- 1Halmos College of Arts and Sciences, Nova Southeastern University, Dania Beach, FL, United States
- 2Centro para la Sostenibilidad Ambiental, Universidad Peruana Cayetano Heredia, Lima, Peru
- 3Chicago Zoological Society, Brookfield, IL, United States
Peru’s coastal waters are characterized by significant environmental fluctuation due to periodic El Niño- La Niña- Southern Oscillation (ENSO) events. This variability results in ecosystem-wide food web changes which are reflected in the tissues of the Peruvian fur seal (Arctocephalus australis). Stable isotope ratios (δ13C and δ15N) in Peruvian fur seal vibrissae (whiskers) are used to infer temporal primary production and dietary variations in individuals. Sea surface temperature anomaly (SSTA) recordings from the Niño 1+2 Index region captured corresponding ENSO conditions. Fluctuations in δ15N values were correlated to SSTA records, indicating that ENSO conditions likely impact the diet of these apex predators over time. Anomalous warm phase temperatures corresponded to decreased δ15N values, whereas cold phase anomalous conditions corresponded to increased δ15N values, potentially from upwelled, nutrient-rich water. Vibrissae δ13C values revealed general stability from 2004 to 2012, a moderate decline during 2013 (La Niña conditions) followed by a period of increased values concurrent with the 2014–2016 El Niño event. Both δ13C and δ15N values were inversely correlated to each other during the strongest El Niño Southern Oscillation event on record (2014–2016), possibly indicating a decline in production leading to an increase in food web complexity. Lower δ13C and δ15N values were exhibited in female compared to male fur seal vibrissae. Findings suggest ENSO conditions influence resource availability, possibly eliciting changes in pinniped foraging behavior as well as food web of the endangered Peruvian fur seal.
Introduction
Large-scale climatic anomalies associated with periodic, alternating El Niño- La Niña-Southern Oscillation (ENSO) conditions are recorded globally through a combination of atmospheric and oceanic teleconnections, resulting in significant, ecosystem-wide impacts (Ropelewski and Halpert, 1987; Trenberth et al., 1998; McPhaden et al., 2006; Sulca et al., 2017). ENSOs are alternating cycles of warm and cold sea surface temperature (SST) in the tropical central and eastern Pacific Ocean; these conditions can last upward of 18 months with reoccurrence approximately every 2–7 years (Gutierrez et al., 2007; Taylor et al., 2008; Grandi et al., 2012). ENSO events are classified by magnitude and are quantified using sea surface temperature anomalies (SSTA; Trenberth, 1997; Oliveira, 2011). Anomalies are deviations from the seasonal average conditions and are anything greater than ± 1°C. Magnitudes of ENSO range from Weak (± 1 to 1.5°C) to Extreme (± 2.5 to 3.0°C; Waluda et al., 2006; Grandi et al., 2012).
The tropical eastern Pacific Ocean is dominated by the Humboldt Current Upwelling Ecosystem, a cold, nutrient-rich coastal current which supports a multitude of ecologically and economically important species (Barber and Chavez, 1983; Ñiquen and Bouchon, 2004; Heileman et al., 2008; Montecino and Lange, 2009; Kluger et al., 2019). Peruvian anchoveta (Engraulis ringens), a keystone species of this ecosystem, comprises the largest single-species fishery in the world, although the species experienced severe stock collapses during the 1971/72, 1982/83, 1997/98 ENSO events (Clark, 1976; Alheit and Ñiquen, 2004; Ñiquen and Bouchon, 2004; Espinoza-Morriberón et al., 2017). South American pinniped mortality events and anchoveta abundance declines coincided with these ENSO periods (Trillmich and Ono, 1991; Arias-Schreiber and Rivas, 1998; Oliveira, 2011; Cárdenas-Alayza, 2012). Other apex predators residing in the eastern Pacific Ocean at the time of ENSO periods have been recorded exhibiting modified behaviors, body mass declines, and unusual mortality events. During the 1982/83 El Niño event, the Galapagos fur seal (Arctocephalus galapagoensis), a top predator found in the direct impact zone of equatorial ENSO conditions, experienced large mortality events and lowered pup production in the following breeding season, likely due to resource limitation (i.e., starvation; Trillmich and Limberger, 1985). Similarly, the 1997/98 El Niño affected behaviors in northern elephant seals (Mirounga angustirostris) off the coast of southern California, whose foraging trip duration and distance traveled increased to compensate for lower foraging success (Crocker et al., 2006). During this same ENSO event (1997/98), South American sea lions (Otaria byronia) off the coast of Peru were recorded foraging at deeper depths evidenced through dietary changes. This apex predators’ diet altered from predominantly anchoveta and squat lobster (Pleuroncodes monodon) to mostly demersal species; interestingly, the only time pelagic squat lobster was absent in this otariid’s diet was during the recorded El Niño event (Soto et al., 2006).
The complex marine food web along the Peruvian coastal margin is influenced by the aforementioned periodic bio-physical changes (Chiu-Werner et al., 2019). The Humboldt Current is inhabited by two fur seal independent evolutionary units of unsettled taxonomic status, the South American fur seal (Arctocephalus australis) and the Peruvian fur seal (A. australis unnamed sp.; Vásquez, 1995; Zavalaga et al., 1998; Arias-Schreiber, 2000, 2003; Cárdenas-Alayza and Oliveira, 2016). Geographical isolation between South American fur seal breeding colonies has led to the genetic variation between these two fur seal taxa (Berta and Churchill, 2012; Nyakatura and Bininda-Emonds, 2012; Oliveira and Brownell, 2014). The Peruvian fur seal, found along the eastern Pacific coastline of South America (Berta and Churchill, 2012; Oliveira and Brownell, 2014; Cárdenas-Alayza and Oliveira, 2016), feed most commonly on pelagic and demersal-pelagic fishes and cephalopods; Peruvian anchoveta and Teuthidae squids comprise 40–60% of their diet (Vásquez, 1995; Zavalaga et al., 1998; Arias-Schreiber, 2000, 2003; Oliveira et al., 2008). The fur seals forage in proximity to their breeding colonies (150 km, Espinoza et al., 2017) and the majority of dives are concentrated at 11–30 m in depth (Gentry and Kooyman, 1986). These seals are subjected to El Niño events in the tropical Pacific, which seem to alter their foraging habits, evidenced in both the 1982/83 and 1997/98 El Niños. During these times adult females foraged at depth averaging 29 to 170 m with trip durations lasting upward of 10 to 20 days searching for prey. These findings suggest pup survival rate was negatively impacted (Gentry and Kooyman, 1986; Majluf, 1987a, b; Cárdenas-Alayza, 2018).
Peruvian pinnipeds are distinctly vulnerable during these strong magnitude ENSO episodes (Oliveira, 2011). These marine mammals serve as sentinel species, indicative of ecosystem health in an environment at the center of fluctuating ENSO conditions (Fossi and Panti, 2017). The feeding ecology and movements of these pinnipeds are of substantial interest as ENSO events continue to intensify in both frequency and magnitude (Sepúlveda et al., 2014). Through a combined analysis of stable isotope profiles and abiotic recordings from El Niño indices, the feeding ecology of the Peruvian fur seal was investigated in Punta San Juan, Peru (Figure 1) over a time series of various ENSO conditions. Stable isotope ratios were used to depict the trophic fluctuations of fur seal individuals and their population. Abiotic recordings from the Niño 1+2 index are reflective of environmental changes due to alternating ENSO conditions along the coast of Peru. By using continuously growing, metabolically inert vibrissae (whiskers) from Peruvian fur seals, it is possible to obtain valuable, multi-year dietary information representative of their foraging environment across fluctuating conditions during overlapping, multiple ENSO events (Hirons, 2001; Cherel et al., 2009; Rea et al., 2015; Edwards, 2018). This is the first study to capture potential ecosystem changes recorded by individual Peruvian fur seals through the assessment of trophic level via vibrissae analyses and relate these changes to multiple ENSO events off the Pacific coast of South America.
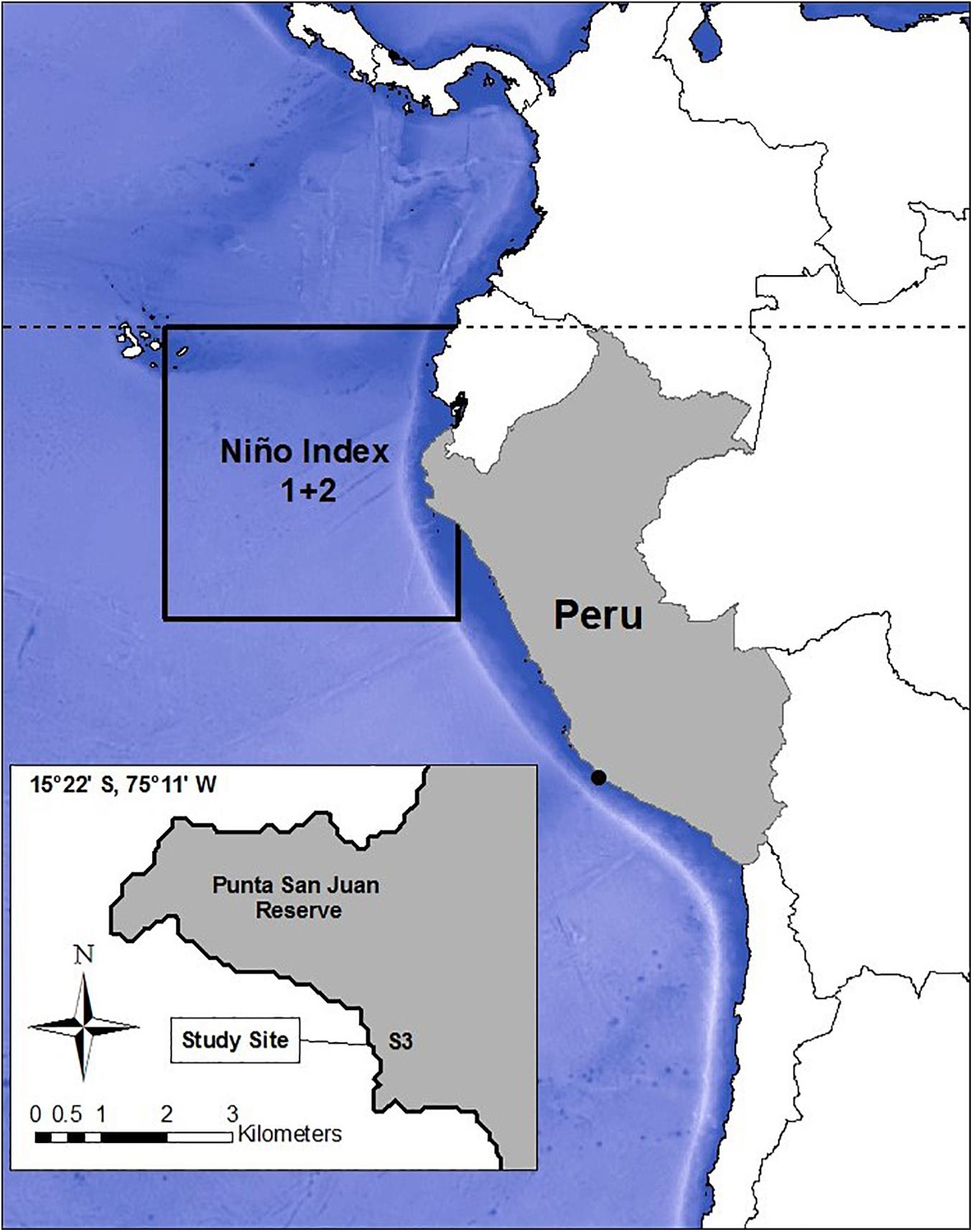
Figure 1. Location of the Punta San Juan reserve (PSJ) in Peru (shaded in gray), and an insert of PSJ with the beach used as the study site (S3), the main breeding colony of Peruvian fur seals. The black square represents the region of coastal waters of Niño Index 1 + 2 found along the equator, characterized by the dashed line. (Map adapted from Cárdenas-Alayza, 2012).
Materials and Methods
Study Area and Sample Collection
Certified personnel of the Punta San Juan Program and Chicago Zoological Society collected pinniped vibrissae during annual health assessments between 2010 and 2016 at the Punta San Juan reserve (PSJ) in southern Peru’s Ica province (15° 22′ S, 75° 11′ W), which forms part of a national natural protected area network called “Reserva Nacional Sistema de Islas, Islotes y Puntas Guaneras,” or RNSIIPG for its Spanish acronym.
To retrieve the most recent growth, mystacial vibrissae were pulled from live animals in November of each year (2010–2012, 2015–2016). Animals were restrained using inhalant anesthetics and/or remotely delivered, multimodal anesthetic drugs as previously described elsewhere (Adkesson et al., 2012, 2019). Vibrissae from sixty-four Peruvian fur seals were collected: 47 adult females and seventeen adult males. The sample years included 2010 (N = 29), 2011 (N = 12), 2012 (N = 11), 2015 (N = 6), and 2016 (N = 6; Table 1). All samples were collected following methods approved under research permits Resolución Jefatural No. 009- 2010-, No. 023- 2011-, No. 022- 2012-, No. 008- 2015-, and No. 019-2016-SERNANP-RNSIIPG issued by the Peruvian National Service of Natural Protected Areas (Spanish acronym SERNANP) and the Peruvian Ministry of the Environment (Spanish acronym MINAM).
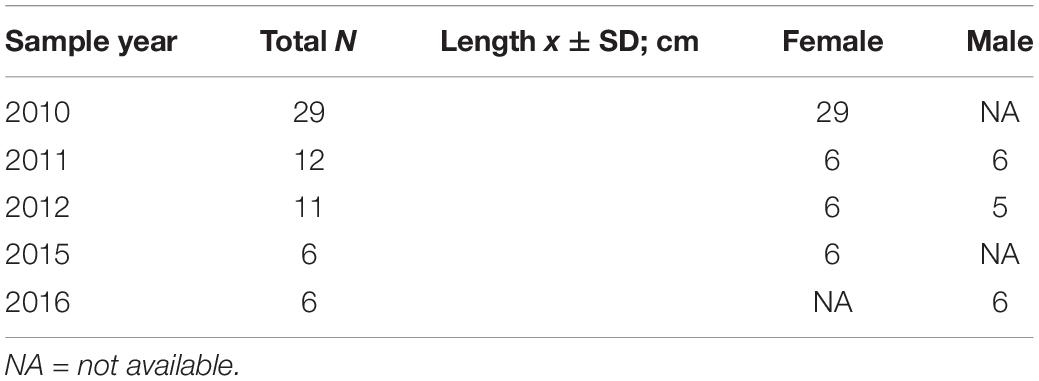
Table 1. Total number of Peruvian fur seal Arctocephalus australis unnamed sp. vibrissae analyzed in this study, including year sampled, mean vibrissae length, and associated sex.
Stable Isotope Analyses
Fur seal vibrissae were cleaned using a scrubbing pad and rinsed with deionized water to remove any surface contaminants. After being thoroughly dried at 60°C for a minimum of 24 h, vibrissae were cut into 0.25 cm sections from base (proximal) to tip (distal) to meet mass requirements for the isotopic analysis (0.6–0.8 mg). Segments were placed in individual tin capsules and pelletized. Samples were combusted and analyzed for δ13C and δ15N values at the Smithsonian Institution’s Museum Conservation Institute (Suitland, MD, United States) using a Thermo Delta V Advantage mass spectrometer in continuous flow mode coupled to a Costech 4010 Elemental Analyzer (EA) via a Thermo Conflo IV (CF-IRMS). A set of standards were run for every 10–12 samples. The standards included USGS40 and USGS41 (L-glutamic acid) as well as Costech acetanilide. All samples and standards were run with the same parameters; this included an expected reproducibility of the standards ≤0.2‰ (1σ) for both δ13C and δ15N. Stable isotope values were expressed in terms of δ and were reported relative to the standard reference material, Vienna Pee Dee Belemnite standard for δ13C and atmospheric air (N2) for δ15N. Following Bond and Hobson (2012), stable isotope values were reported with the standard parts per thousand notation (‰):
where jX is the heavier isotope, such as 13C, and iX the lighter isotope, such as 12C, in the analytical sample (numerator) and the international measurement standard (denominator).
Oceanographic Conditions
Sea surface temperature anomalies (°C) from the 1+2 Niño index, our proxy for ENSO conditions, were obtained from the National Oceanographic and Atmospheric Administration’s (NOAA) Teleconnections ENSO website1. NOAA_ERSST_V5 data were obtained from NOAA/OAR/ESRL PSD, Boulder, Colorado, United States2. Data were collected from Extended Reconstructed Sea Surface Temperature (ERSST.v5) dataset, which is a global, monthly SST analysis derived from the International Comprehensive Ocean-Atmosphere Dataset (ICOADS). The ERSST.v5 dataset includes information from modern buoy observation, Argos-profiling CTD floats, global drift buoys like ICOADS R3.0 (from R2.5), and Hadley Center Ice-SST version 2 (HadISST2) sea ice concentration (Huang et al., 2014, 2015, 2017, 2018a, b; Liu et al., 2014).
Statistical Analyses
The most recent vibrissa growth is located at the base of the whisker, and an individual vibrissa can represent several years’ growth (Hirons et al., 2001; Ginter et al., 2012). The vibrissae growth rate used for this study was based on Kelleher (2016) northern fur seal (Callorhinus ursinus) growth rate of 0.09 mm/day. Each 0.25 cm segment represented approximately 28 days using this growth rate. With an average of 12.31 ± 3.28 cm in length for each adult, individual vibrissa represented on average 45.6 ± 12 months (3.8 ± 1 years). By analyzing monthly means of individual seals’ isotopic values, a mean for both δ13C and δ15N values can be acquired on a monthly scale for the overall population of seals (i.e., month, year). Across the 64 sampled vibrissae, a total of 12 years’ stable isotope data were recorded.
The statistical package R Core Team (2016) was used for all analyses. Both covariance and correlation analyses were calculated between δ13C and δ15N, δ13C and SSTA, and δ15N and SSTA for both fur seal sexes. Correlations tested the strength of relationships between the stable isotope ratios of the vibrissae and ENSO conditions. Pearson’s correlations were used when the parametric assumptions were met; however, non-parametric conditions, for sample sizes greater than 30, used Kendall’s tau correlations when datasets could not be transformed to meet parametric assumptions. Covariances detected how changes in SSTA were associated with changes in vibrissae δ13C and δ15N values.
Linear Mixed Effects models (Pinheiro and Bates, 2000) with Gaussian distributions and identity link functions were applied to test for a significant linear relationship between SSTA, sex and/or their interaction term to explain changes in δ13C and δ15N values in sampled individuals. Mixed effects models were used to account for the repeated measurements in individuals over time. These models had individual identity (i.e., Animal ID) as a random effect to account for repeated measures of each response variable on the different segments of each vibrissa (Franco-Trecu et al., 2014). We constructed linear mixed effects models using nlme (Pinheiro et al., 2017) since we consider that each individual can have a different isotopic signature and variation depending on the month and year of sampling. Model diagnostics were verified to not violate Gaussian assumptions. We ran backwards selection, progressively removing the non-significant terms, and compared sequential models. Best model was selected based on the lowest Akaike’s Information Criterion (AIC) score. We applied a bootstrapping routine (n = 1000) to estimate 95% confidence intervals for the population average for each sex with lme package (Bates et al., 2015) in R statistical environment (R Core Team, 2016).
Results
Similarity in isotopic oscillating patterns occurred across all vibrissae. Both male and female fur seal vibrissae exhibited nearly identical mean stable isotope patterns, but male fur seals exhibited higher values than females in both stable isotopes by up to 0.5‰ (δ13C) and 1.5‰ (δ15N; Figure 2). Overall, δ13C values ranged from −18.13 to −13.17‰ with a mean of −14.31 ± 0.31‰ and δ15N values ranged from 15.83 to 22.31‰ with a mean of 19.08 ± 0.83‰. The δ13C values exhibited minor fluctuations from 2004 to 2009 [−14.08 to −13.59‰ (Δ 0.49‰)]; then δ13C was highly variable from 2010 through 2016 (−15.09 to −13.80‰; Δ 1.29‰). Additionally, from late 2015 through 2016, δ13C values resembled patterns previously recorded in 2004–2009, where δ13C ranged −14.46 to −13.80‰ (Δ 0.66‰). The δ13C values from 2010 to 2014 were the most depleted (−14.42‰) and had the largest range, −15.09 to −13.91‰ (Δ 1.18‰) across all years of the study. In contrast to δ13C, δ15N values had much larger fluctuations. From late 2004, the earliest stable isotope data recorded, through 2009, δ15N values decreased 2.41‰. The δ15N values ranged from 18.40 to 20.81‰. Following this nearly 6-year gradual decline in δ15N values, a rapid, approximately 2‰ increase, occurred between 2010 and 2011, after which δ15N returned to levels recorded in previous years (ca. 2004/2005). A gradual decline of 4.35‰ in δ15N values (20.84‰ decreasing to 16.49‰) took place from the beginning of 2012 until 2016. Data from the remainder of 2016 showed a steep increase from 17.00 to 18.56‰ (Δ 1.56‰).
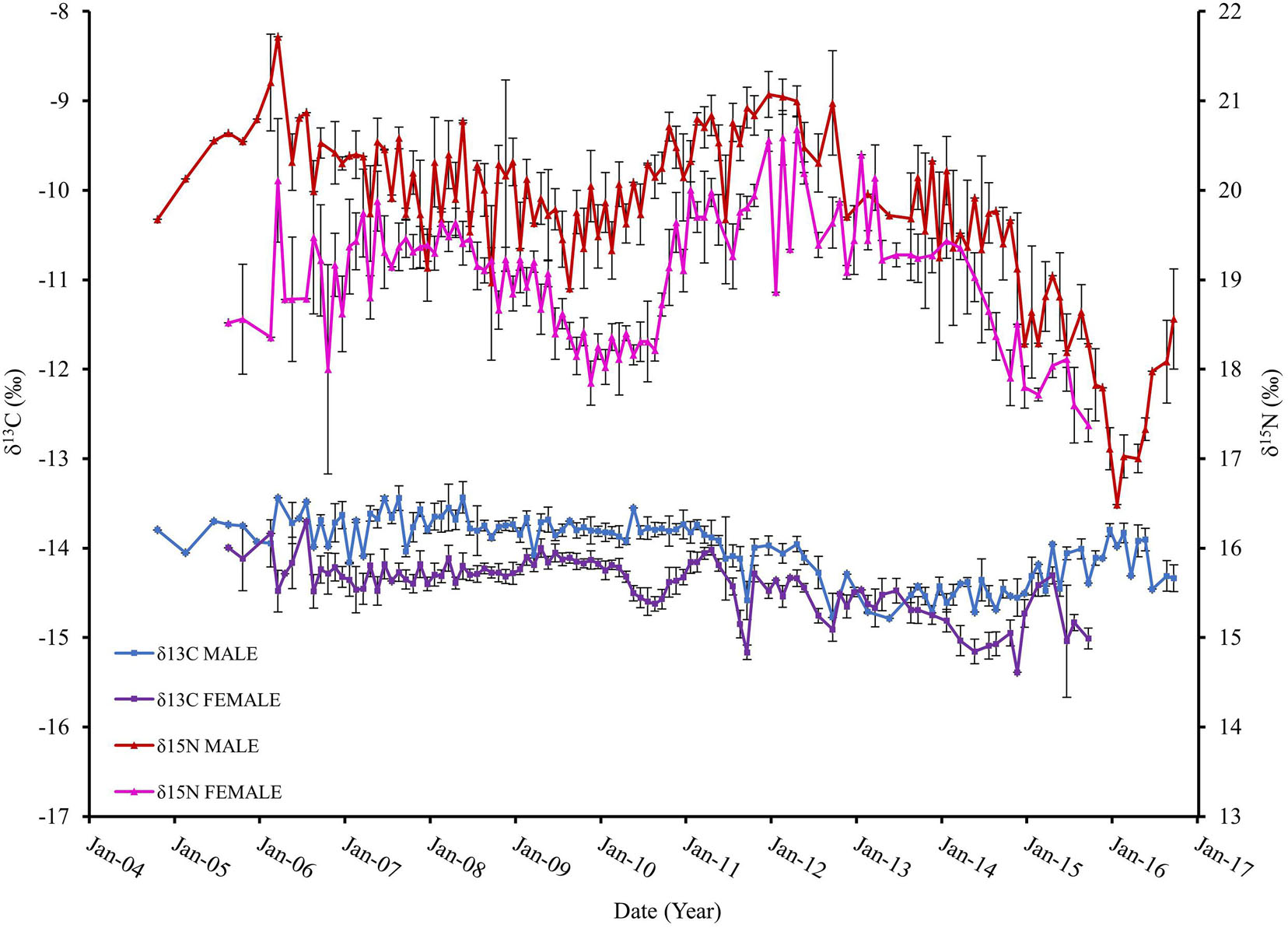
Figure 2. Peruvian fur seal male and female δ13C and δ15N measured in parts per thousand (‰), 2004–2016. Female δ13C is purple and δ15N is pink, while male δ13C is blue and δ15N is red.
A Kendall’s tau correlation assessed the relationship between ever-changing SSTA and stable isotope values across all 12 years from November 2004 through 2016. The number of animals, adult female (N = 47) and adult male (N = 17), represent a monthly mean ranging anywhere from 1 to 47 individuals per month. The fur seals’ mean δ13C did not correlate with SSTA over the 12-year period. However, δ13C values did significantly correlate with SSTA over a shorter period of 7 years, 2009 through 2016 (p = 0.040). A significant inverse correlation existed between mean δ15N values and SSTA (r = −0.254, p < 0.001). Warmer temperature anomalies correlated to lower δ15N values. Peruvian fur seal vibrissae mean δ13C and δ15N values did not correlate over the entire 12-year period; however, a Pearson’s correlation coefficient showed δ13C and δ15N values were significantly inversely correlated during the 2014–2016 ENSO event (p = 0.002).
Two linear mixed effect models were employed to explain changes in Peruvian fur seal δ13C and δ15N values over time with changing environmental conditions (SSTA):
Exploratory plots were accessed for both Models 1 and 2; when year is factored, patterns are visible in relation to SSTA and stable isotope values. Model 1 revealed similar trends in δ13C values throughout the years with a decline around 2013 linked to colder sea surface waters and a change to rapidly warming conditions in the following years (2014–2016) when the trend in δ13C values began to increase (Figure 3). Model 2 revealed dynamic patterns from year to year in comparison to Model 1 (δ13C); however, δ15N values began a declining trend seen as early as 2013 with increasing SSTA over the following years (2013–2016; Figure 4). Linear Mixed Models are presented in Table 2 where the estimates for the fixed factors are reported for each variable. The interaction term of Sex:SSTA was not significant for the δ13C; and sex alone was not sufficient to explain the variation in δ15N values. The best fitting model (lowest AIC score) included the sum of Sex and SSTA as explanatory variables of δ13C and δ15N values (p < 0.01). Prediction plots for both Models 1 and 2 (Figure 5) illustrated sex differences; males generally had more enriched 13C and 15N than females. Best fit values seen in Model 1 showed a slight increase in 13C with increasing SSTA whereas best fit values from Model 2 showed a decrease in 15N with increasing SSTA.
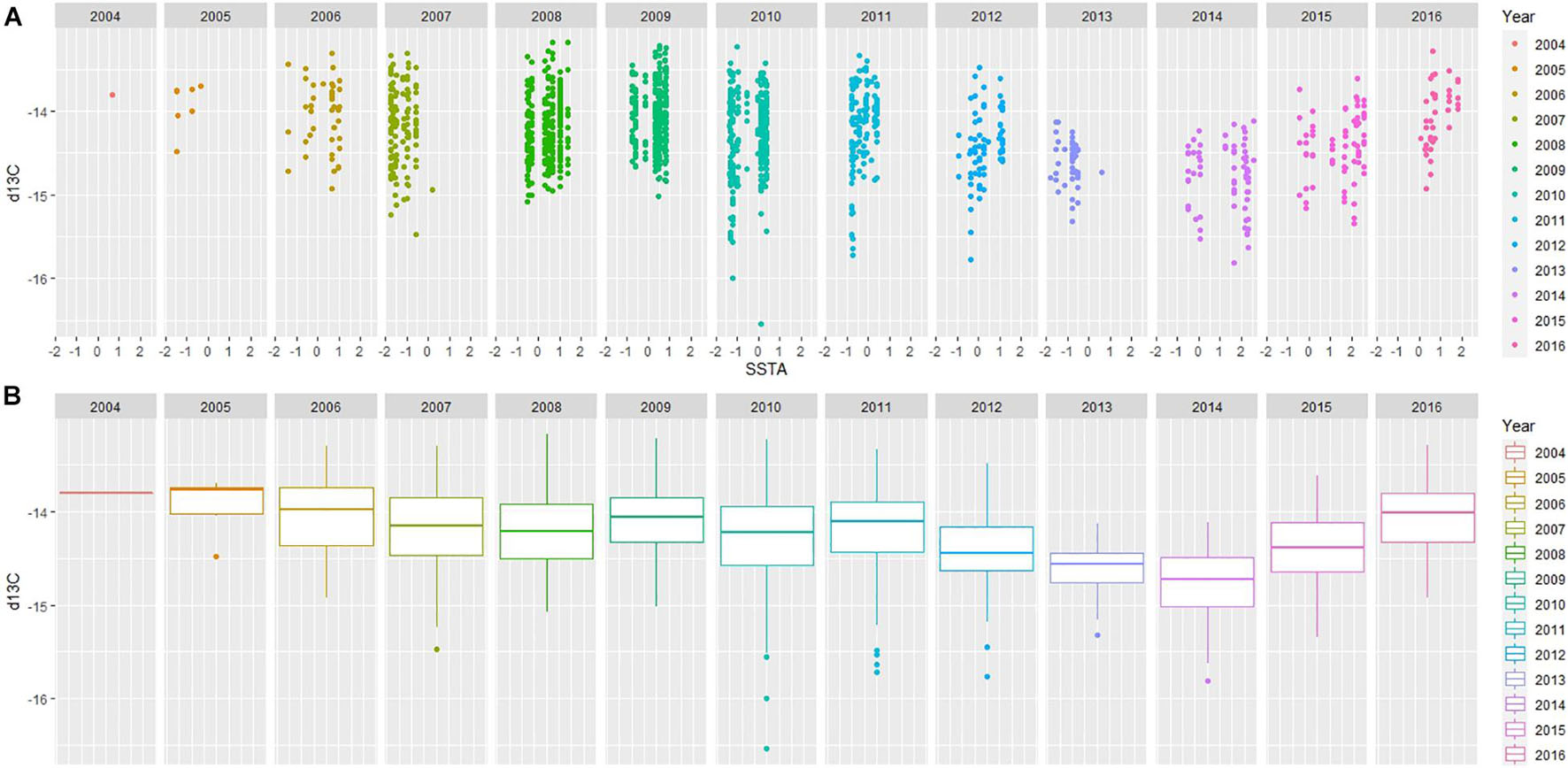
Figure 3. (A) Mixed Linear Effects ggplot of Model 1. δ13C measured in parts per thousand (‰) versus recorded SSTA in degrees Celsius (°C). Year is an additional factor; all years are different colors (see key for reference). (B) A boxplot of δ13C measured in parts per thousand (‰) to visualize distribution of δ13C for each year. Points seen outside the extreme line may or may not represent outliers.
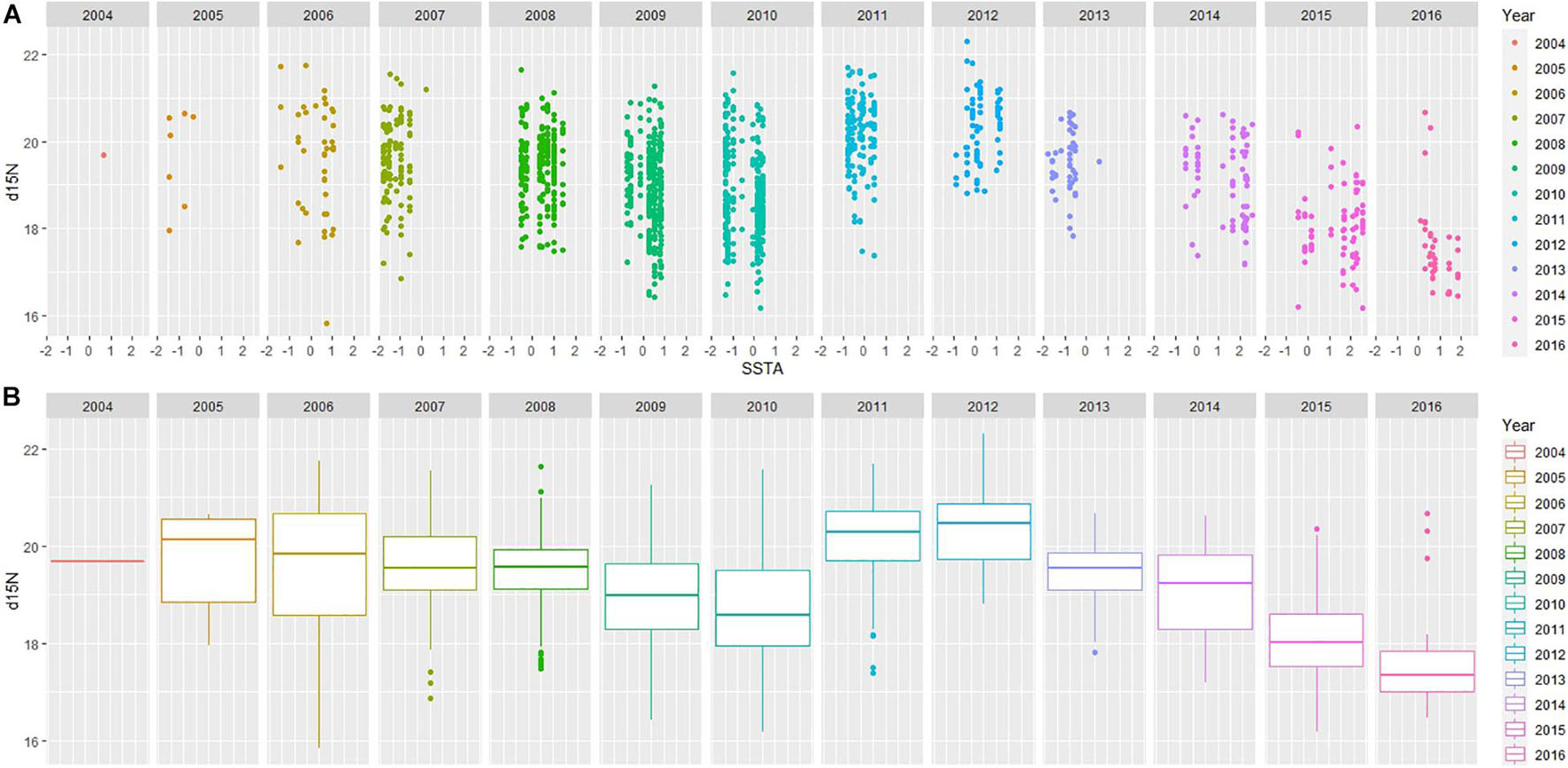
Figure 4. (A) Mixed Linear Effects ggplot of Model 2. δ15N measured in parts per thousand (‰) versus recorded SSTA in degrees Celsius (°C). Year is an additional factor; all years are different colors (see key for reference). (B) A boxplot of δ15N measured in parts per thousand (‰) to visualize distribution of δ15N for each year.
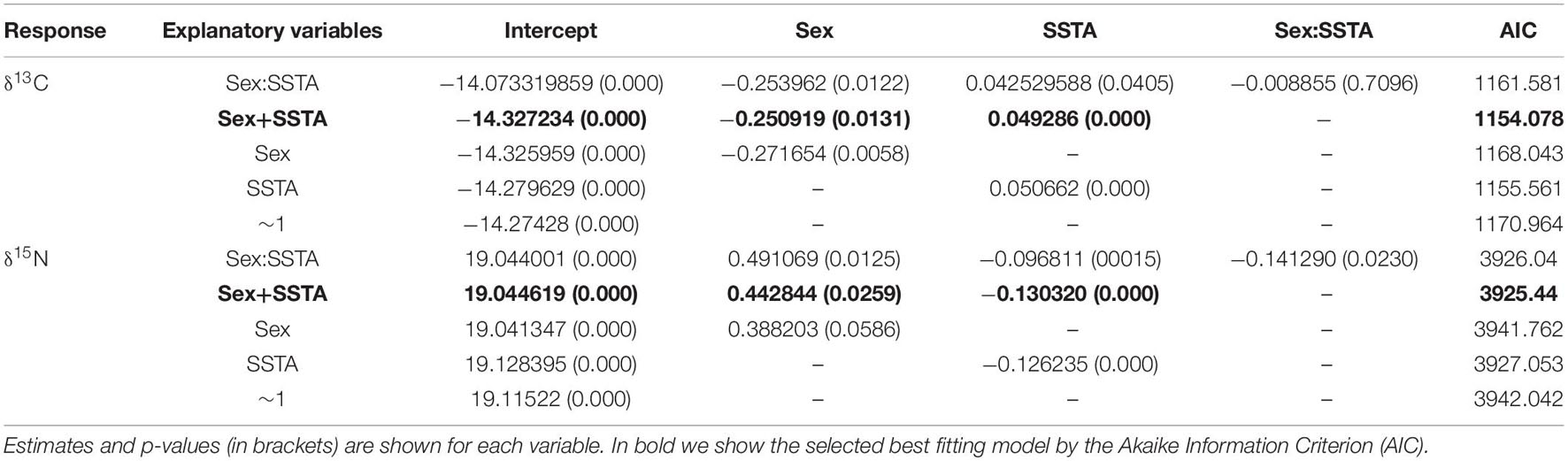
Table 2. Linear mixed models for δ13C and δ15N vibrissae values and sex, SSTA and their interactions as fixed effects.
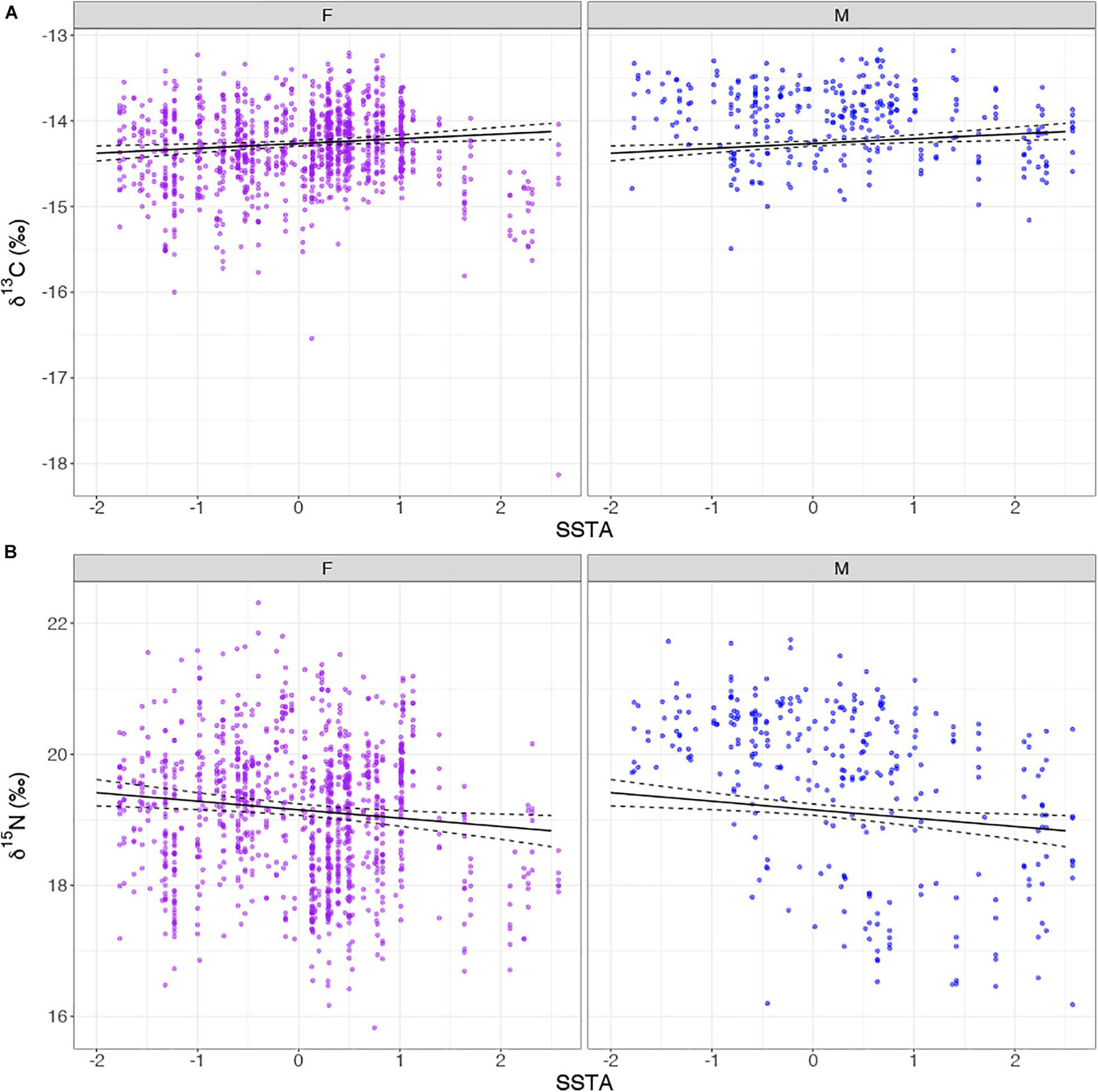
Figure 5. Prediction plot for best fitted values for (A) δ13C and SSTA by Sex and (B) δ15N and SSTA by Sex. The black line represents the model’s estimated average stable isotope value measured in parts per thousand (‰) across various sea surface temperature anomaly (SSTA) recordings (°C). The area within the dashed black lines represents the uncertainty in the average stable isotope value (95% confidence interval). F = Females (purple) and M = Males (blue).
Discussion
Capturing El Niño Southern Oscillation Signals
The proxy for ENSO conditions (e.g., monthly SSTA readings) in this study was collected from the closest Niño index, Niño 1+2, which is located more than 800 km from the Punta San Juan rookery. Although this is a substantial distance, previous ENSO years have shown documented impacts in the coastal Peruvian ecosystem based on the index, including the 1997/98 anchoveta fishery collapse which is believed to have caused a Peruvian fur seal mass mortality event documented in PSJ, Peru (Arias-Schreiber and Rivas, 1998; Cárdenas-Alayza, 2012). The analysis of δ13C and δ15N values from vibrissae in this study provided a timeline of trophic and production changes in this dynamic ecosystem, one where ENSO conditions fluctuate, and organisms must adapt to survive potentially stressful conditions. The PSJ population’s vibrissae recorded significant differences in δ13C and δ15N values with varying SSTA among years. SST is correlated with primary production changes in the ecosystem but may also influence the movement patterns of fishes and cephalopods as well. It is presumed that prey availability played a significant role in these findings; however, primary producer and potential prey stable isotope values are needed for further evaluation.
According to the SSTA from the Niño 1+2 region, the strongest ENSO on record occurred during this study in years 2014–2016, and the biotic results of the event were reflected in vibrissae collected in 2015 and 2016. Results in this study suggest that the 2014–2016 ENSO event impacted the trophic dynamics of the Peruvian coastal ecosystem in ways that had not been seen throughout the prior 9 years evaluated (2004–2013). The significant inverse correlation between δ15N and δ13C values during the 2014–2016 extreme El Niño event demonstrates sharply declining δ15N while δ13C slowly increases. This distinct change in fur seal trophic dynamics may correspond to a dietary shift linked to an influx of oceanic prey species during ENSO conditions. Meanwhile, the incremental increase in carbon enrichment may reflect a change in the dominate primary producers supporting the food web during nutrient limitation. This is evidenced in decreased surface chlorophyll concentrations, more pronounced during temperature extremes (Espinoza-Morriberón et al., 2017). Additionally, alternate foraging grounds may be linked to a weakening and delay in offshore transport of cold waters, as reported in previous ENSO events, may contribute to this isotopic variation (Roy and Reason, 2001; Montecinos and Gomez, 2010). These findings could infer that strong ENSO cycles may alter the specialized diet of the Peruvian fur seal.
Stable Carbon Isotope (δ13C)
A wild animal’s trophic discrimination factor varies according to the species’ diet and body condition, both of which would be potentially altered during ENSO periods. The comparison of consumer tissue δ13C values over time provides information regarding ocean production in foraging grounds throughout fluctuating ENSO conditions (France and Peters, 1997; Hirons et al., 2001; Kurle and Worthy, 2001). From 2014 through 2016 as SSTA increased, declines in primary production were expected, reflected as lower δ13C values. However, the mixed δ13C values observed during this time (Figure 6) may also suggest a change in dominant primary producers in the system as well as use of alternate foraging locations (Cárdenas-Alayza pers comm). However, an investigation into the region’s trophic structure would be necessary to support these inferences. Production variability and behavioral modifications may explain how fur seals are able to survive the extreme food shortages presumed to occur during ENSO events.
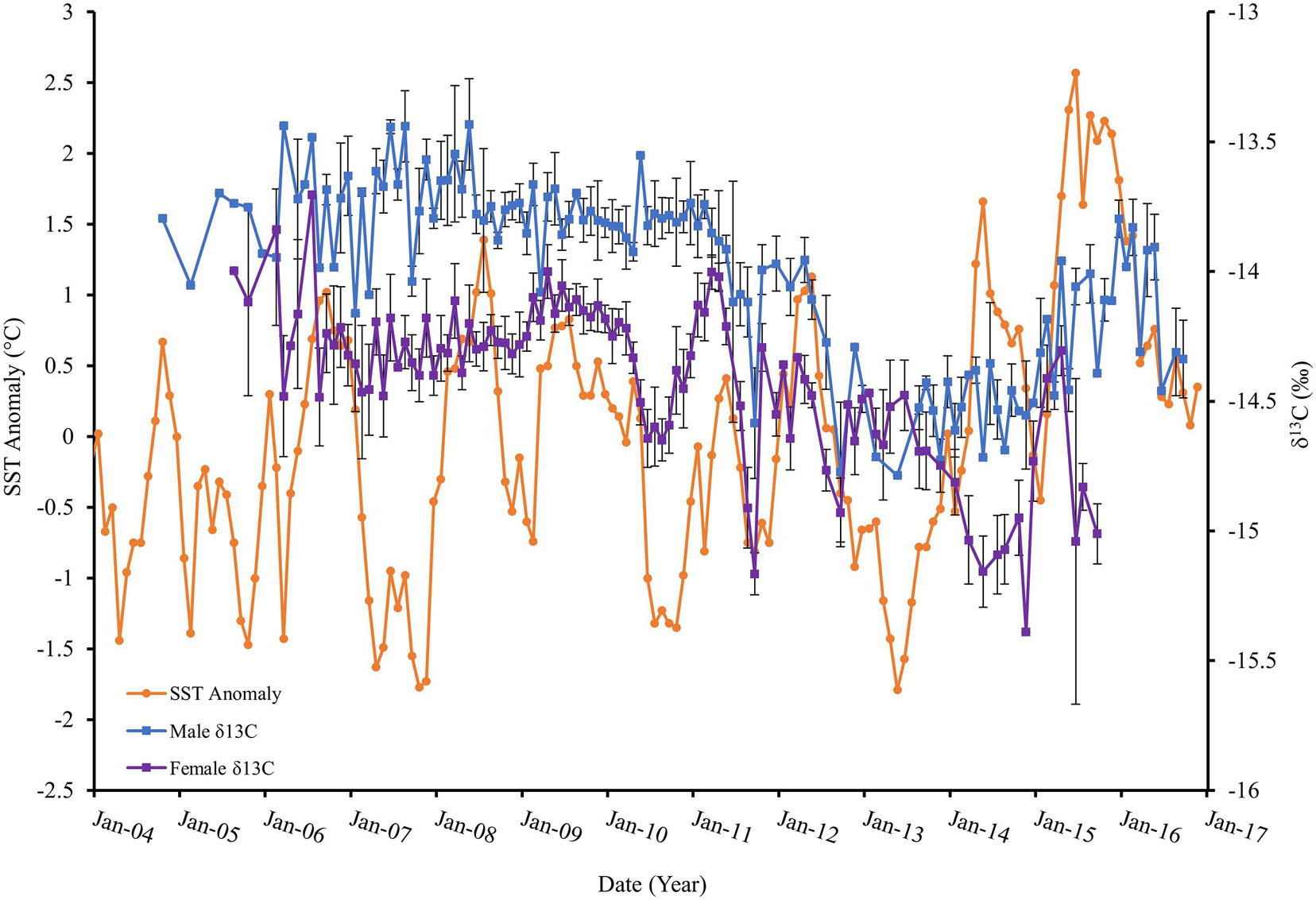
Figure 6. Peruvian fur seal male mean δ13C (blue) and female mean δ13C (purple) measured in parts per thousand (‰) relative to sea surface temperature anomaly (orange) degrees Celsius (°C), 2004–2016.
Related studies with sampling efforts in the Humboldt Current, such as Espinoza et al. (2017), revealed more depleted 13C values further offshore (Sydeman et al., 1997; Hill et al., 2006; Miller et al., 2008). Increased distance from shore corresponded to increased faunal 13C depletion which is common in upwelling ecosystems (Sydeman et al., 1997; Miller et al., 2008; Espinoza-Morriberón et al., 2017). Primary production decreases from coastal to neritic to oceanic waters (France and Peters, 1997; Hill et al., 2006; Espinoza et al., 2017). ENSO conditions could also contribute to fluctuating patterns in primary production during warm and cold phases, subsequently compromising resource availability within an environment.
Stable Nitrogen Isotope (δ15N)
The δ15N in consumer tissues relative to diet provide information regarding potential food web linkages (France and Peters, 1997; Hirons et al., 2001; Kurle and Worthy, 2001). The adult Peruvian fur seals all showed a similar pattern in δ15N values through time, a long-term gradual decline followed by a quick increase. The δ15N values from 2004 to 2009 were lower (Δ−2.41‰), followed by a rapid rise (Δ+2.17‰) from 2010 to 2011. A similar pattern persisted from 2012 through the end of 2016, revealing an oscillation of a 5-year decline (Δ−4.35‰) followed by a 9-month (Δ+1.56‰) increase. Males showed consistently higher δ15N values than females regardless of changes in SSTA recorded in the Nino 1+2 index (Figure 7). Whether this pattern specifically corresponds to ENSO conditions is uncertain; however, as El Niño conditions strengthened and lasted longer, δ15N continually decreased.
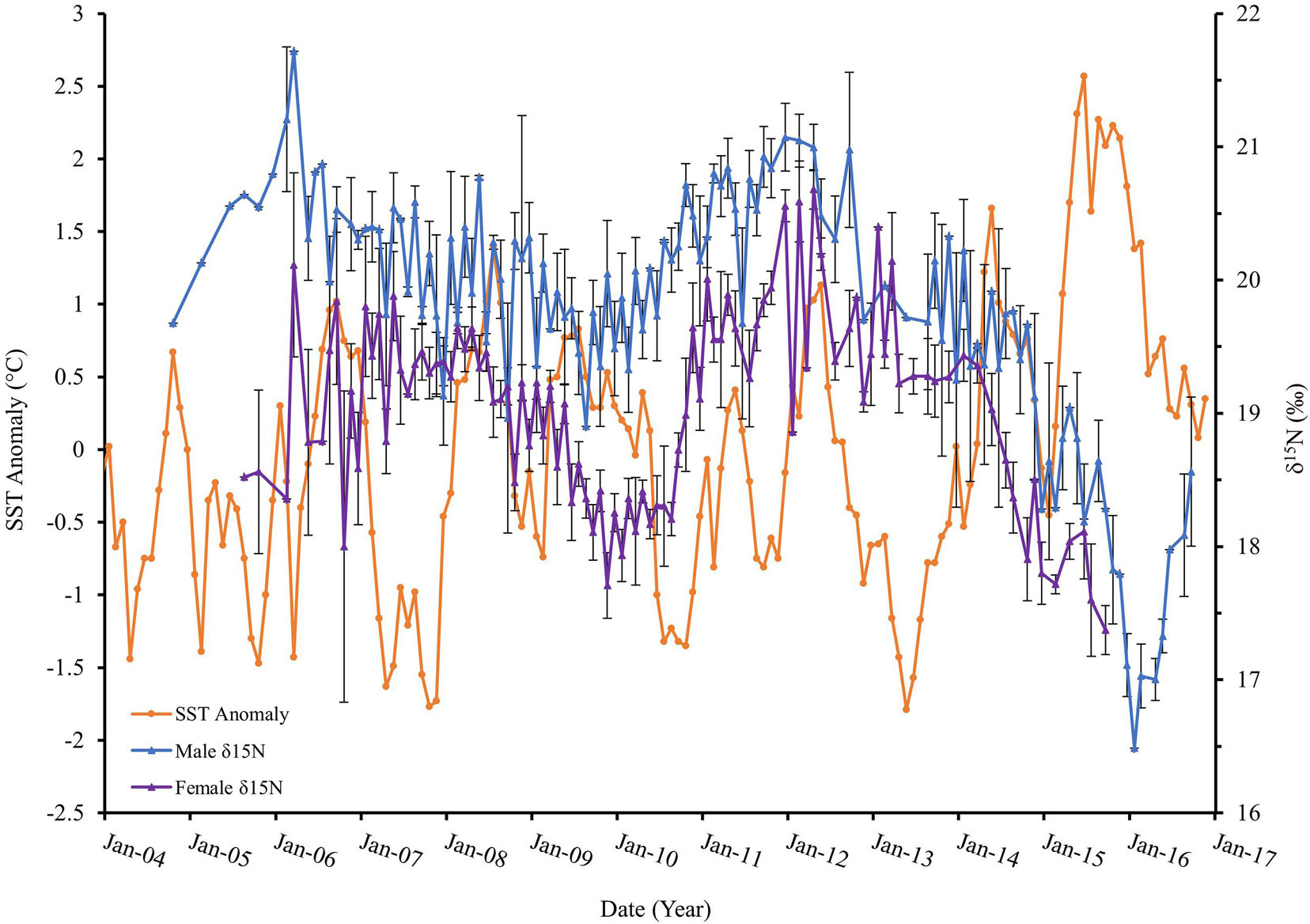
Figure 7. Peruvian fur seal male mean δ15N (blue) and female mean δ15N (purple) measured in parts per thousand (‰) relative to sea surface temperature anomaly (orange) degrees Celsius (°C), 2004–2016.
Warmer SSTs correlated to lower δ15N values. When evaluating ENSO conditions by phase (i.e., cold, normal, and warm), significant correlations between warmer phases and lower δ15N values as well as colder phases and higher δ15N values were identified. During warmer phases, the lowest δ15N values were detected, while consistently higher δ15N values were detected during cold phases. The increasing magnitude of ENSO conditions revealed significant correlation to lower δ15N values; stronger or more abnormally warm conditions revealed below average δ15N values, whereas norm, weak, and moderate magnitudes revealed mean population δ15N values (Edwards, 2018). Previous trophic study methodology on South American fur seal populations in Peru were done via scat analyses. A valuable diet evaluation was done from 1982 to 1988, which similarly to us, encompassed varying cold and warm periods, including two El Niño events. Scat findings in fur seal diet varied as a result of anchoveta availability; a wider range of prey was seen in scat when anchoveta were less available (Majluf, 1989). It can be hypothesized that mean Peruvian fur seal δ15N values could be reflecting these wider prey ranges during periods of anchoveta absence in foraging grounds in relation to warmer SSTA. This adaptive behavior has also been seen in the Peruvian population of South American sea lions during the 1998 El Niño, where they fed on a variety of prey when their primary prey source(s) were scarce (Soto et al., 2006).
Sex Evaluation
Both δ13C and δ15N values were significantly different between adult male and female Peruvian fur seal. Female 13C and 15N values were significantly lower than males so foraging differences between sex can be inferred. Male Peruvian fur seals are known to forage in larger ranges than adult females with pups; females must return to the rookery to nurse their pup, so they have abbreviated forage trips in comparison to males (Punta San Juan Program, unpublished data). While no gender-size and foraging relationships are known for Peruvian fur seals, they have been observed in South American sea lions. Male sea lions from northern Patagonia exploit benthic and deeper foraging grounds than smaller females (Campagna et al., 2001; Drago et al., 2009), although Drago et al. (2009) noted differences in foraging habits between the sexes are not constant over time. The potential for foraging in dissimilar water masses with contrasting prey composition may explain why male δ13C and δ15N values were higher than females (0.51‰, 1.36‰, respectively). Whether this is due to physical ability, metabolic capability, foraging location, depth, or simply different prey, these differences cannot be explained without potential prey source isotope signatures and/or tracking data (Edwards, 2018).
Conclusion
The Punta San Juan rookery located within the Humboldt Current Large Marine Ecosystem is home to the Peruvian fur seal (A. australis) unnamed sp. Environmental conditions within the ecosystem fluctuate frequently due to ENSO conditions, producing ecological consequences for wildlife. Vibrissae yield biochemical, multi-year timelines which allow for the evaluation of long-term trophic fluctuations within this dynamic ecosystem. Stable isotope ratios from Peruvian fur seals revealed temporal variations, possibly in diet, ecosystem production, and/or foraging habitat, related to anomalous ENSO conditions. The δ13C and δ15N values acted as a proxy for sex-related resource and habitat use.
Variability in SSTA was correlated to fluctuations in both δ13C and δ15N values, providing evidence that ENSO conditions alter the foraging of these apex predators, potentially due to changes in surrounding resources over time. Although behavioral adaptations could not be evaluated solely by these pinnipeds’ stable isotope ratios, fluctuations in both stable isotopes across years revealed that the fur seals were still feeding during these stressful conditions. Sex-related stable isotope ratio differences observed during ENSO conditions illustrated that sex-specific adaptations may exist. Further long term, trophic-based studies will be necessary to demonstrate the existence of true adaptations.
The anomalous climatic conditions along the western South American coast may lead Peruvian fur seals to utilize alternative foraging strategies to survive; these data corroborate Soto et al. (2006) that Peruvian fur seals may be utilized as a biological indicator of relative changes in marine resources. With the mean trend of SSTA in the 1+2 Nino region continuously increasing over 1°C during the past decade, culminating in the strongest ENSO event on record (2014–2016), both increased ENSO duration and magnitude threaten this vulnerable species and the coastal marine ecosystem.
Data Availability Statement
The original contributions presented in the study are included in the article/Supplementary Material, further inquiries can be directed to the corresponding author/s.
Ethics Statement
Ethical review and approval was not required for the animal study because sample collection was conducted by MA during a previous project – CZS IACUC approved.
Author Contributions
AH and ME acquired funding for the analyses. MA and SC-A previously collected the samples. ME and MD-A processed all samples. ME, SC-A, and AH performed all data analyses. All authors contributed equally to the project development and manuscript preparation.
Conflict of Interest
The authors declare that the research was conducted in the absence of any commercial or financial relationships that could be construed as a potential conflict of interest.
Publisher’s Note
All claims expressed in this article are solely those of the authors and do not necessarily represent those of their affiliated organizations, or those of the publisher, the editors and the reviewers. Any product that may be evaluated in this article, or claim that may be made by its manufacturer, is not guaranteed or endorsed by the publisher.
Acknowledgments
These data contributed to the completion of Master of Science thesis by ME Nova Southeastern University’s President’s Faculty Research and Development Grant (PFRDG) provided funding to AH. The Southern Florida Chapter of the Explorers Club provided field work funding to ME. J. Coley and L. Alfino provided laboratory assistance. R. Milligan provided analytical assistance. C. France from the Smithsonian Institution’s Museum Support Center provided stable isotope analyses. Chicago Zoological Society and the Punta San Juan Consortium funded the field research and all associated field operations. We thank G. Jankowski, J. Meegan, M. Allender, and the veterinary and field biologist staff that assisted with sample collection. We further acknowledge SERNANP for access to the RNSIIPG-PSJ reserve and AGRORURAL for use of field facilities. All samples were collected in accordance with the SERNANP research permits Resolución Jefatural Nos. 009-2010, 023-2011, 022-2012, and 008-2015-SERNANP-RNSIIPG.
Supplementary Material
The Supplementary Material for this article can be found online at: https://www.frontiersin.org/articles/10.3389/fmars.2021.651212/full#supplementary-material
Footnotes
References
Adkesson, M. J., Chinnadurai, S. K., Balko, J. A., Jankowski, G., Meegan, J. M., Cárdenas-Alayza, S., et al. (2019). “Anesthesia of free-ranging Peruvian fur seals (Arctocephalus australis) using medetomidine, midazolam, and butorphanol,” in Proceedings of the Zoo and Wildlife Health Conference, Kolmarden.
Adkesson, M. J., Jankowski, G., and Cardenas-Alayza, S. (2012). Reversible Immobilization of South American Fur Seals (Arctocephalus australis) and South American Sea Lions (Otaria flavescens) Using Medetomidine, Butorphanol, and Midazolam. Santiago: IAAAM.
Alheit, J., and Ñiquen, M. (2004). Regime shifts in the Humboldt Current ecosystem. Prog. Oceanogr. 60, 201–222. doi: 10.1016/j.pocean.2004.02.006
Arias-Schreiber, M. (2000). Los lobos marinos y su relacion con la abundancia de la anchoveta peruana durante 1979-2000. Boletin del Instituto del Mar del Peru. 19, 133–138.
Arias-Schreiber, M. (2003). Prey Spectrum and Feeding Behaviour of two Sympatric Pinnipeds (Arctocephalus australis and Otaria flavescens) in Relation to the 1997/98 ENSO in Southern Peru. Ph.D. thesis. Bremen: University of Bremen.
Arias-Schreiber, M., and Rivas, C. (1998). Distribución, tamaño poblacional y estructura de las poblaciones de lobos marinos (Arctocephalus australis y Otaria byronia) en el litoral peruano en noviembre de 1996 y Marzo de 1997. Informe Progresivo 73, 16–32.
Barber, R. T., and Chavez, F. P. (1983). Biological consequences of El Niño. Science 222, 1203–1210. doi: 10.1126/science.222.4629.1203
Bates, D., Maechler, M., Bolker, B., and Walker, S. (2015). Fitting linear mixed-effects models using lme4. J. Stat. Softw. 67, 1–48. doi: 10.18637/jss.v067.i01
Berta, A., and Churchill, M. (2012). Pinniped taxonomy: review of species and subspecies currently recognized, and evidence used for their description. Mammal. Rev. 42, 207–234. doi: 10.1111/j.1365-2907.2011.00193
Bond, A., and Hobson, K. (2012). Reporting stable-isotope ratios in ecology: recommended terminology, guidelines and best practices. Waterbirds 35, 324–331. doi: 10.1675/063.035.0213
Campagna, C., Werner, R., Karesh, W., Marin, M. R., Koontz, F., Cook, R., et al. (2001). Movements and location at sea of South American sea lions (Otaria flavescens). J. Zool. 257, 205–220. doi: 10.1017/S0952836901001285
Cárdenas-Alayza, S. (2012). Prey Abundance and Population Dynamics of South American fur seals (Arctocephalus australis) in Peru. Ph.D. thesis. Vancouver, BC: University of British Columbia.
Cárdenas-Alayza, S. (2018). “South American fur seal,” in Encyclopedia of Marine Mammals, 3rd Edn, eds B. Würsig, J. G. M. Thewissen, and K. Kovacs (USA: Academic Press), 905–907.
Cárdenas-Alayza, S., and Oliveira, L. (2016). Arctocephalus australis (Peruvian/Northern Chilean subpopulation). The IUCN Red List of Threatened Species 2016. Available online at: https://www.iucnredlist.org/species/72050476/72050985 (accessed October 31, 2020).
Cherel, Y., Kernaléguen, L., Richard, P., and Guinet, C. (2009). Whisker isotopic signature depicts migration patterns and multi-year intra- and inter-individual foraging strategies in fur seals. Biol. Lett. 5, 830–832. doi: 10.1098/rsbl.2009.0552
Chiu-Werner, A., Ceia, F., Cárdenas-Alayza, S., Cardeña-Mormontoy, M., Adkesson, M., and Xavier, J. (2019). Inter-annual isotopic niche segregation of wild Humboldt penguins through years of different El Nino intensities. Mar. Environ. Res 150:104755.
Clark, W. G. (1976). The Lessons of the Peruvian Anchoveta Fishery. CalCOFI Reports, Vol. 19. Rome: Food and Agriculture Organization of the United Nations (FAO), 57–63.
Crocker, D. E., Costa, D. P., and Le Boeuf, B. (2006). Impact of El Niño on the foraging behavior of northern elephant seals. Mar. Ecol. Prog. Ser. 309, 1–10. doi: 10.3354/meps309001
Drago, M., Crespo, E. A., Aguilar, A., Cardona, L., García, N., Dans, S. L., et al. (2009). Historic diet change of the South American sea lion in Patagonia as revealed by isotopic analysis. Mar. Ecol. Prog. Ser. 384, 273–286. doi: 10.3354/MEPS08017
Edwards, M. R. (2018). Peruvian Pinnipeds as Archivists of ENSO Effects. Master’s Thesis. Fort Lauderdale, FL: Nova Southeastern University.
Espinoza, P., Lorrain, A., Ménard, F., Cherel, Y., Tremblay-Boyer, L., Argüelles, J., et al. (2017). Trophic structure in the Humboldt Current system: new perspectives from stables isotope analysis. Mar. Biol. 164:86. doi: 10.1007/s00227-017-3119-8
Espinoza-Morriberón, D., Echevin, V., Colas, F., Tam, J., Ledesma, J., Vásquez, L., et al. (2017). Impacts of El Niño events on the Peruvian upwelling system productivity. J. Geophys. Res. Oceans 122, 5423–5444. doi: 10.1002/2016JC012439
Fossi, M. C., and Panti, C. (2017). Sentinel Species of Marine Ecosystems. Oxford Research Encyclopedia of Environmental Science. USA: Oxford University Press.
France, R. L., and Peters, R. H. (1997). Ecosystem differences in the trophic enrichment of 13C in aquatic food webs. Can. J. Fish Aquat. Sci. 54, 1255–1258. doi: 10.1139/f97-044
Franco-Trecu, V., Aurioles-Gamboa, D., and Inchausti, P. (2014). Individual trophic specialization and niche segregation explain the contrasting population trends of two sympatric otariids. Mar. Biol. 161, 609–618. doi: 10.1007/s00227-013-2363-9
Gentry, R. L., and Kooyman, G. L. (1986). Fur Seals: Maternal Strategies on Land and at Sea. Princeton, NJ: Princeton University Press.
Ginter, C. C., DeWitt, T. J., Fish, F. E., and Marshall, C. D. (2012). Fused traditional and geometric morphometrics demonstrate pinniped whisker diversity. PLoS One 7:e34481. doi: 10.1371/journal.pone.0034481
Grandi, M. F., Dans, S. L., Crespo, E. A., and García, N. A. (2012). Sexual Maturity and Corporal Growth of the South American Sea Lion, Otaria flavescens, in Patagonia. Estado de Situación del Lobo Marino Común en su Área de Distribución. Valparaíso: Editorial Universidad de Valparaíso, 44.
Gutierrez, M., Swartzman, G., Bertrand, A., and Bertrand, S. (2007). Anchovy (Engraulis ringens) and sardine (Sardinops sagax) spatial dynamics and aggregation patterns in the Humboldt Current ecosystem, Peru, from 1983–2003. Fish. Oceanogr. 16, 155–168.
Heileman, S., Guevara, R., Chavez, F., Bertrand, A., and Soldi, H. (2008). “XVII-56 Humboldt Current LME,” in The UNEP Large Marine Ecosystem Report: A Perspective on Changing Conditions in LMEs of the World’s Regional Seas. UNEP Regional Seas Report and Studies No. 182, eds K. Sherman and G. Hempel (Nairobi: United Nations Environment Programme), 749–762.
Hill, J. M., McQuaid, C. D., and Kaehler, S. (2006). Biogeographic and nearshore-offshore trends in isotope ratios of intertidal mussels and their food sources around the coast of southern Africa. Mar. Ecol. Progr. Ser. 318, 63–73. doi: 10.3354/meps318063
Hirons, A. C. (2001). Trophic Dynamics of Pinniped Populations in Alaska Using Stable Carbon and Nitrogen Isotope Ratios. Ph.D. dissertation. Fairbanks, AK: University of Alaska Fairbanks.
Hirons, A. C., Schell, D. M., and Finney, B. P. (2001). Temporal records of δ13C and δ15N in North Pacific pinnipeds: inferences regarding environmental change and diet. Oecologia 129, 591–601. doi: 10.1007/s004420100756
Huang, B., Angel, W., Boyer, T., Cheng, L., Chepurin, G., Freeman, E., et al. (2018a). Evaluating SST analyses with independent ocean profile observations. J. Clim. 31, 5015–5030. doi: 10.1175/JCLI-D-17-0824.1
Huang, B., Liu, C., Ren, H., Zhang, H., and Zhang, L. (2018b). The role of buoy and Argo observations in two SST analyses in the global and tropical Pacific oceans. J. Clim. 32, 2517–2535. doi: 10.1175/JCLI-D-18-0368.1
Huang, B., Banzon, V. F., Freeman, E., Lawrimore, J., Liu, W., Peterson, T. C., et al. (2014). Extended reconstructed sea surface temperature version 4 (ERSST.v4). Part I: upgrades and intercomparisons. J. Clim. 28, 911–930. doi: 10.1175/JCLI-D-00006.1
Huang, B., Thorne, P., Banzon, V., Boyer, T., Chepurin, G., Lawrimore, J., et al. (2017). NOAA Extended Reconstructed Sea Surface Temperature (ERSST), Version 5. [Nino 1+2]. Washington, DC: NOAA.
Huang, B., Thorne, P., Smith, T., Liu, W., Lawrimore, J., Banzon, V., et al. (2015). Further exploring and quantifying uncertainties for Extended Reconstructed Sea Surface Temperatures (ERSST) version 4 (v4). J. Clim. 29, 3119–3142. doi: 10.1175/JCLI-D-15-0430.1
Kelleher, C. (2016). Vibrissae Growth Rates and Foraging and Migration Patterns for Juvenile Male Northern Fur Seals (Callorhinus ursinus) From St. Paul Island, Alaska Determined With Stable Isotope Analysis. Ph.D. thesis. San Diego, CA: University of California.
Kluger, L., Kochalski, S., Aguirre-Velarde, A., Vivar, I., and Wolff, M. (2019). Coping with abrupt environmental change: the impact of the coastal El Niño 2017 on artisanal fisheries and mariculture in northern Peru. ICES J. Mar. Sci. 76, 1122–1130. doi: 10.1093/icesjms/fsy171
Kurle, C. M., and Worthy, G. A. (2001). Stable isotope assessment of temporal and geographic differences in feeding ecology of northern fur seals (Callorhinus ursinus) and their prey. Oecologia 126, 254–265. doi: 10.1007/s004420000518
Liu, W., Huang, B., Thorne, P. W., Banzon, V. F., Zhang, H., Freeman, E., et al. (2014). Extended reconstructed sea surface temperature version 4 (ERSST.v4): part II. Parametric and structural uncertainty estimations. J. Clim. 28, 931–951. doi: 10.1175/JCLI-D-14-00007.1
Majluf, P. (1987a). Reproductive Ecology of Female South American Fur Seals at Punta San Juan, Peru. Ph.D. thesis. Cambridge: University of Cambridge.
Majluf, P. (1987b). South American Fur Seal, Arctocephalus australis, in Peru. NOAA technical Report NMFS, eds J. P. Croxall and R. L. Gentry (Cambridge: US Dept Commerce, NOAA & NMFS), 33–35.
Majluf, P. (1989). “Reproductive ecology of South American fur seals in Peru,” in The Peruvian Upwelling Ecosystem: Dynamics and Interactions, eds D. Pauly, P. Muck, J. Mendo, and I. Tsukayama (Callao: Instituto Del Mar Del Perú (IMARPE)), 332–343.
McPhaden, M. J., Zebiak, S. E., and Glantz, M. H. (2006). ENSO as an integrating concept in earth science. Science 314, 1740–1745. doi: 10.1126/science.1132588
Miller, T. W., Brodeur, R. D., and Rau, G. H. (2008). Carbon stable isotopes reveal relative contribution of shelf-slope production to the northern California Current pelagic community. Limnol. Oceanogr. 53, 1493–1503. doi: 10.4319/lo.2008.53.4.1493
Montecino, V., and Lange, C. (2009). The Humboldt Current system: ecosystem components and processes, fisheries, and sediment studies. Prog. Oceanogr. 83, 65–79. doi: 10.1016/j.pocean.2009.07.041
Montecinos, A., and Gomez, F. (2010). ENSO modulation of the upwelling season off southern-central Chile. Geophys. Res. Lett. 37:L02708. doi: 10.1029/2009GL041739
Ñiquen, M., and Bouchon, M. (2004). Impact of El Niño events on pelagic fisheries in South American waters. Deep Sea Res II 51, 563–574. doi: 10.1016/j.dsr2.2004.03.001
Nyakatura, K., and Bininda-Emonds, O. R. P. (2012). Updating the evolutionary history of carnivora (Mammalia): a new species-level supertree complete with divergence time estimates. BMC Biol. 10:12. doi: 10.1186/1741-7007-10-12
Oliveira, L. (2011). “Vulnerability of South American pinnipeds under El Niño southern oscillation events,” in Global Warming Impacts-Case Studies on the Economy, Human Health, and on Urban and Natural Environments, ed. S. Casalegno (Rijeka: InTech), 237–252.
Oliveira, L. R., and Brownell, Jr, R. L. (2014). Taxonomic status of two subspecies of South American fur seals: Arctocephalus australis australis vs. A. a. gracilis. Mar. Mamm. Sci. 30, 1258–1263. doi: 10.1111/mms.12098
Oliveira, L. R., Hoffman, J. I., Highst-Zaher, E., Majluf, P., Muelbert, M. M. C., Morgante, J. S., et al. (2008). Morphological and genetic evidence for two evolutionarily significant units (ESUs) in the South American fur seal. Arctocephalus australis. Conserv. Genet. 9, 1451–1466. doi: 10.1007/s10592-007-9473-1
Pinheiro, J., Bates, D., DebRoy, S., Sarkar, D., and R Core Team (2017). nlme: Linear and Nonlinear Mixed Effects Models. R package version 3.1-131. Available online at: https://CRAN.R-project.org/package=nlme (accessed April 20, 2021).
Pinheiro, J. C., and Bates, D. M. (2000). Mixed-Effects Models in S and S-Plus. New York, NY: Springer, 3–56.
R Core Team (2016). R: A Language and Environment for Statistical Computing. Vienna: R Foundation for Statistical Computing.
Rea, L. D., Christ, A. M., Hayden, A. B., Stegall, V. K., Farley, S. D., Striker, C. A., et al. (2015). Age-specific vibrissae growth rates: a tool for determining the timing of ecologically important events in Steller sea lions. Mar. Mamm. Sci. 31, 1213–1233. doi: 10.1111/mms.12221
Ropelewski, C. F., and Halpert, M. S. (1987). Global and regional scale precipitation patterns associated with the El Niño/Southern oscillation. Mon. Weather Rev. 115, 1606–1626. doi: 10.1175/1520-04931987115<1606:GARSPP<2.0.CO;2
Roy, C., and Reason, C. (2001). Enso-related modulation of coastal upwelling in the eastern Atlantic. Prog. Oceanog. 49, 245–255. doi: 10.1016/S0079-6611(01)00025-8
Sepúlveda, M., Olea, D., Carrasco, P., Santos-Carvello, M., Castillo, J., and Quiñones, R. (2014). Latitudinal variation in local productivity influences body condition of South American sea lion pups. Aquat. Biol. 23, 39–47. doi: 10.3354/ab00604
Soto, K. H., Trites, A. W., and Arias-Schreiber, M. (2006). Changes in diet and maternal attendance of South American sea lions indicate changes in the marine environment and prey abundance. Mar. Ecol. Prog. Ser. 312, 277–290. doi: 10.3354/meps312277
Sulca, J., Takahashi, K., Espinoza, J. C., Vuille, M., and Waldo, L. (2017). Impacts of different ENSO flavors and tropical Pacific convection variability (ITCZ, SPCZ) on austral summer rainfall in South America, with a focus on Peru. Int. J. Climatol. 38, 420–435. doi: 10.1002/joc.5185
Sydeman, W. J., Hobson, K. A., Pyle, P., and McLaren, E. B. (1997). Trophic relationships among seabirds in Central California: combined stable isotope and conventional dietary approach. Condor 99, 327–336. doi: 10.2307/1369938
Taylor, M. H., Wolff, M., Mendo, J., and Yamashiro, C. (2008). Changes in trophic flow structure of Independence Bay (Peru) over an ENSO cycle. Prog. Oceanogr. 79, 336–351. doi: 10.1016/j.pocean.2008.10.006
Trenberth, K. E. (1997). The definition of El Niño. Bull. Am. Meteorol. Soc. 78, 2771–2777. doi: 10.1175/1520-04771997078<2771:TDOENO<2.0CO;2
Trenberth, K. E., Branstator, G. W., Karoly, D., Kumar, A., Lau, N., and Ropelewski, C. (1998). Progress during TOGA in understanding and modelling global teleconnections associated with tropical sea surface temperatures. J. Geophys. Res. 103, 14291–14324. doi: 10.1029/97JC01444
Trillmich, F., and Limberger, D. (1985). Drastic effects of El Niño on Galapagos pinnipeds. Oecologia 67, 19–22. doi: 10.1007/BF00378445
Trillmich, F., and Ono, K. O. (1991). Pinnipeds and El Niño: Responses to Environmental Stress. Berlin: Springer.
Vásquez, P. (1995). Determinacion de los Habitos Alimentarios de Arctocephalus australis y Otaria byronia en Punta San Juan de Marcona, Ica, Perú. Ph.D. thesis. Lima: Universidad Nacional Agraria La Molina.
Waluda, C. M., Yamashiro, C., and Rodhouse, P. G. (2006). Influence of the ENSO cycle on the light-fishery for Dosidicus gigas in the Peru Current: an analysis of remotely sensed data. Fish Res. 79, 56–63. doi: 10.1016/j.fishres.2006.02.017
Keywords: Peruvian fur seal, stable isotope ratio, El Niño Southern Oscillation (ENSO), δ13C and δ15N, sea surface temperature anomalies, SECLER
Citation: Edwards MR, Cárdenas-Alayza S, Adkesson MJ, Daniels-Abdulahad M and Hirons AC (2021) Peruvian Fur Seals as Archivists of El Niño Southern Oscillation Effects. Front. Mar. Sci. 8:651212. doi: 10.3389/fmars.2021.651212
Received: 08 January 2021; Accepted: 18 October 2021;
Published: 15 November 2021.
Edited by:
Rob Harcourt, Macquarie University, AustraliaReviewed by:
Richard Reina, Monash University, AustraliaLuis Cardona, University of Barcelona, Spain
Copyright © 2021 Edwards, Cárdenas-Alayza, Adkesson, Daniels-Abdulahad and Hirons. This is an open-access article distributed under the terms of the Creative Commons Attribution License (CC BY). The use, distribution or reproduction in other forums is permitted, provided the original author(s) and the copyright owner(s) are credited and that the original publication in this journal is cited, in accordance with accepted academic practice. No use, distribution or reproduction is permitted which does not comply with these terms.
*Correspondence: Amy C. Hirons, aGlyb25zQG5vdmEuZWR1