- 1Australian Institute of Marine Science, Townsville, QLD, Australia
- 2Australian Institute of Marine Science, Indian Ocean Marine Research Centre, University of Western Australia, Crawley, WA, Australia
Surviving after settlement through the first year of life is a recognised bottleneck in up-scaling reef coral restoration. Incorporating spatial refugia in settlement devices has the potential to alleviate some hazards experienced by young recruits, such as predation and accidental grazing, and can increase the likelihood of survival to size-escape thresholds. Yet optimising the design of microrefugia is challenging due to the complexity of physical and biological processes that occur at fine spatial scales around a recruit. Here, we investigated the effects of microhabitat features on the survival of Acropora tenuis spat in a year-long experimental field deployment of two types of artificial settlement devices—grooved-tiles and lattice-grids—onto three replicate racks on a shallow, central mid-shelf reef of the Great Barrier Reef. Spat survival across device types averaged between 2 and 39% and about half of all devices had at least one surviving coral after a year. While the larvae settled across all micro-habitats available on the devices, there was strong post-settlement selection for corals on the lower edges, lower surfaces, and in the grooves, with 100% mortality of recruits on upper surfaces, nearly all within the first 6 months of deployment. The device type that conferred the highest average survival (39%) was a tile with wide grooves (4 mm) cut all the way through, which significantly improved survival success over flat and comparatively featureless control tiles (13%). We hypothesise that the wide grooves provided protection from accidental grazing while also minimising sediment accumulation and allowing higher levels of light and water flow to reach the recruits than featureless control devices. We conclude that incorporating design features into deployment devices such as wide slits has the potential to substantially increase post-deployment survival success of restored corals.
Introduction
Coral populations are declining globally (Gardner et al., 2003; Bruno and Selig, 2007; De’ath et al., 2012; Hughes et al., 2017), stimulating widespread efforts to mitigate further losses, enhance the recovery of existing populations, and potentially increase reef resilience through coral restoration programs (Boström-Einarsson et al., 2018, 2020; Bay et al., 2019). Seeding a recruitment-limited reef with deployment devices carrying sexually derived and newly settled coral spat (Okamoto et al., 2008; Chamberland et al., 2015, 2017) is one of the interventions being tested and refined (Bay et al., 2019). The benefits of using sexually produced coral propagules in reef restoration include improvements in genetic diversity, scalability and cost (Baria-Rodriguez et al., 2019; Doropoulos et al., 2019; Gibbs et al., 2019; Randall et al., 2020), and if harnessing spawn slicks (Heyward et al., 1999, 2002; Doropoulos et al., 2019), retention of species diversity and community composition (Heyward et al., 1999; Doropoulos et al., 2019). Seeding reefs with already-settled coral spat also, at least temporarily, overcomes challenges associated with the settlement process, including a lack of available substrate or settlement cues (Kuffner et al., 2008; Webster et al., 2011, 2013), and the presence of settlement inhibitors (Kuffner et al., 2006; Arnold et al., 2010; Webster et al., 2015; Speare et al., 2019). Yet post-settlement mortality can be exceedingly high (>99%) in some habitats and under various environmental conditions (Babcock, 1985; Hunt and Scheibling, 1997; Wilson and Harrison, 2005; Vermeij and Sandin, 2008; Penin et al., 2010, 2011; Ritson-Williams et al., 2010; Trapon et al., 2013; Miller, 2014; Suzuki et al., 2018), potentially diminishing the benefits of the seeding technique.
High post-settlement mortality in corals can be caused by accidental grazing by fishes (Baria et al., 2010; Penin et al., 2010, 2011; Trapon et al., 2013; Gallagher and Doropoulos, 2017), competition with other benthic organisms (Box and Mumby, 2007; Hughes et al., 2007; Vermeij and Sandin, 2008; Vermeij et al., 2009), sedimentation (Sato, 1985; Babcock and Smith, 2002; Jones et al., 2015; but see Trapon et al., 2013), and direct corallivory (Gallagher and Doropoulos, 2017). Incorporating structural refugia in settlement devices, and controlling the benthic community composition on those surfaces, has the potential to mitigate these stressors and increase the likelihood of survival to size-escape thresholds (Petersen et al., 2005; Nozawa, 2008, 2012; Okamoto et al., 2008; Doropoulos et al., 2012b, 2016; Edmunds et al., 2014; Whalan et al., 2015; Chamberland et al., 2017; Gallagher and Doropoulos, 2017). Optimising the design of microrefugia is challenging, however, due to the complexity of physical (i.e., light availability, sedimentation rates, and flow dynamics) and biological (i.e., benthic competition and herbivory) processes that occur at fine spatial scales around a recruit, and the species-specific responses to those processes. Furthermore, high variability in post-settlement growth rates among species and growth morphologies (Miller, 2014; Suzuki et al., 2018) means that what may work for one species or growth morphology may not work for another.
Larvae of some coral species preferentially settle in crevices and interstitial spaces (Carleton and Sammarco, 1987; Petersen et al., 2005; Whalan et al., 2015), whereas others prefer edges or undersides of substrata (Maida et al., 1994; Babcock and Mundy, 1996; Baird and Hughes, 2000). Yet, strong post-settlement selection in some habitats for corals on upper surfaces (Babcock and Mundy, 1996; Cameron and Harrison, 2020) and those on the undersides closest to the edges (Maida et al., 1994; Cameron and Harrison, 2020) during the first few months suggests that light availability is critically important for driving post-settlement survival in the long-term (Mundy and Babcock, 1998), either directly through the facilitation of growth from photosynthesis, or indirectly through modification of the competitive benthic community on the surfaces around the spat. Consequently, designing artificial seeding devices that provide microrefugia while also maintaining light availability and limiting sedimentation could be advantageous. To that end, we designed and tested the settlement, survival and growth of two deployment device-design types – grooved-tiles and lattice-grids – on aquarium-settled Acropora tenuis spat over a ∼1-year deployment on a mid-shelf reef in the central Great Barrier Reef. Our objectives were to describe settlement preferences among the microhabitats within each device design and to compare the survival and growth of spat among device designs and microhabitats to their size-escape threshold in a field deployment.
Materials and Methods
Coral Collection, Spawning and Larval Rearing
Gravid Acropora tenuis (Dana 1846) colonies were collected from Backnumbers Reef on the central mid-shelf of the Great Barrier Reef (18°30′S, 147° 08′E, GBRMPA Permit G12/35236.1) on the 25th of November 2018, ahead of the predicted coral spawning. In situ, colonies were visually inspected for pigmented oocytes in sampled branch tips (Wallace 1985) and then fragments of mature colonies were collected via hammer and chisel and transported to the Australian Institute of Marine Science’s (AIMS, Townsville, QLD, Australia) National Sea Simulator (SeaSim1). The corals were maintained in temperature controlled outdoor aquaria emulating mid-shelf ambient reef conditions (27.0 ± 0.2°C) and monitored in the evenings for gamete release. On the 28th November, the fifth night after the full moon, the colonies were observed setting gamete bundles in the polyp mouth (Babcock et al., 1986) and were isolated in 60 L tanks. Six colonies synchronously released buoyant egg-sperm bundles at 19:30, approximately 1 h after sunset, and were skimmed from the surface with a clean cup. The bundles were gently agitated and filtered through a 106 μm mesh sieve to separate eggs and sperm. The oocytes were rinsed with 0.4 μm filtered seawater (FSW) and gametes from all six parent colonies were pooled for cross-fertilisation with approximately 106 sperm mL–1 in a 60 L tank of 4 μm FSW. After 1 h, when embryos were observed cleaving, they were gently rinsed of sperm and transferred into a 500 L flow-through tank for culture. Light aeration was introduced after 24 h (gastrula stage) and was increased after 72 h (swimming planulae) to allow moderate circulation. Larvae were maintained in the culture tank until used in the experiment.
Experimental Device Design
Two types of experimental settlement devices—lattice-grids and grooved-tiles—were designed and manufactured at AIMS to test the effects of various elements of microcrevice design on larval settlement choice and post-settlement survival. The lattice-grids were 90 mm (L) × 90 mm (W) × 12 mm (H) grey polylactic acid (PLA) plastic 3D-printed grids (Figure 1). They had two aperture widths, wide (30 mm) or narrow (10 mm), with 4 × 4 or 2 × 2 grid squares per grid, respectively. On each lattice-grid, four ‘habitats’ were identified: (i) upper inner, (ii) upper outer, (iii) lower inner, and (iv) lower outer regions (Figure 1). We hypothesised that coral recruits on narrow-aperture lattice-grids would be better protected from accidental herbivory damage (hereafter ‘grazing’) than those on wide-aperture lattice-grids, and also, that ‘inner’ and ‘lower’ habitats would be better protected from grazing than ‘outer’ and ‘upper’ habitats, due to the protection that microhabitat features can provide from the bites of corallivorous and herbivorous fishes (Gallagher and Doropoulos, 2017). The aperture features of the lattice-grid devices represent two levels of protection from predation and grazing.
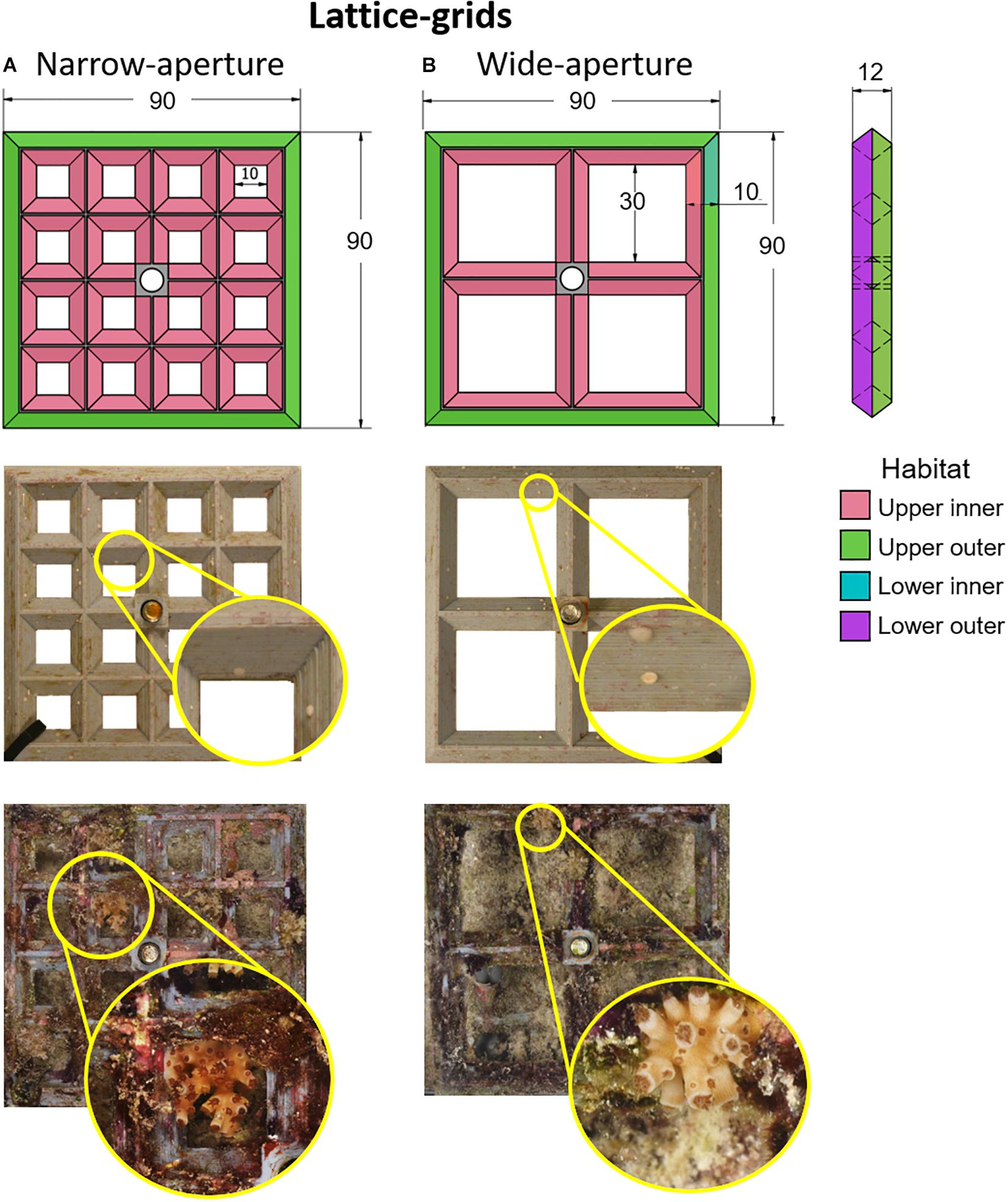
Figure 1. Lattice-grids. Schematic diagrams (top row) with corresponding representative device images immediately after settlement (middle row) and after retrieval from a 1-year field deployment (bottom row) for two lattice-device types: narrow-aperture (A) and wide-aperture (B). Colour shading in the first row indicates settlement habitats; note that only upper and outer surfaces are shown in this diagram. Insets for each image in the middle and bottom rows show close-ups of the Acropora tenuis spat or juvenile colonies, respectively, on each device type. Each image is of the underside of the device and shows the same corals as spat and juveniles. The brightness and contrast of inset images have been adjusted to improve the discrimination of coral spat and juveniles. In the top row, numbers indicate size in mm.
The grooved-tiles were 50 mm (L) × 50 mm (W) mm × 6 mm (H) pieces cut from grey polyvinyl chloride (PVC) sheets (Figure 2). Each tile had four grooves and they were either narrow (2 mm) or wide (4 mm) and were either cut all the way through the tile (6 mm deep) to create slits, or were cut 2 mm deep, giving rise to four combinations of grooved-tile types; (i) open-narrow, (ii) closed-narrow, (iii) open-wide and (iv) closed-wide (Figure 2). A fifth tile with no grooves served as a control. On each tile, five settlement habitats were classified: (i) upper and (ii) lower faces of the tiles, (iii) within the grooves, and along the (iv) upper edge and (v) lower edge of the bevelled corners (Figure 2). We hypothesised that spat settled on the walls of the open grooves would perform better than those settled in closed grooves, due to higher water flow allowing the mass transfer of gases and metabolites (Nakamura, 2010) and a reduced likelihood of smothering from the accumulation of fine sediments (Babcock and Smith, 2002).
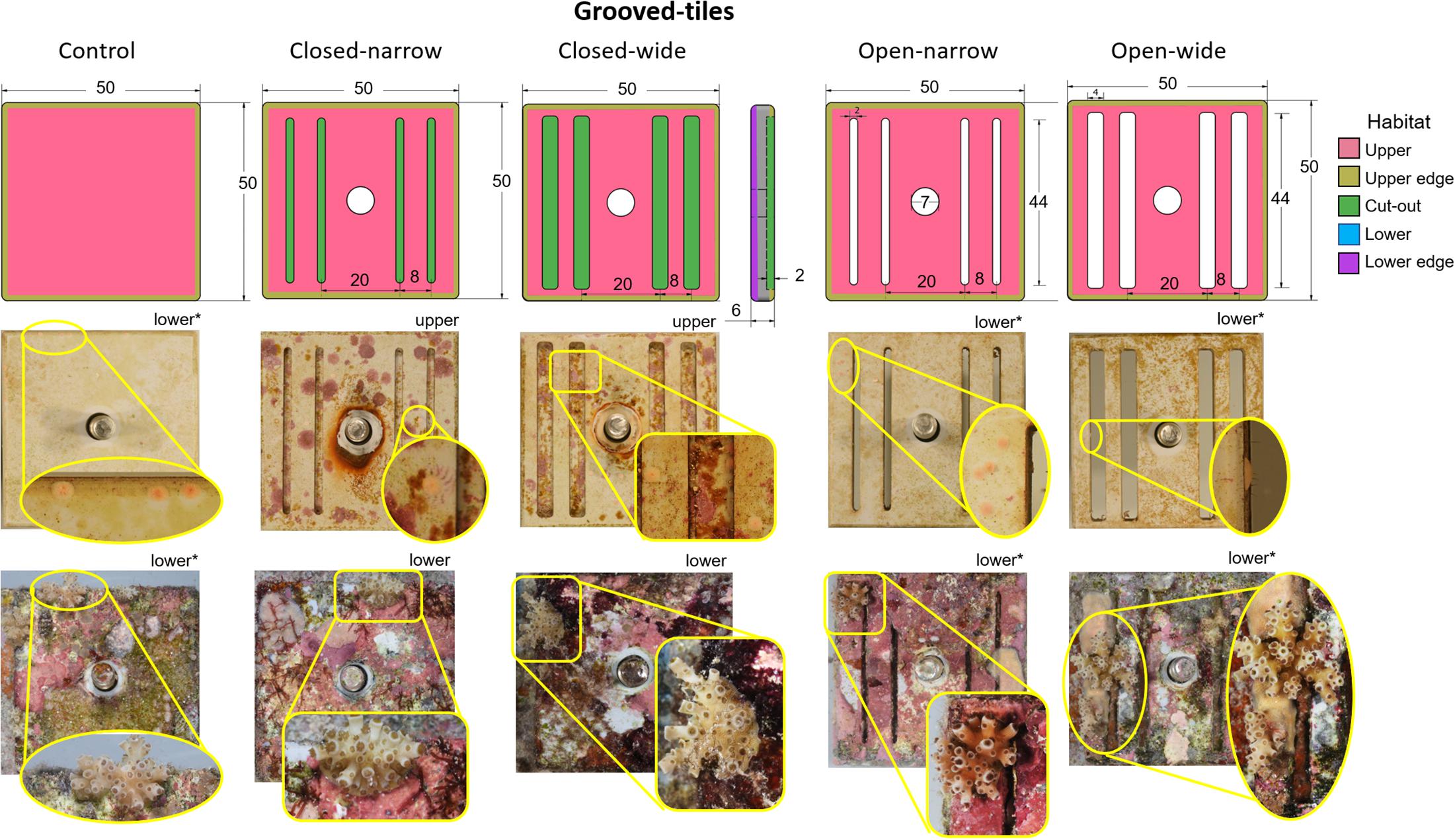
Figure 2. Grooved-tiles. Schematic diagrams (top row) with corresponding representative device images immediately after settlement (middle row) and after retrieval from a 1-year field deployment (bottom row) for five grooved-tile types. Colour shading in the first row indicates settlement habitats; note that only upper and edge surfaces are shown in this row. Insets for each image in the middle and bottom rows show close-ups of the Acropora tenuis spat or juvenile colonies, respectively, on each device type. Whether each image shows the top side or under side of the device is indicated and devices identified by asterisks show the same corals as spat and juveniles. No spat on the top sides of any closed grooved-tiles survived. The brightness and contrast of inset images have been adjusted to improve the discrimination of coral spat and juveniles. In the top row, numbers indicate size in mm.
Larval Settlement
Prior to settlement, all experimental devices were conditioned in the SeaSim for approximately 4 weeks to develop a biofilm and recruit crustose coralline algae (CCA) for larval settlement induction; all shapes had recruited visible CCA prior to settlement. Acrylic settlement tanks (50 L) with 300 mL min–1 flow-through of 4 μm FSW and 112 μm mesh overflow filter, were stocked with either eight replicate lattice-grid devices (four wide and four narrow) or 15 replicate grooved-tile devices (three each of the open-narrow, closed-narrow, open-wide, closed-wide, and control tiles). The lattice-grid devices were each raised slightly (∼1 cm) off the bottom by a central stem and bolt to allow larvae uninhibited access to the device undersides for settlement. Grooved-tile devices were laid directly on the bottom of settlement tanks to promote settlement of larvae in the grooves. There were three replicate settlement tanks for each device experiment, for a total of 24 lattice-grids (n = 12 per device type) and 45 grooved-tiles (n = 9 per device type).
Eight days after fertilisation, A. tenuis larvae were competent to settle, as determined by routine settlement assays in the laboratory (Heyward and Negri, 1999; Nishikawa et al., 2003), and approximately 30 A. tenuis larvae per device were added to the tanks for settlement. The larvae were left to settle on the devices for 4 days with 12:12 h light:dark cycle, then relocated to outdoor holding aquaria (2500 L semi-recirculating system, ambient light with 50% shade cloth) on deployment trays. Three replicate deployment trays for each treatment held devices in a raised position approximately 2 cm from the tray bottom, via threaded 316 stainless steel bar through a central hole and secured with a 316 stainless steel wingnut. The distance between adjacent lattice-grids within a tray was 1–3 cm and the distance between adjacent grooved-tiles within a tray was 2–3 cm.
Upper- and under-side images of the devices were taken 9 and 11 days after larval introduction to the grooved-tiles and lattice-grids, corresponding with approximately 5- and 7-days post settlement, respectively. A cable tie was placed in the corner of each lattice-grid to provide an orientation point for imaging. Pre-deployment images were taken to quantify the number and location of spat settled on each device and habitat; devices were submerged and imaged using a Nikon D810 with a Nikon AF-S 60 mm f/2.8 G Micro ED Lens outfitted with four Ikelite DS160 Strobes mounted on a trolley. For each spat that was mapped, data were recorded on whether that spat resulted from a single larva, or whether an aggregate of larvae had settled together. A spat was classified as an aggregate when there was physical contact between two adjacent larvae at the time of imaging (up to 10 days post settlement). For all subsequent data analyses, aggregated spat were considered as individual recruits. It was not possible to determine whether surviving corals were the result of chimeras or a single, competitively dominant individual, but our focus was on the number of surviving colonies. The trays of devices were maintained in outdoor holding aquaria until deployment.
Deployment and Post-deployment Survival
The lattice-grids and grooved-tiles were transported to Backnumbers Reef (18°29′18.19″S, 147°9′31.31″E) on the 19th December 2018, with spat approximately 1 month old, and deployed onto three replicate fibreglass reinforced plastic (FRP) frames. The deployment site was located on the south-western facing (leeward) side of a northern reef bommie at approximately 6 m depth, in an Acropora dominated community (Supplementary Figure 1). Each frame [1 m (W) × 1.5 m (L)] was secured with star pickets over rubble substrate immediately adjacent to the bommie and supported the trays of devices approximately 20 cm above the seabed (Supplementary Figure 1). Each replicate frame received one tray of lattice-grids and one tray of grooved-tiles.
Survival of A. tenuis recruits was tracked by assessment of in situ images, taken on SCUBA, of the upper- and under-sides of each device. Images were taken at 41, 95, 185, 246, and 311 days and then imaged again upon retrieval from the field on 16th December 2019 at the final time point of 376 days post settlement. The maximum planar diameter of each live juvenile coral was measured from the final images taken upon retrieval using ImageJ2. Detritus and sediments were gently cleared from the devices before imaging. All deployments were undertaken under GBRMPA Permit G18/41046.1.
Statistical Analyses
To compare survival success among device types, within each group of devices (i.e., among grooved-tiles and among lattice-grids), the total numbers of live and dead spat on each device were modelled against device type (fixed effect) using a generalised linear mixed effects model (GLMM), with replicate tray considered as a random effect, using a binomial distribution and a logit-link function. Model assumptions of homogenous variance and normally distributed residuals were verified using the package ‘sjPlot’ (Ludecke, 2021) and in cases where the overall models were significant, least-squares (marginal) means calculated with a Tukey adjustment were estimated using ‘emmeans’ to examine pairwise differences in survival response. Because the method of manufacture (3D printing vs. machining slabs), the material (PLA vs. PVC) and the general size and surface texture of the two devices types differed, grooved-tiles and lattice-grids were modelled separately and the two groups of devices were qualitatively compared. All models were run in R (R Core Team, 2020) using the package ‘lme4’ (Bates et al., 2014) and the data were visualised using the package ‘ggplot2’ (Wickham, 2016).
To compare survival success among micro-habitats on each device, the total numbers of live and dead spat within each habitat were modelled against habitat type (fixed effect), as described above, with device ID considered as a random effect. We note that the ‘upper inner’ and ‘upper outer’ habitats were excluded from the analysis of lattice-grids and that ‘upper’ and ‘upper edge’ habitats were excluded from the analysis of grooved-tiles, respectively, because survival success was 0% on these habitat types across all devices.
To evaluate whether there was a relationship between the initial number (and density) of spat settled on each device and the likelihood of having at least one surviving coral on that device after a year, logistic regressions with a binomial distribution and a logit link function were modelled using ‘glm’ from the ‘stats’ package in R for each of the device types (R Core Team, 2020). The maximum diameters of the juvenile corals after a year of deployment were also compared among device types using a GLM with a Gaussian distribution, and where necessary, maximum diameter data were log-transformed to meet model assumptions.
To determine whether larvae preferentially settled in certain habitats, Pearson’s Chi-squared goodness-of-fit tests were used to compare the observed total counts of spat within each habitat type against the expected numbers of spat in each habitat type, normalised to the surface area of each habitat. Analyses were run separately for each device type. We note that because the grooved-tiles were placed directly on the surface of the tank for settlement, access to the ‘lower’ habitat may have been partially restricted, despite some settlement on that habitat. We also note that because larvae are known to settle gregariously, it is likely that settlement habitats selected by individual larvae were not strictly independent and this should be considered when interpreting the results of this analysis. The numbers of surviving corals were too low to estimate goodness-of-fit across habitat types after a year of deployment, but the relative proportions of spat that settled within each habitat were qualitatively compared with those that survived through data visualisation.
Finally, to test whether aggregated spat were more likely to survive than single spat, survival data were modelled using a GLMM, as described above, with a binomial distribution and a logit link function. The grouping factor (i.e., single or aggregate) was modelled as the fixed effect, and the habitats within each device were treated as random effects.
Results
Lattice-Grids
Larval settlement averaged 38 ± 23 spat (mean ± SD; 0.25 spat cm–2) and 18 ± 20 spat (0.15 spat cm–2) on the narrow and wide lattice-grids, respectively (Figure 1). Generally, settlement was highest in the ‘upper inner’ habitats, followed by ‘lower inner,’ ‘upper outer,’ and ‘lower outer’ habitats, respectively (Figure 3). On the narrow aperture lattice-grids, observed settlement was similar to that expected, based on the relative surface area of each habitat (χ2 = 6.2, df = 3, p = 0.10; Figure 3). On the wide lattice-grids, however, more larvae settled on the upper surfaces and fewer settled on the lower surfaces than expected, given the available surface area in each habitat (χ2 = 25.1, df = 3, p < 0.0001; Figure 3).
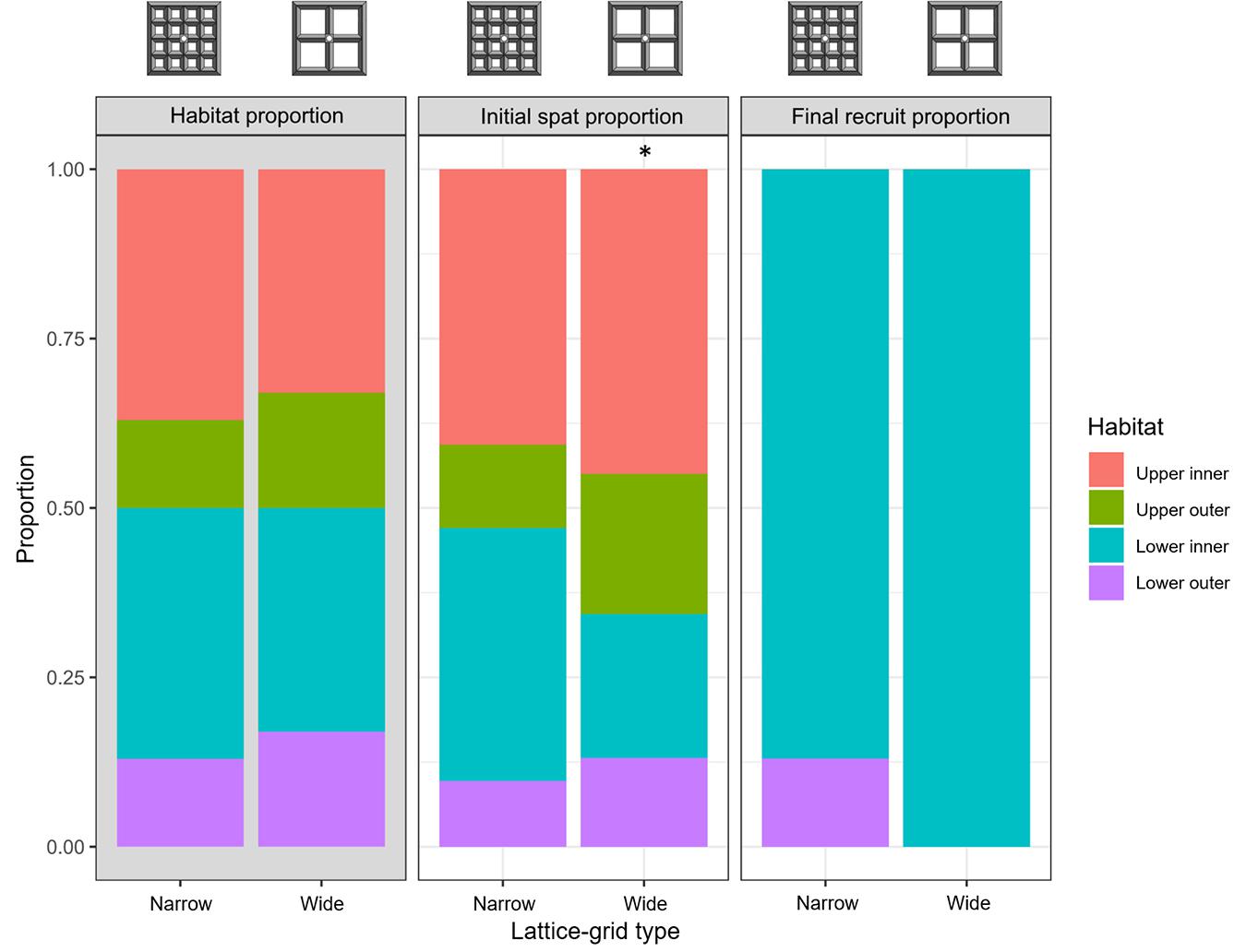
Figure 3. Proportion of space available within each habitat type (grey plot, ‘Habitat proportion’), the proportion of live Acropora tenuis spat within each habitat type immediately after settlement (‘Initial spat proportion’) and the proportion of live A. tenuis juveniles approximately 1 year post-deployment (‘Final recruit proportion’) on two types of lattice-grids. Reference diagrams of each device type are included above the plots. Asterisk indicates a statistically significant difference in the observed and expected settlement proportions based on a Chi-square goodness-of-fit test (p < 0.05).
Spat survival after 376 days averaged 3 ± 5% (mean ± SD) and 2 ± 3% for the narrow and wide aperture lattice-grids, respectively, with no significant differences in survival detected between narrow and wide types (GLMM: z = −0.70, p = 0.48) (Figure 4A). The spat that survived 376 days were almost entirely located on lower inner habitats (Figure 3). No spat survived on any upper surface of either lattice-grid type and were thus excluded from the comparison of survival success among habitat types. Survival was significantly higher for spat on ‘lower inner’ compared with ‘lower outer’ habitats across lattice devices (GLMM: z = −1.97, p = 0.049). A significant rack effect was also observed, such that corals deployed on one rack survived better than those on the other two racks (GLM: z = 2.826, p = 0.005).
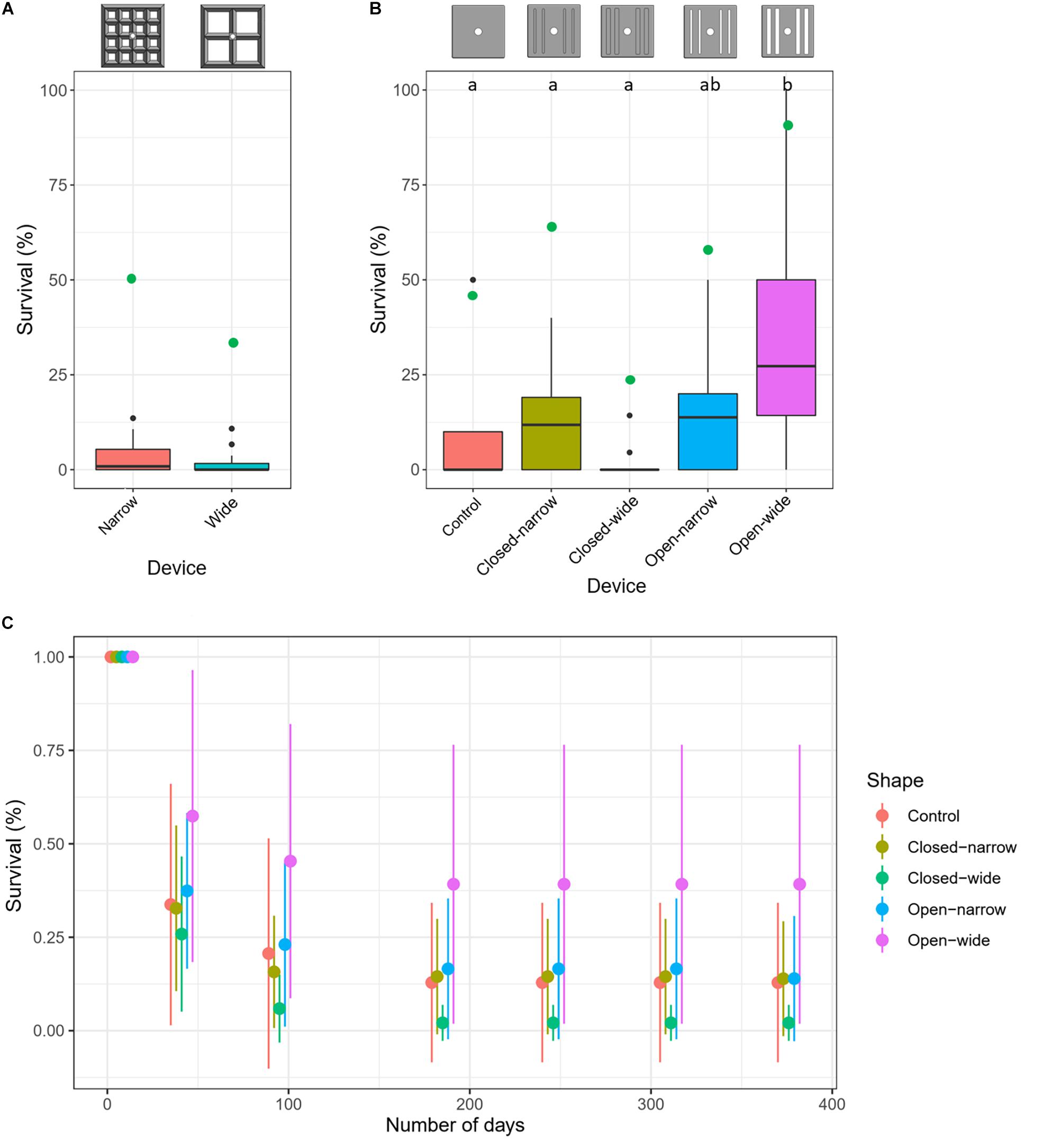
Figure 4. Average survival (%) of Acropora tenuis spat after approximately 1 year deployed in the field on two types of lattice-grids (A) and on five types of grooved-tiles (B). Letters (a and b) in panel (B) indicate statistically significant differences in survival among treatments. (C) Average (±SD) spat survival through time on the five types of grooved-tiles. Reference diagrams of each device type are included above the plots in panels (A) and (B). Overlaid green points in panels (A) and (B) indicate survival (%) at the device-level (i.e., having at least one surviving recruit on the device at the end of deployment).
At the device level, 50% of the narrow aperture lattice-grids had at least one surviving recruit, whereas 31% of the wide aperture lattice-grids had at least one survivor after a year (Figure 4A). When all lattice-grids were considered together, the likelihood of having at least one survivor on a device significantly increased as a function of the initial number of recruits on that device (GLM: z = 2.603, p = 0.0092), with the probability of survival switching from favouring 0 (dead) to 1 (alive) at 27 spat (or 0.2 spat cm–2; Figure 5A). This result was also significant when the model was run using density data, with survival probability favouring one at 0.2 spat cm–2 (GLM: z = 2.600, p = 0.0093). When each lattice-grid type was considered separately, however, the trends were not statistically significant as the sample size was low.
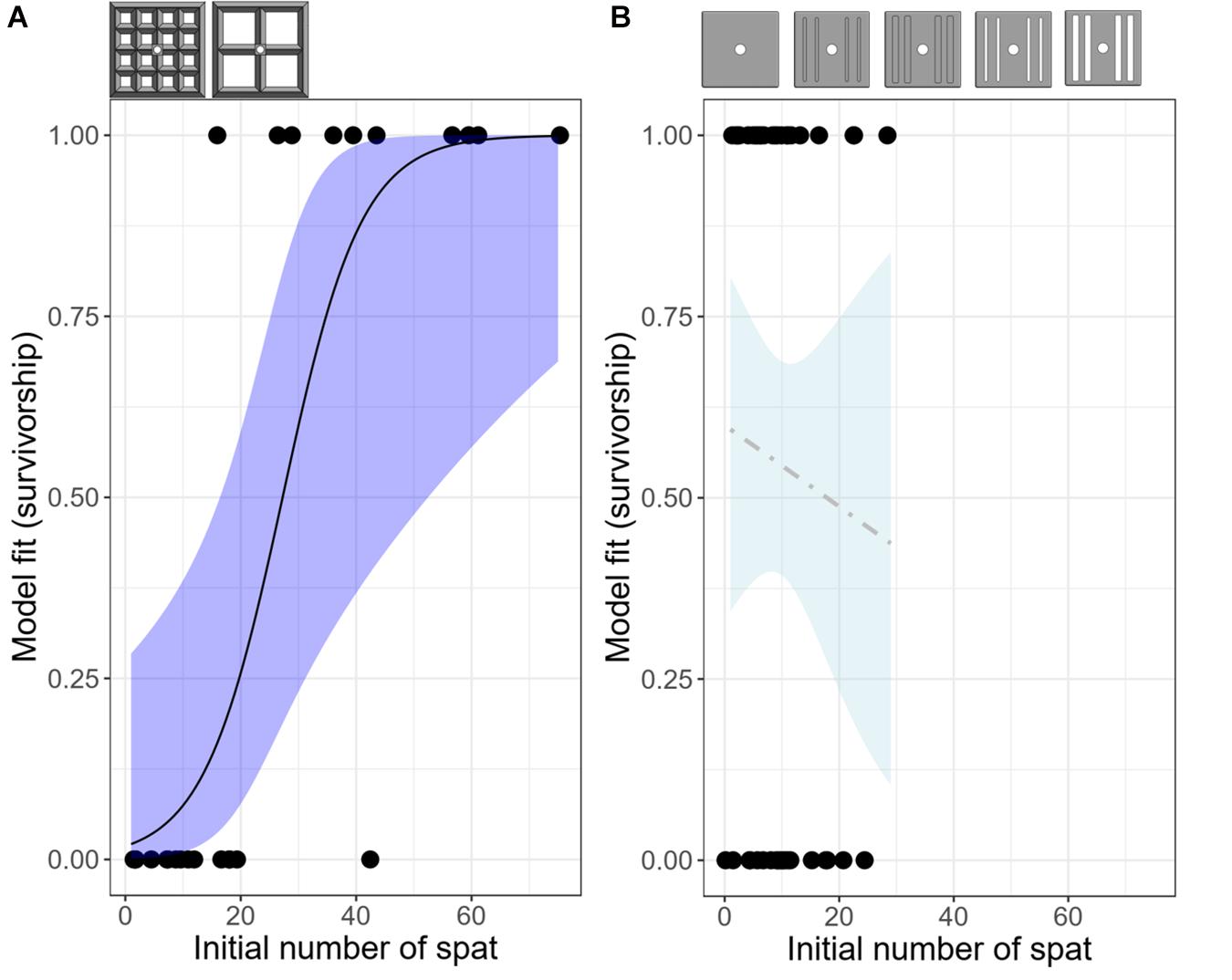
Figure 5. Logistic models predicting the probability of having at least one surviving recruit on each deployed device as a function of the initial number of spat present on that device at the time of deployment, for all lattice-grid (A) and grooved-tile (B) devices. n = 24 for the lattice-grids and n = 44 for the grooved-tiles. Shaded areas indicate confidence intervals; dark blue indicates a statistically significant model whereas light blue indicates a non-significant model. Reference diagrams of device types included in each model are above their respective plots.
Approximately 93% of the spat on the lattice-grids were solitary, whereas 7% of the spat resulted from larvae that settled in aggregations of up to 4, with the vast majority of aggregated spat resulting from two larvae settled together (Figure 6A). Survival probability on the lattice devices was not dependent on whether larvae settled singly or in aggregates (GMLE: z = 0.66, p = 0.51), with only one aggregate surviving the full deployment period (Figure 6C).
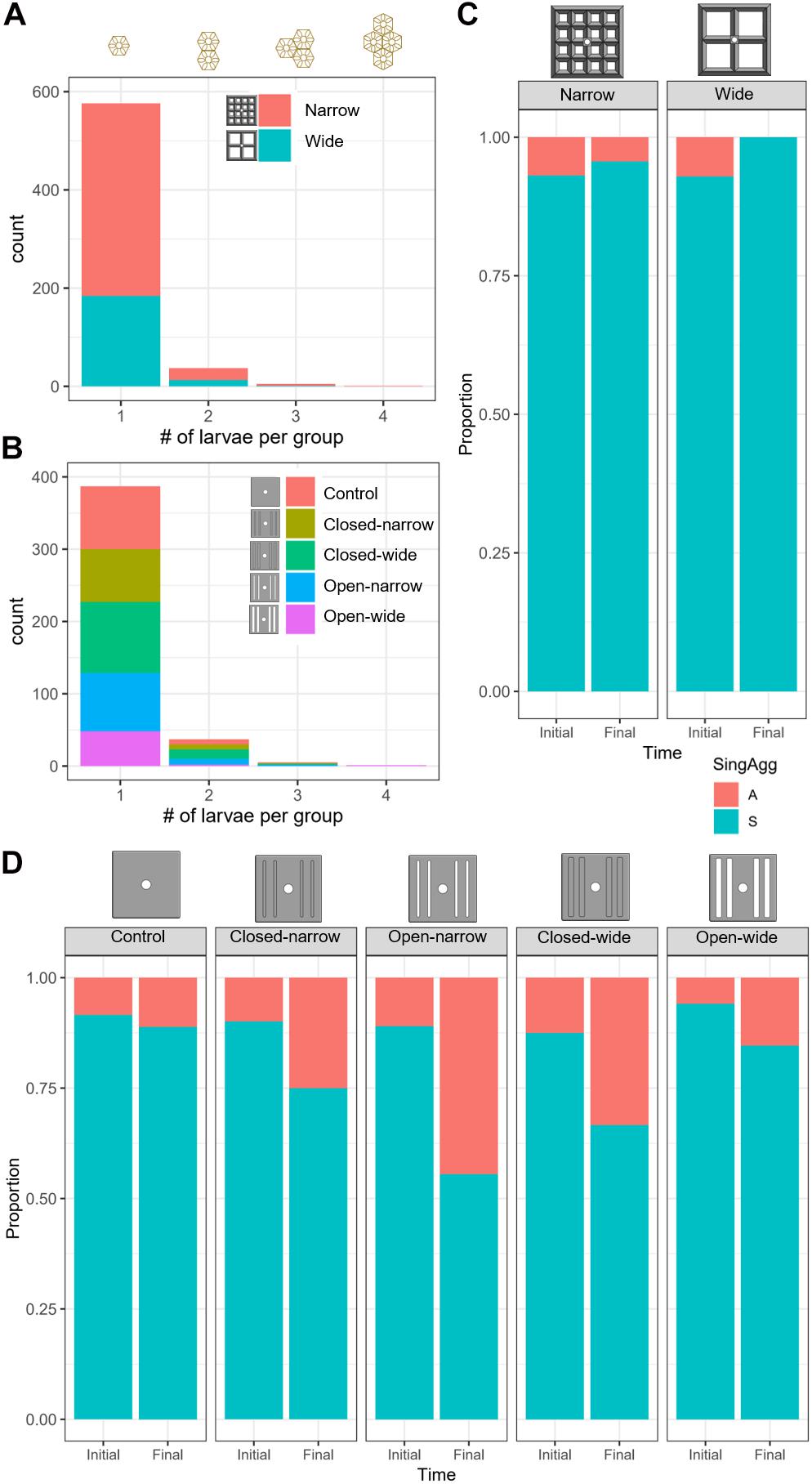
Figure 6. Histograms indicating the distribution of spat that were classified as resulting from 1 (single, “S”), 2, 3, or 4 (aggregated, “A”) larvae for the lattice-grid (A) and grooved-tile (B) devices, by device type (colour). The proportion of spat identified as single spat (“S”) or aggregated spat (“A”) immediately after settlement (Initial) and after approximately 1 year of deployment (Final) on the lattice-grids (C) and the grooved-tiles (D).
Due to the difficulty of censusing the larger lattice-grids in the field with sufficient resolution to observe recruits, only initial and final time points were used in the lattice-device analyses. Qualitatively, however, it appeared that most recruit mortality occurred in the first 3 months post deployment, prior to the first census.
Juvenile corals on the lattice-grids averaged 11.5 ± 4.3 mm (mean ± SD) in maximum diameter after 1 year, ∼20% larger on the narrow aperture lattice-grids (11.9 ± 4.7) than the wide aperture lattice-grids (10.0 ± 2.1), although the trends were not statistically significant (GLM: t = −0.87, p = 0.39).
Grooved-Tiles
Settlement averaged 12 ± 10 spat (mean ± SD; ∼0.25 spat cm–2) per device, and was highest on the closed-narrow grooved-tiles and lowest on the open-wide grooved-tiles, with high variability across device types. In general, settlement was higher in the grooves and on the lower edges than expected, based on available surface area (Figures 2, 7). By contrast, settlement was lower on the upper edges, and lower surfaces than expected, although we note that because the grooved-tiles were placed directly on the surface of the tank for settlement, access to the ‘lower’ habitat was at least partially restricted, resulting in lower-than-expected proportions of settled spat.
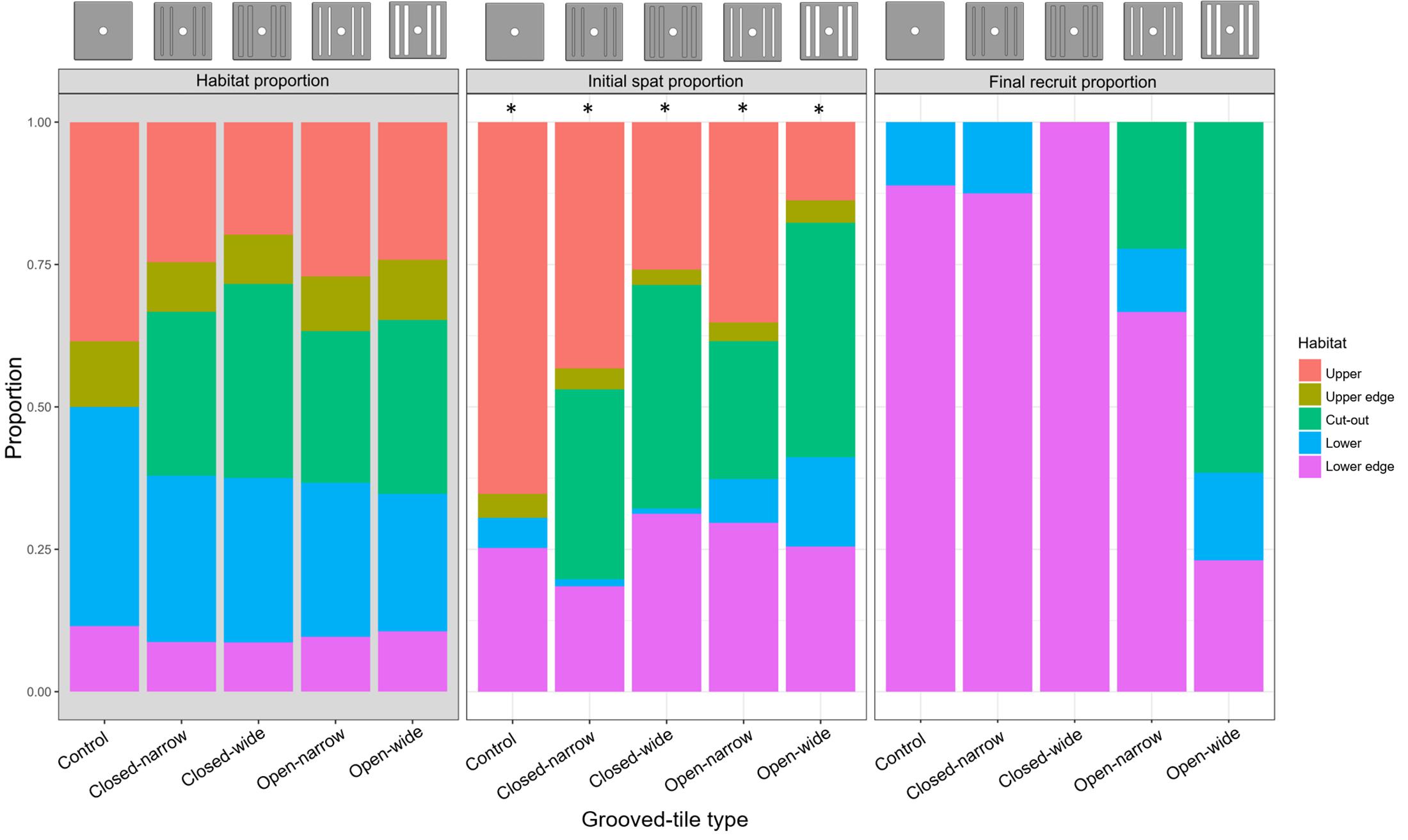
Figure 7. Proportion of space available within each habitat type (‘Habitat proportion’), the proportion of live Acropora tenuis spat within each habitat type immediately after settlement (‘Initial spat proportion’), and the proportion of live A. tenuis juveniles approximately 1 year post-deployment (‘Final recruit proportion’) on five types of grooved-tiles. Note that the control devices did not have any grooves. Reference diagrams of each device type are included above the plots. Asterisks in the ‘Initial spat proportion’ data indicate statistically significant differences in the observed and expected settlement proportions based on a Chi-square goodness-of-fit test (p < 0.05), but we stress that access to the ‘lower’ habitat by the larvae was at least partially restricted.
Spat survival averaged 16 ± 24% (mean ± SD) across all devices but was significantly higher on the devices with wide grooves that went all the way through the tile (open-wide, 39 ± 37%) and was lowest on the devices with wide shallow grooves (closed-wide, 2 ± 5%; Figure 4B). Surviving spat overwhelmingly were located on lower edge habitats across all device types, except when the grooves went all the way through the tiles; on those devices, spat survived both on the lower-edge habitats and in the grooves (Figure 7). No spat survived in any groove that did not go through the tile, and no spat survived on upper or upper-edge habitats on any tile.
The majority of coral mortality occurred within the first 6 months of the deployment (Figure 4C). Across all grooved tiles, 55% had at least one surviving recruit after a year, with the highest survival success achieved for the open-wide tile (89%) and the lowest on the closed-wide grooved-tile (22%) (Figure 4C). The probability of having at least one surviving spat on a device at the end of the deployment was not significantly predicted by the starting number or density of larvae settled on each device, both when considering all grooved-tiles together and when testing each device individually (Figure 5B).
On the grooved-tiles, 90% of settlers were identified as single spat and 10% were classified as aggregates of up to four larvae, with the majority of aggregates composed of two larvae (Figure 6B). On the grooved-tiles, aggregations of spat were significantly more likely to survive than single spat (GLMM: z = −2.3, p = 0.02; Figure 6D), although we note that this does not account for the inherent increase in the probability of survival as a function of the number of individuals in an aggregation. There was no clear relationship between the numbers of larvae in an aggregate and the likelihood of that aggregate surviving, with only 10 aggregates surviving after a year deployed.
Juvenile corals that survived a year of deployment averaged 10.4 ± 4.9 mm at the largest, and 8.2 ± 2.5 at the smallest, on the open-wide grooved-tiles and the control tiles, respectively, although the differences in size were not statistically significant.
Discussion
In this era of coral reef decline (Gardner et al., 2003; De’ath et al., 2012; Hughes et al., 2017), coral populations are fighting an uphill battle toward recovery, contending with reduced cover and a consequent reduction in larval supply exacerbated by Allee effects (Hughes et al., 2019). Of those larvae that do make it to the reef, shifts in the benthic-community composition and the declining condition of the substratum may further impede larval detection of settlement cues, hinder settlement, and reduce post-settlement survival (Albright et al., 2010; Doropoulos et al., 2012a, 2017b; Webster et al., 2013; Fabricius et al., 2015). While difficult to quantify, the mortality of coral settlers can exceed 99% on a healthy reef (Babcock, 1985; Hunt and Scheibling, 1997; Wilson and Harrison, 2005; Vermeij and Sandin, 2008; Penin et al., 2010, 2011; Ritson-Williams et al., 2010; Trapon et al., 2013; Miller, 2014; Suzuki et al., 2018). Seeding reefs with sexually produced coral settlers on deployment devices (Chamberland et al., 2017) is a restoration method with the potential to overcome some of these impediments to recovery, but must achieve unnaturally high levels of post-settlement survival to contend with scalability challenges (Randall et al., 2020). The best performing device tested here, the open-wide grooved-tiles, achieved an average survival of 39% at the level of individual spat—a threefold increase over featureless control tiles—and an even higher 89% at the level of deployment device, suggesting that optimising functional features in deployment devices has the potential to improve spat survival and, consequently, improve the feasibility of larger scale coral seeding.
Patterns of Settlement Within and Among Devices
The settlement patterns observed in this study were complex and somewhat surprising. Coral larvae often preferentially settle in grooves, in microcrevices, and on the undersides of substrates; larvae tend to avoid exposed, upper-facing surfaces (Baird and Hughes, 2000; Petersen et al., 2005; Nozawa, 2008; Vermeij et al., 2009; Whalan et al., 2015; Doropoulos et al., 2016, 2017a; Ricardo et al., 2017; Cameron and Harrison, 2020). While we expected to observe higher settlement on the undersides of the lattice-grids, settlement patterns across those microhabitats roughly reflected the available surface area, suggesting indiscriminate settlement behaviour (Figure 3). The reason for this settlement pattern is unknown but several possible explanations warrant consideration. Firstly, there were no horizontal upward-facing surfaces on the devices; instead, the ‘upper inner’ and ‘upper outer’ surfaces sloped down at a 51° angle. The lattice devices also did not have dedicated microcrevices to offer alternative settlement locations. Secondly, it could be that, because settlement took place in experimental aquaria under artificial lights, the environmental drivers of preferential settlement on downward-facing surfaces, such as natural light gradients (Maida et al., 1994; Mundy and Babcock, 1998; Baird and Hughes, 2000) and sedimentation gradients (Jones et al., 2015; Ricardo et al., 2017) were lacking. Thirdly, because the devices were conditioned in the laboratory and for only 4 weeks, the differential biological communities typical of those microhabitats may have been less pronounced at the time of settlement and different from field-conditioned communities (Doropoulos et al., 2017b) although they were markedly different among microhabitats by the end of the deployment period (Supplementary Figure 1).
Settlement behaviour on the grooved-tiles was difficult to assess due to the positioning of tiles directly on the base of the tank to promote settlement in the grooves, which at least somewhat restricted access to the lower surface. However, there was some selective settlement behaviour—larvae avoided settling on the upper edges of the tiles but settled in all other available microhabitats including upward-facing horizontal surfaces, within the grooves, and on lower edges (Figure 7). Again, the relatively high proportion of spat settling on upward-facing surfaces was surprising but could be due to the immature biological communities on the tiles or the environmental conditions present at the time of settlement.
Given the strong patterns observed in recruit survival, detailed in the next section, investigating ways to direct settlement to the microhabitats that confer the highest survival may further improve the performance of the devices. This could be achieved by modifying the environmental conditions present at the time of settlement (i.e., light or flow), restricting access to upward-facing surfaces during settlement (i.e., using physical barriers or antifoulants), modifying the duration of, or conditions for, benthic-community development prior to settlement, or promoting particular benthic species to induce settlement (i.e., promoting particular crustose coralline algae species).
Patterns of Survival Within and Among Devices
Spat survival averaged between 2 and 39% over 376 days, depending on the device design, and declined most rapidly within the first 6 months, typical of survival on artificial deployment devices (reviewed in Randall et al., 2020). Spat survival is affected by a myriad of factors, which make comparisons of spat survival among studies difficult to interpret. Nevertheless, average spat survival on the open-wide grooved-tiles (39%) was high compared with what has been achieved in similar deployment studies over an equivalent time frame (12 months), which tend to average <10–20% (Chamberland et al., 2015, 2017; dela Cruz and Harrison, 2017, 2020; Baria-Rodriguez et al., 2019). Small variations in the holding period prior to deployment can have significant downstream consequences for survival estimates, however, and thus we avoid making direct comparisons with other studies. Comparing spat survival on artificial surfaces against natural substrates is also difficult, since the larvae often settle in cryptic locations and are extremely difficult to locate in situ during the first 6 months (dela Cruz and Harrison, 2017, 2020). Therefore, our comparisons of spat survival below focus on the factors (i.e., microhabitat features and device designs) that could be quantitatively compared under the deployment conditions.
In this study, spat mortality on the upper surfaces and upper edges was 100% across all device types; the vast majority of surviving recruits were located on lower-edge microhabitats (Figures 3, 7) although many of the survivors had begun to grow up around the sides of the devices by the end of the deployment (Figures 1, 2). We hypothesize that these results were primarily driven by processes related to sedimentation, light attenuation, and grazing.
Firstly, no spat survived in groove microhabitats when they were closed, but the open-wide grooves were the best performing microhabitats (Figure 7). Observations during field deployments indicated that sediment accumulated in the turfs within the grooves when they were not cut all the way through the tiles whereas the open grooves appeared to reduce sediment load (Jones et al., 2015; Ricardo et al., 2017). Interestingly, the grooves seemed to harbour a turfing community (Supplementary Figure 2), perhaps due to restricted herbivory, but the spat were better able to contend with the turf when sediment did not accumulate.
Secondly, survival was higher on the lower inner microhabitats compared with the lower outer microhabitats on the lattice-grids, a result similar to that of Gallagher and Doropoulos (2017) who found that inner crevices protected coral juveniles better than outer crevices. This may be because the inner areas of the lattice-grids had reduced access to herbivores but still allowed the interior underside habitats to function more like ‘edge’ habitats, by allowing light to pass through the lattice-grid and reach the recruits. Maida et al. (1994) documented that mortality of coral spat on the undersides of settlement plates increased with distance from the edge of the plate and attributed this to the decay of light intensity from the outer edge to the centre of the tiles. Indeed, older coral juveniles are often found on exposed edges and upper surfaces reflecting the strong role of light in driving juvenile and adult coral distributions (Maida et al., 1994; Babcock and Mundy, 1996; Baird and Hughes, 2000; Cameron and Harrison, 2020). For example, Cameron and Harrison (2020) documented the proportional change in A. tenuis distribution on settlement tiles over 12 months during a field deployment. Spat were overwhelmingly dominant on underhangs 5 days after settlement but were located almost entirely on the edges of the tiles after 12 months, with more than half of the surviving corals having originally settled on underhangs and subsequently grown onto the edges. We suggest that the wide grooved-tiles and wide lattice-grids promote edge-like conditions throughout the undersides of the shapes. Indeed, there were no differences in the maximum diameter of recruits among microhabitats or device-types in this study, suggesting no obvious limitations to growth (Supplementary Figure 3).
Lastly, it was clear that upper surfaces of the grooved-tiles were heavily grazed by fish, based on field observations of roving acanthurid and scarid schools, the presence of resident pomacentrids, and in situ images that show an abundance of bite marks (Supplementary Figure 2). We hypothesize that this herbivory pressure removed the spat on upper surfaces where fish had access (Penin et al., 2010; Trapon et al., 2013). Indeed, Trapon et al. (2013) undertook an herbivore exclusion experiment of Acropora cytherea recruits on the Great Barrier Reef and found that the exclusion of herbivores significantly increased survival of recruits on the reef crest.
While we documented clear patterns in post-deployment survival of Acropora tenuis among microhabitats on the devices, these patterns may vary among environments with differing light and flow regimes, across depth gradients and among species. For example, Baird and Hughes (2000) documented a significant difference in recruitment of A. hyacinthus in shaded and unshaded environments owing to differential light levels and Miller (2014) identified species-specific responses to deployment orientation in A. palmata and Orbicella faveolata in the Caribbean. Patterns of recruit survival are also likely to differ across ontogeny as the relative influence of various intrinsic and environmental pressures shift (Babcock and Mundy, 1996; Doropoulos et al., 2017a). For example, Babcock and Mundy (1996) identified divergent drivers of mortality during the first 4 months after settlement compared with the subsequent 5 months, suggesting that there are competing pressures that vary in their relative influence through time, and indicating that the method of deployment should consider all the drivers of mortality throughout early development to optimise survival success.
Density Dependence in Spat Survival
Relationships between spat density and recruit survival have been described for several Acropora species and suggest that intermediate densities often confer the best outcome (Suzuki et al., 2012; Edwards et al., 2015; Doropoulos et al., 2017a, 2018; Cameron and Harrison, 2020). Suzuki et al. (2012) examined the impact of larval density in a field seeding trial of A. tenuis and A. muricata in Japan and found that moderate spat densities (∼0.1 cm–2) resulted in a better overall outcome than low (∼0.07 spat cm–2) and high densities (∼0.6 cm–2), as determined by survival at 6 months and genetic diversity endpoints. Similarly, in a 2-year field study of Acropora tenuis, Cameron and Harrison (2020) found that the highest colony abundance and coral cover was achieved on tiles with an intermediate spat density (∼1 cm–2), although the intermediate density was higher than that described by Suzuki et al. (2012), perhaps owing to the differences in the richness of species deployed and the environmental conditions at the deployment sites. Similarly, here we identified a significant increase in the likelihood of having at least one surviving coral on a lattice device when there were at least 0.2 spat cm–2. The highest settlement density achieved in the study was 0.5 spat cm–2 and no negative density-dependent effects were observed. The mechanisms that drive these density-dependent effects, and the variations in those effects observed within and among studies (i.e., Doropoulos et al., 2017a) are complex but likely include space limitation leading to inter- and intra-specific competition and more settlement in sub-optimal microhabitats as density increase (Roughgarden et al., 1985; Cameron and Harrison, 2020), mediated by genetic relatedness of the spat (see next section). Regardless of the mechanism, our results support these previous findings and suggest a minimum density of at least 0.2 spat cm–2 for A. tenuis seeding devices.
Restoration Outcomes With Chimeras
Coral larvae tend to settle gregariously and may occasionally form chimeras, which can increase juvenile survival, size and growth (Raymundo and Maypa, 2004; Amar et al., 2008; Suzuki et al., 2012; dela Cruz and Harrison, 2017; Doropoulos et al., 2017a). Promoting gregarious settlement on seeding devices has been proposed as a method for improving restoration outcomes (Raymundo and Maypa, 2004; Puill-Stephan et al., 2012b) but its usefulness will depend on the level of allorecognition and genetic histocompatibility in the aggregation (Puill-Stephan et al., 2012a, b; reviewed in Randall et al., 2020). Furthermore, the benefit of aggregated settlement is context dependent, and can vary in response to environmental factors and spat settlement location (Doropoulos et al., 2017a). For example, Doropoulos et al. (2017a) identified a positive effect of gregarious settlement on survival of spat on exposed surfaces but not of those that settled in crevices. In this study, the impact of aggregated settlement on the likelihood of survival was also mixed. On the one hand, there were no differences in survival probability between single and aggregated spat on the lattice devices, while on the other hand, aggregations of spat were more likely to survive on the grooved-tiles. However, these analyses compare single and aggregated spat survival directly, and do not account for the inherent increase in the probability of survival as a function of the number of individuals in an aggregation. In other words, there are more ‘chances’ for the coral to survive as the number of spat in an aggregation increases. Therefore, these results likely overestimate the benefit of aggregation in spat survival.
The present study evaluated spat from six contributing parents of one species, which likely led to a large proportion of closely related (half and full sibling) spat. While histocompatibility mechanisms have not been investigated in Acropora tenuis, Puill-Stephan et al. (2012b) found that the expression of allorecognition in the similar species A. millepora took at least 5 and 13 months for half- and full-siblings, respectively, suggesting that our deployment of 376 days may not have been long enough to capture the full allorecognition response in the spat. Thus, it is possible that rejection within some chimeras was yet to take place. Furthermore, chimeric adult A. millepora genotypes show high levels of relatedness suggesting that genetic similarity is required for long-term persistence of the chimera (Puill-Stephan et al., 2009). Thus, while we documented an increase in survival probability of aggregated spat on the grooved-tiles, it may not be advantageous to promote gregarious settlement of spat when a more diverse broodstock contributes to a mass culture used in restoration. We suggest that longer-term research on more genetically diverse spat and on more species is needed to determine the value of promoting aggregated settlement for restoration.
Limitations
While we documented a clear improvement in spat survival associated with specific device-design features, the mechanisms driving this response remain unknown. We hypothesise that protection from fish-feeding behaviours (Trapon et al., 2013), prevention of sediment accumulation (Sato, 1985), and improved light attenuation all may have contributed to improved device function. Yet ascertaining the likely non-linear direct and indirect effects of these features on the mechanisms driving survival requires additional experiments, particularly with devices directly deployed onto the reef framework. Due to permitting limitations, the devices were deployed on fibreglass frames immediately adjacent to (within 1 m of) the bommies over a rubble and sand bottom. Anecdotal observations made during the deployment and census trips suggest that the frames attracted resident and transient grazing fishes, potentially subjecting the shapes to disproportionally high grazing pressure. Yet other strongly site-associated organisms may not have ventured out to the racks, also potentially altering the natural grazing community. Seeding devices directly onto the reef would remove this potential bias and improve our understanding of the drivers of post-deployment survival.
Three-dimensional (3D) printed plastic shapes are cheap and easy to produce to test various design features and to use as proxies for future deployment devices. However, the biofilms and biological communities that develop on plastics during conditioning are different from those on terracotta, ceramic and other materials and can have downstream consequences on benthic community composition, grazing, and microenvironments (Kennedy et al., 2017). The design features tested with 3D printed plastic require validation with acceptable materials for use in reef restoration (Spieler et al., 2001; Randall et al., 2020).
Conclusion
Optimising functional features in deployment devices can improve spat survival and may consequently support the up-scaling of coral seeding. Doubling the survival rate allows for halving the scale of deployment to achieve the same restoration goal, and could potentially lead to substantial reductions in cost per unit area, acknowledging that restoration costs do not scale linearly with restored area (Anthony et al., 2019; Gibbs et al., 2019). In this study, the wide-grooved-tiles achieved three times higher average survival than the comparatively featureless tiles of the same material, showing promise for overcoming bottlenecks of survival to size-escape thresholds and improving scalability. Incorporating grooves in other recently designed deployment substrates, such as ‘coral plug-ins’ (Guest et al., 2014; Tabalanza et al., 2020) and tetrapods (Chamberland et al., 2017) may improve survival and warrant further testing. While the wide grooved-tiles worked best for the Acropora tenuis spat tested here, it is likely that the drivers of mortality differ among species, among coral growth morphologies, and throughout ontogeny; identifying what design features work best in these various scenarios and across environmental gradients requires further investigation. One approach to device design could be to integrate multiple design features into a single device to maximise its effectiveness across a broad cross-section of hermatypic corals. Another approach could be to develop a suite of designs fit for particular growth morphologies, taxa, and receiving environments. A third approach could be to uncouple the settlement device from the deployment device. In this scenario, a small device could be designed with microrefugia, such as wide grooves, to direct settlement and enhance post-settlement survival. The settlement device would then be attached in modular fashion to a larger deployment device designed to optimise deployment logistics while maximising survival and retention on the reef. Regardless of the approach, innovative and fit-for-design devices (Petersen et al., 2005; Nozawa, 2008; Okamoto et al., 2008; Chamberland et al., 2017) may improve restoration outcomes in the future.
Data Availability Statement
The raw data supporting the conclusions of this article will be made available by the authors, without undue reservation.
Author Contributions
CR, AN, and AH conceived of and designed the experiments. CR and CG conducted the experiments. CR analysed the data. CR and CG wrote the manuscript. All authors edited and approved the final draft.
Funding
Funding was provided by the Australian Institute of Marine Science.
Conflict of Interest
The authors declare that the research was conducted in the absence of any commercial or financial relationships that could be construed as a potential conflict of interest.
Acknowledgments
We acknowledge the Bindal and Wulgurukaba People as the traditional owners of the sea country from which the Acropora tenuis corals were collected, and the land on which this research took place. We pay our respects to their elders past, present, and emerging and acknowledge their continuing spiritual connection to their sea country. We thank M. Abdul-Wahab, G. Milton, B. Stephenson, V. Mocellin, N. Webster, T. Whitman and the crews of the RV Cape Ferguson, and the RV Apollo for assistance in the field. We thank the staff of the Sea Simulator for assistance during spawning, larval rearing and mass settlement, and the staff of the AIMS workshop for assistance producing the devices.
Supplementary Material
The Supplementary Material for this article can be found online at: https://www.frontiersin.org/articles/10.3389/fmars.2021.662263/full#supplementary-material
Footnotes
References
Albright, R., Mason, B., Miller, M., and Langdon, C. (2010). Ocean acidification compromises recruitment success of the threatened Caribbean coral Acropora palmata. Proc. Natl. Acad. Sci. U.S.A. 107, 20400–20404. doi: 10.1073/pnas.1007273107
Amar, K.-O., Chadwick, N. E., and Rinkevich, B. (2008). Coral kin aggregations exhibit mixed allogeneic reactions and enhanced fitness during early ontogeny. BMC Evol. Biol. 8:126. doi: 10.1186/1471-2148-8-126
Anthony, K. R. N., Bowen, J., Mead, D., and Hardisty, P. E. (2019). R3: Intervention Analysis and Recommendations. A report provided to the Australian Government by the Reef Restoration and Adaptation Program, Townsville, Qld. Townsville: Reef Restoration and Adaptation Program.
Arnold, S., Steneck, R., and Mumby, P. (2010). Running the gauntlet: inhibitory effects of algal turfs on the processes of coral recruitment. Mar. Ecol. Prog. Ser. 414, 91–105. doi: 10.3354/meps08724
Babcock, R., and Mundy, C. (1996). Coral recruitment: consequences of settlement choice for early growth and survivorship in two scleractinians. J. Exp. Mar. Biol. Ecol. 206, 179–201. doi: 10.1016/s0022-0981(96)02622-6
Babcock, R. C. (1985). “Growth and mortality in juvenile corals (Goniastrea, Platygyra and Acropora): the first year,” in Proceedings of the Fifth International Coral Reef Congress, (Tahiti: French Polynesia), 355–360.
Babcock, R. C., Bull, G. D., Harrison, P. L., Heyward, A. J., Oliver, J. K., Wallace, C. C., et al. (1986). Synchronous spawnings of 105 scleractinian coral species on the Great Barrier Reef. Mar. Biol. 90, 379–394. doi: 10.1007/BF00428562
Babcock, R. C., and Smith, L. (2002). “Effects of sedimentation on coral settlement and survivorship,” in Proceedings of the 9th International Coral Reef Symposium, Bali, 245–248.
Baird, A. H., and Hughes, T. P. (2000). Competitive dominance by tabular corals: an experimental analysis of recruitment and survival of understorey assemblages. J. Exp. Mar. Biol. Ecol. 251, 117–132. doi: 10.1016/s0022-0981(00)00209-4
Baria, M. V. B., Guest, J. R., Edwards, A. J., Aliño, P. M., Heyward, A. J., and Gomez, E. D. (2010). Caging enhances post-settlement survival of juveniles of the scleractinian coral Acropora tenuis. J. Exp. Mar. Biol. Ecol. 394, 149–153. doi: 10.1016/j.jembe.2010.08.003
Baria-Rodriguez, M. V., Cruz, D. W., Dizon, R. M., Yap, H. T., and Villanueva, R. D. (2019). Performance and cost-effectiveness of sexually produced Acropora granulosa juveniles compared with asexually generated coral fragments in restoring degraded reef areas. Aquat. Conserv. Mar. Freshw. Ecosyst. 29, 891–900. doi: 10.1002/aqc.3132
Bates, D., Machler, M., Bolker, B. M., and Walker, S. (2014). Fitting linear mixed-effects models using lme4. arXiv [Preprint]. doi: 10.18637/jss.v067.i01
Bay, L. K., Rocker, M., Boström-Einarsson, L., Babcock, R. C., Buerger, P., Cleves, P. A., et al. (2019). Reef Restoration and Adaptation Program: Intervention Technical Summary. A report provided to the Australian Government by the Reef Restoration and Adaptation Program, Townsville, Qld. Townsville: Reef Restoration and Adaptation Program.
Boström-Einarsson, L., Babcock, R. C., Bayraktarov, E., Ceccarelli, D., Cook, N., Ferse, S. C. A., et al. (2020). Coral restoration – A systematic review of current methods, successes, failures and future directions. PLoS One 15:e0226631. doi: 10.1371/journal.pone.0226631
Boström-Einarsson, L., Ceccarelli, D., Babcock, R. C., Bayraktarov, E., Cook, N., Harrison, P., et al. (2018). Coral Restoration in a Changing World - A Global Synthesis of Methods and Techniques. Cairns: Reef and Rainforest Research Centre Ltd.
Box, S., and Mumby, P. (2007). Effect of macroalgal competition on growth and survival of juvenile Caribbean corals. Mar. Ecol. Prog. Ser. 342, 139–149. doi: 10.3354/meps342139
Bruno, J. F., and Selig, E. R. (2007). Regional decline of coral cover in the indo-pacific: timing, extent, and subregional comparisons. PLoS One 2:e711. doi: 10.1371/journal.pone.0000711
Cameron, K. A., and Harrison, P. L. (2020). Density of coral larvae can influence settlement, post-settlement colony abundance and coral cover in larval restoration. Sci. Rep. 10:5488.
Carleton, J. H., and Sammarco, P. W. (1987). Effects of substratum irregularity on success of coral settlement: quantification by comparative geomorphological techniques. Bull. Mar. Sci. 40, 85–98.
Chamberland, V. F., Petersen, D., Guest, J. R., Petersen, U., Brittsan, M., and Vermeij, M. J. A. (2017). New seeding approach reduces costs and time to outplant sexually propagated corals for reef restoration. Sci. Rep. 7, 1–12.
Chamberland, V. F., Vermeij, M. J. A., Brittsan, M., Carl, M., Schick, M., Snowden, S., et al. (2015). Restoration of critically endangered elkhorn coral (Acropora palmata) populations using larvae reared from wild-caught gametes. Glob. Ecol. Conserv. 4, 526–537. doi: 10.1016/j.gecco.2015.10.005
De’ath, G., Fabricius, K. E., Sweatman, H., and Puotinen, M. (2012). The 27-year decline of coral cover on the Great Barrier Reef and its causes. Proc. Natl. Acad. Sci. U.S.A. 109, 17995–17999. doi: 10.1073/pnas.1208909109
dela Cruz, D. W., and Harrison, P. L. (2017). Enhanced larval supply and recruitment can replenish reef corals on degraded reefs. Sci. Rep. 7, 1–13.
dela Cruz, D. W., and Harrison, P. L. (2020). Enhancing coral recruitment through assisted mass settlement of cultured coral larvae. PLoS One 15:e0242847. doi: 10.1371/journal.pone.0242847
Doropoulos, C., Elzinga, J., ter Hofstede, R., van Koningsveld, M., and Babcock, R. C. (2019). Optimizing industrial-scale coral reef restoration: comparing harvesting wild coral spawn slicks and transplanting gravid adult colonies. Restorat. Ecol. 27, 758–767. doi: 10.1111/rec.12918
Doropoulos, C., Evensen, N. R., Gómez-Lemos, L. A., and Babcock, R. C. (2017a). Density-dependent coral recruitment displays divergent responses during distinct early life-history stages. R. Soc. open Sci. 4:170082. doi: 10.1098/rsos.170082
Doropoulos, C., Roff, G., Visser, M.-S., and Mumby, P. J. (2017b). Sensitivity of coral recruitment to subtle shifts in early community succession. Ecology 98, 304–314. doi: 10.1002/ecy.1663
Doropoulos, C., Gómez-Lemos, L. A., and Babcock, R. C. (2018). Exploring variable patterns of density-dependent larval settlement among corals with distinct and shared functional traits. Coral Reefs 37, 25–29. doi: 10.1007/s00338-017-1629-y
Doropoulos, C., Roff, G., Bozec, Y.-M., Zupan, M., Werminghausen, J., and Mumby, P. J. (2016). Characterising the ecological trade-offs throughout the early ontogeny of coral recruitment. Ecol. Monogr. 1, 20–24.
Doropoulos, C., Ward, S., Diaz-Pulido, G., Hoegh-Guldberg, O., and Mumby, P. J. (2012a). Ocean acidification reduces coral recruitment by disrupting intimate larval-algal settlement interactions: elevated CO2 alters CCA-larval interactions. Ecol. Lett. 15, 338–346. doi: 10.1111/j.1461-0248.2012.01743.x
Doropoulos, C., Ward, S., Marshell, A., Diaz-Pulido, G., and Mumby, P. J. (2012b). Interactions among chronic and acute impacts on coral recruits: the importance of size-escape thresholds. Ecology 93, 2131–2138. doi: 10.1890/12-0495.1
Edmunds, P. J., Nozawa, Y., and Villanueva, R. D. (2014). Refuges modulate coral recruitment in the Caribbean and the Pacific. J. Exp. Mar. Biol. Ecol. 454, 78–84. doi: 10.1016/j.jembe.2014.02.009
Edwards, A., Guest, J., Heyward, A., Villanueva, R., Baria, M., Bollozos, I., et al. (2015). Direct seeding of mass-cultured coral larvae is not an effective option for reef rehabilitation. Mar. Ecol. Prog. Ser. 525, 105–116. doi: 10.3354/meps11171
Fabricius, K. E., Kluibenschedl, A., Harrington, L., Noonan, S., and De’ath, G. (2015). In situ changes of tropical crustose coralline algae along carbon dioxide gradients. Sci. Rep. 5:9537.
Gallagher, C., and Doropoulos, C. (2017). Spatial refugia mediate juvenile coral survival during coral–predator interactions. Coral Reefs 36, 51–61. doi: 10.1007/s00338-016-1518-9
Gardner, T. A., Cote, I. M., Gill, J. A., Grant, A., and Watkinson, A. R. (2003). Long-term region-wide declines in Caribbean corals. Science 301, 958–960. doi: 10.1126/science.1086050
Gibbs, M., Mead, D., Babcock, R. C., Harrison, D., Ristovski, Z., Harrison, P., et al. (2019). Reef Restoration and Adaptation Program: Future Deployment Scenarios and Costing. Townsville: Reef Restoration and Adaptation Program.
Guest, J. R., Baria, M. V., Gomez, E. D., Heyward, A. J., and Edwards, A. J. (2014). Closing the circle: is it feasible to rehabilitate reefs with sexually propagated corals? Coral Reefs 33, 45–55. doi: 10.1007/s00338-013-1114-1
Heyward, A., Smith, L., Rees, M., and Field, S. (2002). Enhancement of coral recruitment by in situ mass culture of coral larvae. Mar. Ecol. Prog. Ser. 230, 113–118. doi: 10.3354/meps230113
Heyward, A. J., and Negri, A. P. (1999). Natural inducers for coral larval metamorphosis. Coral Reefs 18, 273–279. doi: 10.1007/s003380050193
Heyward, A. J., Rees, M., and Smith, L. D. (1999). “Coral spawning slicks harnessed for large-scale coral culture,” in Proceedings of the Program and Abstracts, International Conference on Scientific Aspects of Coral Reef Assessment, Monitoring and Restoration, (Florida: National Coral Reef Institute, Nova Southeastern University), 188–189.
Hughes, T. P., Barnes, M. L., Bellwood, D. R., Cinner, J. E., Cumming, G. S., Jackson, J. B. C., et al. (2017). Coral reefs in the Anthropocene. Nature 546, 82–90.
Hughes, T. P., Kerry, J. T., Baird, A. H., Connolly, S. R., Chase, T. J., Dietzel, A., et al. (2019). Global warming impairs stock–recruitment dynamics of corals. Nature 568, 387–390. doi: 10.1038/s41586-019-1081-y
Hughes, T. P., Rodrigues, M. J., Bellwood, D. R., Ceccarelli, D., Hoegh-Guldberg, O., McCook, L., et al. (2007). Phase shifts, herbivory, and the resilience of coral reefs to climate change. Curr. Biol. 17, 360–365. doi: 10.1016/j.cub.2006.12.049
Hunt, H., and Scheibling, R. (1997). Role of early post-settlement mortality in recruitment of benthic marine invertebrates. Mar. Ecol. Prog. Ser. 155, 269–301. doi: 10.3354/meps155269
Jones, R., Ricardo, G. F., and Negri, A. P. (2015). Effects of sediments on the reproductive cycle of corals. Mar. Pollut. Bull. 100, 13–33. doi: 10.1016/j.marpolbul.2015.08.021
Kennedy, E., Ordoñez, A., Lewis, B., and Diaz-Pulido, G. (2017). Comparison of recruitment tile materials for monitoring coralline algae responses to a changing climate. Mar. Ecol. Prog. Ser. 569, 129–144. doi: 10.3354/meps12076
Kuffner, I., Walters, L., Becerro, M., Paul, V., Ritson-Williams, R., and Beach, K. (2006). Inhibition of coral recruitment by macroalgae and cyanobacteria. Mar. Ecol. Prog. Ser. 323, 107–117. doi: 10.3354/meps323107
Kuffner, I. B., Andersson, A. J., Jokiel, P. L., Rodgers, K. S., and Mackenzie, F. T. (2008). Decreased abundance of crustose coralline algae due to ocean acidification. Nat. Geosci. 1, 114–117. doi: 10.1038/ngeo100
Ludecke, D. (2021). SjPlot: Data Visulization for Statistics in Social Science. Townsville: Reef Restoration and Adaptation Program.
Maida, M., Coll, J. C., and Sammarco, P. W. (1994). Shedding new light on scleractinian coral recruitment. J. Exp. Mar. Biol. Ecol. 180, 189–202. doi: 10.1016/0022-0981(94)90066-3
Miller, M. W. (2014). Post-settlement survivorship in two Caribbean broadcasting corals. Coral Reefs 33, 1041–1046. doi: 10.1007/s00338-014-1177-7
Mundy, C. N., and Babcock, R. C. (1998). Role of light intensity and spectral quality in coral settlement: implications for depth-dependent settlement? J. Exp. Mar. Biol. Ecol. 223, 235–255. doi: 10.1016/s0022-0981(97)00167-6
Nakamura, T. (2010). Importance of water-flow on the physiological responses of reef-building corals. Galaxea J. Coral Reef Stud. 12, 1–14. doi: 10.3755/galaxea.12.1
Nishikawa, A., Katoh, M., and Sakai, K. (2003). Larval settlement rates and gene flow of broadcast-spawning (Acropora tenuis) and planula-brooding (Stylophora pistillata) corals. Mar. Ecol. Prog. Ser. 256, 87–97. doi: 10.3354/meps256087
Nozawa, Y. (2008). Micro-crevice structure enhances coral spat survivorship. J. Exp. Mar. Biol. Ecol. 367, 127–130. doi: 10.1016/j.jembe.2008.09.004
Nozawa, Y. (2012). Effective size of refugia for coral spat survival. J. Exp. Mar. Biol. Ecol. 413, 145–149. doi: 10.1016/j.jembe.2011.12.008
Okamoto, M., Nojima, S., Fujiwara, S., and Furushima, Y. (2008). Development of ceramic settlement devices for coral reef restoration using in situ sexual reproduction of corals. Fish. Sci. 74, 1245–1253. doi: 10.1111/j.1444-2906.2008.01649.x
Penin, L., Michonneau, F., Baird, A., Connolly, S., Pratchett, M., Kayal, M., et al. (2010). Early post-settlement mortality and the structure of coral assemblages. Mar. Ecol. Prog. Ser. 408, 55–64. doi: 10.3354/meps08554
Penin, L., Michonneau, F., Carroll, A., and Adjeroud, M. (2011). Effects of predators and grazers exclusion on early post-settlement coral mortality. Hydrobiologia 663, 259–264. doi: 10.1007/s10750-010-0569-0
Petersen, D., Laterveer, M., and Schuhmacher, H. (2005). Innovative substrate tiles to spatially control larval settlement in coral culture. Mar. Biol. 146, 937–942. doi: 10.1007/s00227-004-1503-7
Puill-Stephan, E., van Oppen, M. J. H., Pichavant-Rafini, K., and Willis, B. L. (2012a). High potential for formation and persistence of chimeras following aggregated larval settlement in the broadcast spawning coral, Acropora millepora. Proc. R. Soc. B Biol. Sci. 279, 699–708. doi: 10.1098/rspb.2011.1035
Puill-Stephan, E., Willis, B., Abrego, D., Raina, J.-B., and van Oppen, M. (2012b). Allorecognition maturation in the broadcast-spawning coral Acropora millepora. Coral Reefs 31, 1019–1028. doi: 10.1007/s00338-012-0912-1
Puill-Stephan, E., Willis, B. L., van Herwerden, L., and van Oppen, M. J. H. (2009). Chimerism in wild adult populations of the broadcast spawning coral Acropora millepora on the Great Barrier Reef. PLoS One 4:e7751. doi: 10.1371/journal.pone.0007751
R Core Team (2020). R: Language and Environment for Statistical Computing. Vienna: R Foundation for Statistical Computing.
Randall, C., Negri, A., Quigley, K., Foster, T., Ricardo, G., Webster, N., et al. (2020). Sexual production of corals for reef restoration in the Anthropocene. Mar. Ecol. Prog. Ser. 635, 203–232. doi: 10.3354/meps13206
Raymundo, L. J., and Maypa, A. P. (2004). Getting bigger faster: mediation of size-specific mortality via fusion in juvenile coral transplants. Ecol. Appl. 14, 281–295. doi: 10.1890/02-5373
Ricardo, G. F., Jones, R. J., Nordborg, M., and Negri, A. P. (2017). Settlement patterns of the coral Acropora millepora on sediment-laden surfaces. Sci. Total Environ. 609, 277–288. doi: 10.1016/j.scitotenv.2017.07.153
Ritson-Williams, R., Paul, V. J., Arnold, S. N., and Steneck, R. S. (2010). Larval settlement preferences and post-settlement survival of the threatened Caribbean corals Acropora palmata and A. cervicornis. Coral Reefs 29, 71–81. doi: 10.1007/s00338-009-0555-z
Roughgarden, J., Iwasa, Y., and Baxter, C. (1985). Demographic theory for an open marine population with space-limited recruitment. Ecology 66, 54–67. doi: 10.2307/1941306
Sato, M. (1985). Mortality and growth of juvenile coral Pocillopora damicornis (Linnaeus). Coral Reefs 4, 27–33. doi: 10.1007/bf00302201
Speare, K. E., Duran, A., Miller, M. W., and Burkepile, D. E. (2019). Sediment associated with algal turfs inhibits the settlement of two endangered coral species. Mar. Pollut. Bull. 144, 189–195. doi: 10.1016/j.marpolbul.2019.04.066
Spieler, R. E., Gilliam, D. S., and Sherman, R. L. (2001). Artificial substrate and coral reef restoration: what do we need to know to know what we need. Bull. Mar. Sci. 29, 1013–1030.
Suzuki, G., Arakaki, S., Suzuki, K., Iehisa, Y., and Hayashibara, T. (2012). What is the optimal density of larval seeding in Acropora corals? Fish. Sci. 78, 801–808. doi: 10.1007/s12562-012-0504-6
Suzuki, G., Wataru, O., Yasutke, Y., Kai, S., Fujikura, Y., Tanita, I., et al. (2018). Interspecific differences in the post-settlement survival of Acropora corals under a common garden experiment. Fish. Sci. 84, 849–856. doi: 10.1007/s12562-018-1230-5
Tabalanza, T. D., Jamodiong, E. A., Diaz, L. A., Tañedo, M. C. S., Leriorato, J. C., Villanueva, R. D., et al. (2020). Successfully cultured and reared coral embryos from wild caught spawn slick in the Philippines. Aquaculture 525:735354. doi: 10.1016/j.aquaculture.2020.735354
Trapon, M. L., Pratchett, M. S., Hoey, A. S., and Baird, A. H. (2013). Influence of fish grazing and sedimentation on the early post-settlement survival of the tabular coral Acropora cytherea. Coral Reefs 32, 1051–1059. doi: 10.1007/s00338-013-1059-4
Vermeij, M. J. A., and Sandin, S. A. (2008). Density-dependent settlement and mortality structure the earliest life phases of a coral population. Ecology 89, 1994–2004. doi: 10.1890/07-1296.1
Vermeij, M. J. A., Smith, J. E., Smith, C. M., Vega Thurber, R., and Sandin, S. A. (2009). Survival and settlement success of coral planulae: independent and synergistic effects of macroalgae and microbes. Oecologia 159, 325–336. doi: 10.1007/s00442-008-1223-7
Webster, F. J., Babcock, R. C., Van Keulen, M., and Loneragan, N. R. (2015). Macroalgae inhibits larval settlement and increases recruit mortality at ningaloo reef, Western Australia. PLoS One 10:e0124162. doi: 10.1371/journal.pone.0124162
Webster, N. S., Soo, R., Cobb, R., and Negri, A. P. (2011). Elevated seawater temperature causes a microbial shift on crustose coralline algae with implications for the recruitment of coral larvae. ISME J. 5, 759–770. doi: 10.1038/ismej.2010.152
Webster, N. S., Uthicke, S., Botté, E. S., Flores, F., and Negri, A. P. (2013). Ocean acidification reduces induction of coral settlement by crustose coralline algae. Glob. Change Biol. 19, 303–315. doi: 10.1111/gcb.12008
Whalan, S., Abdul Wahab, M. A., Sprungala, S., Poole, A. J., and de Nys, R. (2015). Larval settlement: the role of surface topography for sessile coral reef invertebrates. PLoS One 10:e0117675. doi: 10.1371/journal.pone.0117675
Keywords: coral settlement, spat, post-settlement survival, microrefugia, grooves, outplant, deployment, coral restoration
Citation: Randall CJ, Giuliano C, Heyward AJ and Negri AP (2021) Enhancing Coral Survival on Deployment Devices With Microrefugia. Front. Mar. Sci. 8:662263. doi: 10.3389/fmars.2021.662263
Received: 31 January 2021; Accepted: 12 April 2021;
Published: 12 May 2021.
Edited by:
Anastazia T. Banaszak, National Autonomous University of Mexico, MexicoReviewed by:
Alasdair Edwards, Newcastle University, United KingdomCiemon Frank Caballes, ARC Centre of Excellence for Coral Reef Studies, Australia
Copyright © 2021 Randall, Giuliano, Heyward and Negri. This is an open-access article distributed under the terms of the Creative Commons Attribution License (CC BY). The use, distribution or reproduction in other forums is permitted, provided the original author(s) and the copyright owner(s) are credited and that the original publication in this journal is cited, in accordance with accepted academic practice. No use, distribution or reproduction is permitted which does not comply with these terms.
*Correspondence: Carly J. Randall, c.randall@aims.gov.au
†These authors share senior authorship