- 1CSIRO Health and Biosecurity, Floreat, WA, Australia
- 2School of Biological Sciences, The University of Western Australia, Crawley, WA, Australia
- 3Australian Institute of Marine Science, Darwin, NT, Australia
- 4Research Institute for the Environment and Livelihoods, Charles Darwin University, Darwin, NT, Australia
- 5Marine Ecology Consultant, Magnetic Island, QLD, Australia
- 6School of Biological Sciences, Monash University, Melbourne, VIC, Australia
- 7Marine Protected Areas Branch, Parks Australia, Kingston, TAS, Australia
- 8Department of Fisheries, North Beach, WA, Australia
- 9CSIRO Ocean and Atmosphere, Indian Ocean Marine Research Centre, Crawley, WA, Australia
- 10Schools of Architecture, Design and Conservation, The Royal Danish Academy of Fine Arts, Copenhagen, Denmark
- 11School of Biological Science, University of Adelaide, Adelaide, SA, Australia
- 12Department of Biological Sciences, Macquarie University, Sydney, NSW, Australia
- 13Western Australian Biodiversity Science Institute, Perth, WA, Australia
Over the past decade, vertebrate populations globally have experienced significant declines in distribution and abundance. Understanding the reasons behind these population declines is the first step in implementing appropriate management responses to improve conservation outcomes. Uncovering drivers of extirpation events after the fact, however, requires a careful forensic approach to prevent similar declines elsewhere. The once abundant and species-rich sea snake fauna of Ashmore Reef Marine Park, in the Timor Sea, collapsed dramatically in the early 2000s. No such decline has occurred on surrounding reefs. We synthesise the evidence for this collapse and the subsequent slow recovery and evaluate the plausibility of potential drivers for the declines, as well as provide evidence against certain explanations that have been proposed in the past. Our systematic review shows that of seven possible hypotheses considered, at least three are credible and require additional information: (1) stochastic environmental events may have increased the snakes’ susceptibility to pathogens, (2) a resurgence in the abundance of top predators may have induced a localised change in trophic structure, and (3) an acute increase in local boat traffic may have had negative physical impacts. One or more of these factors, possibly acting in combination with as yet other unidentified factors, is the most plausible explanation for the precipitous decline in sea snake populations observed. Based on this position, we identify future research directions with a focus on addressing critical gaps in knowledge to inform and prioritise future management actions.
Introduction
Globally, vertebrate populations across terrestrial, fresh water and marine environments are experiencing precipitous declines in distribution and abundance (Butchart et al., 2010; Hoffmann et al., 2010; Rodrigues et al., 2014; Ceballosa et al., 2017; Sánchez-Bayoa and Wyckhuysb, 2019), such that many species are being pushed toward both local extirpation and global extinction (Wake and Vredenburg, 2008). In many of these cases, the drivers of population declines are complex and multi-faceted. Species extinctions can be directly or indirectly attributed to global environmental change, including localised over-exploitation (Rosser and Mainka, 2002), habitat loss/change (Brooks et al., 2002), invasive alien species (Shine, 2010), disease (Smith et al., 2006), and/or broad-scale threats such as climate change (Urban, 2015). Nonetheless, some population declines have unknown or uncertain causes (Stuart et al., 2004; Spitzen-van der Sluijs et al., 2013). Some of these declines can prove to be truly enigmatic, with extended periods during which there is little scientific consensus on the drivers of decline (e.g., global amphibian declines in the 1980s before the chytrid fungus was identified as a key threat).
In instances where as-yet-unexplained processes threaten focal species, such critical knowledge gaps prevent conservation managers from identifying and implementing appropriate responses. Uncovering the drivers behind such enigmatic declines is an essential first step in designing effective management tools to prevent further biodiversity loss. Management policies designed without a good understanding of key drivers of population declines may result in significant investment of time and money into conservation efforts that do not provide recovery outcomes for populations (Campbell et al., 2020). The process of collecting sufficient information to pinpoint drivers of decline, and the design of effective mitigation policy requires a collaborative partnership between information providers (e.g., researchers, specialists) and end-users (e.g., conservation estate managers) to maximise the success of conservation outcomes (Sunderland et al., 2009).
One such instance of significant declines in marine vertebrates in recent times is the inexplicable extirpation of sea snakes from the shallow reef systems of the Ashmore Reef Marine Park in the Timor Sea (Figure 1). This is a region once renowned as the global hotspot for sea snake diversity (Guinea and Whiting, 2005). The population collapsed in the early 2000s, despite other regional reef populations apparently remaining stable, and is recognised as one of the most significant losses of vertebrate species in recent times (Lukoschek et al., 2013). As yet there has been no evidence-based explanation identified for the decline, although multiple ideas have been hypothesised in the past. In this study, we use the sea snake decline at Ashmore reef as an example system to demonstrate (i) how broad engagement with knowledge holders and synthesis of disparate data can be an effective mechanism to identify and prioritise knowledge shortfalls relating to drivers of vertebrate declines, (ii) how this insight can be then used to identify ways to improve management programs to underpin improved conservation outcomes. To achieve this aim we (a) bring together the existing literature with previously unavailable datasets and expertise from researchers and managers working at Ashmore Reef Marine Park to pinpoint key drivers of declines in sea snake populations, and (b) provide recommendations for actionable mitigation tools to prevent further declines in similar systems.
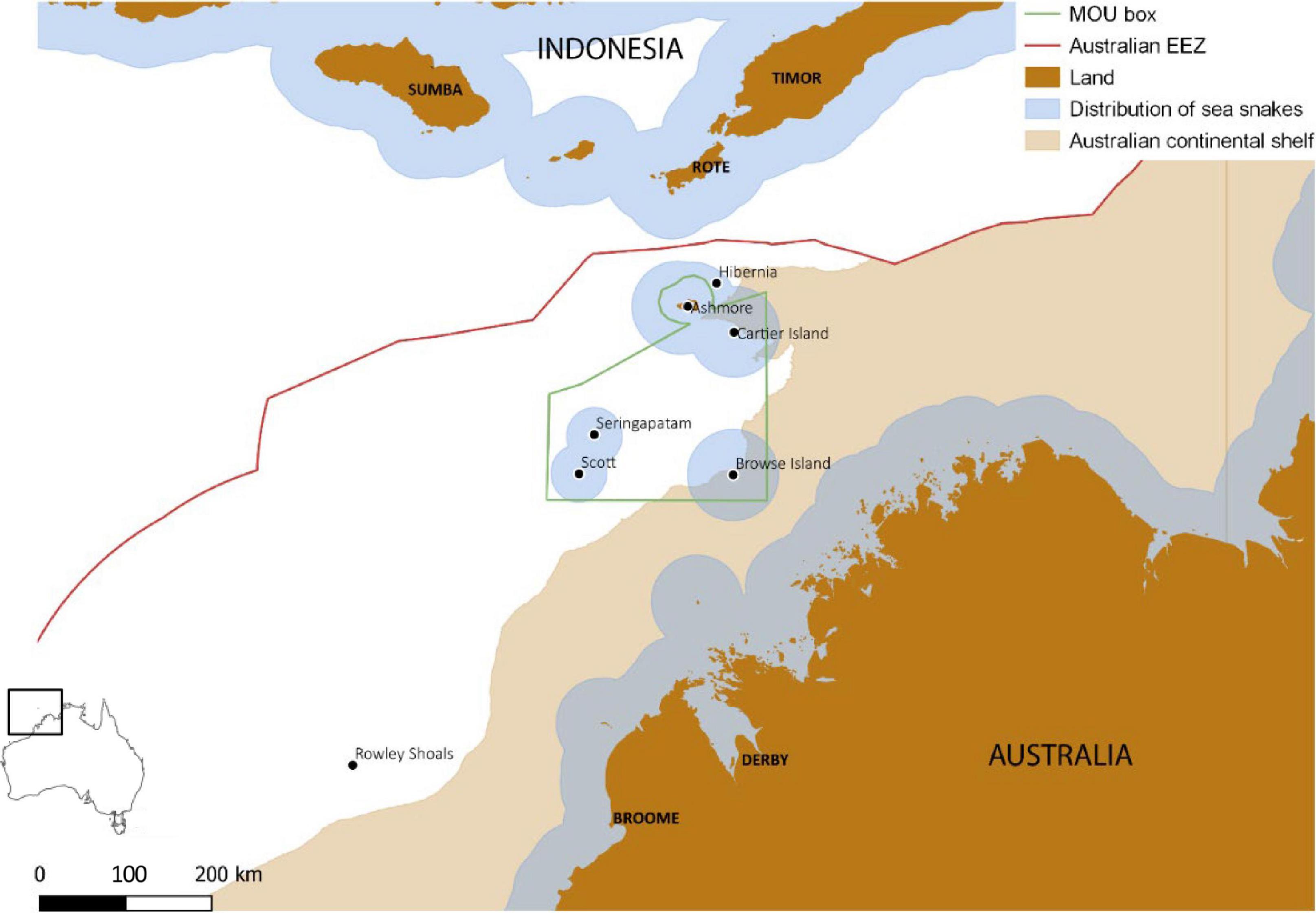
Figure 1. Location of Ashmore Reef in relation to other reefs and islands in the Timor sea, and administrative boundaries including the Memorandum Of Understanding (MOU) Box (ATNS, 2004), Australian Exclusive Economic Zone (EEZ), Indonesia and Australia. Estimated potential distribution of benthic-foraging sea snakes is based on habitat preferences and presence records generated by the IUCN sea snakes specialists’ group for the IUCN Red List of Threatened Species (downloaded on 1 October 2019).
Sea Snakes at Ashmore Reef Marine Park
“True” sea snakes (subfamily Hydrophiinae; hereafter referred to as sea snakes) are a diverse group of air-breathing, live-bearing marine reptiles that have a completely marine life cycle. Some 71 species of sea snake inhabit tropical and subtropical waters globally (Rasmussen et al., 2011b), with the highest diversity of species in the tropical coastal waters of Australia and the Indo-Malay region (Elfes et al., 2013; Cogger, 2018). Within this region, species diversity of sea snakes is particularly high in parts of the Timor Sea, extending from the southern coast of Timor to Australia’s north-west coast. This group of marine reptiles are considered mesopredators within most reef systems, and display a range of dietary preferences and specialisations, but are largely piscivores (Voris and Voris, 1983). This relatively small region supports at least 19 species, representing c. 27% of the global species diversity of sea snakes (Minton and Heatwole, 1975; Guinea and Whiting, 2005; Mirtschin et al., 2017), second only to the species diversity documented from the South China Sea (25 known species: Rasmussen et al., 2011a).
Totalling 583 km2 in extent and comprising four small islands with surrounding reef flats of 174 km2, the Ashmore Reef Marine Park (“Ashmore” hereafter) is the largest managed reef system of the isolated reefs in the Timor Sea (Ceccarelli et al., 2011). Ashmore experiences a confluence of seasonal currents from the Indian and Pacific Oceans (Hale and Butcher, 2013). The oceanic productivity resulting from these currents supports numerous marine species, some of which are endemic to the reef (Edgar et al., 2017). Over 750 species of fish (including eight species of sharks), 30 species of pelagic birds (with over 100,000 breeding individuals), dugongs and three species of sea turtle feed on the seagrass beds and reef or utilise terrestrial areas as nesting grounds at Ashmore (Allen, 1993; Russell et al., 2001; Clarke et al., 2011; Guinea, 2013). The islands and reefs of the Timor Sea are historically, culturally, economically, and strategically important for both Indonesia and Australia. Since the 1800’s, the reefs, lagoons and islands of Ashmore have been used by traditional fishermen, by Europeans to mine guano in the latter half of the 19th century, by at least one Australian commercial fisher in the early 1900’s, for military exercises, for weather observations, and as a camp location for oil and gas exploration (Wilkinson, 1908; Russell, 2005). Since its establishment as a Marine Park in 1983, the majority of Ashmore is classified as a Sanctuary Zone where visitation is strictly controlled, although some visitation by traditional Indonesian fishers is still allowed. All vessels however are prohibited from entering the Sanctuary Zone without approval from the Director of National Parks. A small region in the north-west of the Marine Park is classed as a Recreational Use Zone, where visitors and recreational fishing activities are permitted. The remote nature of Ashmore restricts the numbers of people visiting, with the majority of activities within the reef and lagoons now largely restricted to national security monitoring and border control activities.
Seventeen species of sea snakes have been recorded at Ashmore, nine as breeding residents and eight as potential waifs (Minton and Heatwole, 1975; Guinea and Whiting, 2005; Cogger, 2018). Historical records suggest that sea snakes were abundant at Ashmore since at least early last century. In 1926, Dr. Malcolm Smith [who was then the physician to the Court of Siam: (Smith, 1947)] negotiated with Malay navigators to collect reptiles throughout South-East Asia. His collectors provided 100 specimens of sea snakes belonging to five species from Ashmore and indicated that many more specimens could have been obtained easily (Smith, 1926). In 1949, Mr. B. Shipway from the Council of Scientific and Industrial Research, while aboard the fisheries research vessel Wareen, collected a leaf-scaled sea snake (Aipysurus foliosquama) from Ashmore for the Western Australian Museum. In 1973, researchers from the research vessel Alpha Helix from the Scripps Institute collected more than 350 sea snakes of nine species in less than 2 weeks at Ashmore and noted that “many more were observed” (Minton and Heatwole, 1975). Subsequent surveys in the 1990s reinforced the observation that Ashmore supported exceptional abundance and globally significant sea snake diversity (Guinea, 1995), with an estimated standing stock of almost 40,000 sea snakes on the 174 km2 reef area (Guinea and Whiting, 2005). For example, mark and recapture studies over 3 years indicated that between 94 and 192 turtle-headed sea snakes (Emydocephalus annulatus) frequented a single coral head 30 m in diameter (Guinea and Whiting, 2005).
A Dramatic Decline
Surveys conducted within the shallow reefs of Ashmore from the early 2000s onward documented a collapse in sea snake populations, to the point that few or no snakes were detected after 2006 (Guinea, 2013; Lukoschek et al., 2013). Although numerous methods were used to survey snake abundance over time, the underlying pattern of decline followed by continued rarity over the past three decades is clear and cannot be an artefact of differences in survey methods. The numbers of sea snakes recorded at Ashmore declined from 46 snakes per day in 1973 to 21 snakes per day three decades later in 2002, to zero in the inner reef by 2013; this near absence has remained consistent through until 2017 (Table 1). Sightings of some species reduced drastically before others; for example, the horned sea snake (Hydrophis peronii) was among the first to decline, followed by the turtle-headed sea snake. Sightings of the olive sea snake (Aipysurus laevis) was the last to decline (by 2010) and were not sighted for 7 years thereafter (Guinea and Mason, 2017).
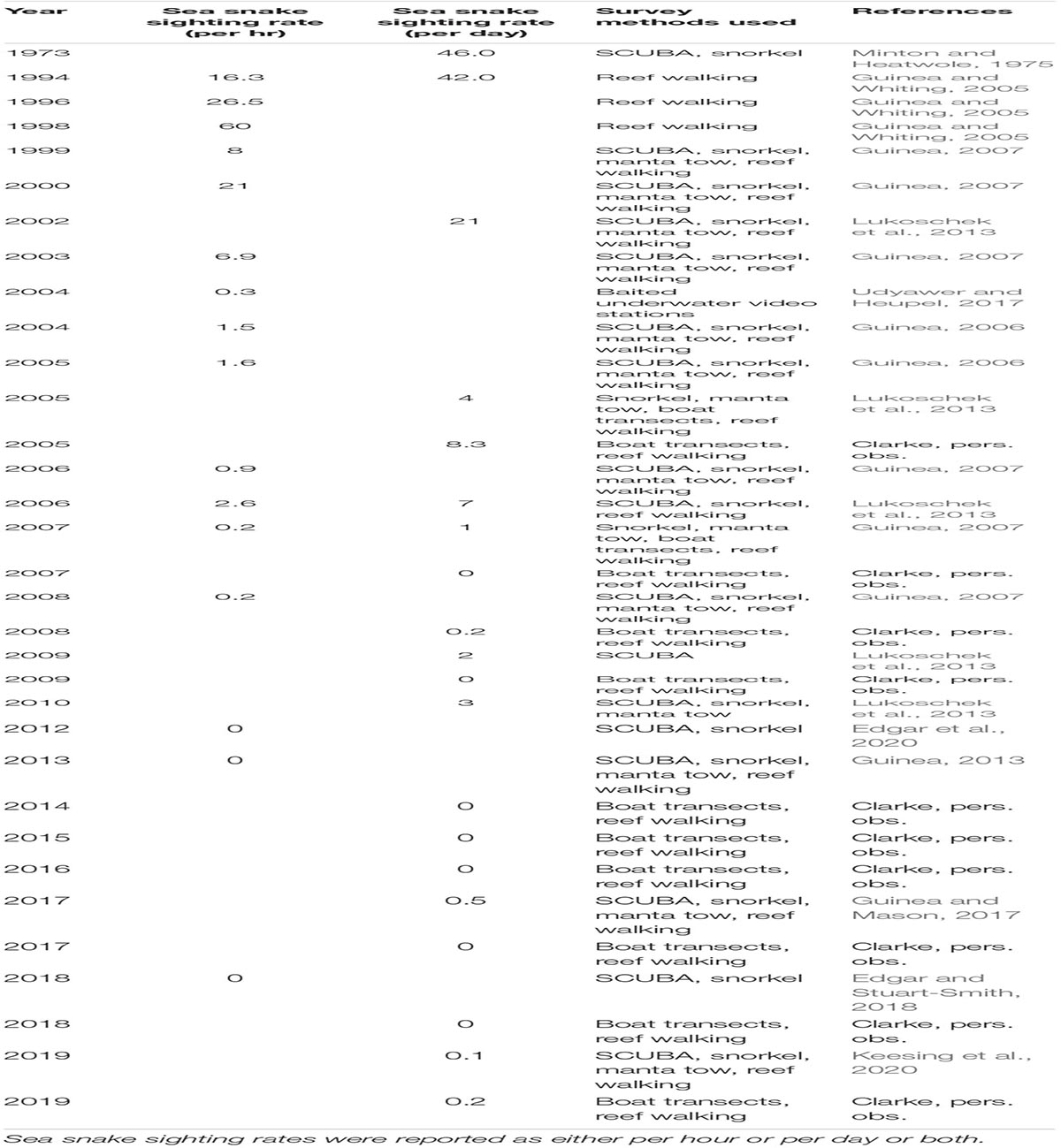
Table 1. Scientific survey history and sea snake abundance at the inner reef at Ashmore Reef Marine Park.
When sea snakes were abundant at Ashmore, they were readily observed on the surface from boats, as is the case in other regions where they are abundant. Between 2013 and 2017, Ashmore has been visited by terrestrial ecologists and ornithologists on an annual basis to survey for birds, and no sea snakes were observed in the inner reef during local operations (Clarke, pers. obs). However, in 2016, three olive sea snakes were recorded by baited camera traps set at 21–33 m depth in the soft sediments outside the reef to the west of Ashmore (Speed, pers. comm). In April 2017, a 10-day survey detected four olive sea snakes at the extreme south-east outer reef and another one in the West Island channel (Guinea and Mason, 2017). Later in 2017, two olive sea snakes were seen within the West Island channel (Guinea and Mason, 2017). However, a 5 day marine biodiversity survey in December 2018 found no sea snakes despite diving at 76 sites within Ashmore (Edgar and Stuart-Smith, 2018), while numerous specimens from three species were observed during surveys at nearby Scott, Seringapatam, Hibernia and Cartier reefs on the same voyage. A 4 day ecological survey in March 2019 opportunistically observed a single unidentified sea snake near the western tip of the outer reef (R. Clarke pers. obs). A 12-day targeted sea snake survey undertaken by CSIRO in May and June 2019, and led by four of the authors, reported a single olive sea snake within the reef system over 12 days of search effort (Keesing et al., 2020). In October 2019, personnel from an Australian Boarder Force patrol vessel photographed a Cogger’s sea snake (Hydrophis coggeri; photographs verified by RS, VU and KS) swimming on the surface near West Island. These recent observations confirms that more than one species is now present at the reef.
Potential Drivers of Sea Snake Decline
Although significant effort has been expended in collecting information on sea snake presence, abundance and diversity patterns, the difficulty in collecting long-term empirical data on environmental conditions, community dynamics and animal health at Ashmore means a forensic approach is needed to identify ecological processes and anthropogenic drivers that may have contributed to sea snake declines. Only once this has happened can we begin to understand the processes behind these declines, and in turn what opportunities there are for management actions that may improve conservation outcomes in the future. Here, we summarise seven hypothesised processes that are potentially threatening sea snakes at Ashmore and discuss their plausibility as drivers of the localised declines. Some of these drivers have been suggested previously (e.g., Lukoschek et al., 2013), however, here we synthesise available insight, including from new sources where available, to take a structured approach in assessing the confidence in each proposed hypothesis. In addition to the ideas previously proposed, we add three novel hypotheses based on new information that may help explain the disappearance of sea snakes at Ashmore and may be relevant in other systems: change in predator abundance, role of pathogenic organisms, and increased maritime activity.
Assessing Plausibility in Hypothesised Drivers of Decline
Each potential driver of decline was framed as a hypothesis, and each was tested using empirical data (where available), expert knowledge of the system, and comparisons with other closely related systems. We drew on expertise, available evidence and theory to qualify (i) the available evidence base for each driver, (ii) the strength, and (iii) direction of evidence for each specific hypothesised driver of decline. Qualitative measures of strength and direction of evidence were broadly categorised into four levels; Strongly supported (+ +), Weakly supported (+), Mixed support (± ), Weakly refuted (−), and Strongly refuted (−−) (Table 2). The availability of sufficient evidence to assess each driver was also qualified broadly into three categories; Direct or sufficient circumstantial evidence, Some circumstantial evidence and Little or none (Table 2). The plausibility of each driver in causing population decline was assessed using the measures of availability, strength and direction of evidence using a framework adapted from Salafsky et al. (2019); Table 2. In the following sections, we assess the evidence and plausibility of each driver from which qualitative measures were obtained from Ashmore and discuss the role of these processes in broader declines of sea snakes in other systems.
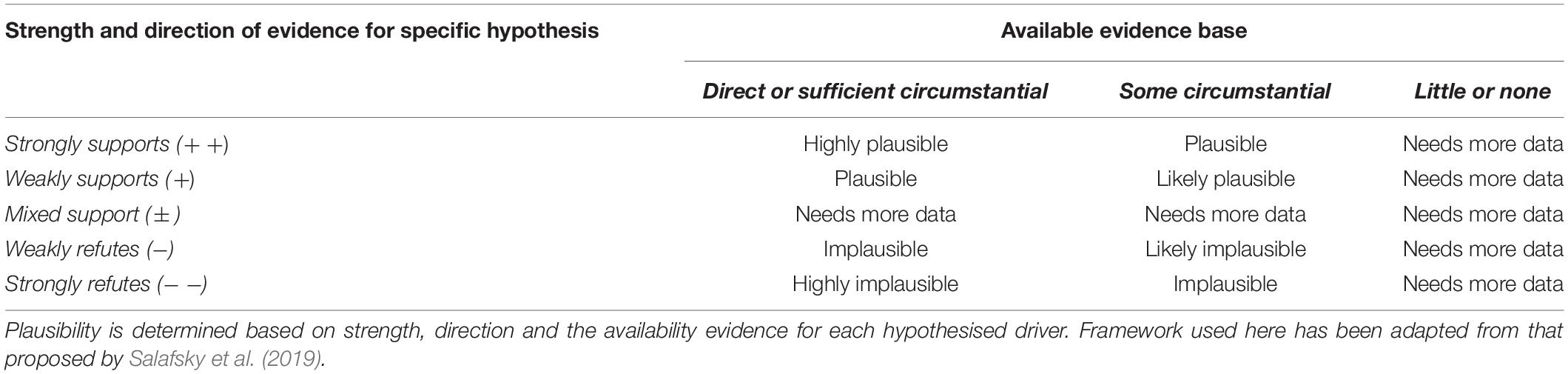
Table 2. Framework used to assess plausibility of each hypothesis explaining driver of decline in sea snake populations using data from Ashmore Reef Marine Park as a case study.
Coral Bleaching
Hypothesis: Historic coral bleaching and mortality events directly impacted sea snake populations causing the observed declines at Ashmore.
Available evidence base: Direct or sufficient circumstantial
Strength and direction of evidence: Weakly refutes (−)
Plausibility: Implausible
The widespread coral mortality event in 1998 that affected most reef systems in the Timor Sea had no noticeable impact on Ashmore (Richards et al., 2009; Ceccarelli et al., 2011). Degradation of coral habitats due to bleaching did not occur until 2003 (Rees et al., 2003), well after the onset of pronounced decline in abundance of sea snakes (Guinea, 2008). We therefore consider it implausible that a decline in reef quality due to coral mortality caused the decline in sea snakes at Ashmore. Although in this case, coral bleaching events at Ashmore were not identified as a likely driver of declines due to mismatch in timing, the strong associations between many species within the sea snake group (e.g., Aipysurus spp.) and coral reefs ecosystems means that populations are likely to be highly sensitive to large-scale and long-term coral mortality.
Extreme Disturbance Events
Hypothesis: Historic acute disturbance events such as cyclones, reduction in fresh water availability and marine heatwaves severely impacted sea snake populations and drove declines at Ashmore.
Available evidence base: Direct or sufficient circumstantial
Strength and direction of evidence: Weakly refutes (−)
Plausibility: Implausible
Cyclones
Sea snakes are thought to be capable of detecting approaching cyclones (Liu et al., 2010) and could presumably find refuge in deeper waters during the peak of a storm. Cyclones occur regularly in the Timor Sea, and we are not aware of any specific cyclone trajectories that might have had an unusually severe impact on Ashmore during the critical period of sea snake decline (Figure 2). Moreover, the nearby Scott Reef has been heavily impacted by repeated cyclones in the 1980 and 1990s yet no change in sea snake numbers was detected (Guinea, 2013; Udyawer and Heupel, 2017).
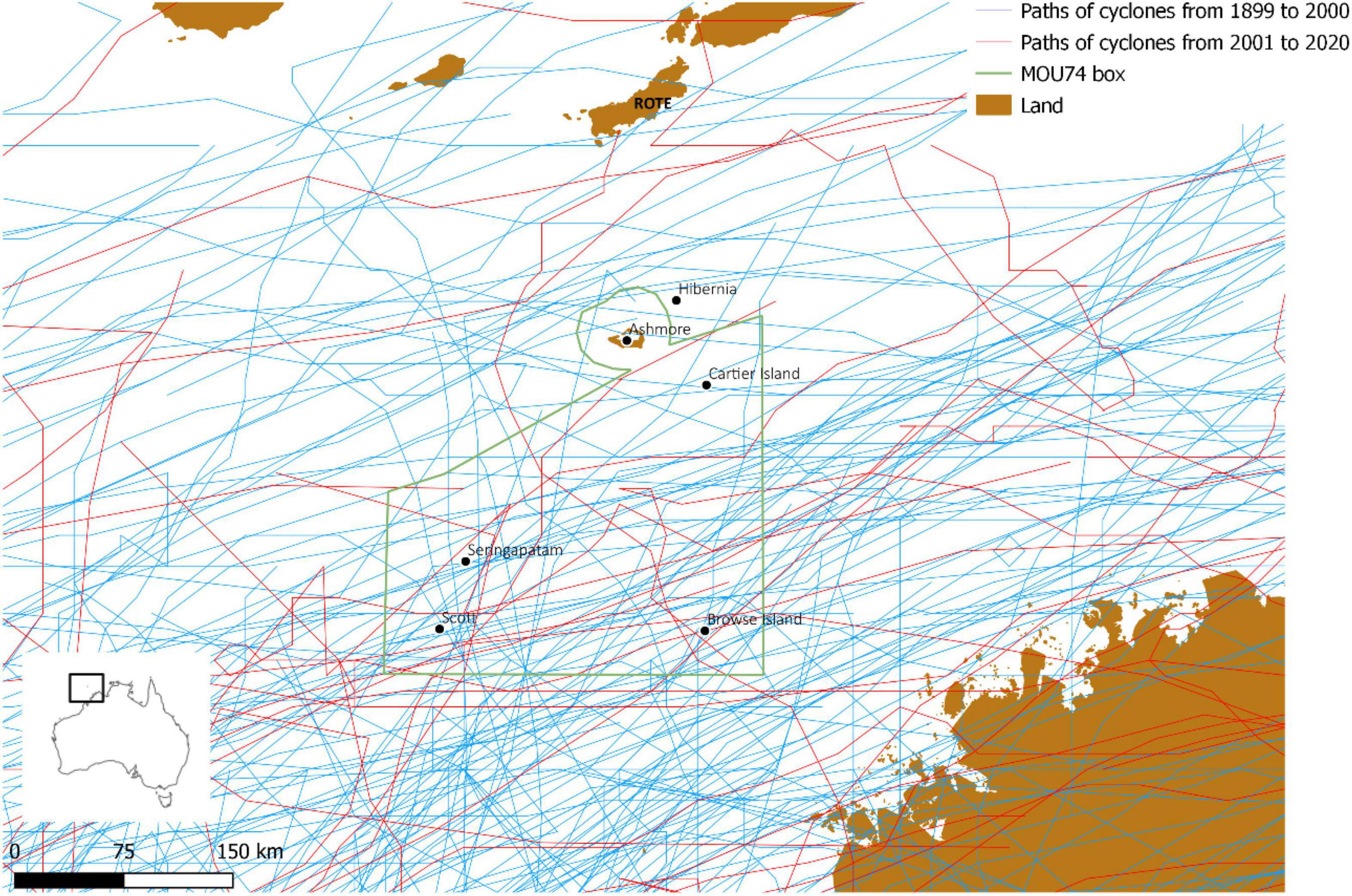
Figure 2. Paths of cyclones over the Timor Sea between 1899 and 2020 and the location of reefs. Tropical Cyclone Linda in 1976 was the only cyclone that was mapped with a direct path over Ashmore Reef Marine Park.
Fresh Water Availability
Cyclones and severe weather may also affect fresh water availability in these remote systems. Although they possess salt-secreting glands, sea snakes require fresh water for metabolic processes (Lillywhite et al., 2019), thus a reduction or lack of fresh water may stress sea snakes. Sea snakes are known to obtain drinking water from the temporary fresh water or dilute brackish-water lens formed on the sea-surface during intense precipitation over the ocean (Lillywhite et al., 2014, 2015, 2019). Long-term seasonal trends and variability in rainfall records at Ashmore and the wider North West Shelf indicates no evidence of significant change compared to the trends across Australia over the time period of sea snake decline (Shi et al., 2008). Cyclones and severe weather, or the rainfall they produce are therefore, unlikely to account for the decline of sea snakes at Ashmore.
Marine Heat Waves
Marine heat waves are periods when water temperatures at a particular location exceed a seasonally varying threshold (usually the 90th percentile over the last 5 years), for at least 5 consecutive days (Hobday et al., 2016). Ashmore experienced several marine heat wave events between 1995 and 2018, with increasing durations over time (Figure 3). Most events were brief, however, and fell below the upper thermal thresholds of sea snakes (39–40°C: Heatwole et al., 2012). Based on the available information, we conclude that marine heat waves have had limited direct impact on sea snakes at Ashmore. Marine heatwaves, however, may have significant indirect influences on sea snake population stability via coral bleaching events and reductions in the overall health of local ecosystems.
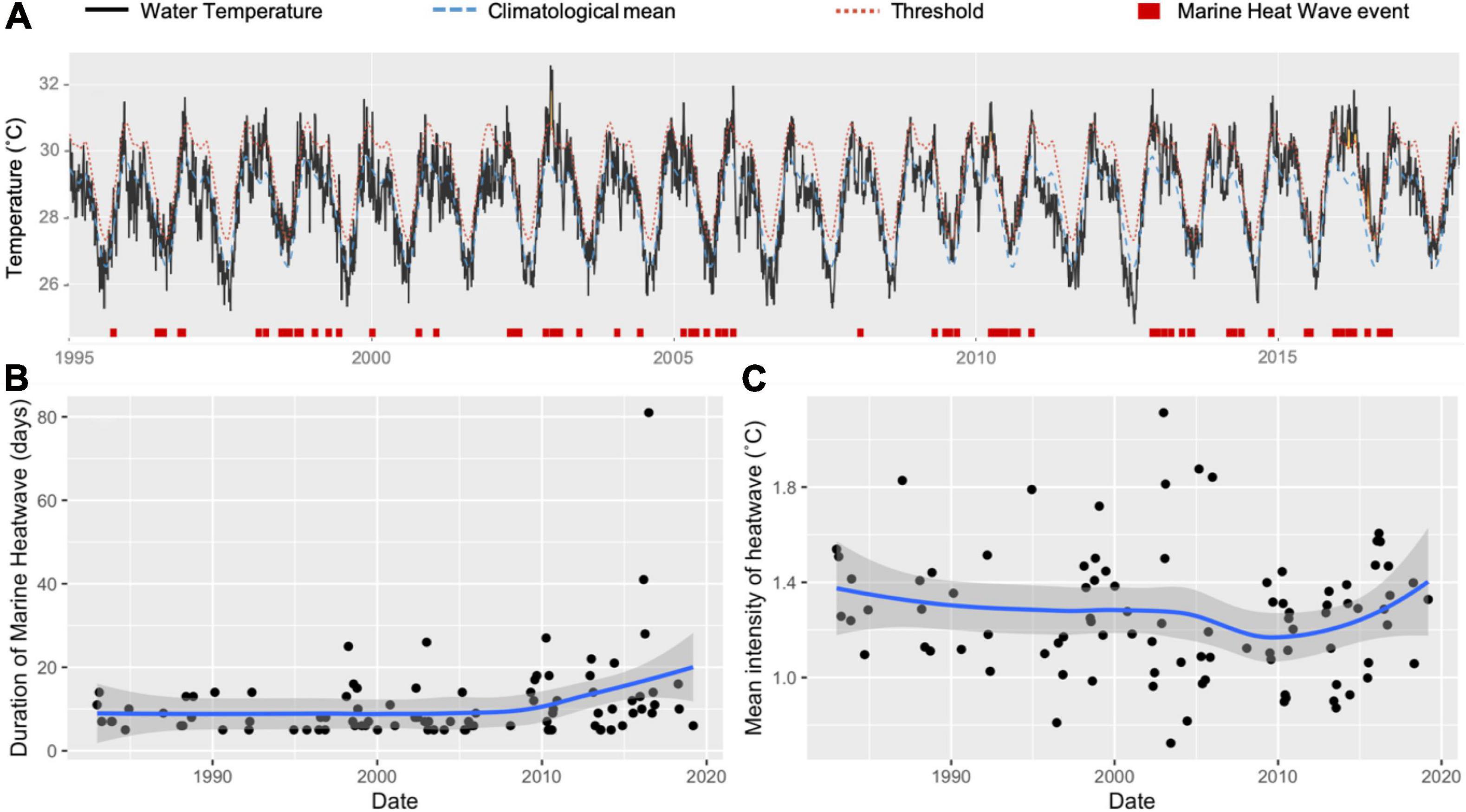
Figure 3. Chronology of water temperature records from Ashmore obtained from remote sensing data between 1995 and 2019 (A). Climatological means (broken blue lines) and thresholds (broken red lines) were calculated using the Marine Heatwave Tracker (Schlegel, 2018). Marine heat wave events are identified in red boxes as periods that exceed seasonally varied thresholds for at least 5 consecutive days (Hobday et al., 2016). (B) shows the historic trend in duration of marine heatwaves (days), while (C) shows the intensity of heatwaves (°C) at Ashmore between 1982 and 2019. Trendlines estimated using Generalised Additive Models with standard error estimate bounds represented as grey polygons.
Sediment Build-Up
Hypothesis: Chronic changes in oceanography and geomorphology at Ashmore impacted sea snake population persistence and drove declines.
Available evidence base: Some circumstantial
Strength and direction of evidence: Weakly refutes (−)
Plausibility: Likely implausible
Two major tsunamis that took place in the Indian ocean due to earthquakes off the northwest coast of Sumatra in December 2004 and the south coast of Java in July 2006 (Drushka et al., 2008) postdate the time of significant sea snake declines at Ashmore. We are unaware of any substantial changes in oceanic currents over the last two decades at Ashmore that could have led to sustained sediment build-up across the full range of sea snake habitat. If this has taken place however, increased siltation and suspended sediments can reduce foraging, growth and condition of fish (Newcombe and MacDonald, 1991; Miller et al., 2002). The first sea snake species reported to disappear from Ashmore (the horned sea snake) forages on small gobies and eels on the sand flats, while the next species to disappear (the turtle-headed sea snake) specialises on fish eggs. Both these food types are likely affected by siltation. However, observation of siltation during the time of sea snake disappearance has been limited to only one site (M. Guinea, pers. obs.). Guinea and Mason (2017) over the period 1994–2003 observed increased levels of siltation near the inner mooring where sea snakes used to be numerous. In 2005, the seagrasses adjacent to the West Island channel leading to the inner mooring were described as stressed and growing in suboptimal conditions (Brown and Skewes, 2001), and the observable reduction in seagrass at the channel in recent years may be a result of sediment movement along the channel. Nevertheless, more recently, siltation has been shown to be more extensive and likely implicated in the disappearance of large aggregations of the sea cucumber (Holothuria leucospilota) at Ashmore. This species was extremely abundant and found in densities of up to 224,000 per hectare on reef flats in 2005 and 2006 (Ceccarelli et al., 2007), but was absent in 2019 when the same sites had transformed into sand flat habitats (Keesing et al., 2020). The formation of the vegetated Splittgerber Cay through siltation has developed a permanent feature since 2010 to the east of East Island and is likely to have influenced currents and water movements within the reef flats. Whilst sand movement can have profound impacts on marine biota, substantial documented changes at a small number of sites took place well after the decline in sea snakes. Furthermore, given sea snakes occupied a diversity of niches at Ashmore prior to decline, including an abundance in other channels and outer reef fringes where sediment build-up has not been observed, this hypothesis is unlikely to explain the disappearance of sea snakes across the reef system.
Targeted Harvest and Fishing Bycatch
Hypothesis: Targeted harvests and retention of bycaught sea snakes by traditional and commercial fishers drove declines in sea snake populations at Ashmore.
Available evidence base: Some circumstantial
Strength and direction of evidence: Strongly refutes (−−)
Plausibility: Implausible
With the exception of a single documented historic collection for the scientific specimen trade (Smith, 1926), there is no evidence that Indonesian fishers have targeted sea snakes at Ashmore; however, bycatch or incidental catches (including sea snakes) may form a significant source of income for fishers who may retain them for sale (Branch et al., 2013). Since record-keeping began in 1986, there are no reports of sea snakes aboard Indonesian vessels inspected by staff of Australian Customs and Australian Fisheries in their routine surveillance examinations (Guinea, 2008). A common superstition amongst fishers that interfering with or damaging sea snakes will lead to an early and mysterious death or ill fortune on the return trip to Indonesia (Guinea, 2008) may in part explain the dearth of records. Trawl fisheries can be detrimental to sea snakes (Fry et al., 2001; Milton et al., 2009) but have not operated and do not currently operate at or close to Ashmore. Based on available knowledge, it is very unlikely that bycatch or incidental catches of sea snakes drove the decline of all sea snake species once present at Ashmore.
Increased Predator Abundance
Hypothesis: Increased abundance of top order predators and a subsequent modification of trophic structure at Ashmore directly or indirectly drove declines in sea snakes.
Available evidence base: Direct or sufficient circumstantial
Strength and direction of evidence: Weakly supports (+)
Plausibility: Plausible
Eight species of sharks are known to occur at Ashmore, with reef sharks being the dominant group by species richness and biomass (Speed et al., 2018, 2019). In the early and mid-1900’s, sharks have been a common feature of the northern Australia shelf, including Ashmore, with Indonesian fishers venturing to the Australian coastline to fish for sharks (Witt, 1951; Campbell and Wilson, 1993; Macknight, 2013). The financial impetus for shark product increased markedly in the 1970s and early 1980 due to market demand in China and South East Asia (Macknight, 2013; Stacey, 2017). In the 1980s, prior to the establishment of the marine park, sharks were extensively fished at Ashmore by Indonesian fishermen, especially for their fins (Russell and Vail, 1988; Figure 4). After interviewing the Indonesian fishermen at Ashmore, Russell and Vail (1988) reported that by 1987 sharks were reduced in number, comprised just 3.8% of the catch, and were harder to catch at Ashmore relative to other areas. At least one crew member of the 13 interviewed considered other reefs to be better than Ashmore for shark fishing at the time. An intensive field survey over 3 weeks in September and October 1998 showed that reef-associated sharks were extremely rare (<1 ha–1) throughout the MOU box—an area in which Indonesian traditional fishers are allowed to continue their customary practices and traditional harvest of fish, trepang, trochus, abalone and other marine food resources (Susanti et al., 2021; Figure 1). In 1998, underwater visual census (UVC) at 231 reef-edge sites across the MOU box recorded just three grey reef sharks (Carcharhinus amblyrhynchos) and 28 whitetip reef sharks (Triaenodon obesus), while 24 grey reef sharks and one silvertip shark (C. albimarginatus) were caught in gill nets set overnight in shallow waters (<50 m) at Seringapatam Reef (Dennis et al., 2005). These low numbers are atypical for reef-sharks on oceanic reefs (Long et al., 1997; Dennis et al., 2005), and may be the result of targeted fishing of carcharhinid sharks for their fins and flesh (Russell and Vail, 1988). Wallner and McLoughlin (1995) also noted a decline in catches of carcharhinid sharks by traditional Indonesian fisherman within the MOU during the early 1990s.
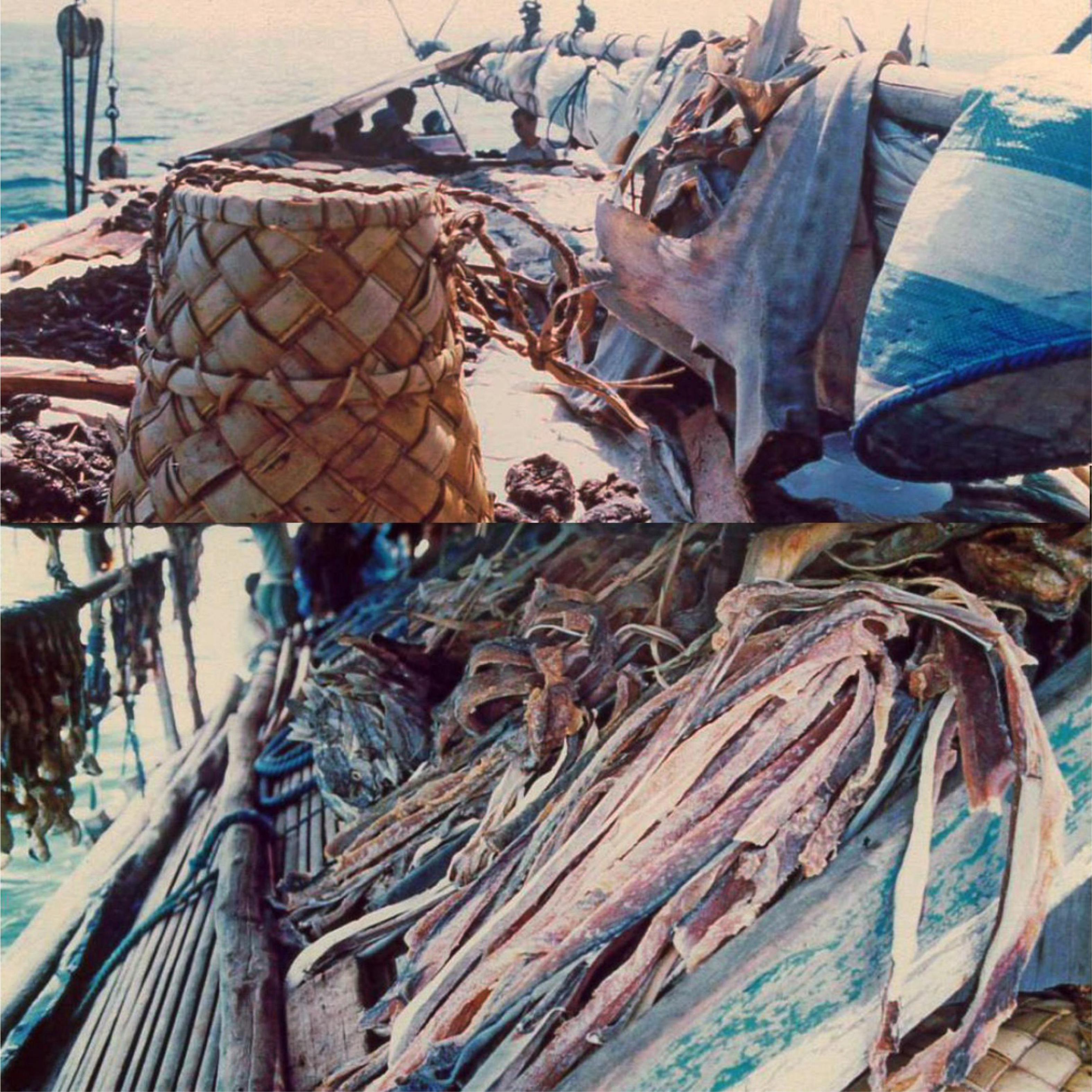
Figure 4. Sharks’ fins (top panel) and strips of shark meat (bottom panel) being died on a traditional Indonesian fishing boat at Ashmore in 1987 [Photo Barry Russell, Northern Territory Museum; used with permission from Plate 4 of Russell and Vail (1988)].
Visual comparisons of sightings of sharks suggest an approximate threefold increase in the abundance of sharks between 2009 and 2019 (Figure 5). Studies by Kospartov et al. (2006) in 2005, Richards et al. (2009) in 2009, and Keesing et al. (2020) in 2019 estimated the abundance of finfish and sharks in shallow water (<30 m) at Ashmore using UVC surveys at 16, 8, 8, and 216 sites, respectively, at Ashmore (Figure 5). These studies recorded a general increase in the rate of sightings of grey reef sharks from 0.07 per hour (1998) to 0.5 per hour (2019). In 2004 the mean relative abundance per hour of the grey reef shark was almost identical to that of Scott Reef (Speed et al., 2018) reaffirming the conclusion by Meekan et al. (2006) that the numbers of whitetip reef sharks and grey reef sharks were similar in shallow habitats of Scott Reef (currently fished), Ashmore Reef, Cartier Island (historically fished) and Rowley Shoals (negligible fishing impacts). In a recent study, Speed et al. (2018) compared the abundance of sharks at Ashmore using baited remote underwater video (BRUV) in 2004 and then again in 2016. The assemblage of sharks shifted considerably between the two surveys, with the proportion of shark species in higher trophic positions (e.g., tiger sharks, Galeocerdo cuvier) and mid-trophic level reef sharks (e.g., grey reef sharks) increasing from 7.1 to 11.9% and from 28.6 to 57.6%, respectively. The relative mean abundance of grey reef sharks increased from 0.16 ± 0.06 individuals per hour (MaxN) in 2004 to 0.74 ± 0.11 MaxN in 2016, a nearly fivefold increase (Figure 5). Speed et al. (2018) concluded that a reduction of fishing pressure at Ashmore as a result of Australian Border Force/Australian Navy presence on a near-permanent basis (DIBP, 2017) since 2008, may have contributed to the increase in shark numbers. However, the speed of recovery of sharks at Ashmore is debatable (Guinea, 2020a; Meekan et al., 2020). More recently, manta tow surveys conducted at 230 reef-edge sites in 2019 recorded 17 individual reef sharks (12 whitetip reef sharks, 1 blacktip reef shark and 4 grey reef sharks; equalling a mean abundance of grey reef sharks of 0.52 ± 0.31 individuals per hour (Figure 5; Keesing et al., 2020). Although survey methods varied between studies (i.e., UVC, BRUVs, fishing surveys), and sighting rates are susceptible to biases associated with each method (Jennings and Polunin, 1995; Ward-Paige et al., 2010), trends from both UVC and BRUVS surveys indicate an increase in shark sighting rates since 2009 at Ashmore (Figure 5).
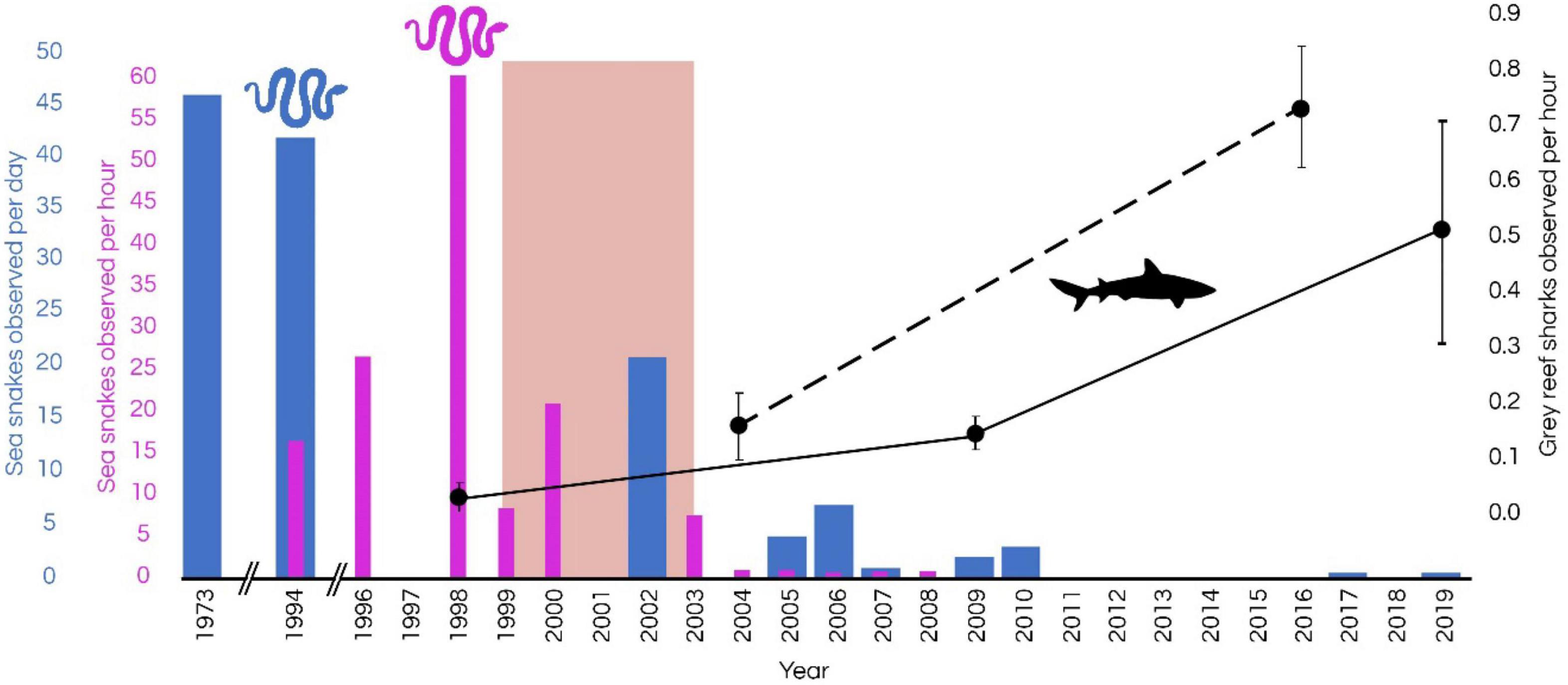
Figure 5. Trends in cumulative sighting rates of sea snakes (up to 17 species; blue and purple bars) and relative sighting rate of grey reef sharks (Carcharhinus amblyrhynchos; black points) at Ashmore Reef Marine Park. Shark observations based on Skewes et al. (1999), Kospartov et al. (2006), Richards et al. (2009), Edgar and Stuart-Smith (2018), Speed et al. (2018), and Keesing et al. (2020). The dashed line shows trends in baited remote underwater video (BRUV) surveys and solid lines show trends in underwater visual census (UVC) surveys. The pink band shows the time of marked decline in sea snake numbers and diversity at Ashmore. Error bars show SE. Metrics of sighting rates of sea snakes and reef sharks have been standardised across studies that utilised multiple methods at multiple sites within reef-edge habitats.
The recovery of sharks, and the resulting increased predation pressure, may have reduced the abundance of small mesopredatory fishes (≤50 cm Total Length) in reef habitats at Ashmore, with an associated increase in large mesopredatory fishes (≥100 cm TL) such as groupers (Plectropomus spp.) and bluefin trevally (Caranx melampygus) (Speed et al., 2019). In the 1990s, three independent surveys observed that larger predatory reef fish were absent from Ashmore (Berry, 1993; Hutchins, 1998; Russell and Vail, 1988), likely due to fishing pressure or possibly environmental factors. Another suggestion—that then-abundant sea snakes consumed the fish (Berry, 1993)–cannot be valid, because large mesopredatory fish species do not comprise a significant proportion of the diets of sea snakes at Ashmore (McCosker, 1975; Voris and Voris, 1983).
Logic of causality dictates the putative cause precedes the effect, given the period of decline in sea snakes took place around 2000 and shark numbers have been only substantially recovering much later (Figure 5). If shark or other top-order predator numbers began to increase earlier than has been recorded at Ashmore, that change might have increased top-down pressures. The subsequent trophic cascade following increased shark abundance may have contributed to the ongoing absence of sea snakes from Ashmore (Figure 5). Unfortunately, critical data on shark abundance at Ashmore prior to 1998, in particular historical baselines and the early decline years (i.e., 1995–1998), does not exist. Below we explore the evidence, logic and mechanisms that may explain the process connecting these two trends.
Lethal Effects of Increased Predator Abundance
Several species of sharks prey on sea snakes (Heatwole et al., 1974; Tuma, 1976; Lyle, 1987; Cliff and Dudley, 1991; Simpfendorfer, 1992; Lowe et al., 1996; Fergusson et al., 2000; Heithaus, 2001; Kerford, 2005). Notably, tiger sharks are important in this respect (Dicken et al., 2017; Ferreira et al., 2017; Aines et al., 2018; Figure 6). In coastal waters of Queensland, Australia, Heatwole (1974) found that 31% of the 637 tiger sharks that contained food had at least one sea snake in their stomachs. Heatwole (1999) examined data on stomach contents of over 7,000 specimens of sharks belonging to 19 species in Queensland and found that six species ate sea snakes. Some tiger sharks had up to four sea snakes in their stomachs. In the southern Ryukyus in Japan, 48 of 343 tiger sharks with prey remains had consumed sea snakes (Masunaga et al., 2008). Although recent surveys of sharks at Ashmore in 2018 and 2019 indicate low densities of tiger sharks (<0.1 per ha: Edgar and Stuart-Smith, 2018; Keesing et al., 2020), their role in regulating the abundance of sea-snakes and other large teleosts including groupers (Heatwole, 1975; Berry, 1986), bill fish (Paulson, 1967), moray eels (Herre, 1942), and large pufferfish (Pickwell et al., 1983) cannot be discounted.
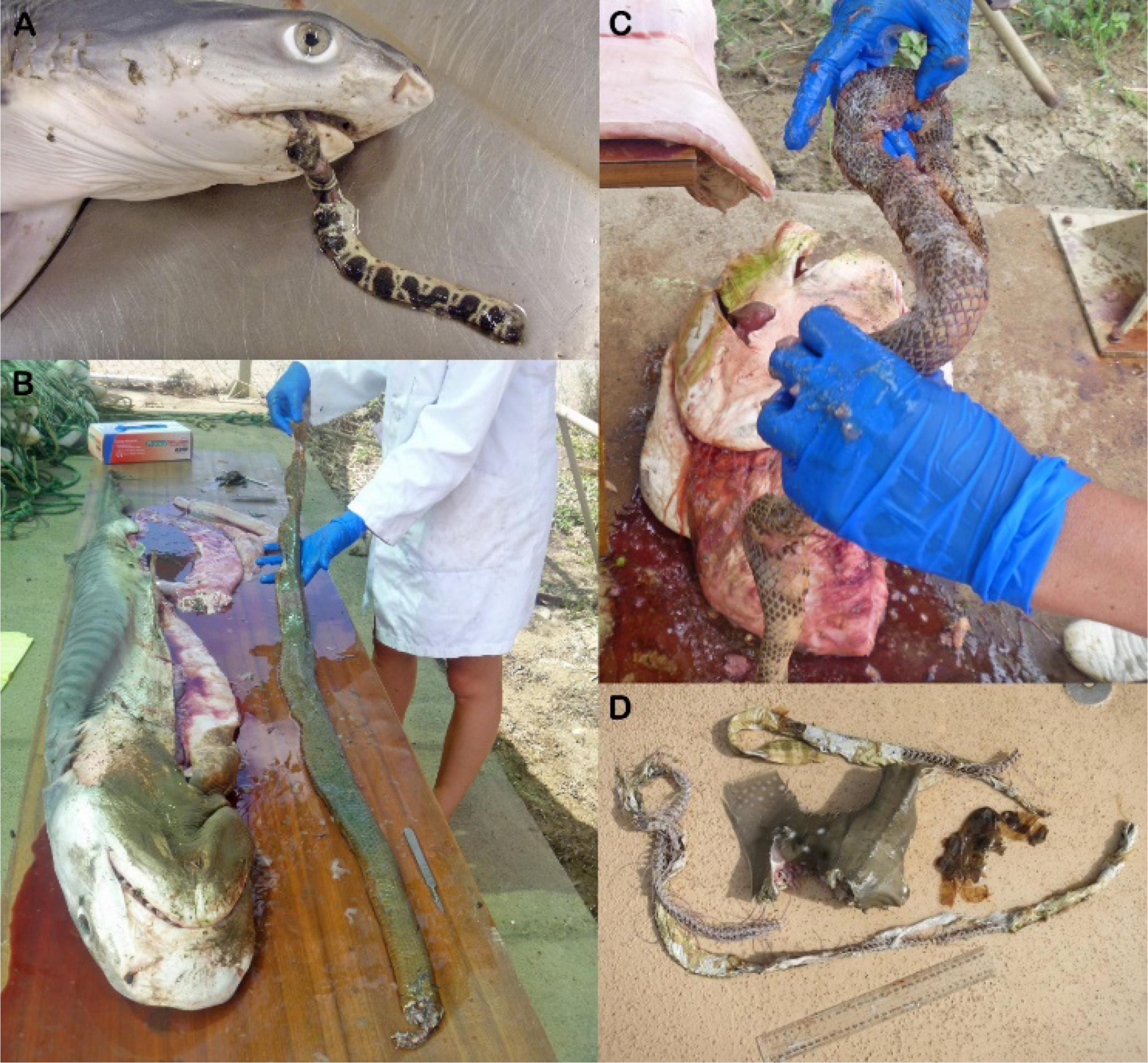
Figure 6. Evidence of predation of sea snakes by tiger sharks (Galeocerdo cuvier): (A) Olive-headed sea snake Hydrophis major, (B) Olive sea snake Aipysurus laevis, (C) Dubois’ sea snake Aipysurus duboisii, and (D) Elegant sea snake Hydrophis elegans. Photos: (A–C) from Queensland, Australia by Bonnie Holmes, D from Western Australia, Australia provided by Department of Primary Industries and Regional Development.
Non-lethal Effects of Increased Predator Abundance
In addition to direct predation of sea snakes by sharks, increased presence of predators might have significant non-consumptive effects, including increased stress and disruption of feeding and mating behaviours (Mitchell and Harborne, 2020). Seasonal abundance of tiger sharks in Shark Bay, Australia increases during the warm period (September–May) when there is also more sea snake activity (Heithaus, 2001). Olive-headed sea snakes (Hydrophis major) in this area avoid the reef edges (which are preferred by sharks) when sharks are abundant, yet feed at these same sites when sharks are temporally scarce (Wirsing and Heithaus, 2009). Speed et al. (2019) showed a decline in smaller fish at Ashmore associated with the increase of sharks and larger predatory fish, suggesting that prey availability for sea snakes may also be affected by the abundance of sharks. The risk of predation by sharks and other large fish may also alter the behaviour, physiology, and morphology of smaller fish (Mitchell and Harborne, 2020) which may have indirect effects on prey availability for sea snakes. A study from reefs in proximity to Ashmore showed that on reefs where sharks were rare, five species of mesopredatory teleosts consumed smaller fish, whereas on reefs with abundant sharks, the same mesopredatory teleosts consumed more benthic invertebrates (Barley et al., 2017). The shift in diet due to the presence of top order predators is likely to disproportionately affect mesopredators with highly specialised diets (e.g., turtle-headed sea snakes, horned sea snakes), as compared to generalist species (e.g., olive sea snakes) that target a range of benthic fish and invertebrates and are likely able to switch prey and persist longer. This hypothesis is consistent with the observed order of declines of sea snake species at Ashmore (Guinea, 2013).
Although trophic cascades induced by changing shark populations are rare in coral reef ecosystems (Frisch et al., 2016; Roff et al., 2016), it is possible that the change in abundance of predators has had a significant top-down influence at Ashmore. A suspected increased abundance of sharks and larger predatory teleosts may be a contributing factor in the decline and ongoing absence of sea snakes at Ashmore via direct predation pressure and indirect stresses. Most species of sea snakes inhabiting remote oceanic reefs have small home ranges in shallow waters and display strong site fidelity (Guinea and Whiting, 2005; Lukoschek and Shine, 2012), suggesting that changes in the abundance of predators, especially in shallow waters, might affect localised populations of sea snakes.
Comparisons With Nearby Sites
Records from reef systems close to Ashmore (Figure 1) supports the “increased predation” hypothesis. The Scott Reefs (three large atolls: Seringapatam, Scott Reef North and Scott Reef South) south of Ashmore have been fished for sharks and teleosts by Indonesian fishers from at least the 1800s (Russell and Vail, 1988), with fishing pressure remaining high to the present day (McCauley et al., 2004; Meekan et al., 2006; Woodside, 2009; Prescott et al., 2017; Stacey, 2017). The abundance of shallow water shark species at the Scott Reefs was approximately equal to those at Cartier Island, Rowley Shoals and Ashmore in 2004 (Meekan et al., 2006). Five species of sea snakes are known from the Scott Reef system, and no declines in populations of those snakes have been noted over the period that sea snakes declined at Ashmore (Guinea, 2013). Similar patterns in lack of decline exist at Hibernia Reef (c. 42 km from Ashmore: Guinea, 2013) and the North West Shoals (c. 150 km from Ashmore: Udyawer and Heupel, 2017), where commercial fishing (but not traditional Indonesian fishing) takes place, and sea snakes remain common (Guinea, 2013). Waters within the MOU-box, between Ashmore and Browse Island are areas still accessed by traditional Indonesian fishers (Stacey, 2007), and include areas fished by a number of federal and state-managed commercial fisheries that target finfish, prawns and elasmobranchs using trap, line and trawl fishing methods (Patterson et al., 2018; Gaughan et al., 2019).
In contrast, the Rowley Shoals (comprising three large reefs: Imperieuse, Clerke and Mermaid) have been protected since 1990 (extended in 2004), 2 years after Ashmore Reef Marine Park was established, and are subject to very low levels of fishing pressure mainly by fishing tour operators (DEC, 2007). Despite several marine biodiversity surveys, no sea snakes have been detected at the Rowley Shoals (Berry, 1986; Bryce, 2009; Edgar et al., 2017), although several sea snake species are common further south in Australian waters (Heatwole and Cogger, 1994; Cogger, 2018). Recent habitat modelling of the Western Australian coastline by Udyawer et al. (2020) identified habitat conditions in the Rowley Shoals as highly suitable for sea snakes despite the lack of their occurrence there. It is possible, therefore, that the large predator populations at the relatively unfished Rowley Shoals (Bryce, 2009) may limit the establishment of sea snakes (Kerford, 2005).
Similar and unexplained declines in turtle-headed sea snakes have been recorded at a protected marine reserve in Noumea, New Caledonia after fishing ceased in 1989 (Goiran and Shine, 2013). If this hypothesis for the observed decline at Ashmore holds true as the putative cause (i.e., cessation of fishing) preceded the decline in sea snakes, it may be that the Noumea decline was due also to recovery of large predators (e.g., sharks) and subsequent trophic cascades (Goiran and Shine, 2013). What remains lacking in terms of evidence to more conclusively support or refute this hypothesis is insight on the historical baseline for shark and sea snake numbers before there was any risk that fishing was impacting direct and indirect interactions between the two groups. One possibility worth considering is that the historical abundance of sea snakes at Ashmore reef was simply an artefact of historical fishing pressure on sharks in the area combined with the traditional beliefs of local fishers minimising any sea snake take. Unfortunately, there are few regions in the world where fishing impacts are absent and where such understanding could be generated now, either via comparison or manipulation.
Increased Maritime Activity
Hypothesis: Increased acute maritime activity and subsequent upsurge in pollution at Ashmore significantly impacted sea snake populations and drove declines.
Available evidence base: Some circumstantial
Strength and direction of evidence: Mixed supports (±)
Plausibility: Needs more data
Since at least the late 19th century, Indonesian fishers have frequently visited the Ashmore islands (Clark, 2000); visits by Indonesian vessels to this region in the period 1900–1940 are also well documented (Crawford, 1969). These visits by Indonesian vessels resumed after World War II (Serventy, 1952), and continue to the current day. Increasing numbers of Suspected Illegal Entry Vessels (SIEVs) carrying asylum seekers entered Australia’s maritime borders between 1999 and 2001 (Phillips and Spinks, 2013; Figure 7). As a response to an influx of SIEVs and a step toward protecting its borders, in 2001 the Australian Government removed several territorial outposts including Ashmore from the country’s “migration zone” (Magner, 2004; Fox, 2010). This saw an increased presence of larger patrolling vessels (e.g., ACV Wauri, ACV Ashmore Guardian) and smaller response vessels to intervene and escort SIEVs around Ashmore, as well as vessels to temporally house the personnel that were onboard SIEVs around year 2000 (Whiting, 2000; Russell et al., 2004; Guinea, 2020a). Because many of the SIEVs at Ashmore were in poor condition, heavily fouled and required constant bailing due to leaking, Whiting (2000) and Russell et al. (2004) recommended the removal of bilge oil and fuel from SIEVs and removal of these vessels from the marine park area before their destruction. Several SIEVs succumbed to their poor condition and sank in the marine park during rough weather (Domaschenz and Pike, Park Managers pers. comm).
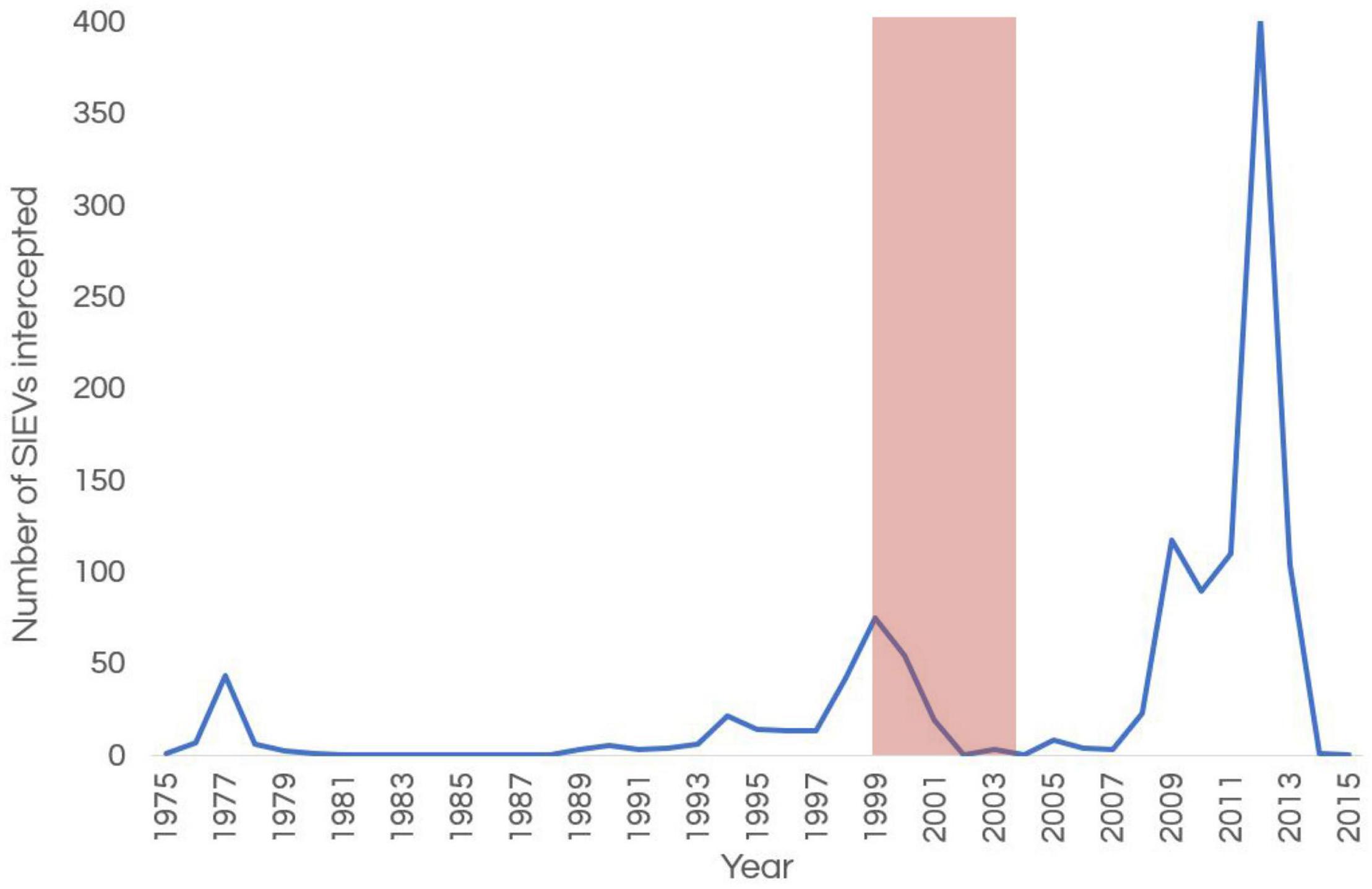
Figure 7. Number of Suspected Illegal Entry Vessels (SIEVs) entering Australia’s border at Ashmore over time. The pink band shows the time of marked decline in sea snake numbers and diversity at Ashmore. The increased number of SIEVs arriving after 2007 was due a change of policy by the then government and not a sudden increase of numbers of refugees.
Therefore, it is possible that increased activity of large boats within the restricted habitats and channels of Ashmore between 2000 and 2002 may have triggered higher mortality rates for sea snakes locally, limited the possibility for populations replenishment from surrounding reefs and caused local populations to decline (Guinea, 2020b). Below we explore the evidence and logic underlying our hypothesis.
Hydrocarbon spills
Naturally occurring hydrocarbon seeps are a common feature of the sea floor in the Timor Sea (O’Brien and Glenn, 2005). These seeps are mostly gas such as methane that is trapped below the thermocline and consumed by bacteria and reef-building biota to produce banks and mounds (O’Brien and Glenn, 2005). Surface hydrocarbons are relatively rare and provide a challenge to marine biota. There is likely to have been a number of petroleum hydrocarbon (PHC) releases in the water from both the increased boat traffic and also from the bilge pumps used on confiscated SIEVs anchored at Ashmore that were awaiting destruction outside the marine protected area. It is likely that animals that move on the surface are more at risk of being affected by exposure to PHC than fish for example. Few data are available on the effects of PHC exposure on sea snakes, although these animals have been identified as vulnerable to oil slicks (Tawfiq and Olsen, 1993; Watson et al., 2009; Short, 2011). While the exact pathway by which hydrocarbon exposure kills sea snakes is not known, it could be via direct inhaling or ingesting of PHCs (Gagnon, 2010) or through secondary exposure to PHCs via prey (Gagnon and Rawson, 2010). Ecotoxicological studies suggest that PHCs can bio-accumulate in sea snakes via food chains (Mote et al., 2015) and that some species are more susceptible than others (Sereshk and Bakhtiari, 2014). It is also possible that oils covering the skin could affect cutaneous respiration in sea snakes. An environmental impact assessment following the blowout of the Montara oil well (c. 250 km SE from Ashmore) in 2009 detected more sea snakes in oil-affected waters than in non-oil-affected waters (Watson et al., 2009). One dead horned sea snake was found floating in oil-affected waters, but the cause of death could not be determined (Watson et al., 2009; Guinea, 2013). In 2012 and 2013, Guinea (2013) conducted follow up surveys to evaluate the potential impacts of the Montara oil spill on marine reptiles in the broader area. Potentially impacted areas surveyed included Ashmore, Cartier Island, and Hibernia Reef, whilst Scott and Seringapatam Reefs and Browse Island were surveyed as control sites. Guinea concluded that there had been no detectable post-spill impact of hydrocarbon release on marine reptiles (sea turtles and sea snakes) at the reefs surveyed (Guinea, 2013). Collectively, there is a significant lack of information on the toxicity of hydrocarbons, their impact on sea snakes and if so, the mechanism of impact. Should surface oil slicks associated with boating and the decommissioning of the SIEVs had affected sea snakes, its impact would have been largely restricted to the West Island Channel at Ashmore. A further consideration could be that elevated boat traffic in the region may reduce the ability of sea snakes to recover from oil spill incidents, however, there is limited data to support this. Currently, there is no evidence to show that hydrocarbons alone caused a reef-wide decline in sea snakes at Ashmore and no evidence of ongoing spills to explain the ongoing absence of sea snakes.
Noise Pollution
The soundscape at Ashmore is likely to have changed since the 2001/2002 period when an increase in regular boat traffic took place at Ashmore. Increased environmental noise can affect the behaviour and population viability of marine animals (Peng et al., 2015). Whether this is due to mechanical vibrations or direct mechanical damage is not clear. Although published information on the impact of noise on sea snakes is very limited, anecdotal observations by the authors suggest that sea snakes may show mixed reactions to regular boat traffic. While some locations with high boat traffic such as Broome Port have very few sea snakes, others such as Shark Bay and Darwin Harbour support large populations of sea snakes. Sea snakes are sensitive to mechanical vibrations (Romanov, 1991) and to low-frequency sounds (peak sensitivity at 60 Hz) but have lower sensitivity than bony fishes and marine turtles (Chapuis et al., 2019). Collectively, very little is known on the impact of vibrations and sound on sea snakes. Therefore, it is not possible, based on current understanding and available data, to assess the likelihood of noise pollution causing a decline of sea snakes at Ashmore.
Plastic Pollution
The proliferation of micro- and macro-plastic pollution in the marine environment has emerged as an important factor affecting marine wildlife (Derraik, 2002; Gregory, 2009). The deleterious effect of entanglement and ingestion of plastics on marine turtles is well known (Nelms et al., 2016) but no information exists about its effects on sea snakes despite extensive reviews of the impact of plastics and other marine debris on marine animals (Kühn et al., 2015). It is known, however, that sea snakes can become fatally entangled in other forms of marine litter (Udyawer et al., 2013). Very little is known on the level of marine debris at Ashmore in the past (Whiting and Guinea, 1998). Except for its prevalence in seabird nests (Lavers et al., 2013), no quantitative data on the extent of plastic pollution at Ashmore has been collected in recent times. However, the large amounts of plastic in the water column, especially fragments of soft plastic bags and food packaging originating from Indonesia, has been remarked on (Keesing et al., 2020). At this stage there is no evidence that can be cited to attribute plastic pollution as causing the decline of sea snakes observed at Ashmore, but this warrants further examination given the well documented effects on other wildlife.
Pathogens
Hypothesis: Increased exposure to chronic or acute marine pathogens at Ashmore significantly impacted sea snake population health and drove declines.
Available evidence base: Some circumstantial
Strength and direction of evidence: Strongly supports (+ +)
Plausibility: Plausible
Many infectious agents, including viruses, bacteria, fungi, and parasites, can cause morbidity and mortality in wild reptiles (Herbst, 1994; Lovich et al., 1996; Smith et al., 1998; Schumacher, 2006). Some diseases cause significant declines in wild populations of reptiles, including terrestrial snakes (Cheatwood et al., 2003; Clark et al., 2011). Increased susceptibility to pathogens in reptiles is often exacerbated by other stochastic environmental events such as disturbances that drive habitat change, or exposure to pollution (Gibbons et al., 2000). Larger seasonal variation in water temperatures may increase rates of disease spread and prevalence in aquatic systems (Karvonen et al., 2010). Rising temperatures and the consequent rise in disease risk have caused notable declines in reptile populations globally. The increasing occurrence and duration of elevated sea surface temperatures at Ashmore (Figure 4) is a factor that may influence the prevalence and spread of pathogens that affect sea snakes (e.g., microsporidia, nematodes: Gillett et al., 2017).
While we are not aware of any reports of disease outbreaks in sea snakes at Ashmore, the lack of sampling and testing for diseases renders it impossible to dismiss disease as a potential contributor to the sea snake decline. Pathogens and their impacts on populations are a known driver of local extinction events in marine systems (Dulvy et al., 2003). For example, the spread of a host-specific water-borne pathogen in 1983 resulted in mass mortalities and local extinctions of the black sea urchin (Diadema antillarum) that was once abundant across the Caribbean (Lessios, 1988). Although the cause of these declines was pinpointed to an unidentified pathogen that spread though the regional current systems, the significant loss of this keystone species has seen ongoing impacts on the wider marine ecosystem in the region (Lessios, 2005). The incidences of marine disease and frequency of outbreaks in seemingly pristine habitats is on the increase due to the effects of climate change, increased urbanisation and other anthropogenic pressures (Ruiz et al., 2000; Harvell et al., 2002). An increase in boat traffic in and around Ashmore (detailed above) presents as a potential pathway for introduction and spread of marine pathogens, as shown elsewhere (Murray et al., 2002; Anderson et al., 2014).
The impact of many viral infections on snakes remains unknown (Shortridge, 1989; Marschang, 2011), but it is possible that viral infections could drive down reproductive output without any direct mortality (e.g., lead to sterile populations or populations where reproduction rates do not meet replacement needs). Whilst it is difficult to monitor for mortality events in remote locations such as Ashmore (low observer density but also rapid decomposition and rapid consumption of remains in tropical reef systems), pathogens that drive populations to extinction without direct mortality would be even more difficult to detect. Infections and inflammations caused by endoparasites are a significant cause of stranding in sea snakes along the Queensland coastline in Australia (Gillett, 2017). Disease risk in sea snakes might be higher in males during the breeding season, due to the higher stress levels associated with such activity. Increased levels of testosterone in reptiles can reduce immune reactivity, thus increasing vulnerability to pathogenic organisms (Zapata et al., 1992; Zimmerman et al., 2010; Gillett, 2017). Additional data are required to assess if this is a likely pathway to reduced population health and fecundity in sea snakes at Ashmore and other locations. The possibility of pathogens affecting sea snake populations has attracted little research and is a key area where more empirical information is required (Gillett et al., 2017). Given that the disappearance of sea snakes at Ashmore took place across all ecological and behavioural cohorts of the sea snake community, a pathogen remains the most plausible mechanism for the rapid decline.
The Way Forward: From Inference to Understanding
The ongoing persistence of sea snakes at reef systems nearby to Ashmore argues for the drivers of observed local extirpation in recent decades being local factors rather than broader regional or global drivers. The apparently dramatic disappearance of sea snakes from Ashmore is inconsistent with hypotheses that invoke some of the single catastrophic events (such as prolong drought, coral bleaching or a cyclone). Moreover, such events did not occur during the time period that sea snakes declined, nor has there been any subsequent increase in numbers that would be expected after pressures from catastrophic events relax. Likewise, some plausible hypotheses put forward in the past (such as fishing pressure) are inconsistent with the full set of evidence and theory synthesised in this review. Some hypotheses remain plausible explanations for the observed decline, either on their own or acting in combination. However, we currently lack the evidence to evaluate them, or to recommend changes to management that could allow for the recovery of sea snakes at Ashmore.
Explaining the Decline
To guide the resourcing of future research efforts, we prioritise key knowledge shortfalls with respect to the seven explanations for sea snake decline explored in this review, presented in order of decreasing priority.
Pathogenic Organisms
Given the general lack of knowledge on disease of sea snakes, there is a need for improved understanding of the role of pathogens in sea snake population stability and resilience. Additional specific recommendations to better inform management of the Ashmore situation are as follows: Screening specimens collected during the period of significant sea snake decline (around year 2000), and now deposited in Australian Museum collections, for pathogens and diseases is arguably the quickest and most efficient way to inform more detailed research programs. In this case, both examination for lesions and molecular screening if the storage medium allows, is recommended. Concurrently, examining the reproductive status of these specimens would enable comparison with conditions elsewhere to evaluate whether reproduction output has been affected. Furthermore, it is highly recommended that procedures are placed in collaboration with Australian Border Force vessels to collect and rapidly freeze any dead sea snakes found for screening purposes. In designing studies, consideration of the following questions will be important to address:
• If a disease or pathogen contributed to a widespread and mass mortality of sea snakes secondarily, why have no dead sea snakes been reported by the many border force and navy vessels patrolling the area? Are potentially infected individuals more susceptible to predators, rendering impacts of pathogens inconspicuous?
• Could it be possible that the mortality occurs over months or several years, especially at a time when sea snakes were abundant, such that a non-specialist wouldn’t think anything of a dead snake?
Increased Predator Abundance
Understanding trophic interactions between sea snakes and apex predators is key to identifying if the increased predator abundance may have had an impact on snake populations at Ashmore, and more generally in other similar reef systems (Edgar et al., 2020). In the context of understanding this process at Ashmore specifically, a standardised survey and monitoring protocol for sea snakes and sharks at Ashmore and comparative sites at other Timor Sea reefs would be of high priority. To be logistically feasible and scientifically justifiable, this program should be consistent in methodology to build on existing knowledge and focus on systematic surveys using standard and validated methods at sites. Surveys should encompass diverse marine habitats of the marine park where monitoring can be repeated consistently across multiple years to build a reliable time-series dataset. Priority questions to address include:
• Was the historically high abundance of sea snakes at Ashmore representative of long term (i.e., multi-century) patterns, or was it an “artificial” and unbalanced situation with regard to predator pressure due to anthropogenic offtake?
• Has the decline in sea snakes at Ashmore been driven either directly or indirectly by a change in shark abundance, including determining if the current absence is a new steady state, or if the ecosystem will continue to change to allow sea snakes to return in time?
Increased Maritime Activity
Targeted data collection would be able to quickly generate insight as to whether there is a valid justification for pursuing this line of explanation further. Recording and monitoring the soundscape at Ashmore will identify the respective contributions made by persistent engine noise of the ACVs, jet-propelled tender vessels and propeller powered dinghies with different engine configurations and at different speeds. In designing studies, consideration of the following questions will be important to address:
• If increased motor traffic affected the sea snakes, how have sea snake populations in some areas with much higher presence and abundance of large vessels (e.g., Shark Bay, Darwin Harbour) managed to survive there?
• Are some species more sensitive to oil slicks and noise pollution than others? Are naïve populations, or species not exposed to chronic marine pollution (like those at Ashmore) more sensitive to short-term increases in maritime activity?
Extreme Disturbance Events
While evidence show that direct impacts of extreme disturbance events may not have had an impact on the sea snakes at Ashmore, we currently have little understanding of the indirect impacts of such events. Ecological communities achieve their stability and resilience from a complex web of direct and indirect interactions among species (Montoya et al., 2006), and the importance of these unexpected indirect effects challenges the validity of a priori predictions about ecological trends (Worm and Paine, 2016). Therefore, extreme events such as cyclones and heat waves may have had cascading indirect impacts at Ashmore. Only long-term studies monitoring a wide range of species would shed light on these trends. Simultaneously, there is a need for establishment and deployment of data loggers to monitor abiotic conditions such as sedimentation and siltation, entrained particulate matter, sea surface temps, wave height etc. which can arguably affect a range of species including sea snakes.
Prioritising Future Management
The near complete loss of sea snakes from the shallows of the Ashmore reef system, previously recognised as a global biodiversity hotspot for these taxa, represents one of the most significant losses of vertebrate species in recent times. That the drivers of this loss remain enigmatic highlights the need for further targeted research to identify causal mechanisms. Only when causal mechanisms have been identified can robust management decisions for sea snake conservation be made. Around the world, anthropogenic impacts loom large on sea snake community collapse, as well as likely future outcomes. Furthermore, increasing global environmental change drivers, such as sea temperature and acidity increases, sea level rise, invasive alien species establishment and maritime activity will only increase the challenges of achieving effective conservation outcomes for Ashmore. Currently, it remains unclear what the most appropriate conservation actions are for sea snakes at Ashmore.
To get to the point where such decisions are possible, it will first be important to understand how the hypothesised changes in the biological community and physical ecosystem, as outlined in this work, directly and indirectly affect sea snake populations. In particular the work will need to explicitly consider how recolonisation, either natural or assisted, would be possible if and when the drivers of past decline have been identified and appropriately mitigated. Having a better understanding of the pathways of impact at Ashmore also might enable us to detect, identify and mitigate future declines in other regions. Our work presents a comprehensive review of the plausible mechanisms for decline of sea snakes at Ashmore and highlights the long-term comparative regional-scale surveys and experiments needed to further resolve these questions. For the future management of sea snakes at Ashmore in particular, and global sea snake conservation more broadly, we hope that this work provides a platform for future research and knowledge implementation in the years ahead.
Author Contributions
RuS and VU devised the main conceptual idea, proof outline, and prepared the figures. RuS, VU, MLG, RC, RiS, and BW made substantial contributions to drafting the manuscript, acquiring literature, and interpreting results. DC, RC, MH, JK, MG, AR, KS, and DT developed specific sections with revisions, literature, and comments. All authors participated in drafting the final version of the manuscript and gave final approval of the version submitted and any revised versions.
Funding
The publication of this research was funded by the Marine Biodiversity Hub through funding from the Australian Government’s National Environmental Science Program. The 2019 surveys conducted by CSIRO at Ashmore Reef Marine Park and the work of DC, JK, RuS, DT, and BW were funded by the Director of National Parks. The views expressed in this publication do not necessarily represent the views of the Director of National Parks or the Australian Government.
Conflict of Interest
The authors declare that the research was conducted in the absence of any commercial or financial relationships that could be construed as a potential conflict of interest.
Acknowledgments
We thank Bonnie Holmes, Daniela Waltrick, Lyle Vail and the Western Australian Department of Primary Industries and Regional Development for the assistance with images. Margaret Miller and Conrad Speed provided assisted in obtaining past survey reports and data.
References
Aines, A. C., Carlson, J. K., Boustany, A., Mathers, A., and Kohler, N. E. (2018). Feeding habits of the tiger shark, Galeocerdo cuvier, in the northwest Atlantic Ocean and Gulf of Mexico. Environ. Biol. Fishes. 101, 403–415. doi: 10.1007/s10641-017-0706-y
Allen, G. (1993). Part 7. Fishes of ashmore reef and cartier Island. Rec. West. Aust. Mus. 44, 67–91.
Anderson, L. G., White, P. C., Stebbing, P. D., Stentiford, G. D., and Dunn, A. M. (2014). Biosecurity and vector behaviour: evaluating the potential threat posed by anglers and canoeists as pathways for the spread of invasive non-native species and pathogens. PLoS One 9:e92788. doi: 10.1371/journal.pone.0092788
ATNS (2004). Memorandum of Understanding of Timor Sea Arrangement. Available online at: https://www.atns.net.au/agreement.asp?EntityID=2438 (accessed March 1, 2019).
Barley, S. C., Meekan, M. G., and Meeuwig, J. J. (2017). Diet and condition of mesopredators on coral reefs in relation to shark abundance. PLoS One 12:e0165113. doi: 10.1371/journal.pone.0165113
Berry, P. (1986). Faunal surveys of the Rowley Shoals, Scott Reef and Seringapatam Reef, north-western Australia. Perth, WA: Western Australian Museum.
Berry, P. (1993). Marine Faunal Surveys of Ashmore Reef and Cartier Island North-Western Australia. Perth, WA: Western Australian Museum.
Branch, T. A., Lobo, A. S., and Purcell, S. W. (2013). Opportunistic exploitation: an overlooked pathway to extinction. Trends Ecol. Evol. 28, 409–413. doi: 10.1016/j.tree.2013.03.003
Brooks, T. M., Mittermeier, R. A., Mittermeier, C. G., Da Fonseca, G. A., Rylands, A. B., Konstant, W. R., et al. (2002). Habitat loss and extinction in the hotspots of biodiversity. Conserv. Biol. 16, 909–923. doi: 10.1046/j.1523-1739.2002.00530.x
Brown, K., and Skewes, T. (2001). “A preliminary assessment of the ecology of seagrasses at Ashmore reef, understanding the cultural and natural heritage values and management challenges of the ashmore region,” in Proceedings of a Symposium organised by the Australian Marine Sciences Association and the Museum and Art Gallery of the Northern Territory, Darwin, 4–6.
Bryce, C. W. (2009). Marine Biodiversity Survey of Mermaid Reef (Rowley Shoals), Scott and Seringapatam Reef, Western Australia, 2006 Rec. West. Aust. Mus. Supplement No. 77. Perth, WA: Western Australian Museum.
Butchart, S. H., Walpole, M., Collen, B., Van Strien, A., Scharlemann, J. P., Almond, R. E., et al. (2010). Global biodiversity: indicators of recent declines. Science 328, 1164–1168.
Campbell, B. C., and Wilson, B. V. (1993). The politics of Exclusion: Indonesian Fishing in the Australian Fishing Zone. Canberra, ACT: Australian Centre for International Agricultural Research.
Campbell, M. A., Connell, M. J., Collett, S. J., Udyawer, V., Crewe, T. L., McDougall, A., et al. (2020). The efficacy of protecting turtle nests as a conservation strategy to reverse population decline. Biol. Conserv. 251, 108769. doi: 10.1016/j.biocon.2020.108769
Ceballosa, G., Ehrlich, P. R., and Dirzob, R. (2017). Biological annihilation via the ongoing sixth mass extinction signaled by vertebrate population losses and declines. Proc. Natl. Acad. Sci. U.S.A. 17, E6089–E6096.
Ceccarelli, D., Choat, J., Ayling, A., Richards, Z., Beger, M., and Birrell, C. (2007). Survey of the Distribution and Abundance of Trochus, Holothurians and Tridacnid clams at Ashmore Reef in 2006, to Assess the Impact of Recent Illegal Fishing on Target Invertebrate Stocks, C&R Consulting and James Cook University for the Department of Environment, Water, Heritage and the Arts, QLD: Douglas.
Ceccarelli, D., Richards, Z., Pratchett, M., and Cvitanovic, C. (2011). Rapid increase in coral cover on an isolated coral reef, the Ashmore Reef National Nature Reserve, north-western Australia. Mar. Freshw. Res. 62, 1214–1220. doi: 10.1071/mf11013
Chapuis, L., Kerr, C. C., Collin, S. P., Hart, N. S., and Sanders, K. L. (2019). Underwater hearing in sea snakes (Hydrophiinae): first evidence of auditory evoked potential thresholds. J. Exp. Biol. 222:jeb198184.
Cheatwood, J. L., Jacobson, E. R., May, P. G., Farrell, T. M., Homer, B. L., Samuelson, D. A., et al. (2003). An outbreak of fungal dermatitis and stomatitis in a free-ranging population of pigmy rattlesnakes (Sistrurus miliarius barbouri) in Florida. J. Wildl. Dis. 39, 329–337. doi: 10.7589/0090-3558-39.2.329
Clark, P. (2000). Ashmore Reef: archaeological evidence of past visitation. Bull. Austr. Instit. Mar. Archaeol. 24, 1–8.
Clark, R. W., Marchand, M. N., Clifford, B. J., Stechert, R., and Stephens, S. (2011). Decline of an isolated timber rattlesnake (Crotalus horridus) population: interactions between climate change, disease, and loss of genetic diversity. Biol. Conserv. 144, 886–891. doi: 10.1016/j.biocon.2010.12.001
Clarke, R. H., Carter, M., Swann, G., and Thomson, J. (2011). The status of breeding seabirds and herons at Ashmore Reef, off the Kimberley coast, Australia. J. R. Soc. West. Aust. 94, 171–182.
Cliff, G., and Dudley, S. (1991). Sharks caught in the protective gill nets off Natal, South Africa. 4. The bull shark Carcharhinus leucas Valenciennes. S. Afr. J. Mar. Sci. 10, 253–270. doi: 10.2989/02577619109504636
Crawford, I. M. (1969). Late Prehistoric Changes in Aboriginal Cultures in Kimberley, Western Australia. London: University College London.
DEC (2007). Rowley Shoals Marine Park Management Plan 2007–2017 Department of Environment and Conservation. Perth, WA: DEC.
Dennis, D., Milton, D., Skewes, T., Taranto, T., and Haywood, M. (2005). A rapid assessment of the fin-fish and shark resources on the shallow reefs in the Timor Sea MOU74 Box. Beagle 1, 185–198. doi: 10.1201/9780429305955-10
Derraik, J. G. (2002). The pollution of the marine environment by plastic debris: a review. Mar. Pollut. Bull. 44, 842–852. doi: 10.1016/s0025-326x(02)00220-5
DIBP (2017). Australian Border Force Cutter (ABFC) Thaiyak. Belconnen, ACT: Department of Immigration and Border Protection.
Dicken, M. L., Hussey, N. E., Christiansen, H. M., Smale, M. J., Nkabi, N., Cliff, G., et al. (2017). Diet and trophic ecology of the tiger shark (Galeocerdo cuvier) from South African waters. PLoS One 12:e0177897. doi: 10.1371/journal.pone.0177897
Drushka, K., Sprintall, J., Gille, S. T., and Pranowo, W. S. (2008). Observations of the 2004 and 2006 Indian Ocean tsunamis from a pressure gauge array in Indonesia. J. Geophys. Res. Oceans 8:113.
Dulvy, N. K., Sadovy, Y., and Reynolds, J. D. (2003). Extinction vulnerability in marine populations. Fish Fish. 4, 25–64. doi: 10.1046/j.1467-2979.2003.00105.x
Edgar, G. J., Ceccarelli, D., Stuart-Smith, R. D., and Cooper, A. T. (2017). Reef Life Survey Assessment of Coral Reef Biodiversity in the North-West Commonwealth Marine Reserves Network Incorporated. Battery Point, TAS: Reef Life Survey Foundation.
Edgar, G. J., Cooper, A., Baker, S. C., Barker, W., Barrett, N. S., Becerro, M. A., et al. (2020). Reef Life Survey: establishing the ecological basis for conservation of shallow marine life. Biol. Conserv. 252, 108855. doi: 10.1016/j.biocon.2020.108855
Edgar, G., and Stuart-Smith, R. (2018). Reef Life Survey (RLS): Global Reef Fish Dataset. Battery Point, TAS: Institute for Marine and Antarctic Studies (IMAS).
Elfes, C. T., Livingstone, S. R., Lane, A., Lukoschek, V., Sanders, K. L., Courtney, A. J., et al. (2013). Fascinating and forgotten: the conservation status of the world’s sea snakes. Herpetol. Conserv. Biol 8, 37–52.
Fergusson, I. K., Compagno, L. J., and Marks, M. A. (2000). Predation by white sharks Carcharodon carcharias (Chondrichthyes: Lamnidae) upon chelonians, with new records from the Mediterranean Sea and a first record of the ocean sunfish Mola mola (Osteichthyes: Molidae) as stomach contents. Environ. Biol. Fishes 58, 447–453. doi: 10.1023/a:1007639324360
Ferreira, L. C., Thums, M., Heithaus, M. R., Barnett, A., Abrantes, K. G., Holmes, B. J., et al. (2017). The trophic role of a large marine predator, the tiger shark Galeocerdo cuvier. Sci. Rep. 7:7641.
Fox, P. D. (2010). International Asylum and Boat People: the Tampa Affair and Australia’s Pacific Solution. Md. J. Int’l L. 25:356.
Frisch, A. J., Ireland, M., Rizzari, J. R., Lönnstedt, O. M., Magnenat, K. A., Mirbach, C. E., et al. (2016). Reassessing the trophic role of reef sharks as apex predators on coral reefs. Coral Reefs 35, 459–472. doi: 10.1007/s00338-016-1415-2
Fry, G., Milton, D., and Wassenberg, T. (2001). The reproductive biology and diet of sea snake bycatch of prawn trawling in northern Australia: characteristics important for assessing the impacts on populations. Pac. Conserv. Biol 7, 55–73. doi: 10.1071/pc010055
Gagnon, M. M. (2010). Report on Biopsy Collections from Specimens Collected from the Surrounds of the West Atlas Oil Leak-Sea Snake Specimen. Perth, WA: Curtin University.
Gagnon, M. M., and Rawson, C. (2010). Montara Well Release: Report on Necropsies From a Timor Sea Horned Sea Snake. Perth, WA: Curtin University.
Gaughan, D. J., Molony, B., and Santoro, K. (2019). Status Reports of the Fisheries and Aquatic Resources of Western Australia 2017/18: The State of the Fisheries. Kensington, WA: Department of Primary Industries and Regional Development.
Gibbons, J. W., Scott, D. E., Ryan, T. J., Buhlmann, K. A., Tuberville, T. D., Metts, B. S., et al. (2000). The global decline of reptiles, déjà vu amphibians. Bioscience 50, 653–666.
Gillett, A. K. (2017). An Investigation into the Stranding of Australian Sea Snakes. St Lucia, QLD: The University of Queensland.
Gillett, A. K., Ploeg, R., Flint, M., and Mills, P. C. (2017). Postmortem examination of Australian sea snakes (Hydrophiinae): anatomy and common pathologic conditions. J. Vet. Diagn. Investig. 29, 593–611. doi: 10.1177/1040638717710056
Goiran, C., and Shine, R. (2013). Decline in sea snake abundance on a protected coral reef system in the New Caledonian Lagoon. Coral Reefs 32, 281–284. doi: 10.1007/s00338-012-0977-x
Gregory, M. R. (2009). Environmental implications of plastic debris in marine settings—entanglement, ingestion, smothering, hangers-on, hitch-hiking and alien invasions. Philos. Trans. R. Soc. Lond. B Biol. Sci. 364, 2013–2025. doi: 10.1098/rstb.2008.0265
Guinea, M. L. (1995). The Sea Turtles and Sea Snakes of Ashmore Reef National Nature Reserve. Darwin, NT: Northern Territory University.
Guinea, M. L. (2006). Final Report Survey 2005: Sea Snakes of Ashmore Reef, Hibernia Reef and Cartier island. Darwin, NT: Charles Darwin University.
Guinea, M. L. (2007). Sea Snakes of Ashmore Reef, Hibernia Reef and Cartier Island with Comments on Scott Reef, DEWHA Final Report Survey 2007. Darwin, NT: Charles Darwin University, 1–20.
Guinea, M. L. (2008). Sea snakes of Ashmore Reef and Cartier Island: Final Report Survey 2008 to DEWHA Canberra. Darwin, NT: Charles Darwin University.
Guinea, M. L. (2013). Surveys of the Sea Snakes and Sea Turtles on Reefs of the Sahul Shelf, Monitoring Program for the Montara Well Release Timor Sea. Darwin, NT: Charles Darwin University.
Guinea, M. L. (2020a). Comments on “Evidence for rapid recovery of shark populations within a coral reef marine protected area”. Speed et al., 2018 220: 308–319. Biol. Conserv. 243:108457. doi: 10.1016/j.biocon.2020.108457
Guinea, M. L. (2020b). “Sea snakes of the Sahul Shelf: spatial, temporal changes and threats, 1993 to 2017,” in Proceedings of the 9th World Congress of Herpetology, Dunedin.
Guinea, M., and Mason, E.-M. (2017). Surveys of Sea Snakes and Marine Turtles and Assessment of Nesting Success of Marine Turtles at Ashmore reef Commonwealth Marine Reserve (CMR) with Comments on Tropical Fire Ants. Palmerston, NT: Report to Parks Australia and Department of the Environment and Energy.
Guinea, M., and Whiting, S. (2005). Insights into the distribution and abundance of sea snakes at Ashmore Reef. The Beagle 1, 199–206.
Hale, J., and Butcher, R. (2013). Ashmore Reef Commonwealth Marine Reserve Ramsar Site Ecological Character Description, A Report to the Department of the Environment. Canberra, ACT: Department of the Environment.
Harvell, C. D., Mitchell, C. E., Ward, J. R., Altizer, S., Dobson, A. P., Ostfeld, R. S., et al. (2002). Climate warm- ing and disease risks for terrestrial and marine biota. Science 296, 2158–2162. doi: 10.1126/science.1063699
Heatwole, H. (1975). “Sea snakes of the Gulf of Carpentaria,” in The Biology of Sea Snakes, ed. W. A. Dunson (Baltimore, MY: University Park Press), 145–149.
Heatwole, H., Grech, A., Monahan, J. F., King, S., and Marsh, H. (2012). Thermal biology of sea snakes and sea kraits. Integr. Comp. Biol 52, 257–273. doi: 10.1093/icb/ics080
Heatwole, H., Heatwole, E., and Johnson, C. R. (1974). Shark predation on sea snakes. Copeia 1974, 780–781. doi: 10.2307/1442694
Heithaus, M. R. (2001). The biology of tiger sharks, Galeocerdo cuvier, in Shark Bay, Western Australia: sex ratio, size distribution, diet, and seasonal changes in catch rates. Environ. Biol. Fishes 61, 25–36. doi: 10.1023/a:1011021210685
Herbst, L. H. (1994). Fibropapillomatosis of marine turtles. Annu. Rev. Fish Dis. 4, 389–425. doi: 10.1016/0959-8030(94)90037-x
Hobday, A. J., Alexander, L. V., Perkins, S. E., Smale, D. A., Straub, S. C., Oliver, E. C., et al. (2016). A hierarchical approach to defining marine heatwaves. Prog. Oceanogr. 141, 227–238. doi: 10.1016/j.pocean.2015.12.014
Hoffmann, M., Hilton-Taylor, C., Angulo, A., Böhm, M., Brooks, T. M., Butchart, S. H., et al. (2010). The impact of conservation on the status of the world’s vertebrates. Science 330, 1503–1509.
Jennings, S., and Polunin, N. (1995). Biased underwater visual census biomass estimates for target-species in tropical reef fisheries. J. Fish Biol. 47, 733–736. doi: 10.1111/j.1095-8649.1995.tb01938.x
Karvonen, A., Rintamäki, P., Jokela, J., and Valtonen, E. T. (2010). Increasing water temperature and disease risks in aquatic systems: climate change increases the risk of some, but not all, diseases. Int. J. Parasitol. 40, 1483–1488. doi: 10.1016/j.ijpara.2010.04.015
Keesing, J. K., Webber, B. L., and Hardiman, L. K. (2020). Ashmore Reef Marine Park environmental assessment. Report to Parks Australia. Crawley: CSIRO.
Kerford, M. (2005). The Ecology of the Bar Bellied Sea Snake (Hydrophis elegans) in Shark Bay, Western Australia. Burnaby, BC: Biological Sciences Department-Simon Fraser University.
Kospartov, M., Beger, M., Ceccarelli, D., and Richards, Z. (2006). An Assessment of the Distribution and Abundance of Sea Cucumbers, Trochus, Giant Clams, Coral, Fish and Invasive Marine Species at Ashmore Reef National Nature Reserve and Cartier Island Marine Reserve. Canberra, ACT: Department of the Environment and Heritage.
Kühn, S., Bravo Rebolledo, E., and Van Franeker, J. A. (2015). “Deleterious effects of litter on marine life,” in Marine Anthropogenic Litter, eds M. Bergmann, L. Gutow, and M. Klages (Cham: Springer International Publishing).
Lavers, J. L., Hodgson, J. C., and Clarke, R. H. (2013). Prevalence and composition of marine debris in Brown Booby (Sula leucogaster) nests at Ashmore Reef. Mar. Pollut. Bull. 77, 320–324. doi: 10.1016/j.marpolbul.2013.09.026
Lessios, H. A. (1988). Mass mortality of Diadema antillarum in the Caribbean: what have we learned? Annu. Rev. Ecol. Evol. Syst. 19, 371–393. doi: 10.1146/annurev.es.19.110188.002103
Lessios, H. A. (2005). Diadema antillarum populations in Panama twenty years following mass mortality. Coral Reefs 24, 125–127. doi: 10.1007/s00338-004-0443-5
Lillywhite, H. B., Sheehy, C. M. III, Brischoux, F., and Grech, A. (2014). Pelagic sea snakes dehydrate at sea. Proc. Roy. Soc. B. 281:20140119. doi: 10.1098/rspb.2014.0119
Lillywhite, H. B., Sheehy, C. M. III, Sandfoss, M. R., Crowe-Riddell, J., and Grech, A. (2019). Drinking by sea snakes from oceanic fresh water lenses at first rainfall ending seasonal drought. PLoS One 14:e0212099. doi: 10.1371/journal.pone.0212099
Lillywhite, H., Heatwole, H., and Sheehy, C. III (2015). Dehydration and drinking behavior in true sea snakes (Elapidae: Hydrophiinae: Hydrophiini). J. Zool. 296, 261–269. doi: 10.1111/jzo.12239
Liu, Y.-L., Lillywhite, H., and Tu, M.-C. (2010). Sea snakes anticipate tropical cyclone. Mar. Biol. 157, 2369–2373. doi: 10.1007/s00227-010-1501-x
Long, B., Skewes, T., Taranto, T., Jacobs, D., and Dennis, D. (1997). Torres Strait reef Resource Inventory and Reef Habitat Mapping. Cleveland, OH: CSIRO, 23.
Lovich, J. E., Gotte, S. W., Ernst, C. H., Harshbarger, J. C., Laemmerzahl, A. F., and Gibbons, J. W. (1996). Prevalence and histopathology of shell disease in turtles from Lake Blackshear, Georgia. J. Wildl. Dis. 32, 259–265. doi: 10.7589/0090-3558-32.2.259
Lowe, C. G., Wetherbee, B. M., Crow, G. L., and Tester, A. L. (1996). Ontogenetic dietary shifts and feeding behavior of the tiger shark, Galeocerdo cuvier, in Hawaiian waters. Environ. Biol. Fishes 47, 203–211. doi: 10.1007/bf00005044
Lukoschek, V., and Shine, R. (2012). Sea snakes rarely venture far from home. Ecol. Evol. 2, 1113–1121. doi: 10.1002/ece3.256
Lukoschek, V., Beger, M., Ceccarelli, D., Richards, Z., and Pratchett, M. (2013). Enigmatic declines of Australia’s sea snakes from a biodiversity hotspot. Biol. Conserv. 166, 191–202. doi: 10.1016/j.biocon.2013.07.004
Lyle, J. (1987). Observations on the biology of Carcharhinus cautus (Whitley), C. melanopterus (Quoy & Gaimard) and C. fitzroyensis (Whitley) from northern Australia. Mar. Freshw. Res. 38, 701–710. doi: 10.1071/mf9870701
Macknight, C. (2013). Studying trepangers. Macassan History and Heritage. Journ. Encount. Influen. 13, 19–40.
Magner, T. (2004). A less than ‘Pacific’solution for asylum seekers in Australia. Int. J. Refug. Law 16, 53–90. doi: 10.1093/ijrl/16.1.53
Masunaga, G., Kosuge, T., Asai, N., and Ota, H. (2008). Shark predation of sea snakes (Reptilia: Elapidae) in the shallow waters around the Yaeyama Islands of the southern Ryukyus, Japan. Mar. Biodivers. Rec. 1:e96.
McCauley, R., Cappo, M. M., Perry, M., and Harvey, E. (2004). Traditional fishing puts the bite on sharks. Australas. Sci. 25:29.
Meekan, M. G., Cappo, M., and Speed, C. W. (2020). Response to comments on “Evidence for rapid recovery of shark populations within a coral reef marine protected area”. Speed et al., 2018 220: 308–319. Biol. Con. 224:108490. doi: 10.1016/j.biocon.2020.108490
Meekan, M. M., Cappo, M. M., Carleton, J. J., and Marriott, R. R. (2006). Surveys of Shark and Fin-Fish Abundance on Reefs Within the MOU74 Box and Rowleys Shoals Using Baited Remote Underwater Video Systems. Prepared for the Australian Government Department of the Environment and Heritage. Canberra, ACT: Australian Government Department of the Environment and Heritage.
Miller, D. C., Muir, C. L., and Hauser, O. A. (2002). Detrimental effects of sedimentation on marine benthos: what can be learned from natural processes and rates? Ecol. Eng. 19, 211–232. doi: 10.1016/s0925-8574(02)00081-2
Milton, D. A., Fry, G. C., and Dell, Q. (2009). Reducing impacts of trawling on protected sea snakes: by-catch reduction devices improve escapement and survival. Mar. Freshw. Res. 60, 824–832. doi: 10.1071/mf08221
Minton, S. A., and Heatwole, H. (1975). “Sea snakes from reefs of the Sahul Shelf,” in The Biology of Sea Snakes, ed. W. A. Dunson (Baltimore, MY: University of Maryland Press), 141–144.
Mirtschin, P., Rasmussen, A., and Weinstein, S. (2017). Australia’s Dangerous Snakes: Identification, Biology and Envenoming. Canberra, ACT: CSIRO Publishing.
Mitchell, M. D., and Harborne, A. R. (2020). Non-consumptive effects in fish predator–prey interactions on coral reefs. Coral Reefs 20, 1–18.
Montoya, J. M., Pimm, S. L., and Solé, R. V. (2006). Ecological networks and their fragility. Nature 442:259. doi: 10.1038/nature04927
Mote, S., Kumar, R., Naik, B., and Ingole, B. S. (2015). Polycyclic aromatic hydrocarbons (PAHs) and n-Alkanes in beaked sea snake Enhydrina schistose (Daudin, 1803) from the Mandovi Estuary. Goa. Bull. Environ. Contam. Toxicol. 94, 171–177. doi: 10.1007/s00128-014-1439-7
Murray, A. G., Smith, R. J., and Stagg, R. M. (2002). Shipping and the spread of infectious salmon anemia in Scottish aquaculture. Emerg. Infect. Dis. 8, 1–5. doi: 10.3201/eid0801.010144
Nelms, S. E., Duncan, E. M., Broderick, A. C., Galloway, T. S., Godfrey, M. H., Hamann, M., et al. (2016). Plastic and marine turtles: a review and call for research. ICES J. Mar. Sci. 73, 165–181. doi: 10.1093/icesjms/fsv165
Newcombe, C. P., and MacDonald, D. D. (1991). Effects of suspended sediments on aquatic ecosystems. N. Am. J. Fish Manage. 11, 72–82. doi: 10.1577/1548-8675(1991)011<0072:eossoa>2.3.co;2
O’Brien, G., and Glenn, K. (2005). Natural hydrocarbon seepage, sub-sea floor geology and eustatic sea-level variations as key determiners on the nature and distribution of carbonate build-ups and other benthic habitats in the Timor Sea, Australia. The Beagle. Supplement 1, 31–42.
Patterson, H., Larcombe, J., Nicol, S., and Curtotti, R. (2018). Fishery Status REPORTS 2018. Canberra, ACT: Australian Bureau of Agricultural and Resource Economics and Sciences.
Peng, C., Zhao, X., and Liu, G. (2015). Noise in the sea and its impacts on marine organisms. Int. J. Environ. Res. Public Health 12, 12304–12323. doi: 10.3390/ijerph121012304
Phillips, J., and Spinks, H. (2013). Boat arrivals in Australia since 1976. Canberra, ACT: Parliament of Australia, Department of Parliamentary Services.
Pickwell, G., Bezy, R., and Fitch, J. (1983). Northern occurrences of the sea-snake, Pelamis platurus, in the eastern Pacific, with a record of predation on the species. Calif. Fish Game 69, 172–177.
Prescott, J., Riwu, J., Prasetyo, A. P., and Stacey, N. (2017). The money side of livelihoods: Economics of an unregulated small-scale Indonesian sea cucumber fishery in the Timor Sea. Mar. Policy 82, 197–205. doi: 10.1016/j.marpol.2017.03.033
Rasmussen, A. R., Elmberg, J., Gravlund, P., and Ineich, I. (2011a). Sea snakes (Serpentes subfamilies Hydrophiinae and Laticaudinae) in Vietnam: a comprehensive checklist and an updated identification key. Zootaxa 11, 1–20. doi: 10.11646/zootaxa.2894.1.1
Rasmussen, A. R., Murphy, J. C., Ompi, M., Gibbons, J. W., and Uetz, P. (2011b). Marine reptiles. PLoS One 6:e27373.
Rees, M. M., Colquhoun, J. J., Smith, L. L., and Heyward, A. A. (2003). Survey of Trochus, Holothuria, Giant Clams and the Coral Communities at Ashmore, Cartier Reef and Mermaid Reef, Northwestern Australia. Darwin, NT: Environment Australia, 64.
Richards, Z., Beger, M., Hobbs, J.-P., Bowling, T., Chong-Seng, K., and Pratchett, M. (2009). Ashmore reef National Nature Reserve and Cartier Island Marine Reserve Marine Survey 2009. ARC Centre of Excellence for Coral Reef Studies. Sydney, NSW: Department of the Environment, Water, Heritage and the Arts.
Rodrigues, A. S., Brooks, T. M., Butchart, S. H., Chanson, J., Cox, N., Hoffmann, M., et al. (2014). Spatially explicit trends in the global conservation status of vertebrates. PLoS One 9:e113934. doi: 10.1371/journal.pone.0113934
Roff, G., Doropoulos, C., Rogers, A., Bozec, Y.-M., Krueck, N. C., Aurellado, E., et al. (2016). The ecological role of sharks on coral reefs. Trends Ecol. Evol. 31, 395–407.
Romanov, S. (1991). The Biological Effect of Vibration and Sound: Paradoxes and Problems of the 20th Century. Leningrad: Nauka.
Rosser, A. M., and Mainka, S. A. (2002). Overexploitation and species extinctions. Conserv. Biol. 16, 584–586. doi: 10.1046/j.1523-1739.2002.01635.x
Ruiz, G. M., Rawlings, T. K., Dobbs, F. C., Drake, F. C., Mullady, T., Huq, A., et al. (2000). Global spread of micro-organisms by ships – ballast water discharged from vessels harbours a cocktail of potential pathogens. Nature 408, 49–50.
Russell, B. C., and Vail, L. L. (1988). Report on Traditional Indonesian Fishing Activities at Ashmore Reef Nature Reserve. Australian National Parks and Wildlife Service and Northern Territory Museum. Darwin, NT: Northern Territory Museum.
Russell, B. C., Neil, K., and Hilliard, R. (2004). Ashmore Reef National Nature Reserve and Cartier Island Marine Reserve: Marine and Terrestrial Introduced Species Prevention and Management Strategy: Report for Department of Environment and Heritage. Sydney, NSW: Department of Environment and Heritage.
Russell, B., Larson, H., Hutchins, J., and Allen, G. (2001). “Reef fishes of the Sahul Shelf, understanding the cultural and natural heritage values and management challenges of the ashmore region,” in Proceedings of a Symposium Organised by the Australian Marine Sciences Association and the Museum and Art Gallery of the Northern Territory, Darwin, Darwin, NT, 4–6.
Salafsky, N., Boshoven, J., Burivalova, Z., Dubois, N. S., Gomez, A., Johnson, A., et al. (2019). Defining and using evidence in conservation practice. Conserv. Sci. Pract. 1:e27. doi: 10.1111/csp2.27
Sánchez-Bayoa, F., and Wyckhuysb, K. A. G. (2019). Worldwide decline of the entomofauna: a review of its drivers. Biol. Conserv. 232, 8–27.
Schlegel, R. W. (2018). Marine Heatwave Tracker: The App to See When and Where Marine Heatwaves Are Happening Around the World. Available online at: http://www.marineheatwaves.org/tracker (accessed March 1, 2019).
Schumacher, J. (2006). Selected infectious diseases of wild reptiles and amphibians. J. Exot. Pet Med. 15, 18–24. doi: 10.1053/j.jepm.2005.11.004
Sereshk, Z. H., and Bakhtiari, A. R. (2014). Distribution patterns of PAHs in different tissues of annulated sea snake (Hydrophis cyanocinctus) and short sea snake (Lapemis curtus) from the Hara Protected Area on the North Coast of the Persian Gulf, Iran. Ecotoxicol. Environ. Saf. 109, 116–123. doi: 10.1016/j.ecoenv.2014.06.004
Serventy, D. L. (1952). The bird islands of the sahul shelf: with remarks on the nesting seasons of Western Australian Sea-birds. Emu 52, 33–59. doi: 10.1071/mu952033
Shi, G., Cai, W., Cowan, T., Ribbe, J., Rotstayn, L., and Dix, M. (2008). Variability and trend of North West Australia rainfall: observations and coupled climate modeling. J. Clim. 21, 2938–2959. doi: 10.1175/2007jcli1908.1
Shine, R. (2010). The ecological impact of invasive cane toads (Bufo marinus) in Australia. Q. Rev. Biol. 85, 253–291. doi: 10.1086/655116
Short, M. (2011). Pacific Adventurer Oil Spill: Big Birds, Sea Snakes and a Couple of Turtles, International Oil Spill Conference Proceedings (IOSC). Washington, DC: American Petroleum Institute.
Simpfendorfer, C. (1992). Biology of tiger sharks (Galeocerdo cuvier) caught by the Queensland shark meshing program off Townsville, Australia. Mar. Freshw. Res. 43, 33–43. doi: 10.1071/mf9920033
Skewes, T., Dennis, D., Jacobs, D., Gordon, S., Taranto, T., Haywood, M., et al. (1999). Survey and stock size estimates of the shallow reef (0–15 m deep) and shoal area (15–50 m deep) marine resources and habitat mapping within the Timor Sea MOU74 Box. Canberra: CSIRO Division of Marine Research.
Smith, K. F., Sax, D. F., and Lafferty, K. D. (2006). Evidence for the role of infectious disease in species extinction and endangerment. Conserv. Biol. 20, 1349–1357. doi: 10.1111/j.1523-1739.2006.00524.x
Smith, M. A. (1926). Monograph of the Sea-Snakes (Hydrophiidae). London: Trustees of the British museum.
Smith, R. B., Seigel, R. A., and Smith, K. R. (1998). Occurrence of upper respiratory tract disease in gopher tortoise populations in Florida and Mississippi. J. Herpetol. 32, 426–430. doi: 10.2307/1565458
Speed, C. W., Cappo, M., and Meekan, M. G. (2018). Evidence for rapid recovery of shark populations within a coral reef marine protected area. Biol. Conserv. 220, 308–319. doi: 10.1016/j.biocon.2018.01.010
Speed, C. W., Rees, M. J., Cure, K., Vaughan, B., and Meekan, M. G. (2019). Protection from illegal fishing and shark recovery restructures mesopredatory fish communities on a coral reef. Ecol. Evol. 9, 10553–10566. doi: 10.1002/ece3.5575
Spitzen-van der Sluijs, A., Spikmans, F., Bosman, W., de Zeeuw, M., van der Meij, T., Goverse, E., et al. (2013). Rapid enigmatic decline drives the fire salamander (Salamandra salamandra) to the edge of extinction in the Netherlands. Amphib Reptilia. 34, 233–239.
Stacey, N. (2007). Boats to Burn: Bajo Fishing Activity in the Australian Fishing Zone. Canberra, ACT: ANU E Press.
Stacey, N. (2017). Transboundary small-scale fisheries in the Timor and Arafra Seas region of Northern Australia. J. Aust. Stud. 30, 71–78.
Stuart, S. N., Chanson, J. S., Cox, N. A., Young, B. E., Rodrigues, A. S., Fischman, D. L., et al. (2004). Status and trends of amphibian declines and extinctions worldwide. Science 306, 1783–1786. doi: 10.1126/science.1103538
Sunderland, T., Sunderland-Groves, J., Shanley, P., and Campbell, B. (2009). Bridging the gap: how can information access and exchange between conservation biologists and field practitioners be improved for better conservation outcomes? Biotropica 41, 549–554. doi: 10.1111/j.1744-7429.2009.00557.x
Susanti, T. A. F., Muhdar, M., and Erawaty, R. (2021). Indonesian traditional fishing rights in ashmore reef area an international law perspective, Mulawarman Natural Resources and Environmental Law 1, 1–18.
Tawfiq, N., and Olsen, D. A. (1993). Saudi Arabia’s response to the 1991 Gulf oil spill. Mar. Pollut. Bull. 27, 333–345. doi: 10.1016/0025-326x(93)90041-h
Tuma, R. E. (1976). “An investigation of the feeding habits of the bull shark, Carcharhinus leucas, in the Lake Nicaragua-Rio San Juan system,” in Investigations of the Ichthyofauna of Nicaraguan Lakes, ed. T. B. Thorson (Lincoln, NE: University of Nebraska-Lincoln), 533–538.
Udyawer, V., and Heupel, M. (2017). Spatial and Temporal Patterns in Sea Snake Populations on the North West Shelf, Report to the National Environmental Science Programme, Marine Biodiversity Hub. Townsville, OLD: Australian Institute of Marine Science, 27.
Udyawer, V., Read, M. A., Hamann, M., Simpfendorfer, C. A., and Heupel, M. R. (2013). First record of sea snake (Hydrophis elegans, Hydrophiinae) entrapped in marine debris. Mar. Pollut. Bull. 73, 336–338.
Udyawer, V., Somaweera, R., Nitschke, C., d’Anastasi, B., Sanders, K., Webber, B. L., et al. (2020). Prioritising search effort to locate previously unknown populations of endangered marine reptiles. Glob. Ecol. Conserv. 22:e01013. doi: 10.1016/j.gecco.2020.e01013
Urban, M. C. (2015). Accelerating extinction risk from climate change. Science 348, 571–573. doi: 10.1126/science.aaa4984
Voris, H. K., and Voris, H. H. (1983). Feeding strategies in marine snakes: an analysis of evolutionary, morphological, behavioral and ecological relationships. Am. Zool. 23, 411–425. doi: 10.1093/icb/23.2.411
Wake, D. B., and Vredenburg, V. T. (2008). Are we in the midst of the sixth mass extinction? A view from the world of amphibians. Proc. Natl. Acad. Sci. U.S.A. 105, 11466–11473. doi: 10.1073/pnas.0801921105
Wallner, B., and McLoughlin, K. (1995). Review of Indonesian Fishing in the Australian Fishing Zone Bureau of Resource Sciences. Canberra. ACT: Department of Primary Industries and Energy.
Ward-Paige, C., Flemming, J. M., and Lotze, H. K. (2010). Overestimating fish counts by non-instantaneous visual censuses: consequences for population and community descriptions. PLoS One 5:e11722.
Watson, J. E., Joseph, L. N., and Watson, A. W. (2009). A Rapid Assessment of the Impacts of the Montara Oil Leak on Birds, Cetaceans and Marine Reptiles. A Report Commissioned by the Department of the Environment, Water, Heritage and the Arts (DEWHA). Brisbane, QLD: DEWHA.
Whiting, S. (2000). Management and Research Issues at Ashmore Reef National Nature Reserve. Darwin: Biomarine International, 29.
Whiting, S. D., and Guinea, M. L. (1998). Surveys of Marine Debris in Northern Australia. Project Report to Keep Australia Beautiful Council & Territory Anti-Litter Committee, Darwin. Darwin, NT: Sea Turtle Research Unit, Northern Territory University, 9.
Wilkinson, J. J. (1908). The Ashmore Islands, Mr J.J. Wilkinson’s venture guano and beche de mere. Reg. Adel. 1908:7.
Wirsing, A. J., and Heithaus, M. R. (2009). Olive-headed sea snakes Disteria major shift seagrass microhabitats to avoid shark predation. Mar. Ecol. Prog. Ser. 387, 287–293. doi: 10.3354/meps08127
Witt, W. (1951). Exploring a Coral Patch in the Timor Sea. Osborne Park, WA: The West Australian, 21.
Woodside. (2009). Scott Reef Status Report 2008, Australian Institute of Marine Science. Perth, WA: Western Australian Museum & Woodside.
Worm, B., and Paine, R. T. (2016). Humans as a hyperkeystone species. Trends. Ecol. Evol. 31, 600–607. doi: 10.1016/j.tree.2016.05.008
Zapata, A., Varas, A., and Torroba, M. (1992). Seasonal variations in the immune system of lower vertebrates. Immunol. Today 13, 142–147. doi: 10.1016/0167-5699(92)90112-k
Keywords: apex predator, extinction, hydrophiids, pathogen, shark, species decline, trophic cascade
Citation: Somaweera R, Udyawer V, Guinea ML, Ceccarelli DM, Clarke RH, Glover M, Hourston M, Keesing J, Rasmussen AR, Sanders K, Shine R, Thomson DP and Webber BL (2021) Pinpointing Drivers of Extirpation in Sea Snakes: A Synthesis of Evidence From Ashmore Reef. Front. Mar. Sci. 8:658756. doi: 10.3389/fmars.2021.658756
Received: 26 January 2021; Accepted: 23 April 2021;
Published: 01 June 2021.
Edited by:
Rachel Ann Hauser-Davis, Oswaldo Cruz Foundation (Fiocruz), BrazilReviewed by:
Harvey B. Lillywhite, University of Florida, United StatesMark E. Bond, Florida International University, United States
Copyright © 2021 Somaweera, Udyawer, Guinea, Ceccarelli, Clarke, Glover, Hourston, Keesing, Rasmussen, Sanders, Shine, Thomson and Webber. This is an open-access article distributed under the terms of the Creative Commons Attribution License (CC BY). The use, distribution or reproduction in other forums is permitted, provided the original author(s) and the copyright owner(s) are credited and that the original publication in this journal is cited, in accordance with accepted academic practice. No use, distribution or reproduction is permitted which does not comply with these terms.
*Correspondence: Ruchira Somaweera, ruchira.somaweera@stantec.com
†Present address: Ruchira Somaweera, Stantec Australia, Perth, WA, Australia