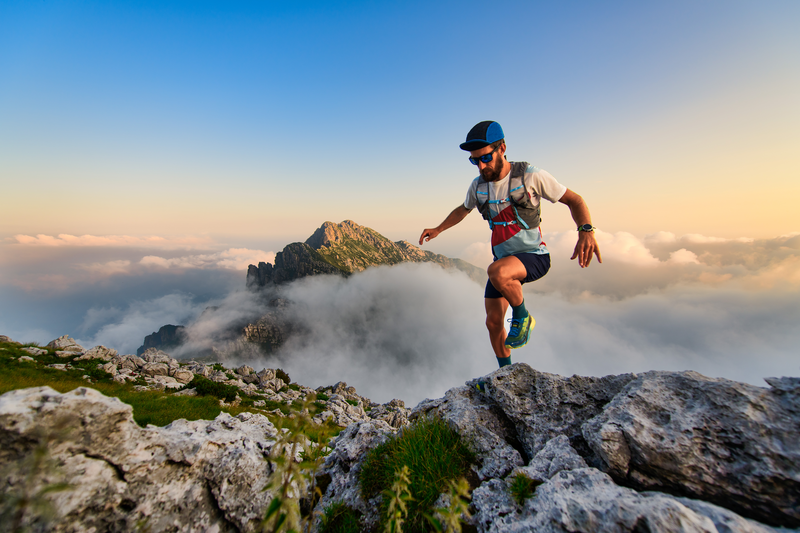
95% of researchers rate our articles as excellent or good
Learn more about the work of our research integrity team to safeguard the quality of each article we publish.
Find out more
ORIGINAL RESEARCH article
Front. Mar. Sci. , 06 April 2021
Sec. Marine Fisheries, Aquaculture and Living Resources
Volume 8 - 2021 | https://doi.org/10.3389/fmars.2021.643347
This article is part of the Research Topic The Approach to Complex Systems in Fisheries View all 9 articles
The fishing bycatch of the loggerhead sea turtle (Caretta caretta) epitomizes the challenges of designing fisheries management strategies to protect highly migratory, endangered species. We present here the case of turtle bycatch in the Gulf of Ulloa, Mexico, in which conservation advocacy groups requested the United States Government to apply the legal provisions for preventing fishing bycatch of protected living marine resources (PLMR). Because these provisions implied the possibility of trade sanctions, the Mexican government had to devise policies equivalent to those imposed on the United States’ fleet. While conservation advocacy groups claimed that the effect of fishing bycatch was proven, the federal fisheries agency disregarded the facts for political reasons. Evidently, there was a need for a practical approach to address this highly contested policy-making problem characterized by limited data, deep uncertainties, and urgency for results. Our goal here is to present the implementation of an exploratory modeling rationale to tackle this sort of complex socio-ecological technological problem. We focused on identifying the bycatch level at which the environmental authorities would be compelled by law to act in protecting the loggerheads. We combined ecological risk analysis and area-oriented multiple-use framework to evaluate a wide range of plausible scenarios consistent with the available data. Results identified the bycatch level that indicated a potential critical transition to a low resilience state of the loggerhead population, and the proper multiple-use management scheme. Our findings were used to formulate regulations aimed to set a bycatch cap and a refuge area for the loggerhead population in the region.
The case of the North Pacific population segment of the loggerhead sea turtle (Caretta caretta) epitomizes the challenges of designing conservation strategies for highly migratory, endangered species. It also problematizes the imperative of transdisciplinary research for addressing complex social-ecological-technological-institutional problems—a central tenet of sustainability science. Loggerheads perform one of the longest trans-oceanic migrations (~12,000 km) of any marine vertebrate. From the nesting areas in Japan, loggerheads are transported by the Kuroshio, North Pacific, and California Currents to the nursery habitat in the Gulf of Ulloa, a biological hotspot off the south-eastern coast of the Baja California Peninsula, Mexico (Figure 1A). Here, upwelling provides rich feeding grounds for large aggregations of juvenile loggerheads year-round, with peaks from April to September. After spending years foraging in the Gulf of Ulloa, they return through the Northern Equatorial Current to their natal nesting beaches for reproduction and remain in the Western Pacific until death (Conant et al., 2009).
Figure 1. (A) Location of Gulf of Ulloa and management zones (GU); (B) overlap between zones and the fishing refuge designed in 2015; (C) overlap between zones and the extended fishing refuge designed in 2016; (D) overlap between zones and the seafloor mining project.
Sea turtles are endangered species protected by multilateral agreements. Incidental fishing bycatch has been documented as one major threat to sea turtles around the world. North Pacific loggerheads are incidentally caught by pelagic longline fisheries in international waters, mainly by Japanese, Korean, and Taiwanese fleets, and by longline and gillnet fishing fleets in the U.S. Pacific, and Gulf of Ulloa (Lewison et al., 2004; Peckham et al., 2008; Conant et al., 2009). Regulations for the Hawaii-based fishery have resulted in significant reductions in loggerhead bycatch (Gilman et al., 2007). However, the development of regulations in Mexico has been a rather elusive process.
Ideally, tackling problems of the sort of the bycatch of loggerhead requires the implementation of transdisciplinary inquiry to enable collaborative policymaking. Yet, addressing power-sensitive issues through collaboration typically faces conceptual, institutional, and social barriers (Lawrence, 2015). In our case, barriers to regulating incidental bycatch in the Gulf of Ulloa involved harsh inter-governmental struggles between the fisheries and environmental agencies (Bojórquez-Tapia et al., 2017). CONAPESCA (National Commission on Fisheries and Aquaculture) officially maintained that incidental bycatch was nil and thus that the fishing permits should not be amended in any way. CONAPESCA also posited that poisoning (perhaps caused by toxic algal blooms or red tides) and disease could explain the carcasses washed ashore. In contrast, SEMARNAT (Secretary of the Environment and Natural Resources) was compelled by law to end fishing practices that jeopardize the loggerhead population. Unsurprisingly, this institutional conflict led to an impasse in policymaking that stemmed from the lack of legitimacy and credibility of the contending positions. The need to respond to possible trade sanctions announced by the United States government compelled the Mexican government to break the deadlock and to arrive at a sensible regulatory framework to reduce loggerhead bycatch.
Here, we present the approach implemented for developing regulations for loggerhead bycatch in the Gulf of Ulloa. Our goal is to demonstrate the advantages of using exploratory modeling (Bankes, 1993, 2002) to address the challenges of uncertainty in a contested policymaking context (Burgman, 2005; Krupnick et al., 2006). The approach entailed the combination of ecological risk assessment (ERA, Suter, 2007) and area-oriented multiple-use analysis (Brooks et al., 1991). These techniques were implemented in a series of consultation workshops with representatives of authorities, academics, the fishing cooperatives, and conservation advocacy organizations, which took place within the context of the marine spatial planning of the North Pacific Region in Mexico (MSP-NPMR; Díaz-de-León and Díaz-Mondragón, 2013; Bojórquez-Tapia et al., 2017; Pedroza-Páez et al., 2020). As a result, a critical ecological threshold of a possible decrease of the loggerhead population was identified which was translated into a maximum allowable bycatch rate of turtles per fishing season. This case study shows that exploratory modeling was essential for achieving a logical and non-biased treatment of the available information and knowledge, which led to an interactive dialogue amongst stakeholders and a systematic examination of policy alternatives.
Policymaking problems of the sort of fishing bycatch of loggerhead sea turtles involve multi-stakeholder, multilevel socio-environmental systems in which non-equilibrium behavior emerges endogenously. Under these conditions, the development of regulations inevitably confronts unmeasurable or “Knightian” uncertainty (after Knight, 1921) that results from the impossibility of knowing all the information needed to set accurate odds of the possible outcomes. Nevertheless, Knightian uncertainty not only implies a lack of agreement between the stakeholders on the alternative courses of action or “solutions” to a problem, but also that available scientific knowledge concerning cause and effect mechanisms is open to criticism and subject to improvement (Balint et al., 2011; Kwakkel et al., 2016).
Because Knightian uncertainty precludes attaining a true representation of reality, the analysis of policymaking problems must rely on explanations of the behavior of complex socio-environmental systems. As posited by Bunge (2004), these explanations entail conjecturing the scientific mechanisms behind the observed facts, rather than the mindless accumulation of data and the mindless search for statistical correlations among them.
Exploratory modeling is an integrated analytical approach that has proved useful for such a task (Bankes, 1993, 2002; Kwakkel and Pruyt, 2013). In our case, it entailed the implementation of computational experiments to systematically elicit the implication of varying conjectures, assumptions, and hypotheses concerning the response of a socio-environmental system to alternative policy interventions. Our goal was to encapsulate the complex interplay between the stakeholders’ cognition and the real socio-environmental dynamics that together shape policymaking.
In the development of exploratory models in support of policymaking, it is convenient to disaggregate uncertainty in terms of sources, type, and level of deviation from deterministic and complete information and knowledge (Lempert, 2002; Regan et al., 2002; Walker et al., 2003; Lane and Maxfield, 2005; Ascough et al., 2008; Carey and Burgman, 2008; Kwakkel et al., 2010; Balint et al., 2011). Accordingly, we identified as sources of uncertainty the spatial and temporal boundaries of the loggerhead population-halibut (Paralichtys californicus) fishery system, the assumptions regarding the loggerhead population size, the nonlinear response of the loggerhead population size to incidental bycatch, and the estimation of both the population size and the bycatch rate.
We also identify four types of uncertainties in our approach: epistemic, linguistic, politically induced, and ontological uncertainties. Epistemic uncertainty refers to the limitations of the knowledge of the state of a system. In our case, it was prevalent in both the measurement error and the random variation of the loggerhead population over time and space, as well as the error in the extrapolation of the population parameters.
Linguistic uncertainty refers to the ambiguity and vagueness of the discursive interactions amongst the stakeholders. Typically, people tend to handle even scientific evidence according to the rules of language rather than numerically or using the rules of probability. It is thus related to the human capacity for receiving, processing, remembering, and transmitting information and knowledge. It can be of two types, semantic or predicate. The former involves polysemous terms, such as “environmental risk,” whereas the latter involves ambiguous expressions, such as “high risk.” In our case, linguistic uncertainty related to the imprecise, qualitative evidence regarding the bycatch rate (alarming for a conservationist, and irrelevant for fisheries) and the aggregation phenomenon of loggerheads in the Gulf of Ulloa (in jeopardy for conservationists, and natural for fisheries).
Politically induced uncertainty (Balint et al., 2011) refers to the “deliberate ignorance” of public agencies when dealing with precarious and controversial circumstances. Through technical and procedural hurdles, these agencies deemphasize the inherent uncertainty in decision-making by intentionally limiting the scope of the required assessments. As explained in detail below, fishing agencies in our case deemphasized the inherent uncertainty of the incidental bycatch of loggerheads in the Gulf of Ulloa.
Ontological uncertainty refers to the ignorance of the entities and relationships of the real world. Because it impedes the formation of propositions about relevant future outcomes, ontological uncertainty prevents foreseeing the consequences of the social agents’ actions. In our case, ontological uncertainty manifested itself in the hidden assumptions of the representation of loggerhead population-incidental bycatch system in the Gulf of Ulloa. More precisely, our model ignored the long-term effect of incidental bycatch on the structure of the Eastern Pacific loggerhead population in the long term, as well as the potential synergist effect of bycatch by pelagic longline fisheries in international waters, and by subsuming any other causes of mortality in the intrinsic growth rate parameter.
With regards to the level, it is useful to contrast shallow from deep uncertainties. Shallow uncertainty refers to the statistical description that involves reasonably good data and the possibility of generating the proper probability functions regarding some phenomenon. It includes sampling error and the inherent variability of socio-environmental systems. In our case, we identify shallow uncertainty in the characterization of the halibut fishing cooperatives. For its part, deep uncertainty is non-probabilistic and closely associated with ontological uncertainty. It emerges whenever planners or stakeholders cannot agree on the probability distributions for key parameters nor on the model structure to represent possible future events. Addressing deep uncertainty thus entails the implementation of scenarios as plausible descriptions of how the system and its driving forces may evolve. This approach generates future “states of the world” to identify robust and satisficing courses of action. In our case, deep uncertainty implied the simulation of multiple scenarios to determine the bycatch level that justified imposing regulations on the halibut fishery in the Gulf of Ulloa.
Gulf of Ulloa is a small shallow sea (∼10,000 km2) that has been cataloged as one “Biological Action Center” (Lluch-Belda et al., 2000) of the Mexican Marine North Pacific Region (Figure 1A). Its exceptionally high productivity originates from the seasonal variation of the California Current. Productivity is the highest from March to June when the California Current and wind-induced upwelling are the strongest (González-Rodríguez et al., 2012). During this period, the Gulf of Ulloa turns into a rich feeding ground for large aggregations of juvenile loggerheads, especially closer to shore where they feed predominantly on the pelagic red crab “langostilla” (Pleuroncodesplanipes), the most abundant nekton species, and on a variety of other invertebrate species, including gelatinous prey. The California halibut fishery coincides with the movement of P. planipes to the shallower continental shelf (Balart, 1996a, b; Wingfield et al., 2011). Thus, loggerheads seeking out favorable foraging conditions are exposed to bycatch by the bottom gillnets of the halibut fishery (Nichols et al., 2000).
Loggerhead carcasses have been found stranded along 43 km of shoreline in the southern portion of the Gulf of Ulloa since 2003 (Peckham et al., 2008). After reports of 438 loggerhead carcasses found stranded in August 2012, the United States National Marine Fisheries Service (NMFS) acted under legal provisions to certify whether Mexico had regulations comparable to those of the United States for preventing fishing bycatch of protected living marine resources (PLMR) and whether Mexican nationals were conducting fishing operations in a way that diminishes the effectiveness of international conservation programs (National Oceanic and Atmospheric Administration Fisheries, 2013). In compliance with the Moratorium Protection Act, NMFS listed Mexico as a country involved in bycatch of PLMR and requested from the Mexican government information about the regulatory programs of gillnet fisheries in December 2012. Because the response did not mention how loggerheads’ bycatch was prevented, the NMFS concluded that Mexican regulations were not equivalent to those of the Hawaiian longline fleet, and the United States government considered imposing trade sanctions on Mexico (National Oceanic and Atmospheric Administration Fisheries, 2013).
The Mexican policymaking context involved two opposing positions. On one side, CONAPESCA didn’t want to affect the fishing cooperatives of the region with the imposition of fishing restrictions and, on the other side, SEMARNAT had the institutional mandate to protect an endangered species and to respond to the non-governmental conservation organizations’ claims. These conflictual positions manifested themselves as deep and politically induced uncertainties. Deep uncertainty can be summarized into (1) lack of agreement on “the problem,” (2) contradictory descriptions and disagreement about the evidence about the “real causes” of the problem, (3) adversarial attitudes by the stakeholders that amplified the effects of divergent ways of knowing and existing power imbalances, and (4) incomplete understanding of the potential outcomes of alternative policies. For its part, politically induced uncertainty can be summarized in the harsh disagreement over the scientific explanation for the observed carcasses washed ashore. On one hand, non-governmental organizations advocating loggerhead conservation (conservation, hereafter) estimated bycatch rates of 1,500 and 3,000 individuals year-1 for 2005 and 2006 (Peckham et al., 2008). On the other, the cooperatives operating the halibut fishery (fishing, hereafter) argued that gillnets had been authorized by fishing permits and that any additional regulation would jeopardize their fishery’s yields, productivity, and the achievement of their cooperatives’ social goals. Local fishing cooperatives and CONAPESCA, moreover, claimed that the published research about bycatch only represented a biased viewpoint from conservationists. Through verbal testimony, some of the fishers who allegedly had assisted in that scientific research asserted that the conclusions were, in rough translation, “bogus.” They also claimed that researchers did not consider that mortality of loggerheads continued even when fishing had been temporally closed.
Our research team began to intervene in the problem by providing technical support for MSP-NPMR in 2013. Along the MSP-NPMR process, the bycatch of loggerheads was identified as one critical issue regarding the incompatible uses in the region. Following federal regulations, the MSP-NPMR entailed the implementation of a collaborative planning framework through a series of workshops. In the workshops, our research team led the participation of the stakeholders in the development of the suitability analysis and the exploratory model described in section “Materials and Methods.”
The mandate in the Mexican Constitution establishes that any act of authority must be duly grounded and reasoned had important implications for our inquiry. It meant that arguments underpinning loggerhead bycatch regulations in the Gulf of Ulloa had to be strictly founded on the law and thoroughly justified by facts. We thus had to ensure that results of exploratory modeling corresponded to the powers and legal attributions of both SEMARNAT (to act in the protection of the endangered loggerhead population) and CONAPESCA (to enforce fishing regulations). Hence, rather than addressing whether the use of gillnets by the halibut fishery was the main cause of the loggerhead carcasses washed ashore, our exploratory modeling rationale centered on (1) identifying the level of bycatch at which SEMARNAT would be compelled by law to protect the long-term viability of large aggregations of juvenile loggerheads in Gulf of Ulloa, and (2) optimally allocating the sectoral activities (i.e., fishing and conservation) across marine space.
Critical transition theorists (Scheffer et al., 2009; Dakos et al., 2015) have postulated that low resilience (or high sensitivity) denotes a strongly nonlinear response in the internal dynamics of a system to a small change in forcing (incidental bycatch in this case). Accordingly, one key premise of our exploratory modeling was that the response of the loggerhead population-halibut fishery system implied a catastrophic threshold (without a switch to an alternative attractor) into a persistent state in which the large aggregation of juvenile loggerheads phenomenon would no longer be possible. From this basic premise, three definitions followed: “abundance,” referring to the population size of juvenile loggerheads during periods of large aggregation in the Gulf of Ulloa, “severity,” to the magnitude of abundance reduction, and “critical ecological threshold,” (CET) to a severity level that would cause a sharp decrease of abundance.
Key assumptions underlying the simulation were:
(a) The carrying capacity for the juvenile loggerhead population in the Gulf of Ulloa cannot exceed the maximum observed abundance reported in the literature.
(b) The CET corresponded to a 10% probability of reaching a severity level equivalent to a decline of one-quarter of the initial abundance. This threshold tacitly represented the “unacceptable risk” tolerable to both SEMARNAT and the non-governmental organizations advocating loggerhead conservation.
(c) The intrinsic growth rate of the loggerhead population considers all the causes of mortality by other factors along the migratory route (including bycatch by other fisheries) except for halibut fishery bycatch in the Gulf of Ulloa (i.e., births + immigration − deaths − emigration).
(d) There was not a delay in the effect of halibut fishery bycatch mortality over the loggerhead population. This implied that the outcome of fishing bycatch may manifest itself after 25 years.
Bunge’s (2004) definitions-axioms-theorems framework was a necessary first step in crafting an early warning indicator of low resilience. The axioms (self-evident statements) included:
(A1) Loggerheads are incidentally caught in gillnets of the halibut fishery.
(A2) The probability of bycatch is related to the fishing effort.
(A3) There is a carrying capacity for the juvenile loggerhead population in the Gulf of Ulloa.
(A4) Bycatch in the Gulf of Ulloa is an additional mortality factor.
The theorems (statements proven on axioms, or other theorems and logical connectives) included the following:
(T1) An increase in fishing effort increases the bycatch rate (the combination of A1, and A2).
(T2) An increase in the bycatch rate results in an increase in the mortality of the juvenile loggerhead population in the Gulf of Ulloa (the combination of assumption d., and T1).
(T3) At some non-catastrophic threshold, an increase in the bycatch rate results in an unacceptable risk of low resilience of the abundance (the combination of assumptions a., b, and c., A3 and A4, and T2).
The biomass model (Figure 2) simulates the change in abundance as the difference between the natural growth of the loggerhead turtle population and the level of bycatch; formally (Schaefer, 1954; Hilborn and Walters, 1992; Acevedo, 2012):
where r is the intrinsic growth rate, B is the biomass (or abundance), K is the carrying capacity, c is the bycatch rate, and t is time.
The bycatch rate, c, is assumed to be proportional to the fishing effort, f, and B (as stated in theorem 1); formally:
where q is the proportionality constant or “catchability coefficient,” representing the fraction of the population caught by a unit of fishing effort.
The fishing effort, f, or the total number of fishing trips in a year, resulted from the number of fishing boats, e, fishing days per month, v, and duration of the fishing season in months, s:
Both B and f at t = 0 were empirically determined. Hence, different catchability coefficients were computed to simulate the number of individuals incidentally caught per fishing boat, given a specific unitary bycatch rate, c′ = {1, 2, …, n}:
Accordingly, the annual bycatch rate, κ (individuals year–1), was assumed to be proportional to the fishing effort and abundance (as stated in theorem 1)—the proportionality constant or catchability coefficient, q, represents the fraction of the population caught by a unit of fishing effort (Arreguín-Sánchez, 1996). The fishing effort, in turn, represents the total number of trips per boat in a fishing season. Change in abundance was simulated as the difference between the natural growth of the loggerhead turtle population and κ (as stated in theorem 2).
Next, we implemented ERA (Suter, 2007) to elicit both the severity and the probability of a low resilience state of the loggerhead population (as stated in theorem 3), rather than directly addressing the actual magnitude of bycatch in the Gulf of Ulloa. The approach entailed the use of Monte Carlo simulation (through the software Crystal Ball®) to allow for random variation of the parameters of the dynamic catch equilibrium model (Table 1). Each simulation was iterated 10,000 times for a simulation time of 100 years.
Table 1. Range of values and random distributions for the Monte Carlo simulation of the loggerhead population-incidental bycatch system in the Gulf of Ulloa.
One key parameter in the construction of scenarios for ERA was the catchability coefficient, q. In fishery science, this parameter theoretically represents the probability of any single individual (or unit of stock) being caught by a unit of fishing effort, or fish caught per fish available per effort unit and per time unit (Arreguín-Sánchez, 1996; Jul-Larsen et al., 2003). Given that in our case the value ranges of both fishing effort and abundance could be empirically determined, we could construct scenarios by varying the number of loggerhead individuals incidentally caught by one boat in a fishing season, what we referred to as the unitary bycatch rate, c′ (individuals boat–1 year–1), for different values of loggerhead abundance, effort unit, and time units.
Marine space was conceptualized in the MSP-NPMR as a complex socio-environmental system that coupled a set of biophysical attributes with the values, needs, and interests of multiple stakeholders (Pedroza-Páez et al., 2020). the MSP-NPMR entailed the implementation of suitability assessment through Spatial Multi-criteria Evaluation (SMCE), a widely used approach for tackling geographic decision-making problems (Malczewski and Rinner, 2015; Porporato et al., 2020). Operationally, SMCE involved carrying out a series of expert consultation workshops in which representatives of each sector were convened to develop geospatial models. The experts included academics, civil society groups (e.g., fishing cooperatives, conservation advocacy groups, industry, and business associations, etc.), and municipal, state, and federal authorities. The output was a suitability map for each sector.
As required by SMCE, the set geographic attributes associated with specific sectoral activity were normalized, weighted, and combined linearly so that a [0,1] suitability score was obtained of each basic unit of observation (i.e., polygons or pixels), 1 indicating the highest suitability. Next, a zoning approach was applied through the multivariate numerical classification of the output of SMCE to delineate the “environmental management units” (EMU) of the North Pacific. Mean suitability per sectoral activity was obtained for every EMU.
Area-oriented multiple-use (AOMU)was implemented to optimally allocate the sectoral activities across the EMU of the MSP-NPMR—AOMU refers to the production of a mix of products and amenities from a given set of management units across a territory (Ridd, 1965; Brooks et al., 1991). The optimization objectives were (1) maximizing consensus by identifying the most suitable EMU for each activity, (2) minimizing conflict by segregating the sectoral activities, and (3) minimizing the “banning cost” caused by precluding a sectoral activity in an EMU of high suitability.
The analysis centered on the four EMU in the Gulf of Ulloa of high suitability for both fishing and conservation (Figure 1): GU-03a (300 km2) and GU-03b (1,026 km2), inshore, GU-04 (2,380 km2), in between the others, and GU-05 (5,676 km2), offshore. We examined two AOMU options to ascertain the implications of the CET on the allocation of fishing and conservation. The selection of an option depended on whether κ exceeded the CET. The first option concerned the geographic separation of activities, that is, putting each EMU to the single-use to which it was most suited. It implied that κ did not exceed the CET. Operationally, it entailed using the output of SMCE to compute for each EMU the mean and the relative suitability (Bojórquez-Tapia et al., 2001), as well as the asymmetry index as a proxy of the banning cost (Bojórquez-Tapia et al., 2017).
The second option was alternating single-use, that is, rotating the use of an EMU from fishing to conservation. It implied that κexceeded the CET. Operationally, this option entailed the implementation of the analytic network process (ANP), a multicriteria model with feedback (Saaty, 2001), using the freeware Super Decisions v. 1.6.01. The ANP model consisted of two “clusters,” each containing as “elements” either activities or EMU. These elements were interconnected by feedback loops (Figure 3): the forward path entailed pairwise comparisons of zones concerning activities, whereas the backward path entailed pairwise comparisons of activities to zones. The pairwise comparisons were carried out using the “fundamental scale,” a 1–9 scale, where 1 represents equal importance and 9 indicates that the first criterion is extremely more important than the second one (see Appendix).
Figure 3. Multicriteria model with feedback implemented through ANP (analytical network process) to analyze a change in activity allocation depending on the level of incidental bycatch.
Results showed that mean abundance decreased with time for every scenario (Table 2). To provide an overall picture of the general behavior of the system, we examined in detailed the results for c′ = {1,2,3,4,5}and B0 = 25,000 (i.e., the mean initial abundance resulting for all scenarios). Likewise, mean severity intersected the CET (Bt = 18,750) before year 25 for c′ = {3,4,5}, before year 50 for c′ = 2, and at no time during the simulation for c′ = 1. Sensitivity analysis showed that unitary bycatch and duration of the fishing season were the most influential variables for the change in abundance.
Table 2. Results of exploratory modeling scenarios for the loggerhead population-incidental bycatch system in the Gulf of Ulloa, Mexico (Risk = probability of reaching the critical ecological threshold).
Dispersion plots of abundance against κ provided a direct clue of a potential critical transition of abundance across a catastrophic threshold (Figure 4). In general, the response severity tended to increase with simulation time and was intensified by low intrinsic population growth rates, r. It tended to cross the CET when r < 0.05 for c′ = {1,2,3}.
Figure 4. Dispersion plots of abundance against annual bycatch rate; (A) 25 years, (B) 50 years; (C) 75 years; (D) 100 years; dot size: r = {0.04, 0.07, 0.1}; color indicates c′: purple = 1, violet = 2, red = 3, orange = 4, yellow = 5; red dotted line: critical ecological threshold; black dots and lines indicate the mean and confidence intervals for c′ = {1,2,3,4,5}, respectively.
An examination of the coefficients of variation (CV) revealed the range in which abundance was distinctively sensitive to relatively small changes in κforcing, ct. In general, the dispersion of abundance increased with both c′ and simulation time. CV for c′ = 1 was six times lower than for c′ = 5; between years 25 and 100, CV ranged from 4 to 11% for c′ = 1, from 9 to 24% for c′ = 2, from 13 to 36% for c′ = 3, from 18 to 49% for c′ = 4, and from 23 to 62% for c′ = 5.
Regarding the κ, dispersion with time tended to be relatively small for c′ = {1,2}, but increased considerably for c′ = {3, 4, 5}: CV between years 25 and 100 ranged from 28 to 32% for c′ = {1,2}, from 23 to 43% for c′ = {3,4}, and from 21 to 52% for c′ = 5.
The ratio of the CV of abundance to the CV of ct provided a normalized measure of the system’s response to an alternative c′. Further analyses of the relative change of these ratios with incremental forcing showed that the system’s response peaked when c′ = 1 increased to c′ = 2 (230% on average; for increments involving 2 ≤ c′ ≤ 5, the relative change of ratios ranged from 105 to 169%). This result suggested a potential catastrophic threshold corresponding to ct≈ 200 individuals year–1 (Figure 4). This value separated the cases corresponding to c′ = 1 from the rest, which exceeded the unacceptable risk of low resilience (Table 2).
The suitability map for fishing included two attributes, depth and sea bottom type, which reflected both the habitat utilization of the California halibut and the characteristics of the fishing gear. Increasing suitability corresponded to depths from 0 to 40 m (being 20 m the best condition) and soft sea bottom. Mean suitability (Table 3) showed an inshore-offshore suitability gradient in which GU-03a and GU-03b were twice as suitable as GU-04 and GU-05.
Table 3. Mean suitability, relative suitability, and asymmetry index results for the environmental management units (EMU) in the Gulf of Ulloa.
The suitability map for conservation was based on the overlap of the areas of peak marine primary productivity and areas of a high probability of loggerheads’ aggregation (p≥0.75) depicted from Wingfield et al. (2011). Mean suitability (Table 3) showed that GU-04 was the most suitable EMU, followed by GU-03b (20% less suitable), GU-03a (30% less suitable), and GU-05 (50% less suitable).
Regarding the geographic separation, single-use option to minimize conflict, relative suitability showed that fishing should be allocated to GU-03a and GU-03b, and conservation to GU-04 and GU-05. Nevertheless, results of the asymmetry index revealed that such a geographic scheme would be unacceptable because of the high banning cost for conservation in both GU-03a and GU-03b. In contrast, the banning cost for fishing was 60% higher in GU-03a than in GU-03b. A comparison of the two activities per EMU, moreover, showed that the cost was slightly higher for fishing than for conservation in GU-03a and substantially higher for conservation than for fishing in GU-03b, GU-04, and GU-05 (Table 3).
Given the combination of high suitability and high banning costs led to the application of the alternating single-use option for GU-03a and GU-03b. Accordingly, the geographic separation option was considered as the base condition for the implementation of the ANP (Figure 3).
The responses to the question “Where is the suitability of an EMU more important for conservation (or fishing), GU-03a or GU-03b?” of the forward path considered the mean suitability of the EMU. It was judged that the suitability for conservation was “strongly more important” in GU-03b than in GU-03a and the suitability for fishing was “very strongly more important” GU-03a than in GU-03b.
The response to the question of the backward path “Which activity has a higher banning cost in GU-03a (or GU-03b), conservation or fishing?” considered whether κsurpassed the CET. For κbelow the CET, it was judged that the cost in GU-03a was “strongly more important” for fishing than for conservation, whereas that the cost in of GU-03b was “between equally to moderately more important” for conservation than for fishing. Results indicated that these EMU should be allocated to fishing (Table 4).
Table 4. Analytical Network Process’s results. Assessment of the relative impact of the banning costs from activity allocation in GU-03.
For κ above the CET, the judgment of the cost in GU-03a changed to “between equally to moderately more important” for fishing than for conservation, whereas in GU-03b to “moderately more important” for conservation than for fishing. Results indicated that these EMU should be allocated to conservation (Table 4).
In summary, results showed that the best course of action would be an alternating multiple-use depending on the observed bycatch rate (Table 4): With κ below the CET, fishing should be allocated to GU-03a without restrictions and to GU-03b with restrictions during the loggerhead aggregation period, as a precautionary measure; however, whenever the bycatch rate surpassed the CET, conservation should be allocated to, and fishing proscribed from the two EMU.
Our results shed light on the advantages of combining the area-oriented multiple-use framework and exploratory modeling to address the challenges of uncertainty in a contested policymaking context. From a practical point of view, it allowed the best use of the available knowledge and the consideration of the different positions. In particular, we have shown how our collaborative approach was useful to cope with Knightian uncertainty and allowed us to (1) determine which features of the real world should be considered (to address system boundaries uncertainty), (2) how the variables and relationships should be examined (to address conceptual uncertainty), and (3) what values should be used to portray the variables and parameters (to address input data uncertainty). The approach also highlighted the critical point of debate, which in this case was crafting regulations for fishing bycatch that were equivalent to those in the United States. Moreover, the definitions-axioms-theorems approach strengthened transparency according to the postulates of collaborative planning.
One key premise of our approach was that duly grounded and reasoned policymaking could be taken as objective only in a restrictive sense. In other words, the challenge was to convey meaningful information to the stakeholders. This implied that exploratory modeling needed to be appropriate in terms of what Kinoshita (2005) refers to as bounded or “procedural rationality” (conforming to the capacity of the stakeholders for processing information) and “expressive rationality” (conforming to their badges of identity). In this regard, the area-oriented multiple-use framework was particularly effective for conveying meaningful spatial information to the stakeholders in a way that enhanced the credibility and legitimacy of the policy results. Suitability analysis helped ensure that the allocation of the two activities in the Gulf of Ulloa was congruent with the specific requirements and preferences expressed by the stakeholders. Furthermore, GIS-MCDA identified procedural and expressive rationalities as a set of decision criteria corresponding to the intangible “projected-world” (Bojórquez-Tapia et al., 2011), which could be linked to concrete geographical attributes of the Gulf of Ulloa.
Methodologically, exploratory modeling was effective for establishing the quantitative metrics needed to ascertain the feasible policy alternatives within a context of deep and politically induced uncertainties. Rather than trying to obtain an account of “scientific truth” to adjudicate responsibilities regarding the cause of turtle mortality, we used exploratory modeling to elaborate explanations for the possible effects of bycatch upon the loggerhead population and justify the resulting policies in terms of the welfare of the fishermen-loggerhead population system as a whole.
Results highlight two important points concerning the unavoidable political dimensions of transdisciplinary inquiry and, by extension, of the exploratory modeling rationale. The first one has to do with one paradoxical reality: Collaboration relies on credible judgment and trust, which are often lacking in complex, problem-driven policymaking (Stein and Harper, 2003). The second point expands this line of argument and pertains to the effect of both deep and politically induced uncertainties in policymaking. Together, these two points help explain why, at the beginning of our intervention, we found a lack of trust amongst the stakeholders, which dwarfed any possibility of consensus about goals, desirable outcomes, and acceptable risks. Besides, the available scientific studies lacked legitimacy and were perceived by the stakeholders as self-serving notions of both the “real causes” and the “acceptable evidence” concerning loggerhead carcasses stranded ashore. This led to a state-of-affairs that exacerbated the effect of deep uncertainty and resulted in a policymaking impasse that no stakeholder had the power to shift. Under this circumstance, the use of exploratory modeling allowed us to re-focus the scope of the debate regarding loggerhead bycatch in the Gulf of Ulloa. This not only confirms previous claims about the capacity of exploratory modeling for achieving a systemic, nonbiased treatment of available information (Lempert, 2002; Singh et al., 2015), it also highlights the advantages of exploratory modeling for widening the scope of appraisals and enabling a sensible debate of the relevant issues and concerns, despite an initial gridlock in policymaking.
The implementation of Bunge’s (2004) definitions-axioms-theorems approach helped ensure the coherence and coreference of the model’s predicates, prevented the inclusion of irrelevant issues and concerns into the analyses, and made the assumptions, structure, and underlying logic self-explanatory. It was thus fundamental for simplifying the involvement of the stakeholders in the development of the logical structure of the exploratory model, and the justification of the resultant regulations. Concerning the early warning indicator, moreover, the simulated response of the loggerhead population to incremental bycatch rates conveyed the idea that the effect of the halibut fishery may not be gradual, predictable, and perhaps reversible. On the contrary, our results showed that small forcing (changes in the bycatch rate) could produce abrupt changes in abundance within a range in which the loggerhead population seemed to be very sensitive. Yet, the first reaction of the stakeholders to the notion of a potential catastrophic threshold corresponding to ≈ 200 individuals year–1 was a combination of skepticism and criticism, although for different reasons. While the representatives of conservation advocacy groups maintained that the CET would be insufficient to protect the loggerhead population, the representatives of the fishing cooperatives complained that our research overestimated the effect of bycatch mortality. Nevertheless, we demonstrated that the CET was 20–40 times lower than the bycatch-induced mortality reported in the literature (40 ≤ c′ ≤ 80) (see Appendix). Therefore, it seemed illogical for them to oppose a regulation based upon a comparatively very low unitary bycatch rate (c′ = 2). Regarding the views of the halibut fishery, the CET matched their own estimate under current conditions (c′ = 2) at which existing levels of fishing effort would not be affected.
Our results corroborate the assertion that designing a model in the absence of an analytical strategy, the designers leave themselves open to an unending process of adding ever more detail. This is not to say that the stakeholders readily accepted our final results. They were indeed very surprised and bewildered when confronted with the results for the first time. Nevertheless, they adopted a more sensible attitude later as they understood the implications better. Our approach enabled a structured interpretation of the stakeholders’ positions, which was particularly useful for exposing inconsistencies in the bycatch-induced mortality estimates. Both ERA and AOMU provided a means for achieving coherence in policymaking despite the opposing systems of beliefs of the stakeholders.
We have shown how exploratory modeling can support pragmatic-collaborative policymaking, as proposed by Harper and Stein (2006). Both the ERA and AOMU provided an analytical platform that was rigorous, transparent, and open to debate. Our goal was to construe a robust and “satisficing” (sensu Simon, 1997) bycatch policy. Exploratory modeling provided a sound means for ascertaining the reliability of the stakeholders’ judgments in a way that considered their respective systems of belief. In the end, the resulting adaptive (Walters, 1986) geospatial scheme for the activities’ allocation was optimal and evenhanded. It provided the required justification for attaining consensus on broadly acceptable and implementable policy, following the normative tenets of Habermasian collaboration.
We acknowledge that collaborative planning presupposes a capacity for addressing the dynamic, uncertain, and politicized circumstances faced by authorities, scientists, and stakeholders. Accordingly, it is important to highlight that the implementation of our approach required a “safe space” (Emerson et al., 2012; Clark et al., 2016) within which different views regarding the conflict could be critically and rationally debated. In our case, the MSP-NPMR provided the conditions needed to develop the collaborative scheme required to tackle the highly political and contested problem fishing bycatch of loggerheads sea turtle in the Gulf of Ulloa. Besides, the versatility of our research team proved to be essential for translating and communicating complex model inputs and outputs back to the stakeholders. The research team effectively handled the technical specifications and theoretical content in each disciplinary domain into common language, and geospatial representation. In this regard, we argue that exploratory modeling played a central role in supporting an open debate, undistorted by imbalances of power and knowledge, and thus allowed us to fulfill the central tenant of Habermasian collaboration. While we concur that integrating diverse forms of knowledge with inherently differential power associations is one major challenge of transdisciplinary inquiry (MacMynowski, 2007; Godemann, 2008; Hoffmann et al., 2017), our results show how exploratory modeling can be used to constructively confront the relationship between power and knowledge. Initially, CONAPESCA and SEMARNAT exercised power through their respective positions regarding the relevance of knowledge and research on characterizing loggerhead bycatch as a policy issue. They accepted disciplinary scientific knowledge if it suited their predefined accounts of “the issue” and rejected any other alternative account of loggerhead mortality.
The policymaking outcome of our inquiry was the response that Mexico presented to the United States. The results of our analysis were used to formulate regulations aimed concerning a bycatch cap and a temporary refuge area (Figure 1B) to protect the loggerhead population in the region. CONAPESCA established a zoning scheme with restrictions on the use of fishing gears and other additional measures to reduce the possible interaction of the fishing activities and the loggerhead turtles in the Gulf of Ulloa. Based on the precautionary criterion of the Code of Conduct for Responsible Fisheries (Food and Agriculture Organization of the United Nations, 2011), CONAPESCA established a lower limit on turtle mortality and decided to ban the use of gillnets for the rest of the loggerhead’s aggregation period, if the incidental bycatch reaches 90 individuals year–1.
In August 2015, NMFS determined that Mexico should receive a negative PLMR certification. This decision was justified in that, although Mexico had established the fishing refuge, it failed to account for how enforcement of the bycatch cap would be implemented outside the fishing refuge (National Oceanic and Atmospheric Administration Fisheries, 2015). CONAPESCA then expanded the fishing restriction area to cover all important and contested spaces in the Gulf of Ulloa (Figure 1C). After reviewing these new measures, NMFS concluded that CONAPESCA had considered the conditions that could bear on the feasibility and efficacy of the bycatch cap and, therefore, that the proposed measures were comparable in effectiveness to that of the United States. In 2016, Mexico received a positive certification (National Oceanic and Atmospheric Administration Fisheries, 2016).
It is worth mentioning that the CET has been used to resolve other sustainability problems. On April 16, 2016, SEMARNAT issued a “resolvent negative” of the environmental impact statement (EIS) of a seafloor mining project to be undertaken in the Gulf of Ulloa (Figure 1D). By analyzing the bycatch mortality rate in isolation from other causes of mortality (considered in the intrinsic growth rate r), the definition of the CET extends to human activities in the Gulf of Ulloa other than fishing. That is, mortality rates above 200 individuals year–1 should not be exceeded by any human-induced mortality factor. Accordingly, the legal argument rested on the need for knowing the possible organism-level sublethal effects of the mining project. SEMARNAT concluded that the EIS failed to address how the project would avoid those effects, including behavioral change and physiological costs, in addition to possible direct disturbance of the loggerhead’s nurturing habitat.
We conclude that, despite of the deep and politically induced uncertainties that had previously led to an impasse in policymaking, our modeling rationale effectively addressed the lack of legitimacy and credibility of the two contending positions. Exploratory modeling indeed enabled us to maintain an agnostic stance regarding the positions in conflict and to provide quantitative metrics of the potential effect of bycatch on the loggerhead population, as well as an spatial pattern that maximized consensus and minimized conflict amongst the stakeholders. Legitimacy was attained by openly examining the relative priority of protecting the juvenile population and its nursery habitat versus the socioeconomic concerns of the small-scale fishing, whereas credibility was achieved through a rigorous, transparent, and systematic quantitative decision analysis framework of the potential effect of bycatch on the loggerhead population.
The original contributions presented in the study are included in the article/supplementary material, further inquiries can be directed to the corresponding author/s.
LB-T, GP-D, and DP-P researched design and performed research. LB-T, GP-D, DP-P, AD-D-L, and FA-S analyzed the data. LB-T wrote the manuscript. DP-P, GP-D, AD-D-L, and FA-S reviewed and approved the submitted manuscript. All authors contributed to the article and approved the submitted version.
This research was conducted as part of the Ecological Ordinance of the North Pacific Marine Region with the financial support of SEMARNAT (DGRMIS-DAC-DGPAIRS No. 020/2011).
AD-D-L is President of ICES Consulting.
The remaining authors declare that the research was conducted in the absence of any commercial or financial relationships that could be construed as a potential conflict of interest.
GP-D thanks to EDI and COFAA-IPN.
Acevedo, M. F. (2012). Simulation of Ecological and Environmental Models. Boca Raton, FL: CRC Press.
Arreguín-Sánchez, F. (1996). Catchability: a key parameter for fish stock assessment. Rev. Fish Biol. Fish. 6, 221–242.
Ascough, J. C., Maier, H. R., Ravalico, J. K., and Strudley, M. W. (2008). Future research challenges for incorporation of uncertainty in environmental and ecological decision-making. Ecol. Model. 219, 383–399. doi: 10.1016/j.ecolmodel.2008.07.015
Balart, E. (1996a). “Pesquería de lenguados,” in Estudio del Potencial Pesquero y Acuícola de Baja California Sur, eds M. Casas Valdez and Y. G. Ponce Díaz (Rome: Organización de las Naciones Unidas para la Agricultura y la Alimentación), 273–285.
Balart, E. (1996b). “Recurso langostilla,” in Estudio del Potencial Pesquero y Acuícola de Baja California Sur, eds M. Casas Valdez and Y G. Ponce Díaz (Rome: Organización de las Naciones Unidas para la Agricultura y la Alimentación).
Balint, P. J., Stewart, R. E., and Desai, A. (2011). Wicked Environmental Problems: Managing Uncertainty and Conflict. Washington, DC: Island Press.
Bankes, S. C. (2002). Tools and techniques for developing policies for complex and uncertain systems. Proc. Natl. Acad. Sci.U.S.A. 99 (suppl 3), 7263–7266.
Bojórquez-Tapia, L. A., Díaz-Mondragón, S., and Ezcurra, E. (2001). GIS-based approach for participatory decision making and land suitability assessment. Int. J. Geogr. Inf. Sci. 15, 129–151. doi: 10.1080/13658810010005534
Bojórquez-Tapia, L. A., Luna-González, L., Cruz-Bello, G. M., Gómez-Priego, P., Juárez-Marusich, L., and Rosas-Pérez, I. (2011). Regional environmental assessment for multiagency policy making: implementing an environmental ontology through GIS-MCDA. Environ. Plann. B Plann. Des. 38, 539–563. doi: 10.1068/b36129
Bojórquez-Tapia, L. A., Pedroza, D., Ponce-Díaz, G., Díaz-de-León, A. J., and Lluch-Belda, D. (2017). A continual engagement framework to tackle wicked problems: curtailing loggerhead sea turtle fishing bycatch in Gulf of Ulloa, Mexico. Sustain. Sci. 12, 535–548. doi: 10.1007/s11625-016-0405-1
Brooks, K. N., Ffolliott, P. F., Gregersen, H. M., and Thames, J. (1991). Hydrology and the Management of Watersheds, 4edn Edn. Ames, IA: Iowa State University Press.
Bunge, M. (2004). How does it work? The search for explanatory mechanisms. Philos. Soc. Sci. 34, 182–210.
Burgman, M. (2005). Risks and Decisions for Conservation and Environmental Management. Cambridge: NY: Cambridge University Press.
Carey, J. M., and Burgman, M. A. (2008). Linguistic uncertainty in qualitative risk analysis and how to minimize it. Annals of the New York Academy of Sciences. 1128, 13–17.
Clark, W. C., van Kerkhoff, L., Lebel, L., and Gallopin, G. C. (2016). Crafting usable knowledge for sustainable development. Proc. Natl. Acad. Sci.U.S.A. 113, 4570–4578. doi: 10.1073/pnas.1601266113
Conant, T. A., Dutton, P. H., Eguchi, T., Epperly, S. P., Fahy, C. C., Godfrey, M. H., et al. (2009). Loggerhead Sea turtle (Caretta caretta) 2009 Status Review Under the U.S. Endangered Species Act. Report of the Biological Review Team to the National Marine Fisheries Service. Silver Spring, MD: National Marine Fisheries Service, 222.
Dakos, V., Carpenter, S. R., van Nes, E. H., and Scheffer, M. (2015). Resilience indicators: prospects and limitations for early warnings of regime shifts. Philos. Trans. R. Soc. B Biol. Sci. 370:20130263. doi: 10.1098/rstb.2013.0263
Díaz-de-León, A. J., and Díaz-Mondragón, S. (2013). “Marine Spatial Planning and LMEs in Mexico,” in 2013. Stress, Sustainability, and Development of Large Marine Ecosystems During Climate Change: Policy and Implementation: Large Marine Ecosystems, 1st Edn, Vol. 18, ed. K. Sherman (New York, NY: UNDP).
Emerson, K., Nabatchi, T., and Balogh, S. (2012). An integrative framework for collaborative governance. J. public Adm. Res. Theory 22, 1–29. doi: 10.1093/jopart/mur011
Food and Agriculture Organization of the United Nations (2011). Code of Conduct for Responsible Fisheries. Rome: Food and Agriculture Organization of the United Nations.
Gilman, E., Kobayashi, D., Swenarton, T., Brothers, N., Dalzell, P., and Kinan-Kelly, I. (2007). Reducing sea turtle interactions in the Hawaii-based longline swordfish fishery. Biol. Conserv. 139, 19–28. doi: 10.1016/j.biocon.2007.06.002
Godemann, J. (2008). Knowledge integration: a key challenge for transdisciplinary cooperation. Environ. Educ. Res. 14, 625–641. doi: 10.1080/13504620802469188
González-Rodríguez, E., Trasviña-Castro, A., Gaxiola-Castro, G., Zamudio, L., and Cervantes-Duarte, R. (2012). Net primary productivity, upwelling and coastal currents in the Gulf of Ulloa, Baja California, México. Ocean Sci. 8, 703–711. doi: 10.5194/osd-8-1979-2011
Harper, T. L., and Stein, S. M. (2006). Dialogical Planning in a Fragmented Society: Critically Liberal, Pragmatic, Incremental. New Jersey, NJ: Center for Urban Policy Research, Rutgers, The State University of New Jersey.
Hilborn, R., and Walters, C. J. (1992). Quantitative Fisheries Stock Assessment: Choice, Dynamics, and Uncertainty. California, CA: Chapman & Hall, Inc.
Hoffmann, S., Pohl, C., and Hering, J. G. (2017). Exploring transdisciplinary integration within a large research program: Empirical lessons from four thematic synthesis processes. Res. Policy. 46, 678–692. doi: 10.1016/j.respol.2017.01.004
Jul-Larsen, E., Kolding, J., Overa, R., Nielsen, J. R., and van Zwieten, P. A. M. (eds) (2003). Management, co-management, or no management? Major Dilemmas in Southern African Freshwater Fisheries. Case Studies. Fisheries Technical Paper No. 426/2. Rome: Food and Agriculture Organization of the United Nations.
Kinoshita, E. (2005). “Why we need AHP/ANP instead of utility theory in today’s complex World-AHP from the perspective of bounded rationality,” in Proceedings of the 8th International Symposium on the Analytic Hierarchy Process, Hawaii, 1–9.
Krupnick, A., Morgenstern, R., Batz, M., Nelson, P., Burtraw, D., Shih, J. S., et al. (2006). Not a Sure Thing: Making Regulatory Choices Under Uncertainty. Washington, DC: Resources for the Future.
Kwakkel, J. H., and Pruyt, E. (2013). Exploratory modeling and analysis, an approach for model-based foresight under deep uncertainty. Technol. Forecast. Soc. Change 80, 419–431. doi: 10.1016/j.techfore.2012.10.005
Kwakkel, J. H., Walker, W. E., and Haasnoot, M. (2016). Coping with the wickedness of public policy problems: approaches for decision making under deep uncertainty. J. Water Res. Plann. Manag. 142:01816001. doi: 10.1061/(ASCE)WR.1943-5452.0000626
Kwakkel, J. H., Walker, W. E., and Marchau, V. A. W. J. (2010). Classifying and communicating uncertainties in model-based policy analysis. Int. J. Technol. Policy Manag. 10:299. doi: 10.1504/IJTPM.2010.036918
Lane, D. A., and Maxfield, R. R. (2005). Ontological uncertainty and innovation. J. Evol. Econ. 15, 3–50. doi: 10.1007/s00191-004-0227-7
Lawrence, R. J. (2015). Advances in transdisciplinarity: Epistemologies, methodologies, and processes. Futures 65, 1–9. doi: 10.1016/j.futures.2014.11.007
Lempert, R. J. (2002). A new decision science for complex systems. Proc. Natl. Acad. Sci.U.S.A. 99(Suppl. 3), 7309–7313. doi: 10.1073/pnas.082081699
Lewison, R. L., Freeman, S. A., and Crowder, L. B. (2004). Quantifying the effects of fisheries on threatened species: The impact of pelagic longlines on loggerhead and leatherback sea turtles. Ecol. Lett. 7, 221–231. doi: 10.1111/j.1461-0248.2004.00573.x
Lluch-Belda, D., Elorduy-Garay, J., Lluch-Cota, S., and Ponce-Díaz, G. (eds) (2000). BAC. Centros de Actividad Biológica Del Pacífico Mexicano. Mexicano: CIBNOR-CICIMAR-CONACyT.
MacMynowski, D. (2007). Pausing at the brink of interdisciplinarity: power and knowledge at the meeting of social and biophysical science. Ecol. Soc. 12:20.
Malczewski, J., and Rinner, C. (2015). Multicriteria Decision Analysis in Geographic Information Science (Advances in Geographic Information Science). Berlin: Springer.
National Oceanic and Atmospheric Administration Fisheries (2013). Improving International Fisheries Management: Report to Congress Pursuant to Section 403(a) of the Magnuson-Stevens Fishery Conservation and Management Reauthorization Act of 2006. Washington, D.C: U.S. Department of Commerce.
National Oceanic and Atmospheric Administration Fisheries (2015). Addendum to the Biennial Report to Congress Pursuant to Section 403(a) of the Magnuson-Stevens Fishery Conservation and Management Reauthorization Act of 2006. Certification determination for Mexico’s 2013 identification for bycatch of north pacific loggerhead sea turtles. Washington, D.C: U.S. Department of Commerce.
National Oceanic and Atmospheric Administration Fisheries (2016). Addendum to the Biennial Report to Congress Pursuant to Section 403(a) of the Magnuson-Stevens Fishery Conservation and Management. Reauthorization Act of 2006. Positive certification determination for Mexico’s 2013 identification for bycatch of north pacific loggerhead sea turtles. Washington, D.C: U.S. Department of Commerce.
Nichols, W. J., Resendiz, A., Seminoff, J. A., and Resendiz, B. (2000). Transpacific migration of a loggerhead turtle monitored by satellite telemetry. Bull. Mar. Sci. 67, 937–947.
Peckham, S. H., Maldonado-Diaz, D., Koch, V., Mancini, A., Gaos, A., Tinker, M. T., et al. (2008). High mortality of loggerhead turtles due to bycatch, human consumption, and strandings at Baja California Sur, Mexico, 2003 to 2007. Endanger. Species Res. 5, 171–183. doi: 10.3354/esr00123
Pedroza Páez, D., Bojórquez-Tapia, L. A., Delgado, G. C., and Lazos, E. (2020). Understanding translation: Co-production of knowledge in marine spatial planning. Ocean Coast. Manag. 190:105163. doi: 10.1016/j.ocecoaman.2020.105163
Porporato, E., Pastres, R., and Brigolin, D. (2020). Site suitability for finfish marine aquaculture in the central Mediterranean Sea. Front. Mar. Sci. 6:772. doi: 10.3389/fmars.2019.00772
Regan, H. M., Colyvan, M., and Burgman, M. A. (2002). A taxonomy and treatment of uncertainty for ecology and conservation biology. Ecol. Appl. 12, 618–628.
Ridd, M. K. (1965). Area-Oriented Multiple-Use Analysis. USDA Forest Service, Research Paper INT-21. Boise, ID: USDA Forest Service, 14.
Saaty, T. (2001). Decision Making With Dependence and Feedback: The Analytic Network Process. Pittsburgh, PA: RWS Publications.
Schaefer, M. B. (1954). Some aspects of the dynamics of populations important to the management of commercial marine fisheries. Int. Am. Tropical Tuna Commission Bull. 1, 23–56.
Scheffer, M., Bascompte, J., Brock, W. A., Brovkin, V., Carpenter, S. R., Dakos, V., et al. (2009). Early-warning signals for critical transitions. Nature 461, 53–59. doi: 10.1038/nature08227
Seminoff, J. A., Eguchi, T., Carretta, J., Allen, C. D., Prosperi, D., Rangel, R., et al. (2014). Loggerhead sea turtle abundance at a foraging hotspot in the eastern Pacific Ocean: implications for at-sea conservation. Endanger. Species Res. 24, 207–220. doi: 10.3354/esr00601
Seminoff, J. A., Peckham, H., Eguchi, T., Sarti-Martinez, A. L., Rangel, R., Forney, K., et al. (2006). “Loggerhead turtle density and abundance along the Pacific coast of the Baja California Peninsula, Mexico, determined through aerial surveys: a preliminary assessment,” in Book of Abstracts, 26th Annual Symposium on Sea Turtle Biology and Conservation, eds M. Frick, A. Pnagopoulou, A. F. Rees, and K. Williams (Athens: International Sea Turtle Society), 321.
Simon, H. A. (1997). Models of Bounded Rationality: Empirically Grounded Economic Reason, Vol. III. Cambridge, MA: MIT Press.
Singh, R., Reed, P. M., and Keller, K. (2015). Many-objective robust decision making for managing an ecosystem with a deeply uncertain threshold response. Ecol. Soc. 20, 1–32. doi: 10.5751/ES-07687-200312
Stein, S. M., and Harper, T. L. (2003). Power, trust, and planning. J. Plann. Educ. Res. 23, 125–139. doi: 10.1177/0739456X03258636
Walker, W. E., Harremoës, P., Rotmans, J., Van Der Sluijs, J. P., Van Asselt, M. B. A., and Janssen, P. (2003). Defining uncertainty. a conceptual basis for uncertainty management in model-based decision support. Integr. Assess. 4, 1–13. doi: 10.1076/iaij.4.1.5.16466
Walters, C. J. (1986). Adaptive Management of Renewable Resources. New York, NY: Macmillan Publishers Ltd.
Wingfield, D. K., Peckham, S. H., Foley, D. G., Palacios, D. M., Lavaniegos, B. E., Durazo, R., et al. (2011). The making of a productivity hotspot in the coastal ocean. PloS One 6:e27874. doi: 10.1371/journal.pone.0027874
The ANP is a generalized model of Saaty’s (2001) analytic hierarchy process (AHP). The ANP extends the AHP by replacing hierarchies with networks, thus incorporating dependence and feedbacks among decision criteria and alternatives. Similar to the AHP, an ANP is based on pairwise comparisons to derive ratio scale measurements of the importance of one criterion to another.
Once all the pertinent pairwise comparisons are completed, the results are analyzed mathematically to generate the importance weights for all elements in the network. A pairwise comparison matrix is generated to rate the relative preferences. The pairwise comparison matrices are reciprocal, so for matrix A = [aij], each entry aij = 1/aij and thus the number of necessary pairwise comparisons is n(n−1)/2. The measurement scale of the comparisons involves the following numerical rating and corresponding verbal judgment of importance: 1 = equally; 3 = moderately; 5 = strongly; 7 = very strongly; and 9 = extremely strongly (odd values represent intermediate degrees of importance).
Mathematically, the set of Nclusters that form an ANP network is represented as N = {Ca,Cb,Cc,…Cn}. Each cluster is composed of a set of elements, or Ci = {ei1,ei2,…,eini}. Every cluster, Ci, can have a linkage on itself or some or any other components, so L = {{Ca,Ca},{Ca,Cb},{Ca,Cc},…{Cn,Cn}} represents the set of all possible linkages or pairwise comparisons between clusters. Linkages within clusters (e.g., {Ca,Ca},…{Cb,Cb}, etc.), is known as “inner dependence,” and between clusters (e.g., {Ca,Cb}, {Cb,Cc}, etc.) is known as “outer dependence. The ANP is solved through a supermatrix approach. This involves arranging the set of clusters, N, as the block matrices, Wij, of supermatrix W:
The elements that make up the columns of each Wij are the eigenvector solutions within the cluster and thus each of this column sums to one (if there are no linkages between elements of two clusters Wij = 0). In the supermatrix, however, each column may include several block matrices and thus the sum of the column is higher than one. In such cases, the supermatrix must be normalized and synthesized to account for the overall component’s influence by column. This process makes the supermatrix column stochastic.
The final priority weights, which account for element interactions, are derived by multiplying the supermatrix by itself until the columns stabilize, which occurs when the supermatrix entries become identical across each row or cycles in blocks in which case one uses what is known as Cesary summability and the result is known as the limiting matrix. The final priority weights are extracted from this limiting matrix, .
Keywords: uncertainty, fishery interactions, GIS-MCDA, marine spatial planning, transdisciplinary inquiry, ecological risk, area-oriented multiple-use
Citation: Bojórquez-Tapia LA, Ponce-Díaz G, Pedroza-Páez D, Díaz-de-León AJ and Arreguín-Sánchez F (2021) Application of Exploratory Modeling in Support of Transdisciplinary Inquiry: Regulation of Fishing Bycatch of Loggerhead Sea Turtles in Gulf of Ulloa, Mexico. Front. Mar. Sci. 8:643347. doi: 10.3389/fmars.2021.643347
Received: 17 December 2020; Accepted: 10 March 2021;
Published: 06 April 2021.
Edited by:
Nancy Louise Shackell, Bedford Institute of Oceanography (BIO), CanadaReviewed by:
Jeffrey Aleksandr Seminoff, Southwest Fisheries Science Center (NOAA), United StatesCopyright © 2021 Bojórquez-Tapia, Ponce-Díaz, Pedroza-Páez, Díaz-de-León and Arreguín-Sánchez. This is an open-access article distributed under the terms of the Creative Commons Attribution License (CC BY). The use, distribution or reproduction in other forums is permitted, provided the original author(s) and the copyright owner(s) are credited and that the original publication in this journal is cited, in accordance with accepted academic practice. No use, distribution or reproduction is permitted which does not comply with these terms.
*Correspondence: Luis A. Bojórquez-Tapia, Ym9qb3JxdWV6QGVjb2xvZ2lhLnVuYW0ubXg=
Disclaimer: All claims expressed in this article are solely those of the authors and do not necessarily represent those of their affiliated organizations, or those of the publisher, the editors and the reviewers. Any product that may be evaluated in this article or claim that may be made by its manufacturer is not guaranteed or endorsed by the publisher.
Research integrity at Frontiers
Learn more about the work of our research integrity team to safeguard the quality of each article we publish.