- 1MaREI Centre, Environmental Research Institute, University College Cork, Cork, Ireland
- 2School of Biological, Earth, and Environmental Sciences, University College Cork, Cork, Ireland
- 3Department of Mathematics and Statistics, Washington State University, Vancouver, WA, United States
- 4Marine Institute, Foras na Mara, Oranmore, Ireland
- 5Centre for Ecology and Conservation, University of Exeter, Penryn, United Kingdom
- 6School of Biological Sciences, University of Aberdeen, King’s College, Aberdeen, United Kingdom
- 7School of Biology, University of Leeds, Leeds, United Kingdom
- 8Lyell Centre, Heriot-Watt University, Edinburgh, United Kingdom
Sex differences in diet and foraging behaviour are common in sexually dimorphic species, often driven by differences in the cost of locomotion or ability to exploit different ecological niches. However, sex-specific foraging strategies also occur in monomorphic or slightly dimorphic species where the drivers are poorly understood. Here, we study sex differences in foraging of northern gannets (Morus bassanus), where females are only slightly heavier than males. Using concurrently tracked gannets (298 full foraging trips from 81 individuals) and fishing vessels across 5 years, we quantify individual-based vessel-associated putative foraging, and relate this to discard consumption. We found a significant positive relationship between time spent in vessel-associated foraging and discard consumption for both sexes. However, while females showed greater proportions of vessel-associated foraging than males, discarded fish contributed less to the diet of females in all years. These results contrast with previous suggestions that female gannets interact with vessels less often than males, and are consistent with competitive exclusion of females from trawler-associated discards. Our findings give insight into sexual differences in foraging behaviour in the absence of dimorphism that are necessary to predict their response to environmental and anthropogenic changes.
Introduction
Understanding trophic decisions in marine predators is necessary to predict their response to environmental and anthropogenic changes that alter prey availability and composition (Tait et al., 2014; Hays et al., 2016). Yet, these decisions vary substantially across species (e.g., Church et al., 2019) and sexes (e.g., Navarro et al., 2010). In general, sex-specific foraging strategies emerge in size-dimorphic species (e.g., Wearmouth and Sims, 2008; Gianuca et al., 2019). However, this variation also occurs in monomorphic species (Peck and Congdon, 2006). A number of factors contribute to sex differences in foraging, including differing nutritional requirements, parental care, sensitivity to offspring condition, and differences in foraging efficiency (Kato et al., 2000; Gray and Hamer, 2001; Lewis et al., 2002; Quillfeldt et al., 2004; Navarro et al., 2010; Stauss et al., 2012; Tait et al., 2014). When intersexual competition is present, competitive exclusion may arise and, in some groups, this is overcome by the differentiation of trophic niches between the sexes (Lewis et al., 2002; Peck and Congdon, 2006; Elliott et al., 2010).
Human activities can create opportunities for trophic and spatial differentiation within wildlife populations (Ellis, 2015; Robertson et al., 2015). In the marine environment, fisheries discards are one of the major human activities shaping marine species’ behaviour and conservation (Oro et al., 2013). Seabirds are important consumers of fisheries waste, and arguably the best-studied marine scavengers (Hudson and Furness, 1989; Furness, 2003; Oro et al., 2013). Discards are an important food source for many seabirds around the world (i.e., 52% of seabird species exploit discards to different degrees) in the form of undersized fish, offal or non-commercial species (Furness, 2003; Oro et al., 2013). For example, in the North Sea, fisheries discards support around three million seabirds (Sherley et al., 2020), enabling access to prey species otherwise beyond the diving ability of many species (Furness et al., 1988; Navarro et al., 2009). Changes in discard availability affect several ecological processes and trophic levels, and thus can have effects that propagate through the whole ecosystem (Votier et al., 2004; Oro et al., 2013). For scavenging seabirds, discard availability can alter their movement patterns (Bartumeus et al., 2010; Bodey et al., 2014, 2018), as well as population dynamics (Oro et al., 2004; Louzao et al., 2006), community ecology (Votier et al., 2004; Almaraz and Oro, 2011; Wagner and Boersma, 2011), breeding biology (Oro et al., 1996), and foraging behaviour (Navarro et al., 2010; Votier et al., 2010). Recent reform of the European Union (EU) Common Fisheries Policy include a landings obligation (or discard ban) for all regulated species in EU waters (Article 15, EU Regulation 1380/2013). The intention is to enforce the landing of unwanted catch, promoting economically and environmentally sustainable fisheries (Bicknell et al., 2013). The policy was implemented in a phased approach with full implementation across all fleets in January 2019. At the ecosystem level, simulation studies have predicted only limited or slight recovery of ecosystem structure (Pennino et al., 2019), making its implementation controversial due to socio-economic implications (Borges, 2015; Villasante et al., 2016).
The northern gannet (Morus bassanus), hereafter gannet, is a piscivorous predator that feeds on a wide range of fish including both pelagic and benthopelagic species with high caloric content, as well as demersal prey discarded by fishing boats (Montevecchi, 2007; Garthe et al., 2011; Votier et al., 2013). The consumption of discards can potentially reduce adult body condition as a result of increased foraging effort for less profitable food (Le Bot et al., 2019), as well as reducing reproductive success (Grémillet et al., 2008; Le Bot et al., 2019). As analytical techniques advance and more data become available, there is a need to investigate how species such as gannets interact with fisheries and exploit discards in order to understand population-level responses to changes in fisheries policy. Gannets show a wide variety of individual behaviours (Votier et al., 2010; Patrick et al., 2014; Bodey et al., 2018), and some sexual, individual, and colony variation in discard consumption has also been reported (Votier et al., 2013; Clark et al., 2019, 2021; Le Bot et al., 2019). Female gannets are slightly heavier than males, but there are no significant differences in the length of the tarsus, bill, or wing between sexes, yet female gannets are reported to undertake longer foraging trips, dive to deeper depths, and consume fewer fisheries discards than males (Stauss et al., 2012; Cleasby et al., 2015), although this varies among years (Clark et al., 2021).
The combination of stable isotope analysis (SIA), GPS tracking data and vessel monitoring system (VMS) data is of great use for understanding the at-sea-behaviour of seabirds in response to fishing activity (Votier et al., 2010; Bodey et al., 2014). Simultaneous tracking of fishing vessels and seabirds, combined with new approaches for identifying periods when animals are actively following moving attractors (Pirotta et al., 2018), allow us to move towards a finer decomposition of tracks, including vessel attraction and vessel-associated foraging behaviour. Here, we link gannet foraging behaviour inferred from tracking data and diet estimated from SIA of blood samples, with concurrently tracked fishing vessels to: (1) determine whether there is a positive relationship between vessel-associated foraging and the amount of discards consumed; and (2) quantify inter-annual and sex-based differences in discard use. Understanding seabird-vessel interactions and any sex-based differences in foraging strategy is important for predicting impacts on populations following changes in fisheries policy and practices.
Materials and Methods
All capture, tagging and sampling procedures underwent ethical approval and were conducted under the relevant regional permits issued by the British Trust for Ornithology (BTO), National Parks and Wildlife Service (NPWS), and Health Products Regulatory Authority (HPRA).
Gannet Capture and Handling
Gannets breeding on Great Saltee Island, Co. Wexford, Ireland (52.10933°N, 6.62213°W) were caught using a metal crook or snare fitted to a 10-m pole whilst attending 3–9 week-old chicks in July 2010, 2011, 2017, 2018, and 2019. Birds were weighed (±0.1 kg) and ringed with a BTO metal leg ring and uniquely numbered plastic ring for identification, before being fitted with a GPS logger. GPS devices [iGot-U GT-200 (2010-2011) or GT-120 (2017-2019), MobileAction®, Taipei, Taiwan] were temporarily attached to the tail feathers of breeding gannets using tape (Tesa® 4651, Hamburg, Germany) in 2010 (n = 19), 2011 (n = 16), 2017 (n = 11), 2018 (n = 21), and 2019 (n = 14). Deployment weight represented approximately 0.7% of average adult body weight (range: 0.57–0.80%). The devices were set to record one position every 2 min (2010–2011 data) or 3 min (2017–2019 data). GPS-equipped birds were recaptured after 4–13 days, and location data were downloaded using the manufacturer’s software. A small volume (<2 ml) of blood was sampled from the tarsal vein for SIA using non-heparinised needles, syringes and Eppendorf tubes, and 2–3 breast feathers were plucked for genetic sexing prior to release. In 2010 and 2011, an additional blood sample was taken during tag recovery to assess consistency. Handling time was typically less than 10 min and all birds flew off strongly following release, with no negative behavioural effects observed. Work within the colony was conducted during day trips, with time ashore generally limited to 11 am–3 pm.
Predator and Prey Isotopic Sample Collection
Gannet blood samples were separated, generally within 4–5 h, into red blood cells (RBC) and plasma using a centrifuge (10,000 rpm for 10 min), and stored frozen at −20°C until laboratory analysis. We used RBC for subsequent analyses, because plasma was not available for all years due to small sample volumes taken from some individuals (see section “Consistency in Stable Isotopes Values”). Lipids were removed using sequential extractions with chloroform:methanol solution (2:1), because high lipid concentration can skew isotopic values by decreasing the 13C content (DeNiro and Epstein, 1978; Bearhop et al., 2000).
Prey stable isotope values were obtained from a published dataset of Celtic Sea fish samples collected during 2010 (Jennings and Cogan, 2015). These samples did not undergo lipid extraction, and, as such, samples with high lipid content may affect the δ13C values (Post et al., 2007; Skinner et al., 2016). Samples with high lipid content (>5%) were thus identified by a C:N ratio higher than 3.5, and δ13C values were corrected following the mathematical correction proposed by Logan et al. (2008). A reduced prey dataset was used in the mixing models, as recommended by Phillips et al. (2014), including only those prey detected in the diet of Great Saltees gannets (Lewis et al., 2003) with more than 3% of occurrence. These species consist of three pelagic species (Atlantic herring Clupea harengus, European sprat Sprattus sprattus, and Atlantic mackerel Scomber scombrus), one benthopelagic species (Lesser sandeel Ammodytes marinus), two species from the Gadidae family (Whiting Merlangius merlangus and Norway Pout Trisopterus esmarkii) and four demersal species (European plaice Pleuronectes platessa, European hake Merluccius merluccius, Red gurnard Chelidonichthys cuculus, and Dragonet Callionymus lyra).
Stable Isotope Analysis
Gannet blood samples were dried at 60°C for 24 h and homogenised using mortar and pestle. A subsample of approximately 1 mg was weighed into a tin cup. SIA was performed using a continuous-flow isotope ratio mass spectrometry using a Costech ECS 4010 Elemental Analyser linked to a Thermo Electron Delta Plus XP Mass Spectrometer for 2010 and 2011 samples. 2017–2019 samples were analysed at Elemtex (Stable Isotope and & Elemental Analysis Expertise) in Cornwall, United Kingdom, using a Thermoquest EA1110 Elemental Analyser linked to a Sercon 2020 stable isotope ratio mass spectrometer (IRMS) running in continuous flow mode. Stable isotope ratios were expressed using a conventional notation as δ values, defined as parts per thousand (‰). Internal standards were routinely calibrated against International Atomic Energy Agency and National Institute of Standards and Technology stable isotope reference materials. A two-way ANOVA was used to detect the effect of year and sex on stable isotope values.
Consistency in Stable Isotopes Values
In 2010 and 2011, samples for SIA were taken at GPS deployment and at recovery of the tracking device. Paired t-tests were conducted for individual gannets to determine whether δ13C and δ15N differed within RBC between the first and second measurement.
Consistency between RBC and plasma samples was also assessed, as plasma samples were not available for all years. In order to maximise sample size and use RBC in subsequent analyses, we estimated the consistency in stable isotope analyses. Specifically, we regressed stable isotope ratios in plasma as a function of stable isotope ratios in RBCs. For δ13C, in order to tease apart the inshore/offshore, benthic/pelagic information given by δ13C from the trophic component, we used the residuals of the relationship with δ15N for the repeatability analysis. Because the two values are repeated measurements of the same individual, we also calculated the repeatability using the intra-class coefficient (ICC).
Standard Ellipses Areas
Isotopic niche breadth, as a proxy of trophic niche breadth, and isotopic niche overlap was evaluated through Bayesian standard ellipses areas (SEAB) based on multivariate ellipse-based metrics (Jackson et al., 2011). SEAB is the area occupied by the “typical” members (encompassing ca. 40% of the data) of a group and it is robust to outliers (Jackson et al., 2011; Syväranta et al., 2013). SEAB was calculated using 10,000 posterior draws to statistically compare niche overlap between groups (sex and years). For visualization, standard ellipses areas corrected for small samples sizes (SEAc) were computed. Analyses were performed with the R package “SIBER” (Jackson et al., 2011).
Mass Balanced Bayesian Mixing Models
Mass-balanced Bayesian mixing models were fitted using the R package “MixSIAR” (Stock and Semmens, 2016a). MixSIAR was built as a unified framework that embraces all previous advances in mixing models. It can include covariates (fixed and random effects) explaining variability in mixture proportions, and calculate relative support for multiple models via information criteria (Stock et al., 2018). Sex and year were included in the models as fixed covariates, and individual ID was included either as a fixed or as a random variable (see Supplementary Table 1). The multiplicative residual × process error structure was used in all cases, except when bird ID was set as a fixed effect. In this latter case, only a residual error structure was used, as recommended by Stock and Semmens (2016b). All models were run on “long” settings (chains = 3, length = 300 000, burn-in = 2,000,000, thinning = 100). Gannet and fish SIA data were included as raw input values, while diet-to-tissue discrimination factors (DTDFs) were provided to the model as means and standard deviations. No gannet-specific DTDFs are available, so here we used a DTDF of 2.25 ± 0.61‰ for δ15N and 0.24 ± 0.79‰ for δ15C, reflecting average values across studies of piscivorous birds (Hobson and Clark, 1992; Thompson et al., 1999; Bearhop et al., 2002; Forero et al., 2002; Cherel et al., 2005) and previously used to estimate gannet diet through isotopic mixing models (Stauss et al., 2012; Bodey et al., 2018; Le Bot et al., 2019). The DTDF was applied equally to all prey sources. Model convergence was assessed with the Gelman-Rubin diagnostic, which compares estimates of variance between and within Markov chains, with values <1.01 indicating convergence (Gelman et al., 2013). The function compare_models from the “MixSIAR” package (Stock and Semmens, 2016a) was used to compare the predictive accuracy of the models constructed. This function uses the “loo” package to compute leave-one-out cross-validation (LOO) for different fitted models to assess the prediction accuracy of the fitted Bayesian model (Vehtari et al., 2017). The relative support for each model was calculated using LOO weights. As the focus was on whether gannets were consuming fishery discards, we grouped the reduced prey dataset into two different groups with the function combine_sources of “MixSIAR” package (Stock and Semmens, 2016a). This a posteriori aggregation strategy does not require that the stable isotope values of the combined sources be similar, so a question-driven aggregation is possible (Stock et al., 2018). Here, we grouped dragonet, red gurnard, whiting, European hake, European plaice, and Norway Pout as discard sources and Atlantic herring, European sprat, Atlantic mackerel, and Lesser sandeel as non-discard sources (Table 1). While pelagic species including mackerel can be caught and discarded in demersal fisheries, their overall contribution to discards is relatively small (i.e., 1% for mackerel in demersal trawlers) and very similar between trawlers and demersal seiners operating in the area (Anon, 2011). Since the isotopic signature of a discarded and naturally foraged mackerel and herring is the same, these cannot be differentiated in mixing models, so we assumed that any contribution from these pelagic species was from natural foraging. Finally, the mixing model was evaluated by constructing a MixPolygon using the script provided by Smith et al. (2013). The routine calculates the probability that the proposed mixing model can estimate source contributions to explain a consumer’s isotopic signature, considering the distributions of the proposed dietary sources and trophic enrichment factors. So, it quantifies if consumers isotope values fall inside the mixing polygon (point-in-polygon assumption) considering the uncertainty associated.
GPS Data Processing
Only complete trips, defined here as GPS tracks that had consecutive data of more than 10 GPS relocations and returned to the colony without breaks of greater than twice the average tag resolution, were used in the analyses (n = 298). GPS fixes that occurred within 250 m of the colony were removed to exclude colony-associated behaviours like rafting and bathing (McSorley et al., 2003; Carter et al., 2016). While more than 85% of the processed tracks collected by the GPS devices had consecutive 2- or 3-min relocation intervals, further analyses required data at regular time intervals that was achieved through linear interpolation via the “adehabitatLT” package (Calenge, 2006).
Behavioural State Classification
Hidden Markov Models (HMM) are an effective method of inferring putative behavioural states based on the features of an animal’s movement (Michelot et al., 2016; Bennison et al., 2018). Typically, seabird movements are classified into three underlying states, defined by state-dependent distributions of step length and turning angle between consecutive locations. These statistical states are then interpreted as resting, transiting and putative foraging; the latter represented by area restricted search (ARS). For central-place foragers, this method has recently been expanded to discern processes that may attract animals, using an individual’s bearing and distance from the closest attractor at each time step (Pirotta et al., 2018). Here, we utilised the frequentist implementation of the method by Pirotta et al. (2018) in the R package “momentuHMM” (McClintock and Michelot, 2020). This original formulation results in six different states, which correspond to transit or ARS behaviour either in the outward phase of a trip, during the return to the colony and while following an attractor (the nearest fishing vessel, in this application). We added a 7th state to capture resting behaviour on the surface (Supplementary Table 2). As in the original formulation, we used a Weibull distribution for the step lengths, a von Mises distribution for the bearing and a log-normal distribution for the distance to the nearest vessel. The transition probabilities to vessel-associated states were affected by distance to the nearest vessel. Because the probability of an individual returning to the colony increases as the trip progresses, transition probabilities to “colony” states were modelled as a function of the proportion of time elapsed across each trip (Pirotta et al., 2018; McClintock and Michelot, 2020). The model assumptions discussed in Pirotta et al. (2018) were retained, although model constraints differ slightly in the frequentist implementation (see McClintock and Michelot, 2020 for details). The addition of the “rest” state required only one further constraint to be added to the approach outlined in McClintock and Michelot (2020). Specifically, the scale of the Weibull distribution for step length was set to be smallest while resting, in order to capture the very small steps performed in this state.
The location (distance and bearing) of the nearest vessel was obtained from VMS data. VMS data were collected at 2-h resolution and linearly interpolated to the same temporal resolution (2 or 3 min) as the seabird tracking data, and the nearest fishing vessel coordinates were extracted for each of the bird locations using SQL. While such interpolation can result in deviation from the actual vessel track (Skaar et al., 2011; Lambert et al., 2012), this uncertainty has been shown to have no detectable effect on the conclusions drawn from the HMM model at these scales (Pirotta et al., 2018). Initial values of the parameters of the state-dependent distributions of step length and bearing were chosen based on k-means clustering, while the initial parameter values for the distributions of distance to the nearest vessel were selected based on the histogram of the observations, and on previous knowledge of the distance at which gannets respond to vessels (approximately 11 km; Bodey et al., 2014). The Viterbi algorithm was used to estimate the most likely sequence of behavioural states across tracks based on the fitted model. As foraging during the transit towards a vessel cannot be ruled out, we explored if patterns remained consistent when considering both vessel-associated behaviours (transit and ARS) together. Finally, a multiple linear regression was used to estimate the relationship between the proportion of vessel-associated ARS behaviour and the discard proportions obtained through SIA, using sex as a fixed effect.
Behavioural Repeatability
We assessed repeatability in foraging trip metrics to infer the extent to which observations were consistent across an individual’s trips. Unadjusted repeatability (R) scores in the proportion of time spent in vessel-associated behaviours, trip distance, and maximum distance from colony were calculated using the R package “rptR” (Stoffel et al., 2017). Bird ID was included as a random effect in repeatability models. The addition of fixed effects of sex and year had no influence on the repeatability scores, so these are not reported here. Repeatability models were run using a Poisson error structure as they were not normally distributed (Shapiro–Wilk test p-values of <0.05) and show overdispersion that would not be accounted for in Gaussian models. In “rptR,” repeatability results for Poisson models are reported on the log link scale (Stoffel et al., 2017), uncertainty is calculated via parametric bootstrapping (1000 times) and the statistical significance of the repeatability is assessed using likelihood ratio tests.
Results
Stable Isotope Analysis
No statistical differences were found either for δ15N (t = −0.91, df = 28, p-value = 0.37) or for δ13C (t = 0.18, df = 28, p-value = 0.86) between RBC samples taken at GPS deployment and recovery (Supplementary Figure 1). In addition, we found a positive relationship between δ15N from plasma and δ15N from RBC (F1,40 = 28.4, p-value < 0.001, R2 = 0.41). δ15N values presented repeatability between plasma and RBC (ICC: r = 0.57, F41,42 = 3.65, p < 0.001). For δ13C, we used the residuals of the relationship with δ15N (RBC: F1,40 = 28.51, R2 = 0.42, p-value < 0.001; Plasma: F1,40 = 24.5, R2 = 0.38, p-value < 0.001). There was a positive relationship between δ13C residual values in plasma and RBC (F1,40 = 3.92, p-value = 0.05, R2 = 0.09). δ13C residual values presented less repeatability between plasma and RBC than δ15N (ICC: r = 0.26, F41,42 = 1.68, p = 0.05). See Supplementary Figure 2.
Red blood cells samples were obtained from 135 individuals during GPS deployment (74 females and 61 males) (Table 1). Values of δ13C ranged from −20.77 to −16.50‰ and values of δ15N from 11.89 to 16.58‰, with males having significantly higher δ13C values compared to females (F1,125 = 8.35, p < 0.0012). There were also differences between years: 2011 and 2017 showed the highest mean values in δ15N, while for δ13C 2010 and 2011 showed the highest mean values (F4,125 = 30.05, p < 0.001) (Table 1 and Figure 1). Isotopic niche breadth (as represented by SEAB) increased through the study period and was higher for males than for females in all years (Supplementary Table 3 and Figure 1). Generally, little to medium overlap (i.e., 2.99–38.97%) occurred between the sexes (see Supplementary Table 4 and Figure 1). Within years, overlap between males and females was smaller in 2010 and 2011 (see Supplementary Table 4).
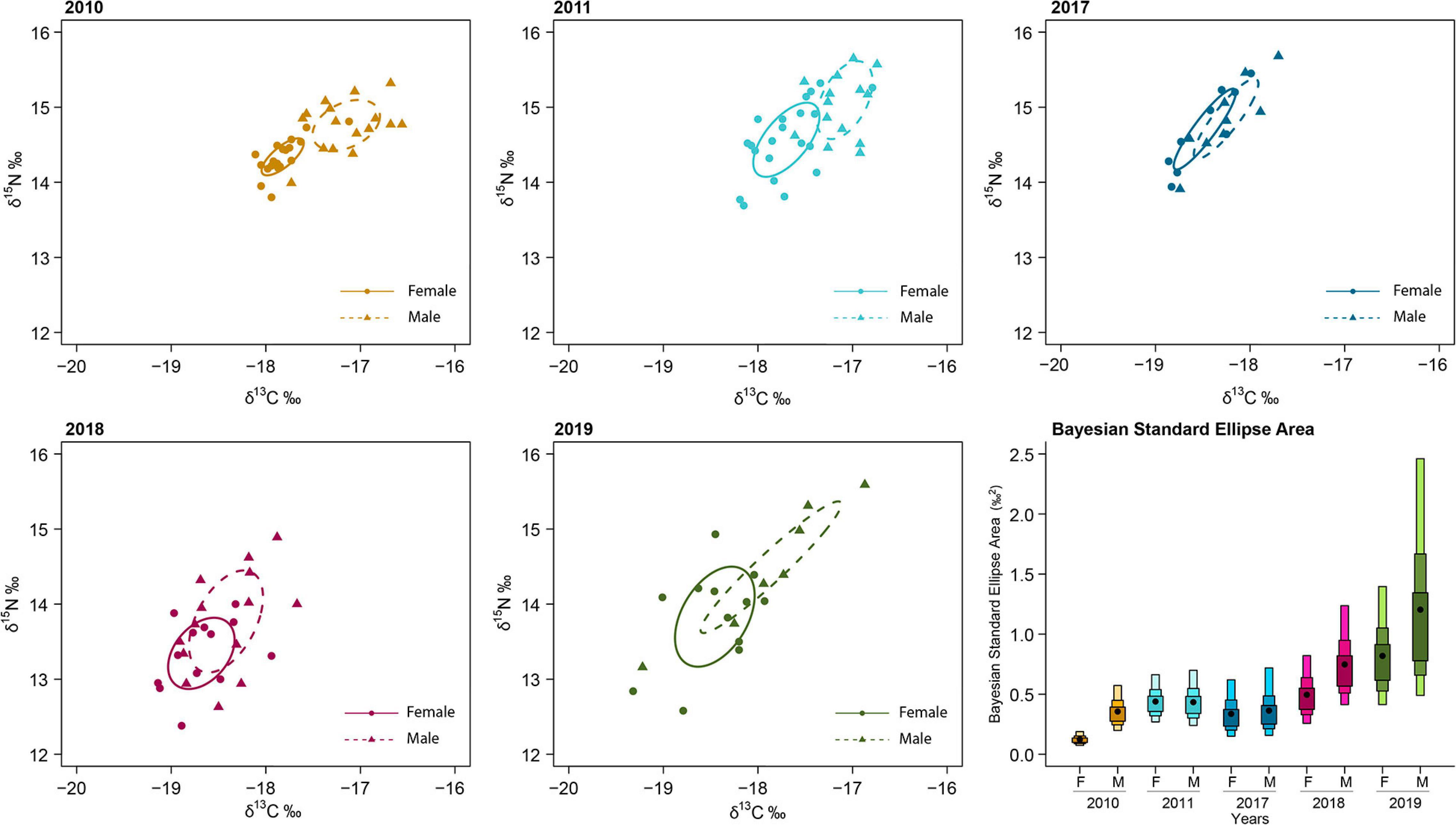
Figure 1. Stable isotope ellipses in RBC samples corrected for small samples size (SEAc) for 2010, 2011, 2017, 2018, and 2019. Isotopic niche breath [as represented by Bayesian standard ellipses areas (SEAb)] are also provided in the last plot for each year and sex.
Diet Reconstruction
All gannet isotopic data were inside the 95% mixing region delimited by the mixing polygon of potential prey adjusted by the DTDF, validating the mixing models fitted (Figure 2). Among the seven models tested (see Supplementary Table 1), the best model included Sex and Year as covariates (model weight: 100%). According to the best model, the mean contribution of discarded fish was higher for males than for females in all years considered. In 2010 and 2011, discard consumption was higher than in the rest of the years (Figure 3A).
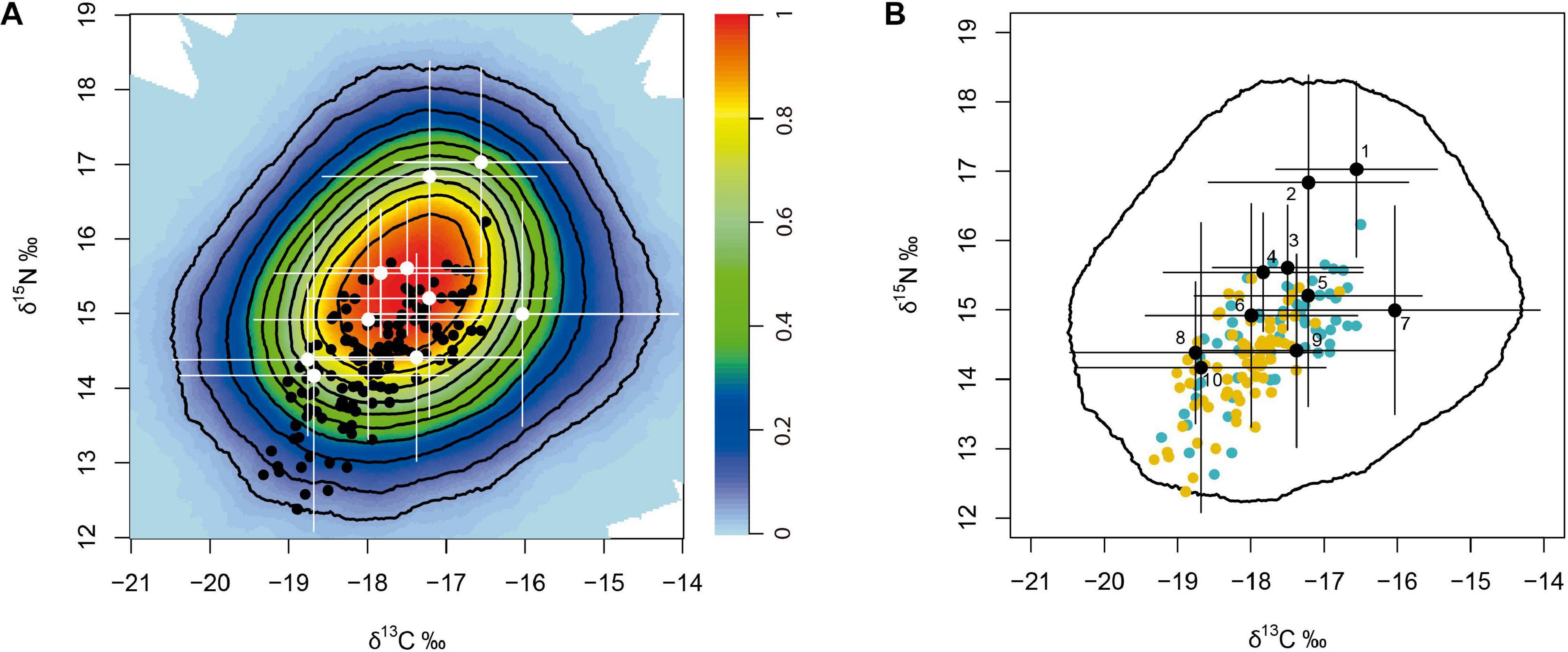
Figure 2. MixPolygon results. (A) Gannets’ isotopic data (black dots) and the potential prey (white dots and bars; mean ± SD). The colour background displays the probability that a consumer’s isotopic signature is explained by the proposed mixing model. Probability contours are printed every 10%. Prey species included are detailed in Table 1. (B) Gannet data coloured by sex: orange, females; blue, males. Prey data numbered: 1, Trisopterus esmarkii; 2, Merlangius merlangus; 3, Merluccius merluccius; 4, Ammodytes spp.; 5, Chelidonichthys cuculus; 6, Clupea harengus; 7, Pleuronectes platessa; 8, Sprattus sprattus; 9, Callionymus spp.; 10, Scomber scombrus.
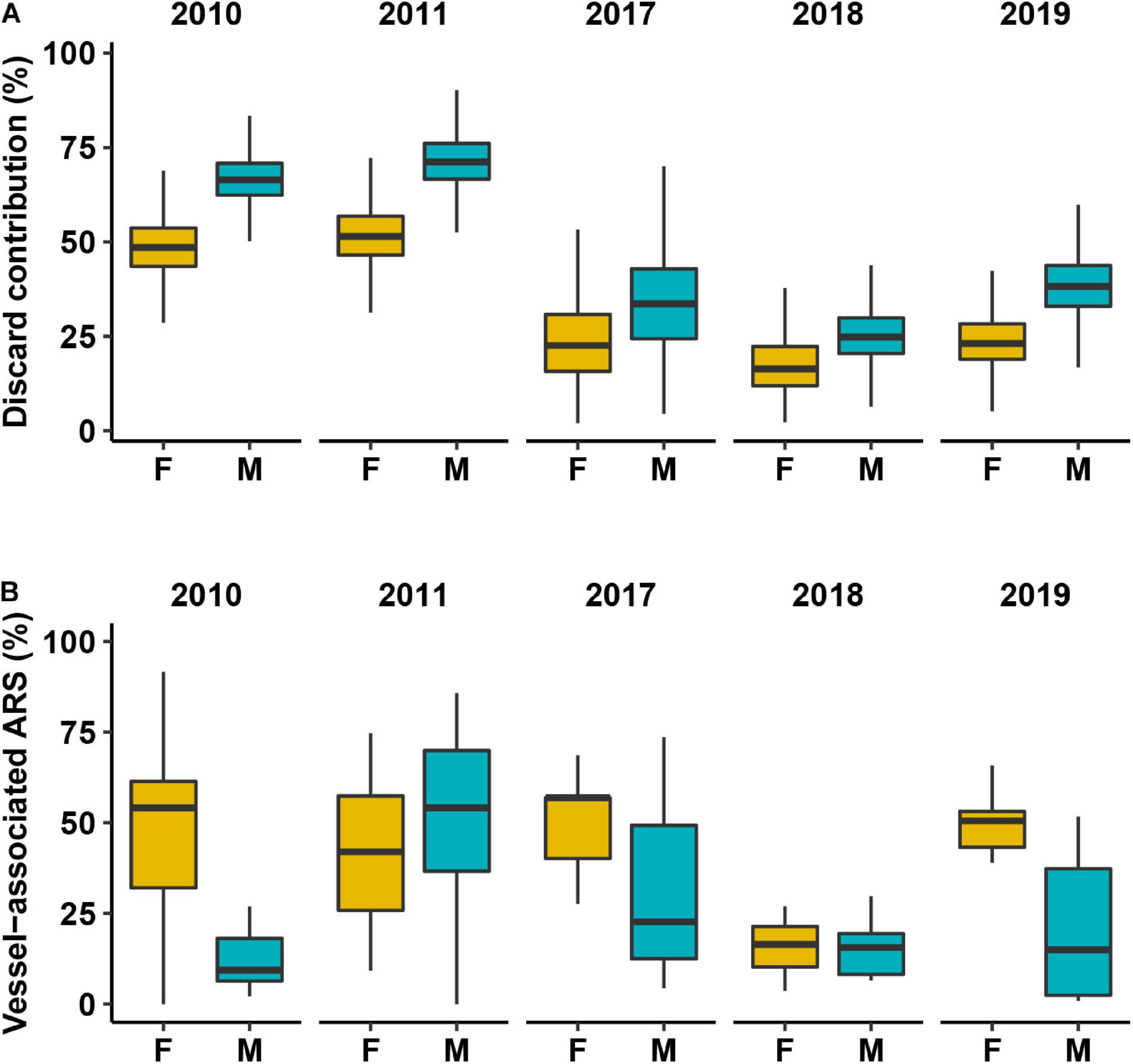
Figure 3. (A) Discard contribution to the diet of northern gannets in Saltee Island and (B) vessel-associated area-restricted search (ARS). Orange, females; blue, males. Black horizontal line depicts the median value.
Movement Analysis and Hidden Markov Models
We recorded 298 complete foraging trips from 81 individuals (40 females and 41 males, Supplementary Figure 3). Females carried out longer trips, travelled further distances and had higher mean trip duration than males except in 2011 and 2017 (Figure 3). ARS behaviour within the different phases of a trip showed that approximately 50% of female ARS was associated with vessels, except for 2018 where natural foraging was the predominant ARS mode. Males had a more variable foraging strategy across the years (Figure 2B) and, despite the higher contribution of discards to the diet, showed less vessel-associated ARS than females in all years except 2011. In 3 of the 5 years, the median proportion of ARS that was associated with vessels in females was twice that of males. This finding remained consistent when considering both vessel-associated behaviours (transit and ARS) (see Supplementary Figure 4). Males were also less likely to switch to vessel-associated foraging [probability = 0.124 (95% CI: 0.120–0.128)] than females [probability = 0.140 (95% CI: 0.135–0.145)]. The state-dependent distributions of the movement variables and associated parameter estimates, the estimates of the transition probabilities between states, and the residual diagnostic plots are reported in Supplementary Figures 6–9 and Supplementary Tables 5, 6.
The repeatability analysis showed that for the individual gannets for which repeat trips were recorded (n = 60; mean trips/individual = 4.7 ± 2.8 SD), the proportion of time spent in vessel-associated behaviours was consistent [R = 0.248, CI = (0.028, 0.437), p = 0.02], as well as broader foraging trip metrics, including total trip distance [R = 0.5, CI = (0.335, 0.612), p = 0.001] and maximum distance travelled from the colony [R = 0.57, CI = (0.347, 0.613), p = 0.001].
Vessel-Associated Foraging and Discards Consumption
Individual gannets associated with a range of vessels likely to provide discards. A total of 42.4% of vessel-associated foraging occurred around demersal trawlers, 32.5% around demersal seines, and 10.3% around static nets/longlines. Females attended proportionately more to demersal seine vessels (35%), compared to males (19%), while males attended proportionately more to demersal trawlers (females 39%, males 63%), X2(4, 13044) = 781.4, p ≤ 0.001 (Supplementary Figure 5). Differences in vessel associations were relatively consistent between sexes across all years of the study (Supplementary Figure 5). There was a significant positive relationship between individual discards consumption (i.e., mixing model output) and vessel-associated foraging (i.e., track analysis output) (R2 = 0.17, p-value = 0.001) with a significant effect of sex (p-value < 0.001) (Figure 4). Despite females engaging in more vessel-associated putative foraging than males (Figure 3B), estimated discards consumption was lower for females than males (Figure 5).
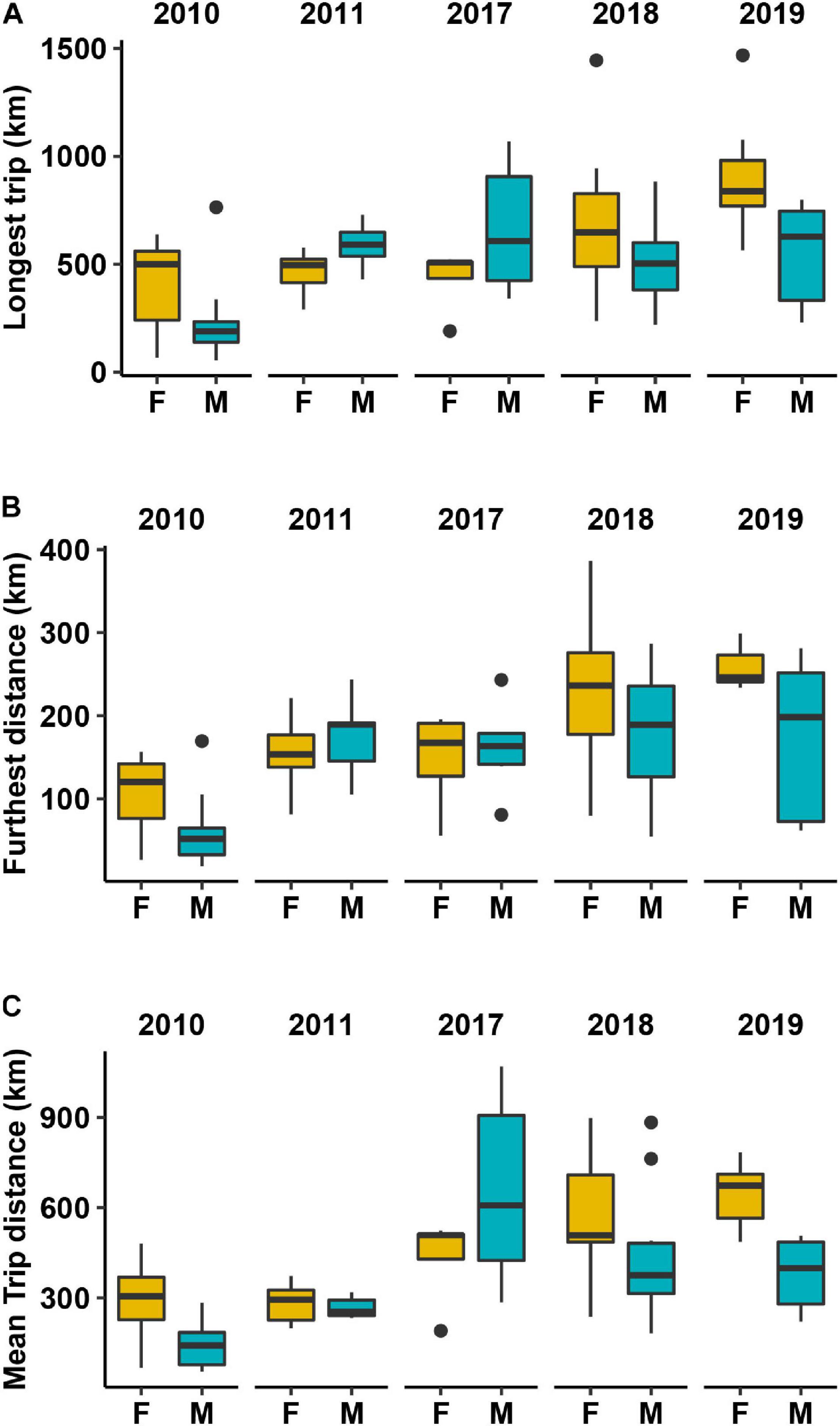
Figure 4. Movement descriptors showing the: (A) longest trip (km), (B) furthest distance travelled from the colony (km) and (C) mean trip distance (km) per individual. Orange, females; blue, males. Black horizontal line depicts the median value.
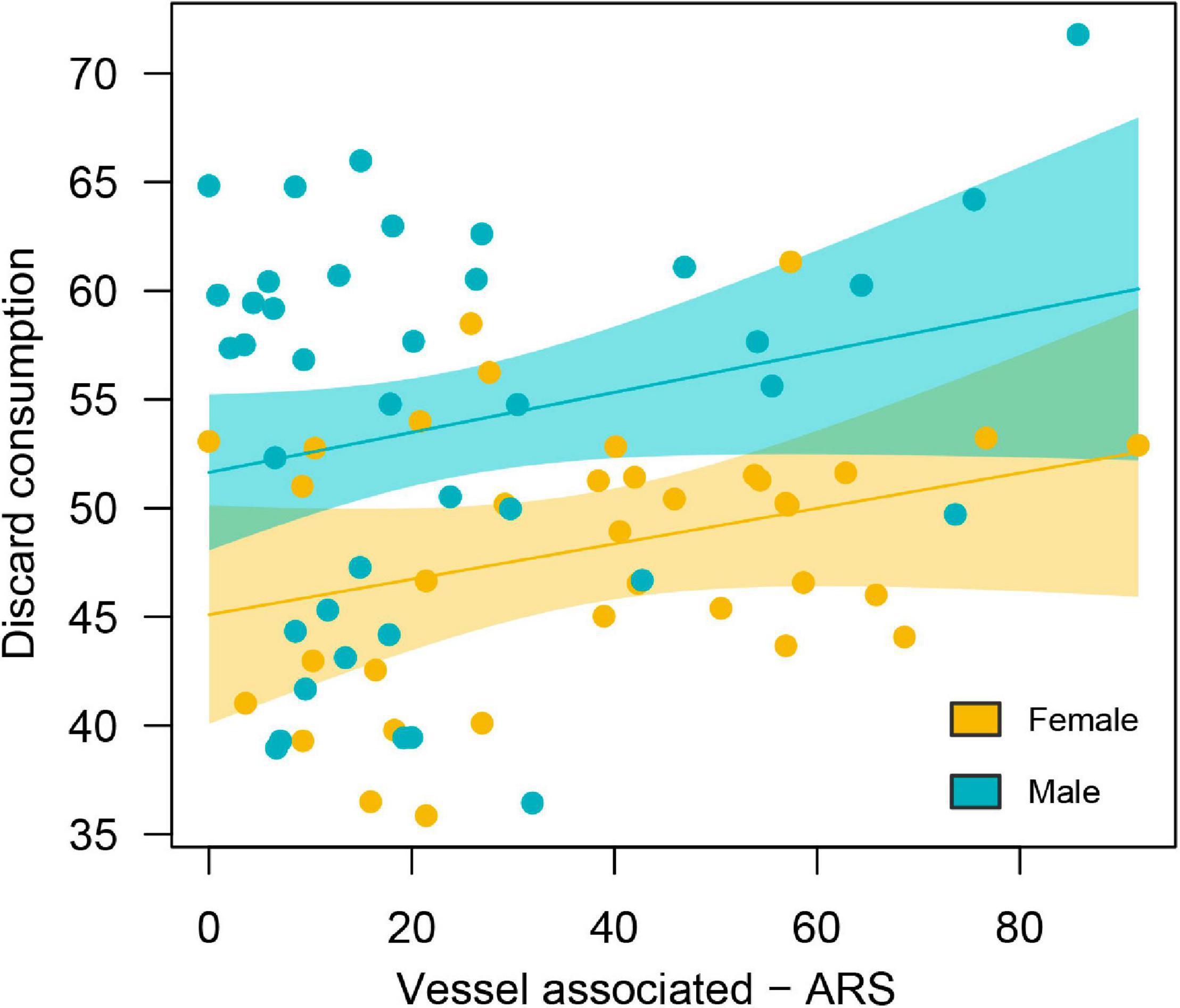
Figure 5. Percentage of area-restricted search (ARS) associated with vessels versus percentage of discard consumption from the same individual. Orange, females; blue, males.
Discussion
We demonstrate a logical, but previously unreported positive relationship between time spent in vessel-associated foraging behaviour and discards consumption in a marine predator. The presence of sexual differences in discards consumption noted in this study is also consistent with other gannet colonies (Stauss et al., 2012; Bodey et al., 2018). However, despite higher discards consumption in males, females actually spent more time in vessel-associated foraging than males. While we do not know the actual mechanism for this mismatch between vessel attendance and discards consumption, a number of hypotheses may explain our results; (1) that females select higher proportions of pelagic species within the discarded catch; (2) that sexes may target different fishing gears as a consequence of foraging habitat preferences; (3) that isotopes and tracking data are not directly comparable because they represent different temporal windows; or (4) that males might be out-competing females at vessels for discards.
The discrepancy between vessel attendance and discards consumption in females may be the result of females selecting a different component of discarded fish compared with males. While such differences in diet or foraging strategies are typically more apparent in highly sexually dimorphic species (Catry et al., 2006), they also occur in monomorphic or only slightly dimorphic species (e.g., Sims et al., 2001; Ruckstuhl and Neuhaus, 2006; Pinet et al., 2012). Gannets are only slightly sexually dimorphic, with females being marginally heavier than males (in our dataset, on average 170 g, or 5–6% of adult body weight), and previous studies have found no significant difference in length of the tarsus, bill, or wing of breeding gannets (Stauss et al., 2012; Malvat et al., 2020). Female gannets also make a greater contribution to chick provisioning (Montevecchi et al., 1984) than males, and are likely to have a calcium (and other nutrients) deficit from egg production, which may require them to target different food, particularly micronutrients compared to males (Tait et al., 2014). Data on discards in our study area shows that the demersal seine vessels, attended proportionately more by females, tend to discard low proportions of pelagic species, and significantly less discards overall than trawlers (Anon, 2011). Coupled with the knowledge that gannets are less selective in their consumption of discards than other birds (Hudson and Furness, 1988), it seems unlikely that females have greater access to pelagic species when scavenging at demersal seine vessels. Furthermore, while our results are consistent with previous studies demonstrating that females undertake longer trips and travel greater cumulative distance than males (Stauss et al., 2012; Cleasby et al., 2015; Bodey et al., 2018; Le Bot et al., 2019), there is considerable overlap in the foraging range of both sexes in all 5 years (Supplementary Figure 3), highlighting the importance of multi-year studies (Clark et al., 2021).
Blood samples for SIA and the estimates of discards consumption represent diet over a longer temporal scale than the corresponding tracking data (weeks versus days). In addition, samples were collected prior to the start of foraging trips. However, we found no significant differences in carbon or nitrogen values for those birds sampled at both deployment and recovery of tracking devices (Supplementary Figure 1). Our analyses also show a high level of individual consistency in diet and foraging behaviour, suggesting that the relationship between vessel-associated foraging and discards consumption is robust to temporal mismatches at the scale studied. However, the temporal mismatch between tracking and stable isotope data could result in some birds with an isotopic signature consistent with discards consumption but no vessel associations captured in the tracking data. The estimated relationship between vessel attendance and discards consumption is therefore likely to reflect the overall tendency of an individual on their feeding habits but should not be used to make predictions of absolute discards consumption based on the small window of available tracking data alone.
An alternative hypothesis for the sexual mismatch between vessel attendance and discards consumption is competitive exclusion of females by males at more discards-profitable trawlers. Observations of discard scavenging seabirds have shown gannets to be a highly aggressive competitor and successful kleptoparasite of discarded fish (Garthe and Hüppop, 1994; Garthe and Hüppop, 1998) at the top of clear species dominance hierarchies (Wahl and Heinemann, 1979; Arcos et al., 2001). The intersexual competition hypothesis suggests that the dominant sex may forage more efficiently, displacing the other sex spatially or into different niches (Elliott et al., 2010). This has been found in several species (e.g., Peck and Congdon, 2006; Elliott et al., 2010; Biggerstaff et al., 2017; Galezo et al., 2018). Nelson (1965) states that male gannets are particularly aggressive and that females show “astonishingly high tolerance of punishment from males.” Aggressiveness of male gannets has previously been proposed as a putative driving force of female displacement in northern gannet foraging distributions (Lewis et al., 2002; Stauss et al., 2012), and longer trips and greater distance travelled by females is also consistent with competitive exclusion from trawlers to fisheries that are lower contributors to total discards, such as demersal seines (Anon, 2011; see Supplementary Table 7).
Identifying the mismatch between discard consumption and vessel-associated foraging was only possible through the use of complementary techniques. However, a number of assumptions and limitations must be acknowledged. Identifying vessel-associated behaviours is dependent on contemporaneous tracking data of vessels and birds at appropriate spatio-temporal scales. While vessel tracking data were collected at a coarser resolution than gannet tracking data, interpolation of vessel tracks to the similar resolution of gannet data was previously shown to not affect behavioural model outputs (see supplementary material in Pirotta et al., 2018). A further assumption is that we have tracking data from all vessels that gannets are likely to interact with. VMS data were only available for vessels >15 m length in 2010 and 2011, and >12 m in 2017–2019. Cameras deployed on gannets show that they typically associate with these larger vessels (Votier et al., 2013), so we are confident that we are not substantially underestimating vessel-associated behaviours due to interactions at smaller vessels. In addition, vessel-associated ARS may not indicate foraging on discards specifically. While there is a good agreement between ARS and prey capture attempts (Bennison et al., 2018), gannets may simply be targeting the same locations as vessels to forage on natural prey. However, the behavioural transition probabilities suggest that vessel-associated foraging is for the purpose of discards, as switching to ARS becomes increasingly more likely with increasing proximity to vessels at very fine spatio-temporal scales (Bodey et al., 2014). While the transition probabilities were low, the marginally higher transition probabilities for females tracked in this study suggest that they are more likely to engage in foraging behaviour around vessels. The same relationship holds when including vessel-associated transit behaviour, which may indicate anticipation of discards availability. Finally, spatio-temporal variability in isotopic baselines that can affect prey isotopic values has not been accounted for. If such baseline variability exists, dietary differences between sexes within years would remain unaffected, but comparisons across years could be affected. Despite these assumptions and limitations, we are confident that the relationship between vessel attendance and discards consumption, as well as the observed sex differences in this relationship, are robust.
While other studies have either detected no evidence for sexual differences in spatio-temporal overlap with fisheries (Garthe et al., 2007; Patrick et al., 2015; Clark et al., 2021) or greater vessel-association in males than females (Votier et al., 2013), we find females actually engaged in more vessel-associated foraging behaviour than males. Breeding gannets have highly consistent foraging departure bearings (Hamer et al., 2007) and foraging routes (Votier et al., 2017) therefore the lower consistency between trips in vessel attendance observed in this study may suggest that vessels are encountered more opportunistically. Fishing vessels are conspicuous visual cues, particularly when attended by large numbers of seabirds and when associated with the provision of discards. Tremblay et al. (2014) suggest that foraging seabirds are not looking directly for prey, but instead search for indicators of the presence of prey. They found that seabird foraging movements were related to visual stimuli, including other predators and fishing boats. If vessels are being used as a foraging cue in a similar way to the presence of other predators (Hoffman et al., 1981; Thiebault et al., 2014), the resulting recruitment of other birds in the area may result in a positive feedback loop, reducing the occurrence of “natural” foraging away from vessels. Large aggregations of seabirds at vessels create a highly competitive environment with numerous aggressive interactions. Our results are more consistent with either lower female success or competitive exclusion of female gannets behind more profitable trawlers than other hypotheses. However, further research should be carried out to understand the causal mechanism for the mismatch between vessel attendance and discards consumption, as well as any individual or population level effects on bird health or breeding success. Such sex differences in vessel attendance and discards consumption is important for assessing population-level impacts (Gianuca et al., 2017), particularly in light of reductions in discards through fisheries reforms. With this in mind, our study also highlights interannual patterns in discards consumption, and the first evidence of continued reliance on discards following implementation of the EU Common Fisheries Policy Landings Obligation. While discarding is not completely banned under the Landing Obligation, one would expect a reduction in discards availability, which may explain the reduced reliance on discards between the 2010–2011 and 2017–2019 sampling periods. The limited sample of tracked individuals in any year may have had contrasting foraging tactics, but interannual differences in vessel attendance and discard consumption may also suggest that gannets, like a range of other seabirds, have a flexible foraging strategy reflecting changing prey availability (Oro and Ruiz, 1997; Montevecchi et al., 2009; Garthe et al., 2014). The increase in isotopic niche breadth through the study period may also indicate an increased consumption of prey with dissimilar isotopic composition over time. This may be the result of more individuals becoming more generalist, or a wider range of individual specialisms sampled within the population (Bolnick et al., 2002). Flexibility in the use of discards and natural prey among years could potentially buffer fluctuating natural resource availability, and make discards-utilising species more resilient to environmental changes (Oro and Ruiz, 1997; Hamer et al., 2007; Grandgeorge et al., 2008; Bicknell et al., 2013). Longer-term studies should be undertaken to explore the effects of the reduction in this subsidised feeding resource on body condition, reproductive success and population trends.
Data Availability Statement
The seabird tracking dataset analysed in this study can be found at www.seabirdtracking.org (Birdlife International) by request (Dataset IDs: 722, 723, 1543, and 1638). SIA dataset is available at Figshare (doi: 10.6084/m9.figshare.13744147).
Ethics Statement
The animal study was reviewed and approved by British Trust for Ornithology (BTO), National Parks and Wildlife Service (NPWS), and Health Products Regulatory Authority (HPRA). The study was also reviewed and approved by the UCC Animal Ethics Committee.
Author Contributions
MJ, JG, and GA conceived the study. KH, SV, SB, TB, AB, and MJ collected the data. HG processed and anonymised the VMS data. GA and EP developed the tracking modelling framework. JG and GA performed the analysis. JG led the writing of the manuscript with input from GA and MJ. All authors contributed to subsequent revisions and approved the final version.
Funding
Funding for 2010 and 2011 data was provided by the National Environment Research Council Standard Grant NE/H007466/1, and Beaufort Marine Research Award funded under the Marine Research Sub-Programme of the National Development Plan 2007–2013. Funding for 2017–2019 data was provided by the Irish Research Council (GOIPG/2016/503), and the Department of Agriculture, Fisheries, and the Marine FishKOSM project under grant (15/S/744). JG was funded by the Department of Agriculture, Fisheries, and the Marine FishKOSM project. GA was funded by the MarPAMM project supported by the EU’s INTERREG VA Programme, managed by the Special EU Programmes Body (SEUPB).
Conflict of Interest
The authors declare that the research was conducted in the absence of any commercial or financial relationships that could be construed as a potential conflict of interest.
Acknowledgments
We would like to thank all those involved in fieldwork as well as the Neale family for permitting work on Great Saltee.
Supplementary Material
The Supplementary Material for this article can be found online at: https://www.frontiersin.org/articles/10.3389/fmars.2021.636468/full#supplementary-material
References
Almaraz, P., and Oro, D. (2011). Size-mediated non-trophic interactions and stochastic predation drive assembly and dynamics in a seabird community. Ecology 92, 1948–1958. doi: 10.1890/11-0181.1
Anon (2011). Atlas of Demersal Discarding: Scientific Observations and Potential Solutions. Rinville: Marine Institute. doi: 10.1017/CBO9781107415324.004
Arcos, J. M., Oro, D., and Sol, D. (2001). Competition between the yellow-legged gull Larus cachinnans and Audouin’s gull Larus audouinii associated with commercial fishing vessels: the influence of season and fishing fleet. Mar. Biol. 139, 807–816. doi: 10.1007/s002270100651
Bartumeus, F., Giuggioli, L., Louzao, M., Bretagnolle, V., Oro, D., and Levin, S. A. (2010). Fishery discards impact on seabird movement patterns at regional scales. Curr. Biol. 20, 215–222. doi: 10.1016/j.cub.2009.11.073
Bearhop, S., Teece, M. A., Waldron, S., and Furness, R. W. (2000). Influence of lipid and uric acid on δ13C and δ15N values of avian blood: implications for trophic studies. Auk 117, 504–507.
Bearhop, S., Waldron, S., Votier, S. C., and Furness, R. W. (2002). Factors that influence assimilation rates and fractionation of nitrogen and carbon stable isotopes in avian blood and feathers. Physiol. Biochem. Zool. 75, 451–458. doi: 10.1086/342800
Bennison, A., Bearhop, S., Bodey, T. W., Votier, S. C., Grecian, W. J., Wakefield, E. D., et al. (2018). Search and foraging behaviors from movement data: a comparison of methods. Ecol. Evol. 8, 13–24. doi: 10.1002/ece3.3593
Bicknell, A. W. J., Oro, D., Camphuysen, K. C. J., and Votier, S. C. (2013). Potential consequences of discard reform for seabird communities. J. Appl. Ecol. 50, 649–658. doi: 10.1111/1365-2664.12072
Biggerstaff, M. T., Lashley, M. A., Chitwood, M. C., Moorman, C. E., and DePerno, C. S. (2017). Sexual segregation of forage patch use: support for the social-factors and predation hypotheses. Behav. Processes 136, 36–42. doi: 10.1016/j.beproc.2017.01.003
Bodey, T. W., Cleasby, I. R., Votier, S. C., Hamer, K. C., Newton, J., Patrick, S. C., et al. (2018). Frequency and consequences of individual dietary specialisation in a wide-ranging marine predator, the northern gannet. Mar. Ecol. Prog. Ser. 604, 251–262. doi: 10.3354/meps12729
Bodey, T. W., Jessopp, M. J., Votier, S. C., Gerritsen, H. D., Cleasby, I. R., Hamer, K. C., et al. (2014). Seabird movement reveals the ecological footprint of fishing vessels. Curr. Biol. 24, R514–R515. doi: 10.1016/j.cub.2014.04.041
Bolnick, D. I., Yang, L. H., Fordyce, J. A., and Davis, J. M. (2002). Measuring individual-level resource specialization. Ecology 83, 2936–2941.
Borges, L. (2015). The evolution of a discard policy in Europe. Fish Fish. 16, 534–540. doi: 10.1111/faf.12062
Calenge, C. (2006). The package “adehabitat” for the R software: a tool for the analysis of space and habitat use by animals. Ecol. Modell. 197, 516-519. doi: 10.1016/j.ecolmodel.2006.03.017
Carter, M. I. D., Cox, S. L., Scales, K. L., Bicknell, A. W. J., Nicholson, M. D., Atkins, K. M., et al. (2016). GPS tracking reveals rafting behaviour of Northern Gannets (Morus bassanus): implications for foraging ecology and conservation. Bird Study 63, 83–95. doi: 10.1080/00063657.2015.1134441
Catry, P., Phillips, R. A., Croxall, J. P., Ruckstuhl, K., and Neuhaus, P. (2006). “Sexual segregation in birds: patterns, processes and implications for conservation,” in Sexual Segregation in Vertebrates: Ecology of the Two Sexes, eds K. E. Ruckstuhl and P. Neuhaus (Cambridge: Cambridge University Press), 351–378.
Cherel, Y., Hobson, K. A., and Hassani, S. (2005). Isotopic discrimination between food and blood and feathers of captive penguins: implications for dietary studies in the wild. Physiol. Biochem. Zool. 78, 106–115.
Church, G. E., Furness, R. W., Tyler, G., Gilbert, L., and Votier, S. C. (2019). Change in the North Sea ecosystem from the 1970s to the 2010s: great skua diets reflect changing forage fish, seabirds, and fisheries. ICES J. Mar. Sci. 76, 925–937. doi: 10.1093/icesjms/fsy165
Clark, B. L., Cox, S. L., Atkins, K. M., Bearhop, S., Bicknell, A. W., Bodey, T. W., et al. (2021). Sexual segregation of gannet foraging over 11 years: movements vary but isotopic differences remain stable. Mar. Ecol. Prog. Ser. doi: 10.3354/meps13636 [Epub ahead of print].
Clark, B. L., Vigfúsdóttir, F., Jessopp, M. J., Burgos, J. M., Bodey, T. W., and Votier, S. C. (2019). Gannets are not attracted to fishing vessels in Iceland — potential influence of a discard ban and food availability. ICES J. Mar. Sci. 77, 692–700. doi: 10.1093/icesjms/fsz233
Cleasby, I. R., Wakefield, E. D., Bodey, T. W., Davies, R. D., Patrick, S. C., Newton, J., et al. (2015). Sexual segregation in a wide-ranging marine predator is a consequence of habitat selection. Mar. Ecol. Prog. Ser. 518, 1–12. doi: 10.3354/meps11112
DeNiro, M. J., and Epstein, S. (1978). Influence of diet on the distribution of carbon isotopes in animals. Geochim. Cosmochim. Acta 42, 495–506.
Elliott, K. H., Gaston, A. J., and Crump, D. (2010). Sex-specific behavior by a monomorphic seabird represents risk partitioning. Behav. Ecol. 21, 1024–1032. doi: 10.1093/beheco/arq076
Ellis, E. C. (2015). Ecology in an anthropogenic biosphere. Ecol. Monogr. 85, 287–331. doi: 10.1890/14-2274.1
Forero, M. G., Hobson, K. A., Bortolotti, G. R., Donázar, J. A., Bertellotti, M., and Blanco, G. (2002). Food resource utilisation by the Magellanic penguin evaluated through stable-isotope analysis: segregation by sex and age and influence on offspring quality. Mar. Ecol. Prog. Ser. 234, 289–299.
Furness, R., Hudson, A., and Ensor, K. (1988). “Interactions between scavenging seabirds and commercial fisheries around the British Isles,” in Seabirds and Other Marine Vertebrates. Competition, Predation and Other Interactions, ed. J. Burger (New York, NY: Columbia University Press), 240–268.
Galezo, A. A., Krzyszczyk, E., and Mann, J. (2018). Sexual segregation in Indo-Pacific bottlenose dolphins is driven by female avoidance of males. Behav. Ecol. 29, 377–386. doi: 10.1093/beheco/arx177
Garthe, S., Guse, N., Montevecchi, W. A., Rail, J. F., and Grégoire, F. (2014). The daily catch: flight altitude and diving behavior of northern gannets feeding on Atlantic mackerel. J. Sea Res. 85, 456–462. doi: 10.1016/j.seares.2013.07.020
Garthe, S., and Hüppop, O. (1994). Distribution of ship-following seabirds and their utilization of discards in the North Sea in summer. Mar. Ecol. Prog. Ser. 106, 1–10.
Garthe, S., and Hüppop, O. (1998). Foraging success, kleptoparasitism and feeding techniques in scavenging seabirds: does crime pay? Helgolander Meeresuntersuchungen 52, 187–196. doi: 10.1007/BF02908747
Garthe, S., Montevecchi, W. A., Chapdelaine, G., April, J. R., Rail, J. F., and Hedd, A. (2007). Contrasting foraging tactics by northern gannets (Sula bassana) breeding in different oceanographic domains with different prey fields. Mar. Biol. 151, 687–694. doi: 10.1007/s00227-006-0523-x
Garthe, S., Montevecchi, W. A., and Davoren, G. K. (2011). Inter-annual changes in prey fields trigger different foraging tactics in a large marine predator. Limnol. Oceanogr. 56, 802–812. doi: 10.4319/lo.2011.56.3.0802
Gelman, A., Carlin, J. B., Stern, H. S., and Rubin, D. B. (2013). Bayesian Data Analysis. New York,NY: Chapman and Hall. doi: 10.1080/01621459.2014.963405
Gianuca, D., Phillips, R. A., Townley, S., and Votier, S. C. (2017). Global patterns of sex- and age-specific variation in seabird bycatch. Biol. Conserv. 205, 60–76. doi: 10.1016/j.biocon.2016.11.028
Gianuca, D., Votier, S. C., Pardo, D., Wood, A. G., Sherley, R. B., Ireland, L., et al. (2019). Sex-specific effects of fisheries and climate on the demography of sexually dimorphic seabirds. J. Anim. Ecol. 88, 1366–1378. doi: 10.1111/1365-2656.13009
Grandgeorge, M., Wanless, S., Dunn, T. E., Maumy, M., Beaugrand, G., and Grémillet, D. (2008). Resilience of the British and Irish seabird community in the twentieth century. Aquat. Biol. 4, 187–199. doi: 10.3354/ab00095
Gray, C. M., and Hamer, K. C. (2001). Food-provisioning behaviour of male and female Manx shearwaters, Puffinus puffinus. Anim. Behav. 62, 117–121. doi: 10.1006/anbe.2001.1717
Grémillet, D., Pichegru, L., Kuntz, G., Woakes, A. G., Wilkinson, S., Crawford, R. J. M., et al. (2008). A junk-food hypothesis for gannets feeding on fishery waste. Proc. R. Soc. B Biol. Sci. 275, 1149–1156. doi: 10.1098/rspb.2007.1763
Hamer, K. C., Humphreys, E. M., Garthe, S., Hennicke, J., Peters, G., Grémillet, D., et al. (2007). Annual variation in diets, feeding locations and foraging behaviour of gannets in the North Sea: flexibility, consistency and constraint. Mar. Ecol. Prog. Ser. 338, 295–305. doi: 10.3354/meps338295
Hays, G. C., Ferreira, L. C., Sequeira, A. M. M., Meekan, M. G., Duarte, C. M., Bailey, H., et al. (2016). Key questions in marine megafauna movement ecology. Trends Ecol. Evol. 31, 463–475. doi: 10.1016/j.tree.2016.02.015
Hobson, K. A., and Clark, R. G. (1992). Assessing avian diets using stable isotope II: factors influencing diet-tissue fractionation. Condor 94, 189–197.
Hoffman, W., Heinemann, D., and Wiens, J. (1981). The ecology of seabird feeding flocks in Alaska. Auk 98, 437–456. doi: 10.1093/auk/98.3.437
Hudson, A. V., and Furness, R. W. (1988). Utilization of discarded fish by scavenging seabirds behind whitefish trawlers in Shetland. J. Zool. 215, 151–166. doi: 10.1111/j.1469-7998.1988.tb04890.x
Hudson, A. V., and Furness, R. W. (1989). The behaviour of seabirds foraging at fishing boats around Shetland results. IBIS 131, 225–237.
Jackson, A. L., Inger, R., Parnell, A. C., and Bearhop, S. (2011). Comparing isotopic niche widths among and within communities: SIBER – stable isotope Bayesian Ellipses in R. J. Anim. Ecol. 80, 595–602. doi: 10.1111/j.1365-2656.2011.01806.x
Jennings, S., and Cogan, S. M. (2015). Nitrogen and carbon stable isotope variation in northeast Atlantic fishes and squids. Ecology 96, 2568–2568. doi: 10.1890/15-0299.1
Kato, A., Watanuki, Y., Nishiumi, I., Kuroki, M., Shaughnessy, P., and Naito, Y. (2000). Variation in foraging and parental behavior of King cormorants. Auk 117, 718–730. doi: 10.1093/auk/117.3.718
Lambert, G. I., Jennings, S., Hiddink, J. G., Hintzen, N. T., Hinz, H., Kaiser, M. J., et al. (2012). Implications of using alternative methods of vessel monitoring system (VMS) data analysis to describe fishing activities and impacts. ICES J. Mar. Sci. 69, 682–693.
Le Bot, T., Lescroël, A., Fort, J., Péron, C., Gimenez, O., Provost, P., et al. (2019). Fishery discards do not compensate natural prey shortage in Northern gannets from the English channel. Biol. Conserv. 236, 375–384. doi: 10.1016/j.biocon.2019.05.040
Lewis, S., Benvenuti, S., Dall’Antonia, L., Griffiths, R., Money, L., Sherratt, T. N., et al. (2002). Sex-specific foraging behaviour in a monomorphic seabird. Proc. R. Soc. B Biol. Sci. 269, 1687–1693. doi: 10.1098/rspb.2002.2083
Lewis, S., Harris, M. P., Wanless, S., Sherratt, T. N., and Hamer, K. C. (2003). Contrasting diet quality of northern gannets Morus bassanus at two colonies. Ardea 91, 167–176.
Logan, J. M., Jardine, T. D., Miller, T. J., Bunn, S. E., Cunjak, R. A., and Lutcavage, M. E. (2008). Lipid corrections in carbon and nitrogen stable isotope analyses: comparison of chemical extraction and modelling methods. J. Anim. Ecol. 77, 838–846. doi: 10.1111/j.1365-2656.2008.01394.x
Louzao, M., Igual, J. M., McMinn, M., Aguilar, J. S., Triay, R., and Oro, D. (2006). Small pelagic fish, trawling discards and breeding performance of the critically endangered Balearic shearwater: improving conservation diagnosis. Mar. Ecol. Prog. Ser. 318, 247–254. doi: 10.3354/meps318247
Malvat, Z., Lynch, S. A., Bennison, A., and Jessopp, M. (2020). Evidence of links between haematological condition and foraging behaviour in northern gannets (Morus bassanus). R. Soc. Open Sci. 7:192164. doi: 10.1098/rsos.192164
McClintock, B. T., and Michelot, T. (2020). momentuHMM: R Package for Analysis of Telemetry Data Using Generalized Multivariate Hidden Markov Models of Animal Movement. Version 1.5.1. doi: 10.1111/2041-210X.12995
McSorley, C. A., Dean, B. J., Webb, A., and Reid, J. B. (2003). Seabird Use of Waters Adjacent to Colonies: Implications for Seaward Extensions to Existing Breeding Seabird Colony Special Protection Areas JNCC Report No. 329. Peterborough: JNCC.
Michelot, T., Langrock, R., and Patterson, T. A. (2016). moveHMM: an R package for the statistical modelling of animal movement data using hidden Markov models. Methods Ecol. Evol. 7, 1308–1315. doi: 10.1111/2041-210X.12578
Montevecchi, W. A. (2007). Binary dietary responses of northern gannets Sula bassana indicate changing food web and oceanographic conditions. Mar. Ecol. Prog. Ser. 352, 213–220. doi: 10.3354/meps07075
Montevecchi, W. A., Benvenuti, S., Garthe, S., Davoren, G. K., and Fifield, D. (2009). Flexible foraging tactics by a large opportunistic seabird preying on forage-and large pelagic fishes. Mar. Ecol. Prog. Ser. 385, 295–306. doi: 10.3354/meps08006
Montevecchi, W. A., Ricklefs, R. E., Kirkham, I. R., and Gabaldon, D. (1984). Growth energetics of nestling Northern Gannets (Sula bassanus). Auk 101, 334–341.
Navarro, J., Louzao, M., Igual, J. M., Oro, D., Delgado, A., Arcos, J. M., et al. (2009). Seasonal changes in the diet of a critically endangered seabird and the importance of trawling discards. Mar. Biol. 156, 2571–2578. doi: 10.1007/s00227-009-1281-3
Navarro, J., Oro, D., Bertolero, A., Genovart, M., Delgado, A., and Forero, M. G. (2010). Age and sexual differences in the exploitation of two anthropogenic food resources for an opportunistic seabird. Mar. Biol. 157, 2453–2459. doi: 10.1007/s00227-010-1509-2
Oro, D., Cam, E., Pradel, R., and Martínez-Abraín, A. (2004). Influence of food availability on demography and local population dynamics in a long-lived seabird. Proc. R. Soc. B Biol. Sci. 271, 387–396. doi: 10.1098/rspb.2003.2609
Oro, D., Genovart, M., Tavecchia, G., Fowler, M. S., and Martínez-Abraín, A. (2013). Ecological and evolutionary implications of food subsidies from humans. Ecol. Lett. 16, 1501–1514. doi: 10.1111/ele.12187
Oro, D., Jover, L., and Ruiz, X. (1996). Influence of trawling activity on the breeding ecology of a threatened seabird, Audouin’s gull Larus audouinii. Mar. Ecol. Prog. Ser. 139, 19–29. doi: 10.3354/meps139019
Oro, D., and Ruiz, X. (1997). Exploitation of trawler discards by breeding seabirds in the north-western Mediterranean: differences between the Ebro Delta and the Balearic Islands areas. ICES J. 54, 695–707.
Patrick, S. C., Bearhop, S., Bodey, T. W., Grecian, W. J., Hamer, K.C., Lee, J., et al. (2015). Individual seabirds show consistent foraging strategies in response to predictable fisheries discards. J. Avian Biol. 46: 431–440. doi: 10.1111/jav.00660
Patrick, S. C., Bearhop, S., Grémillet, D., Lescroël, A., Grecian, W. J., Bodey, T. W., et al. (2014). Individual differences in searching behaviour and spatial foraging consistency in a central place marine predator. Oikos 123, 33–40. doi: 10.1111/j.1600-0706.2013.00406.x
Peck, D. R., and Congdon, B. C. (2006). Sex-specific chick provisioning and diving behaviour in the wedge-tailed shearwater Puffinus pacificus. J. Avian Biol. 37, 245–251. doi: 10.1111/j.2006.0908-8857.03558.x
Pennino, M. G., Bevilacqua, A. H., Torres, M. A., Bellido, J. M., Sole, J., Steenbeek, J., et al. (2019). Discard ban: a simulation-based approach combining hierarchical Bayesian and food web spatial models. Mar. Policy 116:103703. doi: 10.1016/j.marpol.2019.103703
Phillips, D. L., Inger, R., Bearhop, S., Jackson, A. L., Moore, J. W., Parnell, A. C., et al. (2014). Best practices for use of stable isotope mixing models in food web studies. Can. J. Zool. 92, 823–835.
Pinet, P., Jaquemet, S., Phillips, R. A., and Le Corre, M. (2012). Sex-specific foraging strategies throughout the breeding season in a tropical, sexually monomorphic small petrel. Anim. Behav. 83, 979–989. doi: 10.1016/j.anbehav.2012.01.019
Pirotta, E., Edwards, E. W. J., New, L., and Thompson, P. M. (2018). Central place foragers and moving stimuli: a hidden-state model to discriminate the processes affecting movement. J. Anim. Ecol. 87, 1116–1125. doi: 10.1111/1365-2656.12830
Post, D. M., Layman, C. A., Arrington, D. A., Takimoto, G., Quattrochi, J., and Montana, C. G. (2007). Getting to the fat of the matter: models, methods and assumptions for dealing with lipids in stable isotope analyses. Oecologia 152, 179–189. doi: 10.1007/s00442-006-0630-x
Quillfeldt, P., Masello, J. F., and Hamer, K. C. (2004). Sex differences in provisioning rules and honest signalling of need in Manx shearwaters, Puffinus puffinus. Anim. Behav. 68, 613–620. doi: 10.1016/j.anbehav.2003.12.002
Robertson, A., McDonald, R. A., Delahay, R. J., Kelly, S. D., and Bearhop, S. (2015). Resource availability affects individual niche variation and its consequences in group-living European badgers Meles meles. Oecologia 178, 31–43. doi: 10.1007/s00442-014-3202-5
Ruckstuhl, K., and Neuhaus, P. (2006). Sexual Segregation in Vertebrates. Cambridge: Cambridge University Press.
Sherley, R. B., Ladd-Jones, H., Garthe, S., Stevenson, O., and Votier, S. C. (2020). Scavenger communities and fisheries waste: North Sea discards support 3 million seabirds, 2 million fewer than in 1990. Fish Fish. 21, 132–145. doi: 10.1111/faf.12422
Sims, D., Nash, J., and Morritt, D. (2001). Movements and activity of male and female dogfish in a tidal sea lough: alternative behavioural strategies and apparent sexual segregation. Mar. Biol. 139, 1165–1175. doi: 10.1007/s002270100666
Skaar, K. L., Jørgensen, T., Ulvestad, B. K. H., and Engås, A. (2011). Accuracy of VMS data from Norwegian demersal stern trawlers for estimating trawled areas in the Barents Sea. ICES J. Mar. Sci. 68, 1615–1620. doi: 10.1093/icesjms/fsr091
Skinner, M. M., Martin, A. A., and Moore, B. C. (2016). Is lipid correction necessary in the stable isotope analysis of fish tissues? Rapid Commun. Mass Spectrom. 30, 881–889. doi: 10.1002/rcm.7480
Smith, J. A., Mazumder, D., Suthers, I. M., and Taylor, M. D. (2013). To fit or not to fit: evaluating stable isotope mixing models using simulated mixing polygons. Methods Ecol. Evol. 4, 612–618. doi: 10.1111/2041-210X.12048
Stauss, C., Bearhop, S., Bodey, T. W., Garthe, S., Gunn, C., Grecian, W. J., et al. (2012). Sex-specific foraging behaviour in northern gannets Morus bassanus: incidence and implications. Mar. Ecol. Prog. Ser. 457, 151–162. doi: 10.3354/meps09734
Stock, B. C., Jackson, A. L., Ward, E. J., Parnell, A. C., Phillips, D. L., and Semmens, B. X. (2018). Analyzing mixing systems using a new generation of Bayesian tracer mixing models. PeerJ 6:e5096. doi: 10.7717/peerj.5096
Stock, B. C., and Semmens, B. X. (2016a). MixSIAR GUI User Manual. Version 3.1. doi: 10.5281/zenodo.56159
Stock, B. C., and Semmens, B. X. (2016b). Unifying error structures in commonly used biotracer mixing models. Ecology 97, 2562–2569. doi: 10.1002/ecy.1517
Stoffel, M. A., Nakagawa, S., and Schielzeth, H. (2017). rptR: repeatability estimation and variance decomposition by generalized linear mixed-effects models. Methods Ecol. Evol. 8, 1639–1644. doi: 10.1111/2041-210X.12797
Syväranta, J., Lensu, A., Marjomäki, T. J., Oksanen, S., and Jones, R. I. (2013). An empirical evaluation of the utility of convex hull and standard ellipse areas for assessing population niche widths from stable isotope data. PLoS One 8:e56094. doi: 10.1371/journal.pone.0056094
Tait, A. H., Raubenheimer, D., Stockin, K. A., Merriman, M., and Machovsky-Capuska, G. E. (2014). Nutritional geometry and macronutrient variation in the diets of gannets: the challenges in marine field studies. Mar. Biol. 161, 2791–2801. doi: 10.1007/s00227-014-2544-1
Thiebault, A., Mullers, R. H. E., Pistorius, P. A., and Tremblay, Y. (2014). Local enhancement in a seabird: reaction distances and foraging consequence of predator aggregations. Behav. Ecol. 25, 1302–1310. doi: 10.1093/beheco/aru132
Thompson, D. R., Lilliendahl, K., Solmundsson, J., and Furness, R. W. (1999). Trophic relationships among six species of Icelandic seabirds as determined through stable isotope. Condor 101, 898–903.
Tremblay, Y., Thiebault, A., Mullers, R., and Pistorius, P. (2014). Bird-borne video-cameras show that seabird movement patterns relate to previously unrevealed proximate environment, not prey. PLoS One 9:e88424. doi: 10.1371/journal.pone.0088424
Vehtari, A., Gelman, A., and Gabry, J. (2017). Practical Bayesian model evaluation using leave-one-out cross-validation and WAIC. Stat. Comput. 27, 1413–1432. doi: 10.1007/s11222-016-9696-4
Villasante, S., Pita, C., Pierce, G. J., Pazos Guimeráns, C., Garcia Rodrigues, J., Antelo, M., et al. (2016). To land or not to land: how do stakeholders perceive the zero discard policy in European small-scale fisheries? Mar. Policy 71, 166–174. doi: 10.1016/j.marpol.2016.05.004
Votier, S. C., Bearhop, S., Witt, M. J., Inger, R., Thompson, D., and Newton, J. (2010). Individual responses of seabirds to commercial fisheries revealed using GPS tracking, stable isotopes and vessel monitoring systems. J. Appl. Ecol. 47, 487–497. doi: 10.1111/j.1365-2664.2010.01790.x
Votier, S. C., Bicknell, A., Cox, S. L., Scales, K. L., and Patrick, S. C. (2013). A bird’s eye view of discard reforms: bird-borne cameras reveal seabird/fishery interactions. PLoS One 8:e57376. doi: 10.1371/journal.pone.0057376
Votier, S. C., Fayet, A. L., Bearhop, S., Bodey, T. W., Clark, B. L., Grecian, J., et al. (2017). Effects of age and reproductive status on individual foraging site fidelity in a long-lived marine predator. Proc. R. Soc. B Biol. Sci. 284:20171068. doi: 10.1098/rspb.2017.1068
Votier, S. C., Furness, R. W., Bearhop, S., Crane, J. E., Caldow, R. W. G., Catry, P., et al. (2004). Changes in fisheries discard rates and seabird communities. Nature 427, 727–730. doi: 10.1038/nature02315
Wagner, E. L., and Boersma, P. D. (2011). Effects of fisheries on seabird community ecology. Rev. Fish. Sci. 19, 157–167. doi: 10.1080/10641262.2011.562568
Wahl, T. R., and Heinemann, D. (1979). Seabirds and fishing vessels: co-occurrence and attraction. Condor 81, 390–396.
Keywords: diet, fisheries, northern gannets, stable isotopes, tracking data
Citation: Giménez J, Arneill GE, Bennison A, Pirotta E, Gerritsen HD, Bodey TW, Bearhop S, Hamer KC, Votier S and Jessopp M (2021) Sexual Mismatch Between Vessel-Associated Foraging and Discard Consumption in a Marine Top Predator. Front. Mar. Sci. 8:636468. doi: 10.3389/fmars.2021.636468
Received: 01 December 2020; Accepted: 19 February 2021;
Published: 15 April 2021.
Edited by:
Alastair Martin Mitri Baylis, South Atlantic Environmental Research Institute, Falkland IslandsReviewed by:
Melinda Grace Conners, Stony Brook University, United StatesTommy Clay, University of California, Santa Cruz, United States
Caitlin Kroeger, Farallon Institute, United States
Copyright © 2021 Giménez, Arneill, Bennison, Pirotta, Gerritsen, Bodey, Bearhop, Hamer, Votier and Jessopp. This is an open-access article distributed under the terms of the Creative Commons Attribution License (CC BY). The use, distribution or reproduction in other forums is permitted, provided the original author(s) and the copyright owner(s) are credited and that the original publication in this journal is cited, in accordance with accepted academic practice. No use, distribution or reproduction is permitted which does not comply with these terms.
*Correspondence: Joan Giménez, Z2ltZW5lei52ZXJkdWdvQGdtYWlsLmNvbQ==
†These authors have contributed equally to this work