- 1Leibniz Centre for Tropical Marine Research (ZMT), Bremen, Germany
- 2University of Bremen, Faculty Biology/Chemistry (FB02), Bremen, Germany
Within the past decade, microplastic (MP) particles (<5 mm in size) became the focus of both scientific and public attention, on one hand due to their cumulative industrial application and global presence, on the other hand due to their size range allowing the interaction with organisms at the base of the marine food web. Along with the growing evidence of their ubiquitous distribution, the ingestion of MP fibers and fragments has been verified for a variety of marine biota, with fish species being among the group of organisms most intensively studied both in the laboratory and in the field. While the gross of scientific literature focuses on the quantification and chemical characterization of MP in the gastrointestinal tract of fish, in-depth investigations on the impacts or a contextualization of ingestion are rarely accomplished. Yet, the constant media-coverage and omnipresence of the topic present a (threat) scenario among civil society which might lack a solid scientific foundation. This review, therefore, analyses the scientific output of 90 field studies covering 487 different fish taxa with due regard to explanatory variables for MP ingestion. Additionally, it highlights persistent knowledge gaps in relation to the examination of in-situ ingestion effects and proposes measures how to approach them in future research initiatives. Moreover, the potential existence of a publication bias and a consequent distortion of the perception of the topic is evaluated.
Introduction
The History of Field Studies on MP Uptake in Fish
Among the diverse anthropogenically caused environmental issues threatening coastal and oceanic ecosystems, plastic litter along with its potential detrimental effects on marine biota has been gaining particular attention and awareness both in the scientific community and civil society (Cunningham and Sigwart, 2019; Völker et al., 2019). A concomitant of the growing research effort to describe the distribution and abundance of plastic pollution across the marine realm is the application of different size classifications in the scientific literature (Andrady, 2015; Thompson, 2015); a common, rigorous definition of the different size classes of plastic litter, as proposed by GESAMP (2015; Figure 1), remains to be formally adopted by the scientific community.
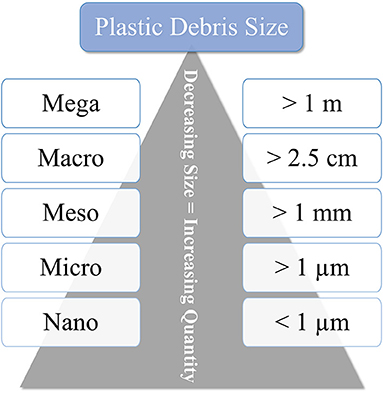
Figure 1. Classification of plastic litter size ranges proposed by GESAMP (2015). With a decrease in size class, an increase in abundance (and, thus, bioavailability) is expected (Wright et al., 2013; Song et al., 2014).
With size being identified as one of the key factors determining the bioavailability of MP in the ocean (Wright et al., 2013), standardization of the size class definitions of MP is considered a top priority to holistically report and evaluate debris abundances in the marine realm and ingestion rates by different organisms on various levels of the trophic food web (Galgani et al., 2015). Irrespective of a lacking coherent categorization, especially micro-sized particles and fibers (commonly defined to range between 5 mm and 1 μm in, e.g., Arthur et al., 2009; GESAMP, 2015, 2016) are of growing interest and concern: on the one hand, this is due to their increasing quantities in freshwater and marine environments as a result of cumulative industrial application of primary microplastics (Ryan, 2015) along with the fragmentation of bigger-sized particles into secondary microplastics. On the other hand, due to their size range making them available for ingestion by a wide range of organisms at the base of the food web (Cole et al., 2013).
As early as in the 1970's, along with the onset of reports on MP in environmental samples (Buchanan, 1971), early life stages of fish, as part of the marine zooplankton community, have been observed to take up MP. In a case study in the Northern Atlantic Ocean (Carpenter et al., 1972), the researchers collected fourteen different fish species by oblique plankton tows, of which eight were found to have ingested white, opaque polystyrene spherules. In investigations from the Severn Estuary (United Kingdom) by Kartar et al. (1973), the analysis of the stomach content of 0+ and 1+ year class flounder Platichthys flesus also revealed the presence of polystyrene particles in the guts. Upon publishing a progress report 3 years later, however, Kartar et al. (1976) stated that the same species of flounder (among other fish species investigated) collected over several years in the same area, was found to have varying levels of MP ingestion, including no uptake at all in some years. During the same time period, Colton et al. (1974) used a combined approach of large-scale field surveys and laboratory feeding experiments in an initial attempt to investigate the potential effects of MP uptake on larval and juvenile fish of different species. In more than half of the samples collected with a neuston net in ichthyoplankton surveys from Cape Cod to the Caribbean, plastic particles were found with varying abundances and plastic compositions. Yet, upon analyzing the guts of over 500 larval and juvenile fish of 22 species collected in the respective areas, the authors did not find ingested plastic particles in any of the fish. Published in the same report, the outcomes of the feeding experiments with larval and juvenile fish of different species, which were offered polystyrene and acrylonitrile-butadiene-styrene suspension beads, likewise showed no MP uptake, as well as no MP-related mortality or detrimental effects (Colton et al., 1974). Following these early investigations, studies were published that highlighted the facts that the presence of MP in fish guts might be caused by post capture ingestion (Lancraft and Robison, 1980) and that not all fish species and age groups living in MP-contaminated environments are prone to ingest fragments or spherules to a high degree (Hoss and Settle, 1990).
Since the start of investigations on MP ingestion by fish in the 1970's, the number of research articles on this subject has been growing continuously (de Sá et al., 2018). Nowadays, MP ingestion has been verified for a multitude of different fish species from all around the world, including the North Pacific Central Gyre (e.g., Boerger et al., 2010; Choy and Drazen, 2013), the North-Atlantic (e.g., Liboiron et al., 2016; Lusher et al., 2016) and South-Atlantic (e.g., Possatto et al., 2011; Pegado et al., 2018), the Mediterranean (e.g., Anastasopoulou et al., 2013; Güven et al., 2017), the North and Baltic Sea (e.g., Rummel et al., 2016; Beer et al., 2018) and even the polar regions (Kühn et al., 2018). Comparable to the early investigations from the 1970's to the 1990's, recent studies often tend to assess species assemblages rather than an individual taxon or life stage when examining the degree to which fish ingest MP (e.g., Davison and Asch, 2011; Lusher et al., 2013; Jabeen et al., 2017; McGoran et al., 2018).
Reviews on MP Ingestion by Marine Fish
To assemble and sort the growing number of reports from all over the world, several review articles have to date been published, covering MP ingestion by marine biota in general (Laist, 1997) as well as by fish in particular. The first comprehensive list of plastic-ingesting marine fish species was collated almost twenty years after the early reports on this matter were published (Hoss and Settle, 1990); this review included both scientific articles and anecdotal references of larval and juvenile stages of twelve fish species from nine different families which were reported to ingest MP in varying intensities. Reports on MP presence in the gastrointestinal tract (GIT) of adult fish were considered subsidiary results of dietary studies rather than specific investigations on MP uptake.
Within the past decade, publications of field-investigations on MP uptake by fish regularly incorporated topically limited literature reviews to put the obtained results into context: in a study on plastic ingestion by Atlantic cod, the authors reviewed 97 publications for plastic ingestion rates by different fish species, deliberately excluding species with an ingestion rate of 0% in their summary (Liboiron et al., 2016). In a baseline study on plastic ingestion by fish in the southern hemisphere, the researchers conducted a literature review of 15 studies with a special focus on methodologies used to establish a standardized sampling protocol for future data collections (Cannon et al., 2016). As part of their study on MP occurrence in commercial fish from a Portuguese estuary, a methodological review by Bessa et al. (2018b) took into account 22 different publications. In addition to the uptake rates among the number of different species investigated, the authors also compared the method of extraction and polymer verification. In a further MP ingestion study with Atlantic chub mackerel Scomber colias, the researchers also conducted a brief literature review with the ISI Web of Science search, listed the respective outcomes of 31 studies in tabular form in the publication and put their obtained results in terms of MP uptake rate and predominant MP type and color into perspective (Herrera et al., 2019).
The latest stand-alone review on field studies on plastic ingestion by marine fish systematically analyzed 93 publications (Markic et al., 2020) and gave special attention to sample sizes and analytical methods. The review synthesized the information available on the number of marine fish species reported to ingest plastic and aimed to investigate patterns in plastic ingestion related to habitat, feeding strategy and geographical distribution. According to the authors, plastic ingestion was demonstrated for 65% of the fish species (n = 494) investigated up to January 2019, and the detection of plastic in the gastrointestinal tract (GIT) of a fish was stated to be highly dependent on adequate sampling sizes, as well as on the analytical methods applied.
Until today, however, methodological ambiguities remain regarding the detection and identification of MP and smaller sized particles (e.g., nanoplastics, Figure 1) both in environmental samples and in marine biota (Käppler et al., 2016; Markic et al., 2020). The interpretation and comparison of the outcomes of different studies in terms of presence or absence of MP need to be done with caution, under consideration of the potential methodological bias resulting from different laboratory protocols (e.g., mesh sizes used for sampling and filtering, contamination control measures, inclusion or exclusion of fibers in MP reporting) and analytical approaches (e.g., naked-eye, infrared spectroscopy or mass spectrometry for identification and quantification).
Aim of This Study
Beyond substantiating the mere presence of one or several MP particles in the GIT of marine fish, information on the actual ecological and physiological implications of MP ingestion remains scarce, despite the long history of MP-uptake investigations. Previously published literature reviews, both stand-alone articles as well as incorporated summaries in scientific field reports, primarily involved the collation of fish species, the respective frequency of MP-feeding and MP particles in the GIT, the representation of geographical locations and the appraisal of methodological approaches. To thoroughly evaluate the significance of MP ingestion by marine fish, however, these findings need to be contextualized (Figure 2) and correlated with oceanographic properties (e.g., temperature, salinity, oxygen), environmental surveys (e.g., prey and MP abundance), GIT content analysis (e.g., abundance or volume ratio of natural prey items to MP particles) and an investigation of the potential impacts (e.g., assessment of the condition of the fish by various approaches).
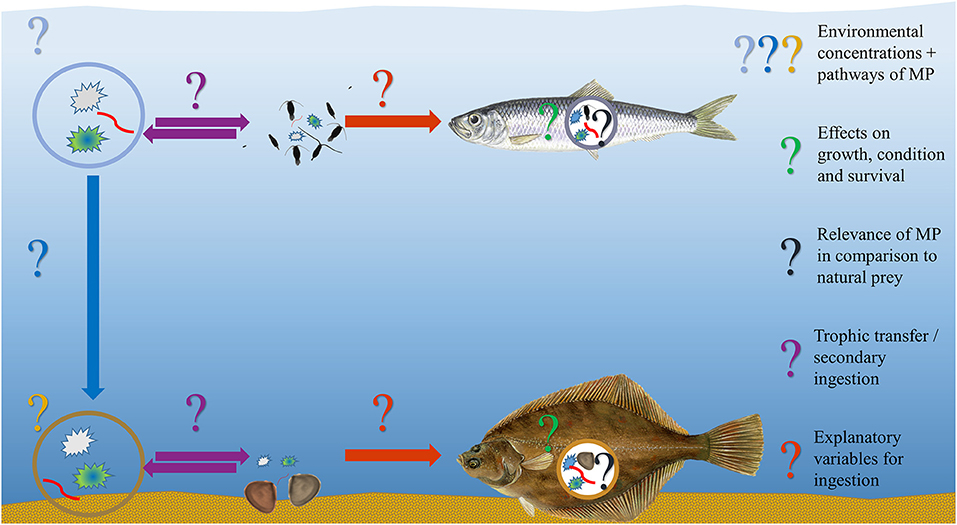
Figure 2. Graphical overview displaying the challenges and open questions related to in-situ microplastic ingestion studies by showcasing two exemplary fish species, the pelagic Clupea harengus and the demersal Platichthys flesus, along with their potential MP exposure and ingestion, the latter being reported by several studies included in this review. Fish graphics ©Scandposters.com.
The constant increase in media coverage of the topic of marine (micro-)litter along with the increasing number of scientific studies reporting uptake and consequent detrimental effects for fish and a variety of marine biota, might lead to the assumption that ingestion is the natural corollary of MP presence in the ocean. Upon closer examination of the literature published within the past decades, however, it becomes obvious that there has been evidence proving the contrary: the scientific reports do not exclusively refer to fish species taking up MP. Among the MP-feeding species, there are frequently also those, which were found without any plastic at all in the GIT (Carpenter et al., 1972; Kartar et al., 1976; Steer et al., 2017; Vendel et al., 2017). Yet these “negative” findings or even entire studies reporting a strikingly low or even zero-uptake along with minor or no effects observed both in the field and in laboratory set-ups appear to be rather unnoticed, underrepresented or even unpublished (Liboiron et al., 2018). This phenomenon, called (positive) publication bias is known across all scientific disciplines (Fanelli, 2012; Mlinarić et al., 2017) and could potentially affect scientific and civil society's perception of the actual extent of MP ingestion by fish.
As fish are among the group of organisms potentially affected by MP ingestion which are most intensively investigated both in the field and in laboratory set-ups (de Sá et al., 2018), the aim of this study is three-fold:
1. it will provide a brief quantitative and qualitative summary of the fish taxa examined in in-situ MP ingestion studies.
2. it will assess the current level of knowledge regarding driving factors of MP ingestion, along with the ecological and physiological implications of MP-uptake by fish to identify persistent knowledge gaps, future research projects should address.
3. it will investigate the potential existence of a publication bias in almost five decades of research on MP uptake to contribute to a sound evaluation of the extent and impact of MP-ingestion by marine fish.
Materials and Methods
Bibliographic Research
A review of scientific papers on in-situ reports only was conducted to obtain a representative number of reports on fish and MP uptake along with the potential effects. The research was performed by using major scientific databases, including Google Scholar and ISI Web of Knowledge. Two key research terms were entered to identify relevant scientific papers: plastic and fish. The focus of the bibliographic research was laid on microplastic materials, i.e., anthropogenic particles and fibers of <5 mm in at least one dimension (Galgani et al., 2015). Studies focusing on this particular size range were considered relevant for this review; results reported on meso- or macroplastic even within the same studies were (if possible) deliberately excluded, the same holds true for studies in which a clear size attribution of the MP particles found was lacking. Studies on freshwater fish species were also not included.
Based on the ISI Web of Science data base inquiry (“Web of Science Core Collection”) with the keywords plastic and fish for TOPIC (TOPIC: (plastic fish); Timespan: All years. Indexes: SCI-EXPANDED, SSCI, A&HCI, CPCI-S, CPCI-SSH, BKCI-S, BKCI-SSH, ESCI, CCR-EXPANDED, IC.), a total of 2,608 studies were listed (status: 3rd July, 2019). Upon consulting the abstract of the listed publications, a total of 116 publications were preselected as potentially relevant. Combined with the results of a Google Scholar database inquiry and a meta-analysis of cited publications in the preselected literature, a total of 90 papers based on in-situ investigations was considered relevant for the objectives of this review.
Data Analysis
To provide a broad perspective on in-situ MP uptake by fish, two databases were set up, focusing either on the fish taxa under investigation (Supplementary Table 1) or on the publications and their respective characteristics under review (Supplementary Table 2). The following main categories were assessed (if available) for each publication and analyzed by descriptive means:
1. Fish-related information (family, species, life-stage, trophic guild, habitat, commercial importance for fisheries)
2. Sampling-related information (geographic region and location of the sampling, sampling time, environmental samples, and parameters)
3. Output-related information (sample size, GIT analysis incl. prey ID or abundance, percentage of MP-feeding individuals, mean number of ingested MP per individual, impacts of ingestion).
The classification to either of the three life stages (adult = ad; sub-adult = sub-ad; larval/juvenile = juv) was based on the information given in the respective publication. If this information was lacking, the categories were derived from comparing the length measurements listed in the publication—if available–to the description accessed on FishBase (Froese and Pauly, 2019). In case neither the life stage category in the actual publication nor the maturity record in FishBase were available for individual species, the term “not applicable” (n/a) was entered. A classification into trophic guilds, habitats and potential interest for commercial fisheries was also based on the data available on Fishbase (Froese and Pauly, 2019). Furthermore, the number of citations was included; if accessible, the citation history as well (i.e., number of citations year-1) based on the ISI Web of Knowledge or Scopus database, assessed on the 06th September 2019.
All statistical analyses included in this review, along with the visual representation of results, have been computed using a combination of Microsoft Office 365 Excel and RStudio, Version 1.2.1335. Level of significance was set to p < 0.05.
An in-depth review of the methodologies applied to detect and identify MP particles in fish GIT was not in the focus of this review as it has been comprehensively discussed in, e.g., Cannon et al. (2016), Bessa et al. (2018b) and Markic et al. (2020).
Results and Discussion
Bibliographic Research
This study reviews a total of 90 papers based on in-situ investigations. Although the number and content of the research articles assessed were considered representative for a review on in-situ MP uptake by fish, this study does not intend to be an exhaustive collection of all reports published, especially given the growth of peer-reviewed publications over the past 10 years. In previously published reviews, the number of reports assessed ranged between 8 and 97. To the best of our knowledge, the only other comparable stand-alone review published so far included 93 reports (Markic et al., 2020), thus, the number of publications included here was considered adequately representative. The results of the data compilation are given in Supplementary Tables 1, 2.
While the earliest report on MP ingestion in fish dates back to 1972 (Carpenter et al., 1972), followed by studies from 1974 and 1976 (Colton et al., 1974; Kartar et al., 1976), the majority of studies reviewed here were published within the last 4 years (Figure 3). Noteworthy is the gap between the years 1976 and 2010: a review on MP ingestion (Hoss and Settle, 1990) as well as several dietary studies (e.g., Manooch and Hogarth, 1983; Young et al., 1997; Joyce et al., 2002) were published in between those years, however, their content (i.e., the size spectrum of MP particles detected was not clearly defined) was not matching the criteria for this review and, therefore, not included in this bar chart. However, the general tendency of an increasing publication effort during the past 5 to 10 years—as reflected by the selection of manuscripts in this review—was also attested by other reviews (Barboza and Gimenez, 2015; Cunningham and Sigwart, 2019; Markic et al., 2020).
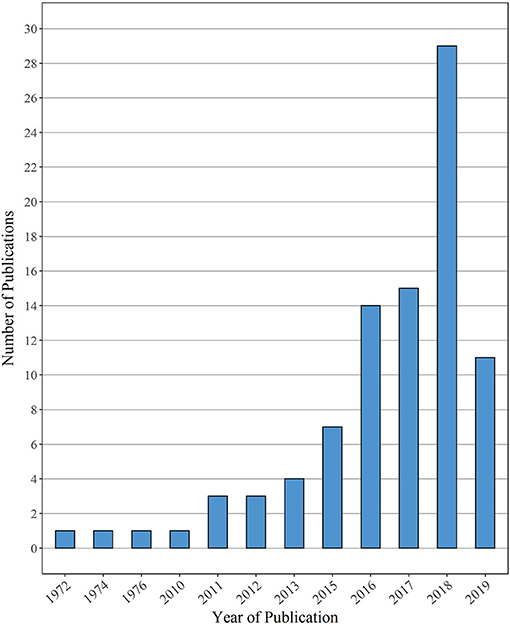
Figure 3. Overview of the number of publications reviewed here, sorted by the respective publication year. Papers published until 3 July 2019 are considered in this review.
Quantitative and Qualitative Representation of Fish Taxa
Number of Taxa per Study and Sample Sizes
The MP uptake of a single species was assessed by 29 of the 90 studies reviewed here (e.g., Gassel et al., 2013; Naidoo et al., 2016; Smith, 2018), the majority of field studies, thus, covering more than one species. Among those studies, 29 others dealt with up to five species, nine investigated six to ten species and nine others examined eleven to twenty different species. A total of 14 studies dealt with more than twenty different species and the highest number of species included in one report was 69 (Vendel et al., 2017). Several different studies investigated the same species (e.g., Kartar et al., 1976; Rummel et al., 2016; McGoran et al., 2017; Liboiron et al., 2019): upon examining those species, which were reported in more than one publication, the results in terms of MP uptake as well as in terms of mean number of particles per individual GIT vary greatly (Supplementary Table 1). To provide a brief overview of those variations, the results for three frequently assessed species are exemplarily listed in Table 1, reporting on the minimum and maximum sample sizes given in one study, along with the corresponding minimum and maximum percentages of MP-feeding individuals as well as minimum and maximum number of particles detected in the GIT of these species.
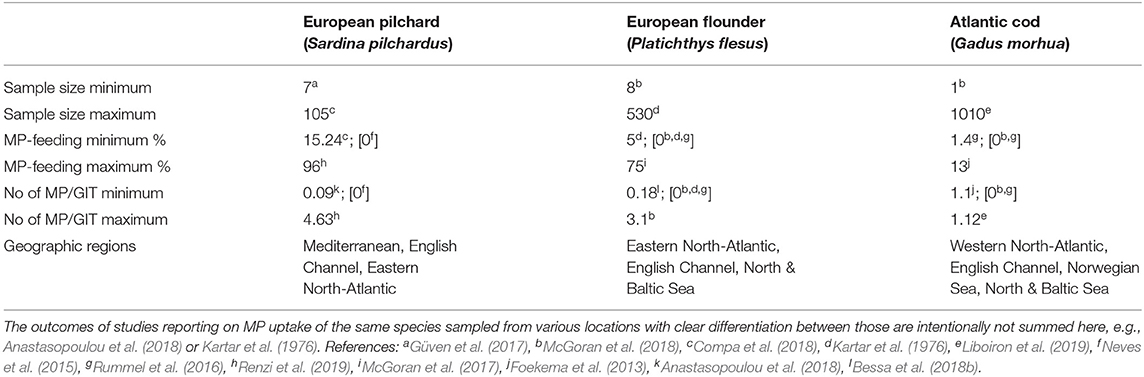
Table 1. Exemplary overview on the MP-uptake results for three fish species, investigated in several different studies from different geographic areas (see referencesa−l).
As can be seen from this exemplary list for three species only, the range in terms of sample sizes, frequency of MP-feeding, and MP particle load per individual fish seems to be quite substantial, without considering the variability in detection methodologies, particles under investigation, size ranges of both particles and fish as well as the life stages under investigation. Assessing all entries for individual taxa and life stages, collected across distinct geographic sampling locations, around one third of the fish were found with MP in their GIT (28.9 ± 33.91%), with an average particle load of around 2.2 ± 4.02 fibers and/or fragments among the MP-feeding individuals. These results are largely consistent with the findings of another stand-alone review that found a slightly higher frequency of plastic-feeding fish 37.6 ± 0.6%, with a comparable ingestion rate of 2.6 ± 0.2 pieces per individual (Markic et al., 2020).
Apart from this, the average sample size per species and study was calculated: in 28 of the studies included in this review, the average sample size per individual species was below thirty, in eight studies it was lower than ten (Supplementary Table 2). Studies reporting on a single species tended to have higher sample sizes per taxon (e.g., Bråte et al., 2016; Ferreira et al., 2016, 2018; Alomar et al., 2017) than studies that investigated species assemblages (e.g., Davison and Asch, 2011; Rochman et al., 2015; Miranda and de Carvalho-Souza, 2016; Pegado et al., 2018). According to the outcomes of the only other known stand-alone review of this dimension by Markic et al. (2020), the recommended sample size with a level of confidence of 95% and a 10% margin of error was n ≥ 91. Applying this recommendation to the results included here, only 16 of 90 studies had an average sample size per species matching this criterion. Accepting a margin of error of 20%, the sample size recommended by Markic et al. (2020) was n ≥ 23; 49 of the 90 studies reviewed here dealt with an average number of at least 23 individuals per species. With sample sizes below ten individuals per species in some studies, the significance of these results in terms of species-specific MP-uptake rates appears to be rather debatable (Liboiron et al., 2018; Markic et al., 2020). Moreover, comparing the results of different assessments to identify baselines of ingestion frequencies or patterns related to the uptake of MP per species is also not considered reasonable (Supplementary Table 1), without even taking into account the variances in analyses, detection methodologies or sampling sites (Collicutt et al., 2019; Liboiron et al., 2019). Thus, the following results (Section Representation of Fish Taxa and Life Stages) are considered to provide an overview rather than to quantitatively compare the results collated.
Representation of Fish Taxa and Life Stages
Representation of Fish Families
In total, the field studies assessed here were concerned with 142 different families, including both bony fish and cartilaginous fish taxa (Supplementary Table 1). Members of the family Myctophidae (nspecies = 32), Sciaenidae (nspecies = 26), Carangidae (nspecies = 24) were investigated most frequently, followed by Clupeidae (nspecies = 19) and Sparidae (nspecies = 17) (Figure 4). Overall, 62 different families were represented by a single species only, e.g., Blenniidae, Chaetodontidae, Nemichthyidae, Pomacanthidae, and Scomberesocidae. Two taxa were investigated in 28 different families, and 16 more families were represented by three taxa each.
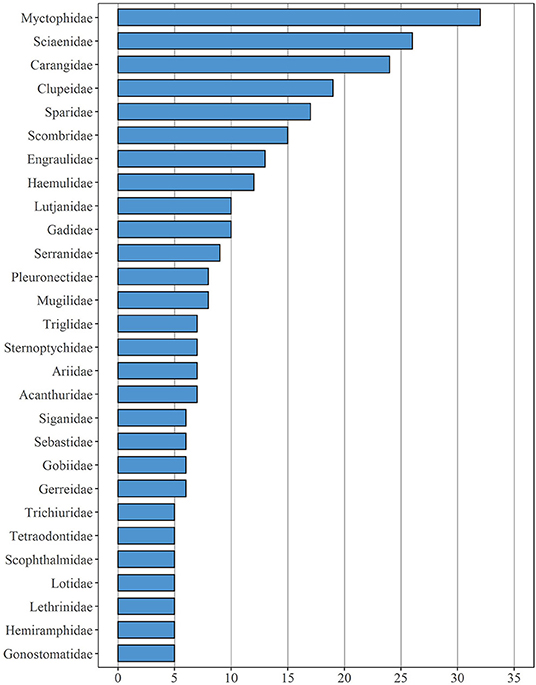
Figure 4. Fish families which are represented by at least five different species mentioned in the 90 studies under review here.
Representation of Fish Species and Commercial Importance
The present review collates information on a total of 487 different marine fish species, MP uptake was verified for around 67% of them (n = 327). The majority of species (80%; n = 393) was mentioned in only one study each. Among the remaining 95 species which were included in more than one publication, were several taxa of commercial importance (Supplementary Table 1) such as the European pilchard Sardina pilchardus, the flathead grey mullet Mugil cephalus and the European flounder Platichthys flesus. Of the 487 species included in this review, about 75% (n = 367) were considered commercially important for the fisheries sector based on the information accessed on FishBase (Froese and Pauly, 2019). Data were not available for 86 species, with an additional 35 species rated as not relevant for the fisheries sector. According to FishBase (Froese and Pauly, 2019), ~7,400 finfish species are used by humans (i.e., in fisheries and aquaculture, as bait or game fish, as well as in ornamental trade), 4,657 of these freshwater and marine taxa are considered relevant for industrial and artisanal fisheries. Thus, this review collates information on 7.9% of the species of importance for the total fisheries sector. Irrespective of the individual outcomes of the MP-feeding studies on commercial fish taxa listed here, the overall significance of MP as a threat to the living marine resources is hardly assessable.
Representation of Life Stages
For the majority of the species listed (286 of 487), no clear classification of life stage was possible. This lack of data in this category may be explained by two major reasons: first of all, the missing data in the original publication—in terms of division to either of the categories or in terms of size dimension which would have been subsequently used here to derive the classification from the data accessible on FishBase (Froese and Pauly, 2019)—or the lack of data on maturity size ranges for particular species in the online database itself. In a few cases, the results of different life stages were grouped in the publication and could not be assessed individually, which resulted in a combination of different life stages, e.g., juveniles + adults of Allosa fallax in Skóra et al. (2012) or in Anchoa januaria in Vendel et al. (2017); sub-adults + adults of Engraulis encrasicolus and Sardina pilchardus in Compa et al. (2018). For a total of 121 species, early life stages were considered with varying sample sizes across the different studies, ranging from one individual only, e.g., Trisopterus minutus in McGoran et al. (2018) to a maximum of 1,090 individuals of Platichthys flesus in Kartar et al. (1976). For another 81 species, only adult individuals were investigated for MP ingestion.
Drivers of MP Ingestion
Along with establishing growing evidence for MP-ingestion by a wide range of marine fish species, researchers seek to elucidate the driving factors of MP uptake. One of the most frequently investigated hypotheses correlates the size of the fish (e.g., standard length or total length) with the amount or size of MP particles taken up (e.g., Boerger et al., 2010; Foekema et al., 2013; Güven et al., 2017; McGoran et al., 2018), while two other prominent hypotheses aim to identify patterns related to the trophic guild/feeding mode of the fish (e.g., Anastasopoulou et al., 2013; Vendel et al., 2017; Sun et al., 2019) as well as the preferred habitat (e.g., Güven et al., 2017; Markic et al., 2018).
Body Dimensions (Size)
In fishery biology surveys and studies, the assessment of the size and weight of a fish has been routinely performed for decades (Smith, 2002)—the same routine seems to be applied for studies on MP ingestion in fish. Frequently, measurements of standard length (SL) (e.g., Boerger et al., 2010; Dantas et al., 2012), total length (TL) (e.g., Anastasopoulou et al., 2013; Ory et al., 2018) or fork length (FL) (e.g., Battaglia et al., 2016; Chagnon et al., 2018) were taken upon collecting or dissecting the fish. In other cases, the lengths of the fish were recorded, but no specification of the measurement was given in the publication (e.g., Neves et al., 2015; Peters et al., 2017) while in some investigations, no length was assessed at all (e.g., Davison and Asch, 2011; Wesch et al., 2016) or the data are not provided in the text even though the researchers reported measuring size and weight of the fish (Miranda and de Carvalho-Souza, 2016; Su et al., 2019). Due to these inconsistencies or entire absence of data on fish body dimensions, the results obtained are difficult to compare across all studies under review here. Notwithstanding, within the individual studies that collected these size-related data, the measurements were occasionally used to investigate a potential correlation between MP uptake (i.e., number of particles taken up, size of particles ingested) and body size of the fish. Comparable to the outcomes of impact-assessments, the results of these correlations turn out to be rather inconsistent. In their study on seven North Sea fish taxa, Foekema et al. (2013) were not able to relate the size of the MP particles ingested to the size of the MP-feeding fish beyond doubt. Likewise, the size of the ingested MP (fibers and non-fibers) by fish collected in Turkish territorial waters was not correlated to the size of the MP-feeding fish itself (Güven et al., 2017). Contrastingly, Sun et al. (2019) found a positive correlation between the length of MP particles detected in the GIT of fish from the Yellow Sea and the fish length.
The existence of a potential correlation between the number of MP particles detected in the GIT and the size of the fish was investigated in several of the studies reviewed here, again with partially divergent outcomes: in a study on 60 lemon damselfish Pomacentrus moluccensis from two locations of the Great Barrier Reef, Jensen et al. (2019) did not find a correlation between the number of MP ingested and the fish TL. Giani et al. (2019) were also not able to detect a correlation between the TL or weight of the examined 132 red mullet Mullus barbatus barbatus and 97 European hake Merluccius merluccius and MP ingestion—neither in terms of occurrence of MP ingestion by fish (in %) nor in terms of particles per individual. For some elasmobranch species, the observations made by Alomar and Deudero (2017) and Bernardini et al. (2018) suggest that early life stages (i.e., juvenile or immature individuals) show a higher probability to ingest MP particles than adults.
As opposed to the abovementioned results, Cheung et al. (2018) found a positive correlation between the abundance of MP and the FL of flathead grey mullet Mugil cephalus, suggesting larger individuals tend to take up MP particles to a higher extent. Similar observations were made by McGoran et al. (2018) in their comparative study of MP ingestion by fish from the Thames estuary and the Firth of Clyde: according to their results, larger fish (based on SL) ingested more MP particles than smaller ones. The same positive correlation between SL of the fish and the number of MP particles ingested was found for various fish species from the Amazon River estuary (Pegado et al., 2018) and the North Pacific Central Gyre (Boerger et al., 2010). For both Atlantic herring Clupea harengus and European sprat Sprattus sprattus, Beer et al. (2018), furthermore, substantiated the same correlation for fish TL and the number of MP particles ingested.
A different connection was investigated by Azad et al. (2018): in their study, the researchers aimed to identify a potential correlation between the mouth size of the fish and the frequency of occurrence of MP in the GIT as well as the size of the MP particles detected. No significant relationship could be attested between the mouth size and either of the MP characteristics. However, as the 24 different fish species under examination showed contrasting tendencies, both positive and negative correlations were found between mouth size and MP frequency of occurrence.
Reasons for the lack of a definite tendency on how body size and MP particle ingestion potentially correlate may be seen for one part in the—to some extent–insufficient data basis of the in-situ studies: comparisons of various taxa with (partially) small sample sizes (Vendel et al., 2017; Pegado et al., 2018), little variation in terms of size classes (Boerger et al., 2010), differing sampling sites (Alomar and Deudero, 2017) and variation in the residence time of prey and/or MP in the GIT related to the feeding mode of the fish (Gassel et al., 2013; Ory et al., 2018) may contribute to the vagueness of results. For the other part, secondary ingestion or trophic transfer along the food chain may play an important role, currently underestimated in the scientific literature (Peters et al., 2017). Furthermore, shifts in dietary preferences and changes of foraging strategy related to ontogenetic development, with younger individuals showing a tendency for more opportunistic feeding strategies (Balon, 1986; Rønnestad et al., 2013; Critchell and Hoogenboom, 2018) may confound the relationship between body size and MP ingestion. Lastly, the possibility of a non-existence of a correlation for all fish taxa alike should not be precluded. Repetitive analyses of the same species or populations from the same sampling site, sampled in adequate numbers over a sufficiently long timespan, may establish evidence for or against the existence of a correlation of body size and MP ingestion on a species-specific level.
Trophic Guild and Feeding Strategies
Different feeding strategies in fish may involve various expenditures of time for satiation (i.e., feeding) and also gastric evacuation; to cover their energy demand, filter feeding, detrivorous, or herbivorous taxa for instance spend more time collecting (numerically more but less nutritious) prey items than predatory, carnivorous taxa which rely only on few, substantial meals (Pandian and Vivekanandan, 1985). These higher temporal costs of feeding and, thus, the potentially higher probability to encounter MP particles and fibers while feeding, however, may be counterbalanced by shorter digestion rates for herbivorous taxa than carnivorous ones (Fänge and Grove, 1979). The trophic guild may, therefore, represent an important explanatory factor for MP presence in the GIT of a fish.
The fish taxa assessed in this review were divided into different feeding categories, following the data accessed on FishBase (Froese and Pauly, 2019) on preferred prey items ingested; the entries can be assessed for each species in Supplementary Table 1. The different categories were defined as follows: herbivore (feeding on plant material exclusively), planktivore (including filter feeders, ingesting both zoo-, and phytoplankton), carnivore (preying on other animals), and omnivore (taking up plant-based materials and other animals alike). According to the data available on FishBase, two species were rated exclusively piscivorous (Caranx papuensis and Scomberoides tol). The majority of taxa was classified as carnivorous (n = 254), followed by planktivorous (n = 61), omnivorous (n = 26), and herbivorous (n = 20)—two taxa were classified piscivorous. Moreover, due to the lack of information available, a number of species (n = 124) could not be attributed to any of the abovementioned categories.
The proportion of MP-feeding individuals across the different trophic guilds was compared by taking into account those entries which included both the MP uptake given for the respective fish taxa in the publication as well as the trophic guild listed according to FishBase (Figure 5)—this figure includes also the different entries made for the same taxa by various studies which explains the variation in total numbers given in the boxplot as compared to the listing above. The median proportion of MP-feeding individuals ranged between 11.25% (omnivorous taxa) and 20.0% (planktivorous taxa). The proportion of MP feeding individuals, represented by the interquartile ranges, is less variable in the herbivorous guild compared to the three others. Moreover, except for two outliers, the overall range in the proportion of MP-feeding individuals in this guild is also smaller. However, this feeding guild was also represented by the lowest number of taxa. The Kruskal–Wallis rank sum test was applied as the data were not normally distributed (Shapiro-Wilk normality test, p < 0.01): the tendencies described above were not substantial and no significant difference in the proportion of MP feeding individuals across the different trophic guilds was verified (p = 0.7316; df = 3). A comparison of the mean number of ingested MP particles per individual likewise revealed no significant difference between the MP uptake rate of the four feeding guilds (Kruskal-Wallis rank sum test, p = 0.4949; df = 3).
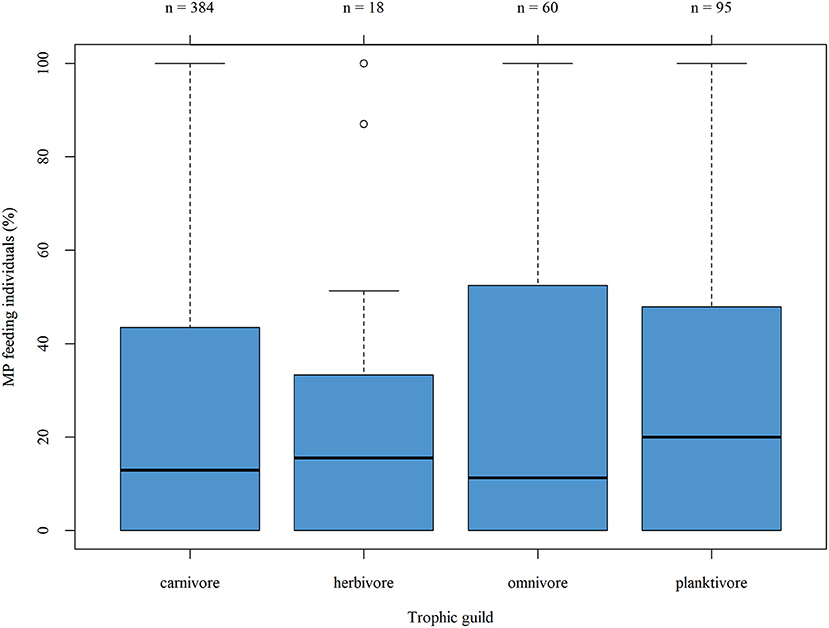
Figure 5. Boxplot showing the proportion of MP-feeding individuals per trophic guild. Piscivorous taxa are included in the carnivorous guild, as only two taxa were classified as piscivorous according to FishBase.
In the only other stand-alone review of comparable dimensions, the authors also compared the representation of feeding guilds among MP-feeding and MP-non-feeding taxa—likewise, no correlation between MP ingestion and feeding mode was ascertained (Markic et al., 2020).
Taking into consideration the results collated at a study-specific level, without interference of different sampling efforts and detection methodologies, the potential existence of correlations between MP ingestion and feeding mode or trophic level was investigated in several publications under review here. Vendel et al. (2017) chose a comparable approach to the one applied here, by analyzing the prey items identified in the full GITs of the fish, and subsequently identifying five different feeding guilds to which the fish were classified: generalist species, benthivorous, zoobenthivorous, algae eating, or zooplanktivorous species. Although MP was found in all the feeding guilds, the highest MP ingestion was detected in zoobenthivorous species (A. lineatus and D. auratus). A different categorization was chosen by Markic et al. (2018), describing not only the preferred prey category but also partially incorporating the feeding mode: grazers, omnivores, planktivores, benthic predators, and pelagic predators. The study found a significant difference among the five trophic guilds with benthic predatory fish showing the lowest MP uptake rate and omnivorous fish showing the highest, even though the authors acknowledged that only two fish species were classified as omnivorous, potentially limiting the significance of results obtained. Omnivorous fish species were also reported by Mizraji et al. (2017) to display higher MP ingestion rates in comparison to herbivorous or carnivorous species. These findings, however, are contradicted by the study of Jabeen et al. (2017), who detected the lowest MP uptake rate in omnivores, with higher rates detected in carnivores and planktivores. Following the categorization applied by Bour et al. (2018), the results indicate a significantly lower number of MP particles ingested by filter-feeders in comparison to predators, though the results concerning the frequency of MP-feeders among the different guilds did not differ significantly. As opposed to those tendencies, research by Güven et al. (2017) and Sun et al. (2019) as well as the review provided by Markic et al. (2020), did not establish significant evidence for a correlation between feeding mode/trophic guild and MP uptake rates.
The relationship between feeding mode and the type of MP taken up was assessed for instance by Anastasopoulou et al. (2013) who found that nektobenthic opportunistic feeders, represented by G. melastomus, ingested all identified plastic debris categories, whereas pelagic and bathypelagic feeders ingested either plastic bags and hard plastics, respectively. Another observation by Markic et al. (2018) highlighted the tendency of omnivorous and grazing fish species to ingest predominantly fibers, with ingestion of MP fragments more important in benthic and pelagic predators.
According to the majority of studies, there is no statistically significant correlation between the trophic level of a fish species and the quantity of MP particles taken up (Güven et al., 2017; Bour et al., 2018; Markic et al., 2018; Pegado et al., 2018; Sun et al., 2019). Only in a single study did results indicate that organisms at higher trophic levels also show a higher abundance of MP particles in the GIT, supporting the idea of trophic transfer of particles (Rios-Fuster et al., 2019). Evidence for trophic transfer of MP was, furthermore, found in 10 out of 57 fish examined by Markic et al. (2018), indicating an indirect uptake of MP from prey to predator, previously described on the basis of laboratory feeding experiments (Farrell and Nelson, 2013; Setälä et al., 2014).
As the GIT content features only a current snapshot of the recent food intake, the rates at which marine fish taxa ingest synthetic particles and the extent to which they are exposed to potentially detrimental effects of this ingestion should be contemplated in the context of species- and life stage-specific GIT retention times (Beer et al., 2018; Markic et al., 2018) or in the special context of prey availability and environmental parameters (see Section Language bias and Duplicate/multiple publication bias).
Habitat
As essential feeding, spawning, and nursery habitats of fish overlap with input sources and fluxes of MP which, in return, facilitates biota-MP-interactions (Figure 6), categorizing fish taxa according to their preferred habitat is another approach commonly used in the scientific literature to identify potential explanatory variables for MP ingestion. As the marine realm can be divided horizontally (e.g., inshore, neritic, oceanic) and vertically (e.g., benthic, epipelagic), it seems advisable to combine both to thoroughly investigate potential patterns in MP uptake related to the choice of habitats.
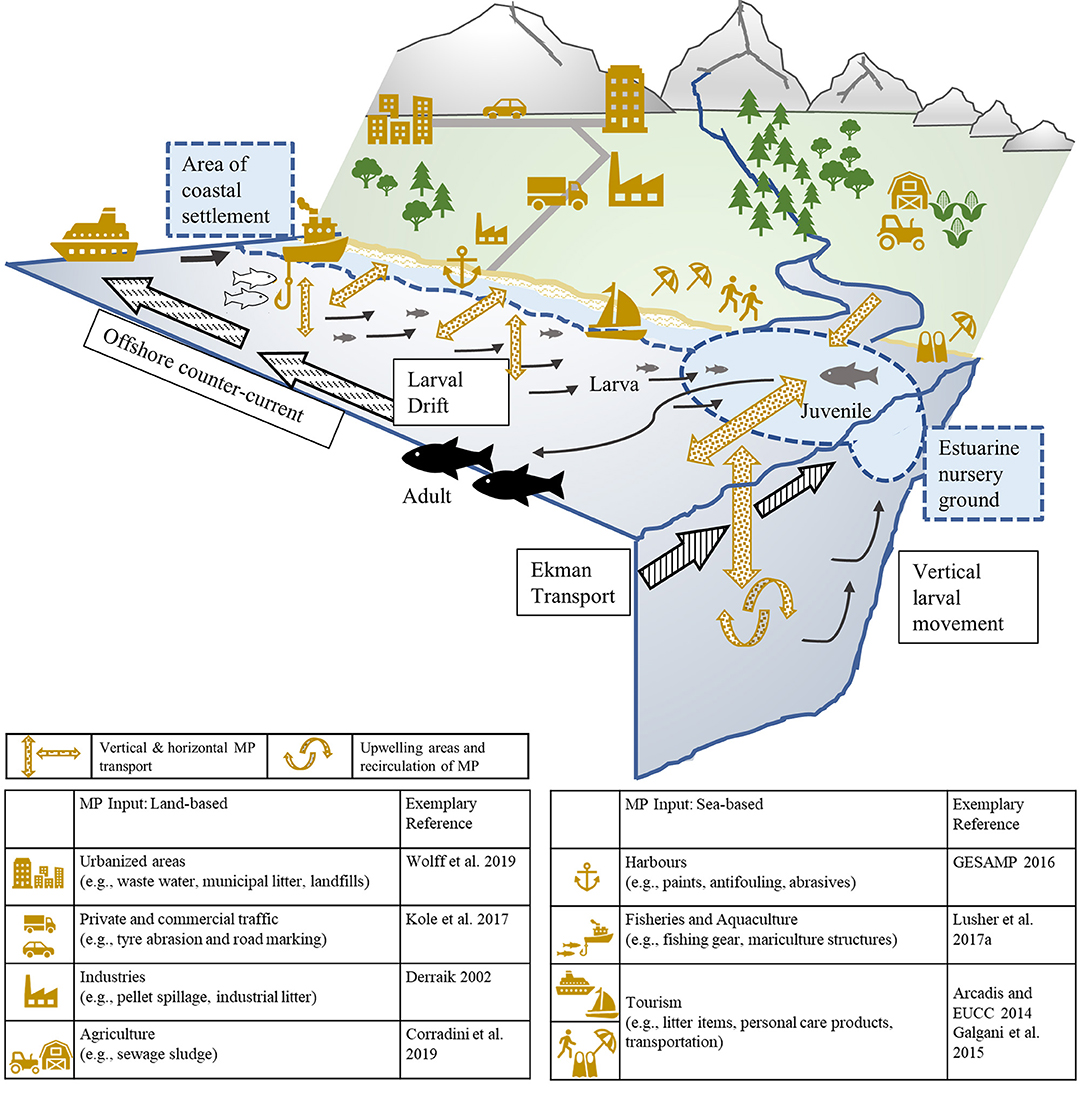
Figure 6. Schematic representation of the transportation and movement processes of marine fish larvae (spawned offshore) to inshore, estuarine nursery grounds [after Boehlert and Mundy (1988)] in combination with the illustration of the spatial distribution of micro-sized plastic particles and fibers from land- and sea-based sources (e.g., Derraik, 2002; Arcadis and European Commission, 2014; Galgani et al., 2015; GESAMP, 2016; Kole et al., 2017; Lusher et al., 2017a; Corradini et al., 2019; Wolff et al., 2019) into different estuarine and marine compartments which overlap with vital fish habitats.
The only study in which a consistent classification to both zones was applied, found a tendency related to the vertical distribution of fish: benthopelagic fish showed the highest uptake rates in comparison to pelagic or demersal taxa (Markic et al., 2018). The study, however, did not detect any statistically significant horizontal patterns. In contrast to these results, a study from Turkish territorial waters, in which a vertical-horizontal combination of categories was applied that was based on the entries available on FishBase (Froese and Pauly, 2019), detected slightly higher ingestion rates in fish species from the pelagic-neritic zone than in taxa from the benthopelagic, reef-associated, demersal, or pelagic-oceanic zones (Güven et al., 2017). Due to the overall lack of a consistent classification scheme in the literature reviewed here along with the variability in fieldwork approaches and laboratory methodologies applied for analyzing MP uptake in fish, a quantitative evaluation and assessment of the results collated was not considered expedient. A similar conclusion was obtained in another stand-alone review on in-situ studies of MP ingestion in fish (Markic et al., 2020). Therefore, a brief overview of the reported outcomes and tendencies will be given in the following.
Horizontal Habitat Classification
As the majority of marine MP originates from land-based sources (GESAMP, 2016), the horizontal distribution and pathways of plastic litter across neritic and oceanic habitats may represent a relevant explanatory variable of MP ingestion; however, only few studies applied a horizontal habitat classification to identify potential driving factors for MP ingestion. Murphy et al. (2017) found a tendency for coastal species to ingest significantly higher amounts of MP than offshore species. Sun et al. (2019) also reported lower numbers of MP per fish, if the fish was collected with greater distance to the coast and in the central Yellow Sea. In comparison to the amount of published MP research conducted in surface waters and accumulation zones (e.g., Boerger et al., 2010; Gassel et al., 2013; Ory et al., 2017), there is an apparent knowledge-gap concerning the exposure and uptake of MP across horizontal scales, especially in estuarine and coastal environments which are of special ecological, economic, and social importance (Martínez et al., 2007). Moreover, the connectivity between coastal and open-ocean areas in relation to fish abundances and MP uptake by various life stages along horizontal gradients (Figure 6) should be prioritized by prospective research initiatives to enhance the assessment of potential vulnerabilities of specific fish taxa or vital life stages.
Vertical Habitat Classification
The vertical distribution and pathways of plastic particles and fibers in the water column have been investigated by various studies (e.g., Kukulka et al., 2012; Enders et al., 2015; Cole et al., 2016; Choy et al., 2019). Similarly, published research included in this review more commonly categorized fish taxa according to their distribution across vertical marine zones. A broad allocation to either the benthic or pelagic realm was chosen by Neves et al. (2015): in their study of 26 commercial fish species sampled off the Portuguese coast, 63.5% of the benthic fish species and 36.5% of the pelagic species ingested MP. A comparable categorization was chosen by Zhu et al. (2019), who found significantly higher MP uptake in demersal fish than in pelagic fish from the Maowei Sea. In line with these observations, Murphy et al. (2017) reported significantly higher numbers of ingested MP in demersal fish species than in pelagic ones. In contrast, in a comparison of MP ingestion across three fish species, a significantly higher MP number per gram of GIT content (wet weight) was found for fish from the pelagic zone in comparison to demersal fish (Digka et al., 2018). In the Mondego estuary (Portugal) significantly lower ingestion rates were detected again for demersal fish species D. labrax and P. flesus in comparison to the benthopelagic D. vulgaris (Bessa et al., 2018b). No significant difference in MP uptake was verified, however, between five demersal and five pelagic fish species collected in the English Channel (Lusher et al., 2013).
Comparable to the attempt to link MP uptake to feeding modes, illustrated in Section Trophic guild and feeding strategies, the abovementioned brief collection of outcomes from different studies of various geographic regions and fish taxa highlights the inconsistency of results as well the remaining ambiguities in establishing habitat selection as an explanatory variable for MP ingestion in fish. Conceivable reasons for the (currently) missing links between habitat selection and MP ingestion may be the lack of environmental samples collected along with the fish-samples for contextualization (Section Environmental samples) as well as the rarity of investigations on early life stages (ELS) of fish (Supplementary Table 1), as part of the plankton community potentially representing a proxy for horizontal MP fluxes. Besides the classification of fish to specific feeding modes or preferred habitats, studies also investigated the relevance of other internal and external influencing factors of MP uptake and ingestion (Supplementary Material).
MP Ingestion Put Into Context
Scientific research efforts (especially based on laboratory experiments) have been substantiating a range of ecological, behavioral, and physiological implications for freshwater and marine fish taxa, though the effects of exposure and ingestion appear to vary across species and life stages, including even no measurable negative impact (Jovanović, 2017; Foley et al., 2018). Given the broad research area of MP ingestion and MP-related effects, this review paper does not aim to reproduce the various impacts that have been verified to date. To give a brief summary, however, some of the detrimental implications of MP ingestion for fish, evidenced predominantly by laboratory studies, should be mentioned here: histopathological intestinal alterations (Pedà et al., 2016), translocation of particles (Avio et al., 2015) and consequent hepatic stress (Rochman et al., 2013), metabolic changes (Cedervall et al., 2012; Lu et al., 2016), and neurotoxic effects (Oliveira et al., 2013), reduction of predatory performance (de Sá et al., 2015), as well as increasing mortality of ELS (Mazurais et al., 2015). By presenting the descriptive measures commonly applied in field studies, the aim of the present review is rather to focus on what in-situ studies on marine fish species have so far substantiated along with suggestions regarding the advancement and amendments of the state-of-the-art procedures.
Impact of Ingestion
The detrimental effects of intentional, accidental, or secondary ingestion of plastic debris by marine biota are manifold and both, physical (Goldstein and Goodwin, 2013; Lusher et al., 2013) and chemical hazards (Teuten et al., 2009; Rochman et al., 2013), have been shown to directly or indirectly impact growth, condition, and survival of different life stages of fish (Supplementary Material). At present, the investigation of MP-uptake related impacts on fish are predominantly based on laboratory feeding experiments (Morgana et al., 2018), which in many cases lack consistency between natural conditions and experimental set-ups (Phuong et al., 2016; Lusher et al., 2017a). As the focus of this literature review is entirely on in-situ studies, it will report exclusively on the MP-related impacts investigated in those studies under consideration; the analysis of potential effects of MP-ingestion was, however, performed rather infrequently (in 11 of 90 studies). The majority of these studies investigated the impact of MP in the GIT on the condition of the fish based on morphometrics (Supplementary Material): eight studies assessed the condition based on Fulton's condition factor K (Fulton, 1904; Foekema et al., 2013; Rummel et al., 2016; Mizraji et al., 2017; Cardozo et al., 2018; Compa et al., 2018; Kazour et al., 2018; McGoran et al., 2018; Arias et al., 2019), two more studies (Ramos et al., 2012; Dantas et al., 2019) made use of the formula of Richardson's condition factor CF (Richardson et al., 2011). The outcomes of these investigations are equivocal; taking into consideration only the seven reports in which Fulton's K was calculated, one found a statistical significant difference between MP-feeding and non-feeding individuals (Cardozo et al., 2018). In their study on Atlantic bigeye Priacanthus arenatus, Cardozo et al. (2018) observed that the ingestion of plastic fragments affected the condition of these fish with MP non-feeding individuals showing a significantly higher condition factor than the MP-feeding individuals in their study. The authors hypothesized that the presence of plastic particles in the GIT might ultimately lead to reduced body weight (and, thus, a lower condition factor) due to a decrease in feeding activity as a result of the false saturation feeling. Contrasting these findings, Mizraji et al. (2017) verified a significant negative relationship between the ingestion of MP and the condition of the omnivorous Girella laevifrons, which was interpreted by the authors as an indicator for a potential negative effect of MP uptake on the health status of an individual.
According to the results of a model by Compa et al. (2018) for Sardina pilchardus from the Mediterranean, fish with lower condition factors were more likely to ingest MP particles. However, in the same study, the model predictions for Engraulis encrasicolus showed the opposite: individuals with higher condition factors would rather take up MP than those with lower body condition. The authors state, however, that no significant relationship between the MP uptake rate and the body condition of a fish could be established by their model. In their study of seven different North Sea fish species, Foekema et al. (2013) were not able to verify any fundamental effect of MP ingestion on the condition of the MP-feeding fish, originating from five of the seven species under investigation. In an assessment of MP uptake by wild and caged juvenile European flounder Platichthys flesus, Kazour et al. (2018) also found no significant correlation between the condition and the number of MP particles taken up at different sampling sites in the eastern English Channel. In an Argentinian estuary, a similar observation was made by Arias et al. (2019) who did not detect statistically significant differences in the condition of individuals of the whitemouth croaker Micropogonias furnieri with plastic in their GIT in comparison to individuals without MP particles. Comparably, the condition factor of MP-feeding and MP non-feeding individuals did not differ significantly in the demersal bigeye sculpin Triglops nybelini and the pelagic polar cod Boreogadus saida collected off Northeast Greenland by Morgana et al. (2018). Rummel et al. (2016) also did not detect any effect of MP ingestion on the condition of different demersal and pelagic fish species collected in the North Sea and Baltic Sea.
The results obtained by calculating Richardson's condition factor appear to be equally inconclusive: according to Dantas et al. (2019), a higher condition factor for juvenile and adult fish without MP fragments in the GIT was found in their study. Following the interpretation of the authors, this result indicates that the ingestion of plastic could cause a reduced body condition. In contrast, Ramos et al. (2012) could not establish any evidence for a lower condition in MP-feeding members of the family Gerreidae in their study from the Goiana estuary.
While the assessment of condition factors is a commonly used tool to describe the physical state of individual fish or a fish population, potential differences in condition based on morphometric measurements should be evaluated with care. According to Froese (2006), the intraspecific variation in weight-length relationships may be significant, depending on the population itself, the season or varying environmental conditions between years. Depending on the group of organisms to compare, Froese (2006) suggests making use of different condition factors such as the Le Cren (1951) relative condition factor for comparing the relative condition of individuals within a sample. For studies of the relative condition across populations or taxa, relative weight in relation to mean weight was advised. One constraint in the evaluation based on condition factors, however, is their dependence on body length and weight of the fish, which on the one hand can be easily assessed in all routine sampling procedures making them available without additional handling effort, yet on the other hand, these morphometrics display different responses to varying environmental pressures (e.g., MP exposure or uptake) rather slowly (Markic et al., 2018). Unless the ingested MP particles accumulate over a long period of time in the individual, leading to a reduced food-uptake due to false saturation feeling and consequent reduced fitness and condition of the individual, the indicative meaning of condition factors based on body dimensions might bear only limited significance.
Another option to assess the potential impact of MP exposure and uptake is to analyze only specific organs such as the liver. Due to its detoxification function and the potential impact of contamination exposure to the size and weight dimensions of this organ, the hepatosomatic index (HSI) was calculated in one study (Arias et al., 2019). Even though their assessment of Fulton's condition factor did not establish evidence for a reduced body condition of MP-feeding individuals, the HSI was significantly higher in fish collected at one sampling site which also was positively correlated with a higher MP ingestion rate in individuals from this specific site.
An enzymatic approach to assess the effect of MP ingestion was chosen by Alomar et al. (2017): the researchers analyzed the antioxidant and detoxification system of fish by measuring different enzymatic activities in samples of the liver of MP-feeding and non-feeding red mullet Mullus surmuletus. The authors did not find signs of oxidative stress or cellular damage in the fish liver of MP-feeding fish. Nevertheless, they assume an induction of the detoxification system of the liver based on their findings of a small increase in the activity of GST (glutathione S-transferase).
These partly contrasting results highlight the challenges inherent to in-situ studies and impact assessments based on snapshot samplings: to effectively evaluate the impact of MP ingestion on the condition of a fish—either assessed via morphometric indices or by investigations on molecular, cellular, or organ level—long-term studies under controlled laboratory conditions across all life stages and trophic guilds are necessary (Morgana et al., 2018). Another major obstacle in examining the effect of MP ingestion based on in-situ studies is the ascertainment of a baseline for comparison, which hypothetically would involve fish growing up in an MP-free environment with no possibility of ingesting MP particles at any given point in time (Markic et al., 2018). Studies comparing the condition of MP feeding individuals with MP non-feeding ones face the uncertainty of former MP-uptake (and consequent egestion) by the latter. Thus, the informative value of these comparisons may be partially limited due to potential effects originating from previous feeding incidences in the now detected MP non-feeding fish. Nevertheless, future research should be strongly encouraged to focus on impact assessments based on field investigations, by continuing to calculate adequate condition factors with reference to the data available. As substantiated by Ryan (2016), the physical impacts of MP ingestion could include injuries of the GIT (i.e., ruptures, abrasions, lesions), thus, the examination of potential damages of the GIT prior to content analysis should be done by default. Both measures are comparably cost-efficient in terms of labor as well as financial input and could potentially contribute toward an enhanced understanding of MP uptake effects, considering the current underrepresentation of such studies and the lack of conclusive results. Another more labor-intensive still financially reasonable approach may involve the reading of otolith microstructures as an indicator for fish condition. The measurements of growth increment widths could offer insights into environmental pressures experienced by the fish with a higher accuracy than body dimensions (Suthers et al., 1992), even though a definite identification of MP exposure or uptake as the causative environmental pressure may prove difficult. Beyond, it appears expedient to further advance the analysis of the ratio of RNA:DNA as a proxy for fish condition in in-situ investigations (Vasconcelos et al., 2009), as well as the examination of inflammatory responses or enzymatic activities in response to MP exposure and ingestion. In summary, it is worth mentioning that the proxies for fish condition frequently applied in ichthyology (i.e., somatic growth, otolith microstructure, and RNA:DNA ratio) are known to heterogeneously reflect recent growth history patterns (Peck et al., 2015), and that this varying degree of suitability in detecting responses to MP exposure and ingestion in fish should be envisioned prior to identifying research objectives.
GIT Content: Natural Prey Items and MP
Besides the exclusive verification of MP in the GIT of the fish, a limited number of studies included reports on natural prey items (e.g., Gassel et al., 2013; Chagnon et al., 2018; Halstead et al., 2018), both qualitatively (i.e., identification of prey items) and quantitatively (e.g., volume or weight of natural prey items in comparison to MP particles). This lack of information in the majority of studies (79 of 90) may be predominantly due to the analytical method applied, which often includes the digestion of organic material as an initial step in MP-GIT-studies.
Accounting for the fact that the in-depth analysis of prey items in fish GIT may be time consuming and potentially susceptible to misidentification, the assessment of stomach fullness may be considered a feasible approach for many studies under time-constraints. Stomach fullness, i.e., the presence of food in the stomach or GIT, could represent a relevant factor in predicting the presence of ingested plastic. In their study on Atlantic cod Gadus morhua, Atlantic salmon Salmo salar and capelin Mallotus villosus, Liboiron et al. (2019) found varying levels of food presence (i.e., stomach fullness) across the three taxa under investigation which was linked to the probability of MP ingestion. A comparable observation was made by Alomar and Deudero (2017) who found a significantly positive correlation between the stomach fullness of Galeus melastomus and the amount of MP (particles gFW-1) in the stomach of these catsharks. In contrast to these findings, Wieczorek et al. (2018) did not detect a significant difference between the median stomach fullness of MP-feeding fish and MP-non-feeding fish in their study of seven fish species from North Atlantic waters, indicating no correlation between stomach fullness and MP ingestion.
Nevertheless, analyzing the entire GIT content should be considered beneficial, as it could yield essential information on the importance of MP as targeted or accidentally ingested prey items. In dietary studies, the relative importance of a specific prey item is frequently inferred from the proportion of all GITs included in the study containing each prey item (Baker et al., 2014). This leads to the questions, concerning why MP as a “prey item” is treated significantly different from natural prey items and how outcomes of MP ingestion studies should be interpreted and evaluated thoroughly without this essential information. By including the analysis of the entire GIT content, researchers will be able to deduce information on the feeding preferences of the fish population or species under investigation—insights that could be subsequently used to further investigate patterns of MP ingestion. This approach was chosen for instance in a study on six different fish species collected off the Texas Gulf coast (Peters et al., 2017): based on their assessment of prey items in the GIT of the fish, the authors were able to statistically correlate MP uptake with the ingestion of specific prey items in each fish species (e.g., shellfish, crabs, vegetation), enabling the researchers to identify patterns in MP ingestion related to foraging modes. Peters et al. (2017), thus, found a tendency for higher MP ingestion related to a generalist foraging mode and methods of prey capture in comparison to selective (invertebrate) foraging, as displayed by grunt (pigfish) Orthopristis chrysoptera in their study.
The assessment of the entire GIT content may imply other valuable insights into modes of MP ingestion in fish, such as trophic transfer and bioaccumulation which are currently rather neglected by most of the scientific literature (Wright et al., 2013; Lusher et al., 2017b; Chagnon et al., 2018). Upon chemically or enzymatically digesting the entire GIT content for MP analysis, information about feeding preferences are lost, which could be relevant for investigating the probability of trophic transfer. Obviously, the size relation between the prey or predator and the MP particles and fibers represents an essential constraint, as the prey organisms need to be capable of ingesting MP particles and fibers >1 μm in size to fall into the category of MP. Only few studies included in this review investigated the potential existence of MP transfer from prey to predator organisms. In a study on different fish species (i.e., predators) and shrimp (i.e., potential prey), McGoran et al. (2018) found higher MP ingestion rates in pelagic fish and flatfish species (MP-feeding fish: 36%) than in the invertebrate taxon (MP-feeding shrimp: 6%). This observation was considered to indicate bioaccumulation of MP from prey to predator. In a study by Chagnon et al. (2018), MP were found in flying fish Cheilopogon rapanouiensis preyed upon by yellowfin tuna Thunnus albacares, thus, the authors argued that MP may be transferred from prey to predator. Rios-Fuster et al. (2019) found undigested Sardina pilchardus in the GIT of Trachurus mediterraneus, and upon analyzing the GIT of the prey fish, anthropogenic particles were found, supporting the hypothesis of trophic transfer in fish. The extent to which the secondary ingestion of MP contributes toward bioaccumulation in fish remains to be critically evaluated on the basis of more in-depth studies on a variety of fish taxa, especially as many of the higher trophic species are consumed by humans. Particular consideration should be devoted, however, to the potential capability of fish taxa to egest small-sized particles so that MP may not necessarily accumulate inside the GIT over time (Foekema et al., 2013; Chagnon et al., 2018).
Environmental Samples
Establishing a link between the content analysis of the fish GIT and the environment in which the fish were sampled is complex; the majority of in-situ studies (73/90) did not include water, plankton or benthos samples as a reference for MP abundance. In the studies which did so, however, the resemblance between the environmental MP and the ingested MP varied greatly. Concerning the abundance of MP in environmental samples and in fish GIT, Lusher et al. (2016) were not able to detect any correlation between the MP numbers in subsurface waters and the amount of MP ingested by fish in the same area. A similar observation was made by Collicutt et al. (2019) comparing the GIT MP concentrations in juvenile chinook salmon to environmental (water and sediment) MP concentrations. In contrast to this, Güven et al. (2017) detected a different pattern in fish from Turkish territorial waters: individuals with higher MP loads in their GIT originated from sampling sites with higher MP particles abundances, thus mirroring the local conditions in terms of MP pollution.
Concerning the color and particle types (i.e., fragments or fibers) of MP detected in the environment and also in the fish GIT, the outcomes of the few studies that took environmental samples into account seem to be equally divergent. Ory et al. (2017) for example found a significantly different representation of the most common colored MP particles ingested by Decapterus muroadsi in comparison to the representation of colored MP detected most frequently in water samples. Specifically, the researchers verified a selective feeding for blue MP consistent with a higher prey selectivity for transparent-blue copepods in the diet of the fish, both more commonly ingested than expected based on their relative representation in the surrounding environment. Consistent with the observation of this selective feeding, the damselfish Pomacentrus moluccensis was found to ingest white or transparent fibers, potentially reflecting their natural prey items, with a higher frequency than this observed in the corresponding surface water samples collected in the habitat of the fish (Jensen et al., 2019).
In contrast, other studies clearly demonstrated the mirroring of the assemblage of MP sampled from surface waters or the water column and the MP assemblage found in the fish GIT, thus, indicating non-selective or random feeding. In mesopelagic fish species from the North-western Atlantic, for instance, no evidence for a color-selective feeding was established, as the MP detected in the alimentary tract were similar to those sampled from surface waters (Wieczorek et al., 2018). Supporting this observation, fish larvae from the western English Channel ingested predominantly blue fibers, corresponding to the particle category most represented in the water column (Steer et al., 2017). In a time series analysis of MP in plankton samples and fish from the Baltic Sea, the GIT content of herring Clupea harengus and sprat Sprattus sprattus also mirrored the composition and abundance of MP in the water column, both in terms of MP types (i.e., fibers, fragments) and size classes of MP over the years (Beer et al., 2018). Juvenile chinook salmon Oncorhynchus tshawytscha were also reported to non-selectively ingest MP of various colors and types (i.e., fibers) with reference to water and sediment samples assessed from the respective sampling sites (Collicutt et al., 2019).
The inconsistent findings and apparent mismatches between ambient samples and fish GIT both in terms of MP abundance and MP categories and colors may be explained on the one hand by the mobile and often migrating behavior of fish on horizontal and vertical scales (Lusher et al., 2016), and on the other by the spatial and temporal heterogeneous distribution of MP in the marine environment (Barnes et al., 2009; Goldstein et al., 2013; Antunes et al., 2018). Moreover, fish species showing specific feeding preferences with regards to sub-surface or benthic prey items may, further, distort the interpretation of results due to varying probabilities of MP encounter and ingestion across certain local, regional, or even seasonal scales (Lusher et al., 2016; Silva et al., 2018a). Thus, considerations of the ecology of the study organism as well as the hydrography of the sampling site are essential to holistically evaluate the correlation between MP in fish GIT and in the ambient environment. As laboratory experiments verified harmful effects of adsorbed toxins (Rochman et al., 2013), the collected MP from environmental samples should be considered for analysis of ambient toxins as well as harmful microorganisms adsorbed or for chemical additives inherent to the particles; the outcomes of such assessments may shed light on the potential implications of MP ingestion for fish.
Environmental Parameters
Environmental parameters may have significant impacts on the physiological and ecological performance of fish. Related to the uptake of prey and, thus, the potential presence of MP particles in the GIT of a fish, the following abiotic factors may be considered relevant: oxygen availability determines, for instance, the swimming and feeding activity (Fry, 1971), temperature is known to impact the feeding and the egestion rates (Fänge and Grove, 1979; Pandian and Vivekanandan, 1985; Beer et al., 2018), light intensity has been shown to also affect activity levels, swimming speed, and visual capacity relevant for near-field location and predation success (Downing and Litvak, 2000; Trippel and Neil, 2003), salinity may shape fish assemblage distribution and with that the probability of MP exposure (e.g., within estuaries; Blaber and Blaber, 1980). Furthermore, current velocity or tidal currents could also influence not only the activity, distribution, and feeding behavior of fish (Stoner, 2004), but also the distribution of MP particles and fibers in the ambient waters (Kanhai et al., 2017; Welden and Lusher, 2017; Zhang, 2017). Concerning the distribution and abundance of MP, rainfall (i.e., seasonality) may be also considered a relevant factor especially in estuarine-based studies, as increased precipitation leads to higher MP abundance caused by increased river discharges (Lima et al., 2014) which in return also affects levels of salinity or temperature in the estuarine environment. All of the above highlight the importance of recording abiotic variables along with prey/MP availability in in-situ MP ingestion studies. However, only a minor part of the studies investigated here (4/90) explicitly reported on any environmental factors (i.e., Ferreira et al., 2016, 2018; Vendel et al., 2017; Silva et al., 2018a).
In studies based on sampling campaigns during the dry and rainy season in the Goiana estuary, Brazil, a spatio-temporal pattern of filament ingestion was revealed for different life stages of Cynoscion acoupa (Ferreira et al., 2016, 2018). According to the authors, the highest ingestion of filaments was recorded for adult individuals during the rainy season; the increased availability of filaments during this time of the year was hypothesized to be related to the peak in fishing activities acting as the origin of the filaments. From the same estuary, a spatio-temporal pattern of MP ingestion was also reported for different life stages of Pomadasys ramosus and Haemulopsis corvinaeformis (Silva et al., 2018a). All of the three studies recorded a variety of different environmental parameters, including salinity, dissolved oxygen, Secchi depth, and rainfall rates obtained from local meteorological stations. A significant impact of any of the assessed environmental parameters on the MP uptake of the fish species studied was, however, neither investigated nor substantiated in either of the studies. The same holds true for a comparative study performed during rainy and dry season along the salinity gradient in two other Brazilian estuaries subjected to varying levels of anthropogenic pressures (Vendel et al., 2017): the authors did not relate MP uptake to the seasonality, the salinity gradient assessed, or any other environmental factor. Notwithstanding the above, it may be expected, however, that a larger number of studies has information on abiotic data available for correlation analyses, especially those studies conducted on board research cruises as part of continuous monitoring campaigns (e.g., Bellas et al., 2016; Hermsen et al., 2017; Beer et al., 2018; Pellini et al., 2018b). As the metabolic demands and, thus, the feeding and egestion rates vary not only with life stage and species but also with prevalent abiotic factors, past, present, and future studies should (re-)evaluate the presence of prey or MP particles in the fish GIT with primary emphasis on temperature and oxygen, and secondary with regards to light intensity, salinity, current velocity, and seasonality.
Publication Biases
Within the previously mentioned broad category of publication biases, different sub-categories were suggested for further differentiation (Figure 7), of which several of the delineated above may be recognized in MP research on fish on the basis of this review.
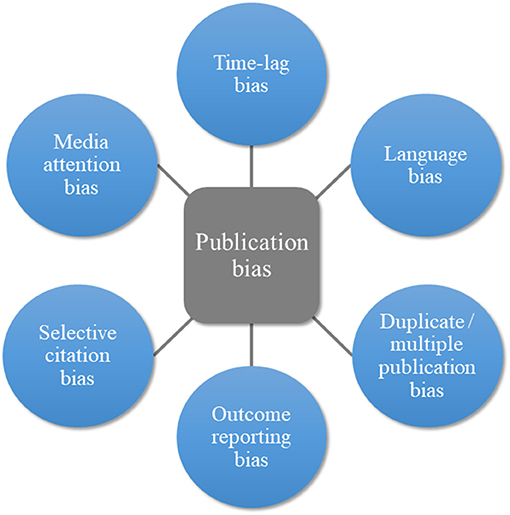
Figure 7. Different types of publication bias (Ekmekci, 2017).
Time-Lag Bias
According to Song et al. (2013), studies reporting significant or major results may be prone to more timely publishing than those stating insignificant outcomes. Taking into account the time-consuming procedures associated with alimentary tract analysis and MP particle identification [e.g., Fourier-transform infrared spectroscopy (FTIR) or Pyrolysis gas-chromatography mass-spectrometry (Py-GC/MS)] used in many studies, and consequently the small amount of samples that can be processed in a short period of time (Käppler et al., 2016, 2018; Silva et al., 2018b), a time lag between the sampling campaign and the consequent publication is inevitable, irrespective of the potential outcomes. In cases where only month and year were given, the last day of the respective month was chosen to calculate the time lag between sampling campaign and publication date online. Moreover, publications which indicated only a season (e.g., spring) as a reference for the sampling campaign were excluded for this analysis. As the entire submission and publication process (including the precise dates) was not uniformly trackable for all publications under review and due to the varying reporting schemes for MP feeding across fish taxa, the number of studies considered for the time lag analyses varies. Based on the accessible information for 67 of the 90 studies under review, 28.21 ± 18.92 months is the average time between the actual sampling campaign and the online publication of the study. Looking at the submission and publication time lag separately, the average time between the last month of sampling and the submission of the manuscript was 24.43 ± 19.34 months (n = 58), whereas after the submission, the review and publication process took on average 4.12 ± 2.26 months (n = 57). Studies reporting an uptake rate of <10% (n = 16) across all individuals investigated took 23.13 ± 12.27 months to be published online, whereas studies with 10–100% MP ingestion (n = 48) across individuals were published within 27.19 ± 15.51 months after the last month in the field. There was no correlation between the proportion of MP feeding individuals in a study and the total time lag between the last month of field work and the online publication (data not normally distributed, Spearman rank correlation coefficient n = 64, R = −0.013, p = 0.92). As opposed to the hypothesis by Song et al. (2013), according to which studies with major results might be published faster, no significant correlation was found between the proportion of MP feeding individuals and the time lag between submission and publication (Spearman rank correlation coefficient n = 55, R = −0.019, p = 0.89). Furthermore, no significant correlation was found between the number of fish taxa included and the total time lag between field work and publication (Spearman rank correlation coefficient n = 67, R = −0.15, p = 0.22) nor between the number of individuals assessed and the total time lag (Spearman rank correlation coefficient n = 66, R = 0.062, p = 0.62). The existence of a time-lag bias may, thus, be considered incidental. Given the overall low number of insignificant, low, or negative reports in terms of MP-feeding currently published, the existence of this bias should be, nonetheless, re-evaluated in the future.
Language Bias
The language in which a report is written may pose a significant obstacle to the thorough and holistic evaluation of the current state of knowledge in a specific research area (Ekmekci, 2017). The literature reviewed here was exclusively written in English and published in peer-reviewed journals, in which the publication-language is English. Reasons for this may be seen in the data-base inquiry itself (Mongeon and Paul-Hus, 2016), which was based on English keywords (plastic and fish), as well as on the fact, that most peer-reviewed, high impact journals in natural sciences are published in English (Meneghini and Packer, 2007; Mongeon and Paul-Hus, 2016). The limitation of a review to English-language literature only may lead to adding a certain tendency—or bias—to the outcome of the review. Beyond the actual language in which a report is published, the framing of titles, abstracts or results using certain phrases or terms may also contribute to a distorted perception of MP ingestion across fish taxa (Section Outcome reporting bias). From the perspective of scientific ethics, the impact of a language bias in the field of biological/natural sciences may be valued differently in comparison with the other biases discussed hereinafter. Notwithstanding the above, researchers, editors, and reviewers should be equally encouraged to pay special attention to the compliance and usage of neutral language in scientific reports. Moreover, researchers may always consider the publication of results for a non-scientific audience in a transparent, easily understandable and potentially local language.
Besides the actual publication language, the areas under investigation may also add to a certain language bias: considering the geographic areas under investigation by the studies reviewed here (Figure 8), certain regions such as the Mediterranean, the Baltic and/or North Sea as well as the Western Atlantic seem to be represented to a greater extent than others, i.e., the Southern Ocean, the Persian Gulf or the Arctic Ocean, are all represented by a single study only (Cannon et al., 2016; Abbasi et al., 2018; Kühn et al., 2018). Other regions, such as the Red Sea or the Indian Ocean are not included at all. It cannot be excluded, that references for the abovementioned areas and beyond do exist and have simply not been assessed by this literature review and data base inquiry. Nonetheless, collating, mapping, and evaluating the knowledge on MP distribution and extent of exposure and ingestion may be substantially impaired by the heterogeneous distribution of geographical areas examined as well as by the existence of unsampled spots on the world ocean map. International collaboration between researchers and institutes should, therefore, promote investigations in areas currently underrepresented to contribute to a holistic overview on the global extent of MP distribution and the potential impact of ingestion.
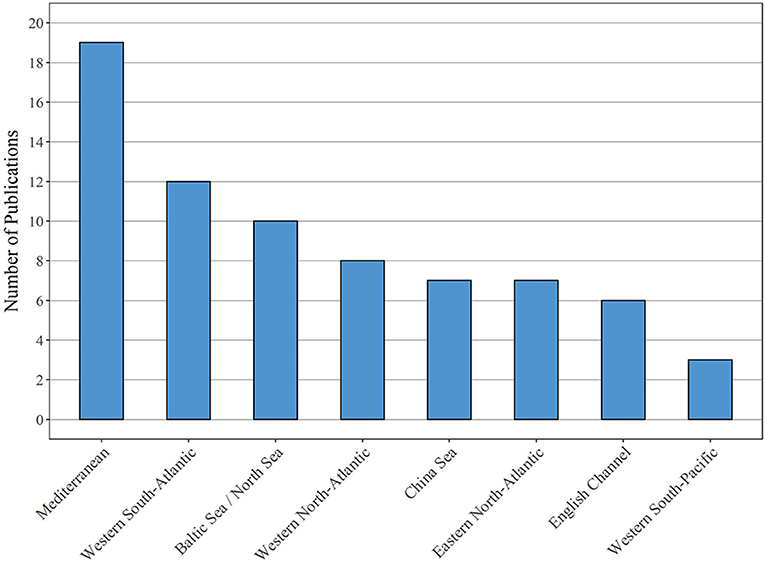
Figure 8. Representation of geographic regions which were covered by at least three or more studies included in this review. Studies from the Baltic and the North Sea were combined for this graphical illustration.
Duplicate/Multiple Publication Bias
Publishing the same research or data set twice, with substantial analogies in both manuscripts in relation to the underlying scientific theories, methodologies, outcomes, or conclusions, may lead to an erroneous perception of the significance of results both among the scientific community and potentially also among civil society (Ekmekci, 2017). In many cases, the same researchers authored similar manuscripts, cross-referencing to both publications happens infrequently, and duplicate publication of results goes beyond abbreviated reports for the media or for a narrow audience, or communicating outcomes in different languages (Johnson, 2006). Even though brief abstracts in conference proceedings are considered acceptable (Committee on Publication Ethics, 2003), the admissibility of publishing the entire study in a conference proceeding may be questioned when later on published as a stand-alone study in another journal. Upon going through the results of the data base inquiry for this review, the following example stood out: Pellini et al. (2018a) published their study on MP ingestion by common soles in the Adriatic Sea within the Proceedings of the International Conference on Microplastic Pollution in the Mediterranean Sea. In Pellini et al. (2018b), MP in the gastrointestinal tract of common soles from the Adriatic Sea was published in the journal Environmental Pollution. Cross-checking both publications revealed a considerable duplication of the area under investigation, the number of individuals examined as well as the results obtained and discussed. Yet, these almost identical studies were listed separately by the means of the data base research, and a cross-reference to each other was not found. Comparably, Bessa et al. (2018a) published a brief version of their study on MP uptake in three commercially important fish species (namely Dicentrarchus labrax, Diplodus vulgaris and Platichthys flesus), likewise, within the Proceedings of the International Conference on Microplastic Pollution in the Mediterranean Sea, with the extended version reporting on the same species, sample sizes and results, however, is published separately as Bessa et al. (2018b) in the journal Marine Pollution Bulletin. In another case, papers by Ferreira et al. (2016) and Ferreira et al. (2018) on acoupa weakfish appear to report on the same data set for a publication on dietary habits (incl. MP) and a more detailed study exclusively on MP ingestion. Even though the objectives of both publications are slightly different from each other, it seems that almost the identical data set of biological samples was used for two reports on MP ingestion by this fish species. These examples obtained by a single data base inquiry exemplify how the significance of MP ingestion by fish might be overemphasized through repetitive publications. Besides the mere increase in published literature on the topic, the waste of resources and ethical implications for the authors, editors, and journals involved, the repetitive release of fundamentally the same information may lead to the misperception of environmental pollution. This, in turn, could ultimately lead to the loss of scientific credibility, which might affect future research initiatives, funding availability, and policy making.
Outcome Reporting Bias
The publication of findings from scientific investigations with multiple outcomes is considered biased if “positive” outcomes have a higher probability to be included in the report than “negative” ones (Song et al., 2013)—this selective editing of results is also called “within-study publication bias.” The following examples were identified from the results of the literature review conducted here: in a study by Steer et al. (2017), the authors only mention those fish species explicitly for which MP uptake was found. In comparison to a total of 23 different species investigated, MP-feeding was reported for five species, which were given in the report by scientific names and abundances investigated. Information on the remaining 18 non-MP feeding species cannot be accessed on the basis of the actual publication. Upon personal request, however, Steer et al. provided original data on the investigated species for this review. Vendel et al. (2017) also reported on only a sub-set (n = 27) of the total number of species investigated (n = 69), explaining this selection by the inadequate sampling sizes for the other species (i.e., below 10 individuals per species) which could potentially impair statistical analysis. A record of the remaining 42 species with minor abundances is not included in the report. In a study by McGoran et al. (2018), the authors also do not mention the non-feeding species in the publication text. However, details on these species are accessible via the table included in the accompanying Supplementary Material. Taking not only recent studies into consideration, it becomes obvious that the existence of this outcome reporting bias dates back almost five decades: Carpenter et al. (1972) explicitly mentioned only those species with common names, scientific names and abundances that were found with MP spheres in their gastrointestinal tract.
Another instance of outcome reporting bias is the non-publication of entire studies if a specific outcome could not be inferred from the data analyzed, i.e., no MP fibers or particles present in the GIT of a fish species examined (Liboiron et al., 2018). A conceivable additional source of bias for MP ingestion studies might be the “relabeling” of a MP-uptake analysis as a dietary analysis of a fish species, which was not found (but initially suspected) to ingest MP particles. The consequent lack of explicit specification of absence of MP in the GIT by focusing on reporting of natural prey items may further distort the perception of MP exposure and ingestion by fish.
Selective Citation Bias
According to Leimu and Koricheva (2005), several different factors may contribute to a selective citation of ecological papers: apart from the journal's impact factor, the number and consortium of researchers and institutions involved (including their nationalities), results supportive of a widely accepted hypothesis are cited more often. Following Ekmekci's (2017) reasoning, studies with statistically significant and “positive” results are cited more frequently than others. Identifying this bias on the basis of the literature reviewed here is hardly possible due to the lack of reports on minor or none uptake of MP in fish (Liboiron et al., 2018). However, due to the high potential of the existence of an outcome reporting bias (see above), the existence of a consequent selective citation bias seems plausible. By assessing the citation history of all articles included in this review (conducted on the 6th September 2019), the potential existence of a selective citation bias was investigated. The number of citations in the year 2019 were excluded, moreover, the respective first year in which a paper was published was not considered either. By this, a mean number of citations per year was computed. If the paper was published in 2017, citations for 2017 and 2019 were not taken into consideration as the calculation of a citation rate per year would have been then based on 1 year only (2018). Therefore, these references were also excluded. Due to the high number of papers published within the past 2 years, the citation per year was, thus, calculated for a subset of 33 manuscripts. Figure 9 shows the computed correlation based on Spearman rank correlation coefficient for not normally distributed data. The correlation coefficient between the citation rate per year and the proportion of MP-feeding individuals shows a marginal trend toward a significant positive relationship (R = 0.3379; p = 0.054), yet with the minority of data within the 95% confidence interval. Based on this analysis, the existence of a selective citation bias was not verified beyond doubt, although given the slight tendencies detected for a subset of papers included in this review, the potential occurrence of this bias may not be completely ruled out.
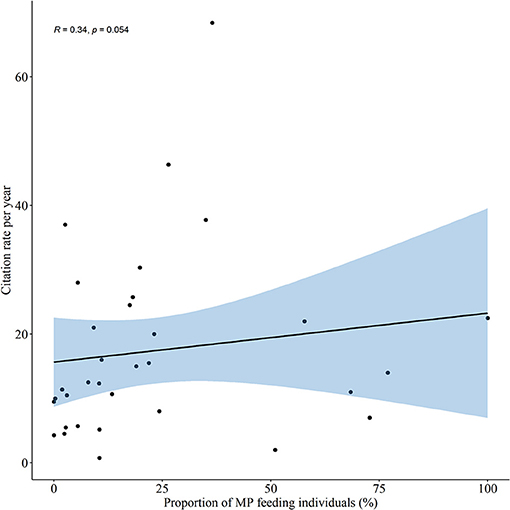
Figure 9. Scatter plot showing the relationship between the citation rate per year and the proportion of MP feeding fish (%) for a subset of studies (n = 33) included in this review. Spearman's rank correlation coefficient (R) was computed, along with the corresponding significance level (p-value) of the correlation. Light-blue shaded area represents the 95% confidence interval.
Media Attention Bias
Online and print media play an important role in distributing information on global environmental concerns such as marine (micro-)plastic pollution, impinging public awareness and political actions alike (Science Advice for Policy by European Academies, 2019). The increase in scientific and media coverage of the topic of MP litter is indubitable (Lusher, 2015; Phuong et al., 2016; de Sá et al., 2018; Liboiron et al., 2018; Cunningham and Sigwart, 2019). Irrespective of the fact that the actual implications of MP presence in the oceans have not been clarified beyond doubt, public perception of marine MP appears to be rather unequivocal. A recent study by Völker et al. (2019) confirmed the media framing of the topic by showing that the minority of scientific studies (24%) actually considers the risk of MP as evidenced beyond doubt, whereas the majority of media coverage (93%) seems to take the existence of detrimental effects of MP for granted. Derived from this, a media attention bias and consequent framing can be ascertained for both the topic of marine MP in general and for MP ingestion by marine biota in particular.
Conclusion and Recommendations
Focus of Future Studies
In the 90 studies under review here, approximately one third of the individual fish assessed were found with MP particles or fibers in their GIT, with an average plastic load of around 2 particles. Yet, comparing the outcomes of different in-situ MP uptake investigations in marine and estuarine fish is challenging due to a lack of harmonized methodologies and standardized protocols as well as to the fact that only few species are examined in sufficient sample sizes. Furthermore, MP identification was frequently conducted exclusively by visual means. Individual ecological parameters (e.g., body dimensions, trophic level, feeding guild, preferred habitat) do not seem to explain the frequency of MP-feeding or the variation of MP ingestion rates beyond doubt. Thus, scientific efforts should go beyond the commonly applied categories to further investigate determining factors for MP ingestion, bearing in mind the following guidelines for in-situ studies on MP ingestion by fish (Figure 10).
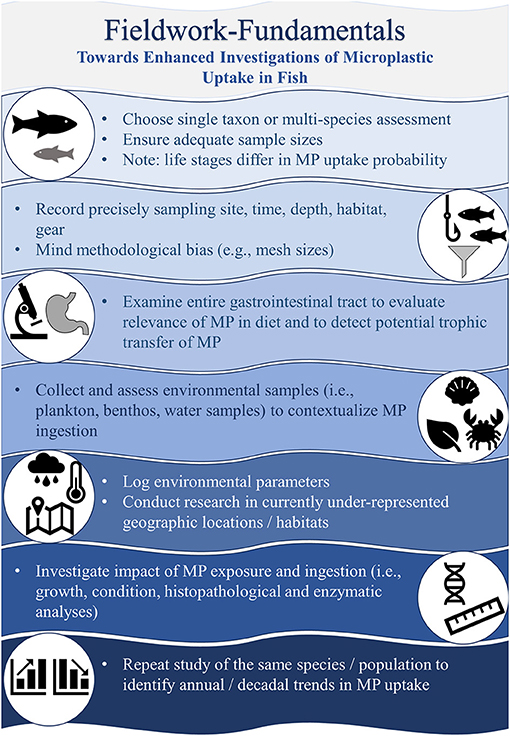
Figure 10. Recommendations of essential details future in-situ studies on MP ingestion by fish to assure effective contributions toward an integrated assessment and holistic evaluation of the extent and impact of MP uptake.
On the basis of the present review and the accompanying Supplementary Tables 1, 2, the following fundamentals for conducting in-situ assessments of MP uptake in marine and estuarine fish are suggested:
Selection of Relevant Fish Taxa und Life Stages
Based on the outcomes of this review, a number of fish species and families can be identified relevant for future research initiatives, as either these taxa have not been studied yet or have been found to vary in terms of MP uptake across different studies and geographic regions. Moreover, ELS (especially of commercially important fish taxa) should be targeted by prospective scientific efforts as they are hypothesized to show a high vulnerability to the ingestion and impact of MP. To significantly contribute toward an enhanced understanding of MP pollution as a threat for marine and estuarine fish, adequate sample sizes (i.e., >20 individuals per taxon) need to be taken and analyzed—prioritization should be given to single-or “few-species” -studies with sufficient sample sizes over multi-species assessments with <10 individuals per taxon.
Publication of Field Data
To ensure comparability of studies and a sound evaluation of the outcomes, essential details of the sampling-strategy and fieldwork need to be recorded and shared with the scientific community as well as civil society. Key information on the precise sampling date, time, location, and depth are just as relevant as specifications about the sampling gear applied (e.g., mesh sizes). Furthermore, the assessment of a potential methodological bias, e.g., stemming from consequent laboratory procedures, is greatly enhanced if details on the sampling and processing protocols are shared.
Investigation of the Gastrointestinal Tract
As demonstrated by this review, future research efforts should take an essential step toward the integrated evaluation of the hazardous potential of MP ingestion by analyzing the entire content of the GIT and, thus, quantitatively and qualitatively compare the uptake of natural prey items to MP fragments and fibers. Thereby, also the importance of trophic transfer of MP along the food chain could be further elucidated.
Contextualization of MP Ingestion
To identify potential MP uptake selectivity across fish taxa, feeding strategies, or life stages, environmental samples (e.g., water, plankton, or benthos collections) should be taken in the respective habitat or at the specific fish-sampling site and, consequently, compared to the outcomes of the GIT analysis. By integrating ambient samples into the assessment of MP uptake by fish, in-depth knowledge is gained on the distribution and abundance of MP in different compartments which may contribute also to an enhanced understanding of the vulnerability of individual taxa or life stages depending on specific habitats or prey items.
Correlation of MP Exposure and Ingestion With Abiotic Parameters
As environmental parameters and local conditions may significantly affect the physiological and ecological performance of a fish and consequently also the ingestion (as well as egestion) of MP particles and fibers, future investigations should aim at detecting links between the uptake of MP and abiotic environmental parameters such as temperature, oxygen, light intensity, or local weather conditions. To comprehensively analyze the horizontal and vertical pathways of MP distribution and the resulting implications for complex food-webs, more research is needed in currently under-represented geographic areas as well as in ecosystems which exhibit specific hydrographic and oceanographic properties (e.g., coastal habitats, marine upwelling ecosystems).
Investigation of MP-Uptake Related Physiological and Ecological Effects
To further unravel the underlying drivers for and impacts of MP ingestion across marine and estuarine fish, the precise recording of morphological/anatomical features is considered essential (e.g., standard length, height, eviscerated weight, mouth gape). The assessment of fish condition and potential differences in growth between MP-feeding and MP-non-feeding individuals may be considered expedient in determining the impact of MP uptake on a species- or population-specific scale. Enzymatic, toxicological as well as histopathological approaches should be also considered by prospective research initiatives to holistically evaluate the physiological as well as ecological effects of MP ingestion across fish species and families.
Verification of Spatio-Temporal Trends in MP Exposure and Uptake
An extension of short-term investigations of MP uptake toward long-term monitoring initiatives should be implemented by future scientific investigations. Therefore, repetitive sampling campaigns in the same area on key indicator species will assist in understanding the progression of marine plastic litter as a potential threat over the years to come and to evaluate the effectiveness of applied ecosystem conservation and litter reduction measures. In this respect, a de novo analysis of already existing samples collected by annual (or at least continuous) monitoring campaigns may be advisable with special focus on MP detection to clarify previous trends in MP abundances and distribution.
Publications Biases
Irrespective of the reasons for why the non-feeding individuals/taxa are not included in the actual publication or for why studies reporting minor or zero uptake rates seem to be underrepresented, one or several biases may be attested for the reporting on MP uptake by fish, both in the past and in recent scientific investigations. With regards to the continuously growing research area, the incidence of these biases needs to be reduced to accurately inform both the scientific and public community about the extent and impact of MP ingestion on fish. Considering the high media coverage along with the easy comprehensibility and accessibility of the topic for a broad audience in comparison to other more complex environmental threats (e.g., ocean acidification), more attention should be devoted to critically examining and reporting about MP and its consequences to preserve the public's trust in scientific research. Scientists and editors should, thus, be encouraged to consider studies contradicting the common notion of an environmentally relevant topic for publication and may even contemplate a re-evaluation of scientific outcomes beyond the mere statistical significance. Moreover, both editors and authors should take steps toward preventing publication biases such as duplicate publication bias.
Data Availability Statement
The original contributions presented in the study are included in the article/Supplementary Material, further inquiries can be directed to the corresponding author/s.
Author Contributions
The author confirms being the sole contributor of this work and has approved it for publication.
Funding
The author thankfully acknowledges the financial support through a Scholarship for Doctoral Studies awarded by the Heinrich-Böll-Foundation, Berlin, Germany.
Conflict of Interest
The author declares that the research was conducted in the absence of any commercial or financial relationships that could be construed as a potential conflict of interest.
Acknowledgments
The author gratefully acknowledges the time dedicated by her two supervisors, Drs. Werner Ekau and Karim Erzini, who discussed this manuscript from the initial idea to the final completion, provided feedback and valuable input on the aim and content of the study and thereby helped to significantly improve this review. Thanks are extended to Drs. Gerrit Nanninga, Tim Dudeck, and Hauke Reuter for exchange of ideas and input on the content of this review. Dr. Simon Jungblut, Dr. Viola Liebich, and Lena Heel are sincerely thanked for their continuous support, input and patience while collating all ICYMARE-related manuscripts. The author is also thankful to the reviewers for their constructive comments on the manuscript. Furthermore, thanks are extended to all researchers who contributed to this review by providing additional information upon request.
Supplementary Material
The Supplementary Material for this article can be found online at: https://www.frontiersin.org/articles/10.3389/fmars.2021.672768/full#supplementary-material
References
Abbasi, S., Soltani, N., Keshavarzi, B., Moore, F., Turner, A., and Hassanaghaei, M. (2018). Microplastics in different tissues of fish and prawn from the Musa Estuary, Persian Gulf. Chemosphere 205, 80–87. doi: 10.1016/j.chemosphere.2018.04.076
Alomar, C., and Deudero, S. (2017). Evidence of microplastic ingestion in the shark Galeus melastomus Rafinesque, 1810 in the continental shelf off the western Mediterranean Sea. Environ. Pollut. 223, 223–229. doi: 10.1016/j.envpol.2017.01.015
Alomar, C., Sureda, A., Capó, X., Guijarro, B., Tejada, S., and Deudero, S. (2017). Microplastic ingestion by Mullus surmuletus Linnaeus, 1758 fish and its potential for causing oxidative stress. Environ. Res. 159, 135–142. doi: 10.1016/j.envres.2017.07.043
Anastasopoulou, A., Kovač Viršek, M., Bojanić Varezić, D., Digka, N., Fortibuoni, T., Koren, S., et al. (2018). Assessment on marine litter ingested by fish in the Adriatic and NE Ionian Sea macro-region (Mediterranean). Mar. Pollut. Bull. 133, 841–851. doi: 10.1016/j.marpolbul.2018.06.050
Anastasopoulou, A., Mytilineou, C., Smith, C. J., and Papadopoulou, K. N. (2013). Plastic debris ingested by deep-water fish of the Ionian Sea (Eastern Mediterranean). Deep Sea Res. 74, 11–13. doi: 10.1016/j.dsr.2012.12.008
Andrady, A. L. (2015). “Persistence of plastic litter in the oceans,” in Marine Anthropogenic Litter, eds M. Bergmann, L. Gutow, M. Klages M (Cham: Springer), 57–72. doi: 10.1007/978-3-319-16510-3_3
Antunes, J., Frias, J., and Sobral, P. (2018). Microplastics on the Portuguese coast. Mar. Pollut. Bull. 131, 294–302. doi: 10.1016/j.marpolbul.2018.04.025
Arcadis European Commission. (2014). Marine Litter Study to Support the Establishment of an Initial Headline Reduction Target. SFRA0025. European commission/DG ENV, project number BE0113.000668, 127. Available online at: https://ec.europa.eu/environment/marine/good-environmental-status/descriptor-10/pdf/final_report.pdf (accessed April 6, 2021).
Arias, A. H., Ronda, A. C., Oliva, A. L., and Marcovecchio, J. E. (2019). Evidence of microplastic ingestion by fish from the bahía blanca estuary in Argentina, South America. B Environ. Contam. Tox. 102, 750–756. doi: 10.1007/s00128-019-02604-2
Arthur, C., Baker, J., and Bamford, H. (2009). Proceedings of the International Research Workshop on the Occurrence, Effects, and Fate of Microplastic Marine Debris. NOAA Marine Debris Program. Technical memorandum NOS-OR&R-30. Available online at: https://marinedebris.noaa.gov/proceedings-second-research-workshop-microplastic-marine-debris
Avio, C. G., Gorbi, S., and Regoli, F. (2015). Experimental development of a new protocol for extraction and characterization of microplastics in fish tissues: first observations in commercial species from Adriatic Sea. Mar. Environ. Res. 111, 18–26. doi: 10.1016/j.marenvres.2015.06.014
Azad, S. M. O., Towatana, P., Pradit, S., Patricia, B. G., Hue, H. T. T., and Jualaong, S. (2018). First evidence of existence of microplastics in stomach of some commercial fishes in the lower Gulf of Thailand. Appl. Ecol. Env. Res. 16, 7345–7360. doi: 10.15666/aeer/1606_73457360
Baker, R., Buckland, A., and Sheaves, M. (2014). Fish gut content analysis. Robust measures of diet composition. Fish 15, 170–177. doi: 10.1111/faf.12026
Balon, E. K. (1986). “Types of feeding in the ontogeny of fishes and the life-history model,” in Contemporary Studies on Fish Feeding: The Proceedings of GUTSHOP '84. Developments in Environmental Biology of fishes, Vol. 7, eds C. A. Simenstad, G. M. Cailliet (Dordrecht: Springer), 11–24. doi: 10.1007/978-94-017-1158-6_1–12
Barboza, L. G. A., and Gimenez, B. C. G. (2015). Microplastics in the marine environment: current trends and future perspectives. Mar. Pollut. Bull. 97, 5–12. doi: 10.1016/j.marpolbul.2015.06.008
Barnes, D. K. A., Galgani, F., Thompson, R. C., and Barlaz, M. (2009). Accumulation and fragmentation of plastic debris in global environments. Phil. Trans. R. Sock. B 364, 1985–1998. doi: 10.1098/rstb.2008.0205
Battaglia, P., Pedà, C., Musolino, S., Esposito, V., Andaloro, F., and Romeo, T. (2016). Diet and first documented data on plastic ingestion of Trachinotus ovatus L. 1758 (Pisces. Carangidae) from the strait of messina (central Mediterranean sea). Ital. J. Zool. 83, 121–129. doi: 10.1080/11250003.2015.1114157
Beer, S., Garm, A., Huwer, B., Dierking, J., and Nielsen, T. G. (2018). No increase in marine microplastic concentration over the last three decades - a case study from the Baltic sea. Sci. Total Environ. 621, 1272–1279. doi: 10.1016/j.scitotenv.2017.10.101
Bellas, J., Martínez-Armental, J., Martínez-Cámara, A., Bescada, V., and Martínez-Gómez, C. (2016). Ingestion of microplastics by demersal fish from the Spanish Atlantic and Mediterranean coasts. Mar. Pollut. Bull. 109, 55–60. doi: 10.1016/j.marpolbul.2016.06.026
Bernardini, I., Garibaldi, F., Canesi, L., Fossi, M. C., and Baini, M. (2018). First data on plastic ingestion by blue sharks (Prionace glauca) from the Ligurian sea (North-Western Mediterranean sea). Mar. Pollut. Bull. 135, 303–310. doi: 10.1016/j.marpolbul.2018.07.022
Bessa, F., Barría, P., Neto, J. M., Frias, J. P. G. L., Otero, V., Sobral, P., et al. (2018a). “Microplastics in juvenile commercial fish from an estuarine environment,” in Proceedings of the International Conference on Microplastic Pollution in the Mediterranean Sea Springer Water, eds M. Cocca, E. Di Pace, M. Errico (Cham: Springer), 131–135. doi: 10.1007/978-3-319-71279-6_18
Bessa, F., Barría, P., Neto, J. M., Frias, J. P. G. L., Otero, V., Sobral, P., et al. (2018b). Occurrence of microplastics in commercial fish from a natural estuarine environment. Mar. Pollut. Bull. 128:75–584. doi: 10.1016/j.marpolbul.2018.01.044
Blaber, S. J. M., and Blaber, T. G. (1980). Factors affecting the distribution of juvenile estuarine and inshore fish. J. Fish Biol. 17, 143–162. doi: 10.1111/j.1095-8649.1980.tb02749.x
Boehlert, G. W., and Mundy, W. (1988). Roles of behaviour and physical factors in larval and juvenile fish recruitment to estuarine nursery area. Am. Fish Soc. Symposium 3, 51–67.
Boerger, C. M., Lattin, G. L., Moore, S. L., and Moore, C. (2010). Plastic ingestion by planktivorous fishes in the North Pacific central gyre. Mar. Pollut. Bull. 60, 2275–2278. doi: 10.1016/j.marpolbul.2010.08.007
Bour, A., Avio, C. G., Gorbi, S., Regoli, F., and Hylland, K. (2018). Presence of microplastics in benthic and epibenthic organisms. Influence of habitat, feeding mode and trophic level. Environ. Pollut. 243, 1217–1225. doi: 10.1016/j.envpol.2018.09.115
Bråte, I. L. N., Eidsvoll, D. P., Steindal, C. C., and Thomas, K. V. (2016). Plastic ingestion by Atlantic cod (Gadus morhua) from the Norwegian coast. Mar. Pollut. Bull. 112, 105–110. doi: 10.1016/j.marpolbul.2016.08.034
Buchanan, J. B. (1971). Pollution by synthetic fibres. Mar. Pollut. Bull. 2, 23. doi: 10.1016/0025-326X(71)90136-6
Cannon, S. M. E., Lavers, J. L., and Figueiredo, B. (2016). Plastic ingestion by fish in the Southern Hemisphere. A baseline study and review of methods. Mar. Pollut. Bull. 107, 286–291. doi: 10.1016/j.marpolbul.2016.03.057
Cardozo, A. L. P., Farias, E. G. G., Rodrigues-Filho, J. L., Moteiro, I. B., Scandolo, T. M., and Dantas, D. V. (2018). Feeding ecology and ingestion of plastic fragments by Priacanthus arenatus. What's the fisheries contribution to the problem? Mar. Pollut. Bull. 130, 19–27. doi: 10.1016/j.marpolbul.2018.03.010
Carpenter, E. J., Anderson, S. J., Harvey, G. R., Miklas, H. P., and Peck, B. B. (1972): Polystyrene spherules in coastal waters. Science 178, 749–750. doi: 10.1126/science.178.4062.749
Cedervall, T., Hansson, L. A., Lard, M., Frohm, B., and Linse, S. (2012). Food chain transport of nanoparticles affects behaviour and fat metabolism in fish. PLoS ONE 7:e32254. doi: 10.1371/journal.pone.0032254
Chagnon, C., Thiel, M., Antunes, J., Ferreira, J. L., Sobral, P., and Ory, N. C. (2018). Plastic ingestion and trophic transfer between Easter Island flying fish (Cheilopogon rapanouiensis) and yellowfin tuna (Thunnus albacares) from Rapa Nui (Easter Island). Environ. Pollut. 243, 127–133. doi: 10.1016/j.envpol.2018.08.042
Cheung, L. T. O., Lui, C. Y., and Fok, L. (2018). Microplastic contamination of wild and captive flathead grey mullet (Mugil cephalus). Int. J. Env. Res. Pub. Health 15:597. doi: 10.3390/ijerph15040597
Choy, C. A., and Drazen, J. C. (2013). Plastic for dinner? Observations of frequent debris ingestion by pelagic predatory fishes from the central North Pacific. Mar. Ecol. Prog. Ser. 485, 155–163. doi: 10.3354/meps10342
Choy, C. A., Robison, B. H., Gagne, T. O., Erwin, B., Firl, E., Halden, R. U., et al. (2019). The vertical distribution and biological transport of marine microplastics across the epipelagic and mesopelagic water column. Sci. Rep. 9:7843. doi: 10.1038/s41598-019-44117-2
Cole, M., Lindeque, P., Fileman, E., Halsband, C., Goodhead, R., Moger, J., et al. (2013). Microplastic ingestion by zooplankton. Environ. Sci. Technol. 47, 6646–6655. doi: 10.1021/es400663f
Cole, M., Lindeque, P. K., Fileman, E., Clark, J., Lewis, C., Halsband, C., et al. (2016). Microplastics alter the properties and sinking rates of zooplankton faecal pellets. Environ. Sci. Technol. 50, 3239–3246. doi: 10.1021/acs.est.5b05905
Collicutt, B., Juanes, F., and Dudas, S. E. (2019). Microplastics in juvenile chinook salmon and their nearshore environments on the east coast of Vancouver island. Environ. Pollut. 244, 135–142. doi: 10.1016/j.envpol.2018.09.137
Colton, J. B., Knapp, F., and Burns, B. R. (1974). Plastic particles in surface waters of the Northwestern Atlantic. Science 185, 491–497. doi: 10.1126/science.185.4150.491
Committee on Publication Ethics (2003). Guidelines on Good Publication Practice. London. Available online at: https://publicationethics.org/files/u7141/1999pdf13.pdf. (accessed November 27, 2019).
Compa, M., Ventero, A., Iglesias, M., and Deudero, S. (2018). Ingestion of microplastics and natural fibres in Sardina pilchardus (Walbaum, 1792) and Engraulis encrasicolus (Linnaeus, 1758) along the Spanish Mediterranean coast. Mar. Pollut. Bull. 128:89–96. doi: 10.1016/j.marpolbul.2018.01.009
Corradini, F., Meza, P., Eguiluz, R., Casado, F., Huerta-Lwanga, E., and Geissen, V. (2019). Evidence of microplastic accumulation in agricultural soils from sewage sludge disposal. Sic. Total Environ. 671, 411–420. doi: 10.1016/j.scitotenv.2019.03.368
Critchell, K., and Hoogenboom, M. O. (2018). Effects of microplastic exposure on the body condition and behaviour of planktivorous reef fish (Acanthochromis polyacanthus). PloS ONE 13:e0193308. doi: 10.1371/journal.pone.0193308
Cunningham, E. M., and Sigwart, J. D. (2019). Environmentally accurate microplastic levels and their absence from exposure studies on aquatic taxa. Integr. Comp. Biol. 59, 1485–1496. doi: 10.1093/icb/icz068
Dantas, D. V., Barletta, M., and da Costa, M. F. (2012). The seasonal and spatial patterns of ingestion of polyfilament nylon fragments by estuarine drums (Sciaenidae). Environ. Sci. Pollut. R 19, 600–606. doi: 10.1007/s11356-011-0579-0
Dantas, D. V., Ribeiro, C. I. R., Frischknecht Cd, CA, Machado, R., and Farias, E. G. G. (2019). Ingestion of plastic fragments by the Guri sea catfish Genidens genidens (Cuvier, 1829) in a subtropical coastal estuarine system. Environ. Sci. Pollut. R 26, 8344–8351. doi: 10.1007/s11356-019-04244-9
Davison, P., and Asch, R. G. (2011). Plastic ingestion by mesopelagic fishes in the North Pacific Subtropical gyre. Mar. Ecol. Prog. Ser. 432, 173–180. doi: 10.3354/meps09142
de Sá, L. C., Luís, L. G., and Guilhermino, L. (2015). Effects of microplastics on juveniles of the common goby (Pomatoschistus microps): confusion with prey, reduction of the predatory performance and efficiency, and possible influence of developmental conditions. Environ. Pollut. 196, 359–362. doi: 10.1016/j.envpol.2014.10.026
de Sá, L. C., Oliveira, M., Ribeiro, F., Rocha, T. L., and Futter, M. N. (2018). Studies of the effects of microplastics on aquatic organisms. What do we know and where should we focus our efforts in the future? Sci. Total Environ. 645, 1029–1039. doi: 10.1016/j.scitotenv.2018.07.207
Derraik, J. G. B. (2002). The pollution of the marine environment by plastic debris: a review. Mar. Pollut. Bull. 44, 842–852. doi: 10.1016/S0025-326X(02)00220-5
Digka, N., Tsangaris, C., Torre, M., Anastasopoulou, A., and Zeri, C. (2018). Microplastics in mussels and fish from the Northern Ionian Sea. Mar. Pollut. Bull. 135, 30–40. doi: 10.1016/j.marpolbul.2018.06.063
Downing, G., and Litvak, M. K. (2000). The effect of photoperiod, tank colour and light intensity on growth of larval haddock. Aquacult. Int. 7, 369–382. doi: 10.1023/A:1009204909992
Ekmekci, P. E. (2017). An increasing problem in publication ethics. Publication bias and editors' role in avoiding it. Med. Health Care Philos. 20, 171–178. doi: 10.1007/s11019-017-9767-0
Enders, K., Lenz, R., Stedmon, C. A., and Nielsen, T. G. (2015). Abundance, size and polymer composition of marine microplastics ≥ 10 μm in the Atlantic ocean and their modelled vertical distribution. Mar. Pollut. Bull. 15, 70–81. doi: 10.1016/j.marpolbul.2015.09.027
Fanelli, D. (2012). Negative results are disappearing from most disciplines and countries. Scientometrics 90, 891–904. doi: 10.1007/s11192-011-0494-7
Fänge, R., and Grove, D. (1979). “Digestion,” in Fish Physiology VIII Bioenergetics and Growth, eds W. S. Hoar, D. J. Randall, J. R. Brett JR (New York, NY: Academic Press), 161–260.
Farrell, P., and Nelson, K. (2013). Trophic level transfer of microplastic Mytilus edulis (L.) to Carcinus maenas (L.). Environ. Pollut. 177, 1–3. doi: 10.1016/j.envpol.2013.01.046
Ferreira, G. V. B., Barletta, M., Lima, A. R. A., Dantas, D. V., Justino, A. K. S., and Costa, M. F. (2016). Plastic debris contamination in the life cycle of acoupa weakfish (Cynoscion acoupa) in a tropical estuary. ICES J. Mar. Sci. 73, 2695–2707. doi: 10.1093/icesjms/fsw108
Ferreira, G. V. B., Barletta, M., Lima, A. R. A., Morley, S. A., Justino, A. K. S., and Costa, M. F. (2018). High intake rates of microplastics in a Western Atlantic predatory fish, and insights of a direct fishery effect. Environ. Pollut. 236, 706–717. doi: 10.1016/j.envpol.2018.01.095
Foekema, E. M., de Gruijter, C., Mergia, M. T., van Franeker, J. A., Murk, A. J., and Koelmans, A. A. (2013). Plastic in North sea fish. Environ. Sci. Technol. 47, 8818–8824. doi: 10.1021/es400931b
Foley, C. J., Feiner, Z. S., Malinich, T. D., and Höök, T. O. (2018). A meta-analysis of the effects of exposure to microplastics on fish and aquatic invertebrates. Sci. Total Environ. 631–632, 550–559. doi: 10.1016/j.scitotenv.2018.03.046
Froese, R. (2006). Cube law, condition factor and weight-length relationships. History, meta-analysis and recommendations. J. Appl. Ichthyol. 22, 241–253. doi: 10.1111/j.1439-0426.2006.00805.x
Froese, R., and Pauly, D. (2019). FishBase. Available online at: www.fishbase.org (accessed October 24, 2019).
Fry, F. E. J. (1971). The effect of environmental factors on the physiology of fish. Fish Physiol. 6, 1–98.
Fulton, T. W. (1904). The rate of growth of fishes. 22nd Annual Report of the Fishery Board of Scotland (Edinburgh), 141–241.
Galgani, F., Hanke, G., and Maes, T. (2015). “Global distribution, composition and abundance of marine litter,” in Marine Anthropogenic Litter, eds M. Bergmann, L. Gutow, M. Klages (Cham: Springer), 29–56. doi: 10.1007/978-3-319-16510-3_2
Gassel, M., Harwani, S., Park, J. S., and Jahn, A. (2013). Detection of nonylphenol and persistent organic pollutants in fish from the North Pacific Central Gyre. Mar. Pollut. Bull. 73, 231–242. doi: 10.1016/j.marpolbul.2013.05.014
GESAMP (2015). “Sources, fate and effects of microplastics in the marine environment: a global assessment,” in Joint Group of Experts on the Scientific Aspects of Marine Environmental Protection (IMO/FAO/UNESCO-IOC/UNIDO/WMO/IAEA/UN/UNEP/UNDP), ed P. J. Kershaw (London: Reports and Study Series GESAMP No. 90).
GESAMP (2016). “Sources, fate and effects of microplastics in the marine environment: part two of a global assessment,” in Joint Group of Experts on the Scientific Aspects of Marine Environmental Protection (IMO/FAO/UNESCO-IOC/UNIDO/WMO/IAEA/UN/UNEP/UNDP), eds P. J. Kershaw, C. Rochmann (London: Reports and Study Series GESAMP No. 93).
Giani, D., Baini, M., Galli, M., Casini, S., and Fossi, M. C. (2019). Microplastics occurrence in edible fish species (Mullus barbatus and Merluccius merluccius) collected in three different geographical sub-areas of the Mediterranean Sea. Mar. Pollut. Bull, 140, 129–137. doi: 10.1016/j.marpolbul.2019.01.005
Goldstein, M. C., and Goodwin, D. S. (2013). Gooseneck barnacles (Lepas spp.) ingest microplastic debris in the North Pacific subtropical gyre. PeerJ 1:e184. doi: 10.7717/peerj.184
Goldstein, M. C., Titmus, A. J., and Ford, M. (2013). Scales of spatial heterogeneity of plastic marine debris in the northeast Pacific ocean. PLoS ONE 8:e80020. doi: 10.1371/journal.pone.0080020
Güven, O., Gökdag, K., Jovanović, B., and Kideyş, A. E. (2017). Microplastic litter composition of the turkish territorial waters of the Mediterranean sea, and its occurrence in the gastrointestinal tract of fish. Environ. Pollut. 223, 286–294. doi: 10.1016/j.envpol.2017.01.025
Halstead, J. E., Smith, J. A., Carter, E. A., Lay, P. A., and Johnston, E. L. (2018). Assessment tools for microplastics and natural fibres ingested by fish in an urbanised estuary. Environ. Pollut. 234, 552–561. doi: 10.1016/j.envpol.2017.11.085
Hermsen, E., Pompe, R., Besseling, E., and Koelmans, A. A. (2017). Detection of low numbers of microplastics in North Sea fish using strict quality assurance criteria. Mar. Pollut. Bull. 122, 253–258. doi: 10.1016/j.marpolbul.2017.06.051
Herrera, A., Stindlov, á A., Martínez, I, Rapp, J., Romero-Kutzner, V., Samper, M. D., et al. (2019). Microplastic ingestion by Atlantic chub mackerel (Scomber colias) in the Canary islands coast. Mar. Pollut. Bull. 139, 127–135. doi: 10.1016/j.marpolbul.2018.12.022
Hoss, D. E., and Settle, L. R. (1990). “Ingestion of plastics by teleost fishes,” in Proceedings of the Second International Conference of Marine Debris, eds R. S. Shomura and M. L. Godfrey (Honolulu: U.S. Department of Commerce, NOAA Tech Memo, NMFS), 693–709.
Jabeen, K., Su, L., Li, J., Yang, D., Tong, C., Mu, J., et al. (2017). Microplastics and mesoplastics in fish from coastal and fresh waters of China. Environ. Pollut. 221, 141–149. doi: 10.1016/j.envpol.2016.11.055
Jensen, L. H., Motti, C. A., Garm, A. L., Tonin, H., and Kroon, F. (2019): Sources, distribution fate of microfibres on the great barrier reef, Australia. Sci. Rep. 9:9021. doi: 10.1038/s41598-019-45340-7
Johnson, C. (2006). Repetitive, duplicate, and redundant publications. A review for authors and readers. J. Manip. Physiol. Ther. 29, 505–509. doi: 10.1016/j.jmpt.2006.07.001
Jovanović, B. (2017). Ingestion of microplastics by fish and its potential consequences from a physical perspective. Integr. Environ. Assess Manag. 13, 510–515. doi: 10.1002/ieam.1913
Joyce, W. N., Campana, S. E., Natanson, L. J., Kohler, N. E., Pratt, H. L. Jr., and Jensen, C. F. (2002). Analysis of stomach contents of the porbeagle shark (Lamna nasus Bonnaterre) in the northwest Atlantic. ICES J. Mar. Sci. 59, 1263–1269. doi: 10.1006/jmsc.2002.1286
Kanhai, D. K., Officer, R., Lyashevska, O., Thompson, R. C., and O'Connor, I. (2017). Microplastic abundance, distribution and composition along a latitudinal gradient in the Atlantic ocean. Mar. Pollut. Bull. 115, 307–314. doi: 10.1016/j.marpolbul.2016.12.025
Käppler, A., Fischer, D., Oberbeckmann, S., Schernewski, G., Labrenz, M., Eichhorn, K. J., et al. (2016). Analysis of environmental microplastics by vibrational microspectroscopy. FTIR, Raman or both? Anal. Bioanal. Chem. 408, 8377–8391. doi: 10.1007/s00216-016-9956-3
Käppler, A., Fischer, M., Scholz-Böttcher, B. M., Oberbeckmann, S., Labrenz, M., Fischer, D., et al. (2018). Comparison of μ-ATR-FTIR spectroscopy and py-GCMS as identification tools for microplastic particles and fibers isolated from river sediments. Anal. Bioanal. Chem. 410, 5313–5327. doi: 10.1007/s00216-018-1185-5
Kartar, S., Abou-Seedo, F., and Sainsbury, M. (1976). Polystyrene spherules in the severn estuary - a progress report. Mar. Pollut. Bull. 7:52. doi: 10.1016/0025-326X(76)90092-8
Kartar, S., Milne, R. A., and Sainsbury, M. (1973). Polystyrene waste in the severn estuary. Mar. Pollut. Bull. 4:144. doi: 10.1016/0025-326X(73)90010-6
Kazour, M., Jemaa, S., El Rakwe, M., Duflos, G., Hermabassiere, L., Dehaut, A., et al. (2018). Juvenile fish caging as a tool for assessing microplastics contamination in estuarine fish nursery grounds. Environ. Sci. Pollut. R 27, 3548–3559. doi: 10.1007/s11356-018-3345-8
Kole, P. J., Löhr, A. J., Van Belleghem, F. G. A. J., and Ragas, A. M. J. (2017). Wear and tear of tyres: a stealthy source of microplastics in the environment. Int. J. Environ. Res. Public Health 14:1265. doi: 10.3390/ijerph14101265
Kühn, S., Schaafsma, F. L., van Werven, B., Flores, H., Bergmann, M., Egelkraut-Holtus, M., et al. (2018). Plastic ingestion by juvenile polar cod (Boreogadus saida) in the Arctic ocean. Polar Biol. 41, 1269–1278. doi: 10.1007/s00300-018-2283-8
Kukulka, T., Proskurowski, G., Morét-Ferguson, S., Meyer, D. W., and Law, K. L. (2012). The effect of wind mixing on the vertical distribution of buoyant plastic debris. Geophys. Res. Lett. 39:L07601. doi: 10.1029/2012GL051116
Laist, D. W. (1997). “Impacts of marine debris - entanglement of marine life in marine debris including a comprehensive list of species with entanglement and ingestion records,” in Marine Debris, eds J. M. Coe, D. B. Rogers (New York, NY: Springer), 99–139. doi: 10.1007/978-1-4613-8486-1_10
Lancraft, T. M., and Robison, B. H. (1980). Evidence of postcapture ingestion by midwater fishes in trawl nets. Fish B-NOAA 77, 713–715
Le Cren, E. D. (1951). The length-weight relationship and seasonal cycle in gonad weight and condition in the perch (Perca fluviatilis). J. Anim. Ecol. 20, 201–219. doi: 10.2307/1540
Leimu, R., and Koricheva, J. (2005). What determines the citation frequency of ecological papers? Trends Ecol. Evol. 20, 28–32. doi: 10.1016/j.tree.2004.10.010
Liboiron, F., Ammendolia, J., Saturno, J., Melvin, J., Zahara, A., Richard, N., et al. (2018). A zero percent plastic ingestion rate by silver hake (Merluccius bilinearis) from the south coast of Newfoundland, Canada. Mar. Pollut. Bull. 131, 267–275. doi: 10.1016/j.marpolbul.2018.04.007
Liboiron, M., Liboiron, F., Wells, E., Richárd, N., Zahara, A., Mather, C., et al. (2016). Low plastic ingestion rate in Atlantic cod (Gadus morhua) from newfoundland destined for human consumption collected through citizen science methods. Mar. Pollut. Bull. 113, 428–437. doi: 10.1016/j.marpolbul.2016.10.043
Liboiron, M., Melvin, J., Richárd, N., Saturno, J., Ammendolia, J., Liboiron, F., et al. (2019). Low incidence of plastic ingestion among three fish species significant for human consumption on the island of Newfoundland, Canada. Mar. Pollut. Bull. 141, 244–248. doi: 10.1016/j.marpolbul.2019.02.057
Lima, A. R. A., Costa, M. F., and Barletta, M. (2014). Distribution patterns of microplastics within the plankton of a tropical estuary. Environ. Res. 132, 146–155. doi: 10.1016/j.envres.2014.03.031
Lu, Y., Zhang, Y., Deng, Y., Jiang, W., Zhao, Y., Geng, J., et al. (2016). Uptake and accumulation of polystyrene microplastics in zebrafish (Danio rerio) and toxic effects in liver. Environ. Sci. Technol. 50, 4054–4060. doi: 10.1021/acs.est.6b00183
Lusher, A. L. (2015). “Microplastics in the marine environment: distribution, interactions and effects,” in Marine Anthropogenic Litter, eds M. Bergmann, L. Gutow, M. Klages (Cham: Springer), 245–307. doi: 10.1007/978-3-319-16510-3_10
Lusher, A. L., Hollman, P. C. H., and Mendoza-Hill, J. J. (2017a). Microplastics in Fisheries and Aquaculture: Status of Knowledge on Their Occurrence and Implications for Aquatic Organisms and Food Safety. Rome: FAO Fisheries and Aquaculture Technical Paper No. 615.
Lusher, A. L., McHugh, M., and Thompson, R. C. (2013). Occurrence of microplastics in the gastrointestinal tract of pelagic and demersal fish from the English Channel. Mar. Pollut. Bull. 67, 94–99. doi: 10.1016/j.marpolbul.2012.11.028
Lusher, A. L., O'Donnell, C., Officer, R., and O'Connor, I. (2016). Microplastic interactions with North Atlantic mesopelagic fish. ICES J. Mar. Sci. 73, 1214–1225. doi: 10.1093/icesjms/fsv241
Lusher, A. L., Welden, N. A., Sobral, P., and Cole, M. (2017b). Sampling, isolating and identifying microplastics ingested by fish and invertebrates. Anal. Methods 9, 1346–1360. doi: 10.1039/C6AY02415G
Manooch, C. S., and Hogarth, W. T. (1983). Stomach contents and giant trematodes from wahoo, Acanthocybium solanderi, collected along the South Atlantic and Gulf coasts of the United States. Bull. Mar. Sci. 33, 227–238.
Markic, A., Gaertner, J., Gaertner-Mazouni, N., and Koelmans, A. (2020). Plastic ingestion by marine fish in the wild. Crit. Rev. Env. Sci. Technol. 50, 657–697. doi: 10.1080/10643389.2019.1631990
Markic, A., Niemand, C., Bridson, J. H., Mazouni-Gaertner, N., Gaertner, J., Eriksen, M., et al. (2018). Double trouble in the South Pacific subtropical gyre. Increased plastic ingestion by fish in the oceanic accumulation zone. Mar. Pollut. Bull. 136, 547–564. doi: 10.1016/j.marpolbul.2018.09.031
Martínez, M. L., Intralawan, A., Vázquez, G., Pérez-Maqueo, O., Sutton, P., and Landgrave, R. (2007). The coasts of our world: ecological, economic and social importance. Ecol. Econ. 63, 254–272. doi: 10.1016/j.ecolecon.2006.10.022
Mazurais, D., Ernande, B., Quazuguel, P., Severe, A., Huelvan, C., Madec, L., et al. (2015). Evaluation of the impact of polyethylene microbeads ingestion in European sea bass (Dicentrarchus labrax) larvae. Mar. Environ. Res. 112, 78–85. doi: 10.1016/j.marenvres.2015.09.009
McGoran, A. R., Clark, P. F., and Morritt, D. (2017). Presence of microplastic in the digestive tracts of European flounder, Platichthys flesus, and European smelt, Osmerus eperlanus, from the river thames. Environ. Pollut. 220, 744–751. doi: 10.1016/j.envpol.2016.09.078
McGoran, A. R., Cowie, P. R., Clark, P. F., McEvoy, J. P., and Morritt, D. (2018). Ingestion of plastic by fish. A comparison of thames estuary and firth of clyde populations. Mar. Pollut. Bull. 137, 12–23. doi: 10.1016/j.marpolbul.2018.09.054
Meneghini, R., and Packer, A. L. (2007). Is there science beyond English? Initiatives to increase the quality and visibility of non-English publications might help to break down language barriers in scientific communication. EMBO Rep. 8, 112–116. doi: 10.1038/sj.embor.7400906
Miranda, D. D. A., and de Carvalho-Souza, G. F. (2016). Are we eating plastic-ingesting fish? Mar. Pollut. Bull. 103, 109–114. doi: 10.1016/j.marpolbul.2015.12.035
Mizraji, R., Ahrendt, C., Perez-Venegas, D., Vargas, J., Pulgar, J., Aldana, M., et al. (2017). Is the feeding type related with the content of microplastics in intertidal fish gut? Mar. Pollut. Bull. 116, 498–500. doi: 10.1016/j.marpolbul.2017.01.008
Mlinarić, A., Horvat, M., and Šupak Smolči,ć, V. (2017). Dealing with the positive publication bias. Why you should really publish your negative results. Biochem. Med. 27:30201. doi: 10.11613/BM.2017.030201
Mongeon, P., and Paul-Hus, A. (2016). The journal coverage of web of science and scopus. A comparative analysis. Scientometrics 106, 213–228. doi: 10.1007/s11192-015-1765-5
Morgana, S., Ghigliotti, L., Estévez-Calvar, N., Stifanese, R., Wieckzorek, A., Doyle, T., et al. (2018). Microplastics in the Arctic. A case study with sub-surface water and fish samples off Northeast greenland. Environ. Pollut. 242, 1078–1086. doi: 10.1016/j.envpol.2018.08.001
Murphy, F., Russell, M., Ewins, C., and Quinn, B. (2017). The uptake of macroplastic & microplastic by demersal & pelagic fish in the Northeast Atlantic around Scotland. Mar. Pollut. Bull. 122, 353–359. doi: 10.1016/j.marpolbul.2017.06.073
Naidoo, T., Smit, A. J., and Glassom, D. (2016). Plastic ingestion by estuarine mullet Mugil cephalus (Mugilidae) in an urban harbour, KwaZulu-Natal, South Africa. Afr. J. Mar. Sci. 38, 145–149. doi: 10.2989/1814232X.2016.1159616
Neves, D., Sobral, P., Ferreira, J. L., and Pereira, T. (2015). Ingestion of microplastics by commercial fish off the Portuguese coast. Mar. Pollut. Bull. 101, 119–126. doi: 10.1016/j.marpolbul.2015.11.008
Oliveira, M., Ribeiro, A., Hylland, K., and Guilhermino, L. (2013). Single and combined effects of microplastics and pyrene on juveniles (0+ group) of the common goby Pomatoschistus microps (Teleostei, Gobiidae). Ecol. Indic. 34, 641–647. doi: 10.1016/j.ecolind.2013.06.019
Ory, N., Chagnon, C., Felix, F., Fernández, C., Ferreira, J. L., Gallardo, C., et al. (2018). Low prevalence of microplastic contamination in planktivorous fish species from the southeast Pacific ocean. Mar. Pollut. Bull. 127, 211–216. doi: 10.1016/j.marpolbul.2017.12.016
Ory, N., Sobral, P., Ferreira, J. L., and Thiel, M. (2017). Amberstripe scad Decapterus muroadsi (Carangidae) fish ingest blue microplastics resembling their copepod prey along the coast of rapa nui (Easter island) in the South Pacific subtropical gyre. Sci. Total Environ. 586, 430–437. doi: 10.1016/j.scitotenv.2017.01.175
Pandian, T. J., and Vivekanandan, E. (1985). “Energetics of feeding and digestion,” in Fish Energetics, eds P. Tytler, P. Calow (Dordrecht: Springer), 99–124.
Peck, M. A., Baumann, H., Clemmesen, C., Herrmann, J. P., Moyano, M., and Temming, A. (2015). Calibrating and comparing somatic-, nucleic acid-, and otolith-based indicators of growth and condition in young juvenile European sprat (Sprattus sprattus). J. Exp. Mar. Biol. Ecol. 471, 217–225. doi: 10.1016/j.jembe.2015.06.011
Pedà, C., Caccamo, L., Fossi, M. C., Gai, F., Andaloro, F., Genovese, L., et al. (2016). Intestinal alterations in European sea bass Dicentrarchus labrax (Linnaeus, 1758) exposed to microplastics: preliminary results. Environ. Pollut. 212, 251–256. doi: 10.1016/j.envpol.2016.01.083
Pegado, S., Schmid, K., Winemiller, K. O., Chelazzi, D., Cincinelli, A., Dei, L., et al. (2018). First evidence of microplastic ingestion by fishes from the Amazon River estuary. Mar. Pollut. Bull. 133, 814–821. doi: 10.1016/j.marpolbul.2018.06.035
Pellini, G., Gomiero, A., Fortibuoni, T., Fabi, G., Grati, F., Tassetti, A. N., et al. (2018a). “Plastic soles: microplastic litter in the gastrointestinal tract of Solea solea from the Adriatic sea,” in Proceedings of the International Conference on Microplastic Pollution in the Mediterranean Sea, Springer Water, eds M. Cocca, E. Di Pace, M. Errico (Cham: Springer), 137–149. doi: 10.1007/978-3-319-71279-6_19
Pellini, G., Gomiero, A., Fortibuoni, T., Ferrà, C., Grati, F., and Tassetti, A. N. (2018b): Characterization of microplastic litter in the gastrointestinal tract of Solea solea from the Adriatic Sea. Environ. Pollut. 234, 943–952. doi: 10.1016/j.envpol.2017.12.038
Peters, C. A., Thomas, P. A., Rieper, K. B., and Bratton, S. P. (2017). Foraging preferences influence microplastic ingestion by six marine fish species from the texas gulf coast. Mar. Pollut. Bull. 124. 82–88. doi: 10.1016/j.marpolbul.2017.06.080
Phuong, N. N., Zalouk-Vergnoux, A., Poirier, L., Kamari, A., Châtel, A., Mouneyrac, C., et al. (2016). Is there any consistency between the microplastics found in the field and those used in laboratory experiments? Environ. Pollut. 211, 111–123. doi: 10.1016/j.envpol.2015.12.035
Possatto, F. E., Barletta, M., Costa, M. F., do Sul, J. A. I., and Dantas, D. V. (2011). Plastic debris ingestion by marine catfish. An unexpected fisheries impact. Mar. Pollut. Bull. 62, 1098–1102. doi: 10.1016/j.marpolbul.2011.01.036
Ramos, J. A. A., Barletta, M., and Costa, M. F. (2012). Ingestion of nylon threads by Gerreidae while using a tropical estuary as foraging grounds. Aquat. Biol. 17, 29–34. doi: 10.3354/ab00461
Renzi, M., Specchiulli, A., Blaškovi,ć, A., Manzo, C., Mancinelli, G., and Cilenti, L. (2019). Marine litter in stomach content of small pelagic fishes from the Adriatic sea. Sardines (Sardina pilchardus) and anchovies (Engraulis encrasicolus). Environ. Sci. Pollut. R 26, 2771–2781. doi: 10.1007/s11356-018-3762-8
Richardson, N., Gordon, A. K., Muller, W. J., and Whitfield, A. K. (2011). A weight-of-evidence approach to determine estuarine fish health using indicators from multiple levels of biological organization. Aquat. Conserv. 21, 423–432. doi: 10.1002/aqc.1207
Rios-Fuster, B., Alomar, C., Compa, M., Guijarro, B., and Deudero, S. (2019). Anthropogenic particles ingestion in fish species from two areas of the western Mediterranean sea. Mar. Pollut. Bull. 144, 325–333. doi: 10.1016/j.marpolbul.2019.04.064
Rochman, C. M., Hoh, E., Kurobe, T., and Teh, S. J. (2013). Ingested plastic transfers hazardous chemicals to fish and induces hepatic stress. Sci. Rep. 3:3263. doi: 10.1038/srep03263
Rochman, C. M., Tahir, A., Williams, S. L., Baxa, D. V., Lam, R., Miller, J. T., et al. (2015). Anthropogenic debris in seafood. Plastic debris and fibers from textiles in fish and bivalves sold for human consumption. Sci. Rep. 5:14340. doi: 10.1038/srep14340
Rønnestad, I., Yúfera, M., Ueberschär, B., Ribeiro, L., Saele, Ø., and Boglione, C. (2013). Feeding behaviour and digestive physiology in larval fish. Current knowledge, and gaps and bottlenecks in research. Rev. Aquacult. 5, 59–98. doi: 10.1111/raq.12010
Rummel, C. D., Löder, M. G. J., Fricke, N. F., Lang, T., Griebeler, E. M., Janke, M., et al. (2016). Plastic ingestion by pelagic and demersal fish from the North sea and Baltic sea. Mar. Pollut. Bull. 102, 134–141. doi: 10.1016/j.marpolbul.2015.11.043
Ryan, P. G. (2015). “A brief history of marine litter research. marine anthropogenic litter,” in Marine Anthropogenic Litter, eds M. Bergmann, L. Gutow, M. Klages (Cham: Springer), 1–25. doi: 10.1007/978-3-319-16510-3_1
Ryan, P. G. (2016). “Ingestion of plastics by marine organisms,” in Hazardous Chemicals Associated with Plastics in the Marine Environment, eds H. Takada, H. Karapanagioti (Cham: Springer), 235–266. doi: 10.1007/698_2016_21
Science Advice for Policy by European Academies (2019). A Scientific Perspective on Microplastics in Nature and Society. Berlin: SAPEA. doi: 10.26356/microplastics
Setälä, O., Fleming-Lehtinen, V., and Lehtiniemi, M. (2014). Ingestion and transfer of microplastics in the planktonic food web. Environ. Pollut. 185, 77–83. doi: 10.1016/j.envpol.2013.10.013
Silva, A. B., Bastos, A. S., Justino, C. I. L., da Costa, J. P., Duarte, A. C., and Rocha-Santos, T. A. P. (2018b). Microplastics in the environment. Challenges in analytical chemistry - a review. Anal. Chim. Acta. 1017, 1–19. doi: 10.1016/j.aca.2018.02.043
Silva, J. D. B., Barletta, M., Lima, A. R. A., and Ferreira, G. V. B. (2018a). Use of resources and microplastic contamination throughout the life cycle of grunts (Haemulidae) in a tropical estuary. Environ. Pollut. 242, 1010–1021. doi: 10.1016/j.envpol.2018.07.038
Skóra, M., Sapota, M., Skóra, K., and Pawelec, A. (2012). Diet of the twaite shad Alosa fallax (Lacépède, 1803) (Clupeidae) in the Gulf of Gdansk, the Baltic sea. Oceanol. Hydrobiol. Stud. 41:141. doi: 10.2478/s13545-012-0024-0
Smith, L. E. (2018). Plastic ingestion by Scyliorhinus canicula trawl captured in the North sea. Mar. Pollut. Bull. 130, 6–7. doi: 10.1016/j.marpolbul.2018.03.001
Smith, T. D. (2002). “A history of fisheries and their science management,” in Handbook of Fish Biology and Fisheries, Vol. 2, eds P. J. B. Hart, J. D. Reynolds (New York, NY: Blackwell Science Ltd), 61–82.
Song, F., Hooper, L., and Loke, Y. K. (2013). Publication bias. What is it? How do we measure it? How do we avoid it? Open Access J. Clin. Trials 5, 71–81. doi: 10.2147/OAJCT.S34419
Song, Y. K., Hong, S. H., Jang, M., Kang, J. H., Kwon, O. Y., Han, G. M., et al. (2014). Large accumulation of micro-sized synthetic polymer particles in the sea surface microlayer. Environ. Sci. Technol. 48, 9014–9021. doi: 10.1021/es501757s
Steer, M., Cole, M., Thompson, R. C., and Lindeque, P. K. (2017). Microplastic ingestion in fish larvae in the western english channel. Environ. Pollut. 226, 250–259. doi: 10.1016/j.envpol.2017.03.062
Stoner, A. W. (2004). Effects of environmental variables on fish feeding ecology. Implications for the performance of baited fishing gear and stock assessment. J. Fish Biol. 65, 1445–1471. doi: 10.1111/j.0022-1112.2004.00593.x
Su, L., Deng, H., Li, B., Chen, Q., Pettigrove, V., Wu, C., et al. (2019). The occurrence of microplastic in specific organs in commercially caught fishes from coast and estuary area of east China. J. Hazard Mater. 365, 716–724. doi: 10.1016/j.jhazmat.2018.11.024
Sun, X., Li, Q., Shi, Y., Zhao, Y., Zheng, S., Liang, J., et al. (2019). Characteristics and retention of microplastics in the digestive tracts of fish from the yellow sea. Environ. Pollut. 249, 878–885. doi: 10.1016/j.envpol.2019.01.110
Suthers, I. M., Fraser, A., and Frank, K. T. (1992). Comparison of lipid, otolith and morphometric condition indices of pelagic juvenile cod Gadus morhua from the Canadian Atlantic. Mar. Ecol. Prog. Ser. 84, 31–40.
Teuten, E. L., Saquing, J. M., Knappe, D. R. U., Barlaz, M. A., Jonsson, S., Bjorn, A., et al. (2009). Transport and release of chemicals from plastics to the environment and to wildlife. Phil. Trans. R. Soc. B 364, 2027–2045. doi: 10.1098/rstb.2008.0284
Thompson, R. C. (2015). “Microplastics in the marine environment: sources, consequences and solutions,” in Marine Anthropogenic Litter, eds M. Bergmann, L. Gutow, M. Klages (Cham: Springer), 185–200. doi: 10.1007/978-3-319-16510-3_7
Trippel, E. A., and Neil, S. R. E. (2003). Effects of photoperiod and light intensity on growth and activity of juvenile haddock (Melanogrammus aeglefinus). Aquaculture 217, 633–645. doi: 10.1016/S0044-8486(02)00198-9
Vasconcelos, R. P., Reis-Santos, P., Fonseca, V., Ruano, M., Tanner, S., Costa, M. J., et al. (2009). Juvenile fish condition in estuarine nurseries along the Portuguese coast. Estuar. Coast Shelf Sci. 82, 128–138. doi: 10.1016/j.ecss.2009.01.002
Vendel, A. L., Bessa, F., Alves, V. E. N., Amorim, A. L. A., Patrício, J., and Palma, A. R. T. (2017). Widespread microplastic ingestion by fish assemblages in tropical estuaries subjected to anthropogenic pressures. Mar. Pollut. Bull. 117, 448–455. doi: 10.1016/j.marpolbul.2017.01.081
Völker, C., Kramm, J., and Wagner, M. (2019). On the creation of risk. Framing of microplastics risks in science and media. Glob. Challen. 50:1900010. doi: 10.1002/gch2.201900010
Welden, N. A., and Lusher, A. L. (2017). Impacts of changing ocean circulation on the distribution of marine microplastic litter. Integr. Environ. Assess. Manag. 13, 483–487. doi: 10.1002/ieam.1911
Wesch, C., Barthel, A. K., Braun, U., Klein, R., and Paulus, M. (2016). No microplastics in benthic eelpout (Zoarces viviparus). An urgent need for spectroscopic analyses in microplastic detection. Environ. Res. 148, 36–38. doi: 10.1016/j.envres.2016.03.017
Wieczorek, A. M., Morrison, L., Croot, P. L., Allcock, A. L., MacLoughlin, E., Savard, O., et al. (2018). Frequency of microplastics in mesopelagic fishes from the Northwest Atlantic. Front. Mar. Sic. 5:1985. doi: 10.3389/fmars.2018.00039
Wolff, S., Kerpen, J., Prediger, J., Barkmann, L., and Müller, L. (2019). Determination of the microplastics emission in the effluent of a municipal waste water treatment plant using Raman microspectroscopy. Water Res. 2:100014. doi: 10.1016/j.wroa.2018.100014
Wright, S. L., Thompson, R. C., and Galloway, T. S. (2013). The physical impacts of microplastics on marine organisms. A review. Environ. Pollut. 178, 483–492. doi: 10.1016/j.envpol.2013.02.031
Young, J. W., Lamb, T. D., Le, D, Bradford, R. W., and Whitelaw, A. W. (1997). Feeding ecology and interannual variations in diet of southern Bluefin tuna, Thunnus maccoyii, in relation to coastal and oceanic waters off eastern Tasmania, Australia. Environ. Biol. Fishes 50, 275–291.
Zhang, H. (2017). Transport of microplastics in coastal seas. Estuar. Coast Shelf Sci. 199, 74–86. doi: 10.1016/j.ecss.2017.09.032
Keywords: marine litter, anthropogenic particles, ingestion, marine fish, publication bias
Citation: Müller C (2021) Not as Bad as It Seems? A Literature Review on the Case of Microplastic Uptake in Fish. Front. Mar. Sci. 8:672768. doi: 10.3389/fmars.2021.672768
Received: 26 February 2021; Accepted: 24 March 2021;
Published: 21 April 2021.
Edited by:
Viola Liebich, Bremen Society for Natural Sciences, GermanyReviewed by:
Anne K. S. Justino, Federal Rural University of Pernambuco, BrazilRaqueline Monteiro, Federal University of Pará, Brazil
Copyright © 2021 Müller. This is an open-access article distributed under the terms of the Creative Commons Attribution License (CC BY). The use, distribution or reproduction in other forums is permitted, provided the original author(s) and the copyright owner(s) are credited and that the original publication in this journal is cited, in accordance with accepted academic practice. No use, distribution or reproduction is permitted which does not comply with these terms.
*Correspondence: Carolin Müller, carolin.mueller@leibniz-zmt.de