- 1Department of Bioscience & Biotechnology, Fakir Mohan University, Balasore, India
- 2Centre of Excellence, Khallikote University, Berhampur, India
- 3Department of Food Technology and Biochemical Engineering, Faculty of Engineering and Technology, Jadavpur University, Kolkata, India
- 4Malda Polytechnic, West Bengal State Council of Technical Education, Government of West Bengal, Malda, India
- 5Faculty of Fisheries and Food Science, Universiti Malaysia Terengganu, Kuala Nerus, Malaysia
- 6Department of Bioengineering and Technology, Gauhati University, Guwahati, India
- 7Department of Chemistry, C.V. Raman Global University, Bhubaneswar, India
- 8Research Divisions, Association for Biodiversity Conservation and Research, Balasore, India
- 9Aquamarina Research Foundation, Dona Paula, India
- 10Forensic Science Programme, School of Health Sciences, Universiti Sains Malaysia, Kelantan, Malaysia
- 11Institute of Tropical Biodiversity and Sustainable Development, Universiti Malaysia Terengganu, Kuala Nerus, Malaysia
The γ-irradiated horseshoe crab chitosan was used as food coating to extend the shelf life of marine shrimp and fish. Fourier-transform infrared spectroscopy (FTIR), field emission scanning electron microscopy (FE-SEM), and X-ray diffraction (XRD) were used to characterize the γ-irradiated chitosan. After employing control (untreated seafood samples/no preservatives), chemical preservation (treated with 2% glacial acetic acid) and 2% chitosan (0, 10, and 20 kGy γ-irradiation) for the assessment assay, the shrimp (Penaeus merguiensis), pomfret (Pampus argenteus), and hilsa fish (Tenualosa ilisha) samples were examined for pH, thiobarbituric acid reactive substance (TBARS), total viable counts (TVC), and sensory evaluation changes while under 15-day refrigeration at 4°C. The results of FT-IR, XRD, and FE-SEM analysis revealed that irradiated chitosan possessed a crystalline structure with smooth texture on its surface. Analysis of pH, TBARS, TVC, and sensory evaluation demarcated irradiated chitosan with the ability to delay microbial growth and this prolonged the shelf life of refrigerated shrimp and fish. With novelty on γ-irradiated horseshoe crab chitosan use as natural preserving agent, fisheries industries and food packaging practitioners would benefit from its microbial-inert abilities particularly for long distant cold storage transport of packaged marine meats.
Introduction
Seafood contains fatty acids such as omega-3, proteins and minerals which altogether contribute to an ideal diet (Cheung et al., 2010; Kwan et al., 2019). However, these nutritional components are sustenance for microbes during food oxidation (spoilage). Rapid spoilage of food is mainly due to the high moisture and the decomposition of unsaturated fatty acids and free amino acids (Binsi et al., 2015). In fact, unsaturated fatty acids are chemically unstable and easily oxidized (Kilincceker et al., 2009). In the presence of oxygen and temperatures above 4°C, aerobic microorganisms are provided with optimum metabolic conditions to reproduce (Özogul et al., 2004; Li et al., 2020). Therefore, additives that can limit microbial growth are certain to delay the decomposition (fatty acid and lipid oxidation) process and thus, extend the shelf life of food. At present, industries involved with marine produce rely on freezing, salting, chemical treatments and nitrogen or vacuum packaging for long-term storage of their products (Gokoglu, 2019; Hazra et al., 2020; Sarkar et al., 2021).
Freezing (cold storage) will delay microbial growth because it suppresses their enzymatic activities which therefore, locks nutrition in food (Jiang and Lee, 2005; Gonçalves and Gindri Junior, 2009). Yet, freezing alone causes surface dehydration, protein denaturation, and lipid oxidation which negatively impacts flavor, odor, color, and texture of the stored foods (Gao et al., 2014; Duan et al., 2019). Although shelf life of frozen foods is extended with synthetic and artificial additives, these chemicals may cause allergy, health complications or require a secondary natural compound to become edible-safe (Matuska et al., 2006; Gultekin and Doguc, 2013). With such shortcomings, researchers resort to identify suitable biological sources that function as both food additives and preservation (Cao et al., 2012). After a series of extract preparations, chitosan (molecular weight: 50,000–190,000 Da) from crustaceans, insects, and fungi were discovered with antimicrobial ability (Shahidi et al., 1999; Prashanth and Tharanathan, 2007; Friedman and Juneja, 2010). In addition, chitosan derived from natural sources has different viscousness in room temperature, has low toxicity and is biodegradable and biocompatible to various foods (USFDA, 2001; Prashanth and Tharanathan, 2007; Soares et al., 2013).
While heating cleaves the glycosidic bonds of chitosan, reducing its molecular weight can enhance the antimicrobial capabilities (Hao et al., 2021). Therefore, heat-treated or increasing the concentration of naturally sourced chitosan has been an industrial practice to preserve raw (Ouattar et al., 2000; Sagoo et al., 2002; Rabea et al., 2003; Soultos et al., 2008; Raafat and Sahl, 2009; Friedman and Juneja, 2010; Bonilla et al., 2013; Duan et al., 2019; Hu and Gänzle, 2019; Lee et al., 2019) and processed meats on the shelf (Darmadji and Izumimoto, 1994; Georgantelis et al., 2007; Gómez-Estaca et al., 2007; Kim and Thomas, 2007; Kanatt et al., 2008) with additional applications that include long distance transport (Casariego et al., 2008; Cerqueira et al., 2009; Souza et al., 2009; Fernández-Saiz et al., 2013; Zarandona et al., 2021). Yet, the antimicrobial properties of naturally sourced chitosan is selective, depending on its molecular weight, temperature of the storage environment and the pH of the coated food (Devlieghere et al., 2004; Kong et al., 2010; He et al., 2016; Xing et al., 2016). For instance, shrimp chitosan with higher molecular weight (2.3–3.5 × 105 gmol–1) offered weaker antimicrobial capabilities than the lower molecular weight horseshoe crab chitosan (1.83 × 105 gmol–1) in comparative assays on food oxidation (Zhao et al., 2010; de Queiroz Antonino et al., 2017; Krisfalusi-Gannon et al., 2018; Boudouaia et al., 2019; Pati et al., 2020a).
Researchers learnt that chitosan from marine sources such as shrimp, crab, lobster, krill, and squid possessed different molecular sizes and this influenced the number of functional groups available for antimicrobial capabilities (Younes and Rinaudo, 2015; Tamzi et al., 2020). Since not all marine resources are available throughout the year and their yields vary with catch efforts, researchers explored on the use of heating to reduce the molecular weight of the derived chitosan. It is learnt that heating will de-alkyl chitosan and expose more functional groups whereby, the now less viscous chitosan offers better antimicrobial efficacy (Souza et al., 2010; Ji et al., 2014). Other methods include altering the pH of preserved products to arrest the oxidation process, but this method is less economical and harmful to consumers (Fan et al., 2009). By far, the promising method to reduce chitosan molecular weight is heat irradiation after which several studies made successful comparison between treated and non-treated chitosan to prolong the preservation of frozen meats (Abdeldaiem, 2014; Pati et al., 2016, 2020b; Xing et al., 2016; Hassanzadeh et al., 2017; Lyu et al., 2017; Zhang et al., 2019).
Meanwhile, the mangrove horseshoe crab Carcinoscorpius rotundicauda has no commercial importance in India except for indigenous (Noida) preparation into health tonics and pain-relief ointment and the sparring collection for biomedical research (John et al., 2018). Elsewhere with similar opinions, the dried carapace of C. rotundicauda is used for bioactive compound research (Alam et al., 2015; Luo et al., 2020; Wardiatno et al., 2021; Xu et al., 2021). With horseshoe crab chitosan, specifically sourced from the dried carapace of C. rotundicauda claimed to have the lowest molecular weight than other marine sources, the present study explores on the use of heat radiation [via gamma (γ) irradiation] to further reduce the molecular weight of the derived chitosan for enhanced antimicrobial capability. The chitosan of different molecular weights, recognized as irradiated and non-irradiated, are then coated onto shrimp (Penaeus merguiensis), pomfret (Pampus argenteus) and hilsa fish (Tenualosa ilisha) which have high commercial value throughout Asia (AlMomin et al., 2016; De et al., 2019; Hoang et al., 2020). With novelty on horseshoe crab chitosan being able to display better antimicrobial capabilities after irradiation, the shelf life extension of refrigerated shrimp and fish is assessed using lipid oxidation values, changes to pH and sensory scores within the 15-day storage period as carried out in the present study.
Materials and Methods
Materials
A total of three seafood species namely, shrimp (P. merguiensis) (each weighing about 30 g, average length 33–38 cm), pomfret (P. argenteus) (each weighing about 650 g, average length 30–35 cm) and hilsa fish (T. ilisha) (each weighing about 800 g, average length 30–39 cm) were selected due to their high market and nutritional values (Figure 1). All samples were randomly selected and purchased from 3 vendors certified with ISO 9001/HACCP (hazard analysis at critical control point) and seafood good manufacturing practices (SGMP). All the three vendors were located at a fish market in Naya Bazar, Balasore (21.5077° N, 86.9279° E). Simple random sampling was carried out by collecting a total of 342 shrimp samples out of a harvesting population of 50 kg (approximately 1,600 shrimp). In terms of fish, 218 pomfret and 152 hilsa fish were collected out of approximately 550 and 250, respectively. The simple random sampling used for sample collection was based on the margin of error (ME) which was considered as ±0.05 with 95% confidence level (C) at a sample proportion (SP) of 50%. The sample size was determined using the following formula:
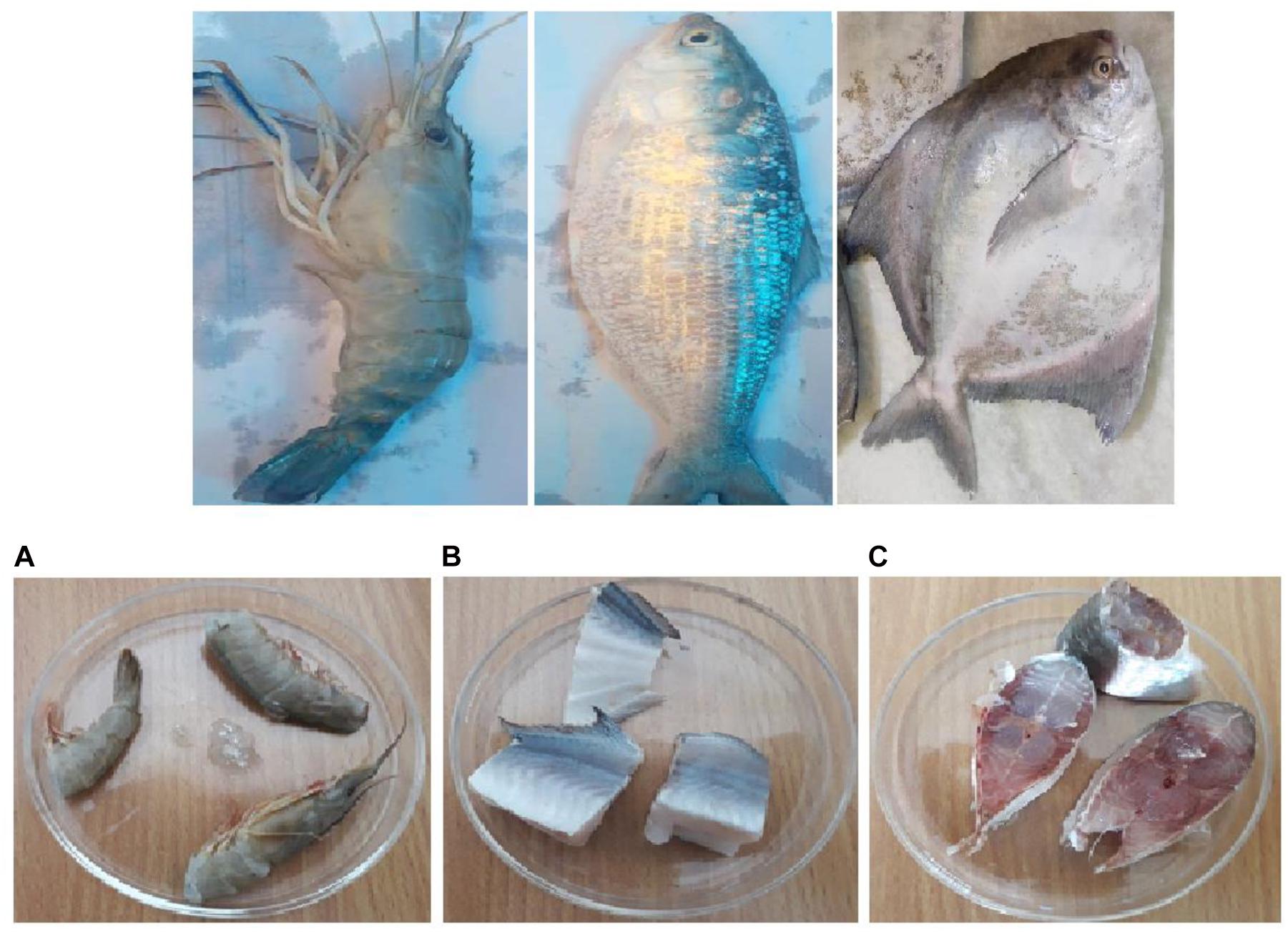
Figure 1. Raw fresh shrimp and fish samples. (A) Penaeus merguiensis, (B) Pampus argenteus, and (C) Tenualosa ilisha.
Where,
Mangrove horseshoe cab carapace (length 14.4 ± 2.1 cm; width 14.7 ± 1.8 cm) samples were obtained from Bichitrapur mangrove sanctuary (21°35′09.3″N and 87°25′21.3″E) estuary (Balasore, Odisha, India). Each shrimp/fish sample was further divided into five equal parts for the later part of the experimentation. Acetic acid (food grade), hydrochloric acid (HCl), sodium hydroxide (NaOH), potassium bromide (KBr), 1-butanol, and thiobarbituric acid (TBA) were purchased from Merck Life Science Pvt. Ltd. (Mumbai, India). The peptone water was purchased from HiMedia Laboratories Pvt. Ltd. (Mumbai, India), waterproof polyvinyl dichloride (PVDC) bags (50–70 micron of thickness) were purchased from Caprihans India Ltd. (Mumbai, India), while double-distilled (DD) water was prepared in the laboratory.
Preparation of Seafood Samples
The specimens were purchased alive and brought into the laboratory in live condition and were killed by placing the samples in chilled water at 4°C to avoid rigor mortise. A thorough cleaning was carried out using DD H2O. Water was then allowed to drain and the samples were left to dry prior to dipping in the chitosan solution. Freshly cleaned samples (500 g) of meat/flesh/muscle tissue was cut, weighed and placed separately (Cao et al., 2020).
Pre-processing of Shrimp Samples
The freshly harvested shrimp samples were drained thrice with potable water to reduce its microbial load and the mud adhered 45 min prior to the initiation of the experiment. After draining, the shrimp was processed manually to remove catgut and deshelling. The resulted fleshy part was washed thoroughly with DD water (Tayel et al., 2020). The processing utensils and equipment used were cleaned with high-pressure water jet to maintain the sanitation standard operating procedures (SSOP).
Chitosan Solution Preparation
Chitosan was prepared from the waste carapace of mangrove horseshoe crab (C. rotundicauda) according to previous studies (Pati et al., 2018, Pati et al., 2020a) and dried at ambient temperature (30 ± 2°C). Irradiation of chitosan was performed using cobalt (Co-60) source at doses of 10 and 50 kGy. The dose rate used was 10 kGy/h in a gamma cell PX-30 irradiation facility. The chitosan solutions were prepared by taking 10 g of horseshoe crab chitosan and dissolving in 500 ml 2% acetic acid. Each of the above solutions was prepared by stirring the samples for 10 min at 60°C for 1 h and the pH was adjusted to five using NaOH (Xuan and Xuan, 2019).
Sample Dipping
Before coating the samples with chitosan, the collected shrimp/fish samples were sub-grouped into five batches each containing 100 g of sample. Batch A was used as control and consisted of untreated seafood samples (no preservatives). Batch B comprised of samples treated with 2% glacial acetic acid as a chemical preservative method. Batches C, D, and E were treated with different types of 2% chitosan solutions (0, 10, and 20 kGy). Preservation was carried out by dipping the samples in respective chitosan solutions for 1 h. After dipping, samples were allowed to dry for 30 min in an aseptic condition before packaging.
Samples Packing and Storage
Samples were packed into air-sealed PVDC bags before they were numbered and marked with the packaging date. The samples were stored in a refrigerator at 4°C and observed over a 15-day period (Bharathi et al., 2019).
Characterization
Fourier-Transform Infrared Spectroscopy Analysis
The FT-IR spectra of dried samples of irradiated and non-irradiated chitosan biopolymer were recorded using Thermo NicoletTM 6700; Thermo Fisher Scientific, United States. The observation of the compound was performed by preparing the powdered samples into KBr pellet and dried before subjection to ATR-attenuated FT-IR at 4,000–500 cm–1 with sixteen scans being taken at 2 cm–1 resolution. Different functional groups against the specific inverse peak of wavenumber (cm–1) had been calculated for the identification of specific functional groups present in the structure of non-irradiated and irradiated samples of the chitosan compound (Jayadevan et al., 2018).
X-ray Diffraction Analysis
The powdered samples of irradiated and non-irradiated chitosan compound were prepared by lyophilization and then subjected to X-ray diffraction (XRD) (Panalytical Diffractometer, X’Pert Pro, United States) at 0.025° (2θ) angle and 52°–45° range with 1.25 s scan time for 1 h with Cu Kα (λ = 1.5406 nm), 45 kV, 30 mA. The crystalline structure and the lattice planes for the changes in the irradiated compound were determined. Different lattice planes against specific diffraction angles were calculated and observed for the identification of the intrinsic details of the structure from the relevant diffractogram (Baran et al., 2015; Sarkar et al., 2020).
Field Emission Scanning Electron Microscopy Analysis
The irradiated and non-irradiated chitosan solutions were prepared at 0, 10, and 20 kGy, the cast was then dropped over the coverslip and visualized under scanning electron microscopy (SEM) (Carl ZEISS SMT, Germany) at 20 kV (Bharathi et al., 2019; Lahiri et al., 2021).
Shelf Life Analysis
Shelf life evaluation was conducted based on physio-chemical and biological indicators. Physio-chemical indicators used were pH and thiobarbituric acid reactive substance (TBARS). Total viable counts (TVC) of microbes was adopted as a biological indicator, while sensory evaluation was employed to evaluate the product acceptability and correlate it with physiochemical and biological parameters. Sampling was carried out on days 0, 10, and 15 for all irradiated and non-irradiated samples.
pH Analysis
The pH of the sample was measured using the GB/T method (GB/T 5009.45-2003) with slight modifications. Approximately, 10 g seafood sample was added to 90 ml of distilled water and filtered. Samples were then incubated for 30 min at room temperature. A digital pH meter was used to measure the pH value (Khodanazary, 2019; Lee et al., 2019).
Thiobarbituric Acid Reactive Substance Analysis
Seafood samples (200 mg) were placed in a flask containing 1 mL 1-butanol. Then, 5 mL reagent (200 mg 2-TBA in 100 mL 1-butanol) was added to the sample before gravity-filtering through Whatman No. 1 filter paper. Test tubes containing the assay mixture were vortexed and placed at 95°C for 120 min in a water bath before cooling using air temperature. Development of a pink colored solution indicated that malondialdehyde (MDA) reacted with TBA (Paparella et al., 2016). A spectrophotometer was used to analyze the samples at 532 nm. TBA was calculated as mg MDA/kg sample using Equation (3):
where As = absorbance of sample and Ab = absorbance of blank.
Total Viable Counts Evaluation
The TVC of microbes were calculated using the pour-plate method (AOAC, 2012) where 25 g of each seafood sample (in triplicates) were aseptically weighed and homogenized with 225 ml of sterilized 0.1% peptone water for 1 min. The homogenized samples were diluted (1:10) in 0.1% peptone water before 1 ml of the samples were plated onto plate count agar and incubated for 48 h at 35–37°C. Result interpretations were made after colony-forming unit data (CFU/g) were log-transformed (Zhang et al., 2019).
Sensory Evaluation
The sensory analysis of all the batches of meat samples was carried out by 10 semi-trained panelists whom were requested to score every batch of samples. Each panelist scored the characteristics from 1 to 9 (9-point hedonic scale) in terms of color, texture, flavor and overall acceptability of samples (9 = like extremely, 5 = do not like or dislike 1 = dislike extremely) (Ehsani et al., 2019).
Statistical Analysis
All experiments were run in triplicate and the data obtained were subjected to statistical analysis (one-way ANOVA for comparing results from the five different sample batches). Differences were considered significant at p < 0.05. The difference between the mean graphs was constructed using OriginPro8.5.
Results and Discussion
Characterization
Fourier-Transform Infrared Spectroscopy Analysis
Functional groups were visualized from the wavelengths of 3,435 cm–1 (OH and NH2), 2,922 and 2,871 cm–1 (–CH), 1,835 cm–1 (–CONH amide I), 1,656 cm–1 (NH2), 1,603 cm–1 (–NH), 1,550 cm–1 (–NH), 1,245 cm–1 (amide-III), 1,160 cm–1 (–O), 1,085 cm–1 (–CO and –OH), and 1,030 cm–1 (–CO and –OH) after FT-IR analysis on irradiated horseshoe crab chitosan (Figure 2). Comparatively, the FT-IR analysis revealed functional groups at 3,000–3,800 cm–1 (OH, NH2), 2,850 cm–1 (–CH), 1,645 cm–1 (–CONH–, amide I), 1,550 cm–1 (–NH, amide II), 1,570 cm–1 (CN), 1,300 cm–1 (amide III), 1,103 cm–1 (CO), and 800 cm–1 (alkyl) wavelengths. Merging the present and previous opinions (Wang et al., 2016), non-irradiated chitosan has less functional groups and all functional groups are easily detected by the FT-IR. Overall, irradiated chitosan possesses additional –OH (3,070–3,750 cm–1), -NH (3,435.81 cm–1) and –CH (2,988.94 cm–1) functional groups after γ-irradiation instead of –NH groups at the peak wavelengths of FT-IR. With the exposure of γ-irradiation on shrimp chitosan, the deacetylation or degradation of polysaccharides allows free radicals to cross-link and produce new functional groups within a crystalline matrix (Ocloo et al., 2011; Younes and Rinaudo, 2015; Li and Zhuang, 2020). This outcome causes the molecular weight of chitosan to become less (Taşkın et al., 2014). While deacetylation of chitosan is proportional and its molecular weight becomes reduced with the increased dose of γ-irradiation from 10 to 20 kGy, the irradiated chitosan of C. rotundicauda (and also Tachypleus gigas – c.a. Pati et al., 2020b) was assumed to have a lower viscosity, have increased solubility and therefore possess better antimicrobial capabilities (Ocloo et al., 2011; Xing et al., 2016; Pati et al., 2020a).
X-ray Diffraction and FE-SEM Analysis
Horseshoe crab chitosan generally has a crystalline lattice structure with 10.5°, 20°, and 27° angles (Muley et al., 2019; Pati et al., 2020a). After 10 kGy irradiation, the horseshoe crab chitosan has a constant 10.5° angled crystal lattice and additional curvature, demarcated with 2θ = 10.5°, will become apparent after 20 kGy irradiation (Figure 3). While chitosan is a biopolymer, γ-irradiation reduces fibrillar curvatures and shifts the paradigm of its crystalline structures into a fixed 10.5° angle which corresponds to the chitosan becoming compact (Rajeswari et al., 2020). The angling from θ = 10.5° after 10 kGy irradiation into 2θ = 10.5° with 20 kGy irradiation symbolizes the effect of a crystalline structure becoming steep and re-structured, whereby the increasing compaction results to reduction of its molecular weight while this molecule develops into a much smoother and defect-free form (Ling et al., 2018; Pati et al., 2020b). Through a visual inspection, non-irradiated chitosan appears much larger and coarser than irradiated chitosan because of its molecular folding (Figures 4A–C). The exposure of γ-irradiation is certain to reorganize chemical bond lengths, giving rise to smaller pore size between the molecules (Muley et al., 2019). However, since the horseshoe crab chitosan becomes compact after γ-irradiation, it possesses more alkyl (–NH) and hydroxyl (–OH) groups, whereby having bonds made between functional groups depicts a stable form of crystal lattice (Ling et al., 2018; Rajeswari et al., 2020).
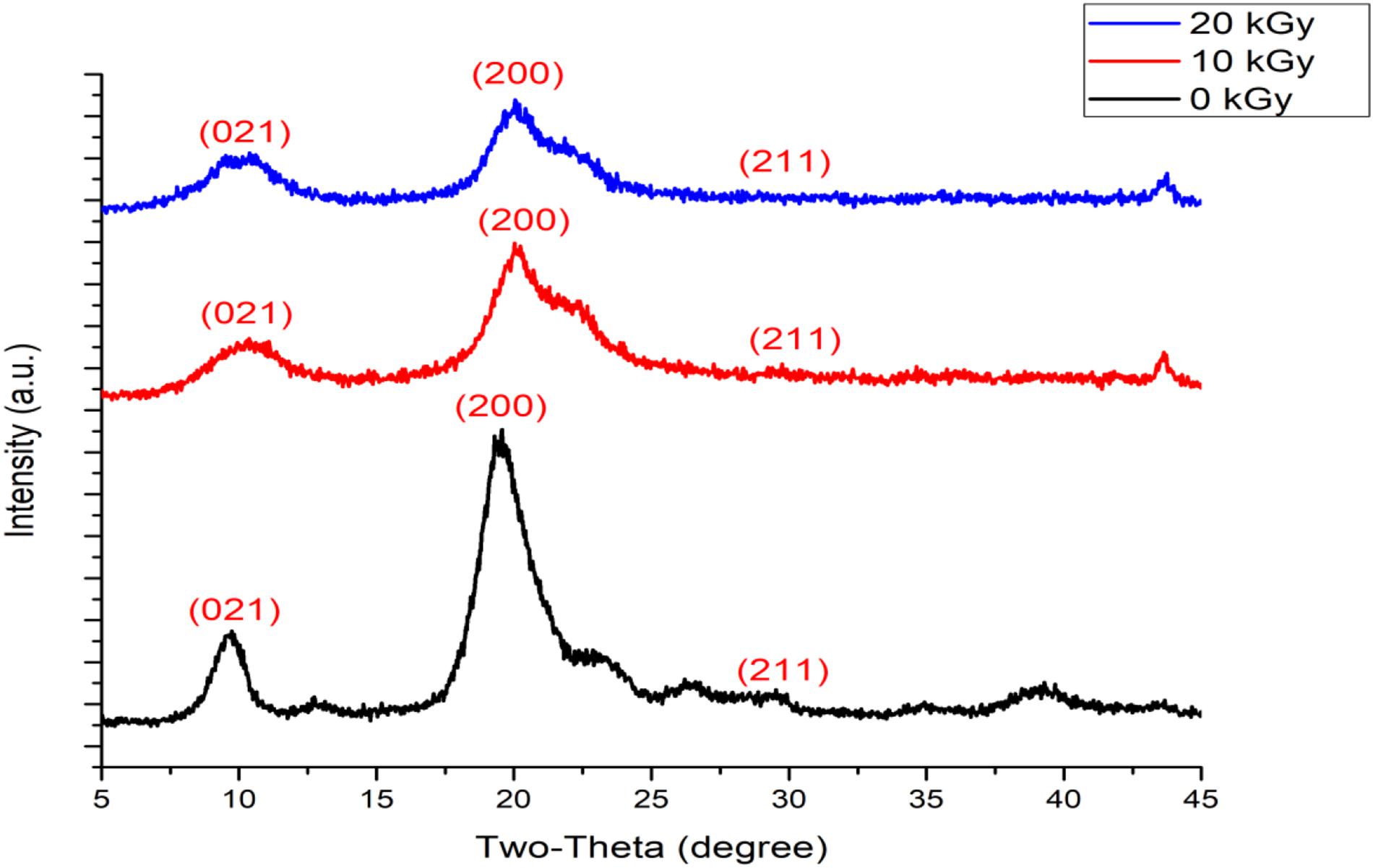
Figure 3. X-ray diffraction (XRD) of chitosan with irradiation at three different irradiations (0–20 kGy).
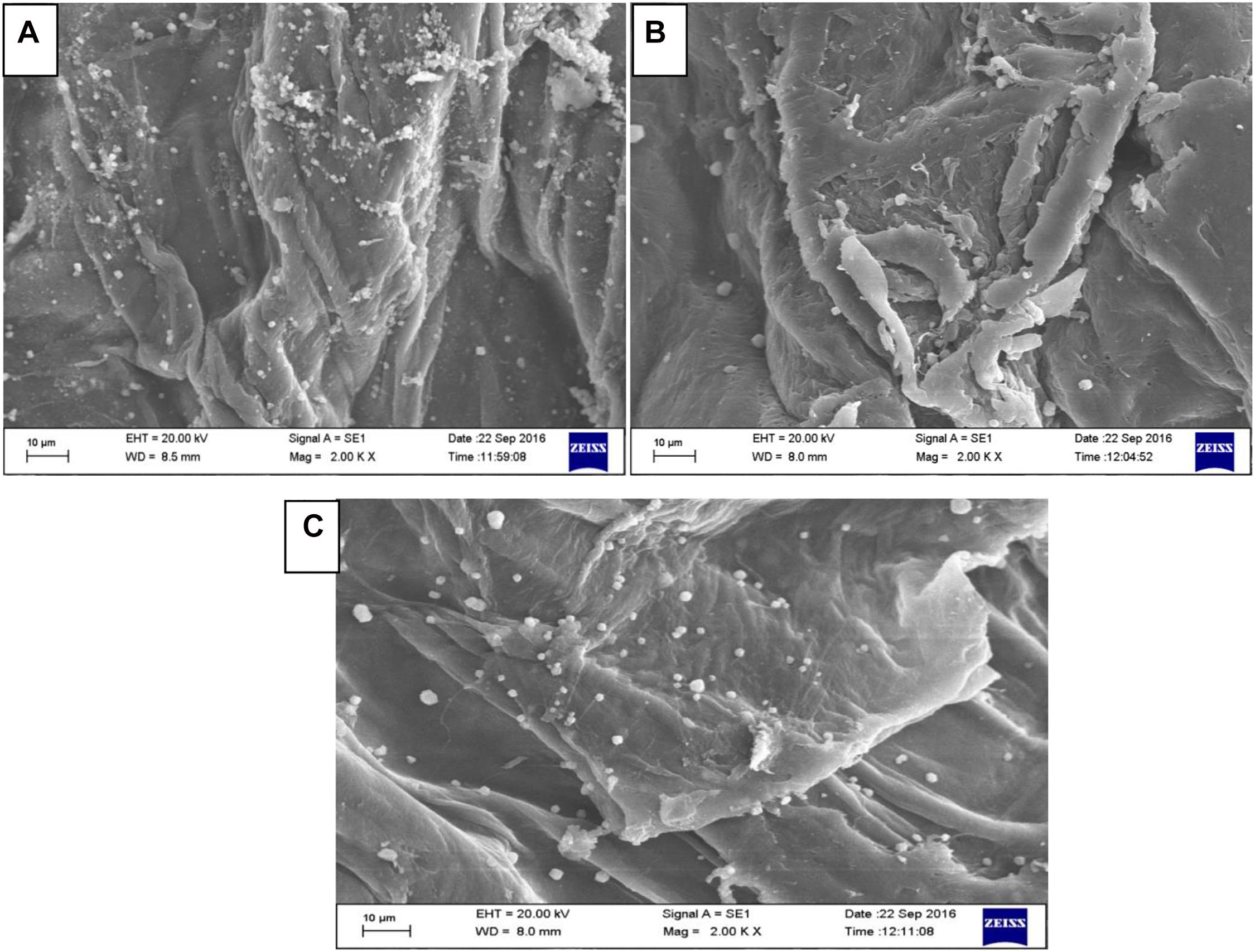
Figure 4. Field emission scanning electron microscopy of chitosan irradiated at three different irradiation ranges (A) 0 kGy, (B) 10 kGy, and (C) 20 kGy irradiation source.
Shelf Life Analysis
pH Analysis
The exposure conditions for chitosan were essential for its chemical stability, particularly to arrest any underlying microbial enzymatic activities. With the varying 0.1–0.5 pH (p < 0.05) in refrigerated conditions, hilsa fish meat appeared to be slightly acidic with pH 6.3 ± 0.9, the lowest compared to pomfret (pH ∼6.3) and shrimp meat (pH ∼6.9). Within 15 days after the 20 kGy irradiated chitosan coating, the pH of shrimp meat increased by 23.18%, 18.75% in pomfret, and 26.98% in hilsa fish (Figure 5). Comparatively, without chitosan treatment (control), the pH of shrimp meat increased by 40.58%, 43.75% in pomfret, and 55.56% in hilsa fish. All refrigerated samples showed an increasing trend of pH for the first 5 days, an observation that conforms to the dissolution of CO2 (Manju et al., 2007; da Silva Santos et al., 2017; Cao et al., 2020). However, the increasing trend of pH leveled off after 10 days of refrigeration suggests that metabolic enzymatic activities within the meat and among microbes have reached an inactive plateau (López-Caballero et al., 2005). Therefore, the pH of meats would increase very minutely after 10 days of cold-storage, regardless with or without the addition of chitosan (Figure 5). However, the freshness, an attribute of moisture and taste locking, pertains to the type of preserving method.
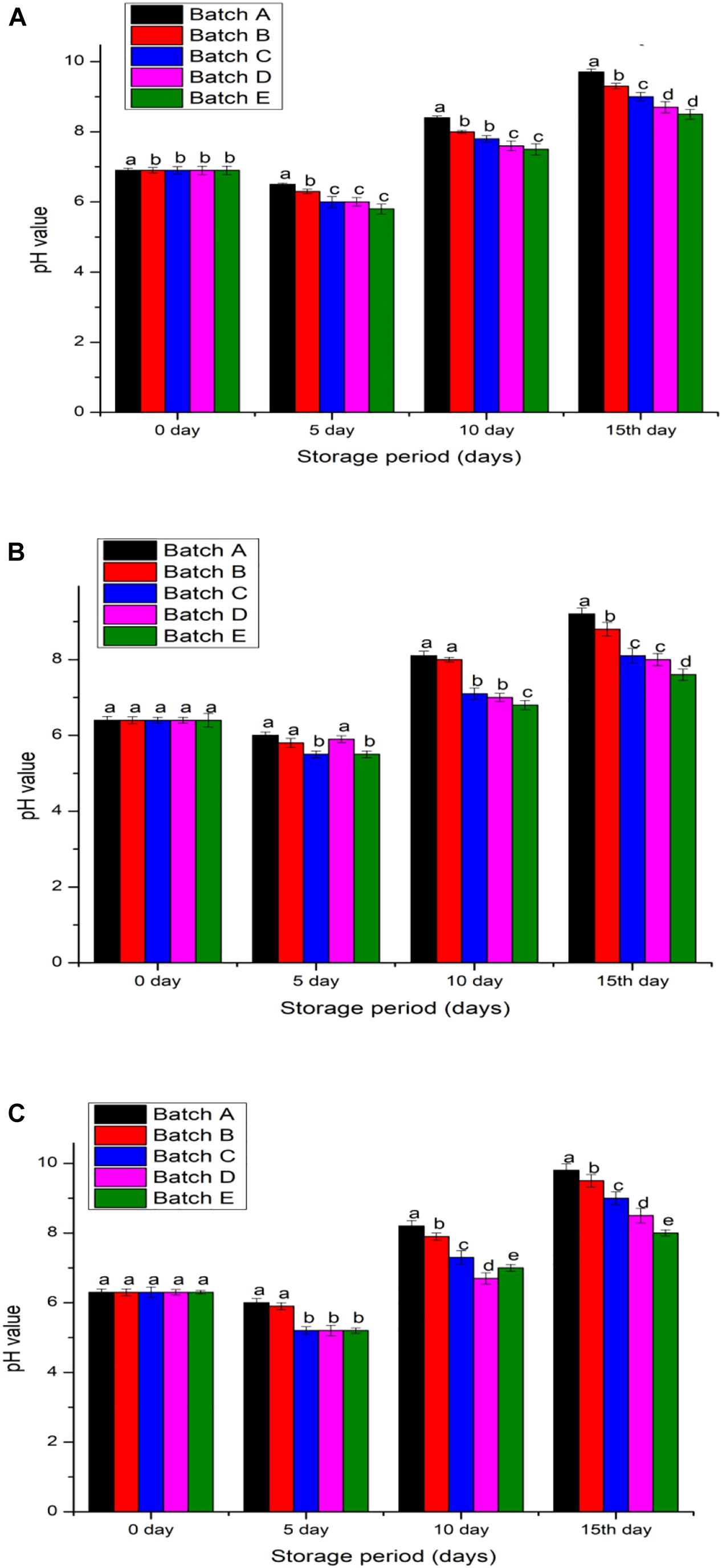
Figure 5. pH value of samples (A) Penaeus merguiensis, (B) Pampus argenteus, and (C) Tenualosa ilisha. Batch A: normal untreated, Batch B: treated with 2% glacial acetic acid, Batches C, D, and E were treated with different types of 2% chitosan solutions (0, 10, and 20 kGy). Value is provided as mean ± SE (n = 3). The same letters (a–e) over the bar plot stands for insignificant difference (p > 0.05).
Thiobarbituric Acid Analysis
The oxidation of fatty acids with three or more double bonds results in malonaldehyde production and this compound negatively impacts color, flavor, and odor food when stored for long periods (Jeon et al., 2002). The detection of high TBA beyond 2 mg malonaldehyde/kg in fish meat symbolizes the effects of spoilage (Jeyakumari et al., 2016). In the present study, all meat products without the chitosan coating and with acetic acid coating registered more than 2 mg/kg TBA on day 0 while with the γ-irradiated chitosan coating, shrimp meat contained 0.6 ± 0.03 mg/kg TBA, pomfret contained 0.26 ± 0.01 mg/kg TBA and hilsa fish contained 0.31 ± 0.01 mg/kg TBA (Figure 6). After 15 days, the control groups (acetic acid preservation and without any treatment) recorded more than 2 mg/kg TBA in the meat. Meanwhile, samples coated with 20 kGy chitosan had less (0.38 mg/kg) TBA readings than the meats coated with 10 kGy chitosan (1.02 mg/kg TBA) after 15 days of refrigeration (Figures 5A–C; p < 0.05). This analysis indicated that partial dehydration from 15-day refrigeration allows some fatty acids to oxidize in the meats but, the selection of appropriate preserving agents such as natural (irradiated chitosan) against chemical (acetic acid) is crucial to increase the shelf life quality of the refrigerated meats (Fan et al., 2009; Guizani et al., 2014; Hassanzadeh et al., 2017). The present findings thus show that cold-storage alone as depicted with the control group (without chitosan coating) is proven ineffective to reduce lipid oxidation and therefore, reduces the quality of meat when stored for long durations (Sneddon, 2009).
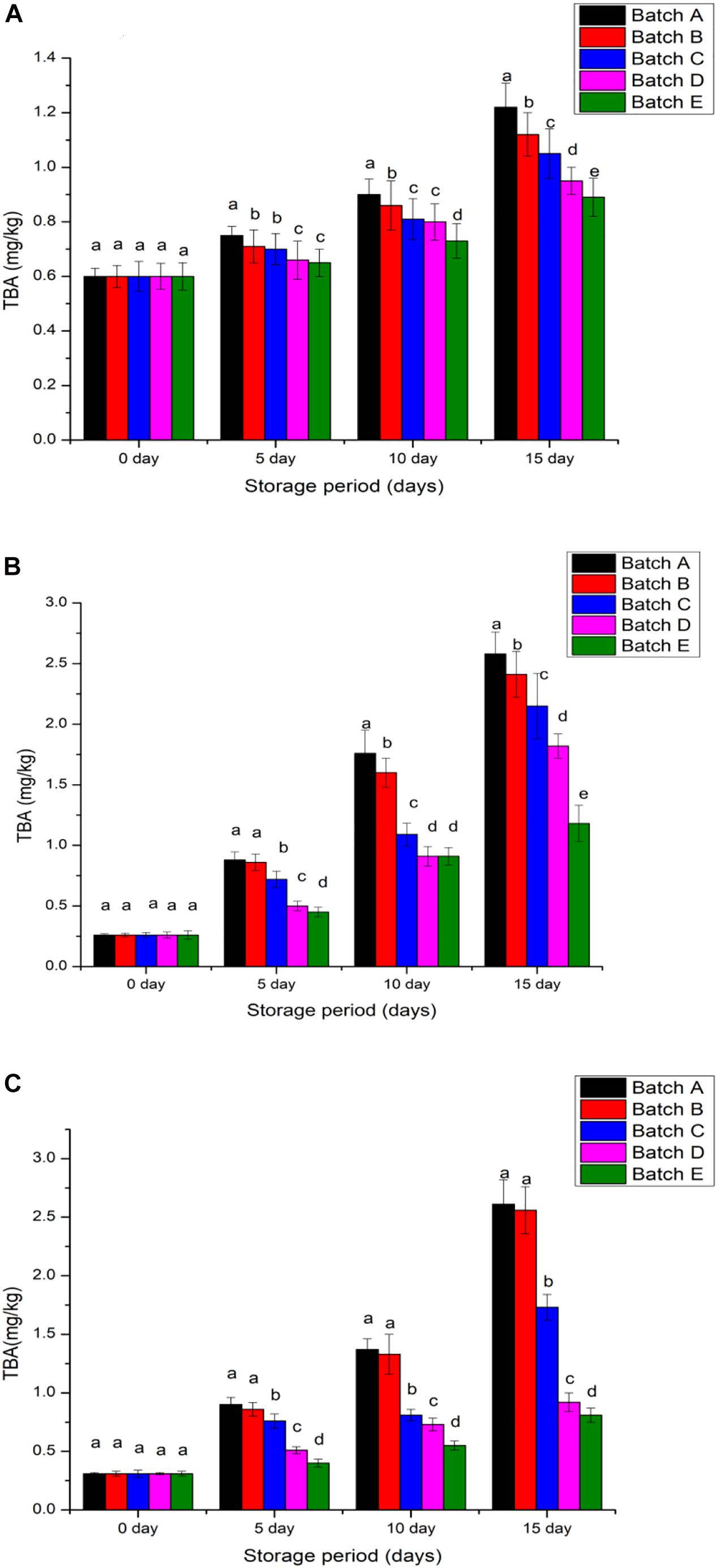
Figure 6. Thiobarbituric acid value of each samples (A) Penaeus merguiensis, (B) Pampus argenteus, and (C) Tenualosa ilisha. Batch A: normal untreated, Batch B: treated with 2% glacial acetic acid, Batches C, D, and E were treated with different types of 2% chitosan solutions (0, 10, and 20 kGy). Value is provided as mean ± SE (n = 3). The same letters (a–e) over the bar plot stands for insignificant difference (p > 0.05).
Total Viable Count
Non-treated meats would attract microbial inhabitants which aid the rotting process. In the food industry, raw salmon (Salmo salar), grass carp (Ctenopharyngodon idellus), and Wuchang bream (Megalobrama amblycephala) filets intended for long-term storage are added with chitosan so that delay to microbial metabolism would decrease their proliferation rate (López-Caballero et al., 2005; Gómez-Estaca et al., 2010; Souza et al., 2010; Fernández-Saiz et al., 2013; Yu et al., 2017). This practice, by using 10 and 20 kGy irradiated chitosan, maintained the TVC of pomfret, and hilsa fish below 6.3 log CFU/g, an acceptable food safety benchmark (Jeyakumari et al., 2016; Figure 7). It was difficult to sustain minimum viable counts in shrimp because on day 0, non-treated samples recorded 4.3 log CFU/g, acetic acid treated samples reached 5.7 log CFU/g, while chitosan treated samples exhibited 4.36 log CFU/g viable counts. All chitosan treated and non-treated shrimps had viable counts that gradually increased within the 15-day refrigeration period but, samples treated with chitosan (10 and 20 kGy) did not exceed 8 log CFU/g in comparison to non-treated samples that exceeded 10 log CFU/g (p < 0.05). Moreover, the rate (days) of viable count growth was delayed in 20 kGy irradiated chitosan than when 10 kGy chitosan was used to coat the meats, which suggest that additional –NH and –OH groups in the further-compacted crystal lattice of 20 kGy chitosan demanded more microbial metabolic energy to break the bonds (Tsai et al., 2004; Song et al., 2011).
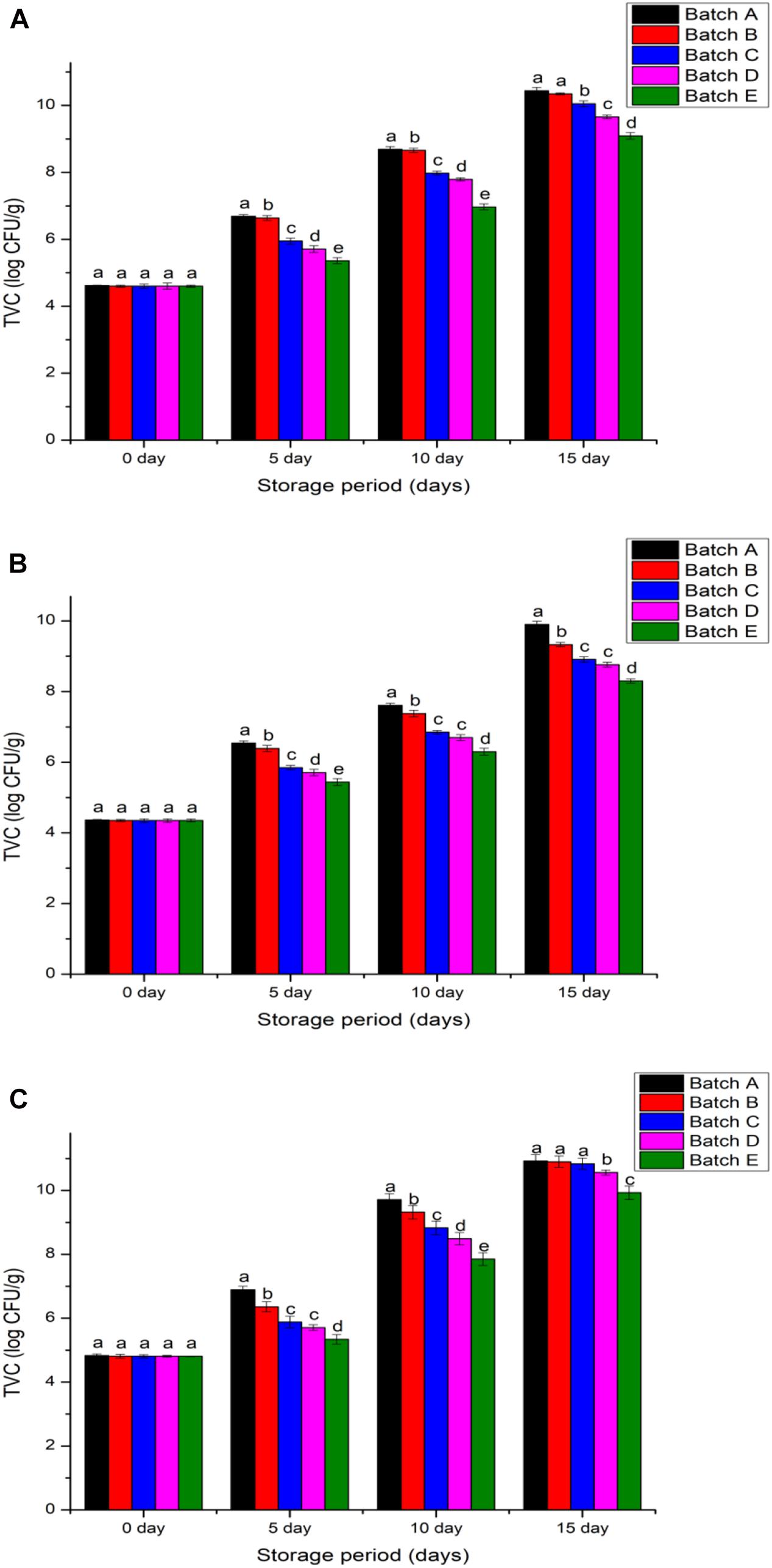
Figure 7. Total viable counts of samples (A) Penaeus merguiensis, (B) Pampus argenteus, and (C) Tenualosa ilisha. Batch A: normal untreated, Batch B: treated with 2% glacial acetic acid, Batches C, D, and E were treated with different types of 2% chitosan solutions (0, 10, and 20 kGy). Value is provided as mean ± SE (n = 3). The same letters (a–e) over the bar plot stands for insignificant difference (p > 0.05).
Sensory Evaluation
The sensory quality of food products revolves with texture, odor, gloss and an appeal (non-stale) to reach customer satisfaction. During refrigeration, the non-treated and acetic acid treated meats changed their color from gray to gray–white with an odor score that immediately reduced and ranged 4.71–4.92 in 5 days before greatly reducing to <1.0 in 15 days (Figure 8). It was different for hilsa fish, shrimp and pomfret that received the chitosan coat because after 5 days, their odor scores were 6.39–6.63 and the score only reduced to 5.04 (for 20 kGy irradiated chitosan) and 4.83 (for 10 kGy irradiated chitosan) within 15 days of refrigeration. It is understood that refrigeration alone is ineffective after 5 days because microbial action to decay the meat will produce a putrid odor (Yu et al., 2017). However, adding a preservative such as (non- or irradiated) chitosan is sufficiently promising to delay the decay of meats and this maintains their odor score above five, which is the baseline score for safe-to-consume shrimp and fish (Yao et al., 2015).
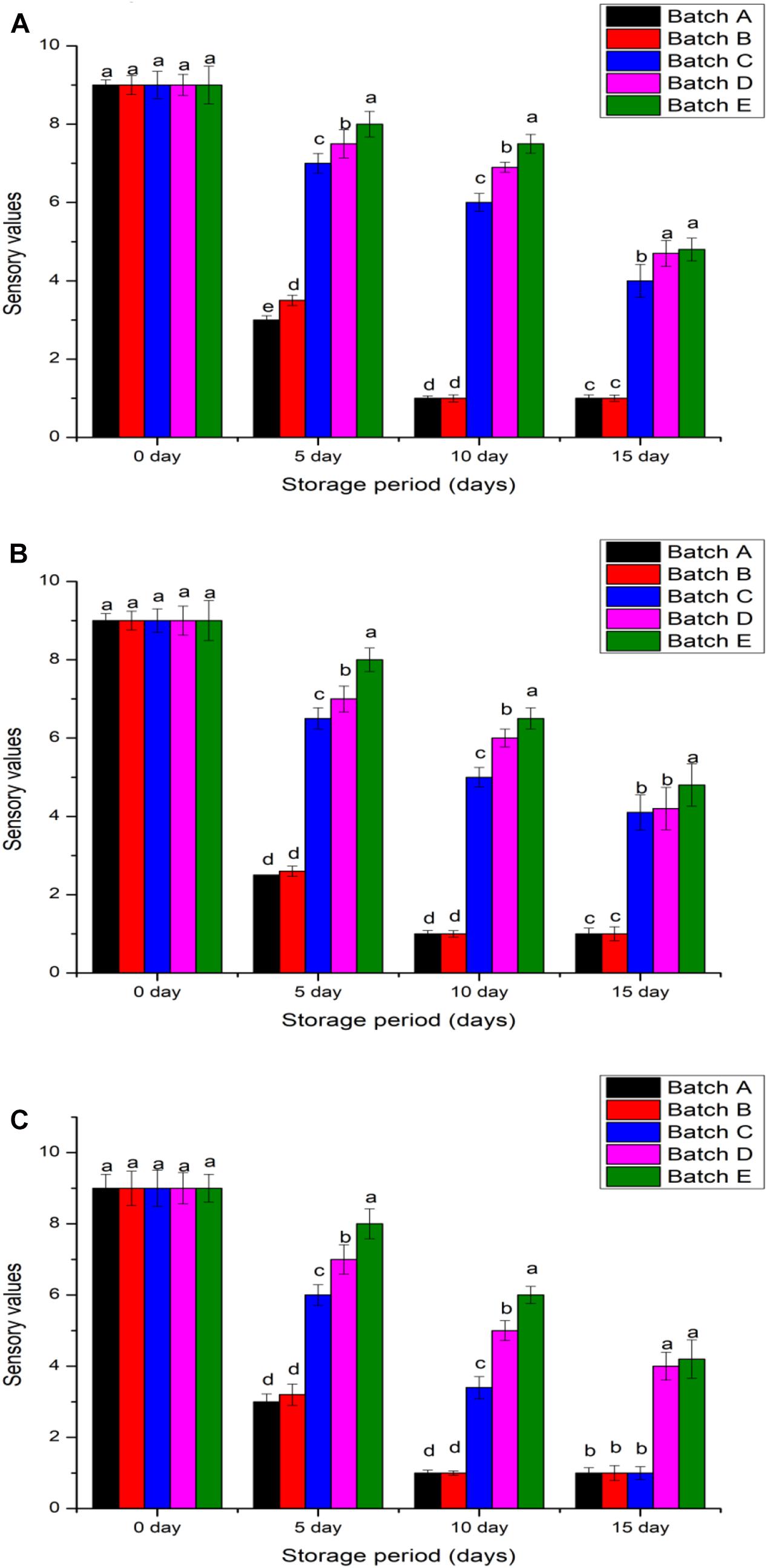
Figure 8. Sensory scores of samples (A) Penaeus merguiensis, (B) Pampus argenteus, and (C) Tenualosa ilisha. Batch A: normal untreated, Batch B: treated with 2% glacial acetic acid, Batches C, D, and E were treated with different types of 2% chitosan solutions (0, 10, and 20 kGy). Value is provided as mean ± SE (n = 3). The same letters (a–d) over the bar plot stands for insignificant difference (p > 0.05).
Conclusion
The effects of γ-irradiated horseshoe crab chitosan on the shelf life of fish and shrimp were evaluated based on microbial activity, pH, TBA, and sensory qualities. It is understood that (10 and 20 kGy) irradiated chitosan had the lowest molecular weight (∼1.83 × 105 gmol–1), possessed additional –NH and –OH functional groups and the 10.5° angles between bonds in the crystal lattice maintained the chitosan within a compact structure and assured this new polymer resistance against microbial action. Overall, high dose (20 kGy) irradiation developed a more effective chitosan polymer for preserving marine meats to successfully extend the shelf life of marine meats beyond the capabilities of refrigeration. The present findings indicate that horseshoe crab chitosan benefits the postharvest industry during long-term transportation of raw meats because coating foods with the irradiated version of this polymer can maintain the freshness and prolong the food shelf life for at least 15 days.
Data Availability Statement
The raw data supporting the conclusions of this article will be made available by the authors, without undue reservation.
Author Contributions
SP designed and performed the experiments and participated in the interpretation of the results and the writing of the manuscript. BN, TS, HE, HS, SB, PM, and KB interpreted the results and edited the manuscript. AC and BD supervised and discussed the research and edited the manuscript. All authors contributed to the realization of the manuscript, have read and agreed to the published version of the manuscript.
Funding
This research was funded by board of Research in Nuclear Sciences (BRNS), Department of Atomic Energy (DAE), Government of India, and Sanction number 2012/35/26/BRNS.
Conflict of Interest
The authors declare that the research was conducted in the absence of any commercial or financial relationships that could be construed as a potential conflict of interest.
Acknowledgments
We acknowledge the research facilities provided by Fakir Mohan University and also Universiti Malaysia Terengganu and Universiti Sains Malaysia for financial support related to the APC. Also, the authors especially thank Sweetie Kanatt, for moral support and encouragement.
References
Abdeldaiem, M. (2014). Using of combined treatment between edible coatings containing ethanolic extract of papaya (Carica Papaya L.) leaves and gamma irradiation for extending shelf-life of minced chicken meat. Am. J. Food Sci. Technol. 2, 6–16.
Alam, H., Chinnari, S., Pati, S., Dash, B. P., and Chatterji, A. (2015). A horseshoe crab peri- vitelline fluid triggers the human bone marrow stem cell differentiation into cardiomyocyte in vitro. Cell Dev. Biol. 4, 3–8.
AlMomin, S., Kumar, V., Al-Amad, S., Al-Hussaini, M., Dashti, T., Al-Enezi, K., et al. (2016). Draft genome sequence of the silver pomfret fish, Pampus argenteus. Genome 59, 51–58. doi: 10.1139/gen-2015-0056
AOAC (2012). Appendix J: AOAC International Methods Committee Guidelines for Validation of Microbiological Methods for Food and Environmental Surfaces. Rockville, MD: AOCA.
Baran, T., Menteş, A., and Arslan, H. (2015). Synthesis and characterization of water soluble O-carboxymethyl chitosan Schiff bases and Cu(II) complexes. Int. J. Biol. Macromol. 72, 94–103. doi: 10.1016/j.ijbiomac.2014.07.029
Bharathi, D., Ranjithkumar, R., Chandarshekar, B., and Bhuvaneshwari, V. (2019). Preparation of chitosan coated zinc oxide nanocomposite for enhanced antibacterial and photocatalytic activity: as a bionanocomposite. Int. J. Biol. Macromol. 129, 989–996. doi: 10.1016/j.ijbiomac.2019.02.061
Binsi, P. K., Viji, P., Visnuvinayagam, S., Ninan, G., Sangeeta, G., Triveni, A., et al. (2015). Microbiological and shelf life characteristics of eviscerated and vacuum packed freshwater catfish (Ompok pabda) during chill storage. J. Food Sci. Technol. 52, 1424–1433. doi: 10.1007/s13197-013-1165-x
Bonilla, J., Atarés, L., Vargas, M., and Chiralt, A. (2013). Properties of wheat starch film-forming dispersions and films as affected by chitosan addition. J. Food Eng. 114, 303–312. doi: 10.1016/j.jfoodeng.2012.08.005
Boudouaia, N., Bengharez, Z., and Jellali, S. (2019). Preparation and characterization of chitosan extracted from shrimp shells waste and chitosan film: application for Eriochrome black T removal from aqueous solutions. Appl. Water Sci. 9:91. doi: 10.1007/s13201-019-0967-z
Cao, R., Liu, Q., Yin, B., and Wu, B. (2012). Chitosan extends the shelf-life of filleted tilapia (Oreochromis niloticus) during refrigerated storage. J. Ocean Univ. China 11, 408–412. doi: 10.1007/s11802-012-1923-9
Cao, X., Islam, M. N., Chitrakar, B., Duan, Z., Xu, W., and Zhong, S. (2020). Effect of combined chlorogenic acid and chitosan coating on antioxidant, antimicrobial, and sensory properties of snakehead fish in cold storage. Food Sci. Nutr. 8, 973–981. doi: 10.1002/fsn3.1378
Casariego, A., Souza, B. W. S., Vicente, A. A., Teixeira, J. A., Cruz, L., and Díaz, R. (2008). Chitosan coating surface properties as affected by plasticizer, surfactant and polymer concentrations in relation to the surface properties of tomato and carrot. Food Hydrocoll. 22, 1452–1459. doi: 10.1016/j.foodhyd.2007.09.010
Cerqueira, M. A., Lima, ÁM., Teixeira, J. A., Moreira, R. A., and Vicente, A. A. (2009). Suitability of novel galactomannans as edible coatings for tropical fruits. J. Food Eng. 94, 372–378. doi: 10.1016/j.jfoodeng.2009.04.003
Cheung, S. G., Wai, H. Y., and Shin, P. K. S. (2010). Fatty acid profiles of benthic environment associated with artificial reefs in subtropical Hong Kong. Mar. Pollut. Bull. 60, 303–308. doi: 10.1016/j.marpolbul.2009.12.001
da Silva Santos, F. M., da Silva, A. I. M., Vieira, C. B., de Araújo, M. H., da Silva, A. L. C., Carneiro-da-Cunha, M., et al. (2017). Use of chitosan coating in increasing the shelf life of liquid smoked Nile tilapia (Oreochromis niloticus) fillet. J. Food Sci. Technol. 54, 1304–1311. doi: 10.1007/s13197-017-2570-3
Darmadji, P., and Izumimoto, M. (1994). Effect of chitosan in meat preservation. Meat Sci. 38, 243–254. doi: 10.1016/0309-1740(94)90114-7
De, D., Mukherjee, S., Anand, P. S. S., Kumar, P., Suresh, V. R., and Vijayan, K. K. (2019). Nutritional profiling of hilsa (Tenualosa ilisha) of different size groups and sensory evaluation of their adults from different riverine systems. Sci. Rep. 9:19306. doi: 10.1038/s41598-019-55845-w
de Queiroz Antonino, R. S. C. M., Lia Fook, B. R. P., de Oliveira Lima, V. A., de Farias Rached, R. Í, Lima, E. P. N., and da Silva Lima, R. J. (2017). Preparation and characterization of chitosan obtained from shells of shrimp (Litopenaeus vannamei Boone). Mar. Drugs 15:141. doi: 10.3390/md15050141
Devlieghere, F., Vermeulen, A., and Debevere, J. (2004). Chitosan: antimicrobial activity, interactions with food components and applicability as a coating on fruit and vegetables. Food Microbiol. 21, 703–714. doi: 10.1016/j.fm.2004.02.008
Duan, C., Meng, X., Meng, J., Khan, M. I. H., Dai, L., Khan, A., et al. (2019). Chitosan as a preservative for fruits and vegetables: a review on chemistry and antimicrobial properties. J. Bioresour. Bioprod. 4, 11–21. doi: 10.21967/jbb.v4i1.189
Ehsani, A., Hashemi, M., Aminzare, M., Raeisi, M., Afshari, A., Mirza Alizadeh, A., et al. (2019). Comparative evaluation of edible films impregnated with sage essential oil or lactoperoxidase system: Impact on chemical and sensory quality of carp burgers. J. Food Process. Preserv. 43:e14070. doi: 10.1111/jfpp.14070
Fan, W., Sun, J., Chen, Y., Qiu, J., Zhang, Y., and Chi, Y. (2009). Effects of chitosan coating on quality and shelf life of silver carp during frozen storage. Food Chem. 115, 66–70. doi: 10.1016/j.foodchem.2008.11.060
Fernández-Saiz, P., Sánchez, G., Soler, C., Lagaron, J. M., and Ocio, M. J. (2013). Chitosan films for the microbiological preservation of refrigerated sole and hake fillets. Food Control. 34, 61–68. doi: 10.1016/j.foodcont.2013.03.047
Friedman, M., and Juneja, V. K. (2010). Review of antimicrobial and antioxidative activities of chitosans in food. J. Food Prot. 73, 1737–1761. doi: 10.4315/0362-028X-73.9.1737
Gao, M., Feng, L., Jiang, T., Zhu, J., Fu, L., Yuan, D., et al. (2014). The use of rosemary extract in combination with nisin to extend the shelf life of pompano (Trachinotus ovatus) fillet during chilled storage. Food Control. 37, 1–8. doi: 10.1016/j.foodcont.2013.09.010
Georgantelis, D., Ambrosiadis, I., Katikou, P., Blekas, G., and Georgakis, S. A. (2007). Effect of rosemary extract, chitosan and α-tocopherol on microbiological parameters and lipid oxidation of fresh pork sausages stored at 4°C. Meat Sci. 76, 172–181. doi: 10.1016/j.meatsci.2006.10.026
Gokoglu, N. (2019). Novel natural food preservatives and applications in seafood preservation: a review. J. Sci. Food Agric. 99, 2068–2077. doi: 10.1002/jsfa.9416
Gómez-Estaca, J., López de Lacey, A., López-Caballero, M. E., Gómez-Guillén, M. C., and Montero, P. (2010). Biodegradable gelatin-chitosan films incorporated with essential oils as antimicrobial agents for fish preservation. Food Microbiol. 102, 278–283. doi: 10.1016/j.fm.2010.05.012
Gómez-Estaca, J., Montero, P., Giménez, B., and Gómez-Guillén, M. C. (2007). Effect of functional edible films and high pressure processing on microbial and oxidative spoilage in cold-smoked sardine (Sardina pilchardus). Food Chem. 130, 797–813. doi: 10.1016/j.foodchem.2007.04.006
Gonçalves, A. A., and Gindri Junior, C. S. G. (2009). The effect of glaze uptake on storage quality of frozen shrimp. J. Food Eng. 90, 285–290. doi: 10.1016/j.jfoodeng.2008.06.038
Guizani, N., Rahman, M. S., Al-Ruzeiqi, M. H., Al-Sabahi, J. N., and Sureshchandran, S. (2014). Effects of brine concentration on lipid oxidation and fatty acids profile of hot smoked tuna (Thunnus albacares) stored at refrigerated temperature. J. Food Sci. Technol. 51:577. doi: 10.1007/s13197-011-0528-4
Gultekin, F., and Doguc, D. K. (2013). Allergic and immunologic reactions to food additives. Clin. Rev. Allergy Immunol. 45, 6–29. doi: 10.1007/s12016-012-8300-8
Hao, G., Hu, Y., Shi, L., Chen, J., Cui, A., Weng, W., et al. (2021). Physicochemical characteristics of chitosan from swimming crab (Portunus trituberculatus) shells prepared by subcritical water pretreatment. Sci. Rep. 11:1646. doi: 10.1038/s41598-021-81318-0
Hassanzadeh, P., Tajik, H., Rohani, S. M. R., Moradi, M., Hashemi, M., and Aliakbarlu, J. (2017). Effect of functional chitosan coating and gamma irradiation on the shelf-life of chicken meat during refrigerated storage. Radiat. Phys. Chem. 141, 103–109. doi: 10.1016/j.radphyschem.2017.06.014
Hazra, S. K., Sarkar, T., Salauddin, M., Sheikh, H. I., Pati, S., and Chakraborty, R. (2020). Characterization of phytochemicals, minerals and in vitro medicinal activities of bael (Aegle marmelos L.) pulp and differently dried edible leathers. Heliyon 6:e05382. doi: 10.1016/j.heliyon.2020.e05382
He, X., Li, K., Xing, R., Liu, S., Hu, L., and Li, P. (2016). The production of fully deacetylated chitosan by compression method. Egypt. J. Aquat. Res. 42, 75–81. doi: 10.1016/j.ejar.2015.09.003
Hoang, T., Ho, H. C., Le, N. P. T., and Bui, T. H. H. (2020). Effects of high temperature on survival and feed consumption of banana shrimp Penaeus merguiensis. Aquaculture 522:735152. doi: 10.1016/j.aquaculture.2020.735152
Hu, Z., and Gänzle, M. G. (2019). Challenges and opportunities related to the use of chitosan as a food preservative. J. Appl. Microbiol. 126, 1318–1331. doi: 10.1111/jam.14131
Jayadevan, J., Alex, R., and Gopalakrishnapanicker, U. (2018). Deproteinised natural rubber latex grafted poly(dimethylaminoethyl methacrylate) - poly(vinyl alcohol) blend membranes: synthesis, properties and application. Int. J. Biol. Macromol. 107, 1821–1834. doi: 10.1016/j.ijbiomac.2017.10.042
Jeon, Y. J., Kamil, J. Y. V. A., and Shahidi, F. (2002). Chitosan as an edible invisible film for quality preservation of herring and Atlantic cod. J. Agric. Food Chem. 50, 5167–5178. doi: 10.1021/jf011693l
Jeyakumari, A., Ninan, G., Joshy, C. G., Parvathy, U., Zynudheen, A. A., and Lalitha, K. V. (2016). Effect of chitosan on shelf life of restructured fish products from pangasius (pangasianodon hypophthalmus) surimi during chilled storage. J. Food Sci. Technol. 53, 2099–2107. doi: 10.1007/s13197-016-2174-3
Ji, J., Wang, L., Yu, H., Chen, Y., Zhao, Y., Zhang, H., et al. (2014). Chemical modifications of chitosan and its applications. Polym. Plast. Technol. Eng. 53, 1494–1505. doi: 10.1080/03602559.2014.909486
Jiang, S. T., and Lee, T. C. (2005). “Freezing seafood and seafood products: principles and applications,” in Handbook of Food Science, Technology, and Engineering - 4 Volume Set, eds Y. H. Hui and F. Sherkat (CRC Press).
John, B. A., Nelson, B. R., Sheikh, H. I., Cheung, S. G., Wardiatno, Y., Dash, B. P., et al. (2018). A review on fisheries and conservation status of Asian horseshoe crabs. Biodivers. Conserv. 27, 3573–3598. doi: 10.1007/s10531-018-1633-8
Kanatt, S. R., Chander, R., and Sharma, A. (2008). Chitosan and mint mixture: a new preservative for meat and meat products. Food Chem. 107, 845–852. doi: 10.1016/j.foodchem.2007.08.088
Khodanazary, A. (2019). Freshness assessment of shrimp Metapenaeus affinis by quality index method and estimation of its shelf life. Int. J. Food Prop. 22, 309–319. doi: 10.1080/10942912.2019.1580719
Kilincceker, O., Dogan, I. S., and Kucukoner, E. (2009). Effect of edible coatings on the quality of frozen fish fillets. LWT Food Sci. Technol. 42, 868–873. doi: 10.1016/j.lwt.2008.11.003
Kim, K. W., and Thomas, R. L. (2007). Antioxidative activity of chitosans with varying molecular weights. Food Chem. 101, 308–313. doi: 10.1016/j.foodchem.2006.01.038
Kong, M., Chen, X. G., Xing, K., and Park, H. J. (2010). Antimicrobial properties of chitosan and mode of action: a state of the art review. Int. J. Food Microbiol. 144, 51–63. doi: 10.1016/j.ijfoodmicro.2010.09.012
Krisfalusi-Gannon, J., Ali, W., Dellinger, K., Robertson, L., Brady, T. E., Goddard, M. K. M., et al. (2018). The role of horseshoe crabs in the biomedical industry and recent trends impacting species sustainability. Front. Mar. Sci. 5:185. doi: 10.3389/fmars.2018.00185
Kwan, B. K. Y., Hu, M., Wang, Y., Cheung, S. G., and Shin, P. K. S. (2019). Fatty acids from controlled feeding as dietary markers of juvenile Chinese horseshoe crab, Tachypleus tridentatus. J. Mar. Biol. Assoc. U. K. 99, 421–428. doi: 10.1017/S0025315418000279
Lahiri, D., Nag, M., Sarkar, T., Dutta, B., and Ray, R. R. (2021). Antibiofilm activity of α-Amylase from Bacillus subtilis and prediction of the optimized conditions for biofilm removal by response surface methodology (RSM) and Artificial Neural Network (ANN). Appl. Biochem. Biotechnol. 193, 1–20. doi: 10.1007/s12010-021-03509-9
Lee, J. S., Jahurul, M. H. A., Pua, V. C., Shapawi, R., and Chan, P. T. (2019). Effects of chitosan and ascorbic acid coating on the chilled tilapia fish (Oreochromis niloticus) fillet. J. Phys. Conf. Ser. 1358:12009. doi: 10.1088/1742-6596/1358/1/012009
Li, J., and Zhuang, S. (2020). Antibacterial activity of chitosan and its derivatives and their interaction mechanism with bacteria: current state and perspectives. Eur. Polym. J. 138:109984. doi: 10.1016/j.eurpolymj.2020.109984
Li, M., Xu, C., Ma, Y., Ye, R., Chen, H., Xie, D., et al. (2020). Effects of dietary n-3 highly unsaturated fatty acids levels on growth, lipid metabolism and innate immunity in juvenile golden pompano (Trachinotus ovatus). Fish Shellf. Immunol. 105, 177–185. doi: 10.1016/j.fsi.2020.06.060
Ling, S., Chen, W., Fan, Y., Zheng, K., Jin, K., Yu, H., et al. (2018). Biopolymer nanofibrils: structure, modeling, preparation, and applications. Prog. Polym. Sci. 85, 1–56. doi: 10.1016/j.progpolymsci.2018.06.004
López-Caballero, M. E., Gómez-Guillén, M. C., Pérez-Mateos, M., and Montero, P. (2005). A chitosan-gelatin blend as a coating for fish patties. Food Hydrocoll. 19, 303–311. doi: 10.1016/j.foodhyd.2004.06.006
Luo, Z., Miao, F., Hu, M., and Wang, Y. (2020). Research development on horseshoe crab: a 30-Year bibliometric analysis. Front. Mar. Sci. 7:41. doi: 10.3389/fmars.2020.00041
Lyu, F., Zhang, J., Wei, Q., Gao, F., Ding, Y., and Liu, S. (2017). Gamma radiation combined with cinnamon oil to maintain fish quality. Radiat. Phys. Chem. 141, 220–222. doi: 10.1016/j.radphyschem.2017.06.005
Manju, S., Jose, L., Srinivasa Gopal, T. K., Ravishankar, C. N., and Lalitha, K. V. (2007). Effects of sodium acetate dip treatment and vacuum-packaging on chemical, microbiological, textural and sensory changes of Pearlspot (Etroplus suratensis) during chill storage. Food Chem. 102, 27–35. doi: 10.1016/j.foodchem.2006.04.037
Matuska, M., Lenart, A., and Lazarides, H. N. (2006). On the use of edible coatings to monitor osmotic dehydration kinetics for minimal solids uptake. J. Food Eng. 72, 85–91. doi: 10.1016/j.jfoodeng.2004.11.023
Muley, A. B., Ladole, M. R., Suprasanna, P., and Dalvi, S. G. (2019). Intensification in biological properties of chitosan after γ-irradiation. Int. J. Biol. Macromol. 131, 435–444. doi: 10.1016/j.ijbiomac.2019.03.072
Ocloo, F. C. K., Quayson, E. T., Adu-Gyamfi, A., Quarcoo, E. A., Asare, D., Serfor-Armah, Y., et al. (2011). Physicochemical and functional characteristics of radiation-processed shrimp chitosan. Radiat. Phys. Chem. 80, 837–841. doi: 10.1016/j.radphyschem.2011.03.005
Ouattar, B., Simard, R. E., Piett, G., Bégin, A., and Holley, R. A. (2000). Inhibition of surface spoilage bacteria in processed meats by application of antimicrobial films prepared with chitosan. Int. J. Food Microbiol. 62, 139–148. doi: 10.1016/S0168-1605(00)00407-4
Özogul, F., Polat, A., and Özogul, Y. (2004). The effects of modified atmosphere packaging and vacuum packaging on chemical, sensory and microbiological changes of sardines (Sardina pilchardus). Food Chem. 85, 49–57. doi: 10.1016/j.foodchem.2003.05.006
Paparella, A., Mazzarrino, G., Chaves-López, C., Rossi, C., Sacchetti, G., Guerrieri, O., et al. (2016). Chitosan boosts the antimicrobial activity of Origanum vulgare essential oil in modified atmosphere packaged pork. Food Microbiol. 59, 23–31. doi: 10.1016/j.fm.2016.05.007
Pati, S., Chatterji, A., and Dash, B. P. (2018). Chitosan from the carapace of Indian horseshoe crab (Tachypleus gigas, müller): isolation and its characterization. Adv. Biores. 9, 52–64. doi: 10.15515/abr.0976-4585.9.4.5264
Pati, S., Chatterji, A., Dash, B. P., Nelson, B. R., Sarkar, T., Shahimi, S., et al. (2020a). Structural characterization and antioxidant potential of chitosan by γ-irradiation from the carapace of horseshoe crab. Polymers 12:2361. doi: 10.3390/polym12102361
Pati, S., Jena, P., Shahimi, S., Nelson, B. R., Acharya, D., Dash, B. P., et al. (2020b). Characterization dataset for pre- and post-irradiated shrimp waste chitosan. Data Br. 30, 1–7. doi: 10.1016/j.dib.2020.106081
Pati, S., Sumedha, C., Chatterji, A., and Dash, B. P. (2016). A novel food formulation for the juveniles of penaeusmonodon (Fabricius). Turk. J. Fish. Aquat. Sci. 16, 481–488.
Prashanth, H. K. V., and Tharanathan, R. N. (2007). Chitin/chitosan: modifications and their unlimited application potential-an overview. Trends Food Sci. Technol. 18, 117–131. doi: 10.1016/j.tifs.2006.10.022
Raafat, D., and Sahl, H. G. (2009). Chitosan and its antimicrobial potential - A critical literature survey. Microb. Biotechnol. 2, 186–201. doi: 10.1111/j.1751-7915.2008.00080.x
Rabea, E. I., Badawy, M. E. T., Stevens, C. V., Smagghe, G., and Steurbaut, W. (2003). Chitosan as antimicrobial agent: applications and mode of action. Biomacromolecules 4, 1457–1465. doi: 10.1021/bm034130m
Rajeswari, A., Stobel, J., Christy, E., Gopi, S., Jayaraj, K., and Pius, A. (2020). “9 - Characterization studies of polymer-based composites related to functionalized filler-matrix interface,” in Woodhead Publishing Series in Composites Science and Engineering, eds K. L. Goh, A. M. Kampton, R. T. De Silva, and F. R. C. Thomas (Cambridge: Woodhead Publishing), 219–250. doi: 10.1016/B978-0-08-102665-6.00009-1
Sagoo, S., Board, R., and Roller, S. (2002). Chitosan inhibits growth of spoilage micro-organisms in chilled pork products. Food Microbiol. 19, 175–182. doi: 10.1006/fmic.2001.0474
Sarkar, T., Salauddin, M., Kumar Hazra, S., and Chakraborty, R. (2020). A novel data science application approach for classification of nutritional composition, instrumental colour, texture and sensory analysis of bael fruit (Aegle marmelos (L) correa). Int. J. Intell. Netw. 1, 59–66. doi: 10.1016/j.ijin.2020.07.003
Sarkar, T., Salauddin, M., Pati, S., Sheikh, H. I., and Chakraborty, R. (2021). Application of raw and differently dried Pineapple (Ananas comosus) pulp on Rasgulla (sweetened Casein Ball) to enhance its phenolic profile, shelf life, and in-vitro digestibility characteristics. J. Food Process. Preserv. 45:e15233. doi: 10.1111/jfpp.15233
Shahidi, F., Arachchi, J. K. V., and Jeon, Y. J. (1999). Food applications of chitin and chitosans. Trends Food Sci. Technol. 10, 37–51. doi: 10.1016/S0924-2244(99)00017-5
Sneddon, L. U. (2009). Pain perception in fish: Indicators and endpoints. ILAR J. 50, 338–342. doi: 10.1093/ilar.50.4.338
Soares, N. M., Mendes, T. S., and Vicente, A. A. (2013). Effect of chitosan-based solutions applied as edible coatings and water glazing on frozen salmon preservation - a pilot-scale study. J. Food Eng. 119, 316–323. doi: 10.1016/j.jfoodeng.2013.05.018
Song, Y., Liu, L., Shen, H., You, J., and Luo, Y. (2011). Effect of sodium alginate-based edible coating containing different anti-oxidants on quality and shelf life of refrigerated bream (Megalobrama amblycephala). Food Control. 22, 608–615. doi: 10.1016/j.foodcont.2010.10.012
Soultos, N., Tzikas, Z., Abrahim, A., Georgantelis, D., and Ambrosiadis, I. (2008). Chitosan effects on quality properties of Greek style fresh pork sausages. Meat Sci. 80, 1150–1156. doi: 10.1016/j.meatsci.2008.05.008
Souza, B. W. S., Cerqueira, M. A., Casariego, A., Lima, A. M. P., Teixeira, J. A., and Vicente, A. A. (2009). Effect of moderate electric fields in the permeation properties of chitosan coatings. Food Hydrocoll. 23, 2110–2115. doi: 10.1016/j.foodhyd.2009.03.021
Souza, B. W. S., Cerqueira, M. A., Martins, J. T., Casariego, A., Teixeira, J. A., and Vicente, A. A. (2010). Influence of electric fields on the structure of chitosan edible coatings. Food Hydrocoll. 24, 330–335. doi: 10.1016/j.foodhyd.2009.10.011
Tamzi, N., Faisal, M., Sultana, T., and Ghosh, S. (2020). Extraction and properties evaluation of chitin and chitosan prepared from different crustacean waste. Bangladesh J. Vet. Anim. Sci. 8, 69–76.
Taşkın, P., Canısaǧ, H., and Şen, M. (2014). The effect of degree of deacetylation on the radiation induced degradation of chitosan. Radiat. Phys. Chem. 94, 236–239. doi: 10.1016/j.radphyschem.2013.04.007
Tayel, A. A., Elzahy, A. F., Moussa, S. H., Al-Saggaf, M. S., and Diab, A. M. (2020). Biopreservation of shrimps using composed edible coatings from chitosan nanoparticles and cloves extract. J. Food Qual. 2020, 8878452. doi: 10.1155/2020/8878452
Tsai, G. J., Zhang, S. L., and Shieh, P. L. (2004). Antimicrobial activity of a low-molecular-weight chitosan obtained from cellulase digestion of Chitosan. J. Food Prot. 67, 396–398. doi: 10.4315/0362-028X-67.2.396
USFDA (2001). Primex Ingredients ASA. GRAS claim notification: Primex Chitosan Available Online at: http://www.accessdata.fda.gov/scripts/fcn/gras_notices/grn000073.pdf (accessed March 11, 2021).
Wang, X., Tang, R., Zhang, Y., Yu, Z., and Qi, C. (2016). Preparation of a novel chitosan based biopolymer dye and application in wood dyeing. Polymers 8:338. doi: 10.3390/polym8090338
Wardiatno, Y., Riyanto, B., Iskandar, N. A., Kleinertz, S., Funch, P., and Kurniawan, F. (2021). A New marine biomaterial: the shell of mangrove horseshoe crabs, Carcinoscorpius rotundicauda (Latreille, 1802) emphasizing its physico-chemical characteristics. Front. Mar. Sci. 8:612016. doi: 10.3389/fmars.2021.612016
Xing, Y., Xu, Q., Li, X., Chen, C., Ma, L., Li, S., et al. (2016). Chitosan-based coating with antimicrobial agents: preparation, property, mechanism, and application effectiveness on fruits and vegetables. Int. J. Polym. Sci. 2016:4851730. doi: 10.1155/2016/4851730
Xu, P., Bai, H., Xie, X., Wang, C.-C., Huang, X., Wang, X., et al. (2021). Tri-Spine horseshoe crab aquaculture, ranching and stock enhancement: perspectives and challenges. Front. Mar. Sci. 8:608155. doi: 10.3389/fmars.2021.608155
Xuan, D., and Xuan, B. (2019). Study on preparation of water-soluble chitosan with varying molecular weights and its antioxidant activity. Adv. Mater. Sci. Eng. 2019:8781013. doi: 10.1155/2019/8781013
Yao, X. C., Chang, C. F., and Wu, S. J. (2015). Effect of peach gum polysaccharides on quality changes of white shrimp. Int. J. Biol. Macromol. 72, 1076–1080. doi: 10.1016/j.ijbiomac.2014.10.024
Younes, I., and Rinaudo, M. (2015). Chitin and chitosan preparation from marine sources. Structure, properties and applications. Mar. Drugs 13, 1133–1174. doi: 10.3390/md13031133
Yu, D., Li, P., Xu, Y., Jiang, Q., and Xia, W. (2017). Physicochemical, microbiological, and sensory attributes of chitosan-coated grass carp (Ctenopharyngodon idellus) fillets stored at 4°C. Int. J. Food Prop. 20, 390–401. doi: 10.1080/10942912.2016.1163267
Zarandona, I., López-Caballero, M. E., Montero, M. P., Guerrero, P., de la Caba, K., and Gó́mez-Guillén, M. C. (2021). Horse mackerel (Trachurus trachurus) fillets biopreservation by using gallic acid and chitosan coatings. Food Control. 120:107511. doi: 10.1016/j.foodcont.2020.107511
Zhang, Z., Xia, G., Yang, Q., Fan, X., and Lyu, S. (2019). Effects of chitosan-based coatings on storage quality of Chinese shrimp. Food Sci. Nutr. 7, 4085–4094. doi: 10.1002/fsn3.1275
Keywords: natural preservative, cold storage, food coating, chitosan, fish, shrimp, sensory evaluation
Citation: Pati S, Sarkar T, Sheikh HI, Bharadwaj KK, Mohapatra PK, Chatterji A, Dash BP, Edinur HA and Nelson BR (2021) γ-Irradiated Chitosan From Carcinoscorpius rotundicauda (Latreille, 1802) Improves the Shelf Life of Refrigerated Aquatic Products. Front. Mar. Sci. 8:664961. doi: 10.3389/fmars.2021.664961
Received: 06 February 2021; Accepted: 13 April 2021;
Published: 05 May 2021.
Edited by:
Paul Shin, City University of Hong Kong, Hong KongReviewed by:
Menghong Hu, Shanghai Ocean University, ChinaWang Yueqi, South China Sea Fisheries Research Institute (CAFS), China
Copyright © 2021 Pati, Sarkar, Sheikh, Bharadwaj, Mohapatra, Chatterji, Dash, Edinur and Nelson. This is an open-access article distributed under the terms of the Creative Commons Attribution License (CC BY). The use, distribution or reproduction in other forums is permitted, provided the original author(s) and the copyright owner(s) are credited and that the original publication in this journal is cited, in accordance with accepted academic practice. No use, distribution or reproduction is permitted which does not comply with these terms.
*Correspondence: Siddhartha Pati, cGF0aXNpZGRoYXJ0aGFAZ21haWwuY29t; Hisham Atan Edinur, ZWRpbnVyQHVzbS5teQ==; Bryan Raveen Nelson, YnJ5YW4ubmVsc29uQHVtdC5lZHUubXk=
†These authors have contributed equally to this work