- Marine Ecology Laboratory, Department of Life Sciences, Presidency University, Kolkata, India
In estuarine sediment, meiobenthos serve as an excellent candidate to perform a range of ecosystem services. However, even though the taxonomic sufficiency of meiobenthos in detecting spatiotemporal gradients is well recognized, very little is known about their functional attributes in response to environmental descriptors. To bridge this knowledge gap, the taxonomic structure and trait-based functional diversity patterns of meiobenthic assemblage, focusing on nematode species composition, were assessed for the first time from the unexplored central sector of Sundarbans Estuarine System (SES). Sediment samples were collected seasonally (monsoon, winter, spring, and summer) selecting a total of eight stations across River Matla (the widest and longest river of SES). Distinct seasonal successional patterns had been observed in meiobenthic abundance modulated by seasonal alteration in the sedimentary environment (PERMANOVA, p < 0.05). Our study revealed a strong preponderance of meiobenthic density in spring (2978 ± 689.98 ind. 10 cm–2) and lowest during monsoon (405 ± 51.22 ind. 10 cm–2). A total of 11 meiobenthic taxa were identified with the dominance of nematodes. Altogether, 79 species of nematode belonging to 22 families had been identified across the entire study area and nematode assemblage was dominated by Sabatieria praedatrix, Sphaerolaimus balticus, Desmodora communis, Dorylaimopsis punctata, and Daptonema hirsutum. Principal component analysis depicted a distinct separation of seasons with reference to environmental variables. Distance-based redundancy analysis delineated that meiobenthic communities were mainly structured by organic matter, salinity, and dissolved oxygen concentration. Biological trait analyses of nematode assemblages were applied to gather the ecological information based on morphological and ecological characteristics. The most common morphotypes were slender body shape, clavate tail shape, striated cuticle pattern, and non-selective deposit feeders with colonizing abilities of 2–3 (c–p score in a scale from 1 to 5). Results from BIO-ENV showed that sediment organic matter and dissolved oxygen played overriding roles in shaping the functional compositions of nematodes. Thus, the characterization of meiobenthos and nematode assemblages in the context of taxonomic as well as functional attributes represents a first step toward building of baseline data which could help to evaluate the ecological status of SES and direct future research priorities concurrently.
Introduction
Meiobenthos represents a highly diverse biocenosis that is made up of microscopically small-sized aquatic invertebrates (Giere, 2009; Curini-Galletti et al., 2012). In aquatic habitats, they serve as excellent candidates to perform a range of ecosystem services. They play a pivotal role in biomineralization of detrital matter and enhance the nutrient regeneration by breaking down detritus (Coull, 1999; Nascimento et al., 2012). Furthermore, they act as key components of energy provision to higher trophic levels (macrobenthos, juvenile demersal fishes, and bottom-feeding shore birds, etc.), thereby constituting an integral part in the benthic food web (Schmid-Araya et al., 2016; Schratzberger and Ingels, 2018). Notably, they can be used as a promising auxiliary to monitor environmental changes due to their small sizes, ubiquitous distribution, higher abundance and taxonomic diversity, fast generation cycles, direct benthic development, and limited mobility (Pusceddu et al., 2007; Vanaverbeke et al., 2011; Semprucci and Balsamo, 2012; Zeppilli et al., 2015). Therefore, a long-term monitoring program using meiobenthic animals could explicitly provide better and accurate information about their surrounding environment.
Within the meiobenthic network of organisms, free-living nematodes are a numerically dominant and species-rich group, constituting more than 90% of the fauna (Lambshead and Boucher, 2003). Their density and diversity vary with the availability of sediment pore water oxygen, organic matter deposition, sediment texture, and other interacting environmental variables (Heip et al., 1985). Recently, attention has been paid to investigate marine ecosystem functioning by analyzing nematode communities. Ecosystem functioning can be summarized as biological, physical, and geochemical processes that operate within an ecosystem, performing by individual ecosystem components or in combination (Jax, 2005; Edwards et al., 2014). It is now well known that higher functional biodiversity contributes to a stable and resilient ecosystem. Thus, species identification and their functional patterns appear to be essential for the maintenance of a healthy ecosystem (Radwell and Brown, 2008). In general, the functional structure or diversity of a community is defined as a combination of species distribution and their abundances in the functional space (Mouillot et al., 2013) and biological traits are those characteristics that define species in the context of their ecological roles (Diaz and Cabido, 2001). Biological trait analysis (BTA) is used to assess the functional diversity of any assemblages based on multiple traits or characteristics (Bremner et al., 2003, 2006). The traditional taxon-based descriptors of nematode community structure analyses from species abundance data, however, may not account their autecological information. Species in functional groups that share morphological or ecological traits are thought to improve our mechanistic understanding of different habitat conditions along with environmental gradients (Chalcraft and Resetarits, 2003; Ristau et al., 2015). Species-level identification may not provide comprehensive knowledge regarding ecosystem functions as closely related species may differ in their functional roles (Maurer, 2000). Nematode assemblages represent numerous morphological characteristics which are considered to play important ecological roles: buccal morphology (a proxy to assess trophic relationships, Wieser, 1953), tail shape (crucial for locomotion, reproduction, and sediment retention, Thistle and Sherman, 1985; Thistle et al., 1995), body shape (important adaptive feature to different sedimentary environments, Soetaert et al., 2002; Vanaverbeke et al., 2003, 2004), and cuticle morphology (a protective covering of the body to avoid intoxication of organisms through diffusion, Soetaert et al., 2002). Moreover, ecological traits such as life history strategy (Bongers, 1990) can also be informative of the habitat condition. The assemblage patterns of marine nematode functional diversity and their spatiotemporal variations in response to environmental settings remain still poorly resolved. Thus, owing to such immense importance of BTA in ecosystem functioning, their studies need to be approached in a proper systematic manner that has never been attempted from any estuaries belonging to the eastern coast of India.
Estuaries are the most hydrodynamically active and pervasive transitional zones, where crucial biogeochemical processes take place (Costanza et al., 1997). They are considered as vital conduits for the transport of nutrients, organic materials, and sediments from rivers to oceans, making them an essential carbon sink. The amenities estuaries offer render them as the most precious national treasures, which may impinge on the health and vibrancy of human society and economy. Due to their irreplaceable ecological functions, such as high productivity, nutrient enrichment, and ephemeral habitats for reproduction, feeding, and nurseries for a variety of animals (Beck et al., 2001; Gili, 2002), estuaries are consequently subjected to fishing, harbor, and dredging activities. It is now widely acknowledged that these naturally stressed ecosystems are characterized by the continuum gradient of environmental factors, which might play an overriding role in shaping the heterogeneous distribution of meiobenthic communities (Adão et al., 2009; Alves et al., 2009, 2013, 2014; Sroczyńska et al., 2020).
From Indian estuaries, meiobenthic organisms have garnered extensive attention in the field of taxonomy and diversity accompanying their environmental descriptors. Along the eastern coastal belt of India, majority of the studies were carried out from the Tamil Nadu (Chinnadurai and Fernando, 2007; Ansari et al., 2014), Kerala (Chinnadurai and Fernando, 2006), and Odisha coasts (Baliarsingh et al., 2015). As yet, monitoring of the status of estuarine meiobenthic components and their key ecosystem functions from Sundarbans Estuarine System (SES) still remains discrete and limited to selected sites, which are not sufficient to exhaustively ensuring ecological goals of sustainability. Meager amount of studies are available on meiofauna inhabiting the western (Rao and Misra, 1983; Dey et al., 2012; Ghosh et al., 2018) and eastern parts (Sen et al., 2016) of Indian Sundarbans, howbeit there is a dearth of information about their composition along the central sector of SES. At the central tract of SES, River Matla divides Indian Sundarbans into Reserved Forest on the west and Sundarban Tiger Reserve on the east and large portions of it are quite inaccessible to human intervention. Therefore, our primary goal was to define the current state of scientific knowledge regarding meiobenthic fauna in a systematic manner to fill the knowledge gap and to provide useful information for future researchers and environmental policymakers. To fulfill that aim, the following questions were addressed: (i) how does meiobenthic community structure, especially nematode species composition, in concert with trait modalities change throughout the year along the same spatial axis and (ii) how do seasonally governed environmental factors influence their distribution pattern?
Materials and Methods
Study Area
Sundarbans, the largest contiguous deltaic mangrove ecosystem of the world, is situated on the delta created by the Ganges, Meghna, and Brahmaputra rivers covering the coastal belt of India and Bangladesh (Papa et al., 2010). This coastal mangrove ecosystem is interconnected by hundreds of islands and numerous creeks, inlets, and canals. For the present investigation, we selected the estuary of Matla River, the widest (26 km) and longest (125 km) (Chatterjee et al., 2013) river of SES, situated at the central part of Indian Sundarbans (Figure 1). This river originates at the confluence of Bidyadhari, Khuratya, and Rampur Khal close to the town of Canning in South 24 Parganas and flows to the Bay of Bengal. This tidally fed river has lost its original freshwater connections with the Ganges due to continuous neotectonic shifts of the Bengal Basin and heavy siltation, resulting in significant changes in the hydrology, sedimentation pattern, and salinity dynamics of the central tract of SES as compared to the western sector (Banerjee, 2013; Ghosh et al., 2015). Our study area includes a total of eight stations (designated as Matla or M1 to M8) along a salinity gradient at the north–south direction starting from Herobhanga Reserve Forest toward Bulchery Island situated near the mouth of the estuary. The detailed GPS locations of the sampling stations are shown in Table 1. The funnel-shaped estuary lies adjacent to the Sundarban Tiger Reserve; howbeit, the northern part of the estuary is notably affected by urbanization and other anthropogenic activities along its river bank. On the other hand, the downstream stretch of this estuary is pristine with luxuriant growth of dense mangrove patches. The study period extended from September 2016 to May 2017, including four different seasons: monsoon (September), winter (December), spring (February), and summer (May).
Sample Collection
The observation of all concerned parameters was carried out during the aforementioned four seasons selecting eight stations from Matla River. Triplicate sediment samples were taken for meiobenthos analyses by a van Veen grab (0.04 m2) from each study site, and from each grab sample two subsamples were collected using a hand corer (5.6-cm internal diameter). One core of the subsample was preserved immediately in 4% buffered Rose Bengal (0.5 g L–1) formalin (Danovaro et al., 2004) and subsequently returned to the laboratory for further analyses. Another core was stored for environmental parameter analyses.
Environmental Parameter Analyses
Sediment temperature was measured by a handheld mercury thermometer in situ. The Niskin water sampler (5 L) was used to collect bottom water for analyses of pH, dissolved oxygen (DO), and salinity according to standard protocols of Strickland and Parsons (1972). The microphytobenthos or chlorophyll a (Chl a) concentration of sediment was estimated by 90% acetone extraction method (Strickland and Parsons, 1972). Organic matter and sediment granulometry were analyzed using the chromic acid oxidation method followed by titration with 0.2 N ammonium ferrous sulfate (El Wakeel and Riley, 1957) and following the sieve and pipette method (Folk, 1968) correspondingly. Suspended particulate matter (SPM) was determined by subsequent literature of Harrison et al. (1997).
Meiofaunal Analyses
Sediment subsamples were washed through a set of sieves (500 and 63 μm) to segregate macro- and meiobenthic organisms, respectively (Pfannkuche and Thiel, 1988). The sediment retained in the 63-μm sieve was then decanted to extract meiofauna following the method of Armenteros et al. (2008). Collected organisms were sorted and enumerated under a stereo zoom microscope (Nikon SMZ745T). The abundance was expressed in individuals 10 cm–2 ± SD.
Nematofaunal Analyses
A total of 2283 nematode specimens were picked and mounted with the help of anhydrous glycerin (Seinhorst, 1959) onto glass slides for identification up to species level under a compound microscope fitted with a camera (Nikon ECLIPSE Ci) based on standard pictorial keys (Platt and Warwick, 1983, 1988; Warwick et al., 1998) and online identification key: NeMys (Bezerra et al., 2021).
For BTA, five traits were selected: four morphological (buccal cavity structure, adult body shape, tail shape, and cuticle morphology) and one ecological (life-history strategy) (Table 2). On the basis of buccal morphology, feeding guilds of each nematode species were assigned according to Wieser’s (1953) classification: 1A—selective deposit feeders, 1B—non-selective deposit feeders, 2A—epistrate feeders, and 2B—carnivorous/omnivorous. Three groups of adult body shapes of nematodes were obtained from the literature of Soetaert et al. (2002)—stout, slender, and long/thin. Individual nematodes were categorized into four tail shapes following the classification of Thistle et al. (1995)—short/round, elongated/filiform, conical, and clavate. Based on cuticle morphology, nematode species were categorized into four groups: smooth, striated, covered with dots, and ornamented (Soetaert et al., 2002). According to Bongers et al. (1991, 1995), each nematode specimen was classified one of the five c–p (colonizer–persister) categories: 1—colonizers (opportunistic species; r-strategists); 2—tolerant to stress; 3—intermediate; 4—highly sensitive to stress; and 5—persisters (k-strategists).
Statistical Analyses
Principal component analysis (PCA) was performed to identify essential environmental variables that characterize the study area. A two-way permutational multivariate analysis of variance (PERMANOVA) was also applied to examine the role of seasonal effects on meiobenthos across Matla River. This analysis was performed using two fixed factors “seasons” and “stations” with four seasons (monsoon, winter, spring, and summer) and eight stations [M1 to M8], respectively. The relationship between meiobenthic communities and environmental parameters was investigated using distance-based linear modeling (DistLM) and visualized using distance-based redundancy analysis (dbRDA) to derive an ordination plot (Anderson et al., 2008). A stepwise selection procedure was used in DistLM with the AICc model selection criteria to permit the fitting of best explanatory environmental factors and to model the meiobenthic community data (Bray Curtis resemblance matrix) against the normalized environmental variables. From Bray–Curtis similarity matrices, the cluster plot and NMDS ordination plot were derived following nematode species abundance in each season and biological traits of nematode species, respectively, after Log (X + 1) transformation. A SIMPROF (similarity profile) test was done to detect the significantly different stations in each season using the default of 1000 permutations for the mean similarity profile and 999 permutations for the simulated profile with a significance level of 0.05. Furthermore, similarity of percentage (SIMPER) analysis was conducted to identify the% contribution of major nematode species responsible for the total dissimilarity between groups. The following biotic indices were also analyzed based on nematode species composition: Shannon–Wiener diversity (H′ loge), Margalef’s species richness (d), Pielou’s evenness (J′) and Simpson index (1 –λ′). The relationships of nematode functional traits with environmental parameters were explored by means of BIOta ENVironmental matching (BIO-ENV) analysis (Clarke and Ainsworth, 1993). All statistical and biological trait analyses were performed using PRIMER (version 6) software (Clarke and Gorley, 2006; Clarke et al., 2008) equipped with add-on package PERMANOVA (Anderson et al., 2008) and SigmaPlot 11.0 correspondingly. Canonical correspondence analysis (CCA) (ter Braak, 1986) was performed in order to visualize the trend of nematode species composition with different environmental variables using the MVSP program (version 3.1) (Kovach, 1998).
Results
Environmental Factors
A suit of environmental factors such as temperature, pH, DO, salinity, Chl a, SPM, organic matter, and sediment texture were measured during each sampling occasion (Table 3). Sediment temperature showed seasonal variability indicating highest in summer [varied between 31 (M3 and M4) and 32.5°C (M1)] and lowest in winter [varied between 21.5 (M5 and M6) and 23.5°C (M8)]. pH was slightly alkaline and relatively constant all over the study period (7.38–8.16). A moderate DO concentration was observed during the course of the sample collection, being highest in monsoon (8.24 mg L–1) and lowest in spring (4.21 mg L–1). A typical estuarine gradient of salinity of bottom water from low to high toward the mouth of the estuary was observed (Table 3). The highest salinity values were recorded in summer [minimum 21.06 (M6) and maximum 35.04 PSU (M4)] and lowest in monsoon [minimum 6.41 (M5) and maximum 16.68 PSU (M3)]. The maximum SPM concentration was detected in summer (433.8 mg L–1), minimum in spring (17 mg L–1). Microphytobenthos biomass (Chl a) was evidenced with seasonal disparity being highest in spring [minimum 2.24 (M2) and maximum 7.61 μg g–1 (M5)] and lowest in winter [minimum 0.18 (M1) and maximum 5.63 μg g–1 (M4)]. Organic matter concentrations were ranged from 0.08 (M7) to 5.28% (M1) in monsoon, 1.29 (M1) to 5.61% (M8) in winter, 1.30 (M4) to 1.91% (M8) in spring, and 1.28 (M4) to 2.06% (M8) in summer. A classic gradient of estuarine sediment was observed as silt and clay fractions increased from upstream toward downstream with some exception. Overall, the sediment samples were characterized by a predominance of clayey silt with a few variations (Table 3).
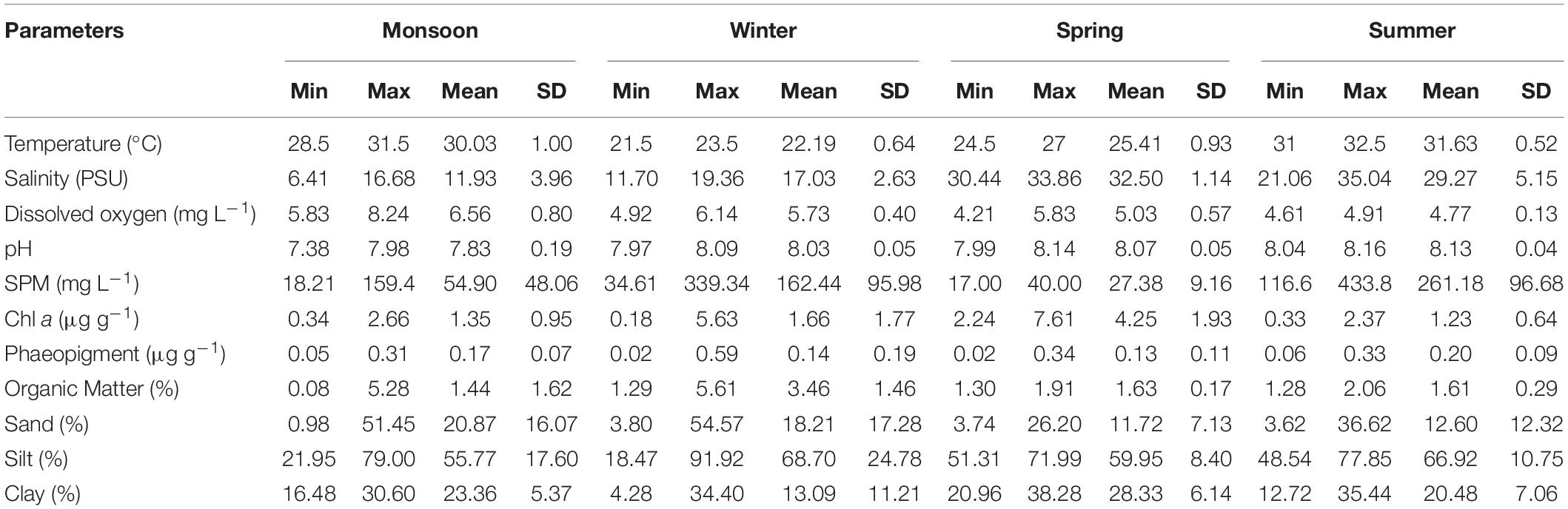
Table 3. Minimum (Min), maximum (Max), mean and standard deviation (SD) of biotic and abiotic parameters of eight stations of Matla River sampled during four seasons.
Meiobenthic Structure
Total meiofaunal abundance differed significantly among seasons (PERMANOVA, p < 0.05) with the highest number (±SD) in spring (2978 ± 689.98 ind. 10 cm–2) followed by 1493 ± 301.39 ind. 10 cm–2 (winter) and 832 ± 203.64 ind. 10 cm–2 (summer) and lowest in monsoon (405 ± 51.22 ind. 10 cm–2) (Figure 2). The taxonomic composition of meiofauna was constituted by 11 taxa throughout the eight stations of Matla during sampling periods with the dominance of free-living nematodes representing 74% of total assemblages. The densities of nematodes were higher in spring and winter than those of summer and monsoon. Harpacticoid copepods contributed 15% to the total fauna, and their densities remained constant in all four seasons. The other abundant groups were kinorhyncha (4%), polychaete juveniles (2%), and benthic foraminifera (1%), while the occurrence of halacarid mites, ostracods, turbellaria, oligochaetes, bivalve settlers, and gastropod juveniles were present in low densities. Turbellaria and gastropod juveniles were found only in monsoon and winter. In order to visualize the impact of crucial abiotic variables, PCA was performed with 11 environmental factors, which depicted a distinct separation of seasons with reference to environmental variables (Figure 3). Stations from monsoon and winter were associated with higher DO, organic matter, and silt and clay fractions of sediment, while higher values of salinity and temperature characterized spring and summer stations. In turn, spatial discrepancy was not evident, although the seasonal gradient appeared distinct. DistLM analysis allowed identifying those abiotic variables that were best correlated with the seasonal distribution patterns of the meiobenthic community. DistLM indicated that the 11 abiotic variables related to the variation in meiofaunal community structure, even though not all variables were significant. Variables such as DO, temperature, and salinity showed the highest correlations (p < 0.05) with meiofaunal assemblages. In the dbRDA ordination diagram, the best explanatory model included DO and temperature in monsoon, whereas salinity, microphytobenthos, and organic matter characterized summer and winter, respectively (Figure 4).
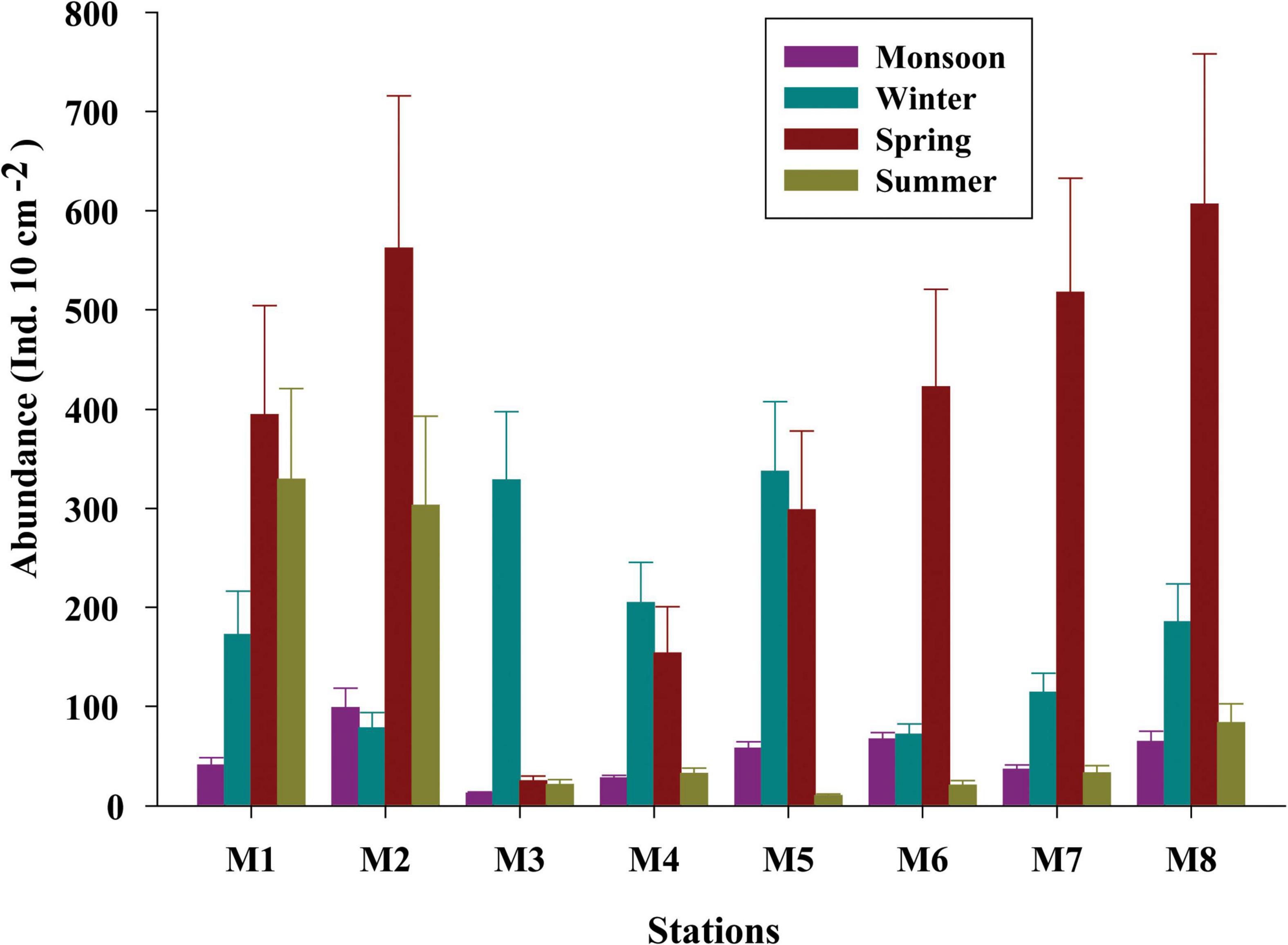
Figure 2. Seasonal variations of the meiobenthic population across eight stations (M1 to M8) of Matla (M) River.
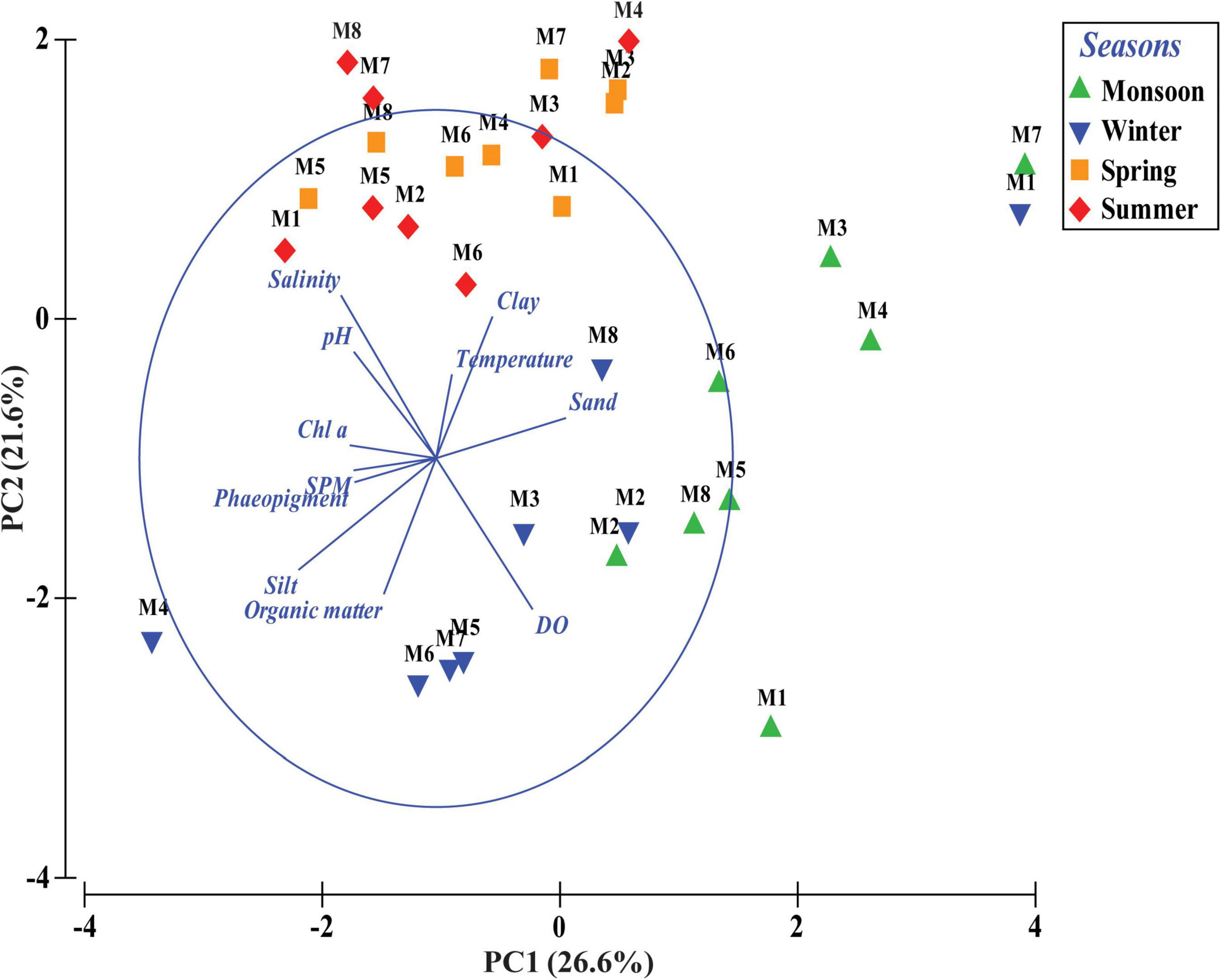
Figure 3. Principal component analysis (PCA) based on abiotic variables of four different seasons across eight stations (M1 to M8) from Matla (M) River.
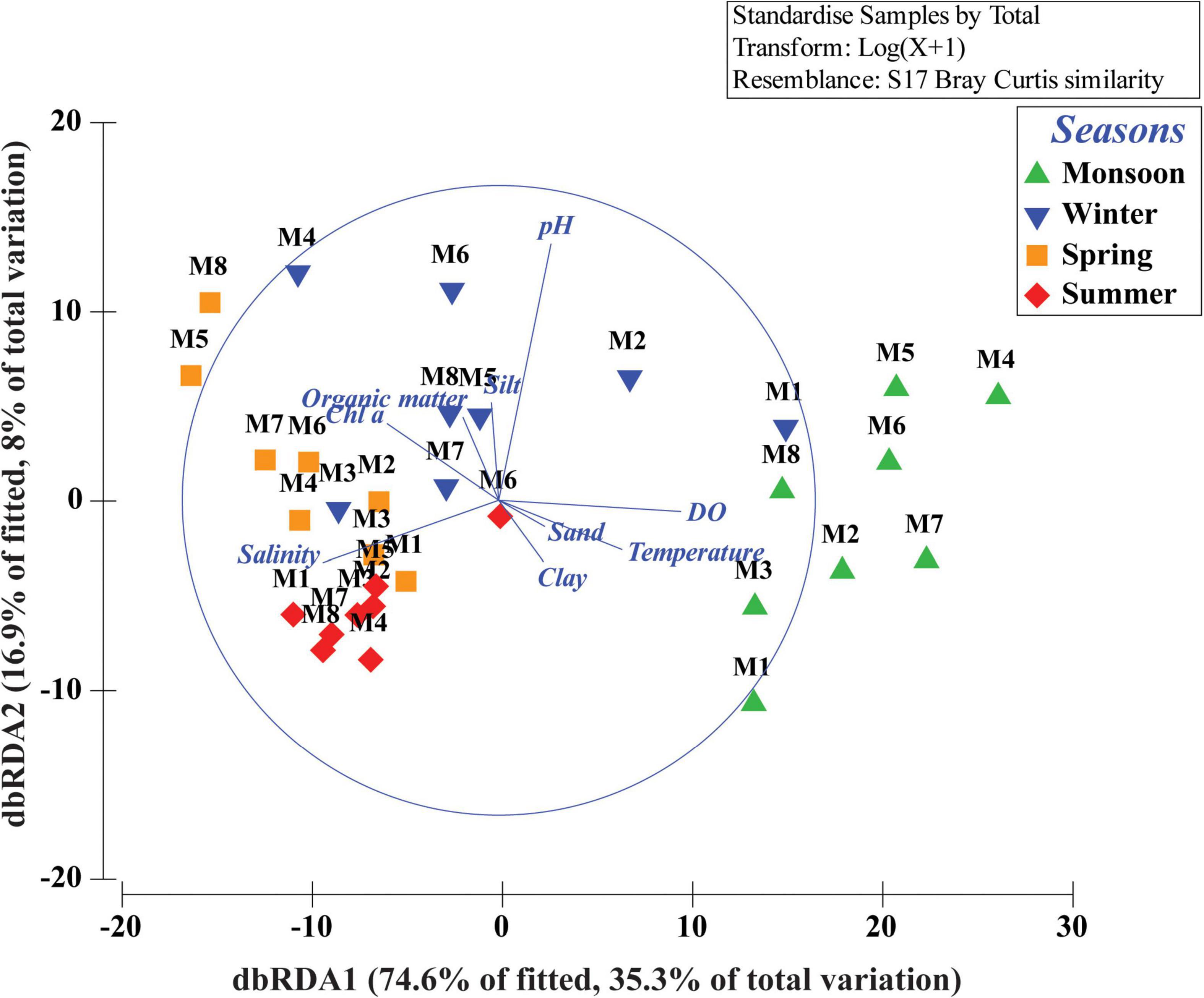
Figure 4. Distance-based redundancy analysis (dbRDA) ordination plot of fitted best environmental variables illustrating meiobenthic community patterns.
Nematode Assemblages
Altogether, 79 species of free-living nematodes belonging to 22 families were identified for the first time from Matla River (Table 4). There was a strong dominance with 19 species contributing individually to greater than 1% to total density. The most spatially widespread species were Sabatieria praedatrix (8%), Sphaerolaimus balticus (6%), Desmodora communis (6%), Dorylaimopsis punctata (5%), Daptonema hirsutum and D. procerum (5% each), Monoposthia costata (5%), and Terschellingia longicaudata (4%). Several species appeared to be characteristic of particular seasons: Camacolaimus barbatus, Eleutherolaimus minutus, Comesa sp., Nemanema sp., and Parasphaerolaimus sp. (winter); Sabatieria alata, Viscosia cobbi, and Pontonema sp. (spring); and Eurystomina sp., Longicyatholaimus sp., and Trissonchulus sp. (summer). In addition, certain species showed a strong patchy distribution, i.e., appeared only in one station and one season, for instance Sabatieria alata (station M6 in spring) and Trissonchulus sp. (station M4 in summer). Biotic indices based on nematode communities are shown in Table 5. A typical seasonal pattern was observed in Shannon–Wiener index (H′ loge), with the highest value (3.24 bit⋅ind–1) in winter (station M6) and lowest (1.01 bit⋅ind–1) in monsoon. Margalef’s species richness (d) was high during winter (7.17) and low during monsoon (1.12), while Pielou’s evenness index (J′) was extended from 0.83 (monsoon) to 0.97 (summer).
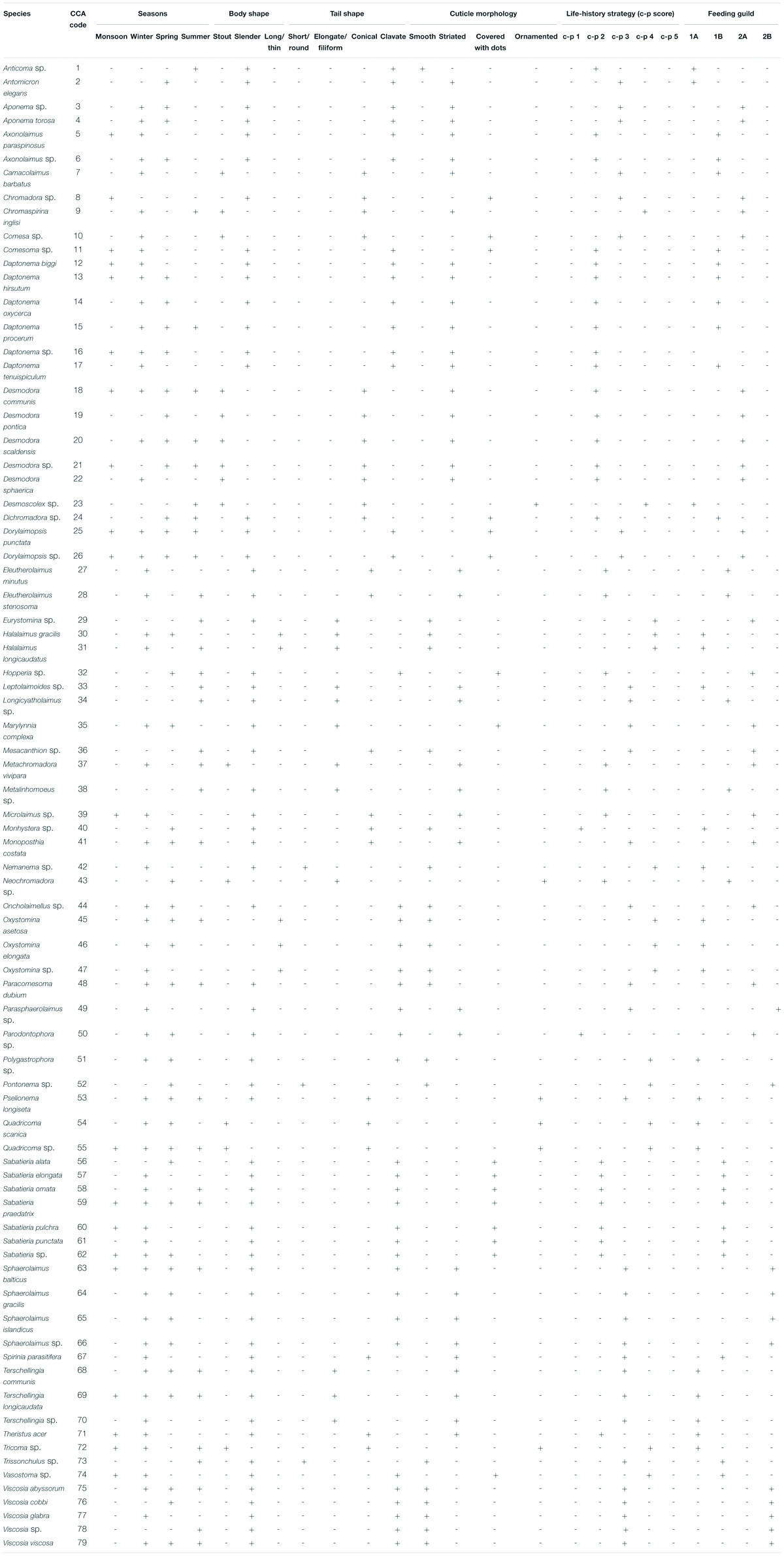
Table 4. List of free-living marine nematode species identified from Matla River along with their CCA codes and biological traits. “+” and “-” indicate present and absent, respectively.
Cluster analyses of the Bray–Curtis similarity matrix based on free-living nematode species delineated two groups and two separate stations (SIMPROF test p < 0.05) during monsoon (Figure 5A). Stations 3 and 7 were segregated due to the presence of some exclusive species: Tricoma sp. in station 3 and Quadricoma sp. at station 7. According to SIMPER analysis, S. balticus and Sabatieria sp. contributed 13.93% and 31.88% in group 1 (56.34% average similarity) and group 2 (60.27% average similarity) formation, respectively (Table 6). In winter, two major groups have been identified: D. procerum (12.84%) and S. praedatrix (10.19%) as major contributors behind the formation of group 1 (52.50% average similarity) and group 2 (56.37% average similarity), correspondingly (Figure 5B). Moreover, stations 1, 4, and 8 were separated from these two groups due to unique species compositions like Aponema torosa in M1, Pselionema longiseta in M4, and Chromaspirina inglisi in M8. Only one group was observed during the spring and summer seasons (Figures 5C,D). Upstream stations, for example, M1, M2, and M3 in spring and M1, M3, and M4 in summer, completely diverged from their downstream counterparts. D. hirsutum in spring and S. praedatrix in summer contributed 9.84% and 10.65%, respectively, in the construction of the groups (SIMPER analysis, Table 6).
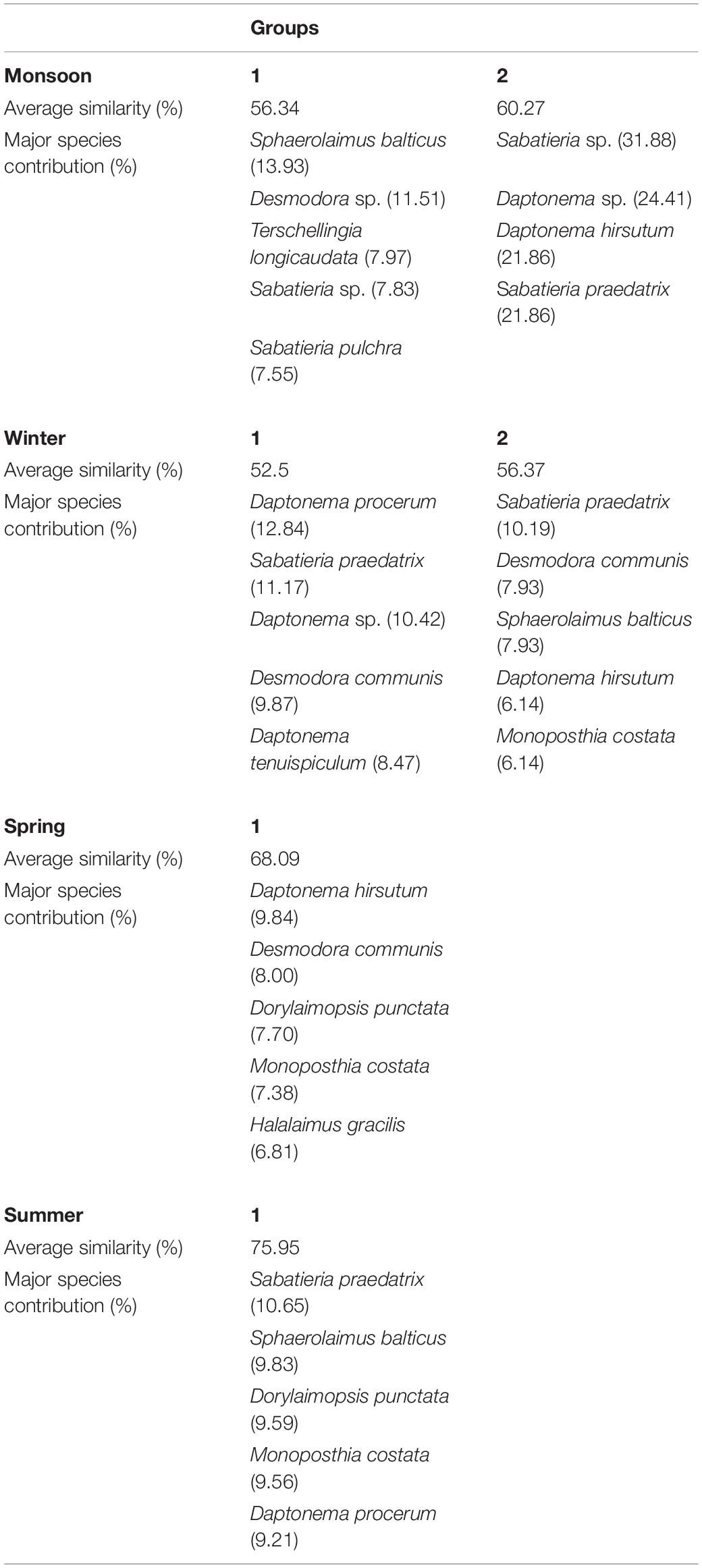
Table 6. SIMPER analysis of free-living nematode assemblages determined by cluster plot considering each season: average similarity (%) and major species contribution (%).
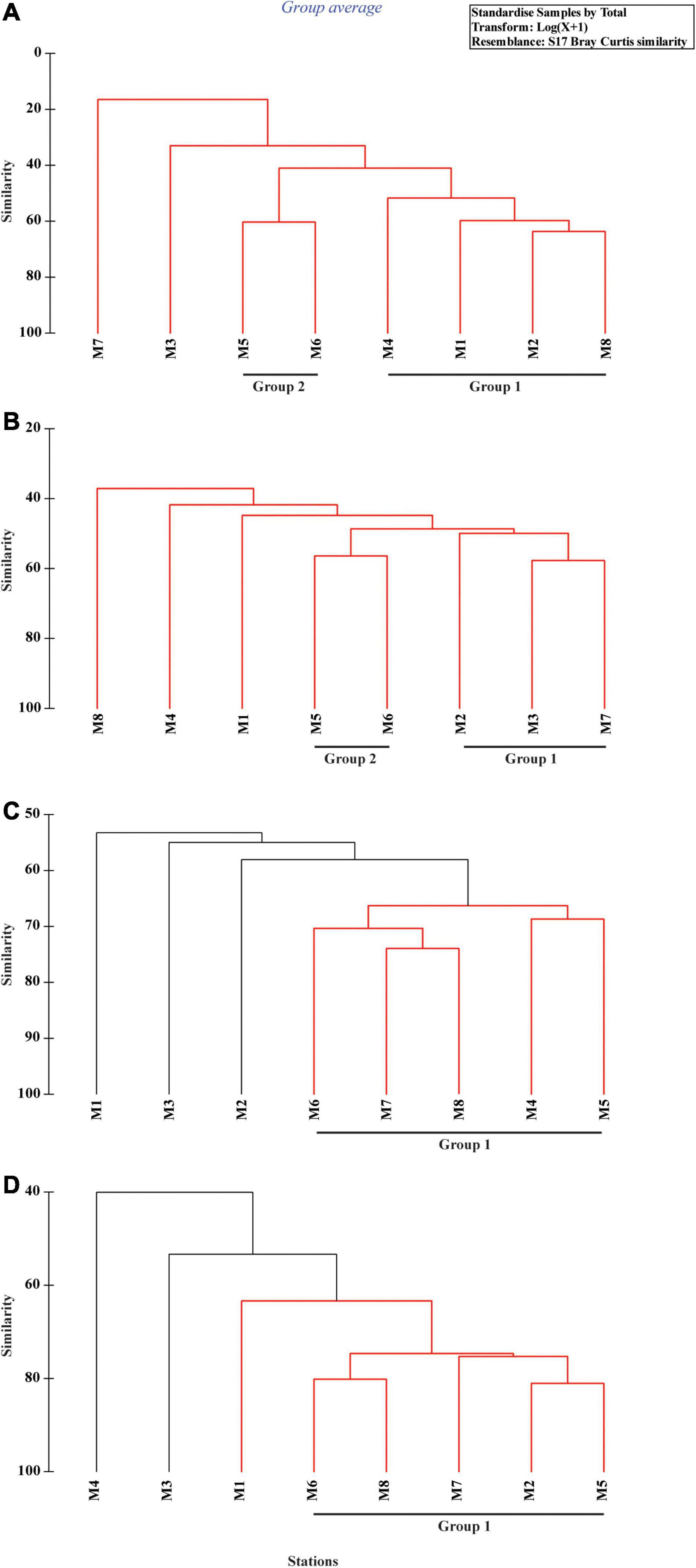
Figure 5. Cluster plot based on Bray–Curtis similarity coefficient of free-living nematode species during four distinct seasons: (A) monsoon, (B) winter, (C) spring, and (D) summer.
Ordination resulting from the CCA biplot for 79 nematode species showed five axes representing 75.42% cumulative constrain percentage. Sediment texture, organic matter, and Chl a were the most important controlling environmental factors in shaping the nematode species composition during four seasons (Figure 6A). In the species biplot, Daptonema biggi, D. oxycerca, Desmodora sphaerica, Sabatieria praedatrix, and Terschellingia sp. have shown a positive correlation with organic matter. Dorylaimopsis punctata, Sphaerolaimus islandicus, and Chromaspirina inglisi were connected with the silty substratum of sediment, whereas Monoposthia costata, Daptonema procerum, and Sabatieria alata associated with clayey sediment texture. Viscosia viscosa, Marylynnia complexa, Oxystomina elongata, and Sphaerolaimus gracilis profited by microphytobenthos (Chl a). Being influenced by the temperature vector, most of the stations in summer occupied the upper left quadrant, except station 4. Maximum stations from spring were favored by Chl a and clay % vectors. During winter, all the stations were positioned toward the organic matter percentage vector except station 8 which showed affinity toward silt percentage. On the other hand, all the stations from the monsoon were not influenced by any other any other environmental factors (Figure 6B).
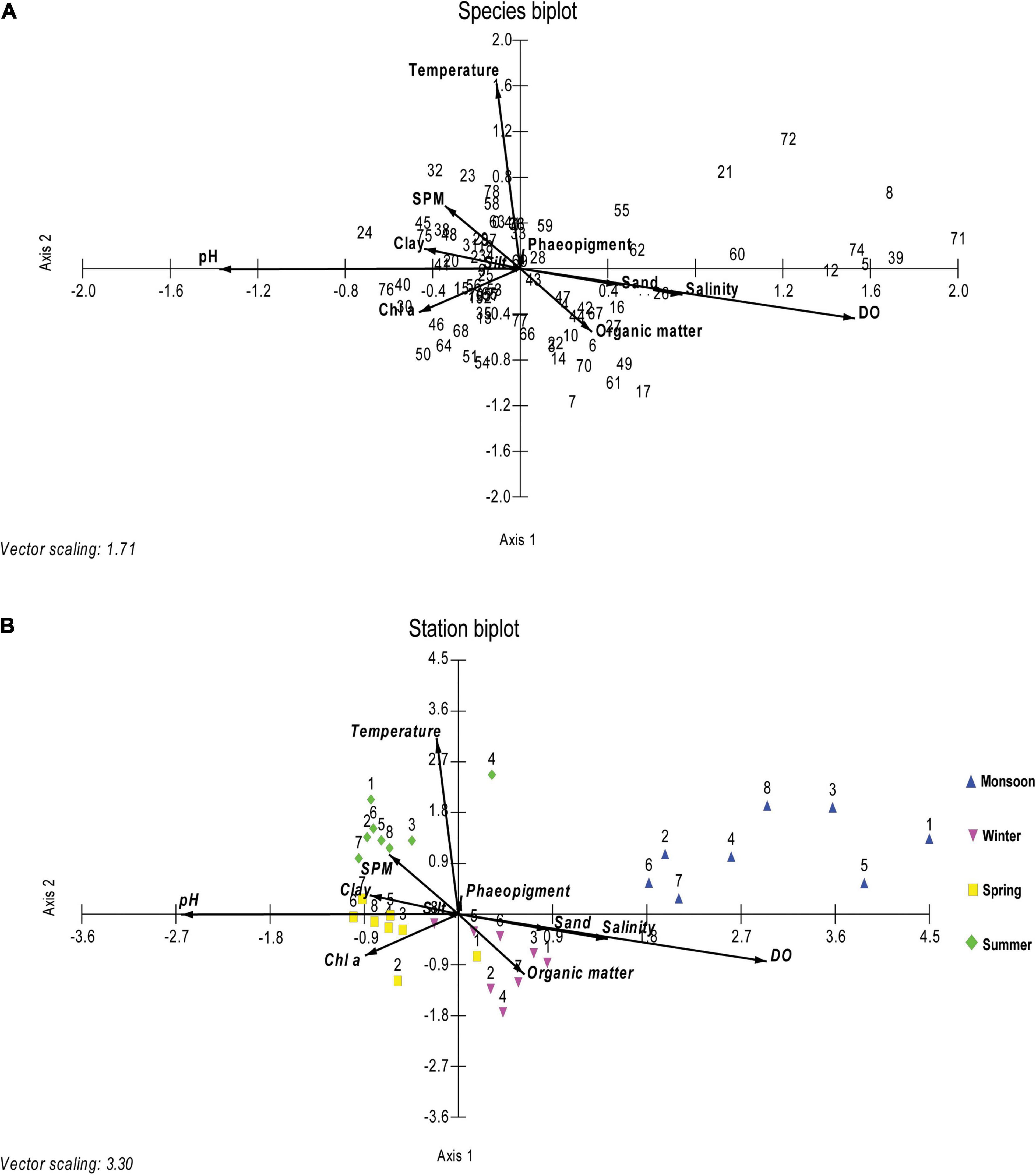
Figure 6. Ordination diagrams for species (A) and stations (B) based on canonical correspondence analysis (CCA) of the nematode community. The environmental factors (temperature, salinity, pH, DO, SPM, sand %, silt %, clay %, chlorophyll a or Chl a, pheopigment, and organic matter) are indicated by arrows. Species codes are given in Table 4.
With regard to the functional diversity, different traits were strongly linked to seasonally fluctuating environmental gradients (Figure 7). A strong predominance of slender body shape was observed among nematode communities across the study periods, whereas proportions of stout and long/thin were comparatively low. Surprisingly, in monsoon, there was absence of long/thin-bodied nematodes (Figure 7A). From the four tail shapes analyzed, clavate tails were the most prevalent at all the seasons and stations and conical and elongate ranked in second and third position, respectively. Stout tail-shaped nematodes were found occasionally. Remarkably, stout accompanied by elongated tails were completely absent in monsoon (Figure 7B). Overall, nematode assemblages were dominated by non-selective deposit feeders (1B), followed by epistrate feeders (2A), selective deposit feeders (1A), and carnivorous/omnivorous (2B) (Figure 7C). Most nematodes attained a colonizer–persister score of 2 followed by 3; at the same time, a score of 1 was rare (Figure 7D). Another trait for cuticle morphology showed differences in the contribution to nematode assemblage structure, which were mainly characterized by striated cuticle. On the other hand, cuticles covered with dots, smooth, and ornamented ranked second, third, and fourth, respectively (Figure 7E). The seasonal separation of sampling stations was less pronounced in the ordinations derived from functional attributes of nematode communities; albeit the spring and summer seasons were clustered into groups, winter and monsoon stations were randomly distributed (Figure 8). Results of BIO-ENV analyses revealed several notable relationships of biological traits with environmental variables. For example, nematode body shapes had the best correlation with DO and organic matter. Tail shapes and cuticle patterns were well correlated with temperature and organic matter, whereas c–p scores and feeding types were significantly associated with organic matter (Table 7).
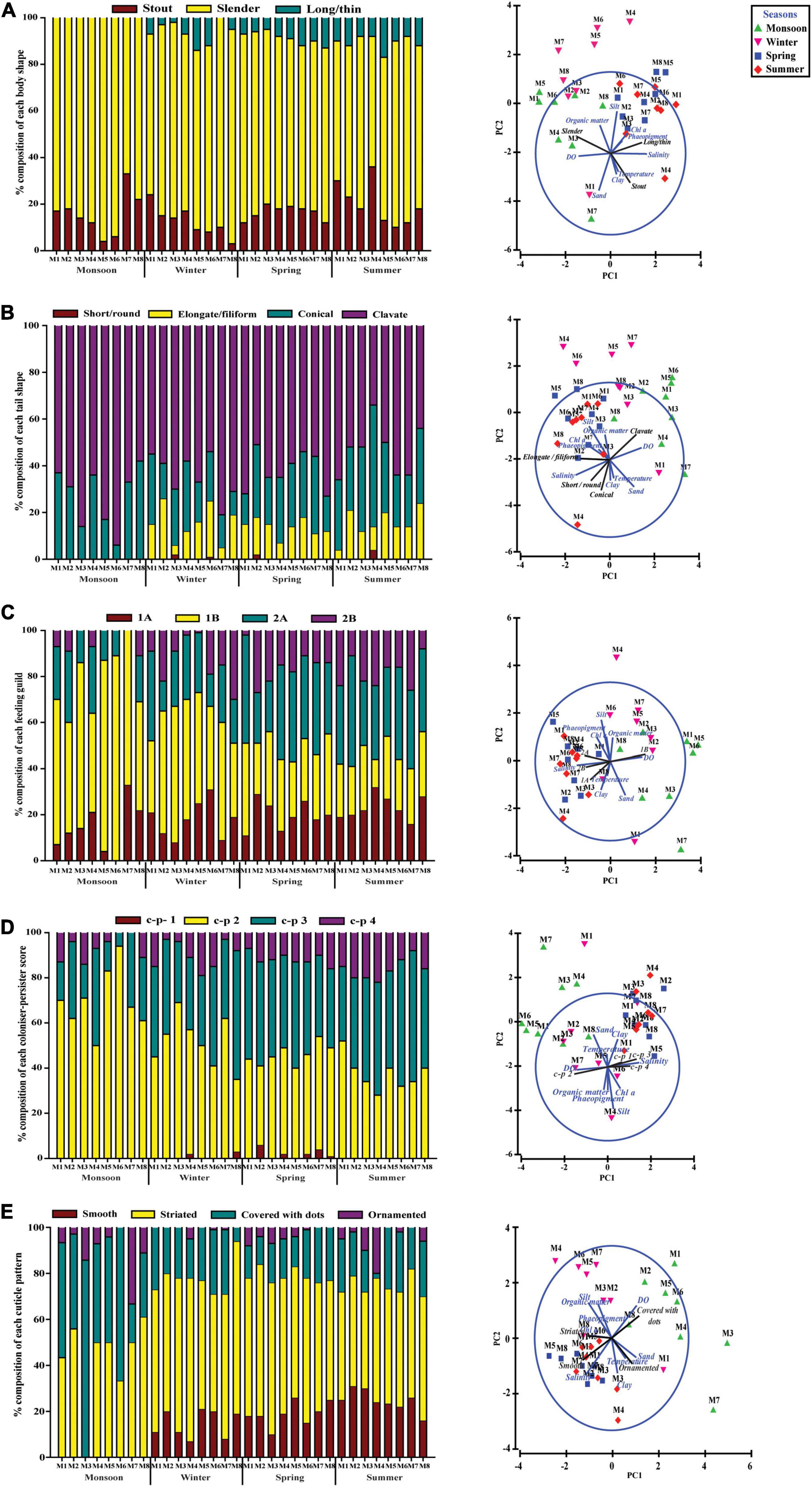
Figure 7. Percentage of trait categories for nematode assemblages and their relationship with environmental factors across eight stations for four seasons. (A) Body shape, (B) tail shape, (C) feeding guild, (D) c–p score, and (E) cuticle morphology.
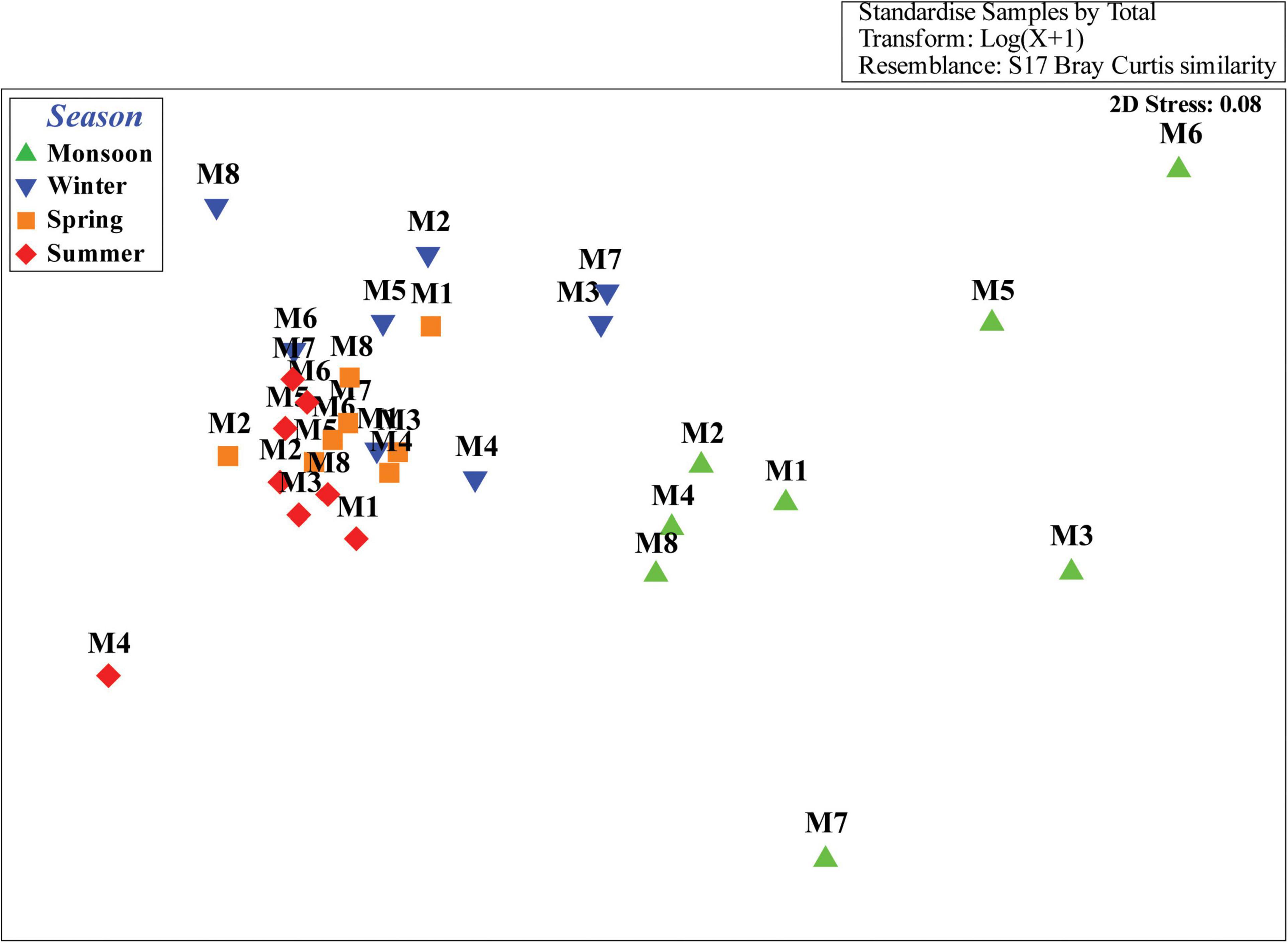
Figure 8. NMDS ordination plot based on nematode species’ functional traits according to the Bray–Curtis similarity matrix.
Discussion
In a transitional ecosystem, unraveling species assembly and the processes that govern their diversity is a major challenge. The estuarine biota is often challenged by different physical, chemical, and biological gradients in this environment. In our study, the environmental descriptors followed a clear pattern of seasonal succession; apart from that, estuarine gradients were also noticed in salinity, microphytobenthos, and sediment texture (Table 3). In general, estuaries provide a natural salinity gradient, which is often strongly linked to other physicochemical characteristics and productivity patterns that can change appreciably throughout the year (Newell, 1982). The present observation showed a clear seasonal pattern in bottom water salinity with maximum during the dry summer period and minimum during the monsoonal wet period. However, spatially our study areas attained varying degrees of salinity even in monsoon (6.41–16.68 PSU), which seemed to be a little higher compared to the western sector of SES (Banerjee, 2013). This is because Matla River is mainly driven by tidal actions as the river basin has lost the freshwater flow from Himalayan River systems due to heavy siltation and clogging in recent times (Ghosh et al., 2015; Dhame et al., 2016). Sediment composition together with organic matter and microphytobenthic contents are considered as governing factors to characterize meiobenthic abundance and diversity patterns (Coull, 1985; Steyaert et al., 2003). From upstream areas, silt and clay fractions tend to increase toward the mouth of the estuary and fine sediments with a higher surface area can retain the highest organic matter as well (Parsons et al., 1990). According to our study, sediment textural properties revealed an overall dominance of muddy (clayey silt) substrates across the sampling locations with few exceptions. The dominance of mud suggested low fluvial discharge with an intense estuarine mixing, which could have resulted in settling of finer suspended particles and flocculation (Antizar-Ladislao et al., 2015). The average values of organic matter percentages of four different seasons in our study (Table 3) were in agreement with other previously reported studies from the western sector of Indian Sundarbans (Dey et al., 2012; Antizar-Ladislao et al., 2015). A typical seasonal growth pattern of microphytobenthos, as estimated by elevated Chl a and pheopigment concentrations, was observed in all stations. Their biomass increased steadily from monsoon through winter up to spring and thereafter dropped sharply and ultimately reached at a minimum value in summer.
The interplay of these crucial factors (salinity dynamics, sediment composition, and organic matter) determining the discrepancy in the distribution of meiobenthic communities is well recognized at several estuarine benthic habitats around the globe (Heip et al., 1985; Soetaert et al., 1995; Moens and Vincx, 2000; Derycke et al., 2007; Ferrero et al., 2008; Adão et al., 2009; Alves et al., 2009, 2013). Along estuaries, their distribution follows a strong heterogeneous and horizontal patchiness as a result of seasonal variation in abiotic conditions (Phillips and Fleeger, 1985). Our investigations revealed a strong preponderance of meiobenthic density in spring, followed by winter, which is likely due to ample amount of food resource availability in the estuarine complex. In tropical estuaries, due to high nutrient load and organic matter input, monsoon is regarded as by high seasonal primary production (Madhu et al., 2007), which slowly settles down to the sea floor, in turn supporting a rich benthic productivity. At the same time, the photosynthetic activity of benthic microalgae can modulate oxygen balance of the overlying sediment–water interface and improve aerobic bacterial degradation of sediment organic matter (Barranguet, 1997; Underwood and Kromkamp, 1999). Additionally, microphytobenthos represent an essential food source for deposit feeding (Montagna et al., 1995; Manini et al., 2000) as well as for suspension feeding meiofauna (Miller et al., 1996). A similar observation was found in a recent study by Ghosh et al. (2018), where intra-monsoonal variability ascribing meiofaunal density was clearly detected. However, this winter–spring peak in food availability was also more pronounced in our study, which subsequently increased faunal abundance in those periods of the year. Moreover, monsoon is generally considered as a breeding period for most of the tropical benthic entities and drop of salinity in monsoon can trigger their gonadal release (Kinne, 1977; Broom, 1982). As a consequence, higher numbers of polychaete juveniles and bivalve settlers were found in spring and winter, respectively. Additionally, as documented by Alongi (1990) and Ghosh et al. (2018), both juvenile and adult populations are more susceptible to monsoonal wash-off owing to lack of any pelagic larval stages in their life cycles. Indeed, the monsoonal density of meiofauna, in the present research, is also a reflection to these scientific evidences. Furthermore, higher temperature, salinity, and low DO concentration might be major limiting factors for the lowest meiofaunal density in summer. Temperature may play a triggering role in regulating reproductive activities of meiobenthos, especially nematodes. In general, higher ambient sedimentary temperature results in a shorter development period, leading to an adult-dominated population (Moens and Vincx, 2000). Our results corroborate these observations, when no bivalve and gastropod juveniles were observed in meiobenthic samples during summer. Therefore, the environmental factors were characteristically variable in each season and were mostly reflected in meiobenthic composition from eight sampling stations.
In marine habitat, nematodes and copepods are generally two of the most abundant groups (Nozais et al., 2005; Moreno et al., 2011). The integration of the present dataset revealed the quantitative description of free-living nematode diversity in spatial and temporal (seasonal) scales. Nematode communities were predominantly composed of Sabatieria, Sphaerolaimus, Dorylaimopsis, Daptonema, Desmodora, Monoposthia, and Terschellingia, which have been reported the most common genera inhabiting estuarine mudflats worldwide (Soetaert et al., 1995; Wetzel et al., 2002; Rzeznik-Orignac et al., 2003; Schratzberger et al., 2006; Steyaert et al., 2007; Adão et al., 2009; Ghosh and Mandal, 2018, 2019). It is noteworthy that some habitat types such as naturally stressed estuarine muddy bottoms normally host certain tolerant species (Elliott and Quintino, 2007). Several species of Sabatieria, Daptonema, and Terschellingia are considered as “opportunistic” or tolerant to “hypoxia” and simultaneously used as “bioindicators” (Moreno et al., 2008; Alves et al., 2014). They are presumed to successfully thrive in fluctuating oxygen concentrations by decreasing their respiration rate and mobility, which sequentially reduce oxygen demand (Warwick and Price, 1979). The high abundance of these genera might be also associated with seasonal differences in sedimentary phytoplankton and organic matter deposition (Hourston et al., 2009). The correlation between species distribution patterns and sedimentary properties might thus point toward a species-specific response to the nutritional quality of the sediment. Seasonal turnover of fresh phytodetritus input was closely coupled with the abundance of many epistrate-feeding nematode species, e.g., Desmodora communis, D. scaldensis, Dorylaimposis punctata, Monoposthia costata, Paracomesoma dubium, and Parodontophora sp. A considerable number of predator nematodes (Sphaerolaimus, Viscosia) were also present throughout the annual cycle as they could be able to exploit a wide range of food resources (Moens and Vincx, 1997). Based on CCA station biplot analysis, four seasons were clearly separated from each other with organic matter, sediment chlorophyll, and textural properties contributing most importantly in structuring the nematode community (Figure 6). On the whole, the nematode community structure displayed a gradual seasonal transition in abundance, being mostly influenced by the sedimentary properties of the environment as well as the overall productivity of the system. Harpacticoid copepod showed a similar trend as nematodes: the winter–spring peak of copepod nauplii also confirmed food availability, particularly deposition of organic matter after late monsoon.
The functional diversity of nematodes based on five biological traits or characteristics [feeding type, body and tail shape, cuticle morphology and life strategy, or colonizer–persister (c–p) score] was evaluated to understand multiple aspects of ecosystem functioning (Figure 7). A significant number of slender body and clavate tail shape nematodes in each season were observed as an adaptation to higher silt and clay fraction of the sediment (Schratzberger et al., 2007). Body shape is associated with available food and sediment biogeochemical condition (Soetaert et al., 2002; Vanaverbeke et al., 2004; Losi et al., 2013). It is well recognized that in changing oxygen concentrations and silty clay sediment, body elongation is an adaptive feature resulting in higher epidermal uptake of oxygen as well as organic matter (Jensen, 1987; Singh and Ingole, 2016). In our case, the PCA biplot revealed a correlation of slender body shape with DO and organic matter of sediment (Figure 7A). In contrast, long/thin trait was found during all seasons, except monsoon, which could be hypothesized that a drop in salinity during monsoon prevented the long/thin population to flourish.
Nematode tails play a significant role in locomotion, feeding, and reproduction which are characteristics of a specific environment (Thistle and Sherman, 1985). A strong dominance of clavate tails followed by conical was observed across the sampling stations at each season. These two tail shapes have been suggested by several authors to be typical inhabitants of muddy sediment (Schratzberger et al., 2007; Armenteros et al., 2009). In agreement with their observations, the present study areas are also characterized by silt-rich sediment. The elongated or filiform tail-shaped nematodes, found in low number, are known as partly sessile in nature (Riemann, 1974; Schratzberger et al., 2007). The authors suggested that this type of tail morphology has been a special adaptation enabling them to retract from narrow interstitial sediment systems and forage for food. Most of the nematodes encountered throughout the study were non-selective deposit feeders (1B), which generally prevailed in detritus-rich muddy sediment due to plasticity of their diet (bacteria and organic detritus) (Tietjen, 1969; Heip et al., 1985). Moreover, dissolved oxygen concentration also seemed to be a crucial driver for this functional feeding group (1B) as mirrored by PCA in our study. Thus, dominance of non-selective deposit feeders could be a potential indicator of low oxygen levels related to different types of disturbances. Similar findings were documented by Alves et al. (2014) and Sroczyńska et al. (2020) for nematode communities in the Mondego and Sado estuaries of Portugal, correspondingly. Beside this, a marked increase in the population of epistrate feeders Desmodora communis, D. scaldensis, Dorylaimopsis punctata, Monoposthia costata, and Paracomesoma dubium after monsoon further reinforces the premise of a microalgal peak in winter and spring (Figure 7C). Microalgae, on the one hand, may alter the sedimentary environment by producing exopolymers that modulate sediment cohesiveness, at the same time affecting sediment the geochemistry and distribution pattern of oxygen to which nematodes show differential responses with adaptation in traits (Ritter, 2012; Alves et al., 2014). Some authors have reported a straightforward pattern of seasonal fluctuation of nematode species with selective deposit feeders and epistrate feeders significantly increasing after the spring phytoplankton bloom (Vanaverbeke et al., 2004; Lampadariou and Eleftheriou, 2018). In a similar way, the feeding type analysis in the present study showed that the high concentrations of Chl a during the winter–spring period were followed mainly by an increase in the abundance of non-selective deposit and epistrate feeders as they might be effectively benefited from an increased amount of fresh organic matter deposition. Life strategy characterization revealed a dominance of colonizers or opportunistics (c–p 2 followed by c–p 3), suggesting a stressful environmental condition (Bongers et al., 1991). The increase in c–p value along with an increase in grain size might be favored by high abundance of Quadricoma and Tricoma (c–p 4), which, as noted in our study, are correlated with higher sand content (Semprucci et al., 2018). Likewise, a differential response of individual nematode species has been revealed previously during one annual cycle where food availability and life-history strategy best explained the observed pattern (Hourston et al., 2009; Lampadariou and Eleftheriou, 2018). Very limited information is available to date on the relationship between the body cuticle structure of nematodes with their habitat types and hydrodynamic condition. The cuticle represents a barrier between organisms and their outer environment. It also confers their body shape and supports locomotion along with the help of body muscle and pseudocoel (Cesaroni et al., 2017). Nematode assemblages in the present study area showed a preponderance of striated cuticle throughout the seasons with the lowest abundance of ornamented cuticle. Cuticle with ornamentations had proven to be related with sandy sediment, which was also reflected in PCA (Figure 7E). At the same time, ornamented cuticle might bestow advantages to thrive in physically harsh environmental conditions of the estuary (Vanreusel et al., 2010).
In this fashion, biological trait analysis added a new dimension in biodiversity study over solely taxonomical information, thereby enhancing our knowledge on ecological gradients existing within an estuary. Environmental variables, in concert with a prevalence of nematode species with non-selective deposit feeders, clavate tail, slender body, and striated cuticular structure with high colonization potential in combination, were able to sustain throughout the study period. Seasonal patterns in the functional structure of nematode assemblages were primarily related to differences in sedimentary conditions. In conjunction with dominant species with combination among different traits, low-abundance species were also contributed to the context of their ecological roles. With respect to their capabilities, they respond differentially to stressful environmental conditions, thereby corroborating resilience to community structure. Nevertheless, a 1-year temporal observation might not be enough to obtain a definite conclusion and obviously need more than a 1-year assessment if the same seasonal succession pattern is repeated annually.
Presently, SES is subjected to multiple anthropogenic environmental changes, e.g., mean sea level rise, salinity intrusion, and land loss., which rapidly transform the community structure and functioning of the estuarine benthic ecosystem. As a consequence, the entire Matla estuarine system is affected by reduced freshwater input, increased salinity, and siltation. In addition, acceleration of various extreme climatic events increases the vulnerability of estuarine biodiversity and causes local extinction of many species (Ghosh et al., 2015). Hence, a complete database about the meiobenthic, especially nematode diversity is essential before many species might get extinct due to loss of biodiversity and ecosystem stability. Seasonal macrobenthic dynamics across Matla River have been revealed in a very recent study by Bhowmik and Mandal (2020), howbeit knowledge of meiobenthic composition is presently lacking from this region. As meiobenthos occupy an important trophic position in the aquatic food web and in maritime countries like India, a large percentage of people rely on their coastal environment for their food security and livelihood; therefore, enhancement of biodiversity knowledge using BTA is indispensable to understand the health of this rapidly changing ecosystem in SES.
Conclusion
The assimilation of our results provided a first-time systematic description of biodiversity patterns of meiobenthic assemblages, exclusively free-living nematodes from pristine subtidal sediment of Matla River of SES in both spatial and temporal (seasonal) scales. This study highlighted that seasonally governed biotic and abiotic factors like salinity, dissolved oxygen concentration, and sediment properties play crucial roles in meiobenthic distribution. Our study also revealed diverse morpho-functional and ecological traits characterized by notable microhabitat and niche heterogeneity that leads to development of a wide array of adaptation in order to inhabit them. Finally, this work represents a reliable dataset on meiobenthic diversity and ecology, which will act as a roadmap for the future researchers and environmental policymakers for the design and implementation of sustainable management blueprints for this ecologically fragile ecosystem. In this respect, it would be fascinating to further investigate seasonal succession in meiobenthic diversity for a longer time frame with addition of more traits.
Data Availability Statement
The original contributions presented in the study are included in the article/supplementary material, further inquiries can be directed to the corresponding author.
Author Contributions
MG: conceptualization, field work, sample collection, laboratory analysis, statistical analysis, data interpretation, writing – original draft, and editing. SM: conceptualization, field work, sample collection, data interpretation, resources, and writing – review and editing, supervision, project administration, and funding acquisition. Both authors contributed to the article and approved the submitted version.
Funding
This work was supported by the Ministry of Earth Sciences, Government of India (Sanction No. MoES/36/OOIS/Extra/24/2013 dated 11.04.2016) and FRPDF grant to SM.
Conflict of Interest
The authors declare that the research was conducted in the absence of any commercial or financial relationships that could be construed as a potential conflict of interest.
Acknowledgments
The authors wish to express their gratitude to the Vice Chancellor, Presidency University, for providing facilities and encouragement to carry out the above research work. MG thanks Presidency University, Kolkata, for University Research Fellowship. Data in this article is a part of a doctoral thesis of MG to be submitted in partial fulfillment of the requirements of a degree of Doctor of Philosophy from Presidency University, Kolkata. Finally, the authors would also like to express their appreciation to all the members of the Marine Ecology Laboratory for their excellent teamwork.
References
Adão, H., Alves, A., Patrício, J., Neto, J. M., Costa, M. J., and Marques, J. C. (2009). Spatial distribution of subtidal Nematoda communities along the salinity gradient in two Southern European estuaries (Portugal). Acta Oecol. 35, 287–300. doi: 10.1016/j.actao.2008.11.007
Alongi, D. M. (1990). The ecology of tropical soft-bottom benthic ecosystem. Oceanogr. Mar. Biol. Annu. Rev. 28, 381–496.
Alves, A. S., Adão, H., Ferrero, T., Marques, J., Costa, M. J., and Patrício, J. (2013). Benthic meiofauna as indicator of ecological changes in estuarine ecosystems: the use of nematodes in ecological quality assessment. Ecol. Indic. 24, 462–475. doi: 10.1016/j.ecolind.2012.07.013
Alves, A. S., Adão, H., Patrício, J., Neto, J. M., Costa, M. J., and Marques, J. C. (2009). Spatial distribution of subtidal meiobenthos along estuarine gradients in two southern European estuaries (Portugal). J. Mar. Biol. Assoc. U.K. 89, 1529–1540. doi: 10.1017/S0025315409000691
Alves, A. S., Veríssimo, H., Costa, M. J., and Marques, J. C. (2014). Taxonomic resolution and Biological Traits Analysis (BTA) approaches in estuarine free-living nematodes. Estuar. Coast. Shelf Sci. 138, 69–78. doi: 10.1016/j.ecss.2013.12.014
Anderson, M. J., Gorley, R. N., and Clarke, K. R. (2008). PERMANOVA+ for PRIMER: Guide to Software and Statistical Methods. Plymouth, UK: PRIMER-E.
Ansari, K. G. M. T., Manokaran, S., Raja, S., Lyla, P. S., and Khan, S. A. (2014). Interaction of free-living marine nematodes in the artificial mangrove environment (Southeast Coast of India). Environ. Monit. Assess. 186, 293–305. doi: 10.1007/s10661-013-3374-1
Antizar-Ladislao, B., Mondal, P., Mitra, S., and Sarkar, S. K. (2015). Assessment of trace metal contamination level and toxicity in sediments from coastal regions of west Bengal, eastern part of India. Mar. Pollut. Bull. 101, 886–894. doi: 10.1016/j.marpolbul.2015.11.014
Armenteros, M., Perez-Garcıa, J. A., Perez-Angulo, A., and Williams, J. P. (2008). Efficiency of extraction of meiofauna from sandy and muddy marine sediments. Rev. Investig. Mar. 29, 113–118.
Armenteros, M., Ruiz-Abierno, A., Fernández-Garcés, R., Pérez-García, J. A., Díaz-Asencio, L., Vincx, M., et al. (2009). Biodiversity patterns of free-living marine nematodes in a tropical bay: cienfuegos, Caribbean Sea. Estuar. Coast. Shelf Sci. 85, 179–189. doi: 10.1016/j.ecss.2009.08.002
Baliarsingh, S. K., Srichandan, S., Sahu, K. C., and Lotliker, A. A. (2015). First record of Desmoscolex falcatus (Nematoda: Adenophorea: Desmoscolecida: Desmoscolecidae) from Rushikulya estuary, Odisha, India. Indian J. Mar. Sci. 44, 519–521.
Banerjee, K. (2013). Decadal change in the surface water salinity profile of indian sundarbans: a potential indicator of climate change. J. Mar. Sci. Res. Dev. S 11:002. doi: 10.4172/2155-9910.s11-002
Barranguet, C. (1997). The role of microphyto benthic primary production in a Mediterranean mussel culture area. Estuar. Coast. Shelf Sci. 44, 753–765. doi: 10.1006/ecss.1996.0153
Beck, M. W., Heck, K., Able, K., Childers, D., Egglestone, D., Gillanders, B., et al. (2001). The identification, conservation and management of estuarine and marine nurseries for fish and invertebrates: a better understanding of the habitats that serve as nurseries for marine species and the factors that create site-specific variability in nursery quality will improve conservation and management of these areas. Bioscience 51, 633–641. doi: 10.1641/0006-3568(2001)051[0633:ticamo]2.0.co;2
Bezerra, T. N., Decraemer, W., Eisendle-Flöckner, U., Hodda, M., Holovachov, O., Leduc, D., et al. (2021). Nemys: World Database of Nematodes. Available online at: http://nemys.ugent.be (accessed January 05, 2021).
Bhowmik, M., and Mandal, S. (2020). Do seasonal dynamics influence traits and composition of macrobenthic assemblages of Sundarbans Estuarine System, India? Oceanologia 63, 80–98. doi: 10.1016/j.oceano.2020.10.002
Bongers, T. (1990). The maturity index: an ecological measure of environmental disturbance based on nematode species composition. Oecologia 83, 14–19. doi: 10.1007/BF00324627
Bongers, T., Alkemade, R., and Yeates, G. W. (1991). Interpretation of disturbance-induced maturity decrease in marine nematode assemblages by means of the Maturity Index. Mar. Ecol. Prog. Ser. 76, 135–142. doi: 10.3354/meps076135
Bongers, T., de Goede, R. G. M., Korthals, G. W., and Yeates, G. W. (1995). Proposed changes of c-p classification for nematodes. Russ. J. Nematol. 3, 61–62.
Bremner, J., Rogers, S. I., and Frid, C. L. J. (2003). Assessing functional diversity in marine benthic ecosystems: a comparison of approaches. Mar. Ecol. Prog. Ser. 254, 11–25. doi: 10.3354/meps254011
Bremner, J., Rogers, S. I., and Frid, C. L. J. (2006). Methods for describing ecological functioning of marine benthic assemblages using biological traits analysis (BTA). Ecol. Indic. 6, 609–622. doi: 10.1016/j.ecolind.2005.08.026
Broom, M. J. (1982). Structure and seasonality in a Malaysia mudflat community. Estuar. Coast. Shelf Sci. 15, 135–150. doi: 10.1016/0272-7714(82)90024-5
Cesaroni, L., Guidi, L., Balsamo, M., and Semprucci, F. (2017). Scanning electron microscopy in the taxonomical study of free-living marine nematodes. Microscopie 28, 31–38.
Chalcraft, D. R., and Resetarits, W. J. (2003). Mapping functional similarity of predators on the basis of trait similarities. Am. Nat. 162, 390–402. doi: 10.1086/378210
Chatterjee, M., Shankar, D., Sen, G. K., Sanyal, P., Sundar, D., Michael, G., et al. (2013). Tidal variations in the Sundarbans Estuarine System, India. J. Earth Syst. Sci. 122, 899–933. doi: 10.1007/s12040-013-0314-y
Chinnadurai, G., and Fernando, O. J. (2006). Meiobenthos of Cochin mangroves (Southwest coast of India) with emphasis of free-living marine nematode assemblages. Russ. J. Nematol. 14, 127–137.
Chinnadurai, G., and Fernando, O. J. (2007). Meiofauna of mangroves of the southeast coast of India with special reference to the free-living marine nematode assemblage. Estuar. Coast. Shelf Sci. 72, 329–336. doi: 10.1016/j.ecss.2006.11.004
Clarke, K. R., and Ainsworth, M. (1993). A method of linking multivariate community structure to environmental variables. Mar. Ecol. Prog. Ser. 92, 205–219. doi: 10.3354/meps092205
Clarke, K. R., Somerfield, P. J., and Chapman, M. G. (2008). Testing of null hypotheses in explanatory community analyses: similarity profiles and biota-environment linkage. J. Exp. Mar. Biol. Ecol. 366, 56–69. doi: 10.1016/j.jembe.2008.07.009
Costanza, R., d‘Arge, R., de Groot, R., Farber, S., Grasso, M., Hannon, B., et al. (1997). The value of the world‘s ecosystem services and natural capital. Nature 387, 253–260. doi: 10.1038/387253a0
Coull, B. C. (1985). Long-term variability of estuarine meiobenthos: an 11 years study. Mar. Ecol. Prog. Ser. 24, 205–218. doi: 10.3354/meps024205
Coull, B. C. (1999). Role of meiobenthos in estuarine soft-bottom habitats. Austral. J. Ecol. 24, 327–343. doi: 10.1046/J.1442-9993.1999.00979.X
Curini-Galletti, M., Artois, T., Delogu, V., De Smet, W. H., Fontaneto, D., Jondelius, U., et al. (2012). Patterns of diversity in soft-bodied meiofauna: dispersal ability and body size matter. PLoS One 7:e33801. doi: 10.1371/journal.pone.0033801
Danovaro, R., Gambi, C., Mirto, S., Sandulli, R., and Ceccherelli, V. U. (2004). Meiofauna. Biol. Mar. Mediterr. 11, 55–97.
Derycke, S., Hendrickx, F., Backeljau, T., D’Hondt, S., Camphijn, L., Vincx, M., et al. (2007). Effects of sublethal abiotic stressors on population growth and genetic diversity of Pellioditis marina (Nematoda) from the Westerschelde estuary. Aquat. Toxicol. 82, 110–119. doi: 10.1016/j.aquatox.2007.02.002
Dey, M., Ganguly, D., Chowdhury, C., Majumder, N., and Jana, T. K. (2012). Intra-annual variation of modern foraminiferal assemblage in a tropical mangrove ecosystem. Wetlands 32, 813–826. doi: 10.1007/s13157-012-0312-x
Dhame, S., Kumar, A., Ramanathan, A. L., and Chaudhuri, P. (2016). Elemental composition, distribution and control of biogenic silica in the anthropogenically disturbed and pristine zone inter-tidal sediments of Indian Sundarbans mangrove-estuarine complex. Mar. Pollut. Bull. 111, 68–85. doi: 10.1016/j.marpolbul.2016.07.027
Diaz, S., and Cabido, M. (2001). Vive la différence: plant functional diversity matters to ecosystem processes. Trends Ecol. Evol. 16, 646–655. doi: 10.1016/S0169-5347(01)02283-2
Edwards, D. P., Tobias, J. A., Sheil, D., Meijaard, E., and Laurance, W. F. (2014). Maintaining ecosystem function and services in logged tropical forests. Trends Ecol. Evol. 29, 511–520. doi: 10.1016/j.tree.2014.07.003
El Wakeel, S. K., and Riley, J. P. (1957). The determination of organic carbon in the marine muds. ICES J. Mar. Sci. 22, 180–183. doi: 10.1093/icesjms/22.2.180
Elliott, M., and Quintino, V. (2007). The estuarine quality paradox, environmental homeostasis and the difficulty of detecting anthropogenic stress in naturally stressed areas. Mar. Pollut. Bull. 54, 640–645. doi: 10.1016/j.marpolbul.2007.02.003
Ferrero, T. J., Debenham, N. J., and Lambshead, P. J. D. (2008). The nematodes of the Thames estuary: assemblage structure and biodiversity, with a test of Attrill’s linear model. Estuar. Coast. Shelf Sci. 79, 409–418. doi: 10.1016/j.ecss.2008.04.014
Ghosh, A., Schmidt, S., Fickert, T., and Nüsser, M. (2015). The Indian Sundarban mangrove forests: history, utilization, conservation strategies and local perception. Diversity 7, 149–169. doi: 10.3390/d7020149
Ghosh, M., and Mandal, S. (2018). Free-living marine nematode diversity from the Indian coasts. Mar. Biodiv. 48, 179–194. doi: 10.1007/s12526-016-0551-9
Ghosh, M., and Mandal, S. (2019). Does vertical distribution of meiobenthic community structure differ among various mangrove habitats of Sundarban Estuarine system? Reg. Stud. Mar. Sci. 31:100778. doi: 10.1016/j.rsma.2019.100778
Ghosh, M., Mandal, S., and Chatterjee, M. (2018). Impact of unusual monsoonal rainfall in structuring meiobenthic assemblages at Sundarban estuarine system, India. Ecol. Indic. 94, 139–150. doi: 10.1016/j.ecolind.2018.06.067
Giere, O. (2009). Meiobenthology. The Microscopic Motile Fauna of Aquatic Sediments, 2nd Edn. Berlin: Springer-Verlag.
Gili, J. M. (2002). Towards a transitory or ephemeral key habitat concept. Trends Ecol. Evol. 17:453. doi: 10.1016/S0169-5347(02)02606-X
Harrison, P. J., Khan, N., Yin, K., Saleem, M., Bano, N., Nisa, M., et al. (1997). Nutrient and phytoplankton dynamics in two mangrove tidal creeks of the Indus river delta Pakistan. Mar. Ecol. Prog. Ser. 157, 13–19. doi: 10.3354/MEPS157013
Heip, C. H., Vincx, M., and Vranken, G. (1985). The ecology of marine Nematoda. Oceanogr. Mar. Biol. 23, 399–489.
Hourston, M., Potter, I. C., Warwick, R. M., Valesini, F. J., and Clarke, K. R. (2009). Spatial and seasonal variations in the ecological characteristics of the free-living nematode assemblages in a large microtidal estuary. Estuar. Coast. Shelf Sci. 82, 309–322. doi: 10.1016/j.ecss.2009.01.018
Jax, K. (2005). Function and “functioning” in ecology: what does it mean? Oikos 111, 641–648. doi: 10.1111/j.1600-0706.2005.13851.x
Jensen, P. (1987). Feeding ecology of free-living aquatic nematodes. Mar. Ecol. Prog. Ser. 35, 87–196. doi: 10.3354/MEPS035187
Kinne, O. (1977). “Environmental factors—salinity: animal—invertebrates,” in Marine Ecology, ed. O. Kinne (New York, NY: John Wiley), 821–996.
Lambshead, P., and Boucher, G. (2003). Marine nematode deep sea biodiversity – hyperdiverse or hype? J. Biogeogr. 30, 475–485. doi: 10.1046/j.1365-2699.2003.00843.x
Lampadariou, N., and Eleftheriou, A. (2018). Seasonal dynamics of meiofauna from the oligotrophic continental shelf of Crete (Aegean Sea, eastern Mediterranean). J. Exp. Mar. Biol. Ecol. 502, 91–104. doi: 10.1016/j.jembe.2017.12.014
Losi, V., Moreno, M., Gaozza, L., Vezzulli, L., Fabiano, M., and Albertelli, G. (2013). Nematode biomass and allometric attributes as indicators of environmental quality in a Mediterranean harbour (Ligurian Sea, Italy). Ecol. Indic. 30, 80–89. doi: 10.1016/j.ecolind.2013.01.034
Madhu, N. V., Jyothibabu, R., Balachandran, K. K., Honey, U. K., Martin, G. D., Vijay, J. G., et al. (2007). Monsoonal impact on planktonic standing stock and abundance in a tropical estuary (Cochin backwaters—India). Estuar. Coast. Shelf Sci. 73, 54–64. doi: 10.1016/j.ecss.2006.12.009
Manini, E., Gambi, C., Danovaro, R., and Fabiano, M. (2000). Meiobenthic grazing rates on bacteria and microphytobenthos in the northern Adriatic Sea: preliminary results. Biol. Mar. Mediterr. 7, 233–238.
Maurer, D. (2000). The dark side of the taxonomic sufficiency (TS). Mar. Pollut. Bull. 40, 98–101. doi: 10.1016/S0025-326X(99)00235-0
Miller, D. C., Geider, R. J., and Macintyre, H. L. (1996). Microphytobenthos: the ecological role of the secret garden of unvegetated, shallow-water marine habitats. II. Role in sediment stability and shallow-water food webs. Estuaries 19, 202–212. doi: 10.2307/1352225
Moens, T., and Vincx, M. (1997). Observations on the feeding ecology of estuarine nematodes. J. Mar. Biol. Assoc. U.K. 77, 211–227. doi: 10.1017/S0025315400033889
Moens, T., and Vincx, M. (2000). Temperature and salinity constraints on the life cycle of two brackish-water nematode species. J. Exp. Mar. Biol. Ecol. 243, 115–135. doi: 10.1016/S0022-0981(99)00113-6
Montagna, P. A., Blanchard, F. G., and Dinet, A. (1995). Effect of production and biomass of intertidal microphytobenthos on meiofaunal grazing rates. J. Exp. Mar. Biol. Ecol. 185, 149–165. doi: 10.1016/0022-0981(94)00138-4
Moreno, M., Ferrero, T. J., Gallizia, I., Vezzulli, L., Albertelli, G., and Fabiano, M. (2008). An assessment of the spatial heterogeneity of environmental disturbance within an enclosed harbor through the analysis of meiofauna and nematode assemblages. Estuar. Coast. Shelf Sci. 77, 565–576. doi: 10.1016/j.ecss.2007.10.016
Moreno, M., Semprucci, F., Vezzulli, L., and Balsamo, M. (2011). The use of nematodes in assessing ecological quality status in the Mediterranean coastal ecosystems. Ecol. Indic. 11, 328–336. doi: 10.1016/j.ecolind.2010.05.011
Mouillot, D., Graham, N. A. J., Ville’ger, S., Mason, N. W. H., and Bellwood, D. R. (2013). A functional approach reveals community responses to disturbances. Trends Ecol. Evol. 28, 167–177. doi: 10.1016/j.tree.2012.10.004
Nascimento, F. J. A., Naslund, J., and Elmgren, R. (2012). Meiofauna enhances organic matter mineralization in soft sediment ecosystems. Limnol. Oceanogr. 57, 338–346. doi: 10.4319/lo.2012.57.1.0338
Newell, R. C. (1982). “The energetics of detritus utilization in coastal lagoons and near shore waters,” in Proceedings of the Int. Symp. on Coastal Lagoons, Bordeaux.
Nozais, C., Perissinotto, R., and Tita, G. (2005). Seasonal dynamics of meiofauna in a South African temporarily open/closed estuary (Mdloti Estuary, Indian Ocean). Estuar. Coast. Shelf Sci. 62, 325–338. doi: 10.1016/j.ecss.2004.09.020
Papa, F., Durand, F., Rossow, W. B., Rahman, A., and Bala, S. K. (2010). Satellite altimeter derived monthly discharge of the Ganga-Brahmaputra River and its seasonal to inter annual variations from 1993 to 2008. J. Geophys. Res. 115:C12013. doi: 10.1029/2009JC006075
Parsons, T. R., Takahashi, M., and Hargrave, B. (1990). Biological Oceanographic Processes, 3rd Edn. Oxford: Pergamon Press.
Pfannkuche, O., and Thiel, H. (1988). “Sample processing,” in Introduction to the Study of Meiofauna, eds R. P. Higgins and H. Thiel (Washington, DC: Smithsonian Institute Press), 134–145.
Phillips, F. E., and Fleeger, J. W. (1985). Meiofauna meso-scale variability in two estuarine habitats. Estuar. Coast. Shelf Sci. 21, 745–756. doi: 10.1016/0272-7714(85)90070-8
Platt, H. M., and Warwick, R. M. (1983). “Free living marine nematodes, Part I, British Enoplids, Pictorial key to world genera and notes for the identification of British species,” in Synopses of the British Fauna, Vol. 28, eds D. M. Kermack and R. S. K. Barnes (Cambridge: Cambridge University Press), 1–307. doi: 10.7591/9781501728419-002
Platt, H. M., and Warwick, R. M. (1988). “Free living marine nematodes, Part II, British Chromodorids, Pictorial key to world genera and notes for the identification of British species,” in Synopses of the British Fauna (new series), Vol. 38, eds D. M. Kermack and R. S. K. Barnes (Leiden: Brill/Backhuys), 1–502. doi: 10.7591/9781501728419-002
Pusceddu, A., Gambi, C., Manini, E., and Danovaro, R. (2007). Trophic state, ecosystem efficiency and biodiversity of transitional aquatic ecosystems: analysis of environmental quality based on different benthic indicators. Chem. Ecol. 23, 505–515. doi: 10.1080/02757540701760494
Radwell, A. J., and Brown, A. V. (2008). Benthic meiofauna assemblage structure of headwater streams: density and distribution of taxa relative to substrate size. Aquat. Ecol. 42, 405–414. doi: 10.1007/s10452-007-9108-0
Rao, G. C., and Misra, A. (1983). Studies on the meiofauna of Sagar Island. Proc. Indian Acad. Sci. 92, 73–85. doi: 10.1007/BF03186173
Riemann, F. (1974). On hemisessile nematodes with flagilliform tails living in marine soft bottoms and on microtubes found in deep sea sediments. Mikrofauna Meeresbod. 40, 1–15.
Ristau, K., Spann, N., and Traunspurger, W. (2015). Species and trait compositions of fresh water nematodes as indicative descriptors of lake eutrophication. Ecol. Indic. 53, 196–205. doi: 10.1016/j.ecolind.2015.01.010
Ritter, A. N. (2012). Effect of Eutrophication on Benthic Microalgae. Vermont: Department of Biology, Middlebury College. Master thesis.
Rzeznik-Orignac, J., Fichet, D., and Boucher, G. (2003). Spatio-temporal structure of the nematode assemblages of the Brouage mudflat (Marennes Ole’ron, France). Estuar. Coast. Shelf Sci. 58, 77–88. doi: 10.1016/S0272-7714(03)00061-1
Schmid-Araya, J. M., Schmid, P. E., Tod, S. P., and Esteban, G. F. (2016). Trophic positioning of meiofauna revealed by stable isotopes and food web analyses. Ecology 97, 3099–3109. doi: 10.1002/ecy.1553
Schratzberger, M., and Ingels, J. (2018). Meiofauna matters: the roles of meiofauna in benthic ecosystems. J. Exp. Mar. Biol. Ecol. 502, 12–25. doi: 10.1016/j.jembe.2017.01.007
Schratzberger, M., Warr, K., and Rogers, S. I. (2006). Patterns of nematode populations in the southwestern North Sea and their link to other components of the benthic fauna. J. Sea Res. 55, 113–127. doi: 10.1016/j.seares.2005.07.002
Schratzberger, M., Warr, K., and Rogers, S. I. (2007). Functional diversity of nematode community in the south-western North Sea. Mar. Environ. Res. 63, 368–389. doi: 10.1016/j.marenvres.2006.10.006
Seinhorst, J. W. (1959). A rapid method for the transfer of nematodes from fixative toanhydrous glycerine. Nematologica 4, 67–69. doi: 10.1163/187529259x00381
Semprucci, F., and Balsamo, M. (2012). “Key role of free-living nematodes in the marineecosystem,” in Nematodes: Morphology, Functions and Management Strategies, eds F. Boeri and A. C. Jordan (Hauppauge: NOVA Science Publ., Inc.), 109–134. doi: 10.1515/9783110274257.109
Semprucci, F., Cesaroni, L., Guidi, L., and Balsamo, M. (2018). Do the morphological and functional traits of free-living marine nematodes mirror taxonomical diversity? Mar. Environ. Res. 135, 114–122. doi: 10.1016/j.marenvres.2018.02.001
Sen, A., Ghosh, M., Khanderao, P., Das, S. K., Roy Chowdhury, D., Sarkar, P. K., et al. (2016). Modern benthic foraminiferal assemblages from the world‘s largest deltaic mangrove ecosystem, the Sundarbans. Mar. Biodiv. 46, 421–431. doi: 10.1007/s12526-015-0383-z
Singh, R., and Ingole, B. S. (2016). Structure and function of nematode communities across the Indian western continental margin and its oxygen minimum zone. Biogeosciences 13, 191–209. doi: 10.5194/bg-13-191-2016
Soetaert, K., Muthumbi, A., and Heip, C. (2002). Size and shape of ocean margin nematodes: morphological diversity and depth-related patterns. Mar. Ecol. Prog. Ser. 242, 179–193. doi: 10.3354/meps242179
Soetaert, K., Vincx, M., Wittoeck, J., and Tulkens, M. (1995). Meiobenthic distribution and nematode community structure in five European estuaries. Hydrobiologia 311, 185–206. doi: 10.1007/BF00008580
Sroczyńska, K., Chainho, P., Vieira, S., and Adào, H. (2020). What makes a better indicator? Taxonomic vs functional response of nematodes to estuarine gradient. Ecol. Indic. 2020:107113. doi: 10.1016/j.ecolind.2020.107113
Steyaert, M., Moodley, L., Nadong, T., Moens, T., Soetaert, K., and Vincx, M. (2007). Responses of intertidal nematodes to short-term anoxic events. J. Exp. Mar. Biol. Ecol. 345, 175–184. doi: 10.1016/j.jembe.2007.03.001
Steyaert, M., Vanaberbeke, J., Vanreusel, A., Barranguet, C., Lucas, C., and Vincx, M. (2003). The importance of fine-scale, vertical profiles in characterizing nematode community structure. Estuar. Coast. Shelf Sci. 58, 353–366. doi: 10.1016/S0272-7714(03)00086-6
Strickland, J. D. H., and Parsons, T. R. (1972). A practical handbook of seawater analysis. Br. Fish. Res. Board Can. 167, 1–310.
ter Braak, C. J. F. (1986). Canonical correspondence analysis: a new eigen vector technique for multivariate direct gradient analysis. Ecology 67, 1167–1179. doi: 10.2307/1938672
Thistle, D., Lambshead, P. J. D., and Sherman, K. M. (1995). Nematode tail-shape groups respond to environmental differences in the deep sea. Vie Milieu 45, 107–115.
Thistle, D., and Sherman, K. M. (1985). The nematode fauna of a deep-sea site exposed to strong near-bottom currents. Deep Sea Res. Part I Oceanogr. Res. Pap. 32, 1077–1088. doi: 10.1016/0198-0149(85)90063-9
Tietjen, J. H. (1969). The ecology of shallow water meiofauna in two New England estuaries. Oecologia 2, 251–291. doi: 10.1007/BF00386114
Underwood, G. J. C., and Kromkamp, J. C. (1999). Primary production by phytoplankton and microphytobenthos in estuaries. Adv. Ecol. Res. 29, 93–153. doi: 10.1016/S0065-2504(08)60192-60190
Vanaverbeke, J., Merckx, B., Degraer, S., and Vincx, M. (2011). Sediment-related distribution patterns of nematodes and macrofauna: two sides of the benthic coin? Mar. Environ. Res. 71, 31–40. doi: 10.1016/j.marenvres.2010.09.006
Vanaverbeke, J., Steyaert, M., Soetaert, K., Rousseau, V., Van Gansbeke, D., Parent, J. Y., et al. (2004). Changes in structural and functional diversity of nematode communities during a spring phytoplankton bloom in the southern North Sea. J. Sea Res. 52, 281–292. doi: 10.1016/j.seares.2004.02.004
Vanaverbeke, J., Steyaert, M., Vanreusel, A., and Vincx, M. (2003). Nematode biomass spectra as descriptors of functional changes to human and natural impact. Mar. Ecol. Prog. Ser. 249, 17–157. doi: 10.3354/MEPS249157
Vanreusel, A., Fonseca, G., Danovaro, R., Cristina da Silva, M., Esteves, A. M., Ferrero, T., et al. (2010). The contribution of deep-sea macrohabitat heterogeneity to global nematode diversity. Mar. Ecol. 31, 6–20. doi: 10.1111/j.1439-0485.2009.00352.x
Warwick, R. M., Platt, H. M., and Somerfield, P. J. D. (1998). “Free-living marine nematodes, Part III, Monhysterids, Pictorial key to world genera and notes for the identification of British species,” in Synopses of the British Fauna (New series), eds D. M. Kermack and R. S. K. Barnes (Shrewsbury, UK: Field Studies Council), 1–296. doi: 10.7591/9781501728419-002
Warwick, R. M., and Price, R. (1979). Ecological and metabolic studies on free-living marine nematodes from an estuarine mud-flat. Estuar. Coast. Shelf Sci. 9, 257–271. doi: 10.1016/0302-3524(79)90039-2
Wetzel, M. A., Weber, A., and Giere, O. (2002). Re-colonization of anoxic/sulfidic sediments by marine nematodes after experimental removal of macroalgal cover. Mar. Biol. 141, 679–689. doi: 10.1007/s00227-002-0863-0
Wieser, W. (1953). Die BeziehungzwischenMundhöhlengestalt, Ernährungswiese und 1000 VorkommenbeifreilebendenmarinenNematoden. Ark. fürZool. 4, 439–484.
Keywords: meiobenthos, free-living nematodes, functional diversity, biological trait analysis, taxonomic sufficiency, Sundarbans
Citation: Ghosh M and Mandal S (2021) Disentangling the Effect of Seasonal Dynamics on Meiobenthic Community Structure From River Matla of Sundarbans Estuarine System, India. Front. Mar. Sci. 8:671372. doi: 10.3389/fmars.2021.671372
Received: 23 February 2021; Accepted: 19 April 2021;
Published: 01 June 2021.
Edited by:
Mandar Nanajkar, National Institute of Oceanography, Council of Scientific and Industrial Research (CSIR), IndiaReviewed by:
Gabriel-Ionut Plavan, Alexandru Ioan Cuza University, RomaniaFehmi Boufahja, Carthage University, Tunisia
Copyright © 2021 Ghosh and Mandal. This is an open-access article distributed under the terms of the Creative Commons Attribution License (CC BY). The use, distribution or reproduction in other forums is permitted, provided the original author(s) and the copyright owner(s) are credited and that the original publication in this journal is cited, in accordance with accepted academic practice. No use, distribution or reproduction is permitted which does not comply with these terms.
*Correspondence: Sumit Mandal, sumit.dbs@presiuniv.ac.in