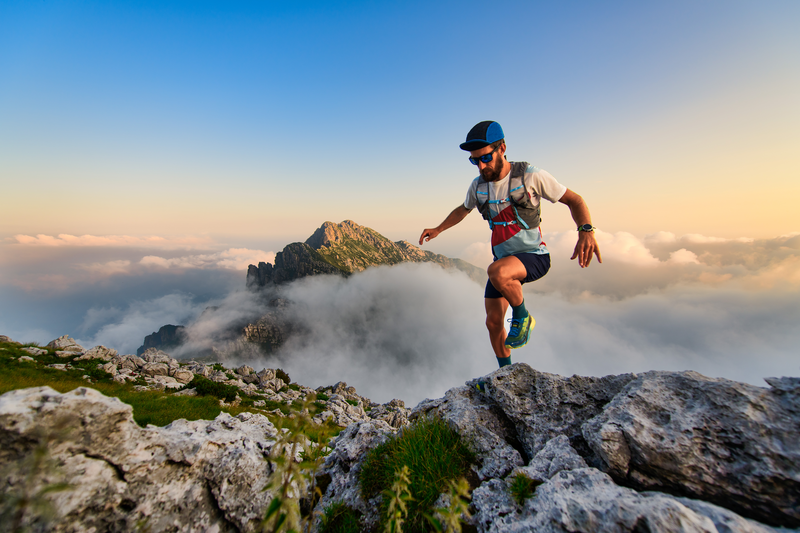
94% of researchers rate our articles as excellent or good
Learn more about the work of our research integrity team to safeguard the quality of each article we publish.
Find out more
REVIEW article
Front. Mar. Sci. , 25 June 2021
Sec. Marine Megafauna
Volume 8 - 2021 | https://doi.org/10.3389/fmars.2021.678173
This article is part of the Research Topic Sociality in the Marine Environment View all 14 articles
Numerous species of cetaceans have been recorded in mixed-species groups (MSGs). By forming groups with individuals of different species, cetaceans may reduce predation risk, improve foraging, and gain social benefits. Most accounts of cetacean MSGs, however, are descriptive and little is known about their functions. Furthermore, research has been hindered by inconsistent use of terminology and the lack of a conceptual framework to guide investigations. We reviewed the cetacean literature to compare how MSGs have been termed and defined, to assess their characteristics, to evaluate what is known about their potential functions, and to provide directions for future study. In total, we reviewed 203 studies reporting observations of cetacean MSGs. These MSGs involved 54 different species, predominantly delphinids, that formed 216 different species pairs with varied morphologies and levels of relatedness. Cetacean MSGs occurred across the globe, from tropical to cold temperate seas, from shallow coastal waters to the open ocean, and varied in characteristics such as group size and frequency of occurrence. Only 27 of the reviewed studies proposed and discussed the potential functions of cetacean MSGs, suggesting reduced predation risk (5 species pairs), improved foraging (17 species pairs), and social benefits (12 species pairs) as the main drivers. In most cases, however, the factors that drive the formation of cetacean MSGs remain unknown. Amongst the reviewed studies, MSGs were referred to by various terms, often with no explicit definitions. To reduce this inconsistency, we recommend that future studies use only the term mixed-species group which we define as individuals of two or more species found in close spatial proximity due to mutual or unreciprocated attraction derived from evolutionary grouping benefits. There were also few structured investigations to confirm MSG occurrence and to analyse their potential causes and consequences. To facilitate the study of cetacean MSGs, we developed a conceptual framework that establishes diverse approaches to, firstly, distinguish MSGs from chance encounters and aggregations and to, secondly, investigate their potential functions. This is necessary if we are to advance this field of study and improve our understanding of the role that MSGs play in species and community ecology.
Group living is fundamental to numerous species of animals as it conveys various benefits and costs (Alexander, 1974; Krause and Ruxton, 2002; Majolo and Huang, 2018). By forming groups, individuals may decrease the risk of predation, improve foraging, increase their reproductive chances, and decrease the energetic cost of movement (Krause and Ruxton, 2002; Majolo and Huang, 2018). Individuals may also incur costs including increased competition for resources, increased probability of detection by predators, inbreeding, and increased risk of disease transmission among group members (Alexander, 1974; Krause and Ruxton, 2002; Majolo and Huang, 2018). Studies on the costs and benefits of group living have provided a comprehensive understanding of the principles underlying group formation, particularly for groups composed of individuals of the same species. Much less is known, however, about the dynamics of groups composed of multiple species (Morse, 1977; Stensland et al., 2003; Goodale et al., 2017). Mixed-species groups (MSGs), also termed interspecific, polyspecific, or heterospecific groups or associations (Whitesides, 1989; Heymann and Buchanan-Smith, 2000; Stensland et al., 2003), are broadly defined as sets of individuals of two or more species that are seen in such close association that they can be regarded as members of the same group (Stensland et al., 2003). As such, MSGs occur when there is an attraction between heterospecific individuals (Stensland et al., 2003; Cords and Würsig, 2014). This attraction can be either mutual or unreciprocated, as long as the presence of the attracted species is tolerated by the other (Stensland et al., 2003). MSGs should be distinguished from aggregations of animals that are attracted to a common resource or that respond in a similar way to environmental stimuli and from chance encounters that result from the coincidental meeting of co-occurring species (Table 1; Waser, 1982, 1984; Cords and Würsig, 2014). MSGs are thought to occur because they provide evolutionary benefits over individuals, populations, or species that do not mix (Stensland et al., 2003; Whitehead, 2008; Ward and Webster, 2016; Goodale et al., 2017). These benefits form the basis of the three principal functional explanations for the formation of MSGs: reduced predation risk, improved foraging, and social advantages (Whitesides, 1989; Stensland et al., 2003; Cords and Würsig, 2014; Sridhar and Guttal, 2018). Participation in MSGs can lead to changes in behaviour and habitat use of one or more of the species involved as individuals alter their ecology in response to the presence of heterospecifics (Peres, 1992; Wolters and Zuberbühler, 2003; Porter and Garber, 2007; Sridhar et al., 2009). Thus, assessing the underlying causes and functions of MSGs is important to better understand the dynamics of ecological communities (Veit and Harrison, 2017; Zou et al., 2018).
MSGs have been recorded amongst closely and distantly related species including fishes (Lukoschek and McCormick, 2000), birds (Sridhar et al., 2009), and mammals (Stensland et al., 2003). Amongst mammals, MSGs have been most commonly documented in ungulates, primates, and cetaceans (Morse, 1977; Heymann and Buchanan-Smith, 2000; Stensland et al., 2003; Cords and Würsig, 2014; Heymann and Hsia, 2015). Cetaceans, particularly delphinids, are known to form MSGs with other cetaceans relatively frequently (Frantzis and Herzing, 2002; Stensland et al., 2003; Bearzi, 2005b; Cords and Würsig, 2014), as well as with other marine mammal species including pinnipeds (Bearzi, 2006; Bacon et al., 2017) and sirenians (Kiszka, 2007). Despite the apparent widespread occurrence of cetacean MSGs (reviewed in Stensland et al., 2003; Cords and Würsig, 2014), large gaps remain in our understanding of their function and the mechanisms underlying their formation. This is largely due to the lack of dedicated studies on the potential drivers and associated costs and benefits of cetacean MSGs (Stensland et al., 2003). The development of such studies is, in turn, hindered by inconsistent terminology and the absence of a conceptual framework to guide the development of cetacean MSG studies. In the literature, several terms, including association, aggregation, and mixed-species group, are used interchangeably with varying definitions (Stensland et al., 2003). Moreover, there is no clear outline of how to distinguish cetacean MSGs from chance encounters and aggregations and how to subsequently investigate their function. Consistent terminology and clear conceptual frameworks are essential when studying ecological phenomena to enable clear communication and to allow comparisons across taxa and regions (Fauth et al., 1996; Hall et al., 1997). Thus, the development and utilisation of such terminology and frameworks is key to the advancement and understanding of ecological topics.
Here, we review the literature on cetacean MSGs to: (1) address any inconsistencies in terminology and definitions; (2) assess their characteristics (i.e., the species involved, occurrence, and distribution); (3) evaluate what is known about their potential functions; and (4) use the results to propose standardised terminology and a conceptual framework to assist future studies with characterising their dynamics and functions.
We used the databases Scopus, ScienceDirect, and Web of Science and the search engine Google Scholar to search for relevant journal articles, book chapters, reports, and theses that contained records of cetacean MSGs. As various terms, including association and aggregation, are often used interchangeably to refer to MSGs, we included these terms in our literature search. More specifically, we combined each of several adjectives, including mixed-species, interspecific, heterospecific, and polyspecific with each of the key terms, including, but not limited to, group, aggregation, association, and interaction to form 40 phrases that refer to MSGs (see Supplementary Table 1 for a full list of the search terms). These phrases were combined with the English names of relevant taxa (i.e., cetacean, whale, dolphin, and porpoise) to create the full search queries. Each of the search queries was entered into the databases and search engine and the citation information of all the results (e.g., titles, abstracts, authors) was downloaded. The titles and abstracts of the results were then read and analysed. Studies on captive animals were removed as they do not represent natural grouping patterns. Studies that were based entirely on data that was obtained remotely (e.g., passive acoustics) or from isotopic or genetic analyses were also removed as they do not contain the observations required to analyse grouping dynamics. The remaining studies were reviewed and those that described multiple species of cetaceans as forming aggregations, associations, groups, or a term that is often considered synonymous (e.g., school, herd, and pod) were included for further analysis. Studies that used any term with an explicit definition that clearly distinguished it from a MSG, however, were not included, as were studies that simply recorded species in the same area with no clear indication of MSG formation. Finally, the reference lists of the included studies were searched to find any additional publications that were missed by the initial searches.
The studies that met the criteria for inclusion were then reviewed to produce a comprehensive compilation of records of cetacean MSGs. The taxonomic classification of all cetaceans involved in MSGs was recorded to the lowest taxonomic level following the 2020 Society for Marine Mammalogy Committee on Taxonomy (Committee on Taxonomy, 2020). Subsequent analyses of species composition were conducted on a species and family level. Where possible, we noted any additional information regarding group size, behaviour, and frequency of MSGs to provide a more detailed understanding of the dynamics of cetacean MSGs. All the terms used to describe MSGs, as well as any explicit definitions of those terms, were also recorded.
To gain insights into the distribution and frequency of cetacean MSGs across the major ocean basins we mapped their geographical distribution using QGIS (QGIS Development Team, 2019) and Plotly (Plotly Technologies Inc., 2015). As the precise geographical locations of the MSGs were often unavailable, we assigned a location value (i.e., ocean basin) to each study based on the study area. Additionally, to understand and visualise the spatial distribution of and the relationships between the cetacean species that most often form MSGs, we constructed a social network diagram where each node represents a species and each edge the occurrences of a species pair in MSGs. The edges were weighted according to the total number of studies reporting each species pair while the sizes of the nodes were made proportional to the total number of partner species that each species had. The average values of each species’ distribution in terms of water depth and latitude were obtained from the Encyclopedia of Marine Mammals (Würsig et al., 2018) and used to position each species’ node along the x and y axes, respectively, in the network diagram.
Finally, to determine the level to which cetacean MSGs have been researched, the studies were separated into those that simply reported the occurrence of cetacean MSGs and those that used observations or investigations to propose functional explanations for them. Details of these investigations and their conclusions were then compiled. Analysis of the data was conducted using Python (Python Software Foundation, 2016) and all figures were created using Plotly (Plotly Technologies Inc., 2015) in Python.
The literature search returned 2154 results, of which 98 were studies that met our criteria for inclusion. Additionally, 94 studies were added by tracing cited studies and a further 11 studies were obtained from a bibliography of publications on cetacean MSGs (Rowley, 2020), amounting to a total of 203 studies. Cetacean MSGs appear to have been first reported in the literature in 1961, with the majority of reports having been published since 1990 (Figure 1).
Out of the 203 studies obtained from the literature review, 116 studies (57.1%) referred to situations where multiple species of cetaceans were observed in close spatial proximity as groups, 95 (46.8%) as associations, 42 (20.7%) as schools, 26 (12.8%) as aggregations, while 11 studies (5.4%) used other terms such as assemblage, encounter, and herd (Figure 2). Over a third of the studies (36.0%) used multiple terms synonymously. Of those studies that used the term group, only 39 (33.6%) provided either a specific definition of a MSG or a definition of group that was applied to both single-species groups (SSGs) and MSGs. This trend was similar for the terms association (18 definitions, 19.0% of studies) and aggregation (8 definitions, 30.8% of studies), while only 3 (7.1%) studies that used the term school provided an explicit definition of this term (Figure 2). Furthermore, only five studies utilised some technique (e.g., analysis of interspecies association patterns or a minimum time limit) to confirm that potential MSGs were indeed MSGs and not simply chance encounters or aggregations.
Figure 2. Number of reviewed studies published between 1961 and 2020 that used several terms (i.e., group, association, school, and aggregation), with or without an explicit definition, to refer to situations where multiple species of cetaceans were observed in close spatial proximity. Other terms, including assemblage, encounter, and herd, are combined in the final bar. These terms were typically preceded by a variety of adjectives including mixed-species, interspecific, and heterospecific. The sum of the bars is greater than the total number of studies found by the review as over a third of the studies employed multiple terms.
The reviewed studies revealed that 54 species of cetaceans belonging to five families of Odontocetes (Delphinidae, Kogiidae, Phocoenidae, Physeteridae, and Ziphiidae) and three families of Mysticetes (Balaenidae, Balaenopteridae, and Eschrichtiidae) were reported to form groups with other cetacean species (see Supplementary Table 2 for full list). Of these species, 43 were Odontocetes and 11 were Mysticetes. The Odontocetes most commonly reported in MSGs belonged to the family Delphinidae, with 197 studies reporting participation in MSGs for almost all known species. The only species of the family Physeteridae, the sperm whale (Physeter macrocephalus), was also well represented with 25 studies reporting its occurrence in MSGs. In contrast, species of the remaining Odontocete families (i.e., Phocoenidae, Ziphiidae, and Kogiidae) were rarely reported in MSGs. Amongst the Mysticetes, the family Balaenopteridae accounted for most of the records (48 studies and 7 species), followed by the single Eschrichtiidae species—the grey whale (Eschrichtius robustus) (9 studies), and the family Balaenidae (6 studies and 3 species).
At the species level, the common bottlenose dolphin (Tursiops truncatus), the common dolphin (Delphinus delphis), and Risso’s dolphin (Grampus griseus) were the three most commonly reported cetacean species in MSGs (Figure 3). These same three species also had the greatest diversity of partner species in MSGs, with the common bottlenose dolphin associating with a total of 34 different species, the common dolphin with 27, and Risso’s dolphin with 22 (Figures 3, 4). Among Mysticetes, the humpback whale (Megaptera novaeangliae) was the most often reported species in MSGs and also the one with the highest diversity of partner species (Figure 3). Other delphinid species, along with several species of Balaenopteridae, made up the majority of the 33 cetacean species that were reported in MSGs by more than five studies, with only four species representing the remaining cetacean families: the sperm whale, Dall’s porpoise (Phocoenoides dalli), the grey whale, and Longman’s beaked whale (Indopacetus pacificus) (Figure 3).
Figure 3. The 33 species of cetaceans that were reported in mixed-species groups (MSGs) by five or more studies published between 1961 and 2020 that were obtained from a literature review on cetacean MSGs. The bar heights represent the number of studies reporting each species’ participation in MSGs as displayed on the y axis. The bar widths are proportional to the total number of species that each species has been observed with in MSGs, i.e., the number of partner species, which is written above each bar. The bars are coloured according to the species’ family.
Figure 4. Social network diagram showing the 47 species pairs (edges) that were reported together in mixed-species groups by five or more of the studies reviewed. The width of each edge is proportional to the total number of studies that reported that pair of species. The shape of each node indicates if the species is a Mysticete (diamond) or an Odontocete (circle) while the colours represent the species’ family. The size of each node is proportional to the total number of partner species that each species has. Each species’ node is placed approximately according to its average distribution with the x axis representing water depth and the y axis representing latitude. An interactive version of this network containing all 216 species pairs is available in Supplementary Figure 1.
The reported cetacean MSGs typically contained only two species, yet groups composed of up to four species were also observed (e.g., Ballance and Pitman, 1998; Kinzey et al., 1999; Anderson, 2005; Anderson et al., 2006; Weir, 2011; Bacon et al., 2017; Alves et al., 2018). We found records for 216 different species pairs observed within MSGs (Supplementary Figure 1 and Supplementary Table 2), 47 of which were recorded by 5 or more studies (Figure 4). Almost all species pairs (91.7%) were from different genera yet the majority belonged to the same family (56.9%) and suborder (i.e., Odontoceti or Mysticeti) (73.2%). In particular, the majority of species pairs in cetacean MSGs consisted of two delphinid species (50.9% of reported species pairs). Furthermore, of the 47 species pairs with five or more records, 35 (74.5%) comprised two delphinid species and all but two involved at least one delphinid (45 species pairs, 95.7%) (Figure 4). The most commonly reported species pairs in MSGs were: common dolphin—striped dolphin (Stenella coeruleoalba) (34 studies), Risso’s dolphin—common bottlenose dolphin (32 studies), and spinner dolphin (Stenella longirostris)—pantropical spotted dolphin (Stenella attenuata) (27 studies) (Figure 4). Many species pairs were found together much less frequently, with the majority (169 pairs) being reported by fewer than 5 studies. Marine mammal surveys across a variety of habitats and spatial scales typically gauged the proportion of cetacean groups that were mixed to be under 10% (Table 2). Dedicated studies of certain cetacean species that are known to form MSGs, on the other hand, reported higher frequencies, with MSGs accounting for up to a third of all groups sighted in some populations (Table 3; Frantzis and Herzing, 2002; Acevedo-Gutiérrez et al., 2005; Thompson, 2010; Kiszka et al., 2011).
Table 2. Cetacean mixed-species groups as a percentage of all groups (i.e., single- and mixed-species groups) that were observed during surveys for species belonging to the target taxa.
Table 3. Mixed-species groups (MSGs) involving delphinids recorded as a percentage of all groups (i.e., single- and mixed-species groups) of particular populations of the listed species as obtained through dedicated studies of MSGs.
Cetacean MSGs were observed from tropical to cold temperate waters in all the major ocean basins, except for the Southern Ocean (Figure 5). Furthermore, they were observed across a range of depths and at varying distances to shore, including: shallow coastal waters (<20 m; e.g., Acevedo-Gutiérrez et al., 2005; Hunt, 2018), over the continental shelf (20–200 m; e.g., Gowans and Whitehead, 1995; Mullin et al., 2004), around oceanic islands (e.g., Anderson, 2005; Quérouil et al., 2008; Gannier, 2009; Kiszka et al., 2011), and in the open ocean (<2,000 m; e.g., Scott and Cattanach, 1998; Jackson et al., 2008).
Figure 5. Geographical distribution of the location of the studies reviewed that reported cetacean mixed-species groups (MSGs) across the major ocean basins from 1961 to 2020. Numbers inside circles represent the number of reviewed studies reporting the occurrence of cetacean MSGs in each ocean basin and the number of cetacean species observed in those MSGs.
Of the 203 studies reviewed, 27 discussed potential functional explanations based on specific observations or investigations of cetacean MSGs (Table 4). These studies covered 25 species pairs of cetaceans, 7 of which had multiple proposed functional explanations. In total, 5 species pairs were hypothesised to form MSGs to reduce predation risk, 17 to improve foraging, and 12 to gain social benefits (Table 4). These hypotheses, rather than conclusions, rely on inferences drawn from behavioural observations and spatial variations in the distribution of SSGs and MSGs. Few studies (n = 5) determined that observed groupings were MSGs rather than mere chance encounters or aggregations and no study, to our knowledge, has directly tested whether participation in cetacean MSGs provides antipredator, foraging, or social benefits to group members. Nevertheless, the results provide an indication of the factors that may potentially drive cetacean MSG formation.
Table 4. Pairs of cetacean species observed in mixed-species groups (MSGs) for which the potential functional explanations have been proposed.
One of the most common functional explanations for the formation of MSGs is that participating individuals benefit from a reduced risk of predation (Whitesides, 1989; Stensland et al., 2003). The presence of heterospecifics with a greater ability to detect predators or better defensive capabilities can lead to the formation of MSGs (Whitesides, 1989; Heymann and Buchanan-Smith, 2000; Stensland et al., 2003; Kiszka et al., 2011; Cords and Würsig, 2014). In addition, an increase in group size as a result of forming a MSG can have similar effects and can also dilute the risk of predation on individual group members (Gygax, 2002b; Cords and Würsig, 2014; Goodale et al., 2017).
In the oceanic eastern tropical Pacific, pantropical spotted and spinner dolphins often form MSGs (e.g., Au and Perryman, 1985; Reilly, 1990; Scott and Cattanach, 1998; Oswald et al., 2008). Long-term observations show no evidence of foraging when in MSGs, likely due to interspecific differences in foraging behaviour, thus making foraging benefits an unlikely driver of these MSGs (Norris and Dohl, 1980; Scott and Cattanach, 1998). Instead, given the potential high risk of predation faced by these oceanic dolphins from pelagic sharks, killer whales (Orcinus orca) and other large delphinids (e.g., false killer whales, Pseudorca crassidens), it has been suggested that these species form MSGs to reduce predation risk (Scott and Cattanach, 1998). More specifically, spinner dolphins, which feed on the deep scattering layer at night and rest during the day (Norris and Dohl, 1980), may seek refuge amongst groups of pantropical spotted dolphins, which are active and more alert during the day, and thus benefit from their vigilance (Scott and Cattanach, 1998). These species also form MSGs around the island of Mayotte in the Indian Ocean (Gross et al., 2009; Kiszka et al., 2011). Here, the absence of feeding and social interactions between the two species does not support the foraging and social benefits hypotheses, respectively. Instead, these MSGs form when spinner dolphins shift habitat to deeper waters where pantropical spotted dolphins preferentially occur, suggesting that spinner dolphins initiate these MSGs, possibly to reduce predation risk while transiting between resting areas (Kiszka et al., 2011).
Foraging benefits gained from MSGs may take the form of an improved ability to detect, herd, and/or utilise food resources (Stensland et al., 2003) and may be obtained by mutual or non-mutual information exchange and coordinated foraging (Whitesides, 1989; Sridhar et al., 2009). Gatherings of different cetacean species at prey aggregations are fairly common and may also include seabirds, sharks, and large predatory fishes (Würsig and Würsig, 1979; Evans, 1982; Scott and Cattanach, 1998; Markowitz, 2004; Kiszka et al., 2015; Veit and Harrison, 2017). Although these gatherings involve the presence of different species in close spatial proximity, and so may be considered MSGs, it is not always clear if their formation is due to a mutual attraction to common prey or an attraction between species (Quérouil et al., 2008).
MSGs of common bottlenose dolphins and false killer whales have been observed in numerous locations (e.g., Scott and Chivers, 1990; Anderson, 2005; Maze-Foley and Mullin, 2006; Baird et al., 2008), including off the coast of New Zealand where they seem to engage in cooperative foraging (Zaeschmar et al., 2013). During foraging, both species feed on the same species of fishes after herding and driving them toward the surface (Zaeschmar et al., 2013). The apparent cooperative nature of the foraging suggests that these MSGs may provide mutualistic benefits. Nonetheless, the degree of cooperation is unknown and the possibility of social parasitism cannot be disregarded (Zaeschmar et al., 2014).
Common bottlenose dolphins, particularly the offshore ecotype, in tropical and warm temperate waters of the Pacific and Atlantic Oceans also regularly form MSGs with short-finned pilot whales (Globicephala macrorhynchus) (e.g., Scott and Chivers, 1990; Mangels and Gerrodette, 1994; Gannier, 2000; Weir, 2006) and with Risso’s dolphins (Grampus griseus) (e.g., Scott and Chivers, 1990; Bearzi, 2005b; Maze-Foley and Mullin, 2006; Weir, 2011; Bacon et al., 2017; Viana, 2019), particularly when the latter species are foraging (Norris and Prescott, 1961; Shane, 1994). The benefit here, however, may not be mutual. Common bottlenose dolphins tend to initiate the formation of MSGs and short-finned pilot whales sometimes display avoidance behaviour when common bottlenose dolphins approach. This suggests that the common bottlenose dolphins seek out the short-finned pilot whales and Risso’s dolphins to improve foraging success, although it is unknown if the other species benefit from these MSGs (Shane, 1994; Bacon et al., 2017). Common bottlenose dolphins have also been frequently observed with long-finned pilot whales (Globicephala melas) around New Zealand (Markowitz, 2004; Zaeschmar, 2014), in the North Atlantic (Gowans and Whitehead, 1995; Weir et al., 2001), and in the Mediterranean (Cañadas et al., 2002), possibly for similar reasons, although these MSGs have not been investigated in detail.
Off the coast of New Zealand, Bryde’s whales (Balaenoptera edeni) often follow feeding common dolphin groups and it has been hypothesised that the Bryde’s whales benefit from the common dolphins’ ability to herd and concentrate epipelagic fish schools (O’Callaghan and Baker, 2002; Burgess, 2006; Stockin et al., 2009). Similarly, in Norway, humpback whales (Megaptera novaeangliae) lunge feed on herring schools that are herded by killer whales (Jourdain and Vongraven, 2017). It is hypothesised that in this case, humpback whales benefit from the foraging effort of killer whales who may, in turn, be negatively affected by the interspecific competition for prey (Burgess, 2006; Jourdain and Vongraven, 2017).
Cetaceans, particularly delphinids, are highly social animals with often complex social structures (Mann et al., 2000; Gowans et al., 2007). Accordingly, there are multiple contrasting social motives that have been hypothesised to lead to both agonistic and affiliative social MSGs involving aggressive, sexual, playful, and caring behaviours (e.g., Herzing and Johnson, 1997; Stensland et al., 2003; Acevedo-Gutiérrez et al., 2005; Parra, 2005; Herzing and Elliser, 2013).
Common bottlenose and Atlantic spotted dolphins (Stenella frontalis) in the Bahamas are often observed in MSGs, with members engaging in socio-sexual behaviours (Herzing and Johnson, 1997; Melillo et al., 2009; Herzing and Elliser, 2013). These sexual interactions are typically initiated by common bottlenose dolphins, mostly subadults, who may seek copulations with Atlantic spotted dolphins as they are unable to copulate with conspecifics due to their lower intraspecific social status (Melillo et al., 2009). Alternatively, these dolphins may use sexual behaviour to reduce levels of aggression by replacing aggressive interactions with sexual ones (Melillo et al., 2009). Furthermore, male Atlantic spotted and male common bottlenose dolphins have also been observed forming interspecific coalitions (defined as “the joining of forces by two or more parties during a conflict of interest with other parties”: de Waal and Harcourt, 1992; Herzing and Johnson, 1997), to both chase away other males and pursue females of both species, although subsequent copulation is only intraspecific (Herzing and Johnson, 1997). Additionally, males of these species often engage in sexual interactions with each other (Herzing and Johnson, 1997; Herzing and Elliser, 2013). In these cases, sexual-aggressive behaviours between males and shared pursuits of females may form bonds that later provide a benefit when they form interspecific coalitions during aggressive encounters (Herzing and Johnson, 1997).
Aggressive and sexual behaviours are also typical of MSGs that involve common bottlenose dolphins and Guiana dolphins (Sotalia guianensis) along Costa Rica’s Caribbean coast (Acevedo-Gutiérrez et al., 2005; May-Collado, 2010). Male common bottlenose dolphins exhibit aggressive behaviours toward Guiana dolphins, such as biting, body slamming, and chasing, seemingly in order to separate female Guiana dolphins from their conspecifics to mate with them (May-Collado, 2010). These observations are supported by photographic evidence and sightings of putative hybrids, although genetic confirmation of hybridisation is required (Acevedo-Gutiérrez et al., 2005). Common and Indo-Pacific (Tursiops aduncus) bottlenose dolphins have been observed exhibiting aggressive-sexual behaviours toward Australian (Sousa sahulensis) and Indian Ocean humpback dolphins (Sousa plumbea) (Saayman et al., 1972; Baldwin et al., 2004; Minton et al., 2010; Ansmann, 2011; Cerchio et al., 2015). Off the coast of Zanzibar, young male Indo-Pacific bottlenose dolphins harass female Indian Ocean humpback dolphins, possibly as a means of practising and developing skills involved in social behaviours in order to increase their social status (Stensland et al., 2003).
Similar interactions occur in northern Australia between Australian humpback dolphins and Australian snubfin dolphins (Orcaella heinsohni). In Cleveland Bay, North Queensland, Australian humpback and Australian snubfin dolphins live in sympatry, have overlapping ranges and exhibit interspecies affiliative and aggressive interactions (Parra, 2005, 2006). Aggressive interactions are more frequently observed and are mainly initiated by adult male Australian humpback dolphins, who pursue and seek physical contact with adult female Australian snubfin dolphins. The female Australian snubfin dolphins, often accompanied by calves, attempt to avoid these interactions and flee (Parra, 2005). It is hypothesised that male Australian humpback dolphins may use these interactions as opportunities for physical training or skill development, a function that would have beneficial effects for interactions with female conspecifics (Parra, 2005). This is similar to what has been suggested for incidences of male common bottlenose dolphins attacking and killing harbour porpoises (Phocoena phocoena) in Scotland and California (Patterson et al., 1998; Cotter et al., 2012).
Affiliative behaviours, including interspecific alloparenting are also occasionally recorded. Herzing and Johnson (1997), for example, reported two cases from the Bahamas of adult female Atlantic spotted dolphins swimming with common bottlenose dolphin calves. In New Zealand, Markowitz (2004) observed a short-term association of a calf common dolphin and an adult dusky dolphin (Lagenorhynchus obscurus) while Stensland et al. (2003) refer to an observation of an Indian Ocean humpback dolphin calf that travelled for several hours with a large group of Indo-Pacific bottlenose dolphin mothers and calves in Zanzibar. It is not known, however, whether these social behaviours are isolated events or if they represent broader patterns of interspecific behaviour.
Mixed-species social behaviours may also be driven by a lack of conspecifics. In the Mediterranean Sea, common dolphins regularly form MSGs with striped dolphins (e.g., Forcada et al., 1994; Cañadas and Hammond, 2008; Bearzi et al., 2011; Santoro et al., 2015; Santostasi et al., 2016) and, on occasion, Risso’s dolphins (e.g., Cañadas et al., 2002; Frantzis and Herzing, 2002; Bearzi et al., 2016) and common bottlenose dolphins (e.g., Ryan et al., 2014; Pace et al., 2015; Espada et al., 2019). Common dolphin populations in the Mediterranean Sea have declined dramatically since the 1980s (Bearzi et al., 2003) and, in areas where they are now uncommon, the frequency of MSGs is higher (Frantzis and Herzing, 2002). It has, therefore, been suggested that the lack of interactions with conspecifics might be an important driver of the formation of MSGs in this region. Interactions between common dolphins and other species appear to be mainly socially driven (García et al., 2000; Frantzis and Herzing, 2002) and there are numerous records of hybrid individuals between common and striped dolphins (Bearzi et al., 2011; Santostasi et al., 2016; Antoniou et al., 2018; Bonizzoni et al., 2019) and between common and common bottlenose dolphins (Espada et al., 2019).
Many species of cetaceans in a variety of habitats have been reported to form MSGs, potentially due to the evolutionary benefits (antipredator, foraging, and social) they may gain. The studies on cetacean MSGs reviewed here often used terminology inconsistently and most did not confirm that observed sets of individuals did indeed form MSGs, rather than mere chance encounters or aggregations. Thus, we cannot be certain that all the records in this review truly represent MSGs, however, because they are potentially MSGs, they were included and treated as such. Furthermore, many studies lacked the thorough testing of hypotheses that is required to determine the potential functional explanations of the observed MSGs.
To better understand the incidence and ecological role of cetacean MSGs, we need to go beyond descriptive accounts and investigate the behavioural and ecological drivers of their formation (Stensland et al., 2003). We reviewed the literature on cetacean MSGs to: address inconsistencies in terminology; assess their characteristics (e.g., species involved, location, frequency); and evaluate what is known about their functional role. Finally, we discuss the results of this review and propose a standardised terminology and a conceptual framework to assist future research (Figure 6).
Figure 6. A conceptual framework for studying cetacean mixed-species groups (MSGs). The framework details the steps required to determine that the species form a MSG (Boxes 1b, 1d, and 1f) and to establish the potential function(s) of the MSG (Boxes 2a and 2f).
Amongst the cetacean species that have been reported in MSGs, delphinids are the most frequently involved and the ones with the most diversity of partner species. The dynamic and fluid social structure of many delphinid species could potentially facilitate the formation of MSGs (Stensland et al., 2003) as could a higher risk of predation when compared to the larger cetaceans, such as the baleen whales. Cetacean species with broad distributions centred on the sub-tropics and the warm temperate zone, such as the common bottlenose dolphin, also appear to be disproportionately represented, with more records of participation in MSGs and more partner species. This is possibly a result of their abundance and widespread distribution bringing them often into contact with a range of other cetacean species. These species are also, however, amongst the most studied (Wells and Scott, 2009), while many of those that are rarely, or never, reported in MSGs, such as the beaked whales, are poorly studied (MacLeod, 2018). Consequently, these results may not reflect the true composition and diversity of cetacean MSGs and may be influenced by the greater research effort dedicated to certain species.
Most of the 216 species pairs that were reported in MSGs were composed of two delphinids, however, there was considerable variation in the relatedness, morphology, and behaviour of partner species. Some MSGs were composed of pairs of closely related and morphologically similar species, such as pantropical spotted and spinner dolphins (e.g., Scott and Cattanach, 1998; Kiszka et al., 2011), while others consisted of distant and dissimilar species, such as common dolphins and Bryde’s whales (e.g., Burgess, 2006; Stockin et al., 2009; Penry et al., 2011). Furthermore, some species pairs were frequently reported together (e.g., common dolphin—striped dolphin and spinner dolphin—pantropical spotted dolphin) (Table 3), while others (e.g., common bottlenose dolphin—dusky dolphin) have been rarely, if ever, observed together in MSGs (Würsig and Würsig, 1979; Markowitz, 2004). Most cetacean species pairs belonged to different genera but the same family and suborder, suggesting that a moderate level of dissimilarity between cetacean species is favourable to MSG formation. This is in accordance with research on other taxa, including primates and birds, where it has been shown that optimum levels of dissimilarity in characteristics such as diet, habitat use, and body size increase the frequency of MSG occurrence (Heymann and Buchanan-Smith, 2000; Sridhar et al., 2009; Heymann and Hsia, 2015; Sridhar and Guttal, 2018). Currently, however, it is not well understood which are the biological factors that determine if and how often cetacean species form MSGs, but future analysis of the similarities and dissimilarities in corresponding characteristics between pairs of cetacean species could provide insight into this question.
It is apparent that MSGs are formed by both inshore and offshore species of cetaceans in a variety of habitats, however, the lack of available information regarding the distribution and grouping dynamics of cetacean MSGs makes it challenging to establish any detailed patterns. We can, nonetheless, observe several potential trends pertaining to the influence of environmental factors. Water depth and distance to shore appear to affect MSGs in the same way they do SSGs (Wells et al., 1980), with coastal species often forming small groups of 5–20 individuals (e.g., Herzing and Johnson, 1997; Acevedo-Gutiérrez et al., 2005; Thompson, 2010) and oceanic species forming large groups of hundreds and even thousands (e.g., Hill and Barlow, 1992; Wade and Gerrodette, 1993; Scott and Cattanach, 1998; Appler et al., 2004; Dulau-Drouot et al., 2008). For some species, such as the common bottlenose dolphin, MSGs have been shown to be more common in oceanic waters (Scott and Chivers, 1990). Potentially higher predation risk offshore may drive certain species to form larger groups (Gygax, 2002a) including MSGs when faced with a low abundance of conspecifics. However, some species that inhabit shallow coastal waters also frequently form MSGs (e.g., Herzing and Johnson, 1997; Acevedo-Gutiérrez et al., 2005; Thompson, 2010), so the drivers of MSG formation likely vary across taxa and habitats. Cetacean MSGs were most commonly reported in the North Pacific and North Atlantic, potentially due to the higher species diversity within those areas (Kaschner et al., 2011; Pompa et al., 2011), although this result may also be influenced by uneven research effort (Kaschner et al., 2012).
To facilitate future studies, we use the results of this review to propose a standardised terminology and a conceptual framework that (1) defines and characterises cetacean MSGs (Figure 6, Boxes 1a-1i) and (2) details how to investigate their functions by testing relevant hypotheses (Figure 6, Boxes 2a-2g).
After evaluating the terms and definitions used in the reviewed studies and the underlying processes involved in the formation of MSGs, we propose to expand on the definition provided by Stensland et al. (2003) (Table 1). This definition considered a MSG to be a set of individuals of two or more species that are seen in such close association that they can be regarded as members of the same group.
Firstly, the application of this definition to MSGs requires an explicit definition of group that contains rules that can be applied in field research settings (Figure 6, Box 1d). Definitions of group should be biologically meaningful and consistent across species and studies (Krause and Ruxton, 2002; Whitehead, 2008). However, what defines a cetacean group remains a contentious and unresolved issue (Connor et al., 1998, 2000; Gibson and Mann, 2009). The spatial proximity of individuals is the most often used criterion for determining group membership along with behaviour and directionality, although there is considerable variation in how each of these criteria is applied (Whitehead, 2008; Gibson and Mann, 2009). In the studies obtained by this literature review the threshold distances for delimiting group membership ranged from 10 to 1,000 m. In the broader cetacean literature, commonly used definitions are equally varied and include: a 10 m chain rule (Smolker et al., 1992); a 100 m fixed point rule (Irvine et al., 1981); and individuals “in apparent association, moving in the same direction and often, but not always, engaged in the same activity” (Shane, 1990). The inconsistency in definitions of group causes confusion and weakens comparisons between studies, yet, as it stands, there is no clear solution to this problem. Dedicated work on this subject is needed to, firstly, determine how groups are defined in the cetacean literature and to, secondly, formulate biologically meaningful definitions via quantitative analyses of parameters such as inter-individual distances or the coordination between individuals (Krause and Ruxton, 2002; Croft et al., 2008; Whitehead, 2008). Until such work is done, we recommend that studies of cetacean MSGs explicitly state the definition used and any justification for their choice.
Secondly, we emphasise that, as a type of group, MSGs provide evolutionary benefits to at least some participants and are consequently formed and maintained by a mutual or unreciprocated attraction between individuals. Therefore, they should be distinguished from chance encounters that occur at random and aggregations of individuals that are attracted toward a common resource or that respond to the same environmental stimuli (Waser, 1982; Powell, 1985; Whitesides, 1989; Goodale et al., 2017; Figure 6, Box 1f). Null models can be used to assess whether sightings of multiple species in close proximity correspond to non-random patterns. For example, gas models and computer simulations recreate the movement of individuals in their environment and can be used to test whether encounter rates and durations occur at random or not (Figure 6, Box 1f; Waser, 1982; Whitesides, 1989; Hutchinson and Waser, 2007). Such approaches are all but absent from studies of cetaceans, likely because they require input data regarding the travel speed and diameter of groups that may be difficult to acquire (Cords and Würsig, 2014). Nevertheless, these data could be obtained through dedicated studies that incorporate group focal follows and technologies that facilitate their acquisition such as unmanned aerial vehicles (UAVs), satellite tracking, and theodolite observations.
Analyses of individual association patterns can be conducted on multiple species, provided that individuals of each species can be feasibly identified (Farine et al., 2012; Zaeschmar et al., 2014; Elliser and Herzing, 2016b). Null models can be used to analyse these networks in order to determine if individuals display non-random patterns of association and, therefore, are not found together by chance (Whitehead, 2008; Farine, 2017). Additionally, by accounting for alternate factors that may bring individuals together, such as spatial overlap or shared resource use, it is possible to determine the influence that social preference (i.e., attraction and avoidance) has on observed patterns of association (Whitehead, 2008; Farine, 2017). This has been done for single-species social networks and could be done on a mixed-species basis (Frère et al., 2010; Farine et al., 2012; Strickland et al., 2017; Zanardo et al., 2018; Hunt et al., 2019; Diaz-Aguirre et al., 2020). In this case, evidence of strong and/or preferential associations between individuals of different species after alternate factors are taken into account would be indicative of attraction between individuals, suggesting that the species form groups and not aggregations (Figure 6, Box 1f).
Alternative modelling approaches include occupancy modelling based on presence/absence data, which can be used to analyse species co-occurrence patterns to determine if there is avoidance or attraction between co-occurring species (Richmond et al., 2010; MacKenzie et al., 2017). Where such modelling is not practical, alternative criteria can be used. For example, situations where species are observed together for less than a minimum time limit can be considered to have occurred by chance (Kiszka et al., 2011; Jourdain and Vongraven, 2017). Such time limits may be arbitrary, but, alternatively, could be based on a comparison of the duration of single-species groups (SSGs) and MSGs.
Thirdly, although the overall attraction amongst participants in a MSG may be mutual or unreciprocated, the presence of each species must be tolerated by the other (Stensland et al., 2003). Consequently, there are several interspecific relationships that we do not consider to fall within the scope of MSGs because they exclusively involve agonistic interactions where species do not congregate for the purpose of group formation (Figure 6, Box 1e). More specifically, we exclude predator-prey relationships, competition-based relationships (e.g., Shane, 1995), and incidences where heterospecifics are used as “objects” in object-oriented play (e.g., Baird, 1998; Patterson et al., 1998; Cotter et al., 2012). MSGs that, on occasion, involve agonistic behaviours, but that also involve affiliative and neutral behaviours (e.g., Herzing and Johnson, 1997; Acevedo-Gutiérrez et al., 2005) are not excluded. Finally, we recommend the use of the term mixed-species group rather than other terms such as interspecific, polyspecific, or heterospecific group or association, as it was the most commonly employed in the studies that we reviewed and is also widely applied in the study of other taxa (Stensland et al., 2003; Goodale et al., 2017).
In summary, we define a MSG as individuals of two or more species found in close spatial proximity due to a mutual or unreciprocated attraction derived from evolutionary grouping benefits. MSGs may involve affiliative, neutral, and agonistic behaviours excluding instances of predation, competition, and heterospecific “object” play.
Once it has been established that the occurrence of different species of cetaceans in close proximity does represent a MSG, and not a chance encounter nor an aggregation, the next step should be to investigate what drives species to group (Figure 6, Box 2a). Throughout any investigation, all three functional explanations (Figure 6, Boxes 2b-2d) should ideally be considered for each species involved as the functional explanations are not mutually exclusive and each species will not necessarily obtain the same benefits and costs (Stensland et al., 2003; Goodale et al., 2017). Furthermore, it is best to independently consider and compare MSGs to SSGs of each species because the differences between them will demonstrate how participation in MSGs affects each species’ biology, in turn revealing what drives them to form MSGs (Sridhar and Guttal, 2018).
To investigate the function of cetacean MSGs, one needs to identify which benefits each species may obtain by analysing ecological, behavioural, and group characteristic data that are relevant to each hypothesis being tested. This should begin with reviewing the existing knowledge of each species’ ecology and grouping dynamics through the perspective of the theory on MSG formation to evaluate which functional explanations are more probable. Data should then be obtained directly from the study populations, including species distributions and abundance, group characteristics (e.g., size, composition, and cohesiveness), encounter rates, and behaviour (e.g., behavioural states, events, and transitions) of both SSGs and MSGs. These data should be combined with relevant environmental (e.g., depth, habitat, and distance to shore) (Scott and Chivers, 1990; Kiszka et al., 2011), food availability (e.g., distribution, abundance, and prey biomass), predation risk (e.g., predator distribution and abundance), and temporal data (e.g., time of day, season, and group duration). This will enable the identification of important factors for MSG formation and can be indicative of the functional explanations for MSGs formation, as detailed in the following sections.
Data on cetacean distribution, abundance, grouping dynamics, and behaviour may be obtained by dedicated boat- and land-based surveys with appropriate sampling protocols and, potentially, the integration of new technologies such as UAVs, acoustic recording, and biologging (Nowacek et al., 2016; Andrews et al., 2019). These technologies provide great potential to acquire data that are relevant to investigations of potential functional explanations but remain underutilised in the study of cetacean MSGs. For example, radio-tags have been used to record the location and diving patterns of dolphins and the tuna that associate with them to track and compare the movements and behaviours of the different species (Scott et al., 2012). Innovative approaches and new technologies, such as those listed above (Nowacek et al., 2016), may also allow the direct measurement of parameters that are indicative of any benefits (e.g., predation attempts and successes, prey capture success rate, food intake rate, reproductive success, and social standing). This would make it possible to confirm that a benefit is gained by determining if these parameters are affected by MSG participation. Here, we discuss several analytical approaches and present potential results which lend support to each of the three principal hypotheses for MSG formation. Each of these approaches presents its own unique challenges that may make them practically and financially unfeasible in certain situations. Therefore, we provide a range of suggestions and entrust to researchers the decision of which approaches are most suitable to be implemented in their studies.
Individuals are more likely to form MSGs for antipredator benefits (Figure 6, Box 2b) when the perceived risk of predation is high and when other avoidance tactics cannot be used (e.g., use of safer habitats, formation of large SSGs). Increased MSG size can be indicative of increased group vigilance and, therefore, decreased predation risk for individuals involved in MSGs (Herzing and Johnson, 1997; Scott and Cattanach, 1998; Gygax, 2002b; Majolo et al., 2008; Melillo et al., 2009; Kiszka et al., 2011). A shift to habitat with a higher perceived predation risk when in MSGs compared to SSGs also supports the antipredator benefits hypothesis, as does increased travelling and resting behaviours (Kiszka et al., 2011). Additionally, a predation risk landscape, based on the distribution of predators or bite scars on each species, could be employed to determine how predation risk, potentially combined with environmental factors, influences the prevalence and characteristics of MSGs. Playback experiments and biologging have been employed to record the reactions of SSGs of cetaceans to the simulated presence of potential predators (Curé et al., 2012, 2019; Bowers et al., 2018) and similar experiments could be undertaken to determine if simulated predator presence increases the propensity of species to form MSGs, as has been done for primates (Noë and Bshary, 1997). Finally, and although it is undoubtedly a logistical challenge, the level of predation risk would ideally be compared between SSGs and MSGs by recording failed and successful predation attempts or by analysing individual vigilance rates as a measure of perceived predation risk (Wolters and Zuberbühler, 2003; Sridhar et al., 2009; Stojan-Dolar and Heymann, 2010).
Co-occurring species may form MSGs in order to gain potential foraging benefits (Figure 6, Box 2c; Smultea et al., 2014; Sridhar and Guttal, 2018). A high or increased prevalence of feeding when in MSGs argues in favour of this hypothesis (Quérouil et al., 2008; Zaeschmar et al., 2014; Bacon et al., 2017), as do observations of species foraging together or following foraging heterospecifics (Shane, 1994; Burgess, 2006; Zaeschmar et al., 2013; Smultea et al., 2014; Bacon et al., 2017; Jourdain and Vongraven, 2017). Evidence, from playback experiments incorporating biologging or UAVs, that a species is attracted to vocalisations of foraging heterospecifics could also be indicative of a foraging benefit (Suzuki and Kutsukake, 2017). Rates of prey capture success and energy intake have been used in studies of primates and birds to determine whether the presence of heterospecifics increases feeding success (Peres, 1992; Sridhar et al., 2009). The use of underwater video of feeding events, potentially recorded with a camera integrated into a biologger (Pearson et al., 2019; Linsky et al., 2020), could allow this, although the difficulties and costs would be considerable. For species that are assumed to improve foraging when in MSGs, one could record and compare the time that individuals spend searching for food when in SSGs and MSGs to see if search time is reduced when heterospecifics are present.
Species that may gain social benefits (Figure 6, Box 2d) typically exhibit high or increased levels of social activity when in MSGs compared to when they are in SSGs (Herzing and Johnson, 1997; García et al., 2000; Acevedo-Gutiérrez et al., 2005; Melillo et al., 2009), while a lack of social interactions argues against this hypothesis (Quérouil et al., 2008; Kiszka et al., 2011). Additionally, the presence of aggressive (e.g., tail slaps and open-mouth postures), sexual (e.g., erections, mating, and presence of hybrids), and affiliative behaviours (e.g., alloparental care, play, and non-aggressive body contact) provides evidence of social benefits and may also assist in unravelling the complexity of social interactions by providing insight into their nature (Herzing and Johnson, 1997; Acevedo-Gutiérrez et al., 2005; Parra, 2005; Melillo et al., 2009). Increased group size of MSGs can also be related to social behaviour (Acevedo-Gutiérrez et al., 2005) while a preferential attraction to the vocalisations of socialising heterospecifics (demonstrated with playback experiments) would be expected from species that form MSGs to obtain social benefits. Social advantages are more difficult to quantify and measure (Stensland et al., 2003), however, from a long-term study, it may be possible to record and compare the mating and reproductive success or the social status of individuals with regard to how often they are observed interacting with heterospecifics.
Our findings show that cetacean MSGs are potentially diverse and complex in various aspects: the species involved, the habitats where they occur, their frequency, and their ecological functions. There are, however, two main impediments to the advancement of our understanding of cetacean MSGs: (1) inconsistent terminology and the lack of approaches to distinguish them from mere aggregations and chance encounters and (2) the lack of studies designed to investigate their dynamics and function. We believe that our proposed terminology and conceptual framework can aid in overcoming these impediments by serving as a guide for future studies of cetacean MSGs. Thus, we strongly encourage our colleagues to employ this framework and to improve upon it as new information and technological developments become available. Research on MSGs of terrestrial species has, so far, led the way by developing the theoretical basis for MSG formation, by detailing ideal approaches for their investigation, and by revealing the broader influence that MSGs can have on the behaviour and ecology of the species involved (Stensland et al., 2003; Sridhar et al., 2009; Goodale et al., 2020). By conducting detailed and structured investigations of cetacean MSGs, we will likewise be able to further unravel their ecological functions and improve our understanding of the role that they play in community ecology.
JS conducted the literature review and data analysis with advice from GP and JK. JS wrote the manuscript with contributions to drafting, critical review, and editorial input from GP and JK. All authors conceived and designed the study.
JS was the recipient of a Flinders University Postgraduate Scholarship while conducting this work.
The authors declare that the research was conducted in the absence of any commercial or financial relationships that could be construed as a potential conflict of interest.
We would like to thank the reviewers for their comments which helped to improve this work and Flinders University for providing funding. This is contribution #274 of the Coastal and Oceans Division within the Institute of Environment at Florida International University.
The Supplementary Material for this article can be found online at: https://www.frontiersin.org/articles/10.3389/fmars.2021.678173/full#supplementary-material
Acevedo-Gutiérrez, A., DiBerardinis, A., Larkin, S., Larkin, K., and Forestell, P. (2005). Social interactions between tucuxis and bottlenose dolphins in Gandoca-Manzanillo, Costa Rica. Lat. Am. J. Aquat. Mamm. 4, 49–54. doi: 10.5597/lajam00069
Alves, F., Ferreira, R., Fernandes, M., Halicka, Z., Dias, L., and Dinis, A. (2018). Analysis of occurrence patterns and biological factors of cetaceans based on long-term and fine-scale data from platforms of opportunity: Madeira Island as a case study. Mar. Ecol. 39:e12499. doi: 10.1111/maec.12499
Anderson, R. C. (2005). Observations of cetaceans in the Maldives, 1990-2002. J. Cetacean Res. Manag. 7, 119–135.
Anderson, R. C., Clark, R., Madsen, P. T., Johnson, C., Kiszka, J., and Breysse, O. (2006). Observations of Longman’s beaked whale (Indopacetus pacificus) in the Western Indian Ocean. Aquat. Mamm. 32, 223–231. doi: 10.1578/am.32.2.2006.223
Andrews, R. D., Baird, R. W., Calambokidis, J., Goertz, C. E. C., Gulland, F. M. D., Heide-Jørgensen, M. P., et al. (2019). Best practice guidelines for cetacean tagging. J. Cetacean Res. Manag. 20, 27–66. doi: 10.47536/JCRM.V20I1.237
Ansmann, I. C. (2011). Fine-Scale Population Structure of Indo-Pacific Bottlenose Dolphins, Tursiops aduncus, in Moreton Bay, Queensland, Australia. Doctor of Philosophy. Australia: The University of Queensland.
Antoniou, A., Frantzis, A., Alexiadou, P., Paschou, N., and Poulakakis, N. (2018). Evidence of introgressive hybridization between Stenella coeruleoalba and Delphinus delphis in the Greek Seas. Mol. Phylogenet. Evol. 129, 325–337. doi: 10.1016/j.ympev.2018.09.007
Appler, J., Barlow, J., and Rankin, S. (2004). Marine mammal data collected during the Oregon, California, and Washington Line-Transect Expedition (ORCAWALE) conducted aboard the NOAA ships McArthur and David Starr Jordan, July - December 2001. La Jolla, CA: National Oceanic and Atmospheric Administration.
Au, D., and Perryman, W. (1985). Dolphin habitats in the eastern Tropical Pacific. Fish. Bull. 83, 623–644.
Bacon, C. E., Smultea, M. A., Fertl, D., Würsig, B., Burgess, E. A., and Hawks-Johnson, S. (2017). Mixed-species associations of marine mammals in the Southern California bight, with emphasis on Risso’s dolphins (Grampus griseus). Aquat. Mamm. 43, 177–184. doi: 10.1578/AM.43.2.2017.177
Baird, R. W. (1998). An interaction between Pacific white-sided dolphins and a neonatal harbor porpoise. Mammalia 62, 129–134.
Baird, R. W., Gorgone, A. M., McSweeney, D. J., Webster, D. L., Salden, D. R., Deakos, M. H., et al. (2008). False killer whales (Pseudorca crassidens) around the main Hawaiian Islands: Long-term site fidelity, inter-island movements, and association patterns. Mar. Mamm. Sci. 24, 591–612. doi: 10.1111/j.1748-7692.2008.00200.x
Baldwin, R. M., Collins, M., Van Waerebeek, K., and Minton, G. (2004). The Indo-Pacific Humpback Dolphin of the Arabian Region: A Status Review. Aquat. Mamm. 30, 111–124. doi: 10.1578/am.30.1.2004.111
Ballance, L. T., and Pitman, R. L. (1998). Cetaceans of the western tropical Indian Ocean: Distribution, relative abundance, and comparisons with cetacean communities of two other tropical ecosystems. Mar. Mamm. Sci. 14, 429–459. doi: 10.1111/j.1748-7692.1998.tb00736.x
Bearzi, G., Bonizzoni, S., Agazzi, S., Gonzalvo, J., and Currey, R. J. C. (2011). Striped dolphins and short-beaked common dolphins in the Gulf of Corinth, Greece: Abundance estimates from dorsal fin photographs. Mar. Mamm. Sci. 27, E165-E184. doi: 10.1111/j.1748-7692.2010.00448.x
Bearzi, G., Bonizzoni, S., Santostasi, N. L., Furey, N. B., Eddy, L., Valavanis, V. D., et al. (2016). Dolphins in a Scaled-Down Mediterranean: The Gulf of Corinth’s Odontocetes. Adv. Mar. Biol. 75, 297–331. doi: 10.1016/bs.amb.2016.07.003
Bearzi, G., Reeves, R. R., Notarbartolo-Di-Sciara, G., Politi, E., Canadas, A., Frantzis, A., et al. (2003). Ecology, status and conservation of short-beaked common dolphins Delphinus delphis in the Mediterranean Sea. Mamm. Rev. 33, 224–252. doi: 10.1046/j.1365-2907.2003.00032.x
Bearzi, M. (2005a). Aspects of the ecology and behaviour of bottlenose dolphins (Tursiops truncatus) in Santa Monica Bay, California. J. Cetacean Res. Manag. 7, 75–83.
Bearzi, M. (2005b). Dolphin sympatric ecology. Mar. Biol. Res. 1, 165–175. doi: 10.1080/17451000510019132
Bearzi, M. (2006). California sea lions use dolphins to locate food. J. Mamm. 87, 606–617. doi: 10.1644/04-MAMM-A-115R4.1
Bearzi, M., and Saylan, C. A. (2011). Cetacean ecology for Santa Monica Bay and nearby areas, California, in the context of the newly established MPAs. Bull. South. Calif. Acad. Sci. 110, 35–51. doi: 10.3160/10-12.1
Black, N. A. (1994). Behavior and ecology of Pacific white-sided dolphins (Lagenorhynchus obliquidens) in Monterey Bay, California. Master of Science. California: San Francisco State University.
Bonizzoni, S., Furey, N. B., Santostasi, N. L., Eddy, L., Valavanis, V. D., and Bearzi, G. (2019). Modelling dolphin distribution within an important marine mammal area in Greece to support spatial management planning. Aquat. Conserv. Mar. Freshw. Ecosyst. 29, 1665–1680. doi: 10.1002/aqc.3148
Bowers, M. T., Friedlaender, A. S., Janik, V. M., Nowacek, D. P., Quick, N. J., Southall, B. L., et al. (2018). Selective reactions to different killer whale call categories in two delphinid species. J. Exp. Biol. 221, 1–12. doi: 10.1242/jeb.162479
Burgess, E. A. (2006). Foraging Ecology of Common Dolphins (Delphinus sp.) in the Hauraki Gulf, New Zealand. Master of Science. Auckland: Massey University.
Cañadas, A., and Hammond, P. S. (2008). Abundance and habitat preferences of the short-beaked common dolphin Delphinus delphis in the southwestern Mediterranean: Implications for conservation. Endanger. Species Res. 4, 309–331. doi: 10.3354/esr00073
Cañadas, A., Sagarminaga, R., and García-Tiscar, S. (2002). Cetacean distribution related with depth and slope in the Mediterranean waters off southern Spain. Deep. Res. Part I Oceanogr. Res. Pap. 49, 2053–2073. doi: 10.1016/S0967-0637(02)00123-1
Cerchio, S., Andrianarivelo, N., and Andrianantenaina, B. (2015). “Ecology and conservation status of indian ocean humpback dolphins (Sousa plumbea) in Madagascar,” in Advances in Marine Biology, eds T. A. Jefferson and B. E. Curry (San Diego, California: Academic Press), 163–199. doi: 10.1016/bs.amb.2015.09.004
Committee on Taxonomy (2020). List of marine mammal species and subspecies. Available Online at: https://marinemammalscience.org/science-and-publications/list-marine-mammal-species-subspecies/ [Accessed December 11, 2020].
Connor, R. C., Mann, J., Tyack, P. L., and Whitehead, H. (1998). Social evolution in toothed whales. Trends Ecol. Evol. 13, 228–232. doi: 10.1016/S0169-5347(98)01326-3
Connor, R., Wells, R., Mann, J., and Read, A. (2000). “The bottlenose dolphin: Social relationships in a fission-fusion society” in Cetacean Societies: Field Studies of Whales and Dolphins, eds J. Mann, R. C. Connor, P. L. Tyack, and H. Whitehead (Chicago, IL: The University of Chicago Press), 91–126.
Cords, M., and Würsig, B. (2014). “A Mix of species: associations of heterospecifics among primates and dolphins,” in Primates and Cetaceans: Field Research and Conservation of Complex Mammalian Societies, eds J. Yamagiwa and L. Karczmarski (Tokyo: Springer Japan), 409–431. doi: 10.1007/978-4-431-54523-1_21
Corkeron, P. J. (1990). “Aspects of the behavioral ecology of inshore dolphins Tursiops truncatus and Sousa chinensis in Moreton Bay, Australia,” in The Bottlenose Dolphin, eds S. Leatherwood and R. R. Reeves (San Diego, California: Academic Press, Inc.), 285–293. doi: 10.1016/b978-0-12-440280-5.50018-4
Cotter, M. P., Maldini, D., and Jefferson, T. A. (2012). “Porpicide” in California: killing of harbor porpoises (Phocoena phocoena) by coastal bottlenose dolphins (Tursiops truncatus). Mar. Mamm. Sci. 28, E1–E15. doi: 10.1111/j.1748-7692.2011.00474.x
Croft, D. P., James, R., and Krause, J. (2008). Exploring Animal Social Networks. Princeton: Princeton University Press.
Curé, C., Antunes, R., Samarra, F., Alves, A. C., Visser, F., Kvadsheim, P. H., et al. (2012). Pilot whales attracted to killer whale sounds: acoustically-mediated interspecific interactions in cetaceans. PLoS One 7:e52201. doi: 10.1371/journal.pone.0052201
Curé, C., Isojunno, S. I. Vester, H., Visser, F., Oudejans, M., Biassoni, N., et al. (2019). Evidence for discrimination between feeding sounds of familiar fish and unfamiliar mammal-eating killer whale ecotypes by long-finned pilot whales. Anim. Cogn. 22, 863–882. doi: 10.1007/s10071-019-01282-1
de Waal, F. B. M., and Harcourt, A. H. (1992). Coalitions and Alliances in Humans and Other Animals. eds A. H. Harcourt and F. B. M. de Waal Oxford: Oxford University Press.
Di Tullio, J. C., Gandra, T. B. R., Zerbini, A. N., and Secchi, E. R. (2016). Diversity and distribution patterns of cetaceans in the subtropical Southwestern Atlantic outer continental shelf and slope. PLoS One 11:e0155841. doi: 10.1371/journal.pone.0155841
Diaz-Aguirre, F., Parra, G. J., Passadore, C., and Möller, L. (2020). Kinship and reproductive condition correlate with affiliation patterns in female southern Australian bottlenose dolphins. Sci. Rep. 10:1891. doi: 10.1038/s41598-020-58800-2
Dulau-Drouot, V., Boucaud, V., and Rota, B. (2008). Cetacean diversity off La Réunion Island (France). J. Mar. Biol. Assoc. U.K. 88, 1263–1272. doi: 10.1017/S0025315408001069
Elliser, C. R., and Herzing, D. L. (2016a). Changes in interspecies association patterns of Atlantic bottlenose dolphins, Tursiops truncatus, and Atlantic spotted dolphins, Stenella frontalis, after demographic changes related to environmental disturbance. Mar. Mamm. Sci. 32, 602–618. doi: 10.1111/mms.12289
Elliser, C. R., and Herzing, D. L. (2016b). Long-term interspecies association patterns of Atlantic bottlenose dolphins, Tursiops truncatus, and Atlantic spotted dolphins, Stenella frontalis, in the Bahamas. Mar. Mamm. Sci. 32, 38–56. doi: 10.1111/mms.12242
Espada, R., Olaya-Ponzone, L., Haasova, L., Martín, E., and García-Gómez, J. C. (2019). Hybridization in the wild between Tursiops truncatus (Montagu 1821) and Delphinus delphis (Linnaeus 1758). PLoS One 14:e0215020. doi: 10.1371/journal.pone.0215020
Evans, P. (1982). Associations between seabirds and cetaceans: a review. Mamm. Rev. 12, 187–206. doi: 10.1111/j.1365-2907.1982.tb00015.x
Farine, D. R. (2017). A guide to null models for animal social network analysis. Methods Ecol. Evol. 8, 1309–1320. doi: 10.1111/2041-210X.12772
Farine, D. R., Garroway, C. J., and Sheldon, B. C. (2012). Social network analysis of mixed-species flocks: Exploring the structure and evolution of interspecific social behaviour. Anim. Behav. 84, 1271–1277. doi: 10.1016/j.anbehav.2012.08.008
Fauth, J. E., Bernardo, J., Camara, M., Resetarits, W. J., Van Buskirk, J., and McCollum, S. A. (1996). Simplifying the jargon of community ecology: A conceptual approach. Am. Nat. 147, 282–286. doi: 10.1086/285850
Forcada, J., Aguilar, A., Hammond, P. S., Pastor, X., and Aguilar, R. (1994). Distribution and numbers of striped dolphins in the western Mediterranean Sea after the 1990 epizootic outbreak. Mar. Mamm. Sci. 10, 137–150. doi: 10.1111/j.1748-7692.1994.tb00256.x
Frantzis, A., and Herzing, D. (2002). Mixed-species associations of striped dolphins (Stenella coeruleoalba), short-beaked common dolphins (Delphinus delphis), and Risso’s dolphins (Grampus griseus) in the Gulf of Corinth (Greece, Mediterranean Sea). Aquat. Mamm. 28, 188–197.
Frère, C. H., Krützen, M., Mann, J., Watson-Capps, J. J., Tsai, Y. J., Patterson, E. M., et al. (2010). Home range overlap, matrilineal and biparental kinship drive female associations in bottlenose dolphins. Anim. Behav. 80, 481–486. doi: 10.1016/j.anbehav.2010.06.007
Gannier, A. (2000). Distribution of cetaceans off the Society Islands (French Polynesia) as obtained from dedicated surveys. Aquat. Mamm. 26, 111–126.
Gannier, A. (2009). Comparison of odontocete populations of the Marquesas and Society Islands (French Polynesia). J. Mar. Biol. Assoc. U.K. 89, 931–941. doi: 10.1017/S0025315408002713
García, S., Knouse, D., Sagarminaga, R., and Cañadas, A. (2000). An Insight on the biological significance of mixed groups of common dolphins (Delphinus delphis) and striped dolphins (Stenella coeruleoalba) in the Alboran Sea. Eur. Res. Cetaceans 14, 135–137.
Gibson, Q. A., and Mann, J. (2009). Do sampling method and sample size affect basic measures of dolphin sociality? Mar. Mammal Sci. 25, 187–198. doi: 10.1111/j.1748-7692.2008.00242.x
Goodale, E., Beauchamp, G., and Ruxton, G. D. (2017). Mixed-Species Groups of Animals: Behavior, Community Structure, and Conservation. Cambridge, MA: Academic Press.
Goodale, E., Sridhar, H., Sieving, K. E., Bangal, P., Colorado, Z. G. J., Farine, D. R., et al. (2020). Mixed company: a framework for understanding the composition and organization of mixed−species animal groups. Biol. Rev. 95, 889–910. doi: 10.1111/brv.12591
Gowans, S., and Whitehead, H. (1995). Distribution and habitat partitioning by small odontocetes in the Gully, a submarine canyon on the Scotian Shelf. Can. J. Zool. 73, 1599–1608. doi: 10.1139/z95-190
Gowans, S., Würsig, B., and Karczmarski, L. (2007). The social structure and strategies of Delphinids: predictions based on an ecological framework. Adv. Mar. Biol. 53, 195–294. doi: 10.1016/S0065-2881(07)53003-8
Gross, A., Kiszka, J., Van Canneyt, O., Richard, P., and Ridoux, V. (2009). A preliminary study of habitat and resource partitioning among co-occurring tropical dolphins around Mayotte, southwest Indian Ocean. Estuar. Coast. Shelf Sci. 84, 367–374. doi: 10.1016/j.ecss.2009.05.017
Gygax, L. (2002a). Evolution of group size in the dolphins and porpoises: Interspecific consistency of intraspecific patterns. Behav. Ecol. 13, 583–590. doi: 10.1093/beheco/13.5.583
Gygax, L. (2002b). Evolution of group size in the superfamily Delphinoidea (Delphinidae, Phocoenidae and Monodontidae): A quantitative comparative analysis. Mamm. Rev. 32, 295–314. doi: 10.1046/j.1365-2907.2002.00114.x
Hall, L. S., Krausman, P. R., and Morrison, M. L. (1997). The habitat concept and a plea for standard terminology. Wildl. Soc. Bull. 25, 173–182.
Herzing, D. L., and Elliser, C. R. (2013). Directionality of sexual activities during mixed-species encounters between atlantic spotted dolphins (Stenella frontalis) and bottlenose dolphins (Tursiops truncatus). Int. J. Comp. Psychol. 26, 124–134. doi: 10.5811/westjem.2011.5.6700
Herzing, D. L., and Johnson, C. M. (1997). Interspecific interaction between Atlantic spotted dolphins and bottlenose dolphins in the Bahamas, 1985-1995. Aquat. Mamm. 23, 85–99.
Herzing, D. L., Moewe, K., and Brunnick, B. J. (2003). Interspecies interactions between Atlantic spotted dolphins, Stenella frontalis and bottlenose dolphins, Tursiops truncatus, on Great Bahama Bank, Bahamas. Aquat. Mamm. 29, 335–341. doi: 10.1578/01675420360736505
Heymann, E. W., and Buchanan-Smith, H. M. (2000). The behavioural ecology of mixed-species troops of callitrichine primates. Biol. Rev. 75, 169–190. doi: 10.1111/j.1469-185X.1999.tb00044.x
Heymann, E. W., and Hsia, S. S. (2015). Unlike fellows - a review of primate-non-primate associations. Biol. Rev. Camb. Philos. Soc. 90, 142–156. doi: 10.1111/brv.12101
Hill, P. S., and Barlow, J. (1992). Report of a marine mammal survey of the California coast aboard the research vessel McArthur, July 28-November 5, 1991. La Jolla, CA: National Oceanic and Atmospheric Administration.
Hodgins, N. K., Dolman, S. J., and Weir, C. R. (2014). Potential hybridism between free-ranging Risso’s dolphins (Grampus griseus) and bottlenose dolphins (Tursiops truncatus) off north-east Lewis (Hebrides, UK). Mar. Biodivers. Rec. 7, 1–7. doi: 10.1017/S175526721400089X
Hunt, T. (2018). Demography, habitat use and social structure of Australian humpback dolphins (Sousa sahulensis) around the North West Cape, Western Australia: Implications for conservation and management. Doctor of Philosophy. Bedford Park, Australia: Flinders University.
Hunt, T. N., Allen, S. J., Bejder, L., and Parra, G. J. (2019). Assortative interactions revealed in a fission–fusion society of Australian humpback dolphins. Behav. Ecol. 30, 914–927. doi: 10.1093/beheco/arz029
Hutchinson, J. M. C., and Waser, P. M. (2007). Use, misuse and extensions of “ideal gas” models of animal encounter. Biol. Rev. 82, 335–359. doi: 10.1111/j.1469-185X.2007.00014.x
Irvine, A. B., Scott, M. D., Wells, R., and Kaufmann, J. (1981). Movements and activities of the Atlantic bottlenose dolphin, Tursiops truncatus, Near Sarasota, Florida. Fish. Bull. 79, 671–688.
Jackson, A., Gerrodette, T., Chivers, S., Lynn, M., Rankin, S., and Mesnick, S. (2008). Marine Mammal Data Collected during a Survey in the Eastern Tropical Pacific Ocean Aboard NOAA Ships David Starr Jordan and McArthur II, July 28-December 7, 2006. La Jolla, CA: National Oceanic and Atmospheric Administration.
Jourdain, E., and Vongraven, D. (2017). Humpback whale (Megaptera novaeangliae) and killer whale (Orcinus orca) feeding aggregations for foraging on herring (Clupea harengus) in Northern Norway. Mamm. Biol. 86, 27–32. doi: 10.1016/j.mambio.2017.03.006
Kaschner, K., Quick, N. J., Jewell, R., Williams, R., and Harris, C. M. (2012). Global coverage of cetacean line-transect surveys: status Quo, data gaps and future challenges. PLoS One 7:e44075. doi: 10.1371/journal.pone.0044075
Kaschner, K., Tittensor, D. P., Ready, J., Gerrodette, T., and Worm, B. (2011). Current and future patterns of global marine mammal biodiversity. PLoS One 6:e19653. doi: 10.1371/journal.pone.0019653
Kinzey, D., Gerrodette, T., Barlow, J., Dizon, A., Perryman, W., and Olson, P. (2000). Marine Mammal Data Collected during a Survey in the Eastern Tropical Pacific Ocean Aboard the NOAA Ships McArthur and David Starr Jordan, July 28-December 9, 1999. La Jolla, CA: National Oceanic and Atmospheric Administration.
Kinzey, D., Gerrodette, T., Barlow, J., Dizon, A., Perryman, W., Olson, P., et al. (1999). Marine Mammal Data Collected during a Survey in the Eastern Tropical Pacific Ocean Aboard the NOAA Ships McArthur and David Starr Jordan and the UNOLS Ship Endeavor, July 31-December 9, 1998. La Jolla, CA: National Oceanic and Atmospheric Administration.
Kiszka, J., Perrin, W. F., Pusineri, C., and Ridoux, V. (2011). What drives island-associated tropical dolphins to form mixed-species associations in the southwest Indian Ocean? J. Mammal. 92, 1105–1111. doi: 10.1644/10-MAMM-A-376.1
Kiszka, J. J. (2007). Atypical associations between dugongs (Dugong dugon) and dolphins in a tropical lagoon. J. Mar. Biol. Assoc. U.K. 87, 101–104. doi: 10.1017/S0025315407055129
Kiszka, J. J., Heithaus, M. R., and Wirsing, A. J. (2015). Behavioural drivers of the ecological roles and importance of marine mammals. Mar. Ecol. Prog. Ser. 523, 267–281. doi: 10.3354/meps11180
Koper, R. P., and Plön, S. (2016). Interspecific interactions between cetacean species in Algoa Bay, South Africa. Aquat. Mamm. 42, 454–461. doi: 10.1578/AM.42.4.2016.454
Linsky, J. M. J., Wilson, N., Cade, D. E., Goldbogen, J. A., Johnston, D. W., and Friedlaender, A. S. (2020). The scale of the whale: using video-tag data to evaluate sea-surface ice concentration from the perspective of individual Antarctic minke whales. Anim. Biotelem. 8, 1–12. doi: 10.1186/s40317-020-00218-8
Lukoschek, V., and McCormick, M. I. (2000). A review of multi-species foraging associations in fishes and their ecological significance. Proc. Ninth Int. Coral Reef Symp. I, 467–474.
MacKenzie, D. I., Nichols, J. D., Royle, J. A., Pollock, K. H., Bailey, L. L., and Hines, J. E. (2017). Occupancy Estimation and Modeling: Inferring Patterns and Dynamics of Species Occurrence. Second Edition. London, England: Academic Press.
MacLeod, C. D. (2018). “Beaked Whales, Overview,” in Encyclopedia of Marine Mammals, eds B. Würsig, J. G. M. Thewissen, and K. Kovacs (Cambridge, MA: Academic Press), 80–83. doi: 10.1016/b978-0-12-804327-1.00062-5
Majolo, B., de Bortoli Vizioli, A., and Schino, G. (2008). Costs and benefits of group living in primates: group size effects on behaviour and demography. Anim. Behav. 76, 1235–1247. doi: 10.1016/j.anbehav.2008.06.008
Majolo, B., and Huang, P. (2018). “Group Living,” in Encyclopedia of Animal Cognition and Behavior, eds J. Vonk and T. Shackelford (Cham: Springer International Publishing), 1–12.
Mangels, K. F., and Gerrodette, T. (1994). Report of cetacean sightings during a marine mammal survey in the eastern tropical Pacific Ocean and the Gulf of California aboard the NOAA ships McArthur and David Starr Jordan, July 28-November 6, 1993. La Jolla, CA: National Oceanic and Atmospheric Administration.
Mann, J., Connor, R. C., Tyack, P. L., and Whitehead, H. eds (2000). Cetacean Societies: Field Studies of Dolphins and Whales. Chicago: The University of Chicago Press.
Markowitz, T. M. (2004). Social organization of the New Zealand dusky dolphin. Doctor of Philosophy. College Station, TX: Texas A&M University.
May-Collado, L. J. (2010). Changes in whistle structure of two dolphin species during interspecific associations. Ethology 116, 1065–1074. doi: 10.1111/j.1439-0310.2010.01828.x
Maze-Foley, K., and Mullin, K. D. (2006). Cetaceans of the oceanic Northern Gulf of Mexico: distributions, group sizes and interspecific associations. J. Cetacean Res. Manag. 8, 2003–2013.
Melillo, K. E., Dudzinski, K. M., and Cornick, L. A. (2009). Interactions between Atlantic spotted (Stenella frontalis) and bottlenose (Tursiops truncatus) dolphins off Bimini, The Bahamas, 2003-2007. Aquat. Mamm. 35, 281–291. doi: 10.1578/AM.35.2.2009.281
Minton, G., Collins, T., Findlay, K., and Baldwin, R. (2010). Cetacean distribution in the coastal waters of the Sultanate of Oman. J. Cetacean Res. Manag. 11, 301–313.
Morse, D. H. (1977). Feeding behavior and predator avoidance in heterospecific groups. Bioscience 27, 332–339. doi: 10.2307/1297632
Mullin, K. D., Hoggard, W., and Hansen, L. J. (2004). Abundance and seasonal occurence of cetaceans in outer continental shelf and slope waters of the north-central and northwestern Gulf of Mexico. Gulf Mex. Sci. 22, 62–73. doi: 10.18785/goms.2201.06
Noë, R., and Bshary, R. (1997). The formation of red colobus-diana monkey associations under predation pressure from chimpanzees. Proc. R. Soc. B Biol. Sci. 264, 253–259. doi: 10.1098/rspb.1997.0036
Norris, K. S., and Dohl, T. P. (1980). Behavior of the Hawaiian spinner dolphin, Stenella longirostris. Fish. Bull. 77, 821–849.
Norris, K. S., and Prescott, J. H. (1961). Observations on Pacific cetaceans of Californian and Mexican Waters. Univ. Calif. Publ. Zool. 63, 291–402.
Nowacek, D. P., Christiansen, F., Bejder, L., Goldbogen, J. A., and Friedlaender, A. S. (2016). Studying cetacean behaviour: new technological approaches and conservation applications. Anim. Behav. 120, 235–244. doi: 10.1016/j.anbehav.2016.07.019
O’Callaghan, T. M., and Baker, C. S. (2002). Summer cetacean community, with particular reference to Bryde’s whales, in the Hauraki Gulf, New Zealand. New Zealand: Department of Conservation.
Oswald, J. N., Rankin, S., and Barlow, J. (2008). To whistle or not to whistle? geographic variation in the whistling behavior of small odontocetes. Aquat. Mamm. 34, 288–302. doi: 10.1578/AM.34.3.2008.288
Pace, D. S., Mussi, B., Airoldi, S., Alessi, J., Arcangeli, A., Atzori, F., et al. (2015). New insights on the presence and distribution of the short-beaked common dolphin, Delphinus delphis, in Italian Waters. Biol. Mar. Mediterr. 22, 262–263.
Parra, G. J. (2005). Behavioural ecology of Irrawaddy, Orcaella brevirostris (Owen in Grey 1866), and Indo-Pacific Humpback dolphins, Sousa chinensis (Osbeck, 1765), in northwest Queensland, Australia: A comparative study. Doctor of Philosophy. Douglas: James Cook University.
Parra, G. J. (2006). Resource partitioning in sympatric delphinids: Space use and habitat preferences of Australian snubfin and Indo-Pacific humpback dolphins. J. Anim. Ecol. 75, 862–874. doi: 10.1111/j.1365-2656.2006.01104.x
Patterson, I. A. P., Reid, R. J., Wilson, B., Grellier, K., Ross, H. M., and Thompson, P. M. (1998). Evidence for infanticide in bottlenose dolphins: An explanation for violent interactions with harbour porpoises? Proc. R. Soc. B Biol. Sci. 265, 1167–1170. doi: 10.1098/rspb.1998.0414
Pearson, H. C., Jones, P. W., Brandon, T. P., Stockin, K. A., and Machovsky-Capuska, G. E. (2019). A biologging perspective to the drivers that shape gregariousness in dusky dolphins. Behav. Ecol. Sociobiol. 73:155. doi: 10.1007/s00265-019-2763-z
Penry, G. S., Cockcroft, V. G., and Hammond, P. S. (2011). Seasonal fluctuations in occurrence of inshore Bryde’s whales in Plettenberg Bay, South Africa, with notes on feeding and multispecies associations. Afr. J. Mar. Sci. 33, 403–414. doi: 10.2989/1814232X.2011.637617
Peres, C. A. (1992). Prey-capture benefits in a mixed-species group of Amazonian tamarins, Saguinus fuscicollis and S. mystax. Behav. Ecol. Sociobiol. 31, 339–347. doi: 10.1007/BF00177774
Pompa, S., Ehrlich, P. R., and Ceballos, G. (2011). Global distribution and conservation of marine mammals. Proc. Natl. Acad. Sci. U. S. A. 108, 13600–13605. doi: 10.1073/pnas.1101525108
Porter, L. M., and Garber, P. A. (2007). Niche expansion of a cryptic primate, Callimico goeldii, while in mixed species troops. Am. J. Primatol. 69, 1340–1353. doi: 10.1002/ajp.20441
Powell, G. V. N. (1985). Sociobiology and adaptive significance of interspecific foraging flocks in the neotropics. Ornithol. Monogr. 36, 713–732. doi: 10.2307/40168313
Psarakos, S., Herzing, D. L., and Marten, K. (2003). Mixed-species associations between Pantropical spotted dolphins (Stenella attenuata) and Hawaiian spinner dolphins (Stenella longirostris) off Oahu, Hawaii. Aquat. Mamm. 29, 390–395. doi: 10.1578/01675420360736578
Python Software Foundation (2016). Python Language Reference, version 3.8.0. Available Online at: https://www.python.org/.
QGIS Development Team (2019). QGIS Geographic Information System, version 3.8.3 Zanzibar. Available Online at: http://qgis.osgeo.org.
Quérouil, S., Silva, M. A., Cascão, I., Magalhães, S., Seabra, M. I., Machete, M. A., et al. (2008). Why do dolphins form mixed-species associations in the Azores? Ethology 114, 1183–1194. doi: 10.1111/j.1439-0310.2008.01570.x
Reilly, S. (1990). Seasonal changes in distribution and habitat differences among dolphins in the eastern tropical Pacific. Mar. Ecol. Prog. Ser. 66, 1–11. doi: 10.3354/meps066001
Richmond, O. M. W., Hines, J. E., and Beissinger, S. R. (2010). Two-species occupancy models: A new parameterization applied to co-occurrence of secretive rails. Ecol. Appl. 20, 2036–2046. doi: 10.1890/09-0470.1
Rowley, K. (2020). Mixed-Species Cetacean Groups. Maryland: National Oceanic and Atmospheric Administration.
Ryan, C., Cucknell, A., Romagosa, M., Boisseau, O., Moscrop, A., Frantzis, A., et al. (2014). A Visual and Acoustic Survey for Marine Mammals in the Eastern Mediterranean Sea during Summer 2013. Kelvedon, UK: Marine Conservation Research International.
Ryan, C., Macleod, G., Dinsdale, C., and Cook, S. (2017). Long-term association between a solitary common dolphin (Delphinus delphis delphis) and a harbour porpoise (Phocoena phocoena). Aquat. Mamm. 43, 113–115. doi: 10.1578/AM.43.1.2017.113
Saayman, G. S., Bower, D., and Tayler, C. K. (1972). Observations on inshore and pelagic Dolphins on the South-Eastern Cape coast of South Africa. Koedoe 15, 1–24. doi: 10.4102/koedoe.v15i1.664
Santoro, R., Sperone, E., Tringali, M. L., Pellegrino, G., Giglio, G., Tripepi, S., et al. (2015). Summer distribution, relative abundance and encounter rates of cetaceans in the Mediterranean waters off southern Italy (western Ionian Sea and southern Tyrrhenian Sea). Mediterr. Mar. Sci. 16, 613–620. doi: 10.12681/mms.1007
Santostasi, N. L., Bonizzoni, S., Bearzi, G., Eddy, L., and Gimenez, O. (2016). A robust design capture-recapture analysis of abundance, survival and temporary emigration of three Odontocete species in the Gulf of Corinth, Greece. PLoS One 11:e0166650. doi: 10.1371/journal.pone.0166650
Scott, M. D., and Cattanach, K. L. (1998). Diel patterns in aggregations of pelagic dolphins and tunas in the eastern Pacific. Mar. Mammal Sci. 14, 401–422. doi: 10.1111/j.1748-7692.1998.tb00735.x
Scott, M. D., and Chivers, S. J. (1990). “Distribution and herd structure of bottlenose dolphins in the eastern tropical pacific ocean,” in The Bottlenose Dolphin, eds S. Leatherwood and R. R. Reeves (San Diego: Academic Press), 387–402. doi: 10.1016/b978-0-12-440280-5.50026-3
Scott, M. D., Chivers, S. J., Olson, R. J., Fiedler, P. C., and Holland, K. (2012). Pelagic predator associations: Tuna and dolphins in the eastern tropical Pacific Ocean. Mar. Ecol. Prog. Ser. 458, 283–302. doi: 10.3354/meps09740
Shane, S. (1994). Occurrence and habitat use of marine mammals at Santa Catalina Island, California from 1983-91. Bull. South. Calif. Acad. Sci. 93:13.
Shane, S. H. (1990). “Behavior and ecology of the bottlenose dolphin at Sanibel Island, Florida,” in The Bottlenose Dolphin, eds S. Leatherwood and R. R. Reeves (San Diego, CA: Academic Press), 245–265. doi: 10.1016/b978-0-12-440280-5.50016-0
Shane, S. H. (1995). Relationship between pilot whales and Risso’s dolphins at Santa Catalina Island, California, USA. Mar. Ecol. Prog. Ser. 123, 5–12. doi: 10.3354/meps123005
Smolker, R. A., Richards, A. F., Connor, R. C., and Pepper, J. W. (1992). Sex differences in patterns of association among Indian Ocean bottlenose dolphins. Behaviour 123, 38–69. doi: 10.1163/156853992X00101
Smultea, M. A., Bacon, C. E., Lomac-MacNair, K., Visser, F., and Bredvik, J. (2014). Rare mixed-species associations between sperm whales and Risso’s and northern right whale dolphins off the southern california bight: Kleptoparasitism and social parasitism? Northwest. Nat. 95, 43–49. doi: 10.1898/nwn13-11.1
Sridhar, H., Beauchamp, G., and Shanker, K. (2009). Why do birds participate in mixed-species foraging flocks? A large-scale synthesis. Anim. Behav. 78, 337–347. doi: 10.1016/j.anbehav.2009.05.008
Sridhar, H., and Guttal, V. (2018). Friendship across species borders: Factors that facilitate and constrain heterospecific sociality. Philos. Trans. R. Soc. B Biol. Sci. 373:20170014. doi: 10.1098/rstb.2017.0014
Stensland, E., Angerbjörn, A., and Berggren, P. (2003). Mixed species groups in mammals. Mamm. Rev. 33, 205–223. doi: 10.1046/j.1365-2907.2003.00022.x
Stockin, K. A., Binedell, V., Wiseman, N., Brunton, D. H., and Orams, M. B. (2009). Behavior of free-ranging common dolphins (Delphinus sp.) in the Hauraki Gulf, New Zealand. Mar. Mammal Sci. 25, 283–301. doi: 10.1111/j.1748-7692.2008.00262.x
Stojan-Dolar, M., and Heymann, E. W. (2010). Vigilance of mustached tamarins in single-species and mixed-species groups-the influence of group composition. Behav. Ecol. Sociobiol. 64, 325–335. doi: 10.1007/s00265-009-0848-9
Strickland, K., Levengood, A., Foroughirad, V., Mann, J., Krzyszczyk, E., and Frère, C. H. (2017). A framework for the identification of long-term social avoidance in longitudinal datasets. R. Soc. Open Sci. 4:170641. doi: 10.1098/rsos.170641
Suzuki, T. N., and Kutsukake, N. (2017). Foraging intention affects whether willow tits call to attract members of mixed-species flocks. R. Soc. Open Sci. 4:170222. doi: 10.1098/rsos.170222
Thompson, L. M. (2010). Long-term inter- and intra- species interactions of marine tucuxi (Sotalia guianensis) and common bottlenose (Tursiops truncatus) dolphins in Gandoca-Manzanillo, Costa Rica. Master of Science. Hempstead, NY: Hofstra University.
Veit, R. R., and Harrison, N. M. (2017). Positive interactions among foraging seabirds, marine mammals and fishes and implications for their conservation. Front. Ecol. Evol. 5:121. doi: 10.3389/fevo.2017.00121
Viana, Y. (2019). Are Tursiops truncatus modulating the whistles parameters in different mixed groups contexts? Master of Science. Juiz de Fora: MG Universidade Federal de Juiz de Fora.
Wade, P. R., and Gerrodette, T. (1993). Estimates of cetacean abundance and distribution in the eastern tropical Pacific. Rep. Int. Whal. Comm. 43, 477–493.
Ward, A., and Webster, M. (2016). Sociality: The Behaviour of Group-Living Animals. Cham: Springer International Publishing.
Waser, P. M. (1982). Primate polyspecific associations: do they occur by chance? Anim. Behav. 30, 1–8. doi: 10.1016/S0003-3472(82)80230-3
Waser, P. M. (1984). “Chance” and mixed-species associations. Behav. Ecol. Sociobiol. 15, 197–202. doi: 10.1007/BF00292975
Weir, C. R. (2006). Occurrence and distribution of cetaceans off northern Angola, 2004/05. J. Cetacean Res. Manag. 9, 225–240.
Weir, C. R. (2011). Distribution and seasonality of cetaceans in tropical waters between Angola and the Gulf of Guinea. Afr. J. Mar. Sci. 33, 1–15. doi: 10.2989/1814232X.2011.572333
Weir, C. R., Pollock, C., Cronin, C., and Taylor, S. (2001). Cetaceans of the Atlantic frontier, north and west of Scotland. Cont. Shelf Res. 21, 1047–1071. doi: 10.1016/S0278-4343(00)00124-2
Wells, R. S., Irvine, A. B., and Scott, M. D. (1980). “The social ecology of inshore Odontocetes,” in Cetacean Behavior: Mechanisms & Functions, ed. L. M. Herman (New York: Wiley), 263–317.
Wells, R. S., and Scott, M. D. (2009). “Common bottlenose dolphin: Tursiops truncatus,” in Encyclopedia of Marine Mammals, eds B. Würsig, J. G. M. Thewissen, and K. M. Kovacs (Amsterdam: Elsevier), 249–255.
Whitehead, H. (2008). Analyzing Animal Societies: Quantitative Methods for Vertebrate Social Analysis. Chicago, IL: University of Chicago Press.
Whitesides, G. H. (1989). Interspecific associations of Diana monkeys, Cercopithecus diana, in Sierra Leone, West Africa: biological significance or chance? Anim. Behav. 37, 760–776. doi: 10.1016/0003-3472(89)90062-6
Wilson, A. D. M., and Krause, J. (2013). Repeated non-agonistic interactions between a bottlenose dolphin (Tursiops truncatus) and sperm whales (Physeter macrocephalus) in Azorean Waters. Aquat. Mamm. 39, 89–96. doi: 10.1578/AM.39.1.2013.89
Wolters, S., and Zuberbühler, K. (2003). Mixed-species associations of Diana and Campbell’s monkeys: The costs and benefits of a forest phenomenon. Behaviour 140, 371–385. doi: 10.1163/156853903321826684
Würsig, B., Thewissen, J. G. M., and Kovacs, K. M. (2018). Encyclopedia of Marine Mammals. San Diego: Academic Press.
Würsig, B., and Würsig, M. (1979). Behavior and ecology of the bottlenose dolphin, Tursiops truncatus, in the South Atlantic. Fish. Bull. 77, 871–890.
Zaeschmar, J. R. (2014). False killer whales (Pseudorca crassidens) in New Zealand Waters. Master of Science. New Zealand: Massey University.
Zaeschmar, J. R., Dwyer, S. L., and Stockin, K. A. (2013). Rare observations of false killer whales (Pseudorca crassidens) cooperatively feeding with common bottlenose dolphins (Tursiops truncatus) in the Hauraki Gulf, New Zealand. Mar. Mammal Sci. 29, 555–562. doi: 10.1111/j.1748-7692.2012.00582.x
Zaeschmar, J. R., Visser, I. N., Fertl, D., Dwyer, S. L., Meissner, A. M., Halliday, J., et al. (2014). Occurrence of false killer whales (Pseudorca crassidens) and their association with common bottlenose dolphins (Tursiops truncatus) off northeastern New Zealand. Mar. Mammal Sci. 30, 594–608. doi: 10.1111/mms.12065
Zanardo, N., Parra, G. J., Diaz-Aguirre, F., Pratt, E. A. L., and Möller, L. M. (2018). Social cohesion and intra-population community structure in southern Australian bottlenose dolphins (Tursiops sp.). Behav. Ecol. Sociobiol. 72, 1–13. doi: 10.1007/s00265-018-2557-8
Keywords: grouping, interspecific association, interaction, antipredator tactics, foraging, social benefits, cetacean, mixed-species group
Citation: Syme J, Kiszka JJ and Parra GJ (2021) Dynamics of Cetacean Mixed-Species Groups: A Review and Conceptual Framework for Assessing Their Functional Significance. Front. Mar. Sci. 8:678173. doi: 10.3389/fmars.2021.678173
Received: 09 March 2021; Accepted: 25 May 2021;
Published: 25 June 2021.
Edited by:
Darren Croft, University of Exeter, United KingdomReviewed by:
Mia Lykbær Kronborg Nielsen, University of Exeter, United KingdomCopyright © 2021 Syme, Kiszka and Parra. This is an open-access article distributed under the terms of the Creative Commons Attribution License (CC BY). The use, distribution or reproduction in other forums is permitted, provided the original author(s) and the copyright owner(s) are credited and that the original publication in this journal is cited, in accordance with accepted academic practice. No use, distribution or reproduction is permitted which does not comply with these terms.
*Correspondence: Jonathan Syme, am9uYXRoYW4uc3ltZUBmbGluZGVycy5lZHUuYXU=
Disclaimer: All claims expressed in this article are solely those of the authors and do not necessarily represent those of their affiliated organizations, or those of the publisher, the editors and the reviewers. Any product that may be evaluated in this article or claim that may be made by its manufacturer is not guaranteed or endorsed by the publisher.
Research integrity at Frontiers
Learn more about the work of our research integrity team to safeguard the quality of each article we publish.