- 1School of Medical Technology, Tianjin Medical University, Tianjin, China
- 2State Key Laboratory of Experimental Hematology, National Clinical Research Center for Blood Diseases, Institute of Hematology & Blood Diseases Hospital, Chinese Academy of Medical Sciences & Peking Union Medical College, Tianjin, China
- 3Clinical Laboratory, First Teaching Hospital of Tianjin University of Traditional Chinese Medicine, Tianjin, China
- 4Clinical Laboratory, Zhu Xianyi Memorial Hospital of Tianjin Medical University, Tianjin, China
- 5Shandong Wan'an Pharmaceutical Co., Ltd, Shandong, China
The environmental oxygen concentration is a crucial factor affecting cell proliferation. Owing to the reversible binding property of hemoglobin to oxygen, it can be utilized to regulate the oxygen concentration in vitro, and its ability to reduce apoptosis can be evaluated. In this study, a process comprising isolation, purification, and extraction was used to obtain hemoglobin from Perinereis aibuhitensis, a polychaete invertebrate. Extracts were separated and characterized using sodium dodecyl sulfate-polyacrylamide gel electrophoresis. Extract component identity was confirmed by matrix-assisted laser desorption time-of-flight mass spectrometry analysis, with the molecular weight determined as 412,216.6875 Da. The oxygen carrying capacity of P. aibuhitensis hemoglobin was comparable with that of human hemoglobin. P. aibuhitensis hemoglobin remarkably downregulated the apoptosis rate. Reactive oxygen species (ROS) assays confirmed the reduction in ROS production, enabling a better elucidation of the mechanism underlying the decrease in apoptosis. These results suggested that P. aibuhitensis hemoglobin is a natural oxygen carrier, that, owing to its low-cost and accessibility, can be considered a candidate for culture medium supplement to reduce the apoptosis rate.
Introduction
Perinereis aibuhitensis (PA) is a polychaete invertebrate that inhabits the estuaries, mangroves, and mud flats common in tropical and subtropical Asia. It is widely distributed along the coasts and estuaries of the Northwest Pacific. Adult worms, about 17–30 cm in length, mainly inhabit muddy sediments (Tian et al., 2019). Recently, PA has been increasingly used in marine ecotoxicological studies (Zhang et al., 2017; Jiang et al., 2019), a resultant of its important role in the fate of chemicals in the sediments owing to its biological distribution, roles in particle mixing and irrigation, and influence on metal forms (Cong et al., 2011; Tong et al., 2019).
Biological agents extracted from PA have been used for antithrombotic food development (Li et al., 2017). Extracts exhibit obvious inhibition of the intrinsic coagulation pathway and a slight inhibition of the extrinsic coagulation pathway. In addition, PA can effectively inhibit fibrinogen to fibrin conversion, which is induced by thrombin. Hemoglobin (Hb) from PA comprise single-chain globin (a), disulfide bridged globin trimer (A-b-B), disulfide bridged non-globin dimers (or linkers; L1–L1, L2–L2, and L1–L2), and oligomers of L1-L2 [(L1–L2)n] (Yamaki et al., 1996). Polypeptide chains of Hb comprise four types of globin chains (a, A, b, and B) and two types of non-heme chains (L1, L2) (Yamaki et al., 1998). Transmission electron microscopy (TEM) measurements to monitor the hemoglobin structure have revealed that it contains 12 similar units (called submultiples), forming a double layer of two sets of six hexagonally arranged submultiples (Ebina et al., 1995; Gotoh et al., 1998). Besides studying the structure, researchers in this study also investigated hemoglobin function. The light absorption spectra of oxy, deoxy, CO, met, and cyanomet derivatives of PA hemoglobin were like those for human hemoglobin. Oxygen affinity and cooperativity of the PA hemoglobin are pH dependent. P50 of oxygen equilibrium curve is 15.5 mmHg at pH 7.4 (Tsuneshige et al., 1989). The activation energy of the autoxidation reaction of the hemoglobin was calculated to be 30.5 kcal/mol at pH 7.2 (Suzuki et al., 1983).
Chinese hamster ovary (CHO) is one of the most widely used host cell lines of eukaryotic expression systems, owing to its high recombinant protein expression ability (Dingermann, 2008). Reactive oxygen species (ROS) are generated during aerobic metabolism. The production of excessive ROS damages the cell membrane structure and leads to apoptosis. Apoptosis occurs in large-scale cell culture and usually causes a decline in cell viability.
Hemoglobin acts as an oxygen carrier, which can reversibly combine with oxygen, depending on different oxygen pressures. Several attempts have been made to apply oxygen carriers, such as expired human erythrocyte or cord or bovine erythrocyte, to trauma surgery, organ preservation, and the treatment of ischemia-reperfusion (Wu et al., 2011; Matton et al., 2018; Yang et al., 2018). Compared with mammalian hemoglobin, PA hemoglobin was stable and resistant to autooxidation (Carvalho et al., 2019). Animal experiments indicated that purified hemoglobin from Lumbricus terrestris could be used for oxygen therapeutic applications (Savla et al., 2020). The extracellular hemoglobin of Arenicola marina, with its advantage of having high oxygen affinity and low immunoreactivity, was regarded as a potential blood substitute (Rousselot et al., 2006). Moreover, multiple studies have demonstrated the extensive application of invertebrate hemoglobin on oxygen carriers.
This study focused on the feasibility of using hemoglobin from PA as a cell culture supplement, owing to its characteristics and oxygen binding properties being comparable with those of human hemoglobin. The study aimed to investigate changes that occur in the cell apoptosis process by adding this hemoglobin extract to culture medium. PA hemoglobin is easily available and can be extracted via a simple process. High molecular weight, the absence of cell membranes, and the high oxygen carrying capacity enable PA hemoglobin to act as a cell culture medium supplement by maintaining an adequate oxygen environment.
Materials and Methods
Materials
P. aibuhitensis was collected from the Eastern Coast of Shandong Peninsula, China, gifted by Shandong Wan'an Pharmaceutical Co., Ltd. The length, width, and weight of PA is ~12.4 cm, 0.8 cm, and 3.2 g, respectively. All animal experiments complied with the ARRIVE guidelines and were conducted under the U.K. Animals (Scientific Procedures) Act 1986, and associated guidelines. Worms were kept alive in local seawater between 4 and 10°C during transport. CHO cell line was purchased from the American Type Culture Collection (ATCC) and cultured in F12-K (HyClone, SH30526.01) supplemented with 10% fetal bovine serum (FBS;10099141, Gibco). Cells were incubated at 37°C in a humidified incubator (HERAcell 240i, Thermo Fisher Scientific, CA, USA) containing 5% carbon dioxide (CO2) in air. Dialysis CE membrane (31–1000 kD) was purchased from SPECTRUMLABS (CA, USA), and the Sephadex G25 gel was purchased from Macklin Biochemical Co., Ltd (S822634, Shanghai, China). Annexin-V-fluorescein isothiocyanate (FITC) apoptosis detection kit (KGA108), Express Plus TM PAGE gels (4–20%) (KGMG420W10), and MTT [3-(4,5-dimethylthiazol-2-yl)-2,5-diphenyl tetrazolium bromide] cell proliferation and cytotoxicity test kit (KGA311) were purchased from KeyGEN Biotech Co., Ltd (Nanjing, China). ROS assay kit (S0033) was purchased from Beyotime Biotech Co., Ltd (Shanghai, China). Bicinchoninic acid (BCA) protein assay kit (PA115) was purchased from TIANGEN Biotech Co., Ltd (Beijing, China).
Isolation and Purification
Extraction was conducted within 24 h after transport. The homogenate was prepared by adding 100 g of worms and an equal-volume phosphate buffer saline (PBS) (pH 7.2) in a blender, and the homogenate was immediately centrifuged at 12,000 rpm (~16,000 × g) for 30 min, after which the supernatant was collected and the cell debris discarded.
After adding 0.1 mol/L of protease inhibitor phenylmethylsulfonyl fluoride (PMSF) to the samples extracted in the previous step, the supernatant was recentrifuged under the same conditions, followed by ammonium sulfate fractionation. Saturated ammonium sulfate was added to the samples while continuously stirring until a flocculent precipitate appeared. The mixture was centrifuged at 12,000 rpm (~16,000 × g) for 40 min. The supernatant was discarded and the red-colored precipitate resuspended in PBS. Following the supernatant dialysis with PBS (pH 7.2), using the dialysis membrane for 48 h, the Hb solution was purified by gel filtration on a 1 × 20 cm Sephadex G-25 column. The column was equilibrated with PBS. The flow was standardized to be smooth and was conducted at 0.5 mL/min. The red eluate was collected. The final product obtained in the steps is a PA hemoglobin solution.
The solution concentration was measured using BCA protein assay kit according to the instructions of the manufacturer. Hb solution absorption was measured at 450 nm by using a microplate reader (Synergy 2, Biotek, VT, USA) and bovine serum albumin as the standard.
Matrix-Assisted Laser Desorption Time-of-Flight Mass Spectrometry
Mass spectrometric analyses were conducted using AB 5800 Matrix-assisted laser desorption time-of-flight mass spectrometry (MALDI-TOF/TOF; AB SCIEX, USA) set in linear mode. Mass range was set between 3,000 and 500,000. Ion generation was achieved using a 60 Hz N2-cartridge-laser, operated at 337 nm. A matrix comprising 2,5-dihydroxy-benzoic acid (DHB) and α-cyano-4-hydroxycinnamic acid (CHCA) were provided by Bruker, and used to prepare a saturated solution of 50% acetonitrile with added 0.1% methanoic acid.
Molecular masses were based on the atomic masses of the elements. Raw mass spectrum data were processed with isotope pattern and data base system using the Data ExplorerTM analysis software.
Sodium Dodecyl Sulfate–Polyacrylamide Gel Electrophoresis
Sodium dodecyl sulfate–polyacrylamide gel electrophoresis (SDS-PAGE) was conducted on the slab gels using the discontinuous buffer system. The gel was purchased from KeyGEN Biotech (4–20%). Samples were added into the loading buffer with and without β-mercaptoethanol. Samples with β-mercaptoethanol were heated at 97°C for 5 min. Both types of samples were loaded on the gel and were run for 30 min at 80 V. After the samples reached the resolving gel, the conversion voltage was changed to 120 V and the samples were electrophoresed for 70 min for protein separation. The protein-containing gel was placed in Coomassie Blue dye R-250 and stained for 2 h. The gel was then decolorized with alcohol and scanned using the BioRad Gel Doc XR+.
Oxygen Carrying Capacity
Human EDTA-K2 anticoagulated blood was centrifuged at 2,000 rpm (~450 g) for 3 min to separate out the plasma, and the erythrocytes were washed twice using PBS. Erythrocytes were resuspended in 10 × volume of ddH2O to lyse the cells. Cell debris was then separated by centrifugation at 12,000 rpm (~16,000 × g) for 3 min. PA hemoglobin was also extracted and purified using the same method. The O2 carrying capacity of blood was quantified using a blood gas analyzer (ABL80, Radiometer, Copenhagen, Denmark).
MTT Assay
In vitro cell proliferation and cytotoxic activity were evaluated using the colorimetric MTT assay (Mosmann, 1983). Briefly, CHO cells were seeded into a 96-well plate at a 1 × 104 per well density. After 24 h incubation, the culture medium was removed from the plate and fresh medium, with and without the extracted hemoglobin, was added and the cells cultured for 24 h. After 24 h stimulation, 100 μL fresh medium without FBS was replaced in the wells and 10 μL MTT reagent was simultaneously added to each well. The plate was placed in a humidified incubator for 3 h, causing the MTT to be cleaved into formazan by the mitochondrial enzyme succinate-dehydrogenase, following which the MTT solution was discarded. The resultant formazan crystal was dissolved in dimethyl sulfoxide (150 μL) for absorbance measurement at 490 nm. Absorption spectra of the wells were recorded using a microplate reader (SynergyTM 2, Biotek, USA).
Determination of Apoptosis Rate by FACS Analysis
Flow cytometric analysis was performed to examine the apoptosis reducing potential of PA hemoglobin. The apoptosis rate was analyzed using FITC Annexin-V and Propidium Iodide (PI) detection kit according to instructions of the manufacturer. Briefly, CHO cells were seeded into a 12-well plate at a 1 × 105 per well density. After cultivation for 24 h, the culture medium was removed and fresh medium with or without corresponding hemoglobin was added to continue culture for 48 or 72 h. After stimulation, cells were washed twice with PBS and harvested using the cell scraper, followed by incubation in 500 μL binding buffer containing 5 μL of FITC Annexin-V and 5 μL of PI for dyeing at 25°C in the dark. Cells were then vortexed adequately and analyzed by flow cytometric analysis (FACS Verse, BD Biosciences, Franklin Lakes, USA) within 1 h, and data were analyzed by FlowJo V10 (De Novo Software, Glendale, CA, USA). Ten thousand events were analyzed in each run.
Determination of ROS by FACS Analysis
Flow cytometric analysis was performed to examine ROS levels in cells. ROS was determined using the ROS kit according to the instructions of the manufacturer. CHO cells were treated with and without hemoglobin according as mentioned above. Cells were then washed twice with serum-free medium and incubated with 100 μM (final concentration) 2,7-dichlorodi-hydrofluorescein diacetate (DCFH-DA) ROS reagent for 1 h. Cells were then washed gently, harvested, and resuspended using serum-free medium. Intracellular ROS generation was detected using flow cytometric analysis (FACS Verse, BD Biosciences, Franklin Lakes, USA), and FITC channel was chosen. A total of 10,000 cells were recorded. Data were analyzed by FlowJo V10 (De Novo Software, Glendale, CA, USA). Ten thousand events were analyzed in each run.
Statistical Analysis
Each experiment was repeated at least twice to verify data reproducibility. Data were analyzed using GraphPad Prism 6.0 software according to the protocol of the manufacturer. Student's t-test was performed on the blood gas analysis data, MTT, and the FACS results to compare the oxygen carrying capacities of PA hemoglobin and human hemoglobin. Cell proliferation, apoptosis rate, and ROS production of the hemoglobin group were compared to the control group using the Student's t-test. A p-value < 0.05 was accepted as statistically significant. Data are presented as mean ± SEM.
Results
Purification of PA Hemoglobin and SDS-PAGE
The centrifuged supernatant containing PA hemoglobin was purified using dialysis and chromatography, presenting a red transparent eluate. Solution concentration was calculated to be 10 μg/μL. The apparent molecular weight (MW) of proteins could be determined by the protein migration distance after being complexed with SDS, a strong cationic detergent, and being separated by SDS-PAGE (Matsumoto et al., 2019). The protein was separated under non-reducing and reducing conditions created by the absence and presence of β-mercaptoethanol, respectively, before loading the samples for electrophoresis. Figure 1 shows the SDS-PAGE image of PA Hb. In line 2 (denoting the non-reduced Hb) the major band appeared near 43 kD, whereas the light band appeared near 13 kD. In line 3, the major band appeared near 17 kD, whereas the light band appeared near 32 kD.
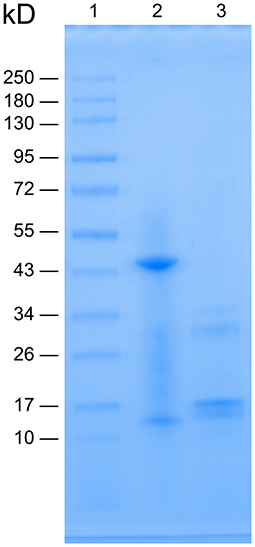
Figure 1. SDS-PAGE image of Perinereis aibuhitensis hemoglobin. Line 1: molecular weight standards; Line 2: non-reduced Hb without β-mercaptoethanol treatment and heating at 97°C for 5 min; Line 3: reduced Hb treated with β-mercaptoethanol and heating at 97°C for 5 min.
Confirmation of Identity and Molecular Weight of PA Hemoglobin by MALDI-TOF-MS
MS spectra of the hemoglobin solution were visually analyzed using Data ExplorerTM analysis software. Figure 2 shows spectra for MALDI-TOF-MS analysis of PA hemoglobin. The extents between mass values 390,000–417,000 is shown as the peak could be observed distinctly. The centroid mass representing the MW was 412,216.6875 Da.
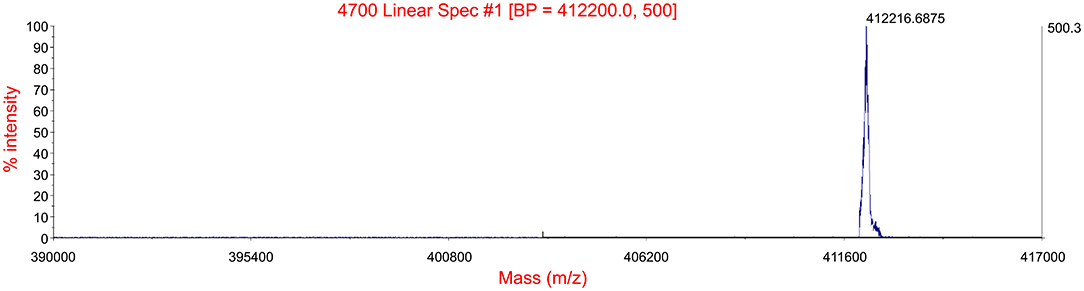
Figure 2. Molecular weight validation using MALDI-TOF-MS. Intact mass of PA hemoglobin analyzed by MALDI-TOF mass spectrometry. The image shows spectrums between mass values from 390,000 to 417,000. The single peak has a mass of 412,216.6875.
PA Hemoglobin Oxygen Carrying Capacity
Hemoglobin extracted at three times from human and PA were analyzed with a blood gas analyzer for the oxygen carrying capacity (Table 1). Oxygen saturation of human Hb was 99.50 ± 0.10%, whereas PA Hb was 99.60 ± 0.06%. The temperature-corrected oxygen partial pressure of human Hb was 190.70 ± 19.37 mmHg whereas PA Hb was 186.00 ± 13.43 mmHg. Therefore, PA Hb was inferred to have a high oxygen carrying capacity, similar to that of human Hb (p ≥ 0.05).
Effect of PA Hemoglobin on CHO Cells Proliferation and Cytotoxicity
P. aibuhitensis Hb effects on CHO cells were estimated by the MTT assay over a wide range of concentration. Hemoglobin was diluted using a double dilution method and added to the cell culture medium at the concentration range from 160 to 0.625 μg/mL. Results in Figure 3 indicate there was no significant change observed in cell viability at a dilution of 1:12800 as compared with the untreated group, proving that cell proliferation capacity is independent of exposure to PA Hb at this concentration. Therefore, 0.625 μg/mL was selected as the appropriate concentration of PA Hb for the subsequent experiment performed to analyze cell apoptosis and detect ROS.
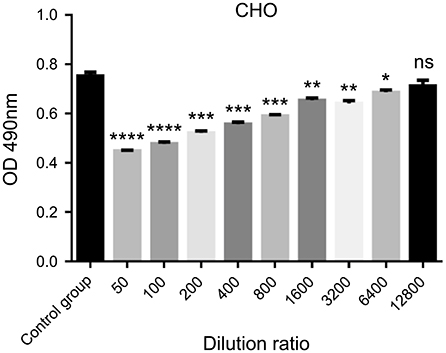
Figure 3. Cell proliferation and cytotoxicity test of Perinereis aibuhitensis hemoglobin. Results are expressed as means ± SEM. ****p < 0.0001; ***p < 0.001; **p < 0.01; *p < 0.05; ns, non-significant. Statistical analysis was performed using the Student's t-test and the control group was used for comparison.
PA Hemoglobin Decreases the Rate of Apoptosis
After FITC Annexin-V and PI dual staining, the late apoptotic cells were seen to be distributed in the upper-right quadrant, and the early apoptotic cells in the lower-right quadrant. As shown in Figure 4, late-stage apoptosis rate of cells treated with PA Hb for 48 h (19.65 ± 0.15%) was significantly decreased compared with the untreated group (25.05 ± 0.65%) (p < 0.05). This effect was more obvious at 72 h in the PA Hb group (20.65 ± 1.05%) compared with the untreated group (39.75 ± 0.95%) (p < 0.01). These results showed that PA hemoglobin decreases the cell apoptosis rate.
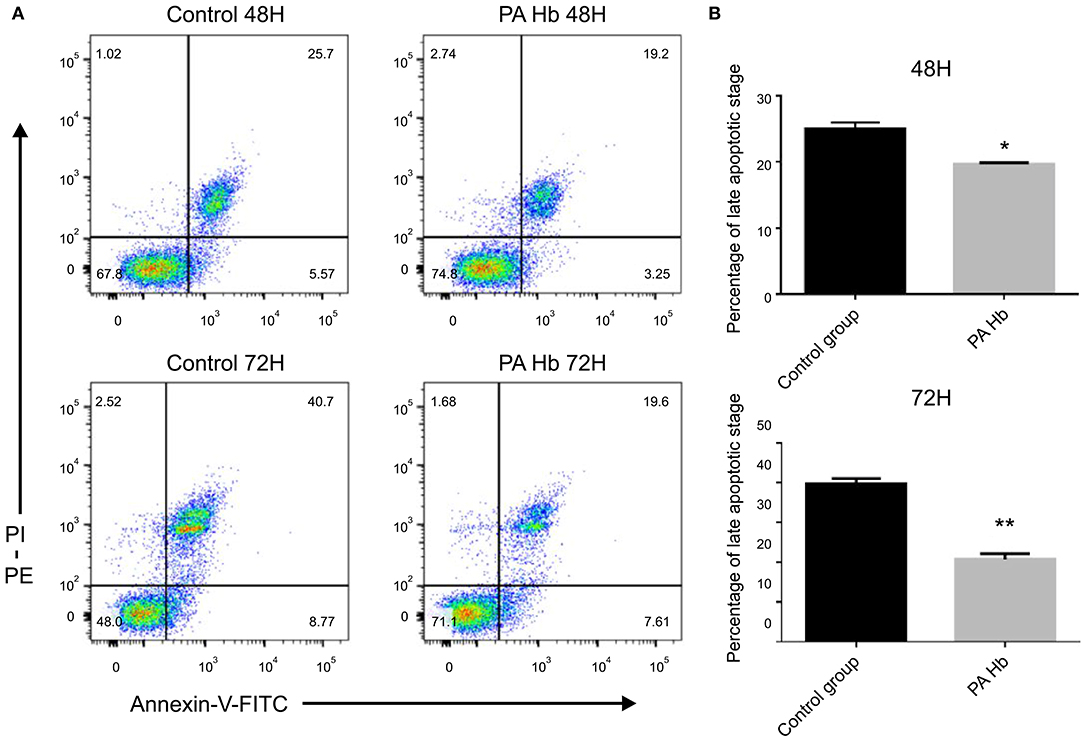
Figure 4. PA hemoglobin decreases the rate of apoptosis. Cells were treated with and without PA Hb for 48 or 72 h at the concentration of 0.625μg/mL, and analyzed by Annexin-V-FITC/PI staining. Apoptosis rate was presented as a percentage of total nucleic number. (A) Representative dot blot image of stained cells. (B) Statistical analysis of the late apoptotic cells proportion. **p < 0.01; *p < 0.05. Results are expressed as means ± SEM. Statistical analysis was performed using the Student's t-test and the control group was used for comparison.
PA Hemoglobin Reduces Apoptosis Through ROS
Activation of apoptosis is associated with ROS generation (Matsumoto et al., 2019). This led to the speculation that PA hemoglobin-mediated reduction of apoptosis might be attributed to ROS reduction. This hypothesis was verified by treating CHO cells with and without PA hemoglobin for 72 h, resulting in obvious late apoptotic changes used to measure ROS rate by flow cytometric analysis (Figure 5). ROS levels in the cell were assessed using the dihydrodichlorofluorescein (DCF) fluorescence method (LeBel et al., 1992; Walrand et al., 2003). Data indicated that the ROS rate in the hemoglobin treated group (5.36 ± 0.11%) was suppressed compared with that in the hemoglobin untreated group (10.35 ± 0.15%).
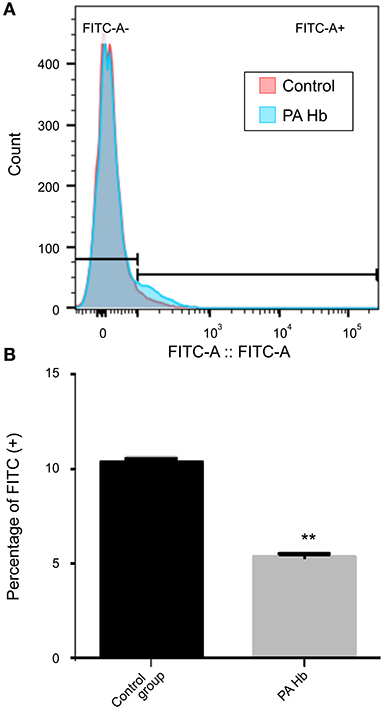
Figure 5. PA Hemoglobin decreases apoptosis through reduction in ROS generation. Cells were treated with and without PA Hb for 72 h at the concentration of 0.625μg/mL. (A) Representative histogram layout image of stained cells. (B) Percent cell population of FITC positive cells. **p < 0.01. Results are expressed as means ± SEM. Statistical analysis was performed using the Student's t-test and the control group was used for comparison. ROS, reactive oxygen species.
Discussion
In this study, hemoglobin was successfully isolated and purified from the marine organism PA. SDS-PAGE results showed that under non-reducing conditions, the major band appeared near 43 kD, whereas the light band appeared near 13 kD. According to previous research (Ebina et al., 1995; Matsubara et al., 1996; Yamaki et al., 1996), the major band is the fragment A-b-B, whereas the light band is the fragment “a.” Under reducing conditions, the major band appeared near 17 kD, whereas the light band appeared near 32 kD, a fragment L2 (linker). Thus, the electrophoresis image is like previous research reported. MALDI-TOF-MS is a modern and fast alternative to traditional proteomic techniques that can identify biological polymers (Bozik et al., 2018). Without prior chromatographic separation, biomolecules being analyzed are mixed with an organic matrix that absorbs laser radiation and facilitates the desorption/ionization process. The positively charged substance introduced into the gas phase reflects relative abundance and determines biomolecule mass-to-charge ratio (m/z) in the selected range (Hajduk et al., 2016). The molecular mass of intact PA hemoglobin was determined by MALDI-TOF-MS (Figure 2) as 412,216.6875 Da. Based on these results, it is proved that the separated extract is relatively clarified PA hemoglobin.
PA hemoglobin contains approximately about 200 polypeptide chains but no heme group. However, it has a special “carbohydrate gluing” structure that organizes a two-tiered hexagonal form, which is beneficial to its oxygen binding capacity (Ebina et al., 1995). Previous reports have shown that the optimal oxygen affinity of PA hemoglobin was not affected by temperature and cofactors (Tsuneshige et al., 1989). Analysis of the oxygen carrying capacity showed that hemoglobin extracted from PA has an ability of oxygen transport similar to human hemoglobin. Oxygen saturation of the PA Hb was 99.6 ± 0.058%, whereas that of the human Hb was 99.5 ± 0.1%. The functional ability of PA hemoglobin results from its high oxygen affinity (Tsuneshige et al., 1989). Human hemoglobin is unstable and likely to lose its oxygen carrying capacity by decomposition and oxidation. Therefore, chemical modification is necessary if human hemoglobin is to be used in vitro. PA hemoglobin is extracted easily, and its extraction procedure skips the lysis of cell membranes. Meanwhile, PA hemoglobin is stable with respect to the basic features of oxygen carriers and more suitable for application in vitro.
Cell culture is widely used in bioengineering and genetic engineering. The conditions and parameters required for cell culture are relatively complicated, including culture media, osmotic pressure, temperature, partial pressure of oxygen, and pH variations (Cao and Zhang, 2017). Among various physical conditions involved in cell culture, environmental oxygen concentration is thought to be one of the most critical factors for cell proliferation (Zhang et al., 2016). Cells need an optimum delivery of gases (O2 and CO2) to maintain a suitable growing environment. At present, air is directly bubbled along with oxygen feeding into the culture atmosphere, which sometimes results in oxygen overload (Le Pape et al., 2015), a phenomenon reported to induce oxidative stress, leading to a secretion of ROS (Zhou et al., 2018). The utilization of molecular oxygen by aerobic organisms inevitably helps to form oxygen-containing reactive species, collectively called ROS (Li et al., 2016), including superoxide anions (O), hydroxyl radical (OH), and hydrogen peroxide (H2O2; Thannickal and Fanburg, 2000). Prooxidants and redox cycling agents, such as H2O2 (Gardner et al., 1997); diamide (Sato et al., 1995); etoposide (Bustamante et al., 1997); and semiquinones (Sun and Ross, 1996), can induce apoptosis. ROS, the main molecule generated during oxidative stress in the body, has been recognized as an important regulator in the apoptosis process (Circu, 2010). Increasing ROS levels disrupt intracellular redox homeostasis, which can activate oxidative stress-induced apoptotic signaling.
CHO, a widely used cell line in cell biology, has a rapid growth rate. In this study, CHO cell lines were cultured for 48 or 72 h to ensure cells had reached a certain density and stayed in the culture environment of oxygen overload for a considerable period. It is speculated that PA hemoglobin can reduce the apoptosis rate of cells by equilibrating the oxygen distribution in the medium. Thus, we selected a large concentration range of PA hemoglobin as the cell culture supplement, trying to determine the optimal maximum concentration that could reduce apoptosis and without cytotoxic effects. Eventually, 0.625 μg/mL was selected as the appropriate concentration of PA Hb, which was added to the culture medium to analyze cell apoptosis and detect ROS. The time points, 48 and 72 h, were selected to detect the apoptosis because the cells were in the middle and late stage of growth, and are prone to apoptosis at those times. Thus, the apoptosis ratio of untreated cells was relatively high. This ratio increased from 25.05 ± 0.65% to 39.75 ± 0.95% over time from 48 to 72 h. Meanwhile, PA hemoglobin was used to reduce apoptosis in the CHO cell line. The apoptosis ratio of the cells with PA hemoglobin decreased to 19.65 ± 0.15% at 48 h and to 20.65 ± 1.05% at 72 h, that is, with no obvious increasing trend over time. Moreover, this hemoglobin successfully reduced ROS levels; the ROS levels in the cells with PA hemoglobin decreased to 5.36 ± 0.11%, whereas the levels in the cells without PA hemoglobin were ~10.35 ± 0.15%. This study provides evidence to support that PA hemoglobin helps to suitably redistribute oxygen in cell culture systems. As the materials required for the hemoglobin extraction procedure are easily available and economical and the hemoglobin dose required for cell culture is low, reduction of apoptosis by the use of PA hemoglobin as a cell-culture supplement may provide a convenient approach to improve the outcome in various types of cell cultures.
Conclusion
Our study innovatively demonstrated that PA Hb addition can act as a cell culture supplement to inhibit cell apoptosis, and the mechanism responsible for this is possibly related to a reduction of ROS overproduction by PA Hb. It is possible to achieve a precise control of the required oxygen concentration in the culture environment and to optimize cell culture by adding PA Hb. Further studies aimed at improving the extraction method and modifying the extract to reduce cell side effects and lower the safe concentration are however warranted.
Data Availability Statement
The raw data supporting the conclusions of this article will be made available by the authors, without undue reservation.
Ethics Statement
Ethical review and approval was not required for the animal study because this study was not approved by an ethics committee, but all animal experiments complied with the ARRIVE guidelines and were conducted under the U.K. Animals (Scientific Procedures) Act 1986, and associated guidelines.
Author Contributions
QH and JL designed and conducted most experiments and wrote the manuscript. YC isolated and validated the hemoglobin. Perinereis aibuhitensis was provided by BC and ML. YL was responsible for supervision and manuscript revision. All authors contributed to the article and approved the submitted version.
Conflict of Interest
BC and ML were employed by the Shandong Wan'an Pharmaceutical Co., Ltd.
The remaining authors declare that the research was conducted in the absence of any commercial or financial relationships that could be construed as a potential conflict of interest.
Publisher's Note
All claims expressed in this article are solely those of the authors and do not necessarily represent those of their affiliated organizations, or those of the publisher, the editors and the reviewers. Any product that may be evaluated in this article, or claim that may be made by its manufacturer, is not guaranteed or endorsed by the publisher.
References
Bozik, M., Cejnar, P., Saskova, M., Pavel, N., Marsik, P., Kloucek, P., et al. (2018). Stress response of Escherichia coli to essential oil components - insights on low-molecular-weight proteins from MALDI-TOF. Sci. Rep. 8:13042. doi: 10.1038/s41598-018-31255-2
Bustamante, J., Tovar, B. A., Montero, G., and Boveris, A. (1997). Early redox changes during rat thymocyte apoptosis. Arch. Biochem. Biophys. 337, 121–128. doi: 10.1006/abbi.1996.9754
Cao, T. T., and Zhang, Y. Q. (2017). The potential of silk sericin protein as a serum substitute or an additive in cell culture and cryopreservation. Amino Acids 49, 1029–1039. doi: 10.1007/s00726-017-2396-3
Carvalho, F. A. O., Caruso, C. S., Nascimento, E. D., Oliveira, T. M. B. F., Bachega, J. F. R., and Tabak, M. (2019). Oligomeric stability of Glossoscolex paulistus hemoglobin as a function of the storage time. Int. J. Biol. Macromol. 133, 30–36. doi: 10.1016/j.ijbiomac.2019.04.072
Circu, M. L. (2010). Reactive oxygen species, cellular redox systems, and apoptosis. Free. Radic. Biol. Med. 48, 749–762. doi: 10.1016/j.freeradbiomed.2009.12.022
Cong, Y., Banta, G. T., Selck, H., Berhanu, D., Valsami-Jones, E., Forbes, V. E., et al. (2011). Toxic effects and bioaccumulation of nano-, micron- and ionic-Ag in the polychaete, Nereis diversicolor. Aquat. Toxicol. 105, 403–411. doi: 10.1016/j.aquatox.2011.07.014
Dingermann, T. (2008). Recombinant therapeutic proteins: production platforms and challenges. Biotechnol. J. 3, 90–97. doi: 10.1002/biot.200700214
Ebina, S., Matsubara, K., Nagayama, K., Yamaki, M., and Gotoh, T. (1995). Carbohydrate gluing, an architectural mechanism in the supramolecular structure of an annelid giant hemoglobin. Proc. Natl. Acad. Sci. U. S. A. 92, 7367–7371. doi: 10.1073/pnas.92.16.7367
Gardner, A. M., Xu, F. H., Fady, C., Jacoby, F. J., Duffey, C. D., Tu, Y., et al. (1997). Apoptotic vs. non-apoptotic cytotoxicity induced by hydrogen peroxide. Free. Radic. Biol. Med. 22, 73–83. doi: 10.1016/S0891-5849(96)00235-3
Gotoh, T., Sano, T., Shibuya, A., Yamaki, M., Imai, K., Ebina, S., et al. (1998). Hexagonal bilayer structuring activity of linker chains of an annelid giant hemoglobin from the polychaete Perinereis aibuhitensis. Arch. Biochem. Biophys. 360, 75–84. doi: 10.1006/abbi.1998.0924
Hajduk, J., Matysiak, J., and Kokot, Z. J. (2016). Challenges in biomarker discovery with MALDI-TOF MS. Clin. Chim. Acta. 458, 84–98. doi: 10.1016/j.cca.2016.04.033
Jiang, X., Tian, L., Ma, Y., and Ji, R. (2019). Quantifying the bioaccumulation of nanoplastics and PAHs in the clamworm Perinereis aibuhitensis. Sci. Total. Environ. 655, 591–597. doi: 10.1016/j.scitotenv.2018.11.227
Le Pape, F., Bossard, M., Dutheil, D., Rousselot, M., Polard, V., Ferec, C., et al. (2015). Advancement in recombinant protein production using a marine oxygen carrier to enhance oxygen transfer in a CHO-S cell line. Artif. Cells. Nanomed. Biotechnol. 43, 186–195. doi: 10.3109/21691401.2015.1029632
LeBel, C. P., Ischiropoulos, H., and Bondy, S. C. (1992). Evaluation of the probe 2′,7′-dichlorofluorescin as an indicator of reactive oxygen species formation and oxidative stress. Chem. Res. Toxicol. 5, 227–231. doi: 10.1021/tx00026a012
Li, R., Jia, Z., and Trush, M. A. (2016). Defining ROS in biology and medicine. React. Oxyg. Species 1, 9–21. doi: 10.20455/ros.2016.803
Li, Y., Li, J., Liu, T., Wang, Y., Zhou, Z., Cheng, F., et al. (2017). Preparation and antithrombotic activity identification of Perinereis aibuhitensis extract: a high temperature and wide pH range stable biological agent. Food. Funct. 8, 3533–3541. doi: 10.1039/C7FO00987A
Matsubara, K., Yamaki, M., Nagayama, K., Imai, K., Ishii, H., Gotoh, T., et al. (1996). Wheat germ agglutinin-reactive chains of giant hemoglobin from the polychaete Perinereis aibuhitensis. Biochim. Biophys. Acta. 1290, 215–223. doi: 10.1016/0304-4165(96)00010-4
Matsumoto, H., Haniu, H., and Komori, N. (2019). Determination of protein molecular weights on SDS-PAGE. Methods Mol. Biol. 1855, 101–105. doi: 10.1007/978-1-4939-8793-1_10
Matton, A., Burlage, L., Van, R. R., et al. (2018). Normothermic machine perfusion of donor livers without the need for human blood products. Liver Transpl. 24, 528–538. doi: 10.1002/lt.25005
Mosmann, T. (1983). Rapid colorimetric assay for cellular growth and survival: application to proliferation and cytotoxicity assays. J. Immunol. Methods. 65, 55–63. doi: 10.1016/0022-1759(83)90303-4
Rousselot, M., Delpy, E., Drieu La Rochelle, C., et al. (2006). Arenicola marina extracellular hemoglobin: a new promising blood substitute. Biotechnol J. 1, 333–345. doi: 10.1002/biot.200500049
Sato, N., Iwata, S., Nakamura, K., Hori, T., Mori, K., Yodoi, J., et al. (1995). Thiol-mediated redox regulation of apoptosis. Possible roles of cellular thiols other than glutathione in T cell apoptosis. J. Immunol. 154, 3194–3203.
Savla, C., Munoz, C., Hickey, R., Belicak, M., Gilbert, C., Cabrales, P., et al. (2020). Purification of Lumbricus terrestris mega-hemoglobin for diverse oxygen therapeutic applications. ACS Biomater. Sci. Eng. 6, 4957–4968. doi: 10.1021/acsbiomaterials.0c01146
Sun, X., and Ross, D. (1996). Quinone-induced apoptosis in human colon adenocarcinoma cells via DT-diaphorase mediated bioactivation. Chem. Biol. Interact. 100, 267–276. doi: 10.1016/0009-2797(96)03704-0
Suzuki, T., Koboyashi, M., Furukohri, T., and Gotoh, T. (1983). Autoxidation of extracellular oxyhaemoglobin from the polychaete Perinereis brevicirris (Grube). Comp. Biochem. Physiol. B Comp. Biochem. 75, 17–21. doi: 10.1016/0305-0491(83)90033-0
Thannickal, V. J., and Fanburg, B. L. (2000). Reactive oxygen species in cell signaling. Am. J. Physiol. Lung. Cell. Mol. Physiol. 279, L1005–L1028. doi: 10.1152/ajplung.2000.279.6.L1005
Tian, S., Tong, Y., and Hou, Y. (2019). The effect of bioturbation by polychaete Perinereis aibuhitensis on release and distribution of buried hydrocarbon pollutants in coastal muddy sediment. Mar. Pollut. Bull. 149:110487. doi: 10.1016/j.marpolbul.2019.110487
Tong, Y., Li, J., Cheng, Q., Tong, Y., Li, J., Cheng, Q., et al. (2019). Enhanced removal of sediment-associated total petroleum hydrocarbons under bioturbation by polychaete Perinereis aibuhitensis. J. Environ. Sci. Health A. Tox. Hazard. Subst. Environ. Eng. 54, 391–397. doi: 10.1080/10934529.2018.1558894
Tsuneshige, A., Imai, K., Hori, H., Tyuma, I., Gotoh, T., et al. (1989). Spectrophotometric, electron paramagnetic resonance and oxygen binding studies on the hemoglobin from the marine polychaete Perinereis aibuhitensis (Grube): comparative physiology of hemoglobin. J. Biochem. 106, 406–417.
Walrand, S., Valeix, S., Rodriguez, C., Ligot, P., Chassagne, J., Vasson, M.-P., et al. (2003). Flow cytometry study of polymorphonuclear neutrophil oxidative burst: a comparison of three fluorescent probes. Clin. Chim. Acta. 331, 103–110. doi: 10.1016/S0009-8981(03)00086-X
Wu, W., Li, T., Liu, J., et al. (2011). Pretreatment before ischemia induction with polymerized human placenta hemoglobin (PolyPHb) attenuates ischemia/reperfusion injury-induced myocardial apoptosis. Artif. Cells Blood Substit. Immobil. Biotechnol. 39, 3–6. doi: 10.3109/10731199.2010.494581
Yamaki, M., Kubota, K., Matsubara, K., Ebina, S., and Gotoh, T. (1998). Carbohydrate gluing is a strategy for supramolecular clamping of submultiples in annelid extracellular multi-subunit hemoglobin. Arch. Biochem. Biophys. 355, 119–123. doi: 10.1006/abbi.1998.0705
Yamaki, M., Matsubara, K., Shibuya, A., Gotoh, T., and Ebina, S. (1996). Identification of the subunit loci in the extracellular multisubunit hemoglobin from annelid Perinereis aibuhitensis. Arch. Biochem. Biophys. 335, 23–31. doi: 10.1006/abbi.1996.0478
Yang, J., Li, W., Luo, L., Jiang, M., Zhu, C., Qin, B., et al. (2018). Hypoxic tumor therapy by hemoglobin-mediated drug delivery and reversal of hypoxia-induced chemoresistance. Biomaterials 182, 145–156. doi: 10.1016/j.biomaterials.2018.08.004
Zhang, K., Zhao, T., Huang, X., He, Y., Zhou, Y., Wu, L., et al. (2016). Dissolved oxygen concentration in the medium during cell culture: defects and improvements. Cell. Biol. Int. 40, 354–360. doi: 10.1002/cbin.10570
Zhang, L. J., Li, Y., Chen, P., MeiLi, X., GanChen, Y., YuHang, Y., et al. (2017). A study of genotoxicity and oxidative stress induced by mercuric chloride in the marine polychaete Perinereis aibuhitensis. Environ. Toxicol. Pharmacol. 56, 361–365. doi: 10.1016/j.etap.2017.10.009
Keywords: hemoglobin, Perinereis aibuhitensis, MALDI-TOF-MS, FACS, cell apoptosis, reactive oxygen species
Citation: Huo Q, Liu J, Cheng Y, Cao B, Lei M and Liu Y (2021) Hemoglobin Extracted From Perinereis aibuhitensis Acts as a Cell Culture Medium Supplement to Reduce Apoptosis. Front. Mar. Sci. 8:659934. doi: 10.3389/fmars.2021.659934
Received: 12 February 2021; Accepted: 05 July 2021;
Published: 10 August 2021.
Edited by:
Roland Wohlgemuth, Lodz University of Technology, PolandCopyright © 2021 Huo, Liu, Cheng, Cao, Lei and Liu. This is an open-access article distributed under the terms of the Creative Commons Attribution License (CC BY). The use, distribution or reproduction in other forums is permitted, provided the original author(s) and the copyright owner(s) are credited and that the original publication in this journal is cited, in accordance with accepted academic practice. No use, distribution or reproduction is permitted which does not comply with these terms.
*Correspondence: Yunde Liu, bHlkQHRtdS5lZHUuY24=
†These authors have contributed equally to this work