- 1Department for Innovation in Biological, Agro-Food and Forest Systems, University of Tuscia, Viterbo, Italy
- 2Department of Ecological and Biological Sciences, University of Tuscia, Viterbo, Italy
- 3Bioscience Research Center, Orbetello, Italy
- 4Department of Life Sciences, University of Trieste, Trieste, Italy
- 5Department of Biological, Geological and Environmental Sciences, University of Catania, Catania, Italy
- 6Department of Biology and Evolution of Marine Organisms, Stazione Zoologica “Anton Dohrn,” Napoli, Italy
- 7Federazione Italiana Pesca Sportiva e Attività Subacquee (FIPSAS), Rome, Italy
Biological invasions result in negative and unpredictable impacts on oceans worldwide. Non-indigenous macrophytes often synthesize secondary metabolites for defensive purposes and increased competition efficiency: this is the case of Caulerpa cylindracea, which has entered the Mediterranean Sea in 1990 and competed against local flora and fauna since. It was demonstrated that the white seabream Diplodus sargus (i) has included the algae into its diet, (ii) is subject to the peculiar Abnormally Tough Specimen (ATS) condition post-cooking, and (iii) suffers physiological and behavioral disturbances from caulerpin, one of the three major algal secondary metabolites. This paper confirms a feeding relationship between the fish and the algae, quantifies caulerpin accumulation in the liver, suggests a possible mollusk- and echinoderm-driven biomagnification, and highlights the fact that all ATS specimens were males. Multivariate analyses on a multi-biomarker panel reveals differential correlations to key cellular processes such as oxidative stress, metabolism, neurotoxicity, and lipid peroxidation as well as to condition indexes.
Introduction
The introduction of non-indigenous species has greatly increased in recent decades (Roques et al., 2016; Seebens et al., 2017). Despite the fact that some positive outcomes have been theorized from the colonization of coral reefs by alien species (Bellwood and Robert Goatley, 2017), biological invasions generally cause serious biodiversity losses and have been consequently regarded as one of the main anthropogenic threats in all oceans. The increasing awareness depends on the fact that such an issue reflects on multiple levels, i.e., evolutionary, ecological, economic, and social (Sax et al., 2007; Azzurro et al., 2019).
Invasive species may exert their effects through defensive secondary metabolites (Mollo et al., 2008), against which autochthonous populations lack any evolutionary adaptation (Terlizzi et al., 2011). Exotic species are provided with a higher chemical uniqueness than non-invasive/native counterparts (Cappuccino and Arnason, 2006 and references therein), and these molecules were demonstrated to bioaccumulate (Baležentienė, 2015) and biomagnificate (Costa et al., 2017) along the food web. Because bio-invaders are described once they have established close relationships with native wildlife, the assessment of their impact is unpredictable and rarely knowledgeable at an early phase (Felline et al., 2017). Also, the actual long-term extent of the damage of such metabolites on key biological/physiological features such as feeding, behavioral, and reproductive habits may be underestimated at the ecosystem level (Langkilde et al., 2017).
In the last few decades, the Mediterranean Sea has suffered from the introduction and spreading of many non-indigenous species (NIS): 265 were overall described, with Macrophyta accounting for the most numerous taxon (65 species, 84% of which established at least in one sub-region). Among them is the order Caulerpales, class Chlorophyceae. Particularly relevant are Caulerpa taxifolia and the Caulerpa racemosa complex, the latter being characterized by high morphological plasticity and therefore unclearly taxonomically classified (Klein and Verlaque, 2008). The C. cylindracea, formerly known as C. racemosa var. cylindracea (Sonder) Verlaque, Huisman, and Boudouresque (a new taxonomic combination proposed by Verlaque et al., 2003), was first considered a “Lessepsian migrant” (Alongi et al., 1993; Giaccone and Di Martino, 1995), but Belton et al. (2014) clarified its southwestern Australian origin. This species has spread to the entire Mediterranean basin since 1990 (Nizamuddin, 1991; Klein and Verlaque, 2008) and was reported in Italian waters for the first time in 1993 at the Island of Lampedusa and at Baia di San Panagia (Alongi et al., 1993). C. cylindracea colonizes photophilic algae and Posidonia oceanica seagrass meadows (Infantes et al., 2011) and algal turfs (Bonifazi et al., 2017), exerting profound impacts on local biological communities (Vázquez-Luis et al., 2009; Deudero et al., 2011; Rizzo et al., 2017) as it decreases α- and β-diversity (Piazzi and Balata, 2008). Its higher invasive potential compared to Mediterranean macrophytes was tentatively explained with a more efficient antioxidant capacity (Cavas and Yurdakoc, 2005). Because of such invasiveness features, C. cylindracea is now considered an invasive alien species (IAS) (Klein and Verlaque, 2008; Katsanevakis et al., 2014) and represents a serious threat to biodiversity and the functioning of the natural Mediterranean ecosystem.
The trophic relationship between C. cylindracea and the white seabream Diplodus sargus (Linnaeus, 1758) was brought to the notice of the scientific community in 2011 (Terlizzi et al., 2011). However, Mediterranean fishermen had witnessed a peculiar condition of the white seabream for over three decades, consisting in the hardening of the meat and the consequent decrease in edibility following the cooking of apparently healthy adult specimens. This is known as the Abnormally Tough Specimen (ATS) phenomenon, and the underlying causes were hypothesized to be pollution (organic and chemical) and a change in feeding habits (Casadevall et al., 2020). In addition to grazing on the algae, the white seabream accumulates the red pigment caulerpin in the liver, red and white muscle, and skin tissues through the diet. By using condition indices and ecotoxicological biomarkers as proxies of general health status, D. sargus was demonstrated to be negatively affected by the algae at the transcriptional and enzymatic levels (Terlizzi et al., 2011; Felline et al., 2012, 2017; Gorbi et al., 2014). Further controlled feeding experiments using caulerpin-enriched food demonstrated lipid metabolism and behavioral disturbances (Magliozzi et al., 2017; Del Coco et al., 2018) and elucidated the molecular pathway underneath such cellular and physiological alterations (Vitale et al., 2018; Magliozzi et al., 2019).
The present study employed a chemo-ecological approach with the aim of assessing (i) the establishment and extent of a feeding relationship between D. sargus and C. cylindracea; (ii) the incidence of the ATS phenomenon; (iii) the accumulation of the main algal secondary metabolite in a fish tissue; and (iv) potential physiological impacts thereof through a multi-biomarker panel indicative of key cellular processes. With regard to the ATS condition, we related our findings to the ex post fish cooking response and visually document the phenomenon. At last, the reader is supplied with the invasive seaweed phenology and percentage coverage data in a Site of Community Importance (European Commission Habitats Directive 92/43/EEC). Importantly, our approach might be applied across a broad range of marine fish species to characterize their physiological response to alike stressors.
Materials and Methods
Fish Sampling
Diplodus sargus specimens were sampled by accredited personnel of University of Tuscia on November 1, 2019, during a FIPSAS (Federazione Italiana Pesca Sportiva e Attività Subacquee) spearfishing competition in the area of Civitavecchia (Italy) (Figure 1). Fish were maintained in ice to avoid tissue proteolysis until in situ processing. Total length (L), total weight (W), eviscerated weight (EW), gonad weight (GW), and liver weight (LW) were recorded with an electronic scale (0.01 g precision) (Table 1). Liver and white muscle were immediately stored in dry ice. Stomachs were removed by severing the esophagus near the buccal cavity and intestine, weighed, and preserved in 75% ethanol. All analyses were performed on a subset of 10 individuals selected for size greater than 300 g (Terlizzi et al., 2011). All individuals were maintained at a constant temperature in ice and cooked within 12 h from capture. Fish were seasoned with water, oil, white wine, tomato, and spices and oven-cooked for 40 min at 180°C. Individuals were identified as “altered” or “non-altered” based on filet appearance and organoleptic tests.
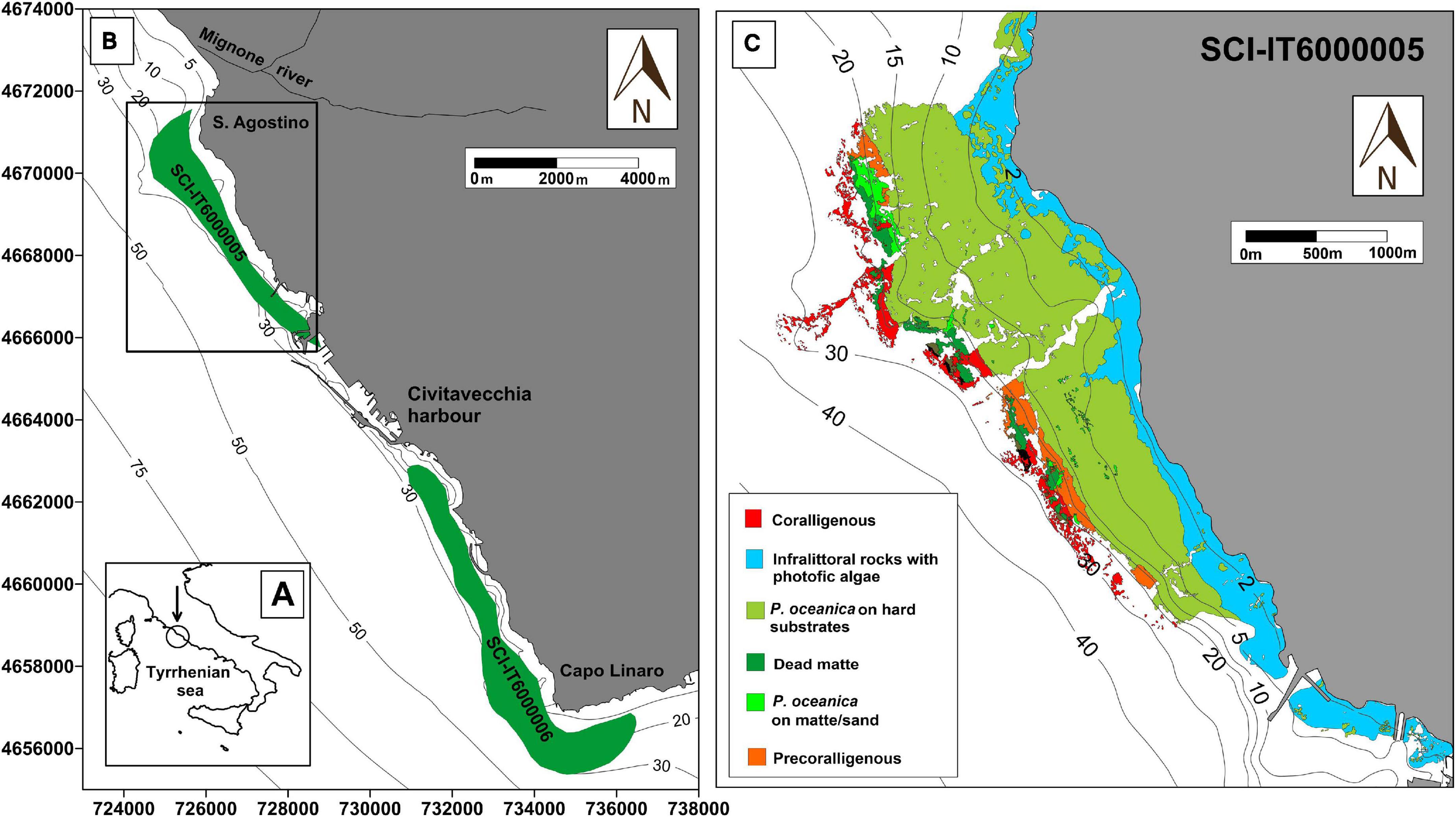
Figure 1. Study area of the CAR project. Map of the sampling location at low (A) and high (B) magnification. All fish and algae sampling occurred exclusively within SCI 6000005 (black frame), which extends from Punta S. Agostino to Punta della Mattonara. SCI 6000006, because of its proximity to the area, is also indicated on the map. (C) Thematic map of the distribution of benthic biocenosis in the study area.
Caulerpa cylindracea Sampling
Caulerpa cylindracea was sampled following the sampling plan and technical details were reported by Piazzi and Ceccherelli (2006) and Ruitton et al. (2005). Monitoring and sampling operations were performed by SCUBA surveys in July and October 2019 in the same area of the spearfishing competition. An area delimited by a 400-cm2 metal reference quadrat was scraped in triplicate after having been photographed/filmed for in-lab image analysis, and C. cylindracea specimens were stored in 80% ethanol-filled plastic bottles. For each replicate, the following phenological variables were measured: thickness of the horizontal stolons, length of the erect axes (fronds), length of lateral branchlets (ramuli), and diameter of the lateral pinnules (terminal vesicles) (Buia et al., 2001). Cover was expressed as percentage of seabed surface covered by vertically projected vegetative material (Boudouresque, 1971).
Condition Biomarkers
Fulton’s K condition factor (CF), hepatosomatic index (HSI), and gonadosomatic index (GSI) were calculated according to Lloret et al. (2014). In particular, CF = [(EW/L3) × 100], HSI = [(LW/EW) × 100], and GSI = [(GW/EW) × 100]. EW was used in order not to account for viscera and gonad contributions.
Stomach Content Analysis
Stomach contents were analyzed under a Leica APO 8 stereomicroscope. The entire content was sorted and identified to the finest taxonomic level possible depending on the level of digestion of the prey. All prey categories were enumerated and their wet weight recorded with a 0.001 g accuracy after superficial drying with absorbing paper.
Chemical Analysis
Caulerpin accumulation was quantified in the liver samples of the subset in triplicate. Twenty-five milligrams of samples was lyophilized with a mortar in liquid nitrogen, resuspended with 0.10 ml of ice-cold ultra-pure water (18 MΩ), and sonicated five times for 10 s each. Thermal shock was applied by alternating exposure at 4°C and 37°C for 30 s. Ice-cold methanol (0.6 ml) and chloroform (0.4 ml) were added. After mixing at a 5-min interval for 30 min, samples were added with 0.10 ml of ice-cold pH-adjusted ultra-pure water and transferred to −20°C for 2–8 h. After thawing, tubes were centrifuged at 13,500 × g for 10 min at 4°C, supernatants were dried to visible pellets, resuspended in 0.1 ml of water containing 5% formic acid, and transferred to glass autosampler vials for LC/MS analysis. The metabolite was identified and quantified using Ultra High Performance Liquid Chromatography system and was coupled with a Q Exactive mass spectrometer (Thermo Scientific). The system was operated in positive ion mode. The system was calibrated against positive or negative ion mode calibration mixes (Pierce, Thermo Fisher, Rockford, IL). Metabolite assignment was performed using the open source MAVEN 5.2 package1, upon conversion of raw files into an mzXML. Caulerpin was quantified relatively to a standard calibration curve (0.0002–2 mg ml–1, R2 = 0.9996). The caulerpin used as standard was purified according to Magliozzi et al. (2017) and its 1H-NMR spectrum was assessed against Ferramosca et al. (2016).
Biochemical Analyses
Biochemical analyses were performed on liver and white muscle homogenate supernatants. One unit (U) is defined as the amount of enzyme that catalyzes the reaction of 1 μmol of substrate per minute. Total protein content was quantified following the BCA method.
Catalase (CAT, EC 1.11.1.6) activity was investigated in terms of peroxidatic function by spectrophotometrically measuring the formaldehyde product at 540 nm with 4-amino-3-hydrazino-5-mercapto1,2,4-triazole as chromogen. Tissues were homogenized in 50 mM potassium phosphate pH 7.0 containing 1 mM EDTA (1:10, w/v) and 20 μl of supernatant was assayed with 100 μl of 100 mM potassium phosphate (pH 7.0) and 30 μl of methanol. Reactions were initiated by adding 20 μl of 35.28 mM hydrogen peroxide and incubated for 20 min at room temperature (RT); they were terminated with 30 μl of 10 M potassium hydroxide, 30 μl of chromogen, a 10-min incubation at RT, and 10 μl potassium periodate in 0.5 M potassium hydroxide. A formaldehyde standard curve was generated with seven points (0–75 μM, R2 = 0.995). Bovine liver catalase was included as positive control. Standards, positive control, and unknown samples were assayed in duplicate. Activity is reported as mU mg protein–1.
The level of glutathione peroxidase (GPx, EC 1.11.1.9), the biotransformation of glutathione S-transferase (GST, EC 2.5.1.18), and the activity of superoxide dismutase (SOD, EC 1.15.1.1) were determined on the cellular fraction S9. To extract it, a variable volume of a 50 mM phosphate buffer containing 2 mM EDTA was added to liver and muscle samples (Vidal-Liñán and Bellas, 2013). Samples were homogenized using an UltraTurrax® and centrifuged at 12,000 × g for 12 min at 4°C. The supernatant was aliquoted in 2-ml test tubes and stored in liquid nitrogen.
GPx was quantified following the method of Badary et al. (2005). Samples were added to a reaction mix of 10 mM GSH, 2.4 U/ml GSSG reductase and 1.5 mM NADPH. The reaction was started by adding 2 mM hydrogen peroxide and absorbance was monitored at 340 nm for 2 min. Enzyme activity was determined using the extinction coefficient of 6.22 mM–1 cm–1 and results were expressed in nmol mg protein–1.
GST activity was determined following the method described by Habig et al. (1974). GSTs catalyzes the conjugation of the substrate 1-chloro-2,4-dinitrobenzene (60 mM CDNB) with 10 mM GSH. The samples were added to a reaction mix (phosphate buffer + GST + CDNB) and absorbance was measured at 340 nm for 5 min. Enzyme activity was determined using the extinction coefficient of 9.6 mM–1 cm–1 for CDNB and results expressed in mU mg protein–1.
SOD activity was quantified following the method of Gao et al. (1998). This is based on the ability of the enzyme to inhibit the autoxidation of pyrogallol, which, in the presence of EDTA at the specific pH used, is 50%. Absorbance was measured at 420 nm. SOD activities were expressed as U/ml; one unit is defined as the amount of enzyme required to cause 50% inhibition of pyrogallol autoxidation.
Citrate synthase (CS, EC 2.3.3.16) activity was assessed by colorimetrically measuring the production of glutathione (GSH) at 412 nm. Homogenization occurred in ice-cold lysis buffer (1:10, w/v) and 50 μl of supernatants were analyzed with 5 μl of 0.1 mM DTNB, 2 μl of 0.3 mM acetyl-coenzyme A, and 0.5 mM oxaloacetate. Absorbance was read at a 5-min interval until the value of the most active sample exceeded the linear range of the standard curve. A GSH standard curve was generated with six points (0–40 nmol, R2 = 0.979 and 0.938 for liver and muscle samples, respectively). Sample blanks were included. Standards, unknown samples, and samples blanks were assayed in duplicate. Activity is reported as mU mg protein–1.
Acetylcholinesterase (AChE, EC 3.1.1.7) activity was measured according to Wilson and Henderson (2007), a variant of the Ellman assay. Briefly, tissues were homogenized on ice in 0.1 M sodium phosphate pH 8.0 containing 0.5% Triton X-100 (1:10, w/v). Supernatants were diluted 1:50 and 30 μl was used in the reaction mix together with 250 μl of 0.1 M sodium phosphate, pH 8.0, 10 μl of 10.3 mM DTNB, and 30 μl of 21.4 mM acetylthiocholine (ATCh substrate, 2 mM final concentration in total reaction volume). A tissue blank and a substrate blank lacking the substrate and the sample, respectively, were included for each sample. The optimal substrate concentration was determined by generating a concentration curve and plotting AChE activity versus the log substrate concentration. A free sulfhydryl standard curve was generated with eight points (0–50 nmol, R2 = 0.999). Standard, samples, and blanks were run in triplicate. Absorbance was read at 412 nm at a 2-min interval for 12 min. Activity is reported as U mg protein–1.
The extent of polyunsaturated lipid peroxidation was determined by the reaction of the natural by-product malondialdehyde (MDA) with thiobarbituric acid (TBA) at 532 nm. Briefly, tissues were homogenized in ice-cold MDA lysis buffer containing 3 μl of butylated hydroxytoluene (BHT) 100 × (1:30, w/v). Two hundred microliters of supernatant was developed by adding three volumes of TBA solution, and the mixture incubated at 95°C for 60 min. Vials were cooled to RT in an ice bath for 10 min and 200 μl of the total volume was read at 25°C. An MDA standard curve was generated with seven points (0–40 nmol, R2 = 0.998). Standards and samples were run in duplicate. MDA concentration is reported as nmol mg tissue–1.
The following chemicals were purchased from Merck (Darmstadt, Germany): glacial acetic acid (cat. A6283); L-Glutathione reduced > 98% (cat. G4251); 5,5′-Dithiobis(2-nitrobenzoic acid), DTNB (cat. D8130); catalase activity assay kit (cat. 219265); citrate synthase activity assay kit (cat. MAK193); TBARS assay kit (cat. MAK085); and BCA protein assay kit (cat. BCA1).
Univariate Statistical Analyses
Caulerpin accumulation in the liver of individual specimens was analyzed with a one-way ANOVA. The difference in caulerpin accumulation between altered/non-altered specimens was tested for significance with a Student’s unpaired t-test assuming homoscedasticity. Both datasets were first verified to fulfill parametric conditions with a Shapiro–Wilk test. Univariate analyses were performed with GraphPad Prism v.8.0.2 (San Diego, CA, United States).
Multivariate Statistical Analyses
Dietary data were standardized by individual total sample biomass to achieve relative percentage composition and square root transformed as pre-treatment. Hierarchical cluster analysis with the group average cluster mode and a non-metric multidimensional scaling ordination (MDS) with 50 restarts were generated using a Bray–Curtis resemblance similarity matrix to display the grouping of samples and represent the samples in a low-dimensional space. The dendrogram was overlaid on the ordination by displaying the 40, 60, and 80% resemblance levels. Statistical significance was explored with a one-way analysis of similarity (ANOSIM) test and the null hypothesis of no differences in diet due to either alteration status or sex within the 10-sample subset or between size groups within the full dataset.
Condition indices and chemical/biochemical markers were first log-transformed and normalized (i.e., values were subtracted of the mean and divided by standard deviation) as pre-treatment and then entered into a principal components analysis (PCA) routine with the aim of visualizing the relatedness of samples while reducing the multidimensionality of data. The 2-d PCA eigenvector plot was superimposed with three biotic variables of the standardized dietary data as bubble plots, namely, Caulerpa sp., Gastropoda, and Echinodermata, to verify the contribution of individual stomach content fractions to abiotic markers.
A Euclidean distance matrix was calculated from the normalized biomarker dataset previously removed from the caulerpin accumulation variable and analyzed with a Canonical Analyses of Principal Coordinates routine to discriminate between a priori ATS-defined groups. To identify the contribution of each biomarker in discriminating between groups, the same matrix was employed in a Similarity Percentage routine by setting a 90% cut off for low contributions.
All datasets were analyzed using the non-parametric multivariate statistical software PRIMER v. 6.1.16 with the PERMANOVA + add-in package v. 1.0.62 (Clarke and Gorley, 2006).
Results
Cooking Analysis
Among the cooking responses of all specimens, FR_03, FR_08, and FR_10 displayed the ATS condition, were inedible, and therefore classified as altered (Figure 2). During the cooking, the skin tore off from the body edges and the meat gradually detached from the skeletal structure producing a crackling sound. Once cooked, several signs of alteration were evident: dryness of the meat, complete detachment of the filet from the bones, stripped appearance of the head, skin retracted to the body center, and a rubbery texture to the touch. The fish filets were dry and chewy, with a bitter and persistent taste.
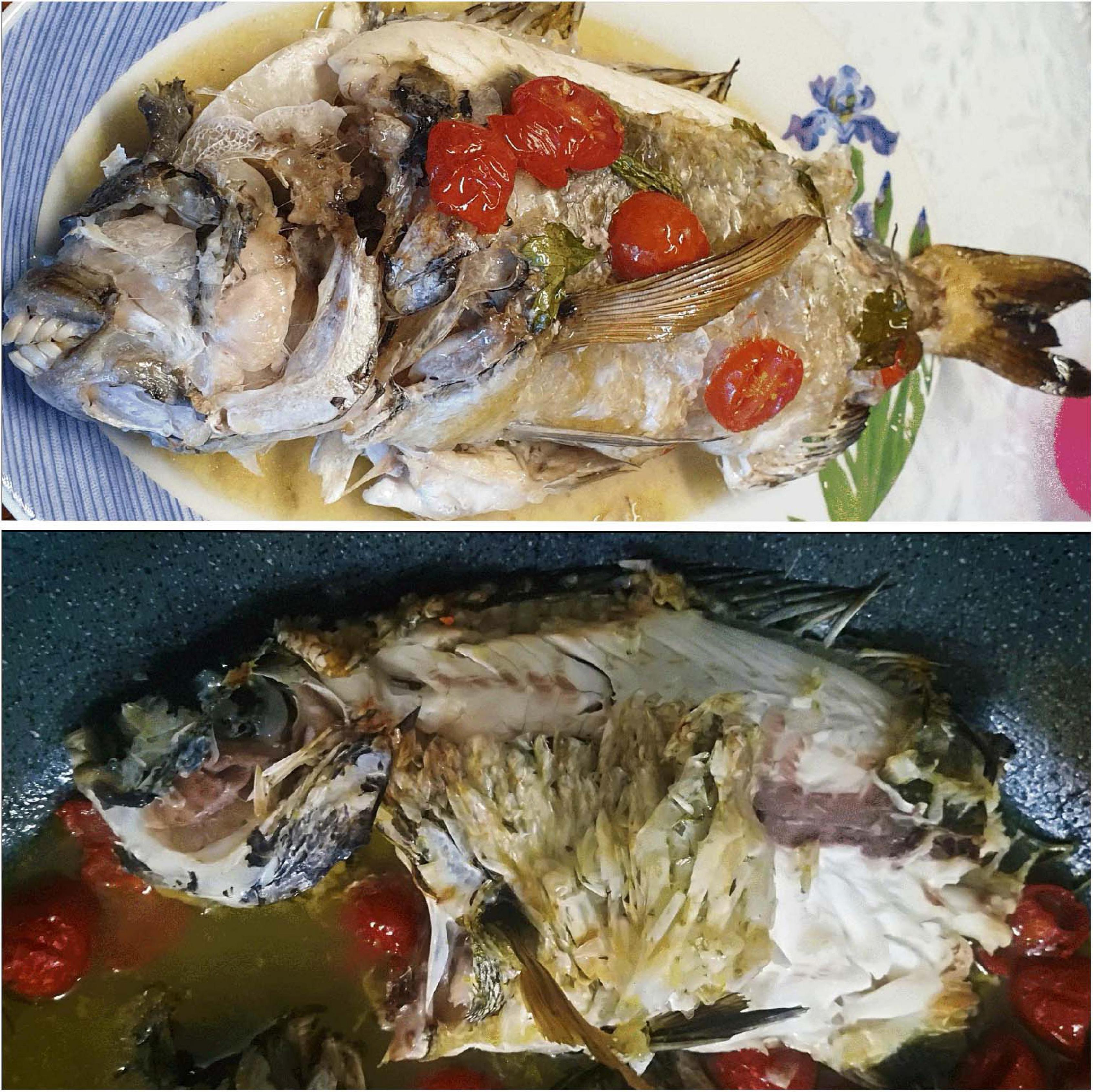
Figure 2. The Abnormally Tough Specimen condition. Adult D. sargus exhibiting the ATS condition as a cooking response. For a detailed description of the filet appearance and organoleptic tests refer to Section 3.1.
Diet Analysis
The stomach contents of D. sargus specimens (n = 10) were analyzed in terms of prey biomass and relative percentage composition (Table 2). The full dataset is supplied as supplementary information (Supplementary Table 1). The most abundant items in terms of relative prey composition were represented by gastropoda, decapoda, echinodermata, algae, and inorganic particles.
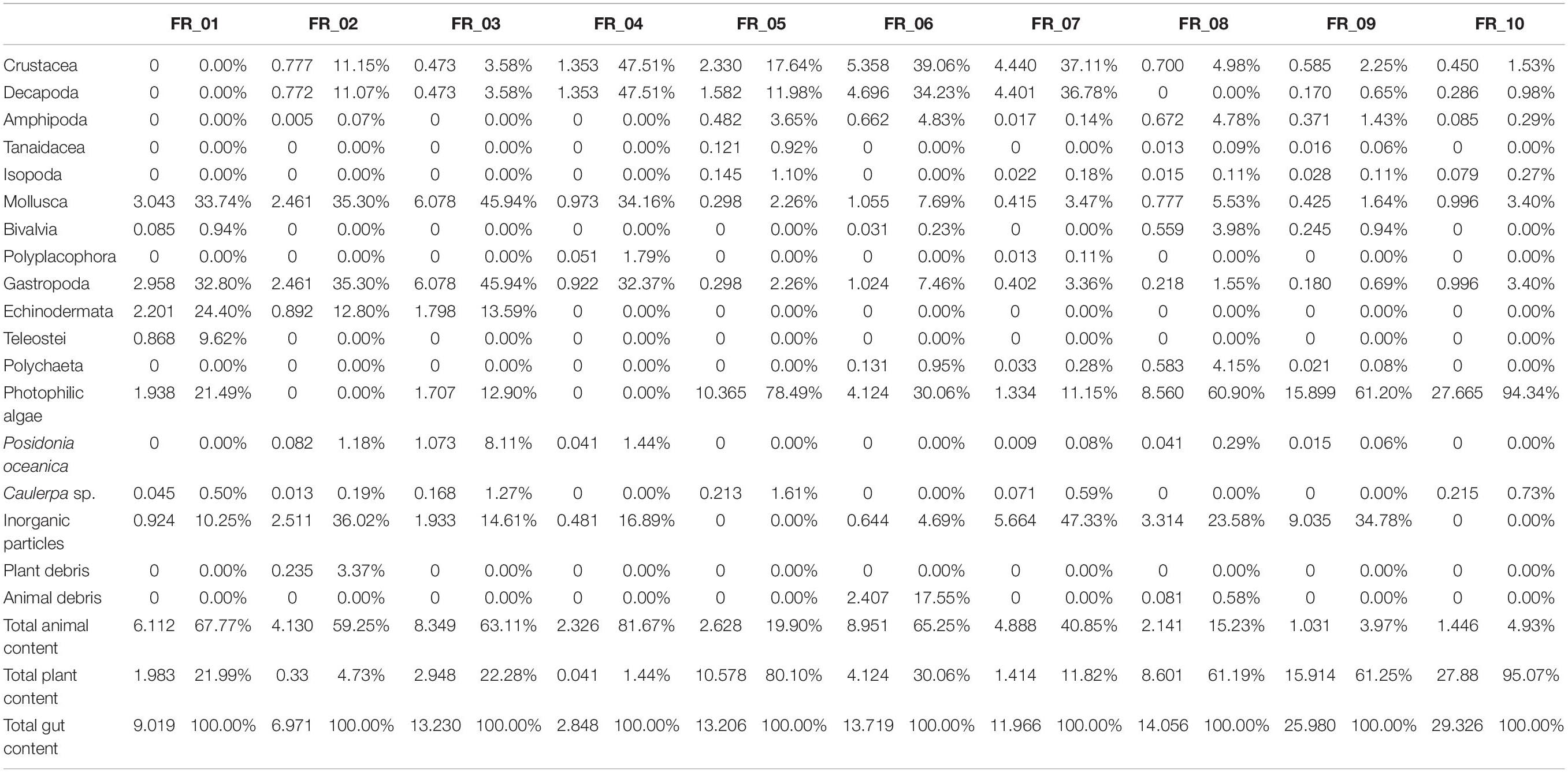
Table 2. Diet composition in terms of biomass (g, left) and relative percentage composition (right) for each of the 10 Diplodus sargus individuals analyzed.
Algae on their own accounted for 78.5% (FR_05) and 94.3% (FR_10) of total stomach contents. The most abundant algae was the Rhodophyta Ellisolandia elongata (J. Ellis and Solander), which in some specimens (FR_08, FR_09, FR_10) represented the 95% of the total vegetal content. The most frequent vegetal species were the algae Halopteris scoparia, E. elongata, and the phanerogam P. oceanica, found in 80%, 70%, and 60% of fish stomach content, respectively. Caulerpa sp. was also identified in 60% of specimens but in extremely little amounts ranging from 0.19% (FR_02) to 1.61% (FR_05) of the total amount (g) of stomach content. Total content of animal origin widely ranged from 3.9% (FR_ 09) to 81.7% (FR_04).
The ingested animal pool was represented by a total of 320 individuals belonging to 42 species. The most abundant taxa were crustacea (53.13% of total abundance, 90% of frequency) and mollusca (35.94% of total abundance, 100% of frequency). Amphipoda, with 119 individuals and nine species, accounted for the 37.19% of total abundance and 70% frequency, and was the most abundant taxonomic group. Ampithoe ramondi (Audouin, 1826) resulted as the most abundant species in the animal pool, with 65 individuals overall contributing to 20.31% of total fauna. Gastropoda was the second class in terms of abundance (27.5%) and was observed in all D. sargus analyzed. It included 88 individuals and 13 species: the rissoid Alvania discors (Brown, 1818) and the cerithiidae Bittium spp. were the dominant ones with 51 and 15 individuals, respectively. In terms of abundance, the tanaidacean Tanais sp. (16 individuals), the bivalve Musculus costulatus (Risso, 1826) (14 ind.), and the isopod Sphaeroma serratum (Fabricius, 1787) (11 ind.) were also significant.
Specimens were allocated to two groups sharing a 40% resemblance level in a 2-d nMDS ordination (Figure 3A; stress 0.07). A 60% similarity was defined among the diets of specimens FR_01–03, 04–07, and 5–10, while those of FR_08 and FR_09 were 80% alike. Differences in nMDS sample allocation were better explained by the relative fractions of gastropoda, echinodermata, or photophilic algae (data not shown) than Caulerpa sp. (Figure 3B). The diets of FR_01-FR_10 did not statistically vary among sexes or altered/non-altered samples (R statistic: −0.071, p < 0.667). Altered and unaltered specimens grouped together, overall defining three sub-populations characterized by varying degrees of similarity.
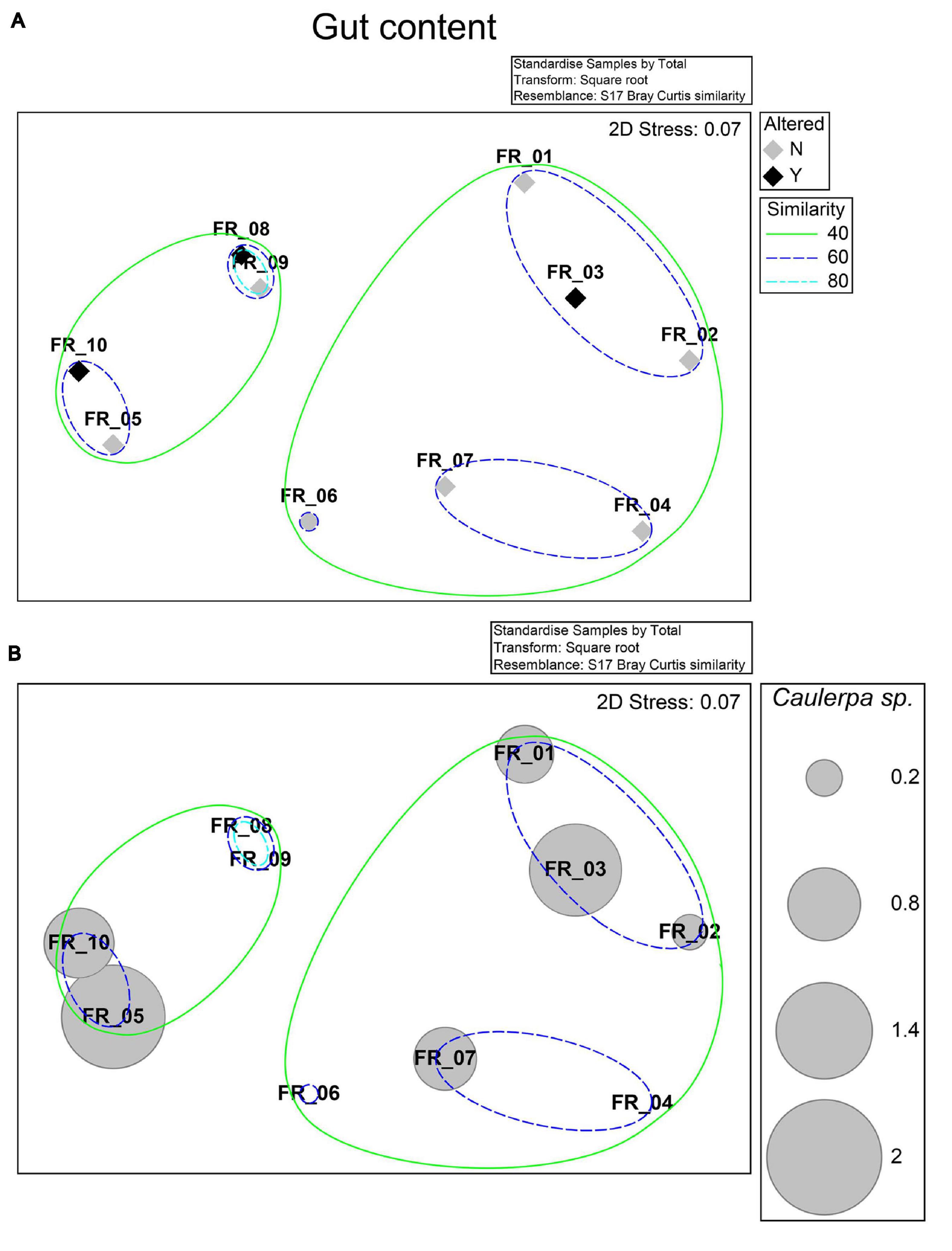
Figure 3. nMDS ordination. Bray–Curtis similarity matrix from standardized and square root-transformed stomach content analysis was superimposed of the levels of the “Altered” factor and 40, 60, and 80% resemblances from a hierarchical cluster analysis (A) or the standardized relative stomach content percentage of Caulerpa sp. (B).
On the other hand, dietary differences between individuals smaller or bigger than 300 g of BW were statistically significant, as indicated by the one-way ANOSIM ran on the full dataset with fish size as factor (R statistic: 0.25, p < 0.029, number of permutations 999) (Supplementary Table 2).
Caulerpa cylindracea Phenology and Percentage of Bottom Cover
The sampling area was characterized by infralittoral rocks colonized by photophilic algae, P. oceanica and Cymodocea nodosa. The percentage of surface cover of Caulerpa cylindracea was approximately 2.3-fold greater in July than in October, when the D. sargus sampling occurred, accounting to 87.5 and 38.3%, respectively (Figure 4A). Morphometric parameters are depicted in Figure 4B. All parameters showed greater values in July than October, in accordance with previous results. These data testify to the seasonal dynamics of C. cylindracea in SCI IT6000005.
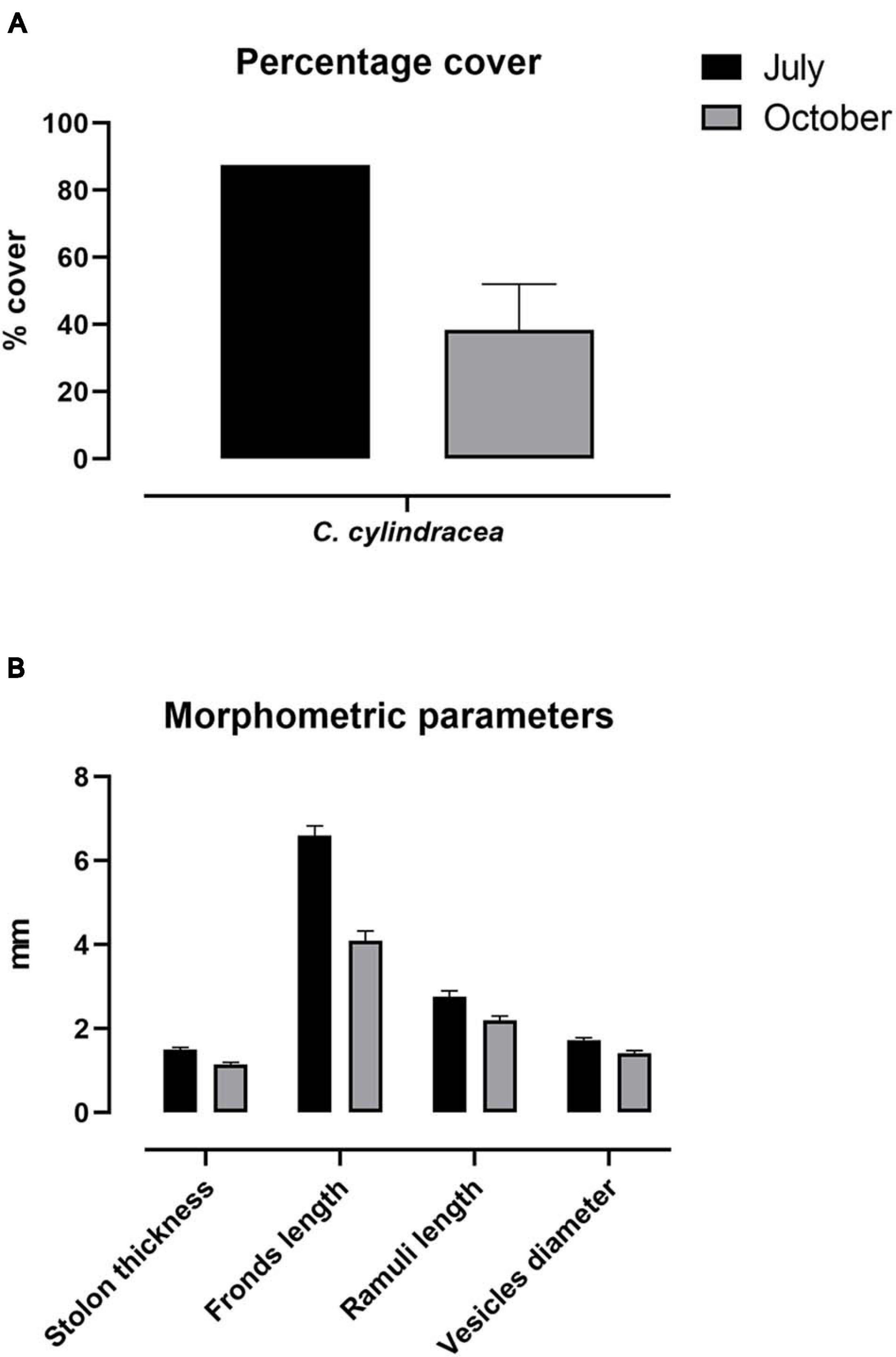
Figure 4. Phenological parameters. Percentage cover (A) and phenology (B) of C. cylindracea sampled in July and October 2019. Morphological parameters are expressed as mean ± SEM.
Chemo-Ecological Panel
Results concerning caulerpin quantification are reported in Table 3. The metabolite was found in the liver of all specimens in the range of 2.441–3.512 μg g–1 dry weight. Statistically significant differences in individual accumulation existed among specimens (F9,20 = 16.80, p < 0.0001). When averaging caulerpin accumulation based on the ATS outcome, altered specimens had a slightly higher concentration of caulerpin than unaltered fish but differences were not statistically significant (t8 = 0.2198; p = 0.8315).
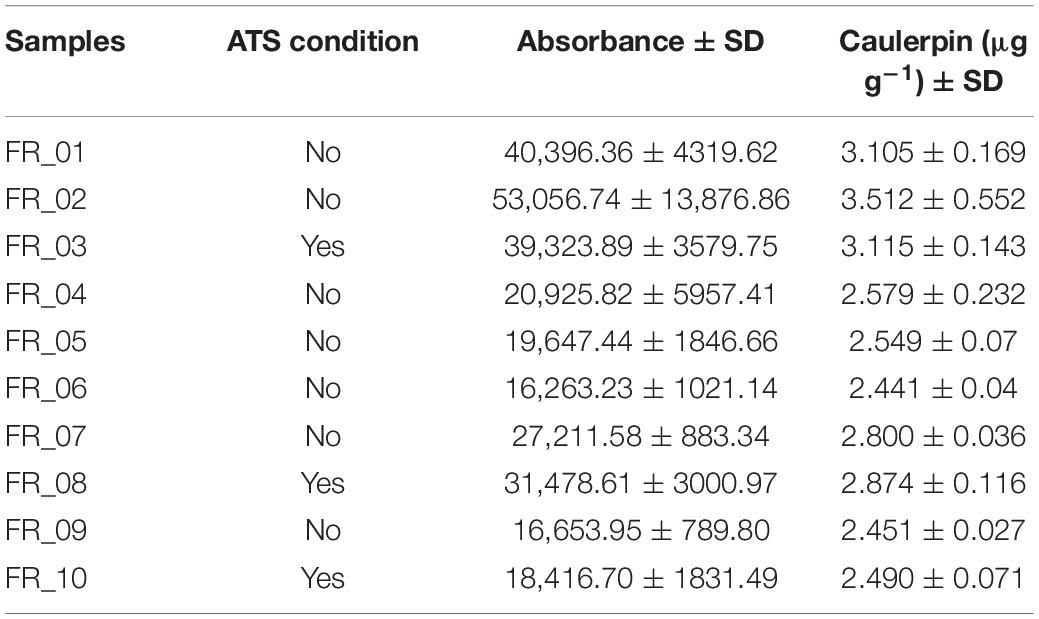
Table 3. Caulerpin bioaccumulation in D. sargus livers expressed as μg g–1 dry weight and indication of the ATS condition. Standard deviation was calculated on n = 3.
The response trends of biomarkers selected to evaluate the physiological conditions of D. sargus are shown in Figure 5. Liver data are always reported on the left y-axis and muscle on the right one. Data are presented for each specimen individually and enzyme activity as well as MDA concentration were normalized either on protein content or weight of the tissue, respectively. The difference in the activities averaged on the basis of the ATS outcome were never significant for any of the biomarkers considered.
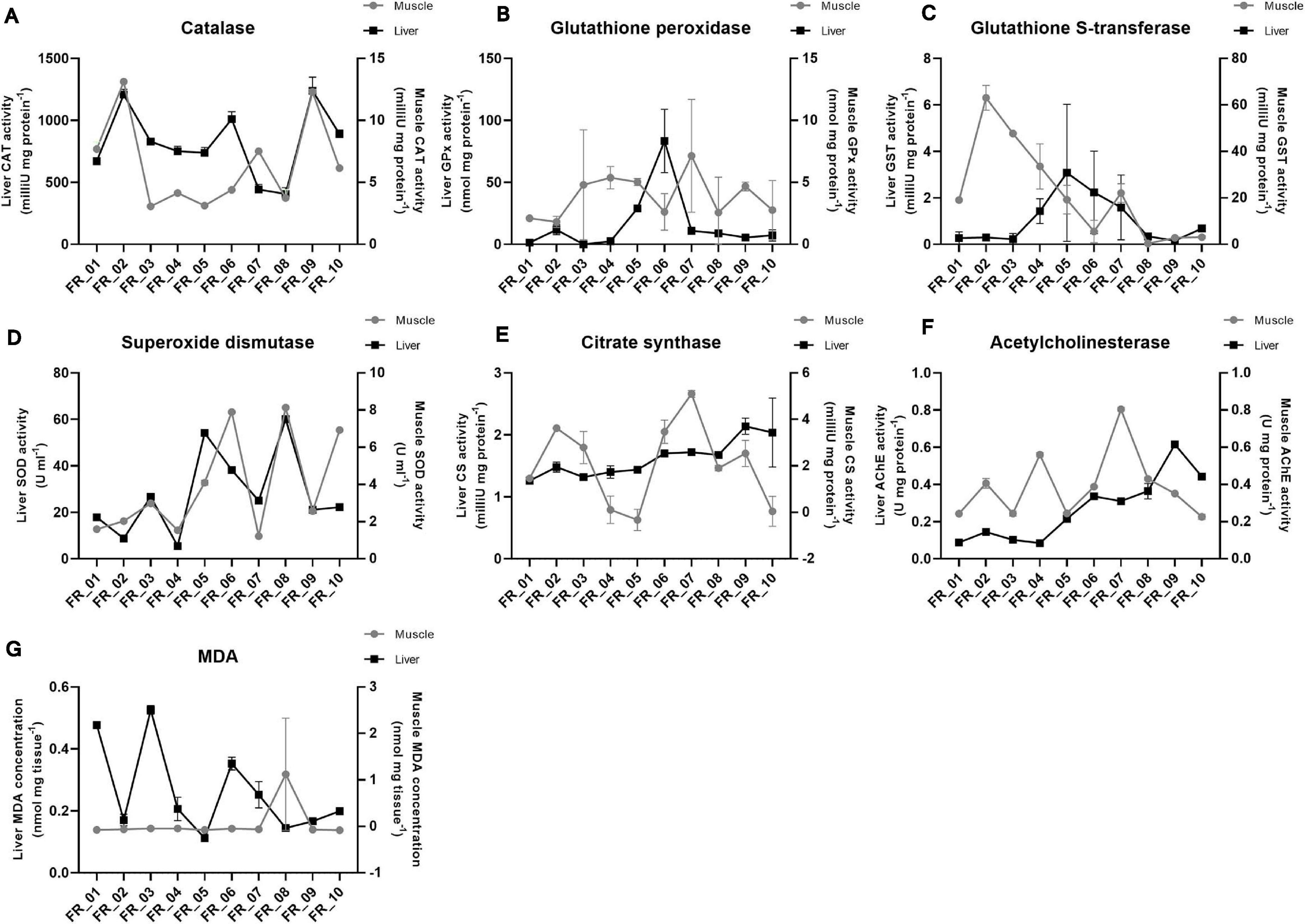
Figure 5. Fish physiological responses. Catalase (A), glutathione peroxidase (B), glutathione S-transferase (C), superoxide dismutase (D), citrate synthase (E), acetylcholinesterase (F), and malondialdehyde (G). Enzyme activities are expressed as either mU or U. MDA is expressed as nmol. All data were normalized to tissue protein content (mg) or weight (mg) except for superoxide dismutase. Liver and muscle data are plotted on the left and right y-axis, respectively, as mean ± SEM.
Catalase was approximately 100-fold more active in the liver than muscle; however, the general pattern seemed maintained between tissues, except for FR_07. The lowest activities were found in FR_08 liver (406.66 mU mg protein–1) and FR_03 muscle (3.07 mU mg protein–1) (Figure 5A).
In most samples, glutathione peroxidase (GPx) activities did not differ substantially between tissues. However, FR_02, FR_05, and FR_06 displayed approximately 10-, 6-, and 40-fold higher GPx activities in the liver than in muscle, respectively (Figure 5B).
A reversed situation was evident for glutathione S-transferase (GST). Activities in the liver were never elevated and the highest value accounted for 3.08 mU mg protein–1 (FR_05). Much higher activities were found in the muscle in six specimens, with FR_01-FR_04 exhibiting the largest fold-change differences (71, 210, 212, and 23, respectively) (Figure 5C).
Superoxide dismutase (SOD) activities matched almost perfectly between tissues in all samples, with liver being the most active of the two. FR_08 had the highest liver activity with 60.07 U ml–1 and FR_03 was one of the four most active samples with 26.66 U ml–1, together with FR_05 and FR_06 (Figure 5D).
Citrate synthase exhibited a higher between-tissue variability, and only samples FR_01, FR_08, and FR_10 displayed similar activities in liver and muscle; with regard to muscle, variability also occurred within sample replicates, as reflected by higher standard error of means (Figure 5E).
AChE trends generally matched between tissues, except for FR_04, FR_07, and FR_09. AChE activity was always greater in muscle than in liver except for FR_09 and FR_10 (Figure 5F).
Malondialdehyde (MDA) was appreciated in the liver, with the highest value accounting to 0.52 nmol mg tissue–1 in FR_03. MDA in muscle was probably below the kit detection limit (Figure 5G).
In the best-fitting plane, PC1 and PC2 explained 27.8 and 17.4% of variation, respectively, equaling a cumulative variability of 45.2%. An additional 14.8% was explained by PC3 (data not shown). The loading plot shows that CF, HSI, AChE_liver, LPO_liver, Caulerpin_liver, GST_liver, GST_muscle, SOD_liver, and SOD_muscle have their main contribution to the first axis, with CF, HSI, AChE_liver, GST_liver, SOD_liver, and SOD_muscle increasing from right to left. GSI, CAT_liver, CAT_muscle, AChE_muscle, CS_liver, CS_muscle, LPO_muscle, GPx_liver, and GPx_muscle are predominantly represented on PC2, with LPO_muscle increasing from top to bottom of the diagram (Figure 6A).
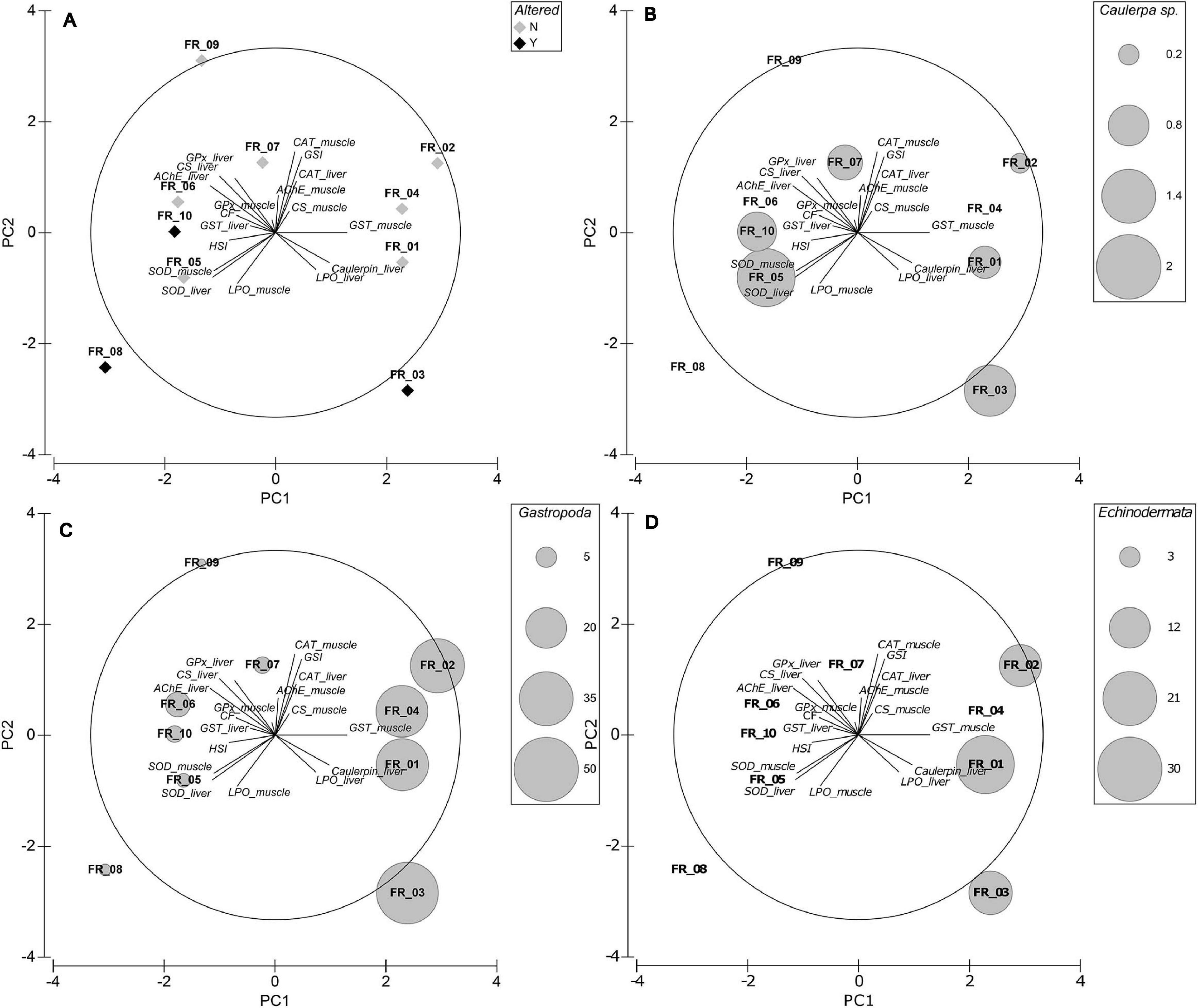
Figure 6. Exploratory MVA analysis. Principal components analyses of biomarker data superimposed on the eigenvector plot (A), Caulerpa sp. relative stomach content fraction (B), Gastropoda relative stomach content fraction (C), and Echinodermata relative stomach content fraction (D). PC1 and PC2 explain 27.8 and 17.4% of variation, respectively.
ATS-wise, FR_03 was associated with high Caulerpin_liver and LPO_liver but low CF, GPx_muscle, and GST_liver (among others). FR_10 was displayed in an almost opposite situation to FR_03. FR_08 correlated mainly with high LPO_muscle and low GSI, CAT, AChE_muscle, and CS_muscle. Although with lower concentrations, samples FR_05–FR_10 accumulated caulerpin in their liver but FR_06, FR_08, and FR_09 did not present any C. cylindracea content in their stomach (Figure 6B). Worthy of note, samples FR_05, FR_06, and FR_10 were similarly located in the 2-d space.
The spatial segregation between altered/non-altered specimens plotted by the sex factor is shown in Figure 7. The model had a large canonical correlation of 0.9597.
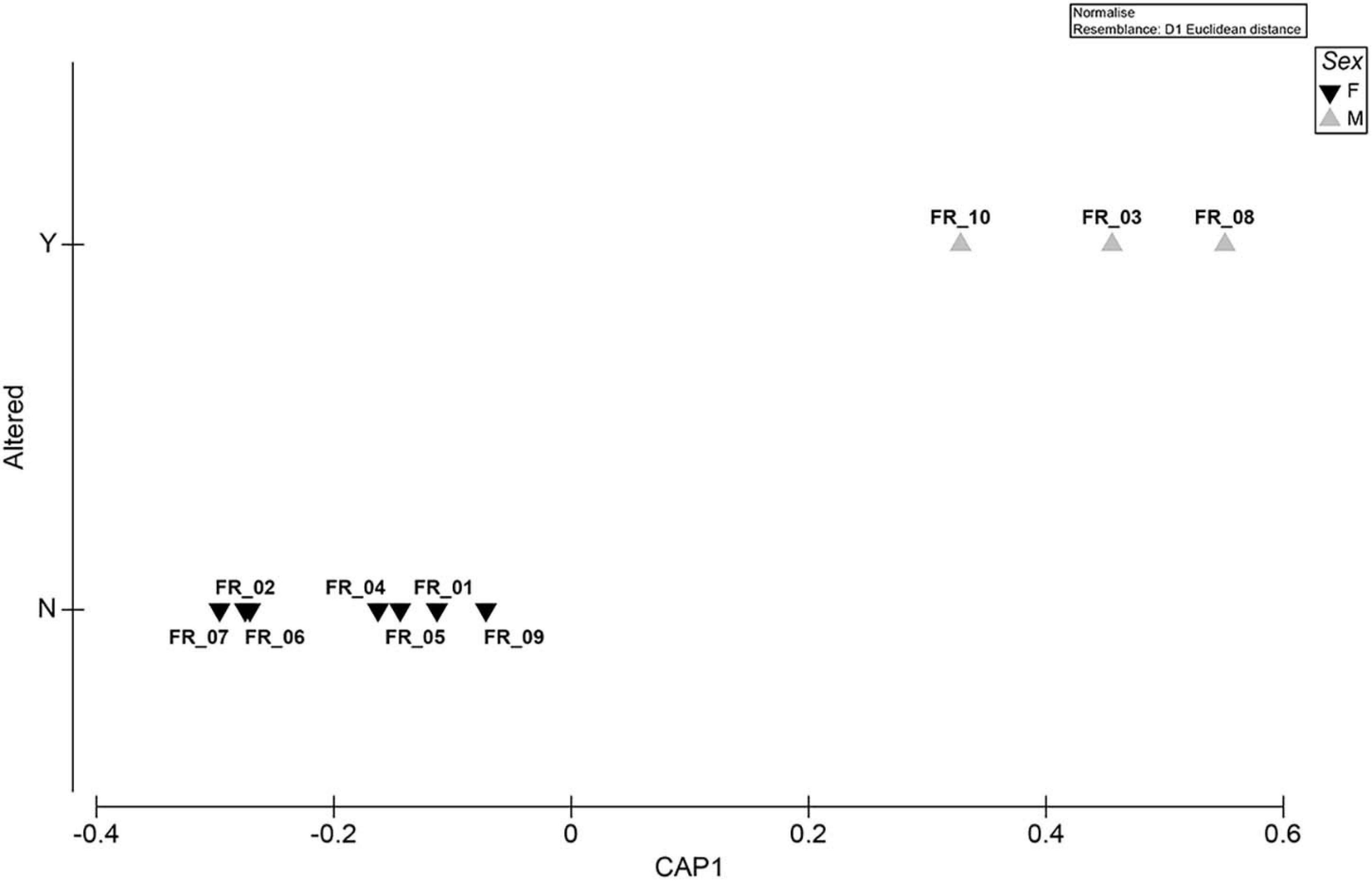
Figure 7. Exploratory MVA analysis. Canonical analysis of principal coordinates run on a Euclidean distance biomarker matrix. The “Sex” factor is superimposed as plot symbols. Correlation equaled 0.9597.
Discussion
This study identified the presence of the invasive C. cylindracea in the northern Tyrrhenian Sea and, by means of diet analysis, phenology, as well as biological and chemo-ecological biomarkers, multidisciplinarily elucidated its impacts on the local white seabream D. sargus population in light of the ATS anomaly. Although much ecological research has been conducted on C. cylindracea in the Mediterranean Sea (Piazzi et al., 2001b; Capiomont et al., 2005; Ruitton et al., 2005; Piazzi and Ceccherelli, 2006; Klein and Verlaque, 2008; Piazzi and Balata, 2008), the biological consequences have so far been explored only in D. sargus individuals originating from the Adriatic Sea, Ionian Sea, and Atlantic Ocean. To the best of our knowledge and ATS-wise, this is the first study to expand the reporting and investigate the physiological status of white seabream in the Tyrrhenian Sea by compa ring the responses of altered and unaltered fish.
In the Mediterranean Sea, the opportunistic C. cylindracea is capable of forming continuous dense meadows in various photophilic and sciaphilic benthic assemblages such as rocky substrate, dead P. oceanica “matte,” coralligenous, and detritic assemblages (Klein and Verlaque, 2008), causing a decrease in the total number of species and total macrophyte cover (Ceccherelli and Piazzi, 2001; Piazzi and Cinelli, 2003; Balata et al., 2004; Piazzi and Ceccherelli, 2006; Cinelli et al., 2007; Klein, 2007; Klein and Verlaque, 2007; Piazzi et al., 2001a, b, 2005, 2007). In our study area, the percent coverage and phenological parameters were greater in July than in October, contrarily to Ruitton et al. (2005). However, morpho-structural characters of the algae were reported to fluctuate pronouncedly over spatial and temporal scales (Piazzi et al., 2001a; Capiomont et al., 2005; Mezgui et al., 2007). As for the seasonal variation in our study area, the regression observed between the period of July and October is likely a phase within a larger vegetative cycle, which reaches its peak in November. Furthermore, sampling carried out in July 2020 showed a lower coverage of C. cylindracea compared to 2019 (our data, unpublished), in line with the typical life cycle (Ruitton et al., 2005).
The order Caulerpales synthesizes 18 secondary metabolites, the most common of which are caulerpenyne (a sesquiterpenoid), caulerpicin (a mixture of hydroxy amides), and caulerpin (a bisindolic alkaloid red pigment) (Higa and Kuniyoshi, 2000; Yang et al., 2015). Methanolic extracts of whole C. cylindracea were proven cytotoxic to a human breast cancer cell line (Chia et al., 2015). Crude extracts as well as purified caulerpenyne had antiproliferative, growth-inhibitory and apoptotic effects on cancerous cell lines (Fischel et al., 1995; Barbier et al., 2001; Cavas and Yurdakoc, 2005). Caulerpenyne also interfered with the sperm flagellar motility and fertilization rates as well as with the timing of first cleavage of sea urchin Lytechinus pictus and Paracentrotus lividus, respectively (Paul and Fenical, 1986; Pesando et al., 1996). Research on the effects of caulerpin has not been as thorough: a lack of acute toxicity was indicated (Vidal et al., 1984; Higa and Kuniyoshi, 2000) and antitumoral and nucleic acid fragmentation potentialities were reported in vitro (Schröder et al., 1998). In general terms, sesquiterpenes/sesquiterpenoids exert their toxicity by GSH and protein alkylation; DNA alkylation and epigenetic machinery deregulation leading to genetic mutations and transcriptional changes; increased oxidative stress; and disruption of mitochondrial homeostasis by inhibition of the SERCA pump (Amorim et al., 2013). Alkaloids compromise enzymatic activities, inhibit DNA synthesis, repair mechanisms and mitochondrial complex II, and affect the nervous system (Matsuura and Fett-Neto, 2015; Ferramosca et al., 2016). Recently, Vitale et al. (2018) and Del Coco et al. (2018) demonstrated that lipid metabolism is directly disrupted by caulerpin too.
Following the methods of Terlizzi et al. (2011), we selected fish larger than 300 g BW to populate our working subset (Table 1). Indeed, a growing body of evidence indicates that the ATS anomaly only occurs in specimens longer than 15–20 cm (Casadevall et al., 2020), a size that, according to length–weight relationships modeled from Mediterranean catches (Verdiell-Cubedo et al., 2006), corresponds to a BW of 189 g. Regardless of body size, stomach samples of the entire dataset were analyzed in their content to attest for ontogenetic diet shifts.
Caulerpa cylindracea is consumed by vertebrate and invertebrate species, either directly or indirectly and regardless of their feeding strategy: Aplysia sp., Ascobulla fragilis (Jeffreys, 1856), Elysia tomentosa (Jensen, 1997), Lobiger serradifalci (Calcara, 1840), Oxynoe olivacea (Rafinesque, 1814), P. lividus (Lamarck, 1816), Sphaerechinus granularis (Lamarck, 1816), Boops boops (Linnaeus, 1758), Coris julis (Linnaeus, 1758), D. sargus (Linnaeus, 1758), Diplodus vulgaris (Geoffroy Saint-Hilaire, 1817), Pagellus acarne (Risso, 1827), Sarpa salpa (Linnaeus, 1758), Siganus luridus (Rüppell, 1829), and Spondyliosoma cantharus (Linnaeus, 1758) (Klein and Verlaque, 2008; Box et al., 2009; Felline et al., 2017; and references therein; Sureda et al., 2006). Our data confirmed a trophic relationship between D. sargus and C. cylindracea, although the percentage by gut content weight was extremely low when not null, contrarily to Felline et al. (2017, 2012) and Terlizzi et al. (2011). Sixty percent of our samples prevalently displayed benthic carnivorous dietary habits (FR_01–FR_04 and FR_06–FR_07), but total plant content reached high relative percentage compositions in the remainder (FR_05 and FR_08–FR_10) (Table 2). Gastropoda, decapoda, echinodermata, photophilic algae, and inorganic particles were highly present in terms of gut relative prey composition in fish larger than 300 g BW. The widespread presence of C. cylindracea in the study area may be the reason why Crustacea and Mollusca were the most abundant stomach content taxa (89.07% of the overall total abundance): in fact, the algal-driven replacement of P. oceanica meadows foster changes in abundance, dominance, and diversity within local macrozoobenthic communities (Vázquez-Luis et al., 2009, 2012; Deudero et al., 2011; Pacciardi et al., 2011).
Mollusks were present in all specimens but had the greatest abundance in FR_01–FR_04 and echinoderms were present exclusively in FR_01–FR_03. Direct physiological interactions between mollusks and echinoderms with Caulerpa spp. were reported: Tejada et al. (2013) demonstrated that P. lividus feeds on C. cylindracea and that the algae induces antioxidant adaptations in the sea urchin; Sureda et al. (2009) highlighted changes in the antioxidant responses of both the gastropod Bittium reticulatum and C. taxifolia following grazing. It must be noted that the cerithiid B. reticulatum was the second most abundant gastropod in our stomach content data, together with Fissurella spp. and Haliotis spp., which are all known to prey on Caulerpa spp. (Poore, 1972; Franz, 1990). In the PCA plot, the correlation of samples FR_01–FR_04 with the marker Caulerpin_liver was evident and sample allocation was better explained by the relative percentage of ingested Gastropoda (Figure 6C) and Echinodermata (Figure 6D) rather than the actual Caulerpa sp. stomach content biomass (Figure 6B). The present study lacks data on caulerpin bioaccumulation in local mollusks and echinoderms and further investigations are needed to clarify this relationship; however, we believe that it is highly possible that D. sargus bioaccumulates caulerpin in the liver due to the consumption of detritivores, which assimilate Caulerpa-derived compounds from the sediment, and generalist herbivores, which actively feed on Caulerpa sp.
According to professional and recreational fishermen, the ATS anomaly is not exclusive of D. sargus but also affects congeners D. puntazzo (Cetti, 1777), D. cervinus (Lowe, 1838), and D. vulgaris (Geoffrey Saint-Hilaire, 1817) as well as other Sparidae species (Casadevall et al., 2020). In our case, 30% of tested specimens displayed the ATS condition (Figure 2). We could not reject the null hypothesis of no differences among diet compositions of fish > 300 g BW on the basis of the Altered factor, meaning that dietary habits of ATS fish were not dissimilar from those of fish not displaying the ATS condition, and Figure 3 further indicates clustering similarities independently of an ATS response. On the other hand, stomach contents of fish larger than 300 g BW differed statistically from those of fish smaller than 300 g BW, whose stomach was almost entirely occupied by Decapoda (Crustacea) and devoid of any plant content (except for FR_SI_03) (Supplementary Tables 1, 2). This is likely due to ontogenetic shifts in the feeding habits of the white sea bream. Similar findings were already reported in the Atlantic Ocean and Western Mediterranean Sea: Figueiredo et al. (2005) statistically demonstrated a shift in the feeding preferences of D. sargus at a length of approximately 25 cm; Merciai et al. (2018) found that the diet of “large” individuals (23.1–32.9 cm L) was not correlated to that of either “small” (4.9–15 cm L) or “medium” (15.1–23 cm L) ones. It is important to highlight that our samples ranged from 24.3 to 33.5 cm in length and that small fish never exhibited the ATS condition following cooking. Given the peculiar phenological parameters presented in Figure 4 and considering past evidence (Figueiredo et al., 2005; Osman and Mahmoud, 2009), we cannot exclude the fact that D. sargus in the northern Tyrrhenian Sea displays seasonal dietary changes too. Our data revealed that the degree of trophic exposure of the fish to the invasive alga, and the overall diet composition of adult specimens, cannot on its own explain the occurrence of the ATS anomaly. It is also unknown whether greater C. cylindracea cover or fronds and ramuli lengths recorded in July contribute to aggravating the incidence of such a phenomenon. Future efforts might be directed at enlarging the sample size for increasing the power of statistical analysis and investigating fish dietary habits, ATS rates, and algal secondary metabolite content also at the peak of the seaweed proliferation period. Because (i) caulerpin accumulation in the liver of D. sargus fluctuates seasonally (Gorbi et al., 2014), (ii) caulerpenyne production varies temporally along the year and spatially within thallus parts (Box et al., 2010), and (iii) algae can promptly transform secondary metabolites into more toxic and deterrent compounds as a response to mechanical wounding (Jung et al., 2002), it is possible that biological responsiveness changes too, even though these aspects have not been studied yet.
The UHPLC analysis identified caulerpin in all liver samples, confirming that caulerpin, as lipophilic metabolites, can be detected even when the animal does not show any sign of direct Caulerpa sp. ingestion (Table 3). Contrarily, Felline et al. (2017) detected the metabolite only in S. cantharus and S. salpa even though the vast majority of the species they investigated had presented algal fragments in their stomachs. Rates/efficiency of detoxification pathways are probably the underlying cause of such a discrepancy. Samples FR_01–FR_03 displayed slightly higher caulerpin concentrations than FR_04–FR_10 but the overall range was 2.441–3.512 μg g–1 dry weight. These values are much lower and less dispersed (mean ± SD: 2.792 ± 0.36 μg g–1) than the liver contents reported by Gorbi et al. (2014) and Terlizzi et al. (2011). Such a small variance might be due to (i) highly homogenous fish sizes in the working subset, (ii) limited direct consumption of C. cylindracea due to reduced algal coverage and morphometric parameters (as per our data), and (iii) adaptive phenotypic plasticity driven by abiotic features and/or biotic factors of the ecological environment resulting in improved metabolic detoxification efficiency (e.g., Ghalambor et al., 2007). The third hypothesis would be the most complex to verify.
The multi-biomarker panel was built with select proxies of antioxidant defenses, metabolic activity, lipid peroxidation, neurotoxicity, and energy availability. It provided information about a possible active response to oxidative stress, the proper sustainment of metabolic processes, the impairment of cell structural integrity, disturbance of the nervous transmission, or the specimen suitability to a successful reproductive investment, respectively. Such an integrative approach is recommended for maximizing the significance of each biomarker and investigating the ecological implications of exposure to toxic metabolites at various levels of biological organization (sub-individual, individual, and population) (Moore et al., 2004). Particular attention was called on oxidative stress biomarkers because Caulerpa sp. bioactive compounds are considered to be a possible source of reactive oxygen species (Sureda et al., 2006, 2009; Felline et al., 2012). The comparison of the responses between altered and unaltered specimens did not uniquely or predictively explain the ATS anomaly (Figure 6A). FR_03, FR_08, and FR_10 was each associated with different physiological markers: those related to the liver were predominantly represented by the first axis of the PCA. Catalase did not seem to be associated with the anomaly as the eigenvector points opposite to ATS fish in the 2-d space; this may be justifiable by its typical biphasic response (Regoli et al., 2011), according to which the marker displays enhanced activity at low concentrations of the contaminant but is inhibited at higher ones. GPx activities in both tissues were associated to FR_08 and FR_10: this may represent an antioxidant compensatory mechanism, with glutathione peroxidase replacing catalase in the removal of hydrogen peroxide (Regoli et al., 2011). Specimens FR_08 and FR_10 were also correlated with high HSI, which may be explained by a liver hypertrophy condition caused by the need for increased biotransformation of lipophilic compounds (Terlizzi et al., 2011). A negative correlation between GSI and ATS fish was evident: this is in line with what was reported by Felline et al. (2012), who found higher GSI values in individuals not accumulating the algal metabolite. Lipid peroxidation of liver and muscle correlated well with FR_03 and FR_08–FR_10, respectively.
All ATS specimens were males (Figure 7). Despite the fact that larger sample numbers are needed to unequivocally confirm the data, to our knowledge, this is the first report of the anomaly being found exclusively in D. sargus of a specific sex. This species was reported to be gonochoric (Bauchot and Hureau, 1986) or have an hermaphroditic proterandry biology (Micale and Perdichizzi, 1994), with sexual inversion occurring at a length of approximately 22 cm (Mouine et al., 2007) even though the range of 25–34 cm was reported for different sub-species populations (e.g., Mann and Buxton, 1998). The constrained approach followed to discriminate among a priori groups was supported by a large canonical correlation and showed a clear separation of the data clouds (Figure 7). The SIMPER routine performed on the same matrix revealed a low within-group distance for both sexes and identified GSI as the variable that contributed the most to differentiating the groups (Supplementary Table 3). The fact that such differential allocation did not emerge from the unconstrained ordination was likely due to the complexity of the data, whose total variation could not be captured by the first two axes of the PCA plots (Anderson et al., 2008). In addition to lipid metabolism and behavioral disturbances (Magliozzi et al., 2017; Del Coco et al., 2018), could algal metabolites also impair fish steroidogenesis?
Taking into consideration the complex results we presented, it is not easy to detect a general trend or provide an unambiguous explanation for the occurrence of the ATS anomaly. Through a correlative study, Casadevall et al. (2020) recently suggested copper in the form of antifouling paints and aquaculture-related copper sulfate rather than C. cylindracea ingestion as the causative agent of the ATS anomaly. It is difficult to discriminate between the effects of caulerpin and copper because the latter does not bioaccumulate, as evidenced by the lack of any correlation between Cu concentration and fish length (Merciai et al., 2018). To clarify the potential C. cylindracea–ATS link, the physiology of AT individuals will be investigated with RNA-seq and metabolomics approaches.
Conclusion
The present paper highlights direct trophic interactions between the white seabream D. sargus and the invasive C. cylindracea in the Tyrrhenian Sea. For the first time, photographic evidence of ATS post cooking is presented. Although further studies are needed to establish whether the algae or its metabolites are directly responsible of the anomaly, the novelty of our approach was to compare altered and unaltered specimens to better elucidate possible underlying differences in their physiological responses.
Data Availability Statement
The original contributions presented in the study are included in the article/Supplementary Material, further inquiries can be directed to the corresponding author/s.
Ethics Statement
Ethical review and approval was not required for this study because the animals were not subject to any experimental manipulation. Fish sampling was performed in strict accordance to Directive 2010/63/EU.
Author Contributions
EM, SB, and MM: funding acquisition. AM and EM: data curation. AM, EM, MB, FP, VL, and FT: investigation. EM and AT: methodology. AM: formal analysis. AM, EM, and MM: resources. MR, AT, and MM: supervision. AM, EM, and MB: visualization. AM, EM, MB, VL, and FT: writing (draft). MR, AT, and SB: writing (revision). All authors contributed to the article and approved the submitted version.
Funding
This work was supported by the project PO-FEAMP 2014-2020 “CAR—Study of the effects of the invasive alien species Caulerpa racemosa on Natura 2000 sites and impact on fishing activities” coordinated by CURSA and funded by Lazio region, the “Departments of Excellence-2018” Program (Dipartimenti di Eccellenza) of the Italian Ministry of Education, University and Research, and DIBAF Department of University of Tuscia, Project “Landscape 4.0—food, wellbeing and environment.”
Conflict of Interest
The authors declare that the research was conducted in the absence of any commercial or financial relationships that could be construed as a potential conflict of interest.
Acknowledgments
We are extremely thankful to Mollo and Cutignano of the Institute of Biomolecular Chemistry (National Research Council of Italy) for providing the pure caulerpin needed for absolute quantification. We would also like to thank the Federazione Italiana Pesca Sportiva e Attività Subacquee (FIPSAS) for providing fish samples for all analyses.
Supplementary Material
The Supplementary Material for this article can be found online at: https://www.frontiersin.org/articles/10.3389/fmars.2021.680787/full#supplementary-material
Footnotes
References
Alongi, G., Cormaci, M., Furnari, G., and Giaccone, G. (1993). Prima segnalazione di Caulerpa racemosa (Chlorophyceae, Caulerpales) per le coste italiane. Boll. dell’Accad. Gioenia di Sci. Nat. Catania 26, 9–53.
Amorim, M. H. R., Gil, Da Costa, R. M., Lopes, C., and Bastos, M. M. S. M. (2013). Sesquiterpene lactones: adverse health effects and toxicity mechanisms. Crit. Rev. Toxicol. 43, 559–579. doi: 10.3109/10408444.2013.813905
Anderson, M., Gorley, R., and Clarke, K. (2008). PERMANOVA+ for PRIMER: Guide to Software and Statistical Methods. Plymouth: PRIMER-E.
Azzurro, E., Sbragaglia, V., Cerri, J., Bariche, M., Bolognini, L., Ben Souissi, J., et al. (2019). Climate change, biological invasions, and the shifting distribution of Mediterranean fishes: a large-scale survey based on local ecological knowledge. Glob. Chang. Biol. 25, 2779–2792. doi: 10.1111/gcb.14670
Badary, O. A., Abdel-Maksoud, S., Ahmed, W. A., and Owieda, G. H. (2005). Naringenin attenuates cisplatin nephrotoxicity in rats. Life Sci. 76, 2125–2135. doi: 10.1016/j.lfs.2004.11.005
Balata, D., Piazzi, L., and Cinelli, F. (2004). A comparison among assemblages in areas invaded by Caulerpa taxifolia and C. racemosa on a subtidal Mediterranean rocky bottom. Mar. Ecol. 25, 1–13. doi: 10.1111/j.1439-0485.2004.00013.x
Baležentienė, L. (2015). Secondary metabolite accumulation and phytotoxicity of invasive species Solidago Canadensis l. During the growth period. Allelopath. J. 35, 217–226.
Barbier, P., Guise, S., Huitorel, P., Amade, P., Pesando, D., Briand, C., et al. (2001). Caulerpenyne from Caulerpa taxifolia has an antiproliferative activity on tumor cell line SK-N-SH and modifies the microtubule network. Life Sci. 70, 415–429. doi: 10.1016/S0024-3205(01)01396-0
Bauchot, M.-L., and Hureau, J.-C. (1986). “Sparidae,” in Fishes of the North-Eastern Atlantic and the Mediterranean, Vol. 2, eds A. Wheeler, P. J. P. Whitehead, M.-L. Bauchot, J.-C. Hureau, J. Nielsen, and E. Tortonese (Paris: UNESCO), 883–907.
Bellwood, D. R., and Robert Goatley, C. H. (2017). Can biological invasions save Caribbean coral reefs? Curr. Biol. 27, R13–R14. doi: 10.1016/j.cub.2016.11.018
Belton, G. S., van Reine, W. F., Huisman, J. M., Draisma, S. G. A., and Gurgel, C. F. D. (2014). Resolving phenotypic plasticity and species designation in the morphologically challenging Caulerpa racemosa-peltata complex (Chlorophyta, Caulerpaceae). J. Phycol. 50, 32–54. doi: 10.1111/jpy.12132
Bonifazi, A., Ventura, D., Gravina, M. F., Lasinio, G. J., Belluscio, A., and Ardizzone, G. D. (2017). Unusual algal turfs associated with the rhodophyta Phyllophora crispa: benthic assemblages along a depth gradient in the Central Mediterranean Sea. Estuar. Coast. Shelf Sci. 185, 77–93. doi: 10.1016/j.ecss.2016.12.013
Boudouresque, C.-F. (1971). Méthodes d’étude qualitative et quantitative du benthos (en particulier du phytobenthos). Tethys 3, 79–104.
Box, A., Deudero, S., Sureda, A., Blanco, A., Alòs, J., Terrados, J., et al. (2009). Diet and physiological responses of Spondyliosoma cantharus (Linnaeus, 1758) to the Caulerpa racemosa var. cylindracea invasion. J. Exp. Mar. Bio. Ecol. 380, 11–19. doi: 10.1016/j.jembe.2009.08.010
Box, A., Sureda, A., Tauler, P., Terrados, J., Marbà, N., Pons, A., et al. (2010). Seasonality of caulerpenyne content in native caulerpa prolifera and invasive C. Taxifolia and C. Racemosa Var. Cylindracea in the Western Mediterranean Sea. Bot. Mar. 53, 367–375. doi: 10.1515/BOT.2010.034
Buia, M. C., Gambi, M. C., Terlizzi, A., and Mazzella, L. (2001). Colonization of Caulerpa racemosa along the southern Italian coasts: I. Distribution, phenological variability and ecological role. GJS Posidonie Publ. 2001, 352–360.
Capiomont, A., Breugnot, E., Den Haan, M., and Meinesz, A. (2005). Phenology of a deep-water population of Caulerpa racemosa var. cylindracea in the northwestern Mediterranean Sea. Bot. Mar. 48, 80–83. doi: 10.1515/BOT.2005.006
Cappuccino, N., and Arnason, J. T. (2006). Novel chemistry of invasive exotic plants. Biol. Lett. 2, 189–193. doi: 10.1098/rsbl.2005.0433
Casadevall, M., Rodríguez-Prieto, C., Pueyo, J., Martí, C., Merciai, R., Verlaque, M., et al. (2020). The strange case of tough white seabream (Diplodus sargus, Teleostei: Sparidae): a first approach to the extent of the phenomenon in the mediterranean. Front. Mar. Sci. 7:387. doi: 10.3389/fmars.2020.00387
Cavas, L., and Yurdakoc, K. (2005). A comparative study: assessment of the antioxidant system in the invasive green alga Caulerpa racemosa and some macrophytes from the Mediterranean. J. Exp. Mar. Biol. Ecol. 321, 35–41. doi: 10.1016/j.jembe.2004.12.035
Ceccherelli, G., and Piazzi, L. (2001). “Caulerpa racemosa e Caulerpa taxifolia in Mediterraneo: effetto della complessità dei popolamenti macroalgali autoctoni e dei loro rapporti competitivi,” in Proceedings of the Congresso SIBM, Ischia, 45.
Chia, Y. Y., Kanthimathi, M. S., Khoo, K. S., Rajarajeswaran, J., Cheng, H. M., and Yap, W. S. (2015). Antioxidant and cytotoxic activities of three species of tropical seaweeds. BMC Complement. Altern. Med. 15:339. doi: 10.1186/s12906-015-0867-1
Cinelli, F., Balata, D., and Piazzi, L. (2007). “Threats to coralligenous assemblages: sedimentation and biological invasions,” in Proceedings of the 3rd Mediterranean Symposium on Marine Vegetation, Marseille, 27–29.
Costa, P. R., Martins, J. C., and Chainho, P. (2017). “Impact of invasions on water quality in marine and freshwater environments,” in Impact of Biological Invasions on Ecosystem Services, eds M. Vilà and P. E. Hulme (New York, NY: Springer International Publishing), 221–234.
Del Coco, L., Felline, S., Girelli, C. R., Angilè, F., Magliozzi, L., Almada, F., et al. (2018). 1 H NMR spectroscopy and MVA to evaluate the effects of caulerpin-based diet on diplodus sargus lipid profiles. Mar. Drugs 16, 1–12. doi: 10.3390/md16100390
Deudero, S., Box, A., Alós, J., Arroyo, N. L., and Marbà, N. (2011). Functional changes due to invasive species: food web shifts at shallow Posidonia oceanica seagrass beds colonized by the alien macroalga Caulerpa racemosa. Estuar. Coast. Shelf Sci. 93, 106–116. doi: 10.1016/j.ecss.2011.03.017
Felline, S., Caricato, R., Cutignano, A., Gorbi, S., Lionetto, M. G., Mollo, E., et al. (2012). Subtle effects of biological invasions: cellular and physiological responses of fish eating the exotic pest Caulerpa racemosa. PLoS One 7:e038763. doi: 10.1371/journal.pone.0038763
Felline, S., Mollo, E., Cutignano, A., Grauso, L., Andaloro, F., Castriota, L., et al. (2017). Preliminary observations of caulerpin accumulation from the invasive Caulerpa cylindracea in native Mediterranean fish species. Aquat. Biol. 26, 27–31. doi: 10.3354/ab00671
Ferramosca, A., Conte, A., Guerra, F., Felline, S., Rimoli, M. G., Mollo, E., et al. (2016). Metabolites from invasive pests inhibit mitochondrial complex II: a potential strategy for the treatment of human ovarian carcinoma? Biochem. Biophys. Res. Commun. 473, 1133–1138. doi: 10.1016/j.bbrc.2016.04.028
Figueiredo, M., Morato, T., Barreiros, J. P., Afonso, P., and Santos, R. S. (2005). Feeding ecology of the white seabream, Diplodus sargus, and the ballan wrasse, Labrus bergylta, in the Azores. Fish. Res. 75, 107–119. doi: 10.1016/j.fishres.2005.04.013
Fischel, J. L., Lemee, R., Formento, P., Caldani, C., Moll, J. L., Pesando, D., et al. (1995). Cell growth inhibitory effects of caulerpenyne, a sesquiterpenoid from the marine algae Caulerpa Taxifolia. Anticancer Res. 15, 2155–2160.
Franz, C. J. (1990). Differential algal consumption by three species of Fissurella (Mollusca: Gastropoda) at Isla de Margarita, Venezuela. Bull. Mar. Sci. 46, 735–748.
Gao, R., Yuan, Z., Zhao, Z., and Gao, X. (1998). Mechanism of pyrogallol autoxidation and determination of superoxide dismutase enzyme activity. Bioelectrochem. Bioenerg. 45, 41–45. doi: 10.1016/S0302-4598(98)00072-5
Ghalambor, C. K., McKay, J. K., Carroll, S. P., and Reznick, D. N. (2007). Adaptive versus non-adaptive phenotypic plasticity and the potential for contemporary adaptation in new environments. Funct. Ecol. 21, 394–407. doi: 10.1111/j.1365-2435.2007.01283.x
Giaccone, G., and Di Martino, V. (1995). La vegetazione a Caulerpa racemosa (Forsskål) J. Agardh nella Baia di S. Panagia (Sicilia sud-orientale). Boll. dell’Accad. Gioenia di Sci. Nat. Catania 28, 59–73.
Gorbi, S., Giuliani, M. E., Pittura, L., d’Errico, G., Terlizzi, A., Felline, S., et al. (2014). Could molecular effects of Caulerpa racemosa metabolites modulate the impact on fish populations of Diplodus sargus? Mar. Environ. Res. 96, 2–11. doi: 10.1016/j.marenvres.2014.01.010
Habig, W. H., Pabst, M. J., and Jakoby, W. B. (1974). Glutathione S transferases. The first enzymatic step in mercapturic acid formation. J. Biol. Chem. 249, 7130–7139. doi: 10.1016/S0021-9258(19)42083-8
Higa, T., and Kuniyoshi, M. (2000). Toxins associated with medicinal and edible seaweeds. J. Toxicol. Toxin Rev. 19, 119–137. doi: 10.1081/TXR-100100317
Infantes, E., Terrados, J., and Orfila, A. (2011). Assessment of substratum effect on the distribution of two invasive Caulerpa (Chlorophyta) species. Estuar. Coast. Shelf Sci. 91, 434–441. doi: 10.1016/j.ecss.2010.11.005
Jung, V., Thibaut, T., Meinesz, A., and Pohnert, G. (2002). Comparison of the wound-activated transformation of caulerpenyne by invasive and noninvasive Caulerpa species of the Mediterranean. J. Chem. Ecol. 28, 2091–2105. doi: 10.1023/A:1020710230532
Katsanevakis, S., Coll, M., Piroddi, C., Steenbeek, J., Lasram, F. B. R., Zenetos, A., et al. (2014). Invading the mediterranean sea: biodiversity patterns shaped by human activities. Front. Mar. Sci. 1:32. doi: 10.3389/fmars.2014.00032
Klein, J. (2007). Impact of Caulerpa racemosa var. Cylindracea (Caulerpales, Chlorophyta) on Macrophyte Assemblages of the North-Western Mediterranean Sea. PhD thesis.
Klein, J., and Verlaque, M. (2007). “Caulerpa racemosa effect on macrophyte assemblages of dead Posidonia beds,” in Proceedings of the 3rd Mediterranean Symposium on Marine Vegetation, Marseille, 27–29.
Klein, J., and Verlaque, M. (2008). The Caulerpa racemosa invasion: a critical review. Mar. Pollut. Bull. 56, 205–225. doi: 10.1016/j.marpolbul.2007.09.043
Langkilde, T., Thawley, C. J., and Robbins, T. R. (2017). “Behavioral adaptations to invasive species: benefits, costs, and mechanisms of change,” in Advances in the Study of Behavior, eds M. Naguib, J. Podos, L. W. Simmons, L. Barrett, S. Healy, and M. Zuk (Cambridge, MA: Academic Press Inc.), 199–235.
Lloret, J., Shulman, G., and Love, R. M. (2014). Condition and Health Indicators of Exploited Marine Fishes. Hoboken, NJ: Wiley Blackwell.
Magliozzi, L., Almada, F., Robalo, J., Mollo, E., Polese, G., Gonçalves, E. J., et al. (2017). Cryptic effects of biological invasions: reduction of the aggressive behaviour of a native fish under the influence of an “invasive” biomolecule. PLoS One 12:e0185620. doi: 10.1371/journal.pone.0185620
Magliozzi, L., Maselli, V., Almada, F., Di Cosmo, A., Mollo, E., and Polese, G. (2019). Effect of the algal alkaloid caulerpin on neuropeptide Y (NPY) expression in the central nervous system (CNS) of Diplodus sargus. J. Comp. Physiol. Neuroethol. Sens. Neural Behav. Physiol. 205, 203–210. doi: 10.1007/s00359-019-01322-8
Mann, B. Q., and Buxton, C. D. (1998). The reproductive biology of Diplodus sargus capensis and D. cervinus hottentotus (Sparidae) off the South-East Cape coast. South Africa. Cybium 22, 31–47.
Matsuura, H. N., and Fett-Neto, A. G. (2015). “Plant alkaloids: main features, toxicity, and mechanisms of action,” in Plant Toxins, P. Gopalakrishnakone, C.R. Carlini, R. Ligabue-Braun, eds (Dordrecht: Springer), 1–15.
Merciai, R., Rodríguez-Prieto, C., Torres, J., and Casadevall, M. (2018). Bioaccumulation of mercury and other trace elements in bottom-dwelling omnivorous fishes: the case of Diplodus sargus (L.) (Osteichthyes: Sparidae). Mar. Pollut. Bull. 136, 10–21. doi: 10.1016/j.marpolbul.2018.08.061
Mezgui, Y., Djellouli, A. S., and Ben Chikh, and Almi, I. (2007). “Etude biométrique (biomasse et phénologie) des populations à Caulerpa racemosa dans la region de Bizerte (Tunisie),” in Proceedings of the 3rd Mediterranean Symposium on Marine Vegetation, Marseille, 111–116.
Micale, V., and Perdichizzi, F. (1994). Further studies on the sexuality of the hermaphroditic teleost Diplodus sargus, with particular reference to protandrous sex inversion. J. Fish Biol. 45, 661–670. doi: 10.1111/j.1095-8649.1994.tb00932.x
Mollo, E., Gavagnin, M., Carbone, M., Castelluccio, F., Pozone, F., Roussis, V., et al. (2008). Factors promoting marine invasions: a chemoecological approach. Proc. Natl. Acad. Sci. U.S.A. 105, 4582–4586. doi: 10.1073/pnas.0709355105
Moore, M. N., Depledge, M. H., Readman, J. W., and Paul Leonard, D. R. (2004). An integrated biomarker-based strategy for ecotoxicological evaluation of risk in environmental management. Mutat. Res. 552, 247–268. doi: 10.1016/j.mrfmmm.2004.06.028
Mouine, N., Francour, P., Ktari, M. H., and Chakroun-Marzouk, N. (2007). The reproductive biology of Diplodus sargus sargus in the Gulf of Tunis (central Mediterranean). Sci. Mar. 71, 461–469. doi: 10.3989/scimar.2007.71n3461
Osman, A. M., and Mahmoud, H. H. (2009). Feeding biology of Diplodus sargus and Diplodus vulgaris (Teleostei, Sparidae) in egyptian mediterranean waters. World J. Fish Mar. Sci. 1, 290–296.
Pacciardi, L., De Biasi, A. M., and Piazzi, L. (2011). Effects of Caulerpa racemosa invasion on soft-bottom assemblages in the Western Mediterranean Sea. Biol. Invasions 13, 2677–2690. doi: 10.1007/s10530-011-9938-5
Paul, V., and Fenical, W. (1986). Chemical defense in tropical green algae, order Caulerpales. Mar. Ecol. Prog. Ser. 34, 157–169. doi: 10.3354/meps034157
Pesando, D., Lemée, R., Ferrua, C., Amade, P., and Girard, J.-P. (1996). Effects of caulerpenyne, the major toxin from Caulerpa taxifolia on mechanisms related to sea urchin egg cleavage. Aquat. Toxicol. 35, 139–155. doi: 10.1016/0166-445X(96)00013-6
Piazzi, L., and Balata, D. (2008). The spread of Caulerpa racemosa var. cylindracea in the Mediterranean Sea: an example of how biological invasions can influence beta diversity. Mar. Environ. Res. 65, 50–61. doi: 10.1016/j.marenvres.2007.07.002
Piazzi, L., Balata, D., Ceccherelli, G., and Cinelli, F. (2005). Interactive effect of sedimentation and Caulerpa racemosa var. cylindracea invasion on macroalgal assemblages in the Mediterranean Sea. Estuar. Coast. Shelf Sci. 64, 467–474. doi: 10.1016/j.ecss.2005.03.010
Piazzi, L., Balata, D., and Cinelli, F. (2007). Invasions of alien macroalgae in Mediterranean coralligenous assemblages. Cryptogam. Algol. 28, 289–301.
Piazzi, L., and Ceccherelli, G. (2006). Persistence of biological invasion effects: recovery of macroalgal assemblages after removal of Caulerpa racemosa var. cylindracea. Estuar. Coast. Shelf Sci. 68, 455–461. doi: 10.1016/j.ecss.2006.02.011
Piazzi, L., Ceccherelli, G., and Cinelli, F. (2001a). “Effet de Caulerpa racemosa sur la structure des communaute’s algales benthiques,” in Proceedings of the 4th International Workshop on Caulerpa taxifolia, eds V. Gravez, S. Ruitton, C. F. Boudouresque, L. Le Direach, A. Meinesz, G. Scabbia, et al. (Marseille: GIS Posidonie Publisher), 371–375.
Piazzi, L., Ceccherelli, G., and Cinelli, F. (2001b). Threat to macroalgal diversity: effects of the introduced green alga Caulerpa racemosa in the Mediterranean. Mar. Ecol. Prog. Ser. 210, 149–159. doi: 10.3354/meps210149
Piazzi, L., and Cinelli, F. (2003). Evaluation of benthic macroalgal invasion in a harbour area of the western Mediterranean Sea. Eur. J. Phycol. 38, 223–231. doi: 10.1080/1364253031000136358
Poore, G. C. B. (1972). Ecology of New Zealand abalones, haliotis species (Mollusca: Gastropoda): 3. Growth. N. Z. J. Mar. Freshw. Res. 6, 534–559. doi: 10.1080/00288330.1972.9515445
Regoli, F., Giuliani, M. E., Benedetti, M., and Arukwe, A. (2011). Molecular and biochemical biomarkers in environmental monitoring: a comparison of biotransformation and antioxidant defense systems in multiple tissues. Aquat. Toxicol. 105(Suppl. 3–4), 56–66. doi: 10.1016/j.aquatox.2011.06.014
Rizzo, L., Pusceddu, A., Stabili, L., Alifano, P., and Fraschetti, S. (2017). Potential effects of an invasive seaweed (Caulerpa cylindracea, Sonder) on sedimentary organic matter and microbial metabolic activities. Sci. Rep. 7:12113. doi: 10.1038/s41598-017-12556-4
Roques, A., Auger-Rozenberg, M.-A., Blackburn, T. M., Garnas, J., Pyšek, P., Rabitsch, W., et al. (2016). Temporal and interspecific variation in rates of spread for insect species invading Europe during the last 200 years. Biol. Invasions 18, 907–920. doi: 10.1007/s10530-016-1080-y
Ruitton, S., Verlaque, M., and Boudouresque, C.-F. (2005). Seasonal changes of the introduced Caulerpa racemosa var. cylindracea (Caulerpales, Chlorophyta) at the northwest limit of its mediterranean range. Aquat. Bot. 82, 55–70. doi: 10.1016/j.aquabot.2005.02.008
Sax, D. F., Stachowicz, J. J., Brown, J. H., Bruno, J. F., Dawson, M. N., Gaines, S. D., et al. (2007). Ecological and evolutionary insights from species invasions. Trends Ecol. Evol. 22, 465–471. doi: 10.1016/j.tree.2007.06.009
Schröder, H. C., Badria, F. A., Ayyad, S. N., Batel, R., Wiens, M., Hassanein, H. M. A., et al. (1998). Inhibitory effects of extracts from the marine alga Caulerpa taxifolia and of toxin from Caulerpa racemosa on multixenobiotic resistance in the marine sponge Geodia cydonium. Environ. Toxicol. Pharmacol. 5, 119–126. doi: 10.1016/S1382-6689(97)10067-9
Seebens, H., Blackburn, T. M., Dyer, E. E., Genovesi, P., Hulme, P. E., Jeschke, J. M., et al. (2017). No saturation in the accumulation of alien species worldwide. Nat. Commun. 8:14435. doi: 10.1038/ncomms14435
Sureda, A., Box, A., Deudero, S., and Pons, A. (2009). Reciprocal effects of caulerpenyne and intense herbivorism on the antioxidant response of Bittium reticulatum and Caulerpa taxifolia. Ecotoxicol. Environ. Saf. 72, 795–801. doi: 10.1016/j.ecoenv.2007.12.007
Sureda, A., Box, A., Enseñat, M., Alou, E., Tauler, P., Deudero, S., et al. (2006). Enzymatic antioxidant response of a labrid fish (Coris julis) liver to environmental caulerpenyne. Comp. Biochem. Physiol. C Toxicol. Pharmacol. 144, 191–196. doi: 10.1016/j.cbpc.2006.08.001
Tejada, S., Deudero, S., Box, A., and Sureda, A. (2013). Physiological response of the sea urchin Paracentrotus lividus fed with the seagrass Posidonia oceanica and the alien algae Caulerpa racemosa and Lophocladia lallemandii. Mar. Environ. Res. 83, 48–53. doi: 10.1016/j.marenvres.2012.10.008
Terlizzi, A., Felline, S., Lionetto, M. G., Caricato, R., Perfetti, V., Cutignano, A., et al. (2011). Detrimental physiological effects of the invasive alga Caulerpa racemosa on the mediterranean white seabream Diplodus sargus. Aquat. Biol. 12, 109–117. doi: 10.3354/ab00330
Vázquez-Luis, M., Borg, J. A., Sanchez-Jerez, P., and Bayle-Sempere, J. T. (2012). Habitat colonisation by amphipods: comparison between native and alien algae. J. Exp. Mar. Biol. Ecol. 432–433, 162–170. doi: 10.1016/j.jembe.2012.07.016
Vázquez-Luis, M., Sanchez-Jerez, P., and Bayle-Sempere, J. T. (2009). Comparison between amphipod assemblages associated with Caulerpa racemosa var. cylindracea and those of other Mediterranean habitats on soft substrate. Estuar. Coast. Shelf Sci. 84, 161–170. doi: 10.1016/j.ecss.2009.04.016
Verdiell-Cubedo, D., Oliva-Paterna, F. J., and Torralva, M. (2006). Length-weight relationships for 22 fish species of the Mar Menor coastal lagoon (western Mediterranean Sea). J. Appl. Ichthyol. 22, 293–294. doi: 10.1111/j.1439-0426.2006.00738.x
Verlaque, M., Durand, C., Huisman, J. M., Boudouresque, C. F., and Le Parco, Y. (2003). On the identity and origin of the Mediterranean invasive Caulerpa racemosa (Caulerpales, Chlorophyta). Eur. J. Phycol. 38, 325–339. doi: 10.1080/09670260310001612592
Vidal, J. P., Laurent, D., Kabore, S. A., Rechencq, E., Boucard, M., Girard, J. P., et al. (1984). Caulerpin, Caulerpicin, Caulerpa scalpelliformis: comparative acute toxicity study. Bot. Mar. 27, 533–537. doi: 10.1515/botm.1984.27.12.533
Vidal-Liñán, L., and Bellas, J. (2013). Practical procedures for selected biomarkers in mussels, Mytilus galloprovincialis - implications for marine pollution monitoring. Sci. Total Environ. 46, 56–64. doi: 10.1016/j.scitotenv.2013.04.079
Vitale, R. M., D’Aniello, E., Gorbi, S., Martella, A., Silvestri, C., Giuliani, M. E., et al. (2018). Fishing for targets of alien metabolites: a novel peroxisome proliferator-activated receptor (PPAR) agonist from a marine pest. Mar. Drugs 16:431. doi: 10.3390/md16110431
Wilson, B. W., and Henderson, J. D. (2007). Determination of cholinesterase in blood and tissue. Curr. Protoc. Toxicol. Chapter 12:Unit 12.13. doi: 10.1002/0471140856.tx1213s34
Keywords: Abnormally Tough Specimen, antioxidant enzymes, biomarker, CAR project, ecotoxicology, metabolites, multivariate analysis, white seabream
Citation: Miccoli A, Mancini E, Boschi M, Provenza F, Lelli V, Tiralongo F, Renzi M, Terlizzi A, Bonamano S and Marcelli M (2021) Trophic, Chemo-Ecological and Sex-Specific Insights on the Relation Between Diplodus sargus (Linnaeus, 1758) and the Invasive Caulerpa cylindracea (Sonder, 1845). Front. Mar. Sci. 8:680787. doi: 10.3389/fmars.2021.680787
Received: 15 March 2021; Accepted: 13 May 2021;
Published: 16 June 2021.
Edited by:
Cataldo Pierri, University of Bari Aldo Moro, ItalyReviewed by:
Daniele Ventura, Sapienza University of Rome, ItalyPere Ferriol, University of the Balearic Islands, Spain
Copyright © 2021 Miccoli, Mancini, Boschi, Provenza, Lelli, Tiralongo, Renzi, Terlizzi, Bonamano and Marcelli. This is an open-access article distributed under the terms of the Creative Commons Attribution License (CC BY). The use, distribution or reproduction in other forums is permitted, provided the original author(s) and the copyright owner(s) are credited and that the original publication in this journal is cited, in accordance with accepted academic practice. No use, distribution or reproduction is permitted which does not comply with these terms.
*Correspondence: Andrea Miccoli, YW5kcmVhLm1pY2NvbGlAdW5pdHVzLml0; Emanuele Mancini, ZS5tYW5jaW5pQHVuaXR1cy5pdA==
†These authors have contributed equally to this work and share first authorship