- 1School of Biosciences and Veterinary Medicine, University of Camerino, Camerino, Italy
- 2Blu Marine Service Soc. Coop., San Benedetto del Tronto, Italy
Tritia mutabilis (Linnaeus, 1758) represents a marine gastropod species of ecological and economical importance especially in coastal areas of the central Adriatic Sea (Italy). T. mutabilis encloses its fertilized eggs within capsules which play a protective role in the embryo defense against adverse environmental factors. Egg capsules are attached to any hard substrates and the availability of adequate substrates for oviposition represents therefore a major determinant of reproductive output of this species. The aim of the present study was to provide the first comprehensive monitoring of T. mutabilis egg capsule deposition and intracapsular embryonic patterns using specific artificial substrates and innovative analytical approaches. Square-based pyramid structures were placed within an area subjected to small-scale fishing activity along the Italian coastal waters of the central Adriatic Sea and monitored from March to June 2019. In addition, a machine learning-based approach was developed in order to speed up and automate time-consuming counting procedures of egg capsules attached on each pyramid. Overall, egg capsules were deposited on the almost totality of the artificial substrates reaching values of the surface coverage per site ranging from 31 to 97%. These findings lead to a quantitative estimation of egg number per site included between 27,060 and 62,940 capsules. Our results demonstrate that using these artificial substrates together with monitoring of both egg capsule deposition patterns and intracapsular developmental stages may be useful to improve the T. mutabilis stock management.
Introduction
Many marine invertebrates, mostly gastropod families, enclose their fertilized eggs within capsules that play a protective role in the embryo defense against adverse environmental factors (Pechenik, 1983; Rawlings, 1994, 1999; von Dassow and Strathmann, 2005; Przeslawski and Davis, 2007). Egg capsules are attached to hard substrates including submerged gear used for small-scale fishing and waste materials (Grati et al., 2018; Kaviarasan et al., 2020; Ganias et al., 2021). Given the energetic cost of capsule production in these organisms (Rawlings, 1999), the availability of adequate substrates for oviposition represents a major determinant of reproductive output of a population. In fact, species living in marine soft-bottom environments with limited availability of hard substrates must adopt different oviposition strategies such as attaching their egg capsules to living organisms (e.g., algae or bivalves; von Dassow and Strathmann, 2005; Carrasco and Phillips, 2014; Aguilar et al., 2017; Corte et al., 2019). Communal egg-laying is one of the most frequent behavior observed in several neogastropod species (Carrasco and Phillips, 2014). This behavior is considered a typical strategy for ensuring stable substrata for egg capsule deposition (Yokoyama and Amaral, 2011) or for providing protection against predators (Dumont et al., 2008).
Artificial substrates have proved to be highly suitable for favoring egg deposition of gastropods living on sandy or muddy habitats (Caprioli and Giansante, 2018). In the central Adriatic Sea, for example, the use of specifically designed artificial substrates was found to facilitate the natural deposition of Tritia mutabilis egg capsules in coastal areas (Caprioli and Giansante, 2018). T. mutabilis (Linnaeus, 1758), a common species belonging to the family of Nassariidae, lives in coastal waters from 2 to 15 m deep on sandy-muddy bottoms (Cespuglio et al., 1999; Grati et al., 2010; Polidori et al., 2015) and is intensively exploited by small-scale fisheries (Polidori et al., 2015). In the central Italian Adriatic coastal waters, the breeding season of T. mutabilis starts in the late winter and ceases in the late spring resulting in massive event of egg capsules deposition on any available hard object (Polidori et al., 2015; Caprioli and Giansante, 2018). The limited literature regarding the reproductive behavior of T. mutabilis reports algae and seagrass leaves as favorite oviposition substrates followed by rocks, gravels, sponges, or shells (Barash and Zenziper, 1980; Bakır et al., 2012). As described by D’Asaro (1993), T. mutabilis egg capsules have a complex morphology characterized by a dome-shaped vault that encloses the embryos and shows spinose lateral ridges on the outer surface. An opening occluded by plugs is positioned eccentrically toward one side. Each capsule contains a variable number of embryos ranging from 8 to 22 and hatching takes place at the veliger stage, after about 3 weeks of encapsulated development (Caprioli and Giansante, 2018). T. mutabilis is by far the most important target species for small-scale coastal fisheries of the western (Italian) side of the central and northern Adriatic Sea. In the Mediterranean, this marine snail is considered an important constituent of seafood diets due to its favorable sterol profile and mineral content (Fiordelmondo et al., 2020). Previous reports have indicated that T. mutabilis fishery accounts for more than 35% of total fishing effort resulting in an annual catch of about 2,000 t (Grati et al., 2010; Caprioli and Giansante, 2018). In the last decade, however, catch rate data demonstrated a constant decrease of the target species that was accompanied by a parallel increase in the capture of another gastropod species, the long sea snail, Tritia reticulata (Linnaeus, 1758; Fabi et al., 2006). These data are the result of a combination of several factors which include fishing pressure associated to catches of undersized individuals due to the inappropriate mesh size of traps, habitat loss/degradation causing reduced availability of spawning substrates and natural predation. All the above factors are potentially responsible for the decline of changeable nassa stocks along the north-central coast of the Adriatic Sea.
The present study aims to monitor T. mutabilis egg capsule deposition and intracapsular embryonic patterns using artificial substrates and innovative analytical approaches. In this regard, an application of machine learning for automatic counting of T. mutabilis egg capsules using digital images has been provided as well as an evaluation of using artificial substrates to enhance the reproductive success of changeable nassa in this coastal area. Such information is essential for promoting restocking interventions and improving the sustainable use and management of natural resources with a direct involvement of fisheries operators.
Methods
Study Area and Artificial Substrates
The study was carried out in the Southern Adriatic coastal area of the Marche region between the cities of San Benedetto del Tronto and Cupra Marittima (Province of Ascoli Piceno, Italy; Figure 1) using specific artificial substrates (i.e., pyramid structures with a square base measuring 40 cm in height and 50 cm in width, a lateral surface area of 0.1 m2/side and covered by a high strength nylon net) provided by the Blu Marine Service Soc. Coop. (San Benedetto del Tronto, AP, Italy) and previously tested by Caprioli and Giansante (2018). During the beginning of the T. mutabilis spawning period (i.e., February 2019), a total of 30 pyramids were placed onto the sea bottom within ten different sites (Figure 1) at a distance from the coast ranging from 0.7 to 4 nautical miles. The different sites were selected according to local small-scale fishermen which participated to the research activities placing the artificial substrates within their favorite fishing spots along with their fishing gears (i.e., basket traps). In each site, three pyramids were placed at about 30-m distance from each other in order to facilitating both inspection and monitoring procedures. Values of temperature, salinity, dissolved oxygen, and pH were recorded within the study area at each monitoring campaign (Supplementary Table 1).
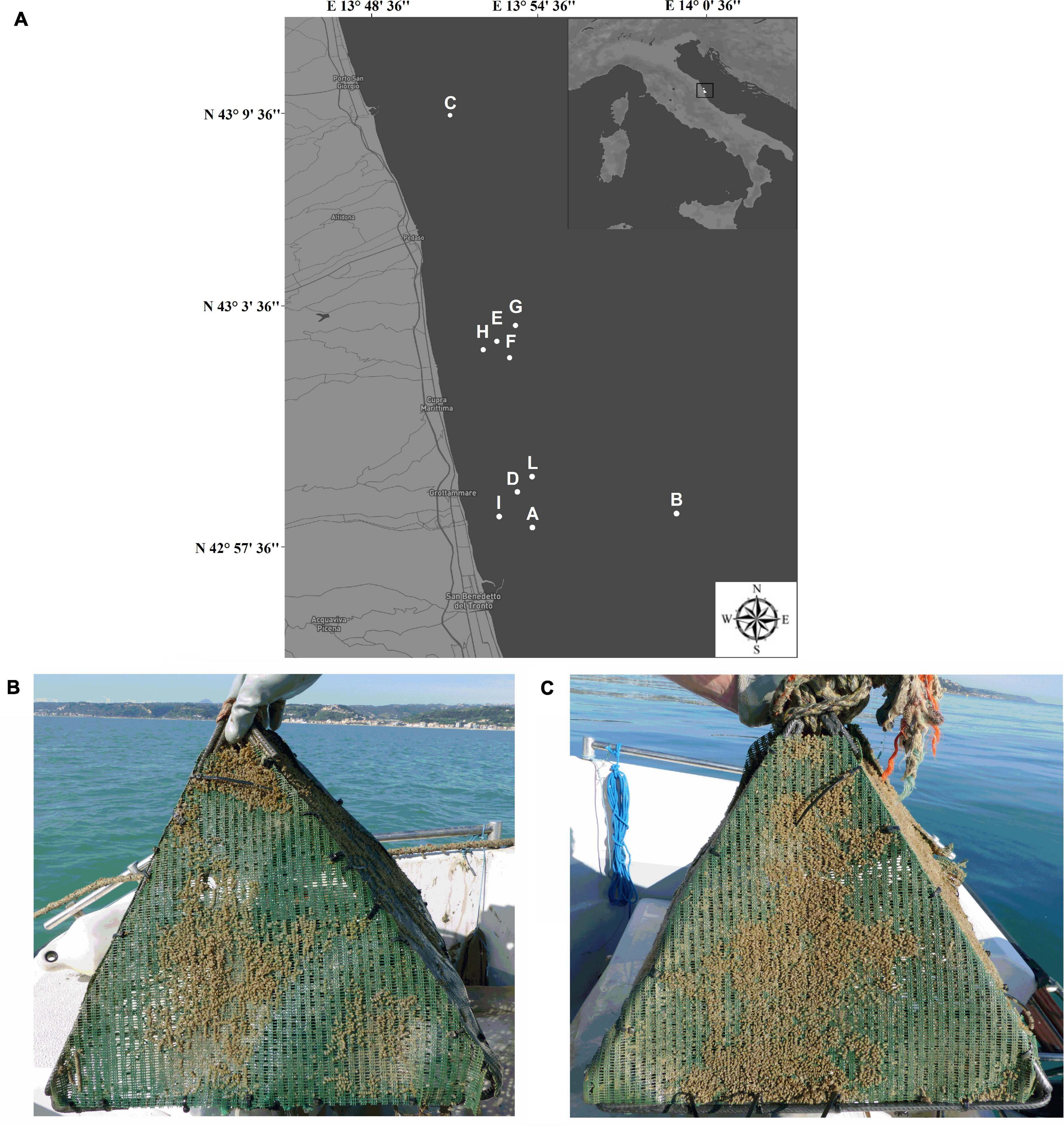
Figure 1. Map of the study area and monitoring locations (A). Representative images of artificial substrates during the first (B) and the second monitoring (C).
Data Collection
In each site, the status of artificial substrates was examined monthly from March to May 2019, by trained fishermen to remove dirt and other unwanted matter. The analysis of surface coverage per site was performed two times during the study period: the first in April and the second during May 2019. Deposition of egg capsules on each side of the pyramids was accurately analyzed and each structure was photographed using a digital camera (Cyber-shot DSC-HX300; Sony). Monitoring activities were integrated by monthly cutting of nylon net squares (50 mm × 50 mm) from each pyramid. In order to maintain structural integrity of pyramids during the entire experimental period, this sampling procedure was exclusively carried out within site B, selected as a representative fishing spot of the overall study area. These cuttings of net were taken to the laboratory for stereomicroscopic analysis (Carl Zeiss StemiTM) of capsule number/size and intracapsular embryonic development. Ethical review and approval were not required for this study because samples were opportunistically collected from substrates and referred to an observational monitoring.
Species Identification
Tritia mutabilis egg capsules were identified on the basis of their morphology that accords with the classification for the family Nassariidae proposed by D’Asaro (1993). Visual identification of capsules from other two cryptic species, T. reticulata (Linnaeus, 1758) and Tritia nitida (Jeffreys, 1867), was further confirmed by molecular analysis as described by Couceiro et al. (2012). Briefly, total DNA was extracted from two egg capsules with TriFastTM reagent following manufacturer’s instructions (VWR Company). Amplification was performed in a 25 μL solution containing 2 μL DNA, 0.4 μM of each primer (FW-AACAGCTCACGCTTTCGTAAT; REV-AGGAAGACGCTCAAAT; Couceiro et al., 2012) and 2X PCR Taq MasterMix (Applied Biological Materials Inc.). Thermocycling condition was for 3 min at 95°C following 40 cycles of 40 s at 95°C, 40 s at 54°C, and 60 s at 72°C. Amplicons were electrophoresed through 1% agarose gel (TopVision, Thermo ScientificTM) and visualized by staining with SafeViewTM Classic dye (Abm) to confirm the presence of 373 bp fragment. Samples were then digested with the appropriate restriction enzyme (10 U DdeI; ThermoFisher Scientific) and with 1 × Buffer Tango (ThermoFisher Scientific). The digested samples were then analyzed on 2.5% agarose gels (TopVision, Thermo ScientificTM) after SafeViewTM Classic dye (Abm) staining (Supplementary Figure 1). According to Couceiro et al. (2012), there are three DdeI recognition sites in T. reticulata and one in T. nitida.
Data Analysis
Color images (300 ppi) of T. mutabilis egg capsules deposited on the surface of each artificial substrate were processed using two distinct approaches: homography-based identification (HI) and deep learning neural networks identification (DLNNI). Homography python program was used to analyze the color images of layered eggs [program: homography.py, using Spyder 3.3.3 (python 3.7), libraries: cv2, numpy, argparse, FLANN based matcher]. A set (N = 240) of individual images showing T. mutabilis egg capsules deposited on pre-cut net squares (see section “Data collection”) was identified and used as a training set for the neural network. The sample images were labeled and converted into the format compatible with YOLOv3. YOLO (You Only Look Once) is a state-of-the-art, real-time object detection system, to train the object detector and it applies a single neural network to the full image for both classification and localization. YOLOv3 uses a custom variant of the Darknet architecture, darknet-53. The YOLO configuration parameters include batch = 24 to use 24 images for every training step and subdivisions = 8 to subdivide the batch by 8 to speed up the training process and encourage generalization. YOLO python implementation was used for the image detector training and image analysis [spyder 3.3.3 (python 3.7), libraries: cv2, numpy, darknet]. Additionally, the developed method was further validated by stereomicroscopic examination and manual counting of egg capsules deposited on the same pre-cut net squares. Data on egg capsules deposition are shown as both mean percentage of covered surface per site and total number per site. Hatching rate was calculated as the percentage of egg capsules showing apical opening. Number of embryos per egg capsule was calculated by stereomicroscopic (Carl Zeiss StemiTM) observation of random egg capsule samples from each site. Analysis of variance was used to test for differences in mean coverage percentages of pyramids among sites. Differences with p < 0.05 were considered statistically significant.
Stereomicroscopic Analysis of T. mutabilis Egg Capsules During Intracapsular Development
To investigate T. mutabilis intracapsular development, egg capsules deposited on nylon net squares sampled from the pyramids located into the site B were analyzed at stereomicroscope (Carl Zeiss Stemi) and classified on the basis of embryonic development. According to common phases that occur during marine gastropods intracapsular embryonic development, three stages have been identified: Stage 1: fertilized round eggs; Stage 2: initial development of the larvae; Stage 3: larvae with distinct protoconch, velum, and digestive tract (Yokoyama and Amaral, 2011). In addition, hatched capsules were distinguished for the presence of an apical opening.
Results and Discussion
In this work, deposition of T. mutabilis egg capsules along the Italian coastal waters of the Central Adriatic Sea (Marche region, Italy) was monitored using artificial substrates and innovative analytical approaches. The artificial substrates employed in the present study were square pyramids covered by a high strength nylon net and anchored on the sandy seabed in order to leave available for deposition a surface of about 0.4 m2 per structure (Figure 1). The usefulness of these types of structures in favoring deposition of T. mutabilis egg capsules was partially supported by recent pilot activities carried out along the coastal waters of the Abruzzi region (Caprioli and Giansante, 2018). In this last regard, our results confirm and integrate previous preliminary observations by providing a quantitative analysis of T. mutabilis spawning capacity during one breeding season within an area subjected to small-scale fishing activity.
Monitoring and counting of T. mutabilis egg capsules were carried out processing digital images of the artificial substrates through both HI and DLNNI approaches (Figures 2A,B). The two proposed methods have positively detected individual eggs in a complex eggs-layer showing small differences in egg count/cm2 values (n = 10; mean ± SD: HI, 10.2 ± 1.1; and DLNN1, 11.4 ± 1.0). In this regard, while HI has been demonstrated to be useful in detecting objects after roto-translation/perspective change, the DLNNI approach has proven to be useful in detecting egg capsules characterized by distorted morphology. Capsules are layered onto the surface with different roto-translational values, and both perspective angles (due in part by the observer) and egg shapes differ slightly within all images analyzed. This latter factor can in part influence the detection capability of HI.
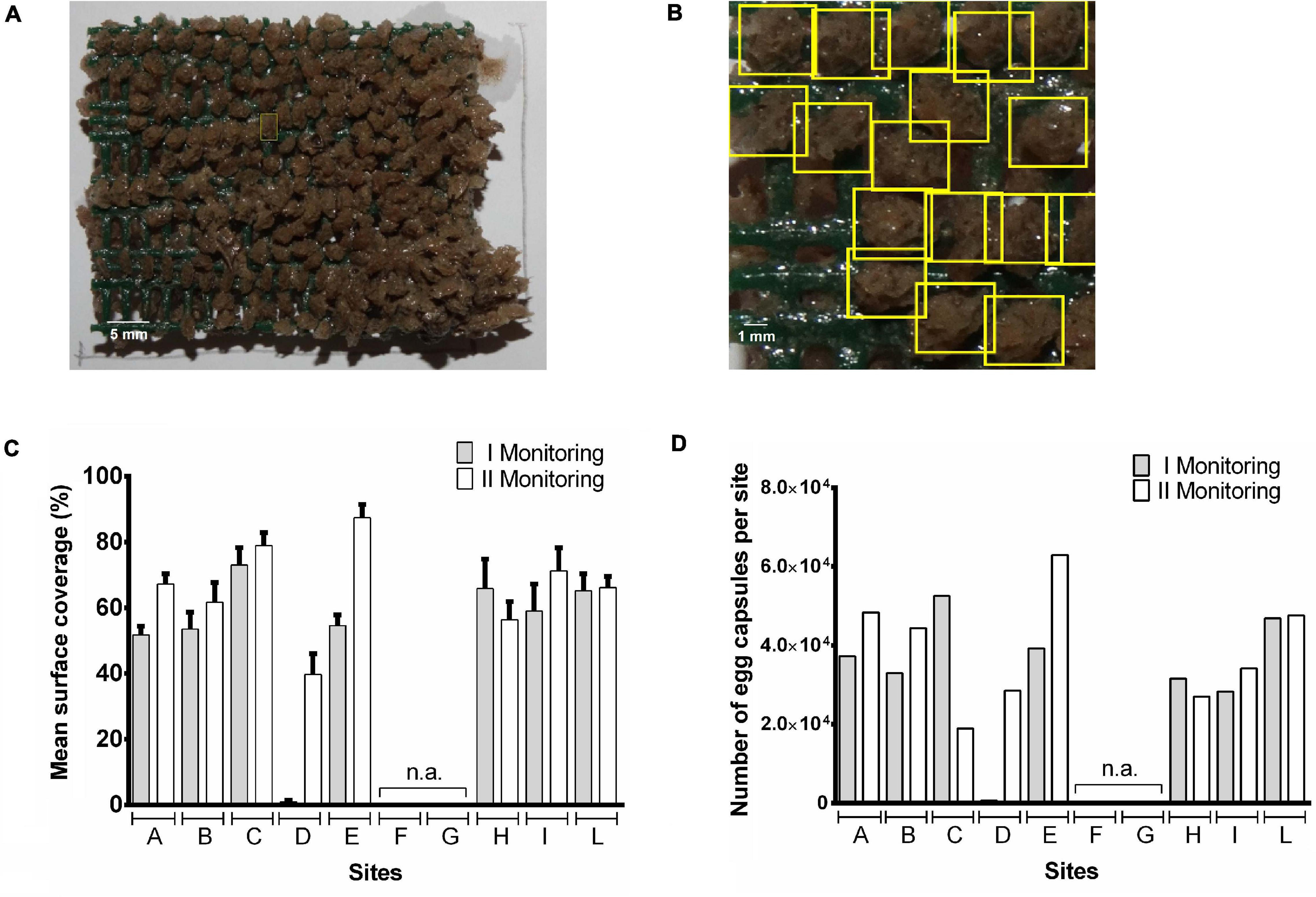
Figure 2. Automatic counting of Tritia mutabilis egg capsules deposited on artificial substrates by Digital Image Processing techniques associated with machine learning methods. Color images of T. mutabilis egg capsules deposited on the surface of each pyramid were processed using two distinct approaches: Homography-based Identification (HI; A) and Deep Learning Neural Networks Identification (DLNNI; B). Results are shown as both mean percentage of covered surface per site (C) and total number of egg capsules per site (D).
During the first monitoring (i.e., April 2019), egg capsules were deposited on the almost totality of the artificial substrates showing, however, a wide variability in the width of covered surface on each pyramid (Supplementary Table 2). This latter may be due to the meteorological conditions since some of the artificial substrates (e.g., within sites F, G, H, and I) suffered heavy damage from adverse marine conditions thus showing a reduction in the surface available for deposition. Low amounts of egg capsules were found on the pyramids belonging to the site D. Analyzing the data per site, the mean% of covered surface ranged from <1% (D site) to 73% (C site; Figure 2C). An overall coverage area close to 50% was observed in sites A, B, E, I with the highest coverage achieved in site C (73%), H (66%), L (65%), and the lowest in site D (<1%). The low coverage value observed at site D is most likely due to a delayed positioning of the structure that occurred in late March. For that reason, these data were not used for statistical comparison of monitoring sites. The number of egg capsules per site ranged from 690 (D site) to 52560 (C site) with a number of embryos per capsule included between 8 and 23 (mean number: 15.6 ± 3.5; n = 300; Figure 2D). With the exclusion of site D, there were no significant differences in the mean coverage percentage of pyramids among all other sites.
During the second monitoring (i.e., May 2019) two artificial substrates placed in the site C got lost after being wrecked by a sea storm. Thus, data collected from site C have been shown but not included in statistical analysis. However, our recorded data showed a general increase in the covered surface and egg capsules were found on each of the available artificial substrates (Supplementary Table 2). The mean coverage of pyramid surface per monitoring site reached values included between 40 and 87% while the number of egg capsules per site ranged from 27,060 (H site) to 62,940 (E site) with an overall increase with respect to the first monitoring ranging from 7% (L site) to 63% (E site; Figures 2C,D). Again, there were no statistically significant differences in% of covered surface per pyramid among sites. However, if we compare the percentage of overall covered area per site between the two monitoring periods, the highest increases were detectable in the A, B, D, and E sites which showed lower (<55%) mean percentage of covered area in April (Figure 2C). Given the substantial environmental homogeneity of the study area, this finding suggests that the rapid achievement of about 50% coverage of the artificial substrates is likely to slow further colonization of the structure (Figure 2C).
During the second monitoring, most of the pyramids presented hatched egg capsules indicating that the majority of larval release was occurred. In line with this observation, a recent study from Caprioli and Giansante (2018), carried out using similar approaches, reported very low levels of T. mutabilis egg capsule deposition between May and June. In this regard, the much higher coverage percentage observed in our study is probably due to a perfect matching between timing of substrate positioning and starting of T. mutabilis breeding season. Similar values were instead reported about the% of hatched capsules per each substrate. Caprioli and Giansante (2018) found that at least 87% of egg capsules hatched in mid-June. In our work, the calculated hatching rate was over 90% at 19.8°C during the early June and about 61% at 17.2°C in late May (Figure 3). These data suggest that the timing of hatching is strongly determined by water temperatures showing a specific range able to increase the developmental rates. However, the role of developing embryos themselves in directing hatching from inside the encapsulating structure remains unexplored in this species. There is evidence that both growth rate and embryo size are key influencing factors affecting the timing of hatching in many marine gastropod species (Andrade-Villagran et al., 2018). In the present work, the intracapsular embryonic development of T. mutabilis was recorded during the whole monitoring period by monitoring the frequency of three developmental stages (Figure 3). Results demonstrated that abundance of each stage was month-related with stage 1 and 2 more abundant in March and April, respectively. On the contrary, embryos at stage 3 development were largely found during May. These findings suggest the presence of a well-defined spawning season, with a deposition of egg capsules that starts in late February, reaches a peak in March/April and disappears in June.
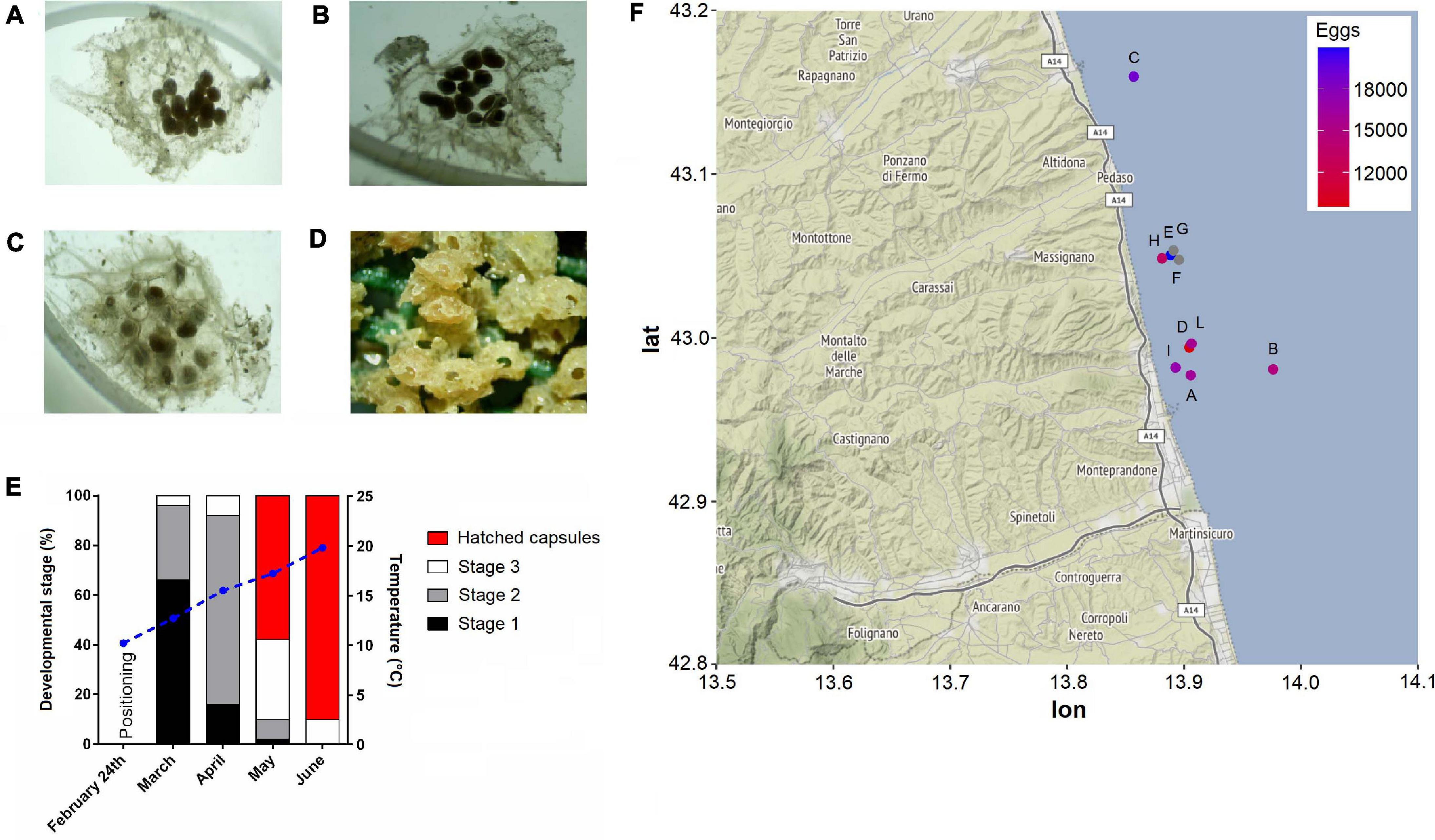
Figure 3. Tritia mutabilis intracapsular embryonic development. Representative light microscope images of stage 1 (A), stage 2 (B), stage 3 (C), and hatchling (D). Monthly percentage of each developmental stage according to the temperature variation (blue straight line; E). Heatmap of egg capsule amount (mean per pyramid) within each monitoring site (F). Gray dots represent sites (i.e., F, G) that were not analyzed.
Our field monitoring also demonstrated that beside T. mutabilis egg capsules, which constitute the vast majority, artificial substrates could favor oviposition of different species (e.g., cuttlefish, squid, and octopus), including other common marine snails, particularly T. reticulata (Figure 4). To assure identification of closely related snail species, the morphological analysis was integrated with validated molecular tools which have recently proved to be highly useful for estimating hidden cryptic biodiversity (Couceiro et al., 2012). Molecular data confirmed the presence of T. reticulata, but not T. nitida egg capsules. We found the presence of T. reticulata egg capsules on three sites (i.e, A, B, E) with the highest coverage of pyramid surface close to 2% (site B). This result supports previous observations which have reported a progressive increase in the capture rate of T. reticulata along the Italian coastal waters of the central Adriatic Sea (Felici et al., 2020). T. reticulata was found to occupy the same ecological niche of T. mutabilis, living on the same habitat and sharing similar feeding behavior (Fabi and Grati, 2005). However, this is a species of much lower commercial value compared to the common sea snail. Despite the overall low amount of T. reticulata egg capsules found attached on the surface of artificial substrates, it is worth mentioning that this species showed a mean number of embryos per capsule much higher than T. mutabilis: 90 ± 10 vs 15.6 ± 3.5 (mean ± SD). These findings highlight the importance of further studies investigating the potential ecological impact of this species.
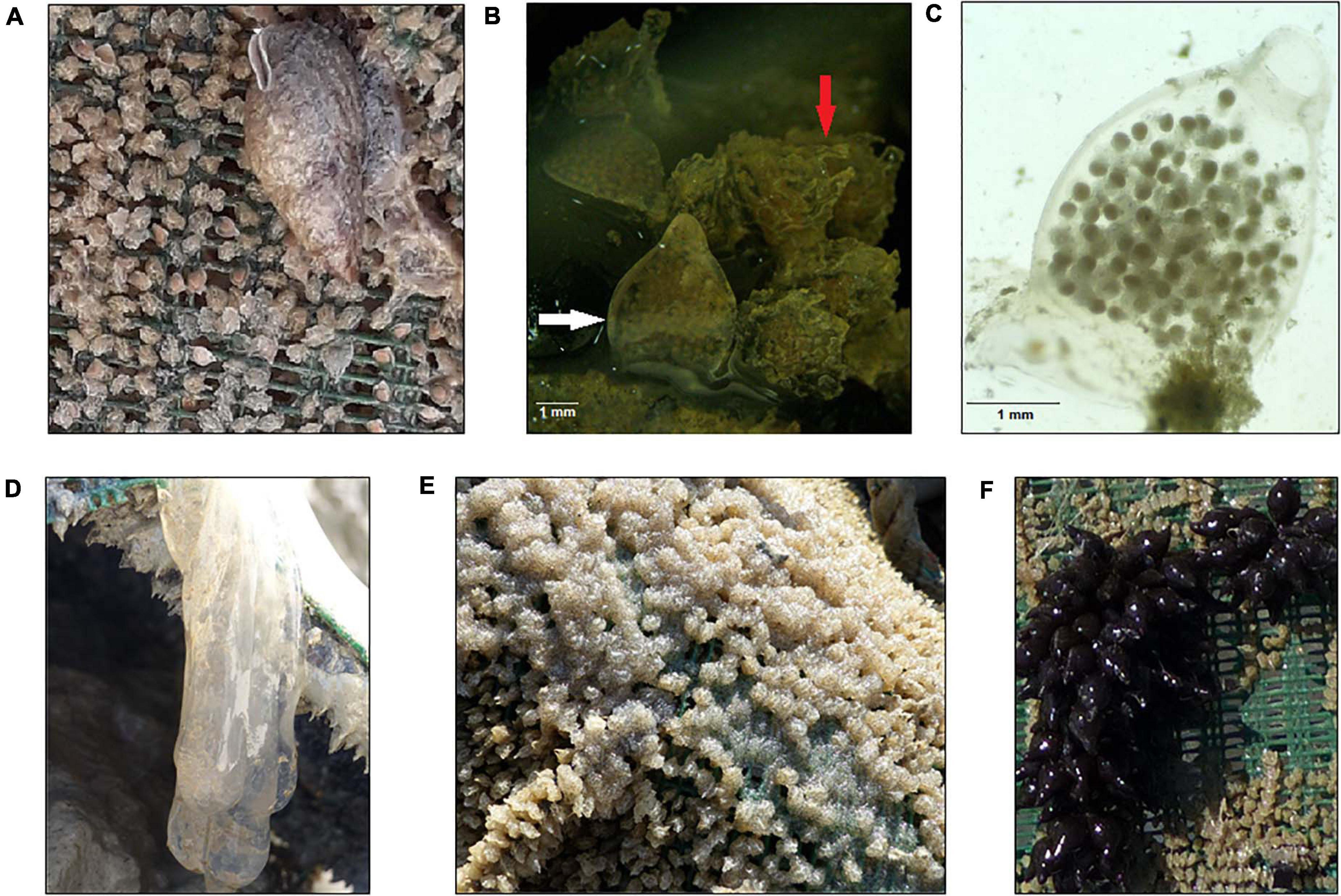
Figure 4. Artificial substrates favor egg deposition by different species, including other common marine snails. Tritia reticulata specimen (A), comparison between Tritia reticulata (white arrow) and Tritia mutabilis (red arrow) egg capsules (B), stereomicroscopic image of Tritia reticulata egg capsule (C), squid (D), octopus (E), and cuttlefish eggs (F).
Conclusion
The main findings of the present study help to enhance our knowledge on temporal trends in T. mutabilis egg capsules deposition pointing out the potential of artificial substrates in facilitating oviposition. Indeed, the study has demonstrated the high efficiency of these pyramids as egg collectors suggesting that additions of such structures to nearshore environments may contribute to improve spawning possibilities for T. mutabilis and favor restocking interventions. Given cost-effectiveness, easy handling and reduced maintenance of the proposed structures, this approach can be continually implemented with the direct involvement of local fishermen. In this regard, the Fisheries Local Action Group “Marche Sud” is taking action to provide free of charge these artificial structures to local small-scale fishermen in order to promote co-management approaches to natural resources.
In addition, we have also developed a deep learning-based method that can speed out egg capsule counting and reduce time-consuming stereomicroscopic analysis during long-term field monitoring activities. Identification of egg capsule deposition patterns together with monitoring of intracapsular developmental stages may be useful to improve the resource management measures. However, a scientific evaluation of restocking efficacy using these artificial substrate types as well as their role in contributing to the spread of other species, including invasive alien species, are still warranted.
Data Availability Statement
The original contributions presented in the study are included in the article/Supplementary Material, further inquiries can be directed to the corresponding author/s.
Author Contributions
PC and FP designed the study and wrote the manuscript. PC and MA performed the data analysis. ET provided the artificial substrates and supported activities at sea. All authors reviewed and approved the final version of the manuscript.
Funding
This work was supported by the Fisheries Local Action Group (FLAG) “Marche Sud” – Action Plan: 2.A.2 – Risorsa ittica.
Conflict of Interest
ET was employed by the company Blu Marine Service Soc. Coop.
The remaining authors declare that the research was conducted in the absence of any commercial or financial relationships that could be construed as a potential conflict of interest.
Supplementary Material
The Supplementary Material for this article can be found online at: https://www.frontiersin.org/articles/10.3389/fmars.2021.661389/full#supplementary-material
References
Aguilar, R., Perry, A., and López, J. (2017). “Conservation and Management of Vulnerable Marine Benthic Ecosystems,” in Marine Animal Forests, eds S. Rossi, L. Bramanti, A. Gori, and C. Orejas (Cham: Springer).
Andrade-Villagran, P. V., Baria, K. S., Montory, J. A., Pechenik, J. A., and Chaparro, O. R. (2018). The influence of encapsulated embryos on the timing of hatching in the brooding gastropod Crepipatella dilatata. Journal of Sea Research 131, 69–78. doi: 10.1016/j.seares.2017.11.004
Bakır, B. B., Öztürk, B., Doğan, A., and Önen, M. (2012). Mollusc Fauna of Iskenderun Bay with a Checklist of the Region. Turkish Journal of Fisheries and Aquatic Sciences 12, 171–184.
Barash, A., and Zenziper, Z. (1980). Egg masses of Mollusca from Mediterranean waters ofIsrael and notes on reproduction of the freshwater species Theodoxus jordani and Melanoides tubercula. Veliger 22, 299–317.
Caprioli, R., and Giansante, C. (2018). Preliminary investigation on the use of artificial substrates to favor Tritia mutabilis (Linnaeus, 1758) spawning in Central Adriatic Sea: a possible contribution to stock maintenance. Acta Adriatica 59, 141–148. doi: 10.32582/Aa.59.1.12
Carrasco, S. A., and Phillips, N. E. (2014). Encapsulation and development of three New Zealand neogastropods with contrasting embryo packaging and maternal provisioning. New Zealand Journal of Zoology 41, 171–186. doi: 10.1080/03014223.2014.921205
Cespuglio, G., Piccinetti, C., and Longinelli, A. (1999). Oxygen and carbon isotope profiles from Nassa mutabilis shells (Gastropoda): accretion rates and biological behaviour. Marine Biology 135, 627–634. doi: 10.1007/s002270050663
Corte, G. N., Yokoyama, L. Q., Denadai, M. R., Bessa, E., Salles, M. C. Z., and Turra, A. (2019). Egg-capsule deposition of the marine gastropod Olivella minuta: the importance of an interspecific relationship with the soft-bottom bivalve Tivela mactroides. Journal of Molluscan Studies 85, 126–132. doi: 10.1093/mollus/eyy060
Couceiro, L., Lopez, L., Sotka, E. E., Ruiz, J. M., and Barreiro, R. (2012). Molecular data delineate cryptic Nassarius species and characterize spatial genetic structure of N. nitidus. Journal of the Marine Biological Association of the United Kingdom 92, 1175–1182. doi: 10.1017/S0025315411000816
D’Asaro, C. N. (1993). Gunnar Thorson’s worldwide collection of Prosobranch egg capsules: Nassariidae. Ophelia. 38, 149–215. doi: 10.1080/00785326.1993.10429896
Dumont, C. P., Roy, J. S., and Himmelman, J. H. (2008). Predation by the sea urchin Strongylocentrotus droebachiensis on capsular egg masses of the whelk Buccinum undatum. Journal of the Marine Biological Association of the United Kingdom 88, 1025–1031. doi: 10.1017/S0025315408001628
Fabi, G., and Grati, F. (2005). Small-scale fisheries in the maritime department of Ancona (Central Northern Adriatic Sea). AdriaMed Technical Documents 15, 85–97.
Fabi, G., Grati, F., De Mauro, M., and Polidori, P. (2006). Distribuzione Spaziale e Densità di Nassarius mutabilis (L.) e Nassarius reticularis (L.) nel Compartimento Marittimo di Ancona. Biologia Marina Mediterranea 13, 240–241.
Felici, A., Bilandzic, N., Magi, G. E., Iaffaldano, N., Fiordelmondo, E., Doti, G., et al. (2020). Evaluation of Long Sea Snail Hinia reticulata (Gastropod) from the Middle Adriatic Sea as a Possible Alternative for Human Consumption. Foods 9, 905. doi: 10.3390/Foods9070905
Fiordelmondo, E., Roncarati, A., Vincenzetti, S., Pinzaru, S. C., and Felici, A. (2020). Sterol and Mineral Profiles of the Common Sea Snail Hinia reticulata and the Long Sea Snail Nassarius mutabilis (Gastropods) Collected from the Middle Adriatic Sea. Current Research in Nutrition and Food Science 8, 757–764. doi: 10.12944/Crnfsj.8.3.08
Ganias, K., Perri, R., and Moditsis, A. (2021). Cuttlefish and squid egg deposition patterns on artificial devices and trap-like gears: implications for offspring survival and population management. ICES Journal of Marine Science. doi: 10.1093/icesjms/fsab062 ∗∗QVPG
Grati, F., Fabi, G., Scarcella, G., Guicciardi, S., Penna, P., Scanu, M., et al. (2018). Artificial spawning substrates and participa-tory research to foster cuttlefish stock recovery: a pilot study inthe Adriatic Sea. PLoS One 13:e0205877. doi: 10.1371/journal.pone.0205877
Grati, F., Polidori, P., Scarcella, G., and Fabi, G. (2010). Estimation of basket trap selectivity for changeable nassa (Nassarius mutabilis) in the Adriatic Sea. Fisheries Research 101, 100–107. doi: 10.1016/j.fishres.2009.09.012
Kaviarasan, T., Sivasankar, R., and Tenjing, S. Y. (2020). Structure and Mechanical Properties of Gastropod Egg Capsules from Southeast Coast of India. Proceedings of the Zoological Society 73, 192–197. doi: 10.1007/s12595-018-0286-7
Pechenik, J. A. (1983). Egg capsules of Nucella lapillus (L.) protect against low-salinity stress. Journal of Experimental Marine Biology and Ecology 71, 165–179. doi: 10.1016/0022-0981(93)90071-u
Polidori, P., Grati, F., Bolognini, L., Domenichetti, F., Scarcella, G., and Fabi, G. (2015). Towards a better management of Nassarius mutabilis (Linnaeus, 1758): biometric and biological integrative study. Acta Adriatica 56, 233–244.
Przeslawski, R., and Davis, A. R. (2007). Does spawning behavior minimize exposure to environmental stressors for encapsulated gastropod embryos on rocky shores? Marine Biology 152, 991–1002. doi: 10.1007/s00227-007-0748-3
Rawlings, T. A. (1994). Encapsulation of Eggs by Marine Gastropods - Effect of Variation in Capsule Form on the Vulnerability of Embryos to Predation. Evolution 48, 1301–1313. doi: 10.2307/2410387
Rawlings, T. A. (1999). Adaptations to physical stresses in the intertidal zone: The egg capsules of neogastropod molluscs. American Zoologist 39, 230–243. doi: 10.1093/icb/39.2.230
von Dassow, Y. J., and Strathmann, R. R. (2005). Full of eggs and no place to lay them: hidden cost of benthic development. Marine Ecology Progress Series 294, 23–34. doi: 10.3354/Meps294023
Keywords: Adriatic Sea, biomonitoring, artificial substrate, Tritia mutabilis, machine learning
Citation: Cocci P, Troli E, Angeletti M and Palermo FA (2021) Field Monitoring of Tritia mutabilis (Linnaeus, 1758) Egg Capsule Deposition and Intracapsular Embryonic Patterns Using Artificial Substrates and Machine Learning-Based Approaches. Front. Mar. Sci. 8:661389. doi: 10.3389/fmars.2021.661389
Received: 30 January 2021; Accepted: 28 May 2021;
Published: 18 June 2021.
Edited by:
Morten Omholt Alver, Norwegian University of Science and Technology, NorwayReviewed by:
Katerina Vasileiadou, Hellenic Centre for Marine Research (HCMR), GreecePierluigi Strafella, National Research Council (CNR), Italy
Jan A. Pechenik, Tufts University, United States
Copyright © 2021 Cocci, Troli, Angeletti and Palermo. This is an open-access article distributed under the terms of the Creative Commons Attribution License (CC BY). The use, distribution or reproduction in other forums is permitted, provided the original author(s) and the copyright owner(s) are credited and that the original publication in this journal is cited, in accordance with accepted academic practice. No use, distribution or reproduction is permitted which does not comply with these terms.
*Correspondence: Paolo Cocci, paolo.cocci@unicam.it