- 1Seascape Consultants Ltd., Romsey, United Kingdom
- 2Okeanos Research Centre, Universidade dos Açores, Horta, Portugal
- 3IMAR Instituto do Mar, Universidade dos Açores, Horta, Portugal
Highly specialised biota occurring at hydrothermally active vents on the northern Mid-Atlantic Ridge (nMAR: from south of Iceland to the Equator) have been the subject of numerous research projects over the 36-year period since these habitats were first discovered in the region. When hydrothermal activity ceases, biota endemic to hydrothermally active habitats are lost, and a new biota colonise these sites. Little is known about the biota colonising hydrothermally inactive sulphide habitats on the nMAR, although these sites may be the target of deep-sea mining within the next decade. In this review, we seek to clarify the current knowledge of biological communities colonising hydrothermally active habitats and inactive sulphide habitats on the nMAR. To achieve this, we (1) used a systematic review process to update the species list of benthic invertebrates associated with hydrothermally active habitats, (2) conducted a regional biogeographic analysis of hydrothermally active vent fields on the nMAR, (3) undertook a comprehensive literature review to provide a descriptive account of biological communities, and (4) identified key knowledge gaps in the current understanding of nMAR hydrothermally active and inactive ecosystems. Our updated species list increases the number of benthic invertebrates recorded from hydrothermally active habitats on the nMAR to 158 taxa. Our regional biogeographic analysis separates nMAR hydrothermal vent fields into distinct clusters based on depth/latitude and chimney composition. Vent fields close to the Azores (Menez Gwen, Lucky Strike, Rainbow) formed a separate cluster from those at greater depths south of the Azores (Broken Spur, TAG, Snake Pit, Logatchev, and Ashadze-1). Moytirra, located north of the Azores, clustered separately, as did Lost City with its unique carbonate chimneys. We present detailed information on the biological communities at hydrothermally active and inactive habitats in this region, and discuss the information available on the diversity, ecosystem function, trophic relationships, connectivity, temporal variability, and resilience and recovery of these communities. Many knowledge gaps still exist, with detailed information needed on all aspects of the biological communities at hydrothermally active habitats and inactive sulphide habitats on the nMAR to understand and predict impacts from natural and human-induced disturbances in the region.
Introduction
Hydrothermal habitats1 form along the mid-ocean ridges and back-arc spreading centres of the global ocean. Where hydrothermal activity is sustained, this can support highly productive biological communities dependent on the chemosynthetic activity of microbes at the base of the food web. Despite the discovery of more than 300 confirmed active vent fields to date (Beaulieu and Szafranski, 2020), the global surface area of hydrothermally active habitats is small, representing <0.00001% of the surface area of the planet (Van Dover et al., 2018). Globally, more than 500 species have been described from hydrothermally active habitats, dominated by benthic invertebrate megafaunal and macrofaunal taxa with some meiofaunal taxa and fishes (Desbruyères et al., 2006). These hydrothermally active habitats and their diverse associated biota are considered to have considerable scientific and societal value (Van Dover et al., 2018).
Hydrothermal activity does not continue at a site indefinitely. Over a timescale of years to centuries, hydrothermal activity wanes and eventually ceases, leaving hydrothermally inactive sulphide sites and fields (Jamieson and Gartman, 2020). Where hydrothermal activity has ceased, the supply of reduced compounds essential to chemosynthesis is removed, and biota endemic to hydrothermally active habitats are subsequently lost. Although little is known about colonisation steps for inactive sulphide substrata, it is presumed that biota endemic to hydrothermally active habitats is gradually replaced by organisms which are not reliant on hydrothermal activity but are able to cope with weathered sulphide substrata.
Hydrothermal activity supports highly specialised biota, which have been the subject of numerous research projects over the 36-year period since the initial discovery of these habitats on the Mid-Atlantic Ridge (MAR) in 1985 (Kong et al., 1985; Rona et al., 1986). Many of the species recorded from hydrothermally active habitats on the northern MAR (nMAR: from south of Iceland to the Equator) are highly adapted and only occur in this region, such as Bathymodiolus azoricus and B. puteoserpentis mussels, and Rimicaris exoculata shrimp. The occurrence of species endemic to the nMAR has led to this region being identified as a separate biogeographic province (Bachraty et al., 2009; Moalic et al., 2012) with some analyses further dividing this region into two provinces (Van Dover et al., 2002). The location of 13 confirmed active vent fields where biological community information is available is provided in Figure 1. Where hydrothermal activity has been “inferred” on the nMAR from water column chemistry (Beaulieu and Szafranski, 2020), further visual surveys may confirm the occurrence of additional hydrothermal vent fields with thriving biological communities.
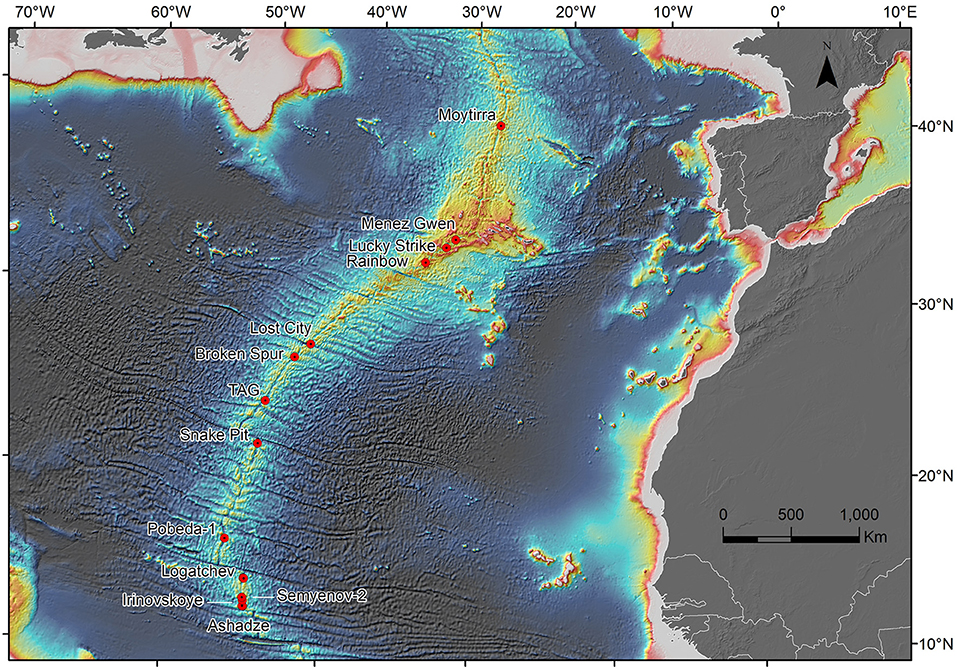
Figure 1. The location of 13 hydrothermally active vent fields on the northern Mid-Atlantic Ridge. Map produced using the ETOPO1 1 Arc-Minute Global Relief Model: Procedures, Data Sources and Analysis. NGDC, NESDIS, NOAA, U.S. Dept. of Commerce, Boulder, CO. Escartin, J., M. Cannat, G. Pouliquen, A. Rabain and J. Lin. 2001. Lourenço, N., J.M. Miranda, J.F. Luis, A. Ribeiro, L.A.Mendes Victor, J. Madeira, and H.D. Needham. 1998.
There are few records of hydrothermally inactive sulphide habitats along the nMAR, for example Mag Mell in the Moytirra vent field between the Azores and Iceland (Wheeler et al., 2013); and some chimneys within the Eiffel Tower sulphide complex in the Lucky Strike vent field near the Azores (Cuvelier et al., 2009). Whilst there are additional occurrences of inactive sulphide substrata along the nMAR, such as the Mir and Alvin zones of the TAG vent field (Rona et al., 1993; Krasnov et al., 1995; Lalou et al., 1998) and the Yubileinoe and Surprise vent fields (Bel'tenev et al., 2017), these commonly have little or no record of any associated biological communities. The distribution of hydrothermally inactive sulphide habitats along the MAR has probably been under-reported to date, with more occurrences waiting to be discovered in off-axis locations or along the ridge axis at sites more distant from active venting (Hannington et al., 2011).
Alongside the biological wealth of hydrothermally active habitats and inactive sulphide habitats on the nMAR, there is growing interest in the potential mineral wealth associated with some of these locations. Hydrothermal fluids can contain high concentrations of metal sulphides, and in some locations, these may form polymetallic sulphides (PMS) of sufficient grade and tonnage to be of economic interest. However, there are currently no exploitation (commercial mining) activities occurring on the MAR, and such activities may not be anticipated for several years, given that exploration contracts currently in place on the nMAR beyond national jurisdiction each have at least 6 years remaining (ISA, 2021).
In this paper, we review the available literature for the biological communities of nMAR hydrothermally active habitats and inactive sulphide habitats. In reviewing this information, we have four aims, to: (1) update the species list of benthic invertebrates associated with hydrothermally active habitats; (2) conduct a regional biogeographic analysis of the known hydrothermally active vent fields on the nMAR; (3) provide a multi-disciplinary descriptive account of the biological communities of nMAR hydrothermally active habitats and inactive sulphide habitats; and (4) discuss the results of our review and identify knowledge gaps in the current understanding of nMAR hydrothermally active and inactive ecosystems. By presenting this information, we hope to clarify the knowledge status for nMAR hydrothermal habitats, particularly in the context for environmental management of human activities on the ridge, including future deep-sea mining.
Materials and Methods
Species List and Abundance Information for Benthic Invertebrates at Hydrothermally Active Habitats
To generate the updated species list and taxa abundance at the different vent fields, we searched the biological literature for studies that reported biodiversity from the hydrothermal vents on the northern section of the Mid Atlantic Ridge (nMAR: between south of Iceland and north of the Equator, see Figure 1). For this systematic review, we followed a PRISMA approach (Figure 2; Supplementary Table 1). Literature searches were conducted using the ISI Web of Science Database (featuring combinations of the keywords: hydrothermal vents; North Atlantic ridge biodiversity; Moytirra, Menez Gwen, Lucky Strike, Rainbow, Lost City, Broken Spur, Snake Pit, TAG, Logatchev, Pobeda, Irinovskoye, Semyenov, and Ashadze) and by following reference lists in papers. The “Handbook of deep-sea hydrothermal vent fauna” edited by Desbruyères et al. (2006) compiles all the available literature prior to 2006 to create the most comprehensive species list for vent invertebrates recorded from hydrothermally active habitats along the MAR to date. Consequently, we focused our literature compilation on all available literature between 2005 and 2020 (June), including all peer-reviewed studies.
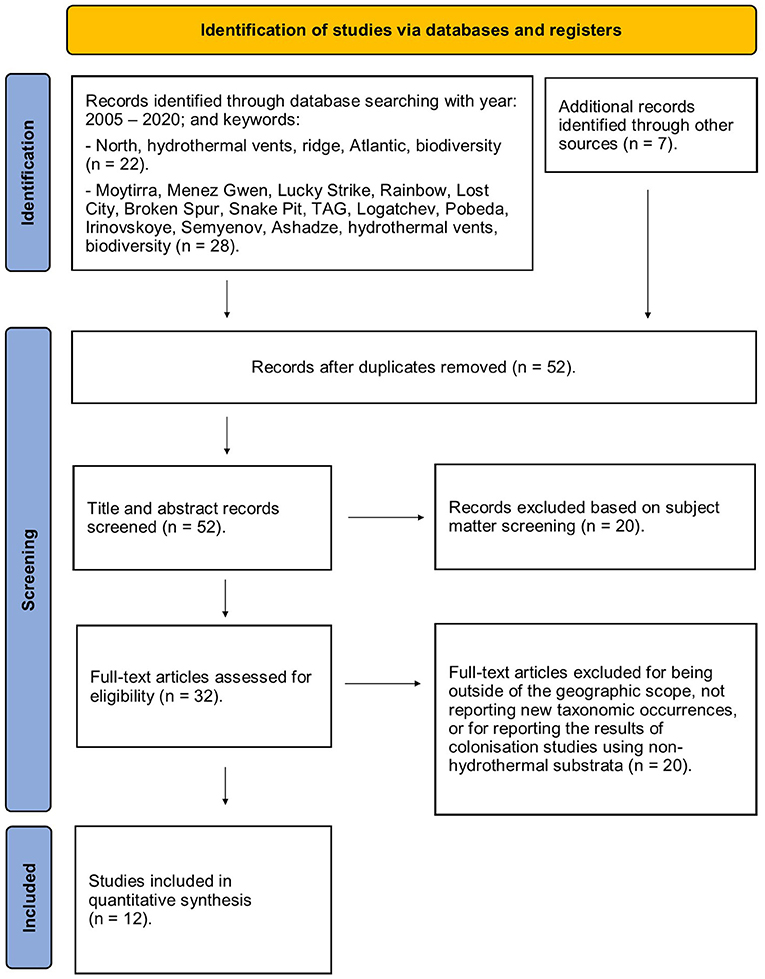
Figure 2. The systematic review protocol to obtain records for quantitative synthesis was conducted according to PRISMA guidelines, adapted from Page et al. (2020). See PRISMA checklist in Supplementary Table 1.
All studies were screened for relevance to by reading the title and abstract; this step identified 32 studies as being relevant to the generation of the updated species list and associated abundance information. Full text was obtained for the 32 studies that were selected by the subject-based screening. The full text of these papers was examined and studies were excluded from our analyses if they presented the results of colonisation studies at hydrothermal vent fields using substrata of non-hydrothermal origin. The decision to exclude these studies was taken to avoid addition of taxa that would not usually be recorded from hydrothermally active habitats. Studies were also excluded if they occurred outside of the geographic scope for the study, or if they did not document additional species or location information when compared to Desbruyères et al. (2006). The taxonomic status of all taxa was checked and updated using the World Register of Marine Species (World Register of Marine Species, 2020). The final taxa list with accompanying abundance information was created using Desbruyères et al. (2006) and a further 12 studies (Stöhr and Segonzac, 2005; Ivanenko et al., 2006, 2012; Zekely et al., 2006a; Fabri et al., 2011; Wheeler et al., 2013; Sarrazin et al., 2015; Tchesunov, 2015; Plum et al., 2017; Yahagi et al., 2019).
The taxonomic and abundance information available for generating the revised species list and abundance dataset is potentially limited by differences in sampling effort amongst sites, and sampling biases introduced by differences in sampling methods or focus on certain taxonomic groups. To account for differences in abundance that may occur because of these potential biases, we restricted our quantitative analyses to the use of presence-absence data. Vent fields where there was insufficient information to provide reliable presence-absence data were excluded from quantitative analyses (see section Regional biogeographic analysis for hydrothermally active vent fields). The potential for these limitations to influence observed trends in diversity along the nMAR was carefully considered in the interpretation of our results (see section Species list for benthic invertebrates at hydrothermally active habitats).
Regional Biogeographic Analysis for Hydrothermally Active Vent Fields
Using the updated taxa list and abundance records described in section Species list and abundance information for benthic invertebrates at hydrothermally active habitats (see Supplementary Table 2), the biological information recorded from 10 of the 13 nMAR vent fields (Motirra, Menez Gwen, Lucky Strike, Rainbow, Lost City, Broken Spur, Snake Pit, TAG, Logatchev, and Ashadze-1) was used for multivariate analysis. There was insufficient biological information to include three of the vent fields (Pobeda-1, Irinovskoye, and Semyenov-2) in the analysis.
Presence-absence data for taxa recorded from the 10 vent fields were analysed using multivariate routines in the statistical software package PRIMER 7. Hierarchical Cluster Analysis (CLUSTER) was performed on the Bray-Curtis resemblance matrix, with a SIMPROF test (at p = 0.05) to determine sample group structure i.e., which vent fields had similar assemblage structure based on the presence-absence of taxa. The Bray-Curtis of a presence-absence matrix is directly equivalent of a Sorensen resemblance matrix (Clarke et al., 2014). In this type of resemblance matrix, joint absences have no effect on similarity amongst samples, instead similarity is determined by the presence of species amongst samples. Inclusion of additional samples does not affect the similarity amongst existing samples (Clarke et al., 2014). The cluster mode was group average with 9,999 permutations.
Non-metric Multidimensional Scaling (nMDS) plots and CLUSTER diagrams were produced to visualise patterns in the grouping of vent fields. For the nMDS, test parameters included minimum and maximum dimensions of 2 and 3 respectively; 50 restarts; a minimum stress of 0.01; and Kruskal fit scheme 1. To determine the taxa that contributed the most to similarity amongst SIMPROF groups, a SIMPER test was performed on the presence-absence data with a cut-off of 100%. The maximum SIMPER cut-off value of 100% was chosen to fully characterise the similarities amongst vent fields within SIMPROF groups.
To explore the relationship between the SIMPROF groups and potential environmental drivers, a BEST test (BioEnv & Stepwise) was performed using the BioENV setting. The test used 9,999 permutations and Spearman Rank Correlation, performed using the presence-absence Bray Curtis resemblance matrix and a Euclidean environmental data matrix. The environmental data matrix featured eight variables for each vent field (Table 1), which were grouped into four indicators: location (latitude); depth (maximum reported depth); host rock (basalt or ultramaphic); tectonic setting (tectonic or magmatic); and chimney type (sulphides or carbonates). These environmental variables were normalised to account for different units (latitude in decimal degrees; depth in metres; host rock, tectonic setting, and chimney type in presence-absence). A PCA plot was produced to illustrate the direction of effect that environmental variables had on the similarities amongst vent fields.
Descriptive Account of Biological Communities
To provide a detailed descriptive account of the biological communities at hydrothermally active habitats and inactive sulphide habitats on the nMAR, we reviewed a wide range of multidisciplinary literature, including biodiversity studies, species descriptions, ecological and geological studies, and studies relating to the function, trophic structure, connectivity, and resilience of these communities. We used more than 100 published literature sources to provide detailed accounts of the regional diversity of biological communities at hydrothermally active and inactive habitats, including microorganisms, benthic invertebrates, and benthic and benthopelagic fishes; ecosystem function; trophic relationships; connectivity; temporal variability; and resilience and recovery.
Results
Species List for Benthic Invertebrates at Hydrothermally Active Habitats
The updated list for benthic invertebrates colonising hydrothermally active habitats on the nMAR features 158 taxa (see Supplementary Table 2). These taxa are phyletically diverse, including Foraminifera (1 taxon), Porifera (3 taxa), Cnidaria (15 taxa), Mollusca (25 taxa), Nematoda (30 taxa), Annelida (13 taxa), Arthropoda (64 taxa), Echinodermata (6 taxa), and Chaetognatha (1 taxon). The vent field with the highest taxonomic richness was Lucky Strike, followed by Snake Pit, Menez Gwen, Rainbow, Logatchev, Ashadze-1, Broken Spur, TAG, Lost City, and Moytirra (Table 2).

Table 2. Number of taxa recorded from each of the 10 vent fields included in the study, from North to South: Moytirra, Menez Gwen, Lucky Strike, Rainbow, Lost City, Broken Spur, TAG, Snake Pit, Logatchev, and Ashadze 1.
Regional Biogeographic Analysis for Hydrothermally Active Vent Fields
Northern MAR hydrothermal vent fields formed four clusters, according to CLUSTER and SIMPROF analyses and visualised by CLUSTER diagram (Figure 3A) and nMDS (Figure 3B). Group c contained most of the vent fields (Ashadze-1, Logatchev, Snake Pit, Broken Spur, TAG, and Rainbow). Group d contained Menez Gwen and Lucky Strike, which occur closer to the Azores. Moytirra and Lost City each formed their own cluster in the analysis.
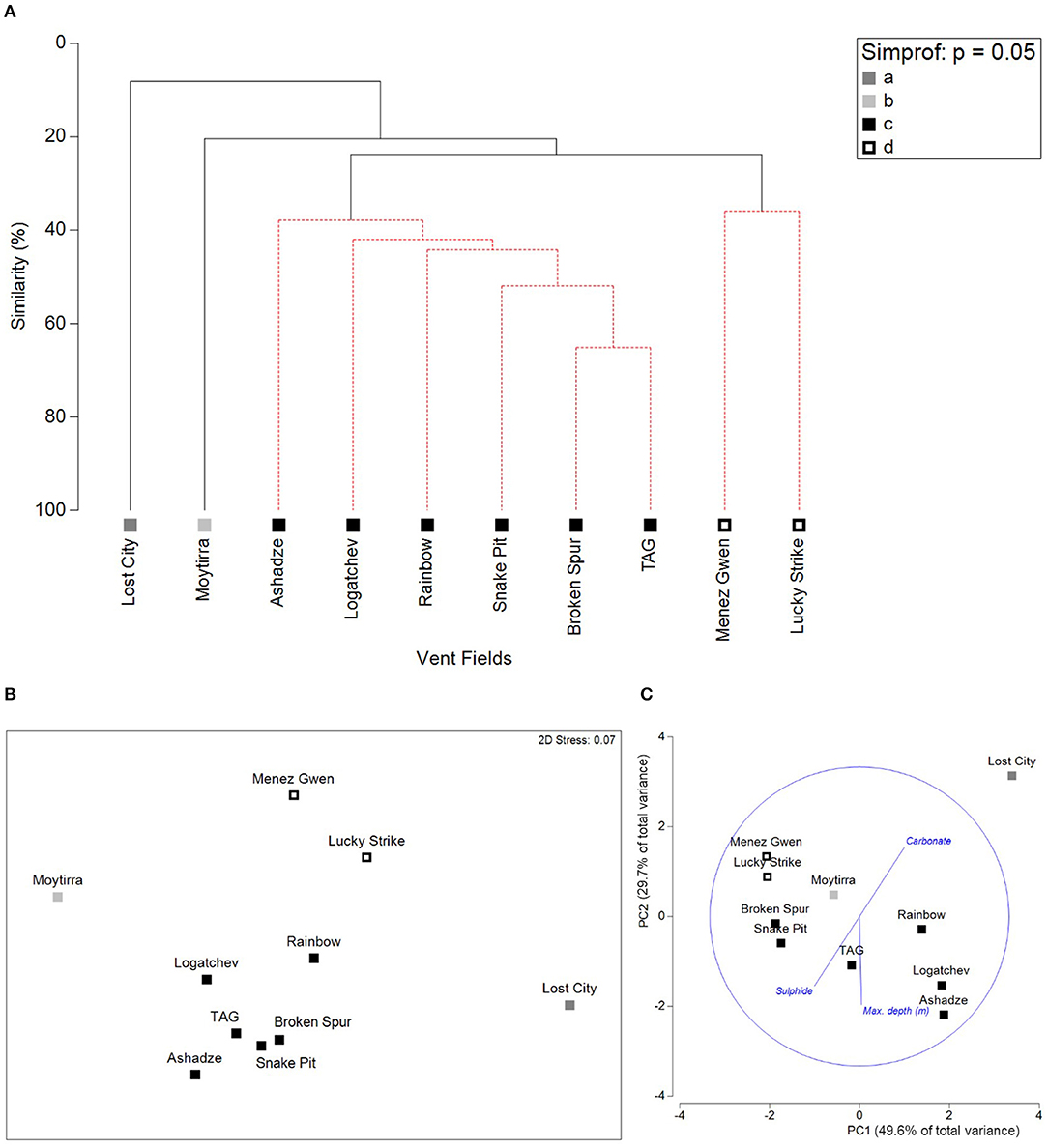
Figure 3. (A) CLUSTER diagram of vent field similarity; (B) Non-metric Multidimensional Scaling (nMDS) plot of vent field similarity; (C) PCA of environmental variables, vectors displayed are the environmental variables (identified by the BEST test) with the strongest correlation with the presence-absence of taxa at the different vent fields.
From the SIMPER test, group d had an average similarity of 35.94%. Within group d, each of the 23 taxa present at both vent fields contributed equally (4.35%) to the similarity between vent fields (Supplementary Table 3), largely due to the use of presence-absence data and the existence of only two vent fields within this group. Group c had an average similarity of 43.93%. Within group c, only 10 taxa contributed more than 4% to the similarity amongst the six vent fields (Supplementary Table 4).
The clustering of nMAR hydrothermal vent fields by taxonomic composition was correlated with environmental variables. The BEST test with variables grouped by indicator determined that the SIMPROF clusters of vent fields had the strongest correlation with a combination of depth and chimney type (Rho = 0.767, p < 0.05). Inclusion of additional environmental variable groups, such as location, host rock or tectonic setting reduced the strength of correlation between the faunal presence-absence data and the environmental variables. Pearson correlation tests to check for confounding variables indicated that depth and latitude were strongly correlated (–0.69), with depth increasing with decreasing latitude, meaning that the influence of depth and latitude cannot be distinguished. However, including latitude in the model in addition to depth did not increase the explanatory power of the model. The PCA plot (Figure 3C) displays the three individual environmental variable vectors (maximum reported depth, carbonate chimneys, and sulphide chimneys) that contributed to the BEST test result with the strongest correlation with vent field taxa presence-absence data. Principle Component (PC) axes 1 and 2 explain 49.6 and 29.7% of the total variance respectively, explaining 79.3% of the total variance collectively.
Regional Diversity of Biological Communities at Hydrothermally Active Habitats and Hydrothermally Inactive Sulphide Habitats
Microorganisms
The local distribution patterns of methanotrophic and chemoautolithotrophic Archaea and Bacteria on the nMAR is linked to the chemistry of hydrothermal fluids, including the relative abundance of methane and sulphide, and the geological setting, such as basalt or ultramaphic rocks (Perner et al., 2007). On the nMAR, different archaeal and bacterial communities were associated with the different geological settings of ultramaphic-hosted Rainbow and basalt-hosted Lucky Strike vent fields (Flores et al., 2011). Comparisons between three ultramaphic-hosted vent fields (Rainbow, Ashadze-1, and Lost City) identified a diverse archaeal community heterogeneously distributed between the hydrothermal sites and types of samples analysed (seawater, hydrothermal fluid, chimney, and sediment) (Roussel et al., 2011). Of these sites, Lost City hosted a distinct microbial diversity, reflecting the highly alkaline warm fluids unique to this location (Roussel et al., 2011). Microbial mats from basalt-hosted Lucky Strike hydrothermal vent field had low diversity of archaeal genetic sequences, compared to ultramaphic-hosted sites, with just a single archaeal group (Crepeau et al., 2011). Conversely, Lucky Strike microbial mats have a diverse and active chemoautolithotrophic bacterial community, including methanotrophic and thiotrophic symbionts associated with bathymodiolid vent mussels (Crepeau et al., 2011).
Some protist lineages appear to occur in hydrothermal areas in multiple oceans, however some lineages have only been detected in the Atlantic (Lopez-Garcia et al., 2003). Microcoloniser experimental systems deployed on the hydrothermally active Eiffel Tower chimney in Lucky Strike indicated that bodonid and ciliate protists may be pioneers in the colonisation process of bare hydrothermally active substrata (Lopez-Garcia et al., 2003). There are no studies characterising the microbial communities of inactive sulphide habitats on the nMAR.
Benthic Invertebrates
According to the literature reviewed, the relative abundance of different megafaunal taxa varies between nMAR vent fields, with differences in abundance potentially being linked to depth or latitude. Starting at Moytirra the dominant megafaunal species in high temperature fluid areas are Peltospira sp. limpets and Mirocaris sp. shrimp, whilst bathymodiolid mussels are absent from the vent field (Wheeler et al., 2013). Moving south, as summarised by Rybakova and Galkin (2015), Menez Gwen and Lucky Strike are dominated by the vent shrimp Mirocaris fortunata in high temperature (20–40°C) habitats, with the vent mussel Bathymodiolus azoricus dominant in lower temperature (2–20°C) habitats. Continuing south and into deeper water, high temperature habitats at Rainbow, Broken Spur, TAG, Snake Pit, and Logatchev are not dominated by M. fortunata, as observed for more northerly sites; instead, these vent fields are dominated by the shrimp Rimicaris exoculata (Van Dover et al., 1988a; Copley et al., 2007; Rybakova and Galkin, 2015). Low temperature habitats at Rainbow remain dominated by B. azoricus, but there is a switch in the dominant mussel species to Bathymodiolus puteoserpentis at Broken Spur, through Snake Pit, and Logatchev (Rybakova and Galkin, 2015). Broken Spur represents a hybridisation zone between B. azoricus and B. puteoserpentis, with both species present and apparently able to interbreed (O'Mullan et al., 2001). The hydrothermal vent fauna of Lost City is sparse, comprising of a few “extremely rare” B. azoricus mussels (Kelley and Shank, 2010). Bathymodiolid mussels are absent from TAG vent field, with low temperature habitats dominated by the sea anemone Maractis rimicarivora, and on hydrothermal sediments, the chaetopterid polychaete Phyllochaetopterus polus (Galkin and Moskalev, 1990; Van Dover, 1995; Morineaux et al., 2010; Fabri et al., 2011). Moving to the most southern vent field on the nMAR, Ashadze-1, bathymodiolid mussels are also absent and the hydrothermally active hard substrata are dominated by M. rimicarivora (Fabri et al., 2011). Vent shrimp are also present at Ashadze-1, with M. fortunata having the highest abundance (Fabri et al., 2011). Illustrative examples of the biological communities at nMAR hydrothermally active habitats are provided in Figure 4.
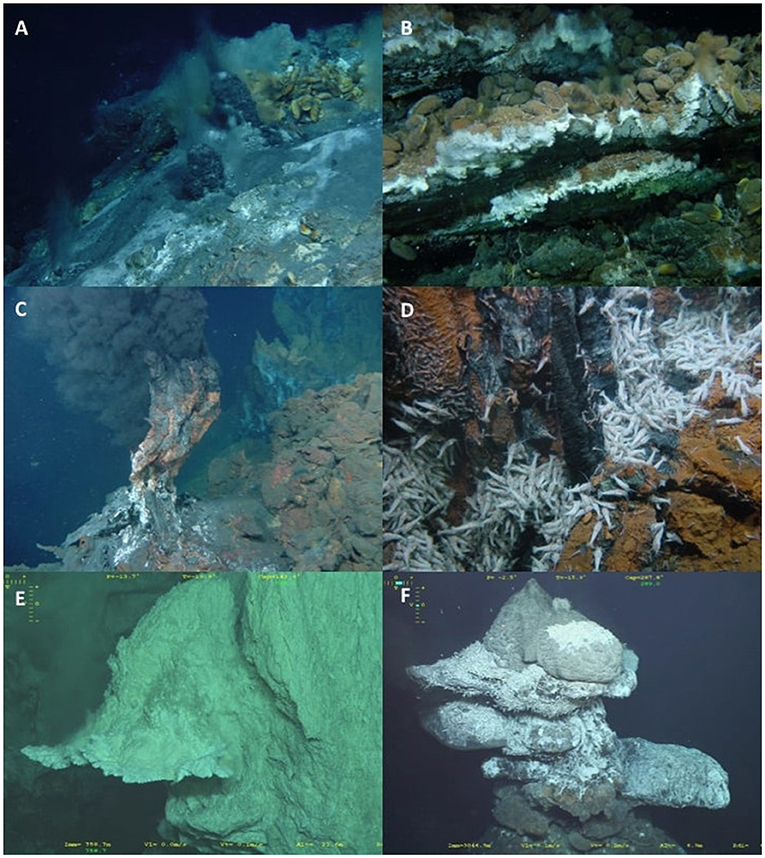
Figure 4. (A) Menez Gwen hydrothermal vent field; (B) Lucky Strike hydrothermal vent field with Bathymodiolus azoricus mussel beds; (C,D) Rainbow vent field with swarms of the vent shrimp Rimicaris exoculata; (E) Carbonate chimney flange at Lost City vent field; (F) Chimney at the Broken Spur vent field with swarms of R. exoculata. Images (A–D) reproduced with permission: ©SEAHMA – PDCTM/MAR/15281/1999. Images (E,F) reproduced with permission: ©Ifremer – Mission Transect (Le Bris, 2018).
From the quantitative studies conducted on meiofauna at hydrothermally active habitats on the nMAR, meiofaunal diversity appears to be low, with 15 meiofauna species found associated with B. puteoserpentis mussel beds at Snake Pit (Zekely et al., 2006a) and 25 meiofaunal taxa identified during a 2-year colonisation experiment on B. azoricus mussel beds at Lucky Strike (Cuvelier et al., 2014). At Snake Pit, metazoan meiofauna consisted of seven nematode species (63% of sampled individuals), six copepod species (35% of sampled individuals), one ostracod species, and one mite species (Zekely et al., 2006a). Meiofaunal taxa at Lucky Strike also included nematodes, copepods, and a mite species, with nematodes and copepods being the most and second-most dominant species, respectively (Cuvelier et al., 2014). Tchesunov (2015) determined that nematodes were the most abundant (700 individuals) and diverse (16 morpho-species) at Lucky Strike, whilst Lost City had the lowest abundance (172 individuals) but similar diversity (14 morpho-species) of nematodes. Sites further North at Menez Gwen, and further South at Snake Pit, were characterised by low diversity with high dominance of single species (Zekely et al., 2006b; Tchesunov, 2015).
The most common copepods recorded from hydrothermally active habitats on the nMAR are dirivultid copepods, a family of copepods which occurs almost exclusively at hydrothermal vents (Gollner et al., 2010a). The majority of the 12 dirivultid species recorded from MAR vents only occur along the MAR, although four species have been recorded from at least one Pacific Ocean region (Gollner et al., 2010a). Harpacticoid copepod species have also been recorded from the nMAR, including from B. azoricus mussel beds at Lucky Strike (Ivanenko and Defaye, 2004; Ivanenko et al., 2012; Cuvelier et al., 2014) and from Snake Pit and Lucky Strike (Zekely et al., 2006a; Gollner et al., 2010b; Cuvelier et al., 2014).
For most hydrothermally inactive sulphide occurrences reported along the nMAR, there are no descriptions of associated biological communities (Reviewed by Krasnov et al., 1995; Lalou et al., 1998; Gablina et al., 2012; Bel'tenev et al., 2017; Van Dover, 2019; Rona et al., 1993). Small patches of inactive sulphide substratum on the Eiffel Tower edifice, Lucky Strike, were colonised by scavenging bythorgraeid crabs and hydroids, as identified from imagery surveys (Cuvelier et al., 2009). A similar occurrence of mobile scavengers, including bythograeid crabs, was reported from the inactive sulphide edifice Mag Mell in the Moytirra vent field (Wheeler et al., 2013). Preliminary data from biological collections made along the MAR between 12°58′N and 13°31′N using geological sampling gear suggested that fauna is scarce at inactive sulphide habitats (Molodtsova et al., 2014). The benthic invertebrates collected were mostly represented by sessile suspension feeders, such as Porifera (sponges), Alcyonacea (soft corals), Antipatharia (black corals), Hydrozoa (hydroids), Cirripedia (barnacles), and Bryozoa (bryozoans) (Molodtsova et al., 2014). Some of the species collected by Molodtsova et al. (2014), such as the black coral Alternatipathes alternata, golden coral Metallogorgia melanotrichos, and its associated basket star Ophiocreas oedipus, are widely distributed, lower bathyal species. According to Molodtsova et al. (2014), there did not appear to be any benthic invertebrate species endemic to hydrothermally inactive sulphide habitats. Preliminary studies from within the Russian Exploration Area along the MAR indicated that seafloor massive sulphide outcrops were characterised by low densities of megafauna, whereas non-hydrothermal rocky habitats were colonised by assemblages of larger suspension feeders, especially at elevated locations (Galkin et al., 2019).
Benthic and Benthopelagic Fishes
There is limited information available on the benthic and benthopelagic fishes at hydrothermally active habitats or inactive sulphide habitats along the nMAR. Ten species of fish were recorded from hydrothermally active habitats in Desbruyères et al. (2006) (Table 3). Four of these species were considered endemic to hydrothermally active areas, whilst the remaining six species were known from other habitats in the North Atlantic or from other oceans.
Almost nothing is known about the benthic and benthopelagic fishes occurring at inactive sulphide habitats on the nMAR, with only one record of a Pachycara sp. zoarcid fish observed at the hydrothermally inactive chimneys of Mag Mell, an edifice in the Moytirra vent field (Wheeler et al., 2013). These mobile predators were also recorded elsewhere within the vent field and were not considered endemic to inactive sulphide habitats.
Ecosystem Function
Vent fields on the nMAR with different geochemistry host functionally different microbial communities, for example, the absence of methanogens at certain sites (Flores et al., 2011). So far, only the following vent fields have been studied for microbial function - Logatchev, Rainbow, TAG, and Snake Pit. At the Irina II site within Logatchev vent field, two important groups of primary producers, Epsilonproteobacteria and Gammaproteobacteria, were discovered to use different pathways for sulphur oxidation and carbon fixation (Hügler et al., 2010). A range of sulphate-reducing prokaryotes were also identified from the Irina II site, including Deltaproteobacteria, Thermodesulfobacteria, and Archaeoglobales. The latter two prokaryotes are thermophyllic and hyperthermophyllic, respectively and are thought to inhabit the higher temperature environment of the seafloor subsurface, being subsequently carried to the seafloor within vent fluids (Hügler et al., 2010). Chemolithoautotrophic iron oxidising Zetaproteobacteria are thought to play an essential role in the global iron cycle and have been identified from Rainbow, TAG, and Snake Pit vent fields (Scott et al., 2015). Zetaproteobacteria at these sites are rare if an iron source is not readily available, suggesting that this organism is likely locally restricted to iron-rich marine environments but may exhibit wide-scale geographic distribution (Scott et al., 2015). Zetaproteobacteria have also been reported in association with the gill chamber of the hydrothermal vent shrimp Rimicaris exoculata from the Rainbow vent field (Zbinden et al., 2004; Jan et al., 2014).
There is no published information available on the ecosystem function of microbial communities colonising hydrothermally inactive sulphide habitats along the nMAR. Other than trophic interactions (see section Trophic Relationships), the functional roles of benthic invertebrates and fishes found at hydrothermally active habitats or inactive sulphide habitats on the nMAR are also unknown.
Trophic Relationships
According to Polar Lipid Fatty Acid (PLFA) profiles, benthic invertebrate species at nMAR hydrothermally active habitats can be divided into two feeding groups: the first group, consisting of mussels, commensal polychaetes, and gastropods, is more dependent on sulphur-oxidising bacteria; the second group, comprising shrimps and crabs, has more anaerobic sulphate-reducing bacteria biomarkers (Colaço et al., 2007). Other benthic invertebrate species fall between the two groups, presenting a mixed feeding strategy that includes feeding on bacteria in addition to other types of food. Of the benthic invertebrates studied, the ampharetid polychaete Amathys lutzi had the most diversified bacterial diet, presenting fatty acid biomarkers from both S-oxidising and S-reducing bacteria (Colaço et al., 2007).
The complex trophic linkages between microbial communities and the fauna at nMAR hydrothermally active habitats were initially determined using the isotopic composition of 24 invertebrate taxa, bacterial mats, and filamentous bacteria from seven vent fields (Menez Gwen, Lucky Strike, Rainbow, Broken Spur, TAG, Snake Pit, and Logatchev) (Colaço et al., 2002). As is typical for hydrothermal vent ecosystems, chemolithotrophic bacteria (both symbiotic and free-living) formed the basis of the food web at the nMAR vent fields. These bacteria support primary consumers through symbioses with nMAR benthic invertebrates or through the grazing of free-living bacteria in warmer vent habitats. Well-documented examples of these symbiotic relationships include the nMAR vent mussels Bathymodiolus puteoserpentis and B. azoricus, which harbour both sulphuroxidising and methane-oxidising bacteria in specialised organs, bacteriocytes, within their gill tissue (Fiala-Médioni et al., 2002; Duperron et al., 2016); and the nMAR vent shrimp Rimicaris exoculata, which harbours two different bacterial epibionts on specialised appendages and on the inner surface of its gill chamber (Petersen et al., 2010). Rimicaris exoculata also benefits from free-living bacteria, which it grazes from the warmer vent habitats (Colaço et al., 2002). The most recently proposed symbiosis on the nMAR occurs with a new species of nematode described from Lucky Strike, Oncholaimus dyvae, which may harbour symbiotic sulphur-oxidising bacteria (Bellec et al., 2018; Zeppilli et al., 2019).
According to stable isotope ratios, many benthic invertebrates at the Menez Gwen, Lucky Strike, Rainbow, Broken Spur, TAG, Snake Pit, and Logatchev vent fields have mixed feeding strategies (Colaço et al., 2002; De Busserolles et al., 2009; Portail et al., 2018). The initial food web description for nMAR hydrothermally active habitats provided by Colaço et al. (2002) indicates that small invertebrates living within the byssus threads of bathymodiolid mussel colonies feed on bacteria or detritus trapped within the byssus net. The shrimp Rimicaris chacei and Mirocaris fortunata are mixotrophic, feeding on bacteria, shrimps, and other small invertebrates. Other shrimp species are predatory, such as Alvinocaris markensis, which consumes other shrimps. The main predators that are restricted to nMAR hydrothermally active habitats include the gastropod snail Phymorhynchus sp., which feeds almost exclusively on the bathymodiolid mussels, and the crab Segonzacia mesatlantica, which eats shrimps and small invertebrates. The top predators consist of common bathyal species, such as the crab Chaceon affinis and some fishes, which make incursions into nMAR vent fields to feed, particularly at shallower sites where the phase separation of fluids and depletion in metals makes these environments less inhospitable to non-vent predators (Colaço et al., 2002).
Stable isotope ratios have also been measured for the four visually most-dominant species of benthic invertebrates at Ashadze-1 vent field: the gastropod snail Phymorhynchus moskalevi, sea anemone Maractis rimicarivora, shrimp Mirocaris fortunata, and chaetopterid polychaete worm Phyllochaetopterus polus (Fabri et al., 2011). All four species were discovered to be heterotrophic, with comparisons between the of δ13C values from Ashadze-1 and other vent fields along the nMAR suggesting that they have the same food source at all vent sites (Fabri et al., 2011). The generally high δ15N values for these four species indicated that their nitrogen source came from recycled organic matter, supporting the concept that these species are all secondary consumers in the Ashadze-1 ecosystem (Fabri et al., 2011). Based on the mean δ34S values for these species, it was considered that the Ashadze-1 food web is based on locally produced organic matter, such as vent sulphides, pore-water sulphides, and thiosulphate (Fabri et al., 2011). The Sulphur isotopic values and high trophic level of the four species investigated were considered evidence for the existence of a chemosynthetic ecosystem at Ashadze-1, despite the visual absence of species known to exhibit chemosynthetic symbiosis during the survey (Fabri et al., 2011).
The food webs of hydrothermal vent fields along the nMAR can also exhibit intra- and inter-field variation. Whilst the dominant basal organic matter sources of food webs at Menez Gwen, Lucky Strike and Rainbow vent fields are similar, there are intra- and inter-field variations in their relative contributions, relating to the availability of reduced compounds to support methanotrophy and chemoautotrophy (Portail et al., 2018). The contribution of photosynthetic-derived organic matter was negligible in all three of these vent fields, irrespective of vent field depth. All the species shared amongst sites exhibited high trophic flexibility, suggesting that differences in the basal organic matter source amongst sites may not be a structuring factor (Portail et al., 2018). Despite variation in environmental conditions and community structure, functional structure was similar across assemblages, and was thought to reflect both the trophic flexibility of vent heterotrophs and the ability of vent fauna to adapt to several geological contexts (Portail et al., 2018).
Smaller scale variation in trophic relationships can also occur at hydrothermally active habitats on the nMAR, as described for the Eiffel Tower edifice within the Lucky Strike vent field (De Busserolles et al., 2009). Bathymodiolus azoricus mussel bed communities were sampled from 12 different locations on the Eiffel Tower edifice, with three species (A. lutzi, B. azoricus and its associated polychaete Bathynoe seepensis) exhibiting significant spatial variability in isotopic signatures. Environmental conditions were at least partially responsible for this variability, with vent fluid characteristics thought to be key factors in the variation of local carbon sources, through influences on microbial production (De Busserolles et al., 2009). In support of previous work conducted at Lucky Strike (Colaço et al., 2002, 2007), De Busserolles et al. (2009) also found two apparently independent trophic groups, the symbiont-bearing fauna (B. azoricus and associated polychaete Bathynoe seepensis), and the heterotrophic fauna (bacterivores, detritivores, scavengers, and predators).
The feeding guilds of meiofaunal taxa collected from Snake Pit were investigated by Zekely et al. (2006a) who determined that primary consumers, mostly deposit feeders, comprised more than 95% of the total meiobenthos at the site, followed by parasitic copepods and mites. All the nematodes and 80% of copepod individuals were primary consumers, with 20% of the copepod community being parasitic. Meiofaunal predators were absent in all samples collected from Snake Pit (Zekely et al., 2006a). Three meiofaunal taxa were also included within the work of Portail et al. (2018), where the isotopic signatures of suspension-feeding ostracods were consistent with bacteriovores or detritivores/scavengers, and the isotopic signatures of deposit-feeding Aphotopontius sp. copepods were consistent with bacteriovores or commensals. The deposit feeding Oncholaimus dyvae nematodes were either detritivore/scavengers or predators according to their stable isotope signatures (Portail et al., 2018).
The non-vent endemic benthopelagic fauna of the Broken Spur vent field exhibited isotopic signatures closer to the chemosynthetic vent fauna than might be expected (Vereshchaka et al., 2000), which was interpreted to indicate some degree of coupling between benthopelagic shrimp and fish and the chemosynthetic communities more typical of hydrothermally active habitats. The trophic structure of the biota colonising inactive sulphide habitats along the nMAR is unknown, largely because the biota of these habitats has not yet been characterised.
Connectivity
Hydrothermal vents along the nMAR can exist in a steppingstone manner along the ridge, with vent fields separated by distances of a few to hundreds of km distance (Murton et al., 1994; German et al., 1996; Beaulieu and Szafranski, 2020). The steppingstone model of connectivity has been suggested for Bathymodiolus mussels along the MAR, where mussel populations are contemporarily isolated and population connectivity can only be maintained in a stepwise manner (Breusing et al., 2016). It has been further suggested that, despite a long pelagic larval duration for Bathymodiolus spp. (Arellano and Young, 2009), the connectivity patterns observed on the MAR would indicate the existence of additional, yet uncharacterised (“phantom”), steppingstones to maintain the isolated populations (Breusing et al., 2017).
Bathymodiolus species on the MAR also exhibit a mid-ocean hybridisation zone, with B. azoricus occurring at the shallower (850–2,251 m) northern localities, B. puteoserpentis occurring at the deeper (3,080–3,650 m) southern localities (Maas et al., 1999), and both species occurring and hybridising at an intermediate latitude (O'Mullan et al., 2001). Examination of DNA sequences from seven nuclear loci and a mitochondrial locus suggests that these closely related species may have split <1 million years ago (Faure et al., 2009), with the genetically mixed population at intermediate latitudes providing no evidence for hybrid incompatibilities (Breusing et al., 2017).
Other species at hydrothermally active habitats on the nMAR have population connectivity patterns more akin to panmixia, with high rates of gene flow and limited evidence of population genetic structure. The shrimp R. exoculata exhibits extraordinarily high rates of gene flow along the MAR (Teixeira et al., 2010), thought to result from the life history traits of this species, including the production of large lecithotrophic eggs and planktotrophic larvae that feed in the photic zone before settling as juveniles (Dixon and Dixon, 1996). Shinkailepas briandi limpets are common in several vent fields on the nMAR, including Menez Gwen and Ashadze-1, with this species exhibiting panmixia throughout its geographic and bathymetric distribution (Yahagi et al., 2019). From the retention of eye pigmentation in newly settled S. briandi juveniles, along with genetic panmixia, it has been suggested that the hatched larvae of S. briandi vertically migrate to the surface waters to take advantage of richer food supplies and stronger currents for dispersal (Yahagi et al., 2019).
Some species on the nMAR are found both at hydrothermally active habitats and at other chemosynthetic habitats in the North Atlantic and further afield. The clam Abyssogena southwardae has been recorded from Logatchev on the nMAR, Clueless vent field on the southern MAR (north and south of the Romanche Fracture Zone, respectively), and from cold seep sites on the West Florida Escarpment, the Barbados Accretionary Prism, and the Lobes of the Congo (Krylova et al., 2010; LaBella et al., 2017). The A. southwardae populations on the MAR were included within the same lineage as the West Florida Escarpment, Barbados Accretionary Prism, and Lobes of the Congo populations, whilst an additional lineage was restricted to the Lobes of the Congo population (LaBella et al., 2017). Haplotypic evidence suggests that there is no major hydrographic barrier for A. southwardae populations north and south of the Romanche Fracture Zone (Van der Heijden et al., 2012; LaBella et al., 2017), with multiple lines of genetic evidence supporting connectivity between the Logatchev, Clueless, and Barbados Accretionary Prism populations of A. southwardae (LaBella et al., 2017).
It has been suggested that some nMAR meiofaunal taxa, such as dirivultid copepods, may have high dispersal potential and thus population connectivity (Gollner et al., 2011). Further research confirmed high genetic connectivity of copepods at hydrothermally active habitats and highlighted the high diversity and expansive population growth of this group (Gollner et al., 2016). Connectivity between hydrothermally active habitats for these copepods may be further facilitated using intermediate non-hydrothermal chemosynthetic habitats as steppingstones (Gollner et al., 2016), a concept which has also been proposed for bathymodiolid mussels (Breusing et al., 2016) and the clam A. southwardae (LaBella et al., 2017).
Little is known about the population connectivity of symbiotic bacteria at hydrothermally active habitats on the nMAR. The two dominant thiotroph- and methanotroph-related symbionts found in B. azoricus and B. puteoserpentis mussels collected from vent sites along the MAR shared highly similar or identical 16S rRNA phylotypes (Duperron et al., 2006), suggesting that populations of symbionts may show different connectivity patterns from their invertebrate hosts. Full population genetics studies of nMAR symbionts have not yet been undertaken. There are no studies on the connectivity of biota colonising inactive sulphide habitats along the nMAR.
Temporal Variability
Temporal variability in biological communities at hydrothermally active habitats along the nMAR has been assessed using a variety of methods. These include repeat surveys of the same site over multiple months or years (Copley et al., 2007; Cuvelier et al., 2011b); fixed-term deployment of in-situ monitoring equipment, such as the TEMPO module deployed at Lucky Strike (Sarrazin et al., 2014); and long-term monitoring through fixed observatories, such as the EMSO-Azores non-cabled seafloor multidisciplinary observatory, also at Lucky Strike.
Some nMAR microbial communities demonstrate potential for rapid colonisation of new suitable substrata, as observed in the Snake Pit vent field where deployment of an in-situ growth chamber (vent cap) resulted in the formation of a white microbial mat following only 5 days of deployment (Reysenbach et al., 2000). This new microbial community contained a mix of hyperthermophiles, moderate thermophiles, and mesophiles, reflecting the gradual reduction in temperature within the chamber (70–20°C) during the 5 days of deployment (Reysenbach et al., 2000). Microbial communities on the nMAR can also respond to episodic events, such as the natural de-gassing events between 2009 and 2010 at the Eiffel Tower site, Lucky Strike, which promoted the development of thermophilic/anaerobic Archaea and Bacteria (Archaeoglobales, Nautiliales, Nitratiruptoraceae) (Rommevaux et al., 2019). The periodicity of natural de-gassing events at Lucky Strike is not known; the frequency of this disturbance and related shift in microbial community is equally unknown. However, these studies demonstrate that microbial communities at high temperature hydrothermally active habitats on the nMAR can respond (at a community level) to rapid changes in environmental parameters, including episodic events.
Shifts in archaeal and bacterial communities at the nMAR Lost City vent field can occur in response to environmental changes over 1,000-year timescales (Brazelton et al., 2009). Lost City presents a different environment for microbial communities, with its carbonate chimneys forming through highly alkaline vent fluid, in contrast to the acidic vent fluids occurring at all other known vent fields along the MAR. “Rare” members of the Lost City microbial community can become dominant when environmental conditions change, on 1,000-year timescales, with the long history of chimney growth cycles at Lost City resulting in numerous closely related microbial “species,” each preadapted to a particular set of reoccurring environmental conditions (Brazelton et al., 2009).
There is evidence from Lucky Strike vent field that relatively diverse assemblages of macrofauna and meiofauna (20 and 25 taxa, respectively) can inhabit newly-available adjacent substrata within a short time (2 years) (Cuvelier et al., 2014). Temporal variation of these groups at shorter or longer time periods is unknown.
The nMAR benthic invertebrate megafauna demonstrates multiple scales of temporal variability, including changes in feeding behaviour corresponding to semi-diurnal tidal cycles (Cuvelier et al., 2017), and seasonality in reproduction for some species (Colaço et al., 2006; Dixon et al., 2006). Different benthic invertebrates may demonstrate different patterns in temporal variation, for example, B. azoricus mussels from Menez Gwen vent field demonstrate seasonal patterns in reproduction linked to the timing of the winter-spring phytoplankton bloom (Dixon et al., 2006; Colaço et al., 2009), whereas there was no evidence for seasonal reproduction in R. exoculata shrimp from TAG vent field (Copley et al., 2007).
Some nMAR benthic invertebrate communities at hydrothermally active habitats demonstrate considerable stability, over multiple time scales. Daily observations of B. azoricus from Lucky Strike during a 48-day period demonstrated the stability of vent mussel assemblages during this time, reflecting the relative stability of environmental conditions (Sarrazin et al., 2014). Although tidal signals could be detected at this site, there was no evidence that the vent mussels, shrimp, crabs, or bucciniform gastropods responded to tidal periodicity. However, polynoid worms did exhibit significant multi-day periodicities, suggesting that the harmonics of tidal cycles may influence their distribution (Cuvelier et al., 2017). Over intermediate time periods at Lucky Strike, only small changes in B. azoricus coverage and density were observed, with an 11% increase in mussel densities between 2006 and 2008 (Sarrazin et al., 2014). Stable patterns of the vent shrimp M. fortunata and crab Segonzacia mesatlantica were also observed at Lucky Strike over a 9-month period (Matabos et al., 2015).
Northern MAR benthic invertebrate communities can also demonstrate stability over multiple years, even decadal periods (Copley et al., 2007; Cuvelier et al., 2011b). Vent shrimp populations at Broken Spur were stable over a 15-month period (Copley et al., 1997), whilst mature vent communities at TAG (Copley et al., 2007) and Lucky Strike (Cuvelier et al., 2011b) demonstrated decadal stability in the abundance of dominant species at the edifice scale. Within the Eiffel Tower edifice at Lucky Strike, significant differences in assemblage coverage and distribution were linked to localised fluctuations in hydrothermal activity (Cuvelier et al., 2011a). Within the Logatchev vent field, significant changes in the population density of predatory gastropods Phymorchynchus spp. and the disappearance of a live vesicomyid clam population over a 10-year period were similarly linked to fluctuations in hydrothermal activity (Gebruk et al., 2010).
There is also some evidence for changes in local biological assemblages linked to alterations in the hydrothermal activity of habitats patches on the nMAR. Changes in the coverage of hydrothermally inactive sulphide habitats patches (“Substratum 1a”: Cuvelier et al., 2009) on the Eiffel Tower edifice within Lucky Strike over a 14-year period were accompanied by increasing colonisation of these patches by B. azoricus mussels, consistent with hydrothermal fluid flux increase (Cuvelier et al., 2011b). There are no studies on the nMAR specifically assessing the temporal variability of biota at inactive sulphide habitats.
Resilience and Recovery
On the nMAR, high-intensity illumination associated with Human-Occupied Vehicles (HOVs) and Remotely Operated Vehicles (ROVs) has been implicated in photoreceptor damage in Rimicaris exoculata shrimp that aggregate at high-temperature hydrothermal vents (Van Dover, 2014). The visual pigment (rhodopsin) of the thoracic eyes in adult R. exoculata is sensitive to light, with studies demonstrating that it degrades rapidly with light exposure (Johnson et al., 1995; Herring et al., 1999). The thoracic eyes of R. exoculata are thought to be an adaptation for detecting the dim light generated by high-temperature venting as a near-field remote-sensing means of avoiding thermal stress and mortality (Van Dover et al., 1989). Behavioural consequences of damage to photoreceptors in vent shrimp are unknown, although there is no evidence for negative impacts on shrimp populations repeatedly exposed to high-intensity illumination (Copley et al., 2007).
Based on physical and genetic studies, the recolonisation of disturbed sites by Bathymodiolus mussels (foundation species where they are present on the MAR) is only expected to occur by chance (Breusing et al., 2017), indicating that populations of these species have a low resilience to impact. A recent small scale disturbance experiment assessing recovery after an artificial disturbance inside the Lucky Strike hydrothermal vent field demonstrated that after 2 years there was a major change in faunal composition, only a partial recovery of faunal densities, and that the species composition of the neighbouring faunal assemblages strongly affects the species composition of the recolonising community (Marticorena et al., 2021).
Discussion
Species List for Benthic Invertebrates at Hydrothermally Active Habitats
The global taxa list for hydrothermal vent invertebrates presented by Desbruyères et al. (2006) included 100 benthic invertebrate taxa sampled from the MAR, representing nearly 20% of the global hydrothermal vent fauna diversity known at that time. Most benthic invertebrate taxa listed by Desbruyères et al. (2006) were megafauna and macrofauna, reflecting that many studies have focused on megafauna, with few studies on macrofauna and even fewer on meiofauna. The megafauna is the only group that can be readily studied through imagery, however abundances of these taxa generated from imagery are often lower compared to abundances generated from physical samples (Cuvelier et al., 2012). Physical samples are needed to fully characterise nMAR communities, especially for macrofaunal taxa such as smaller polychaetes and gastropods, pycnogonids, ostracods, actinids, halacarids, nematodes, copepods, tanaids, and amphipods (Cuvelier et al., 2012).
Further survey and research efforts along the nMAR have discovered new species, including smaller meiofaunal species, which do not feature in the Desbruyères et al. (2006) taxa list. The updated taxa list presented here features 158 taxa and is expected to be refined following ongoing scientific expeditions to the nMAR. The updated list includes a greater number of recorded taxa at nMAR vent fields than was reported previously (Fabri et al., 2011; Sun et al., 2020), reflecting ongoing species discovery in the region. The most northern, shallowest nMAR vent fields (Menez Gwen, Lucky Strike, Rainbow) were reported by Fabri et al. (2011) as having the highest species richness, followed by Snake Pit. This general pattern also occurs in the present analysis, although the deeper Snake Pit vent field is the second most taxonomically diverse, after Lucky Strike, and before Menez Gwen and Rainbow. As in Fabri et al. (2011), the deeper more southerly vent fields (Ashadze-1, Logatchev, TAG, and Broken Spur) all have lower taxonomic diversity than the more northerly, shallower vent fields. Lost City has very low taxonomic diversity in both studies. The vent field with the lowest taxonomic diversity in the present study was Moytirra, which was discovered after Fabri et al. (2011) was published.
It is possible that the apparent trends in diversity may be linked to differences in sampling effort amongst sites. However, given that similar patterns in diversity have been observed in the present study and Fabri et al. (2011), despite the present study having almost twice the number of taxa compared to Fabri et al. (2011) (158 and 88 taxa, respectively), the observed differences in taxonomic diversity may represent true trends in diversity distribution along the nMAR. Further targeted sampling programs across vent fields, using standard methods and spanning whole communities (microbes to megafauna), would be needed to confirm this apparent trend in diversity distribution along the nMAR.
Regional Biogeographic Analysis for Hydrothermally Active Vent Fields
Apart from the earlier work of Desbruyères et al. (2000, 2001), there have been few studies on the regional differences in vent fauna along the nMAR. Global biogeographic analyses have identified the nMAR as a separate biogeographic province (Bachraty et al., 2009; Moalic et al., 2012). Some analyses have further divided this province into two additional provinces, with the boundary between the north and south nMAR provinces occurring just south of the Azores (Van Dover et al., 2002). Various statistical methods have been used in previous studies to compare vent communities between segments on the same ridge (Gebruk et al., 1997; Van Dover et al., 2002; Tsurumi and Tunnicliffe, 2003) however, these studies were rarely able to include presence-absence data for taxa from all vent fields in the region because the available faunal data were insufficient to be used in statistical or biological and ecological studies (Van Dover, 1995; Jollivet, 1996; Gebruk et al., 1997; Tsurumi and Tunnicliffe, 2003).
The analysis in the present study is the first quantitative regional (meso-scale) biogeography analysis to include presence-absence data for taxa at the recently discovered Moytirra vent field (Wheeler et al., 2013) and the unusual geochemical environment occurring at Lost City. These multivariate results suggest that, based on presence-absence data, the regional biogeography of benthic invertebrate vent fauna on the nMAR is structured by depth/latitude and chimney type (sulphide or carbonate), with the shallower vent fields closer to the Azores (Menez Gwen and Lucky Strike) having a different taxonomic composition from those occurring at greater depths further south (Ashadze-1, Logatchev, Snake Pit, Broken Spur, TAG, and Rainbow). These results are similar to those obtained by Van Dover et al. (2002) and Fabri et al. (2011), where vent fields on the nMAR also formed two groups: the shallower vent fields close to the Azores and the deeper vent fields further south. As for these previous studies, our analysis was unable to separate the covarying effects of latitude and depth in influencing regional biogeography. Our results differ from Fabri et al. (2011) in the clustering of Rainbow vent field (2,300 m depth) with the deeper vent fields of Broken Spur, Snake Pit, TAG, Logatchev, and Ashadze-1 (3,050–4,200 m depth), instead of clustering with the shallower Menez Gwen and Lucky Strike vent fields (850–1,700 m depth).
Moytirra falls within a separate cluster, which may result from its higher latitude location, with the Azores potentially acting as a barrier to faunal dispersal for some species. The biological information available for Moytirra is less detailed, with only nine taxa reported from this vent field, which may contribute to it clustering separately. Lost City also forms its own cluster which, according to the environmental analyses results, is probably due to the different geochemical environment, with serpentinisation leading to hydrogen- and methane-rich vent fluids and the precipitation of its unique carbonate chimneys. Future analyses may be able to include biological information from additional nMAR vent fields, such as Pobeda-1, Irinovskoye, and Semyenov-2, when these have been better characterised through further survey efforts.
It is also important to note that in the present analysis, taxonomic similarity is not 100% within the same vent field cluster, i.e., not all taxa occur at each vent field. Vent fields within the same cluster are taxonomically more similar compared to vent fields in other clusters. However, vent fields within each cluster do not have exactly the same taxonomic composition, which is an important consideration for environmental management. Clustering of nMAR vent fields according to faunal presence-absence data provides useful information on the regional biogeography for these taxa, and in combination with environmental analyses, indicates the environmental variables that may be the cause of these clusters. However, presence-absence data do not offer any information on the dominance of species at different vent fields, only their occurrence at any given location. More detailed quantitative datasets would be needed to compare all vent fields based on their abundance-weighted community structure.
Biological Communities at Hydrothermally Active Habitats and Hydrothermally Inactive Sulphide Habitats and Key Knowledge Gaps
Despite more than three decades of scientific research, our detailed literature review of nMAR biological communities highlights that key knowledge gaps still need to be addressed. The most information is available on the biodiversity and distribution of nMAR hydrothermally active ecosystems, with considerably less known about the temporal variation, connectivity, ecosystem function (including trophic relationships), and resilience and recovery of these ecosystems. Almost nothing is known about the biological communities colonising inactive sulphide habitats on the nMAR. Inactive sulphide habitats are poorly characterised in most oceans (Van Dover, 2019), yet are considered to have specific environmental management needs (Van Dover et al., 2020). Here, we discuss the key knowledge gaps for nMAR hydrothermally active and inactive ecosystems to spur collaborative research in the region, and to support regional-scale environmental management of human activities on the nMAR, including future deep-sea mining.
Biodiversity and Distribution
Existing data on the biodiversity and distributions of biota at hydrothermally active habitats relate mainly to benthic invertebrate megafauna. Considerably less information is available for smaller organisms, such as the macrofauna, meiofauna, and microorganisms at these habitats. Some vent fields, such as Moytirra, have been less studied than other fields, such as Lucky Strike, with the consequence that their biological communities are not as comprehensively characterised. More detailed studies are needed, replicated across all vent fields with standard methods, to determine the regional biodiversity and distributions for the biota. This is particularly the case for smaller organisms not identifiable from imagery. Further visual surveys may confirm the occurrence of additional hydrothermal vent fields supporting biological communities, particularly where hydrothermal activity has been “inferred” on the nMAR from water column chemistry (Beaulieu and Szafranski, 2020). Continued survey and research efforts are also needed to review and update the species list for benthic and benthopelagic fishes occurring at hydrothermally active habitats and inactive sulphide habitats on the nMAR.
Little is known about the biota colonising inactive sulphide habitats on the nMAR. Historically, there are few records of benthic invertebrates colonising nMAR hydrothermally inactive sulphide habitats, although this apparent absence of biological communities may reflect the geological focus of earlier studies and not a true absence of biota at these habitats. Preliminary data from more recent studies suggest that fauna may be scarce at nMAR inactive sulphide habitats (Molodtsova et al., 2014; Galkin et al., 2019), although these results may in part reflect limitations of the sampling gear used. Of the organisms observed, most of the benthic invertebrates were suspension feeders (Molodtsova et al., 2014). Studies in other regions support these findings, for example, some inactive sulphide chimneys in the southwest Pacific were devoid of benthic invertebrate megafauna, although other chimneys supported elevated abundances (Boschen et al., 2016a). Suspension feeders also dominated the invertebrate assemblages occurring at inactive sulphide habitats in the southwest Pacific (Galkin, 1997; Collins et al., 2012; Sen et al., 2014; Boschen et al., 2016a) and Indian Ocean (Gerdes et al., 2019). There is currently no evidence for benthic invertebrate species that are endemic to the weathered sulphide environment on the nMAR. Invertebrate-symbiont associations, similar to those seen at hydrothermally active habitats, have not been reported from inactive sulphide habitats (Van Dover, 2019). Most benthic invertebrates observed at inactive sulphide habitats have also been recorded from non-hydrothermal hard substrata in the region, suggesting a broad regional distribution of taxa occurring at hydrothermally inactive sulphides (reviewed by Van Dover, 2019). Dedicated studies are needed to determine if benthic invertebrate assemblages colonising inactive sulphide habitats on the nMAR occur at other non-hydrothermal habitats in the wider region.
Hydrothermally active habitats on the nMAR support an array of microorganisms, including Archaea, Bacteria, and protozoa, which can occur as free-living on the substrata, within hydrothermal plumes, and in symbiosis with hydrothermal vent fauna (Dick, 2019). Studies on microorganisms colonising hydrothermally active habitats have focussed on free-living and symbiotic chemoautotrophic groups, generally at the site or vent field scale. Comprehensive regional analyses looking at trends in the biodiversity and distribution of microbial communities across all nMAR vent fields have not been reported. Archaea and some Bacteria may be thiotrophic (oxidises Sulphur compounds) or methanotrophic (oxidises methane) and potentially endemic to hydrothermally active habitats (either basalt or ultramaphic rocks) along the nMAR. Other microorganisms, such as protozoa, fungi, and some Bacteria, are heterotrophic (metabolise organic carbon sources) and may occur in other deep-sea habitats along the nMAR. Microscopic eukaryotes are particularly under-studied, although studies from other regions show these can be abundant at hydrothermally active habitats (Van Dover et al., 1988b; Brönnimann et al., 1989). The regional distribution of nMAR symbiotic bacteria is little known. Bacterial symbionts on the MAR do not always share the same regional distribution as their hosts, for example the genealogies of chemoautotrophic and methanotrophic symbionts of Bathymodiolus spp. located on the MAR north and south of the Equator were inconsistent, and different from their mussel hosts, indicating disconnected biogeography patterns (Van der Heijden et al., 2012).
There are no studies characterising the microbial communities of inactive sulphide habitats on the nMAR. The distribution patterns from other oceans (reviewed by Van Dover, 2019) could provide some insight into the nMAR. For example, bacterial density at inactive sulphide habitats along the Juan de Fuca Ridge decreases according to the following mineral sequence: elemental sulphur, chimney sulphide, marcasite, pyrite, sphalerite, chalcopyrite (Edwards et al., 2003). If the same pattern occurs along the nMAR, then this may impact the regional distribution of bacterial communities. It has been suggested that inactive sulphide habitats may host microorganisms not found elsewhere or only detectable in low numbers (Han et al., 2018); others suggest that microbial communities at inactive sulphides may be similar to groups common in other marine sediments (Meier et al., 2019). Dedicated studies are needed to characterise the microbial communities colonising inactive sulphide habitats on the nMAR, and to compare these with microbial communities colonising other habitats in the wider region.
In general, more detailed information is needed on the spatial distribution of species and communities colonising nMAR hydrothermally active habitats and inactive sulphide habitats. To get a complete picture of communities colonising these habitats, biodiversity characterisation needs to span microbes to larger benthic invertebrates and benthopelagic fishes, at suitable spatial scales and resolution. Fully characterising these communities over a wide area of the nMAR would enable quantitative regional biodiversity analyses to be conducted, alongside the identification of ecologically important locations that may require enhanced environmental management measures.
Ecosystem Function, Including Trophic Relationships
Ecosystem function has been defined in multiple ways. For the purpose of this review, ecosystem function was considered to be “the capacity of natural processes and components to provide goods and services that satisfy human needs, either directly or indirectly” (De Groot et al., 2002). Through this definition, ecosystem functions are a subset of ecological processes and ecosystem structures, with each function the result of the natural processes of the ecological system (De Groot et al., 2002). Ecosystem functions in this context include regulatory functions, habitat functions, production functions, and information functions (see Table 4). Regulatory and habitat functions are essential to the maintenance of natural processes and components, and to the delivery of production and information functions.
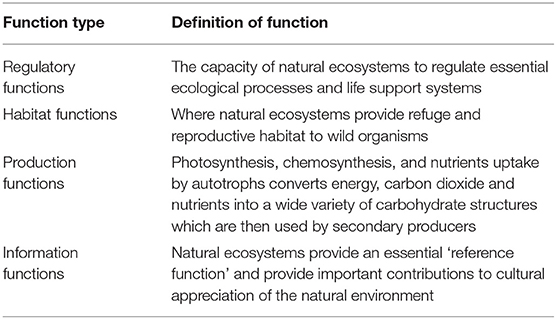
Table 4. Types of ecosystem functions as defined by De Groot et al. (2002).
The functions and services provided by the deep-sea environment are habitat or ecosystem dependent (Thurber et al., 2014; Le et al., 2017). In a general sense, deep-sea hydrothermally active habitats and hydrothermally inactive sulphide habitats provide all four of the ecosystem function groups, making particularly important contributions to the regulatory and habitat functions that are essential to maintaining biological communities at hydrothermally active habitats and inactive sulphide habitats (Turner et al., 2019). Hydrothermally active habitats provide key production functions, as these habitats are biologically more productive and generally support greater biomass relative to surrounding non-hydrothermal deep-sea habitats (Levin et al., 2016; Bell et al., 2017; Turner et al., 2019). Other production functions provided by hydrothermally active habitats include enhanced trophic and structural complexity, which provides the setting for complex trophic interactions (Colaço et al., 2007; Portail et al., 2018, Turner et al., 2019). Hydrothermally active habitats also provide regulatory functions through their role in global biogeochemical cycling and elemental transformation of carbon, sulphur, and nitrogen (Lilley et al., 1995; Petersen et al., 2011; Sievert and Vetriani, 2012).
Microorganisms play a particularly important role in both the regulatory and production functions of hydrothermally active habitats. Chemolithoautotrophic microorganisms use the energy released through the oxidation of reduced compounds within hydrothermal fluids for the fixation of inorganic carbon, thus mediating the transfer of energy from the geothermal source to higher trophic levels and so forming the basis of the unique food webs existing in these environments (Hügler et al., 2010). Microorganisms within hydrothermally active habitats perform numerous functions, including sulphur oxidation and sulphate reduction; ammonia and nitrite oxidation; nitrate reduction; hydrogen and methane oxidation; manganese oxidation; and iron II oxidation and reduction (Ding et al., 2017).
The functional roles of free-living microorganisms colonising hydrothermally active habitats have been studied at Logatchev, Rainbow, TAG, and Snake Pit (Hügler et al., 2010; Scott et al., 2015). However, detailed studies at other nMAR vent fields would be needed to fully appreciate microbial contribution to regional ecosystem function. For hydrothermally inactive sulphide habitats on the nMAR, microbial contribution to ecosystem function is not known, but studies from other regions suggests microbial communities can perform a range of functions, as reviewed by Van Dover (2019). For example, Bacteria and Archaea at inactive sulphide habitats have the metabolic potential for nitrogen (nitrogen fixation, ammonia oxidation, denitrification) and methane cycling, in addition to iron and sulphide oxidation (Zhang et al., 2016; Li et al., 2017). Some of the microbes associated with hydrothermally inactive sulphides may also have the potential to enhance bioleaching of potentially toxic metals (iron, copper, zinc) from sulphides and to accelerate galvanic interactions that increase metal dissolution rates (Fallon et al., 2017).
Ecosystem function studies on the nMAR have largely focussed on the trophic relationships at hydrothermally active habitats in the Menez Gwen, Lucky Strike, Rainbow, Broken Spur, TAG, and Snake Pit vent fields (Colaço et al., 2002; De Busserolles et al., 2009; Portail et al., 2018); and separately at Ashadze-1 vent field (Fabri et al., 2011). However, trophic relationships have not been characterised for Moytirra or Lost City vent fields, and there are no analyses assessing trends in trophic relationships across all ten nMAR vent fields. Most studies conducted to date on the trophic relationships of the benthic invertebrates at hydrothermally active habitats have focused on the megafauna or macrofauna, with comparatively few studies addressing the trophic role of meiofauna.
Trophic relationships for the biota colonising hydrothermally inactive sulphide habitats along the nMAR are unknown, largely because these biological communities have not been characterised. Whilst not demonstrated for the nMAR, there is some evidence from other regions that non-vent suspension feeders colonising inactive sulphide habitats may benefit from secondary production at active vents (Erickson et al., 2009). It is not known whether the microbial community colonising inactive sulphide habitats along the nMAR provides an important nutritional source for any benthic invertebrates in these habitats.
Other than trophic relationships, the functional role of benthic invertebrates and fishes found at hydrothermally active habitats or inactive sulphide habitats on the nMAR has not been reported. The global hydrothermal vent functional traits database produced by Chapman et al. (2019) includes species recorded from the nMAR, and should facilitate future functional studies for those taxa included in the database.
Further studies are needed to appreciate the role of different taxa and groups (microbes to benthic invertebrates and benthopelagic fishes) in ecosystem function, where there is functional overlap, and any cases where species have a unique contribution to ecosystem function. This is essential knowledge in the context of predicting disturbance-induced impacts to ecosystem function, and for implementing the ecosystem approach in environmental management.
Connectivity
The most referred to concept of connectivity is population connectivity, which describes how individuals or groups of individuals from the same species can move between populations, and in the case of genetic connectivity, the extent to which they are able to exchange genetic material. The persistence and maintenance of populations distributed amongst patchy hydrothermally active habitats is thus determined by the balance between the loss of individuals and the provision of new recruits to the population, either from the resident population or from neighbouring populations. Excepting the more mobile crabs and fishes, most species at hydrothermally active habitats are sessile as adults and are reliant on larval dispersal to maintain connectivity amongst sites. Local vent communities are linked by pelagic larval dispersal, together forming regional metacommunities nested within biogeographic provinces (Mitarai et al., 2016; Mullineaux et al., 2018). Connectivity patterns are thus influenced by the larval development mode, where larvae travel within the water column, and the local and regional current regime, amongst other factors (Hilário et al., 2015; Boschen et al., 2016b).
Of the models describing connectivity amongst populations, the two most applicable to populations at hydrothermally active habitats are isolation-by-distance and panmixia (Vrijenhoek, 2010; Boschen et al., 2016b), both of which have been demonstrated for benthic invertebrates colonising nMAR hydrothermally active habitats. Connectivity patterns of hydrothermally active habitats on the nMAR can be complicated in space and time, largely because these habitats are spatially fragmented, and can change over time.
Population connectivity has only been determined for a small number of typically abundant benthic invertebrate species at hydrothermally active habitats along the nMAR; there are still many species where connectivity has not been assessed. Most population connectivity studies on the nMAR have focused on megafaunal and macrofaunal taxa, with very few studies assessing the connectivity of meiofaunal taxa. Even for species where some aspects of connectivity are known, there are still multiple knowledge gaps, including population size, reproductive biology, larval duration, and ocean currents (Hilário et al., 2015). Full population genetics studies of nMAR symbionts have not yet been conducted, however studies from other regions suggest that bacterial symbionts can demonstrate different connectivity patterns from their hosts. For example, a study from the East Pacific Rise, Galápagos Rift, and Pacific-Antarctic Ridge demonstrated that northern and southern bacterial symbionts appeared to be completely isolated, despite mussel species hybridising along the Easter Microplate (Ho et al., 2017). It is not known if a similar pattern occurs in bathymodiolid symbionts at hybridisation zones along the MAR, such as Broken Spur vent field.
The connectivity of the biota colonising hydrothermally inactive sulphide habitats on the nMAR is completely unknown, as is the potential for inactive sulphide habitats to support source populations important for regional population connectivity. In other regions, most species observed at inactive sulphides have been recorded from other habitats (reviewed by Van Dover, 2019).
In terms of environmental management for human activities, such as deep-sea mining, understanding connectivity patterns between the proposed mine site and hydrothermally active habitats in the broader region is key to determining the potential impacts of the proposed mining operation and the potential for recovery at that site (Boschen et al., 2016b). For example, locating a mine site at a source population, which provides recruits to other populations in the region as part of a metapopulation framework, could impact neighbouring populations which are reliant on larval supply from the source population (Boschen et al., 2016b; Mullineaux et al., 2018). If the proposed mine site harbours a self-recruiting population, then extirpation of this population could mean that this species is not able to recolonise the site once mining activities have ceased. A detailed review of connectivity in the context of deep-sea mining of PMS deposits, environmental management, and the role of genetic tools, is provided by Boschen et al. (2016b).
Connectivity assessments need to be undertaken for a greater number of species colonising hydrothermally active habitats on the nMAR, spanning the full range of potential life histories. At a regional level, there needs to be detailed information on population source-sink dynamics and self-recruitment to predict impacts from disturbance to populations, and to inform decisions on potential networks of protected areas. It is also important to consider the potential influence of ocean currents on connectivity patterns. A recent study based on biophysical modelling on the nMAR suggested that there are both persistent zones of connectivity and barriers to dispersal along the ridge; knowledge of such locations could be used to inform environmental management decisions for region (Yearsley et al., 2020).
Temporal Variability
Temporal variability can be related to food availability, breeding and reproductive cycles, ontogenetic shifts, or changes in environmental parameters (Colaço et al., 2009; Glover et al., 2010; Cuvelier et al., 2011b). These factors can lead to variability over multiple time scales, including diurnal, monthly, seasonal, inter-annual, or even decadal time scales (Glover et al., 2010). Temporal variation can be particularly complex for biota at hydrothermally active habitats, with multiple scales of both temporal and spatial variability potentially interacting to influence overall productivity of this community (Le Bris et al., 2019). Less predictable episodic events can also result in temporal variability in such communities, such as volcanic eruptions (reviewed by Le Bris et al., 2019). The slow-spreading MAR axis is considered to be a relatively stable environment for hydrothermally active biological communities, predicted to have distantly spaced vent fields with lower frequency of episodic events than fast-spreading locations, such as the East Pacific Rise and Juan de Fuca Ridge (Vrijenhoek, 2010).
Temporal variability in ecological processes has been documented for microbes and benthic invertebrates at hydrothermally active habitats on the nMAR, using a variety of methods and across a range of temporal scales. Studies on the temporal variability of benthic invertebrates at hydrothermally active habitats have to date focused on the megafauna, with little known about the temporal variability of the macrofauna or meiofauna.
Although not demonstrated for hydrothermal vents with sulphide chimneys on the nMAR, studies in other ocean regions suggest that the microbial communities can stabilise over multiple years (Fortunato et al., 2018). It has also been suggested that microbial communities on the MAR in low-temperature fluids (circa 8°C) may exhibit greater short-term variability (minutes to hours) than those at high-temperature habitats, as has been observed at mussel beds in the Clueless vent field (5°S) in response to short-term hydrothermal fluid variability (Perner et al., 2009). If this also occurs at mussel beds on the nMAR, microbial communities in different hydrothermal fluid environments may have different abilities to respond to changes in environmental conditions associated with both natural and human induced disturbance.
There have not been any dedicated studies addressing temporal variation for microbial communities or benthic invertebrates at hydrothermally inactive sulphide habitats on the nMAR. As hydrothermally active areas along the nMAR become inactive, hydrothermal vent endemic biota will be lost, and over time there may be an increase in microbes and benthic invertebrates who can either tolerate or exploit the inactive sulphide habitats. Although this style of succession has been suggested for other ocean regions (Sen et al., 2014), it has yet to be observed for the nMAR. Some hydrothermally inactive habitats, such as relict or extinct sulphide structures, may provide stable habitats for colonisation by benthic invertebrates, as has been observed in other regions (Boschen et al., 2016a; Gerdes et al., 2019).
Characterising temporal variability is particularly important in the context of environmental management, as it would enable impacts from human activities, such as future deep-sea mining, to be assessed against natural temporal variability. Understanding temporal variability is also key to the development of suitable environmental management measures, to ensure that these measures can accommodate natural temporal variability within the region, and that they will remain effective in the context of longer-term environmental variability, such as climate change.
Ecological information for nMAR hydrothermally active habitats and inactive sulphide habitats needs to be collected over a range of time scales (hours, days, week, months, years, decades) at multiple locations to establish a robust regional baseline for temporal variability against which to measure disturbance-induced changes, and to help predict future responses. More long-term data collection stations or observatories are needed on the nMAR to help provide this information.
Resilience and Recovery
Resilience represents a key concept in ecosystem response to disturbance effects. In the context of this review, recoverability, or resilience, refers to persistence of ecosystems in the face of natural or anthropogenic disturbances (Holling, 1973), and can be quantified as the recovery time to the original state after disturbance (Holling, 1996). Resilience can be further defined as the ability of a system to maintain its overall identity i.e., the same function and structure, in the face of internal change and external perturbations (Walker et al., 2004; Cumming et al., 2013).
Disturbances to hydrothermally active ecosystems can be natural or human induced, across different spatial and temporal scales. The temporal scale of natural disturbances to hydrothermally active ecosystems can range from decades to centuries (Van Dover, 2014). Few human induced disturbances have been studied at hydrothermal vent ecosystems, excepting research conducted on the effect of the high-intensity illumination associated with HOVs and ROVs.
Apart from a recent small-scale disturbance experiment conducted in the Lucky Strike vent field (Marticorena et al., 2021), dedicated resilience and recovery studies have not been conducted for the biological communities colonising hydrothermally active habitats or inactive sulphide habitats on the nMAR. The results from the study by Marticorena et al. (2021) demonstrated the complexity of recovery patterns in response to even small-scale disturbances on the nMAR, highlighting the importance of prior information on connectivity and temporal patterns to fully understand recovery processes after a major disturbance.
Recovery patterns of biological communities colonising hydrothermally active habitats have been assessed in greater detail in the Pacific Ocean. On the East Pacific Rise (EPR), observations on the recovery of biological communities from disturbance due to volcanic activity suggested that most of the diversity and biomass recovered within 5 years (reviewed by Gollner et al., 2017). Recoverability on the EPR varied significantly amongst biological communities from hydrothermally active vents, inactive vents, and within the vent periphery (Gollner et al., 2017). A recent study indicates that faunal succession on the EPR continued a decade after volcanic disturbance, and that there is strong variation amongst and across environmental gradients (Mullineaux et al., 2020). Recovery time on the EPR was considered slower than previously expected, with communities having lower resilience to disturbances, such as deep-sea mining, than initially estimated (Mullineaux et al., 2020). Variable recovery times for hydrothermal vent communities were also predicted in the western Pacific Ocean, based on modelling simulated disturbance for communities in 131 vent fields (Suzuki et al., 2018). The analysis identified substantial differences in recovery time (from <2 years to more than 400 years) due to differences in regional connectivity between known vent fields. In some cases, simultaneous disturbance of a series of vent fields was predicted to delay or wholly prevent recovery (Suzuki et al., 2018).
Predicting the recovery of nMAR hydrothermal vent communities based on the more extensive observations and models from the Pacific Ocean is inherently flawed, given the differences in the frequency of natural disturbance events between these regions and an absence of information on local controls for the nMAR (Mullineaux et al., 2018). There is also limited information on the importance of self-recruitment for populations of nMAR hydrothermal vent fauna. If self-recruitment occurs, a disturbance event at that site could remove or reduce the abundance of eggs and larvae for benthic invertebrate populations, which alongside localised loss or degradation of suitable habitat for colonisation, could severely reduce recovery potential for that population or even result in localised species extinction.
Some of the organisms colonising hydrothermally inactive sulphide habitats may be both sessile and slow growing, such as the hard corals observed on inactive sulphide chimneys along the Kermadec Volcanic Arc in the southwest Pacific, estimated to be at least 160 years old (Boschen et al., 2016a). If the species of black and golden corals recorded from inactive sulphide habitats on the nMAR (Molodtsova et al., 2014) are also slow-growing and long-lived, as has been demonstrated for other species of black corals on the nMAR (Carreiro-Silva et al., 2013), these corals may take decades or centuries to recover from disturbances.
Information is urgently needed on the resilience to disturbance of species and communities colonising hydrothermally active habitats and inactive sulphide habitats on the nMAR. Information is also needed on the biological succession sequence for these habitats following disturbance, and the frequency of natural disturbances on the nMAR. This knowledge is essential for predicting responses to disturbance, assessing environmental impacts, and monitoring recovery from impact. In the absence of natural disturbance events to observe and monitor, controlled disturbance experiments could be used to evaluate biological responses.
Data Availability Statement
The original contributions presented in the study are included in the article/Supplementary Material, further inquiries can be directed to the corresponding authors.
Author Contributions
RB-R and AC jointly conceived the idea for the manuscript. AC generated the updated taxa list, RB-R conducted the statistical analyses. RB-R and AC jointly reviewed the literature and wrote the manuscript. Both authors contributed to the article and approved the submitted version.
Funding
This research was conducted as part of the Atlantic REMP Project (Areas of particular environmental interest in the Atlantic: EASME/EMFF/2017/1.3.1.1 - SI2.775068) funded by the European Commission. AC was also supported through the FunAzores - ACORES 01-0145-FEDER-000123 grant and by FCT and Direção-Geral de Politica do Mar (DGPM) through the project Mining2/2017/005. AC was further supported by Investigadores MarAZ (ACORES-01-0145-FEDER-000140 and by national funds through FCT Foundation for Science and Technology within the scope of UIDB/05634//2020 and UIDP/05634/2020 granted to OKEANOS.
Conflict of Interest
RB-R was employed by the company Seascape Consultants Ltd. during this research.
The remaining author declares that the research was conducted in the absence of any additional commercial or financial relationships that could be construed as a potential conflict of interest.
Publisher's Note
All claims expressed in this article are solely those of the authors and do not necessarily represent those of their affiliated organizations, or those of the publisher, the editors and the reviewers. Any product that may be evaluated in this article, or claim that may be made by its manufacturer, is not guaranteed or endorsed by the publisher.
Acknowledgments
We gratefully acknowledge the support of Prof. Phil Weaver as Project Coordinator for the Atlantic REMP Project, Prof. Nadine Le Bris for allowing us to reproduce the Mission Transect images, Ricardo Medeiros for help with Figure 1, and three reviewers for their constructive comments on the manuscript.
Supplementary Material
The Supplementary Material for this article can be found online at: https://www.frontiersin.org/articles/10.3389/fmars.2021.657358/full#supplementary-material
Footnotes
1. ^For the purpose of this review, “hydrothermal habitats” encompasses (1) “hydrothermally active habitats,” where hydrothermal activity is ongoing and provides seafloor habitats, and (2) “inactive sulphide habitats,” where hydrothermal activity has ceased leaving behind sulphide substrata.
References
Arellano, S. M., and Young, C. M. (2009). Spawning, development, and the duration of larval life in a deep-sea cold-seep mussel. Biol. Bull. 216, 149–162. doi: 10.1086/BBLv216n2p149
Bachraty, C., Legendre, P., and Desbruyères, D. (2009). Biogeographic relationships among deep-sea hydrothermal vent faunas at a global scale. Deep-Sea Res. Part I 56, 1371–1378. doi: 10.1016/j.dsr.2009.01.009
Beaulieu, S. E., and Szafranski, K. M. (2020). InterRidge Global Database of Active Submarine Hydrothermal Vent Fields Version 3.4. PANGEA.
Bell, J. B., Woulds, C., and Oevelen, D. V. (2017). Hydrothermal activity, functional diversity and chemoautotrophy are major drivers of seafloor carbon cycling. Sci. Rep. 7:12025. doi: 10.1038/s41598-017-12291-w
Bellec, L., Cambon Bonavita, M. A., Cueff-Gauchard, V., Durand, L., Gayet, N., and Zeppilli, D. (2018). A nematode of the mid-Atlantic ridge hydrothermal vents harbors a possible symbiotic relationship. Front. Microbiol. 9:2246. doi: 10.3389/fmicb.2018.02246
Bel'tenev, V. E., Lazareva, L. I., Cherkashev, G. A., Ivanov, V. I., Rozhdestvenskaya, I. I., Kuznetsov, V., et al. (2017). New hydrothermal sulphide fields of the Mid-Atlantic Ridge: Yubileinoe (20°09'N) and Surprise (20°45.4'N). Dokl. Earth Sci. 476, 1010–1015. doi: 10.1134/S1028334X17090227
Boschen, R. E., Collins, P. C., Tunnicliffe, V., Carlsson, J., Gardner, J. P. A., Lowe, J., et al. (2016b). A primer for use of genetic tools in selecting and testing the suitability of set-aside sites protected from deep-sea seafloor massive sulfide mining activities. Ocean Coast. Manag. 122, 37–48. doi: 10.1016/j.ocecoaman.2016.01.007
Boschen, R. E., Rowden, A. A., Clark, M. R., Pallentin, A., and Gardner, J. P. A. (2016a). Seafloor massive sulfide deposits support unique megafaunal assemblages: implications for seabed mining and conservation. Mar. Env. Res. 115, 78–88. doi: 10.1016/j.marenvres.2016.02.005
Brazelton, W. J., Ludwig, K. A., Sogin, M. L., Andreishcheva, E. N., Kelley, D. S., Shen, C.-C., et al. (2009). Archaea and bacteria with surprising microdiversity show shifts in dominance over 1,000-year time scales in hydrothermal chimneys. Proc. Natl. Acad. Sci. U. S. A. 107, 1612–1617. doi: 10.1073/pnas.0905369107
Breusing, C., Biastoch, A., Drews, A., Metaxas, A., Jollivet, D., Vrijenhoeck, C., et al. (2016). Biophysical and population genetic models predict the presence of “phantom” stepping stones connecting Mid-Atlantic Ridge vent ecosystems. Curr. Biol. 26, 2257–2267. doi: 10.1016/j.cub.2016.06.062
Breusing, C., Vrijenhoek, R. C., and Reusch, T. B. H. (2017). Widespread introgression in deep-sea hydrothermal vent mussels. BMC Evol. Biol. 17:13. doi: 10.1186/s12862-016-0862-2
Brönnimann, P., Van Dover, C., and Whittaker, J. E. (1989). Abyssotherma pacifica, n. gen., n. sp., a recent remaneicid (Foraminiferida, Remaneicacea) from the East Pacific Rise. Micropaleontology 35, 142–149. doi: 10.2307/1485465
Carreiro-Silva, M., Andrews, A. H., Braga-Henriques, A., Porteiro, F. M., Matos, V., and Santos, R. S. (2013). Variability in growth rates of long-lived black coral Leiopathes sp. from the Azores (NE Atlantic). Mar. Ecol. Prog. Ser. 473, 189–199. doi: 10.3354/meps10052
Chapman, A.S., Beaulieu, S.E., Colaço, A., Gebruk, A.V., Hilario, A., Kihara, T.C., et al. (2019). sFDvent: a global trait database for deep-sea hydrothermal vent fauna. Glob. Ecol. Biogeog. 28, 1538–1551. doi: 10.1111/geb.12975
Clarke, K. R., Gorley, R. N., Somerfield, P. J., and Warwick, R. M. (2014). Change in Marine Communities: An Approach to Statistical Analysis and Interpretation, 3rd Edn. Plymouth: PRIMER-E.
Colaço, A., Desbruyères, D., and Dehairs, F. (2002). Nutritional relations of deep-sea hydrothermal fields at the Mid-Atlantic Ridge: a stable isotope approach. Deep-Sea Res. Part I 49, 395–412. doi: 10.1016/S0967-0637(01)00060-7
Colaço, A., Desbruyères, D., and Guezennec, J. (2007). The use of polar lipid fatty acids to determine trophic links in chemosynthetic communities. Mar. Ecol. 28, 15–24. doi: 10.1111/j.1439-0485.2006.00123.x
Colaço, A., Martins, I., Laranjo, M., Pires, L., Leal, C., Prieto, C., et al. (2006). Annual spawning of the hydrothermal vent mussel, Bathymodiolus azoricus, under controlled aquarium conditions at atmospheric pressure. J. Exp. Mar. Biol. Ecol. 333, 166–171. doi: 10.1016/j.jembe.2005.12.005
Colaço, A., Prieto, C., Martins, A., Figueiredo, M., Lafon, V., Monteiro, M., et al. (2009). Seasonal variations in lipid composition of the hydrothermal vent mussel Bathymodiolus azoricus from the Menez Gwen vent field. Mar. Environ. Res. 67, 146–152. doi: 10.1016/j.marenvres.2008.12.004
Collins, P. C., Kennedy, R., and Van Dover, C. L. (2012). A biological survey method applied to seafloor massive sulphides (SMS) with contagiously distributed hydrothermal-vent fauna. Mar. Ecol. Prog. Ser. 452, 89–107. doi: 10.3354/meps09646
Copley, J. T. P., Jorgensen, P. B. K., and Sohn, R. A. (2007). Assessment of decadal-scale ecological change at a deep Mid-Atlantic hydrothermal vent and reproductive time-series in the shrimp Rimicaris exoculata. J. Mar. Biolog. Assoc. 87, 859–867. doi: 10.1017/S0025315407056512
Copley, J. T. P., Tyler, P. A., Murton, B. J., and Van Dover, C. L. (1997). Spatial and interannual variation in the faunal distribution at Broken Spur vent field (29°N, Mid-Atlantic Ridge). Mar. Biol. 129, 723–733. doi: 10.1007/s002270050215
Crepeau, V., Bonavita, M.-A. C., Lesongeur, F., Randrianalivelo, H., Sarradin, P.-M., Sarrazin, J., et al. (2011). Diversity and function in microbial mats from the Lucky Strike hydrothermal vent field. FEMS Microbiol. Ecol. 76, 524–540. doi: 10.1111/j.1574-6941.2011.01070.x
Cumming, G. S., Olsson, P., Chapin, F. S., and Holling, C. S. (2013). Resilience, experimentation, and scale mismatches in social-ecological landscapes. Landscape Ecol. 28, 1139–1150. doi: 10.1007/s10980-012-9725-4
Cuvelier, D., Beesau, J., Ivanenko, V. N., Zeppilli, D., Sarradin, P.-M., and Sarrazin, J. (2014). First insights into macro- and meiofaunal colonisation patterns on paired wood/slate substrata at Atlantic deep-sea hydrothermal vents. Deep-Sea Res. Part I 87, 70–81. doi: 10.1016/j.dsr.2014.02.008
Cuvelier, D., de Busserolles, F., Lavaud, R., Floc'h, E., Fabri, M. C., Sarradin, P. M., et al. (2012). Biological data extraction from imagery – How far can we go? A case study from the Mid-Atlantic Ridge. Mar. Environ. Res. 82, 15–27. doi: 10.1016/j.marenvres.2012.09.001
Cuvelier, D., Legendre, P., Laes-Huon, A., Sarradin, P. M., and Sarrazin, J. (2017). Biological and environmental rhythms in (dark) deep-sea hydrothermal ecosystems. Biogeosciences 14, 2955–2977. doi: 10.5194/bg-14-2955-2017
Cuvelier, D., Sarradin, P.-M., Sarrazin, J., Colaco, A., Copley, J. T., Desbruyères, D., et al. (2011a). Hydrothermal faunal assemblages and habitat characterisation at the Eiffel Tower edifice (Lucky Strike, Mid-Atlantic Ridge). Mar. Ecol. 32, 243–255. doi: 10.1111/j.1439-0485.2010.00431.x
Cuvelier, D., Sarrazin, J., Colaço, A., Copley, J., Glover, A., Tyler, P., et al. (2011b). Community dynamics over 14 years at the Eiffel Tower hydrothermal edifice on the Mid-Atlantic Ridge. Limnol. Oceanogr. 56, 1624–1640. doi: 10.4319/lo.2011.56.5.1624
Cuvelier, D., Sarrazin, J., Colaco, A., Copley, J. T., Desbruyères, D., Glover, A. G., et al. (2009). Distribution and spatial variation of hydrothermal faunal assemblages at Lucky Strike (Mid-Atlantic Ridge) revealed by high-resolution video image analysis. Deep-Sea Res. Part I 56, 2026–2040. doi: 10.1016/j.dsr.2009.06.006
De Busserolles, F., Sarrazin, J., Gauthier, O., Gelinas, Y., Fabri, M. C., Sarradin, P. M., et al. (2009). Are spatial variations in the diets of hydrothermal fauna linked to local environmental conditions? Deep-Sea Res. Part II 56, 1649–1664. doi: 10.1016/j.dsr2.2009.05.011
De Groot, R. S., Wilson, M. A., and Bourmans, R. M. J. (2002). A typology for the classification, description and valuation of ecosystem functions, goods and services. Ecol. Econ. 41, 393–408. doi: 10.1016/S0921-8009(02)00089-7
Desbruyères, D., Almeida, A., Biscoito, M., Comtet, T., Khripounoff, A., Le Bris, N., et al. (2000). A review of the distribution of hydrothermal vent communities along the northern Mid-Atlantic Ridge: dispersal vs. environmental controls. Hydrobiologia 440, 201–216. doi: 10.1023/A:1004175211848
Desbruyères, D., Biscoito, M., Caprais, J.-C., Colaco, A., Comtet, T., Crassous, P., et al. (2001). Variations in deep-sea hydrothermal vent communities on the Mid-Atlantic Ridge near the Azores plateau. Deep-Sea Res. Part I 48, 1325–1346. doi: 10.1016/S0967-0637(00)00083-2
Desbruyères, D., Segonzac, M., and Bright, M. (2006). Handbook of Deep-Sea Hydrothermal Vent Fauna. Land Oberösterreich, Biologiezentrum der Oberösterreichische Landesmuseen.
Dick, G. J. (2019). The microbiomes of deep-sea hydrothermal vents: distributed globally, shaped locally. Nat. Rev. Microbiol. 17, 271–283. doi: 10.1038/s41579-019-0160-2
Ding, J., Zhang, Y., Wang, H., Jian, H., Leng, H., and Xiao, X. (2017). Microbial community structure of deep-sea hydrothermal vents on the ultraslow spreading Southwest Indian Ridge. Front. Microbiol. 8:1012. doi: 10.3389/fmicb.2017.01012
Dixon, D. R., and Dixon, L. R. J. (1996). Results of DNA analyses conducted on vent shrimp post larvae collected above the Broken Spur vent field during the CD95 cruise, August 1995. BRIDGE Newsletter 11, 9–15.
Dixon, D. R., Lowe, D. M., Miller, P. I., Villemin, G. R., Colaco, A., Serrao-Santos, R., et al. (2006). Evidence of seasonal reproduction in the Atlantic vent mussel Bathymodiolus azoricus, and an apparent link with the timing of photosynthetic primary production. J. Mar. Biolog. Assoc. 86, 1363–1371. doi: 10.1017/S0025315406014391
Duperron, S., Bergin, C., Zielinski, F., Blazejak, A., Pernthaler, A., McKiness, Z. P., et al. (2006). A dual symbiosis shared by two mussel species, Bathymodiolus azoricus and Bathymodiolus puteoserpentis (Bivalvia: Mytilidae), from hydrothermal vents along the northern Mid-Atlantic Ridge. Environ. Microbiol. 8, 1441–1447. doi: 10.1111/j.1462-2920.2006.01038.x
Duperron, S., Quiles, A., Szafranski, K. M., Leger, N., and Shilito, B. (2016). Estimating symbiont abundances and gill surface areas in specimens of the hydrothermal vent mussel Bathymodiolus puteoserpentis maintained in pressure vessels. Front. Mar. Sci. 3:16. doi: 10.3389/fmars.2016.00016
Edwards, K. J., McCollom, T. M., Konishi, H., and Buseck, P. R. (2003). Seafloor bioalteration of sulphide minerals: results from in situ incubation studies. Geochim. Cosmochim. 67, 2843–2856. doi: 10.1016/S0016-7037(03)00089-9
Erickson, K. L., Macko, S. A., and Van Dover, C. L. (2009). Evidence for a chemoautotrophically based food web at inactive hydrothermal vents (Manus Basin). Deep-Sea Res. Part II 56, 1577–1585. doi: 10.1016/j.dsr2.2009.05.002
Fabri, M.-C., Bargain, A., Briand, P., Gebruk, A., Fouquet, Y., Morineaux, M., et al. (2011). The hydrothermal vent community of a new deep-sea field, Ashadze-1, 12°58'N on the Mid-Atlantic Ridge. J. Mar. Biolog. Assoc. 91, 1–13. doi: 10.1017/S0025315410000731
Fallon, E. K., Petersen, S., Brooker, R. A., and Scott, T. B. (2017). Oxidative dissolution of hydrothermal mixed-sulphide ore: an assessment of current knowledge in relation to seafloor massive sulphide mining. Ore Geol. Rev. 86, 309–337. doi: 10.1016/j.oregeorev.2017.02.028
Faure, B., Jollivet, D., Tanguy, A., Bonhomme, F., and Bierne, N. (2009). Speciation in the deep sea: multi-locus analysis of divergence and gene flow between two hybridizing species of hydrothermal vent mussels. PLoS ONE 4:e6485. doi: 10.1371/journal.pone.0006485
Fiala-Médioni, A., McKiness, Z., Dando, P., Boulegue, J., Mariotti, A. A. A. D., Alayse-Danet, A., et al. (2002). Ultrastructural, biochemical, and immunological characterization of two populations of the mytilid mussel Bathymodiolus azoricus from the mid-Atlantic ridge: evidence for a dual symbiosis. Mar. Biol. 141, 1035–1043. doi: 10.1007/s00227-002-0903-9
Flores, G. E., Campbell, J. H., Kirshtein, J. D., Meneghin, J., Podar, M., Steinberg, J. I., et al. (2011). Microbial community structure of hydrothermal deposits from geochemically different vent fields along the Mid-Atlantic Ridge. Environ. Microbiol. 13, 2158–2171. doi: 10.1111/j.1462-2920.2011.02463.x
Fortunato, C. S., Larson, B., Butterfield, A. B., and Huber, J. A. (2018). Spatially distinct, temporally stable microbial populations mediate biogeochemical cycling at and below the seafloor in hydrothermal vent fluids. Environ. Microbiol. 20, 769–784. doi: 10.1111/1462-2920.14011
Gablina, I. F., Dobretsova, I. G., Bel'tenev, V. E., Lyutkevich, A. D., Narkenskii, E. V., and Gustaitis, A. N. (2012). Peculiarities of present-day sulfide mineralization at 19°15'N - 20°08'N, Mid Atlantic Ridge. Dokl. Earth Sci. 442, 163–167. doi: 10.1134/S1028334X1202002X
Galkin, S. V. (1997). Megafauna associated with hydrothermal vents in the Manus Back-arc Basin (Bismark Sea). Mar. Geol. 142, 197–206. doi: 10.1016/S0025-3227(97)00051-0
Galkin, S. V., Molodtsova, T. N., Minin, K. V., and Kobyliansky, S. G. (2019). Ecological studies of the Russian Exploration Area on the Mid-Atlantic Ridge on the 39th cruise of RV Professor Logachev. Oceanology 59, 616–617. doi: 10.1134/S0001437019040040
Galkin, S. V., and Moskalev, L. I. (1990). Hydrothermal fauna of the Mid-Atlantic Ridge. Oceanology 30, 624–627.
Gebruk, A. V., Fabri, M.-C., Briand, P., and Desbruyères, D. (2010). Community dynamics over a decadal scale at Logatchev, 14°45'N, Mid-Atlantic Ridge. Cah. Biol. Mar. 51, 383–388. doi: 10.21411/CBM.A.FB805CDE
Gebruk, A. V., Galkin, S. V., Vereshchaka, A. L., Moskalev, L. I., and Southward, A. J. (1997). Ecology and biogeography of the hydrothermal vent fauna of the Mid-Atlantic Ridge. Adv. Mar. Biol. 32, 93–144. doi: 10.1016/S0065-2881(08)60016-4
Gerdes, K. H., Arbizu, P. M., Schwentner, M., Freitag, R., Schwarz-Schampera, U., Brandt, A., et al. (2019). Megabenthic assemblages at the southern Central Indian Ridge–Spatial segregation of inactive hydrothermal vents from active-, periphery-and non-vent sites. Mar. Environ. Res. 151:104776. doi: 10.1016/j.marenvres.2019.104776
German, C. R., Parson, L. M., Bougalt, H., Coller, D., Critchley, M., Dapoigny, A., et al. (1996). Hydrothermal exploration near the Azores Triple Junction: tectonic control of venting at slow-spreading ridges? Earth Planet. Sci. Lett. 138, 93–104. doi: 10.1016/0012-821X(95)00224-Z
German, C. R., Petersen, S., and Hannington, M. D. (2016). Hydrothermal exploration of mid-ocean ridges: where might the largest sulfide deposits be forming? Chem. Geol. 420, 114–126. doi: 10.1016/j.chemgeo.2015.11.006
Glover, A. G., Gooday, A. J., Bailey, D. M., Billett, D. S. M., Chevaldonné, P., Colaço, A., et al. (2010). Temporal change in deep-sea benthic ecosystems: a review of the evidence from recent time-series studies. Adv. Mar. Biol. 58, 1–95. doi: 10.1016/B978-0-12-381015-1.00001-0
Gollner, S., Fontaneto, D., and Martinez Arbizu, P. (2011). Molecular taxonomy confirms morphological classification of deep-sea hydrothermal vent copepods (Dirivultidae) and suggests broad physiological tolerance of species and frequent dispersal along ridges. Mar. Biol. 158, 221–231. doi: 10.1007/s00227-010-1553-y
Gollner, S., Ivanenko, V. N., Martinez Arbizu, P., and Bright, M. (2010a). Advances in taxonomy, ecology, and biogeography of Dirivultidae (Copepoda) associated with chemosynthetic environments in the deep sea. PLoS ONE 5:e9801. doi: 10.1371/journal.pone.0009801
Gollner, S., Kaiser, S., Menzel, L., Jones, D. O. B., Brown, A., Mestre, N. C., et al. (2017). Resilience of benthic deep-sea fauna to mining activities. Mar. Environ. Res. 129, 76–101. doi: 10.1016/j.marenvres.2017.04.010
Gollner, S., Reimer, B., Martinez-Arbizu, P., Le Bris, N., and Bright, M. (2010b). Diversity of meiofauna from the 9°50'N East Pacific Rise across a gradient of hydrothermal fluid emissions. PLoS ONE 5:e12321. doi: 10.1371/journal.pone.0012321
Gollner, S., Stuckas, H., Kihara, T. C., Laurent, S., Kodami, S., and Arbizu, P. M. (2016). Mitochondrial DNA analyses indicate high diversity, expansive population growth and high genetic connectivity of vent copepods (Dirivultidae) across different oceans. PLoS ONE 11:e0163776. doi: 10.1371/journal.pone.0163776
Han, Y., Gonnella, G., Adam, N., Schippers, A., Burkhardt, L., Kurtz, S., et al. (2018). Hydrothermal chimneys host habitat-specific microbial communities: analogues for studying the possible impact of mining seafloor massive sulfide deposits. Sci. Rep. 8:10386. doi: 10.1038/s41598-018-28613-5
Hannington, M., Jamieson, J., Monecke, T., Petersen, S., and Beaulieu, S. (2011). The abundance of seafloor massive sulfide deposits. Geology 39, 1155–1158. doi: 10.1130/G32468.1
Herring, P. J., Gaten, E., and Shelton, P. M. (1999). Are vent shrimps blinded by science? Nature 398, 116–116. doi: 10.1038/18142
Hilário, A., Metaxas, A., Gaudron, S. M., Howell, K. L., Mercier, A., Mestre, N. C., et al. (2015). Estimating dispersal distance in the deep sea: challenges and applications to marine reserves. Front. Mar. Sci. 2:6. doi: 10.3389/fmars.2015.00006
Ho, P. T., Park, E., Hong, S. G., Kim, E. H., Kim, K., Jang, S. J., et al. (2017). Geographical structure of endosymbiotic bacteria hosted by Bathymodiolus mussels at eastern Pacific hydrothermal vents. BMC Evol. Biol. 17:121. doi: 10.1186/s12862-017-0966-3
Holling, C. S. (1973). Resilience and stability of ecological systems. Ann. Rev. Ecol. Syst. 4, 1–23. doi: 10.1146/annurev.es.04.110173.000245
Holling, C. S. (1996). “Engineering resilience versus ecological resilience,” in Engineering Within Ecological Constraints, ed National Academy of Engineering (Washington, DC: National Academies Press), 31–44.
Hügler, M., Gärtner, A., and Imhoff, J. F. (2010). Functional genes as markers for sulfur cycling and CO2 fixation in microbial communities of hydrothermal bents of the Logatchev field. FEMS Microb. Ecol. 73, 526–537. doi: 10.1111/j.1574-6941.2010.00919.x
ISA (2021). Minerals: Polymetallic Sulphides. Available online at: https://www.isa.org.jm/exploration-contracts/polymetallic-sulphides (accessed January 10, 2021).
Ivanenko, V.N., Martínez Arbizu, P., and Stecher, J. (2006). Copepods of the family Dirivultidae (Siphonostomatoida) from deep-sea hydrothermal vent fields on the Mid-Atlantic Ridge at 14°N and 5°S. Zootaxa 1277, 1–21. doi: 10.11646/zootaxa.1277.1.1
Ivanenko, V. N., Corgosinho, P. H. C., Ferrari, F., Sarradin, P.-M., and Sarrazin, J. (2012). Microhabitat distribution of Smacigastes micheli (Copoepoda: harpacticoida: Tegastidae) from deep-sea hydrothermal vents at the Mid-Atlantic Ridge, 37°N (Lucky Strike), with a morphological description of its Nauplius. Mar. Ecol. 33, 1–11. doi: 10.1111/j.1439-0485.2011.00484.x
Ivanenko, V. N., and Defaye, D. (2004). A new and primitive genus and species of deep-sea Tegastidae (Crustacea, Copepoda, Harpacticoida) from the Mid-Atlantic Ridge, 37°N (Azores Triple Junction, Lucky Strike). Cah. Biol. Mar. 45, 255–268. doi: 10.21411/CBM.A.8BB81F1F
Jamieson, J. W., and Gartman, A. (2020). Defining active, inactive, and extinct seafloor massive sulfide deposits. Mar. Pol. 117:103926. doi: 10.1016/j.marpol.2020.103926
Jan, C., Petersen, J. M., Werner, J., Teeling, H., Huang, S., Golyshina, O. V., et al. (2014). The gill chamber epibiosis of deep-sea shrimp Rimicaris exoculata: an in-depth metagenomic investigation and discovery of Zetaproteobacteria. Environ. Microbiol. 16, 2723–2738. doi: 10.1111/1462-2920.12406
Johnson, M. L., Shelton, P. M., Herring, P. J., and Gardner, S. (1995). Spectral responses from the dorsal organ of a juvenile Rimicaris exoculata from the TAG hydrothermal vent. BRIDGE Newsletter 8, 38–42.
Jollivet, D. (1996). Specific and genetic diversity at deep-sea hydrothermal vents: an overview. Biodivers. Conserv. 5, 1619–1653. doi: 10.1007/BF00052119
Kelley, D. S., and Shank, T. M. (2010). Hydrothermal systems: a decade of discovery in slow spreading environments. Geophys. Monogr. Ser. 188, 369–407. doi: 10.1029/2010GM000945
Kong, L., Ryan, W. B. F., Mayer, L., Detrick, R. S., Fox, P. J., and Manchester, K. (1985). Bare-rock drill sites, ODP Legs 106 and 109: evidence for hydrothermal activity at 23° N in the Mid-Atlantic Ridge. Eos 66:1106.
Krasnov, S. G., Cherkashev, G. A., Stepanova, T. V., Batuyev, B. N., Krotov, A. G., Malin, B. V., et al. (1995). “Detailed geological studies of hydrothermal fields in the North Atlantic,” in Hydrothermal Vents and Processes, eds L. M. Parson, C. L. Walker, and D. R. Dixon (London: Geological Society Special Publication 87), 43–64. doi: 10.1144/GSL.SP.1995.087.01.05
Krylova, E. M., Sahling, H., and Janssen, R. (2010). Abyssogena: a new genus of the family Vesicomyidae (Bivalvia) from deep-water vents and seeps. J. Mollus. Stud. 76, 107–132. doi: 10.1093/mollus/eyp052
LaBella, A. L., Van Dover, C. L., Jollivet, D., and Cunningham, C. W. (2017). Gene flow between Atlantic and Pacific Ocean basins in three lineages of deep-sea clams (Bivalvia: Vesicomyidae: Pliocardiinae) and subsequent limited gene flow within the Atlantic. Deep-Sea Res. Part II 137, 307–317. doi: 10.1016/j.dsr2.2016.08.013
Lalou, C., Reyss, J. L., and Brichet, E. (1998). Age of sub-bottom sulphide samples at the TAG active mound. Proc. Ocean Drilling Prog. Sci. Results 158, 111–117. doi: 10.2973/odp.proc.sr.158.214.1998
Le Bris, N., Yücel, M., Das, A., Sievert, S. M., LokaBharathi, P., and Girguis, P. R. (2019). Hydrothermal energy transfer and organic carbon production at the deep seafloor. Front. Mar. Sci. 5:531. doi: 10.3389/fmars.2018.00531
Le, J. T., Levin, L. A., and Carson, R. T. (2017). Incorporating ecosystem services into environmental management of deep-seabed mining. Deep-Sea Res. Part II 137, 486–503. doi: 10.1016/j.dsr2.2016.08.007
Levin, L. A., Baco, A. R., Bowden, D. A., Colaço, A., Cordes, E. E., Cunha, M. R., et al. (2016). Hydrothermal vents and methane seeps: rethinking the sphere of influence. Front. Mar. Sci. 3:72. doi: 10.3389/fmars.2016.00072
Li, J., Cui, J., Yang, Q., Cui, G., Wei, B., Wu, Z., et al. (2017). Oxidative weathering and microbial diversity of an inactive seafloor hydrothermal sulfide chimney. Front. Microbiol. 8:1378. doi: 10.3389/fmicb.2017.01378
Lilley, M. D., Feely, R. A., and Trefry, J. H. (1995). “Chemical and biochemical transformations in hydrothermal plumes,” in Seafloor Hydrothermal Systems: Physical, Chemical, Biological, and Geological Interactions, eds S. E. Humphris, R. A. Zierenberg, L. S. Mullineaux, and R. E. Thomson (Washington, DC: American Geophysical Union), 369–391. doi: 10.1029/GM091p0369
Lopez-Garcia, P., Philippe, H., Gail, F., and Moreira, D. (2003). Authochthonous eukaryotic diversity in hydrothermal sediment and experimental microcolonizers at the Mid-Atlantic Ridge. Proc. Natl. Acad. Sci. U. S. A. 100, 697–702. doi: 10.1073/pnas.0235779100
Ludwig, K. A., Kelley, D. S., Butterfield, D. A., Nelson, B. K., and Früh-Green, G. (2006). Formation and evolution of carbonate chimneys at the Lost City Hydrothermal Field. Geochim. Cosmochim. Acta 70, 3625–3645. doi: 10.1016/j.gca.2006.04.016
Maas, P. A. Y., O'Mullan, G. D., Lutz, R. A., and Vrijenhoek, R. C. (1999). Genetic and morphometric characterization of mussels (Bivalvia: Mytilidae) from Mid-Atlantic hydrothermal vents. Biol. Bull. 196, 265–272. doi: 10.2307/1542951
Marques, A. F. A., Barriga, F. J., and Scott, S. D. (2007). Sulfide mineralization in an ultramafic-rock hosted seafloor hydrothermal system: from serpentinization to the formation of Cu–Zn–(Co)-rich massive sulfides. Mar. Geol. 245, 20–39. doi: 10.1016/j.margeo.2007.05.007
Marticorena, J., Matabos, M., Ramirez-Llodra, E., Cathalot, C., Laes-Huon, A., Leroux, R., et al. (2021). Recovery of hydrothermal vent communities in response to an induced disturbance at the Lucky Strike vent field (Mid-Atlantic Ridge). Mar. Env. Res. 168:105316. doi: 10.1016/j.marenvres.2021.105316
Matabos, M., Cuvelier, D., Brouard, J., Shillito, B., Ravaux, J., Zbinden, M., et al. (2015). Behavioural study of two hydrothermal crustacean decapods: Mirocaris fortunata and Segonzacia mesatlantica, from the Lucky Strike vent field (Mid Atlantic Ridge). Deep-Sea Res. Part II 121, 146–158. doi: 10.1016/j.dsr2.2015.04.008
Meier, D. V., Pjevac, P., Bach, W., Markert, S., Schweder, T., Jamieson, J., et al. (2019). Microbial metal-sulfide oxidation in inactive hydrothermal vent chimneys suggested by metagenomic and metaproteomic analyses. Environ. Microbiol. 21, 682–701. doi: 10.1111/1462-2920.14514
Mitarai, S., Watanabe, H., Nakajima, Y., Shchepetkin, A. F., and McWilliams, J. C. (2016). Quantifying dispersal from hydrothermal vent fields in the western Pacific Ocean. Proc. Natl. Acad. Sci. U. S. A. 113, 2976–2981. doi: 10.1073/pnas.1518395113
Moalic, Y., Desbruyères, D., Duarte, C. M., Rozenfeld, A., Bachraty, C., and Arnaud-Haond, S. (2012). Biogeography revisited with network theory: Retracing the history of hydrothermal vent communities. Syst. Biol. 61, 127–137. doi: 10.1093/sysbio/syr088
Molodtsova, T. N., Galkin, S. V., and Dobretsova, I. (2014). “Preliminary data on fauna of inactive hydrothermal sulfide fields on the Mid Atlantic Ridge at 12°58'-13°31'N,” in World Conference on Marine Biodiversity (Qingdao).
Morineaux, M., Nishi, E., Ormos, A., and Mouchel, O. (2010). A new species of Phyllochaetopterus (Annelida: Chaetopteridae) from deep-sea hydrothermal Ashadze-1 vent field, Mid-Atlantic Ridge: taxonomical description and partial COI DNA sequence. Cah. Biol. Mar. 51, 239–248. doi: 10.21411/CBM.A.42737680
Mullineaux, L. S., Metaxas, A., Beaulieu, S. E., Bright, M., Gollner, S., Grupe, B. M., et al. (2018). Exploring the ecology of deep-sea hydrothermal vents in a metacommunity framework. Front. Mar. Sci. 5:49. doi: 10.3389/fmars.2018.00049
Mullineaux, L. S., Mills, S. W., Le Bris, N., Beaulieu, S. E., Sievert, S. M., and Dykman, L. N. (2020). Prolonged recovery time after eruptive disturbance of a deep-sea hydrothermal vent community. Proc. R. Soc. London B 287:20202070. doi: 10.1098/rspb.2020.2070
Murton, B. J., Klinkhammer, G., Becker, K., Briais, A., Edge, D., Hayward, N., et al. (1994). Direct evidence for the distribution and occurrence of hydrothermal activity between 27°N – 30°N on the Mid-Atlantic Ridge. Earth Planet. Sc. Lett. 125, 119–128. doi: 10.1016/0012-821X(94)90210-0
O'Mullan, G. D., Maas, P. A. Y., Lutz, R. A., and Vrijenhoek, R. C. (2001). A hybrid zone between hydrothermal vent mussels (Bivalvia: Mytilidae) from the Mid-Atlantic Ridge. Mol. Ecol. 10, 2819–2831. doi: 10.1046/j.0962-1083.2001.01401.x
Page, M. J., McKenzie, J. E., Bossuyt, P. M., Boutron, I., Hoffmann, T. C., Mulrow, C. D., et al. (2020). The PRISMA 2020 statement: an updated guideline for reporting systematic reviews. BMJ 372:n71. doi: 10.1136/bmj.n71
Perner, M., Bach, W., Hentscher, M., Koschinsky, A., Garbe-Schonberg, D., Streit, W. R., et al. (2009). Short-term microbial and physico-chemical variability in low-temperature hydrothermal fluids near 5°S on the Mid-Atlantic Ridge. Environ. Microbiol. 11, 2526–2541. doi: 10.1111/j.1462-2920.2009.01978.x
Perner, M., Kuever, J., Seifert, R., Pape, T., Koschinsky, A., Schmidt, K., et al. (2007). The influence of ultramafic rocks on microbial communities at the Logatchev hydrothermal field, located 15°N on the Mid-Atlantic Ridge. FEMS Microb. Ecol. 61, 97–109. doi: 10.1111/j.1574-6941.2007.00325.x
Petersen, J. M., Ramette, A., Lott, C., Cambon-Bonavita, M. A., Zbinden, M., and Dubilier, N. (2010). Dual symbiosis of the vent shrimp Rimicaris exoculata with filamentous gamma-and epsilonproteobacteria at four Mid-Atlantic Ridge hydrothermal vent fields. Environ. Microbiol. 12, 2204–2218. doi: 10.1111/j.1462-2920.2009.02129.x
Petersen, J. M., Zielinski, F. U., Pape, T., Seifert, R., Moraru, C., Amann, R., et al. (2011). Hydrogen is an energy source for hydrothermal vent symbioses. Nature 476, 176–180. doi: 10.1038/nature10325
Plum, C., Pradillon, F., Fujiwara, Y., and Sarrazin, J. (2017). Copepod colonization of organic and inorganic substrata at a deep-sea hydrothermal vent site on the Mid-Atlantic Ridge. Deep-Sea Res. Part II 137, 335–348. doi: 10.1016/j.dsr2.2016.06.008
Portail, M., Brandily, C., Cathalot, C., Colaço, A., Gelinas, Y., Husson, B., et al. (2018). Food-web complexity across hydrothermal vents on the Azores triple junction. Deep-Sea Res. Part I 131, 101–120. doi: 10.1016/j.dsr.2017.11.010
Reysenbach, A.-L., Longnecker, K., and Kirshtein, J. (2000). Novel bacteria and archaeal lineages from an in-situ growth chamber deployed at a Mid-Atlantic Ridge hydrothermal vent. Appl. Environ. Microbiol. 66, 3798–3806. doi: 10.1128/AEM.66.9.3798-3806.2000
Rommevaux, C., Henri, P., Degboe, J., Chavagnac, V., Lesongeur, F., Godfroy, A., et al. (2019). Prokaryote communities at active chimney and in-site colonization devices after a magmatic degassing event (37°N MAR, EMSO-Azores DeepSea Observatory). Geochem. Geophy. Geosy. 20, 3056–3089. doi: 10.1029/2018GC008107
Rona, P. A., Hannington, M. D., Raman, C. V., Thompson, G., Tivey, M. K., Humphris, S. E., et al. (1993). Active and relict sea-floor hydrothermal mineralization at the TAG hydrothermal field, Mid-Atlantic Ridge. Econ. Geol. 88, 1989–2017. doi: 10.2113/gsecongeo.88.8.1989
Rona, P. A., Klinkhammer, G., Nelsen, T. A., Trefry, J. H., and Elderfield, H. (1986). Black smokers, massive sulphides and vent biota at the Mid-Atlantic Ridge. Nature 321, 33–37. doi: 10.1038/321033a0
Roussel, E. G., Konn, C., Charlou, J.-L., Donval, J.-P., Fouquet, Y., Querellou, J., et al. (2011). Comparison of microbial communities associated with three Atlantic ultramafic hydrothermal systems. FEMS Microbial. Ecol. 77, 647–665. doi: 10.1111/j.1574-6941.2011.01161.x
Rybakova, E., and Galkin, S. (2015). Hydrothermal assemblages associated with different foundation species on the East Pacific Rise and Mid-Atlantic Ridge, with a special focus on mytilids. Mar. Ecol. 36, 45–61. doi: 10.1111/maec.12262
Sarrazin, J., Cuvelier, D., Peton, L., Legendre, P., and Sarradin, P. M. (2014). High-resolution dynamics of a deep-sea hydrothermal vent mussel assemblage monitored by the EMSO-Azores MoMAR observatory. Deep-Sea Res. Part I 90, 62–75. doi: 10.1016/j.dsr.2014.04.004
Sarrazin, J., Legendre, P., De Busserolles, F., Fabri, M. C., Guilini, K., Ivanenko, V. N., et al. (2015). Biodiversity patterns, environmental drivers and indicator species on a high temperature hydrothermal edifice, Mid-Atlantic Ridge. Deep-Sea Res. Pt II 121, 177–192. doi: 10.1016/j.dsr2.2015.04.013
Scott, J. J., Breier, J. A., Luther, I. I. I. G. W, and Emerson, D. (2015). Microbial iron mats at the MidAtlantic Ridge and evidence that Zetaproteobacteria may be restricted to iron-oxidizing systems. PLoS ONE 10:e0119284. doi: 10.1371/journal.pone.0119284
Sen, A., Podowski, E. L., Becker, E. L., Shearer, E. A., Gartman, A., Yucel, M., et al. (2014). Community succession in hydrothermal vent habitats of the Eastern Lau Spreading Center and Valu Fa Ridge, Tonga. Limnol. Oceanogr. 59, 1510–1528. doi: 10.4319/lo.2014.59.5.1510
Sievert, S., and Vetriani, C. (2012). Chemoautotrophy at deep-sea vents: past, present, and future. Oceanography 25, 218–233. doi: 10.5670/oceanog.2012.21
Stöhr, S., and Segonzac, M. (2005). Deep-sea ophiuroids (Echinodermata) from reducing and non-reducing environments in the North Atlantic Ocean. J. Mar. Biol. Assoc. U. K. 85, 383–402. doi: 10.1017/S0025315405011318h
Sun, J., Zhou, Y., Chen, C., Hang Kwan, Y., Sun, Y., Wang, X., et al. (2020). Nearest vent, dearest friend: biodiversity of Tiancheng vent field reveals cross-ridge similarities in the Indian Ocean. R. Soc. Open Sci. 7:200110. doi: 10.1098/rsos.200110
Suzuki, K., Yoshida, K., Watanabe, H., and Yamamoto, H. (2018). Mapping the resilience of chemosynthetic communities in hydrothermal vent fields. Sci. Rep. 8, 1–8. doi: 10.1038/s41598-018-27596-7
Tchesunov, A. V. (2015). Free-living nematode species (Nematoda) dwelling in hydrothermal sites of the North Mid-Atlantic Ridge. Helg. Mar. Res. 69, 343–384. doi: 10.1007/s10152-015-0443-6
Teixeira, S., Cambon-Bonavita, M. A., Serrao, E. A., Desbruyères, D., and Arnaud-Haond, S. (2010). Recent population expansion and connectivity in the hydrothermal shrimp Rimicaris exoculata along the Mid-Atlantic Ridge. J. Biogeogr. 38, 564–574. doi: 10.1111/j.1365-2699.2010.02408.x
Thurber, A. R., Sweetman, A. K., Narayanaswamy, B. E., Jones, D. O., Ingels, J., and Hansman, R. L. (2014). Ecosystem function and services provided by the deep sea. Biogeosciences 11, 3941–3963. doi: 10.5194/bg-11-3941-2014
Tsurumi, M., and Tunnicliffe, V. (2003). Tubeworm-associated communities at hydrothermal vents on the Juan de Fuca Ridge, Northeast Pacific. Deep Sea Res. Part I Oceanogr. Res. Pap. 50, 611–629. doi: 10.1016/S0967-0637(03)00039-6
Turner, P. J., Thaler, A. D., Freitag, A., and Collins, P. C. (2019). Deep-sea hydrothermal vent ecosystem principles: Identification of ecosystem processes, services and communication of value. Mar. Pol. 101, 118–124. doi: 10.1016/j.marpol.2019.01.003
Van der Heijden, K., Petersen, J. M., Dubilier, N., and Borowski, C. (2012). Genetic connectivity between North and South Mid-Atlantic Ridge chemosynthetic bivalves and their symbionts. PLoS ONE 7:e39994. doi: 10.1371/journal.pone.0039994
Van Dover, C. L. (1995). “Ecology of Mid-Atlantic Ridge hydrothermal vents,” in Hydrothermal Vents and Processes, eds L. M. Parson, C. L. Walker, and D. R. Dixon (London: Geological Society Special Publication 87), 257–294. doi: 10.1144/GSL.SP.1995.087.01.21
Van Dover, C. L. (2014). Impacts of anthropogenic disturbances at deep-sea hydrothermal vent ecosystems: a review. Mar. Environ. Res. 102, 59–72. doi: 10.1016/j.marenvres.2014.03.008
Van Dover, C. L. (2019). Inactive sulphide ecosystems in the deep sea: a review. Front. Mar. Sci. 6:461. doi: 10.3389/fmars.2019.00461
Van Dover, C. L., Arnaud-Haond, S., Gianni, M., Helmreich, S., Huber, J. A., Jaeckel, A. L., et al. (2018). Scientific rationale and international obligations for protection of active hydrothermal vent ecosystems from deep-sea mining. Mar. Pol. 90, 20–28. doi: 10.1016/j.marpol.2018.01.020
Van Dover, C. L., Berg, C. J. Jr, and Turner, R. D. (1988b). Recruitment of marine invertebrates at deep-sea hydrothermal vents on the East Pacific Rise and Galapagos spreading center. Deep-Sea Res. Part I 35, 1833–1849. doi: 10.1016/0198-0149(88)90052-0
Van Dover, C. L., Colaço, A., Collins, P. C., Croot, P., Metaxas, A., Murton, B. J., et al. (2020). Research is needed to inform environmental management of hydrothermally inactive and extinct polymetallic sulfide (PMS) deposits. Mar. Pol. 121:104183. doi: 10.1016/j.marpol.2020.104183
Van Dover, C. L., Fry, B., Grassle, J. F., Humphris, S., and Rona, P. A. (1988a). Feeding biology of the shrimp Rimicaris exoculata at hydrothermal vents on the Mid-Atlantic Ridge. Mar. Biol. 98, 209–216. doi: 10.1007/BF00391196
Van Dover, C. L., German, C. R., Speer, K. G., Parson, L. M., and Vrijenhoek, R. (2002). Evolution and biogeography of deep-sea vent and seep invertebrates. Science 295, 1253–1257. doi: 10.1126/science.1067361
Van Dover, C. L., Szuts, E. Z., Chamberlain, S. C., and Cann, J. R. (1989). A novel eye in 'eyeless' shrimp from hydrothermal vents of the Mid-Atlantic Ridge. Nature 337, 458–460. doi: 10.1038/337458a0
Vereshchaka, A. L., Vinogradov, G. M., Lein, A. Y., Dalton, S., and Dehairs, F. (2000). Carbon and nitrogen isotopic composition of the fauna from the Broken Spur hydrothermal vent field. Mar. Biol. 136, 11–17. doi: 10.1007/s002270050002
Vrijenhoek, R. C. (2010). Genetic diversity and connectivity of deep-sea hydrothermal vent metapopulations. Mol. Ecol. 19, 4391–4111. doi: 10.1111/j.1365-294X.2010.04789.x
Walker, B., Holling, C. S., Carpenter, S. R., and Kinzig, A. (2004). Resilience, adaptability and transformability in social–ecological systems. Ecol. Soc. 9:5. doi: 10.5751/ES-00650-090205
Wheeler, A. J., Murton, B., Copley, J. T., Lim, A., Carlsson, J., Collins, P. C., et al. (2013). Moytirra: discovery of the first known deep-sea hydrothermal vent field on the slow-spreading Mid-Atlantic Ridge north of the Azores. Geochem. Geophy. Geosys. 14, 4170–4184. doi: 10.1002/ggge.20243
World Register of Marine Species (2020). Available online at: http://www.marinespecies.org at VLIZ (accessed July 22, 2020).
Yahagi, T., Fukumori, H., Warén, A., and Kano, Y. (2019). Population connectivity of hydrothermal vent limpets along the northern Mid-Atlantic Ridge (Gastropoda: Neritimorpha: Phenacolepadidae). J. Mar. Biolog. Assoc. 99, 179–185. doi: 10.1017/S0025315417001898
Yearsley, J. M., Salmanidou, D. M., Carlsson, J., Burns, D., and Van Dover, C. L. (2020). Biophysical models of persistent connectivity and barriers on the northern Mid-Atlantic Ridge. Deep-Sea Res. Part II 180:104819. doi: 10.1016/j.dsr2.2020.104819
Zbinden, M., Le Bris, N., Gaill, F., and Compere, P. (2004). Distribution of bacteria and associated minerals in the gill chamber of the vent shrimp Rimicaris exoculata and related biogeochemical processes. Mar. Ecol. Prog. Ser. 284, 237–251. doi: 10.3354/meps284237
Zekely, J., Sørensen, M. V., and Bright, M. (2006b). Three new nematode species (Monhysteridae) from deep-sea hydrothermal vents. Meiofauna Marina 15, 25–42. Available online at: https://pfeil-verlag.de/publikationen/meiofauna-marina-band-15/
Zekely, J., Van Dover, C. L., Nemeschkal, H. L., and Bright, M. (2006a). Hydrothermal vent meiobenthos associated with mytilid mussel aggregations from the Mid-Atlantic Ridge and the East Pacific Rise. Deep-Sea Res. Part I 53, 1363–1378. doi: 10.1016/j.dsr.2006.05.010
Zeppilli, D., Bellec, L., Cambon-Bonavita, M.-A., Decraemer, W., Fontaneto, D., Fuchs, S., et al. (2019). Ecology and trophic role of Oncholaimus dyvae sp. nov. (Nematoda: Oncholaimidae) from the lucky strike hydrothermal vent field (Mid-Atlantic Ridge). BMC Zool. 4, 1–15. doi: 10.1186/s40850-019-0044-y
Keywords: active hydrothermal vent, inactive sulphide habitats, polymetallic sulphide, North Atlantic, deep-sea mining, biodiversity, biogeography, ecology
Citation: Boschen-Rose RE and Colaço A (2021) Northern Mid-Atlantic Ridge Hydrothermal Habitats: A Systematic Review of Knowledge Status for Environmental Management. Front. Mar. Sci. 8:657358. doi: 10.3389/fmars.2021.657358
Received: 22 January 2021; Accepted: 27 July 2021;
Published: 20 August 2021.
Edited by:
David Billett, Deep Seas Environmental Solutions Ltd., United KingdomReviewed by:
Michael Vecchione, National Oceanic and Atmospheric Administration (NOAA), United StatesMustafa Yucel, Middle East Technical University, Turkey
Elva G. Escobar-Briones, National Autonomous University of Mexico, Mexico
Copyright © 2021 Boschen-Rose and Colaço. This is an open-access article distributed under the terms of the Creative Commons Attribution License (CC BY). The use, distribution or reproduction in other forums is permitted, provided the original author(s) and the copyright owner(s) are credited and that the original publication in this journal is cited, in accordance with accepted academic practice. No use, distribution or reproduction is permitted which does not comply with these terms.
*Correspondence: Rachel E. Boschen-Rose, reboschen-rose@outlook.com; Ana Colaço, maria.aa.colaco@uac.pt
†Present address: Rachel E. Boschen-Rose, Ocean and Earth Science, University of Southampton, Southampton, United Kingdom