- 1Zhejiang Key Laboratory of Aquatic Germplasm Resources, College of Biological and Environmental Sciences, Zhejiang Wanli University, Ningbo, China
- 2School of Marine Sciences, Ningbo University, Ningbo, China
Beyond its role as an oxygen transport protein, the homodimer hemoglobin of blood clam Tegillarca granosa (Tg-HbI) has been found to possess antibacterial activity. However, the mechanism of antibacterial activity of Tg-HbI remain to be investigated. In this study, we investigated the effects of Cu2+ on the structure, peroxidase activity, and antibacterial ability of Tg-HbI. Tg-HbI was significantly inactivated by Cu2+ in a non-competitive inhibition manner, following first-order reaction kinetics. The Spectroscopy results showed that Cu2+ changed the iron porphyrin ring and the coordination of heme with proximal histidine of Tg-HbI, and increased the hydrophobicity of heme pocket. We found that proline could stabilize the heme pocket structure of Tg-HbI, hence, protect peroxidase activity and antimicrobial activity of Tg-HbI against damage by Cu2+. Our results suggest that Cu2+ inhibits the peroxidase and antibacterial activity of Tg-HbI by destroying its heme pocket structure and Tg-HbI probably plays an antibacterial role through its peroxidase activity. This result could provide insights into the antibacterial mechanism of Tg-HbI.
Introduction
The blood clam (Tegillarca granosa), a marine invertebrate of important economic value, inhabits the sandy muds of the intertidal zone in the east coast of China and Southeast Asia. T. granosa belongs to the family Arcidae of the class Bivalvia (phylum Mollusca), one of a few invertebrate groups that have hemoglobin-containing red hemocytes in the hemolymph (Bao et al., 2011a,b). So far, two kinds of hemoglobin (Hb), Tg-HbI (homodimer), and Tg-HbII (tetramer), have been purified from T. granosa hemocytes. Tg-HbI is made up of a single type of polypeptide chain, which differs from two chains of heterotetramer in molecular weight and isoelectric point (Bao et al., 2013, 2016; Wang et al., 2014). Homodimer Hb exists in only a few invertebrates (Weber and Vinogradov, 2001), unlike tetramer Hb, which exists in both vertebrates, and invertebrates.
Several studies have shown that Hb from more than one species, such as human, snake, mouse, horse, not only transports oxygen and carbon dioxide, but also has antibacterial effects (Hodson and Hirsch, 1958; Parish et al., 2001; Jiang et al., 2007; Du et al., 2010). In recent years, the antibacterial activity of Hb has been reported in Arcidae like T. granosa and Scapharca subcrenata (Wang et al., 2014, 2017; Xu et al., 2015; Bao et al., 2016). The mechanisms underlying the antibacterial activity of Hb still remain poorly perceived.
The peroxidase activity of hemoglobin also has been detected in several species, such as human, bacteria Vitreoscilla, and the invertebrate T. granosa (Kawano et al., 2002; Kvist et al., 2007; Wang et al., 2017). Why the hemoglobin possess peroxidase activity remains to be investigated. In addition, some researchers have hypothesized that this peroxidase activity is essential for Hb's antimicrobial activity (Jiang et al., 2007; Du et al., 2010), however, in-depth research has not been carried out in this area.
Preliminary research done by us showed that Tg-HbI has stronger peroxidase activity and antibacterial ability than Tg-HbII (Wang et al., 2014). It is plausible to speculate that Tg-HbI may play an important role in immune defense through peroxidase activity. It is of great significance to study the antibacterial mechanism of Tg-HbI, given that homodimer Hb only exist in invertebrates.
Moreover, in preliminary research we found that Cu2+ can inhibit peroxidase activity of Tg-HbI (Wang et al., 2017). The aim of the current work was to study the effects of Cu2+ on Tg-HbI with respect to changes in structure and antibacterial activity, which would be helpful in exploring the association of structure, peroxidase activity, and antibacterial activity, and could provide useful avenues for further research on the antibacterial mechanism of Tg-HbI.
Materials and Methods
Peroxidase Activity Assay of Tg-HbI
Tg-HbI, which produces a single band on dodecyl sulfate sodium salt polyacrylamide gel electrophoresis(SDS-PAGE), was purified from T. granosa with phosphate buffered saline (PBS) buffer using a method that has been reported in our earlier work (Wang et al., 2014). Peroxidase activity was measured spectrophotometrically, as reported previously (Wang et al., 2017); the substrate buffer used was 50 mM acetate buffer (pH 5.0) and the detection time was 0.5 min. The activity was measured with ultraviolet-visible spectrophotometer (UV-1800, Shimadzu, Japan) connected with a recorder. The final concentration of Tg-HbI in the peroxidase activity measurement system was 30 μg/mL.
Cu2+ dissolved in 50 mM acetic acid buffer (pH 5.0) at different concentrations were incubated with purified Tg-HbI for 2 h at 25°C, and then peroxidase activity was measured. To test the protection from proline (Pro) to Tg-HbI, the Tg-HbI was incubated with 0.2 mM Cu2+ and different concentrations of Pro for 2 h at 25°C, and then the activity was measured. All measurements were done in triplicate.
Spectroscopy Assay of Tg-HbI
Tg-HbI was incubated in 50 mM acetic acid buffer (pH 5.0) with various concentrations of Cu2+ and Pro for 2 h at 25°C. The final concentration of Tg-HbI was 300 μg/mL. The structure changes of Tg-HbI were detected by UV-visible spectra, intrinsic fluorescence emission spectra and 1, 8-anilinonaphthalenesulfonate (ANS)-binding fluorescence spectra.
The conformational change of Tg-HbI was determined with UV-visible spectrophotometer (UV-1800, Shimadzu, Japan) at 200–700 nm. The change in the microenvironment around tryptophan (Trp) was detected with spectrofluorometer (F-2500, Hitach, Japan), and the excitation and emission wavelengths were 280 nm and 300–400 nm, respectively. ANS dye can bind to hydrophobic amino acid residues and use for probing the hydrophobic surface. Fluorescence was measured after incubation of 40 μM ANS with the samples for 40 min in the dark, and the excitation and emission wavelengths were 390 nm and 420–600 nm, respectively.
Antibacterial Ability Assay of Tg-HbI
The agarose diffusion method was used to explore the effect of Cu2+ on the antibacterial ability of Tg-HbI (Wang et al., 2014). Tg-HbI in group A was mixed with 50 mM PBS (pH 7.2). Tg-HbI in group B was mixed with 50 mM PBS (pH 7.2) containing Cu2+. Tg-HbI in group C was mixed with 50 mM PBS (pH 7.2) containing Cu2+ and Pro. The inhibition circle size reflects the antibacterial ability.
Results
The Effect of Cu2+ on the Peroxidase Activity of Tg-HbI
Pure Tg-HbI was assayed after incubation with different concentrations of Cu2+ for 2 h at 25°C. Tg-HbI was inactivated by Cu2+ in a dose-dependent manner. When the Cu2+ concentration was higher than 0.5 mM, Tg-HbI activity was completely abolished. The IC50 (half maximal inhibitory concentration) value was measured as 0.103±0.007 mM (n = 3) (Figure 1A). A Lineweaver-Burk plot analysis was performed to evaluate the mode of inhibition. The apparent Vmax (velocity maximum) changed while the Km (michaelis constant) did not, indicating that Cu2+ induced non-competitive inhibition for guaiacol (Figure 1B). To evaluate the inactivation kinetics, time interval measurements were performed. The different time courses of Tg-HbI in the presence of 0.02, 0.05, 0.1, 0.15, 0.2, and 0.25 mM Cu2+, were recorded (Figure 1C). The results indicated that catalytic activity changed detectably with time from the lowest (0.02 mM) to the highest (0.25 mM) Cu2+ concentration. The enzyme activity gradually decreased in a time-dependent manner with first-order reaction kinetics.
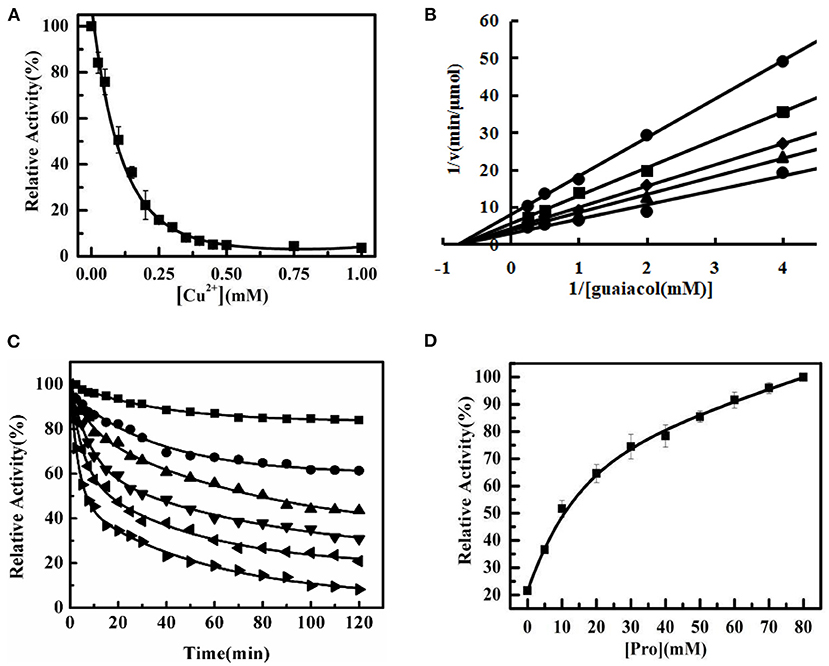
Figure 1. The inactivation of Tg-HbI induced by Cu2+. (A) The effect of Cu2+ on the peroxidase activity of Tg-HbI. Tg-HbI was assayed after incubation with different concentrations of Cu2+ in 50 mM acetate buffer (pH 5.0) for 2 h at 25°C. The data are presented as means (n = 3) with standard deviations. (B) Lineweaver-Burk plot for Tg-HbI in the presence of Cu2+.The concentration of Cu2+ from top to bottom is 0.3, 0.2, 0.1, 0.05, 0 mM. (C) Time interval kinetic measurements of Tg-HbI inactivation. Cu2+ concentration is 0.02 (■), 0.05 (•), 0.1 (▴), 0.15 (▾), 0.2 (◂), 0.25 (▸) mM. (D) The peroxidase activity of Tg-HbI after adding proline. The Tg-HbI was incubated with 0.2 mM Cu2+ and different concentrations of proline at 25°C for 2 h and then the activity was measured. The final concentration of Tg-HbI incubating with Cu2+ was 300 μg/mL.
After Tg-HbI was incubated with 0.2 mM Cu2+ and different concentrations of Pro for 2 h at 25°C, the peroxidase activity of Tg-HbI was measured. The peroxidase activity of Tg-HbI was significantly protected with increasing Pro concentrations. When the concentration of Pro was higher than 30 mM, more than 70% of the activity of Tg-HbI remained (Figure 1D). These results indicate that Pro could prevent Tg-HbI from inactivation by Cu2+.
The Change in Tertiary Structure of Tg-HbI Induced by Cu2+
Ultraviolet-visible absorption spectroscopy was used to investigate the conformational changes in Tg-HbI. The Soret band near 420 nm is formed by the coordination of heme and histidine, and is often used to characterize the changes between the porphyrin ring and conformation of protein, whereas the Q band, two weak absorption peaks appearing in the range of 500–600 nm, is the characteristic peak of iron porphyrin (Basak et al., 2015). The results showed that the Soret band of Tg-HbI had a blue shift, and the Q band of Tg-HbI gradually disappeared, with an increase in Cu2+ concentration, thereby indicating that the iron porphyrin ring of Tg-HbI and the coordination of heme and histidine had changed (Figures 2A,B).
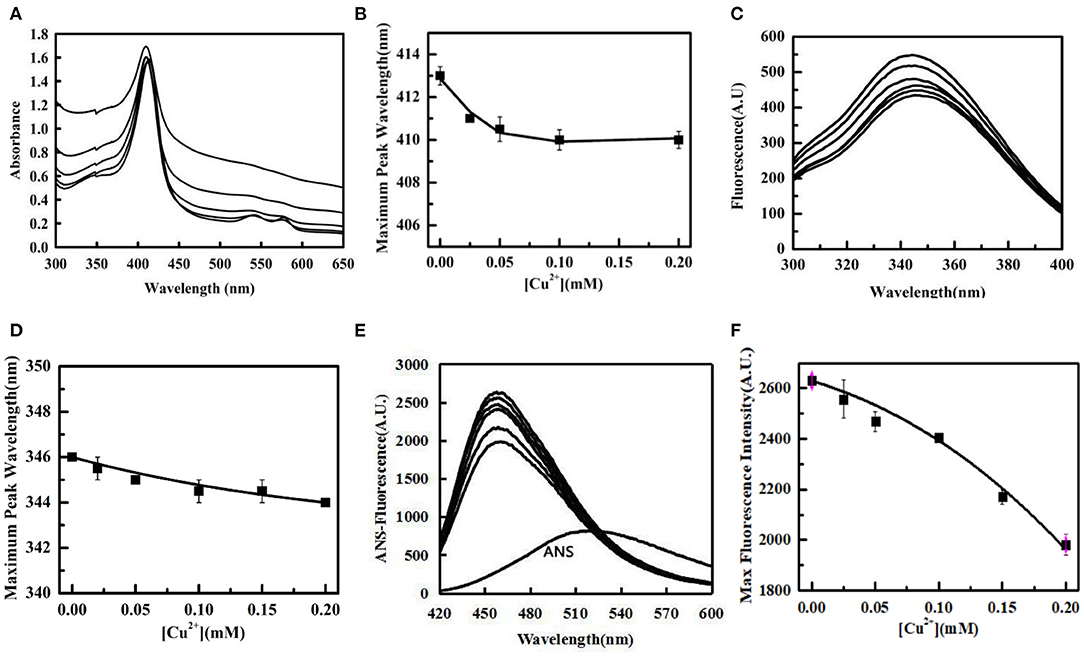
Figure 2. The change of tertiary structure of Tg-HbI induced by Cu2+. (A) UV absorption spectrum of Tg-HbI. The concentration of Cu2+ from bottom to top is 0, 0.02, 0.05, 0.1, 0.2 mM. (B) Plot of maximum peak wavelength vs. [Cu2+]. The data were obtained from (A). (C) Intrinsic fluorescence changes of Tg-HbI. The concentration of Cu2+ from top to bottom is 0, 0.02, 0.05, 0.1, 0.15, 0.2 mM. (D) Plot of maximum fluorescence peak wavelength vs. [Cu2+]. The data were obtained from (C). (E) ANS-binding fluorescence changes of Tg-HbI. The concentration of Cu2+ from bottom to top is 0.2, 0.15, 0.1, 0.05, 0.02, 0.0 mM. (F) Plot of maximum fluorescence intensity vs. [Cu2+]. The data were obtained from (E). The final concentration of Tg-HbI was 300 μg/mL.
Trp has intrinsic fluorescence and is usually used as a probe to detect the conformation change of protein. The results showed that not only fluorescence intensity increased with increase in Cu2+ concentration in linear mode, but also the maximum emission wavelength showed a blue shift (Figures 2C,D). This indicated that Cu2+ caused an increase in non-polarity in the microenvironment around it. The ANS fluorescence was used to monitor the tertiary structural changes of Tg-HbI. The results showed that the ANS fluorescence intensity of Tg-HbI reduced with increase in Cu2+ concentration in a concentration-dependent manner (Figures 2E,F). This indicated that Cu2+ reduced the hydrophobic surfaces of Tg-HbI.
The change in tertiary structure of Tg-HbI were detected after Tg-HbI was incubated with 0.2 mM Cu2+ and different concentrations of Pro for 2 h at 25°C, correspondingly. The results of UV-visible absorption spectroscopy showed that Soret band of Tg-HbI had a red shift and Q band gradually manifested with increase in Pro (Figures 3A,B). It is evident that the addition of Pro protects the structure of the iron porphyrin ring and heme pocket structure of Tg-HbI from damage by Cu2+.
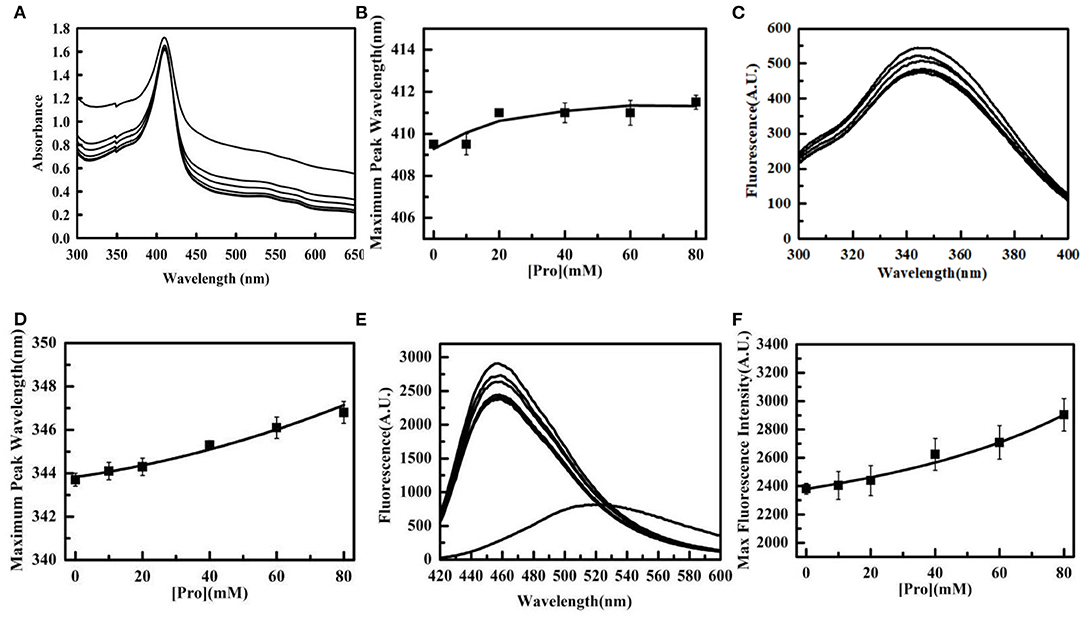
Figure 3. The protection of Pro to the tertiary structure of Tg-HbI. (A) UV absorption spectrum of Tg-HbI in the presence of proline. The concentration of Pro from top to bottom is 0, 10, 20, 40, 60, 80 mM. (B) Plot of maximum peak wavelength vs. [Pro]. The data were obtained from (A). (C) Intrinsic fluorescence changes of Tg-HbI in the presence of Pro. The concentration of Pro from bottom to top is 80, 0, 60, 10, 20, 40 mM. (D) Plot of maximum peak wavelength vs. [Pro].The data were obtained from (C). (E) ANS-binding fluorescence changes of Tg-HbI in the presence of proline. The concentration of Pro from bottom to top is 0, 10, 20, 40, 60, 80 mM. (F) Plot of maximum fluorescence intensity vs. [Pro]. The data were obtained from (E). The final concentrations of Tg-HbI and Cu2+ were 300 μg/mL and 0.2 mM, respectively.
The intrinsic fluorescence intensity decreased with increase in Pro until Pro concentration reached 30 mM, and the maximum emission wavelength showed a red shift with increase in Pro concentration, ranging from 0 to 80 mM (Figures 3C,D). These results showed that Pro can stabilize the polar microenvironment around Trp. The intrinsic fluorescence intensity increased with increase in Pro, when Pro concentration was higher than 30 mM. The reason was possibly because Pro is a non-polar amino acid, causing an increase in non-polarity when its concentration was high.
Pro reduced the effect of hydrophobic structure inside the Tg-HbI induced by 0.2 mM Cu2+ in a dose-dependent manner, as measured by ANS binding fluorescence (Figures 3E,F). Similar to the protection of peroxidase activity shown in Figure 1D, Pro acted as a stabilizer for protecting the tertiary structure of Tg-HbI.
The Effect of Cu2+ on the Antibacterial Activity of Tg-HbI
The size of the antibacterial circle outside the Oxford cup can reflect antibacterial ability. The results showed that the antibacterial ability of Tg-HbI disappeared in the presence of 0.2 mM Cu2+ and was restored when 80 mM Pro was added (Figure 4). This was consistent with the fact that Cu2+ could destroy the structure and peroxidase activity of Tg-HbI and Pro could protect the structure and peroxidase activity from being destroyed by Cu2+.
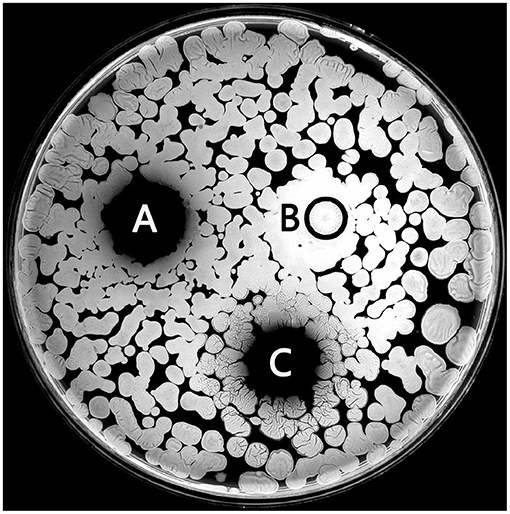
Figure 4. The antibacterial activity of Tg-HbI. (A) Tg-HbI; (B) Tg-HbI mixing with Cu2+; (C) Tg-HbI mixing with Cu2+ and proline. The final concentrations of Tg-HbI, Cu2+, and proline were 1 mg/ml, 0.2 mM, and 80 Mm, respectively.
Discussion
In this study, Cu2+ induced a non-competitive inhibition for guaiacol and inhibited the peroxidase activity of Tg-HbI in a dose-dependent manner. The enzyme activity gradually decreased in a time-dependent manner with first-order reaction kinetics, and inactivation of Tg-HbI accelerated with increase in Cu2+ concentration.
During protein unfolding, hydrophobic amino acids embedded deep in the protein are gradually exposed, resulting in the increase of hydrophobic surface of protein and the enhancement of ANS fluorescence intensity. Try is usually used as an intrinsic fluorescence probe to detect the conformation change of protein due to its high quantum yield, relatively sensitive to environmental changes and low content in protein molecule. The exposure of Try to the aqueous solution will result in decreased fluorescence intensity and red shift of the maximum emission wavelength. There are two Trys in one heme pocket of Tg-HbI. In this study, the intrinsic fluorescence results showed that Cu2+ increased the intrinsic fluorescence intensity of Tg-HbI and caused blue shift of the maximum emission wavelength. This indicated that Cu2+ didn′t unfold Tg-HbI, and even enhanced the hydrophobicity around Try, which was further verified by the result that Cu2+ reduced the hydrophobic surface of Tg-HbI. UV absorption spectroscopy showed that Cu2+ changed the iron porphyrin ring and the coordination of heme and histidine of Tg-HbI.
It has been reported that Cu2+ was located within 10Å of the heme iron of the β subunit in human hemoglobin, coordinating with the imidazole nitrogen of proximal histidine in heme pocket and forming a copper-imidazole-iron configuration (Antholine et al., 1984, 1985). The heme pocket, containing heme, proximal histidine and distal histidine, is the key structures needed for peroxidase activity (Wang et al., 2017). The proximal histidine formed coordination adducts with the heme iron atom, whereas the corresponding distal coordination site above the plane of the heme was vacant. The cavity can bind H2O2, the substrate of peroxidase (Mika et al., 2008; Wang et al., 2017). Therefore, we speculated that Cu2+ changed the microenvironment of the cavity and increased the hydrophobicity of Tg-HbI heme pocket through forming a copper-imidazole-iron configuration. This was not conducive to H2O2 bonding with heme, and thereby inhibited the peroxidase activity of Tg-HbI. This was further verified by the result that the inactivation of peroxidase activity was synchronized with the conformational change of Tg-HbI.
The protective effects of Pro on the structure and activity of some proteins have been reported, but the exact mechanism is unknown (Ignatova and Gierasch, 2006; Lü et al., 2009; Wang et al., 2013). In this study, Tg-HbI retained its peroxidase activity when Pro stabilized of heme pocket in prensence of Cu2+. Pro maybe prevent Cu2+ from forming a copper-imidazole-iron configuration with Tg-HbI or maintain the microenvironment of heme pocket even after forming a copper-imidazole-iron configuration, however, the specific mechanism remains to be further studied. The protective effect of glycine on the structure and activity of Tg-HbI was similar to that of Pro (not shown).
Tg-HbI lost its antimicrobial activity when it lost its peroxidase activity on account of damage to the active site by Cu2+, whereas Tg-HbI retained its antimicrobial activity when Pro was used to protect its peroxidase activity. So, we speculated that Tg-HbI played an antibacterial role by virtue of its peroxidase activity. In fact, it is widely reported that some peroxidases have antimicrobial activity (Edwin and Thomas, 1978; Borelli et al., 2003; Wojciechowski et al., 2005; Almagro et al., 2009). Myeloperoxidase plays an important role in microbicidal action of phagocytes by oxidizing Cl− to form HOCl (Klebanoff, 1999, 2005; Malle et al., 2007), and lactoperoxidase is responsible for antimicrobial properties of bovine milk (Boots and Floris, 2006; Bafort et al., 2015). The peroxidase in Arabidopsis is a major component of pattern-triggered immunity (Daudia et al., 2012). Jiang et al. (2007) and Du et al. (2010) have also proposed that human hemoglobin can generate microbicidal free radicals by its peroxidase activity. It is reasonable, therefore, to conclude that Tg-HbI plays an antimicrobial role through its intrinsic peroxidase activity, similar to some peroxidases.
In conclusion, Tg-HbI lost peroxidase activity and antibacterial ability in presence of Cu2+, because Cu2+ changed the iron porphyrin ring and microenvironment of the heme pocket of Tg-HbI. Pro could stabilize the heme pocket of Tg-HbI, and protect peroxidase and antimicrobial activity of Tg-HbI against damage by Cu2+, accordingly. Our results indicate that Cu2+ inhibits the peroxidase and antibacterial activity of Tg-HbI by destroying its heme pocket structure, and Tg-HbI perhaps play an antibacterial role through its peroxidase activity. It is worth further evaluating the antibacterial activity in vivo and antibacterial mechanism of Tg-HbI in future studies.
Data Availability Statement
The raw data supporting the conclusions of this article will be made available by the authors, without undue reservation.
Author Contributions
SW and YB: conceptualization. XY and SZ: methodology. XY and SW: data curation. XY: writing—original draft preparation. SW, ZC, and ZL: writing—review and editing. All authors have read and agreed to the published version of the manuscript.
Funding
This research was supported by the National Natural Science Foundation of China (31672678), Natural Science Foundation of Zhejiang (LY19C190002), Natural Science Foundation of Ningbo (2018A620226), Key Natural Science Key Foundation of Zhejiang (LZ20C190001), Demonstration Project for Innovative Development of Marine Economy (NBHY-2017-S4), Zhejiang Provincial Top Key Discipline of Biological Engineering (KF2020005), and The Basic Scientific Research Operating Expense Project of Zhejiang Provincial Universities.
Conflict of Interest
The authors declare that the research was conducted in the absence of any commercial or financial relationships that could be construed as a potential conflict of interest.
Acknowledgments
We thank International Science Editing (http://www.internationalscienceediting.com) for editing this manuscript.
References
Almagro, L., Gómez Ros, L. V., Belchi-Navarro, S., Bru, R., Ros Barceló, A., and Pedreño, M. A. (2009). Class III peroxidases in plant defence reactions. J. Exp. Bot. 60, 377–390. doi: 10.1093/jxb/ern277
Antholine, W. E., Basosi, R., Hyde, J. S., and Taketa, F. (1984). Interaction between low-affinity cupric ion and human methemoglobin. J. Inorg. Biochem. 21, 125–136. doi: 10.1016/0162-0134(84)85045-X
Antholine, W. E., Taketa, F., Wang, J. T., Manoharan, P. T., and Rifkind, J. M. (1985). Interaction between bound cupric ion and spin-labeled cysteine β-93 in human and horse hemoglobins. Jo. Inorg. Biochem. 25, 95–108. doi: 10.1016/0162-0134(85)80018-0
Bafort, F., Parisi, O., Perraudin, J. P., and Jijakli, M. H. (2015). Mode of action of lactoperoxidase as related to its antimicrobial activity: a review. Enzyme Res. 2014:517164. doi: 10.1155/2014/517164
Bao, Y., Wang, J., Li, C., Li, P., and Lin, Z. (2016). A preliminary study on the antibacterial mechanism of Tegillarca granosa hemoglobin by derived peptides and peroxidase activity. Fish Shellfish Immunol. 51, 9–16. doi: 10.1016/j.fsi.2016.02.004
Bao, Y., Wang, Q., and Lin, Z. (2011a). Hemoglobin of the bloody clam Tegillarca granosa (Tg-HbI) is involved in the immune response against bacterial infection. Fish Shellfish Immunol. 31, 517–523. doi: 10.1016/j.fsi.2011.05.029
Bao, Y. B., Wang, Q., Guo, X. M., and Lin, Z. H. (2013). Structure and immune expression analysis of hemoglobin genes from the blood clam Tegillarca granosa. Genet. Mol. Res. 12, 3110–3123. doi: 10.4238/2013.February.28.5
Bao, Y. B., Wang, Q., Liu, H. M., and Lin, Z. H. (2011b). A small HSP gene of bloody clam (Tegillarca granosa) involved in the immune response against Vibrio parahaemolyticus and lipopolysaccharide. Fish Shellfish Immunol. 30, 729–733. doi: 10.1016/j.fsi.2010.12.002
Basak, P., Kundu, N., Pattanayak, R., and Bhattacharyya, M. (2015). Denaturation properties and folding transition states of leghemoglobin and other heme proteins. Biochemistry 80, 463–472. doi: 10.1134/S0006297915040100
Boots, J. W., and Floris, R. (2006). Lactoperoxidase: from catalytic mechanism to practical applications. Int. Dairy J. 16, 1272–1276. doi: 10.1016/j.idairyj.2006.06.019
Borelli, V., Vita, F., Shankar, S., Soranzo, M. R., Banfi, E., Scialino, G., et al. (2003). Human eosinophil peroxidase induces surface alteration, killing, and lysis of mycobacterium tuberculosis. Infect. Immun. 71, 605–613. doi: 10.1128/IAI.71.2.605-613.2003
Daudia, A., Cheng, Z., O'Brien, J. A., Mammarella, N., Khan, S., Ausubel, F. M., et al. (2012). The apoplastic oxidative burst peroxidase in Arabidopsis is a major component of pattern-triggered immunity. Plant Cell 24, 275–287. doi: 10.1105/tpc.111.093039
Du, R., Ho, B., and Ding, J. L. (2010). Rapid reprogramming of hemoglobin structure-function exposes multiple dual-antimicrobial potencies. EMBO J. 29, 632–642. doi: 10.1038/emboj.2009.380
Edwin, L., and Thomas, M. (1978). Cofactor role of iodide in peroxidase antimicrobial action against Escherichia coli. Antimicrob. Agents Chemother. 13, 1000–1005. doi: 10.1128/AAC.13.6.1000
Hodson, D., and Hirsch, J. (1958). The antibacterial activity of hemoglobin. J. Exp. Med. 107, 167–183. doi: 10.1084/jem.107.2.167
Ignatova, Z., and Gierasch, L. M. (2006). Inhibition of protein aggregation in vitro and in vivo by a natural osmoprotectant. Proc. Natl. Acad. Sci. U.S.A. 103, 13357–13361. doi: 10.1073/pnas.0603772103
Jiang, N., Tan, N. S., Ho, B., and Ding, J. L. (2007). Respiratory protein-generated reactive oxygen species as an antimicrobial strategy. Nat. Immunol. 8, 1114–1121. doi: 10.1038/ni1501
Kawano, T., Pinontoan, R., Hosoya, H., and Muto, S. (2002). Monoamine-dependent production of reactive oxygen species catalyzed by pseudoperoxidase activity of human hemoglobin. J. Agric. Chem. Soc. Jpn. 66, 1224–1232. doi: 10.1271/bbb.66.1224
Klebanoff, S. (1999). Myeloperoxidase. Proc. Assoc. Am. Physicians 111, 383–389. doi: 10.1111/paa.1999.111.5.383
Klebanoff, S. (2005). Myeloperoxidase: friend and foe. J. Leukoc. Biol. 77, 598–625. doi: 10.1189/jlb.1204697
Kvist, M., Ryabova, E. S., Nordlander, E., and Bülow, L. (2007). An investigation of the peroxidase activity of vitreoscilla hemoglobin. J. Biol. Inorg. Chem. 12, 324–334. doi: 10.1007/s00775-006-0190-x
Lü, Z. R., Wang, Y. J., Lee, D. Y., Park, Y. D., Zou, H. C., and Zou, F. (2009). The effect of Zn2+ on human brain creatine kinase: unfolding and aggregation studies. J. Biomol. Struct. Dyn. 26, 567–574. doi: 10.1080/07391102.2009.10507272
Malle, E., Furtmüller, P. G., Sattler, W., and Obinger, C. (2007). Myeloperoxidase: a target for new drug development? Br. J. Pharmacol. 152, 838–854. doi: 10.1038/sj.bjp.0707358
Mika, A., Buck, F., and Luethje, S. (2008). Membrane-bound class III peroxidases: identification, biochemical properties and sequence analysis of isoenzymes purified from maize (Zea maysL.) roots. Proteomics 71, 412–424. doi: 10.1016/j.jprot.2008.06.006
Parish, C. A., Jiang, H., Tokiwa, Y., Berova, N., Nakanishi, K., McCabe, D., et al. (2001). Broad-spectrum antimicrobial activity of hemoglobin. Bioorg. Med. Chem. 9, 377–382. doi: 10.1016/S0968-0896(00)00263-7
Wang, S. F., Bao, Y. B., Shi, M. J., Zheng, D. N., Yang, T. T., and Lin, Z. H. (2014). Purification and antibacterial activity of hemoglobin from Tegillarca granosa. Acta Oceanol. Sin. 36, 67–73. doi: 10.3969/j.issn.0253-4193
Wang, S. F., Lee, J., Wang, W., Si, Y. X., Li, C. Y., Tae-Rae Kim, T. R., et al. (2013). The effect of Zn2+ on human brain creatine kinase: unfolding and aggregation studies. J. Biomol. Struct. Dyn. 31, 572–590. doi: 10.1080/07391102.2012.706074
Wang, S. F., Yu, X. P., Lin, Z. H., Zhang, S. Q., Bao, Y. B., and Xue, Q. G. (2017). Hemoglobins likely function as peroxidase in blood clam Tegillarca granosa hemocytes. J. Immunol. Res. 2017:7125084. doi: 10.1155/2017/7125084
Weber, R. E., and Vinogradov, S. N. (2001). Nonvertebrate hemoglobins: functions and molecular adaptations. Physiol. Rev. 81, 569–628. doi: 10.1152/physrev.2001.81.2.569
Wojciechowski, G., and Huang, L. S., and Montellano, P. (2005). Autocatalytic modification of the prosthetic heme of horseradish but not lactoperoxidase by thiocyanate oxidation products, a role for hemeprotein covalent cross-linking. J. Am. Chem. Soc. 127, 15871–15879. doi: 10.1021/ja054084t
Keywords: tegillarca granosa, homodimer hemoglobin, Cu2+, structure, peroxidase activity, antibacterial activity
Citation: Wang S, Yu X, Zhang S, Jin H, Chen Z, Lin Z and Bao Y (2021) Cu2+ Inhibits the Peroxidase and Antibacterial Activity of Homodimer Hemoglobin From Blood Clam Tegillarca granosa by Destroying Its Heme Pocket Structure. Front. Mar. Sci. 8:635210. doi: 10.3389/fmars.2021.635210
Received: 30 November 2020; Accepted: 15 January 2021;
Published: 10 February 2021.
Edited by:
Xiaotong Wang, Ludong University, ChinaReviewed by:
Hetron Mweemba Munang'andu, Norwegian University of Life Sciences, NorwayXiaoting Huang, Ocean University of China, China
Copyright © 2021 Wang, Yu, Zhang, Jin, Chen, Lin and Bao. This is an open-access article distributed under the terms of the Creative Commons Attribution License (CC BY). The use, distribution or reproduction in other forums is permitted, provided the original author(s) and the copyright owner(s) are credited and that the original publication in this journal is cited, in accordance with accepted academic practice. No use, distribution or reproduction is permitted which does not comply with these terms.
*Correspondence: Zhihua Lin, zhihua9988@126.com; Yongbo Bao, bobbao2001@gmail.com
†These authors have contributed equally to this work