- 1Office of Protected Resources, National Marine Fisheries Service, National Oceanic and Atmospheric Administration, Pascagoula, MS, United States
- 2Division of Sea Turtle Science and Recovery, Padre Island National Seashore, National Park Service, Corpus Christi, TX, United States
Knowledge of green turtle (Chelonia mydas) foraging ecology in the northwestern Gulf of Mexico (GOM) is critical as populations begin to recover from heavy harvesting in prior centuries. We present a comprehensive long-term assessment of green turtle diets from carcasses salvaged from 1987 to 2014 along the Texas coast. Digestive tract contents were examined from 420 green turtles, ranging in size from 7.3 to 86.0 cm in straight carapace length (SCLmax). Green turtles as small as 16.2 cm SCLmax recruit from the oceanic environment to nearshore foraging habitat in the northwestern GOM and consume macroalgae principally. A successive shift in diet and habitat to inshore seagrasses was evident by the seagrass-dominated diet of turtles larger than 30 cm SCLmax. Animal matter remained a frequently ingested diet item suggesting these immature green turtles are better classified as omnivores. The overall evidence indicates that Texas’ recovering green turtle assemblage is exhibiting foraging plasticity within seagrass meadows changing species composition and density.
Introduction
Green turtles (Chelonia mydas) were once so abundant in Texas waters that they supported a commercial fishery, with a peak annual landing of approximately 265,350 kg of turtles in 1890 (Hildebrand, 1982). Pressures on this marine turtle assemblage were inexorable. By 1903 the green turtle fishery had virtually collapsed due to the commercial harvest and severe hypothermic stunning events in the winters of 1894–1895 and 1899, which decimated the turtle population (Hildebrand, 1982; Doughty, 1984). Since green turtles were listed under the protection of the U.S. Endangered Species Act in 1978 and Mexico banned the commercial harvest of all sea turtles in 1990, Texas waters once again support a rapidly growing immature green turtle aggregation (Shaver et al., 2013). In-water research indicates increased green turtle population growth, with a catch per unit of effort on the lower Texas coast at 10 times greater in 2002–2010 than in 1991–1994 (Metz and Landry, 2013). Furthermore, the Sea Turtle Stranding and Salvage Network (STSSN) provides evidence of an increased Texas green turtle population. The STSSN reports increasingly high stranding numbers of immature green turtles (Stacy et al., 2020) and record-breaking stranding events from cold weather. From 1980 through 2015, the largest totals (>450 turtles) of turtles cold-stunned were during the winters of 2009–2010, 2010–2011, 2013–2014, and 2014–2015 (Shaver et al., 2017).
Immature green turtles in Texas primarily originate from Mexican rookeries in the western Gulf of Mexico (GOM) (Shamblin et al., 2016). They disperse from their nesting beaches as hatchlings and occupy an oceanic stage in the offshore waters of the GOM (Bolten, 2003). During this life-history stage, young green turtles reside and feed within the large Sargassum mats floating in the GOM (Witherington et al., 2012). The aforementioned algae mats break away in massive segments and wash ashore in the spring and summer seasons in massive wracks along the Texas gulf coast (Gherskiere et al., 2006; Gower et al., 2006; Webster and Linton, 2013). Large recruitment pulses of immature oceanic green turtles frequently accompany these Sargassum mats (Shaver et al., 2017; Stacy et al., 2020). Post-oceanic recruits often reside at neritic granite rock jetties designed to stabilize channels that connect to inshore bays and lagoons (Manzella et al., 1990; Renaud et al., 1992; Williams and Manzella, 1992; Coyne, 1994; Renaud et al., 1994; Shaver, 1994; Renaud and Williams, 1997; Williams and Renaud, 1998). These structures provide protection and abundant invertebrate and macroalgae food sources, with over 80 macroalgae species reported at the Port Mansfield jetty in south Texas (Edwards and Kapraun, 1973; Kaldy et al., 1995). Green turtles have been documented to inhabit jetty channels for up to 1,100 days (Shaver, 2000), where they consume macroalgae (Coyne, 1994). Some of the smallest daily movements recorded for juvenile green turtles were at a jettied pass in South Texas (Renaud et al., 1995), further evidence of the green turtles’ dependency on this habitat.
Texas green turtles exhibit a size-based transition, typically at 25–45 cm SCL, from jetty inhabitation to residency in inshore seagrass beds (Gorga, 2010; Howell, 2012). Among these beds, there are macroalgal communities (Breuer, 1962; Hildebrand and King, 1978). Data on the green turtle foraging habits in the northern GOM are historically limited. While Atlantic green turtles are known to maintain and selectively feed within seagrass plots (Bjorndal, 1985), only recently was this identified for the first time in the northern GOM (Rodriguez and Heck, 2020). Research suggests seagrasses are the dominant diet item for turtles captured in Texas’ lagoon and bay systems (Landry et al., 1992; Coyne, 1994). The three most common species of seagrass in Texas waters are shoal (Halodule beaudettei), Gulf manatee (Cymodocea filiformis), and turtle (Thalassia testudinum) grass (Quammen and Onuf, 1993; Withers, 2002). The 209-km long Laguna Madre accounts for 81% of the Texas coast’s entire seagrass coverage (Mendelssohn et al., 2017). Consequentially, the Laguna Madre supports the greatest abundance of inshore green turtles in the state (Doughty, 1984; Metz and Landry, 2013).
Ecological succession in seagrass beds typically starts with the colonization of shoal grass in disturbed or barren areas and climaxes with turtle grass (Patriquin, 1975). Historically, shoal grass dominated Texas’ estuaries. However, from the mid-1960s to 1998, bare regions increased, and shoal grass acreage declined by 36%, with partial replacement by turtle and manatee grass (Quammen and Onuf, 1993; Onuf, 1996; Pulich and Onuf, 2007; Gutierrez et al., 2010; Hobson and Whisenant, 2018). The changes in seagrass coverage and composition are attributed to maintenance dredging, propeller scarring, brown tide algae blooms, and natural processes (Onuf, 1994; Pulich and Calnan, 1999; Dunton et al., 2002). With the drafting of a Seagrass Conservation Plan for Texas in 1999, efforts to protect and enhance Texas seagrass beds’ health and quality were implemented. They were expected to be effective at seagrass restoration within 2 years once high-priority actions were accomplished (Pulich and Calnan, 1999). Although seagrass distribution and density are essential to Texas’s green turtle occurrence (Shaver et al., 2017), the impact of changes in seagrass composition and coverage on turtles’ foraging habits is unknown.
The Texas coast is temperate to a subtropical system, with long hot summers and short, mild winters. Temperature frequently drives the macroalgal seasonality in warm-water regions like Texas (Mathieson and Penniman, 1986). Seagrass beds in Texas have displayed similar growth and biomass changes from the seasons’ light and temperature-dependent fluctuations (Kowalski et al., 2009). Variability in resource availability can bring about variation in turtle foraging habits (López-Mendilaharsu et al., 2008; Guebert-Bartholo et al., 2011). For these reasons, it is critical to understand Texas green turtle foraging habits within their year-round range and how the diet might change with seasonal environmental fluctuations.
Describing the diet of green turtles found stranded in Texas for nearly three decades may discern any food-related changes due to seagrass composition and abundance variations. Further, examining the diet of multiple life-history stages in this rapidly increasing assemblage will provide a baseline for future diet studies. The significance of conventional gut contents analyses (GCA) to improve nutrition interpretation is highlighted in the sea turtle literature (Parker et al., 2005; Hatase et al., 2006; Revelles et al., 2007; Casale et al., 2008; Hoarau et al., 2014; Behera et al., 2015). We used GCA to examine the dietary composition of all size ranges of green turtles stranding on the Texas coast and to explore ontogenetic, temporal, and seasonal diet trends.
Materials and Methods
Study Area
Seven major estuaries are covering 2.6 million acres along the Texas coast (Figure 1). Across the 350 miles long Texas coast, nine federally maintained jettied ship channels (Army Corps of Engineers, 2021) provide access to the bays and the Laguna Madre. Red algae species are most numerous in the jetty habitat, followed by brown and green algae (Fikes and Lehman, 2010). There are five species of seagrass in Texas, including widgeon (Ruppia maritima), star (Halophila engelmannii), shoal (Halodule beaudettei), Gulf manatee (Cymodocea filiformis), and turtle (Thalassia testudinum) grass. Amongst the seagrass beds, there are macroalgal communities dominated by Cladophora sp., Digenea simplex, Gracilaria spp., Hypnea musciformis, Ulva lactuca, and Yuzurua poiteaui (Breuer, 1962; Hildebrand and King, 1978).
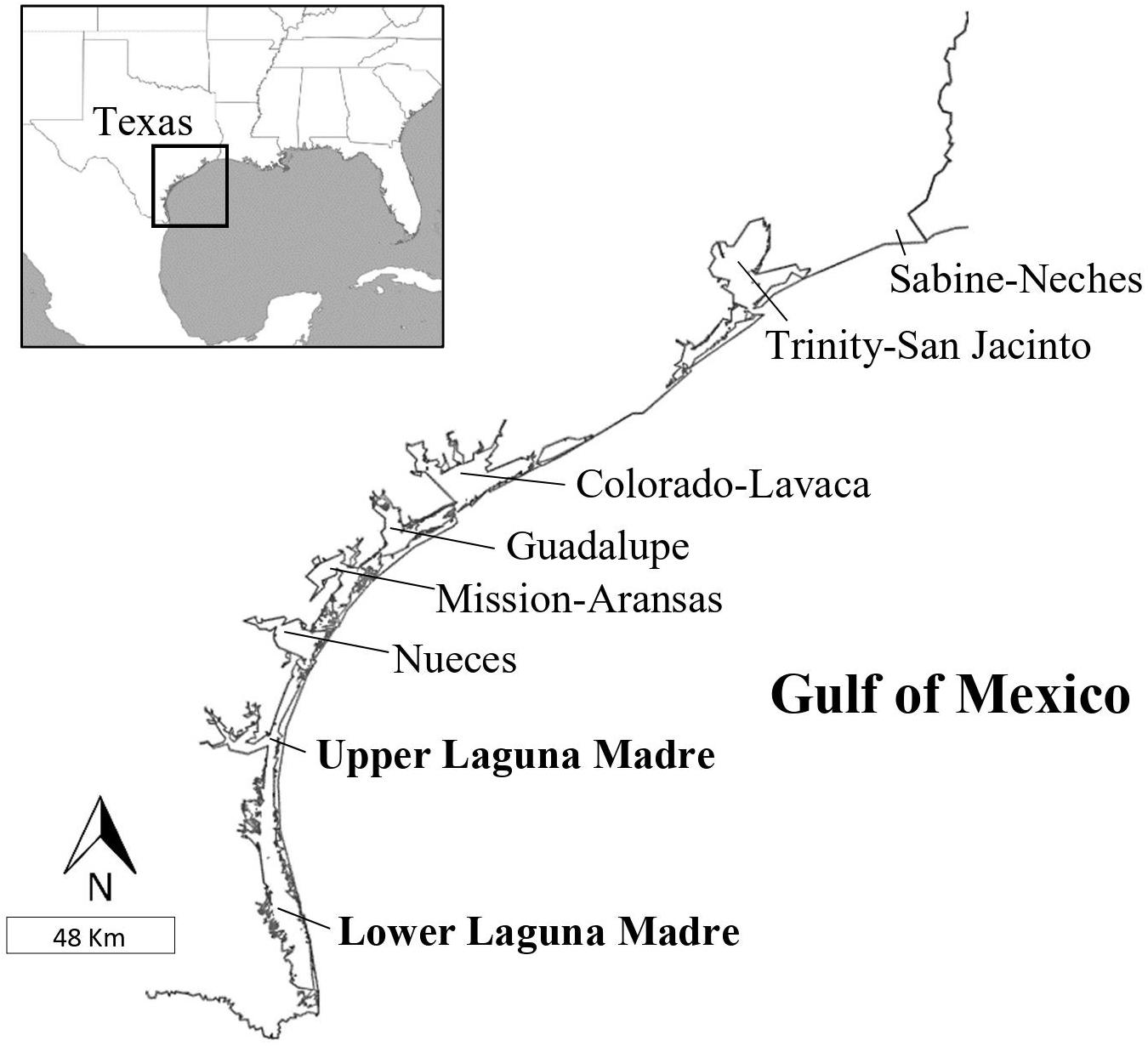
Figure 1. Map of the Texas, United States, and coast, showing the major estuaries and the geographic area where green turtles (Chelonia mydas) were stranded from 1987 to 2014. The Laguna Madre is one estuary but is labeled upper and lower for illustrative purposes and is given in bold. Inset shows the location of Texas in the western Gulf of Mexico.
Sample collection. We collected diet samples from 306 green turtles stranded from 1987 to 2014 from the Texas coast’s inshore and offshore waters, and we incorporated the unpublished weight data from 114 Texas green turtles (Howell et al., 2016); for a total of 420 samples from individual turtles (Table 1.) All measurements presented are maximum straight carapace length (SCLmax); ±0.1 cm, measured from carapace notch to the posterior-most tip. Turtles sampled for this study ranged in size from 7.3 to 86.0 cm SCLmax, and their body condition suggested normal behaviors (i.e., actively foraging) prior to death. Data were grouped into four size classes, based on previous size-based distribution studies in Texas, to improve our assessments of size-based diet and habitat differences (Coyne, 1994; Shaver, 2000; Shaver et al., 2013; Howell et al., 2016). These size classes are referred to herein as oceanic (≤20.0 cm), recruit (20.1–30.0 cm), transitional (30.1–40.0 cm), and inshore (≥40.1 cm).
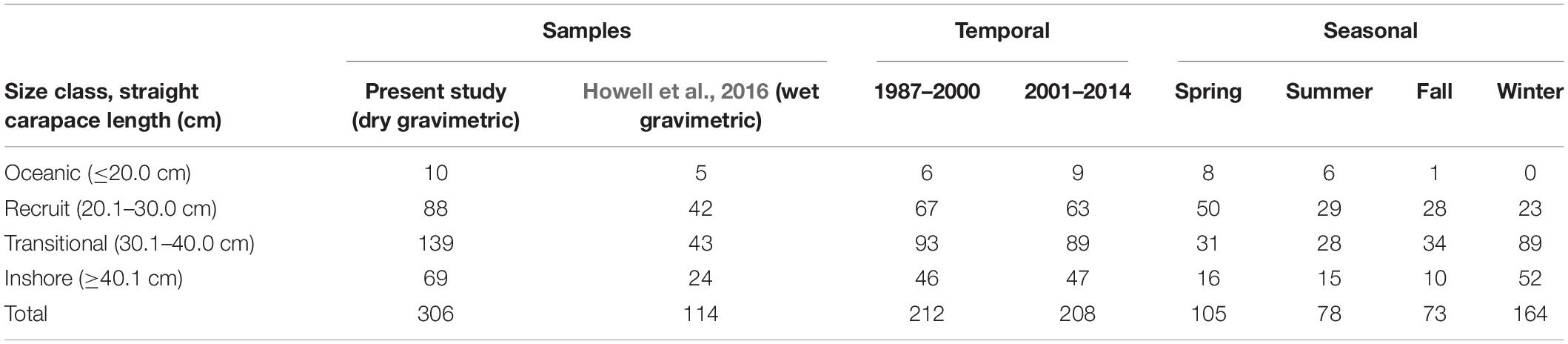
Table 1. The number of stranded green sea turtles (Chelonia mydas) analyzed temporally and seasonally in this study (n = 306) and from previously unreported data from Howell et al. (2016) (n = 114), within each size class.
Necropsies were performed on carcasses following standard sampling procedures (Wyneken, 2001), and the entire digestive tracts were extracted and frozen for subsequent analysis. Diet items were removed from the whole gastrointestinal tract and identified to the lowest taxon possible with a dissecting microscope. Diet items were quantified by dry (n = 306) and wet (n = 114) gravimetric analyses (Hyslop, 1980; Bigg and Perez, 1985; Forbes, 1999). Dry weights were obtained by drying identified taxa samples for 24–48 h at 60°C and measuring the cooled samples to the nearest hundredth of a gram. The wet mass of each identified taxa was weighed to the nearest hundredth of a gram.
Sample Analysis
Cumulative prey curves were employed to determine if an adequate number of samples had been collected to describe Texas’s green turtle diet (Ferry and Cailliet, 1996). The order in which the samples were analyzed was randomized 10 times to reduce bias to construct the prey curve. The cumulative number of prey types was plotted against the cumulative number of guts analyzed. The number of samples analyzed is considered sufficient for describing dietary habits when the resulting curve reaches an asymptote.
The percent weight (Wi) by individual diet taxon was used as a more effective measure to reduce variance, minimize bias from the difference in individual sample weights, and standardize amongst quantification methods. The Wi calculated by dividing each diet taxon’s weight in a given sample by the total weight of that turtle’s foregut contents (×100). The gravimetric method may overestimate the relative importance of slowly digested hard-bodied items (George and Hadley, 1979); accordingly, additional metrics are useful to interpretations (Amundsen and Sánchez-Hernández, 2019). The frequency of occurrence (Fi) for each diet item was determined by dividing the number of samples containing each food item by the total number of samples examined (×100). The relative importance of each item in the diet was determined using an index of relative importance (IRI; Bjorndal et al., 1997):
where F is the frequency of occurrence of the target taxon i, and W is the mean percent taxon weight in all individual turtles (Wi) for the collective gravimetric methods.
The IRI is a compound index incorporating frequency of occurrence and weight into a single numerical measure to estimate dietary importance. While single metrics aid diet interpretations, compound indices can additionally provide a general picture of prey items’ importance in predators’ diets (Liao et al., 2001). Higher IRI values indicate a less diverse diet. Depending on the gravimetric method employed, hard-bodied prey can bias prey importance outcomes by contributing more to the Wi (Hyslop, 1980). The IRI values for each gravimetric method were calculated independently and collectively to explore differences between them. There were no differences detected amongst the principal diet groups’ estimated dietary importance. Therefore, all gravimetric data were collectively combined and analyzed.
Food habits were analyzed in relation to the size class, the season of stranding (northern meteorological seasons of winter, spring, summer, fall), and two time periods (1987–2000, 2001–2014). The year divisions of 1987–2000 and 2001–2014 were used to compare general diet information after the 2-year implementation of the 1999 Texas Seagrass Conservation Plan. The frequency of occurrence approach provides a comprehensive and reliable account of diet composition, is unaffected by the diet item’s condition, and can be used to make comparisons across studies (Baker et al., 2014). With a large sample size for this study, the frequency of occurrence allows for population-wide assessments with minimal bias. The frequency of occurrence was the consistent metric used for statistical analyses. Chi-square tests were conducted using the software package IBM SPSS 25.0 to examine the relationship between size class and whether a particular diet taxon was consumed. The Cochran-Armitage test of trend was used to determine whether there was a linear trend in the primary diet group chosen within each life-history stage over the study period. The Cochran-Armitage test was additionally conducted to examine any linear trends in seagrass species selected by all combined size classes. The binomial logistic regression procedure in SPSS Statistics was used to generate the result of the Cochran-Armitage test of trend. The Goodman and Kruskal’s lambda test was used to measure the strength of the association between the diet items selected by each size class within the stranding season. For all analyses, α = 0.05.
Results
The cumulative prey curve reached asymptote indicating most major prey items had been collected to describe green turtles’ diet in Texas waters (Figure 2). Green turtles consumed 73 unique species, comprised of five seagrasses, 33 red algae, 12 green algae, eight brown algae, and 15 animals (Supplementary Table 1). Food items were classified as six major diet groups based on an overall F ≥ 25%: seagrasses, animal matter, anthropogenic debris, red, green, and brown macroalgae. All F values presented refer to the frequency of occurrence from all 420 turtles. Results were collectively presented on Sargassum species and Gelidium species, which intermix in their respective habitat.
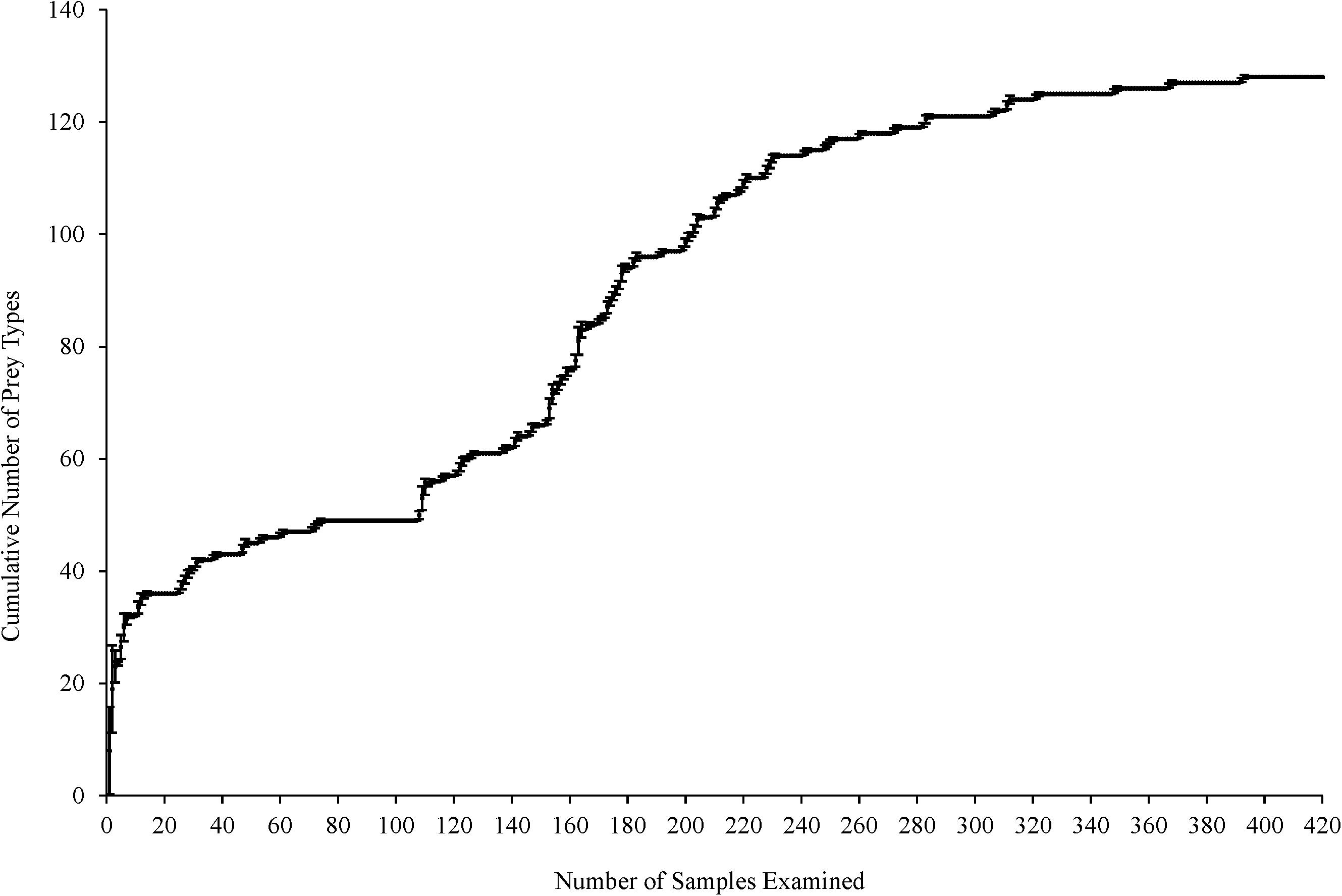
Figure 2. Cumulative prey curve (prey taxa per gastrointestinal tract) for green turtles (Chelonia mydas) (n = 420) collected along the Texas coast. Error bars represent one standard deviation.
Ontogenetic Shifts
Multiple size-based diet shifts were evident in the IRI values (Figure 3). There was a significant association between the ingestion of brown macroalgae and size class [χ2(3) = 70.149, p < 0.0001]. Brown macroalgae, specifically Sargassum spp., were the principal diet item of oceanic stage turtles (F = 93.3%, IRI = 73.9%). Red macroalgae and the size class feeding on them were significantly associated [χ2(3) = 21.538, p < 0.0001]. The highest IRI values for recruits demonstrated red and brown macroalgae were the most important diet groups (IRI = 24.2%, IRI = 27.4%, respectively). The relationship between the frequency of green algae consumption and size class was significant [χ2(3) = 9.563, p = 0.022]. The food items with the highest frequency of occurrence in the recruits were Sargassum spp. (63.0%), turtle grass (38.5%), mollusks (36.9%), shoal grass (33.1%), red algae Gelidium spp. (29.2%), and green algae Ulva spp. (23.1%). A secondary size-based diet shift was apparent with significant differences in seagrass consumption among the size classes [χ2(3) = 46.0047, p < 0.0001]. Seagrass was the principal diet item for the transitional class (IRI = 51.2%) and inshore class (IRI = 83.6%). The highest frequency of occurrence among food items in the transitional turtles was shoal grass (44.5%), turtle grass (43.9%), Gelidium spp. (41.7%), mollusks (38.4%), manatee seagrass (24.2%), and Ulva spp. (21.3%). Turtles of the inshore class most frequently consumed turtle grass (62.4%), shoal grass (51.6%), mollusks (36.56%), manatee grass (33.3%), and star grass (Halophila englemannii) (33.3%). Foraging on anthropogenic debris occurred most frequently (F = 60.0%) in oceanic class turtles with significant variation amongst all size classes [χ2(3) = 19.521, p = 0.0002]. In all collective size classes, ≥53.3% of individuals ingested animal matter. There was not a significant association between animal matter consumption and the size class feeding on it [χ2(3) = 2.965, p = 0.397].
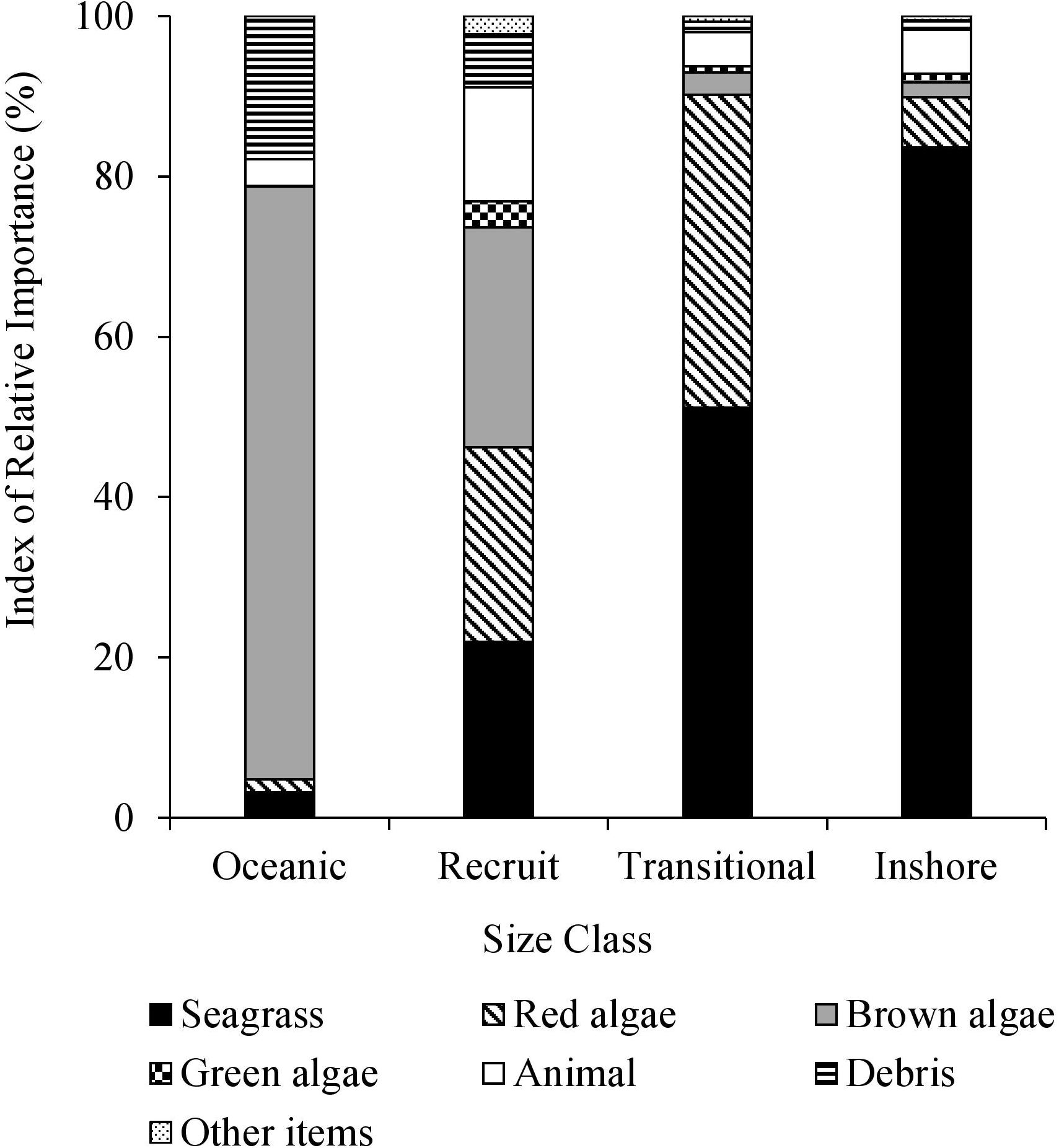
Figure 3. Percent index of relative importance (IRI) (%) of major diet items from size classes of stranded green sea turtles (Chelonia mydas) in Texas.
Temporal Trends
There was a significant decrease in the frequency of seagrass consumption in recruits (p < 0.0001) and transitional turtles (p = 0.031) between the two study periods (Figures 4B,C). Ingestion of red macroalgae by recruits increased significantly (p = 0.028) over time while there was no significant change in transitional turtles (p = 0.131). The presence of anthropogenic debris in gastrointestinal tracts decreased significantly between the two time periods for oceanic and recruits (p < 0.05) (Figures 4A,B). Inshore class turtles consumed red algae (p = 0.045) and brown algae (p < 0.0001) less frequently over time (Figure 4D). Among all size classes combined, turtle grass consumption decreased significantly over time, from F = 50.0% to 40.0% (p = 0.023). The ingestion of shoal grass decreased across the collective size classes, from F = 50.9% to 31.8% between the two periods (p < 0.0001). Manatee grass consumption did not change over time (F = 21.6–22.2%, p = 0.989).
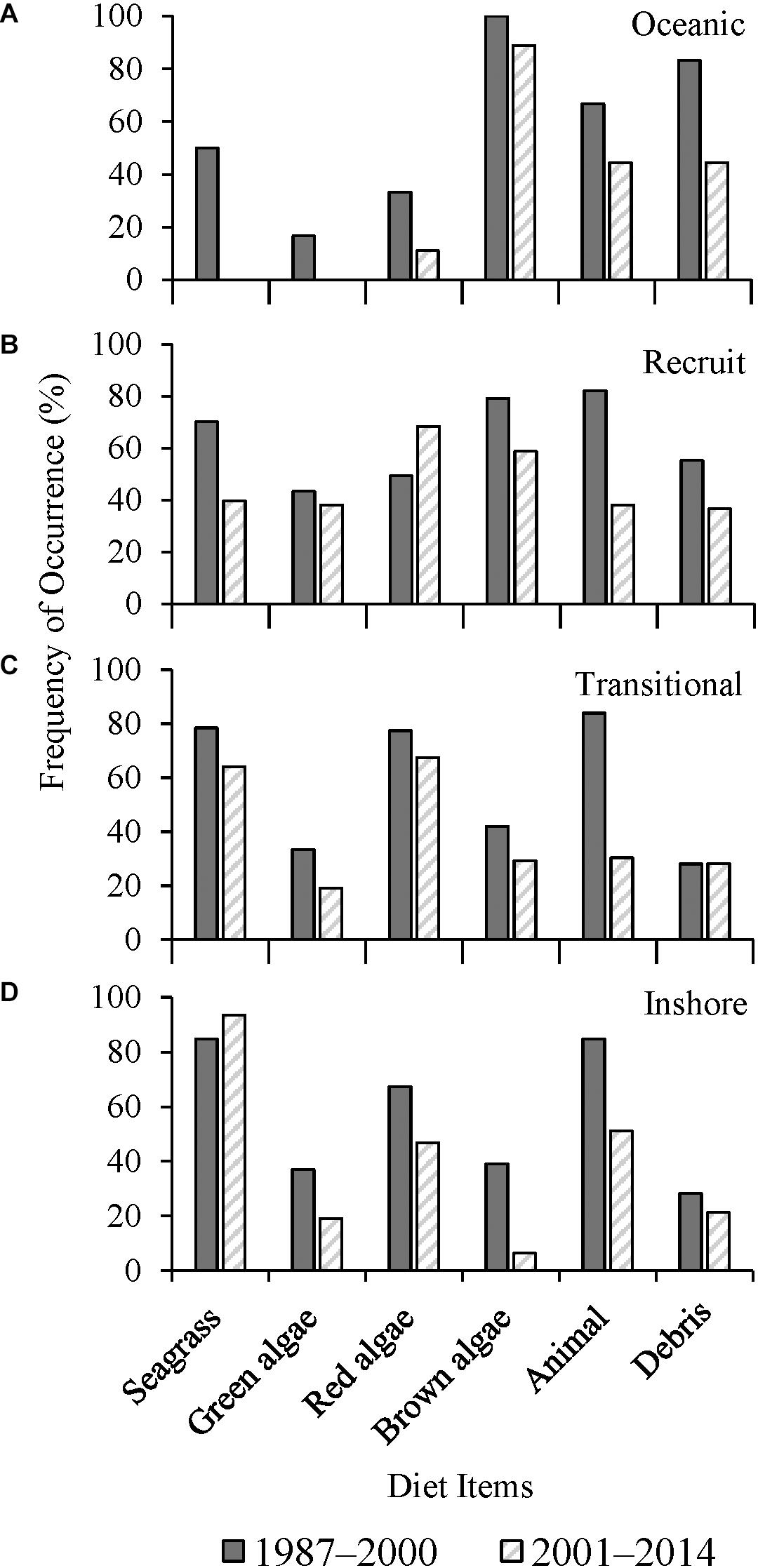
Figure 4. Frequency of occurrence (F) (%) of major diet items from (A) oceanic (1987–2000, n = 6; 2001–2014, n = 9), (B) recruit (1987–2000, n = 67; 2001–2014, n = 63), (C) transitional (1987–2000, n = 93; 2001–2014, n = 89), and (D) inshore (1987–2000, n = 46; 2001–2014, n = 47) size classes of stranded green sea turtles (Chelonia mydas) in Texas, grouped by years (1987–2000, n = 212; 2001–2014, n = 208).
Seasonal Differences
The interseasonal differences in oceanic turtles’ diet were not statistically analyzed as samples were unavailable for all seasons (Figure 5A). The proportion of recruits consuming red algae was significantly associated with the time of year of stranding (λ = 0.022, p = 0.037), with red algae consumption documented the least in the spring months (Figure 5B). The proportion of brown algae recorded in recruits was predicted by the season (λ = 0.150, p = 0.006), present in 92% of the spring turtles. In the winter and spring months, recruits ingested animal matter proportionally more than the other seasons (λ = 0.020, p = 0.050). There was a significant association between seagrass consumption and season of stranding in transitional turtles (λ = 0.060, p = 0.0005), wherein seagrass was documented more frequently in the winter months (Figure 5C). The presence of red algae in the diet was significantly associated with the season in which transitional turtles were stranded (λ = 0.026, p = 0.003). It was documented less frequently in the winter months than any other season. The consumption of green algae by the inshore class was significantly associated with the time of year (λ = 0.039, p = 0.014). Inshore size class turtles ingested green macroalgae most frequently in the spring (Figure 5D).
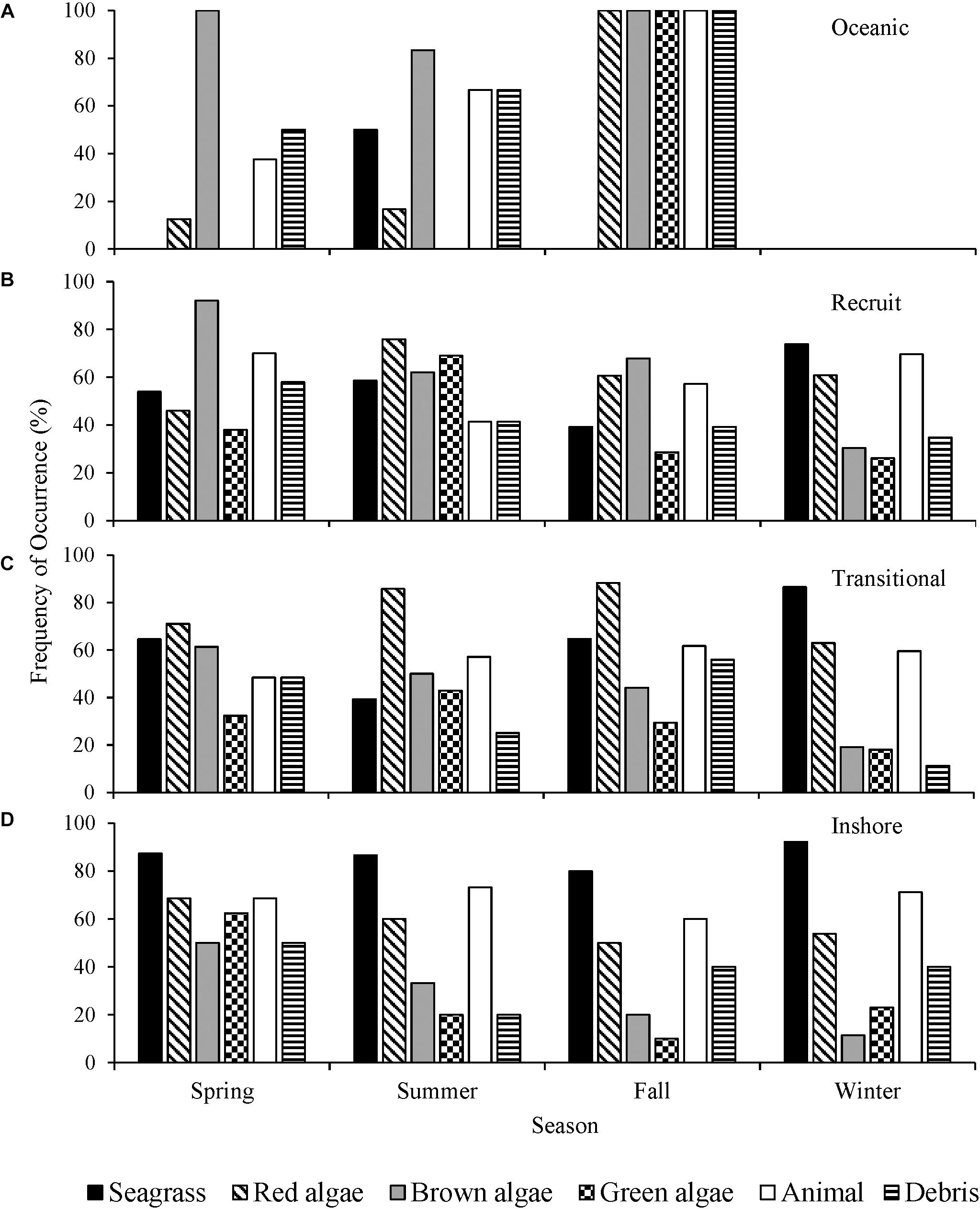
Figure 5. Frequency of occurrence (F) (%) of major diet items from (A) oceanic, (B) recruit, (C) transitional, and (D) inshore size classes of stranded green sea turtles (Chelonia mydas) in Texas, grouped by northern meteorological seasons.
Discussion
The green turtle population in Texas is increasing rapidly, and, as such, understanding the diet of this growing assemblage is critical to endangered species management. Conservation managers can then prioritize preservation areas and policies designed to protect these essential green sea turtle foraging grounds. We characterized the diet of green turtles stranding along the Texas coast for 28 years to evaluate changes over time. This first long-term diet study of Texas green turtles demonstrated turtles ingest a diversity of food items across size classes with foraging differences observed seasonally and temporally.
Diet of the smallest size class suggested this group was mostly representative of the oceanic life-history stage. The frequency of brown macroalgae (F = 93.3%), specifically the Sargassum spp., in oceanic turtles diet, was similar to the esophageal and fecal samples (F = 86, 87%, respectively) from oceanic size green turtles captured in pelagic Sargassum habitat off Florida, United States (Witherington et al., 2012). The authors suggested the ingestion of Sargassum was incidental to foraging on sessile, epiphytic animals on the brown algae. Furthermore, the high occurrence of Sargassum in the oceanic size class is similar to studies that have reported turtles foraging at the surface (Carr, 1987b; Morais et al., 2014). Stable isotope results (δ15N values) from the scutes of some of our oceanic-sized green turtles indicate they are not assimilating the Sargassum-dominated diet. Instead, they had tissue isotope values reflective of the animals that frequent the floating algae mats (Howell et al., 2016). Primarily the animals ingested by this size class are organisms known to be closely associated with the Sargassum community (e.g., Cnidarians, Schypozoans, Teleosts) (Witherington, 2006; Boyle and Limpus, 2008; Jones and Seminoff, 2013).
Convergence zones are oceanographic features that collect Sargassum and marine debris (Carr, 1987a), and as such, anthropogenic debris consumption was highest in oceanic turtles (F = 60%). Anthropogenic rubbish ingestion by marine turtles has been linked to numerous health issues, including blockage and compaction of the digestive tract, ultimately with lethal effects (Bjorndal et al., 1994; Tourinho et al., 2010; Gonzalez Carman et al., 2014; Wilcox et al., 2016). Our findings were similar to a study on oceanic green turtles captured in the Sargassum habitat, where synthetic material was the third most frequently ingested diet item (Witherington et al., 2012). Reduction of marine debris is identified as a recovery action in the Atlantic green turtle’s ESA Recovery Plan (NMFS, 1991), and prioritization should continue.
Previous diet studies in Texas showed seagrass was not present in turtles ≤20 cm SCLmax in Texas (Howell, 2012); therefore, our finding of seagrass in the diet of ≥16.2 cm–20 cm SCLmax turtles (F = 20.0%) was unexpected. These oceanic size class turtles also consumed macroalgae frequently found along the Texas coast (Kaldy et al., 1995; Agan and Lehman, 2002; Fikes and Lehman, 2008, 2010), suggesting nearshore occupancy for them. Young green turtles inhabiting jetty channels feed on flotsam, including seagrass blades detached from nearby inshore grass beds (L. N. Howell, D. J. Shaver, per. obs.). Our diet data suggest these individuals were likely foraging on benthic algae and floating seagrass blades in the nearshore environment before death. There are inherent variabilities in obtaining these smallest turtles, and our interpretations are based on a limited sample size. Nonetheless, the presence of multiple benthic species of neritic diet items in the oceanic class demonstrates recruitment at ≤20 cm SCLmax to Texas’ nearshore waters. Marine turtles are vulnerable to being taken as bycatch in coastal fishery operations and other anthropogenic threats (Magnuson et al., 1990). An explicit understanding of size ranges occupying Texas nearshore waters is critical to protected species management, as threats vary depending on the size class.
While green turtles in neritic foraging grounds typically consume benthic items (Redfoot, 1997; Holloway-Adkins, 2001; Gilbert, 2005; Makowski et al., 2006; Foley et al., 2007), floating Sargassum spp. were the most frequently ingested item in recruits and dominated the diet of turtles found in spring months. The mean digestive passage time for a food item consumed by immature green turtles was determined to be 23.3 ± 6.6 days (Amorocho and Reina, 2008). The size at recruitment to the neritic zone varies for oceanic stage green turtles (summarized in Avens and Snover, 2013). Consequently, the Sargassum-dominated diet of oceanic stage turtles could still be present in newly recruited turtles’ gastrointestinal tracts. Alternatively, it is equally plausible that Texas recruits are resourcefully foraging inside the massive wracks of Sargassum that enter the channel passes in the spring months (Breuer, 1962) while continuing to feed benthically within the jetty environment. Immature neritic Atlantic green turtles foraging amongst artificial structures in Florida, United States, were noted to opportunistically consume flotsam in addition to their benthic macroalgae-dominated diet (Holloway-Adkins and Hanisak, 2017). The stomach contents of multiple recruits contained jetty algae (e.g., Gelidium spp. and Ulva spp.) and Sargassum spp., indicating this size class inhabits the jetty habitat. Dedicated surveys of turtle feeding behavior within the channel environment when Sargassum mats are present would help elucidate the foraging activity of turtles resident in these channels. Collectively, benthic macroalgae found on the Texas coast (Wynne, 2008) dominated the recruits’ diet indicating this size group is in the jetty habitat.
Several recruits (F = 23.3%) ingested all three primary species of seagrasses, suggestive of foraging in seagrass beds and not on the floating matter in the jetty channels. Tracking data revealed that turtles occupying the jetty environment made brief expeditions into the nearby inshore habitat but returned to jettied-channels (Shaver, 2000). Recruitment may not represent a distinct unidirectional shift from one habitat to the next. As an alternative, younger turtles may display an intermediate stage sampling on macroalgae and seagrasses in diverse environments (Arthur et al., 2008). Considerably, the fluctuation in density and concentration of macroalgae on jetty structures (Kaldy et al., 1995; Renaud et al., 1995; Fikes and Lehman, 2008) could force turtles to feed at alternative sites. Studies incorporating fecal analysis and esophageal lavage may provide a more precise understanding of recruitment size and principal diet.
Green turtles in the western Atlantic are frequently considered obligate seagrass consumers (Mendonca and Ehrhart, 1982; Bjorndal, 1997), with omnivory reported in the Atlantic southwest (Bugoni et al., 2003) and the Pacific (Bjorndal, 1997; Seminoff et al., 2006; Fukuoka et al., 2019). More than half the individuals in all collective size classes ingested animal matter, indicating that Texas’s immature green turtles are omnivores in these developmental foraging grounds. This finding is similar to other studies that have demonstrated animal consumption persisting through ontogeny (Amorocho and Reina, 2008; Cardona et al., 2010; Carrión-Cortez et al., 2010; Morais et al., 2014). The relative importance of animal matter varied across the size classes, with the highest collective IRI value noted in recruits, primarily due to mollusk consumption. Mollusc ingestion may occur incidentally to foraging on macroalgae in the jetty habitat or represent a selective effort. The more frequent ingestion of animals in the winter and spring months by the recruit size class could be proportional to the seasonal variation in the macroalgal abundance documented in the Texas jetty habitat (Fikes et al., 2010). In a previous study in the northern GOM, immature green turtles consumed animal matter more in the winter months due to the seasonal fluctuations in seagrass and algae biomass (Williams et al., 2014). Furthermore, green turtle digestive efficiency decreases when water temperatures drop (Bjorndal, 1980); and animal matter is easier to digest than plant material for green turtles (Bjorndal, 1985). Conceivably, the recruit class selects easier to digest animal matter more frequently during the cooler months when jetty algae composition changes. Regardless of seasonal environmental variations, we recommend that juvenile green turtles foraging in Texas be described as omnivores. Multisource stable isotope mixing models have highlighted invertebrate consumption and assimilation among omnivorous green turtles (Lemons et al., 2011). Therefore, future diet studies in Texas should incorporate isotope mixing models to gain supplemental information on the nutritional contribution and importance of animal matter to all size classes’ diets.
The recent seagrass conservation efforts in Texas have achieved some success. Seagrass acreage was reported to have increased to cover a mean area of 87.7 ± 25.5% in the upper and 50.0 ± 38.4% in the Lower Laguna Madre (Dunton et al., 2013). However, transitional turtles consumed seagrass less frequently over time, indicating that this size class utilizes different habitat niches. Any beneficial effects of recovering seagrass meadows may be negated by an exponentially growing turtle population (Shaver et al., 2017). Plausibly driven by resource competition from turtles in the seagrass beds, transitional turtles incorporate a varied diet of seagrasses and algae. Satellite tracking data revealed green turtles (mean SCL 37.9 ± 5.2 cm) migrate in and out of the Laguna Madre seagrass beds via the jetty habitats (Shaver et al., 2013). The high frequency of ingestion of the typical jetty red algae, Gelidium spp. by transitional turtles indicates turtles supplement their seagrass diet with benthic macroalgae as they transit from inshore waters to the GOM. The concept above is further supported by analyzing digesta boluses throughout the gastrointestinal tract, which indicate separate feeding sessions. Some transitional turtles alternated their diets between seagrass (e.g., Cymodocea sp.) and jetty algae (e.g., Gelidium spp.).
In contrast to transitional turtles, the more frequent consumption of seagrasses by the inshore size class indicates these larger turtles have become highly reliant on the seagrasses with time. Immature green turtles are more susceptible to cold stunning in Texas as they overwinter and show strong site fidelity for inshore habitats (Arms, 1996). Cold stunning is the most significant cause of stranding events in Texas (Shaver et al., 2017) and cold-stunned turtles comprised most winter samples for transitional and inshore size classes. The high frequency of seagrasses in the diet of the two largest classes of turtles stranded in the winter months most certainly reflects this strong seagrass bed dependency.
Shoal grass in the lower Laguna Madre has declined in abundance and increased in bed fragmentation, while the upper Laguna Madre has indicated some expansion in coverage (Onuf, 2007; Wilson and Dunton, 2017). Previous research revealed green turtles in the Laguna Madre selected shoal grass over the other seagrasses (Coyne, 1994). Interestingly, we documented a significant decrease of shoal grass in all size classes’ diet over time with no parallel increase in the frequency of other seagrass species consumed. Turtle grass was still frequently consumed and dominated the diet of our inshore size classes. Conceivably, immature turtles are exhibiting foraging plasticity within their changing seagrass meadows by consuming a variety of macroalgae and animal matter species. Overall, seagrass collectively dominated the diet of turtles larger than 30 cm SCLmax, illustrating the necessity of conserving and monitoring seagrass habitats along the northwestern GOM coast. Potential impacts of changes in seagrass composition and distribution on this green turtle assemblage’s diet should be rigorously monitored through future diet studies.
Management and Conservation Implications
Marine turtles inhabiting the Texas coast face a variety of threats, both human-related and natural. As this green turtle population continues to increase rapidly, it is critical to incorporate foraging ecology studies into conservation management decisions that strengthen the species and habitat protection. Size-related variation in sea turtle foraging habits necessitates integrated management strategies that reduce impacts to immature turtles in Texas jetty and seagrass habitats. Gear modifications in commercial fisheries could be implemented to reduce the incidental take in our nearshore waters, hence providing increased protection of this threatened species. Additionally, it is essential to manage the seagrass beds fundamental to the survival of this exponentially growing green turtle assemblage that will eventually recruit to the breeding population.
Data Availability Statement
The original contributions presented in the study are included in the article/Supplementary Material, further inquiries can be directed to the corresponding author/s.
Ethics Statement
Ethical review and approval was not required for the animal study because the animals used in this study were deceased upon discovery.
Author Contributions
DS and LH conceived and designed the study. LH performed the statistical analysis, created the figures, and wrote the manuscript’s first draft. Both authors contributed to manuscript revision, read and approved the submitted version.
Funding
This work was supported by the National Park Service and NOAA Fisheries. The funders provided support in salaries for the authors (DS and LH) and supplies.
Disclaimer
The statements and findings are those of the authors. They do not necessarily reflect the views of NOAA Fisheries, the U.S. Department of Commerce, the National Park Service, or the Department of the Interior.
Conflict of Interest
The authors declare that the research was conducted in the absence of any commercial or financial relationships that could be construed as a potential conflict of interest.
Acknowledgments
We thank the many participants in the Sea Turtle Stranding and Salvage Network for collecting the stranded sea turtles used in this study. B. Stacy, B. Schroeder, C. Gredzens, and S. Walker provided suggestions that improved the manuscript. We thank the journal reviewers whose critical review helped improve and clarify this manuscript.
Supplementary Material
The Supplementary Material for this article can be found online at: https://www.frontiersin.org/articles/10.3389/fmars.2021.658368/full#supplementary-material
References
Agan, J. C., and Lehman, R. L. (2002). Species composition and seasonal periodicity of macroalgal species in Corpus Christi bay. Texas. J. Phycol. 36:1. doi: 10.1046/j.1529-8817.1999.00001-2.x
Amorocho, D. F., and Reina, R. D. (2008). Intake passage time, digesta composition and digestibility in East Pacific green turtles (Chelonia mydas agassizii) at Gorgona National Park. Colombian Pacific. J. Exp. Mar. Biol. Ecol. 360, 117–124. doi: 10.1016/j.jembe.2008.04.009
Amundsen, P., and Sánchez-Hernández, J. (2019). Feeding studies take guts – critical review and recommendations of methods for stomach contents analysis in fish. J. Fish. Biol. 95, 1364–1373. doi: 10.1111/jfb.14151
Arms, S. (1996). Overwintering Behavior and Movement of Immature Green Sea Turtles in South Texas Waters. M.S. College Station, TX: Texas A&M University.
Army Corps of Engineers (2021). Navigation. Available online at: https://www.swg.usace.army.mil/ (accessed March 6, 2021).
Arthur, K. E., Boyle, M. C., and Limpus, C. J. (2008). Ontogenetic changes in diet and habitat use in green sea turtle (Chelonia mydas) life history. Mar. Ecol. Prog. Ser. 362, 303–311. doi: 10.3354/meps07440
Avens, L., and Snover, M. (2013). “Age and age estimation in sea turtles,” in The Biology of Sea Turtles, Vol.III, eds J. Wyneken, K. Lohmann, and J. Musick (Boca Raton, FL: CRC Press), 97–134. doi: 10.1201/b13895-6
Baker, R., Buckland, A., and Sheaves, M. (2014). Fish gut content analysis: robust measures of diet composition. Fish Fish. 15, 170–177. doi: 10.1111/faf.12026
Behera, S., Tripathy, B., Sivakumar, K., and Choudhury, B. (2015). Stomach Contents of Olive Ridley Turtles (Lepidochelys Olivacea) occurring in gahirmatha, Odisha Coast of India. P. Zool. Soc. 68, 91–95. doi: 10.1007/s12595-014-0100-0
Bigg, M. A., and Perez, M. A. (1985). “Modified volume: a frequency–volume method to assess marine mammal food habits,” in Marine Mammals and Fisheries, eds J. R. Beddington, R. J. H. Beverton, D. M. Lavigne, and D. Martin (London: Allen & Unwin), 277–283.
Bjorndal, K. A. (1980). Nutrition and grazing behavior of the green turtle, Chelonia mydas. Mar. Biol. 56, 147–154. doi: 10.1007/bf00397131
Bjorndal, K. A. (1985). Nutritional ecology of sea turtles. Copeia 1985, 736–751. doi: 10.2307/1444767
Bjorndal, K. A. (1997). “Foraging ecology and nutrition of sea turtles,” in The Biology of Sea Turtles, Vol. I, eds P. Lutz and J. Musick (Boca Raton, FL: CRC Press), 199–231. doi: 10.1201/9780203737088
Bjorndal, K. A., Bolten, A. B., and Lagueux, C. J. (1994). Ingestion of marine debris by juvenile sea turtles in coastal Florida habitats. Mar. Pollut. Bull. 28, 154–158. doi: 10.1016/0025-326X(94)90391-3
Bjorndal, K. A., Bolten, A. B., Lagueux, C. J., and Jackson, D. R. (1997). Dietary overlap in three sympatric congeneric freshwater turtles (Pseudemys) in Florida. Chelonian Conserv. Biol. 2, 430–433.
Bolten, A. B. (2003). “Variation in sea turtle life history patterns: neritic vs. oceanic developmental stages,” in The Biology of Sea Turtles, Vol.II, eds P. Lutz, J. Musick, and J. Wyneken (Boca Raton, FL: CRC Press), 243–257. doi: 10.1201/9781420040807.CH9
Boyle, M. C., and Limpus, C. J. (2008). The stomach contents of post-hatchling green and loggerhead sea turtles in the southwest Pacific: an insight into habitat association. Mar. Biol. 155, 233–241. doi: 10.1007/s00227-008-1022-z
Breuer, J. P. (1962). An ecological survey of the lower laguna madre of texas, 1953-1959. Publ. Inst. Mar. Sci. 8, 153–183.
Bugoni, L., Krause, L., and Petry, M. V. (2003). Diet of sea turtles in southern Brazil. Chelonian Conserv. Biolo. 4, 685–688.
Cardona, L., Campos, P., Levy, Y., Demetropoulos, A., and Margaritoulis, D. (2010). Asynchrony between dietary and nutritional shifts during the ontogeny of green turtles (Chelonia mydas) in the Mediterranean. J. Exp. Mar. Biol. Ecol. 393, 83–89. doi: 10.1016/j.jembe.2010.07.004
Carr, A. (1987a). Impact of nondegradable marine debris on the ecology and survival outlook of sea turtles. Mar. Pollut. Bull. 18, 352–356. doi: 10.1016/S0025-326X(87)80025-5
Carr, A. (1987b). New perspectives on the pelagic stage of sea turtle development. Conserv. Biol. 1, 103–121. doi: 10.1111/j.1523-1739.1987.tb00020.x
Carrión-Cortez, J. A., Zárate, P., and Seminoff, J. A. (2010). Feeding ecology of the green sea turtle (Chelonia mydas) in the Galapagos Islands. J. Mar. Biol. Assoc. UK. 90, 1005–1013. doi: 10.1017/s0025315410000226
Casale, P., Abbate, G., Freggi, D., Conte, N., Oliverio, M., and Argano, R. (2008). Foraging ecology of loggerhead sea turtles Caretta caretta in the central Mediterranean Sea: evidence for a relaxed life history model. Mar. Ecol. Prog. Ser. 372, 265–276. doi: 10.3354/meps07702
Coyne, M. S. (1994). Feeding Ecology of Subadult Green Sea Turtles in South Texas Waters. M.S. College Station TX: Texas A&M University.
Dunton, K., Onuf, C. P., and Schonberg, S. (2002). Assessment of propeller scarring in seagrass beds of the south texas coast. J. Coastal Res. 37, 100–110. doi: 10.1515/BOT.2008.015
Dunton, K. H., Wilson, C. J., Wilson, S. S., and Whiteaker, T. L. (2013). Assessment of Seagrass Habitat quality and Plant Condition in Texas Coastal Waters: 2011–2012. Final Report. Austin, TX: Texas General Land Office, 42.
Edwards, P., and Kapraun, D. F. (1973). Benthic marine algae ecology in the port aransas. Texas Area. Contr. Mar. Sci. 17, 5–52. doi: 10.3989/scimar.2002.66s35
Ferry, L. A., and Cailliet, G. M. (1996). “Sample size and data analysis: are we characterizing and comparing diet properly?,” in Feeding Ecology and Nutrition in Fish, eds D. MacKinlay and K. Shearer (San Francisco, CA: American Fisheries Society), 71–80.
Fikes, R., and Lehman, R. L. (2008). The occurrence of Agardhiella ramosissima (Gigartinales) and Acanthophora spicifera (Ceramiales) in the Texas coastal bend. Tex. J. Sci. 60, 221–224.
Fikes, R., and Lehman, R. L. (2010). Recruitment and colonization of macroalgae to a newly constructed rocky intertidal habitat in the northwest Gulf of Mexico. Gulf Caribbean Rese. 22, 9–20. doi: 10.18785/gcr.2201.02
Fikes, R. L., Lehman, R. L., and Klootwyk, K. V. (2010). An update on the benthic algae of Mansfield pass. Texas. Tex. J. Sci. 62, 183–194.
Foley, A. M., Singel, K. E., Dutton, P. H., Summers, T. M., Redlow, A. E., and Lessman, J. (2007). Characteristics of a green turtle (Chelonia mydas) assemblage in northwestern Florida determined during a hypothermic stunning event. Gulf Mexico Sci. 25, 131–143. doi: 10.18785/GOMS.2502.04
Forbes, G. A. (1999). “Diet sampling and diet component analysis,” in Research and Management Techniques for the Conservation of Sea Turtles, eds K. Eckert, K. Bjorndal, F. A. Abreu-Grobois, and M. Donnelly (Gland: IUCN Publication).
Fukuoka, T., Narazaki, T., Kinoshita, C., and Sato, K. (2019). Diverse foraging habits of juvenile green turtles (Chelonia mydas) in a summer-restricted foraging habitat in the northwest Pacific ocean. Mar. Biol. 166:25. doi: 10.1007/s00227-019-3481-9
George, E. L., and Hadley, W. F. (1979). Food and habitat partitioning between rock bass (Ambloplites rupestris) and smallmouth bass (Micropterus dolomieui) young of the year. Trans. Am. Fish. Soc. 108, 253–261. doi: 10.1577/1548-8659(1979)108<253:fahpbr>2.0.co;2
Gherskiere, T., Magda, V., Greet, P., and Steve, D. (2006). Are strandline meiofaunal assemblages affected by a once-only mechanical beach cleaning? Exp. Findings. Mar. Environ. Res. 61, 245–264. doi: 10.1016/J.MARENVRES.2005.10.003
Gilbert, E. I. (2005). Juvenile green turtle (Chelonia mydas) foraging ecology: Feeding selectivity and forage nutrient analysis. M.S. Orlando FL: University of Central Florida
Gonzalez Carman, V., Acha, E. M., Maxwell, S. M., Albareda, D., Campagna, C., and Mianzan, H. (2014). Young green turtles, Chelonia mydas, exposed to plastic in a frontal area of the SW Atlantic. Mar. Pollut. Bull. 78, 56–62. doi: 10.1016/j.marpolbul.2013.11.012
Gorga, C. C. T. (2010). Foraging Ecology of Green Turtles (Chelonia mydas) on the Texas Coast, as Determined by Stable Isotope Analysis. M.S. College Station TX: Texas A&M University.
Gower, J., Hu, C., Borstad, G., and King, S. (2006). Ocean color satellites show extensive lines of floating Sargassum in the Gulf of Mexico. IEEE T. Geosci. Remote 44, 3619–3625. doi: 10.1109/TGRS.2006.882258
Guebert-Bartholo, F. M., Barletta, M., Costa, M. F., and Monteiro-Filho, E. L. A. (2011). Using gut contents to assess foraging patterns of juvenile green turtles Chelonia mydas in the Paranaguá Estuary, Brazil. Endanger. Species Res. 13, 131–143. doi: 10.3354/esr00320
Gutierrez, M. A., Cardona, A. A., and Smee, D. L. (2010). Growth patterns of shoal grass Halodule wrightii and manatee grass Syringodium filiforme in the western Gulf of Mexico. Gulf Caribbean Res. 22, 71–75. doi: 10.18785/gcr.2201.09
Hatase, H., Sato, K., Yamaguchi, M., Takahashi, K., and Tsukamoto, K. (2006). Individual variation in feeding habitat use by adult female green sea turtles (Chelonia mydas): Are they obligate neritic herbivores? Oecologia 149, 52–64. doi: 10.1007/s00442-006-0431-
Hildebrand, H. H. (1982). “A historical review of the status of sea turtle populations in the western Gulf of Mexico,” in Biology and Conservation of Sea Turtles, ed. K. A. Bjorndal (Washington, D. C: Smithsonian Institution Press), 447–453.
Hildebrand, H. H., and King, D. (1978). A Biological Study of the Caro del Aso and the Pita Island area of the Laguna Madre, 1976-1977. Corpus Christi, TX: Report to Central Power and Light, Co.
Hoarau, L., Ainley, L., Jean, C., and Ciccione, S. (2014). Ingestion and defecation of marine debris by loggerhead sea turtles, Caretta caretta, from by-catches in the South-West Indian Ocean. Mar. Pollut. Bull. 84, 90–96. doi: 10.1016/j.marpolbul.2014.05.031
Hobson, C., and Whisenant, A. (2018). Seagrass monitoring in San Antonio Bay, Texas with implications for management. Tex. J. Sci. 70:1. doi: 10.32011/txjsci_70_1_Article1
Holloway-Adkins, K. G. (2001). A Comparative Study of the Feeding Ecology of Chelonia Mydas (Green Turtle) and the Incidental Ingestion of Prorocentrum Spp. M.S. Orlando FL: University of Central Florida.
Holloway-Adkins, K. G., and Hanisak, D. M. (2017). Macroalgal foraging preferences of juvenile green turtles (Chelonia mydas) in a warm temperate/subtropical transition zone. Mar. Biol. 164:161. doi: 10.1007/s00227-017-3191-0
Howell, L. N. (2012). Ontogenetic shifts in diet and habitat by juvenile green sea turtles (Chelonia mydas) along the middle and lower Texas coast. M.S. College Station TX: Texas A&M University.
Howell, L. N., Reich, K. J., Shaver, D. J., Landry, A. M. Jr., and Gorga, C. C. T. (2016). Ontogenetic shifts in diet and habitat of juvenile green sea turtles in the northwestern Gulf of Mexico. Mar. Ecol. Prog. Ser. 559, 217–229. doi: 10.3354/meps11897
Hyslop, E. J. (1980). Stomach contents analysis - A review of methods and their application. J. Fish Biol. 17, 411–429. doi: 10.1111/j.1095-8649.1980.tb02775.x
Jones, T. T., and Seminoff, J. A. (2013). “Feeding biology: advances from field-based observations, physiological studies, and molecular techniques,” in The Biology of Sea Turtles, eds J. Musick, J. Wyneken, and K. Lohman (Boca Raton, FL: CRC Press), 211–248. doi: 10.1201/b13895
Kaldy, J. E., Dunton, K. H., and Czerny, A. B. (1995). Variation in macroalgal species composition and abundance on a rock jetty in the northwest Gulf of Mexico. Bot. Mar. 38, 519–528. doi: 10.1515/botm.1995.38.1-6.519
Kowalski, J. L., DeYoe, H. R., and Allison, T. C. (2009). Seasonal production and biomass of the Seagrass, Halodule wrightii Aschers. (Shoal Grass), in a subtropical Texas lagoon. Estuar. Coast. 32, 467–482. doi: 10.1007/s12237-009-9146-z
Landry, A. M. Jr., Costa, D. T., Williams, B. B., and Coyne, M. S. (1992). Sea Turtle Capture and Habitat Characterization Study. Washington, D.C: National Oceanic and Atmospheric Administration.
Lemons, G., Lewison, R., Komoroske, L., Gaos, A., Lai, C., Dutton, et al. (2011). Trophic ecology of green sea turtles in a highly urbanized bay: insights from stable isotopes and mixing models. J. Exp. Mar. Biol. Ecol. 405, 25–32. doi: 10.1016/j.jembe.2011.05.012
Liao, H., Pierce, C. L., and Larscheid, J. G. (2001). Empirical assessment of indices of prey importance in the diets of predacious fish. Trans. Am. Fish. Soc. 130, 583–591. doi: 10.1577/1548-8659(2001)130<0583:eaoiop>2.0.co;2
López-Mendilaharsu, M., Gardner, S. C., Riosmena-Rodrigues, R., and Seminoff, J. A. (2008). Diet selection by immature green turtles (Chelonia mydas) at bahía magdalena foraging ground in the pacific coast of the baja California Peninsula, México. J. Mar. Biol. Assoc. UK. 88, 641–647. doi: 10.1017/S0025315408001057
Magnuson, J., Bjorndal, K., Dupaul, W., Graham, G., Owens, D., Peterson, C., et al. (1990). Decline of the Sea Turtle; Causes and Prevention. Committee on Sea turtle Conservation. Washington, DC: National Academy Press, doi: 10.17226/1536
Manzella, S. A., Williams, J. A., and Caillouet, C. W. Jr. (1990). “Radio and Sonic Tracking of Juvenile Sea Turtles in Inshore Waters of Louisiana and Texas” in Tenth Annual Workshop on Sea Turtle Biology and Conservation, Vol. 278. Washington, D.C: NOAA, 115.
Mathieson, A. C., and Penniman, C. A. (1986). A phytogeographic interpretation of the marine flora from the Isles of Shoals. U.S.A. Bot. Mar. 29, 413–434.
Makowski, C., Seminoff, J. A., and Salmon, M. (2006). Home range and habitat use of juvenile Atlantic green turtles (Chelonia mydas L.) on shallow reef habitats in Palm Beach, Florida, USA. Mar. Biol. 148, 1167–1179. doi: 10.1007/s00227-005-0150-y
Mendelssohn, I. A., Byrnes, M. R., Kneib, R. T., and Vittor, B. A. (2017). “Coastal habitats of the Gulf of Mexico,” in Habitats and Biota of the Gulf of Mexico: Before the Deepwater Horizon Oil Spill: Volume 1: Water Quality, Sediments, Sediment Contaminants, Oil and Gas Seeps, Coastal Habitats, Offshore Plankton and Benthos, and Shellfish, ed. C. H. Ward (New York, NY: Springer), 359–640. doi: 10.1007/978-1-4939-3447-8_6
Mendonca, M. T., and Ehrhart, L. M. (1982). Activity, population size and structure of immature Chelonia mydas and Caretta caretta in Mosquito Lagoon, Florida. Copeia 1982, 161–167. doi: 10.2307/1444280
Metz, T. L., and Landry, A. M. Jr. (2013). An assessment of green turtle (Chelonia mydas) stocks along the Texas coast, with emphasis on the lower Laguna Madre. Chelonian Conser. Biol. 12, 293–302. doi: 10.2744/CCB-1046.1
Morais, R. A., dos Santos, R. G., Longo, G. O., Yoshida, E. T., Stahelin, G. D., Horta, P. A., et al. (2014). Direct evidence for gradual ontogenetic dietary shift in the green turtle, Chelonia mydas. Chelonian Conserv. Biol. 12, 260–266. doi: 10.2744/CCB-1058.1
NMFS (1991). Recovery Plan for U.S. Population of Atlantic Green Turtle (Chelonia mydas). Washington, D. C: National Oceanic and Atmospheric Administration, National Marine Fisheries Service, Office of Protected Resources.
Onuf, C. P. (1994). Seagrasses, dredging, and light in Laguna Madre, Texas, USA. Estuarine. Coastal Shelf Sci. 39, 75–91. doi: 10.1006/ecss.1994.1050
Onuf, C. P. (1996). Seagrass responses to long-term light reduction by brown tide in upper Laguna Madre, Texas: distribution and biomass patterns. Mar. Ecol. Prog. Ser. 138, 219–231. doi: 10.3354/meps138219
Onuf, C. P. (2007). “Laguna Madre,” in Seagrass Status and Trends in the Northern Gulf of Mexico: 1940–2002 Scientific Investigations Report 2006-5287 and U.S. Environmental Protection Agency 855–R–04–003, eds L. Handley, D. Altsman, and R. Demay (Washington, D.C: U.S. Geological Survey), 29–40.
Parker, D. M., Cooke, W. J., and Balazs, G. H. (2005). Diet of oceanic loggerhead sea turtles (Caretta caretta) in the central North Pacific. Fish. Bull. 103, 142–152.
Patriquin, D. G. (1975). Migration of blowouts in seagrass beds at Barbados and Carriacou, West Indies, and its ecological and geological implications. Aquat. Bot. 1, 163–189. doi: 10.1016/0304-3770(75)90021-2
Pulich, W. M., and Calnan, T. (1999). Seagrass Conservation Plan for Texas” Resource Protection Division. Austin, TX: Texas Parks and Wildlife Department, 67.
Pulich, W. M., and Onuf, C. P. (2007). “Statewide Summary for Texas,” in Seagrass Status and Trends in the Northern Gulf of Mexico: 1940–2002, Scientific Investigations Report 2006-5287 and U.S. Environmental Protection Agency 855–R–04–003, eds L. Handley, D. Altsman, and R. Demay (Washington, D.C: U.S. Geological Survey), 7–16.
Quammen, M. L., and Onuf, C. P. (1993). Laguna Madre: seagrass changes continue decades after salinity reduction. Estuaries 16, 302–310. doi: 10.2307/1352503
Redfoot, W. E. (1997). Population Structure and Feeding Ecology of Green Turtles Utilizing the Trident Submarine Basin, Cape Canaveral, Florida as Developmental Habitat. M.S. Orlando FL: University of Central Florida.
Renaud, M. L., Carpenter, J. A., Manzella, S. A., and Williams, J. A. (1992). Telemetric Tracking of Green sea Turtles (Chelonia mydas) in Relation to Dredged Channels at South Padre Island, Texas July through September 1992”. Final Report. National Oceanic and Atmospheric Administration. Silver Spring, MA: National Marine Fisheries Service, 56.
Renaud, M. L., Carpenter, J. A., Manzella, S. A., and Williams, J. A. (1994). “Radio and sonic telemetric monitoring of immature green sea turtles in the Brazos-Santiago Pass area, South Padre Island, Texas,” in Proceeding of the 13th Annual Symposium on Sea Turtle Biology and Conservation, eds B. E. Witherington and B. A. Schroeder (Miami, FL: National Oceanographic and Atmospheric Administration), 148.
Renaud, M. L., Carpenter, J. A., Williams, J. A., and Manzella-Tirpak, S. A. (1995). Activities of juvenile green turtles, Chelonia mydas, at a jettied pass in South Texas. Fish. Bull. 93, 586–593.
Renaud, M. L., and Williams, J. A. (1997). Movements of Kemp’s ridley (Lepidochelys kempii) and Green (Chelonia mydas) Sea Turtles Using Lavaca Bay and Matagorda Bay 1996-1997”. Final Report. Washington, D.C: Environmental Protection Agency, 62.
Revelles, M., Cardona, L., Aguilar, A., and Fernandez, G. (2007). The diet of pelagic loggerhead sea turtles (Caretta caretta) off the Balearic archipelago (western Mediterranean): Relevance of long-line baits. J. Mar. Biol. Assoc. UK. 87, 805–813. doi: 10.1017/s0025315407054707
Rodriguez, A., and Heck, K. (2020). Green turtle herbivory and its effects on the warm, temperate seagrass meadows of St. Joseph Bay, Florida (USA). Mar. Ecol. Prog. Ser. 693, 37–51. doi: 10.3354/meps13285
Seminoff, J. A., Jones, T. T., and Marshall, G. J. (2006). Underwater behaviour of green turtles monitored with video-time-recorders: what’s missing from dive profiles? Mar. Ecol. Prog. Ser. 322, 269–280. doi: 10.3354/meps322269
Shamblin, B. M., Dutton, P. H., Shaver, D. J., Bagley, D. A., Putman, N. F., Mansfield, K. L., et al. (2016). Mexican origins for the Texas green turtle foraging aggregation: A cautionary tale of incomplete baselines and poor marker resolution. J. Exp. Mar. Biol. Ecol. 488, 111–120. doi: 10.1016/j.jembe.2016.11.009
Shaver, D. J. (1994). Relative abundance, temporal patterns, and growth of sea turtles at the Mansfield Channel. Texas. J. Herpetol. 28, 491–497. doi: 10.2307/1564963
Shaver, D. J. (2000). Distribution, Residency, and Seasonal Movements of the Green Sea Turtle, Chelonia mydas (Linnaeus, 1758), in Texas. Ph.D. Thesis. College Station TX: Texas A&M University.
Shaver, D. J., Hart, K. M., Fujisaki, I., Rubio, C., and Sartain, A. R. (2013). “Movement mysteries unveiled: spatial ecology of juvenile green sea turtles,” in Reptiles in Research, ed. W. I. Lutterschmidt (Hauppauge, NY: Nova Science Publishers), 463–484.
Shaver, D. J., Tissot, P. E., Streich, M. M., Walker, J. S., Rubio, C., Amos, A. F., et al. (2017). Hypothermic stunning of green sea turtles in a western Gulf of Mexico foraging habitat. PLoS One 12:e0173920. doi: 10.1371/journal.pone.0173920
Stacy, B., Hardy, R., Shaver, D., Purvin, C., Howell, L., Wilson, H. M., et al. (2020). 2019 Sea Turtle Strandings in Texas: A summary of findings and analyses. NOAA Techn. Memorandum NMFS OPR 66:64.
Tourinho, P. S., Ivar Do Sul, J. A., and Fillmann, G. (2010). Is marine debris ingestion still a problem for the coastal marine biota of southern Brazil? Mar. Pollut. Bull. 60, 396–401. doi: 10.1016/j.marpolbul.2009.10.013
Webster, R., and Linton, T. (2013). Development and implementation of Sargassum Early Advisory System (SEAS). Shore Beach 81, 1–6.
Wilcox, C., Mallos, N. J., Leonard, G. H., Rodriguez, A., and Hardesty, B. D. (2016). Using expert elicitation to estimate the impacts of plastic pollution on marine wildlife. Mar. Policy 65, 107–114. doi: 10.1016/j.marpol.2015.10.014
Williams, J. A., and Manzella, S. A. (1992). “Sea turtle sightings at passes on the Texas Gulf Coast,” in Eleventh Annual Workshop on Sea Turtle Biology and Conservation, eds M. Salmon and J. Wyneken (Washington, D.C: NOAA).
Williams, J. A., and Renaud, M. L. (1998). “Tracking of kemp’s ridley (lepidochelys kempii) and green (chelonia mydas) sea turtles in the matagorda bay system, texas,” in Proceedings of the 17th Annual Sea Turtle Symposium, eds S. Epperly and J. Braun (Orlando, FL: NOAA).
Williams, N. C., Bjorndal, K. A., Lamont, M. M., and Carthy, R. R. (2014). Winter diets of immature green turtles (Chelonia mydas) on a northern feeding ground: Integrating stomach contents and stable isotope analyses. Estuaries Coasts 37, 986–994. doi: 10.1007/s12237-013-9741-x
Wilson, S. S., and Dunton, K. H. (2017). Hypersalinity during regional drought drives mass mortality of the seagrass Syringodium filiforme in a subtropical lagoon. Estuaries Coasts 41, 855–865. doi: 10.1007/s12237-017-0319-x
Witherington, B. (2006). The Mystery of Lost Little Turtles: Where do Sea Turtles Spend Their First Years of life? SWOT Report, Vol. II. Arlington: State of the World’s Sea Turtles (SWOT), 7–8.
Witherington, B., Hirama, S., and Hardy, R. (2012). Young sea turtles of the pelagic Sargassum-dominated drift community: habitat use, population density, and threats. Mar. Ecol. Prog. Ser. 463, 1–22. doi: 10.3354/meps09970
Withers, K. (2002). “Red and brown tides,” in the Laguna Madre of Texas and Tamaulipas, eds F. W. Judd and J. W. Tunnell (College Station, TX: Texas A&M University Press), 255–258.
Keywords: sea turtle, Chelonia mydas, gut content analysis, foraging ecology, Texas
Citation: Howell LN and Shaver DJ (2021) Foraging Habits of Green Sea Turtles (Chelonia mydas) in the Northwestern Gulf of Mexico. Front. Mar. Sci. 8:658368. doi: 10.3389/fmars.2021.658368
Received: 25 January 2021; Accepted: 29 March 2021;
Published: 15 April 2021.
Edited by:
Michele Thums, Australian Institute of Marine Science (AIMS), AustraliaReviewed by:
Jeffrey Aleksandr Seminoff, Southwest Fisheries Science Center (NOAA), United StatesKaren Holloway-Adkins, East Coast Biologists, Inc., United States
Jeffrey Schmid, Conservancy of Southwest Florida, United States
Copyright © 2021 Howell and Shaver. This is an open-access article distributed under the terms of the Creative Commons Attribution License (CC BY). The use, distribution or reproduction in other forums is permitted, provided the original author(s) and the copyright owner(s) are credited and that the original publication in this journal is cited, in accordance with accepted academic practice. No use, distribution or reproduction is permitted which does not comply with these terms.
*Correspondence: Lyndsey N. Howell, THluZHNleS5Ib3dlbGxAbm9hYS5nb3Y=