- 1Center of Marine Sciences, CCMAR, University of Algarve, Faro, Portugal
- 2Oceana, Madrid, Spain
Identifying vulnerable marine habitats is fundamental to promote evidence-based management and a sustainable use of our oceans. Although progress in the mapping of marine benthic habitats has been made, complex ecosystems such as submarine canyons remain largely unexplored, hampering evidence-based management from taking place in these ubiquitous features worldwide. Data from a remotely operated vehicle (ROV) survey in the upper São Vicente Submarine Canyon in southwestern Portugal from 2011, was analysed to carry out a comprehensive assessment of megabenthic habitats, particularly those of conservation concern occuring between 90 and 560 m. Underwater videos from three exploratory dives, covering a total of 8.8 kilometers, were analyzed through multivariate and visual assessments, and the identified habitats were assessed against a set of criteria derived from current definitions of priority habitats. Thirteen potential priority habitats were characterized, out of which four distinct “coral gardens” and one “deep-sea sponge aggregation” are aligned with OSPAR definitions of priority habitats, and one corresponds to the habitat of a strictly protected sea urchin listed in the Habitats Directive. Three circalittoral sponge aggregations were also suggested based on the abundance of indicator species. The specific composition and diversity of priority habitats as described here, has not yet been described in any other canyon in the NE Atlantic region. Depth was revealed to be particularly important for the distribution of habitats, followed by relief and substratum. The approach presented in this study contributes to improve the understanding about submarine canyons, which is paramount to implement knowledge-based protection measures. Furthermore, it can be used to support future mapping efforts of deep-sea environments such as submarine canyons.
Introduction
Identifying and mapping benthic habitats is fundamental for a sustainable use of our oceans (Buhl-Mortensen et al., 2015). Nearshore habitats have been more extensively studied in comparison to offshore and deeper areas, mainly due to their easier access. Exploration at depths below the range of SCUBA divers was long restricted to soft substrata due to the constraints of traditional sampling tools (Joint Nature Conservation Committee (JNCC), 2018), limiting our knowledge about the deep-water communities colonizing hard substrata. It was not until the relatively recent development of modern tools such as remotely operated vehicles (ROVs), that we could start exploring more complex substrata at greater depths. Consequently, physiographically complex ecosystems, such as submarine canyons, are only just beginning to be unraveled (Fernandez-Arcaya et al., 2017).
Deep-water habitats characterized by ecosystem engineers (sensu Jones et al., 1994) such as corals and sponges (Buhl-Mortensen et al., 2010; Miller et al., 2012) have been increasingly studied and recognized as important (e.g., Freiwald et al., 2011; Beazley et al., 2013; Maldonado et al., 2016 among others). That has resulted in their incorporation into several regional legal frameworks, such as the OSPAR (Agreement 2008-7) and (Bern Convention, 1996), and into the global Deep-sea Fisheries Guidelines developed by the Food and Agriculture Organization (FAO) of the United Nations, which particularly targets ecosystems and habitats vulnerable to bottom-contact fishing gears (Food and Agriculture Organization (FAO), 2016). The physical structures created by sponges and corals provide shelter, feeding opportunities, settlement and nursery opportunities for other species (e.g., Bell, 2008; Marliave et al., 2009; Baillon et al., 2012; Folkers and Rombouts, 2020), enhancing their abundance and general biodiversity (Buhl-Mortensen et al., 2010; Beazley et al., 2013; Maldonado et al., 2016). Sponge and coral based habitats have also been shown to play an instrumental role in the benthic-pelagic coupling by providing a trophic link between the benthos and the overlying water column (e.g., Cathalot et al., 2015; Coppari et al., 2016). Recent studies revealed their significant role in the blue carbon budget, by acting as carbon sinks and immobilizing carbon in long-living structures (e.g., CoralFISH, 2013; Coppari et al., 2019). Altogether, these habitats seem to perform a fundamental role in supporting secondary production, ultimately leading to the provision of biomass (read: finfish and shellfish) for human consumption (CoralFISH, 2013). Lastly, their slow growth, late maturity and low chances of recovery when impacted (Althaus et al., 2009) are characteristics which call for their global precautionary protection.
Submarine canyon formations facilitate the occurrence of currents that intercept the otherwise dominating isobath-parallel currents on the shelf and slope (Jiang and Garwood, 1998; Matsumura and Hasumi, 2011). Consequently, these complex hydrodynamic and geomorphic systems are likely to enhance primary productivity and the benthic-pelagic coupling (Ryan et al., 2005), laying ground for the theory that canyons may act as hotspots for biomass production in the deep sea (De Leo et al., 2010; Vetter et al., 2010). The topographical complexity and heterogenous seascapes present within canyon systems provide environmental conditions for the establishment of a great diversity of megabenthic communities, often dominated by filter-feeding organisms such as sponges and corals (e.g., McClain and Barry, 2010; Miller et al., 2012; Davies et al., 2014; Pierdomenico et al., 2019). This suggests that they may benefit from the enhanced current exposure, and the specific sedimentation and microcurrent regimes located within canyons (Huvenne et al., 2011). It has furthermore been shown that habitats sustained by canyons attract and support sperm whales and a wide range of other cetaceans (e.g., Ruano et al., 2007; Claro et al., 2020), suggesting these ecosystems are also important for top predators. Priority habitats which have so far been reported to occur in submarine canyons include coral gardens, deep-sea sponge aggregations, Lophelia pertusa reefs and sea-pen and burrowing megafauna communities; the first three being listed as threatened/declining in OSPAR region IV (Agreement 2008-6), where the study area is found.
Exploitation of fishery resources on the continental shelf and slope is recognized as one of the main drivers of the degradation of vulnerable marine habitats (Ramirez-Llodra et al., 2011; Ramalho et al., 2017). As exploitation within canyon ecosystems has usually been hampered by the complex geomorphology, canyons can offer a last refuge for vulnerable species which are threatened by such activities (Huvenne et al., 2011). Additionally, they may potentially act as larval sources and aid in the recolonization of disturbed sites elsewhere on the continental margin (Vetter et al., 2010; Huvenne et al., 2011). Nonetheless, fishing pressure along the head and flanks of submarine canyons may be seriously threatening the integrity of these ecosystems (Paradis et al., 2017), as these marginal areas are preferential fishing targets in certain areas (Würtz, 2012; Martín et al., 2014). Collecting baseline information on habitats and their faunal composition, population structure and health status, is an essential step toward developing evidence-based and effective planning and management of the marine environment (Sardá et al., 2012; Rossi, 2013; Buhl-Mortensen et al., 2015). A broader knowledge of seabed habitats is also critical to develop comprehensive classification systems that support the production of seabed habitat maps that are comparable at regional and global scales. The European Nature Information System (EUNIS) is underdeveloped for canyon habitats and the southern European Atlantic region. Similarly, the OSPAR database poorly represents habitats on the Iberian Atlantic coast (OCEANA, 2011). This highlights the need for habitat descriptions to support evidence-based management in the region. Furthermore, quantitative studies are necessary for monitoring the status and impacts of anthropogenic activity, regardless of the protective measures taken.
The benthic assemblages of the São Vicente Canyon (SVC) off southwestern Portugal remained unexplored until an exploratory ROV survey took place in 2011, conducted by OCEANA in collaboration with CCMAR/MeshAtlantic (OCEANA, 2011). The present study aims to (1) identify all the megabenthic assemblages observed during the survey in 2011 that are listed as priority habitats by international conventions, or that potentially merit protection due to their suggested valuable ecosystem functions; (2) give a complete quantitative and qualitative characterization of these priority habitats; (3) explore the environmental variables influencing the distribution of priority habitats in the São Vicente Canyon.
Materials and Methods
Study Area
The São Vicente Submarine Canyon (SVC; Figure 1) is located off the southwestern coast of mainland Portugal and is one of the most prominent geomorphological features in the area, extending for over 120 km in length and 20 km in width (Vizcaino et al., 2005; Valadares et al., 2009). The semi-circular canyon head starts about 12 km off the southwestern tip of Portugal, at a depth around 70 m, and proceeds to cut deeply through the shelf and slope, creating a wide corridor between the Portuguese continental shelf and the Horseshoe abyssal plain at around 4,900 m depth. The canyon floor is dominated by complex hard substrata, and active sediment erosion and transport (Terrinha et al., 2009). Gullies, landslide headscarps and mass wasting deposits are common near the base of the canyon flanks, while soft substrata become increasingly dominating when moving further out on the flanks (Vizcaino et al., 2005; Terrinha et al., 2009). Close to the shore, near the head of the canyon, episodical wind-induced upwelling conditions are reported to occur during spring (Relvas and Barton, 2002). The main sources of sediment in the canyon are the dismantling of canyon flanks and the intersection of sediment fluxes generated by the Mediterranean Overflow Water (MOW; Serra et al., 2020).
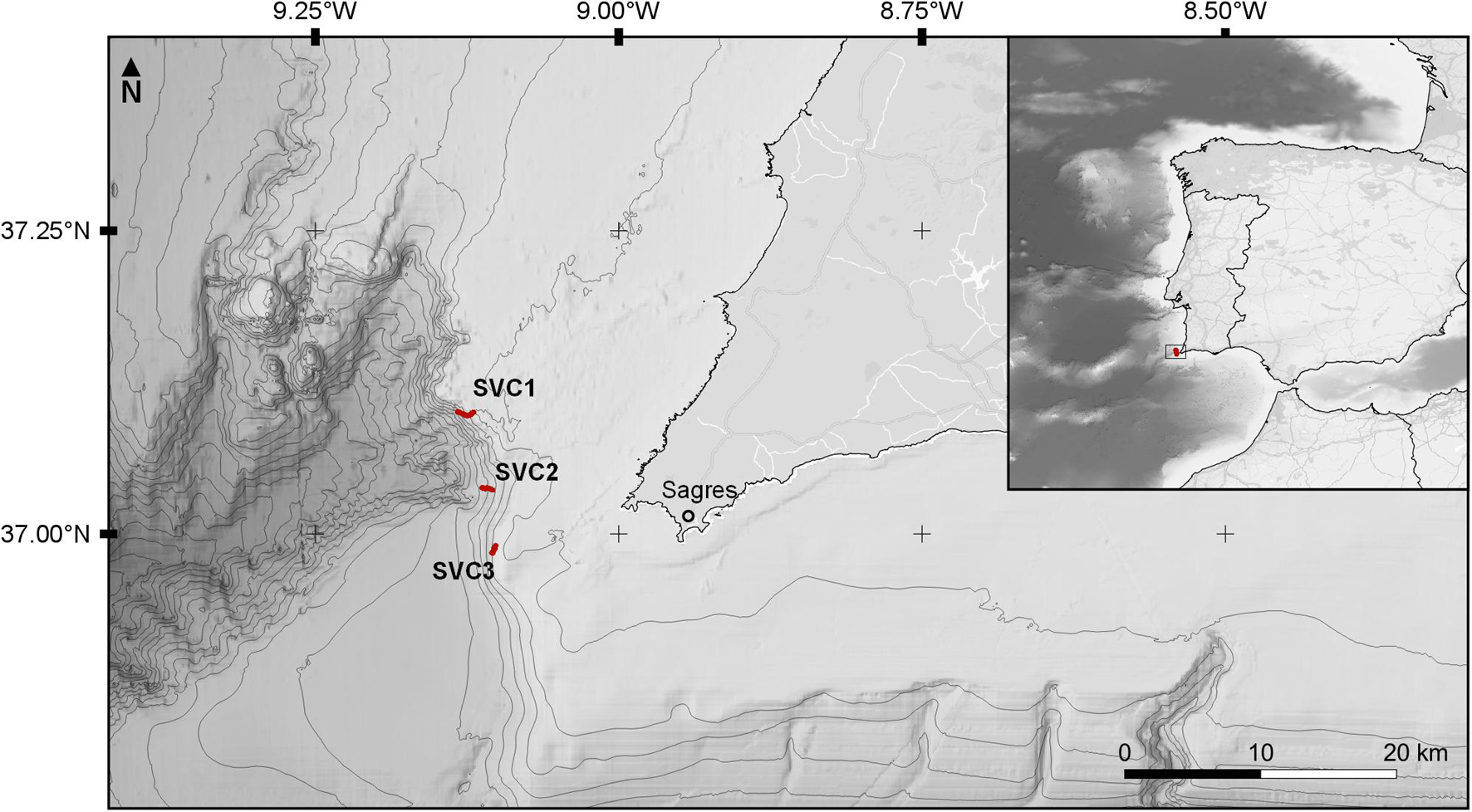
Figure 1. The location of the study area at the head of the São Vicente Submarine Canyon in the southwest of Portugal. The large map shows the location of the three transects located around the head of the canyon (SVC1, SVC2 and SVC3 respectively, from north to south). The map in the right corner shows the location on the Iberian Atlantic coast. Bathymetry lines are drawn for every 200 m; data was obtained from EMODnet Bathymetry.
While trawling in Portugal is legally restricted to grounds outside the six nautical mile buffer (∼11.1 km from the coastline), the study area in the upper SVC is located right off the line where trawling is permitted. Fishing effort within or near the SVC has not yet been quantified, however, Martín et al. (2014) found that the flanks of submarine canyons have been increasingly targeted by bottom-trawling fleets, as part of the general offshore expansion. The Iberian continental margin is reported to be one of the most impacted regions in Europe, with the south and south-west region off Portugal being the main target area for crustacean trawling fisheries in the region (Bueno-Pardo et al., 2017; Ramalho et al., 2017). The main trawling pressure in the area is exerted between 150–700 m (Monteiro et al., 2001; Bueno-Pardo et al., 2017). Long-line fisheries were shown to be the main source of litter in the upper canyon (Oliveira et al., 2015), but the area is also targeted by gill-netting, which is known to have an impact on coral gardens (Dias et al., 2020).
Survey and Data Collection
The data was obtained from three ROV exploratory dives at or near the canyon head in summer 2011, during a survey conducted by OCEANA in collaboration with CCMAR/MeshAtlantic on board of Oceana’s Ranger. The ROV (Saab Seaeye Falcon DR) was equipped with two forward-facing cameras; one standard definition (SD; 480 TV lines, 0.2 lux) and one secondary high-definition camera (HD; 1080i) which was equipped with zoom. The former was continuously recording the transects, while the latter was used to record high quality video in short sequences and to obtain detailed images of specimens considered of interest during the exploratory survey. The ROV was maintained at constant speed, at 0.2–0.3 knots, on a steady heading and close to the seafloor (approx. 50 cm) whenever possible. As there was no multibeam data available, sonar was used to aid in the navigation and, at times, directed the survey for potential complex features (e.g., strong sonar reflections). The average field of view of the ROV was estimated to be 1.75 m (Pardo et al., 2011), and was used to convert linear species densities to densities per m2 in this study. Sections with loss of bottom-view due to ROV maneuvres were considered off-bottom events and were excluded from the analysis and the total lengths of the transects. Navigation data was recorded with an Ultra Short BaseLine (USBL) system (Linkquest TN1510AH with an accuracy of up to 0.25°) and was post-processed and smoothed with a moving average.
Sampling Design
Each of the exploratory dives was regarded as a single continuous transect (SVC1, SVC2 and SVC3, Figure 1). All video records (SD and HD) were reviewed thoroughly. Using the tools available in the annotation software (e.g., pause, frame by frame) and aided by the HD video segments, all visible distinguishable organisms (>5 cm) within the field of view were identified to the lowest possible taxonomic level and counted along the transects. Experienced taxonomists were consulted and illustrated guides (Howell et al., 2017; Oliveira et al., 2017) were used for the identification of species present. Where species could not be inferred from the video material, organisms were given morphotype designations either at a higher taxonomic level, or according to morphologically distinctive traits such as shape and color (Althaus et al., 2013, 2015; Bullimore et al., 2013). For encrusting forms, where individuals are hardly distinguishable, the number of occurrences was used (pseudo-counts). The transects were furthermore continuously classified in terms of substratum type, relief and depth classes (10 m intervals). Following the contours of the canyon and the EUNIS classification, two biological zones were also defined for the study area; circalittoral (<200 m) and deep sea (>200 m; Gage and Tyler, 1991). Substratum types were classified using a simplified Folk scale following Connor et al. (2006), with five categories: Rock, Mixed sediments, Mud, Muddy sand and artificial substratum (fishing net). The CATAMI classification (Althaus et al., 2013) was further used to define the relief categories with an ordinal nature (1 = flat, 2 = low, 3 = moderate, 4 = high, and 5 = steep slope). Brief descriptions of the substratum and relief categories can be found in Supplementary Material 1. Species annotations were made using the COVER software (Carré, 2010) and complemented with manual entries as needed. Changes in substratum, depth class and relief were annotated using BORIS software (Friard and Gamba, 2016).
For the study of the benthic assemblages, and to have comparable units, transects were divided into 10 m long samples (after annotations were completed), following Bullimore et al. (2013). Samples in which a change of substratum type was recorded, that contained off-bottom events or that were < 10 m, were excluded from further analysis. Only megabenthic invertebrate species were considered in the community analysis, and only samples with, at least, one such observation were included. Each sample was therefore characterized by substratum type, relief, depth class, the megabenthic invertebrate fauna and the corresponding coordinates. Samples with more than one relief or depth class received merged classifications (e.g., moderate to high).
Data Analysis
Community Analysis
To identify the main benthic assemblages of the study area, Bray-Curtis similarity indices were calculated on the square-root transformed species abundance matrix, with the addition of a dummy variable to remove the effect of shared zeros (Clarke and Gorley, 2006). Cluster analysis with group-averaged linking was performed on the resulting similarity matrix, and SIMPROF (similarity profile analysis) tested the defined clusters for evidence of significant differences at a 95% confidence level, based on 1,000 permutations and 999 simulations (Clarke and Gorley, 2006). The characteristic species of each of the significantly distinct clusters were identified using the SIMPER (similarity percentage) routine; these were defined as those species with high similarity/standard deviation (Sim/SD) ratio (Clarke et al., 2014) and that contributed >3% to the cluster similarity (Ramos et al., 2016). A high Sim/SD ratio indicates that the species occurs consistently in a high proportion of the samples of the respective cluster. The term “assemblages” is hereafter used to describe the units of similar species composition produced by the cluster analysis. The analyses were performed using PRIMER v.6 (Plymouth Marine Laboratory) and R Studio was used for data handling (RStudio Team, 2020).
Identifying Priority Habitats
With the aim of assigning the resulting assemblages to “priority habitats,” as defined by the literature, agreements and guidelines provided by the OSPAR, FAO, and the EU Habitats Directive, assemblages were evaluated based on their characteristics, and grouped when representing similar priority habitats. To identify qualifying assemblages and to scale up to the “habitats” level, assemblages were assessed against the following set of criteria (i–v):
(i) At least one of the dominant and characteristic species of the assemblage must be recognized as a habitat-forming, vulnerable or protected species, and should preferably fit within any of the taxonomic groups listed as characteristic of priority habitats by OSPAR, FAO (Vulnerable Marine Ecosystem [VME] indicator species) or the EU Habitats Directive (OSPAR Agreement 2008-7; Food and Agriculture Organization (FAO), 2016; Council Directive 92/43/EEC). These species (hereafter “priority/indicator species”) must also have a high Sim/SD ratio.
(ii) If an assemblage consists of samples with a single species occurrence and these samples do not appear consecutively, it is not considered a meaningful assemblage in this study. Assemblages that met the first two criteria were considered assemblages of potential conservation concern and were considered in the further inspection (following criteria).
(iii) Occurrences or assemblages that clearly present dense prominent megafauna despite failing the first criterion, or that are only represented by a single sample, will also be considered and described, but their conservation concern is to be evaluated individually.
(iv) Assemblages that present similar habitat-forming indicator species and very similar traits are to be grouped into priority habitat types, facilitating interpretation and policy application (e.g., gorgonian gardens).
(v) As OSPAR defines that the minimum extent of priority habitats should be of at least 25 m2, a habitat (defined in iv) has to occur at least once in two consecutive [10 m] samples under video inspection (covering an estimated area of 35 m2) to qualify for the priority habitat designation. Habitats that were below this threshold but passed the other criteria, were still described in this study.
For the identification of “coral gardens” (OSPAR Agreement 2008-7), the threshold value of 0.1 individuals per m2 applied by Bullimore et al. (2013), was also adopted, if all the other criteria were met.
A characterization including species composition, densities and depth distribution was given for each of the habitat-forming assemblages, and brief qualitative descriptions of the communities and their environmental conditions were given at the broader habitat level. QGIS (QGIS Development Team, 2019) was used to map the distribution of the habitats and the recorded environmental variables along with the transects.
The Influence of Environmental Variables
The relationship between species composition and environmental variables was explored using DistLM (Distance-based Linear Modeling) combined with dbRDA (Distance-Based Redundancy Analysis). The DistLM procedure, using stepwise selection and 9,999 permutations, allowed to test for the significance of explanatory variables independently (marginal tests), and sequentially when added to the model one by one (sequential tests), giving a quantitative measure of the influence of each variable. The dbRDA was used to obtain an ordination of the fitted DistLM model (McArdle and Anderson, 2001). During these procedures, relief and the 10 m depth classes (using the maximum depth of each class) were treated as continuous variables (Anderson et al., 2008) while substratum type remained categorical. The environmental analyses were performed using PRIMER v.6 (Clarke and Gorley, 2006).
Results
One hundred and fifty-nine morphospecies were identified in total, out of which 111 were megabenthic invertebrates and thus included in the community analysis (a full list of identified morphospecies is available in Supplementary Material 2, including their recorded depth distributions). Forty-one of the megabenthic invertebrates were identified to species level. Overall, 409 samples (of 10 m) were considered valid for analysis, covering a length of 4.09 km, out of a total combined transect length of 8.82 km. The depth range covered was from 90 to 560 m (Table 1). A total of 257 samples were classified as belonging to the circalittoral zone (<200 m) and 152 samples were in the deep sea (>200 m). Rock was the dominant substratum type (52.4%), soft substrata (mud and muddy sand) represented 41.1% of the surveyed area, mixed sediments were less common (6.3%), and a large abandoned fishing net (0.2%) was also recorded (Figure 2). Substratum and relief maps of each transect are shown in Supplementary Material 3.

Table 1. Summary of the three remotely operated vehicle (ROV) recorded transects in São Vicente Canyon.
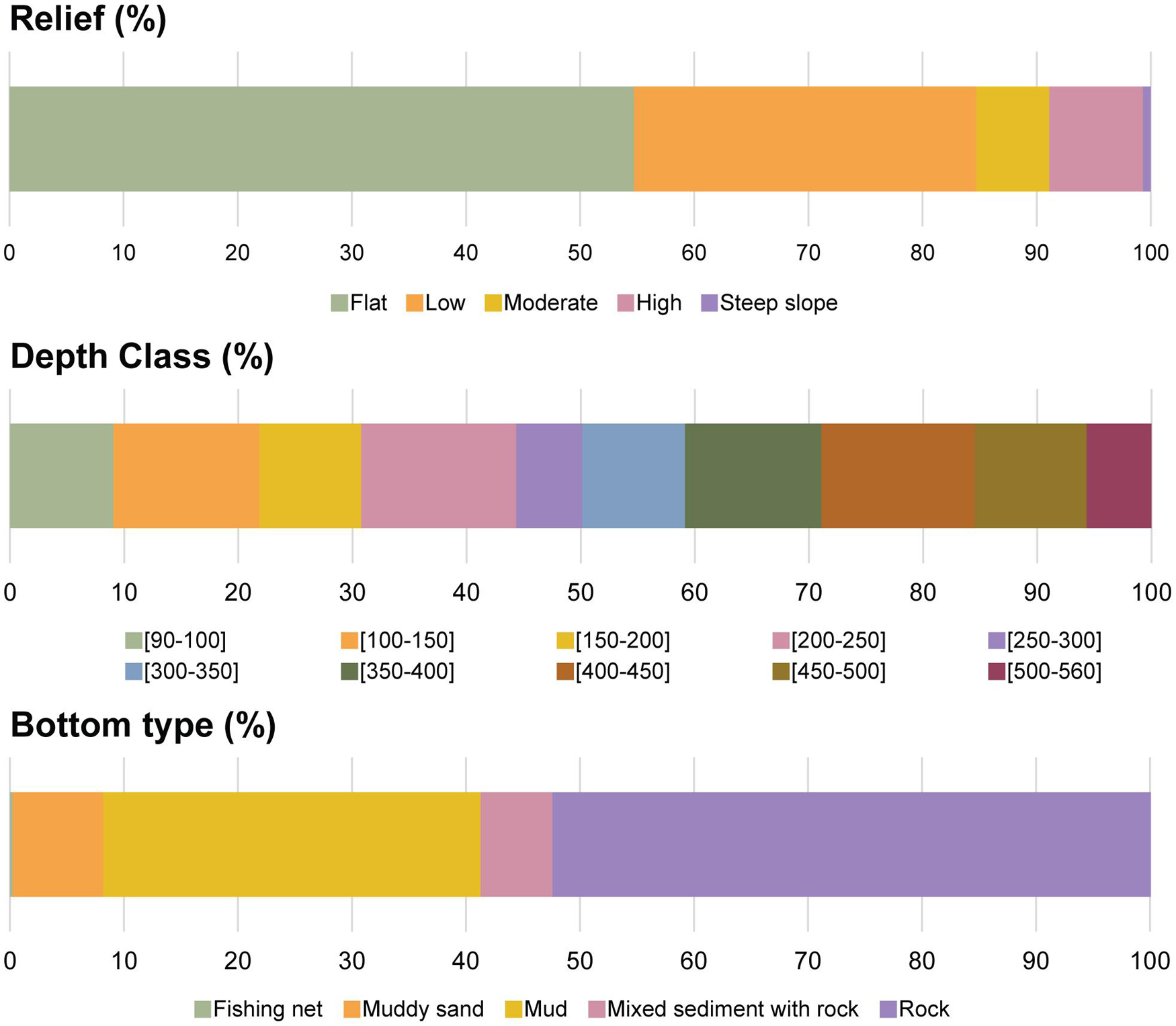
Figure 2. Percentage contribution of the relief, depth class and bottom type (substratum) categories in the surveyed area of the São Vicente Canyon.
Community Analysis and Evaluation of Criteria
The hierarchical cluster analysis resulted in the separation of 39 faunally distinct assemblages (SIMPROF p < 0.05; dashed lines, Figure 3), out of which 25 qualified as assemblages of potential conservation concern based on meeting the first two criteria and hence were considered for further evaluation. Two additional cases were included based on the third criterion, related to rare habitats (1) and to a prominent species of potential conservation interest (2). (1) One single sample, representing a clearly defined and dense sponge aggregation of Artemisina transiens, was not included into any of the significantly distinct assemblages resulting from the cluster analysis, but it was still included for the following evaluation since its exclusion would imply the loss of a clearly distinguishable and rare habitat (assigned SA10, Figure 3). It was also a better representation and denser aggregation of this habitat compared to assemblage SA9, which only covered the fringes of that respective habitat. (2) Three assemblages characterized by the crinoid Leptometra celtica met criterion (ii) but failed to meet criterion (i). These were still included for descriptions in this study because they are characterized by a species of potential interest (LC1, LC2 and LC3, Figure 3). Most of the dismissed assemblages were excluded based on failing the second criterion, as they were represented by very sparse and singular occurrences of species and did not correspond to relevant assemblages at the scale of this study.
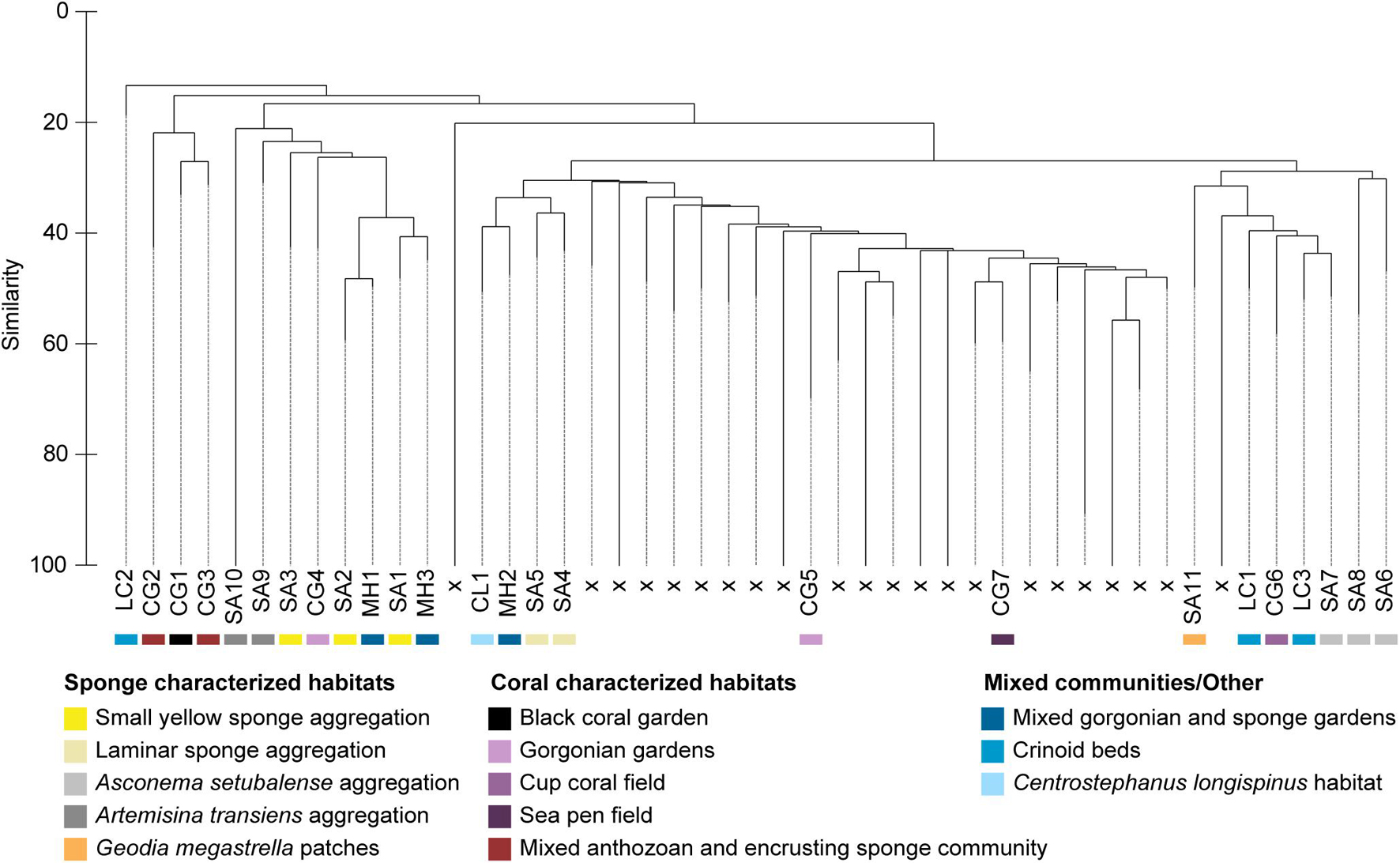
Figure 3. Cluster analysis of the species data with the inclusion of the SIMPROF routine. The 25 assemblages of potential conservation concern resulting from the first two criteria, are indicated with labels and a color code corresponding to the habitat to which they were assigned. Excluded assemblages are labeled “x” and were rejected based on failing criteria (i) and (ii) (dashed lines) or not resulting in significant assemblages according to SIMPROF (solid lines).
The characteristic species of the 25 assemblages (SIMPER routine), the mean density of priority indicator species and the extents and depth ranges of the assemblages are given in Tables 2–4, which separate between assemblages characterized and dominated by sponges (Table 2), corals (Table 3) and mixed communities/other habitats (Table 4). Additional details about each assemblage, including the estimated densities of each characteristic species [mean, standard deviation (SD), max. and min.] and their percentage contribution to SIMPER similarity, are provided in Supplementary Material 4. While merging and assigning assemblages to predefined priority habitats, the 25 assemblages of potential conservation concern were grouped into 13 habitats (Figure 3). Respective habitats are noted in the first column of the assemblages’ tables (Tables 2–4). Representative images of the habitats are included for reference, with their descriptive names captioned (Figure 4).
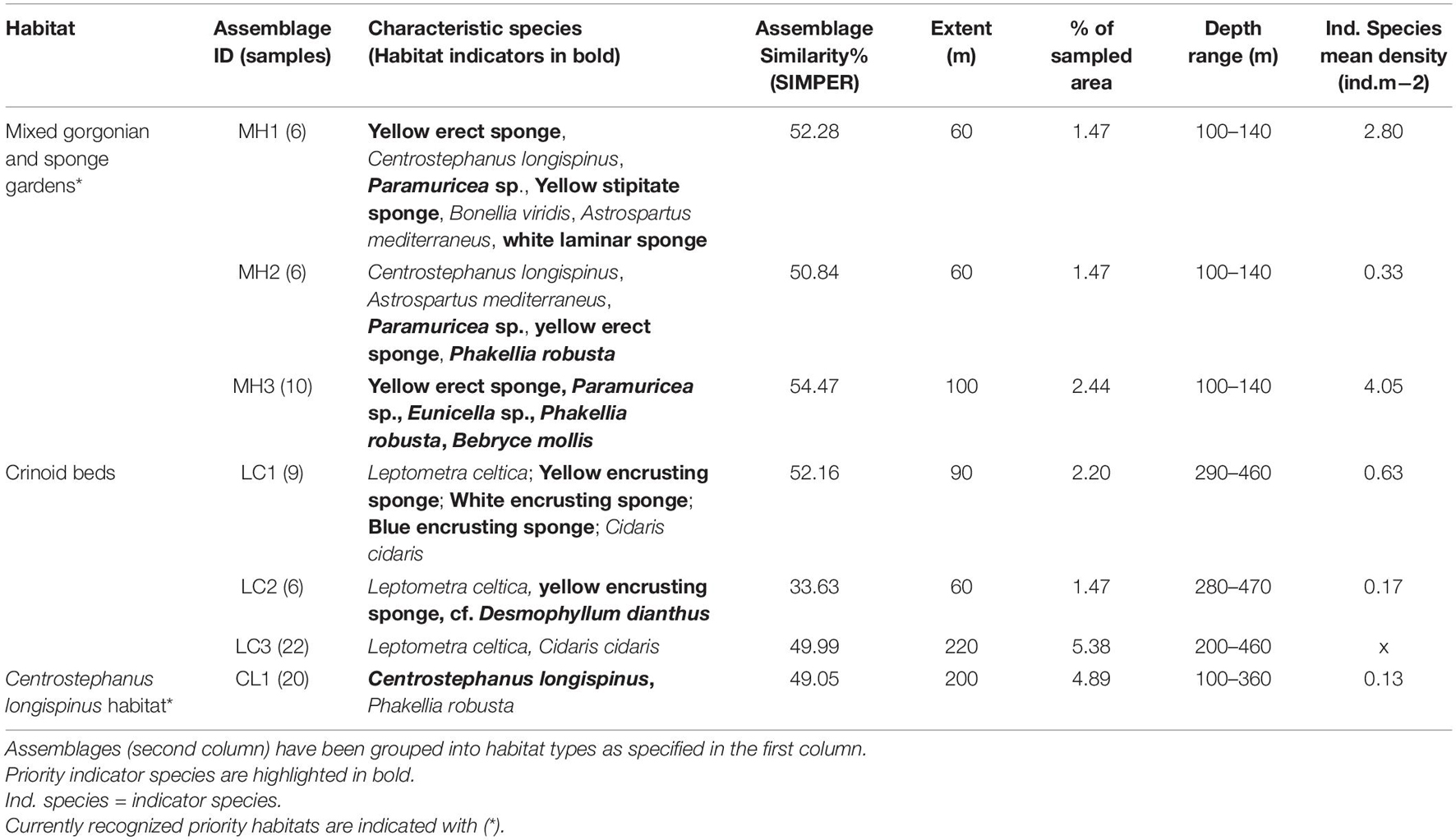
Table 4. Assemblages classified as mixed communities and other habitats of potential conservation concern in the São Vicente Submarine Canyon.
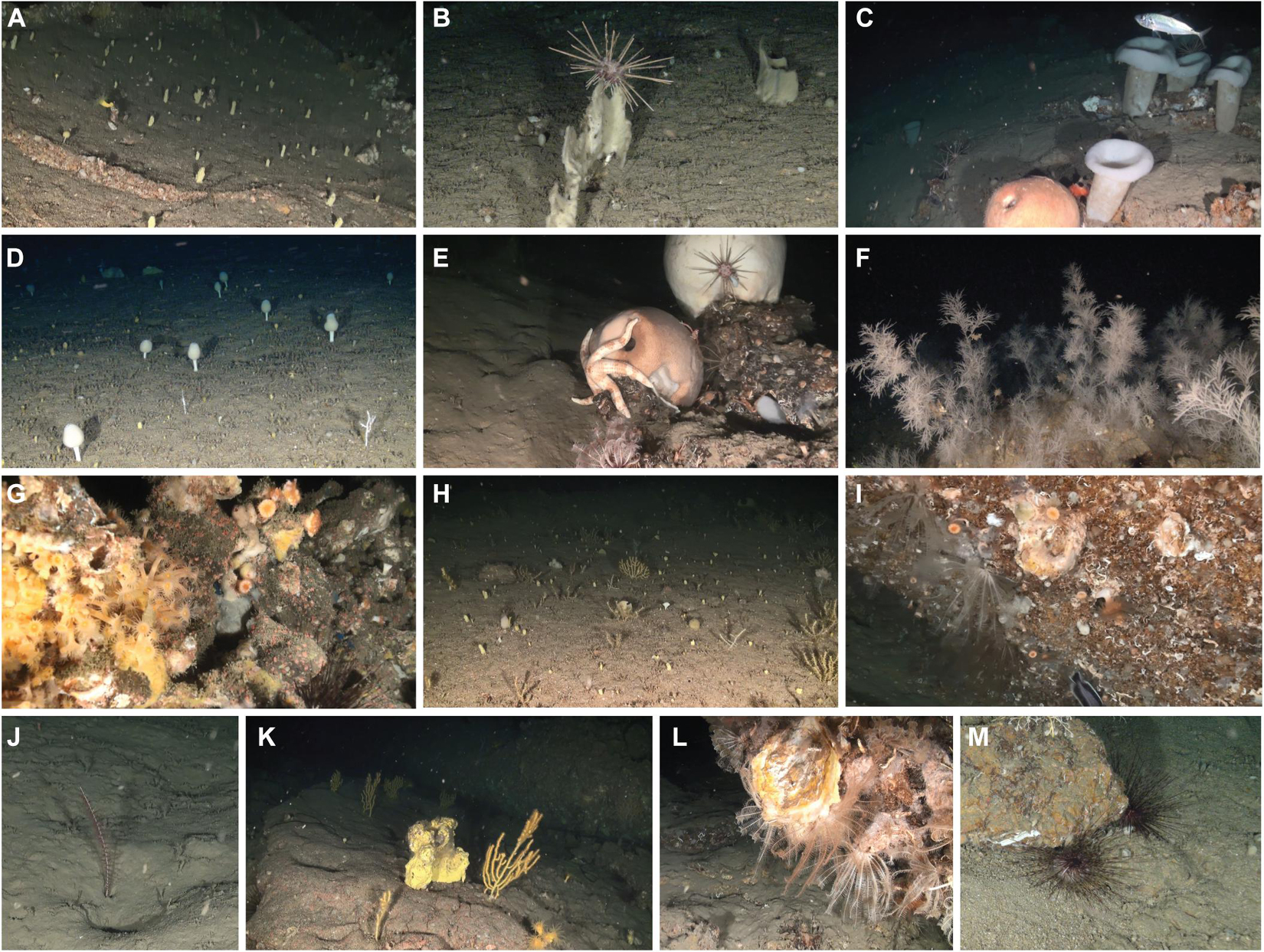
Figure 4. Representative images of the 13 habitats found in the São Vicente Submarine Canyon, identified as containing features of potential conservation interest. From left to right and top to bottom: (A) Small yellow sponge aggregation; (B) Laminar sponge aggregation; (C) Asconema setubalense aggregation; (D) Artemisina transiens aggregation; (E) Geodia megastrella patches; (F) Black coral garden; (G) Mixed anthozoan and encrusting sponge communities; (H) Gorgonian gardens; (I) Cup coral field; (J) Sea pen field; (K) Mixed gorgonian and sponge gardens; (L) Crinoid beds; (M) Centrostephanus longispinus habitat. Images obtained from the surveys conducted by OCEANA in collaboration with CCMAR/MeshAtlantic.
Descriptions of Priority and Potential Priority Habitats
Out of the 13 distinct habitats, 12 were dominated by habitat-forming, vulnerable or a strictly protected species, and one was dominated by a crinoid of particular interest. Six habitats qualified as priority habitats under current definitions and conservation measures established by OSPAR and the EU Habitats Directive and by following the criteria of this study, but several other habitats exhibited dense and prominent occurrences of megafauna with potential conservation value. A summary of the criteria and definitions met by each habitat is provided in Supplementary Material 5. Since samples were clustered based on species composition, the physical environment (substratum, relief and depth) of samples within an assemblage could vary. Brief descriptions of the habitats, their typical associated environmental variables, as well as their current priority status, are provided in this section.
At least three of the coral characterized habitats matched the OSPAR definition of “coral gardens” (black coral garden, mixed anthozoan and encrusting sponge community and gorgonian gardens); four if also counting the “mixed gorgonian and sponge gardens.” The four “coral gardens” occurred on circalittoral rock of varying relief, and together they occupied 17.6% of the total surveyed area.
The “black coral gardens” occurred on rocky bottoms of moderate to high reliefs and were dominated by the black coral Antipathella subpinnata, which formed dense and particularly large three-dimensional structures resembling forests, often obscuring the abundant encrusting fauna living on the rocky substratum below (Figure 4F).
The “mixed anthozoan and encrusting sponge communities” were composed by a mix of anthozoan and encrusting sponge species, densely covering the vertical walls of large boulders. Species such as Corallium rubrum, Dendrophyllia spp., Paramuricea sp., solitary cup-shaped scleractinians and dense aggregations of Parazoanthus axinellae, frequently occurred. The habitat presented the largest extent of the recorded “coral gardens,” occupying 8.3% of the total surveyed area (Figure 4G).
The “gorgonian gardens” were characterized by smaller gorgonians, identified as Bebryce mollis and Eunicella sp., and were most often recorded on flat to low relief rocky substratum covered by sediment veneer. Bebryce mollis often formed relatively dense aggregations within these habitats, while Eunicella sp. occurred more sparsely (Figure 4H).
The “mixed gorgonian and sponge gardens” were characterized and dominated by a combination of gorgonian and yellow erect sponge species, and were found mostly on low to moderate relief rocky substratum. The abundance of large, branched gorgonians identified as Paramuricea sp. varied within the habitat, and some areas presented more scattered colonies of lesser branched gorgonians and were co-dominated by echinoderms (Figure 4K).
The “cup coral field” was recorded in the deep-sea zone (>200 m) and was characterized by solitary cup-shaped scleractinians growing densely on rocky outcrops of low and moderate relief. The habitat was also occupied by a rich community of crustaceans, polychaetes and echinoderms, aside from the characterizing taxa revealed by SIMPER (Figure 4I). It classifies as a VME habitat type, but it did not qualify as a “de facto” priority habitat in this study as it failed the fifth criterion (not represented by two valid consecutive samples). It is, nevertheless, a small patch of indicator species of a recognized priority habitat (ICES, 2019).
The “sea pen field” was characterized by scattered and sparse occurrences of the sea pen Protoptilum carpenterii, and occurred solely on flat muddy substrata, most frequently between 430–560 m (with one exception at 200 m depth). The habitat is on the list of OSPAR priority habitats but failed the minimum extent criterion in this study, due to the very sparse occurrences (Figure 4J).
Five distinct sponge dominated habitats were identified, where one met the definition of the OSPAR priority habitat “deep-sea sponge aggregations” (Asconema setubalense aggregation), another deep-sea aggregation failed the minimum extension criterion (Geodia megastrella patches) and three habitats corresponded to circalittoral sponge aggregations and met all the criteria (small yellow sponge aggregation, laminar sponge aggregation and Artemisina transiens aggregation; <200 m depth, i.e., not considered deep-sea sponge aggregations). Together, the five sponge habitats covered 27.6% of the total surveyed area.
The Asconema setubalense aggregation was characterized and dominated by the conspicuous and structure-forming hexactinellid sponge Asconema setubalense. It typically occurred on rocky substrata of some relief, ranging from low to high. The substratum was often covered by sediment veneer, and the habitat frequently hosted various species of crustaceans, echinoderms and fish (Figure 4C).
Although the second candidate for this priority habitat designation, the “Geodia megastrella patches,” failed on the minimum extent criterion, sightings of this priority species were relatively common in the deep-sea of the SVC. The habitat was mostly recorded on slightly exposed bathyal rocky outcrops, but also in muddy substrata, and often in association with other species such as ophiuroids and crustaceans (Figure 4E).
The “small yellow sponge aggregation” was strongly characterized by small-sized yellow erect as well as stipitate sponges (possibly pertaining to the genera Axinella and Stylocordyla, respectively) and a laminar sponge identified as Phakellia robusta. The habitat often extended over large uninterrupted areas (accounting for 16.87% of the total surveyed area in this study), and typically occurred on flat to low relief rocky substrata. It often presented inclusions of other unidentified massive, caliculate and papillate sponges, as well as some anthozoans (Figure 4A). In some areas, aggregations of the oyster Neopycnodonte cochlear also occurred within this habitat.
The “laminar sponge aggregation” typically occurred near the aforementioned habitat, although it was dominated by regular occurrences of the laminar sponge Phakellia robusta, as well as some other relatively large, structure-forming unidentified sponge species, rather than the small-sized yellow sponges characteristic of the previous habitat (Figure 4B).
The Artemisina transiens aggregation was uniformly characterized by a single conspicuous sponge species, identified as Artemisina transiens, and clearly delimited to one specific area. The monotypic habitat occurred on flat rocky substrata covered with sediment veneer and presented high densities in its core area (5.4 ind. per m2), with only a few contributions of other taxa such as Phakellia robusta and anthozoans (Table 2). The inclusion of this habitat was based on the third criterion (a single sample of a priority habitat).
The study also revealed a habitat strongly characterized by the crinoid Leptometra celtica (fam. Antedonidae). Although it is not considered a priority species, its habitat has been included in this study due to its potential association with Essential Fish Habitats (Colloca et al., 2004), based on the third criterion. Moreover, the species frequently occurred on top of Neopycnodonte zibrowii deep-sea oyster aggregations on deep (>200 m) rocky outcrops, together with a rich community of encrusting sponges, solitary scleractinians (cf. Desmophyllum dianthus), echinoderms such as Cidaris cidaris, and many other unidentified encrusting biota (Figure 4L). Assemblages dominated by this species were found on rocky substrata of all classes of relief and on top of an abandoned fishing net.
The habitat of a strictly protected species of sea urchin (Centrostephanus longispinus) was identified in the SVC. It is cited in the Annex IV of the Habitats Directive, which states that “a strict protection regime must be applied across their entire natural range within the EU.” The habitat was furthermore characterized by sparse aggregations of laminar sponges, and was found between 90–360 m, although 98% of the species occurrences were recorded between 90–240 m. The species was mostly recorded on low relief rocky substrata covered by sediment veneer (Figure 4M).
Included in the figures below are the distribution maps of the habitats along the three transects (Figure 5) and a representation of their vertical depth distribution (Figure 6).
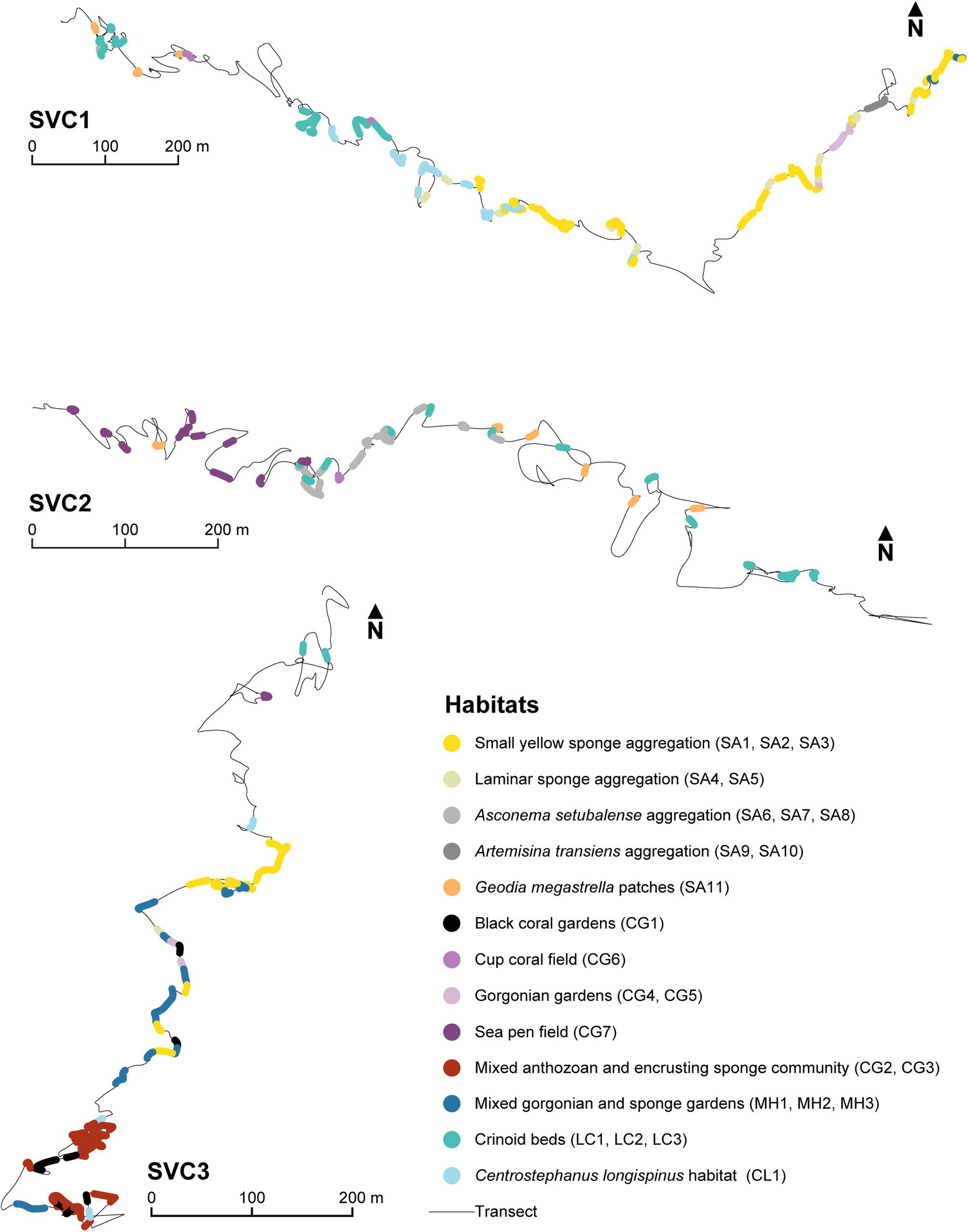
Figure 5. Habitat distribution maps of the 13 potential priority habitats, representing the 25 assemblages (IDs in parenthesis) within each study transect (SVC1, SVC2 and SVC3). Habitats are mapped with different colors as specified in the legend.
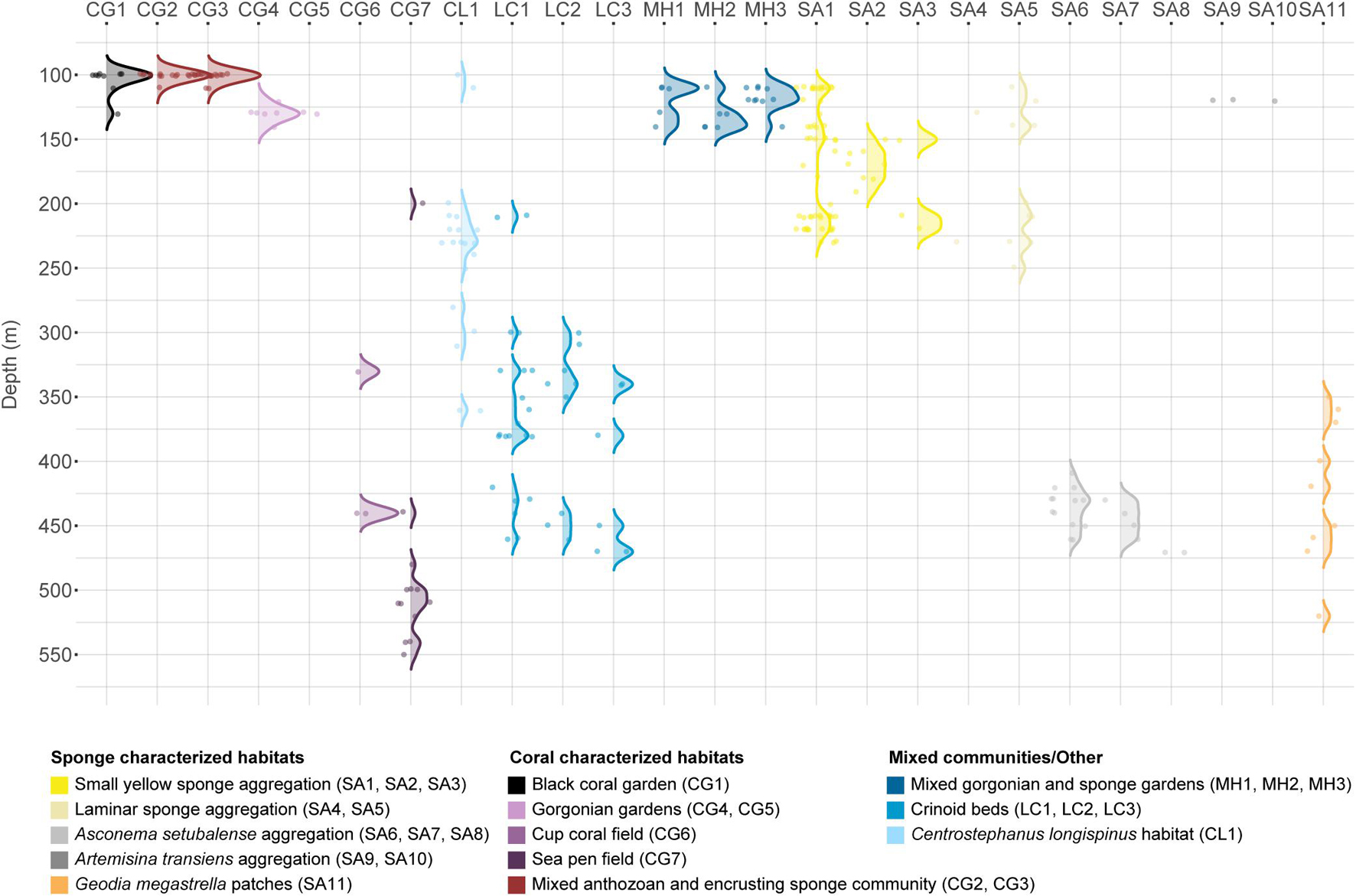
Figure 6. Ridgeline plot of the vertical distribution of assemblages grouped by habitat in the upper S. Vicente submarine canyon (Jittered points represent occurrences). Habitats with two or less data points are represented only by points (occurrences).
Influence of Environmental Drivers
The DistLM marginal tests revealed that substratum type, depth and relief were all significant factors that influence the species composition of habitats (p < 0.001). When tested individually, marginal tests revealed that depth explained the greatest part of the variance, at 13.2%, while relief and substratum explained 7.3 and 6.8%, respectively. Overall, the three environmental variables explained only 25.5% of the variation in the species data (adjusted-R2 = 0.237). When fitted sequentially, depth continued explaining the greatest part of the variance (13.2%), followed by relief (7.4%). Substratum added only 4.9% to the explained variation, once depth and relief had been fitted in the model.
A gradient existed for all three factors. Depth mainly explained the variation in species composition along the first axis, and relief and substratum mostly explained variation along the second axis. The relief gradient was associated with substratum types, with mud and muddy sand solely found on the “flat” and “low” relief end and only rocky substratum on the high relief end. A small gradient for substratum could also be observed along the first axis, potentially indicating some association with depth. The dbRDA plots displaying the ordination of the DistLM model are shown in Supplementary Material 6.
Discussion
The present study identified and characterized 13 relevant habitat types in the upper São Vicente Canyon (SVC), from which 12 were dominated by habitat-forming, vulnerable or a strictly protected species, and one was dominated by a crinoid of particular interest. A pre-existing description of main species (OCEANA, 2011) was now complemented by quantitative and multivariate approaches, providing new insights on species associations, densities and the environmental characteristics of these habitats. The six distinct priority habitats (i.e., OSPAR priority habitats and the strictly protected urchin) that were identified in this study of the upper SVC occupied 27.2% of the total sampled transect length; 48% if also counting the three circalittoral sponge aggregations. The variety and extent of coral and sponge habitats characterizing the upper SVC, suggests that they may sustain and likely enhance biodiversity and ecosystem function in the canyon (McClain and Barry, 2010), and potentially elsewhere on the continental margin (Vetter et al., 2010; Huvenne et al., 2011). A previous study in the nearby Portimão Submarine Canyon did not detect any coral or sponge species besides of tube-dwelling anemones from the genus Cerianthus (Morais et al., 2007), in the upper canyon. Following an exploratory dive in the deeper parts of the same canyon in 2011 (in Portimão), OCEANA reported on the occurrence of bathyal soft bottom communities dominated by the sea pen Kophobelemnon stelliferum. Another sea pen, Pennatula phosphorea, and the solitary scleractinians Flabellum chunii and Caryophyllia smithii var. clavus (the latter living free on the sediments), were also considered abundant in the community (OCEANA, 2011). Studies in other Portuguese canyons like the Nazaré, Cascais and Setúbal Canyons mainly focus on macrofaunal assemblages (Tyler et al., 2009; Cunha et al., 2011; Gomes-Pereira et al., 2015). A study of the Nazaré Canyon briefly reports on megafaunal occurrences, i.e., hard and soft corals, sea fans, anemones and sea urchins, on the steep sides of the upper canyon, while stating that megafauna are sparser in the middle and lower parts of the canyon (Tyler et al., 2009). Nevertheless, very few species are mentioned in the published study, and the assemblages are poorly described. In the upper Cascais Canyon, Gomes-Pereira et al. (2015) found soft-bottom burrowing communities dominated by Nephrops norvegicus, but epibenthic corals were absent (e.g., sea pens and other typical soft-bottom octocorals). To the best of our knowledge, the present study represents the most comprehensive assessment of priority megabenthic fauna in a Portuguese submarine canyon to date. Furthermore, the variety of priority habitats in the SVC appears to be substantial, even when compared to other canyons in the NE Atlantic and Mediterranean region (e.g., Morais et al., 2007; Louzao et al., 2009; Davies et al., 2015; Pierdomenico et al., 2016).
Coral Characterized Habitats
The four distinct habitats that qualified as “coral gardens” under the OSPAR definition (Agreement 2008-7) were represented by a diverse range of non-reef-forming corals (antipatharians, scleractinians, zoanthids and gorgonians and other alcyonaceans). Most habitats were characterized by a mix of coral species, although the dominating taxa varied clearly between the habitats, suggesting that the ecological functions sustained by each of the habitats are likely to differ (Buhl-Mortensen et al., 2010).
The four “coral gardens” detected in the circalittoral zone of the SVC (mixed anthozoan and encrusting sponge community, gorgonian gardens, black coral garden and mixed gorgonian and sponge gardens), between 90–140 m depth, show similarities with other habitats reported in the Mediterranean and NE Atlantic region, not exclusively in canyons. For instance, similar habitats to the gorgonian habitats and the “mixed anthozoan and encrusting sponge community” reported here, have been recorded in the SW Mediterranean (on the Seco de los Olivos Seamount) on similar substrata and depths (De la Torriente et al., 2018). The latter has also been recorded in the NE Atlantic (at the Hatton Bank, Rosemary Bank Seamount and the Wyville–Thomson Ridge), although at much greater depths (average depth 745 m) than in the SVC, and in association with reef-building corals (Howell et al., 2010). However, it has not previously been reported to occur in canyons. A gorgonian identified as belonging to the genus Paramuricea characterized many of the habitats in the SVC. Similar species from this genus have frequently been observed in the Mediterranean, including in canyons in the Ligurian Sea (Giusti et al., 2019). Particularly, the species P. macrospina and P. clavata are commonly reported on, and often in the same areas as Eunicella cavolinii (Fabri et al., 2014), E. verrucosa (De la Torriente et al., 2018), E. singularis, Acanthogorgia hirsuta, Swiftia pallida, Bebryce mollis (Grinyó et al., 2015) and Antipathella subpinnata (Bo et al., 2008), which could resemble some of the habitats observed in the SVC in association with Paramuricea. Although not dominant, several observations of the rare and conspicuous Atlantic-Mediterranean coral species Ellisella paraplexauroides (Angiolillo et al., 2012; Maldonado et al., 2013) were also made within the reported “coral gardens” in the SVC.
The antipatharian observed forms dense forests in the SVC. This species, Antipathella subpinnata, is known to host rich fish communities (Bo et al., 2008) and pertains to an order presenting outstanding maximum longevities, ranging from decades to millennia (Antipatharia; e.g., Wagner et al., 2012). The A. subpinnata has previously been recorded in a few canyons in the western Mediterranean (Fabri et al., 2014; Pierdomenico et al., 2016), but densities from canyons have not been reported. However, studies on A. subpinnata on other geomorphological features, suggest that the population in SVC [mean ∼1 per m2, max. 4.4] is comparable to the densest population on the Italian continental shelf, in Palermo, where 100 colonies were found covering 40 m2 [mean 2.5 per m2] (Bo et al., 2008), and to the densities of the monotypic garden reported on a deep-circalittoral hill top off an Azores island [max. 2.64 m2] (De Matos et al., 2014).
Gorgonians and antipatharians, both of which created important habitats in the SVC, are habitat-forming organisms that are particularly vulnerable to bottom-tending fishing gears and entanglement with lost fishing gear (Sampaio et al., 2012; Oliveira et al., 2015; Giusti et al., 2019; Dias et al., 2020). Such impacts may cause mortality or reduced growth rates and reproductive success (Bavestrello et al., 1997; Tsounis et al., 2012), and physical evidence of impacts may quickly disappear due to taxonomic degradation (Edinger and Sherwood, 2012). It has also been suggested that the histological traits of antipatharians may make them particularly vulnerable to sediment deposition (Wagner et al., 2012 and references therein) explaining why they are typically found in areas where the topography accelerates currents (e.g., Bo et al., 2008; Wagner et al., 2012; Pierdomenico et al., 2019), as occurs in the SVC. Significant changes in the sedimentation rates in the upper reaches of canyons have been revealed as a lateral effect of bottom trawling fisheries occurring along canyon flanks (Paradis et al., 2017). This underlines the importance of managing bottom-tending practices in areas where vulnerable species occur, including in the SVC.
The red coral Corallium rubrum dominated one of the identified habitats; the species is common in the Mediterranean and was reported to still occur along the southern Portuguese coast (OCEANA, 2011; Boavida et al., 2016) where it was thought to be commercially extinct since the early 18th century (da Silva Lopes, 1841). The species has been highly exploited to produce jewelry, and is currently considered endangered by the IUCN red list for the Mediterranean (Garrabou et al., 2015) and protected by a variety of legal frameworks (e.g., Bern Convention, Annex III; Habitats Directive, Annex V; Barcelona Convention; Portuguese National law). In the Atlantic, it is typically found between 60- and 100-meters depth (Boavida et al., 2016).
One of the two coral characterized habitats that were found in the deep sea (>200 m) of the SVC, was dominated by solitary cup-shaped scleractinians (Caryophyllidae), possibly Caryophyllia smithii var. clavus. Comparable habitats at similar densities have been recorded in several canyons and seamounts in the NE Atlantic (Howell et al., 2010; Bullimore et al., 2013; De la Torriente et al., 2018), as well as on continental slopes in the Mediterranean (Davies et al., 2017) where it has been proposed as a “coral garden biotope” (Bullimore et al., 2013; Davies et al., 2017). Following the criteria of this study, it did not qualify as a de facto priority habitat due to the required minimum extent, however, visual observations suggest that larger extensions of this habitat may occur in the SVC. The second deep-sea coral habitat, represented by sparse occurrences of the sea pen Protoptilum carpenterii on muddy substrata, did not reach the required minimum extent either, but it is described here as larger sea pen fields are considered priority habitats by OSPAR (Agreement 2008-7). This species is known to occur on soft substratum throughout a wide bathymetric range, between 400 and 4,270 m in the Atlantic Ocean (Mastrototaro et al., 2014), mostly supported by the findings in this study. In shallower muddy substrata of the SVC (100–120 m) Spinimuricea atlantica was also observed on several occasions.
Altogether, similar coral habitats to those reported here, have previously been described in other areas in the Atlantic-Mediterranean region, revealing the influence from Mediterranean outflow waters (Baringer and Price, 1997). Nevertheless, the specific composition and diversity of coral habitats characterized here has not yet been described in any other canyon in the NE Atlantic region. Furthermore, no match in literature could be found for the mixed habitats that were detected, characterized by a combination of yellow erect sponges and gorgonians such as Paramuricea sp., suggesting that the canyon may also host unique assemblages, possibly due to a mix of biogeographic influences and complex hydrodynamics.
Sponge Aggregations
International mechanisms aiming to protect the deep areas of the ocean (e.g., OSPAR) use the legal definition of the deep sea (>200 m) when associated to sponge aggregations, limiting the consideration of sponge aggregations in other ocean depth zones. However, relatively deep areas of the ocean, such as the lower circalittoral, also include vulnerable and relevant structuring species of sponges with potential interest for conservation; e.g., rare Artemisina transiens aggregations (Gubbay et al., 2016), which were recorded in the SVC, and aggregations of Axinella spp. (ICES, 2019) which were also observed here, and have been reported to exhibit exceptionally slow growth (Fowler and Laffoley, 1993; Hiscock, 2014). Further, optimal depth and density envelopes of certain species have been shown to differ in canyons, when compared to the shelf and slope, likely due to the differing hydrodynamics (Huvenne et al., 2011), requiring more flexibility in habitat definitions when identifying habitats of concern in the outer shelf. Circalittoral sponge aggregations are currently included under the “1,170 Reefs” habitat category of the EU Habitats Directive (Gubbay et al., 2016), for which the conservation status has been assessed as “unfavorable—inadequate” (European Environment Agency (EEA), 2012), offering a conservation incentive.
Deep-Sea Sponge Aggregations
The hexactinellid sponge Asconema setubalense dominated a clear and recurring “deep-sea sponge aggregation.” Habitats dominated by the species have previously been recorded on bathyal depths in several places in the North Atlantic and Mediterranean (e.g., Klitgaard and Tendal, 2004; Guillaumont et al., 2016; Maldonado et al., 2016; De la Torriente et al., 2019; Rodríguez-Basalo et al., 2021). Although the OSPAR definition of this priority habitat refers to occurrences of denser biological aggregations (4–5 per m2 for Hexactinellida and 0.5–1 per m2 for Demospongia), and the current methodology revealed lower mean densities for the indicator species in the SVC, the conservation value of the recorded habitat is not to be neglected. First of all, the OSPAR definition does not detail density criteria at the species level, although research has evidenced large differences in densities between species (e.g., Maldonado et al., 2016). Secondly, large intra-species variability may also occur as a result of environmental differences (e.g., Purser et al., 2013; Duckworth, 2016; Post et al., 2017; Davison et al., 2019). Additionally, very few detailed studies exist on this habitat characterized by A. setubalense, and most do not report on densities (e.g., Klitgaard and Tendal, 2004; Beazley et al., 2013; De la Torriente et al., 2019). This precludes an accurate habitat definition based on minimum densities and justifies highlighting this conspicuous habitat in the SVC. These specific sponge aggregations added substantial three-dimensional structure, and occasional close-up footage revealed crustaceans, echinoderms and fish associated with them, suggesting their physical role in supporting megafaunal assemblages. Furthermore, recent studies show that A. setubalense may reach its maximum size only after a century of growth (Prado et al., 2021), and due to its large structural capacity, the species is also considered a VME indicator for hard-bottom sponge aggregations, a VME habitat type (ICES, 2019).
The patchy habitat characterized and dominated by the deep-sea sponge Geodia megastrella did not qualify as a priority habitat due to the minimum extent criterion established by OSPAR. Yet, the habitats shaped by G. megastrella in the SVC often seemed to host other megabenthic species, and several species in the Geodia genus are listed as representative VME taxa of both soft- and hard-bottom sponge aggregations (ICES, 2019).
Circalittoral Sponge Aggregations
The circalittoral habitat characterized by yellow erect sponges, yellow stipitate sponges, the laminar sponge Phakellia robusta and Centrostephanus longispinus, that occurred over large sections of the surveyed circalittoral zone in the SVC (covering 16.9% of the total surveyed area in this study), appears to have no direct match in literature. This means that the species composition of this sponge aggregation is firstly described here. These multi-species aggregations may in part resemble the communities of other laminar sponge species (identified as Pachastrella monilifera and Poecillastra compressa) and urchins (such as Cidaris cidaris) reported for the Gioia canyon (Tyrrhenian Sea) and Le Danois Bank (Cantabrian Sea) (Pierdomenico et al., 2016; Sánchez et al., 2017), although the presence of the dominant yellow erect and stipitate sponges was not mentioned there. The communities reported in those regions are more similar to the laminar sponge aggregations observed in the SVC.
Species identification based on video material is difficult for some groups where taxonomy is uncertain without up-close and high-quality imagery (e.g., corals), and sometimes impossible without physical samples and microscopic examination of the skeleton (such as sponges). Although the species of the abundant small yellow erect sponges could not be identified with certainty, the morphology and distribution suggest the sponges could pertain to the genus Axinella (e.g., Fowler and Laffoley, 1993; Bo et al., 2008). Aside from the occasionally remarkably high densities of these sponges (yellow erect sponge, max. 13 per m2), their habitat also extended over relatively large areas; sometimes over 100 m without interruption, while covering a wide range of reliefs (flat to moderate). Hence, the potential ecological role of this habitat should not be neglected. This habitat was classified as pertaining to the circalittoral, as it was observed in large extensions at depths shallower than 200 m, but it was also recorded in the upper bathyal zone, with a depth range of 100–250 m. Per definition of biological zones (Howell, 2010), it could also be classified as a “deep-sea sponge aggregation.”
Another clearly distinct circalittoral sponge aggregation was mainly characterized by the conspicuous Artemisina transiens (Demospongiae), forming the most clearly delimited monotypic habitat that was recorded during the survey in this region. Ríos et al. (2018) described the first known aggregations of the species in the north of Spain; hence little is still known about its distribution. This observation in the SVC represents the first finding of such aggregations in a canyon. In the SVC, the habitat was recorded between 100–120 m, as previously reported by Ríos et al. (2018), supporting previous knowledge on the species (Topsent, 1890).
Other Habitats of Potential Conservation Concern
Several habitats were characterized and dominated by the strictly protected sea urchin Centrostephanus longispinus. The higher densities of the species were found in areas with rocky outcrops and some relief, often co-occurring in habitats dominated by sponges and corals, suggesting that these are also important habitats for the protected urchin species. Occurrences of the species were recorded from 90 to 360 m (with most records shallower than 240 m), even though literature suggests its bathymetric range extends from 5 to 208 m (Pawson and Miller, 1983; Templado and Moreno, 1996). Our findings could possibly indicate an extension of its bathymetric range in the SVC.
As an additional feature of interest, we describe a ubiquitous habitat in the deep sea, dominated by the crinoid Leptometra celtica (Antedonidae). Habitats colonized by crinoids from the Antedonidae family may serve as indicators of highly productive areas, where support is given to several commercial fish species at their juvenile and reproductive life stages (i.e., acting as an essential fish habitat; Colloca et al., 2004). In the SVC, aggregations and beds of L. celtica were often observed in association with other deep-sea habitats, and often on features such as Neopycnodonte zibrowii aggregations (deep sea oysters), that were inhabited by other encrusting and filter feeding organisms (e.g., sponges). Crinoid beds of the same species have also been observed on the soft bottoms of a deep sedimentary plateau (450–500 m) off the southern Portuguese coast, where the species was reported to occur near Norway lobster grounds (Fonseca et al., 2014). Sightings of the Norway lobster together with Eledone cirrhosa, the curled octopus, were also commonly recorded in the muddy substrata of the SVC. In some areas of the SVC, muddy substrata were in addition widely covered by the erect foraminifera Pelosina sp., possibly offering structure for smaller species on the seabed, such as polychaetes and other foraminifera (Buhl-Mortensen et al., 2010). Habitat provisioning by Neopycnodonte oysters in circalittoral and deep-sea environments has been demonstrated in previous studies (e.g., Johnson et al., 2013; Cardone et al., 2020), endorsing their potential conservation interest in the SVC.
Influence of Environmental Drivers on Habitat Distribution
Knowledge on the physical drivers for habitat distribution on the shelf and in the deep sea is relatively well established (Allee et al., 2000; Howell, 2010; Woolley et al., 2016), but knowledge on their relative importance in highly dynamic environments such as submarine canyons is still scarce and challenging to obtain (Fernandez-Arcaya et al., 2017; Pierdomenico et al., 2019). Our results suggest that depth, relief and substratum can be related to the distribution of megabenthic assemblages in the study area. The importance of depth as a driver for habitat distribution has previously been documented in other canyons (e.g., Davies et al., 2014; Pierdomenico et al., 2019), and can clearly be observed in the vertical depth distribution of habitats in the SVC. A relatively clear separation exists between habitats located in the circalittoral zone and in the deep sea, splitting the typical deep sea species (such as Asconema setubalense, Geodia megastrella, and Protoptilum carpenterii) from the circalittoral assemblages characterized by a range of gorgonians and laminar and smaller sized sponges. However, Pierdomenico et al. (2019) found that canyon geomorphology constitutes a more important factor than depth. Here, relief and substratum type explained a lower proportion of the variation. Yet, the preference for certain geomorphological features may be due to topographically-driven differences in exposure to water flow and microcurrents (McArthur et al., 2010), which in turn affect the exposure to sedimentation and food particle provisioning. Rocky surfaces coated by sediment often hosted biological assemblages distinct from bare rock. The differential association with substratum types found between habitats is largely controlled by the different attachment mechanisms of the dominant species (e.g., Miller et al., 2012). Biological traits such as reproduction strategies in concert with species specific adaptations, may also play a relevant role in the distribution of habitats (Coelho and Lasker, 2016; De la Torriente et al., 2018), but are beyond the scope of our analyses.
Conclusion
In total, 12 habitats characterized by a wide range of priority species and ecosystem engineers have been identified and described in this study of the São Vicente Canyon, all of which are highly sensitive to the impacts of bottom-fishing activities, and some are also threatened by changes in sedimentation regimes which can follow trawling on the canyon flanks. Although an assessment of the fishing effort in the area is currently not available, the study area is located in a highly productive and intensively fished region. Comparisons with other canyons in the Atlantic-Mediterranean region suggest that a noteworthy diversity of potential priority habitats, of sometimes unique composition, exists in the canyon. The 12 potential priority habitats characterized and dominated by corals and sponges accounted for 54.1% of the surveyed area (3,865 m2). They occurred on a variety of substratum types, reliefs and depths in the upper canyon, suggesting that their overall distribution area in the canyon may moreover be of substantial size. A widespread habitat dominated by crinoids was also identified in the deeper sections of the canyon. Several international agreements and instruments are calling for the protection of the recorded priority habitats (e.g., OSPAR, Habitats Directive, Bern Convention, FAO). The establishment of a SVC Marine Protected Area that accounts for the high diversity and patchy distribution of priority habitats should promptly be discussed, and the effects of sediment resuspension considered in the design. Further, this study provides support in understanding the drivers determining habitat distribution in submarine canyons, which is paramount to improve the mapping of this kind of environments through predictive habitat modeling.
Data Availability Statement
The original contributions presented in the study are included in the article/Supplementary Material, further inquiries can be directed to the corresponding author/s.
Author Contributions
JMSG, RA, BHC, and FO conceived the study. BHC, FO, PM, LB, and JMSG defined the data analyses. FO, NH, PM, RA, and I-MN identified the species in the video. I-MN analyzed the data and wrote the manuscript with BHC, FO, and JMSG. All authors reviewed the manuscript and agreed with its content.
Funding
This study received funding from the MeshAtlantic project (UE INTERREG AA 2.2 2009-1/110) and from FCT—Foundation for Science and Technology through the strategic project to CCMAR—UIDB/04326/2020. BHC was supported by national funds through FCT—Foundation for Science and Technology, I.P., in agreement with University of Algarve, in the scope of Norma Transitória DL57/2016/CP1361/CT0038. FO was supported by project HABMAR (grant no. MAR-01.04.02-FEAMP-0018) and co-financed by the European Maritime and Fisheries Fund (EMFF) of the Operational Program MAR 2020 for Portugal (Portugal 2020). NH was supported by the Ph.D. research fellowship ref. nr: 2020.05583.BD from the FCT—Foundation for Science and Technology, I.P.
Conflict of Interest
The authors declare that the research was conducted in the absence of any commercial or financial relationships that could be construed as a potential conflict of interest.
The reviewer FT declared a past co-authorship with one of the authors BHC to the handling editor.
Publisher’s Note
All claims expressed in this article are solely those of the authors and do not necessarily represent those of their affiliated organizations, or those of the publisher, the editors and the reviewers. Any product that may be evaluated in this article, or claim that may be made by its manufacturer, is not guaranteed or endorsed by the publisher.
Acknowledgments
We would like to acknowledge the OCEANA NGO for inviting CCMAR to join its scientific expeditions in the eastern Atlantic. We would like to thank Helmut Zibrowius for the all the support in the identification of Scleractinia, as far as the collected video material allowed. We would also like to thank the two reviewers and the editor for their constructive criticisms and insightful comments that helped improve the manuscript.
Supplementary Material
The Supplementary Material for this article can be found online at: https://www.frontiersin.org/articles/10.3389/fmars.2021.672850/full#supplementary-material
References
Allee, R. J., Dethier, M., Brown, D., Deegan, L., Glenn, R., Thomas, F., et al. (2000). Marine and Estuarine Ecosystem and Habitat Classification. NOAA Technical Memorandum NMFS-F/SPO-43. Washington, DC: National Oceanic and Atmospheric Administration, 1–43.
Althaus, F., Hill, N. A., Edwards, L., and Ferrari, R. (2013). CATAMI Classification Scheme for Scoring Marine Biota and Substrata in Underwater Imagery – A Pictorial Guide to the Collaborative and Annotation Tools for Analysis of Marine Imagery and Video (CATAMI) Classification Scheme, Vol. 1. Canberra, ACT: National Environmental Research Program, Marine Biodiversity Hub, 1–17.
Althaus, F., Hill, N., Ferrari, R., Edwards, L., Przeslawski, R., Schönberg, C. H., et al. (2015). A standardised vocabulary for identifying benthic biota and substrata from underwater imagery: the CATAMI classification scheme. PLoS One 10:e0141039. doi: 10.1371/journal.pone.0141039
Althaus, F., Williams, A., Schlacher, T., Kloser, R., Green, M., Barker, B., et al. (2009). Impacts of bottom trawling on deep-coral ecosystems of seamounts are long-lasting. Mar. Ecol. Prog. Ser. 397, 279–294. doi: 10.3354/meps08248
Anderson, M., Gorley, R. N., and Clarke, K. R. (2008). PERMANOVA + for PRIMER User Manual, Vol. 1. Plymouth: PRIMER-E Ltd, 1–218.
Angiolillo, M., Bo, M., Bavestrello, G., Giusti, M., Salvati, E., and Canese, S. (2012). Record of ellisella paraplexauroides (Anthozoa: Alcyonacea: Ellisellidae) in Italian waters (Mediterranean Sea). Mar. Biodivers. Rec. 5:E4. doi: 10.1017/S1755267211000972
Baillon, S., Hamel, J. F., Wareham, V. E., and Mercier, A. (2012). Deep cold-water corals as nurseries for fish larvae. Front. Ecol. Environ. 10, 351–356. doi: 10.1890/120022
Baringer, M. O. N., and Price, J. F. (1997). J. Phys. Oceanogr. 27, 1654–1677. doi: 10.1175/1520-04851997027<1654:MASOTM<2.0.CO;2
Bavestrello, G., Cerrano, C., Zanzi, D., and Cattaneo-Vietti, R. (1997). Damage by fishing activities to the Gorgonian coral Paramuricea clavata in the Ligurian Sea. Aquatic Conserv.Mar. Freshw. Ecosyst. 7, 253–262. doi: 10.1002/(SICI)1099-0755(199709)7:33.0.CO
Beazley, L. I., Kenchington, E. L., Murillo, F. J., Sacau, M., and del, M. (2013). Deep-sea sponge grounds enhance diversity and abundance of epibenthic megafauna in the Northwest Atlantic. ICES J. Mar. Sci. 70, 1471–1490. doi: 10.1093/icesjms/fst124
Bell, J. J. (2008). The functional roles of marine sponges. Estuar. Coast. Shelf Sci. 79, 341–353. doi: 10.1016/j.ecss.2008.05.002
Bern Convention (1996). Convention on the Conservation of European Wildlife and Natural Habitats. Standing Committee. Resolution No. 4 Listing Endangered Natural Habitats Requiring Specific Conservation Measures Adopted By the Standing Committee of the Bern Convention on the Conservation of European Wildlife and Natural Habitats (19 September 1979): 1982–96. Strasbourg: Council of Europe Publishing.
Bern Convention on the Conservation of European Wildlife and Natural Habitats Standing Committee (1996). Bern Convention on the Conservation of European Wildlife and Natural Habitats Standing Committee.
Bo, M., Tazioli, S., Spanò, N., and Bavestrello, G. (2008). Antipathella subpinnata (Antipatharia, Myriopathidae) in Italian seas. Ital. J. Zool. 75, 185–195. doi: 10.1080/11250000701882908
Boavida, J., Paulo, D., Aurelle, D., Arnaud-Haond, S., Marschal, C., Reed, J., et al. (2016). A well-kept treasure at depth: precious red coral rediscovered in Atlantic deep coral gardens (SW Portugal) after 300 Years. PLoS One 11:e0147228. doi: 10.1371/journal.pone.0147228
Bueno-Pardo, J., Ramalho, S., García-Alegre, A., Morgado, M., Vieira, R. P., Cunha, M. R., et al. (2017). Deep-sea crustacean trawling fisheries in Portugal: quantification of effort and assessment of landings per unit effort using a Vessel Monitoring System (VMS). Sci. Rep. 7:40795. doi: 10.1038/srep40795
Buhl-Mortensen, L., Buhl-Mortensen, P., Dolan, M. J. F., and Gonzalez-Mirelis, G. (2015). Habitat mapping as a tool for conservation and sustainable use of marine resources: some perspectives from the MAREANO programme, Norway. J. Sea Res. 100, 46–61. doi: 10.1016/j.seares.2014.10.014
Buhl-Mortensen, L., Vanreusel, A., Gooday, A. J., Levin, L. A., Priede, I. G., Buhl-Mortensen, P., et al. (2010). Biological structures as a source of habitat heterogeneity and biodiversity on the deep ocean margins. Mar. Ecol. 31, 21–50. doi: 10.1111/j.1439-0485.2010.00359.x
Bullimore, R. D., Foster, N. L., and Howell, K. L. (2013). Coral-characterized benthic assemblages of the deep Northeast Atlantic: defining “Coral Gardens” to support future habitat mapping efforts. ICES J. Mar. Sci. 70, 511–522. doi: 10.1093/icesjms/fss195
Cardone, F., Corriero, G., Longo, C., Maria, M., Onen Tarantini, S., Gravina, M. F., et al. (2020). Massive bioconstructions built by Neopycnodonte cochlear (Mollusca, Bivalvia) in a mesophotic environment in the central Mediterranean Sea. Sci. Rep. 10:6337. doi: 10.1038/s41598-020-63241-y
Carré, C. (2010). Customizable Observation Video imagE Record (COVER). Ifremer DEEP/LEP User Manual. 255.
Cathalot, C., Van Oevelen, D., Cox, T. J. S., Kutti, T., Lavaleye, M., Duineveld, G., et al. (2015). Cold-water coral reefs and adjacent sponge grounds: hotspots of benthic respiration and organic carbon cycling in the deep sea. Front. Mar. Sci. 2:37. doi: 10.3389/fmars.2015.00037
Clarke, K. R., and Gorley, R. N. (2006). PRIMER v6: User Manual. Plymouth: Plymouth Marine Laboratory.
Clarke, K. R., Gorley, R. N., Somerfield, P. J., and Warwick, R. M. (2014). Change in Marine Communities: An Approach to Statistical Analysis and Interpretation, 3rd Edn. Plymouth: PRIMER-E.
Claro, B., Pérez-Jorge, S., and Frey, S. (2020). Seafloor geomorphic features as an alternative approach into modelling the distribution of cetaceans. Ecol. Inform. 58:101092. doi: 10.1016/j.ecoinf.2020.101092
Coelho, M. A. G., and Lasker, H. R. (2016). “Larval dispersal and population connectivity in anthozoans,” in The Cnidaria, Past, Present and Future, eds S. Goffredo and Z. Dubinsky (Berlin: Springer), 291–315.
Colloca, F., Carpentieri, P., Balestri, E., and Ardizzone, G. D. (2004). A critical habitat for Mediterranean fish resources: shelf-break areas with Leptometra phalangium (Echinodermata: Crinoidea). Mar. Biol. 145, 1129–1142. doi: 10.1007/s00227-004-1405-8
Connor, D. W., Gilliland, P. M., Golding, N., Robinson, P., Todd, D., and Verling, E. (2006). UKSeaMap: The Mapping of Seabed and Water Column Features of UK Seas. Peterborough: Joint Nature Conservation Committee, 1–104.
Coppari, M., Gori, A., Viladrich, N., Saponari, L., Canepa, A., Grinyó, J., et al. (2016). The role of Mediterranean sponges in benthic-pelagic coupling processes: Aplysina aerophoba and Axinella polypoides case studies. J. Exp. Mar. Biol. Ecol. 477, 57–68. doi: 10.1016/j.jembe.2016.01.004
Coppari, M., Zanella, C., and Rossi, S. (2019). The importance of coastal gorgonians in the blue carbon budget. Sci. Rep. 9:13550. doi: 10.1038/s41598-019-49797-4
CoralFISH (2013). Final Report: Assessment of the Interaction Between Corals, Fish and Fisheries, in Order to Develop Monitoring and Predictive Modelling Tools for Ecosystem Based Management in the Deep Waters of Europe and Beyond. Brussels: European Commission.
Cunha, M., Paterson, G. L. J., Amaro, T., Blackbird, S., Stigter, H., Ferreira, C., et al. (2011). Biodiversity of macrofaunal assemblages from three Portuguese submarine canyons (NE Atlantic). Deep Sea Res. II Top. Stud. Oceanogr. 58, 2433–2447. doi: 10.1016/j.dsr2.2011.04.007
da Silva Lopes, J. (1841). Corografia ou Memória Económica, Estatística e Topográfica do Reino do Algarve. Libson: Academia das Ciências de Lisboa.
Davies, J. S., Guillaumont, B., Tempera, F., Vertino, A., Beuck, L., Ólafsdóttir, S. H., et al. (2017). A new classification scheme of European cold-water coral habitats: implications for ecosystem-based management of the deep sea. Deep Sea Res. II Top. Stud. Oceanogr. 145, 102–109. doi: 10.1016/j.dsr2.2017.04.014
Davies, J. S., Howell, K. L., Stewart, H. A., Guinan, J., and Golding, N. (2014). Defining biological assemblages (biotopes) of conservation interest in the submarine canyons of the South West Approaches (offshore United Kingdom) for use in marine habitat mapping. Deep Sea Res. II Top. Stud. Oceanogr. 104, 208–229. doi: 10.1016/j.dsr2.2014.02.001
Davies, J. S., Stewart, H. A., Narayanaswamy, B. E., Jacobs, C., Spicer, J., Golding, N., et al. (2015). Benthic assemblages of the Anton Dohrn Seamount (NE Atlantic): defining deep-sea biotopes to support habitat mapping and management efforts with a focus on vulnerable marine ecosystems. PLoS One 10:e0124815. doi: 10.1371/journal.pone.0124815
Davison, J. J., van Haren, H., Hosegood, P., Piechaud, N., and Howell, K. L. (2019). The distribution of deep-sea sponge aggregations (Porifera) in relation to oceanographic processes in the Faroe-Shetland channel. Deep Sea Res. I Oceanogr. Res. Pap. 146, 55–61. doi: 10.1016/j.dsr.2019.03.005
De la Torriente, A., González-Irusta, J. M., Aguilar, R., Fernández-Salas, L. M., Punzón, A., and Serrano, A. (2019). Benthic habitat modelling and mapping as a conservation tool for marine protected areas: a seamount in the western Mediterranean. Aquat. Conserv. 29, 732–750. doi: 10.1002/aqc.3075
De la Torriente, A., Serrano, A., Fernández-Salas, L. M., García, M., and Aguilar, R. (2018). Identifying epibenthic habitats on the seco de los olivos seamount: species assemblages and environmental characteristics. Deep Sea Res. I Oceanogr. Res. Pap. 135, 9–22. doi: 10.1016/j.dsr.2018.03.015
De Leo, F. C., Smith, C. R., Rowden, A. A., Bowden, D. A., and Clark, M. R. (2010). Submarine canyons: hotspots of benthic biomass and productivity in the deep sea. Proc. R. Soc. B Biol. Sci. 277, 2783–2792. doi: 10.1098/rspb.2010.0462
De Matos, V., Gomes-Pereira, J. N., Tempera, F., Ribeiro, P. A., Braga-Henriques, A., and Porteiro, F. (2014). First record of Antipathella subpinnata (Anthozoa, Antipatharia) in the Azores (NE Atlantic), with description of the first monotypic garden for this species. Deep Sea Res. II Top. Stud. Oceanogr. 99, 113–121. doi: 10.1016/j.dsr2.2013.07.003
Dias, V., Oliveira, F., Boavida, J., Serrão, E. A., Goncalves, J., and Coelho, M. A. (2020). High coral bycatch in bottom-set gillnet coastal fisheries reveals rich coral habitats in southern portugal. Front. Mar. Sci. 7:603438. doi: 10.3389/fmars.2020.603438
Duckworth, A. R. (2016). Substrate type affects the abundance and size of a coral-reef sponge between depths. Mar. Freshw. Res. 67:246. doi: 10.1071/MF14308
Edinger, E., and Sherwood, O. (2012). Applied taphonomy of gorgonian and antipatharian corals in Atlantic Canada: experimental decay rates, field observations, and implications for assessing fisheries damage to deep-sea coral habitats. Neues Jahrb. Geol. Paläontol. Abh. 265, 199–218. doi: 10.1127/0077-7749/2012/0255
European Environment Agency (EEA) (2012). 1170 Reefs Assessment of Conservation Status at the European Biogeographical Level. Copenhagen: European Environment Agency, 1–8.
Fabri, M. C., Pedel, L., Beuck, L., Galgani, F., Hebbeln, D., and Freiwald, A. (2014). Megafauna of vulnerable marine ecosystems in French Mediterranean submarine canyons: Spatial distribution and anthropogenic impacts. Deep Sea Res. II Top. Stud. Oceanogr. 104, 184–207. doi: 10.1016/j.dsr2.2013.06.016
Fernandez-Arcaya, U., Ramirez-Llodra, E., Aguzzi, J., Allcock, A. L., Davies, J. S., Dissanayake, A., et al. (2017). Ecological role of submarine canyonsand need for canyon conservation: a review. Front. Mar. Sci. 4:5. doi: 10.3389/fmars.2017.00005
Folkers, M., and Rombouts, T. (2020). “Sponges revealed: a synthesis of their overlooked ecological functions within aquatic ecosystems,” in YOUMARES 9 - The Oceans: Our Research, Our Future: Proceedings of the 2018 Conference for YOUng MArine RESearcher in Oldenburg, Germany, eds S. Jungblut, V. Liebich, and M. Bode-Dalby (Springer: Cham), 181–193. doi: 10.1007/978-3-030-20389-4_9
Fonseca, P., Abrantes, F., Aguilar, R., Campos, A., Cunha, M., Ferreira, D., et al. (2014). A deep-water crinoid Leptometra celtica bed off the Portuguese south coast. Mar. Biodivers. 44, 223–228. doi: 10.1007/s12526-013-0191-2
Food and Agriculture Organization (FAO) (2016). Vulnerable Marine Ecosystems: Processes and practices in the High Seas. In FAO Fisheries and Aquaculture Technical Paper No 595. Rome: FAO, 185.
Fowler, S., and Laffoley, D. (1993). Stability in Mediterranean-Atlantic sessile epifaunal communities at the northern limits of their range. J. Exp. Mar. Biol. Ecol. 172, 109–127. doi: 10.1016/0022-0981(93)90092-3
Freiwald, A., Fosså, J. H., Grehan, A., Koslow, T., and Roberts, J. M. (2011). Cold-Water Coral Reefs: Out of Sight - No Longer Out of Mind. UNEP-WCMC Biodiversity Series 22. Cambridge: UNEP-WCMC, 1–85.
Friard, O., and Gamba, M. (2016). BORIS: a free, versatile open-source event-logging software for video/audio coding and live observations. Methods Ecol. Evol. 7, 1325–1330. doi: 10.1111/2041-210X.12584
Gage, J. D., and Tyler, P. A. (1991). Deep-Sea Biology: A Natural History of Organisms at the Deep Sea Floor. Cambridge: Cambridge University Press, 504.
Garrabou, J., Bavestrello, G., Cattaneo-Vietti, R., Cerrano, C., Garcia, S., Goffredo, S., et al. (2015). Corallium rubrum (errata Version Published in 2017). The IUCN Red List of Threatened Species 2015, e.T50013405A110609252. Downloaded on 25 January 2021. Gland: IUCN.
Giusti, M., Canese, S., Fourt, M., Bo, M., Innocenti, C., Goujard, A., et al. (2019). Coral forests and derelict fishing gears in submarine canyon systems of the ligurian sea. Prog. Oceanogr. 178:102186. doi: 10.1016/j.pocean.2019.102186
Gomes-Pereira, J. N., Tojeira, I., Ribeiro, L. P., Santos, R. S., Dias, F., and Tempera, F. (2015). First in situ observations of soft bottom megafauna from the Cascais Canyon head. Arquipélago Life Mar. Sci. 32, 67–74.
Grinyó, J., Gori, A., Ambroso, S., Purroy, A., Calatayud, C., Dominguez-Carrió, C., et al. (2015). “Spatial, bathymetrical and size distribution of deep unexpected well preserved mediterranean gorgonian assemblages (menorca channel, western mediterranean sea),” in Proceedings of the ICES Annual Science Conference CM, 2015/D:19, (Copenhagen).
Gubbay, S., Saunders, G., Tyler-Walters, H., Dankers, N., Otero-Ferrer, F., Forde, J., et al. (2016). A4.12 Sponge 952 Communities on Lower Circalittoral Rock. European Red List of Habitats, Marine Habitat Group, 1–9.
Guillaumont, B., Tempera, F., Davies, J., Vertino, A., Beuck, L., Ólafsdóttir, S. H., et al. (2016). CoralFISH Northeast Atlantic and Mediterranean Cold-Water Coral Habitats Catalogue. Geneva: Zenodo.
Hiscock, K. (2014). Marine Biodiversity Conservation: A Practical Approach. London: Routledge, 318. doi: 10.1017/9781315857640
Howell, K. L. (2010). A benthic classification system to aid in the implementation of marine protected area networks in the deep/high seas of the NE Atlantic. Biol. Conserv. 143, 1041–1056. doi: 10.1016/j.biocon.2010.02.001
Howell, K. L., Davies, J. S., and Narayanaswamy, B. E. (2010). Identifying deep-sea megafaunal epibenthic assemblages for use in habitat mapping and marine protected area network design. J. Mar. Biol. Assoc. U. K. 90, 33–68. doi: 10.1017/S0025315409991299
Howell, K. L., Davies, J. S., and van den Beld, I. (2017). Deep-Sea Species Image Catalogue. Plymouth: University of Plymouth.
Huvenne, V. A. I., Tyler, P. A., Masson, D. G., Fisher, E. H., Hauton, C., Hühnerbach, V., et al. (2011). A picture on the wall: innovative mapping reveals cold-water coral refuge in submarine canyon. PLoS One 6:e28755. doi: 10.1371/journal.pone.0028755
ICES (2019). ICES / NAFO joint Working Group on Deep-water Ecology (WGDEC). ICES Sci. Rep. 1:119. doi: 10.17895/ices.pub.5567
Jiang, L., and Garwood, R. W. (1998). Effects of topographic steering and ambient stratification on overflows on continental slopes: a model study. J. Geophys. Res. Oceans 103, 5459–5476. doi: 10.1029/97jc03201
Johnson, M., White, M., Wilson, A., Schwabe, L., Schwabe, E., Folch, H., et al. (2013). A vertical wall dominated by Acesta excavata and Neopycnodonte zibrowii, part of an undersampled group of deep-sea habitats. PLoS One 8:e79917. doi: 10.1371/journal.pone.0079917
Joint Nature Conservation Committee (JNCC) (2018). Remotely Operated Vehicles for Use in Marine Benthic Monitoring. Marine Monitoring Platform Guidelines No.1. Peterborough: Joint Nature Conservation Committee.
Jones, C. G., Lawton, J. H., and Shachak, M. (1994). Organisms as ecosystem engineers. Oikos 69:373. doi: 10.2307/3545850
Klitgaard, A. B., and Tendal, O. S. (2004). Distribution and species composition of mass occurrences of large-sized sponges in the northeast Atlantic. Prog. Oceanogr. 61, 57–98. doi: 10.1016/j.pocean.2004.06.002
Louzao, M., Anadón, N., Arrontes, J., Álvarez-Claudio, C., Fuente, D. M., Ocharan, F., et al. (2009). Historical macrobenthic community assemblages in the Avilés Canyon, N Iberian Shelf: baseline biodiversity information for a marine protected area. J. Mar. Syst. 80, 47–56. doi: 10.1016/j.jmarsys.2009.09.006
Maldonado, M., Aguilar, R., Bannister, R. J., Bell, J. J., Conway, K. W., Dayton, P. K., et al. (2016). “Sponge grounds as key marine habitats: a synthetic review of types, structure, functional roles, and conservation concerns,” in Marine Animal Forests, eds S. Rossi, L. Bramanti, A. Gori, and C. Orejas Saco del Valle (Cham: Springer), 1–39.
Maldonado, M., López-Acosta, M., Sánchez-Tocino, L., and Sitjà, C. (2013). The rare, giant gorgonian Ellisella paraplexauroides: demographics and conservation concerns. Mar. Ecol. Prog. Ser. 479, 127–141. doi: 10.3354/meps10172
Marliave, J. B., Conway, K. W., Gibbs, D. M., Lamb, A., and Gibbs, C. (2009). Biodiversity and rockfish recruitment in sponge gardens and bioherms of southern British Columbia, Canada. Mar. Biol. 156, 2247–2254. doi: 10.1007/s00227-009-1252-8
Martín, J., Puig, P., Palanques, A., and Ribó, M. (2014). Trawling-induced daily sediment resuspension in the flank of a Mediterranean submarine canyon. Deep Sea Res. II Top. Stud. Oceanogr. 104, 174–183. doi: 10.1016/j.dsr2.2013.05.036
Mastrototaro, F., Chimienti, G., Capezzuto, F., Carlucci, R., and Williams, G. (2014). First record of Protoptilum carpenteri (Cnidaria: Octocorallia: Pennatulacea) in the Mediterranean Sea. Ital. J. Zool. 82, 61–68. doi: 10.1080/11250003.2014.982218
Matsumura, Y., and Hasumi, H. (2011). Dynamics of cross-isobath dense water transport induced by slope topography. J. Phys. Oceanogr. 41, 2402–2416. doi: 10.1175/JPO-D-10-05014.1
McArdle, B., and Anderson, M. (2001). Fitting multivariate models to community data: a comment on distance-based redundancy analysis. Ecology 82, 290–297. doi: 10.2307/2680104
McArthur, M. A., Brooke, B. P., Przeslawski, R., Ryan, D. A., Lucieer, V. L., Nichol, S., et al. (2010). On the use of abiotic surrogates to describe marine benthic biodiversity. Estuar. Coast. Shelf Sci. 88, 21–32. doi: 10.1016/j.ecss.2010.03.003
McClain, C. R., and Barry, J. P. (2010). Habitat heterogeneity, disturbance, and productivity work in concert to regulate biodiversity in deep submarine canyons. Ecology 91, 964–976. doi: 10.1890/09-0087.1
Miller, R. J., Hocevar, J., Stone, R. P., and Fedorov, D. V. (2012). Structure-forming corals and sponges and their use as fish habitat in bering sea submarine canyons. PLoS One 7:e33885. doi: 10.1371/journal.pone.0033885
Monteiro, P., Araújo, A., Erzini, K., and Castro, M. (2001). Discards of the Algarve (southern Portugal) crustacean trawl fishery. Hydrobiologia 449, 267–277. doi: 10.1023/A:1017575429808
Morais, P., Borges, T. C., Carnall, V., Terrinha, P., Cooper, C., and Cooper, R. (2007). Trawl-induced bottom disturbances off the south coast of Portugal: direct observations by the ‘Delta’ manned-submersible on the Submarine Canyon of Portimão. Mar. Ecol. 28(Suppl. 1), 112–122. doi: 10.1111/j.1439-0485.2007.00175.x
OCEANA (2011). OSPAR Workshop on the Improvement of the Definitions of Habitats in the OSPAR list: Background Document for Discussion: “Coral Gardens”, “Deep Sea Sponge Aggregations” and “Seapen and Burrowing Megafauna Communities. Bergen: OCEANA.
Oliveira, F., Aguilar, R., Monteiro, P., Bentes, L., Afonso, C. M. L., García, S., et al. (2017). A Photographic Guide of the Species of the Gorringe Bank. Faro: Centro de Ciências do Mar (CCMAR)/Oceana, 312.
Oliveira, F., Monteiro, P., Bentes, L., Henriques, N. S., Aguilar, R., and Gonçalves, J. M. (2015). Marine litter in the upper São Vicente submarine canyon (SW Portugal): abundance, distribution, composition and fauna interactions. Mar. Pollut. Bull. 97, 401–407. doi: 10.1016/j.marpolbul.2015.05.060
Paradis, S., Puig, P., Masqué, P., Juan-Diáz, X., Martín, J., and Palanques, A. (2017). Bottom-trawling along submarine canyons impacts deep sedimentary regimes. Sci. Rep. 7:46903. doi: 10.1038/srep43332
Pardo, E., Aguilar Rubio, R., García, S., Torriente de la, A., and Ubero, J. (2011). Documentación de arrecifes de corales de agua fría en el Mediterráneo occidental (Mar de Alborán). Chronica Naturae 1, 20–34.
Pawson, D. L., and Miller, J. E. (1983). Systematics and ecology of the sea urchin genus Centrostephanus (Echinodermata: Echinoidea) from the Atlantic and Eastern Pacific Oceans. Smithson. Contrib. Mar. Sci. 1–15. doi: 10.5479/si.01960768.20.1
Pierdomenico, M., Cardone, F., Carluccio, A., Casalbore, D., Chiocci, F., Maiorano, P., et al. (2019). Megafauna distribution along active submarine canyons of the central Mediterranean: relationships with environmental variables. Prog. Oceanogr. 171, 49–69. doi: 10.1016/j.pocean.2018.12.015
Pierdomenico, M., Martorelli, E., Dominguez-Carrió, C., Gili, J. M., and Chiocci, F. L. (2016). Seafloor characterization and benthic megafaunal distribution of an active submarine canyon and surrounding sectors: the case of Gioia Canyon (Southern Tyrrhenian Sea). J. Mar. Syst. 157, 101–117. doi: 10.1016/j.jmarsys.2016.01.005
Post, A. L., Lavoie, C., Domack, E. W., Leventer, A., Shevenell, A., and Fraser, A. D. (2017). Environmental drivers of benthic communities and habitat heterogeneity on an East Antarctic shelf. Antarct. Sci. 29, 17–32. doi: 10.1017/S0954102016000468
Prado, E., Basalo, A., Ríos, P., Cristobo, J., Rodríguez-Cabello, C., and Sánchez, F. (2021). In situ growth rate assessment of the hexactinellid sponge asconema setubalense using 3D photogrammetric reconstruction. Front. Mar. Sci. 8: 612613. doi: 10.3389/fmars.2021.612613
Purser, A., Orejas, C., Gori, A., Tong, R., Unnithan, V., and Thomsen, L. (2013). Local variation in the distribution of benthic megafauna species associated with cold-water coral reefs on the Norwegian margin. Cont. Shelf Res. 54, 37–51. doi: 10.1016/j.csr.2012.12.013
QGIS Development Team (2019). QGIS Geographic Information System. Open Source Geospatial Foundation Project. Available online at: http://qgis.osgeo.org
Ramalho, S. P., Lins, L., Bueno-Pardo, J., Cordova, E. A., Amisi, J. M., Lampadariou, N., et al. (2017). Deep-sea mega-epibenthic assemblages from the SW Portuguese Margin (NE Atlantic) subjected to bottom-trawling fisheries. Front. Mar. Sci. 4:350. doi: 10.3389/fmars.2017.00350
Ramirez-Llodra, E., Tyler, P. A., Baker, M. C., Bergstad, O. A., Clark, M. R., Escobar, E., et al. (2011). Man and the last great wilderness: human impact on the deep sea. PLoS One 6:e22588. doi: 10.1371/journal.pone.0022588
Ramos, M., Bertocci, I., Tempera, F., Calado, G., Albuquerque, M., and Duarte, P. (2016). Patterns in megabenthic assemblages on a seamount summit (Ormonde Peak, Gorringe Bank, Northeast Atlantic). Mar. Ecol. 37, 1057–1072. doi: 10.1111/maec.12353
Relvas, P., and Barton, E. D. (2002). Mesoscale patterns in the Cape São Vicente (Iberian Peninsula) upwelling region. J. Geophys. Res. 107, 28–21. doi: 10.1029/2000jc000456
Ríos, P., Aguilar, R., Torriente, A., Muñoz, A., and Cristobo, J. (2018). Sponge grounds of Artemisina (Porifera, Demospongiae) in the Iberian Peninsula, ecological characterization by ROV techniques. Zootaxa 4466, 95–123. doi: 10.11646/zootaxa.4466.1.10
Rodríguez-Basalo, A., Prado, E., Sánchez, F., Ríos, P., Gómez-Ballesteros, M., and Cristobo, J. (2021). High resolution spatial distribution for the hexactinellid sponges Asconema setubalense and Pheronema carpenteri in the Central Cantabrian Sea. Front. Mar. Sci. 8:612761. doi: 10.3389/fmars.2021.612761
Rossi, S. (2013). The destruction of the ‘animal forests’ in the oceans: towards an over-simplification of the benthic ecosystems. Ocean Coast. Manag. 84, 77–85. doi: 10.1016/j.ocecoaman.2013.07.004
Ruano, A., Silva, P., Solano, S., and Naves, J. (2007). Cetáceos del Litoral Asturiano: Áreas de Interés Para la Conservación. Oviedo: Principado de Asturias, 1–130.
Ryan, J. P., Chavez, F. P., and Bellingham, J. G. (2005). Physical-biological coupling in Monterey Bay, California: topographic influences on phytoplankton ecology. Mar. Ecol. Prog. Ser. 287, 23–32. doi: 10.3354/meps287023
Sampaio, Í, Braga-Henriques, A., Pham, C., Ocaña, O., De Matos, V., Morato, T., et al. (2012). Cold-water corals landed by bottom longline fisheries in the Azores (north-eastern Atlantic). J. Mar. Biol. Assoc. U.K. 92, 1547–1555. doi: 10.1017/S0025315412000045
Sánchez, F., Rodríguez Basalo, A., García-Alegre, A., and Gómez-Ballesteros, M. (2017). Hard-bottom bathyal habitats and keystone epibenthic species on Le Danois Bank (Cantabrian Sea). J. Sea Res. 130, 134–153. doi: 10.1016/j.seares.2017.09.005
Sardá, R., Rossi, S., Martí, X., and Gili, J. M. (2012). Marine benthic cartography of the Cap de Creus (NE Catalan Coast, Mediterranean Sea). Sci. Mar. 76, 159–171. doi: 10.3989/scimar.03101.18D
Serra, C., Martinez Loriente, S., Gràcia, E., Urgeles, R., Vizcaino, A., Perea, H., et al. (2020). Tectonic evolution, geomorphology and influence of bottom currents along a large submarine canyon system: the São Vicente Canyon (SW Iberian margin). Mar. Geol. 426:106219. doi: 10.1016/j.margeo.2020.106219
Templado, J., and Moreno, D. (1996). Nuevos datos sobre la distribución de Centrostephanus longispinus (Echinodermata: Echinoidea) en las costas españolas. Graellsia 52, 107–113. doi: 10.3989/graellsia.1996.v52.i0.382
Terrinha, P., Matias, L., Vicente, J., Duarte, J., Luís, J., Pinheiro, L., et al. (2009). Morphotectonics and strain partitioning at the Iberia-Africa plate boundary from multibeam and seismic reflection data. Mar. Geol. 267, 156–174. doi: 10.1016/j.margeo.2009.09.012
Topsent, E. (1890). Notice préliminaire sur les spongiaires recueillis durant les campagnes de l’Hirondelle. Bull. Soc. Zool. Fr. 15, 26–32. doi: 10.5962/bhl.part.18721
Tsounis, G., Martinez, L., Viladrich, N., Bramanti, L., Martinez Quintana, A., Gili, J. M., et al. (2012). Anthropogenic effects on reproductive effort and allocation of energy reserves in the Mediterranean octocoral Paramuricea clavata. Mar. Ecol. Prog. Ser. 449, 161–172. doi: 10.3354/meps09521
Tyler, P., Amaro, T., Arzola, R., Cunha, M., De Stigter, H., Gooday, A., et al. (2009). Europe’s grand canyon: nazaré submarine canyon. Oceanogr. Mar. Biol. 22, 46–57. doi: 10.5670/oceanog.2009.05
Valadares, V., Roque, C., and Terrinha, P. (2009). Tectonic control and mass-wasting processes along S. Vicente Canyon (SW Iberia): evidences from multibeam bathymetry and seismic reflection data. Geophys. Res. Abstr. 11:12685.
Vetter, E. W., Smith, C. R., and De Leo, F. C. (2010). Hawaiian hotspots: enhanced megafaunal abundance and diversity in submarine canyons on the oceanic islands of Hawaii. Mar. Ecol. 31, 183–199. doi: 10.1111/j.1439-0485.2009.00351.x
Vizcaino, A., Gràcia, E., Pallàs, R., Terrinha, P., Diez, S., Dañobeitia, J., et al. (2005). Active Tectonic and Sedimentary Processes along the Sao Vicente Canyon (SW Iberian Margin): High-Resolution Imaging. Barcelona: SARTI, Technical University of Catalonia (UPC).
Wagner, D., Luck, D. G., and Toonen, R. J. (2012). The biology and ecology of black corals (Cnidaria: Anthozoa: Hexacorallia: Antipatharia). Adv. Mar. Biol. 63, 67–132. doi: 10.1016/B978-0-12-394282-1.00002-8
Woolley, S. N. C., Tittensor, D. P., Dunstan, P. K., Guillera-Arroita, G., Lahoz-Monfort, J. J., Wintle, B. A., et al. (2016). Deep-sea diversity patterns are shaped by energy availability. Nature 533, 393–396. doi: 10.1038/nature17937
Keywords: priority habitats, submarine canyon, coral gardens, sponge aggregations, OSPAR, deep-sea and circalittoral conservation, habitat mapping, megabenthic communities
Citation: Nestorowicz I-M, Oliveira F, Monteiro P, Bentes L, Henriques NS, Aguilar R, Horta e Costa B and Gonçalves JMS (2021) Identifying Habitats of Conservation Priority in the São Vicente Submarine Canyon in Southwestern Portugal. Front. Mar. Sci. 8:672850. doi: 10.3389/fmars.2021.672850
Received: 26 February 2021; Accepted: 23 August 2021;
Published: 22 September 2021.
Edited by:
Trevor Willis, Stazione Zoologica Anton Dohrn Napoli, ItalyReviewed by:
Fernando Tempera, Independent Researcher, Brest, FranceJaime Selina Davies, University of Plymouth, United Kingdom
Copyright © 2021 Nestorowicz, Oliveira, Monteiro, Bentes, Henriques, Aguilar, Horta e Costa and Gonçalves. This is an open-access article distributed under the terms of the Creative Commons Attribution License (CC BY). The use, distribution or reproduction in other forums is permitted, provided the original author(s) and the copyright owner(s) are credited and that the original publication in this journal is cited, in accordance with accepted academic practice. No use, distribution or reproduction is permitted which does not comply with these terms.
*Correspondence: Barbara Horta e Costa, bbcosta@ualg.pt
†These authors have contributed equally to this work
‡These authors share senior authorship