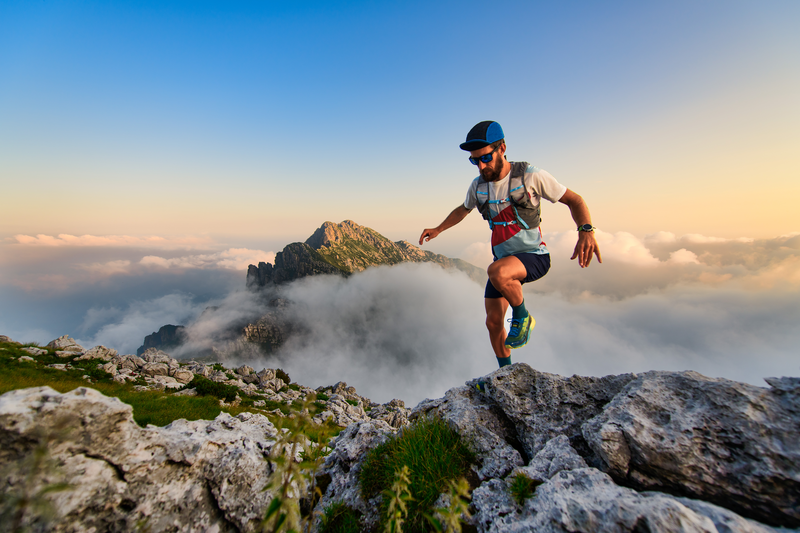
94% of researchers rate our articles as excellent or good
Learn more about the work of our research integrity team to safeguard the quality of each article we publish.
Find out more
ORIGINAL RESEARCH article
Front. Mar. Sci. , 18 March 2021
Sec. Marine Conservation and Sustainability
Volume 8 - 2021 | https://doi.org/10.3389/fmars.2021.633118
This article is part of the Research Topic Evaluating Invasion Processes of Alien and Neonative Species via Impact Assessment Protocols. View all 4 articles
Invasive alien species (IAS) are currently considered one of the greatest threats to global marine ecosystems. Thus, ships and maritime activity have been identified as the main factors responsible for the vast majority of accidental species translocations around the world, implying that prevention should be the core of environmental port policies. Preventive port strategies should include analyzing risks based on traffic origins and volumes, revising port policies for inspections, estimating probabilities of non-indigenous species (NIS) appearance, monitoring routine species within ports, and finally implementing management plans and focused actions. Here, we conducted a comprehensive NIS prediction analysis for the port of Gijon (northern Spain), one of the largest ports in the south Bay of Biscay, as a case study that can be extrapolated to other international seaports. An extensive bibliographic search (1953–2020) was conducted and we identified 380 species that have been transported through hull fouling and ballast water around the world. We evaluated their likelihood of arriving (from 14 years of traffic data) and becoming established (from habitat suitability and demonstrated impacts and invasion ability) within the Gijon port, creating a new NIS Invasion Threat Score (NIS-ITS). This new index could help to identify target species that are likely invaders for early detection and prevention policies within the port. The results showed that 15 NIS had >90% likelihood of becoming a biological invasion problem in Gijon Port. At the same time, we reported morphological and genetic analysis of biota found in two successive annual monitoring surveys of Gijon port and ships (n = 612 individuals) revealing 18 NIS, including 6 of the NIS predicted from high NIS-ITS. Actually, 80% (12 NIS) of those potentially most dangerous species (NIS-ITS > 90%) have already been detected in the Bay of Biscay area. We propose the use of this new tool for a risk-reduction strategy in ports, based on accurate predictions that help in promoting specific early detection tests and specific monitoring for NIS that have a high chance of establishment. All international seaports can adopt this strategy to address the problem of biological invasions and become “blueports” in line with EU policy.
The distribution of marine taxa is limited by natural barriers to their dispersal (Elton, 1958). However, over the past centuries, human activities have modified those barriers (Vemeij, 1978) and, consequently, the distribution of many species (Kotta et al., 2016). At present, based on data from the European Alien Species Information Network (EASIN), there are approximately 14,000 non-indigenous species (NIS) recorded in Europe (EASIN Catalog)1 and invasive alien species (IAS) represent a major threat to the ecological, economic and social values of marine environments (Vanderploeg et al., 2002; Molnar et al., 2008). They are considered one of the greatest threats to global marine ecosystems, affecting their structure and function (Costello et al., 2010), with negative socioeconomic consequences (e.g., Gherardi et al., 2011). Ballast water and hull fouling, associated with maritime transport, are responsible for the vast majority of accidental marine translocations around the world (Carlton, 2001; Hewitt et al., 2009; Seebens et al., 2013), however, there are other human-mediated pathways that can be used by species to spread to new habitats, such as the opening of new corridors that facilitate the spread of species (Steger et al., 2019; Castellanos-Galindo et al., 2020) or escapes from aquaculture facilities (Thorvaldsen et al., 2015; Ju et al., 2020) among others.
At a global scale, ships are widely recognized as the primary vector of aquatic biological invasions (Carlton, 1985; Ruiz et al., 2000; Hewitt et al., 2004; Gollasch, 2006). Additionally, ships contain sub-vectors, including ballast water (Carlton, 1985), ballast sediments (Bailey et al., 2005), hull biofouling (Allen, 1953; Ashton et al., 2016), internal tank biofouling (Drake et al., 2005), sea chests (Coutts, 1999), and internal seawater piping systems (Carlton, 1985; Lewis and Dimas, 2007). As a result, all ships are different in terms of vector risk, and there are differences in species entrainment, transfer and release among different ship types (Davidson et al., 2018).
Risk analyses and management actions to reduce ship-mediated speciesdispersal around the world are being developed and have increased as marine biosecurity concerns grow (Davidson et al., 2018). There are several international legislative frameworks focused on managing the introduction and spread of potential NIS. Within the European Union, the main policy and legislative drivers to target marine NIS are the Marine Strategy Framework Directive (MSFD), the Biodiversity Strategy, and EU Regulation no. 1143/2014 on Aquatic Invasive Species (EC, 2014). Within this legislative framework, the EU blue growth strategy advocates for a blue economy that exploits natural resources in a sustainable way and protects the environment (European Commission, 2012). This includes the Blueports objective, a strategy that aims to create an adequate management of resources along with new strategies to avoid biological invasions and the environmental or economic impacts that these can produce (Dopico Rodríguez and Borrell Pichs, 2020). Recently, the first EU-scale Horizon Scanning (HS) focusing on marine NIS was performed, aiming to deliver a ranked list of species that should be of high priority for risk assessment (Tsiamis et al., 2020). These lists of species with invasive potential can be used to update the EU regulation 1143/2014, which shows a very limited coverage of marine invasive species and, this way, preventive actions against these potentially harmful species can be promoted (Tsiamis et al., 2020). These global initiatives are extremely relevant, although they can lose effectiveness if they are not properly interpolated and adjusted to the characteristics of the different marine areas in a much more concrete or specific way within the European Union (areas, countries, even ports). On the other hand, it is necessary to develop accessible and friendly methodologies for stakeholders (usually not experts in biological invasions) with the responsibility of making decisions that can prevent these events (e.g., port authorities).
Outside of Europe, a set of port monitoring guidelines have been developed to maintain up-to-date records of the distribution of NIS, such as those for Australian ports (Hayes et al., 2019). Similarly, in New Zealand, a series of targeted (species-specific) surveys are now implemented in 11 harbors every 6 months (Arthur et al., 2015). Moreover, in order to avoid species introductions via marine traffic, some Australian ports require arriving ships to be inspected and treated for marine pests before they can enter a marina. These inspections are compulsory for vessels arriving from international ports (DPC, 2013).
Recently, the Ballast Water Management Convention (BWM) entered into force. All international sea-going ships under this convention must comply with standards that require discharging ballast water from the last port and replacing it with new sea water at a minimum of 200 nautical miles from shore before arriving at a new port (IMO, 2004, 2011). Despite this, several studies have indicated that in comparison to ballast water, biofouling likely accounts for more NIS introductions (e.g., Fofonoff et al., 2003; Davidson et al., 2009; Hewitt and Campbell, 2010). In this context, other projects such as the GloFouling partnership project (a collaboration among the International Maritime Organization, the United nations Development Programme and the Global Environment Facility) have been developed with the objective of building capacity in developing countries for implementing the IMO Biofouling and other relevant guidelines for biofouling management and to catalyze overall reductions in the transboundary introduction of biofouling-mediated IAS. Thus, biofouling should be included in a marine NIS risk assessment regardless of the currently low capacity to predict inoculation rates and difficulties associated with sampling (Ware et al., 2014).
Although a management and legislative framework and a marine vector-based risk assessment methodology are well-established in the literature (Campbell and Hewitt, 2011; Keller et al., 2011; Chan et al., 2012; Floerl et al., 2013; Ware et al., 2014), both have many limitations. First, NIS monitoring surveys are not yet performed in all international ports consequently, there is not a global and updated information source about the current state of biodiversity in ports (Blueports Interreg Project Advisory Committee, pers. comm.) and there are not complete baselines for temporal and spatial intra-/inter-port comparisons worldwide. Secondly, vessel arrival details are a poor proxy since the substantial variety in ships and shipping routes make the process stochastic (Verling et al., 2005; Lawrence and Cordell, 2010; Ruiz et al., 2013; Ware et al., 2014).
In this study, an initial biological invasion preventive strategy has been developed for the international port of Gijon, located in the Bay of Biscay, which is the leading port for solid bulk movement in Spain. The approach consisted on a global NIS occurrence likelihood assessment based on an exhaustive review of worldwide previously reported species introductions through shipping in the last 70 years (biofouling and ballast water) and combined with, the habitat suitability for each species in the given port conditions, species invasiveness and traffic data analyses. Together, these factors were considered to produce a specific NIS invasion threat score (NIS-ITS) for the port of Gijon. Two consecutive years of physical monitoring surveys of Gijon Port’s biota were performed, and different hull ships were sampled to analyze the biofouling community with the aim of comparing predicted and actual NIS scenarios.
Located on the Cantabrian coast (5°41.9496′W, 43°33.5120′N), the port of Gijon is one of the main seaports in the Atlantic arc and the leading port for solid bulk movement in Spain2. The port of Gijon covers 415 hectares and has more than 7,000 linear meters of docks. The spaces provided by the port of Gijon are structured in areas divided by each type of traffic (solid bulk, liquid and container terminals, and multipurpose facilities for various types of traffic). The port also has a small marina with recreational boats located outside the main docks (Figure 1).
Figure 1. Map of “El Muse1” international industrial port of Gijon, Bay of Biscay, Spain. (A–D) correspond to the different sampling areas inside the port divided by the type of traffic received.
To identify and control environmental quality, the Port Authority of Gijon has established and implemented an Environmental Surveillance Plan. This plan was developed taking into account the applicable regulations and the Program of Recommendations for Maritime Works (ROM) and based on the standards of the International Standard ISO 14001. For this reason, the Port Authority of Gijon is focused on identifying its most significant environmental impacts and acting on them by continuously improving its management strategies3.
To evaluate the threats of invasions in the port of Gijon, we created a NIS-Invasion Threat Score (NIS-ITS). A review of the scientific literature from 1953 to 2020 was conducted considering reports of species dispersal via maritime traffic. This search was conducted considering all living organisms, from microscopic species such as dinophytes that have been studied traveling in ballast tanks to macroscopic organisms such as bryozoans or ascidians that have been observed traveling via hull fouling. All translocation reports were collected, and a list of species with evidence of using marine traffic as a vector for expansion was generated. This dataset was analyzed for the case study of the port of Gijon and species were categorized as native, NIS, IAS or cryptogenic in the area. These classifications were carried out by using the Invasive Species Specialist Group (ISSG) global database of the International Union for Conservation of Nature4, the DAISIE (Roy et al., 2020) and the AquaNIS (AquaNIS, 2015) databases. Once this categorization was done, the NIS-ITS was calculated for the NIS in the area under study. On the other hand, those species with evidences of using maritime traffic as a vector, but classified as native in the area under study were listed apart in order to facilitate a checklist to other regions where these species could arrive and might become a NIS.
The NIS-ITS was calculated using three criteria. The first criterion was the suitability of the environmental conditions of the port of Gijon for each of the potentially arriving species. This information was obtained from the SeaLifeBase webpage (Palomares and Pauly, 2020). Values for habitat suitability (Hs) range from 0 (totally unsuitable habitat) to 1 (perfect environmental conditions for the species). These values are calculated for each species and geographic location by using estimates of environmental preferences with respect to depth, water temperature, salinity, primary productivity, sea ice concentration and distance to land (Ready et al., 2010). Each species-specific environmental preferences are matched against local environmental conditions to determine the suitability of a given area in the ocean for a particular species.
By combining the information for these parameters, an environmental envelope is generated for each species, which is essentially a response curve that describes the habitat usage of such species or its preferences with respect to certain environmental parameters. If the mean environmental conditions fall within the preferred parameter range of the species, the habitat suitability will be the highest (Hs = 1.00). However, when values fall outside this range, it will decrease linearly toward the species’ absolute minimum or maximum parameter thresholds and it is set to zero (Hs = 0.00) beyond the absolute threshold values (Ready et al., 2010). The suitability is first calculated for each of the individual environmental parameters and the product is then used to determine the overall habitat suitability in a given location, that is:
This multiplicative approach allows each environmental predictor to act as a “knock-out” criterion. For instance, if the salinity in a given location exceeds the salinity preference of a particular species, the probability of occurrence with respect to salinity will be zero (Ssalinity = 0.00). The overall habitat suitability for the species in that given location will then be zero (Hs = 0.00), even if all other environmental attributes are within the preferred range of that species. Habitat suitability data can be found for specific geographic regions and for particular species and it is accessible at https://www.aquamaps.org/search.php. Georeferenced values for the mentioned parameters are provided at 0.5° resolution (Kaschner et al., 2019). For this case study, data from the point 43.75°, −5.75° were employed since the port of Gijon is located inside this area.
The second criterion was the known invasion history of the species. A numeric score is given to each species depending on the following situations: (a) the species is only present in its native habitat and there are no reports of its introduction in any other area, (b) the species has been introduced in areas outside its native range but there is no information on any relevant impact, (c) the species has invaded new areas and causes various impacts of different magnitudes. The score for each of these conditions is set based on the six EICAT (Environmental Impact Classification for Alien Taxa) impact categories from Hawkins et al. (2015) by assigning an ascending numerical value according to the level of impact of the species (Supplementary Table 1). A score of zero was given to those species that are present only in their native habitat without any known introduction event. A score of 0.2 was given to those species that have been introduced in areas out of their native range but do not cause any relevant impact. This score corresponds to the “minimal concern” EICAT impact category which includes species with “small inconsequential changes,” as suggested by Roy et al. (2019),. An invasion history score of 1 was given to those species that have been introduced and produce environmental or economic impacts outside their native areas. This score corresponds to the rest of categories with higher EICAT impact magnitudes (minor, moderate, major and massive) which are all merged due to the lack of specific information to differentiate between these categories with high accuracy.
Finally, the third criterion employed for the calculation of the NIS Invasion Threat Score (ITS) was the maritime traffic. In order to calculate the maritime traffic score for a given species, its native range is determined based on bibliography. Results are reported as presence or absence of the species in each of the seven biogeographic areas defined by Menéndez-Teleña (2019) (see Supplementary Table 2). Once the native range for a species is assessed, it is also checked if it has stablished populations in other biogeographic areas. If so, these areas are also considered as potential donors of species and included in the traffic score calculation. The specific dispersal method that may be employed by the species (e.g., ballast water or hull fouling) is not taken into account. If the species is present in a biogeographic zone, the maritime traffic originating from this area is analyzed and summed to other potential areas where the species is present and therefore may arrive to the port of Gijon using the ships as vectors. The traffic score is calculated by using available traffic data for the port under study. Depending on the case, this data availability may vary. However, this score can be calculated in any case without a minimum data limitation. This means that the score can be calculated, for example, annually in those cases with scarce traffic data, but it can also employ data from a longer period, such as in this case study, in which 14 years of traffic in the port of Gijon were used. Once the global distribution of the species is determined, the relative proportions of traffic from native and invaded areas (the ratio between the volumes of traffic from the area divided by the total traffic arriving at Gijon) are estimated and then summed to obtain a unique total traffic score. Areas with heavy maritime traffic will show a high traffic ratio. Then, species that are present in these areas will show high maritime traffic scores. Values for maritime traffic also range from 0 (total absence of a species in all of the biogeographic areas with marine traffic to Gijon) to 1 (a species that was present in all the areas with maritime traffic to the port of Gijon).
Following the methods used to calculate invasion risk scores by different authors (Bomford, 2008; Gallardo et al., 2016; Tsiamis et al., 2020), the NIS-ITS can be calculated by the sum of the specific criteria, which in this case are the habitat suitability, invasion history and maritime traffic. All of them are considered equally important as in Gallardo et al. (2016) and references therein. This method (unlike multiplicative ones) allows obtaining non-zero scores when one of the parameters is null. In summary, the index can be calculated by the following formula:
The maximum NIS-ITS that a species could obtain after combining all the criteria is 3 (examples of ITS calculations can be seen in Supplementary File 1A). A NIS-ITS = 3 (100% of the maximum ITS) indicates that the port of Gijon presents perfectly suitable environmental conditions for the specific species (habitat suitability = 1), that the species has shown previous capacity to impact ecosystems out of its native range and biologically invade elsewhere (invasion history = 1), and the species is present in all the biogeographic areas from which the port of Gijon receives marine traffic (total traffic risk = 1). On the contrary, a species could also obtain an NIS-ITS = 0 when: environmental conditions are outside the species’ absolute minimum or maximum parameter thresholds on at least one of the environmental parameters (habitat suitability = 0), the species is only present on its native range, without any known introduction or invasion event (invasion history = 0) and when the species is not present in any of the biogeographic areas with maritime traffic with the port under study (maritime traffic = 0).
This method does not take into account if vessels employ techniques for the prevention of biological invasions, such as techniques to release ballast water away from ports or to avoid fouling by using paints that cover the hulls. In these cases where these preventive measures are taken, the NIS-ITS should be lower than in cases where they are not. Due to the lack of data, this method cannot be implemented in this case study, but we provide an example for its calculation that could be considered for future cases (see Supplementary File 1B).
The port of Gijon can also be a donor of species, so it is also important to understand which species that are present within the port can be transferred via maritime traffic. Thus, from the initial list of species containing species with the potential of using maritime traffic as a vector for expansion, those that are native to the area studied in this work (the port of Gijon) were analyzed. These species were categorized as IAS (when the species has invaded new habitats and causes impacts and environmental damages), NIS (when the species has been reported out of its native range, but there is not information about any impact in these habitats) or NA (no alien populations). It is expected that these data can help destination ports complete their own and specific NIS-ITS information with data on species habitat suitability and maritime traffic for their own conditions.
The port biota study was focused on macroinvertebrates attached to port facilities (rocky ground and port structures). Targeting macroinvertebrates is the typical sampling procedure routinely followed in most ports to estimate biotic indices for environmental quality assessments of marine ecosystems (Borja et al., 2000, 2009) and is recommended in both the EU Water Framework Directive (WFD, Directive 2000/60/EC) and the Marine Strategy Framework Directive (MSFD, Directive 2008/56/EC) (Borja et al., 2015). Sampling was performed in two consecutive years (2016 and 2017) during the highest spring tide. Two years of sampling were selected to optimize the surveillance design, in terms of effort vs. resolution, by following the recommendations for sampling aquatic invasive species in Hoffman et al. (2016).
The sampling was divided into the structured areas of the port: A, B, C, D, and marina (Figure 1). In each area, two locations were studied, except for in the marina, where only one place was sampled due to its small area. At each location, sampling occurred at three different tidal levels (high, low and medium). A rapid assessment survey approach (RAS; Minchin, 2007) and the sampling protocol from Miralles et al. (2016) were adapted and conducted on the assemblages living on artificial port structures. A team of five researchers (two postdoc and three predoc) expert in marine science did the 2 years sampling. Before starting the sampling and to avoid biases due to patchy spatial distributions, a visual inspection to determine the different types of organisms present at the sampling site was conducted for approximately 10 min. To standardize the sampling effort, the surface sampled at each site within the area of the port was approximately 200 m2, and the duration of the sampling was 30 min. Roughly 1% of the animals visually detected attached on that surface were collected at random. The number of individuals picked of each morphotype (group of organisms phenotypically different) was approximately proportional to the abundance of such morphotype in the area. A maximum of 50 individuals per location were selected and were placed in buckets with seawater for further morphological, taxonomical and genetic analyses.
All sampled individuals were immediately transported to the laboratory of Genetics of Natural Resources at the University of Oviedo. In the laboratory, individuals were identified based on their morphology to the species or to the lowest possible taxonomic level. Then, a piece of tissue from each individual was preserved in absolute ethanol for further genetic analysis. The taxonomic nomenclature of all identified species was verified on the World Register of Marine Species (WoRMS, 2020).
Apart from the biota on the port structures, species attached on the hulls of ships arriving at the port of Gijon were analyzed. To carry out this effort, during July 2017, 22 ships from 12 different countries were contacted directly through the Port Authority of Gijon to ask them to participate in this study. Sampling consisted of scratching all biofouling organisms from a quadrat of approximately 30 × 30 cm in three different places along the draft of the vessel: stern, mid-ship and bow. Sampling was performed under strict safety procedures with professional divers. All divers were fastened by wiring and had audio and visual connections with the supporting ship where the scientists were placed located. The general procedure of diving was based on the Collective Agreement of Professional Diving and Hyperbaric Means; the Spanish Ministerial Order of October 14, 1997; the Modification to the Collective Agreement of Professional Diving and Hyperbaric Means of October 18, 2016; and the UNE-EN 15333-1. Samples were placed in bags with seawater and transported to the University of Oviedo. In the laboratory, individuals were identified based on morphology, and a piece of tissue from each individual was preserved in absolute ethanol for further genetic analysis. The taxonomic nomenclature of all identified species was verified on the World Register of Marine Species (WoRMS, 2020).
The list of NIS found in these samplings was enhanced by data from previous studies of macroinvertebrates and algae (such as Arias et al., 2014; Miralles et al., 2016) and from metabarcoding data (Borrell et al., 2017; Ibabe et al., 2020a,b) to generate an accurate and complete NIS scenario for the port of Gijon.
Genetic species identification was carried out to confirm the morphological taxonomic identification following Miralles et al. (2016). DNA was extracted from ethanol-preserved individuals using a Chelex® resin (Bio-Rad Laboratories Inc., United States) protocol (Estoup et al., 1996) or the EZNA Mollusc DNA Kit (Omega Bio-Tek Inc., United States). The genetic barcode employed was the mitochondrial cytochrome oxidase subunit I coding region (COI gene), with additional genes (RNAr18s or RNAr16s) to improve resolution or to determine species when the database references were insufficient (Miralles et al., 2016; Pejovic et al., 2016). The obtained DNA sequences were visually inspected and edited with BioEdit v7.2.5 (Hall, 1999). All sequences were compared with online public databases using nBLAST in NCBI5 and Bold Systems6. The sequence with a maximum score and at least a 97% nucleotide identity and an E-value <e-100 was considered the reference for genetic species identification.
All sampled port biota and ship biofouling individuals were classified as native species, NIS, IAS or cryptogenic species. The Invasive Species Specialist Group (ISSG) global database of the International Union for Conservation of Nature (IUCN), the DAISIE (Delivering Alien Invasive Species Inventories from Europe), and the AquaNIS (information system on aquatic non-indigenous and cryptogenic species database) were the references used to confirm the introduced and invasion status of each NIS when sufficient information was available. Species that are unable to be identified as native or NIS were classified as cryptogenic (Carlton, 1996). Once species identity was confirmed, species abundances for statistical analyses were calculated based on the number of individuals with confirmed identification and the total number of species identified in each sampling (2 year separately).
Since samplings were conducted in different port areas and years, a multivariate non-metric multidimensional scaling (nmMDS) analysis was conducted to determine whether the distribution of the species (including both, native and NIS) was strongly clustered by area or year or whether they were more or less scattered. The Bray-Curtis dissimilarity index was applied, and a stress <0.2 was considered acceptable. The Shepard plot was inspected to determine the linearity of the index in the dataset. A SIMPER analysis was conducted (only for the non-indigenous biota) to assess the species that most contributed to the differences from 1 year to the other and detect any potential expansion or population growth. Comparisons between years and areas as well as diversity indexes (Shannon and Simpson), tests and plots were performed with PAST version 3.8 (Hammer et al., 2001).
Eighty research articles recording worldwide evidences of species introductions and translocations through shipping from approximately 70 years of marine research were compiled in this work. These reports were used to create a list of species that were able to travel by ship and have the potential to be future invaders in any port. A large species taxonomical representation was obtained from that list of 380 species. From this dataset, 252 species could be considered as NIS (if found in the port of Gijon), whereas 128 were classified as native in accordance with their natural geographic distributions. NIS habitat suitability data for the port of Gijon were available only for 71 out of the 252 NIS. This information was combined with the invasiveness of each species and the corresponding port of Gijon’s maritime traffic score to estimate the NIS-ITS for each individual species (Supplementary Table 3). Although NIS-ITS cannot be estimated for those species lacking habitat suitability information, scores for maritime traffic and invasion history were also estimated for these species (Supplementary Table 4).
A maximum invasion threat score (NIS-ITS = 3.00) was obtained by 3 out of the 71 species [Bugula neritina (Linnaeus, 1758), Ficopomatus enigmaticus (Fauvel, 1923), and Botryllus schlosseri (Pallas, 1766)]. On the contrary, some species such as Corynactis californica Carlgren, 1936 (NIS-ITS = 0.153) showed low scores, indicating a lower likelihood of being a successful invader in the area (Supplementary Table 3). Most of the analyzed species were crustaceans (Arthropoda), ascidians (Chordata), bivalves and gastropods (Mollusca). All species belonging to Rhodophyta and Ctenophora phyla showed invasion threat scores above 70%. Similarly, four polychaetes showed also more than a 70% score and two out of four bryozoans scored above 90% (Figure 2). A total of fifteen NIS showed more than 90% of invasion likelihood (NIS-ITS > 2.70) (Table 1). Most of them were meroplanktonic animals belonging to classes such as Malacostraca, Hexanauplia, Polychaeta, or Ascidiacea. The only exception was Hypnea musciformis Lamouroux, 1813, which is a seaweed belonging to the class Florideophyceae. All these species with a NIS-ITS higher than 90% showed an invasiveness score of 1, meaning that all of them had previously invaded areas out of their native ranges and produced environmental impacts. Special care and attention should be given to these species to avoid their establishment in the port of Gijon. In fact, most of the species with an invasion history score of 1 showed a NIS-ITS above 70% whilst species that are only known to be present in their native habitat (invasion history score = 0) showed low NIS-ITS values (Figure 3).
Figure 2. Count of species belonging to different phyla grouped based on their invasion threat scores (NIS-ITS)
Table 1. Non-indigenous species (NIS) with more than a 90% invasion threat score (NIS-ITS) in the port of Gijon.
Figure 3. The NIS invasion threat score percentages obtained for each of the groups based on species invasion history score. Group 0 (for those species only present in their native habitat and without known introduction events), group 0.2 (for species that have been introduced outside their native range but do not cause any known impact) and group 1 (for those species that have invaded new areas and cause various impacts of different magnitudes).
On the other hand, 128 species capable of using maritime traffic as a vector were found to be native to Gijon (Supplementary Table 5). Sixteen of these species have already invaded other areas and produced environmental impacts outside their native range. Similarly, 32 species that are native to the area under study, despite having no records of producing environmental impacts, have already been detected outside their native habitats. These species are mainly dinophytes transferred by ballast water or bryozoans transferred by hull fouling (Supplementary Table 5).
A total of 522 individuals were genetically identified to 82 taxa in the 2 years samplings of the port of Gijon. In general, genetic analyses confirmed the previous morphological identification. The observed communities were mainly composed by polychaetes, bivalves and gastropods (GenBank accession numbers MN185333-MN185374 for COI gene, MN164033-MN164046 for 18s RNA gene, and MN164346-MN164348 for 16s RNA gene; Supplementary Table 6).
The global biodiversity in the port of Gijon was not significantly different between 2016 and 2017 (diversity t-test for Shannon index t = −1.108 and p = 0.268; Simpson index t = 0.901 and p = 0.368; Figure 4A). Regarding the observed NIS, IAS and cryptogenic species, significant differences between years were found (diversity t-test for Shannon index t = −2.475 and p = 0.015; Simpson index t = 2.61 and p = 0.010). The rarefaction plot revealed higher NIS richness in 2017 than in 2016 (Figure 4B). SIMPER analysis showed that the NIS that mainly accounted for the differences between 2016 and 2017 communities were Magallana gigas (Thunberg, 1793), Mytilaster minimus (Poli, 1795), Watersipora subtorquata (d’Orbigny, 1852), and Mytilus trossulus (Gould, 1850), which accounted for 70% of the dissimilarities. The first two species decreased, while W. subtorquata (7.74% of the contribution to the dissimilarities between 2016 and 2017), and M. trossulus (5.31% of the contribution to dissimilarities) increased in observed specimens from 2016 to 2017. In fact, W. subtorquata was found at all five stations in 2017 (found only at stations A and B and the marina in 2016), and M. trossulus was found in stations A and C and the marina in 2017 while it was present only at station C in 2016 (Supplementary Table 6).
Figure 4. Diversity rarefaction plots of (A) all observed species and (B) non-indigenous species from the port of Gijon, Bay of Biscay, Spain.
Biodiversity comparisons among the main industrial docks (A, B, C, and D) showed that they clustered together regardless of the sampling year (Figure 5, stress = 0.080). However, the marina always appeared slightly distant from the remainder of the industrial docks both years, and notable differences were found when comparing communities inhabiting the marina in 2016 and 2017 (Figure 5).
Figure 5. Two-dimension non-metric multidimensional scaling (nmMDS) plot of the areas under study in the Port of Gijon, Bay of Biscay, Spain in 2016 and 2017 and its Shepard plot. Crosses represent samples collected in 2016 and dots represent samples from 2017. The name of the area is given (A–D and Marina). Stress value is 0.080.
Apart from analyzing the biota inhabiting the different areas of the port of Gijon, the species attached to the hulls of three ships docked in the port were also studied. Notably, out of the 22 ships contacted, these three ships were the only ones that agreed to participate in the study. To maintain anonymity, the sampled vessels were labeled ships 1, 2, and 3. Ship 1 was an international vessel that arrived to Gijon from Russia and its destiny was United Kingdom. Ship 2 was a dredge vessel that used to work along the Cantabrian Sea (South Bay of Biscay) but it was stopped due to maintenance in Gijon for 13 days before sampling. Finally, Ship 3 was an inactive vessel docked in Gijon for 3 months (details about the study vessels in Supplementary Table 7). A total of 73 specimens and 15 different species, including native but also 5 NIS, were found fouling the hulls of the ships (Table 2). Most of the species were natives already observed in the samplings from other port areas. However, some species were only found on the vessels. For instance, Ship 3 had been docked in Gijon for the longest period, and almost all the fouling species were already described in the sampled areas of the port. Nevertheless, a NIS [Diplosoma listerianum (Milne Edwards, 1841)] that did not appear in the port samplings was collected from this ship. Two new potentially dangerous species (which were not reported in the 2 years previous monitoring surveys) appeared on Ship 2: Monocorophium acherusicum (Costa, 1853) and Spirobranchus taeniatus (Lamarck, 1818), in addition to other NIS, Spirobranchus latiscapus (Marenzeller, 1884) and Dipolydora capensis (Day, 1955) that had already been observed in the port samplings (Table 2). Thus, the risk of being a vector of NIS through biofouling was not the same for the three vessels. Ship 1 could be classified as “no invasion risk” (because there was no attached biota), while ships 2 and 3 could represent a “potential vector of invasions” since new species were present in their hull (and not in the port). In addition, these ships could represent a “vector of dispersion” because they carried several fouling native species that could eventually spread to new areas.
Table 2. The number of non-indigenous (NIS) and invasive (IAS) specimens observed in macroinvertebrates samplings conducted in two consecutive years (2016, 2017) in the port of Gijon, Bay of Biscay, Spain.
Complete data to estimate the proposed NIS-ITS were available only for approximately a quarter (28.2%) of the potential invaders. Four out of the fifteen species (26.7%) with NIS-ITS values above 90% have been already detected in the port of Gijon (Table 1). Two of them [Bugula neritina (Linnaeus, 1758) and Diplosoma listerianum (Milne Edwards, 1841)] were found in the samplings from this study, and the other two were found in samplings from a previous report for the same port [Botryllus schlosseri (Pallas, 1766) (Nydam et al., 2017) and Styela plicata (Lesueur, 1823) (Miralles et al., 2016)]. Moreover, eight other NIS (Ficopomatus enigmaticus (Fauvel, 1923); Diadumene lineata (Verril, 1869); Acartia tonsa Dana, 1849; Callinectes sapidus Rathbun, 1896; Rhithropanopeus harrisii Gould, 1841; Mytilopsis leucophaeata (Conrad, 1831); Mnemiopsis leidyi Agassiz, 1865; and Hypnea musciformis Lamouroux, 1813) have also been reported in the Bay of Biscay in previous studies (Table 1). Therefore, 80% (12 NIS) of the potentially most dangerous species have already been detected in the Bay of Biscay area. In addition, two other NIS [Watersipora subtorquata (d’Orbigny, 1852) ITS = 2.593 (86%) and Balanus trigonus Darwin, 1854 ITS = 2.199 (73%)] that were also included in the expected NIS scenario have also been found in the samplings from this study (Table 2). Globally, from the total list of 252 NIS (including species with and without habitat suitability information), 12 species have already been reported in Gijon (6 were species detected in the samplings from this work) and 52 species (25.6%) in the Bay of Biscay (Supplementary Tables 3, 4).
In this work, a representative case study in one of the main seaports in the Atlantic arc (Port of Gijon) was conducted as a proof of concept to be implemented in other international seaports with the aim of reaching real “blueports” (ports with an adequate management of resources and wastes that put in practice new strategies to avoid biological invasions) according to EU policy (European Commission, 2012). Prevention and early detection of NIS must be at the core of current port policies since they are the most cost-effective strategies for NIS management (CBD, 1992). However, some species may slip through prevention and detection efforts and might establish and become abundant pests (Lodge et al., 2006). Prioritization of efforts for particular “target species,” defined as species previously identified as high invasion risks within a port, is indispensable for the correct management of biological invasions (McGeoch et al., 2016). On the other hand, biological port surveys set a baseline of native and non-indigenous port biodiversity. This knowledge can help in both, early NIS detection avoiding the proliferation and spread of NIS, and in the establishing of effective IAS management strategies (e.g., Ojaveer et al., 2014, 2018; Miralles et al., 2016, 2018; Marraffini et al., 2017).
The present study represents the first step to establish a NIS threat assessment procedure in the Port of Gijon and in all ports connected with it via maritime traffic. This biodiversity baseline can be consulted in future NIS assessments for the port. As a result, a list of potential NIS ranked by their Invasion Threat Scores (NIS-ITS) is now available. The biota list is now ready to be used by stakeholders and researchers, and should be updated from further samplings, and include species detected in the area from other studies. Fixing a specific score to consider a species a threat will always be a raw approximation or a little bit subjective decision. For example, Gallardo et al. (2016) set the threshold in 12 points out of the maximum 16 that a species can obtain. Similarly, Tsiamis et al. (2020) set the limit in 38 points out of 48, so that species above this score will be considered as high-risk or high-likely invaders. Both studies use expert judgment to establish this threshold. In the case of NIS-ITS, authorities should pay special attention to those species over the 90% NIS-ITS (high likely invaders). Even more, most of the species with known invasive potential (invasion history score = 1) showed invasion threat scores over 70% (Figure 3). In fact, a posteriori analyses revealed that those species that according to the Spanish catalog of invasive species (BOE, 2013) are classified as IAS are all above the 70% threshold, except Caulerpa taxifolia Agardh, 1817, which obtained a 60% score due to very low habitat suitability in the Bay of Biscay area (Supplementary Table 3). These results indicate the importance of carefully studying the potential threat of those species with more than 70% NIS-ITS, which show specific traits that can make them especially dangerous.
Given this information, it is expected that specific port prevention strategies would be enriched and environmental monitoring would be developed in advance and thus improved. Currently, in addition to physical surveys, environmental DNA (eDNA) assays could also be a useful alternative for determining the presence of species based on organism vestigial particles that remain in the environment, negating the need to catch or observe the organism (Darling and Mahon, 2011; Borrell et al., 2017). It is also possible to design species-specific primers for the rapid, early detection of certain species of interest within a seawater community using eDNA (e.g., Devloo-Delva et al., 2016; Miralles et al., 2019).
The new NIS-ITS can be implemented and used by any other international port as a first line of defense against biological invasions without the need of experts. The index contains three components, and two of them (habitat suitability and invasiveness/impact) are based on joint efforts by researchers and are currently public and available for all stakeholders concerned about this challenge. The AquaMaps7 and the Global Invasive Species Database8 websites are constantly updated and improved, and they have incorporated relevant tools such as the Hawkins et al. (2015) and Palomares and Pauly (2020) approaches, respectively. The third NIS-ITS component is a relatively easy-to-create port database that compiles all the destinations and cargo origins (traffic) within a port (e.g., 15 years for our case study at the port of Gijon). However, this score can be calculated in any case without a minimum data limitation. This last component could also be improved if the complete, and accurate, itinerary of the cargo were available in all port registries and if treatments of the vessels hulls and ballast water management procedures were known. The full history of ships beyond immediate cargo origin can be important since hull foulers can be entrained in all locations visited. In the case of ballast water, knowing the origin and history or the water being discharged into Gijon is important and its origin is likely not where ships sourced cargo. Unfortunately, complete history of the vessels travels and treatments/procedures are not always available. Despite this, we propose how to incorporate BW and Hull Fouling treatments (if available) in our maritime traffic element when calculating the NIS-ITS in future studies.
This work is consistent with previous studies (Cardeccia et al., 2018) that indicate the need to collect data about the biological and ecological traits of NIS. These data can be used to generate habitat suitability information, which is necessary to calculate indices such as NIS-ITS that may facilitate the prevention of biological invasions. In fact, due to the lack of this information, we obtained only approximately 30% of the complete habitat suitability data for all the potential NIS travelers identified from the literature screening. At the same time, for some species with wide and abundant distributions in the Bay of Biscay, such as Magallana gigas (Thunberg, 1793), very low values of habitat suitability were found (0.110) (Supplementary Table 3). Most likely, aquaculture practices, which could imply diverse sources and sizes of the inoculums, periodic introduction events and differential extents of bottleneck processes in the new environments (Roman and Darling, 2007), could be a modulating factor currently not considered (but relevant) when predicting habitat suitability for those species. In the same way, although the IUCN Environmental Impact Classification for Alien Taxa (EICAT) was developed a few years ago and it is in use, data related to the impacts that are produced in the invaded areas are still lacking for many relevant IAS (Ojaveer and Kotta, 2015). One of the main recommendations that arises from this work is that more efforts and initiatives must be developed (and funded) to collect biological data for the main IAS and NIS around the world and that this information must be integrated into databases that are easily accessible for any type of stakeholder.
Another possible biological invasion risk for the port of Gijon, which was not considered in the newly proposed NIS-ITS, could originate from other surrounding marinas. There have been multiple cases where recreational vessels, yachts and boats (not industrial traffic) have been cited as either the primary introduction vector or secondary spread vector for an invasive species (Floerl and Inglis, 2005; Davidson et al., 2010; Clarke-Murray et al., 2014; Ulman et al., 2019; Iacarella et al., 2020). For large industrial ports, traffic data are often accessible and public but recreational vessel traffic data are hard to collect and compile (Le Tixerant et al., 2018). A relevant tool to integrate this information into biological invasion predictions could be tracking ship movements with an automatic identification system (AIS) (EU, 2002; Merchant et al., 2012; Ulman et al., 2017). Coastal and offshore area data from satellite-based AIS (Loretta, 2016) could provide information about the spatial and temporal distribution of shipping (Shelmerdine, 2015). However, AIS transmitters are only required on larger ships, passenger vessels and large fishing vessels. Thus, millions of recreational vessels worldwide are not required to have an AIS, which makes it difficult to develop AIS-based models (Hermannsen et al., 2019). Despite this issue, it is clear that in the future, NIS Invasion Threat Scores should include recreational vessel traffic data from surrounding marinas when estimating a specific port’s ITS and predicted scenarios.
Despite the previously mentioned limitations, in the predicted port of Gijon NIS scenario based on the new ITS, we found 15 marine species with values above the 90% ITS. After sampling macroinvertebrates on the port structures and in the hulls of vessels (as conducted during routine water quality inspections within ports; see Borja et al., 2015), 26.7% of these high-threat species were observed in the port. The outcome revealed that eight other NIS have also been reported in or near the port of Gijon (south Bay of Biscay) (Table 1). These results increase the percentage of NIS findings with regard to the previously described expected NIS scenario with an 80% coincidence level. This scenario could indicate that expected species arrivals based on the NIS-ITS could be, even with the current limitations, close to reality and should be considered at the time of developing prevention/management port strategies. In the case of Gijon, specific management plans must be developed for the species already present in the port. This is also necessary for those NIS that are not present in the port yet but were classified as high-threat species by the NIS-ITS, indicating their potential to become future invaders that are spread by port industrial activity and traffic (Colautti et al., 2006; Floerl et al., 2009; Bishop and Hutchings, 2011; Ojaveer et al., 2018).
One important discovery here was that three species were found on boat hulls and not in the port where they were sampled in. Considering that only 3 out of 22 ships authorized hull samplings, it seems that Spanish port policies should be updated in correspondence with the great threat that biological invasions are for biological diversity. When vessels move from one port to another, organisms can fall off the hulls and may become introduced into a new host environment (Dobroski et al., 2017). Thus, mandatory routine monitoring, along with periodic inspections on arriving boats should be implemented to detect new arrivals of NIS (Bishop and Hutchings, 2011), and marine stakeholder participation (authorities, ship owners, scientists and citizens) will be fundamental.
The port of Gijon is not only a destination for exotic species, it can also be the origin of new introductions to other regions. For example, native species that commonly inhabit the port structures can be transported to other areas outside their native range where they can display invasive features. This study also provides a list of native species at risk of being inoculum (port of Gijon as a donor) to spread/start new invasion processes overseas, since the results of the bibliographic search showed that they have the ability to use maritime traffic as a vector for expansion. As an example, Carcinus maenas (Linnaeus, 1758) is native to the European coasts (where the port of Gijon is located) but has been listed as one of the “100 worst invasive species” as it has become invasive in areas like the northwest Pacific or the southwest Atlantic because it is a voracious predator that affects epibenthic and infaunal species through predation, competition and burrowing activities (Pollard and Hutchings, 1990; Bravo et al., 2007; Briski et al., 2011; Edgell and Hollander, 2011; Lim et al., 2017). This is why this list can be very useful in other regions and these species can be considered in future risk assessments.
Globalization has increased international marine traffic, particularly the shipping industry (Tournadre, 2014; Halpern et al., 2015), by moving not only people and goods but also NIS around the world (Hulme, 2009). It is urgent to develop effective prevention strategies and management plans of biological invasions. Each international port should develop its expected NIS scenario and prepare themselves for early/rapid detection of high-risk species. Thus, the use of the new NIS-ITS index proposed here (where there is not the need of experts) and the implementation of species-specific detection tests using eDNA or species-specific physical sampling strategies within ports will be of help for that. Finally, annual biota monitoring within ports is fundamental and must be mandatory. These data will help update global data about port biodiversity (and its risks) and to obtain substantially more insights into NIS colonization dynamics and a clearer picture of the real influence of maritime traffic on marine biological invasion processes worldwide.
Together with these ecological studies, it is necessary to put into practice other strategies related to the management of shipping activities that facilitate the spread of NIS, such as the implementation of mandatory periodic hull cleanings, discharges of ballast water at least 200 nautical miles from the nearest land or the implementation of specific policies related to the boat treatments in recreational boating that can avoid the dispersal of species (Anwar, 2016; IMO, 2018). The combination of these methods can lead to a better understanding and management of biological invasions and a significant reduction of environmental risks, leading to a real blue economy.
The datasets generated for this study can be found in the online repositories. The names of the repository/repositories and accession number(s) can be found below: https://www.ncbi.nlm.nih.gov/genbank/, MN185333-MN185374; MN164033-MN164348.
LM: methodology, formal analysis, investigation, writing—original draft, and writing—review and editing. AI: methodology, formal analysis, investigation, visualization, and writing—review and editing. MG: project administration and funding acquisition. EG-V: conceptualization, supervision, project administration, and funding acquisition. YB: methodology, investigation, conceptualization, supervision, project administration, and funding acquisition, and writing—review and editing. All authors contributed to the article and approved the submitted version.
This study has been supported by the Spanish Ministry of Economy and Competitiveness, Project MINECO-17-CGL2016-79209-R.
The authors declare that the research was conducted in the absence of any commercial or financial relationships that could be construed as a potential conflict of interest.
Two reviewers made relevant contributions/comments to improve this manuscript. AI holds a FPI Scholarship awarded by the Spanish Ministry of Economy and Competitiveness. We would like to thank the Authorities from the Port of Gijon for their help, and Carlos Carleos (Department of Statistics and Operations Research and Didactics of Mathematics, UNIOVI) and Andres Arias (Department of Organisms and Systems Biology, Zoology, UNIOVI) for their useful comments on the manuscript. This is a contribution of the Marine Observatory of Asturias (OMA) and the Biotechnology Institute of Asturias (IUBA).
The Supplementary Material for this article can be found online at: https://www.frontiersin.org/articles/10.3389/fmars.2021.633118/full#supplementary-material
Allen, F. E. (1953). Distribution of marine invertebrates by ships. Austral. J. Mar. Freshw. Res. 4, 307–316. doi: 10.1071/MF9530307
Altuna, Á. (2015). Cnidarios (Cnidaria) bentónicos del Golfo de Vizcaya y zonas próximas (Atl ntico NE) (Lista de especies, batimetría y anotaciones).
AquaNIS (2015). Information system on Aquatic Non-indigenous and Cryptogenic species. Geneva: World Wide Web electronic publication.
Aravena, G., Villate, F., Uriarte, I., Iriarte, A., and Ibanez, B. (2009). Response of Acartia populations to environmental variability and effects of invasive congenerics in the estuary of Bilbao, Bay of Biscay. Estuar. Coast. Shelf. Sci. 83, 621–628. doi: 10.1016/j.ecss.2009.05.013
Arias, A., Bañon, R., Almon, B., Anadon, N., Borrell, Y. J., Cremades, J., et al. (2014). “Non-indigenous marine species (NIS) in the Cantabrian sea and adjacent Atlantic (NW-N Iberian peninsula): a first approach for the Marine Strategy Framework Directive in northern Spain waters,” in XVIII Simposio Ibérico de Estudios de Biología Marina. Libro de resúmenes, eds P. Ríos, L. A. Suarez, and J. Cristobo (Gijón: Centro Oceanográfico de Gijón), 252.
Arthur, T., Arrowsmith, A., Parsons, S., and Summerson, R. (2015). Monitoring for Marine Pests: A review of the design and use of Australia’s National Monitoring Strategy and identification of possible improvements. Canberra: Department of Agriculture and Water Resources.
Ashton, G. V., Davidson, I. C., Geller, J., and Ruiz, G. M. (2016). Disentangling the biogeography of ship biofouling: Barnacles in the Northeast Pacific. Glob. Ecol. Biogeogr. 25, 739–750. doi: 10.1111/geb.12450
Bailey, S. A., Duggan, I. C., Jenkins, P. T., and MacIsaac, H. J. (2005). Invertebrate resting stages in residual ballast sediment of transoceanic ships. Can. J. Fisher. Aquat. Sci. 62, 1090–1103. doi: 10.1139/f05-024
Bishop, M. J., and Hutchings, P. A. (2011). How useful are port surveys focused on target pest identification for exotic species management? Mar. Pollut. Bull. 62, 36–42. doi: 10.1016/j.marpolbul.2010.09.014
BOE (2013). Real Decreto 630/2013, de 2 de agosto, por el que se regula el Catálogo español de especies ex óticas invasoras. Boletín Oficial del Estado 185, 56764–56786.
Bomford, M. (2008). Risk assessment models for establishment of exotic vertebrates in Australia and New Zealand. Canberra: Invasive Animals Cooperative Research Centre.
Borja, A., Franco, J., and Pérez, V. (2000). A marine biotic index to establish the ecological quality of soft-bottom benthos within European estuarine and coastal environments. Mar. Pollut. Bull. 40, 1100–1114. doi: 10.1016/s0025-326x(00)00061-8
Borja, A., Marín, S. L., Muxika, I., Pino, L., and Rodríguez, J. G. (2015). Is there a possibility of ranking benthic quality assessment indices to select the most responsive to different human pressures? Mar. Pollut. Bull. 97, 85–94. doi: 10.1016/j.marpolbul.2015.06.030
Borja, A., Miles, A., Occhipinti-Ambrogi, A., and Berg, T. (2009). Current status of macroinvertebrate methods used for assessing the quality of European marine waters: implementing the Water Framework Directive. Hydrobiologia 633, 181–196. doi: 10.1007/s10750-009-9881-y
Borrell, Y. J., Miralles, L., Do Huu, H., Mohammed-Geba, K., and Garcia-Vazquez, E. (2017). DNA in a bottle—Rapid metabarcoding survey for early alerts of invasive species in ports. PLoS One 12:e0183347. doi: 10.1371/journal.pone.0183347
Bravo, M., Metaxas, A., and Cameron, B. (2007). Salinity tolerance in the early larval stages of Carcinus maenas (Decapoda, Brachyura), a recent invader of the Bras d’Or Lakes, Nova Scotia, Canada. Crustaceana 80, 475–490. doi: 10.1163/156854007780440957
Briski, E., Bailey, S. A., and MacIsaac, H. J. (2011). Invertebrates and their dormant eggs transported in ballast sediments of ships arriving to the Canadian coasts and the Laurentian Great Lakes. Limnol. Oceanogr. 56, 1929–1939. doi: 10.4319/lo.2011.56.5.1929
Campbell, M. L., and Hewitt, C. L. (2011). Assessing the port to port risk of vessel movements vectoring non-indigenous marine species within and across Australian borders. Biofouling 27, 631–644. doi: 10.1080/08927014.2011.593715
Cardeccia, A., Marchini, A., Occhipinti-Ambrogi, A., Galil, B., Gollasch, S., Minchin, D., et al. (2018). Assessing biological invasions in European Seas: Biological traits of the most widespread non-indigenous species. Estuar. Coastal Shelf Sci. 201, 17–28. doi: 10.1016/j.ecss.2016.02.014
Carlton, J. T. (1985). Transoceanic and interoceanic dispersal of coastal marine organisms: The biology of ballast water. Oceanogr. Mar. Biol. Annu. Rev. 23, 313–371.
Carlton, J. T. (1996). Marine bioinvasions: The alteration of marine ecosystems by nonindigenous species. Oceanography 9, 36–43. doi: 10.5670/oceanog.1996.25
Carlton, J. T. (2001). Introduced species in US coastal waters: environmental impacts and management priorities. Arlington, VA: Pew Oceans Commission.
Castellanos-Galindo, G. A., Robertson, D. R., and Torchin, M. E. (2020). A new wave of marine fish invasions through the Panama and Suez canals. Nat. Ecol. Evolut. 4, 1444–1446. doi: 10.1038/s41559-020-01301-2
Chan, F. T., Bailey, S. A., Wiley, C. J., and MacIsaac, H. J. (2012). Relative risk assessment for ballast-mediated invasions at Canadian Arctic ports. Biol. Invasions 15, 295–308. doi: 10.1007/s10530-012-0284-z
Clarke-Murray, C., Gartner, H., Gregr, E. J., Chan, K., Pakhomov, E., and Therriault, T. W. (2014). Spatial distribution of marine invasive species: environmental, demographic and vector drivers. Divers. Distribut. 20, 824–836. doi: 10.1111/ddi.12215
Colautti, R. I., Grigorovich, I. A., and MacIsaac, H. J. (2006). Propagule pressure: a null model for biological invasions. Biol. Invasions 8, 1023–1037. doi: 10.1007/s10530-005-3735-y
Costello, M. J., Coll, M., Danovaro, R., Halpin, P., Ojaveer, H., and Miloslavich, P. A. (2010). Census of marine biodiversity knowledge, resources, and future challenges. PLoS One 5:e12110. doi: 10.1371/journal.pone.0012110
Coutts, A. D. M. (1999). Hull fouling as a modern vector for marine bio- logical invasions: Investigation of merchant vessels visiting northern Tasmania. Ph. D. thesis, Launceston: Australian Maritime College.
Darling, J. A., and Mahon, A. R. (2011). From molecules to management: adopting DNAbased methods for monitoring biological invasions in aquatic environments. Environ. Res. 111, 978–988. doi: 10.1016/j.envres.2011.02.001
Davidson, I. C., Scianni, C., Minton, M. S., and Ruiz, G. M. (2018). A history of ship specialization and consequences for marine invasions, management and policy. J. Appl. Ecol. 55, 1799–1811. doi: 10.1111/1365-2664.13114
Davidson, I. C., Sytsma, M., and Ruiz, G. (2009). “Ship fouling: a review of an enduring worldwide vector of nonindigenous species,” in Report to the California State Lands Commission, Marine Invasive Species Program, (California: California State Lands Commission).
Davidson, I. C., Zabin, C. J., Chang, A. L., Brown, C. W., Sytsma, M. D., and Ruiz, G. M. (2010). Recreational boats as potential vectors of marine organisms at an invasion hotspot. Aquat. Biol. 11, 179–191. doi: 10.3354/ab00302
Devloo-Delva, F., Miralles, L., Ardura, A., Borrell, Y. J., Pejovic, I., Tsartsianidou, V., et al. (2016). Detection and characterisation of the biopollutant Xenostrobus securis (Lamarck 1819) Asturian population from DNA Barcoding and eBarcoding. Mar. Pollut. Bull. 105, 23–29. doi: 10.1016/j.marpolbul.2016.03.008
Dobroski, B., Nedelcheva, S., and Thompson. (2017). 2017 Biennial Report on the California Marine Invasive Species Program. California: California State lands Commission.
Dopico Rodríguez, E. V., and Borrell Pichs, Y. J. (2020). Scientific and educational strategies for a sustainable port activity facing biological invasions: from Ports to BluePorts. Is it possible?. Oviedo: Universidad de Oviedo.
Drake, L. A., Meyer, A. E., Forsberg, R. L., Baier, R. E., Doblin, M. A., Heinemann, S., et al. (2005). Potential invasion of micro- organisms and pathogens via ‘interior hull fouling’: Biofilms inside ballast water tanks. Biol. Invasions 7, 969–982. doi: 10.1007/s10530-004-3001-8
Edgell, T. C., and Hollander, J. (2011). “The evolutionary ecology of European green crab, Carcinus maenas, in North America,” in In the Wrong Place-Alien Marine Crustaceans: Distribution, Biology and Impacts, eds B. S. Galil, P. F. Clark, and J. T. Carlton (Dordrecht: Springer), 641–659. doi: 10.1007/978-94-007-0591-3_23
Estoup, A., Largiader, C. R., Perrot, E., and Chourrout, D. (1996). Rapid one-tube DNA extraction protocol for reliable PCR detection of fish polymorphic markers and transgenes. Mol. Mar. Biol. Biotechnol. 5, 295–298.
EU (2002). Directive 2002/59/EC of the European Parliament and of the Council of 27 June 2002 Establishing a Community Vessel Traffic Monitoring and Information System and Repealing Council Directive 93/75/EEC, as amended. France: EU.
European Commission (2012). Blue Growth Opportunities for marine and maritime sustainable growth. France: European Commission.
Floerl, O., and Inglis, G. J. (2005). Starting the invasion pathway: the interaction between source populations and human transport vectors. Biol. Invasions 7, 589–606. doi: 10.1007/s10530-004-0952-8
Floerl, O., Inglis, G. J., Dey, K., and Smith, A. (2009). The importance of transport hubs in stepping-stone invasions. J. Appl. Ecol. 46, 37–45. doi: 10.1111/j.1365-2664.2008.01540.x
Floerl, O., Rickard, G., Inglis, G., and Roulston, H. (2013). Predicted effects of climate change on potential sources of non-indigenous marine species. Divers. Distribut. 19, 257–267. doi: 10.1111/ddi.12048
Fofonoff, P. W., Ruiz, G. M., Steves, B., and Carlton, J. T. (2003). “In ships or on ships? Mechanisms of transfer and invasion for non-native species to the coasts of North America,” in Invasive species: vectors and management strategies, eds G. M. Ruiz and J. T. Carlton (Washington, DC: Island Press), 152–182.
Gallardo, B., Zieritz, A., Adriaens, T., Bellard, C., Boets, P., Britton, J. R., et al. (2016). Trans-national horizon scanning for invasive non-native species: a case study in western Europe. Biol. Invasions 18, 17–30. doi: 10.1007/s10530-015-0986-0
Gherardi, F., Aquiloni, L., Dieguez-Uribeondo, J., and Tricarico, E. (2011). Managing invasive crayfish: is there a hope? Aquat. Sci. 73, 185–200. doi: 10.1007/s00027-011-0181-z
Gollasch, S. (2006). Overview on introduced aquatic species in European navigational and adjacent waters. Helgolander Mar. Res. 60, 84–89. doi: 10.1007/s10152-006-0022-y
Hall, T. A. (1999). BioEdit: a user-friendly biological sequence alignment editor and analysis program for Windows 95/98/NT. Nucleic Acids Symp. Ser. 41, 95–98.
Halpern, B. S., Frazier, M., Potapenko, J., et al. (2015). Spatial and temporal changes in cumulative human impacts on the world’s ocean. Nat. Communicat. 6:7615.
Hammer, Ø, Harper, D. A. T., and Ryan, P. D. (2001). PAST: Paleontological statistics software package for education and data analysis. Palaeontol. Electron. 4, 1–9.
Hawkins, C. L., Bacher, S., Essl, F., Hulme, P. E., Jeschke, J. M., Kühn, I., et al. (2015). Framework and guidelines for implementing the proposed IUCN Environmental Impact Classification for Alien Taxa (EICAT). Divers. Distribut. 21, 1360–1363. doi: 10.1111/ddi.12379
Hayes, K. R., Inglis, G., and Barry, S. C. (2019). The assessment and management of marine pest risks posed by shipping: The Australian and New Zealand experience. Front. Mar. Sci. 6:489. doi: 10.3389/fmars.2019.00489
Hermannsen, L., Mikkelsen, L., Tougaard, J., et al. (2019). Recreational vessels without Automatic Identification System (AIS) dominate anthropogenic noise contributions to a shallow water soundscape. Sci. Rep. 9:15477.
Hewitt, C. L., and Campbell, M. L. (2010). The relative contribution of vectors to the introduction and translocation of invasive marine species. Australia: Prepared for the Department of Agriculture.
Hewitt, C. L., Campbell, M. L., Thresher, R. E., Martin, R. B., Boyd, S., Cohen, B. F., et al. (2004). Introduced and cryptogenic species in Port Philip Bay, Victoria, Australia. Mar. Biol. 144, 183–202.
Hewitt, C. L., Gollasch, S., and Minchin, D. (2009). “The vessel as a vector – Biofouling, ballast water and sediments,” in Biological invasion in marine ecosystems, eds G. Rilov and J. A. Crooks (Heidelberg: Springer-Verlag), 117–131. doi: 10.1007/978-3-540-79236-9_6
Hoffman, J. C., Schloesser, J., Trebitz, A. S., Peterson, G. S., Gutsch, M., Quinlan, H., et al. (2016). Sampling design for early detection of aquatic invasive species in Great Lakes ports. Fisheries 41, 26–37. doi: 10.1080/03632415.2015.1114926
Hulme, P. E. (2009). Trade, transport and trouble: managing invasive species pathways in an era of globalization. J. Appl. Ecol. 46, 10–18. doi: 10.1111/j.1365-2664.2008.01600.x
Iacarella, J. C., Burke, L., Davidson, I. C., DiBacco, C., Therriault, T. W., and Dunham, A. (2020). Unwanted networks: Vessel traffic heightens the risk of invasions in marine protected areas. Biol. Conserv. 245:108553. doi: 10.1016/j.biocon.2020.108553
Ibabe, A., Miralles, L., Carleos, C. E., Soto-López, V., Menéndez-Teleña, D., Bartolomé, M., et al. (2020a). Environmental evaluations in industrial ports using eDNA biota indices including species invasiveness: a new Blue-gAMBI index at the Port of Gijon, Bay of Biscay. Spain: University of Oviedo.
Ibabe, A., Rayón-Viña, F., Martínez, J. L., and Vázquez, E. (2020b). Environmental DNA from plastic and textile marine litter detects exotic and nuisance species nearby ports. PLoS One 15:e0228811. doi: 10.1371/journal.pone.0228811
IMO (2004). International convention for the control and management of ships’ ballast water and sediments. London: IMO, 38.
IMO (2011). Guidelines for the control and management of ships’ biofouling to minimize the transfer of invasive aquatic species. Adoptedby resolution MEPC, 207. London: IMO, 16.
IMO (2018). International Convention for the Control and Management of Ships’ Ballast Water and Sediments (BWM). London: IMO.
Ju, R. T., Li, X., Jiang, J. J., Wu, J., Liu, J., Strong, D. R., et al. (2020). Emerging risks of non-native species escapes from aquaculture: Call for policy improvements in China and other developing countries. J. Appl. Ecol. 57, 85–90. doi: 10.1111/1365-2664.13521
Kaschner, K., Kesner-Reyes, K., Garilao, C., Segschneider, J., Rius-Barile, J., Rees, T., et al. (2019). AquaMaps: Predicted range maps for aquatic species. Geneva: World Wide Web electronic publication.
Keller, R. P., Drake, J. M., Drew, M. B., and Lodge, D. M. (2011). Linking environmental conditions and ship move- ments to estimate invasive species transport across the global shipping network. Divers. Distribut. 17, 93–102. doi: 10.1111/j.1472-4642.2010.00696.x
Kotta, J., Nurkse, K., Puntila, R., and Ojaveer, H. (2016). Shipping and natural environmental conditions determine the distribution of the invasive non-indigenous round goby Neogobius melanostomus in a regional sea. Estuar. Coastal Shelf Sci. 169, 15–24. doi: 10.1016/j.ecss.2015.11.029
Lawrence, D. J., and Cordell, J. R. (2010). Relative contributions of domestic and foreign sourced ballast water to propagule pressure in Puget Sound, Washington, USA. Biol. Conserv. 143, 700–709. doi: 10.1016/j.biocon.2009.12.008
Le Tixerant, D., Le Guyader, F., Gourmelon, B., and Queffelec. (2018). How can Automatic Identification System (AIS) data be used for maritime spatial planning? Ocean Coast Manag. 166, 18–30. doi: 10.1016/j.ocecoaman.2018.05.005
Lewis, J. A., and Dimas, J. (2007). Treatment of biofouling in internal seawater systems-Phase 2 (No. DSTO-TR-2081). Victoria: Defense science and technology organization.
Lim, C. S., Leong, Y. L., and Tan, K. S. (2017). Managing the risk of non-indigenous marine species transfer in Singapore using a study of vessel movement. Mar. Pollut. Bull. 115, 332–344. doi: 10.1016/j.marpolbul.2016.12.009
Lodge, D. M., Williams, S., MacIsaac, H. J., Hayes, K. R., Leung, B., Reichard, S., et al. (2006). Biological invasions: recommendations for U.S. Policy and Management. Ecol. Appl. 16, 2035–2054.
Loretta, A. (2016). AIS: New OG2 Satellites Enable Near Real-Time Vessel Monitoring. Montréal: ORBCOMM Blog.
Marraffini, M. L., Ashton, G. V., Brown, C. W., Chang, A. L., and Ruiz, G. M. (2017). Settlement plates as monitoring devices for non-indigenous species in marine fouling communities. Manage. Biol. Invasions 8, 559–566. doi: 10.3391/mbi.2017.8.4.11
McGeoch, M. A., Genovesi, P., Bellingham, P. J., Costello, M. J., McGrannachan, C., and Sheppard, A. (2016). Prioritizing species, pathways, and sites to achieve conservation targets for biological invasion. Biol. Invasions 18, 299–314. doi: 10.1007/s10530-015-1013-1
Menéndez-Teleña, D. (2019). Contaminación biológica de especies invasoras por agua de lastre e incrustaciones en el Puerto de Gijón. Ph. D. thesis, Marítimo: Universitario en Naútica y Gestión del Transporte Marítimo.
Merchant, N. D., Witt, M. J., Blondel, P., Godley, B. J., and Smith, G. H. (2012). Assessing sound exposure from shipping in coastal waters using a single hydrophone and Automatic Identification System (AIS) data. Mar. Pollut. Bull. 64, 1320–1329. doi: 10.1016/j.marpolbul.2012.05.004
Minchin, D. (2007). Rapid coastal survey for targeted alien species associated with floating pontoons in Ireland. Aquat. Invasions 2, 63–70. doi: 10.3391/ai.2007.2.1.8
Miralles, L., Ardura, A., Arias, A., lusa, L., Opico, E., Ernande de o as, A., et al. (2016). Barcodes of marine invertebrates from north Iberian ports: Native diversity and resistance to biological invasions. Mar. Poll. Bull. 112, 183–188. doi: 10.1016/j.marpolbul.2016.08.022
Miralles, L., Ardura, A., Clusa, L., and Garcia-Vazquez, E. (2018). DNA barcodes of Antipode marine invertebrates in Bay of Biscay and Gulf of Lion ports suggest new biofouling challenges. Sci. Rep. 8:16214.
Miralles, L., Parrondo, M., de Rojas, A. H., Garcia-Vazquez, E., and Borrell, Y. J. (2019). Development and validation of eDNA markers for the detection of Crepidula fornicata in environmental samples. Mar. Pollut. Bull. 146, 827–830. doi: 10.1016/j.marpolbul.2019.07.050
Molnar, J. L., Gamboa, R. L., Revenga, C., and Spalding, M. D. (2008). Assessing the global threat of invasive species to marine biodiversity. Front. Ecol. Environ. 6, 485–492. doi: 10.1890/070064
Nydam, M. L., Giesbrecht, K. B., and Stephenson, E. E. (2017). Origin and dispersal history of two colonial ascidian clades in the Botryllus schlosseri species complex. PLoS One 12:e0169944. doi: 10.1371/journal.pone.0169944
Ojaveer, H., and Kotta, J. (2015). Ecosystem impacts of the widespread non-indigenous species in the Baltic Sea: literature survey evidences major limitations in knowledge. Hydrobiologia 750, 171–185. doi: 10.1007/s10750-014-2080-5
Ojaveer, H., Galil, B. S., Carlton, J. T., Alleway, H., Goulletquer, P., Lehtiniemi, M., et al. (2018). Historical baselines in marine bioinvasions: Implications for policy and management. PLoS One 13:e0202383. doi: 10.1371/journal.pone.0202383
Ojaveer, H., Galil, B. S., Minchin, D., Olenin, S., Amorim, A., Canning-Clode, J., et al. (2014). Ten recommendations for advancing the assessment and management of non-indigenous species in marine ecosystems. Mar. Policy 44, 160–165. doi: 10.1016/j.marpol.2013.08.019
Palomares, M. L. D., and Pauly, D. (2020). SeaLifeBase. Geneva: World Wide Web electronic publication.
Pejovic, I., Ardura, A., Miralles, L., Arias, A., Borrell, Y. J., and Garcia-Vazquez, E. (2016). DNA barcoding for assessment of exotic molluscs associated with maritime ports in northern Iberia. Mar. Biol. Res. 12, 168–176. doi: 10.1080/17451000.2015.1112016
Pollard, D. A., and Hutchings, P. A. (1990). A review of exotic marine organisms introduced to the Australian region. 2. Invertebrates and algae. Asian Fisher. Sci. Metro Manila 3, 223–250.
Ready, J., Kaschner, K., South, A. B., Eastwood, P. D., Rees, T., Rius, J., et al. (2010). Predicting the distributions of marine organisms at the global scale. Ecol. Model. 221, 467–478. doi: 10.1016/j.ecolmodel.2009.10.025
Roman, J., and Darling, J. D. (2007). Paradox lost: genetic diversity and the success of aquatic invasions. Trends Ecol. Evolut. 22, 454–464. doi: 10.1016/j.tree.2007.07.002
Roy, D., Alderman, D., Anastasiu, P., Arianoutsou, M., Augustin, S., Bacher, S., et al. (2020). DAISIE-Inventory of alien invasive species in Europe. Version 1.7. doi: 10.15468/ybwd3x
Roy, H. E., Bacher, S., Essl, F., Adriaens, T., Aldridge, D. C., Bishop, J. D., et al. (2019). Developing a list of invasive alien species likely to threaten biodiversity and ecosystems in the European Union. Glob. Change Biol. 25, 1032–1048.
Ruiz, G. M., Fofonoff, P. W., Ashton, G., Minton, M. S., and Miller, W. A. (2013). Geographic variation in marine invasions among large estuaries: effects of ships and time. Ecol. Applicat. 23, 311–320. doi: 10.1890/11-1660.1
Ruiz, G. M., Fofonoff, P. W., Carlton, J. T., Wonham, M. J., and Hines, A. H. (2000). Invasion of coastal marine communities in North America: Apparent patterns, processes, and biases. Annu. Rev. Ecol. Systemat. 31, 481–531. doi: 10.1146/annurev.ecolsys.31.1.481
Seebens, H., Gastner, M. T., Blasius, B., and Courchamp, F. (2013). The risk of marine bioinvasion caused by global shipping. Ecol. Lett. 16, 782–790. doi: 10.1111/ele.12111
Shelmerdine, R. L. (2015). Teasing out the detail: How our understanding of marine AIS data can better inform industries, developments, and planning. Mar. Policy 54, 17–25. doi: 10.1016/j.marpol.2014.12.010
Steger, J., Galil, B. S., Hinterplattner, A., Zuschin, M., and Albano, P. G. (2019). Massive impacts of the Lessepsian invasion on molluscan communities of the Israeli Mediterranean shelf. In Geophysical Research Abstracts. Munich, 21
Thorvaldsen, T., Holmen, I. M., and Moe, H. K. (2015). The escape of fish from Norwegian fish farms: Causes, risks and the influence of organisational aspects. Mar. Policy 55, 33–38. doi: 10.1016/j.marpol.2015.01.008
Tournadre, J. (2014). Anthropogenic pressure on the open ocean: The growth of ship traffic revealed by altimeter data analysis. Geophys. Res. Lett. 41, 7924–7932. doi: 10.1002/2014gl061786
Tsiamis, K., Azzurro, E., Bariche, M., Çinar, M. E., Crocetta, F., De Clerck, O., et al. (2020). Prioritizing marine invasive alien species in the European Union through horizon scanning. Aquat. Conserv. Mar. Freshw. Ecosyst. 30, 794–845. doi: 10.1002/aqc.3267
Ulman, A., Ferrario, J., Forcada, A., Seebens, H., Arvanitidis, C., Occhipinti−Ambrogi, A., et al. (2019). Alien species spreading via biofouling on recreational vessels in the Mediterranean Sea. J. Appl. Ecol. 56, 2620–2629. doi: 10.1111/1365-2664.13502
Ulman, A., Ferrario, J., Occhpinti-Ambrogi, A., Arvanitidis, C., Bandi, A., Bertolino, M., et al. (2017). A massive update of non-indigenous species records in Mediterranean marinas. PeerJ 5:e3954. doi: 10.7717/peerj.3954
Vanderploeg, H. A., Nalepa, T. F., Jude, D. J., Mills, E. L., Holeck, K. T., Liebig, J. R., et al. (2002). Dispersal and emerging ecological impacts of Ponto-Caspian species in the Laurentian Great Lakes. Can. J. Fisher. Aquat. Sci. 59, 1209–1228. doi: 10.1139/f02-087
Vemeij, G. L. (1978). Biogeography and adaptation: patterns of marine life. Cambridge. Massachusetts: Harvard University Press, 1978.
Verling, E., Ruiz, G. M., Smith, L. D., Galil, B., Miller, A. W., and Murphy, K. R. (2005). Supplyside invasion ecology: charac- terizing propagule pressure in coastal ecosystems. Proc. R. Soc. Lond. Ser. B Biol. Sci. 272, 1249–1257. doi: 10.1098/rspb.2005.3090
Ware, C., Berge, J., Sundet, J. H., Kirkpatrick, J. B., Coutts, A. D., Jelmert, A., et al. (2014). Climate change, non-indigenous species and shipping: assessing the risk of species introduction to a high-A rctic archipelago. Divers. Distribut. 20, 10–19. doi: 10.1111/ddi.12117
Keywords: invasive species, genetic barcoding, ports strategies, blueports, NIS assessment, invasion likelihood
Citation: Miralles L, Ibabe A, González M, García-Vázquez E and Borrell YJ (2021) “If You Know the Enemy and Know Yourself”: Addressing the Problem of Biological Invasions in Ports Through a New NIS Invasion Threat Score, Routine Monitoring, and Preventive Action Plans. Front. Mar. Sci. 8:633118. doi: 10.3389/fmars.2021.633118
Received: 24 November 2020; Accepted: 24 February 2021;
Published: 18 March 2021.
Edited by:
Rochelle Diane Seitz, College of William & Mary, United StatesReviewed by:
Jasmine Ferrario, University of Pavia, ItalyCopyright © 2021 Miralles, Ibabe, González, García-Vázquez and Borrell. This is an open-access article distributed under the terms of the Creative Commons Attribution License (CC BY). The use, distribution or reproduction in other forums is permitted, provided the original author(s) and the copyright owner(s) are credited and that the original publication in this journal is cited, in accordance with accepted academic practice. No use, distribution or reproduction is permitted which does not comply with these terms.
*Correspondence: Yaisel J. Borrell, Ym9ycmVsbHlhaXNlbEB1bmlvdmkuZXM=
†These authors have contributed equally to this work
‡Present address: Laura Miralles, Ecohydros S.L., Polígono Industrial de Cros, Maliaño, Cantabria
Disclaimer: All claims expressed in this article are solely those of the authors and do not necessarily represent those of their affiliated organizations, or those of the publisher, the editors and the reviewers. Any product that may be evaluated in this article or claim that may be made by its manufacturer is not guaranteed or endorsed by the publisher.
Research integrity at Frontiers
Learn more about the work of our research integrity team to safeguard the quality of each article we publish.