- 1British Antarctic Survey, Cambridge, United Kingdom
- 2UK Antarctic Heritage Trust, Cambridge, United Kingdom
- 3Cetacean Ecology Research Group, School of Natural Sciences, Massey University, Auckland, New Zealand
- 4Animal Welfare Science and Bioethics Centre, School of Veterinary Science, Massey University, Palmerston North, New Zealand
- 5Centro de Estudios Avanzados en Zonas Aridas (CEAZA), La Serena, Chile
- 6Oceanswell, Colombo, Sri Lanka
- 7UWA Oceans Institute, University of Western Australia, Crawley, WA, Australia
The study of cetacean strandings was globally recognised as a priority topic at the 2019 World Marine Mammal Conference, in recognition of its importance for understanding the threats to cetacean communities and, more broadly, the threats to ecosystem and human health. Rising multifaceted anthropogenic and environmental threats across the globe, as well as whale population recovery from exploitation in some areas, are likely to coincide with an increase in reported strandings. However, the current methods to monitor strandings are inherently biased towards populated coastlines, highlighting the need for additional surveying tools in remote regions. Very High Resolution (VHR) satellite imagery offers the prospect of upscaling monitoring of mass strandings in minimally populated/unpopulated and inaccessible areas, over broad spatial and temporal scales, supporting and informing intervention on the ground, and can be used to retrospectively analyse historical stranding events. Here we (1) compile global strandings information to identify the current data gaps; (2) discuss the opportunities and challenges of using VHR satellite imagery to monitor strandings using the case study of the largest known baleen whale mass stranding event (3) consider where satellites hold the greatest potential for monitoring strandings remotely and; (4) outline a roadmap for satellite monitoring. To utilise this platform to monitor mass strandings over global scales, considerable technical, practical and environmental challenges need to be addressed and there needs to be inclusivity in opportunity from the onset, through knowledge sharing and equality of access to imagery.
Introduction
Cetacean species are highly mobile, wide-ranging and inhabit relatively inaccessible locations, including the high seas, presenting considerable challenges for monitoring and conservation (Magera et al., 2013; Correia et al., 2015; Borowicz et al., 2019; Betty et al., 2020). Consequently, large areas of the world’s oceans remain un-surveyed (Kaschner et al., 2012). Indeed, the widespread occurrence of cetaceans in remote regions means that for many populations, information on ecology, abundance, distribution and prevailing threats remain poorly understood (Magera et al., 2013; Betty et al., 2020).
Monitoring cetaceans and particularly understanding the threats to cetaceans is critical, since as ecosystem engineers (see Glossary), cetaceans are influential to the structuring of marine ecosystems (Kaschner et al., 2012; Roman et al., 2014). Oceanic conditions that impact cetaceans often affect other less visible species, and there are potential ramifications for human health too (e.g., ingestion of poisons/contaminants and disease risk) (Bossart, 2011). To recognise the complex connections that exist between animals, their ecosystems and humans, the World Health Organisation developed “One Health,” a collaborative multidisciplinary approach to help improve public health (Zinsstag et al., 2011; World Health Organisation [WHO], 2017).
There are growing concerns that marine ecosystem health is deteriorating, due to an increased reporting of disease presence in marine organisms (Gulland and Hall, 2007). As sentinel species, cetacean strandings (see Glossary) act as early warning systems, providing valuable insights into ecosystem health (Maldini et al., 2005; Moore, 2008; Stockin et al., 2009; Pyenson, 2010; Bossart, 2011; Peltier et al., 2012; Betty et al., 2020) by highlighting emerging public health issues (Gulland and Hall, 2007; Bossart, 2011; Waltzek et al., 2012; Bossart and Duignan, 2018). Environmental stressors, biotoxins, cumulative poisons and contaminants are of concern, given that cetaceans and humans sit at similar trophic levels and often consume the same prey, and in certain countries consumption of cetaceans continues, despite protection measures (Van Bressem et al., 2009; Bossart, 2011). Additionally, zoonotics linked to the terrestrial human/animal interface are now emerging within marine ecosystems, with associated health concerns for cetaceans and humans (Woo et al., 2013; Bossart and Duignan, 2018; Wang et al., 2020). With changes to the climate and increasing anthropogenic environmental degradation likely to exacerbate environmental stressors and disease virulence (Van Bressem et al., 2009; Bossart, 2011; Waltzek et al., 2012), understanding and mitigating health concerns linked to the ocean is imperative (Bossart and Duignan, 2018). This is particularly important for remote regions, where environmental threats are poorly monitored, and where strandings may offer the most evident signal of a negative impact. Strandings also present a unique opportunity to understand the extent to which human-induced threats impact local populations of cetaceans and other marine life. Cetaceans are subject to growing human-induced threats (Tin et al., 2009; Leaper and Miller, 2011; Clapham, 2016), including from ship strikes; bycatch and entanglement; ocean noise; chemical contaminants; wildlife tourism and climate induced large-scale oceanic ecosystem changes (Simmonds and Elliott, 2009; Stockin et al., 2010; Leaper and Miller, 2011; Clapham, 2016; de Vos et al., 2016).
The study of and response to stranded marine mammals has been globally recognised as a priority topic for marine mammal research, one of three main goals of the 2019 World Marine Mammal Conference (World Marine Mammal Conference [WMMC], 2019; Gulland and Stockin, 2019). This goal culminated in the formation of the “Global Stranding Network,” an international initiative amongst marine mammal stranding experts, with the aim to enhance global collaborations and the sharing of knowledge and resources to develop science, improve animal welfare and the response to strandings (Gulland and Stockin, 2019). Many international bodies, conventions and agreements, such as the International Whaling Commission (IWC) and the United Nations Environmental Programme’s (UNEP) Convention for the Conservation of Migratory Species of Wild Animals (CMS), also advocate the importance of monitoring marine mammal strandings, to enhance the global capacity to respond to strandings and to conduct research (IWC, 2015; UNEP et al., 2017; Betty et al., 2020).
Despite the strong conservation rationale to study strandings, their occurrence in remote or poorly resourced locations can impede detection, identification of cause and effect of prevailing threats, and implementation of effective monitoring (Magera et al., 2013; Bossart and Duignan, 2018; Fretwell et al., 2019). While strandings are observed at a global scale, networks are geographically biased towards populated coastlines as their capacity to monitor is dependent upon local expertise and resource availability. Given the importance of stranding records as a valuable source of information of the threats to cetacean communities (Geraci and Loundsbury, 2005; Pyenson, 2010) and the ocean health more broadly (Gulland and Hall, 2007), increased emphasis should be put on upscaling stranding monitoring in remote regions.
Whilst individual cetaceans can strand, mass stranding events (MSE), mass mortality events (MME) and unusual mortality events (UME) (see Glossary) are of particular concern for marine conservation and therefore benefit most from increased monitoring efforts. Very High Resolution (VHR) satellites (see Glossary) orbiting the Earth offer an opportunity for mass strandings surveillance in remote regions at scales not possible with traditional approaches, with no location or habitat specific restrictions (Fretwell et al., 2019). Recognising that satellite imagery is presently unable to differentiate species (a factor required for distinguishing MSE, MME and UME events), we will collectively refer to MSE’s, MME’s and UME’s as mass strandings, when referring to strandings in the context of satellite imagery. While satellites are not currently suitable as an emergency response tool to live mass strandings, due to time delays in image collection; there are four main ways in which satellites can support existing mass stranding monitoring methods. These include (1) systematic long term monitoring programmes; which in turn could inform (2) response, directing intervention on the ground; (3) early warning of increased stranding rates, alerting managers to a problem and allowing for appropriate response; and (4) retrospective analysis of past mass stranding events to understand the spatiotemporal patterns. However, there are also significant technical challenges associated with satellites that need to be addressed for this platform to become a viable support tool for long term mass stranding monitoring programmes.
This paper reviews the feasibility of VHR satellite imagery as an emerging, complementary tool to monitor mass strandings by identifying; (1) knowledge gaps for mass strandings through a compilation of global mass strandings information; (2) the opportunities and challenges of using VHR satellite imagery to fill these knowledge gaps; (3) the geographical areas where satellites hold most promise for monitoring; and (4) the advances and adjustments required to make VHR satellite imagery a viable support tool for the remote monitoring of mass strandings.
Identifying Knowledge Gaps for Strandings
To identify the knowledge gaps in the monitoring of mass strandings, we (1) explore the current methods to monitor mass strandings, (2) identify the present challenges of monitoring mass strandings, and (3) suggest priority areas to upscale monitoring efforts in the future.
Current Monitoring Methods: Stranding Networks
Long-term monitoring of strandings can provide critical insights into local marine health and aid marine mammal conservation (Geraci and Loundsbury, 2005; Peltier et al., 2012). In the 1980s, this goal, and the need to provide a live emergency response to stranded marine mammals for animal welfare reasons, provided the momentum for the formation of regional stranding alert networks and monitoring programmes across the globe (Geraci and Loundsbury, 2005). Stranding networks utilise opportunistic public observations, dedicated communication platforms, a network of trained voluntary observers, and in a few cases where logistics and funding allow, aerial and vessel surveys (Evans and Hammond, 2004). The information collected is then archived in a stranding repository/database. Networks and synergised networks of networks continue to grow and develop around the world, and declarations such as that made at the 2019 World Marine Mammal Conference, Barcelona, Spain recognise the increasing need to build capacity to study strandings, and endorse international collaboration and sharing of knowledge, expertise, training and best practices (Geraci and Loundsbury, 2005; Gulland and Stockin, 2019).
Challenges to Monitoring Strandings
Minimally Populated/Unpopulated Coastlines
Remote regions present considerable logistical and economic challenges for monitoring strandings (Magera et al., 2013) resulting in absent or delayed detection (Table 1). Complex coastlines can further enhance the difficulties due to limited access (Table 1). For example, Chile’s extensive coastline is more than 8,000 km long and is home to over 40% of the world’s cetacean species, but is sparsely populated with complex geographical features. So despite its clear significance, few studies of strandings have been conducted in Chile (IWC, 2015; Alvarado-Rybak et al., 2020). Geographical constraints are not the only characteristics defining remote areas (Häussermann et al., 2017; Borowicz et al., 2019). In the context of the terrestrial/marine interface associated with strandings, there are other complexities that can render an area remote, for example geopolitical inaccessibility through war or conflict (Table 1). Such challenges have been recognised by international bodies such as the IWC, who aim to provide targetted support for strandings training and monitoring in minimally populated/unpopulated areas through their IWC Strandings Initiative (Stockin et al., 2019).
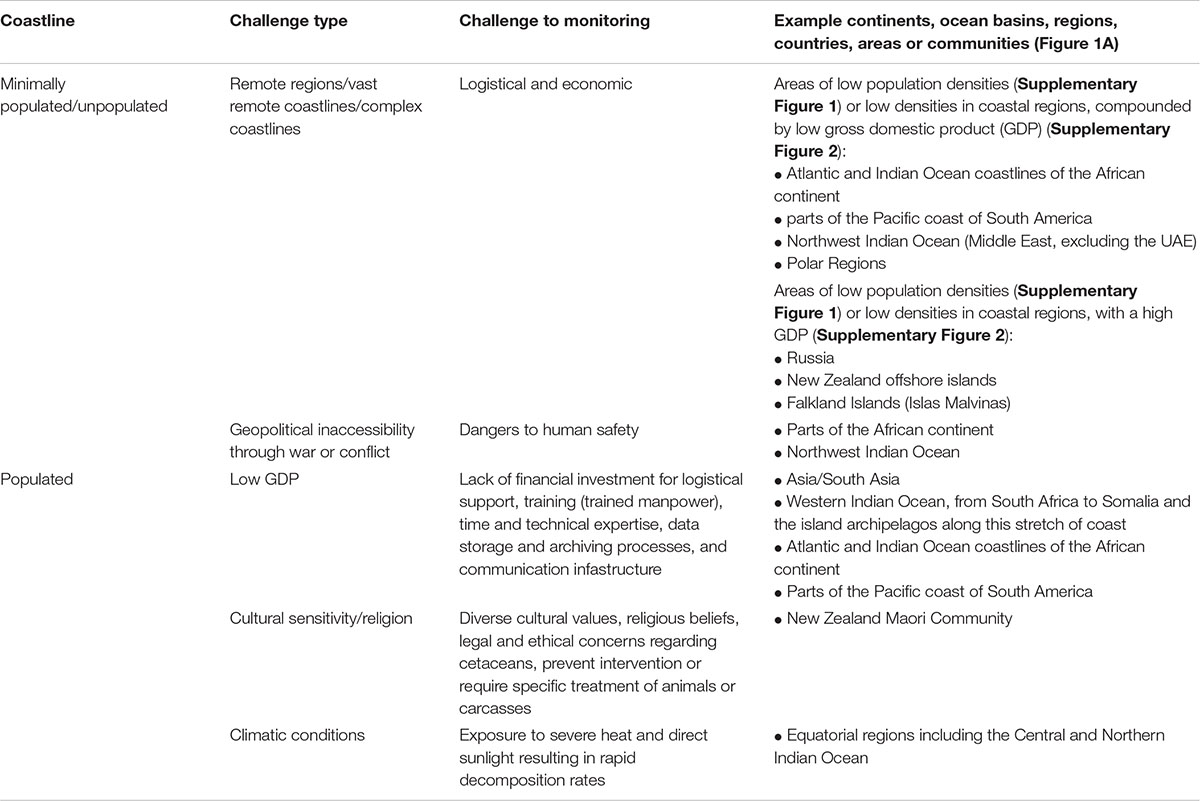
Table 1. A table outlining the current challenges to stranding monitoring programmes through stranding networks, in both minimally populated/unpopulated areas and populated areas, with examples of continents, ocean basins, regions, countries, areas or communities.
Populated Coastlines
Despite the steady expansion of global stranding networks, it is evident from Figure 1A that coastal coverage on a global scale is very uneven (Pyenson, 2011). Stranding networks need trained manpower (Table 1), and therefore coverage is biased towards coastlines where a population resides at or near the coast; and where the community has the experience, logistical and financial capacity and civil rest to monitor and gather data and samples from strandings. While collaboration and sharing knowledge is important for regional or local networks, development beyond rudimentary monitoring to include data collection and to be spatially and temporally sustainable requires significant financial input (Caughlan and Oakley, 2001; Peltier et al., 2014; Gulland et al., 2018). Investment is required for logistical support, training, time and expertise; data storage and archiving processes; and communication infrastructure (Geraci and Loundsbury, 2005). It appears that there is a dependence on capital for building successful stranding networks, given that countries with a higher GDP tend to have mass stranding networks. Figure 1 and Supplementary Figure 2 show a visible concordance between high GDP countries such as the United States, United Kingdom, Australia, and Japan and the reporting of mass stranding events, when compared with lower GDP countries (Table 1).
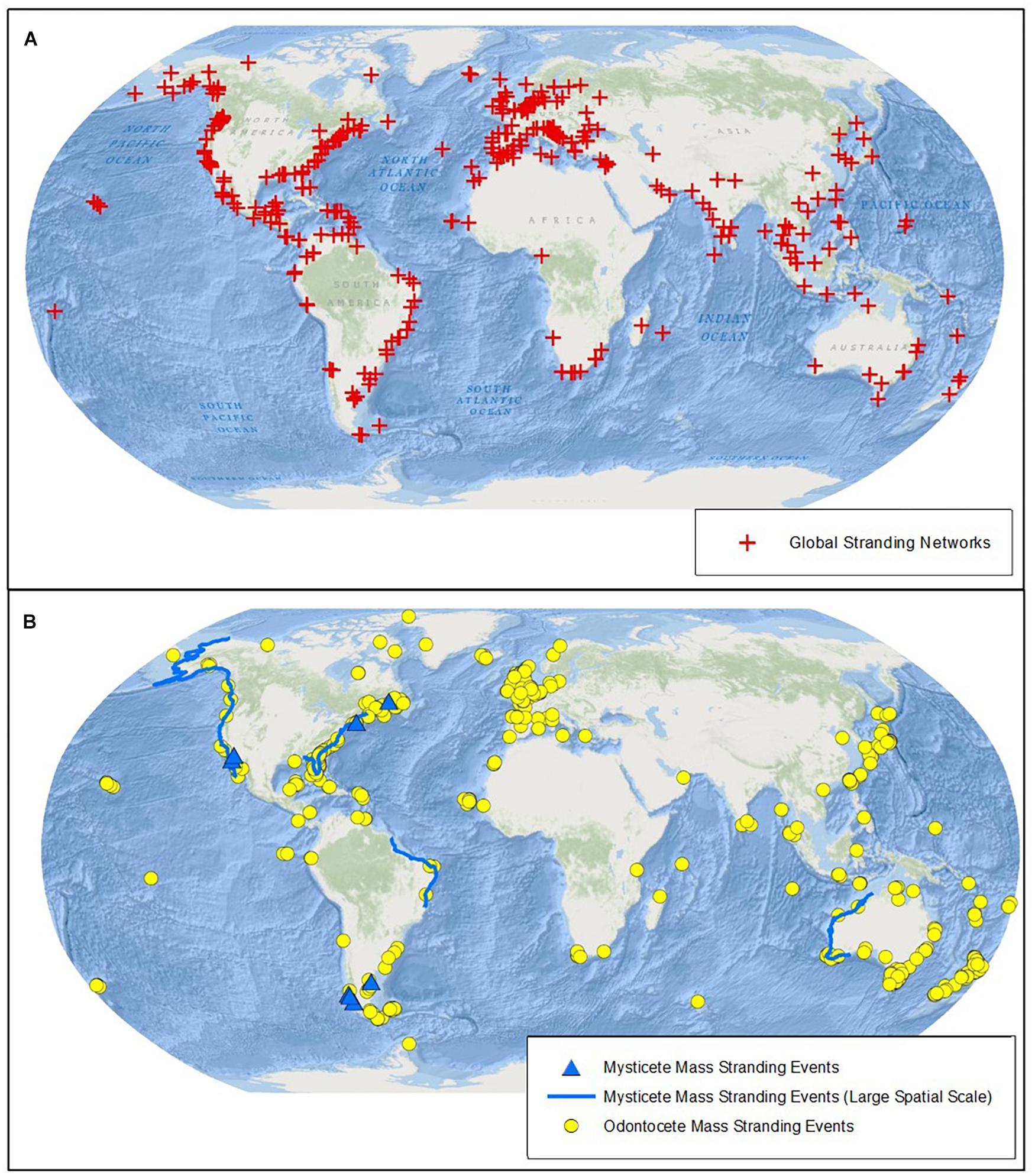
Figure 1. Global stranding patterns and networks, (A) shows global stranding networks (red cross) and (B) shows cetacean mass mortality events globally. Yellow circles show odontocete mass stranding events with 10 or more individuals (summary data reproduced from Hamilton, 2018); Blue triangles and lines show baleen whale mass mortality events (updated from Table 1 in Häussermann et al., 2017). Triangles represent events with a known and specified location and lines represent events with only a known country level location or UME’s that occurred over large coastal areas (a single line can depict several events in the same location, individual event data is shown in Supplementary Table 2). Events of unknown or unspecified locations are not mapped. All data was up to date as of the 2nd September 2020. Base map source: ESRI, GEBCO, NOAA, National Geographic, Gamin, HERE, Geonames.org, and other contributors Esri, Garmin, GEBCO, NOAANGDC, and other contributors.
Under the “One Health” paradigm, insights from strandings in low GDP areas may be particularly important indicators of changes in ocean health, as those areas are often less able to enforce environmental protection laws. Rudimentary monitoring of strandings can point to possible problems at sea, for example pollution events or significant changes in the local foodweb (Schwacke et al., 2014; Peters et al., 2020). Many coastal communities in low GDP areas rely on local fisheries for sustenance, and those areas are also historically under-surveyed for biodiversity (Klein et al., 2015). Considering that 70% of the world’s coastlines are in low GDP countries (de Vos, 2020), this represents a significant area in which more collaboration and knowledge sharing, and infrastructure to monitor strandings would be hugely valuable (Wilkinson and Worthy, 1999). The Asian Marine Mammal Stranding Network (AMMSN) is an excellent non-governmental exemplar of knowledge sharing between countries (Fadela, 2016). This network illustrates that even the most basic observations and baseline data can be incredibly valuable for the study of strandings and understanding of One Health.
Cultural sensitivities and religion can influence the way a network monitors strandings (Gulland and Stockin, 2019; Table 1), with implications for acquiring the necessary permits to establish a monitoring programme (Gulland et al., 2018); successful operations are inclusive of local cultural values. For example, New Zealand is a hotspot for mass stranding events (IWC, 2017; Betty et al., 2020; Figure 1B) and New Zealand Māori (indigenous Polynesian people of mainland New Zealand, Aotearoa) consider cetaceans as taonga or treasure (Table 1). In some indigenous communities, cetaceans are consumed as aquatic bushmeat (Cosentino and Fisher, 2016). In those contexts, networks that monitor strandings in synergy with local communities could offer an opportunity to share knowledge about consumption risks, to minimise possible negative impacts for human health. This has relevance in countries with increasing population size, poverty and famine coupled with declining fish stocks and drought, as is already the case in Mozambique (Welch, 2019). Religious beliefs can further prevent intervention, or require specific treatment of animals or carcasses (Christiansen, 2018).
Climatic conditions can make it difficult to identify the causes of strandings, regardless of monitoring capacity, particularly in equatorial regions. Exposure to severe heat and direct sunlight can result in rapid decomposition rates, limiting the opportunity to make even the most basic observations of stranded cetaceans (Table 1). It is, therefore, important to be mindful of existing climatic conditions when establishing stranding networks, as harsher climates determine what data can be most usefully obtained with the available resources. With climate change, exposure to severe heat is anticipated to become a challenge for a growing number of countries around the world.
Data Gaps and Future Risk Areas for Monitoring Strandings
As human populations grow, bringing with them multifaceted anthropogenic and environmental threats, and whale populations recover from global exploitation, an increase in the frequency and number of cetacean stranding events is anticipated (Tucker et al., 2018). The greatest increases are most likely to result from geographical overlaps between recovering whale populations and increasingly populated, industrial coastlines (Gulland and Stockin, 2019). The effect of these overlaps can also be tele-connected; for example, local pollutant events can lead to strandings elsewhere, depending on animal migration, and regional oceanographic and coastal processes that facilitate or inhibit on-shore drift (Peltier et al., 2012). While increasing human populations pose significant threats for cetaceans in coastal areas, they may also serve to increase the manpower to monitor strandings.
Given that strandings are not geographically constrained, they are important to study on a global scale to understand ocean, animal, and human health. In under resourced locations, where populations reside at or near the coast, the priority should be to increase local technical capacity and incorporate with traditional knowledge, to ensure data from stranding events are appropriately collected, a priority identified also by the IWC. For remote coastlines, emerging, innovative methods may help to upscale monitoring and the initial detection of strandings over broad spatial and temporal scales. Areas which already have rudimentary monitoring may be a good starting point for upscaling, for example across much of the Western Indian Ocean, particularly the coastline from South Africa to Somalia and the island archipelagos along this stretch of coast, and for the AMMSN, South Asia (Gulland and Stockin, 2019; Plön et al., 2020; Table 1).
Other priority areas for strandings monitoring are those where we anticipate increases in the anthropogenic impacts on and consequent strandings of cetaceans in these regions. We recommend stranding monitoring efforts concentrate here, to acquire baseline data to document future change. These future risk areas include; the Northwest Indian Ocean (Middle East), where some coastal regions are rendered inaccessible for monitoring due to regional geopolitics (Table 1), and where significant conservation concerns for multiple cetacean species inhabiting these waters, such as coastal developments and shipping, are rapidly increasing (Minton et al., 2008; Anderson et al., 2020); and the polar regions, particularly the Arctic, where there are predicted changes associated with climate warming and consequent increases in anthropogenic threats associated with greater accessibility to the poles, including shipping and pollution (Reeves et al., 2014; Table 1).
Very High Resolution Satellite Imagery: the Potential to Address the Knowledge Gaps in Mass Stranding Monitoring
VHR satellites orbiting the Earth collect regular images across large, minimally populated/unpopulated and inaccessible areas (Abileah, 2002; Fretwell et al., 2014, 2019; LaRue et al., 2017; Cubaynes et al., 2019; Bamford et al., 2020) and have the potential to significantly improve the monitoring of mass strandings in remote regions, particularly the priority areas identified in section “Data gaps and future risk areas for monitoring strandings” (Fretwell et al., 2019). Since satellite companies also regularly take images across the world, they also hold archives that can be used retrospectively to study past mass stranding events (Fretwell et al., 2019; Figure 2). Here we demonstrate how VHR satellite imagery has retrospectively been applied to count and identify a mass stranding event in a remote region, and discuss the opportunities and challenges of using this technology to address the knowledge gaps in mass stranding monitoring.
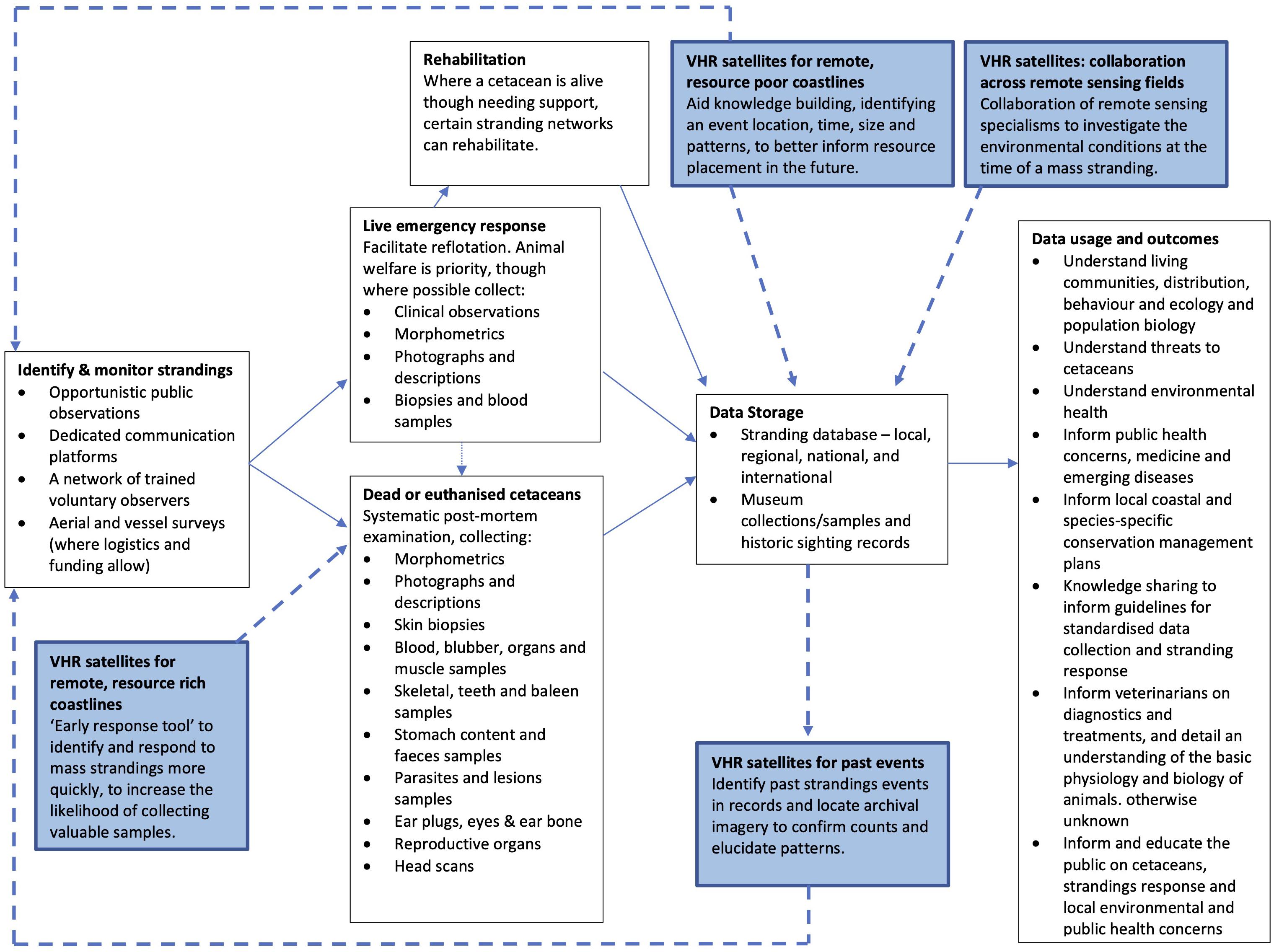
Figure 2. A schema diagram representing how stranding networks monitor strandings (i.e., data collected, what it is used for, and the outcomes), and where/how VHR satellite imagery could assist.
Case Study: Retrospective Identification of a Mass Stranding Event
The Golfo de Penas, Chile, is extremely remote, located 200 km from the nearest human settlement, and has extensive and complex fjord systems (Häussermann et al., 2017). It is one of the most wave-impacted coastlines in the world, and one of the least studied marine regions (Försterra, 2009). For these reasons, it is nearly impossible to monitor whales or strandings using traditional survey methods in this area and as such, in 2015, the largest known mass stranding of baleen whales went undetected for 2 months (Häussermann et al., 2017). Following detection, carcass sampling and aerial surveys suggested that the mass stranding occurred over 3–6 months (starting in February 2015) and was primarily composed of sei whales (Balaenoptera borealis) (Häussermann et al., 2017). Häussermann et al. (2017) applied VHR satellite imagery to quality-check the GPS positioning of strandings identified via aerial survey. This satellite analysis was subsequently expanded, using additional archival satellite imagery to review the timing and extent of the mass stranding (Fretwell et al., 2019; Figure 3A). Although the satellite analysis covered a more limited area than the aerial survey (15% of the aerially surveyed area), Fretwell et al. (2019) upwardly revised the estimate of the total number of stranded whales, and identified that the majority of the mass stranding event had occurred by March 2015.
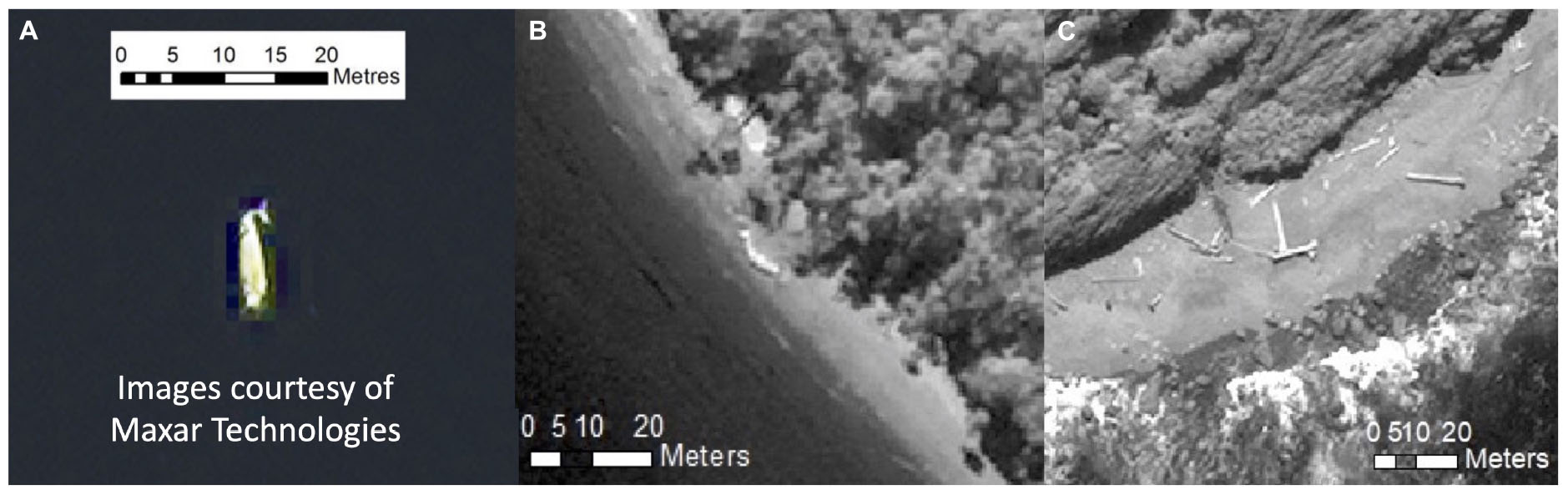
Figure 3. (A) An image of a whale identified using VHR satellite imagery by Fretwell et al. (2019), showing visible whale-like features, e.g., a fluke. (B) An image of the only definite whale identified in the 2019 VHR satellite imagery from Golfo de Penas, displaying whale-like shape and size, but less defined whale-like features when compared with image (A). (C) An image of wood along the coastline in the region of the case study, an example of a confounding feature when identifying cetaceans in the later stages of decomposition. All images are courtesy of Digital Globe Foundation (now Maxar Technologies).
Since 2015, further strandings have been annually reported in Golfo de Penas. Whilst not on the scale of the original event, the recurrence raises concern. In 2016, Häussermann et al. (2017) documented 16 carcasses. Subsequently Olavarría et al. (2019) documented 19, 8, and 27 carcasses in 2017, 2018, and 2019, respectively, with the 2019 mass stranding recorded between late March and April. To establish the timing of the 2019 mass stranding, a search for stranded whales was conducted using VHR satellite imagery, acquired between the 2nd and 18th February 2019, covering the Seno Newman and Estero slight area of the region (Olavarría et al., 2019). Very few whale-like features were identified in the imagery, suggesting that the 2019 sei whale strandings reported by Olavarría et al. (2019) likely occurred during late February/March, after the period when the images were collected.
In 2015, extreme remoteness led to a delay in discovery and assessment, so the advanced state of decomposition restricted pathological sampling and thus, the ability to rule out virulent causes, highlighting the importance of time in understanding a stranding event. Whilst Chile has an established stranding network, remote regions of Patagonia, like the Golfo de Penas, are absent of systematic monitoring, and in the case of these recurring events, such a programme is crucial to understand the prevailing threats for “One Health.” If systematic monitoring using VHR satellite imagery had been in place at the time of this event, then the platform could potentially have acted as an “early response” tool, informing a quicker response and better diagnostics, and in the long-term elucidating patterns and timing to better inform and direct resources (Figure 2).
This case study on strandings in the Golfo de Penas region highlights the potential of VHR satellite imagery as a remote monitoring tool for identifying the timing and spatio-temporal extent of mass strandings. Such an approach can then be linked to environmental health, to better inform and direct resources where available. This is especially the case in the Gulfo de Penas region, an area used by poorly understood sei whales in the austral summer, with several years of mass strandings now identified in this region (Häussermann et al., 2017; Olavarría et al., 2019).
Opportunities and Challenges of Using Very High Resolution Imagery to Monitor Mass Strandings
VHR satellite imagery offers an opportunity to better understand mass strandings and the environmental conditions that precipitate them; to broaden both the geographical region of study and the time span through both annual to historic periods. With regular monitoring the platform has the potential to highlight patterns and act as an “early response” tool, and in turn complement existing stranding monitoring methods (Figure 2). Investment in satellite imagery could offer the conservation benefit of identifying important areas to invest in for in situ data sampling, and highlight patterns which would otherwise remain undocumented, or require costly expeditions to investigate. However, for this to be a viable tool for the long-term monitoring of mass strandings, significant technical, practical and environmental considerations and challenges must be addressed.
Acquiring Tasked or Archival Imagery
VHR satellites are operated by a combination of commercial, government, and military providers (Supplementary Table 1). There are currently 27 operational VHR satellites, of which three are military and not routinely available to researchers [“operational” refers to a satellite that is actively tasking (see Glossary) imagery]. Government-run VHR satellites are limited in number and tend to be accessible only to researchers or institutions within that country. Commercially operated satellites are those most accessible for researchers and NGOs worldwide, and imagery can be acquired either by requesting the operator to task an image or by ordering archival imagery.
Tasked imagery: Revisit rate, Cloud Cover and Prioritisation
Tasking a satellite, in principle, offers a researcher or stranding network the opportunity to choose a time and location for image acquisition. Some VHR satellites, such as Worldview-3, have the capacity to revisit the same location daily (temporal resolution—see Glossary; Supplementary Table 1), thus offering the potential to task imagery regularly. In remote areas where traditional stranding monitoring platforms or tools cannot regularly monitor, VHR imagery could therefore be extremely valuable (Fretwell et al., 2019). However, VHR satellites do not task continuously and images cannot be requested instantaneously. Commercial providers will only acquire imagery on request, and tasking opportunities vary across regions, further compounded by cloud coverage. Cloud cover can obscure detection of features and the presence of shadowing or dense formations can make object detection impossible (Platonov et al., 2013; LaRue et al., 2017; Fretwell et al., 2019; Lennert-Cody et al., 2019). In regions where cloud cover is heavy and persistent, there is therefore less potential for effective long-term monitoring with this approach. Technological advances in synthetic aperture radar sensor resolution (such as ICEYE at sub-metre resolution; ICEYE, 2021) may reduce the challenge of cloud cover in the future, since these sensors can monitor independent of weather.
In certain areas of the globe, even when conditions for acquisition are optimal, tasking is not always possible due to access prioritisation. For example, Maxar Technologies’ Direct Access Programme prioritises defence and commercial customers with reserved access for tasking time (Maxar, 2020a). While prioritisation programmes such as this can lead to missed opportunities for tasking imagery to monitor a specific mass stranding event, these programmes also represent a framework that could be valuable to build from for future systematic long-term monitoring programmes worldwide. As a result of prioritisation programmes, satellite revisit rates, and cloud cover, VHR satellites currently have limited capacity to provide “real time” identification of an unfolding mass stranding event (Fretwell et al., 2019). Rather researchers must request imagery at least one week prior to the date of capture, or request regular image collection from a location of interest (Fretwell et al., 2019). The current lead time of one week for tasking imagery could be too slow in regions with severe heat conditions, as stranded cetaceans would rapidly decompose. However, if regular image collection were in place, it could be possible to identify these events as they happen.
Archival Imagery
Stranding networks and traditional monitoring tools capture data at the time of discovery, but in remote areas delayed detection can lead to under reporting if cetaceans wash offshore or decompose in the intervening time. All images taken by VHR satellites are archived, creating a wealth of data that provides an opportunity to investigate the temporal patterns of mass stranding events prior to the point of detection on the ground. In addition to the 27 operational VHR satellites as of 2020, a further four decommissioned satellites exist (Supplementary Table 1), offering an archive of images for the period they were in operation. For example, Maxar offers access to an extensive imagery archive, the GBDX catalogue1, provided by an array of high resolution earth imaging satellites.
Archival images can provide a historic perspective to retrospectively analyse mass strandings in remote regions (Figure 2), by improving the deduction of timings of an event, as shown in the case study (Häussermann et al., 2017; Fretwell et al., 2019; Olavarría et al., 2019). However, archival imagery is largely available for high demand regions of the globe tasked for commercial use (Borowicz et al., 2019), which tend not to include remote locations, particularly in high latitudes, such as the Arctic. Nevertheless, in some instances archival images may offer great coverage for middle latitude remote locations, or to areas rendered inaccessible due to war and conflict, which can be of high demand for tasking. The growing demand for remotely sensed data for earth observation and environmental monitoring could also facilitate the growth of a more diverse image archive for retrospective analysis of mass stranding patterns in remote locations.
Imagery Cost
Tasked or archived images are accessible at a cost and can be expensive, particularly for newly tasked imagery (Fretwell et al., 2019). Since strandings monitoring is already challenged by a lack of funding, low GDP countries identified as having either rudimentary or absent networks are unlikely to invest in satellite-based monitoring (Supplementary Figure 2). Moreover, in areas of high demand for imagery, including war torn areas (identified above as a priority area for strandings monitoring), costs are substantially higher when tasking, although the large archival repositories may simultaneously offer a cheaper source of past imagery (Fretwell et al., 2019). Many satellite providers now offer concessionary pricing for image collection related to research and education (Fretwell et al., 2014; LaRue et al., 2017). As of May 2021, Planet Labs offers researchers, NGOs and governments access to SkySat 50 cm tasked and archival imagery (Skysat 16–21 launched in 2020 and images contain <15% cloud cover); equal to 15,000 terabytes of data per year (∼30 million km2 of imagery), for $15,000 (USD) per year. Through past collaborations Planet and the European Space Agencies ‘‘Education and Research Programme’’ have offered access to these data for free, and could form a framework for future initiatives to facilitate research2. Costs can also be defrayed through collaborative partnerships between commercial satellite companies and stranding networks, governments and NGOs. Existing successful partnerships and initiatives such as, The European Commission’s Copernicus Programme3, Planet’s NICFI programme4, and the Open Landscape Partnership Platform5; demonstrate the power and potential of partnerships for conservation and planetary research.
Analysis of Satellite Images
Detecting Strandings
To date, detecting mass strandings in VHR satellite imagery has mostly been achieved through a manual approach. Manually scanning satellite imagery to identify stranded whales is time intensive; scanning an area of 100 km2 takes approximately 200 min (Cubaynes et al., 2019; Fretwell et al., 2019; Olavarría et al., 2019). When scanning over broader spatial and temporal scales, this demand exponentially increases (Borowicz et al., 2019; Cubaynes et al., 2019). Therefore, whilst manual scanning currently remains the most accurate method, for regular monitoring of mass strandings, on a global scale, modifications to this approach must be made. The time needed to manually scan imagery can be reduced by applying automated approaches such as object-based image analysis, deep learning, and thresholding (Borowicz et al., 2019; Cubaynes et al., 2019; Fretwell et al., 2019) or using a crowdsourcing approach to analyse images (Rey et al., 2017).
Previous attempts to automate the detection of strandings using VHR satellite imagery utilised a Spectral Angle Mapper procedure (SAM) (Fretwell et al., 2019). SAM returns features that have a similar spectral profile to a set of identified and input target features. However, due to the varying decomposition rates of stranded cetaceans, spectral profiles differed substantially between individuals, resulting in poor accuracy. Therefore, the automatic detection of mass strandings using VHR satellite imagery is optimal when carcasses are fresh. Fresh stranded cetaceans emit a high reflectance in the red and NIR bands and this unique spectral profile may be used as a tool to automatically differentiate strandings from confounding features (Fretwell et al., 2019; see spectral resolution in Glossary; Figure 4). However, this is only applicable for satellites where eight spectral bands are present, as only then are red and NIR bands available. As carcasses decompose, they become increasingly heterogeneous in terms of their visual aspect on satellite images, creating complexity for automated classification (Fretwell et al., 2019). This is further complicated by the positioning of the cetacean on the coast, the proximity of individuals to one another, whether the stranding is partially floating or beachcast and is ventral side up, and the size and shape of the cetacean. In the later stages of decomposition, where appendages and cetacean-like features are non-existent, confounding features such as wood and rock can appear cetacean-like (Figure 3; Fretwell et al., 2019). The resulting errors of omission and commission (see Glossary) are therefore likely to increase as decomposition proceeds, as such VHR satellite imagery is likely to be most useful for automated monitoring of freshly stranded animals.
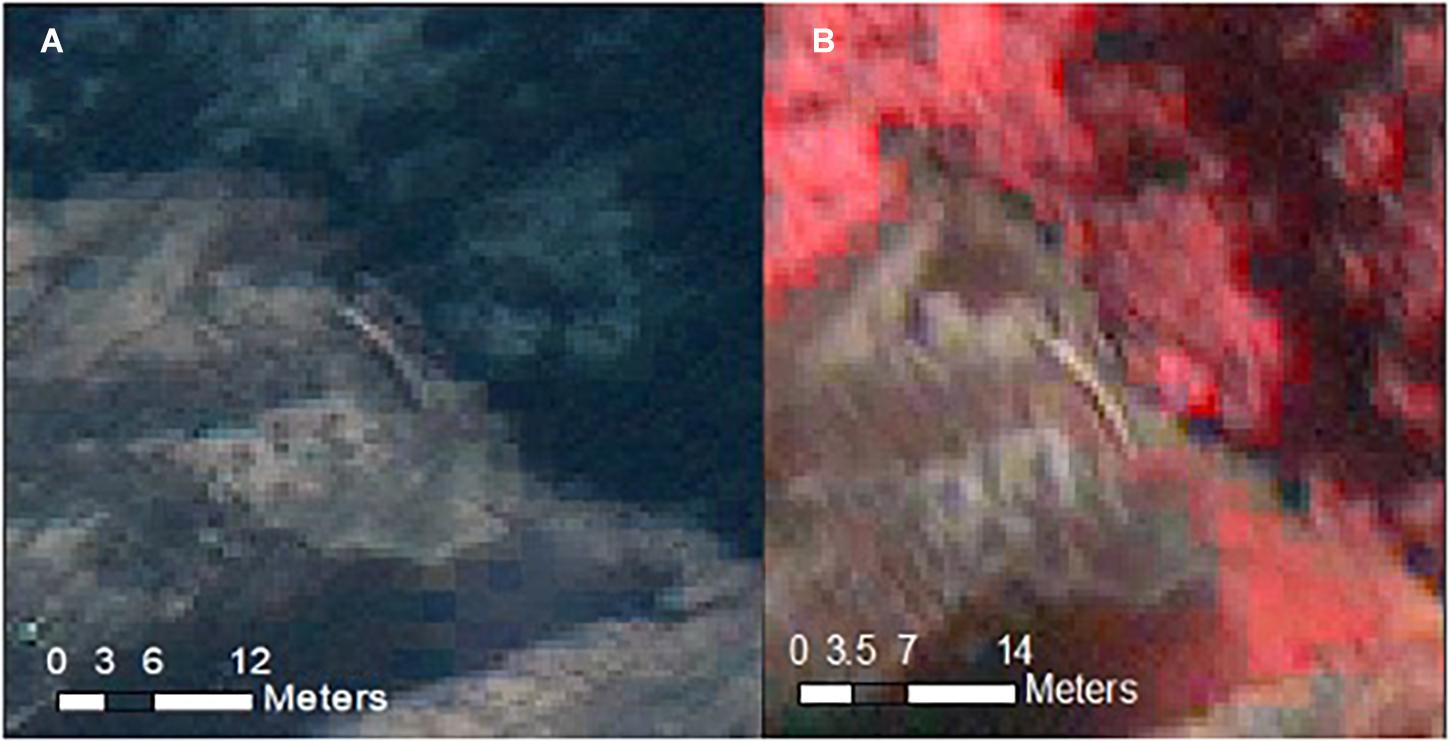
Figure 4. (A) An image of a possible whale-like feature identified in the 2019 VHR satellite imagery, displaying a similar appearance to the confounding wood features in Figure 3C. (B) An image of the same possible whale-like feature displayed in 4 (A), however, the image is displayed in the red and NIR bands and demonstrates the contrast between the high spectral reflectance (pink/cream hue) of the possible whale like feature when compared with the red colour of the surrounding vegetation. All images are courtesy of Digital Globe Foundation (now Maxar Technologies).
Ground Truthing
Ground truthing (see Glossary) in accessible areas, where strandings can be observed on the ground, could allow a greater understanding of how decomposition affects identification in satellite imagery. Ground truthing could also minimise the potential for errors in detection, particularly when local topography, dense vegetation and the orientation of a coastline to the sun, can cast shadows or obscure a target, and lead to the creation of confounding features (Fretwell et al., 2019). In remote regions where there is little or no knowledge of the local environmental conditions and where in situ data or direct field observations are not always possible, confidence in identification could be increased by using multi-swath imaging or repeat visits (image differencing—see Glossary). The high spatial resolution (see Glossary) available with VHR satellite imagery can also increase confidence in detection. In the context of monitoring mass strandings, the identification of cetacean defining characteristics such as a fluke, would increase the confidence of a detection; and this level of detail is only likely to be possible with VHR satellite imagery. For example it has been shown that whale defining features such as a fluke would most likely be detected in images with 30 cm resolution or higher (Cubaynes et al., 2019). Currently only the WorldView-3 satellite, operated by Maxar Technologies, offers such a resolution to non-military users. However, innovative technology now offers the opportunity to intelligently increase the resolution of lower resolution VHR images across archival repositories, to resolutions as high as 15 cm6. This tool could increase the library of suitable imagery greatly, although the costs for this currently limit access. At present, lower resolution VHR satellites remain a useful lower cost tool through Planet Labs to identify areas of concern for the subsequent investment in higher resolution VHR satellite imagery.
Large Area and Global Coverage
The scale of mass stranding events is often hard to identify rapidly; this is exacerbated in more remote and inaccessible areas (Betty et al., 2020). For example, a mass stranding event in Tasmania, Australia in September 2020 was initially thought to comprise of 270 long-finned pilot whales (Globicephala melas), and later identified as Australia’s largest known mass stranding event involving an estimated 470 whales. Using aerial-based surveys, ∼200 extra whales in addition to the original 270 estimate were later identified in three distinct areas up to 12 km apart (Carlyon, DPIPWE, pers. comm). This stranding occurred over a relatively populated coastal area, highlighting that the issue can be significantly more pronounced in more remote areas. In countries where aerial surveys are not available, easily accessible, or where areas are too remote for their rapid deployment; VHR satellite imagery presents an opportunity to validate counts of events. For instance, Worldview-3 has a daily collection capacity of up to 680,000 km2 per day and in one pass of an area of interest, it can collect either 4,716 km2 in a single image or 7,336 km2 through a collection of images (Supplementary Table 1; Maxar, 2020b). Image collection can be used to inform the response where intervention on the ground is possible, and in remote regions it provides a broader scale means of monitoring mass strandings (Figure 2) (species size permitting), allowing for improved knowledge of the spatial extent of these events (Fretwell et al., 2019).
Recurrent UMEs present a unique case for monitoring mass strandings, as they span large geographic areas and time spans. This presents considerable challenges for stranding networks, particularly along remote coastlines. The large geographic extent of gray whale UMEs along the complex and remote western US coastlines (Gulland et al., 2005), the annually recurring sei whale UMEs along the complex fjords of the Chilean Patagonia (Olavarría et al., 2019) and the southern right whale UMEs on their nursing grounds, are all examples of events that are critical to monitor for “One Health” but challenging to manage in practice (Rowntree et al., 2013; Figure 1B). VHR satellites could offer the opportunity to achieve the large spatial extent needed to monitor UME’s, not currently possible using traditional methods.
The global coverage offered by most VHR satellites means there is the potential to monitor mass strandings across the world, as the majority of VHR satellites are sun-synchronous (Liang and Wang, 2019). Sun-synchronous is a type of polar orbit in which the satellite orbits the earth synchronously with the sun, which means that images can be captured for almost anywhere on Earth. This also optimises the light conditions for imagery acquisition and means that a satellite revisits a location at the same time of day, an important consideration for regular monitoring or temporal investigations (The European Space Agency [ESA], 2020b). The majority of VHR satellites also orbit between 600 and 800 km from Earth, in combination providing high spatial and temporal resolution (The European Space Agency [ESA], 2020a). However, this resolution means the swath width (see Glossary) is reduced relative to more distant satellites, and is limited in most cases to tens of kilometres at most (Supplementary Table 1; Rees, 2013).
VHR satellites are able to collect multiple images in a single overhead pass (called the multi-strip collection scenarios), enabling survey coverage of large areas in a short timeframe. In the case study presented in this paper, the Golfo de Penas has an inland extent of 89 km and a north-south extent of 80 km (Encyclopaedia Britannica, 2021). The WorldView-3 satellite, offering the highest commercially available spatial resolution, can cover an area as large as 66.5 km by 112 km in one acquisition using its “large area collect” collection scenario (5 strips of image) (Maxar, 2020b). Worldview-3 currently has a revisit rate of less than one day, and therefore, the capacity to survey the whole of the Golfo de Penas in one day. In order to realise this potential scale of coverage, partnerships with satellite providers are likely to be important, as coverage is currently prioritised for commercial or defence customers, and costs increase with the area tasked (see section “Acquiring Tasked or Archival Imagery”).
Species Differentiation and Size of Stranded Cetaceans
To date, free swimming cetaceans have only been identified on satellite images to species level in areas of homogenous presence (Cubaynes et al., 2019). Without prior knowledge of species presence, spatial resolution alone is too coarse to identify species (Lennert-Cody et al., 2019). In the case of mass strandings, the fact that stranded cetaceans are in differing states of decomposition and many key species features may be absent, make species identification almost impossible via satellite. The lower limit of stranded animal sizes that current satellites can detect has not yet been established. When conducting surveys in remote areas where there is no prior knowledge of species presence, it might be difficult to detect a mass stranding event if the species is small in size. Further work is needed to discern what the smallest identifiable cetacean is using VHR satellite imagery across differing satellite resolutions, applying ground truthing by collecting images from easily accessible hotspots with known mass strandings of small cetaceans.
Priority Areas
Satellites offer an opportunity to enhance our understanding of mass strandings where capacity was previously very limited and where surveys are infrequent, such as geographically remote areas (polar regions, islands or complex fjord systems) and geopolitically remote areas (Northwest Indian Ocean) (Table 1). To establish the cause of a stranding, it is critical to be at the site soon after the event due to the rate of decomposition and the potential for carcasses to drift offshore (Geraci et al., 1989). Consequently, for many stranding events, the cause is inconclusive (Gulland et al., 2005; Rowntree et al., 2013). Whilst satellites cannot directly assist in sample collection, in resource rich remote regions, satellites could form an “early response” tool, aiding a faster response to mass strandings (Figure 2). In regions where cultural and religious practices do not align with stranding network goals (Table 1), VHR satellites could offer a more ethical and sensitive means to monitor mass strandings. By monitoring remotely, VHR satellites allow the collection of valuable data on temporal and spatial stranding patterns, even if more invasive in-person sample collection is prohibited or impossible in that culture or society. In areas of limited resources satellites could form a knowledge building tool to better inform when and where to invest in traditional monitoring methods/in situ sampling in the future (Figure 2). Through partnerships or collaborations with satellite providers, this platform has great potential to build local technical capacity and form sustainable stranding networks in remote regions globally.
In addition to the remoteness discussed in section “Data gaps and future risk areas for monitoring strandings,” “temporary remoteness” can also occur where a usually accessible coastline can become inaccessible for a limited period of time. For example during the COVID-19 pandemic, many populated coastlines were rendered temporarily remote due to travel and work restrictions. These measures have reduced the effectiveness of stranding networks due to forced cancellation of fieldwork associated with stranding monitoring. For areas where UMEs regularly occur (e.g., Northeast Pacific gray whale (Eschrichtius robustus) strandings, Argentine right whale (Eubalaena australis) strandings), reductions in monitoring can stall mitigation or conservation efforts. Satellite imagery offers the prospect of continued basic monitoring during such periods of absence, providing a remote means to quantify and identify mass strandings and to detail the spatio-temporal pattern of an event, that would otherwise remain unreported.
A Road Map for Satellite Monitoring
In remote and inaccessible areas, where very little is known about cetacean populations and where there are challenges in documenting mass stranding events, such as the Golfo de Penas; even the most basic observation data can be useful for conservation management of animals inhabiting remote coastlines (IWC, 2017). For this reason, the opportunity to obtain basic data, such as the identification of an event that would otherwise have remained undetected, highlights the value of information VHR satellites may provide. Moreover, if regularly used in such areas, satellites can identify patterns to better inform when to invest in traditional survey methods to establish species information (Figure 2). Satellites should be considered a complementary monitoring tool to augment data collected through traditional monitoring methods (Hollings et al., 2018). While there are immediate opportunities for VHR satellites to monitor mass strandings, the routine use of this platform to aid systematic long-term monitoring of mass strandings on a global scale would require various technical, geographical and practical challenges to be addressed. With new constellations, technological advancements and adjustments, VHR satellites could become a key supporting tool for future monitoring of mass strandings. In this section we discuss the advances or adjustments necessary to make this possible and propose areas where satellites hold the most promise as a tool for remotely monitoring mass strandings.
Automation of Detection
To minimise imagery processing time and to augment monitoring over larger spatial and temporal scales, developing automated or semi-automated processes is the crucial next step (Fretwell et al., 2019). Machine learning (see Glossary) using Convolutional Neural Networks and a semi-automated approach has been applied to satellite images of whales in the marine environment with promising results (Borowicz et al., 2019), and therefore the viability of this procedure should be investigated for strandings. However, automation is in its infancy and there are challenges such as the need for large and diverse training datasets, required to improve the reliability of this method. While the use of SAM was unsuccessful, other approaches exist and should be tested. Object-based automation should be more promising, as such algorithms identify features based on similarity in size and shape. Rule based analysis may also be a viable automation method, in which features are identified based on delimiting rules, for example a defined size range and reflectance in the red, NIR or other available bands. However, as with spectral profiles, the heterogenous decomposition rates for stranded cetaceans mean that size and shape can differ greatly. The highest resolution VHR satellites yield the most detail, so may offer an increased likelihood of discrimination based on shape. Unlike for SAM and object or rule based analysis, the changes in strandings as they decompose could be useful to explore automated image differencing, which has been successfully applied to study polar bears (Ursus maritimus) (LaRue et al., 2015). The detection of change between images, whether through a change in spectral profiles of pixels or a change in the size or shape of an object, could prove useful for the identification and confidence in detection of carcasses in remote regions.
To automate detection effectively, extensive and complex training datasets are necessary, in order to minimise errors of commission and omission. Presently, there are not currently enough stranding data points in existence (<60 in peer reviewed publication), so immediate effort is required to annotate as many stranded individuals and confounding features as possible. This is highly time consuming and potentially repetitive work, and therefore outside the scope for any single individual or research group to compile. Therefore, this could be achieved by; augmenting existing data; acquiring and down-sampling aerial imagery of stranded whales (i.e., the process of making high resolution drone imagery have the same spatial resolution as VHR satellite imagery; Borowicz et al., 2019), or by using crowdsourcing (where thousands of volunteers annotate imagery; Wege et al., 2020) to initially increase the available annotated imagery (Rey et al., 2017). An important source of information that is available immediately for annotation is archived satellite imagery, which makes a good starting point for review of stranded whales, particularly in areas where strandings regularly occur. For scalability of strandings monitoring using VHR satellite imagery, to large and remote areas, initially a semi-automated approach might be best. Algorithms could identify likely mass strandings, for further investigation or verification using a combination of automated and manual scanning (Fretwell et al., 2019). Crowd-sourced monitoring also holds significant promise (e.g., Maxar’s crowdsourcing platform GeoHIVE7 (formerly known as Tomnod). This approach requires careful planning in order to generate results useful for rapid response (LaRue et al., 2019; Wege et al., 2020).
Data Storage and Sharing
In order to develop a greater understanding of mass strandings in remote areas, large amounts of imagery would need to be analysed, and for future automation of strandings detection, large and complex training datasets are required. Multi-institute collaborations to freely share knowledge and annotated imagery would be important to achieve future automation goals, and compile a large database of annotated imagery. Such databases require vast computational power, storage and infrastructure (Borowicz et al., 2019). To rapidly progress mass stranding monitoring, a global platform to freely share such information would be a crucial first step, and could be formed in conjunction with the existing global hub and aligned aims of the Global Stranding Network. The development of protocols to analyse imagery should also investigate the use of freely available software, such as QGIS, for which Planet has plugins for efficient use, to facilitate replication and sharing of data.
New Satellite Constellations
The launch of additional VHR satellites has the potential to transform the future of mass strandings monitoring, by increasing the frequency of coverage of the earth’s surface and providing greater opportunities for image tasking. In 2021, Maxar plan to launch Worldview Legion, six new satellites into orbit, offering 0.29 m resolution in the panchromatic band (see Glossary) and up to 15 revisits of a location per day (Maxar, 2020c). In 2021, Airbus plan to launch Neo Pléiades, a constellation of four new 0.3 m resolution satellites (Airbus, 2020). In addition, Planet recently launched SkySat-16–21 with 0.57 m resolution (The European Space Agency [ESA], 2020a) and Earth-I will shortly launch Vivid-I constellation, operational by 2022, comprising 15 new satellites with 0.6 m resolution, full colour video, and an initial temporal resolution (see Glossary) of two visits per day (Earth-i, 2020). Such constellations could play an important role in identifying and counting mass stranding events or highlighting areas of concern for later investment in 0.3 m resolution imagery.
Collaboration Across Remote Sensing Fields
Biological sampling or direct observations are important tools to uncover stranding causality, highlighting ecosystem health and emerging public health issues (Gulland and Hall, 2007). For many stranding events there is no single causal factor, rather a complex interplay of a number of contributing elements (Cordes, 2011). To effectively understand and attribute causes to large-scale multifactorial stranding events, a collaborative, transdisciplinary approach is vital, such as that proposed by the World Health Organisation’s One Health approach (IJsseldijk et al., 2018). Satellite monitoring can be used in conjunction with in situ tools to provide a more comprehensive overview of stranding patterns in remote locations, where the capacity for sampling is impeded by logistical and environmental challenges. Remotely sensed earth observations from an array of platforms and sensors can also provide information on local environmental, biogeographic and anthropogenic conditions, at varying levels of resolution. Other remotely sensed data include: sea surface temperatures, upwelling indices, chlorophyll a and phytoplankton blooms and wind direction and intensity (Dohan and Maximenko, 2010; Blondeau-Patissier et al., 2014; Groom et al., 2019). Such data can be accessed freely through NASA’s MODIS8 instruments and the European Space Agency’s Copernicus Programme9 Sentinel satellites. Collaborations across remote sensing (see Glossary) fields could offer another dimension for understanding the environmental conditions prior to and during mass stranding events (Figure 2). For example, combining these data sources could highlight putative environmental or anthropogenic drivers influencing mass stranding patterns. Remotely sensed environmental data could help develop predictive hypotheses that could be used to better respond to future strandings and potentially, support the early detection of risks to public health. These environmental proxies may provide an “early warning” system, to mitigate mass stranding events and to develop more informed and knowledgeable rapid response stranding networks in those regions (LaRue et al., 2017; Fretwell et al., 2019). Such proxies could be an environmental factor such as toxic algal blooms, or habitat degradation or human disturbance such as shipping traffic or offshore oil and gas exploration (Magera et al., 2013; Fretwell et al., 2019).
Another way in which satellite monitoring of strandings could be augmented is by the parallel satellite-based monitoring of whales at sea (Fretwell et al., 2014; Cubaynes et al., 2019), for example to monitor the densities of whales using the local area (Bamford et al., 2020) also in relation to local environmental conditions. This could provide important context to understanding the local oceanic conditions and their impact on local whale densities at the time of stranding.
Accessibility of Satellite Imagery
One of the greatest challenges to stranding monitoring programmes is access to financial resources. Future integration of VHR satellite imagery into regular monitoring of mass strandings will depend on the financial capacity of stranding networks, governments, and NGOs, to meet the costs of image collection by satellite companies. The current tasking costs offered by commercial companies limits access to those platforms (Szantoi et al., 2015; Höschle et al., 2021). These costs in particular prohibit nations with limited resources from utilising this technology to monitor strandings and risks the facilitation of colonial science (de Vos, 2020), where NGOs or research institutes in higher GDP countries conduct research in lower GDP countries. Therefore, novel partnerships between NGOs and satellite companies or agreements to share imagery is crucial for inclusivity. Stranding monitoring on a global scale has potential to provide a powerful tool supporting conservation management of local coastlines and monitoring cetacean, ocean and human health. Collaborative partnerships between satellite companies and stranding networks, governments and NGOs, to support mass strandings monitoring, is an important and inclusive way for this platform to prosper, and is recommended by the IWC Scientific Committee (IWC, in press, p. 119). For commercial satellite providers, such collaborations could help to meet their sustainable development goals, and show leadership within the industry in protecting cetaceans and ultimately ocean ecosystem and human health.
Next Steps
Before applying this technology to remote regions, we recommend conducting pilot studies on mass stranding hotspots, where there is a reliable stranding network present, in order to compare identified strandings with satellite identifications at different resolutions, and assess the range of species, spectral profiles and decomposition levels for which this approach works effectively. By initially applying this tool to areas of known presence, we can test the robustness of this technology and develop working protocols for broader monitoring. Partnerships with existing and effective stranding networks in hotspot areas, such as New Zealand and Tasmania, Australia, provide an important opportunity to ground truth, as well as helping to develop automated detection procedures.
To date, VHR satellites have been successfully used to identify and count mass strandings in a remote region, where a priori knowledge of a mass stranding event exists (Fretwell et al., 2019). The present and future areas of concern for mass stranding risk, outlined in the section “Data gaps and future risk areas for monitoring strandings,” are largely remote areas with little prior strandings information or in some cases even living cetacean communities are unknown. Therefore, the priority, after ground-truthing the approach in well-studied hotspot regions, would be to set up monitoring test sites in these areas, beginning with resource rich-remote areas where there are identified risks and later resource-poor remote areas with identified risks (Table 1). A priority location for monitoring, where satellite based analysis has already begun, is the Chilean Patagonia region; as documented in this paper and described in Fretwell et al. (2019) and Olavarría et al. (2019). Coasts in politically turbulent regions such as the Northwest Indian Ocean (a present and future risk area for strandings) (Table 1), where there is high demand for tasked imagery by government, military and commercial customers, might also be appropriate for initial expansion of the approach, as these areas may have large archival image repositories which can be accessed at a lower cost than tasked imagery.
Conclusion
While several challenges remain for the application of VHR satellite imagery to aid the monitoring of mass strandings, such as the capacity to differentiate species, this platform offers an opportunity to detect and count stranded animals in remote regions, advancing information on cetaceans that would otherwise be unknown (Fretwell et al., 2019). Furthermore, a continuous mass stranding monitoring programme using satellite imagery could better inform spatio-temporal resource placement to acquire valuable in situ data. As emerging forensic and biological sampling approaches become more versatile, with more data gathering now possible from smaller and more degraded samples, it is ever more important to facilitate a more rapid detection and response to mass strandings. Long-term monitoring of mass strandings using satellite imagery presents an opportunity to; develop a broader understanding of cetacean behaviour, foraging and breeding ecology, and migratory pathways (Fretwell et al., 2014) and; significantly enhance the monitoring of mass strandings in remote regions, aiding the future understanding of strandings and more broadly, One Health and cetacean conservation management. For this technology to enhance our global mass stranding monitoring capacity, efforts should focus on devising automated and semi-automated detection algorithms and in addressing the technical, practical and environmental challenges required to make this platform an accessible and efficient tool for monitoring.
Author Contributions
PC: conceived the idea, conceived the manuscript, data curation, formal analysis, writing—original draft, writing—review, and editing. JJ: conceived the idea, conceived the manuscript, data curation, writing—original draft, writing—review, and editing. HC: conceived the manuscript, data curation, formal analysis, writing—original draft, writing—review, and editing. KS: conceived the manuscript, data curation, writing—original draft, writing—review, and editing. PF: formal analysis, writing—original draft, writing—review, and editing. CO and AdV: writing—original draft, writing—review, and editing. All authors contributed to the article and approved the submitted version.
Funding
This study forms part of the Ecosystems component of the British Antarctic Survey Polar Science for Planet Earth Programme, funded by the Natural Environment Research Council.
Conflict of Interest
The authors declare that the research was conducted in the absence of any commercial or financial relationships that could be construed as a potential conflict of interest.
Publisher’s Note
All claims expressed in this article are solely those of the authors and do not necessarily represent those of their affiliated organizations, or those of the publisher, the editors and the reviewers. Any product that may be evaluated in this article, or claim that may be made by its manufacturer, is not guaranteed or endorsed by the publisher.
Acknowledgments
We are grateful to L. J. Hamilton for permission to use their data in this manuscript, to the DigitalGlobe Foundation (now Maxar Technologies) for providing free archival satellite imagery discussed in this manuscript, to Beatrice Mainas of Planet Labs for providing guidance on image acquisition costs, and to Virginia Andrews-Goff and Kris Carlyon for providing updates to the 2020 Tasmanian mass stranding event. We are grateful for the valuable comments of the two reviewers, which greatly improved the manuscript.
Supplementary Material
The Supplementary Material for this article can be found online at: https://www.frontiersin.org/articles/10.3389/fmars.2021.650735/full#supplementary-material
Supplementary Figure 1 | A map showing human population density (people per sq. km of land area) by country (human population density data accessed from the World Bank using: HYPERLINK https://data.worldbank.org/indicator/EN.POP.DNST?end=2019&start=1961&type=points&view=chart&year=2019 and the world map country polygon shapefile accessed using: https://hub.arcgis.com/data sets/UIA::countries-wgs84?geometry=11.250%2C-89.998%2C-11.250%2C-79.513).
Supplementary Figure 2 | A map showing current GDP US$ (millions) for all countries and economies (GDP data accessed from the World Bank using: https://data.worldbank.org/indicator/NY.GDP.MKTP.CD and the world map country polygon shapefile accessed using: https://hub.arcgis.com/datasets/UIA::countries-wgs84?geometry=11.250%2C-89.998%2C-11.250%2C-79.513.
Supplementary Table 1 | Current list of operational and decommissioned VHR satellites, detailing ownership and the technical specification of each satellite.
Supplementary Table 2 | List of baleen whale mass mortality events updated from Table 1 in Häussermann et al. (2017) an updated version of Table 1 in Rowntree et al. (2013). Data from this table is presented in a map in Figure 1(B). An asterix represents data that has been newly inputted or updated from the prior versions.
Glossary
Stranding Terms
Ecosystem Engineers—are animals that create, significantly modify, maintain or destroy habitats, and can have a large impact on species richness and heterogeneity of the environment they inhabit. Cetacean faecal plumes facilitate vertical and latitudinal nutrient transfer throughout the ocean, stimulating phytoplankton growth, which forms the base of all marine food-webs; and cetacean falls (carcasses) sequester carbon to the depths of the ocean, forming nutrient hotspots, that sustain concentrated diverse and rich communities of deep-sea organisms (Roman et al., 2014).
Mass Mortality Event (MME)—is when cetaceans strand either deceased or dying, over extended temporal scales (days to weeks) (Moore et al., 2018).
Mass Stranding Event (MSE)—is when two or more individuals of the same species, not including mother calf pairs, simultaneously strand alive in the same location (Geraci and Loundsbury, 2005; Moore et al., 2018).
Stranding—is a cetacean that falters ashore, debilitated, or is in an environment incompatible with its natural survival; if the cessation of life has occurred prior to deposition, the cetacean is considered beachcast (Geraci and Loundsbury, 2005).
Unusual Mortality Event (UME)—is a stranding event that is considered unexpected for; a given area or time of year for the species in question; a different age or sex assemblage for an area or time of year; the size of an event; an increase in the frequency of an event when compared with previous years; cetaceans exhibiting unusual pathology or behaviour or for the stranding of critically endangered cetacean species. An UME event comprises a substantial number of dead individuals of any cetacean population and necessitates immediate action (Geraci and Loundsbury, 2005; Mazzariol et al., 2020).
Remote Sensing Terms
Errors of Commission—also known as false positive, in the context of strandings, this is when a confounding feature or pixel containing a non-stranded cetacean is misclassified as a stranded cetacean.
Errors of Omission—also known as false negative, in the context of strandings, this is when a stranded cetacean or pixel containing a stranded cetacean is omitted in error and misclassified as another feature.
Ground truthing—is the acquisition of in situ data providing a true representation of a feature or point of reference on the ground, at the exact location of a pixel within a satellite image (Lilliesand and Kiefer, 1979).
Image Differencing—also known as change detection, is the identification of changes between a target image and a reference image collected at different times (LaRue et al., 2015).
Machine Learning—is the use of computational power to apply a diversity of data analysis approaches and algorithms to existing data/information, to learn from, and then accurately predict (Humphries et al., 2018).
Panchromatic and Multispectral imagery—is electromagnetic radiation with emission properties in the visible electromagnetic spectrum that produces greyscale imagery (panchromatic) and colour imagery (multispectral) (Dowman et al., 2012; Rees, 2013).
Remote sensing—also known as “Earth Observation,” is the principle of deriving information about the earth’s surface using a remote device, acquired using a number of electromagnetic radiation sensors such as: microwave, radar, thermal, infrared, ultraviolet and multispectral (Campbell and Wynne, 2011; Rees, 2013).
Spatial Resolution—also known as ground sampling distance, is the length of ground represented by a single pixel, which in turn determines the level of detail visible (Liang and Wang, 2019).
Spectral Resolution—is the number of radiometric sensors aboard a satellite, that are receptive to different ranges of wavelengths (spectral bands) of incoming electromagnetic radiation. These include the panchromatic band, the visible light spectrum, and infrared bands (Rees, 2013; Liang and Wang, 2019).
Swath Width—is the maximum width of the earth’s surface acquired in an image in one acquisition (Rees, 2013).
Tasking—the ability of a satellite image provider to directly send information to a satellite, requesting when and where imagery be collected, and the satellites return transmission of imagery (Bayir, 2003).
Temporal Resolution—also known as the revisit rate, is the time between the acquisition of two subsequent images in the same location on the earth’s surface (Liang and Wang, 2019).
Very High Resolution (VHR)—is the capability of satellite imagery to collect sub-metre spatial resolution images of the earth’s surface (LaRue et al., 2017; Cubaynes et al., 2019).
Footnotes
- ^ https://gbdxdocs.digitalglobe.com
- ^ https://www.planet.com/markets/education-and-research/
- ^ https://www.tandfonline.com/doi/full/10.1080/0143116031000139863
- ^ https://www.planet.com/nicfi/
- ^ https://www.geoconnexion.com/in-depth/open-landscape-partnership-platform
- ^ https://www.euspaceimaging.com/15-cm-hd/
- ^ https://www.maxar.com/products/geohive
- ^ https://modis.gsfc.nasa.gov/data/
- ^ www.esa.int/Enabling_Support/Operations/Sentinels
References
Abileah, R. (2002). Marine mammal census using space satellite imagery. U.S. Navy J. Underwater Acoust. 52, 709–724.
Airbus (2020). Pléiades Neo Trusted Intelligence: The Best of Very-High Resolution Optical Imagery. Available Online at: https://www.intelligence-airbusds.com/en/8671-pleiades-neo-trusted-intelligence [accessed Dec 9, 2020].
Alvarado-Rybak, M., Toro, F., Escobar-Dodero, J., Kinsley, A. C., Sepúlveda, M. A., Capella, J., et al. (2020). 50 years of cetacean strandings reveal a concerning rise in Chilean Patagonia. Sci. Rep. 10:11972. doi: 10.1038/s41598-020-68367-7
Anderson, R. C., Herrera, M., Ilangakoon, A. D., Koya, K. M., Moazzam, M., Mustika, P. L., et al. (2020). Cetacean bycatch in Indian Ocean tuna gillnet fisheries. Endanger. Species Res. 41, 39–53. doi: 10.3354/esr01008
Bamford, C., Kelly, N., Dalla Rosa, L., Cade, D., Cubaynes, H., Mesquita, A., et al. (2020). A comparison of baleen whale density estimates derived from overlapping satellite imagery and a shipborne survey. Sci. Rep. 10:12985. doi: 10.1038/s41598-020-69887-y
Bayir, I. (2003). “IKONOS collection capability with the direct tasking from a regional operation center,” in Proceeding of the 2003 International Conference on Recent Advances in Space Technologies (Istanbul:IEEE), 30–33.
Betty, E. L., Bollard, B., Murphy, S., Ogle, M., Hendriks, H., Orams, M. B., et al. (2020). Using emerging hot spot analysis of stranding records to inform conservation management of a data-poor cetacean species. Biodivers. Conserv. 29, 643–665. doi: 10.1007/s10531-019-01903-8
Blondeau-Patissier, D., Gower, J. F. R., Dekker, A. G., Phinn, S. R., and Brando, V. E. (2014). A review of ocean color remote sensing methods and statistical techniques for the detection, mapping and analysis of phytoplankton blooms in coastal and open oceans. Progr. Oceanogr. 123, 123–144. doi: 10.1016/j.pocean.2013.12.008
Borowicz, A., Le, H., Humphries, G., Nehls, G., Höschle, C., Kosarev, V., et al. (2019). Aerial-trained deep learning networks for surveying cetaceans from satellite imagery. PLoS One 14:e0212532. doi: 10.1371/journal.pone.0212532
Bossart, G. D. (2011). Marine mammals as sentinal species for oceans and human health. Vet. Pathol. 48, 676–690. doi: 10.1177/0300985810388525
Bossart, G. D., and Duignan, P. J. (2018). Emerging viruses in marine mammals. CAB Rev. 13:17. doi: 10.1079/PAVSNNR201913052
Campbell, J. B., and Wynne, R. H. (2011). Introduction to Remote Sensing. New York: The Guilford Press.
Caughlan, L., and Oakley, K. (2001). Cost considerations for long-term ecological monitoring. Ecol. Indic. 1, 123–134. doi: 10.1016/S1470-160X(01)00015-2
Christiansen, P. (2018). Whale Worship: Exploring the Role of Whales in Vietnam’s Coastal Lore. Available Online at: https://saigoneer.com/saigon-culture/13047-whale-worship-exploring-the-role-of-whales-in-vietnam-s-coastal-lore [accessed Jan 2, 2021].
Clapham, P. J. (2016). Managing leviathan: conservation challenges for the great whales in a post-whaling world. Oceanography 29, 214–225. doi: 10.5670/oceanog.2016.70
Cordes, D. O. (2011). The causes of whale strandings. N. Z. Vet. J. 30, 21–24. doi: 10.1080/00480169.1982.34865
Correia, A. M., Tepsich, P., Rosso, M., Caldeira, R., and Sousa-Pinto, I. (2015). Cetacean occurrence and spatial distribution: habitat modelling for offshore waters in the Portuguese EEZ (NE Atlantic). J. Mar. Syst. 143, 73–85. doi: 10.1016/j.jmarsys.2014.10.016
Cosentino, A. M., and Fisher, S. (2016). The utilization of aquatic bushmeat from small cetaceans and manatees in South America and West Africa. Front. Mar. Sci. 3:163. doi: 10.3389/fmars.2016.00163
Cubaynes, H. C., Fretwell, P. F., Bamford, C., Gerrish, L., and Jackson, J. A. (2019). Whales from space: four mysticete species described using new VHR satellite imagery. Mar. Mammal Sci. 35, 466–491. doi: 10.1111/mms.12544
de Vos, A. (2020). The Problem of ‘Colonial Science. Available Online at: https://www.scientificamerican.com/article/the-problem-of-colonial-science/ [accessed Nov 18, 2020].
de Vos, A., Brownell, R. L. Jr., Tershy, B., and Croll, D. (2016). Anthropogenic threats and conservation needs of blue whales, Balaenoptera musculus indica, around Sri Lanka. J. Mar. Biol. 1:12. doi: 10.1155/2016/8420846
Dohan, K., and Maximenko, N. (2010). Monitoring ocean currents with satellite sensors. Oceanography 23, 94–103. doi: 10.5670/oceanog.2010.08
Dowman, I., Jacobsen, K., Konecny, G., and Sandau, R. (2012). High Resolution Optical Satellite Imagery. U.K.: Whittles Publishing.
Earth-i. (2020). Constellations. Available Online at: https://earthi.space/constellations/ [accessed Dec 9, 2020].
Encyclopaedia Britannica (2021). Gulf of Peñas. Available Online at: https://www.britannica.com/place/Chile/Chile-in-the-21st-century [accessed Jan 5, 2021].
Evans, P. G. H., and Hammond, P. S. (2004). Monitoring cetaceans in European waters. Mamm. Rev. 34, 131–156. doi: 10.1046/j.0305-1838.2003.00027.x
Fadela, S. N. (2016). Second Southeast Asian Marine Mammal Stranding Network Symposium. Available Online at: https://www.dugongconservation.org/media/2018/10/Report_SEAMMSN.pdf [accessed Nov 18, 2020].
Försterra, G. (2009). “Ecological and biogeographical aspects of the Chilean fjord region,” in Marine Benthic Fauna of Chilean Patagonia, ed. F. G. Häussermann (Puerto Montt: Nature in Focus), 61–76.
Fretwell, P. T., Jackson, J. A., Ulloa Encina, M. J., Häussermann, V., Perez Alvarez, M. J., Olavarría, C., et al. (2019). Using remote sensing to detect whale strandings in remote areas: the case of sei whales mass mortality in Chilean Patagonia. PLoS One 14:e0225209. doi: 10.1371/journal.pone.0222498
Fretwell, P. T., Staniland, I. J., and Forcada, J. (2014). Whales from space: counting Southern right whales by satellite. PLoS One 9:e88655. doi: 10.1371/journal.pone.0088655
Geraci, J. R., Anderson, D. M., Timperi, R. J., Aubin, D. J. S., Early, G. A., Prescott, J. H., et al. (1989). Humpback whales (Megaptera novaeangliae) fatally poisoned by dinoflagellate toxin. Can. J. Fish. Aquat. Sci. 46, 1895–1898.
Geraci, J. R., and Loundsbury, V. J. (2005). Marine Mammal Ashore: A Field Guide for Strandings. Baltimore: National Aquarium in Baltimore, Inc.
Groom, S., Sathyendranath, S., Ban, Y., Bernard, S., Brewin, R., Brotas, V., et al. (2019). Satellite ocean colour: current status and future perspective. Front. Mar. Sci. 6:485. doi: 10.3389/fmars.2019.00485
Gulland, F. M. D., Dierauf, L. A., and Whitman, K. L. (2018). CRC Handbook of Marine Mammal Medicine. Boca Raton: CRC Press.
Gulland, F. M. D., and Hall, A. J. (2007). Is marine mammal health deteriorating? Trends in the global reporting of marine mammal disease. Eco Health 4, 135–150. doi: 10.1007/s10393-007-0097-1
Gulland, F. M. D., Peìrez-Corteìs, M. H., Urbaìn, J., Rojas-Bracho, L., Ylitalo, G., Weir, J., et al. (2005). Eastern North Pacific Gray whale (Eschrichtius robustus) Unusual Mortality Event, 1999-2000. Washington: United States of America Department of Commerce.
Gulland, F. M. D., and Stockin, K. A. (2019). “Harmonizing global strandings response,” Report from the Society for Marine Mammalogy/European Cetacean Society Conference Workshop. Barcelona: European Cetacean Society.
Hamilton, L. J. (2018). Large mass strandings of selected odontocete species: statistics, locations, and relation to earth processes. J. Cetacean Res. Manag. 19, 57–78.
Häussermann, V., Gutstein, C. S., Bedington, M., Cassis, D., Olavarria, C., Dale, A. C., et al. (2017). Largest baleen whale mass mortality during strong El Niño event is likely related to harmful toxic algal bloom. PeerJ 5:e3123. doi: 10.7717/peerj.3123/table-7
Hollings, T., Burgman, M., Andel, M. V., Gilbert, M., and Robinson, T. (2018). How do you find the green sheep? A critical review of the use of remotely sensed imagery to detect and count animals. Methods Ecol. Evol. 9, 881–892. doi: 10.1111/2041-210x.12973
Höschle, C., Cubaynes, H. C., Clarke, P. J., Humphries, G., and Borowicz, A. (2021). The potential of satellite imagery for surveying whales. Sensors 21:963. doi: 10.3390/s21030963
Humphries, G. R. W., Magness, D. R., and Huettmann, F. (2018). Machine Learning for Ecology and Sustainable Natural Resource Managment. Switzerland: Springer.
ICEYE (2021). Completing the Picture SAR Product Guide. Available Online at: https://www.iceye.com/hubfs/Downloadables/ICEYE_SAR_Product_Guide_2021_V4.0.pdf [accessed Aug 12, 2021].
IJsseldijk, L. L., van Neer, A., Deaville, R., Begeman, L., van de Bildt, M., van den Brand, J. M. A., et al. (2018). Beached bachelors: an extensive study on the largest recorded sperm whale Physeter macrocephalus mortality event in the North Sea. PLoS One 13:e0201221. doi: 10.1371/journal.pone.0201221
IWC (2015). Workshop on Investigations Of Large Mortality Events, Mass Strandings, And International Stranding Response. Paper SC/66b/REP/09 Presented to the IWC Scientific Committee (Unpublished). Available Online at: https://archive.iwc.int/pages/search.php?search=!collection24438”. (accessed May 31, 2021).
IWC (2017). Report of an IWC Workshop Developing Practical Guidance For The Handling of Cetacean Stranding Events. IWC/66/WKM&WI Rep02. Schaffhausen:IWC.
IWC (in press). Report of the IWC Scientific Committee. Available Online at: https://archive.iwc.int/pages/view.php?ref=19276&k= (accessed August 6, 2021).
Kaschner, K., Quick, N. J., Jewell, R., Williams, R., and Harris, C. M. (2012). Global coverage of cetacean line-transect surveys: status quo, data gaps and future challenges. PLoS One 7:e44075. doi: 10.1371/journal.pone.0044075
Klein, C. J., Brown, C. J., Halpern, B. S., Segan, D. B., McGowan, J., Beger, M., et al. (2015). Shortfalls in the global protected area network at representing marine biodiversity. Sci. Rep. 5:17539. doi: 10.1038/srep17539
LaRue, M. A., Ainley, D. G., Pennycook, J., Stamatiou, K., Salas, L., Nur, N., et al. (2019). Engaging ‘the crowd’ in remote sensing to learn about habitat affinity of the Weddell seal in Antarctica. Remote Sens. Ecol. Conserv. 6, 70–78. doi: 10.1002/rse2.124
LaRue, M. A., Stapleton, S., and Anderson, M. (2017). Feasibility of using high-resolution satellite imagery to assess vertebrate wildlife populations. Conserv. Biol. 31, 213–220. doi: 10.1111/cobi.12809
LaRue, M. A., Stapleton, S., Porter, C., Atkinson, S., Atwood, T., Dyck, M., et al. (2015). Testing methods forusing high-resolution satellite imagery to mointor polar bear abundance and distribution. Wildlife Soc. Bull. 39, 772–779. doi: 10.1002/wsb.596
Leaper, R., and Miller, C. (2011). Management of Antarctic baleen whales amid past exploitation, current threats and complex marine ecosystems. Antarc. Sci. 23, 503–529. doi: 10.1017/s0954102011000708
Lennert-Cody, C. E., Buckland, S. T., Gerrodette, T., Webb, A., Barlow, J., Fretwell, P. T., et al. (2019). Review of potential line-transect methodologies for estimating abundance of dolphin stocks in the eastern tropical Pacific. J. Cetacean Res. Manag. 19, 9–21.
Liang, S., and Wang, J. (2019). Advanced Remote Sensing: Terrestrial Information Extraction and Applications. Cambridge: Academic Press.
Lilliesand, T. M., and Kiefer, R. W. (1979). Remote sensing and image interpretation. Geol. Mag. 117:612.
Magera, M., Mills Flemming, J. E., Kaschner, K., Christensen, L. B., and Lotze, H. K. (2013). Recovery trends in marine mammal populations. PLoS One 8:e77908. doi: 10.1371/journal.pone.0077908
Maldini, D., Mazzuca, L., and Atkinson, S. (2005). Odontocete stranding patterns in the main Hawaiian islands (1937-2002): how do they compare with live animal surveys? Pacific Sci. 59, 55–67. doi: 10.1353/psc.2005.0009
Maxar (2020a). Direct Access Programme. Available Online at: https://www.maxar.com/products/direct-access-program [accessed Dec 10, 2020].
Maxar (2020b). Worldview-3. Available Online at: https://resources.maxar.com/data-sheets/worldview-3 [accessed Dec 5, 2020].
Maxar (2020c). Worldview Legion. Available Online at: https://www.maxar.com/splash/it-takes-a-legion [accessed Dec 9, 2020].
Mazzariol, S., Siebert, U., Scheinin, A., Deaville, R., Brownlow, A., Uhart, M., et al. (2020). Summary of Unusual Cetacean Stranding Events Worldwide (2918-2020). Available Online at: https://archive.iwc.int/pages/view.php?search=%21collection29937+&k=&modal=&display=list&order_by=title&offset=0&per_page=240&archive=&sort=DESC&restypes=&recentdaylimit=&foredit=&ref=17280 [accessed May 15, 2020].
Minton, G., Collins, T., Pomilla, C., Findlay, K. P., Rosenbaum, H., Baldwin, R., et al. (2008). Megaptera novaeangliae (Arabian Sea subpopulation). The IUCN Red List of Threatened Species 2008: e.T132835A3464679. Available Online at: https://www.iucnredlist.org/species/132835/3464679 [accessed Dec 31, 2020].
Moore, K. M., Simeone, C. A., and Brownell, R. L. Jr. (2018). “Strandings,” in Encyclopedia of Marine Mammals, eds B. Würsig, J. G. M. Thewissen, and K. M. Kovacs (United Kingdom: Academic Press), 945–951. Third Edition.
Moore, S. E. (2008). Marine mammals as ecosystem sentinals. J. Mammal. 89, 534–540. doi: 10.1644/07-MAMM-S-312R1.1
Olavarría, C., Pashuk, K., Landreth, G., Torres, D., Rodriguez, F., Arrau, F. P., et al. (2019). Preliminary report on a new baleen whale mortality at the Golfo de Penas, southern Chile, that occurred late 2019 summer. Available Online at: https://archive.iwc.int/pages/view.php?ref=11991&k=&search=%21last1000&offset=0&order_by=field74&sort=DESC&archive [accessed Nov 21, 2020].
Peltier, H., Dabin, W., Daniel, P., Van Canneyt, O., Dorémus, G., Huon, M., et al. (2012). The significance of stranding data as indicators of cetacean populations at sea: modelling the drift of cetacean carcasses. Ecol. Indic. 18, 278–290. doi: 10.1016/j.ecolind.2011.11.014
Peltier, H., Jepson, P. D., Dabin, W., Deavillec, R., Daniel, P., Van Canneyt, O., et al. (2014). The contribution of stranding data to monitoring and conservation strategies for cetaceans: developing spatially explicit mortality indicators for common dolphins (Delphinus delphis) in the eastern North-Atlantic. Ecol. Indic. 39, 203–214. doi: 10.1016/j.ecolind.2013.12.019
Peters, K. J., Bury, S. J., Betty, E. L., Parra, G. J., Tezanos-Pinto, G., and Stockin, K. A. (2020). Foraging ecology of the common dolphin Delphinus delphis revealed by stable isotope analysis. Mar. Ecol. Progr. Ser. 652, 173–186. doi: 10.3354/meps13482
Platonov, N., Mordvintsev, I. N., and Rozhnov, V. V. (2013). The possibility of using high resolution satellite images for detection of marine mammals. Biol. Bull. 40, 217–226. doi: 10.1134/S1062359013020106
Plön, S., Andrianarivelo, N., Braulik, G., Bunbury, N., Collins, T., Duffaud, M.-H., et al. (2020). IndoCet Stranding Network- Report on Strandings for the Western Indian Ocean Region. Available Online at: https://archive.iwc.int/pages/view.php?ref=17271&k=&search=%21collection29937+&offset=0&order_by=title&sort=DESC&archive= [accessed Nov 22, 2020].
Pyenson, N. (2011). The high fidelity of the cetacean stranding record: insights into measuring diversity by integrating taphonomy and macroecology. Proc. R. Soc. 278, 3608–3616. doi: 10.1098/rspb.2011.0441
Pyenson, N. D. (2010). Carcasses on the coastline: measuring the ecological fidelity of the cetacean stranding record in the eastern North Pacific Ocean. Paleobiology 36, 453–480. doi: 10.1666/09018.1
Reeves, R. R., Ewins, P. J., Agbayani, S., Heide-Jørgensen, M. P., Kovacs, K. M., Lydersen, C., et al. (2014). Distribution of endemic cetaceans in relation to hydrocarbon development and commercial shipping in a warming Arctic. Mar. Policy 44, 375–389. doi: 10.1016/j.marpol.2013.10.005
Rey, N., Volpi, M., Joost, S., and Tuiabd, D. (2017). Detecting animals in African Savanna with UAVs and the crowds. Rem. Sens. Environ. 200, 341–351. doi: 10.1016/j.rse.2017.08.026
Roman, J., Estes, J. A., Morissette, L., Smith, C., Costa, D., McCarthy, J., et al. (2014). Whales as marine ecosystem engineers. Front. Ecol. Environ. 12, 377–385. doi: 10.1890/130220
Rowntree, V., Uhart, M. M., Sironi, M., Chirife, A., Di Martino, M., La Sala, L., et al. (2013). Unexplained recurring high mortality of Southern right whale Eubalaena australis calves at Península Valdés, Argentina. Mar. Ecol. Progr. Ser. 493, 275–289. doi: 10.3354/meps10506
Schwacke, L. H., Smith, C. R., Townsend, F. I., Wells, R. S., Hart, L. B., Balmer, B. C., et al. (2014). Health of common bottlenose dolphins (Tursiops truncatus) in Barataria Bay, Louisiana, Following the deepwater horizon oil spill. Environ. Sci. Technol. 48, 93–103. doi: 10.1021/es403610f
Simmonds, M. P., and Elliott, W. J. (2009). Climate change and cetaceans: concerns and recent developments. J. Mar. Biol. Assoc. 89, 203–210. doi: 10.1017/S0025315408003196
Stockin, K. A., Duignan, P. J., Roe, W. D., Meynier, L., Alley, M., and Fettermann, T. (2009). Causes of mortality in stranded common dolphins (Delphinus sp.) from New Zealand waters between 1998 and 2008. Pacific Conserv. Biol. 15, 217–227. doi: 10.1071/PC090217
Stockin, K. A., Law, R. J., Roe, W. D., Meynier, L., Martinez, E., Duignan, P. J., et al. (2010). PCBs and organochlorine pesticides in Hector’s (Cephalorhynchus hectori hectori) and Maui’s (Cephalorhynchus hectori maui) dolphins. Mar. Pollut. Bull. 60, 834–842. doi: 10.1016/j.marpolbul.2010.01.009
Stockin, K. A., Mazzariol, S., Chansue, N., Deaville, R., Grover, D., Gulland, F. M. D., et al. (2019). IWC Strandings Initiative Guidelines for International Training and Emergancy Response Requests. Available Online at: https://archive.iwc.int/pages/view.php?search=%21collection29805+&k=&modal=&display=list&order_by=title&offset=0&per_page=240&archive=&sort=DESC&restypes=&recentdaylimit=&foredit=&ref=12012 [accessed May 31, 2021].
Szantoi, Z., Turner, W., Rondinini, C., Pettorelli, N., Mora, B., Leidner, A., et al. (2015). Free and open-access satellite data are key to biodiversity conservation. Biol. Conserv. 182, 173–176. doi: 10.1016/j.biocon.2014.11.048
The European Space Agency [ESA] (2020b). Types of Orbits. Available Online at: https://www.esa.int/Enabling_Support/Space_Transportation/Types_of_orbits#GEO [accessed Dec 5, 2020].
The European Space Agency [ESA] (2020a). SkySat: mission. Available Online at: https://earth.esa.int/eogateway/missions/skysat [accessed Dec 9, 2020].
Tin, T., Fleming, Z. L., Hughes, K. A., Ainley, D. G., Convey, P., Moreno, C. A., et al. (2009). Impacts of local human activities on the Antarctic environment. Antarct. Sci. 21, 3–33. doi: 10.1017/S0954102009001722
Tucker, J. P., Santos, I. R., Crocetti, S., and Butcher, P. (2018). Whale carcass strandings on beaches: management challenges, research needs, and examples from Australia. Ocean Coast. Manag. 163, 323–383. doi: 10.1016/j.ocecoaman.2018.07.006
UNEP, CMS and ASCOBANS (2017). Progress Report of the Agreement on the Conservation of Small Cetaceans of the Baltic, North East Atlantic, Irish and North Seas (ASCOBANS). UNEP/CMS/COP12/Inf.6.3. Nairobi: UNEP.
Van Bressem, M.-F., Raga, J. A., Di Guardo, G., Jepson, P. D., Duignan, P. J., Siebert, U., et al. (2009). Emerging infectious diseases in cetaceans worldwide and the possible role of environmental stressors. Dis. Aquat. Organ. 86, 143–157. doi: 10.3354/dao02101
Waltzek, T. B., Cortés-Hinojosa, G., Wellehan, J. F. X. Jr., and Gray, G. C. (2012). Marine mammal zoonoses: a review of disease manifestations. Zoonoses Public Health 59, 521–535. doi: 10.1111/j.1863-2378.2012.01492.x
Wang, L., Maddox, C., Terio, K., Lanka, S., Fredrickson, R., Novick, B., et al. (2020). Detection and characterization of new coronavirus in bottlenose dolphin, United States, 2019. Emerg. Indect. Dis. 26, 1610–1612. doi: 10.3201/eid2607.200093
Wege, M., Salas, L., and LaRue, M. (2020). Citizen science and habitat modelling facilitates conservation planning for crabeater seals in the Weddell Sea. Divers. Distrib. 26, 1291–1304. doi: 10.1111/ddi.13120
Welch, C. (2019). More People are Eating Marine Animals—With Deadly Results. Available Online at: https://www.nationalgeographic.com/environment/2019/05/people-eating-decaying-dead-sea-creatures-dying/ [accessed Nov 22, 2020].
Wilkinson, D., and Worthy, G. A. J. (1999). “Marine mammal stranding networks,” in Conservation and Management of Marine Mammals, eds J. R. Twiss and R. R. Reeves (Washingnton: Smithsonian Institution Press), 396–411.
Woo, P. C. Y., Lau, S. K. P., Lam, C. S. F., Tsang, A. K. L., Hui, S., Fan, R. Y. Y., et al. (2013). Discovery of a novel bottlenose dolphin coronavirus reveals a distinct species of marine mammal coronavirus in gammacoronavirus. J. Virol. 88, 1318–1331. doi: 10.1128/JVI.02351-13
World Health Organisation [WHO] (2017). One Health. Available Online at: https://www.who.int/news-room/q-a-detail/one-health [accessed July 5, 2020].
World Marine Mammal Conference [WMMC] (2019). Barcelona Declaration. Available Online at: https://www.wmmconference.org/barcelona-declaration/ [accessed May 14, 2020].
Keywords: VHR satellite imagery, mass strandings, cetacean, remote monitoring, One Health, inclusivity
Citation: Clarke PJ, Cubaynes HC, Stockin KA, Olavarría C, de Vos A, Fretwell PT and Jackson JA (2021) Cetacean Strandings From Space: Challenges and Opportunities of Very High Resolution Satellites for the Remote Monitoring of Cetacean Mass Strandings. Front. Mar. Sci. 8:650735. doi: 10.3389/fmars.2021.650735
Received: 07 January 2021; Accepted: 15 September 2021;
Published: 18 November 2021.
Edited by:
Gilles Reverdin, Centre National de la Recherche Scientifique (CNRS), FranceReviewed by:
Christophe Guinet, Centre National de la Recherche Scientifique (CNRS), FranceMichelle Jillian Devlin, Centre for Environment, Fisheries and Aquaculture Science (CEFAS), United Kingdom
Copyright © 2021 Clarke, Cubaynes, Stockin, Olavarría, de Vos, Fretwell and Jackson. This is an open-access article distributed under the terms of the Creative Commons Attribution License (CC BY). The use, distribution or reproduction in other forums is permitted, provided the original author(s) and the copyright owner(s) are credited and that the original publication in this journal is cited, in accordance with accepted academic practice. No use, distribution or reproduction is permitted which does not comply with these terms.
*Correspondence: Penny J. Clarke, clarke.pennyj@gmail.com