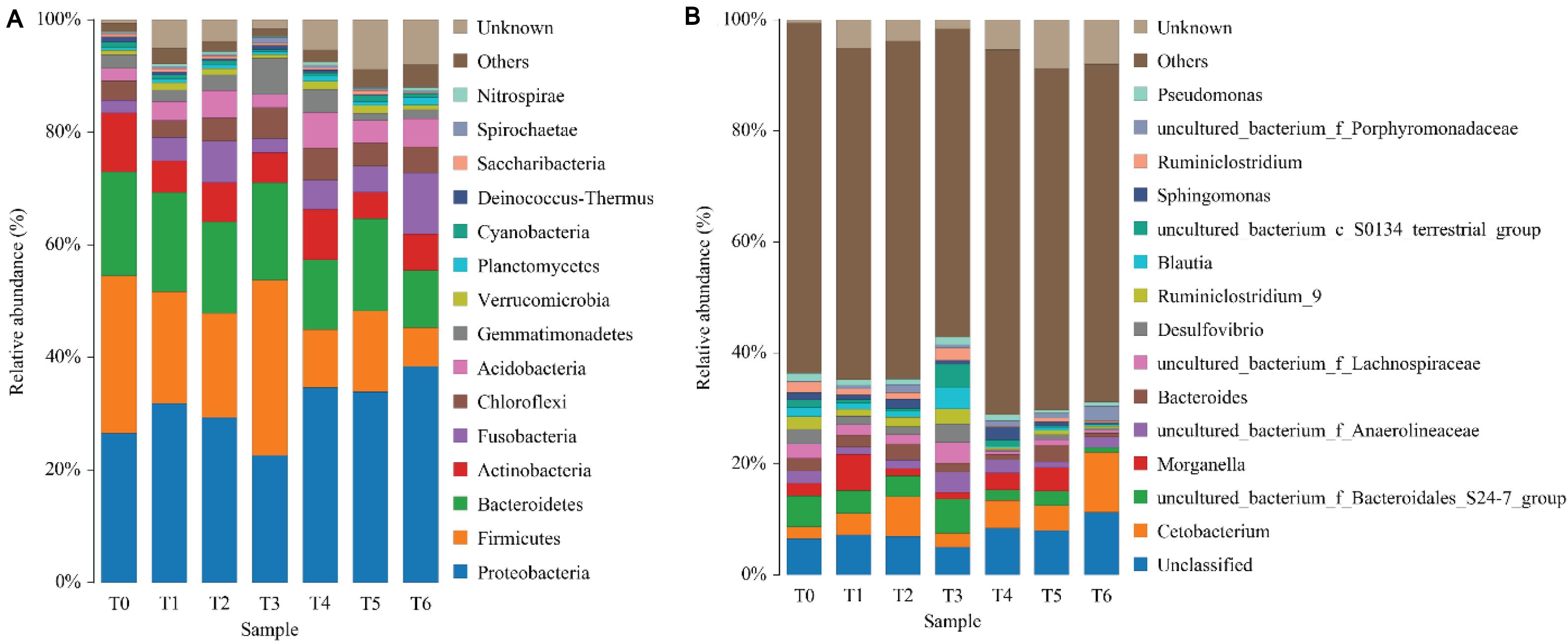
Figure 3. Taxonomy classification of reads from 16 S rRNA V3–V4 regions at the phylum (A) and genus (B) taxonomic levels in intestinal microbiota of grouper E. coioides at day 56. Only top 15 most abundant (based on relative abundance) bacteria phylum and genus were exhibited in (A,B), and other phylum and genus were all classified as “others.”
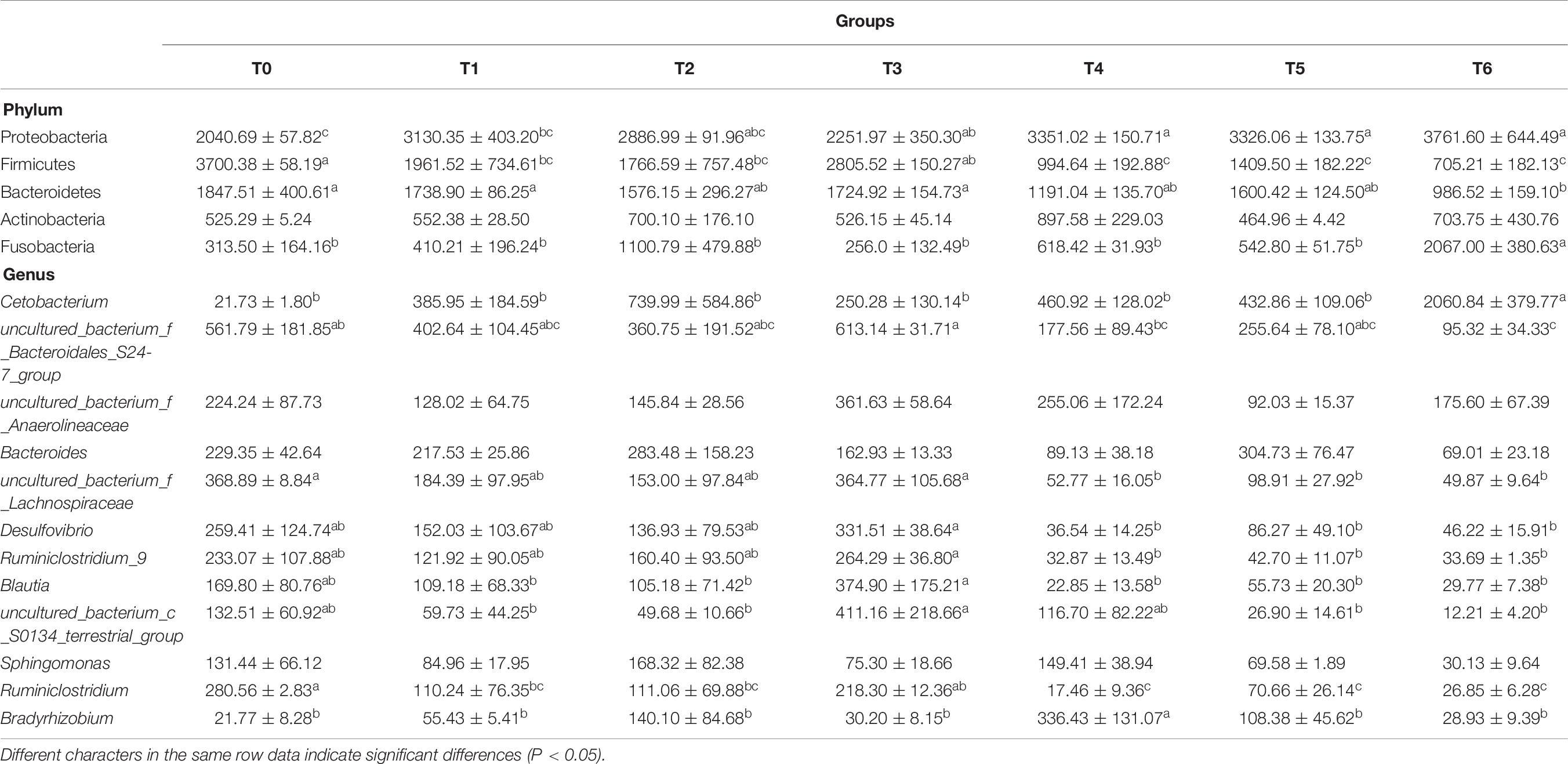
Table 7. MetaStat analysis of the abundance of intestinal bacterial phyla and genera (× 10–4) of grouper at day 56.
The Beta (β)-diversity boxplots and UPGMA phylogenetic tree based on binary_jaccard distance were used to analyze the microbial similarities among different groups. From the data in Figure 4A, a clear separation was observed between histamine treated groups and the control (T0) at phylum level, suggesting that supplementation of histamine changed the intestinal microbial community in E. coioides, especially in groups T5 and T6. However, no significant differences were found for the intestinal microbial β-diversity in different treatments, although apparent difference was observed in groups T5 and T6 (Figure 4B).
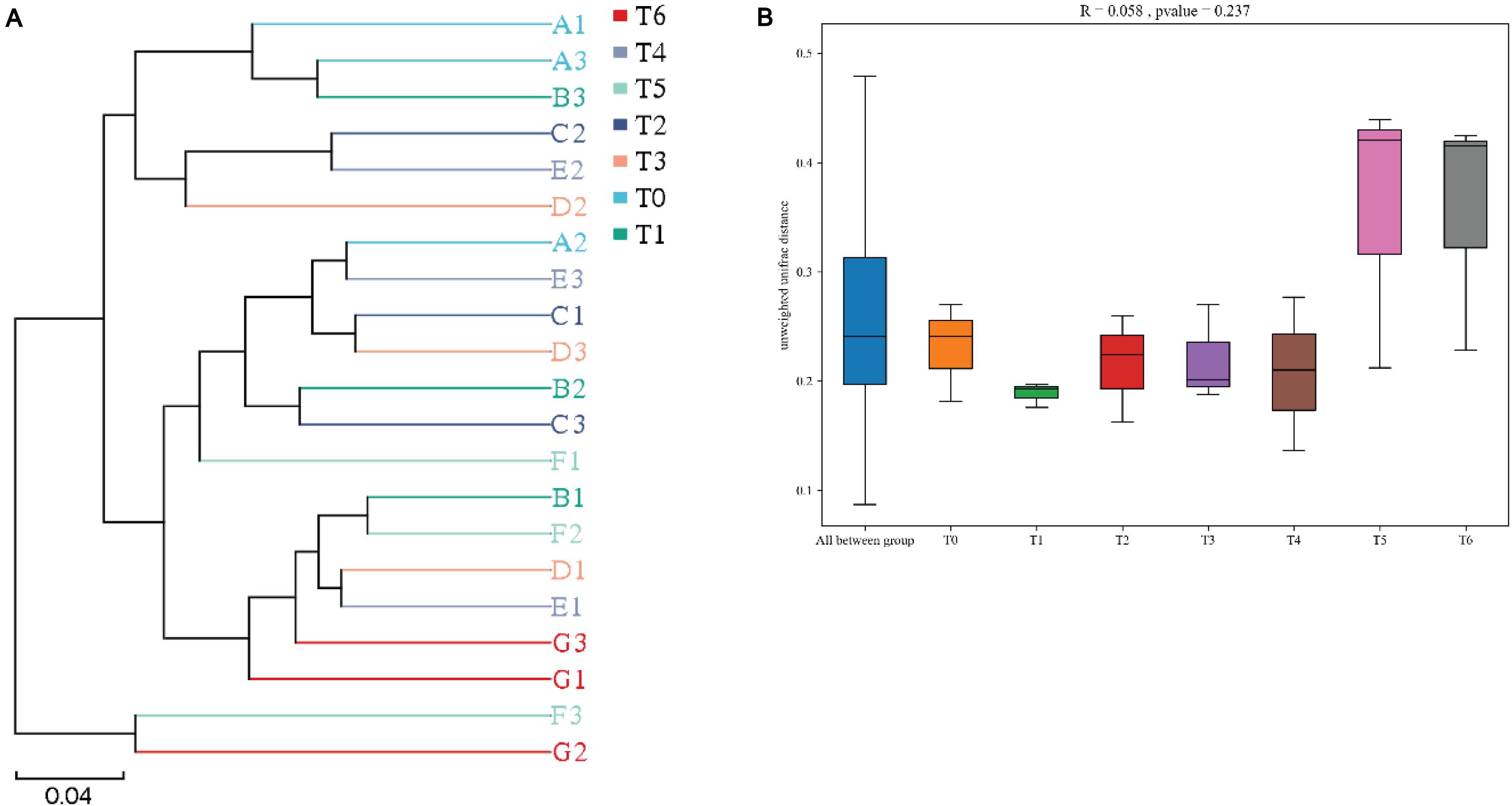
Figure 4. Beta diversity of intestinal microbiota based on binary_jaccard distance of grouper E. coioides at day 56. (A) UPGMA-clustering trees at genus level. (B) Beta (β)-diversity boxplots based on phylum. A1, A2, and A3 refer to triplicates of group T0; B1, B2, and B3 refer to triplicates of group T1; C1, C2, and C3 refer to triplicates of group T2; D1, D2, and D3 refer to triplicates of group T3; E1, E2, and E3 refer to triplicates of group T4; F1, F2, and F3 refer to triplicates of group T5; G1, G2, and G3 refer to triplicates of group T6.
Discussion
An initial objective of the present study was to evaluate the effect of graded levels of dietary histamine on performance of grouper (E. coioides). In the present study, the SR was unaffected by dietary histamine, which is in line with the result of previous studies (Watanabe et al., 1987; Fairgrieve et al., 1994; Tapia-Salazar et al., 2001; Zhai et al., 2020). Furthermore, we have demonstrated that the growth performance was not statistically different among the dietary treatments at initial feeding period (0–28 days), whereas dietary histamine suppressed growth performance at the whole feeding period (0–56 days), especially when histamine supplementary doses were 0.25 and 0.3%. Similar results were reported in Nile tilapia (Oreochromis niloticus) (Reyes-Sosa and Castellanos-Molina, 1995), Atlantic halibut (Hippoglossus hippoglossus) (Aksnes and Mundheim, 1997), Atlantic salmon (Salmo salar L.) (Opstvedt et al., 2000), Japanese seabass (Lateolabrax japonicus) (Hu et al., 2013), and American eel (A. rostrata) (Zhai et al., 2020). In Chinese mitten crab (E. sinensis), however, histamine supplementation (1, 2, 4 g/kg) did not affect the growth performance (Zhao et al., 2016). Tapia-Salazar et al. (2001) studied the effect of dietary histamine supplementation in blue shrimp Litopenaeus stylirostris and observed that a quadratic effect between weight gain and histamine supplementation levels, while the optimum dietary histamine supplementation levels (1,200 and 2,400 mg/kg), had favorable effects on growth performance. He et al. (2018) also reported that optimum dietary histamine level (18 mg/kg) significantly improved growth performance of yellow catfish (Pelteobagrus fulvidraco). Diamine oxidase (DAO) is one of the crucial enzymes in charge of the exogenous histamine metabolizing and scavenging system in organisms (Smolinska et al., 2014). Several studies suggested that DAO activity exists diversity in animals due to different contents of histamine and histidine infeed (Waton, 1963; Yang et al., 2010), which is a major reason for the difference in histamine intolerance. It is possible, therefore, that the sensitivities of fish and shrimp to histamine are highly species-specific, which partly explained the discrepancy of growth performance, although the information is lacking in aquatic animals.
Serum non-specific immune parameters served as important indexes that reflect health status of animals (Yu et al., 2019; Zhai et al., 2020). AKP and ACP, two important phosphatase enzymes, play a key role in clearing extracellular invaders and immune defense (Ellis et al., 2011; Matozzo et al., 2011; Tripathi et al., 2012). In the current study, serum ACP and AKP activities decreased significantly in fish fed 0.25 and 0.3% histamine containing diets, suggesting suppressed immune response. In accord with our results, Zhai et al. (2020) noticed the reduction of serum ACP and AKP activities in American eel (A. rostrata) fed commercial diet (containing 217 mg/kg histamine) supplemented 300 mg/kg histamine. Another study in Chinese mitten crab (E. sinensis) had reported that after 6 h of histamine injection, ACP and AKP activities in the 1 and 50 mg/g (body weight) histamine treated groups were significantly lower than those in the control (Zhao et al., 2012). However, contrary results were reported that improvement of lysozyme activity in histamine treated Tetrahymena (Kovacs and Csaba, 1990) and increased AKP activities were observed in all histamine treated groups (50, 100, and 200 μg/kg) in rabbits (Tripathi et al., 2012). Phosphatase activity enhanced significantly during incubation of Tetrahymena pyriformis in histamine-supplemented cultures, and histamine was identified as a phagocytic stimulus affecting phosphatase synthesis and phagocytosis of hemocytes (Kovacs and Csaba, 1990; Ellis et al., 2011; Matozzo et al., 2011). These inconsistent effects of histamine may be due to the different administration regimes and species specificity. Generally, the serum T-AOC and SOD activities can provide an indication of the antioxidant status of organisms, while higher serum T-AOC and SOD activities might indicate higher antioxidant capacity (Reddy et al., 1991). In the present study, the significant reduction of serum T-AOC and SOD activity might indicate a diminished antioxidant capability by high dose of histamine (0.25 and 0.3%). However, Zhao et al. (2012) reported that injected histamine could increase SOD activity at 6–24 h in E. sinensis. In an in vitro study, enhancement of peroxide activity and superoxide anion production were observed in whole hemolymph of Sydney rock oysters (Saccostrea glomerata) after treatment for 30 min with noradrenaline, another biogenic amine (Aladaileh et al., 2008). These paradoxes may be explained in that short-term histamine stress can rapidly improve the antioxidant capacity by compensatory mechanism; however, long-term dietary histamine damage the ability to respond to reactive oxygen intermediates, which subsequently result in oxidative damage and cause the noticed decline with T-AOC and SOD activities.
Liver biochemical indices provide generally effective information about the function and health status of liver. Malondialdehyde (MDA), an endogenous genotoxic product of enzymatic and oxygen radical-induced lipid peroxidation, is commonly used as a bioindicator of oxidative injury. Additionally, the reduction of liver GPT and GOT activities suggested probable liver damage or dysfunction (Zhang J. Z. et al., 2020). In this study, histamine was noticed to cause higher MDA level and lower activities of GPT and GOT in liver. In accordance with our results, histamine-rich diets resulted in liver injury with a significant improvement in plasma GOT and GPT activities (Li et al., 2018; Zhai et al., 2020). Study done in immunized rabbits observed that histamine may cause hepatic damage at different dosage by determining the characteristic ratios and changes in serum GPT and GOT activities (Tripathi et al., 2012). However, different from the above studies, dietary supplementation with 10 g/kg histamine did not significantly affect the plasma GOT and GPT activities in rainbow trout (Shiozaki et al., 2004). The reason for these controversial results is unclear but it may have something to do with different degree of histamine intolerance in rearing species. Interestingly, grouper fed diets supplemented low dose histamine (0.1, 0.15, and 0.2%) at day 28 showed significantly lower antioxidative capacity (estimating by determining the activity of SOD, the T-AOC, and the level of MDA) compared with those fed the control diet (T0), but no significant differences were observed at day 56. This suggested that grouper had the capacity to adapt their antioxidative physiology changes caused by a long-term exposure to low dose of dietary histamine to some degree.
It has been evidenced extensively that the enhancement of pro-inflammatory factors (e.g., IL-1β, ITF, and FABP2) sharpens the inflammatory extent and results in intestinal and local tissue injury in organisms (Andre et al., 2000; Sharma et al., 2004; Levy et al., 2009; Overland et al., 2009; Skov et al., 2012; Venold et al., 2012; Song et al., 2013; Couto et al., 2014; Lee et al., 2017; Impellizzeri et al., 2018). The present study showed that dietary histamine increased the intestinal ITF and serum IL-1β and FABP2 levels at days 28 and 56, especially in the high dose group (0.3% histamine), illustrating that supplementation of histamine induced inflammation in grouper. In line with our results, Zhai et al. (2020) reported that high level of dietary histamine resulted in liver inflammation and oxidative damage in juvenile American eel (A. rostrata). These results confirmed that histamine is biologically active in fish with regulating the inflammatory response (Mulero et al., 2007). Interestingly, the contents of intestinal ITF and serum IL-1β and FABP2 at day 56 were lower than those at day 28, indicating that grouper adapted to dietary histamine gradually during the long-term feeding period. CRP and SAA are two key acute phase proteins associated with an acute inflammatory response, with a significant enhancement of serum CRP and SAA levels following organism inflammation, injury, or infection (Pepys et al., 1978; Lindhorst et al., 1997; Pepys and Hirschfield, 2003; MacCarthy et al., 2008; Rosani et al., 2016; Franco-Martinez et al., 2019; Bello-Perez et al., 2020; Gursky, 2020; Williams et al., 2020). Derebe et al. (2014) reported that vitamin A deficiency may lead to an immune deficiency in mice with decreasing the abundances of SAAs in small intestine and liver. Another study in mice also demonstrated that animals may against lipopolysaccharides (LPS)-induced inflammation and tissue injury through increasing content of serum acute-phase SAA (Cheng et al., 2018). On the other hand, MacCarthy et al. (2008) reported that serum CRP-like protein level improved several folds in common carp (Cyprinus carpio) challenged with the pathogen Aeromonas hydrophila. A study done in rainbow trout showed that serum CRP concentration rose to a maximum at 6 or 9 days after exposure to formalin for 3.5 h at 300 ppm or 9.5 h at 30 ppm, respectively, and subsequently it began to decline until below normal at day 18 (Kodama et al., 2004). Kodama et al. (2004) also reported that the CRP content enhanced significantly to a maximum at day 3 after exposure to metriphonate (9.9 times higher than normal), then reduced to below normal. Interestingly, the present study showed that dietary histamine did not markedly affect serum CRP and SAA contents at days 28 and 56, although slight enhancement was observed in histamine-supplemented groups. A possible explanation for this might be that serum acute phase proteins (CRP and SAA) contents had recovered in some extent after starvation for 24 h. Unfortunately, serum CRP and SAA contents have not been immediately measured after feeding in this study. Taken together, exogenous high dose histamine not only causes liver oxidative damage but also induces inflammation on the digestive tract in both terrestrial and aquatic animals.
Intestinal morphometrical change is a helpful indicator of gut inflammation and health status (Venold et al., 2012). Intestinal integrity is mainly referred by MT, MFH, and abundance of goblet cells (Garcia-Ortega et al., 2016). Generally, higher MT and MFH indicated healthier intestinal structure, strongly linked to the suppression of enteritis and nutrient transport capacity. In this study, the reduction of MT and MFH demonstrated high doses of histamine (0.25 and 0.3%) exert harmfulness effect on intestinal morphology of grouper. Similar results were reported that high dose of histamine (103.5 mg/kg or more) may result in destruction of gastric and intestinal mucosal barrier in yellow catfish (P. fulvidraco) (He et al., 2018). As far as we know, to date, information about the effects of histamine on intestinal morphology in fish remains limited. Several studies indicated that histological and morphological pathology of digestive systems appeared in both rainbow trout and chicks when dietary histamine content exceeded 2 g/kg (Watanabe et al., 1987; Fairgrieve et al., 1994).
It has been extremely reported that intestinal microbiota might be of crucial importance to host health by improving intestinal morphology (Lee et al., 2014; Sayyaf Dezfuli et al., 2018; Torraca and Mostowy, 2018; Cani et al., 2019; Liu et al., 2019; Ortega et al., 2019), modulating metabolic, physiological, and immunological processes (Al-Fataftah and Abdelqader, 2014; Reda and Selim, 2015; Wang et al., 2018; Heiss and Olofsson, 2019; Deng et al., 2020), and which is highly sensitive to dietary ingredients and components (Ringø et al., 2016). In the current study, the intestinal microbiota of grouper was dominated by Proteobacteria, Firmicutes, Bacteroidetes, and Actinobacteria, which is in keeping with our previous studies in grouper (Yang et al., 2019). Dietary 0.3% histamine significantly enhanced relative abundance of Fusobacteria compared with the control. Bacteria from this phylum includes several common pathogens in human (Tahara et al., 2014; Han, 2015; Harrandah et al., 2021) and aquatic animals (Meng et al., 2021; Wang et al., 2021), which can cause tissue necrosis and inflammatory response of the host. The relative abundances of Firmicutes and Bacteroidetes in histamine supplemented groups were lower than those in the control, and it has been reported that members of these phyla improve the functions of intestinal mucosal barrier and non-specific immunity of fish (Costantini et al., 2017; Duan et al., 2018; Wang et al., 2019; Meng et al., 2021). These results may partly explain why the growth performance and non-specific immunity of grouper in the control group were higher than those in histamine supplemented groups. Although significant difference was not observed in intestinal microbial diversity of grouper, higher histamine-treated diets changed bacterial composition in the intestine and increased its diversity, such as increased relative abundances of Cetobacterium, as well as decreased abundances of Ruminiclostridium, uncultured_bacterium_f_Lachnospiraceae, and uncultured_bacterium_f_ Bacteroidetes_S24-7_group. In accord with our study, Ye et al. (2020) reported that chilled trash fish generally contain high levels of biogenic amines (such as histamine) (Zhao et al., 2012, 2016), led to significantly decreased intestinal beneficial bacteria (e.g., Bacteroidetes_S24-7_group and Lachnospiraceae, etc.) in hybrid grouper (Epinephelus fuscoguttatus ♀ × Epinephelus lanceolatus ♂), paralleled with suppression of growth performance. As for horse study, supplementation of Saccharomyces cerevisiae (S. cerevisiae) modulated positively the overall structure of intestinal microbiota with increased relative abundance of Lachnospiraceae (Garber et al., 2020), which is a family of butyrate-producing bacteria (Nicholson et al., 2012; Hamilton et al., 2020). On the other hand, high fat diet resulted in a reduction of the relative abundance of the Bacteroidetes_S24-7_group and Lachnospiraceae in mice, which might be one of the primary etiological mechanisms underlying obesity (Li et al., 2020). Similar to this study, the lower relative abundance of Cetobacterium is accompanied by greater growth performance in tilapia (O. niloticus) (Standen et al., 2015; Li et al., 2019). Moreover, combined with the poor growth performance and increased relative abundances of Cetobacterium in the soybean meal (SM) group, Wang et al. (2020) speculated that Cetobacterium may exert a harmful effect in bullfrog (Lithobates catesbeianus). However, these results are contrary to that of Meng et al. (2021) who suggested Cetobacterium has a beneficial effect on the production of vitamin B-12 in common carp (Cyprinus carpio L.). In the present study, combined with the results of intestinal morphology and inflammation markers, the increase of intestinal microbial diversity by supplemented histamine may be adverse to maintain homeostasis of intestine, indicating a tendency to induce inflammation, which is in line with previous study (Reveco et al., 2014). The above data suggested that the intestinal microbiota composition of grouper was greatly shaped by supplementation of high dose of histamine, which might negatively affect the growth performance, immune function, and intestinal morphology.
Conclusion
Diet supplemented with no more than 0.2% histamine did not negatively affect the growth performance, immune response, and intestinal health in grouper, while high doses (0.25 and 0.3%) of dietary histamine exert apparently negative effects on growth performance, immune response, and intestinal health in grouper. This study lays the foundation for future studies on exploring effective strategies to eliminate the adverse effects of histamine in marine fish.
Data Availability Statement
The datasets presented in this study can be found in online repositories. The names of the repository/repositories and accession number(s) can be found below: NCBI [accession: PRJNA718150].
Ethics Statement
The animal study was reviewed and approved by the Animal Care and Use Committee of Jimei University.
Author Contributions
Z-YL: investigation and original draft. H-LY: data analysis and writing. L-HH: investigation and data analysis. WY: feed preparation and animal trial. C-XA: review and editing. Y-ZS: supervision, project administration, funding acquisition, review and editing. All authors contributed to the article and approved the submitted version.
Funding
This work was financially supported by the National Natural Science Foundation of China (Grant No. 32072990), Xiamen Marine and Fisheries Development Fund (Grant No. 19CZP018HJ04), Research Foundation of Education Bureau of Fujian Province (Grant No. JAT190351), and the Industry-University Cooperation Project of Fujian Province (Grant No. 2018N5011).
Conflict of Interest
WY was employed by the company Xiamen Jiakang feed Co., Ltd.
The remaining authors declare that the research was conducted in the absence of any commercial or financial relationships that could be construed as a potential conflict of interest.
Footnotes
References
Aksnes, A., Izquierdo, M. S., Robaina, L., Vergara, J. M., and Montero, D. (1997). Influence of fish meal quality and feed pellet on growth, feed efficiency and muscle composition in gilthead seabream (Sparus aurata). Aquaculture 153, 251–261. doi: 10.1016/S0044-8486(97)00046-X
Aksnes, A., and Mundheim, H. (1997). The impact of raw material freshness and processing temperature for fish meal on growth, feed efficiency and chemical composition of Atlantic halibut (Hippoglossus hippoglossus). Aquaculture 149, 87–106. doi: 10.1016/S0044-8486(96)01438-X
Aladaileh, S., Nair, S. V., and Raftos, D. A. (2008). Effects of noradrenaline on immunological activity in Sydney rock oysters. Dev. Comp. Immunol. 32, 627–636. doi: 10.1016/j.dci.2007.10.001
Al-Fataftah, A. R., and Abdelqader, A. (2014). Effects of dietary Bacillus subtilis on heat-stressed broilers performance, intestinal morphology and microflora composition. Anim. Feed Sci. Technol. 198, 279–285. doi: 10.1016/j.anifeedsci.2014.10.012
Anderson, J. S., Higgs, D. A., and Rowshandeli, M. (1997). Fish meal quality assessment for Atlantic salmon (Salmo salar L.) reared in sea water. Aquacult. Nutr. 3, 25–38. doi: 10.1046/j.1365-2095.1997.00067.x
Andre, M., Ando, S., Ballagny, C., Durliat, M., Poupard, G., Briancon, C., et al. (2000). Intestinal fatty acid binding protein gene expression reveals the cephalocaudal patterning during zebrafish gut morphogenesis. Int. J. Dev. Biol. 44, 249–252.
Bello-Perez, M., Pereiro, P., Coll, J., Novoa, B., Perez, L., and Falco, A. (2020). Zebrafish C-reactive protein isoforms inhibit SVCV replication by blocking autophagy through interactions with cell membrane cholesterol. Sci. Rep. 10:566. doi: 10.1038/s41598-020-57501-0
Biji, K. B., Ravishankar, C. N., Venkateswarlu, R., Mohan, C. O., and Gopal, T. K. S. (2016). Biogenic amines in seafood: a review. J. Food Sci. Technol. 53, 2210–2218. doi: 10.1007/s13197-016-2224-x
Caballero, M. J., Lopez-Calero, G., Socorro, J., Roo, F. J., Izquierdo, M. S., and Fernandez, A. J. (1999). Combined effect of lipid level and fish meal quality on liver histology of gilthead seabream (Sparus aurata). Aquaculture 179, 277–290. doi: 10.1016/S0044-8486(99)00165-9
Cani, P. D., Van Hul, M., Lefort, C., Depommier, C., Rastelli, M., and Everard, A. (2019). Microbial regulation of organismal energy homeostasis. Nat. Metab. 1, 34–46. doi: 10.1038/s42255-018-0017-4
Cheng, N., Liang, Y. R., Du, X. P., and Ye, R. D. (2018). Serum amyloid A promotes LPS clearance and suppresses LPS-induced inflammation and tissue injury. EMBO Rep. 19:e45517. doi: 10.15252/embr.201745517
China Fishery and Statistics Yearbook (2020). Bureau of Fisheries, Ministry of Agriculture. Beijing: China Agriculture Press.
Costantini, L., Molinari, R., Farinon, B., and Merendino, N. (2017). Impact of omega-3 fatty acids on the gut microbiota. Int. J. Mol. Sci. 18:2645. doi: 10.3390/ijms18122645
Couto, A., Kortner, T. M., Penn, M., Bakke, A. M., Krogdahl, A., and Oliva-Teles, A. (2014). Effects of dietary phytosterols and soy saponins on growth, feed utilization efficiency and intestinal integrity of gilthead sea bream (Sparus aurata) juveniles. Aquaculture 432, 295–303. doi: 10.1016/j.aquaculture.2014.05.009
Cowey, C. B., and Cho, C. Y. (1992). Failure of dietary putrescine to enhance the growth of rainbow-trout (Oncorhynchus-Mykiss). Can. J. Fish Aquat. Sci. 49, 2469–2473. doi: 10.1139/f92-272
Deng, Z. X., Luo, X. M., and Wang, H. F. (2020). Quorum sensing, biofilm, and intestinal mucosal barrier: involvement the role of probiotic. Front. Cell Infect. Microbiol. 10:538077. doi: 10.3389/fcimb.2020.538077
Derebe, M. G., Zlatkov, C. M., Gattu, S., Ruhn, K. A., Vaishnava, S., Diehl, G. E., et al. (2014). Serum amyloid A is a retinol binding protein that transports retinol during bacterial infection. eLife 3:e03206. doi: 10.7554/eLife.03206
Doeun, D., Davaatseren, M., and Chung, M. S. (2017). Biogenic amines in foods. Food Sci. Biotechnol. 26, 1463–1474. doi: 10.1007/s10068-017-0239-3
Duan, Y. F., Wang, Y., Dong, H. B., Ding, X., Liu, Q. S., Li, H., et al. (2018). Changes in the intestine microbial, digestive, and immune-related genes of Litopenaeus vannamei in response to dietary probiotic Clostridium butyricum supplementation. Front. Microbiol. 9:2191. doi: 10.3389/fmicb.2018.02191
Ellis, R. P., Parry, H., Spicer, J. I., Hutchinson, T. H., Pipe, R. K., and Widdicombe, S. (2011). Immunological function in marine invertebrates: responses to environmental perturbation. Fish Shellfish Immunol. 30, 1209–1222. doi: 10.1016/j.fsi.2011.03.017
Fairgrieve, W. T., Myers, M. S., Hardy, R. W., and Dong, F. M. (1994). Gastric abnormalities in rainbow-trout (Oncorhynchus-Mykiss) fed amine-supplemented diets or chicken gizzard-erosion-positive fish-meal. Aquaculture 127, 219–232. doi: 10.1016/0044-8486(94)90428-6
Franco-Martinez, L., Tvarijonaviciute, A., Mateo, S. V., Ceron, J. J., Romero, D., Oliveira, M., et al. (2019). Evaluation of C-reactive-like protein in Mytilus galloprovincialis. Ecol. Indic. 106:105537. doi: 10.1016/j.ecolind.2019.105537
Garber, A., Hastie, P. M., Farci, V., McGuinness, D., Bulmer, L., Alzahal, O., et al. (2020). The effect of supplementing pony diets with yeast on 2. The faecal microbiome. Animal 14, 2493–2502. doi: 10.1017/S1751731120001512
Garcia-Ortega, A., Kissinger, K. R., and Trushenski, J. T. (2016). Evaluation of fish meal and fish oil replacement by soybean protein and algal meal from Schizochytrium limacinum in diets for giant grouper Epinephelus lanceolatus. Aquaculture 452, 1–8. doi: 10.1016/j.aquaculture.2015.10.020
Gursky, O. (2020). Structural basis for vital function and malfunction of serum amyloid A: an acute-phase protein that wears hydrophobicity on its sleeve. Curr. Atheroscler. Rep. 22:69. doi: 10.1007/s11883-020-00888-y
Hamilton, A. L., Kamm, M. A., De Cruz, P., Wright, E. K., Feng, H., Wagner, J., et al. (2020). Luminal microbiota related to Crohn’s disease recurrence after surgery. Gut Microbes 11, 1713–1728. doi: 10.1080/19490976.2020.1778262
Han, Y. P. W. (2015). Fusobacterium nucleatum: a commensal-turned pathogen. Curr. Opin. Microbiol. 23, 141–147. doi: 10.1016/j.mib.2014.11.013
Harrandah, A. M., Chukkapalli, S. S., Bhattacharyya, I., and Chan, E. K. L. (2021). Fusobacteria modulate oral carcinogenesis and promote cancer progression. J. Oral Microbiol. 13:1849493. doi: 10.1080/20002297.2020.1849493
Harry, E. G., and Tucker, J. F. (1976). The effect of orally administered histamine on the weight gain and development of gizzard lesions in chicks. Vet. Rec. 99, 206–207. doi: 10.1136/vr.99.11.206
Harry, E. G., Tucker, J. F., and Laursen-Jones, A. P. (1975). The role of histamine and fish meal in the incidence of gizzard erosion and pro-ventricular abnormalities in the fowl. Br. Poult. Sci. 16, 69–78. doi: 10.1080/00071667508416161
He, J., Wu, D., Ye, Y., Cai, C., Wu, P., Luo, Q., et al. (2018). Effects of dietary histamine level on growth performance, serum biochemical indexes and gastrointestinal mucosa structure of yellow catfish (Pelteobagrus fulvidraco). Chinese J. Anim. Nutr. 30, 2581–2593. doi: 10.3969/j.issn.1006-267x.2018.07.018
Heiss, C. N., and Olofsson, L. E. (2019). The role of the gut microbiota in development, function and disorders of the central nervous system and the enteric nervous system. J. Neuroendocrinol. 31:e12684. doi: 10.1111/jne.12684
Higgs, D. A., Dosanjh, B. S., Prendergast, A. F., Beames, R. M., Hardy, R. W., Riley, W., et al. (1995). “Use of rapeseed/canola protein products in finfish diets,” in Nutrition and Utilization Technology in Aquaculture, eds C. E. Lim and D. J. Sessa (Champaign, IL: AOCS Press), 130–156.
Hu, L., Yun, B. A., Xue, M., Wang, J., Wu, X. F., Zheng, Y. H., et al. (2013). Effects of fish meal quality and fish meal substitution by animal protein blend on growth performance, flesh quality and liver histology of Japanese seabass (Lateolabrax japonicus). Aquaculture 372, 52–61. doi: 10.1016/j.aquaculture.2012.10.025
Hu, X., Yang, H. L., Yan, Y. Y., Zhang, C. X., Ye, J. D., Lu, K. L., et al. (2019). Effects of fructooligosaccharide on growth, immunity and intestinal microbiota of shrimp (Litopenaeu vannamei) fed diets with fish meal partially replaced by soybean meal. Aquacult. Nutr. 25, 194–204. doi: 10.1111/anu.12843
Impellizzeri, D., Siracusa, R., Cordaro, M., Peritore, A. F., Gugliandolo, E., Mancuso, G., et al. (2018). Therapeutic potential of dinitrobenzene sulfonic acid (DNBS)-induced colitis in mice by targeting IL-1beta and IL-18. Biochem. Pharmacol. 155, 150–161. doi: 10.1016/j.bcp.2018.06.029
Kodama, H., Matsuoka, Y., Tanaka, Y., Liu, Y. C., Iwasaki, T., and Watarai, S. (2004). Changes of C-reactive protein levels in rainbow trout (Oncorhynchus mykiss) sera after exposure to anti-ectoparasitic chemicals used in aquaculture. Fish Shellfish Immunol. 16, 589–597. doi: 10.1016/j.fsi.2003.09.007
Kovacs, P., and Csaba, G. (1990). Impact of histamine treatment and feeding on the hydrolytic enzyme level of Tetrahymena in culture and in conditions of starvation. Comp. Biochem. Phys. B 97, 429–433. doi: 10.1016/0305-0491(90)90139-K
Lee, P. T., Bird, S., Zou, J., and Martin, S. A. M. (2017). Phylogeny and expression analysis of C-reactive protein (CRP) and serum amyloid-P (SAP) like genes reveal two distinct groups in fish. Fish Shellfish Immunol. 65, 42–51. doi: 10.1016/j.fsi.2017.03.037
Lee, S. H., Ingale, S. L., Kim, J. S., Kim, K. H., Lokhande, A., Kim, E. K., et al. (2014). Effects of dietary supplementation with Bacillus subtilis LS 1-2 fermentation biomass on growth performance, nutrient digestibility, cecal microbiota and intestinal morphology of weanling pig. Anim. Feed. Sci. Technol. 188, 102–110. doi: 10.1016/j.anifeedsci.2013.12.001
Levy, E., Menard, D., Delvin, E., Montoudis, A., Beaulieu, J. F., Mailhot, G., et al. (2009). Localization, function and regulation of the two intestinal fatty acid-binding protein types. Histochem. Cell Biol. 132, 351–367. doi: 10.1007/s00418-009-0608-y
Li, H. Q., Zhou, Y., Ling, H. Y., Luo, L., and Feng, L. (2019). The effect of dietary supplementation with Clostridium butyricum on the growth performance, immunity, intestinal microbiota and disease resistance of tilapia (Oreochromis niloticus). PLoS One 14:e0223428. doi: 10.1371/journal.pone.0223428
Li, W., Pan, X. H., Cheng, W. X., Cheng, Y. B., Yin, Y. L., Chen, J. T., et al. (2018). Serum biochemistry, histology and transcriptomic profile analysis reflect liver inflammation and damage following dietary histamine supplementation in yellow catfish (Pelteobagrus fulvidraco). Fish Shellfish Immunol. 77, 83–90. doi: 10.1016/j.fsi.2018.03.036
Li, Y. C., Liu, Q. X., and Ruan, B. (2020). Both gut microbiota and differentially expressed proteins are relevant to the development of obesity. Biomed. Res. Int. 2020:5376108. doi: 10.1155/2020/5376108
Lindhorst, E., Young, D., Bagshaw, W., Hyland, M., and Kisilevsky, R. (1997). Acute inflammation, acute phase serum amyloid A and cholesterol metabolism in the mouse. Biochim. Biophys. Acta 1339, 143–154. doi: 10.1016/S0167-4838(96)00227-0
Liu, Y., Chen, Z. C., Dai, J. H., Yang, P., Xu, W. Q., Ai, Q. H., et al. (2019). Sodium butyrate supplementation in high-soybean meal diets for turbot (Scophthalmus maximus L.): effects on inflammatory status, mucosal barriers and microbiota in the intestine. Fish Shellfish Immunol. 88, 65–75. doi: 10.1016/j.fsi.2019.02.064
MacCarthy, E. M., Burns, I., Irnazarow, I., Polwart, A., Greenhough, T. J., Shrive, A. K., et al. (2008). Serum CRP-like protein profile in common carp Cyprinus carpio challenged with Aeromonas hydrophila and Escherichia coli lipopolysaccharide. Dev. Comp. Immunol. 32, 1281–1289. doi: 10.1016/j.dci.2008.04.004
Matozzo, V., Gallo, C., Monari, M., and Marin, M. G. (2011). Cellular and biochemical parameters in the crab Carcinus aestuarii after experimentally-induced stress: effects of bacterial injection, leg ablation and bacterial injection/leg ablation combination. J. Exp. Mar. Biol. Ecol. 398, 18–25. doi: 10.1016/j.jembe.2010.11.014
Meng, X. L., Wu, S. K., Hu, W. P., Zhu, Z. X., Yang, G. K., Zhang, Y. M., et al. (2021). Clostridium butyricum improves immune responses and remodels the intestinal microbiota of common carp (Cyprinus carpio L.). Aquaculture 530:735753. doi: 10.1016/j.aquaculture.2020.735753
Moghaddam, M. R. M., Janmohammadi, H., Sheikhzade, N., and Moghanloo, K. S. (2015). The effect of histamine resulted from decarboxylase corruption of the diets on non-specific immune response, growth performance and hepatic index of rainbow trout (Oncorhynchus mykiss). Int. J. Biosci. 6, 243–251. doi: 10.12692/ijb/6.1.243-251
Mulero, I., Sepulcre, M. P., Meseguer, J., Garcia-Ayala, A., and Mulero, V. (2007). Histamine is stored in mast cells of most evolutionarily advanced fish and regulates the fish inflammatory response. Proc. Natl. Acad. Sci. U.S.A. 104, 19434–19439. doi: 10.1073/pnas.0704535104
Nicholson, J. K., Holmes, E., Kinross, J., Burcelin, R., Gibson, G., Jia, W., et al. (2012). Host-gut microbiota metabolic interactions. Science 336, 1262–1267. doi: 10.1126/science.1223813
Opstvedt, J., Mundheim, H., Nygard, E., Aase, H., and Pike, I. H. (2000). Reduced growth and feed consumption of Atlantic salmon (Salmo salar L.) fed fish meal made from stale fish is not due to increased content of biogenic amines. Aquaculture 188, 323–337. doi: 10.1016/S0044-8486(00)00343-4
Ortega, C., Canas-Lopez, L., Irgang, R., Fajardo, R., Poblete-Morales, M., Valladares-Carranza, B., et al. (2019). First detection of spring viraemia of carp virus in common carp (Cyprinus carpio L.) affected by a septicaemic disease in Mexico. J. Fish Dis. 42, 667–675. doi: 10.1111/jfd.12969
Overland, M., Sorensen, M., Storebakken, T., Penn, M., Krogdahl, A., and Skrede, A. (2009). Pea protein concentrate substituting fish meal or soybean meal in diets for Atlantic salmon (Salmo salar)-effect on growth performance, nutrient digestibility, carcass composition, gut health, and physical feed quality. Aquaculture 288, 305–311. doi: 10.1016/j.aquaculture.2008.12.012
Pepys, M. B., Dash, A. C., Fletcher, T. C., Richardson, N., Munn, E. A., and Feinstein, A. (1978). Analogues in other mammals and in fish of human plasma proteins, C-reactive protein and amyloid P component. Nature 273, 168–170. doi: 10.1038/273168a0
Pepys, M. B., and Hirschfield, G. M. (2003). C-reactive protein: a critical update. J. Clin. Invest. 111, 1805–1812. doi: 10.1172/Jci200318921
Pike, I. H., and Hardy, R. W. (1997). “Standards for assaying quality of feed ingredients,” in Crustacean Nutrition.: Advances in World Aquaculture, eds L. R. D’Abramo, D. E. Conklin, and D. M. Akiyama (Baton Rouge: Louisiana State University), 473–492.
Reda, R. M., and Selim, K. M. (2015). Evaluation of Bacillus amyloliquefaciens on the growth performance, intestinal morphology, hematology and body composition of Nile tilapia, Oreochromis niloticus. Aquacult. Int. 23, 203–217. doi: 10.1007/s10499-014-9809-z
Reddy, R., Sahebarao, M. P., Mukherjee, S., and Murthy, J. N. (1991). Enzymes of the antioxidant defense system in chronic-schizophrenic patients. Biol. Psychiat. 30, 409–412. doi: 10.1016/0006-3223(91)90298-Z
Reveco, F. E., Overland, M., and Mydland, L. T. (2014). Intestinal bacterial community structure differs between healthy and inflamed intestines in Atlantic salmon (Salmo salar L.). Aquaculture 420, 262–269. doi: 10.1016/j.aquaculture.2013.11.007
Reyes-Sosa, C. F., and Castellanos-Molina, R. (1995). Nutritional evaluation of gizzard erosion positive brown fish meal in starter diets for Nile tilapia, Oreochromis niloticus. Aquaculture 138, 323–329. doi: 10.1016/0044-8486(95)00052-6
Ringø, E., Zhou, Z., Vecino, J. L. G., Wadsworth, S., Romero, J., Krogdahl, A., et al. (2016). Effect of dietary components on the gut microbiota ofaquatic animals. A never-ending story? Aquacult. Nutr. 22, 219–282. doi: 10.1111/anu.12346
Rosani, U., Domeneghetti, S., Gerdol, M., Franzoi, M., Pallavicini, A., and Venier, P. (2016). Serum amyloid A in marine bivalves: an acute phase and innate immunity protein. Dev. Comp. Immunol. 59, 136–144. doi: 10.1016/j.dci.2016.01.019
Sayyaf Dezfuli, B., Castaldelli, G., and Giari, L. (2018). Histopathological and ultrastructural assessment of two mugilid species infected with myxozoans and helminths. J. Fish. Dis. 41, 299–307. doi: 10.1111/jfd.12713
Sharma, M. K., Denovan-Wright, E. M., Degrave, A., Thisse, C., Thisse, B., and Wright, J. M. (2004). Sequence, linkage mapping and early developmental expression of the intestinal-type fatty acid-binding protein gene (fabp2) from zebrafish (Danio rerio). Comp. Biochem. Phys. B 138, 391–398. doi: 10.1016/j.cbpc.2004.05.009
Shiozaki, K., Nakano, T., Yamaguchi, T., Sato, M., and Sato, N. (2004). The protective effect of stevia extract on the gastric mucosa of rainbow trout Oncorhynchus mykiss (Walbaum) fed dietary histamine. Aquacult. Res. 35, 1421–1428. doi: 10.1111/j.1365-2109.2004.01164.x
Skov, J., Kania, P. W., Holten-Andersen, L., Fouz, B., and Buchmann, K. (2012). Immunomodulatory effects of dietary β-1,3-glucan from Euglena gracilis in rainbow trout (Oncorhynchus mykiss) immersion vaccinated against Yersinia ruckeri. Fish Shellfish Immunol. 33, 111–120. doi: 10.1016/j.fsi.2012.04.009
Smolinska, S., Jutel, M., and O’Mahony, L. (2014). Histamine and gut mucosal immune regulation. Allergy 69, 273–281. doi: 10.1111/all.12330
Song, J. L., Qian, Y., Li, G. J., and Zhao, X. (2013). Anti-inflammatory effects of kudingcha methanol extract (Ilex kudingcha C.J. Tseng) in dextran sulfate sodium-induced ulcerative colitis. Mol. Med. Rep. 8, 1256–1262. doi: 10.3892/mmr.2013.1635
Standen, B. T., Rodiles, A., Peggs, D. L., Davies, S. J., and Merrifield, D. L. (2015). Modulation of the intestinal microbiota and morphology of tilapia, Oreochromis niloticus, following the application of a multi-species probiotic. Appl. Microbiol. Biotechnol. 99, 8403–8417. doi: 10.1007/s00253-015-6702-2
Tahara, T., Yamamoto, E., Suzuki, H., Maruyama, R., Chung, W., Garriga, J., et al. (2014). Fusobacterium in colonic flora and molecular features of colorectal carcinoma. Cancer Res. 74, 1311–1318. doi: 10.1158/0008-5472.Can-13-1865
Tao, Z. H., Sato, M., Zhang, H. M., Yamaguchi, T., and Nakano, T. (2011). A survey of histamine content in seafood sold in markets of nine countries. Food Control 22, 430–432. doi: 10.1016/j.foodcont.2010.09.018
Tapia-Salazar, M., Cruz-Suarez, L. E., Ricque-Marie, D., Pike, I. H., Smith, T. K., Harris, A., et al. (2004). Effect of fishmeal made from stale versus fresh herring and of added crystalline biogenic amines on growth and survival of blue shrimp Litopenaeus stylirostris fed practical diets. Aquaculture 242, 437–453. doi: 10.1016/j.aquaculture.2004.02.013
Tapia-Salazar, M., Smith, T. K., Harris, A., Ricque-Marie, D., and Cruz-Suarez, L. E. (2001). Effect of dietary histamine supplementation on growth and tissue amine concentrations in blue shrimp Litopenaeus stylirostris. Aquaculture 193, 281–289. doi: 10.1016/S0044-8486(00)00494-4
Torraca, V., and Mostowy, S. (2018). Zebrafish infection: from pathogenesis to cell biology. Trends Cell Biol. 28, 143–156. doi: 10.1016/j.tcb.2017.10.002
Tripathi, T., Shahid, M., Raza, A., Khan, H. M., Khan, R. A., Mahdi, A. A., et al. (2012). Dose-dependent effect of histamine on liver function markers in immunized rabbits. Exp. Toxicol. Pathol. 64, 875–881. doi: 10.1016/j.etp.2011.03.010
Venold, F. F., Penn, M. H., Krogdahl, A., and Overturf, K. (2012). Severity of soybean meal induced distal intestinal inflammation, enterocyte proliferation rate, and fatty acid binding protein (Fabp2) level differ between strains of rainbow trout (Oncorhynchus mykiss). Aquaculture 364, 281–292. doi: 10.1016/j.aquaculture.2012.08.035
Visciano, P., Schirone, M., Tofalo, R., and Suzzi, G. (2012). Biogenic amines in raw and processed seafood. Front. Microbiol. 3:188. doi: 10.3389/fmicb.2012.00188
Visciano, P., Schirone, M., Tofalo, R., and Suzzi, G. (2014). Histamine poisoning and control measures in fish and fishery products. Front. Microbiol. 5:500. doi: 10.3389/fmicb.2014.00500
Wang, A. R., Ran, C., Ringo, E., and Zhou, Z. G. (2018). Progress in fish gastrointestinal microbiota research. Rev. Aquacult. 10, 626–640. doi: 10.1111/raq.12191
Wang, A. R., Ran, C., Wang, Y. B., Zhang, Z., Ding, Q. W., Yang, Y. L., et al. (2019). Use of probiotics in aquaculture of China-a review of the past decade. Fish Shellfish Immunol. 86, 734–755. doi: 10.1016/j.fsi.2018.12.026
Wang, L., Wang, J., Lu, K. L., Song, K., Mai, K. S., Zhang, C. X., et al. (2020). Total replacement of fish meal with soybean meal in diets for bullfrog (Lithobates catesbeianus): effects on growth performance and gut microbial composition. Aquaculture 524:735236. doi: 10.1016/j.aquaculture.2020.735236
Wang, P., Chen, S. Q., Wei, C. Y., Yan, Q. P., Sun, Y. Z., Yi, G. F., et al. (2021). Monascus purpureus M-32 improves growth performance, immune response, intestinal morphology, microbiota and disease resistance in Litopenaeus vannamei. Aquaculture 530:735947. doi: 10.1016/j.aquaculture.2020.735947
Watanabe, T., Takeuchi, T., Satoh, S., Toyama, K., and Okuzumi, M. (1987). Effect of dietary histidine or histamine on growth and development of stomach erosion in rainbow trout. Nippon Suisan Gakk 53, 1207–1214. doi: 10.2331/suisan.53.1207
Waton, N. G. (1963). Is tissue histamine formation necessary in cat, dog and man? Int. Arch. Allergy Appl. Immunol. 22, 87–101. doi: 10.1159/000229390
Williams, R. D., Moran, J. A., Fryer, A. A., Littlejohn, J. R., Williams, H. M., Greenhough, T. J., et al. (2020). Monomeric C-reactive protein in serum with markedly elevated CRP levels shares common calcium-dependent ligand binding properties with an in vitro dissociated form of C-reactive protein. Front. Immunol. 11:12. doi: 10.3389/fimmu.2020.00115
Yang, H. L., Sun, Y. Z., Hu, X., Ye, J. D., Lu, K. L., Hu, L. H., et al. (2019). Bacillus pumilus SE5 originated PG and LTA tuned the intestinal TLRs/MyD88 signaling and microbiota in grouper (Epinephelus coioides). Fish Shellfish Immunol. 88, 266–271. doi: 10.1016/j.fsi.2019.03.005
Yang, X. Z., Wang, J. F., Fan, P., Zhao, L. L., Cheng, Y. X., Wu, X. G., et al. (2010). Survival, growth, sexual maturity and tissue histamine accumulation of the mysis, Neomysis awatschensis and N. japonica Nakazawa, fed histamine supplemented diets. Aquaculture 302, 256–260. doi: 10.1016/j.aquaculture.2010.02.006
Ye, G. L., Dong, X. H., Yang, Q. H., Chi, S. Y., Liu, H. Y., Zhang, H. T., et al. (2020). A formulated diet improved digestive capacity, immune function and intestinal microbiota structure of juvenile hybrid grouper (Epinephelus fuscoguttatus ♀× Epinephelus lanceolatus ♂) when compared with chilled trash fish. Aquaculture 523:735230. doi: 10.1016/j.aquaculture.2020.735230
Yu, H., Liang, H. L., Ren, M. C., Ji, K., Yang, Q., Ge, X. P., et al. (2019). Effects of dietary fenugreek seed extracts on growth performance, plasma biochemical parameters, lipid metabolism, Nrf2 antioxidant capacity and immune response of juvenile blunt snout bream (Megalobrama amblycephala). Fish Shellfish Immunol. 94, 211–219. doi: 10.1016/j.fsi.2019.09.018
Zhai, S. W., Wang, Y., He, Y. X., and Chen, X. H. (2020). Oligomeric proanthocyanidins counteracts the negative effects of high level of dietary histamine on American Eel (Anguilla rostrata). Front. Mar. Sci. 7:549145. doi: 10.3389/fmars.2020.549145
Zhang, J. J., Yang, H. L., Yan, Y. Y., Zhang, C. X., and Sun, Y. Z. (2020). Effects of fish origin probiotics on growth performance, immune response and intestinal health of shrimp (Litopenaeus vannamei) fed diets with fish meal partially replaced by soybean meal. Aquacult. Nutr. 26, 1255–1265. doi: 10.1111/anu.13081
Zhang, J. Z., Zhong, L., Chi, S. Y., Chu, W. Y., Liu, Y. L., and Hu, Y. (2020). Sodium butyrate supplementation in high-soybean meal diets for juvenile rice field eel (Monopterus albus): effects on growth, immune response and intestinal health. Aquaculture 520:734952. doi: 10.1016/j.aquaculture.2020.734952
Zhao, L. L., Yang, X. Z., Cheng, Y. X., Liang, P., Zhang, J. B., Hong, Y. H., et al. (2012). Effects of histamine on survival and immune parameters of the Chinese Mitten Crab, Eriocheir Sinensis. J. Shellfish Res. 31, 827–834. doi: 10.2983/035.031.0329
Keywords: histamine, growth performance, immune response, intestinal health, Epinephelus coioides
Citation: Liu Z-Y, Yang H-L, Hu L-H, Yang W, Ai C-X and Sun Y-Z (2021) Dose-Dependent Effects of Histamine on Growth, Immunity and Intestinal Health in Juvenile Grouper (Epinephelus coioides). Front. Mar. Sci. 8:685720. doi: 10.3389/fmars.2021.685720
Received: 25 March 2021; Accepted: 10 May 2021;
Published: 14 June 2021.
Edited by:
Fotini Kokou, Wageningen University and Research, NetherlandsReviewed by:
Shuang Zhang, Guangdong Ocean University, ChinaSeyed Hossein Hoseinifar, Gorgan University of Agricultural Sciences and Natural Resources, Iran
Copyright © 2021 Liu, Yang, Hu, Yang, Ai and Sun. This is an open-access article distributed under the terms of the Creative Commons Attribution License (CC BY). The use, distribution or reproduction in other forums is permitted, provided the original author(s) and the copyright owner(s) are credited and that the original publication in this journal is cited, in accordance with accepted academic practice. No use, distribution or reproduction is permitted which does not comply with these terms.
*Correspondence: Chun-Xiang Ai, Y2h1bnhhaUB4bXUuZWR1LmNu; Yun-Zhang Sun, am11c3VueXVuemhhbmdAMTYzLmNvbQ==
†These authors have contributed equally to this work and share first authorship