- 1Scottish Oceans Institute, School of Biology, University of St Andrews, St Andrews, United Kingdom
- 2Marine Laboratory, Marine Scotland Science, Aberdeen, United Kingdom
- 3NatureScot, Oban, United Kingdom
- 4Global Oceans Institute, World Maritime University, Malmö, Sweden
- 5Department of Biology, University of Ottawa, Ottawa, ON, Canada
- 6School of Biology, University of Aberdeen, Aberdeen, United Kingdom
Seasonal and ontogenetic variations in depth use by benthic species are often concomitant with changes in their spatial distribution. This has implications for the efficacy of spatial conservation measures such as marine protected areas (MPAs). The critically endangered flapper skate (Dipturus intermedius) is the designation feature of an MPA in Scotland. This species is generally associated with deeper waters >100 m; however, little is known about its seasonal or ontogenetic variation in habitat use. This study used archival depth data from 25 immature and mature flapper skate tagged in the MPA over multiple years. Time series ranged from 3 to 772 (mean = 246) days. Generalised additive mixed models and highest density intervals were used to identify home (95%) and core (50%) highest density depth regions (HDDRs) to quantify depth use in relation to time of year and body size. Skate used a total depth range of 1–312 m, but home HDDRs typically occurred between 20 and 225 m. Core HDDRs displayed significant seasonal and ontogenetic variations. Summer core HDDRs (100–150 m) suggest high occupancy of the deep trenches in the region by skate of most size classes. There was an inverse relationship between body size and depth use and a seasonal trend of skate moving into shallow water over winter months. These results suggest that flapper skate are not solely associated with deep water, as skate, especially large females, are frequently found in shallow waters (25–75 m). The current management, which protects the entire depth range, is appropriate for the protection of flapper skate through much of its life history. This research demonstrates why collecting data across seasonal scales and multiple ontogenetic stages is needed to assess the effectiveness of spatial management.
Introduction
Marine protected areas (MPAs) are increasingly being established globally to conserve and recover species, habitats, biodiversity, and ecosystem functions (Edgar et al., 2007). MPAs designated for species conservation should decrease mortality and/or increase recruitment, facilitating population maintenance or net increase (Polunin, 2002). For mobile species, such as elasmobranchs (sharks and rays), this aim is generally considered to be more attainable in areas of core activity (Knip et al., 2012; Neat et al., 2014; Thorburn et al., 2015; Doherty et al., 2017). In addition, for strongly K-selected elasmobranchs, survival to maturity is key for population growth, so all age classes should be considered in conservation measures (Cortés, 2002; Stevens, 2002; Frisk et al., 2005; Kinney and Simpfendorfer, 2009).
Elasmobranch habitat use is driven by a combination of abiotic and biotic variables that can impact movement in the short and long-term (Grubbs, 2010; Schlaff et al., 2014). Seasonal changes in habitat use, including depth, are often related to changes in abiotic conditions (e.g., temperature) and seasonal variability in prey or resource abundance (Holden and Horrod, 1979; Bres, 1993; Schlaff et al., 2014; Thorburn et al., 2015, 2019). Intraspecific variation in habitat use may be driven by variation in resource requirements and hunting capabilities between different life-history groups (Economakis and Lobel, 1998; Hurst et al., 1999; Pratt and Carrier, 2001; Grubbs, 2010; Wearmouth and Sims, 2010; Papastamatiou et al., 2015; Thorburn et al., 2019). These variations in habitat use can impact the efficacy of spatial management measures, both seasonally and throughout an individual’s lifetime (Martell et al., 2000; Speed et al., 2010). As a result, an understanding of a species’ spatial ecology, including movement and habitat preferences, is required at ontogenetic and seasonal scales in order to implement effective management strategies (Martell et al., 2000; Speed et al., 2010).
Until recently, the movement patterns of skate (Rajidae) species were poorly understood, and they were assumed to be mainly sedentary and make limited movements. However, the recent use of electronic tags has provided increased insight into their movement (Siskey et al., 2019). Studies have shown fine-scale interspecific depth separation (Humphries et al., 2016) and seasonal offshore movements (Hunter et al., 2005), with extensive migrations through coastal waters reported for some species of skate (King and McFarlane, 2010; Farrugia et al., 2016; Frisk et al., 2019). Site fidelity to coastal areas has also been shown in several species (Ellis et al., 2011; Neat et al., 2014; Sousa et al., 2019), including in relation to nursery grounds (Hunter et al., 2006). In some species, year-long residential behaviour has been reported in localised coastal areas (Clay, 1991; Hunter et al., 2005; Neat et al., 2014; Humphries et al., 2016; Simpson et al., 2020). Ontogenetic variation in movements, depth, and habitat use has also been documented in several skate species (Brickle et al., 2003; Barbini et al., 2018; Sousa et al., 2019).
The flapper skate (Dipturus intermedius) is the largest skate species in European waters. They are currently listed by the IUCN as critically endangered and are a priority marine feature in Scotland due to severe population decreases and historic extirpation from the Irish Sea (Brander, 1981), though the species is now showing signs of recovery (Rindorf et al., 2020). Throughout their range, the probability of occurrence for flapper skate is highest between 100 and 400 m depth (Pinto et al., 2016). In 2014, the Loch Sunart to the Sound of Jura Nature Conservation (LStSoJ) MPA was designated to protect flapper skate. In 2016, the Conservation Order came into force prohibiting suction dredging, mechanical dredging, beam trawling, demersal trawling, and longline fishing throughout most of the site. In certain areas, dredging and trawling without a tickler chain are allowed as this gear modification has been shown to reduce skate bycatch (Kynoch et al., 2015). Previous research investigating depth use of flapper skate in the area has focused on a limited number of individuals, principally females, with few long-term depth time series (n = 3, Neat et al., 2014; n = 4, Pinto and Spezia, 2016; n = 6, Wearmouth and Sims, 2009). These studies suggest that skate <165 cm total length (TL) may show a preference for deeper water (Wearmouth and Sims, 2009; Neat et al., 2014) and that the core depth range for the species is 50–180 m (Neat et al., 2014); however, these studies did not explicitly consider seasonal or ontogenetic variation in depth use. Seasonal and ontogenetic variations in depth use are poorly understood for flapper skate, yet identifying these changes could be essential to implement effective management (Grüss et al., 2011; Doherty et al., 2017).
To address this knowledge gap, this study aimed to investigate the seasonal and ontogenetic depth use of flapper skate within LStSoJ MPA by deploying data storage tags (DSTs) on immature and mature flapper skate over multiple seasons and years. Data were also included from Neat et al. (2014) and Pinto and Spezia (2016) to supplement the sample size. As a dorsoventrally flattened species, adapted for a benthic lifestyle, skate are generally associated as being on, or close to, the seafloor (Hunter et al., 2004) and are often found resting there (Kuhnz et al., 2019). Flapper skate have been shown to spend prolonged periods, up to 30 h, resting on the seafloor (Wearmouth and Sims, 2009) throughout the year (Wearmouth and Sims, 2009; Pinto et al., 2016); thus, depth use is a good proxy for habitat use in flapper skate. Here, we examine the extent to which the current spatial management plan within the MPA represents the depths used by flapper skate.
Materials and Methods
All skate were caught using rod and line. The sex (male, M; female, F) of each skate was recorded, and TL and disc width (DW) were measured. Maturity status was estimated from TL based on lengths at 50% maturity published for the species (F = 197.5 cm and M = 185.5 cm; Iglésias et al., 2010). All skate were fitted with a DST (Star Oddi, Garðabær, Iceland; milli-TD) in the LStSoJ MPA (Figure 1) between 2011 and 2017 (Table 1). In 2016 and 2017, pre-started tags were attached to the dorsal surface of the wing near the leading edge with two stainless steel pins through the wing that were secured on the ventral surface using a base plate. Silicon pads were used on both the dorsal and ventral surface of the wing to cushion the tag and the base plate. Tags were set to record depth and temperature every 2 min, and each tag was marked with an ID number and contact details to allow return via recreational angling. All skate were released at their capture site within the MPA (Figure 1). All tagging work was carried out under a UK Home Office Animals (Scientific Procedures) Project Licence by licenced personnel. Full details of tagging methods for skate caught prior to 2016 can be found in Neat et al. (2014). All data analysis and mapping were undertaken in R (R Core Team, 2013) using base functions and the packages ggplot2 (Wickham, 2016), sf (Pebesma, 2018), rgdal (Bivand et al., 2019), and MuMIn (Barton, 2020). Time series from returned tags were trimmed to remove the first and final hour to exclude depth behaviour associated with capture events. Individual depth and temperature distributions were plotted per month. Not all time series from previous studies had temperature records (tag IDs 6, 8, and 25; Table 1), so these skate were discounted from temperature analyses.
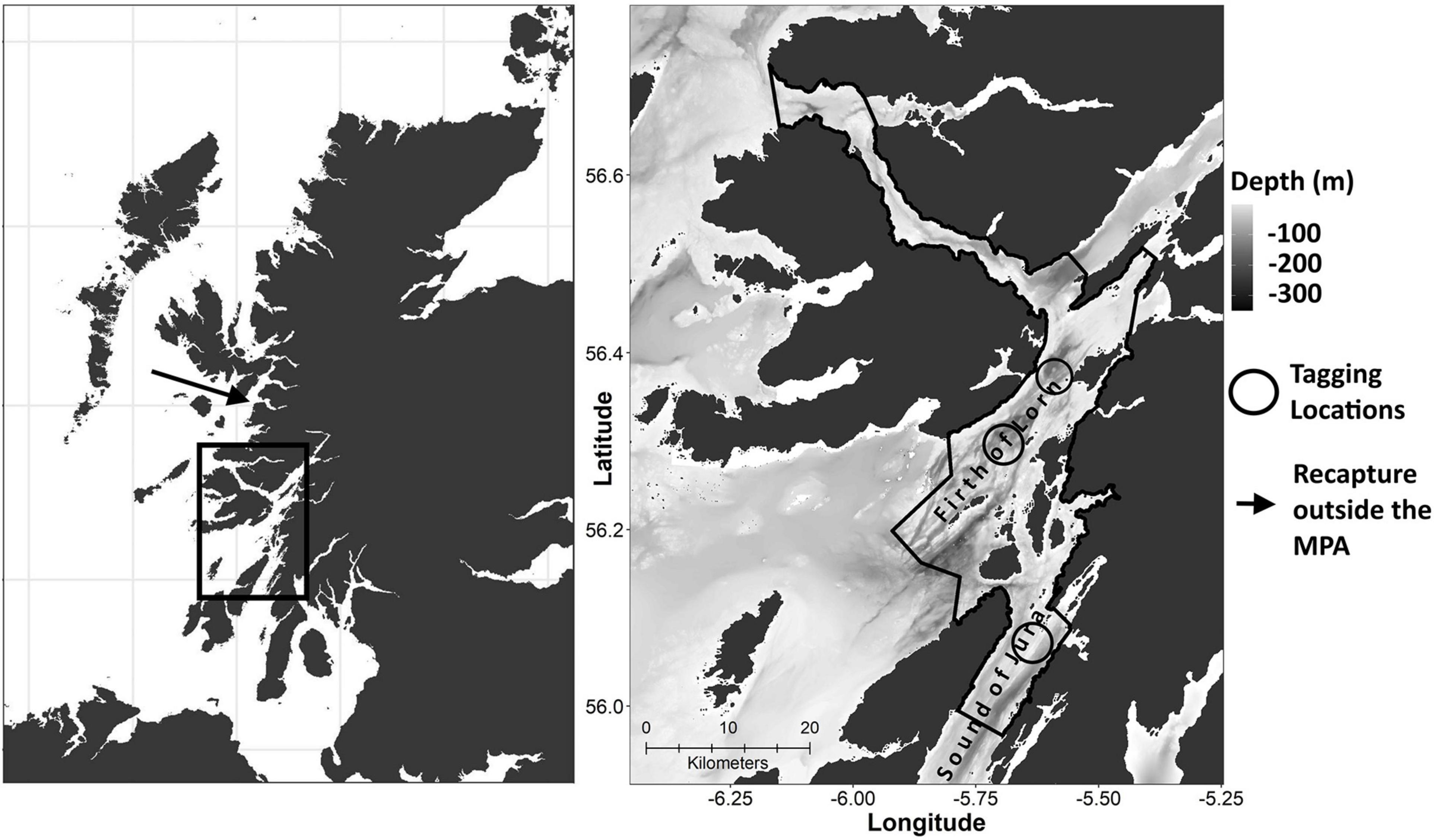
Figure 1. Firth of Lorn and Sound of Jura regions on the west coast of Scotland. The left panel shows the location of the Loch Sunart to the Sound of Jura MPA in relation to Scotland and indicates the recapture location of skate ID 3 (Table 1) off Mallaig. The right panel depicts the boundary of the management area, including the LStSoJ MPA and the Firth of Lorn SAC based on the management measures in the LStSoJ Marine Conservation Order 2016, the bathymetry and tag deployment sites, including the Sound of Jura site from Neat et al. (2014).
Seasonal and Ontogenetic Variations in Depth and Temperature: Generalised Additive Mixed Models
Seasonal and ontogenetic shifts in depth and temperature use were investigated using generalised additive mixed models (GAMMs) with Gaussian error distributions. Both dependent variables were modelled in relation to smooth functions of TL and time of year (Julian day), with individual as a random effect. To focus on trends over seasonal timescales, daily averages for depth, and temperature data were used. Non-linear effects of TL and Julian day were fitted using thin plate regression and cyclic cubic regression splines, respectively. An interaction between TL and Julian day was included in both models using a tensor product smooth. The models were fitted using the gamm function in the mgcv package (Wood, 2017), and an autoregressive order 1 parameter was included to account for serial correlation. Sex was not included in either model due to a lack of data across a suitable range of sizes for each sex, which limited our ability to separate size and sex effects.
Home and Core Highest Density Depth and Temperature Ranges
Home and core ranges, in movement ecology, are usually described as 95 and 50% utilisation distributions (Silva et al., 2008; Vaudo et al., 2017; Logan et al., 2020). We followed this concept to identify the home and core depth and temperature ranges of flapper skate. For analysis, both depth and temperature time series at 2-min resolution were thinned by averaging every five observations and assigning the mean value to the middle (third) time value so that all time series were expressed at a 10-min resolution. These data were grouped into 10 cm length classes (Table 1), and for each length class, monthly depth and temperature distributions were produced. The 95% (home) and 50% (core) highest density intervals (HDI) (Kruschke, 2014) were calculated from these distributions using the HDInterval package (Meredith and Kruschke, 2020). Depth and temperature values between these intervals form either the home or core highest density depth or temperature regions (HDDR or HDTR; example for HDDRs shown in Figure 2). This method accounts for multimodal distributions and allows changes in depth use commonly associated with diurnal migration to be accounted for by identifying the most highly used depths within each time period.
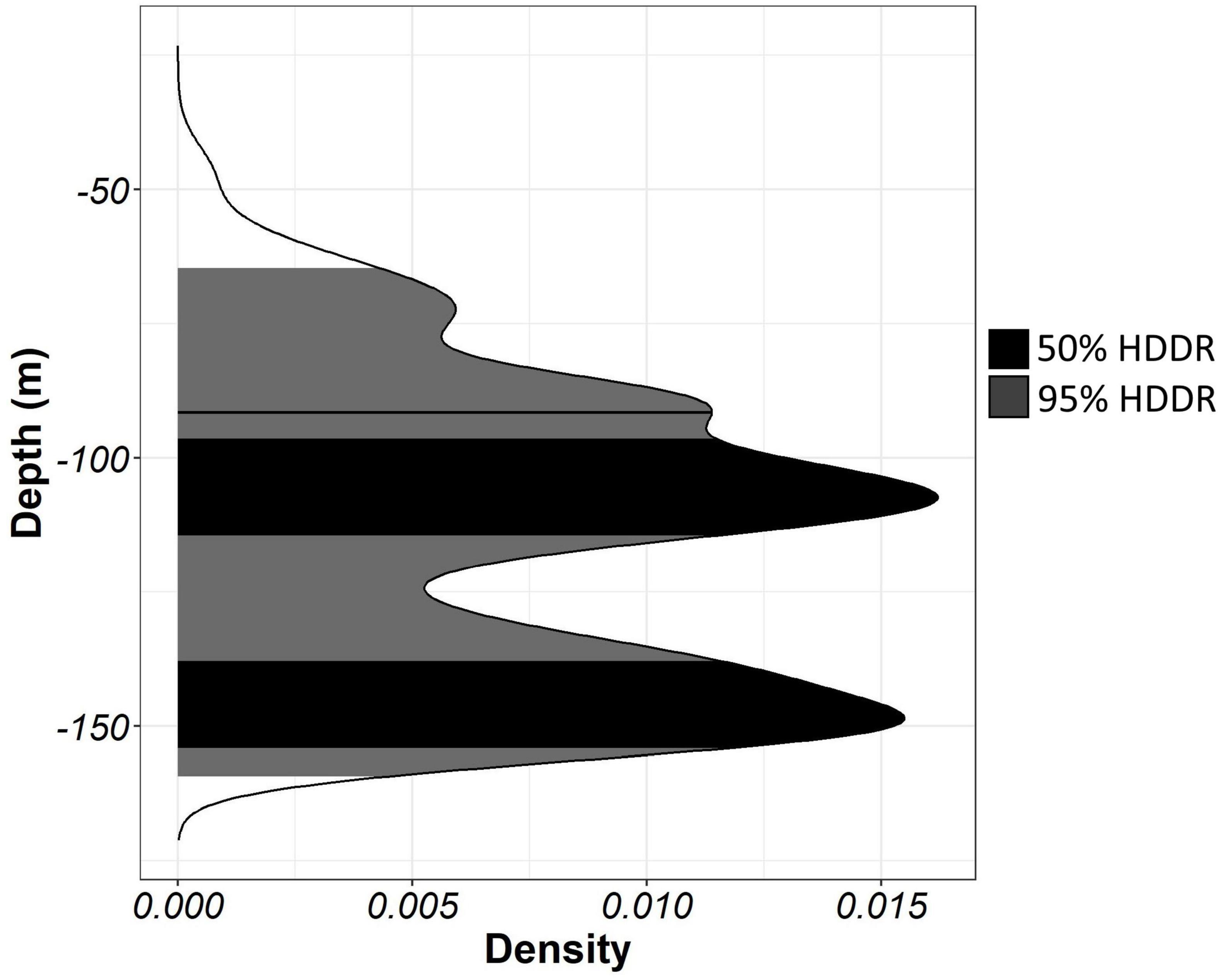
Figure 2. An example monthly depth distribution with 50 and 95% highest density depth regions (HDDRs) identified using highest density intervals (HDIs).
MPA Coverage of Areas Associated With Core and Home Range Depths
The close association of flapper skate with the seafloor suggests that depth is a useful variable to use to identify preferred habitat, and therefore, it is possible to identify preferred habitat on the basis of detailed bathymetry. The extent to which the LStSoJ MPA covers potential habitats associated with preferred depths, how this changes through time, and how this compares with the surrounding region was investigated by assigning HDDRs to bathymetry data for the area. Bathymetry data were derived from two sources. For 553 km2 of the MPA, fine-scale (5 m) bathymetry data from the INIS Hydro (Ireland, Northern Ireland, and Scotland Hydrographic Survey) programme were used (Howe et al., 2015). For the remaining MPA area and surrounding region, bathymetry data were downloaded from the European Marine Observation and Data Network Bathymetry portal1 at approximately 115 m resolution. Bathymetry data were combined and rounded into 1 m depth bands. The resulting bathymetry data covered 94% (717 km2) of the LStSoJ MPA (as defined by the Loch Sunart to the Sound of Jura Marine Conservation Order 2016 which includes the Firth of Lorn Special Area of Conservation—approximately 764 km2 total water surface area). To identify depths used by multiple length classes as part of their HDDRs, for each 1 m depth band in the bathymetry raster, the number of 10 cm length classes that had an associated home or core HDDR that included that band was calculated. This was then converted into a percentage of the total number of length classes that had depth data for that month. Separate maps for monthly HDDRs, with associated percentage of length classes per 1 m depth band, were produced for home and core HDDRs showing the MPA and surrounding region. This allowed both the MPA and the areas beyond the boundaries to be assessed for their association with HDDRs. The area within the MPA boundary with bathymetric depths associated with skate HDDRs was calculated. Based on the total area of bathymetry data within the MPA (717 km2), the area of bathymetric depths associated with skate HDDR depths was converted into a percentage coverage value for the MPA for each length class per month and plotted as a radar plot.
Results
In this study, 45 skate were tagged with DSTs. Of these, 21 tags were recovered (47%). Combined with the four time series from Neat et al. (2014) and Pinto and Spezia (2016), a total of 25 archival depth records, ranging in duration from 3 to 772 (mean = 246) days (Table 1), were analysed. The sexes were unevenly distributed between the 10-cm length classes (Table 1). Linear displacement distances ranged between 0 and 70 (mean = 5.13) km. Only one skate was recaptured outside of the MPA (Figure 1).
Depth and Temperature Use
Depth ranges were 3–312 m for males and 1–301 m for females (Figure 3). Maximum depth for both sexes showed a seasonal trend, being shallower from November to April (M: 220–245 m; F: 224–248 m) and deeper from June to September (M: 280–312 m; F: 265–301 m). The depth range used by all skate encompassed the full depth range available within the MPA throughout the year. There was considerable individual variation observed in depth preferences (Figure 3). Some individuals spent their time within a 50-m range of depths, while others displayed wide depth distributions with no apparent depth preference. High usage of waters shallower than 50 m was observed in all skate larger than 135 cm TL but most apparent in females (Figure 3). Diurnal vertical migration was noted in the depth time series.
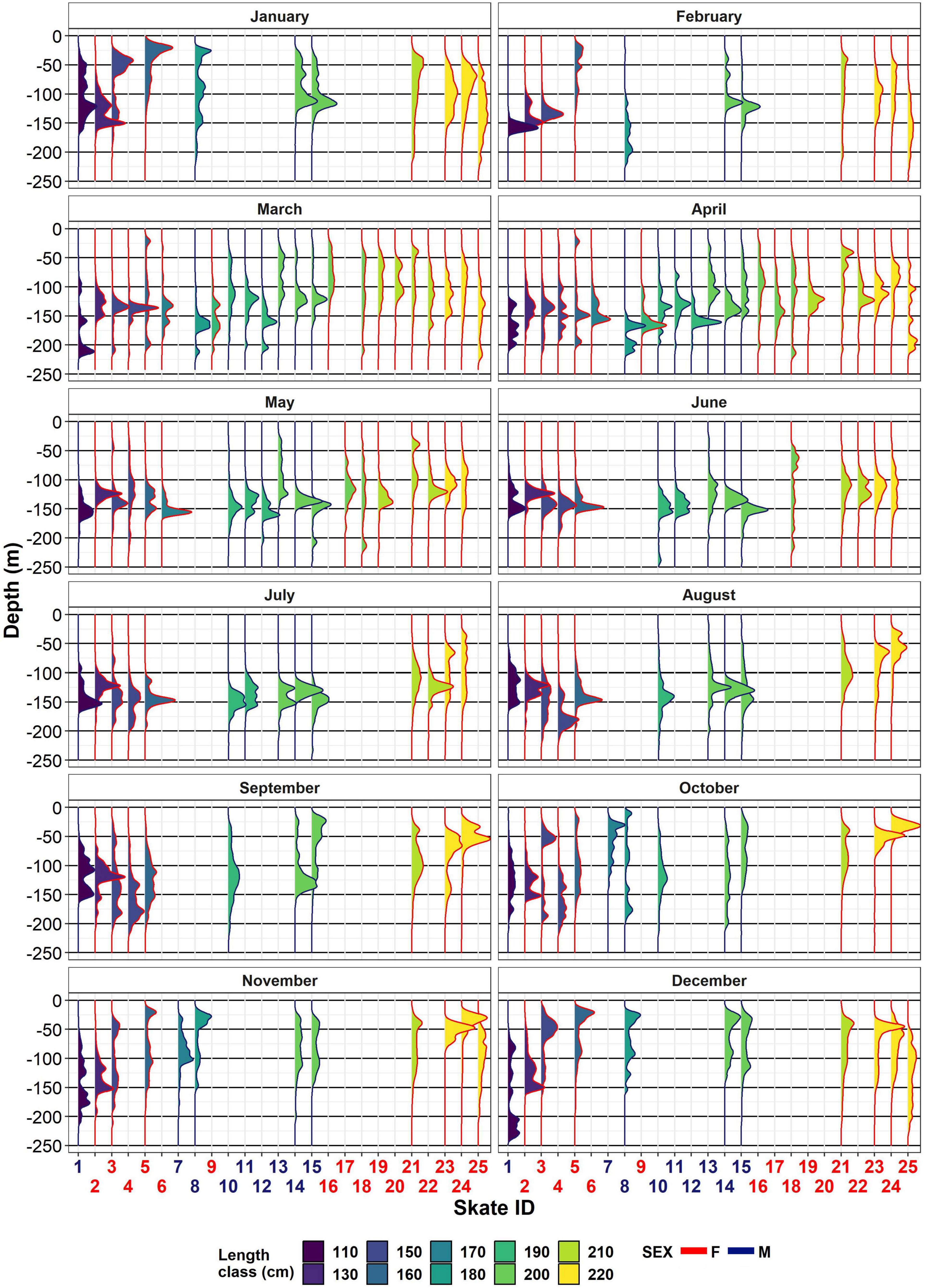
Figure 3. Individual monthly depth distributions. The x-axis shows the skate ID (staggered to ease visualisation) as in Table 1. Length class is colour-coded to facilitate interpretation. Sex is also colour-coded, both in the x-axis label and in the distribution outline: females are outlined in red and males outlined in blue.
Temperatures ranged from 6.3 to 16.37°C (mean = 11.25, SD = 3.03). Individual temperature distributions are shown in Supplementary Material 2. These show that temperature use is dependent on available temperatures at depth; in some months, an individual displayed a bimodal range of temperatures and depths. In other months, temperature distributions were narrow and unimodal, despite the individual displaying a wide range and/or bimodal distribution of depth use.
Generalised Additive Mixed Models
The GAMM for temperature showed a strong seasonal trend (Figure 4), but there were no apparent differences in temperature use with body size. Skate 150 cm and larger were predicted to be in slightly warmer water (14 vs. 13°C) between August and November and skate 180 cm and larger were predicted to be in cooler (8 vs. 9°C) water in February and March; however, in general, size classes occupied similar temperatures. The seasonal temperature model had an adjusted r2 value of 0.967 and a conditional r2 value of 0.887. The random effect of individual was not significant (Table 2) but was left in order to control for possible dependence in the data due to repeated measures or order effects.
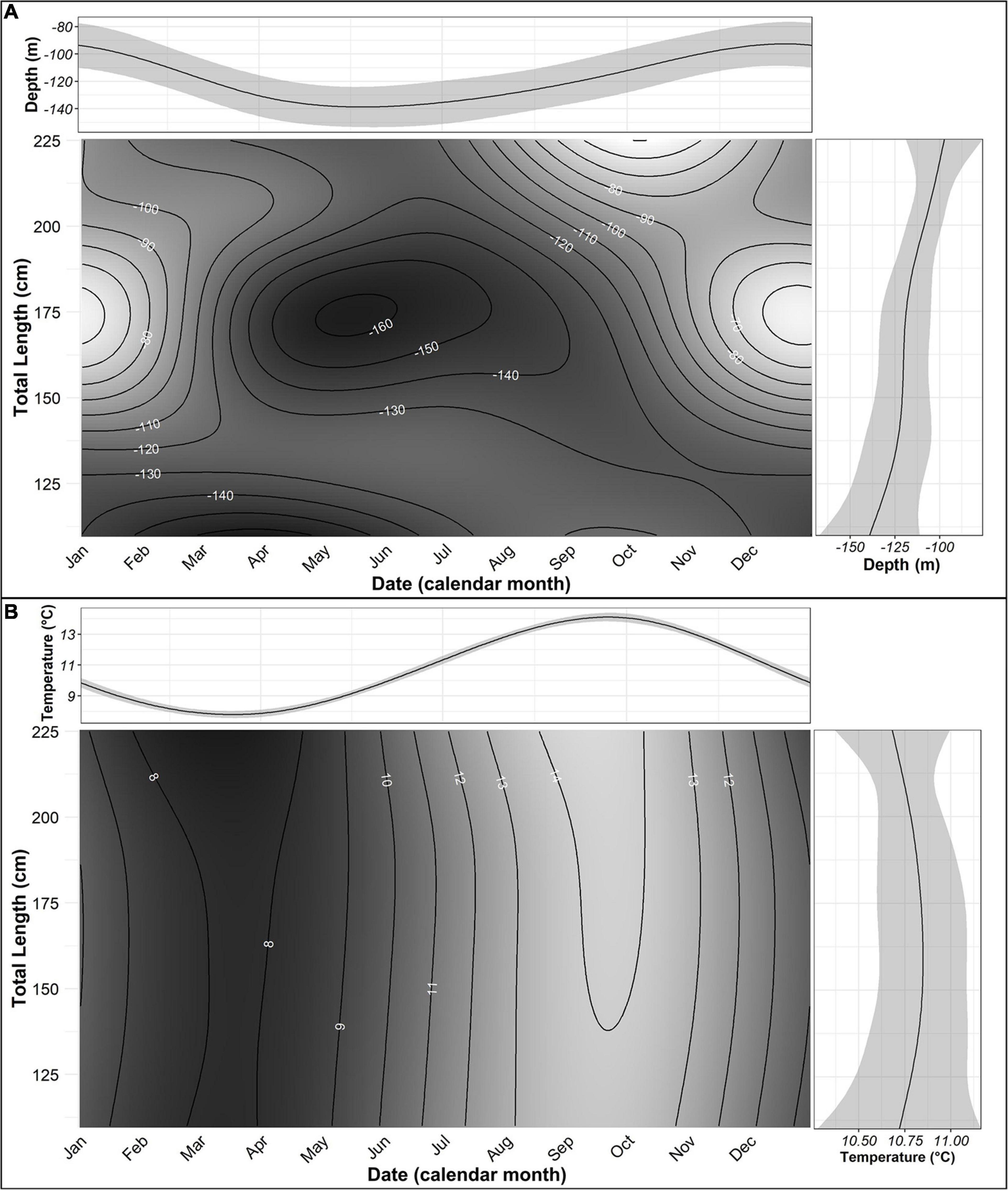
Figure 4. Generalised additive mixed model predictions for depth (A) and temperature (B) in relation to total length and Julian day. In A,B, top and side panels show the expected value of the response in relation to Julian day and total length, respectively, with 95% confidence bands. The main panel shows the expected value in relation to both terms. Model coefficients are given in Table 2.
The GAMM of depth showed an inverse relationship between depth and body size, with expected depths deeper for smaller individuals (140 m for skate 110 cm TL) and shallower for larger individuals (100 m for skate 221 cm TL) (Figure 4). The model showed a strong seasonal pattern in depth use by all size classes above 130 cm TL (Figure 4). Skate 200–225 cm TL showed a similar seasonal pattern of depth use, with depth decreasing with body size. They were predicted to be in shallow waters (between 50 and 90 m) from September to November and in deeper waters (between 120 and 130 m) from April to July (Figure 4). Skate between 130 and 190 cm TL were predicted to be shallower from November to February (between 60 and 120 m) and deeper from April to September (between 130 and 160 m). Skate smaller than 130 cm TL showed less seasonal variation in depth use, with predicted depths deepest from January to May (130–160 m) and shallowest from August to December (120–130 m; Figure 4). Skate 175 cm TL were predicted to have the greatest seasonal range in depth use, from 60 m between December and January to 160 m in May (Figure 4). The depth model had an adjusted r2 value of 0.502 and a conditional r2 of 0.622. All terms were significant (Table 2).
Home and Core Highest Density Temperature and Depth Ranges
Home HDTRs were between 6.24 and 16.25°C (mean = 10.84, SD = 2.20) and core HDTRs were between 6.38 and 16.05°C (mean = 10.81, SD = 2.11). The temperature HDTRs for all size classes showed significant monthly variation (Figure 5). The core and home HDTRs did not vary between skate 130–190 cm TL within each month. Skate in the 200- and 210-cm length classes experienced a wider range of home HDTRs in all months, but most core temperatures used were similar to those experienced by smaller skate (Figure 5). In March and April, home and core HDTRs were multimodel for skate 200 and 210 cm TL, which appears to be caused by water temperatures in 2018 being particular cold in these months. Similarly, warmer waters between July and September in 2018 caused some home HDTRs to be warmer in some larger skate; however, core HDTRs during these months are similar for all size classes (Figure 5).
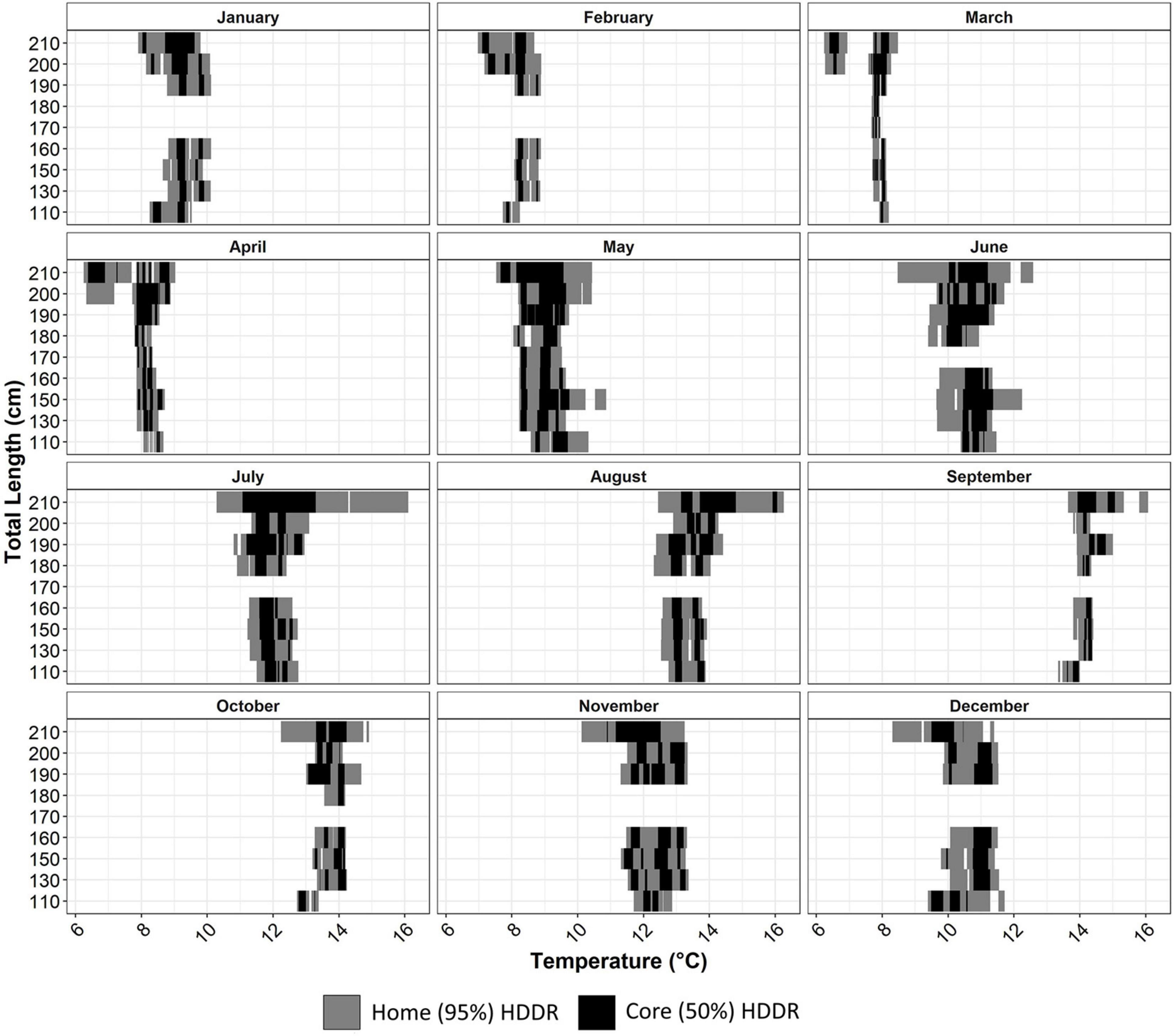
Figure 5. Home (95%) and core (50%) monthly highest density temperature ranges (HDTRs) for each 10-cm length class of skate.
Home (95%) HDDRs were predominantly in water <200 m (Figure 6). There was substantial seasonal variation in the home HDDR occupied for each length class. Home HDDRs were mostly between 20 and 225 m in range; these were generally narrower May–July and wider August–April. Core (50%) HDDRs tended to be more focused around a narrow (<50 m) range of depths for each length class. Similar to the seasonal trend shown by the GAMM, from October to February, the core depths for most length classes were in water <50 m. From March to September, most core HDDRs were below 100 m (Figure 6).
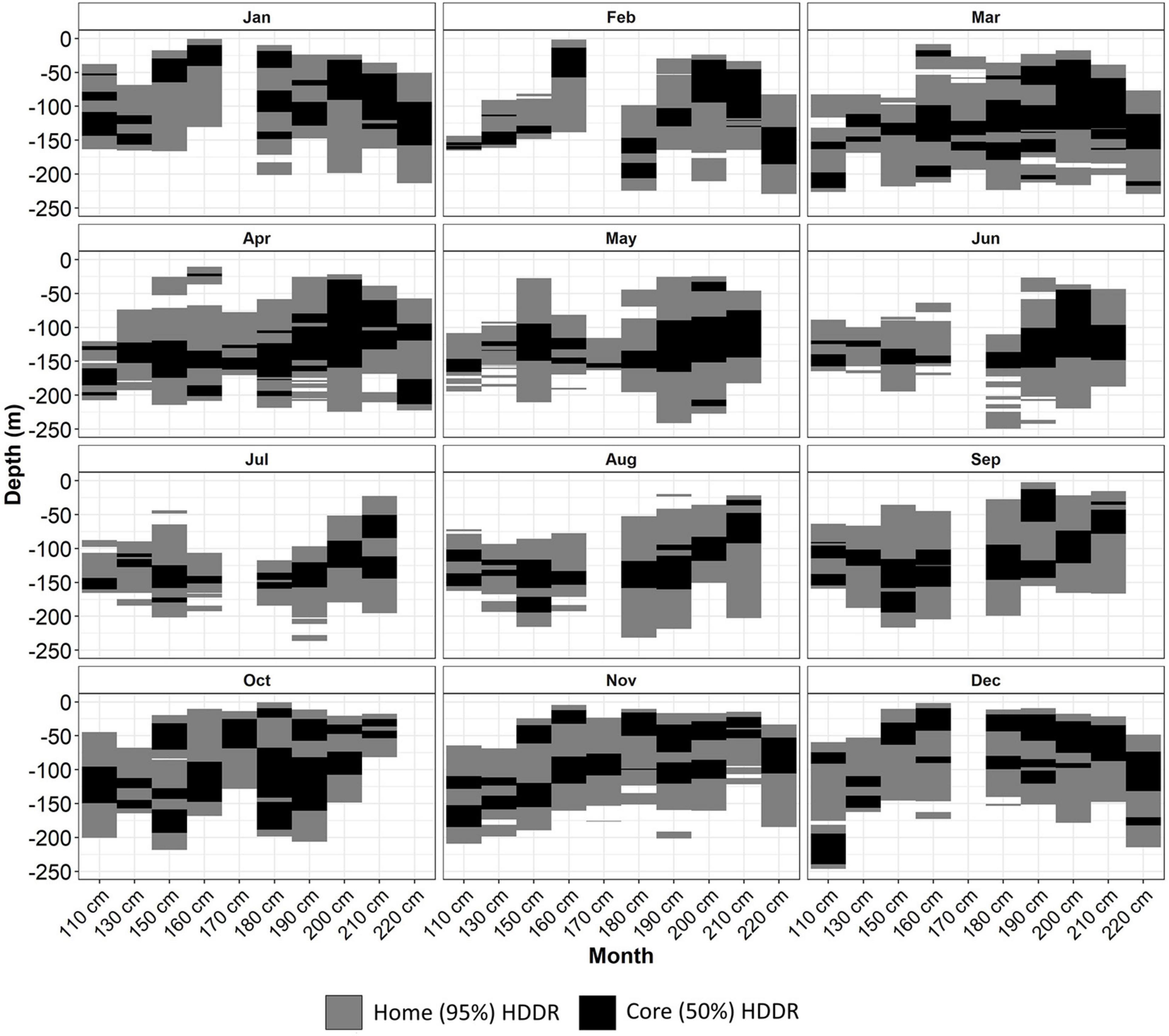
Figure 6. Home (95%) and core (50%) monthly highest density depth regions (HDDRs) for each 10-cm length class of skate.
MPA Coverage of Predicted Space Use
The core HDDR potential habitats for all skate were widespread throughout the region between September and April, most notably between October and January for most length classes (Figure 7). From March to September, the core HDDR for most length classes was restricted to depths associated with the deep trench system in the Firth of Lorn and Sound of Jura (Figure 7). Seasonal variation in depth use resulted in a smaller proportion of the MPA covering both core (Table 3 and Figure 8) and home (Supplementary Material 3) HDDRs over spring and summer, especially between May and August. Ontogenetic variation was also apparent, with the percentage of the MPA covering core HDDRs for large skate (>200 cm TL) higher throughout the year (Table 3 and Figure 8). Home range monthly maps and percentage coverage values for home HDDRs can be found in Supplementary Material 3.
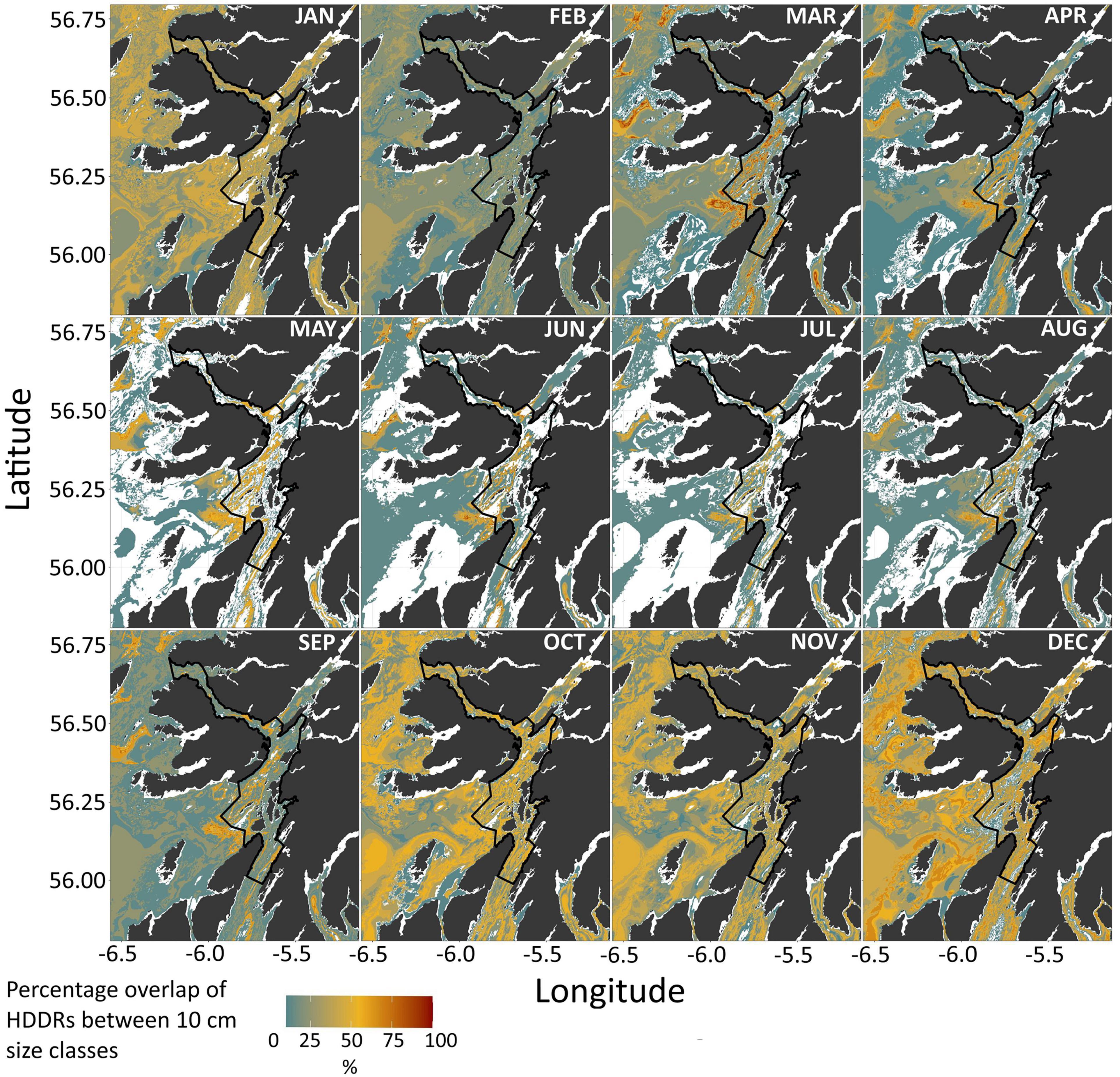
Figure 7. Spatial representation of monthly core (50%) HDDRs across all size classes within the Loch Sunart to the Sound of Jura MPA management area and surrounding region. Colour reflects the number of size classes (as a percentage) whose HDDRs match the depth value in each cell. Darker values represent a higher degree of overlap, with more size classes present at the corresponding depth, while lighter colours represent a lower degree of overlap.
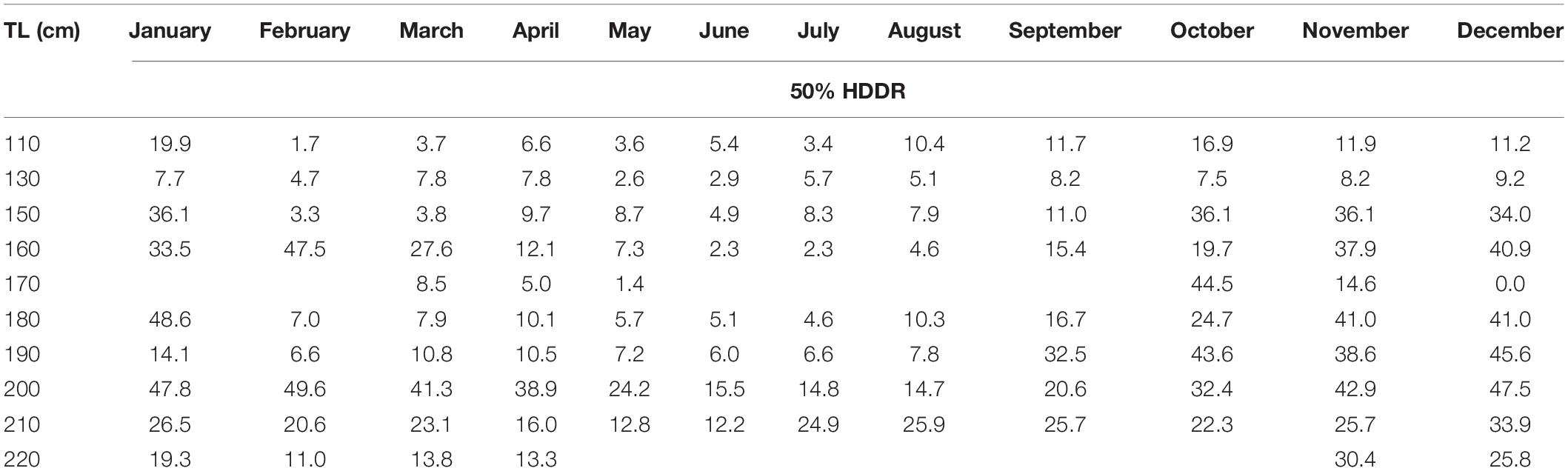
Table 3. The percentage area of the LStSoJ MPA (717 km2) that matches the core (50%) highest density depth regions (HDDRs) for each 10 cm TL class per month.
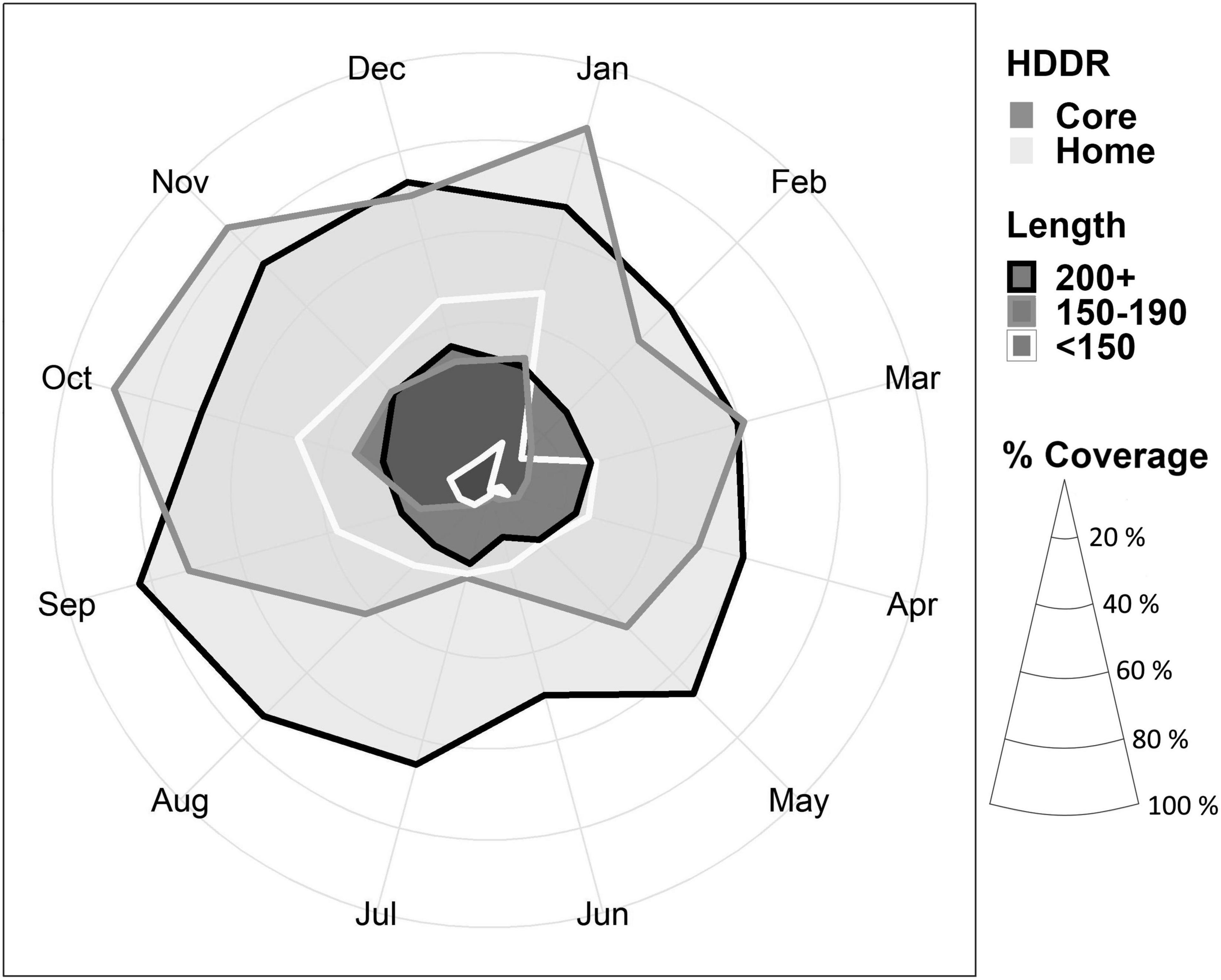
Figure 8. Percentage cover in the MPA of areas associated with home and core HDDRs. Length classes grouped into <150, 150–190, and >200 cm total length to assist visualisation.
Discussion
While past studies report that flapper skate are predominantly found in depths >100 m (Stehmann and Burkel, 1984; Dulvy et al., 2006; Pinto et al., 2016), the present study demonstrates that flapper skate are not solely associated with deep water and make extensive use of shallow-water habitats. Flapper skate ranged across all of the depths available in the region examined, including waters <10 m. Depths between 20 and 75 m were used as frequently as depths between 100 and 175 m, notably by larger females. In addition, there were clear seasonal and ontogenetic variations in depth preference. This has implications for the potential habitats covered by spatial management. The deeper-water trenches appear especially important for all sizes of skate throughout the year and should be the focus for management measures. However, the amount of time spent in waters <50 m complements emerging evidence of the importance of shallow-water habitats to the flapper skate (NatureScot, 2021) and suggests that current management measures, in line with the LStSoJ MPA Conservation Order, are right to incorporate shallow areas.
Wearmouth and Sims (2009) presented limited evidence of larger females using shallower waters, while Neat et al. (2014) found little indication of depth preferences in relation to body size, but both studies were limited by sample sizes and tag data duration. The more comprehensive dataset in this study revealed that larger skate show a preference for shallower depths, while smaller skate frequently use deeper depths. This was most noticeable between August and November, with the core depth range of large (>210 cm TL) female skate being between 20 and 100 m, while skate 130–190 cm TL had a wider range of core depths between 20 and 150, and the skate 110 cm TL had core depths down to 240 m. This inverse relationship between size and depth is important for both the ecology and spatial management of flapper skate, and more data are needed from males and females with a similar distribution of body sizes to fully investigate the effect of sex on depth use.
The home range depths observed in this study are wider than those reported in Wearmouth and Sims (2009) and Neat et al. (2014), with a greater maximum depth, yet shallower than the depth range predicted by Pinto et al. (2016). Due to the different geographic scales of these studies, this suggests that flapper skate use all available depths and that depth use is reflective of the local bathymetric environment. For example, a preference for water <100 m deep, reported by Wearmouth and Sims (2009), may reflect the maximum depth in Loch Sunart (124 m, Gillibrand et al., 1995). Similarly, Neat et al. (2014) reported a preferred depth range of 50–180 m, which is in the range of the maximum depth of their study site in the northern part of the Sound of Jura of 200 m (Howe et al., 2015). This study included a large number of tag records from skate tagged in the Firth of Lorn, an area characterised by three main basins with maximum depths of 290, 225, and 210 m (Howe et al., 2015). Prolonged, localised movements, as reported for flapper skate (Neat et al., 2014), in this bathymetric environment would result in the observed home HDDRs of 20–225 m. However, there were obvious movements into water deeper than 290 m, which suggest some skate moved out of the region, as proved by the recapture of one large female off Mallaig, ∼120 km away from the MPA. The high use of depths <75 m by flapper skate in this study differs from the low probability of species presence in waters <100 m found by Pinto et al. (2016). This may be due to several reasons. It may be an artefact of the survey and deep-water angling data used in Pinto et al. (2016) not being fully representative of shallow-water coastal areas. There may also be a lack of survey data for flapper skate in shallow waters if their preferred habitats at these depths are too rugged to survey with mobile fishing gear. Alternatively, the use of shallower habitats within the MPA may reflect a localised reduction in anthropogenic pressure which, in other areas, may limit the exploitation of shallow waters by skate.
Diet in other skate species has been shown to drive variation in depth use (Grigorov et al., 2015; Simpson et al., 2019). The diet of flapper skate is not well studied, but they are thought to feed predominantly on crustaceans and teleost fish (Dulvy et al., 2006). In the blue skate (Dipturus batis; cf. Dipturus flossada), smaller individuals are benthic specialists, while the diet becomes broader with ontogenetic development, culminating in large adults specialising on teleost fishes (Brown-Vuillemin et al., 2020). Similar ontogenetic diet changes together with seasonal prey availability may be an explanation for the seasonal change in depth use observed. Captured flapper skate in the MPA have been observed regurgitating the langoustine Nephrops norvegicus, proving they form a component of their diet. The deep trenches are characterised by muddy sediments (Howe et al., 2015; Boswarva et al., 2018). This provides suitable habitat for N. norvegicus (Johnson et al., 2013) that may form the basis for the diet of skate populations over the summer when most core HDDRs are associated with these depths. Brooding N. norvegicus tend to remain in burrows over winter (Aguzzi et al., 2007) making them a less available food source. This may drive skate of mid-large size with a more generalist diet to shallow-water habitats that support a different range of prey species (Boswarva et al., 2018), thus reducing the resource competition in deeper waters. This would allow smaller skate with a more specialist diet to remain at depth, resulting in the low seasonal depth variation of smaller skate shown by the GAMM. Variation of depth use based on body size has been observed in other species of skate to reduce resource and predation pressures (Brickle et al., 2003; Humphries et al., 2016). Resource competition between flapper skate is likely to be exacerbated by their residential behaviour (Neat et al., 2014), which will put additional strain on seasonally abundant prey species (Muto et al., 2001). This may promote the individual variation in depth use observed in the larger skate. However, this may also be a product of the increased number of tag records for larger skate. Additional depth data from smaller individuals would allow further investigation into the depth use of earlier life-history stages, while research into the feeding ecology of flapper skate would better inform our understanding of habitat use.
Other drivers for seasonal and ontogenetic depth use in skate species may be related to reproductive events (Hunter et al., 2006). Common skate were thought to lay eggs over the spring and summer (Whitehead et al., 1986), but little is known about the egg-laying behaviour of flapper skate specifically. A preference for depths <50 m was most noticeable in skate over 200 cm TL, all females assumed to be mature. These shallow depth ranges are similar to those of a flapper skate egg nursery identified on the west coast of Scotland (NatureScot, 2021). This suggests that the increased use of shallower depths may be caused by mature females utilising habitats suitable for egg deposition. Unlike viviparous species, female oviparous elasmobranchs need to remain in the vicinity of an egg nursery for prolonged periods while depositing eggs. Egg-laying rates for Rajidae have been reported between 0.24 and 1 egg per day (Holden et al., 1971; Concha et al., 2012). Based on these rates and an estimated fecundity of 40 eggs per year (Brander, 1981), mature female flapper skate may be associated with egg nurseries for between 40 and 160 days. This could result in the extended preference for shallow depths observed in some large females. Variation in the timing of egg deposition among females through an extended egg-laying season, as shown in other skate species (Luer et al., 2007), may account for the individual variation in shallow-water use. Not every female over 200 cm showed prolonged use of shallow water, which could be explained by a biennial reproductive cycle, previously suggested for flapper skate (Little, 1995). It is also possible that females lay eggs at different depths. However, as egg nurseries are thought to be selected based on the provision of optimal conditions for embryo development (Leonard et al., 1999; Hoff, 2008, 2010), variation in egg nursery habitat is likely to be limited.
Reproduction is thought to be controlled, in part, by suitable environmental temperatures (Gelsleichter, 2004). The temperatures used by the flapper skate in this study are similar to those reported throughout its range, 4.96–15.50°C (Frost et al., 2020), but show a higher maximum temperature of 16.37°C. The high core temperature of 16.05°C suggests that skate tolerate these higher temperatures. This suggests that temperature does not strongly influence skate movement and that localised temperature extremes are unlikely to influence skate presence. Temperature is often viewed as an important driver of movement in elasmobranchs (Schlaff et al., 2014); however, if temperature were a driver behind the depth use of flapper skate, we would expect to see a similar relationship between temperature and body size, either positive or negative, as observed for depth and body size. The lack of this relationship, coupled with the apparent lack of correlation between depth and temperature observed in their distributions, suggests that depth use is not driven by a requirement to use specific temperatures. Furthermore, the negligible amount of variation among individual mean temperature (i.e., non-significant individual identity random effect) supports this by indicating that temperature use is similar across all skate in this study. Similarly, core HDTRs of all skate showed significant overlap despite variation in depth use, suggesting core temperatures were available throughout the water column.
As the current management in the MPA protects habitats encompassing all core HDDRs displayed by the skate in this study, it will aid population recovery by safeguarding a range of life-history stages, which is vital in a strongly K-selected elasmobranch (Frisk et al., 2005). The protection of shallower depths is relevant all year, but especially from August to February, when predicted habitats associated with core depth ranges are present throughout the entire management area. Building on Neat et al. (2014), this study also suggests that the deep-water trench system in the Firth of Lorn may be particularly important, especially over spring and summer when the core depth ranges for most body sizes are covered by this region. This may promote local increases in skate presence, escalating the population impacts of damaging activities in these areas. Areas of deep water beyond the MPA boundaries were also associated with core depth ranges, and investigations into skate presence and behaviour in these areas would be beneficial to ensure maximum coverage of core habitats by management measures. Given the wide coverage of core depth ranges between August and February and home depth ranges throughout the year, future work should focus on determining the drivers of flapper skate habitat use in shallower depths <50 m to allow management measures to be fully assessed. This is especially pertinent given shallow-water habitats are favoured by large females, an important feature of a population to protect in order to maintain or improve its reproductive potential (Gallucci et al., 2006), and may be utilised as egg nurseries (NatureScot, 2021).
Humphries et al. (2016) demonstrated that area association with core depth use could be used to illustrate geographic separation between skate species and suggested this could be used to assess the level of protection offered by spatial management. Building on this, we demonstrate that not only can depth use be used to infer area coverage of home and core HDDRs, but it can show how space use may change over seasonal and ontogenetic timescales. While it is important to identify behaviours, such as site fidelity, that promote the use of spatial management, depth data can, in lieu of other data, be used to design or assess management measures at a relatively fine scale based on the area coverage of the core and home HDDRs of multiple ontogenetic stages. Furthermore, management effectiveness can be assessed over the course of a year for multiple life-history stages, allowing for more flexible management approaches to be adopted. This has wide implications for assessing the management of mobile benthic species.
Data Availability Statement
The raw data supporting the conclusions of this article may be made available upon request to the authors.
Ethics Statement
Data use was reviewed and approved by the University of St Andrews Bioethics Committee. All data was collected under a UK Home Office Project License using licensed procedures.
Author Contributions
JT, FN, JD, JM, EL, and PW conceived and designed the project, methodology, and analysis. JT, JD, and FN collected the data. JT, CL, JM, and EL analysed the data. JT and MJ led the writing of the manuscript. All authors contributed critically to the drafts and gave final approval for publication.
Funding
The project was undertaken through the Movement Ecology of the Flapper skate project at St Andrews and received support from the Ecology and Conservation Group, Marine Scotland Science, and Marine Scotland Planning & Policy and NatureScot. It was funded by Marine Scotland projects SP004 and SP02B0 and NatureScot project 015960.
Conflict of Interest
The authors declare that the research was conducted in the absence of any commercial or financial relationships that could be construed as a potential conflict of interest.
Acknowledgments
The authors thank Ian Burrett, Ronnie Campbell, and Roger Eaton for skippering the angling charter vessels used in this research; and Knox Marine Services, Inverlussa staff, Derek Bailey, David Isaacs, Gordon Shearer, Lismore Seafoods, and Jake Crossley for assistance with maintaining the acoustic array. The authors also thank the reviewers, whose comments helped improve this manuscript.
Supplementary Material
The Supplementary Material for this article can be found online at: https://www.frontiersin.org/articles/10.3389/fmars.2021.656368/full#supplementary-material
Footnotes
References
Aguzzi, J., Company, J. B., and Sarda, F. (2007). The activity rhythm of berried and unberried females of Nephrops norvegicus (Decapoda, Nephropidae). Crustaceana 80, 1121–1134. doi: 10.1163/156854007782008577
Barbini, S. A., Sabadin, D. E., and Lucifora, L. O. (2018). Comparative analysis of feeding habits and dietary niche breadth in skates: the importance of body size, snout length, and depth. Rev. Fish Biol. Fish. 28, 625–636. doi: 10.1007/s11160-018-9522-5
Barton, K. (2020). MuMIn: Multi-Model Inference. R Package Version 1.43.17. Available online at: https://CRAN.R-project.org/package=MuMIn (accessed April 22, 2021).
Bivand, R., Keitt, T., and Rowlingson, B. (2019). “rgdal: bindings for the “Geospatial” data abstraction library”, R Package Version 1.4-8. (Accessed September 13, 2020).
Boswarva, K., Butters, A., Fox, C. J., Howe, J. A., and Narayanaswamy, B. (2018). Improving marine habitat mapping using high-resolution acoustic data; a predictive habitat map for the Firth of Lorn, Scotland. Cont. Shelf Res. 168, 39–47. doi: 10.1016/j.csr.2018.09.005
Brander, K. (1981). Disappearance of common skate Raia batis from Irish Sea. Nature 290, 48–49. doi: 10.1038/290048a0
Brickle, P., Laptikhovsky, V., Pompert, J., and Bishop, A. (2003). Ontogenetic changes in the feeding habits and dietary overlap between three abundant rajid species on the Falkland Islands’ shelf. J. Mar. Biolog. Assoc. U. K. 83, 1119–1125. doi: 10.1017/S0025315403008373h
Brown-Vuillemin, S., Barreau, T., Caraguel, J.-M., and Iglésias, S. P. (2020). Trophic ecology and ontogenetic diet shift of the blue skate (Dipturus cf. Flossada). J. Fish Biol. 97, 515–526. doi: 10.1111/jfb.14407
Clay, D. (1991). Seasonal distribution of demersal fish (Osteichthyes) and skates (Chondrichthyes) in the southeastern Gulf of St. Lawrence. Can. Spec. Publ. Fish. Aquat. Sci. 113, 241–259.
Concha, F., Oddone, M. C., Bustamante, C., and Morales, N. (2012). Egg capsules of the yellownose skate Zearaja chilensis (Guichenot 1848) and the roughskin skate Dipturus trachyderma (Krefft and Stehmann 1974)(Rajiformes: Rajidae) from the south-eastern Pacific Ocean. Ichthyol. Res. 59, 323–327. doi: 10.1007/s10228-012-0293-z
Cortés, E. (2002). Incorporating uncertainty into demographic modeling: application to shark populations and their conservation. Conserv. Biol. 16, 1048–1062. doi: 10.1046/j.1523-1739.2002.00423.x
Doherty, P. D., Baxter, J. M., Godley, B. J., Graham, R. T., Hall, G., Hall, J., et al. (2017). Testing the boundaries: seasonal residency and inter-annual site fidelity of basking sharks in a proposed marine protected area. Biol. Conserv. 209, 68–75. doi: 10.1016/j.biocon.2017.01.018
Dulvy, N. K., Notarbartolo di Sciara, G., Serena, F., Tinti, F., Ungaro, N., Mancus, C., et al. (2006). “Dipturus batis”, in The IUCN Red List of Threatened Species 2006. Available online at: http://www.iucnredlist.org (accessed September 13, 2020).
Economakis, A., and Lobel, P. (1998). Aggregation behavior of the grey reef shark, Carcharhinus amblyrhynchos, at Johnston Atoll, Central Pacific Ocean. Environ. Biol. Fish. 51, 129–139. doi: 10.1023/A:1007416813214
Ellis, J. R., Morel, G., Burt, G., and Bossy, S. (2011). Preliminary observations on the life history and movements of skates (Rajidae) around the Island of Jersey, western English Channel. J. Mar. Biolog. Assoc. U. K. 91, 1185–1192. doi: 10.1017/s0025315410001906
Farrugia, T. J., Goldman, K. J., Tribuzio, C., and Seitz, A. C. (2016). First use of satellite tags to examine movement and habitat use of big skates Beringraja binoculata in the Gulf of Alaska. Mar. Ecol. Prog. Ser. 556, 209–221. doi: 10.3354/meps11842
Frisk, M. G., Miller, T. J., and Dulvy, N. K. (2005). Life histories and vulnerability to exploitation of elasmobranchs: inferences from elasticity, perturbation and phylogenetic analyses. J. Northw. Atl. Fish. Sci. 35, 27–45. doi: 10.2960/j.v35.m514
Frisk, M. G., Shipley, O. N., Martinez, C. M., McKown, K. A., Zacharias, J. P., and Dunton, K. J. (2019). First observations of long-distance migration in a large skate species, the winter skate: implications for population connectivity, ecosystem dynamics, and management. Mar. Coast. Fish. 11, 202–212. doi: 10.1002/mcf2.10070
Frost, M., Neat, F. C., Stirling, D., Bendall, V., Noble, L. R., and Jones, C. S. (2020). Distribution and thermal niche of the common skate species complex in the north-east atlantic. Mar. Ecol. Progr. Ser. 656, 65–74. doi: 10.3354/meps13545
Gallucci, V. F., Taylor, I. G., and Erzini, K. (2006). Conservation and management of exploited shark populations based on reproductive value. Can. J. Fish. Aquat. Sci. 63, 931–942. doi: 10.1139/f05-267
Gelsleichter, J. (2004). “Hormonal regulation of elasmobranch physiology,” in Biology of Sharks and Their Relatives, Vol. 20043354, eds J. Musick, J. Carrier, and M. Heithaus (Boca Raton, FL: CRC Press), 287–323. doi: 10.1201/9780203491317.ch11
Gillibrand, P. A., Turrell, W. R., and Elliott, A. J. (1995). Deep-water renewal in the upper basin of Loch Sunart, a Scottish fjord. J. Phys. Oceanogr. 25, 1488–1503. doi: 10.1175/1520-0485(1995)025<1488:dwritu>2.0.co;2
Grigorov, I. V., Orlov, A. M., and Baitalyuk, A. A. (2015). Spatial distribution, size composition, feeding habits, and dynamics of abundance of Alaska skate Bathyraja parmifera in the North Pacific. J. Ichthyol. 55, 644–663. doi: 10.1134/s0032945215050069
Grubbs, R. D. (2010). Ontogenetic Shifts in Movements and Habitat Use. Sharks and Their Relatives II: Biodiversity, Adaptive Physiology, and Conservation. Boca Raton: CRC Press, 319–350.
Grüss, A., Kaplan, D. M., and Hart, D. R. (2011). Relative impacts of adult movement, larval dispersal and harvester movement on the effectiveness of reserve networks. PLoS One 6:e19960. doi: 10.1371/journal.pone.0019960
Hoff, G. R. (2008). A nursery site of the Alaska skate (Bathyraja parmifera) in the eastern Bering Sea. Fish. Bull. 106, 233–244.
Hoff, G. R. (2010). Identification of skate nursery habitat in the eastern Bering Sea. Mar. Ecol. Prog. Ser. 403, 243–254. doi: 10.3354/meps08424
Holden, M. J., and Horrod, R. G. (1979). The migrations of tope, Galeorhinus galeus (L), in the eastern North Atlantic as determined by tagging. J. Conseil 38, 314–317. doi: 10.1093/icesjms/38.3.314
Holden, M. J., Rout, D. W., and Humphreys, C. N. (1971). The rate of egg laying by three species of ray. ICES J. Mar. Sci. 33, 335–339. doi: 10.1093/icesjms/33.3.335
Howe, J. A., Anderton, R., Arosio, R., Dove, D., Bradwell, T., Crump, P., et al. (2015). The seabed geomorphology and geological structure of the Firth of Lorn, western Scotland, UK, as revealed by multibeam echo-sounder survey. Earth Environ. Sci. Trans. R. Soc. Edinb. 105, 273–284. doi: 10.1017/s1755691015000146
Humphries, N. E., Simpson, S. J., Wearmouth, V. J., and Sims, D. W. (2016). Two’s company, three’s a crowd: fine-scale habitat partitioning by depth among sympatric species of marine mesopredator. Mar. Ecol. Prog. Ser. 561, 173–187. doi: 10.3354/meps11937
Hunter, E., Berry, F., Buckley, A. A., Stewart, C., and Metcalfe, J. D. (2006). Seasonal migration of thornback rays and implications for closure management. J. Appl. Ecol. 43, 710–720. doi: 10.1111/j.1365-2664.2006.01194.x
Hunter, E., Buckley, A. A., Stewart, C., and Metcalfe, J. D. (2005). Migratory behaviour of the thornback ray, raja clavata, in the southern north sea. J. Mar. Biolog. Assoc. U. K. 85, 1095–1105. doi: 10.1017/S0025315405012142
Hunter, E., Metcalfe, J. D., O’Brien, C. M., Arnold, G. P., and Reynolds, J. D. (2004). Vertical activity patterns of free-swimming adult plaice in the southern North Sea. Mar. Ecol. Prog. Ser. 279, 261–273. doi: 10.3354/meps279261
Hurst, R. J., Bagley, N. W., McGregor, G. A., and Francis, M. P. (1999). Movements of the New Zealand school shark, Galeorhinus galeus, from tag returns. N. Z. J. Mar. Freshw. Res. 33, 29–48. doi: 10.1080/00288330.1999.9516854
Iglésias, S. P., Toulhoat, L., and Sellos, D. Y. (2010). Taxonomic confusion and market mislabelling of threatened skates: important consequences for their conservation status. Aquat. Conserv. 20, 319–333. doi: 10.1002/aqc.1083
Johnson, M. P., Lordan, C., and Power, A. M. (2013). “Chapter two—habitat and ecology of Nephrops norvegicus,” in Advances in Marine Biology, Vol. 64, eds M. L. Johnson and M. P. Johnson (Cambridge, MA: Academic Press), 27–63. doi: 10.1016/B978-0-12-410466-2.00002-9
King, J. R., and McFarlane, G. A. (2010). Movement patterns and growth estimates of big skate (Raja binoculata) based on tag-recapture data. Fish. Res. 101, 50–59. doi: 10.1016/j.fishres.2009.09.006
Kinney, M. J., and Simpfendorfer, C. A. (2009). Reassessing the value of nursery areas to shark conservation and management. Conserv. Lett. 2, 53–60. doi: 10.1111/j.1755-263x.2008.00046.x
Knip, D. M., Heupel, M. R., and Simpfendorfer, C. A. (2012). Evaluating marine protected areas for the conservation of tropical coastal sharks. Biol. Conserv. 148, 200–209. doi: 10.1016/j.biocon.2012.01.008
Kuhnz, L. A., Bizzarro, J. J., and Ebert, D. A. (2019). In situ observations of deep-living skates in the eastern North Pacific. Deep Sea Res. I Oceanogr. Res. Pap. 152:103104. doi: 10.1016/j.dsr.2019.103104
Kynoch, R. J., Fryer, R. J., and Neat, F. C. (2015). A simple technical measure to reduce bycatch and discard of skates and sharks in mixed-species bottom-trawl fisheries. ICES J. Mar. Sci. 72, 1861–1868. doi: 10.1093/icesjms/fsv037
Leonard, J. B., Summers, A. P., and Koob, T. J. (1999). Metabolic rate of embryonic little skate, Raja erinacea (Chondrichthyes: Batoidea): the cost of active pumping. J. Exp. Zool. 283, 13–18. doi: 10.1002/(sici)1097-010x(19990101)283:1<13::aid-jez3>3.0.co;2-s
Little, W. (1995). Common skate and tope: first results of Glasgow Museum’s tagging study. Glasgow Natural. 22, 455–465.
Logan, R. K., Vaudo, J. J., Sousa, L. L., Sampson, M., Wetherbee, B. M., and Shivji, M. S. (2020). Seasonal movements and habitat use of juvenile smooth hammerhead sharks in the western north atlantic ocean and significance for management. Front. Mar. Sci. 7:566364. doi: 10.3389/fmars.2020.566364
Luer, C. A., Walsh, C. J., Bodine, A. B., and Wyffels, J. T. (2007). “Normal embryonic development in the clearnose skate, Raja eglanteria, with experimental observations on artificial insemination,” in Biology of Skates, eds D. A. Ebert and J. Sulikowski (Berlin: Springer), 133–149. doi: 10.1007/978-1-4020-9703-4_9
Martell, S. J., Walters, C. J., and Wallace, S. S. (2000). The use of marine protected areas for conservation of lingcod (Ophiodon elongatus). Bull. Mar. Sci. 66, 729–743.
Meredith, M., and Kruschke, J. K. (2020). HDInterval: Highest (Posterior) Density Intervals. R Package Version 0.2.2. Available online at: https://CRAN.R-Project.Org/Package=HDInterval
Muto, E. Y., Soares, L. S. H., and Goitein, R. (2001). Food resource utilization of the skates Rioraja agassizii (Müller & Henle, 1841) and Psammobatis extenta (Garman, 1913) on the continental shelf off Ubatuba, South-eastern Brazil. Revista Brasileira de Biologia 61, 217–238. doi: 10.1590/s0034-71082001000200005
NatureScot (2021). Flapper Skate Protection: NatureScot Advice to the Scottish Government. Edinburgh: The Scottish Govement.
Neat, F., Pinto, C., Burrett, I., Cowie, L., Travis, J., Thorburn, J., et al. (2014). Site fidelity, survival and conservation options for the threatened flapper skate (Dipturus cf. Intermedia). Aquat. Conserv. 25, 6–20. doi: 10.1002/aqc.2472
Papastamatiou, Y. P., Meyer, C. G., Kosaki, R. K., Wallsgrove, N. J., and Popp, B. N. (2015). Movements and foraging of predators associated with mesophotic coral reefs and their potential for linking ecological habitats. Mar. Ecol. Prog. Ser. 521, 155–170. doi: 10.3354/meps11110
Pebesma, E. (2018). Simple features for R: standardized support for spatial vector data. R J. 10, 439–446. doi: 10.32614/RJ-2018-009
Pinto, C., and Spezia, L. (2016). Markov switching autoregressive models for interpreting vertical movement data with application to an endangered marine apex predator. Methods Ecol. Evol. 7, 407–417. doi: 10.1111/2041-210x.12494
Pinto, C., Thorburn, J. A., Neat, F., Wright, P. J., Wright, S., Scott, B. E., et al. (2016). Using individual tracking data to validate the predictions of species distribution models. Divers. Distrib. 22, 682–693. doi: 10.1111/ddi.12437
Polunin, N. V. C. (2002). “Marine protected areas, fish and fisheries,” in Handbook of Fish Biology and Fisheries eds P. J. B. Hart and J. D. Reynolds (Hoboken, NJ: Wiley), 293–318. doi: 10.1002/9780470693919.ch14
Pratt, H. L., and Carrier, J. C. (2001). A review of elasmobranch reproductive behavior with a case study on the nurse shark, Ginglymostoma cirratum. Environ. Biol. Fish. 60, 157–188. doi: 10.1007/978-94-017-3245-1_11
R Core Team (2013). R: A Language and Environment for Statistical Computing. Vienna: R Foundation for Statistical Computing.
Rindorf, A., Gislason, H., Burns, F., Ellis, J. R., and Reid, D. (2020). Are fish sensitive to trawling recovering in the Northeast Atlantic? J. Appl. Ecol. 57, 1936–1947. doi: 10.1111/1365-2664.13693
Schlaff, A. M., Heupel, M. R., and Simpfendorfer, C. A. (2014). Influence of environmental factors on shark and ray movement, behaviour and habitat use: a review. Rev. Fish Biol. Fish. 24, 1089–1103. doi: 10.1007/s11160-014-9364-8
Silva, M. A., Prieto, R., Magalhães, S., Seabra, M. I., Santos, R. S., and Hammond, P. S. (2008). Ranging patterns of bottlenose dolphins living in oceanic waters: implications for population structure. Mar. Biol. 156, 179–192. doi: 10.1007/s00227-008-1075-z
Simpson, S. J., Humphries, N. E., and Sims, D. W. (2020). The spatial ecology of Rajidae from mark-recapture tagging and its implications for assessing fishery interactions and efficacy of Marine Protected Areas. Fish. Res. 228:105569. doi: 10.1016/j.fishres.2020.105569
Simpson, S. J., Sims, D. W., and Trueman, C. N. (2019). Ontogenetic trends in resource partitioning and trophic geography of sympatric skates (Rajidae) inferred from stable isotope composition across eye lenses. Mar. Ecol. Prog. Ser. 624, 103–116. doi: 10.3354/meps13030
Siskey, M. R., Shipley, O. N., and Frisk, M. G. (2019). Skating on thin ice: identifying the need for species-specific data and defined migration ecology of Rajidae spp. Fish Fish. 20, 286–302. doi: 10.1111/faf.12340
Sousa, I., Baeyaert, J., Gonçalves, J. M., and Erzini, K. (2019). Preliminary insights into the spatial ecology and movement patterns of a regionally critically endangered skate (Rostroraja alba) associated with a marine protected area. Mar. Freshw. Behav. Physiol. 52, 1–17.
Speed, C. W., Field, I. C., Meekan, M. G., and Bradshaw, C. J. (2010). Complexities of coastal shark movements and their implications for management. Mar. Ecol. Prog. Ser. 408, 275–293. doi: 10.3354/meps08581
Stehmann, M. F., and Burkel, D. L. (1984). “Rajidae,” in Fishes of the North-Eastern Atlantic and Mediterranean, Vol. 1, eds P. J. P. Whitehead, M. L. Bauchot, J. C. Hureau, J. Nielson, and E. Tortonese (Paris: UNESCO), 163–196.
Stevens, J. (2002). The role of protected areas in elasmobranch fisheries management and conservation. Occas. Paper IUCN Species Surviv. Comm. 25, 241–242.
Thorburn, J., Neat, F., Bailey, D. M., Noble, L. R., and Jones, C. (2015). Winter residency and site association in the critically endangered North East Atlantic spurdog Squalus acanthias. Mar. Ecol. Prog. Ser. 526, 113–124. doi: 10.3354/meps11210
Thorburn, J., Neat, F., Burrett, I., Henry, L.-A., Bailey, D., Jones, C., et al. (2019). Ontogenetic and seasonal variation in movements and depth use, and evidence of partial migration in a benthopelagic elasmobranch. Front. Ecol. Evol. 7:353. doi: 10.3389/fevo.2019.00353
Vaudo, J. J., Byrne, M. E., Wetherbee, B. M., Harvey, G. M., and Shivji, M. S. (2017). Long-term satellite tracking reveals region-specific movements of a large pelagic predator, the shortfin mako shark, in the western north atlantic ocean. J. Appl. Ecol. 54, 1765–1775. doi: 10.1111/1365-2664.12852
Wearmouth, V. J., and Sims, D. W. (2009). Movement and behaviour patterns of the critically endangered common skate Dipturus batis revealed by electronic tagging. J. Exp. Mar. Biol. Ecol. 380, 77–87. doi: 10.1016/j.jembe.2009.07.035
Wearmouth, V. J., and Sims, D. W. (2010). Sexual segregation in elasmobranchs. Biol. Mar. Mediterr. 17, 236–239.
Whitehead, P. J., Bauchot, M. L., Hureau, J. C., Neilson, J., and Tortonese, E. (1986). Fishes of the North-Eastern Atlantic and the Mediterranean, Vol. I, II & III. Paris: United Nations Educational, Scientific and Cultural Organisation (UNESCO).
Wickham, H. (2016). ggplot2: Elegant Graphics for Data Analysis. New York, NY: Springer-Verlag. Available online at: https://ggplot2.tidyverse.org
Keywords: Dipturus intermedius, flapper skate, highest density intervals, home and core depth range, marine protected area, Rajidae, spatial ecology
Citation: Thorburn J, Wright PJ, Lavender E, Dodd J, Neat F, Martin JGA, Lynam C and James M (2021) Seasonal and Ontogenetic Variation in Depth Use by a Critically Endangered Benthic Elasmobranch and Its Implications for Spatial Management. Front. Mar. Sci. 8:656368. doi: 10.3389/fmars.2021.656368
Received: 20 January 2021; Accepted: 09 June 2021;
Published: 19 July 2021.
Edited by:
Adrian C. Gleiss, Murdoch University, AustraliaReviewed by:
Samantha Andrzejaczek, Stanford University, United StatesDaniel M. Coffey, Texas A&M University–Corpus Christi, United States
Copyright © 2021 Thorburn, Wright, Lavender, Dodd, Neat, Martin, Lynam and James. This is an open-access article distributed under the terms of the Creative Commons Attribution License (CC BY). The use, distribution or reproduction in other forums is permitted, provided the original author(s) and the copyright owner(s) are credited and that the original publication in this journal is cited, in accordance with accepted academic practice. No use, distribution or reproduction is permitted which does not comply with these terms.
*Correspondence: James Thorburn, amF0MjFAc3QtYW5kcmV3cy5hYy51aw==