- 1Department of Biological Sciences, Old Dominion University, Norfolk, VA, United States
- 2Department of Environment and Sustainability, University at Buffalo, Buffalo, NY, United States
- 3Institute of Environment, Department of Biological Sciences, Florida International University, Miami, FL, United States
Underwater sound is used by many marine larvae to orient to coastal habitats including backreef, sponge-dominated hardbottom habitat in the Florida Keys (FL, United States)—a particularly “noisy” coastal habitat. However, the distance over which acoustic cues are attractive to settlement-stage larvae is generally unknown. We examined this phenomenon in a region of the Florida Keys where mass sponge die-offs have diminished both underwater soundscapes and larval settlement. The absence of pronounced hardbottom-associated sound over such a large area allowed us to experimentally test in situ the response of fish and invertebrate larvae to broadcasted sounds at different distances from their source. We first measured the signal-to-noise ratio of healthy hardbottom habitat soundscapes broadcast from an underwater speaker at seven distances to determine the maximum range of the signal. Based on those results, larval collectors were then deployed at 10, 100, 500, and 1,000 m from speakers broadcasting sounds recorded at either degraded or healthy hardbottom sites for five consecutive nights during each of three new and full moon periods in summer/fall 2019. Larval settlement onto those collectors was affected by lunar phase and soundscape type, but varied among species. In most cases, the effect was small and not likely to be ecologically significant. The absence of a strong larval settlement response to a sound cue lies in contrast to results from other studies. We suspect that the small (<500 m) radius of the broadcasted soundscapes may have limited the magnitude of the larval response to locally available larvae whose abundance may have been low because the experiment was conducted within a large, relatively quiet seascape. If true, it is possible that planktonic larvae may require a series of acoustic “sign-posts,” perhaps in combination with other cues (e.g., chemical), to successfully orient to distant nursery habitats. Although habitat restoration efforts may be able to restore healthy soundscapes, the typically small size and number of restoration sites may limit the range of the acoustic cue and thus larval attraction to restored habitats.
Introduction
Soundscapes, the collection of sounds emanating from landscapes, convey important information about an environment and its biota. In the sea, different habitats have unique acoustic fingerprints (Butler et al., 2016) and within habitat types, soundscapes vary temporally (Radford et al., 2010) and spatially (Kennedy et al., 2010). Marine soundscapes are correlated with environmental (e.g., sea state, habitat depth) and biological parameters (e.g., coral cover, fish diversity and biomass; Piercy et al., 2014; Kaplan et al., 2017), and those acoustic signals are used by pelagic larvae to locate nursery habitats. Larvae that are attracted to soundscapes associated with settlement habitats include mobile species such as fish (Simpson et al., 2004; Parmentier et al., 2015) and decapod crustaceans (Stanley et al., 2012; Hinojosa et al., 2016), as well as sessile species like bivalves (Lillis et al., 2013, 2015) and corals (Lillis et al., 2016, 2018). Sound is different from other settlement cues (i.e., visual or olfactory cues) because it can carry information over long distances and is not affected by currents, turbidity, or light.
For sound to be a useful settlement cue, pelagic larvae must be able to detect it far from the source. Soundscapes of temperate rocky coastal reefs may be detected up to 10–25 km offshore by hydrophones (Cato and McCauley, 2002) and tropical coral reefs have been distinguished up to a few km (Piercy et al., 2014). As distance from the source increases, sounds are homogenized and the signal fades (Piercy et al., 2014). Given the limits of hearing capabilities of larvae, suitable habitats may only be detectable by larvae within a few hundreds of meters (Egner and Mann, 2005; Mann et al., 2007; Wright et al., 2010). Although many studies demonstrate the use of sound cues by fish and invertebrate larvae, the range at which they can detect and discriminate between cues is unclear.
Habitat degradation alters both the characteristics of the soundscape and the range of the signal. Soundscapes of degraded habitats (e.g., low coral cover, low fish diversity and biomass, loss of sponges) are often quieter and less complex than healthy habitats (Butler et al., 2016; Gordon et al., 2018). Lower soundscape amplitudes at the point of origin results in a smaller detection range (Piercy et al., 2014) and degraded soundscapes are less attractive to larval fish and invertebrates than soundscapes of healthy habitats (Butler, 2016; Gordon et al., 2018), likely hindering recovery of degraded areas.
Habitat restoration may restore marine soundscapes that act as settlement cues, but the detection range for cues from small restoration patches may be limited and preclude reestablishment of normal levels of larval settlement. In the Florida Keys, backreef hardbottom habitats are characterized by sponges, octocorals, and macroalgae. But cyanobacteria blooms have caused mass die-offs of sponges in a portion of Florida Bay leaving large swaths of barren habitat with few sponges and associated fauna (Butler et al., 1995; Herrnkind et al., 1997; Stevely et al., 2011). Previous studies have characterized backreef soundscapes and larval settlement in areas of healthy and degraded hardbottom habitat, as well as small patches of restored hardbottom habitats (Butler et al., 2016). Degraded hardbottom soundscapes are quieter and less complex than healthy hardbottom soundscapes and fewer larvae settle there. Although the soundscapes of restored patches of hardbottom are similar to those in healthy hardbottom, the abundance and diversity of larvae settling in restored patches is not the same as in healthy habitats (Butler, 2016). It is possible that the small size of the restoration patches may limit the transmission range of the restored soundscapes such that they do not propagate far enough to attract settling larvae.
In this study, we aimed to examine larval fish and invertebrate settlement at varying distances from broadcasted soundscapes from healthy hardbottom habitats. We hypothesized that healthy soundscapes would increase settlement over degraded soundscapes and settlement would decrease with distance from the sound source. To test this, we first determined the maximum range of detection of the auditory cue broadcast from an underwater speaker (i.e., where the signal to noise ratio was near zero). We then examined larval assemblages that settled on collectors placed at increasing distances from underwater speakers broadcasting healthy soundscapes at natural amplitudes, simulating a small, restored patch of habitat. Those results were compared to similar data wherein we broadcasted degraded soundscapes.
Materials and Methods
Study Area
Our studies were carried out in Florida Bay and nearshore waters just north of the middle Florida Keys (United States) where there is a mixture of seagrass meadows, sandy-mud bottom, mangrove islands, and sponge-dominated karst hardbottom (Figure 1). These habitats vary considerably in their soundscape profiles. Healthy hardbottom produces soundscapes containing large numbers of snapping shrimp snaps (Butler et al., 2016). In contrast, degraded hardbottom sites are significantly quieter than healthy sites with fewer snapping shrimp snaps and soundscape spectra similar to seagrass beds (see Figure 2 in Butler et al., 2016). The present study takes advantage of the now quiet, barren hardbottom areas in a large region in the central Florida Keys affected by sponge die-offs, as a location in which we could broadcast experimental soundscapes with minimal interference from natural soundscapes.
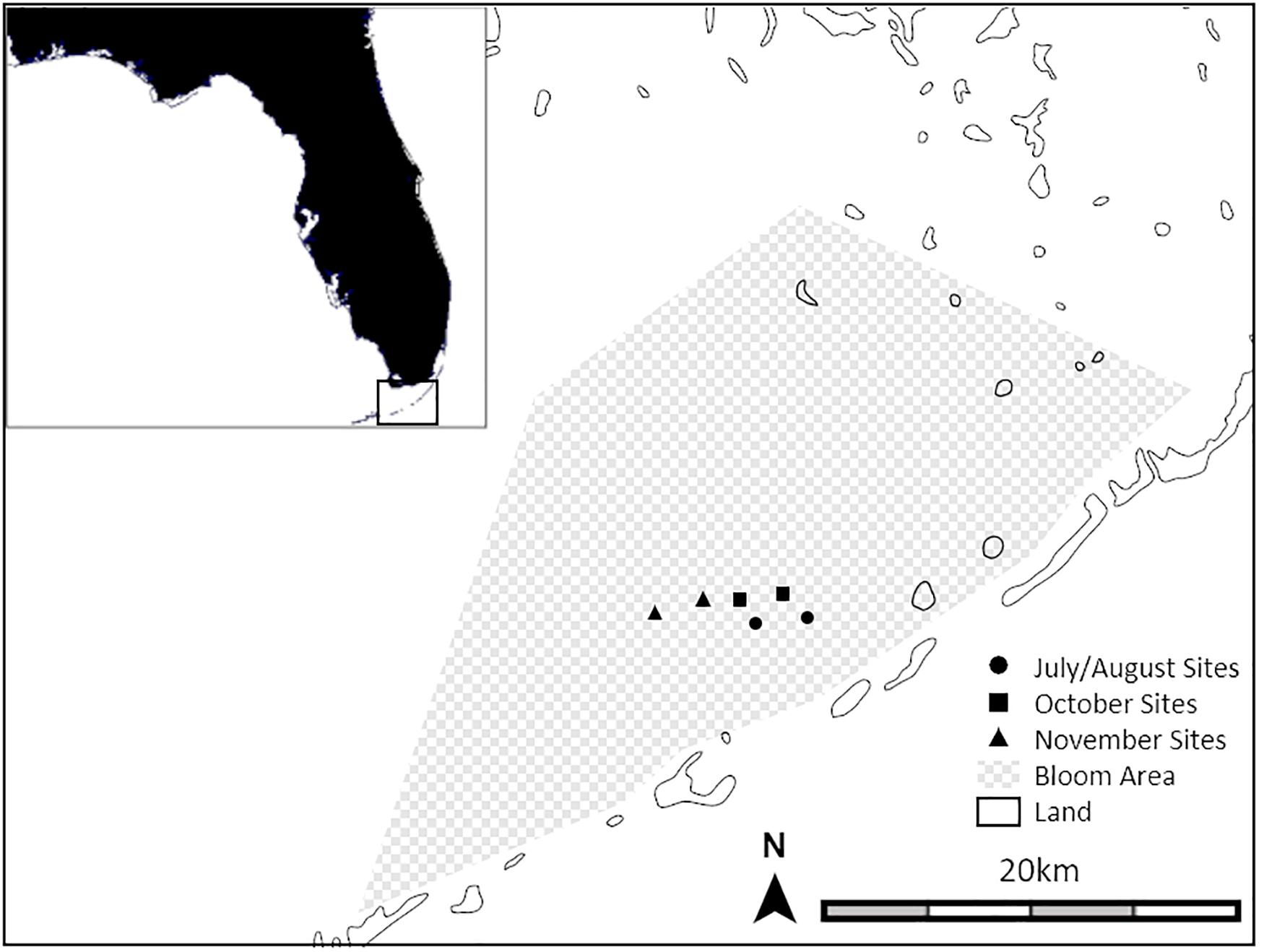
Figure 1. Map showing the six experimental soundscape broadcast locations including the approximate extent of hardbottom habitat degraded by cyanobacteria blooms and sponge die-offs. (map: d-maps.com).
Transmission Loss
Modeling sound propagation in shallow waters, such as Florida Bay, is difficult (Urick, 1983) and many of the necessary physical parameter estimates (e.g., bedrock depth and density) have not been made. Therefore, the easiest method to estimate transmission loss was with empirical measurements. In July 2018, we conducted a transmission loss experiment in degraded hardbottom to determine the approximate distance that an auditory cue from a playback device can be detected above the ambient sound. We defined this point as the distance from the source where the signal can no longer be distinguished from the background noise and the signal to noise ratio (SNR) is approximately 0 dB. SNR is the ratio of the intensity of the signal (IS) to the intensity of the background level (IN), expressed in dB, and calculated as
We deployed an underwater speaker (Lubell Labs 916H underwater loudspeaker; frequency response 200 Hz–20 kHz, 180 db re 1 μPa output @ 1 kHz), connected to a waterproof barrel containing a WAV player (R-07 solid-state WAV recorder; Roland Corporation, Japan; flat frequency response 20 Hz–40 kHz), an amplifier to drive the speaker (TOA CA-160 amplifier; flat frequency response 100 Hz–10 kHz), and a 12 V deep cycle battery to power the speaker and amplifier. Then, we broadcasted overlaid pure tones of known frequency (100, 500, 1,000, 1,500, and 2,000 Hz) and amplitude (115 dB re 1 μPa) in degraded hardbottom habitat. Pure tones were chosen to represent a range of frequencies observed in natural hardbottom soundscapes without the variation in amplitude and frequency inherent to recordings of natural habitats. The tones were recorded using Aquarian Audio H2a omnidirectional hydrophones (Aquarian Audio Products: sensitivity −180 dB re 1V/μPa [+/− 4 dB 20 Hz–4 kHz]; flat frequency response 10 Hz–100 kHz), attached to Roland Edirol R-05 or R-07 solid-state WAV recorders (Roland Corporation, Japan; 48 kHz; 16bit; flat frequency response 20 Hz–40 kHz) contained in waterproof housings at increasing distances (1, 10, 25, 50, 100, 200, and 500 m) from the speaker (Figure 2). Playback and recordings were conducted in two sets within 30 min of each other in late afternoon on July 20, 2018. Four hydrophones were deployed at 1, 10, 25, and 50 m from the speaker system and tones were played for 30 s. The hydrophones were then repositioned at 50, 100, 200, and 500 m and tone playback was repeated. We waited 15 min after the first playback to allow the soundscape to recover from any potential acoustic effect of playing the tones. SNRs were calculated from two 15 s clips from each recording (the 15 s immediately before tone playback, and 15 s during tone playback) at each distance. No filters were applied to the recordings so frequency bandwidth used in the SNR calculations was 0 Hz–24 kHz. The two recordings at 50 m indicated there was no change in SNR due to the delayed recording of the second set.
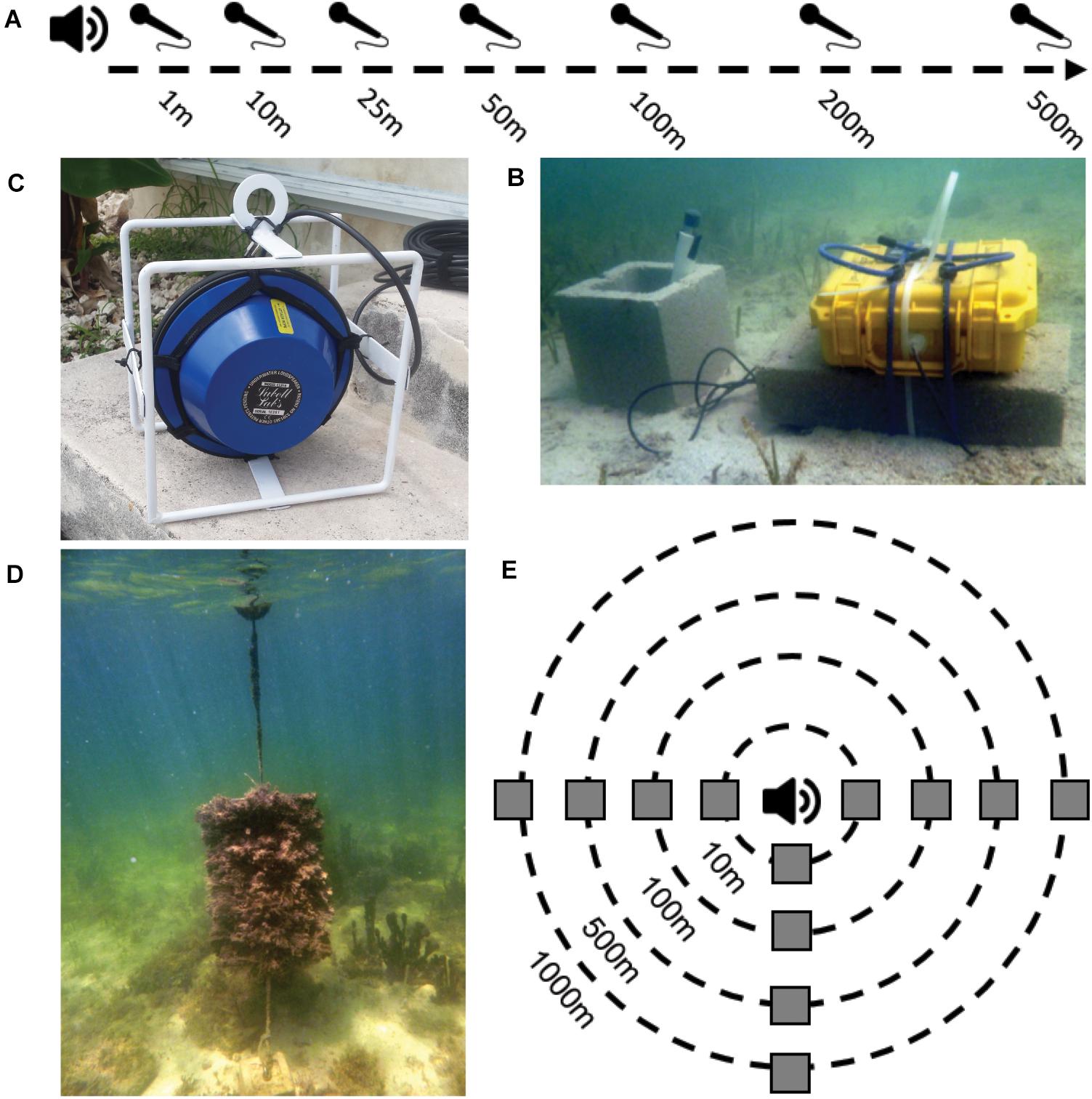
Figure 2. Graphics and photos describing experimental set-ups and equipment. (A) Graphical depiction of the transmission loss experimental set up with hydrophones set up at 1, 10, 25, 50, 100, 200, and 500 m from the sound source. (B) Underwater photo of Aquarian Audio H2a omnidirectional hydrophones attached to Roland Edirol R-05 or R-07 solid-state WAV recorders contained in a waterproof housing (photo credit: Jack Butler). (C) Photo of the underwater speaker. (D) Photo of a larval collector made of frayed rope attached to a mesh back with 3/4 in PVC pipe top and bottom frame and tethered to a buoy and anchor made of cement blocks (photo credit: Jack Butler). (E) Graphical depiction of experimental set-up to test larval attraction to sound at different distances. Three artificial larval collectors (gray squares) were deployed at four distances (10, 100, 500, and 1,000 m) from an omnidirectional underwater speaker system (at center) broadcasting either healthy or degraded hardbottom soundscapes.
Larval Response to Soundscape and Distance
Soundscapes from healthy and degraded hardbottom habitats were broadcasted at degraded sites (i.e., sites devoid of sponges) within Florida Bay using the system described above to test the effects of soundscape type and distance from the source on larval settlement. Prior to the start of the experiment, soundscapes from several haphazardly selected healthy and degraded hardbottom sites were recorded at new and full moons using an omnidirectional hydrophone (described above). Recordings were only used for one trial to avoid pseudoreplication. Root mean square sound pressure level over a 15 s clip was calculated for each recording and used to calculate the required voltage output for the speaker system to broadcast the recordings at approximately the same amplitude at which they were originally recorded. Voltage output was matched by manipulating the volume of the WAV player containing the recording prior to deployment of the speaker. The sound pressure level of the recorded soundscapes used in the experiment ranged from 76 to 80 dB re 1 μPa at 1 kHz for healthy soundscapes and 65–68 dB re 1 μPa at 1 kHz for degraded soundscapes (Figure 3).
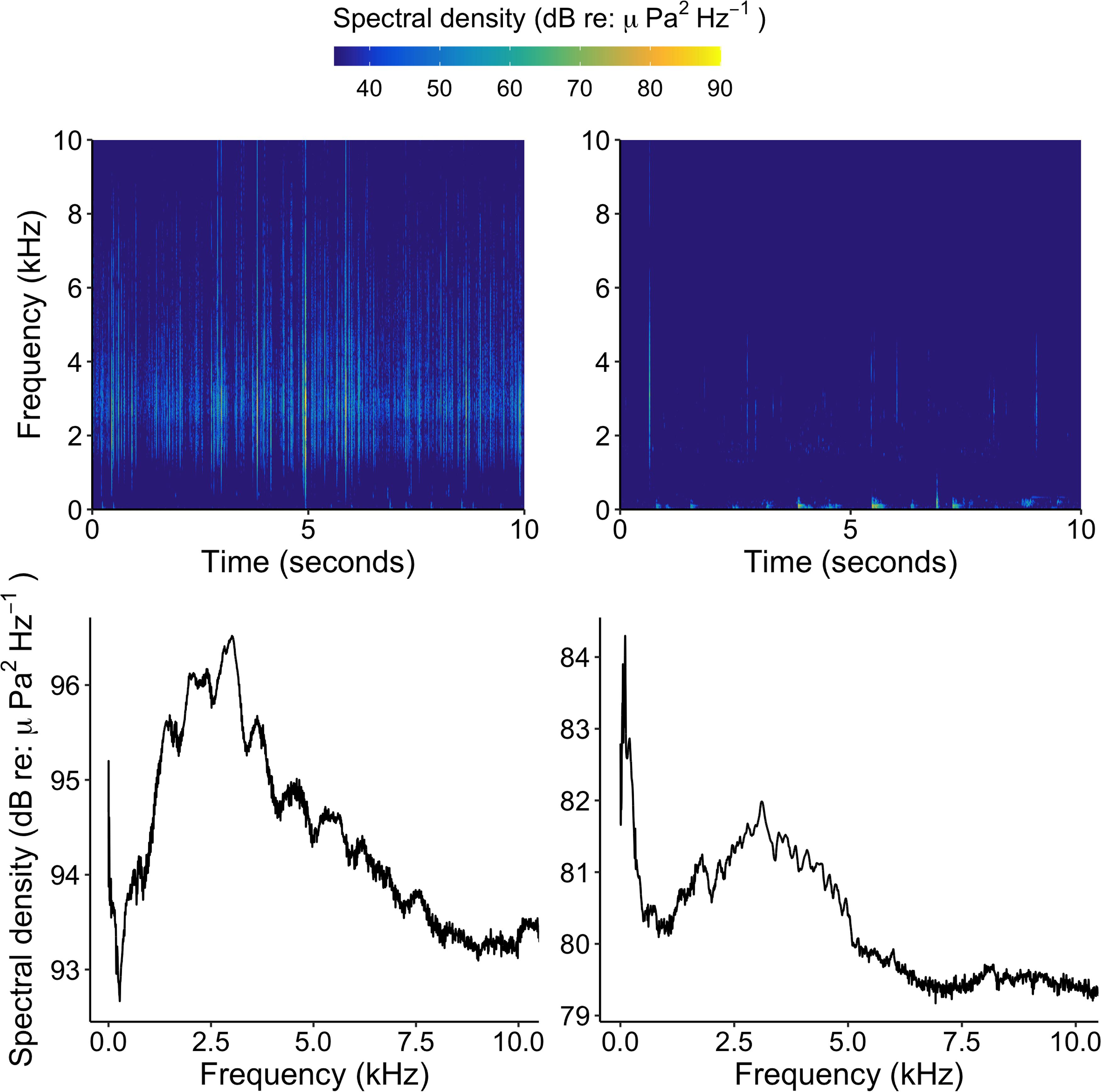
Figure 3. Spectrograms (top panels) and power spectral densities (bottom panels) of healthy hardbottom soundscapes (left panels) and degraded hardbottom soundscapes (right panels) during new moon. Note the y-scale difference between lower left and lower right panels.
Three pairs of sites (wherein a pair of sites consists of one site where healthy hardbottom soundscapes were broadcast and one site where degraded hardbottom soundscapes were broadcast) were haphazardly chosen within the ∼500 km2 area of Florida Bay affected by the sponge die-offs (Figure 1). All sites were at least 3 km apart and thus represented separate sources of sound within a relatively quiet background environment. For each pair of sites, experiments were run twice: once during a full moon and once during a new moon to capture the differences in larval settlement that are common between the two moon phases. All larval collections were made between July and November 2019. Animals caught in the collectors were mostly recently metamorphosed post-settlement juveniles but are hereafter referred to as “larvae.”
At each site, three artificial collectors were placed in opposing directions at four distances from the speaker: ∼1 wavelength (10 m), mid-range (100 m), ∼0 dB SNR (500 m), and out of range (1,000 m, Figure 2E). Wavelength is proportional to frequency, therefore lower frequencies have longer wavelengths. The 10 m distance was chosen as the ∼1 wavelength distance because it was the approximate length of the lowest frequency the speaker could produce given the speaker’s frequency response. Mid-range and ∼0 dB SNR distances were selected based on the transmission loss experiment in hardbottom habitat. Collectors were made of frayed rope attached to a mesh back 50 cm × 100 cm in size with in PVC pipe top and bottom frame, tethered to concrete blocks and suspended in the water column by a surface buoy to prevent colonization by mobile benthic organisms (Figure 2D). These collectors mimic the physical structure of hardbottom vegetation that many settling larvae use and have been successful in previous larval studies in the area (Butler, 2016). Collectors were placed in healthy hardbottom habitat for six weeks prior to the start of the experiment to develop a biofilm and were shaken prior to the start of each trial to remove any larvae that may have settled between trials so that only larvae settling during the trials would be collected.
Trials were run for five nights: two nights before a full or new moon to two nights after the moon phase of interest. During each trial, a speaker was deployed at each site and broadcasted either a healthy or a degraded hardbottom soundscape at approximately the same volume as the original recording. Speakers were deployed each evening of the trial and retrieved the following morning. Collectors were sampled the morning following the last night of the trial. Collectors were unclipped from the mooring and carefully moved into a mesh bag (1 mm2 mesh) before being brought aboard a vessel where they were shaken 10 times to dislodge the larvae into the bag. Larval fish were separated and immediately euthanized by overdose with tricain methanosulfonate (MS-222) following an IACUC approved protocol. Fish and invertebrate larvae were then preserved in 70% ethanol for quantification and identification to the lowest taxonomic level.
Larval community assemblage data were Hellinger transformed prior to analysis, visually represented with two-dimensional non-metric multidimensional scaling (nMDS) and compared using a non-parametric (permutational) multivariate analysis of variance (PERMANOVA). The Hellinger transformation was chosen because it does not give high weights to rare species, which occurred sporadically in this data set, and it makes the data more suitable for ordination techniques (Legendre and Gallagher, 2001). PERMANOVA uses traditional analysis of variance experimental design extended to a matrix of pairwise distances with P-values obtained by permutation (Anderson, 2001). Moon phase, distance, and soundscape were treated as fixed factors, and site was treated as a random factor. Analyses were run using the vegan package (v2.5-6; Oksanen et al., 2019) in R 3.6.3 (R Core Team, 2020).
Results
Transmission Loss
The SNR decreased exponentially with distance underwater and at 500 m was nearly undetectable (∼3 dB, Figure 4). Because the recordings used in the larval settlement experiment (65–80 dB re 1 μPa) were quieter than the tones used in the transmission loss experiment (115 dB re 1μPa), a curve was fit to the transmission loss results (Figure 4) and we calculated the distance where the SNR was equal to 2.6 dB, near the limit of detection. The calculated distance for the broadcasted soundscapes with a SNR of 2.6 dB ranges from approximately 490–497 m for healthy soundscapes and 466–474 m for degraded soundscapes. Therefore, the distances used for collector placement were appropriate for the sound levels of the broadcasted hardbottom recordings.
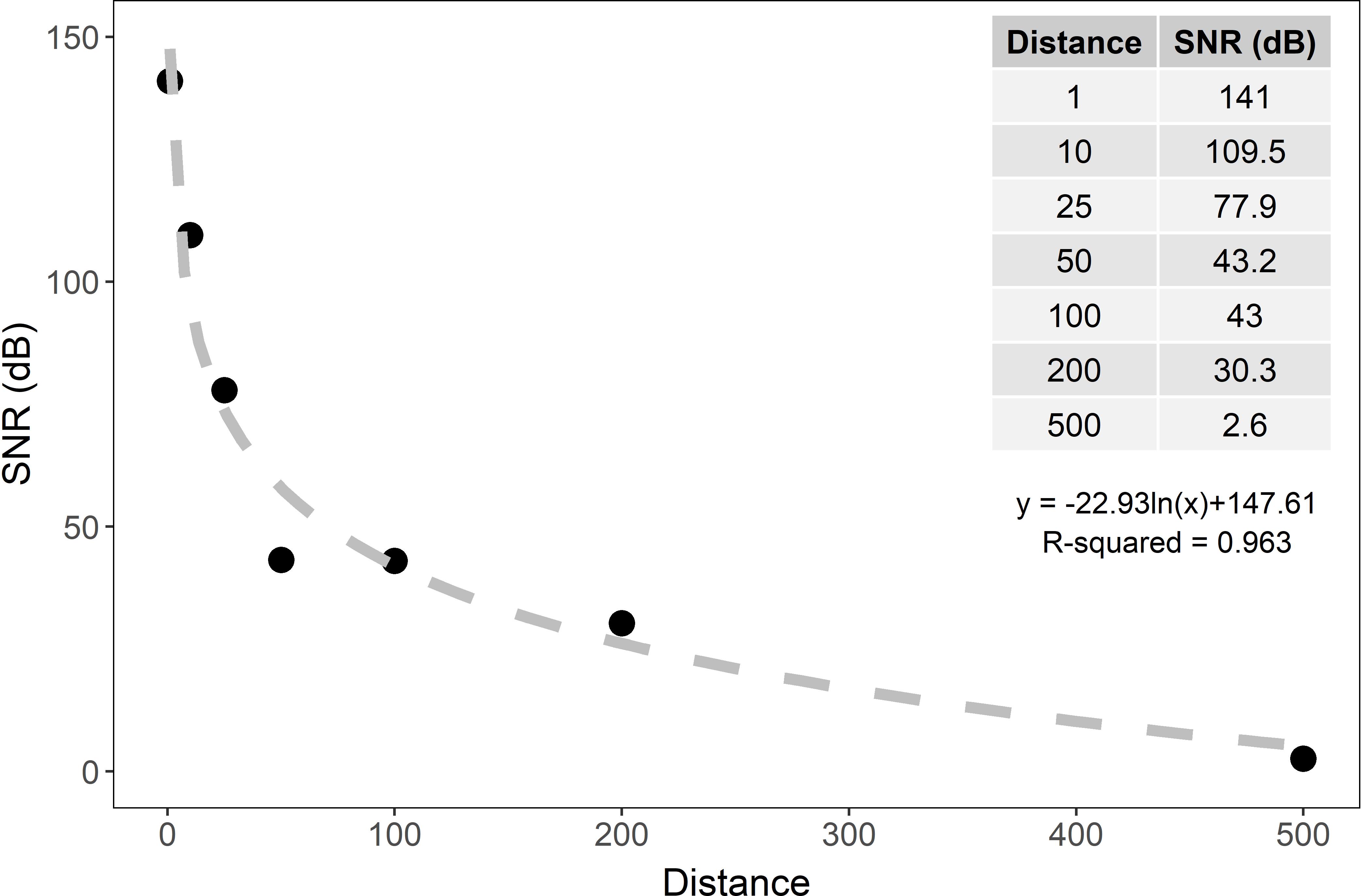
Figure 4. Signal to noise ratio (SNR) and regression line of pure tones broadcasted at maximum volume in degraded hardbottom calculated from hydrophone recordings taken at seven distances (1, 10, 25, 50, 100, 200, and 500 m) from the playback device. At 500 m from the playback device, the signal is nearly undetectable from the background noise (SNR = 2.6 dB).
Larval Response to Soundscape and Distance
Over the course of the experiment 8,551 individual recruits of 41 taxa were collected. Bivalves (50.9%, 4353 individuals, 5 species) and crustaceans (35.3%, 3017 individuals, 6 species) comprised the majority of the catch. Larval assemblages differed between moon phases (PERMANOVA: F1,141 = 6.377, R2 = 0.043, p = 0.001) and mean settlement per collector was 1.3 times higher during full moon than new moon (full moon = 68.4 ± 26.6 95% CI; new moon = 51.2 ± 13.7 95% CI). Larval assemblages also differed between healthy and degraded soundscapes (PERMANOVA: F1, 141 = 1.908, R2 = 0.013, p = 0.001) and 1.4 times as many larvae settled on collectors subject to healthy hardbottom soundscapes (70.5 ± 28.4 95% CI) compared to collectors exposed to degraded soundscapes (49.9 ± 7.6 95% CI).
Overall, distance from the speaker did not affect the settling larval assemblage (PERMANOVA, F2,141 = 0.985, R2 = 0.021, p = 0.085). There was no difference in the mean number of settlers between collectors at 10 and 100 m from the speaker systems (57.2 ± 13.7 95% CI and 57.8 ± 16.4 95% CI), but larval settlement on collectors deployed at 10 and 100 m was 1.3-fold higher than at 500 m (45.6.6 ± 12.4 95% CI). Even more larvae settled on collectors furthest from the sound source (1,000 m = 79.5 ± 55.5 95% CI) as compared to collectors placed at 10, 100, and 500 m. Given that the collectors at 1,000 m were deployed at more than twice the range of the speaker systems, the high mean settlement at this distance was not due to the effects of the broadcasted soundscapes. However, the effects of distance on mean larval settlement are small when separated by moon phase and soundscape (Figure 5).
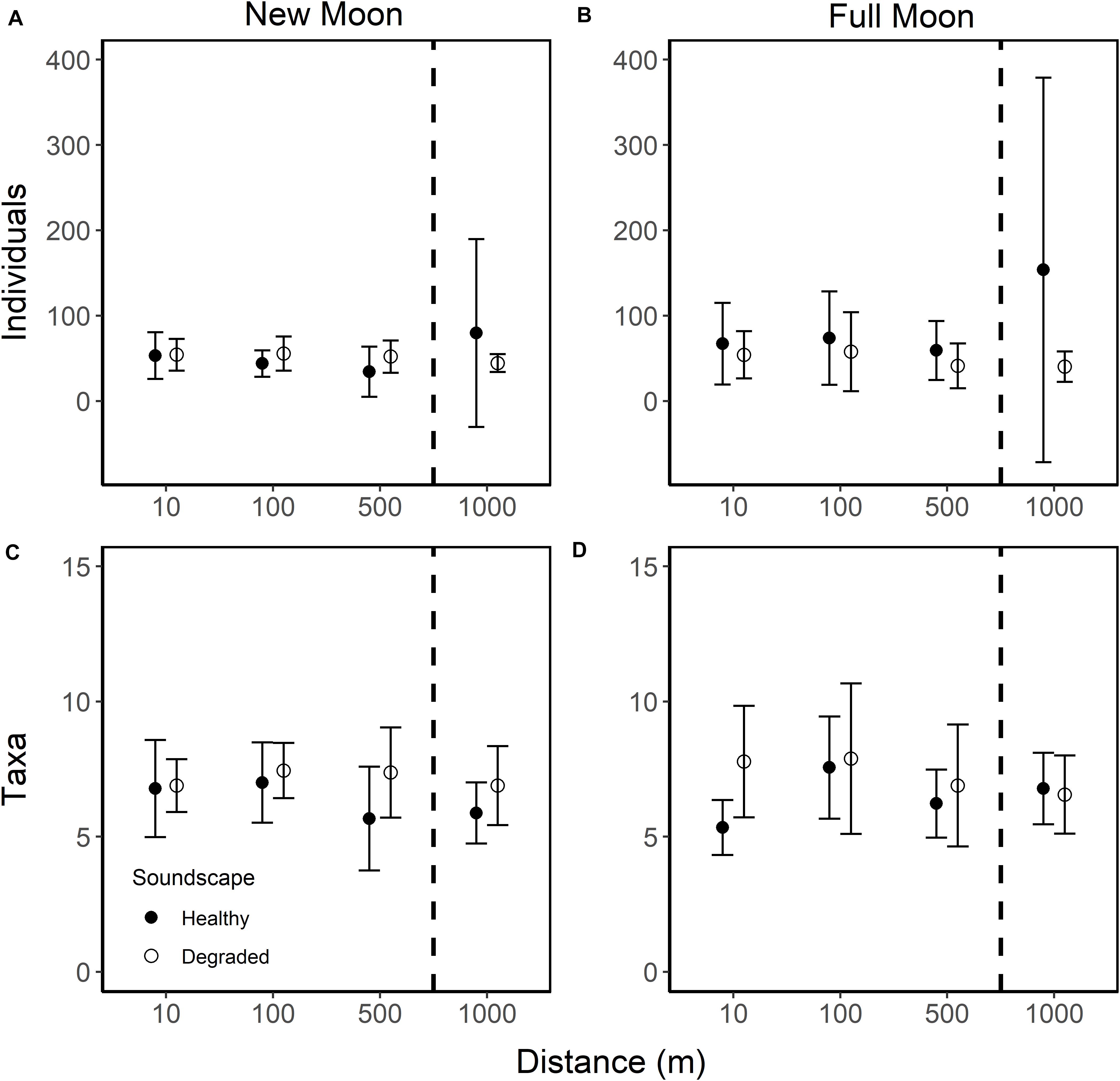
Figure 5. Mean number of individual larvae (A,B) and taxa (C,D) per collector for collectors deployed 10, 100, 500, and 1,000 m from broadcasted healthy (closed circle) and degraded (open circle) soundscapes during new (A,C) and full moon (B,D) phases. All error bars are 95% confidence intervals. Dashed lines indicate collectors that lie outside the detectable sound range (1,000 m).
Deeper inspection of the larval collector data revealed that two of the 35 collectors deployed at 1,000 m were outliers with extremely high settlement, as much as 7.6 times greater than the collector with the next highest number of individuals. Lima clams dominated the settlement on those collectors (4.2 and 1.8 times greater than the next highest collector count). Removing these two collectors removed differences in mean settlement between 500 and 1,000 m (500 m: 45.6 ± 12.4 95% CI, 1,000 m: 44.0 ± 8.9 95% CI) and effect sizes between the distances inside the range and both distances outside the range of the speaker were the same (1.3×). Removal of these outlier collectors altered the effect of the soundscape treatment on larval settlement: settlement was slightly higher on healthy soundscapes than degraded soundscapes (1.1×; 53.3 ± 13.7 95% CI and 49.9 ± 7.6 95% CI, respectively) and the effect of moon phase on mean settlement was minimal (1.2×; 46.7 ± 6.4 95% CI and 56.3 ± 11.2 95% CI).
The nMDS (Figure 6, stress: 0.153, r2 = 0.993) plot places each artificial collector on a two-dimensional ordination plane based on larval assemblage. Figures 6A–C illustrates the effects of moon phase, distance from the speaker system, soundscape, and collection period (one sequential new and full moon phase) on the larval assemblages settling on collectors. Although significant in the PERMANOVA model, the nMDS and the low PERMANOVA R2 values for moon phase and soundscape suggest that those factors account for a small portion of the total variance (Table 1). The nMDS also suggests that site or sampling period, which are correlated because sites were moved each month, accounts for some of the variance in larval assemblage.
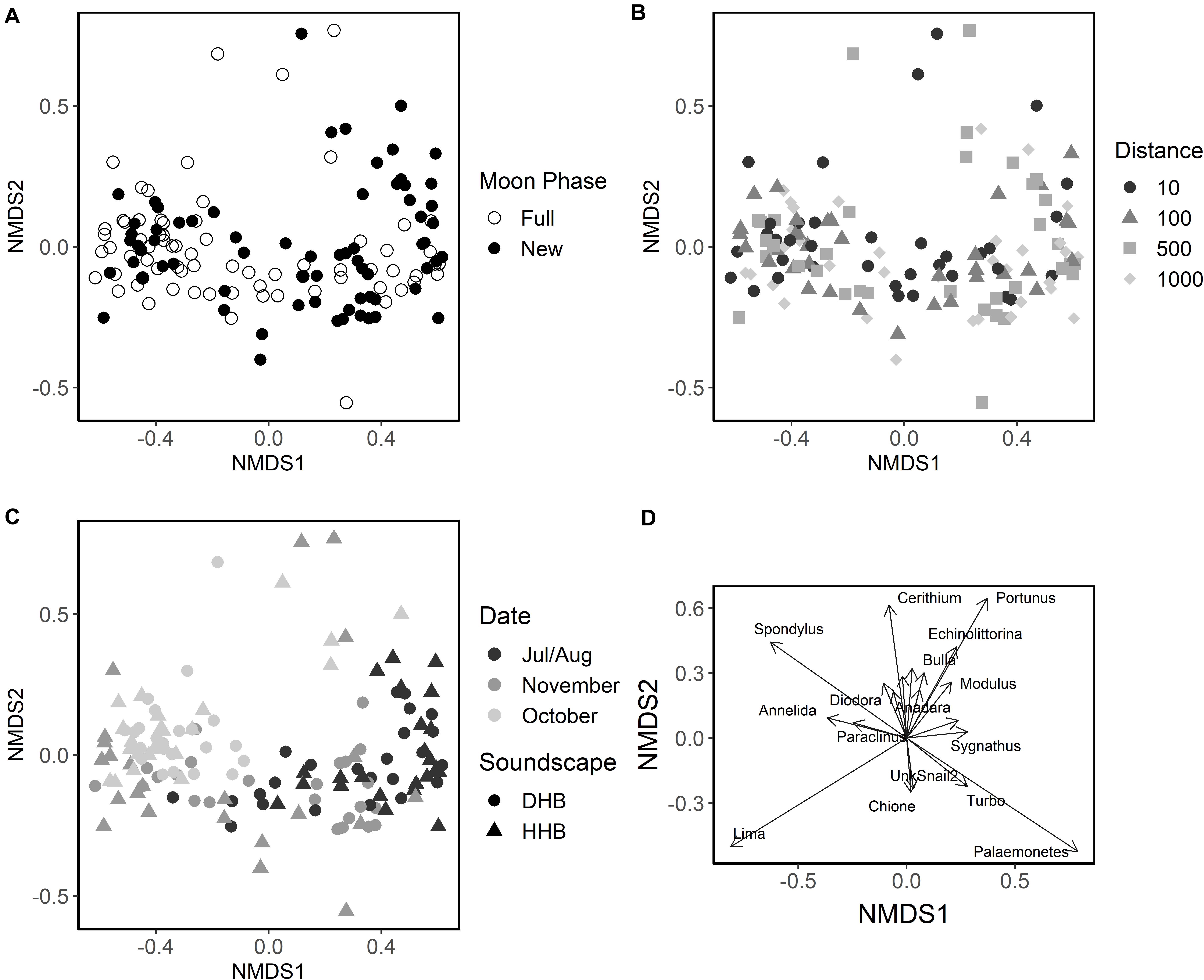
Figure 6. Non-metric Multidimensional Scaling ordination of larval assemblage samples. Each point represents the larval assemblage found on an individual collector. (A) Collector points shaded by the moon phase during collection (open circle = full moon, closed circle = new moon). (B) Shapes indicate the distance of the collector from the playback device (circle = 10 m, triangle = 100 m, square = 500 m, and diamond = 1,000 m). (C) Collector points colored by collection period (black = July/August, dark gray = October, light gray = November) and shape indicates the broadcasted soundscape (circle = degraded hardbottom, triangle = healthy hardbottom). Each collection period includes one full and one new moon sampling session. (D) Plot of influential species vectors, lengths are scaled by their correlation so that strong predictors have longer arrows than weak predictors.
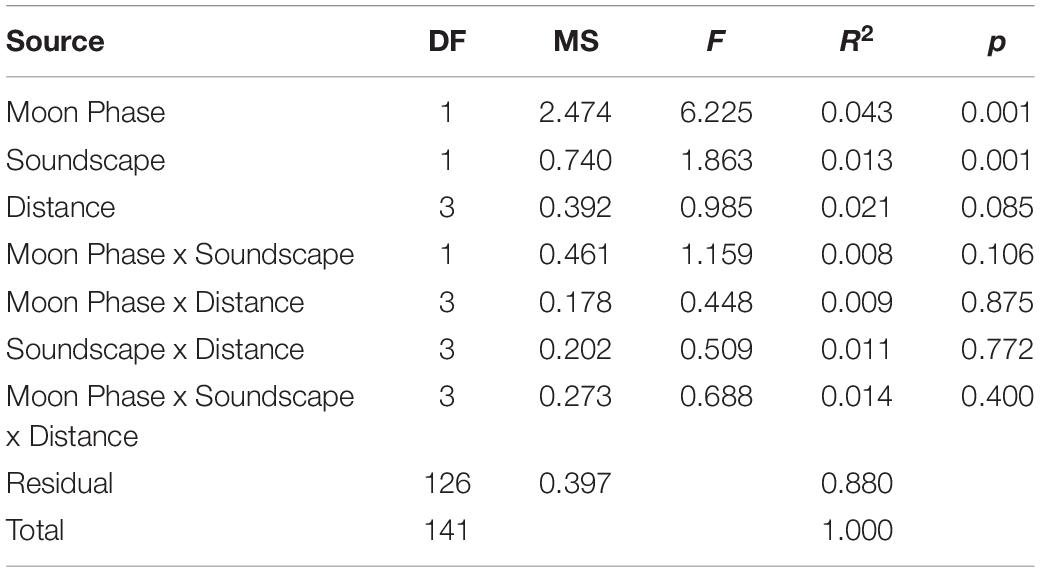
Table 1. PERMANVOA results testing the effects of moon phase, soundscape type, and distance from the underwater speaker on larval community assemblage.
Species richness was similar among treatments (Figure 5), but larval responses to moon phase, soundscape, and distance varied among taxa (Figure 7 and Supplementary Table 1). Overall, 29 taxa had low (<50 total individuals) settlement with no discernable relationship to moon phase, type of broadcasted sound, or distance from sound source (Supplementary Table 2). Only 12 taxa had settlement high enough to look for trends (>50 total individuals; Supplementary Table 1). Of these, two taxa displayed no settlement trends (Portunus sayi, Nudibranchs). The spiny lobster Panulirus argus had higher settlement during new moon than full moon (new moon: 0.8 ± 0.1 SE; full moon: 0.2 ± 0.1 SE) but had no settlement trends for soundscape or distance. Only nine taxa displayed trends for soundscape and distance, six of which also had moon phase trends. For most of these taxa, trends were small and unlikely to be ecologically significant, though a few displayed stronger trends. For example, within full moon samples Lima settlement was 1.9–2.7 times higher on collectors deployed at 10 m (37.9 ± 20.5 SE) and 100 m (26.4 ± 12.2 SE) from speakers broadcasting healthy soundscapes than on collectors outside the range, with the two outliers at 1,000 m removed [500 m: 14.4 ± 8.2 SE; 1,000 m: 13.9 ± 6.6 SE (Figure 7D)]. The blenny genus Paraclinus also had settlement 1.1–1.5 times higher within the range of the speakers broadcasting degraded soundscapes than outside the range during full moon (Figure 7F). The shrimp Palaemonetes, which dwells in quiet seagrass meadows, had opposing settlement patterns with higher settlement on degraded soundscape collectors during new moon and healthy soundscape collectors during full moon (Figures 7G,H).
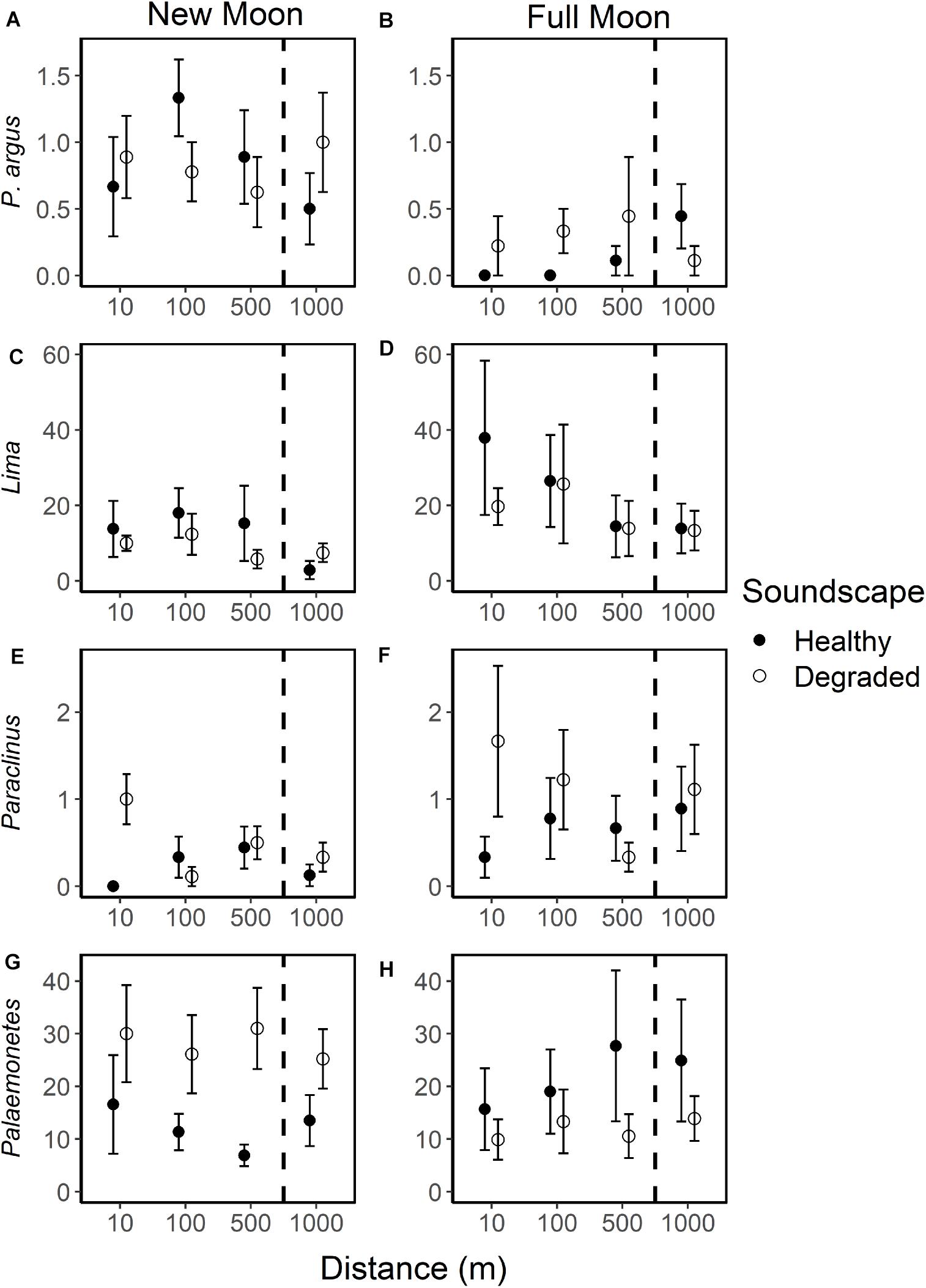
Figure 7. Mean individuals of selected taxa per collector during new and full moon phases on collectors exposed to broadcasted healthy (closed circle) and degraded (open circle) soundscapes at 10, 100, 500, and 1,000 m from the underwater speaker. All error bars are standard error. Selected species are shown: spiny lobster [Panulirus argus (A,B)], clam [Lima (C,D)] with two outliers at 1,000 m removed, blenny [Paraclinus (E,F)], shrimp [Palaemonetes (G,H)]. Dashed lines indicate collectors that lie outside the detectable sound range (1,000 m).
Discussion
Underwater soundscapes are useful settlement cues for many larval fish and invertebrate species, but their usefulness depends on the range at which they can be detected (Radford et al., 2011). In this study, we examined sound propagation from an underwater speaker to determine the maximum potential range of detection by marine larvae that use sound to locate settlement habitat. We then compared the larval assemblages that settled on collectors placed both within and outside the detection radius of broadcasted soundscapes.
Results of the sound propagation experiment suggest that at natural amplitudes and calm surface conditions, healthy hardbottom soundscapes can be detected above natural soundscape conditions up to approximately 500 m from the source. However, under less ideal conditions (e.g., high winds, rainstorms) or where bottom type and depth are different, sound propagation is likely to differ. Collectors deployed at 1,000 m (more than double the ∼0 dB SNR range) were well outside the range of the speaker system, so settlement at those collectors was not affected by the soundscape treatment, as is probably the case for collectors at 500 m. The slightly higher number of larvae settling on collectors at 10 and 100 m from the broadcast source indicates a small effect of the broadcasted soundscape, although this effect is unlikely to be ecologically significant because differences in mean settlement and the composition of those larval assemblages were small (Figure 5 and Table 1).
The PERMANOVA indicated that larval assemblages differed between moon phases and mean settlement was greater during full moon overall, though this was likely driven by a few individual taxa, particularly the clam Lima. Marine organisms such as corals (Harrison et al., 1984; Brady et al., 2016) and fish (Farmer et al., 2017) use the lunar cycle to time the release of larvae, many of which settle around specific moon phases [fishes—bluehead wrasse (Victor, 1986); damselfish and surgeonfish (Sponaugle and Cowen, 1996), decapods—crabs (Cannicci et al., 2019), lobster (Acosta et al., 1997)].
The PERMANOVA results from our study indicated that there were community-level differences in larval assemblage between soundscapes. However, total larval settlement on collectors exposed to healthy soundscapes was only slightly higher than those exposed to degraded soundscapes and there was no effect of distance on larval settlement. Other studies have found soundscapes from nursery habitats are between 1.4 and 6.2 times more attractive to larvae than degraded soundscapes, non-settlement habitat soundscapes, or silent controls (Tolimieri et al., 2000; Leis et al., 2003; Simpson et al., 2004, 2008; Hinojosa et al., 2016). Healthy soundscapes can increase species-specific fish and invertebrate larval settlement by 1.4 to 4.8-fold (Simpson et al., 2005; Vermeij et al., 2010; Lillis et al., 2015, 2016, 2018; Gordon et al., 2018). But many studies on larval response to soundscapes are inherently biased because they were designed to test the response of larval taxa specifically chosen because they were suspected to respond to sound. That does not diminish the value of those studies, but they were not intended to randomly sample the planktonic meroplankton, as is the case for our study. Whether indicative of other habitats or not, we found that approximately 22% of the 41 taxa observed demonstrated a response to acoustic cues. For example, a fish (Paraclinus) and a gastropod (Turbo castanea) had higher settlement in response to broadcasted degraded soundscapes while two other gastropods (Bulla and Echinolittorina) and a bivalve (Lima) responded to healthy hardbottom soundscapes. However, responses were small for most of these taxa and given that approximately 78% of taxa collected had either no response to soundscapes or low abundance, sound may not be a particularly important settlement cue for most species in tropical hardbottom habitats.
Approximately 71% of the observed taxa had low settlement during the experiment (<50 individuals). Low settlement could indicate low larval supply, reflecting the degraded environment in which the experiment was conducted. If few larvae were present, their chances of encountering the small range of the broadcasted soundscapes was low and thus the effects of soundscape over distance were not likely to be detected. Low settlement could also reflect the type of larval collector we deployed. The collectors used in this experiment were designed to mimic benthic algae commonly used as settlement habitat by many organisms within hardbottom habitat, but taxa that prefer different settlement substrates may have avoided the collectors. Additionally, collectors were suspended vertically in the 2–4 m deep water column rather than directly on the bottom to prevent colonization by mobile benthic organisms. Also, highly mobile organisms such as fish, could have abandoned the collector prior to collection. Although the collectors appeared to be adequate for capturing some benthic fish species such as the blenny Paraclinus, other common backreef fishes were rarely caught (e.g., Haemulon, Opsanus) or not caught at all (e.g., Lutjanus, Calamus). Larval fish supply to the Florida Keys is lowest in late fall (D’Alessandro et al., 2007) which could also account for the low numbers of fish larvae that we observed in our summer-fall study period.
Larvae have available to them a variety of settlement cues and some species utilize multiple cues to locate nursery habitat (e.g., Eastern oyster, Crassotrea virginica; Turner et al., 1994; Lillis et al., 2013; blue crab, Callinectes sapidus; Tankersley et al., 1995; horseshoe crab, Limulus polyphemis; Medina and Tankersley, 2010). This experiment may thus have missed larvae that use sound in addition to other settlement cues such as olfaction.
Because the degraded soundscapes were broadcast at natural amplitudes there should be little difference in soundscape (Butler et al., 2016) or settlement between collectors inside and outside the range of the broadcast. Yet, a few genera were collected in greater numbers within the range of degraded soundscapes (e.g., Paraclinus, Turbo) than outside the range of the speaker system. This indicates that there is a difference between broadcasted degraded soundscapes and natural degraded soundscapes for at least some settlers. Although soundscapes were broadcasted at ecologically relevant, natural amplitudes there are artifacts of playback that alter the properties of the soundscapes. For example, the speaker frequency response drops off drastically below 200 Hz, so frequencies below 200 Hz in the hardbottom soundscape would not have been broadcast as loudly as they were recorded.
Taxa found in greater numbers within range of the degraded soundscapes may also prefer different nursery habitat types or were avoiding healthy hardbottom soundscapes. For example, Simpson et al. (2011) found that taxa with pelagic or nocturnally active lifestyles, avoided reef soundscapes. Healthy hardbottom soundscapes are naturally louder and more complex (Butler et al., 2016) and the soundscapes we broadcasted reflected that. Louder, more complex soundscapes could indicate a larger biological community and potentially more predators. Unfortunately, the preferred nursery habitats for most of the more than 40 taxa observed in this study are unknown.
A few taxa had contradictory settlement patterns. For example, the shrimp genus Palaemonetes, had high settlement during new moon on degraded soundscape collectors and on healthy soundscape collectors during full moon (Figures 7G,H). We were unable to identify this genus to species and it is possible that more than one species was collected, each with contrasting settlement patterns. This highlights the need to look more deeply at the settlement preferences of Palaemonetes and other taxa with inconsistent settlement patterns.
Much of the variation in larval settlement, such as the high settlement of Lima at two 1,000 m collectors, may be due to random factors such as local currents, habitat structure, or time period. Though not designed as a factor of interest in the experiment, site and collection date were random factors that were correlated (one pair of sites were used for a consecutive full and new moon period, or one “collection period”) and could have affected larval settlement. As site and date were intrinsically linked in this experiment, it was not possible to explore the individual contributions of these factors, but both are likely to contribute to the observed variation in larval settlement and community assemblage since larval settlement varies temporally (e.g., on diel, lunar, and seasonal cycles) and spatially (O’Beirn et al., 1996; Acosta et al., 1997, Martínez and Navarrete, 2002; D’Alessandro et al., 2007) for many species.
Given the small range of the broadcasted soundscapes that we measured, the soundscapes of small patches of healthy hardbottom habitat likely have a very limited range. This restricted range of sound propagation has implications for restoration efforts. Previous work in Florida Bay found greater larval settlement within healthy habitats than in degraded habitat (Butler, 2016). But within small (25 m × 25 m) hardbottom patches, where the sponge community and soundscape had been restored, larval settlement did not match natural healthy hardbottom levels (Butler, 2016). Though the soundscape had been restored within these small patches, they were essentially a point source and therefore had a much smaller range of detection than the large area of healthy habitat (Radford et al., 2011; Piercy et al., 2014). More larvae were potentially able to detect the sounds of natural healthy habitat at greater distances and could explain why Butler (2016) did not see comparable settlement in restored patches and healthy hardbottom areas. Our results indicate that the range for a small point source of healthy hardbottom soundscape is a few hundred meters but the effective detection range for larvae is likely much smaller.
While it is possible to restore soundscapes to natural levels, the small patch size of many restored sites may limit the range of the acoustic settlement cue, negating its effectiveness as a settlement cue for larvae and hindering ecological recovery. Future work should examine what acoustic range or level of acoustic connectivity between patches is necessary to restore the settling larval assemblage. A larger patch will have a larger detection range but several small patches within range of each other may extend that detection range with fewer resources. Patches close to large areas of healthy hardbottom may benefit from spill-over, being near a potential source of larvae or colonizing animals but the greater detection range may draw more larvae to the natural healthy habitat at the expense of the restored patch. Restoration patch size, patch connectivity, and patch location are well studied concepts in other ecosystems (Schultz and Crone, 2005; Fink et al., 2009; Morrison et al., 2010; Gittman et al., 2018) but have not yet been explored in hardbottom habitats.
The results from this study adds to the growing body of literature investigating soundscapes as settlement cues for marine larvae. Our results suggest that sound alone may not be an important settlement cue for many larvae in hardbottom habitats and that sound emanating from a point source at natural levels has a relatively small range of attraction and may not have the necessary range to draw in larvae over long distances. Single, small point sources, such as small restoration patches, may not be effective at restoring settlement to the same level as large areas of healthy habitat. However, sound is just one cue that larvae use to locate and choose settlement habitat and should be considered in conjunction with other settlement cues.
Data Availability Statement
The raw data supporting the conclusions of this article will be made available by the authors, without undue reservation.
Ethics Statement
The animal study was reviewed and approved by Old Dominion University Institutional Animal Care and Use Committee.
Author Contributions
EA and MB funded the research. EA performed the research and analyses with guidance from MB and JB and wrote the initial manuscript with revisions made by MB and JB. All authors designed the study.
Funding
This research was funded by The Explorer’s Club Mamont Scholars Grant, Women Divers Hall of Fame Marine Conservation Scholarship, Sigma Xi Grants in aid of Research, the Lerner-Gray Marine Research Grant and EPA South Florida Initiative (Grant # X700D41015).
Conflict of Interest
The authors declare that the research was conducted in the absence of any commercial or financial relationships that could be construed as a potential conflict of interest.
Acknowledgments
We are grateful to the many people who provided logistical support for this project, including N. Evans, M. Williams, S. Hagedorn, and other members of M. Butler’s Marine Ecology Lab and Fall 2019 Marine Ecology class at Old Dominion University. We thank T. Birge for comments on the initial manuscript. Permission to conduct research in Florida Bay and the Florida Keys was granted by the Everglades National Park (Permit #: EVER-2019-SCI-0041) and the Florida Keys National Marine Sanctuary (Permit #: FKNMS-2017-062). This is contribution #272 from the Coastlines and Oceans Division of the Institute of Environment at Florida International University.
Supplementary Material
The Supplementary Material for this article can be found online at: https://www.frontiersin.org/articles/10.3389/fmars.2021.663887/full#supplementary-material
References
Acosta, C. A., Matthews, T. R., and Butler, M. J. IV (1997). Temporal patterns and transport processes in recruitment of spiny lobster (Panulirus argus) postlarvae to south Florida. Mar. Biol. 129, 79–85. doi: 10.1007/s002270050148
Anderson, M. J. (2001). A new method for non-parametric multivariate analysis of variance. Austral Ecol. 26, 32–46. doi: 10.1111/j.1442-9993.2001.01070.pp.x
Brady, A. K., Willis, B. L., Harder, L. D., and Vize, P. D. (2016). Lunar phase modulates circadian gene expression cycles in the broadcast spawning coral Acropora millepora. Biol. Bull. 230, 130–142. doi: 10.1086/BBLv230n2p130
Butler, J. R. (2016). Characterization of Soundscapes in Shallow Water Habitats of the Florida Keys (USA) and Their Influence on the Settlement of Larval Fish and Invertebrates. Ph.D. thesis. Norfolk, VA: Old Dominion University.
Butler, J., Stanley, J. A., and Butler, M. J. (2016). Underwater soundscapes in near-shore tropical habitats and the effects of environmental degradation and habitat restoration. J. Exp. Mar. Biol. Ecol. 479, 89–96. doi: 10.1016/j.jembe.2016.03.006
Butler, M., Hunt, J., Herrnkind, W., Childress, M., Bertelsen, R., Sharp, W., et al. (1995). Cascading disturbances in Florida Bay, USA: cyanobacteria blooms, sponge mortality, and implications for juvenile spiny lobsters Panulirus argus. Mar. Ecol. Prog. Ser. 129, 119–125. doi: 10.3354/meps129119
Cannicci, S., Mostert, B., Fratini, S., McQuaid, C., and Porri, F. (2019). Recruitment limitation and competent settlement of sesarmid crab larvae within East African mangrove forests. Mar. Ecol. Prog. Ser. 626, 123–133. doi: 10.3354/meps13062
Cato, D. H., and McCauley, R. D. (2002). Australian research in ambient sea noise. Acoust. Aust. 30, 13–20.
D’Alessandro, E., Sponaugle, S., and Lee, T. (2007). Patterns and processes of larval fish supply to the coral reefs of the upper Florida Keys. Mar. Ecol. Prog. Ser. 331, 85–100. doi: 10.3354/meps331085
Egner, S., and Mann, D. (2005). Auditory sensitivity of sergeant major damselfish Abudefduf saxatilis from post-settlement juvenile to adult. Mar. Ecol. Prog. Ser. 285, 213–222. doi: 10.3354/meps285213
Farmer, N. A., Heyman, W. D., Karnauskas, M., Kobara, S., Smart, T. I., Ballenger, J. C., et al. (2017). Timing and locations of reef fish spawning off the southeastern United States. PLoS One 12:e0172968. doi: 10.1371/journal.pone.0172968
Fink, R. D., Lindell, C. A., Morrison, E. B., Zahawi, R. A., and Holl, K. D. (2009). Patch size and tree species influence the number and duration of bird visits in forest restoration plots in southern Costa Rica. Restor. Ecol. 17, 479–486. doi: 10.1111/j.1526-100X.2008.00383.x
Gittman, R. K., Fodrie, F. J., Baillie, C. J., Brodeur, M. C., Currin, C. A., Keller, D. A., et al. (2018). Living on the edge: increasing patch size enhances the resilience and community development of a restored salt marsh. Estuaries Coasts 41, 884–895. doi: 10.1007/s12237-017-0302-6
Gordon, T. A., Harding, H. R., Wong, K. E., Merchant, N. D., Meekan, M. G., McCormick, M. I., et al. (2018). Habitat degradation negatively affects auditory settlement behavior of coral reef fishes. Proc. Natl. Acad. Sci. U.S.A. 115, 5193–5198. doi: 10.1073/pnas.1719291115
Harrison, P. L., Babcock, R. C., Oliver, J. K., Bull, G. D., Wallace, C. C., and Willis, B. L. (1984). Mass spawning in the Tropical Reef corals. Science 223, 1186–1189. doi: 10.1126/science.223.4641.1186
Herrnkind, W. F., Iv, M. J. B., Hunt, J. H., and Childress, M. (1997). Role of physical refugia: implications from a mass sponge die-off in a lobster nursery in Florida. Mar. Freshw. Res. 48, 759–770. doi: 10.1071/mf97193
Hinojosa, I. A., Green, B. S., Gardner, C., Hesse, J., Stanley, J. A., and Jeffs, A. G. (2016). Reef sound as an orientation cue for shoreward migration by pueruli of the rock lobster. Jasus edwardsii. PLoS One 11:e0157862. doi: 10.1371/journal.pone.0157862
Kaplan, M. B., Lammers, M. O., Zang, E., and Aran Mooney, T. (2017). Acoustic and biological trends on coral reefs off Maui, Hawaii. Coral Reefs 37, 121–133. doi: 10.1007/s00338-017-1638-x
Kennedy, E. V., Holderied, M. W., Mair, J. M., Guzman, H. M., and Simpson, S. D. (2010). Spatial patterns in reef-generated noise relate to habitats and communities: evidence from a Panamanian case study. J. Exp. Mar. Biol. Ecol. 395, 85–92. doi: 10.1016/j.jembe.2010.08.017
Legendre, P., and Gallagher, E. D. (2001). Ecologically meaningful transformations for ordination of species data. Oecologia 129, 271–280. doi: 10.1007/s004420100716
Leis, J. M., Carson-Ewart, B. M., Hay, A. C., and Cato, D. H. (2003). Coral-reef sounds enable nocturnal navigation by some reef-fish larvae in some places and at some times. J. Fish Biol. 63, 724–737. doi: 10.1046/j.1095-8649.2003.00182.x
Lillis, A., Apprill, A., Suca, J. J., Becker, C., Llopiz, J. K., and Mooney, T. A. (2018). Soundscapes influence the settlement of the common Caribbean coral Porites astreoides irrespective of light conditions. R. Soc. Open Sci. 5:181358. doi: 10.1098/rsos.181358
Lillis, A., Bohnenstiehl, D. R., and Eggleston, D. B. (2015). Soundscape manipulation enhances larval recruitment of a reef-building mollusk. PeerJ 3:e999. doi: 10.7717/peerj.999
Lillis, A., Bohnenstiehl, D., Peters, J. W., and Eggleston, D. (2016). Variation in habitat soundscape characteristics influences settlement of a reef-building coral. PeerJ 4:e2557. doi: 10.7717/peerj.2557
Lillis, A., Eggleston, D. B., and Bohnenstiehl, D. R. (2013). Oyster larvae settle in response to habitat-associated underwater sounds. PLoS One 8:e79337. doi: 10.1371/journal.pone.0079337
Mann, D. A., Casper, B. M., Boyle, K. S., and Tricas, T. C. (2007). On the attraction of larval fishes to reef sounds. Mar. Ecol. Prog. Ser. 338, 307–310. doi: 10.3354/meps338307
Martínez, P., and Navarrete, S. A. (2002). Temporal and spatial variation in settlement of the gastropod Concholepas concholepas in natural and artificial substrata. J. Mar. Biol. Assoc. U. K. 82, 257–264. doi: 10.1017/s002531540200543x
Medina, J. M., and Tankersley, R. A. (2010). Orientation of larval and juvenile horseshoe crabs Limulus polyphemus to visual cues: effects of chemical odors. Curr. Zool. 56, 618–633. doi: 10.1093/czoolo/56.5.618
Morrison, E. B., Lindell, C. A., Holl, K. D., and Zahawi, R. A. (2010). Patch size effects on avian foraging behaviour: implications for tropical forest restoration design. J. Appl. Ecol. 47, 130–138. doi: 10.1111/j.1365-2664.2009.01743.x
O’Beirn, F. X., Heffernan, P. B., and Walker, R. L. (1996). Recruitment of the Eastern oyster in coastal Georgia: patterns and recommendations. North Am. J. Fish. Manag. 16, 413–426. doi: 10.1577/1548-86751996016<0413:ROTEOI<2.3.CO;2
Oksanen, J., Blanchet, F. G., Friendly, M., Kindt, R., Legendre, P., McGlinn, D., et al. (2019). vegan: Community Ecology Package. R package version 2.5–6. Available online at: https://CRAN.R-project.org/package=vegan
Parmentier, E., Berten, L., Rigo, P., Aubrun, F., Nedelec, S. L., Simpson, S. D., et al. (2015). The influence of various reef sounds on coral-fish larvae behaviour: reef-sound influence on fish larvae behaviour. J. Fish Biol. 86, 1507–1518. doi: 10.1111/jfb.12651
Piercy, J., Codling, E., Hill, A., Smith, D., and Simpson, S. (2014). Habitat quality affects sound production and likely distance of detection on coral reefs. Mar. Ecol. Prog. Ser. 516, 35–47. doi: 10.3354/meps10986
R Core Team. (2020). R: A Language and Environment for Statistical Computing, Vienna, Austria. Available online at: https://www.R-project.org/.
Radford, C. A., Stanley, J. A., Tindle, C. T., Montgomery, J. C., and Jeffs, A. G. (2010). Localised coastal habitats have distinct underwater sound signatures. Mar. Ecol. Prog. Ser. 401, 21–29. doi: 10.3354/meps08451
Radford, C., Tindle, C., Montgomery, J., and Jeffs, A. (2011). Modelling a reef as an extended sound source increases the predicted range at which reef noise may be heard by fish larvae. Mar. Ecol. Prog. Ser. 438, 167–174. doi: 10.3354/meps09312
Schultz, C. B., and Crone, E. E. (2005). Patch size and connectivity thresholds for butterfly habitat restoration. Conserv. Biol. 19, 887–896. doi: 10.1111/j.1523-1739.2005.00462.x
Simpson, S. D., Meekan, M. G., Jeffs, A., Montgomery, J. C., and McCauley, R. D. (2008). Settlement-stage coral reef fish prefer the higher-frequency invertebrate-generated audible component of reef noise. Anim. Behav. 75, 1861–1868. doi: 10.1016/j.anbehav.2007.11.004
Simpson, S. D., Meekan, M. G., McCauley, R. D., and Jeffs, A. (2004). Attraction of settlement-stage coral reef fishes to reef noise. Mar. Ecol. Prog. Ser. 276, 263–268. doi: 10.3354/meps276263
Simpson, S. D., Meekan, M. G., Montgomery, J., McCauley, R., and Jeffs, A. (2005). Homeward sound. Science 308:221. doi: 10.1126/science.1107406
Simpson, S. D., Radford, A. N., Tickle, E. J., Meekan, M. G., and Jeffs, A. G. (2011). Adaptive avoidance of reef noise. PLoS One 6:e16625. doi: 10.1371/journal.pone.0016625
Sponaugle, S., and Cowen, R. (1996). Larval supply and patterns of recruitment for two Caribbean reef fishes Stegastes partitrus. Mar. Freshw. Res. 47, 433–447. doi: 10.1071/MF9960433
Stanley, J. A., Radford, C. A., and Jeffs, A. G. (2012). “Effects of Underwater Noise on Larval settlement,” in The Effects of Noise on Aquatic Life Advances in Experimental Medicine and Biology, eds A. N. Popper and A. D. Hawkins (New York, NY: Springer), 371–374. doi: 10.1007/978-1-4419-7311-5_84
Stevely, J. M., Sweat, D. E., Bert, T. M., Sim-Smith, C., and Kelly, M. (2011). Sponge mortality at Marathon and long key, Florida: patterns of species response and population recovery. Proc. 63rd Gulf Caribb. Fish. Inst. 63, 384–400.
Tankersley, R. A., McKelvey, L. M., and Forward, R. B. (1995). Responses of estuarine crab megalopae to pressure, salinity and light: implications for flood-tide transport. Mar. Biol. 122, 391–400. doi: 10.1007/BF00350871
Tolimieri, N., Jeffs, A., and Montgomery, J. C. (2000). Ambient sound as a cue for navigation by the pelagic larvae of reef fishes. Mar. Ecol. Prog. Ser. 207, 219–224. doi: 10.3354/meps207219
Turner, E. J., Zimmer−Faust, R. K., Palmer, M. A., Luckenbach, M., and Pentchef, N. D. (1994). Settlement of oyster (Crassostrea virginica) larvae: effects of water flow and a water-soluble chemical cue. Limnol. Oceanogr. 39, 1579–1593. doi: 10.4319/lo.1994.39.7.1579
Vermeij, M. J. A., Marhaver, K. L., Huijbers, C. M., Nagelkerken, I., and Simpson, S. D. (2010). Coral larvae move toward reef sounds. PLoS One 5:e10660. doi: 10.1371/journal.pone.0010660
Victor, B. C. (1986). Larval settlement and juvenile mortality in a recruitment-limited coral reef fish population. Ecol. Monogr. 56, 145–160. doi: 10.2307/1942506
Keywords: underwater sound, larvae, settlement, hardbottom, Florida Bay, marine soundscapes
Citation: Anderson ER, Butler J and Butler MJ (2021) Response of Fish and Invertebrate Larvae to Backreef Sounds at Varying Distances: Implications for Habitat Restoration. Front. Mar. Sci. 8:663887. doi: 10.3389/fmars.2021.663887
Received: 03 February 2021; Accepted: 18 May 2021;
Published: 09 June 2021.
Edited by:
Ilse Catharina Van Opzeeland, Alfred Wegener Institute Helmholtz Centre for Polar and Marine Research (AWI), GermanyReviewed by:
T. Aran Mooney, Woods Hole Oceanographic Institution, United StatesAntoine De Ramon N’Yeurt, University of the South Pacific, Fiji
Copyright © 2021 Anderson, Butler and Butler. This is an open-access article distributed under the terms of the Creative Commons Attribution License (CC BY). The use, distribution or reproduction in other forums is permitted, provided the original author(s) and the copyright owner(s) are credited and that the original publication in this journal is cited, in accordance with accepted academic practice. No use, distribution or reproduction is permitted which does not comply with these terms.
*Correspondence: Emily R. Anderson, eande005@odu.edu