- 1Coastal Survey Solutions LLC, Lajas, PR, United States
- 2Reef Research Inc., Lajas, PR, United States
Mesophotic coral ecosystems (MCEs) are ecologically and functionally vital, as they are Essential Fish Habitats that function as refugia for corals and sponges of shallow-water reefs. Stony Coral Tissue Loss Disease (SCTLD) is a relatively new lethal coral disease, first affecting coral reefs in Florida and has now spread through most of the Caribbean. SCTLD was observed in Puerto Rico in December 2019 in Culebra Island. Since then, SCTLD has appeared along the east coast of Puerto Rico, affecting primarily shallow reefs in San Juan, Culebra and Vieques Island, and Fajardo. During late June and July 2020, four mesophotic reef habitats were surveyed at El Seco (off Vieques Island), on the southeast coast of Puerto Rico. SCTLD was observed at colonized pavement (CPRT – 23–30 m), bank coral reef (BCR – 35–40 m), patch coral reef (PCR – 36–42 m), and rhodolith (Rhodo – 40–50 m) habitats. The mean percent substrate cover by sessile-benthic categories varied significantly between habitats (PERMANOVA, p < 0.001), with a higher mean (± SE) coral cover at BCR (26.95 ± 5.60%), followed by PCR (12.88 ± 3.88%). SCTLD was detected in all habitats, but the disease prevalence was significantly higher at BCR, ranging from 9.70 to 21.13% of colonies infected (Kruskal-Wallis ANOVA, p < 0.007). Even though PCR habitats exhibited less coral cover, SCTLD prevalence was still elevated ranging from 6.66 to 15.07%. The deepest record of SCTLD at El Seco was 40.9 m. The majority (∼98%) of the corals infected with the disease were from the Orbicella complex spp. (faveolata/franksi). However, there were other infected species, such as Agaricia grahamae, A. lamarcki, Montastraea cavernosa, and Porites astreoides. As seen in the surveys conducted in 2011 and 2020, the loss of coral cover allows for the emergence of other benthic “detractors,” such as peyssonnelids, specifically Ramicrusta spp. Ramicrusta spp., an aggressive encrusting red alga known to take over available space and overgrow corals, significantly increased its substrate cover at the impacted reefs. Therefore, the severity and virulence of SCTLD will most likely have severe and long-lasting negative impacts on the coral communities at El Seco mesophotic reef system.
Introduction
Mesophotic Coral Ecosystems (MCEs) are light-depending coral communities occurring in the deepest half of the photic zone from 30 to 150 m in sub-tropical and tropical regions (Hinderstein et al., 2010). The US Caribbean’s MCEs are divided into two broad categories, low-gradient platforms composed of insular shelves, banks and seamounts and high gradient slopes (Armstrong and Singh, 2012; Sherman et al., 2019). High diversity of fish, corals, sponges, and cryptofauna in MCEs occur at rocky discontinuities (outcrops, ledges, etc.) and along the upper depth zone (Pyle and Copus, 2019; Sherman et al., 2019; Artim et al., 2020). These ecosystems are prime residential, foraging, mating, nesting, and seasonal spawning aggregation habitats for a wide variety of commercially valuable fishes and shellfishes (García-Sais et al., 2020).
Mesophotic coral ecosystems are logistically challenging to monitor (Sherman et al., 2013), given their depth range and distance from shore. Therefore, unlike their shallow-water counterparts, there is a lack of information on the distribution and the prevalence and incidence of disturbances at mesophotic depths (Rogers et al., 2015; Andradi-Brown et al., 2016; Weil, 2019). MCEs were once thought to be more stable than shallow-water coral (Lesser et al., 2009; Bridge et al., 2011; Slattery et al., 2011; Armstrong and Singh, 2012; Reardon, 2018). However, recent Caribbean studies show that MCEs are as vulnerable to disturbances such as bleaching, hurricanes and diseases (Calnan et al., 2008; Bongaerts et al., 2010, 2013; Smith et al., 2010, 2019; Rocha et al., 2018; Bongaerts and Smith, 2019; Weil, 2019; García-Sais et al., 2020; Chaves-Fonnegra et al., 2021) as their shallow-water counterparts.
Mesophotic coral ecosystems are impacted by several different coral diseases such as black band, dark spot, white plague, and yellow blotch/band (Calnan et al., 2008; Smith et al., 2008; Andradi-Brown et al., 2016; Reed et al., 2019; Chaves-Fonnegra et al., 2021). The most documented disease on Caribbean MCEs is white plague (WP). Chaves-Fonnegra et al. (2021) observed WP prevalence to be higher on the upper mesophotic coral reefs than shallow-water reefs in the US Virgin Islands. The high prevalence of WP at 30–40 m was due to the high abundance of corals (“hosts”) at the bank habitat (Smith et al., 2010), and environmental factors, such as nutrients and temperature. Many WP outbreaks at MCEs seemed to be positively associated with high thermal anomalies (Menza et al., 2007; Weil, 2019). Corals more susceptible to diseases at MCEs are Agaricia lamarcki, A. agaricites, Mycetophyllia aliciae, Montastraea cavernosa, Stephanocoenia intersepta, Siderastrea siderea, Orbicella franksi, Porites astreoides, A. undata, and A. grahamae (Smith et al., 2010, 2019; Weil and Rogers, 2011; Appeldoorn et al., 2019).
Stony Coral Tissue Loss Disease (SCTLD), a new and aggressive coral disease, was first reported off the coast in southeastern Florida in 2014 (Precht et al., 2016; Muller et al., 2020), with regional coral losses ranging from 30 to 60% (Walton et al., 2018). Since then, SCTLD has spread throughout the Caribbean, affecting more than 20 scleractinian coral species (National National Oceanic and Atmospheric Administration [NOAA], 2018). In Puerto Rico, SCTLD was first reported at shallow reefs in Culebra Island in 2019 (Weil et al., 2019) and is now present in Vieques Island, Fajardo, San Juan, Salinas and Ponce. Originally, SCTLD was first described as a WP disease outbreak (Precht et al., 2016; Walton et al., 2018). However, the gross morphological features of the SCTLD differ from WP, as lesions usually start in the middle of the colonies, not at the base or margin (Aeby et al., 2019; Florida Department of Environmental Protection, 2019). Also, SCTLD is considered a more serious disease because it infects a higher number of species. The rates of lesion progression are fast, and the percent survival of infected species is low (Walton et al., 2018). Coral loss from SCTLD has been high in the Caribbean, with 46 and 62% loss recorded in Cozumel and Turks and Caicos, respectively (Estrada-Saldívar et al., 2020; Heres et al., 2021).
The majority of SCTLD observations in the Caribbean are from shallow-water reefs. There is only one published report (Bloomberg and Holstein, 2021) and further anecdotal observations confirming severe SCTLD infections at an MCE, Marine Conservation District (MCD) in the US Virgin Islands (AGRRA, 2019). In late June and July 2020, high disease prevalence was observed during the monitoring of El Seco Reef, an MCE off the southeast coast of Puerto Rico. Photographs of the diseased corals were sent to several disease experts who confirmed the identification as SCTLD. In this study, we report the SCTLD prevalence between habitats and coral species at El Seco Reef. In addition, we analyzed differences in benthic composition at stations surveyed in 2011 and 2020. Understanding the long-term changes might aid in the future management of this essential mesophotic ecosystem.
Materials and Methods
Site Description
El Seco Reef, a submerged ridge located at the southeastern edge of the Vieques shelf, is the largest continuous coral reef habitat known in Puerto Rico with a surface area of (at least) 3.7 km2. The ridge has an elliptical shape and runs along a north-south axis and rises from the basin at depths of 33–36 m to a mostly flat hard ground reef top at depths of 23–28 m (Figure 1). The mesophotic coral reef system ends as patch reef spurs separated by coralline sand pools at depths between 40 and 45 m. Within the 30–50 m depth range, El Seco Reef system consists of at least four main (biologically colonized) benthic habitats: bank coral reef, patch coral reef, rhodolith beds, and colonized pavement (García-Sais et al., 2011). The colonized pavement habitat was estimated to cover approximately 1.6 km2 or 14.0% of the surveyed area. It was described as a highly heterogeneous seafloor with extensive low-relief hard bottom areas with turf algae and interspersed barrel sponges. Rocky outcrops and low ridges were colonized by scleractinian corals, gorgonians and another reef biota. The bank coral reef habitat was an impressive continuous (aggregated) formation of scleractinian corals, with 17 species identified. However, the coral communities were mainly comprised (84%) of continuous, thick laminar growth forms of Orbicella faveolata/franksi. (hereafter Orbicella complex spp.), growing at depths of 33–41 m throughout the northern and northeastern sections of the study area. The total surface area of the bank coral reef was estimated as 4.6 km2, or 30% of the total surveyed area. Patch coral reefs were separated by coralline sand/coral rubble channels and pools covering an estimated surface area of 1.5 km2, or 10.0% of the surveyed area. Rhodolith habitat was mostly comprised of a flat, homogeneous benthic habitat characterized by deposits of algal rhodolith nodules. Rhodolith habitat was reported along the entire western section of the study area at depths between 35–50 m. Algal rhodoliths were colonized by benthic algae, scleractinian corals, sponges and another encrusting reef biota. The areal extension of the rhodolith habitat was estimated at 7.1 km2, or 48.6% of the surveyed area.
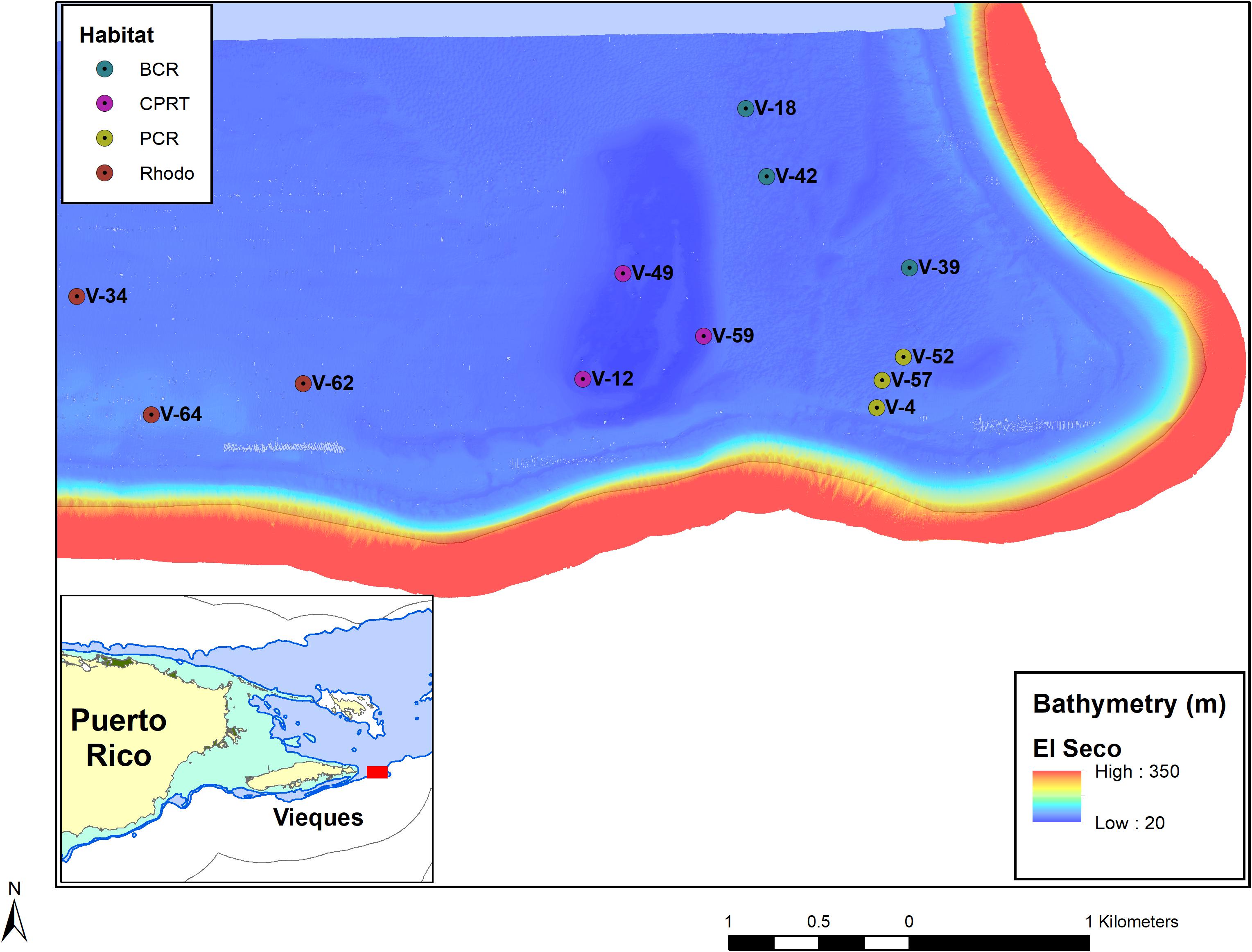
Figure 1. A map of the stations at El Seco Reef, off southeast Vieques Island, Puerto Rico. The stations are labeled and color coded with the different habitats. BCR, bank coral reef; CPRT, colonized pavement; PCR, patch coral reef and Rhodo: rhodolith bed habitat.
Benthic Surveys
The sampling approach and methodologies of the 2020 monitoring survey of El Seco followed the field protocols designed for the original baseline characterizations of sessile-benthic communities in 2011 (García-Sais et al., 2011). Data on the percent reef substrate cover by sessile-benthic categories, including benthic algae, cyanobacteria, stony corals, soft corals, black corals, sponges, and abiotic substrates, were produced from 10 m long photo-transects of the seafloor at stations previously characterized during baseline surveys at each site. Six photo-transects were surveyed from each of the main benthic habitats within the 25–50 m depth range. At least ten non-overlapping photos were taken along transect lines separated approximately 0.5 m from the substrate using a Nikon 500 camera with an Aquatica housing. There was a total of 330 photographs analyzed and the approximate area of each photograph was 1 m2. The digital images of the seafloor were uploaded and analyzed with Coral Point Count (CPCe) software. An array of 25 random points was overlaid on each photo image, and the underlying sessile-benthic categories were identified to species or the lowest taxonomic unit possible. The percent substrate cover was calculated as the fraction of total points overlaid on each species/benthic category divided by the total points overlaid on each transect and multiplied by 100. In addition to calculating coral cover, we calculated the coral abundance in each photograph. Disease prevalence was calculated by the total amount of diseased colonies divided by the total number of stony corals in the photograph. Coral richness was defined by the number of coral species identified in the habitat.
Statistics
Two-way distance Permutational Multivariate Analyses of Variance (PERMANOVA) tests were performed to compare the difference of benthic composition and coral community structure between habitats during 2020 surveys and sampling years (2011, 2020). The data (Supplementary Tables 1, 2) were square-root transformed before any statistical analyses. Each PERMANOVA procedure was based on Bray-Curtis similarity measures. Principal Coordinate Analyses (PCO) were run to visualize the differences of coral community structure between habitats in 2020 surveys and between years at each habitat. Vectors overlaid on the PCO were based on Pearson Correlations. Similarity percentage analysis (SIMPER) with a cut-off of 70%, was carried out to identify the dissimilarities of coral composition between the years and habitats.
A two-way PERMANOVA test was performed to examine the differences in hard coral cover between habitats and sampling years. Lastly, one-way PERMANOVA was performed to examine the difference of Ramicrusta spp. cover between habitats during 2020 surveys. Since these are univariate dependent variables, a similarity matrix based on Euclidean distances was used. Euclidean distance measures for univariate PERMANOVA analyses produce sums-of-square estimates equivalent to parametric ANOVA (Anderson, 2001). PERMANOVA pair-wise assessments were carried out when significant differences were observed. All PERMANOVA SIMPER and PCO procedures were performed using PRIMER-e and PERMANOVA add-on software (Anderson et al., 2008).
A Kruskal-Wallis ANOVA was conducted to examine the differences in disease prevalence between habitats for the 2020 surveys (Supplementary Table 3). Prevalence was calculated by the number of diseased coral colonies divided by the total amount of coral colonies per photograph. This analysis was run in Statistica v7 software. In addition, a one-way PERMANOVA test was performed to assess the differences in disease prevalence between the different coral species at each of the habitats.
Results
Stony Coral Cover
Hard coral cover was significantly higher at BCR than the other habitats in the 2020 monitoring survey (Figure 2), with station means (± SE) ranging from 9.77 ± 0.51% at station 39 to 41.71 ± 0.54% at station 42. PCR displayed the second-highest coral cover (Figure 2), ranging from 1.95 ± 0.96% at station 4 to 20.32 ± 6.19% at station 52. Coral cover was low at CPRT and Rhodo. This same pattern was observed during the 2011 surveys. The magnitude of coral reduction was dependent on habitat type. PCR was the only habitat that did not display a significant decrease in coral cover between the years (Table 1), while at the BCR habitat, the coral cover was significantly reduced by 38.55% between 2011 and 2020.
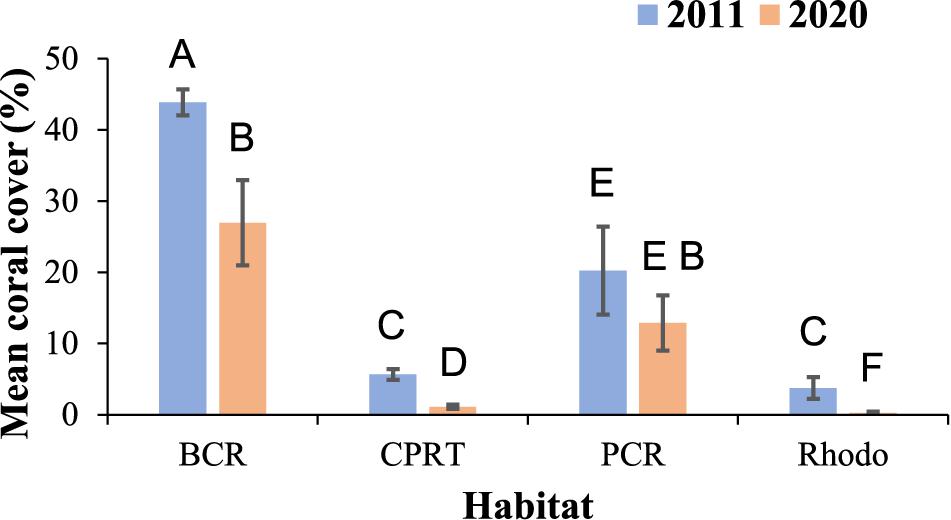
Figure 2. Temporal variations of the mean cover of coral cover (%) at El Seco mesophotic habitats. Bars denote standard errors. BCR, bank coral reef; CPRT, colonized pavement; PCR, patch coral reef and Rhodo: rhodolith bed habitat. Letters signify differences according to the pairwise PERMANOVA test.
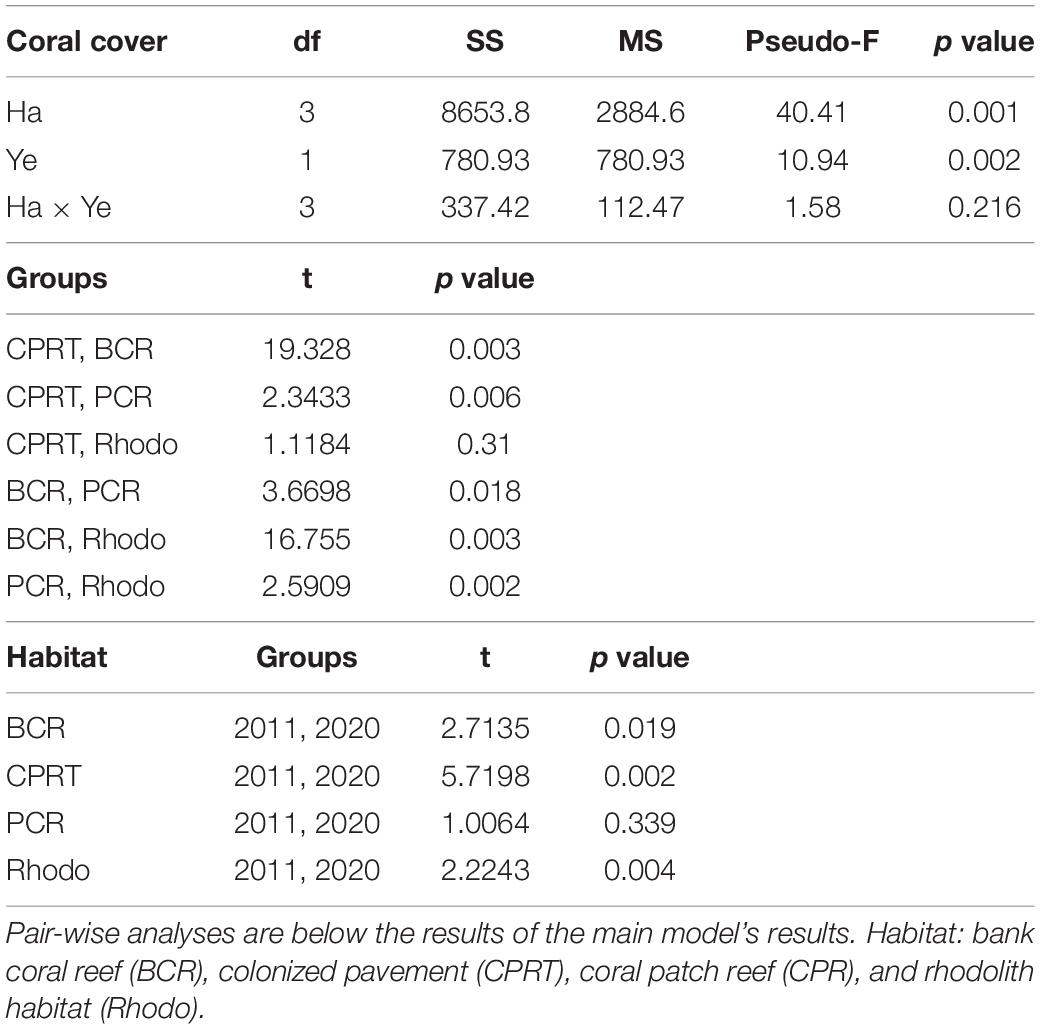
Table 1. The results of the Permutational Multivariate Analyses of Variance (PERMANOVA) tests to compare the differences of coral cover between habitats (Ha) and sampling year (Ye) at El Seco Reef, Puerto Rico.
Hard Coral Composition
A total of 16 stony corals species were identified at El Seco during the 2020 surveys. The BCR habitat exhibited the highest coral richness with 12 species, followed by 10 species at CPRT, 10 species at PCR and 4 species at Rhodo. The taxonomic composition of hard corals varied significantly between habitats during the 2020 surveys (Figure 3; Table 2 and Supplementary Table 1). Orbicella complex spp. dominated the coral composition at BCR (mean: 23.24 ± 6.35%), contributing 86% of the relative coral cover (Table 3). Even though there was a reduction in the cover of Orbicella complex spp. at BCR between 2011 and 2020, the coral community composition did not change. The only habitat that exhibited coral community composition changes between sampling years was CPRT. The two corals that contributed to this dissimilarity between years were Montastraea cavernosa and Orbicella complex spp., contributing 16.86 and 11.40%, respectively (SIMPER). In 2020, Orbicella complex spp. declined in cover by 99.97%, and M. cavernosa was absent from surveys.
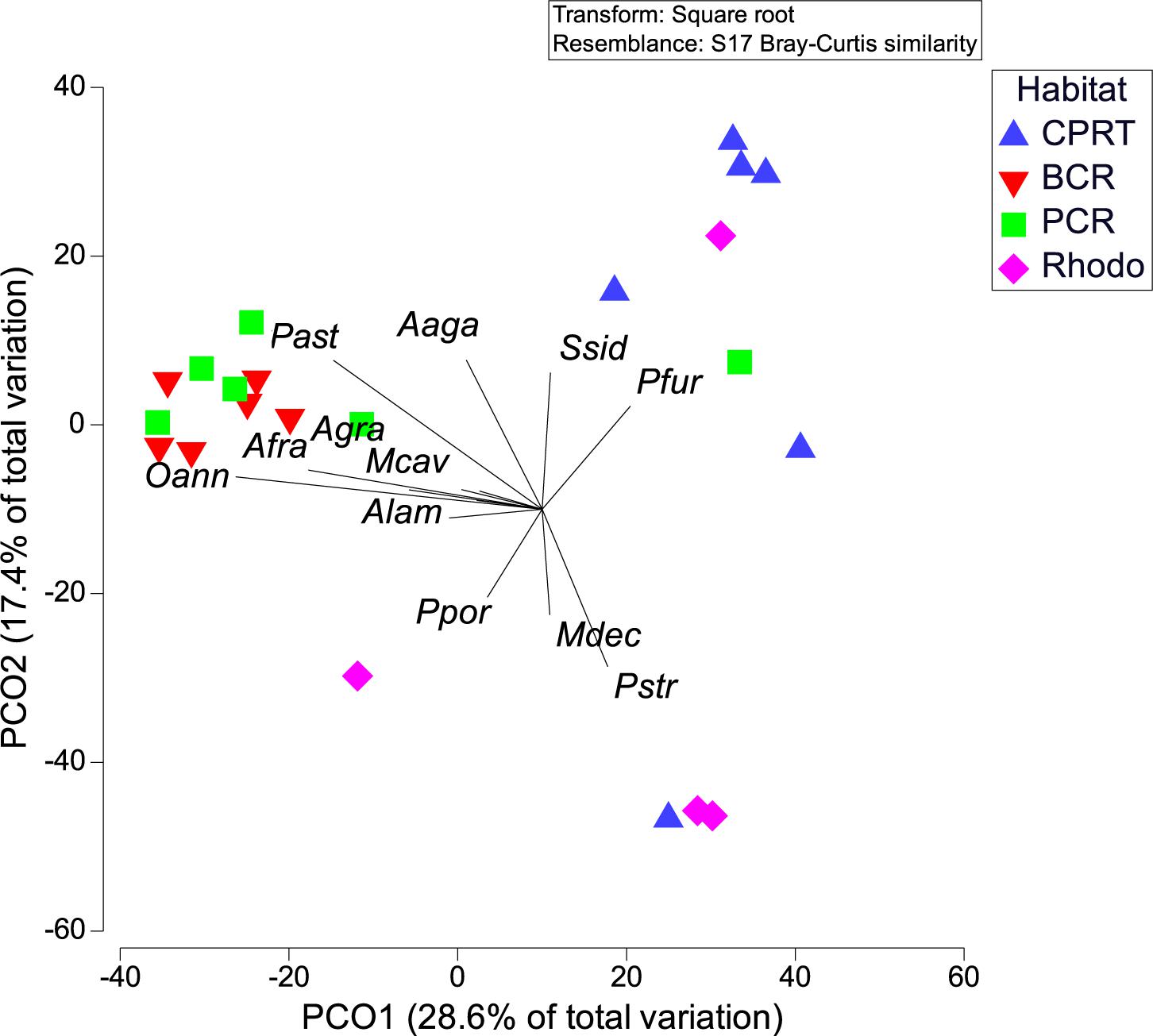
Figure 3. Principal coordinate analyses (PCOs) of a) coral composition between habitats during 2020 survey, at El Seco Reef. Coral species: Agaricia agaricites (Aaga), A. grahamae (Agra), A. lamarcki (Alam), Orbicella faveolata/franksi (Oann), Montastraea cavernosa (Mcav), Porites astreoides (Past), P. furcata (Pfur), P. porites (Ppor), and Siderastrea siderea (Ssid).
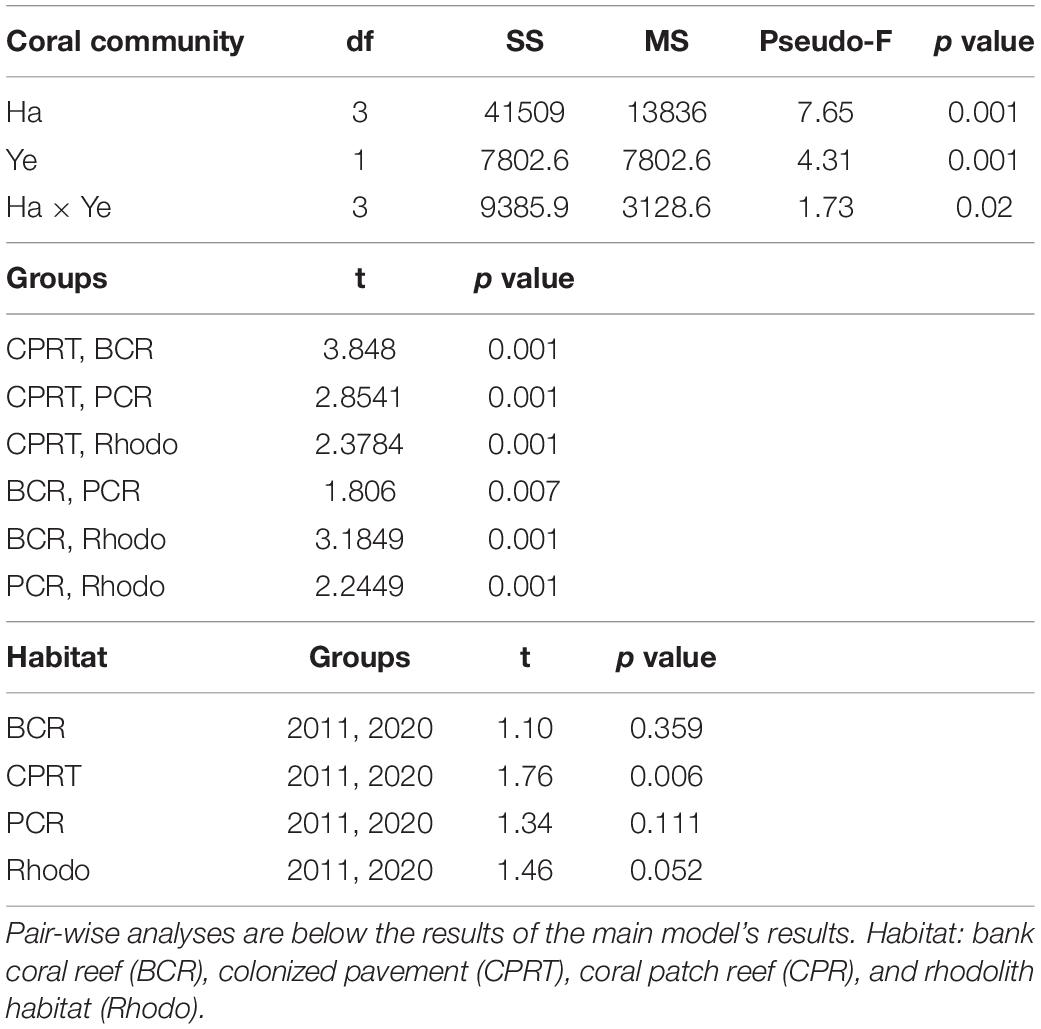
Table 2. The results of the Permutational Multivariate Analyses of Variance (PERMANOVA) tests to compare the differences of coral composition between habitats (Ha) and sampling year (Ye) at El Seco Reef, Puerto Rico.
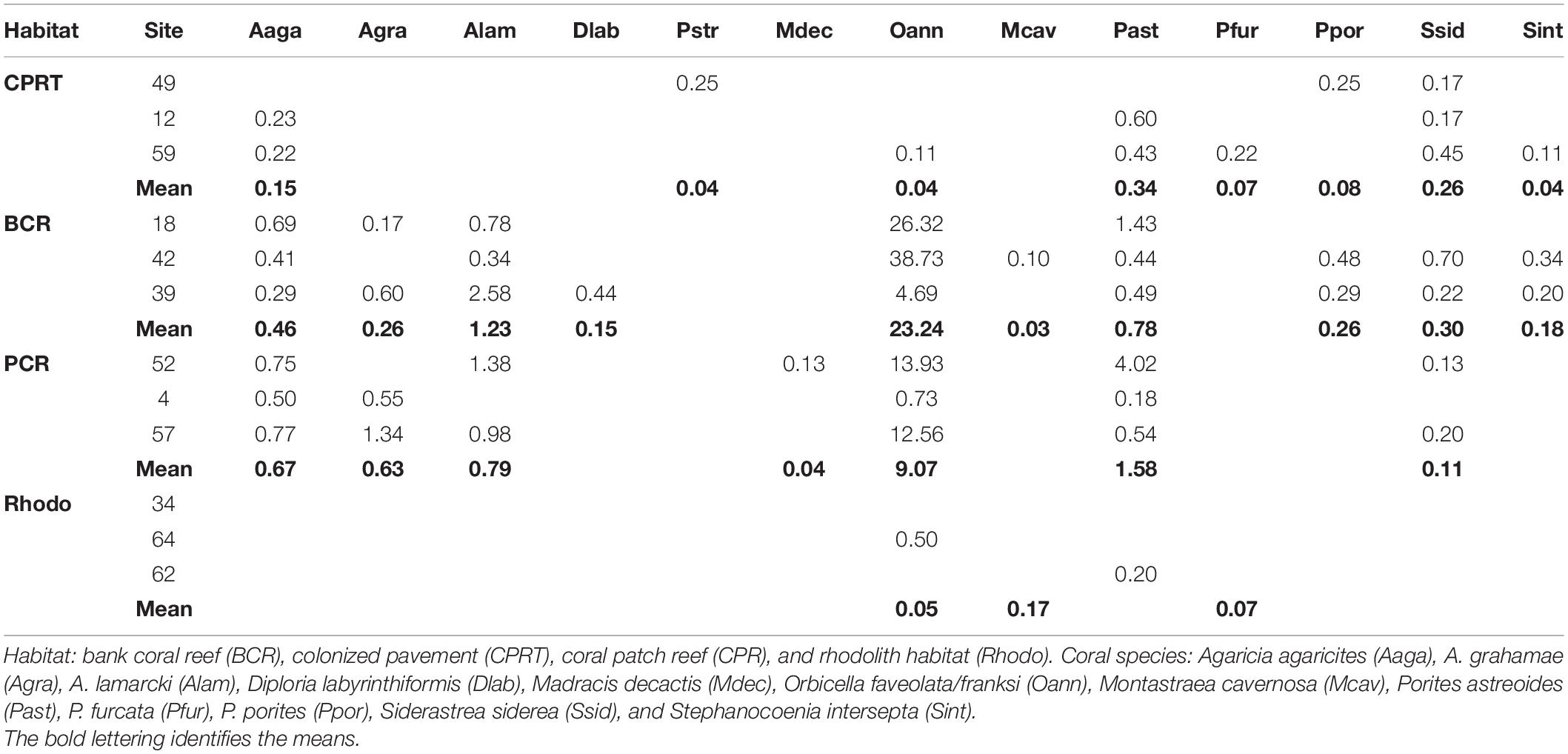
Table 3. Mean percent cover of the different coral species at each site and habitat at El Seco Reef, Puerto Rico.
Disease Prevalence
The deepest record of SCTLD at El Seco was 40.9 m. SCTLD infections were observed on hard corals from all habitats surveyed; however, the prevalence was significantly higher at the BCR, with transect values ranging from 9.70 to 21.13% of colonies infected (Figure 4, Kruskal-Wallis ANOVA, p < 0.007). Even though PCR habitats exhibited less coral cover, SCTLD prevalence was still high, with transects ranging from 6.66 to 15.07% of coral colonies infected. Two out of the three sites in Rhodo habitat did not exhibit any coral disease infection. Composition of the diseased coral species did not vary among habitats (one-way PERMANOVA, Pseudo-F = 3.01, p = 0.07), considering the majority (∼96.77%) of the corals infected were from the Orbicella complex spp. (Table 4). Other species were also observed infected, such as, A. gramahae, A. lamarcki, M. cavernosa, Colpophyllia natans, and Porites astreoides (Table 4). Most of the lesions were in patches or blotches in the middle of the colonies. However, some of the infected Orbicella complex spp. colonies had lesions on the colony’s border (Figure 5). The infected areas were both focal and multifocal in gross morphology. Some colonies exhibited almost complete tissue loss. Recently dead coral colonies were recorded at CPRT, BCR, and PCR habitats.
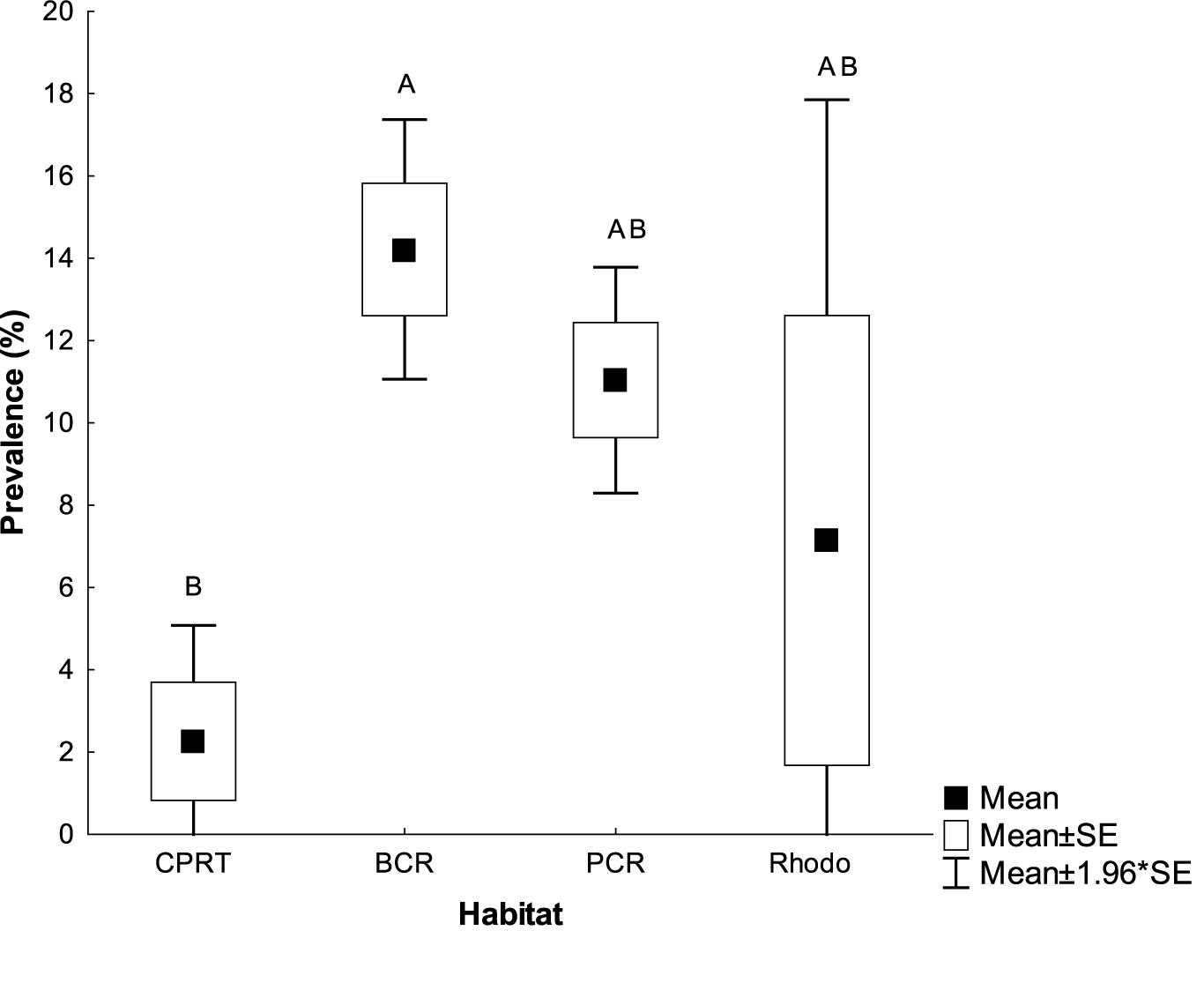
Figure 4. The mean Stony Coral Tissue Loss prevalence (% colonies infected) at the habitats at El Seco mesophotic system, Puerto Rico. Bars denote standard errors. BCR, bank coral reef; CPRT, colonized pavement; PCR, patch coral reef and Rhodo: rhodolith habitat. Letters signify differences according to the pairwise PERMANOVA test.
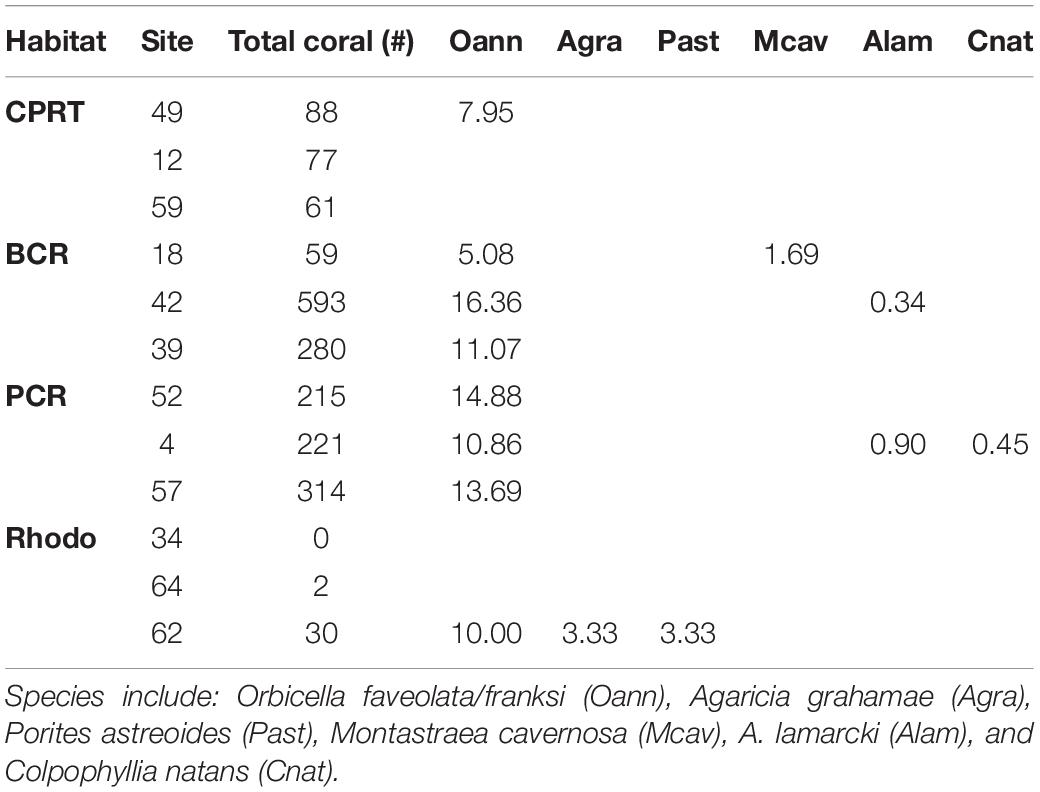
Table 4. Total amount of coral colonies counted (Total coral #) in the photographs and the percent prevalence of Stony Coral Tissue Loss Disease (SCTLD) for the different coral species at each site at El Seco Reef, Puerto Rico.
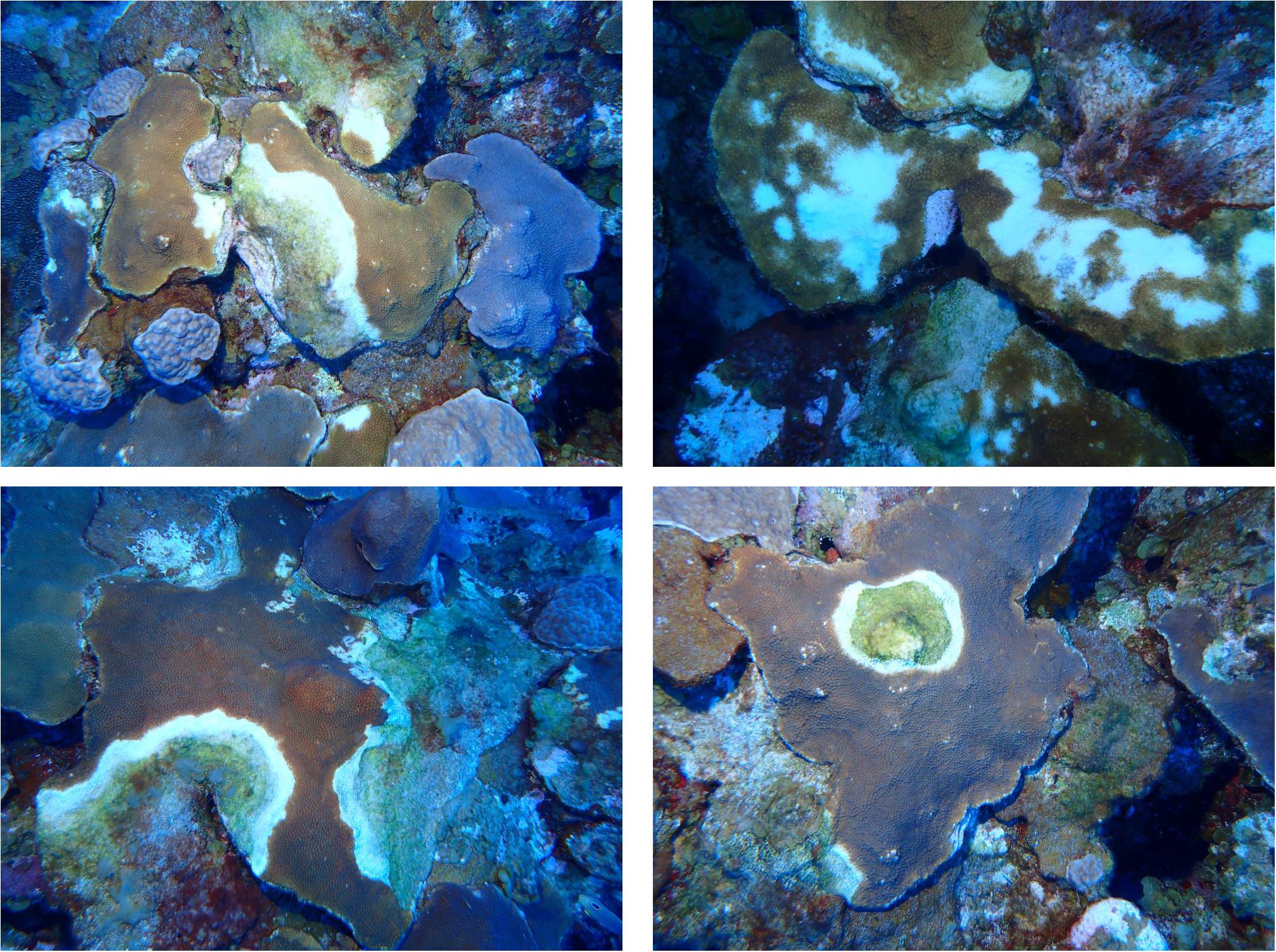
Figure 5. Photographs of diseased Orbicella faveolata/franksi colonies at El Seco Reef, Puerto Rico.
Sessile-Benthic Taxonomic Structure
The sessile-benthic taxonomic structure significantly varied between habitats and survey years at El Seco (Table 5). As seen in the estimates of components of variation of the main model, year contributed more to the variation of sessile-benthic taxonomic structure than year. In the 2020 survey, benthic algae were the main substrate category in terms of substrate cover at all habitats with means (± SE), ranging between 67.77 ± 6.24% at BCR and 91.55 ± 1.65% at CPRT. Lobophora species complex (further referred to as Lobophora spp.) was the dominant benthic alga from all habitats with mean substrate cover ranging between 38.20 ± 6.00% at CPRT and 64.39 ± 3.55% at Rhodo, representative of 41.7 and 72.2% of the total cover by benthic algae, respectively. The increase of cover by benthic algae between 2011 and 2020 was driven by a 133.8% increase of Lobophora spp., and the proliferation of red crustose calcareous algae, peyssonnelids, from 0% in 2011 to 13.89% in 2020 (Figure 6). Mean substrate cover by Ramicrusta spp. (8.17 ± 1.53%) represented more than half of the total cover by peyssonnelid algae. The low Ramicrusta spp. cover at Rhodo (1.10 ± 0.32%) drove the statistical difference between habitats (Table 6). Even though Ramicrusta spp. was found in greater abundance at CPRT (14.83 ± 4.09%) and BCR (7.40 ± 1.07%) habitats, the values did not significantly vary from PCR (5.85 ± 2.09%). Many dead standing coral colonies, particularly Dendrogyra cylindrus, Orbicella complex spp., and S. siderea, were observed completely overgrown by Ramicrusta spp. at CPRT.
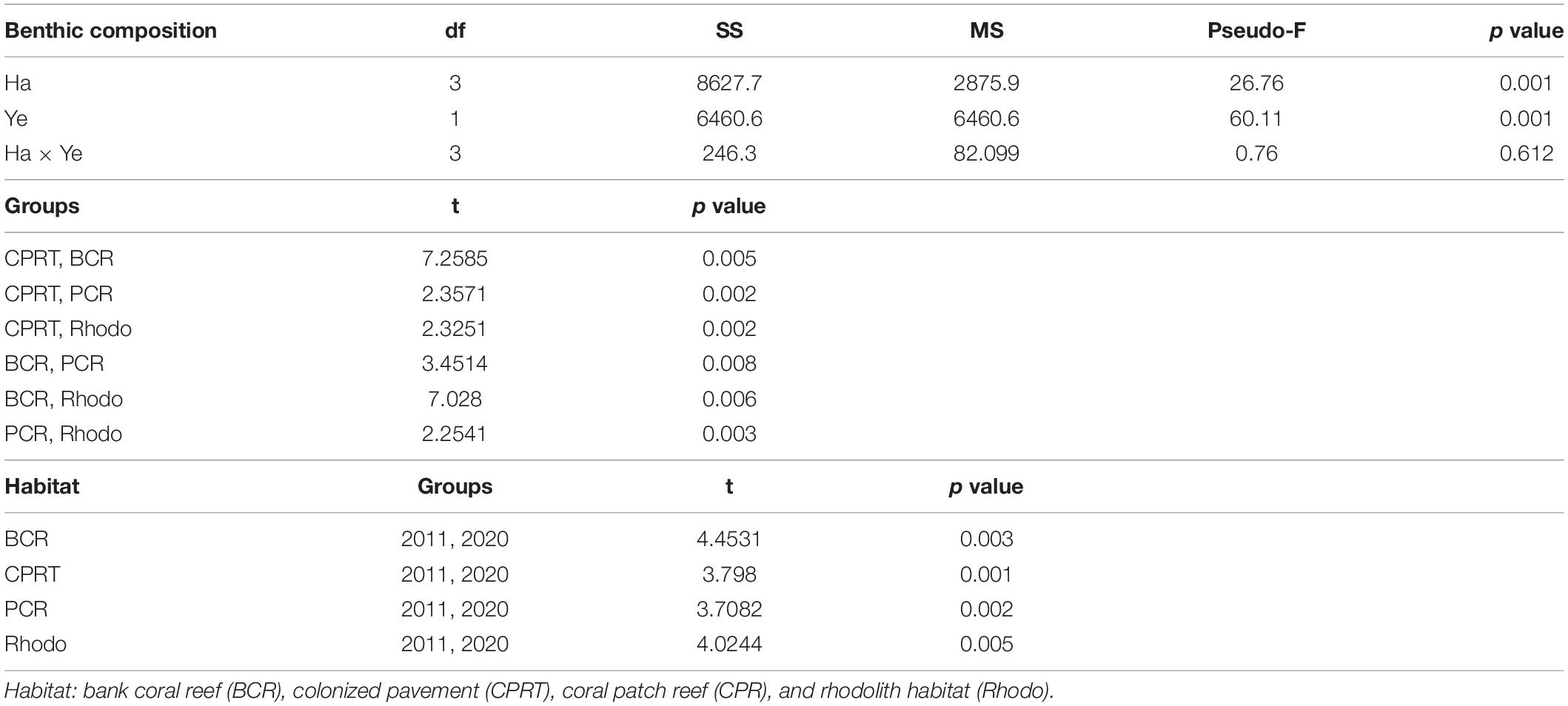
Table 5. The results of the Permutational Multivariate Analyses of Variance (PERMANOVA) tests to compare the differences of benthic taxonomic structure between habitats (Ha) and sampling year (Ye) at El Seco Reef, Puerto Rico.
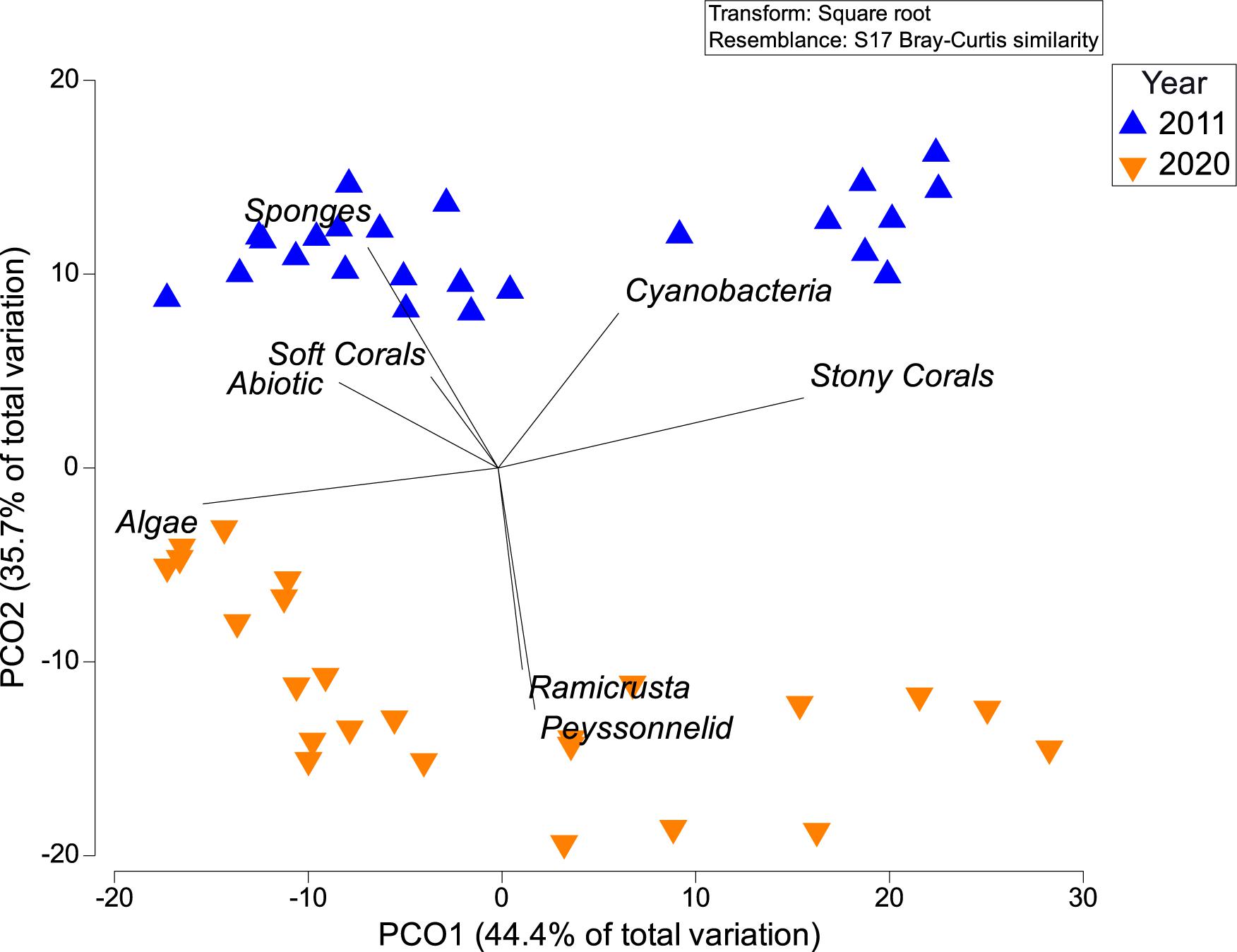
Figure 6. Principal coordinate analyses (PCOs) showing differences of sessile-benthic taxonomic structure at El Seco between the 2011 and 2020 surveys.
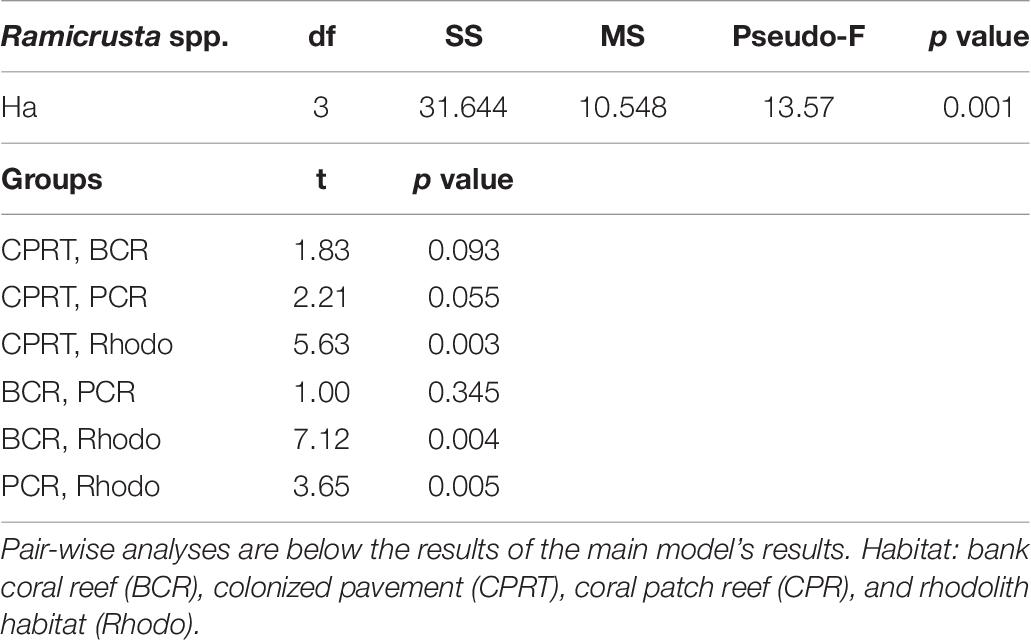
Table 6. The results of the Permutational Multivariate Analyses of Variance (PERMANOVA) tests to compare the differences of Ramicrusta spp. cover between habitats (Ha) at El Seco Reef, Puerto Rico.
Soft corals, sponges, cyanobacteria, and abiotic categories represented relatively minor components of reef substrate cover at El Seco during both survey years. Therefore, even though variations were relatively high in terms of the percent change between surveys, absolute differences of the mean percent cover were small.
Discussion
This study reports the first observation of SCTLD at an MCE in Puerto Rico. Furthermore, it also reports the deepest observation of SCTLD in the Greater Caribbean. As seen in this study and others (Smith et al., 2010, 2016, 2019), MCEs are not resistant to coral disease. The timing of SCTLD infection at El Seco is unknown. However, severe infections of SCTLD were recorded at Marine Conservation District (MCD) in the US Virgin Islands (AGRRA, 2019) in June 2020. SCTLD is a waterborne and highly transmissible disease (Weil et al., 2019), spreading quickly by 7–10 km/month (Lunz et al., 2017; Florida Keys National Marine Sanctuary, 2018; Meyer et al., 2019; Aeby et al., 2019). The MCD is physically connected to El Seco Reef as both reefs share the same shelf. The dominant current flow along the insular shelf at El Seco is toward the southwest, with velocity vectors pointing toward the west at the surface and rotating southerly closer to the bottom (García-Sais et al., 2011). It was only a matter of time before SCTLD reached the east coast of Puerto Rico and the deeper mesophotic reefs off Vieques Island from St. Thomas. SCTLD was concentrated along the north-northeast coasts of Puerto Rico at the end of 2020 (Weil et al., 2019); SM Williams pers comm). However, it was recently reported at reefs along the south coast of Puerto Rico, Salina and Ponce (AGRRA, 2019).
Stony Coral Tissue Loss Disease was present in all habitats at El Seco. However, SCTLD infection was primarily concentrated at the BCR and PCR habitats. Both habitats had a higher coral cover compared to Rhodo and CPRT habitats. BCR habitat exhibited the highest prevalence of SCTLD, and this habitat is characterized by a dense tabular growth formation of Orbicella complex spp. colonies. SCTLD is thought to be transmitted via the water or by direct contact by fish, invertebrates, or other corals (Aeby et al., 2019; Noonan and Childress, 2020). Transmission of SCTLD may be greater at sites with a higher density of corals regardless of the taxa (Bruno et al., 2007; Chaves-Fonnegra et al., 2021; Williams et al., 2021).
The BCR habitat is perhaps the most critical habitat at the El Seco due to its geographical extension and high structural complexity, as it is characterized by a dense and continuous bank of orbicellid corals. Coral reductions were measured from all habitats, but the coral loss was more pronounced at the BCR habitat. The reduction of coral cover in this study is consistent with recent reports from the Puerto Rico Coral Reef Monitoring Program (PRCRMP), which includes a permanent monitoring station in the BCR habitat at El Seco. Coral cover at the permanent station has been on the decline since 2011, with a reduction of 17.8% in 2016 to 33.8% in 2018 (García-Sais et al., 2018). The decline of Orbicella complex spp. measured in the 2020 survey drove the changes in coral cover between surveys. Long-term losses in Orbicella spp. have been observed at many of the shallow-water reefs of Puerto Rico (García-Sais et al., 2017) and the Caribbean (Bruckner and Bruckner, 2006; Edmunds and Elahi, 2007). There is a threat with the demise of Orbicella spp., as these species are the main contributors to the 3D structural complexity and calcium carbonate precipitation (González-Barrios and Álvarez-Filip, 2018). The structural intricacy of the tabular growth formed by Orbicella spp. provides an essential habitat for invertebrate, fish and cryptofauna species (Smith et al., 2010; García-Sais et al., 2011, 2020). The loss of these corals is compounded by increases in unfavorable substrates such as nuisance algae, as seen by Ramicrusta spp. appearance, and bioeroding organisms, which contributes overall decline of structural complexity on an impacted reef (Sheppard et al., 2002; Graham et al., 2006, 2009; Carballo et al., 2013; Magel et al., 2019).
Orbicella colonies have shown signs of stress in past surveys. In 2018, Orbicella colonies were recorded with discoloration and necrotic tissue patches (García-Sais et al., 2018). Many had small, bleached patches in the middle of the colonies. Given the ambiguous nature of the symptoms, they were not related to any coral disease or bleaching event at the time. As seen in this study, SCTLD disease was prevalent in Orbicella faveolata/franksi, and this was not limited by habitat nor depths. Orbicellids are not listed as highly susceptible species to SCTLD (Florida Keys National Marine Sanctuary, 2018). Highly susceptible species include Pseudodiploria strigosa, Dendrogyra cylindrus, Meandrina meandrites, and Dichocoenia stokesii (Precht et al., 2016; Alvarez-Filip et al., 2019; Weil et al., 2019; Precht, 2021). However, Meiling et al. (2021) observed regional differences in the species response to SCTLD for shallow-water reefs. Through laboratory trials, O. annularis showed the greatest susceptibility to SCTLD in the USVI (Meiling et al., 2021). O. annularis, C. natans and S. siderea had a higher risk of lesion development and faster lesion progression rates than other susceptible species listed for Florida. Further monitoring at El Seco will only confirm if the deeper orbicellids show the same patterns of susceptibility as the shallow-water O. annularis. Given the lethality and high prevalence of SCTLD, further coral loss is expected to occur at El Seco. The recovery of Orbicella spp. is unlikely to happen as the sexual recruitment success of Orbicella spp. is limited or non-existent on coral reefs (Rogers et al., 1984; Edmunds and Elahi, 2007; Irizarry-Soto and Weil, 2009; Hernández-Delgado et al., 2014). Therefore, the extensive and chronic loss of these corals could have dramatic impacts not only on the diversity but to the ecosystem function of El Seco, as many fish, invertebrates and cryptofauna use these habitats as refuge (Artim et al., 2020).
Stony Coral Tissue Loss Disease can severely change the coral composition on a coral reef (Alvarez-Filip et al., 2019). As seen in our analyses, this has yet to occur in most habitats, even with the significant reduction in coral cover during the past 9 years. This does not mean the change in the coral composition will not happen in the future. The only habitat that showed yearly dissimilarities in coral composition was CPRT, and this was due to the reduction and loss of Orbicella complex spp. and M. cavernosa. It is unknown if variations between yearly surveys at CPRT were caused by SCTLD or other stress factors, including the impacts associated with the passing of hurricanes Irma and Maria in September 2017 (Smith et al., 2016; Reardon, 2018), the impact of winter storm Riley in March 2018, and/or other disease-induced mortality associated with the increased vulnerability prompted by the 2019 winter bleaching event.
The overall sessile-benthic community structure changed markedly between surveys, however, and changes were noted from all surveyed habitats at El Seco. Hard coral cover decline was associated with an increase of cover by benthic algae. Such coral to algal transition is quite common for many coral reefs in the Caribbean (Hughes, 1994; Jackson et al., 2014; García-Sais et al., 2017; Evensen et al., 2019). The lack of herbivory by both parrotfish and sea urchins, as well as eutrophication are reasons for the persistence of high algal cover (Hughes et al., 1999; Lapointe, 1997; Littler et al., 2006; Bruno et al., 2009; Mumby, 2009; Lesser and Slattery, 2011). Lobophora spp. and Dictyota spp. are prominent components at mesophotic reefs of the Western Atlantic (García-Sais et al., 2011, 2020; Evensen et al., 2019; Spalding et al., 2019). In this study, cover increases of more than 100% by Lobophora spp. were measured at El Seco in a 9-year span. The increases in fleshy macroalgae can possibly be attributed to the nutrient enrichment caused by the passing of major hurricanes, like Maria and Irma. The torrential rainfall caused by Hurricane Maria and Irma caused an increase of colored dissolved organic matter (Ortiz-Rosa et al., 2020), and divergent processes caused by the hurricanes may have influenced a pulse of nutrient upwelling at mesophotic reefs (Walker et al., 2005; García-Sais et al., 2018). In addition, other stressors such as reduction of herbivores and disease outbreaks from thermal anomaly events could also attribute to the increase of fleshy macroalgae (Hughes, 1994; McManus and Polsenberg, 2004; Estrada-Saldívar et al., 2020). Lobophora-dominated reefs are associated with poor reef health (Slattery and Lesser, 2014; Johns et al., 2018; Evensen et al., 2019) because these algae alter microbial communities (Rasher and Hay, 2010; Morrow et al., 2012, 2017) and colonize available space. The high abundance of Lobophora spp. could further affect the resistance of El Seco to recover, as these algae also inhibit coral larvae from settling (Evensen et al., 2019).
Not only was there an increase in fleshy macroalgae, but a substantial increase in peyssonnelids, specifically Ramicrusta spp., from most habitats surveyed at El Seco. Ramicrusta spp. is an encrusting red alga, a member of the peyssonneliacean family (Rhodophyta). Ramicrusta spp. was more prominent in the shallower habitats (CPRT and BCR) than the deeper habitats (CPR and Rhodo). In Puerto Rico, Ramicrusta spp. was first identified in 2011 at a shallow-water reef off Caja de Muerto. It has spread throughout Puerto Rico (Williams and García-Sais, 2020), reaching a cover of ∼60% at shallow-water east coast reefs. Williams and García-Sais (2020) also observed depth variation of Ramicrusta spp. cover, with shallower reefs having a higher cover. Ramicrusta spp. can be detrimental as it is known to overgrow and smother the living tissues of corals (Pueschel and Saunders, 2009; Ballantine et al., 2011, 2016; Eckrich et al., 2011; Ballantine and Ruiz, 2013). The rapid growth of Ramicrusta spp. has the potential to reduce the amount of suitable substrate for the settlement of corals and other benthic organisms (Williams and García-Sais, 2020). As observed in these recent surveys, many of the recently dead coral skeletons were observed colonized by Ramicrusta spp.
Conclusion
Our study observed significant declines in coral cover on MCEs at El Seco over 11 years, corresponding with increases in algal abundance. A newly described coral disease, SCTLD, was also found with relatively high abundance at mesophotic depths. While changes to the coral composition were not seen over the study period, this disease’s impact on the dominant reef-building Orbicella complex spp. could have lasting impacts on reef persistence. Camp et al. (2018) stated that the future structure and ecological functions of a reef will be shaped by the vulnerability of different species to climate change and other anthropogenic stressors. As seen in this study, the coral communities at El Seco reef system are at high risk to disturbances and local stressors, as they are directly affected by coral disease outbreaks and are also vulnerable to extreme water temperature anomalies (García-Sais et al., 2020). The continual downward trend in coral cover and uptrend in algal cover over the years is worrying, especially with the surge in Ramicrusta spp. However, even with the coral decline, the BCR habitat still has high conservation value, as the cover of orbicellids is higher than most shallow-water reefs in Puerto Rico. Long-term monitoring needs to continue at the MCEs to understand how these coral reef communities will react to the cumulative impacts of the different disturbances. This will allow the designation of persistence areas (Smith et al., 2019) for further management and protection.
Data Availability Statement
The original contributions presented in the study are included in the article/Supplementary Material, further inquiries can be directed to the corresponding author.
Author Contributions
SMW analyzed the underwater photographs, ran the statistical tests, and led the writing of the manuscript. JG-S designed the work and edited the manuscript. JS-C edited the manuscript and provided the map and underwater photographs. All authors contributed to the article and approved the submitted version.
Funding
This project was funded by NOAA Coral Reef Conservation Program and the Caribbean Fishery Management Council (NA09NMF4410037 and NA17NMF4410270).
Conflict of Interest
SMW, JG-S, and JS-C were employed by Reef Research.
Publisher’s Note
All claims expressed in this article are solely those of the authors and do not necessarily represent those of their affiliated organizations, or those of the publisher, the editors and the reviewers. Any product that may be evaluated in this article, or claim that may be made by its manufacturer, is not guaranteed or endorsed by the publisher.
Acknowledgments
We would like to thank M. Carlo, R. Esteves, B. Castro, and E. Tuohy for their assistance in the field. We would also like to thank J. Lang, K. Neely, D. Wusinich-Mendez, and M. Brandt for taking their time to look at underwater photographs and help with the identification and confirmation of SCTLD at El Seco. We would also like to thank three reviewers for their helpful comments, which made the manuscript stronger.
Supplementary Material
The Supplementary Material for this article can be found online at: https://www.frontiersin.org/articles/10.3389/fmars.2021.668669/full#supplementary-material
References
Aeby, G. S., Ushijima, B., Campbell, J. E., Jones, S., Williams, G. J., Meyer, J. L., et al. (2019). Pathogenesis of a tissue loss disease affecting multiple species of corals along the Florida Reef Tract. Front. Mar. Sci. 6:679. doi: 10.3389/fmars.2019.00678
AGRRA (2019). Coral Disease Outbreak, Stony Coral Tissue Loss Disease. Available online at: http://www.agrra.org/coral-disease-outbreak/ (accessed July 27, 2021)
Alvarez-Filip, L., Estrada-Saldívar, N., Pérez-Cervantes, E., Molina-Hernández, A., and González-Barrios, F. J. (2019). A rapid spread of the stony coral tissue loss disease outbreak in the Mexican Caribbean. PeerJ 7:e8069. doi: 10.7717/peerj.8069
Anderson, M. J. (2001). Permutation tests for univariate or multivariate analysis of variance and regression. Can. J. Fish. Aquat. Sci. 58, 626–639.
Anderson, M. J., Gorley, R. N., and Clarke, K. R. (2008). PERMANOVA+ for PRIMER: Guide to Software and Statistical Methods, PRIMER-E, Plymouth, UK. Available online at: http://www.nrc.ca/cgi-bin/cisti/journals/rp/rp2_abst_e?cjfas_f01-004_58_ns_nf_cjfas58-01 (accessed July 27, 2021)
Andradi-Brown, D. A., Macaya-Solis, C., Exton, D. A., Gress, E., Wright, G., and Rogers, A. D. (2016). Assessing Caribbean shallow and mesophotic reef fish communities using baited-remote underwater video (BRUV) and diver-operated video (DOV) survey techniques. PLoS One 11:e0168235. doi: 10.1371/journal.pone.0168235
Appeldoorn, R. S., Alfaro, M., Ballantine, D. L., Bejarano, I., Ruíz, H. J., Schizas, N., et al. (2019). “Puerto Rico,” in Mesophotic Coral Ecosystems, eds Y. Loya, K. A. Puglise, and T. Bridge (Cham: Springer Nature), 111–129.
Armstrong, R. A., and Singh, H. (2012). “Mesophotic coral reefs of the Puerto Rico shelf,” in Seafloor Geomorphology as Benthic Habitat, ed. P. T. Harris (Waltham, MA: Elsevier Inc.), 365–374.
Artim, J. M., Nicholson, M. D., Hendrick, G. C., Brandt, M., Smith, T. B., and Sikkel, P. C. (2020). Abundance of a cryptic generalist parasite reflects degradation of an ecosystem. Ecosphere 11:e033268. doi: 10.1002/ecs2.3268
Ballantine, D. L., and Ruiz, H. (2013). A unique red algal reef formation in Puerto Rico. Coral Reefs 32:411. doi: 10.1007/s00338-013-1016-2
Ballantine, D. L., Athanasiadis, A., and Ruiz, H. (2011). Notes on the benthic marine algae of Puerto Rico. X. Additions to the flora. Bot. Mar. 62, 337–344. doi: 10.1515/BOT.2011.039
Ballantine, D. L., Ruiz, H., Lozada-Troche, C., and Norris, J. N. (2016). The genus Ramicrusta (Peyssonneliales, Rhodophyta) in the Caribbean Sea, including Ramicrusta bonairensis sp. Nov. and Ramicrusta monensis sp. Nov. Bot. Mar. 59, 417–431. doi: 10.1515/bot-2016-0086
Bloomberg, J., and Holstein, D. M. (2021). Mesophotic coral refuges following multiple disturbances. Coral Reefs 40, 821–834. doi: 10.1007/s00338-021-02087-w
Bongaerts, P., and Smith, T. B. (2019). “Beyond the ‘deep reef refuge’ hypothesis: a conceptual framework to characterize persistence at depth,” in Mesophotic Coral Ecosystems, eds Y. Loya, K. A. Puglise, and T. Bridge (Cham: Springer Nature), 881–895.
Bongaerts, P., Muir, P., Englebert, N., Bridge, T. C. L., and Hoegh-Guldberg, O. (2013). Cyclone damage at mesophotic depths on Myrmidon Reef (GBR). Coral Reefs 32:935. doi: 10.1007/s00338-013-1052-y
Bongaerts, P., Ridgway, T., Sampayo, E. M., and Hoegh-Guldberg, O. (2010). Assessing the ‘deep reef refugia’ hypothesis: focus on Caribbean reefs. Coral Reefs 29, 309–327. doi: 10.1007/s00338-009-0581-x
Bridge, T., Done, T., Friedman, A., Beaman, R., Williams, S., Pizarro, O., et al. (2011). Variability in mesophotic coral reef communities along the Great Barrier Reef, Australia. Mar. Ecol. Prog. Ser. 428, 63–75. doi: 10.3354/meps09046
Bruckner, A., and Bruckner, R. (2006). Consequences of yellow band disease (YBD) on Montastraea annularis (species complex) populations on remote reefs off Mona Island, Puerto Rico. Dis. Aquat. Organ. 69, 67–73. doi: 10.3354/dao069067
Bruno, J. F., Selig, E. R., Casey, K. S., Page, C. A., Willis, B. L., Harvell, C. D., et al. (2007). Thermal stress and coral cover as drivers of coral disease outbreaks. PLoS Biol. 5:e124. doi: 10.1371/journal.pbio.0050124
Bruno, J. F., Sweatman, H., Precht, W. F., Selig, E. R., and Schutte, V. G. W. (2009). Assessing evidence of phase shifts from coral to macroalgal dominance on coral reefs. Ecology 90, 1478–1484. doi: 10.1890/08-1781.1
Calnan, J. M., Smith, T. B., Nemeth, R. S., Kadison, E., and Blondeau, J. (2008). Coral disease prevalence and host susceptibility on mid-depth and deep reefs in the United States Virgin Islands. Rev. Biol. Trop. 56, 223–234. doi: 10.15517/rbt.v56i0.5589
Camp, E. F., Schoepf, V., Mumby, P. J., Hardtke, L. A., Rodolfo-Metalpa, R., Smith, D. J., et al. (2018). The future of coral reefs subject to rapid climate change: lessons from natural extreme environments. Front. Mar. Sci. 5:4. doi: 10.3389/fmars.2018.00004
Carballo, J. L., Bautista, E., Nava, H., Cruz-Barraza, J. A., and Chávez, J. A. (2013). Boring sponges, an increasing threat for coral reefs affected by bleaching events. Ecol. Evol. 3, 872–886. doi: 10.1002/ece3.452
Chaves-Fonnegra, A., Panassiti, B., Smith, T. B., Brown, E., Clemens, E., Sevier, M., et al. (2021). Environmental and biological drivers of white plague disease on shallow and mesophotic coral reefs. Ecography 44, 1071–1085. doi: 10.1111/ecog.05527
Eckrich, C. E., Engel, M. S., and Peachey, R. B. J. (2011). Crustose, calcareous algal bloom (Ramicrusta sp.) overgrowing scleractinian corals, gorgonians, a hydrocoral, sponges, and other algae in Lac Bay, Bonaire, Dutch Caribbean. Coral Reefs 30:131. doi: 10.1007/s00338-010-0683-5
Edmunds, P. J., and Elahi, R. (2007). The demographics of a 15-year decline in cover of the Caribbean reef coral Montastraea annularis. Ecol. Monogr. 77, 3–18. doi: 10.1890/05-1081
Estrada-Saldívar, N., Molina-Hernández, A., Pérez-Cervantes, E., Medellín-Maldonado, F., González-Barrios, F. J., and Alvarez-Filip, L. (2020). Reef-scale impacts of the stony coral tissue loss disease outbreak. Coral Reefs 39, 861–866. doi: 10.1007/s00338-020-01949-z
Evensen, N. R., Doropoulos, C., Wong, K. J., and Mumby, P. J. (2019). Stage-specific effects of Lobophora on the recruitment success of a reef-building coral. Coral Reefs 38, 489–498. doi: 10.1007/s00338-019-01804-w
Florida Department of Environmental Protection (2019). Florida Reef Tract Coral Disease Outbreak (2014 – Present). Tallahassee, FL: Florida Department Environmental Protection.
Florida Keys National Marine Sanctuary (2018). Case Definition: Stony Coral Tissue Loss Disease (SCTLD). Washington, DC: National Oceanic and Atmospheric Administration.
García-Sais, J. R., Sabater-Clavell, J., Esteves, R., Capella, J., and Carlo, M. (2011). Characterization of Benthic Habitats and Associated Mesophotic Coral Reef Communities at El Seco, Southeast Vieques, Puerto Rico. Final Report Submitted to the Caribbean Fisheries Management Council. Available online at: https://www.drna.pr.gov/documentos/characterization-of-benthic-habitats-and-associated-mesophotic-coral-reef-communities-at-el-seco-southeast-vieques-puerto-rico/ (accessed July 27, 2021).
García-Sais, J. R., Williams, S. M., and Amirrezvani, A. (2017). Mortality, recovery, and community shifts of scleractinian corals in Puerto Rico one decade after the 2005 regional bleaching event. PeerJ 5:e3611. doi: 10.7717/peerj.3611
García-Sais, J. R., Williams, S. M., Sabater-Clavell, J., and Carlo, M. (2018). Puerto Rico Coral Reef Monitoring Program: 2017 – 2018 Survey. Final Report for the Department of Natural and Environmental Resources (DNER). DNER’s Coral Reef Conservation and Management Program. Available online at: https://gcrmn.net/wp-content/uploads/2019/04/DRNA-2018-Final-Report-12-3-18_web1-2.pdf (accessed July 27, 2021).
García-Sais, J. R., Williams, S., Tuohy, E., Sabater-Clavell, J., and Carlo, M. (2020). Monitoring of Mesophotic Habitats and Associated Benthic and Fish/Shellfish Communities from Abrir la Sierra, Bajo de Sico, Tourmaline, Isla Desecheo, El Seco and Boya 4, 2018-20 Survey. San Juan, PR: Caribbean Fisheries Management Council.
González-Barrios, F. J., and Álvarez-Filip, L. (2018). A framework for measuring coral species-specific contribution to reef functioning in the Caribbean. Ecol. Indic. 95, 877–886. doi: 10.1016/j.ecolind.2018.08.038
Graham, N. A. J., Wilson, S. K., Jennings, S., Polunin, N. V. C., Bijoux, J. P., and Robinson, J. (2006). Dynamic fragility of oceanic coral reef ecosystems. Proc. Natl. Acad. Sci. U.S.A. 103, 8425–8429. doi: 10.1073/pnas.0600693103
Graham, N. A. J., Wilson, S. K., Pratchett, M. S., Polunin, N. V. C., and Spalding, M. D. (2009). Coral mortality versus structural collapse as drivers of corallivorous butterflyfish decline. Biodivers. Conserv. 18, 3325–3336. doi: 10.1007/s10531-009-9633-3
Heres, M. M., Farmer, B. H., Elmer, F., and Hertler, H. (2021). Ecological consequences of stony coral tissue loss disease in the Turks and Caicos Islands. Coral Reefs 40, 609–624. doi: 10.1007/s00338-021-02071-4
Hernández-Delgado, E. A., González-Ramos, C. M., and Alejandro-Camis, P. J. (2014). Large-scale coral recruitment patterns on Mona Island, Puerto Rico: evidence of a transitional community trajectory after massive coral bleaching and mortality. Rev. Biol. Trop. 62, 49–64.
Hinderstein, L. M., Marr, J. C. A., Martinez, F. A., Dowgiallo, M. J., Puglise, K. A., Pyle, R. L., et al. (2010). Theme section on mesophotic coral ecosystems: characterization, ecology, and management. Coral Reefs 29, 247–252. doi: 10.1007/s00338-010-0614-5
Hughes, T. P. (1994). Catastrophes, phase shifts, and large-scale degradation of a Caribbean Coral Reef. Science 265, 1547–1551. doi: 10.1126/science.265.5178.1547
Hughes, T., Szmant, A. M., Steneck, R., Carpenter, R., and Miller, S. (1999). Algal blooms on coral reefs: what are the causes? Limnol. Oceanogr. 44, 1583–1586. doi: 10.4319/lo.1999.44.6.1583
Irizarry-Soto, E., and Weil, E. (2009). Spatial and temporal variability in juvenile coral densities, survivorship and recruitment in La Parguera, southwestern Puerto Rico. Caribb. J. Sci. 45, 269–281. doi: 10.18475/cjos.v45i2.a14
Jackson, J. B. C., Donovan, M. K., Cramer, K., and Lam, V. Y. Y. (2014). Status and Trends of Caribbean Coral Reefs, 1970-2012. Available online at: http://books.google.com/books?id=sGLZoAEACAAJ&dq=intitle:Status+and+Trendsof+Caribbean+Coral+Reefs&hl=&cd=1&source=gbs_api (accessed July 27, 2021).
Johns, K. A., Emslie, M. J., Hoey, A. S., Osborne, K., Jonker, M. J., and Cheal, A. J. (2018). Macroalgal feedbacks and substrate properties maintain a coral reef regime shift. Ecosphere 9:e02349. doi: 10.1002/ecs2.2349
Lapointe, B. E. (1997). Nutrient thresholds for bottom-up control of macroalgal blooms and coral reefs. Limnol. Oceanogr. 42, 1119–1131.
Lesser, M. P., and Slattery, M. (2011). Phase shift to algal dominated communities at mesophotic depths associated with lionfish (Pterois volitans) invasion on a Bahamian coral reef. Biol. Invasions, 13, 1855–1868. doi: 10.1007/s10530-011-0005-z
Lesser, M. P., Slattery, M., and Leichter, J. J. (2009). Ecology of mesophotic coral reefs. J. Exp. Mar. Biol. Ecol. 375, 1–8. doi: 10.1016/j.jembe.2009.05.009
Littler, M. M., Littler, D. S., and Brooks, B. L. (2006). Harmful algae on tropical coral reefs: Bottom-up eutrophication and top-down herbivory. Harmful Algae 5, 565–585. doi: 10.1016/j.hal.2005.11.003
Lunz, K., Landsberg, J., Kiryu, Y., and Brinkhuis, V. (2017). Investigation of the Coral Disease Outbreak Affecting Scleractinian Coral Species Along the Florida Reef Tract. Tallahassee, FL: Florida Department of Environmental Protection.
Magel, M. T., Burns, J. H. R., Gates, R. D., and Baum, J. K. (2019). Effects of bleaching-associated mass coral mortality on reef structural complexity across a gradient of local disturbance. Sci. Rep. 9:2512. doi: 10.1038/s41598-018-37713-1
McManus, J. W., and Polsenberg, J. F. (2004). Coral–algal phase shifts on coral reefs: ecological and environmental aspects. Prog. Oceanogr. 60, 263–279. doi: 10.1016/j.pocean.2004.02.014
Meiling, S. S., Muller, E. M., Lasseigne, D., Rossin, A., Veglia, A. J., MacKnight, N., et al. (2021). Variable species responses to experimental stony coral tissue loss disease (SCTLD) exposure. Front. Mar. Sci. 8:464. doi: 10.3389/fmars.2021.670829
Menza, C., Kendall, M., Rogers, C., and Miller, J. (2007). A deep reef in deep trouble. Cont. Shelf Res. 27, 2224–2230. doi: 10.1016/j.csr.2007.05.017
Meyer, J. L., Castellanos-Gell, J., Aeby, G. S., Häse, C. C., Ushijima, B., and Paul, V. J. (2019). Microbial community shifts associated with the ongoing stony coral tissue loss disease outbreak on the Florida reef tract. Front. Microbiol. 10:2244. doi: 10.3389/fmicb.2019.02244
Morrow, K. M., Bromhall, K., Motti, C. A., Munn, C. B., and Bourne, D. G. (2017). Allelochemicals produced by brown macroalgae of the Lobophora genus are active against coral larvae and associated bacteria, supporting pathogenic shifts to Vibrio dominance. Appl. Environ. Microbiol. 83:1. doi: 10.1128/AEM.02391-16
Morrow, K. M., Ritson-Williams, R., Ross, C., Liles, M. R., and Paul, V. J. (2012). Macroalgal extracts induce bacterial assemblage shifts and sublethal tissue stress in Caribbean Corals. PLoS One 7:e44859. doi: 10.1371/journal.pone.0044859
Muller, E. M., Sartor, C., Alcaraz, N. I., and van Woesik, R. (2020). Spatial epidemiology of the stony-coral-tissue-loss disease in Florida. Front. Mar. Sci. 7:163. doi: 10.3389/fmars.2020.00163
Mumby, P. J. (2009). Phase shifts and the stability of macroalgal communities on Caribbean coral reefs. Coral Reefs 28, 761–773. doi: 10.1007/s00338-009-0506-8
National Oceanic and Atmospheric Administration [NOAA] (2018). Stony Coral Tissue Loss Disease Case Definition. Available online at: https://Nmsfloridakeys.blob.core.windows.net/floridakeys-prod/media/docs/20181002-stony-coral-tissue-loss-disease-case-definition.pdf (accessed July 28, 2020).
Noonan, K. R., and Childress, M. J. (2020). Association of butterflyfishes and stony coral tissue loss disease in the Florida Keys. Coral Reefs 39, 1581–1590. doi: 10.1007/s00338-020-01986-8
Ortiz-Rosa, S., Hernández, W. J., Williams, S. M., and Armstrong, R. A. (2020). Water quality anomalies following the 2017 hurricanes in southwestern Puerto Rico: absorption of colored detrital and dissolved material. Remote Sens. 12, 1–15. doi: 10.3390/rs12213596
Precht, W. (2021). Failure to respond to a coral disease epizootic in Florida: causes and consequences. Rethink. Ecol. 6, 1–47. doi: 10.3897/rethinkingecology.6.56285
Precht, W. F., Gintert, B. E., Robbart, M. L., Fura, R., and van Woesik, R. (2016). Unprecedented disease-related coral mortality in Southeastern Florida. Sci. Rep. 6:31374. doi: 10.1038/srep31374
Pueschel, C. M., and Saunders, G. W. (2009). Ramicrusta textilis sp. nov. (Peyssonneliaceae, Rhodophyta), an anatomically complex Caribbean alga that overgrows corals. Phycologia 48, 480–491. doi: 10.2216/09-04.1
Pyle, R. L., and Copus, J. M. (2019). “Mesophotic coral ecosystems: introduction and overview,” in Mesophotic Coral Ecosystems, eds Y. Loya, K. A. Puglise, and T. Bridge (Cham: Springer Nature), 3–27.
Rasher, D. B., and Hay, M. E. (2010). Chemically rich seaweeds poison corals when not controlled by herbivores. Proc. Natl. Acad. Sci. U.S.A. 107, 9683–9688. doi: 10.1073/pnas.0912095107
Reardon, S. (2018). Hurricane Maria’s wrath leaves clues to coral reefs’ future. Nature 560, 421–422. doi: 10.1038/d41586-018-06014-y
Reed, J. K., Farrington, S., David, A., Harter, S., Pomponi, S. A., Cristina Diaz, M., et al. (2019). “Pulley ridge, Gulf of Mexico, USA,” in Mesophotic Coral Ecosystems, eds Y. Loya, K. A. Puglise, and T. Bridge (Cham: Springer Nature), 57–69.
Rocha, L. A., Pinheiro, H. T., Shepherd, B., Papastamatiou, Y. P., Luiz, O. J., Pyle, R. L., et al. (2018). Mesophotic coral ecosystems are threatened and ecologically distinct from shallow water reefs. Science 361, 281–284. doi: 10.1126/science.aaq1614
Rogers, A. D., Kemp, K. M., Davies, A. J., and Lisa Taylor, M. (2015). “The diseases of deep-water corals,” in Diseases of Coral, eds C. M. Woodley, C. A. Downs, A. W. Bruckner, J. W. Porter, and S. B. Galloway (Hoboken, NJ: John Wiley & Sons, Inc), 416–441.
Rogers, C. S., Fitz, H. C., Gilnack, M., Beets, J., and Hardin, J. (1984). Scleractinian coral recruitment patterns at Salt River submarine canyon, St. Croix, U.S. Virgin Islands. Coral Reefs 3, 69–76. doi: 10.1007/BF00263756
Sheppard, C. R. C., Spalding, M., Bradshaw, C., and Wilson, S. (2002). Erosion vs. Recovery of coral reefs after 1998 El Niño: Chagos reefs, Indian Ocean. Ambio 31, 40–48. doi: 10.1579/0044-7447-31.1.40
Sherman, C. E., Locker, S. D., Webster, J. M., and Weinstein, D. K. (2019). “Geology and geomorphology,” in Mesophotic Coral Ecosystems, eds Y. Loya, K. A. Puglise, and T. Bridge (Cham: Springer Nature), 849–878.
Sherman, C., Appeldoorn, R., Ballantine, D., Carlo, M., Kesling, D., Nemeth, M., et al. (2013). “Exploring the mesophotic zone: diving operations and scientific highlights of three research cruises (2010-2012) across Puerto Ric and US Virgin Islands,” in Proceedings of the AAUS Symposium, Curacao, (Dauphin Island, AL: American Academy of Underwater Sciences).
Slattery, M., and Lesser, M. P. (2014). Allelopathy in the tropical algal Lobophora variegata (Phaeophyceae): mechanistic basis for a phase shift on mesophotic coral reefs? J. Phycol. 50, 493–505. doi: 10.1111/jpy.12160
Slattery, M., Lesser, M. P., Brazeau, D., Stokes, M. D., and Leichter, J. J. (2011). Connectivity and stability of mesophotic coral reefs. J. Exp. Mar. Biol. Ecol. 408, 32–41. doi: 10.1016/j.jembe.2011.07.024
Smith, T. B., Blondeau, J., Nemeth, R. S., Pittman, S. J., Calnan, J. M., Kadison, E., et al. (2010). Benthic structure and cryptic mortality in a Caribbean mesophotic coral reef bank system, the Hind Bank Marine Conservation District, U.S. Virgin Islands. Coral Reefs 29, 289–308. doi: 10.1007/s00338-009-0575-8
Smith, T. B., Gyory, J., Brandt, M. E., Miller, W. J., Jossart, J., and Nemeth, R. S. (2016). Caribbean mesophotic coral ecosystems are unlikely climate change refugia. Global Change Biol. 22, 2756–2765. doi: 10.1111/gcb.13175
Smith, T. B., Holstein, D. M., and Ennis, R. S. (2019). “Disturbance in mesophotic coral ecosystems and linkages to conservation and management,” in Mesophotic Coral Ecosystems, eds Y. Loya, K. A. Puglise, and T. Bridge (Cham: Springer Nature), 911–929.
Smith, T. B., Nemeth, R. S., Blondeau, J., Calnan, J. M., Kadison, E., and Herzlieb, S. (2008). Assessing coral reef health across onshore to offshore stress gradients in the US Virgin Islands. Mar. Poll. Bull. 56, 1983–1991. doi: 10.1016/j.marpolbul.2008.08.015
Spalding, H. L., Amado-Filho, G. M., Bahia, R. G., Ballantine, D. L., Fredericq, S., Leichter, J. J., et al. (2019). “Macroalgae,” in Mesophotic Coral Ecosystems, eds Y. Loya, K. A. Puglise, and T. Bridge (Cham: Springer Nature), 507–536.
Walker, N. D., Leben, R. R., and Balasubramanian, S. (2005). Hurricane-forced upwelling and chlorophyll a enhancement within cold-core cyclones in the Gulf of Mexico. Geophys. Res. Lett. 32:L18610. doi: 10.1029/2005GL023716
Walton, C. J., Hayes, N. K., and Gilliam, D. S. (2018). Impacts of a regional, multi-year, multi-species coral disease outbreak in Southeast Florida. Front. Mar. Sci. 5:323. doi: 10.3389/fmars.2018.00323
Weil, E. (2019). “Disease problems,” in Mesophotic Coral Ecosystems, eds Y. Loya, K. A. Puglise, and T. Bridge (Cham: Springer Nature), 779–800.
Weil, E., and Rogers, C. S. (2011). “Coral reef diseases in the Atlantic-Caribbean,” in Coral Reefs: An Ecosystem in Transition, eds Z. Dubinsky and N. Stambler (Dordrecht: Springer Netherlands), 465–491.
Weil, E., Hernández-Delgado, E. A., Gonzalez, M., Williams, S., and Figuerola, M. (2019). Spread of the new coral disease “SCTLD” into the Caribbean?: implications for Puerto Rico. Reef Encount. 34, 38–43.
Williams, S. D., Walter, C. S., and Muller, E. M. (2021). Fine scale temporal and spatial dynamics of the stony coral tissue loss disease outbreak within the lower Florida keys. Front. Mar. Sci. 8:422. doi: 10.3389/fmars.2021.631776
Keywords: Caribbean, Ramicrusta spp., coral decline, Stony Coral Tissue Loss Disease, Mesophotic Coral Ecosystem, SCTLD
Citation: Williams SM, García-Sais J and Sabater-Clavell J (2021) Prevalence of Stony Coral Tissue Loss Disease at El Seco, a Mesophotic Reef System off Vieques Island, Puerto Rico. Front. Mar. Sci. 8:668669. doi: 10.3389/fmars.2021.668669
Received: 16 February 2021; Accepted: 20 July 2021;
Published: 30 August 2021.
Edited by:
Christina A. Kellogg, United States Geological Survey (USGS), United StatesReviewed by:
Paul Carl Sikkel, Arkansas State University, United StatesMichael S. Studivan, University of Miami, United States
Gretchen Goodbody-Gringley, Central Caribbean Marine Institute, Cayman Islands
Copyright © 2021 Williams, García-Sais and Sabater-Clavell. This is an open-access article distributed under the terms of the Creative Commons Attribution License (CC BY). The use, distribution or reproduction in other forums is permitted, provided the original author(s) and the copyright owner(s) are credited and that the original publication in this journal is cited, in accordance with accepted academic practice. No use, distribution or reproduction is permitted which does not comply with these terms.
*Correspondence: Stacey M. Williams, stcmwilliams@gmail.com