- 1Key Laboratory of Tropical Marine Bio-Resources and Ecology, South China Sea Institute of Oceanology, Chinese Academy of Sciences, Guangzhou, China
- 2Department of Ocean Engineering and Marine Sciences, Florida Institute of Technology, Melbourne, FL, United States
- 3St. Johns River Water Management District, Palatka, FL, United States
Harmful blooms of nanophytoplankton (2–20 μm) are increasingly common and sometimes severe, but requirements and controls of such blooms (e.g., water quality constraints, requirements for nutrients, and the presence of different guilds of grazers) are poorly understood. Laboratory grazing experiments were conducted to evaluate the potential for top-down control by the copepod Parvocalanus crassirostris on a small harmful algal species (an unidentified chlorophyte Picochlorum, 1–4 μm) and to test the effects of cell sizes on grazing rates. The Picochlorum sp. is a strain isolated from a long-lasting harmful algal bloom in the Indian River Lagoon that reached high densities (>1 × 106 cells ml–1). Experiments contrasted grazing on Picochlorum sp. with grazing on the palatable prymnesiophyte Isochrysis galbana (4–6 μm) in monocultures and mixed cultures. When presented in monocultures, grazing rates on Picochlorum sp. were lower than grazing rates on the palatable alternative. When Picochlorum sp. were presented alongside I. galbana, copepods essentially ceased feeding on the former. In additional experiments, P. crassirostris were fed plastic beads with diameters of 2.0–17.9 μm to control for differences in taste, toxicity, production of mucilage and shape of potential food. Paracalanus crassirostris fed most efficiently on beads with diameters of 7.0–7.9 μm. Results revealed that P. crassirostris can consume Picochlorum sp., but small size and the presence of palatable cells reduces the likelihood of top-down control of blooms of Picochlorum sp.
Introduction
Harmful Algal Blooms (HABs) are an increasing problem in estuaries around the world (Berry et al., 2015; Phlips et al., 2015; Cao et al., 2017). This increase in the frequency of blooms has been attributed to eutrophication and the effects of climate change (Smayda, 2008). Recent studies have highlighted the influence of such drivers on the structure and dynamics of phytoplanktonic assemblages (Zingone et al., 2010; O’Neil et al., 2012; Phlips et al., 2015). One example of such changes is a shift to nanoplanktonic HABs in the Indian River Lagoon (IRL), Florida. Before 2011, algal blooms in the IRL were dominated by dinoflagellates and diatoms (Howell, 1953; Steidinger et al., 1998; Badylak et al., 2004; Hsia et al., 2006; Phlips et al., 2011). Since a major bloom in 2011, however, blooms have been composed of primarily small cyanobacteria and eukaryotes (IRL Consortium, 2015). The algal species targeted in this study is an unidentified Picochlorum sp., isolated from the 2011 “superbloom.” This Picochlorum sp. is a unicellular, nanoplanktonic, non-flagellated alga, 1–4 μm in diameter. It was present in the lagoon, but not dominant until 2011 (Phlips et al., 2015), when its highest water column density was documented to be >7 million cells ml–1 (IRL Consortium, 2015). Some species in the genus Picochlorum have been studied for biofuel and bioproduct applications (Zhu and Dunford, 2013) and no toxicity has been reported. Although Picochlorum sp. is unlikely to produce toxins, the bloom can be considered a HAB because of its ecological effects. Extremely high cell densities stopped sunlight from reaching seagrass, which led to the loss of tens of thousands of acres of this critical habitat. The occurrence of an intense and long-lasting bloom of small cells leads to questions about constraints on grazing by zooplankton, especially constraints related to size-selective feeding.
Size-selective feeding is a common characteristic of grazing by zooplankton (Wilson, 1973; Berggreen et al., 1988; Hansen et al., 1994). The efficiency of grazing on particles of various sizes varies among taxa (Wilson, 1973; Berggreen et al., 1988; Hansen et al., 1994, 1997). For example, Hansen et al. (1994) found that the length ratio between predator and their optimal food is around 18:1 for copepods, 8:1 for ciliates, and 1:1 for dinoflagellates. A question that arises for the IRL is whether the size of Picochlorum sp. limited the ability of common copepod grazers to capture, sort, and ingest a dominant phytoplankter, thus, limiting the potential for top-down control. In this study, grazing rates of a common herbivorous copepod, known to co-occur with the bloom of Picochlorum sp., were examined to evaluate the potential for top-down control of this small species. Additionally, grazing rates on a slightly larger (4–6 μm) and palatable microalgae (Isochrysis galbana) were examined for comparison (Shields et al., 2005). Thus, this study aims to (1) determine the grazing rates of the copepod (Parvocalanus crassirostris) on Picochlorum sp.; (2) compare those grazing rates with grazing on a palatable alternative (I. galbana); (3) explore the role of selective feeding by copepods offered both the palatable food and the HAB species at the same densities; and (4) isolate the effects of particle size on grazing rates using plastic beads.
Materials and Methods
Culturing Copepods and Algae
The grazer used in this study was P. crassirostris, which was the dominant herbivorous copepod in the region of the superbloom according to a 3-years record of mesozooplanktonic abundances (Sweat et al., unpublished data). It is a small (∼2 mm long), holoplanktonic, calanoid copepod that dwells in the upper 6–20 m of tropical and subtropical estuarine, coastal, and oceanic waters (Milstein, 1979; Turner and Dagg, 1983; Wong et al., 1993; Tang et al., 1994; Almeida et al., 2012; Sun et al., 2012; Liu et al., 2013). It is common for this species and other calanoid copepods to reside permanently in the mid- to upper water column (Ma and Johnson, 2017), where they feed on microalgae and nanoplankton. These cosmopolitan copepods have been shown to tolerate a wide range of temperatures (15–31°C), salinities (20–37), and eutrophic or turbid conditions (Milstein, 1979; Wong et al., 1993; Almeida et al., 2012). Paracalanus crassirostris mainly grazes on nanophytoplankton (Calbet et al., 2000) and is a globally important mesozooplanktonic grazer.
Both copepods (P. crassirostris) and algae (Picochlorum sp. and I. galbana) were cultured by Algagen, LLC (Vero Beach, FL 32961, United States). Copepods were originally isolated from net tows in the IRL. Starter cultures of the algae were isolated from IRL by the E. Phlips at the University of Florida. Cultures were maintained in whole seawater filtered through a 0.45-μm filter, with the addition of Guillard’s F/2 marine enrichment solution. All algae were cultured in 2-l glass jars held at 26°C, with continuous aeration and 1,100 lux of light provided continuously by fluorescent bulbs. Both I. galbana and Picochlorum sp. were cultured at a salinity of 25. Copepods were cultured at 26°C and a salinity of 25 in 2-l glass jars that were aerated gently, and they were fed I. galbana at 15,000 cells ml–1 daily. For 24 h immediately preceding experiments, copepods were maintained without food in gently aerated, 2-l glass jars under a 12/12 light/dark cycle.
Grazing on Algae
Experiments were carried out in filtered seawater and held at 26°C for 20 h under 1,100 lux of artificial light. The experimental setup followed Abu-Rezq et al. (1997) which was an experiment involving relatively high densities of algae. Adult copepods (>200 μm long) were held in 3-ml containers, with algae at specified densities. Controls to estimate algal growth and non-grazing mortality had the same densities of algae and no copepods. Five replicates were prepared for each treatment and the control.
Size Selection
In order to minimize crowding and container effects, size selection experiments were carried out in a larger volume with a lower grazer density (ten adult copepods in 200 ml of filtered seawater). Preliminary experiments showed that copepods proactively grazed on beads for about 6 h, with grazing rates in the same range as that for some algae, and then grazing slowed. For this reason, size selection experiments were limited to a 6-h duration. Therefore, copepods were held for 6 h at temperatures of 25°C and salinities of 25 under 1,100 lux of artificial light. Beakers were gently aerated (one bubble per second) to keep beads suspended. Beads were flavored with water extracted from an I. galbana culture and filtered through a 0.45-μm membrane to encourage grazing.
Copepods were fed six different sizes of polystyrene beads (2.0–2.4, 3.0–3.4, 5.0–5.9, 7.0–7.9, 8.0–12.9, and 13.0–17.9 μm; Spherotech, Inc.). Copepods were fed each size range separately and in a mixture of all sizes. Concentrations were ∼500 beads ml–1 for all experiments. Controls had the same seawater volume and densities of beads but lacked copepods.
Statistical Analyses
The initial and final densities of microalgae or beads were measured via flow cytometry (BD Accuri C6), and copepod survival was scored via stereomicroscopy. Grazing rates (cells copepod–1 h–1) were determined using equations from Frost (1972), with the mean of replicate controls used for comparison (Frost, 1972). Statistically significant differences in grazing rates among different densities of algae were determined via analysis of variance (ANOVA) and post hoc Tukey pairwise comparisons (α = 0.05).
Methodological Considerations
In such laboratory grazing experiments, crowding and container effects are common concerns (Folt and Goldman, 1981; Peters, 1984; Helgen, 1987; Burns, 1995, 2000; Preuss et al., 2009; Lee et al., 2012), but the extreme densities of algae required to mimic conditions in the superbloom (at least 106 cells ml–1), dictated experimental volumes. The goal was to conduct replicated trials using monocultures and 50:50 mixtures of the two algal types at densities of 5.8 × 104, 1.2 × 105, 2.3 × 105, 4.8 × 105, 9.6 × 105, and 1.9 × 106 cells ml–1, but cultures only yielded 9.5 × 105 cells ml–1 for the trials involving mixtures of the Picochlorum sp. and I. galbana. In addition, according to our preliminary experiments, grazer densities of 2.5 individuals ml–1 were necessary in order to observe measurable effects in a reasonable timeframe. To our knowledge, copepod grazing rates have not been tested with cell densities on par with the superbloom. Exploring this unique phenomenon necessitated compromise in the experimental design and it will, therefore, be important to interpret results with due regard for possible container artifacts.
Copepods used in the experiment were mostly adult females. P. crassirostris adult males are non-feeding due to their reduced feeding appendages, so they usually have short lifespan compared to females (Lawson and Grice, 1973). Copepods were kept in batch culture with densities from 1,000 to 2,000 individuals L–1. At these densities, female and male ratio is over 7:1 (Alajmi and Zeng, 2014). Thus, 80–90% of the copepods were females. Female and male copepods are usually morphologically differentiated by fifth pereiopoda and genital somite (Prusova et al., 2012). The body size of P. crassirostris females (up to 450 μm) is larger than that of the males (up to 350 μm). In preparation for experiments, adult copepods were pre-selected using a 200 μm mesh. Then the largest copepods were gently selected using a pasteur pipet under stereomicroscopy. Beyond these selection methods, sex of individual copepods remained unconfirmed because closer scrutiny of the fifth pereiopoda and genital somites might injure the copepods. Therefore, it is acknowledged that a low percentage of non-feeding males were likely included in these experiments. Replication (n = 5) was increased in an attempt to counteract the potential increased variability in grazing rates resulting from the limited inclusion of non-feeding males.
It has been documented that prior experience with a food may influence consumption rates in downstream grazing experiments, such as with the ambush feeder Eucalanus pileatus (Price and Paffenhöfer, 1984). However, filter-feeders display relatively indiscriminate grazing behavior compared to ambush feeders (Gismervik, 2006). As a filter-feeder (Santhosh et al., 2018), P. crassirostris may be less likely to be influenced by previous feeding experience. It is acknowledged, however, that the culturing of P. crassirostris adults on I. galbana prior to the start of the experiments could potentially increase grazing on that same species in short-term experiments (Ayukai, 1987). In order to minimize the influence of previous grazing experience, copepods were starved for 24 h prior to the onset of experiments. However, this common operation used in other studies sometimes inflated estimates of grazing rates (Frost, 1972; Toullec et al., 2019). It is acknowledged that some of the aforementioned methods could result in exaggerated ingestion rates under some conditions.
Results
Parvocalanus crassirostris grazed on monocultures of I. galbana and the Picochlorum sp. without suffering any mortality. Grazing rates increased with increasing densities of both I. galbana (p < 0.001 between the treatments of 5.8 × 104 and 1.9 × 106 cells ml–1) and the Picochlorum sp., although the rates were not statistically different among different Picochlorum sp. treatments (Figure 1A). At densities above 2.3 × 105 cells ml–1, grazing pressure on the Picochlorum sp. was significantly less than that exerted on the more palatable I. galbana (p < 0.05; Figure 1A).
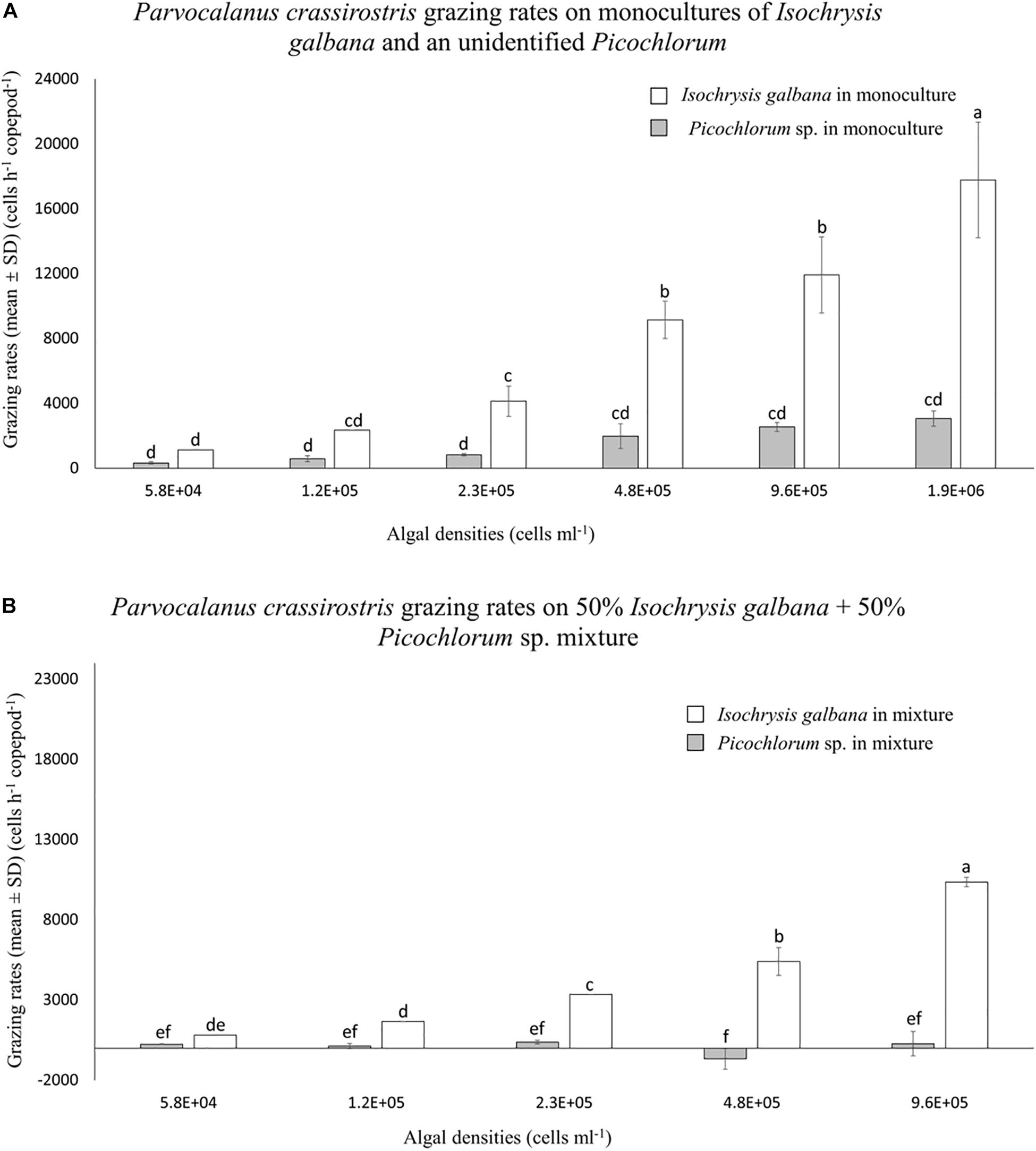
Figure 1. Mean numbers of cells consumed per hour (±standard deviation, SD) by Parvocalanus crassirostris grazing on different densities of Isochrysis galbana and Picochlorum sp. presented as (A) monocultures and as (B) mixtures of 50% I. galbana and 50% Picochlorum sp. Different letters identify significantly different means based on analysis of variance and Tukey’s post hoc pairwise comparisons (α = 0.05).
There was no copepod mortality in experiments employing mixed cultures of I. galbana and the Picochlorum sp. In mixed treatments, grazing rates on I. galbana were lower than when they were presented as a monoculture, but the general pattern of increased grazing at higher densities was similar (Figure 1). In contrast, grazing on the Picochlorum sp. in the mixed culture decreased or ceased altogether, and grazing rates at densities of 1.2 × 105, 4.8 × 105, and 9.6 × 105 cells ml–1 are indistinguishable from zero (Figure 1B).
There was no copepod mortality observed in size selection experiments. Parvocalanus crassirostris grazed differentially on different size beads when fed only one size range (Figure 2A). Copepod grazing rates on 7.0–7.9 μm beads were significantly higher and less variable than consumption rates for the smallest beads (2.0–2.4 μm) and the largest beads (13.0–17.9 μm; p < 0.05; Figure 2A). When fed mixtures of bead sizes, differences in grazing rates disappeared, and the grazing rates on both the smallest beads (2.0–2.4 μm) and the largest beads (13.0–17.9 μm) increased, rendering the rates indistinguishable from rates on mid-sized beads (p > 0.05, Figure 2B).
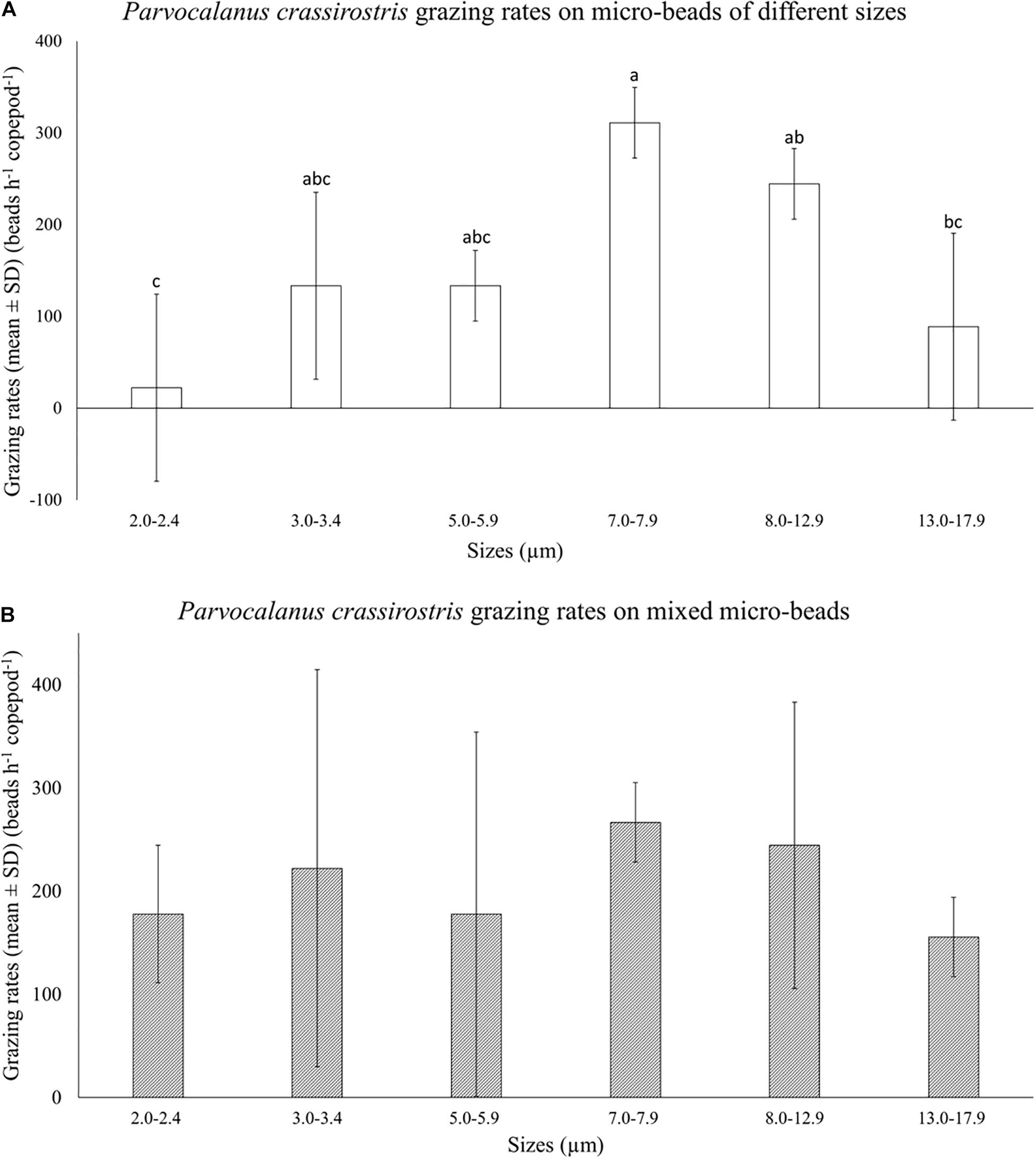
Figure 2. Mean numbers of beads consumed per hour (±standard deviation, SD) by Parvocalanus crassirostris grazing on (A) beads of a single size and (B) a mixture of different sized beads. Different letters identify significantly different means based on analysis of variance and Tukey’s post hoc pairwise comparisons (α = 0.05).
Discussion
Top-down control of algae via grazing varies with grazer characteristics, algal food type, and environmental conditions (Peters, 1984; Hansen et al., 1997; Atkinson, 1998; Nejstgaard et al., 2007; Seuront and Vincent, 2008). Our experiments involved adults of one documented herbivore, which standardized many characteristics (body size, foraging speed, feeding efficiency, and life stage) for these experiments. This study focused on characteristics of phytoplanktonic food, in particular their densities and cell sizes.
Algal densities influenced P. crassirostris grazing rates, regardless of the species of algae being offered or whether they were offered as monocultures or mixed cultures containing HAB species. Mean grazing rates on the palatable I. galbana in monocultures ranged from 1.1 × 103 to 1.8 × 104 cells copepod–1 h–1, which were similar to rates reported for P. crassirostris feeding on nanoplankton (Calbet et al., 2000). P. crassirostris is a filter feeder, with no evidence of raptorial behavior (McKinnon and Klumpp, 1998; Santhosh et al., 2018). Such copepods have been observed to generate small-scale currents that entrain particles, which are subsequently captured by feeding appendages (Koehl and Strickler, 1981). It follows that encounters and resulting grazing rates will, to some degree, depend on algal densities, provided there are no other constraints. Given that many studies have shown positive density-dependent grazing patterns in copepods (Frost, 1972; Abu-Rezq et al., 1997; Anzueto-Sánchez et al., 2014), artifacts due to containment should not have affected HAB grazing substantially or differentially.
Copepods have exhibited size-selective feeding (Wilson, 1973; Berggreen et al., 1988). Parvocalanus crassirostris has been observed to graze on nanophytoplankton with diameters of 2–20 μm (Calbet et al., 2000), but this Picochlorum sp. and other algae at the lower edge of this range may be more difficult for P. crassirostris to detect, capture, or consume. The potential for a grazer to control a harmful bloom is likely influenced by whether the algae are of optimal size. The size of the Picochlorum sp. was 1–4 μm in diameter, which is outside of the optimal size range based upon patterns of feeding on beads (7.0–7.9 μm). Small particle size could contribute to the low grazing rates on Picochlorum sp. Other algal species comprising that same bloom were picocyanobacteria (<1 μm; IRL Consortium, 2015), and these harmful bloom species were smaller than the apparent optimal size of food for P. crassirostris, which may have made them difficult to detect, capture or consume. This copepod species was the dominant mesozooplanktonic herbivore in the IRL, and its inability to exert clear top-down control on this mixed bloom of small-celled algae may have exacerbated the chronic bloom. According to our 3-year (2014–2016) mesozooplankton survey, P. crassirostris populate the IRL year-round, with an average density of 5,452 ± 648 (mean ± SE individuals m–3), which comprised ∼30% of total copepod abundance in the IRL (Sweat et al., unpublished data). Even if applying our highest grazing rates of 3,067 cells hour–1 individual–1, only 4.01E + 08 cells m–3 of Picochlorum sp. would be grazed per day. During the superbloom, Picochlorum sp. density was up to 7 million cells ml–1 (7E + 12 cells m–3), which is 4 orders-of-magnitude higher than the estimated daily grazing capacity from P. crassirostris. Although abundance data were not available for mesozooplankton during the 2011 superbloom, a brown tide occurred during our mesozooplankton survey from late 2015 to early 2016. During the brown tide, numbers of P. crassirostris declined by 97% relative to the same months without a bloom in the preceding year (Sweat et al., unpublished data). Reductions in populations of copepods during algal blooms is a common phenomenon (Badylak and Phlips, 2008). Thus, P. crassirostris may be a poor candidate for top-down control on algal blooms. Some studies have pointed out that nauplii and copepodites are often more abundant than the adult copepods and they can have a significant grazing impact on populations of their foods (Merrell and Stoecker, 1998; Böttjer et al., 2010; Jungbluth et al., 2017). However, since the P. crassirostris nauplius is smaller than 200 μm, it falls out of the scope of the mesozooplankton survey and it is not possible to estimate the grazing potential from other stages of P. crassirostris. Given the reduced numbers of adult P. crassirostris during the brown tide, populations of nauplii and copepodites also may have decreased, which would have minimized grazing pressure on algal blooms. In fact, the average density of total meso-sized copepod nauplii (not identified to species) was only 14 ± 3 (mean ± SE individuals m–3) during the brown tide based on our survey (Sweat et al., unpublished data).
Compared with I. galbana, the diameter of Picochlorum sp. was roughly half. Copepod grazing rates on 2-μm beads, the same size as an average Picochlorum sp., were lower but not significantly different from grazing rates on 5-μm beads, the same size as an average I. galbana cell. These results suggest low grazing rates could be partially due to the size of Picochlorum sp., but they also suggest that other algal characteristics, such as secondary metabolites and structural defenses, could render them less palatable to grazers (Mitra and Flynn, 2006). Leising et al. (2005) found that Calanus pacificus rejected certain Thalassiosira spp. even though they were abundant, the right size, and congeneric with preferred food, because they were producing chemicals that had deleterious effects on reproduction. Little is known, however, about the physiology and ecology of the unidentified Picochlorum sp. used in this study, although potentially related species have not been shown to produce toxins or inhibit grazing by zooplankton (DeBiase et al., 1990). In addition, P. crassirostris always survived when feeding on the Picochlorum sp., which demonstrated that any negative effects are not lethal in the short term, even at very high densities of cells. Further work is needed to confirm the exact mechanism, or combination of mechanisms, that prevent P. crassirostris from exerting strong grazing pressure on Picochlorum sp.
When Picochlorum sp. and I. galbana were offered together in mixed cultures, grazing rates on Picochlorum sp. dropped to nearly zero at most densities, while grazing on I. galbana persisted but at lower rates than in monocultures (Figure 1B). One possible explanation for the absence of grazing on Picochlorum sp. in mixed culture with I. galbana is that P. crassirostris displayed selective grazing. Studies have provided strong evidence that copepods may respond selectively when offered different choices (Donaghay and Small, 1979; Huntley et al., 1983), and this result is even true for some suspension feeders (DeMott, 1989). This phenomenon was attributed to food quality: copepods may distinguish between “food” and “nutritious food” using biochemical differences between foods (Cowles et al., 1988; Butler et al., 1989). However, one recent study demonstrated that this may only be true when food is plentiful. In field studies with limited food and mixed assemblages, the prospect of differentiation based purely on nutrition seems unlikely, and typically factors such as size, motility, and taxonomic composition are considered the major factors driving selectivity by copepods (Isari et al., 2013). In addition, another possible explanation for the absence of grazing on Picochlorum sp. when I. galbana was present is that the latter may have functioned as “background plankton,” that obscured Picochlorum sp. from detection or hindered capture (Johnson and Shanks, 1997, 2003). The size selection experiments also suggested this as a possible explanation. When a mixture of bead sizes was offered to P. crassirostris, differential grazing rates were less distinct (Figure 2B); the smallest and largest beads may have functioned as background plankton, obscuring the intermediate-sized beads or occupying the foraging time of the copepod.
In conclusion, grazing rates on Picochlorum sp. were much lower than grazing rates on I. galbana, and copepods appeared to show selective avoidance of Picochlorum sp. when offered the palatable alternative. Size selection experiments confirmed that P. crassirostris had lower grazing rates when feeding on smaller particles that were in the same size range as the unidentified Picochlorum. Thus, P. crassirostris, a dominant, cosmopolitan, herbivorous copepod present during the bloom of Picochlorum sp., was unlikely to exert effective top-down control on this harmful alga.
Data Availability Statement
The raw data supporting the conclusions of this article will be made available by the authors, without undue reservation.
Author Contributions
XM and KJ conceived the study. XM carried out data collection. XM and CJ analyzed data. All authors contributed to interpretation of the results and preparation and revision of the manuscript. All authors approved the submission.
Funding
The research was funded by contract 27786 from the St. Johns River Water Management District.
Conflict of Interest
The authors declare that the research was conducted in the absence of any commercial or financial relationships that could be construed as a potential conflict of interest.
Acknowledgments
Much appreciation is expressed to E. J. Phlips for supplying the initial HAB cultures. Thanks to J. Trefry, S. Fire, K. Hunsucker, and C. Combs for their advice in developing this project and comments on the manuscript. Thanks to E. Stenn and AlgaGen LLC Culture Company for culturing the copepods and algae. Appreciation also is expressed to L. H. Sweat and H. Kolb for assistance with laboratory work and helpful suggestions.
References
Abu-Rezq, T. S., Yule, A. B., and Teng, S. K. (1997). Ingestion, fecundity, growth rates and culture of the Harpacticoid copepod, Tisbe furcata, in the laboratory. Hydrobiologia 347, 109–118.
Alajmi, F., and Zeng, C. (2014). The effects of stocking density on key biological parameters influencing culture productivity of the Calanoid copepod, Parvocalanus crassirostris. Aquaculture 434, 201–207. doi: 10.1016/j.aquaculture.2014.08.029
Almeida, L. R. D., Costa, I. S., and Eskinazi-Sant’Anna, E. M. (2012). Composition and abundance of zooplankton community of an impacted estuarine lagoon in Northeast Brazil. Braz. J. Biol. 72, 12–24. doi: 10.1590/s1519-69842012000100002
Anzueto-Sánchez, M. A., Barón-Sevilla, B., Cordero-Esquivel, B., and Celaya-Ortega, A. (2014). Effects of food concentration and temperature on development, growth, reproduction and survival of the copepod Pseudodiaptomus euryhalinus. Aquac. Int. 22, 1911–1923. doi: 10.1007/s10499-014-9791-5
Atkinson, A. (1998). Life cycle strategies of epipelagic copepods in the Southern Ocean. J. Mar. Syst. 15, 289–311. doi: 10.1016/s0924-7963(97)00081-x
Ayukai, T. (1987). Discriminate feeding of the Calanoid copepod, Acartia clausi in mixtures of phytoplankton and inert particles. Mar. Biol. 94, 579–587. doi: 10.1007/BF00431404
Badylak, S., Kelley, K., and Phlips, E. J. (2004). A description of Pyrodinium bahamense (Dinophyceae) from the Indian River Lagoon, Florida, USA. Phycologia 43, 653–657. doi: 10.2216/i0031-8884-43-6-653.1
Badylak, S., and Phlips, E. J. (2008). Spatial and temporal distributions of zooplankton in Tampa Bay, Florida, including observations during a HAB event. J. Plankton Res. 30, 449–465. doi: 10.1093/plankt/fbn010
Berggreen, U., Hansen, B., and Kiørboe, T. (1988). Food size spectra, ingestion and growth of the copepod Acartia tonsa during development: implications for determination of copepod production. Mar. Biol. 99, 341–352. doi: 10.1007/bf02112126
Berry, D. L., Goleski, J. A., Koch, F., Wall, C. C., Peterson, B. J., Anderson, O. R., et al. (2015). Shifts in cyanobacterial strain dominance during the onset of harmful algal blooms in Florida Bay, USA. Microb. Ecol. 70, 361–371. doi: 10.1007/s00248-014-0564-5
Böttjer, D., Morales, C. E., and Bathmann, U. (2010). Trophic role of small cyclopoid copepod nauplii in the microbial food web: a case study in the coastal upwelling system off central Chile. Mar. Biol. 157, 689–705. doi: 10.1007/s00227-009-1353-4
Burns, C. W. (1995). Effects of crowding and different food levels on growth and reproductive investment of Daphnia. Oecologia 101, 234–244. doi: 10.1007/bf00317289
Burns, C. W. (2000). Crowding induced changes in growth, reproduction and morphology of Daphnia. Freshw. Biol. 43, 19–29. doi: 10.1046/j.1365-2427.2000.00510.x
Butler, N. M., Suttle, C. A., and Neill, W. E. (1989). Discrimination by freshwater zooplankton between single algal cells differing in nutritional status. Oecologia 78, 368–372. doi: 10.1007/BF00379111
Calbet, C. J., Landry, M. R., and Scheinberg, R. D. (2000). Copepod grazing in a subtropical bay: species-specific responses to a midsummer increase in nanoplankton standing stock. Mar. Ecol. Prog. Ser. 193, 75–84. doi: 10.3354/meps193075
Cao, X., Yu, Z., Wu, Z., Cheng, F., He, L., Yuan, Y., et al. (2017). Environmental characteristics of annual pico/nanophytoplankton blooms along the Qinhuangdao coast. Chin. J. Oceanol. Limnol. 36, 281–292. doi: 10.1007/s00343-017-5216-4
Cowles, T. J., Olson, R. J., and Chisholm, S. W. (1988). Food selection by copepods: discrimination on the basis of food quality. Mar. Biol. 100, 41–49. doi: 10.1007/BF00392953
DeBiase, A. E., Sanders, R. W., and Porter, K. G. (1990). Relative nutritional value of ciliate protozoa and algae as food for Daphnia. Microb. Ecol. 19, 199–210. doi: 10.1007/bf02012100
DeMott, W. R. (1989). Optimal foraging theory as a predictor of chemically mediated food selection by suspension-feeding copepods: optimal foraging by copepods. Limnol. Oceanogr. 34, 140–154. doi: 10.4319/lo.1989.34.1.0140
Donaghay, P. L., and Small, L. F. (1979). Food selection capabilities of the estuarine copepod Acartia clausi. Mar. Biol. 52, 137–146. doi: 10.1007/BF00390421
Folt, C., and Goldman, C. R. (1981). Allelopathy between zooplankton: a mechanism for interference competition. Science 213, 1133–1135. doi: 10.1126/science.213.4512.1133
Frost, B. W. (1972). Effects of size and concentration of food particles on the feeding behavior of the marine planktonic copepod Calanus pacificus. Limnol. Oceanogr. 17, 805–815. doi: 10.4319/lo.1972.17.6.0805
Gismervik, I. (2006). Top-down impact by copepods on ciliate numbers and persistence depends on copepod and ciliate species composition. J. Plankton Res. 28, 499–507. doi: 10.1093/plankt/fbi135
Hansen, B., Bjørnsen, P. K., and Hansen, P. J. (1994). Prey size selection in planktonic zooplankton. Limnol. Oceanogr. 39, 395–403.
Hansen, P. J., Bjørnsen, P. K., and Hansen, B. W. (1997). Zooplankton grazing and growth: scaling within the 2–2,000 μm body size range. Limnol. Oceanogr. 42, 687–704. doi: 10.4319/lo.1997.42.4.0687
Helgen, J. C. (1987). Feeding rate inhibition in crowded Daphnia pulex. Hydrobiologia 154, 113–119. doi: 10.1007/bf00026835
Howell, J. F. (1953). Gonyaulax monilata, sp. nov., the causative dinoflagellate of a red tide on the east coast of Florida in August-September, 1951. Trans. Am. Microsc. Soc. 72, 153–156. doi: 10.2307/3223513
Hsia, M. H., Morton, S. L., Smith, L. L., Beauchesne, K. R., Huncik, K. M., and Moeller, P. D. (2006). Production of goniodomin A by the planktonic, chain-forming dinoflagellate Alexandrium monilatum (Howell) Balech isolated from the gulf coast of the United States. Harmful Algae 5, 290–299. doi: 10.1016/j.hal.2005.08.004
Huntley, M. E., Barthel, K.-G., and Star, J. L. (1983). Particle rejection by Calanus pacificus: discrimination between similarly sized particles. Mar. Biol. 74, 151–160. doi: 10.1007/BF00413918
IRL Consortium (2015). 2011 Superbloom Report: Evaluating Effects and Possible Causes with Available Data. Palatka, FL: St. Johns River Water Management District.
Isari, S., Antü, M., and Saiz, E. (2013). Copepod foraging on the basis of food nutritional quality: can copepods really choose? PLoS One 8:e84742. doi: 10.1371/journal.pone.0084742
Johnson, K. B., and Shanks, A. L. (1997). The importance of prey densities and background plankton in studies of predation on invertebrate larvae. Mar. Ecol. Prog. Ser. 158, 293–296. doi: 10.3354/meps158293
Johnson, K. B., and Shanks, A. L. (2003). Low rates of predation on planktonic marine invertebrate larvae. Mar. Ecol. Prog. Ser. 248, 125–139. doi: 10.3354/meps248125
Jungbluth, M., Selph, K., Lenz, P., and Goetze, E. (2017). Species-specific grazing and significant trophic impacts by two species of copepod nauplii, Parvocalanus crassirostris and Bestiolina similis. Mar. Ecol. Prog. Ser. 572, 57–76. doi: 10.3354/meps12139
Koehl, M. A. R., and Strickler, J. R. (1981). Copepod feeding currents: food capture at low Reynolds number. Limnol. Oceanogr. 26, 1062–1073. doi: 10.4319/lo.1981.26.6.1062
Lawson, T. J., and Grice, G. D. (1973). The developmental stages of Paracalanus crassirostris Dahl, 1894 (Copepoda, Calanoida). Crustaceana 24, 43–56. doi: 10.1163/156854073x00056
Lee, K. W., Rhee, J. S., Han, J., Park, H. G., and Lee, J. S. (2012). Effect of culture density and antioxidants on naupliar production and gene expression of the cyclopoid copepod, Paracyclopina nana. Comp. Biochem. Physiol. A. Mol. Integr. Physiol. 161, 145–152. doi: 10.1016/j.cbpa.2011.10.019
Leising, A. W., Pierson, J. J., Halsband-Lenk, C., Horner, R., and Postel, J. (2005). Copepod grazing during spring blooms: does Calanus pacificus avoid harmful diatoms? Prog. Oceanogr. 67, 384–405. doi: 10.1016/j.pocean.2005.09.008
Liu, H., Li, K., Huang, H., Song, X., Yin, J., and Huang, L. (2013). Seasonal community structure of mesozooplankton in the Daya Bay, South China Sea. J. Ocean Univ. China 12, 452–458. doi: 10.1007/s11802-013-1991-5
Ma, X., and Johnson, K. B. (2017). Comparative phototaxis of calanoid and Harpacticoid copepods. Mar. Biol. 164:26. doi: 10.1007/s00227-016-3054-0
McKinnon, A. D., and Klumpp, D. W. (1998). Mangrove zooplankton of North Queensland, Australia: II. Copepod egg production and diet. Hydrobiologia 362, 145–160. doi: 10.1023/a:1003138718716
Merrell, J. R., and Stoecker, D. K. (1998). Differential grazing on protozoan microplankton by developmental stages of the calanoid copepod Eurytemora affinis Poppe. J. Plankton Res. 20, 289–304. doi: 10.1093/plankt/20.2.289
Milstein, A. (1979). Vertical distribution of Paracalanus crassirostris (Copepoda, Calanoidea): analysis by the general linear model. Bol. Inst. Ocean. 28, 65–78. doi: 10.1590/s0373-55241979000200008
Mitra, A., and Flynn, K. J. (2006). Promotion of harmful algal blooms by zooplankton predatory activity. Biol. Lett. 2, 194–197. doi: 10.1098/rsbl.2006.0447
Nejstgaard, J. C., Tang, K. W., Steinke, M., Dutz, J., Koski, M., Antajan, E., et al. (2007). Zooplankton grazing on Phaeocystis: a quantitative review and future challenges. Biogeochemistry 83, 147–172. doi: 10.1007/s10533-007-9098-y
O’Neil, J. M., Davis, T. W., Burford, M. A., and Gobler, C. J. (2012). The rise of harmful cyanobacteria blooms: the potential roles of eutrophication and climate change. Harmful Algae 14, 313–334. doi: 10.1016/j.hal.2011.10.027
Peters, R. H. (1984). “Methods for the study of feeding, grazing and assimilation by zooplankton,” in Manual on Methods for the Assessment of Secondary Productivity in Fresh Waters (IBP Handbook No. 17), 2nd Edn, eds J. A. Downing and F. H. Rigler (Oxford: Blackwell), 336–412.
Phlips, E. J., Badylak, S., Christman, M., Wolny, J., Brame, J., Garland, J., et al. (2011). Scales of temporal and spatial variability in the distribution of harmful algae species in the Indian River Lagoon, Florida, USA. Harmful Algae 10, 277–290. doi: 10.1016/j.hal.2010.11.001
Phlips, E. J., Badylak, S., Lasi, M. A., Chamberlain, R., Green, W. C., Hall, L. M., et al. (2015). From red tides to green and brown tides: bloom dynamics in a restricted subtropical lagoon under shifting climatic conditions. Estuaries Coasts 38, 886–904. doi: 10.1007/s12237-014-9874-6
Preuss, T. G., Hammers-Wirtz, M., Hommen, U., Rubach, M. N., and Ratte, H. T. (2009). Development and validation of an individual based Daphnia magna population model: the influence of crowding on population dynamics. Ecol. Model. 220, 310–329. doi: 10.1016/j.ecolmodel.2008.09.018
Price, H. J., and Paffenhöfer, G. A. (1984). Effects of feeding experience in the copepod Eucalanus pileatus: a cinematographic study. Mar. Biol. 84, 35–40. doi: 10.1007/BF00394524
Prusova, I., Smith, S. L., and Popova, E. (2012). Calanoid Copepods of the Arabian Sea region. Muscat: Sultan Qaboos University–Academic Publication Board.
Santhosh, B., Anil, M. K., Muhammed Anzeer, F., Aneesh, K. S., Mijo, V. A., Gopakumar, G., et al. (2018). Culture Techniques of Marine Copepods. Kochi: Central Marine Fisheries Research Institute (CMFRI).
Seuront, L., and Vincent, D. (2008). Increased seawater viscosity, Phaeocystis globosa spring bloom and Temora longicornis feeding and swimming behaviours. Mar. Ecol. Prog. Ser. 363, 131–145. doi: 10.3354/meps07373
Shields, R. J., Kotani, T., Molnar, A., Marion, K., Kobashigawa, J., and Tang, L. (2005). “Intensive cultivation of a subtropical paracalanid copepod, Parvocalanus sp., as prey for small marine fish larvae,” in Copepods in Aquaculture, 1st Edn, eds C.-S. Lee, P. O’Bryen, and N. Marcus (Hoboken, NY: Blackwell Publishing), 209–223.
Smayda, T. J. (2008). Complexity in the eutrophication–harmful algal bloom relationship, with comment on the importance of grazing. Harmful Algae 8, 140–151. doi: 10.1016/j.hal.2008.08.018
Steidinger, K. A., Landsberg, J., Truby, E. W., and Roberts, B. S. (1998). First report of Gymnodinium pulchellum (Dinophyceae) in North America and associated fish kills in the Indian River, Florida. J. Phycol. 43, 431–437. doi: 10.1046/j.1529-8817.1998.340431.x
Sun, X., Sun, S., Li, C., and Wang, M. (2012). Seasonal change in body length of important small copepods and relationship with environmental factors in Jiaozhou Bay, China. Chin. J. Oceanol. Limnol. 30, 404–409. doi: 10.1007/s00343-012-1140-9
Tang, K. W., Chen, Q. C., and Wong, C. K. (1994). Diel vertical migration and gut pigment rhythm of Paracalanus parvus, P. crassirostris, Acartia erythraea and Eucalanus subcrassus (Copepoda, Calanoida) in Tolo Harbour, Hong Kong. Hydrobiologia 292–293, 389–396. doi: 10.1007/978-94-017-1347-4_49
Toullec, J., Vincent, D., Frohn, L., Miner, P., Le Goff, M., Devesa, J., et al. (2019). Copepod grazing influences diatom aggregation and particle dynamics. Front. Mar. Sci. 6:751. doi: 10.3389/fmars.2019.00751
Turner, J. T., and Dagg, M. J. (1983). Vertical distributions of continental shelf zooplankton in stratified and isothermal waters. Biol. Oceanogr. 3, 1–40.
Wong, C. K., Chan, A. L. C., and Chen, Q. C. (1993). Planktonic copepods of Tolo Harbour, Hong Kong. Crustaceana 64, 76–84. doi: 10.1163/156854093x00081
Zhu, Y., and Dunford, N. T. (2013). Growth and biomass characteristics of Picochlorum oklahomensis and Nannochloropsis oculata. J. Am. Oil Chem. Soc. 90, 841–849. doi: 10.1007/s11746-013-2225-0
Keywords: top-down control, size selection, background plankton, nanoplankton, Indian River Lagoon
Citation: Ma X, Jacoby CA and Johnson KB (2021) Grazing by the Copepod Parvocalanus crassirostris on Picochlorum sp. at Harmful Bloom Densities and the Role of Particle Size. Front. Mar. Sci. 8:664154. doi: 10.3389/fmars.2021.664154
Received: 04 February 2021; Accepted: 28 June 2021;
Published: 22 July 2021.
Edited by:
Edward J. Phlips, University of Florida, United StatesReviewed by:
Michelle Jungbluth, San Francisco State University, United StatesJelena Godrijan, Rudjer Boskovic Institute, Croatia
Copyright © 2021 Ma, Jacoby and Johnson. This is an open-access article distributed under the terms of the Creative Commons Attribution License (CC BY). The use, distribution or reproduction in other forums is permitted, provided the original author(s) and the copyright owner(s) are credited and that the original publication in this journal is cited, in accordance with accepted academic practice. No use, distribution or reproduction is permitted which does not comply with these terms.
*Correspondence: Xiao Ma, maxiao@scsio.ac.cn