- 1Bahamas Marine Mammal Research Organisation, Abaco, Bahamas
- 2Sea Mammal Research Unit, Scottish Oceans Institute, University of St Andrews, St Andrews, United Kingdom
- 3Ocean Environmental Consulting, Halifax, NS, Canada
- 4Open Ocean Consulting, Petersfield, United Kingdom
As underwater noise from ship traffic increases, profound effects on the marine environment highlight the need for improved mitigation measures. One measure, reduction in ship speed, has been shown to be one of the key drivers in reducing sound source levels of vessels. In 2017, a study began to assess the impacts of increasing commercial shipping traffic on sperm whales in Northwest Providence Channel, northern Bahamas, an international trade route that primarily serves the southeast US. Ship data were collected from an Automatic Identification System (AIS) station combined with recordings from an acoustic recorder to measure underwater sound levels and to detect the presence of sperm whales. Here we analyze a subset of these data to opportunistically investigate potential changes in ship traffic before and during the COVID-19 pandemic. These data span one calendar year from October 2019 to October 2020. A pre-COVID-19 dataset of 121 days, from a recorder approximately 2 km from the shipping route was compared to a 134-day dataset collected during COVID-19 from the same site, comprising 2900 and 3181 ten-minute recordings, respectively. A dramatic decrease in ocean noise levels concurrent with changes in shipping activity occurred during the pandemic. The mean pre-COVID-19 power density level in the 111–140 Hz 1/3-octave band was 88.81 dB re 1 μPa (range 81.38–100.90) and decreased to 84.27 dB re 1 μPa (range 78.60–99.51) during COVID-19, equating to a 41% reduction in sound pressure levels (SPL). After differences in seasonal changes in wind speed were accounted for, SPL decreased during the pandemic by 3.98 dB (37%). The most notable changes in ship activity were significantly reduced vessel speeds for all ship types and fewer ships using the area during the pandemic. Vessel speed was highly correlated to SPL and the only ship-based variable that predicted SPLs. Despite the opportunistic nature [i.e., not a standard before-after-control-impact (BACI) study], this study provides a unique opportunity to assess the effectiveness of ship traffic management strategies, such as slowing ships down, to mitigate impacts on marine life in the study area, including local sperm whale populations.
Introduction
Anthropogenic noise is increasing globally and impacting marine soundscapes (Andrew et al., 2002; Hildebrand, 2009; Erbe et al., 2014; Duarte et al., 2021). Maritime trade is reliant on shipping networks linking all major global economies and where seaborne routes exist, marine soundscapes are altered by vessel noise. This noise pollution is proving detrimental to the lives of a plethora of marine organisms, including commercial fish (Sarà et al., 2007; Stanley et al., 2017) and whale species (Clark et al., 2009; Putland et al., 2017; Cholewiak et al., 2018). The number of commercial vessels, their size and speed, all have upward trends (Frisk, 2012; McKenna et al., 2012), creating ever increasing issues of noise pollution and for large marine animals, greater threat associated with ship strike (Rockwood et al., 2020), highlighting the need for improved mitigation measures to limit impacts on marine life.
The combined use of Automatic Identification System (AIS) and passive acoustic monitoring have revealed evidence of the impact that high volume, fast moving, large vessels have on the marine environments and their occupants (Vanderlaan and Taggart, 2009; van der Hoop et al., 2012; Putland et al., 2017; Joy et al., 2019). Changes in vessel behavior such as reduced speed can decrease sound source levels and the cumulative impact they have on soundscapes (McKenna et al., 2013; Putland et al., 2017; MacGillivray et al., 2019). Additionally, the risk of injury or death of large marine animals due to ship strike can also be reduced by modifications to vessel behavior, such as speed and route alterations (Gende et al., 2011; Conn and Silber, 2013; Crum et al., 2019).
The islands of The Bahamas lie just off the southeastern seaboard of the United States. They span over 1,222 km and consist of 700 islands with a variety of habitats and rich biodiversity including submarine canyons and deep-water channels, the world’s third largest coral-reef system, carbon-sink seagrass beds, diverse marine megafauna with over 24 different marine mammal species, along with four species of turtle and over 40 species of shark. Northwest Providence Channel (NPC), in the northern Bahamas is strategically located, facilitating access of Atlantic shipping and Caribbean cruise ship fleets to Florida’s major seaports and cruise ship hubs (www.MiamiDade.Gov, 2019; Rodrigue and Wang, 2020). The NPC route bisects two shallow-water bank systems, the Great Bahama and Little Bahama Banks, both of which are crucial for commercial and game fisheries in The Bahamas, notably for spiny lobster (Panulirus argus) and bonefish (Albula vulpes) (Fedler, 2010; Adams and Murchie, 2015; Sherman et al., 2018), and home to resident dolphin populations (Herzing, 1997; Durban et al., 2000; Fearnbach et al., 2012). Moreover, at least two locations along the bank edge of NPC are historical spawning aggregation sites of the endangered Nassau grouper (Epinephelus striatus) that may be impacted by increasing ship noise as it overlaps in frequency with sounds produced by this species during reproductive behavior (Scharer et al., 2012). The deep (>1,000 m) waters of the NPC are also utilized by many marine mammal species, with particular philopatry shown by sperm whale (Physeter macrocephalus) clans (Claridge et al., 2015; Joyce et al., 2017). In 2017, the Bahamas Marine Mammal Research Organisation (BMMRO) began a passive acoustic monitoring study in NPC to track sperm whale movement through the area to understand the overlap between sperm whale habitat use and commercial shipping traffic. An AIS station was installed to track ships within the study area.
At the end of December 2019, the World Health Organization (WHO) became aware of a new disease, COVID-19, caused by a new coronavirus called SARS-CoV-2, originating in the People’s Republic of China and by mid-March 2020 the WHO reported the outbreak a pandemic (World Health Organization [WHO], 2020; Poon and Peiris, 2020). Global movement of people and goods altered dramatically, decreasing and even eliminating some commercial vessel-based activities, such as the cruise ship industry (Gössling et al., 2021). In The Bahamas, the first COVID-19 case was reported on 15th March 2020, and the government enacted emergency orders on 19th March 2020 (Government of The Bahamas, 2020). It was anticipated that the combination of restrictions of movement within The Bahamas, the United States and the wider Atlantic region in response to the COVID-19 pandemic would generate a shift in vessel traffic behavior in Bahamian waters. Soundscapes can be altered by both anthropogenic (Duarte et al., 2021) and environmental factors (e.g., wind speed and wave height) (Thomson and Barclay, 2020). Here, we use acoustic and AIS data collected for the sperm whale study, and available environmental data to opportunistically investigate potential changes to shipping activity and the ocean soundscape in the NPC during the COVID-19 pandemic.
Materials and Methods
Acoustic Data Collection and Analysis
A passive acoustic recorder, SoundTrap ST500 from Ocean Instruments, was deployed on the seafloor at the southeastern-most tip of Little Bahama Bank off southern Abaco Island, northern Bahamas (25.803445, −77.112943, depth = 28.65 m) to record underwater sound levels including noise from ship traffic and marine life in NPC (Figure 1). The hydrophone was intentionally placed at <30 m to allow for safe scuba deployment and retrieval, yet with proximity to heavy shipping traffic to investigate use of the area by sperm whales and, as a secondary question, to detect noise that might impact a Nassau grouper spawning aggregation site. The device sampled at a rate of 144 kHz on a duty cycle of 10 min, every 60 min. This schedule was selected based on the recording capacity of the recorders 256 GB internal memory and the speed at which sperm whales traverse this area.
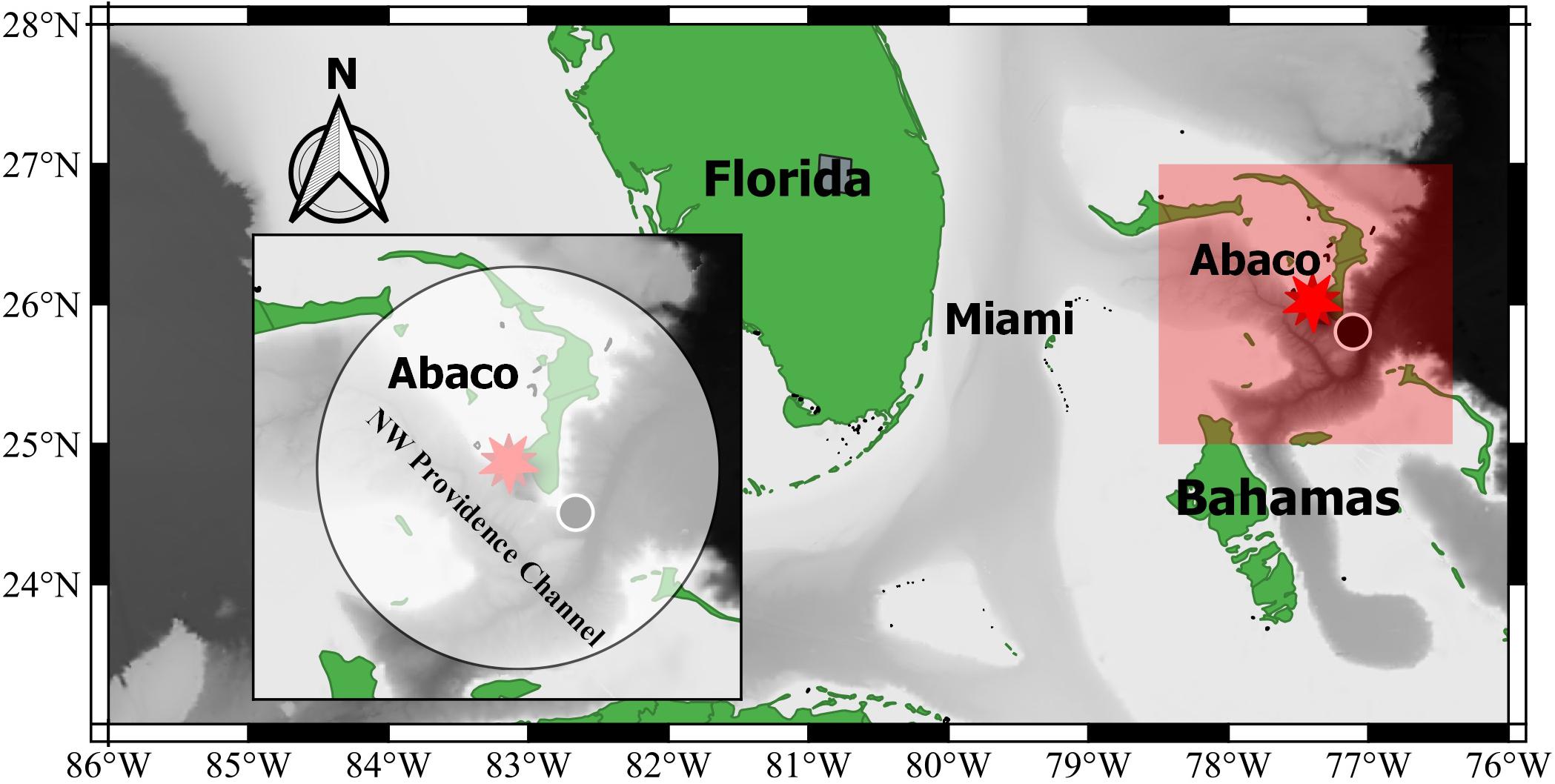
Figure 1. A map of islands in the northern Bahamas, their proximity to the Florida panhandle and the study area, a circle indicating the average 93 km distance messages can be received at the Automatic Identification System (AIS) station, including locations of the AIS station ID 844 (red star) and ST500 hydrophone (black circle) off the southern end of Abaco Island. GEBCO (2020).
The hydrophone recorder was deployed on 15th October 2019, but the dataset does not begin until 29th October, the date our AIS tower was operational following hurricane Dorian, a category 5 storm that hit The Bahamas on 1st September. The recorder was retrieved on 29th February 2020 to download the data, recharge the batteries, and redeploy as soon as possible. However, COVID-19 stay-at-home orders were issued by the Government of The Bahamas soon thereafter which prevented redeployment until 1st June 2020. Despite lack of a continuous acoustic record from the start of the pandemic, these data still provide a unique opportunity to compare underwater sound levels in NPC pre-COVID-19 and during COVID-19.
To measure underwater sound levels, sound pressure level (SPL) measurements were made from the hydrophone recordings using the noise band monitor module in the open-source PAMGuard software version 2.01.05 BETA (Gillespie et al., 2009). Continuous underwater sound levels were measured in nine different standard 1/3-octave bands between 70 and 500 Hz in every 10 s period for each 10-min recording, as peak frequencies produced by commercial ships are typically over 100 Hz (Merchant et al., 2014). Sound levels in the nine different 1/3-octave bands were strongly correlated and therefore just one band was chosen that was representative of the environments sound levels, the 111–140 Hz 1/3-octave band. The binary files generated from PAMGuard were processed with Matlab_R2020b (Mathworks, Nantick, MA) to extract the sound levels as band energy (dB re 1 μPa), and a median sound level was calculated for each 10-min recording.
In situ environmental data at the recording site were not available so archived wind speed data was accessed at the National Data Buoy Center website (NOAA, National Data Buoy Center) for the weather buoy nearest the study site (station 41047 located at 27.5N, −71.5W), 590 km ENE from our hydrophone. Archived wind speeds (m/s) were averaged over an 8-min period by averaging ‘‘the simple scalar average of the wind speed observations1.”
Marine Traffic Data
AIS data were collected using BMMRO’s AIS station, ID 844 (26.0N, −77.4W; elevation 10 m). A MATLAB function was written to decode the AIS messages, and R scripts (R Core Team, 2020) were used to identify the unique number of vessels using the area, their speed, and their locations. The closest point of approach (CPA) of each unique vessel to the hydrophone was calculated using the great-circle-distance with the Vincenty (ellipsoid) method from the R package geosphere (version 1.5-10). Only messages which were complete and without error were considered in this analysis. The Marine Traffic website2 provides information on ship type, size and tonnage (but not load) based on each vessel’s Maritime Mobile Service Identity (MMSI) number. MMSI numbers transmitted by AIS to station 844 were matched to the Marine Traffic database to obtain vessel details. Two percent of the transmitted MMSI numbers did not have an associated entry in the Marine Traffic database, so these records were discarded for analyses that included ship type. AIS data without corresponding hydrophone recordings were also excluded (i.e., when the recorder was not deployed).
Study Area
Marine Traffic summary statistics for AIS station ID 844 showed that during the study period, the average reception distance from which a ship’s message could be received was 93 km. The ST500 acoustic recorder was deployed 37 km from the AIS station, which provided confidence that the recorded acoustic data were representative of the ship traffic detected at the AIS station (Figure 1).
Statistical Analysis
To explore causation of changes in SPL between the pre- and during COVID-19 datasets, a generalized linear model (GLM) was used in the software package R, using the “glm” function in the lme4 package (Bates et al., 2012). A normal (Gaussian) error structure was chosen with SPL as the response variable, and six predictor variables, including continuous variables; number of ships, the log transform of the CPA distance, speed, and wind speed, and categorical variables; pre- or during COVID-19, and day or night. The day or night variable was added to include small recreational fishing vessels that may not have AIS. These vessels do not typically use the area at night, defined here as between 7 p.m. and 7 a.m. local time. Ship data were filtered to include ships within a 20 km radius of the recorder to include only the ships that were most likely affecting the sound levels on the hydrophone recordings, and ships that were present during recording periods (the first 10 min of every hour). Additionally, to focus our investigation on the effect of ship activity on the SPL, we also filtered the data to only include time periods when wind speeds were not greater than a “gentle breeze,” or less than 5.5 m/s as described in Pensieri et al., 2015, as high wind speeds can dominate sound recordings making it difficult to discriminate the contributors to any changes in sound.
The Akaike Information Criterion (AIC) was used to select the best statistical model (Burnham and Anderson, 2002), or which models to average, if more than one model was appropriate. The “dredge” function from the MuMIn package was used for model selection, i.e., those with the smallest AIC values. For all models with an AIC difference of < 3 compared to the best model, model averaging was performed. Summed Akaike weights were used to estimate the relative importance of variables within the model (Burnham and Anderson, 2002).
Results
Acoustic Data Collection and Analysis
The acoustic record spans a full calendar year; the pre-COVID-19 dataset consists of 121 days of recordings from 29th October 2019 to 29th February 2020, comprising 2900 ten-minute duration wav files. This was compared to the during COVID-19 dataset of 134 days of recordings from 1st June 2020 to 12th October 2020, and 3181 ten-minute duration wav files (Table 1).
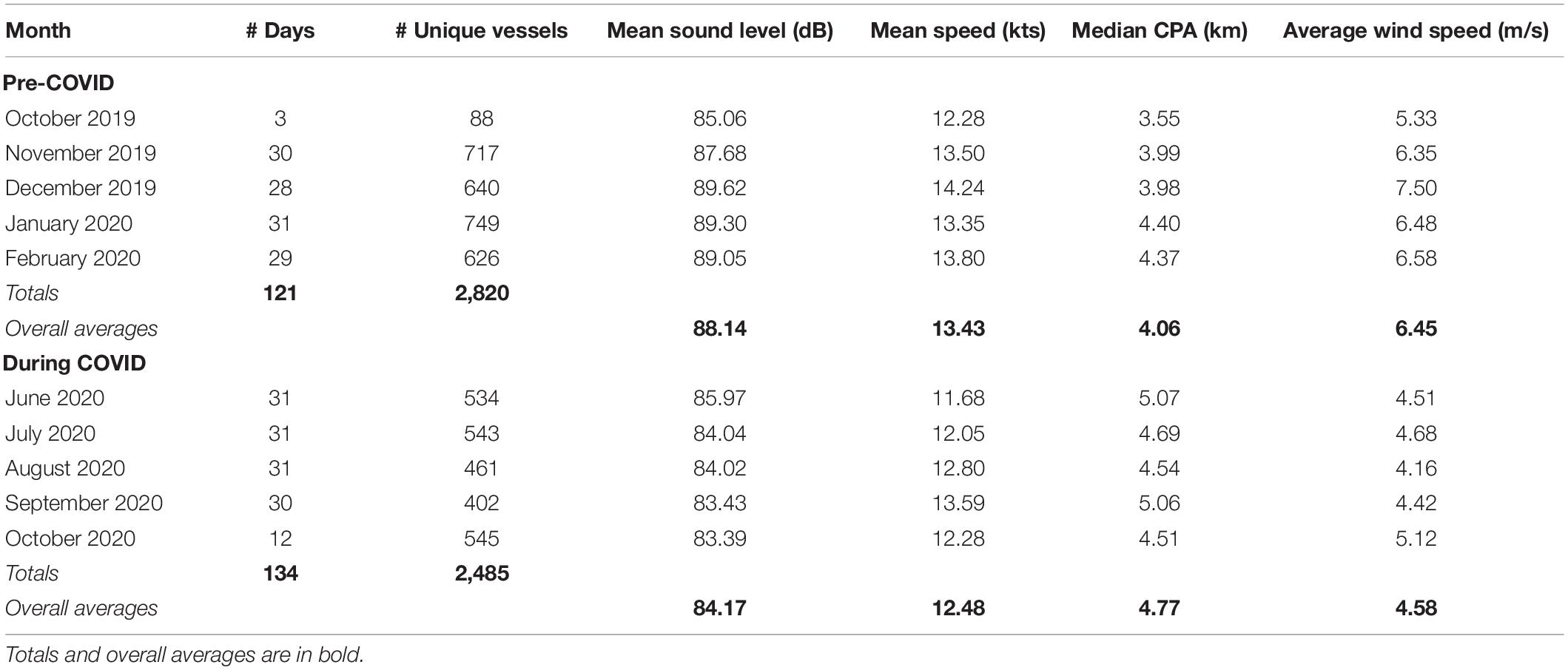
Table 1. A summary of the dataset showing the number of days AIS data were recorded with corresponding hydrophone data, the number of unique vessels per month, the mean sound level detected at the acoustic recorder, the mean ship speed derived from the mean speeds for each vessel per month, the median Closest Point of Approach (CPA) of unique vessels per month and the average wind speed per month.
Comparisons between datasets were calculated from all data, not just using the monthly averages shown in Table 1. The mean pre-COVID-19 power level in the 111–140 Hz 1/3-octave band was 88.81 dB re 1 μPa (range 81.38–100.90), compared to 84.27 dB re 1 μPa (range 78.60–99.51) for the during COVID-19 dataset, a resultant decrease of 4.54 dB. Using methods described by Urick (1983), the reported spectral level decrease of 4.54 dB equates to a soundscape sound pressure reduction of 41% during the pandemic compared to the pre-COVID-19 period. A Welch two-sample t-test showed the reduction in sound levels during the pandemic was highly significant (p < 0.01), with a 95% confidence interval of 4.39–4.68.
To focus the investigation on the effects of ship behavior on changes of SPL, the dataset was filtered to include only ships within 20 km of the recorder, that were present during the recorders’ duty cycle, and wind speed was less than 5.5 m/s (Pensieri et al., 2015). During these time periods, average wind speeds for the pre-COVID dataset, i.e., fall/winter months, were higher (<2 m/s) than during the spring/summer months of the pandemic dataset. This filtering resulted in a 3.98 dB decrease in the spectral level which equates to a 37% soundscape sound pressure reduction, suggesting that although seasonal changes in wind speed during the pandemic contributed to a reduction in overall sound, anthropogenic causes were predominant.
Marine Traffic Data
Using AIS data, a comparison of commercial ship activity in the study area before and during the pandemic showed that while some uses of the area remained the same or were similar throughout the period, some changes did occur. A plot of ship location data shows that the primary route used by ships to traverse the study area did not appear to differ before and during COVID-19 (Figure 2). This assumption was supported by similar CPA distances of ships to the hydrophone during both time periods for all ships (Table 1); the pre-COVID-19 median CPA was 4.09 km (range 0.02–289.98 km) compared to 4.77 km during-COVID-19 (range 0.11–274.41 km). However, the number of unique ships present in the pre-COVID-19 period was higher (n = 2,820) compared to during COVID-19 (n = 2,485).
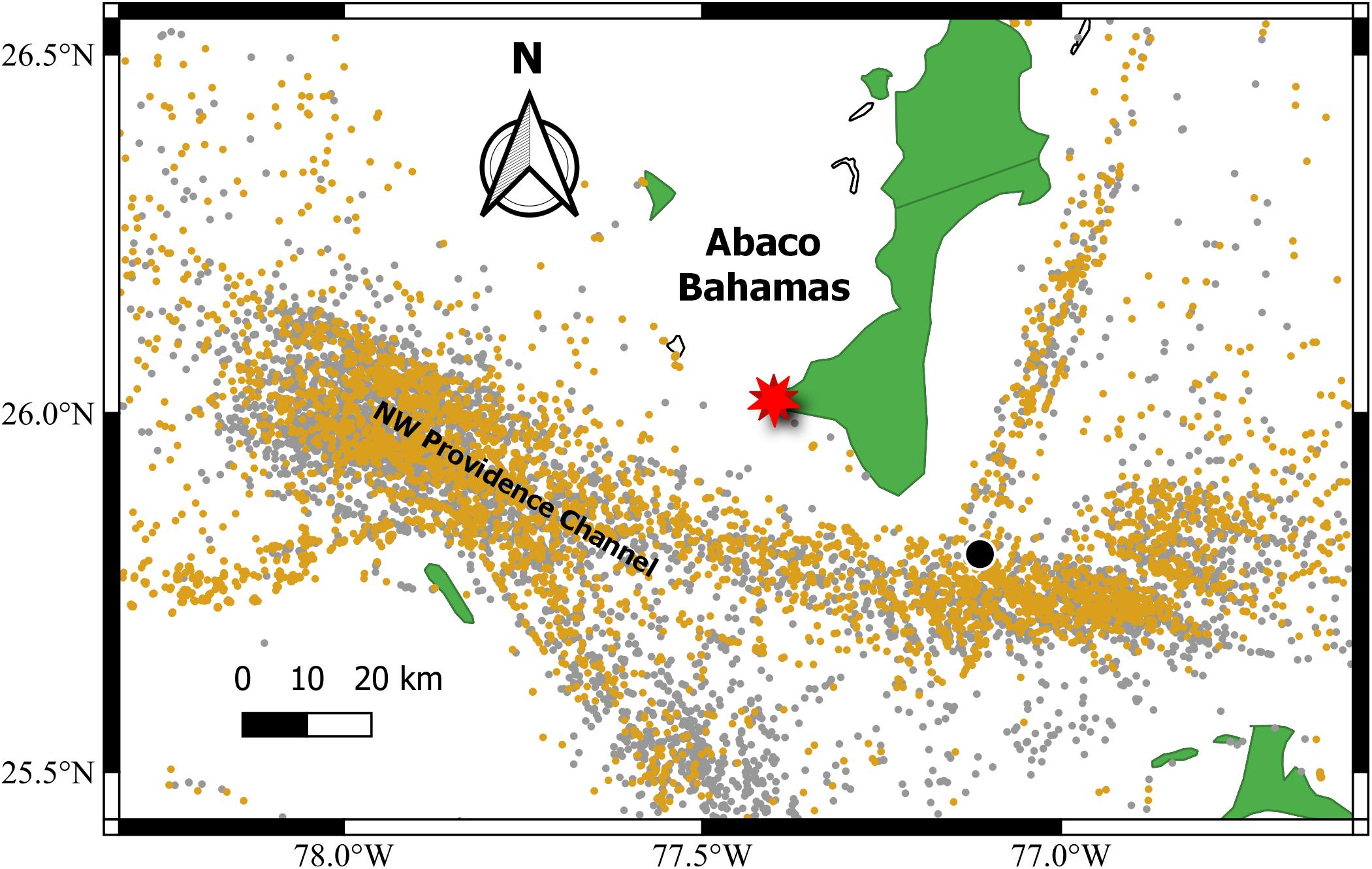
Figure 2. Map of Northwest Providence Channel (NPC) depicting ship location messages transmitted and received by AIS receiver station 844 (red star) for the pre-COVID-19 time period (ship locations in gray, n = 6,449) and during COVID-19 (ship locations in orange, n = 5,692) during corresponding times when the ST500 hydrophone (black circle) was deployed. Each ship is represented by one location per day.
In addition to fewer number of ships present during the pandemic, there were pronounced changes in ship activity between the two periods; the most notable change in ship behavior was that ships traveled at a slower speed as they passed through the study area during the pandemic (Figure 3). The mean speed of vessels using the AIS data in the pre-COVID-19 dataset was 13.77 kts (range 0–36.7), compared to 12.35 kts (range 0–29.2) during COVID-19. A Welch two-sample t-test showed the reduction in speed during the pandemic was highly significant (p < 0.01), with a 95% confidence interval of 1.42–1.41 kts. A simple Pearson correlation showed a strong (0.9) correlation between the ship speeds and sound levels for the pre-COVID-19 dataset and a strong (0.7) correlation during COVID-19.
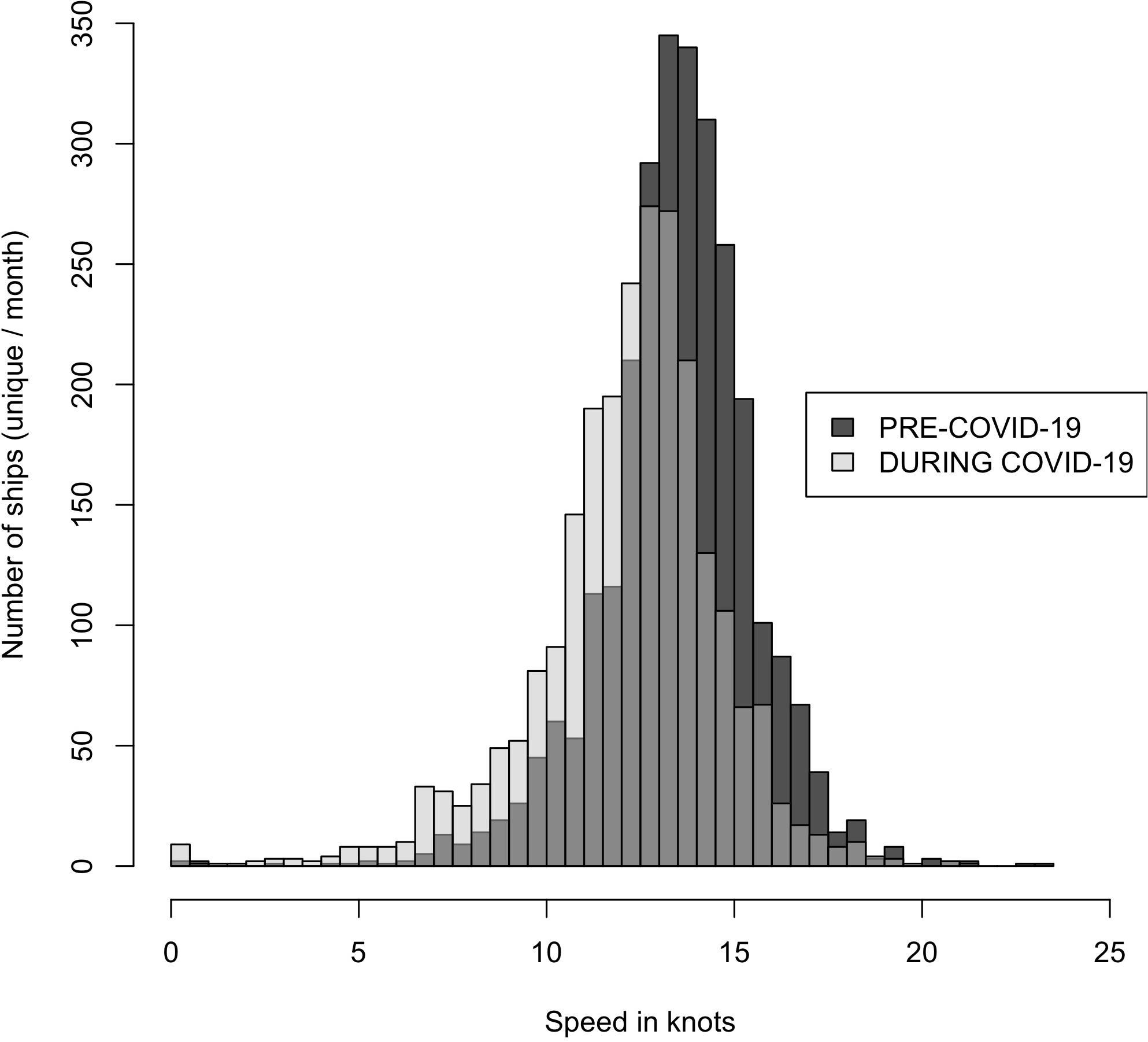
Figure 3. A histogram presenting the number of ships/months and the mean ship speed for each unique ship per month PRE-COVID-19 (dark gray, n = 2,781) and during COVID-19 (light gray, n = 2,439). The gray central area is where the datasets overlap.
Other COVID-related changes in ship activity were noted. Throughout the study AIS transmissions were received from 6 to 10 different ship types per month, with both the lowest and highest number of different ship types per month occurring in the pre-COVID-19 period. However, not all ship types were represented in the study area equally. Ship traffic primarily consisted of tankers and cargo ships (which include container ships), both before and during the pandemic, but both types were recorded more frequently during COVID-19 than in the pre-COVID-19 period (tankers: pre- 33%, during 39% and cargo: pre- 39%, during 44%). Other changes in use of the area by vessel type included “Fishing” and “High Speed Craft” vessels present before the pandemic, but absent during COVID-19, and “Search and Rescue” vessels present during the pandemic but absent in the pre-COVID-19 dataset (Figure 4). Welch two-sample t-tests for each individual ship type (e.g., “Cargo,” “Passenger,” etc.) found vessel speeds were significantly greater in the pre-COVID-19 period (n = 2,569 ships) than during COVID-19 (n = 2,294 ships) regardless of ship type. This dataset only includes records with MMSI numbers which provides the ship type information.
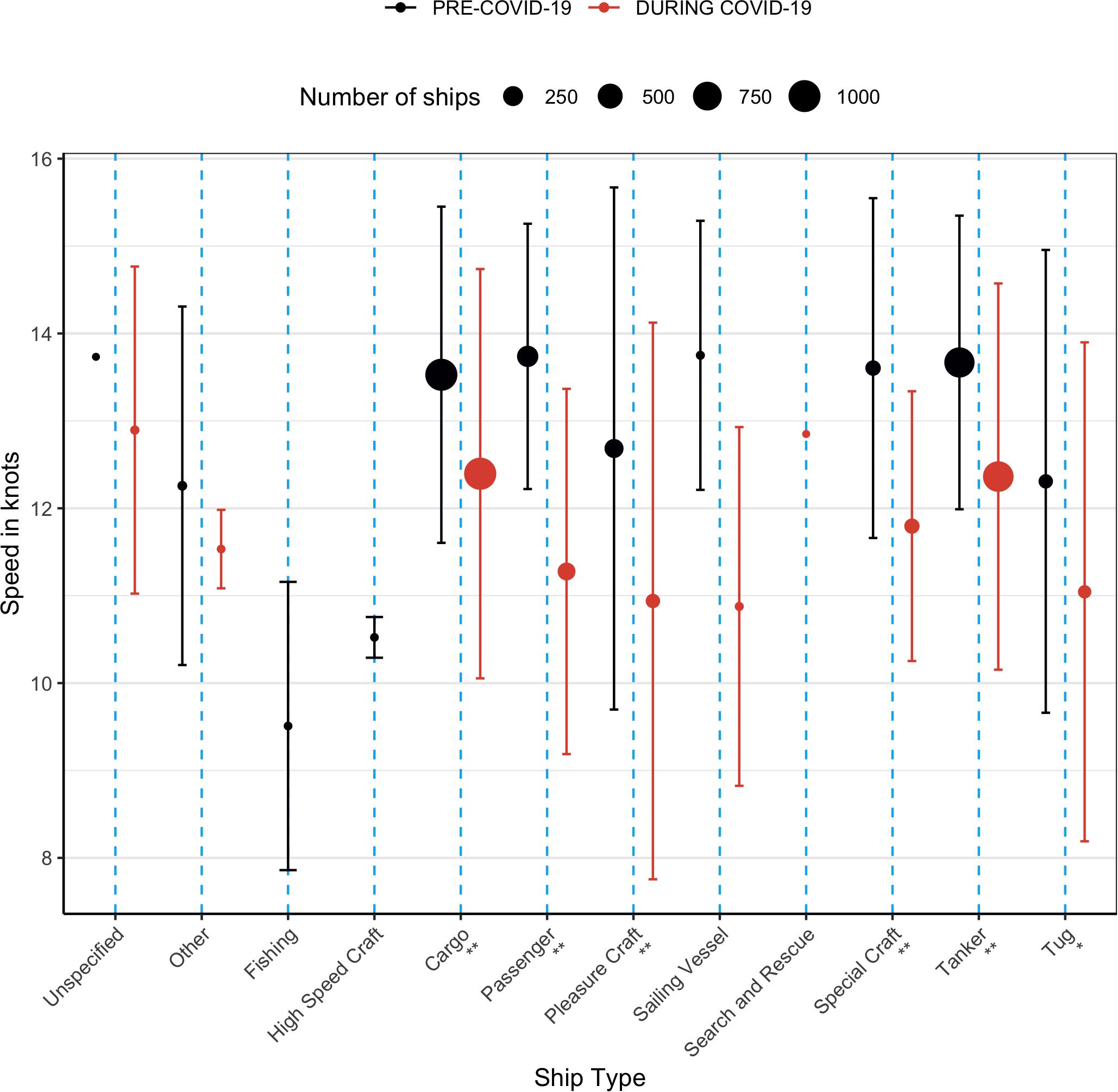
Figure 4. Speed by ship type, pre- and during COVID-19, with the size of the point scaled to represent the number of ships, error bars representing the standard deviation of the mean speeds and the p-value of the t-test between datasets (*p < 0.05; **p < 0.01) for each ship type. Sample sizes were not large enough for Unspecified and Other ships to be included. The ship type “Special Craft” is made up of a variety of types (e.g., fire fighting vessels, supply vessels and landing craft).
To further investigate potential changes in ship activity pre- and during COVID-19, we investigated the amount of time ships spent in the area before and during the pandemic. The amount of time was similar for all types of ship with three exceptions (Figure 5). “Passenger” ships (predominantly cruise ships) initially remained in the study area for longer durations during the beginning of the COVID-19 study period, but then the time in the area declined to pre-COVID levels (pre-COVID-19: median 4, range 1–30; during COVID-19: median 5, range 1–27). The time “Special Craft” (e.g., fire-fighting vessels, supply vessels, and landing craft) spent in the study area followed an opposite pattern, with increasing time spent in the area during the pandemic (pre-COVID-19: median 2, range 1–16; during COVID-19: range 1–17, median 3). The most predominant ship types, tankers and cargo ships, used the area in the same way before and during the pandemic, spending 2 days or less in the study area. Both October datasets were omitted from this analysis, as neither contained a complete record of the entire month’s activity.
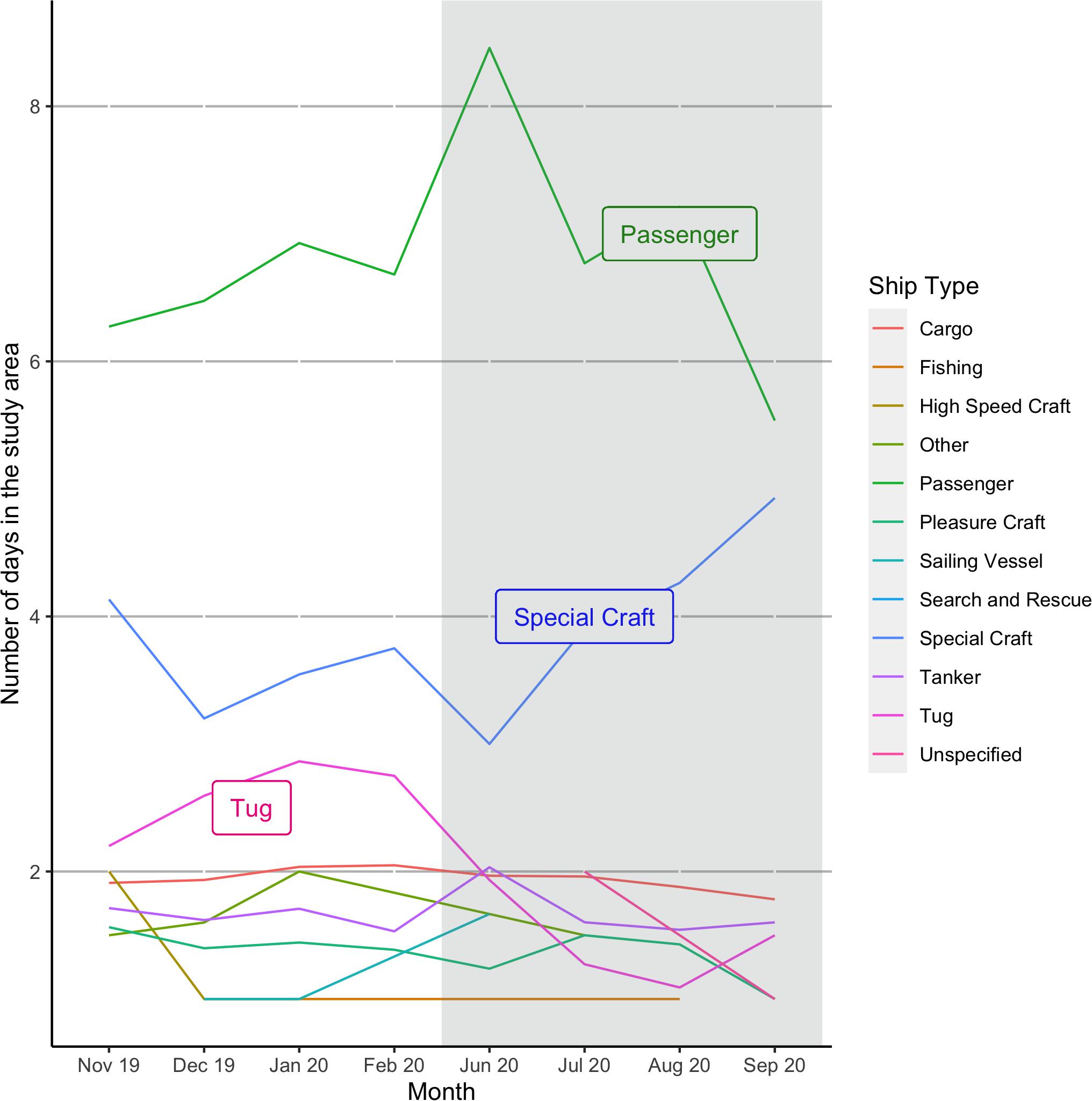
Figure 5. The number of days ship types were present in the study area pre- and during COVID-19 (shaded gray), with the three ship types that had changes in behavior labeled on their respective line.
Statistical Analysis
GLMs were fit to two different datasets. A GLM using CPA (log transformed) and speed from only the ship closest to the hydrophone during each 10-min recording period had a higher AIC than a GLM that used mean CPA (log transformed) and mean speed of all ships present during each recording period. Therefore, the dredge function was performed on the preferred model, i.e., the GLM using mean values. The best model kept all but the day/night variable and the mean CPA (log-transformed), and the remaining four variables were all significant. Six models had an ΔAIC less than 3 from the best model, and the results from these six models were averaged and weighted by each model’s Akaike weight. Model results indicate the most important variables (shown as the summed Akaike weights in Table 2) for predicting SPL were whether it was pre- or during the pandemic and mean ship speed. The positive relationship between ship speed and SPL shows that as ship speed increased, so too did SPL, as seen by the positive slope and standard error (Table 2).
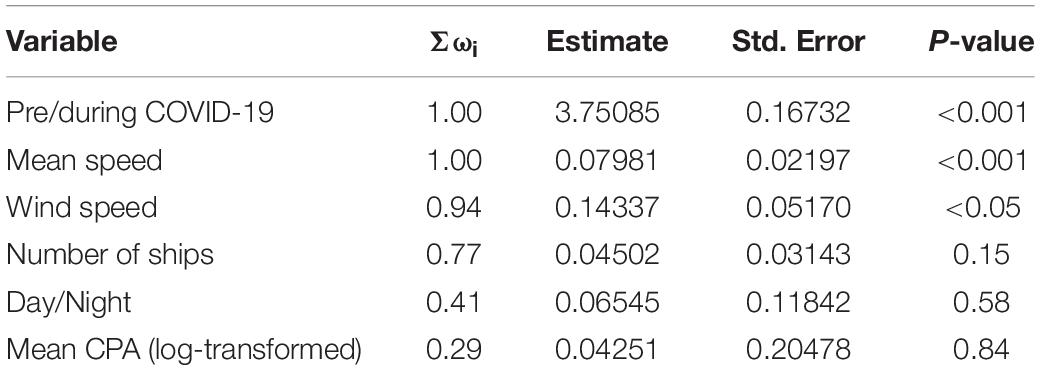
Table 2. Summed Akaike weights (Σωi), model averaged estimates and their standard errors and p-values for AIS and other data variables in the GLM model.
Discussion
The global spread of COVID-19 has had catastrophic impacts on the planet’s human inhabitants. The pandemic’s influence has been far reaching, dramatically altering lives, infrastructure and the fabric of societal norms. Global trade saw a steep downturn due to the pandemic, which in turn reduced and altered the nature of commercial shipping activity. Our study showed changes in commercial ship traffic in Northwest Providence Channel in The Bahamas during the pandemic and dramatically reduced ocean noise levels. After filtering for low wind speeds (because our interest is the effect of anthropogenic changes and not climatic variations during the pandemic), we found SPLs in the study area decreased by 3.98 dB which equates to a soundscape sound pressure reduction of 37% during the pandemic compared to the pre-COVID-19 period. Despite the opportunistic nature of the study [i.e., not a standard before-after-control-impact (BACI) study], we found robust evidence of concurrent changes in commercial shipping activity, most notably reduced vessel speeds and fewer ships using the area during the pandemic. However, our statistical analyses revealed vessel speed was the only ship-based variable that predicted SPLs, which has strong implications for management of ship activities in the area to reduce noise-related impacts on marine life.
In spite of the retrospective approach used in this study, its findings are consistent with other studies, implicating ship activities with changes in soundscapes. Globally ships have become the most widespread and predominant anthropogenic source of noise in the ocean (Erbe et al., 2019). Ship source levels are correlated with vessel speed (McKenna et al., 2012; Leaper, 2019; Ryan et al., 2021), but other characteristics such as vessel type, size, draft, and load can all affect noise emissions from vessels (MacGillivray et al., 2019). To determine the factors influencing reduced SPLs found in our study, we investigated the effect of vessel speed, the number of ships and their distance to the recorder, and explored changes in ship type, and the amount of time ships spent in the study area pre- and during COVID-19. We found a significant reduction in vessel speeds during the pandemic, and multiple lines of evidence suggested slower vessel speed was the most predominant cause of the reduced SPL. Not surprisingly, speed was also strongly correlated to SPL both pre- and during COVID. However, other changes in ship activity also have to be considered.
We documented several other changes in ship activity during the pandemic. For example, there were 335 fewer ships using the area, however, the proportion of cargo ships and tankers, which represented most of the ship traffic (>70%), remained the same during the two periods. Other types of ships using the area and the time ships remained in the study area changed, although only slightly. Voluntary slowdown studies have provided detailed evidence for ship source levels (SL) versus speed for differing vessel categories (Joy et al., 2019). In our study, all ship types slowed down during the pandemic, but we were unable to determine which types had the greatest impact. Although direct correlation of SPL with average ship speed is complex and beyond the limits of this study, our finding of a median speed reduction of 1.42 kts could result in a reduction in SPL of 3.98 dB (e.g., see MacGillivray et al., 2019; Leaper, 2019). Given this and the strong predictive power that speed had on SPL in our study, we believe that slower ship speeds during the pandemic was the predominant anthropogenic cause of a quieter soundscape in NPC.
The opportunistic nature of this study presented some analysis challenges and limited our ability to carry out analyses that would further strengthen our conclusions. Firstly, the hydrophone was intentionally placed at a shallow enough depth that allowed scuba deployment and retrieval, yet with proximity to heavy shipping traffic to investigate use of the area by sperm whales, and, as a secondary question, to determine if it was a grouper spawning aggregation site. As such, we were unable to carry out analysis conducted in other studies, e.g., pairing the acoustic data with individual contributors of noise such as ships, as seen in MacGillivray et al., 2019, where single vessels were isolated and matched to their acoustic data by rejecting recordings when there were other AIS vessels within “six times the measured CPA of the vessel of interest.” Similarly, Putland et al., 2017 rejected AIS vessels from their analyses that were not alone for at least 30 min. Most importantly, we were not able to calculate ship source levels. Extreme bathymetric changes in the study area (ranging from < 5 to > 4,000 m) suggest a complex acoustic environment, but we did not have a sound speed profile for the area, therefore we were not able to pair the hydrophone data with individual ships from the AIS data. However, the median CPA of ∼4 km for both datasets indicates most ships in this study were close enough to the hydrophone to account for the received SPLs but future work should include a sound propagation model.
Similarly, in situ environmental data must also be considered, particularly wind speed and wave height which both influence background sound (Thomson and Barclay, 2020). The data available to us for this study, however, was from a buoy 300 nm away from the recorder, and our datasets were seasonal. As expected, the winter months had higher winds, but the change in average windspeeds between the two periods was small and so was not expected to be a major driver of different sound levels. To limit wind effects we focused on comparing spectral sound levels in the 1/3 octave band from 111 to 140 Hz to avoid sensitivity to wind-generated distant noise (above 1 kHz, see Urick, 1983) and variation in acoustic propagation loss from surface reflection loss (Jones et al., 2009). After filtering out higher wind speeds (as our interest was changes in ship activity, not environmental), we showed a reduction in the SPL of the soundscape from only 41 to 37%. However, the statistical model still weighted the lower (filtered) wind speed as an important variable in predicting SPL. In situ data would better capture local conditions and its variability and should be included in future studies.
The presence or absence of smaller vessels could potentially have a significant influence on the NPC soundscape (see Hermannsen et al., 2019). Of specific concern during our study was that the acoustic recorder was located within a popular sport-fishing site used primarily by smaller vessels which do not possess AIS, and their presence was undocumented due to the opportunistic nature of this investigation. However, an extreme climatic event and the pandemic itself limited the number of non-AIS vessels in the study area. On the 1st September 2019 hurricane Dorian struck The Bahamas as a category 5 storm causing catastrophic damage to the northern Bahamas3. Ninety percent of boats and all marinas in the hurricane’s path were destroyed, drastically decreasing sport-fishing activity. The pandemic further slowed the recovery and rebuilding efforts from hurricane Dorian as the Bahamian government imposed stay-at-home orders and travel restrictions throughout the country. As such, small vessel presence in the study area is expected to have remained low during COVID-19 resulting in little change during the study period. For these reasons, we consider the effect of smaller non-AIS vessels on the observed soundscape sound reduction during the pandemic likely to be negligible, as supported by the GLM analyses which used day/night as a proxy for fishing activity. However, as the area recovers from hurricane Dorian and pandemic restrictions are lifted, future studies will have to consider the influence of non-AIS vessels on noise levels at this site.
Marine environments have seen a steep rise in the degree with which they are altered by anthropogenic influences in recent decades. Noise pollution being non-visible, but dramatic in its effects is of particular concern. Ocean sound is increasing by 3 dB per decade (Wenz, 1962; Andrew et al., 2002), and thus a 3.98 dB reduction in SPLs found in this study is a considerable one, especially when considering the short timeframe in which the change occurred. In a theoretical sense, an ocean environment which relatively quickly became 37% quieter during COVID-19, may have returned the study site to a soundscape that has not existed for over a decade. This dramatic reduction in sound may have had an impact on marine life (Putland et al., 2017; Duarte et al., 2021), broadening communication space and reducing masking effects. For example, the critically endangered and commercially valuable Nassau grouper (Sadovy et al., 2018; Waterhouse et al., 2020) produces pulse trains (mean peak frequency 77.4 ± 30.3 Hz) and tonal sounds (mean peak frequency 99.0 ± 33.6 Hz) for predator avoidance and reproduction (Scharer et al., 2012). The quieter soundscape in NPC during the pandemic has the potential to permit better transmission of alarm sounds to conspecifics, leading to reduced predation risk and higher reproductive success through improved communication during spawning aggregations. Slower vessel speeds are also correlated to reduced collision rates for large marine animals, such as sharks, turtles, and whales (Rowat et al., 2006; Hazel et al., 2007; Casale et al., 2010; Pierce and Norman, 2016) so fewer large animals may have been injured by ship strikes during the pandemic.
Adult female sperm whales are year-round inhabitants of NPC (Claridge et al., 2015) raising concerns about the impact of increasing commercial shipping traffic in the region on this local population and has been the focus of an on-going study to investigate management needs. The reduction in ship speed, number of ships and SPLs during the pandemic provides a unique opportunity to assess the effectiveness of strategies to mitigate impacts, such as slowing ships. These large whales (female length 11 m and weight 15 tons; Whitehead, 2003) produce 10 Hz–30 kHz sounds for communication and foraging (Watkins, 1977; Madsen et al., 2002; Mohl et al., 2003; Whitehead, 2003; Rendell and Whitehead, 2004) and have long surfacing intervals after foraging dives (Gordon et al., 1992). Ship traffic not only presents a threat of ship strikes to large whales, but shipping noise can elevate stress levels (Rolland et al., 2012) while vessel presence can alter surfacing behavior and respiration rates (Gordon et al., 1992). Fewer vessels in NPC during the COVID-19 period (12% less), combined with a quieter environment (37% quieter) and average vessel speed decrease (1.42 kts slower) may allow individual whales to better detect and avoid approaching vessels, reducing the risk of ship strike, especially for naïve calves that spend more time at, or close to, the surface than adults (Gordon et al., 1992). The reduction in sound levels was evident in all bandwidths, including within the frequency range of sperm-whale vocalizations, which will have resulted in increasing the communication space for sperm whales using the study area. One counter influence of ship speed reduction is an increase in vessel residency in the area which may increase the risk of collision for animals, although it is unknown if slower, quieter vessels present a greater collision threat (Erbe et al., 2019). Quantifying how the change in ship residency during the pandemic may have altered the risk of collision presents an interesting aspect for future research. However, maintaining slower ship speeds such as those recorded during the pandemic, but over longer periods could have positive population level effects, e.g., population growth for sperm whales and other marine life through increased survival and reproductive success. This study provides a unique opportunity for the investigation of the potential changes in sperm whale habitat use in the study area during the pandemic that could elucidate the extent to which changes in ship traffic activity impacted sperm whales in NPC, informing effective management directives.
Conclusion
Collecting persistent multi-sensor data such as AIS and acoustic data is greatly beneficial to studies of shifting environmental and anthropogenic change and can even drive policy and management directives, such as seen with the Port of Vancouver’s ECHO Program in which ships voluntarily slowdown. Critically, such data collection regimes provide data acquisition and quantifiable metrics for events that are unplanned or unforeseen, such as the COVID-19 outbreak. These circumstances, although lacking experimental design, allow insights into events that hitherto could not be studied such as impacts of this anthropause, providing a unique opportunity to test prospective management directives. This study found that a relatively small decrease in ship speed (<2 knot) during transit of NPC led to a 37% SPL reduction in the marine soundscape, a level that would not have existed for a decade. These opportunistically collected data provide baseline information for future mitigation measures for the management of shipping through Bahamian waters. Vessel speed reduction will need to consider the trade-off between source level reduction, time spent in a region and if a ship can travel at speeds slower than their operational speed (McKenna et al., 2012). However, effective mitigation such as routing traffic away from prime habitat and slowing ships down could help maintain biodiversity of marine life, increase their resilience to other anthropogenic stressors like climate change, and aid in the recovery of commercial fish stocks and other vulnerable, threatened or endangered species in NPC.
Given the dramatic COVID-related alteration to the soundscape in the study area, it is important to continue data collection and assess whether and for how long these changes will persist. The reduction in vessel speed was likely driven by changes in global consumer demand and the supply infrastructure. Reduced consumer demand and the closure of retail sectors left retailers with large inventories. Increased transit times, longer routes and stowing of excess inventory on slower moving vessels while waiting for markets to reopen benefits importers and retailers, allowing them to delay payment for products paid on delivery (Saul et al., 2020). With the global roll out of vaccination programs, financial markets and global trade is beginning to rebound, directly influencing shipping activity. It is conceivable that as economies endeavor to recover, shipping traffic may increase, potentially to levels beyond historic records. Continued monitoring of vessel activity and acoustic output will provide crucial information to determine if these documented changes are short-lived or not, and to what extent dramatic shifts in soundscapes over periods of months have beneficial implications for soundscapes and the inhabitants of these environments. Reductions in anthropogenic noise such as we describe, but implemented using shipping management initiatives, will aid ecosystem health. Despite the opportunistic nature of this study our findings are useful to inform policy makers and assist governance of the shipping industry to effect protection measures for the marine environment and also provide an important baseline for establishing an acoustic budget in Northwest Providence Channel.
Data Availability Statement
The raw data supporting the conclusions of this article will be made available by the authors, without undue reservation.
Author Contributions
CD and DC: funding acquisition and field work. CD, JT, LH, and DC: conceptualization. CD and JT: data analysis. LH: writing first draft. All authors contributed to the writing—review and editing process.
Funding
Funding for the purchase of SoundTrap hydrophones and associated field work came from Field School, Sabine and Reinhold Probst, M Rogers, The Devereux Ocean Foundation and The Bahamian Environment Protection Foundation.
Conflict of Interest
LH was employed by the company Open Ocean Consulting. JT volunteered with the company Ocean Environmental Consulting. Both authors freely donate their time as board members of the Bahamas Marine Mammal Research Organization.
The remaining authors declare that the research was conducted in the absence of any commercial or financial relationships that could be construed as a potential conflict of interest.
Publisher’s Note
All claims expressed in this article are solely those of the authors and do not necessarily represent those of their affiliated organizations, or those of the publisher, the editors and the reviewers. Any product that may be evaluated in this article, or claim that may be made by its manufacturer, is not guaranteed or endorsed by the publisher.
Acknowledgments
We would like to thank Stephanie Archer for advice with the data plots, Stacy De Ruiter for guidance with modeling, Luke Rendell for help with the AIS parser, and the following for assistance with the deployment and retrieval of hydrophones and visual surveys of sperm whales; Field School staff and volunteers, Thomas Brown, Bahamian students from University of the Bahamas and overseas, Sabine and Reinhold Probst, Mark Kopp and Kim Rody, Tim and Rebekah Higgs, Enie Hensel, Michael Sheratt, and Jeremy Pleydell-Bouverie, The Bahamian Environment Protection Foundation, and the Perry Institute for Marine Science.
Supplementary Material
The Supplementary Material for this article can be found online at: https://www.frontiersin.org/articles/10.3389/fmars.2021.673565/full#supplementary-material
Footnotes
- ^ https://www.ndbc.noaa.gov/wndav.shtml
- ^ www.marinetraffic.com
- ^ https://www.nhc.noaa.gov/archive/2019/DORIAN_graphics.php?product=5day_cone_with_line
References
Adams, A. J., and Murchie, K. J. (2015). Recreation fisheries as conservation tools for mangrove habitats. Am. Fish. Soc. Symp. 83, 43–56.
Andrew, R. K., Howe, B. M., Mercer, J. A., and Dzieciuch, M. A. (2002). Ocean ambient sound: comparing the 1960s with the 1990s for a receiver off the California coast. Acoust. Res. Lett Online 3, 65–70. doi: 10.1121/1.1461915
Bates, D., Machler, M., Bolker, B., and Walker, S. (2012). Fitting linear mixed-effects models using lme4. J. Stat. Softw. 67, 1–48.
Burnham, K. P., and Anderson, D. R. (2002). Information and Likelihood Theory: A Basis for Model Selection and Inference. In: Model Selection and Multimodel Inference: A Practical Approach. New York: Springer-Verlag, 49–79.
Casale, P., Affronte, M., Insacco, G., Freggi, D., Vallini, C., Pino d’Astore, P., et al. (2010). Sea turtle strandings reveal high anthropogenic mortality in Italian waters. Aquat. Conserv. Mar. Freshw. Ecosyst. 20, 611–620. doi: 10.1002/aqc.1133
Cholewiak, D., Clark, C. W., Ponirakis, D., Frankel, A., Hatch, L. T., Risch, D., et al. (2018). Communicating amidst the noise: modeling the aggregate influence of ambient and vessel noise on baleen whale communication space in a national marine sanctuary. Endang. Species Res. 36, 59–75. doi: 10.3354/esr00875
Claridge, D., Dunn, C., Ylitalo, G., Herman, D., Durban, J., and Parsons, K. (2015). Behavioral Ecology of Deep- diving Odontocetes in The Bahamas. SERDP Project Number: RC-2114 Bahamas Marine Mammal Research Organisation, NOAA Alaska Fisheries Science Center – Final Technical Report Vers. 2. Washington, DC: NOAA.
Clark, C. W., Ellison, W. T., Southall, B. L., Hatch, L., Van Parijs, S. M., Frankel, A., et al. (2009). Acoustic masking in marine ecosystems: intuitions, analysis, and implication. Mar. Ecol. Prog. Ser. 395, 201–222. doi: 10.3354/meps08402
Conn, P. B., and Silber, G. K. (2013). Vessel speed restrictions reduce risk of collision-related mortality for North Atlantic right whales. Ecosphere 4, 1–15.
Crum, N., Gowan, T., Krzystan, A., and Martin, J. (2019). Quantifying risk of whale–vessel collisions across space, time, and management policies. Ecosphere 10:e02713. doi: 10.1002/ecs2.2713
Duarte, C. M., Chapuis, L., and Collin, S. P. (2021). The soundscape of the Anthropocene ocean. Science 371;eaba4658. doi: 10.1126/science.aba4658
Durban, J. W., Parsons, K. M., Claridge, D. E., and Balcomb, K. C. (2000). Quantifying dolphin occupancy patterns. Mar. Mamm. Sci. 16, 825–828. doi: 10.1111/j.1748-7692.2000.tb00975.x
Erbe, C., Marley, S. A., Schoeman, R. P., Smith, J. N., Trigg, L. E., and Embling, C. B. (2019). The effects of ship noise on marine mammals-a review. Front. Mar. Sci. 6:606. doi: 10.3389/fmars.2019.00606
Erbe, C., Williams, R., Sandilands, D., and Ashe, E. (2014). Identifying modeled ship noise hotspots for marine mammals of canada’s pacific region. PLoS One 9:e89820. doi: 10.1371/journal.pone.0089820
Fearnbach, H., Durban, J., Parsons, K., and Claridge, D. (2012). Seasonality of calving and predation risk in bottlenose dolphins on Little Bahama Bank. Mar. Mamm. Sci. 28, 402–411. doi: 10.1111/j.1748-7692.2011.00481.x
Fedler, T. (2010). The economic impact of flats fishing in The Bahamas. Nassau: Bahamas Flats Fishing Alliance, 16.
Frisk, G. (2012). Noiseonomics: the relationship between ambient noise levels in the sea and global economic trends. Sci. Rep. 2:437. doi: 10.1038/srep00437
GEBCO (2020). General Bathymetric Chart of Oceans [online]. Available online at: www.gebco.net (accessed February, 2021).
Gende, S. M., Hendrix, A. N., Harris, K. R., Eichenlaub, B., Nielsen, J., and Pyare, S. (2011). A Bayesian approach for understanding the role of ship speed in whale–ship encounters. Ecol. Appl. 21, 2232–2240. doi: 10.1890/10-1965.1
Gillespie, D., Dunn, C., Gordon, J., Claridge, D., Embling, C., and Boyd, I. (2009). Field recordings of Gervais’ beaked whales Mesoplodon europaeus from the Bahamas. J. Acoust. Soc. Am. 125, 3428–3433. doi: 10.1121/1.3110832
Gordon, J., Leaper, R., Hartley, F. G., and Chappell, O. (1992). Effects of whale- watching vessels on the surface and underwater acoustic behaviour of sperm whales off Kaikoura, New Zealand. Conserv. Te Papa Atawhai. 48, 1–64.
Gössling, S., Scott, D., and Hall, M. C. (2021). Pandemics, tourism and global change: a rapid assessment of COVID-19. J. Sustain. Tour. 29, 1–20. doi: 10.1080/09669582.2020.1758708
Government of The Bahamas (2020). Available online at: https://www.bahamas.gov.bs (accessed February, 2021).
Hazel, J., Lawler, I., Marsh, H., and Robson, S. (2007). Vessel speed increases collision risk for the green turtle Chelonia mydas. Endang. Spec. Res. 3, 105–113. doi: 10.3354/esr003105
Hermannsen, L., Mikkelsen, L., Tougaard, J., Beedholm, K., Johnson, M., and Madsen, P. T. (2019). Recreational vessels without Automatic Identification System (AIS) dominate anthropogenic noise contributions to a shallow water soundscape. Sci. Rep. 9:15477. doi: 10.1038/s41598-019-51222-9
Herzing, D. L. (1997). The life history of free-ranging Atlantic spotted dolphins (Stenella frontalis): age classes, color phases and female reproduction. Mar. Mamm. Sci. 13, 576–595. doi: 10.1111/j.1748-7692.1997.tb00085.x
Hildebrand, J. A. (2009). Anthropogenic and natural sources of ambient noise in the ocean. Mar. Ecol. Prog. Ser. 395, 5–20. doi: 10.3354/meps08353
Jones, A., Sendt, J., Duncan, A. J., Clarke, Paul, A., and Maggi, A. (2009). Modelling the Acoustic Re-flection Loss at the Rough Ocean Surface. Acoustics. Sydney: Australian Acoustical Society.
Joy, R., Tollit, D., Wood, J., MacGillivray, A., Li, Z., Trounce, K., et al. (2019). Potential benefits of vessel slowdowns on endangered southern resident killer whales. Front. Mar. Sci. 6:344. doi: 10.3389/fmars.2019.00344
Joyce, T. W., Durban, J. W., Claridge, D. E., Dunn, C. A., Fearnbach, H., Parsons, K. M., et al. (2017). Physiological, morphological, and ecological tradeoffs influence vertical habitat use of deep-diving toothed-whales in the Bahamas. PLoS One 12:e0185113. doi: 10.1371/journal.pone.0185113
Leaper, R. (2019). The role of slower vessel speeds in reducing greenhouse gas emissions, underwater noise and collision risk to whales. Front. Mar. Sci. 6:505. doi: 10.3389/fmars.2019.00505
MacGillivray, A. O., Li, Z., Hannay, D. E., Trounce, K. B., and Robinson, O. M. (2019). Slowing deep-sea commercial vessels reduces underwater radiated noise. J. Acoust. Soc. Am. 146:340. doi: 10.1121/1.5116140
Madsen, P. T., Wahlberg, M., and Mohl, B. (2002). Male sperm whale (Physeter macrocephalus) acoustics in a high-latitude habitat: implications for echolocation and communication. Behav. Ecol. Sociobiol. 53, 31–41. doi: 10.1007/s00265-002-0548-1
McKenna, M., Wiggins, S., and Hildebrand, J. (2012). Relationship between container ship underwater noise levels and ship design, operational and oceanographic conditions. Sci. Rep. 3:1760. doi: 10.1038/srep01760
McKenna, M. F., Katz, S. L., Wiggins, S. M., Ross, D., and Hildebrand, J. A. (2012). A quieting ocean: unintended consequence of a fluctuating economy. J. Acoust. Soc. Am. 132, EL169–EL175. doi: 10.1121/1.4740225
Merchant, N. D., Pirotta, E., Barton, T. R., and Thompson, P. M. (2014). Monitoring ship noise to assess the impact of coastal developments on marine mammals. Mar. Pollut. Bull. 78, 85–89. doi: 10.1016/j.marpolbul.2013.10.058
Mohl, B., Wahlberg, M., and Madsen, P. (2003). The monopulsed nature of sperm whale clicks. J. Acoust. Soc. Am. 114:1143. doi: 10.1121/1.1586258
Pensieri, S., Bozzano, R., Nystuen, J. A., Anagnostou, E. N., Anagnostou, M. N., and Bechini, R. (2015). Underwater acoustic measurements to estimate wind and rainfall in the Mediterranean Sea. Adv. Meteorol. 2015:612512. doi: 10.1155/2015/612512
Pierce, S. J., and Norman, B. (2016). Rhincodon typus. The IUCN Red List of Threatened Species. e.T19488A2365291. Gland: IUCN.
Poon, L. L. M., and Peiris, M. (2020). Emergence of a novel human coronavirus threatening human health. Nat. Med. 26, 317–319. doi: 10.1038/s41591-020-0796-5
Putland, R. L., Merchant, N. D., Farcas, A., and Radford, C. A. (2017). Vessel noise cuts down communication space for vocalizing fish and marine mammals. Glob. Change Biol. 24, 1708–1721. doi: 10.1111/gcb.13996
R Core Team (2020). R: A Language and Environment for Statistical Computing. Vienna: R Foundation for Statistical Computing.
Rendell, L., and Whitehead, H. (2004). Do sperm whales share coda vocalizations? Insights into coda usage from acoustic size measurement. Anim. Behav. 67, 865–874. doi: 10.1016/j.anbehav.2003.04.018
Rockwood, R. C., Adams, J., Silber, G., and Jahncke, J. (2020). Estimating effectiveness of speed reduction measures for decreasing whale-strike mortality in a high risk region. Endang. Species Res. 43, 145–166. doi: 10.3354/est01056
Rodrigue, J., and Wang, G. W. Y. (2020). Cruise shipping supply chains and the impacts of disruptions: the case of the Caribbean. Res. Transp. Bus. Manag. 2020:100551. doi: 10.1016/j.rtbm.2020.100551
Rolland, R. M., Parks, S. E., Hunt, K. E., Castellote, M., Corkeron, P. J., Nowacek, D. P., et al. (2012). Evidence that ship noise increases stress in right whales. Proc. R. Soc. B. 279, 2363–2368. doi: 10.1098/rspb.2011.2429
Rowat, D., Meekan, M. G., Engelhardt, U., Pardigon, B., and Vely, M. (2006). Aggregations of juvenile whale sharks (Rhincodon typus) in the Gulf of Tadjoura, Djibouti. Environ. Biol. Fish. 80, 465–472. doi: 10.1007/s10641-006-9148-7
Ryan, J. P., Joseph, J. E., Margolina, T., Hatch, L. T., Azzara, A., Reyes, A., et al. (2021). Reduction of low-frequency vessel noise in monterey bay national marine sanctuary during the COVID-19 pandemic. Front. Mar. Sci. 8:656566. doi: 10.3389/fmars.2021.656566
Sadovy, Y., Aguilar-Perera, A., and Sosa-Cordero, E. (2018). Epinephelus Striatus: The IUCN Red List of Threatened Species. 2018. Report no. e.T7862A46909843. Gland: International Union for Conservation of Nature and Natural Resources.
Sarà, G., Dean, J., D’Amato, D., Buscaino, G., Oliveri, A., Genovese, S., et al. (2007). Effect of boat noise on the behaviour of bluefin tuna Thunnus thynnus in the Mediterranean Sea. Mar. Ecol. Prog. Ser. 331, 243–253. doi: 10.3354/meps331243
Saul, J., Baertlein, L., and Dowsett, S. (2020). Ocean Shipping Shrinks as Pandemic Pummels Retailers. Available online at: https://www.reuters.com/article/us-health-coronavirus-shipping-retail/ocean-shipping-shrinks-as-pandemic-pummels-retailers-idUSKBN23C0MS (accessed February, 2021).
Scharer, M. T., Rowell, T. J., Nemeth, M. I., and Appeldoorn, R. S. (2012). Sound production associated with reproductive behaviour of Nassau grouper Epinephelus striatus at spawning aggregations. Endang. Species Res. 19, 29–38. doi: 10.3354/esr00457
Sherman, K. D., Shultz, A. D., Dahlgren, C. P., Thomas, C., Brooks, E., Brooks, A., et al. (2018). Contemporary and emerging fisheries in The Bahamas - Conservation and management challenges, achievements and future directions. Fish. Manag. Ecol. 25, 319–331. doi: 10.1111/fme.12299
Stanley, J. A., Van Parijs, S. M., and Hatch, L. T. (2017). Underwater sound from vessel traffic reduces the effective communication range in Atlantic cod and haddock. Sci. Rep. 7:14633. doi: 10.1038/s41598-017-14743-9
Thomson, D. J. M., and Barclay, D. R. (2020). Real-time observations of the impact of COVID-19 on underwater noise. J. Acoust. Soc. Am. 147:3390. doi: 10.1121/10.0001271
van der Hoop, J. M., Vanderlaan, A. S. M., and Taggart, C. T. (2012). Absolute probability estimates of lethal vessel strikes to North Atlantic right whales in Roseway Basin, Scotian Shelf. Ecol. Appl. 22, 2021–2033. doi: 10.1890/11-1841.1
Vanderlaan, A. S., and Taggart, C. T. (2009). Efficacy of a voluntary area to be avoided to reduce risk of lethal vessel strikes to endangered whales. Conserv. Biol. 23, 1467–1474. doi: 10.1111/j.1523-1739.2009.01329.x
Waterhouse, L., Heppell, S. A., Pattengill-Semmens, C. V., McCoy, C., Bush, P., Johnson, B. C., et al. (2020). Recovery of critically endangered Nassau grouper (Epinephelus striatus) in the Cayman Islands following targeted conservation actions. Proc. Natl. Acad. Sci. U.S.A. 117, 1587–1595. doi: 10.1073/pnas.1917132117
Wenz, G. M. (1962). Acoustic ambient noise in the ocean: spectra and sources. J. Acoust. Soc. Am. 34, 1936–1956. doi: 10.1121/1.1909155
Whitehead, H. (2003). Sperm Whales – Social Evolution in the Ocean. London: The University of Chicago Press, Ltd.
World Health Organization [WHO] (2020). Coronavirus Disease (COVID-19) Outbreak. Available online at: https://www.who.int/emergencies/diseases/novel-coronavirus-2019/interactive-timeline/ (accessed February, 2021).
www.MiamiDade.Gov (2019). PortMiami Port Guide 2018-2019. Available online at: https://www.miamidade.gov/portmiami/library/stats-brochure-2019.pdf (accessed February, 2021).
Keywords: COVID-19, noise, sperm whales, ecosystem health, ship speed, marine mammal, shipping, automatic identification system
Citation: Dunn C, Theriault J, Hickmott L and Claridge D (2021) Slower Ship Speed in the Bahamas Due to COVID-19 Produces a Dramatic Reduction in Ocean Sound Levels. Front. Mar. Sci. 8:673565. doi: 10.3389/fmars.2021.673565
Received: 27 February 2021; Accepted: 07 June 2021;
Published: 26 July 2021.
Edited by:
Edward Urban, Scientific Committee on Oceanic Research, United StatesReviewed by:
Sofie Van Parijs, Northeast Fisheries Science Center (NOAA), United StatesWilliam Halliday, Wildlife Conservation Society, Canada
Copyright © 2021 Dunn, Theriault, Hickmott and Claridge. This is an open-access article distributed under the terms of the Creative Commons Attribution License (CC BY). The use, distribution or reproduction in other forums is permitted, provided the original author(s) and the copyright owner(s) are credited and that the original publication in this journal is cited, in accordance with accepted academic practice. No use, distribution or reproduction is permitted which does not comply with these terms.
*Correspondence: Charlotte Dunn, cdunn@bahamaswhales.org