- 1Department of Microbiology and Parasitology, Faculty of Pharmacy, University of Seville, Seville, Spain
- 2Department of Plant Biology and Ecology, Faculty of Biology, University of Seville, Seville, Spain
Pantoea agglomerans RSO7, a rhizobacterium previously isolated from Spartina maritima grown on metal polluted saltmarshes, had demonstrated good plant growth promoting activity for its host halophyte, but was never tested in crops. The aims of this study were: (1) testing PGP activity on a model plant (alfalfa) in vitro; (2) testing a bacterial consortium including RSO7 as biofertilizer in a pilot experiment in urban orchard; and (3) identifying the traits related to PGP activities. RSO7 was able to enhance alfalfa growth in vitro, particularly the root system, besides improving plant survival and protecting plants against fungal contamination. In addition, in a pilot experiment in urban orchard, a consortium of three bacteria including RSO7 was able to foster the growth and yield of several winter crops between 1.5 and 10 fold, depending on species. Moreover, the analysis of chlorophyll fluorescence revealed that photosynthesis was highly ameliorated. Genome analysis of RSO7 depicted the robustness of this bacterial strain which showed resilience to multiple stresses (heat, cold, UV radiation, several xenobiotics). Together with wide metabolic versatility, genes conferring resistance to oxidative stress were identified. Many genes involved in metal resistance (As, Cu, Ni, Co, Zn, Se, Te) and in tolerance toward high osmolality (production of a battery of osmoprotectans) were also found. Regarding plant growth promoting properties, traits for phosphate solubilization, synthesis of a battery of siderophores and production of IAA were detected. In addition, the bacterium has genes related to key processes in the rhizosphere including flagellar motility, chemotaxis, quorum sensing, biofilm formation, plant-bacteria dialog, and high competitiveness in the rhizosphere. Our results suggest the high potential of this bacterium as bioinoculant for an array of crops. However, the classification in biosecurity group 2 prevents its use according to current European regulation. Alternative formulations for the application of the bioinoculant are discussed.
Introduction
Interest in the environment is increasingly present in people’s lives. This concern has evolved so much that climate risks are at the forefront of the planet’s concerns today (World Economic Forum, 2020). Among these attempts, searching for less polluting fertilization methods than the commonly used chemicals is prioritized in order to evolve toward a more sustainable agriculture1. One of the most challenging politics in this new green revolution is the use of biofertilizers, included in the recent regulation of the European Union on fertilizers (2019/1009). Biostimulants were defined by du Jardin (2015) as “any substance or microorganism applied to plants with the aim of improving nutritional efficiency, tolerance to abiotic stress and/or quality traits of the crop, regardless of its nutrient content.”
Of particular interest are plant growth promoting rhizobacteria (PGPR), which are soil bacteria able to establish beneficial relationships with plants (Backer et al., 2018). These bacteria are a real alternative to agrochemicals and are proven to improve plant resilience toward an array of stress situations (drought, salinity, high temperature, poor and degraded soils) together with defense against plant pathogens (Backer et al., 2018; Enebe and Babalola, 2018). In this particular, coastal ecosystems are a source of rhizosphere bacteria with particular properties, since they are able to tolerate an array of stress situations such as high salinity, extreme temperature, irradiation, xenobiotic pollutants, heavy metals, etc. (Mesa-Marín et al., 2019; Rodríguez-Llorente et al., 2019).
There are multiple mechanisms by which PGPRs can promote plant growth, which are classified into direct and indirect mechanisms. Direct mechanisms are those that provide nutrients or regulate plant growth, including phosphate solubilization, nitrogen fixation, iron acquisition or the secretion of growth stimulating phytohormones. Indirect mechanisms are those that protect plants from acquiring infections (biotic stress) or help the plant to grow healthy during a period of environmental stress (abiotic stress) for instance through regulation of ethylene production due to the enzyme aminocyclopropane (ACC) deaminase (Goswami et al., 2016; Singh and Jha, 2017). Some of the most frequent bacterial genera used as PGPR are Pseudomonas, Bacillus, Azospirillum, Azotobacter, Rhizobium, Enterobacter, etc. (Ferreira et al., 2019).
In our case, the study involves the bacterium Pantoea agglomerans RSO7, a Gram negative belonging to the Enterobacteriaceae family. The genus Pantoea comprises 20 species with high diversity including PGPR, xenobiotic degraders, antibiotic producers, biocontrol agents, plant pathogens and opportunistic human pathogens (Walterson and Stavrinides, 2015; Dutkiewicz et al., 2016). Concerning the strain RSO7, it had been previously isolated from the rhizosphere of Spartina maritima, a species present in the marshes of southwestern Spain (Castillo et al., 2010) and other parts of Europe. The bacterium was shown to tolerate high levels of arsenic and heavy metals (Zn, Pb, Cu), it shows high salt tolerance and display very good PGPR properties (Paredes-Páliz et al., 2016a), which are listed in Supplementary Table 1. Furthermore, inoculation of Spartina densiflora plants with a bacterial consortium containing RSO7 improved seed germination, enhanced plant growth and ameliorated the physiological state of the host halophyte in metal polluted soils (Paredes-Páliz et al., 2016b, 2017).
However, the PGP properties of this bacterium were never tested in crops. In this particular, alfalfa (Medicago sativa) was selected for a preliminary study in vitro for several reasons. In the first place, it is a legume or Fabaceae, which is a family of plants with high importance for humans. Legumes are considered as the second most important food for humanity, second only to cereals. Their extensive consumption is justified by their high nutritional value, since they provide essential amino acids, complex carbohydrates, fiber, unsaturated fats, vitamins and minerals (Kouris-Blazos and Belski, 2016; Maphosa and Jideani, 2017). Besides alfalfa, RSO7 has been tested as bioinoculant for an array of five winter crops (lettuce, spinach, winter onion, radish and beet) in a pilot experiment in an urban orchard.
In recent times, studies of the complete genomes of PGPR are elucidating the genes that explain the plant growth promoting activities and the plant-bacteria dialog in the rhizosphere (Song et al., 2012; Usha et al., 2015; Bhattacharyya et al., 2017). Accordingly, the draft genomes of several Pantoea strains with PGPR activities have been compared (Bruto et al., 2014; Palmer et al., 2018; Song et al., 2020). In general these microorganisms show wide versatility and adaptability, together with multiple PGP activities such as phosphate solubilization, nitrogen fixation, ACC deaminase activity, auxins production and secretion of siderophores (Shariati et al., 2017; Chen and Liu, 2019; Luziatelli et al., 2020a).
Taking into account the precedent information, the objectives of this work have been: (1) testing PGP activity on a model plant in vitro (in this case, the legume Medicago sativa, alfalfa); (2) Testing a bacterial consortium including RSO7 as biofertilizer in a pilot experiment in an urban orchard; and (3) identifying the genes underlying the PGP activity as well as metal and salt tolerance in this strain.
Materials and Methods
Cultivation of the Bacterial Strain
The strain P. agglomerans RSO7 (Paredes-Páliz et al., 2016a) was routinely maintained on TSA plates. For the preparation of inocula, a single colony was cultivated in TSB liquid medium and incubated at 28°C for 48 h. Optical density of the cultures at 600 nm was determined and adjusted to 1.0 with sterile TSB medium in order to use always the same bacterial density in all the procedures.
Cultivation of Medicago sativa in Agar Plates
Cultivation of alfalfa in vitro was performed in sterile 20 cm × 20 cm square plates filled with BNM (Buffered Nodulation Medium, Ehrhardt et al., 1992) containing 0.9% agar. This medium is commonly used for studies of rhizobium-legume nodulation and lacks nitrogen. Since, in this case, the alfalfa plants were not inoculated with rhizobia, the medium was supplemented with 2.5 mM ammonium nitrate. The medium was used at normal concentration (1 × BNM) and at half concentration of all nutrients (1/2 × BNM) in order to simulate poor or degraded soils.
Commercial M. sativa seeds were superficially disinfected in 90% alcohol for 5 min, after which they were thoroughly washed with sterile distilled water. Subsequently, they were treated with a sodium hypochlorite solution (commercial bleach diluted 1: 5) for 5 min. Finally, they were exhaustively washed with sterile distilled water to remove traces of bleach that could inhibit germination. Seeds were pre-germinated for 24 h on water-agar plates at 22°C in the darkness. Twelve seeds at the same developmental stage were transferred to each of the agar plates, in duplicate. For each concentration (1 × BNM or 1/2 × BNM) two treatments were performed: inoculated and non-inoculated plates. For inoculation, 100 μL of a culture of RSO7 was deposited on every seed. In the non-inoculated plates 100 μL of TSB sterile medium was applied on every seed. Plates were allowed to stand until the inoculum was embedded in the agar and then sealed with film-tape. The lower part of the plates was covered with black paper to protect roots from light and plates were incubated in a plant growth incubator at 22°C/18°C and 16 h light/8 h darkness (120–130 μE m–2 s–1). The experiment was conducted for 16 days (at longer periods, plant roots and stems reached the bottom and the top of the plate, respectively, so length measurements were not accurate). At day 8th, seeds were inoculated again with 5 μL of an RSO7 culture as before, and the same volume of sterile TSB was applied to each seed of the non-inoculated plates.
Determination of the Effect of Inoculation With RSO7 on Medicago sativa Plant Survival and Growth in vitro
To find out whether inoculation with P. agglomerans RSO7 bacteria affected plant survival, the percentage of viable plants was assessed at day 16th. Percentages per plate were calculated and the mean of the duplicate plates was made. The effect of inoculation on root growth was evaluated every day for the first 8 days, and then at day 12th at final time (day 16th). Roots were measured and the number of lateral roots was consigned. The daily average of the size of the roots and the average number of lateral roots per plate was calculated and plotted against time to establish the kinetics of root growth and development of inoculated and non-inoculated plants in the two media considered. Finally, on day 16th, the stem size was measured and the number of trefoils was recorded.
Design of a Pilot Experiment in Urban Orchard
The pilot experiment was performed in the urban orchard of the College I.E.S. Pablo Neruda, located in Castilleja de la Cuesta (Seville, Spain: 37°23′16.7′′N; 6°03′27.5′′W). The experiment was done in the frame of a collaborative research project called “Doing research together” between the University of Seville and the College I.E.S. Pablo Neruda in an attempt to disseminate the knowledge generated in the university to society and also to foster the interest of students for scientific research.
The orchard had four permaculture terraces for planting (area 2 × 1 m) delimited by rails. Two of them were used for inoculation and another two were kept without inoculation as controls. For inoculation, a consortium of three bacteria was used including Pantoea agglomerans RSO7, Pantoea agglomerans RSO6 and Bacillus aryabathai RSO25 (Paredes-Páliz et al., 2016a). The three bacteria were cultivated individually in 1 L of TSB for 48 h at 28°C and 150 rpm. The three cultures were mixed before inoculation.
The experiment was done in the winter season of 2019. In January, plants of five species were planted in the orchard, namely winter onions, spinach, lettuce, radishes and beets. Commercial local varieties of onions bulbs and seeds of the rest of the plants were submerged in the culture of the three bacteria for 30 min and then planted in the terraces. The rest of the bacterial culture was mixed with 50 L tap water and used to water the inoculated plants. At the same time, analogous number of onion bulbs and seeds was planted in the control terraces (non-inoculated) and they were watered with 50 L tap water. Three inoculations were done, the first one at sowing and then once every month (at the end of February and at the end of March). The experiment was conducted for four months. During all this time, the teachers and the students of the college watered the plants twice a week and removed the weeds for keeping the orchard in adequate conditions. Plants were finally harvested at the end of April 2019.
Determination of the Effect of Inoculation on the Photosynthesis and Yield of Winter Crops
The saturation pulse method was used to determine leaf light and dark-adapted fluorescence parameters at midday (1600 μmol m–2 s–1) using a portable modulated fluorimeter (FMS-2; Hansatech Instruments Ltd., United Kindom) (Maxwell and Johnson, 2000; Baker and Oxborough, 2004). Plants were dark-adapted for 30 min using leaf–clips designed for this purpose. The minimal fluorescence level in the dark-adapted state (F0) was measured using a modulated pulse (< 0.05 μmol m–2 s–1 for 1.8 μs) too small to induce significant physiological changes in the plant. Maximal fluorescence level in this state (Fm) was measured after applying a saturating actinic light pulse of 10000 μmol m–2 s–1 for 0.8 s. Maximum quantum efficiency of PSII photochemistry (Fv/Fm) were calculated from F0 and Fm. The same leaf section of each plant was used to measure light-adapted parameters. For this purpose, steady state fluorescence yield (Fs) was recorded at ambient light conditions and saturating actinic light pulse of 10000 μmol m–2 s–1for 0.8 s was then used to produce the maximum fluorescence yield (Fm′) by temporarily inhibiting PSII photochemistry. Finally, using both parameters the quantum efficiency of PSII (ΦPSII = (Fm′ – Fs)/Fm′) was calculated.
At the end of the experiment plants were harvested and the aerial parts were measured and weighed. The bulbs of onions and tubers of radishes and beets were unearthed and weighed.
Isolation of DNA, Whole Genome Sequencing and Annotation of the RSO7 Genome
Pantoea agglomerans RSO7 was cultivated in 3 mL of liquid TSB medium at 28°C and 150 rpm for 24 h. 1.5 mL of the culture were centrifuged and genomic DNA was extracted using the G-spinTM Genomic DNA Extraction Kit for Bacteria (iNtRON Biotechnology Ltd, Korea) according to the specifications of the supplier. Whole genome sequencing was carried out through the company Sistemas Genómicos, S.A. (Valencia) and by the MicrobesNG company (Birmingham, United Kingdom) using Illumina technology. Kraken was used to identify the closest available reference (Wood and Salzberg, 2014). Quality of data was studied mapping the reads with BWA mem (Li, 2013), de novo assembly of the genome was performed using SPAdes (Bankevich et al., 2012) and, then, more quality parameters were checked with BWA mem. The whole genome was deposited in GenBank/EMBL/DDBJ under the accession number CAJOSF01000000. Genome annotation was performed with PROKKA (Seemann, 2014) and basics statistics about the genome were extracted using RAST server v2.0 (Aziz et al., 2008), QUAST v.4.6.3 software (Gurevich et al., 2013), SignalP 4.1 server (Petersen et al., 2011), TMHMM server v.2.0 (Krogh et al., 2001), and CRISPRFinder (Grissa et al., 2007) and PlasFlow (Krawczyk et al., 2018).
Once annotated, genes have been classified in four categories: genes related to adaptability and resilience under several stress situations; genes related to plant growth promoting activities; genes related to rhizosphere processes and genes involved in toxicity and pathogenesis.
Statistical Analysis
For the in vitro experiment with alfalfa, the results obtained were expressed as the mean ± standard error of 2 independent experiments (2 plates for each condition with n = 10–12 plants each depending on plant survival). For the orchard experiment, the results are the mean ± standard error of 2 independent experiments (2 independent terraces with variable number of plants depending on the crop, n = 10–30). The means were compared using the Student test and significant differences at p < 0.05 between inoculated and non-inoculated plants are indicated in figures and tables.
Statistical analysis of chlorophyll fluorescence was carried out using Statistica v. 10.0 (Statsoft Inc.). Data differences between inoculation treatments for the same vegetable species were recorded by using the Student test (t-test). Data were first tested for normality with the Kolmogorov-Smirnov test and for homogeneity with the Brown-Forsythe test.
Results and Discussion
Effect of Inoculation on Plant Survival in vitro
The effect of inoculation with P. agglomerans RSO7 on alfalfa survival in vitro was studied. Data are shown in Table 1. In the case of the complete medium (1 × BNM), the survival index was 4.16% higher in the inoculated plates with regard to non-inoculated; however, this difference was not statistically significant. In the case of the plates with half the nutrients, the percentage of survival in non-inoculated plants decreased to 58.3%. By contrast, in this case, inoculation had very positive effect and the survival of the inoculated plates increased up to 79.2%. Since all the seeds were selected at the same developmental stage (at emerging root) our results suggest that the bacterium ameliorated plant survival in conditions of nutrient limitation. This effect could be a consequence of biocontrol properties of the strain (for instance secretion of siderophores) since much higher fungal contamination was observed in the non-inoculated plates as compared to the inoculated ones (not shown).
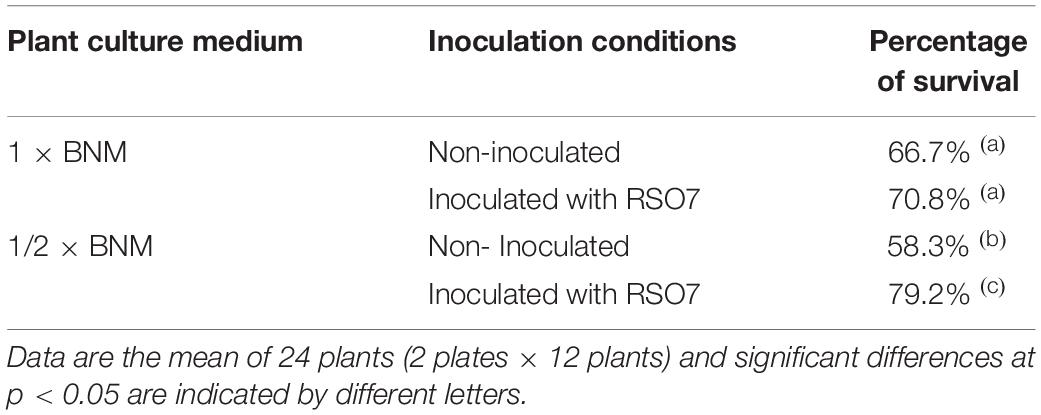
Table 1. Percentage of survival of inoculated and non-inoculated plants at day 16th in complete medium (1 × BNM) and half nutrient medium (1/2 × BNM).
Effect of Inoculation With RSO7 on Medicago sativa Growth in vitro
The effect of inoculation with P. agglomerans RSO7 on root growth was followed every day and, from day 8, every four days. The results are shown in Figure 1. In complete medium (1 × BNM) data showed a slight and late positive effect of inoculation that was observed from day 12th. Finally, on day 16th, the mean of the root in non-inoculated plants was 6.6 cm, while in inoculated plants it was 8.5 cm. The results in deficit medium (1/2 × BNM) were very different. In this case, a marked positive effect of inoculation was observed from the 5th day. The roots became approximately twice as long in inoculated plants as compared to non-inoculated ones. Finally, on day 16th, the mean of the root in non-inoculated plants was 5.48 cm, whereas in the inoculated plants it was 9.41 cm. These results indicated that the effect of bacteria was much greater when the plants were in a situation of lack of nutrients. In the presence of full nutrients all the plants grow well, however, in the case of 1/2 × BNM where the availability of nutrients is more limited, the beneficial effects of bacteria can be better observed, since they can solubilize phosphate, fix nitrogen, synthesize siderophores, etc. (Paredes-Páliz et al., 2016a) allowing better root growth with less supply of nutrients (biofertilizer).
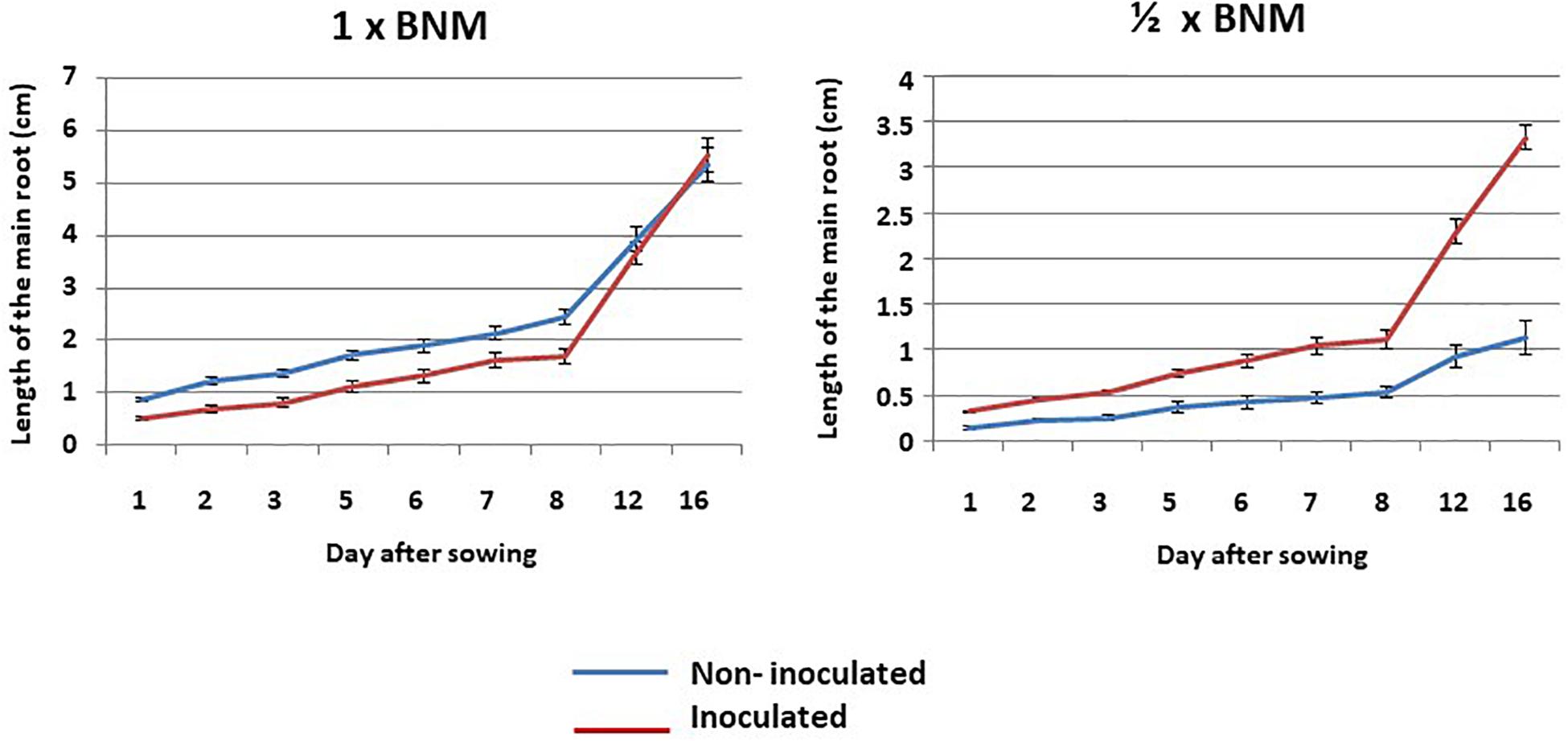
Figure 1. Kinetics of root growth in non-inoculated and inoculated plants of Medicago sativa grown in vitro in square plates. Values represent mean ± SE, n = 24.
Concerning the number of secondary roots, the results are shown in Figure 2. In this case, the data confirmed that the presence of the bacteria increased the number of secondary roots of the plant, regardless of the medium considered (1 × BNM or 1/2 × BNM); in the first case, data showed a notable difference between the number of secondary roots of the non-inoculated plates, with an average of 0, and the number of secondary roots present in the inoculated plates, with an average of 3.54. The results in deficit medium also showed mean number of secondary roots of 0.5 for non-inoculated face to average 2.42 lateral roots in the inoculated plants. These effects could be probably related to the bacterium ability to synthesize auxins (Paredes-Páliz et al., 2016a), since these phytohormones regulate, among other processes, the growth and branching of the root and the development of a greater number of root hairs (Weijers et al., 2018).
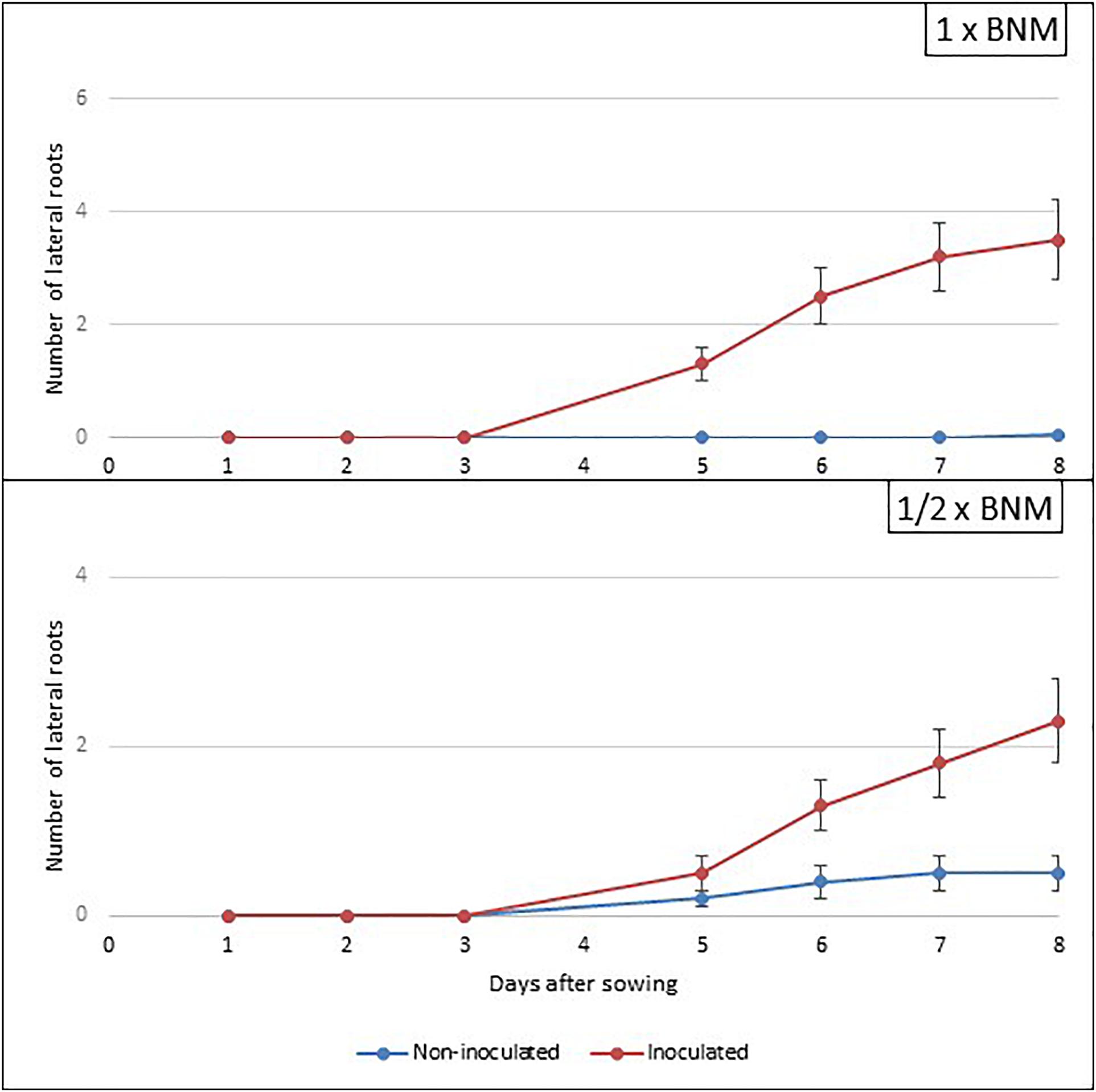
Figure 2. Kinetics of appearance of secondary roots in non-inoculated and inoculated plants of Medicago sativa grown in vitro in square plates. Values represent mean ± SE, n = 24.
The effect of inoculation has also been studied on shoot growth by determining shoot length and the number of trefoils (Table 2). There was an increase in the stem size of the inoculated plants compared to those that were not inoculated in both media. However, the differences were not significant in 1 × BNM (20% increase in shoot length) whereas it was a significant difference in the low-nutrients medium where the increase in shoot length was 53%. When considering the number of trefoils, there were significant differences in both media, with increases of 55%. Again, the results suggest that there is a greater growth promoting effect under conditions of nutrient limitation.

Table 2. Length of the primary root of alfalfa plants grown on 1 × BNM (normal concentration of nutrients) and 1/2 × BNM (half nutrients) and inoculated or non-inoculated with P. agglomerans RSO7.
Determination of the Effect of Inoculation on the Yield of Crop Plants in the Pilot Experiment in Urban Orchard
The effect of inoculation with a consortium of 3 bacterial strains including RSO7 was analyzed on 5 winter crops in a pilot experiment in urban orchad. This bacterial consortium had been previously used for inoculation of the host plant Spartina densiflora (Paredes-Páliz et al., 2017) having demonstrated plant growth promotion and protection of plant against stress by heavy metals (Paredes-Páliz et al., 2017, 2018).
In this opportunity, 5 winter crops were selected to test the effect of the inoculant, namely lettuce, spinach, winter onion, beet and radish. As explained before, the experiment was done within the frame of a collaborative teaching-service project between our research group at the University of Seville and the College I.E.S. Pablo Neruda with professors of Biology and Applied Sciences and students aging 15-16. Supplementary Figure 1 shows several moments of the development of the project. Final data of yield after 4 months are shown in Table 3. The results indicated an enhancement of growth of all the plant species (also observed in Figure 3). The highest effects were found in lettuce and spinach which increased their weights by 8 and 5 fold in average, respectively. Concerning winter onions, the increase was about 15% and it was remarkable that in the case of radish and beet, only inoculated plants gave tubers.
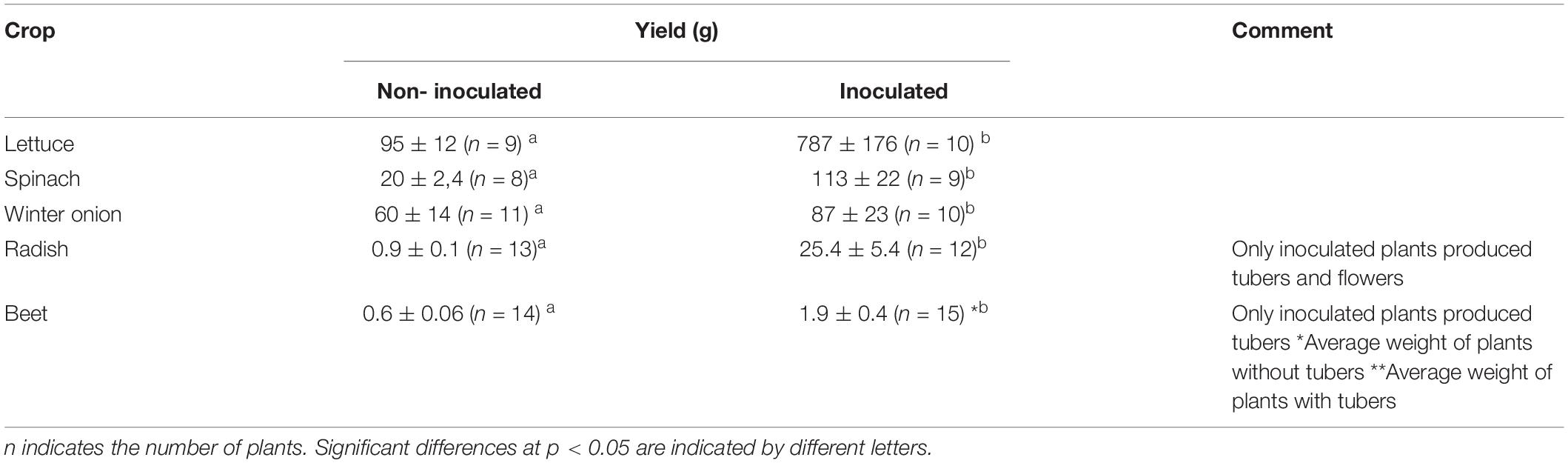
Table 3. Yield of winter crops grown in an urban orchard and inoculated or not with a consortium including the bacterial strains Pantoea agglomerans RSO7, P. agglomerans RSO6, and Bacillus aryabatthai RSO25 (Paredes-Páliz et al., 2016a).
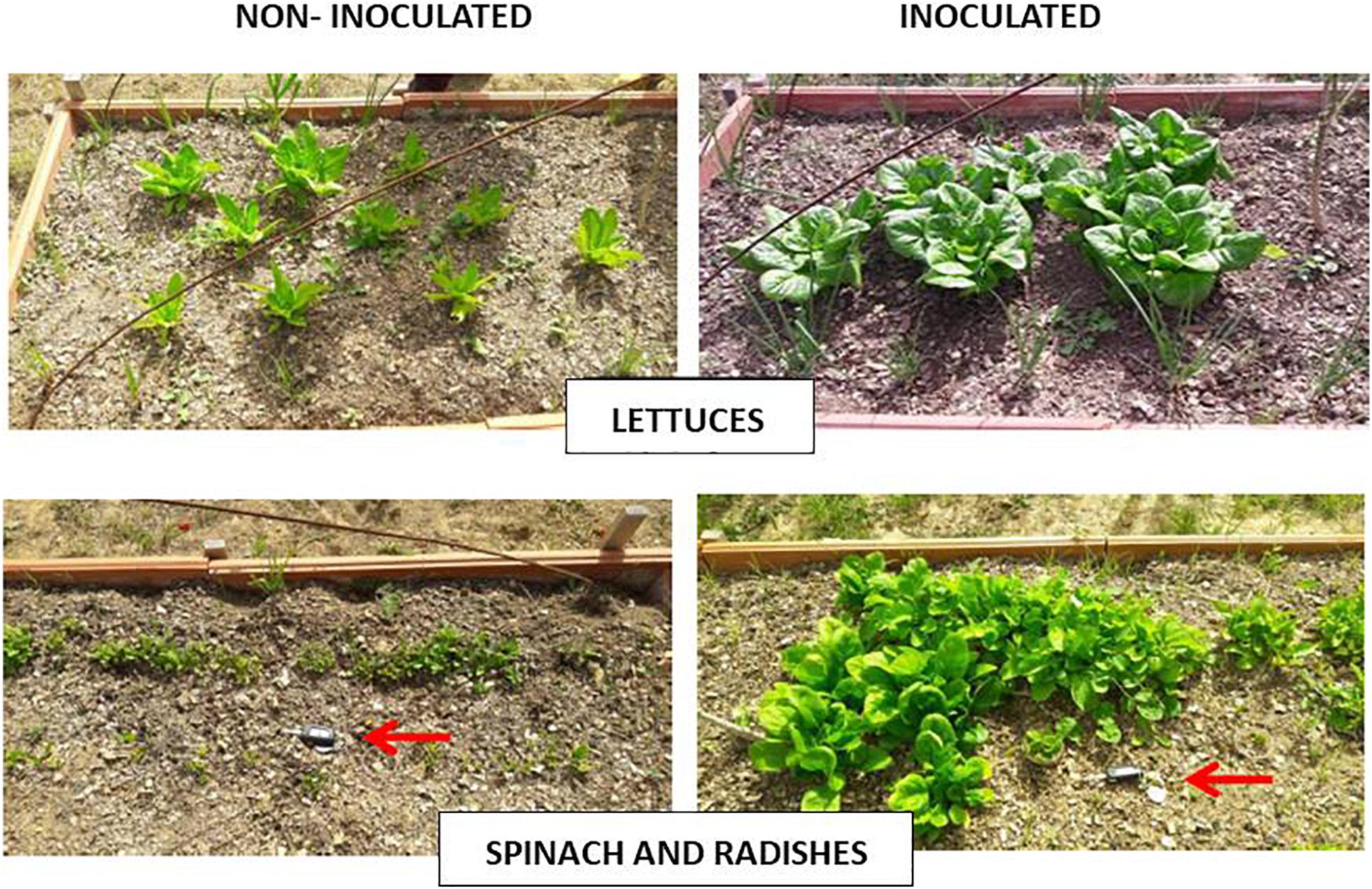
Figure 3. Aspect of lettuces, spinach and radish plants in the orchard one month after planting. Left: non-inoculated; right: inoculated. The red arrow points a key used for size comparison. Values represent mean ± SE, n = 10 (for spinach and lettuces), and n = 50 (for radishes).
These results confirm, in a pilot experiment, the usefulness of the biofertilizer for enhancement of plant growth and yield. The effect is probably due to the plant growth promoting activities of the strains which are able to solubilize phosphate, produce siderophores, and secrete auxins (Paredes-Páliz et al., 2016a). In addition, the survival of plants was much more efficient upon inoculation (not shown) being probably related to the biocontrol properties of RSO7, protecting plants against fungal contamination. Other Pantoea strains also are able to improve the resilience of plants against several stressing environmental conditions and display biocontrol activities (Chen and Liu, 2019; Luziatelli et al., 2020a).
Determination of the Physiological State of Crop Plants in the Pilot Experiment in Urban Orchard
The fluorescence parameters were determined in inoculated and non-inoculated plants. Chlorophyll fluorescence analysis indicated that overall F0 and Fm values were higher in inoculated plants compared with their non-inoculated counterparts (t-test, P < 0.05; Figures 4A,B), which consequently led to greater maximum quantum efficiency of PSII photochemistry (Fv/Fm) for all species (t-test, P < 0.05; Figure 4C). In addition, a very similar trend was recorded for the quantum efficiency of PSII (ΦPSII) for all species except for spinach and beet, which did not show any statistical difference between both inoculation treatments (Figure 4D). These results suggested a positive effect of bacterial inoculation on PSII functionality, in terms of optimization the antenna size to prevent photoinhibition and increase photosynthetic efficiency (Adams and Demmig-Adams, 2004; Ort et al., 2011) with a consequent increment in plant biomass, as was aforementioned.
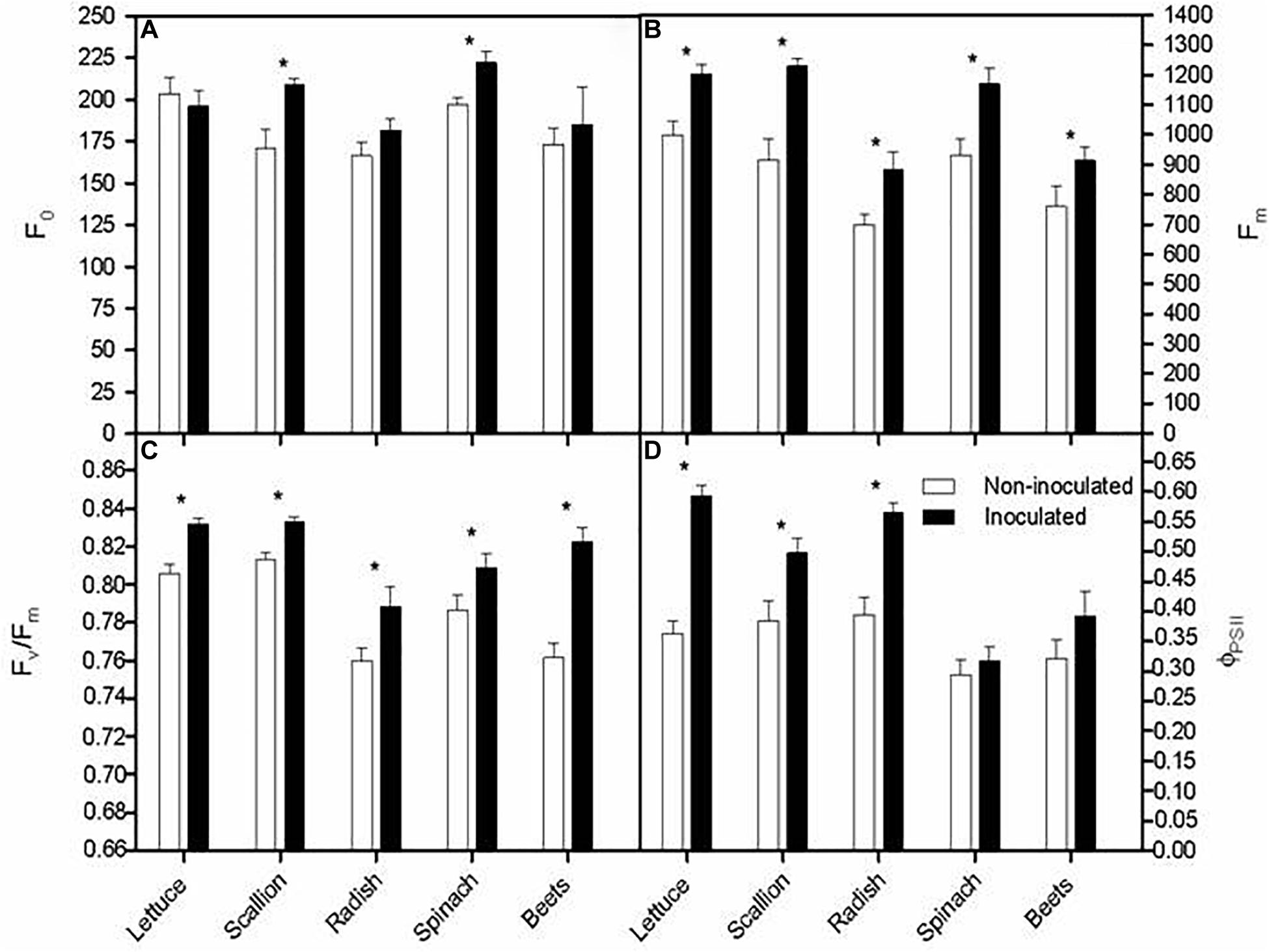
Figure 4. Minimal fluorescence level, F0 (A), maximal fluorescence level, Fm (B); maximum quantum efficiency of PSII photochemistry, Fv/Fm (C) and quantum efficiency of PSII, ΦPSII (D) at midday in randomly selected, fully developed leaves of five winter vegetables in non-inoculated and inoculated plants. Asterisks indicate means that are significantly different between inoculation treatments (t-test, P < 0.05).
Analysis of the RSO7 Genome
The genome of P. agglomerans RSO7 is composed by a chromosome of 4,829,129 bp and it does not contain plasmids (Supplementary Table 2). According to other species of the genus, the G + C content is 55.1%. The analysis depicted 4384 coding sequences from which 616 corresponded to proteins with signal peptides and 1051 to proteins with transmembrane domains. Moreover, the sequence encoded 85 RNAs, from which 11 were rRNA, 73 were tRNA, and 1 tmRNA. Furthermore, 3 CRISPR sequences were identified.
A global analysis of the genome revealed that P. agglomerans RSO7 is a very versatile bacterium capable of adapting to a large number of environmental situations. For example, operons for the use of a large number of C sources (sorbitol, tartrate, citrate, xylulose, galactofuranose, rhamnose, arabinose, galactose, maltose, mannose, etc.) have been found. It can also use various sources of N (nitrate, ammonium, amino acids, even some N2 fixation genes have been detected) and S (sulfate, amino acids like cysteine and methionine together with taurine). The genus Pantoea is known by its robustness and by displaying wide metabolic versatility (Shariati et al., 2017; Luziatelli et al., 2020a). Along with its metabolic versatility, this bacterium shows resistance toward many stresses, not only to heavy metals (Shariati et al., 2017) and salinity as will be disclosed later, but also to heat, cold, UV radiation, acidic conditions, etc. Besides, genes are present for the degradation of diverse xenobiotics including azo compounds (FMN-azoreductase), curcumin (curcumin reductase), compounds with formyl groups (it has formylase and formyl transferase), halogenated compounds (thanks to a dehalogenase), compounds with nitro groups (nitroreductase), etc. Some of these situations produce oxidative stress the bacterium is able to deal with. In this particular, genes for several antioxidant systems (catalase and various peroxidases, as well as superoxide dismutase, glyoxylase, glutathione reductase, glutathione transferase, etc.) were found. On the other hand, the presence of several genes (Syx, crp) indicate that it is naturally competent, being able to incorporate DNA and therefore acquire new capabilities. It can acquire plasmid DNA by conjugation having detected pilin precursor genes, an ATPase involved in pilus retraction and ppdAB genes involved in conjugation. The presence of genes encoding phage integrases (lysogeny) suggests that it may also acquire genetic information by transduction. All this indicates that it displays a high resilience to many environmental variations and is very competitive in the rhizosphere. In particular, among all the genes, three aspects that are fundamental for its application as a bioinoculant will be focused: (a) Resistance to heavy metals and salt (high osmolarity); (b) PGP Properties, and (c) Important rhizosphere processes for colonization and plant-microorganism interaction.
Resistance Toward Heavy Metals and Metalloids
The genome carries operons that encode resistance toward metalloids As, Te and Se, and toward heavy metals such as Cu, Zn, Co, and Ni (Table 4) (The complete list of genes for resistance toward metals and metalloids is displayed in Supplementary Table 3). One of the most frequent mechanisms of resistance of prokaryotes against heavy metals consists in pumping the metal out of the bacterial cell (Nies, 2003). In this particular, several metal efflux pumps have been found, including CopA and YebZ for Cu (Grass and Rensing, 2001); ZntB and ZitB for Zn (Grass et al., 2001); RcnA for Co and Ni (Rodrigue et al., 2005) and the arsenic resistance operon arrHBC (Fekih et al., 2018).
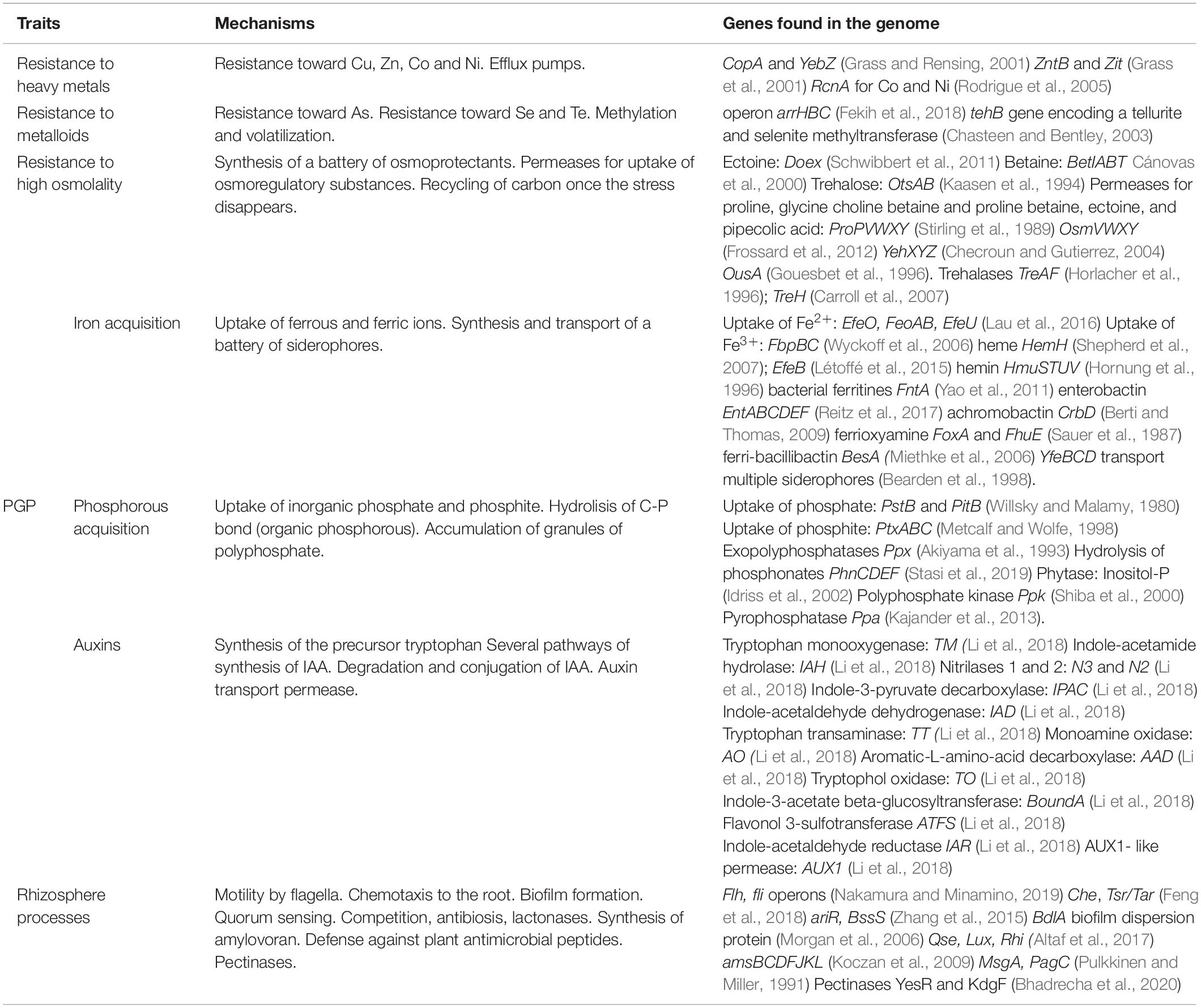
Table 4. Summary of mechanisms (and the corresponding traits) that justify the plant growth promoting properties and resilience of Pantoea agglomerans RSO7.
On this side, the resistance toward metalloids Se and Te (both from group VIB of the periodic table) consists in methylation and volatilization of volatile species such as dimethylselenium and dimethyltellide through the membrane (Chasteen and Bentley, 2003). The tehB gene encoding an enzyme with tellurite methyltransferase and selenite methyltransferase activities has been found in the RSO7 genome.
Resistance to High Osmolality
The RSO7 strain is equipped with a battery of genes that regulate the resistance to salt (osmotic stress) (Table 4) (The complete list of genes for resistance toward metals and metalloids is shown in Supplementary Table 4). Analysis of the genome revealed the presence of genes involved in the synthesis and degradation of a large number of osmoprotectants, such as ectoine (Schwibbert et al., 2011), betaine (Cánovas et al., 2000), trehalose (OtsAB) (Kaasen et al., 1994). Besides, the bacterium has permeases for uptake of osmoregulatory substances such as proline, glycine choline betaine and proline betaine, ectoine and pipecolic acid (Stirling et al., 1989; Gouesbet et al., 1996; Checroun and Gutierrez, 2004; Frossard et al., 2012). Once the osmotic stress conditions disappear, the osmotic metabolites are “recycled” as carbon source by the activity of trehalases (Horlacher et al., 1996; Carroll et al., 2007).
Plant Growth Promotion Traits
Genes involved in promoting plant growth were also carefully extracted, highlighting those involved in iron transport and metabolism, as well as those related to phosphate uptake and solubilization and auxin production (Table 4).
Genes Related to Iron Transport and Metabolism
Iron is a fundamental element for bacteria, being part of many redox enzyme cofactors, electron transporters, etc. For this reason, the bacteria make sure to achieve it and even compete for this element, so production of siderophores is besides a biocontrol trait (The complete list of genes involved in control of osmolality is listed in Supplementary Table 5). The bacterium has low and high affinity transporters for free ion, both in the form of ferrous (EfeO, FeoAB, EfeU, ESA_00329) (Lau et al., 2016) and ferric ions (FbpBC) (Wyckoff et al., 2006). Besides, the bacterium produces and/or transports a great diversity of siderophores including heme (Otto et al., 1992), hemin (Hornung et al., 1996), several bacterial ferritines (Yao et al., 2011), enterobactin (Reitz et al., 2017), achromobactin (Berti and Thomas, 2009), ferrioxyamine (Sauer et al., 1987), and ferri-bacillibactin (Miethke et al., 2006). Finally, several copies of the YfeBCD complex able to transport multiple iron complexes were found (Bearden et al., 1998).
Genes Related to Phosphorous Uptake and Solubilization
The complete list of genes involved in phosphorous uptake and metabolism is shown in Supplementary Table 6. Phosphorous can be captured both in inorganic and organic forms. In its inorganic forms, it is most often found in the form of phosphate (PO4–3) which is captured by low and high affinity transporters of phosphate such as PstB and PitB (Willsky and Malamy, 1980). Besides phosphite (P3+) can be transported by the PtxABC phosphite transporter (Metcalf and Wolfe, 1998).
Referring to organic forms of phosphorus, the bacterium can hydrolyze C-P bonds by exopolyphosphatases (Akiyama et al., 1993) and nucleases. Besides it can use phosphonates (the PhnCDEF operon has been found, Stasi et al., 2019) and inositol-P by the activity of phytase (Matsuhisa et al., 1995). The captured phosphorus can accumulate in polyphosphate granules in the cytoplasm thanks to the Ppk polyphosphate kinase (Shiba et al., 2000) and be mobilized when necessary by means of the inorganic pyrophosphatase Ppa (Kajander et al., 2013).
Genes Related to Auxin Production
Respecting auxins production, RSO7 genome showed genes involved in the tryptophan biosynthesis and indole-3-acetic acid (IAA) biosynthesis. The main precursor in the IAA synthesis is tryptophan and five different pathways to synthetize IAA have been studied: indol-3-acetamide pathway, indole-3-pyruvate pathway, tryptamine pathway, tryptophan side-chain oxidase pathway and indole-3-acetonitrile pathway (Li et al., 2018; Duca and Glick, 2020). Strain RSO7 has genes involved in four of these pathways (indol-3-acetamide pathway, indole-3-pyruvate pathway, tryptamine pathway and indole-3-acetonitrile pathway) (Table 4) (The complete list of genes for auxin biosynthesis is listed in Supplementary Table 7), and all of them are completed from tryptophan to IAA, supporting the IAA production for this strain in results obtained in a previous work (Paredes-Páliz et al., 2016a). These genes have been detected in other strain of P. agglomerans (Morris, 1995; Manulis et al., 1998; Spaepen et al., 2007). Moreover, genes involved in the IAA degradation or conjugation and a transporter have been found (Table 4).
Genes Involved in Rhizosphere Processes Important to Plant Colonization
The correct colonization of the root is an important trait which depends on many factors, such as mobility, the ability to form biofilms and bacterial communication, among others. In this sense, genes involved in rhizosphere processes important for plant-bacterium interaction are displayed in Table 4 (the complete list of genes involved in important rhizosphere processes is displayed in Supplementary Table 8).
The mobility of the bacteria is determined by the presence of flagella (genes for synthesis, rotation and regulation were found; Nakamura and Minamino, 2019). The mobility of the bacteria in the rhizosphere is determined by chemotaxis toward the root, which secretes bacteria-attracting compounds (root exudates). In this particular, genes involved in chemotaxis such as che and Tsr/Tar were identified (Feng et al., 2018). Genes involved in biofilm formation such as ariR and BssS are also present (Zhang et al., 2015). Besides, the BdlA gene encoding a biofilm dispersion protein (Morgan et al., 2006) was identified. For all these rhizosphere processes to occur there must be a minimum cell density, detected by quorum sensing systems such as Qse, Lux, and Rhi (Altaf et al., 2017).
Another group of genes present in RSO7 have to do with plant-bacteria interaction. In order to avoid the initial plant defense (Bordiec et al., 2011), RSO7 synthetizes amilovoran, an extracellular polysaccharide that functions as a virulence factor in the formation of bioflims (Koczan et al., 2009). Once the bacterium attaches and multiplies on the root, it has pectinases that degrade the plant cell wall (Bhadrecha et al., 2020) and allow it to survive in the host (even as an endophyte) and defend against antibacterial peptides produced by the host (Pulkkinen and Miller, 1991; Tu et al., 2016).
Finally, genes were found that ensure the competition of the bacterium in the rhizosphere, for example, it has lactonase able to inhibiting quorum sensing signals of other bacteria (Zhang et al., 2007). It also produces toxins (Shidore and Triplett, 2017) and antibiotics such as colicin V and carbepenem (Kenawy et al., 2019).
Genes Involved in Toxicity and Pathogenicity
From the point of view of biosecurity, Pantoea agglomerans is included in group 2. Only group 1 microorganisms are authorized by European legislation to be used as inoculants (GRAS microorganisms, which stand for Generally Recognized as Safe). The species Pantoea agglomerans causes opportunistic infections, particularly in nosocomial patients with previous pathologies such as cystic fibrosis, cancer, etc. (Cruz et al., 2007). Some plant endophytic PGPR strains have also caused infections in workers such as gardeners who, after being pricked by plants, became infected (Jain et al., 2012). In this particular, it has to be noticed that in our experiment, protocols for working with a microorganism of the group 2 have been followed: students and teachers were previously instructed; no immunocompromised people has participated in the experiment; the microorganism does not transmit via inhalation, but only by punching with branches of plants; all people participating in the experiment wore gloves; the organism was isolated from soil and it has been not modified; the orchard perimeter was delimited by lateral “walls” 25 cm high in order to avoid interference between inoculated plants and not inoculated controls.
In this sense, a search has been made for genes that are related to pathogenicity. The RSO7 strain has several determinants of resistance to multiple antibiotics including polymyxin B, phosphinothricin, chloramphenicol, fumonisin, novobiocin, bicyclomycin, nitroimidazole, and bacitracin. Along with these activities, it has several beta-lactamases that break the beta-lactam ring. On the other hand, the presence of a large number of pumps for efflux of toxic substances increases its resistance to antibiotics including erythomycin, tetracycline, ampicillin and norfloxacin. It is also capable of synthesizing toxins (hemolysins, RNAase). It possesses the YejABEF operon for resistance to host antibacterial defense peptides. Finally, the production of siderophores is also a mechanism that ensures the competitiveness of this bacterium. All these factors are considered as virulence factors.
For these reasons it cannot be used as an inoculant. Strategies have been designed to take advantage of the PGP characteristics in cell-free extracts. In this way, the bacteria are grown and only the culture supernatant is used to inoculate the plants. In this supernatant are the molecules and proteins secreted by the bacteria which exert their effect on the plant without the risk of using a microorganism of the group 2 (Luziatelli et al., 2020b).
Conclusion
The use of biofertilizers rises up as a real alternative for a more sustainable and affordable agriculture, particularly in poor countries. In this context, some strains isolated from the rhizosphere of coastal plants, such as Pantoea agglomerans RSO7 have excellent PGP properties and high resilience toward multiple stresses, together with high competitiveness in the rhizosphere. These properties have been demonstrated when using this bacterium as inoculant both in vitro experiments and pilot experiments in an urban orchard with summer and winter crops. Full genome analysis has allowed the identification of the traits behind this important biofertilization capability. With the previous results, the great potential of this bacterium as a promoter of plant growth can be concluded. Finally, restrictions for the use of microorganisms of the biosafety group 2 as bioinoculants could limit its use, and therefore possible bio-sure alternatives, such as using the supernatant of cultures, are proposed.
Data Availability Statement
The datasets presented in this study can be found in online repositories. The names of the repository/repositories and accession number(s) can be found below: https://www.ebi.ac.uk/ena, CAJOSF01000000.
Author Contributions
EP: design of the work, supervision, funding, pilot experiment, and writing the manuscript. SA: in vitro experiments and genome analysis. IR-L: design of the experiments and pilot experiment. EM-N and SR-G: photosynthesis measurements, pilot experiment, and draft writing. FM: supervision of the work and funding. SN-T: genome analysis, supervision of the work, and writing the manuscript. All authors contributed to the article and approved the submitted version.
Funding
This work was financed by Project P11-RNM-7274-MO (Junta de Andalucía), Project FIUS19/0065 (FIUS, University of Seville), Project US-1262036 (Program I + D + i FEDER 2014-2020, Junta de Andalucía), and AE2-18 of the University of Seville.
Conflict of Interest
The authors declare that the research was conducted in the absence of any commercial or financial relationships that could be construed as a potential conflict of interest.
Publisher’s Note
All claims expressed in this article are solely those of the authors and do not necessarily represent those of their affiliated organizations, or those of the publisher, the editors and the reviewers. Any product that may be evaluated in this article, or claim that may be made by its manufacturer, is not guaranteed or endorsed by the publisher.
Acknowledgments
The professors and students of the College I.E.S. Pablo Neruda (Castilleja de la Cuesta, Seville) are heartily acknowledged, in particular José Juan Pastor Milán.
Supplementary Material
The Supplementary Material for this article can be found online at: https://www.frontiersin.org/articles/10.3389/fmars.2021.685076/full#supplementary-material
Footnotes
References
Adams, W. W., and Demmig-Adams, B. (2004). “Chlorophyll fluorescence as a tool to monitor plant response to the environment” in Chlorophyll Fluorescence: a Signature of Photosynthesis. eds G. C. Papageorgiou and Govindjee (Dordrecht: Springer). 583–604. doi: 10.1007/s11738-016-2113-y
Akiyama, M., Crooke, E., and Kornberg, A. (1993). An exopolyphosphatase of Escherichia coli. The enzyme and its ppx gene in a polyphosphate operon. J. Biol. Chem. 268, 633–639. doi: 10.1016/s0021-9258(18)54198-3
Altaf, M. M., Khan, M. S. A., Abulresh, H. H., and Ahmad, I. (2017). “Quorum Sensing in Plant Growth-Promoting Rhizobacteria and Its Impact on Plant-Microbe Interaction” in Plant-Microbe Interactions in Agro-Ecological Perspectives. eds D. Singh, H. Singh, and R. Prabha (Singapur: Springer). 311–331. doi: 10.1007/978-981-10-5813-4_16
Aziz, R. K., Bartels, D., Best, A. A., DeJongh, M., Disz, T., Edwards, R. A., et al. (2008). The RAST server: rapid annotations using subsystems technology. BMC Genom. 9:75. doi: 10.1186/1471-2164-9-75
Backer, R., Rokem, J. S., Ilangumaran, G., Lamont, J., Praslickova, D., Ricci, E., et al. (2018). Plant Growth-Promoting Rhizobacteria: context, Mechanisms of Action, and Roadmap to Commercialization of Biostimulants for Sustainable Agriculture. Front. Plant Sci. 9:1473. doi: 10.3389/fpls.2018.01473
Baker, N. R., and Oxborough, K. (2004). “Chlorophyll Fluorescence as a Probe of Photosynthetic Productivity” in Chlorophyll a Fluorescence. Advances in Photosynthesis and Respiration. eds G. C. Papageorgiou and Govindjee (Dordrecht: Springer). 65–82. doi: 10.1007/978-1-4020-3218-9_3
Bankevich, A., Nurk, S., Antipov, D., Gurevich, A. A., Dvorkin, M., Kulikov, A. S., et al. (2012). SPAdes: a new genome assembly algorithm and its applications to single-cell sequencing. J. Comput. Biol. 19, 455–477. doi: 10.1089/cmb.2012.0021
Bearden, S. W., Staggs, T. M., and Perry, R. D. (1998). An ABC transporter system of Yersinia pestis allows utilization of chelated iron by Escherichia coli SAB11. J. Bacteriol. 180, 1135–1147. doi: 10.1128/JB.180.5.1135-1147.1998
Berti, A. D., and Thomas, M. G. (2009). Analysis of achromobactin biosynthesis by Pseudomonas syringae pv. syringae B728a. J. Bacteriol. 191, 4594–4604. doi: 10.1128/JB.00457-09
Bhadrecha, P., Bala, M., Khasa, Y. P., Arshi, A., Singh, J., and Kumar, M. (2020). Hippophae rhamnoides L. rhizobacteria exhibit diversified cellulase and pectinase activities. Physiol. Mol. Biol. Plants 26, 1075–1085.
Bhattacharyya, C., Bakshi, U., Mallick, I., Mukherji, S., Bera, B., and Ghosh, A. (2017). Genome-Guided Insights into the Plant Growth Promotion Capabilities of the Physiologically Versatile Bacillus aryabhattai Strain AB211. Front. Microbiol. 8:411. doi: 10.3389/fmicb.2017.00411
Bordiec, S., Paquis, S., Lacroix, H., Dhondt, S., Barka, E. A., Kauffmann, S., et al. (2011). Comparative analysis of defence responses induced by the endophytic plant growth-promoting rhizobacterium Burkholderia phytofirmans strain PsJN and the non-host bacterium Pseudomonas syringae pv. pisi in grapevine cell suspensions. J Exp Bot. 62, 595–603. doi: 10.1093/jxb/erq291
Bruto, M., Prigent-Combaret, C., Muller, D., and Moënne-Loccoz, Y. (2014). Analysis of genes contributing to plant-beneficial functions in plant growth-promoting rhizobacteria and related Proteobacteria. Sci. Rep. 4:6261. doi: 10.1038/srep06261
Cánovas, D., Vargas, C., Kneip, S., Morón, M. J., Ventosa, A., Bremer, E., et al. (2000). Genes for the synthesis of the osmoprotectant glycine betaine from choline in the moderately halophilic bacterium Halomonas elongata DSM 3043, USA. Microbiology 146, 455–463. doi: 10.1099/00221287-146-2-455
Carroll, J. D., Pastuszak, I., Edavana, V. K., Pan, Y. T., and Elbein, A. D. (2007). A novel trehalase from Mycobacterium smegmatis - purification, properties, requirements. FEBS J. 274, 1701–1714. doi: 10.1111/j.1742-4658.2007.05715.x
Castillo, J. M., Ayes, D. R., Leira-Doce, P., Bailey, J., Blum, M., Strong, D. R., et al. (2010). The production of hybrids with high ecological amplitude between exotic Spartina densiflora and native S. maritima in the Iberian Peninsula: new Spartina hybrid in Iberian Peninsula. Divers. Distrib. 16, 547–558. doi: 10.1111/j.1472-4642.2010.00673.x
Chasteen, T. G., and Bentley, R. (2003). Biomethylation of selenium and tellurium: microorganisms and plants. Chem. Rev. 103, 1–25. doi: 10.1021/cr010210+
Checroun, C., and Gutierrez, C. (2004). Sigma(s)-dependent regulation of yehZYXW, which encodes a putative osmoprotectant ABC transporter of Escherichia coli. FEMS Microbiol. Lett. 236, 221–226. doi: 10.1016/j.femsle.2004.05.046
Chen, Q., and Liu, S. (2019). Identification and Characterization of the Phosphate-Solubilizing Bacterium Pantoea sp. S32 in Reclamation Soil in Shanxi, China. Front. Microbiol. 10:2171. doi: 10.3389/fmicb.2019.02171
Cruz, A. T., Cazacu, A. C., and Allen, C. H. (2007). Pantoea agglomerans, a plant pathogen causing human disease. J. Clin. Microbiol. 45, 1989–1992. doi: 10.1128/JCM.00632-07
du Jardin, P. (2015). Plant biostimulants: definition, concept, main categories and regulation. Sci Hortic. 196, 3–14. doi: 10.1016/j.scienta.2015.09.021
Duca, D. R., and Glick, B. R. (2020). Indole-3-acetic acid biosynthesis and its regulation in plant-associated bacteria. App. Microbiol. Biotechnol. 104, 8607–8619. doi: 10.1007/s00253-020-10869-5
Dutkiewicz, J., Mackiewicz, B., Lemieszek, K. M., Golec, M., and Milanowski, J. (2016). Pantoea agglomerans: a mysterious bacterium of evil and good. Part III. Deleterious effects: infections of humans, animals and plants. Ann. Agric. Environ. Med. 23, 197–205. doi: 10.5604/12321966.1203878
Ehrhardt, D. W., Atkinson, E. M., and Long, S. R. (1992). Depolarization of alfalfa root hair membrane potential by Rhizobium meliloti Nod factors. Science 256, 998–1000. doi: 10.1126/science.10744524
Enebe, M. C., and Babalola, O. O. (2018). The influence of plant growth-promoting rhizobacteria in plant tolerance to abiotic stress: a survival strategy. Appl Microbiol Biotechnol. 102, 7821–7835. doi: 10.1007/s00253-018-9214-z
Fekih, B. I., Zhang, C., Li, Y. P., Zhao, Y., Alwathnani, H. A., Saquib, Q., et al. (2018). Distribution of Arsenic Resistance Genes in Prokaryotes. Front. Microbiol. 9:2473. doi: 10.3389/fmicb.2018.02473
Feng, H., Zhang, N., Du, W., Zhang, H., Liu, Y., Fu, R., et al. (2018). Identification of Chemotaxis Compounds in Root Exudates and Their Sensing Chemoreceptors in Plant-Growth-Promoting Rhizobacteria Bacillus amyloliquefaciens SQR9. Mol. Plant Microbe Interact. 31, 995–1005. doi: 10.1094/MPMI-01-18-0003-R
Ferreira, C. M. H., Soares, H. M. V. M., and Soares, E. V. (2019). Promising bacterial genera for agricultural practices: an insight on plant growth-promoting properties and microbial safety aspects. Sci. Total Environ. 682, 779–799. doi: 10.1016/j.scitotenv.2019.04.225
Frossard, S. M., Khan, A. A., Warrick, E. C., Gately, J. M., Hanson, A. D., Oldham, M. L., et al. (2012). Identification of a third osmoprotectant transport system, the osmU system, in Salmonella enterica. J. Bacteriol. 194, 3861–3871. doi: 10.1128/JB.00495-12
Goswami, D., Thakker, C. N., and Dhandhukia, P. C. (2016). Portraying mechanics of plant growth promoting rhizobacteria (PGPR): a review. Cogent Food Agric. 2:1127500. doi: 10.1080/23311932.2015.1127500
Gouesbet, G., Trautwetter, A., Bonnassie, S., Wu, L. F., and Blanco, C. (1996). Characterization of the Erwinia chrysanthemi osmoprotectant transporter gene ousA. J Bacteriol. 178, 447–455. doi: 10.1128/jb.178.2.447-455.1996
Grass, G., and Rensing, C. (2001). CueO is a multi-copper oxidase that confers copper tolerance in Escherichia coli. Biochem. Biophys. Res. Commun. 286, 902–908. doi: 10.1006/bbrc.2001.5474
Grass, G., Fan, B., Rosen, B. P., Franke, S., Nies, D. H., and Rensing, C. (2001). ZitB (YbgR), a member of the cation diffusion facilitator family, is an additional zinc transporter in Escherichia coli. J. Bacteriol. 183, 4664–4667. doi: 10.1128/JB.183.15.4664-4667.2001
Grissa, I., Vergnaud, G., and Pourcel, C. (2007). CRISPRFinder: a web tool to identify clustered regularly interspaced short palindromic repeats. Nucleic Acids Res. 35, W52–W57. doi: 10.1093/nar/gkm360
Gurevich, A., Saveliev, V., Vyahhi, N., and Tesler, G. (2013). QUAST: quality assessment tool for genome assemblies. Bioinformatics 29, 1072–1075. doi: 10.1093/bioinformatics/btt086
Horlacher, R., Uhland, K., Klein, W., Ehrmann, M., and Boos, W. (1996). Characterization of a cytoplasmic trehalase of Escherichia coli. J. Bacteriol. 178, 6250–6257. doi: 10.1128/jb.178.21.6250-6257.1996
Hornung, J. M., Jones, H. A., and Perry, R. D. (1996). The hmu locus of Yersinia pestis is essential for utilization of free haemin and haem–protein complexes as iron sources. Mol. Microbiol. 20, 725–739. doi: 10.1111/j.1365-2958.1996.tb02512.x
Idriss, E. E., Makarewicz, O., Farouk, A., Rosner, K., Greiner, R., Bochow, H., et al. (2002). Extracellular phytase activity of Bacillus amyloliquefaciens FZB45 contributes to its plant-growth-promoting effect. Microbiology 148, 2097–2109. doi: 10.1099/00221287-148-7-2097
Jain, S., Bohra, I., Mahajan, R., Jain, S., and Chugh, T. D. (2012). Pantoea agglomerans infection behaving like a tumor after plant thorn injury: an unusual presentation. Indian J. Pathol. Microbiol. 55, 386–388. doi: 10.4103/0377-4929.101754
Kaasen, I., McDougall, J., and Strøm, A. R. (1994). Analysis of the otsBA operon for osmoregulatory trehalose synthesis in Escherichia coli and homology of the OtsA and OtsB proteins to the yeast trehalose-6-phosphate synthase/phosphatase complex. Gene 145, 9−15. doi: 10.1016/0378-1119(94)90316-6
Kajander, T., Kellosalo, J., and Goldman, A. (2013). Inorganic pyrophosphatases: one substrate, three mechanisms. FEBS Lett. 587, 1863–1869. doi: 10.1016/j.febslet.2013.05.003
Kenawy, A., Dailin, D. J., Abo-Zaid, G., Malek, R. A., Ambehabati, K. K., Zakaria, K. H. N., et al. (2019). “Biosynthesis of Antibiotics by PGPR and Their Roles in Biocontrol of Plant Diseases” in Plant Growth Promoting Rhizobacteria for Sustainable Stress Management 13. ed. R. Z. Sayyed (Singapur: Springer). 1–35. doi: 10.1007/978-981-13-6986-5_1
Koczan, J. M., McGrath, M. J., Zhao, Y., and Sundin, G. W. (2009). Contribution of Erwinia amylovora exopolysaccharides amylovoran and levan to biofilm formation: implications in pathogenicity. Phytopathology 99, 1237–1244. doi: 10.1094/PHYTO-99-11-1237
Kouris-Blazos, A., and Belski, R. (2016). Health benefits of legumes and pulses with a focus on Australian sweet lupins. Asia Pac. J. Clin. Nutr. 25, 1–17. doi: 10.6133/apjcn.2016.25.1.23
Krawczyk, P. S., Lipinski, L., and Dziembowski, A. (2018). PlasFlow: predicting plasmid sequences in metagenomic data using genome signatures. Nucleic Acids Res. 46:e35. doi: 10.1093/nar/gkx1321
Krogh, A., Larsson, B., von Heijne, G., and Sonnhammer, E. L. (2001). Predicting transmembrane protein topology with a hidden Markov model: application to complete genomes. J. Mol. Biol. 305, 567–580. doi: 10.1006/jmbi.2000.4315
Lau, C. K., Krewulak, K. D., and Vogel, H. J. (2016). Bacterial ferrous iron transport: the Feo system. FEMS Microbiol. Rev. 40, 273–298. doi: 10.1093/femsre/fuv049
Létoffé, S., Heuck, G., Delepelaire, P., Lange, N., and Wandersman, C. (2015). Bacteria capture iron from heme by keeping tetrapyrrol skeleton intact. Proc. Natl. Acad. Sci. U.S.A. 106, 11719–11724. doi: 10.1073/pnas.0903842106
Li, H. (2013). Aligning sequence reads, clone sequences and assembly contigs with BWA-MEM. arXiv 1303. doi: 10.6084/M9.FIGSHARE.963153.V1
Li, M., Guo, R., Yu, F., Chen, X., Zhao, H., Li, H., et al. (2018). Indole-3-Acetic Acid Biosynthesis Pathways in the Plant-Beneficial Bacterium Arthrobacter pascens ZZ21. Int. J. Mol. Sci. 19:443. doi: 10.3390/ijms19020443
Luziatelli, F., Ficca, A. G., Bonini, P., Muleo, R., Gatti, L., Meneghini, M., et al. (2020b). A Genetic and Metabolomic Perspective on the Production of Indole-3-Acetic Acid by Pantoea agglomerans and Use of Their Metabolites as Biostimulants in Plant Nurseries. Front. Microbiol. 11:1475. doi: 10.3389/fmicb.2020.01475
Luziatelli, F., Ficca, A. G., Cardarelli, M., Melini, F., Cavalieri, A., and Ruzzi, M. (2020a). Genome Sequencing of Pantoea agglomerans C1 Provides Insights into Molecular and Genetic Mechanisms of Plant Growth-Promotion and Tolerance to Heavy Metals. Microorganisms 8:153. doi: 10.3390/microorganisms8020153
Manulis, S., Haviv-Chesner, A., Brandl, M. T., Lindow, S. E., and Barash, I. (1998). Differential involvement of indole-3-acetic acid biosynthetic pathways in pathogenicity and epiphytic fitness of Erwinia herbicola pv. gypsophilae. Mol. Plant–Microbe Interact. 11, 634–642. doi: 10.1094/MPMI.1998.11.7.634
Maphosa, Y., and Jideani, V. A. (2017). “The Role of Legumes in Human Nutrition” in The Role of Legumes in Human Nutrition. ed. M. Hueda Chavarri (London: InTechOpen). 103–122. doi: 10.5772/intechopen.69127
Matsuhisa, A., Suzuki, N., Noda, T., and Shiba, K. (1995). Inositol monophosphatase activity from the Escherichia coli suhB gene product. J. Bacteriol. 177, 200–205. doi: 10.1128/jb.177.1.200-205.1995
Maxwell, K., and Johnson, G. N. (2000). Chlorophyll fluorescence—a practical guide. J. Exp. Bot. 51, 659–668. doi: 10.1093/jexbot/51.345.659
Mesa-Marín, J., Mateos-Naranjo, E., Rodríguez-Llorente, I. D., Pajuelo, E., and Redondo-Gómez, S. (2019). “Synergic Effects of Rhizobacteria: increasing Use of Halophytes in a Changing World” in International Halophytes and Climate Change: adaptive Mechanisms and Potential Uses. eds M. Hasanuzzaman, S. Shabala, and M. Fujita (United Kingdom: CAB International), 240–254. doi: 10.1079/9781786394330.0240
Metcalf, W. W., and Wolfe, R. S. (1998). Molecular genetic analysis of phosphite and hypophosphite oxidation by Pseudomonas stutzeri WM88. J. Bacteriol. 180, 5547–5558. doi: 10.1128/JB.180.21.5547-5558.1998
Miethke, M., Klotz, O., Linne, U., May, J. J., Beckering, C. L., and Marahiel, M. A. (2006). Ferri-bacillibactin uptake and hydrolysis in Bacillus subtilis. Mol. Microbiol 61, 1413–1427. doi: 10.1111/j.1365-2958.2006.05321.x
Morgan, R., Kohn, S., Hwang, S. H., Hassett, D. J., and Sauer, K. (2006). BdlA, a chemotaxis regulator essential for biofilm dispersion in Pseudomonas aeruginosa. J Bacteriol. 188, 7335–7343. doi: 10.1128/JB.00599-06
Morris, R. O. (1995). “Genes specifying auxin and cytokinin biosynthesis in prokaryotes” in Plant Hormones. ed. P. J. Davies (Dordrecht: Kluwer Academic Publishers). 318–339. doi: 10.1007/978-94-011-0473-9_15
Nakamura, S., and Minamino, T. (2019). Flagella-Driven Motility of Bacteria. Biomolecules 9:279. doi: 10.3390/biom9070279
Nies, D. H. (2003). Efflux-mediated heavy metal resistance in prokaryotes. FEMS Microbiol. Rev. 27, 313–339. doi: 10.1016/S0168-6445(03)00048-2
Ort, D. R., Zhu, X., and Melis, A. (2011). Optimizing antenna size to maximize photosynthetic efficiency. Plant Physiol. 155, 79–85. doi: 10.1104/pp.110.165886
Otto, B. R., Verweij-van Vught, A. M., and MacLaren, D. M. (1992). Transferrins and heme-compounds as iron sources for pathogenic bacteria. Crit. Rev. Microbiol. 18, 217–233. doi: 10.3109/10408419209114559
Palmer, M., Steenkamp, E. T., Coetzee, M. P. A., Blom, J., and Venter, S. N. (2018). Genome-based characterization of biological processes that differentiate closely related bacteria. Front. Microbiol. 9:113. doi: 10.3389/fmicb.2018.00113
Paredes-Páliz, K. I., Caviedes, M. A., Doukkali, B., Mateos-Naranjo, E., Rodriguez-Llorente, I. D., and Pajuelo, E. (2016a). Screening beneficial rhizobacteria from Spartina maritima for phytoremediation of metal polluted salt marshes: comparison of gram-positive and gram-negative strains. Environ. Sci. Pollut. Res. 23, 19825–19837. doi: 10.1007/s11356-016-7184-1
Paredes-Páliz, K. I., Mateos-Naranjo, E., Doukkali, B., Caviedes, M. A., Redondo-Gómez, S., Rodríguez-Llorente, I. D., et al. (2017). Modulation of Spartina densiflora plant growth and metal accumulation upon selective inoculation treatments: a comparison of gram negative and gram positive rhizobacteria. Mar. Pollut. Bull. 125, 77–85. doi: 10.1016/j.marpolbul.2017.07.072
Paredes-Páliz, K. I., Pajuelo, E., Doukkali, B., Caviedes, M. A., Rodríguez-Llorente, I. D., and Mateos-Naranjo, E. (2016b). Bacterial inoculants for enhanced seed germination of Spartina densiflora: implications for restoration of metal polluted areas. Mar. Pollut. Bull. 110, 396–400. doi: 10.1016/j.marpolbul.2016.06.036
Paredes-Páliz, K., Rodr guez-Vázquez, R., Duarte, B., Caviedes, M. A., Mateos-Naranjo, E., Redondo-G mez, S., et al. (2018). Investigating the mechanisms underlying phytoprotection by plant growth-promoting rhizobacteria in Spartina densiflora under metal stress. Plant Biol. 20, 497–506. doi: 10.1111/plb.12693
Petersen, T. N., Brunak, S., von Heijne, G., and Nielsen, H. (2011). SignalP 4.0: discriminating signal peptides from transmembrane regions. Nat. Methods 8, 785–786. doi: 10.1038/nmeth.1701
Pulkkinen, W. S., and Miller, S. I. (1991). A Salmonella typhimurium virulence protein is similar to a Yersinia enterocolitica invasion protein and a bacteriophage lambda outer membrane protein. J. Bacteriol. 173, 86–93. doi: 10.1128/jb.173.1.86-93.1991
Reitz, Z. L., Sandy, M., and Butler, A. (2017). Biosynthetic considerations of triscatechol siderophores framed on serine and threonine macrolactone scaffolds. Metallomics 9, 824–839. doi: 10.1039/c7mt00111h
Rodrigue, A., Effantin, G., and Mandrand-Berthelot, M. A. (2005). Identification of rcnA (yohM), a nickel and cobalt resistance gene in Escherichia coli. J. Bacteriol. 187, 2912–2916. doi: 10.1128/JB.187.8.2912-2916.2005
Rodríguez-Llorente, I. D., Pajuelo, E., Navarro-Torre, S., Mesa-Marín, J., and Caviedes, M. A. (2019). “Bacterial endophytes from halophytes: how do they help plants to alleviate salt stress?” in Saline Soil-based Agriculture by Halotolerant Microorganisms. eds M. Kumar, H. Etesami, and V. Kumar (Singapore: Springer). 147–160. doi: 10.1007/978-981-13-8335-9_6
Sauer, M., Hantke, K., and Braun, V. (1987). Ferric-coprogen receptor FhuE of Escherichia coli: processing and sequence common to all TonB-dependent outer membrane receptor proteins. J. Bacteriol. 169, 2044–2049. doi: 10.1128/jb.169.5.2044-2049.1987
Schwibbert, K., Marin-Sanguino, A., Bagyan, I., Heidrich, G., Lentzen, G., Seitz, H., et al. (2011). A blueprint of ectoine metabolism from the genome of the industrial producer Halomonas elongata DSM 2581 T. Environ. Microbiol. 13, 1973–1994. doi: 10.1111/j.1462-2920.2010.02336.x
Seemann, T. (2014). Prokka: rapid prokaryotic genome annotation. Bioinformatics 30, 2068–2069. doi: 10.1093/bioinformatics/btu153
Shariati, J. V., Malboobi, M. A., Tabrizi, Z., Tavakol, E., Owlia, P., and Safari, M. (2017). Comprehensive genomic analysis of a plant growth-promoting rhizobacterium Pantoea agglomerans strain P5. Sci. Rep. 7:15610. doi: 10.1038/s41598-017-15820-9
Shepherd, M., Heath, M. D., and Poole, R. K. (2007). NikA binds heme: a new role for an Escherichia coli periplasmic nickel-binding protein. Biochemistry 46, 5030–5037. doi: 10.1021/bi700183u
Shiba, T., Tsutsumi, K., Ishige, K., and Noguchi, T. (2000). Inorganic polyphosphate and polyphosphate kinase: their novel biological functions and applications. Biochemistry 65, 315–323.
Shidore, T., and Triplett, L. R. (2017). Toxin-antitoxin systems: implications for plant disease. Annu. Rev. Phytopathol. 55, 161–179. doi: 10.1146/annurev-phyto-080516-035559
Singh, R. P., and Jha, P. N. (2017). The PGPR Stenotrophomonas maltophilia SBP-9 augments resistance against biotic and abiotic stress in wheat plants. Front. Microbiol. 8:1945. doi: 10.3389/fmicb.2017.01945
Song, J.-Y., Kim, H.-A., Kim, J.-S., Kim, S.-Y., Jeong, H., Kang, S.-G., et al. (2012). Genome sequence of the plant growth-promoting rhizobacterium Bacillus sp. strain JS. J. Bacteriol. 194, 3760–3761. doi: 10.1128/JB.00676-12
Song, Z., Lu, Y., Liu, X., Wei, C., Oladipo, A., and Fan, B. (2020). Evaluation of Pantoea eucalypti FBS135 for pine (Pinus massoniana) growth promotion and its genome analysis. J. Appl. Microbiol. 129, 958–970. doi: 10.1111/jam.14673
Spaepen, S., Vanderleyden, J., and Remans, R. (2007). Indole-3-acetic acid in microbial and microorganism-plant signaling. FEMS Microbiol. Rev. 31, 425–448. doi: 10.1111/j.1574-6976.2007.00072.x
Stasi, R., Neves, H. I., and Spira, B. (2019). Phosphate uptake by the phosphonate transport system PhnCDE. BMC Microbiol. 19:79. doi: 10.1186/s12866-019-1445-3
Stirling, D. A., Hulton, C. S., Waddell, L., Park, S. F., Stewart, G. S., Booth, I., et al. (1989). Molecular characterization of the proU loci of Salmonella typhimurium and Escherichia coli encoding osmoregulated glycine betaine transport systems. Mol Microbiol. 3, 1025–1038. doi: 10.1111/j.1365-2958.1989.tb00253.x
Tu, J., Huang, B., Zhang, Y., Xue, T., Li, S., and Qi, K. (2016). Modulation of virulence genes by the two-component system PhoP-PhoQ in avian pathogenic Escherichia coli. Pol. J. Vet. Sci. 19, 31–40. doi: 10.1515/pjvs-2016-0005
Usha, D., Indu, K., Reena, V. S., Lalit, K., Devender, S., Aditi, G., et al. (2015). Genomic and Functional Characterization of a Novel Burkholderia sp. Strain AU4i from Pea Rhizosphere Conferring Plant Growth Promoting Activities. Adv. Genet. Eng. 4:129. doi: 10.4/2169-0111.1000129
Walterson, A. M., and Stavrinides, J. (2015). Pantoea: insights into a highly versatile and diverse genus within the Enterobacteriaceae. FEMS Microbiol. Rev. 39, 968–984. doi: 10.1093/femsre/fuv027
Weijers, D., Nemhauser, J., and Yang, Z. (2018). Auxin: small molecule, big impact. J. Exp. Bot. 69, 133–136. doi: 10.1093/jxb/erx463
Willsky, G. R., and Malamy, M. H. (1980). Characterization of two genetically separable inorganic phosphate transport systems in Escherichia coli. J. Bacteriol. 144, 356–365. doi: 10.1128/JB.144.1.356-365.1980
Wood, D. E., and Salzberg, S. L. (2014). Kraken: ultrafast metagenomic sequence classification using exact alignments. Genome Biol. 15:R46. doi: 10.1186/gb-2014-15-3-r46
Wyckoff, E. E., Mey, A. R., Leimbach, A., Fisher, C. F., and Payne, S. M. (2006). Characterization of ferric and ferrous iron transport systems in Vibrio cholerae. J. Bacteriol. 188, 6515–6523. doi: 10.1128/JB.00626-06
Yao, H., Jepkorir, G., Lovell, S., Nama, P. V., Weeratunga, S., Bataile, K. P., et al. (2011). Two distinct ferritin-like molecules in Pseudomonas aeruginosa: the product of the bfrA gene is a bacterial ferritin (FtnA) and not a bacterioferritin (Bfr). Biochemistry 50, 5236–5248. doi: 10.1021/bi2004119
Zhang, H. B., Wang, L. H., and Zhang, L. H. (2007). Detection and analysis of quorum-quenching enzymes against acyl homoserine lactone quorum-sensing signals. Curr. Protoc. Microbiol. Chapter 1, Unit 1C.3. doi: 10.1002/9780471729259.mc01c03s05
Keywords: Pantoea agglomerans RSO7, saltmarshes, PGPR, bioinoculant, genome analysis, PGP traits, metabolic versatility, resilience
Citation: Pajuelo E, Arjona S, Rodríguez-Llorente ID, Mateos-Naranjo E, Redondo-Gómez S, Merchán F and Navarro-Torre S (2021) Coastal Ecosystems as Sources of Biofertilizers in Agriculture: From Genomics to Application in an Urban Orchard. Front. Mar. Sci. 8:685076. doi: 10.3389/fmars.2021.685076
Received: 24 March 2021; Accepted: 28 July 2021;
Published: 19 August 2021.
Edited by:
Bernardo Duarte, Center for Marine and Environmental Sciences (MARE), PortugalReviewed by:
Ricardo Cruz de Carvalho, University of Lisbon, PortugalIsabel Caçador, Center for Marine and Environmental Sciences (MARE), Portugal
Copyright © 2021 Pajuelo, Arjona, Rodríguez-Llorente, Mateos-Naranjo, Redondo-Gómez, Merchán and Navarro-Torre. This is an open-access article distributed under the terms of the Creative Commons Attribution License (CC BY). The use, distribution or reproduction in other forums is permitted, provided the original author(s) and the copyright owner(s) are credited and that the original publication in this journal is cited, in accordance with accepted academic practice. No use, distribution or reproduction is permitted which does not comply with these terms.
*Correspondence: Salvadora Navarro-Torre, snavarro1@us.es