- 1Key Laboratory of Tropical Marine Bio-Resources and Ecology, South China Sea Institute of Oceanology, Chinese Academy of Sciences, Guangzhou, China
- 2Department of Ocean Engineering and Marine Sciences, Florida Institute of Technology, Melbourne, FL, United States
- 3St. Johns River Water Management District, Palatka, FL, United States
Harmful algal blooms (HABs) are increasing in frequency and severity, underscoring the importance of understanding potential top-down controls of blooms. In the Indian River Lagoon (IRL), a shallow subtropical estuary, one harmful bloom was co-dominated by an unresolved prochlorophyte in the Family Prochlorothricaceae, which reached densities of > 106 cells ml–1 engendering the term “Superbloom.” Experiments were conducted to evaluate grazing rates and the potential for top-down control by an abundant herbivorous copepod, Parvocalanus crassirostris, on the prochlorophyte. Those grazing rates were lower than the rates on a palatable alternative algal food, Isochrysis galbana, when both algal species were presented in monocultures with identical densities. Grazing on the prochlorophyte decreased or ceased at densities over 4.8 × 105 cells ml–1. When the prochlorophyte and the palatable alternative each comprised half of the total density, both species were consumed, but grazing on I. galbana was reduced compared to the grazing rates in a monoculture of this species, especially at higher cell densities. Copepod mortality was observed in treatments with high concentrations of the prochlorophyte, and these treatments contained mucilage. Experiments simulating viscosities produced by prochlorophyte mucilage yielded results consistent with the original grazing experiments (i.e., copepods showed lower grazing rates and higher mortality rates in higher viscosity treatments). Results reveal potential limitations of top-down controls by this grazer on prochlorophyte blooms and HABs that produce mucilage.
Introduction
Harmful algal blooms (HABs) are increasing in estuaries around the world (Smayda, 2008; Berry et al., 2015; Phlips et al., 2015; Cao et al., 2017). Synchronicity at the global scale has been attributed to climate change and eutrophication brought on by increased loads of nutrients (Smayda, 2008), whereas important local influences on the magnitude and composition of HABs include longevity, toxicity, and differential losses due to grazing (Turner, 2006, 2010; Smayda, 2008; Phlips et al., 2010, 2015; Berry et al., 2015; Cao et al., 2017).
An unprecedented sequence of intense and long-lasting algal blooms have afflicted the Indian River Lagoon (IRL) in Florida, starting in 2011 (Phlips et al., 2010, 2011, 2015; Lapointe et al., 2015), with the initial bloom being dubbed the “superbloom” because of its ∼12-month duration and concentrations of chlorophyll-a that reached 136 μg L–1 (2–4 × the concentrations recorded from 1996 to 2010). In contrast to blooms dominated by dinoflagellates or diatoms (Phlips et al., 2015), the superbloom was co-dominated by cyanobacteria and a nanoplanktonic chlorophyte. Both taxa were documented at > 1 million cells ml–1 during the event (IRL Consortium, 2015). The bloom caused harm by decreasing light penetration for a duration that led to the loss of tens of thousands of acres of seagrass, and the resulting decreased competition for nutrients may have promoted subsequent blooms of phytoplankton (Phlips et al., 2015). In addition to bottom-up stimulation by nutrients, the relatively small size and unusually high densities of the species in the superbloom led to questions about top-down control by herbivores like zooplankton.
The alga targeted in this study was an unresolved prochlorophyte in the Family Prochlorothricaceae, which was isolated from the 2011 superbloom. The prochlorophyta are a group of cyanobacteria abundant in nutrient-poor tropical waters and possessing a light-harvesting apparatus composed of the higher plant pigments chlorophylls a and b (Partensky et al., 1993; Post and Bullerjahn, 1994; Lewin, 2002). Studies of prochlorophytes are sparse, but no toxic species have been reported. The strain used in this study was an oval-shaped unicellular cyanobacterium, with cells approximately ∼5 μm in diameter. Heavy mucus production was observed during the bloom and in monocultures of the prochlorophyte. During the superbloom, concentrations of the prochlorophyte exceeded 5 × 106 cells ml–1. This exceptionally high density is unusual for a natural bloom, even a HAB, and it raises concerns about how such densities might impact grazers. Grazing on the prochlorophyte was evaluated to determine the potential for a key herbivore to exert top-down control. Grazing on Isochrysis galbana, a palatable microalga, was examined to contrast with grazing on the prochlorophyte. I. galbana is roughly spherical, with a diameter of 4–6 μm, and it is commonly used as a food for culturing copepods (Shields et al., 2005).
The grazer selected for this study is Parvocalanus crassirostris, a small (∼2 mm long), holoplanktonic, calanoid copepod that dwells in the upper 6–20 m of tropical and subtropical estuarine, coastal, and oceanic waters (Milstein, 1979; Turner and Dagg, 1983; Wong et al., 1993; Tang et al., 1994; Almeida et al., 2012; Sun et al., 2012; Liu et al., 2013). It is common for calanoid copepods to reside permanently in the mid- to upper water column (Ma and Johnson, 2017), where they feed on microalgae and nanoplankton. P. crassirostris is a cosmopolitan copepod and tolerates a wide range of temperatures (15–31°C), salinities (20–37), and eutrophic or turbid conditions (Milstein, 1979; Wong et al., 1993; Almeida et al., 2012). P. crassirostris mainly grazes on nanophytoplankton (Calbet et al., 2000) and, according to a 3-year record of mesozooplanktonic abundances, it was the dominant herbivorous copepod in the region of the aforementioned superbloom (Sweat unpublished data).
This study aims to (1) determine the grazing rates of P. crassirostris on the prochlorophyte, (2) compare grazing rates on the HAB species with those on a palatable alternative (I. galbana), and (3) examine selective grazing when the HAB species is presented together with the palatable alternative at equal densities. Additionally, when it was discovered that the prochlorophyte produced heavy mucilage, altering culture media, this study investigated potential impact of that mucilage on copepod grazing rates and mortality.
Materials and Methods
Culturing Copepods and Algae
Copepods (Parvocalanus crassirostris) and algae (The prochlorophyte and Isochrysis galbana) were cultured by AlgaGen LLC (Vero Beach, FL, United States). P. crassirostris were initially isolated from the IRL. Starter cultures of the prochlorophyte were isolated from the IRL superbloom by the Phlips Laboratory (University of Florida). Cultures used seawater filtered through a 0.45-μm filter. All algae were cultured in 2-L glass jars held at 26°C, with continuous aeration and 1,100 lux of light provided continuously by fluorescent bulbs. Guillard’s (F/2) marine water enrichment solution was used as culture medium. The best yield of the prochlorophyte came from cultures held at a salinity of 32. Around 1,000–2,000 adult P. crassirostris were cultured at 26°C and a salinity of 25 in 2-L glass jars that were aerated gently. Pre-experiment cultures of copepods were fed I. galbana at 1.5 × 104 cells ml–1 (Hoff and Snell, 2007). The sizes of the algal cells were measured through a microscope with scales.
For 24 h immediately preceding experiments, copepods were maintained without food in gently aerated, 2-L glass jars under a 12/12 light/dark cycle. To reduce stress in the experiments involving the prochlorophyte, P. crassirostris, and I. galbana were gently acclimated to a salinity of 32 in advance of feeding experiments according to the plankton Culture Manual (Hoff and Snell, 2007).
Grazing Experiments
To our knowledge, copepod grazing has not been tested with cell densities on par with the IRL superbloom. Exploring this unique phenomenon necessitated a compromise with chamber size in order to have target algal densities acclimated to appropriate salinities while constrained by the limits of culture volume production. The challenge was to conduct replicated trials using monocultures and 50:50 mixtures of the appropriate algae at densities of 5.8 × 104, 1.2 × 105, 2.3 × 105, 4.8 × 105, 9.6 × 105, and 1.9 × 106 cells ml–1. Five adult copepods were placed in a 3-ml incubation chamber (with 2-ml incubation volume) along with algae at a variety of densities, including superbloom densities of 106 cells ml–1. According to our preliminary experiments, grazer densities of 2.5 individuals ml–1 were necessary in order to observe measurable effects in a reasonable timeframe. Copepods used in the experiment were adult females. Adult males of P. crassirostris do not feed due to their reduced feeding appendages (Lawson and Grice, 1973). Morphologically, the body size of P. crassirostris females (up to 450 μm) is larger than that of the males (up to 350 μm). In preparation for experiments, adult copepods were pre-selected using a 200 μm mesh. Then the large active females were gently selected using a pasteur pipet under stereomicroscopy. Although individuals of different ages could have an influence on the results, replication (n = 5) was increased in an attempt to counteract this potential impact. Controls to estimate algal growth and mortality not due to grazing had the same densities, but lacked copepods. Experiments were conducted in filtered seawater held at 26°C for 20 h under 1,100 lux of artificial light.
Behavioral artifacts due to containment always have been a concern in laboratory feeding experiments (Elmore, 1982; Johnson and Shanks, 1997, 2003), but the extreme algal densities required to mimic observed superbloom conditions, near and exceeding 106 cells ml–1, dictated final experimental volumes. In fact, standard culture procedures (Hoff and Snell, 2007) did not produce the required densities, a testament to the extreme nature of the IRL superbloom. AlgaGen LLC, a company specializing in high-density production, used a proprietary culture procedure to produce the required densities of the prochlorophyte and I. galbana.
Simulating the Effects of Mucilage
In cultures and during the experiments, the prochlorophyte produced mucilage that affected the viscosity of the water. Therefore, an additional experiment was designed wherein P. crassirostris were fed palatable algae (I. galbana) in water with different viscosities to test for a physical effect of mucilage on grazing. In the mucilage effect experiment, the algal densities were held constant at 1.8 × 104 cells ml–1, a common density used in culturing copepods (Hoff and Snell, 2007). The viscosity of the water was manipulated by adding polyvinyl pyrrolidone (PVP, average Mw 360,000). This polymer was chosen because it has been used in analogous experiments with no detectable toxicity to delicate planktonic larvae (Podolsky and Emlet, 1993). The polymer was added until the water reached the desired kinematic viscosity as measured using a falling-ball viscometer (Gilmont model # GV-2100). The experimental viscosities were 0.923, 1.45, 1.98, 2.50, 3.03, and 3.56 × 10–6 m2 s–1, with untreated water (seawater at the same temperature and salinity) having a viscosity of 0.923 × 10–6 m2 s–1.
Mucilage experiments were not constrained by the need for extreme phytoplankton densities, so they were carried out in larger volumes with lower densities of grazers (i.e., ten adult copepods within 200 ml of filtered seawater) and prey algae. This approach is comparable to other laboratory experiments (Abu-Rezq et al., 1997; Jungbluth et al., 2017), and should reduce artifacts, if such were occurring. These experiments were conducted at 25 ± 0.1°C (controlled by a micro-temperature controller) and a salinity of 25 for 11 h under 1,100 lux artificial light with gentle aeration. Preliminary work showed that a minimum of 11 h was required to generate reliable reductions due to grazing. Controls involved the same sized containers, algal density and viscosities as the treatments, but they lacked copepods. Five replicate treatments and corresponding controls were prepared for each experiment (n = 5).
Analysis of Data
The initial and final densities of microalgae were measured via flow cytometry (BD Accuri C6), and copepod survival was scored via stereo microscopy. Grazing rates (cells copepod–1 h–1) were determined using equations from Frost (1972), with the mean of replicate controls used for comparison. Statistically significant differences in grazing rates among treatments were determined via analysis of variance (ANOVA) and post hoc Tukey pairwise comparisons (α = 0.05). When mortality of copepods occurs, grazing rates for all densities were calculated using the assumption that they had lived for half of the experiment (Turner et al., 2012), since we do not know when ailing copepods ceased grazing or died during the experiment.
Results
When copepods were fed the prochlorophyte, some mortality was observed (Figure 1). Copepod mortality rates (less than 20%) were similar between less dense monocultures of the prochlorophyte (less than 2.3 × 105 cells ml–1) and mixed treatments (Figure 1). However, mortality rates were over 40% in monocultures containing the prochlorophyte at high densities (≥4.8 × 105 cells ml–1), and the rates increased substantially with densities ≥ 4.8 × 105 cells ml–1 (Figure 1A). Copepod mortality was not observed in monocultures of I. galbana.
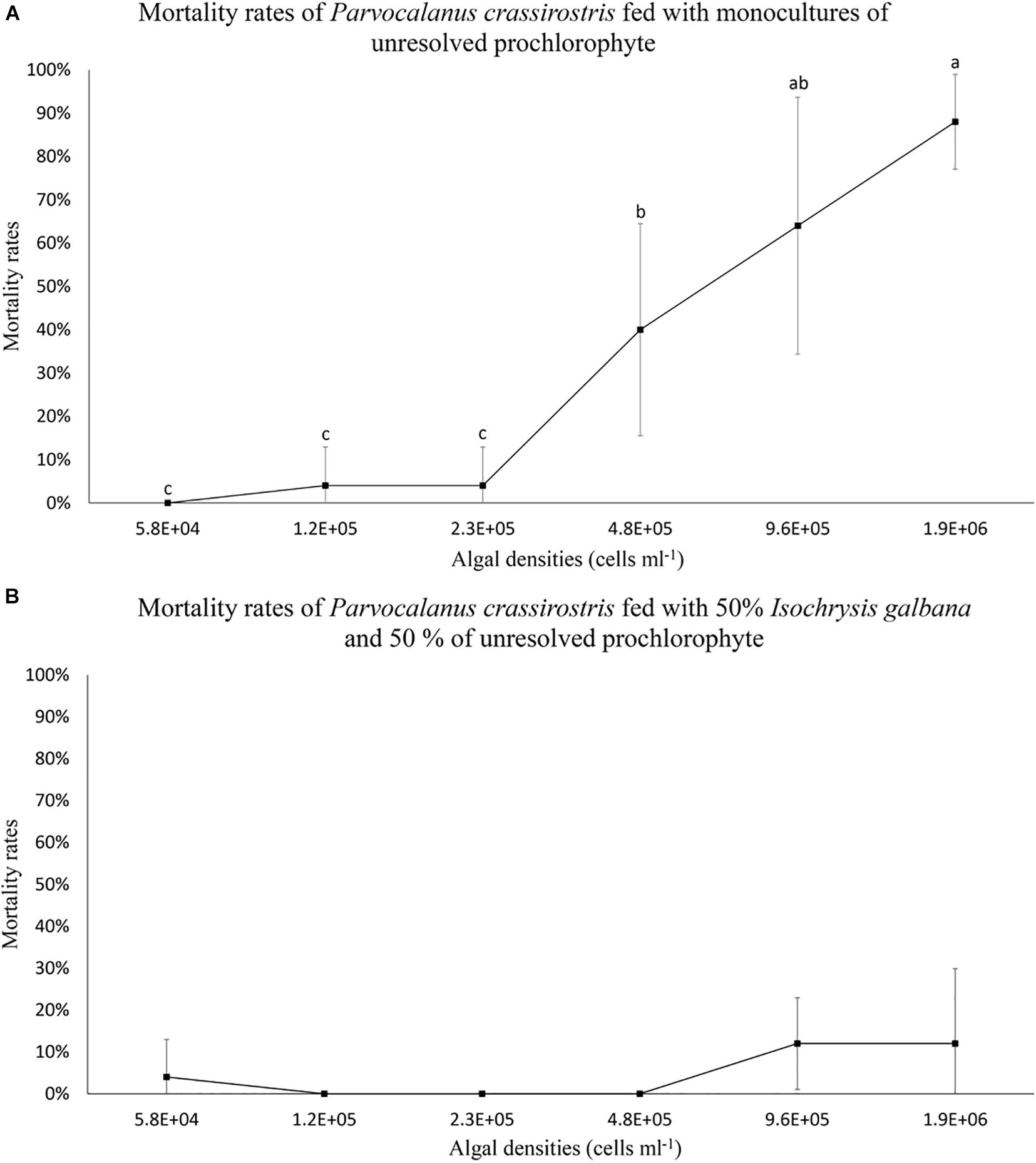
Figure 1. Mean mortality rates (± standard deviation, SD) for Parvocalanus crassirostris exposed to different densities of an unresolved prochlorophyte presented (A) as a monoculture and (B) as a mixture of 50% Isochrysis galbana and 50% the prochlorophyte. Different letters identify significantly different means based on analysis of variance and Tukey’s post hoc pairwise comparisons (α = 0.05). Mean mortality rates in the mixed culture (B) were statistically equivalent and not significantly different from zero.
Copepods grazed on the prochlorophyte in treatments with ≤ 4.8 × 105 cells ml–1; grazing rates on the denser prochlorophyte at treatments of 9.6 × 105 and 1.9 × 106 cells ml–1 were indistinguishable from zero (Figure 2A). Compared to grazing rates on monocultures of I. galbana, rates on the prochlorophyte were significantly lower in treatments with ≥ 2.3 × 105 cells ml–1 (p < 0.05) (Figure 2A). Again, water that contained the prochlorophyte was more viscous than filtered seawater alone due to the production of mucilage.
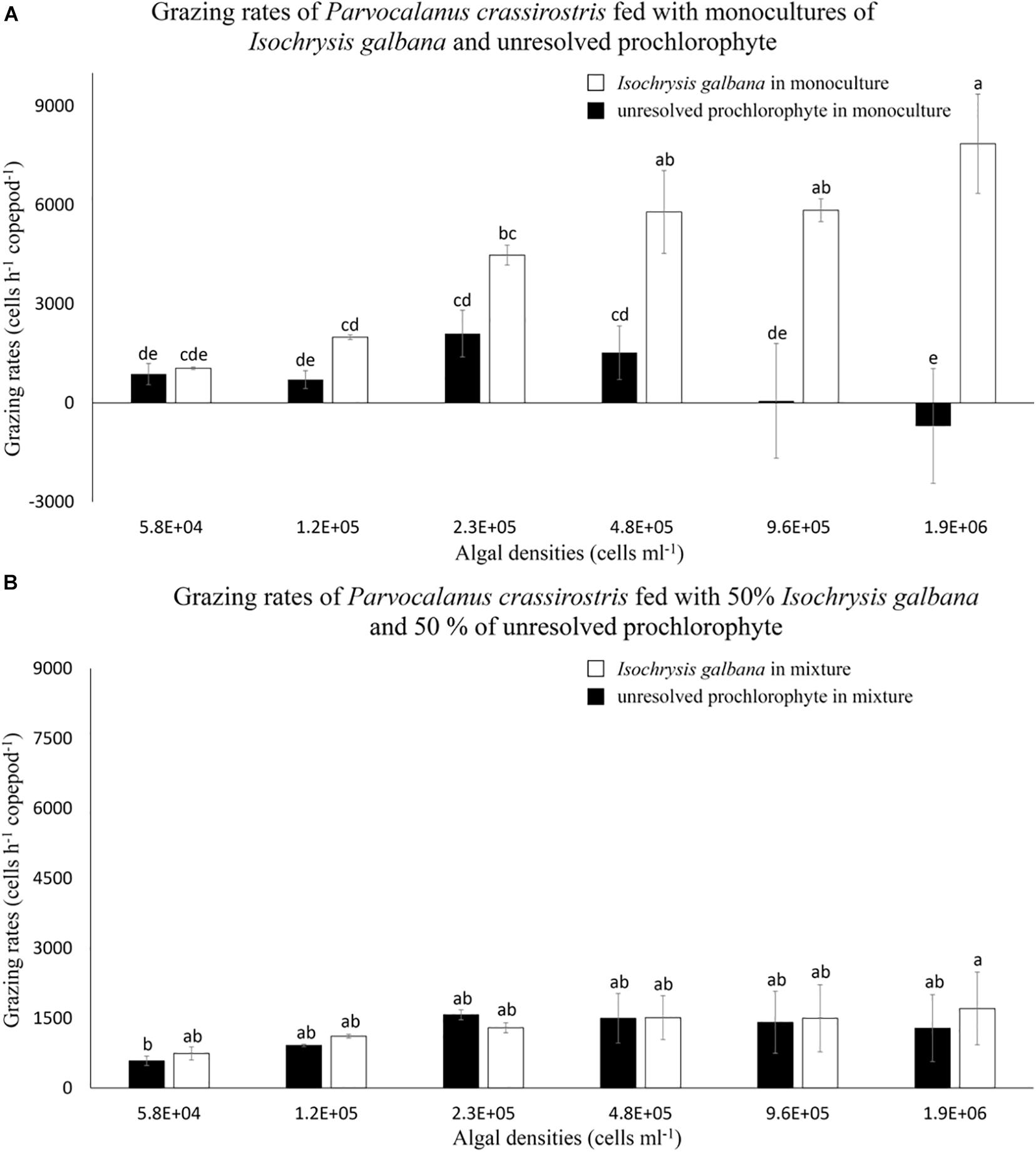
Figure 2. Mean numbers of cells consumed per hour (± standard deviation, SD) by Parvocalanus crassirostris grazing on different densities of Isochrysis galbana and an unresolved prochlorophyte presented (A) as monocultures and (B) as mixtures of 50% I. galbana and 50% the prochlorophyte. Different letters identify significantly different means based on analysis of variance and Tukey’s post hoc pairwise comparisons (α = 0.05).
In experiments with mixed cultures, grazing on I. galbana was markedly reduced compared to grazing recorded in monocultures (Figure 2), with grazing rates on I. galbana decreasing by 80% in the highest density mixed treatment as compared to the monoculture of I. galbana at the same density. In mixed cultures, the grazing rates on I. galbana were statistically indistinguishable from those on the prochlorophyte (Figure 2B). Conversely, grazing rates on the prochlorophyte in mixed cultures were equal to or higher than rates on the same species in monoculture (Figure 2). This was especially true for the highest algal density treatments (≥9.6 × 105 cells ml–1).
When exposed to I. galbana in PVP treated water, copepod mortality increased at higher viscosities (Figure 3A). Copepods grazed on I. galbana in low viscosity treatments, but grazing rates declined significantly with increasing viscosity, dropping from 6.8 × 103 cells copepod–1 h–1 to zero (Figure 3B).
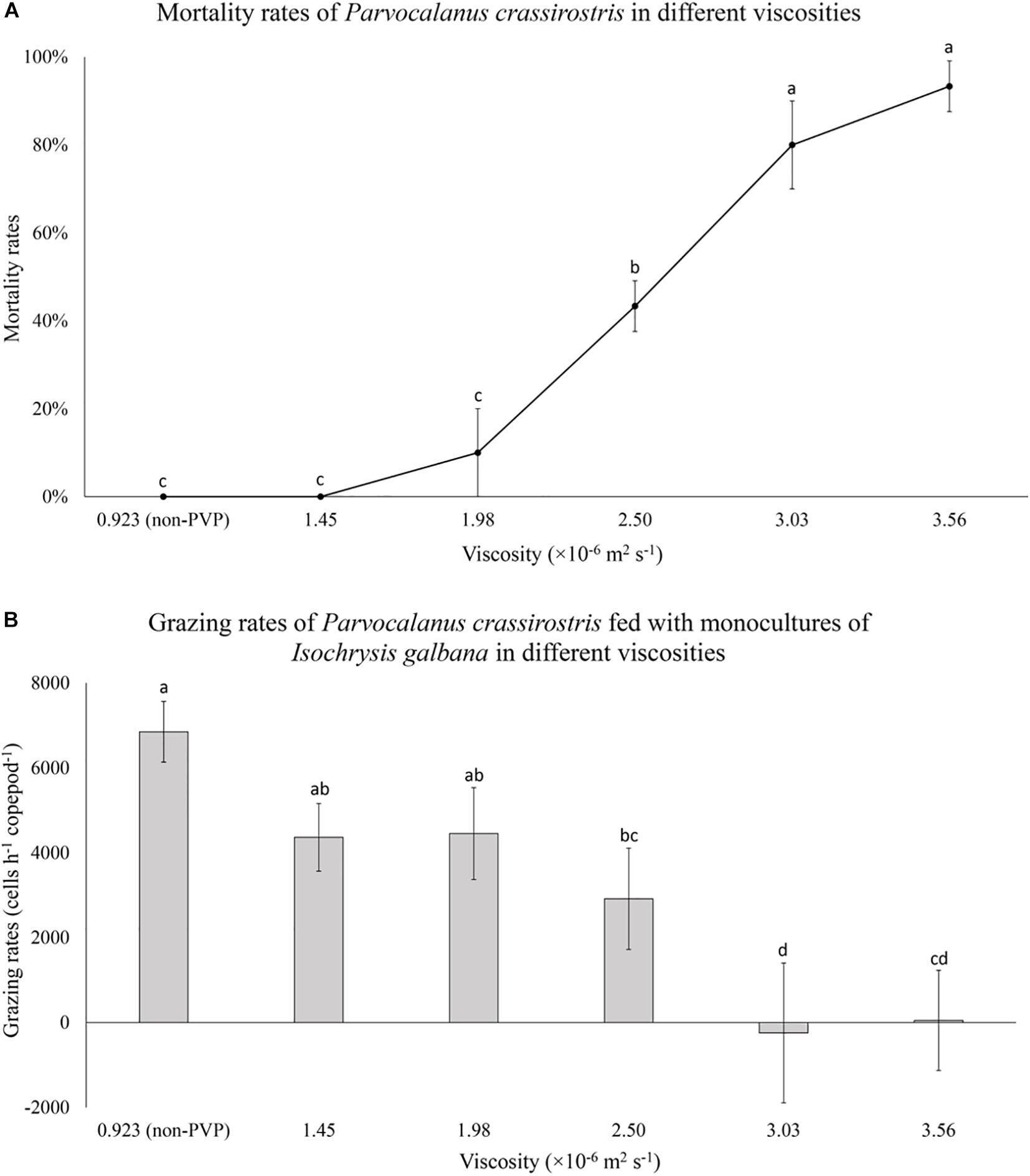
Figure 3. (A) Mean mortality rates (± standard deviation, SD) for Parvocalanus crassirostris exposed to water with different viscosities and (B) mean numbers of cells consumed per hour (± standard deviation, SD) by P. crassirostris grazing on 1.8 × 104 cells ml–1 of Isochrysis galbana in water with different viscosities. Different letters identify significantly different means based on analysis of variance and Tukey’s post hoc pairwise comparisons (α = 0.05). Non-PVP, unaltered algal culture.
Discussion
Top-down control via grazing has been shown to vary with the characteristics of grazers, their algal food, and environmental conditions. Our experiments involved consistent environmental conditions and life stage (adult) of one grazer, which limited the influence of body size, foraging speed, feeding efficiency, and life history stage (Peters, 1984; Hansen et al., 1997; Atkinson, 1998; Nejstgaard et al., 2007; Seuront and Vincent, 2008). Our focus was on characteristics of phytoplankton, including cell density, size, and apparent palatability (Peters, 1984; Hansen et al., 1994; Kiørboe et al., 1996; Norberg and DeAngelis, 1997; Calbet, 2001; Seuront and Vincent, 2008).
Algal density had a substantial influence on grazing by P. crassirostris, regardless of the species of algae being offered or whether they were offered as monocultures or mixed cultures containing the prochlorophyte. Mean grazing rates on I. galbana in monocultures ranged from 1.1 × 103 to 1.8 × 104 cells copepod–1 h–1, and are similar to rates reported for P. crassirostris feeding on other nanoplankton (Calbet et al., 2000), Tisbe furcata feeding on a flagellated haptophyte and a flagellated cryptophyte of similar size (Abu-Rezq et al., 1997), and Pseudodiaptomus euryhalinus feeding on an Isochrysis sp. (Anzueto-Sánchez et al., 2014). P. crassirostris has been documented to be a suspension feeder, with no evidence of raptorial behavior (McKinnon and Klumpp, 1998).
Such copepods typically generate small-scale currents to entrain particles, which subsequently are trapped by feeding appendages (Koehl and Strickler, 1981). It follows that encounter rates and the resulting grazing rates would, to some degree, depend on algal densities, provided there were no other constraints. Given that other studies have also shown correlations of grazing rates with higher algal densities (Frost, 1972), that general pattern does not appear to have been altered by experimental conditions, neither for I. galbana nor the prochlorophyte.
Consumption of the prochlorophyte presented as a monoculture or mixed with I. galbana varied from 0 to 3.0 × 103 cells copepod–1 h–1 at densities from 5.8 × 104 to 1.9 × 106 cells ml–1. These rates were low compared to consumption in monocultures of I. galbana, especially at algal densities of 9.6 × 105–1.9 × 106 cells ml–1. Grazing on I. galbana in mixed cultures also was depressed, which in combination with the relatively consistent rate of grazing on the prochlorophyte in all cultures, suggests that copepods did not select prey based on cell taste.
Copepod feeding mechanics are predominantly governed by viscous forces (Koehl and Strickler, 1981; Yen, 2000; van Duren and Videler, 2003). Viscosity indicates how well a fluid can resist deformation under stress and is sometimes equated with fluid “thickness.” Mucilage produced by the prochlorophyte in this study may have reduced grazing rates. Mucilage was unlikely to have been an artifact of containment, because cyanobacteria have been shown to produce it in natural assemblages found in Florida Bay (Berry et al., 2015) and off the coast of Venezuela (Ramos and Reyes-Vazquez, 1990). In previous work, polymeric exudates (a.k.a. mucilage) reduced copepod grazing rates, either via chemical inhibition or mechanical interference with filtration or particle capture (Malej and Harris, 1993; Dilling et al., 1998; Nejstgaard et al., 2007). The effects of mucilage were most obvious above a threshold cell density, because copepods grazed on low densities of the prochlorophyte at rates that were not significantly different from grazing on I. galbana. The presence of a threshold has been shown for Acartia clausi grazing on the toxic dinoflagellate Alexandrium minutum, with consumption decreasing dramatically when A. minutum was abundant and producing more toxin (Guisande et al., 2002). In fact, the apparent increase in grazing on the prochlorophyte in higher density mixed cultures could have been due to actual densities of cyanobacteria cells being half of those in monocultures. In general, mucilage may have interfered with handling of cells, with the increased viscosity rendering the delicate work of particle sorting and selection more difficult. The diameters of I. galbana and the prochlorophyte were similar (∼ 5 μm), making sorting based solely on size challenging. In addition, the experiments involving PVP indicated that viscosity had a substantial influence on P. crassirostris grazing rates. Copepods grazed effectively on I. galbana in low viscosity treatments, but grazing rates fell with increasing viscosity (Figure 3B). Feeding behaviors of suspension feeders have been shown to be influenced by water viscosity (Podolsky, 1994; Bolton and Havenhand, 1998). In fact, Podolsky (1994) found that high viscosities cut grazing by sand dollar larvae in half, and higher viscosities shifted ingestion to larger particles. “Brown tide,” Aureoumbra lagunensis, is another harmful algal bloom species that secretes extracellular polymeric substances (EPS), doing so mainly under hypersaline conditions (Liu and Buskey, 2000). Some species of protozoa had difficulty feeding on high densities of A. lagunensis, but they grew faster on cells separated from their EPS exudates via gentle centrifugation (Liu and Buskey, 2000). Given these reports and our results, it seems likely that depressed grazing reflects a natural consequence of increased viscosity.
Mortality was observed when P. crassirostris was fed the prochlorophyte, especially in high-density monocultures (Figure 1). This result may have been due to toxicity or physical effects associated with mucilage. Although toxic cyanobacteria species are common (Martins et al., 2005, 2007; Valério et al., 2010), many other cyanobacteria may serve as food for copepods (Koski and Klein-Breteler, 2003; Kâ et al., 2012). Lower mortality in mixed cultures of the prochlorophyte and I. galbana, even at higher total densities, suggested that acute toxicity is unlikely. High mortality rates also were observed when P. crassirostris was feeding on palatable algae in water with high viscosity (Figure 3B), and PVP has been used in analogous experiments with no evidence of toxicity to delicate zooplankton (Podolsky and Emlet, 1993; Larsen et al., 2008). One possible explanation for copepod mortality is that oxygen diffuses more slowly in viscous water creating a risk of suffocation. Copepods have no gills, and oxygen uptake and carbon dioxide release take place through the integument and in the hindgut (Blaxter et al., 1998). Mucilage and PVP may have interfered with effective exchange of gases. In addition, I. galbana occasionally formed aggregates when PVP was added, and aggregates may have been difficult for copepods to grasp or masticate, which may cause stress, or occupy the copepod’s time examining non-consumable aggregate particles. Mortality from starvation was unlikely, however, due to the short duration of the experiments. The presence of non-consumable particles, however, could be considered a form of background plankton that reduces overall grazing rates (Johnson and Shanks, 1997, 2003).
Crowding and container effects have been a common concern when conducting laboratory grazing studies (Folt and Goldman, 1981; Peters, 1984; Helgen, 1987; Burns, 1995, 2000; Preuss et al., 2009; Lee et al., 2012). Potential artifacts can arise from physical interference among zooplankton, interactions between zooplankton and vessel walls or boundary layers (Peters, 1984), or chemically-mediated interactions among competitors (Folt and Goldman, 1981). Some studies demonstrate that crowding or container effects can lead to depression of zooplankton feeding rates (Folt and Goldman, 1981; Helgen, 1987; Burns, 1995, 2000; Preuss et al., 2009; Lee et al., 2012). However, Mullin (1963) found that container size was not a significant influence on feeding rates when specifically testing copepods. Small containers also can crowd grazers, and we must acknowledge that the influence of such effects cannot be known with certainty. However, Lee et al. (2012) demonstrated that some copepod species could be raised at densities as high as 20 individuals ml–1. In fact, numerous copepod grazing studies have utilized small containers or high densities of grazers (Hong et al., 2013; Motwani and Gorokhova, 2013; Boersma et al., 2016; Ger et al., 2016; Rangel et al., 2016; Svensen and Vernet, 2016; Mathews et al., 2018). Overall, evidence suggests the present experiments yielded useful insights into HAB grazing by copepods.
In conclusion, grazing on the prochlorophyte by P. crassirostris declined when algal densities were high and the water was viscous, whereas grazing on palatable algae increased with algal density. Copepod mortality increased with increasing viscosity, whether created by mucilage or a polymer. Copepod mortality and lower grazing rates in the presence of cultured prochlorophyte densities, meant to reflect in situ superbloom densities, suggest that P. crassirostris, a dominant herbivorous copepod, could be prevented from exerting top-down control in bloom conditions.
Data Availability Statement
The raw data supporting the conclusions of this article will be made available by the authors, without undue reservation.
Author Contributions
XM and KJ conceived the study. XM carried out data collection. XM and CJ analyzed data. All authors contributed to interpretation of the results, preparation and revision of the manuscript, and approved the submission.
Funding
This research was funded by contract 27786 from the St. Johns River Water Management District.
Conflict of Interest
The authors declare that the research was conducted in the absence of any commercial or financial relationships that could be construed as a potential conflict of interest.
Publisher’s Note
All claims expressed in this article are solely those of the authors and do not necessarily represent those of their affiliated organizations, or those of the publisher, the editors and the reviewers. Any product that may be evaluated in this article, or claim that may be made by its manufacturer, is not guaranteed or endorsed by the publisher.
Acknowledgments
We would like to thank E. J. Phlips for supplying the initial HAB cultures. We would also like to thank J. Trefry, S. Fire, K. Hunsucker, and C. Combs for their advice in developing this project and comments on the manuscript. We would further like to thank E. Stenn and AlgaGen LLC Culture Company for culturing the copepods and algae. We would also further like to thank L. H. Sweat and H. Kolb for assistance with laboratory work and helpful suggestions.
References
Abu-Rezq, T. S., Yule, A. B., and Teng, S. K. (1997). Ingestion, fecundity, growth rates and culture of the harpacticoid copepod Tisbe furcata, in the laboratory. Hydrobiologia 347, 109–118. doi: 10.1023/A:1003071318933
Almeida, L. R. D., Costa, I. S., and Eskinazi-Sant’Anna, E. M. (2012). Composition and abundance of zooplankton community of an impacted estuarine lagoon in Northeast Brazil. Braz. J. Biol. 72, 12–24. doi: 10.1590/S1519-69842012000100002
Anzueto-Sánchez, M. A., Barón-Sevilla, B., Cordero-Esquivel, B., and Celaya-Ortega, A. (2014). Effects of food concentration and temperature on development, growth, reproduction and survival of the copepod Pseudodiaptomus euryhalinus. Aquac. Int. 22, 1911–1923. doi: 10.1007/s10499-014-9791-5
Atkinson, A. (1998). Life cycle strategies of epipelagic copepods in the Southern Ocean. J. Mar. Syst. 15, 289–311. doi: 10.1016/S0924-7963(97)00081-X
Berry, D. L., Goleski, J. A., Koch, F., Wall, C. C., Peterson, B. J., Anderson, O. R., et al. (2015). Shifts in cyanobacterial strain dominance during the onset of harmful algal blooms in Florida Bay. USA. Microb. Ecol. 70, 361–371. doi: 10.1007/s00248-014-0564-5
Blaxter, J. H., Douglas, B., Tyler, P. A., and Mauchline, J. (1998). Advances in marine biology, the biology of calanoid copepod. Cambridge, MA: Academic Press.
Boersma, M., Mathew, K. A., Niehoff, B., Schoo, K. L., Franco−Santos, R. M., and Meunier, C. L. (2016). Temperature driven changes in the diet preference of omnivorous copepods: no more meat when it’s hot? Ecol. Lett. 19, 45–53. doi: 10.1111/ele.12541
Bolton, T. F., and Havenhand, J. N. (1998). Physiological versus viscosity-induced effects of an acute reduction in water temperature on microsphere ingestion by trochophore larvae of the serpulid polychaete Galeolaria caespitosa. J. Plankton Res. 20, 2153–2164. doi: 10.1093/plankt/20.11.2153
Burns, C. W. (1995). Effects of crowding and different food levels on growth and reproductive investment of Daphnia. Oecologia 101, 234–244. doi: 10.1007/BF00317289
Burns, C. W. (2000). Crowding−induced changes in growth, reproduction and morphology of Daphnia. Freshw. Biol. 43, 19–29. doi: 10.1046/j.1365-2427.2000.00510.x
Calbet, A. (2001). Mesozooplankton grazing effect on primary production: a global comparative analysis in marine ecosystems. Limnol. Oceanogr. 46, 1824–1830. doi: 10.4319/lo.2001.46.7.1824
Calbet, C. J., Landry, M. R., and Scheinberg, R. D. (2000). Copepod grazing in a subtropical bay: species-specific responses to a midsummer increase in nanoplankton standing stock. Mar. Ecol. Prog. Ser. 193, 75–84. doi: 10.3354/meps193075
Cao, X., Yu, Z., Wu, Z., Cheng, F., He, L., Yuan, Y., et al. (2017). Environmental characteristics of annual pico/nanophytoplankton blooms along the Qinhuangdao coast. Chin. J. Oceanol. Limnol. 2017, 1–12.
Dilling, L., Wilson, J., Steinberg, D., and Alldredge, A. (1998). Feeding by the euphausiid Euphausia pacifica and the copepod Calanus pacificus on marine snow. Mar. Ecol. Prog. Ser. 170, 189–201. doi: 10.3354/meps170189
Elmore, J. L. (1982). The influence of food concentration and container volume on life history parameters of Diaptomus dorsalis Marsh from subtropical Florida. Hydrobiologia 89, 215–223. doi: 10.1007/BF00005707
Folt, C., and Goldman, C. R. (1981). Allelopathy between zooplankton: a mechanism for interference competition. Science 213, 1133–1135. doi: 10.1126/science.213.4512.1133
Frost, B. W. (1972). Effects of size and concentration of food particles on the feeding behavior of the marine planktonic copepod Calanus pacificus. Limnol. Oceanogr. 17, 805–815. doi: 10.4319/lo.1972.17.6.0805
Ger, K. A., Faassen, E. J., Pennino, M. G., and Lürling, M. (2016). Effect of the toxin (microcystin) content of Microcystis on copepod grazing. Harmful Algae 52, 34–45. doi: 10.1016/j.hal.2015.12.008
Guisande, C., Frangópulos, M., Maneiro, I., Vergara, A. R., and Riveiro, I. (2002). Ecological advantages of toxin production by the dinoflagellate Alexandrium minutum under phosphorus limitation. Mar. Ecol. Prog. Ser. 225, 169–176. doi: 10.3354/meps225169
Hansen, B., Bjørnsen, P. K., and Hansen, P. J. (1994). Prey size selection in planktonic zooplankton. Limnol. Oceanogr. 39, 395–403. doi: 10.4319/lo.1994.39.2.0395
Hansen, P. J., BjØrnsen, P. K., and Hansen, B. W. (1997). Zooplankton grazing and growth: Scaling within the 2–2,000 μm body size range. Limnol. Oceanogr. 42, 687–704. doi: 10.4319/lo.1997.42.4.0687
Helgen, J. C. (1987). Feeding rate inhibition in crowded Daphnia pulex. Hydrobiologia 154, 113–119. doi: 10.1007/BF00026835
Hoff, F. H., and Snell, T. W. (2007). Plankton Culture Manual, 6th Edn. Florida: Florida Aqua Farms Inc.
Hong, Y., Burford, M. A., Ralph, P. J., Udy, J. W., and Doblin, M. A. (2013). The cyanobacterium Cylindrospermopsis raciborskii is facilitated by copepod selective grazing. Harmful Algae 29, 14–21. doi: 10.1016/j.hal.2013.07.003
IRL Consortium (2015). 2011 Superbloom Report: Evaluating Effects and Possible Causes with Available Data. Florida: St. Johns River Water Management District.
Johnson, K. B., and Shanks, A. L. (1997). The importance of prey densities and background plankton in studies of predation on invertebrate larvae. Mar. Ecol. Prog. Ser. 158, 293–296. doi: 10.3354/meps158293
Johnson, K. B., and Shanks, A. L. (2003). Low rates of predation on planktonic marine invertebrate larvae. Mar. Ecol. Prog. Ser. 248, 125–139. doi: 10.3354/meps248125
Jungbluth, M. J., Selph, K. E., Lenz, P. H., and Goetze, E. (2017). Incubation duration effects on copepod naupliar grazing estimates. J. Exp. Mar. Biol. Ecol. 494, 54–62. doi: 10.1016/j.jembe.2017.05.005
Kâ, S., Mendoza-Vera, J. M., Bouvy, M., Champalbert, G., N’Gom-Kâ, R., and Pagano, M. (2012). Can tropical freshwater zooplankton graze efficiently on cyanobacteria? Hydrobiologia 679, 119–138. doi: 10.1007/s10750-011-0860-8
Kiørboe, T., Saiz, E., and Viitasalo, M. (1996). Prey switching behaviour in the planktonic copepod Acartia tonsa. Mar. Ecol. Prog. Ser. 143, 65–75. doi: 10.3354/meps143065
Koehl, M. A. R., and Strickler, J. R. (1981). Copepod feeding currents: food capture at low Reynolds number. Limnol. Oceanogr. 26, 1062–1073. doi: 10.4319/lo.1981.26.6.1062
Koski, M., and Klein-Breteler, W. C. M. (2003). Influence of diet on copepod survival in the laboratory. Mar. Ecol. Prog. Ser. 264, 73–82. doi: 10.3354/meps264073
Lapointe, B. E., Herren, L. W., Debortoli, D. D., and Vogel, M. A. (2015). Evidence of sewage-driven eutrophication and harmful algal blooms in Florida’s Indian River Lagoon. Harmful Algae 43, 82–102. doi: 10.1016/j.hal.2015.01.004
Larsen, P. S., Madsen, C. V., and Riisgård, H. U. (2008). Effect of temperature and viscosity on swimming velocity of the copepod Acartia tonsa, brine shrimp Artemia salina and rotifer Brachionus plicatilis. Aquat. Biol. 4, 47–54. doi: 10.3354/ab00093
Lawson, T. J., and Grice, G. D. (1973). The Developmental Stages of Paracalanus Crassirostris Dahl, 1894 (Copepoda, Calanoida). Crustaceana 24, 43–56. doi: 10.1163/156854073X00056
Lee, K. W., Rhee, J. S., Han, J., Park, H. G., and Lee, J. S. (2012). Effect of culture density and antioxidants on naupliar production and gene expression of the cyclopoid copepod, Paracyclopina nana. Comp. Biochem. Physiol. A. Mol. Integr. Physiol. 161, 145–152. doi: 10.1016/j.cbpa.2011.10.019
Liu, H., and Buskey, E. J. (2000). The exopolymer secretions (EPS) layer surrounding Aureoumbra lagunensis cells affects growth, grazing, and behavior of protozoa. Limnol. Oceanogr. 45, 1187–1191. doi: 10.4319/lo.2000.45.5.1187
Liu, H., Li, K., Huang, H., Song, X., Yin, J., and Huang, L. (2013). Seasonal community structure of mesozooplankton in the Daya Bay, South China Sea. J. Ocean Univ. China 12, 452–458. doi: 10.1007/s11802-013-1991-5
Ma, X., and Johnson, K. B. (2017). Comparative phototaxis of calanoid and harpacticoid copepods. Mar. Biol. 164:3054. doi: 10.1007/s00227-016-3054-0
Malej, A., and Harris, R. (1993). Inhibition of copepod grazing by diatom exudates: A factor in the development of mucus aggregates? Mar. Ecol. Prog. Ser. 96, 33–42. doi: 10.3354/meps096033
Martins, R., Fernandez, N., Beiras, R., and Vasconcelos, V. (2007). Toxicity assessment of crude and partially purified extracts of marine Synechocystis and Synechococcus cyanobacterial strains in marine invertebrates. Toxicon 50, 791–799. doi: 10.1016/j.toxicon.2007.06.020
Martins, R., Pereira, P., Welker, M., Fastner, J., and Vasconcelos, V. M. (2005). Toxicity of culturable cyanobacteria strains isolated from the Portuguese coast. Toxicon 46, 454–464. doi: 10.1016/j.toxicon.2005.06.010
Mathews, L., Faithfull, C. L., Lenz, P. H., and Nelson, C. E. (2018). The effects of food stoichiometry and temperature on copepods are mediated by ontogeny. Oecologia 188, 75–84. doi: 10.1007/s00442-018-4183-6
McKinnon, A. D., and Klumpp, D. W. (1998). Mangrove zooplankton of North Queensland, Australia: II. Copepod egg production and diet. Hydrobiologia 362, 145–160. doi: 10.1023/A:1003138718716
Milstein, A. (1979). Vertical distribution of Paracalanus crassirostris (Copepoda, Calanoidea): analysis by the general linear model. Bol. Inst. Ocean. 28, 65–78. doi: 10.1590/S0373-55241979000200008
Motwani, N. H., and Gorokhova, E. (2013). Mesozooplankton grazing on picocyanobacteria in the Baltic Sea as inferred from molecular diet analysis. PLoS One 8:e79230. doi: 10.1371/journal.pone.0079230
Mullin, M. M. (1963). Some factors affecting the feeding of marine copepods of genus Calanus. Limnol. Oceanogr. 8, 239–250. doi: 10.4319/lo.1963.8.2.0239
Nejstgaard, J. C., Tang, K. W., Steinke, M., Dutz, J., Koski, M., Antajan, E., et al. (2007). Zooplankton grazing on Phaeocystis: a quantitative review and future challenges. Biogeochemistry 83, 147–172. doi: 10.1007/s10533-007-9098-y
Norberg, J., and DeAngelis, D. (1997). Temperature effects on stocks and stability of a phytoplankton-zooplankton model and the dependence on light and nutrients. Ecol. Model. 95, 75–86. doi: 10.1016/S0304-3800(96)00033-6
Partensky, F., Hoepffner, N., Li, W. K. W., Ulloa, O., and Vaulot, D. (1993). Photoacclimation of Prochlorococcus sp. (Prochlorophyta) Strains Isolated from the North Atlantic and the Mediterranean Sea. Plant Physiol. 101, 285–296. doi: 10.1104/pp.101.1.285
Peters, R. H. (1984). “Methods for the study of feeding, grazing and assimilation by zooplankton, in Downing,” A manual on methods for the assessment of secondary productivity in freshwaters, IBP Handbook 17, 2nd edition eds J. A. Downing and F. H. Rigler (Blackwell, Oxford) 336–412.
Phlips, E. J., Badylak, S., Christman, M., Wolny, J., Brame, J., Garland, J., et al. (2011). Scales of temporal and spatial variability in the distribution of harmful algae species in the Indian River Lagoon, Florida, USA. Harmful Algae 10, 277–290. doi: 10.1016/j.hal.2010.11.001
Phlips, E. J., Badylak, S., Christman, M. C., and Lasi, M. A. (2010). Climatic trends and temporal patterns of phytoplankton composition, abundance, and succession in the Indian River Lagoon, Florida, USA. Estuaries Coasts 33, 498–512. doi: 10.1007/s12237-009-9166-8
Phlips, E. J., Badylak, S., Lasi, M. A., Chamberlain, R., Green, W. C., Hall, L. M., et al. (2015). From red tides to green and brown tides: bloom dynamics in a restricted subtropical lagoon under shifting climatic conditions. Estuaries Coasts 38, 886–904. doi: 10.1007/s12237-014-9874-6
Podolsky, R. D. (1994). Temperature and water viscosity: physiological versus mechanical effects on suspension feeding. Science 265, 100–103. doi: 10.1126/science.265.5168.100
Podolsky, R. D., and Emlet, R. B. (1993). Separating the effects of temperature and viscosity on swimming and water movement by sand dollar larvae (Dendraster excentricus). J. Exp. Biol. 176, 207–222. doi: 10.1242/jeb.176.1.207
Post, A. F., and Bullerjahn, G. S. (1994). The photosynthetic machinery in prochlorophytes: Structural properties and ecological significance. FEMS Microbiol. Rev. 13, 393–413. doi: 10.1111/j.1574-6976.1994.tb00059.x
Preuss, T. G., Hammers-Wirtz, M., Hommen, U., Rubach, M. N., and Ratte, H. T. (2009). Development and validation of an individual based Daphnia magna population model: the influence of crowding on population dynamics. Ecol. Model. 220, 310–329. doi: 10.1016/j.ecolmodel.2008.09.018
Ramos, D. J. R., and Reyes-Vazquez, G. R. (1990). Efecto de la concentracion de NaCl sobre el crecimiento y morfologia de Synechococcus sp. A0185001PS (Cyanobacteria) aislado de salina artificial de Araya, Araya, Venezuela. Boletin Inst. Oceanogr. Venezuela Cumana 29, 153–159.
Rangel, L. M., Ger, K. A., Silva, L. H., Soares, M. C. S., Faassen, E. J., and Lürling, M. (2016). Toxicity overrides morphology on Cylindrospermopsis raciborskii grazing resistance to the calanoid copepod Eudiaptomus gracilis. Microb. Ecol. 71, 835–844. doi: 10.1007/s00248-016-0734-8
Seuront, L., and Vincent, D. (2008). Increased seawater viscosity, Phaeocystis globosa spring bloom and Temora longicornis feeding and swimming behaviours. Mar. Ecol. Prog. Ser. 363, 131–145. doi: 10.3354/meps07373
Shields, R. J., Kotani, T., Molnar, A., Marion, K., Kobashigawa, J., and Tang, L. (2005). Intensive cultivation of a subtropical paracalanid copepod, Parvocalanus sp., as prey for small marine fish larvae in Copepods in Aquaculture. Hoboken, NJ: Blackwell Publishing, 209–223. doi: 10.1002/9780470277522.ch16
Smayda, T. J. (2008). Complexity in the eutrophication–harmful algal bloom relationship, with comment on the importance of grazing. Harmful Algae 8, 140–151. doi: 10.1016/j.hal.2008.08.018
Sun, X., Sun, S., Li, C., and Wang, M. (2012). Seasonal change in body length of important small copepods and relationship with environmental factors in Jiaozhou Bay. China. Chin. J. Oceanol. Limnol. 30, 404–409. doi: 10.1007/s00343-012-1140-9
Svensen, C., and Vernet, M. (2016). Production of dissolved organic carbon by Oithona nana (Copepoda: Cyclopoida) grazing on two species of dinoflagellates. Mar. Biol. 163:237. doi: 10.1007/s00227-016-3005-9
Tang, K. W., Chen, Q. C., and Wong, C. K. (1994). Diel vertical migration and gut pigment rhythm of Paracalanus parvus, P. crassirostris, Acartia erythraea and Eucalanus subcrassus (Copepoda, Calanoida) in Tolo Harbour, Hong Kong. Hydrobiologia 292/293, 389–396.
Turner, J. T. (2006). Harmful algae interactions with marine planktonic grazers in Ecology of harmful algae. Berlin: Springer, 259–270. doi: 10.1007/978-3-540-32210-8_20
Turner, J. T. (2010). Zooplankton community grazing impact on a bloom of Alexandrium fundyense in the Gulf of Maine. Harmful Algae 9, 578–589. doi: 10.1016/j.hal.2010.04.008
Turner, J. T., and Dagg, M. J. (1983). Vertical distributions of continental shelf zooplankton in stratified and isothermal waters. Biol. Oceanogr. 3, 1–40.
Turner, J. T., Roncalli, V., Ciminiello, P., Dell’Aversano, C., Fattorusso, E., Tartaglione, L., et al. (2012). Biogeographic effects of the Gulf of Mexico red tide dinoflagellate Karenia brevis on Mediterranean copepods. Harmful Algae 16, 63–73. doi: 10.1016/j.hal.2012.01.006
Valério, E., Chaves, S., and Tenreiro, R. (2010). Diversity and Impact of Prokaryotic Toxins on Aquatic Environments: A Review. Toxins 2, 2359–2410. doi: 10.3390/toxins2102359
van Duren, L. A., and Videler, J. J. (2003). Escape from viscosity: the kinematics and hydrodynamics of copepod foraging and escape swimming. J. Exp. Biol. 206, 269–279. doi: 10.1242/jeb.00079
Wong, C. K., Chan, A. L. C., and Chen, Q. C. (1993). Planktonic copepods of Tolo Harbour. Hong Kong. Crustaceana 64, 76–84. doi: 10.1163/156854093X00081
Keywords: prochlorophyte, harmful algal blooms, mucilage, top-down control, Indian River Lagoon
Citation: Ma X, Jacoby CA and Johnson KB (2021) High Densities of a Prochlorophyte (Unresolved Species) Inhibit Grazing by the Herbivorous Copepod Parvocalanus crassirostris. Front. Mar. Sci. 8:664153. doi: 10.3389/fmars.2021.664153
Received: 04 February 2021; Accepted: 20 September 2021;
Published: 10 November 2021.
Edited by:
Edward J. Phlips, University of Florida, United StatesReviewed by:
Mie Hylstofte Sichlau Winding, Greenland Climate Research Centre, GreenlandAndrea Pain, University of Maryland Center for Environmental Science (UMCES), United States
Copyright © 2021 Ma, Jacoby and Johnson. This is an open-access article distributed under the terms of the Creative Commons Attribution License (CC BY). The use, distribution or reproduction in other forums is permitted, provided the original author(s) and the copyright owner(s) are credited and that the original publication in this journal is cited, in accordance with accepted academic practice. No use, distribution or reproduction is permitted which does not comply with these terms.
*Correspondence: Xiao Ma, bWF4aWFvQHNjc2lvLmFjLmNu