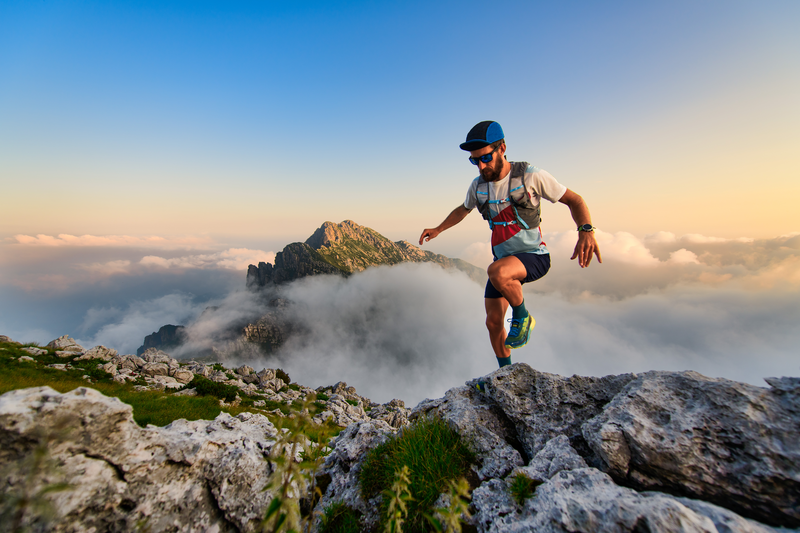
94% of researchers rate our articles as excellent or good
Learn more about the work of our research integrity team to safeguard the quality of each article we publish.
Find out more
ORIGINAL RESEARCH article
Front. Mar. Sci. , 07 July 2021
Sec. Global Change and the Future Ocean
Volume 8 - 2021 | https://doi.org/10.3389/fmars.2021.672574
This article is part of the Research Topic Environmental Threats to the State of Florida—Climate Change and Beyond View all 8 articles
Over the past three decades, coral populations have declined across the tropical and subtropical oceans because of thermal stress, coral diseases, and pollution. Restoration programs are currently attempting to re-establish depauperate coral populations along the Florida reef tract. We took an integrated Bayesian approach to determine which Florida reefs ranked highest based on the survival of outplanted colonies of Acropora cervicornis from 2012 to 2018. Survival of A. cervicornis outplants was highly variable in the upper Florida Keys with some reefs showing the highest likelihood of survival (e.g., North Dry Rocks, Carysfort, Key Largo Dry Rocks, and Conch Reef), whereas some adjacent reefs showed the lowest likelihood of survival (e.g., an Unnamed Reef, Pickles Reef, and U47 Patch Reef). Similarly, survival was highly variable in the middle and lower Florida Keys and in the Broward-Miami subregions. Survival was high and less variable in Biscayne Bay and low and less variable in the Marquesas subregions. The reefs that ranked lowest for outplant survival were exposed to high wave energy. Partitioning out the spatial effects of reefs and subregions from the model, we detected spatial latent effects of low survival that were most evident in the middle and the upper Florida Keys, particularly between 2015 and 2017. The overall high spatial and temporal variability in survival among adjacent reefs highlights a need to outplant nursery-reared colonies strategically, in order to optimize coral-population recovery efforts in Florida.
Coral populations have declined globally in the past three decades (Hoegh-Guldberg et al., 2007; Edwards and Gomez, 2007; Hughes et al., 2018; Lough et al., 2018). Two acroporid species, Acropora cervicornis and Acropora palmata, which previously dominated the Caribbean, and were major reef builders through millennia (Agassiz, 1885; Vaughan, 1919; Goldberg, 1973; Marszalek et al., 1977; Precht and Miller, 2007), have experienced some of the largest declines. Diseases and thermal anomalies have been the main causes of coral population declines in the Caribbean (Porter and Meier, 1992; Aronson and Precht, 2001; Toth et al., 2014; Precht et al., 2016; Walton et al., 2018). A major outbreak of white-band disease in the 1970s caused a 95% decline in Caribbean acroporids (Aronson and Precht, 2001; Miller et al., 2002; Gardner et al., 2003). Since then, A. cervicornis and A. palmata have been listed as “threatened” under the US Endangered Species Act in 2006 (National Marine Fisheries Service, 2006) and “critically endangered” on the World Conservation Union (IUCN) Red List in 2008 (Aronson et al., 2008). Coral restoration efforts are now attempting to restore acroporid populations along the Florida reef tract.
Reef-restoration programs first appeared in Florida and the wider Caribbean in the 1990s. Acropora species have been targeted in recent restoration efforts because of their: (i) threatened status (National Marine Fisheries Service, 2006), (ii) low recruitment rates (van Woesik et al., 2014), (iii) rapid growth rates (Tunnicliffe, 1981), (iv) importance in reef-building framework (Jackson, 1992), and (v) reproductive strategies through fragmentation (Highsmith, 1982). These factors make acroporids ideal candidates for coral restoration programs. However, restoration through propagation and outplanting techniques alone will not be enough if the benchmarks set by the Paris Agreement are not met (Hoegh-Guldberg et al., 2014).
Restoration techniques primarily rely on either securing corals that have been naturally fragmented (Bruckner and Bruckner, 2001), rescuing and relocating colonies from habitats threatened by local stressors (Gayle et al., 2005; Seguin et al., 2010; Young et al., 2012), or outplanting nursery-based corals (Ware et al., 2020). Most recent efforts have focused on outplanting nursery-reared corals and these methods are evolving rapidly (Edwards and Gomez, 2007; Rinkevich, 2014; Forsman, 2015; Page et al., 2015) and include both land-based and marine programs (Rinkevich, 1995; Oren and Benayahu, 1997; van Treeck and Schuhmacher, 1999). Coral-restoration practitioners are also attempting to identify coral genotypes that are most tolerant to disease and thermal stress (Baums et al., 2019), essentially accelerating natural selection, with the goal of using those robust individuals to repopulate reefs (van Oppen and Gates, 2006; Baums, 2008; van Oppen et al., 2015; Pausch et al., 2018).
One of the more difficult hurdles of coral restoration, however, is developing models that can accurately predict optimal localities for coral outplanting at a variety of spatial scales (Wirt et al., 2013; van Woesik et al., 2020a). Such models aim to determine which subregions (at the 100-km scale), reefs (at the 10-km scale), or habitats (at the 0.1–1-km scale) are most favorable for Acropora survival along the Florida reef tract. Indeed, there is an urgent need for hierarchical models that inform restoration practitioners and managers which coral species to outplant, and where to outplant them, at a range of spatial scales along the Florida reef tract.
There are, however, some analytical barriers preventing accurate predictions, primarily because acroporids along the Florida reef tract are scarce. Most models that estimate the spatial distribution of Acropora species in Florida have had high specificity (i.e., skilled at predicting true negatives) but low sensitivity (i.e., not skilled at predicting true positives) (van Woesik et al., 2020b). In other words, regional models have a low accuracy of predicting where an acroporid species is likely to occur because the frequency and intensity of recent disturbances makes that niche space highly dynamic and unpredictable. Therefore, developing models that can perform with limited presence data, incorporate environmental predictors, and accurately predict optimal restoration sites are urgently needed at all spatial scales along the Florida reef tract.
At the local, habitat scale, several studies have recently made considerable progress at predicting the presence of A. cervicornis. For example, A. cervicornis colonies are most commonly found growing close to reef edges, where water-flow rates are high (D’Antonio et al., 2016), and in habitats with moderate wave energy, between 0.5 and 1.5 kJ/m2 (van Woesik et al., 2020b). In addition, A. cervicornis outplant survival appears highest in back-reef and fore-reef habitats (van Woesik et al., 2020a). There is, however, little information on the survival of acroporids at the reef scale, even though the reef scale has been identified as the scale of highest variability in Florida (Murdoch and Aronson, 1999). To optimize restoration efforts and improve A. cervicornis outplant survival we developed a spatio-temporal Integrated Nested Laplace Approximation (INLA) survival model, within a Bayesian framework, which used survival data from A. cervicornis outplants along the Florida reef tract from 2012 to 2018. The objectives of this study were to: (i) rank 67 reefs in terms of survival of outplanted colonies of the coral A. cervicornis along the Florida reef tract, and (ii) determine whether outplant survival was geographically consistent through time.
The data were a compilation of work conducted by six different coral restoration programs that examined the survival of a total of 22,634 A. cervicornis colonies raised in nurseries and outplanted to reef habitats along the Florida reef tract between 2012 and 2018 (van Woesik et al., 2020a). The restoration programs were conducted by: (1) The Nature Conservancy, (2) the Mote Marine Laboratory, (3) the Florida Fish and Wildlife Conservation Commission, (4) the Coral Restoration Foundation, (5) the University of Miami, and (6) Nova Southeastern University (Supplementary Document). All six coral restoration programs monitored the survival of A. cervicornis outplants after 1-month and 1-year, and several programs continued to monitor colony survival annually for 4 years (i.e., The Nature Conservancy, the Mote Marine Laboratory, the Florida Fish and Wildlife Conservation Commission, and the University of Miami). Each outplanted colony was visually assessed to determine whether it was alive or dead at each monitoring interval. An A. cervicornis colony was considered censored when the colony was still alive at the last monitoring interval. For analysis purposes, censored individuals were given a value of 0. By contrast, individuals that died within the timeframe of the study were given a value of 1, at the time of death. All outplanted A. cervicornis colonies analyzed in this study were considered as shallow (<8 m) outplants. All the data are available at: https://github.com/rvanwoesik/Acropora_survival.
We used Integrated Nested Laplace Approximation (INLA) (Rue et al., 2009) within a Bayesian framework to examine spatial differences in coral survival (η) at a given site on a reef, i, expressed as:
where γ is an intercept coefficient, β is the fixed-effect coefficient vector, Z is a matrix of covariates at the location of the data points si, ξ(si) is the spatial random effect in a spatial Gaussian Markov Random Field (GMRF), and ε(si) is the measurement error defined by a Gaussian white-noise process [∼ N (0,σ2ε)]. The GMRF combines the Gaussian field with Matérn covariance functions using stochastic partial differential equations, which in turn use a finite element representation to define the Matérn field by triangulation of the spatial domain (Lindgren et al., 2011; Figure 1). The GMRF computational properties have been recently enhanced by using INLA (Rue et al., 2009) for Bayesian inference, which is a computationally effective algorithm that produces fast and accurate approximations of posterior distributions (Blangiardo and Cameletti, 2015). Here we are particularly interested in the coefficients of the covariates, to determine how much of the variance is explained by the covariates reef and subregion, and the variation in the spatial latent effect explained by the variance-covariance matrix calculated using the Matérn correlation function. Model selection was based on the lowest Deviance Information Criterion (DIC) and the lowest Watanabe-Akaike Information Criterion (WAIC). To validate the model, we used spatial leave-one-out cross validation, to assess the root mean squared error (Le Rest et al., 2014). All the models were coded in R (R Core Team, 2018) and are available at: https://github.com/rvanwoesik/INLA_Florida.
Figure 1. Study sites (red dots) and a constrained refined Delaunay triangulation mesh (outlined in blue) used in the Integrated Nested Laplace Approximation (INLA) model for 22,634 Acropora cervicornis outplants in 7 subregions and 67 reefs along the Florida reef tract, United States from 2012 to 2018. The mesh uses the A. cervicornis critical habitat as the inner boundary and a buffer around that critical boundary to avoid edge effects.
In conjunction with the INLA coral survival model, we used a binary logistic regression model to examine the relationship between the probability of survival of A. cervicornis outplants along the Florida reef tract and wave energy. Mean wave energy was derived from site location and fetch, at a 1-km resolution (from van Woesik et al., 2020b; Supplementary Document) and used as the predictive covariate. For the response covariates, we assigned 33 reefs (ranked 1–33) that had the highest likelihood of outplant survival (Table 1), based on the INLA results, a value of 1, and 34 reefs (ranked 34–67) that had the lowest likelihood of outplant survival (Table 1), based on the INLA results, a value of 0.
Table 1. Posteriori mean (Mean); standard deviation (SD); 0.025, and 0.975% Quantile values; and Mode of the posteriori mean values using 67 reefs as the fixed effect in the Integrated Nested Laplace Approximation (INLA) model for 22,634 Acropora cervicornis outplants in 7 subregions along the Florida reef tract, United States from 2012 to 2018. The 7 subregions (from north east to south west) include: 1) Broward-Miami, 2) Biscayne, 3) Upper Keys, 4) Middle Keys, 5) Lower Keys, 6) Marquesas, and 7) Dry Tortugas. The reefs are listed from highest to lowest likelihood of survival of A. cervicornis outplants. Carysfort Reef, ranked # 4 and marked in bold, is included in the “Iconic Reefs” initiative.
The INLA coral survival model examining “reefs” as fixed effects showed considerable variability in outplant survival across the study region. Indeed, the top ranked reefs, where the likelihood of survival of outplants was highest, were widely distributed along the Florida reef tract (Figure 2). There was also considerable variability in outplant survival within each of the seven subregions (Table 1). Survival of Acropora cervicornis outplants was highly variable in the upper Florida Keys with some reefs showing high survival (e.g., North Dry Rocks, Carysfort, Key Largo Dry Rocks, and Conch Reef), whereas some adjacent or nearby reefs showed low survival (e.g., an Unnamed Reef, Pickles Reef, and U47 Patch Reef) (Figure 2). Similarly, survival of A. cervicornis was highly variable in the middle and lower Florida Keys and in the Broward-Miami subregions, with some reefs showing high survival, whereas some adjacent or nearby reefs in the same subregion showed low survival (Table 1). By contrast, survival of A. cervicornis outplants in the Biscayne subregion showed low variability, but survival was consistently high (Table 1). Similarly, survival of A. cervicornis outplants was consistent within the Marquesas and the Dry Tortugas subregions, although survival was moderate to low (Table 1). Notably, the Marquesas and the Dry Tortugas had comparably fewer outplanting sites (3 and 4, respectively) than the other subregions.
Figure 2. Location of the top-ranked 33 reefs (depicted by yellow circles) with the highest likelihood of survival of Acropora cervicornis outplants, and the bottom-ranked 34 reefs (depicted by black circles) with the lowest likelihood of survival for A. cervicornis outplants in 7 subregions along the Florida reef tract, United States from 2012 to 2018—based on the posteriori mean values using “reef” as the fixed effect in the Integrated Nested Laplace Approximation (INLA) model.
Outplant survival on reefs was significantly (p = 0.0124) related to mean wave energy. Reefs exposed to moderate levels of mean wave energy (2–4 kJ m–2) had a high probability of outplant survival. Conversely, reefs exposed to high levels of mean wave energy (>4 kJ m–2) had a relatively low probability of outplant survival (Figure 3).
Figure 3. Logistic regression (black line) showing the effect of wave energy (kJ m– 2) on the probability of survival of Acropora cervicornis outplants in 7 subregions along the Florida reef tract, United States from 2012 to 2018—based on reefs with the highest likelihood of survival (green dots) and the lowest likelihood of survival (red dots). The 95% confidence intervals are shown in blue.
After removing the effects of “reefs” and “subregions” from the spatio-temporal model, spatial latent effects were evident for each sampling period (Figure 4). These spatial latent effects showed consistently low survival of A. cervicornis outplants in the middle Florida Keys, which were consistent through time (Figure 4). There were also spatial latent effects from 2015 through to 2017 in the upper Florida Keys, suggesting lower survival of A. cervicornis outplants in these years. There were no obvious spatial latent effects northeast of the upper Florida Keys and west of the lower Florida Keys, although survival of A. cervicornis outplants was lower between 2015 and 2017 than for 2012 and 2014 in both of those subregions (Figure 2).
Figure 4. Posteriori mean values of the spatial random effect for the years 2012, and 2014 to 2017 (note that 2013 and 2018 had insufficient data), obtained by the Integrated Nested Laplace Approximation (INLA) model, for 22,634 Acropora cervicornis outplants in 7 subregions along the Florida reef tract, United States—after considering “reef” and “subregion” as fixed effects. High survival of A. cervicornis outplants is highlighted in blue and low survival in red.
This study showed considerable variability in A. cervicornis outplant survival across the Florida reef tract from 2012 to 2018. Reefs within the upper and middle Florida Keys had high variability in survival of A. cervicornis outplants, supporting some of the best, but also some of the worst, reefs for outplant survival. For example, North Dry Rocks, Carysfort, Key Largo Dry Rocks, and Conch Reef, in the upper Florida Keys, and Coffins Patch and Tropical Rocks, in the middle Florida Keys were among the best reefs for outplant survival. Yet, some reefs that were immediately adjacent to or nearby these high-survival reefs were among the worst reefs for outplant survival (Figure 2). Such results suggest that although the environmental conditions within a subregion influence outplant survival (Toth et al., 2018; van Woesik et al., 2020a), variation among reefs within a given subregion, and variation of habitats within reefs also play major roles in the probability of outplant survival.
High variability among reefs within subregions agrees with Murdoch and Aronson (1999), who examined variation in coral cover across the Florida reef tract. They suggested that coral cover on a given reef did not predict coral cover on the adjacent or nearby reefs, because reefs are disproportionately exposed to stressors and disturbances. Some of the stressors include differential exposure to the inclement waters from Florida Bay that have long been variable in temperature, salinity, nutrients, and sediment loads (Ginsburg and Shinn, 1994). Murdoch and Aronson (1999) found that most variation along the Florida reef tract occurred among reefs at the 10–20-km scale. These results from Florida contrast with a study along the Great Barrier Reef, in Australia, by Hughes et al. (1999) who showed that the highest variation in coral cover occurred among habitats at the 0.5–3-km scale. Understanding variation in coral cover and outplant survival is critical for restoration practitioners, because such variation reflects differences in key processes, such as differential recruitment and post-settlement mortality, that can influence restoration success. In addition, outplant survival does not always suggest an increase in coral cover as growth can be independent of survival. Similarly, outplant mortality does not necessarily result in a decline in coral cover as coral colonies can fragment, move, and fuse. Monitoring outplant survival is the cornerstone of monitoring restoration success, but to improve the overall understanding of restoration success, colony growth and fragmentation records should be also examined.
Coral reef restoration and monitoring is performed by various agencies throughout the Florida Keys, with each agency generally self-restricted to a particular subregion for outplanting and monitoring. Therefore, intra-regional variation in survival is likely not a consequence of outplanting technique, as the same agency uses the same technique across each subregion. We did find that differences in reef exposure to water flow and wave energy influenced outplant survival, which warrants further investigation. Based on previous findings (D’Antonio et al., 2016; van Woesik et al., 2020b) A. cervicornis seems to prefer reef substrate that is exposed to moderate levels of water flow and wave energy—a finding which is supported in the present study. For example, the reefs that ranked highest for outplant survival were exposed to moderate wave energy, whereas nearby reefs that ranked lowest for outplant survival were exposed to moderate to high wave energy (Figure 3). These results point to a potential wave-energy threshold, where high wave energy is not conducive to A. cervicornis survival. No outplants were positioned on reefs with low wave-energy exposure, and therefore we do not know the wave-energy threshold where waters may become stagnant and thus harmful for A. cervicornis outplant survival.
Since suitable habitat for A. cervicornis outplant survival is limited throughout the Florida reef tract (van Woesik et al., 2020b), reefs showing high survival of outplants, in subregions with low overall survival, deserve special attention. Such bright spots, within subregions that are less conducive to survival, have been referred to as microrefugia and have played major roles in genetic preservation and population recovery during glacial-interglacial cycles in the past (Mosblech et al., 2011). With the continuation of climate change and ocean warming, protecting such microrefugia should be prioritized in the hope of sustaining coral populations. For example, microrefugia in the middle Florida Keys could be vital stepping-stones in maintaining connectivity among coral populations between the lower and upper Florida Keys (Frys et al., 2020). Additionally, with A. cervicornis and A. palmata listed as “threatened” and “critically endangered,” microrefuges throughout the Florida reef tract are of great importance in protecting and restoring the acroporids. Unfortunately, the genetic identity for all of the 22,634 outplants in this study were not available and prevented the assessment of individual genotypes as a confounding variable. However, we encourage the inclusion of individual genotypes as a variable in further analyses because it could reveal valuable insights into the performance of coral outplants under current and future stressors.
While the present study highlights reefs where A. cervicornis had the highest and lowest likelihood of survival, other coral species may have broader or narrower tolerances. Therefore, this study does not suggest abandoning coral-restoration practices in the middle Florida Keys, nor does it serve as a conduit for judging the success of other coral species. Conservation initiatives and restoration programs are only in their infancy in Florida. One such recent 2020 initiative, called “Iconic Reefs” (NOAA Fisheries, 2019), was designed to conserve and restore seven reefs along the Florida reef tract. The “Iconic Reefs” mission is an emergency restoration plan focused on providing rapid restoration efforts to seven reefs in the Florida Keys (i.e., Carysfort Reef, Horseshoe Reef, Cheeca Rocks, Sombrero Reef, Newfound Harbor, Looe Key Reef, and Eastern Dry Rocks). Carysfort Reef (north and south) is scheduled to receive 36,554 A. cervicornis outplants during phases 1 and 2—a notable amount of outplants, and higher than the number of outplants scheduled for the other “Iconic Reefs” (NOAA Fisheries, 2019). Carysfort Reef was ranked fourth among the highest-ranking reefs in the present study (Table 1). While we cannot comment on whether all seven reefs of the “Iconic Reefs” program will support A. cervicornis populations (because we did not have any outplant data on 6 of the 7 chosen reefs), the variability within our study suggests that the seven “Iconic Reefs” will also show variable responses in outplant success, and future studies may reveal that the seven reefs vary in which coral populations they can best support.
Contemporary restoration efforts take place in a dynamic backdrop of global, regional, and local stressors (van Hooidonk et al., 2017). Coral populations in Florida have been heavily impacted by diseases and thermal-stress events (Porter and Meier, 1992; Toth et al., 2014; Precht et al., 2016; Walton et al., 2018). For example, the Stony Coral Tissue Loss Disease (SCTLD)—a particularly aggressive disease affecting more than 19 coral species throughout the Florida reef tract and wider Caribbean (Muller et al., 2020)—recently changed the composition of reefs in Florida (Muller et al., 2020). Similarly, the effects of thermal stress was evident in our study in the years 2015–2017 (Figure 4), which coincided with a global El Niño event. A. cervicornis outplant survival was, in general, lower between 2015 and 2017 than it was for 2012 and 2014 in the upper and lower Florida Keys. Therefore, we suggest that A. cervicornis restoration efforts along the Florida reef tract should: (i) continue to strive for breeding diverse yet thermally tolerant and disease resistant colonies (Baums et al., 2013; van Oppen et al., 2015), and (ii) evaluate outplant success and the dynamics of that success on a reef-by-reef basis.
The INLA approach helped us examine temporal consistency in A. cervicornis outplant survival, but it also helped us detect spatially latent effects that were not explicit variables in our analysis. In particular, spatially latent effects were observed in the middle Florida Keys that were independent of reef, subregion, and year of monitoring. We also noticed lower survival of A. cervicornis outplants in the upper Florida Keys from 2015 through to 2017 than in the other years of the study—that was most likely associated with thermal-stress related effects. In addition, the effects of Hurricane Irma may have had an effect on the survival of colonies outplanted immediately prior to its overpass in 2017 (van Woesik et al., 2020a).
In conclusion, A. cervicornis outplant survival was highly variable in the middle and lower Florida Keys and Broward-Miami subregions. By contrast, A. cervicornis outplant survival was relatively high and less variable in Biscayne Bay, and low and less variable in the Marquesas subregions. The highest variability in A. cervicornis outplant survival, in general, was evident among adjacent or nearby reefs, as reefs appear disproportionately exposed to stressors. Such spatial and temporal differences in survival of A. cervicornis outplants at a “reef” scale highlight a need for strategic research to enhance our understanding of processes that influence growth and survival of A. cervicornis outplants in order to optimize population recovery along the Florida reef tract.
The original contributions presented in the study are included in the article/Supplementary Material, further inquiries can be directed to the corresponding author.
RBB and RvW analyzed the data and wrote the manuscript. RvW conceptualized the study and provided funding. Both authors contributed to the article and approved the submitted version.
Florida Fish and Wildlife Conservation Commission provided funds to conduct the research (award 16008).
The authors declare that the research was conducted in the absence of any commercial or financial relationships that could be construed as a potential conflict of interest.
We thank extend to all the six agencies that collected the field data: The Nature Conservancy, the Mote Marine Laboratory, the Florida Fish and Wildlife Conservation Commission, the Coral Restoration Foundation, the University of Miami, and Nova Southeastern University. We would like to thank the Florida Fish and Wildlife Commission, award 16008 to RvW that partially supported this research. We would like to thank Sandra J. van Woesik for her editorial comments on the manuscript, and Lynnette Roth for her database expertise.
The Supplementary Material for this article can be found online at: https://www.frontiersin.org/articles/10.3389/fmars.2021.672574/full#supplementary-material
Agassiz, A. (1885). Explorations of the surface fauna of the Gulf Stream, under the auspices of the United States Coast Survey II. Mem. Am. Acad. Arts Sci. 11, 106–133.
Aronson, R. B., Bruckner, A., Moore, J., Precht, B., and Weil, E. (2008). Acropora cervicornis and A. palmata. The IUCN Red List of Threatened Species. Available online at: https://dx.doi.org/10.2305/IUCN.UK.2008.RLTS.T133006A3536699.en (accessed May 19, 2020).
Aronson, R. B., and Precht, W. F. (2001). White-band disease and the changing face of Caribbean coral reefs. Hydrobiologia 460, 25–38. doi: 10.1007/978-94-017-3284-0_2
Baums, I. B. (2008). A restoration genetics guide for coral reef conservation. Mol. Ecol. 17, 2796–2811. doi: 10.1111/j.1365-294x.2008.03787.x
Baums, I. B., Baker, A. C., Davies, S. W., Grottoli, A. G., Kenkel, C. D., Kitchen, S. A., et al. (2019). Considerations for maximizing the adaptive potential of restored coral populations in the western Atlantic. Ecol. Appl. 29:e01978. doi: 10.1002/eap.1978
Baums, I. B., Devlin-Durante, M. K., Polato, N. R., Xu, D., Giri, S., Altman, N. S., et al. (2013). Genotypic variation influences reproductive success and thermal stress tolerance in the reef building coral, Acropora palmata. Coral Reefs 32, 703–717. doi: 10.1007/s00338-013-1012-6
Blangiardo, M., and Cameletti, M. (2015). Spatial and Spatio-temporal Bayesian Models with R – INLA, eds M. Blangiardo and M. Cameletti (Hoboken, NJ: John Wiley & Sons), 259–302.
Bruckner, A., and Bruckner, R. (2001). Condition of restored Acropora palmata fragments off Mona Island, Puerto Rico, 2 years after the Fortuna Reefer ship grounding. Coral Reefs 20, 235–243. doi: 10.1007/s003380100164
D’Antonio, N. L., Gilliam, D. S., and Walker, B. K. (2016). Investigating the spatial distribution and effects of nearshore topography on Acropora cervicornis abundance in Southeast Florida. PeerJ 4:e2473. doi: 10.7717/peerj.2473
Edwards, A., and Gomez, E. (2007). “Reef restoration concepts and guidelines: making sensible management choices in the face of uncertainty,” in Coral Reef Targeted Research & Capacity Building for Management Programme, ed. W. F. Precht (Newcastle upon Tyne: Newcastle University), 38.
Forsman, A. (2015). Rethinking phenotypic plasticity and its consequences for individuals, populations and species. Heredity 115, 276–284. doi: 10.1038/hdy.2014.92
Frys, C., Saint-Amand, A., Le Hénaff, M., Figueiredo, J., Kuba, A., Walker, B., et al. (2020). Fine-scale coral connectivity pathways in the florida reef tract: implications for conservation and restoration. Front. Mar. Sci. 7:312. doi: 10.3389/fmars.2020.00312
Gardner, T. A., Côte’, I. M., Gill, J. A., Grant, A., and Watkinson, A. R. (2003). Long-term region-wide declines in Caribbean corals. Science 301, 958–960. doi: 10.1126/science.1086050
Gayle, P. M. H., Wilson-Kelly, P., and Green, S. (2005). Transplantation of benthic species to mitigate impacts of coastal development in Jamaica. Rev. Biol. Trop. 53, 105–115.
Ginsburg, R. N., and Shinn, E. A. (1994). “Preferential distribution of reefs in the Florida reef tract: the past is the key to the present,” in Proceedings of the Colloquium on Global Aspects of Coral Reefs: Health, Hazards and History, ed. R. N. Ginsburg (Miami, FL: University of Miami), 21–26.
Goldberg, W. M. (1973). The ecology of the coral-octocoral communities off the southeast Florida coast: geomorphology, species composition, and zonation. Bull. Mar. Sci. 23, 465–488.
Highsmith, R. C. (1982). Reproduction by fragmentation in corals. Mar. Ecol. Prog. Ser. 7, 207–226. doi: 10.3354/meps007207
Hoegh-Guldberg, O., Cai, R., Poloczanska, E. S., Brewer, P. G., Sundby, S., Hilmi, K., et al. (2014). “The Ocean,” in Climate Change 2014: Impacts, Adaptation, and Vulnerability. Part B: Regional Aspects. Contribution of Working Group II to the Fifth Assessment Report of the Intergovernmental Panel on Climate Change, eds V. R. Barros, C. B. Field, D. J. Dokken, M. D. Mastrandrea, K. J. Mach, T. E. Bilir, et al. (Cambridge: Cambridge University Press), 1655–1731.
Hoegh-Guldberg, O., Mumby, P. J., Hooten, A. J., Steneck, R. S., Greenfield, P., Gomezet, E., et al. (2007). Coral reefs under rapid climate change and ocean acidification. Science 318, 1737–1742.
Hughes, T. P., Anderson, K. D., Connolly, S. R., Heron, S. F., Kerry, J. T., Lough, J. M., et al. (2018). Spatial and temporal patterns of mass bleaching of corals in the Anthropocene. Science 359, 80–83. doi: 10.1126/science.aan8048
Hughes, T. P., Baird, A. H., Dinsdale, E. A., Moltschaniwskyj, N. A., Pratchett, M. S., Tanner, J. E., et al. (1999). Patterns of recruitment and abundance of corals along the Great Barrier Reef. Nature 397, 59–63. doi: 10.1038/16237
Jackson, J. B. C. (1992). Pleistocene perspectives on coral reef community structure. Am. Zool. 32, 719–731. doi: 10.1093/icb/32.6.719
Le Rest, K., Pinaud, D., Monestiez, P., Chadoeuf, J., and Bretagnolle, V. (2014). Spatial leave-one-out cross-validation for variable selection in the presence of spatial autocorrelation. Glob. Ecol. Biogeogr. 23, 811–820. doi: 10.1111/geb.12161
Lindgren, F., Rue, H., and Lindström, J. (2011). An explicit link between Gaussian fields and Gaussian Markov random fields: the stochastic partial differential equation approach. J. R. Stat. Soc. Ser. B Stat. Methodol. 73, 423–498. doi: 10.1111/j.1467-9868.2011.00777.x
Lough, J. M., Anderson, K. D., and Hughes, T. P. (2018). Increasing thermal stress for tropical coral reefs: 1871-2017. Sci. Rep. 8:6079.
Marszalek, D. S., Babashoff, G. Jr., Noel, M. R., and Worley, D. R. (1977). “Reef distribution in south Florida 2,” in Proceedings of 3rd International Coral Reef Symposium, ed. D. L. Taylor (Miami Beach, FL: University of Miami), 223–230.
Miller, M., Bourque, A., and Bohnsack, J. (2002). An analysis of the loss of acroporid corals at Looe Key, Florida, USA: 1983–2000. Coral Reefs 21, 179–182. doi: 10.1007/s00338-002-0228-7
Mosblech, N. A. S., Bush, M. B., and van Woesik, R. (2011). On metapopulations and microrefugia: palaeoecological insights. J. Biogeogr. 38, 419–429. doi: 10.1111/j.1365-2699.2010.02436.x
Muller, E. M., Sartor, C., Alcaraz, N. I., and van Woesik, R. (2020). Spatial epidemiology of the stony-coral-tissue-loss disease in Florida. Front. Mar. Sci. 7:163. doi: 10.3389/fmars.2020.00163
Murdoch, T. J. T., and Aronson, R. B. (1999). Scale-dependent spatial variability of coral assemblages along the Florida Reef Tract. Coral Reefs 18, 341–351. doi: 10.1007/s003380050210
National Marine Fisheries Service (2006). Endangered and Threatened Species: Final Listing Determinations for Elkhorn Coral and Staghorn Coral, 71(FR26852): 26852-26872. Silver Spring, MD: National Marine Fisheries Service (NMFS).
NOAA Fisheries (2019). Restoring Seven Iconic Reefs: A Mission to Recover the Coral Reefs of the Florida Keys. Mission: Iconic Reefs–Summary. Available online at: https://media.fisheries.noaa.gov/dam-migration/restoring_seven_iconic_reefs_-_a_mission_to_recover_the_coral_reefs_of_the_florida_keys.pdf (accessed June 4, 2021).
Oren, U., and Benayahu, Y. (1997). Transplantation of juvenile corals: a new approach for enhancing colonization of artificial reefs. Mar. Biol. 127, 499–505. doi: 10.1007/s002270050038
Page, A. J., Cummins, C. A., Hunt, M., Wong, V. K., Reuter, S., Holden, M. T., et al. (2015). Roary: rapid large-scale prokaryote pan genome analysis. Bioinformatics 31, 3691–3693. doi: 10.1093/bioinformatics/btv421
Pausch, R. E., Williams, D. E., and Miller, M. W. (2018). Impacts of fragment genotype, habitat, and size on outplanted elkhorn coral success under thermal stress. Mar. Ecol. Prog. Ser. 592, 109–117. doi: 10.3354/meps12488
Porter, J. W., and Meier, O. W. (1992). Quantification of loss and change in Floridian reef coral populations. Am. Zool. 32, 625–640. doi: 10.1093/icb/32.6.625
Precht, W. F., Gintert, B. E., Robbart, M. L., Fura, R., and van Woesik, R. (2016). Unprecedented disease-related coral mortality in southeastern Florida. Sci. Rep. 6:31374.
Precht, W. F., and Miller, S. L. (2007). “Ecological Shifts along the Florida reef tract: the past as a key to the future,” in Geological Approaches to Coral Reef Ecology, ed. R. B. Aronson (New York, NY: Springer), 442.
R Core Team (2018). R: A Language and Environment for Statistical Computing. Vienna: R Foundation for Statistical Computing.
Rinkevich, B. (1995). Restoration strategies for coral reefs damaged by recreational activities: the use of sexual and asexual recruits. Restor. Ecol. 3, 241–251. doi: 10.1111/j.1526-100x.1995.tb00091.x
Rinkevich, B. (2014). Rebuilding coral reefs: Does active reef restoration lead to sustainable reefs? Curr. Opin. Environ. Sustain. 7, 28–36. doi: 10.1016/j.cosust.2013.11.018
Rue, H., Martino, S., and Chopin, N. (2009). Approximate Bayesian inference for latent Gaussian models by using integrated nested Laplace approximations. J. R. Stat. Soc. Ser. B Stat. Methodol. 71, 319–392. doi: 10.1111/j.1467-9868.2008.00700.x
Seguin, F., Le Brun, O., Hirst, R., Al-Thary, I., and Dutrieux, E. (2010). “Large coral transplantation in Bal Haf (Yemen): an opportunity to save corals during the construction of a liquefied natural gas plant using innovative techniques,” in Proceedings of the 11th International Coral Reef Symposium, Fort Lauderdale, FL, 1267–1270.
Toth, L. T., Kuffner, I. B., Stathakopoulos, A., and Shinn, E. A. (2018). A 3,000-year lag between the geological and ecological shutdown of Florida’s coral reefs. Glob. Change Biol. 24, 5471–5483. doi: 10.1111/gcb.14389
Toth, L. T., van Woesik, R., Smith, S. R., Murdoch, T. J. T., Ogden, J. C., Precht, W. F., et al. (2014). Do no-take reserves benefit Florida’s corals? 14 years of change and stasis in the Florida Keys National Marine Sanctuary. Coral Reefs 33, 565–577. doi: 10.1007/s00338-014-1158-x
Tunnicliffe, V. (1981). Breakage and propagation of the stony coral Acropora cervicornis. Proc. Natl. Acad. Sci. U.S.A. 78, 2427–2431.
van Hooidonk, R., Maynard, J., Tamelander, J., Gove, J., Ahmadia, G., Raymundo, L., et al. (2017). Coral Bleaching Futures: Downscaled Projections of Bleaching Conditions for the World’s Coral Reefs, Implications of Climate Policy and Management Responses. Available online at: https://stg-wedocs.unep.org/bitstream/handle/20.500.11822/22048/Coral_Bleaching_Futures.pdf?sequence=1&isAllowed=y (accessed January 2021).
van Oppen, M. J. H., and Gates, R. D. (2006). Conservation genetics and the resilience of reef-building corals. Mol. Ecol. 15, 3863–3883. doi: 10.1111/j.1365-294x.2006.03026.x
van Oppen, M. J. H., Oliver, J. K., Putnam, H. M., and Gates, R. D. (2015). Building coral reef resilience through assisted evolution. Proc. Natl. Acad. Sci. U.S.A. 112, 2307–2313. doi: 10.1073/pnas.1422301112
van Treeck, P., and Schuhmacher, H. (1999). Mass diving tourism – a new dimension calls for new management approaches. Mar. Pollut. Bull. 37, 499–504. doi: 10.1016/s0025-326x(99)00077-6
van Woesik, R., Banister, R. B., Bartels, E., Gilliam, D. S., Goergen, E. A., Lustic, C., et al. (2020a). Differential survival of nursery-reared Acropora cervicornis outplants along the Florida reef tract. Restor. Ecol. 29:e13302.
van Woesik, R., Roth, L. M., Brown, E. J., McCaffrey, K. R., and Roth, J. R. (2020b). Niche space of corals along the Florida reef tract. PLoS One 15:e0231104. doi: 10.1371/journal.pone.0231104
van Woesik, R., Scott, W. J. I. V., and Aronson, R. B. (2014). Lost opportunities: coral recruitment does not translate to reef recovery in the Florida Keys. Mar. Pollut. Bull. 88, 110–117. doi: 10.1016/j.marpolbul.2014.09.017
Walton, C. J., Hayes, N. K., and Gilliam, D. S. (2018). Impacts of a regional, multi-year, multi-species coral disease outbreak in southeast Florida. Front. Mar. Sci. 5:323. doi: 10.3389/fmars.2018.00323
Ware, M., Garfield, E. N., Nedimyer, K., Levy, J., Kaufman, L., Precht, W., et al. (2020). Survivorship and growth in staghorn coral (Acropora cervicornis) outplanting projects in the Florida Keys National Marine Sanctuary. PLoS One 15:e0231817. doi: 10.1371/journal.pone.0231817
Wirt, K. E., Hallock, P., Palandro, D., and Daly, K. L. (2013). Potential habitat of Acropora spp. on Florida reefs. Appl. Geogr. 39, 118–127. doi: 10.1016/j.apgeog.2012.12.009
Keywords: coral, restoration, outplants, survival, Acropora cervicornis, Florida reef tract
Citation: Banister RB and van Woesik R (2021) Ranking 67 Florida Reefs for Survival of Acropora cervicornis Outplants. Front. Mar. Sci. 8:672574. doi: 10.3389/fmars.2021.672574
Received: 26 February 2021; Accepted: 15 June 2021;
Published: 07 July 2021.
Edited by:
Frank S. Gilliam, University of West Florida, United StatesReviewed by:
Joshua Patterson, University of Florida, United StatesCopyright © 2021 Banister and van Woesik. This is an open-access article distributed under the terms of the Creative Commons Attribution License (CC BY). The use, distribution or reproduction in other forums is permitted, provided the original author(s) and the copyright owner(s) are credited and that the original publication in this journal is cited, in accordance with accepted academic practice. No use, distribution or reproduction is permitted which does not comply with these terms.
*Correspondence: Robert van Woesik, cnZ3QGZpdC5lZHU=
Disclaimer: All claims expressed in this article are solely those of the authors and do not necessarily represent those of their affiliated organizations, or those of the publisher, the editors and the reviewers. Any product that may be evaluated in this article or claim that may be made by its manufacturer is not guaranteed or endorsed by the publisher.
Research integrity at Frontiers
Learn more about the work of our research integrity team to safeguard the quality of each article we publish.