- Faculty of Exact and Natural Sciences, Institute of Biology, Universidad de Antioquia, Medellín, Colombia
Mangroves are fragmented habitats thriving in human-dominated coastalscapes worldwide. They provide refuge to threatened plant species, such as the Neotropical Piñuelo Mangrove (formerly the monotypic Pelliciera genus, recently split into two species: P. rhizophorae and P. benthamii). However, little is known about the relationship between urbanization and the spatial configuration of mangrove habitat and how it would drive ecological and evolutionary changes in the small populations of Pelliciera spp. We used open data (e.g., land cover, mangrove cover) for 107 locations of Pelliciera spp. in Colombia (extant populations) to assess coastalscape structure and urbanization. We described coastalscape composition dynamics (for 2000 and 2010) and mangrove habitat configuration along a human-domination gradient, using landscape metrics. We computed an urban index to compare urbanization intensity among the study areas along the Caribbean coast and compared coastalscape structure and mangrove spatial metrics between basins (Caribbean and Pacific). The proportion of artificial surfaces was greater in the Caribbean than in the Pacific, but no temporal difference was found. The Caribbean basin exhibited a greater Urban Index, particularly in Cartagena and Urabá. Mangrove fragmentation was also greater in the Caribbean and it was influenced by the degree of urbanization. Mangrove area and core area were smaller in the Caribbean than in the Pacific, while cohesion was significantly lower in Cartagena than in other urban areas of the Caribbean. We propose a conceptual eco-evolutionary framework for linking mangrove patch reduction and isolation to demographic variables of Pelliciera spp. Edge effects are expected to affect birth and mortality rates in small patches. Small patches and small effective populations are expected to be more sensitive to novel biotic interactions, extreme weather, and gradual climate change. Isolation will also influence both emigration and immigration rates of propagules. We propose: (1) Assessing the conservation status of the two species, (2) setting monitoring programs of their populations of Pelliciera spp. and their surrounding coastalscapes (particularly in the Caribbean, (3) declaring protected areas, in wild and urban settings, to reduce mangrove fragmentation and urbanization.
Introduction
Mangrove tree species are threatened by anthropogenic drivers operating at the landscape level (Polidoro et al., 2010), promoting cascading effects mediated by eco-evolutionary dynamics that are poorly understood. Mangroves, as habitats for vulnerable plant species, have been cleared or degraded during many decades due to residential and commercial development, expansion of agricultural frontiers and aquaculture districts (Friess et al., 2019; Goldberg et al., 2020). For instance, between 2000 and 2016, human activity was the primary driver of mangrove area loss, with a 47% due to commodities such as rice, shrimp, and oil palm cultivation (Goldberg et al., 2020). In addition, natural hazards such as coastal erosion, extreme atmospheric and oceanographic events, and sea level rise are further threats to mangrove fringes worldwide, responsible for 38% of loss between 2000 and 2016 (Goldberg et al., 2020). Despite the fact that only 3% of mangrove loss was due to conversion to human settlements, it is still an important driver at local scales, particularly in geographic areas of accelerated urban expansion (Branoff, 2017; Tuholske et al., 2017; Goldberg et al., 2020).
These anthropogenic and natural pressures are more critical for a dozen of true-mangrove species and mangrove associates listed as threatened by the International Union for the Conservation of Nature (hereafter, IUCN). Their conservation status is assessed based on population size, quantitative analysis of probability of extinction and/or geographic range (extent of occurrence and/or area of occupancy), among other variables (IUCN, 2012). In addition, coastal wetland deterioration and loss are determinant processes for the fate of endangered species because they depend on habitat stability, a result of interacting anthropogenic and natural threats, under the current sea level rise rates (Kirwan and Megonigal, 2013). In mangroves, the remaining small patches in many regions are more prone to disappear due to deforestation (Bryan-Brown et al., 2020) while those located in the proximity to populated areas and paved roads experience greater anthropogenic edge effects (e.g., Blanco-Libreros and Estrada-Urrea, 2015; Blanco-Libreros et al., 2016; Zamprogno et al., 2016; Branoff, 2017; Hayashi et al., 2019), similar to the reported for tropical terrestrial forests (Laurance et al., 2002, 2011; Taubert et al., 2018; Hansen et al., 2020).
Global mangrove species diversity is divided into two realms, the Atlantic East Pacific (AEP) and the Indo-West Pacific (IWP) (Duke et al., 1998). All families of the AEP are represented in the IWP, but Tetrameristaceae (formerly Pellicieraceae) only occurs in the AEP, where the highest proportion of threatened mangrove species is found (Duke et al., 1998; Polidoro et al., 2010; Saenger et al., 2019). Pelliciera Planch. & Triana (Magnoliophyta: Tetrameristaceae) is the only mangrove genus endemic to the Neotropics and it was formerly recognized as a monotypic genus. It was solely represented by P. rhizophorae Planch. & Triana, but a substantial amount of evidence (Castillo-Cárdenas et al., 2005, 2012, 2015a,b, 2016) suggested morphological and genetic differences among P. rhizophorae populations, particularly at both sides of the Panama Isthmus. In 2020, two independent publications suggested a redefined P. rhizophorae and proposed a new species: P. benthamii (Planch. & Triana) Cornejo (Cornejo and Bonifaz, 2020), and P. benthamii (Planch. & Triana) Duke (2020). However, both publications likely refer to different entities. It is now clear that what it was known before as a single species are two species (and probably some intermediates or hybrids) that will require further basic biology and ecology studies, conservation status assessments, and active conservation programs.
P. rhizophorae was listed as Vulnerable [B2ab (iii)] by the IUCN, due to its reduced range and isolated populations, its estimated area of occupancy between 500 and 2,000 km2, and the decline of the mangrove area and the quality of the habitat in its range of occurrence (Ellison et al., 2010). But its recent split into P. rhizophorae and P. benthamii likely make them more threatened than previously thought. According to Duke (2020), P. rhizophorae is the most widely distributed, occurring mainly along the American Pacific coast, with small populations in the Caribbean coast of Central America (Jimenez, 1984; Ellison et al., 2010; Duke, 2020). In contrast, P. benthamii only occurs on the Panamanian Pacific and the Colombian Caribbean (Duke, 2020). Ellison et al. (2010) suggested that the majority of Pelliciera populations are not found in protected areas, and the situation is likely worse for each species considered separately. They recommended continued monitoring and research, to better assess the conservation status of these rare mangrove. However, given the complex logistics required to research in wild and isolated areas within the geographical range, and due to the limited funding to set long-term monitoring programs, it is necessary to provide alternatives to monitor the conservation status of Pelliciera spp. and other endangered mangrove plant species elsewhere.
One alternative, to update assessments of conservation status of Pelliciera spp. and other threatened species, is the use of spatial proxies such as landscape metrics (Fernandes et al., 2011; Millán-Aguilar et al., 2019). Several studies have shown the relationship between landscape structure (assessed by landscape metrics), and the diversity, status and/or density of a particular taxon (Uuemaa et al., 2009). These metrics seem to be a cost-effective solution to identify potentially threatened habitats (Hale et al., 2004) and to identify geographical disparities between loss and fragmentation, even in mangrove ecosystems (Taubert et al., 2018; Bryan-Brown et al., 2020). Besides, the anthromes, the biomes built or modified by humans, can give a global classification of drivers of habitat change (i.e., dense human settlements, croplands, used forests, rangelands) at the coastalscape level (Ellis and Ramankutty, 2008).
Northern South America, with coasts on both the Pacific Ocean and the Caribbean Sea, provides a unique opportunity to study the effect of human activities on the coastalscape structure and dynamics, and consequently on mangrove habitats. The Pacific coast of Colombia stands almost continuously covered with extensive mangroves (194,880 ha) and non-tidal wetlands, while the Caribbean coast (with almost 90,170 ha of mangroves) has witnessed a dramatic clearing and transformation of mangroves and coastal dry forests at expense of high-density settlements and extensive agricultural and pastoral areas since 1800s (Etter et al., 2008; Blanco et al., 2012; López-Angarita et al., 2016; Mejía-Rentería et al., 2018; Urrego et al., 2018; official mangrove extent data for 2011 in Blanco-Libreros and Álvarez-León, 2019). Global mapping efforts have pointed to the importance of the extensive wilderness areas along the Pacific coast as blue carbon hotspots, while selected extensive deltas along the Caribbean seemly remain as important areas for wildlife conservation and ecosystem services (Aldana-Domínguez et al., 2017; Hamilton and Friess, 2018; Simard et al., 2019; Sandoval-Londoño et al., 2020).
The Antilles have been a model to understand urban sprawl and its consequences on mangroves, but little is known about coastal cities in South America (Martinuzzi et al., 2009; Branoff and Martinuzzi, 2020). Cartagena, in the central Caribbean, is the largest city in Colombian Caribbean and it has experienced great transformation since the Spanish invasion ca. 1502 (Etter and van Wyngaarden, 2000; Etter et al., 2008). In fact, Cartagena surpasses the numbers of inhabitants of most cities in the Antilles, except Santo Domingo and La Habana. To the south of Cartagena, there are various towns that show a gradient of urban size (in area and population) (i.e., from Tolú and Coveñas in Morrosquillo Gulf to Necoclí and Turbo in Urabá Gulf). This gradient provides an opportunity for studying marine urban ecology, in the context of small towns and medium-sized cities, where even less research has been done. These areas are important because they have a greater perimeter/area ratio, then higher interface with other ecosystems, such as mangroves (Aguayo et al., 2007).
Urbanization processes in Northern South America likely have significant impacts on biodiversity-rich areas that are not fully understood. Cities are areas where rapid eco-evolutionary dynamics on animal and plant populations are driven by anthropogenic processes (e.g., habitat loss and fragmentation; Liu et al., 2016; Dubois and Cheptou, 2017; Alberti et al., 2020). Humans in cities are driving ecological and micro-evolutionary changes that alter biodiversity and ecosystem function in different timescales (Alberti, 2015; Alberti et al., 2020). For instance, change rates of survival or reproductive success have resulted in altered genetic frequencies within populations or even in species shifts in communities (Hermansen et al., 2015; Alberti et al., 2017). Urbanization also promotes expansion of existing species or colonization by new species through the processes of niche alteration and niche construction (Numbere, 2018). As a result of the new species assemblage, novel community-level interactions may emerge thus promoting further changes in species composition and ecosystem function.
Thus, cities are areas where populations, communities, and ecosystems are experiencing turning points, but less information is available for the marine realm of cities located in the Tropics. For these reasons, the objectives of this study were as follows: (1) Describe coastalscape composition (i.e., land cover classes) dynamics within the geographic range of Pelliciera spp. in northern South America, (2) describe the urbanization intensity along the human domination gradient in the Caribbean, (3) compare mangrove habitat configuration (i.e., area, shape and aggregation of mangrove patches) among urban intensities, and (4) set a conceptual framework linking coastalscape structure in urban and peri-urban settings with potential drivers of eco-evolutionary dynamics of Pelliciera spp.
Results
We analyzed 107 coastalscapes in Colombia, 82 in the Pacific basin and 25 in the Caribbean basin (Figure 1), using only extant populations despite the extensive fossil record. We defined coastalscapes as circular buffers (500 m, 1 km, and 5 km in radius) point-centered on records of Pelliciera rhizophorae Tr&Pl in the literature. However, due to the difficulty of knowing the identity of the Pelliciera species, all the records were taken as genus records. The Pelliciera coastalscapes were evenly distributed along all the Pacific basin but they were restricted to three regions in the Caribbean basin: Urabá Gulf, Morrosquillo Gulf, and two neighboring embayments Barbacoas and Cartagena (the two later will be named as Cartagena hereafter). Coastalscapes were predominantly natural, forested or wild along the Pacific coast (likely P. rhizophorae distribution range), but they exhibited different degrees of human domination within the three regions in the Caribbean coast (likely the P. benthamii distribution range, except Urabá Gulf).
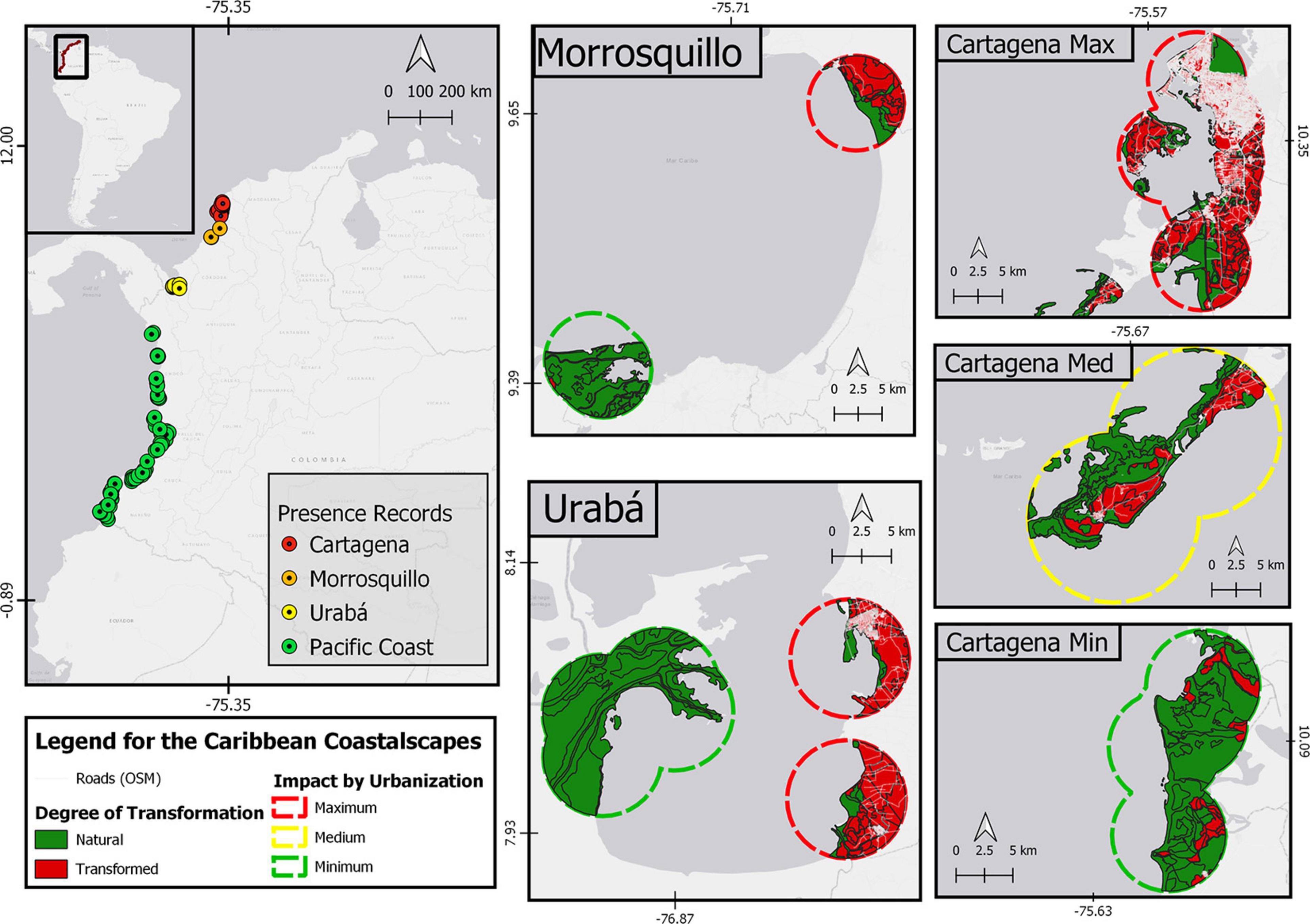
Figure 1. Study area in Northern South America. On the left panel, the records of Pelliciera spp. in Colombia. In the right and central panel, the detail of Pelliciera spp. coastalscapes in the Caribbean are shown as dissolved circular buffers of 5 km radius. In addition, the degree of transformation is detailed (natural or transformed) and the roads are shown in white. For the Cartagena region, the areas with the least impact due to urbanization (Canal del Dique area of influence), with medium impact (Barú peninsula) and with high impact (the city of Cartagena itself) are shown. The map was made in QGIS Desktop 3.10.7 with data from IDEAM, Instituto Alexander von Humboldt, IGAC, INVEMAR, and Ministerio de Ambiente y Desarrollo Sostenible (2017) and OpenStreetMap contributors, 2020.
Coastalscape Composition
We defined the composition of the coastalscape as the proportion of land cover classes (i.e., artificial surfaces, agricultural areas, forest and seminatural areas, wetlands, and water bodies) inside the circular buffers. We conducted a Permutational Analysis of Variance (PERMANOVA hereafter) to compare coastalscape composition of Pelliciera spp. between periods and basins, and between periods and Caribbean regions (Cartagena, Morrosquillo, and Urabá). We used two different land cover layers, comprising the first decade of the 21st century: 2000–2002, and 2010–2012. These layers represent the official benchmark for recent land cover dynamics, also matching the timeframe of most of the Pelliciera spp. records used in this analysis.
Comparison Between Basins
The PERMANOVA showed that coastalscape composition was significantly different between the Caribbean and Pacific basins in northern South America (P ≤ 0.01; Supplementary Figure 1 and Supplementary Table 2), but it was not between periods nested within basins. Moreover, multivariate dispersion was also significantly different between basins (F = 33.67; df1:1; df2:184; P(perm): ≤ 0.01). The proportion of artificial surfaces in the coastalscape was greater for the Caribbean (mean ± SD: 0.172 ± 0.324) than for the Pacific (0.033 ± 0.130). For agricultural areas, there were slight differences between Caribbean (0.064 ± 0.122) and Pacific basins (0.090 ± 0.181). But the proportion of forest and seminatural areas was greater for the Pacific (0.766 ± 0.221) than for the Caribbean (0.610 ± 0.352).
Comparison Among Caribbean Regions
According to PERMANOVA, there were significant differences in coastalscape composition among Caribbean regions (P ≤ 0.01; Figure 2 and Table 1) but not between years nested within regions. Furthermore, there was no homogeneity in the multivariate dispersion between Caribbean regions (P ≤ 0.01), as assessed by PERMDISP. The proportion of artificial surfaces was greater for the coastalscapes in Cartagena ( = 0.3; Median = 0.06; s = 0.39), followed by Urabá ( = 0.01; Med. = 0; s = 0.02). The coastalscapes of Pelliciera spp. in Morrosquillo did not exhibit artificial surfaces. The coastalscapes in Caribbean regions exhibited a reduced proportion of agricultural surfaces [Cartagena ( = 0.01; Med = 0; s = 0.02); Urabá ( = 0; Med = 0; s = 0); Morrosquillo ( = 0.03; Med = 0; s = 0.05)]. Coastalscapes in Urabá and Morrosquillo exhibited greater proportion of forest (Urabá: = 0.92, Med = 0.92, s = 0.05; Morrosquillo: = 0.85, Med = 0.92, s = 0.19) compared to the coastalscapes in Cartagena ( = 0.01; Med = 0; s = 0.02).
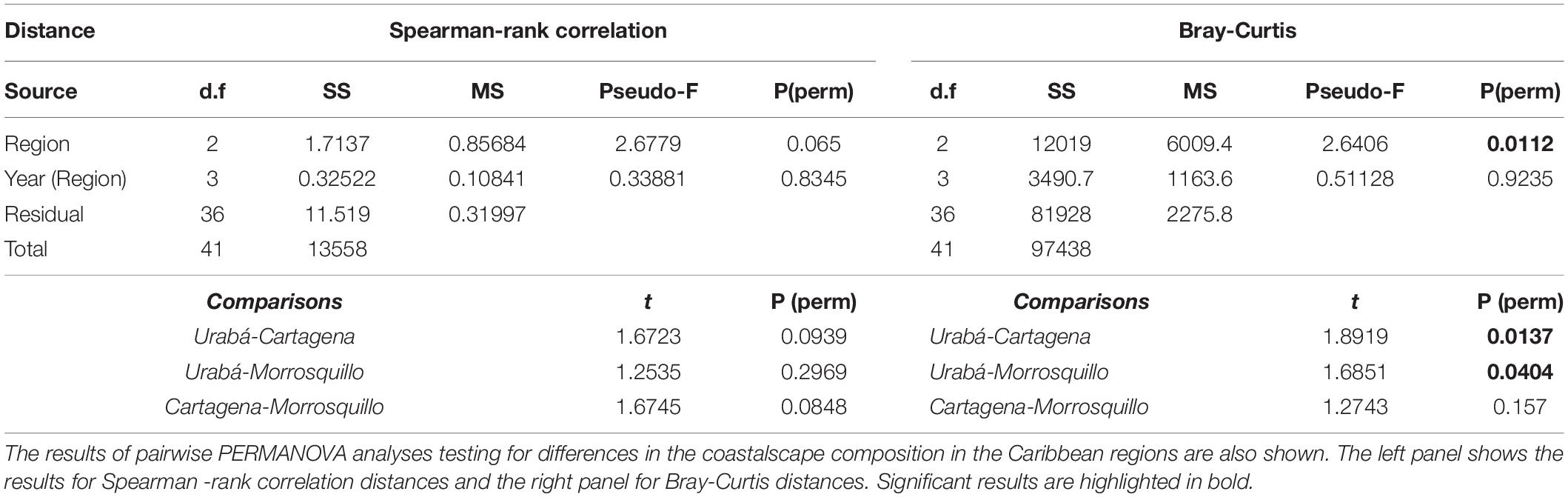
Table 1. Results of PERMANOVA analyses testing for the effects of Region (Cartagena, Morrosquillo and Urabá) and year (2000 and 2010) on coastalscape composition of Pelliciera spp. in the Caribbean coast of Colombia.
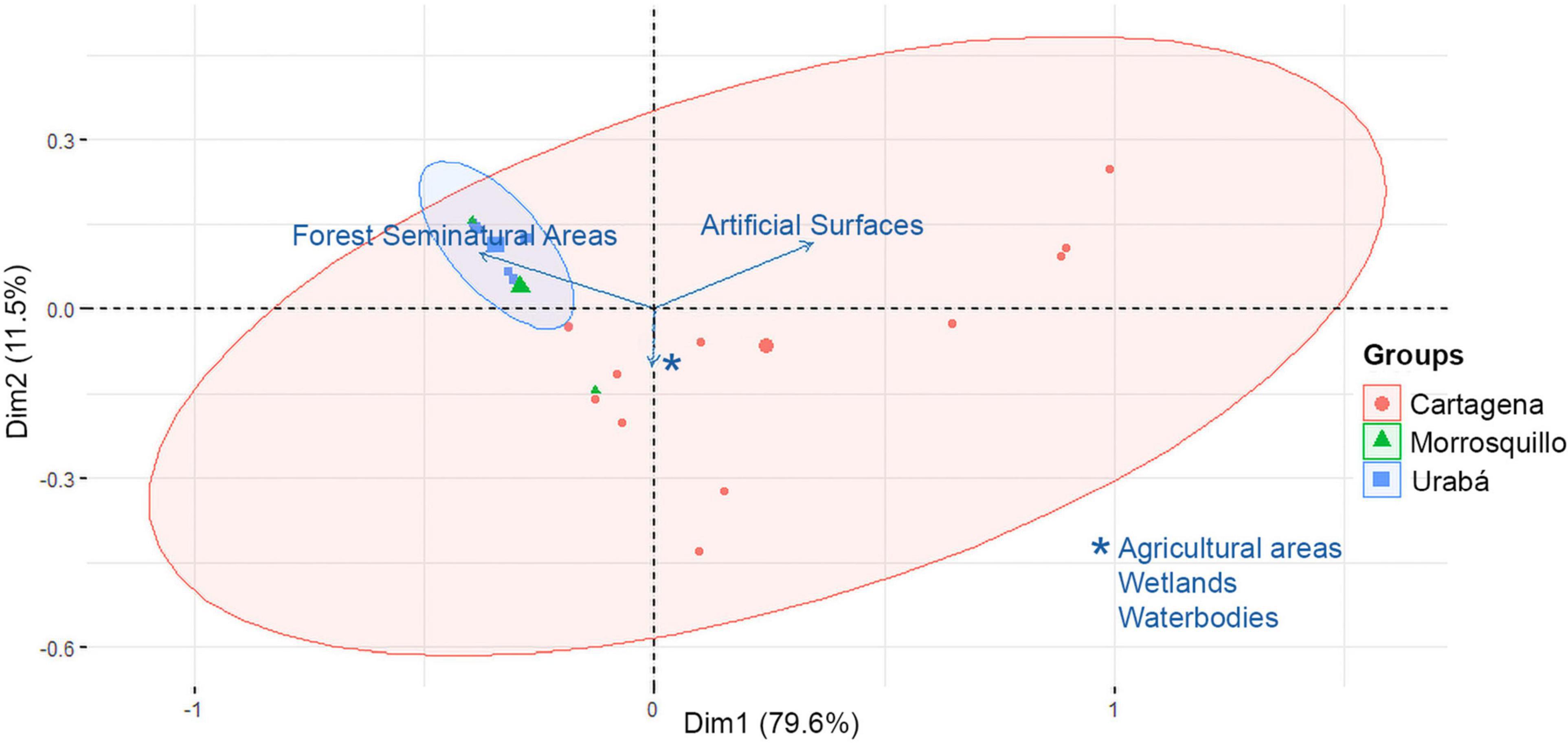
Figure 2. Biplot of principal components analysis (PCA) for the coastalscapes composition of Pelliciera spp. in the three Caribbean regions. Arrows indicate direction of increasing value for each land cover variable.
Urban Index
The extent of urban areas was remarkable in some coastalscapes within each of the three Caribbean regions (see Figure 1 and Supplementary Figure 2). A Local Urban Index (LUI) was computed to indicate an absolute difference among Caribbean regions (Table 2). As expected, Cartagena scored the highest LUI (most urban), due to the greatest road length, transformed area, and artificial surface per square kilometer. Contrary to the expected, Morrosquillo was the least urbanized region, at least within the coastalscapes of Pelliciera spp. In Morrosquillo, occurrences were only reported within or near marine protected areas (Cispatá Bay Regional District of Integrated Management and Boca de Guacamaya Natural Regional Park) but mangroves also occur in the proximity of two small populated centers (Tolú and Coveñas). Low urbanization was expected within the coastalscapes in Urabá, however, the presence of Pelliciera spp. in the vicinity of Turbo, a high-density populated center with a highly compact urbanization, offsets the wild coastalscape observed in the Atrato River Delta where additional presences were recorded (see Supplementary Figure 2). Therefore, the LUI was greater in Urabá than in Morrosquillo.
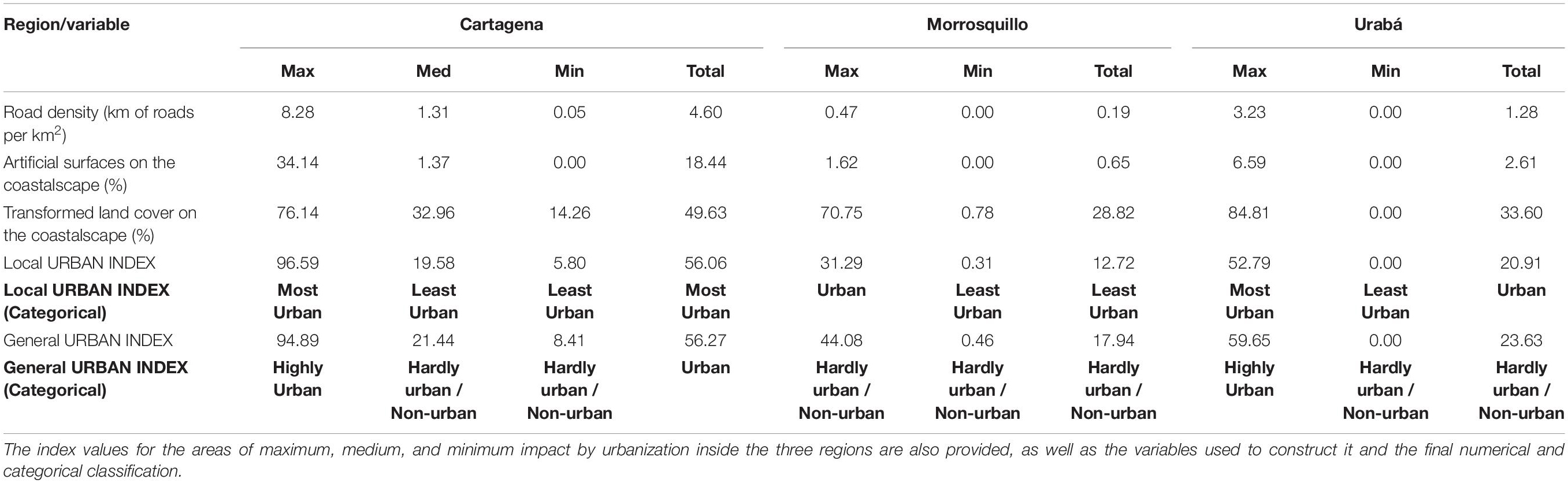
Table 2. Summary of the urban index values for the coastalscapes of Pelliciera spp. in the three occupied coastalscapes in the Colombian Caribbean.
Prior to the analysis, we visually defined areas of maximum and minimum urban extent within each region of the Caribbean. The LUI was consistent with the preliminary classification of minimum and maximum urban extent, except in the Barú Peninsula, where low urbanization rather than high was observed. It was probably because only two small towns are present, and secondary roads are almost absent, while there is only a two-lane primary road connecting the area to Cartagena (boat transportation has been historically prevalent).
We also calculated a General Urban Index that describes the coastalscapes according to theoretical maximums and minimums of anthropogenic land covers in the coastalscape. It classified Cartagena, and Turbo as Highly Urban. Highly compact areas in the seaside within these cities may be comparable to other heavily urbanized areas in the Caribbean region. Coastalscapes in Morrosquillo were classified as Moderately Urban or Not Urban.
Mangrove Habitat Configuration
Comparison Between Basins
For the coastalscapes of 5-km radius, the Mann-Whitney-Wilcoxon tests indicated that median patch area (AREA_MN) was greater for the Pacific than for the Caribbean (Medians = 70 and 15.2 ha, respectively; U = 612, p < 0.01). The total area of mangroves (CA) was greater for the Pacific than for the Caribbean basin (Medians = 2034 and 502 ha, respectively; U = 676, p < 0.05). The core area (CPLAND) with a 100-m edge was greater for the Pacific than for the Caribbean (Medians = 46.7 and 35.8%, respectively; U = 685, p < 0.05). We also found differences in PAFRAC (U = 1027, p < 0.05), being slightly greater in the Caribbean than in the Pacific (Medians: 1.38 and 1.36, respectively), indicating more irregular shapes in the Caribbean (see Supplementary Table 5 for details). At 5 km radius, COHESION was not homoscedastic, and the Mann-Whitney-Wilcoxon tests indicated no significant differences between the Pacific and the Caribbean neither for COHESION, nor for CPLAND (500-m edge) and ENN_MN. For the coastalscapes of 500 m and 1 km in radius, the Mann-Whitney-Wilcoxon test indicated that there were no significant differences between basins for AREA_MN, CA, COHESION, CPLAND (with a 100-m edge) and ENN_MN.
Comparison Among Caribbean Regions
For the coastalscapes of 5-km radius, the Kruskal-Wallis test showed significant differences among regions in the Caribbean in terms of COHESION (Chi square = 6.05, df = 2, p ≤ 0.05), and CPLAND with a 100-m edge (Chi square = 7.29, df = 2, p < 0.05) (see Figure 3 to inspect the boxplots of the metrics significantly different among regions). Pair-wise comparisons showed significant differences between Cartagena and Morrosquillo in terms of both COHESION (Observed difference = 11.12; Critical difference = 10.84) and CPLAND (100-m edge) (observed = 11.97; critical = 10.84). No significant differences were found for AREA_MN, CA, ENN_MN and PAFRAC at this spatial scale (see Table 3 for details). CPLAND (500 m edge) did not meet the assumptions of the test and we did not run pair-wise comparisons. No significant differences were found among the three regions for any metric at smaller spatial scales.
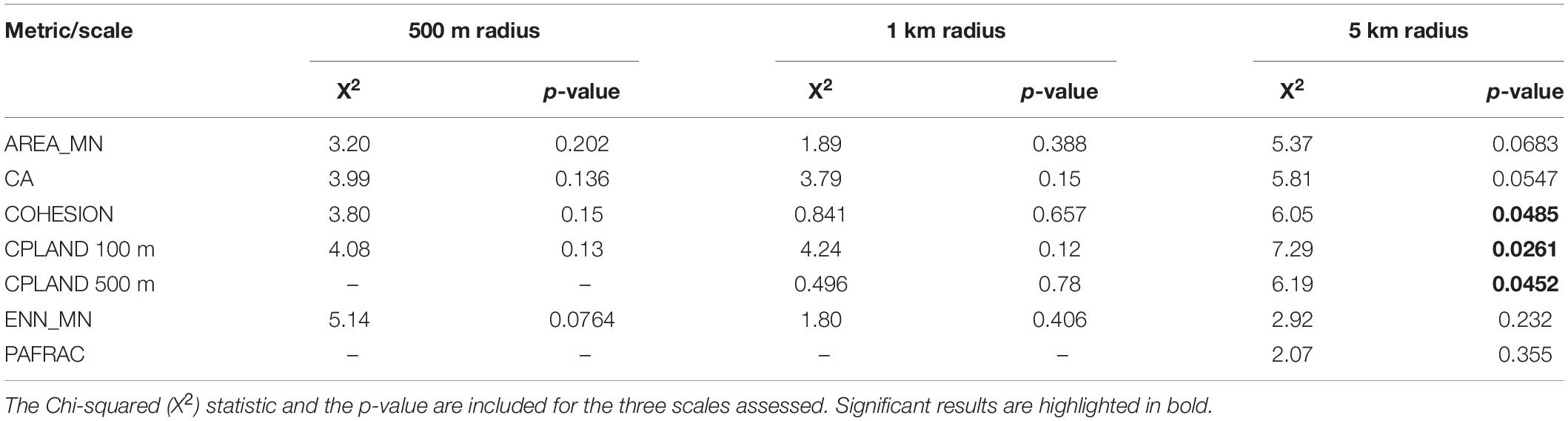
Table 3. Kruskal-Wallis test results comparing coastalscape configuration metrics among the three Caribbean regions (Urabá, Cartagena and Morrosquillo).
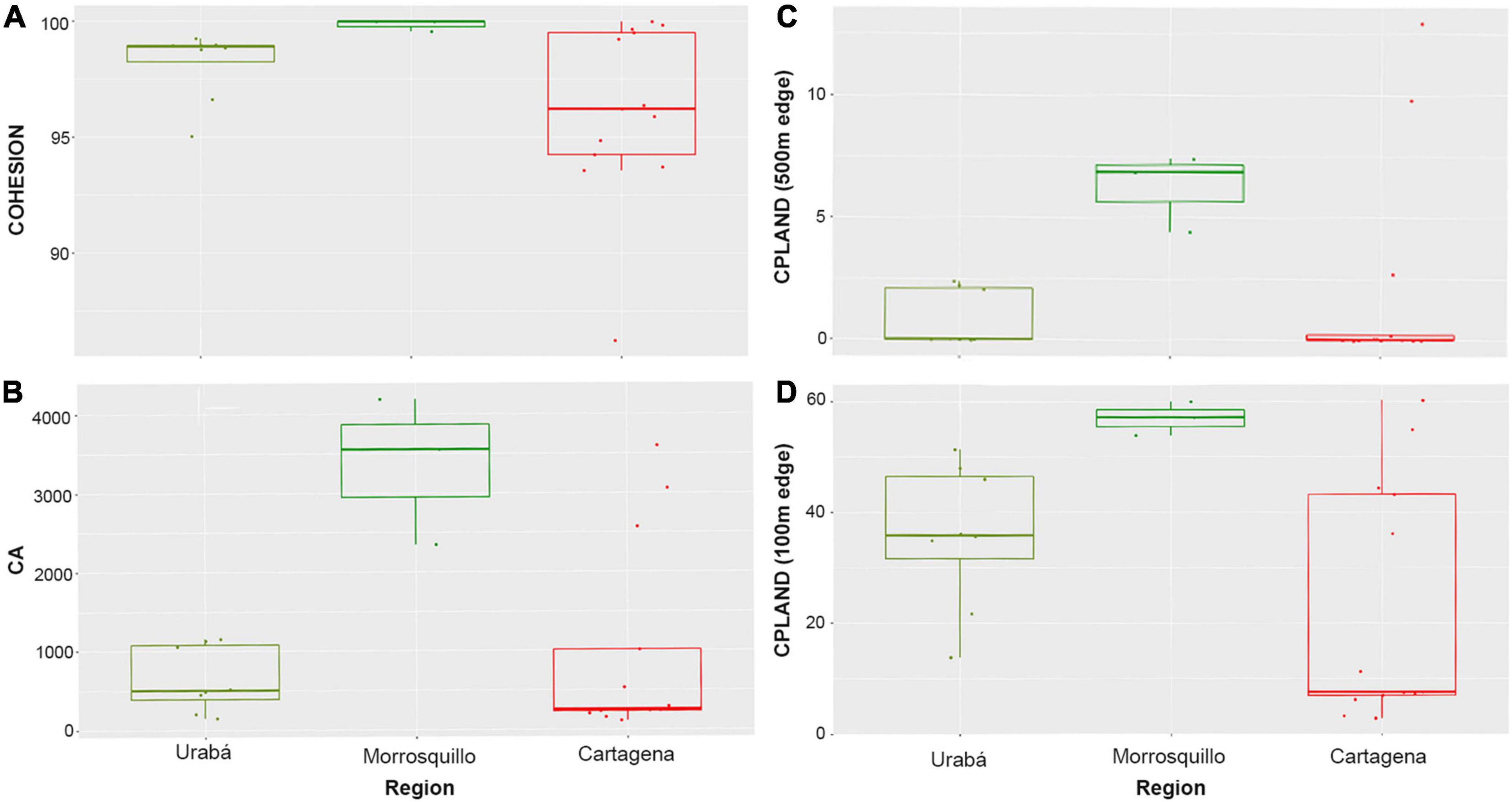
Figure 3. Boxplots for mangrove configuration metrics on the three Caribbean regions (Urabá, Morrosquillo, or Cartagena). The color code follows the anthromes (Ellis et al., 2010). Each panel shows significantly different metrics between regions: (A) Patch Cohesion Index. (B) Mangrove area. (C) Core Area Percentage of Mangrove in the coastalscape with an edge of 500 m. (D) Core Area Percentage of Mangrove in the coastalscape with an edge of 100 m. The coastalscapes are defined as circular buffers of 5 km radius.
Discussion
Coastalscape in the Anthropocene: Human Domination and Urbanization
This study reports the gradient in human-domination along the coasts of northern South America. Our results support previous studies indicating that the Pacific coast of Colombia remains dominated by forested biomes with low extents of agricultural and pastoral lands (Etter et al., 2008; Sánchez-Cuervo et al., 2012). On the contrary, the Caribbean coastalscapes were dominated by extensive rangelands (despite shrimp aquaculture was also observed in the proximity to Cartagena, a pattern consistent with previous national-scale studies; Larsson et al., 1994; Etter et al., 2008; Sánchez-Cuervo et al., 2012). These findings are also consistent with the reports using the anthrome framework (Ellis and Ramankutty, 2008) and the human footprint index for Colombia (Correa-Ayram et al., 2020).
The lack of significant temporal variability in coastalscape composition in the study area suggests a reduction in the deforestation rates and, in cases, an increase in afforestation rates, as observed in Latin America during the first decade of the 21st century (Sánchez-Cuervo et al., 2012; Graesser et al., 2015). Specifically, mangrove deforestation rates worldwide have been slower since the 2000s (Friess et al., 2019; Goldberg et al., 2020). The lack of temporal variation in coastalscape composition may also suggest the reach of an advanced stage in the deforestation cycle where prime lands for agriculture and other types of exploitation have been already transformed and monopolized (Meyfroidt and Lambin, 2011). Besides, further stages in the economic development of different countries in Latin American and the Caribbean, have implied conversion of former agricultural areas into urban and suburban areas, but not a reduction in forest cover (e.g., Grau et al., 2003; López-Marrero et al., 2012).
A significant landmark of the Anthropocene in northern South America is the urban development in various spots along the Caribbean coast. While high scores of local and general urban indices were found in Cartagena and Urabá, additional urban settlements are present within the distribution range of Pelliciera spp., according to the present study. Moreover, if an urban index would be computed for the entire mangrove habitat regardless of the presence of vulnerable species, a south-north gradient would be also evident. On the contrary, there was no urbanization gradient along the Pacific and only two major port cities are found (Buenaventura and Tumaco) but immersed in coastalscapes dominated by forested lands (agriculture to a lower extent) consistent with previous reports (López-Angarita et al., 2018; Fagua et al., 2019). While the low degree of urbanization in the Pacific is an exception of the generality in the Tropics, the compact urbanization in Turbo (Urabá) and Cartagena is more alike to the wider Caribbean. Turbo is the typical case of a small and compact commercial port city that experienced rapid population growth between 1980 and 2000s (like others in the Antilles; Parés-Ramos et al., 2008; López-Marrero et al., 2012). Cartagena is a typical example of a coastal metropolis experiencing rapid urban sprawl into neighbor rural areas, similar to the observed elsewhere in the Caribbean, Brazil, West Africa, and Southeast Asia (Martinuzzi et al., 2009; Nfotabong-Atheull et al., 2013; Friess et al., 2019). Morrosquillo is an example of low density and dispersed urban development (Tolú and Coveñas) found in tourism districts in the Antilles and the Caribbean basin of Central America and Mexico (e.g., Hirales-Cota et al., 2010; Díaz-Gallegos et al., 2011).
Therefore, the spatial template offered by urbanization in the Caribbean basin of South America provides an opportunity for studying the ecological and evolutionary consequences of urbanization on mangrove ecosystems. Despite the biogeographic differences between the Pacific and the Caribbean basins, it would be interesting to study the ecological consequences of specific forms of human domination on the coastalscapes. We recommend focal studies on urban and peri-urban mangroves (e.g., Buenaventura and Tumaco) and coastalscape analyses in the South Pacific where rapid landscape transformation occurred in coastal watersheds since the 2000s as the consequence of armed conflicts and proliferation of illicit cash crops (Quintero-Angel et al., 2021). Finally, we also recommend the use of innovative remote sensing techniques such as city lights and LIDAR for high-resolution analyses of urban sprawl into coastal wetland areas, particularly in Cartagena. The suburbanization and compaction processes in Cartagena seem to be similar to those of large cities in the northern Andes and the Caribbean, where the application of such techniques has been instrumental for improving scientific understanding, nature conservation, and urban planning (Parés-Ramos et al., 2013; Álvarez-Berríos et al., 2013; Martinuzzi et al., 2018; Branoff and Martinuzzi, 2020).
Mangroves as Fragmented Habitats in the Anthropocene
Mangrove configuration, at 5-km radius coastalscapes, was different between basins. The Caribbean exhibited higher fragmentation with a greater fractal dimension of patches and lower median patch size, mangrove area, and core area, as previously reported for terrestrial forests and mangroves worldwide (Taubert et al., 2018; Bryan-Brown et al., 2020). The differences in mangrove configuration related to the degree of human domination in the coastalscapes and could represent differences in the rates and drivers of deforestation in peripheral areas (as in Etter et al., 2006). A greater mangrove area reflects the wild condition of the Pacific coastalscapes and their low deforestation rates (Hamilton and Friess, 2018; López-Angarita et al., 2018; Mejía-Rentería et al., 2018; Simard et al., 2019). Therefore, this study suggests that urbanization translates into a patchy configuration of the remaining mangrove areas.
However, we still need to better understand the patterns derived from the fragmentation process through multi-temporal analyses and high-resolution mapping efforts, which are urgently needed. These efforts would benefit from employing open-access satellite imagery, cloud-computing (e.g., Bhargava et al., 2020), or the available open access multitemporal global layers (e.g., Thomas et al., 2017; Bunting et al., 2018; Bryan-Brown et al., 2020; Richards et al., 2020). Efforts must concentrate around protected, urban and peri-urban areas, as well as on to assess the effectiveness of conservation efforts and to identify threats and deforestation hotspots.
In the Caribbean, the urban intensity was related to higher fragmentation, a pattern previously reported by Blanco-Libreros and Estrada-Urrea (2015) in the Urabá Gulf. The loss rate related to proximity to an urban center and patch density, while patch density and mean patch area were related to human disturbance (Blanco-Libreros and Estrada-Urrea, 2015). Increased patch density, due to trampling and logging, also promoted species shifts at the community level (Blanco et al., 2012; Blanco-Libreros and Estrada-Urrea, 2015). Small-scale deforestation and logging have been reported in the proximity of human settlements in many rural and peri-urban locations world-wide (e.g., Blanco et al., 2012; Nfotabong-Atheull et al., 2013; Palacios and Cantera, 2017; Scales and Friess, 2019). Thus, we recommend field surveys of Pelliciera spp. in different areas along the distribution range, but particularly comparing areas of maximum and minimum urbanization, for understanding the effects of patch geometry and mangrove habitat configuration on demographic variables. We also recommend using the protected versus non-protected contrast to achieve this objective and to further understand the ecological service of habitat provision to these and other species of plants and animals relative to patch size. Finally, we need to better understand why differences in mangrove configuration were not observed at smaller spatial scales.
Potential Effects of Coastalscape Structure on Eco-Evolutionary Dynamics
Mangroves are the most threatened ecosystems across tropical coastalscapes, with the highest urban population density per habitat area, and this trend will seemingly continue until 2050 (McDonald et al., 2013). Urbanization has the potential to alter the quantity, quality, and spatio-temporal arrangement of resources for mangrove plant species and populations, similarly to the observed in other systems (Parris, 2016; Dubois and Cheptou, 2017). A main consequence of the urbanization process is the simultaneous decrease in habitat amount and the increase in habitat fragmentation over time (Liu et al., 2016). Fragmentation usually provides a better descriptor of trait variation than urbanization per se (Dubois and Cheptou, 2017). The main direct effects of fragmentation are the creation of smaller patches and the increased isolation of patches (sensu Wilcove et al., 1986).
In small forest patches, there is less habitat and resources, while more area is susceptible to edge effects, thus supporting smaller populations than large patches (Gascon et al., 2000; Laurance et al., 2002; Hobbs and Yates, 2003). In addition, small mangrove populations are more vulnerable to local extinction caused by environmental and demographic stochastic events and are less likely to be rescued if they are very isolated in areas of high cyclonic activity, such as the Caribbean region (Krauss and Osland, 2020). Besides, increased fragmentation can reduce the survival rate of seedlings, the production, and quality of fruits, and pollinator abundance, even in naturally-patchy habitats such as mangroves and in species such as Pelliciera spp. interacting with winged pollinators (birds, moths, and/or bats; Hermansen et al., 2014, 2017; Duke, 2020). This can result in reduced gene diversity and higher genetic structure in mangrove plant species (Arnaud-Haond et al., 2006). In addition, small Pelliciera populations in the Caribbean (presumably P. benthamii) have low intrapopulation genetic diversity and they are probably affected by genetic drift, inbreeding, and bottlenecks (Castillo-Cárdenas et al., 2012). Genetic drift can reduce the ability of populations to cope with novel environments and small populations can also experience fitness reductions as population size declines (Allee effects; Gilpin and Soule, 1986; Keller and Waller, 2002). Fitness reductions from drift, inbreeding depression, and Allee effects can reduce population size, creating a dangerous feedback loop (Young et al., 1996; Cheptou and Avendaño, 2006; Cheptou et al., 2017).
We propose that mangrove patch shrinking and isolation, due to fragmentation, will interact with environmental changes thus contributing to lower effective population size, affecting birth and death rates, as well as immigration and emigration, for northern South America. The different pathways of interaction are illustrated in our theoretical framework shown in Figure 4. The conceptual framework is partly based on Hobbs and Yates (2003), Parris (2016), and Cheptou et al. (2017), and in a review of the literature on urban mangroves (see Supplementary Table 6 for details). In our study, we used Mean Patch Size (AREA_MN) and Patch Cohesion Index (COHESION) as spatial proxies of decreased patch size and increased patch isolation, respectively. We used Core Area Percentage of Landscape (CPLAND), Mangrove (Class) Area (CA), and Perimeter-Area Fractal Dimension (PAFRAC) as proxies of greater area susceptible to edge effects. We propose that Pelliciera spp. populations in the Caribbean (particularly the recently reported P. benthamii) are at greater risk of extinction due to their low genetic diversity (Castillo-Cárdenas et al., 2012), the small number of propagules that survive each year (Dangremond, 2015), and the high amount of area susceptible to edge effects (smaller total mangrove area, smaller core area, and more irregular patches when compared to the coastalscapes along the Pacific). For populations in Cartagena, we predict the highest susceptibility induced by the smallest core area (related to edge effects) observed across the study area, and their location in the limit of the distribution range (likely having lower gene flow; Eckert et al., 2008; Castillo-Cárdenas et al., 2012).
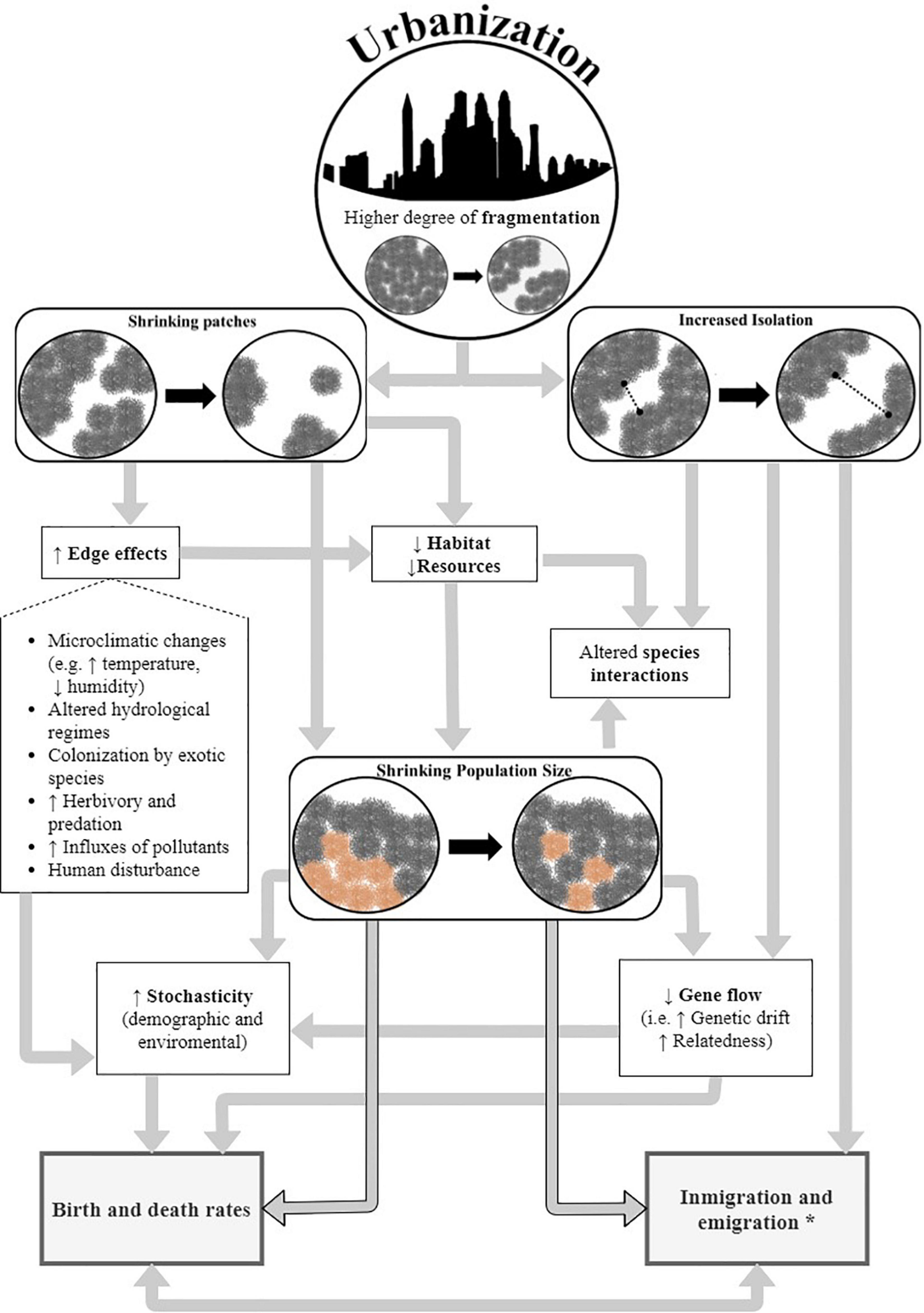
Figure 4. A conceptual framework of urban eco-evolutionary dynamics in the coastalscape of Pelliciera spp. and other endangered mangrove species in urban environments. *Influx and outflux of propagules.
Recommendations for Conservation
• Create monitoring programs for the populations of P. rhizophorae and P. benthamii, primarily in protected areas (e.g., The Sanctuary of Fauna and Flora “El Corchal del Mono Hernández” in southern Cartagena) and in urban and peri-urban areas (e.g., Cartagena and Turbo in the Caribbean, and Tumaco and Buenaventura in the Pacific).
• Include a genetic perspective in mangrove conservation and restoration programs. If fragmentation induces the evolution of life-history trait changes, restoration efforts would benefit from appropriate source populations (i.e., locally adapted, and high dispersive capacity; Cheptou et al., 2017). It is also important to enrich local genetic diversity by artificial propagule translocation, as suggested by Castillo-Cárdenas et al. (2012). However, it is crucial to avoid mixing the genetic material of P. rhizophorae and P. benthamii in restoration efforts, particularly in non-sympatric patches, since the hybrid intermediates are less viable and likely infertile (Duke, 2020).
• Include a landscape perspective in mangrove conservation and restoration programs. Pelliciera spp. would benefit from increasing the size of the existing mangrove patches as they would be exposed to narrow edge effects, and they could potentially support larger populations. It is also important to maximize the area-perimeter ratio of protected patches, to further reduce edge effects (e.g., leaving a natural buffer zone to avoid sharp edges). Creating a network of spatially-continuous protected (or restored) areas would maintain or rebuild connectivity among adjacent populations.
• Elevate regional protected areas with occurrence of Pelliciera spp. to national-level protection categories. While some coastalscapes of Pelliciera spp. in the Caribbean are inside regional protected areas, they are administered by departmental environmental agencies (e.g., Cispatá and Guacamaya in Morrosquillo Gulf). In Colombia, regional protected areas (mixed-use) seem to be ineffective to reduce deforestation rates due to poor law enforcement, but collective lands and national protected areas (strict-use) have been reported to be more effective (López-Angarita et al., 2018; Bonilla-Mejía and Higuera-Mendieta, 2019).
• Declare new protected areas, particularly in the wilderness forests Atrato River Delta in Urabá and in the urban areas of Turbo and Cartagena. The Atrato River Delta exhibits the longest mangrove coastline where discrete populations of Pelliciera spp. (likely P. rhizophorae) thrive seemingly isolated from others along the Caribbean coasts of Colombia and Panamá. Other populations within the Urabá Gulf in urban and rural settings also need urgent protection from illegal logging and mangrove clearing (Blanco-Libreros et al., 2016). P. benthamii is known to occur in less than 15 sites and it likely occurs in Cartagena’s urban mangroves (Duke, 2020), therefore setting urban conservation schemes may reduce the local extinction risk by combining private, government, and citizen efforts.
• Finally, we urge the IUCN Mangrove Specialist Group and the Colombian Ministry of Environment to assess the conservation status and risk of extinction of P. rhizophorae and P. benthamii. It is urgent to implement a management plan for the species, particularly in Colombia. Moreover, it is important to evaluate how Pelliciera spp. would be affected by regional climate change, invasions by exotic species, and the occurrence of multiple human disturbances within the entire geographical range of distribution. Species distribution modeling and population genetics studies are urgently needed for the only neotropical endemic mangrove genus before this eco-evolutionary unique is locally or regionally lost.
Materials and Methods
Study Location
The study area covered the Pacific and Caribbean coasts of Colombia (1°23′29.4″N – 12°27′30.2″N, 71°07′18.6″W – 79°00′31.8″W), the area of distribution of Pelliciera spp. (Planchon and Triana Silva, 1863) in northern South America (Figure 1). Presence records of Pelliciera spp. were collected from the Colombian National Mangrove Assessment (HELIO_SP.CO v1; Blanco-Libreros and Álvarez-León, 2018), the Global Biodiversity Information Facility (GBIF.org, 2020), technical reports, regional experts, and scientific papers (For a detailed description of the occurrence data used and sources, see Supplementary Table 1). The presence records were georeferenced using QGIS Desktop 3.10.7. Only one record per square kilometer was chosen randomly to reduce spatial autocorrelation. A total of 107 records of Pelliciera spp. were compiled: 82 in the Pacific and 25 in the Caribbean.
Since the Caribbean basin exhibits a greater human-domination in the coastalscapes (Álvarez-León and Polanía-Vorenberg, 1996; Álvarez-León, 2003), the occurrences of Pelliciera spp. were pooled into three Caribbean regions (Urabá Gulf, Morrosquillo Gulf and two bays in the vicinity of Cartagena City). Within the three regions of the Caribbean, areas of maximum and minimum urban extension were defined to quantitatively evaluate urbanization as a driver of mangrove change, as proposed by Branoff (2020).
Coastalscape Composition and Dynamics
The composition of the coastalscapes was calculated using National Land Cover maps (Scale 1:100,000) for two different periods 2000–2002 and 2010–2012 (IDEAM, 2010b, 2014; available in http://www.siac.gov.co/catalogo-de-mapas). These vector layers are based on the Corine Land Cover Methodology adapted for Colombia and they included five land cover classes: (1) artificial surfaces (including ponds for marine and continental aquaculture), (2) agricultural areas, (3) forest and seminatural areas, (4) wetlands and (5) water bodies.
The coastalscapes were defined as circular buffers of 1 km radius, centered on the presence points of the species. The land cover layers were rasterized using a pixel size of 60 m × 60 m. R 4.0.2 (R Core Team, 2020) and the landscapemetrics package (Hesselbarth et al., 2019) were used to calculate the absolute and relative extent (CA and PLAND) of Forest and seminatural areas as proxies of potential habitat for Pelliciera spp., and Artificial surfaces and Agricultural areas as proxies of anthropogenic drivers of loss and degradation. The wetlands category was not used as a proxy of potential habitat for Pelliciera spp. because the floristic composition of wetlands comprises Pleustophyta, Rizophyta, and Haptophyta but not true mangrove plant species, according to the layer authors (IDEAM, 2010a).
A PERMANOVA (Anderson, 2001; McArdle and Anderson, 2001) was performed to test for differences in the composition of the Pelliciera coastalscapes between basins (the Caribbean versus Pacific) or among Caribbean regions (Urabá, Morrosquillo, and Cartagena). In addition, years (2000 versus 2010) were nested within basins or regions, respectively. Pairwise comparisons were employed when significant differences were detected. PERMDISP was used to detect differences in multivariate dispersions among groups. Bray-Curtis similarity index and Spearman rank correlation were used as proximity metrics, and the analysis was conducted in PERMANOVA+ for PRIMER v7 (Anderson et al., 2008; Clarke and Gorley, 2015).
Quantifying Urbanization
Spatial datasets used for quantification of urban variables and the urban indexes are described in the Supplementary Table 3. All spatial analyses were performed in QGIS Desktop 3.10.7 using Group Stats plugin (QGIS Development Team, 2020; Szostok et al., 2020). The urban index proposed by Branoff (2020) was used to calculate a Local Urban Index (LUI) and a General Urban Index (GUI) for the three regions in the Caribbean (Cartagena, Morrosquillo and Urabá), and in the areas of maximum and minimum urban extent within each one. The urban indices are a representation of the relative intensity of urbanization, in which 100 is the most urbanized site and 1 is the least urbanized site. The index was calculated using the following equation:
where n is the number of variables used in the index and Yi represents the variables normalized to a range of 0 to 100 through the following equation in which Y and X represent the normalized and raw values, respectively:
For the LUI, we included road density, percentage of transformed land cover and artificial surfaces in the coastalscapes. The variables were normalized according to the distribution of the data. For the GUI, we excluded road density, and the variables were normalized according to an ideal distribution with a maximum of 100 and a minimum of 0. We expected to have a measurement of urbanization allowing comparison in the degree of urbanization of the Pelliciera spp. coastalscapes in our study area, with those in other urbanized coastalscapes within the range of occurrence. The regions were also classified into categories based on their quartiles of the urban index. For the LUI, the “Most Urban” sites were those with an urban index greater than the third quartile, “Urban” sites were those in the interquartile range and the “Least Urban” sites were those with an urban index lower than the first quartile. For the GUI, the “Highly Urban” sites were those with an urban index greater than the third quartile (more than 75), “Urban” sites were those in the interquartile range (between 25 and 75) and the “Hardly urban or Non-urban” sites were those with an urban index lower than the first quartile (less than 25).
Habitat Configuration of Pelliciera spp.
The coastalscapes were defined as circular buffers of 500-m, 1-km, or 5-km radius to assess mangrove habitat configuration for Pelliciera spp. A pixel size of 35 × 35 m was used to calculate fragmentation metrics at the mangrove class, using the national mangrove layer. This layer was mapped from 2005 to 2009, with scales ranging from 1: 10,000 to 1: 50,000 (INVEMAR, 2009; available in https://gis.invemar.org.co/arcgis/rest/services/SIGMA/MANGLARES_COLOMBIA/MapServer).
All spatial and statistical analyses were conducted using R version 4.0.2 (R Core Team, 2020) using rgeos, rgdal, raster and landscapemetrics (Hesselbarth et al., 2019; Bivand and Rundel, 2020; Robert, 2020). PAFRAC, ENN_MN and AREA_MN were calculated, following Bryan-Brown et al. (2020), using Queen’s case contiguity when needed. CA, COHESION and CPLAND (with edges of 100 m and 500 m) were also calculated. Differences in configuration between Pacific and Caribbean basins were explored through Mann-Whitney test with wilcox.test function in R. In testing differences between Most Urban, Urban, and Least urban sites in the Caribbean, defined by the Local Urban Index, the Kruskal-Wallis rank sum test was used through the kruskal.test function of base R. The subsequent post-hoc differences were identified through Multiple Comparison Test After Kruskal-Wallis by the kruskalmc function of pgirmess package (Giraudoux, 2018).
Data Availability Statement
The original contributions presented in the study are included in the article/Supplementary Material, further inquiries can be directed to the corresponding author/s.
Author Contributions
JB-L conceived this manuscript. KR-R assembled the database, conducted statistical analyses, and constructed figures and tables with input from JB-L. Both authors analyzed the data and wrote the manuscript.
Conflict of Interest
The authors declare that the research was conducted in the absence of any commercial or financial relationships that could be construed as a potential conflict of interest.
Acknowledgments
The authors are thankful to María Fernanda Peña, Carlos Reyes, Faidith Bracho Altamiranda, Ricardo Álvarez-León, and Juan Luis Parra for discussions on theoretical and methodological aspects of the study. This article reports partial results of the honors thesis by KR-R. The authors greatly acknowledge the valuable comments provided by the two reviewers that improved significantly the manuscript. To the memory of Heliodoro Sánchez-Páez.
Supplementary Material
The Supplementary Material for this article can be found online at: https://www.frontiersin.org/articles/10.3389/fmars.2021.670354/full#supplementary-material
References
Aguayo, M. I., Wiegand, T., Azócar, G. D., Wiegand, K., and Vega, C. E. (2007). Revealing the driving forces of mid-cities urban growth patterns using spatial modeling: a case study of Los Ángeles. Chile. Ecol. Soc. 12:13.
Alberti, M. (2015). Eco-evolutionary dynamics in an urbanizing planet. Trends Ecol. Evol. 30, 114–126. doi: 10.1016/j.tree.2014.11.007
Alberti, M., Correa, C., Marzluff, J. M., Hendry, A. P., Palkovacs, E. P., Gotanda, K. M., et al. (2017). Global urban signatures of phenotypic change in animal and plant populations. Proc. Natl. Acad. Sci. U.S.A. 114, 8951–8956. doi: 10.1073/pnas.1606034114
Alberti, M., Palkovacs, E. P., Roches, S. D., Meester, L. D., Brans, K. I., Govaert, L., et al. (2020). The complexity of urban eco-evolutionary dynamics. Bioscience 70, 772–793. doi: 10.1093/biosci/biaa079
Aldana-Domínguez, J., Montes, C., Martínez, M., Medina, N., Hahn, J., and Duque, M. (2017). Biodiversity and ecosystem services knowledge in the Colombian caribbean. Trop. Conserv. Sci. 10:194008291771422. doi: 10.1177/1940082917714229
Álvarez-Berríos, N. L., Parés-Ramos, I. K., and Aide, T. M. (2013). Contrasting patterns of urban expansion in Colombia, Ecuador, Peru, and Bolivia between 1992 and 2009. Ambio 42, 29–40. doi: 10.1007/s13280-012-0344-8
Álvarez-León, R. (2003). Los manglares de Colombia y la recuperación de sus áreas degradadas: revisión bibliográfica y nuevas experiencias. Rev. Madera Bosques. 9, 3–25. doi: 10.21829/myb.2003.911286
Álvarez-León, R., and Polanía-Vorenberg, J. H. (1996). Los manglares del Caribe colombiano: síntesis de su conocimiento. Rev. Acad. Colomb. Ciencias Exact. Físicas Nat. 20, 447–464.
Anderson, M. J. (2001). A new method for non-parametric multivariate analysis of variance. Aust. Ecol. 26, 32–46. doi: 10.1111/j.1442-9993.2001.01070.pp.x
Anderson, M. J., Gorley, R. N., and Clarke, K. R. (2008). PERMANOVA+ for PRIMER: Guide to Software and Statistical Methods.
Arnaud-Haond, S., Teixeira, S., Massa, S. I., Billot, C., Saenger, P., Coupland, G., et al. (2006). Genetic structure at range edge: Low diversity and high inbreeding in Southeast Asian mangrove (Avicennia marina) populations. Mol. Ecol. 15, 3515–3525. doi: 10.1111/j.1365-294X.2006.02997.x
Bhargava, R., Sarkar, D., and Friess, D. A. (2020). A cloud computing-based approach to mapping mangrove erosion and progradation: Case studies from the Sundarbans and French Guiana. Estuar. Coast. Shelf Sci. 248:106798. doi: 10.1016/j.ecss.2020.106798
Blanco-Libreros, J., and Álvarez-León, R. (2018). HELIO_SP.CO v.1: Hierarchical, Entity-based and Landscape-level Information Observatory for mangrove SPecies in Colombia, version 1. doi: 10.7910/DVN/GGLRXW
Blanco-Libreros, J., and Estrada-Urrea, E. (2015). Mangroves on the edge: anthrome-dependent fragmentation influences ecological condition (Turbo, Colombia, Southern Caribbean). Diversity 7, 206–228. doi: 10.3390/d7030206
Blanco-Libreros, J. F., and Álvarez-León, R. (2019). Mangroves of Colombia revisited in an era of open data, global changes, and socio-political transition: homage to Heliodoro Sánchez-Páez. Rev. Acad. Ciencias Exact. Fis. Nat. 43, 84–97. doi: 10.18257/raccefyn.780
Blanco-Libreros, J. F., Estrada-Urrea, E. A., Pérez-Montalvo, R. J., Taborda-Marín, A., and Álvarez-León, R. (2016). Anthropic influence on the landscape of Pelliciera rhizophorae (Ericales: Tetrameristaceae) Southern-most Caribbean populations (Turbo, Colombia). Rev. Biol. Trop. 64, 79–94.
Blanco, J. F., Estrada, E. A., Ortiz, L. F., and Urrego, L. E. (2012). Ecosystem-wide impacts of deforestation in mangroves: the urabá Gulf (Colombian Caribbean) case study. Int. Sch. Res. Not. 2012, 1–14. doi: 10.5402/2012/958709
Bonilla-Mejía, L., and Higuera-Mendieta, I. (2019). Protected areas under weak institutions: evidence from Colombia. World Dev. 122, 585–596. doi: 10.1016/j.worlddev.2019.06.019
Branoff, B. L. (2017). Quantifying the influence of urban land use on mangrove biology and ecology: a meta-analysis. Glob. Ecol. Biogeogr. 26, 1339–1356. doi: 10.1111/geb.12638
Branoff, B. L. (2020). The role of urbanization in the flooding and surface water chemistry of Puerto Rico’s mangroves. Hydrol. Sci. J. 65, 1326–1343. doi: 10.1080/02626667.2020.1747620
Branoff, B. L., and Martinuzzi, S. (2020). The structure and composition of Puerto Rico’s urban mangroves. Forests 11:1119. doi: 10.3390/f11101119
Bryan-Brown, D. N., Connolly, R. M., Richards, D. R., Adame, F., Friess, D. A., and Brown, C. J. (2020). Global trends in mangrove forest fragmentation. Sci. Rep. 10:7117. doi: 10.1038/s41598-020-63880-1
Bunting, P., Rosenqvist, A., Lucas, R., Rebelo, L.-M., Hilarides, L., Thomas, N., et al. (2018). The Global Mangrove Watch—a new 2010 global baseline of mangrove extent. Remote Sens. 10:1669. doi: 10.3390/rs10101669
Castillo-Cárdenas, M. F., Toro-Perea, N., and Cárdenas-Henao, H. (2005). Population genetic structure of neotropical mangrove species on the Colombian Pacific coast: Pelliciera rhizophorae (Pellicieraceae). Biotropica 37, 266–273. doi: 10.1111/j.1744-7429.2005.00036.x
Castillo-Cárdenas, M. F., Díaz-González, F., and Toro-Perea, N. (2012). Low genetic diversity within Caribbean patches of Pelliciera rhizophorae, a neotropical mangrove species with reduced distribution. Aquat. Bot. 96, 48–51. doi: 10.1016/j.aquabot.2011.09.011
Castillo-Cárdenas, M. F., Díaz-Gonzales, F., Cerón-Souza, I., Sanjur, O., and Toro-Perea, N. (2015a). Jumping a geographic barrier: diversification of the mangrove species Pelliciera rhizophorae (Tetrameristaceae) across the central american isthmus. Tree Genet. Genomes 11, 822. doi: 10.1007/s11295-014-0822-1
Castillo-Cárdenas, M. F., Ramirez-Silva, J. A., Sanjur, O., and Toro-Perea, N. (2015b). Evidence of incipient speciation in the neotropical mangrove Pelliciera rhizophorae (Tetrameristaceae) as revealed by molecular, morphological, physiological and climatic characteristics. Bot. J. Linn. Soc. 179, 499–510. doi: 10.1111/boj.12337
Castillo-Cárdenas, M. F., Sanjur, O., and Toro-Perea, N. (2016). Differences in sculpture and size of pollen grains: new morphological evidence of diversification in Pelliciera rhizophorae, an ancient neotropical mangrove species. Palynology 40, 302–307. doi: 10.1080/01916122.2015.1045050
Cheptou, P.-O., Hargreaves, A. L., Bonte, D., and Jacquemyn, H. (2017). Adaptation to fragmentation: evolutionary dynamics driven by human influences. Phil. Trans. R. Soc. B 372:20160037. doi: 10.1098/rstb.2016.0037
Cheptou, P. O., and Avendaño, V. L. G. (2006). Pollination processes and the Allee effect in highly fragmented populations: Consequences for the mating system in urban environments. New Phytol. 172, 774–783. doi: 10.1111/j.1469-8137.2006.01880.x
Cornejo, X., and Bonifaz, C. (2020). Pelliciera benthamii (Tetrameristaceae): New status and lectotypification of an overlooked neotropical mangrove. Harvard Pap. Bot. 25, 47. doi: 10.3100/hpib.v25iss1.2020.n5
Correa-Ayram, C. A., Etter, A., Díaz-Timoté, J., Rodríguez Buriticá, S., Ramírez, W., and Corzo, G. (2020). Spatiotemporal evaluation of the human footprint in Colombia: Four decades of anthropic impact in highly biodiverse ecosystems. Ecol. Indic. 117:106630. doi: 10.1016/j.ecolind.2020.106630
Dangremond, E. M. (2015). Propagule predation by crabs limits establishment of an endemic mangrove. Hydrobiologia 755, 257–266. doi: 10.1007/s10750-015-2238-9
Díaz-Gallegos, J., Acosta-Velázquez, J., Rodríguez-Zúñiga, M., Cruz, I., Vázquez-Lule, A. D., Troche, C., et al. (2011). “The mangrove forests of mexico: transformation, conservation and threats,” in Frontiers in Biodiversity Studies, eds D. Thangadurai, S. Jayabalan, C. A. Busso, and L. G. Abarca Arena (Daryaganj: I. K International), 15.1–15.32.
Dubois, J., and Cheptou, P. O. (2017). Effects of fragmentation on plant adaptation to urban environments. Philos. Trans. R. Soc. B Biol. Sci. 372, 20160038. doi: 10.1098/rstb.2016.0038
Duke, N., Ball, M., and Ellison, J. (1998). Factors influencing biodiversity and distributional gradients in mangroves. Glob. Ecol. Biogeogr. Lett. 7, 27–47. doi: 10.1111/J.1466-8238.1998.00269.X
Duke, N. C. (2020). A systematic revision of the vulnerable mangrove genus Pelliciera (Tetrameristaceae) in equatorial America. Blumea 65, 107–120. doi: 10.3767/blumea.2020.65.02.04
Eckert, C. G., Samis, K. E., and Lougheed, S. C. (2008). Genetic variation across species’ geographical ranges: the central-marginal hypothesis and beyond. Mol. Ecol. 17, 1170–1188. doi: 10.1111/j.1365-294X.2007.03659.x
Ellis, E. C., and Ramankutty, N. (2008). Putting people in the map: Anthropogenic biomes of the world. Front. Ecol. Environ. 6:439–447. doi: 10.1890/070062
Ellis, E. C., Klein Goldewijk, K., Siebert, S., Lightman, D., and Ramankutty, N. (2010). Anthropogenic transformation of the biomes, 1700 to 2000. Glob. Ecol. Biogeogr. 19, 589–606. doi: 10.1111/j.1466-8238.2010.00540.x
Ellison, A., Farnsworth, E., and Moore, G. (2010). Pelliciera rhizophorae. IUCN Red List Threat. Species 2010:8235.
Etter, A., McAlpine, C., Phinn, S., Pullar, D., and Possingham, H. (2006). Unplanned land clearing of Colombian rainforests: Spreading like disease? Landsc. Urban Plan. 77, 240–254. doi: 10.1016/j.landurbplan.2005.03.002
Etter, A., McAlpine, C., and Possingham, H. (2008). Historical patterns and drivers of landscape change in Colombia since 1500: A regionalized spatial approach. Ann. Assoc. Am. Geogr. 98, 2–23. doi: 10.1080/00045600701733911
Etter, A., and van Wyngaarden, W. (2000). Patterns of landscape transformation in Colombia, with emphasis in the andean region. AMBIO A J. Hum. Environ. 29, 432–439. doi: 10.1579/0044-7447-29.7.432
Fagua, J. C., Baggio, J. A., and Ramsey, R. D. (2019). Drivers of forest cover changes in the Chocó-Darien global ecoregion of South America. Ecosphere 10:e02648. doi: 10.1002/ecs2.2648
Fernandes, M. R., Aguiar, F. C., and Ferreira, M. T. (2011). Assessing riparian vegetation structure and the influence of land use using landscape metrics and geostatistical tools. Landsc. Urban Plan. 99, 166–177. doi: 10.1016/j.landurbplan.2010.11.001
Friess, D. A., Rogers, K., Lovelock, C. E., Krauss, K. W., Hamilton, S. E., Lee, S. Y., et al. (2019). The state of the World’s mangrove forests: Past, present, and future. Annu. Rev. Environ. Resour. 44, 1–27. doi: 10.1146/annurev-environ-101718-033302
Gascon, C., Williamson, G. B., and da Fonseca, G. A. B. (2000). Receding forest edges and vanishing reserves. Science 288, 1356–1358. doi: 10.1126/science.288.5470.1356
GBIF.org (2020). GBIF Occurrence Download. Available online at : https://doi.org/10.15468/dl.hhgdjs. (accessed February 11, 2020).
Gilpin, M. E., and Soule, M. E. (1986). “Minimum viable populations: processes of species extinction,” in Conservation Biology: The Science of Scarcity and Diversity, eds M. E. Gilpin and M. E. Soule (Sunderland, MA: Sinsuer Associates. Inc).
Giraudoux, P. (2018). pgirmess: Spatial analysis and data mining for field ecologists R package version 1.6.9. https://CRAN.R-project.org/package=pgirmess
Goldberg, L., Lagomasino, D., Thomas, N., and Fatoyinbo, T. (2020). Global declines in human-driven mangrove loss. Glob. Chang. Biol. 26, 5844–5855. doi: 10.1111/gcb.15275
Graesser, J., Aide, T. M., Grau, H. R., and Ramankutty, N. (2015). Cropland/pastureland dynamics and the slowdown of deforestation in Latin America. Environ. Res. Lett. 10:34017. doi: 10.1088/1748-9326/10/3/034017
Grau, H. R., Aide, T. M., Zimmerman, J. K., Thomlinson, J. R., Helmer, E., and Zou, X. (2003). The ecological consequences of socioeconomic and land-use changes in postagriculture Puerto Rico. Bioscience 53, 1159–1168. doi: 10.1641/0006-3568(2003)053[1159:tecosa]2.0.co;2
Hale, S. S., Paul, J. F., and Heltshe, J. F. (2004). Watershed landscape indicators of estuarine benthic condition. Estuaries 27, 283–295. doi: 10.1007/BF02803385
Hamilton, S. E., and Friess, D. A. (2018). Global carbon stocks and potential emissions due to mangrove deforestation from 2000 to 2012. Nat. Clim. Chang. 8, 240–244. doi: 10.1038/s41558-018-0090-4
Hansen, M. C., Wang, L., Song, X. P., Tyukavina, A., Turubanova, S., Potapov, P. V., et al. (2020). The fate of tropical forest fragments. Sci. Adv. 6, 8574–8585. doi: 10.1126/sciadv.aax8574
Hayashi, S. N., Souza-Filho, P. W. M., Nascimento, W. R., and Fernandes, M. E. B. (2019). The effect of anthropogenic drivers on spatial patterns of mangrove land use on the Amazon coast. PLoS One 14:e0217754. doi: 10.1371/journal.pone.0217754
Hermansen, T. D., Ayre, D. J., and Minchinton, T. E. (2014). Effects of stand size on pollination in temperate populations of the mangrove Avicennia marina. Plant Ecol. 215, 1153–1162. doi: 10.1007/s11258-014-0374-3
Hermansen, T. D., Minchinton, T. E., and Ayre, D. J. (2017). Habitat fragmentation leads to reduced pollinator visitation, fruit production and recruitment in urban mangrove forests. Oecologia 185, 221–231. doi: 10.1007/s00442-017-3941-1
Hermansen, T. D., Roberts, D. G., Toben, M., Minchinton, T. E., and Ayre, D. J. (2015). Small urban stands of the mangrove Avicennia marina are genetically diverse but experience elevated inbreeding. Estuar. Coasts 38, 1898–1907. doi: 10.1007/s12237-015-9955-1
Hesselbarth, M. H. K., Sciaini, M., With, K. A., Wiegand, K., and Nowosad, J. (2019). Landscapemetrics: an open-source R tool to calculate landscape metrics. Ecography (Cop.). 42, 1648–1657. doi: 10.1111/ecog.04617
Hirales-Cota, M., Espinoza-Avalos, J., Schmook, B., Ruiz-Luna, A., and Ramos-Reyes, R. (2010). Drivers of mangrove deforestation in Mahahual–Xcalak, Quintana roo, Southeast Mexico. Ciencias Mar. 36, 147–159. doi: 10.7773/cm.v36i2.1653
Hobbs, R. J., and Yates, C. J. (2003). Impacts of ecosystem fragmentation on plant populations: Generalising the idiosyncratic. Aust. J. Bot. 51, 471–488. doi: 10.1071/BT03037
IDEAM (2010a). Legend for National Land Cover: CORINE Land Cover Methodology adapted for Colombia. Scale 1: 100,000 (in Spanish). Bogotá, D. C: Instituto de Hidrología, Meteorología y Estudios Ambientales.
IDEAM (2010b). Land Cover Map of Colombia 2000-2002 (in Spanish). Available online at: http://www.siac.gov.co/catalogo-de-mapas (accessed November 10, 2019).
IDEAM (2014). Land Cover Map of Colombia 2010-2012 (in Spanish). Available online at: http://www.siac.gov.co/catalogo-de-mapas (accessed November 10, 2019).
IDEAM, Instituto Alexander von Humboldt, IGAC, INVEMAR, and Ministerio de Ambiente y Desarrollo Sostenible (2017). Map of Continental, Marine and Coastal Ecosystems of Colombia. Version 2.1, Scale 1:100.000 (in Spanish). Bogota D. C: Instituto de Hidrología, Meteorología y Estudios Ambientales.
INVEMAR (2009). Mangroves of Colombia layer (in Spanish). Available online at: https://gis.invemar.org.co/arcgis/rest/services/SIGMA/MANGLARES_COLOMBIA/MapServer (accessed February 7, 2020).
Jimenez, J. A. (1984). A Hypothesis to explain the reduced distribution of the mangrove Pelliciera rhizophorae Tr. & Pl. Biotropica 16:304. doi: 10.2307/2387939
Keller, L. F., and Waller, D. M. (2002). Inbreeding effects in wild populations. Trends Ecol. Evol. 17, 230–241. doi: 10.1016/S0169-5347(02)02489-8
Kirwan, M. L., and Megonigal, J. P. (2013). Tidal wetland stability in the face of human impacts and sea-level rise. Nature 504, 53–60. doi: 10.1038/nature12856
Krauss, K. W., and Osland, M. J. (2020). Tropical cyclones and the organization of mangrove forests: a review. Ann. Bot. 125, 213–234. doi: 10.1093/aob/mcz161
Larsson, J., Folke, C., and Kautsky, N. (1994). Ecological limitations and appropriation of ecosystem support by shrimp farming in Colombia. Environ. Manage 18:663. doi: 10.1007/BF02394632
Laurance, W. F., Camargo, J. L. C., Luizão, R. C. C., Laurance, S. G., Pimm, S. L., Bruna, E. M., et al. (2011). The fate of Amazonian forest fragments: A 32-year investigation. Biol. Conserv. 144, 56–67. doi: 10.1016/j.biocon.2010.09.021
Laurance, W. F., Lovejoy, T. E., Vasconcelos, H. L., Bruna, E. M., Didham, R. K., Stouffer, P. C., et al. (2002). Ecosystem decay of Amazonian forest fragments: a 22-year investigation. Conserv. Biol. 16, 605–618. doi: 10.1046/j.1523-1739.2002.01025.x
Liu, Z., He, C., and Wu, J. (2016). The relationship between habitat loss and fragmentation during urbanization: An empirical evaluation from 16 world cities. PLoS One 11:e0154613. doi: 10.1371/journal.pone.0154613
López-Angarita, J., Roberts, C. M., Tilley, A., Hawkins, J. P., and Cooke, R. G. (2016). Mangroves and people: Lessons from a history of use and abuse in four Latin American countries. For. Ecol. Manage 368, 151–162. doi: 10.1016/j.foreco.2016.03.020
López-Angarita, J., Tilley, A., Hawkins, J. P., Pedraza, C., and Roberts, C. M. (2018). Land use patterns and influences of protected areas on mangroves of the Eastern Tropical Pacific. Biol. Conserv. 227, 82–91. doi: 10.1016/j.biocon.2018.08.020
López-Marrero, T., Yamane, K., Heartsill Scalley, T., and Villanueva Colón, N. (2012). The various shapes of the insular Caribbean: Population and environment. Caribb. Stud. 40, 17–37. doi: 10.1353/crb.2012.0024
Martinuzzi, S., Gould, W. A., Lugo, A. E., and Medina, E. (2009). Conversion and recovery of Puerto Rican Mangroves: 200 years of change. For. Ecol. Manage. 257, 75–84. doi: 10.1016/j.foreco.2008.08.037
Martinuzzi, S., Ramos-González, O. M., Muñoz-Erickson, T. A., Locke, D. H., Lugo, A. E., and Radeloff, V. C. (2018). Vegetation cover in relation to socioeconomic factors in a tropical city assessed from sub-meter resolution imagery. Ecol. Appl. 28, 681–693. doi: 10.1002/eap.1673
McArdle, B. H., and Anderson, M. J. (2001). Fitting multivariate models to community data: a comment on distance-based redundancy analysis. Ecology 82, 290–297. doi: 10.1890/0012-9658(2001)082[0290:fmmtcd]2.0.co;2
McDonald, R. I., Marcotullio, P. J., and Güneralp, B. (2013). “Urbanization and global trends in biodiversity and ecosystem services,” in Urbanization, Biodiversity and Ecosystem Services: Challenges and Opportunities: A Global Assessment, eds T. Elmqvist et al. (Netherlands: Springer), 31–52. doi: 10.1007/978-94-007-7088-1_3
Mejía-Rentería, J. C., Castellanos-Galindo, G. A., Cantera-Kintz, J. R., and Hamilton, S. E. (2018). A comparison of Colombian Pacific mangrove extent estimations: implications for the conservation of a unique Neotropical tidal forest. Estuar. Coast. Shelf Sci. 212, 233–240. doi: 10.1016/j.ecss.2018.07.020
Meyfroidt, P., and Lambin, E. F. (2011). Global forest transition: Prospects for an end to deforestation. Annu. Rev. Environ. Resour. 36, 343–371. doi: 10.1146/annurev-environ-090710-143732
Millán-Aguilar, O., Nettel-Hernanz, A., Hurtado-Oliva, M. Á, Dodd, R. S., Flores-Cárdenas, F., and Manzano-Sarabia, M. (2019). Landscape metrics and conservation status of five mangrove wetlands in the eastern Gulf of California margin. J. Coast. Res. 36:94. doi: 10.2112/jcoastres-d-18-00060.1
Nfotabong-Atheull, A., Din, N., and Dahdouh-Guebas, F. (2013). Qualitative and quantitative characterization of mangrove vegetation structure and dynamics in a peri-urban setting of Douala (Cameroon): an approach using air-borne imagery. Estuar. Coasts 36, 1181–1192. doi: 10.1007/s12237-013-9638-8
Numbere, A. O. (2018). “The impact of oil and gas exploration: Invasive nypa palm species and urbanization on mangroves in the niger river delta, Nigeria,” in Threats to Mangrove Forests. Coastal Research Library, eds C. Makowski and C. Finkl (Cham: Springer), 247–266. doi: 10.1007/978-3-319-73016-5_12
Open StreetMap Contributors (2020). OpenStreetMap. Planet Dump. Availablity online at: https://planet.osm.org (accessed August 28, 2020).
Palacios, M. L., and Cantera, J. R. (2017). Mangrove timber use as an ecosystem service in the Colombian Pacific. Hydrobiologia 803, 345–358. doi: 10.1007/s10750-017-3309-x
Parés-Ramos, I., Álvarez-Berríos, N., and Aide, T. M. (2013). Mapping urbanization dynamics in major cities of Colombia, Ecuador, Perú, and Bolivia using night-time satellite imagery. Land 2, 37–59. doi: 10.3390/land2010037
Parés-Ramos, I., Gould, W. A., and Aide, T. M. (2008). Suburban growth and forest expansion following agricultural abandonment in Puerto Rico (1991-2000). Ecol. Soc. 13, 1–24.
Parris, K. M. (2016). “Population- and species-level responses to urbanization,” in Ecology of Urban Environments, (Hoboken NJ: John Wiley & Sons Ltd), 41–80.
Planchon, J.-É, and Triana Silva, J. J. (1863). Prodromus Florae Novo-Granatensis [Phanérogamie]. Paris: Victor Masson et fils, Place de l’École-de-Médecine Imprimerie de E. Martinet, rue Mignon. 2.
Polidoro, B. A., Carpenter, K. E., Collins, L., Duke, N. C., Ellison, A. M., Ellison, J. C., et al. (2010). The loss of species: Mangrove extinction risk and geographic areas of global concern. PLoS One 5:e10095. doi: 10.1371/journal.pone.0010095
QGIS Development Team, (2020). QGIS Geographic Information System. Open Source Geospatial Foundation Project. http://qgis.osgeo.org
Quintero-Angel, M., Coles, A., and Duque-Nivia, A. A. (2021). A historical perspective of landscape appropriation and land use transitions in the Colombian South Pacific. Ecol. Econ. 181:106901. doi: 10.1016/j.ecolecon.2020.106901
R Core Team. (2020). R: A language and environment for statistical computing. R Foundation for Statistical Computing, Vienna, Austria. https://www.R-project.org/
Robert J. Hijmans (2020). raster: Geographic data analysis and modeling. R package version 3.3-6. Available online at: https://CRAN.R-project.org/package=raster
Richards, D. R., Thompson, B. S., and Wijedasa, L. (2020). Quantifying net loss of global mangrove carbon stocks from 20 years of land cover change. Nat. Commun. 11:4260. doi: 10.1038/s41467-020-18118-z
Saenger, P., Ragavan, P., Sheue, C.-R., López-Portillo, J., Yong, J. W. H., and Mageswaran, T. (2019). “Mangrove Biogeography of the Indo-Pacific,” in Sabkha Ecosystems Tasks for Vegetation Science, eds B. Gul, B. Böer, M. Khan, M. Clüsener-Godt, and A. Hameed (Cham: Springer), 379–400. doi: 10.1007/978-3-030-04417-6_23
Sánchez-Cuervo, A. M., Aide, T. M., Clark, M. L., and Etter, A. (2012). Land cover change in colombia: Surprising forest recovery trends between 2001 and 2010. PLoS One 7, 1–14. doi: 10.1371/journal.pone.0043943
Sandoval-Londoño, L. A., Leal-Flórez, J., and Blanco-Libreros, J. F. (2020). Linking mangroves and fish catch: a correlational study in the southern Caribbean Sea (Colombia). Bull. Mar. Sci. 96, 415–429. doi: 10.5343/bms.2019.0022
Scales, I. R., and Friess, D. A. (2019). Patterns of mangrove forest disturbance and biomass removal due to small-scale harvesting in southwestern Madagascar. Wetl. Ecol. Manag. 27, 609–625. doi: 10.1007/s11273-019-09680-5
Simard, M., Fatoyinbo, L., Smetanka, C., Rivera-Monroy, V. H., Castañeda-Moya, E., Thomas, N., et al. (2019). Mangrove canopy height globally related to precipitation, temperature and cyclone frequency. Nat. Geosci. 12, 40–45. doi: 10.1038/s41561-018-0279-1
Szostok, R., Jurgiel, B., and Spångmyr, H. (2020). Group Stats: Stats and analysis for vector layers data for QGIS 3. https://plugins.qgis.org/plugins/GroupStats/ (accessed July 15, 2020).
Taubert, F., Fischer, R., Groeneveld, J., Lehmann, S., Müller, M. S., Rödig, E., et al. (2018). Global patterns of tropical forest fragmentation. Nature 554, 519–522. doi: 10.1038/nature25508
Thomas, N., Lucas, R., Bunting, P., Hardy, A., Rosenqvist, A., and Simard, M. (2017). Distribution and drivers of global mangrove forest change, 1996-2010. PLoS One 12, 1–14. doi: 10.1371/journal.pone.0179302
Tuholske, C., Tane, Z., López-Carr, D., Roberts, D., and Cassels, S. (2017). Thirty years of land use/cover change in the Caribbean: Assessing the relationship between urbanization and mangrove loss in Roatán, Honduras. Appl. Geogr. 88, 84–93. doi: 10.1016/j.apgeog.2017.08.018
Urrego, L. E., Correa-Metrio, A., and González-Arango, C. (2018). Colombian Caribbean mangrove dynamics: Anthropogenic and environmental drivers. Bol. Soc. Geol. Mex. 70, 133–145. doi: 10.18268/BSGM2018v70n1a8
Uuemaa, E., Antrop, M., Roosaare, J., Marja, R., and Mander, Ü (2009). Landscape metrics and indices: an overview of their use in landscape research. Living Rev. Landsc. Res. 3, 1–28. doi: 10.12942/lrlr-2009-1
Wilcove, D. S., McLellan, C. L., and Dobson, A. P. (1986). “Habitat fragmentation in the temperate zone,” in Conservation Biology: The Science of Scarcity and Diversity, ed. M. E. Soulé (Sunderland, MA: Sinsuer Associates. Inc), 237–256.
Young, A., Boyle, T., and Brown, T. (1996). The population genetic consequences of habitat fragmentation for plants. Trends Ecol. Evol. 11, 413–418. doi: 10.1016/0169-5347(96)10045-8
Zamprogno, G. C., Tognella, M. M. P., Quaresma, V., da, S., da Costa, M. B., Pascoalini, S. S., et al. (2016). The structural heterogeneity of an urbanised mangrove forest area in southeastern Brazil: Influence of environmental factors and anthropogenic stressors. Brazilian J. Oceanogr. 64, 157–171. doi: 10.1590/S1679-87592016111706402
Keywords: Pelliciera rhizophorae, urban mangroves, Colombia, coastalscape composition, habitat configuration metrics, Piñuelo Mangrove, Pelliciera benthamii
Citation: Blanco-Libreros JF and Ramírez-Ruiz K (2021) Threatened Mangroves in the Anthropocene: Habitat Fragmentation in Urban Coastalscapes of Pelliciera spp. (Tetrameristaceae) in Northern South America. Front. Mar. Sci. 8:670354. doi: 10.3389/fmars.2021.670354
Received: 21 February 2021; Accepted: 06 May 2021;
Published: 02 June 2021.
Edited by:
Guillem Chust, Technological Center Expert in Marine and Food Innovation (AZTI), SpainReviewed by:
Emily Dangremond, Roosevelt University, United StatesNorman C. Duke, James Cook University, Australia
Copyright © 2021 Blanco-Libreros and Ramírez-Ruiz. This is an open-access article distributed under the terms of the Creative Commons Attribution License (CC BY). The use, distribution or reproduction in other forums is permitted, provided the original author(s) and the copyright owner(s) are credited and that the original publication in this journal is cited, in accordance with accepted academic practice. No use, distribution or reproduction is permitted which does not comply with these terms.
*Correspondence: Juan F. Blanco-Libreros, anVhbi5ibGFuY29AdWRlYS5lZHUuY28=
†These authors have contributed equally to this work