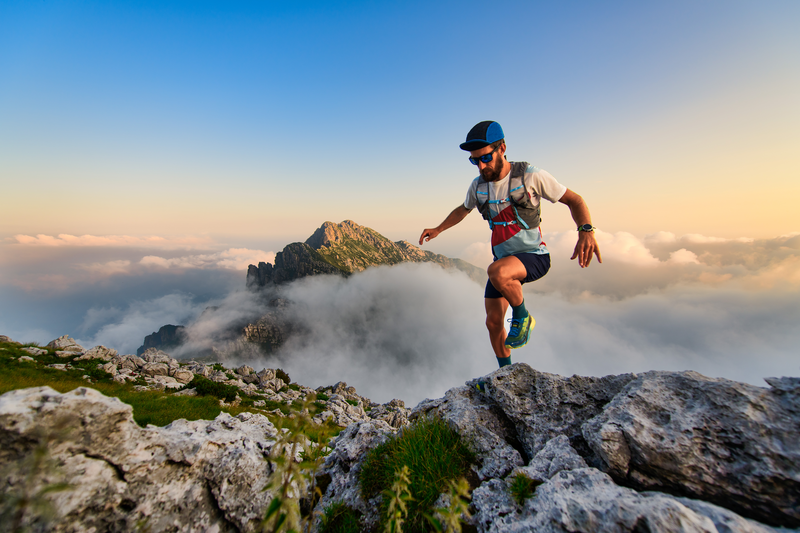
94% of researchers rate our articles as excellent or good
Learn more about the work of our research integrity team to safeguard the quality of each article we publish.
Find out more
ORIGINAL RESEARCH article
Front. Mar. Sci. , 29 April 2021
Sec. Marine Evolutionary Biology, Biogeography and Species Diversity
Volume 8 - 2021 | https://doi.org/10.3389/fmars.2021.656757
This article is part of the Research Topic Benthic Biodiversity of the Indian Ocean View all 14 articles
The huge riverine influx and associated processes decrease the ambient salinity, stratify the water column, modulate the oxygen-deficient zone, and are also responsible for the recent acidification in the Bay of Bengal. Here, we have studied the effect of these riverine influx-dominated ecological parameters on living benthic foraminifera in the west-central Bay of Bengal. We report that the pH below 7.6 in front of the Krishna river, reduces the diversity and the richness of living benthic foraminifera on the adjacent shelf and the slope. A similar decreased diversity and richness is also observed in front of the Godavari River. We delineate three prominent assemblages, representing different depth zones with associated distinct physico-chemical conditions. The shallow water assemblage (∼27–100 m) is represented by Nonionella labradorica, Hanzawaia nipponica, Brizalina dilatata, Ammonia tepida, and Nonionella limbato-striata. These species are adapted to relatively warmer temperatures and more oxygenated waters. The deepwater assemblage (∼1,940–2,494 m) includes Bulimina cf. delreyensis, Bulimina marginata, Hormosinella guttifera, Cassidulina laevigata, and Gyroidinoides subzelandica and can tolerate a relatively colder temperature. The intermediate-depth assemblage (∼145–1,500 m) dominated by Eubuliminella exilis, Bolivinellina earlandi, Fursenkoina spinosa, Bolivinellina lucidopunctata, Globobulimina globosa, Fursenkoina spinosa, Eubuliminella cassandrae, Uvigerina peregrina, Rotaliatinopsis semiinvoluta, and Cassidulina laevigata, represents oxygen-deficient and organic carbon-rich environment. Besides the pH, temperature, dissolved oxygen and organic matter, we also report a strong influence of bathymetry, coarse fraction (CF) and the type of organic matter on a few living benthic foraminifera. The ecological preferences of 40 such dominant living benthic foraminifera, each representing a specific environment, have also been reported for site-specific proxy. We conclude that although the huge riverine influx affects living benthic foraminifera on the shelf, the dissolved oxygen and organic carbon mostly control benthic foraminiferal distribution in the deeper west-central Bay of Bengal.
The huge riverine influx creates a unique environment in the Bay of Bengal. A few of the world’s largest snow and rainfall-fed rivers (Irrawaddy, Ganga, Brahmaputra, Mahanadi, Krishna, and Godavari) drain freshwater and sediments into the Bay of Bengal, creating a specific environment with associated physical and chemical parameters. The riverine influx reduces the salinity, warms the seawater and creates strong stratification (Sengupta et al., 2006). The associated sediment influx contributes enormous nutrients, sustaining the primary productivity (Prasanna Kumar et al., 2002). In addition to the riverine influx, the anthropogenic input (sulfate and nitrogen aerosols) to the Bay of Bengal significantly affects its biogeochemistry (Sarma et al., 2016). Therefore, the riverine influx primarily modulates the ambient physico-chemical conditions in the northern Bay of Bengal. Additionally, the dissolved oxygen, a critical requirement of a large fraction of marine biota, is also perennially low at the Bay of Bengal’s intermediate depths (Sarma et al., 2013). As suggested by the acidification and a possibility of the bay turning into the foremost nitrogen contributor soon (Bristow et al., 2017), the increasing stress implies that it is essential to understand the effect of these critical ecological parameters on the marine biota.
Benthic foraminifera are the dominant marine biota in the continental margin sediments and are the primary component of the global carbon cycling. The large abundance, combined with the sensitivity to the ambient conditions and long-term preservation in sediments, makes benthic foraminifera a widely used proxy to reconstruct paleoceanographic changes (Saraswat, 2015; Saraswat et al., 2017). The precise knowledge of the influence of ambient environmental parameters on abundance and distribution, is a prerequisite to use benthic foraminiferal characteristics for paleoceanographic and paleoclimatic interpretation (Murray, 2001; Gooday, 2003; Jorissen et al., 2007; Gooday and Jorissen, 2012; Saraswat and Nigam, 2013). Various environmental parameters, including food, oxygen concentration, salinity, pH, temperature, substrate, water depth and others, influence the diversity and abundance of benthic foraminifera (Lutze and Coulbourn, 1984; Kaminski et al., 1988; Altenbach, 1992; Mackensen et al., 1995; Gooday and Rathburn, 1999; Van der Zwaan et al., 1999; Ernst et al., 2002; Altenbach et al., 2003; Lei et al., 2017, 2019). The relative influence of various factors on faunal distribution, however, varies from region to region. The salinity and temperature are the major factors in riverine influx dominated continental shelves (Manasa et al., 2016). However, the deeper depth continental slope assemblages are mainly controlled by the availability of food and dissolved oxygen concentration (Gooday, 1986; Corliss and Chen, 1988; Mackensen and Douglas, 1989; Corliss and Emerson, 1990; Barmawidjaja et al., 1992; Jorissen et al., 1992; Rosoff and Corliss, 1992; Rathburn and Corliss, 1994; Nisha and Singh, 2012; Singh et al., 2015a, 2018; Verma et al., 2018). Benthic foraminiferal abundance, diversity, species richness and evenness also depend on ambient conditions (Gibson and Buzas, 1973; Buzas and Culver, 1991; Murray, 2006; Singh et al., 2015a, b). The overall benthic foraminiferal abundance and diversity decreases, whereas the abundance of abnormal or stress-tolerant specimen increases, under both naturally and anthropogenically stressed environment (Alve, 1995; Saraswat et al., 2004; Nigam et al., 2007). As the extent of stress varies regionally, the benthic foraminiferal response has to be assessed from different regions.
The fossil benthic foraminiferal assemblage is often biased by the biological and taphonomical processes, including transport, test disintegration or dissolution (Murray, 1991; Jorissen and Wittling, 1999; Berkeley et al., 2014). Therefore, living benthic foraminifera (rose-Bengal stained) provide a comparatively more reliable response to the ecological conditions. The previous studies on the distribution and ecology of living benthic foraminifera (rose-Bengal stained) from the northern Bay of Bengal are rare (Boltovskoy, 1978). The few studies documenting living benthic foraminifera from the Bay of Bengal cover a limited spatial extent, concentrating only on nearshore-beaches and estuaries (maximum 19 m water depth) (Gandhi et al., 2007; Gandhi and Solai, 2010). The influence of deep water ecological conditions on benthic foraminifera from the Bay of Bengal is rarely documented. Recently, Suokhrie et al. (2020), compared the living benthic foraminifera of the western Bay of Bengal’s oxygen-deficient zone with that from a similar region in the Arabian Sea. A distinctly different benthic foraminiferal assemblage was reported from the Bay of Bengal oxygen deficient waters. However, the effect of other stressors, including salinity, temperature, pH, riverine influx on benthic foraminifera, both above and below the oxygen-deficient zone of the western Bay of Bengal, was not addressed. Therefore, we study the combined effect of multiple ambient parameters on the living (stained) benthic foraminiferal assemblage from the shallow inner shelf to the slope and further deeper region of the west-central Bay of Bengal. The main objective of this study is to establish representative living (rose-Bengal stained) benthic foraminifera assemblages characterizing a particular set of ecological niches on the shallow continental shelf to the abyssal depth in the west-central Bay of Bengal, where the freshwater influx and associated processes primarily influence the physico-chemical parameters. The baseline information will be beneficial to understand the foraminiferal response to climatic changes in the future. The precise information about benthic foraminifera’s ecological preferences, will also be useful for a better reconstruction of the past environmental conditions.
The west-central Bay of Bengal is strongly influenced by the riverine influx. Some of the world’s largest rivers drain into the western Bay of Bengal, with an average runoff being Ganga-11,892 m3s–1, Brahmaputra-16,186 m3s–1, Godavari-3,180 m3s–1, Krishna-1,730 m3s–1, Pennar-95 m3s–1, and Cauveri-664 m3s–1 (Rao, 1975; Subramanian, 1979; Dai and Trenberth, 2002). The lithogenic components’ contribution is ∼39% in the northern bay and ∼12.6% in the southern bay (Unger et al., 2003). The riverine influx is most intense during the summer monsoon and is also responsible for the salinity-controlled stratification (Gomes et al., 2000; Unger et al., 2003; Sarma et al., 2016) and surface circulation in the Bay of Bengal. The southwestern Bay of Bengal receives significant precipitation during the winter monsoon. The surface circulation (East India Coastal Current) reverses seasonally along the bay’s western boundary, flowing southward during the winter season and northward during the summer season (Vinayachandran et al., 1999; Shankar et al., 2002). The precipitation resulting from the seasonal reversal of winds in the Bay of Bengal is maximum during the summer monsoon (318 mm/month) as compared to the winter monsoon (88 mm/month) (Ramesh Kumar and Prasad, 1997). The summer precipitation causes substantial spatial variation in the salinity and temperature in the bay. The sea surface salinity varies from 29.1 psu closer to the continental margin on the inner shelf to 33.7 psu toward the open ocean. The warmer water input from the rivers is evident by the relatively higher sea surface temperature (29–30°C) in the river mouths’ vicinity (Durand et al., 2011; Locarnini et al., 2013). The dissolved oxygen is low at intermediate depths (∼100–1,000 m), with concentration being as low as 5 μM (Wyrtki, 1971; Rao et al., 1994; Sardessai et al., 2007; Bristow et al., 2017). The shallower inner shelf water in the vicinity of the river has a higher dissolved oxygen. The chlorophyll-a concentration varies from ∼0.3 mg/m3 to 2.0 mg/m3 in the western Bay of Bengal, with higher concentration closer to the river mouth (NASA Goddard Space Flight Center, Ocean Ecology Laboratory, Ocean Biology Processing Group, 2014). A strong wind-induced upwelling spread over ∼40 km wide band, all along the eastern margin of India, is observed in the western Bay of Bengal, during the summer season (Shetye et al., 1991). As a consequence of this upwelling, salinity increases coastward (Shetye et al., 1991). The modern-day lysocline depth is at 2,000–2,600 m, shallowing from south to north in the Bay of Bengal (Cullen and Prell, 1984).
A total of 46 surface sediment samples (35 multi-core and 11 spade-core) were collected in 2014 and 2012, during the 308th cruise of ORV Sagar Kanya (SK 308) and RV Sindhu Sankalp (SSK 35) from the west-central Bay of Bengal, respectively (Figure 1 and Supplementary Table 1). The samples were collected along the coast perpendicular transects at regular depth intervals, starting from the continental shelf to the slope (27–2,494 m), during the pre-summer monsoon season of 2012 and 2014 (more details in Suokhrie et al., 2020). After retrieval, the top 10 cm (0–10 cm) of the sediment collected by using Ocean Science Industries Limited (OSIL) – Maxi multi-corer was sub-sampled at 1 cm interval. Half of the sediment from each section was immediately preserved in ethanol rose-Bengal solution (2 g rose-Bengal per liter of ethanol), to stain living benthic foraminifera. The top two sections (0–2 cm) were analyzed, as they contain the majority of the living benthic foraminifera in the northern Indian Ocean (Singh et al., 2018). The use of rose-Bengal stained living benthic foraminifera has been widely discussed since its introduction by Walton (1952). A study by Walker et al. (1974) compared rose-Bengal and Sudan Black B stains and reported that rose-Bengal stain identified 70% of the specimens containing protoplasm. The concern also remains in differentiating the protoplasm living at the time of sample collection with the recently dead protoplasm (Bernhard, 1988). However, a majority of the tests lying in the sediments are usually devoid of protoplasm because of reproduction, predation, or growth stages (Murray and Bowser, 2000). Also, the living protoplasm (those living at the time of staining) tend to acquire deeper and denser stain up to one additional chamber. So, if the pattern of staining can be understood thoroughly, the error of over-estimating the stained living fauna can be reduced (Linshy, 2010).
Figure 1. The location of surface sediment samples in the west-central Bay of Bengal. Multicore stations are marked with red circles and green squares represent spade core stations. The major rivers draining in the area are marked by thin black lines. The bathymetry is marked by colored contours.
The overlying bottom water in the multi-cores was collected at each station, and its salinity, pH and temperature were measured onboard by using a hand-held multimeter (S47-K/“SevenMulti”) (Supplementary Table 2). The bottom water sample was not retrieved at a few multi-core stations. For these stations, salinity, temperature, dissolved oxygen, and also all the environmental data for the 11 spade core stations were downloaded from the World Ocean Atlas 2013 (Garcia et al., 2013; Locarnini et al., 2013; Zweng et al., 2013) by using the Ocean Data View software (Schlitzer, 2016, Ocean Data View)1 (Figure 2).
Figure 2. Bottom water environmental parameters measured onboard from the water collected in multicores along with data downloaded from the World Ocean Atlas (Garcia et al., 2013; Locarnini et al., 2013; Zweng et al., 2013), and analyzed sediment characteristics in the western Bay of Bengal. (A) Salinity (psu), (B) dissolved oxygen (ml/l), (C) temperature (°C), (D) pH (The pH data in the southern part of the region is not available as we could not collect samples from the sediment-water interface), (E) coarse fraction (CF%), (F) organic carbon (%Corg), (G) organic carbon/total nitrogen (Corg/TN) and (H) calcium carbonate (CaCO3%). The stations are marked by black inverted triangles. The thin black lines mark the bathymetric contours.
The stained sediments were stored in cold-storage (4°C) for a minimum of 2 weeks and were then processed following the standard procedure (Manasa et al., 2016; Singh et al., 2018; Suokhrie et al., 2020). The excess ethanol rose-Bengal was removed, and the sediments were freeze-dried. The dried samples were then weighed, and ∼2 g of the sediment was finely powdered for total carbon, nitrogen and inorganic carbon analysis. The rest of the sample was wet sieved by using a 63 μm sieve. The >63 μm material coarse fraction (CF) was dried, weighed and then transferred into plastic vials. A representative aliquot of the CF was weighed to pick a minimum of 300 living benthic foraminifera, wherever possible. All available living benthic foraminifera were picked from a fixed 0.1 g CF, in samples where a limited number of specimens were found. The picked specimens were mounted on the micropaleontological slides for counting and identification. Only those specimens with precise pink coloration with more than one chamber completely stained were picked and counted for living benthic foraminifera. All specimens were identified by using the previously published literature. The foraminiferal treatise (Loeblich and Tappan, 1988) was followed for genus confirmation, and for species confirmation, we have referred to the foraminiferal catalog (Ellis and Messina, 1940–2015). The genus of a few species have been shifted, as per the taxonomic shifts. Bolivinellina has been shifted from Bolivina following Saidova (1975). One species (Eubuliminella cassandrae) was also shifted from genus Bulimina to Eubuliminella following Revets (1993). Only those references mentioned in the main text of the paper have been added to the reference list. All other species identification references are mentioned in Supplementary Table 4 for further reading.
The sedimentary characteristics, namely total inorganic carbon (IC), total nitrogen (TN), and total carbon (TC), were analyzed by using dried and finely powdered sediment (∼10 mg weight). TIC was analyzed by using UIC CM 5015 CO2 Coulometer and TC, TN by CNS elemental analyzer (Thermo Fisher Scientific). Calcium carbonate (CaCO3) was calculated from IC and organic carbon (Corg) was estimated by subtracting TIC from TC.
To draw meaningful inferences from the large dataset of the relative abundance of all benthic foraminifera species identified from the western Bay of Bengal, 54 dominant species (≥3% abundance at a minimum of two stations) (Figures 3–6), were used for the Cluster analysis. Bray Curtis similarity index was applied through Q-mode cluster analysis by using Primer 6.1.10 software. The major clusters were identified with similar species having a particular percentage contribution in each cluster. The dominant assemblage representing a cluster and the relative contribution of each species to the respective cluster was identified by using the Simple Percentage (SIMPER) program at 100%. The canonical correspondence analysis (CCA) was done to understand the influence of multiple parameters on the dominant species. The multivariate statistical package version 3.1 (Kovach, 1998) was used for CCA. The CCA provides a better insight into the cumulative effect of a set of ambient environmental parameters on the abundance of species.
Figure 3. The dominant living benthic foraminifera (relative abundance ≥ 3% at atleast three stations) in the west-central Bay of Bengal (1) Hormosinella distans (Brady, 1881); (2–4) Hormosinella guttifera (Brady, 1881); (5) Reophax scottii (Chaster, 1892); (6) Ammobaculites agglutinans (d’Orbigny 1846); (7) Ammoglobigerina globigeriniformis (Parker and Jones, 1865); (8) Trochamminopsis quadriloba (Hoglund, 1947); (a) Ventral view (b) Dorsal view; (9) Eggerella propinqua (Brady, 1884); (10) Textularia oceanica Cushman, 1932; (11) Spiroloculina convexa Said, 1949, (a–c); (12) Quinqueloculina bicarinata (d’Orbigny, 1878); (a) 4-chambered view (b) Apertural view (c) 3-chambered view; (13, 14) Bolivinellina earlandi (Parr, 1950); (15) Bolivinellina lucidopunctata (Conato, 1964); (16) Bolivinellina pacifica (Cushman and McCulloch, 1942); (17) Bolivinellina translucens (Phleger and Parker, 1951); (18, 19) Brizalina dilatata (Reuss, 1850); (20) Bolivina mekranensis (Haque, 1970); (21) Brizalina spathulata (Williamson, 1858); (22) Latibolivina lepida; (23) Latibolivina persiensis (Lutze, 1974); (24) Cassidulina carinata Silvestri, 1896; (a) Ventral view showing aperture (b) Dorsal view; (25) Cassidulina laevigata (d’Orbigny, 1826), (a) Dorsal view, (b) Ventral view showing aperture. Scale bar is 100 μm.
Figure 4. (1) Globocassidulina oblonga (Reuss, 1850); (a) Ventral view (b) Dorsal view; (2, 3) Islandiella cushmani (Stewart and Stewart, 1930); (a,b) Side views (c) Apertural view; (3) Eubuliminella cassandrae (Revets, 1993); (4, 5) Eubuliminella exilis (Brady, 1884); (6) Hopkinsinella glabra (Millett, 1903); (7) Bulimina cf. delreyensis (Cushman and Galliher, 1934); (8) Bulimina marginata (d’Orbigny, 1826); (9) Globobulimina globosa (Leroy, 1944) (10); Protoglobobulimina pupoides (d’Orbigny 1846); (11) Uvigerina globulosa (Egger, 1895); (12, 13) Neouvigerina ampullacea (Brady, 1884); (14) Uvigerina peregrina (Cushman, 1923); (15) Fursenkoina pauciloculata (Brady, 1884); (16) Fursenkoina spinosa (Heron-Allen and Earland, 1932); (17) Fursenkoina schreibersiana (Czjzek, 1848); (18) Rutherfordoides rotundiformis (McCulloch, 1977); (19) Suggrunda alata (Seguenza 1862); (a) Side view (b) Lateral view showing aperture; (20) Suggrunda semiclara (McCulloch 1977); (a) Side view (b) Lateral view showing aperture; (21) Baggina irregularis (McCulloch, 1977); (a) Ventral view (b) Dorsal view. Scale bar is 100 μm.
Figure 5. (1) Cancris oblongus (Williamson, 1858); (a) Ventral view (b) Dorsal view; (2) Eponides cribrorepandus (Asano and Uchio, 1951); (a) Ventral view (b) Dorsal view; (3) Gavelinopsis mira (Cushman, 1922); (a) Ventral view (b) Lateral view (c) Dorsal view; (4) Pseudoeponides japonicum (Uchio, 1950); (a) Dorsal view (b) Ventral view; (5) Nonionella auris (d’Orbigny, 1839); (a) Side view (b) Apertural view (c) Side view showing the last chamber flap covering the umbilicus; (6) Nonionella limbato-striata (Cushman 1931); (7) Nonionellina labradorica (Dawson, 1860); (a,c) Side views (b) Apertural view; (8) Melonis (?) chathamensis (McCulloch, 1977); (a,c) Side views (b) Apertural view; (9) Pullenia bulloides (d’Orbigny, 1846); (a,b) Side views (c) Apertural view. Scale bar is 100 μm.
Figure 6. (1) Chilostomella oolina Schwager 1878; (2) Gyroidinoides subzelandica Hornibrook, 1961; (a) Dorsal view (b) Apertural view (c) Ventral view; (3) Rotaliatinopsis semiinvoluta (Germeraad, 1946); (a,c) Side views (b) Apertural view; (4) Hanzawaia nipponica Asano, 1944; (a) Ventral view (b) Apertural view (c) Dorsal view; (5) Ammonia tepida (Cushman, 1926); (a) Dorsal view (b) Ventral view. Scale bar is 100 μm.
The salinity at the sediment-water interface was higher in the shallow regions than at the deeper regions. The salinity ranged from ∼32.15 psu to 35.2 psu (Figure 2A). The dissolved oxygen varies from ∼0.3 ml/l to a maximum of ∼4.9 ml/l. The dissolved oxygen is low within the depth range of ∼100–1,000 m (Figure 2B). The previous reports suggest excessively low oxygen concentration (as low as 18 nM) at ∼150–450 m (Bristow et al., 2017). The temperature at the sediment-water interface was relatively warmer (>23°C) in the shallow coastal zone as compared to the deeper region (<17°C) (Figure 2C). The seawater pH at the spade core stations was not available, and hence the data from the multicore stations only are plotted. The measured pH at the sediment-water interface in the study area ranges from 7.5 to 8.1. The region off Godavari and Pennar rivers has higher pH than off the Krishna river (Figure 2D).
The fraction retained on the sieve (>63 μm) is considered as the CF and comprises both biogenic remains (shells and tests), and terrigenous material (quartz, mica, feldspars, and others). A relatively more CF is observed in the southern segment and at the shallower and deeper stations (Figure 2E). Overall, the upper slope stations (∼250–1,000 m) have a comparatively higher finer fraction. CF varied from ∼0.5% to an enormously high percentage upto ∼90% at the southern stations. The influence of riverine influx on CF, by contributing terrigenous material, is evident from the increase in CF in front of the river mouths, especially in the southern part of the study area (SC42, SC43, and SC44). Interestingly, the increased CF is also observed in the deeper region and is attributed to increased contribution from biogenic remains, including foraminiferal tests (Figure 2E).
The organic carbon varied from 0.3 to 1.8% in the west-central Bay of Bengal (Supplementary Table 2). The maximum %Corg was at the deeper depths. The influence of riverine discharge by contributing terrigenous organic matter and the fine fraction is also evident (Figure 2F). The organic carbon comes both from the terrigenous influx as well as marine productivity. These two sources’ relative contribution is delineated by the organic carbon to nitrogen ratio of the organic matter (Corg/TN). Generally, Corg/TN > 6.0 is considered to be of terrestrial input, as vascular plants have nitrogen depleted organic matter (Müller and Mathesius, 1999; Ramaswamy et al., 2008), and values < 6.0 are used to infer marine input. A majority of the Corg/TN values are >6.0 and hence suggests that the organic matter is mainly contributed by terrestrial sources rather than by marine productivity. Expectedly, the maximum Corg/TN is infront of the Godavari, Krishna, and Cauvery rivers (SC37, SC38, MC30, MC31, SC40, SC39, MC27, and MC28) (Figure 2G).
The biogenic skeletal remains mainly contribute CaCO3 to the sediments. CaCO3 concentration in the west-central Bay of Bengal ranged from 1.3 to 87.2%. CaCO3 concentration increases toward the southern part of the west-central Bay of Bengal. Additionally, CaCO3 is low at a few stations on the lower slope (depth >1000 m), likely due to a reduction in the total foraminiferal abundance, which mainly contributes to the CaCO3 in the sediments at these depths (Figure 2H).
A total of 263 living benthic foraminifera have been identified (Supplementary Tables 3, 4). The cluster analysis groups similar objects together to draw meaningful inferences from a large dataset like the number of species and their relative abundance in the western Bay of Bengal (Kaufman and Rousseeuw, 2009; Khare et al., 2017). Based on the distribution at different stations, 54 dominant species were selected for the cluster analysis. We delineate four major clusters at 20% similarity index, other than a few insignificant ones (Figure 7). The clusters were named as Cluster I, II, III, and IV. The dominant assemblage representing a cluster and each species’ relative contribution to the respective cluster is described below.
Figure 7. Cluster analysis using Bray-Curtis similarity at 20% similarity level. The four identified clusters have been shaded in different colors as Cluster I, II, III, and IV.
This cluster is represented by six stations, namely SC45, MC36, MC32, SC36, MC24, and MC09. The stations in this cluster are from a shallow depth (107–274 m) except for one station MC36 (1,511 m). The similarity percentage (SIMPER) analysis shows that this cluster has an average similarity of 20.59% consisting of 12 species. The dominant species are Eubuliminella exilis (24.88%), Bolivinellina earlandi (19.89%), Fursenkoina spinosa (12.54%), Bolivinellina lucidopunctata (8.76%), and Globobulimina globosa (6.48%).
Fursenkoina spinosa (15.64%), Eubuliminella cassandrae (11.41%), Rotaliatinopsis semiinvoluta (11.07%), Uvigerina peregrina (10.4%), and Cassidulina laevigata (8.26%) are the dominant species in this cluster having an average similarity of 51.31%. This cluster represents a depth range of 500–1,518 m.
This cluster is represented by five stations (MC15, MC2, MC37, MC18, and MC38) representing a depth range of ∼1,940–2,494 m with a similarity of 47.62%. Twelve species comprise of this cluster and the five most dominant ones are Bulimina cf. delreyensis (18.59%), Bulimina marginata (18.14%), Hormosinella guttifera (9.05%), Cassidulina laevigata (8.02%), and Gyroidinoides subzelandica (6.18%).
The SIMPER analysis shows that the average similarity of this cluster is 34.53%. The representative benthic foraminiferal assemblage of this cluster includes nine species of which the five most dominant ones are listed here, Nonionellina labradorica (37.85%), Hanzawaia nipponica (17.58%), Brizalina dilatata (17.31%), Ammonia tepida (4.71%), and Nonionella limbato-striata (4.08%).
From the spatial distribution of various clusters, it is clear that Cluster I, Cluster II and Cluster III, and Cluster IV follow a depth zonation (Figure 8). Cluster IV is confined to the shallow water depth. Cluster I represents a shallow to intermediate depth zone covering the upper slope region. Cluster II mainly comprising of angular asymmetrical foraminifera, represents a wide range of intermediate water depth to deep water depth locations. The assemblages in this cluster consist of species that are indicative of an oxygen-deficient region. The species in Cluster III represent the deep-water species.
Figure 8. Distribution of the four clusters in the western Bay of Bengal. A clear depth preference of the clusters is evident. Cluster I and II, intermediate water; Cluster III, deep water; and Cluster IV, shallow water. The black triangles represent the sampling stations.
The dominant effect of one or a combination of several parameters, out of a set of multiple parameters in the field, on living benthic foraminiferal species, can be identified from CCA. Out of the 54 dominant species, only 40 species showed a significant relationship with atleast one of the ambient environmental parameters (Figure 9). The rest of the species do not show a significant relationship with any particular parameter, as evident from the close proximity of these species to the centroid in the CCA biplot (Ter Braak, 1986). From CCA, it is evident that the dissolved oxygen, CF, and temperature, are the most significant parameters controlling the living benthic foraminifera species distribution in the west-central Bay of Bengal. Besides these dominant factors, Corg, salinity, and Corg/TN also influence the distribution of living benthic foraminifera.
Figure 9. Canonical correspondence analysis (CCA) between species abundance and environmental parameters. The arrows represent environmental parameters-Corg, organic carbon; Corg/TN, organic carbon/total nitrogen; CF, coarse fraction; DO, dissolved oxygen; T, temperature; S, salinity; D, depth. The triangles represent the species (modified from Suokhrie et al., 2020).
Ammobaculites cf. agglutinans, Quinqueloculina bicarinata, Bolivinellina pacifica, Brizalina dilatata, Latibolivina lepida, Latibolivina persiensis, Fursenkoina pauciloculata, Eponides cribrorepandus, Nonionella auris, Nonionella limbato-striata, Nonionellina labradorica, Hanzawaia nipponica, and Ammonia tepida were positively correlated with dissolved oxygen and organic carbon, whereas negatively correlated with temperature (Figure 9). Ammobaculites cf. agglutinans, an agglutinated benthic foraminifer, was present only at four stations, all shallower than ∼60 m. Quinqueloculina bicarinata was present at three stations, all shallower than 56 m. Even Bolivinellina pacifica was present only upto 76 m. Brizalina dilatata was found at stations mostly within depths of ∼300 m, beyond which <1% abundance was observed at two stations. Latibolivina lepida was found only at two stations with abundance varying from 4.2% (53 m) to 6.2% (52 m). Likewise, Latibolivina persiensis was also present at shallow depths between 40 and 76 m, beyond which it was absent. Fursenkoina pauciloculata was abundant over a wider depth range (∼30–1,000 m) but the highest abundance was at the shallower depths. Eponides cribrorepandus was present only at three stations with relative abundance varying from 0.15% (500 m water depth) to 25% (107 m water depth). It was totally absent at stations deeper than ∼500 m. Nonionella auris was present at most of the stations and abundant between ∼200 m and ∼1,000 m. Nonionellina labradorica was present upto a depth of ∼750 m. Hanzawaia nipponica, Ammonia tepida and Nonionella limbato-striata were abundant at stations shallower than ∼150 m.
The species having a negative correlation with dissolved oxygen and a positive correlation with temperature and salinity were Reophax scottii, Eggerella propinqua, B. earlandii, B. lucidopunctata, Bolivinellina translucens, Brizalina spathulata, Eubuliminella cassandrae, E. exilis, Hopkinsinella glabra, Protoglobobulimina pupoides, Uvigerina globulosa, Uvigerina peregrina, Fursenkoina spinosa, Rutherfordoides rotundiformis, Suggrunda semiclara, Baggina irregularis, Cancris oblongus, Pullenia bulloides, Chilostomella oolina, and Rotaliatinopsis semiinvoluta (Figure 9).
Agglutinated benthic foraminifera, Reophax scottii was abundant only at four stations with abundance ranging from 0.14% (1,069 m) to 40.96% (259 m). Other agglutinated foraminifer, Eggerella propinqua was abundant at depths varying from ∼200 m to ∼700 m except for two shallow stations at 27 m and 31 m. The species of genus Bolivinellina reported in the west-central Bay of Bengal include B. translucens, B. earlandi, and B. lucidopunctata. The abundance of these three species was influenced by bathymetry, with B. translucens and B. lucidopunctata being the most abundant between 76 m and ∼500 m, while B. earlandi was abundant from 76 m upto ∼2,000 m. Brizalina spathulata was the most abundant between ∼100 m and 270 m. Eubuliminella cassandrae was abundant at depths >∼150 up to ∼1,000 m, whereas E. exilis was present only upto a depth of 274 m. Hopkinsinella glabra was present only at 11 stations, with most of the stations being within ∼200–900 m. Protoglobobulimina pupoides was present only at depth >∼250 m. Uvigerina globulosa was present at depths >∼750 m except at one shallow station (55 m). Uvigerina peregrina was also dominant at deeper than 190 m with a maximum abundance of 21%. Fursenkoina spinosa was also abundant at stations >200 m deep, with a relative abundance ranging from 0 to a maximum of 36%. Rutherfordoides rotundiformis was present at stations deeper than ∼100 m, except for one station at ∼40 m. Suggrunda semiclara was present at seven stations, of which four stations were on the upper slope, within a depth range of ∼390–500 m. Baggina irregularis, Pullenia bulloides and Rotaliatinopsis semiinvoluta were abundant at stations deeper than 500 m. Cancris oblongus was present only at nine stations with the highest abundance of 4.3% at 500 m depth. Similarly, Chilostomella oolina with a maximum relative abundance of 6.2% was present at stations deeper than ∼200 m. Interestingly the maximum abundance of all the species listed above was within the oxygen deficient zone (∼100–1,000 m).
Ammoglobigerina globigeriniformis, Trochammina quadriloba, Islandiella cushmani, Bulimina marginata, Bulimina delreyensis, Gavelinopsis mira, and Gyroidinoides subzelandica were positively correlated with CF and Corg/TN (Figure 9). Ammoglobigerina globigeriniformis, an agglutinated foraminifer was present at almost all the stations with the highest abundance (∼19%) at ∼1,980 m. Trochammina quadriloba, another agglutinated foraminifer, was abundant at stations deeper than ∼250 m, except for three shallower stations with abundance ≤2%. Islandiella cushmani was present at most of the stations in the study area but mostly abundant at stations deeper than ∼200 m. Bulimina marginata, Bulimina delreyensis, Gavelinopsis mira, and Gyroidinoides subzelandica were abundant at the deeper stations (>∼250 m) in the west-central Bay of Bengal. Although the CCA biplot shows influence of both CF as well as Corg/TN on these species, the influence of CF was more significant. Interestingly, Hormosina guttifera shows a positive correlation only with CF.
Interestingly, the abundance of living (stained) benthic foraminifera between ∼200 m and ∼1,000 m was higher (∼1,300 specimen/g sediment), as compared to the continental shelf (∼300 specimen/g sediment upto ∼200 m) as well as at depths deeper than ∼1,500 m (∼46 specimen/g sediment) (Suokhrie et al., 2020). We suggest that the lower dissolved oxygen concentration with an average of ∼0.5 ml/l within the depth range of ∼100–1,000 m and corresponding high organic matter content, dominantly control the living benthic foraminiferal population at these depths in the western Bay of Bengal. The high %Corg concentration between ∼250 m and 1,500 m water depth is attributed to the relatively finer sediments at these depths. The finer sediments can preserve more Corg (Mayer, 1994). Additionally, there is an enormous influx of both freshwater and sediments in the northern Bay of Bengal. The riverine influx is also a source of organic carbon. The immense terrestrial organic carbon contribution is further confirmed by a clear pattern of very high Corg/TN (∼20.0) close to the river mouth as compared to low Corg/TN, indicating marine organic carbon, away from the river mouth (>6.0).
The foraminiferal abundance is low at stations shallower than ∼100 m water depth with sediments dominated by terrestrially sourced organic matter. This low abundance is attributed to the taphonomic processes, including more disturbance or turbulence from the riverine influx leading to less proliferation and diversification of benthic foraminifera (Berkeley et al., 2014). Besides, the %Corg at the shallow depths is also lower than the intermediate water depth, except in front of the Godavari river mouth. The enormous freshwater runoff in the bay results in ocean surface stratification and an oligotrophic condition and thus the low organic matter on the shelf. This is also a factor for the low living benthic foraminiferal abundance at the shallow depth stations as the organic carbon is the food for benthic foraminifera. The lack or low organic carbon content in sediments is detrimental to benthic foraminifera (Duffield et al., 2014). The coarser sediments close to the river mouth are one reason for the lower %Corg despite the high primary productivity at these depths, as evident from the chlorophyll-a content (NASA Goddard Space Flight Center, Ocean Ecology Laboratory, Ocean Biology Processing Group, 2014). The intermediate depths within ∼100–1,000 m have abundant organic matter, which leads to reduced bottom water DO, and hence the abundance of total living abundance is a positive response to the organic matter supply. The increased foraminiferal abundance at these depths is also due to the significantly reduced predatory pressure as the macrofauna are comparatively more susceptible to oxygen-deficient environments. These depths are dominated by opportunistic species that thrive well in low DO concentrations. With a decrease in organic matter, the DO concentration increases beyond ∼1,000 m water depth. A drastic decrease in foraminiferal abundance is seen below ∼1,000 m water depth in the study area, and is attributed to the increase in predatory pressure. Additionally, the environment is more food limiting than oxygen and leads to a significant reduction in the total living fauna.
The low diversity and species richness in front of the major rivers suggests a strong riverine influx. Both the diversity and species richness are low in the southern part, suggesting a restricted environment facilitating a select few species to survive (Suokhrie et al., 2020). Incidentally, the seawater pH is very low in front of the major river mouths. Although benthic foraminifera can tolerate a wide range of pH, comparatively less alkaline pH is detrimental to calcareous tests (Saraswat et al., 2015). Therefore, the low diversity in low pH regions is attributed to the thin-walled benthic foraminifera’s inability to survive in adverse conditions. In contrast, more diverse microenvironments in the northern part are inferred from the high diversity and richness. From our earlier work based on CCA between benthic foraminiferal abundance and ambient parameters in the oxygen-deficient zone of this region, we inferred that the increased abundance of a few angular benthic foraminifera thriving as opportunistic species (F. spinosa, E. cassandrae, U. peregrina, R. semiinvoluta, S. semiclara, E. propinqua, and B. irregularis) contributes to the overall more population of living benthic foraminifera in the oxygen-deficient zones on the slope. The dominance of a few opportunistic species is supported by low diversity and richness at these depths (Suokhrie et al., 2020). The present study’s cluster analysis groupings also indicate the abundance of angular forms at the depths where dissolved oxygen is low. Such a decrease in diversity of living benthic foraminifera in a low dissolved oxygen environment has also been reported from the oxygen minimum zone (Jannink et al., 1998; Gooday et al., 2000; Schumacher et al., 2007; Suokhrie et al., 2020).
From cluster analysis, we delineate three faunal assemblages based on significant correlation of the assemblages to a similar set of environmental parameters in the study area. Incidentally, the assemblages dominate different depth zones as well. The details of the representative assemblages are discussed below.
This assemblage comprises of Cluster IV. The dominant species are N. labradorica, H. nipponica, B. dilatata, A. tepida, and N. limbato-striata with minor contributions from F. pauciloculata, B. pacifica, L. persiensis, and N. auris (Figure 10). These species are abundant on the continental shelf (<∼100 m water depth). The Assemblage I includes only calcareous benthic foraminifera. Nonionellina labradorica is a dominant species in this assemblage and has also been reported from the Corg rich fjords in the Arctic (Shetye et al., 2011). This species has been referred to as Arctic-boreo species (Seidenkrantz, 1993). Its high abundance is also reported from the oxygen-depleted environments where it sequesters chloroplast as it enables it to survive even in the anoxic conditions (Cedhagen, 1991; Bernhard and Bowser, 1999). In our study area, however, the abundant presence of N. labradorica is associated with the shallow inner shelf warm well-oxygenated waters with low organic carbon. We report another species belonging to the Assemblage I, namely H. nipponica, with its preference for shallow, well-oxygenated waters. From the Bay of Bengal, this species has been reported as a ‘turbidity sensitive species’ (Jayaraju et al., 2010).
Figure 10. Surface distribution (relative abundance) of dominant living benthic foraminifera from Cluster IV representing Assemblage I and having a significant positive correlation with dissolved oxygen and temperature. Nonionella labradorica, Hanzawaia nipponica, Brizalina dilatata, Ammonia tepida, and Nonionella limbato-striata. The stations are marked by black inverted triangles. The thin black lines mark the bathymetric contours.
Similarly, B. dilatata is also abundant on the shallow shelf, with a rare presence at a few stations upto ∼700 m. Its abundance in shallow to intermediate water depth is also reported by Gooday (1993) and Bernhard and Gupta (1999). Brizalina dilatata is also commonly reported from low oxygenated and high organic carbon-rich environments (Gupta and Machain-Castillo, 1993; Rouchy et al., 1998; Kuhnt et al., 2007). In the present study, this species correlates well with the shallow and warmer waters. The characteristic shallow-water species A. tepida is also abundant on the shelf of the present study. Ammonia tepida is widely distributed over estuaries, shelf and salt marshes (Murray, 1991; Nisha and Singh, 2012). Most workers have reported A. tepida as a pollution-sensitive species (Bergin et al., 2006; Burone et al., 2007; Frontalini and Coccioni, 2008; Elshanawany, 2011; Debenay et al., 2015; Schintu et al., 2016; Saalim et al., 2017). Ammonia tepida is also reported as a dominant species in the modern intertidal and estuarine areas (Nigam, 1984; Adarsh and Rajeshwara Rao, 2010). We also report A. tepida being abundant in shallow and warm water with a moderate organic matter supply in the study area. Nonionella limbato-striata is another dominant species in this assemblage, preferring the shallow and well-oxygenated waters.
Fursenkoina pauciloculata is a minor species in this assemblage. Bolivinellina pacifica, another dominant species of this assemblage, was earlier reported from the shallow water depths (Saidova, 2010), same as that in this study area. Latibolivina persiensis is reported from shallow depth (Sliter, 1969, 1970; Panchang and Nigam, 2014). It has been found to prefer oligotrophic and well-oxygenated water (Abu-Zied, 2013), similar to its distribution in this study, where this species is present only in shallow, well-oxygenated water (upto 76 m). Another minor species is N. auris in this assemblage.
The species belonging to Assemblage I representing the shallow locations indicate that they are positively correlated with dissolved oxygen, temperature and Corg. The dissolved oxygen concentration is high (>3.0 ml/l) and the organic matter content is low (∼0.9%) with warmer temperature on the shelf. Thus, Assemblage I represents a shallow, well-oxygenated, warm environment with relatively high organic matter in the sediments.
The dominant species in this assemblage are E. exilis, B. earlandi, F. spinosa, B. lucidopunctata, G. globosa from Cluster I and F. spinosa, E. cassandrae, U. peregrina, R. semiinvoluta, C. laevigata from Cluster II (Figure 11).
Figure 11. Relative abundance of species representing intermediate depth assemblage (Assemblage II), having significant negative correlation with dissolved oxygen and positive correlation with organic carbon. Eubuliminella exilis, Bolivinellina earlandi, Fursenkoina spinosa, Bolivinellina lucidopunctata, Globobulimina globosa, Fursenkoina spinosa, Eubuliminella cassandrae, Uvigerina peregrina, Rotaliatinopsis semiinvoluta, and Cassidulina laevigata. The stations are marked by black inverted triangles. The thin black lines mark the bathymetric contours.
Eubuliminella exilis has been reported as deep infaunal taxa (Jorissen, 1999) and an indicator of low oxygenated environments and unchanged supply of organic matter (Caralp, 1989; Jannink et al., 1998; Schumacher et al., 2007). Amongst the dominant species belonging to Assemblage II, F. spinosa is a shallow infaunal species inhabiting waters upto a depth of ∼120 m (Phleger, 1963). In the present study, F. spinosa is abundant at depths deeper than ∼200 m, indicating less depth dependence and a large influence of dissolved oxygen. The relative abundance of infaunal foraminifera is controlled by the availability of both the metabolizable organic matter and dissolved oxygen. The infaunal foraminifera are abundant in organic matter rich but oxygen-deficient environments (Jorissen et al., 1995; Van der Zwaan et al., 1999). The higher relative abundance of F. spinosa at depths >200 m within the low DO concentration in the western Bay of Bengal, is more in line with its infaunal nature. The species belonging to Bolivinellina have been reported from shallow regions (Nigam and Chaturvedi, 2006; Nagendra et al., 2011; Panchang and Nigam, 2014; Gandhi et al., 2016). Both B. earlandi and B. lucidopunctata have been recorded from the upper slope region in the study area. G. globosa is another dominant species in this assemblage. Generally, rectilinear foraminifera comprising of genera like Bolivina, Brizalina, Bulimina, Fursenkoina, and Uvigerina have previously been reported as a common indicator of low oxygenated environments by several workers (Gupta and Machain-Castillo, 1993; Kaiho, 1994; Murray, 2006; Nigam et al., 2009; Mazumder and Nigam, 2014; Das et al., 2017; Verma et al., 2018). In the study area, these species are abundant at the stations falling within a depth range of ∼>100 to ∼1,500 m where DO concentration is minimum (0.5 ml/l). All the major species of this assemblage are significantly negatively correlated with the dissolved oxygen, suggesting that they are well adapted to low dissolved oxygen (Suokhrie et al., 2020). The type species of E. cassandrae is E. exilis (Revets, 1993). The ecology of E. cassandrae has been rarely described. However, like its type species, E. cassandrae is also abundant at the intermediate depth locations with the low oxygenated environment. Uvigerina peregrina is also reported from this assemblage, suggesting its preference to low dissolved oxygen at these depths, which is in line with its reported dominance within OMZ (Hermelin and Shimmield, 1990). It has also been described as a shallow dysoxic-infaunal species (Das et al., 2017). Rotaliatinopsis semiinvoluta has been well documented as a low oxygen tolerant species reported from the Arabian Sea OMZ at intermediate water depths (den Dulk et al., 1998; Jannink et al., 1998; Caulle et al., 2015), and a similar preference to low dissolved oxygen is also found in our study. Cassidulina laevigata is another intermediate to deep-water fauna and is a dominant species in this assemblage. Amongst the other minor species of this assemblage, R. scottii is predominantly infaunal (Kaminski et al., 1988) and a characteristic fauna of muddy substrate, sometimes present in low oxygenated fjords (Murray, 1970; Filipsson and Nordberg, 2004). The substrate seems to be a less influential factor for this species in the west-central Bay of Bengal. Here, R. scottii is more indicative of low oxygenated conditions as its abundance increases at the stations with low DO values. Bolivina spathulata has been described as a deep infaunal and dysoxic species occurring in high organic flux environments (Bernhard and Gupta, 1999; Das et al., 2017). Furthermore, B. spathulata has been associated with a more saline environment (Eris et al., 2011), as is also seen in the study area. Brizalina spathulata is often the last species to disappear before oxygen deficiency starts to inhibit any benthic life (Jonkers, 1984). In the west-central Bay of Bengal, B. spathulata is most common at stations between ∼120 m and ∼250 m. A significant drop in DO is observed at these depths. Uvigerina peregrina and E. cassandrae are minor species in this assemblage. Trochamminopsis quadriloba is more abundant at ∼2,000 m. Alve et al. (2011) reported the abundant presence of T. quadriloba at ∼100 m. However, the depth does not seem to significantly control its abundance (Buzas et al., 1993).
The ecology of S. semiclara is rarely described. Here, its abundance in the intermediate waters suggests its preference for low oxygen and relatively more saline environment. Pullenia bulloides was earlier reported as a part of the assemblage representing the Antarctic Bottom Water (AABW) (Corliss, 1979). In the present study, P. bulloides prefers lower levels of oxygen, high food supply and deeper depth, as also reported by other workers [north Atlantic-Schnitker, 1979; southwestern Indian Ocean- Corliss, 1983; Gooday, 1994; south Atlantic-Mackensen et al., 1995; Off Pakistan (Arabian Sea)-Schumacher et al., 2007; southeastern Indian Ocean-Verma et al., 2013; Arabian Sea-Caulle et al., 2015]. Although the type and preservation status of the organic matter also matters (Nomaki et al., 2005; Duffield et al., 2014), the organic carbon (Corg) is a good indicator of the food availability for foraminifera. Some foraminifera prefer the “easily metabolizable food particles” while the infaunal forms mainly tolerate more refractory organic matter (Gooday, 1993; Jorissen et al., 1995). Rutherfordoides rotundiformis was found in extremely low oxygen conditions and in sapropelic sequences (Rohling et al., 1997; Mercone et al., 2001; Abu-Zied et al., 2008). Rutherfordoides rotundiformis is the most abundant at the stations with minimum DO concentration in the present study. Chilostomella oolina is a deep infaunal species abundant in low oxygenated environments similar to its generic preferences (Corliss, 1991; Gooday and Rathburn, 1999; Jorissen, 1999; Schmiedl et al., 2003; Schumacher et al., 2007; Kuhnt et al., 2013). The survival and abundance of C. oolina in low oxygenated environment is attributed to its nitrate respiration strategy (Kuhnt et al., 2013). Cassidulina carinata and G. globosa are also part of this intermediate assemblage. Cassidulina carinata is part of the assemblage preferring high food supply (Gupta and Thomas, 1999), while G. globosa is often described as an abyssal species (Takata et al., 2013). Bolivina earlandi have been described as a deep infaunal and dysoxic species occurring in high organic flux environments (Bernhard and Gupta, 1999; Das et al., 2017). Genus Chilostomella with the characteristic unornamented and thin-walled tests was an abundant genus among organic carbon-rich and less oxygenated sediments (Kaiho and Nishimura, 1992). Trochamminopsis quadriloba is another minor species in this assemblage as well as the previous assemblage. Other minor species also include F. pauciloculata abundant in the low oxygenated regions of the Indian waters (Mazumder and Nigam, 2014) and N. auris is an indicator of high organic carbon rich environments (Wefer et al., 1994; Bhaumik and Gupta, 2007). In the west-central Bay of Bengal, B. marginata is a deep infaunal species (Jorissen, 1999). Both B. marginata and B. cf. delreyensis, are abundant at depths deeper than ∼2,000 m, on the slope. Although B. marginata is often used as oxygen-deficient water proxy, we report a strong negative influence of temperature on the abundance of both B. marginata and B. cf. delreyensis, in the study area. Baggina irregularis is also part of the same assemblage that prefers less oxygenated waters. This species’ ecology is not well known, although they are reported from the Bay of Bengal (Panchang and Nigam, 2014). In short, all the species described above are abundant in more saline, organic matter rich and poorly oxygenated environment.
A set of 12 species from Cluster III (B. cf. delreyensis, B. marginata, H. guttifera, C. laevigata, G. subzelandica, C. carinata, G. mira, N. ampullacea, P. bulloides, U. peregrina, A. globigeriniformis, and T. quadriloba) has been clubbed together in Assemblage III. The dominant species of this assemblage are B. cf. delreyensis, B. marginata, H. guttifera, C. laevigata, and G. subzelandica (Figure 12). Bulimina marginata is a deep infaunal species (Jorissen, 1999). Both B. marginata and B. cf. delreyensis, are abundant at depths deeper than ∼2,000 m, on the slope. Although, B. marginata is often used as oxygen deficient water proxy, the abundance of these two species at deeper colder waters (>1,500 m) is suggestive of a strong negative influence of temperature on the abundance of both B. marginata and B. cf. delreyensis. The dominant agglutinating species in this assemblage is H. guttifera, which represents deep-water assemblages (Bernhard et al., 2009; Enge et al., 2012). C. laevigata is a shallow to deep infaunal species (Singh et al., 2012). Gyroidinoides subzelandica is a part of the same assemblage. All these species are significantly negatively correlated with the ambient temperature as the deeper waters have colder temperature. Additionally, the species in Assemblage III are also influenced by the type of organic matter (marine origin) and sediment texture, evidenced from the relatively lower Corg content in the samples at these depths.
Figure 12. Surface distribution (relative abundance) of dominant living benthic foraminifera representing deep water assemblage (Assemblage III), having a significant negative correlation with CF and Corg/TN, in the west-central Bay of Bengal. Bulimina cf. delreyensis, Bulimina marginata, Hormosinella guttifera, Cassidulina laevigata, and Gyroidinoides subzelandica. The stations are marked by black inverted triangles. The thin black lines mark the bathymetric contours.
The other minor species in this assemblage like C. carinata, U. peregrina and P. bulloides also represent Assemblage III indicating that these species have a wide range of abundance from the intermediate to the deep waters in the study area. U. peregrina is a dominant species in Assemblage III which suggests its preference for the environmental conditions prevalent at the intermediate waters rather than the deep waters. Gavelinopsis mira, N. ampullacea in Assemblage III, also have a significant preference for colder water with lower CF abundance and marine organic matter.
The spatial distribution of living benthic foraminifera and its comparison with the ambient parameters in the riverine influx dominated west-central Bay of Bengal, suggests a strong influence of multiple ecological parameters, mainly the dissolved oxygen, CF, and temperature to some extent. The organic carbon content is high (∼1.4% on average) at intermediate depths (∼100–1,000 m) where bottom water dissolved oxygen is at its lowest (∼0.5 ml/l on average). The maximum living benthic foraminiferal abundance at these depths with limited diversity indicates high abundance of a few opportunistic species. The high Corg/TN suggests that Corg% is mostly of terrestrial origin, especially closer to the river mouths. Three significant faunal assemblages delineated from the statistical analysis give more insight on the ecological preferences of foraminifera in the west-central Bay of Bengal. The shallow water (∼27–100 m) assemblage comprising of N. labradorica, H. nipponica, B. dilatata, A. tepida, and N. limbato-striata represents well oxygenated warmer waters with low Corg% and coarser sediment. The intermediate water (∼145–1,500 m) assemblage comprising of E. exilis, B. earlandi, F. spinosa, B. lucidopunctata, G. globosa, F. spinosa, E. cassandrae, U. peregrina, R. semiinvoluta, and C. laevigata assemblage represents poorly oxygenated, colder waters with high Corg%. The deep water (∼1,940–2,494 m water depth) assemblage comprising of B. cf. delreyensis, B. marginata, H. guttifera, C. laevigata, and G. subzelandica represents high Corg% but relatively higher bottom water dissolved oxygen than the intermediate water depth. We also report the specific preference of 40 dominant species each influenced by a set of environmental parameters. The ecological preferences of foraminiferal species and foraminiferal assemblages delineated from this study can be utilized as proxies in sub-surface samples to understand paleo-ecology and paleoclimatic changes from the west-central Bay of Bengal.
The original contributions presented in the study are included in the article/Supplementary Material, further inquiries can be directed to the corresponding author/s.
TS curated the data and wrote the first draft of the manuscript. RS conceptualized and investigated the research work. RN helped in the funding acquisition needed to carry out the work. All authors contributed to the article and approved the submitted version.
The authors declare that the research was conducted in the absence of any commercial or financial relationships that could be construed as a potential conflict of interest.
We are thankful to the Director, CSIR-National Institute of Oceanography, India for the permission and support. We acknowledge the financial support by the Ministry of Earth Sciences and the Department of Science and Technology, Government of India. TS acknowledge University Grants Commission (UGC), New Delhi, for the financial assistance in the form of Junior and Senior Research Fellowship. Dr. B. N. Nath is acknowledged for providing the spade core samples and Dr. C. Prakash Babu for his technical assistance with the organic carbon analysis. We also thank Mr. Shashikant Velip, for the help in collecting multi-core samples.
The Supplementary Material for this article can be found online at: https://www.frontiersin.org/articles/10.3389/fmars.2021.656757/full#supplementary-material
Supplementary Table 1 | The details of the sampling locations for multicore as well as spadecore.
Supplementary Table 2 | The details of stations and ambient physico-chemical parameters at each station. Also given are the measured sedimentary characteristics (CF, Corg, Corg/TN, and CaCO3). The salinity and pH at the sediment water interface were measured from the water retrieved in multi-core tops. The DO and other data for the spade core station were retrieved from the World Ocean Atlas 2013 (Garcia et al., 2013; Locarnini et al., 2013; Zweng et al., 2013).
Supplementary Table 3 | The relative abundance of the dominant species having atleast >3% abundance at a minimum of two stations.
Supplementary Table 4 | Check-list of living benthic foraminiferal species found in the surface sediments of the western Bay of Bengal. The maximum relative abundance of the species and the depth of the respective station is also provided.
Abu-Zied, R. H. (2013). Effect of the Red Sea brine-filled deeps (Shaban and Kebrit) on the composition and abundance of benthic and planktonic foraminifera. Arabian J. Geosci. 6, 3809–3826. doi: 10.1007/s12517-012-0641-3
Abu-Zied, R. H., Rohling, E. J., Jorissen, F. J., Fontanier, C., Casford, J. S., and Cooke, S. (2008). Benthic foraminiferal response to changes in bottom-water oxygenation and organic carbon flux in the eastern mediterranean during LGM to Recent times. Mar. Micropaleontol. 67, 46–68. doi: 10.1016/j.marmicro.2007.08.006
Adarsh, P., and Rajeshwara Rao, N. (2010). Utility of sub-surface benthic foraminiferal assemblages to decipher the paleoenvironment of the north-west Kerala coast. Int. J. Environ. Sci. 2, 1771–1782.
Altenbach, A. V. (1992). Short term processes and patterns in the foraminiferal response to organic flux rates. Mar. Micropaleontol. 19, 119–129. doi: 10.1016/0377-8398(92)90024-e
Altenbach, A. V., Lutze, G. F., Schiebel, R., and Schönfeld, J. (2003). Impact of interrelated and interdependent ecological controls on benthic foraminifera: an example from the Gulf of Guinea. Palaeogeogr. Palaeoclimatol. Palaeoecol. 197, 213–238. doi: 10.1016/s0031-0182(03)00463-2
Alve, E. (1995). Benthic foraminiferal responses to estuarine pollution; a review. J. Foraminifer. Res. 25, 190–203. doi: 10.2113/gsjfr.25.3.190
Alve, E., Murray, J. W., and Skei, J. (2011). Deep-sea benthic foraminifera, carbonate dissolution and species diversity in Hardangerfjord, Norway: an initial assessment. Estuar. Coast. Shelf Sci. 92, 90–102. doi: 10.1016/j.ecss.2010.12.018
Barmawidjaja, D. M., Jorissen, F. J., Puskaric, S., and Van der Zwaan, G. J. (1992). Microhabitat selection by benthic foraminifera in the northern Adriatic Sea. J. Foraminifer. Res. 22, 297–317. doi: 10.2113/gsjfr.22.4.297
Bergin, F., Kucuksezgin, F., Uluturhan, E., Barut, I. F., Meric, E., Avsar, N., et al. (2006). The response of benthic foraminifera and ostracoda to heavy metal pollution in Gulf of Izmir (Eastern Aegean Sea). Estuar. Coast. Shelf Sci. 66, 368–386. doi: 10.1016/j.ecss.2005.09.013
Berkeley, A., Perry, C. T., Smithers, S. G., and Hoon, S. (2014). Towards a formal description of foraminiferal assemblage formation in shallow-water environments: qualitative and quantitative concepts. Mar. Micropaleontol. 112, 27–38. doi: 10.1016/j.marmicro.2014.08.005
Bernhard, J. M. (1988). Postmortem vital staining in benthic foraminifera; duration and importance in population and distributional studies. J. Foraminifer. Res. 18, 143–146. doi: 10.2113/gsjfr.18.2.143
Bernhard, J. M., and Bowser, S. S. (1999). Benthic foraminifera of dysoxic sediments: chloroplast sequestration and functional morphology. Earth Sci. Rev. 46, 149–165. doi: 10.1016/s0012-8252(99)00017-3
Bernhard, J. M., and Gupta, B. K. S. (1999). “Foraminifera of oxygen-depleted environments,” in Modern Foraminifera, ed. B. K. S. Gupta (Berlin: Springer), 201–216. doi: 10.1007/0-306-48104-9_12
Bernhard, J. M., Barry, J. P., Buck, K. R., and Starczak, V. R. (2009). Impact of intentionally injected carbon dioxide hydrate on deep-sea benthic foraminiferal survival. Glob. Chang. Biol. 15, 2078–2088. doi: 10.1111/j.1365-2486.2008.01822.x
Bhaumik, A. K., and Gupta, A. K. (2007). Evidence of methane release from blake ridge ODP Hole 997A during the Plio-pleistocene: benthic foraminifer fauna and total organic carbon. Curr. Sci. 92, 192–199.
Boltovskoy, E. (1978). Late cenozoic benthonic foraminifera of the ninetyeast ridge (Indian Ocean). Elsevier Oceanogr. Ser. 21, 139–175. doi: 10.1016/0025-3227(78)90050-6
Bristow, L. A., Callbeck, C. M., Larsen, M., Altabet, M. A., Dekaezemacker, J., Forth, M., et al. (2017). N2 production rates limited by nitrite availability in the Bay of Bengal oxygen minimum zone. Nat. Geosci. 10, 24–29. doi: 10.1038/ngeo2847
Burone, L., Pires-Vanin, P., Sousa, S., Mahiques, M., and Braga, E. (2007). Benthic foraminiferal variability on a monthly scale in a subtropical bay moderately affected by urban sewage. Sci. Mar. 71, 775–792. doi: 10.3989/scimar.2007.71n4775
Buzas, M. A., and Culver, S. J. (1991). Species diversity and dispersal of benthic foraminifera. BioScience 41, 483–489. doi: 10.2307/1311806
Buzas, M. A., Culver, S. J., and Jorissen, F. J. (1993). A statistical evaluation of the microhabitats of living (stained) infaunal benthic foraminifera. Mar. Micropaleontol. 20, 311–320. doi: 10.1016/0377-8398(93)90040-5
Caralp, M. H. (1989). Abundance of Bulimina exilis and Melonis barleeanum: relationship to the quality of marine organic matter. Geo Mar. Lett. 9, 37–43. doi: 10.1007/bf02262816
Caulle, C., Mojtahid, M., Gooday, A. J., Jorissen, F. J., and Kitazato, H. (2015). Living (Rose-Bengal-stained) benthic foraminiferal faunas along a strong bottom-water oxygen gradient on the Indian margin (Arabian Sea). Biogeosciences 12, 5005–5019. doi: 10.5194/bg-12-5005-2015
Cedhagen, T. (1991). Retention of chloroplasts and bathymetric distribution in the sublittoral foraminiferan Nonionellina labradorica. Ophelia 33, 17–30. doi: 10.1080/00785326.1991.10429739
Corliss, B. H. (1979). Recent deep-sea benthonic foraminiferal distributions in the southeast Indian Ocean: inferred bottom-water routes and ecological implications. Mar. Geol. 31, 115–138. doi: 10.1016/0025-3227(79)90059-8
Corliss, B. H. (1983). Distribution of holocene deep-sea benthonic foraminifera in the southwest Indian Ocean. Deep Sea Res. A. Oceanogr. Res. Papers 30, 95–117. doi: 10.1016/0198-0149(83)90064-x
Corliss, B. H. (1991). Morphology and microhabitat preferences of benthic foraminifera from the northwest Atlantic Ocean. Mar. Micropaleontol. 17, 195–236. doi: 10.1016/0377-8398(91)90014-w
Corliss, B. H., and Chen, C. (1988). Morphotype patterns of Norwegian sea deep-sea benthic foraminifera and ecological implications. Geology 16, 716–719. doi: 10.1130/0091-7613(1988)016<0716:mponsd>2.3.co;2
Corliss, B. H., and Emerson, S. (1990). Distribution of Rose Bengal stained deep-sea benthic foraminifera from the Nova Scotian continental margin and Gulf of Maine. Deep Sea Res. A Oceanogr. Res. Papers 37, 381–400. doi: 10.1016/0198-0149(90)90015-n
Cullen, J. L., and Prell, W. L. (1984). Planktonic foraminifera of the northern Indian Ocean: distribution and preservation in surface sediments. Mar. Micropaleontol. 9, 1–52. doi: 10.1016/0377-8398(84)90022-7
Dai, A., and Trenberth, K. E. (2002). Estimates of freshwater discharge from continents: latitudinal and seasonal variations. J. Hydrometeorol. 3, 660–687. doi: 10.1175/1525-7541(2002)003<0660:eofdfc>2.0.co;2
Das, M., Singh, R. K., Gupta, A. K., and Bhaumik, A. K. (2017). Holocene strengthening of the oxygen minimum zone in the northwestern Arabian Sea linked to changes in intermediate water circulation or Indian monsoon intensity? Palaeogeogr. Palaeoclimatol. Palaeoecol. 483, 125–135. doi: 10.1016/j.palaeo.2016.10.035
Debenay, J. P., Marchand, C., Molnar, N., Aschenbroich, A., and Meziane, T. (2015). Foraminiferal assemblages as bioindicators to assess potential pollution in mangroves used as a natural biofilter for shrimp farm effluents (New Caledonia). Mar. Pollut. Bull. 93, 103–120. doi: 10.1016/j.marpolbul.2015.02.009
den Dulk, M., Reichart, G. J., Memon, G. M., Roelofs, E. M. P., Zachariasse, W. J., and Van der Zwaan, G. J. (1998). Benthic foraminiferal response to variations in surface water productivity and oxygenation in the northern Arabian Sea. Mar. Micropaleontol. 35, 43–66. doi: 10.1016/s0377-8398(98)00015-2
Duffield, C. J., Edvardsen, B., Eikrem, W., and Alve, E. (2014). Effects of different potential food sources on upper-bathyal benthic foraminifera: an experiment with propagules. J. Foraminifer. Res. 44, 416–433. doi: 10.2113/gsjfr.44.4.416
Durand, F., Papa, F., Rahman, A., and Bala, S. K. (2011). Impact of Ganges-Brahmaputra interannual discharge variations on Bay of Bengal salinity. J. Earth Syst. Sci. 120, 859–872. doi: 10.1007/s12040-011-0118-x
Ellis, B. F., and Messina, A. (1940–2015). Catalogue of Foraminifera. New York: Micropaleontology Press.
Elshanawany, R. (2011). Anthropogenic impact on benthic foraminifera. Abu-qir bay. J. Foraminifer. Res. 41, 326–348. doi: 10.2113/gsjfr.41.4.326
Enge, A. J., Kucera, M., and Heinz, P. (2012). Diversity and microhabitats of living benthic foraminifera in the abyssal Northeast Pacific. Mar. Micropaleontol. 96, 84–104. doi: 10.1016/j.marmicro.2012.08.004
Eriş, K. K., Çağatay, M. N., Akçer, S., Gasperini, L., and Mart, Y. (2011). Late glacial to Holocene sea-level changes in the sea of Marmara: new evidence from high-resolution seismics and core studies. Geo Mar. Lett. 31, 1–18. doi: 10.1007/s00367-010-0211-1
Ernst, S., Duijnstee, I., and van der Zwaan, B. (2002). The dynamics of the benthic foraminiferal microhabitat: recovery after experimental disturbance. Mar. Micropaleontol. 46, 343–361. doi: 10.1016/s0377-8398(02)00080-4
Filipsson, H. L., and Nordberg, K. (2004). A 200-year environmental record of a low-oxygen fjord, Sweden, elucidated by benthic foraminifera, sediment characteristics and hydrographic data. J. Foraminifer. Res. 34, 277–293. doi: 10.2113/34.4.277
Frontalini, F., and Coccioni, R. (2008). Benthic foraminifera for heavy metal pollution monitoring: a case study from the central Adriatic Sea coast of Italy. Estuar. Coast. Shelf Sci. 76, 404–417. doi: 10.1016/j.ecss.2007.07.024
Gandhi, M. S., and Solai, A. (2010). Statistical studies and ecology of benthic foraminifera from the depositional environment. a case study between Mandapam and Tuticorin, south east coast of India. Int. J. Res. Rev. Appl. Sci. 5, 86–94.
Gandhi, M. S., Rajeshwara Rao, N., Raja, M., and Suresh, N. (2016). Benthic foraminiferal distribution and its ecological studies in a shallow shelf setting off Colachel, Kanyakumari District, Tamil Nadu. Indian J. Geo Mar. Sci. 45, 742–748.
Gandhi, M. S., Solai, A., and Mohan, S. P. (2007). Benthic foraminiferal and its environmental degradation studies between the tsunamigenic sediments of Mandapam and Tuticorin, south east coast of India. Sci. Tsunami Hazards 26:115.
Garcia, H. E., Locarnini, R. A., Boyer, T. P., Antonov, J. I., Mishonov, A. V., Baranova, O. K., et al. (2013). World Ocean Atlas 2013, Vol.3: Dissolved Oxygen, Apparent Oxygen Utilization, and Oxygen Saturation. eds S. Levitus and A. Mishonov. Washington, DC: NOAA.
Gibson, T. G., and Buzas, M. A. (1973). Species diversity: patterns in modern and Miocene foraminifera of the eastern margin of North America. Geol. Soc. Am. Bull. 84, 217–238. doi: 10.1130/0016-7606(1973)84<217:sdpima>2.0.co;2
Gomes, H. R., Goes, J. I., and Saino, T. (2000). Influence of physical processes and freshwater discharge on the seasonality of phytoplankton regime in the Bay of Bengal. Cont. Shelf Res. 20, 313–330. doi: 10.1016/s0278-4343(99)00072-2
Gooday, A. J. (1986). Soft-shelled foraminifera in meiofaunal samples from the bathyal northeast Atlantic. Sarsia 71, 275–287. doi: 10.1080/00364827.1986.10419697
Gooday, A. J. (1993). Deep-sea benthic foraminiferal species which exploit phytodetritus: characteristic features and controls on distribution. Mar. Micropaleontol. 22, 187–205. doi: 10.1016/0377-8398(93)90043-w
Gooday, A. J. (1994). The biology of deep-sea foraminifers: a review of some advances and their applications in paleooceanography. Palaios 9, 14–31. doi: 10.2307/3515075
Gooday, A. J. (2003). Benthic foraminifera (Protista) as tools in deep-water palaeoceanography: environmental influences on faunal characteristics. Adv. Mar. Biol. 46, 1–90. doi: 10.1016/s0065-2881(03)46002-1
Gooday, A. J., and Jorissen, F. J. (2012). Benthic foraminiferal biogeography: controls on global distribution patterns in deep-water settings. Annu. Rev. Mar. Sci. 4, 237–262. doi: 10.1146/annurev-marine-120709-142737
Gooday, A. J., and Rathburn, A. E. (1999). Temporal variability in living deep-sea benthic foraminifera: a review. Earth Sci. Rev. 46, 187–212. doi: 10.1016/s0012-8252(99)00010-0
Gooday, A. J., Bernhard, J. M., Levin, L. A., and Suhr, S. B. (2000). Foraminifera in the Arabian Sea oxygen minimum zone and other oxygen-deficient settings: taxonomic composition, diversity, and relation to metazoan faunas. Deep Sea Res. II: Top. Stud. Oceanogr. 47, 25–54. doi: 10.1016/s0967-0645(99)00099-5
Gupta, A. K., and Thomas, E. (1999). Latest miocene-pleistocene productivity and deep-sea ventilation in the northwestern Indian ocean (Deep Sea Drilling Project Site 219). Paleoceanography 14, 62–73. doi: 10.1029/1998pa900006
Gupta, B. K. S., and Machain-Castillo, M. L. (1993). Benthic foraminifera in oxygen-poor habitats. Mar. Micropaleontol. 20, 183–201. doi: 10.1016/0377-8398(93)90032-s
Hermelin, J. O. R., and Shimmield, G. B. (1990). The importance of the oxygen minimum zone and sediment geochemistry in the distribution of recent benthic foraminifera in the northwest Indian Ocean. Mar. Geol. 91, 1–29. doi: 10.1016/0025-3227(90)90130-c
Jannink, N. T., Zachariasse, W. J., and Van der Zwaan, G. J. (1998). Living (Rose Bengal stained) benthic foraminifera from the Pakistan continental margin (northern Arabian Sea). Deep Sea Res. I Oceanogr. Res. Papers 45, 1483–1513. doi: 10.1016/s0967-0637(98)00027-2
Jayaraju, N., Raja, B. S., and Reddy, K. R. (2010). Impact of dredging on sediment of Krishnapatnam Port, east coast of India: implications for marine biodiversity. J. Environ. Sci. Eng. 4:66.
Jonkers, H. A. (1984). “Morphological study on bolivina.” in Proceedings of the Koninklke Nederlandse Akademie van Wetenschappen. Series B. Palaeontology, Geology, Physics and Chemistry. Amsterdam
Jorissen, F. J. (1999). Benthic foraminiferal successions across late quaternary mediterranean sapropels. Mar. Geol. 153, 91–101. doi: 10.1016/s0025-3227(98)00088-7
Jorissen, F. J., and Wittling, I. (1999). Ecological evidence from live–dead comparisons of benthic foraminiferal faunas off Cape Blanc (Northwest Africa). Palaeogeogr. Palaeoclimatol. Palaeoecol. 149, 151–170. doi: 10.1016/s0031-0182(98)00198-9
Jorissen, F. J., Barmawidjaja, D. M., Puskaric, S., and Van der Zwaan, G. J. (1992). Vertical distribution of benthic foraminifera in the northern Adriatic sea: the relation with the organic flux. Mar. Micropaleontol. 19, 131–146. doi: 10.1016/0377-8398(92)90025-f
Jorissen, F. J., de Stigter, H. C., and Widmark, J. G. (1995). A conceptual model explaining benthic foraminiferal microhabitats. Mar. Micropaleontol. 26, 3–15. doi: 10.1016/0377-8398(95)00047-x
Jorissen, F. J., Fontanier, C., and Thomas, E. (2007). Chapter seven: Paleoceanographical proxies based on deep-sea benthic foraminiferal assemblage characteristics. Dev. Mar. Geol. 1, 263–325. doi: 10.1016/s1572-5480(07)01012-3
Kaiho, K. (1994). Benthic foraminiferal dissolved-oxygen index and dissolved-oxygen levels in the modern ocean. Geology 22, 719–722. doi: 10.1130/0091-7613(1994)022<0719:bfdoia>2.3.co;2
Kaiho, K., and Nishimura, A. (1992). Distribution of holocene benthic foraminifers in the izu-bonin arc1. Proc. Ocean Drill. Prog. Sci. Results 126, 311–320.
Kaminski, M. A., Grassle, J. F., and Whitlatch, R. B. (1988). Life history and recolonization among agglutinated foraminifera in the Panama Basin. Abhandlungen der geologischen Bundesanstalt 41, 229–243.
Kaufman, L., and Rousseeuw, P. J. (2009). Finding Groups in Data: an Introduction to Cluster Analysis. John Wiley & Sons, 344.
Khare, N., Nigam, R., Mayenkar, D. N., and Saraswat, R. (2017). Cluster analysis of benthic foraminiferal morpho-groups from the western margin of India reflects its depth preference. Cont. Shelf. Res. 151, 72–83. doi: 10.1016/j.csr.2017.10.011
Kuhnt, T., Friedrich, O., Schmiedl, G., Milker, Y., Mackensen, A., and Lückge, A. (2013). Relationship between pore density in benthic foraminifera and bottom-water oxygen content. Deep Sea Res. I Oceanogr. Res. Papers 76, 85–95. doi: 10.1016/j.dsr.2012.11.013
Kuhnt, T., Schmiedl, G., Ehrmann, W., Hamann, Y., and Hemleben, C. (2007). Deep-sea ecosystem variability of the Aegean Sea during the past 22 kyr as revealed by Benthic Foraminifera. Mar. Micropaleontol. 64, 141–162. doi: 10.1016/j.marmicro.2007.04.003
Lei, Y., Li, C., Li, T., and Jian, Z. (2019). The effect of temperature and food concentration on ingestion rates of Quinqueloculina seminula on the diatom Nitzschia closterium. J. Foraminifer. Res. 49, 3–10. doi: 10.2113/gsjfr.49.1.3
Lei, Y., Li, T., Jian, Z., and Nigam, R. (2017). Taxonomy and distribution of benthic foraminifera in an intertidal zone of the Yellow Sea, PR China: correlations with sediment temperature and salinity. Mar. Micropaleontol. 133, 1–20. doi: 10.1016/j.marmicro.2017.04.005
Linshy, V. N. (2010). Laboratory Culture Experiments on Benthic Foraminifera. Unpublished thesis. Goa: Goa University.
Locarnini, R. A., Mishonov, A. V., Antonov, J. I., Boyer, T. P., Garcia, H. E., Baranova, O. K., et al. (2013). World Ocean Atlas 2013, Volume 1: Temperature. Washington, DC: NOAA.
Loeblich, A. R., and Tappan, H. (1988). Foraminiferal Genera and their Classification. New York: Van Nostarnd Reinhold
Lutze, G. F., and Coulbourn, W. T. (1984). Recent benthic foraminifera from the continental margin of northwest Africa: community structure and distribution. Mar. Micropaleontol. 8, 361–401. doi: 10.1016/0377-8398(84)90002-1
Mackensen, A., and Douglas, R. G. (1989). Down-core distribution of live and dead deep-water benthic foraminifera in box cores from the Weddell Sea and the California continental borderland. Deep Sea Res. A. Oceanogr. Res. Papers 36, 879–900. doi: 10.1016/0198-0149(89)90034-4
Mackensen, A., Schmiedl, G., Harloff, J., and Giese, M. (1995). Deep-sea foraminifera in the South Atlantic Ocean: ecology and assemblage generation. Micropaleontology 41, 342–358. doi: 10.2307/1485808
Manasa, M., Saraswat, R., and Nigam, R. (2016). Assessing the suitability of benthic foraminiferal morpho-groups to reconstruct paleomonsoon from Bay of Bengal. J. Earth Syst. Sci. 125, 571–584. doi: 10.1007/s12040-016-0677-y
Mayer, L. M. (1994). Surface area control of organic carbon accumulation in continental shelf sediments. Geochim. Cosmochim. Acta 58, 1271–1284. doi: 10.1016/0016-7037(94)90381-6
Mazumder, A., and Nigam, R. (2014). Bathymetric preference of four major genera of rectilinear benthic foraminifera within oxygen minimum zone in Arabian Sea off central west coast of India. J. Earth Syst. Sci. 123, 633–639. doi: 10.1007/s12040-014-0419-y
Mercone, D., Thomson, J., Abu-Zied, R. H., Croudace, I. W., and Rohling, E. J. (2001). High-resolution geochemical and micropalaeontological profiling of the most recent eastern Mediterranean sapropel. Mar. Geol. 177, 25–44. doi: 10.1016/s0025-3227(01)00122-0
Müller, A., and Mathesius, U. (1999). The palaeoenvironments of coastal lagoons in the southern Baltic Sea, I. the application of sedimentary Corg/N ratios as source indicators of organic matter. Palaeogeogr. Palaeoclimatol. Palaeoecol. 145, 1–16. doi: 10.1016/s0031-0182(98)00094-7
Murray, J. W. (1970). Foraminifers of the western approaches to the English Channel. Micropaleontology 16, 471–485. doi: 10.2307/1485074
Murray, J. W. (2001). The niche of benthic foraminifera, critical thresholds and proxies. Mar. Micropaleontol. 41, 1–7. doi: 10.1016/s0377-8398(00)00057-8
Murray, J. W. (2006). Ecology and Applications of Benthic Foraminifera. New York: Cambridge University Press.
Murray, J. W., and Bowser, S. S. (2000). Mortality, protoplasm decay rate, and reliability of staining techniques to recognize ‘living’foraminifera: a review. J. Foraminifer. Res. 30, 66–70. doi: 10.2113/0300066
Nagendra, R., Prakash, T. N., Jayamurugan, K., Gayathri, R., and Reddy, A. N. (2011). A preliminary report on benthic foraminiferal assemblage in the Ashtamudi Estuary, Kerala. J. Palaeontol. Soc. India 56:137.
NASA Goddard Space Flight Center, Ocean Ecology Laboratory, Ocean Biology Processing Group (2014). Sea-viewing Wide Field-of-view Sensor (SeaWiFS) Ocean Color Data, NASA OB. DAAC. doi: 10.5067/ORBVIEW-2/SEAWIFS/L2/OC/2014
Nigam, R. (1984). Living benthonic foraminifera in a tidal environment: gulf of Khambhat (India). Mar. Geol. 58, 415–425. doi: 10.1016/0025-3227(84)90211-1
Nigam, R., and Chaturvedi, S. K. (2006). Do inverted depositional sequences and allochthonous foraminifers in sediments along the Coast of Kachchh, NW India, indicate palaeostorm and/or tsunami effects? Geo Mar. Lett. 26, 42–50. doi: 10.1007/s00367-005-0014-y
Nigam, R., Mazumder, A., Henriques, P. J., and Saraswat, R. (2007). Benthic foraminifera as proxy for oxygen-depleted conditions off the central west coast of India. J. Geol. Soc. India 70, 1047–1054.
Nigam, R., Prasad, V., Mazumder, A., Garg, R., Saraswat, R., and Henriques, P. J. (2009). Late holocene changes in hypoxia off the west coast of India: micropalaeontological evidences. Curr. Sci. 96, 708–713.
Nisha, N. R., and Singh, A. D. (2012). Benthic foraminiferal biofacies on the shelf and upper continental slope off North Kerala (Southwest India). J. Geol. Soc. India 80, 783–801. doi: 10.1007/s12594-012-0208-7
Nomaki, H., Heinz, P., Hemleben, C., and Kitazato, H. (2005). Behavior and response of deep-sea benthic foraminifera to freshly supplied organic matter: a laboratory feeding experiment in microcosm environments. J. Foraminifer. Res. 35, 103–113. doi: 10.2113/35.2.103
Panchang, R., and Nigam, R. (2014). Benthic ecological mapping of the Ayeyarwady delta shelf off Myanmar, using foraminiferal assemblages. J. Palaeontolol. Soc. India 59, 121–168.
Phleger, F. B. (1963). Depth patterns of benthonic foraminifera in the eastern Pacific. Prog. Oceanogr. 3, 273–287. doi: 10.1016/0079-6611(65)90023-6
Prasanna Kumar, S., Muraleedharan, P. M., Prasad, T. G., Gauns, M., Ramaiah, N., de Souza, S. N., et al. (2002). Why is the Bay of Bengal less productive during summer monsoon compared to the Arabian Sea? Geophys. Res. Lett. 29, 881–884.
Ramaswamy, V., Gaye, B., Shirodkar, P. V., Rao, P. S., Chivas, A. R., Wheeler, D., et al. (2008). Distribution and sources of organic carbon, nitrogen and their isotopic signatures in sediments from the Ayeyarwady (Irrawaddy) continental shelf, northern Andaman Sea. Mar. Chem. 111, 137–150. doi: 10.1016/j.marchem.2008.04.006
Ramesh Kumar, M. R., and Prasad, T. G. (1997). Annual and interannual variation of precipitation over the tropical Indian Ocean. J. Geophys. Res. 102, 18519–18527. doi: 10.1029/97jc00979
Rao, C. K., Naqvi, S. W. A., Kumar, M. D., Varaprasad, S. J. D., Jayakumar, D. A., George, M. D., et al. (1994). Hydrochemistry of the Bay of Bengal: possible reasons for a different water-column cycling of carbon and nitrogen from the Arabian Sea. Mar. Chem. 47, 279–290. doi: 10.1016/0304-4203(94)90026-4
Rathburn, A. E., and Corliss, B. H. (1994). The ecology of living (stained) deep sea benthic foraminifera from the Sulu sea. Paleoceanography 9, 87–150. doi: 10.1029/93pa02327
Revets, S. A. (1993). The revision of the genus Buliminellita Cushman and Stainforth, 1947, and Eubuliminella gen. nov. J. Foraminifer. Res. 23, 141–151. doi: 10.2113/gsjfr.23.2.141
Rohling, E. J., Jorissen, F. J., and De Stigter, H. C. (1997). 200-year interruption of Holocene sapropel formation in the Adriatic Sea. J. Micropalaeontol. 16, 97–108. doi: 10.1144/jm.16.2.97
Rosoff, D. B., and Corliss, B. H. (1992). An analysis of recent deep-sea benthic foraminiferal morphotypes from the Norwegian and Greenland seas. Palaeogeogr. Palaeoclimatol. Palaeoecol. 91, 13–20. doi: 10.1016/0031-0182(92)90028-4
Rouchy, J. M., Taberner, C., Blanc-Valleron, M. M., Sprovieri, R., Russell, M., Pierre, C., et al. (1998). Sedimentary and diagenetic markers of the restriction in a marine basin: the Lorca Basin (SE Spain) during the Messinian. Sediment. Geol. 121, 23–55. doi: 10.1016/s0037-0738(98)00071-2
Saalim, S. M., Saraswat, R., Suokhrie, T., Bhadra, S. R., Kurtarkar, S. R., and Nigam, R. (2017). Benthic foraminiferal response to changes in mining pattern: a case study from the Zuari estuary, Goa, India. Environ. Earth Sci. 76:706.
Saidova, K. M. (1975). Bentosnye Foraminifery Tikhogo Okeana [Benthonic foraminifera of the Pacific Ocean], Institut Okeanologii P. P. Shirshova. Moscow: Akademiya Nauk SSSR.
Saidova, K. M. (2010). Benthic foraminifer communities of the Persian Gulf. Oceanology 50, 61–66. doi: 10.1134/s0001437010010078
Saraswat, R. (2015). Non-destructive foraminiferal paleoclimatic proxies: a brief insight. Proc. Indian Natl. Sci. Acad. 81, 381–395.
Saraswat, R., and Nigam, R. (2013). Benthic Foraminifera. Encyclopaedia of Quaternary Science ed. Elias, S.A. Amsterdam: Elsevier.
Saraswat, R., Kouthanker, M., Kurtarkar, S. R., Nigam, R., Naqvi, S. W. A., and Linshy, V. N. (2015). Effect of salinity induced pH/alkalinity changes on benthic foraminifera: a laboratory culture experiment. Estuar. Coast. Shelf Sci. 153, 96–107. doi: 10.1016/j.ecss.2014.12.005
Saraswat, R., Kurtarkar, S. R., Mazumder, A., and Nigam, R. (2004). Foraminifers as indicators of marine pollution: a culture experiment with Rosalina leei. Mar. Pollut. Bull. 48, 91–96. doi: 10.1016/s0025-326x(03)00330-8
Saraswat, R., Manasa, M., Suokhrie, T., Saalim, S. M., and Nigam, R. (2017). Abundance and ecology of endemic Asterorotalia trispinosa from the Western Bay of Bengal: implications for its application as a paleomonsoon proxy. Acta Geol. Sinica 91, 2268–2282. doi: 10.1111/1755-6724.13463
Sardessai, S., Ramaiah, N., Kumar, P., and Sousa, D. (2007). Inluuence of environmental forcings on the seasonality of dissolved oxygen and nutrients in the Bay of Bengal. J. Mar. Res. 65, 301–316. doi: 10.1357/002224007780882578
Sarma, V. V. S. S., Krishna, M. S., Viswanadham, R., Rao, G. D., Rao, V. D., Sridevi, B., et al. (2013). Intensified oxygen minimum zone on the western shelf ofBay of Bengal during summer monsoon: influence of river discharge. J. Oceanogr. 69, 45–55. doi: 10.1007/s10872-012-0156-2
Sarma, V. V. S. S., Rao, G. D., Viswanadham, R., Sherin, C. K., Salisbury, J., Omand, M. M., et al. (2016). Effects of freshwater stratification on nutrients, dissolved oxygen and phytoplankton in the Bay of Bengal. Oceanography 29, 126–135.
Schintu, M., Marrucci, A., Marras, B., Galgani, F., Buosi, C., Ibba, A., et al. (2016). Heavy metal accumulation in surface sediments at the port of Cagliari (Sardinia, western Mediterranean): environmental assessment using sequential extractions and benthic foraminifera. Mar. Pollut. Bull. 111, 45–56. doi: 10.1016/j.marpolbul.2016.07.029
Schlitzer, R. (2016). Ocean Data View. Available online at: http://odv.awi.de (accessed February 1, 2016).
Schmiedl, G., Mitschele, A., Beck, S., Emeis, K. C., Hemleben, C., Schultz, H., et al. (2003). Benthic foraminiferal record of ecosystem variability in the eastern mediterranean Sea during times of sapropel S5 and S6 deposition. Paleogeogr. Paleoclimatol. Plaeoecol. 190, 139–164. doi: 10.1016/s0031-0182(02)00603-x
Schnitker, D. (1979). The deep waters of the western North Atlantic during the past 24,000 years and the re-initiation of the western boundary undercurrent. Mar. Micropaleontol. 4, 265–280. doi: 10.1016/0377-8398(79)90020-3
Schumacher, S., Jorissen, F. J., Dissard, D., Larkin, K. E., and Gooday, A. J. (2007). Live (Rose Bengal stained) and dead benthic foraminifera from the oxygen minimum zone of the Pakistan continental margin (Arabian Sea). Mar. Micropaleontol. 62, 45–73. doi: 10.1016/j.marmicro.2006.07.004
Seidenkrantz, M. S. (1993). Benthic foraminiferal and stable isotope evidence for a “Younger Dryas-style” cold spell at the Saalian-Eemian transition, Denmark. Palaeogeogr. Palaeoclimatol. Palaeoecol. 102, 103–120. doi: 10.1016/0031-0182(93)90008-7
Sengupta, D., Bharath Raj, G. N., and Shenoi, S. S. C. (2006). Surface freshwater from Bay of Bengal runoff and Indonesian throughflow in the tropical Indian Ocean. Geophys. Res. Lett. 33:L22609.
Shankar, D., Vinayachandran, P. N., and Unnikrishnan, A. S. (2002). The monsoon currents in the north Indian Ocean. Prog. Oceanogr. 52, 63–120. doi: 10.1016/s0079-6611(02)00024-1
Shetye, S. R., Shenoi, S. S. C., Gouveia, A. D., Michael, G. S., Sundar, D., and Nampoothiri, G. (1991). Wind-driven coastal upwelling along the western boundary of the Bay of Bengal during the southwest monsoon. Cont. Shelf Res. 11, 1397–1408. doi: 10.1016/0278-4343(91)90042-5
Shetye, S., Mohan, R., Shukla, S. K., Maruthadu, S., and Ravindra, R. (2011). Variability of Nonionellina labradorica Dawson in surface sediments from Kongsfjorden, West Spitsbergen. Acta Geol. Sinica English Edition 85, 549–558. doi: 10.1111/j.1755-6724.2011.00450.x
Singh, R. K., Gupta, A. K., and Das, M. (2012). Paleoceanographic significance of deep-sea benthic foraminiferal species diversity at southeastern Indian Ocean Hole 752A during the Neogene. Palaeogeogr. Palaeoclimatol. Palaeoecol. 361, 94–103. doi: 10.1016/j.palaeo.2012.08.008
Singh, A. D., Rai, A. K., Tiwari, M., Naidu, P. D., Verma, K., Chaturvedi, M., et al. (2015a). Fluctuations of mediterranean outflow water circulation in the Gulf of cadiz during MIS 5 to 7: evidence from benthic foraminiferal assemblage and stable isotope records. Glob. Planet. Chang. 133, 125–140. doi: 10.1016/j.gloplacha.2015.08.005
Singh, A. D., Rai, A. K., Verma, K., Das, S., and Bharti, S. K. (2015b). Benthic foraminiferal diversity response to the climate induced changes in the eastern Arabian Sea oxygen minimum zone during the last 30 ka BP. Quat. Int. 374, 118–125. doi: 10.1016/j.quaint.2014.11.052
Singh, D. P., Saraswat, R., and Kaithwar, A. (2018). Changes in standing stock and vertical distribution of benthic foraminifera along a depth gradient (58–2750 m) in the south-eastern Arabian Sea. Mar. Biodivers. 48, 73–88. doi: 10.1007/s12526-017-0823-z
Sliter, W. V. (1969). Three Inner-Neritic Recent Foraminifers from Southern California. Kansas: Paleontological Contributions, The University of Kansas.
Sliter, W. V. (1970). Inner neritic bolivinitidae from the eastern Pacific margin. Micropaleontology 16, 155–174. doi: 10.2307/1485111
Subramanian, V. (1979). Chemical and suspended-sediment characteristics of rivers of India. J. Hydrol. 44, 37–55. doi: 10.1016/0022-1694(79)90145-8
Suokhrie, T., Saraswat, R., and Nigam, R. (2020). Lack of denitrification causes a difference in benthic foraminifera living in the oxygen deficient zone of the Bay of Bengal and the Arabian Sea. Mar. Pollut. Bull. 153:110992. doi: 10.1016/j.marpolbul.2020.110992
Takata, H., Nomura, R., Tsujimoto, A., Khim, B. K., and Chung, I. K. (2013). Abyssal benthic foraminifera in the eastern equatorial Pacific (IODP Exp 320) during the middle Eocene. J. Paleontol. 87, 1160–1185. doi: 10.1666/12-107
Ter Braak, C. J. (1986). Canonical correspondence analysis: a new eigenvector technique for multivariate direct gradient analysis. Ecology 67, 1167–1179. doi: 10.2307/1938672
Unger, D., Ittekkot, V., Schäfer, P., Tiemann, J., and Reschke, S. (2003). Seasonality and interannual variability of particle fluxes to the deep Bay of Bengal: influence of riverine input and oceanographic processes. Deep Sea Res. II Top. Stud. Oceanogr. 50, 897–923. doi: 10.1016/s0967-0645(02)00612-4
Van der Zwaan, G. J., Duijnstee, I. A. P., Den Dulk, M., Ernst, S. R., Jannink, N. T., and Kouwenhoven, T. J. (1999). Benthic foraminifers: proxies or problems? a review of paleocological concepts. Earth Sci. Rev. 46, 213–236. doi: 10.1016/s0012-8252(99)00011-2
Verma, K., Bharti, S. K., and Singh, A. D. (2018). Late glacial–holocene record of benthic foraminiferal morphogroups from the eastern Arabian Sea OMZ: paleoenvironmental implications. J. Earth Syst. Sci. 127:21.
Verma, S., Gupta, A. K., and Singh, R. K. (2013). Variations in deep-sea benthic foraminifera at ODP Hole 756B, southeastern Indian Ocean: evidence for changes in deep ocean circulation. Palaeogeogr. Palaeoclimatol. Palaeoecol. 376, 172–183. doi: 10.1016/j.palaeo.2013.02.034
Vinayachandran, P. N., Masumoto, Y., Mikawa, T., and Yamagata, T. (1999). Intrusion of the southwest monsoon current into the Bay of Bengal. J. Geophys. Res. Oceans 104, 11077–11085. doi: 10.1029/1999jc900035
Walker, D. A., Linton, A. E., and Schafer, C. T. (1974). Sudan black B; a superior stain to Rose Bengal for distinguishing living from non-living foraminifera. J. Foraminifer. Res. 4, 205–215.
Walton, W. R. (1952). Techniques for recognition of living foraminifera. Cushman Foundation Foraminifer. Res. Contribution 3, 56–60.
Wefer, G., Heinze, P. M., and Berger, W. H. (1994). Clues to ancient methane release. Nature 369, 282–282. doi: 10.1038/369282a0
Wyrtki, K. (1971). Oceanographic Atlas of the International Indian Ocean Expedition. Washington, DC: National Science Foundation.
Keywords: foraminifera, ecology, organic matter, pH, Bay of Bengal, riverine influx
Citation: Suokhrie T, Saraswat R and Nigam R (2021) Multiple Ecological Parameters Affect Living Benthic Foraminifera in the River-Influenced West-Central Bay of Bengal. Front. Mar. Sci. 8:656757. doi: 10.3389/fmars.2021.656757
Received: 21 January 2021; Accepted: 08 April 2021;
Published: 29 April 2021.
Edited by:
Gustavo Fonseca, Federal University of São Paulo, BrazilCopyright © 2021 Suokhrie, Saraswat and Nigam. This is an open-access article distributed under the terms of the Creative Commons Attribution License (CC BY). The use, distribution or reproduction in other forums is permitted, provided the original author(s) and the copyright owner(s) are credited and that the original publication in this journal is cited, in accordance with accepted academic practice. No use, distribution or reproduction is permitted which does not comply with these terms.
*Correspondence: Thejasino Suokhrie, dHN1b2tocmllQG5pby5vcmc=
Disclaimer: All claims expressed in this article are solely those of the authors and do not necessarily represent those of their affiliated organizations, or those of the publisher, the editors and the reviewers. Any product that may be evaluated in this article or claim that may be made by its manufacturer is not guaranteed or endorsed by the publisher.
Research integrity at Frontiers
Learn more about the work of our research integrity team to safeguard the quality of each article we publish.